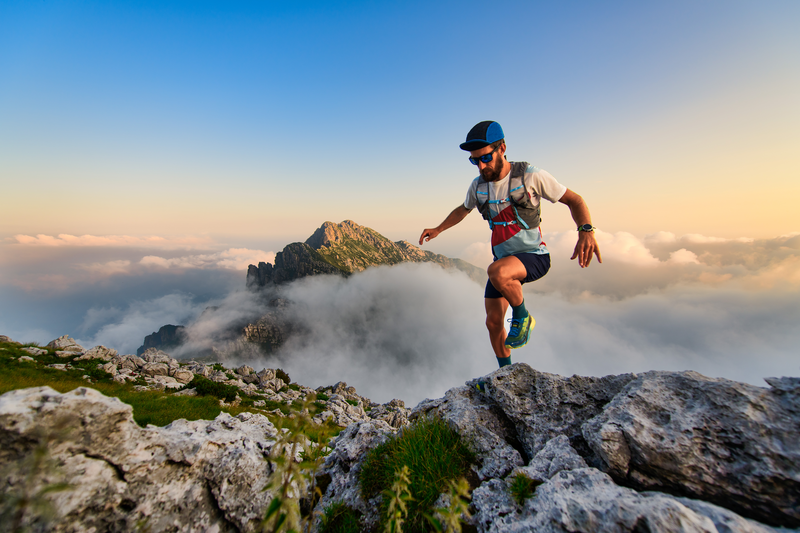
94% of researchers rate our articles as excellent or good
Learn more about the work of our research integrity team to safeguard the quality of each article we publish.
Find out more
ORIGINAL RESEARCH article
Front. Pharmacol. , 03 October 2022
Sec. Experimental Pharmacology and Drug Discovery
Volume 13 - 2022 | https://doi.org/10.3389/fphar.2022.938760
Emerging evidence suggests that butyrate, a short-chain fatty acid, may have beneficial effects on obesity and its associated metabolic comorbidities, but the related molecular mechanism is largely unknown. This study aims to investigate the role of butyrate in diet-induced obesity and metabolic disorders and the relevant regulatory mechanisms. Here, dietary supplementation with Sodium butyrate (NaB) was carried out in mice fed with a high-fat diet (HFD) or chow diet. At week 14, mice on HFD displayed an obese phenotype and down-regulated expression of thermogenic regulators including Ucp-1 and Pgc-1α in adipose tissue. Excitingly, NaB add-on treatment abolished these detrimental effects. Moreover, the obesity-induced insulin resistance, inflammation, fatty liver, and intestinal dysfunction were also attenuated by NaB administration. Mechanistically, NaB can promote fat thermogenesis via the increased local sympathetic innervation of adipose tissue, and blocking the β3-adrenergic signaling pathway by 6-hydroxydopamine abolished NaB-induced thermogenesis. Our study reveals a potential pharmacological target for NaB to combat obesity and metabolic disorders.
In recent years, along with the change in dietary habits and sedentary lifestyles, the prevalence of obesity significantly increased (Soltani et al., 2021). Obesity is associated with many chronic diseases such as diabetes, fatty liver, and cancer (Park et al., 2020), which arises from an imbalance between food intake and energy expenditure that eventually leads to an excessive accumulation of fat mass (Kim et al., 2020). Mammalian adipose tissue is generally classified into white and brown adipose tissues. White adipose tissue (WAT) stores energy in the form of triglycerides, and excessive WAT results in obesity and insulin resistance (An et al., 2021). Meanwhile, brown adipose tissue (BAT) consumes energy depending on the mitochondrial uncoupling protein 1 (UCP-1), which dissipates the mitochondrial proton gradient leading to heat generation (Shamsi et al., 2020). Recently, a new type of thermogenic adipocyte, known as beige adipocyte, has emerged in WAT upon cold exposure or β3-adrenergic receptor (β3AR) agonists (Liu et al., 2019; Becher et al., 2021). This process is the so-called “WAT browning” (Becher et al., 2021). Multiple studies have shown that increasing brown and beige fat activity can effectively increase whole-body energy expenditure, which can be used therapeutically to reduce diet-induced obesity and the associated complications (Balazova et al., 2021; Cui et al., 2021).
Adipose tissue is innervated by the sympathetic nervous system (SNS), which plays a key role in controlling its lipolytic and thermogenic functions (Bartness et al., 2014). When exposed to lower temperatures, cold stimulates sympathetic outflow to adipose depots, which acts through secreting neurotransmitter norepinephrine (NE) to adipocytes (Seki et al., 2016). Mechanically, NE binds to β3AR, then activates cyclic adenosine monophosphate (cAMP)-dependent protein kinase A (PKA) (Harms and Seale, 2013), which ultimately promotes the activation of transcriptional factors such as cAMP-response element binding protein (Creb), thermogenic markers such as peroxisome proliferator-activated receptor γ coactivator-1α (Pgc-1α) and uncoupling protein 1 (Ucp-1) (Altarejos and Montminy, 2011). Surgical or pharmacologic destruction of these sympathetic fibers inhibits the leptin-stimulated lipolytic response and cold-induced beiging process of WAT(Zeng et al., 2015; Jiang et al., 2017). Meanwhile, optogenetic activation of sympathetic fibers in fat has been reported to induce lipolysis and reduce fat mass (Zeng et al., 2015). Thus, targeting the sympathetic neurons in fat has the potential to be a novel anti-obesity strategy.
