- Department of Pharmacology, Faculty of Pharmacy, Osaka Medical and Pharmaceutical University, Osaka, Japan
Nicotine induces kinetic tremor, which resembles pharmacological features of essential tremors, via activating the inferior olive (IO) neurons. Since nicotine is known to enhance dopamine release by stimulating α4β2 and/or α6 nACh receptors, we examined the effects of various dopamine receptor ligands on nicotine-induced tremor to clarify the role of the dopaminergic system in modulating nicotine tremor. A tremorgenic dose of nicotine increased the dopamine level in the pons and medulla oblongata (P/MO), and the levels of dopamine metabolites in the hippocampus, P/MO, and striatum. Treatment of animals with the D1/5 agonist SKF-38393 inhibited the induction of nicotine tremor, whereas the D3 agonist PD-128,907 facilitated nicotine-induced tremor. The D2 agonist sumanirole showed no effect. In addition, nicotine tremor was significantly enhanced by the D1/5 antagonist SCH-23390 and inhibited by the D3 antagonist U-99194. Neither the D2 (L-741,626) nor D4 (L-745,870) antagonist affected the generation of nicotine tremor. Furthermore, microinjection of U-99194 into the cerebellum significantly inhibited nicotine-induced tremor, whereas its injection into IO or the striatum did not affect tremor generation. Although intrastriatal injection of SCH-23390 showed no effects, its injection into IO tended to enhance nicotine-induced tremor. The present study suggests that dopamine D3 and D1/5 receptors regulate the induction of nicotine tremor in an opposite way, D3 receptors facilitately and D1/5 receptors inhibitorily. In addition, the cerebellar D3 receptors may play an important role in modulating the induction of nicotine tremor mediated by the olivo-cerebellar system.
Introduction
Nicotine, an active ingredient of tobacco products, produces a variety of pharmacological effects, including addictive actions (Le Foll and Goldberg, 2009; Besson et al., 2012; Harrington et al., 2016), antidepressant effects (Vieyra-Reyes et al., 2008; Mineur and Picciotto, 2010; Haj-Mirzaian et al., 2015), cognitive enhancement (Swan and Lessov-Schlaggar, 2007; Wood et al., 2016), and motor disturbances (e.g., tremor and seizures) (Lippold et al., 1980; Shiffman et al., 1983; Miner et al., 1985; Louis, 2007; Lin et al., 2012; Gilley and Beno, 2020). These actions are generally considered to be mediated by nicotinic acetylcholine (nACh) receptors, particularly α7 and α4β2 receptors, which are major subtypes in the brain (Tuesta et al., 2011; Besson et al., 2012; Koukouli and Maskos, 2015; Wood et al., 2016). In addition, it has been shown that nicotine facilitates synaptic dopamine release via activating α4β2 receptors and/or nACh receptors containing α6 subunits, which are abundantly expressed in the mesolimbic and nigrostriatal dopaminergic neurons (Damsma et al., 1988; Haikala and Ahtee, 1988; Wonnacott, 1997; Wonnacott et al., 2000; Exley et al., 2008; Quik et al., 2011). The activation of dopaminergic neurotransmission by nicotine is closely associated with its psychoemotional actions (e.g., addictive and antidepressant effects); however, the role of the dopaminergic system in modulating nicotine-induced motor disturbances (e.g., tremor) remains unknown.
Tremor is an involuntary and rhythmic movement disorder which appears in different parts of the body. According to the clinical manifestation, tremor can be classified into several types: 1) resting tremor occurring in the resting state of the limb, 2) kinetic tremor which appears during movement of parts of the body, 3) postural tremor which occurs when the body part is voluntarily maintained against gravity (holding a specific posture) (Puschmann and Wszolek, 2011; Kosmowska and Wardas, 2021). Resting tremor is one of the typical symptoms of Parkinson’s disease, while kinetic and postural tremors are frequently manifested in patients with essential tremor. In addition, several drugs, discretionary products (e.g., coffee and cigarettes), and toxins are known to induce tremor.
Previous studies reported that cigarette smoking and oral nicotine induce hand tremor (Lippold et al., 1980; Shiffman et al., 1983; Louis, 2007; Lin et al., 2012) and worsen essential tremor (Marshall and Schnieden, 1966). We previously showed that nicotine at relatively low doses (0.5–1 mg/kg, i.p.) evokes kinetic tremor in rodents (Kunisawa et al., 2016; Kunisawa et al., 2017; Kunisawa et al., 2018). Immunohistochemical analysis of Fos protein expression, a biological marker of neural excitation (Iha et al., 2016), revealed that tremorgenic doses of nicotine region-specifically increased the activities of the inferior olive (IO) neurons (Kunisawa et al., 2016) similarly to the rat model of essential tremor (Ohno et al., 2015a). In addition, electrical lesion of IO suppressed the induction of nicotine tremor, illustrating that nicotine induces kinetic tremor by activating IO neurons. Since excitation of the olivo-cerebellar neural pathway is reportedly involved in generation of essential tremor in humans (Puschmann and Wszolek, 2011; Kosmowska and Wardas, 2021; Welton et al., 2021), our findings suggest that nicotine-induced tremor has the same neural basis as essential tremor. In fact, nicotine tremor was significantly alleviated by drugs effective for human essential tremor (e.g., propranolol, diazepam, and phenobarbital), but was unaffected by medications for Parkinson’s disease tremor (i.e., trihexyphenidyl) (Kunisawa et al., 2018). Thus, nicotine-induced tremor may serve as an animal model of essential tremor. Interestingly, we found several factors which can modulate the intensity of nicotine tremor, including 5-HT1A and 5-HT2 receptors, adrenergic β receptors, Nav1 channels, Cav3 channels, and GABAA receptors. (Ohno et al., 2015a; Kunisawa et al., 2017; Kunisawa et al., 2018).
In the present study, we examined the effects of various dopamine receptor ligands on nicotine-induced tremor to explore the role and mechanism of the dopaminergic system in the generation of nicotine tremor. Since blockade of D3 receptors or stimulation of D1/5 receptors was found to reduce nicotine-induced tremor, D3 and D1/5 receptors may be involved in regulation of the induction of kinetic tremor.
Materials and Methods
Animals
Male ddY mice and SD rats (Japan SLC, Shizuoka, Japan) at 7–11 weeks of age were used. The animals were given food and water ad libitum and kept in air-conditioned rooms (24 ± 2°C and 55 ± 10% relative humidity) under a 12 h light/dark cycle (light on at 8:00 a.m.). The animal care and housing methods complied with the Guide for the Care and Use of Laboratory Animals of the Ministry of Education, Science, Sports and Culture of Japan. The experimental protocols were approved by the Experimental Animal Research Committee at Osaka Medical and Pharmaceutical University.
Analysis of Brain Dopamine and its Metabolite Levels
A tremorgenic dose (1 mg/kg, i.p.) of nicotine was injected into mice and, 15 min later, brains were removed from the skull under anesthesia (pentobarbital: 80 mg/kg, i.p.). Each brain was immediately washed with ice-cold saline and dissected into 8 regions (cerebral cortex, hippocampus, striatum, thalamus, hypothalamus, midbrain, pons and medulla oblongata (P/MO), and cerebellum) on an ice-cold petri dish. Tissue samples were then weighed, suspended in a 20% (w/v) volume of 0.1 M perchloric acid solution containing 1 ng/μl isoproterenol (internal standard), and homogenized (1,500 rpm × 10 strokes). After centrifugation (20,000 g × 15 min) at 0°C, the supernatant was filtered, and 35 μl of 1 M sodium acetate solution was added to 200 μl of the filtrate. Dopamine and its metabolites, 3,4-Dihydroxyphenylacetic acid (DOPAC) and homovanillic acid (HVA), in the extracts were measured using the HPLC-ECD system (Eicom, Kyoto, Japan). Namely, 1 μl of each sample were diluted with 99 μl 0.02 M acetic acid, and 10 μl of diluted samples were injected by an auto injector (M-500, Eicom, Kyoto, Japan). Dopamine, DOPAC, and HVA were separated by 3.0φ × 150 mm reversed-phase column (EICOMPAK SC-5ODS, Eicom). The column temperature was constantly held at 25°C with a column oven (ATC-300, Eicom). The mobile phase consisted of 0.1 M acetate-citrate buffer, 190 mg/L sodium 1-octanesulfonate, 5 mg/L EDTA, pH 3.5, and 17% methanol pumped at a flow rate of 500 μl/min by a reciprocal dual piston pump (EP-700, Eicom). The detector potential was +750 mV vs. Ag/AgCl (ECD-700A, Eicom). All data were analyzed by using a data processor (EPC-500, Eicom) and PowerChrom software (eDAQ Pty Ltd, Denistone East, NSW, Australia). Dopamine, DOPAC, and HVA were identified based on their retention times.
