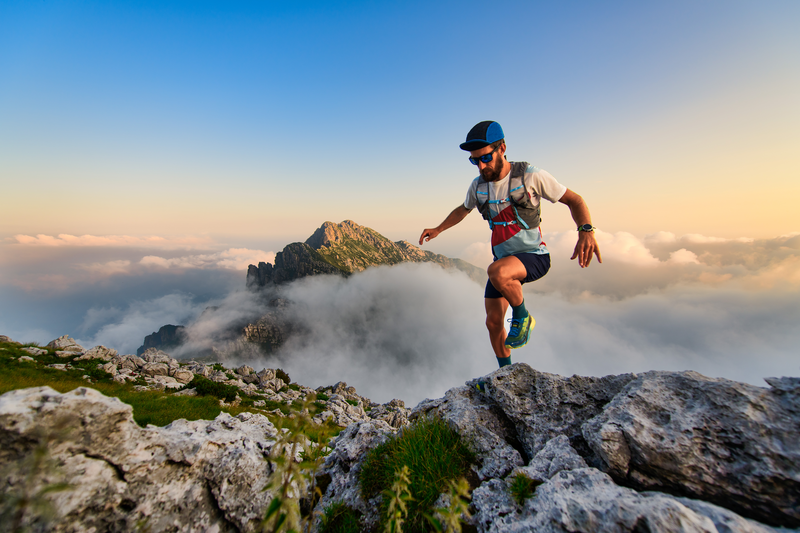
94% of researchers rate our articles as excellent or good
Learn more about the work of our research integrity team to safeguard the quality of each article we publish.
Find out more
MINI REVIEW article
Front. Pharmacol. , 15 July 2022
Sec. Pharmacology of Anti-Cancer Drugs
Volume 13 - 2022 | https://doi.org/10.3389/fphar.2022.935553
This article is part of the Research Topic New Anti-Cancer Strategies Targeting Epigenetic Modifications and Associated Metabolism Reprogramming View all 10 articles
Metabolic reprogramming is an emerging hallmark of tumor cells. In order to survive in the nutrient-deprived environment, tumor cells rewire their metabolic phenotype to provide sufficient energy and build biomass to sustain their transformed state and promote malignant behaviors. Amino acids are the main compositions of protein, which provide key intermediate substrates for the activation of signaling pathways. Considering that cells can synthesize arginine via argininosuccinate synthase 1 (ASS1), arginine is regarded as a non-essential amino acid, making arginine depletion as a promising therapeutic strategy for ASS1-silencing tumors. In this review, we summarize the current knowledge of expression pattern of ASS1 and related signaling pathways in cancer and its potential role as a novel therapeutic target in cancer. Besides, we outline how ASS1 affects metabolic regulation and tumor progression and further discuss the role of ASS1 in arginine deprivation therapy. Finally, we review approaches to target ASS1 for cancer therapies.
A key characteristics of tumor metabolism is the capability to hijack and remodel existing metabolic pathways to obtain sufficient nutrients from a nutrient-deprived environment and use these nutrients to sustain cell survival and build cellular material (Pavlova and Thompson, 2016). Amino acids serve as the primary compositions of protein, which provide important intermediate substrates for the activation of signaling pathways. Therefore, therapies of amino acid depletion that impair amino acid utilization via targeting key enzymes engaged in amino acid metabolism have been extensively studied (Tabe et al., 2019). Arginine is utilized by various metabolic pathways to mediate a series of cellular processes including protein synthesis and production of nitric oxide (NO), creatine phosphate, agmatine, polyamines, ornithine, and citrulline (Tong and Barbul, 2004) (Figure 1). Given that cells can synthesize arginine from citrulline and aspartate via argininosuccinate synthase 1 (ASS1) and argininosuccinate lyase (ASL), arginine is considered as a non-essential amino acid (Chen et al., 2021). Thus, arginine depletion may be a promising therapeutic strategy for cancer management.
FIGURE 1. Illustration of arginine metabolism in cells. ADC, arginine decarboxylase; ADI, arginine deiminase; ARG, arginase; ASL, arginine-succinate lyase; ASS1, arginine-succinate synthetase 1; GAMT, guanidinoacetate-N-methyltransferase; NO: nitric oxide; NOS: nitric oxide synthase; OCT, Ornithine carbamoyl transferase.
ASS1 is the enzyme that catalyzes the conversion of nitrogen from ammonia and aspartate from glutamine to form argininosuccinate. The somatic silence of ASS1 expression is commonly observed in a wide range of tumors, such as mesothelioma, non-small-cell lung cancer, myxofibrosarcomas (Huang et al., 2013; Szlosarek et al., 2017; Giatromanolaki et al., 2021). Moreover, low ASS1 expression levels in tumor tissues are associated with unfavorable clinical outcomes in a wide variety of malignancies. ASS1 loss not only confers tumor cells with lack of tumor suppressor functions but also endows tumor cells to be more reliant on arginine supplement. As illustrated in previous review, ASS1 and arginine metabolism represent compelling molecular targets for cancer management. In this review, we summarize the current knowledge of expression pattern of ASS1 and related signaling pathways in cancer and the potential role as a novel therapeutic target in cancer. Besides, we outline how ASS1 affects metabolic regulation and tumor progression and further discuss the role of ASS1 in arginine deprivation therapy. Finally, we review approaches to target ASS1 for cancer therapies.
