Corrigendum: Clostridium butyricum protects against pancreatic and intestinal injury after severe acute pancreatitis via downregulation of MMP9
- 1Department of Gastroenterology, Guangzhou First People’s Hospital, South China University of Technology, Guangzhou, China
- 2Department of Gastroenterology, The Second Affiliated Hospital, School of Medicine, South China University of Technology, Guangzhou, China
- 3Department of Gastroenterology, The First Affiliated Hospital of Nan Chang University, Nanchang, China
- 4Department of Gastroenterology, Changhai Hospital, Shanghai, China
Background: Evidence have shown that gut microbiota plays an important role in the development of severe acute pancreatitis (SAP). In addition, matrix metalloproteinase-9 (MMP9) plays an important role in intestinal injury in SAP. Thus, we aimed to determine whether gut microbiota could regulate the intestinal injury during SAP via modulating MMP9.
Methods: In this study, the fecal samples of patients with SAP (n = 72) and healthy controls (n = 32) were analyzed by 16S rRNA gene sequencing. In addition, to investigate the association between gut microbiota and MMP9 in intestinal injury during SAP, we established MMP9 stable knockdown Caco2 and HT29 cells in vitro and generated a MMP9 knockout (MMP9−/−) mouse model of SAP in vivo.
Results: We found that the abundance of Clostridium butyricum (C. butyricum) was significantly decreased in the SAP group. In addition, overexpression of MMP9 notably downregulated the expressions of tight junction proteins and upregulated the expressions of p-p38 and p-ERK in Caco2 and HT29 cells (p < 0.05). However, C. butyricum or butyrate treatment remarkably upregulated the expressions of tight junction proteins and downregulated the expressions of MMP9, p-p38 and p-ERK in MMP9-overexpressed Caco2 and HT29 cells (p < 0.05). Importantly, C. butyricum or butyrate could not affect the expressions of tight junction proteins, and MMP9, p-p38 and p-ERK proteins in MMP9-knockdown cells compared with MMP9-knockdown group. Consistently, C. butyricum or butyrate could not attenuate pancreatic and intestinal injury during SAP in MMP9−/− mice compared with the SAP group.
Conclusion: Collectively, C. butyricum could protect against pancreatic and intestinal injury after SAP via downregulation of MMP9 in vitro and in vivo.
Introduction
Acute pancreatitis (AP) is a common severe inflammatory disease of the pancreas characterized by immune infiltration, necrosis, abscess, hemorrhage, and pain (Leung, 2010; García-Alonso et al., 2012; Lankisch et al., 2015). According to the disease severity, AP is classified into three types: mild AP, moderately severe AP, and severe AP (SAP). SAP is the most serious subtype of AP with a high morbidity and mortality rate (Zerem, 2014). During the AP, excessive inflammatory cytokines were released into the bloodstream (Liang et al., 2021). These released cytokines induced the injury in intestinal barrier and intestinal microbiota dysbiosis and gut bacterial translocation (Liang et al., 2021). Once gut bacteria pass through the intestinal wall and enter the blood, it can affect multiple organs of the human body (Cen et al., 2018; Liang et al., 2021). A previous study showed that gut bacterial translocation is a key process for aggravating AP (Jia et al., 2020). Thus, gut microbiota may play an important role in the development of SAP.
Human gut microbiota plays an important role on the host’s health via interfering multiple intestinal functions (Nishijima et al., 2016; Meng et al., 2018). In addition, a healthy gut microbiota is characterized by large bacterial taxonomic richness and diversity, which has more than 1,500 species including Firmicutes, Bacteroidetes and Proteobacteria (Qin et al., 2010; Lozupone et al., 2012; Li X. Y. et al., 2020). Evidence has shown that gut microbiota in SAP is characterized by reduced bacterial diversity (Tan et al., 2015). Patients with SAP have a lower proportion of Bifidobacterium and a higher proportion of Enterococcus and Enterobacteriaceae in fecal microbiota (Tan et al., 2015). Thus, the use of gut microbiota therapies might be a potential approach for the treatment of SAP. In this study, 16s rRNA gene sequencing was used to analyze differences in the gut microbiota in fecal samples from patients with SAP and healthy controls, and we found that the abundance of Clostridiales was significantly decreased in the SAP group. Meanwhile, our previous study has found that Clostridium butyricum (C. butyricum) and its major metabolite butyrate could reduce intestinal injury in a rat model of SAP with intra-abdominal hypertension (Zhao et al., 2020). However, the mechanism by which C. butyricum regulates the intestinal barrier function in a mice model of SAP remains unclear.
Matrix metallopeptidase 9 (MMP9), a member of MMPs family, has been implicated in a variety of biological processes, such as inflammation and cell proliferation (Kou et al., 2020; Zhang et al., 2020; Ringland et al., 2021). Mechanically, MMP9 can degrade many extracellular matrix (ECM) proteins and remodel the dynamic balance of ECM (Huang, 2018). Evidence have shown that MMPs play an important role in the pathogenesis of gastrointestinal diseases by affecting matrix degradation, mucosal destruction, and inflammatory cell migration (Cervinková et al., 2014; Jiang et al., 2020). Pan et al. (2017) found that MMP9 expression was upregulated in intestinal tissues in a rat model of SAP. In addition, MMP9 overexpression could promote the loss of intestinal villous (Kocael et al., 2016). Conversely, intestinal tissues from MMP9−/− mice displayed reduced intestinal epithelial injury compared with wild type (WT) mice (Santana et al., 2006). These data indicated that MMP9 may play an important role in the development of intestinal injury. Thus, in this study, we aimed to investigate whether C. butyricum could exert protective roles on intestinal injury during SAP via modulating MMP9.
Materials and Methods
Human Subjects
Seventy-four patients who underwent SAP and admitted to Guangzhou First People’s Hospital and Changhai Hospital, from June 2018 to July 2020, were recruited in this study. In addition, thirty-two healthy controls were enrolled in this study. The inclusion criteria for healthy controls: participants had no prior history of illness and were in good mental health. The inclusion criteria for patients with SAP were based on the 2012 revised Atlanta criteria (Banks et al., 2013), and the exclusion criteria included chronic pancreatitis, cancer, diabetes, severe liver disease and inflammatory bowel disease. Approximately 1–2 g of fresh fecal samples from all participants were collected and then stored at −80°C. Written informed consent was obtained from all participants. This study has been approved by the Medical Ethics Committee of the South China University of Technology.
16S rRNA Gene Sequencing
Microbial genomic DNA was extracted from fecal samples with the HiPure Stool DNA Kits (Magen, Guangzhou, China). Next, the 16S rDNA target region of the ribosomal RNA gene was amplified by PCR and the cycling was as follows: 95°C for 5 min, followed by 30 cycles for 60 s at 95°C, 72°C for 60 s and 72°C for 7 min. After that, amplicons were purified using the AMPure XP Beads (Beckman Coulter, Inc., Union City, CA, Unites States). Subsequently, the purified amplicons were sequenced on an Illumina Novaseq 6,000 sequencing platform (Illumina Inc.) according to the protocol by Gene Denovo Biotechnology Co., Ltd.
The alpha diversity, including Chao1, ACE, Shannon and Simpson, was analyzed by Kruskal–Wallis test and Dunn’ test. In addition, Anosim test was used to evaluate the differences in microbiota abundance between SAP and control groups. Meanwhile, the indicator species analysis was conducted to screen the biomarker between SAP and control groups using R language labdsv package.
Cell Culture and Transfection
The human colorectal adenocarcinoma cell lines (Caco-2 and HT-29) were purchased from Shanghai Zhongqiao Xinzhou Biotechnology Co., Ltd. and maintained in DMEM medium (BasalMedia, Shanghai, China) supplemented with 10% FBS (Thermo Fisher Scientific, Waltham, MA, United States) and incubated at 5% CO2 at 37°C.
The MMP9 sequence was synthesized by General Biol and then inserted into the pCDH-CMV-MCS-EF1-copGFP-T2A-Puro lentiviral expression vector plasmids (General Biol, Anhui, China), called MMP9 OE plasmids. In addition, lentivirus-containing shRNA targeting MMP9 (MMP9 shRNA1: 5′-GGAATACCTGTACCGCTATGG-3′, MMP9 shRNA2: 5′- GCAGACATCGTCATCCAGTTTC-3′ and MMP9 shRNA3: 5′-GCTTAGATCATTCCTCAGTGC-3′) were synthesized by GenePharma (Shanghai, China). After that, 293T cells were transfected with indicated lentiviral plasmids, packaging plasmids (pAX2) and envelope plasmid (pMD2. G) for 72 h. Next, virus-containing supernatants was filtered through a filter membrane (0.22 μm pore size), and then transduced into Caco-2 and HT-29 cells. Later on, the infected cells were selected by puromycin.