Evaluation of Nicotine-Induced Tremor in Mice
Nicotine-induced tremor was evaluated as reported previously (Kunisawa et al., 2018). Briefly, mice were treated with a tremorgenic dose (1 mg/kg, i.p.) of nicotine and individually placed in an observation cage (16 × 24 × 12 cm). The intensity and duration of nicotine-induced tremor were measured in a time-sampling manner over 1-2, 3-4, 5-6, 7-8, and 9–10 min (each for 1-min measurement) after the nicotine injection. The tremor intensity was measured using 4-point ranked scoring (0: no tremor, 1: mild tremor in head and tail, and straub tail, 2: moderate tremor in upper trunk, 3: marked tremor in whole body). The total tremor score and tremor duration were calculated as a sum of the tremor score or duration time per point, respectively.
To evaluate the effects of dopamine receptor agonists on nicotine tremor, the following agents were administered intraperitoneally 15 min before the nicotine injection: the D1/5 receptor agonist SKF-38393 (3, 10 mg/kg), selective D2 receptor agonist sumanirole (1, 3 mg/kg), or selective D3 receptor agonist PD-128,907 (1, 3 mg/kg). The D1/5 receptor antagonist SCH-23390 (1, 3 mg/kg), selective D2 receptor antagonist L-741,626 (3, 10 mg/kg), selective D3 receptor antagonist U-99194 (30 mg/kg), or selective D4 receptor antagonist L-745,870 (0.3, 1 mg/kg) were also administered intraperitoneally as dopamine antagonists 15 min before the nicotine treatment. The dosage of each dopamine agonist or antagonist was set to effectively act on each dopamine receptor according to previous reports (SKF-38393 and SCH-23390; Ohno et al., 1997, sumanirole; Crans et al., 2020; PD-128,907; Boulay et al., 1999; L-741,626; Manvich et al., 2019; U-99194; Barth et al., 2013, L-745,870; Bernaerts and Tirelli, 2003).
Microinjection Experiments With Dopamine Antagonists in Rats
Microinjection experiments were performed in rats as reported previously (Shimizu et al., 2013; 2014). Briefly, rats were anesthetized with pentobarbital (45 mg/kg, i.p.) and isoflurane inhalation, and the brain was fixed to a stereotaxic apparatus (Narishige, Tokyo, Japan). For the intraolivar or intrastriatal microinjection, two small holes were made in the skull, and bilateral stainless guide cannulae were implanted 1 mm above IO (AP: –12.4 mm, LM: ±0.5 mm, DV: +6.8 mm) or the striatum (AP: +1.0 mm, LM: ±3.5 mm, DV: +2.5 mm), respectively (Paxinos and Watson, 2007). Thereafter, guide cannulae were fixed to the skull with dental cement. For the intracerebellar microinjection, one guide cannula was implanted 1 mm above lobe IX of the cerebellum (AP: –13.1 mm, LM: ±0.0 mm, DV: +3.0 mm) (Paxinos and Watson, 2007).
After a recovery period of about 1 week, microinjection experiments with dopamine antagonists were performed. Under freely moving conditions, injection cannulae filled with SCH-23390 (3 μg/μl), U-99194 (30 μg/μl), or saline solution (control) were inserted into IO, the striatum, or cerebellum via guide cannulae, and 1 μl/site of the drug solution was gradually injected into the target site at a flow rate of 0.25 μl/min for 4 min using a microinfusion pump (KDS220, KD scientific, Holliston, MA, United States). Fifteen minutes after finishing the microinjection, the rats were treated with nicotine (1 mg/kg, i.p.) and behavioral observations of nicotine-induced tremor were performed during 5–16 min after the nicotine treatment, as described previously. The microinjection dosage of each dopamine antagonist was set to effectively act on each dopamine receptor according to previous reports (SCH-23390; Pardey et al., 2013, U-99194; Shimizu et al., 2014).
Drugs
(–)-Nicotine and R(+)-SCH-23390 hydrochloride were purchased from Sigma-Aldrich (St. Louis, MO, United States). L-741,626, L-745,870 trihydrochloride, and (±)-SKF-38393 hydrochloride were purchased from Abcam (Cambridge, United Kingdom). U-99194 maleate, sumanirole maleate, and (+)-PD-128,907 hydrochloride were purchased from Tocris Bioscience (Bristol, United Kingdom). L-741,626 and L-745,870 were first dissolved in 1% lactate, and then they were diluted with distilled water. Other drugs were dissolved in saline.
Statistical Analyses
Data are presented as the mean ± S.E.M. Significant differences on two-group comparisons were determined by Student’s t-test (parametric analysis) or the Mann-Whitney’s U-test (non-parametric analysis). For multigroup comparisons, the Kruskal-Wallis test followed by Steel-Dwass post-hoc multiple comparison test was performed. When the p-value was less than 0.05, it was considered significant.
Results
Effects of Tremorgenic Nicotine on Brain Dopamine and its Metabolite Levels
To investigate the changes in brain dopamine and its metabolite levels by nicotine, we treated mice with a tremorgenic dose (1 mg/kg, i.p.) of nicotine and measured the levels of dopamine, DOPAC, and HVA in 8 brain regions.
Nicotine significantly increased the dopamine level in P/MO as compared with control animals (t = 5.1374, df = 12, p = 0.0002) (Table 1). The dopamine level also tended to increase with nicotine in the striatum and hippocampus (Table 1). The level of DOPAC was significantly elevated in the hippocampus (t = 3.9544, df = 12, p = 0.0019) and P/MO (t = 2.3037, df = 12, p = 0.0399) (Table 1). Moreover, the HVA level was significantly increased in the striatum (t = 3.7921, df = 12, p = 0.0026) (Table 1).
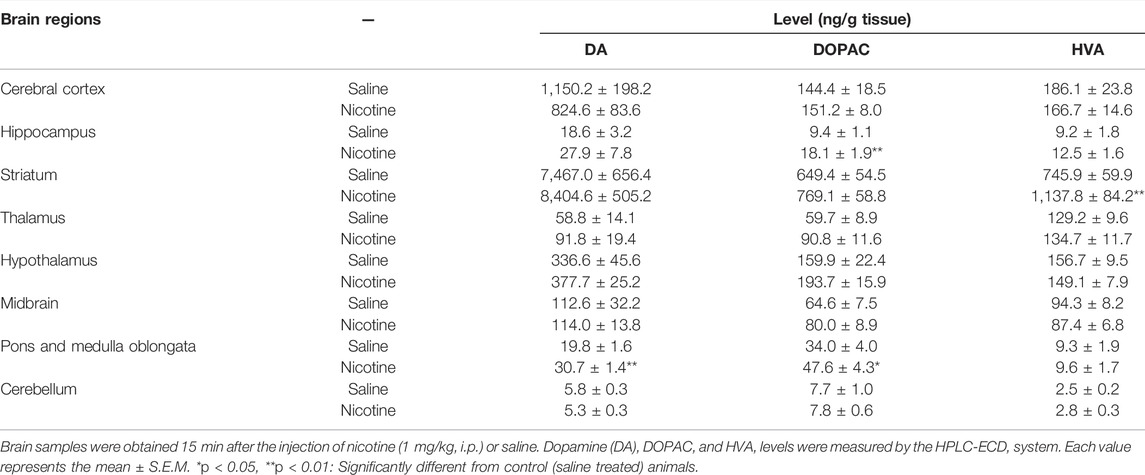
TABLE 1. Changes in brain dopamine and its metabolite levels by a tremorgenic dose of nicotine in mice.