ASS1 was initially identified in the liver, and functions as a rate-limiting enzyme for arginine metabolism. Dysregulated promoter methylation is regarded as a key feature of tumors via downregulating tumor suppressor genes (Kulis and Esteller, 2010). It is worth noticing that ASS1 silencing is resulted from the epigenetic silencing of the ASS1 promoter via methylation of the CpG islands, which has been observed in multiple tumor types (Syed et al., 2013). For instance, ASS1 promoter is frequently hypermethylated in myxofibrosarcoma, resulting in the aberrant loss of ASS1 expression to mediate tumor aggressiveness (Huang et al., 2013). Methylation landscape in cisplatin-resistant bladder cancer has shown that ASS1 is hypermethylated, leading to downregulated expression (Yeon et al., 2018). Aberrant methylation in the promoter of ASS1 makes ovarian tumor cells more resistant to platinum-induced cell death (Nicholson et al., 2009). Tumoral expression levels of ASS1 are also regulated when encountering external factors from the tumor microenvironment to metabolically benefit tumor cell survival. Under acidic and hypoxic conditions, it has been demonstrated that hypoxia inducible factor alpha (HIF1α) binds to ASS1 and downregulates the expression levels of ASS1, providing tumor cells with a metabolic advantage for survival (Silberman et al., 2019). Besides, arginine and glutamine starvation therapies can downregulate HIF1α to upregulate ASS1 expression (Long et al., 2017). Transcription factor c-Myc could directly bind to the promoter of ASS1, mediating arginine deiminase resistance in melanoma cells (Long et al., 2013). Methyltransferase 14 (METTL14), a RNA N6-adenosine methyltransferase, participates in tumor development via modulating RNA function (Chen et al., 2020). ASS1 is a target of METTL14-mediated N6-methyladenosine modification (Miao et al., 2022). Specifically, METTL14 upregulation increases mRNA m6A modification of ASS1 and suppresses ASS1 transcriptional expression. Additionally, miRNAs are also essential for the expression of ASS1. In renal cancer cell, miR-34a-5p directly binds to the 3′ untranslated region of ASS1 to reduce its protein expression, while ASS1P3 serves as a competing endogenous RNA for miR-34a-5p to modulate ASS1 expression (Wang et al., 2019). Similarly, lncRNA 00312 attenuates tumor proliferation and invasion by functioning as a competitive endogenous RNA binding to miR34a-5p, making miR34a-5p unable binding to ASS1 to reduce ASS1 expression (Zeng et al., 2020). Considering that post-translational modification is essential for the stability of protein, it mediates diverse cellular processes. For instance, the TRAF2 E3 ubiquitin ligase binds to ASS1, leading to increased ubiquitination and degradation of ASS1 to reduce arginine biosynthesis. The diverse regulation pattern of ASS1 transcriptional and protein expression makes it a promising therapeutic target, and it is necessary to investigate the underlying mechanisms engaged in the expression of ASS1.
Arginine is a precursor for a wide variety of molecules engaged in the regulation of tumor initiation and development (Zhang et al., 2021). Tumoral downregulation of ASS1 confers tumor cells to be more dependent on extracellular arginine since ASS1-negative cells fail to mediate arginine biosynthesis for tumor survival. The reliance on extracellular arginine has been regarded as arginine auxotrophy, which has been exploited as an’‘Achilles’ heel for cancer management. Thus, ASS1 has been established as a key indicator of arginine auxotrophy. Network analysis of the metabolomics revealed that ASS1-negative glioblastoma cells exhibit altered arginine and citrulline metabolism (Mörén et al., 2018). In ASS1-negative glioblastoma cells, levels of alanine and glutamate are reduced, whereas levels of α-ketoglutarate and pyruvate are increased, indicating that ASS1-negative glioblastoma cells are converting less pyruvate to alanine. Multiple pathways for citrulline production are upregulated, and degradation of arginine in ASS negative cells is decreased. In addition, ASS1 functions as an indicator for glutamine-deprivation response. ASS1 inhibition leads to increased sensitivity to both arginine and glutamine deprivation, whereas ASS1 overexpression increases resistance to both arginine and glutamine deprivation (Long et al., 2017). Depletion of extracellular arginine in arginine-auxotrophic cancer cells causes mitochondrial distress and transcriptional reprogramming. Mechanistically, arginine starvation induces asparagine synthetase (ASNS), depleting these cancer cells of aspartate, and disrupting their malate-aspartate shuttle (Cheng et al., 2018). A metabolite profiling of arginine depletion by pharmacological inhibition exhibits elevated serine biosynthesis, glutamine anaplerosis, oxidative phosphorylation, and impaired aerobic glycolysis (Kremer et al., 2017).
Pyrimidines play key role in mediating tumor cell survival and proliferation through providing the nucleic acids and other precursors for cell membrane synthesis (Mollick and Laín, 2020; Siddiqui and Ceppi, 2020). During the synthesis process, the pyrimidine ring structure is formed through a multi-step pathway with glutamine and aspartate as main precursors, which is conversed to dihydroorotate by the three activities of the multifunctional enzyme carbamoyl-phosphate synthase 2, aspartate transcarbamylase, dihydroorotase complex (CAD) (Del Caño-Ochoa et al., 2019; Li et al., 2021). It has been found that tumoral ASS1 expression determines aspartate availability for pyrimidine synthesis (Rabinovich et al., 2015). Intracellular aspartate functions as a substrate for both ASS1 and the enzymatic complex CAD. Tumoral ASS1 loss increases cytosolic aspartate availability for CAD for the synthesis of pyrimidine nucleotides to promote proliferation (Rabinovich et al., 2015). In renal cellular carcinoma, loss of ASS1 and ASL makes aspartate flux towards pyrimidine synthesis to support tumor proliferation (Khare et al., 2021).
