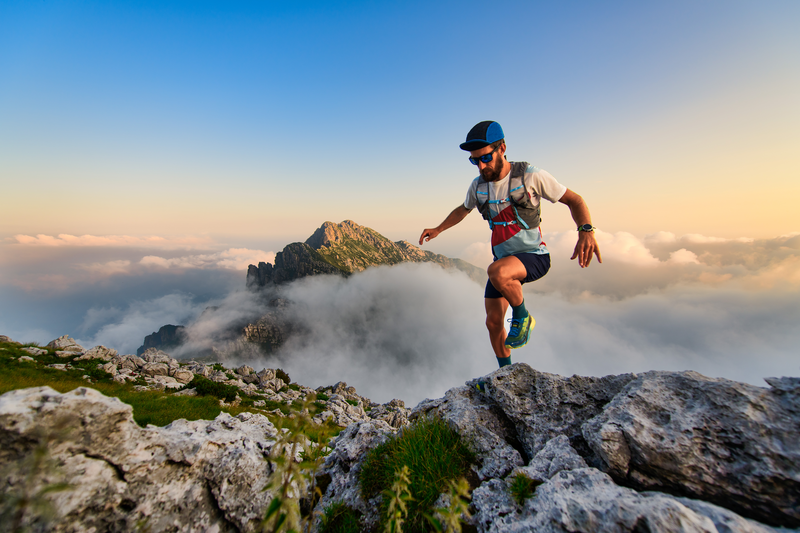
94% of researchers rate our articles as excellent or good
Learn more about the work of our research integrity team to safeguard the quality of each article we publish.
Find out more
REVIEW article
Front. Pharmacol. , 27 June 2022
Sec. Pharmacology of Infectious Diseases
Volume 13 - 2022 | https://doi.org/10.3389/fphar.2022.917184
This article is part of the Research Topic Reviews in Pharmacology of Infectious Diseases: 2022 View all 7 articles
Helicobacter pylori (H. pylori) is a notorious, recalcitrant and silent germ, which can cause a variety of debilitating stomach diseases, including gastric and duodenal ulcers and gastric cancer. This microbe predominantly colonizes the mucosal layer of the human stomach and survives in the inhospitable gastric microenvironment, by adapting to this hostile milieu. In this review, we first discuss H. pylori colonization and invasion. Thereafter, we provide a survey of current curative options based on polypharmacy, looking at pharmacokinetics, pharmacodynamics and pharmaceutical microbiology concepts, in the battle against H. pylori infection.
The Nobel Prize in medicine or physiology 2005 was jointly awarded to the Australian-born scientists, Robin Warren and Barry J. Marshall, for the discovery of Helicobacter pylori (H. pylori), as a fastidious Gram-negative microaerophilic spiral bacterium (Warren and Marshall, 1983; Arora et al., 2012). This germ is 2.5–5 μm long and 0.5–1.0 μm wide, with six unipolar sheathed flagella visible under electron microscopy (Goodwin and Armstrong, 1990; Geis et al., 1993; Zhao et al., 2014). In 1994, the international agency for research on cancer (IARC) classified H. pylori as a type І carcinogen and also declared that there was a strong relation between gastric cancer and this potentially deadly pathogen (Li et al., 2019). Over the years, H. pylori has been discovered to cause a variety of gastric diseases such as peptic ulcer, gastric ulcer and gastric cancer (Figure 1) (Hunt, 1996; Umamaheshwari and Jain, 2003; Hussein et al., 2008; Garza-González et al., 2014; Liu et al., 2021).
FIGURE 1. Representation of healthy stomach compartments, and different gastric disorders induced by H. pylori. (a) normal body. (b) normal antrum. (c) normal duodenum. (d) antral gastritis. (e) duodenal ulcer. (f) gastric ulcer. (g) gastric cancer. (h) esophagitis. (i) polypoid lesion in the antrum.
Moreover, H. pylori can be associated with some non-gastric diseases, such as cardiovascular, respiratory, neurologic, hematologic, dermatologic and allergic disorders (Gravina et al., 2018; Attaran et al., 2021). As one example, it was discovered that there was a correlation between H. pylori infection and problems in the digestive tract, such as abdominal pain and diarrhea in Coronavirus disease of 2019 (COVID-19) patients (Balamtekin et al., 2021). As time goes on, despite valuable experience in predicting the best treatment protocols for elimination of this pathogen from the human stomach, until now the treatment of H. pylori infection remains a major challenge for clinicians (Zhao et al., 2014). It has been reported that this bacterial pathogen has contributed to almost 75% of the gastric cancer burden worldwide (Amieva and Peek, 2016). Incredibly, in the United States the rate of infection remained consistent during the years 2000–2010, while the rate of eradication dramatically decreased (Thung et al., 2016; Gong and Yuan, 2018). Also the rate of incidence in developing and developed countries was 50.8% and 34.7%, respectively (Peng et al., 2018; Zamani et al., 2018). This incidence is maximum during childhood (11–16 years old), under poor socioeconomic conditions, and also with poor overall hygiene (Mishra et al., 2008; Arora et al., 2012; Mahmoudi et al., 2017). Regarding this bacterium, one of the key principles to take into account is its transmission routes. Despite some discrepancies in the exact mode of transmission (Ng et al., 2017), person-to-person is the major route of transmission (Jabbari Moghaddam et al., 2009; Eusebi et al., 2014). However, environmental transmission, such as consumption of polluted water, vegetables, animal products such as milk and meat contaminated with feces can transmit H. pylori into the human body (Vale and Vítor, 2010; Ghanbari et al., 2020). Unfortunately, this pathogen can survive in contaminated water and food products for long periods of time (Quaglia and Dambrosio, 2018; Ghanbari et al., 2020). In order to understand the emerging foodborne and waterborne diseases associated with H. pylori, Monno et al. (2019) investigated the relation between dietary habits and H. pylori infection status in Apulia (Southern of Italy). The authors reported that there was a significant relationship between H. pylori positivity and consumption of some foods, such as raw mussels and other molluscs, which had been extensively consumed by local people of that area. Despite older studies, which could not culture H. pylori from polluted water or food products, several pioneering microbiological studies confirmed that H. pylori could indeed be isolated and cultured from contaminated water and food (Hemmatinezhad et al., 2016; Ranjbar et al., 2016b; Ranjbar et al., 2016a). The current literature shows that there is a difference in the % prevalence of H. pylori infection in the world population, due to variations in socioeconomic and hygiene conditions (Hu et al., 2017; Saxena et al., 2020). Moreover, according to the World Health Organization (WHO), stomach cancer associated with H. pylori caused up to 0.5 million deaths in the world population (Arora et al., 2012). There are several comprehensive reviews which have discussed the pathogenesis and pharmacotherapy of H. pylori infection (Georgopoulos and Papastergiou, 2020; Alexander et al., 2021; Tshibangu-Kabamba and Yamaoka, 2021; Ansari and Yamaoka, 2022; Sousa et al., 2022). To our knowledge, there has been little attention paid to the potential use of antibiotics and other antimicrobial agents in terms of the biopharmaceutical implications. In this review, we first discuss H. pylori colonization and invasion in an easy-to-understand manner. Thereafter, we provide a survey of current curative options based on polypharmacy, looking at pharmacokinetics, pharmacodynamics and pharmaceutical microbiology concepts, in the battle against H. pylori infection.
Generally H. pylori resides deeply in the antrum area of the human stomach where the viscous mucus layer protects the bacteria from the effects of the lower pH (Kusters et al., 2006; Zhao et al., 2014; Worku et al., 2015). Generally speaking, the steps of the H. pylori-invasion pathway can be categorized as four consecutive processes: 1) survival; 2) swimming; 3) adherence; 4) tissue destruction (Walton, 2016). The steps of H. pylori invasion are depicted in Figure 2.
Upon bacterial entry into the stomach, the obstacles standing in the way of H. pylori include, gastric acidity, motility, peristaltic movement of the stomach, and mucosal materials secreted by the glands of the epithelial cells. All these factors intrinsically operate against the pathogen to retard its colonization (Arora et al., 2012). However, it is also known that H. pylori is an adaptable germ and can escape from host defense mechanisms (Karkhah et al., 2019; Mao et al., 2021). Colonization of the gastric mucosa is made possible by exploiting a unique arsenal of weapons, including production of a potent urease enzyme, possessing rapid motility, and expressing a number of adherence molecules on its cell membrane (Arora et al., 2012). It should be pointed out that non-motile flagellated or non-flagellated H. pylori bacteria are less able (or even unable) to initiate cellular infection (Skene et al., 2007). In general, H. pylori colonization occurs in the gastric mucus layer close to the epithelial cells of the stomach, but the bacteria usually do not directly colonize the epithelial cells themselves (Arora et al., 2012; Zhao et al., 2014). However, it has been proposed that H. pylori bacteria can be localized within the intracellular or intercellular space of gastric epithelial cells (Necchi et al., 2007; Chu et al., 2010; Arora et al., 2012; Kao et al., 2016; Sit et al., 2020). The epithelial cells are shielded by a thick layer of mucus as a barrier against foreign invasion, therefore H. pylori has to employ its flagellae to penetrate towards the underlying epithelial cells (Walton, 2016; Charitos et al., 2021). In addition, a variety of outer membrane proteins are expressed by H. pylori strains, such as H. pylori-outer membrane protein (Hop) and Hop-related proteins (Hor) (Feige et al., 2018). The Hop protein category act as virulence factors, including blood-group antigen binding adhesion (BabA), sialic acid-binding adhesion (SabA), H. pylori-outer membrane protein Q (HopQ), adherence-associated lipoproteins A and B (AlpA and AlpB) which are responsible for adhesion to the gastric mucosal layer (Oleastro and Ménard, 2013; Salama et al., 2013; Feige et al., 2018). Indeed, these factors permit the bacteria to gain a foothold in mucosal niches (Couturier, 2013). During adhesion to the gastric mucosa, the urease enzyme metabolizes urea to ammonia and bicarbonate, elevating the pH towards neutral (Arora et al., 2012; Piscione et al., 2021; Serrano et al., 2021). Hence, H. pylori provides the appropriate conditions in the gastric mucus to survive, and develop gastric disease and malignancies (Arora et al., 2012; Piscione et al., 2021). Given this situation, some advanced investigations have focused on the bioinorganic mechanism of urease as a nickel-containing metalloenzyme (Dixon et al., 1980; Jabri et al., 1995; Karplus et al., 1997; Pearson et al., 1997). As a matter of fact, Ni is considered to be an essential co-factor for urease activity (Maier and Benoit, 2019; Mazzei et al., 2020; Maroney and Ciurli, 2021). One bioinorganic mechanism was proposed by Dixon et al. (1980). At the first step, water and hydroxyl-containing molecules are coordinated by one of the two active sites of Ni (І) or Ni (ІІ) ions (coordination involves binding of ligands to a central metal atom). At the beginning of this process, the water molecule is replaced with urea. At the second step, a nucleophilic attack occurs on the carbonyl C atom by Ni (ІІ) to produce a tetrahedral intermediate. At the third step, this intermediate becomes unstable at basic pH and is easily disintegrated. Next, a carbamate ester and ammonium cation are generated. Following this reaction, water molecules can easily cause the release of the carbamate ester, and finally all of the mentioned cycles are repeated again. Figure 3 illustrates the proposed mechanism.
