- 1Department of Immunology, National Health Commission (NHC) Key Laboratory of Medical Immunology (Peking University), School of Basic Medical Sciences, Peking University, Beijing, China
- 2Department of Pharmacology, Jilin University, Changchun, China
Immune abnormality involves in various diseases, such as infection, allergic diseases, autoimmune diseases, as well as transplantation. Several signal pathways have been demonstrated to play a central role in the immune response, including JAK/STAT, NF-κB, PI3K/AKT-mTOR, MAPK, and Keap1/Nrf2/ARE pathway, in which multiple targets have been used to develop immunosuppressive agents. In recent years, varieties of immunosuppressive agents have been approved for clinical use, such as the JAK inhibitor tofacitinib and the mTOR inhibitor everolimus, which have shown good therapeutic effects. Additionally, many immunosuppressive agents are still in clinical trials or preclinical studies. In this review, we classified the immunosuppressive agents according to the immunopharmacological mechanisms, and summarized the phase of immunosuppressive agents.
Introduction
Immunosuppressive agents are a class of drugs that inhibit the abnormal immune response of the body and suppress the proliferation and function of cells related to the immune response (macrophages such as T cells and B cells), thereby reducing the antibody immune response, and are now mainly used in organ transplantation anti-rejection reactions and autoimmune diseases. Undoubtedly, immunosuppressive agents have achieved great progress and success in the treatment of these diseases, thus further demonstrating the great research and development prospects of immunosuppressive agents. In 1949, Edward and Philip successfully extracted the adrenocorticotropic hormone cortisone from animals and elucidated its structure and biological effects. Since then, immunosuppressive agents have been on the stage of history, and glucocorticoids have been widely used in clinical practice, and two great scientists were awarded the Nobel Prize in 1950 for this discovery. However, medical problems abound, and the quest of our ancestors never stops. In the 1950s, the anti-rejection reactions present in organ transplants could only be controlled by radiation, and patient survival could not be guaranteed. Subsequently, medical personnel applied glucocorticoids to organ transplants, but the quality of patient survival was delayed due to the highly toxic side effects of hormones, and the development of immunosuppressive agents was imminent. It was not until 1953 when George Hitchings and his associate Gertrude Elion successfully developed the anti-cancer drug 6-mercaptopurine and structurally modified it to develop mercaptopurine, which was used in combination with hormonal drugs for organ transplantation, that the life span of transplanted organs was greatly extended, but research into the use of new drugs for organ transplantation is still progressing at a rapid pace today. Although the problems of organ transplantation have improved, new problems have arisen. We all know that the use of immunosuppressive drugs is a very effective way to treat autoimmune diseases, but the drugs used need constant innovation. In the early days, autoimmune diseases were mainly treated with glucocorticoids and cytotoxic drugs, but it was found that these two types of drugs were too selective for cells, thus easily injuring normal cells by mistake. As a result, the demand for highly selective immunosuppressive drugs has gradually increased. Cyclosporine A has received much attention since its successful use in the treatment of organ anti-rejection, and researchers have again used cyclosporine A in animal studies and found it to be effective in human-like autoimmune myasthenia gravis in rats. Since then, cyclosporine A has been used clinically to treat human autoimmune diseases. However, mankind has never stopped for the use of immunosuppressive drugs to treat diseases. In 2019, a neo-coronavirus outbreak was reported in Wuhan, and studies on patients can reveal that granulocyte colony factor, interferon-inducible protein 10, monocyte chemotactic protein 1, macrophage inflammatory protein 1α, and TNF-α levels are higher in patients with severe COVID-19 than in those without severe disease, demonstrating that cytokine storms can have an impact on the extent of COVID-19 disease (Huang et al., 2020). The use of glucocorticoids for the treatment of refractory cytokine storms is now well documented and widely accepted, and researchers have put methylprednisolone into clinical use and found it to be effective in patients with COVID-19, with a mild discontinuation response. But then researchers conducted a retrospective study that included 309 patients with severe MERS and found that glucocorticoids, while suppressing the cytokine storm, also interfered with the immune response, resulting in reduced clearance of the pathogen (Arabi et al., 2018). Therefore, for patients with severe COVID-19, the principle of no glucocorticoid therapy for patients not meeting the indications for glucocorticoid application is currently adopted. Of course, seeing the dawn of immunosuppressive therapy for COVID-19, researchers will devote more time and effort to the development and use of immunosuppressive agents in the future.
Since the successful completion of the first kidney transplantation in the United States in the 1950s, immunosuppression has received more attention. In 1978, cyclosporine A was first used in clinical renal transplantation in the United Kingdom, and its combined application with hormonal drugs and azathioprine was called “triple therapy”, which greatly improved the 1-year survival rate of transplanted kidneys, which was a new milestone in the history of immunosuppressant development, from which immunosuppressive agents began to develop formally in the 1970s. By now, various types of immunosuppressive agents have been put into clinical application, and the immunosuppressive agents commonly used in clinical practice can be broadly classified into the following categories: 1). Glucocorticoids; 2). Cytotoxic drugs (e.g., cyclophosphamide); 3). Calmodulin inhibitors (e.g., cyclosporine, tacrolimus); 4). Macrolamines (e.g., macrolimus ethyl ester); 5). Chinese herbal immunosuppressive agents (e.g., total ginseng). Glucocorticoids have a wide range of pharmacological mechanisms of action and are the most commonly used immunosuppressive agents in clinical practice, and they can have inhibitory effects on multiple aspects of the immune response. Studies have shown that it inhibits the production of initial T lymphocytes and monocytes mainly by affecting the differentiation maturation, phenotype and function of dendritic cells in order to induce clonal incompetence or apoptosis of T lymphocytes, followed by the acquisition of immune tolerance to specific antigens. However, because of their excessive adverse effects, they are currently used mainly for the combination treatment of various diseases. In order to minimize the adverse effects caused by glucocorticosteroids during treatment, researchers are working to develop new synthetic immunosuppressive agents to reduce the amount of glucocorticosteroids. In the 1960s, azathioprine was successfully modified and first used successfully in organ transplantation (Nordham and Ninokawa, 2022), but after a long period of use it was found to have serious teratogenic and carcinogenic adverse effects. In addition to this, it is less selective for cells and still has a killing effect on cells that are proliferating faster. In order to find a solution to the strong organ toxicity of azathioprine, researchers have conducted studies on antimetabolic immunosuppressive agents and have succeeded in extracting mycophenolate esters from Penicillium spp. fungi, which inhibit the production of antibodies and control the rejection reactions that occur during organ transplantation. In 1995, mycophenolate was approved by the US FDA as an adjunct to cyclosporine A for the prevention of acute renal transplant rejection. There are still studies in China that can show that mycophenolate, as a highly selective immunosuppressant, can replace azathioprine in combination with hormones, and that the toxic effects in the treated organ are greatly reduced. Although mycophenolate esters have shown good improvements in their toxic effects, they still have a degree of significant gastrointestinal irritation (Omair et al., 2015), which limits their widespread use. Meanwhile, researchers succeeded in synthesizing cyclosporine in 1980 and then tacrolimus in 1984, and these mTOR inhibitors showed good immunosuppressive effects and greatly reduced adverse effects such as myelosuppression. In order to maximize the pharmacological effects of cyclosporine, researchers subsequently extracted and discovered sirolimus and imidazolibine, which were combined with cyclosporine, and found that the former and the latter had increased efficacy due to synergistic effects (Kahan, 1999). In the 21st century, more attention has been given to the development of monoclonal antibodies in order to further improve the targeting and duration of drug delivery. As a result, baximab and daximab were introduced. It was found that monoclonal drugs have a longer half-life, which can improve the dosing time to some extent.Because cyclosporine is widely used, but its nephrotoxic reactions are very obvious, so in recent years, researchers have tried to modify its structure, and finally obtained a new generation of calmodulin inhibitors - a cyclosporine derivative, vincristine, developed by Isotechnika, a Canadian company. This derivative has a better immunosuppressive effect and fewer adverse effects than cyclosporine and tacrolimus, and is widely used in autoimmune diseases such as psoriasis. In March 2021, it was also approved for the treatment of systemic lupus erythematosus in the US. In addition, studies are currently underway to investigate its use in COVID-19.
However, despite our previous adequate development of immunosuppressive drugs, there is still confusion and blind spots regarding the clinical use of immunosuppressive drugs. To date, the mechanism of efficacy of immunosuppressive agents has relied on their inhibition of lymphocyte proliferation and suppression of immune system-associated cytokine production. However, it is clear that there are many signaling pathways in the body that regulate cytokine production, thus complicating the impact of immunosuppressive agents at the molecular level. For example, as a common immune-related cytokine, its gene expression is regulated by many pathways, such as PP2A-GSK3β-MCL-1, PI3K-AKT-mTOR, MAPK and so on. On top of this, there are many targets on each signaling pathway, including proteins, kinases, DNA, etc. This complexity of organismal pathways greatly calls for a more refined classification of immunosuppressive agents, which can lead to greater clarity in drug use. To date, a number of immunosuppressive agents based on targets in signaling pathways have been introduced to the market. The first immunosuppressant to enter the public eye and clinical trials targeting the JAK-STAT pathway was tofacitinib, which is now approved by the FDA for autoimmune diseases such as rheumatoid arthritis. In addition to tofacitinib, rheumatoid arthritis has been pursued for targeted therapies for the past decades, and the advent of JAK inhibitors such as Filgotinib and Upadacitinib has led to promising treatment results in randomized controlled trials for this disease (Biggioggero et al., 2019). In the treatment of rheumatoid arthritis, sulforaphane can also exert its effects by inhibiting the MAPK pathway as well as the NF-κB pathway (see the MAPK pathway section in the main text for the specific mechanism of this part). Subsequently, studies on other autoimmune diseases on the JAK-STAT pathway and the development of targets have gradually increased, and a large amount of experimental data as well as clinical evidence support the possibility of developing immunosuppressive agents on this pathway, and clinical trials for some other indications, such as graft-versus-host rejection, transplantation, asthma, and lupus (Dowty et al., 2014; Okiyama et al., 2014; Furumoto et al., 2017), have been successfully conducted and obtained satisfactory results. While the JAK-STAT pathway has been methodically studied, many discoveries have been made in other pathways, for example, sirolimus, which acts in the PI3K-AKT-mTOR pathway, has long been approved as an immunosuppressant for the prevention of immune rejection of organ transplantation; berberine and curcumin, which act in the Keap1/ARE-Nrf2 pathway, have also demonstrated their anti-inflammatory efficacy, providing a basis for future The development of immunosuppressive agents has laid the foundation for future development. At present, there are already a variety of drugs that act on the target in the clinic, and the drugs that can act on the same target show similar physicochemical properties and conformational relationships, which to a certain extent has facilitated the development of new drugs and the transformation of old drugs. This new focus on further clarification of the pharmacological mechanisms as well as the targets of action of immunosuppressive drugs can be attributed in part to the prevalent disease complexity and the growing need for precision therapy and combination drug use. This article focuses on reviewing the latest research advances in immunosuppressive drugs, which will facilitate the clinical use of immunosuppressive drugs and improve the status of combinations. In addition, this review will introduce the common immune-related signaling pathways in the body, including JAK-STAT, NF-κB, PI3K-AKT-mTOR, MAPK, Keap1-Nrf2-ARE, and for each specific pathway, summarize the targets that immunosuppressive drugs can act on, and list the representative drugs that have been marketed in the clinic and in clinical trials.
JAK-STAT Pathway
The JAK-STAT pathway was discovered by Darwell when he studied the signaling molecules required for the activation of target genes after the action of interferon (Darnell, 1998), and it is one of the main mechanisms regulating the production of cytokines. More than 50 cytokines, growth factors and hormones, such as interleukins, interferons, granulocytes/macrophages colony-stimulating factor, erythropoietin, and thrombopoietin, etc., by intercalating with transmembrane receptors, this brings them spatially close to JAK kinase, which changes the spatial conformation of JAK kinase and makes it susceptible to trans-activation. Activated JAK kinases promote STAT monomer phosphorylation and further dimerization, nuclear translocation, and binding to specific enhancer sequences of target genes in dimeric or more complex oligomeric forms, thus functioning as classical transcription factors. For example, STAT is involved in three types of transcription in immune cells, namely 1) general transcription, such as acetyltransferase, methyltransferase, p300, RNA polymerase, etc.; 2) transcription of some basic inflammation-related substances, such as IRF, NF-κB family transcription factors, etc.; 3) major transcription factors that are critical to follow the specification (Harrison, 2012; Villarino et al., 2015). The main three negative regulators involved in the negative regulation of JAK-STAT are: cytokine signaling inhibitory protein, activated STATs protein inhibitor, and protein tyrosine phosphatase. Among them, cytokine signaling inhibitory proteins negatively regulate JAK-STAT through three main mechanisms, including 1) binding to phosphorylated tyrosine on the receptor, which physically blocks the binding of STATs to the receptor; 2) binding to JAKs or the receptor, which blocks the activity of JAKs; 3) interaction of the SOCS box with the elonginB/C complex, which results in the degradation of JAKs and STATs, etc., are degraded via the ubiquitination pathway (Trengove and Ward, 2013). Activated STATs protein inhibitors achieve inhibition of STATs through two pathways: 1) binding to dimerized STATs and masking the DNA-binding region of STATs; and 2) binding to STATs monomers thereby hindering their dimerization.Protein tyrosine phosphatases block the activity of JAKs by dephosphorylating them through binding to JAKs and receptors, in addition to negatively regulating STATs (Quintás-Cardama and Verstovsek, 2013). CBP/p300 is a histone acetyltransferase that regulates the acetylation of STATs (Wieczorek et al., 2012), which would affect the signaling of NF-κB pathway, transcriptional activity and stability of STATs homodimers, and apoptosis (Ginter et al., 2013; Zhuang, 2013) (Figure 1). It was found that IL-6, IL-13, IL-22, granulocyte colony-stimulating factor and IFN exert their biological functions mainly by binding to JAK1, while IL-2, IL-4, IL-17, IL-15 and IL-21 exert their biological functions mainly by binding to JAK3 (O’shea et al., 2002). The pathways generally work together to regulate the cell through interactions such as mutual synergy, with large and small connections arising between each pathway. For example, because both STAT2 and PI3K proteins have SH2 structural domains on them, both can bind to these phosphorylated receptors and function when JAK proteins are activated and tyrosine residues on the receptors are phosphorylated. That is, the STAT2 protein on the JAK-STAT pathway and the PI3K protein on the PI3K-AKT-mTOR pathway have a synergistic effect, and they can jointly regulate signaling between cells (Ma et al., 2010). In addition, the JAK-STAT pathway can also interact with the MAPK/ERK pathway. A protein called Grb2, which plays an important role in the MAPK/ERK pathway, also has an SH2 structural domain and can also act on the phosphorylated receptor, thus acting synergistically with the two outer pathways (Xu and Qu, 2008). JAK-STAT can also indirectly activate the MAPK pathway through SOCS3, which can bind RasGAP, a negative regulator of the MAPK pathway, and thus exert a role in promoting the MAPK pathway (Herranz et al., 2012). Numerous studies have demonstrated that the activation of STATs is mostly accomplished not by JAKs but by receptor tyrosine kinases, by two mechanisms. One is that activation of some RTKs, including epidermal growth factor receptor and platelet-derived growth factor receptor, leads to the completion of STATs tyrosine phosphorylation via Src kinase. The other is that activation of the RTK/Ras pathway causes upregulation of mitogen-activated protein kinase activation, with MAPK specifically phosphorylating a serine (Ser) at the C-terminus of most STATs, and Ser phosphorylation greatly enhances the transcriptional activity of STATs (Coskun et al., 2013). Because of its involvement in the pathogenesis of many diseases, such as solid tumors, leukemia, lymphoma, and inflammatory diseases, a large number of studies on targeted therapies for this pathway have proliferated, with JAKs and STATs as the most common targets. For example, the STAT inhibitor Fludarabine has been approved for the treatment of B-cell chronic lymphocytic leukemia; the JAK inhibitor Upadacitinib has been approved for the treatment of moderately to severely active rheumatoid arthritis or active psoriatic arthritis; and the STAT inhibitor Stattic can exert an inhibitory effect on the auto-inflammatory response in myeloid, lymphatic and neuronal tissue compartments by inhibiting STAT3 (Alhazzani et al., 2021). In addition, this pathway can be inhibited by inhibiting the binding of STAT to DNA. For example, Rabies virus P protein can downregulate type I IFN production by inhibiting STAT1 binding to the DNA structural domain (Vidy et al., 2007); Phosphotyrosyl Peptides PY*LKTK,PY*L,AY*L (where Y* represents phosphotyrosine) can block this pathway by inhibiting STAT1 or STAT3 binding to DNA (You et al., 2020). CBP and p300 are essential transcriptional co-activators and histone acetyltransferases in cells, and overexpression or mutation of these two may cause the development of related diseases such as cancer, so inhibitors targeting them can also block the JAK-STAT pathway and thus play a therapeutic role. For example, Y08197 is a new inhibitor of this target with an indication of activity for the treatment of prostate cancer (Zou et al., 2019). Some of the JAK inhibitors and STAT inhibitors have been approved for marketing, while most of the drugs are still in the process of clinical trials or even animal studies, as shown in Table 1, which lists some of the drugs targeting the JAK-STAT pathway and their targets, indications, and stages of study.
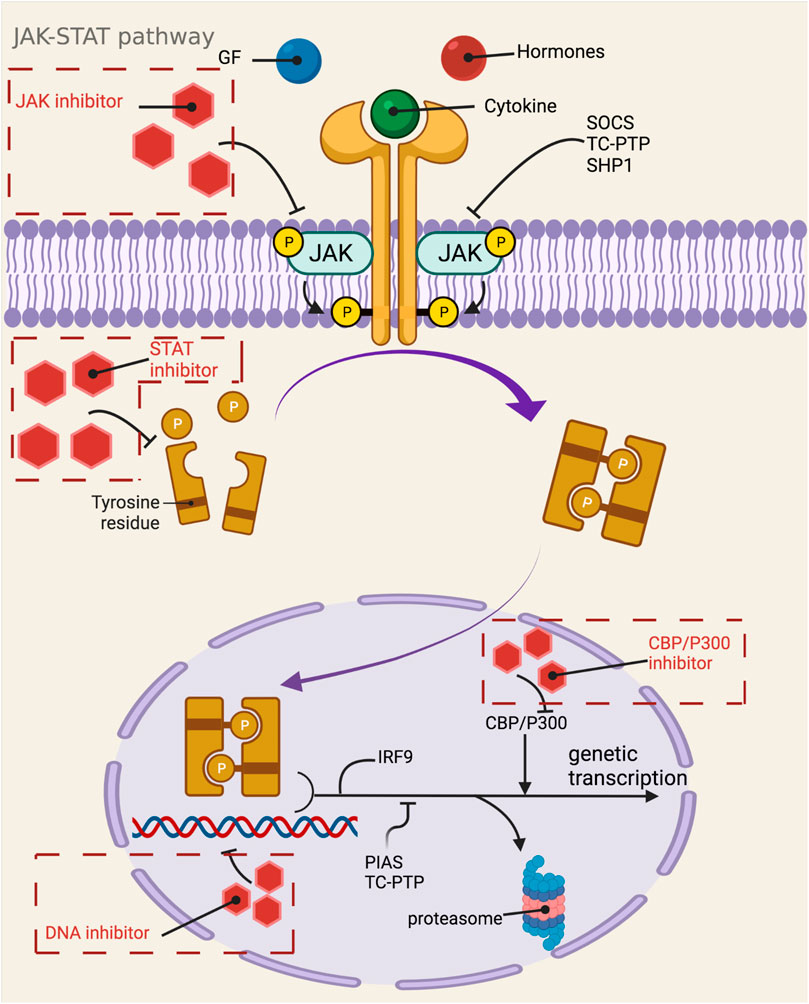
FIGURE 1. Mechanistic map of the JAK-STAT signaling pathway. CBP, calmodulin-binding peptide; GF, growth factor; IRF9, interferon regulatory factor 9; PIAS, protein inhibitor of activated STAT; SHP1, Src homology region 2 domain-containing phosphatase 1; SOCS, suppressor of cytokine siganaling; TC-PTP, T-cell protein tyrosine phosphatase. The figure is created with BioRender.com.
Tofacitinib, developed by Pfizer, selectively inhibits JAK1 kinase, JAK2 kinase, and JAK3 kinase, and in a study of its stereochemical structure, Meyer et al. found that the chiral structure of tofacitinib determines its binding to the JAK receptor (Meyer et al., 2010). In their study, O’shea et al. found that the drug inhibited JAK1 kinase and JAK3 kinase to a greater extent than JAK2 kinase (O’Shea et al., 2015). In addition to this, the researchers found that tofacitinib had negligible activity against TYK2 (Zerbini and Lomonte, 2012). In 2020, the FDA approved it for the treatment of chronic idiopathic arthritis (including rheumatoid arthritis, psoriatic arthritis, ankylosing spondylitis, and juvenile idiopathic arthritis) through a risk assessment and mitigation strategy. In addition to rheumatoid arthritis and ulcerative colitis, tofacitinib can also be used to prevent immune reactions to organ transplants, as well as baldness and psoriasis, for which the application is still in clinical trials.After oral administration of tofacitinib, its absolute bioavailability is 74%, peak blood concentration is reached 1–2 h after dosing, and half-life is about 3 h. A high-fat diet does not affect AUC, but it decreases Cmax by 32%. When tofacitinib was administered intravenously, Vd = 87L and the drug was distributed to an equal extent in red blood cells and plasma. The metabolism of tofacitinib is mainly metabolized by the hepatic drug enzymes CYP3A4 and CYP2C19, and 30% is excreted by the kidneys in the form of the prototype drug. Kostovic et al. found that the pharmacological activity of the metabolites of tofacitinib was less than 10% of that of the prototype drug, proving that the pharmacologically active form of the drug is the prototype drug (Kostovic et al., 2017).
JAK inhibitors have been shown to increase the risk of herpes virus infection in the treatment of ulcerative colitis and psoriasis, demonstrating that JAK inhibitors have the ability to The side effect of reducing the immunity of the body and thus inducing infection. In addition, while tofacitinib has shown good efficacy, studies have statistically found that it also shows a risk of venous embolism. Although no thorough studies have shown that JAK inhibitors are harmful to pregnant or lactating women, long-term statistical observations have shown that they are teratogenic and should be avoided in pregnant and lactating women. In addition, the use of JAK inhibitors can also promote the course of hyperlipidemia, malignancy, and gastrointestinal perforation, thus suggesting the need for more caution in drug use (Agrawal et al., 2020). In addition to JAK inhibitors, STAT inhibitors can also exhibit side effects similar to those of JAK inhibitors, and Wong et al. found that two patients developed unusual infections with symptoms of herpes virus infection as well as acute epididymitis during a clinical trial of the novel STAT3 inhibitor OPB-51602, warning of the safety of its use (Wong et al., 2015).
