- 1College of Traditional Chinese Medicine, Changchun University of Chinese Medicine, Changchun, China
- 2College of Integration Science, Yanbian University, Yanji, China
- 3Anorectal Diagnosis and Treatment Center, Affiliated Hospital of Changchun University of Chinese Medicine, Changchun, China
Objective: Colon cancer is a malignant neoplastic disease that seriously endangers the health of patients. Pulsatilla decoction (PD) has some therapeutic effects on colon cancer. This study is based on the analytical methods of network pharmacology and molecular docking to study the mechanism of PD in the treatment of colon cancer.
Methods: Based on the Traditional Chinese Medicine Systems Pharmacology Database, the main targets and active ingredients in PD were filtered, and then, the colon cancer-related targets were screened using Genecards, OMIM, PharmGKB, and Drugbank databases. Then, the screened drug and disease targets were Venn analyzed to obtain the intersection targets. Cytoscape software was used to construct the “Components–Targets–Pathway” map, and the String database was used to analyze the protein interaction network of the intersecting targets and screen the core targets, and then, the core targets were analyzed using Gene Ontology (GO) and Kyoto Encyclopedia of Genes and Genomes (KEGG) analyses. Molecular docking was implemented using AutoDockTools to predict the binding capacity for the core targets and the active components in PD.
Results: Sixty-five ingredients containing 188 nonrepetitive targets were screened and 180 potential targets of PD anticolon cancer were identified, including 10 core targets, namely, MAPK1, JUN, AKT1, TP53, TNF, RELA, MAPK14, CXCL8, ESR1, and FOS. The results of GO analysis showed that PD anticolon cancer may be related to cell proliferation, apoptosis, energy metabolism, immune regulation, signal transduction, and other biological processes. The results of KEGG analysis indicated that the PI3K-Akt signaling pathway, MAPK signaling pathway, proteoglycans in cancer, IL-17 signaling pathway, cellular senescence, and TNF signaling pathway were mainly involved in the regulation of tumor cells. We further selected core targets with high degree values as receptor proteins for molecular docking with the main active ingredients of the drug, including MAPK1, JUN, and AKT1. The docking results showed good affinity, especially quercetin.
Conclusion: This study preliminarily verified that PD may exert its effect on the treatment of colon cancer through multi-ingredients, multitargets, and multipathways. This will deepen our understanding of the potential mechanisms of PD anticolon cancer and establish a foundation for further basic experimental research.
1 Introduction
Colon cancer is a common malignant tumor of the gastrointestinal tract that originates in the mucosal epithelium of the colon. In recent years, the incidence rate of colon cancer has been increasing year by year, and the incidence rate in young people has increased rapidly (Benson et al., 2018). According to the data, the incidence and mortality rate of human colon cancer are 10.2 and 9.2%, respectively, ranking second in the new incidence and mortality rate of cancer (Siegel et al., 2017; Sung et al., 2021). There are many factors in the development of colon cancer, and its clinical symptoms may not show up in the early stage, but in the middle and late stages, there may be abdominal pain, mucopurulent stool, constipation, intestinal obstruction, lymphatic metastasis, etc. (Gupta et al., 2019). The present treatment for colon cancer is mainly based on surgery, chemotherapy, and biological antibody therapy (Yaghoubi et al., 2020). Related adjuvant therapies are also emerging in an endless stream, mainly fluorouracil, capecitabine, and oxaliplatin but with obvious side effects, such as neutropenia, neuropathy, diarrhea, and drug resistance, as well as other related symptoms (Gelibter et al., 2019). Therefore, it is necessary to develop more effective and safer therapeutic strategies for the treatment of colon cancer that reduce toxic side effects and complications and complement the aforementioned therapeutic limitations.
In Traditional Chinese medicine (TCM), colon cancer is called “Locked Anus Hemorrhoids.” Before treating colon cancer, TCM physicians will comprehensively understand the patient’s physical condition according to the patient’s pulse, tongue, symptoms, signs, and relevant modern laboratory examination. The treatment scheme varies for patients with different physical statuses, but the overall treatment is based on clearing heat and dampness, cooling the blood, and detoxification. Pulsatilla decoction (PD) comes from the classical Chinese medicine book “Shang Han Treatise.” It is a herbal formula composed of four herbs, namely, Phellodendron, Pulsatilla, Coptis Chinensis, and Cortex Fraxini, which have the effect of clearing heat and dampness, cooling the blood, and detoxification. Contemporary pharmacological research has proven that PD has immune function regulation, bactericidal, anti-inflammatory, antitumor, and other effects (Song et al., 2022). It is commonly used for the treatment of amebic dysentery, ulcerative colitis, bacterial dysentery, etc. (Wang L. et al., 2020; Wang X. et al., 2022). In recent years, our research team has been focusing on the regulatory role of mixed and single components of PD in colon tumor cells.
Pulsatilla saponin, an extract of Pulsatilla in PD, demonstrated its antitumor effect by inducing DNA damage and apoptosis and causing G2 receptor arrest in liver, pancreatic, and colon cancer cells (Liu et al., 2014; Xu et al., 2017). Nexrutine, an extract from Phellodendron, has been shown to exhibit antitumor effects in a variety of cancers, such as prostate, gastric, and pancreatic (Gong et al., 2017; Hussain et al., 2018; Zhuang et al., 2020). Berberine, an extract of Coptis Chinensis, inhibited the pernicious behavior of CRC by targeting the MAPK or TGF-β1/Smad signaling pathways to induce the cell cycle, promote apoptosis, and inhibit the growth factor signaling pathway in cancer cells (Zhao et al., 2021). Its chemical component epiberberine can regulate apoptotic in tumor cells via the P53 signaling pathway (Yu et al., 2020). Fraxetin, the chemical component of Cortex Fraxini, has been shown to inhibit prostate cancer. Its chemical composition escin can inhibit the effects of ovarian and colorectal cancer (Wang et al., 2018a; Kenny et al., 2021; Ma et al., 2022).
From the perspective of modern pharmacological studies, the extracted components of individual Chinese herbs in PD are candidates for the treatment of colon cancer; however, because of the multicomponent nature of Chinese herbs, the results of the study of PD in the treatment of colon cancer are not sufficient; thus, we need a more comprehensive research theory to systematically explore the relevant biomechanics of PD anticolon cancer. This study quotes the theory of “Network Pharmacology” put forward by pharmacologist Andrew L. Hopkins (Hopkins, 2008) and the theory of “Correlation between TCM and Biomolecular Network” put forward by Professor Li Shao (Li S. et al., 2020b), an expert of traditional Chinese medicine. As an analytical system based on the combination of pharmacology and systems biology theory, network pharmacology is particularly suitable for TCM with complex composition.
In this research, the method of network pharmacology was applied to construct the “Components–Targets–Pathway” network and validated the core targets and pathways using Gene Ontology (GO) enrichment analysis, Kyoto Encyclopedia of Genes and Genomes (KEGG) pathway analysis, and molecular docking techniques to explore the potential mechanisms of PD anticolon cancer. The flow chart for this study is shown in Figure 1.
2 Methods
2.1 Screening of components and target prediction of pulsatilla decoction
The keywords “Phellodendron, Pulsatilla, Coptis Chinensis, and Cortex Fraxini” were searched accordingly on the Traditional Chinese Medicine Systems Pharmacology Database (TCMSP) (https://lsp.nwu.edu.cn/tcmsp.php) (Yang et al., 2017; Zeng et al., 2022) to obtain all the chemical components of PD. Moreover, all chemical components were screened for active ingredients according to the screening criteria of bioactive components with oral bioavailability (OB) ≥ 30% and drug-likeness (DL) ≥ 0.18. Using TCMSP to get the potential targets of active components of PD. UniProt database (https://www.uniprot.org/) (Xu X. et al., 2022) was used to limit the species to “Homosapiens” and standardize the annotation of the target genes.
2.2 Prediction of colon cancer targets
Colon cancer was searched in the GeneCards (https://www.genecards.org/) (Xiang et al., 2022), OMIM (https://www.omim.org/) (Li et al., 2021), PharmGkb (https://www.pharmgkb.org/) (Zhang et al., 2020), and DrugBank (https://go.drugbank.com/) (Li et al., 2021) databases, respectively, restricted the species to human and selected genes; then, the gene contents of the four databases were combined to remove duplicate values, and the results obtained were the relevant targets for colon cancer.
2.3 Target prediction of pulsatilla decoction anticolon cancer
The compound targets of drugs and diseases are the potential targets of drugs acting on diseases (Huang et al., 2022). In this study, the targets of PD and colon cancer were taken to intersect using R language, and the common targets of both are the relevant targets of PD against colon cancer.