The reaction catalyzed by ASS1 is essential for the citrulline-NO cycle. NO is a crucial regulator of multiple cellular processes, such as tumor cell proliferation and angiogenesis (Somasundaram et al., 2019). Decreased levels of NO metabolites and nitric oxide synthase expression have been observed in renal cellular carcinomas and tumor cells lacking ASS1 and ASL (Khare et al., 2021). Combined ASS1 and ASL downregulation significantly reduces aspartate level to impair NO production via decreased substrate availability or enzymatic activity. Therefore, ASS1 downregulation influences NO metabolism and promotes tumor cell survival and proliferation via mitigation of cytotoxic effects of NO accumulation. Under glucose deprivation, ASS1 expression is induced by c-MYC, therefore promoting tumor cell survival by upregulating NO production and activating the gluconeogenic enzymes via S-nitrosylation (Keshet et al., 2020). This metabolic rewiring leads to enhanced gluconeogenesis to increase serine, glycine and purine synthesis. In this ASS1-expressed tumors, purine synthesis inhibition is effective and sensitizes these tumors to immune checkpoint inhibition therapy.
ASS1 loss is associated with polyamine metabolic reprogramming. Results from transcriptomic and metabolomic profiling illustrates that ASS1-lacked cells exhibit reduced accumulation of acetylated polyamine metabolites and therefore a compensatory elevation in the expression of polyamine biosynthetic enzymes (Locke et al., 2016).
Numerous studies have shown that tumoral ASS1 functions as a tumor suppressor to sustain the anti-tumor function in a wide variety of tumors (Huang et al., 2013; Allen et al., 2014). Downregulation of ASS1 has been found to play a tumor suppressor role in multiple malignancies. ASS1 exerts its role mainly through its arginine metabolism-dependent mechanisms. It is gradually recognized that ASS1 play different role according to different tumor types. Renal tumors exhibit downregulated ASS1, and loss of ASS1 redirects aspartate towards pyrimidine synthesis and regulates NO production to support enhanced proliferation, uncovering promising metabolic vulnerabilities in renal cellular carcinoma. Besides, it has also been demonstrated that ASS1 may function as a tumor suppressor via metabolism–independent mechanism. In hepatic cell carcinoma cells, ASS1 induces cell death by upregulating ER stress response, independent of arginine metabolism. Specifically, ASS1 overexpression effectively inhibits tumor growth by activating PERK/eIF2α/ATF4/CHOP axis in Huh7 and SNU475 cells, indicating upregulating tumoral ASS1 expression as a promising strategy in tumors with low ASS1 expression (Kim et al., 2021). In renal cellular carcinoma, androgen receptor could reduce ASS1 expression to promote SW-839 and OSRC-3 cell proliferation via ASS1P3. Thus, this androgen receptor-induced ASS1 downregulation as a therapeutic target for treatment.
In contrast to its tumor-inhibiting effects, ASS1 has a pro-tumor role in tumor proliferation and metastasis, and the mechanisms seem to vary and differ depending on the specific type of tumor. Based on the differential transcriptional expression analyses of colorectal cancer (CRC) tumors, urea cycle enzymes including ASS1 has been identified to be transcriptionally upregulated in KRAS-mutant primary CRC. Bateman et al. elucidated that ASS1 inhibition impairs CRC survival and proliferation. Metabolomic profiling has pointed that ASS1 inhibition reduces the levels of oncogenic metabolite fumarate, resulting in impaired glycolytic phenotype and reduced CRC progression (Bateman et al., 2017). Snail is a master regulator and transcriptional repressor of epithelial-mesenchymal transition. In colorectal cancer, Snail mediates tumor cell metastasis by preventing non-coding RNA LOC113230-induced degradation of ASS1. Snail regulates arginine biosynthesis by suppressing LOC113230-induced LRPPRC/TRAF2/ASS1 axis (Jia et al., 2021). In gastric cancer, ASS1 knockdown leads to impaired tumor cell invasion by promoting autophagy-lysosome machinery to degrade Snail and Twist (Tsai et al., 2018).
Numerous studies have reported that ASS1 loss is correlated with the development of chemotherapeutic resistance in tumors. For instance, epigenetic silencing of ASS1 confers ovarian tumor cells resistance to platinum chemotherapy. Thus, the expression level of ASS1 has also been regarded as a predictor of clinical outcome in ovarian cancer patients treated with platinum-based chemotherapy (Nicholson et al., 2009). In hepatocellular carcinoma, ASS1 loss has been found to be associated with resistance to cisplatin. Moreover, ASS1 overexpression effectively improves the anti-tumor effect of chemotherapy by activating the PERK/eIF2α/ATF4/CHOP axis. Treatment with decitabine, a hypomethylating agent to increase ASS1 promoter activity, can effectively reduce cisplatin resistance in SNU449 and Huh7 cells. Apoptosis level indicated by cleaved PARP and caspase-3 is significantly upregulated in SNU449 and Huh7 cells after combination treatment with cisplatin and decitabine (Kim et al., 2021).
