- 1Outpatient and Emergency Management Office, National Children’s Medical Center, Children’s Hospital of Fudan University, Shanghai, China
- 2Department of Pediatrics, Tongji Hospital, School of Medicine, Tongji University, Shanghai, China
- 3Department of Hematology and Oncology, National Children’s Medical Center, Children’s Hospital of Fudan University, Shanghai, China
Cyclosporine (CsA) is a component of the first-line treatment for acquired aplastic anemia (acquired AA) in pediatric patients. This study aimed to develop a population pharmacokinetic (PK) model of CsA in Chinese pediatric patients with acquired AA to inform individual dosage regimens. A total of 681 CsA whole blood concentrations and laboratory data of 157 pediatric patients with acquired AA were retrospectively collected from two hospitals in Shanghai. A nonlinear mixed-effect model approach was used to build the population PK model. Potential covariate effects of age, body weight, and biochemical measurements (renal and liver functions) on CsA PK disposition were evaluated. Model fit was assessed using the basic goodness of fit and a visual predictive check. The CsA concentration data were accurately described using a two-compartment disposition model with first-order absorption and elimination. Body weight value was implemented as a fixed allometric function on all clearance and volume of distribution parameters. Total bilirubin level was identified as a significant covariate on apparent clearance (CL/F), with a 1.07% reduction per 1 nmol/L rise in total bilirubin level. The final estimates for CL/F and central volume (Vc/F) were 29.1 L/h and 325 L, respectively, for a typical 28 kg child. Other covariates (e.g., gender, age, albumin, hemoglobin, hematocrit, serum creatinine, and concomitant medication) did not significantly affect the PK properties of CsA. This population PK model, along with a maximum a posteriori Bayesian approach, could estimate individual PK parameters in pediatric patients with acquired AA to conduct individual CsA therapy.
Introduction
Acquired aplastic anemia (acquired AA) is a rare heterogeneous disorder characterized by peripheral pancytopenia and bone marrow aplasia or hypoplasia. Most patients with acquired AA (70–80%) are idiopathic because their primary etiology remains unknown (Marsh et al., 2009; Shallis et al., 2018). The annual incidence of acquired AA in Asians is 2- to 3-fold higher than that in the Western population, which has been reported to be approximately 2–2.3 patients per million (Issaragrisil et al., 1991; Montane et al., 2008; Young and Kaufman, 2008). The median age at disease diagnosis among children is around 9 years (Jeong et al., 2011). Acquired AA diagnosis was divided into three subtypes according to the related clinical guidance (Camitta et al., 1975; Camitta et al., 1976; Bacigalupo et al., 1988; Brodsky and Jones, 2005), including nonsevere AA (NSAA), severe AA (SAA), and very severe AA (vSAA). As per current clinical guidelines, immunosuppressive therapy (IST) using antithymocyte globulin (ATG) combined with cyclosporine A (CsA) is the standard first-line treatment for patients with SAA or vSAA without a suitable donor and for those with NSAA who are transfusion-dependent or experienced bleeding (Speck et al., 1977; Bacigalupo et al., 1988; Marsh et al., 1999; Rosenfeld et al., 2003; Viollier et al., 2005; Yoshida and Kojima, 2018). The overall survival rate of patients treated with IST was reported to be 68–90%, and the response rate ranged from 58 to 90% (Frickhofen et al., 1991; Rosenfeld et al., 1995; Frickhofen and Rosenfeld, 2000; Rosenfeld et al., 2003; Führer et al., 2005; Locasciulli et al., 2007; Pongtanakul et al., 2008; Saracco et al., 2008; Deyell et al., 2011; Samarasinghe et al., 2012; Dufour et al., 2015).
CsA, a classic calcineurin inhibitor, has been widely used in IST for decades (Yoshida and Kojima, 2018). CsA is mainly metabolized via cytochrome P450 isoenzymes (CYP) 3A4 and 3A5 in the liver and is also a substrate of P-glycoprotein (Kronbach et al., 1988; Aoyama et al., 1989; von Richter et al., 2004; Wojnowski, 2004; Patel and Wairkar, 2019). CsA has been reported to have high variability in its pharmacokinetic (PK) disposition (Ptachcinski et al., 1986), especially for oral dosing (Lindholm et al., 1988; Patel and Wairkar, 2019). CsA has a narrow therapeutic window for immunosuppressive purposes, usually with a whole blood trough concentration of 100–200 ng/ml for clinical indications (Marsh et al., 2009; Jain et al., 2019). Suboptimal concentration results in an insufficient clinical response, and high exposure raises patient safety concerns. The serious adverse effects included dyslipidemia, posttransplant diabetes mellitus, hypertension, intermittent renal hypoperfusion, and both reversible acute toxicity and irreversible tubulointerstitial fibrosis (Dunn et al., 2001; Olyaei et al., 2001). Its effects are different in children compared to adults because of the developmental processes, and the ontogeny of enzymes and body size could affect the disposition of the drug in the body. Thus, according to clinical guidelines, routine therapeutic drug monitoring (TDM) is strongly recommended for CsA, particularly in pediatric patients.
Population PK properties of CsA in pediatric patients have been investigated in several clinical trials, mainly targeting stem cell transplantation, posttransplantation, and nephrotic syndrome conditions (Irtan et al., 2007; Willemze et al., 2008; Kim et al., 2015; Li et al., 2019; Zhao et al., 2022). To date, population PK analysis of CsA in children with acquired AA has seldom been reported (Ni et al., 2013). Considering the remarkable difference in the physiopathology between transplantation and acquired AA, PK extrapolation across indications would have high uncertainties. This study aimed to establish a population model to characterize the PK of CsA in Chinese pediatric patients with acquired AA and to explore the potential covariate effects. The proposed population PK model can provide guidance for individual CsA therapy in pediatric patients with acquired AA.
Materials and Methods
Study Population
The eligibility criteria for the study population were pediatric patients (≤18 years old) diagnosed with acquired AA who received CsA treatment at two hospitals in Shanghai (Children’s Hospital of Fudan University and Tongji Hospital of Tongji University) from January 2014 to December 2021. The diagnosis of acquired AA was based on several critical criteria (e.g., peripheral blood investigations, bone marrow smear, and biopsy) and excluded other disease conditions such as autoimmune disease, congenital bone marrow failure, acute myeloid leukemia, acute lymphoblastic leukemia, myelodysplastic syndrome, and other malignant hematological tumors. Patients with acquired AA who received stem cell transplantation were also excluded from this study. The study protocol and the data collection were approved by the hospital’s research ethics committee.