2.4 Construction of “components–targets–pathway” network
The Cytoscape software (version 3.8.2) was used to correlate the drugs, active components, overlapping targets, pathways, and diseases and construct the interactive network diagram between the components and the targets, in which the edge represents the interrelationship between nodes and the node stands for the active ingredients and targets; then, the main active ingredients of drugs were analyzed.
2.5 Screening core targets
The overlapping targets of colon cancer and PD were imported to the String database (https://string-db.org/) (Xu X. et al., 2022; Xiang et al., 2022), and the species was selected as human to obtain protein interaction relationship. The results were exported in TSV format and imported into Cytoscape software to obtain protein–protein interaction (PPI) diagrams, and the CytoNCA plug-in was used to analyze the topology of this network based on the conditions of Betweenness, Closeness, Degree, Eigenvector, Local Average Connectivity, and Network. Then, the importance of nodes in the PPI network was measured based on the classical “degree” value.
2.6 Gene Ontology and Kyoto Encyclopedia of Genes and Genomes pathway enrichment analysis
To investigate the biological process and signaling pathway transduction process of PD anticolon cancer, the R language and colorspace, stringi, ggplot2, ClusterProfiler, enrichplot, DOSE, and pathview packages in Bioconductor software were used, and the filtering condition was set to p < 0.05 to obtain the enrichment analysis results of GO and KEGG; the results were displayed in barplot or bubble.
2.7 Molecular docking
The core target protein screened using PPI network topology analysis was selected as the core receptor protein, and the target protein name was input to the Protein Data Bank (PDB) (http://www.rcsb.org/pdb/) (Tu et al., 2021), and the species was configured with “Homosapiens,” and the high-resolution three-dimensional target protein structure was selected. By using PyMOL software to remove water molecules and original ligand molecules from the PDB file of the original receptor protein, the PDB file of the treated receptor and ligand was imported into AutoDockTools for routine processing and saved as the format file of pdbqt. Then, AutogGrid was used to obtain the docking site parameters, run AutoDockVina for molecular docking, and then take the lowest binding energy as the docking results of the target protein and ligand; PyMOL software was used for analysis and visualization.
3 Results
3.1 Collection of pulsatilla decoction active components and overlapping targets
A total of 65 active ingredients of four drugs in PD were identified by searching the TCMSP under the conditions of OB ≥ 30% and DL ≥ 18%, including 37 Phellodendron, 11 Pulsatilla, 14 Coptis Chinensis, and 3 Cortex Fraxini. Afterward, 53 active ingredients of PD were obtained after removing the repeated ingredients (details in Table 1). Then, 36 active ingredients were obtained after removing the ingredients that were not screened for human-related targets. According to the TCMSP, there were 443 potential targets of active components in PD, including 183 Phellodendron, 66 Pulsatilla, 163 Coptis Chinensis, and 31 Cortex Fraxini. Then, 188 potential targets were obtained after removing the repeated targets. By using GeneCards, OMIM, PharmGkb, and DrugBank databases to integrate data and delete duplicate targets, a total of 12,391 targets linked with colon cancer were identified. Overlapping targets between PD and colon cancer were inputted into the Venn diagram to obtain 180 overlapping targets, which may be potential targets of PD against colon cancer (displayed in Figure 2).
3.2 Construction of “components–targets–pathway” network
The compound targets of Drug and Disease and the active ingredients of drugs were imported into Cytoscape software to construct the “Components–Targets–Pathway” network, which shows the complex relationship between PD and colon cancer. There are 216 nodes and 516 edges in the network, of which green represents Coptis Chinensis, dark blue represents Phellodendron, red represents Pulsatilla, orange represents Cortex Fraxini, and pink represents genes (Figure 3). Among them, quercetin, isorhamnetin, and beta-sitosterol have the highest connectivity, ranking in the top three (structure in Figure 4). Their degree values were 135, 27, and 26, respectively. In the therapy of colon cancer, quercetin may be the most critical component.
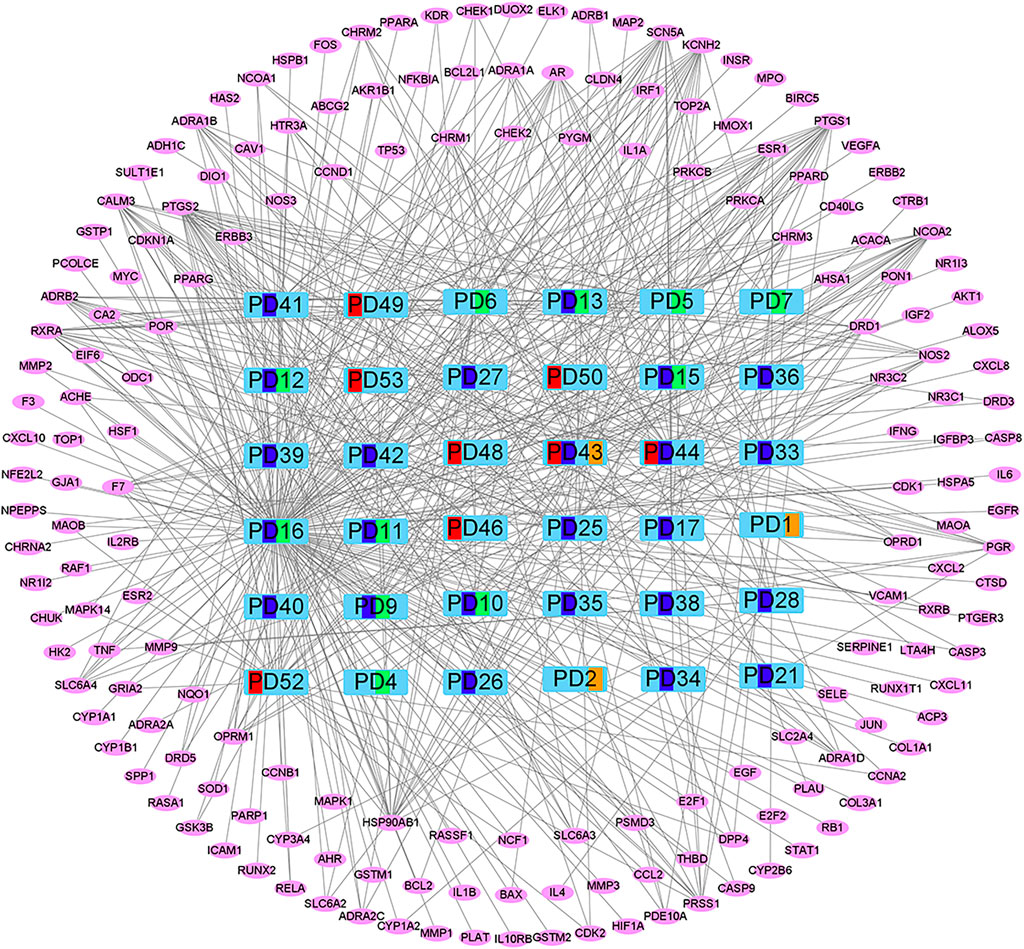
FIGURE 3. “Components–Targets–Pathway” network. Green represents Coptis Chinensis, dark blue represents Phellodendron, red represents Pulsatilla, orange represents Cortex Fraxini, and pink represents genes.
3.3 Screening core targets
The common targets of colon cancer and PD were imported into the String database, and 154 target proteins and 676 protein interactions were obtained. The results were transferred to Cytoscape software to obtain PPI maps and topological analysis (Figure 5). To enhance its reliability, a confidence score of ≥0.9 was set. The results showed that the top 10 core proteins were MAPK1, JUN, AKT1, TP53, TNF, RELA, MAPK14, CXCL8, ESR1, and FOS (Table 2).
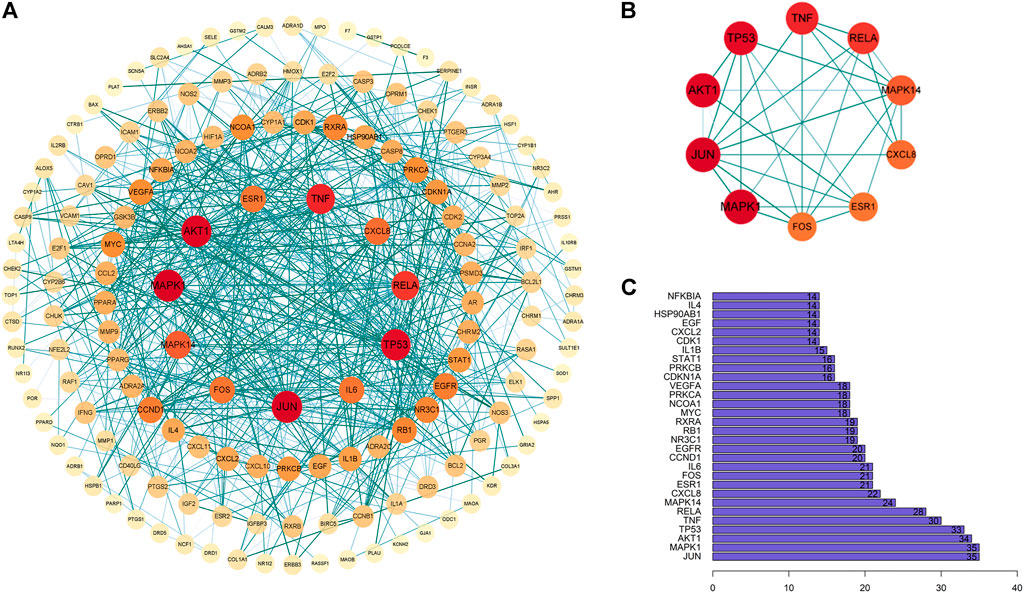
FIGURE 5. Interaction network diagram of PD target proteins against colon cancer. (A) PPI network of 154 duplicate targets. (B) network of the top 10 core proteins. (C) top 30 targets ranked by the degree value.
