- 1Yueyang Hospital of Integrated Traditional Chinese and Western Medicine, Shanghai University of Traditional Chinese Medicine, Shanghai, China
- 2Shanghai Frontiers Science Center of TCM Chemical Biology, Institute of Interdisciplinary Integrative Medicine Research, Shanghai University of Traditional Chinese Medicine, Shanghai, China
Oxidative stress contributes greatly to initiation and progression of liver injury. Activation of nuclear-factor erythroid 2-related factor 2 (Nrf2) has been considered as an attractive strategy for preventing and treating the oxidative damage related to liver injury. This study aimed to find an efficacious agent to activate Nrf2/HO-1 signaling pathway from clinically used therapeutic agents and to characterize the usefulness for preventing and treating CCl4-induced acute liver injury. For this purpose, a series of clinically used therapeutic agents were collected and their activation potentials on Nrf2 were assayed by using 293T-Nrf2-luc cell line. Among all tested therapeutic agents, midazolam was found with good Nrf2 activation effect and this agent could significantly ameliorate CCl4-induced damage to HepG2 cells. In vivo animal tests showed that pretreatment with midazolam reduced the liver pathological tissue damage and the serum levels of ALT and AST in CCl4-induced liver injury mice. Further investigations showed that midazolam could strongly up-regulate the expression of both Nrf2 and HO-1 in the mice liver, accompanied by increasing of the levels of antioxidant enzyme SOD and reducing the production of MDA, as well as reducing the pro-inflammatory cytokines (IL-6, TNF-α) secretion. Collectively, our results clearly demonstrate that midazolam can ameliorate CCl4-induced acute liver injury and oxidative stress via activating the Nrf2 signaling pathway.
Introduction
Oxidative stress is a state of imbalance between oxidation and antioxidant effects in human body, which could result in the generation of a large number of reactive oxygen species (ROS) in cells. Under physiological conditions, excessive ROS will affect a series of cell transduction pathways, influence the production and secretion of oxidative stress molecules, and regulate the balance between proliferation and apoptosis of cells. Meanwhile, it can also affect the function of mitochondria, leading to cell dysfunction, and eventually damage various tissues and organs (Giustarini et al., 2009). Particularly, for perioperative and severe patients, oxidative stress can cause serious complications (e.g., inflammation, bleeding, postoperative cognitive dysfunction and organ dysfunction), whose incidence of these complications is as high as 30% (Toro-Pérez and Rodrigo, 2021).
More and more attention has been paid to the methods of reducing perioperative oxidative stress, such as endoscopic surgery (Arsalani-Zadeh et al., 2011), reducing the concentration of inhaled oxygen (Oldman et al., 2021) and giving antioxidant drugs (Ali- Hasan- Al- Saegh et al., 2016) and other methods. However, due to the limitations of surgical methods and patients’ own conditions, the clinical application of the first and second method are limited. The application of antioxidants signifies a rational curative strategy to prevent and cure liver diseases involving oxidative stress. Although conclusions drawn from clinical studies remain uncertain, animal studies have revealed the promising in vivo therapeutic effect of antioxidants on liver diseases. Particularly, some sedative and analgesic anesthetics have been widely concerned as treatment methods to reduce oxidative stress and protect important organ functions. Studies have shown that propofol can reduce the release of ROS and malondialdehyde (MDA) from the liver after ischemia-reperfusion, and contribute to the recovery of lung function (Chan et al., 2008). In the model of hepatic ischemia/reoxygenation, sufentanil increased superoxide dismutase (SOD), decreased MDA, reduced oxidative stress, and played a protective role in liver (Lian et al., 2019).
A large number of studies indicated that oxidative stress underlies the pathophysiology of various etiologies of liver disease, including chronic hepatitis C (Koike, 2007), alcoholic liver disease and non-alcoholic fatty liver disease (NAFLD)(Cederbaum et al., 2009), and contributes to the development of hepatocarcinogenesis (Cichoż-Lach and Michalak, 2014). Nuclear-factor erythroid 2-related factor 2 (Nrf2), known as the “main regulator” of antioxidant response, regulates a series of antioxidant and cytoprotective dependent protein genes by interacting with the antioxidant response element (ARE) sequences of antioxidant and cytoprotective genes (Kaspar et al., 2009), for example, heme oxygenase-1 (HO-1), quinone oxidoreductase1 (NQO1), glutathione-S-transferase (GST) (Tang et al., 2014). The enhanced activation of Nrf2 by pharmacologic molecules or genetic engineering has been shown to protect the liver in different oxidative stress models (Feng et al., 2021; Lim et al., 2021).
This study aimed to find drugs that effectively ameliorates acute liver injury through screen Nrf2 agonist from the commonly used analgesic. The selected positive Nrf2 agonist was applied to CCl4 liver injury model mice to determine its antioxidant and anti-inflammatory effects by detecting inflammatory factors and oxidative stress products. The results of this study can provide a new treatment strategy for perioperative and severe patients to reduce oxidative stress.
Materials and Methods
Construction of Stable Transfection Cells
293T-Nrf2-luc cell line was developed via lentiviral stable transfection of pGMLV-Nrf2-luc. pGMLV-Nrf2-luc is a kind of plasmid that designed by Genomeditech (Shanghai, China), which harbors Nrf2 promoter-luciferase reporter gene construct driven by multiple dioxin response elements. After that, 293T-Nrf2-luc cell line was obtained through antibiotic screening.
Cell Culture
Both of 293T-Nrf2-luc cell lines and HepG2 cell lines were grown in DMEM/high glucose medium (Hyclone, United States) containing 10% fetal bovine serum (Hyclone, United States) and 1% penicillin-streptomycin solution (Hyclone, United States). The culture condition was constant temperature of 37°C, a humidified atmosphere of 95% air and 5% CO2. The fresh media were replaced every 2 days.
Nrf2 Reporter Assay
293T-Nrf2-luc cell lines were first seeded in 96-well plates at a density of 8 × 103 cells per well and grown overnight. Baicalin and iso-dihydromyricetin were prepared in our laboratory (Xiong et al., 2021; Zhao et al., 2021). Propofol (Fresenius Kabi, China), midazolam (Enhua, China), sufentanil (Humanwell, China), remifentanil (Humanwell, China), dexmedetomidine (Enhua, China), ketamine (Hengrui, China), dopamine (Fenghe, China),norepinephrine (Fenghe, China), epinephrine (Fenghe, China), diazepam (Xinyi, China), estazolam (Zhongxin, China), alprazolam (Enhua, China), rocuronium (Xianju, China), wogonin, liquiritin, anhydroicaritin, silybin A-SILYBIN-A, isoginkgetin, wogonoside, baicalein, scutellarein, scutellarin, hyperoside, liquiritigenin, glutamate, myricetin, dihydromyricetin, procyanidin (They were all purchased from Chengdu Purfield Biotechnology Co., Ltd. China), Baicalin and iso-dihydromyricetin were added at the final concentration of 10 μM, while sulforaphane (Sigma-Aldrich, United States) was used as a positive control (Russo et al., 2018). The negative control group was treated with the vehicle DMSO alone. The final concentration of DMSO in all experiments was below 1% (V/V).
Following 24 h incubation, luciferase activity was measured by the Steady-Glo® Reagent (Promega, United States) according to the manufacturer’s directions. Luciferase activity was measured on Spectramax M3 (Molecular devices, United States) with luciferase production as readout. Data was shown as the percentage of fold induction of the test compound against the vehicle control group.