The short-chain fatty acids (SCFAs) including acetate, propionate, and butyrate are products of dietary fiber metabolization by gut microbiota (Luu et al., 2019). SCFAs are widely thought to serve multiple roles in host homeostasis and mediate the beneficial effects of the microbiota community (Zhang et al., 2020). Among SCFAs, butyrate has been studied extensively in immunomodulatory and gut barrier function (Luu et al., 2019). Comparisons of microbiota composition have shown lower abundance of a butyrate-producing bacterium in diabetic or obese patients, while dietary supplementation with butyrate ameliorates inflammation and insulin resistance (Li et al., 2020; Gart et al., 2021). Thus, increasing attention has been paid to butyrate for its protective role in obesity (Coppola et al., 2021). In this study, Sodium butyrate (NaB) was found to protect against diet-induced-obesity (DIO) by activating brown fat and white fat browning via the increased sympathetic innervation of adipose tissue, thus attenuated the consequent insulin resistance and inflammation which is expected to provide an opportunity for combating obesity and metabolic diseases.
C57BL/6J male mice, 6 weeks old, 20–22 g (HFK Co. Ltd., Beijing, China) were used in this study. All mice were housed in temperature-controlled (23 ± 2°C), pathogen-free barrier facility on a 12 h/12 h light/dark cycle and had access to food and water ad libitum. After 1 week of acclimatization, all mice were randomly divided into three groups, six mice per group: control group that received normal chow diet (CD, 1022); obese group that received high-fat diet (HFD, D12492, 60 kcal% fat); NaB-treated group that received HFD with 0.4% w/w of NaB (HNaB). The whole experiment lasted for 14 weeks, then mice were fasted overnight and euthanized under terminal anesthesia. Blood samples, adipose tissue, liver and gut were collected for further experiment. All animal studies were conducted in compliance with the National Institutes of Health guidelines and were approved by the Animal Care and Use Committee of Southwest Medical University.
Mouse blood samples were allowed to clot at room temperature followed by extraction of serum via centrifugation. Serum triglycerides, total cholesterol and glucose were measured using standard enzymatic methods. For serum tumor necrosis factor-α(TNF-α), Lipopolysaccharide (LPS) and insulin measurement, the ELISA kits were used according to the manufacturer’s instructions.
Mouse colon, liver and adipose tissues were fixed with 10% neutral buffered formalin for 24 h, embedded in paraffin blocks. All slices were dewaxed with xylene for 10 min, anhydrous ethanol for 10 min, 90% ethanol for 5 min, 80% ethanol for 5 min and 70% ethanol for 5 min. Tissue histology was evaluated using hematoxylin-eosin staining. Image acquisition was carried out by using a microscope (Olympus America Inc. Melville, NY, United States). Adipocyte cross-sectional area and distribution were determined using ImageJ software (NIH image software).
The waxing and dewaxing of the tissue samples were performed as described previously. Deparaffinized sections were rehydrated and boiled in 10 mM citric acid at 98°C for 10 min, then treated for 20 min with 0.3% H2O2 in PBS for antigen retrieval. Tissue sections were incubated with 10% normal goat serum for 30 min to block non-specific antibody binding. After washing, primary antibodies were incubated overnight at 4°C (anti-UCP-1, 1:500). The following day, the sections were incubated with the appropriate secondary antibodies. The 3,3-diaminobenzidine (DAB) solution was used as a chromogen. Images were acquired with a microscope connected to a camera (Olympus America Inc. Melville, NY, United States).
The liver, gut and adipose tissues were homogenized using TissueLyzer and total RNA was isolated using TRIzol Reagent (Vazyme, Nanjing, China). 2–5 ng of cDNA was amplified using Bio-Rad CFX ConnectTM Real-Time System (CFX ConnectTM Real-time system, Bio-Rad, United States) with universal SYBR green mix (Vazyme, Nanjing, China). The thermal profile employed one cycle of initial denaturation at 95°C for 5 min, 39 cycles of denaturation at 95°C for 20 s, annealing at 60°C for 20 s and extension at 72°C for 20 s. Relative expression levels of target genes were converted using the 2−ΔΔCT method against the internal control 18 s, and data were presented as fold change relative to control groups. For primer sequences, refer to Supplementary Table S1.
Adipose tissues were homogenized with 1×loading lysis buffer. Protein concentrations were detected by a Bradford protein assay kit (Thermo Scientific, United States). Equal amounts of protein were loaded on 10% SDS-PAGE gel and transferred onto a polyvinylidene difluoride (PVDF) membrane (Millipore, Billerica, MA). The membranes were blocked by 5% skim milk for 60 min, and then incubated overnight at 4°C with primary antibody. Membranes were washed and incubated in secondary antibody for 1 h. The ECL chromogenic substrate was used to visualize the bands (Epizyme Biotech, China). Protein bands were quantified using ImageJ software. For primary antibodies, refer to Supplementary TableS2.
All statistical analyses were performed using GraphPad Prism eight software (San Diego, CA, United States). All data are presented as mean ± SE. The data were analyzed using one-way analysis of variance (ANOVA) followed by post hoc Tukey’s tests and paired sample t test for comparisons between multiple groups. p < 0.05 was considered as statistically significant.
To explore the effect of dietary supplementation of NaB on diet-induced obesity, the body weight of mice was measured weekly in this study. As shown in Figure 1A, the HFD mice gradually showed a difference in body weight after 3 weeks of feeding compared to the mice on chow diet. At 14 weeks, mice in the HFD group displayed an obese phenotype, dietary supplementation of NaB protected mice from diet-induced obesity (Figure 1A). Then, all mice were sacrificed and the adipose tissues were isolated. The weight of inguinal subcutaneous adipose tissue (sWAT), epididymal adipose tissue (eWAT) and brown adipose tissue in mice from the CD and NaB groups was significantly lower than that from the HFD group (Figure 1B). Obesity is characterized by an excessive accumulation of WAT, which expands by adipocyte hypertrophy and hyperplasia. Therefore, to evaluate the effect of NaB on white fat, HE staining was performed. Compared with control group, adipocytes in the HFD group exhibited round cell borders and larger cell area (Figures 1C,D). However, this phenotype was effectively ameliorated by NaB treatment, and multilocular beige adipocytes was observed in sWAT and eWAT from mice in the NaB group (Figures 1C,D). Moreover, NaB-treated mice exhibited higher proportion of small size adipocytes than that of mice on HFD (Figures 1E,F), which suggested that NaB might have potential effects on dietary obesity and fat deposition.