Tumors with ASS1 loss fail to mediate the arginine biosynthesis, making these cells to be more reliant on extracellular arginine for tumor survival. Thus, arginine depletion therapy may be a promising therapeutic strategy for ASS1-negative tumors. In nearly 70% of tumors, ASS1 loss has been observed, resulting in more efforts to exploit the metabolic vulnerability for development of arginine deprivation therapy. Arginine deprivation therapy is considered to be more effective in ASS1-negative tumors than tumors with low level of ASS1 expression. Arginine deprivation can induce signal alteration. For example, arginine deprivation impairs mTOR and p70S6K activation with consequent inactivation of PI3K/Akt pathway (Wang et al., 2020). PEGylated arginine deiminase (ADI-PEG20) is an arginine-metabolizing enzyme to mediate arginine degradation, which is currently being tested in many clinical trials. ADI-PEG20 has been verified to exhibit anti-tumor effect in a wide range of tumors, including primary acute myeloid leukemia, metastatic melanoma, hepatocellular carcinoma, thoracic cancer (Izzo et al., 2004; Ascierto et al., 2005; Miraki-Moud et al., 2015; Beddowes et al., 2017). ADI-PEG 20 treatment increases T cell infiltration in the low PD-L1 tumor microenvironment to enhance the anti-tumor effect of PD-1 inhibition (Chang et al., 2021). ADI-PEG20 also significantly improves progression-free survival in patients with ASS1-loss mesothelioma (Szlosarek et al., 2017). In pancreatic tumor, ADI-PEG20 can also augment the anti-tumor effect of radiation via the activation of ER stress signaling pathway (Singh et al., 2019). ADI-PEG 20 has also been found to be specifically effective in MYC-driven tumors (Chalishazar et al., 2019). ADI-PEG20 can disrupt pyrimidine pools in ASS1-lacked high-grade gliomas to increase tumor sensitivity to the antifolate and pemetrexed (Hall et al., 2019). Although promising results from the preclinical and clinical trials, there are tumors exhibiting resistance to ADI-PEG20. It has been well-established that the re-expression of ASS1 is a main reason for tumor resistance to ADI-PEG20. In mesothelioma cells exhibiting ADI-PEG20 resistance, it is believed that this resistance is induced by regain of ASS1 expression by demethylation of the ASS1 promoter. Considering the efficacy of ADT as a single agent therapy is limited due to frequently observed resistance, combined arginine deprivation and other therapeutic targets may be an answer to improve the therapeutic effect. For instance, HDAC inhibition has been found to induce degradation of a key DNA repair enzyme C-terminal-binding protein interacting protein (CtIP), leading to DNA damage and apoptosis. Arginine deprivation and HDAC inhibition can synergistically mediate DNA damage and degradation of CtIP, resulting in apoptosis (Kim et al., 2020). In addition, a metabolic synthetic lethal strategy has been developed to combine ADI-PEG20 with chloroquine to induce cell death in ASS1-deficient sarcomas (Bean et al., 2016). More importantly, combination treatment of ADI-PEG 20 with chemotherapeutic drugs has been extensively studied. Several clinical trials indicated that this combination treatment has acceptable safety profiles and anti-tumour activity against ASS1-deficient solid tumors (Hall et al., 2019; Szlosarek et al., 2021; Yao et al., 2021; Chan et al., 2022). Summary of ADI-PEG20 utilization in tumor management has been illustrated in Table 1.
The safety and anti-tumor activities of a newly developed arginine depleting drug pegylated recombinant human arginase (PEG-BCT-100) has been verified in patients with advanced arginine auxotrophic tumors (Cheng et al., 2021). PEG-BCT-100 has been tested in chemo naïve post-sorafenib hepatocellular carcinoma, showing well-tolerated with moderate disease control rate (Chan et al., 2021). More clinical studies should be designed to further verify the safety and anti-tumor efficacy, and the potential effects of combination therapy with other chemotherapeutic and targeted agents should also be further explored.
In non-small-cell lung cancer, lack of expression of ASS1 in tumor cells is associated with high angiogenesis. Patients with ASS1 expression in tumor cells exhibit a favorable prognosis, which may be related to high density of iNOS-expressing tumor-infiltrating lymphocytes in tumor cells with ASS1 expression (Giatromanolaki et al., 2021). ASS1 is lower in renal cell carcinomas compared with paired normal tissues, and a lower ASS1 expression is correlated with a worse prognosis in patients with renal cell carcinoma (Wang et al., 2019).
Considering the tumor suppressor role of ASS1 in tumor cells, exploring potent ASS1 agonist to activate ASS1 expression and activity in tumor cells with low ASS1 expression is a promising therapeutic strategy. It has been demonstrated that spinosyn A and its derivative LM-2I exhibit anti-tumor function, which is regulated by activation of ASS1. Mechanistically, spinosyn A binds to ASS1 at the 97th cysteine site in tumor cells, thus increasing ASS1 enzymatic activity and anti-tumor effect (Zou et al., 2021). Currently, the investigation on the development of ASS1 activators is still limited, it is still required to explore deeper to identify the safety and efficacy of ASS1 activators.
ASS1 is an enzyme that catalyzes the conversion of nitrogen from ammonia and aspartate from glutamine to form argininosuccinate. The down-regulation of ASS1 expression are very common in various tumors, and associated with clinicopathological factors and prognosis. For precision therapeutic purposes, it is important to understand the mechanisms driving malignant diseases to identify the most promising therapy for individual patients. Currently, the down-regulation of ASS1 expression is extensively studied in mesothelioma, non-small-cell lung cancer, myxofibrosarcomas and others. Arginine deprivation leads to immunosuppression, and the removal of L-arginine can improve the elimination of arginine-auxotrophic tumors. However, the removal of L-arginine has no inhibition on the development of non-auxotrophic tumors, given that these tumors synthesize L-arginine from citrulline by expressing ASS1. Therefore, it is necessary to identify the auxotrophic and non-auxotrophic tumors. To date, numerous studies of phase 1–3 clinic trials are being conducted to evaluate the efficacy of ADI-PEG20 therapy in diverse tumor types for its anti-tumor activity, it is still required to explore deeper to identify the cancer types that can be effectively treated with ADI-PEG20 therapy. In addition, combination of ASS1 activators with anti-tumor drugs like chemotherapy and TKIs could augment the anti-tumor effect of traditional regimens. Given that ASS1 is commonly downregulated in multiple tumor types and participates in the regulation of tumor development depending on diverse mechanisms, it may be a robust potential therapeutic target for cancer management.