Cell Viability Assay
HepG2 cells were seeded in 96-well culture plates (1.0 × 104 cells/well) and grown overnight. To verify the effects of midazolam on HepG2 viability, cells were treated with midazolam at different concentrations (0, 1.25, 2.5, 5, 10, 20, 40 μM) for 24 h. The cells were incubated with CCK-8 (DOJINDO Laboratories, Japan) solution (20 μl/well) and cultured at 37°C for another 1 h. The absorbance of the dissolved solutions was detected at 450 nm on Spectramax M3.
In order to verify the protective effect of midazolam on CCl4-induced HepG2 cells activity, different concentrations of CCl4 (0, 0.5, 1, 2, 4, 6%) were added to culture for 24 h, and HepG2 cells activity was detected by CCK-8 to determine the IC50 value of CCl4. After 24 h of intervention with different concentrations of midazolam, IC50 concentration of CCl4 was added for another 24 h of intervention, and finally CCK-8 was performed to detect cell activity.
Animals Assay
The antioxidant effect of midazolam was tested in adult C57BL/6 mice (male, 6–8 weeks, 18–22 g) provided by Shanghai Slake Experimental Animal Co., Ltd. All mice were fed in the laboratory at a temperature of about 22°C and a relative humidity of 50%, with a light-dark cycle for 12 h. A 1-week acclimatization period was adopted before the experiment, during which the mice also had free access to food and water. All animal experiments were approved by the animal ethics committee of Yueyang Hospital of integrated traditional Chinese and Western Medicine Affiliated to Shanghai University of traditional Chinese medicine (ethics No.: YYLAC-2020-0801).
Next, 32 mice were randomly divided into the following groups (n = 8 in each group): control, CCl4 group (CCl4, Sinopharm Chemical Reagent, China), CCl4 plus midazolam 4 mg/kg/day (CCl4 + M4 group); CCl4 plus midazolam 8 mg/kg/day (CCl4 + M8 group). In the CCl4 group, mice were intraperitoneally (i.p.) injected with 0.5% (v/v) CCl4 (10 ml/kg, dissolved in corn oil). In the CCl4 + M4 group or CCl4 + M8 group, midazolam was respectively i. p. injected at 4 or 8 mg/kg for 3 days, and 0.5% CCl4 was i. p. injected at 2 h after the last dose of midazolam. Mice were sacrificed 24 h after CCl4 injection, and blood and liver samples were collected. A part of the liver was immediately fixed in 10% formaldehyde for histopathological observation, while the rest was frozen in liquid nitrogen and stored at −80°C for other experiments.
In order to explore the effect of midazolam on CCl4-induced lethality, 40 mice were used and randomly divided into the CCl4 group and CCl4 + midazolam group (n = 20). Midazolam was i. p. injected at 8 mg/kg for 3 days, and 50% CCl4 (2.6 ml/kg, dissolved in corn oil) were i.p. injected at 2 h after the last dose of midazolam. The mortality of mice in each group was recorded up to 36 h after CCl4 administration.
Histological Examination of Liver Damage
The liver of each mouse (n = 8) was fixed in 10% neutral buffered formalin for 48 h and embedded in paraffin. Then 4 μm thick sections were cut and stained with hematoxylin and eosin (H&E) according to standard procedure. The samples were observed and images were obtained using an optical microscope (Olympus, Japan).
Biochemical Analysis for Blood and Liver
Blood samples were centrifuged at 3000 × g for 10 min at 4°C and the serum was collected to measure the activity of aspartate aminotransferase (AST) and alanine transaminase (ALT), which were measured by kits (Nanjing Jiancheng Bioengineering Institute, China). Liver tissue homogenization was performed using tissue homogenizer (FSH-2A, XFK, China). The homogenates were centrifuged (1,000 × g, 15 min) at 4°C, and the obtained supernatant was used for subsequent measurement of MDA and SOD by kits (Nanjing Jiancheng Bioengineering Institute, China). The levels of TNF-α and IL-6 in the liver tissues were measured using ELISA kits according to the manufacturer’s instructions (Elabscience, China).
Western Blot Assay
Total proteins were isolated from the liver tissues and cells using protein extraction kit (Beyotime Biotechnology, China). The cell nucleus proteins were extracted using Nuclear and Cytoplasmic Protein Extraction Kit (Beyotime Biotechnology, China). Protein concentrations were measured using BCA protein assay kit (Beyotime Biotechnology, China). Equal amounts of protein from each sample were subjected to 10% sodium dodecyl sulfate-polyacrylamide gel electrophoresis (SDS-PAGE), and the separated proteins were transferred to PVDF membranes. Then, the membranes were blocked with 1 × TBST containing 5% nonfat milk for 2 h at room temperature and continued to incubate with primary antibodies against mice Nrf2 (1:1000, Abcam, United Kingdom), HO-1 (1:1000, Abcam, United Kingdom), β-actin (1:1000, Beyotime Biotechnology, China) overnight at 4°C. Subsequently, the membranes were washed three times with TBST and incubated with secondary antibody at room temperature for 2 h. Blots were visualized using a hypersensitive chemiluminescence kit (Biotechnology, China). The results were quantified by densitometry using ImageJ software.
Molecular Docking Simulations
The 3D structure of Kelch-like ECH-associated protein 1(Keap1) was downloaded from the Protein Data Bank (PDB Code:1ZGK) (Beamer et al., 2005). AutoDockTools 1.5.6 was applied to pre-process of coordinate files of the Keap1 and ligand by adding polar hydrogens, calculating Kollman charges and assigning AD4 atomic type. Docking site of Keap1 was situated at the kelch-like site (KS) as well as the functional site of Keap1 described by Beamer et al. (Beamer et al., 2005). The grid box was placed at KS, and the docking simulations was conducted by AutoDock Vina (1.1.2). Further analyses of the docking result was conducted by Discovery Studio Visualizer (BIOVIA Discovery Studio 2019; Dassault Systèmes, San Diego, United States).
Statistical Analysis
The data were analyzed with GraphPad Prism 7.0 (San Diego, CA, United States) and presented as the means ± standard deviation (S.D.). One-way ANOVA analysis was used for differences between groups. The survival rate was analyzed by the log-rank test p-values less than 0.05 were considered statistically significant.
Results
Screening of Therapeutic Drugs on Nrf2 Activation
Using the Nrf2 luciferease reporter construct, the active effect of Nrf2 by 30 kinds of therapeutic agents (10 μM, final concentration) were evaluated. The results showed that midazolam up-regulated the activity of Nrf2 more than 2-fold compared with the negative control group (p < 0.01) (Figure 1).