FIGURE 1. Effects of NaB on body weight under HFD. (A) The growth curves of mice under HFD (n = 5–6); (B) the weight of brown adipose tissue, subcutaneous adipose tissue and epididymal adipose tissue (n = 5–6); (C,D) H&E staining of subcutaneous adipose tissue and epididymal adipose tissue sections, scale bar = 50 μm; (E,F) Frequency distribution of adipocyte cell size in subcutaneous adipose tissue and epididymal adipose tissue (n = 5–6); (G) Daily calorie intake in mice (n = 5–6); (H) Infrared imaging of core body temperature in mice at 22°C (n = 5–6); (I) Core body temperature in mice at 4°C cold exposure (n = 5–6). Values are represented as the mean ± SE. One-way ANOVA with Tukey’s post hoc test, ∗p < 0.05, ∗∗p < 0.01 respectively compared with the HFD group.
We next performed experiments to investigate the relevant mechanisms. As shown in Figure 1G, slight or no changes was observed in food intake among groups, meanwhile, NaB-treated mice exhibited a higher core body temperature at room temperature compared with mice on HFD (Figure 1H). Furthermore, compared with mice on chow diet, HFD-fed mice showed a rapid decrease in temperature when subjected to acute cold exposure, but this trend was diminished by NaB (Figure 1I). Together, these data suggested that the decreased adiposity in NaB-treated mice might be due to the enhanced energy expenditure.
Given that adipose tissue is a major organ for energy expenditure, we then performed a chain of experiments to investigate the effects of NaB on fat metabolism. qRT-PCR analysis of sWAT showed that the mRNA expression of genes involved in thermogenesis including Ucp-1 and Pgc-1α, specific beige-selective genes including Hoxa9 and Tmem 26, were significantly increased in sWAT from NaB-treated mice (Figure 2A). In addition, the decreased expression of Tfam (an important mitochondrial biogenesis marker) induced by HFD was also abolished largely by NaB treatment (Figure 2A). Immumohistochemical staining and Western blot analysis further identified that UCP-1 and PGC-1α protein levels were also upregulated by NaB in sWAT (Figures 2B,C). Despite not being considered to be the main organ for thermogenesis, eWAT is also shown to play a regulatory role in energy metabolism (Wan et al., 2014). Consistently, the protein levels of UCP-1 and PGC-1α in eWAT were also upregulated by NaB treatment (Supplementary FigureS1). These results suggested that NaB can promote the browning of WAT and energy expenditure in DIO.
FIGURE 2. Effects of NaB on white adipose tissue in DIO. (A) mRNA expression of thermogenic genes and specific beige-selective genes (n = 5–6); (B) immunohistochemical staining of UCP-1 in subcutaneous adipose tissue and epididymal adipose tissue, scale bar = 50 μm; (C) Protein levels of PGC-1α and UCP-1 in subcutaneous adipose tissue (n = 3, representative of three biological replicates for each group). Relative intensity was calculated by using ImageJ software. Values are represented as the mean ± SE. One-way ANOVA with Tukey’s post hoc test, ∗p < 0.05, ∗∗p < 0.01 respectively compared with the HFD group.
It is well documented that obesity is usually accompanied with disorders of adipose tissue function, lipid accumulation often results in the “whitening” of brown fat and impaired thermogenesis (Lee C. C. et al., 2019). We next performed experiments to determine whether the function of brown adipose tissue can be influenced by NaB. Indeed, HE staining analysis showed that compared with mice on CD, the area of brown adipocyte in mice from HFD group was increased obviously. Meanwhile, similar to white adipocytes, multilocular brown adipocytes were remodeled into unilocular adipocytes (Figure 3A). In order to further assess the mechanism of NaB on brown fat, we detected the mRNA expression of thermogenic genes. Compared with the CD group, thermogenic genes including Ucp-1, Prdm16 and Pgc-1α were down-regulated significantly. However, NaB significantly restored these expression abnormalities (Figure 3B). Moreover, NaB treatment also promoted the protein expression of UCP-1 and PGC-1α of brown fat (Figures 3C,D). These data indicated that the activity of brown fat was enhanced by NaB treatment.
FIGURE 3. Effects of NaB on brown adipose tissue in DIO. (A) H&E staining, scale bar = 50 μm; (B) mRNA expression of thermogenic genes in brown adipose tissue (n = 5–6); (C) immunohistochemical staining of UCP-1 in interscapular brown adipose tissue, scale bar = 50 μm; (D) Protein levels of PGC-1α, UCP-1 in brown adipose tissue (n = 3, representative of three biological replicates for each group). Relative intensity was calculated by using ImageJ software. Values are represented as the mean ± SE. One-way ANOVA with Tukey’s post hoc test, ∗p < 0.05, ∗∗p < 0.01 respectively compared with the HFD group.
It has been shown that fat lipolysis and thermogenesis is mediated by sympathetic neurons that innervate adipocytes. To assess whether the effect of NaB on thermogenesis was attributable to sympathetic outputs onto the adipose tissue, we performed Elisa analysis and found the levels of key sympathetic neurotransmitters NE in both sWAT and BAT were significantly increased in NaB-treated mice (Figure 4A and Figure 4C). mRNA and protein levels of tyrosine hydroxylase (TH), a marker for sympathetic neurons, were upregulated in both sWAT and BAT from NaB-treated mice (Figure 4B and Figure 4D). Additionally, the expression of ubiquitin carboxyl-terminal esterase L1 (Uchl1/PGP9.5), another pan-neuronal marker, was also increased by NaB treatment (Figure 4B and Figure 4D). Moreover, dietary supplementation of NaB led to higher mRNA levels of dopamine β-hydroxylase (Dbh, an enzyme required for catecholamine synthesis) compared with HFD mice (Figure 4B and Figure 4D). Together, the results provide evidence that activity of adipose sympathetic nerves was enhanced by NaB. Effects of the SNS on fat metabolism is mainly mediated via cAMP-PKA signaling pathway, which induces a chain of cascade reactions likely the phosphorylation of CREB, ultimately enhances the transcriptional activity of Pgc-1α and Ucp-1. Here, we observed increased phosphorylation of PKA substrate and CREB in sWAT and BAT from NaB -treated mice (Figures 4E,F). Accordingly, the activation of BAT and browning of WAT induced by NaB might be, at least partly, via the increased intro-adipose sympathetic innervation.