NF-κB Pathway
NF-κB pathway is an important potential target pathway for drug treatment of diseases in human body. Since nuclear factor-κB is a common class of pro-inflammatory factors, this pathway is closely related to invasive response, inflammatory response, angiogenic and metastatic response. With the gradual research, it reflects the association between NF-κB pathway and many cancer and inflammatory diseases, such as viral infection, AIDS, arthritis, atherosclerosis, asthma, diarrhea, etc. (Gupta et al., 2010b). In mammals, NF-κB can be divided into five species, namely RelA (p65), RelB, c-Rel, NF-κB1 (p50) and NF-κB2 (p52). These five parts ensure their interconnection through the conserved Rel homology structural domains and even further form heterodimers or homodimers (Prescott and Cook, 2018). In the unactivated state, the NF-κB dimer binds tightly to the kappa B protein inhibitor in order to maintain the stability of the NF-κB dimer and inhibit its entry into the nucleus to interact with DNA. Activation of the NF-κB pathway can be divided into typical and atypical pathways, with the former involving IKK (a heterodimer composed of IKKα, IKKβ, IKKγ, and NEMO, of which IKKβ is the catalytic subunit), IκB, and NF-κB (e.g., p65/p50 heterodimer), including the TNF-α pathway, the IL-1β pathway, and the cellular stress pathway (Lin et al., 2010b). Among the classical pathways, the TNF-α pathway is the most well studied, and its activation pathway is as follows: after TNF-α and TNFR binding, IKK is aggregated in TNFR1 with the help of TRAF2/5 and receptor-interacting protein kinase, and then, receptor-interacting protein kinase mediates IKK phosphorylation to activate it, in which MEKK3 or TAK1 is also involved in the phosphorylation process (Devin et al., 2000). The catalytic subunit IKKβ is re-activated and then activates serine residues 32 and 36 of IκB, and further polyubiquitinates and degrades IκB by the proteasome. After this process, the NLS signal on p65 and p50 is exposed, promoting the p65/p50 nuclear translocation. Subsequently, p65/p50 binds to DNA and undergoes transcription, which is regulated by phosphorylation and acetylation of the p65 subunit, resulting in Bcl, p100, IL-8, IL-1β, TNF-α, A20, COX2, MIP-2, etc (Yang et al., 2001b) (Figure 2). The non-classical pathway includes CD40 pathway, LTβ pathway, etc. Unlike the classical pathway, the non-classical pathway mainly relies on NF-κB-inducible kinase (NIK) to activate IKKα, which in turn triggers the cleavage of p100 to p52. P52 in turn binds to RelB in a complex and undergoes nuclear translocation, binding to DNA and thus enhancing gene expression (Chen, 2005). In addition, through the cIAP protein, the classical and non-classical pathways can in turn be regulated by each other (Zarnegar et al., 2008). The current common targets and corresponding drugs are listed in Table 2.
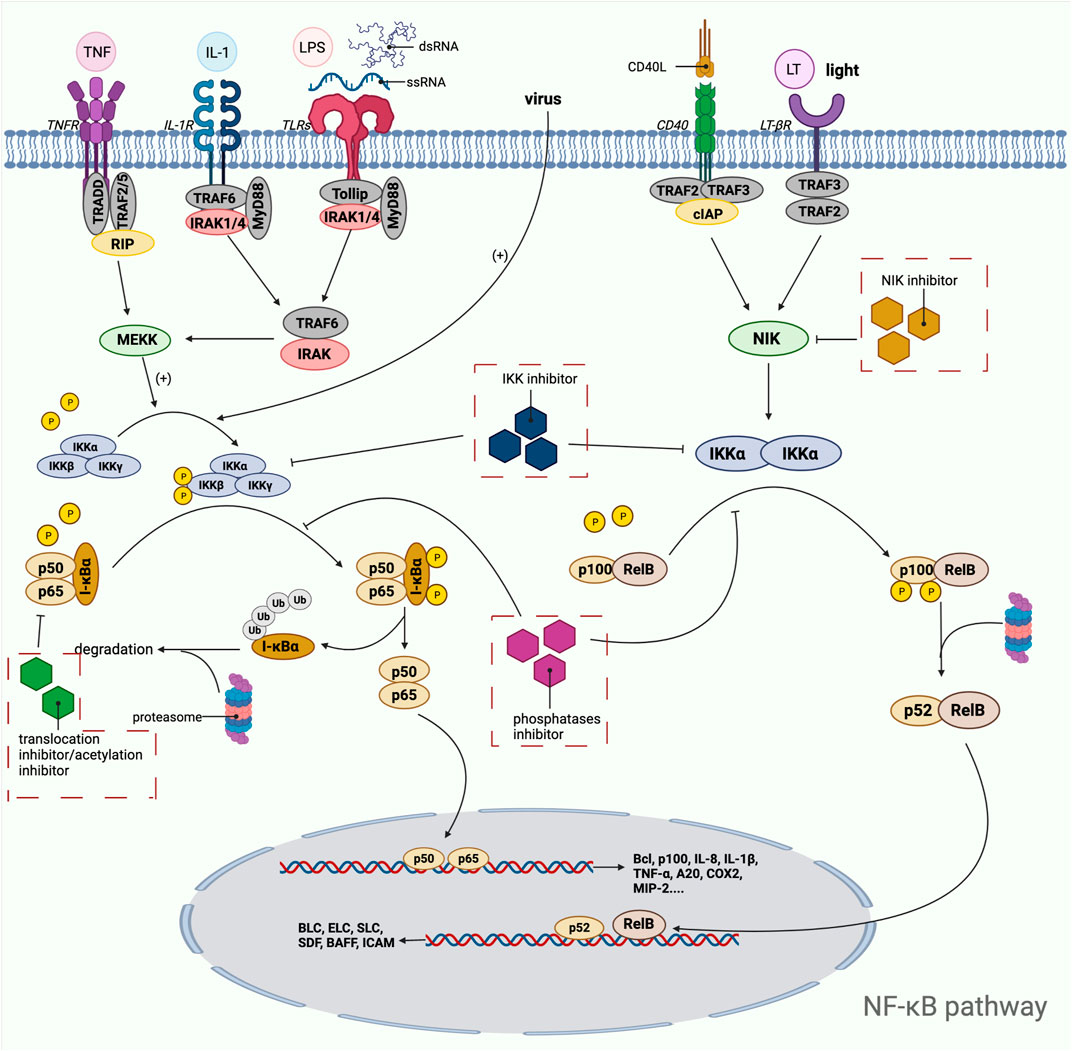
FIGURE 2. Mechanistic map of the NF-κB signaling pathway. BAFF, B-lymphocyte activating factor; Bcl, B-cell lymphoma; BLC, blood lactic acid; cIAP, cytoplasmic inhibitor apoptosisprotein; COX2, cyclooxygenase 2; ELC, ELongin C; ICAM, intercellular cell adhesion molecule; IL, interleukin; IRAK, interleukin-1 receptor-associated kinase; LPS, lipopolysaccharide; LT, Leukotriene; MIP, macrophage inflammatory protein; MyD: myeloid differentiation factor; RIP, receptor-interacting protein; SDF, stromal cell-derived factor; SLC, secondary lymphoid tissue chemokine; TNF, tumor necrosis factor; TRADD, TNF receptor-associated death domain; TRAF, tumor necrosis factor receptor associated factor The figure is created with BioRender.com.
Artemisinin is now publicly recognized as an effective drug for the treatment of malaria and has helped many countries around the world that are afflicted by the malaria disease. Mechanistically, artemisinin inhibits TNF-induced phosphorylation of NF-κB reporter factor I-κBα and its degradation by the proteasome, nuclear translocation of p65, and kinases upstream of IKK thereby achieving inhibition of the NF-κB pathway, thereby regulating genes related to cell proliferation, survival, invasion, and angiogenesis, such as Bcl, COX-2, MMP9, VEGF (Awasthee et al., 2019). As early as 2017, Wang et al. should have speculated on the possibility of artemisinin for the treatment of inflammation and cancer (Wang et al., 2017), and corresponding clinical trials are actively underway. In September 2021, clinical trials on the safety and efficacy of the herbal agent artemisinin for use in COVID-19 subjects were conducted, further demonstrating that its research on inflammation due to viral infections is still being explored.
The most common target in the NF-κB pathway is IKKβ, but IKKβ inhibitors are still less widely used in the clinic and are currently being developed at a lower rate, which has more to do with their safety profile. In fact, the NF-κB pathway is often considered as a “double-edged sword”, its anti-inflammatory response varies with the condition, for example, immune cells in tumors can play a dual role under the regulation of the pathway, which can be anti-inflammatory and anti-tumor response, but also can promote the development of tumor immune escape response (Ben-Neriah and Karin, 2011; Taniguchi and Karin, 2018). In addition, IKKβ inhibitors in the NF-κB pathway have more pronounced host differences, which may result from species variation or human host dependence (Prescott and Cook, 2018), which also makes it difficult to analyze the correlation between preclinical studies and clinical trials, and the difficulty of further drug development. More surprisingly, it was found that inhibition of IKKβ in certain cells or tissues exacerbates inflammation spontaneously, making drug use less safe and certain.Charles et al. found that IKKβ inhibited tumor growth in Colla2-expressing fibroblasts in a CAC model, but this was strictly dependent on increased secretion of HGF, and their speculation is that IKKβ/NF-κB may have different functions in different subpopulations of fibroblasts (Pallangyo et al., 2015). Therefore, it is important to have sufficient risk-taking ability when developing drugs for this pathway, as the good therapeutic effects of drugs in preclinical studies in animal models of disease may not be applicable to humans. Even if host differences are small, large individual differences may arise due to differences in the physiopathological conditions of the organism.
PI3K-AKT-mTOR Pathway
PI3K/AKT/mTOR pathway is one of the most important signaling pathways in human body, which plays a crucial role in the activation of various downstream effector molecules and is involved in the regulation of cell proliferation, differentiation, apoptosis, autophagy, invasion and metastasis, etc. In response to stimulating factors such as growth factors and cytokines, residues on the transmembrane phosphorylated tyrosine kinase interact with the SH2 structural domain on PI3K, relieving the inhibitory effect of p58 on p110, i.e., the dimeric conformation is altered, leading to activation of PI3K (Osaki et al., 2004). In addition, PI3K activation can be accomplished by direct recognition and binding of Ras to p110. PI3K activation leads to the conversion of 3,4-bisphosphatidylinositol (PIP2) to 3,4,5-trisphosphatidylinositol (PIP3). The generated PIP3 recognizes each other with the PH structural domain of AKT, which results in the transfer of AKT from the cytoplasm to the cytosol, along with a conformational change of AKT, exposing threonine proteins as well as serine proteins. AKT is activated by the co-activation of Thr308 phosphorylation in the presence of PDK1 and Ser743 phosphorylation in the presence of PDK2 (Ma and Blenis, 2009). AKT is activated and translocated to the cytoplasm or nucleus, where it targets and regulates downstream signaling molecules, including mTOR. The nodular sclerosis complex-1 (TSC-1) and nodular sclerosis complex-2 (TSC-2) can form a dimeric complex that further inhibits the GTPase Rheb, which is required to stimulate mTOR activation, so the TSC-1/TSC-2 complex has an inhibitory effect on mTOR activation. However, the activation of AKT can release the inhibition of mTOR by TSC-1/TSC-2, thus allowing the smooth activation of mTOR. In addition, AKT can also act directly on mTOR1 to activate mTOR (Huang and Manning, 2008). Phosphorylated mTOR further regulates ribosomal S6 protein kinase (S6K) and the eukaryotic initiation factor 4E-binding protein 1 (4E-BP1), which promotes phosphorylation of ribosomal S6 proteins and inactivates 4E-BP1 by phosphorylation. This leads to the synthesis of ribosomal proteins and the initiation of protein translation processes, respectively (Wan and Helman, 2007) (Figure 3). Since this pathway is shown to be dysregulated in various tumors and inflammatory diseases, this shows great promise for the study of this pathway. Researchers have identified and developed a number of drugs that target this pathway, which can be broadly classified as PI3K inhibitors, AKT inhibitors, mTOR inhibitors, and dual PI3K/mTOR inhibitors. These inhibitors inhibit the activation of PI3K, AKT, and mTOR by inhibiting these three targets, which in turn is closely linked to the degree of tumor metastasis and related disease progression. A large number of preclinical studies and clinical trials on these inhibitors exist today to ensure safety and efficacy during drug use. Table 3 provides a summary of some of the inhibitors for different targets, with information about their indications.
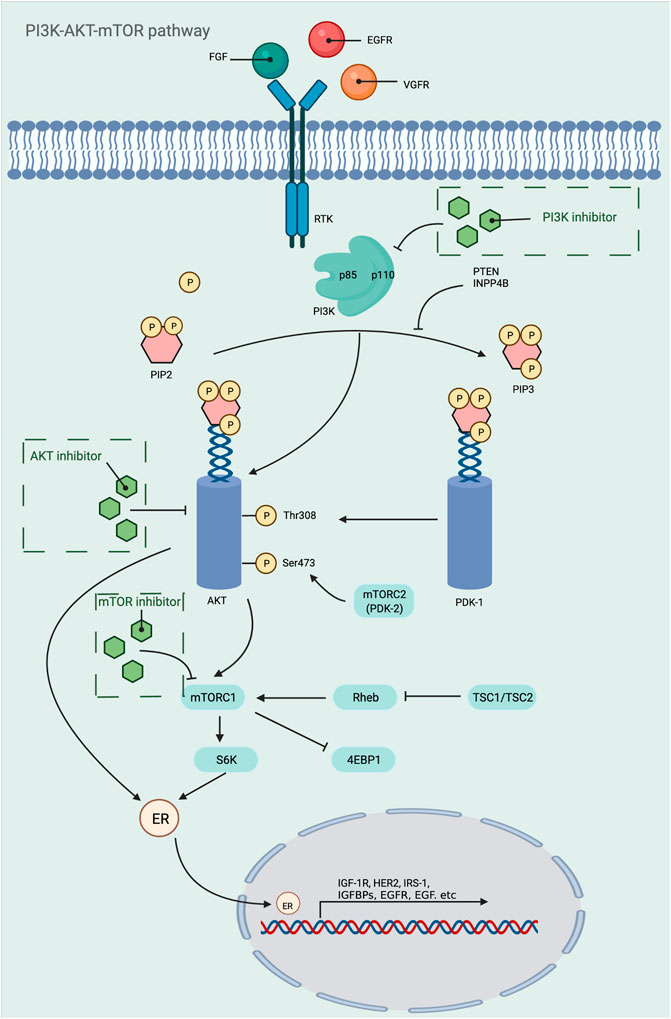
FIGURE 3. Mechanistic map of the PI3K-AKT-mTOR signaling pathway. EGF, epidermal growth factor; EGFR, epidermal growth factor receptor; FGF, fibroblast growth factor; HER2, human epidermalgrowth factor receptor-2; IGFBP, insulin-like growth factor-binding protein; IGF-1R, insulin-like growth factor 1 receptor; INPP4B, inositol polyphosphate 4-phosphatase typeⅡ; IRS-1, insulin receptor substrate 1; PDK, phosphoinositide-dependent protein kinase; PTEN, Phosphatase and tensin homologue deleted on chromosome 10; Rheb, Ras homolog enriched in brain; TSC, tumor stem cell; VGFR, vascular endothelial growth factor; 4EBP1, e IF4E-binding protein 1. The figure is created with BioRender.com.
The PI3K/AKT/mTOR pathway is associated with many diseases, and there are many factors that affect the physiological status of the human body in addition to the three critical targets of PI3K, AKT, and mTOR. For example, PTEN, a tumor suppressor, blocks the activation of PI3K/AKT/mTOR pathway by inhibiting the transition from PIP2 to PIP3. It was found that PTEN knockout mice had abnormally high levels of PIP3 compared to normal mice, and AKT remained continuously activated, which in turn induced tumorigenesis (Papa et al., 2014; Haddadi et al., 2018). In addition, the knockout mice also showed hypoglycemia, suggesting that it also has some effect on blood glucose regulation in vivo (Nguyen et al., 2006).
Sirolimus is a first-generation mTOR inhibitor targeting mTORC1, which specifically acts on mTORC1, causing phosphorylation of the carboxy terminus of mTOR and loss of catabolic activity, blocking the immune response triggered by interleukin-2, interleukin-15 and CD28/B7 co-stimulatory pathway to activate mTOR. It can inhibit the growth and proliferation of immune cells by keeping them in the G1/S phase, in addition to inhibiting the synthesis of immune molecules such as interleukin-1. Nowadays, it is mainly used in clinical practice to prevent rejection after organ transplantation and to treat autoimmune diseases (Weichhart, 2018; Li et al., 2019). Nowadays, indications for sirolimus are gradually being developed. For example, in primary immune thrombocytopenia, decreased Treg cell levels are the main cause of refractory/recurrent ITP, which provides a theoretical basis for sirolimus treatment of ITP. After a randomized group trial by Li (Cuker and Neunert, 2016) et al. showed that sirolimus significantly improved remission rates as well as platelet counts in patients with ITP (Li et al., 2013). Jasinski et al. included 12 patients with ITP and switched to sirolimus combined with hormone therapy after conventional treatment failed and found that the patients’ cure rate was greatly improved and no significant adverse effects were observed during the use of the drug (Jasinski et al., 2017), further establishing the possibility of sirolimus for the treatment of immune thrombocytopenia. Blood levels peaked after 1 h in healthy subjects after administration of sirolimus. In stable renal transplant patients, the half-life of sirolimus can be monitored to be approximately 46–78 h. The study demonstrated that the mean bioavailability of sirolimus tablets was 27% higher compared to the solution and that the plasma protein binding of sirolimus was 92%, with approximately 97% being bound to serum proteins. The metabolism of sirolimus is mainly carried out by CYP3A4 enzyme, and after hepatic metabolism, it is excreted out of the body mainly in the feces, and only a small amount is excreted out of the urine. Regarding the safety of sirolimus, the most common side effect was found to be grade 1–2 mucositis, in addition to many other adverse reactions such as stomatitis, oral ulcers, hyperlipidemia, infection and hepatic impairment, but it was found that most of these adverse reactions are dose dependent and the symptoms of these adverse reactions can be alleviated when the blood concentration of sirolimus decreases (Bride et al., 2016; Long et al., 2018; Chen et al., 2020b; Feng et al., 2020). In addition to mTOR inhibitors, other targets on the PI3K-AKT-mTOR pathway still exist, such as PI3K. Alpelisib was the first PI3Kα inhibitor identified and is currently approved and widely used for the treatment of breast cancer, in addition to being approved by the FDA in 2020 as a fast track for the treatment of the PIK3CA-associated overgrowth disease spectrum. During preclinical modeling, it was found to inhibit two common mutation sites of PI3K (H1047R and E545K) (Fritsch et al., 2014), in addition to having a dual mechanism of action, i.e., simultaneous inhibition of PI3K as well as induction of p110α degradation (Chang et al., 2021), and these highlight the possibility of its development as an immunosuppressive agent.
Keap1-Nrf2-ARE Pathway
The Keap-Nrf2-ARE pathway is a key pathway for cellular resistance to oxidative stress and has neutralizing, antioxidant as well as detoxifying effects because it regulates antioxidant enzymes and phase II detoxifying enzymes. This pathway is often used as a drug target to treat a variety of diseases, including neurodegenerative diseases, cancer, cardiovascular diseases, respiratory diseases, and various inflammatory conditions. For example, quercetin can enhance brain function in learning memory by upregulating the expression of Nrf2 and the antioxidant gene OH-1 thereby reducing oxidative stress in the brain (Silva et al., 2017), and resveratrol can effectively protect against oxidative damage due to renal hyperglycemia mediated by upregulating antioxidant genes including catalase (CAT), GSH-Px, SOD and HO-1 (Muselin and Cristina, 2019). The Keap1-Nrf2-ARE pathway consists of three core components, Keap1, Nrf2, and ARE, which are activated to transcribe and express downstream antioxidant genes through various targets in this pathway. Under normal physiological conditions, most of Nrf2 couples to the Neh2 structural domain on Keap1 in an overall stable intracellular environment and anchors to the cytoplasm in conjunction with cytoplasmic agonist proteins. In contrast, under oxidative stress as well as stimulation by electrophile substances, the electrically sensitive cysteine structure on Keap1 protein is mutated, resulting in a conformational change of Keap1 (Wakabayashi et al., 2004). Changes in the structure of Keap1 cause dissociation between it and Nrf2, and the activated Nrf2 translocates into the nucleus and binds to Maf proteins in the nucleus to form a heterodimer, which in turn binds to the ARE and regulates transcription of downstream target genes (Magesh et al., 2012). Besides, the variation of Keap1 structure can also reduce the degradation of Nrf2 ubiquitination, i.e., it can make the Nrf2 protein more stable (Kensler et al., 2007). The increased stability of Nrf2 protein is also associated with the phosphorylation of its degron region under oxidative stress and the resulting conformational change, which prevents its recognition by E3 ubiquitinylation ligase, thereby weakening the recognition of Nrf2 by the protease and activating the intrinsic transcriptional activity of Nrf2.It has been shown that Nrf2, upon dissociation from Keap1, also synergistically promotes activation of the intrinsic transcriptional activity of Nrf2 by its two active regions, Neh4 and Neh5 coactivators, CBP proteins (Nguyen et al., 2005). The downstream target proteins regulated by Nrf2 have now been shown to fall into several categories: phase II metabolic enzymes, antioxidant proteins/enzymes, proteasomal/molecular partners, anti-inflammatory factors, and phase III metabolic enzymes (i.e., drug transporters). Among them, the main ones of wide interest are quinone oxidoreductase-1 (NQO1), heme oxygenase-1 (HO-1) and γ-glutamylcysteine synthetase (γ-GCS), which exert antioxidant effects and thus treat related diseases (Figure 4). Nrf2 and NF-κB pathway are key factors sensitive to redox homeostasis, and the interaction mechanism between the two may lead to a variety of diseases, such as pharmacogenic liver diseases. When a drug stimulates oxidative stress in cells, Nrf2 increases the expression of antioxidant enzymes and GSH, neutralizes ROS in hepatocytes, helps to reduce the degree of oxidative stress, and inhibits NF-κB expression; on the contrary, if Nrf2 expression is absent, NF-κB is more active at this time, leading to the accumulation of inflammatory factors (Ganesh Yerra et al., 2013). Therefore, the use of Nrf2 activators and Nrf2 inhibitors affects not only the operation of the Keap1/ARE-Nrf2 pathway, but also the NF-κB pathway. Currently, a large number of drugs targeting this pathway have entered clinical trials, while some of them only have pharmacological indications, Table 4 provides a summary of some of the drugs targeting the Keap1-Nrf2-ARE pathway, with a brief overview of their indications and stages of study, etc.