Effects of Dopamine Receptor Agonists on Nicotine-Induced Tremor
To clarify the role of dopamine receptor subtypes in modulating nicotine tremor, we first examined the effects of various dopamine receptor agonists on nicotine-induced tremor. As shown in Figure 1, treatment of mice with the D1/5 receptor agonist SKF-38393 (3, 10 mg/kg, i.p.) inhibited nicotine (1 mg/kg, i.p.)-induced tremor in a dose-dependent manner. The total tremor duration was markedly reduced by 10 mg/kg of SKF-38393 (χ2 = 13.9752, df = 2, p = 0.0009). In contrast, the selective D3 receptor agonist PD-128,907 significantly increased the total tremor score and duration of nicotine tremor (total tremor score: χ2 = 10.0783, df = 2, p = 0.0065; total tremor duration: χ2 = 8.8997, df = 2, p = 0.0117). The D2 selective agonist sumanirole did not affect the induction of nicotine tremor (Figure 1B).
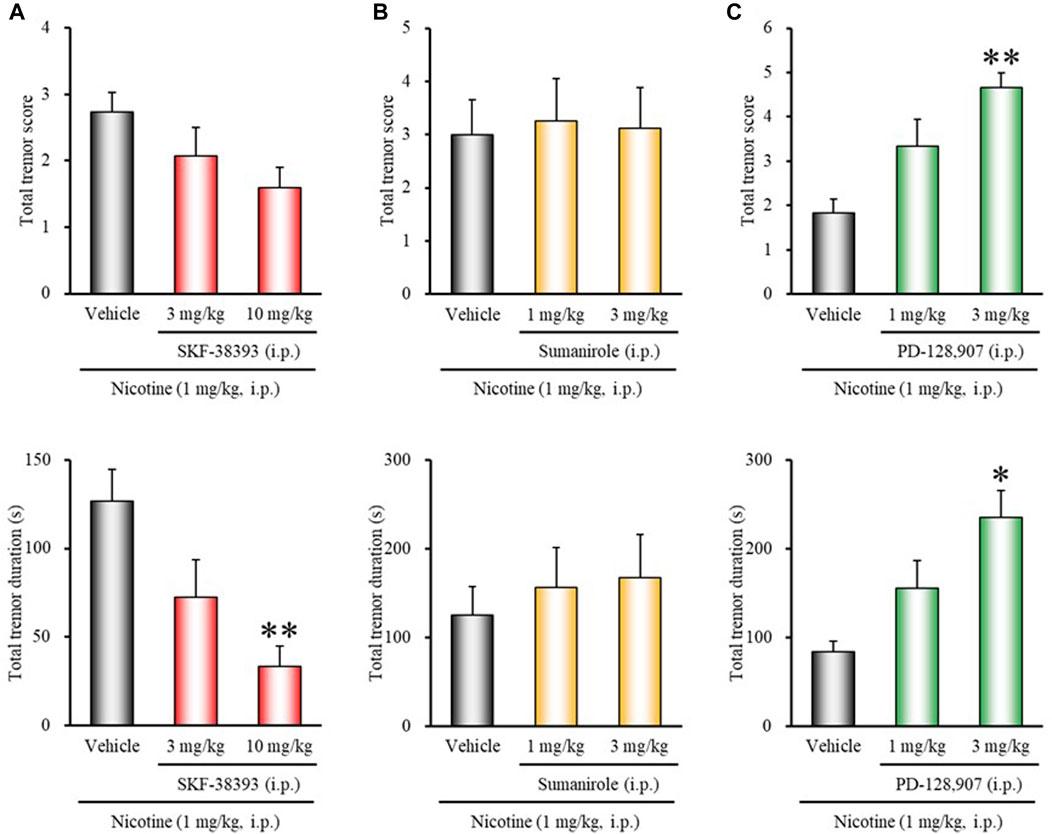
FIGURE 1. Effects of dopamine receptor agonists on nicotine-induced tremor in mice. Mice were treated with: (A) the D1/5 agonist SKF-38393 (3, 10 mg/kg, i.p.), (B) the D2 agonist sumanirole (1, 3 mg/kg, i.p.), (C) the D3 agonist PD-128,907 (1, 3 mg/kg, i.p.), or vehicle (control) 15 min before the nicotine (1 mg/kg, i.p.) injection. Data show the total tremor score (intensity) and duration of nicotine-induced tremor over the 10 min observation period. Each column represents the mean ± S.E.M. *p < 0.05, **p < 0.01: Significantly different from control animals.
Effects of Dopamine Receptor Antagonists on Nicotine-Induced Tremor
To determine whether endogenous dopamine is involved in modulating nicotine tremor, we examined the direct effects of dopamine receptor antagonists on nicotine-induced tremor. As shown in Figure 2A, treatment of mice with the D1/5 antagonist SCH-23390 (3 mg/kg, i.p.) significantly potentiated nicotine-induced tremor (total tremor score: χ2 = 8.4150, df = 2, p = 0.0149; total tremor duration: χ2 = 8.5796, df = 2, p = 0.0137). Conversely, the D3 selective antagonist U-99194 (30 mg/kg, i.p.) significantly suppressed the nicotine tremor (total tremor score: z = 1.9646, p = 0.0495; total tremor duration: z = 2.1704, p = 0.0300) (Figure 2B). Nicotine-induced tremor, however, was unaffected either with the D2 selective antagonist L-741,626 or the D4 selective antagonist L-745,870 (Figure 3).
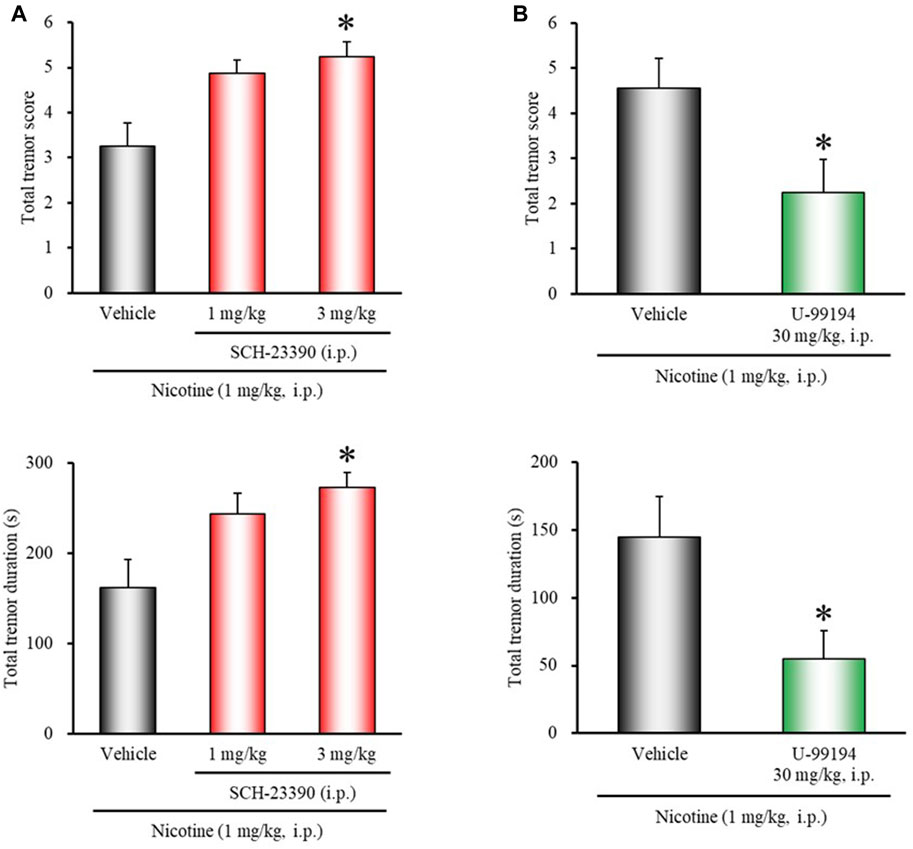
FIGURE 2. Effects of D1/5 or D3 receptor antagonists on nicotine-induced tremor in mice. Mice were treated with: (A) the D1/5 antagonist SCH-23390 (1, 3 mg/kg, i.p.), (B) the D3 antagonist U-99194 (30 mg/kg, i.p.), or vehicle (control) 15 min before the nicotine (1 mg/kg, i.p.) injection. Data show the total tremor score (intensity) and duration of nicotine-induced tremor over the 10 min observation period. Each column represents the mean ± S.E.M. *p < 0.05: Significantly different from control animals.