3.4 Gene Ontology and Kyoto Encyclopedia of Genes and Genomes enrichment analyses
To further investigate the mechanism of the anticolon cancer effect of PD, we performed the GO function and KEGG pathway by using the R language and Bioconductor software package. In the GO enrichment analysis results, the top 10 elements were picked from biological process (BP), cellular component (CC), and molecular function (MF) for visual analysis (Figure 6). In the BP items, the anticolon cancer effect of PD is mainly concentrated in response to drug, response to oxidative stress, reactive oxygen species, metallic process, response to molecular of bacterial origin, response to reactive oxygen species, response to metal ion, response to lipopolysaccharide, response to oxygen levels, response to steroid hormone, and cellular response to chemical stress. The MF project mainly includes nuclear receptor activity, DNA-binding transcription factor binding, G protein-coupled amine receptor activity, ligand activated, steroid hormone receptor activity, ubiquitin-like protein ligase binding, RNA polymerase II-specific DNA-binding transcription factor binding, catecholamine binding, transcription factor activity, drug binding, and ubiquitin–protein ligase binding. A variety of biological processes also show that PD could be utilized to treat colon cancer and other illnesses.
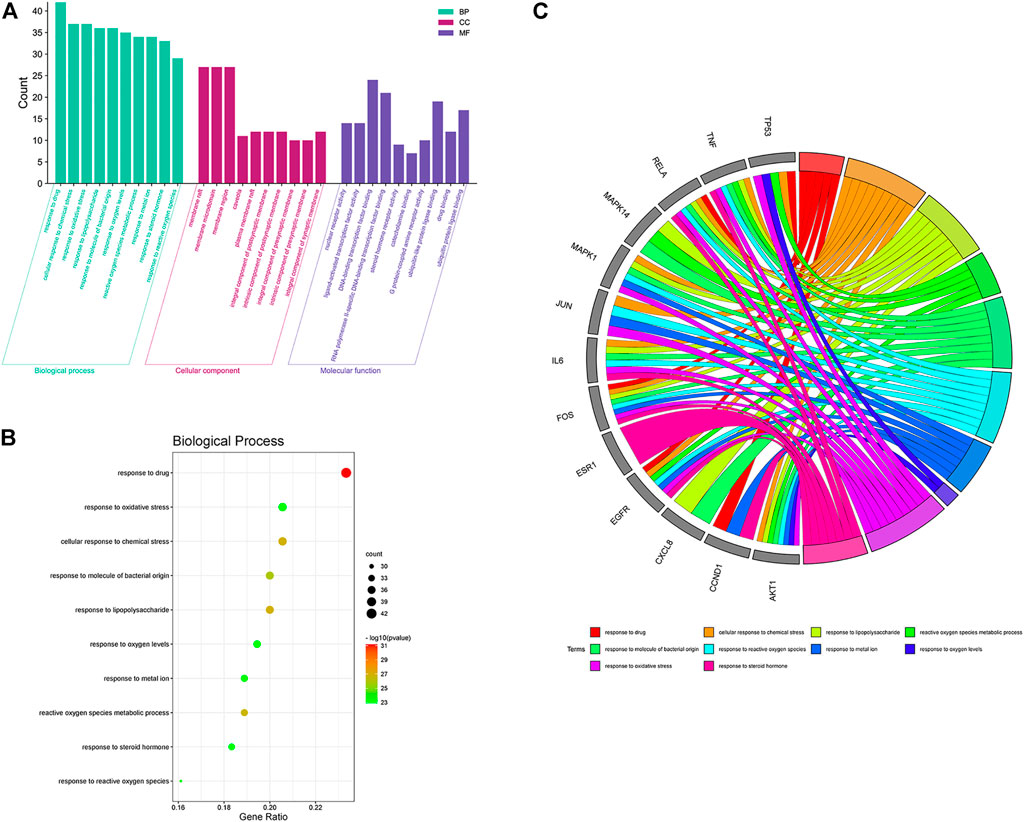
FIGURE 6. Gene Ontology (GO) function enrichment analysis of PD in the treatment of colon cancer. (A) GO function analysis, including BP, CC, and MF. (B) bubble diagram of BP enrichment. (C) GO chord diagram of the top 10 BP in the PD anticolon cancer.
In the KEGG pathway analysis (Figure 7), the size of the node indicates the number of targets converged to the pathway, and the greater the node, the greater the quantity of targets enriched. The color of the node ranges from green to red indicates the p-value from large to small, so the larger the red node indicates the higher the significance of the signaling pathway and the more important the role, which mainly includes lipid and atherosclerosis, PI3K-Akt signaling pathway, fluid shear stress and atherosclerosis, AGE-RAGE signaling pathway in diabetic complications, hepatitis B, cellular senescence, MAPK signaling pathway, pathways of neurodegeneration-multiple diseases, human cytomegalovirus infection, Epstein–Barr virus infection, proteoglycans in cancer, human T-cell leukemia virus 1 infection, human papillomavirus infection, prostate cancer, IL-17 signaling pathway, hepatocellular carcinoma, TNF signaling pathway, influenza A, Kaposi sarcoma-associated herpesvirus infection, and hepatitis C. We found that many enrichment pathways are associated with additional pathological effects, possibly caused by homologous molecular targets in various diseases. Among them, the PI3K-Akt signaling pathway, MAPK signaling pathway, IL-17 signaling pathway, TNF signaling pathway, cellular senescence, and proteoglycans in cancer were most closely associated with the mechanism of PD anticolon cancer (Figure 8).
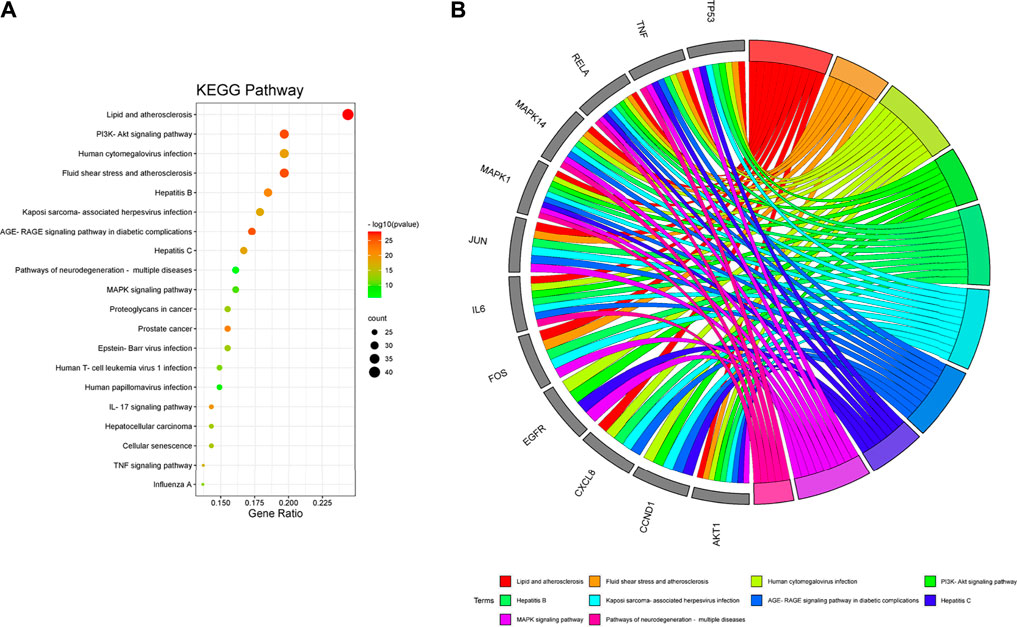
FIGURE 7. Kyoto Encyclopedia of Genes and Genomes (KEGG) pathway enrichment analysis of PD in the treatment of colon cancer. (A) bubble diagram of KEGG pathway enrichment. (B) GO chord diagram of the top 10 pathways in PD anticolon cancer.