Western Blot Assay
Cells were lysed in RIPA buffer (Beyotime, Beijing, China) and the concentration of the proteins were measured by a BCA protein assay kit (Sangon biotech, Shanghai, China). After that, proteins were subjected to SDS-PAGE gels and then transferred onto polyvinylidene fluoride (PVDF) membranes (Millipore, Billerica, MA, United States). The membranes were then incubated with primary antibodies targeting ZO-1 (1:1,000, Abcam Cambridge, MA, United States), MMP9 (1:1,000, Abcam, Claudin3 (1:1,000, Abcam), Claudin5 (1:1,000, Abcam), Occludin (1:1,000, Abcam), p-p38 (1:1,000, Cell Signaling Technology, Danvers, MA, United States), p38 (1:1,000, Cell Signaling Technology), p-ERK (1:1,000, Cell Signaling Technology), ERK (1:2,000, Cell Signaling Technology), α-tubulin (1:8,000, proteintech, Rosemont, IL, United States), and GAPDH (1:8000, proteintech) at 4°C overnight. Later on, the membranes were incubated with corresponding horseradish peroxidase (HRP)-conjugated secondary antibodies (Beyotime) at room temperature for 1 h. Subsequently, the blots were visualized by an ECL kit (Yeasen, Shanghai, China).
Cell-Counting Kit-8 Assay
The Caco-2 and HT-29 cells were added into 96-well plates with 1 × 104 cells per well and cultured overnight at 37°C. After that, cells were treated with different concentrations of butyrate (Sigma Aldrich, St. Louis, MO, United States) and incubated for 48 h. Later on, 10 µl of CCK-8 solution (Beyotime) added to each well and cells were then incubated for another 3 h. Next, the absorbance was detected with a microplate reader (Tecan M1000, Tecan, Switzerland) at 450 nm.
Immunofluorescence Assay
Cells were fixed in 4% paraformaldehyde for 30 min at room temperature. After blocking with 1% BSA for 30 min, cells were incubated with the following primary antibodies: ZO-1 (1: 400, Abcam), Occludin (1: 400, Abcam), Claudin 3 (1: 400, Abcam), Claudin 5 (1: 400, Abcam) at 4°C overnight. Later on, cells were incubated with the Alexa Fluor 594 goat anti-rabbit IgG (H + L) secondary antibody (Yeasen) for 60 min at room temperature. Subsequently, images were captured with a fluorescence microscope. Nuclei were stained with DAPI for 5 min.
Culture of C. butyricum
The C. butyricum was obtained from Shanghai qingdayingke Medical Technology Co., Ltd, and was cultured in Reinforced Clostridial Medium (Beijing Land Bridge Technology Co., Ltd, Beijing, China) at 37°C for 30 h in an anaerobic environment. After that, C. butyricum were washed 3 times with ice-cold PBS and resuspended with saline. The Caco-2 and HT-29 cells were co-cultured with C. butyricum for 6 h in an anaerobic environment.
Animal Study
A total of 24 6-week-old C57BL/6-Mmp9em1Smoc mice (MMP9 gene knockout mice; males, SPF level) were obtained from Shanghai Model Organisms (Shanghai, China) and were then randomly assigned into four groups: control, SAP, SAP + C. butyricum and SAP + butyrate groups. All experimental protocols were performed according to the National Institutes of Health Laboratory Animal Guidelines and were approved by the Animal Ethics Committee of the South China University of Technology. Mice in the control or SAP group were orally gavaged with 0.9% saline (200 µl) for 1 week once a day; mice in the SAP + C. butyricum and SAP + butyrate groups were orally gavaged with C. butyricum (2 × 108 CFU, 200 µl) or butyrate (200 mg/kg, 200 µl) respectively for 1 week once a day. After that, mice in the control group were injected intraperitoneally by 0.9% saline (200 µl) once an hour for 9 h. Mice in the SAP, SAP + C. butyricum and SAP + butyrate groups were intraperitoneally (i.p.) treated with caerulein (50 μg/kg) once an hour for 8 h. At 1 h after the final caerulein injection, mice were injected with LPS (10 mg/kg, i.p.) (Liu et al., 2017; Kong et al., 2021). After 1 h of LPS injection, all mice were sacrificed.
Histological Analysis
The pancreatic and small intestine tissues were paraffin embedded and cut into 5 μM sections. After that, hematoxylin and eosin (H and E) were used to stain the sections. Subsequently, morphological changes were observed by using a light microscope.
Immunohistochemistry Analysis
The paraffin-embedded sections were deparaffinized and rehydrated in graded alcohol series. Later on, the sections were boiled in pressure cooker for 3 min to retrieve antigen. After blocking with 1% BSA for 30 min at room temperature, the sections were incubated at 4°C overnight with the following primary antibodies: ZO-1 (1: 400, Abcam), Occludin (1: 400, Abcam), Claudin 3 (1: 400, Abcam), Claudin 5 (1: 400, Abcam). After that, the sections were incubated with the HRP-conjugated secondary antibody (Beyotime) for 50 min at 37°C. Subsequently, images were visualized with a light microscope.
Transmission Electron Microscope Analysis
The small intestine tissues were fixed in 2.5% glutaraldehyde at 4°C overnight, and then fixed in 1% osmium tetroxide. After that, the samples were dehydrated in graded ethanol, embedded in epoxy resin, and then cut into ultra-thin sections (80 nm). Subsequently, the samples were observed in a HT7800 TEM (HITACHI, Japan).
ELISA Assay
The levels of IL-1β (cat. no. ab197742; Abcam), IL-6 (cat. no. ab100712; Abcam), TNF-α (cat. no. ab208348; Abcam) and diamine oxidase (DAO; Fine Test, Wuhan, China) in serum samples were measured by ELISA kits. In addition, the levels of α-amylase (cat. no. H012; Beijing Leadman Biochemistry Co., Ltd., Beijing, China) and lipase (cat. no. H427; Beijing Leadman Biochemistry Co., Ltd., Beijing, China) in serum samples were detected by a HITACHI 7180 automatic biochemical analyzer (HITACHI).
Statistical Analysis
Data are presented as the mean ± standard deviation (S.D.) Differences between three or more groups were analyzed by One-way analysis of variance (ANOVA) and Tukey’s tests. p values of <0.05 were considered statistically significant. All data were repeated independently at least three times.
Results
Difference in Species of Bacteria Between Control and Severe Acute Pancreatitis Groups
To explore gut microbiome linked to the progression of SAP, the abundance and composition of gut microbiota in fecal samples between SAP and control groups were analyzed by 16s rRNA gene sequencing. First, Shannon and Simpson analyses were used to measure intestinal microbial diversity and ACE and Chao analyses were used to determine species richness. The data showed that microbial richness and diversity in the fecal samples was slightly, but not significantly, lower in the SAP group than that in the control group (p > 0.05, Figure 1A), indicating that the two groups had similar microbial alpha diversity. In addition, Anosim test (beta diversity) showed significant differences in bacterial community composition between SAP and control groups (Figure 1B). Collectively, there are significant changes in microbial communities between the two groups.
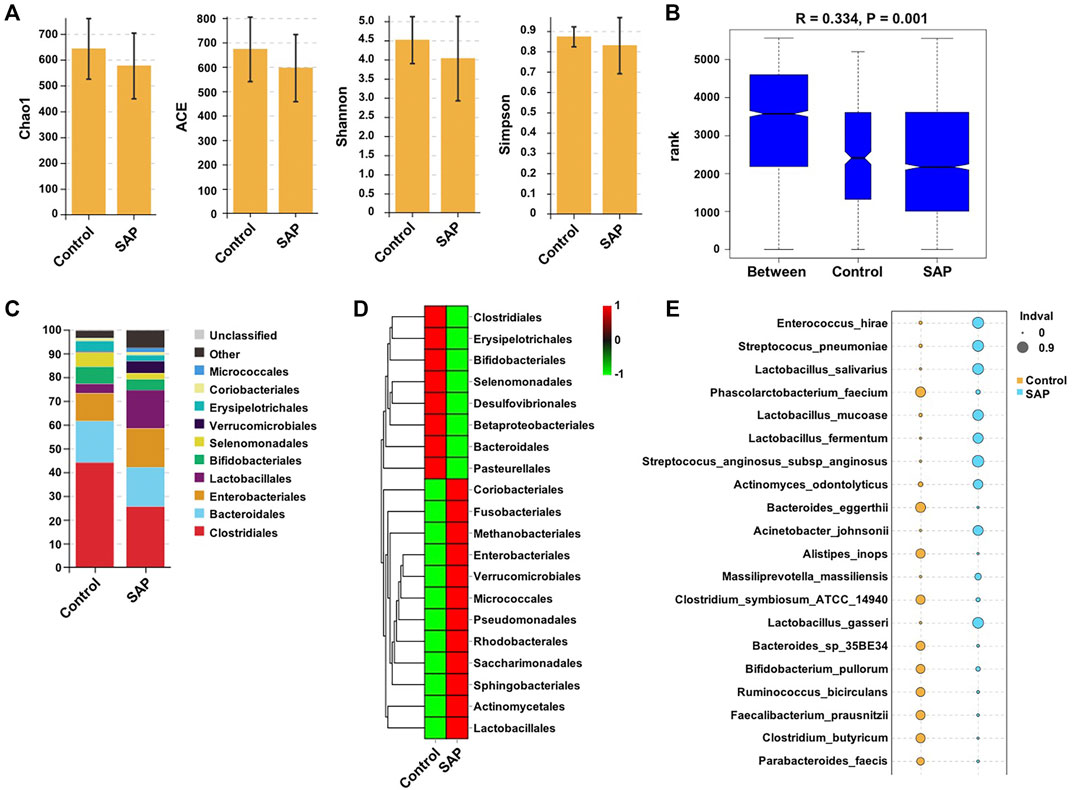
FIGURE 1. Difference in species of bacteria between control and SAP groups. 16s rRNA gene sequencing was used to determine the abundance and composition of gut microbiota in fecal samples from patients with SAP and controls. (A) Alpha diversity (Chao1, ACE, Shannon, Simpson) and (B) Beta diversity (Anosim) comparison between control and SAP groups. (C) The relative abundance of the microbiota at the order level was displayed using the stacked bar chart. (D) Heatmap showed the differences in microbiota abundance at genus level between SAP and control groups. (E) The indicator species analysis was conducted to screen the biomarker between SAP and control groups. SAP, severe acute pancreatitis.