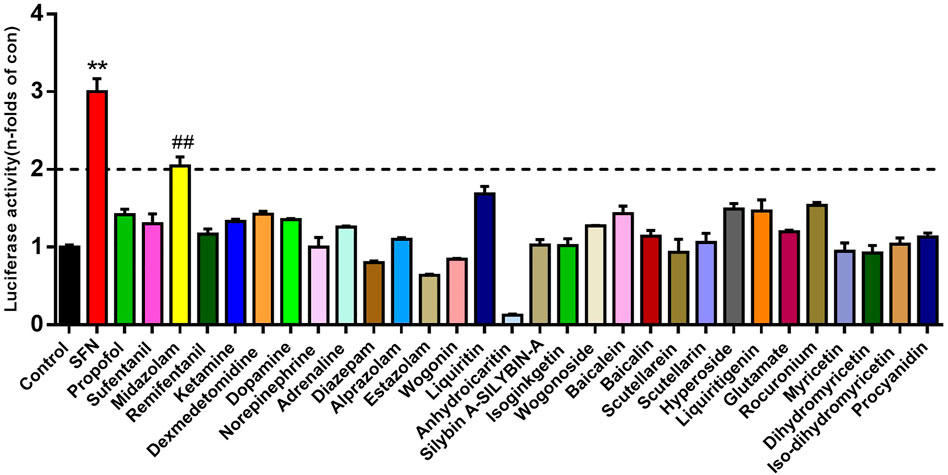
FIGURE 1. The activation effects of different therapeutic drugs on Nrf2. Luciferase report assay analyzed the activity of Nrf2 in 293T-Nrf2-luc cells after 24 h treatment with different therapeutic agents (10 μM, final concentration). Results were presented as the mean ± S.D. of six independent experiments. SFN, sulforaphane. The results were shown as mean ± S.D. **p < 0.01 vs. the control group; ##p < 0.01 vs. the SFN group.
Midazolam Dose-Dependently Enhances Nrf2 Expression
The expression of Nrf2 activity in 293T-Nrf2-luc cells increased with increasing midazolam concentration, and was dose-dependent (p < 0.01) (Figure 2A). To determine the effect of midazolam on cell viability, cells were treated with different concentrations of midazolam for 24 h. Cell viability was measured by CCK-8 assay. As shown in Figure 2B, even if treatment with high-dose midazolam (40 μM), there was no significant difference compared with the control group.
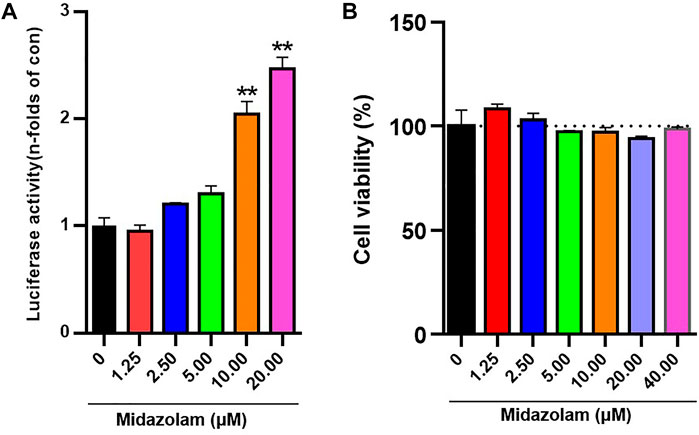
FIGURE 2. Effects of different concentrations of midazolam on Nrf2 activation and cell viability. (A) Luciferase report assay analyzed the activity of 293T-Nrf2-luc cells after 24 h treatment with different concentrations of midazolam (0, 1.25, 2.5, 5, 10, 20 μM); (B) CCK-8 assay analyzed the effect of different concentrations of midazolam (0, 1.25, 2.5, 5, 10, 20, 40 μM) on 293T-Nrf2-luc cells viability. The results were shown as mean ± S.D. **p < 0.01 vs. the control group.
To further verify the inductive effect of midazolam on Nrf2, the protein levels of Nrf2 in 293T-Nrf2-luc cells with or without midazolam treatment were determined. As shown in Figure 3A, Western blotting analysis showed that the protein expression of Nrf2 was elevated with the increasing concentrations of midazolam. It is well-known that Nrf2 is a transcriptional factor that needs to migrate to the nucleus to exert its function (Dinkova-Kostova et al., 2002). Under basal conditions Nrf2 is largely bound in the cytoplasm to Keap1, which is anchored to the actin cytoskeleton. When inducers disrupt the Keap1–Nrf2 complex, Nrf2 migrates to the nucleus where, in heterodimeric combinations with other transcription factors, it binds to antioxidant response element (ARE) regions of phase two genes and accelerates their transcription (Dinkova-Kostova et al., 2002). After the proteins in the nucleus and cytoplasm were extracted respectively, western blot analysis showed that with the increase of midazolam concentration, the Nrf2 protein level in the nucleus increased (Figure 3B) and the Nrf2 protein level in the cytoplasm decreased (Figure 3C). Midazolam promoted the translocation of Nrf2 to the nucleus.
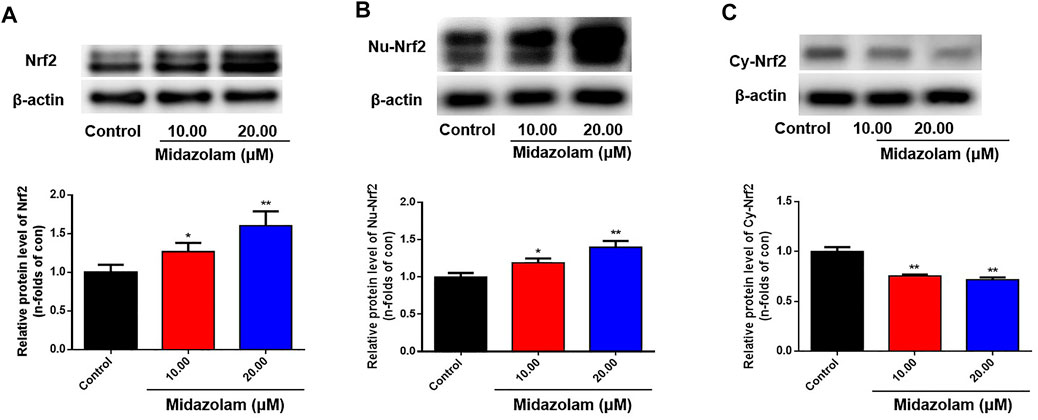
FIGURE 3. Effects of different concentrations of midazolam on Nrf2 protein level. 293T-Nrf2-luc cells were treated with different concentrations of midazolam (0, 10, 20 μM) for 24 h, and then using western blot to measure the level of Nrf2 protein. (A) Total Nrf2 protein level in cells; (B) Nrf2 protein level in the nucleus; (C) Nrf2 protein level in the cytoplasm. β-actin was used as an internal control for total protein. The results were shown as mean ± S.D. *p < 0.05 and **p < 0.01 vs. the control group.
Midazolam Reduces the Damage of Hepatocytes Induced by CCl4
Next, the protective effects of midazolam on hepatocytes were investigated by using CCl4-induced HepG2 cells were used as model cells. After pretreatment of HepG2 cells with different concentrations of midazolam for 24 h, CCl4 (IC50 = 1.5%, Supplementary Figure S1) was added to induce cell damage. As shown in Figure 4, CCl4 significantly decreased HepG2 cells activity by more than 50% compared with the control group (p < 0.01). By contrast, midazolam could significantly improve HepG2 cells activity compared with the CCl4 group (p < 0.01) even at low concentration (2.50 μM), as depicted in Figure 4. These findings clearly demonstrated that midazolam had good protective effects on CCl4-induced hepatocytes.