FIGURE 4. Effects of NaB on sympathetic innervation of adipose tissue. (A–C) Levels of norepinephrine in brown adipose tissue and subcutaneous adipose tissue (n = 5–6); (B–D) mRNA expression of Dbh, Th, and Uchl1/Pgp9.5 in brown adipose tissue and subcutaneous adipose tissue (n = 5–6); (E,F) Protein levels of TH, Uchl1/PGP9.5, CREB, p-CREB, and p-PKA-substrate in brown adipose tissue and subcutaneous adipose tissue (n = 3, representative of three biological replicates for each group). Relative intensity was calculated by using ImageJ software. Values are represented as the mean ± SE. One-way ANOVA with Tukey’s post hoc test, ∗p < 0.05, ∗∗p < 0.01 respectively compared with the HFD group.
To further comfirm the observed phenotype is related to enhanced sympathetic innervation, we applied the “unilateral denervation model”. Selective local sympathetic denervation is accomplished by intra-sWAT microinjections of the catecholamine-specific neurotoxin 6-hydroxy-dopamine (6-OHDA) (Rooks et al., 2005; Foster and Bartness, 2006; Giordano et al., 2006). We found that the increased protein levels of thermogenic regulators induced by NaB were abolished when blocking the β3-adrenergic signaling pathway by 6-OHDA (Figures 5A,B). In addition, it has been indicated that Brain-derived neurotrophic factor (BDNF) neurons in hypothalamus can drive thermogenesis by regulating sympathetic innervation of adipose tissue (An et al., 2015; Wang P. et al., 2020). By performing western blot analysis, NaB treatment significantly enhanced the protein levels of BDNF in hypothalamus (Figure 5C). These data indicated that the upregulated BDNF in hypothalamus might be involved in the increased intro-adipose sympathetic innervation induced by NaB.
FIGURE 5. NaB enhances sympathetic nerve activity. Subcutaneous adipose tissue of mice under HFD was locally treated with saline or 6-OHDA. (A) Western blotting analysis of TH, Uchl1/PGP9.5, CREB, p-CREB in subcutaneous adipose tissue (n = 3, representative of three biological replicates for each group); (B) Western blotting analysis of PGC-1α, UCP-1in subcutaneous adipose tissue (n = 3, representative of three biological replicates for each group); (C) Western blotting analysis of BDNF in hypothalamus (n = 3, representative of three biological replicates for each group). Values are represented as the mean ± SE. One-way ANOVA with Tukey’s post hoc test, ∗p < 0.05, ∗∗p < 0.01 respectively compared with the HFD group.
Obesity is characterized by insulin resistance and low-grade chronic inflammation, we next measured the levels of plasma glucose, triglyceride and total cholesterol, and found that dietary supplementation of NaB can effectively improve the status of hyperglycemia and hypertriglyceridemia in obese mice (Figures 6A,B). To explore the effect of NaB on insulin sensitivity, we evaluated fasting insulin levels and calculated the value of homeostasis model assessment of insulin resistance (HOMA-IR) in all mice, and found that NaB treatment significantly reversed the hyperinsulinemia induced by HFD and improved insulin resistance (Figure 6B).
FIGURE 6. Effects of NaB on inflammation and insulin resistance in DIO. (A) Serum levels of triglyceride (n = 5–6); (B) Plasma glucose, insulin and the value of HOMA-IR (n = 5–6); (C) Serum TNF-α levels (n = 5–6); (D) mRNA expression of inflammatory cytokines including F4/80, Il-6, and Tnf-α in subcutaneous adipose tissue (n = 5–6). Values are represented as the mean ± SE. n = 5–6. One-way ANOVA with Tukey’s post hoc test, ∗p < 0.05, ∗∗p < 0.01 respectively compared with the HFD group.
To further address the role of NaB on inflammation, we next performed Elisa analysis to detect the pro-inflammatory factors. The results showed that compared with the control mice, the serum TNF-α levels of mice on HFD were increased, while this effect was diminished by NaB(Figure 6C). Moreover, increased adiposity, especially in the visceral, is reported to directly cause an increase in systemic inflammation. We then performed qRT-PCR analysis on subcutaneous white fat and found that the mRNA expression levels of inflammatory cytokines such as Tnf-α, F4/80, and IL-6 were distinctly increased in DIO. However, dietary supplementation of NaB effectively inhibited these aberrant expressions (Figure 6D). All these data indicated that NaB can effectively attenuate inflammation and insulin resistance in DIO.
Fat metabolism disorders are shown to cause fatty liver and intestinal barrier dysfunction. In our results, the hepatocytes in the HFD group were swollen and exhibited increased lipid vacuoles, and dietary addition of NaB can effectively attenuate fat deposition in the liver (Figure 7A). Meanwhile, total liver lipids were determined and hepatic triglyceride and total cholesterol levels were found to be decreased by NaB (Figure 7B). Fat accumulation in the liver results in oxidative stress and the release of inflammatory cytokines. By performing qRT-PCR analysis of liver, add-on treatment of NaB significantly decreased the expression of pro-inflammatory gene (Tnf-α and F4/80) and increased the expression of anti-inflammatory gene (Il-10) compared with mice on HFD (Figure 7C).
