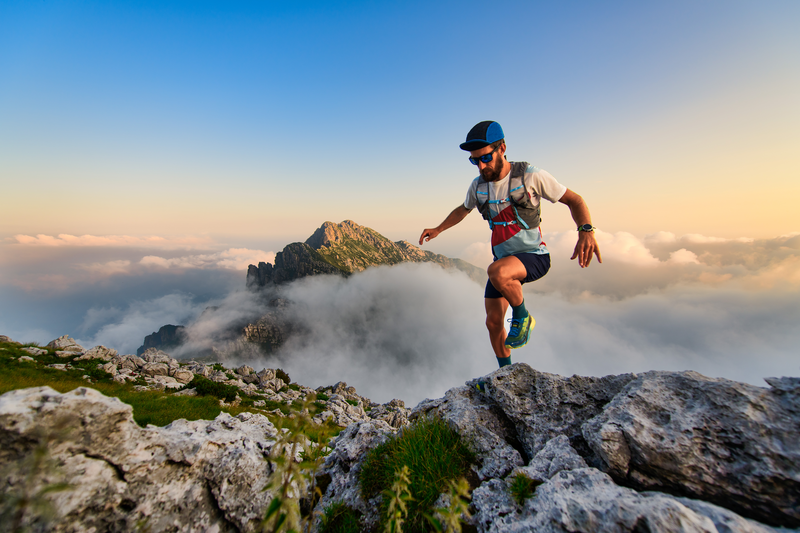
94% of researchers rate our articles as excellent or good
Learn more about the work of our research integrity team to safeguard the quality of each article we publish.
Find out more
ORIGINAL RESEARCH article
Front. Pharmacol. , 01 August 2022
Sec. Drugs Outcomes Research and Policies
Volume 13 - 2022 | https://doi.org/10.3389/fphar.2022.926711
This article is part of the Research Topic Advances in Drug-induced Diseases View all 20 articles
Objectives: There have been limited studies concerning the safety and efficacy of linezolid (LZD) in children. This study aimed to evaluate the association between LZD exposure and clinical safety and efficacy in Chinese pediatric patients.
Methods: This retrospective cross-sectional study included patients ≤18 years of age who received ≥3 days of LZD treatment between 31 January 2015, and 31 December 2020. Demographic characteristics, medication information, laboratory test information, and bacterial culture results were collected from the Hospital Information System (HIS). Exposure was defined as AUC24 and calculated by the non-linear mixed-effects modeling program (NONMEM), version 7.2, based on two validated population pharmacokinetic models. Binary logistic regression analyses were performed to analyze the associations between AUC24 and laboratory adverse events, and receiver operating characteristic curves were used to calculate the cut-off values. Efficacy was evaluated by bacterial clearance.
Results: A total of 413 paediatric patients were included, with an LZD median (interquartile range) dose, duration, clearance and AUC24 of 30.0 (28.1-31.6) mg/kg/day, 8 (4‒15) days,1.31 (1.29-1.32) L/h and 81.1 (60.6-108.7) mg/L·h, respectively. Adverse events associated with TBil, AST, ALT, PLT, hemoglobin, WBC, and neutrophil count increased during and after LZD treatment when compared with before medication (p < 0.05), and the most common adverse events were thrombocytopaenia (71/399, 17.8%) and low hemoglobin (61/401, 15.2%) during the LZD treatment. Patients with AUC24 higher than 120.69 mg/L h might be associated with low hemoglobin 1–7 days after the end of the LZD treatment, and those with an AUC24 higher than 92.88 mg/L∙h might be associated with thrombocytopaenia 8–15 days after the end of the LZD treatment. A total of 136 patients underwent bacterial culture both before and after LZD treatment, and the infection was cleared in 92.6% (126/136) of the patients, of whom 69.8% (88/126) had AUC24/MIC values greater than 80.
Conclusion: Hematological indicators should be carefully monitored during LZD treatment, especially thrombocytopaenia and low hemoglobin, and a continuous period of monitoring after LZD withdrawal is also necessary. Since the AUC24 cut-off values for laboratory adverse events were relatively low, a trade-off is necessary between the level of drug exposure required for treatment and safety, and the exposure target (AUC24/MIC) in pediatric patients should be further studied, especially for patients with complications and concomitant medications.