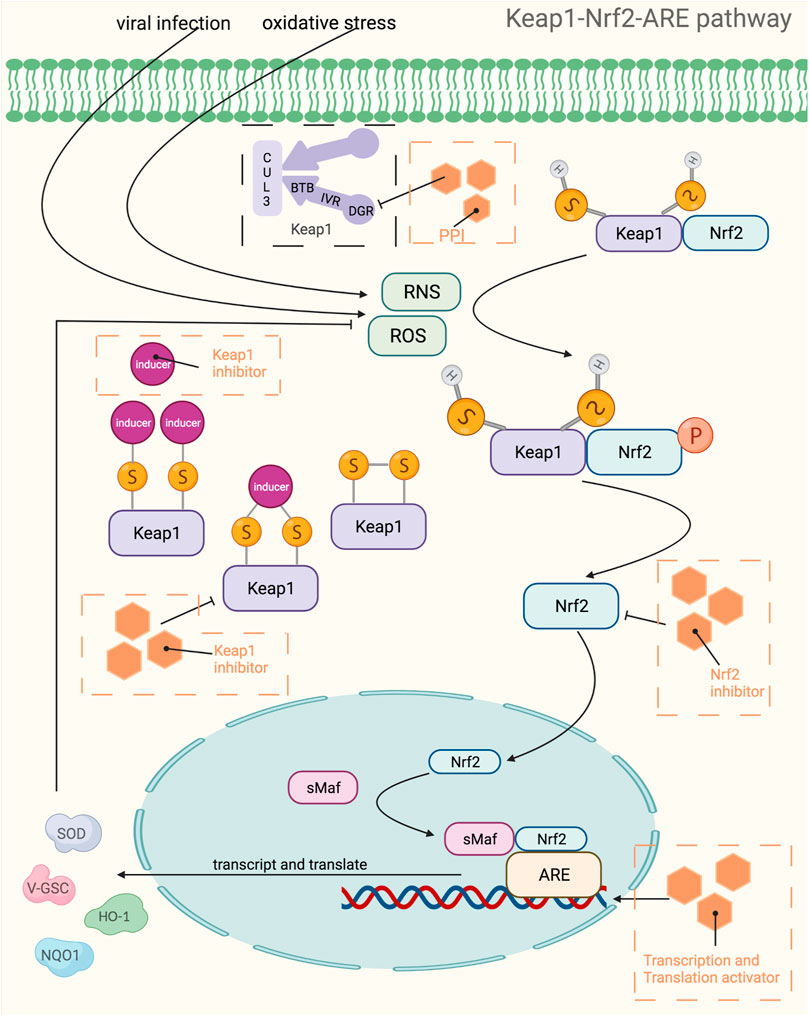
FIGURE 4. Mechanistic map of the Keap1-Nrf2-ARE signaling pathway. BTB, broad complex, tramtrack and bric-à-Brac; CUL3, cullin 3; DGR, double glycine repeat; HO-1, Heme Oxygenase-1; IVR, intervening region; NQO1, NAD(P)H:quinone oxidoreductase 1; PPI, proton pump inhibitors; RNS, reactive nitrogen species; ROS, reactive oxygen species; sMaf, specific macrophage activation factor; SOD, superoxide dismutase; GSC, glioblastoma stem cell. The figure is created with BioRender.com.
All-trans retinoic acid is an agonist of retinoic acid receptor alpha, which is a nuclear receptor agonist that inhibits the transcriptional activity of Nrf2 (Natalia et al., 2019). It was shown that all-trans retinoic acid could interfere with the dimerization between bZIP factors and small Maf proteins, which would severely affect the binding between Nrf2 and DNA (Nioi et al., 2003). In addition to this, all-trans retinoic acid can also bind to RARs as a ligand for RARs, further leading to the subnuclear relocalization of Nrf2 and affecting the delocalization of transcriptional intermediate factor 1 to the centromeric heterochromatin region (Cammas et al., 2002), all of which would demonstrate the inhibitory effect of all-trans retinoic acid on Nrf2. The treatment of acute promyelocytic leukemia is its most common use today and has been approved by the FDA for clinical treatment as early as a decade ago. All-trans retinoic acid exerts its therapeutic effect by inducing terminal dichotomization in leukemic cell lines as well as APL cells, and the first study in France found that patients with acute promyelocytic leukemia using all-trans retinoic acid had few reactions such as primary resistance and alopecia (Degos and Wang, 2001). In 2021, the FDA approved all-trans retinoic acid in combination with benzoyl peroxide in cream form for the treatment of acne vulgaris in patients 9 years of age and older, demonstrating its new use and enabling a wider range of applications. In addition to the topical treatment of acne vulgaris, it can also be used to treat psoriasis, congenital ichthyosis, ichthyosis vulgaris, lamellar ichthyosis, phyllodes keratoses and other skin conditions as well as to improve fine lines, hyperpigmentation, roughness and symptoms associated with photodamage. However, to ensure the safety and efficacy of the treatment, the related diseases are still in the clinical trial or recruitment stage. When treating skin diseases, only 1%–31% of the drug is absorbed by the skin, and when combined with benzoyl peroxide, the degree of absorption is again influenced by age. All-trans retinoic acid has a half-life of 0.5–2 h and is metabolized in the body mainly by the liver, with the end product being retinoic β-glucuronide. In safety studies of all-trans retinoic acid, it has been found that some patients develop leukocyte activation syndrome (Castaigne et al., 1990) and drug resistance (Frankel et al., 1992) after treatment of acute promyelocytic leukemia, and to minimize the occurrence of side effects, all-trans retinoic acid is now commonly used in combination with intensive chemotherapy or by high-dose injections of glucocorticoids to resist side effects (Degos and Wang, 2001).
Since the Keap1-Nrf2-ARE pathway is closely related to the body’s resistance to oxidative stress, its use as a therapeutic target can achieve considerable efficacy while excessive activation of the pathway makes it difficult to avoid adverse effects and side effects. Although this pathway can be used in the treatment of cancer, over-activation of this pathway can, on the contrary, greatly increase the chance of cancer induction and, in addition, may cause diseases such as atherosclerosis (Gonzalez-Donquiles et al., 2017; Lignitto et al., 2019). Over-activation of Nrf2 can increase the survival advantage of tumors or even develop chemotherapy resistance, therefore, another idea for tumor treatment can be adopted, namely, targeted inhibition of Nrf2 and thus sensitization therapy. Besides, there are few natural compounds such as opium bitter alcohol and lignan that can be used as Nrf2 inhibitors in clinical practice. These would suggest that Nrf2 is a double-edged sword, and when conducting treatment, a reasonable individualized treatment plan should be designed and the correct dosing regimen should be chosen according to the patient’s condition and individual circumstances.
MAPK Pathway
MAPKs are a class of threonine or serine protein kinases that are expressed in all eukaryotic cells. Activation of MAPK shows a typical three-stage enzymatic cascade reaction, namely MAP3K-MAP2K-MAPK chain. Upon activation of the upstream protein by cytokines, cellular stress, hormones, and neurotransmitters, the chain shows a cascade phosphorylation, thus transmitting the upstream signal to the downstream response molecules, which in turn are involved in the cellular anti-stress and anti-inflammatory responses. MAPKs signaling pathways play an important role in mediating cellular responses, and are widely involved in cell growth and reproduction, apoptosis, and a variety of cellular biochemical reactions. The pathways mediated by these four isoforms (JNK pathway, p38MAPK pathway, MEK5/ERK5 pathway, and ERK1/ERK2 pathway) are widely involved in the inflammatory, oxidative stress, and extracellular metabolic responses of cells in the body. ERK is one of the first MAPK isoforms to be identified and has five isoforms (ERK1 to ERK5), among which ERK1 and ERK2 are the most intensively studied and have a high degree of homology (Guo et al., 2008). The signaling pathway mediated by ERK1/ERK2 is mainly a signaling axis consisting of Ras, Raf, MEK, and ERK, through which upstream signals are transmitted step by step, leading to the regulation of multiple downstream genes, ultimately leading to the regulation of multiple genes downstream. In normal resting cells, Ras binds to GDP in an inactive state, while when the cell is stimulated by the outside world, Ras binds to GTP, which has one more phosphate group than GDP, and converts to an active state. The extra phosphate group puts the two switches (threonine-35 and glycine-60) in a “load spring” state, and when the phosphate group is released, the switch site shifts back to the inactive state (Santarpia et al., 2012). Ras-GTP induces Raf binding to Ras, mobilizes inactive proteins in the cytoplasm, and causes Raf kinase to accumulate at the cytosolic membrane (Chong et al., 2003; Wellbrock et al., 2004). When the Ras-Raf complex reaches the cell membrane, Ras can activate the function of the Raf isoform of serine/threonine kinase. When the Ras-Raf complex reaches the cell membrane, Ras activates the function of the Raf isoform of serine/threonine kinase. Activated Raf with its C-terminal catalytic region binds to MEK, phosphorylating the two serines (Ser221 and Ser217) in its subregion and activating MEK. Activated MEK can in turn phosphorylate the dual threonine and tyrosine sites on ERK, which activates ERK (Tyr183 and Tyr185 for ERK1 phosphorylation sites and Tyr202 and Tyr204 for ERK2 phosphorylation sites). ERK1 and ERK2 are activated for nuclear translocation and regulate many effector genes that will be relevant to cell proliferation, differentiation, survival, growth, and angiogenesis (Crews et al., 1992; Meloche and Pouysségur, 2007; Mebratu and Tesfaigzi, 2009). The ERK5 pathway, also known as the BMK1 pathway, can be activated by epidermal growth factors and a variety of extracellular stimuli, including hyperosmolarity, hypoxia, oxidants, and fluid shear stress. Tyr218 and Tyr220 on ERK5 are activated by regulation of the upstream protein kinase MAPKK5, which, like ERK1 and ERK2, undergoes nuclear translocation and regulates the corresponding genes regulation (Figure 5). The ERK5 pathway is also important for cell proliferation and differentiation and organogenesis. For example, Sohn et al. showed that it is ERK5, but not ERK1/2, that plays a key role in the developmental maturation of thymocytes, revealing that ERK5 has a role in mediating the differentiation of T lymphocytes (Sohn et al., 2008). The c-Jun amino-terminal transferase (JNK) was discovered during the study of a series of biological processes (UV responses) in cells exposed to ultraviolet radiation (UV), and it mainly regulates the phosphorylation of activated proteins such as c-Jun (Devary et al., 1992). The JNK pathway is activated after cells are exposed to various biotic or abiotic stress events, such as infection, inflammation, oxidative and other stresses, DNA damage, osmotic stress, or cytoskeletal changes (Zeke et al., 2016). In addition, G proteins such as Rac, CDC-42, tumor necrosis factor receptor-associated factor-based bridging proteins, and death-effector domain-containing proteins can also regulate JNK activation (Schattenberg et al., 2012). MAPK is activated by MAPK kinases (MKKs, MEKs, JNKKs, MAP2Ks), which are activated by MKK kinases (MEKKs, MAPKKKs, MAP3Ks). The first MAP3K found to activate JNK was MEKK1 (Minden et al., 1994). Subsequently, MEKK2 and MEKK3, MEKK4, mixed family kinases 2 and 3 (MLK2, MLK3), double leucine pull chain kinase (DLK), tumor transposon-2 (Tpl-2), TGF-β activating kinase (TAK1), apoptosis signaling regulatory proteases 1 and 2 (ASK1, ASK2), and 1001 amino acid kinases 1 and 2 (Tao1, Tao2) were identified (Karin and Gallagher, 2005). The two MAP2Ks specific to the JNK pathway are MKK4 and MKK7, with MKK4 more likely to phosphorylate the 185th tyrosine residue of JNK, while MKK7 prefers the 183rd tyrosine residue (Lawler et al., 1998). After JNK is activated, it then activates numerous downstream substrates that are involved in numerous intracellular functions, including apoptosis, cytoskeletal reorganization, transcriptional activity, and universal proteinization (Chen et al., 2001). The most common p38MAPK activators are lipopolysaccharides, in addition to osmotic stress, oxidative stress, UV exposure, heat shock, hypoxia, ischemia, interleukin-1β (IL-1β), tumor necrosis factor-α (TNF-α), and transforming growth factor-β (TGF-β), and neuropathic pain (Koul et al., 2013). Unlike the JNK pathway, p38 is mainly activated by two MAPKKs, MKK3 and MKK6, and activation of p38 requires simultaneous dual phosphorylation of threonine and tyrosine.As mentioned before G proteins such as Rac, CDC-42 can activate the JNK pathway, and its activation of p38 is also promoted. Rac1 can bind to MEKK1 or MLK1, while Cdc42 can only bind to MLK1, both of which can lead to the activation of p38 through MAP3Ks (Tibbles et al., 1996; Hirai et al., 1997). p38 then nuclear translocates and acts on downstream substrates, which include a large number of transcription factors such as activated transcription factors 1, 2 and 6 (ATF-1/2/6), SRF accessory protein (Sap1), CHOP (growth arrest and DNA damage inducible gene 153, or GADD153), p53, C/EBPβ, myocyte enhancer factor 2C (MEF2C) MEF2A, MITF1, DDIT3, ELK1, NFAT, and high mobility histone box protein 1 (HBP1) (Zarubin and Han, 2005) (Figure 6). Due to the complexity of the MAPK signaling pathway, resulting in a wealth of targets for its action, researchers have so far conducted a lot of research and development on inhibitors of various targets on this pathway. Table 5 shows a summary of some of the targeted inhibitors of the MAPK pathway, with a list of their indications and stages of research.
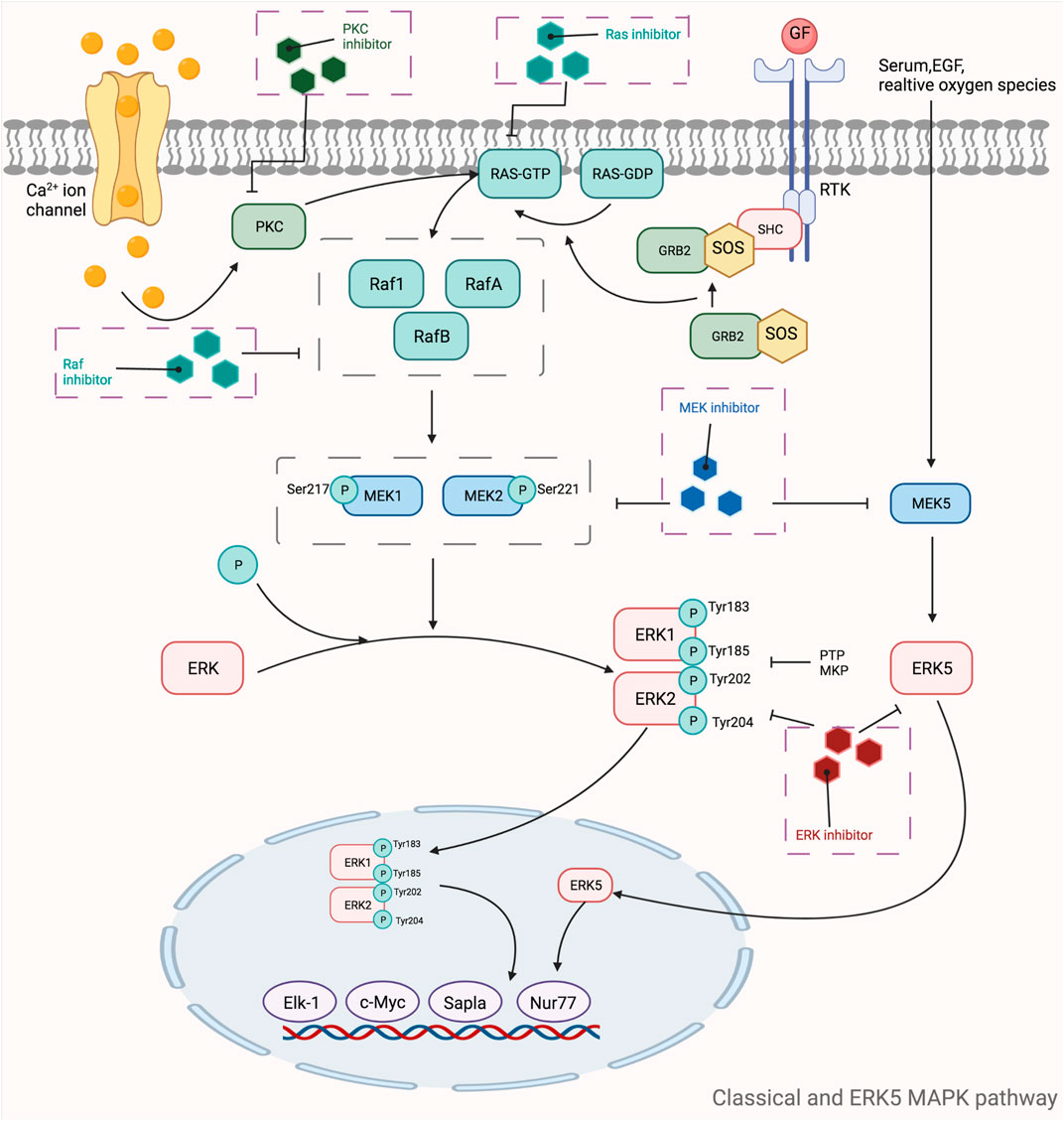
FIGURE 5. Mechanistic map of the classical and ERK5 MAPK signaling pathway. c-Myc, cell-myc; EGF, epidermal growth factor; Elk-1, ETS domain-containing protein Elk-1; ERK, extracellular regulated protein kinases; GDP, guanosine diphosphate; GTP, guanosinetriphosphate; GRB2, growth factor receptor-bound protein 2; MEK, mitogen-activated protein kinase kinase; MKP, mitogen-activated protein kinase phosphatase1; Nur77, nerve growthfactor-induced gene B; PKC, protein kinase C; PTP, protein tyrosine phosphatase; Ras, rennin angiotensin system; RTK, receptor tyrosine kinases; SHC, Src homology 2 domain containing; SOS, son of sevenless The figure is created with BioRender.com.
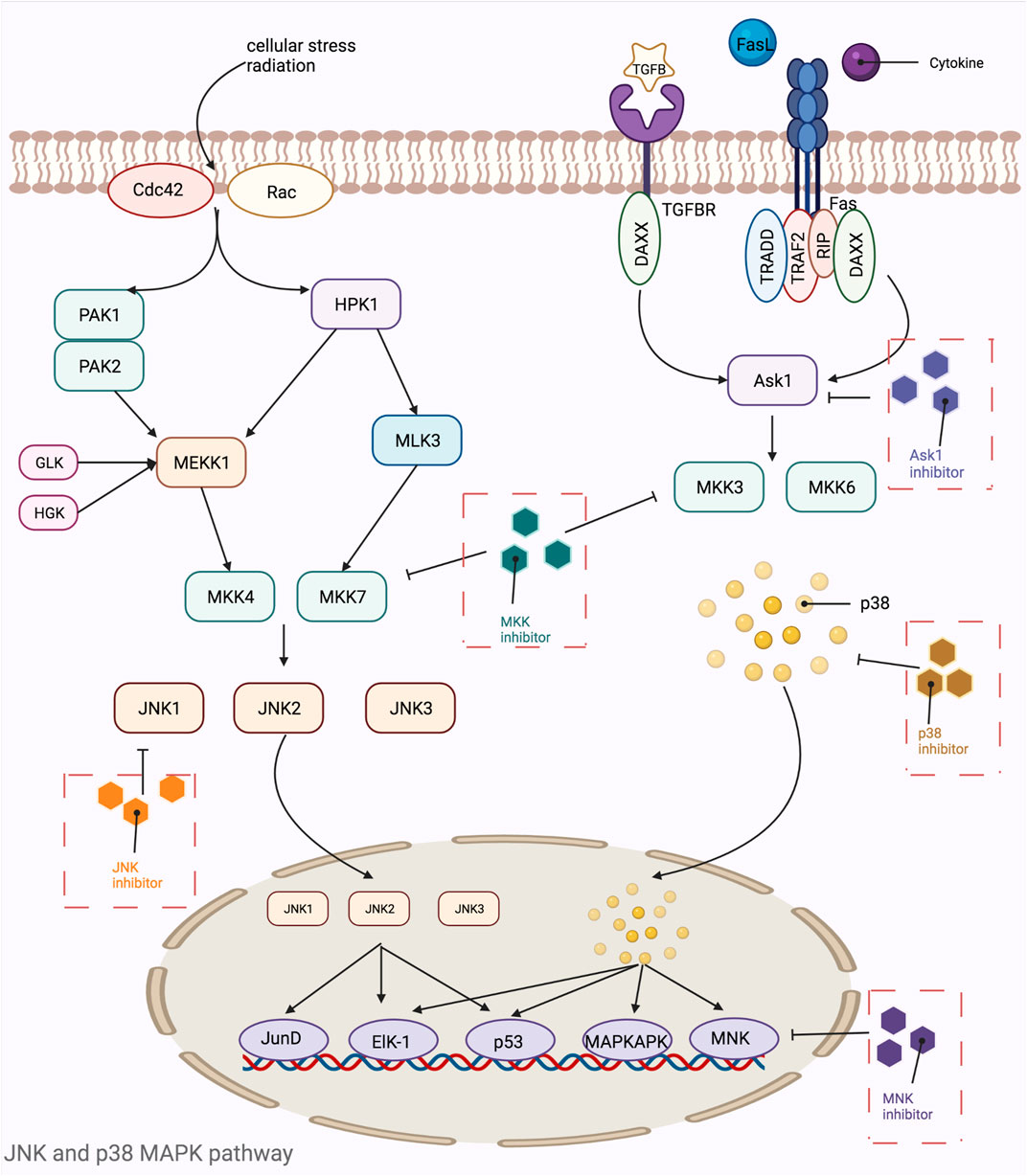
FIGURE 6. Mechanistic map of the JNK and p38 MAPK signaling pathway. Ask1, apoptosis signal-regulating kinase 1; Cdc42, cell division cycle 42; DAXX, death domain-associated protein; Elk-1, ETS domain-containing protein Elk-1; Fas, fatty acid synthase; GLK, glucokinase; HGK, human glandular kallikrein; HPK, histidine protein kinases; JNK, c-Jun N-terminal kinase; MAPKAPK, mitogen activated protein kinase activated protein kinase; MKK, mitogen-activated protein kinase kinase; MLK, mixed lineage kinase; MNK, mitogen-activated protein kinase interacting kinase; PAK, p21-activated kinase; Rac, Ras-related C3 botulinum substrate; RIP, receptor-interacting protein; TGFB, transforming growth factor; TRADD, TNF receptor-associated death domain; TRAF, tumor necrosis factor receptor associated factor. The figure is created with BioRender.com.