Acquired Aplastic Anemia Treatment Protocol
The acquired AA treatment regimen was in accordance with the suggestions in the clinical guidance, including IST-containing treatment (e.g., ATG + CsA, CsA + androgen, or CsA monotherapy) and supportive care measures (transfusions, protective isolation, antibiotics, and others). The initial oral dosing regimen of CsA was 5 mg/kg/day orally, twice daily. In general, the dose was adjusted from 5 to 8 mg/kg/day and could even to 10 mg/kg/day, depending on CsA concentration, to ensure that the concentration is within the therapeutic window. As per a clinical guideline for childhood acquired AA in China (The Society of Pediatrics, 2014), it was recommended that the therapeutic windows for CsA be 100–200 ng/ml and 300–400 ng/ml for trough and peak concentration, respectively. The first whole blood concentration of CsA at a steady state was monitored after 2 weeks of administration, and then every 3–6 months afterward, if indicated. The dose was reduced only after the concentration was maintained at these levels for at least 12 months. The dose was tapered slowly (e.g., 10–20% of the original dosage was tapered once every 3 months). The clinical physician closely monitored the complete blood count, liver and renal functions, and whole blood CsA concentration at each time of dose adjustment and carefully reduced the amount if there was any fluctuation. All treatments were in accordance with the diagnosis and treatment recommendations of the pediatric society of the Chinese Medical Association.
Pharmacokinetic Sampling and Measurement
For TDM purposes, only sparse whole blood samples were routinely collected for CsA concentration measurement according to clinical practice in the two study hospitals. The PK samples were collected at or around the peak (2–4 h postdosing) and predose (within 1 h prior to dosing). CsA whole blood concentrations were determined using an Emit® 2000 Cyclosporine Specific assay (6R079UL; Siemens Healthcare Diagnostics, Inc., Newark, NJ, United States) in accordance with the procedures in the manual.
Data Collection
All relevant clinical data for the present population PK analysis were collected from patients’ medical records in the hospital information system. The data mainly contained: 1) Demographic data (gender, age at treatment, and body weight at treatment); 2) laboratory tests, including but not limited to complete blood count (red blood cell, white blood cell, platelets, hemoglobin [Hb], hematocrit [HCT]), liver function (aspartate transaminase [AST], alanine transaminase [ALT], alkaline phosphatase [ALP], direct bilirubin, total bilirubin [TBIL]), and renal function (serum creatinine [SCr] levels); 3) concomitant medications (e.g., granulocyte colony-stimulating factor, rabbit ATG [r-ATG], glucocorticoids, and testosterone undecanoate) during the therapy; and 4) PK data, such as the date and time of CsA administration, and CsA concentration readout.
Population Pharmacokinetic Analysis Approach
Population PK analysis was conducted using a nonlinear mixed-effect modeling approach using NONMEM® software (version 7.4, ICON Development Solutions, Ellicott City, MD, United States) with a gFortran compiler (version 4.6.0). PsN (version 4.6.0) and the R language (version 3.4.0) were used to summarize and visualize the modeling outputs. First-order conditional estimation with the η-ε interaction algorithm (FOCE-I) was utilized throughout the model-building procedures. Discrimination between hierarchical models was based on the objective function value (OFV), which was proportional to twice the log-likelihood (-2LL). A decrease in OFV (∆OFV) of 3.84 was considered a statistically significant improvement in model fitting (p < 0.05) between the two hierarchical models after the inclusion of one additional parameter (df = 1).
CsA concentrations were logarithmically converted for modeling analysis. A base model was selected without any covariates capable of appropriately capturing the concentration–time data. During the base model selection stage, all possible structural compartments (i.e., one- and two-compartment disposition models) were investigated.
Interindividual variability (IIV) was modeled as an exponential function on all PK parameters, where applicable (Eq. 1).
where
Covariate Modeling
Body weight was implemented in the model as a simultaneous inclusion of an allometric function for all clearance and distribution volume parameters (Eqs 2, 3, respectively).
where BWi is the individual body weight for the ith individual and BWmedian is the median body weight of the study population (28 kg).
In addition to body weight, other potential covariates, such as age, gender, laboratory tests of liver and renal function, and concomitant drugs, were investigated for all model parameters, except absorption-rate constant, using a forward selection (p = 0.05) and followed a strict backward elimination (p = 0.01) procedure.
Model Evaluation
Basic goodness-of-fit plots, such as the conditional weighted residue versus population prediction, conditional weighted residue versus time, observation versus population prediction, and individual prediction, were used to evaluate systematic discrepancies and model misspecification if it exists. The sampling importance resampling approach was employed to derive parameter uncertainties for the final population PK model with the options of sample = 2,000 and resample = 1,000. The overall predictive performance of the final population PK model was evaluated using prediction-corrected visual predictive checks [(Bergstrand et al., 2011), n = 1,000 simulations].
In Silico Simulation
Based on the final population PK model, in silico simulations were conducted according to different clinical scenarios, such as body weight, significant covariates, and dosing regimens (n = 1,000 for each scenario). The simulated PK exposure parameters (e.g., trough concentration) for each scenario were summarized and visualized.
Results
In total, 681 whole blood CsA concentrations of samples from 157 pediatric patients were included in the current population PK modeling analysis. The basic demographic characteristics of patients are presented in Table 1. Overall, the baseline demographic data of the patients were comparable between the two hospitals.
A one-compartment model with first-order absorption and elimination processes offered an OFV of 299.011. Utilizing a two-compartment model indicated a significant improvement in the model fit (∆OFV = −249.597). However, the peripheral volume of distribution estimate was implausible (6350 L); therefore, this parameter and intercompartment clearance were fixed at 496 and 5 L/h, respectively, according to the reported literature value (Eljebari et al., 2012). This model still resulted in a superior model fit compared with the one-compartment model (∆OFV = −136.676).
Body weight implemented as an allometric function on all clearance and volume of distribution parameters in the model did not lead to a worse model (∆OFV = −1.363).