LZD is an oxazolidinone antibiotic that inhibits bacterial protein synthesis and prevents bacterial reproduction by binding to the bacterial 23S ribosomal RNA of the 50S subunit blocking the formation of a functional 70S initiation complex (Daniel and Ronald, 2001). The absolute oral bioavailability of LZD is approximately 100%, with good tissue penetration and non-susceptibility to drug resistance (Roger et al., 2018). It is commonly used to treat severe Gram-positive bacterial infections. It is considered clinically effective but is usually difficult to manage because of its large individual pharmacokinetic differences and related adverse events, especially in pediatric patients, and it is an antibiotic with a narrow therapeutic window and dose-dependent toxicity (Sotgiu et al., 2012; Peyrani et al., 2014).
A meta-analysis showed that approximately one out of every two patients experienced adverse events due to LZD (4), but the incidence of LZD-related adverse reactions in Chinese children has rarely been reported. Hematological toxicity, hyperlacticaemia, and optic neuropathy are the main adverse reactions to LZD (3, 4, 5), and thrombocytopaenia is a significant adverse drug reaction with the highest risk in the clinic (Han et al., 2021). The incidence of LZD-induced thrombocytopaenia varies from 3.8% to 15.7% in children worldwide (Meissner et al., 2003; Garazzino et al., 2011; Garazzino and Tovo, 2011), which is lower than that in adults (range 16.7–60.5%) but higher than the drug label reported (2.4% in children) (Natsumoto et al., 2014; Hirano et al., 2014; U.S. Department of Health and Human Services, 2021). In addition, the risk of adverse reactions increased with exposure and duration of LZD treatment (Matsumoto et al., 2014; Rao et al., 2020), the incidence of thrombocytopaenia in adult patients was significantly higher when the trough concentration was greater than 7.5 mg/L (Nukui et al., 2013), and children with thrombocytopaenia had a significantly higher average trough concentration than those without thrombocytopaenia (19.8 vs. 6.8 mg/L) (Ogami et al., 2019), but the relationship between LZD exposure and adverse reactions in Chinese children has not been studied.
Population pharmacokinetic models of LZD in children have been widely established and have been used to calculate drug exposure, and their extrapolated predictive performance has been confirmed (Vinks, 2002; Jungbluth et al., 2003; Rao et al., 2020; Ogami et al., 2021). Therefore, in this study, we aimed to calculate LZD exposure using population pharmacokinetic models of LZD in children and then evaluated the relationship between drug exposure and adverse events in Chinese pediatric patients. The efficacy of LZD was also evaluated for personalized drug therapy using LZD and risk assessment in clinical therapy.
We conducted a retrospective cross-sectional study of hospitalized children who received LZD treatment in the Children’s Hospital of Chongqing Medical University (Chongqing, China) from 31 January 2015, to 31 December 2020. This study was approved by the Ethics Committee of the Children’s Hospital of Chongqing Medical University with an informed consent exemption considering the observational and retrospective nature of the study, and the data were collected without identifiers (Approval No. 2020–282). We used the STROBE checklist as the main reference in reports of this cross-sectional study.
Patients younger than 18 years of age that were intravenously or orally administered LZD for at least three consecutive days were included. The criteria for patient exclusion were a lack of demographic data, LZD medication information, or baseline laboratory data for safety assessment.
Medical records in the hospital information system (HIS) database of the patients who matched the inclusion criteria were extracted by an information centre engineer, and then, two of the authors manually screened the information for inclusion and identified and recorded reasons for exclusion. Any disagreements were resolved through discussion or by consulting a third author. The HIS database is a comprehensive, integrated information system includes detailed clinical and demographic information about all pediatric patients, and the information from the HIS database are derived from daily notes recorded by clinicians of all the patients, which helps to improve patient care by assessing data and making recommendations for care. The following information of the included patients was extracted and recorded.
1) Demographic parameters, medication information, and serum creatinine concentration (Scr): sex, age, body weight, height, clinical diagnosis, LZD medication route, dosage, administration time and duration of LZD treatment, and serum creatinine concentrations measured during LZD medication. These data were used for the LZD exposure calculations.
2) Data for safety assessment: total bilirubin (TBil), aspartate aminotransferase (AST), alanine aminotransferase (ALT), Scr, blood urea nitrogen (BUN), platelet (PLT), hemoglobin, white blood cell count (WBC), and neutrophil count measured before LZD medication, during treatment, and 1–7 days and 8–15 days after the last dose of LZD administration.
3) Data for efficacy assessment: bacterial culture results and the measured LZD minimal inhibitory concentration (MIC) values.