Sulindac is an FDA-approved drug for the treatment of autoimmune diseases such as rheumatoid arthritis that blocks the MAPK pathway and whose primary target is HRAs (one of four highly homologous proteins encoded by the Ras gene) (O'Bryan Pharmacological targeting of RAS, 2019). Subsequently, it was shown that sulforaphane sulfide, a metabolite of sulforaphane, could directly block Ras activation of Raf and reduce Ras-mediated transformation in vitro (Herrmann et al., 1998). Of course, sulforaphane does not only act on one pathway, but it can also exert its effects by inhibiting the NF-κB pathway, which has been shown to inhibit NF-κB activation by binding to the ATP-binding site of IKK and regulating RelA nuclear translocation (Berman et al., 2002). Approximately 93% of sulindac and the prototype drug and 98% of the sulfated metabolites are bound to serum albumin after oral administration, with the liver being an important elimination pathway. Currently, approximately 50% of sulindac is excreted in the urine, and studies have found that sulindac can be excreted from rat milk, while it is debatable whether it can be excreted from human milk. Between the complexity of Ras targeting and the fact that the Ras family is one of the most commonly mutated genes in tumor cancers, researchers have put a great deal of effort into the family. Until a decade ago, researchers had not found an effective Ras inhibitor, so much so that Ras was used as an ineffective therapeutic target. However, after nearly 3 decades of research, a breakthrough point in Ras inhibitor research has emerged and tremendous progress has been made to date, with tremendous scope for research. The first Ras inhibitor to enter clinical trials was AMG510, which has now been cleared by the FDA for marketing. It was found that the target of action is mainly KRAS-G12C, which can be covalently inhibited by cysteine at codon 12 of the gene, whereas wild-type KRAS does not have covalently bindable cysteine specifically, so AMG510 is a specific target drug for this commonly mutated region of G12C (Moore et al., 2020). Subsequently, researchers have identified other Ras inhibitors that are partially in clinical trials, such as Adagrasib, JNJ-74699157, and LY3499446, while some are still in the preclinical study evaluation stage and have not entered clinical trials or marketing, such as ARS-853 and ARS-1620. In addition to the target KRAS-G12C, Shokat et al. identified and defined for the first time a metamorphic binding pocket located in the switch-II region of the G12C mutation, for which they designed a series of irreversible inhibitory compounds that resulted in a good inhibitory effect on the pathway (Ostrem et al., 2013), thus showing a new research direction and a good research prospect for Ras inhibitors.
Although the treatment of cancer patients or patients with neurodegenerative lesions with inhibitors targeting this pathway has shown a good trend of prolonged survival and good improvement of lesion symptoms, these inhibitors still inevitably cause side effects. For example, Stephnie et al. reported that patients treated with the MEK inhibitor trametinib for melanoma experienced prolonged visual loss that did not completely resolve after discontinuation of the drug and could even progress to extensive uveitis and multiple plasmacytoid retinal detachments (Sarny et al., 2017). The mechanism of the complication of this ocular side effect cannot be elucidated at this time, but physicians and pharmacists are cautioned to use the medication carefully and to adjust it within the therapeutic window. In addition to this, in a study of the patient population when trametinib was combined with dabrafenib in the treatment of non-small cell lung cancer, it was found that most patients experienced systemic adverse effects such as fever, skin inflammation, mouth ulcers, diarrhea, and loss of appetite (Chalmers et al., 2019), but this was mainly less related to the inhibition of the MAPK pathway and more due to drug metabolites or drug. This is less related to MAPK pathway inhibition and more to pathological changes caused by drug metabolites or drugs themselves. However, this is also a warning to physicians and pharmacists to master the balance between the therapeutic effects of drugs and adverse drug reactions.
Conclusion
This article reviews representative targets and their inhibitors on the JAK-STAT, NF-κB, PI3K-AKT-mTOR, MAPK, and Keap1-Nrf2-ARE pathways, and indicates their current research stages and indications, thus facilitating researchers to conduct in-depth comparative studies on drugs with the same targets. A large number of studies and clinical observations have demonstrated the efficacy of targeted immunosuppressive agents in chronic inflammatory diseases, but a variety of adverse effects or ethical issues have resulted in relatively few marketed drugs for human use, and most of the drugs found to be effective have been forced to end up in clinical trials or preclinical studies. Researchers are now working to find commonalities between immunosuppressive agents of the same target and to study the structural similarities of the drugs, thus facilitating further development work on the target molecules. In addition to this, researchers face the challenge of studying the targeting of drugs to specific cells or tissues, i.e. the detailed study of the pharmacokinetics of a particular drug. It is hoped that the therapeutic potential and safety of small molecule immunosuppressive agents/targeted therapy immunosuppressive agents will be further demonstrated and evaluated to achieve more interventions, improvements and treatments for chronic diseases.
Although the widespread use of immunosuppressive agents has solved many problems in autoimmune diseases and organ transplantation, among others, the road to immunosuppression has never stopped. Currently, the world is still facing a shortage of donor organs for transplantation, which will mean that we will need to find xenogeneic donors, such as pigs, thus alleviating the lack of donors. For example, today porcine xenografts and hepatocyte transplants are gradually being classified into human treatment options for liver diseases. However, it has been found that xenografted porcine grafts can cause many adverse reactions such as rejection, coagulation disorders, and thrombocytopenia while performing a liver support role (Li et al., 2022). Even with porcine modified donor livers (PERV-KO/3-KO/9-TG), humoral rejection, interstitial hemorrhage, and inflammatory injury still occur. Therefore, the focus of transplantation is now more towards porcine allogeneic hepatocyte transplantation. The lower immunogenicity of genetically modified porcine hepatocytes has led to a much higher success rate of transplantation, and it is hypothesized that the key to successful cellular xenotransplantation is related to the source of blood for liver perfusion. In contrast to whole organs that are perfused by the donor’s vessels, the blood supply for cellular grafts originates from the recipient (Parker et al., 1996; Cascalho and Platt, 2001). Although the success rate of surgery is gradually improving, there is still a need to pursue more efficient immunosuppression combined with more excellent genetic modification protocols, which can not only solve the problem from the donor, but also improve the prognostic quality of the recipient in the postoperative period.This raises thoughts and requirements for in-depth development and flexible combination applications of immunosuppressive agents.
Author Contributions
Literature review and writing—original draft preparation: ZX. Writing—review and editing: MC. Supervision and funding acquisition: MC. All authors have read and agreed to the published version of the manuscript.
Funding
This work was supported by National Natural Science Foundation of China (81603119) and Natural Science Foundation of Beijing Municipality (7174316). This work was sponsored by the interdisciplinary medicine Seed Fund of Peking University BMU2021MX021; supported by the Fundamental Research Funds for the Central Universities. The figure in this manuscript was created with BioRender.com.
Conflict of Interest
The authors declare that the research was conducted in the absence of any commercial or financial relationships that could be construed as a potential conflict of interest.
Publisher’s Note
All claims expressed in this article are solely those of the authors and do not necessarily represent those of their affiliated organizations, or those of the publisher, the editors and the reviewers. Any product that may be evaluated in this article, or claim that may be made by its manufacturer, is not guaranteed or endorsed by the publisher.
References
Abe, K., Saitoh, T., Horiguchi, Y., Utsunomiya, I., and Taguchi, K. (2005). Synthesis and Neurotoxicity of Tetrahydroisoquinoline Derivatives for Studying Parkinson's Disease. Biol. Pharm. Bull. 28 (8), 1355–1362. doi:10.1248/bpb.28.1355
Agrawal, M., Kim, E. S., and Colombel, J. F. (2020). JAK Inhibitors Safety in Ulcerative Colitis: Practical Implications. J. Crohns Colitis 14, S755–S760. doi:10.1093/ecco-jcc/jjaa017
Alfonso, L., Ai, G., Spitale, R. C., and Bhat, G. J. (2014). Molecular Targets of Aspirin and Cancer Prevention. Br. J. Cancer 111, 61–67. doi:10.1038/bjc.2014.271
Alhazzani, K., Ahmad, S. F., Al-Harbi, N. O., Attia, S. M., Bakheet, S. A., Sarawi, W., et al. (2021). Pharmacological Inhibition of STAT3 by Stattic Ameliorates Clinical Symptoms and Reduces Autoinflammation in Myeloid, Lymphoid, and Neuronal Tissue Compartments in Relapsing-Remitting Model of Experimental Autoimmune Encephalomyelitis in SJL/J Mice. Pharmaceutics 13 (7), 925. doi:10.3390/pharmaceutics13070925
Aminzadeh, Z., Ziamajidi, N., and Abbasalipourkabir, R. (2021). Anticancer Effects of Cinnamaldehyde through Inhibition of ErbB2/HSF1/LDHA Pathway in 5637 Cell Line of Bladder Cancer. Anticancer Agents Med. Chem. doi:10.2174/1871520621666210726142814
Amos, L. A., Ma, F. Y., Tesch, G. H., Liles, J. T., Breckenridge, D. G., Nikolic-Paterson, D. J., et al. (2018). ASK1 Inhibitor Treatment Suppresses P38/JNK Signalling with Reduced Kidney Inflammation and Fibrosis in Rat Crescentic Glomerulonephritis. J. Cell. Mol. Med. 22 (9), 4522–4533. doi:10.1111/jcmm.13705
An, J. Y., Pang, H. G., Huang, T. Q., Song, J. N., Li, D. D., Zhao, Y. L., et al. (2018). AG490 Ameliorates Early Brain Injury via Inhibition of JAK2/STAT3-Mediated Regulation of HMGB1 in Subarachnoid Hemorrhage. Exp. Ther. Med. 15 (2), 1330–1338. doi:10.3892/etm.2017.5539
Arabi, Y. M., Mandourah, Y., Al-Hameed, F., Sindi, A. A., Almekhlafi, G. A., Hussein, M. A., et al. (2018). Corticosteroid Therapy for Critically Ill Patients with Middle East Respiratory Syndrome. Am. J. Respir. Crit. Care Med. 197 (6), 757–767. doi:10.1164/rccm.201706-1172OC
Arlt, A., Sebens, S., Krebs, S., Geismann, C., Grossmann, M., Kruse, M. L., et al. (2013). Inhibition of the Nrf2 Transcription Factor by the Alkaloid Trigonelline Renders Pancreatic Cancer Cells More Susceptible to Apoptosis through Decreased Proteasomal Gene Expression and Proteasome Activity. Oncogene 32 (40), 4825–4835. doi:10.1038/onc.2012.493
Asano, Y., Kitamura, S., Ohra, T., Itoh, F., Kajino, M., Tamura, T., et al. (2008). Discovery, Synthesis and Biological Evaluation of Isoquinolones as Novel and Highly Selective JNK Inhibitors (2). Bioorg Med. Chem. 16 (8), 4699–4714. doi:10.1016/j.bmc.2008.02.028
Avci, A. B., Feist, E., and Burmester, G. R. (2021). The Role of Upadacitinib in the Treatment of Moderate-To-Severe Active Rheumatoid Arthritis. Ther. Adv. Musculoskelet. Dis. 13, 131759720X211047662. doi:10.1177/1759720X211047662
Awasthee, N., Rai, V., Chava, S., Nallasamy, P., Kunnumakkara, A. B., Bishayee, A., et al. (2019). Targeting IκappaB Kinases for Cancer Therapy. Semin. Cancer Biol. 56, 12–24. doi:10.1016/j.semcancer.2018.02.007
Azuma, M., Yamashita, T., Aota, K., Tamatani, T., and Sato, M. (2001). 5-Fluorouracil Suppression of NF-KappaB Is Mediated by the Inhibition of IKappab Kinase Activity in Human Salivary Gland Cancer Cells. Biochem. Biophys. Res. Commun. 282 (1), 292–296. doi:10.1006/bbrc.2001.4571
Ba, W., Xu, Y., Yin, G., Yang, J., Wang, R., Chi, S., et al. (2019). Metformin Inhibits Pro-inflammatory Responses via Targeting Nuclear Factor-Κb in HaCaT Cells. Cell. Biochem. Funct. 37 (1), 4–10. doi:10.1002/cbf.3367
Bagley, M. C., Davis, T., Murziani, P. G., Widdowson, C. S., and Kipling, D. (2010). Use of P38 MAPK Inhibitors for the Treatment of Werner Syndrome. Pharm. (Basel) 3 (6), 1842–1872. doi:10.3390/ph3061842
Ballou, L. M., and Lin, R. Z. (2008). Rapamycin and mTOR Kinase Inhibitors. J. Chem. Biol. 1, 27–36. doi:10.1007/s12154-008-0003-5
Banerjee, A. A., Shen, H., Hautman, M., Anwer, J., Hong, S., Kapetanovic, I. M., et al. (2013). Enhanced Oral Bioavailability of the Hydrophobic Chemopreventive Agent (SR13668) in Beagle Dogs. Curr. Pharm. Biotechnol. 14 (4), 464–469. doi:10.2174/1389201011314040012
Banerjee, A., Koziol-White, C., and Panettieri, R. (2012). p38 MAPK Inhibitors, IKK2 Inhibitors, and TNFα Inhibitors in COPD. Curr. Opin. Pharmacol. 12 (3), 287–292. doi:10.1016/j.coph.2012.01.016
Barrett, S. D., Bridges, J., Dudley, D. T., Saltiel, A. R., Fergus, J. H., Flamme, C. M., et al. (2008). The Discovery of the Benzhydroxamate MEK Inhibitors CI-1040 and PD 0325901. Bioorg. Med. Chem. Lett. 18, 6501–6504. doi:10.1016/j.bmcl.2008.10.054
Bassères, D. S., Ebbs, A., Cogswell, P. C., and Baldwin, A. S. (2014). IKK Is a Therapeutic Target in Kras-Induced Lung Cancer with Disrupted P53 Activity. Genes. Cancer 5, 41–55. doi:10.18632/genesandcancer.5
Ben-Neriah, Y., and Karin, M. (2011). Inflammation Meets Cancer, with NF-Κb as the Matchmaker. Nat. Immunol. 12 (8), 715–723. doi:10.1038/ni.2060
Berdeja, J., Palandri, F., Baer, M. R., Quick, D., Kiladjian, J. J., Martinelli, G., et al. (2018). Phase 2 Study of Gandotinib (LY2784544) in Patients with Myeloproliferative Neoplasms. Leuk. Res. 71, 82–88. doi:10.1016/j.leukres.2018.06.014
Berman, K. S., Verma, U. N., Harburg, G., Minna, J. D., Cobb, M. H., and Gaynor, R. B. (2002). Sulindac Enhances Tumor Necrosis Factor-Alpha-Mediated Apoptosis of Lung Cancer Cell Lines by Inhibition of Nuclear Factor-kappaB. Clin. Cancer Res. 8 (2), 354–360.
Bernier, M., Kwon, Y. K., Pandey, S. K., Zhu, T. N., Zhao, R. J., Maciuk, A., et al. (2006). Binding of Manumycin a Inhibits IkappaB Kinase Beta Activity. J. Biol. Chem. 281, 2551–2561. doi:10.1074/jbc.M511878200
Beydoun, T., Deloche, C., and Perino, J. (2015). Subconjunctival Injection of XG-102, a JNK Inhibitor Peptide, in Patients with Intraocular Inflammation: a Safety and Tolerability Study. J. Ocul. Pharmacol. Ther. 31, 93–99. doi:10.1089/jop.2013.0247
Bhagwat, S. V., Gokhale, P. C., Crew, A. P., Cooke, A., Yao, Y., Mantis, C., et al. (2011). Preclinical Characterization of OSI-027, a Potent and Selective Inhibitor of mTORC1 and mTORC2: Distinct from Rapamycin. Mol. Cancer Ther. 10, 1394–1406. doi:10.1158/1535-7163.MCT-10-1099
Bhat, S. S., Prasad, S. K., Shivamallu, C., Prasad, K. S., Syed, A., Reddy, P., et al. (2021). Genistein: A Potent Anti-breast Cancer Agent. Curr. Issues Mol. Biol. 43 (3), 1502–1517. doi:10.3390/cimb43030106
Biggioggero, M., Becciolini, A., Crotti, C., Agape, E., and Favalli, E. G. (2019). Upadacitinib and Filgotinib: the Role of JAK1 Selective Inhibition in the Treatment of Rheumatoid Arthritis. Drugs Context 8, 212595. doi:10.7573/dic.212595
Birrell, M. A., Wong, S., Hardaker, E. L., Catley, M. C., McCluskie, K., Collins, M., et al. (2006). IkappaB Kinase-2-independent and -dependent Inflammation in Airway Disease Models: Relevance of IKK-2 Inhibition to the Clinic. Mol. Pharmacol. 69, 1791–1800. doi:10.1124/mol.105.019521
Block, M., Fister, S., Emons, G., Seeber, S., Gründker, C., and Günthert, A. R. (2010). Antiproliferative Effects of Antiestrogens and Inhibitors of Growth Factor Receptor Signaling on Endometrial Cancer Cells. Anticancer Res. 30, 2025–2031.