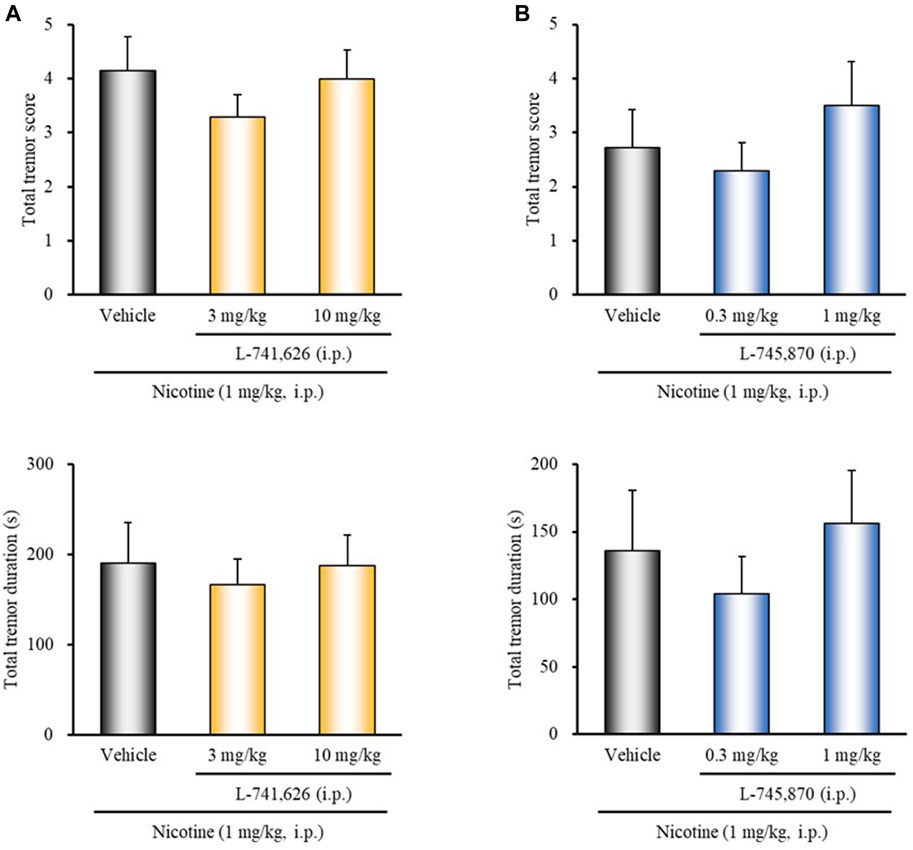
FIGURE 3. Effects of D2 or D4 receptor antagonists on nicotine-induced tremor in mice. Mice were treated with: (A) the D2 antagonist L-741,626 (3, 10 mg/kg, i.p.), (B) the D4 antagonist L-745,870 (0.3, 1 mg/kg, i.p.), or vehicle (control) 15 min before the nicotine (1 mg/kg, i.p.) injection. Data show the total tremor score (intensity) and duration of nicotine-induced tremor over the 10 min observation period. Each column represents the mean ± S.E.M.
Microinjection Studies With Dopamine Receptor Antagonists
To explore the regions of D1/5 and D3 receptors for regulation of nicotine-induced tremor, we conducted microinjection experiments using D1/5 (SCH-23390) and D3 (U-99194) antagonists in rats. Since IO and the striatum are important sites for the induction of nicotine tremor (Kunisawa et al., 2016) and parkinsonian tremor (Ohno et al., 2013; 2015b), respectively, SCH-23390 or U-99194 was injected into these two regions. In addition, as D3 receptors are highly expressed in the cerebellum and known to regulate locomotion and extrapyramidal movement disorders (Barik and de Beaurepaire, 1996, 2005; Kolasiewicz et al., 2008; Shimizu et al., 2014), U-99194 was also injected into the cerebellum (lobe IX).
Microinjection of SCH-23390 (3 μg/injection site) into IO tended to enhance nicotine-induced tremor, although the changes did not reach significance (total tremor score: z = 1.7400, p = 0.0819; total tremor duration: z = 1.6963, p = 0.0898) (Figure 4A). Microinjection of SCH-23390 (3 μg/injection site) into the striatum did not affect the induction of nicotine tremor (Figure 4B). Furthermore, although microinjection of U-99194 into IO or the striatum failed to affect nicotine-induced tremor, its injection into the cerebellum markedly inhibited the induction of nicotine tremor (total tremor score: z = 2.4488, p = 0.0143; total tremor duration: z = 1.9393, p = 0.0525) (Figure 5).
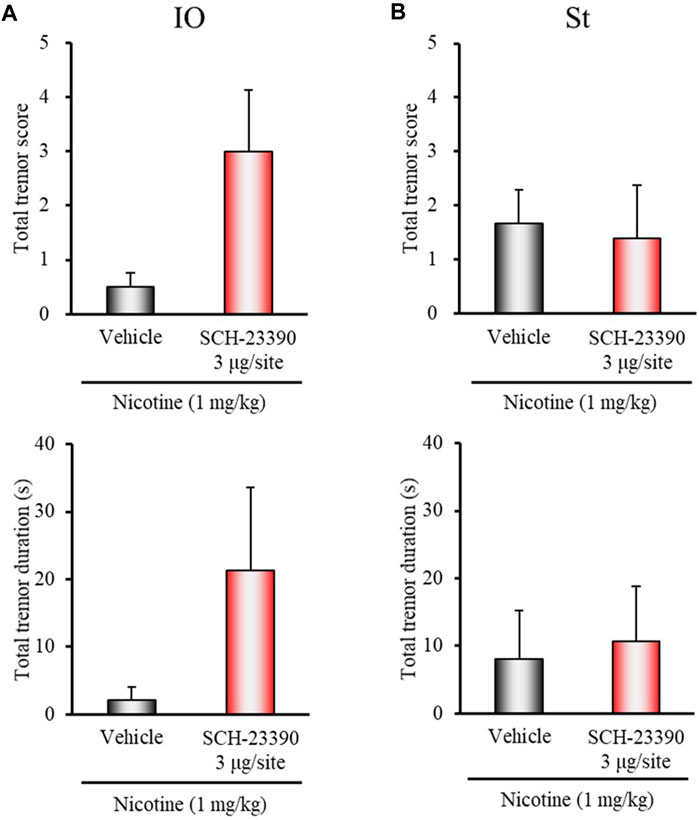
FIGURE 4. Effects of brain microinjection of SCH-23390 on nicotine-induced tremor in rats. The D1/5 antagonist SCH-23390 (3 μg/injection site) or vehicle (control) was injected into: (A) IO or (B) the striatum (St) in rats 15 min before the nicotine (1 mg/kg, i.p.) injection. Data show the total tremor score (intensity) and duration of nico tine-induced tremor during the 5–16 min observation period. Each column represents the mean ± S.E.M.