Two population pharmacokinetic models established by Garcia-Prats AJ et al. (Garcia-Prats et al., 2019) and Si-Chan Li et al. (Li et al., 2019) were chosen to calculate the exposure of LZD in this study, and the predictive performance of the two models has been validated and used in our hospitals. The NONMEM, version 7.2 (Icon Development Solutions, Columbia, MD, United States), was used to perform the simulations and calculate the LZD exposure. The related formulas of the two models are shown in Supplementary Table S1. Exposure to LZD was defined as a 24-h area under the concentration-time curve (AUC24) in the steady state, and AUC24 = daily dose/clearance.
In this study, the safety of LZD was evaluated by laboratory adverse events, which were defined based on the Food and Drug Administration label for LZD (13), see Table 1. Efficacy was evaluated by comparing the bacterial culture results before and after LZD treatment, and the proportion of AUC24/MIC values greater than 80 was also calculated, as previous studies have shown that higher success rates for LZD might occur at AUC24/MIC values greater than 80 (22,23,24,25).
TABLE 1. The definition of laboratory adverse eventsa.
The Shapiro–Wilk test was performed to assess whether the data were normally distributed. Continuous outcomes with abnormal distributions are expressed as medians and interquartile ranges. Categorical outcomes are reported as counts and percentages. AUC24 was calculated to indicate the in vivo exposure of LZD, and patients were divided into four groups according to the interquartile range (IQR) of AUC24: quartile 1, quartile 2, quartile 3, and quartile 4. A generalized estimating equation (GEE) was used to analyze the incidence of changes in the laboratory adverse events over time before and after LZD treatment. Binary logistic regression analyses were performed to analyze the associations between AUC24 and safety, and age, sex, and laboratory parameters measured before the LZD medication were considered potential confounding factors based on the preliminary analysis and a literature review (Chang et al., 2013; Mullins et al., 2013; Chuang et al., 2014) and were included for adjustment. The covariates were evaluated continuously, and by the quartile of exposure, odds ratios (ORs) and 95% confidence intervals (CIs) were calculated. Receiver operating characteristic (ROC) curves were used to estimate the exposure cut-off values for the laboratory adverse events. The sensitivity and specificity and the maximum Youden’s index of the ROC curve were calculated, and the maximum Youden’s index was selected as the optimal exposure cut-off value. Youden’s index equals the result of subtracting one from the sum of sensitivity and specificity (Rui et al., 2016). p values less than 0.05 were considered to be statistically significant. Data were gathered using the Microsoft Excel software (Redmond, WA, United States), and all analyses were performed using the IBM SPSS statistical software package, version 22.0 (SPSS, Chicago, IL, United States).
Medical records of 865 patients who received LZD therapy were extracted and screened, and 413 patients who met our inclusion criteria were included in this study (Figure 1). Demographic characteristics, medication information, and exposure to LZD after drug administration are shown in Table 2. Counts and percentages of the laboratory adverse events are shown in Table 3. The most common adverse events were thrombocytopaenia (71/399, 17.8%) and low hemoglobin (61/401, 15.2%) during LZD treatment.
TABLE 2. Demographic characteristics, medication information, and exposure of linezolid according to the AUC24 quartile (n = 413).
TABLE 3. Counts and percentages of the laboratory adverse events and the change over time before and after linezolid treatment according to the AUC24 quartile.
The incidence of changes in the laboratory adverse events over time, before, and after medication is shown in Table 3. Adverse events associated with TBil, AST, ALT, PLT, hemoglobin, WBC, and neutrophil count increased during and after LZD treatment when compared with previous medication (p < 0.05). The association between LZD exposure and laboratory adverse events is shown in Table 4. The AUC24 quartile four group was associated with increased odds of low hemoglobin 1–7 days after LZD treatment compared with the quartile one group (adjusted OR: 4.768, 95% CI: 1.323-17.184, p = 0.017), and the AUC24 quartile three and quartile four groups were associated with increased odds of thrombocytopaenia 8–15 days after LZD treatment compared with the quartile one group (adjusted OR: 3.306, 95% CI: 1.126-9.709, p = 0.030 and adjusted OR: 3.770, 95% CI: 1.079-13.171, p = 0.038, respectively).
TABLE 4. Association between AUC24 and the laboratory adverse events during treatment, and 1–7 days, 8–15 days after the end of linezolid administrationa.