Bollong, M. J., Yun, H., Sherwood, L., Woods, A. K., Lairson, L. L., and Schultz, P. G. (2015). A Small Molecule Inhibits Deregulated NRF2 Transcriptional Activity in Cancer. ACS Chem. Biol. 10 (10), 2193–2198. doi:10.1021/acschembio.5b00448
Boonlarppradab, C., Suriyachadkun, C., Rachtawee, P., and ChoowongSaccharosporones A, W. B. and. C. (2013). Saccharosporones A, B and C, Cytotoxic Antimalarial Angucyclinones from Saccharopolyspora Sp. BCC 21906. J. Antibiot. (Tokyo) 66 (6), 305–309. doi:10.1038/ja.2013.16
Bors, L., Bajza, Á., Kocsis, D., and Erdő, F. (2018)., 159. Hungarian, 384–390. doi:10.1556/650.2018.30993Koffein: Hagyományos És Új Terápiás Indikációk, Valamint Felhasználás Dermatológiai Modellvegyületként [Caffeine: Traditional and New Therapeutic Indications and Use as a Dermatological Model Drug]Orv. Hetil.10
Bride, K. L., Vincent, T., Smith-Whitley, K., Lambert, M. P., Bleesing, J. J., Seif, A. E., et al. (2016). Sirolimus Is Effective in Relapsed/refractory Autoimmune Cytopenias: Results of a Prospective Multi-Institutional Trial. Blood 127 (1), 17–28. doi:10.1182/blood-2015-07-657981
Brimson, J. M., Onlamoon, N., Tencomnao, T., and Thitilertdecha, P. (2019). Clerodendrum Petasites S. Moore: The Therapeutic Potential of Phytochemicals, Hispidulin, Vanillic Acid, Verbascoside, and Apigenin. Biomed. Pharmacother. 118, 109319. doi:10.1016/j.biopha.2019.109319
Budas, G. R., Boehm, M., Kojonazarov, B., Viswanathan, G., Tian, X., Veeroju, S., et al. (2018). ASK1 Inhibition Halts Disease Progression in Preclinical Models of Pulmonary Arterial Hypertension. Am. J. Respir. Crit. Care Med. 197 (3), 373–385. doi:10.1164/rccm.201703-0502OC
Bunn, P. A., Chan, D. C., Earle, K., Zhao, T. L., Helfrich, B., Kelly, K., et al. (2002). Preclinical and Clinical Studies of Docetaxel and Exisulind in the Treatment of Human Lung Cancer. Semin. Oncol. 29 (1 Suppl. 4), 87–94. doi:10.1053/sonc.2002.31529
Buontempo, F., Chiarini, F., Bressanin, D., Tabellini, G., Melchionda, F., Pession, A., et al. (2012). Activity of the Selective IκB Kinase Inhibitor BMS-345541 against T-Cell Acute Lymphoblastic Leukemia: Involvement of FOXO3a. Cell. Cycle 11, 2467–2475. doi:10.4161/cc.20859
Butt, S. S., Khan, K., Badshah, Y., Rafiq, M., and Shabbir, M. (2021). Evaluation of Pro-apoptotic Potential of Taxifolin against Liver Cancer. PeerJ 9, e11276. doi:10.7717/peerj.11276
Cammas, F., Oulad-Abdelghani, M., Vonesch, J. L., Huss-Garcia, Y., Chambon, P., and Losson, R. (2002). Cell Differentiation Induces TIF1beta Association with Centromeric Heterochromatin via an HP1 Interaction. J. Cell. Sci. 115 (Pt 17), 3439–3448. doi:10.1242/jcs.115.17.3439
Canon, J., Rex, K., Saiki, A. Y., Mohr, C., Cooke, K., Bagal, D., et al. (2019). The Clinical KRAS(G12C) Inhibitor AMG 510 Drives Anti-tumour Immunity. Nature 575 (7781), 217–223. doi:10.1038/s41586-019-1694-1
Cascalho, M., and Platt, J. L. (2001). The Immunological Barrier to Xenotransplantation. Immunity 14 (4), 437–446. doi:10.1016/s1074-7613(01)00124-8
Castaigne, S., Chomienne, C., Daniel, M. T., Ballerini, P., Berger, R., Fenaux, P., et al. (1990). Blood, 76, 1704 ± 1713.doi:10.1182/blood.v76.9.1704.1704
Catlett, I. M., Hu, Y., Gao, L., Banerjee, S., Gordon, K., and Krueger, J. G. (2021). Molecular and Clinical Effects of Selective Tyrosine Kinase 2 Inhibition with Deucravacitinib in Psoriasis. J. Allergy Clin. Immunol. (21), S0091–S6749. doi:10.1016/j.jaci.2021.11.001
Ceccarelli, M., D’Andrea, G., Micheli, L., Gentile, G., Cavallaro, S., Merlino, G., et al. (2021). Tumor Growth in the High Frequency Medulloblastoma Mouse Model Ptch1+/−/Tis21KO Has a Specific Activation Signature of the PI3K/AKT/mTOR Pathway and Is Counteracted by the PI3K Inhibitor MEN1611. Front. Oncol. 11, 692053. doi:10.3389/fonc.2021.692053
Cerbone, A., Toaldo, C., Minelli, R., Ciamporcero, E., Pizzimenti, S., Pettazzoni, P., et al. (2012). Rosiglitazone and AS601245 Decrease Cell Adhesion and Migration through Modulation of Specific Gene Expression in Human Colon Cancer Cells. PLoS One 7 (6), e40149. doi:10.1371/journal.pone.0040149
Cerbone, A., Toaldo, C., and Pizzimenti, S. (20122012). AS601245, an Anti-inflammatory JNK Inhibitor, and Clofibrate Have a Synergistic Effect in Inducing Cell Responses and in Affecting the Gene Expression Profile in CaCo-2 Colon Cancer Cells. PPAR Res., 1–16. doi:10.1155/2012/269751
Challa, S., Guo, J. P., Ding, X., Xu, C. X., Li, Y., Kim, D., et al. (2016). IKBKE Is a Substrate of EGFR and a Therapeutic Target in Non-small Cell Lung Cancer with Activating Mutations of EGFR. Cancer Res. 76 (15), 4418–4429. doi:10.1158/0008-5472.CAN-16-0069
Chandar, J., Abitbol, C., Montané, B., and Zilleruelo, G. (2007). Angiotensin Blockade as Sole Treatment for Proteinuric Kidney Disease in Children. Nephrol. Dial. Transpl. 22 (5), 1332–1337. doi:10.1093/ndt/gfl839
Chang, D. Y., Ma, W. L., and Lu, Y. S. (2021). Role of Alpelisib in the Treatment of PIK3CA-Mutated Breast Cancer: Patient Selection and Clinical Perspectives. Ther. Clin. Risk Manag. 17, 193–207. doi:10.2147/TCRM.S251668
Chen, B., Ning, M., and Yang, G. (2012). Effect of Paeonol on Antioxidant and Immune Regulatory Activity in Hepatocellular Carcinoma Rats. Molecules 17 (4), 4672–4683. doi:10.3390/molecules17044672
Chen, H., Li, M., Ng, N., Yu, E., Bujarski, S., Yin, Z., et al. (2021). Ruxolitinib Reverses Checkpoint Inhibition by Reducing Programmed Cell Death Ligand-1 (PD-L1) Expression and Increases Anti-tumour Effects of T Cells in Multiple Myeloma. Br. J. Haematol. 192 (3), 568–576. doi:10.1111/bjh.17282
Chen, J., Yin, W., Tu, Y., Wang, S., Yang, X., Chen, Q., et al. (0012). A Novel Multifunctional ROCK Inhibitor, Suppresses Neuroinflammation In Vitro and In Vivo: Involvement of NF-Κb Inhibition and Nrf2 Pathway Activation. Eur. J. Pharmacol. 806, 1–9. doi:10.1016/j.ejphar.2017.03.025
Chen, Y., Bi, X., Zhang, F., Sun, Z., Xu, P., Jiang, H., et al. (2020). Design, Synthesis, and Biological Evaluation of Tetrahydroquinolin Derivatives as Potent Inhibitors of CBP Bromodomain. Bioorg Chem. 101, 103991. doi:10.1016/j.bioorg.2020.103991
Chen, Y. L., Zeng, M., Liu, Y., Xu, Y., Bai, Y., Cao, L., et al. (2020). CHA2DS2-VASc Score for Identifying Patients at High Risk of Postoperative Atrial Fibrillation after Cardiac Surgery: A Meta-Analysis. Ann. Thorac. Surg. 109 (4), 1210–1216. doi:10.1016/j.athoracsur.2019.07.084
Chen, Z., Liu, X., Chen, M., Yang, C., and Han, B. (2020). Successful Sirolimus Treatment of Patients with Pure Red Cell Aplasia Complicated with Renal Insufficiency. Ann. Hematol. 99 (4), 737–741. doi:10.1007/s00277-020-03946-2
Chen, Z., Gibson, T. B., Robinson, F., Silvestro, L., Pearson, G., Xu, B., et al. (2001). MAP Kinases. Chem. Rev. 101 (8), 2449–2476. doi:10.1021/cr000241p
Chen, Z. J. (2005). Ubiquitin Signalling in the NF-kappaB Pathway. Nat. Cell. Biol. 7 (8), 758–765. doi:10.1038/ncb0805-758
Cheng, Y., and Tian, H. (2017). Current Development Status of MEK Inhibitors. Molecules 22 (10), 1551. doi:10.3390/molecules22101551
Chertow, G. M., Pergola, P. E., Chen, F., Kirby, B. J., Sundy, J. S., and Patel, U. D. (2019). Effects of Selonsertib in Patients with Diabetic Kidney Disease. J. Am. Soc. Nephrol. 30 (10), 1980–1990. doi:10.1681/ASN.2018121231
Chew, J., Biswas, S., Shreeram, S., Humaidi, M., Wong, E. T., Dhillion, M. K., et al. (2009). WIP1 Phosphatase Is a Negative Regulator of NF-kappaB Signalling. Nat. Cell. Biol. 11, 659–666. doi:10.1038/ncb1873
Chien, J. Y., Chou, Y. Y., Ciou, J. W., Liu, F. Y., and Huang, S. P. (2021). The Effects of Two Nrf2 Activators, Bardoxolone Methyl and Omaveloxolone, on Retinal Ganglion Cell Survival during Ischemic Optic Neuropathy. Antioxidants (Basel) 10 (9), 1466. doi:10.3390/antiox10091466
Choi, E. J., Jung, B. J., Lee, S. H., Yoo, H. S., Shin, E. A., Ko, H. J., et al. (2017). A Clinical Drug Library Screen Identifies Clobetasol Propionate as an NRF2 Inhibitor with Potential Therapeutic Efficacy in KEAP1 Mutant Lung Cancer. Oncogene 36 (37), 5285–5295. doi:10.1038/onc.2017.153
Choi, K. C., Lee, Y. H., Jung, M. G., Kwon, S. H., Kim, M. J., Jun, W. J., et al. (2009). Gallic Acid Suppresses Lipopolysaccharide-Induced Nuclear Factor-kappaB Signaling by Preventing RelA Acetylation in A549 Lung Cancer Cells. Mol. Cancer Res. 7, 2011–2021. doi:10.1158/1541-7786.MCR-09-0239
Choi, S. I., Lee, S. Y., Jung, W. J., Lee, S. H., Lee, E. J., Min, K. H., et al. (2016). The Effect of an IκB-Kinase-Β(ikkβ) Inhibitor on Tobacco Smoke-Induced Pulmonary Inflammation. Exp. Lung Res. 42, 182–189. doi:10.1080/01902148.2016.1174749
Chong, H., Vikis, H. G., and Guan, K. L. (2003). Mechanisms of Regulating the Raf Kinase Family. Cell. Signal 15 (5), 463–469. doi:10.1016/s0898-6568(02)00139-0
Chorner, P. M., and Moorehead, R. A. (674563201). A-674563, a Putative AKT1 Inhibitor that Also Suppresses CDK2 Activity, Inhibits Human NSCLC Cell Growth More Effectively Than the Pan-AKT Inhibitor, MK-2206. PLoS One 13 (2), e0193344. doi:10.1371/journal.pone.0193344
Chresta, C. M., Davies, B. R., Hickson, I., Harding, T., Cosulich, S., Critchlow, S. E., et al. (2010). AZD8055 Is a Potent, Selective, and Orally Bioavailable ATP-Competitive Mammalian Target of Rapamycin Kinase Inhibitor with In Vitro and In Vivo Antitumor Activity. Cancer Res. 70, 288–298. doi:10.1158/0008-5472.CAN-09-1751
Cicenas, J., Zalyte, E., Rimkus, A., Dapkus, D., and Noreika, R. (2017). Urbonavicius, S. JNK, P38, ERK, and SGK1 Inhibitors in Cancer. Cancers (Basel) 10 (1), 1. doi:10.3390/cancers10010001
Coffey, G. P., Feng, J., Betz, A., Pandey, A., Birrell, M., Leeds, J. M., et al. (2019). Cerdulatinib Pharmacodynamics and Relationships to Tumor Response Following Oral Dosing in Patients with Relapsed/Refractory B-Cell Malignancies. Clin. Cancer Res. 25 (4), 1174–1184. doi:10.1158/1078-0432.CCR-18-1047
Coker-Gurkan, A., Can, E., Sahin, S., Obakan-Yerlikaya, P., and Arisan, E. D. (2021). Atiprimod Triggered Apoptotic Cell Death via Acting on PERK/eIF2α/ATF4/CHOP and STAT3/NF-Κb axis in MDA-MB-231 and MDA-MB-468 Breast Cancer Cells. Mol. Biol. Rep. 48 (6), 5233–5247. doi:10.1007/s11033-021-06528-1
Coldewey, S. M., Rogazzo, M., Collino, M., Patel, N. S., and Thiemermann, C. (2013). Inhibition of IκB Kinase Reduces the Multiple Organ Dysfunction Caused by Sepsis in the Mouse. Dis. Model. Mech. 6, 1031–1042. doi:10.1242/dmm.012435
Coskun, M., Salem, M., Pedersen, J., and Nielsen, O. H. (2013). Involvement of JAK/STAT Signaling in the Pathogenesis of Inflammatory Bowel Disease. Pharmacol. Res. 76, 1–8. doi:10.1016/j.phrs.2013.06.007
Costa-Rodrigues, J., Pinho, O., and Monteiro, P. R. R. (2018). Can Lycopene Be Considered an Effective Protection against Cardiovascular Disease? Food Chem. 245, 1148–1153. doi:10.1016/j.foodchem.2017.11.055
Crews, C. M., Alessandrini, A., and Erikson, R. L. (1992). The Primary Structure of MEK, a Protein Kinase that Phosphorylates the ERK Gene Product. Science 258 (5081), 478–480. doi:10.1126/science.1411546
Cui, P., Wei, F., Hou, J., Su, Y., Wang, J., and Wang, S. (2020). STAT3 Inhibition Induced Temozolomide-Resistant Glioblastoma Apoptosis via Triggering Mitochondrial STAT3 Translocation and Respiratory Chain Dysfunction. Cell. Signal 71, 109598. doi:10.1016/j.cellsig.2020.109598
Cuker, A., and Neunert, C. E. (2016). How I Treat Refractory Immune Thrombocytopenia. Blood 128 (12), 1547–1554. doi:10.1182/blood-2016-03-603365
D'Amico, F., Fiorino, G., Furfaro, F., Allocca, M., and Danese, S. (2018). Janus Kinase Inhibitors for the Treatment of Inflammatory Bowel Diseases: Developments from Phase I and Phase II Clinical Trials. Expert Opin. Investig. Drugs 27 (7), 595–599. doi:10.1080/13543784.2018.1492547
Darnell, J. E. (1998). Studies of IFN-Induced Transcriptional Activation Uncover the Jak-Stat Pathway. J. Interferon cytokine Res. 18 (8), 549–554. doi:10.1089/jir.1998.18.549
Das, R., Rauf, A., Akhter, S., Islam, M. N., Emran, T. B., Mitra, S., et al. (2021). Role of Withaferin A and its Derivatives in the Management of Alzheimer's Disease: Recent Trends and Future Perspectives. Molecules 26 (12), 3696. doi:10.3390/molecules26123696
de Frias, M., Iglesias-Serret, D., Cosialls, A. M., Coll-Mulet, L., Santidrián, A. F., González-Gironès, D. M., et al. (2009). Akt Inhibitors Induce Apoptosis in Chronic Lymphocytic Leukemia Cells. Haematologica 94 (12), 1698–1707. doi:10.3324/haematol.2008.004028
de Oliveira, M. R. (2018). Carnosic Acid as a Promising Agent in Protecting Mitochondria of Brain Cells. Mol. Neurobiol. 55 (8), 6687–6699. doi:10.1007/s12035-017-0842-6
De Vries, L. C. S., Wildenberg, M. E., De Jonge, W. J., and D'Haens, G. R. (20171201). The Future of Janus Kinase Inhibitors in Inflammatory Bowel DiseaseErratum in. J. Crohns ColitisJ Crohns Colitis 1111 (78), 8851028–8851893. doi:10.1093/ecco-jcc/jjx003
Degos, L., and Wang, Z. (2001). All Trans Retinoic Acid in Acute Promyelocytic Leukemia. Oncogene 20, 7140–7145. doi:10.1038/sj.onc.1204763
Del Rosso, J. Q. (2020). Topical Corticosteroid Therapy for Psoriasis-A Review of Clobetasol Propionate 0.025% Cream and the Clinical Relevance of Penetration Modification. J. Clin. Aesthet. Dermatol 13 (2), 22–29.
Deng, C., Lipstein, M., Rodriguez, R., Serrano, X. O., McIntosh, C., Tsai, W. Y., et al. (2015). The Novel IKK2 Inhibitor LY2409881 Potently Synergizes with Histone Deacetylase Inhibitors in Preclinical Models of Lymphoma through the Downregulation of NF-Κb. Clin. Cancer Res. 21, 134–145. doi:10.1158/1078-0432.CCR-14-0384
Desir, S., O’Hare, P., and Vogel, R. I. (2018). Chemotherapy-induced Tunneling Nanotubes Mediate Intercellular Drug Efflux in Pancreatic Cancer. Sci. Rep. 8, 9484. doi:10.1038/s41598-018-27649-x
Devary, Y., Gottlieb, R. A., Smeal, T., and Karin, M. (1992). The Mammalian Ultraviolet Response Is Triggered by Activation of Src Tyrosine Kinases. Cell. 71 (7), 1081–1091. doi:10.1016/s0092-8674(05)80058-3
Devin, A., Cook, A., Lin, Y., Rodriguez, Y., Kelliher, M., and Liu, Z. (2000). The Distinct Roles of TRAF2 and RIP in IKK Activation by TNF-R1: TRAF2 Recruits IKK to TNF-R1 while RIP Mediates IKK Activation. Immunity 12 (4), 419–429. doi:10.1016/s1074-7613(00)80194-6
Dhillon, S. (2020). Delgocitinib: First Approval. Drugs 80 (6), 609–615. doi:10.1007/s40265-020-01291-2
Dhillon, S., and Keam, S. J. (2020). Filgotinib: First Approval. Drugs 80 (18), 1987–1997. doi:10.1007/s40265-020-01439-0
Dolly, S. O., Wagner, A. J., Bendell, J. C., Kindler, H. L., Krug, L. M., Seiwert, T. Y., et al. (2016). Phase I Study of Apitolisib (GDC-0980), Dual Phosphatidylinositol-3-Kinase and Mammalian Target of Rapamycin Kinase Inhibitor, in Patients with Advanced Solid Tumors. Clin. Cancer Res. 22 (12), 2874–2884. doi:10.1158/1078-0432.CCR-15-2225
Dong, N., Liu, X., Zhao, T., Wang, L., Li, H., Zhang, S., et al. (2018). Apoptosis-inducing Effects and Growth Inhibitory of a Novel Chalcone, in Human Hepatic Cancer Cells and Lung Cancer Cells. Biomed. Pharmacother. 105, 195–203. doi:10.1016/j.biopha.2018.05.126
Dong, Q., Dougan, D. R., Gong, X., Halkowycz, P., Jin, B., Kanouni, T., et al. (2011). Discovery of TAK-733, a Potent and Selective MEK Allosteric Site Inhibitor for the Treatment of Cancer. Bioorg. Med. Chem. Lett. 21, 1315–1319. doi:10.1016/j.bmcl.2011.01.071
Dong, T., Li, C., Wang, X., Dian, L., Zhang, X., Li, L., et al. (2015). Ainsliadimer A Selectively Inhibits IKKα/β by Covalently Binding a Conserved Cysteine. Nat. Commun. 6, 6522. doi:10.1038/ncomms7522
Dowty, M. E., Jesson, M. I., Ghosh, S., Lee, J., Meyer, D. M., Krishnaswami, S., et al. (2014). Preclinical to Clinical Translation of Tofacitinib, a Janus Kinase Inhibitor, in Rheumatoid Arthritis. J. Pharmacol. Exp. Ther. 348 (1), 165–173. doi:10.1124/jpet.113.209304
Drew, B. A., Burow, M. E., and Beckman, B. S. (2012). MEK5/ERK5 Pathway: the First Fifteen Years. Biochim. Biophys. Acta 1825 (1), 37–48. doi:10.1016/j.bbcan.2011.10.002
Du, Z., Whitt, M. A., Baumann, J., Garner, J. M., Morton, C. L., Davidoff, A. M., et al. (2012). Inhibition of Type I Interferon-Mediated Antiviral Action in Human Glioma Cells by the IKK Inhibitors BMS-345541 and TPCA-1. J. Interferon Cytokine Res. 32, 368–377. doi:10.1089/jir.2012.0002
Eapen, M. S., Kota, A., Vindin, H., McAlinden, K. D., Xenaki, D., Oliver, B. G., et al. (2018). Apoptosis Signal-Regulating Kinase 1 Inhibition Attenuates Human Airway Smooth Muscle Growth and Migration in Chronic Obstructive Pulmonary Disease. Clin. Sci. 132 (14), 1615–1627. doi:10.1042/CS20180398
Ebelt, N. D., Kaoud, T. S., and Edupuganti, R. (2017). A C-Jun N-Terminal Kinase Inhibitor, JNK-IN-8, Sensitizes Triple Negative Breast Cancer Cells to Lapatinib. Oncotarget 8, 104894–104912. doi:10.18632/oncotarget.20581
Ekanem, T. I., Tsai, W. L., Lin, Y. H., Tan, W. Q., Chang, H. Y., Huang, T. C., et al. (2020). Identification of the Effects of Aspirin and Sulindac Sulfide on the Inhibition of HMGA2-Mediated Oncogenic Capacities in Colorectal Cancer. Molecules 25 (17), 3826. doi:10.3390/molecules25173826
Eleutherakis-Papaiakovou, E., Gavriatopoulou, M., Ntanasis-Stathopoulos, I., Kastritis, E., Terpos, E., and Dimopoulos, M. A. (2019). Elotuzumab in Combination with Pomalidomide and Dexamethasone for the Treatment of Multiple Myeloma. Expert Rev. Anticancer Ther. 19 (11), 921–928. doi:10.1080/14737140.2019.1685879
Eleutherakis-Papaiakovou, E., Kanellias, N., Kastritis, E., Gavriatopoulou, M., Terpos, E., and Dimopoulos, M. A. (2020). Efficacy of Panobinostat for the Treatment of Multiple Myeloma. J. Oncol. 2020, 20207131802. doi:10.1155/2020/7131802
Eynott, P. R., Salmon, M., Huang, T. J., Oates, T., Nicklin, P. L., and Chung, K. F. (2003). Effects of Cyclosporin A and a Rapamycin Derivative (SAR943) on Chronic Allergic Inflammation in Sensitized Rats. Immunology 109 (3), 461–467. doi:10.1046/j.1365-2567.2003.01672.x
Feldman, M. E., Apsel, B., Uotila, A., Loewith, R., Knight, Z. A., Ruggero, D., et al. (2009). Active-site Inhibitors of mTOR Target Rapamycin-Resistant Outputs of mTORC1 and mTORC2. PLoS Biol. 7, e38. doi:10.1371/journal.pbio.1000038
Feng, Y., Xiao, Y., Yan, H., Wang, P., Zhu, W., Cassady, K., et al. (2020). Sirolimus as Rescue Therapy for Refractory/Relapsed Immune Thrombocytopenia: Results of a Single-Center, Prospective, Single-Arm Study. Front. Med. (Lausanne) 7, 110. doi:10.3389/fmed.2020.00110
Ferreira, S., Guttman-Yassky, E., and Torres, T. (2020). Selective JAK1 Inhibitors for the Treatment of Atopic Dermatitis: Focus on Upadacitinib and Abrocitinib. Am. J. Clin. Dermatol 21 (6), 783–798. doi:10.1007/s40257-020-00548-6
Francis, M., Gopinathan, G., Salapatas, A., Nares, S., Gonzalez, M., Diekwisch, T. G. H., et al. (2020). SETD1 and NF-Κb Regulate Periodontal Inflammation through H3K4 Trimethylation. J. Dent. Res. 99 (13), 1486–1493. doi:10.1177/0022034520939029
Frankel, S. R., Eardley, A., Lauwers, G., Weiss, M., and Warrell, R. P. (1992). Ann. Intern. Med., 117, 293 ± 296.doi:10.7326/0003-4819-117-8-698_2
Franza, L., Carusi, V., Nucera, E., and Pandolfi, F. (2021). Luteolin, Inflammation and Cancer: Special Emphasis on Gut Microbiota. Biofactors 47 (2), 181–189. doi:10.1002/biof.1710
Frelin, C., Imbert, V., Griessinger, E., Peyron, A. C., Rochet, N., Philip, P., et al. (2005). Targeting NF-kappaB Activation via Pharmacologic Inhibition of IKK2-Induced Apoptosis of Human Acute Myeloid Leukemia Cells. Blood 105 (2), 804–811. doi:10.1182/blood-2004-04-1463
Frew, I. J., and Krek, W. (2008). pVHL: a Multipurpose Adaptor Protein. Sci. Signal 1 (24), pe30. doi:10.1126/scisignal.124pe30
Fritsch, C., Huang, A., Chatenay-Rivauday, C., Schnell, C., Reddy, A., Liu, M., et al. (2014). Characterization of the Novel and Specific PI3Kα Inhibitor NVP-Byl719 and Development of the Patient Stratification Strategy for Clinical Trials. Mol. Cancer Ther. 13 (5), 1117–1129. doi:10.1158/1535-7163.MCT-13-0865
Furumoto, Y., Smith, C. K., Blanco, L., Zhao, W., Brooks, S. R., Thacker, S. G., et al. (2017). Tofacitinib Ameliorates Murine Lupus and its Associated Vascular Dysfunction. Arthritis Rheumatol. 69 (1), 148–160. doi:10.1002/art.39818
Ganesh Yerra, V., Negi, G., Sharma, S. S., and Kumar, A. (2013). Potential Therapeutic Effects of the Simultaneous Targeting of the Nrf2 and NF-Κb Pathways in Diabetic Neuropathy. Redox Biol. 1 (1), 394–397. doi:10.1016/j.redox.2013.07.005
Gao, Y. J., Cheng, J. K., and Zeng, Q. (2009). Selective Inhibition of JNK with a Peptide Inhibitor Attenuates Pain Hypersensitivity and Tumor Growth in a Mouse Skin Cancer Pain Model. Exp. Neurol. 219, 146–155. doi:10.1016/j.expneurol.2009.05.006
García-Martínez, J. M., Moran, J., Clarke, R. G., Gray, A., Cosulich, S. C., Chresta, C. M., et al. (2009). Ku-0063794 Is a Specific Inhibitor of the Mammalian Target of Rapamycin (mTOR). Biochem. J. 421, 29–42. doi:10.1042/BJ20090489
Gerczuk, P. Z., Breckenridge, D. G., Liles, J. T., Budas, G. R., Shryock, J. C., Belardinelli, L., et al. (2012). An Apoptosis Signal-Regulating Kinase 1 Inhibitor Reduces Cardiomyocyte Apoptosis and Infarct Size in a Rat Ischemia-Reperfusion Model. J. Cardiovasc Pharmacol. 60 (3), 276–282. doi:10.1097/FJC.0b013e31825ea0fa
Geuna, E., Milani, A., Martinello, R., Aversa, C., Valabrega, G., Scaltriti, M., et al. (2015). Buparlisib , an Oral Pan-Pi3k Inhibitor for the Treatment of Breast Cancer. Expert Opin. Investig. Drugs 24 (3), 421–431. doi:10.1517/13543784.2015.1008132
Ghasemzadeh, R. M., and Hosseinzadeh, H. (2020). Effects of Rosmarinic Acid on Nervous System Disorders: an Updated Review. Naunyn Schmiedeb. Arch. Pharmacol. 393 (10), 1779–1795. doi:10.1007/s00210-020-01935-w
Gheorgheosu, D., Duicu, O., Dehelean, C., Soica, C., and Muntean, D. (2014). Betulinic Acid as a Potent and Complex Antitumor Phytochemical: a Minireview. Anticancer Agents Med. Chem. 14 (7), 936–945. doi:10.2174/1871520614666140223192148
Gieroba, B., Arczewska, M., Swika-Brych, A., Rzeski, W., Stepulak, A., and Gago, M. (2020). Prostate and Breast Cancer Cells Death Induced by Xanthohumol Investigated with Fourier Transform Infrared Spectroscopy. Spectrochim. Acta A Mol. Biomol. Spectrosc. 231, 118112. doi:10.1016/j.saa.2020.118112
Ginter, T., Heinzel, T., and Krämer, O. H. (2013). Acetylation of Endogenous STAT Proteins. Methods Mol. Biol. 967, 167–178. doi:10.1007/978-1-62703-242-1_12
Giuli, M. V., Hanieh, P. N., Giuliani, E., Rinaldi, F., Marianecci, C., Screpanti, I., et al. (2020). Current Trends in ATRA Delivery for Cancer Therapy. Pharmaceutics 12 (8), 707. doi:10.3390/pharmaceutics12080707
Gong, L., Tan, Y. C., and Boice, G. (2012). Discovery of a Novel Series of 4-quinolone JNK Inhibitors. Bioorg Med. Chem. Lett. 22, 7381–7387. doi:10.1016/j.bmcl.2012.10.066
Gonzalez-Donquiles, C., Alonso-Molero, J., Fernandez-Villa, T., Vilorio-Marqués, L., Molina, A. J., and Martín, V. (2017). The NRF2 Transcription Factor Plays a Dual Role in Colorectal Cancer: A Systematic Review. PLoS One 12 (5), eO177549. doi:10.1371/journal.pone.0177549
Govindaraj, J., Emmadi, P., and Puvanakrishnan, R. (2011). Therapeutic Effects of Proanthocyanidins on the Pathogenesis of Periodontitis-Aan Overview. Indian J. Exp. Biol. 49 (2), 83–93.