In order to facilitate the adhesion process, some components of the bacterial type IV secretion system (T4SS) apparatus, such as CagI, CagL, CagY and CagA (Cag: Cytotoxin-associated gene) are able to interact with integrin β1 (Kwok et al., 2007; Backert et al., 2011; Salama et al., 2013). For more information on the T4SS system, interested readers are referred to the following review (Costa et al., 2021). The role of adhesion factors is apparent in the elegant work by Jan et al. (2020). In brief, the major concept involves the activity of the pathogenicity island 6′-O-acyl-α-D-glucopyranoside (CAG) in the bacteria, through hijacking of cholesterol from human gastric epithelial cells thereby forming a lipid-raft cluster, and promoting bacterial attachment to human gastric cells (Figure 4A). During the acquisition of cholesterol, the Glucosyltransferase (CGT) enzyme catalyzes the conversion of cholesterol to Cholesteryl α-D-glucopyranoside (CG). Afterward, further functionalization on the O6′ group of glucose of CG occurs by the action of the Cholesteryl α-D-glucopyranoside 6′-acyltransferase (CGAT) enzyme. CGAT can produce human lipid-containing CAGs with different fatty acid acyl side chains, including myristic acid (14:0), palmitic acid (16:0), stearic acid (18:0) or oleic acid (18:1), as shown in Figure 4B. Confocal microscopy has been used to visualize CAGs with different acyl side chains Figure 4C. Upon treatment with CG or CAG for 1 h, lipid rafts in AGS cells were labeled with AlexaFluor 594-conjugated cholera toxin subunit b (red fluorescence). The imaging revealed that the amount of bacterial adhesion was directly related to the presence of different acyl side chains on CAG (Figures 4D,E). This study showed that lipid-raft clustering with an increase in the length of the acyl-side chains of CAG, increased the delivery of CagA into gastric host cells.
FIGURE 4. H. pylori attachment in the stomach. (A) The major steps of H. pylori attachment. (a) The secretion of OMV (outer membrane vesicle) to deliver CGAT (cholesteryl-D-glucopyranoside acyltransferase) and CGT (cholesterol α-glucosyltransferase) enzymes to human gastric cells. (b) The formation of lipid-raft clustering to facilitate bacterial adhesion. (c) Localization of integrin and Lewis antigens at the site of adhesion, leading to bacterial adhesion. (B) The scheme for production of CAG (6′-O-acyl-α-D-glucopyranoside). (C) Confocal images of CAG with different acyl side chains. (D) and (E) Effect of different acyl-side chains of CAG on the amount of H. pylori adherence to gastric epithelial cells. Reproduced from (Jan et al., 2020). Copyright 2020 with permission from “Springer Nature”.
The flagellar sheath of H. pylori acts as a shelter to protect against acidic stress conditions (Geis et al., 1993; Sit et al., 2020). Other hallmarks of H. pylori infection involve the expression of two heat-hock proteins (Hsps), GroES-like HspA (Hsp10) and GroEL-like HspB (Hsp60). The Hsps help to protect against the harsh gastric milieu (Roncarati and Scarlato, 2018; Sit et al., 2020). After adhering to the gastric mucosa, H. pylori begins to release additional virulence factors, which can damage host cells either directly or by inducing inflammatory responses (Couturier, 2013). The neutrophil activating protein (NAP) in H. pylori can attract neutrophils, monocytes and dendritic cells into the infected area (Couturier, 2013; Peng et al., 2018). Afterwards, the cytokine IL-10 secreted from dendritic cells can induce Treg cells, which can promote persistent colonization by suppressing inflammatory responses induced by IL-17 (Kabir, 2011; Narayana et al., 2015). Also, the secretion of IL-8 can be triggered by the high level secretion of IL-17, and IL-8 recruits neutrophils into infected regions, reinforcing the stimulation of inflammation induced by H. pylori (Kabir, 2011; Narayana et al., 2015). During inflammation, pro-inflammatory cytokines such as IL-1β, TNF-α, IL-8, IL-6 and IL-17 are upregulated (Kabir, 2011). Interestingly, H. pylori demonstrates resistance against attack by these immune cells even if it is recognized by them (Zhao et al., 2014). Finally, several protein/toxin co-factors such as CagA and vacuolating cytotoxin A (VacA) can act as hijackers by disturbing vital cell processes (cell cycle progression, cell junction integrity, cell polarity, cytoskeletal structure, and mitogenic gene regulation) (Lima et al., 2011; Couturier, 2013). In addition, other virulence factors such as duodenal ulcer promoting A (DupA) and outer inflammatory protein A (OipA) are involved in pathogenesis, as inducers of inflammatory response during the colonization process (Baker, 2020; El-Sayed et al., 2020). Moreover, it is possible that the morphology of the bacterium can affect its motility, which is known to be an important factor in the virulence of H. pylori (Ansari and Yamaoka, 2019). It appears that helical-shaped H. pylori bacteria have faster motility than those with precoccoidal or coccoidal morphology, therefore, bacteria with a helical shape can better prepare themselves to colonize the gastric mucosa, and those bacteria with precoccoidal or coccoidal structures are more easily cleared from the gastric mucosa (Worku et al., 2015; Salama, 2020).
The Scottish-born scientist, Sir Alexander Fleming is credited for the discovery of “penicillin” as the first antibiotic in 1923, and along with Howard Florey has been called the “father of the antibiotic era” (Gaynes, 2017; Liu et al., 2019). Nowadays, antibiotics are extensively used by clinicians throughout the world to treat bacterial infections (Xiong et al., 2014). Currently, the conventional antibiotics generally utilized in the treatment of H. pylori infection include, clarithromycin, metronidazole, amoxicillin, levofloxacin, tetracycline and rifampicin (Arora et al., 2012; Katzung, 2012; Xiong et al., 2014; Talebi Bezmin Abadi, 2017). A simple glance at the current treatment recommendations shows that mono-antibiotic therapy to fight H. pylori infection is not recommended by gastroenterologists, due to instability in the gastric environment, low bioavailability, and the short half-life of these drugs (Talebi Bezmin Abadi, 2014; Xiong et al., 2014; Talebi Bezmin Abadi, 2017). To improve therapeutic efficacy, antibiotics can be combined with proton pump inhibitors (PPIs) to reduce acid secretion during first-line triple and second-line quadruple antibiotic regimens (Talebi Bezmin Abadi, 2014; Fallone et al., 2019). Some well-known PPIs are, omeprazole, rabeprazole and lansoprazole (Arora et al., 2012). The beneficial effects of these drugs is ascribed to increasing the pH of the stomach to protect the antibiotic structure within the acidic environment, which leads to increased stability, better tissue penetration, and absorption of the antibiotics (Arora et al., 2012). Although many investigations have tended to use conventional PPIs for H. pylori eradication (Talebi Bezmin Abadi, 2014; Oppong et al., 2015), the unwanted condition of atrophic gastritis can emerge after long-term consumption of PPIs (Hagiwara et al., 2015). In a very recent review study, Talebi Bezmin Abadi and Ierardi evaluated the literature before February 2019 concerning the application of vonoprazan as a novel acid-blocker, which could be a suitable alternative to conventional PPIs (Abadi and Ierardi, 2019). The authors confirmed that vonoprazan could potentiate the onset of action of antibiotics, while showing, safety, tolerability, and effective inhibition of gastric acid secretion without any undesirable side effects. The authors suggested that new standard antibiotic regimens containing vonoprazan could be used for future treatment of H. pylori infection.
Conventionally, the term “polypharmacy” refers to concurrent use of five (or more than five) separate drugs in adults, which can often produce unfavorable drug-drug interactions (Frahm et al., 2019; Payne, 2020; Sugioka et al., 2020; Turnbull et al., 2020; Weng et al., 2020). In 2018, Bakaki et al. (2018) suggested that this term could also be defined as the prescription of two (or more than two) drugs to pediatric patients. Over recent decades, data obtained from clinical studies has proved that polypharmacy based on triple combination therapy is a suitable regimen for H. pylori eradication. It consists of a PPI (standard dose), clarithromycin (500 mg), and amoxicillin (1 g) over 10–14 days treatment course (Katzung, 2006; Talebi Bezmin Abadi, 2016). Since the first isolation of H. pylori from human gastric tissue, the overall cure rate using this regimen has consistently decreased. Therefore, clinicians have used a quadruple therapy regiment for the same 14 days as a treatment course. Quadruple therapy consists of standard dose of PPI twice a day, metronidazole 500 mg three times daily, bismuth subsalicylate 525 mg, and tetracycline 250–500 mg four times daily (Katzung, 2012). Saracino’s group suggested that 10 days of bismuth-based quadruple therapy under the name of “Pylera®” which contains in one capsule, 140 mg bismuth subcitrate, 125 mg metronidazole, 125 mg tetracycline, prescribed as three tablets four times a day, along with esomeprazole 20 mg twice day could be useful for eradication of H. pylori. In this study, the Pylera® regimen was administered to 153 patients with an overall cure rate of 88.3% (Saracino et al., 2020).