FIGURE 7. Effects of NaB on fatty liver and gut barrier function in DIO. (A) H&E staining of the liver, scale bar = 100 μm; (B) levels of triglyceride and total cholesterol in liver (n = 5–6). (C) mRNA expression of proinflammatory genes (Tnf-α, F4/80) and anti-inflammatory gene (Il-10) in liver; (D) H&E staining of colon (n = 5–6); (E) mRNA expression of Z O -1, Occludin, and Muc-2 in colon; (F) Serum LPS levels (n = 5–6); (G) mRNA expression of inflammatory gene (F4/80 and Il-6) in colon (n = 5–6). Values are represented as the mean ± SE. One-way ANOVA with Tukey’s post hoc test, ∗p < 0.05, ∗∗p < 0.01 respectively compared with the HFD group.
HFD has once been shown to damage the intestinal epithelial barrier and trigger intestinal leakage. In this study, H&E staining demonstrated that mice on HFD displayed blunted colon villi and elongated crypts (Figure 7D), moreover, the expression of tight junction protein zonula occludens-1 (ZO-1), mucoprotein-2 (MUC-2) and Occludin in the colon from HFD mice was decreased, while NaB treatment ameliorated these phenotypes (Figure 7E). Impaired barrier function increases intestinal permeability and thereby facilitates the translocation of microbiota-derived endotoxins such as LPS into the systemic circulation (Ma et al., 2020). Here, increased serum LPS induced by HFD was abolished by NaB treatment (Figure 7F). qRT-PCR analysis further showed that NaB treatment decreased the abnormal upregulated expression of inflammatory factors (F4/80 and Il-6) in colon induced by HFD (Figure 7G). All these data demonstrated that dietary supplementation of NaB can ameliorate fatty liver and gut barrier dysfunction in DIO.
Obesity is a high risk factor of multiple metabolic and cardiovascular diseases (Merry et al., 2020). In recent years, flora-metabolites such as SCFAs and bile acids that are reported to be beneficial for metabolic disorders (Zhang et al., 2021). As a major component of SCFAs, butyrate has been shown to effectively improve glucose and lipid metabolism in diet-induced obesity (Beisner et al., 2021). In Beisner et al’study, the combination of inulin and NaB can improve barrier function induced by western diet and antimicrobial peptide function in the ileum, and ultimately reduce weight gain and liver weight (Beisner et al., 2021). Meanwhile, Fang et al reported that NaB treatment effectively remodeled intestinal microflora structure and attenuated HFD-induced intestinal barrier, leading to decreased serum LPS levels (Fang et al., 2019). In this study, we observed that NaB can promote the activation of brown adipocytes and browning of white adipocytes, which ultimately leads to increased energy expenditure and improvement in insulin resistance, fatty liver, and intestinal dysfunction. Furthermore, these beneficial effects of NaB on thermogenesis is mediated in part through the sympathetic innervation of adipose tissue.
In previous studies, NaB have been thought to function principally in the gut. However, other study has shown that long-term dietary supplementation of NaB can facilitate this little molecule entering the blood circulation, and act directly on brain or adipose tissue (Dalile et al., 2019). Li et al further demonstrated that butyric acid is able to participate in the tricarboxylic acid cycle of brown and white fat (Li et al., 2019), which provides the possibility that NaB directly regulates the fat metabolism. Additionally, a recent study revealed that butyrate gavage effectively reversed the thermogenic functional impairment in antibiotic cocktail (ABX) -treated mice, which acted mainly through the activation of lysine specific demethylase in BAT and sWAT(Wang D. et al., 2020). In the current study, we found that the thermogenic effect of NaB is associated with the action of intro-adipose sympathetic nerve fibers, which uncovered a novel regulatory mechanism of butyrate in glucose and lipid metabolism. It is worth noting that besides adipose tissue, other metabolic tissues such as skeletal muscle and pancreas are also involved in the regulation of energy homeostasis (Jouvet and Estall, 2017; Balakrishnan and Thurmond, 2022), whether these tissues play a regulatory role in NaB-induced metabolic benefits remain to be determined.
Butyrate entered in systemic circulation can be distributed to the brain, and influence the expression of multiple neurotrophic factors to play regulatory roles (Petry et al., 2016; Sun et al., 2016). For instance, NaB stimulates cell proliferation and differentiation in the dentate gyrus, and enhances the expression of BDNF, prevents the impairing effects of hippocampal gastrin-releasing peptide receptor antagonism on memory consolidation and extinction (Lee H. J. et al., 2019). BDNF is also reported to be a key regulator of energy balance, and BDNF neurons in medial and posterior paraventricular hypothalamus drive thermogenesis by projecting to spinal cord and forming polysynaptic connections to brown adipose tissues (An et al., 2015). Meanwhile, a leptin-BDNF pathway was also reported to regulate sympathetic innervation of adipose tissue (Wang P. et al., 2020). In this study, the protein levels of BDNF in hypothalamus was significantly enhanced by NaB treatment. Thus, we can speculate that NaB might promote sympathetic innervation of adipose tissue, at least partly, via the increased expression of BDNF in hypothalamus, and further experiments are needed to explore the intricate mechanisms.
It is well documented that obesity is a chronic and complex metabolic disease that involves multiple organs and tissues, and is associated with numerous health risks including cardiovascular disease, diabetes, fatty liver and gut dysfunction (Patterson et al., 2016; Wu and Ballantyne, 2020). Insulin resistance and inflammation play a vital role in the development of above obesity-related diseases (Wu and Ballantyne, 2020). Meanwhile, it has been shown that increased fat thermogenesis can improve insulin sensitivity and metabolic disease by regulating glucose and lipid metabolism (Brandão et al., 2021). In this study, we found that administration of NaB led to an activation of brown adipocytes and browning of white adipocytes, which contributed to the improvement of insulin resistance and inflammation. This may, at least partially explain the NaB-induced benefits in liver and gut function. However, whether the increased intro-adipose sympathetic activity directly affect liver or gut remain to be determined.