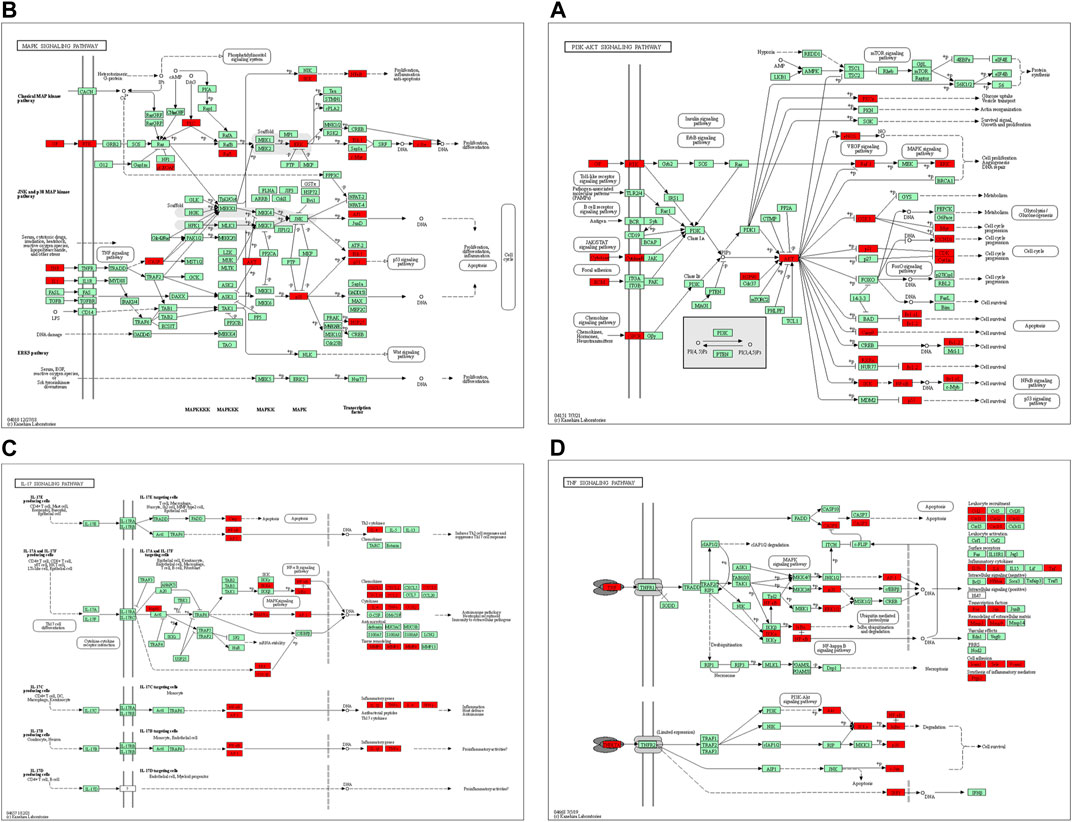
FIGURE 8. The primary pathways were colored using the KEGG mapper. Red nodes represent the targets regulated by PD in colon cancer. (A) PI3K-Akt signaling pathway. (B) MAPK signaling pathway. (C) IL-17 signaling pathway. (D) TNF signaling pathway.
3.5 Molecular docking findings
The “Components–Targets–Pathway” network diagram revealed that the quercetin node is the main active component of PD. Topological analysis shows that MAPK1, JUN, and AKT1 were the main targets. Therefore, the molecular docking of MAPK1, JUN, and AKT1 with quercetin was carried out by using Auto Dock Vina software. The results are shown in Figures 9A–C. An index of ≤ −5.0 kcal/mol (Xiang et al., 2022), which represents two molecules with a standard binding capacity, is used. It can be found that quercetin interacts with MAPK1 through three amino acid residues, namely, ASN-45, ARG-22, and ARG-351, with a hydrogen bonding energy of −5.0 kcal/mol; with JUN through three amino acid residues, namely, ARG-270, DA-309, and LYS-273, with a hydrogen bonding energy of −8.9 kcal/mol; and with AKT1 through six amino acid residues, namely, GLY-1227, VAL-1181, GLN-1180, ALA-1178, SER-1177, and LYS-1077, with a hydrogen bonding energy of −9.0 kcal/mol.
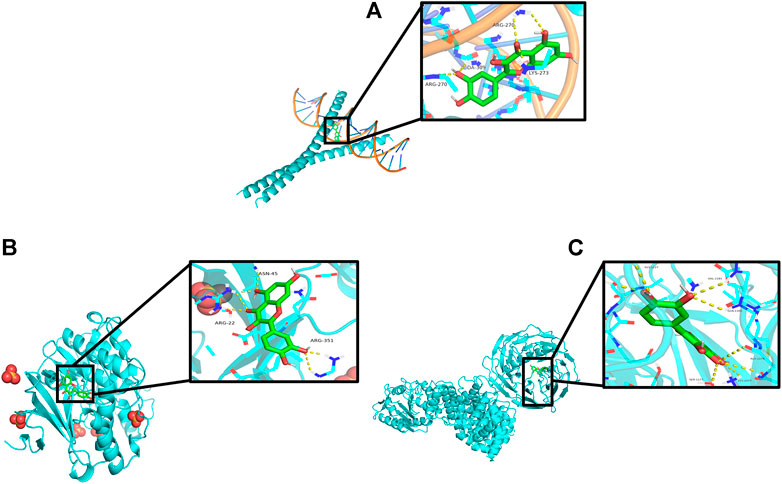
FIGURE 9. Schematic diagram of quercetin docking with core targets. (A) JUN docking with quercetin. (B) MAPK1 docking with quercetin. (C) AKT1 docking with quercetin.
4 Discussion
Colon cancer is a common malignant neoplastic disease that often occurs at the intersection between the sigmoid colon and colon. Its pathogenesis is complex. Smoking, alcohol consumption, red meat consumption, and family history of colon cancer are all considered to be the risk factors for this cancer (Young et al., 2010; Chan et al., 2011; Fedirko et al., 2011). With the gradual increase in morbidity rate in recent years and the high tumor recurrence rate in some patients after surgery, the health and quality of life of patients have been seriously and adversely affected (Mejri et al., 2017; Ramphal et al., 2018; Gupta et al., 2019). Issues like side effects and tolerance to anticolon cancer medications have led researchers to reconsider new alternatives to treat colon cancer. Research shows that TCM has unique advantages in cancer treatment, such as high efficiency and fewer side effects (Liu et al., 2020). Because of the complexity of TCM components, many TCM components have been proven to have certain curative effects in the laboratory (Qi et al., 2015). Individual TCM component has been shown to be effective in the treatment of tumor diseases, but the results of studies on TCM compound component are relatively few. However, the TCM treatment of diseases mostly appears in the form of a whole, so the research of TCM compounds for the treatment of illnesses needs to be further confirmed (Ling et al., 2014; Liu et al., 2019; Wang J. et al., 2020).
With the advancement of modern science and technology, medical research is becoming more and more important with the help of computer technology and other research tools. As new research technology means integrating system biology, bioinformatics, and network science, network pharmacology can analyze the molecular association between drugs and therapeutic objects and reveal the systemic pharmacological mechanism of drugs from the overall perspective of systemic level and biological network. Its characteristics of multichannel and multitarget are very suitable for research with this kind of complex component of TCM (Societies, 2021). Therefore, this study systematically revealed the mechanism of PD in the treatment of colon cancer.
According to the “Components–Targets–Pathway” network, quercetin, isorhamnetin, and beta-sitosterol may be the most important potential components of PD anticolon cancer. It has been reported that quercetin can bind to kinases such as PI3K-Akt/PKB, SEK1, and MAPK to induce tumor cell cycle arrest and inhibit growth and metastasis, antioxidant replication, and angiogenesis by participating in the induction and expression of phosphorylation of key intracellular targets (Darband et al., 2018). Cosan et al. (2011) demonstrated the mechanism of quercetin anticolon cancer by targeting telomerase activity and inhibiting Akt phosphorylation to induce cell senescence. Li C et al. pointed out that isorhamnetin inhibited the proliferation of SW480, HCT116, and HT-29cells by inhibiting the PI3K-Akt-MTOR signaling pathway; reduced the phosphorylation level of Akt, phosph-p70s6 kinase, and phosph-4e-bp1 (T37/46) proteins; and enhanced the expression of Cyclin B1 protein, thereby inhibiting the progression of colon cancer cells (Li et al., 2014). Suho S et al. verified the antioxidant effect of isorhamnetin, which proved its antimetastasis of colon cancer cells by inhibiting ROS-mediated HIF1α accumulation (Seo et al., 2016). Beta-sitosterol can reduce β-catenin and proliferating cell nuclear antigen with proliferative activity in colon cancer cells, to regulate the apoptosis and multiplication in tumor cells (Bin Sayeed and Ameen, 2015). Furthermore, beta-sitosterol can regulate the p53/NF-κB/BCRP signal axis to adjust the response of colorectal cancer to chemotherapy (Wang Z. et al., 2020). In addition, there are some biological processes of other active components of PD anticolon cancer, which need to be further explored.