Grimshaw, K. M., Hunter, L. J., Yap, T. A., Heaton, S. P., Walton, M. I., Woodhead, S. J., et al. (2010). AT7867 Is a Potent and Oral Inhibitor of AKT and P70 S6 Kinase that Induces Pharmacodynamic Changes and Inhibits Human Tumor Xenograft Growth. Mol. Cancer Ther. 9 (5), 1100–1110. doi:10.1158/1535-7163.MCT-09-0986
Guo, H., Kuang, S., Song, Q. L., Liu, M., Sun, X. X., and Yu, Q. (2018). Cucurbitacin I Inhibits STAT3, but Enhances STAT1 Signaling in Human Cancer Cells In Vitro through Disrupting Actin Filaments. Acta Pharmacol. Sin. 39 (3), 425–437. doi:10.1038/aps.2017.99
Guo, N., and Peng, Z. (2013). MG132, a Proteasome Inhibitor, Induces Apoptosis in Tumor Cells. Asia Pac J. Clin. Oncol. 9 (1), 6–11. doi:10.1111/j.1743-7563.2012.01535.x
Guo, X., Harada, C., Namekata, K., Matsuzawa, A., Camps, M., Ji, H., et al. (2010). Regulation of the Severity of Neuroinflammation and Demyelination by TLR-ASK1-P38 Pathway. EMBO Mol. Med. 2 (12), 504–515. doi:10.1002/emmm.201000103
Guo, Y. J., Pan, W. W., and Liu, S. B. (2008). ERK/MAPK Signalling Pathway and tumorigenesisCJ]. Exp. Ther. Med»2020 19 (3), 1997–2007. doi:10.3892/etm.2020.8454
Gupta, P., Wright, S. E., Kim, S. H., and Srivastava, S. K. (2014). Phenethyl Isothiocyanate: a Comprehensive Review of Anti-cancer Mechanisms. Biochim. Biophys. Acta 1846 (2), 405–424. doi:10.1016/j.bbcan.2014.08.003
Gupta, S. C., Sundaram, C., Reuter, S., and Aggarwal, B. B. (2010). Inhibiting NF-Κb Activation by Small Molecules as a Therapeutic Strategy. Biochim. Biophys. Acta 1799 (10-12), 775–787. doi:10.1016/j.bbagrm.2010.05.004
Gupta, S. C., Sundaram, C., Reuter, S., and Aggarwal, B. B. (2010). Inhibiting NF-Κb Activation by Small Molecules as a Therapeutic Strategy. Biochim. Biophys. Acta 1799, 775–787. doi:10.1016/j.bbagrm.2010.05.004
Haarberg, K. M., Li, J., Heinrichs, J., Wang, D., Liu, C., Bronk, C. C., et al. (2013). Pharmacologic Inhibition of PKCα and PKCθ Prevents GVHD while Preserving GVL Activity in Mice. Blood 122 (14), 2500–2511. doi:10.1182/blood-2012-12-471938
Haddadi, N., Lin, Y., Travis, G., Simpson, A. M., Nassif, N. T., and McGowan, E. M. (2018). PTEN/PTENP1: 'Regulating the Regulator of RTK-dependent PI3K/Akt Signalling', New Targets for Cancer Therapy. Mol. Cancer 17, 37. doi:10.1186/s12943-018-0803-3
Hallin, J., Engstrom, L. D., Hargis, L., Calinisan, A., Aranda, R., Briere, D. M., et al. (2020). The KRASG12C Inhibitor MRTX849 Provides Insight toward Therapeutic Susceptibility of KRAS-Mutant Cancers in Mouse Models and Patients. Cancer Discov. 10 (1), 54–71. doi:10.1158/2159-8290.CD-19-1167
Hammitzsch, A., Tallant, C., Fedorov, O., O'Mahony, A., Brennan, P. E., Hay, D. A., et al. (2015). CBP30, a Selective CBP/p300 Bromodomain Inhibitor, Suppresses Human Th17 Responses. Proc. Natl. Acad. Sci. U. S. A. 112 (34), 10768–10773. doi:10.1073/pnas.1501956112
Han, E. K., Leverson, J. D., McGonigal, T., Shah, O. J., Woods, K. W., Hunter, T., et al. (2007). Akt Inhibitor A-443654 Induces Rapid Akt Ser-473 Phosphorylation Independent of mTORC1 Inhibition. Oncogene 26 (38), 5655–5661. doi:10.1038/sj.onc.1210343
Hancox, U., Cosulich, S., Hanson, L., Trigwell, C., Lenaghan, C., Ellston, R., et al. (2015). Inhibition of PI3Kβ Signaling with AZD8186 Inhibits Growth of PTEN-Deficient Breast and Prostate Tumors Alone and in Combination with Docetaxel. Mol. Cancer Ther. 14 (1), 48–58. doi:10.1158/1535-7163.MCT-14-0406
Handley, M. E., Rasaiyaah, J., Chain, B. M., and Katz, D. R. (2007). Mixed Lineage Kinases (MLKs): a Role in Dendritic Cells, Inflammation and Immunity? Int. J. Exp. Pathol. 88 (2), 111–126. doi:10.1111/j.1365-2613.2007.00531.x
Hao, G., Zhai, J., Jiang, H., Zhang, Y., Wu, M., Qiu, Y., et al. (2020). Acetylshikonin Induces Apoptosis of Human Leukemia Cell Line K562 by Inducing S Phase Cell Cycle Arrest, Modulating ROS Accumulation, Depleting Bcr-Abl and Blocking NF-Κb Signaling. Biomed. Pharmacother. 122, 109677. doi:10.1016/j.biopha.2019.109677
Hapner, C. D., Deuster, P., and Chen, Y. (2010). Inhibition of Oxidative Hemolysis by Quercetin, but Not Other Antioxidants. Chem. Biol. Interact. 186 (3), 275–279. doi:10.1016/j.cbi.2010.05.010
Harder, B. G., Peng, S., Sereduk, C. P., Sodoma, A. M., Kitange, G. J., Loftus, J. C., et al. (2019). Inhibition of Phosphatidylinositol 3-kinase by PX-866 Suppresses Temozolomide-Induced Autophagy and Promotes Apoptosis in Glioblastoma Cells. Mol. Med. 25 (1), 49. doi:10.1186/s10020-019-0116-z
Harrison, D. A. (2012). The Jak/STAT Pathway. Cold Spring Harb. Perspect. Biol. 4 (3), a011205. doi:10.1101/cshperspect.a011205
Hatzivassiliou, G., Haling, J. R., Chen, H., Song, K., Price, S., Heald, R., et al. (2013). Mechanism of MEK Inhibition Determines Effificacy in Mutant KRAS-Versus BRAF-Driven Cancers. Nature 501, 232–236. doi:10.1038/nature12441
Haynes, N. E., Scott, N. R., Chen, L. C., Janson, C. A., Li, J. K., Lukacs, C. M., et al. (2012). Identification of an Adamantyl Azaquinolone JNK Selective Inhibitor. ACS Med. Chem. Lett. 3 (9), 764–768. doi:10.1021/ml300175c
He, P., Zeng, B., Zhang, X. L., Fang, D. L., Zhou, X. Q., Wan, K. Q., et al. (2016). Protective Effect of Apoptosis Signal-Regulating Kinase 1 Inhibitor against Mice Liver Injury. Asian Pac J. Trop. Med. 9 (3), 283–287. doi:10.1016/j.apjtm.2016.01.029
He, Y., Duckett, D., and Chen, W. (2014). Synthesis and SAR of Novel Isoxazoles as Potent C-Jun N-Terminal Kinase (JNK) Inhibitors. Bioorg Med. Chem. Lett. 24, 161–164. doi:10.1016/j.bmcl.2013.11.052
He, Y., Kamenecka, T. M., and Shin, Y. (2011). Synthesis and SAR of Novel Quinazolines as Potent and Brain-Penetrant C-Jun N-Terminal Kinase (JNK) Inhibitors. Bioorg Med. Chem. Lett. 21, 1719–1723. doi:10.1016/j.bmcl.2011.01.079
He, Z. X., Wei, B. F., Zhang, X., Gong, Y. P., Ma, L. Y., and Zhao, W. (2021). Current Development of CBP/p300 Inhibitors in the Last Decade. Eur. J. Med. Chem. 209, 112861. doi:10.1016/j.ejmech.2020.112861
Hedvat, M., Huszar, D., Herrmann, A., Gozgit, J. M., Schroeder, A., Sheehy, A., et al. (2009). The JAK2 Inhibitor AZD1480 Potently Blocks Stat3 Signaling and Oncogenesis in Solid Tumors. Cancer Cell. 16, 487–497. doi:10.1016/j.ccr.2009.10.015
Heffron, T. P., Berry, M., Castanedo, G., Chang, C., Chuckowree, I., Dotson, J., et al. (2010). Identification of GNE-477, a Potent and Efficacious Dual PI3K/mTOR Inhibitor. Bioorg Med. Chem. Lett. 20, 2408–2411. doi:10.1016/j.bmcl.2010.03.046
Hemshekhar, M., Sebastin Santhosh, M., Kemparaju, K., and Girish, K. S. (2012). Emerging Roles of Anacardic Acid and its Derivatives: a Pharmacological Overview. Basic Clin. Pharmacol. Toxicol. 110 (2), 122–132. doi:10.1111/j.1742-7843.2011.00833.x
Herranz, H., Hong, X., Hung, N. T., Voorhoeve, P. M., and Cohen, S. M. (2012). Oncogenic Cooperation between SOCS Family Proteins and EGFR Identified Using a Drosophila Epithelial Transformation Model. Genes. Dev. 26 (14), 1602–1611. doi:10.1101/gad.192021.112
Herrmann, C., Block, C., Geisen, C., Haas, K., Weber, C., Winde, G., et al. (1998). Sulindac Sulfide Inhibits Ras Signaling. Oncogene 17 (14), 1769–1776. doi:10.1038/sj.onc.1202085
Hideshima, T., Chauhan, D., Richardson, P., Mitsiades, C., Mitsiades, N., Hayashi, T., et al. (2002). NF-kappa B as a Therapeutic Target in Multiple Myeloma. J. Biol. Chem. 277, 16639–16647. doi:10.1074/jbc.M200360200
Hideshima, T., Neri, P., Tassone, P., Yasui, H., Ishitsuka, K., Raje, N., et al. (2006). MLN120B, a Novel IkappaB Kinase Beta Inhibitor, Blocks Multiple Myeloma Cell Growth In Vitro and In Vivo. Clin. Cancer Res. 12, 5887–5894. doi:10.1158/1078-0432.CCR-05-2501
Hillig, R. C., Sautier, B., Schroeder, J., Moosmayer, D., Hilpmann, A., Stegmann, C. M., et al. (2019). Discovery of Potent SOS1 Inhibitors that Block RAS Activation via Disruption of the RAS-SOS1 Interaction. Proc. Natl. Acad. Sci. U. S. A. 116 (7), 2551–2560. doi:10.1073/pnas.1812963116
Hirai, H., Sootome, H., Nakatsuru, Y., Miyama, K., Taguchi, S., Tsujioka, K., et al. (2010). MK-2206, an Allosteric Akt Inhibitor, Enhances Antitumor Efficacy by Standard Chemotherapeutic Agents or Molecular Targeted Drugs In Vitro and In Vivo. Mol. Cancer Ther. 9, 1956–1967. doi:10.1158/1535-7163.MCT-09-1012
Hirai, Si., Katoh, M., Terada, M., Kyriakis, J. M., Zon, L. I., Rana, A., et al. (1997). MST/MLK2, a Member of the Mixed Lineage Kinase Family, Directly Phosphorylates and Activates SEK1, an Activator of C-Jun N-Terminal Kinase/stress-Activated Protein Kinase. J. Biol. Chem. 272 (24), 15167–15173. doi:10.1074/jbc.272.24.15167
Hoffmann, G., Breitenbücher, F., Schuler, M., and Ehrenhofer-Murray, A. E. (2014). A Novel Sirtuin 2 (SIRT2) Inhibitor with P53-dependent Pro-apoptotic Activity in Non-small Cell Lung Cancer. J. Biol. Chem. 289 (8), 5208–5216. doi:10.1074/jbc.M113.487736
Hou, J., Lam, F., Proud, C., and Wang, S. (2012). Targeting Mnks for Cancer Therapy. Oncotarget 3 (2), 118–131. doi:10.18632/oncotarget.453
Huang, C., Wang, Y., Li, X., Ren, L., Zhao, J., Hu, Y., et al. (2020). Clinical Features of Patients Infected with 2019 Novel Coronavirus in Wuhan, China. Lancet 395 (10 223), 497–506. doi:10.1016/S0140-6736(20)30183-5
Huang, J., and Manning, B. D. (2008). The TSC1-TSC2 Complex: a Molecular Switchboard Controlling Cell Growth. Biochem. J. 412 (2), 179–190. doi:10.1042/BJ20080281
Huo, H. Z., Zhou, Z. Y., Wang, B., Qin, J., Liu, W. Y., and Gu, Y. (2014). Dramatic Suppression of Colorectal Cancer Cell Growth by the Dual mTORC1 and mTORC2 Inhibitor AZD-2014. Biochem. Biophys. Res. Commun. 443 (2), 406–412. doi:10.1016/j.bbrc.2013.11.099
Huo, S., Liu, X., Zhang, S., Lyu, Z., Zhang, J., Wang, Y., et al. (2021). p300/CBP Inhibitor A-485 Inhibits the Differentiation of Osteoclasts and Protects against Osteoporotic Bone Loss. Int. Immunopharmacol. 94, 107458. doi:10.1016/j.intimp.2021.107458
Huynh, D. L., Ngau, T. H., Nguyen, N. H., Tran, G. B., and Nguyen, C. T. (2020). Potential Therapeutic and Pharmacological Effects of Wogonin: an Updated Review. Mol. Biol. Rep. 47 (12), 9779–9789. doi:10.1007/s11033-020-05972-9
Imran, M., Rauf, A., Shah, Z. A., Saeed, F., Imran, A., Arshad, M. U., et al. (2019). Chemo-preventive and Therapeutic Effect of the Dietary Flavonoid Kaempferol: A Comprehensive Review. Phytother. Res. 33 (2), 263–275. doi:10.1002/ptr.6227
Isshiki, Y., Kohchi, Y., Iikura, H., Matsubara, Y., Asoh, K., Murata, T., et al. (2011). Design and Synthesis of Novel Allosteric MEK Inhibitor CH4987655 as an Orally Available Anticancer Agent. Bioorg. Med. Chem. Lett. 21, 1795–1801. doi:10.1016/j.bmcl.2011.01.062
Ivanov, V. N., and Hei, T. K. (2013). Induction of Apoptotic Death and Retardation of Neuronal Differentiation of Human Neural Stem Cells by Sodium Arsenite Treatment. Exp. Cell. Res. 319 (6), 875–887. doi:10.1016/j.yexcr.2012.11.019
Iverson, C., Larson, G., Lai, C., Yeh, L. T., Dadson, C., Weingarten, P., et al. (2009). RDEA119/BAY 869766: A Potent, Selective, Allosteric Inhibitor of MEK1/2 for the Treatment of Cancer. Cancer Res. 69, 6839–6847. doi:10.1158/0008-5472.CAN-09-0679
Izutsu, K., Kinoshita, T., Takizawa, J., Fukuhara, S., Yamamoto, G., Ohashi, Y., et al. (2021). A Phase II Japanese Trial of Fludarabine, Cyclophosphamide and Rituximab for Previously Untreated Chronic Lymphocytic Leukemia. Jpn. J. Clin. Oncol. 51 (3), 408–415. doi:10.1093/jjco/hyaa215
Jabbarzadeh Kaboli, P., Salimian, F., Aghapour, S., Xiang, S., Zhao, Q., Li, M., et al. (2020). Akt-targeted Therapy as a Promising Strategy to Overcome Drug Resistance in Breast Cancer - A Comprehensive Review from Chemotherapy to Immunotherapy. Pharmacol. Res. 156, 104806. doi:10.1016/j.phrs.2020.104806
Jain, P. P., Zhao, T., Xiong, M., Song, S., Lai, N., Zheng, Q., et al. (2021). Halofuginone, a Promising Drug for Treatment of Pulmonary Hypertension. Br. J. Pharmacol. 178 (17), 3373–3394. doi:10.1111/bph.15442
Jaiswal, N., Akhtar, J., Singh, S. P., and BadruddeenAhsan, F. (2019). An Overview on Genistein and its Various Formulations. Drug Res. (Stuttg). 69 (6), 305–313. doi:10.1055/a-0797-3657
Jamieson, D., Griffifin, M. J., Sludden, J., Drew, Y., Cresti, N., Swales, K., et al. (2016). A Phase I Pharmacokinetic and Pharmacodynamic Study of the Oral Mitogen-Activated Protein Kinase Kinase (MEK) Inhibitor, WX-554, in Patients with Advanced Solid Tumours. Eur. J. Cancer 68, 1–10. doi:10.1016/j.ejca.2016.08.026
Janes, M. R., Zhang, J., Li, L. S., Hansen, R., Peters, U., Guo, X., et al. (2018). Targeting KRAS Mutant Cancers with a Covalent G12C-specific Inhibitor. Cell. 172 (3), 578–589. doi:10.1016/j.cell.2018.01.006
Jasinski, S., Weinblatt, M. E., and Glasser, C. L. (2017). Sirolimus as an Effective Agent in the Treatment of Immune Thrombocytopenia (ITP) and Evans Syndrome (ES): A Single Institution's Experience. J. Pediatr. Hematol. Oncol. 39 (6), 420–424. doi:10.1097/MPH.0000000000000818
Ji, N., Yang, Y., Cai, C. Y., Lei, Z. N., Wang, J. Q., Gupta, P., et al. (2019). Selonsertib (GS-4997), an ASK1 Inhibitor, Antagonizes Multidrug Resistance in ABCB1- and ABCG2-Overexpressing Cancer Cells. Cancer Lett. 440– 441, 82–93. doi:10.1016/j.canlet.2018.10.007
Jimi, E., Aoki, K., Saito, H., D'Acquisto, F., May, M. J., Nakamura, I., et al. (2004). Selective Inhibition of NF-Kappa B Blocks Osteoclastogenesis and Prevents Inflammatory Bone Destruction In Vivo. Nat. Med. 10 (6), 617–624. doi:10.1038/nm1054
Jnoff, E., Albrecht, C., Barker, J. J., Barker, O., Beaumont, E., Bromidge, S., et al. (2014). Binding Mode and Structure-Activity Relationships Around Direct Inhibitors of the Nrf2-Keap1 Complex. ChemMedChem 9 (4), 699–705. doi:10.1002/cmdc.201300525
Johnson, F. L., Patel, N. S. A., Purvis, G. S. D., Chiazza, F., Chen, J., Sordi, R., et al. (2017). Inhibition of IκB Kinase at 24 Hours after Acute Kidney Injury Improves Recovery of Renal Function and Attenuates Fibrosis. J. Am. Heart Assoc. 6, e005092. doi:10.1161/JAHA.116.005092
Johnson, J., Shi, Z., Liu, Y., and Stack, M. S. (2014). Inhibitors of NF-kappaB Reverse Cellular Invasion and Target Gene Upregulation in an Experimental Model of Aggressive Oral Squamous Cell Carcinoma. Oral Oncol. 50, 468–477. doi:10.1016/j.oraloncology.2014.02.004
Johra, F. T., Bepari, A. K., Bristy, A. T., and Reza, H. M. (2020). A Mechanistic Review of 尾-Carotene, Lutein, and Zeaxanthin in Eye Health and Disease. Antioxidants (Basel) 9 (11), 1046. doi:10.3390/antiox9111046
Ju, W., Li, N., Wang, J., Yu, N., Lei, Z., Zhang, L., et al. (2021). Design and Synthesis of Novel Mitochondria-Targeted CDDO Derivatives as Potential Anti-cancer Agents. Bioorg Chem. 115, 105249. doi:10.1016/j.bioorg.2021.105249
Kadri, H., Lambourne, O. A., and Mehellou, Y. (2018). Niclosamide, a Drug with Many (Re)purposes. ChemMedChem 13 (11), 1088–1091. doi:10.1002/cmdc.201800100
Kahan, B. D. (1999). Sirolimus Is More Effective Than Azathioprine to Reduce Incidence of Acute Allograft Rejection Episodes when Used in Combination with Cyclosprine and Predinsone:a Phase Ⅲ US Multicenter Study. J].Transplant. 68 (10), 1056. doi:10.1097/00007890-199911270-00016
Kanduri, M., Tobin, G., Aleskog, A., Nilsson, K., and Rosenquist, R. (2011). The Novel NF-Κb Inhibitor IMD-0354 Induces Apoptosis in Chronic Lymphocytic Leukemia. Blood Cancer J. 1, e12. doi:10.1038/bcj.2011.9
Kapahi, P., Takahashi, T., Natoli, G., Adams, S. R., Chen, Y., Tsien, R. Y., et al. (2000). Inhibition of NF-Kappa B Activation by Arsenite through Reaction with a Critical Cysteine in the Activation Loop of Ikappa B Kinase. J. Biol. Chem. 275 (46), 36062–36066. doi:10.1074/jbc.M007204200
Karin, M., and Gallagher, E. (2005). From JNK to Pay Dirt: Jun Kinases, Their Biochemistry, Physiology and Clinical Importance. IUBMB Life 57 (4-5), 283–295. doi:10.1080/15216540500097111
Karoulia, Z., Wu, Y., Ahmed, T. A., Xin, Q., Bollard, J., Krepler, C., et al. (2016). An Integrated Model of RAF Inhibitor Action Predicts Inhibitor Activity against Oncogenic BRAF Signaling. Cancer Cell. 30 (3), 485–498. doi:10.1016/j.ccell.2016.06.02410.1016/j.ccell.2016.08.008
Kensler, T. W., Wakabayashi, N., and Biswal, S. (2007). Cell Survival Responses to Environmental Stresses via the Keap1-Nrf2-ARE Pathway. Annu. Rev. Pharmacol. Toxicol. 47, 89–116. doi:10.1146/annurev.pharmtox.46.120604.141046
Kersting, S., Behrendt, V., and Kersting, J. (2013). The Impact of JNK Inhibitor D-JNKI-1 in a Murine Model of Chronic Colitis Induced by Dextran Sulfate Sodium. J. Inflamm. Res. 6, 71–81. doi:10.2147/JIR.S40092
Khalesi, N., Korani, S., Korani, M., Johnston, T. P., and Sahebkar, A. (2021). Bortezomib: a Proteasome Inhibitor for the Treatment of Autoimmune Diseases. Inflammopharmacology 29 (5), 1291–1306. doi:10.1007/s10787-021-00863-2
Ki, S. H., Cho, I. J., Choi, D. W., and Kim, S. G. (2005). Glucocorticoid Receptor (GR)-associated SMRT Binding to C/EBPbeta TAD and Nrf2 Neh4/5: Role of SMRT Recruited to GR in GSTA2 Gene Repression. Mol. Cell. Biol. 25 (10), 4150–4165. doi:10.1128/MCB.25.10.4150-4165.2005
Kidger, A. M., Sipthorp, J., and Cook, S. J. (2018). ERK1/2 Inhibitors: New Weapons to Inhibit the RAS-Regulated RAF-Mek1/2-Erk1/2 Pathway. Pharmacol. Ther. 187, 45–60. doi:10.1016/j.pharmthera.2018.02.007
Kim, T. W. (2019). Belvarafenib, a Novel Pan-RAF Inhibitor, in Solid Tumor Patients Harboring BRAF,KRAS, or NRAS Mutations: Phase I Study. J. Clin. Oncol. 37, 3000. doi:10.1200/jco.2019.37.15_suppl.3000
Kimura, A., Toyoda, T., Iwasaki, M., Hirama, R., and Osafune, K. (2020). Combined Omics Approaches Reveal the Roles of Non-canonical WNT7B Signaling and YY1 in the Proliferation of Human Pancreatic Progenitor Cells. Cell. Chem. Biol. 27 (12), 1561–1572. doi:10.1016/j.chembiol.2020.08.018
Kishore, N., Sommers, C., Mathialagan, S., Guzova, J., Yao, M., Hauser, S., et al. (2003). A Selective IKK-2 Inhibitor Blocks NF-Kappa B-dependent Gene Expression in Interleukin-1 Beta-Stimulated Synovial Fibroblasts. J. Biol. Chem. 278, 32861–32871. doi:10.1074/jbc.M211439200
Knapper, S., Russell, N., Gilkes, A., Hills, R. K., Gale, R. E., Cavenagh, J. D., et al. (2017). A Randomized Assessment of Adding the Kinase Inhibitor Lestaurtinib to First-Line Chemotherapy for FLT3-Mutated AML. Blood 129 (9), 1143–1154. doi:10.1182/blood-2016-07-730648
Komoto, T. T., Lee, J., Lertpatipanpong, P., Ryu, J., Marins, M., Fachin, A. L., et al. (2021). Trans-chalcone Suppresses Tumor Growth Mediated at Least in Part by the Induction of Heme Oxygenase-1 in Breast Cancer. Toxicol. Res. 37 (4), 485–493. doi:10.1007/s43188-021-00089-y
Komrokji, R., Wadleigh, M., Seymour, J. F., Roberts, A. W., To, L. B., Zhu, H. J., et al. (2011). Results of a Phase 2 Study of Pacritinib (SB1518), a Novel Oral JAK2 Inhibitor, in Patients with Primary, Post-polycythemia Vera, and Post-essential Thrombocythemia Myelofifibrosis. Blood 118. doi:10.1182/blood.v118.21.282.282
Kopp, E., and Ghosh, S. (1994). Inhibition of NF-Kappa B by Sodium Salicylate and Aspirin. Science 265, 956–959. doi:10.1126/science.8052854
Kostovic, K., Gulin, S. J., Mokos, Z. B., and Ceovic, R. (2017). Tofacitinib, an Oral Janus Kinase Inhibitor: Perspectives in Dermatologyperspectives in Dermatology[J]. Curr. Med. Chem. 24 (11), 1158–1167. doi:10.2174/1874467210666170113104503
Koul, H. K., Pal, M., and Koul, S. (2013). Role of P38 MAP Kinase Signal Transduction in Solid Tumors. Genes. Cancer 4 (9-10), 342–359. doi:10.1177/1947601913507951
Krajka-Kuniak, V., Paluszczak, J., and Baer-Dubowska, W. (2013). Xanthohumol Induces Phase II Enzymes via Nrf2 in Human Hepatocytes In Vitro. Toxicol Vitro 27 (1), 149–156. doi:10.1016/j.tiv.2012.10.008
Kurasawa, K., Arai, S., Namiki, Y., Tanaka, A., Takamura, Y., Owada, T., et al. (2018). Tofacitinib for Refractory Interstitial Lung Diseases in Anti-melanoma Differentiation-Associated 5 Gene Antibody-Positive Dermatomyositis. Rheumatol. Oxf. 57 (12), 2114–2119. doi:10.1093/rheumatology/key188
Labetoulle, R., Paul, S., and Roblin, X. (2018). Filgotinib for the Treatment of Crohn's Disease. Expert Opin. Investig. Drugs 27 (3), 295–300. doi:10.1080/13543784.2018.1442433
Lam, L. T., Davis, R. E., Pierce, J., Hepperle, M., Xu, Y., Hottelet, M., et al. (2005). Small Molecule Inhibitors of IkappaB Kinase Are Selectively Toxic for Subgroups of Diffuse Large B-Cell Lymphoma Defined by Gene Expression Profiling. Clin. Cancer Res. 11, 28–40.