An ROC analysis was subsequently performed to calculate the cut-off points of AUC24 for low hemoglobin and thrombocytopenia. The ROC curves and the associated results are shown in Figure 2. The cut-off with the largest Youden index of low hemoglobin 1–7 days after the end of LZD treatment was 120.69 mg/L h with a sensitivity of 83.8% and a specificity of 67.3%, and the cut-off with the largest Youden index of thrombocytopenia 8–15 days after the end of LZD treatment was 92.88 mg/L h with a sensitivity of 75.7% and a specificity of 72.5%.
FIGURE 2. Receiver operating characteristic (ROC) curve of linezolid-induced adverse effect. (A) ROC curve of low hemoglobin 1–7 days after the end of linezolid administration. (Youden index = 0.511, cut-off values = 120.69, area under the ROC curve = 0.783, 95% confidence interval=(0.706-0.860), p < 0.001, sensitivity = 0.838, specificity = 0.673). (B) ROC curve of thrombocytopenia 8–15 days after the end of linezolid administration. (Youden index = 0.482, cut-off values = 92.88, area under the ROC curve = 0.756, 95% confidence interval=(0.693-0.820), p < 0.001, sensitivity = 0.757, specificity = 0.725).
The most common site of infection was pulmonary [254 (61.5%)], followed by skin [99 (24.0%)], blood [90 (21.8%)], and endocarditis [83 (20.1%)](Supplementary Table S2). A total of 86.4% (357/413) of the included patients underwent bacterial culture before LZD treatment, and bacteria were found in 56.0% (200/357) of the patients. The species and MIC distributions of the bacterial strains isolated from patients before LZD treatment are presented in Table 5. Bacterial culture was performed in 68% (136/200) of the aforementioned patients by the end of the LZD therapy, and infections of 92.6% (126/136) of the patients were cleared, of whom 69.8% (88/126) of the patients had AUC24/MIC values greater than 80. Bacterial infections in 7.4% (10/136) of the patients were not cleared, of whom 90.0% (9/10) of the patients had AUC24/MIC values greater than 80.
TABLE 5. The species and MIC distribution of bacterial strains isolated from patients before linezolid treatment (n = 200).
In this study, the patients who were intravenously or orally administered with LZD were all included, and the pharmacokinetic models of the corresponding route of administration were used to calculate the drug exposures. Studies have shown that there were no significant racial differences in the pharmacokinetic process of LZD in pediatrics (Jungbluth et al., 2003), so, we chose the pharmacokinetic model of oral administration, which was established based on a multiracial population, with race not considered a significant covariant (Garcia-Prats et al., 2019). Moreover, the pharmacokinetic model of intravenous administration of LZD was established based on Chinese pediatrics (Li et al., 2019).
We included patients with no “normal baseline values” in our study, as no “normal baseline values” does not mean it has reached the level of adverse events as defined in the study, and by comparing the incidence of associated adverse events before and after medication, we could see if there was a statistically significant increase in the incidence of related adverse events after medication. In fact, we did not find that patients with no “normal baseline values” were more prone to develop adverse events from our data. When analyzing the association between AUC24 and safety, laboratory parameters measured before LZD were considered as a potential confounding factor and were included as covariates in the binary logistic regression analyses, to avoid the influence of parameter differences between individuals before LZD medication on the statistical analysis, and to keep the validity of the results.
The hematological toxicity of LZD is widely known (Sasaki et al., 2011; Bayram et al., 2017), and in this study, thrombocytopenia and low hemoglobin were particularly significant. Pediatric patients with the treatment duration more than 28 days were more likely to have laboratory adverse events of low hematological indicators after using LZD. Therefore, hematological indicators should be carefully monitored during LZD treatment, especially for patients with long-term treatment (Dong et al., 2016). A previous study reported that one patient developed grade 4 neutropoenia 7 days after the end of LZD administration (Yasu et al., 2021), but the other influencing factors were unclear. In our study, low hemoglobin, thrombocytopenia, low WBC, and low neutrophil count occurred after the end of the LZD treatment in a significant proportion of the patients. The related mechanisms and other influencing factors deserve further study, but it seems a continuous period of monitoring after LZD withdrawal is also necessary.
Studies have reported that an adequate exposure to LZD was an AUC24 ranging between 160 and 300 mg/L h in adults (Pea et al., 2012; Cojutti et al., 2019), but the AUC24 cut-off value of LZD-associated thrombocytopenia was 280.7 mg/L h in adult patients (Pea et al., 2012) and 93.4 mg/L h for mitochondrial toxicity in infants and toddlers (Srivastava et al., 2016). In our study, we calculated the AUC24 cut-off values of 120.69 and 92.88 mg/L h for low hemoglobin and thrombocytopenia, respectively. It is suggested that for patients, especially pediatric patients, a trade-off is necessary between the level of drug exposure required for treatment and safety since an AUC24/MIC value greater than 80 is commonly recommended in clinics (Andes et al., 2002; Rayner et al., 2003; Pea et al., 2010; Li et al., 2019).