The PPI network analysis of the duplicate targets of PD and colon cancer revealed that the core targets were MAPK1, JUN, AKT1, TP53, TNF, RELA, MAPK14, CXCL8, ESR1, and FOS. There are abundant interactions with other targets, indicating a higher degree of hub, to complete specific molecular functions. It was demonstrated that MARK1 and MAPK14 can affect the multiplication and apoptosis in cancer cells (Fang and Richardson, 2005; Madkour et al., 2021). Yong Y et al. significantly inhibited the invasion and metastasis in colon tumor cells by interfering with the expression of MAPK1 (Yang et al., 2018). Felipe PM et al. discovered that MAPK14 expression was higher in colorectal tumors (Wang D. et al., 2022) and that tumor cell progression and apoptosis could be induced by inhibiting MAPK14 expression in colon cancer samples (Chiacchiera and Simone, 2008). Ryan W S et al. verified that JUN could bind to and participate in the KRAS-mediated transcriptional activation of the USP28 promoter, followed by KRAS inducing transcriptional silencing of the repressor gene TSG to affect colon cancer cells (Serra et al., 2014). Overexpression of Akt can be detected in the early stages of colon cancer (Roy et al., 2002). Diminished Akt signaling, inhibition of phosphorylation processes, and downregulation of expression are implicated in the differentiation, apoptosis, and metastasis of colon cancer cells (Agarwal et al., 2013; Minnee and Faller, 2021). Colorectal carcinogenesis is closely associated with TP53 gene mutations, which can be a trigger for cancer, and its high expression in colorectal cancer is often indicative of poor survival (Li et al., 2018; Li L. et al., 2020a). TNF can cause apoptosis and is also an inflammatory cytokine (Dash et al., 2021). Inflammation contributes to tumor development, and overproduction of the pro-inflammatory factor TNF can both induce tumor cell progression (Ma et al., 2017; Cruceriu et al., 2020). Chen T et al. detected that by preventing REAL deacetylation, RELA acetyl-dependent expression was promoted, which in turn affected the migration and invasion of colon tumor cells (Chen et al., 2017). The expression of TNF-β and TNF-α and their receptors can induce the activation of NF-κB and NF-κB-related genes, thus regulating the survival, metastasis, etc. of colon tumors (Buhrmann et al., 2019).
The results of GO enrichment analysis showed that the anticolon cancer effect of PD may be related to a variety of biological processes, mainly including response to molecule of bacterial origin, response to oxidative stress, response to reactive oxygen species, response to lipopolysaccharide, cellular response to chemical stress, G protein-coupled amine receptor activity, membrane raft, drug binding, steroid hormone receptor activity, ubiquitin-like protein ligase binding. Moreover, the results reflect the involvement of multiple changes in cell proliferation, apoptosis, energy metabolism, signal transduction, and immune regulation in the process of PD against colon cancer.
The results of KEGG enrichment analysis indicated that numerous different illnesses were also enriched besides colorectal cancer, probably because of the existence of the same molecular targets in different disease pathologies processes. We selected signaling pathways closely connected with colorectal cancer for the analysis. We found that “PI3K-Akt signaling pathway,” “MAPK signaling pathway,” “Proteoglycans in cancer,” “IL-17 signaling pathway,” “Cellular senescence,” and “TNF signaling pathway” may be the potentially critical mechanism of PD anticolon cancer. The PI3K-Akt signaling pathway is an important signal transduction pathway for colon tumors. PI3K activation produces phosphatidylinositol triphosphate, which then leads to the activation of Akt through a series of signaling cascades. Akt activation can inhibit or activate a series of downstream substrates through phosphorylation, further regulating cell differentiation, proliferation, migration, and apoptosis (Danielsen et al., 2015). Activation of PI3K/Akt signal significantly promoted β-catenin nuclear translocation, cell proliferation, and apoptosis in colorectal cancer epithelial cells (Bishnupuri et al., 2019). Huang et al. (2020) found that different concentrations of quercetin could downregulate the protein levels of PI3K, Akt, p-Akt, and BCL2 and upregulate the protein level of Bax, to inhibit the proliferation and induce apoptosis of HCT116 colon cancer cells and HT29 colon cancer cells through PI3K-Akt signaling pathway. Further studies showed that quercetin inhibited the activity of Akt by blocking the phosphorylation level of Akt, thus regulating colon cancer through the PI3K-Akt signaling pathway (Refolo et al., 2015; Yang et al., 2016; Raja et al., 2017). Alterations in the MAPK signaling pathway are strongly associated with the progression of colon cancer cells (Burotto et al., 2014). Xu C et al. demonstrated that altering the expression of ERK1/2 and Akt genes downstream of the MAPK signaling pathway regulates DNA synthesis, proliferation, and apoptosis in colon cancer cells (Xu C. et al., 2022). Ma W et al. downregulated the phosphorylated expression of MEK1/2, ERK1/2, and RAF1 in the MAPK signaling pathway as a way to regulate the malignant proliferating phenotype of colon tumors (Ma et al., 2021). Wang Z et al. used Baicalin to upregulate the decidual protein induced by progesterone to activate the Ras and Raf signals to induce senescence in human colon cancer cells, (Wang et al., 2018b). It was found that quercetin could upregulate JNK, c-Jun, p-P38, and P53 and downregulate p-ERK in the MAPK signaling pathway, to promote apoptosis of colon cancer Caco-2, DLD-1, and HCT-15 and cause SW620 cell cycle arrest (Dihal et al., 2008; Kim et al., 2013; Refolo et al., 2015; Kee et al., 2016; Zhang et al., 2017). IL-17 signaling pathway and TNF signaling pathway may be mainly involved in inflammation and immune regulation. Dong P et al. inhibited the proliferation of colon cancer cells and reduced inflammatory cytokines by inhibiting the expression of IL17f mRNA in the IL-17 signaling pathway (Pan et al., 2022). Xun W et al. pointed out that the IL-17 signal pathway and TNF signal pathway regulate PD-L1 expression in colorectal cancer HCT116 cells, and the expression of PD-L1 is closely related to colon cancer treatment (Wang et al., 2017; Yaghoubi et al., 2019). Proteoglycans are complex molecule existing in the extracellular matrix and cell membrane, which plays an important role in cell growth, metastasis, and invasion. Yip g et al. found that the imbalance of the expression of biosynthesis and degradation enzymes of heparan sulfate proteoglycan can affect various stages of tumorigenesis (Yip et al., 2006). Zang YQ et al. promoted cancer progression by targeting the miR200 family with lumican to promote epithelial cell transformation into mesenchymal cells. Therefore, the regulation of proteoglycan expression is an important approach to treating colorectal cancer (Zang et al., 2021). Cellular senescence is a state of growth arrest, and induction of cellular senescence can inhibit the development of cancer (Sun et al., 2007). Hou Z et al. downregulated the expression of p21 through the interaction between Tribbles homolog 2 and AP4 to inhibit cellular senescence and thus regulate colon tumor cell progression (Hou et al., 2018). With the above results, it was considered that PD against colon cancer achieves its effects through various pathways such as tumor metabolic pathway, apoptotic pathway, and inflammatory pathway. In addition, many other signaling pathways have been shown, and their mechanisms of action need to be further explored.
5 Conclusion
Our study utilized network pharmacology theory and relevant software to systematically elaborate the potential mechanism of PD anticolon cancer. A variety of components of PD could exert an effective role in the treatment of colon cancer through multiple pathways and multiple targets. The accuracy of the outcomes was further verified using subsequent molecular docking. The results showed that PD could regulate the proliferation, metastasis, differentiation, senescence, apoptosis, and other biological processes of tumor cells by regulating the expression of related genes in colon cancer cells, reflecting the anticolon cancer effect of PD. However, the research results still need to be further corroborated using animal experiments and other relevant experiments to ensure the reliability of the study results. In summary, our research provides a new basis for further exploration and subsequent experimental verification of PD in the treatment of colon cancer.
Data availability statement
The original contributions presented in the study are included in the article/Supplementary Material; further inquiries can be directed to the corresponding authors.
Author contributions
Study design: CL and LH, Data collection: BQ and YH, Data analysis: YH and HL, Manuscript writing: HL, Manuscript revision: CY and LW, Graphs presentation: YZ.
Funding
This work was supported by the Science and Technology Development Foundation of Jilin Province (No.20200403126SF).
Acknowledgments
We sincerely appreciate the support provided by TCMSP, Uniport, GeneCards, OMIM, PharmGkb, DrugBank, String, Bioconductorand database, and Cytoscape, PyMOL, R language, AutoDockTools Software.