Additionally, it has been proposed that polypharmacy based on a non-bismuth quadruple regimen could be also used when bismuth is not available, which was called concomitant therapy (Gisbert and Calvet, 2011; Talebi Bezmin Abadi, 2014; Wang et al., 2017). This regimen includes PPI (standard dose), amoxicillin (1 g), metronidazole (500 mg), and clarithromycin (500 mg) (Gisbert and Calvet, 2011; Talebi Bezmin Abadi, 2014; Wang et al., 2017). Indeed, triple therapy becomes concomitant therapy when 500 mg of metronidazole or tinidazole is added twice daily to improve therapeutic efficacy (Gisbert and Calvet, 2011; Molina-Infante and Gisbert, 2014; Talebi Bezmin Abadi, 2014; Wang et al., 2017). Recent innovations led to development of polypharmacy based on sequential therapy (as an alternative to triple therapy) which includes PPI (standard dose) and amoxicillin (1 g) for first 5 days of therapy, and then PPI (standard dose), clarithromycin (500 mg) and metronidazole (500 mg) for the remaining 5 days (Gisbert and Calvet, 2011; Talebi Bezmin Abadi, 2014; Roszczenko-Jasińska et al., 2020).
The pharmacy literature has shown that specific routes of drug administration, such as oral, nasal, vaginal, pulmonary, ocular, and buccal have all been proposed for individual applications (Bae and Park, 2020). Also, it is known that oral administration of antibiotics can have more long term problems than other routes of administration, such as parenteral and Intravenous (IV) (Kelly et al., 2020). Despite the problems associated with oral administration of antibiotics, oral antibiotic therapy has been traditionally prescribed by clinicians due to the need for good patient compliance, its cost-effectiveness, non-invasiveness, and ease of use (Ahadian et al., 2020; Laffleur and Keckeis, 2020). Moreover, the intraluminal pathway has been used to decrease the duration of treatment as well as boosting the antibiotic activity. Liou et al. (2019) used three types of antibiotics, including a single-dose regimen of amoxicillin (3 g), metronidazole (2 g) and crushed enteric-coated clarithromycin (1 g) which were mixed with a sucralfate suspension for intraluminal administration. They reported that the eradication rate of H. pylori with standard triple therapy plus intraluminal administration of antibiotics was 87%, higher than that for 1-week standard triple therapy which was 81.2%.
Although considerable efforts have been made to discover or design novel candidate antibiotics, most of them are never approved due to poor biopharmaceutical performance. A review by Bae and Park (2020) discussed the biopharmaceutical aspects of biomaterials. They critically asked an important question: “If a capable scientist makes a new polymer that can treat cancer in humans, isn’t it required for the scientist to know the basic pharmacokinetics or how drugs work in humans?” The answer is clearly “yes, it is urgently required for both conventional drugs and new biomaterials”. In 2019, the global antibiotic research and development partnership (GARD) held a workshop to evaluate the role of PK and PD in determining the required dosage regimens to prevent the antibiotic resistance crisis of pathogens (Theuretzbacher et al., 2020). Thus, it is of the utmost importance to understand the general concepts and biopharmaceutical principles of antibacterial agents (Bergman et al., 2007). The term “PK” is defined as the time course of drug movement within the body (Neely and Reed, 2018; Khoruts et al., 2021). The main factors in PK methodology are known as ADME (absorption, distribution, metabolism, and excretion) (Bergman et al., 2007; Asín-Prieto et al., 2015; Yılmaz and Özcengiz, 2017; Neely and Reed, 2018; Udy et al., 2018). The term “PD” is defined as the pharmacological properties of a drug within the body (Bergman et al., 2007; Khoruts et al., 2021). In systemic absorption, in order to reach the bloodstream, the drug must remain intact when it passes through the stomach, resist metabolism, and avoid liver excretion (Bergman et al., 2007; Neely and Reed, 2018). The term “bioavailability” is defined as the amount of drug absorbed into the bloodstream. It is calculated as:
The parameters
The term
IV drug administration does not require drug absorption from the gastrointestinal tract (GIT) to show therapeutic efficacy (Neely and Reed, 2018). Drugs with lower molecular weight have better absorption than drugs with higher molecular weight (Bergman et al., 2007). Also, drugs with higher solubility and permeability will show better systematic absorption (Neely and Reed, 2018). After absorption of the drug in the body, it will distribute to various tissues (Bergman et al., 2007; Neely and Reed, 2018). Biodistribution refers to the drug spreading through the blood circulation into all the accessible anatomic compartments of the body. The best biodistribution occurs for small, non-protein-bound, non-ionized, lipid-soluble drugs (Bergman et al., 2007; Neely and Reed, 2018). Moreover, factors including age, diet, lifestyle, and diseases can influence the biodistribution (Neely and Reed, 2018). The key parameter in biodistribution is the volume of distribution (
Where,
where
In modern microbiological research, the recent development of pharmaceutical products based on microorganisms represents an emerging branch of pharmacy and microbiology namely “pharmaceutical microbiology” (Sandle, 2016; Li et al., 2020). In 2016, Sandle described the potential of field to produce new pharmaceuticals based on microbiological concepts (Sandle, 2016). According to Magdy (2014) the main role of a microbiologist in pharmaceutical companies is to assure the safety and sterility of raw biomaterials before manufacturing. However other major concepts in pharmaceutical microbiology can be exploited not only for treatment of H. pylori infection, but also for other recalcitrant bacterial infections (Figure 6).
FIGURE 6. Schematic representation of concepts in pharmaceutical microbiology relevant to the treatment of bacterial infections (FTIR: Fourier Transform Infrared Spectroscopy, NMR: Nuclear Magnetic Resonance, PET: Positron emission tomography, SPECT: Single photon emission computed tomography, PK/PD: Pharmacokinetics/Pharmacodynamics, GIT: Gastrointestinal tract and FMT: Fecal microbiota transplantation).
Before discussing in detail the major concepts of pharmaceutical microbiology, it is necessary to review information on the microbiota. The term “microbiota” is defined as a collection of microbial communities which live on or in a particular biological system (Abdelsalam et al., 2020). The term was preceded by the use of “flora” or “microflora” (Abdelsalam et al., 2020). The gut microbiota is also considered as a virtual organ in modern biological science (Carlucci et al., 2016). The normal human gut microbiota is composed of harmless microorganisms ranging from Gram-positive Firmicutes to several Gram-negative Bacteroidetes such as Bacteroides, Prevotella, Parabacteroides, and Alistipes (Roy and Trinchieri, 2017; Toor et al., 2019). Their normal functions include the control of host metabolism, nutrition, homeostasis, inflammation, and immunity. An appropriate balance between these microbes is pivotal to keep the human body in a healthy condition (Dzutsev et al., 2015; Roy and Trinchieri, 2017; Visconti et al., 2019). Importantly, the composition of host gut microbiota can be affected by metabolic, inflammatory bowel disease, physiological, and immune-related diseases (Roy and Trinchieri, 2017; Chung et al., 2018). In order to choose a good animal model for microbiota research, a review by Zheng et al. (2018) suggested that honey bees could be appropriate because of similarities between honey bee gut microbiota and human gut microbiota. Such research can provide an understanding of microbe-microbe and microbe-host interactions, which take place in the human body.
Moreover, a great deal of attention has recently been paid to pharmacogenomics, and this has also led to a surge of interest in emerging pharmacomicrobiomics (Weersma et al., 2020). This term was first introduced by Rizkallah et al. (2010). While pharmacogenomics concentrates on interactions between drugs and the human genome, pharmacomicrobiomics focuses on interactions between drugs and the host gut microbiota, which can influence the PK/PD of pharmaceuticals (Aziz et al., 2018; Doestzada et al., 2018; Sharma et al., 2019; Scher et al., 2020). Given the lack of specific pharmacogenomics and pharmacomicrobiomics testing for many drugs, Aziz et al. (2020) asked the question: “Has not the time come for routine microbiome testing and establishing pharmacomicrobiomic guidelines, at least for some drugs, in 2020?”. Therefore there is a strong need to determine clinical guidelines, at least for those drugs which have well-identified pharmacomicrobiomic interactions (Aziz et al., 2020). Equally important is the fact that the association between microbial metabolites and drug responses should be further investigated (Sharma et al., 2019). This is applicable in two related fields, pharmacometabolomics and pharmacometabonomics (Sharma et al., 2019). The major point in pharmacometabolomics is the metabotypes resulting from environmental, chemical, genetic, and physiological factors, while pharmacometabonomics involves the measurement of metabolic profiles in bio-fluids (Everett, 2015; Balasopoulou et al., 2016). For the detection of such metabolites, mass spectrometry and NMR spectroscopy are mainly used for analyzing metabolic data (Everett, 2015; Van Der Hooft et al., 2020). Another term used in pharmaceutical microbiology is toxicomicrobiomics. This involves the interaction between host gut microbiota and xenobiotics (foods, drugs, and environmental chemicals) in terms of toxicology (Aziz, 2018; Abdelsalam et al., 2020). Some of these interactions may increase or decrease the toxicity of antibiotics. Thus, these interactions should be comprehensively explored in pharmaceutical microbiology. To date, the use of pharmaceutical microbiology concepts is still lacking in many studies. If mechanistic data in this field can be more widely appreciated, many of the challenges associated with antibiotic treatment could be addressed.