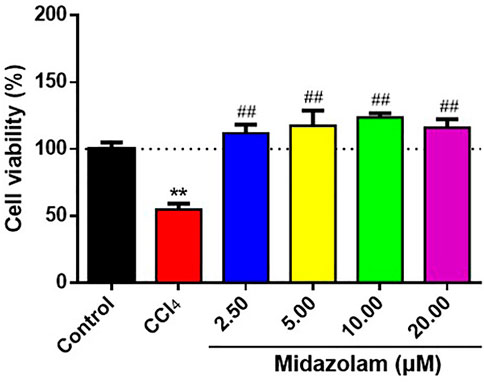
FIGURE 4. Effects of different concentrations of midazolam on HepG2 cells activity induced by CCl4. HepG2 cells were pretreated with different concentrations of midazolam (2.5, 5, 10, 20 Μm) for 24 h. CCl4 ((IC50 = 1.5%) induced HepG2 cells injury for 24 h. CCK-8 was used to analyze the effects of different concentrations of midazolam on cell viability induced by CCl4. The results were shown as mean ± S.D. **p < 0.01 vs. the control group; ##p < 0.01 vs. the CCl4 group.
Midazolam Attenuates CCl4-Induced Acute Liver Injury in Mice
Next, we evaluated the protective effects of midazolam (4 and 8 mg/kg/day, lasting for 3 days, i. p. injection) on CCl4 induced acute liver injury in mice. As shown in Figure 5, compared with the normal mice group, the activity levels of ALT and AST in serum of the CCl4 group were significantly increased (p < 0.01). In sharp contrast, the activity levels of ALT and AST in serum collected from midazolam pretreatment group were significantly decreased (p < 0.01), when compared with that from the CCl4 group (Figures 5A,B). Furthermore, H&E staining also suggested that the hepatocytes of the CCl4 group were swollen, while the liver cells were necrosis and a large number of inflammatory cells infiltrated. By contrast, following Midazolam pretreatment, the hepatocyte necrosis and the inflammatory cell infiltration were significantly reduced (Figure 5C). The histopathological scores showed a significant lower in both 4 and 8 mg/kg midazolam pretreatment compared to the CCl4 group (p < 0.01, Figure 2S). These findings clearly demonstrated that midazolam could attenuate CCl4 induced acute liver injury in mice.
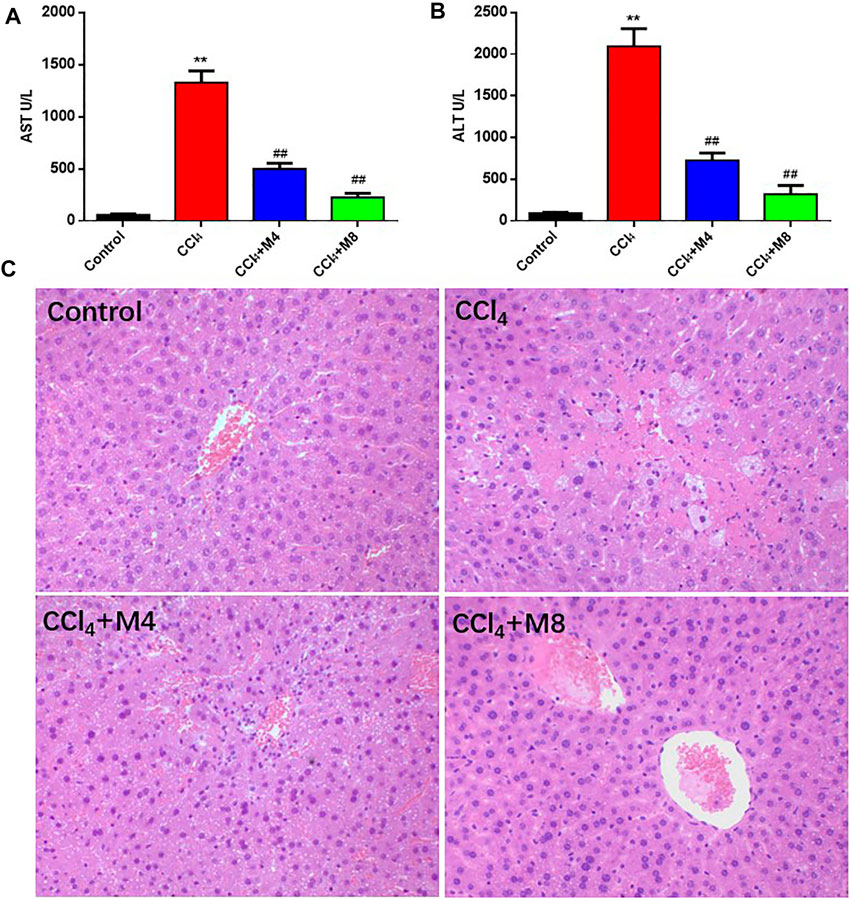
FIGURE 5. Protective effects of midazolam on CCl4-induced hepatic injury in mice. (A,B) The levels of AST and ALT in serum were detected at 24 h after CCl4 exposure. (C) Pathological images of H&E stained sections of liver in control, CCl4 and CCl4 plus midazolam treated mice (magnification, ×200). The results were shown as mean ± S.D. **p < 0.01 vs. the control group; ##p < 0.01 vs. the CCl4 group.
Midazolam Up-Regulates the Expression of Nrf2 and HO-1 in Mice Liver
As shown in Figure 6, Western blot analysis showed that the expression levels of Nrf2 and HO-1 in the CCl4 group were down-regulated evidently when compared to that in the control group. However, following administration of midazolam, the protein levels of both Nrf2 and HO-1 were significantly enhanced. These findings clearly suggested that Nrf2-related signaling pathway in mice liver could be enhanced following administration of midazolam.
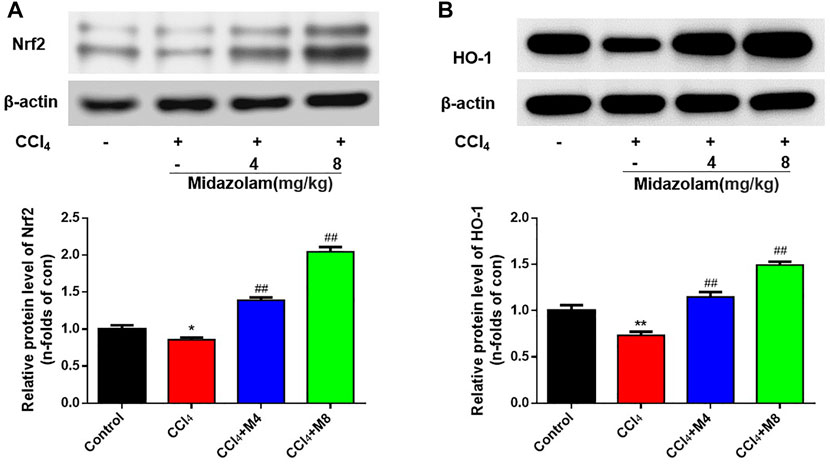
FIGURE 6. Effect of midazolam on the expression of Nrf2 and HO-1 in mice liver. The protein levels of Nrf2 (A) and HO-1 (B) were examined at 24 h after CCl4 exposure. (n = 8 in each group). **p < 0.01 vs. the control group; ##p < 0.01 vs. the CCl4 group.
Midazolam Ameliorates CCl4-Induced Oxidative Stress and Inflammation in Liver Tissue
It is well-known that SOD and MDA are typical biomarkers of antioxidation and oxidative stress, respectively. As shown in Figure 7, compared with the control group, MDA levels in liver were increased (p < 0.05), while SOD activity levels were decreased at 24 h after CCl4 treatment (p < 0.05). Compared with CCl4 group, midazolam pretreatment group reduced MDA levels (p < 0.05) and increased SOD activity levels (p < 0.05) (Figures 7A,B). Meanwhile, it was also found that CCl4 treatment significantly increased TNF-α and IL-6 expression levels compared with the control group (p < 0.01). As expected, midazolam dose-dependently reduced IL-6 expression levels (p < 0.01) when compared with that in the CCl4 group, while the serum levels of TNF-α were also significantly reduced following administration with high dose midazolam (8 mg/kg) (p < 0.05) (Figures 7C,D).