In accordance with the drug labels, the dosage of LZD was approximately 30.0 mg/kg/day for both intravenous and oral administrations in this study (U.S. Department of Health and Human Services, 2021), and we found that, although the infections in 92.6% (126/136) of the patients were cleared, 30.2% (38/126) of the patients had an AUC24/MIC value lower than 80. Since patient characteristics, peculiar pathophysiological conditions (e.g., cystic fibrosis, burn injuries, and sepsis), and combination medications could all affect the drug pharmacokinetic process and the apparent pharmacokinetic parameters (Di Paolo et al., 2010), and clearance of LZD in children younger than 12 years of age was greater than adults, with a correspondingly lower AUC24 (Jungbluth et al., 2003; Principi and Esposito, 2019), a previous study suggested that the LZD exposure target was an AUC24/MIC ratio of 62 with combination therapy (faropenem, LZD, and moxifloxacin) for disseminated and intrathoracic tuberculosis in infants and toddlers (Srivastava et al., 2016). Although concomitant medication was not a significant covariant in either of the two population pharmacokinetic models, when considering the efficacy, the exposure target of LZD in pediatrics might require further study, especially for pediatric patients with complications and concomitant medications.
This study had several limitations. First, the results were potentially only biased by the LZD that we analyzed being used at a limited centre. Second, our study only included patients younger than 13 years of age, limiting our ability to comprehensively assess LZD’s safety. Additionally, this study had a short follow-up period; therefore, large-scale, randomized clinical trials with longer follow-ups are still needed to further verify the safety and clinical efficacy of LZD.
Hematological indicators should be carefully monitored during LZD treatment, especially thrombocytopenia and low hemoglobin, and a continuous period of monitoring after LZD withdrawal is also necessary. Since the AUC24 cut-off values for laboratory adverse events were relatively low, a trade-off is necessary between the level of drug exposure required for treatment and safety, and the exposure target (AUC24/MIC) in pediatrics should be further studied, especially for patients with complications and concomitant medications.
The original contributions presented in the study are included in the article/Supplementary Material; further inquiries can be directed to the corresponding authors.
B-NH and Y-EW contributed equally to the manuscript. LS and Y-TJ drafted the study protocol. B-NH, LS, R-QZ, and Q-BL performed the study and obtained the data. Y-EW performed the pharmacometric analysis. B-NH and LS made a statistical analysis of the data. All authors contributed to the data, revised the manuscript critically for content, and approved the final version.
This research was supported by the following research grants: Chongqing Clinical pharmacy Key Specialty Construction Project; the National Natural Science Foundation of China (82173897); Young Taishan Scholars Program of Shandong Province; Distinguished Young and Middle-aged Scholar of Shandong University.
The authors declare that the research was conducted in the absence of any commercial or financial relationships that could be construed as a potential conflict of interest.
All claims expressed in this article are solely those of the authors and do not necessarily represent those of their affiliated organizations, or those of the publisher, the editors, and the reviewers. Any product that may be evaluated in this article, or claim that may be made by its manufacturer, is not guaranteed or endorsed by the publisher.
The Supplementary Material for this article can be found online at: https://www.frontiersin.org/articles/10.3389/fphar.2022.926711/full#supplementary-material
ALT, alanine aminotransferase; AST, aspartate aminotransferase; AUC24, 24 h area under the concentration-time curve; BUN, blood urea nitrogen; CIs, confidence intervals; HIS, hospital information system; IQR, interquartile range; LZD, linezolid; MIC, minimal inhibition concentration; NONMEM, nonlinear mixed-effects modeling program; ORs, odds ratios; PLT, platelet; ROC, receiver operating characteristic; TBil, total bilirubin; WBC, white blood cell count.