The antibacterial properties of a drug are strongly dependent on the affinity of the pharmaceutical agent to the targeted microbes, and provision of a sufficient drug dose into the infected area (Neely and Reed, 2018). According to microbiological in vitro tests, the minimum inhibitory concentration (MIC) and minimum bactericidal concentration (MBC) values, are the gold standards for determining the biocidal activity of pharmaceuticals (Bergman et al., 2007; Feng et al., 2019). As its name suggests, MIC is the lowest concentration of an antibiotic to inhibit bacterial growth, whereas MBC is lowest concentration of an antibiotic to kill a certain number of logs of a particular bacterium (Andrews, 2001). Many efforts have been made to overcome the limitations of bacterial eradication, by an accurate selection of the PK/PD properties of pharmaceuticals. Antibiotics are generally divided into two categories, time-dependent antibiotics (beta-lactams, tetracyclines, clindamycin and macrolides, except for azalides such as azithromycin) and concentration-dependent antibiotics (such as vancomycin, aminoglycosides, azithromycin, ketolides, and quinolones) (Bergman et al., 2007; Pelgrift and Friedman, 2013; Neely and Reed, 2018). The PK/PD ratio is the major factor used to evaluate the efficacy of antibiotics: Tmax:MIC, Cmax:MIC, and
There is an urgent need to discover new alternative antibiotics for rescuing clinical failures. A study by Andrei et al. (2019) pointed out that the number of FDA-approved antibacterial drugs (FDA: United.States. Food and Drug Administration) was strikingly lower than drugs for other indications, over more than a quarter-century. In one study, Jin et al. (2018) suggested that standard triple therapy containing ilaprazole could be used for the future treatment of H. pylori. They showed that there was no significant change in PK drug interactions. Although they reported the PK parameters of ilaprazole, including
Over the years, H. pylori has been discovered to be as major causative agent of a variety of gastric diseases such as peptic ulcer, gastric ulcer and gastric cancer. As stated earlier, triple and quadruple antibiotic therapy regimens are by far the preferred option for clinicians. Statistical analysis from recent microbiological studies has confirmed that the antibiotic resistance crisis is becoming critical for healthcare, and this also applies to H. pylori. Due to the sky-rocketing development of antibiotic resistance, there is an ever-increasing need to improve current clinical approaches. If nothing changes, we will probably encounter a new pandemic of extremely resistant bacteria in the near future. To overcome the challenges associated with antibiotic therapy, it is necessary to consider biopharmaceutical principles of antibiotics such as PK/PD studies and concepts of pharmaceutical microbiology. Despite undeniable challenges, there is still a hope for improving current antibiotic therapy in the near future.
AHM: Conceptualization, Investigation, Methodology, Project administration, Resources, Supervision, Validation, Visualization, Writing—original draft and Writing—review and editing; MK: Conceptualization and Software; AL-L, CL, MGW, IH, and HAA: Conceptualization; MRH, AY, and MR-M: Conceptualization, Supervision and Writing—review and editing; MRZ: Supervision.
The authors declare that the research was conducted in the absence of any commercial or financial relationships that could be construed as a potential conflict of interest.
All claims expressed in this article are solely those of the authors and do not necessarily represent those of their affiliated organizations, or those of the publisher, the editors and the reviewers. Any product that may be evaluated in this article, or claim that may be made by its manufacturer, is not guaranteed or endorsed by the publisher.
We would like to thank Department of Pharmaceutical Biomaterials of Tehran University of Medical Sciences for their supports in our investigations. Also, we are grateful to Foodborne and Waterbone Diseases Research Center for affording the essential facilities in carrying out the works.
Abadi, A. T. B., and Ierardi, E. (2019). Vonoprazan and Helicobacter pylori Treatment: A Lesson from Japan or a Limited Geographic Phenomenon? Front. Pharmacol. 10, 316. doi:10.3389/fphar.2019.00316
Abdelsalam, N. A., Ramadan, A. T., Elrakaiby, M. T., and Aziz, R. K. (2020). Toxicomicrobiomics: The Human Microbiome vs. Pharmaceutical, Dietary, and Environmental Xenobiotics. Front. Pharmacol. 11, 390. doi:10.3389/fphar.2020.00390
Ahadian, S., Finbloom, J. A., Mofidfar, M., Diltemiz, S. E., Nasrollahi, F., Davoodi, E., et al. (2020). Micro and Nanoscale Technologies in Oral Drug Delivery. Adv. Drug Deliv. Rev. 157, 37–62. doi:10.1016/j.addr.2020.07.012
Alexander, S. M., Retnakumar, R. J., Chouhan, D., Devi, T. N. B., Dharmaseelan, S., Devadas, K., et al. (2021). Helicobacter pylori in Human Stomach: The Inconsistencies in Clinical Outcomes and the Probable Causes. Front. Microbiol. 12, 713955. doi:10.3389/fmicb.2021.713955
Amieva, M., and Peek, R. M. (2016). Pathobiology of helicobacter Pylori-Induced Gastric Cancer. Gastroenterology 150, 64–78. doi:10.1053/j.gastro.2015.09.004
Andrei, S., Droc, G., Droc, G., and Stefan, G. (2019). FDA Approved Antibacterial Drugs: 2018-2019. Discov. (Craiova) 7, e102. doi:10.15190/d.2019.15
Andrews, J. M. (2001). Determination of Minimum Inhibitory Concentrations. J. Antimicrob. Chemother. 48 (Suppl. 1), 5–16. doi:10.1093/jac/48.suppl_1.5
Ansari, S., and Yamaoka, Y. (2019). Helicobacter pylori Virulence Factors Exploiting Gastric Colonization and its Pathogenicity. Toxins (Basel) 11, 677. doi:10.3390/toxins11110677
Ansari, S., and Yamaoka, Y. (2022). Helicobacter pylori Infection, its Laboratory Diagnosis, and Antimicrobial Resistance: a Perspective of Clinical Relevance. Clin. Microbiol. Rev. 35, e0025821. doi:10.1128/cmr.00258-21
Arora, S., Bisen, G., and Budhiraja, R. (2012). Mucoadhesive and Muco-Penetrating Delivery Systems for Eradication of helicobacter Pylori. Asian J. Pharm. 6, 18–30. doi:10.4103/0973-8398.100127
Asín-Prieto, E., Rodríguez-Gascón, A., and Isla, A. (2015). Applications of the Pharmacokinetic/pharmacodynamic (PK/PD) Analysis of Antimicrobial Agents. J. Infect. Chemother. 21, 319–329. doi:10.1016/j.jiac.2015.02.001
Attaran, B., Salehi, N., Ghadiri, B., Esmaeili, M., Kalateh, S., Tashakoripour, M., et al. (2021). The Penicillin Binding Protein 1A of Helicobacter pylori, its Amoxicillin Binding Site and Access Routes. Gut Pathog. 13, 43. doi:10.1186/s13099-021-00438-0
Aziz, R. K., Hegazy, S. M., Yasser, R., Rizkallah, M. R., and Elrakaiby, M. T. (2018). Drug Pharmacomicrobiomics and Toxicomicrobiomics: from Scattered Reports to Systematic Studies of Drug-Microbiome Interactions. Expert Opin. Drug Metab. Toxicol. 14, 1043–1055. doi:10.1080/17425255.2018.1530216
Aziz, R. K., Rizkallah, M. R., Saad, R., and Elrakaiby, M. T. (2020). Translating Pharmacomicrobiomics: Three Actionable Challenges/Prospects in 2020. OMICS 24, 60–61. doi:10.1089/omi.2019.0205
Aziz, R. K. (2018). Toxicomicrobiomics: Narrowing the Gap Between Environmental and Medicinal Toxicogenomics. OMICS 22, 788–789. doi:10.1089/omi.2018.0183
Backert, S., Clyne, M., and Tegtmeyer, N. (2011). Molecular Mechanisms of Gastric Epithelial Cell Adhesion and Injection of CagA by Helicobacter pylori. Cell Commun. Signal. 9, 28. doi:10.1186/1478-811X-9-28
Bae, Y. H., and Park, K. (2020). Advanced Drug Delivery 2020 and beyond: Perspectives on the Future. Adv. Drug Deliv. Rev. 158, 4–16. doi:10.1016/j.addr.2020.06.018
Bakaki, P. M., Horace, A., Dawson, N., Winterstein, A., Waldron, J., Staley, J., et al. (2018). Defining Pediatric Polypharmacy: A Scoping Review. PLoS One 13, e0208047. doi:10.1371/journal.pone.0208047
Baker, D. A. (2020). Plants against Helicobacter pylori to Combat Resistance: An Ethnopharmacological Review. Biotechnol. Rep. (Amst) 26, e00470. doi:10.1016/j.btre.2020.e00470
Balamtekin, N., Artuk, C., Arslan, M., and Gülşen, M. (2021). The Effect of helicobacter Pylori on the Presentation and Clinical Course of Coronavirus Disease 2019 Infection. J. Pediatr. Gastroenterol. Nutr. 72, 511–513. doi:10.1097/MPG.0000000000003005
Balasopoulou, A., Patrinos, G. P., and Katsila, T. (2016). Pharmacometabolomics Informs Viromics toward Precision Medicine. Front. Pharmacol. 7, 411. doi:10.3389/fphar.2016.00411
Bergman, S. J., Speil, C., Short, M., and Koirala, J. (2007). Pharmacokinetic and Pharmacodynamic Aspects of Antibiotic Use in High-Risk Populations. Infect. Dis. Clin. North Am. 21, 821–846. doi:10.1016/j.idc.2007.07.004
Cao, S., Zhou, G., Ou-Yang, D. S., Wu, H. Z., Xiao, K., Chen, Y., et al. (2012). Pharmacokinetic Interactions between Ilaprazole and Clarithromycin Following Ilaprazole, Clarithromycin and Amoxicillin Triple Therapy. Acta Pharmacol. Sin. 33, 1095–1100. doi:10.1038/aps.2012.64
Carlucci, C., Petrof, E. O., and Allen-Vercoe, E. (2016). Fecal Microbiota-Based Therapeutics for Recurrent Clostridium difficile Infection, Ulcerative Colitis and Obesity. EBioMedicine 13, 37–45. doi:10.1016/j.ebiom.2016.09.029
Charitos, I. A., D’Agostino, D., Topi, S., and Bottalico, L. (2021). 40 Years of Helicobacter pylori: A Revolution in Biomedical Thought. Gastroenterol. Insights 12, 111–135. doi:10.3390/gastroent12020011
Chen, B. R., Li, W. M., Li, T. L., Chan, Y. L., and Wu, C. J. (2022). Fucoidan from Sargassum Hemiphyllum Inhibits Infection and Inflammation of Helicobacter pylori. Sci. Rep. 12, 429. doi:10.1038/s41598-021-04151-5
Chen, M. J., Wu, M. S., Chen, C. C., Chen, C. C., Fang, Y. J., Bair, M. J., et al. (2017). Impact of Amoxicillin Resistance on the Efficacy of Amoxicillin-Containing Regimens for Helicobacter pylori Eradication: Analysis of Five Randomized Trials. J. Antimicrob. Chemother. 72, 3481–3489. doi:10.1093/jac/dkx320
