- 1Department of Neurosurgery, Shanghai General Hospital, Shanghai Jiao Tong University School of Medicine, Shanghai, China
- 2Department of Neurosurgery, Second Affiliated Hospital, School of Medicine, Zhejiang University, Zhejiang, China
- 3Clinical Research Center for Neurological Diseases of Zhejiang Province, Hangzhou, China
- 4Department of Neurosurgery, Huashan Hospital, Shanghai Medical College, Fudan University, Shanghai, China
Post-stroke depression (PSD) is the most frequent and important neuropsychiatric consequence of stroke. It is strongly associated with exacerbated deterioration of functional recovery, physical and cognitive recoveries, and quality of life. However, its mechanism is remarkably complicated, including the neurotransmitters hypothesis (which consists of a monoaminergic hypothesis and glutamate-mediated excitotoxicity hypothesis), inflammation hypothesis, dysfunction of the hypothalamic-pituitary-adrenal (HPA) axis, and neurotrophic hypothesis and neuroplasticity. So far, the underlying pathogenesis of PSD has not been clearly defined yet. At present, selective serotonin reuptake inhibitors (SSRIs) have been used as the first-line drugs to treat patients with PSD. Additionally, more than SSRIs, a majority of the current antidepressants complied with multiple side effects, which limits their clinical application. Currently, a wide variety of studies revealed the therapeutic potential of natural products in the management of several diseases, especially PSD, with minor side effects. Accordingly, in our present review, we aim to summarize the therapeutic targets of these compounds and their potential role in-clinic therapy for patients with PSD.
1 Introduction
Stroke is the leading cause of death and disability worldwide (Donnan et al., 2008). According to the World Health Organization, approximately 15 million people suffer a stroke annually (Mackay et al., 2004). As a major contributor to long-term disability in adults, stroke results in a need for long-term rehabilitation care and imposes an economic burden (Lin et al., 2014; Aoki et al., 2019; Wang et al., 2020a; Katzan et al., 2021). In addition, stroke always brings great psychological stress, which makes stroke patients vulnerable to depression.
Among the many neuropsychiatric effects of stroke, post-stroke depression (PSD) is the most prevalent and serious one. Over half of stroke survivors suffer from depression at some point (Ayerbe et al., 2013). Depression is strongly associated with compromised functional, physical, and cognitive recovery as well as poor quality of life (Li et al., 2017). The main clinical symptoms of PSD include depressed mood, apathy, weight loss or gain, sleep changes, a sense of worthlessness, anhedonia, and fatigue, the first two of which are the core symptoms (Feng et al., 2014). PSD is linked to an increased risk of short-term and long-term mortality (Bartoli et al., 2013). Moreover, clinical management of depressive symptoms has been demonstrated to be related to better functional recovery and more favorable outcomes (Chemerinski et al., 2001).
To date, the underlying pathophysiological mechanisms of PSD have not been elucidated (Lin et al., 2020). Numerous studies have suggested that the possible mechanisms include a change in ascending monoamine pathways, excess proinflammatory cytokines, dysfunction of the hypothalamic-pituitary-adrenal (HPA) axis, and alterations in neuroplasticity (Villa et al., 2018). Based on the classic monoamine hypothesis, selective serotonin reuptake inhibitors (SSRIs), serotonin and noradrenaline reuptake inhibitors (SNRIs), and some other antidepressants have been extensively developed and studied for the treatment of PSD (Villa et al., 2018; Effects of fluoxetine on functional, 2019). However, these antidepressants have various side effects, such as dizziness, sedation, and anticholinergic side effects (Sarko, 2000; Choi-Kwon et al., 2006). Furthermore, there is some controversy regarding the effectiveness of antidepressants in improving quality of life, function, and cognitive outcomes in patients with neurological disorders (Price et al., 2011). To minimize the side effects and maximize the therapeutic outcomes, alternative treatment strategies are urgently needed.
Compounds inspired by natural products may provide highly promising new resources for the treatment of PSD. Medicinal plants contain a wide variety of phytochemicals recognized for their therapeutic potential in the treatment of several diseases, including PSD (Zeng et al., 2017; Zhang et al., 2021a). In recent years, various phytochemical compounds with strong anti-depressant activity have been reported to exist in various herbal medicines (Shah et al., 2021). More importantly, natural products are often seen as ecologically sound and readily available resources with few side effects (Joshi et al., 2020). The potential of natural products in the treatment of PSD is a matter that warrants attention.
In this review, we focus on the potential pathogenesis of PSD and treatments in the context of recent evidence regarding available therapeutic procedures for depression. Then, we summarize the active compounds in natural products for the potential treatment of PSD and explore the novel therapeutic targets of these compounds. Finally, the perspectives of future research are reviewed in the outlook.
The following search terms were used: post-stroke depression, depression, natural products, Traditional Chinese medicine, treatments. Once we found the target article, we continued our search in the similar articles section of Pubmed. We evaluated studies in English that investigated interventions for natural products in depression. The current literatures on treatments with natural products for PSD were summarized in this review.
2 Pathophysiological Mechanisms of PSD
The pathophysiology of PSD is remarkably complicated and, at present, poorly understood (Villa et al., 2018; Chen et al., 2020a); factors including both biological and psychosocial factors are likely to be involved. No single pathophysiological mechanism fully explains PSD (Loubinoux et al., 2012). Further research is needed to develop a better pathophysiological understanding of PSD to develop targeted interventions for prevention and treatment. Research supports the hypothesis that the disease process may be characterized by the reciprocal modulation of neurotransmitter systems, neuroinflammation, neuroendocrine activation, and neuroplasticity (Loubinoux et al., 2012). The main pathophysiological mechanisms of PSD are described in this section and illustrated in Figure 1.
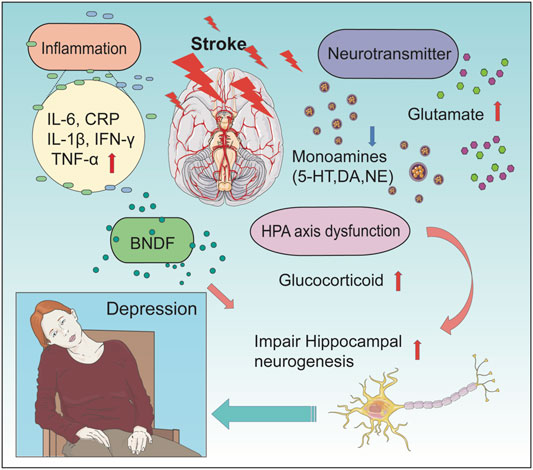
FIGURE 1. Pathophysiological mechanisms of PSD. The picture shows the association of the main pathophysiological mechanisms of PSD. There are four main mechanisms leading to PSD (including alterations in the neurotransmitter system, change in levels of inflammatory cytokine, dysfunction of the HPA axis, and influence of neuroplasticity). After a stroke, alterations of the neurotransmitter system include deduced level of monoamines (5-HT, DA, and NE) and an increased level of glutamate. Changes in levels of inflammatory cytokine include an increased level of IL-6, CRP, IL-1β, IFN-γ, and TNF-α. In PSD patients, the concentration of BNDF in the hippocampal was found to be decreased, which lead to impairment of hippocampal neurogenesis. At the same time, HPA axis dysfunction can lead to an increased concentration of glucocorticoid, which further impairs hippocampal neurogenesis. These changes can finally result in PSD.
Depressive behavior tests of forced swim test (FST) and tail suspension test (TST) were used in most of the research articles we included in the present review. FST and TST are commonly used to test for depression-like behavior, and screen for antidepressant properties in drugs, since antidepressants can cause immobile behavior to shorten (Porsolt et al., 1977; Steru et al., 1985; Toyoda, 2017). However, they are also usually used as animal models of depression (Gomez and Barros, 2000; Shinde et al., 2015). In fact, the majority of the studies that identify the antidepressants used TST and FST as animal models, including the articles we selected. In order to solve the problem that there are no animal models which both resemble depression and are selectively sensitive to clinically effective antidepressants, FST was reported as the first animal model for depression (Porsolt et al., 1977). TST as an animal model for screening antidepressants in mice was first recommended by Steru et al., in 1985 (Steru et al., 1985), which shares a common theoretical basis and behavioral measure with the FST. Although some researchers regarded FST and TST as only behavioral tests for screening antidepressants (AD) activity (Yan et al., 2010), some scholars hold that both of them are animal models of depression (Duman, 2010; Yi et al., 2011). According to previous research, we classify TST and FST as acute stress model (Toyoda, 2017). Besides FST and TST, some other animal models such as Chronic unpredictable mild stress (CUMS), Learned helplessness (LH) model, Olfactory bulbectomy (OBX) model, and Chronic social defeat stress (CSDS) model are also used in the articles we included.
2.1 Neurotransmitter Hypotheses
The neurotransmitter hypotheses have been regarded as the most significant set of mechanisms in PSD (Spalletta et al., 2006). At present, there are two leading concepts regarding the role of neurotransmitters in PSD: the monoaminergic hypothesis and glutamate-mediated excitotoxicity.
2.1.1 Monoaminergic Hypothesis
For decades, studies have linked depression to monoamine levels (Moret and Briley, 2011). The monoamine hypothesis of depression is a classic model for understanding its pathogenesis (Tiemeier, 2003). In the 1960s, it was reported that monoamine deficiency in patients treated with reserpine for hypertension caused adverse reactions leading to depression, leading to the proposal of a neurochemical model of depression, which revealed that monoamine dysfunction in the central nervous system (CNS) has an association with depression (Schildkraut, 1965). Previous studies have firmly established an association between PSD and monoamine levels (Li et al., 2014). And monoamines are regarded as an important factor in PSD (Loubinoux et al., 2012; Espárrago Llorca et al., 2015). Monoamines, which mainly include 5-hydroxytryptamine (5-HT, or serotonin), dopamine (DA), and norepinephrine (NE), play a crucial role in the central nervous system (Chávez-Castillo et al., 2019). Previous studies have shown that 5-HT, NE, and DA neurons are interconnected in the CNS (Hamon and Blier, 2013). This phenomenon was first proposed by Robinson and Bloom in the 1970s, who also observed an association between decreased catecholamine concentrations and abnormal activity in rats (Robinson and Bloom, 1977). Additionally, a considerable number of studies have indicated that monoamine levels are lower in patients with depression than in those without depression. Some studies found that the brain 5-HT and NE levels of patients with depression were lower than those of patients without depression (Maurer-Spurej et al., 2007; Du et al., 2016). However, depressive symptoms can be effectively alleviated by increasing 5-HT bioavailability in synapses (Delgado et al., 1990).
Monoamines (5-HT, dopamine, and NE) are broadly distributed in the brain (Blier and de Montigny, 1994; Ji et al., 2014). Multiple antidepressant drugs (fluoxetine and tranylcypromine) can promote the release of dopamine and increase the expression of dopamine receptors to enhance dopaminergic function (Lammers et al., 2000). These increases in dopamine receptor expression are controlled by brain-derived neurotrophic factor (BDNF) (Guillin et al., 2001).
2.1.2 Glutamate-Mediated Excitotoxicity Hypothesis
Glutamate-mediated excitotoxicity is another hypothesis of PSD. Although the role of glutamate concentrations in depression has been less extensively investigated than that of monoamines at both preclinical and clinical stages, there is still a great deal of research evidence on the role of glutamate in depression. There are studies indicating that glutamate is implicated in mood disorders (Veldic et al., 2019). Glutamate excitotoxicity is one of the main causes of neurodegeneration (Olivares-Bañuelos et al., 2019). Excitotoxicity caused by glutamate is reported to be important in several neurological and psychiatric disorders, including PSD (Mehta et al., 2013; Villa et al., 2018).
Several studies have shown that astrocyte cells can release gliotransmitters, including glutamate (Padmashri et al., 2015). In addition, astroglia expresses various glutamate receptors to respond to synaptically released glutamate (Morel et al., 2014). In stroke patients, high serum glutamate levels on admission are associated with stroke severity and poor prognosis. Additionally, high levels of glutamate after admission are independently associated with a worse disease course during the hospital stay (Hervella et al., 2020), which includes an impact on depression. Previous research has shown that alterations in glutamate recycling and glutamate receptors might be viable new possibilities for treating depression (Choudary et al., 2005), which provides potential ideas for the treatment of PSD patients.
2.2 The Inflammation Hypothesis
A growing body of convincing evidence shows that the inflammatory response, a vital biological event, has a strong relationship with depression (Ferrucci and Fabbri, 2018; Leonard, 2018). Increased inflammatory activation of the immune system is associated with stroke and depression, which negatively impacts health in both conditions (Wijeratne and Sales, 2021). The levels of multiple inflammatory factors in serum or plasma were frequently observed to increase in patients with PSD; examples include IL-6, CRP, IL-1, IFN-γ, IL-1β, and TNF-α (Yang et al., 2010; Su et al., 2012; Bensimon et al., 2014; Jiao et al., 2016; Kang et al., 2016). Thus, researchers suspected that inflammation was related to the occurrence of PSD and confirmed this hypothesis through animal experiments (Gibney et al., 2013).
Some researchers have even proposed the concept of depression as a dysfunction of the immune system (Leonard, 2010). The activated inflammatory response is hypothesized to be responsible for the high prevalence of depression following stroke (Pascoe et al., 2011). Cell death, including apoptosis and necrosis, might also be regulated by inflammatory cytokines (Gamdzyk et al., 2020). The growth of cerebral infarcts in vulnerable brain regions such as the hippocampus can be attributed to increased cell death in these areas. Depressive symptoms are also linked to increased cell death in these areas. (Kubera et al., 2011). As a result of cell death, large amounts of inflammatory cytokines are released (Rock and Kono, 2008). Consequently, inflammatory cytokines and cell death influence each other, further exacerbating the pathological process of depression.
This well-characterized cascade of inflammatory events occurs both in stroke and depression, which emphasizes the importance of proinflammatory cytokines (Adzic et al., 2018; Aleem and Tohid, 2018). The first prospective study on inflammatory cytokines and PSD has been conducted by Yang et al. (Yang et al., 2010). The authors found that an increased serum IL-18 concentration at the acute stage of stroke can be an independent risk factor for PSD. This was corroborated by reports that higher levels of TNF-α, IL-1B, IL-6, and IL-18 also independently predicted PSD occurrence in acute and subacute periods (Spalletta et al., 2006; Spalletta et al., 2013). A meta-analysis by Chen et al. showed that interleukin-6 (IL-6) and tumor necrosis factor-alpha (TNF-α) serum concentrations were higher in the PSD group than in the non-PSD group (Chen et al., 2020b).