Next, we assessed whether there are changes in the relative abundance of the taxa. The results showed that the gut microbiota from individuals with SAP harbored a lower relative abundance of Clostridiales, Bacteroidales, Bifidobacteriales, Erysipelotrichales, Selenomonalales and a higher relative abundance of Enterobacteriales, Lactobacillales, Verrucomicrobiales, Coriobacteriales, Micrococcales compared with that from healthy controls (Figure 1C). In addition, the heatmap analysis showed that among the top 20 order with significant difference, the abundance of Clostridiales was notably decreased in the SAP group compared with the control group (Figure 1D).
The indicator species analysis was used to further compare the microbiota between SAP and control groups. As shown in Figure 1E and Supplementary Figure S1, the relative abundances of the species Clostridium butyricum (C. butyricum) were found to be lower in the fecal samples in patients with SAP compared with healthy controls.
C. butyricum or Butyrate Treatment Reduced MMP9 Expression in Caco-2 and HT-29 Cells
To investigate whether the protective role of C. butyricum in intestinal injury is associated with MMP9, we detected MMP9 expression in C. butyricum-treated Caco-2 and HT-29 cells. As indicated in Figures 2A,B butyricum (1 × 108 CFU/mL) treatment remarkably downregulated the expression of MMP9 in Caco-2 and HT-29 cells. In addition, 2 and 1 mM butyrate had very limited effect on the viability of Caco-2 and HT-29 cells respectively (Supplementary Figures S2A,B). Thus, 2 and 1 mM of butyrate were used to treat Caco-2 and HT-29 cells in the following experiment respectively.
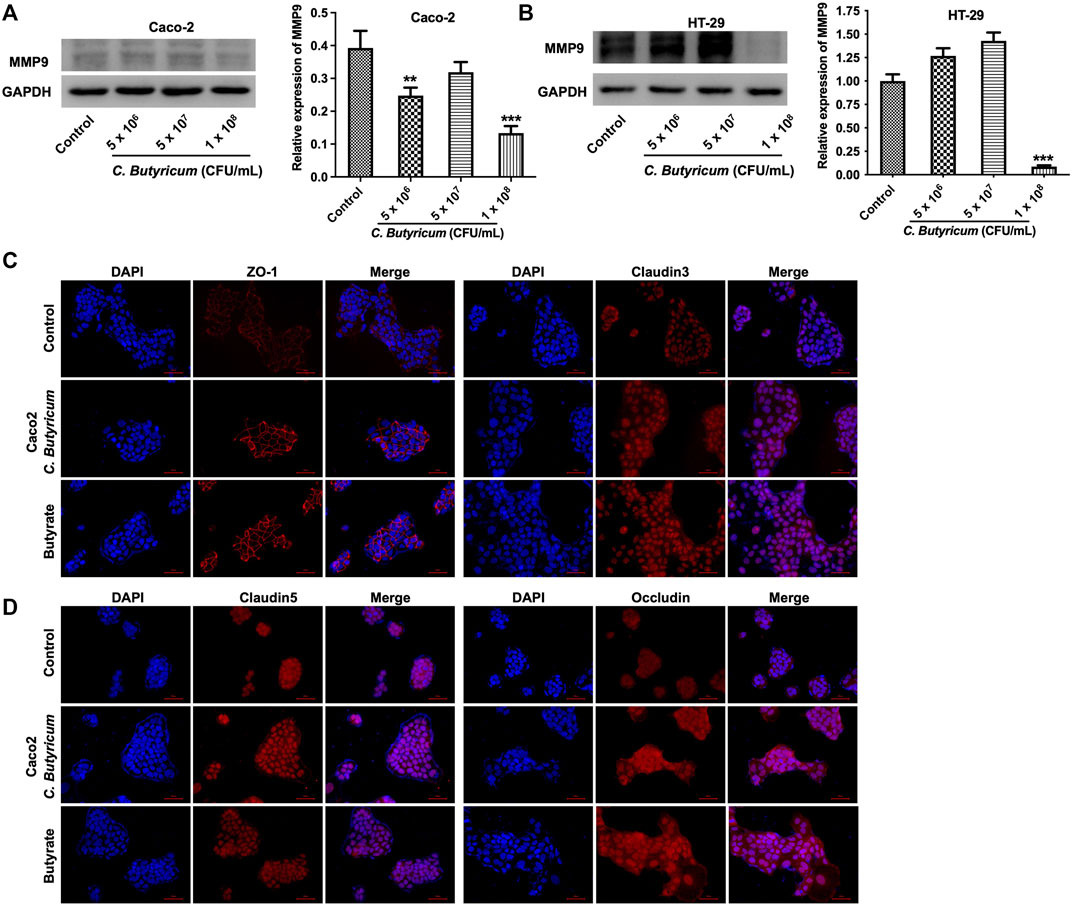
FIGURE 2. Clostridium butyricum or butyrate treatment reduced MMP9 expression in Caco-2 and HT-29 cells. (A) The Caco-2 and (B) HT-29 cells were co-cultured with (C) butyricum (5 × 106, 5 × 107, or 1 × 108 CFU/ml) for 6 h in an anaerobic environment. After that, the supernatant was removed, and cells were then cultured in normoxia environment for 48 h. Western blot assay was used to determine the expression of MMP9 in cells. (C,D) Caco-2 cells were treated with 2 mM butyrate for 48 h. Meanwhile, cells were co-cultured with C. butyricum (1 × 108 CFU/ml) for 6 h in an anaerobic environment. After that, the supernatant was removed, and cells were then cultured in normoxia environment for 48 h. IF assay was used to determine the expressions of ZO-1, Claudin3, Claudin5, Occludin in cells. **p < 0.01, ***p < 0.001 vs. control group. MMP9, matrix metallopeptidase 9; ZO-1, Zona occludens 1.
Furthermore, IF and western blot assays results showed that C. butyricum or butyrate treatment notably increased the expressions of tight junction proteins ZO-1, Claudin3, Claudin5, Occludin in Caco-2 and HT-29 cells compared with the control group (Figures 2C,D, 3A,B and Supplementary Figures S3A,B). Meanwhile, C. butyricum or butyrate treatment remarkably reduced the expressions of MMP9, p-p38 and p-ERK in Caco-2 and HT-29 cells compared to the control group (Figures 3A,B). To sum up, C. butyricum or butyrate treatment could reduce MMP9 expression and upregulate the expressions of tight junction proteins in Caco-2 and HT-29 cells.