Chu, Y. T., Wang, Y. H., Wu, J. J., and Lei, H. Y. (2010). Invasion and Multiplication of helicobacter Pylori in Gastric Epithelial Cells and Implications for Antibiotic Resistance. Infect. Immun. 78, 4157–4165. doi:10.1128/IAI.00524-10
Chung, H. J., Nguyen, T. T. B., Kim, H. J., and Hong, S. T. (2018). Gut Microbiota as a Missing Link between Nutrients and Traits of Human. Front. Microbiol. 9, 1510. doi:10.3389/fmicb.2018.01510
Costa, T. R. D., Harb, L., Khara, P., Zeng, L., Hu, B., and Christie, P. J. (2021). Type IV Secretion Systems: Advances in Structure, Function, and Activation. Mol. Microbiol. 115, 436–452. doi:10.1111/mmi.14670
Couturier, M. R. (2013). The Evolving Challenges of helicobacter Pylori Disease, Diagnostics, and Treatment, Part I. Clin. Microbiol. Newsl. 35, 19–24. doi:10.1016/j.clinmicnews.2013.01.001
Di Fermo, P., Di Lodovico, S., Amoroso, R., De Filippis, B., D’Ercole, S., Di Campli, E., et al. (2020). Searching for New Tools to Counteract the helicobacter Pylori Resistance: The Positive Action of Resveratrol Derivatives. Antibiotics 9, 891. doi:10.3390/antibiotics9120891
Di Stefano, M., Pagani, E., Pesatori, E. V., Bergonzi, M., Figura, N., Corazza, G. R., et al. (2019). Polysorbate 80 Add-On Therapy in the Treatment of Helicobacter pylori Infection: Polysorbate 80 and HP Antibiotic Resistance. Clin. Nutr. ESPEN 34, 101–103. doi:10.1016/j.clnesp.2019.08.005
Dixon, N. E., Riddles, P. W., Gazzola, C., Blakeley, R. L., and Zerner, B. (1980). Jack Bean Urease (EC 3.5.1.5). V. On the Mechanism of Action of Urease on Urea, Formamide, Acetamide, N-Methylurea, and Related Compounds. Can. J. Biochem. 58, 1335–1344. doi:10.1139/o80-181
Doestzada, M., Vila, A. V., Zhernakova, A., Koonen, D. P. Y., Weersma, R. K., Touw, D. J., et al. (2018). Pharmacomicrobiomics: a Novel Route towards Personalized Medicine? Protein Cell 9, 432–445. doi:10.1007/s13238-018-0547-2
Dzutsev, A., Goldszmid, R. S., Viaud, S., Zitvogel, L., and Trinchieri, G. (2015). The Role of the Microbiota in Inflammation, Carcinogenesis, and Cancer Therapy. Eur. J. Immunol. 45, 17–31. doi:10.1002/eji.201444972
Efrati, C., Nicolini, G., Cannaviello, C., O'Sed, N. P., and Valabrega, S. (2012). Helicobacter pylori Eradication: Sequential Therapy and Lactobacillus Reuteri Supplementation. World J. Gastroenterol. 18, 6250–6254. doi:10.3748/wjg.v18.i43.6250
El-Sayed, M. S., Musa, N., Eltabbakh, M., Abdelhamid, D. H., Mostafa, S. M. I., Salah, M. M., et al. (2020). Detection of Helicobacter pylori oipA and dupA Genes Among Dyspeptic Patients with Chronic Gastritis. Alexandria J. Med. 56, 105–110. doi:10.1080/20905068.2020.1780675
Eusebi, L. H., Zagari, R. M., and Bazzoli, F. (2014). Epidemiology of Helicobacter pylori Infection. Helicobacter 19, 1–5. doi:10.1111/hel.12165
Everett, J. R. (2015). Pharmacometabonomics in Humans: A New Tool for Personalized Medicine. Pharmacogenomics 16, 737–754. doi:10.2217/pgs.15.20
Fallone, C. A., Moss, S. F., and Malfertheiner, P. (2019). Reconciliation of Recent helicobacter Pylori Treatment Guidelines in a Time of Increasing Resistance to Antibiotics. Gastroenterology 157, 44–53. doi:10.1053/j.gastro.2019.04.011
Feige, M. H., Sokolova, O., Pickenhahn, A., Maubach, G., and Naumann, M. (2018). HopQ Impacts the Integrin α5β1-independent NF-κB Activation by Helicobacter pylori in CEACAM Expressing Cells. Int. J. Med. Microbiol. 308, 527–533. doi:10.1016/j.ijmm.2018.05.003
Feng, Z. H., Fan, L., Yang, J., Huo, X. Y., Guo, Y., Zhang, Y., et al. (2019). Mutant Selection Window of Clarithromycin for Clinical Isolates of Helicobacter pylori. BMC Microbiol. 19, 176–177. doi:10.1186/s12866-019-1558-8
Frahm, N., Hecker, M., and Zettl, U. K. (2019). Multi-drug Use Among Patients with Multiple Sclerosis: A Cross-Sectional Study of Associations to Clinicodemographic Factors. Sci. Rep. 9, 3743. doi:10.1038/s41598-019-40283-5
Garza-González, E., Perez-Perez, G. I., Maldonado-Garza, H. J., and Bosques-Padilla, F. J. (2014). A Review of helicobacter Pylori Diagnosis, Treatment, and Methods to Detect Eradication. World J. Gastroenterol. 20, 1438–1449. doi:10.3748/wjg.v20.i6.1438
Gaynes, R. (2017). The Discovery of Penicillin-New Insights After More Than 75 Years of Clinical Use. Emerg. Infect. Dis. 23, 849–853. doi:10.3201/eid2305.161556
Geis, G., Suerbaum, S., Forsthoff, B., Leying, H., and Opferkuch, W. (1993). Ultrastructure and Biochemical Studies of the Flagellar Sheath of Helicobacter pylori. J. Med. Microbiol. 38, 371–377. doi:10.1099/00222615-38-5-371
Georgopoulos, S., and Papastergiou, V. (2020). An Update on Current and Advancing Pharmacotherapy Options for the Treatment of H. pylori Infection. Expert Opin. Pharmacother. 22, 729–741. doi:10.1080/14656566.2020.1845649
Ghanbari, F., Vaez, H., Taheri, R. A., Sahebkar, A., Behshod, P., and Khademi, F. (2020). Helicobacter pylori in Water, Vegetables and Foods of Animal Origin: A Systematic Review and Meta-Analysis on the Prevalence, Antibiotic Resistance and Genotype Status in Iran. Gene Rep. 21, 100913. doi:10.1016/j.genrep.2020.100913
Gisbert, J. P., and Calvet, X. (2011). Review Article: Non-bismuth Quadruple (Concomitant) Therapy for Eradication of Helicobater Pylori. Aliment. Pharmacol. Ther. 34, 604–617. doi:10.1111/j.1365-2036.2011.04770.x
Gong, Y., and Yuan, Y. (2018). Resistance Mechanisms of Helicobacter pylori and its Dual Target Precise Therapy. Crit. Rev. Microbiol. 44, 371–392. doi:10.1080/1040841X.2017.1418285
González, A., Salillas, S., Velázquez-Campoy, A., Espinosa Angarica, V., Fillat, M. F., Sancho, J., et al. (2019). Identifying Potential Novel Drugs against Helicobacter pylori by Targeting the Essential Response Regulator HsrA. Sci. Rep. 9, 11294. doi:10.1038/s41598-019-47746-9
Goodwin, C. S., and Armstrong, J. A. (1990). Microbiological Aspects of Helicobacter pylori (Campylobacter pylori). Eur. J. Clin. Microbiol. Infect. Dis. 9, 1–13. doi:10.1007/BF01969526
Gravina, A. G., Zagari, R. M., De Musis, C., Romano, L., Loguercio, C., and Romano, M. (2018). Helicobacter pylori and Extragastric Diseases: A Review. World J. Gastroenterol. 24, 3204–3221. doi:10.3748/wjg.v24.i29.3204
Hagiwara, T., Mukaisho, K., Nakayama, T., Hattori, T., and Sugihara, H. (2015). Proton Pump Inhibitors and helicobacter Pylori-Associated Pathogenesis. Asian Pac J. Cancer Prev. 16, 1315–1319. doi:10.7314/apjcp.2015.16.4.1315
Hemmatinezhad, B., Momtaz, H., and Rahimi, E. (2016). VacA, cagA, iceA and oipA Genotypes Status and Antimicrobial Resistance Properties of Helicobacter pylori Isolated from Various Types of Ready to Eat Foods. Ann. Clin. Microbiol. Antimicrob. 15, 2. doi:10.1186/s12941-015-0115-z
Hu, Y., Zhu, Y., and Lu, N. H. (2017). Novel and Effective Therapeutic Regimens for Helicobacter pylori in an Era of Increasing Antibiotic Resistance. Front. Cell. Infect. Microbiol. 7, 168. doi:10.3389/fcimb.2017.00168
Hunt, R. H. (1996). The Role of Helicobacter Pylori in Pathogenesis: The Spectrum of Clinical Outcomes. Scand. J. Gastroenterol. Suppl. 220, 3–9. Available at: http://www.ncbi.nlm.nih.gov/pubmed/8898429. doi:10.3109/00365529609094743
Hussein, N. R., Mohammadi, M., Talebkhan, Y., Doraghi, M., Letley, D. P., Muhammad, M. K., et al. (2008). Differences in Virulence Markers between Helicobacter pylori Strains from Iraq and Those from Iran: Potential Importance of Regional Differences in H. Pylori-Associated Disease. J. Clin. Microbiol. 46, 1774–1779. doi:10.1128/JCM.01737-07
Jabbari Moghaddam, Y., Rafeey, M., and Radfar, R. (2009). Comparative Assessment of Helicobacter pylori Colonization in Children Tonsillar Tissues. Int. J. Pediatr. Otorhinolaryngol. 73, 1199–1201. doi:10.1016/j.ijporl.2009.05.005
Jabri, E., Carr, M. B., Hausinger, R. P., and Karplus, P. A. (1995). The Crystal Structure of Urease from Klebsiella Aerogenes. Science 268, 998–1004. doi:10.1126/science.7754395
Jan, H. M., Chen, Y. C., Yang, T. C., Ong, L. L., Chang, C. C., Muthusamy, S., et al. (2020). Cholesteryl α-D-glucoside 6-acyltransferase Enhances the Adhesion of Helicobacter pylori to Gastric Epithelium. Commun. Biol. 3, 120–213. doi:10.1038/s42003-020-0855-y
Jia, J., Zhang, C., Liu, Y., Huang, Y., Bai, Y., Hang, X., et al. (2022). Armeniaspirol A: a Novel Anti-Helicobacter pylori Agent. Microb. Biotechnol. 15, 442–454. doi:10.1111/1751-7915.13807
Jin, B. H., Yoo, B. W., Park, J., Kim, J. H., Lee, J. Y., Shin, J. S., et al. (2018). Pharmacokinetic Drug Interaction and Safety after Coadministration of Clarithromycin, Amoxicillin, and Ilaprazole: a Randomised, Open-Label, One-Way Crossover, Two Parallel Sequences Study. Eur. J. Clin. Pharmacol. 74, 1149–1157. doi:10.1007/s00228-018-2489-2
Kabir, S. (2011). The Role of Interleukin-17 in the Helicobacter pylori Induced Infection and Immunity. Helicobacter 16, 1–8. doi:10.1111/j.1523-5378.2010.00812.x
Kao, C. Y., Sheu, B. S., and Wu, J. J. (2016). Helicobacter pylori Infection: An Overview of Bacterial Virulence Factors and Pathogenesis. Biomed. J. 39, 14–23. doi:10.1016/j.bj.2015.06.002
Karkhah, A., Ebrahimpour, S., Rostamtabar, M., Koppolu, V., Darvish, S., Vasigala, V. K. R., et al. (2019). Helicobacter pylori Evasion Strategies of the Host Innate and Adaptive Immune Responses to Survive and Develop Gastrointestinal Diseases. Microbiol. Res. 218, 49–57. doi:10.1016/j.micres.2018.09.011
Karplus, P. A., Pearson, M. A., and Hausinger, R. P. (1997). 70 Years of Crystalline Urease: What Have We Learned? Acc. Chem. Res. 30, 330–337. doi:10.1021/ar960022j
Katzung, B. G. (2006). Basic and Clinical Pharmacology,. Available at: http://www.usdoj.gov/dea/pubs/scheduling.html (Accessed April 25, 2019).