There is evidence that antidepressants may reduce depressive symptoms by affecting immune markers and mood (Wijeratne and Sales, 2021). For instance, SSRI and SNRI administration was associated with increased levels of IL-10 (Ma et al., 2017). Similarly, an animal study demonstrated that IL-10 was increased after treatment with amitriptyline and fluoxetine (Qiu et al., 2017). In addition, the inflammation hypothesis is associated with the HPA axis. The HPA axis plays an important role in inflammation (Mahmood et al., 2020). HPA axis activation occurs in peripheral inflammation, leading to chronic stress-associated anxiety and depression (Sun et al., 2019). Several studies have shown that cytokines might induce hypercortisolism and glucocorticoid resistance through the inhibition of glucocorticoid receptors (Pace et al., 2011).
2.3 Dysfunction of the Hypothalamic-Pituitary-Adrenal (HPA) Axis
In psychiatry, abnormalities of the HPA axis have consistently been linked to depression, and the HPA axis is hyperactive in people who suffer from melancholic depression (Juruena et al., 2018). The HPA axis is a major component of the stress system that is involved in regulating mood (Glynn et al., 2013). When the HPA axis is activated, glucocorticoids are produced by the adrenal cortex (Li et al., 2016). HPA axis dysregulation, leading to high cortisol reactivity, has been regarded as contributing to the somatic symptoms of depression (Chaplin et al., 2021).
Following a stroke, HPA axis activation, which is characterized by hypercortisolism, is quite common. (Johansson et al., 1997). Fassbander et al. found that acute ischemic stroke acts as a stressor and thus stimulates the HPA axis resulting in increased glucocorticoid levels (Fassbender et al., 1994). And studies have proved that serum cortisol levels were increased in PSD mice (Zhang et al., 2015a). Thus, we think that the Dysfunction of the HPA axis is one of the mechanisms of PSD. However, the specific mechanism of hypercortisolism-related depression is still unclear (Feng et al., 2014). There are insights that depression is associated with hypercortisolism and glucocorticoid receptor dysfunction (Carvalho and Pariante, 2008), while Weidenfeld et al. argue that the effect of glucocorticoids on stroke might improve neurological outcomes (Weidenfeld et al., 2011).
Moreover, the hippocampus is a key component linked to the HPA axis (Jacobson and Sapolsky, 1991). Corticosterone, the final product of the HPA axis, has a major target in the hippocampus, and the action of this hormone on the hippocampus is correlated with the pathogenesis and progression of depression (Saveanu and Nemeroff, 2012). According to the experiment of Zhang et al., we hypothesized that drugs used for inhibition of corticosterone-induced neurotoxicity in the hippocampus can be applied for clinical treatment of PSD (Zhang et al., 2021b).
2.4 Neurotrophic Hypothesis and Neuroplasticity
The neurogenesis hypothesis is relatively new when compared with other hypotheses about depression. According to the neurogenesis hypothesis, depression is largely the result of impaired nerve growth in the brain and the impairment of the brain’s ability to promote neurogenesis is the root cause of depression (Yan et al., 2016).
A number of correlative studies support the hypothesis that new neurons of the hippocampus play an essential role in mood control and the efficacy of antidepressants. Researchers have discovered that patients with depression suffer from lower neurogenesis and hippocampal volume, whereas antidepressants can increase neurogenesis in the hippocampus (Eisch and Petrik, 2012). It appears that hippocampal alterations play a critical role in the pathogenesis of depression, according to a variety of studies (Frodl et al., 2002). Hippocampal volume loss is a hallmark of clinical depression and restoration of adult hippocampal neurogenesis leads to recovery in depression patients (Sheline et al., 1996; Jacobs et al., 2000). The effects of chronic stress-induced depression on adult hippocampal neurogenesis have been demonstrated in rodent studies (Sahay and Hen, 2007). Additionally, rodents with impaired hippocampal neurogenesis leads to the development of depression-like behaviors, partly due to the fact that hippocampal neurogenesis buffers the over-reactivity of the HPA axis when stress is present (Snyder et al., 2011; Egeland et al., 2015).
Nevertheless, antidepressants and alternative antidepressant interventions stimulate adult hippocampal neurogenesis, which could play a role in treatment outcomes. Fang et al. presumed that potential therapeutic strategies for depression may include increased neurogenesis (Fang et al., 2020). Neurogenesis and synaptic plasticity in the hippocampus can be impaired by chronic excess glucocorticoids (Stranahan et al., 2008; Anacker et al., 2013). Reduced neurogenesis is linked to stress and depression, but antidepressant therapy exhibits anti-depressive effects and increases neurogenesis (Mahar et al., 2014). Depression is always accompanied by decreased hippocampal neurogenesis, while chronic antidepressant treatments (such as fluoxetine) can upregulate hippocampal neurogenesis (Wang et al., 2008a).
At the same time, some studies found that hippocampal volume decreased in stroke patients compared with healthy controls. Khlif et al. found that Atrophy rates for first-ever strokes, especially those with ipsi-lesional hippocampal atrophy, are much higher than for healthy controls and contra-lesional strokes (Khlif et al., 2019). Werden et al. found a smaller hippocampal volume in first-ever stroke patients than in healthy controls, and the hippocampal volume was smaller in patients with recurrent strokes than in controls and patients with their first stroke (Werden et al., 2017). These studies suggest that upregulating hippocampal neurogenesis might be a potential treatment for PSD patients.
Brain-derived neurotrophic factor (BDNF), as an endogenous neurotrophic factor, is necessary to modulate neuronal plasticity, inhibiting cell death cascades and increasing cell survival proteins. In the central nervous system, it maintains and promotes the proliferation of neurons (Benarroch, 2015). BDNF is an important regulator of synaptogenesis, neurogenesis, synaptic plasticity, and cell survival underlying memory and learning (Sairanen et al., 2005). The rate of hippocampal neurogenesis was increased by BDNF. Nevertheless, it has been observed that blood and hippocampal BDNF levels drop in patients with depression (Aydemir et al., 2006; Castrén et al., 2007). Antidepressant drug effects correlate with increased BDNF synthesis and activity in the hippocampus (Chen et al., 2001; Vásquez et al., 2014). Accordingly, BDNF has been proposed as one of the major candidate targets for the treatment of the antidepressant response.
In summary, the pathophysiology of PSD is complex and likely influenced by both biological and psychosocial factors. As previously described, the pathophysiology of PSD does not exist in isolation in the pathogenic process. For example, inflammation and the HPA axis influence each other. The HPA axis is one major regulator of inflammation, and a stress-induced release of cytokines, like IL-6, activates the HPA axis, which leads to rapid increases in adrenocorticotropic hormone (ACTH) and cortisol (Bethin et al., 2000; Mahmood et al., 2020). Dopamine receptors are strongly associated with the inflammasome signaling pathway (Wang et al., 2020b). Moreover, it was discovered that dopamine receptors are critical therapeutic targets in immunoregulation and inflammation (Taylor et al., 2003). Some monoamine reuptake inhibitors applied in clinical practice have been found to increase the expression of BDNF in depression-like rodent models (Molteni et al., 2006). A better understanding of PSD pathophysiology is essential to developing targeted prevention and treatment interventions.
3 Traditional Treatments for PSD
Although its precise mechanisms remain to be delineated, the treatment of PSD has made great progress. Traditional methods of treating PSD remain pharmacotherapy and psychological therapy. The most effective method of treating PSD is pharmacotherapy because of its high efficacy. In general, SSRIs are the first line of treatment for PSD, and tricyclic drugs are the second line of treatment (Castilla-Guerra et al., 2020).
Previous studies have indicated that antidepressants improve the recovery from the disability of patients treated with them than those not treated with antidepressants, and cognitive skills and functional recovery can improve with antidepressants in patients with PSD. Antidepressant treatment initiated early in non-depressed stroke patients can improve their cognitive and functional recovery and reduce their risk of developing post-stroke depression (Mead et al., 2015).
Several studies have examined the efficacy of pharmacotherapy or psychotherapy to prevent PSD. The introduction of TCAs and MAOIs in the 1950s revolutionized the treatment of depression (Pacher and Kecskemeti, 2004). With the development of newer agents, SSRIs have become the first-line drugs for the treatment of depression among several other indications (Dremencov et al., 2009). In animal models of stroke, SSRIs have shown convincing benefits (McCann et al., 2014). Among them, duloxetine (a serotonin and norepinephrine reuptake inhibitor) has been found to be an effective antidepressant when compared to a placebo and to be equally effective as various SSRIs (Girardi et al., 2009). Multiple clinical trials have been conducted since then to evaluate the effectiveness of antidepressants in PSD. The studies above indicated that all pharmacological treatments are SSRIs (e.g., escitalopram), SNRIs (e.g., duloxetine or venlafaxine), and tricyclic drugs (e.g., nortriptyline). Recently, a network meta-analysis that included all RCTs published between February 1984 and October 2016 assessed and ranked the efficacy and tolerability of antidepressants for PSD. The most acceptable treatments are paroxetine, placebo, sertraline, and nortriptyline based on the acceptability ranking (Deng et al., 2017). Nevertheless, a recent network meta-analysis, including 14 RCTs, examined the efficacy, acceptability, and tolerability of antidepressants in the treatment of PSD. The results showed that the major antidepressants did not display a significantly increased effectiveness when the efficacy of different antidepressants was compared (Qin et al., 2018). Some drugs, such as agomelatine, have a rapid antidepressant action and a high degree of safety and tolerability that probably enhances compliance with treatment. In brain areas such as the hippocampus and prefrontal cortex, agomelatine improves neuroplasticity mechanisms as well as adult neurogenesis (Pompili et al., 2013).
For the moment, there is still controversy about the best drug to treat PSD from those currently available. PSD treatment still leaves many questions unanswered, such as the most useful medications and the best timing for treatment. Additionally, the adverse effects of current pharmacotherapy cannot be ignored. Although antidepressants showed therapeutic effects in patients with PSD, we must also take into account other issues. For example, obvious complications often occur, such as addiction, toxicity, reduced effectiveness over time, relapse, and recurrence concerns (Sarko, 2000).
4 Potential and Mechanisms of Natural Products in the Treatment of PSD
Natural products are a potential source of drugs for nervous system-related disorders, including PSD (Zhang et al., 2021a). Being easily accessible and having few side effects, natural products are seen as having the potential for the therapeutic use of PSD (Joshi et al., 2020). Thus, it becomes imperative to search for novel therapies without side effects based on natural products. Even though drug design and discovery rely heavily on synthetic chemistry, the contribution of natural products cannot be ignored. In this part, we aim to identify the active compounds from natural products for the promising treatment of PSD by different types of mechanisms. The therapeutic effects of some natural products occur through a variety of mechanisms.
4.1 Therapeutic Strategy of Modulation in Neurotransmitters
As described previously, the neurotransmitter hypothesis has been regarded as the most significant mechanism in PSD, while monoamines are the most studied and mainly include 5-HT, DA, and NE. Anti-neurotransmitter therapy has been widely found in many natural products. Numerous studies have indicated that numerous natural products exert antidepressant effects by acting on neurotransmitters; however, most of those studies could not draw firm conclusions. Further studies are needed to reveal the exact antidepressant mechanism.
Monoamine levels were lower in patients with depression than in patients without depression, which is partly due to monoamine oxidase. Monoamine oxidase (MAO) degrades several monoamine neurotransmitters (Chen et al., 2011). When individuals suffer from depression, the level of monoamine oxidase enzyme in the brain is increased, which in turn reduces levels of monoamines and aggravates the symptoms of depression. Enzyme inhibition has already led to the discovery of a wide variety of useful natural products in the treatment of PSD. Several studies have found that some natural products produce antidepressant effects through the inhibition of MAO. All of the animal models used in this research involved one or both the FST and TST models. In a study by Zhu et al., they found that baicalin reduced immobility time in the FST and TST in mice. Baicalin, at doses of 12.5, 25, and 50 mg/kg (p.o.), also decreased immobility time in the FST in rats, which may act through MAO-A and MAO-B inhibition (Zhu et al., 2006). Kaempferol, apigenin, and chrysin proved to be potent monoamine oxidases (MAOIs); however, they produced more pronounced inhibition of MAO-A than MAO-B and exerted monoamine catabolism and neuroprotection on the rat brain. Sloley et al. found that kaempferol reduces the immobility time in the FST and TST when administered at a dose of 30 mg/kg (o.p.), possibly acting via MAO inhibition (Sloley et al., 2000). Similar studies have been abundant in the last few decades. For example, methanol extract of the roots of Sophora flavescens, curcumin, fisetin, trans-resveratrol, punarnavine, total glycosides of peony (TGP), isorhynchophylline (IRN), and cocoa polyphenolic extract have been found to inhibit MAO (Hwang et al., 2005; Mao et al., 2008a; Kulkarni et al., 2008; Messaoudi et al., 2008; Xu et al., 2010; Zhen et al., 2012; Dhingra and Bhankher, 2014; Xian et al., 2017).
Some studies have found that some natural products exert their antidepressant effects by upregulating the level of 5-HT or 5-HT receptors; some such natural products act entirely through a single mechanism. Depending on an interaction with the serotonergic 5-HT1A receptors, hesperidin decreased the immobility time in the FST and TST without affecting locomotor activity in the open-field test (OFT) (Souza et al., 2013). Neferine has also been found to have antidepressant activities by stimulating 5-HT1A receptors (Sugimoto et al., 2010). Through direct or indirect facilitation of central serotonergic transmission, extract of Tagetes lucida and the butanol and chloroform fractions from Hypericum canariense and Hypericum glandulosum exert antidepressant-like effects in mice (Sánchez-Mateo et al., 2005; Guadarrama-Cruz et al., 2008; Sánchez-Mateo et al., 2009). In addition, by stimulating neuronal 5-HT2A receptors, 1-(m-chlorophenyl) piperazine (1 mg/kg, i. p.) exhibited depressant-like effects in the FST and TST (in mice) without affecting locomotor status (Rajkumar et al., 2009).
Moreover, one study had an opposite finding, in which the downregulation of the 5-HT2A receptor might underlie the observed antidepressant effect. Immobility time in the FST and TST was significantly reduced in animals treated with ethanol extract of Marsilea minuta, and Marsilea minuta (400 mg/kg, p. o.) significantly downregulated the 5-HT2A receptor in the frontal cortex, which is considered a potential antidepressant mechanism (Bhattamisra et al., 2008).