Further inclusion of albumin (ALB) on clearance with a linear function resulted in a significant decrease in OFV (∆OFV = −11.425). However, the parameter estimate had poor precision (RSE = 56%); therefore, ALB was not retained in the model. Inclusion of TBIL on clearance in a linear manner led to a significant improvement in the model fit (∆OFV = −8.762) with a good precision of estimate (RSE = 20.4%) and therefore was retained in the model. Other covariates had no statistically significant effects on PK parameters.
The final parameter estimates had a good precision (RSE<30%) and confirmed the stability of the model (Table 2). The basic goodness-of-fit diagnostic plots (Figure 1) did not show any evident systematic discrepancies. Overall, the predictive-corrected visual predictive checks showed good consistency between the model-predicted and observed CsA concentration versus time profiles, although the maximum concentrations were slightly underestimated (Figure 2).
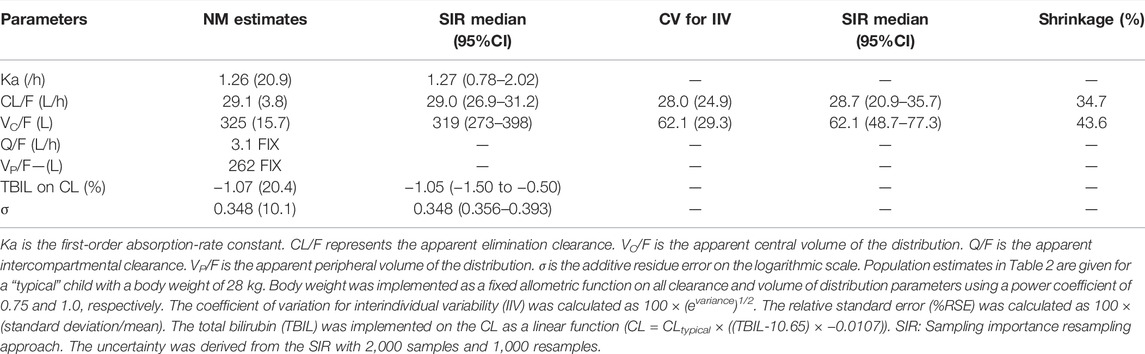
TABLE 2. Pharmacokinetic parameter estimates from the final population model of cyclosporine A in children with acquired aplastic anemia.
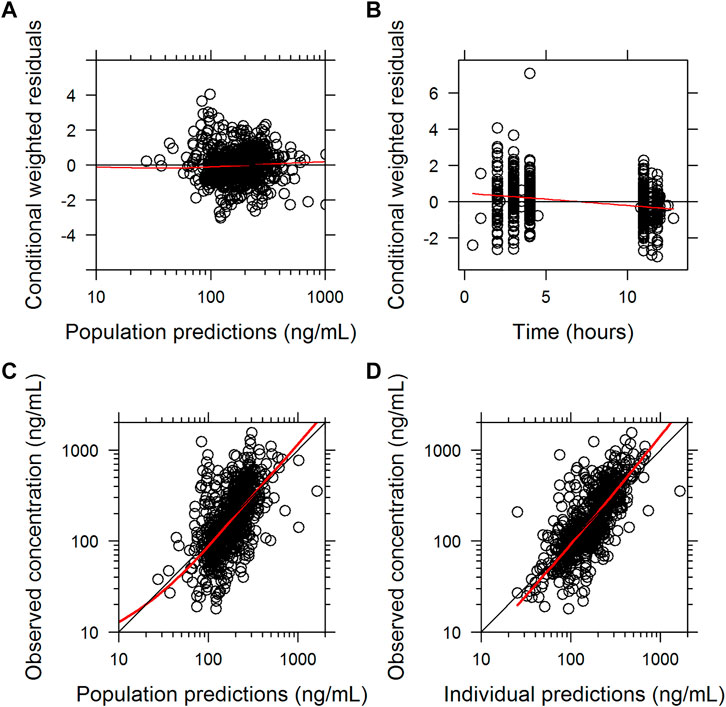
FIGURE 1. Basic goodness of fit of the final population pharmacokinetic model of cyclosporine A. (A) conditionally weighted residuals vs. population-predicted concentrations. (B) conditionally weighted residuals vs. time. (C) observed plasma concentrations vs. population-predicted concentrations. (D) observed plasma concentrations vs. individually predicted concentrations; solid red lines represent locally weighted least-squares regressions.
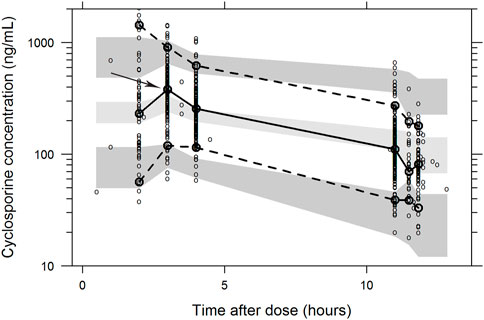
FIGURE 2. Visual predictive check of the final population pharmacokinetic model of cyclosporine A. The visual predictive check was based on 1,000 stochastic simulations. Open circles are the observations and solid lines represent the 5th, 50th, and 95th percentiles of the observed data. The shaded areas represent 95% prediction intervals around the simulated 5th, 50th, and 95th percentiles. The Cmax was slightly underestimated.
In Silico Simulation
After orally administering a 5 mg/kg daily dose of CsA, the model predicted a trough concentration at a steady state by body weight and TBIL levels, as shown in Figure 3. Considering the proposed dosing regimen, the exposure in pediatric patients with low body weight bands (<30 kg) was below the therapeutic windows (100–200 ng/ml), suggesting that an increase in dosage must be considered to achieve sufficient exposure. Moreover, pediatric patients with higher TBIL levels appeared to have higher exposure, and dose reduction in these patients was deemed necessary.