Katzung, B. G. (2017). Tetracyclines, Macrolides, Clindamycin, Chloramphenicol, Streptogramins, and Oxazolidinones Basic Clin. Pharmacol. 14e. McGraw Hill. Available at: https://accesspharmacy.mhmedical.com/content.aspx?bookid=2249§ionid=175222953
Kelly, S. A., Rodgers, A. M., O'Brien, S. C., Donnelly, R. F., and Gilmore, B. F. (2020). Gut Check Time: Antibiotic Delivery Strategies to Reduce Antimicrobial Resistance. Trends Biotechnol. 38, 447–462. doi:10.1016/j.tibtech.2019.10.008
Khoruts, A., Staley, C., and Sadowsky, M. J. (2021). Faecal Microbiota Transplantation for Clostridioides Difficile: Mechanisms and Pharmacology. Nat. Rev. Gastroenterol. Hepatol. 18, 67–80. doi:10.1038/s41575-020-0350-4
Klesiewicz, K., Karczewska, E., Budak, A., Marona, H., and Szkaradek, N. (2016). Anti-Helicobacter pylori Activity of Some Newly Synthesized Derivatives of Xanthone. J. Antibiot. (Tokyo) 69, 825–834. doi:10.1038/ja.2016.36
Kusters, J. G., van Vliet, A. H., and Kuipers, E. J. (2006). Pathogenesis of Helicobacter pylori Infection. Clin. Microbiol. Rev. 19, 449–490. doi:10.1128/CMR.00054-05
Kwok, T., Zabler, D., Urman, S., Rohde, M., Hartig, R., Wessler, S., et al. (2007). Helicobacter Exploits Integrin for Type IV Secretion and Kinase Activation. Nature 449, 862–866. doi:10.1038/nature06187
Laffleur, F., and Keckeis, V. (2020). Advances in Drug Delivery Systems: Work in Progress Still Needed? Int. J. Pharm. 590, 119912. doi:10.1016/j.ijpharm.2020.119912
Li, H., Yang, T., Tang, H., Tang, X., Shen, Y., Benghezal, M., et al. (2019). Helicobacter pylori Infection Is an Infectious Disease and the Empiric Therapy Paradigm Should Be Changed. Precis. Clin. Med. 2, 77–80. doi:10.1093/pcmedi/pbz009
Li, S., Jiang, W., Zheng, C., Shao, D., Liu, Y., Huang, S., et al. (2020). Oral Delivery of Bacteria: Basic Principles and Biomedical Applications. J. Control. Release 327, 801–833. doi:10.1016/j.jconrel.2020.09.011
Lima, V. P., Silva-Fernandes, I. J., Alves, M. K., and Rabenhorst, S. H. (2011). Prevalence of Helicobacter pylori Genotypes (vacA, cagA, cagE and virB11) in Gastric Cancer in Brazilian's Patients: an Association with Histopathological Parameters. Cancer Epidemiol. 35, e32. doi:10.1016/j.canep.2011.02.017
Liou, T. C., Liao, P. H., Lin, Y. C., Chu, C. H., and Shih, S. C. (2019). Intraluminal Therapy for Helicobacter pylori Infection. J. Gastroenterol. Hepatol. 34, 1337–1343. doi:10.1111/jgh.14627
Lista, A. D., and Sirimaturos, M. (2021). Pharmacokinetic and Pharmacodynamic Principles for Toxicology. Crit. Care Clin. 37, 475–486. doi:10.1016/j.ccc.2021.03.001
Liu, W., Tian, J., Hui, W., Kong, W., Feng, Y., Si, J., et al. (2021). A Retrospective Study Assessing the Acceleration Effect of Type I Helicobacter pylori Infection on the Progress of Atrophic Gastritis. Sci. Rep. 11, 4143. doi:10.1038/s41598-021-83647-6
Liu, Y., Shi, L., Su, L., van der Mei, H. C., Jutte, P. C., Ren, Y., et al. (2019). Nanotechnology-based Antimicrobials and Delivery Systems for Biofilm-Infection Control. Chem. Soc. Rev. 48, 428–446. doi:10.1039/C7CS00807D
Magdy, M. (2014). Brief Introduction to Pharmaceutical Microbiologist. J. microbiol. exp. 1, 165–166. doi:10.15406/jmen.2014.01.00029
Mahmoudi, S., Mamishi, S., Banar, M., Keshavarz Valian, S., Bahador, A., Najafi, M., et al. (2017). Antibiotic Susceptibility of Helicobacter pylori Strains Isolated from Iranian Children: High Frequency of A2143G Point Mutation Associated with Clarithromycin Resistance. J. Glob. Antimicrob. Resist. 10, 131–135. doi:10.1016/j.jgar.2017.04.011
Maier, R., and Benoit, S. (2019). Role of Nickel in Microbial Pathogenesis. Inorganics 7, 80. doi:10.3390/inorganics7070080
Mao, F. Y., Lv, Y. P., Hao, C. J., Teng, Y. S., Liu, Y. G., Cheng, P., et al. (2021). Helicobacter Pylori-Induced Rev-Erbα Fosters Gastric Bacteria Colonization by Impairing Host Innate and Adaptive Defense. Cell. Mol. Gastroenterol. Hepatol. 12, 395–425. doi:10.1016/j.jcmgh.2021.02.013
Maroney, M. J., and Ciurli, S. (2021). Nickel as a Virulence Factor in the Class I Bacterial Carcinogen, Helicobacter pylori. Semin. Cancer Biol. 76, 143–155. doi:10.1016/j.semcancer.2021.04.009
Mazzei, L., Musiani, F., and Ciurli, S. (2020). The Structure-Based Reaction Mechanism of Urease, a Nickel Dependent Enzyme: Tale of a Long Debate. J. Biol. Inorg. Chem. 25, 829–845. doi:10.1007/s00775-020-01808-w
Mishra, S., Singh, V., Rao, G. R., Dixit, V. K., Gulati, A. K., and Nath, G. (2008). Prevalence of Helicobacter pylori in Asymptomatic Subjects-Aa Nested PCR Based Study. Infect. Genet. Evol. 8, 815–819. doi:10.1016/j.meegid.2008.08.001
Molina-Infante, J., and Gisbert, J. P. (2014). Optimizing Clarithromycin-Containing Therapy for Helicobacter pylori in the Era of Antibiotic Resistance. World J. Gastroenterol. 20, 10338–10347. doi:10.3748/wjg.v20.i30.10338
Monno, R., De Laurentiis, V., Trerotoli, P., Roselli, A. M., Ierardi, E., and Portincasa, P. (2019). Helicobacter pylori Infection: Association with Dietary Habits and Socioeconomic Conditions. Clin. Res. Hepatol. Gastroenterol. 43, 603–607. doi:10.1016/j.clinre.2018.10.002
Naghibzadeh, N., Salmani, F., Nomiri, S., and Tavakoli, T. (2022). Investigating the Effect of Quadruple Therapy with Saccharomyces Boulardii or Lactobacillus Reuteri Strain (DSMZ 17648) Supplements on Eradication of Helicobacter pylori and Treatments Adverse Effects: a Double-Blind Placebo-Controlled Randomized Clinical Trial. BMC Gastroenterol. 22, 107. doi:10.1186/s12876-022-02187-z
Narayana, J. L., Huang, H. N., Wu, C. J., and Chen, J. Y. (2015). Epinecidin-1 Antimicrobial Activity: In Vitro Membrane Lysis and in Vivo Efficacy against Helicobacter pylori Infection in a Mouse Model. Biomaterials 61, 41–51. doi:10.1016/j.biomaterials.2015.05.014
Necchi, V., Candusso, M. E., Tava, F., Luinetti, O., Ventura, U., Fiocca, R., et al. (2007). Intracellular, Intercellular, and Stromal Invasion of Gastric Mucosa, Preneoplastic Lesions, and Cancer by Helicobacter pylori. Gastroenterology 132, 1009–1023. doi:10.1053/j.gastro.2007.01.049
Neely, M. N., and Reed, M. D. (2018). “Pharmacokinetic-pharmacodynamic Basis of Optimal Antibiotic Therapy,” in Principles and Practice of Pediatric Infectious Diseases. Fifth Edition (Netherlands: Elsevier), 1478. doi:10.1016/B978-0-323-40181-4.00291-7
Ng, C. G., Loke, M. F., Goh, K. L., Vadivelu, J., and Ho, B. (2017). Biofilm Formation Enhances Helicobacter pylori Survivability in Vegetables. Food Microbiol. 62, 68–76. doi:10.1016/j.fm.2016.10.010
Oleastro, M., and Ménard, A. (2013). The Role of Helicobacter pylori Outer Membrane Proteins in Adherence and Pathogenesis. Biol. (Basel) 2, 1110–1134. doi:10.3390/biology2031110
Oppong, P., Majumdar, D., Atherton, J., and Bebb, J. (2015). Helicobacter pylori Infection and Peptic Ulcers. Medicine 43, 215–222. doi:10.1016/j.mpmed.2015.01.008
Payne, R. A. (2020). Polypharmacy and Deprescribing. Medicine 48, 468–471. doi:10.1016/j.mpmed.2020.04.003