Conflict of interest
The authors declare that the research was conducted in the absence of any commercial or financial relationships that could be construed as potential conflicts of interest.
Publisher’s note
All claims expressed in this article are solely those of the authors and do not necessarily represent those of their affiliated organizations, or those of the publisher, the editors, and the reviewers. Any product that may be evaluated in this article, or claim that may be made by its manufacturer, is not guaranteed or endorsed by the publisher.
Supplementary material
The Supplementary Material for this article can be found online at: https://www.frontiersin.org/articles/10.3389/fphar.2022.940508/full#supplementary-material
References
Agarwal, E., Brattain, M. G., and Chowdhury, S. (2013). Cell survival and metastasis regulation by Akt signaling in colorectal cancer. Cell. Signal. 25, 1711–1719. doi:10.1016/j.cellsig.2013.03.025
Benson, A. B., Venook, A. P., Al-Hawary, M. M., Cederquist, L., Chen, Y. J., Ciombor, K. K., et al. (2018). NCCN guidelines insights: Colon cancer, version 2.2018. J. Natl. Compr. Canc. Netw. 16, 359–369. doi:10.6004/jnccn.2018.0021
Bin Sayeed, M. S., and Ameen, S. S. (2015). Beta-sitosterol: A promising but orphan nutraceutical to fight against cancer. Nutr. Cancer 67, 1214–1220. doi:10.1080/01635581.2015.1087042
Bishnupuri, K. S., Alvarado, D. M., Khouri, A. N., Shabsovich, M., Chen, B., Dieckgraefe, B. K., et al. (2019). Ido1 and kynurenine pathway metabolites activate PI3K-Akt signaling in the neoplastic colon epithelium to promote cancer cell proliferation and inhibit apoptosis. Cancer Res. 79, 1138–1150. doi:10.1158/0008-5472.Can-18-0668
Buhrmann, C., Yazdi, M., Popper, B., Shayan, P., Goel, A., Aggarwal, B. B., et al. (2019). Evidence that TNF-β induces proliferation in colorectal cancer cells and resveratrol can down-modulate it. Exp. Biol. Med. 244, 1–12. doi:10.1177/1535370218824538
Burotto, M., Chiou, V. L., Lee, J. M., and Kohn, E. C. (2014). The MAPK pathway across different malignancies: A new perspective. Cancer 120, 3446–3456. doi:10.1002/cncr.28864
Chan, D. S., Lau, R., Aune, D., Vieira, R., Greenwood, D. C., Kampman, E., et al. (2011). Red and processed meat and colorectal cancer incidence: Meta-analysis of prospective studies. PLoS One 6, e20456. doi:10.1371/journal.pone.0020456
Chen, T., Li, J., Xu, M., Zhao, Q., Hou, Y., Yao, L., et al. (2017). PKCε phosphorylates MIIP and promotes colorectal cancer metastasis through inhibition of RelA deacetylation. Nat. Commun. 8, 939. doi:10.1038/s41467-017-01024-2
Chiacchiera, F., and Simone, C. (2008). Signal-dependent regulation of gene expression as a target for cancer treatment: Inhibiting p38alpha in colorectal tumors. Cancer Lett. 265, 16–26. doi:10.1016/j.canlet.2008.02.061
Cosan, D. T., Soyocak, A., Basaran, A., Degirmenci, I., Gunes, H. V., Sahin, F. M., et al. (2011). Effects of various agents on DNA fragmentation and telomerase enzyme activities in adenocarcinoma cell lines. Mol. Biol. Rep. 38, 2463–2469. doi:10.1007/s11033-010-0382-x
Cruceriu, D., Baldasici, O., Balacescu, O., and Berindan-Neagoe, I. (2020). The dual role of tumor necrosis factor-alpha (TNF-α) in breast cancer: Molecular insights and therapeutic approaches. Cell. Oncol. 43, 1–18. doi:10.1007/s13402-019-00489-1
Danielsen, S. A., Eide, P. W., Nesbakken, A., Guren, T., Leithe, E., Lothe, R. A., et al. (2015). Portrait of the PI3K/AKT pathway in colorectal cancer. Biochim. Biophys. Acta 1855, 104–121. doi:10.1016/j.bbcan.2014.09.008
Darband, S. G., Kaviani, M., Yousefi, B., Sadighparvar, S., Pakdel, F. G., Attari, J. A., et al. (2018). Quercetin: A functional dietary flavonoid with potential chemo-preventive properties in colorectal cancer. J. Cell. Physiol. 233, 6544–6560. doi:10.1002/jcp.26595
Dash, S., Sahu, A. K., Srivastava, A., Chowdhury, R., and Mukherjee, S. (2021). Exploring the extensive crosstalk between the antagonistic cytokines- TGF-β and TNF-α in regulating cancer pathogenesis. Cytokine 138, 155348. doi:10.1016/j.cyto.2020.155348
Dihal, A. A., Van Der Woude, H., Hendriksen, P. J., Charif, H., Dekker, L. J., Ijsselstijn, L., et al. (2008). Transcriptome and proteome profiling of colon mucosa from quercetin fed F344 rats point to tumor preventive mechanisms, increased mitochondrial fatty acid degradation and decreased glycolysis. Proteomics 8, 45–61. doi:10.1002/pmic.200700364
Fang, J. Y., and Richardson, B. C. (2005). The MAPK signalling pathways and colorectal cancer. Lancet. Oncol. 6, 322–327. doi:10.1016/s1470-2045(05)70168-6
Fedirko, V., Tramacere, I., Bagnardi, V., Rota, M., Scotti, L., Islami, F., et al. (2011). Alcohol drinking and colorectal cancer risk: An overall and dose-response meta-analysis of published studies. Ann. Oncol. 22, 1958–1972. doi:10.1093/annonc/mdq653
Gelibter, A. J., Caponnetto, S., Urbano, F., Emiliani, A., Scagnoli, S., Sirgiovanni, G., et al. (2019). Adjuvant chemotherapy in resected colon cancer: When, how and how long? Surg. Oncol. 30, 100–107. doi:10.1016/j.suronc.2019.06.003
Gong, J., Muñoz, A. R., Pingali, S., Payton-Stewart, F., Chan, D. E., Freeman, J. W., et al. (2017). Downregulation of STAT3/NF-κB potentiates gemcitabine activity in pancreatic cancer cells. Mol. Carcinog. 56, 402–411. doi:10.1002/mc.22503
Gupta, R., Bhatt, L. K., Johnston, T. P., and Prabhavalkar, K. S. (2019). Colon cancer stem cells: Potential target for the treatment of colorectal cancer. Cancer Biol. Ther. 20, 1068–1082. doi:10.1080/15384047.2019.1599660
Hopkins, A. L. (2008). Network pharmacology: The next paradigm in drug discovery. Nat. Chem. Biol. 4, 682–690. doi:10.1038/nchembio.118
Hou, Z., Guo, K., Sun, X., Hu, F., Chen, Q., Luo, X., et al. (2018). TRIB2 functions as novel oncogene in colorectal cancer by blocking cellular senescence through AP4/p21 signaling. Mol. Cancer 17, 172. doi:10.1186/s12943-018-0922-x
Huang, D., Wen, X., Lu, C., Zhang, B., Fu, Z., Huang, Y., et al. (2022). Investigating the molecular mechanism of Compound Danshen Dropping Pills for the treatment of epilepsy by utilizing network pharmacology and molecular docking technology. Ann. Transl. Med. 10, 216. doi:10.21037/atm-22-195
Huang, S., Zhang, Z., Li, W., Kong, F., Yi, P., Huang, J., et al. (2020). Network pharmacology-based prediction and verification of the active ingredients and potential targets of zuojinwan for treating colorectal cancer. Drug Des. devel. Ther. 14, 2725–2740. doi:10.2147/dddt.S250991
Hussain, S. S., Huang, S. B., Bedolla, R. G., Rivas, P., Basler, J. W., Swanson, G. P., et al. (2018). Suppression of ribosomal protein RPS6KB1 by Nexrutine increases sensitivity of prostate tumors to radiation. Cancer Lett. 433, 232–241. doi:10.1016/j.canlet.2018.07.009
Kee, J. Y., Han, Y. H., Kim, D. S., Mun, J. G., Park, J., Jeong, M. Y., et al. (2016). Inhibitory effect of quercetin on colorectal lung metastasis through inducing apoptosis, and suppression of metastatic ability. Phytomedicine 23, 1680–1690. doi:10.1016/j.phymed.2016.09.011
Kenny, H. A., Hart, P. C., Kordylewicz, K., Lal, M., Shen, M., Kara, B., et al. (2021). The natural product β-escin targets cancer and stromal cells of the tumor microenvironment to inhibit ovarian cancer metastasis. Cancers (Basel) 13, 3931. doi:10.3390/cancers13163931
Kim, G. T., Lee, S. H., and Kim, Y. M. (2013). Quercetin regulates sestrin 2-AMPK-mTOR signaling pathway and induces apoptosis via increased intracellular ROS in HCT116 colon cancer cells. J. Cancer Prev. 18, 264–270. doi:10.15430/jcp.2013.18.3.264
Li, C., Bu, J., Liao, Y., Zhang, J., Han, J., Zhang, H., et al. (2018). High expressions of CUL4A and TP53 in colorectal cancer predict poor survival. Cell. Physiol. biochem. 51, 2829–2842. doi:10.1159/000496013
Li, C., Yang, X., Chen, C., Cai, S., and Hu, J. (2014). Isorhamnetin suppresses colon cancer cell growth through the PI3K-Akt-mTOR pathway. Mol. Med. Rep. 9, 935–940. doi:10.3892/mmr.2014.1886
Li, D., Liu, L., Yang, S., Xing, Y., Pan, L., Zhao, R., et al. (2021). Exploring the therapeutic mechanisms of huzhang-shanzha herb pair against coronary heart disease by network pharmacology and molecular docking. Evid. Based. Complement. Altern. Med. 2021, 5569666. doi:10.1155/2021/5569666
Li, L., Li, M., and Wang, X. (2020a). Cancer type-dependent correlations between TP53 mutations and antitumor immunity. DNA Repair (Amst) 88, 102785. doi:10.1016/j.dnarep.2020.102785
Li, S., Niu, M., Zhang, S., Zhang, B., and Yang, K. (2020b). Interpretation of network pharmacology evaluation method guidance. TCM Drug 52, 4119–4129.