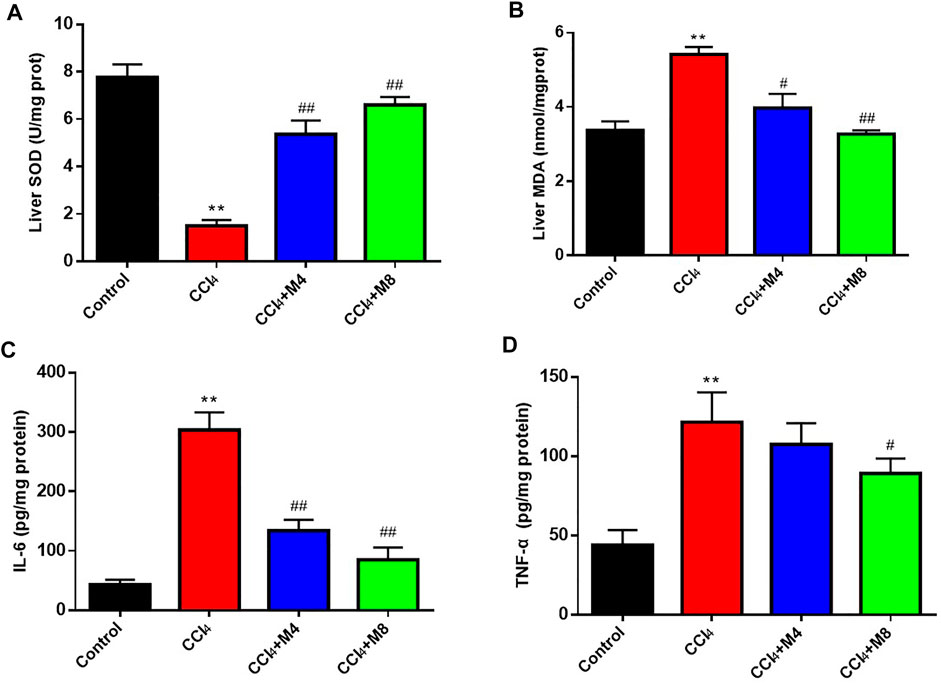
FIGURE 7. The effects of midazolam on the levels of SOD (A), MDA (B), IL-6 (C) and TNF-α (D) in mice liver. (n = 8 in each group). **p < 0.01 vs. the control group; #p < 0.05 and ##p < 0.01 vs. CCl4 group.
Midazolam Increases the Survival Rate of Mice Treated With Lethal Dose of CCl4
To further verify the hepatoprotective effect of midazolam, we investigated the ability of midazolam to affect the survival rate of mice treated with lethal dose of CCl4. For this purpose, a lethal dose of CCl4 for mice (2.6 ml/kg, 50%, i. p.) was used, while midazolam (8 mg/kg) was administrated for three consecutive days (once per day). As shown in Figure 8, midazolam significantly improved the survival rate of mice when compared with the CCl4 group (p < 0.05).
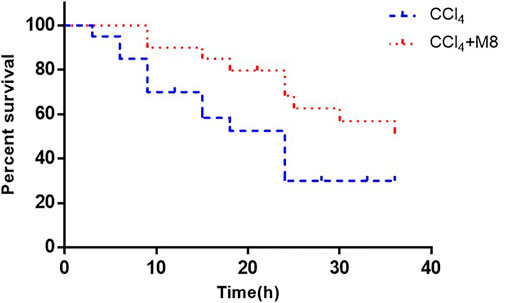
FIGURE 8. The effect of midazolam pretreatment on the survival rate of mice in which liver injury was induced by CCl4. Midazolam was i. p. injected at 8 mg/kg for 3 days 50% CCl4 (2.6 ml/kg) were i. p. injected at 2 h after the last dose of midazolam (n = 20). The results were analyzed using the log-rank test and expressed as Kaplan-Meier survival curves.
Molecular Docking Simulations
Finally, to decipher the binding modes and the molecular mechanism of midazolam in enhancing of Nrf2 signaling pathway, molecular docking simulations of midazolam into Keap1 were performed. Under homeostatic conditions, Nrf2 is kept inactive being bound to its endogenous inhibitor Keap1 (Bellezza et al., 2018). As shown in Figure 9, midazolam could be well-docked into the kelch-like site (KS) of Keap1, forming strong hydrophobic interactions, electrostatic and hydrogen bonding interactions with several key residuals in the inner wall of KS. Remarkably, the unsaturated and aromatic functional groups of midazolam interacted with Ala556 and Tyr525 via strong hydrophobic interactions including Alkyl, Pi-Alkyl and Pi-Pi stacked interactions (Figure 9). It was particular that three phenyl containing lipophilic residues (Phe577, Tyr572 and Tyr525) stretched the side chains orienting the sheer center direction of KS, resulting a regional hydrophobic surface of KS inner wall (Figure 9). In addition, the group of chlorobenzene and fluorobenzene were vital for the binding of midazolam. The group of chlorobenzene interacted with Arg415 via Pi-Cation interactions, while the fluorobenzene could interact with Gln530 via hydrogen bonding. These observations clearly suggest that midazolam could stably and tightly bind to Keap1, which in turn, blocking the coalition between Keap1 and Nrf2.
Discussion
Nrf2 is a key member of cap-n-collar basic leucine zipper transcription factor family, and is also a key regulator of mammalian genes involved in cell protection and detoxification by preventing oxidative stress (Martin et al., 1998). Under physiological conditions, Nrf2 mainly binds to Keap1 in cytoplasm (Yamamoto et al., 2018). After exposure to oxidative stress, Nrf2 and Keap1 are isolated and transferred to the nucleus. By interacting with the antioxidant response element (ARE) sequence of antioxidant and cytoprotective genes, Nrf2 induces the expression of a variety of antioxidant enzymes and phase II drug metabolism enzymes, including HO-1 and NQO1, and inhibits oxidative stress (Yamamoto et al., 2018)(Bellezza et al., 2018). Therefore, looking for Nrf2 activators has become a research hotspot in the prevention and treatment of oxidative stress-related diseases. Studies have shown that sedative and analgesic anesthetics commonly used in clinic can reduce the perioperative oxidative stress level. For example, in the acetaminophen induced liver injury animal model (Kostopanagiotou et al., 2009) and ischemia-reperfusion liver injury animal model (Chan et al., 2008), propofol can significantly reduce MDA, an index of oxidative stress in the liver. Another study has shown that ketamine can rapidly reduce the production of ROS and reduce protein damage, and play an antidepressant role in the hippocampus of ketamine treated mice through metabonomics and proteomics analysis (Weckmann et al., 2017). However, there is no comparative study on the effects of various common anesthetics on antioxidant stress. In our study, based on Nrf2 target, 30 kinds of therapeutic agents were screened by 293T-Nrf2-luc cell line to evaluate the inductive effect on Nrf2. The results showed that the inductive effect of midazolam on Nrf2 was more than 2 times, and the high dose of midazolam (20 μM) did not affect cell activity. This finding leads us to speculate that midazolam exerts stronger antioxidative stress ability than other anesthetics through Nrf2.