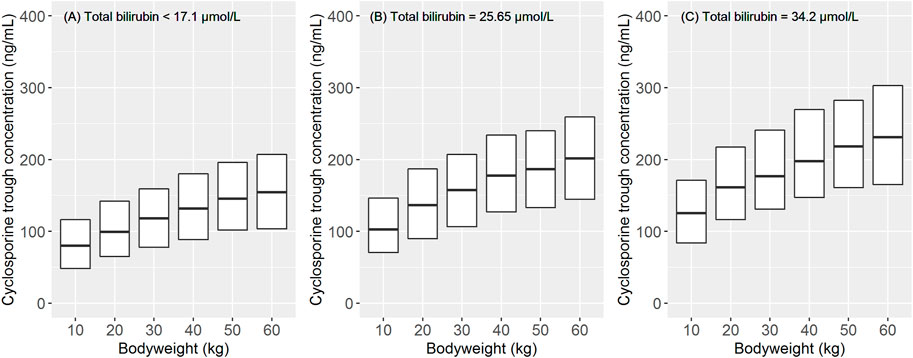
FIGURE 3. Impact of body weight and total bilirubin level on the pharmacokinetic exposure at steady state after the oral administration of cyclosporine A (5 mg/kg/d). The data of 1,000 children were used for the simulation for each body weight band. The simulation was stratified by different total bilirubin levels: (A) the normal level (<17.1 μmol/L). (B) and (C) 1.5 and 2 times the upper limits of the normal level (25.65 and 34.2 μmol/L), respectively. The total simulated exposure was presented as median values (25th–75th percentiles).
Discussion
This is a pooled population PK analysis of CsA in pediatric patients with acquired AA in two study hospitals. The proposed population PK model could accurately describe the PK properties of CsA in the target population, and TBIL could affect clearance.
As CsA has high variability in PK profiles, routine TDM is mandatory in clinical practice for individual therapy. The population PK approach combined with Bayesian estimates for individual PK parameters offers a powerful tool to achieve this purpose. Regarding the structural model in population analyses for CsA, the one-compartment deposition model was commonly used for sparse PK data (Xiaoli and Qiang, 2009; Ni et al., 2013; Li et al., 2019; Albitar et al., 2020). The current modeling analysis using CsA trough and peak concentrations suggested an appropriate two-compartment disposition model, which was consistent with several published CsA population analyses (Wilhelm et al., 2012; Okada et al., 2017). In the current investigation, the apparent clearance (CL/F) estimate for a typical 28 kg child was 29.1 L/h, which was higher than that reported in a previous study including 102 children with AA (15.1 L in a child weighing 29.8 kg) (Ni et al., 2013). The discrepancy in CL/F might be attributed to the difference in sampling strategy (trough and peak concentrations in our study) and the sequential utilization of different structural models. However, the CL/F estimate in the present study was within the range of that of published population PK models for pediatric patients receiving transplantation. The CL/F was 23.1 L/h in 98 pediatric renal transplant patients weighing 35.2 kg (Irtan et al., 2007) and 29.3 L/h in 17 pediatric patients receiving stem cell transplantation with an average body weight of 32.4 kg (Willemze et al., 2008). In the present study, the central volume (Vc/F) estimate was 325 L for a typical 28 kg child, which was higher than that reported in a previous pediatric AA study (89.1 L for a 29.8 kg child) (Ni et al., 2013), pediatric patients who received renal transplants (70.3 L for a 35.2 kg child) (Irtan et al., 2007), and pediatric patients who received stem cell transplantation (42.7 L for a 32.4 kg child) (Willemze et al., 2008) and lower than that reported in a recent study of Chinese pediatric patients with nephrotic syndrome (2320 L for a typical 25 kg child) (Zhao et al., 2022). The Vc/F estimate was still within the range of reported values from literature. Moreover, the total V/F (Vc + Vp) normalized to a 70 kg adult was 1,468 L, which was similar to those reported in some studies with adult patients (1,080 and 1,010 L for Chinese adults (Zhou et al., 2012; Wang et al., 2022), and 1,990 L for Korean adults (Ji et al., 2011)).
Considering the principles of allometry in pediatrics, the body weight was highly suggested to be included in the model (Holford et al., 2013). Fanta et al. (2007) conducted a population PK analysis for 162 pediatric patients before a transplant and found that young patients (<8 years) had approximately 25% higher body weight normalized clearance than older children. In a population PK study of pediatric patients with acquired AA, the body weight was found to correlate with CL/F and V/F (Ni et al., 2013). In the present study, we applied the body weight value in all the clearance and volume parameters as an allometric function with the fixed exponent of 0.75 and 1.0, respectively, which did not result in a poor model. In addition, a few studies included body surface area (BSA) (Okada et al., 2017) in the model; however, this variable was not investigated in the present study since some height data were missing, and we were unable to calculate the BSA.
Since CsA undergoes liver metabolism and renal elimination, laboratory tests for liver and renal function show that PK disposition is seemingly affected in the body. Fanta et al. (2007) suggested that total plasma cholesterol level was correlated with CL/F in pediatric patients undergoing renal transplantation, with a 5.4% reduction per 1 mmol/L increase in cholesterol level. A population PK analysis of Chinese patients who underwent allogeneic hematopoietic stem cell transplantation showed that the plasma albumin level was inversely correlated with CL/F, with a 2.89% drop per 1 g/L increase in albumin level (Zhou et al., 2012). In the present study, total plasma cholesterol level was not routinely measured during outpatient visits; therefore, this covariate was not investigated. Moreover, we had a similar finding on the albumin covariate, but considering the poor precision of the estimate (>50%), the albumin value was removed from the final model. We identified TBIL as a significant covariate on CL/F, with a 1.07% reduction per 1 nmol/L rise in TBIL. Several studies on patients with transplantation have reported that TBIL, a biomarker of liver function, was relevant to CL/F (Wu et al., 2005; Ji et al., 2011). This finding suggested that dose adjustment may be required in patients with elevated TBIL levels.
Ni et al. (2013) found that SCr levels were a significant covariate on CL/F in pediatric patients with AA, with an 8.1% decrease per 1 μmol/L increase in SCr. Fanta et al. (2007) indicated that SCr levels were significantly correlated with CL/F in children undergoing renal transplantation, although the covariate effect size was small. A population PK analysis of CsA in Chinese pediatric patients receiving hematopoietic stem cell transplantation suggested a nonlinear relationship between estimated glomerular filtration rates and CL/F, with an exponent of 0.545 in power function (Li et al., 2019). In the present study, we did not identify a significant effect of SCr levels; the most likely explanation was that the majority of children had normal renal functions during the treatment period. Moreover, CsA was highly bound to erythrocytes and plasma proteins, and its distribution in the blood was reported to be approximately 41–58% in erythrocytes (Han et al., 2013). HCT was considered a significant covariate in a few previous studies (Wu et al., 2005; Yin et al., 2006; Fanta et al., 2007; Zhou et al., 2012). However, we did not find such a relationship in this study. Considering transfusion was needed in some patients, the relationship between Hb and Vc/F has been assessed with no statistical significance in the present study. However, a power relationship was suggested, with the exponent estimate of 0.159. Again, the Vc/F estimate was 290 L for a typical child with a Hb level of 85 g/L. The impact of Hb on Vc/F was not substantial.