Pearson, M. A., Michel, L. O., Hausinger, R. P., and Karplus, P. A. (1997). Structures of Cys319 Variants and Acetohydroxamate-Inhibited Klebsiella Aerogenes Urease. Biochemistry 36, 8164–8172. doi:10.1021/bi970514j
Pelgrift, R. Y., and Friedman, A. J. (2013). Nanotechnology as a Therapeutic Tool to Combat Microbial Resistance. Adv. Drug Deliv. Rev. 65, 1803–1815. doi:10.1016/j.addr.2013.07.011
Peng, X., Zhang, R., Duan, G., Wang, C., Sun, N., Zhang, L., et al. (2018). Production and Delivery of Helicobacter pylori NapA in Lactococcus Lactis and its Protective Efficacy and Immune Modulatory Activity. Sci. Rep. 8, 6435. doi:10.1038/s41598-018-24879-x
Piscione, M., Mazzone, M., Di Marcantonio, M. C., Muraro, R., and Mincione, G. (2021). Eradication of helicobacter Pylori and Gastric Cancer: A Controversial Relationship. Front. Microbiol. 12, 630852. doi:10.3389/fmicb.2021.630852
Quaglia, N. C., and Dambrosio, A. (2018). Helicobacter pylori: A Foodborne Pathogen? World J. Gastroenterol. 24, 3472–3487. doi:10.3748/wjg.v24.i31.3472
Ranjbar, R., Khamesipour, F., Jonaidi-Jafari, N., and Rahimi, E. (2016a). Helicobacter pylori in Bottled Mineral Water: Genotyping and Antimicrobial Resistance Properties. BMC Microbiol. 16, 40. doi:10.1186/s12866-016-0647-1
Ranjbar, R., Khamesipour, F., Jonaidi-Jafari, N., and Rahimi, E. (2016b). Helicobacter pylori Isolated from Iranian Drinking Water: vacA, cagA, iceA, oipA and babA2 Genotype Status and Antimicrobial Resistance Properties. FEBS Open Bio 6, 433–441. doi:10.1002/2211-5463.12054
Rao, G. G., and Landersdorfer, C. B. (2021). Antibiotic Pharmacokinetic/pharmacodynamic Modelling: MIC, Pharmacodynamic Indices and beyond. Int. J. Antimicrob. Agents 58, 106368. doi:10.1016/j.ijantimicag.2021.106368
Riahizadeh, S., Malekzadeh, R., Agah, S., Zendehdel, N., Sotoudehmanesh, R., Ebrahimi-Dariani, N., et al. (2010). Sequential Metronidazole-Furazolidone or Clarithromycin-Furazolidone Compared to Clarithromycin-Based Quadruple Regimens for the Eradication of Helicobacter pylori in Peptic Ulcer Disease: A Double-Blind Randomized Controlled Trial. Helicobacter 15, 497–504. doi:10.1111/j.1523-5378.2010.00798.x
Risandiansyah, R., and Yahya, A. (2022). Antibiotic Activity of Actinomycetes Isolated from Young Tectona Grandis (L.) Wood and Pith. Biointerface Res. Appl. Chem. 12, 8174–8183. doi:10.33263/BRIAC126.81748183
Rizkallah, M., Saad, R., and Aziz, R. (2010). The Human Microbiome Project, Personalized Medicine and the Birth of Pharmacomicrobiomics. Cppm 8, 182–193. doi:10.2174/187569210792246326
Roncarati, D., and Scarlato, V. (2018). The Interplay between Two Transcriptional Repressors and Chaperones Orchestrates Helicobacter pylori Heat-Shock Response. Int. J. Mol. Sci. 19, 1702. doi:10.3390/ijms19061702
Ropponen, H. K., Richter, R., Hirsch, A. K. H., and Lehr, C. M. (2021). Mastering the Gram-Negative Bacterial Barrier - Chemical Approaches to Increase Bacterial Bioavailability of Antibiotics. Adv. Drug Deliv. Rev. 172, 339–360. doi:10.1016/j.addr.2021.02.014
Roszczenko-Jasińska, P., Wojtyś, M. I., and Jagusztyn-Krynicka, E. K. (2020). Helicobacter pylori Treatment in the Post-antibiotics Era-Searching for New Drug Targets. Appl. Microbiol. Biotechnol. 104, 9891–9905. doi:10.1007/s00253-020-10945-w
Roy, S., and Trinchieri, G. (2017). Microbiota: A Key Orchestrator of Cancer Therapy. Nat. Rev. Cancer 17, 271–285. doi:10.1038/nrc.2017.13
Salama, N. R. (2020). Cell Morphology as a Virulence Determinant: Lessons from Helicobacter pylori. Curr. Opin. Microbiol. 54, 11–17. doi:10.1016/j.mib.2019.12.002
Salama, N. R., Hartung, M. L., and Müller, A. (2013). Life in the Human Stomach: Persistence Strategies of the Bacterial Pathogen Helicobacter pylori. Nat. Rev. Microbiol. 11, 385–399. doi:10.1038/nrmicro3016
Sandle, T. (2016). Introduction to Pharmaceutical Microbiology. Pharm. Microbiol., 1–14. doi:10.1016/b978-0-08-100022-9.00001-3
Saracino, I. M., Pavoni, M., Zullo, A., Fiorini, G., Saccomanno, L., Lazzarotto, T., et al. (2020). Rifabutin-based Triple Therapy or Bismuth-Based Quadruple Regimen as Rescue Therapies for Helicobacter pylori Infection. Eur. J. Intern. Med. 81, 50–53. doi:10.1016/j.ejim.2020.06.029
Sasahara, G. L., Gouveia Júnior, F. S., Rodrigues, R. O., Zampieri, D. S., Fonseca, S. G. D. C., Gonçalves, R. C. R., et al. (2020). Nitro-imidazole-based Ruthenium Complexes with Antioxidant and Anti-inflammatory Activities. J. Inorg. Biochem. 206, 111048. doi:10.1016/j.jinorgbio.2020.111048
Saxena, A., Mukhopadhyay, A. K., and Nandi, S. P. (2020). Helicobacter pylori: Perturbation and Restoration of Gut Microbiome. J. Biosci. 45, 1–15. doi:10.1007/s12038-020-00078-7
Scheff, J. D., Almon, R. R., Dubois, D. C., Jusko, W. J., and Androulakis, I. P. (2011). Assessment of Pharmacologic Area under the Curve when Baselines Are Variable. Pharm. Res. 28, 1081–1089. doi:10.1007/s11095-010-0363-8
Scher, J. U., Nayak, R. R., Ubeda, C., Turnbaugh, P. J., and Abramson, S. B. (2020). Pharmacomicrobiomics in Inflammatory Arthritis: Gut Microbiome as Modulator of Therapeutic Response. Nat. Rev. Rheumatol. 16, 282–292. doi:10.1038/s41584-020-0395-3
Serrano, C., Harris, P. R., Smith, P. D., and Bimczok, D. (2021). Interactions between H. pylori and the Gastric Microbiome: Impact on Gastric Homeostasis and Disease. Curr. Opin. Physiology 21, 57–64. doi:10.1016/j.cophys.2021.04.003
Shah, C. P., Purushothaman, G., Thiruvenkatam, V., Kirubakaran, S., Juvale, K., and Kharkar, P. S. (2019). Design, Synthesis and Biological Evaluation of Helicobacter pylori Inosine 5'-monophosphate Dehydrogenase (HpIMPDH) Inhibitors. Further Optimization of Selectivity towards HpIMPDH over Human IMPDH2. Bioorg. Chem. 87, 753–764. doi:10.1016/j.bioorg.2019.04.001
Shao, Q. Q., Yu, X. C., Yu, M., Ma, J., Zhao, J. B., Yuan, L., et al. (2022). Rabeprazole Plus Amoxicillin Dual Therapy Is Equally Effective to Bismuth-Containing Quadruple Therapy for Helicobacter pylori Eradication in Central China: A Single-Center, Prospective, Open-Label, Randomized-Controlled Trial. Helicobacter 27, e12876. doi:10.1111/hel.12876
Sharma, A., Buschmann, M. M., and Gilbert, J. A. (2019). Pharmacomicrobiomics: The Holy Grail to Variability in Drug Response? Clin. Pharmacol. Ther. 106, 317–328. doi:10.1002/cpt.1437
Sit, W. Y., Chen, Y. A., Chen, Y. L., Lai, C. H., and Wang, W. C. (2020). Cellular Evasion Strategies of Helicobacter pylori in Regulating its Intracellular Fate. Semin. Cell Dev. Biol. 101, 59–67. doi:10.1016/j.semcdb.2020.01.007
Skene, C., Young, A., Every, A., and Sutton, P. (2007). Helicobacter pylori Flagella: Antigenic Profile and Protective Immunity. FEMS Immunol. Med. Microbiol. 50, 249–256. doi:10.1111/j.1574-695X.2007.00263.x
Sousa, C., Ferreira, R., Azevedo, N. F., Oleastro, M., Azeredo, J., Figueiredo, C., et al. (2022). Helicobacter pylori Infection: from Standard to Alternative Treatment Strategies. Crit. Rev. Microbiol. 48, 376–396. doi:10.1080/1040841x.2021.1975643
Sugioka, M., Tachi, T., Mizui, T., Koyama, A., Murayama, A., Katsuno, H., et al. (2020). Effects of the Number of Drugs Used on the Prevalence of Adverse Drug Reactions in Children. Sci. Rep. 10, 21341. doi:10.1038/s41598-020-78358-3