As the first step of metabolism, the liver is often exposed to high concentrations of exogenous and endogenous metabolites, especially vulnerable to oxidative damage caused by intermediate active substances. Nrf2/ARE, as one of the important mechanisms to protect the liver from oxidative stress, can play a protective role in liver inflammation, ischemia/reperfusion, fibrosis and regeneration by inducing target genes (Cuadrado et al., 2019; G; Bardallo et al., 2021). The activators of Nrf2, such as glycyrrhizic acid (Chen et al., 2013), sulforaphane (Wu et al., 2014), and curcumin (Peng et al., 2018), can induce the expression of antioxidant enzymes and reduce oxidative stress and liver injury. CCl4 is a strong hepatoxic and prooxidant agent widely used to induce hepatotoxicity, and its animal model has been widely used to screen the anti-hepatotoxicity and/or hepatoprotective activity of drugs (Yang et al., 2015; Cong et al., 2017; Zou et al., 2017). CCl4 causes a decrease in liver cells, in the activities of antioxidant enzymes and increases the protein carbonyl content, which is a protein oxidation product, at the oxidative stress biomarker MDA level (Sun et al., 2018). The purpose of this study was to evaluate the antioxidant capacity of midazolam in vivo, different doses of midazolam pretreatment were given to mice with acute liver injury induced by CCl4. Our results indicated that Midazolam pretreatment could significantly reduce the level of MDA which were markers of oxidative stress in liver tissue, and increased the activities of SOD antioxidant enzymes. Liver ALT, AST and pathological sections showed the protective effect of midazolam on liver. And we found that the higher the dose of midazolam, the greater the effect of reducing liver damage. Therefore, we chose a higher dose of midazolam to test the survival rate of mice given a lethal dose of CCl4. Midazolam significantly improved the survival rate of mice.
Midazolam is a benzodiazepine sedative, which is commonly used in clinical anesthesia and intensive care unit (ICU) (Lazzaroni and Bianchi Porro, 2003; Garcia et al., 2021). Clinical research shows that the sedation of critical patients with midazolam 48 h continuous infusion can significantly reduce the level of IL-1β, IL-6 and TNF- α in blood (Helmy and Al-Attiyah, 2001). In LPS and galactosamine induced acute liver injury model mice, midazolam can reduce serum ALT and liver TNF-α levels, reduce liver injury and protect liver function (Li J. et al., 2018). Consistently, our results suggested that midazolam could also reduce the hepatic TNF-α and IL-6 induced by CCl4. Previous studies on the antioxidant stress of midazolam have mainly focused on the nervous system. Midazolam can inhibit oxidative stress, reduce the level of ROS, reduce the death of neurons, and play a neuroprotective role (Liu et al., 2017; Li GZ. et al., 2018). To conclude from the observation from molecular docking simulations, midazolam could bind to Keap1 stably, and hinder the coalition between Keap1 and Nrf2. This may be an important mechanism for midazolam to exert its anti-oxidative stress (Figure 10).
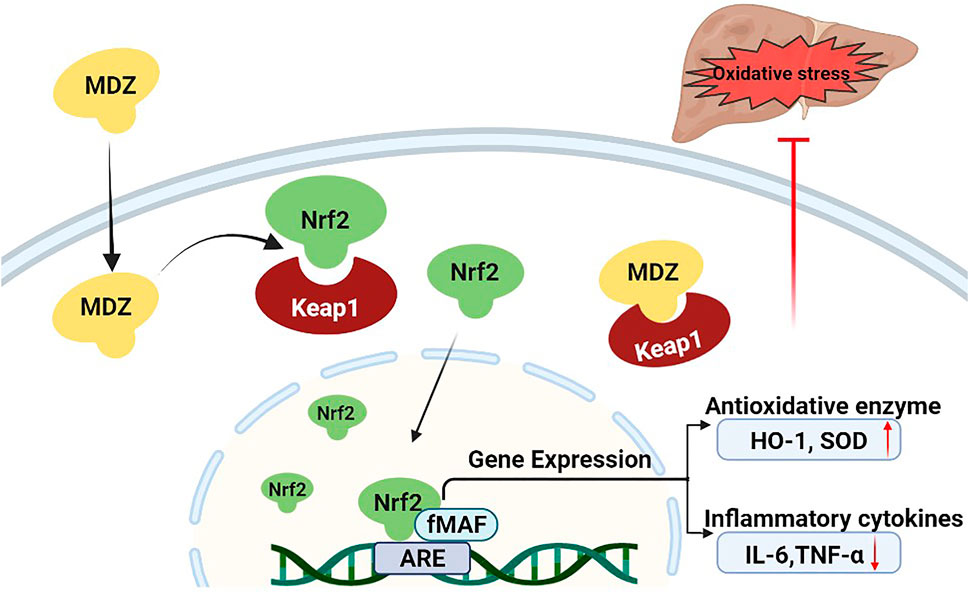
FIGURE 10. Proposed mechanism of midazolam for ameliorating acute liver injury and related oxidative stress induced by CCL4. Under normal physiological conditions, the Nrf2 protein level is tightly controlled by Keap1. Upon oxidative stress treatment, midazolam can bind to Keap1 stably, and hinder the coalition between Keap1 and Nrf2. Nrf2 is released and translocation to the nucleus to bind to AREs and initiate the anti-oxidative response by upregulating the expression of downstream target genes including HO-1 and SOD, which then decreases intracellular ROS production and alleviates oxidative stress-induced cytotoxicity. Midazolam ameliorates acute liver injury induced by carbon tetrachloride via enhancing Nrf2 signaling pathway.
In summary, this study found that midazolam acted as an efficacious agent to activate Nrf2/HO-1 signaling pathway, which could enhance the levels of several key antioxidant proteins both in vitro and in vivo. Cell assays demonstrated that midazolam dose-dependently enhanced Nrf2 expression and showed good protective effects on CCl4-induced HepG2 cells. Animal tests showed that midazolam could significantly reduce the liver pathological tissue damage and the serum levels of both ALT and AST in CCl4-induced liver injury, accompanied by increasing of the levels of antioxidant enzyme SOD and reducing the production of MDA, as well as reducing the pro-inflammatory cytokines (IL-6, TNF-α) secretion. Molecular docking simulations suggested that midazolam could stably bind to Keap1 and hinder the binding of Keap1 to Nrf2. Collectively, our findings suggested that midazolam may be a good protector for alleviating CCl4-induced acute liver injury via enhancing Nrf2 signaling pathway, which offered a new pharmacotherapy for preventing and treating toxin-induced organ injury.
Data Availability Statement
The original contributions presented in the study are included in the article/Supplementary Material, further inquiries can be directed to the corresponding authors.
Ethics Statement
The animal study was reviewed and approved by Yueyang Hospital of integrated traditional Chinese and Western Medicine Affiliated to Shanghai University of traditional Chinese medicine (ethics No.:YYLAC-2020-0801).
Author Contributions
YoZ and YaZ contributed this work equally.
Funding
This study was supported by grants from The National Natural Science Funds (82074228, 81922070 and 81973286).
Conflict of Interest
The authors declare that the research was conducted in the absence of any commercial or financial relationships that could be construed as a potential conflict of interest.