In summary, our study shows that chronic NaB treatment attenuated DIO and its associated metabolic disorders by promoting fat thermogenesis via the increased intro-adipose sympathetic innervation, which provides a new perspective for further investigating the pharmacological action of NaB in energy metabolism.
The raw data supporting the conclusions of this article will be made available by the authors, without undue reservation.
The animal study was reviewed and approved by the Animal Care and Use Committee of Southwest Medical University.
Conceptualization, YH and XY; methodology, XY, WZ, and KP; software, YZ; validation, WZ, CX, and KP; formal analysis, WZ, CX, KP, XT, and YL; investigation, WZ, KP, CX, and YZ; resources, XY and YL; data curation, WZ, CX, KP, YZ, XT, and YL writing-original draft preparation, WZ and KP; writing-review and editing, YH and XY; visualization, WZ and CX; supervision, YH and XY. All authors have read and agreed to the published version of the manuscript.
This study was supported by research funding from National Natural Science Foundation of China (No.82104251). Science and Technology Planning Project of Sichuan Province (No.2019YFS0180). Doctoral Research Initiation Fund of Affiliated Hospital of Southwest Medical University (No.21032). Foundation for Young Scholars of Southwest Medical University (No.2019ZQN151, 2020ZRQNB030).
The authors declare that the research was conducted in the absence of any commercial or financial relationships that could be construed as a potential conflict of interest.
All claims expressed in this article are solely those of the authors and do not necessarily represent those of their affiliated organizations, or those of the publisher, the editors and the reviewers. Any product that may be evaluated in this article, or claim that may be made by its manufacturer, is not guaranteed or endorsed by the publisher.
The Supplementary Material for this article can be found online at: https://www.frontiersin.org/articles/10.3389/fphar.2022.938760/full#supplementary-material
Altarejos, J. Y., and Montminy, M. (2011). CREB and the CRTC co-activators: Sensors for hormonal and metabolic signals. Nat. Rev. Mol. Cell Biol. 12, 141–151. doi:10.1038/nrm3072
An, J. J., Liao, G. Y., Kinney, C. E., Sahibzada, N., and Xu, B. (2015). Discrete BDNF neurons in the paraventricular hypothalamus control feeding and energy expenditure. Cell Metab. 22, 175–188. doi:10.1016/j.cmet.2015.05.008
An, M., Park, Y. H., and Lim, Y. H. (2021). Antiobesity and antidiabetic effects of the dairy bacterium Propionibacterium freudenreichii MJ2 in high-fat diet-induced obese mice by modulating lipid metabolism. Sci. Rep. 11, 2481. doi:10.1038/s41598-021-82282-5
Balakrishnan, R., and Thurmond, D. C. (2022). Mechanisms by which skeletal muscle myokines ameliorate insulin resistance. Int. J. Mol. Sci. 23, 4636. doi:10.3390/ijms23094636
Balazova, L., Balaz, M., Horvath, C., Horváth, Á., Moser, C., Kovanicova, Z., et al. (2021). GPR180 is a component of TGFβ signalling that promotes thermogenic adipocyte function and mediates the metabolic effects of the adipocyte-secreted factor CTHRC1. Nat. Commun. 12, 7144. doi:10.1038/s41467-021-27442-x
Bartness, T. J., Liu, Y., Shrestha, Y. B., and Ryu, V. (2014). Neural innervation of white adipose tissue and the control of lipolysis. Front. Neuroendocrinol. 35, 473–493. doi:10.1016/j.yfrne.2014.04.001
Becher, T., Palanisamy, S., Kramer, D. J., Eljalby, M., Marx, S. J., Wibmer, A. G., et al. (2021). Brown adipose tissue is associated with cardiometabolic health. Nat. Med. 27, 58–65. doi:10.1038/s41591-020-1126-7
Beisner, J., Filipe Rosa, L., Kaden-Volynets, V., Stolzer, I., Günther, C., and Bischoff, S. C. (2021). Prebiotic inulin and sodium butyrate attenuate obesity-induced intestinal barrier dysfunction by induction of antimicrobial peptides. Front. Immunol. 12, 678360. doi:10.3389/fimmu.2021.678360
Brandão, B. B., Poojari, A., and Rabiee, A. (2021). Thermogenic fat: Development, physiological function, and therapeutic potential. Int. J. Mol. Sci. 22, 5906. doi:10.3390/ijms22115906
Coppola, S., Avagliano, C., Calignano, A., and Berni Canani, R. (2021). The protective role of butyrate against obesity and obesity-related diseases. Mol. (Basel, Switz. 26, 682. doi:10.3390/molecules26030682
Cui, X., Jing, J., Wu, R., Cao, Q., Li, F., Li, K., et al. (2021). Adipose tissue-derived neurotrophic factor 3 regulates sympathetic innervation and thermogenesis in adipose tissue. Nat. Commun. 12, 5362. doi:10.1038/s41467-021-25766-2
Dalile, B., Van Oudenhove, L., Vervliet, B., and Verbeke, K. (2019). The role of short-chain fatty acids in microbiota-gut-brain communication. Nat. Rev. Gastroenterol. Hepatol. 16, 461–478. doi:10.1038/s41575-019-0157-3
Fang, W., Xue, H., Chen, X., Chen, K., and Ling, W. (2019). Supplementation with sodium butyrate modulates the composition of the gut microbiota and ameliorates high-fat diet-induced obesity in mice. J. Nutr. 149, 747–754. doi:10.1093/jn/nxy324