Concomitant drugs, such as anabolic steroids (Ni et al., 2013) and triazole antifungal agents (Zhou et al., 2012; Li et al., 2019; Ling et al., 2021), have been reported to affect CsA PK exposure. Children undergoing IST often receive steroids as a concomitant drug (Ettenger, 1998; Benfield et al., 1999; Clucas et al., 2019). In theory, steroids could reduce CYP450 3A metabolism in CsA via competitive inhibition (Nakamura et al., 2002). The effects of steroids (methylprednisolone, prednisolone, or prednisone) on CsA PK exposure were further assessed in adult patients (Lam et al., 2008). In the present study, five types of steroids (Table 1) were comedicated with CsA in the treatment; however, the most frequently used steroids (testosterone, prednisone, and methylprednisolone) did not significantly influence CL/F according to the modeling analysis. Moreover, only a few patients (<5%) received triazole antifungal agent treatment, and its effect on CL/F was not further investigated.
This study has several limitations. 1) The CsA data were retrospectively collected from two centers, and a prospective clinical study would improve the data accuracy. 2) Enzyme polymorphisms have been demonstrated to contribute to the PK variability in CsA. However, relevant CYP3A and ABCB1 polymorphisms were not detected in this study, which may reduce the chance of finding polymorphism-related covariates. 3) In the present study, only two covariates were included in the final model; other significant covariates such as albumin were not retained due to the poor precision of parameter estimation. This model should, in the future, be updated with emerging data, which will allow the assessment of the covariates in a broader population and improve the goodness of fit accordingly, especially for population/individual predictions versus observation plots.
Conclusion
In this study, we developed a population PK model to describe the PK property of CsA in Chinese pediatric patients with acquired AA. Body weight and TBIL level were significant covariates for the PK disposition of CsA. The proposed model could inform precision medicine in CsA therapy for pediatric patients with acquired AA.
Data Availability Statement
The original contributions presented in the study are included in the article/Supplementary Materials; further inquiries can be directed to the corresponding authors.
Ethics Statement
The studies involving human participants were reviewed and approved by the Research Ethics Committee of the Children’s Hospital of Fudan University. Written informed consent to participate in this study was provided by the participants’ legal guardian/next of kin.
Author Contributions
X-WZ and H-SW conceived and designed the study. XG, Z-LB, X-HQ, X-WQ, JL, G-MS, HM, YY, J-HM, X-HZ, J-YJ, JL, and LY collected the data, whereas X-WZ and H-SW evaluated the data. XG, Z-LB, and X-HQ built the model and wrote the manuscript. X-WZ and H-SW have reviewed and edited the manuscript. All the authors have read and approved the final manuscript.
Funding
This work was supported by the Science and Technology Commission of Shanghai Municipality (21Y31900302) and Cyrus Tang Foundation (ZSBK0070).
Conflict of Interest
The authors declare that the research was conducted in the absence of any commercial or financial relationships that could be construed as potential conflicts of interest.
Publisher’s Note
All claims expressed in this article are solely those of the authors and do not necessarily represent those of their affiliated organizations or those of the publisher, the editors, and the reviewers. Any product that may be evaluated in this article, or claim that may be made by its manufacturer, is not guaranteed or endorsed by the publisher.
Acknowledgments
We would like to pay our sincere and kind respect to pediatric patients for their participation, to the doctors and nurses at the Children’s Hospital of Fudan University and Tongji Hospital of Tongji University for taking care of the patients, and to the lab and the Department of Pharmacy for measurements.
References
Albitar, O., Ballouze, R., Harun, S. N., Mohamed Noor, D. A., and Sheikh Ghadzi, S. M. (2020). Population Pharmacokinetic Modeling of Cyclosporine Among Malaysian Renal Transplant Patients: An Evaluation of Methods to Handle Missing Doses in Conventional Drug-Monitoring Data. J. Clin. Pharmacol. 60, 1474–1482. doi:10.1002/jcph.1670
Aoyama, T., Yamano, S., Waxman, D. J., Lapenson, D. P., Meyer, U. A., Fischer, V., et al. (1989). Cytochrome P-450 hPCN3, a Novel Cytochrome P-450 IIIA Gene Product that Is Differentially Expressed in Adult Human Liver. cDNA and Deduced Amino Acid Sequence and Distinct Specificities of cDNA-Expressed hPCN1 and hPCN3 for the Metabolism of Steroid Hormones and Cyclosporine. J. Biol. Chem. 264, 10388–10395. doi:10.1016/s0021-9258(18)81632-5
Bacigalupo, A., Hows, J., Gluckman, E., Nissen, C., Marsh, J., Van Lint, M. T., et al. (1988). Bone Marrow Transplantation (BMT) versus Immunosuppression for the Treatment of Severe Aplastic Anaemia (SAA): a Report of the EBMT SAA Working Party. Br. J. Haematol. 70, 177–182. doi:10.1111/j.1365-2141.1988.tb02460.x