Talebi Bezmin Abadi, A. (2017). Helicobacter pylori Treatment: New Perspectives Using Current Experience. J. Glob. Antimicrob. Resist. 8, 123–130. doi:10.1016/j.jgar.2016.11.008
Talebi Bezmin Abadi, A. (2014). Therapy of helicobacter Pylori: Present Medley and Future Prospective. Biomed. Res. Int. 2014, 124607. doi:10.1155/2014/124607
Talebi Bezmin Abadi, A. (2016). Vaccine against Helicobacter pylori: Inevitable Approach. World J. Gastroenterol. 22, 3150–3157. doi:10.3748/wjg.v22.i11.3150
Theuretzbacher, U., Barbee, L., Connolly, K., Drusano, G., Fernandes, P., Hook, E., et al. (2020). Pharmacokinetic/pharmacodynamic Considerations for New and Current Therapeutic Drugs for Uncomplicated Gonorrhoea-Challenges and Opportunities. Clin. Microbiol. Infect. 26, 1630–1635. doi:10.1016/j.cmi.2020.08.006
Thung, I., Aramin, H., Vavinskaya, V., Gupta, S., Park, J. Y., Crowe, S. E., et al. (2016). Review Article: The Global Emergence of Helicobacter pylori Antibiotic Resistance. Aliment. Pharmacol. Ther. 43, 514–533. doi:10.1111/apt.13497
Toor, D., Wsson, M. K., Kumar, P., Karthikeyan, G., Kaushik, N. K., Goel, C., et al. (2019). Dysbiosis Disrupts Gut Immune Homeostasis and Promotes Gastric Diseases. Int. J. Mol. Sci. 20, 2432. doi:10.3390/ijms20102432
Tshibangu-Kabamba, E., and Yamaoka, Y. (2021). Helicobacter pylori Infection and Antibiotic Resistance - from Biology to Clinical Implications. Nat. Rev. Gastroenterol. Hepatol. 18, 613–629. doi:10.1038/s41575-021-00449-x
Turnbull, A. J., Donaghy, E., Salisbury, L., Ramsay, P., Rattray, J., Walsh, T., et al. (2020). Polypharmacy and Emergency Readmission to Hospital after Critical Illness: a Population-Level Cohort Study. Br. J. Anaesth. 126, 415–422. doi:10.1016/j.bja.2020.09.035
Udy, A. A., Roberts, J. A., Lipman, J., and Blot, S. (2018). The Effects of Major Burn Related Pathophysiological Changes on the Pharmacokinetics and Pharmacodynamics of Drug Use: An Appraisal Utilizing Antibiotics. Adv. Drug Deliv. Rev. 123, 65–74. doi:10.1016/j.addr.2017.09.019
Umamaheshwari, R. B., and Jain, N. K. (2003). Receptor Mediated Targeting of Lectin Conjugated Gliadin Nanoparticles in the Treatment of Helicobacter pylori. J. Drug Target. 11, 415–424. doi:10.1080/10611860310001647771
Vale, F. F., and Vítor, J. M. (2010). Transmission Pathway of Helicobacter pylori: Does Food Play a Role in Rural and Urban Areas? Int. J. Food Microbiol. 138, 1–12. doi:10.1016/j.ijfoodmicro.2010.01.016
Van Der Hooft, J. J. J., Mohimani, H., Bauermeister, A., Dorrestein, P. C., Duncan, K. R., and Medema, M. H. (2020). Linking Genomics and Metabolomics to Chart Specialized Metabolic Diversity. Chem. Soc. Rev. 49, 3297–3314. doi:10.1039/d0cs00162g
Visconti, A., Le Roy, C. I., Rosa, F., Rossi, N., Martin, T. C., Mohney, R. P., et al. (2019). Interplay between the Human Gut Microbiome and Host Metabolism. Nat. Commun. 10, 4505. doi:10.1038/s41467-019-12476-z
Walton, E. L. (2016). Helicobacter pylori's Road to Colonization. Biomed. J. 39, 1–4. doi:10.1016/j.bj.2016.03.001
Wang, Z. J., Chen, X. F., Zhang, Z. X., Li, Y. C., Deng, J., Tu, J., et al. (2017). Effects of Anti-Helicobacter pylori Concomitant Therapy and Probiotic Supplementation on the Throat and Gut Microbiota in Humans. Microb. Pathog. 109, 156–161. doi:10.1016/j.micpath.2017.05.035
Warren, J. R., and Marshall, B. (1983). Unidentified Curved Bacilli on Gastric Epithelium in Active Chronic Gastritis. Lancet 1, 1273–1275. doi:10.1016/s0140-6736(83)92719-8
Weersma, R. K., Zhernakova, A., and Fu, J. (2020). Interaction between Drugs and the Gut Microbiome. Gut 69, 1510–1519. doi:10.1136/gutjnl-2019-320204
Weng, Y. A., Deng, C. Y., and Pu, C. (2020). Targeting Continuity of Care and Polypharmacy to Reduce Drug-Drug Interaction. Sci. Rep. 10, 21279. doi:10.1038/s41598-020-78236-y
Worku, M. L., Sidebotham, R. L., Walker, M. M., Keshavarz, T., and Karim, Q. N. (2015). The Relationship between Helicobacter pylori Motility, Morphology and Phase of Growth: Implications for Gastric Colonization and Pathology. Microbiol. Read. 145, 2803–2811. doi:10.1099/00221287-145-10-2803
Xiong, M. H., Bao, Y., Yang, X. Z., Zhu, Y. H., and Wang, J. (2014). Delivery of Antibiotics with Polymeric Particles. Adv. Drug Deliv. Rev. 78, 63–76. doi:10.1016/j.addr.2014.02.002
Yılmaz, Ç., and Özcengiz, G. (2017). Antibiotics: Pharmacokinetics, Toxicity, Resistance and Multidrug Efflux Pumps. Biochem. Pharmacol. 133, 43–62. doi:10.1016/j.bcp.2016.10.005
Zamani, M., Ebrahimtabar, F., Zamani, V., Miller, W. H., Alizadeh-Navaei, R., Shokri-Shirvani, J., et al. (2018). Systematic Review with Meta-Analysis: The Worldwide Prevalence of Helicobacter pylori Infection. Aliment. Pharmacol. Ther. 47, 868–876. doi:10.1111/apt.14561
Zhao, S., Lv, Y., Zhang, J. B., Wang, B., Lv, G. J., and Ma, X. J. (2014). Gastroretentive Drug Delivery Systems for the Treatment of Helicobacter pylori. World J. Gastroenterol. 20, 9321–9329. doi:10.3748/wjg.v20.i28.9321
Keywords: H. pylori, antibiotic therapy, biopharmaceutical principles, pharmaceutical microbiology, pharmacokinetics, pharmacodynamics
Citation: Miri AH, Kamankesh M, Llopis-Lorente A, Liu C, Wacker MG, Haririan I, Asadzadeh Aghdaei H, Hamblin MR, Yadegar A, Rad-Malekshahi M and Zali MR (2022) The Potential Use of Antibiotics Against Helicobacter pylori Infection: Biopharmaceutical Implications. Front. Pharmacol. 13:917184. doi: 10.3389/fphar.2022.917184
Received: 10 April 2022; Accepted: 31 May 2022;
Published: 27 June 2022.
Edited by:
Mitsushige Sugimoto, Tokyo Medical University Hospital, JapanReviewed by:
Sonia M. Rodrigues Oliveira, University of Aveiro, PortugalCopyright © 2022 Miri, Kamankesh, Llopis-Lorente, Liu, Wacker, Haririan, Asadzadeh Aghdaei, Hamblin, Yadegar, Rad-Malekshahi and Zali. This is an open-access article distributed under the terms of the Creative Commons Attribution License (CC BY). The use, distribution or reproduction in other forums is permitted, provided the original author(s) and the copyright owner(s) are credited and that the original publication in this journal is cited, in accordance with accepted academic practice. No use, distribution or reproduction is permitted which does not comply with these terms.
*Correspondence: Michael R. Hamblin, aGFtYmxpbi5sYWJAZ21haWwuY29t; Abbas Yadegar, YS55YWRlZ2FyQHNibXUuYWMuaXI=; Mazda Rad-Malekshahi, bS1yYWRtYWxla3NoYWhpQHNpbmEudHVtcy5hYy5pcg==; Mohammad Reza Zali, bm56YWxpQGhvdG1haWwuY29t
Disclaimer: All claims expressed in this article are solely those of the authors and do not necessarily represent those of their affiliated organizations, or those of the publisher, the editors and the reviewers. Any product that may be evaluated in this article or claim that may be made by its manufacturer is not guaranteed or endorsed by the publisher.
Research integrity at Frontiers
Learn more about the work of our research integrity team to safeguard the quality of each article we publish.