Andes, D., van Ogtrop, M. L., Peng, J., and Craig, W. A. (2002). In Vivo pharmacodynamics of a New Oxazolidinone (Linezolid). Antimicrob. Agents Chemother. 46, 3484–3489. doi:10.1128/AAC.46.11.3484-3489.2002
Bayram, N., Düzgöl, M., Kara, A., Özdemir, F. M., and Devrim, I. (2017). Linezolid-related Adverse Effects in Clinical Practice in Children. Arch. Argent. Pediatr. 115, 470–475. doi:10.5546/aap.2017.eng.470
Chang, K. C., Yew, W. W., Tam, C. M., and Leung, C. C. (2013). WHO Group 5 Drugs and Difficult Multidrug-Resistant Tuberculosis: a Systematic Review with Cohort Analysis and Meta-Analysis. Antimicrob. Agents Chemother. 57, 4097–4104. doi:10.1128/AAC.00120-13
Chuang, Y. C., Wang, J. T., Lin, H. Y., and Chang, S. C. (2014). Daptomycin versus Linezolid for Treatment of Vancomycin-Resistant Enterococcal Bacteremia: Systematic Review and Meta-Analysis. BMC Infect. Dis. 14, 687. doi:10.1186/s12879-014-0687-9
Cojutti, P., Maximova, N., Crichiutti, G., Isola, M., and Pea, F. (2019). Pharmacokinetic/pharmacodynamic Evaluation of Linezolid in Hospitalized Paediatric Patients: a Step toward Dose Optimization by Means of Therapeutic Drug Monitoring and Monte Carlo Simulation. J. Antimicrob. Chemother. 70, 198–206. doi:10.1093/jac/dku337
Daniel, J. D., and Ronald, N. J. (2001). Oxazolidinone Antibiotics. Lancet 358, 1975–1982. doi:10.1016/S0140-6736(01)06964-1
Di Paolo, A., Malacarne, P., Guidotti, E., Danesi, R., and Del Tacca, M. (2010). Pharmacological Issues of Linezolid: an Updated Critical Review. Clin. Pharmacokinet. 49, 439–447. doi:10.2165/11319960-000000000-00000
Dong, H., Xie, J., Wang, T., Chen, L., Zeng, X., Sun, J., et al. (2016). Pharmacokinetic/pharmacodynamic Evaluation of Linezolid for the Treatment of Staphylococcal Infections in Critically Ill Patients. Int. J. Antimicrob. agents 48, 259–264. doi:10.1016/j.ijantimicag.2016.05.009
Garazzino, S., Krzysztofiak, A., Esposito, S., Castagnola, E., Plebani, A., Galli, L., et al. (2011). Use of Linezolid in Infants and Children: a Retrospective Multicentre Study of the Italian Society for Paediatric Infectious Diseases. J. Antimicrob. Chemother. 66, 2393–2397. doi:10.1093/jac/dkr285
Garazzino, S., and Tovo, P. A. (2011). Clinical Experience with Linezolid in Infants and Children. J. Antimicrob. Chemother. 66 (Suppl. 4), iv23–iv41. doi:10.1093/jac/dkr074
Garcia-Prats, A. J., Schaaf, H. S., Draper, H. R., Garcia-Cremades, M., Winckler, J., Wiesner, L., et al. (2019). Pharmacokinetics, Optimal Dosing, and Safety of Linezolid in Children with Multidrug-Resistant Tuberculosis: Combined Data from Two Prospective Observational Studies. PLoS Med. 16, e1002789. doi:10.1371/journal.pmed.1002789
Han, X., Wang, J., Zan, X., Peng, L. R., and Peng, X. (2021). Risk Factors for Linezolid-Induced Thrombocytopenia in Adult Inpatients. Int. J. Clin. Pharm. 44, 330–338. doi:10.1007/s11096-021-01342-y
Hirano, R., Sakamoto, Y., Tachibana, N., and Ohnishi, M. (2014). Retrospective Analysis of the Risk Factors for Linezolid-Induced Thrombocytopenia in Adult Japanese Patients. Int. J. Clin. Pharm. 36, 795–799. doi:10.1007/s11096-014-9961-6
Jungbluth, G. L., Welshman, I. R., and Hopkins, N. K. (2003). Linezolid Pharmacokinetics in Pediatric Patients: an Overview. Pediatr. Infect. Dis. J. 22, S153–S157. doi:10.1097/01.inf.0000086954.43010.63
Li, S. C., Ye, Q., Xu, H., Zhang, L., and Wang, Y. (2019). Population Pharmacokinetics and Dosing Optimization of Linezolid in Pediatric Patients. Antimicrob. Agents Chemother. 63, e02387–18. doi:10.1128/AAC.02387-18
Matsumoto, K., Shigemi, A., Takeshita, A., Watanabe, E., Yokoyama, Y., Ikawa, K., et al. (2014). Analysis of Thrombocytopenic Effects and Population Pharmacokinetics of Linezolid: a Dosage Strategy According to the Trough Concentration Target and Renal Function in Adult Patients. Int. J. Antimicrob. Agents 44, 242–247. doi:10.1016/j.ijantimicag.2014.05.010
Meissner, H. C., Townsend, T., Wenman, W., Kaplan, S. L., Morfin, M. R., Edge-padbury, B., et al. (2003). Hematologic Effects of Linezolid in Young Children. Pediatr. Infect. Dis. J. 22, S186–S192. doi:10.1097/01.inf.0000087021.20838.d9
Mullins, C. D., Yang, K., Onukwugha, E., Eisenberg, D. F., Myers, D. E., Huang, D. B., et al. (2013). Rehospitalizations and Direct Medical Costs for cSSSI: Linezolid versus Vancomycin. Am. J. Pharm. Benefits 5, 258–267.