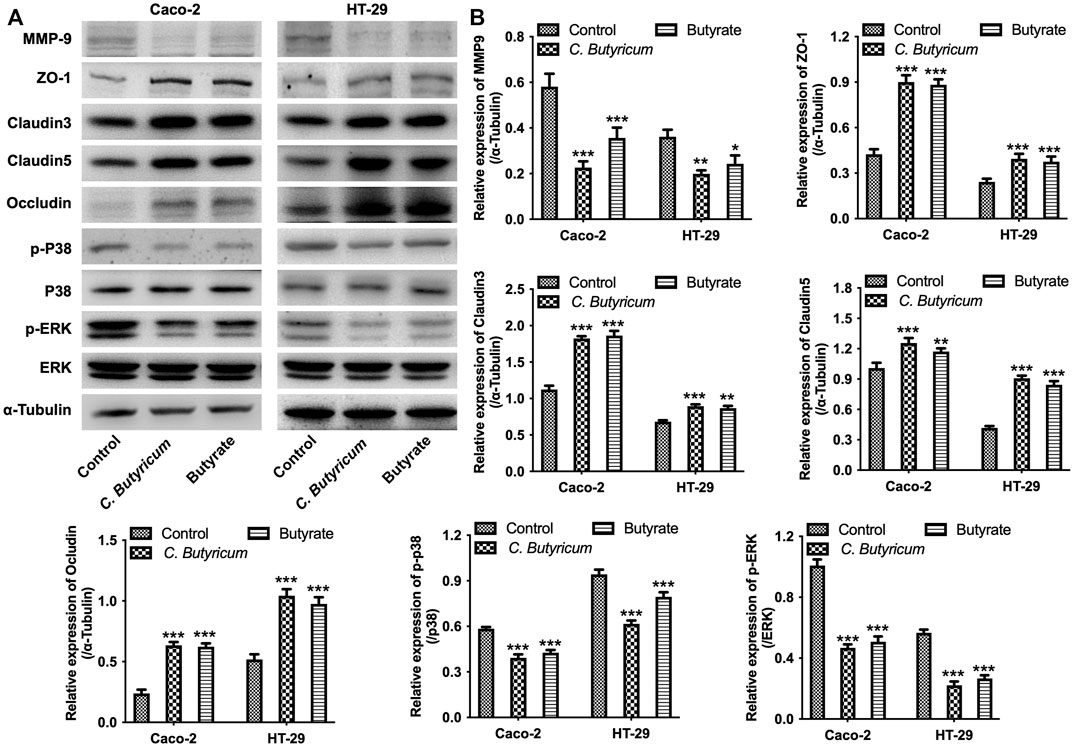
FIGURE 3. Clostridium butyricum or butyrate treatment reduced MMP9 expression and upregulated the expressions of tight junction proteins in Caco-2 and HT-29 cells. (A,B) Caco-2 and HT-29 cells were treated with butyrate (2 mM for Caco-2 cell and 1 mM for HT-29 cell) for 48 h. Meanwhile, Caco-2 and HT-29 cells were co-cultured with C. butyricum (1 × 108 CFU/ml) for 6 h in an anaerobic environment. After that, the supernatant was removed, and cells were then cultured in normoxia environment for 48 h. Western blot assay was used to determine the expressions of MMP9, ZO-1, Claudin3, Claudin5, Occludin, p-p38, p-ERK in cells. *p < 0.05, **p < 0.01, ***p < 0.001 vs. control group. MMP9, matrix metallopeptidase 9; ZO-1, Zona occludens 1.
C. butyricum or Butyrate Treatment Protected MMP9-Induced the Destruction of Intercellular Tight Junction in Caco-2 and HT-29 Cells
To further investigate whether MMP9 participate in C. butyricum-mediated protective effects of intestinal injury, we overexpressed the expression of MMP9 in Caco-2 and HT-29 cells (Figures 4A,B). Meanwhile, three different shRNAs (MMP9 shRNA1, MMP9 shRNA2, and MMP9 shRNA3) was used to reduce the expression of MMP9 in Caco-2 and HT-29 cells (Figures 4C,D). Since MMP9 shRNA3 exhibited the best inhibitory effect on MMP9 expression comparing with MMP9 shRNA1 or MMP9 shRNA2, MMP9 shRNA3 was used in the next experiments.
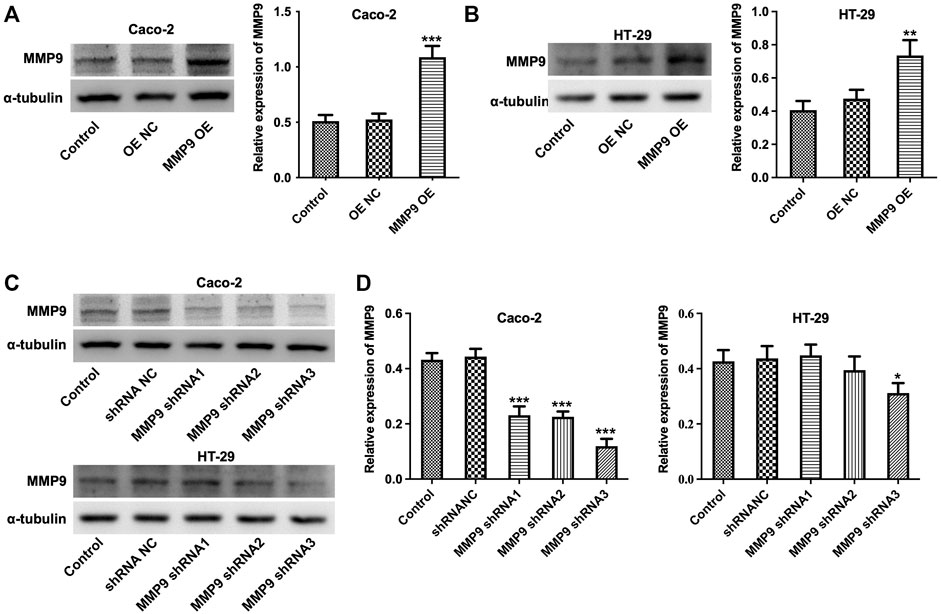
FIGURE 4. Establishment of MMP9 overexpressing and MMP9 knockdown Caco-2 and HT-29 cells. (A,B) Caco-2 and HT-29 cells were transfected with MMP9 OE plasmids. Western blot assay was used to determine the expression of MMP9 in cells. **p < 0.01, ***p < 0.001 vs. OE NC group. (C,D) Caco-2 and HT-29 cells were transfected with MMP9 shRNA1, MMP9 shRNA2 or MMP9 shRNA3 plasmids. Western blot assay was used to determine the expression of MMP9 in cells. *p < 0.05, ***p < 0.001 vs. shRNA NC group. MMP9, matrix metallopeptidase 9; OE NC, overexpression negative control; shRNA NC, short hairpin RNA negative control.
Furthermore, the results of western blot assay showed that upregulation of MMP9 markedly elevated the expressions of MMP9, p-p38 and p-ERK, and downregulated the expressions of ZO-1, Claudin3, Claudin5 and Occludin in Caco-2 and HT-29 cells; however, these changes were notably reversed by C. butyricum or butyrate treatment (Figures 5A–D). In addition, downregulation of MMP9 significantly decreased the expressions of MMP9, p-p38 and p-ERK, and increased the expressions of ZO-1, Claudin3, Claudin5, Occludin in Caco-2 and HT-29 cells. (Figures 6A–D). However, C. butyricum or butyrate treatment caused no differences in the expressions of MMP9, ZO-1, Claudin3, Claudin5, Occludin, p-p38 and p-ERK in MMP9 knockdown Caco2 and HT29 cells compared with MMP9 shRNA3 group (Figures 6A–D). Collectively, C. butyricum or butyrate treatment protected MMP9-induced the destruction of intercellular tight junction in Caco2 and HT29 cells.
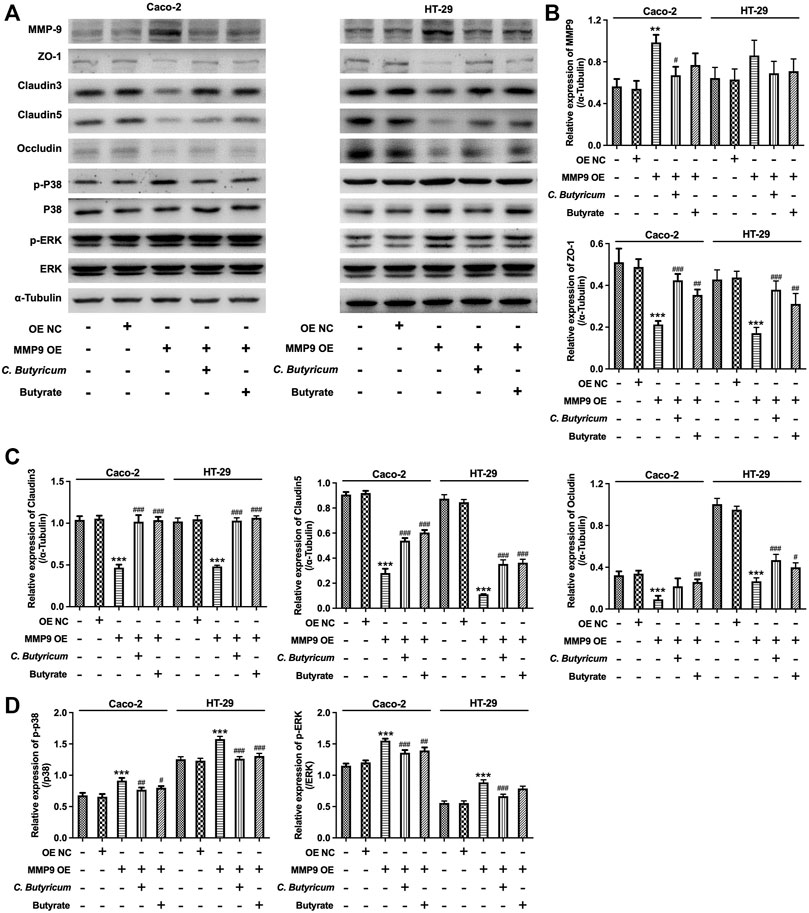
FIGURE 5. Clostridium butyricum or butyrate treatment protected MMP9-induced the destruction of intercellular tight junction in Caco-2 and HT-29 cells. (A–D) Caco-2 and HT-29 cells were transfected with MMP9 OE plasmids. The transfected Caco-2 and HT-29 cells were then treated with butyrate (2 mM for Caco-2 cell and 1 mM for HT-29 cell) for 48 h. Meanwhile, the transfected Caco-2 and HT-29 cells were co-cultured with C. butyricum (1 × 108 CFU/ml) for 6 h in an anaerobic environment. After that, the supernatant was removed, and cells were then cultured in normoxia environment for 48 h. Western blot assay was used to determine the expressions of MMP9, ZO-1, Claudin3, Claudin5, p-p38, p-ERK in cells. **p < 0.01, ***p < 0.001 vs. control group; #p < 0.05, ##p < 0.01, ###p < 0.001 vs. MMP9 OE group. MMP9, matrix metallopeptidase 9; OE NC, overexpression negative control; ZO-1, Zona occluden 1.