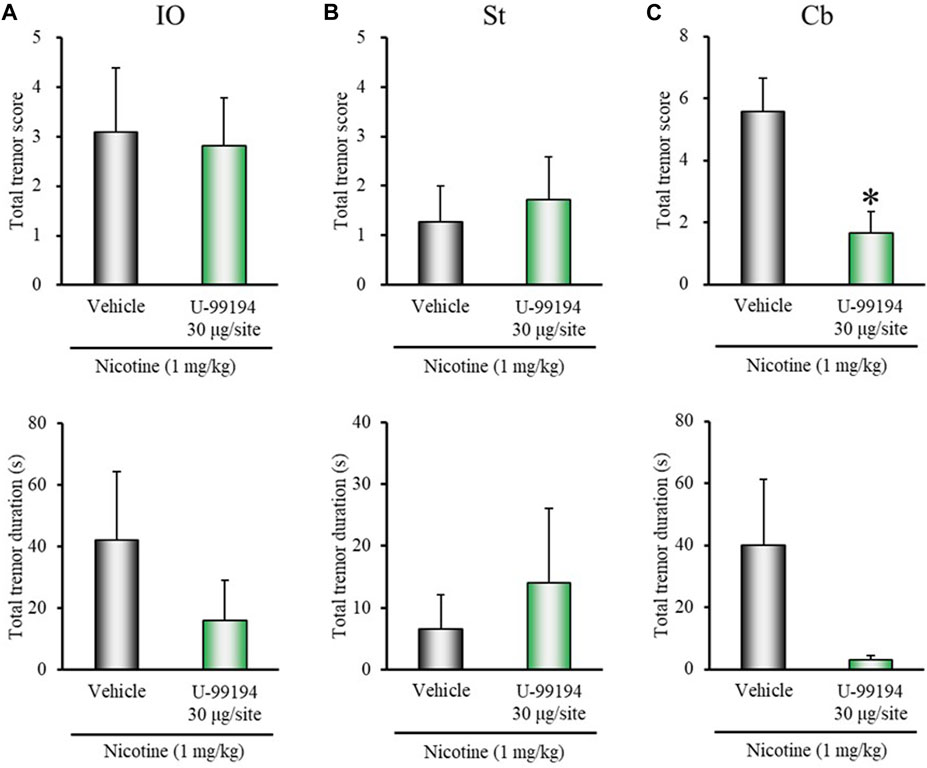
FIGURE 5. Effects of brain microinjection of U-99194 on nicotine-induced tremor in rats. The D3 antagonist U-99194 (30 μg/injection site) or vehicle (control) was injected into: (A) IO, (B) the striatum (St), or (C) the cerebellum lobe IX (Cb) in rats 15 min before the nicotine (1 mg/kg, i.p.) injection. Data show the total tremor score (intensity) and duration of nicotine-induced tremor during the 5–16 min observation period. Each column represents the mean ± S.E.M. *p < 0.05: Significantly different from control animals.
Discussion
Nicotine increases dopamine release and enhances dopaminergic neurotransmission in several brain regions (e.g., striatum, nucleus accumbens, and ventral tegmental area), which are suggested to be mediated by α4β2, α6β2, and partly α7 nACh receptors (Damsma et al., 1988; Haikala and Ahtee, 1988; Wonnacott, 1997; Wonnacott et al., 2000; Exley et al., 2008; Quik et al., 2011; Besson et al., 2012; Harrington et al., 2016). Previous studies showed that nicotine weakly increase the level of dopamine metabolites (e.g., DOPAC) by about 20–30% without affecting DA levels (Tani et al., 1997; Suemaru et al., 2006). The present study confirmed that nicotine at a tremorgenic dose increased dopamine and its metabolite levels in several parts of the brain (e.g., striatum, hippocampus, and P/MO), suggesting activation of the dopaminergic system by nicotine in these regions. Our results of nicotine actions on the striatal and hippocampal dopamine metabolism are similar to those in above previous studies. Meanwhile, in P/MO, nicotine elevated both dopamine and DOPAC levels by about 40–50%. Although the reason why nicotine elevated the DA level in P/MO remains uncertain, the effects of nicotine on dopamine neurons may involve its interactions with the serotonergic and/or noradrenergic systems (Gros et al., 2021; Pierucci et al., 2022). Although we did not specifically measure the dopamine level in IO, it is of interest that nicotine increased the dopaminergic tone in the P/MO region containing IO that forms the olivo-cerebellar neural circuit regulating tremor induction (Puschmann and Wszolek, 2011; Ohno et al., 2015a; Kosmowska and Wardas, 2021).
The present study using selective dopamine receptor ligands revealed that activation of D3 receptors facilitates the induction of nicotine tremor, whereas D1/5 receptor stimulation negatively regulates nicotine-induced tremor. Although D3 receptors belong to the D2 receptor family (Prieto, 2017; Kosmowska and Wardas, 2021), selective stimulation of D2 receptors by sumanirole showed no effects. Furthermore, since D1/5 and D3 antagonists per se enhanced and inhibited nicotine-induced tremor, respectively, activation of these receptors by endogenous dopamine may be involved in the regulation of nicotine tremor induction. It was confirmed that selective block of D2 or D4 receptors did not affect the induction of nicotine tremor, indicating that only D3 receptors in the D2 receptor family actively modulate the induction of kinetic tremor. Although we cannot completely deny the possibility that multiple actions of dopamine ligands used (e.g., interaction of SCH-23390 with 5-HT2 receptors and partial antagonism of SKF-38393 at D1/5 receptors) (Hoyer et al., 1989; Desai et al., 2005) also affected nicotine-induced tremor, our results suggest that dopamine D3 and D1/5 receptors regulate the induction of nicotine tremor in an opposite way, D3 receptors facilitately and D1/5 receptors inhibitorily.
We previously demonstrated that electrical lesion of IO suppressed nicotine-induced tremor (Kunisawa et al., 2016). Consistent with the fact that IO is closely involved in the pathogenesis of essential tremor in humans (Puschmann and Wszolek, 2011; Kosmowska and Wardas, 2021; Welton et al., 2021), our results suggest that the IO-cerebellar system plays a crucial role in the generation of nicotine-induced kinetic tremor. IO receives dopaminergic innervation from the ventral tegmental and prerubral parafascicular areas (Fallon et al., 1984; Toonen et al., 1998; Kitahama et al., 2000), suggesting a potential role of dopaminergic neurons in modulating tremor induction. Although microinjection of D1/5 and D3 antagonists into IO did not significantly affect nicotine tremor, the D1/5 antagonist markedly increased the intensity and duration of nicotine tremor. Our results suggest that D1/5 receptors in IO may be partly involved in modulation of nicotine tremor induction. Other D1/5 receptor regions involved in regulation of nicotine tremor remain to be clarified.
It is well-known that striatal dopamine receptors, particularly D2 receptors, play a crucial role in regulation of extrapyramidal motor functions and induction of parkinsonian symptoms, including resting tremor (Ohno et al., 2013; Ohno et al., 2015b). In the present study, however, the selective D2 receptor agonist sumanirole did not affect the generation of nicotine-induced kinetic tremor. In addition, neither microinjection of D1/5 nor D3 antagonist into the striatum altered the induction of nicotine tremor. Thus, our results indicate that, unlike parkinsonian resting tremor, striatal dopamine receptors are not involved in regulation of nicotine-induced kinetic tremor.
In the D2 receptor family, D3 receptors are highly expressed in the cerebellum in addition to cerebral cortex, limbic regions, and striatum (Sokoloff et al., 1990; Bouthenet et al., 1991; Lévesque et al., 1992; Diaz et al., 2000; Kim et al., 2009). The cerebellum receives dopaminergic neurons from the red nucleus, substantia nigra, and ventral tegmental area (Kizer et al., 1976; Kosmowska and Wardas, 2021), and contains a high density of D3 receptors whereas the levels of D1, D2, D4, and D5 receptors are low (Diaz et al., 2000; Kosmowska and Wardas, 2021). We and other groups showed that cerebellar D3 receptors regulated spontaneous locomotor activity and the induction of extrapyramidal movement disorders (i.e., catalepsy) (Barik and de Beaurepaire, 1996, 2005; Kolasiewicz et al., 2008; Shimizu et al., 2014). Interestingly, the present study demonstrated that microinjection of the D3 antagonist U-99194 suppressed nicotine-induced tremor generation. Our results strongly suggest that cerebellar D3 receptors positively control the induction of nicotine tremor mediated by the olivo-cerebellar neural pathway.