Natsumoto, B., Yokota, K., Omata, F., and Furukawa, K. (2014). Risk Factors for Linezolid-Associated Thrombocytopenia in Adult Patients. Infection 42, 1007–1012. doi:10.1007/s15010-014-0674-5
Nukui, Y., Hatakeyama, S., Okamoto, K., Yamamoto, T., Hisaka, A., Suzuki, H., et al. (2013). High Plasma Linezolid Concentration and Impaired Renal Function Affect Development of Linezolid-Induced Thrombocytopenia. J. Antimicrob. Chemother. 68, 2128–2133. doi:10.1093/jac/dkt133
Ogami, C., Tsuji, Y., To, H., and Yamamoto, Y. (2019). Pharmacokinetics, Toxicity and Clinical Efficacy of Linezolid in Japanese Pediatric Patients. J. Infect. Chemother. 25, 979–986. doi:10.1016/j.jiac.2019.05.025
Ogami, C., Tsuji, Y., Nishi, Y., Kawasuji, H., To, H., and Yamamoto, Y. (2021). External Evaluation of Population Pharmacokinetics and Pharmacodynamics in Linezolid-Induced Thrombocytopenia: The Transferability of Published Models to Different Hospitalized Patients. Ther. Drug Monit. 43, 271–278. doi:10.1097/FTD.0000000000000816
Pea, F., Furlanut, M., Cojutti, P., Cristini, F., Zamparini, E., Franceschi, L., et al. (2010). Therapeutic Drug Monitoring of Linezolid: a Retrospective Monocentric Analysis. Antimicrob. Agents Chemother. 54, 4605–4610. doi:10.1128/AAC.00177-10
Pea, F., Viale, P., Cojutti, P., Del Pin, B., Zamparini, E., and Furlanut, M. (2012). Therapeutic Drug Monitoring May Improve Safety Outcomes of Long-Term Treatment with Linezolid in Adult Patients. J. Antimicrob. Chemother. 67, 2034–2042. doi:10.1093/jac/dks153
Peyrani, P., Wiemken, T. L., Kelley, R., Zervos, M. J., Kett, D. H., File, T. M., et al. (2014). Higher Clinical Success in Patients with Ventilator-Associated Pneumonia Due to Methicillin-Resistant Staphylococcus aureus Treated with Linezolid Compared with Vancomycin: Results from the IMPACT-HAP Study. Crit. Care 18, R118. doi:10.1186/cc13914
Principi, N., and Esposito, S. (2019). Antibiotic-related Adverse Events in Paediatrics: Unique Characteristics. Expert Opin. Drug Saf. 18, 795–802. doi:10.1080/14740338.2019.1640678
Rao, G. G., Konicki, R., Cattaneo, D., Alffenaar, J. W., Marriott, D. J. E., and Neely, M. (2020). Therapeutic Drug Monitoring Can Improve Linezolid Dosing Regimens in Current Clinical Practice: A Review of Linezolid Pharmacokinetics and Pharmacodynamics. Ther. Drug Monit. 42, 83–92. doi:10.1097/FTD.0000000000000710
Rayner, C. R., Forrest, A., Meagher, A. K., Birmingham, M. C., and Schentag, J. J. (2003). Clinical Pharmacodynamics of Linezolid in Seriously Ill Patients Treated in a Compassionate Use Programme. Clin. Pharmacokinet. 42, 1411–1423. doi:10.2165/00003088-200342150-00007
Roger, C., Roberts, J. A., and Muller, L. (2018). Clinical Pharmacokinetics and Pharmacodynamics of Oxazolidinones. Clin. Pharmacokinet. 57, 559–575. doi:10.1007/s40262-017-0601-x
Rui, G. U. O., Yiqin, W. A. N. G., Jianjun, Y. A. N., and Haixia, Y. A. N. (2016). “A Recurrence Quantification Analysis of Traditional Chinese Medicine Pulse and its Application in Assessment of Coronary Artery Disease,” in International Conference on Biological Engineering and Pharmacy 2016 (BEP 2016) (Atlantis: Atlantis Press), 187–192.