Benfield, M. R., Stablein, D., and Tejani, A. (1999). Trends in Immunosuppressive Therapy: a Report of the North American Pediatric Renal Transplant Cooperative Study (NAPRTCS). Pediatr. Transpl. 3, 27–32. doi:10.1034/j.1399-3046.1999.00001.x
Bergstrand, M., Hooker, A. C., Wallin, J. E., and Karlsson, M. O. (2011). Prediction-corrected Visual Predictive Checks for Diagnosing Nonlinear Mixed-Effects Models. Aaps J. 13, 143–151. doi:10.1208/s12248-011-9255-z
Brodsky, R. A., and Jones, R. J. (2005). Aplastic Anaemia. Lancet 365, 1647–1656. doi:10.1016/s0140-6736(05)66515-4
Camitta, B. M., Rappeport, J. M., Parkman, R., and Nathan, D. G. (1975). Selection of Patients for Bone Marrow Transplantation in Severe Aplastic Anemia. Blood 45, 355–363. doi:10.1182/blood.v45.3.355.bloodjournal453355
Camitta, B. M., Thomas, E. D., Nathan, D. G., Santos, G., Gordon-Smith, E. C., Gale, R. P., et al. (1976). Severe Aplastic Anemia: a Prospective Study of the Effect of Early Marrow Transplantation on Acute Mortality. Blood 48, 63–70. doi:10.1182/blood.V48.1.63.63
Clucas, D. B., Fox, L. C., Wood, E. M., Hong, F. S., Gibson, J., Bajel, A., et al. (2019). Revisiting Acquired Aplastic Anaemia: Current Concepts in Diagnosis and Management. Intern Med. J. 49, 152–159. doi:10.1111/imj.14140
Deyell, R. J., Shereck, E. B., Milner, R. A., and Schultz, K. R. (2011). Immunosuppressive Therapy without Hematopoietic Growth Factor Exposure in Pediatric Acquired Aplastic Anemia. Pediatr. Hematol. Oncol. 28, 469–478. doi:10.3109/08880018.2011.568043
Dufour, C., Pillon, M., Sociè, G., Rovò, A., Carraro, E., Bacigalupo, A., et al. (2015). Outcome of Aplastic Anaemia in Children. A Study by the Severe Aplastic Anaemia and Paediatric Disease Working Parties of the European Group Blood and Bone Marrow Transplant. Br. J. Haematol. 169, 565–573. doi:10.1111/bjh.13297
Dunn, C. J., Wagstaff, A. J., Perry, C. M., Plosker, G. L., and Goa, K. L. (2001). Cyclosporin: an Updated Review of the Pharmacokinetic Properties, Clinical Efficacy and Tolerability of a Microemulsion-Based Formulation (Neoral)1 in Organ Transplantation. Drugs 61, 1957–2016. doi:10.2165/00003495-200161130-00006
Eljebari, H., Gaies, E., Fradj, N. B., Jebabli, N., Salouage, I., Trabelsi, S., et al. (2012). Population Pharmacokinetics and Bayesian Estimation of Cyclosporine in a Tunisian Population of Hematopoietic Stem Cell Transplant Recipient. Eur. J. Clin. Pharmacol. 68, 1517–1524. doi:10.1007/s00228-012-1275-9
Ettenger, R. B. (1998). New Immunosuppressive Agents in Pediatric Renal Transplantation. Transpl. Proc. 30, 1956–1958. doi:10.1016/s0041-1345(98)00493-x
Fanta, S., Jönsson, S., Backman, J. T., Karlsson, M. O., and Hoppu, K. (2007). Developmental Pharmacokinetics of Ciclosporin-Aa Population Pharmacokinetic Study in Paediatric Renal Transplant Candidates. Br. J. Clin. Pharmacol. 64, 772–784. doi:10.1111/j.1365-2125.2007.03003.x
Frickhofen, N., Kaltwasser, J. P., Schrezenmeier, H., Raghavachar, A., Vogt, H. G., Herrmann, F., et al. (1991). Treatment of Aplastic Anemia with Antilymphocyte Globulin and Methylprednisolone with or without Cyclosporine. The German Aplastic Anemia Study Group. N. Engl. J. Med. 324, 1297–1304. doi:10.1056/nejm199105093241901
Frickhofen, N., and Rosenfeld, S. J. (2000). Immunosuppressive Treatment of Aplastic Anemia with Antithymocyte Globulin and Cyclosporine. Semin. Hematol. 37, 56–68. doi:10.1016/s0037-1963(00)90030-1
Führer, M., Rampf, U., Baumann, I., Faldum, A., Niemeyer, C., Janka-Schaub, G., et al. (2005). Immunosuppressive Therapy for Aplastic Anemia in Children: a More Severe Disease Predicts Better Survival. Blood 106, 2102–2104. doi:10.1182/blood-2005-03-0874
Han, K., Pillai, V. C., and Venkataramanan, R. (2013). Population Pharmacokinetics of Cyclosporine in Transplant Recipients. AAPS J. 15, 901–912. doi:10.1208/s12248-013-9500-8
Holford, N., Heo, Y. A., and Anderson, B. (2013). A Pharmacokinetic Standard for Babies and Adults. J. Pharm. Sci. 102, 2941–2952. doi:10.1002/jps.23574
Irtan, S., Saint-Marcoux, F., Rousseau, A., Zhang, D., Leroy, V., Marquet, P., et al. (2007). Population Pharmacokinetics and Bayesian Estimator of Cyclosporine in Pediatric Renal Transplant Patients. Ther. Drug Monit. 29, 96–102. doi:10.1097/FTD.0b013e3180310f9d
Issaragrisil, S., Sriratanasatavorn, C., Piankijagum, A., Vannasaeng, S., Porapakkham, Y., Leaverton, P. E., et al. (1991). Incidence of Aplastic Anemia in Bangkok. The Aplastic Anemia Study Group. Blood 77, 2166–2168. doi:10.1182/blood.v77.10.2166.bloodjournal77102166
Jain, R., Trehan, A., Bansal, D., and Varma, N. (2019). Aplastic Anemia in Children: How Good Is Immunosuppressive Therapy? Pediatr. Hematol. Oncol. 36, 211–221. doi:10.1080/08880018.2019.1621970
Jeong, D. C., Chung, N. G., Kang, H. J., Koo, H. H., Kook, H., Kim, S. K., et al. (2011). Epidemiology and Clinical Long-Term Outcome of Childhood Aplastic Anemia in Korea for 15 Years: Retrospective Study of the Korean Society of Pediatric Hematology Oncology (KSPHO). J. Pediatr. Hematol. Oncol. 33, 172–178. doi:10.1097/MPH.0b013e31820826a8
Ji, E., Kim, M. Y., Yun, H. Y., Kim, K. I., Kang, W., Kwon, K. I., et al. (2011). Population Pharmacokinetics of Cyclosporine in Korean Adults Undergoing Living-Donor Kidney Transplantation. Pharmacotherapy 31, 574–584. doi:10.1592/phco.31.6.574