We previously showed that nicotine-induced tremor was of IO origin and alleviated by medications for essential tremor (e.g., propranolol, diazepam, and phenobarbital) (Kunisawa et al., 2016; 2018), suggesting that nicotine-induced tremor is useful as a model of essential tremor. Essential tremor is one of the common neurological disorders affecting approximately 1% of people worldwide (Puschmann and Wszolek, 2011; Kosmowska and Wardas, 2021). Although several drugs are applicable in its treatment, there are no specific drugs for essential tremor, and approximately one third of patients are treatment-resistant. The present study suggests that the selective antagonists for D3 receptors or agonists for D1/5 receptors may have a therapeutic potential. Interestingly, linkage analysis of genes associated with essential tremor identified four loci: chromosome 3q13 (ETM1) (Gulcher et al., 1997), 2p22-p25 (ETM2) (Higgins et al., 1997), 6p23 (ETM3) (Shatunov et al., 2006), and 5q35 (Hicks et al., 2016), among which ETM1 contains the DRD3 gene encoding D3 receptors (Gulcher et al., 1997). In addition, DRD3-Gly (Ser9Gly variant), which shows a greater affinity for dopamine than the non-mutated form, is reportedly associated with the risk of essential tremor (Jeanneteau et al., 2006). Thus, dopamine D3 receptors may be involved in the modulation of essential tremor. Taken together with the present results, dopamine D3 receptor antagonists may be useful for the treatment of essential tremor. Further studies are required to validate this hypothesis and clarify the role of dopaminergic neurons in the pathogenesis of essential tremor.
Data Availability Statement
The raw data supporting the conclusion of this article will be made available by the authors, without undue reservation.
Ethics Statement
The animal study was reviewed and approved by Osaka Medical and Pharmaceutical University Experimental Animal Research Committee.
Author Contributions
YO designed the research. MK, NK, SS, HI, and YO performed experiments, analyzed data, and wrote the article.
Funding
This study was supported in part by a Grant-in-Aid for Scientific Research from the Ministry of Education, Culture, Sports, Science and Technology of Japan (YO: 21K06587, NK: 21K15278, SS: 19K16384), by a research grant from the Ministry of Education, Science, Sports and Culture, and by a research grant from the Smoking Research Foundation.
Conflict of Interest
The authors declare that the research was conducted in the absence of any commercial or financial relationships that could be construed as a potential conflict of interest.
Publisher’s Note
All claims expressed in this article are solely those of the authors and do not necessarily represent those of their affiliated organizations, or those of the publisher, the editors and the reviewers. Any product that may be evaluated in this article, or claim that may be made by its manufacturer, is not guaranteed or endorsed by the publisher.
Acknowledgments
We thank Y. Ishikura, N. Hirata, M. Yasunaga, C. Takashima, K. Tsujimura, K. Horikawa for their excellent technical assistance in this study.
References
Barik, S., and de Beaurepaire, R. (2005). Dopamine D3 Modulation of Locomotor Activity and Sleep in the Nucleus Accumbens and in Lobules 9 and 10 of the Cerebellum in the Rat. Prog. Neuropsychopharmacol. Biol. Psychiatry 29, 718–726. doi:10.1016/j.pnpbp.2005.04.020
Barik, S., and de Beaurepaire, R. (1996). Evidence for a Functional Role of the Dopamine D3 Receptors in the Cerebellum. Brain Res. 737, 347–350. doi:10.1016/0006-8993(96)00964-x
Barth, V., Need, A. B., Tzavara, E. T., Giros, B., Overshiner, C., Gleason, S. D., et al. (2013). In Vivo occupancy of Dopamine D3 Receptors by Antagonists Produces Neurochemical and Behavioral Effects of Potential Relevance to Attention-Deficit-Hyperactivity Disorder. J. Pharmacol. Exp. Ther. 344, 501–510. doi:10.1124/jpet.112.198895
Bernaerts, P., and Tirelli, E. (2003). Facilitatory Effect of the Dopamine D4 Receptor Agonist PD168,077 on Memory Consolidation of an Inhibitory Avoidance Learned Response in C57BL/6J Mice. Behav. Brain Res. 142, 41–52. doi:10.1016/s0166-4328(02)00371-6
Besson, M., David, V., Baudonnat, M., Cazala, P., Guilloux, J. P., Reperant, C., et al. (2012). Alpha7-nicotinic Receptors Modulate Nicotine-Induced Reinforcement and Extracellular Dopamine Outflow in the Mesolimbic System in Mice. Psychopharmacol. Berl. 220, 1–14. doi:10.1007/s00213-011-2422-1
Boulay, D., Depoortere, R., Rostene, W., Perrault, G., and Sanger, D. J. (1999). Dopamine D3 Receptor Agonists Produce Similar Decreases in Body Temperature and Locomotor Activity in D3 Knock-Out and Wild-type Mice. Neuropharmacology 38, 555–565. doi:10.1016/s0028-3908(98)00213-5
Bouthenet, M. L., Souil, E., Martres, M. P., Sokoloff, P., Giros, B., and Schwartz, J. C. (1991). Localization of Dopamine D3 Receptor mRNA in the Rat Brain Using In Situ Hybridization Histochemistry: Comparison with Dopamine D2 Receptor mRNA. Brain Res. 564, 203–219. doi:10.1016/0006-8993(91)91456-b
Crans, R. A. J., Wouters, E., Valle-León, M., Taura, J., Massari, C. M., Fernández-Dueñas, V., et al. (2020). Striatal Dopamine D2-Muscarinic Acetylcholine M1 Receptor-Receptor Interaction in a Model of Movement Disorders. Front. Pharmacol. 11, 194. doi:10.3389/fphar.2020.00194
Damsma, G., Westerink, B. H., de Vries, J. B., and Horn, A. S. (1988). The Effect of Systemically Applied Cholinergic Drugs on the Striatal Release of Dopamine and its Metabolites, as Determined by Automated Brain Dialysis in Conscious Rats. Neurosci. Lett. 89, 349–354. doi:10.1016/0304-3940(88)90551-4
Desai, R. I., Terry, P., and Katz, J. L. (2005). A Comparison of the Locomotor Stimulant Effects of D1-like Receptor Agonists in Mice. Pharmacol. Biochem. Behav. 81, 843–848. doi:10.1016/j.pbb.2005.06.006
Diaz, J., Pilon, C., Le Foll, B., Gros, C., Triller, A., Schwartz, J. C., et al. (2000). Dopamine D3 Receptors Expressed by All Mesencephalic Dopamine Neurons. J. Neurosci. 20, 8677–8684. doi:10.1523/JNEUROSCI.20-23-08677.2000
Exley, R., Clements, M. A., Hartung, H., McIntosh, J. M., and Cragg, S. J. (2008). Alpha6-containing Nicotinic Acetylcholine Receptors Dominate the Nicotine Control of Dopamine Neurotransmission in Nucleus Accumbens. Neuropsychopharmacology 33, 2158–2166. doi:10.1038/sj.npp.1301617
Fallon, J. H., Schmued, L. C., Wang, C., Miller, R., and Banales, G. (1984). Neurons in the Ventral Tegmentum Have Separate Populations Projecting to Telencephalon and Inferior Olive, Are Histochemically Different, and May Receive Direct Visual Input. Brain Res. 321, 332–336. doi:10.1016/0006-8993(84)90188-4
Gao, Z. G., Cui, W. Y., Zhang, H. T., and Liu, C. G. (1998). Effects of Nicotine on 1-Methyl-4-Phenyl-1,2,5,6-Tetrahydropyridine-Induced Depression of Striatal Dopamine Content and Spontaneous Locomotor Activity in C57 Black Mice. Pharmacol. Res. 38, 101–106. doi:10.1006/phrs.1998.0337
Gilley, M., and Beno, S. (2020). Vaping Implications for Children and Youth. Curr. Opin. Pediatr. 32, 343–348. doi:10.1097/MOP.0000000000000889
Gros, A., Lavenu, L., Morel, J. L., and De Deurwaerdère, P. (2021). Simulated Microgravity Subtlety Changes Monoamine Function across the Rat Brain. Int. J. Mol. Sci. 22, 11759. doi:10.3390/ijms222111759
Gulcher, J. R., Jónsson, P., Kong, A., Kristjánsson, K., Frigge, M. L., Kárason, A., et al. (1997). Mapping of a Familial Essential Tremor Gene, FET1, to Chromosome 3q13. Nat. Genet. 17, 84–87. doi:10.1038/ng0997-84