NS writing and elaborating the figures. XZ writing and reviewing the final version.
This work was supported by the Project of Liaoning Province Natural Science Foundation (2021-BS-117).
The authors declare that the research was conducted in the absence of any commercial or financial relationships that could be construed as a potential conflict of interest.
All claims expressed in this article are solely those of the authors and do not necessarily represent those of their affiliated organizations, or those of the publisher, the editors and the reviewers. Any product that may be evaluated in this article, or claim that may be made by its manufacturer, is not guaranteed or endorsed by the publisher.
Abou-Alfa, G. K., Qin, S., Ryoo, B. Y., Lu, S. N., Yen, C. J., Feng, Y. H., et al. (2018). Phase III randomized study of second line ADI-PEG 20 plus best supportive care versus placebo plus best supportive care in patients with advanced hepatocellular carcinoma. Ann. Oncol. 29 (6), 1402–1408. doi:10.1093/annonc/mdy101
Allen, M. D., Luong, P., Hudson, C., Leyton, J., Delage, B., Ghazaly, E., et al. (2014). Prognostic and therapeutic impact of argininosuccinate synthetase 1 control in bladder cancer as monitored longitudinally by PET imaging. Cancer Res. 74 (3), 896–907. doi:10.1158/0008-5472.CAN-13-1702
Ascierto, P. A., Scala, S., Castello, G., Daponte, A., Simeone, E., and Ottaiano, A. (2005). Pegylated arginine deiminase treatment of patients with metastatic melanoma: Results from phase I and II studies. J. Clin. Oncol. 23 (30), 7660–7668. doi:10.1200/JCO.2005.02.0933
Bateman, L. A., Ku, W. M., Heslin, M. J., Contreras, C. M., Skibola, C. F., Nomura, D. K., et al. (2017). Argininosuccinate synthase 1 is a metabolic regulator of colorectal cancer pathogenicity. ACS Chem. Biol. 12 (4), 905–911. doi:10.1021/acschembio.6b01158
Bean, G. R., Kremer, J. C., Prudner, B. C., Schenone, A. D., Yao, J. C., Schultze, M. B., et al. (2016). A metabolic synthetic lethal strategy with arginine deprivation and chloroquine leads to cell death in ASS1-deficient sarcomas. Cell Death Dis. 7 (10), e2406. doi:10.1038/cddis.2016.232
Beddowes, E., Spicer, J., Chan, P. Y., Khadeir, R., Corbacho, J. G., Repana, D., et al. (2017). Phase 1 dose-escalation study of pegylated arginine deiminase, cisplatin, and pemetrexed in patients with argininosuccinate synthetase 1-deficient thoracic cancers. J. Clin. Oncol. 35 (16), 1778–1785. doi:10.1200/JCO.2016.71.3230
Chalishazar, M. D., Wait, S. J., Huang, F., Ireland, A. S., Mukhopadhyay, A., Lee, Y., et al. (2019). MYC-driven small-cell lung cancer is metabolically distinct and vulnerable to arginine depletion. Clin. Cancer Res. 25 (16), 5107–5121. doi:10.1158/1078-0432.CCR-18-4140
Chan, P. Y., Phillips, M. M., Ellis, S., Johnston, A., Feng, X., Arora, A., et al. (2022). A phase 1 study of ADI-PEG20 (pegargiminase) combined with cisplatin and pemetrexed in ASS1-negative metastatic uveal melanoma. Pigment. Cell Melanoma Res. 35, 461–470. doi:10.1111/pcmr.13042
Chan, S. L., Cheng, P. N. M., Liu, A. M., Chan, L. L., Li, L., Chu, C. M., et al. (2021). A phase II clinical study on the efficacy and predictive biomarker of pegylated recombinant arginase on hepatocellular carcinoma. Invest. New Drugs 39 (5), 1375–1382. doi:10.1007/s10637-021-01111-8
Chang, K. Y., Chiang, N. J., Wu, S. Y., Yen, C. J., Chen, S. H., Yeh, Y. M., et al. (2021). Phase 1b study of pegylated arginine deiminase (ADI-PEG 20) plus Pembrolizumab in advanced solid cancers. Oncoimmunology 10 (1), 1943253. doi:10.1080/2162402X.2021.1943253
Chen, C. L., Hsu, S. C., Ann, D. K., Yen, Y., and Kung, H. J. (2021). Arginine signaling and cancer metabolism. Cancers 13 (14), 3541. doi:10.3390/cancers13143541
Chen, X., Xu, M., Xu, X., Zeng, K., Liu, X., Pan, B., et al. (2020). METTL14-mediated N6-methyladenosine modification of SOX4 mRNA inhibits tumor metastasis in colorectal cancer. Mol. Cancer 19 (1), 106. doi:10.1186/s12943-020-01220-7
Cheng, C. T., Qi, Y., Wang, Y. C., Chi, K. K., Chung, Y., Ouyang, C., et al. (2018). Arginine starvation kills tumor cells through aspartate exhaustion and mitochondrial dysfunction. Commun. Biol. 1, 178. doi:10.1038/s42003-018-0178-4
Cheng, P. N. M., Liu, A. M., Bessudo, A., and Mussai, F. (2021). Safety, PK/PD and preliminary anti-tumor activities of pegylated recombinant human arginase 1 (BCT-100) in patients with advanced arginine auxotrophic tumors. Invest. New Drugs 39 (6), 1633–1640. doi:10.1007/s10637-021-01149-8