Publisher’s Note
All claims expressed in this article are solely those of the authors and do not necessarily represent those of their affiliated organizations, or those of the publisher, the editors and the reviewers. Any product that may be evaluated in this article, or claim that may be made by its manufacturer, is not guaranteed or endorsed by the publisher.
Supplementary Material
The Supplementary Material for this article can be found online at: https://www.frontiersin.org/articles/10.3389/fphar.2022.940137/full#supplementary-material
Abbreviations
ALT, alanine transaminase; ARE, antioxidant response element; AST, aspartate aminotransferase; GST, glutathione-S-transferase; H&E, hematoxylin and eosin; HO-1, heme oxygenase-1; Keap1, Kelch-like ECH-associated protein 1; KS, kelch-like site; MDA, malondialdehyde; NAFLD, non-alcoholic fatty liver disease; NQO1, quinone oxidoreductase1; Nrf2, nuclear-factor erythroid 2-related factor 2; ROS, reactive oxygen species; SFN, sulforaphane; SOD, superoxide dismutase.
References
Ali- Hasan- Al- Saegh, S., Mirhosseini, S. J., Tahernejad, M., Mahdavi, P., Shahidzadeh, A., Karimi-Bondarabadi, A. A., et al. (2016). Impact of Antioxidant Supplementations on Cardio-Renal Protection in Cardiac Surgery: an Updated and Comprehensive Meta-Analysis and Systematic Review. Cardiovasc Ther. 34, 360–370. doi:10.1111/1755-5922.12207
Arsalani-Zadeh, R., Ullah, S., Khan, S., and MacFie, J. (2011). Oxidative Stress in Laparoscopic versus Open Abdominal Surgery: a Systematic Review. J. Surg. Res. 169, e59–68. doi:10.1016/j.jss.2011.01.038
Bardallo, R. G., Panisello‐Roselló, A., Sanchez‐Nuno, S., Alva, N., Roselló‐Catafau, J., and Carbonell, T. (2021). Nrf2 and Oxidative Stress in Liver Ischemia/reperfusion Injury. FEBS J. doi:10.1111/febs.16336
Beamer, L. J., Li, X., Bottoms, C. A., and Hannink, M. (2005). Conserved Solvent and Side-Chain Interactions in the 1.35 Angstrom Structure of the Kelch Domain of Keap1. Acta Crystallogr. D. Biol. Crystallogr. 61, 1335–1342. doi:10.1107/S0907444905022626
Bellezza, I., Giambanco, I., Minelli, A., and Donato, R. (2018). Nrf2-Keap1 Signaling in Oxidative and Reductive Stress. Biochim Biophys Acta Mol Cell ResMolecular Cell Res. 1865, 721–733. doi:10.1016/j.bbamcr.2018.02.010
Cederbaum, A. I., Lu, Y., and Wu, D. (2009). Role of Oxidative Stress in Alcohol-Induced Liver Injury. Arch. Toxicol. 83, 519–548. doi:10.1007/s00204-009-0432-0
Chan, K. C., Lin, C. J., Lee, P. H., Chen, C. F., Lai, Y. L., Sun, W. Z., et al. (2008). Propofol Attenuates the Decrease of Dynamic Compliance and Water Content in the Lung by Decreasing Oxidative Radicals Released from the Reperfused Liver. Anesth. Analg. 107, 1284–1289. doi:10.1213/ane.0b013e318181f4e6
Chen, S., Zou, L., Li, L., and Wu, T. (2013). The Protective Effect of Glycyrrhetinic Acid on Carbon Tetrachloride-Induced Chronic Liver Fibrosis in Mice via Upregulation of Nrf2. PloS one 8, e53662. doi:10.1371/journal.pone.0053662
Cichoż-Lach, H., and Michalak, A. (2014). Oxidative Stress as a Crucial Factor in Liver Diseases. World J. Gastroenterol. 20, 8082–8091. doi:10.3748/wjg.v20.i25.8082
Cong, M., Zhao, W., Liu, T., Wang, P., Fan, X., Zhai, Q., et al. (2017). Protective Effect of Human Serum Amyloid P on CCl4-Induced Acute Liver Injury in Mice. Int. J. Mol. Med. 40, 454–464. doi:10.3892/ijmm.2017.3028
Cuadrado, A., Rojo, A. I., Wells, G., Hayes, J. D., Cousin, S. P., Rumsey, W. L., et al. (2019). Therapeutic Targeting of the NRF2 and KEAP1 Partnership in Chronic Diseases. Nat. Rev. Drug Discov. 18, 295–317. doi:10.1038/s41573-018-0008-x
Dinkova-Kostova, A. T., Holtzclaw, W. D., Cole, R. N., Itoh, K., Wakabayashi, N., Katoh, Y., et al. (2002). Direct Evidence that Sulfhydryl Groups of Keap1 Are the Sensors Regulating Induction of Phase 2 Enzymes that Protect against Carcinogens and Oxidants. Proc. Natl. Acad. Sci. U. S. A. 99, 11908–11913. doi:10.1073/pnas.172398899
Feng, X.-H., Xu, H.-Y., Wang, J.-Y., Duan, S., Wang, Y.-C., and Ma, C.-M. (2021). In Vivo hepatoprotective Activity and the Underlying Mechanism of Chebulinic Acid from Terminalia Chebula Fruit. Phytomedicine 83, 153479. doi:10.1016/j.phymed.2021.153479
Garcia, R., Salluh, J. I. F., Andrade, T. R., Farah, D., da Silva, P. S. L., Bastos, D. F., et al. (2021). A Systematic Review and Meta-Analysis of Propofol versus Midazolam Sedation in Adult Intensive Care (ICU) Patients. J. Crit. Care 64, 91–99. doi:10.1016/j.jcrc.2021.04.001
Giustarini, D., Dalle-Donne, I., Tsikas, D., and Rossi, R. (2009). Oxidative Stress and Human Diseases: Origin, Link, Measurement, Mechanisms, and Biomarkers. Crit. Rev. Clin. Lab. Sci. 46, 241–281. doi:10.3109/10408360903142326
Helmy, S. A., and Al-Attiyah, R. J. (2001). The Immunomodulatory Effects of Prolonged Intravenous Infusion of Propofol versus Midazolam in Critically Ill Surgical Patients. Anaesthesia 56, 4–8. doi:10.1046/j.1365-2044.2001.01713.x
Kaspar, J. W., Niture, S. K., and Jaiswal, A. K. (2009). Nrf2:INrf2 (Keap1) Signaling in Oxidative Stress. Free Radic. Biol. Med. 47, 1304–1309. doi:10.1016/j.freeradbiomed.2009.07.035
Koike, K. (2007). Pathogenesis of HCV-Associated HCC: Dual-Pass Carcinogenesis through Activation of Oxidative Stress and Intracellular Signaling. Hepatol. Res. 37 Suppl 2 (Suppl. 2), S115–S120. doi:10.1111/j.1872-034X.2007.00173.x
Kostopanagiotou, G. G., Grypioti, A. D., Matsota, P., Mykoniatis, M. G., Demopoulos, C. A., Papadopoulou-Daifoti, Z., et al. (2009). Acetaminophen-induced Liver Injury and Oxidative Stress: Protective Effect of Propofol. Eur. J. Anaesthesiol. 26, 548–553. doi:10.1097/EJA.0b013e32831c8a01