Ling, C.-Q., Yue, X.-Q., and Ling, C. (2014). Three advantages of using traditional Chinese medicine to prevent and treat tumor. J. Integr. Med. 12, 331–335. doi:10.1016/s2095-4964(14)60038-8
Liu, C., Yang, S., Wang, K., Bao, X., Liu, Y., Zhou, S., et al. (2019). Alkaloids from traditional Chinese medicine against hepatocellular carcinoma. Biomed. Pharmacother. 120, 109543. doi:10.1016/j.biopha.2019.109543
Liu, Q., Chen, W., Jiao, Y., Hou, J., Wu, Q., Liu, Y., et al. (2014). Pulsatilla saponin A, an active molecule from Pulsatilla chinensis, induces cancer cell death and inhibits tumor growth in mouse xenograft models. J. Surg. Res. 188, 387–395. doi:10.1016/j.jss.2014.01.026
Liu, Y., Yang, S., Wang, K., Lu, J., Bao, X., Wang, R., et al. (2020). Cellular senescence and cancer: Focusing on traditional Chinese medicine and natural products. Cell. Prolif. 53, e12894. doi:10.1111/cpr.12894
Ma, W., Chen, Y., Xiong, W., Li, W., Xu, Z., Wang, Y., et al. (2021). STOML2 interacts with PHB through activating MAPK signaling pathway to promote colorectal Cancer proliferation. J. Exp. Clin. Cancer Res. 40, 359. doi:10.1186/s13046-021-02116-0
Ma, Y., Ren, Y., Dai, Z. J., Wu, C. J., Ji, Y. H., Xu, J., et al. (2017). IL-6, IL-8 and TNF-α levels correlate with disease stage in breast cancer patients. Adv. Clin. Exp. Med. 26, 421–426. doi:10.17219/acem/62120
Ma, Z., Sun, Y., and Peng, W. (2022). Fraxetin down-regulates polo-like kinase 4 (PLK4) to inhibit proliferation, migration and invasion of prostate cancer cells through the phosphatidylinositol 3-kinase (PI3K)/protein kinase B (Akt) pathway. Bioengineered 13, 9345–9356. doi:10.1080/21655979.2022.2054195
Madkour, M. M., Anbar, H. S., and El-Gamal, M. I. (2021). Current status and future prospects of p38α/MAPK14 kinase and its inhibitors. Eur. J. Med. Chem. 213, 113216. doi:10.1016/j.ejmech.2021.113216
Mejri, N., Dridi, M., Labidi, S., El Benna, H., Daoud, N., Boussen, H., et al. (2017). Annual hazard rate of relapse of stage II and III colorectal cancer after primary therapy. Clin. Transl. Oncol. 19, 1524–1530. doi:10.1007/s12094-017-1696-0
Minnee, E., and Faller, W. J. (2021). Translation initiation and its relevance in colorectal cancer. Febs J. 288, 6635–6651. doi:10.1111/febs.15690
Pan, D., Huang, B., Gan, Y., Gao, C., Liu, Y., Tang, Z., et al. (2022). Phycocyanin ameliorates colitis-associated colorectal cancer by regulating the gut microbiota and the IL-17 signaling pathway. Mar. Drugs 20, 260. doi:10.3390/md20040260
Qi, F., Zhao, L., Zhou, A., Zhang, B., Li, A., Wang, Z., et al. (2015). The advantages of using traditional Chinese medicine as an adjunctive therapy in the whole course of cancer treatment instead of only terminal stage of cancer. Biosci. Trends 9, 16–34. doi:10.5582/bst.2015.01019
Raja, S. B., Rajendiran, V., Kasinathan, N. K., P, A., Venkatabalasubramanian, S., Murali, M. R., et al. (2017). Differential cytotoxic activity of Quercetin on colonic cancer cells depends on ROS generation through COX-2 expression. Food Chem. Toxicol. 106, 92–106. doi:10.1016/j.fct.2017.05.006
Ramphal, W., Boeding, J. R. E., Gobardhan, P. D., Rutten, H. J. T., De Winter, L., Crolla, R., et al. (2018). Oncologic outcome and recurrence rate following anastomotic leakage after curative resection for colorectal cancer. Surg. Oncol. 27, 730–736. doi:10.1016/j.suronc.2018.10.003
Refolo, M. G., D'alessandro, R., Malerba, N., Laezza, C., Bifulco, M., Messa, C., et al. (2015). Anti proliferative and pro apoptotic effects of flavonoid quercetin are mediated by CB1 receptor in human colon cancer cell lines. J. Cell. Physiol. 230, 2973–2980. doi:10.1002/jcp.25026
Roy, H. K., Olusola, B. F., Clemens, D. L., Karolski, W. J., Ratashak, A., Lynch, H. T., et al. (2002). AKT proto-oncogene overexpression is an early event during sporadic colon carcinogenesis. Carcinogenesis 23, 201–205. doi:10.1093/carcin/23.1.201
Seo, S., Seo, K., Ki, S. H., and Shin, S. M. (2016). Isorhamnetin inhibits reactive oxygen species-dependent hypoxia inducible factor (HIF)-1α accumulation. Biol. Pharm. Bull. 39, 1830–1838. doi:10.1248/bpb.b16-00414
Serra, R. W., Fang, M., Park, S. M., Hutchinson, L., and Green, M. R. (2014). A KRAS-directed transcriptional silencing pathway that mediates the CpG island methylator phenotype. Elife 3, e02313. doi:10.7554/eLife.02313
Siegel, R. L., Miller, K. D., and Jemal, A. (2017). Cancer statistics, 2017. Ca. Cancer J. Clin. 67, 7–30. doi:10.3322/caac.21387
Societies, W. F. O. C. M. (2021). Network pharmacology evaluation MethodGuidance. Wor CN Med. 16, 527–532. doi:10.3969/j.issn.1673-7202.2021.04.001
Song, J., Gong, Y., and Liu, Q. (2022). Effects of baitouweng decoction on expressions of intestinal flora, inflammatory factors and HER-2 in mice with colorectal cancer. Infor TCM 39, 20–24. doi:10.19656/j.cnki.1002-2406.20220304
Sun, P., Yoshizuka, N., New, L., Moser, B. A., Li, Y., Liao, R., et al. (2007). PRAK is essential for ras-induced senescence and tumor suppression. Cell. 128, 295–308. doi:10.1016/j.cell.2006.11.050
Sung, H., Ferlay, J., Siegel, R. L., Laversanne, M., Soerjomataram, I., Jemal, A., et al. (2021). Global cancer statistics 2020: GLOBOCAN estimates of incidence and mortality worldwide for 36 cancers in 185 countries. Ca. Cancer J. Clin. 71, 209–249. doi:10.3322/caac.21660
Tu, Y., Wu, Q., He, J., Xu, J., Yu, S., Wang, Q., et al. (2021). Exploring the potential molecular mechanism of scutellaria baicalensis georgi in the treatment of gastric cancer based on network pharmacological analysis and molecular docking technology. Front. Pharmacol. 12, 697704. doi:10.3389/fphar.2021.697704
Wang, D., Peng, L., Hua, L., Li, J., Liu, Y., Zhou, Y., et al. (2022a). Mapk14 is a prognostic biomarker and correlates with the clinicopathological features and immune infiltration of colorectal cancer. Front. Cell. Dev. Biol. 10, 817800. doi:10.3389/fcell.2022.817800
Wang, J., Qi, F., Wang, Z., Zhang, Z., Pan, N., Huai, L., et al. (2020a). A review of traditional Chinese medicine for treatment of glioblastoma. Biosci. Trends 13, 476–487. doi:10.5582/bst.2019.01323
Wang, L., Tao, X., Ou, Y., and Yang, Z. (2020b). Bacteriostasis in vitro effect of baitouweng decoction on Shigella dysenteriae. Infor TCM 37, 49–53. doi:10.19656/j.cnki.1002-2406.200130
Wang, X., Xu, L., Wang, T., Xu, J., Fan, F., Zhang, Y., et al. (2022b). Pulsatilla decoction alleviates colitis by enhancing autophagy and regulating PI3K-Akt-mTORC1 signaling pathway. Mol. Med. Rep. 25, 108. doi:10.3892/mmr.2022.12624