Kim, M. G., Kim, I. W., Choi, B., Han, N., Yun, H. Y., Park, S., et al. (2015). Population Pharmacokinetics of Cyclosporine in Hematopoietic Stem Cell Transplant Patients: Consideration of Genetic Polymorphisms. Ann. Pharmacother. 49, 622–630. doi:10.1177/1060028015577798
Kronbach, T., Fischer, V., and Meyer, U. A. (1988). Cyclosporine Metabolism in Human Liver: Identification of a Cytochrome P-450III Gene Family as the Major Cyclosporine-Metabolizing Enzyme Explains Interactions of Cyclosporine with Other Drugs. Clin. Pharmacol. Ther. 43, 630–635. doi:10.1038/clpt.1988.87
Lam, S., Partovi, N., Ting, L. S., and Ensom, M. H. (2008). Corticosteroid Interactions with Cyclosporine, Tacrolimus, Mycophenolate, and Sirolimus: Fact or Fiction? Ann. Pharmacother. 42, 1037–1047. doi:10.1345/aph.1K628
Li, T. F., Hu, L., Ma, X. L., Huang, L., Liu, X. M., Luo, X. X., et al. (2019). Population Pharmacokinetics of Cyclosporine in Chinese Children Receiving Hematopoietic Stem Cell Transplantation. Acta Pharmacol. Sin. 40, 1603–1610. doi:10.1038/s41401-019-0277-x
Lindholm, A., Henricsson, S., Lind, M., and Dahlqvist, R. (1988). Intraindividual Variability in the Relative Systemic Availability of Cyclosporin after Oral Dosing. Eur. J. Clin. Pharmacol. 34, 461–464. doi:10.1007/bf01046702
Ling, J., Yang, X. P., Dong, L. L., Jiang, Y., Zou, S. L., Hu, N., et al. (2021). Population Pharmacokinetics of Ciclosporin in Allogeneic Hematopoietic Stem Cell Transplant Recipients: C‐reactive Protein as a Novel Covariate for Clearance. Clin. Pharm. Ther. 47, 483–492. doi:10.1111/jcpt.13569
Locasciulli, A., Oneto, R., Bacigalupo, A., Socié, G., Korthof, E., Bekassy, A., et al. (2007). Outcome of Patients with Acquired Aplastic Anemia Given First Line Bone Marrow Transplantation or Immunosuppressive Treatment in the Last Decade: a Report from the European Group for Blood and Marrow Transplantation (EBMT). Haematologica 92, 11–18. doi:10.3324/haematol.10075
Marsh, J., Schrezenmeier, H., Marin, P., Ilhan, O., Ljungman, P., Mccann, S., et al. (1999). Prospective Randomized Multicenter Study Comparing Cyclosporin Alone versus the Combination of Antithymocyte Globulin and Cyclosporin for Treatment of Patients with Nonsevere Aplastic Anemia: A Report from the European Blood and Marrow Transplant (EBMT) Severe Aplastic Anaemia Working Party. Blood 93, 2191–2195. doi:10.1182/blood.V93.7.2191
Marsh, J. C. W., Ball, S. E., Cavenagh, J., Darbyshire, P., Dokal, I., Gordon-Smith, E. C., et al. (2009). Guidelines for the Diagnosis and Management of Aplastic Anaemia. Br. J. Haematol. 147, 43–70. doi:10.1111/j.1365-2141.2009.07842.x
Montané, E., Ibáñez, L., Vidal, X., Ballarín, E., Puig, R., García, N., et al. (2008). Epidemiology of Aplastic Anemia: a Prospective Multicenter Study. Haematologica 93, 518–523. doi:10.3324/haematol.12020
Nakamura, H., Nakasa, H., Ishii, I., Ariyoshi, N., Igarashi, T., Ohmori, S., et al. (2002). Effects of Endogenous Steroids on CYP3A4-Mediated Drug Metabolism by Human Liver Microsomes. Drug Metab. Dispos. 30, 534–540. doi:10.1124/dmd.30.5.534
Ni, S. Q., Zhao, W., Wang, J., Zeng, S., Chen, S. Q., Jacqz-Aigrain, E., et al. (2013). Population Pharmacokinetics of Ciclosporin in Chinese Children with Aplastic Anemia: Effects of Weight, Renal Function and Stanozolol Administration. Acta Pharmacol. Sin. 34, 969–975. doi:10.1038/aps.2013.9
Okada, A., Ushigome, H., Kanamori, M., Morikochi, A., Kasai, H., Kosaka, T., et al. (2017). Population Pharmacokinetics of Cyclosporine A in Japanese Renal Transplant Patients: Comprehensive Analysis in a Single Center. Eur. J. Clin. Pharmacol. 73, 1111–1119. doi:10.1007/s00228-017-2279-2
Olyaei, A. J., De Mattos, A. M., and Bennett, W. M. (2001). Nephrotoxicity of Immunosuppressive Drugs: New Insight and Preventive Strategies. Curr. Opin. Crit. Care 7, 384–389. doi:10.1097/00075198-200112000-00003
Patel, D., and Wairkar, S. (2019). Recent Advances in Cyclosporine Drug Delivery: Challenges and Opportunities. Drug Deliv. Transl. Res. 9, 1067–1081. doi:10.1007/s13346-019-00650-1
Pongtanakul, B., Das, P. K., Charpentier, K., and Dror, Y. (2008). Outcome of Children with Aplastic Anemia Treated with Immunosuppressive Therapy. Pediatr. Blood Cancer 50, 52–57. doi:10.1002/pbc.21377
Ptachcinski, R. J., Venkataramanan, R., and Burckart, G. J. (1986). Clinical Pharmacokinetics of Cyclosporin. Clin. Pharmacokinet. 11, 107–132. doi:10.2165/00003088-198611020-00002
Rosenfeld, S., Follmann, D., Nunez, O., and Young, N. S. (2003). Antithymocyte Globulin and Cyclosporine for Severe Aplastic Anemia: Association between Hematologic Response and Long-Term Outcome. Jama 289, 1130–1135. doi:10.1001/jama.289.9.1130
Rosenfeld, S. J., Kimball, J., Vining, D., and Young, N. S. (1995). Intensive Immunosuppression with Antithymocyte Globulin and Cyclosporine as Treatment for Severe Acquired Aplastic Anemia. Blood 85, 3058–3065. doi:10.1182/blood.v85.11.3058.bloodjournal85113058
Samarasinghe, S., Steward, C., Hiwarkar, P., Saif, M. A., Hough, R., Webb, D., et al. (2012). Excellent Outcome of Matched Unrelated Donor Transplantation in Paediatric Aplastic Anaemia Following Failure with Immunosuppressive Therapy: a United Kingdom Multicentre Retrospective Experience. Br. J. Haematol. 157, 339–346. doi:10.1111/j.1365-2141.2012.09066.x