Lawler, S., Fleming, Y., Goedert, M., and Cohen, P. (1998). Synergistic Activation of SAPK1/JNK1 by Two MAP Kinase Kinases In Vitro. Curr. Biol. 8 (25), 1387–1390. doi:10.1016/s0960-9822(98)00019-0
Le, M., Berman-Rosa, M., Ghazawi, F. M., Bourcier, M., Fiorillo, L., Gooderham, M., et al. (2021). Systematic Review on the Efficacy and Safety of Oral Janus Kinase Inhibitors for the Treatment of Atopic Dermatitis. Front. Med. 8, 682547. doi:10.3389/fmed.2021.682547
Lee, C. S., and Duesbery, N. S. (2010). Highly Selective MEK Inhibitors. Curr. Enzym. Inhib. 6, 146–157. doi:10.2174/157340810793384124
Leung, E. L., Luo, L. X., Li, Y., Liu, Z. Q., Li, L. L., Shi, D. F., et al. (2019). Identification of a New Inhibitor of KRAS-Pdeδ Interaction Targeting KRAS Mutant Nonsmall Cell Lung Cancer. Int. J. Cancer 145 (5), 1334–1345. doi:10.1002/ijc.32222
Leung, K. H., Liu, L. J., Lin, S., Lu, L., Zhong, H. J., Susanti, D., et al. (2015). Discovery of a Small-Molecule Inhibitor of STAT3 by Ligand-Based Pharmacophore Screening. Methods 71, 38–43. doi:10.1016/j.ymeth.2014.07.010
Leung, K. H., Liu, L. J., Lin, S., Lu, L., Zhong, H. J., Susanti, D., et al. (2015). Discovery of a Small-Molecule Inhibitor of STAT3 by Ligand-Based Pharmacophore Screening. Methods 71, 38–43. doi:10.1016/j.ymeth.2014.07.010
Levy, B., Spira, A., Becker, D., Evans, T., Schnadig, I., Camidge, D. R., et al. (2014). A Randomized, Phase 2 Trial of Docetaxel with or without PX-866, an Irreversible Oral Phosphatidylinositol 3-kinase Inhibitor, in Patients with Relapsed or Metastatic Non-small-cell Lung Cancer. J. Thorac. Oncol. 9 (7), 1031–1035. doi:10.1097/JTO.0000000000000183
Levy, D. S., Kahana, J. A., and Kumar, R. (2009). AKT Inhibitor, GSK690693, Induces Growth Inhibition and Apoptosis in Acute Lymphoblastic Leukemia Cell Lines. Blood 113, 1723–1729. doi:10.1182/blood-2008-02-137737
Li, J., Wang, Z., Dai, L., Cao, L., Su, J., Zhu, M., et al. (2013). Effects of Rapamycin Combined with Low Dose Prednisone in Patients with Chronic Immune Thrombocytopenia. Clin. Dev. Immunol. 2013, 548085. doi:10.1155/2013/548085
Li, M., Wang, S., Li, X., Kou, R., Wang, Q., Wang, X., et al. (2018). Diallyl Sulfide Treatment Protects against Acetaminophen-/carbon Tetrachloride-Induced Acute Liver Injury by Inhibiting Oxidative Stress, Inflammation and Apoptosis in Mice. Toxicol. Res. (Camb). 8 (1), 67–76. doi:10.1039/c8tx00185e
Li, S., Li, J., Shen, C., Zhang, X., Sun, S., Cho, M., et al. (2014). tert-Butylhydroquinone (tBHQ) Protects Hepatocytes against Lipotoxicity via Inducing Autophagy Independently of Nrf2 Activation. Biochim. Biophys. Acta 1841 (1), 22–33. doi:10.1016/j.bbalip.2013.09.004
Li, X., Gao, Q., Feng, Y., and Zhang, X. (2019). Developing Role of B Cells in the Pathogenesis and Treatment of Chronic GVHD. Br. J. Haematol. 184 (3), 323–336. doi:10.1111/bjh.15719
Li, X., Zhang, Y., Walana, W., Zhao, F., Li, F., and Luo, F. (2020). GDC-0941 and CXCL8 (3-72) K11R/G31P Combination Therapy Confers Enhanced Efficacy against Breast Cancer. Future Oncol. 16 (14), 911–921. doi:10.2217/fon-2020-0035
Li, X., Wang, Y., Yang, H., and Dai, Y. (2022). Liver and Hepatocyte Transplantation: What Can Pigs Contribute? Front. Immunol. 12, 802692. doi:10.3389/fimmu.2021.802692
Liang, M. C., Bardhan, S., Pace, E. A., Rosman, D., Beutler, J. A., Porco, J. A., et al. (2006). Inhibition of Transcription Factor NF-kappaB Signaling Proteins IKKbeta and P65 through Specific Cysteine Residues by Epoxyquinone A Monomer: Correlation with its Anti-cancer Cell Growth Activity. Biochem. Pharmacol. 71 (5), 634–645. doi:10.1016/j.bcp.2005.11.013
Liang, W., Huang, X., and Chen, W. (2017). The Effects of Baicalin and Baicalein on Cerebral Ischemia: A Review. Aging Dis. 8 (6), 850–867. doi:10.14336/AD.2017.0829
Lignitto, L., eBoeuf, I., and Homer, H. (2019). Nrf2 Activation Promotes Lung Cancer Metastasis by Inhibiting the Degradation of Bachl. J. Cell. 178 (2), 316–329. doi:10.1016/j.cell.2019.06.003
Liles, J. T., Corkey, B. K., Notte, G. T., Budas, G. R., Lansdon, E. B., HinojosaKirschenbaum, F., et al. (2018). ASK1 Contributes to Fibrosis and Dysfunction in Models of Kidney Disease. J. Clin. Investig. 128 (10), 4485–4500. doi:10.1172/JCI99768
Limonciel, A., and Jennings, P. (2014). A Review of the Evidence that Ochratoxin A Is an Nrf2 Inhibitor: Implications for Nephrotoxicity and Renal Carcinogenicity. Toxins (Basel) 6 (1), 371–379. doi:10.3390/toxins6010371
Lin, L., Hutzen, B., Li, P. K., Ball, S., Zuo, M., DeAngelis, S., et al. (2010). A Novel Small Molecule, LLL12, Inhibits STAT3 Phosphorylation and Activities and Exhibits Potent Growth-Suppressive Activity in Human Cancer Cells. Neoplasia 12, 39–50. doi:10.1593/neo.91196
Lin, Y., Bai, L., Chen, W., and Xu, S. (2010). The NF-kappaB Activation Pathways, Emerging Molecular Targets for Cancer Prevention and Therapy. Expert Opin. Ther. Targets 14 (1), 45–55. doi:10.1517/14728220903431069
Lin, Y., Ukaji, T., Koide, N., and Umezawa, K. (2018). Inhibition of Late and Early Phases of Cancer Metastasis by the NF-Κb Inhibitor DHMEQ Derived from Microbial Bioactive Metabolite Epoxyquinomicin: A Review. Int. J. Mol. Sci. 19 (3), 729. doi:10.3390/ijms19030729
Lito, P., Solomon, M., Li, L. S., Hansen, R., and Rosen, N. (2016). Allele-specific Inhibitors Inactivate Mutant KRAS G12C by a Trapping Mechanism. Science 351 (6273), 604–608. doi:10.1126/science.aad6204
Liu, Q., Wu, H., Chim, S. M., Zhou, L., Zhao, J., Feng, H., et al. (2013). SC-514, a Selective Inhibitor of IKKβ Attenuates RANKL-Induced Osteoclastogenesis and NF-Κb Activation. Biochem. Pharmacol. 86, 1775–1783. doi:10.1016/j.bcp.2013.09.017
Lo Cascio, F., Marzullo, P., Kayed, R., and Palumbo Piccionello, A. (2021). Curcumin as Scaffold for Drug Discovery against Neurodegenerative Diseases. Biomedicines 9 (2), 173. doi:10.3390/biomedicines9020173
Logie, E., and Vanden Berghe, W. (2020). Tackling Chronic Inflammation with Withanolide Phytochemicals-A Withaferin a Perspective. Antioxidants (Basel) 9 (11), 1107. doi:10.3390/antiox9111107
Long, Z., Yu, F., Du, Y., Li, H., Chen, M., Zhuang, J., et al. (2018). Successful Treatment of Refractory/relapsed Acquired Pure Red Cell Aplasia with Sirolimus. Ann. Hematol. 97 (11), 2047–2054. doi:10.1007/s00277-018-3431-5
Loomba, R., Lawitz, E., Mantry, P. S., Jayakumar, S., Caldwell, S. H., Arnold, H., et al. (2018). The ASK1 Inhibitor Selonsertib in Patients with Nonalcoholic Steatohepatitis: a Randomized, Phase 2 Trial. Hepatology 67 (2), 549–559. doi:10.1002/hep.29514
Lu, H., Lu, Q., Gaddipati, S., Kasetti, R. B., Wang, W., Pasparakis, M., et al. (2014). IKK2 Inhibition Attenuates Laser-Induced Choroidal Neovascularization. PLoS ONE 9, e87530. doi:10.1371/journal.pone.0087530
Lun, M., Zhang, P. L., Siegelmann-Danieli, N., Blasick, T. M., and Brown, R. E. (2005). Intracellular Inhibitory Effects of Velcade Correlate with Morphoproteomic Expression of Phosphorylated-Nuclear Factor-kappaB and P53 in Breast Cancer Cell Lines. Ann. Clin. Lab. Sci. 35, 15–24.
Lv, L., Cui, H., Ma, Z., Liu, X., and Yang, L. (2021). Recent Progresses in the Pharmacological Activities of Caffeic Acid Phenethyl Ester. Naunyn Schmiedeb. Arch. Pharmacol. 394 (7), 1327–1339. doi:10.1007/s00210-021-02054-w
Ma, L., Gao, J. S., Guan, Y., Shi, X., Zhang, H., Ayrapetov, M. K., et al. (2010). Acetylation Modulates Prolactin Receptor Dimerization. Proc. Natl. Acad. Sci. U. S. A. 107 (45), 19314–19319. doi:10.1073/pnas.1010253107
Ma, X. M., and Blenis, J. (2009). Molecular Mechanisms of mTOR-Mediated Translational Control. Nat. Rev. Mol. Cell. Biol. 10 (5), 307–318. doi:10.1038/nrm2672
Macejová, M., Sačková, V., Hradická, P., Jendželovský, R., Demečková, V., and Fedoročko, P. (2020). Combination of Photoactive Hypericin and Manumycin A Exerts Multiple Anticancer Effects on Oxaliplatin-Resistant Colorectal Cells. Toxicol. Vitro 66, 104860. doi:10.1016/j.tiv.2020.104860
MacMaster, J. F., Dambach, D. M., Lee, D. B., Berry, K. K., Qiu, Y., Zusi, F. C., et al. (2003). An Inhibitor of IkappaB Kinase, BMS-345541, Blocks Endothelial Cell Adhesion Molecule Expression and Reduces the Severity of Dextran Sulfate Sodium-Induced Colitis in Mice. Inflamm. Res. 52, 508–511. doi:10.1007/s00011-003-1206-4
Madeo, F., Eisenberg, T., Pietrocola, F., and Kroemer, G. (2018). Spermidine in Health and Disease. Science 359 (6374), eaan2788. doi:10.1126/science.aan2788
Magesh, S., Chen, Y., and Hu, L. (2012). Small Molecule Modulators of Keap1-Nrf2-ARE Pathway as Potential Preventive and Therapeutic Agents. Med. Res. Rev. 32 (4), 687–726. doi:10.1002/med.21257
Mahajan, S., Hogan, J. K., Shlyakhter, D., Oh, L., Salituro, F. G., Farmer, L., et al. (2015). VX-509 (Decernotinib) Is a Potent and Selective Janus Kinase 3 Inhibitor that Attenuates Inflammation in Animal Models of Autoimmune Disease. J. Pharmacol. Exp. Ther. 353 (2), 405–414. doi:10.1124/jpet.114.221176
Maira, S. M., Stauffer, F., Brueggen, J., Furet, P., Schnell, C., Fritsch, C., et al. (2008). Identification and Characterization of NVP-Bez235, a New Orally Available Dual Phosphatidylinositol 3-kinase/mammalian Target of Rapamycin Inhibitor with Potent In Vivo Antitumor Activity. Mol. Cancer Ther. 7, 1851–1863. doi:10.1158/1535-7163.MCT-08-0017
Manna, A., De Sarkar, S., De, S., Bauri, A. K., Chattopadhyay, S., and Chatterjee, M. (2015). The Variable Chemotherapeutic Response of Malabaricone-A in Leukemic and Solid Tumor Cell Lines Depends on the Degree of Redox Imbalance. Phytomedicine 22 (7-8), 713–723. doi:10.1016/j.phymed.2015.05.007
Manrique-Gil, I., Sánchez-Vicente, I., Torres-Quezada, I., and Lorenzo, O. (2021). Nitric Oxide Function during Oxygen Deprivation in Physiological and Stress Processes. J. Exp. Bot. 72 (3), 904–916. doi:10.1093/jxb/eraa442
Marcotte, D., Zeng, W., Hus, J. C., McKenzie, A., Hession, C., Jin, P., et al. (2013). Small Molecules Inhibit the Interaction of Nrf2 and the Keap1 Kelch Domain through a Non-covalent Mechanism. Bioorg Med. Chem. 21 (14), 4011–4019. doi:10.1016/j.bmc.2013.04.019
Marín-Ramos, N. I., Balabasquer, M., Ortega-Nogales, F. J., Torrecillas, I. R., Gil-Ordóñez, A., Marcos-Ramiro, B., et al. (2019). A Potent Isoprenylcysteine Carboxylmethyltransferase (ICMT) Inhibitor Improves Survival in Ras-Driven Acute Myeloid Leukemia. J. Med. Chem. 62 (13), 6035–6046. doi:10.1021/acs.jmedchem.9b00145
Mateo, J., Ganji, G., Lemech, C., Burris, H. A., Han, S. W., Swales, K., et al. (2017). A First-Time-In-Human Study of GSK2636771, a Phosphoinositide 3 Kinase Beta-Selective Inhibitor, in Patients with Advanced Solid Tumors. Clin. Cancer Res. 23 (19), 5981–5992. doi:10.1158/1078-0432.CCR-17-0725
Mathas, S., Lietz, A., Janz, M., Hinz, M., Jundt, F., Scheidereit, C., et al. (2003). Inhibition of NF-kappaB Essentially Contributes to Arsenic-Induced Apoptosis. Blood 102 (3), 1028–1034. doi:10.1182/blood-2002-04-1154
May, M. J., D'Acquisto, F., Madge, L. A., Glöckner, J., Pober, J. S., and Ghosh, S. (2000). Selective Inhibition of NF-kappaB Activation by a Peptide that Blocks the Interaction of NEMO with the IkappaB Kinase Complex. Science 289 (5484), 1550–1554. doi:10.1126/science.289.5484.1550
Mazgaj, R., Szudzik, M., Lipiński, P., Jończy, A., Smuda, E., Kamyczek, M., et al. (2020). Effect of Oral Supplementation of Healthy Pregnant Sows with Sucrosomial Ferric Pyrophosphate on Maternal Iron Status and Hepatic Iron Stores in Newborn Piglets. Anim. (Basel) 10 (7), 1113. doi:10.3390/ani10071113
Mbalaviele, G., Sommers, C. D., Bonar, S. L., Mathialagan, S., Schindler, J. F., Guzova, J. A., et al. (2009). A Novel, Highly Selective, Tight Binding IkappaB Kinase-2 (IKK-2) Inhibitor: A Tool to Correlate IKK-2 Activity to the Fate and Functions of the Components of the Nuclear Factor-kappaB Pathway in Arthritis-Relevant Cells and Animal Models. J. Pharmacol. Exp. Ther. 329, 14–25. doi:10.1124/jpet.108.143800
McIntyre, K. W., Shuster, D. J., Gillooly, K. M., Dambach, D. M., Pattoli, M. A., Lu, P., et al. (2003). A Highly Selective Inhibitor of I Kappa B Kinase, BMS-345541, Blocks Both Joint Inflammation and Destruction in Collagen-Induced Arthritis in Mice. Arthritis Rheum. 48, 2652–2659. doi:10.1002/art.11131
Mebratu, Y., and Tesfaigzi, Y. (2009). How ERK1/2 Activation Controls Cell Proliferation and Cell Death: Is Subcellular Localization the Answer? Cell. Cycle 8 (8), 1168–1175. doi:10.4161/cc.8.8.8147
Meloche, S., and Pouysségur, J. (2007). The ERK1/2 Mitogen-Activated Protein Kinase Pathway as a Master Regulator of the G1- to S-phase Transition. Oncogene 26 (22), 3227–3239. doi:10.1038/sj.onc.1210414
Meng, T., Xiao, D., Muhammed, A., Deng, J., Chen, L., and He, J. (2021). Anti-Inflammatory Action and Mechanisms of Resveratrol. Molecules 26 (1), 229. doi:10.3390/molecules26010229
Mengarda, A. C., Mendonça, P. S., Morais, C. S., Cogo, R. M., Mazloum, S. F., Salvadori, M. C., et al. (2020). Antiparasitic Activity of Piplartine (Piperlongumine) in a Mouse Model of Schistosomiasis. Acta Trop. 205, 105350. doi:10.1016/j.actatropica.2020.105350
Meyer, D. M., Jesson, M. I., Li, X., Elrick, M. M., Funckes-Shippy, C. L., Warner, J. D., et al. (2010). Anti-inflammatory Activity and Neutrophil Reductions Mediated by the JAK1/JAK3 Inhibitor, CP-690,550, in Rat Adjuvant-Induced Arthritis. J. Inflamm. (Lond) 7, 741. doi:10.1186/1476-9255-7-41
Milite, C., Feoli, A., Sasaki, K., La Pietra, V., Balzano, A. L., Marinelli, L., et al. (2015). A Novel Cell-Permeable, Selective, and Noncompetitive Inhibitor of KAT3 Histone Acetyltransferases from a Combined Molecular Pruning/classical Isosterism Approach. J. Med. Chem. 58 (6), 2779–2798. doi:10.1021/jm5019687
Minden, A., Lin, A., McMahon, M., Lange-Carter, C., Dérijard, B., Davis, R. J., et al. (1994). Differential Activation of ERK and JNK Mitogen-Activated Protein Kinases by Raf-1 and MEKK. Science 266 (5191), 1719–1723. doi:10.1126/science.7992057
Mirahmadi, M., Azimi-Hashemi, S., Saburi, E., Kamali, H., Pishbin, M., and Hadizadeh, F. (2020). Potential Inhibitory Effect of Lycopene on Prostate Cancer. Biomed. Pharmacother. 129, 110459. doi:10.1016/j.biopha.2020.110459
Miryan, M., Soleimani, D., Askari, G., Jamialahmadi, T., Guest, P. C., Bagherniya, M., et al. (2021). Curcumin and Piperine in COVID-19: A Promising Duo to the Rescue? Adv. Exp. Med. Biol. 1327, 197–204. doi:10.1007/978-3-030-71697-4_16
Mohmmad Abdul, H., and Butterfield, D. A. (2005). Protection against Amyloid Beta-Peptide (1-42)-induced Loss of Phospholipid Asymmetry in Synaptosomal Membranes by Tricyclodecan-9-Xanthogenate (D609) and Ferulic Acid Ethyl Ester: Implications for Alzheimer's Disease. Biochim. Biophys. Acta 1741 (1-2), 140–148. doi:10.1016/j.bbadis.2004.12.002
Molckovsky, A., and Siu, L. L. (2008). First-in-class, First-In-Human Phase I Results of Targeted Agents: Highlights of the 2008 American Society of Clinical Oncology Meeting. J. Hematol. Oncol. 1, 20. doi:10.1186/1756-8722-1-20
Monaco, K. A. (2019). RAF Inhibitor LXH254 Effectively Inhibits B-And-CRAF, but Not ARAF [abstract]. Cancer ResLB– 79 (Suppl. 13), 144.