Some natural products can exert antidepressant effects by merely activating dopamine receptors. Carvacrol, given daily for seven consecutive days (12.5 mg/kg p. o.), has been shown to increase levels of dopamine and serotonin in the prefrontal cortex and hippocampus, which may operate via action on dopamine D1 and D2 receptors (Zotti et al., 2013). Through activation of dopamine D1 and D2 receptors, pretreatment of mice with ursolic acid (0.1 mg/kg, p. o.) was able to prevent the antidepressant-like effect (Colla et al., 2014).
Indeed, some natural products exert antidepressant effects by more than one mechanism. Most of them function via multiple mechanisms. Li et al. found that naringenin (10, 20, and 50 mg/kg) possessed antidepressant-like activity in the tail suspension test by elevating NA, 5-HT, and GR levels in the hippocampal region (Yi et al., 2010). The antidepressant-like effect of nobiletin has been proven by Li et al., who found that nobiletin (25, 50, and 100 mg/kg, p. o.) decreased the immobility time in both the FST and TST without locomotor alterations in the OFT, which seems to be mediated by an interaction with the serotonergic (5-HT1A and 5-HT2 receptors), noradrenergic (α1-adrenoceptor) and dopaminergic (D1 and D2 receptors) systems (Yi et al., 2011). Through interaction with the 5-HT2 receptor and α1-and α2-adrenoceptors, amentoflavone significantly (p < 0.001) reduced the duration of immobility in the FST and TST, with peak effects observed at 100 and 50 mg/kg, respectively, in comparison to control treatment (Ishola et al., 2012). With the involvement of the serotonergic and noradrenergic and/or dopaminergic systems, rutin (0.three to three mg/kg, p. o.) reduced the immobility time in the TST (Machado et al., 2008). In addition, liquiritin and isoliquiritin, berberine, Hedyosmum brasiliense, podoandin, genipin, bacopaside I, Ptychopetalum olacoides ethanol extract (POEE), punarnavine, Ceratonia siliqua, total ethanolic extract of Convolvulus pluricaulis, and Terminalia bellirica Roxb. fruits have been proven to function via multiple mechanisms, including but not limited to neurotransmitter mechanisms (Irie et al., 2004; Dhingra and Valecha, 2007a; Dhingra and Valecha, 2007b; Peng et al., 2007; Kulkarni and Dhir, 2008; Piato et al., 2009; Agrawal et al., 2011; Zhang et al., 2011; Gonçalves et al., 2012; Liu et al., 2013; Dhingra and Valecha, 2014a; Wang et al., 2014).
Certainly, in addition to the anti-depressant natural products mentioned above, there are several other natural products that exert antidepressant effects by interacting with neurotransmitter systems. The main natural products with this property are shown in Table 1.
4.2 Anti-inflammation Therapy
Although much convincing evidence has established that inflammatory responses, as important biological events, have a strong relationship with depression, few existing studies have found the exact anti-inflammatory effects of natural products in the treatment of PSD. In a study of an olfactory bulbectomy (OBX) rat model, researchers found that after a surgical recovery period of 2 weeks, treatment with quercetin (40, 80 mg/kg; p. o., 14 days) significantly prevented OBX-induced behavioral, biochemical, molecular, and histopathological alterations associated with suppression of the oxidative-nitrosative stress-mediated neuroinflammation-apoptotic cascade (Rinwa and Kumar, 2013). The main natural products are shown in Table 2.
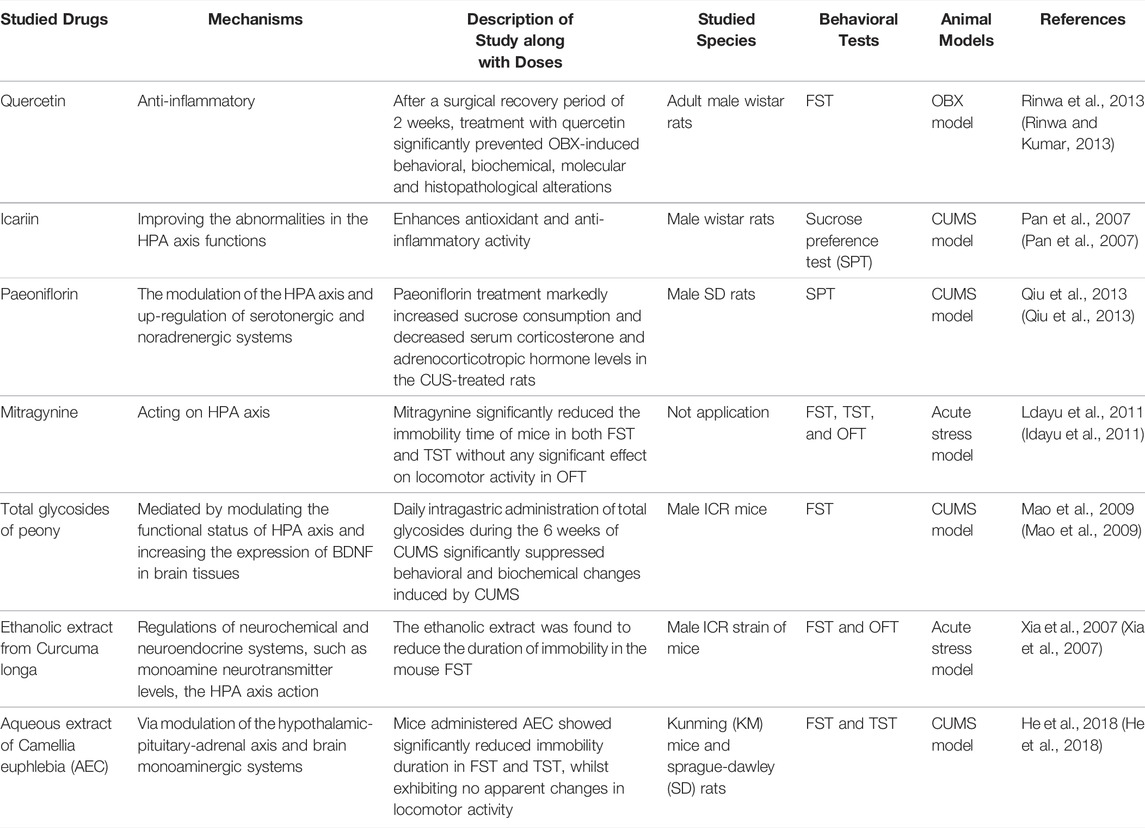
TABLE 2. Potential natural products for the treatment of PSD by anti-inflammation and modulating HPA axis.
4.3 Therapeutic Strategy of Modulation of the HPA Axis
HPA axis activation is quite common after stroke and leads to elevated glucocorticoid levels. Several types of research proved that some natural products create anti-depressant activity via the modulation of the HPA axis, to speak exactly, downregulating the level of HPA. Pan et al. found that icariin, a major constituent of flavonoids isolated from Epimedium brevicornum, possessed potent anti-inflammatory activity at a dose of 20 or 40 mg/kg, which was in part mediated by improving abnormalities in HPA axis functions (Pan et al., 2007). Acting on the HPA axis, mitragynine at a dose of 10 mg/kg and 30 mg/kg i. p. injected extremely reduced the immobility time of mice in both the FST and TST and significantly reduced the release of corticosterone (Idayu et al., 2011). In addition, paeoniflorin, total glycosides of peony, ethanolic extract from Curcuma longa, and aqueous extract of Camellia euphlebia (AEC), which act via multiple mechanisms, have also been investigated previously (Xia et al., 2007; Mao et al., 2009; Qiu et al., 2013; He et al., 2018). The main natural products are shown in Table 2.
4.4 Therapeutic Strategy for Modulating Central Neuroplasticity
Previous studies proved that an impairment in nerve growth is largely responsible for depression, and the impairment of the brain’s ability to promote neurogenesis underlies depression (Yan et al., 2016). According to this hypothesis, new neuronal connections in the hippocampus contribute to mood regulation and the pharmaceutical effect of antidepressants. BDNF, as an endogenous neurotrophic factor, is necessary to modulate neuronal plasticity. Several antidepressant effects of natural products correlate with increased BDNF synthesis and activity in the hippocampus.
In previous studies, Walker et al. found that the PI3K/Akt/mTOR pathway plays a critical role in neuroprotection (Walker et al., 2019). On this basis, Zhang et al. further demonstrated that Sinisan (SNS) protects neurons from corticosterone-induced injury by inhibiting autophagy through induction of the PI3K/AKT/mTOR pathway (Zhang et al., 2021b). In addition, other natural products produce an anti-depressant effect by inducing BDNF, which further promotes neurogenesis. Lee et al. proved the potential for Angelica gigas extract (AGN) to effectively treat repeated injection of corticosterone (CORT)-related depression and anxiety-like symptoms, possibly by modulating the central noradrenergic system and regulating the expression of BDNF (Lee et al., 2015). Zhang et al. found that ethanol extract of Gardenia jasminoides exerts rapid antidepressant effects that are associated with a rapid increase in BDNF expression in the hippocampus (Zhang et al., 2015b). Additionally, hyperoside, baicalein, albiflorin, tetrandrine, cannabidiol, hyperforin, protopanaxadiol (PPD), ginsenoside Rg1, and eugenol have been proven to produce antidepressant effects by elevating the expression of BDNF (Irie et al., 2004; Zanelati et al., 2010; Xiong et al., 2011; Jiang et al., 2012; Zheng et al., 2012; Gao et al., 2013; Gibon et al., 2013; Wang et al., 2016; Jiang et al., 2019; Silote et al., 2019). The main natural products in this section are shown in Table 3.
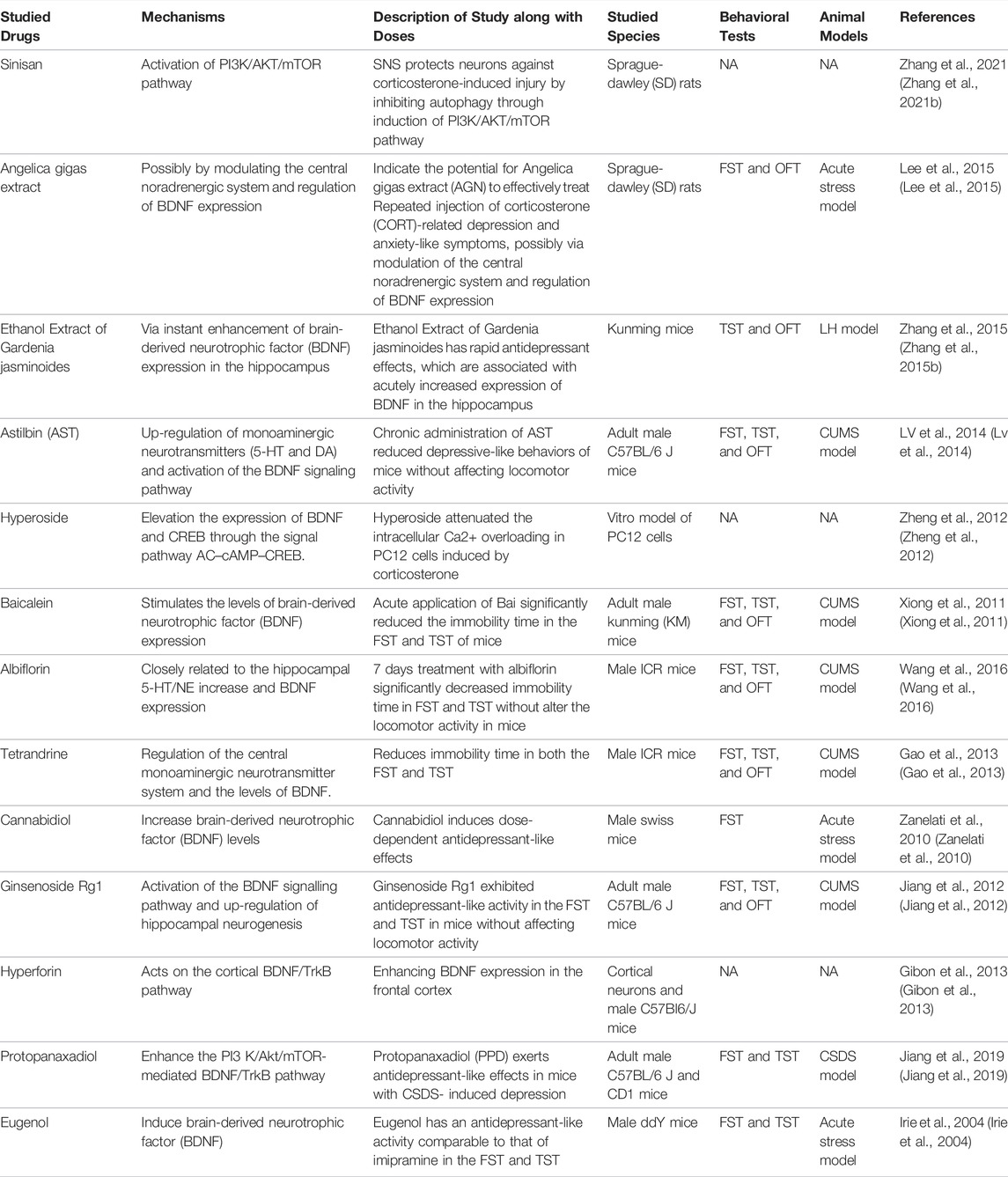
TABLE 3. Potential natural products for the treatment of PSD by modulating the central neuroplasticity.
4.5 Therapeutic Strategies Targeting Other Mechanisms
Apart from the main mechanisms described above, natural products can also produce anti-depressant effects via other mechanisms. Some studies did not find an exact mechanism for natural products in anti-depressant procession. Through an inverse-agonistic mechanism located in benzodiazepine receptors, the treatment of animals with harmane (5–15 mg/kg, i. p.), norharmane (2.5–10 mg/kg, i. p.), and harmine (5–15 mg/kg, i. p.) reduced the immobility time in a dose-dependent manner (Farzin and Mansouri, 2006). Chen et al. found that a single bilateral intra-ventrolateral orbital cortex (VLO) infusion of sanguinarine (2.5, 5, or 10 g/0.5 L per side) significantly reduced immobility time in the FST in a dose-dependent fashion, which may act through a decrease in the expression of Mkp-1 and an increase in ERK activation (Chen et al., 2012). By decreasing the expression of Mkp-1 and increasing ERK activation, a single bilateral intra-ventrolateral orbital cortex (VLO) infusion of sanguinarine (2.5, 5, or 10 g/0.5 L per side) significantly reduced immobility time in the FST in a dose-dependent fashion (Chen et al., 2012). Action on cannabinoid receptor subtype 2, β-caryophyllene, at doses of 25, 50, and 100 mg/kg mitigates stress-related changes in the hippocampal region (Hwang et al., 2020). Mediated by both the k-opioid and endocannabinoid systems, salvinorin A, given s. c. (0.001–1,000 mg·kg-1), exhibited both anxiolytic- and antidepressant-like effects (Braida et al., 2009). Blocking the activities of high mobility group box 1 (HMGB1) may be one of the principal ways in association with the antidepressant effect of glycyrrhizin (Wang et al., 2018). The aqueous root extract of Securidaca longipedunculata (Polygalaceae) produced a significant naloxone-reversible antidepressant-like effect in the FST, with possible involvement of opioidergic pathways (Adebiyi et al., 2006).