Del Caño-Ochoa, F., Moreno-Morcillo, M., and Ramón-Maiques, S. (2019). CAD, A multienzymatic protein at the head of de Novo pyrimidine biosynthesis. Subcell. Biochem. 93, 505538. doi:10.1007/978-3-030-28151-9_17
Giatromanolaki, A., Harris, A. L., and Koukourakis, M. I. (2021). The prognostic and therapeutic implications of distinct patterns of argininosuccinate synthase 1 (ASS1) and arginase-2 (ARG2) expression by cancer cells and tumor stroma in non-small-cell lung cancer. Cancer Metab. 9 (1), 28. doi:10.1186/s40170-021-00264-7
Glazer, E. S., Piccirillo, M., Albino, V., Di Giacomo, R., Palaia, R., Mastro, A. A., et al. (2010). Phase II study of pegylated arginine deiminase for nonresectable and metastatic hepatocellular carcinoma. J. Clin. Oncol. 28 (13), 2220–2226. doi:10.1200/JCO.2009.26.7765
Hall, P. E., Lewis, R., Syed, N., Shaffer, R., Evanson, J., Ellis, S., et al. (2019). A phase I study of pegylated arginine deiminase (pegargiminase), cisplatin, and pemetrexed in argininosuccinate synthetase 1-deficient recurrent high-grade glioma. Clin. Cancer Res. 25 (9), 2708–2716. doi:10.1158/1078-0432.CCR-18-3729
Huang, H. Y., Wu, W. R., Wang, Y. H., Wang, J. W., Fang, F. M., Tsai, J. W., et al. (2013). ASS1 as a novel tumor suppressor gene in myxofibrosarcomas: Aberrant loss via epigenetic DNA methylation confers aggressive phenotypes, negative prognostic impact, and therapeutic relevance. Clin. Cancer Res. 19 (11), 2861–2872. doi:10.1158/1078-0432.CCR-12-2641
Izzo, F., Marra, P., Beneduce, G., Castello, G., Vallone, P., De Rosa, V., et al. (2004). Pegylated arginine deiminase treatment of patients with unresectable hepatocellular carcinoma: Results from phase I/II studies. J. Clin. Oncol. 22 (10), 1815–1822. doi:10.1200/JCO.2004.11.120
Jia, H., Yang, Y., Li, M., Chu, Y., Song, H., Zhang, J., et al. (2021). Snail enhances arginine synthesis by inhibiting ubiquitination-mediated degradation of ASS1. EMBO Rep. 22 (8), e51780. doi:10.15252/embr.202051780
Keshet, R., Lee, J. S., Adler, L., Iraqi, M., Ariav, Y., Lim, L. Q. J., et al. (2020). Targeting purine synthesis in ASS1-expressing tumors enhances the response to immune checkpoint inhibitors. Nat. Cancer 1 (9), 894–908. doi:10.1038/s43018-020-0106-7
Khare, S., Kim, L. C., Lobel, G., Doulias, P. T., Ischiropoulos, H., Nissim, I., et al. (2021). ASS1 and ASL suppress growth in clear cell renal cell carcinoma via altered nitrogen metabolism. Cancer Metab. 9 (1), 40. doi:10.1186/s40170-021-00271-8
Kim, S., Lee, M., Song, Y., Choi, I., Park, I. S., Kim, J., et al. (2021). Argininosuccinate synthase 1 suppresses tumor progression through activation of PERK/eIF2α/ATF4/CHOP axis in hepatocellular carcinoma. J. Exp. Clin. Cancer Res. 40 (1), 127. doi:10.1186/s13046-021-01912-y
Kim, S. S., Xu, S., Cui, J., Poddar, S., Le, T. M., Hayrapetyan, H., et al. (2020). Histone deacetylase inhibition is synthetically lethal with arginine deprivation in pancreatic cancers with low argininosuccinate synthetase 1 expression. Theranostics 10 (2), 829–840. doi:10.7150/thno.40195
Kremer, J. C., Prudner, B. C., Lange, S. E. S., Bean, G. R., Schultze, M. B., Brashears, C. B., et al. (2017). Arginine deprivation inhibits the warburg effect and upregulates glutamine anaplerosis and serine biosynthesis in ASS1-deficient cancers. Cell Rep. 18 (4), 991–1004. doi:10.1016/j.celrep.2016.12.077
Kulis, M., and Esteller, M. (2010). DNA methylation and cancer. Adv. Genet. 70, 27–56. doi:10.1016/B978-0-12-380866-0.60002-2
Li, G., Li, D., Wang, T., and He, S. (2021). Pyrimidine biosynthetic enzyme CAD: Its function, regulation, and diagnostic potential. Int. J. Mol. Sci. 22 (19), 10253. doi:10.3390/ijms221910253
Locke, M., Ghazaly, E., Freitas, M. O., Mitsinga, M., Lattanzio, L., Lo Nigro, C., et al. (2016). Inhibition of the polyamine synthesis pathway is synthetically lethal with loss of argininosuccinate synthase 1. Cell Rep. 16 (6), 1604–1613. doi:10.1016/j.celrep.2016.06.097
Long, Y., Tsai, W. B., Wang, D., Hawke, D. H., Savaraj, N., Feun, L. G., et al. (2017). Argininosuccinate synthetase 1 (ASS1) is a common metabolic marker of chemosensitivity for targeted arginine- and glutamine-starvation therapy. Cancer Lett. 388, 54–63. doi:10.1016/j.canlet.2016.11.028