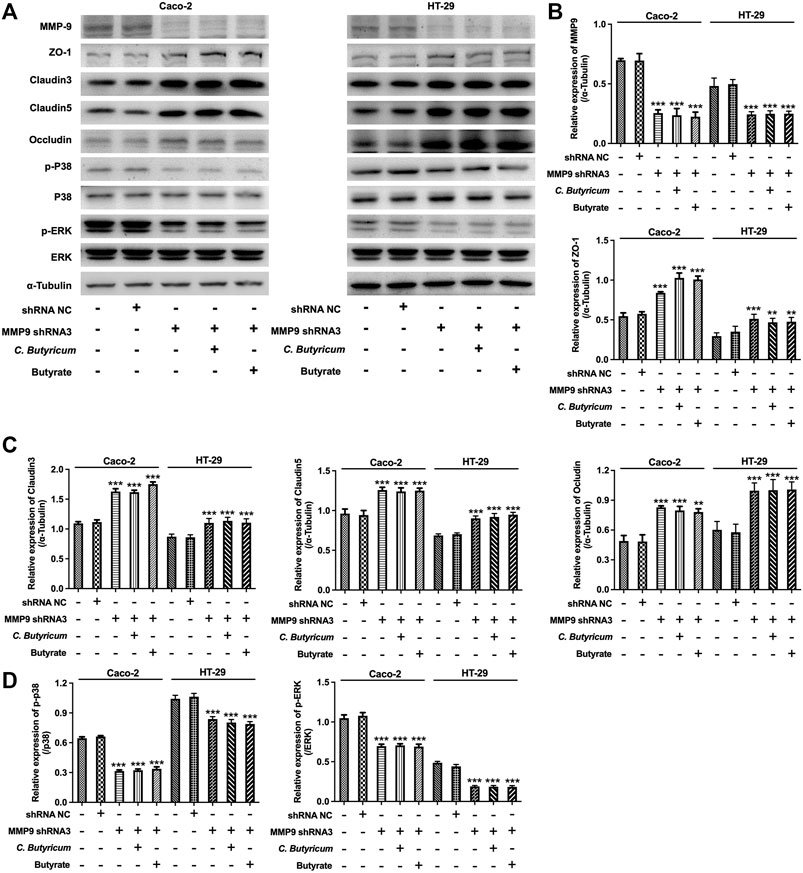
FIGURE 6. Clostridium butyricum or butyrate could not exert protective roles on intercellular tight junction in MMP9-knockdown Caco-2 and HT-29 cells. (A–D) Caco-2 and HT-29 cells were transfected with MMP9 shRNA3 plasmids. The transfected Caco-2 and HT-29 cells were then treated with butyrate (2 mM for Caco-2 cell and 1 mM for HT-29 cell) for 48 h. Meanwhile, the transfected Caco-2 and HT-29 cells were co-cultured with C. butyricum (1 × 108 CFU/ml) for 6 h in an anaerobic environment. After that, the supernatant was removed, and cells were then cultured in normoxia environment for 48 h. Western blot assay was used to determine the expressions of MMP9, ZO-1, Claudin3, Claudin5, p-p38, p-ERK in cells. **p < 0.01, ***p < 0.001 vs. control group. MMP9, matrix metallopeptidase 9; shRNA NC, short hairpin RNA negative control; ZO-1, Zona occludens 1.
C. butyricum or Butyrate Could Not Exert Protective Roles on Intestinal Injury During Severe Acute Pancreatitis in MMP9−/− Mice
To further investigate whether C. butyricum or butyrate could exert protective roles on intestinal inflammation and barrier integrity during SAP via modulating MMP9, MMP9−/− mice were subjected to caerulein and LPS. The results of HE staining assay showed that the pancreas tissues of MMP9−/− mice from SAP group displayed increased intracellular vacuoles in acinar cells, interstitial edema and acinar cell necrosis, hemorrhage, compared with the control group; however, C. butyricum or butyrate treatment did not restore the damaged pancreas tissues compared with the SAP group (Figure 7A). In addition, the levels of α-amylase and lipase in serum samples were significantly increased in the SAP group compared with the control group (Figure 7B). However, C. butyricum or butyrate treatment had no effects on the levels of α-amylase and lipase in serum samples compared with the SAP group (Figure 7B). Collectively, C. butyricum or butyrate could not attenuate pancreas injury during SAP in MMP9−/− mice.
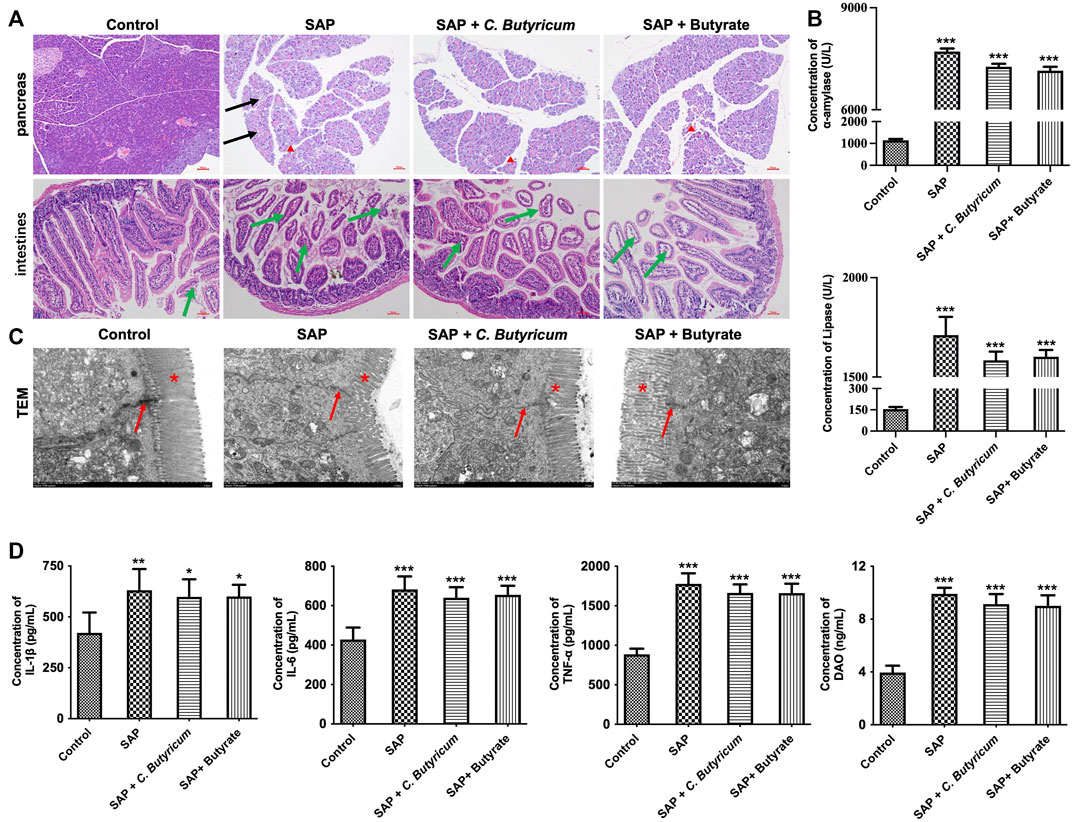
FIGURE 7. Clostridium butyricum or butyrate could not exert protective roles on intestinal injury during SAP in MMP9−/− mice. (A) H and E staining showed the pathological changes in the pancreas and small intestine tissues. The black arrows denote acinar cells and the red triangle denote interstitial edema in pancreas tissues. The green arrows denote crypt atrophy in small intestine tissues. (B) ELISA assay was used to detect the levels of α-amylase and lipase in serum samples of MMP9−/− mice. (C) The intestinal epithelial villi in MMP9−/− mice was observed by TEM. The red arrow represents tight junctions; the red star represents intestinal microvilli. (D) The levels of IL-1β, IL-6, TNF-α and DAO in serum samples of MMP9−/− mice was detected by ELISA. *p < 0.05, **p < 0.01, ***p < 0.001 vs. control group. diamine oxidase, DAO; MMP9, matrix metallopeptidase 9; SAP, severe acute pancreatitis; TEM, transmission electron microscope.