In addition, guarana, hydroethanolic of extract of Aloysia polystachya (CEAp), Lafoensia pacari A. St.-Hil. (Lythraceae), D-004, asiaticoside, hydroalcoholic extract of Gastrodia elata, hydroethanolic and dichloromethanic extracts of Sonchus oleraceus, and ethanolic extract of Hypericum perforatum produce anti-depressant effects with no clear mechanism (Kumar et al., 2000; Bach-Rojecky et al., 2004; Campos et al., 2005; Zhou et al., 2006; Hellión-Ibarrola et al., 2008; Liang et al., 2008; Carbajal et al., 2009; Galdino et al., 2009; Vilela et al., 2010). The main natural products in this section are shown in Table 4.
5 Conclusion and Perspectives
PSD is a major disease affecting patients’ life quality (Bai and Wang, 2019; Sutoko et al., 2020). It is also a critical problem for patients because PSD increases suicide and mortality (Mitchell et al., 2017; Tang et al., 2020). For the last few decades, antidepressants such as monoamine oxidase inhibitors, tricyclic antidepressants, and selective serotonin reuptake inhibitors have been used clinically (Li et al., 2018). However, most of these antidepressants have many serious adverse side effects (Sarko, 2000; Ferguson, 2001; Coupland et al., 2011). Therefore, many alternative therapeutic strategies for the treatment of depression have been reported. In particular, many studies have reported that natural products with antioxidant and anti-inflammatory effects could improve neurodegeneration. In recent years, strong evidence from different scientific studies has supported the idea that natural products may be new therapeutic tools against depression due to more advantages than synthetic prescription drugs.
The literature review summarizes the current literature on treatments with natural products for PSD. And the purpose of this review is to prospect the potential of natural products in the treatment of PSD and make more specific requests for subsequent research. However, our literature review is not without limitations. Firstly, the mechanisms of some of the original studies we included are merely at the hypothesis stage and are not very clear. Secondly, tremendous studies are still in the experimental stage, and there is still a lack of clinical studies to assess the safety and potency of phytochemicals with prospective antidepressant activities. Thirdly, we have emphasized the adverse effects of traditional treatment for PSD, however, we did not address any potential side effects of the reported natural compounds. Being “natural” does not necessarily mean that they are free of toxicity or side effects. In future studies, a large number of studies that confirm the safety and effectiveness of natural products in the treatment of PSD should be designed. Those natural products that have been proved safe and effective may be used in clinical applications widely.
Author Contributions
CF, ZZ, and HX designed the review and wrote the manuscript. YL, XW, ZZ, LY conceived the artwork and performed the bibliographical research. YX, AZ, AS, and ML supervised the writing. All the authors revised and approved the final version of the manuscript.
Funding
This work was supported by grants from the Project Supported by Zhejiang Provincial Natural Science Foundation of China (LY22H090020).
Conflict of Interest
The authors declare that the research was conducted in the absence of any commercial or financial relationships that could be construed as a potential conflict of interest.
Publisher’s Note
All claims expressed in this article are solely those of the authors and do not necessarily represent those of their affiliated organizations, or those of the publisher, the editors and the reviewers. Any product that may be evaluated in this article, or claim that may be made by its manufacturer, is not guaranteed or endorsed by the publisher.
References
Adebiyi, R. A., Elsa, A. T., Agaie, B. M., and Etuk, E. U. (2006). Antinociceptive and Antidepressant like Effects of Securidaca Longepedunculata Root Extract in Mice. J. Ethnopharmacol. 107, 234–239. doi:10.1016/j.jep.2006.03.017
Adzic, M., Brkic, Z., Mitic, M., Francija, E., Jovicic, M. J., Radulovic, J., et al. (2018). Therapeutic Strategies for Treatment of Inflammation-Related Depression. Curr. Neuropharmacol. 16, 176–209. doi:10.2174/1570159X15666170828163048
Agrawal, A., Mohan, M., Kasture, S., Foddis, C., Frau, M. A., Loi, M. C., et al. (2011). Antidepressant Activity of Ceratonia Siliqua L. Fruit Extract, a Source of Polyphenols. Nat. Prod. Res. 25, 450–456. doi:10.1080/14786419.2010.527447
Aleem, D., and Tohid, H. (2018). Pro-inflammatory Cytokines, Biomarkers, Genetics and the Immune System: A Mechanistic Approach of Depression and Psoriasis. Rev. Colomb. Psiquiatr. 47, 177–186. doi:10.1016/j.rcp.2017.03.002
Anacker, C., Cattaneo, A., Luoni, A., Musaelyan, K., Zunszain, P. A., Milanesi, E., et al. (2013). Glucocorticoid-related Molecular Signaling Pathways Regulating Hippocampal Neurogenesis. Neuropsychopharmacology 38, 872–883. doi:10.1038/npp.2012.253
Aoki, J., Iguchi, Y., Urabe, T., Yamagami, H., Todo, K., Fujimoto, S., et al. (2019). Acute Aspirin Plus Cilostazol Dual Therapy for Noncardioembolic Stroke Patients within 48 hours of Symptom Onset. J. Am. Heart Assoc. 8, e012652. doi:10.1161/JAHA.119.012652
Aydemir, C., Yalcin, E. S., Aksaray, S., Kisa, C., Yildirim, S. G., Uzbay, T., et al. (2006). Brain-derived Neurotrophic Factor (BDNF) Changes in the Serum of Depressed Women. Prog. Neuropsychopharmacol. Biol. Psychiatry 30, 1256–1260. doi:10.1016/j.pnpbp.2006.03.025
Ayerbe, L., Ayis, S., Crichton, S., Wolfe, C. D., and Rudd, A. G. (2013). The Natural History of Depression up to 15 Years after Stroke: the South London Stroke Register. Stroke 44, 1105–1110. doi:10.1161/STROKEAHA.111.679340
Bach-Rojecky, L., Kalodjera, Z., and Samarzija, I. (2004). The Antidepressant Activity of Hypericum perforatum L. Measured by Two Experimental Methods on Mice. Acta Pharm. 54, 157–162.
Bai, Z. F., and Wang, L. Y. (2019). Efficacy of Sertraline for Post-stroke Depression: A Systematic Review Protocol of Randomized Controlled Trial. Med. Baltim. 98, e15299. doi:10.1097/MD.0000000000015299
Bartoli, F., Lillia, N., Lax, A., Crocamo, C., Mantero, V., Carrà, G., et al. (2013). Depression after Stroke and Risk of Mortality: a Systematic Review and Meta-Analysis. Stroke Res. Treat. 2013, 862978. doi:10.1155/2013/862978
Benarroch, E. E. (2015). Brain-derived Neurotrophic Factor: Regulation, Effects, and Potential Clinical Relevance. Neurology 84, 1693–1704. doi:10.1212/WNL.0000000000001507
Bensimon, K., Herrmann, N., Swardfager, W., Yi, H., Black, S. E., Gao, F. Q., et al. (2014). Kynurenine and Depressive Symptoms in a Poststroke Population. Neuropsychiatr. Dis. Treat. 10, 1827–1835. doi:10.2147/NDT.S65740
Bethin, K. E., Vogt, S. K., and Muglia, L. J. (2000). Interleukin-6 Is an Essential, Corticotropin-Releasing Hormone-independent Stimulator of the Adrenal axis during Immune System Activation. Proc. Natl. Acad. Sci. U. S. A. 97, 9317–9322. doi:10.1073/pnas.97.16.9317
Bhattamisra, S. K., Khanna, V. K., Agrawal, A. K., Singh, P. N., and Singh, S. K. (2008). Antidepressant Activity of Standardised Extract of Marsilea Minuta Linn. J. Ethnopharmacol. 117, 51–57. doi:10.1016/j.jep.2008.01.012
Binfaré, R. W., Rosa, A. O., Lobato, K. R., Santos, A. R., and Rodrigues, A. L. (2009). Ascorbic Acid Administration Produces an Antidepressant-like Effect: Evidence for the Involvement of Monoaminergic Neurotransmission. Prog. Neuropsychopharmacol. Biol. Psychiatry 33, 530–540. doi:10.1016/j.pnpbp.2009.02.003
Blier, P., and de Montigny, C. (1994). Current Advances and Trends in the Treatment of Depression. Trends Pharmacol. Sci. 15, 220–226. doi:10.1016/0165-6147(94)90315-8
Braida, D., Capurro, V., Zani, A., Rubino, T., Viganò, D., Parolaro, D., et al. (2009). Potential Anxiolytic- and Antidepressant-like Effects of Salvinorin A, the Main Active Ingredient of Salvia Divinorum, in Rodents. Br. J. Pharmacol. 157, 844–853. doi:10.1111/j.1476-5381.2009.00230.x
Campos, A. R., Barros, A. I., Albuquerque, F. A., M Leal, L. K., and Rao, V. S. (2005). Acute Effects of Guarana (Paullinia Cupana Mart.) on Mouse Behaviour in Forced Swimming and Open Field Tests. Phytother. Res. 19, 441–443. doi:10.1002/ptr.1471
Capra, J. C., Cunha, M. P., Machado, D. G., Zomkowski, A. D., Mendes, B. G., Santos, A. R., et al. (2010). Antidepressant-like Effect of Scopoletin, a Coumarin Isolated from Polygala Sabulosa (Polygalaceae) in Mice: Evidence for the Involvement of Monoaminergic Systems. Eur. J. Pharmacol. 643, 232–238. doi:10.1016/j.ejphar.2010.06.043
Carbajal, D., Ravelo, Y., Molina, V., Mas, R., and Arruzazabala, Mde. L. (2009). D-004, a Lipid Extract from Royal Palm Fruit, Exhibits Antidepressant Effects in the Forced Swim Test and the Tail Suspension Test in Mice. Pharmacol. Biochem. Behav. 92, 465–468. doi:10.1016/j.pbb.2009.01.008
Carvalho, L. A., and Pariante, C. M. (2008). In Vitro modulation of the Glucocorticoid Receptor by Antidepressants. Stress 11, 411–424. doi:10.1080/10253890701850759
Castilla-Guerra, L., Fernandez Moreno, M. D. C., Esparrago-Llorca, G., and Colmenero-Camacho, M. A. (2020). Pharmacological Management of Post-stroke Depression. Expert Rev. Neurother. 20, 157–166. doi:10.1080/14737175.2020.1707666
Castrén, E., Võikar, V., and Rantamäki, T. (2007). Role of Neurotrophic Factors in Depression. Curr. Opin. Pharmacol. 7, 18–21. doi:10.1016/j.coph.2006.08.009
Chaplin, A. B., Jones, P. B., and Khandaker, G. M. (2021). Sexual and Physical Abuse and Depressive Symptoms in the UK Biobank. BMC Psychiatry 21, 248. doi:10.1186/s12888-021-03207-0
Chávez-Castillo, M., Núñez, V., Nava, M., Ortega, Á., Rojas, M., Bermúdez, V., et al. (2019). Depression as a Neuroendocrine Disorder: Emerging Neuropsychopharmacological Approaches beyond Monoamines. Adv. Pharmacol. Sci. 2019, 7943481.
Chemerinski, E., Robinson, R. G., and Kosier, J. T. (2001). Improved Recovery in Activities of Daily Living Associated with Remission of Poststroke Depression. Stroke 32, 113–117. doi:10.1161/01.str.32.1.113
Chen, B., Dowlatshahi, D., MacQueen, G. M., Wang, J. F., and Young, L. T. (2001). Increased Hippocampal BDNF Immunoreactivity in Subjects Treated with Antidepressant Medication. Biol. Psychiatry 50, 260–265. doi:10.1016/s0006-3223(01)01083-6
Chen, B., Zhao, M., Chen, B., Yang, Z., Yu, X., Lin, X., et al. (2020). Effectiveness and Safety of Acupuncture in Post-stroke Depression (PSD): Protocol for a Bayesian Analysis. Med. Baltim. 99, e18969. doi:10.1097/MD.0000000000018969
Chen, K., Ou, X. M., Wu, J. B., and Shih, J. C. (2011). Transcription Factor E2F-Associated Phosphoprotein (EAPP), RAM2/CDCA7L/JPO2 (R1), and Simian Virus 40 Promoter Factor 1 (Sp1) Cooperatively Regulate Glucocorticoid Activation of Monoamine Oxidase B. Mol. Pharmacol. 79, 308–317. doi:10.1124/mol.110.067439
Chen, Y., Pu, J., Liu, Y., Tian, L., Chen, X., Gui, S., et al. (2020). Pro-inflammatory Cytokines Are Associated with the Development of Post-stroke Depression in the Acute Stage of Stroke: A Meta-Analysis. Top. Stroke Rehabil. 27, 1–10. doi:10.1080/10749357.2020.1755813
Chen, Y., Wang, H., Zhang, R., Wang, H., Peng, Z., Sun, R., et al. (2012). Microinjection of Sanguinarine into the Ventrolateral Orbital Cortex Inhibits Mkp-1 and Exerts an Antidepressant-like Effect in Rats. Neurosci. Lett. 506, 327–331. doi:10.1016/j.neulet.2011.11.038
Choi-Kwon, S., Han, S. W., Kwon, S. U., Kang, D. W., Choi, J. M., and Kim, J. S. (2006). Fluoxetine Treatment in Poststroke Depression, Emotional Incontinence, and Anger Proneness: a Double-Blind, Placebo-Controlled Study. Stroke 37, 156–161. doi:10.1161/01.STR.0000190892.93663.e2
Choudary, P. V., Molnar, M., Evans, S. J., Tomita, H., Li, J. Z., Vawter, M. P., et al. (2005). Altered Cortical Glutamatergic and GABAergic Signal Transmission with Glial Involvement in Depression. Proc. Natl. Acad. Sci. U. S. A. 102, 15653–15658. doi:10.1073/pnas.0507901102
Colla, A. R., Oliveira, A., Pazini, F. L., Rosa, J. M., Manosso, L. M., Cunha, M. P., et al. (2014). Serotonergic and Noradrenergic Systems Are Implicated in the Antidepressant-like Effect of Ursolic Acid in Mice. Pharmacol. Biochem. Behav. 124, 108–116. doi:10.1016/j.pbb.2014.05.015
Coupland, C., Dhiman, P., Morriss, R., Arthur, A., Barton, G., and Hippisley-Cox, J. (2011). Antidepressant Use and Risk of Adverse Outcomes in Older People: Population Based Cohort Study. Bmj 343, d4551. doi:10.1136/bmj.d4551
Delgado, P. L., Charney, D. S., Price, L. H., Aghajanian, G. K., Landis, H., and Heninger, G. R. (1990). Serotonin Function and the Mechanism of Antidepressant Action. Reversal of Antidepressant-Induced Remission by Rapid Depletion of Plasma Tryptophan. Arch. Gen. Psychiatry 47, 411–418. doi:10.1001/archpsyc.1990.01810170011002
Deng, L., Sun, X., Qiu, S., Xiong, Y., Li, Y., Wang, L., et al. (2017). Interventions for Management of Post-stroke Depression: A Bayesian Network Meta-Analysis of 23 Randomized Controlled Trials. Sci. Rep. 7, 16466. doi:10.1038/s41598-017-16663-0
Dhingra, D., and Bhankher, A. (2014). Behavioral and Biochemical Evidences for Antidepressant-like Activity of Palmatine in Mice Subjected to Chronic Unpredictable Mild Stress. Pharmacol. Rep. 66, 1–9. doi:10.1016/j.pharep.2013.06.001
Dhingra, D., and Valecha, R. (2007). Evaluation of Antidepressant-like Activity of Aqueous and Ethanolic Extracts of Terminalia Bellirica Roxb. Fruits in Mice. Indian J. Exp. Biol. 45, 610–616.