Long, Y., Tsai, W. B., Wangpaichitr, M., Tsukamoto, T., Savaraj, N., Feun, L. G., et al. (2013). Arginine deiminase resistance in melanoma cells is associated with metabolic reprogramming, glucose dependence, and glutamine addiction. Mol. Cancer Ther. 12 (11), 2581–2590. doi:10.1158/1535-7163.MCT-13-0302
Lowery, M. A., Yu, K. H., Kelsen, D. P., Harding, J. J., Bomalaski, J. S., Glassman, D. C., et al. (2017). A phase 1/1B trial of ADI-PEG 20 plus nab-paclitaxel and gemcitabine in patients with advanced pancreatic adenocarcinoma. Cancer 123 (23), 4556–4565. doi:10.1002/cncr.30897
Miao, Y. Q., Chen, W., Zhou, J., Shen, Q., Sun, Y., Li, T., et al. (2022). N(6)-adenosine-methyltransferase-14 promotes glioma tumorigenesis by repressing argininosuccinate synthase 1 expression in an m6A-dependent manner. Bioengineered 13 (1), 1858–1871. doi:10.1080/21655979.2021.2018386
Miraki-Moud, F., Ghazaly, E., Ariza-McNaughton, L., Hodby, K. A., Clear, A., Anjos-Afonso, F., et al. (2015). Arginine deprivation using pegylated arginine deiminase has activity against primary acute myeloid leukemia cells in vivo. Blood 125 (26), 4060–4068. doi:10.1182/blood-2014-10-608133
Mollick, T., and Laín, S. (2020). Modulating pyrimidine ribonucleotide levels for the treatment of cancer. Cancer Metab. 8, 12. doi:10.1186/s40170-020-00218-5
Mörén, L., Perryman, R., Crook, T., Langer, J. K., Oneill, K., Syed, N., et al. (2018). Metabolomic profiling identifies distinct phenotypes for ASS1 positive and negative GBM. BMC Cancer 18 (1), 167. doi:10.1186/s12885-018-4040-3
Nicholson, L. J., Smith, P. R., Hiller, L., Kimberley, C., Sehouli, J., Koensgen, D., et al. (2009). Epigenetic silencing of argininosuccinate synthetase confers resistance to platinum-induced cell death but collateral sensitivity to arginine auxotrophy in ovarian cancer. Int. J. Cancer 125 (6), 1454–1463. doi:10.1002/ijc.24546
Ott, P. A., Carvajal, R. D., Pandit-Taskar, N., Jungbluth, A. A., Hoffman, E. W., Wu, B. W., et al. (2013). Phase I/II study of pegylated arginine deiminase (ADI-PEG 20) in patients with advanced melanoma. Invest. New Drugs 31 (2), 425–434. doi:10.1007/s10637-012-9862-2
Pavlova, N. N., and Thompson, C. B. (2016). The emerging hallmarks of cancer metabolism. Cell Metab. 23 (1), 27–47. doi:10.1016/j.cmet.2015.12.006
Rabinovich, S., Adler, L., Yizhak, K., Sarver, A., Silberman, A., Agron, S., et al. (2015). Diversion of aspartate in ASS1-deficient tumours fosters de novo pyrimidine synthesis. Nature 527 (7578), 379–383. doi:10.1038/nature15529
Siddiqui, A., and Ceppi, P. (2020). A non-proliferative role of pyrimidine metabolism in cancer. Mol. Metab. 35, 100962. doi:10.1016/j.molmet.2020.02.005
Silberman, A., Goldman, O., Boukobza Assayag, O., Jacob, A., Rabinovich, S., Adler, L., et al. (2019). Acid-induced downregulation of ASS1 contributes to the maintenance of intracellular pH in cancer. Cancer Res. 79 (3), 518–533. doi:10.1158/0008-5472.CAN-18-1062
Singh, P. K., Deorukhkar, A. A., Venkatesulu, B. P., Li, X., Tailor, R., Bomalaski, J. S., et al. (2019). Exploiting arginine auxotrophy with pegylated arginine deiminase (ADI-PEG20) to sensitize pancreatic cancer to radiotherapy via metabolic dysregulation. Mol. Cancer Ther. 18 (12), 2381–2393. doi:10.1158/1535-7163.MCT-18-0708
Somasundaram, V., Basudhar, D., Bharadwaj, G., No, J. H., Ridnour, L. A., Cheng, R. Y. S., et al. (2019). Molecular mechanisms of nitric oxide in cancer progression, signal transduction, and metabolism. Antioxid. Redox Signal. 30 (8), 1124–1143. doi:10.1089/ars.2018.7527
Syed, N., Langer, J., Janczar, K., Singh, P., Lo Nigro, C., Lattanzio, L., et al. (2013). Epigenetic status of argininosuccinate synthetase and argininosuccinate lyase modulates autophagy and cell death in glioblastoma. Cell Death Dis. 4 (1), e458. doi:10.1038/cddis.2012.197
Szlosarek, P. W., Steele, J. P., Nolan, L., Gilligan, D., Taylor, P., Spicer, J., et al. (2017). Arginine deprivation with pegylated arginine deiminase in patients with argininosuccinate synthetase 1-deficient malignant pleural mesothelioma: A randomized clinical trial. JAMA Oncol. 3 (1), 58–66. doi:10.1001/jamaoncol.2016.3049