Sasaki, T., Takane, H., Ogawa, K., Isagawa, S., Hirota, T., Higuchi, S., et al. (2011). Population Pharmacokinetic and Pharmacodynamic Analysis of Linezolid and a Hematologic Side Effect, Thrombocytopenia, in Japanese Patients. Antimicrob. Agents Chemother. 55, 1867–1873. doi:10.1128/AAC.01185-10
Sotgiu, G., Centis, R., D'Ambrosio, L., Alffenaar, J. W., Anger, H. A., Caminero, J. A., et al. (2012). Efficacy, Safety and Tolerability of Linezolid Containing Regimens in Treating MDR-TB and XDR-TB: Systematic Review and Meta-Analysis. Eur. Respir. J. 40, 1430–1442. doi:10.1183/09031936.00022912
Srivastava, S., Deshpande, D., Pasipanodya, J., Nuermberger, E., Swaminathan, S., and Gumbo, T. (2016). Optimal Clinical Doses of Faropenem, Linezolid, and Moxifloxacin in Children with Disseminated Tuberculosis: Goldilocks. Clin. Infect. Dis. 63, S102–S109. doi:10.1093/cid/ciw483
Takahashi, Y., Takesue, Y., Nakajima, K., Ichiki, K., Tsuchida, T., Tatsumi, S., et al. (2011). Risk Factors Associated with the Development of Thrombocytopenia in Patients Who Received Linezolid Therapy. J. Infect. Chemother. 17, 382–387. doi:10.1007/s10156-010-0182-1
Tang, S., Yao, L., Hao, X., Zhang, X., Liu, G., Liu, X., et al. (2015). Efficacy, Safety and Tolerability of Linezolid for the Treatment of XDR-TB: a Study in China. Eur. Respir. J. 45, 161–170. doi:10.1183/09031936.00035114
U.S. Department of Health and Human Services (2021). LINEZOLID Labels for NDA 021130. Available at: www.accessdata.fda.gov/drugsatfda_docs/label/2021/021130s042,021131s038,021132s041lbl.pdf (Accessed November 17, 2021).
Vinks, A. A. (2002). The Application of Population Pharmacokinetic Modeling to Individualized Antibiotic Therapy. Int. J. Antimicrob. Agents 19, 313–322. doi:10.1016/S0924-8579(02)00023-7
Keywords: linezolid, exposure, safety, efficacy, paediatric
Citation: Huo B-N, Wu Y-E, Shu L, Zhang R-Q, Xiao J-W, Li Q-B, Zhao W, Jia Y-T and Song L (2022) Relationship Between Linezolid Exposure and the Typical Clinical Laboratory Safety and Bacterial Clearance in Chinese Pediatric Patients. Front. Pharmacol. 13:926711. doi: 10.3389/fphar.2022.926711
Received: 23 April 2022; Accepted: 23 June 2022;
Published: 01 August 2022.
Edited by:
Patricia Moriel, State University of Campinas, BrazilReviewed by:
Marília Berlofa Visacri, University of São Paulo, BrazilCopyright © 2022 Huo, Wu, Shu, Zhang, Xiao, Li, Zhao, Jia and Song. This is an open-access article distributed under the terms of the Creative Commons Attribution License (CC BY). The use, distribution or reproduction in other forums is permitted, provided the original author(s) and the copyright owner(s) are credited and that the original publication in this journal is cited, in accordance with accepted academic practice. No use, distribution or reproduction is permitted which does not comply with these terms.
*Correspondence: Yun-Tao Jia, amlheXVudGFvbWFpbEBob3NwaXRhbC5jcW11LmVkdS5jbg==; Lin Song, c29uZ2xpbkBob3NwaXRhbC5jcW11LmVkdS5jbg==
†These authors have contributed equally to this work
Disclaimer: All claims expressed in this article are solely those of the authors and do not necessarily represent those of their affiliated organizations, or those of the publisher, the editors and the reviewers. Any product that may be evaluated in this article or claim that may be made by its manufacturer is not guaranteed or endorsed by the publisher.
Research integrity at Frontiers
Learn more about the work of our research integrity team to safeguard the quality of each article we publish.