Dhingra, D., and Valecha, R. (2007). Evaluation of the Antidepressant-like Activity of Convolvulus Pluricaulis Choisy in the Mouse Forced Swim and Tail Suspension Tests. Med. Sci. Monit. 13, Br155–61.
Dhingra, D., and Valecha, R. (2014). Evidence for Involvement of the Monoaminergic System in Antidepressant-like Activity of an Ethanol Extract of Boerhaavia Diffusa and its Isolated Constituent, Punarnavine, in Mice. Pharm. Biol. 52, 767–774. doi:10.3109/13880209.2013.870583
Dhingra, D., and Valecha, R. (2014). Punarnavine, an Alkaloid Isolated from Ethanolic Extract of Boerhaavia Diffusa Linn. Reverses Depression-like Behaviour in Mice Subjected to Chronic Unpredictable Mild Stress. Indian J. Exp. Biol. 52, 799–807. doi:10.3109/13880209.2013.870583
do Rego, J. C., Benkiki, N., Chosson, E., Kabouche, Z., Seguin, E., and Costentin, J. (2007). Antidepressant-like Effect of Hyperfoliatin, a Polyisoprenylated Phloroglucinol Derivative from Hypericum Perfoliatum (Clusiaceae) Is Associated with an Inhibition of Neuronal Monoamines Uptake. Eur. J. Pharmacol. 569, 197–203. doi:10.1016/j.ejphar.2007.05.008
Donnan, G. A., Fisher, M., Macleod, M., and Davis, S. M. (2008). Stroke. Lancet 371, 1612–1623. doi:10.1016/s0140-6736(08)60694-7
Dremencov, E., El Mansari, M., and Blier, P. (2009). Effects of Sustained Serotonin Reuptake Inhibition on the Firing of Dopamine Neurons in the Rat Ventral Tegmental Area. J. Psychiatry Neurosci. 34, 223–229.
Du, H., Wang, K., Su, L., Zhao, H., Gao, S., Lin, Q., et al. (2016). Metabonomic Identification of the Effects of the Zhimu-Baihe Saponins on a Chronic Unpredictable Mild Stress-Induced Rat Model of Depression. J. Pharm. Biomed. Anal. 128, 469–479. doi:10.1016/j.jpba.2016.06.019
Duman, C. H. (2010). Models of Depression. Hormones Limbic Syst. 1, 1–21. doi:10.1016/s0083-6729(10)82001-1
Effects of Fluoxetine on Functional Outcomes after Acute Stroke (FOCUS): a Pragmatic, Double-Blind, Randomised, Controlled Trial. Lancet 393 (2019) 265–274.doi:10.1016/S0140-6736(18)32823-X
Egeland, M., Zunszain, P. A., and Pariante, C. M. (2015). Molecular Mechanisms in the Regulation of Adult Neurogenesis during Stress. Nat. Rev. Neurosci. 16, 189–200. doi:10.1038/nrn3855
Eisch, A. J., and Petrik, D. (2012). Depression and Hippocampal Neurogenesis: a Road to Remission? Science 338, 72–75. doi:10.1126/science.1222941
Espárrago Llorca, G., Castilla-Guerra, L., Fernández Moreno, M. C., Ruiz Doblado, S., and Jiménez Hernández, M. D. (2015). Post-stroke Depression: an Update. Neurologia 30, 23–31.
Fang, Y., Shi, B., Liu, X., Luo, J., Rao, Z., Liu, R., et al. (2020). Xiaoyao Pills Attenuate Inflammation and Nerve Injury Induced by Lipopolysaccharide in Hippocampal Neurons In Vitro. Neural Plast. 2020, 8841332. doi:10.1155/2020/8841332
Farzin, D., and Mansouri, N. (2006). Antidepressant-like Effect of Harmane and Other Beta-Carbolines in the Mouse Forced Swim Test. Eur. Neuropsychopharmacol. 16, 324–328. doi:10.1016/j.euroneuro.2005.08.005
Fassbender, K., Schmidt, R., Mössner, R., Daffertshofer, M., and Hennerici, M. (1994). Pattern of Activation of the Hypothalamic-Pituitary-Adrenal axis in Acute Stroke. Relation to Acute Confusional State, Extent of Brain Damage, and Clinical Outcome. Stroke 25, 1105–1108. doi:10.1161/01.str.25.6.1105
Feng, C., Fang, M., and Liu, X. Y. (2014). The Neurobiological Pathogenesis of Poststroke Depression. ScientificWorldJournal 2014, 521349. doi:10.1155/2014/521349
Ferguson, J. M. (2001). SSRI Antidepressant Medications: Adverse Effects and Tolerability. Prim. Care Companion J. Clin. Psychiatry 3, 22–27. doi:10.4088/pcc.v03n0105
Ferrucci, L., and Fabbri, E. (2018). Inflammageing: Chronic Inflammation in Ageing, Cardiovascular Disease, and Frailty. Nat. Rev. Cardiol. 15, 505–522. doi:10.1038/s41569-018-0064-2
Freitas, A. E., Budni, J., Lobato, K. R., Binfaré, R. W., Machado, D. G., Jacinto, J., et al. (2010). Antidepressant-like Action of the Ethanolic Extract from Tabebuia Avellanedae in Mice: Evidence for the Involvement of the Monoaminergic System. Prog. Neuropsychopharmacol. Biol. Psychiatry 34, 335–343. doi:10.1016/j.pnpbp.2009.12.010
Frodl, T., Meisenzahl, E. M., Zetzsche, T., Born, C., Groll, C., Jäger, M., et al. (2002). Hippocampal Changes in Patients with a First Episode of Major Depression. Am. J. Psychiatry 159, 1112–1118. doi:10.1176/appi.ajp.159.7.1112
Galdino, P. M., Nascimento, M. V., Sampaio, B. L., Ferreira, R. N., Paula, J. R., and Costa, E. A. (2009). Antidepressant-like Effect of Lafoensia Pacari A. St.-Hil. Ethanolic Extract and Fractions in Mice. J. Ethnopharmacol. 124, 581–585. doi:10.1016/j.jep.2009.05.001
Gamdzyk, M., Doycheva, D. M., Kang, R., Tang, H., Travis, Z. D., Tang, J., et al. (2020). GW0742 Activates miR-17-5p and Inhibits TXNIP/NLRP3-mediated Inflammation after Hypoxic-Ischaemic Injury in Rats and in PC12 Cells. J. Cell Mol. Med. 24, 12318–12330. doi:10.1111/jcmm.15698
Gao, S., Cui, Y. L., Yu, C. Q., Wang, Q. S., and Zhang, Y. (2013). Tetrandrine Exerts Antidepressant-like Effects in Animal Models: Role of Brain-Derived Neurotrophic Factor. Behav. Brain Res. 238, 79–85. doi:10.1016/j.bbr.2012.10.015
Gibney, S. M., McGuinness, B., Prendergast, C., Harkin, A., and Connor, T. J. (2013). Poly I:C-induced Activation of the Immune Response Is Accompanied by Depression and Anxiety-like Behaviours, Kynurenine Pathway Activation and Reduced BDNF Expression. Brain Behav. Immun. 28, 170–181. doi:10.1016/j.bbi.2012.11.010
Gibon, J., Deloulme, J. C., Chevallier, T., Ladevèze, E., Abrous, D. N., and Bouron, A. (2013). The Antidepressant Hyperforin Increases the Phosphorylation of CREB and the Expression of TrkB in a Tissue-specific Manner. Int. J. Neuropsychopharmacol. 16, 189–198. doi:10.1017/S146114571100188X
Girardi, P., Pompili, M., Innamorati, M., Mancini, M., Serafini, G., Mazzarini, L., et al. (2009). Duloxetine in Acute Major Depression: Review of Comparisons to Placebo and Standard Antidepressants Using Dissimilar Methods. Hum. Psychopharmacol. 24, 177–190. doi:10.1002/hup.1005
Glynn, L. M., Davis, E. P., and Sandman, C. A. (2013). New Insights into the Role of Perinatal HPA-axis Dysregulation in Postpartum Depression. Neuropeptides 47, 363–370. doi:10.1016/j.npep.2013.10.007
Gomez, R., and Barros, H. M. (2000). Ethopharmacology of the Antidepressant Effect of Clonazepam in Diabetic Rats. Pharmacol. Biochem. Behav. 66, 329–335. doi:10.1016/s0091-3057(00)00221-5
Gonçalves, A. E., Bürger, C., Amoah, S. K., Tolardo, R., Biavatti, M. W., and de Souza, M. M. (2012). The Antidepressant-like Effect of Hedyosmum Brasiliense and its Sesquiterpene Lactone, Podoandin in Mice: Evidence for the Involvement of Adrenergic, Dopaminergic and Serotonergic Systems. Eur. J. Pharmacol. 674, 307–314.
Guadarrama-Cruz, G., Alarcon-Aguilar, F. J., Lezama-Velasco, R., Vazquez-Palacios, G., and Bonilla-Jaime, H. (2008). Antidepressant-like Effects of Tagetes Lucida Cav. In the Forced Swimming Test. J. Ethnopharmacol. 120, 277–281. doi:10.1016/j.jep.2008.08.013
Guillin, O., Diaz, J., Carroll, P., Griffon, N., Schwartz, J. C., and Sokoloff, P. (2001). BDNF Controls Dopamine D3 Receptor Expression and Triggers Behavioural Sensitization. Nature 411, 86–89. doi:10.1038/35075076
Hamon, M., and Blier, P. (2013). Monoamine Neurocircuitry in Depression and Strategies for New Treatments. Prog. Neuropsychopharmacol. Biol. Psychiatry 45, 54–63. doi:10.1016/j.pnpbp.2013.04.009
He, D., Sai, X., Wang, N., Li, X., Wang, L., and Xu, Y. (2018). Camellia Euphlebia Exerts its Antidepressant-like Effect via Modulation of the Hypothalamic-Pituitary-Adrenal axis and Brain Monoaminergic Systems. Metab. Brain Dis. 33, 301–312. doi:10.1007/s11011-017-0167-1
Hellión-Ibarrola, M. C., Ibarrola, D. A., Montalbetti, Y., Kennedy, M. L., Heinichen, O., Campuzano, M., et al. (2008). The Antidepressant-like Effects of Aloysia Polystachya (Griseb.) Moldenke (Verbenaceae) in Mice. Phytomedicine 15, 478–483.