Saracco, P., Quarello, P., Iori, A. P., Zecca, M., Longoni, D., Svahn, J., et al. (2008). Bone Marrow Failure Study Group of the, ACyclosporin A Response and Dependence in Children with Acquired Aplastic Anaemia: a Multicentre Retrospective Study with Long-Term Observation Follow-Up. Br. J. Haematol. 140, 197–205. doi:10.1111/j.1365-2141.2007.06903.x
Shallis, R. M., Ahmad, R., and Zeidan, A. M. (2018). Aplastic Anemia: Etiology, Molecular Pathogenesis, and Emerging Concepts. Eur. J. Haematol. 101, 711–720. doi:10.1111/ejh.13153
Speck, B., Gluckman, E., Haak, H. L., and Van Rood, J. J. (1977). Treatment of Aplastic Anaemia by Antilymphocyte Globulin with and without Allogeneic Bone-Marrow Infusions. Lancet 2, 1145–1148. doi:10.1016/s0140-6736(77)91537-9
The Society of Pediatrics, C. M. A. (2014). Recommendations on Diagnosis and Treatment for Childhood Acquired Aplastic Anemia. Chin. J. Pediatr. 52, 103–106. doi:10.3760/cma.j.issn.0578-1310.2014.02.006
Viollier, R., Passweg, J., Gregor, M., Favre, G., Kühne, T., Nissen, C., et al. (2005). Quality-adjusted Survival Analysis Shows Differences in Outcome after Immunosuppression or Bone Marrow Transplantation in Aplastic Anemia. Ann. Hematol. 84, 47–55. doi:10.1007/s00277-004-0930-3
Von Richter, O., Burk, O., Fromm, M. F., Thon, K. P., Eichelbaum, M., and Kivistö, K. T. (2004). Cytochrome P450 3A4 and P-Glycoprotein Expression in Human Small Intestinal Enterocytes and Hepatocytes: a Comparative Analysis in Paired Tissue Specimens. Clin. Pharmacol. Ther. 75, 172–183. doi:10.1016/j.clpt.2003.10.008
Wang, D. D., He, S. M., Yang, Y., Mao, Y. Z., Yin, D., Zheng, Z. Q., et al. (2022). Effects of Cimetidine on Ciclosporin Population Pharmacokinetics and Initial Dose Optimization in Aplastic Anemia Patients. Eur. J. Pharm. Sci. 174, 106183. doi:10.1016/j.ejps.2022.106183
Wilhelm, A. J., De Graaf, P., Veldkamp, A. I., Janssen, J. J., Huijgens, P. C., and Swart, E. L. (2012). Population Pharmacokinetics of Ciclosporin in Haematopoietic Allogeneic Stem Cell Transplantation with Emphasis on Limited Sampling Strategy. Br. J. Clin. Pharmacol. 73, 553–563. doi:10.1111/j.1365-2125.2011.04116.x
Willemze, A. J., Cremers, S. C., Schoemaker, R. C., Lankester, A. C., Den Hartigh, J., Burggraaf, J., et al. (2008). Ciclosporin Kinetics in Children after Stem Cell Transplantation. Br. J. Clin. Pharmacol. 66, 539–545. doi:10.1111/j.1365-2125.2008.03217.x
Wojnowski, L. (2004). Genetics of the Variable Expression of CYP3A in Humans. Ther. Drug Monit. 26, 192–199. doi:10.1097/00007691-200404000-00019
Wu, K. H., Cui, Y. M., Guo, J. F., Zhou, Y., Zhai, S. D., Cui, F. D., et al. (2005). Population Pharmacokinetics of Cyclosporine in Clinical Renal Transplant Patients. Drug Metab. Dispos. 33, 1268–1275. doi:10.1124/dmd.105.004358
Xiaoli, D., and Qiang, F. (2009). Population Pharmacokinetic Study of Cyclosporine in Patients with Nephrotic Syndrome. J. Clin. Pharmacol. 49, 782–788. doi:10.1177/0091270009337132
Yin, O. Q., Lau, S. K., and Chow, M. S. (2006). Population Pharmacokinetics of Cyclosporine in Chinese Cardiac Transplant Recipients. Pharmacotherapy 26, 790–797. doi:10.1592/phco.26.6.790
Yoshida, N., and Kojima, S. (2018). Updated Guidelines for the Treatment of Acquired Aplastic Anemia in Children. Curr. Oncol. Rep. 20, 67. doi:10.1007/s11912-018-0716-8
Young, N. S., and Kaufman, D. W. (2008). The Epidemiology of Acquired Aplastic Anemia. Haematologica 93, 489–492. doi:10.3324/haematol.12855
Zhao, Y., He, H., Zang, Y., Zhao, L., and Wang, X. (2022). Population Pharmacokinetics and Dose Simulation of Cyclosporine in Chinese Children with Nephrotic Syndrome: Effects of Weight and Total Cholesterol. Int. J. Clin. Pharmacol. Ther. 60, 87–96. doi:10.5414/cp203921
Keywords: cyclosporine, population pharmacokinetics, NONMEM, acquired aplastic anemia, pediatric patients
Citation: Gao X, Bian Z-L, Qiao X-H, Qian X-W, Li J, Shen G-M, Miao H, Yu Y, Meng J-H, Zhu X-H, Jiang J-Y, Le J, Yu L, Wang H-S and Zhai X-W (2022) Population Pharmacokinetics of Cyclosporine in Chinese Pediatric Patients With Acquired Aplastic Anemia. Front. Pharmacol. 13:933739. doi: 10.3389/fphar.2022.933739
Received: 01 May 2022; Accepted: 22 June 2022;
Published: 26 July 2022.
Edited by:
Yang Zhou, Brown University, United StatesReviewed by:
Wei Zhao, Shandong University, ChinaJignesh D. Dalal, Case Western Reserve University, United States
Copyright © 2022 Gao, Bian, Qiao, Qian, Li, Shen, Miao, Yu, Meng, Zhu, Jiang, Le, Yu, Wang and Zhai. This is an open-access article distributed under the terms of the Creative Commons Attribution License (CC BY). The use, distribution or reproduction in other forums is permitted, provided the original author(s) and the copyright owner(s) are credited and that the original publication in this journal is cited, in accordance with accepted academic practice. No use, distribution or reproduction is permitted which does not comply with these terms.
*Correspondence: Xiao-Wen Zhai, xwzhai@fudan.edu.cn; Hong-Sheng Wang, honswang@hotmail.com
†These authors have contributed equally to this work and share first authorship