Haikala, H., and Ahtee, L. (1988). Antagonism of the Nicotine-Induced Changes of the Striatal Dopamine Metabolism in Mice by Mecamylamine and Pempidine. Naunyn Schmiedeb. Arch. Pharmacol. 338, 169–173. doi:10.1007/BF00174865
Haj-Mirzaian, A., Kordjazy, N., Haj-Mirzaian, A., Ostadhadi, S., Ghasemi, M., Amiri, S., et al. (2015). Evidence for the Involvement of NMDA Receptors in the Antidepressant-like Effect of Nicotine in Mouse Forced Swimming and Tail Suspension Tests. Psychopharmacol. Berl. 232, 3551–3561. doi:10.1007/s00213-015-4004-0
Harrington, L., Viñals, X., Herrera-Solís, A., Flores, A., Morel, C., Tolu, S., et al. (2016). Role of β4* Nicotinic Acetylcholine Receptors in the Habenulo-Interpeduncular Pathway in Nicotine Reinforcement in Mice. Neuropsychopharmacology 41, 1790–1802. doi:10.1038/npp.2015.346
Hicks, J. E., Konidari, I., Scott, B. L., Stajich, J. M., Ashley-Koch, A. E., Gilbert, J. R., et al. (2016). Linkage of Familial Essential Tremor to Chromosome 5q35. Mov. Disord. 31, 1059–1062. doi:10.1002/mds.26582
Higgins, J. J., Pho, L. T., and Nee, L. E. (1997). A Gene (ETM) for Essential Tremor Maps to Chromosome 2p22-P25. Mov. Disord. 12, 859–864. doi:10.1002/mds.870120605
Hoyer, D., Waeber, C., Schoeffter, P., Palacios, J. M., and Dravid, A. (1989). 5-HT1C Receptor-Mediated Stimulation of Inositol Phosphate Production in Pig Choroid Plexus. A Pharmacological Characterization. Naunyn Schmiedeb. Arch. Pharmacol. 339, 252–258. doi:10.1007/BF00173573
Iha, H. A., Kunisawa, N., Tokudome, K., Mukai, T., Kinboshi, M., Shimizu, S., et al. (2016). “Immunohistochemical Analysis of Fos Protein Expression for Exploring Brain Regions Related to Central Nervous System Disorders and Drug Actions.” in In VivoNeuropharmacology and Neurophysiology, ed. A. Philippu (New York, NY: Springer), 389–408. doi: doi:10.1007/978-1-4939-6490-1_17
Jeanneteau, F., Funalot, B., Jankovic, J., Deng, H., Lagarde, J. P., Lucotte, G., et al. (2006). A Functional Variant of the Dopamine D3 Receptor Is Associated with Risk and Age-At-Onset of Essential Tremor. Proc. Natl. Acad. Sci. U. S. A. 103, 10753–10758. doi:10.1073/pnas.0508189103
Kim, Y. S., Shin, J. H., Hall, F. S., and Linden, D. J. (2009). Dopamine Signaling Is Required for Depolarization-Induced Slow Current in Cerebellar Purkinje Cells. J. Neurosci. 29, 8530–8538. doi:10.1523/JNEUROSCI.0468-09.2009
Kitahama, K., Nagatsu, I., Geffard, M., and Maeda, T. (2000). Distribution of Dopamine-Immunoreactive Fibers in the Rat Brainstem. J. Chem. Neuroanat. 18, 1–9. doi:10.1016/s0891-0618(99)00047-2
Kizer, J. S., Palkovits, M., and Brownstein, M. J. (1976). The Projections of the A8, A9 and A10 Dopaminergic Cell Bodies: Evidence for a Nigral-Hypothalamic-Median Eminence Dopaminergic Pathway. Brain Res. 108, 363–370. doi:10.1016/0006-8993(76)90192-x
Kolasiewicz, W., Maj, J., and Ossowska, K. (2008). The Involvement of Cerebellar Dopamine D3 Receptors in Locomotor Activity of Rats. J. Neural Transm. (Vienna) 115, 677–681. doi:10.1007/s00702-007-0009-0
Kosmowska, B., and Wardas, J. (2021). The Pathophysiology and Treatment of Essential Tremor: The Role of Adenosine and Dopamine Receptors in Animal Models. Biomolecules 11, 1813. doi:10.3390/biom11121813
Koukouli, F., and Maskos, U. (2015). The Multiple Roles of the α7 Nicotinic Acetylcholine Receptor in Modulating Glutamatergic Systems in the Normal and Diseased Nervous System. Biochem. Pharmacol. 97, 378–387. doi:10.1016/j.bcp.2015.07.018
Kunisawa, N., Iha, H. A., Nomura, Y., Onishi, M., Matsubara, N., Shimizu, S., et al. (2017). Serotonergic Modulation of Nicotine-Induced Kinetic Tremor in Mice. J. Pharmacol. Sci. 134, 131–138. doi:10.1016/j.jphs.2017.06.001
Kunisawa, N., Iha, H. A., Shimizu, S., Tokudome, K., Mukai, T., Kinboshi, M., et al. (2016). Nicotine Evokes Kinetic Tremor by Activating the Inferior Olive via α7 Nicotinic Acetylcholine Receptors. Behav. Brain Res. 314, 173–180. doi:10.1016/j.bbr.2016.08.013
Kunisawa, N., Shimizu, S., Kato, M., Iha, H. A., Iwai, C., Hashimura, M., et al. (2018). Pharmacological Characterization of Nicotine-Induced Tremor: Responses to Anti-tremor and Anti-epileptic Agents. J. Pharmacol. Sci. 137, 162–169. doi:10.1016/j.jphs.2018.05.007
Le Foll, B., and Goldberg, S. R. (2009). Effects of Nicotine in Experimental Animals and Humans: an Update on Addictive Properties. Handb. Exp. Pharmacol., 335–367. doi:10.1007/978-3-540-69248-5_12
Lévesque, D., Diaz, J., Pilon, C., Martres, M. P., Giros, B., Souil, E., et al. (1992). Identification, Characterization, and Localization of the Dopamine D3 Receptor in Rat Brain Using 7-[3H]hydroxy-N,n-Di-N-Propyl-2-Aminotetralin. Proc. Natl. Acad. Sci. U. S. A. 89, 8155–8159. doi:10.1073/pnas.89.17.8155
Lin, C. Y., Yeh, C. H., Chang, T. T., Kao, C. H., and Tsai, S. Y. (2012). Smoking, Dopamine Transporter, and Hand Tremor. Clin. Nucl. Med. 37, 35–38. doi:10.1097/RLU.0b013e318238f12d
Lippold, O. C., Williams, E. J., and Wilson, C. G. (1980). Finger Tremor and Cigarette Smoking. Br. J. Clin. Pharmacol. 10, 83–86. doi:10.1111/j.1365-2125.1980.tb00505.x
Louis, E. D. (2007). Kinetic Tremor: Differences between Smokers and Non-smokers. Neurotoxicology 28, 569–575. doi:10.1016/j.neuro.2006.12.006
Manvich, D. F., Petko, A. K., Branco, R. C., Foster, S. L., Porter-Stransky, K. A., Stout, K. A., et al. (2019). Selective D2 and D3 Receptor Antagonists Oppositely Modulate Cocaine Responses in Mice via Distinct Postsynaptic Mechanisms in Nucleus Accumbens. Neuropsychopharmacology 44, 1445–1455. doi:10.1038/s41386-019-0371-2
Marshall, J., and Schnieden, H. (1966). Effect of Adrenaline, Noradrenaline, Atropine, and Nicotine on Some Types of Human Tremor. J. Neurol. Neurosurg. Psychiatry 29, 214–218. doi:10.1136/jnnp.29.3.214
Miner, L. L., Marks, M. J., and Collins, A. C. (1985). Relationship between Nicotine-Induced Seizures and Hippocampal Nicotinic Receptors. Life Sci. 37, 75–83. doi:10.1016/0024-3205(85)90628-9
Mineur, Y. S., and Picciotto, M. R. (2010). Nicotine Receptors and Depression: Revisiting and Revising the Cholinergic Hypothesis. Trends Pharmacol. Sci. 31, 580–586. doi:10.1016/j.tips.2010.09.004
Ohno, Y., Ishida-Tokuda, K., Ishibashi, T., and Nakamura, M. (1997). Effects of Perospirone (SM-9018), a Potential Atypical Neuroleptic, on Dopamine D1 Receptor-Mediated Vacuous Chewing Movement in Rats: a Role of 5-HT2 Receptor Blocking Activity. Pharmacol. Biochem. Behav. 57, 889–895. doi:10.1016/s0091-3057(96)00468-6
Ohno, Y., Shimizu, S., Tatara, A., Imaoku, T., Ishii, T., Sasa, M., et al. (2015a). Hcn1 Is a Tremorgenic Genetic Component in a Rat Model of Essential Tremor. PLoS One 10, e0123529. doi:10.1371/journal.pone.0123529
Ohno, Y., Shimizu, S., Tokudome, K., Kunisawa, N., and Sasa, M. (2015b). New Insight into the Therapeutic Role of the Serotonergic System in Parkinson's Disease. Prog. Neurobiol. 134, 104–121. doi:10.1016/j.pneurobio.2015.09.005
Ohno, Y., Shimizu, S., and Tokudome, K. (2013). Pathophysiological Roles of Serotonergic System in Regulating Extrapyramidal Motor Functions. Biol. Pharm. Bull. 36, 1396–1400. doi:10.1248/bpb.b13-00310
Pardey, M. C., Kumar, N. N., Goodchild, A. K., and Cornish, J. L. (2013). Catecholamine Receptors Differentially Mediate Impulsive Choice in the Medial Prefrontal and Orbitofrontal Cortex. J. Psychopharmacol. 27, 203–212. doi:10.1177/0269881112465497
Paxinos, G., and Watson, C. (2007). The Rat Brain in Stereotaxic Coordinates. San Diego, CA: Academic Press.