Hervella, P., Rodríguez-Castro, E., Rodríguez-Yáñez, M., Arias, S., Santamaría-Cadavid, M., López-Dequidt, I., et al. (2020). Intra- and Extra-hospital Improvement in Ischemic Stroke Patients: Influence of Reperfusion Therapy and Molecular Mechanisms. Sci. Rep. 10, 3513. doi:10.1038/s41598-020-60216-x
Hwang, E. S., Kim, H. B., Lee, S., Kim, M. J., Kim, K. J., Han, G., et al. (2020). Antidepressant-like Effects of β-caryophyllene on Restraint Plus Stress-Induced Depression. Behav. Brain Res. 380, 112439. doi:10.1016/j.bbr.2019.112439
Hwang, J. S., Lee, S. A., Hong, S. S., Lee, K. S., Lee, M. K., Hwang, B. Y., et al. (2005). Monoamine Oxidase Inhibitory Components from the Roots of Sophora Flavescens. Arch. Pharm. Res. 28, 190–194. doi:10.1007/BF02977714
Idayu, N. F., Hidayat, M. T., Moklas, M. A., Sharida, F., Raudzah, A. R., Shamima, A. R., et al. (2011). Antidepressant-like Effect of Mitragynine Isolated from Mitragyna Speciosa Korth in Mice Model of Depression. Phytomedicine 18, 402–407. doi:10.1016/j.phymed.2010.08.011
Irie, Y., Itokazu, N., Anjiki, N., Ishige, A., Watanabe, K., and Keung, W. M. (2004). Eugenol Exhibits Antidepressant-like Activity in Mice and Induces Expression of Metallothionein-III in the hippocampus. Brain Res. 1011, 243–246. doi:10.1016/j.brainres.2004.03.040
Ishola, I. O., Chatterjee, M., Tota, S., Tadigopulla, N., Adeyemi, O. O., Palit, G., et al. (2012). Antidepressant and Anxiolytic Effects of Amentoflavone Isolated from Cnestis Ferruginea in Mice. Pharmacol. Biochem. Behav. 103, 322–331. doi:10.1016/j.pbb.2012.08.017
Jacobs, B. L., van Praag, H., and Gage, F. H. (2000). Adult Brain Neurogenesis and Psychiatry: a Novel Theory of Depression. Mol. Psychiatry 5, 262–269. doi:10.1038/sj.mp.4000712
Jacobson, L., and Sapolsky, R. (1991). The Role of the hippocampus in Feedback Regulation of the Hypothalamic-Pituitary-Adrenocortical axis. Endocr. Rev. 12, 118–134. doi:10.1210/edrv-12-2-118
Ji, X. W., Wu, C. L., Wang, X. C., Liu, J., Bi, J. Z., and Wang, D. Y. (2014). Monoamine Neurotransmitters and Fibroblast Growth Factor-2 in the Brains of Rats with Post-stroke Depression. Exp. Ther. Med. 8, 159–164. doi:10.3892/etm.2014.1674
Jiang, B., Xiong, Z., Yang, J., Wang, W., Wang, Y., Hu, Z. L., et al. (2012). Antidepressant-like Effects of Ginsenoside Rg1 Are Due to Activation of the BDNF Signalling Pathway and Neurogenesis in the hippocampus. Br. J. Pharmacol. 166, 1872–1887. doi:10.1111/j.1476-5381.2012.01902.x
Jiang, N., Lv, J. W., Wang, H. X., Wang, Q., Lu, C., Yang, Y. J., et al. (2019). Antidepressant-like Effects of 20(S)-protopanaxadiol in a Mouse Model of Chronic Social Defeat Stress and the Related Mechanisms. Phytother. Res. 33, 2726–2736. doi:10.1002/ptr.6446
Jiao, J. T., Cheng, C., Ma, Y. J., Huang, J., Dai, M. C., Jiang, C., et al. (2016). Association between Inflammatory Cytokines and the Risk of Post-stroke Depression, and the Effect of Depression on Outcomes of Patients with Ischemic Stroke in a 2-year Prospective Study. Exp. Ther. Med. 12, 1591–1598. doi:10.3892/etm.2016.3494
Jin, L., Wu, F., Li, X., Li, H., Du, C., Jiang, Q., et al. (2013). Anti-depressant Effects of Aqueous Extract from Acanthopanax Senticosus in Mice. Phytother. Res. 27, 1829–1833. doi:10.1002/ptr.4938
Johansson, A., Olsson, T., Carlberg, B., Karlsson, K., and Fagerlund, M. (1997). Hypercortisolism after Stroke-Ppartly Cytokine-Mediated? J. Neurol. Sci. 147, 43–47. doi:10.1016/s0022-510x(96)05308-7
Joshi, B., Panda, S. K., Jouneghani, R. S., Liu, M., Parajuli, N., Leyssen, P., et al. (2020). Antibacterial, Antifungal, Antiviral, and Anthelmintic Activities of Medicinal Plants of Nepal Selected Based on Ethnobotanical Evidence. Evid. Based Complement. Altern. Med. 2020, 1043471. doi:10.1155/2020/1043471
Juruena, M. F., Bocharova, M., Agustini, B., and Young, A. H. (2018). Atypical Depression and Non-atypical Depression: Is HPA axis Function a Biomarker? A Systematic Review. J. Affect Disord. 233, 45–67. doi:10.1016/j.jad.2017.09.052
Kang, H. J., Bae, K. Y., Kim, S. W., Kim, J. T., Park, M. S., Cho, K. H., et al. (2016). Effects of Interleukin-6, Interleukin-18, and Statin Use, Evaluated at Acute Stroke, on Post-stroke Depression during 1-year Follow-Up. Psychoneuroendocrinology 72, 156–160. doi:10.1016/j.psyneuen.2016.07.001
Katzan, I., Schuster, A., and Kinzy, T. (2021). Physical Activity Monitoring Using a Fitbit Device in Ischemic Stroke Patients: Prospective Cohort Feasibility Study. JMIR Mhealth Uhealth 9, e14494. doi:10.2196/14494
Khlif, M. S., Werden, E., Egorova, N., Boccardi, M., Redolfi, A., Bird, L., et al. (2019). Assessment of Longitudinal Hippocampal Atrophy in the First Year after Ischemic Stroke Using Automatic Segmentation Techniques. Neuroimage Clin. 24, 102008. doi:10.1016/j.nicl.2019.102008
Kubera, M., Obuchowicz, E., Goehler, L., Brzeszcz, J., and Maes, M. (2011). In Animal Models, Psychosocial Stress-Induced (Neuro)inflammation, Apoptosis and Reduced Neurogenesis Are Associated to the Onset of Depression. Prog. Neuropsychopharmacol. Biol. Psychiatry 35, 744–759. doi:10.1016/j.pnpbp.2010.08.026
Kulkarni, S. K., Bhutani, M. K., and Bishnoi, M. (2008). Antidepressant Activity of Curcumin: Involvement of Serotonin and Dopamine System. Psychopharmacol. Berl. 201, 435–442. doi:10.1007/s00213-008-1300-y
Kulkarni, S. K., and Dhir, A. (2008). On the Mechanism of Antidepressant-like Action of Berberine Chloride. Eur. J. Pharmacol. 589, 163–172. doi:10.1016/j.ejphar.2008.05.043
Kumar, V., Jaiswal, A. K., Singh, P. N., and Bhattacharya, S. K. (2000). Anxiolytic Activity of Indian Hypericum perforatum Linn: an Experimental Study. Indian J. Exp. Biol. 38, 36–41.
Kwon, S., Lee, B., Kim, M., Lee, H., Park, H. J., and Hahm, D. H. (2010). Antidepressant-like Effect of the Methanolic Extract from Bupleurum Falcatum in the Tail Suspension Test. Prog. Neuropsychopharmacol. Biol. Psychiatry 34, 265–270. doi:10.1016/j.pnpbp.2009.11.015
Lammers, C. H., Diaz, J., Schwartz, J. C., and Sokoloff, P. (2000). Selective Increase of Dopamine D3 Receptor Gene Expression as a Common Effect of Chronic Antidepressant Treatments. Mol. Psychiatry 5, 378–388. doi:10.1038/sj.mp.4000754
Lee, B., Sur, B., Shim, I., Lee, H., and Hahm, D. H. (2015). Angelica gigas Ameliorate Depression-like Symptoms in Rats Following Chronic Corticosterone Injection. BMC Complement. Altern. Med. 15, 210. doi:10.1186/s12906-015-0746-9
Leonard, B. E. (2018). Inflammation and Depression: a Causal or Coincidental Link to the Pathophysiology? Acta Neuropsychiatr. 30, 1–16. doi:10.1017/neu.2016.69
Leonard, B. E. (2010). The Concept of Depression as a Dysfunction of the Immune System. Curr. Immunol. Rev. 6, 205–212. doi:10.2174/157339510791823835
Li, S., Wang, C., Li, W., Koike, K., Nikaido, T., and Wang, M. W. (2007). Antidepressant-like Effects of Piperine and its Derivative, Antiepilepsirine. J. Asian Nat. Prod. Res. 9, 421–430. doi:10.1080/10286020500384302
Li, T., Wang, D., Zhao, B., and Yan, Y. (2018). Xingnao Jieyu Decoction Ameliorates Poststroke Depression through the BDNF/ERK/CREB Pathway in Rats. Evid. Based Complement. Altern. Med. 2018, 5403045. doi:10.1155/2018/5403045
Li, W., Ling, S., Yang, Y., Hu, Z., Davies, H., and Fang, M. (2014). Systematic Hypothesis for Post-stroke Depression Caused Inflammation and Neurotransmission and Resultant on Possible Treatments. Neuro Endocrinol. Lett. 35, 104–109.
Li, Y., Wu, Y., Zhai, L., Wang, T., Sun, Y., and Zhang, D. (2017). Longitudinal Association of Sleep Duration with Depressive Symptoms Among Middle-Aged and Older Chinese. Sci. Rep. 7, 11794. doi:10.1038/s41598-017-12182-0
Li, Y. C., Liu, Y. M., Shen, J. D., Chen, J. J., Pei, Y. Y., and Fang, X. Y. (2016). Resveratrol Ameliorates the Depressive-like Behaviors and Metabolic Abnormalities Induced by Chronic Corticosterone Injection. Molecules 21, 1. doi:10.3390/molecules21101341
Liang, X., Yan Ni Huang, H., Si Wei Chen, C., Wen Juan Wang, W., Xu, N., Cui, S., et al. (2008). Antidepressant-like Effect of Asiaticoside in Mice. Pharmacol. Biochem. Behav. 89, 444–449. doi:10.1016/j.pbb.2008.01.020
Lin, K. H., Chen, C. H., Chen, Y. Y., Huang, W. T., Lai, J. S., Yu, S. M., et al. (2014). Bidirectional and Multi-User Telerehabilitation System: Clinical Effect on Balance, Functional Activity, and Satisfaction in Patients with Chronic Stroke Living in Long-Term Care Facilities. Sensors (Basel) 14, 12451–12466. doi:10.3390/s140712451
Lin, S., Luan, X., He, W., Ruan, Y., Yuan, C., Fan, A., et al. (2020). Post-stroke Depression and Estimated Glomerular Filtration Rate: A Prospective Stroke Cohort. Neuropsychiatr. Dis. Treat. 16, 201–208. doi:10.2147/NDT.S225905
Liu, X., Liu, F., Yue, R., Li, Y., Zhang, J., Wang, S., et al. (2013). The Antidepressant-like Effect of Bacopaside I: Possible Involvement of the Oxidative Stress System and the Noradrenergic System. Pharmacol. Biochem. Behav. 110, 224–230. doi:10.1016/j.pbb.2013.07.007
Loubinoux, I., Kronenberg, G., Endres, M., Schumann-Bard, P., Freret, T., Filipkowski, R. K., et al. (2012). Post-stroke Depression: Mechanisms, Translation and Therapy. J. Cell Mol. Med. 16, 1961–1969. doi:10.1111/j.1582-4934.2012.01555.x
Lv, Q. Q., Wu, W. J., Guo, X. L., Liu, R. L., Yang, Y. P., Zhou, D. S., et al. (2014). Antidepressant Activity of Astilbin: Involvement of Monoaminergic Neurotransmitters and BDNF Signal Pathway. Biol. Pharm. Bull. 37, 987–995. doi:10.1248/bpb.b13-00968
Ma, M., Ren, Q., Yang, C., Zhang, J. C., Yao, W., Dong, C., et al. (2017). Antidepressant Effects of Combination of Brexpiprazole and Fluoxetine on Depression-like Behavior and Dendritic Changes in Mice after Inflammation. Psychopharmacol. Berl. 234, 525–533. doi:10.1007/s00213-016-4483-7
Machado, D. G., Bettio, L. E., Cunha, M. P., Capra, J. C., Dalmarco, J. B., Pizzolatti, M. G., et al. (2009). Antidepressant-like Effect of the Extract of Rosmarinus Officinalis in Mice: Involvement of the Monoaminergic System. Prog. Neuropsychopharmacol. Biol. Psychiatry 33, 642–650. doi:10.1016/j.pnpbp.2009.03.004
Machado, D. G., Bettio, L. E., Cunha, M. P., Santos, A. R., Pizzolatti, M. G., Brighente, I. M., et al. (2008). Antidepressant-like Effect of Rutin Isolated from the Ethanolic Extract from Schinus Molle L. In Mice: Evidence for the Involvement of the Serotonergic and Noradrenergic Systems. Eur. J. Pharmacol. 587, 163–168. doi:10.1016/j.ejphar.2008.03.021
Machado, D. G., Kaster, M. P., Binfaré, R. W., Dias, M., Santos, A. R., Pizzolatti, M. G., et al. (2007). Antidepressant-like Effect of the Extract from Leaves of Schinus Molle L. In Mice: Evidence for the Involvement of the Monoaminergic System. Prog. Neuropsychopharmacol. Biol. Psychiatry 31, 421–428. doi:10.1016/j.pnpbp.2006.11.004
Mackay, J., Mensah, G. A., and Greenlund, K. (2004). The Atlas of Heart Disease and Stroke. World Health Organization.
Mahar, I., Bambico, F. R., Mechawar, N., and Nobrega, J. N. (2014). Stress, Serotonin, and Hippocampal Neurogenesis in Relation to Depression and Antidepressant Effects. Neurosci. Biobehav Rev. 38, 173–192. doi:10.1016/j.neubiorev.2013.11.009
Mahmood, Z., Davidsson, A., Olsson, E., Leanderson, P., Lundberg, A. K., and Jonasson, L. (2020). The Effect of Acute Exercise on Interleukin-6 and Hypothalamic-Pituitary-Adrenal axis Responses in Patients with Coronary Artery Disease. Sci. Rep. 10, 21390. doi:10.1038/s41598-020-78286-2
Mao, Q., Huang, Z., Ip, S., and Che, C. (2008). Antidepressant-like Effect of Ethanol Extract from Paeonia Lactiflora in Mice. Phytother. Res. 22, 1496–1499. doi:10.1002/ptr.2519
Mao, Q. Q., Ip, S. P., Ko, K. M., Tsai, S. H., and Che, C. T. (2009). Peony Glycosides Produce Antidepressant-like Action in Mice Exposed to Chronic Unpredictable Mild Stress: Effects on Hypothalamic-Pituitary-Adrenal Function and Brain-Derived Neurotrophic Factor. Prog. Neuropsychopharmacol. Biol. Psychiatry 33, 1211–1216. doi:10.1016/j.pnpbp.2009.07.002
Mao, Q. Q., Ip, S. P., Tsai, S. H., and Che, C. T. (2008). Antidepressant-like Effect of Peony Glycosides in Mice. J. Ethnopharmacol. 119, 272–275. doi:10.1016/j.jep.2008.07.008
Maurer-Spurej, E., Pittendreigh, C., and Misri, S. (2007). Platelet Serotonin Levels Support Depression Scores for Women with Postpartum Depression. J. Psychiatry Neurosci. 32, 23–29.