Wang, X., Yang, L., Huang, F., Zhang, Q., Liu, S., Ma, L., et al. (2017). Inflammatory cytokines IL-17 and TNF-α up-regulate PD-L1 expression in human prostate and colon cancer cells. Immunol. Lett. 184, 7–14. doi:10.1016/j.imlet.2017.02.006
Wang, Z., Chen, Q., Li, B., Xie, J. M., Yang, X. D., Zhao, K., et al. (2018a). Escin-induced DNA damage promotes escin-induced apoptosis in human colorectal cancer cells via p62 regulation of the ATM/γH2AX pathway. Acta Pharmacol. Sin. 39, 1645–1660. doi:10.1038/aps.2017.192
Wang, Z., Ma, L., Su, M., Zhou, Y., Mao, K., Li, C., et al. (2018b). Baicalin induces cellular senescence in human colon cancer cells via upregulation of DEPP and the activation of Ras/Raf/MEK/ERK signaling. Cell. Death Dis. 9, 217. doi:10.1038/s41419-017-0223-0
Wang, Z., Zhan, Y., Xu, J., Wang, Y., Sun, M., Chen, J., et al. (2020c). β-Sitosterol reverses multidrug resistance via BCRP suppression by inhibiting the p53-MDM2 interaction in colorectal cancer. J. Agric. Food Chem. 68, 3850–3858. doi:10.1021/acs.jafc.0c00107
Xiang, C., Liao, Y., Chen, Z., Xiao, B., Zhao, Z., Li, A., et al. (2022). Network pharmacology and molecular docking to elucidate the potential mechanism of ligusticum chuanxiong against osteoarthritis. Front. Pharmacol. 13, 854215. doi:10.3389/fphar.2022.854215
Xu, C., Chen, Y., Long, F., Ye, J., Li, X., Huang, Q., et al. (2022a). Prognostic value and biological function of LRRN4 in colorectal cancer. Cancer Cell. Int. 22, 158. doi:10.1186/s12935-022-02579-x
Xu, L., Cheng, G., Lu, Y., and Wang, S. (2017). An active molecule from Pulsatilla chinensis, Pulsatilla saponin A, induces apoptosis and inhibits tumor growth of human colon cancer cells without or with 5-FU. Oncol. Lett. 13, 3799–3802. doi:10.3892/ol.2017.5884
Xu, X., Fang, C., Wang, Y., Lu, F., and Liu, S. (2022b). Integrating network pharmacology and metabolomics to elucidate the mechanism of action of huang qin decoction for treament of diabetic liver injury. Front. Pharmacol. 13, 899043. doi:10.3389/fphar.2022.899043
Yaghoubi, A., Khazaei, M., Avan, A., Hasanian, S. M., and Soleimanpour, S. (2020). The bacterial instrument as a promising therapy for colon cancer. Int. J. Colorectal Dis. 35, 595–606. doi:10.1007/s00384-020-03535-9
Yaghoubi, N., Soltani, A., Ghazvini, K., Hassanian, S. M., and Hashemy, S. I. (2019). PD-1/PD-L1 blockade as a novel treatment for colorectal cancer. Biomed. Pharmacother. 110, 312–318. doi:10.1016/j.biopha.2018.11.105
Yang, L., Liu, Y., Wang, M., Qian, Y., Dong, X., Gu, H., et al. (2016). Quercetin-induced apoptosis of HT-29 colon cancer cells via inhibition of the Akt-CSN6-Myc signaling axis. Mol. Med. Rep. 14, 4559–4566. doi:10.3892/mmr.2016.5818
Yang, Y., Li, X. J., Li, P., and Guo, X. T. (2018). MicroRNA-145 regulates the proliferation, migration and invasion of human primary colon adenocarcinoma cells by targeting MAPK1. Int. J. Mol. Med. 42, 3171–3180. doi:10.3892/ijmm.2018.3904
Yang, Y., Li, Y., Wang, J., Sun, K., Tao, W., Wang, Z., et al. (2017). Systematic investigation of ginkgo biloba leaves for treating cardio-cerebrovascular diseases in an animal model. ACS Chem. Biol. 12, 1363–1372. doi:10.1021/acschembio.6b00762
Yip, G. W., Smollich, M., and Götte, M. (2006). Therapeutic value of glycosaminoglycans in cancer. Mol. Cancer Ther. 5, 2139–2148. doi:10.1158/1535-7163.Mct-06-0082
Young, R. L., Page, A. J., Cooper, N. J., Frisby, C. L., and Blackshaw, L. A. (2010). Sensory and motor innervation of the crural diaphragm by the vagus nerves. Gastroenterology 138, 1091–1101. e1-5. doi:10.1053/j.gastro.2009.08.053
Yu, M., Ren, L., Liang, F., Zhang, Y., Jiang, L., Ma, W., et al. (2020). Effect of epiberberine from Coptis chinensis Franch on inhibition of tumor growth in MKN-45 xenograft mice. Phytomedicine 76, 153216. doi:10.1016/j.phymed.2020.153216
Zang, Y., Dong, Q., Lu, Y., Dong, K., Wang, R., Liang, Z., et al. (2021). Lumican inhibits immune escape and carcinogenic pathways in colorectal adenocarcinoma. Aging (Albany NY) 13, 4388–4408. doi:10.18632/aging.202401
Zeng, P., Su, H. F., Ye, C. Y., Qiu, S. W., Shi, A., Wang, J. Z., et al. (2022). A tau pathogenesis-based network pharmacology approach for exploring the protections of chuanxiong rhizoma in alzheimer's disease. Front. Pharmacol. 13, 877806. doi:10.3389/fphar.2022.877806
Zhang, L., Shi, X., Huang, Z., Mao, J., Mei, W., Ding, L., et al. (2020). Network pharmacology approach to uncover the mechanism governing the effect of radix achyranthis bidentatae on osteoarthritis. BMC Complement. Med. Ther. 20, 121. doi:10.1186/s12906-020-02909-4
Zhang, Y., Guo, Y., Wang, M., Dong, H., Zhang, J., Zhang, L., et al. (2017). Quercetrin from Toona sinensis leaves induces cell cycle arrest and apoptosis via enhancement of oxidative stress in human colorectal cancer SW620 cells. Oncol. Rep. 38, 3319–3326. doi:10.3892/or.2017.6042
Zhao, Z., Zeng, J., Guo, Q., Pu, K., Yang, Y., Chen, N., et al. (2021). Berberine suppresses stemness and tumorigenicity of colorectal cancer stem-like cells by inhibiting m(6)A methylation. Front. Oncol. 11, 775418. doi:10.3389/fonc.2021.775418
Keywords: pulsatilla decoction, colon cancer, quercetin, network pharmacology, molecular docking
Citation: Liu H, Hu Y, Qi B, Yan C, Wang L, Zhang Y and Chen L (2022) Network pharmacology and molecular docking to elucidate the mechanism of pulsatilla decoction in the treatment of colon cancer. Front. Pharmacol. 13:940508. doi: 10.3389/fphar.2022.940508
Received: 19 May 2022; Accepted: 28 June 2022;
Published: 08 August 2022.
Edited by:
Balasubramani Ravindran, Kyonggi University, South KoreaReviewed by:
Sumei He, Affiliated Suzhou Science and Technology Town Hospital of Nanjing Medical University, ChinaYaling Deng, Jiangxi University of Traditional Chinese Medicine, China
Copyright © 2022 Liu, Hu, Qi, Yan, Wang, Zhang and Chen. This is an open-access article distributed under the terms of the Creative Commons Attribution License (CC BY). The use, distribution or reproduction in other forums is permitted, provided the original author(s) and the copyright owner(s) are credited and that the original publication in this journal is cited, in accordance with accepted academic practice. No use, distribution or reproduction is permitted which does not comply with these terms.
*Correspondence: Yuting Hu, huyuting0921@163.com; Liang Chen, chenliangczd@163.com