Furthermore, MMP9−/− mice subjected to caerulein and LPS displayed increased intestinal injury, as determined by reduction of goblet cells, crypt atrophy, villi necrosis and edema (Figure 7A). However, C. butyricum or butyrate treatment caused no major histopathological changes in the intestine tissues of MMP9−/− mice compared with the SAP group (Figure 7A). Moreover, TEM observation showed dysfunctional cell junctions and villous shedding and disordered arrangement in intestines tissues of MMP9−/− mice from SAP group compared with the control group; however, C. butyricum or butyrate treatment did not change these effects in MMP9−/− mice (Figure 7C). Meanwhile, the levels of IL-1β, IL-6, TNF-α and DAO were notably increased in serum samples in MMP9−/− mice from SAP group; however, C. butyricum or butyrate treatment failed to reduce their levels in MMP9−/− mice compared with the SAP group (Figure 7D). Significantly, the expressions of ZO-1, Claudin3, Claudin5, Occludin were notably downregulated in intestines tissues from MMP9−/− mice during SAP, compared with the control group; however, C. butyricum or butyrate treatment did not change their expressions in MMP9−/− mice (Figures 8A–C). Additionally, the expressions of p-p38 and p-ERK were markedly upregulated in intestines tissues from MMP9−/− mice during SAP, whereas these changes were reversed by C. butyricum or butyrate treatment (Figures 8A,B). Collectively, C. butyricum or butyrate could not attenuate intestinal injury during SAP in MMP9−/− mice.
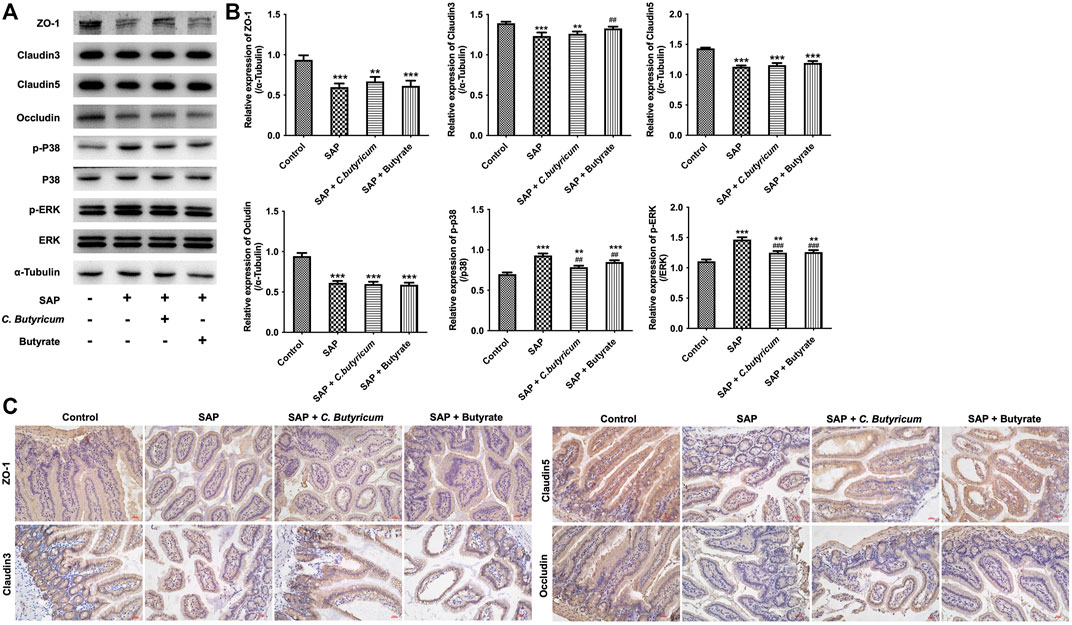
FIGURE 8. Clostridium butyricum or butyrate could not exert protective roles on intestinal injury during SAP in MMP9−/− mice via regulation of p38 and ERK MAPK signaling pathways. (A,B) Western blot analysis of the expressions of ZO-1, Claudin3, Claudin5, Occludin, p-p38, p-ERK in small intestine tissues of MMP9−/− mice. (C) IHC analysis of the expressions of ZO-1, Claudin3, Claudin5, Occludin in small intestine tissues of MMP9−/− mice. **p < 0.01, ***p < 0.001 vs. control group; ##p < 0.01, ###p < 0.001 vs. SAP group. MMP9, matrix metallopeptidase 9; ZO-1, Zona occludens 1.
Discussion
Evidence have shown that SAP is an acute inflammatory disease of the pancreas that is associated with intestinal barrier dysfunction (Tu et al., 2014; Zhang et al., 2015). In addition, the intestinal epithelial barrier is strongly interacted with the richness and diversity of gut microbial community (Takiishi et al., 2017). Gut microbiota dysbiosis could affect the intestinal barrier function, which in turn affect the development of SAP (Takiishi et al., 2017; Wan et al., 2019; Li J. et al., 2020). Zhu et al. (2019) found that patients with SAP have a lower proportion of beneficial bacteria such as Blautia in fecal samples compared with patients with mild AP and moderately SAP. Yu et al. (2020) reported that Enterococcus was significantly higher and Eubacterium hallii was remarkedly lower in abundance in rectal swab samples from patients with SAP. Thus, maintaining the balance of gut microbiota might be a potential approach for the treatment of SAP (Tang et al., 2019). In this study, we studied the gut microbial community between patients with SAP and healthy controls by sequencing 16S rRNA gene of the microbiota. Our data showed that the abundance of C. butyricum was significantly decreased in the SAP group. In addition, C. butyricum or its major metabolite butyrate treatment significantly upregulated the expressions of tight junction proteins (ZO-1, claudin-3, claudin-5, occludin) in Caco-2 and HT-29 cells, suggesting that C. butyricum or butyrate could enhance the intercellular tight junction. Moreover, our previous study showed that C. butyricum or butyrate treatment could attenuate intestinal injury caused by SAP in rats via maintaining the intestinal barrier function (Zhao et al., 2020). Consistent with our results, Pan et al. (2019) reported that C. butyricum treatment markedly attenuated intestinal inflammation and barrier dysfunction in mice during SAP. However, the underlying mechanism by which C. butyricum or butyrate protects intestinal barrier function remains unclear.
It has been shown that MMPs could increase endothelial cell permeability via disruption of tight junction proteins (Apostolidou et al., 2012). In addition, Yang et al. (2016) found that inhibition of MMP9 could attenuate IL-1β-induced tight junction proteins disruption. In addition, MMP9 has been found to play an important role in the development of the intestinal injury (Lucafò et al., 2019; Wu et al., 2019). Overexpression of MMP9 could facilitate the loss of intestinal villous (Kocael et al., 2016). In the present study, our results showed that overexpression of MMP9 significantly downregulated the expressions of tight junction proteins in Caco-2 and HT-29 cells, whereas MMP9 knockdown exhibited the opposite effects, suggesting that MMP9 could induce the disruption of the intercellular tight junction in vitro. Thus, we further investigated whether C. butyricum or butyrate could exert protective roles on intestinal injury during SAP via downregulating MMP9 expression. Our results showed that C. butyricum or butyrate significantly upregulated the expressions of tight junction proteins in MMP9-overexpressed Caco-2 and HT-29 cells, suggesting that C. butyricum or butyrate treatment could protect MMP9-induced the destruction of intercellular tight junction in Caco-2 and HT-29 cells. However, C. butyricum or butyrate could not affect intercellular tight junction in MMP9 knockdown Caco-2 and HT-29 cells. Consistently, C. butyricum or butyrate could not improve intestinal injury during SAP in MMP9−/− mice. However, in our previous study, a rat model with SAP was established and injuries of intestinal tissues were evaluated in C. butyricum-treated mice, and we found that C. butyricum or butyrate could attenuate intestinal injury caused by SAP in wild type rats (Zhao et al., 2020). These data indicated that the protective effect of C. butyricum or butyrate on intestinal injury during SAP was absent in MMP9−/− mice, demonstrating the importance of MMP9 for C. butyricum or butyrate-mediated protective effects on intestinal injury. Collectively, C. butyricum or butyrate could exert protective roles on intestinal injury during SAP via downregulation of MMP9.