Foster, M. T., and Bartness, T. J. (2006). Sympathetic but not sensory denervation stimulates white adipocyte proliferation. Am. J. Physiol. Regul. Integr. Comp. Physiol. 291, R1630–R1637. doi:10.1152/ajpregu.00197.2006
Gart, E., Van Duyvenvoorde, W., Toet, K., Caspers, M. P. M., Verschuren, L., Nielsen, M. J., et al. (2021). Butyrate protects against diet-induced NASH and liver fibrosis and suppresses specific non-canonical TGF-β signaling pathways in human hepatic stellate cells. Biomedicines 9, 1954. doi:10.3390/biomedicines9121954
Giordano, A., Song, C. K., Bowers, R. R., Ehlen, J. C., Frontini, A., Cinti, S., et al. (2006). White adipose tissue lacks significant vagal innervation and immunohistochemical evidence of parasympathetic innervation. Am. J. Physiol. Regul. Integr. Comp. Physiol. 291, R1243–R1255. doi:10.1152/ajpregu.00679.2005
Harms, M., and Seale, P. (2013). Brown and beige fat: Development, function and therapeutic potential. Nat. Med. 19, 1252–1263. doi:10.1038/nm.3361
Jiang, H., Ding, X., Cao, Y., Wang, H., and Zeng, W. (2017). Dense intra-adipose sympathetic arborizations are essential for cold-induced beiging of mouse white adipose tissue. Cell Metab. 26, 686–692. e683. doi:10.1016/j.cmet.2017.08.016
Jouvet, N., and Estall, J. L. (2017). The pancreas: Bandmaster of glucose homeostasis. Exp. Cell Res. 360, 19–23. doi:10.1016/j.yexcr.2017.03.050
Kim, K., Nam, K. H., Yi, S. A., Park, J. W., Han, J. W., and Lee, J. (2020). Ginsenoside Rg3 induces browning of 3T3-L1 adipocytes by activating AMPK signaling. Nutrients 12, 427. doi:10.3390/nu12020427
Lee, C. C., Shih, Y. C., Kang, M. L., Chang, Y. C., Chuang, L. M., Devaraj, R., et al. (2019a). Naa10p inhibits beige adipocyte-mediated thermogenesis through N-α-acetylation of Pgc1α. Mol. Cell 76, 500–515. e508. doi:10.1016/j.molcel.2019.07.026
Lee, H. J., Son, Y., Lee, M., Moon, C., Kim, S. H., Shin, I. S., et al. (2019b). Sodium butyrate prevents radiation-induced cognitive impairment by restoring pCREB/BDNF expression. Neural Regen. Res. 14, 1530–1535. doi:10.4103/1673-5374.255974
Li, B., Li, L., Li, M., Lam, S. M., Wang, G., Wu, Y., et al. (2019). Microbiota depletion impairs thermogenesis of Brown adipose tissue and browning of white adipose tissue. Cell Rep. 26, 2720–2737. e2725. doi:10.1016/j.celrep.2019.02.015
Li, Q., Chang, Y., Zhang, K., Chen, H., Tao, S., and Zhang, Z. (2020). Implication of the gut microbiome composition of type 2 diabetic patients from northern China. Sci. Rep. 10, 5450. doi:10.1038/s41598-020-62224-3
Liu, P., Huang, S., Ling, S., Xu, S., Wang, F., Zhang, W., et al. (2019). Foxp1 controls Brown/beige adipocyte differentiation and thermogenesis through regulating β3-AR desensitization. Nat. Commun. 10, 5070. doi:10.1038/s41467-019-12988-8
Luu, M., Pautz, S., Kohl, V., Singh, R., Romero, R., Lucas, S., et al. (2019). The short-chain fatty acid pentanoate suppresses autoimmunity by modulating the metabolic-epigenetic crosstalk in lymphocytes. Nat. Commun. 10, 760. doi:10.1038/s41467-019-08711-2
Ma, L., Ni, Y., Wang, Z., Tu, W., Ni, L., Zhuge, F., et al. (2020). Spermidine improves gut barrier integrity and gut microbiota function in diet-induced obese mice. Gut microbes 12, 1–19. doi:10.1080/19490976.2020.1832857
Merry, T. L., Hedges, C. P., Masson, S. W., Laube, B., Pöhlmann, D., Wueest, S., et al. (2020). Partial impairment of insulin receptor expression mimics fasting to prevent diet-induced fatty liver disease. Nat. Commun. 11, 2080. doi:10.1038/s41467-020-15623-z
Park, E., Lee, C. G., Jeong, H., Yeo, S., Kim, J. A., and Jeong, S. Y. (2020). Antiadipogenic effects of mixtures of cornus officinalis and ribes fasciculatum extracts on 3T3-L1 preadipocytes and high-fat diet-induced mice. Mol. (Basel, Switz. 25, 2350. doi:10.3390/molecules25102350
Patterson, E., Ryan, P. M., Cryan, J. F., Dinan, T. G., Ross, R. P., Fitzgerald, G. F., et al. (2016). Gut microbiota, obesity and diabetes. Postgrad. Med. J. 92, 286–300. doi:10.1136/postgradmedj-2015-133285
Petry, F. S., Dornelles, A. S., Lichtenfels, M., Valiati, F. E., De Farias, C. B., Schwartsmann, G., et al. (2016). Histone deacetylase inhibition prevents the impairing effects of hippocampal gastrin-releasing peptide receptor antagonism on memory consolidation and extinction. Behav. Brain Res. 307, 46–53. doi:10.1016/j.bbr.2016.03.041
Rooks, C. R., Penn, D. M., Kelso, E., Bowers, R. R., Bartness, T. J., and Harris, R. B. (2005). Sympathetic denervation does not prevent a reduction in fat pad size of rats or mice treated with peripherally administered leptin. Am. J. Physiol. Regul. Integr. Comp. Physiol. 289, R92–R102. doi:10.1152/ajpregu.00858.2004
Seki, T., Hosaka, K., Lim, S., Fischer, C., Honek, J., Yang, Y., et al. (2016). Endothelial PDGF-CC regulates angiogenesis-dependent thermogenesis in beige fat. Nat. Commun. 7, 12152. doi:10.1038/ncomms12152
Shamsi, F., Xue, R., Huang, T. L., Lundh, M., Liu, Y., Leiria, L. O., et al. (2020). FGF6 and FGF9 regulate UCP1 expression independent of Brown adipogenesis. Nat. Commun. 11, 1421. doi:10.1038/s41467-020-15055-9