Lazzaroni, M., and Bianchi Porro, G. (2003). Preparation, Premedication and Surveillance. Endoscopy 35, 103–111. doi:10.1055/s-2003-37012
Li, G. Z., Tao, H. L., Zhou, C., Wang, D. D., and Peng, C. B. (2018a). Midazolam Prevents Motor Neuronal Death from Oxidative Stress Attack Mediated by JNK-ERK Pathway. Hum. Cell 31, 64–71. doi:10.1007/s13577-017-0184-8
Li, J., Tan, H., Zhou, X., Zhang, C., Jin, H., Tian, Y., et al. (2018b). The Protection of Midazolam against Immune Mediated Liver Injury Induced by Lipopolysaccharide and Galactosamine in Mice. Front. Pharmacol. 9, 1528. doi:10.3389/fphar.2018.01528
Lian, Y. H., Fang, J., Zhou, H. D., Jiang, H. F., and Xie, K. J. (2019). Sufentanil Preconditioning Protects against Hepatic Ischemia-Reperfusion Injury by Suppressing Inflammation. Med. Sci. Monit. 25, 2265–2273. doi:10.12659/MSM.913145
Lim, J.-Y., Lee, J.-H., Yun, D.-H., Lee, Y.-M., and Kim, D.-K. (2021). Inhibitory Effects of Nodakenin on Inflammation and Cell Death in Lipopolysaccharide-Induced Liver Injury Mice. Phytomedicine 81, 153411. doi:10.1016/j.phymed.2020.153411
Liu, J. Y., Guo, F., Wu, H. L., Wang, Y., and Liu, J. S. (2017). Midazolam Anesthesia Protects Neuronal Cells from Oxidative Stress-Induced Death via Activation of the JNK-ERK Pathway. Mol. Med. Rep. 15, 169–179. doi:10.3892/mmr.2016.6031
Martin, F., van Deursen, J. M., Shivdasani, R. A., Jackson, C. W., Troutman, A. G., and Ney, P. A. (1998). Erythroid Maturation and Globin Gene Expression in Mice with Combined Deficiency of NF-E2 and Nrf-2. Blood 91, 3459–3466. doi:10.1182/blood.V91.9.3459
Oldman, A. H., Martin, D. S., Feelisch, M., Grocott, M. P. W., and Cumpstey, A. F. (2021). Effects of Perioperative Oxygen Concentration on Oxidative Stress in Adult Surgical Patients: a Systematic Review. Br. J. Anaesth. 126, 622–632. doi:10.1016/j.bja.2020.09.050
Peng, X., Dai, C., Liu, Q., Li, J., and Qiu, J. (2018). Curcumin Attenuates on Carbon Tetrachloride-Induced Acute Liver Injury in Mice via Modulation of the Nrf2/HO-1 and TGF-β1/Smad3 Pathway. Molecules 23, 215. doi:10.3390/molecules23010215
Russo, M., Spagnuolo, C., Russo, G. L., Skalicka-Woźniak, K., Daglia, M., Sobarzo-Sánchez, E., et al. (2018). Nrf2 Targeting by Sulforaphane: A Potential Therapy for Cancer Treatment. Crit. Rev. Food Sci. Nutr. 58, 1391–1405. doi:10.1080/10408398.2016.1259983
Sun, J., Wu, Y., Long, C., He, P., Gu, J., Yang, L., et al. (2018). Anthocyanins Isolated from Blueberry Ameliorates CCl(4) Induced Liver Fibrosis by Modulation of Oxidative Stress, Inflammation and Stellate Cell Activation in Mice. Food Chem. Toxicol. 120, 491–499. doi:10.1016/j.fct.2018.07.048
Tang, W., Jiang, Y. F., Ponnusamy, M., and Diallo, M. (2014). Role of Nrf2 in Chronic Liver Disease. World J. Gastroenterol. 20, 13079–13087. doi:10.3748/wjg.v20.i36.13079
Toro-Pérez, J., and Rodrigo, R. (2021). Contribution of Oxidative Stress in the Mechanisms of Postoperative Complications and Multiple Organ Dysfunction Syndrome. Redox Rep. 26, 35–44. doi:10.1080/13510002.2021.1891808
Weckmann, K., Deery, M. J., Howard, J. A., Feret, R., Asara, J. M., Dethloff, F., et al. (2017). Ketamine's Antidepressant Effect Is Mediated by Energy Metabolism and Antioxidant Defense System. Sci. Rep. 7, 15788. doi:10.1038/s41598-017-16183-x
Wu, Q., Zhang, D., Tao, N., Zhu, Q. N., Jin, T., Shi, J. S., et al. (2014). Induction of Nrf2 and Metallothionein as a Common Mechanism of Hepatoprotective Medicinal Herbs. Am. J. Chin. Med. 42, 207–221. doi:10.1142/S0192415X14500141
Xiong, Y., Zhu, G. H., Zhang, Y. N., Hu, Q., Wang, H. N., Yu, H. N., et al. (2021). Flavonoids in Ampelopsis Grossedentata as Covalent Inhibitors of SARS-CoV-2 3CL(pro): Inhibition Potentials, Covalent Binding Sites and Inhibitory Mechanisms. Int. J. Biol. Macromol. 187, 976–987. doi:10.1016/j.ijbiomac.2021.07.167
Yamamoto, M., Kensler, T. W., and Motohashi, H. (2018). The KEAP1-NRF2 System: a Thiol-Based Sensor-Effector Apparatus for Maintaining Redox Homeostasis. Physiol. Rev. 98, 1169–1203. doi:10.1152/physrev.00023.2017
Yang, B. Y., Zhang, X. Y., Guan, S. W., and Hua, Z. C. (2015). Protective Effect of Procyanidin B2 against CCl4-Induced Acute Liver Injury in Mice. Molecules 20, 12250–12265. doi:10.3390/molecules200712250
Zhao, D. F., Fan, Y. F., Wang, F. Y., Hou, F. B., Gonzalez, F. J., Li, S. Y., et al. (2021). Discovery and Characterization of Naturally Occurring Potent Inhibitors of Catechol-O-Methyltransferase from Herbal Medicines. RSC Adv. 11, 10385–10392. doi:10.1039/d0ra10425f
Keywords: oxidative stress, Nrf2, midazolam, acute liver injury, molecular docking simulations
Citation: Zhang Y, Zhu Y, Li Y, Ji F, Ge G and Xu H (2022) Midazolam Ameliorates Acute Liver Injury Induced by Carbon Tetrachloride via Enhancing Nrf2 Signaling Pathway. Front. Pharmacol. 13:940137. doi: 10.3389/fphar.2022.940137
Received: 10 May 2022; Accepted: 20 June 2022;
Published: 08 July 2022.
Edited by:
Guo Ma, Fudan University, ChinaReviewed by:
Milton Prabu, Annamalai University, IndiaLan Tang, Southern Medical University, China
Xuan Qin, Baylor College of Medicine, United States
Copyright © 2022 Zhang, Zhu, Li, Ji, Ge and Xu. This is an open-access article distributed under the terms of the Creative Commons Attribution License (CC BY). The use, distribution or reproduction in other forums is permitted, provided the original author(s) and the copyright owner(s) are credited and that the original publication in this journal is cited, in accordance with accepted academic practice. No use, distribution or reproduction is permitted which does not comply with these terms.
*Correspondence: Guangbo Ge, Z2VndWFuZ2JvQGRpY3AuYWMuY24=; Hua Xu, cHNoaHVheHVAMTYzLmNvbQ==
†These authors have contributed equally to this work