McCann, S. K., Irvine, C., Mead, G. E., Sena, E. S., Currie, G. L., Egan, K. E., et al. (2014). Efficacy of Antidepressants in Animal Models of Ischemic Stroke: a Systematic Review and Meta-Analysis. Stroke 45, 3055–3063. doi:10.1161/STROKEAHA.114.006304
Mead, G., Hackett, M. L., Lundström, E., Murray, V., Hankey, G. J., and Dennis, M. (2015). The FOCUS, AFFINITY and EFFECTS Trials Studying the Effect(s) of Fluoxetine in Patients with a Recent Stroke: a Study Protocol for Three Multicentre Randomised Controlled Trials. Trials 16, 369. doi:10.1186/s13063-015-0864-1
Mehta, A., Prabhakar, M., Kumar, P., Deshmukh, R., and Sharma, P. L. (2013). Excitotoxicity: Bridge to Various Triggers in Neurodegenerative Disorders. Eur. J. Pharmacol. 698, 6–18. doi:10.1016/j.ejphar.2012.10.032
Messaoudi, M., Bisson, J. F., Nejdi, A., Rozan, P., and Javelot, H. (2008). Antidepressant-like Effects of a Cocoa Polyphenolic Extract in Wistar-Unilever Rats. Nutr. Neurosci. 11, 269–276. doi:10.1179/147683008X344165
Mitchell, A. J., Sheth, B., Gill, J., Yadegarfar, M., Stubbs, B., Yadegarfar, M., et al. (2017). Prevalence and Predictors of Post-stroke Mood Disorders: A Meta-Analysis and Meta-Regression of Depression, Anxiety and Adjustment Disorder. Gen. Hosp. Psychiatry 47, 48–60. doi:10.1016/j.genhosppsych.2017.04.001
Molteni, R., Calabrese, F., Bedogni, F., Tongiorgi, E., Fumagalli, F., Racagni, G., et al. (2006). Chronic Treatment with Fluoxetine Up-Regulates Cellular BDNF mRNA Expression in Rat Dopaminergic Regions. Int. J. Neuropsychopharmacol. 9, 307–317. doi:10.1017/S1461145705005766
Morel, L., Higashimori, H., Tolman, M., and Yang, Y. (2014). VGluT1+ Neuronal Glutamatergic Signaling Regulates Postnatal Developmental Maturation of Cortical Protoplasmic Astroglia. J. Neurosci. 34, 10950–10962. doi:10.1523/JNEUROSCI.1167-14.2014
Moret, C., and Briley, M. (2011). The Importance of Norepinephrine in Depression. Neuropsychiatr. Dis. Treat. 7, 9–13. doi:10.2147/NDT.S19619
Olivares-Bañuelos, T. N., Chí-Castañeda, D., and Ortega, A. (2019). Glutamate Transporters: Gene Expression Regulation and Signaling Properties. Neuropharmacology 161, 107550.
Pace, T. W., Hu, F., and Miller, A. H. (2011). Activation of cAMP-Protein Kinase A Abrogates STAT5-Mediated Inhibition of Glucocorticoid Receptor Signaling by Interferon-Alpha. Brain Behav. Immun. 25, 1716–1724. doi:10.1016/j.bbi.2011.07.227
Pacher, P., and Kecskemeti, V. (2004). Trends in the Development of New Antidepressants. Is There a Light at the End of the Tunnel? Curr. Med. Chem. 11, 925–943. doi:10.2174/0929867043455594
Padmashri, R., Suresh, A., Boska, M. D., and Dunaevsky, A. (2015). Motor-Skill Learning Is Dependent on Astrocytic Activity. Neural Plast. 2015, 938023. doi:10.1155/2015/938023
Pan, Y., Kong, L. D., Li, Y. C., Xia, X., Kung, H. F., and Jiang, F. X. (2007). Icariin from Epimedium Brevicornum Attenuates Chronic Mild Stress-Induced Behavioral and Neuroendocrinological Alterations in Male Wistar Rats. Pharmacol. Biochem. Behav. 87, 130–140. doi:10.1016/j.pbb.2007.04.009
Pascoe, M. C., Crewther, S. G., Carey, L. M., and Crewther, D. P. (2011). Inflammation and Depression: Why Poststroke Depression May Be the Norm and Not the Exception. Int. J. Stroke 6, 128–135. doi:10.1111/j.1747-4949.2010.00565.x
Peng, W. H., Lo, K. L., Lee, Y. H., Hung, T. H., and Lin, Y. C. (2007). Berberine Produces Antidepressant-like Effects in the Forced Swim Test and in the Tail Suspension Test in Mice. Life Sci. 81, 933–938. doi:10.1016/j.lfs.2007.08.003
Piato, A. L., Rizon, L. P., Martins, B. S., Nunes, D. S., and Elisabetsky, E. (2009). Antidepressant Profile of Ptychopetalum Olacoides Bentham (Marapuama) in Mice. Phytother. Res. 23, 519–524. doi:10.1002/ptr.2664
Pompili, M., Serafini, G., Innamorati, M., Venturini, P., Fusar-Poli, P., Sher, L., et al. (2013). Agomelatine, a Novel Intriguing Antidepressant Option Enhancing Neuroplasticity: a Critical Review. World J. Biol. Psychiatry 14, 412–431. doi:10.3109/15622975.2013.765593
Porsolt, R. D., Le Pichon, M., and Jalfre, M. (1977). Depression: a New Animal Model Sensitive to Antidepressant Treatments. Nature 266, 730–732. doi:10.1038/266730a0
Posser, T., Kaster, M. P., Baraúna, S. C., Rocha, J. B., Rodrigues, A. L., and Leal, R. B. (2009). Antidepressant-like Effect of the Organoselenium Compound Ebselen in Mice: Evidence for the Involvement of the Monoaminergic System. Eur. J. Pharmacol. 602, 85–91. doi:10.1016/j.ejphar.2008.10.055
Price, A., Rayner, L., Okon-Rocha, E., Evans, A., Valsraj, K., Higginson, I. J., et al. (2011). Antidepressants for the Treatment of Depression in Neurological Disorders: a Systematic Review and Meta-Analysis of Randomised Controlled Trials. J. Neurol. Neurosurg. Psychiatry 82, 914–923. doi:10.1136/jnnp.2010.230862
Qin, B., Chen, H., Gao, W., Zhao, L. B., Zhao, M. J., Qin, H. X., et al. (2018). Efficacy, Acceptability, and Tolerability of Antidepressant Treatments for Patients with Post-stroke Depression: a Network Meta-Analysis. Braz J. Med. Biol. Res. 51, e7218. doi:10.1590/1414-431x20187218
Qiu, F. M., Zhong, X. M., Mao, Q. Q., and Huang, Z. (2013). Antidepressant-like Effects of Paeoniflorin on the Behavioural, Biochemical, and Neurochemical Patterns of Rats Exposed to Chronic Unpredictable Stress. Neurosci. Lett. 541, 209–213. doi:10.1016/j.neulet.2013.02.029
Qiu, W., Wu, M., Liu, S., Chen, B., Pan, C., Yang, M., et al. (2017). Suppressive Immunoregulatory Effects of Three Antidepressants via Inhibition of the Nuclear Factor-Κb Activation Assessed Using Primary Macrophages of Carp (Cyprinus carpio). Toxicol. Appl. Pharmacol. 322, 1–8. doi:10.1016/j.taap.2017.03.002
Rajkumar, R., Pandey, D. K., Mahesh, R., and Radha, R. (2009). 1-(m-Chlorophenyl)piperazine Induces Depressogenic-like Behaviour in Rodents by Stimulating the Neuronal 5-HT(2A) Receptors: Proposal of a Modified Rodent Antidepressant Assay. Eur. J. Pharmacol. 608, 32–41. doi:10.1016/j.ejphar.2009.02.041
Rinwa, P., and Kumar, A. (2013). Quercetin Suppress Microglial Neuroinflammatory Response and Induce Antidepressent-like Effect in Olfactory Bulbectomized Rats. Neuroscience 255, 86–98. doi:10.1016/j.neuroscience.2013.09.044
Robinson, R. G., and Bloom, F. E. (1977). Pharmacological Treatment Following Experimental Cerebral Infarction: Implications for Understanding Psychological Symptoms of Human Stroke. Biol. Psychiatry 12, 669–680.
Rock, K. L., and Kono, H. (2008). The Inflammatory Response to Cell Death. Annu. Rev. Pathol. 3, 99–126. doi:10.1146/annurev.pathmechdis.3.121806.151456
Sahay, A., and Hen, R. (2007). Adult Hippocampal Neurogenesis in Depression. Nat. Neurosci. 10, 1110–1115. doi:10.1038/nn1969
Sairanen, M., Lucas, G., Ernfors, P., Castrén, M., and Castrén, E. (2005). Brain-derived Neurotrophic Factor and Antidepressant Drugs Have Different but Coordinated Effects on Neuronal Turnover, Proliferation, and Survival in the Adult Dentate Gyrus. J. Neurosci. 25, 1089–1094. doi:10.1523/JNEUROSCI.3741-04.2005
Sánchez-Mateo, C. C., Bonkanka, C. X., Prado, B., and Rabanal, R. M. (2005). Antidepressant Properties of Some Hypericum Canariense L. And Hypericum Glandulosum Ait. Extracts in the Forced Swimming Test in Mice. J. Ethnopharmacol. 97, 541–547. doi:10.1016/j.jep.2004.12.019
Sánchez-Mateo, C. C., Bonkanka, C. X., and Rabanal, R. M. (2009). Hypericum Grandifolium Choisy: a Species Native to Macaronesian Region with Antidepressant Effect. J. Ethnopharmacol. 121, 297–303. doi:10.1016/j.jep.2008.10.031
Sarko, J. (2000). Antidepressants, Old and New. A Review of Their Adverse Effects and Toxicity in Overdose. Emerg. Med. Clin. North Am. 18, 637–654. doi:10.1016/s0733-8627(05)70151-6
Saveanu, R. V., and Nemeroff, C. B. (2012). Etiology of Depression: Genetic and Environmental Factors. Psychiatr. Clin. North Am. 35, 51–71. doi:10.1016/j.psc.2011.12.001
Schildkraut, J. J. (1965). The Catecholamine Hypothesis of Affective Disorders: a Review of Supporting Evidence. Am. J. Psychiatry 122, 509–522. doi:10.1176/ajp.122.5.509
Shah, A. J., Mir, R. H., Mohi-Ud-Din, R., Pottoo, F. H., Masoodi, M. H., and Bhat, Z. A. (2021). Depression: An Insight into Heterocyclic and Cyclic Hydrocarbon Compounds Inspired from Natural Sources. Curr. Neuropharmacol. 19, 2020–2037. doi:10.2174/1570159x19666210426115234
Sheline, Y. I., Wang, P. W., Gado, M. H., Csernansky, J. G., and Vannier, M. W. (1996). Hippocampal Atrophy in Recurrent Major Depression. Proc. Natl. Acad. Sci. U. S. A. 93, 3908–3913. doi:10.1073/pnas.93.9.3908
Shinde, V., Yegnanarayan, R., Shah, P., Gupta, A., and Pophale, P. (2015). Antidepressant-like Activity of Flunarizine in Modified Tail Suspension Test in Rats. N. Am. J. Med. Sci. 7, 100–103. doi:10.4103/1947-2714.153921
Silote, G. P., Sartim, A., Sales, A., Eskelund, A., Guimarães, F. S., Wegener, G., et al. (2019). Emerging Evidence for the Antidepressant Effect of Cannabidiol and the Underlying Molecular Mechanisms. J. Chem. Neuroanat. 98, 104–116. doi:10.1016/j.jchemneu.2019.04.006
Sloley, B. D., Urichuk, L. J., Morley, P., Durkin, J., Shan, J. J., Pang, P. K., et al. (2000). Identification of Kaempferol as a Monoamine Oxidase Inhibitor and Potential Neuroprotectant in Extracts of Ginkgo Biloba Leaves. J. Pharm. Pharmacol. 52, 451–459. doi:10.1211/0022357001774075
Snyder, J. S., Soumier, A., Brewer, M., Pickel, J., and Cameron, H. A. (2011). Adult Hippocampal Neurogenesis Buffers Stress Responses and Depressive Behaviour. Nature 476, 458–461. doi:10.1038/nature10287
Souza, L. C., de Gomes, M. G., Goes, A. T., Del Fabbro, L., Filho, C. B., Boeira, S. P., et al. (2013). Evidence for the Involvement of the Serotonergic 5-HT(1A) Receptors in the Antidepressant-like Effect Caused by Hesperidin in Mice. Prog. Neuropsychopharmacol. Biol. Psychiatry 40, 103–109. doi:10.1016/j.pnpbp.2012.09.003
Spalletta, G., Bossù, P., Ciaramella, A., Bria, P., Caltagirone, C., and Robinson, R. G. (2006). The Etiology of Poststroke Depression: a Review of the Literature and a New Hypothesis Involving Inflammatory Cytokines. Mol. Psychiatry 11, 984–991. doi:10.1038/sj.mp.4001879
Spalletta, G., Cravello, L., Imperiale, F., Salani, F., Bossù, P., Picchetto, L., et al. (2013). Neuropsychiatric Symptoms and Interleukin-6 Serum Levels in Acute Stroke. J. Neuropsychiatry Clin. Neurosci. 25, 255–263. doi:10.1176/appi.neuropsych.12120399
Steru, L., Chermat, R., Thierry, B., and Simon, P. (1985). The Tail Suspension Test: a New Method for Screening Antidepressants in Mice. Psychopharmacol. Berl. 85, 367–370. doi:10.1007/BF00428203
Stranahan, A. M., Arumugam, T. V., Cutler, R. G., Lee, K., Egan, J. M., and Mattson, M. P. (2008). Diabetes Impairs Hippocampal Function through Glucocorticoid-Mediated Effects on New and Mature Neurons. Nat. Neurosci. 11, 309–317. doi:10.1038/nn2055
Su, J. A., Chou, S. Y., Tsai, C. S., and Hung, T. H. (2012). Cytokine Changes in the Pathophysiology of Poststroke Depression. Gen. Hosp. Psychiatry 34, 35–39. doi:10.1016/j.genhosppsych.2011.09.020
Subarnas, A., Tadano, T., Nakahata, N., Arai, Y., Kinemuchi, H., Oshima, Y., et al. (1993). A Possible Mechanism of Antidepressant Activity of Beta-Amyrin Palmitate Isolated from Lobelia Inflata Leaves in the Forced Swimming Test. Life Sci. 52, 289–296. doi:10.1016/0024-3205(93)90220-w
Sugimoto, Y., Furutani, S., Nishimura, K., Itoh, A., Tanahashi, T., Nakajima, H., et al. (2010). Antidepressant-like Effects of Neferine in the Forced Swimming Test Involve the serotonin1A (5-HT1A) Receptor in Mice. Eur. J. Pharmacol. 634, 62–67. doi:10.1016/j.ejphar.2010.02.016