Szlosarek, P. W., Wimalasingham, A. G., Phillips, M. M., Hall, P. E., Chan, P. Y., Conibear, J., et al. (2021). Phase 1, pharmacogenomic, dose-expansion study of pegargiminase plus pemetrexed and cisplatin in patients with ASS1-deficient non-squamous non-small cell lung cancer. Cancer Med. 10 (19), 6642–6652. doi:10.1002/cam4.4196
Tabe, Y., Lorenzi, P. L., and Konopleva, M. (2019). Amino acid metabolism in hematologic malignancies and the era of targeted therapy. Blood 134 (13), 1014–1023. doi:10.1182/blood.2019001034
Tong, B. C., and Barbul, A. (2004). Cellular and physiological effects of arginine. Mini Rev. Med. Chem. 4 (8), 823–832. doi:10.2174/1389557043403305
Tsai, C. Y., Chi, H. C., Chi, L. M., Yang, H. Y., Tsai, M. M., Lee, K. F., et al. (2018). Argininosuccinate synthetase 1 contributes to gastric cancer invasion and progression by modulating autophagy. FASEB J. 32 (5), 2601–2614. doi:10.1096/fj.201700094R
Tsai, H. J., Jiang, S. S., Hung, W. C., Borthakur, G., Lin, S. F., Pemmaraju, N., et al. (2017). A phase II study of arginine deiminase (ADI-PEG20) in relapsed/refractory or poor-risk acute myeloid leukemia patients. Sci. Rep. 7 (1), 11253. doi:10.1038/s41598-017-10542-4
Wang, H., Li, Q. F., Chow, H. Y., Choi, S. C., and Leung, Y. C. (2020). Arginine deprivation inhibits pancreatic cancer cell migration, invasion and EMT via the down regulation of Snail, Slug, Twist, and MMP1/9. J. Physiol. Biochem. 76 (1), 73–83. doi:10.1007/s13105-019-00716-1
Wang, K., Sun, Y., Guo, C., Liu, T., Fei, X., and Chang, C. (2019). Androgen receptor regulates ASS1P3/miR-34a-5p/ASS1 signaling to promote renal cell carcinoma cell growth. Cell Death Dis. 10 (5), 339. doi:10.1038/s41419-019-1330-x
Yang, T. S., Lu, S. N., Chao, Y., Sheen, I. S., Lin, C. C., Wang, T. E., et al. (2010). A randomised phase II study of pegylated arginine deiminase (ADI-PEG 20) in Asian advanced hepatocellular carcinoma patients. Br. J. Cancer 103 (7), 954–960. doi:10.1038/sj.bjc.6605856
Yao, S., Janku, F., Subbiah, V., Stewart, J., Patel, S. P., Kaseb, A., et al. (2021). Phase 1 trial of ADI-PEG20 plus cisplatin in patients with pretreated metastatic melanoma or other advanced solid malignancies. Br. J. Cancer 124 (9), 1533–1539. doi:10.1038/s41416-020-01230-8
Yeon, A., You, S., Kim, M., Gupta, A., Park, M. H., Weisenberger, D. J., et al. (2018). Rewiring of cisplatin-resistant bladder cancer cells through epigenetic regulation of genes involved in amino acid metabolism. Theranostics 8 (16), 4520–4534. doi:10.7150/thno.25130
Zeng, J., Li, Y., Wang, Y., Xie, G., Feng, Q., Yang, Y., et al. (2020). lncRNA 00312 attenuates cell proliferation and invasion and promotes apoptosis in renal cell carcinoma via miR-34a-5p/ASS1 Axis. Oxid. Med. Cell Longev. 2020, 5737289. doi:10.1155/2020/5737289
Zhang, Y., Chung, S. F., Tam, S. Y., Leung, Y. C., and Guan, X. (2021). Arginine deprivation as a strategy for cancer therapy: An insight into drug design and drug combination. Cancer Lett. 502, 58–70. doi:10.1016/j.canlet.2020.12.041
Keywords: metabolic reprogramming, arginine, amino acid, resistance, prognosis
Citation: Sun N and Zhao X (2022) Argininosuccinate synthase 1, arginine deprivation therapy and cancer management. Front. Pharmacol. 13:935553. doi: 10.3389/fphar.2022.935553
Received: 04 May 2022; Accepted: 29 June 2022;
Published: 15 July 2022.
Edited by:
Ke-Wu Zeng, Peking University, ChinaReviewed by:
Linchong Sun, Guangdong Academy of Medical Sciences, ChinaCopyright © 2022 Sun and Zhao. This is an open-access article distributed under the terms of the Creative Commons Attribution License (CC BY). The use, distribution or reproduction in other forums is permitted, provided the original author(s) and the copyright owner(s) are credited and that the original publication in this journal is cited, in accordance with accepted academic practice. No use, distribution or reproduction is permitted which does not comply with these terms.
*Correspondence: Xing Zhao, emhhb3hpbmdfZGxAMTI2LmNvbQ==
Disclaimer: All claims expressed in this article are solely those of the authors and do not necessarily represent those of their affiliated organizations, or those of the publisher, the editors and the reviewers. Any product that may be evaluated in this article or claim that may be made by its manufacturer is not guaranteed or endorsed by the publisher.
Research integrity at Frontiers
Learn more about the work of our research integrity team to safeguard the quality of each article we publish.