Pierucci, M., Delicata, F., Colangeli, R., Marino Gammazza, A., Pitruzzella, A., Casarrubea, M., et al. (2022). Nicotine Modulation of the Lateral Habenula/ventral Tegmental Area Circuit Dynamics: An Electrophysiological Study in Rats. Neuropharmacology 202, 108859. doi:10.1016/j.neuropharm.2021.108859
Prieto, G. A. (2017). Abnormalities of Dopamine D3 Receptor Signaling in the Diseased Brain. J. Cent. Nerv. Syst. Dis. 9, 1179573517726335. doi:10.1177/1179573517726335
Puschmann, A., and Wszolek, Z. K. (2011). Diagnosis and Treatment of Common Forms of Tremor. Semin. Neurol. 31, 65–77. doi:10.1055/s-0031-1271312
Quik, M., Perez, X. A., and Grady, S. R. (2011). Role of α6 Nicotinic Receptors in CNS Dopaminergic Function: Relevance to Addiction and Neurological Disorders. Biochem. Pharmacol. 82, 873–882. doi:10.1016/j.bcp.2011.06.001
Shatunov, A., Sambuughin, N., Jankovic, J., Elble, R., Lee, H. S., Singleton, A. B., et al. (2006). Genomewide Scans in North American Families Reveal Genetic Linkage of Essential Tremor to a Region on Chromosome 6p23. Brain 129, 2318–2331. doi:10.1093/brain/awl120
Shiffman, S. M., Gritz, E. R., Maltese, J., Lee, M. A., Schneider, N. G., and Jarvik, M. E. (1983). Effects of Cigarette Smoking and Oral Nicotine on Hand Tremor. Clin. Pharmacol. Ther. 33, 800–805. doi:10.1038/clpt.1983.109
Shimizu, S., Mizuguchi, Y., Tatara, A., Kizu, T., Andatsu, S., Sobue, A., et al. (2013). 5-HT1A Agonist Alleviates Serotonergic Potentiation of Extrapyramidal Disorders via Postsynaptic Mechanisms. Prog. Neuropsychopharmacol. Biol. Psychiatry 46, 86–91. doi:10.1016/j.pnpbp.2013.06.016
Shimizu, S., Tatara, A., Sato, M., Sugiuchi, T., Miyoshi, S., Andatsu, S., et al. (2014). Role of Cerebellar Dopamine D(3) Receptors in Modulating Exploratory Locomotion and Cataleptogenicity in Rats. Prog. Neuropsychopharmacol. Biol. Psychiatry 50, 157–162. doi:10.1016/j.pnpbp.2013.12.013
Sokoloff, P., Giros, B., Martres, M. P., Bouthenet, M. L., and Schwartz, J. C. (1990). Molecular Cloning and Characterization of a Novel Dopamine Receptor (D3) as a Target for Neuroleptics. Nature 347, 146–151. doi:10.1038/347146a0
Suemaru, K., Yasuda, K., Cui, R., Li, B., Umeda, K., Amano, M., et al. (2006). Antidepressant-like Action of Nicotine in Forced Swimming Test and Brain Serotonin in Mice. Physiol. Behav. 88, 545–549. doi:10.1016/j.physbeh.2006.05.007
Swan, G. E., and Lessov-Schlaggar, C. N. (2007). The Effects of Tobacco Smoke and Nicotine on Cognition and the Brain. Neuropsychol. Rev. 17, 259–273. doi:10.1007/s11065-007-9035-9
Tani, Y., Saito, K., Tsuneyoshi, A., Imoto, M., and Ohno, T. (1997). Nicotinic Acetylcholine Receptor (nACh-R) Agonist-Induced Changes in Brain Monoamine Turnover in Mice. Psychopharmacol. Berl. 129, 225–232. doi:10.1007/s002130050184
Toonen, M., van Dijken, H., Holstege, J. C., Ruigrok, T. J., Koekkoek, S. K., Hawkins, R. K., et al. (1998). Light Microscopic and Ultrastructural Investigation of the Dopaminergic Innervation of the Ventrolateral Outgrowth of the Rat Inferior Olive. Brain Res. 802, 267–273. doi:10.1016/s0006-8993(98)00593-9
Tuesta, L. M., Fowler, C. D., and Kenny, P. J. (2011). Recent Advances in Understanding Nicotinic Receptor Signaling Mechanisms that Regulate Drug Self-Administration Behavior. Biochem. Pharmacol. 82, 984–995. doi:10.1016/j.bcp.2011.06.026
Vieyra-Reyes, P., Mineur, Y. S., Picciotto, M. R., Túnez, I., Vidaltamayo, R., and Drucker-Colín, R. (2008). Antidepressant-like Effects of Nicotine and Transcranial Magnetic Stimulation in the Olfactory Bulbectomy Rat Model of Depression. Brain Res. Bull. 77, 13–18. doi:10.1016/j.brainresbull.2008.05.007
Welton, T., Cardoso, F., Carr, J. A., Chan, L. L., Deuschl, G., Jankovic, J., et al. (2021). Essential Tremor. Nat. Rev. Dis. Prim. 7, 83. doi:10.1038/s41572-021-00314-w
Wonnacott, S., Kaiser, S., Mogg, A., Soliakov, L., and Jones, I. W. (2000). Presynaptic Nicotinic Receptors Modulating Dopamine Release in the Rat Striatum. Eur. J. Pharmacol. 393, 51–58. doi:10.1016/s0014-2999(00)00005-4
Wonnacott, S. (1997). Presynaptic Nicotinic ACh Receptors. Trends Neurosci. 20, 92–98. doi:10.1016/s0166-2236(96)10073-4
Keywords: nicotine, tremor, dopamine receptors, D3 receptors, inferior olive, cerebellum
Citation: Kato M, Kunisawa N, Shimizu S, Iha HA and Ohno Y (2022) Mechanisms Underlying Dopaminergic Regulation of Nicotine-Induced Kinetic Tremor. Front. Pharmacol. 13:938175. doi: 10.3389/fphar.2022.938175
Received: 07 May 2022; Accepted: 30 May 2022;
Published: 16 June 2022.
Edited by:
Philippe De Deurwaerdere, Université de Bordeaux, FranceReviewed by:
Anna Carolyna Lepesteur Gianlorenco, Federal University of São Carlos, BrazilAlexandre Gironell, Hospital de la Santa Creu i Sant Pau, Spain
Copyright © 2022 Kato, Kunisawa, Shimizu, Iha and Ohno. This is an open-access article distributed under the terms of the Creative Commons Attribution License (CC BY). The use, distribution or reproduction in other forums is permitted, provided the original author(s) and the copyright owner(s) are credited and that the original publication in this journal is cited, in accordance with accepted academic practice. No use, distribution or reproduction is permitted which does not comply with these terms.
*Correspondence: Yukihiro Ohno, eXVraWhpcm8ub2hub0BvbXB1LmFjLmpw