Sun, Y., Bai, L., Niu, X., Wang, Z., Yin, B., Bai, G., et al. (2019). Elevated Serum Levels of Inflammation-Related Cytokines in Mild Traumatic Brain Injury Are Associated with Cognitive Performance. Front. Neurol. 10, 1120. doi:10.3389/fneur.2019.01120
Sutoko, S., Atsumori, H., Obata, A., Funane, T., Kandori, A., Shimonaga, K., et al. (2020). Lesions in the Right Rolandic Operculum Are Associated with Self-Rating Affective and Apathetic Depressive Symptoms for Post-stroke Patients. Sci. Rep. 10, 20264. doi:10.1038/s41598-020-77136-5
Tang, W. K., Wang, L., Kwok Chu Wong, G., Ungvari, G. S., Yasuno, F., Tsoi, K. K. F., et al. (2020). Depression after Subarachnoid Hemorrhage: A Systematic Review. J. Stroke 22, 11–28. doi:10.5853/jos.2019.02103
Taylor, B. K., Joshi, C., and Uppal, H. (2003). Stimulation of Dopamine D2 Receptors in the Nucleus Accumbens Inhibits Inflammatory Pain. Brain Res. 987, 135–143. doi:10.1016/s0006-8993(03)03318-3
Tiemeier, H. (2003). Biological Risk Factors for Late Life Depression. Eur. J. Epidemiol. 18, 745–750. doi:10.1023/a:1025388203548
Toyoda, A. (2017). Social Defeat Models in Animal Science: What We Have Learned from Rodent Models. Anim. Sci. J. 88, 944–952. doi:10.1111/asj.12809
Vásquez, C. E., Riener, R., Reynolds, E., and Britton, G. B. (2014). NMDA Receptor Dysregulation in Chronic State: a Possible Mechanism Underlying Depression with BDNF Downregulation. Neurochem. Int. 79, 88–97. doi:10.1016/j.neuint.2014.09.007
Veldic, M., Millischer, V., Port, J. D., Ho, A. M., Jia, Y. F., Geske, J. R., et al. (2019). Genetic Variant in SLC1A2 Is Associated with Elevated Anterior Cingulate Cortex Glutamate and Lifetime History of Rapid Cycling. Transl. Psychiatry 9, 149. doi:10.1038/s41398-019-0483-9
Viana, A., do Rego, J. C., von Poser, G., Ferraz, A., Heckler, A. P., Costentin, J., et al. (2005). The Antidepressant-like Effect of Hypericum Caprifoliatum Cham & Schlecht (Guttiferae) on Forced Swimming Test Results from an Inhibition of Neuronal Monoamine Uptake. Neuropharmacology 49, 1042–1052. doi:10.1016/j.neuropharm.2005.06.002
Vilela, F. C., Padilha, Mde. M., Alves-da-Silva, G., Soncini, R., and Giusti-Paiva, A. (2010). Antidepressant-like Activity of Sonchus Oleraceus in Mouse Models of Immobility Tests. J. Med. Food 13, 219–222. doi:10.1089/jmf.2008.0303
Villa, R. F., Ferrari, F., and Moretti, A. (2018). Post-stroke Depression: Mechanisms and Pharmacological Treatment. Pharmacol. Ther. 184, 131–144. doi:10.1016/j.pharmthera.2017.11.005
Walker, C. L., Wu, X., Liu, N. K., and Xu, X. M. (2019). Bisperoxovanadium Mediates Neuronal Protection through Inhibition of PTEN and Activation of PI3K/AKT-mTOR Signaling after Traumatic Spinal Injuries. J. Neurotrauma 36, 2676–2687. doi:10.1089/neu.2018.6294
Wang, B., Lian, Y. J., Dong, X., Peng, W., Liu, L. L., Su, W. J., et al. (2018). Glycyrrhizic Acid Ameliorates the Kynurenine Pathway in Association with its Antidepressant Effect. Behav. Brain Res. 353, 250–257. doi:10.1016/j.bbr.2018.01.024
Wang, J. W., David, D. J., Monckton, J. E., Battaglia, F., and Hen, R. (2008). Chronic Fluoxetine Stimulates Maturation and Synaptic Plasticity of Adult-Born Hippocampal Granule Cells. J. Neurosci. 28, 1374–1384. doi:10.1523/JNEUROSCI.3632-07.2008
Wang, Q. S., Tian, J. S., Cui, Y. L., and Gao, S. (2014). Genipin Is Active via Modulating Monoaminergic Transmission and Levels of Brain-Derived Neurotrophic Factor (BDNF) in Rat Model of Depression. Neuroscience 275, 365–373. doi:10.1016/j.neuroscience.2014.06.032
Wang, W., Hu, X., Zhao, Z., Liu, P., Hu, Y., Zhou, J., et al. (2008). Antidepressant-like Effects of Liquiritin and Isoliquiritin from Glycyrrhiza Uralensis in the Forced Swimming Test and Tail Suspension Test in Mice. Prog. Neuropsychopharmacol. Biol. Psychiatry 32, 1179–1184. doi:10.1016/j.pnpbp.2007.12.021
Wang, X., Wu, D., and Zhong, P. (2020). Serum Bilirubin and Ischaemic Stroke: a Review of Literature. Stroke Vasc. Neurol. 5, 198–204. doi:10.1136/svn-2019-000289
Wang, Y. H., Lv, H. N., Cui, Q. H., Tu, P. F., Jiang, Y., and Zeng, K. W. (2020). Isosibiricin Inhibits Microglial Activation by Targeting the Dopamine D1/D2 Receptor-dependent NLRP3/caspase-1 Inflammasome Pathway. Acta Pharmacol. Sin. 41, 173–180. doi:10.1038/s41401-019-0296-7
Wang, Y. L., Wang, J. X., Hu, X. X., Chen, L., Qiu, Z. K., Zhao, N., et al. (2016). Antidepressant-like Effects of Albiflorin Extracted from Radix Paeoniae Alba. J. Ethnopharmacol. 179, 9–15. doi:10.1016/j.jep.2015.12.029
Weidenfeld, J., Leker, R. R., Gai, N., Teichner, A., Bener, D., and Ovadia, H. (2011). The Function of the Adrenocortical axis in Permanent Middle Cerebral Artery Occlusion: Effect of Glucocorticoids on the Neurological Outcome. Brain Res. 1407, 90–96. doi:10.1016/j.brainres.2011.06.035
Werden, E., Cumming, T., Li, Q., Bird, L., Veldsman, M., Pardoe, H. R., et al. (2017). Structural MRI Markers of Brain Aging Early after Ischemic Stroke. Neurology 89, 116–124. doi:10.1212/WNL.0000000000004086
Wijeratne, T., and Sales, C. (2021). Understanding Why Post-Stroke Depression May Be the Norm rather Than the Exception: The Anatomical and Neuroinflammatory Correlates of Post-Stroke Depression. J. Clin. Med. 10, 1. doi:10.3390/jcm10081674
Xia, X., Cheng, G., Pan, Y., Xia, Z. H., and Kong, L. D. (2007). Behavioral, Neurochemical and Neuroendocrine Effects of the Ethanolic Extract from Curcuma Longa L. In the Mouse Forced Swimming Test. J. Ethnopharmacol. 110, 356–363. doi:10.1016/j.jep.2006.09.042
Xian, Y. F., Fan, D., Ip, S. P., Mao, Q. Q., and Lin, Z. X. (2017). Antidepressant-Like Effect of Isorhynchophylline in Mice. Neurochem. Res. 42, 678–685. doi:10.1007/s11064-016-2124-5
Xiong, Z., Jiang, B., Wu, P. F., Tian, J., Shi, L. L., Gu, J., et al. (2011). Antidepressant Effects of a Plant-Derived Flavonoid Baicalein Involving Extracellular Signal-Regulated Kinases Cascade. Biol. Pharm. Bull. 34, 253–259. doi:10.1248/bpb.34.253
Xu, Y., Wang, Z., You, W., Zhang, X., Li, S., Barish, P. A., et al. (2010). Antidepressant-like Effect of Trans-resveratrol: Involvement of Serotonin and Noradrenaline System. Eur. Neuropsychopharmacol. 20, 405–413. doi:10.1016/j.euroneuro.2010.02.013
Yan, H. C., Cao, X., Das, M., Zhu, X. H., and Gao, T. M. (2010). Behavioral Animal Models of Depression. Neurosci. Bull. 26, 327–337. doi:10.1007/s12264-010-0323-7
Yan, L., Hu, Q., Mak, M. S., Lou, J., Xu, S. L., Bi, C. W., et al. (2016). A Chinese Herbal Decoction, Reformulated from Kai-Xin-San, Relieves the Depression-like Symptoms in Stressed Rats and Induces Neurogenesis in Cultured Neurons. Sci. Rep. 6, 30014. doi:10.1038/srep30014
Yang, L., Zhang, Z., Sun, D., Xu, Z., Zhang, X., and Li, L. (2010). The Serum Interleukin-18 Is a Potential Marker for Development of Post-stroke Depression. Neurol. Res. 32, 340–346. doi:10.1179/016164110X12656393665080
Yao, C. Y., Wang, J., Dong, D., Qian, F. G., Xie, J., and Pan, S. L. (2009). Laetispicine, an Amide Alkaloid from Piper Laetispicum, Presents Antidepressant and Antinociceptive Effects in Mice. Phytomedicine 16, 823–829. doi:10.1016/j.phymed.2009.02.008
Yi, L. T., Li, C. F., Zhan, X., Cui, C. C., Xiao, F., Zhou, L. P., et al. (2010). Involvement of Monoaminergic System in the Antidepressant-like Effect of the Flavonoid Naringenin in Mice. Prog. Neuropsychopharmacol. Biol. Psychiatry 34, 1223–1228. doi:10.1016/j.pnpbp.2010.06.024
Yi, L. T., Xu, H. L., Feng, J., Zhan, X., Zhou, L. P., and Cui, C. C. (2011). Involvement of Monoaminergic Systems in the Antidepressant-like Effect of Nobiletin. Physiol. Behav. 102, 1–6. doi:10.1016/j.physbeh.2010.10.008
Yin, C., Gou, L., Liu, Y., Yin, X., Zhang, L., Jia, G., et al. (2011). Antidepressant-like Effects of L-Theanine in the Forced Swim and Tail Suspension Tests in Mice. Phytother. Res. 25, 1636–1639. doi:10.1002/ptr.3456
Zanelati, T. V., Biojone, C., Moreira, F. A., Guimarães, F. S., and Joca, S. R. (2010). Antidepressant-like Effects of Cannabidiol in Mice: Possible Involvement of 5-HT1A Receptors. Br. J. Pharmacol. 159, 122–128. doi:10.1111/j.1476-5381.2009.00521.x
Zeng, L. F., Cao, Y., Wang, L., Dai, Y. K., Hu, L., Wang, Q., et al. (2017). Role of Medicinal Plants for Liver-Qi Regulation Adjuvant Therapy in Post-stroke Depression: A Systematic Review of Literature. Phytother. Res. 31, 40–52. doi:10.1002/ptr.5740
Zhang, G., Chen, L., Yang, L., Hua, X., Zhou, B., Miao, Z., et al. (2015). Combined Use of Spatial Restraint Stress and Middle Cerebral Artery Occlusion Is a Novel Model of Post-stroke Depression in Mice. Sci. Rep. 5, 16751. doi:10.1038/srep16751
Zhang, H., Xue, W., Wu, R., Gong, T., Tao, W., Zhou, X., et al. (2015). Rapid Antidepressant Activity of Ethanol Extract of Gardenia Jasminoides Ellis Is Associated with Upregulation of BDNF Expression in the Hippocampus. Evid. Based Complement. Altern. Med. 2015, 761238. doi:10.1155/2015/761238
Zhang, H., Li, M., and Xu, T. (2021). Therapeutic Effect of Chinese Herbal Medicines for Post-stroke Depression. Med. Baltim. 100, e24173. doi:10.1097/md.0000000000024173
Zhang, M., Zhang, Y., Sun, H., Ni, H., Sun, J., Yang, X., et al. (2021). Sinisan Protects Primary Hippocampal Neurons against Corticosterone by Inhibiting Autophagy via the PI3K/Akt/mTOR Pathway. Front. Psychiatry 12, 627056. doi:10.3389/fpsyt.2021.627056
Zhang, Y. J., Huang, X., Wang, Y., Xie, Y., Qiu, X. J., Ren, P., et al. (2011). Ferulic Acid-Induced Anti-depression and Prokinetics Similar to Chaihu-Shugan-San via Polypharmacology. Brain Res. Bull. 86, 222–228. doi:10.1016/j.brainresbull.2011.07.002
Zhen, L., Zhu, J., Zhao, X., Huang, W., An, Y., Li, S., et al. (2012). The Antidepressant-like Effect of Fisetin Involves the Serotonergic and Noradrenergic System. Behav. Brain Res. 228, 359–366. doi:10.1016/j.bbr.2011.12.017
Zheng, M., Liu, C., Pan, F., Shi, D., and Zhang, Y. (2012). Antidepressant-like Effect of Hyperoside Isolated from Apocynum Venetum Leaves: Possible Cellular Mechanisms. Phytomedicine 19, 145–149. doi:10.1016/j.phymed.2011.06.029
Zhou, B. H., Li, X. J., Liu, M., Wu, Z., and Ming Hu, X. (2006). Antidepressant-like Activity of the Gastrodia Elata Ethanol Extract in Mice. Fitoterapia 77, 592–594. doi:10.1016/j.fitote.2006.06.016
Zhu, W., Ma, S., Qu, R., Kang, D., and Liu, Y. (2006). Antidepressant Effect of Baicalin Extracted from the Root ofScutellaria Baicalensis. In Mice and Rats. Pharm. Biol. 44, 503–510. doi:10.1080/13880200600878684
Keywords: natural products, post-stroke depression, treatment, HPA, hypothalamic-pituitary-adrenal, inflammation
Citation: Fang C, Zhang Z, Xu H, Liu Y, Wang X, Yuan L, Xu Y, Zhu Z, Zhang A, Shao A and Lou M (2022) Natural Products for the Treatment of Post-stroke Depression. Front. Pharmacol. 13:918531. doi: 10.3389/fphar.2022.918531
Received: 12 April 2022; Accepted: 10 May 2022;
Published: 30 May 2022.
Edited by:
Hansen Chen, Stanford University, United StatesReviewed by:
Chong Gao, Zhejiang University City College, ChinaGianluca Serafini, San Martino Hospital (IRCCS), Italy
Copyright © 2022 Fang, Zhang, Xu, Liu, Wang, Yuan, Xu, Zhu, Zhang, Shao and Lou. This is an open-access article distributed under the terms of the Creative Commons Attribution License (CC BY). The use, distribution or reproduction in other forums is permitted, provided the original author(s) and the copyright owner(s) are credited and that the original publication in this journal is cited, in accordance with accepted academic practice. No use, distribution or reproduction is permitted which does not comply with these terms.
*Correspondence: Anke Zhang, theanke@163.com; Anwen Shao, shaoanwen@zju.edu.cn; Meiqing Lou, Meiqing_Lou2020@163.com
†These authors have contributed equally to this work