Soltani, S., Abdollahi, S., Aune, D., and Jayedi, A. (2021). Body mass index and cancer risk in patients with type 2 diabetes: A dose-response meta-analysis of cohort studies. Sci. Rep. 11, 2479. doi:10.1038/s41598-021-81671-0
Sun, J., Wang, F., Hong, G., Pang, M., Xu, H., Li, H., et al. (2016). Antidepressant-like effects of sodium butyrate and its possible mechanisms of action in mice exposed to chronic unpredictable mild stress. Neurosci. Lett. 618, 159–166. doi:10.1016/j.neulet.2016.03.003
Wan, Z., Root-Mccaig, J., Castellani, L., Kemp, B. E., Steinberg, G. R., and Wright, D. C. (2014). Evidence for the role of AMPK in regulating PGC-1 alpha expression and mitochondrial proteins in mouse epididymal adipose tissue. Obesity 22, 730–738. doi:10.1002/oby.20605
Wang, D., Liu, C. D., Li, H. F., Tian, M. L., Pan, J. Q., Shu, G., et al. (2020a). LSD1 mediates microbial metabolite butyrate-induced thermogenesis in Brown and white adipose tissue. Metabolism. 102, 154011. doi:10.1016/j.metabol.2019.154011
Wang, P., Loh, K. H., Wu, M., Morgan, D. A., Schneeberger, M., Yu, X., et al. (2020b). A leptin-BDNF pathway regulating sympathetic innervation of adipose tissue. Nature 583, 839–844. doi:10.1038/s41586-020-2527-y
Wu, H., and Ballantyne, C. M. (2020). Metabolic inflammation and insulin resistance in obesity. Circ. Res. 126, 1549–1564. doi:10.1161/circresaha.119.315896
Zeng, W., Pirzgalska, R. M., Pereira, M. M., Kubasova, N., Barateiro, A., Seixas, E., et al. (2015). Sympathetic neuro-adipose connections mediate leptin-driven lipolysis. Cell 163, 84–94. doi:10.1016/j.cell.2015.08.055
Zhang, H. Y., Tian, J. X., Lian, F. M., Li, M., Liu, W. K., Zhen, Z., et al. (2021). Therapeutic mechanisms of traditional Chinese medicine to improve metabolic diseases via the gut microbiota. Biomed. Pharmacother. 133, 110857. doi:10.1016/j.biopha.2020.110857
Zhang, Z. J., Pedicord, V. A., Peng, T., and Hang, H. C. (2020). Site-specific acylation of a bacterial virulence regulator attenuates infection. Nat. Chem. Biol. 16, 95–103. doi:10.1038/s41589-019-0392-5
NaB Sodium butyrate
HFD high-fat diet
RT-PCR quantitative real-time polymerase chain reaction analyses
UCP-1 uncoupling protein-1
PGC- 1αperoxisome proliferator-activated receptor gamma coactivator-1α
Prdm16 positive regulatory domain containing 16
WAT white adipose tissue
BAT brown adipose tissueβ3AR, β3‐adrenergic receptor
SNS sympathetic nervous system
NE norepinephrine
cAMP cyclic adenosine monophosphate
PKA cAMP-dependent protein kinase A
CREB cAMP-response element binding protein
SCFAs short-chain fatty acids
CD chow diet
TNF-α tumor necrosis factor-α
LPS Lipopolysaccharide
PBS phosphate-buffered salineTfammitochondrial transcription factor A
DIO diet-induced obesity
sWAT inguinal subcutaneous adipose tissue
eWAT epididymal adipose tissue
6-OHDA 6-hydroxy-dopamine
BDNF brain-derived neurotrophic factor
HOMA-IR homeostasis model assessment of insulin resistance
IL-6 Interleukin- 6
ABX antibiotic cocktail
IR insulin resistance
TH tyrosine hydroxylase
Uchl1/PGP9.5 ubiquitin carboxyl-terminal esterase L1
Dbh dopamine β-hydroxylase
ZO-1 zonula occludens-1
MUC- mucoprotein-2.
Keywords: sodium butyrate, obesity, adipose tissue, thermogenesis, sympathetic innervation
Citation: Zhu W, Peng K, Zhao Y, Xu C, Tao X, Liu Y, Huang Y and Yang X (2022) Sodium butyrate attenuated diet-induced obesity, insulin resistance and inflammation partly by promoting fat thermogenesis via intro-adipose sympathetic innervation. Front. Pharmacol. 13:938760. doi: 10.3389/fphar.2022.938760
Received: 08 May 2022; Accepted: 12 September 2022;
Published: 03 October 2022.
Edited by:
Fabiana Piscitelli, National Research Council (CNR), ItalyReviewed by:
Cuiqing Liu, Zhejiang Chinese Medical University, ChinaCopyright © 2022 Zhu, Peng, Zhao, Xu, Tao, Liu, Huang and Yang. This is an open-access article distributed under the terms of the Creative Commons Attribution License (CC BY). The use, distribution or reproduction in other forums is permitted, provided the original author(s) and the copyright owner(s) are credited and that the original publication in this journal is cited, in accordance with accepted academic practice. No use, distribution or reproduction is permitted which does not comply with these terms.
*Correspondence: Yilan Huang, bHp5eHl5eHAxMjNAMTYzLmNvbQ==; Xuping Yang, eWFuZ3h1cGluZzY1NTJAMTYzLmNvbQ==
†These authors have contributed equally to this work.
Disclaimer: All claims expressed in this article are solely those of the authors and do not necessarily represent those of their affiliated organizations, or those of the publisher, the editors and the reviewers. Any product that may be evaluated in this article or claim that may be made by its manufacturer is not guaranteed or endorsed by the publisher.
Research integrity at Frontiers
Learn more about the work of our research integrity team to safeguard the quality of each article we publish.