ERK and p38 signaling pathways can mediate intestinal epithelial barrier permeability through affecting intestinal epithelial tight junctions and cell injury (Tang et al., 2021; Zhang et al., 2021). Mao et al. (2020) found that probiotic Lactobacillus rhamnosus could improve intestinal barrier function in LPS-treated piglets via inhibition of ERK and p38 signalings. In addition, Al-Sadi et al. (2019) reported that MMP9 could increase the intestinal epithelial tight permeability via activation of p38 MAPK signaling pathway. In this study, we found that overexpression of MMP9 significantly increased the expressions of p-p38 and p-ERK in Caco-2 and HT-29 cells; however, these changes were reversed by C. butyricum or butyrate treatment. These data showed that C. butyricum or butyrate could attenuate intestinal injury during SAP by downregulating MMP9 through p38 and ERK signaling pathways.
A limitation of the current study is that we used the commercial product of C. butyricum not the isolated C. butyricum strain to investigate whether C. butyricum could exert protective roles on intestinal injury during SAP via modulating MMP9. In the future, we aimed to determine the strain of the isolated C. butyricum and investigate the effect of the isolated C. butyricum on intestinal barrier functions.
Conclusion
In this study, we found that C. butyricum or butyrate treatment could protect against pancreatic and intestinal Injury after SAP via downregulation of MMP9 in vitro and in vivo. Thus, C. butyricum or butyrate might be a possible therapeutic drug to diminish intestinal injury during SAP.
Data Availability Statement
The raw data supporting the conclusion of this article will be made available by the authors, without undue reservation.
Ethics Statement
This study has been approved by the Medical Ethics Committee of the South China University of Technology. Written informed consent was obtained from all participants.
Author Contributions
QY and LJ made major contributions to the conception, design and manuscript drafting of this study. LJ, BW, YW, YZ, and QW were responsible for data acquisition, data analysis, data interpretation and manuscript revision. LJ made substantial contributions to conception and design of the study and revised the manuscript critically for important intellectual content. All authors agreed to be accountable for all aspects of the work. All authors read and approved the final manuscript.
Funding
This study was supported by grants from The National Natural Science Foundation of China (No. 81870438) and the Guangzhou Technology Projects (No. 202102080099).
Conflict of Interest
The authors declare that the research was conducted in the absence of any commercial or financial relationships that could be construed as a potential conflict of interest.
Publisher’s Note
All claims expressed in this article are solely those of the authors and do not necessarily represent those of their affiliated organizations, or those of the publisher, the editors and the reviewers. Any product that may be evaluated in this article, or claim that may be made by its manufacturer, is not guaranteed or endorsed by the publisher.
Supplementary Material
The Supplementary Material for this article can be found online at: https://www.frontiersin.org/articles/10.3389/fphar.2022.919010/full#supplementary-material
References
Al-Sadi, R., Youssef, M., Rawat, M., Guo, S., Dokladny, K., Haque, M., et al. (2019). MMP-9-induced Increase in Intestinal Epithelial Tight Permeability Is Mediated by P38 Kinase Signaling Pathway Activation of MLCK Gene. Am. J. Physiol. Gastrointest. Liver Physiol. 316 (2), G278–g290. doi:10.1152/ajpgi.00126.2018
Apostolidou, E., Paraskeva, E., Gourgoulianis, K., Molyvdas, P. A., and Hatzoglou, C. (2012). Matrix Metalloproteinases 2 and 9 Increase Permeability of Sheep Pleura In Vitro. BMC Physiol. 12, 2. doi:10.1186/1472-6793-12-2
Banks, P. A., Bollen, T. L., Dervenis, C., Gooszen, H. G., Johnson, C. D., Sarr, M. G., et al. (2013). Classification of Acute Pancreatitis--2012: Revision of the Atlanta Classification and Definitions by International Consensus. Gut 62 (1), 102–111. doi:10.1136/gutjnl-2012-302779
Cen, M. E., Wang, F., Su, Y., Zhang, W. J., Sun, B., and Wang, G. (2018). Gastrointestinal Microecology: a Crucial and Potential Target in Acute Pancreatitis. Apoptosis 23 (7-8), 377–387. doi:10.1007/s10495-018-1464-9
Cervinková, M., Horák, P., Kanchev, I., Matěj, R., Fanta, J., Sequens, R., et al. (2014). Differential Expression and Processing of Matrix Metalloproteinase 19 Marks Progression of Gastrointestinal Diseases. Folia Biol. (Praha) 60 (3), 113–122.
García-Alonso, F. J., Garrido Gómez, E., Botella-Carretero, J. I., Pérez-Lasala, J., Cano Ruiz, A., and Moreira Vicente, V. (2012). Nutrition Acute Pancreatitis. Nutr. Hosp. 27 (2), 333–340. doi:10.1590/s0212-16112012000200002
Huang, H. (2018). Matrix Metalloproteinase-9 (MMP-9) as a Cancer Biomarker and MMP-9 Biosensors: Recent Advances. Sensors (Basel) 18 (10), 3249. doi:10.3390/s18103249
Jia, L., Chen, H., Yang, J., Fang, X., Niu, W., Zhang, M., et al. (2020). Combinatory Antibiotic Treatment Protects against Experimental Acute Pancreatitis by Suppressing Gut Bacterial Translocation to Pancreas and Inhibiting NLRP3 Inflammasome Pathway. Innate Immun. 26 (1), 48–61. doi:10.1177/1753425919881502
Jiang, X. E., Yang, S. M., Zhou, X. J., and Zhang, Y. (2020). Effects of Mesalazine Combined with Bifid Triple Viable on Intestinal Flora, Immunoglobulin and Levels of Cal, MMP-9, and MPO in Feces of Patients with Ulcerative Colitis. Eur. Rev. Med. Pharmacol. Sci. 24 (2), 935–942. doi:10.26355/eurrev_202001_20079
Kocael, A., Inal, B. B., Guntas, G., Kelten, C., Inal, H., Topac, H. I., et al. (2016). Evaluation of Matrix Metalloproteinase, Myeloperoxidase, and Oxidative Damage in Mesenteric Ischemia-Reperfusion Injury. Hum. Exp. Toxicol. 35 (8), 851–860. doi:10.1177/0960327115607946
Kong, L., Deng, J., Zhou, X., Cai, B., Zhang, B., Chen, X., et al. (2021). Sitagliptin Activates the P62-Keap1-Nrf2 Signalling Pathway to Alleviate Oxidative Stress and Excessive Autophagy in Severe Acute Pancreatitis-Related Acute Lung Injury. Cell Death Dis. 12 (10), 928. doi:10.1038/s41419-021-04227-0
Kou, M., Guo, D., Liu, L., Gao, X., Xing, G., Zha, A., et al. (2020). Expression Pattern and Association Analysis of Porcine Matrix Metallopeptidase 9 (MMP9) with Diarrhea and Performance Traits in Piglets. Res. Vet. Sci. 129, 53–58. doi:10.1016/j.rvsc.2019.12.017
Lankisch, P. G., Apte, M., and Banks, P. A. (2015). Acute Pancreatitis. Lancet 386 (9988), 85–96. doi:10.1016/s0140-6736(14)60649-8
Leung, P. S. (2010). Common Pancreatic Disease. Adv. Exp. Med. Biol. 690, 29–51. doi:10.1007/978-90-481-9060-7_3
Li, J., Han, J., Lv, J., Wang, S., Qu, L., and Jiang, Y. (2020). Saikosaponin A-Induced Gut Microbiota Changes Attenuate Severe Acute Pancreatitis through the Activation of Keap1/Nrf2-ARE Antioxidant Signaling. Oxid. Med. Cell Longev. 2020, 9217219. doi:10.1155/2020/9217219
Li, X. Y., He, C., Zhu, Y., and Lu, N. H. (2020). Role of Gut Microbiota on Intestinal Barrier Function in Acute Pancreatitis. World J. Gastroenterol. 26 (18), 2187–2193. doi:10.3748/wjg.v26.i18.2187
Liang, X. Y., Jia, T. X., and Zhang, M. (2021). Intestinal Bacterial Overgrowth in the Early Stage of Severe Acute Pancreatitis Is Associated with Acute Respiratory Distress Syndrome. World J. Gastroenterol. 27 (15), 1643–1654. doi:10.3748/wjg.v27.i15.1643
Liu, Y., Chen, X. D., Yu, J., Chi, J. L., Long, F. W., Yang, H. W., et al. (2017). Deletion of XIAP Reduces the Severity of Acute Pancreatitis via Regulation of Cell Death and Nuclear Factor-κB Activity. Cell Death Dis. 8 (3), e2685. doi:10.1038/cddis.2017.70
Lozupone, C. A., Stombaugh, J. I., Gordon, J. I., Jansson, J. K., and Knight, R. (2012). Diversity, Stability and Resilience of the Human Gut Microbiota. Nature 489 (7415), 220–230. doi:10.1038/nature11550
Lucafò, M., Pugnetti, L., Bramuzzo, M., Curci, D., Di Silvestre, A., Marcuzzi, A., et al. (2019). Long Non-Coding RNA GAS5 and Intestinal MMP2 and MMP9 Expression: A Translational Study in Pediatric Patients with IBD. Int. J. Mol. Sci. 20 (21), 5280. doi:10.3390/ijms20215280
Mao, J., Qi, S., Cui, Y., Dou, X., Luo, X. M., Liu, J., et al. (2020). Lactobacillus Rhamnosus GG Attenuates Lipopolysaccharide-Induced Inflammation and Barrier Dysfunction by Regulating MAPK/NF-κB Signaling and Modulating Metabolome in the Piglet Intestine. J. Nutr. 150 (5), 1313–1323. doi:10.1093/jn/nxaa009
Meng, C., Bai, C., Brown, T. D., Hood, L. E., and Tian, Q. (2018). Human Gut Microbiota and Gastrointestinal Cancer. Genomics Proteomics Bioinforma. 16 (1), 33–49. doi:10.1016/j.gpb.2017.06.002
Nishijima, S., Suda, W., Oshima, K., Kim, S. W., Hirose, Y., Morita, H., et al. (2016). The Gut Microbiome of Healthy Japanese and its Microbial and Functional Uniqueness. DNA Res. 23 (2), 125–133. doi:10.1093/dnares/dsw002
Pan, L. Y., Chen, Y. F., Li, H. C., Bi, L. M., Sun, W. J., Sun, G. F., et al. (2017). Dachengqi Decoction Attenuates Intestinal Vascular Endothelial Injury in Severe Acute Pancreatitis In Vitro and In Vivo. Cell Physiol. Biochem. 44 (6), 2395–2406. doi:10.1159/000486155
Pan, L. L., Niu, W., Fang, X., Liang, W., Li, H., Chen, W., et al. (2019). Clostridium Butyricum Strains Suppress Experimental Acute Pancreatitis by Maintaining Intestinal Homeostasis. Mol. Nutr. Food Res. 63, e1801419. doi:10.1002/mnfr.201801419
Qin, J., Li, R., Raes, J., Arumugam, M., Burgdorf, K. S., Manichanh, C., et al. (2010). A Human Gut Microbial Gene Catalogue Established by Metagenomic Sequencing. Nature 464 (7285), 59–65. doi:10.1038/nature08821
Ringland, C., Schweig, J. E., Eisenbaum, M., Paris, D., Ait-Ghezala, G., Mullan, M., et al. (2021). MMP9 Modulation Improves Specific Neurobehavioral Deficits in a Mouse Model of Alzheimer's Disease. BMC Neurosci. 22 (1), 39. doi:10.1186/s12868-021-00643-2
Santana, A., Medina, C., Paz-Cabrera, M. C., Díaz-Gonzalez, F., Farré, E., Salas, A., et al. (2006). Attenuation of Dextran Sodium Sulphate Induced Colitis in Matrix Metalloproteinase-9 Deficient Mice. World J. Gastroenterol. 12 (40), 6464–6472. doi:10.3748/wjg.v12.i40.6464
Takiishi, T., Fenero, C. I. M., and Câmara, N. O. S. (2017). Intestinal Barrier and Gut Microbiota: Shaping Our Immune Responses throughout Life. Tissue Barriers 5 (4), e1373208. doi:10.1080/21688370.2017.1373208
Tan, C., Ling, Z., Huang, Y., Cao, Y., Liu, Q., Cai, T., et al. (2015). Dysbiosis of Intestinal Microbiota Associated with Inflammation Involved in the Progression of Acute Pancreatitis. Pancreas 44 (6), 868–875. doi:10.1097/mpa.0000000000000355
Tang, Q., Wang, H., Wang, X., Fang, M., and Zhang, H. (2019). Effect of PI3K/PKB Signal Pathway Inhibitor Wortmannin Pretreatment on Intestinal Barrier Function in Severe Acute Pancreatitic Rats. Adv. Clin. Exp. Med. 28 (8), 1059–1066. doi:10.17219/acem/99910
Tang, M., Yuan, D., and Liao, P. (2021). Berberine Improves Intestinal Barrier Function and Reduces Inflammation, Immunosuppression, and Oxidative Stress by Regulating the NF-κB/MAPK Signaling Pathway in Deoxynivalenol-Challenged Piglets. Environ. Pollut. 289, 117865. doi:10.1016/j.envpol.2021.117865
Tu, X. H., Huang, S. X., Li, W. S., Song, J. X., and Yang, X. L. (2014). Mesenchymal Stem Cells Improve Intestinal Integrity during Severe Acute Pancreatitis. Mol. Med. Rep. 10 (4), 1813–1820. doi:10.3892/mmr.2014.2453
Wan, Y. D., Zhu, R. X., Bian, Z. Z., and Pan, X. T. (2019). Improvement of Gut Microbiota by Inhibition of P38 Mitogen-Activated Protein Kinase (MAPK) Signaling Pathway in Rats with Severe Acute Pancreatitis. Med. Sci. Monit. 25, 4609–4616. doi:10.12659/msm.914538
Wu, X. X., Huang, X. L., Chen, R. R., Li, T., Ye, H. J., Xie, W., et al. (2019). Paeoniflorin Prevents Intestinal Barrier Disruption and Inhibits Lipopolysaccharide (LPS)-Induced Inflammation in Caco-2 Cell Monolayers. Inflammation 42 (6), 2215–2225. doi:10.1007/s10753-019-01085-z
Yang, F., Zhao, K., Zhang, X., Zhang, J., and Xu, B. (2016). ATP Induces Disruption of Tight Junction Proteins via IL-1 Beta-Dependent MMP-9 Activation of Human Blood-Brain Barrier In Vitro. Neural Plast. 2016, 8928530. doi:10.1155/2016/8928530
Yu, S., Xiong, Y., Xu, J., Liang, X., Fu, Y., Liu, D., et al. (2020). Identification of Dysfunctional Gut Microbiota through Rectal Swab in Patients with Different Severity of Acute Pancreatitis. Dig. Dis. Sci. 65 (11), 3223–3237. doi:10.1007/s10620-020-06061-4
Zerem, E. (2014). Treatment of Severe Acute Pancreatitis and its Complications. World J. Gastroenterol. 20 (38), 13879–13892. doi:10.3748/wjg.v20.i38.13879
Zhang, J. W., Zhang, G. X., Chen, H. L., Liu, G. L., Owusu, L., Wang, Y. X., et al. (2015). Therapeutic Effect of Qingyi Decoction in Severe Acute Pancreatitis-Induced Intestinal Barrier Injury. World J. Gastroenterol. 21 (12), 3537–3546. doi:10.3748/wjg.v21.i12.3537
Zhang, H., Liu, L., Jiang, C., Pan, K., Deng, J., and Wan, C. (2020). MMP9 Protects against LPS-Induced Inflammation in Osteoblasts. Innate Immun. 26 (4), 259–269. doi:10.1177/1753425919887236
Zhang, Z., Zhang, Q., Li, F., Xin, Y., and Duan, Z. (2021). Contributions of HO-1-Dependent MAPK to Regulating Intestinal Barrier Disruption. Biomol. Ther. Seoul. 29 (2), 175–183. doi:10.4062/biomolther.2020.112
Zhao, H. B., Jia, L., Yan, Q. Q., Deng, Q., and Wei, B. (2020). Effect of Clostridium Butyricum and Butyrate on Intestinal Barrier Functions: Study of a Rat Model of Severe Acute Pancreatitis with Intra-Abdominal Hypertension. Front. Physiol. 11, 561061. doi:10.3389/fphys.2020.561061
Keywords: severe acute pancreatitis, Clostridium butyricum, gut microbiota, matrix metallopeptidase 9, intestinal injury
Citation: Yan Q, Jia L, Wen B, Wu Y, Zeng Y and Wang Q (2022) Clostridium butyricum Protects Against Pancreatic and Intestinal Injury After Severe Acute Pancreatitis via Downregulation of MMP9. Front. Pharmacol. 13:919010. doi: 10.3389/fphar.2022.919010
Received: 13 April 2022; Accepted: 17 June 2022;
Published: 18 July 2022.
Edited by:
Stefania Tacconelli, University of Studies G d'Annunzio Chieti and Pescara, ItalyReviewed by:
Miguel Angel Plaza, University of Zaragoza, SpainMarcos Edgar Herkenhoff, University of São Paulo, Brazil
Jinbiao Zhao, China Agricultural University, China
Copyright © 2022 Yan, Jia, Wen, Wu, Zeng and Wang. This is an open-access article distributed under the terms of the Creative Commons Attribution License (CC BY). The use, distribution or reproduction in other forums is permitted, provided the original author(s) and the copyright owner(s) are credited and that the original publication in this journal is cited, in accordance with accepted academic practice. No use, distribution or reproduction is permitted which does not comply with these terms.
*Correspondence: Lin Jia, 13925012853@139.com