Montilla, A. M., Gómez-García, F., Gómez-Arias, P. J., Gay-Mimbrera, J., Hernández-Parada, J., Isla-Tejera, B., et al. (2019). Scoping Review on the Use of Drugs Targeting JAK/STAT Pathway in Atopic Dermatitis, Vitiligo, and Alopecia Areata. Dermatol Ther. (Heidelb) 9 (4), 655–683. doi:10.1007/s13555-019-00329-y
Moore, A. R., Rosenberg, S. C., McCormick, F., and Malek, S. (2020). RAS-Targeted Therapies: Is the Undruggable Drugged?. Nat. Rev. Drug Discov. 19 (8), 533–552. doi:10.1038/s41573-020-0068-6
Moss, N. C., Stansfield, W. E., Willis, M. S., Tang, R. H., and Selzman, C. H. (2007). IKKbeta Inhibition Attenuates Myocardial Injury and Dysfunction Following Acute Ischemia-Reperfusion Injury. Am. J. Physiol. Heart Circ. Physiol. 293, H2248–H2253. doi:10.1152/ajpheart.00776.2007
Munoz, J., Follows, G. A., and Nastoupil, L. J. (2021). Copanlisib for the Treatment of Malignant Lymphoma: Clinical Experience and Future Perspectives. Target Oncol. 16 (3), 295–308. doi:10.1007/s11523-021-00802-9
Muselin, F. Z., and Cristina, R. T. (2019). Homeostatic Changes of Some Trace Elements in Geriatric Rats in the Condition of Oxidative Stress Induced by Aluminum and the Beneficial Role of Resveratrol [J]. J. Trace Elem. Med. Biol. 55, 136–142. doi:10.1016/j.jtemb.2019.06.013
Muthiah, D., and Callaghan, R. (2017). Dual Effects of the PI3K Inhibitor ZSTK474 on Multidrug Efflux Pumps in Resistant Cancer Cells. Eur. J. Pharmacol. 815, 127–137. doi:10.1016/j.ejphar.2017.09.001
Nagini, S., Nivetha, R., and Palrasu, M.Neem Limonoid (2021). Nimbolide, a Neem Limonoid, Is a Promising Candidate for the Anticancer Drug Arsenal. J. Med. Chem. 64 (7), 3560–3577. doi:10.1021/acs.jmedchem.0c02239
Nakamura, A., Arita, T., Tsuchiya, S., Donelan, J., Chouitar, J., Carideo, E., et al. (2013). Antitumor Activity of the Selective Pan-RAF Inhibitor TAK-632 in BRAF Inhibitor-Resistant Melanoma. Cancer Res. 73 (23), 7043–7055. doi:10.1158/0008-5472.CAN-13-1825
Nan, J., Du, Y., Chen, X., Bai, Q., Wang, Y., Zhang, X., et al. (2014). TPCA-1 Is a Direct Dual Inhibitor of STAT3 and NF-Κb and Regresses Mutant EGFR-Associated Human Non-small Cell Lung Cancers. Mol. Cancer Ther. 13, 617–629. doi:10.1158/1535-7163.MCT-13-0464
Natalia, R-A., Raquel, F-G., Gina, M., and Antonio, C. (2019). Activators and Inhibitors of NRF2: A Review of Their Potential for Clinical Development. Oxidative Med. Cell. Longev. 2019.
Negi, G., and Sharma, S. S. (2015). Inhibition of IκB Kinase (IKK) Protects against Peripheral Nerve Dysfunction of Experimental Diabetes. Mol. Neurobiol. 51, 591–598. doi:10.1007/s12035-014-8784-8
Neitz, R. J., Konradi, A. W., and Sham, H. L. (2011). Highly Selective C-Jun N-Terminal Kinase (JNK) 3 Inhibitors with In Vitro CNS-like Pharmacokinetic Properties II. Central Core Replacement. Bioorg Med. Chem. Lett. 21, 3726–3729. doi:10.1016/j.bmcl.2011.04.074
Nelson, E. A., Walker, S. R., Kepich, A., Gashin, L. B., Hideshima, T., Ikeda, H., et al. (2008). Nifuroxazide Inhibits Survival of Multiple Myeloma Cells by Directly Inhibiting STAT3. Blood 112 (13), 5095–5102. doi:10.1182/blood-2007-12-129718
Nelson, E. A., Walker, S. R., Weisberg, E., Bar-Natan, M., Barrett, R., Gashin, L. B., et al. (2011). The STAT5 Inhibitor Pimozide Decreases Survival of Chronic Myelogenous Leukemia Cells Resistant to Kinase Inhibitors. Blood 117 (12), 3421–3429. doi:10.1182/blood-2009-11-255232
Ngoei, K. R., Catimel, B., and Church, N. (2011). Characterization of a Novel JNK (C-Jun N-Terminal Kinase) Inhibitory Peptide. Biochem. J. 434, 399–413. doi:10.1042/BJ20101244
Nguyen, K. T., Tajmir, P., Lin, C. H., Liadis, N., Zhu, X. D., Eweida, M., et al. (2006). Essential Role of Pten in Body Size Determination and Pancreatic Beta-Cell Homeostasis In Vivo. Mol. Cell. Biol. 26, 4511–4518. doi:10.1128/MCB.00238-06
Nguyen, T., Sherratt, P. J., Nioi, P., Yang, C. S., and Pickett, C. B. (2005). Nrf2 Controls Constitutive and Inducible Expression of ARE-Driven Genes through a Dynamic Pathway Involving Nucleocytoplasmic Shuttling by Keap1. J. Biol. Chem. 280 (37), 32485–32492. doi:10.1074/jbc.M503074200
Nichols, R. J., Haderk, F., Stahlhut, C., Schulze, C. J., Hemmati, G., Wildes, D., et al. (2018). RAS Nucleotide Cycling Underlies the SHP2 Phosphatase Dependence of Mutant BRAF-, NF1- and RAS-Driven Cancers. Nat. Cell. Biol. 20 (9), 1064–1073. doi:10.1038/s41556-018-0169-1
Nioi, P., McMahon, M., Itoh, K., Yamamoto, M., and Hayes, J. D. (2003). Identification of a Novel Nrf2-Regulated Antioxidant Response Element (ARE) in the Mouse NAD(P)H:quinone Oxidoreductase 1 Gene: Reassessment of the ARE Consensus Sequence. Biochem. J. 374 (Pt 2), 337–348. doi:10.1042/BJ20030754
Nitulescu, G. M., Margina, D., Juzenas, P., Peng, Q., Olaru, O. T., Saloustros, E., et al. (2016). Akt Inhibitors in Cancer Treatment: The Long Journey from Drug Discovery to Clinical Use (Review). Int. J. Oncol. 48 (3), 869–885. doi:10.3892/ijo.2015.3306
Nogueira, M., Puig, L., and Torres, T. (2020). JAK Inhibitors for Treatment of Psoriasis: Focus on Selective TYK2 Inhibitors. Drugs 80 (4), 341–352. doi:10.1007/s40265-020-01261-8
Nordham, K. D., and Ninokawa, S. (2022). The History of Organ Transplantation. Proc. (Bayl Univ. Med. Cent. 35 (1), 124–128. doi:10.1080/08998280.2021.1985889
Nygaard, G., Di Paolo, J. A., Hammaker, D., Boyle, D. L., Budas, G., Notte, G. T., et al. (2018). Regulation and Function of Apoptosis Signal-Regulating Kinase 1 in Rheumatoid Arthritis. Biochem. Pharmacol. 151, 282–290. doi:10.1016/j.bcp.2018.01.041
O'Bryan, J. P. (2019). Pharmacological Targeting of RAS: Recent Success With Direct Inhibitors. Pharmacol. Res. 139, 503–511. doi:10.1016/j.phrs.2018.10.021
Ochiai, T., Saito, Y., Saitoh, T., Dewan, M. Z., Shioya, A., Kobayashi, M., et al. (2008). Inhibition of IkappaB Kinase Beta Restrains Oncogenic Proliferation of Pancreatic Cancer Cells. J. Med. Dent. Sci. 55, 49–59.
Ogut, D., Reel, B., Gonen Korkmaz, C., Arun, M. Z., Cilaker Micili, S., and Ergur, B. U. (2016). Doxycycline Down-Regulates Matrix Metalloproteinase Expression and Inhibits NF-Κb Signaling in LPS-Induced PC3 Cells. Folia Histochem Cytobiol. 54 (4), 171–180. doi:10.5603/FHC.a2016.0022
Okada, M., Kuramoto, K., and Takeda, H. (2016). The Novel JNK Inhibitor AS602801 Inhibits Cancer Stem Cells In Vitro and In Vivo. Oncotarget 7, 27021–27032. doi:10.18632/oncotarget.8395
Okaniwa, M., Hirose, M., Arita, T., Yabuki, M., Nakamura, A., Takagi, T., et al. (2013). Discovery of a Selective Kinase Inhibitor (TAK-632) Targeting Pan-RAF Inhibition: Design, Synthesis, and Biological Evaluation of C-7-Substituted 1,3-benzothiazole Derivatives. J. Med. Chem. 56 (16), 6478–6494. doi:10.1021/jm400778d
Okiyama, N., Furumoto, Y., Villarroel, V. A., Linton, J. T., Tsai, W. L., Gutermuth, J., et al. (2014). Reversal of CD8 T-Cell-Mediated Mucocutaneous Graft-versus-host-like Disease by the JAK Inhibitor Tofacitinib. J. Investig. Dermatol 134 (4), 992–1000. doi:10.1038/jid.2013.476
Olsen, L. S., Hjarnaa, P. J., Latini, S., Holm, P. K., Larsson, R., Bramm, E., et al. Anticancer Agent CHS 828 Suppresses Nuclear Factor-Kappa B Activity in Cancer Cells through Downregulation of IKK Activity. Int. J. Cancer 2004;111:198 ^ 205.doi:10.1002/ijc.20255
Omair, M. A., Alahmadi, A., and Johnson, S. R. (2015). Safety and Effectiveness of Mycophenolate in Systemic Sclerosis. A Systematic Review. PLoS One 10 (5), e0124205. doi:10.1371/journal.pone.0124205
Onimoe, G. I., Liu, A., Lin, L., Wei, C. C., Schwartz, E. B., Bhasin, D., et al. (2012). Small Molecules, LLL12 and FLLL32, Inhibit STAT3 and Exhibit Potent Growth Suppressive Activity in Osteosarcoma Cells and Tumor Growth in Mice. Investig. New Drugs 30 (3), 916–926. doi:10.1007/s10637-011-9645-1
Osaki, M., Oshimura, M., and Ito, H. (2004). PI3K-Akt Pathway: its Functions and Alterations in Human Cancer. Apoptosis 9 (6), 667–676. doi:10.1023/B:APPT.0000045801.15585.dd
O’shea, J. J., Gadina, M., and Schreiber, R. D. (2002). Cytokine Signaling in 2002new Surprises in the Jak/Stat pathway[J], 109 SupplS121–S131.
O’Shea, J. J., Schwartz, D. M., Villarino, A. V., Gadina, M., McInnes, I. B., and Laurence, A. (2015). The JAK-STAT Pathway: Impact on Human Disease and Therapeutic Intervention. Annu. Rev. Med. 66, 311–328. doi:10.1146/annurev-med-051113-024537
Ostrem, J. M., Peters, U., Sos, M. L., Wells, J. A., and Shokat, K. M. K- (2013). Ras(G12C) Inhibitors Allosterically Control GTP Affinity and Effector Interactions. Nature 503 (7477), 548–551. doi:10.1038/nature12796
Page, B. D., Khoury, H., Laister, R. C., Fletcher, S., Vellozo, M., Manzoli, A., et al. (2012). Small Molecule STAT5-SH2 Domain Inhibitors Exhibit Potent Antileukemia Activity. J. Med. Chem. 55, 1047–1055. doi:10.1021/jm200720n
Pal, A., Asad, Y., Ruddle, R., Henley, A. T., Swales, K., Decordova, S., et al. (2020). Metabolomic Changes of the Multi (-AGC-) Kinase Inhibitor AT13148 in Cells, Mice and Patients Are Associated with NOS Regulation. Metabolomics 16 (4), 50. doi:10.1007/s11306-020-01676-0
Pallangyo, C. K., Ziegler, P. K., and Greten, F. R. (2015). IKKβ Acts as a Tumor Suppressor in Cancer-Associated Fibroblasts during Intestinal Tumorigenesis. J. Exp. Med. 212 (13), 2253–2266. doi:10.1084/jem.20150576
Pancholi, S., Leal, M. F., Ribas, R., Simigdala, N., Schuster, E., Chateau-Joubert, S., et al. (20194202). Combination of mTORC1/2 Inhibitor Vistusertib Plus Fulvestrant In Vitro and In Vivo Targets Oestrogen Receptor-Positive Endocrine-Resistant Breast cancerErratum in. Breast Cancer ResBreast Cancer Res. 2122 (11), 13514. doi:10.1186/s13058-019-1222-0
Pandey, M. K., Sung, B., Kunnumakkara, A. B., Sethi, G., Chaturvedi, M. M., and Aggarwal, B. B. (2008). Berberine Modifies Cysteine 179 of IkappaBalpha Kinase, Suppresses Nuclear Factor-kappaB-Regulated Antiapoptotic Gene Products, and Potentiates Apoptosis. Cancer Res. 68, 5370–5379. doi:10.1158/0008-5472.CAN-08-0511
Pandey, M. K., Sandur, S. K., Sung, B., Sethi, G., Kunnumakkara, A. B., and Aggarwal, B. B. (2007). Butein, a Tetrahydroxychalcone, Inhibits Nuclear Factor (NF)-κB and NF-Κb-Regulated Gene Expression through Direct Inhibition of IκBα Kinase β on Cysteine 179 Residue. J. Biol. Chem. 282 (24), 17340–17350. doi:10.1074/jbc.m700890200
Papa, A., Wan, L., Bonora, M., Salmena, L., Song, M. S., Hobbs, R. M., et al. (2014). Cancer-associated PTEN Mutants Act in a Dominant-Negative Manner to Suppress PTEN Protein Function. Cell. 157, 595–610. doi:10.1016/j.cell.2014.03.027
Park, H. M., Cho, J. M., Lee, H. R., Shim, G. S., and Kwak, M. K. (2008). Renal Protection by 3H-1,2-Dithiole-3-Thione against Cisplatin through the Nrf2-Antioxidant Pathway. Biochem. Pharmacol. 76 (5), 597–607. doi:10.1016/j.bcp.2008.06.021
Parker, W., Saadi, S., Lin, S. S., Holzknecht, Z. E., Bustos, M., and Platt, J. L. (1996). Transplantation of Discordant Xenografts: a Challenge Revisited. Immunol. Today 17 (8), 373–378. doi:10.1016/0167-5699(96)10028-1
Patel, C. G., Rangachari, L., Patti, M., Griffin, C., Shou, Y., and Venkatakrishnan, K. (2019). Characterizing the Sources of Pharmacokinetic Variability for TAK-117 (Serabelisib), an Investigational Phosphoinositide 3-Kinase Alpha Inhibitor: A Clinical Biopharmaceutics Study to Inform Development Strategy. Clin. Pharmacol. Drug Dev. 8 (5), 637–646. doi:10.1002/cpdd.613
Patricelli, M. P., Janes, M. R., Li, L. S., Hansen, R., Peters, U., Kessler, L. V., et al. (2016). Selective Inhibition of Oncogenic KRAS Output with Small Molecules Targeting the Inactive State. Cancer Discov. 6 (3), 316–329. doi:10.1158/2159-8290.CD-15-1105
Paul, S., Roy, D., Pati, S., and Sa, G. (2021). The Adroitness of Andrographolide as a Natural Weapon against Colorectal Cancer. Front. Pharmacol. 12, 731492. doi:10.3389/fphar.2021.731492
Peng, S. B. (2015). Inhibition of RAF Isoforms and Active Dimers by LY3009120 Leads to Anti-tumor Activities in RAS or BRAF Mutant Cancers. Cancer Cell. 28, 384–398. doi:10.1016/j.ccell.2015.08.002
Pheneger, J., Wallace, E., Marlow, A., Hurley, B., Lyssikatos, J., Bendele, A. M., et al. (2006). “Characterization of ARRY-438162, a Potent MEK Inhibitor in Combination with Methotrexate or Ibuprofen in In Vivo Models of Arthritis,” in Proceedings of the 2006 Annual Scientifific Meeting (Boston, MA, USA, 794.
Picaud, S., Fedorov, O., Thanasopoulou, A., Leonards, K., Jones, K., Meier, J., et al. (2015). Generation of a Selective Small Molecule Inhibitor of the CBP/p300 Bromodomain for Leukemia Therapy. Cancer Res. 75 (23), 5106–5119. doi:10.1158/0008-5472.CAN-15-0236
Pierpaoli, E., Cirioni, O., Simonetti, O., Orlando, F., Giacometti, A., Lombardi, P., et al. (2021). Potential Application of Berberine in the Treatment of Escherichia coli Sepsis. Nat. Prod. Res. 35 (22), 4779–4784. doi:10.1080/14786419.2020.1721729
Pileri, A., Delfino, C., Grandi, V., and Pimpinelli, N. (2013). Role of Bexarotene in the Treatment of Cutaneous T-Cell Lymphoma: the Clinical and Immunological Sides. Immunotherapy 5 (4), 427–433. doi:10.2217/imt.13.15
Ping, H., Yang, F., Wang, M., Niu, Y., and Xing, N. (2016). IKK Inhibitor Suppresses Epithelial-Mesenchymal Transition and Induces Cell Death in Prostate Cancer. Oncol. Rep. 36, 1658–1664. doi:10.3892/or.2016.4915
Plantevin Krenitsky, V., Nadolny, L., and Delgado, M. (2012). Discovery of CC-930, an Orally Active Anti-fibrotic JNK Inhibitor. Bioorg Med. Chem. Lett. 22, 1433–1438. doi:10.1016/j.bmcl.2011.12.027
Podolin, P. L., Callahan, J. F., Bolognese, B. J., Li, Y. H., Carlson, K., Davis, T. G., et al. (2005). Attenuation of Murine Collagen-Induced Arthritis by a Novel, Potent, Selective Small Molecule Inhibitor of IkappaB Kinase 2, TPCA-1 (2-[(aminocarbonyl)amino]-5-(4-Fluorophenyl)-3-Thiophenecarboxamide), Occurs via Reduction of Proinflammatory Cytokines and Antigen-Induced T Cell Proliferation. J. Pharmacol. Exp. Ther. 312, 373–381. doi:10.1124/jpet.104.074484
Posthumadeboer, J., van Egmond, P. W., and Helder, M. N. (2012). Targeting JNK-Interacting-Protein-1 (JIP1) Sensitises Osteosarcoma to Doxorubicin. Oncotarget 3, 1169–1181. doi:10.18632/oncotarget.600
Prescott, J. A., and Cook, S. J. (2018). Targeting IKKβ in Cancer: Challenges and Opportunities for the Therapeutic Utilisation of IKKβ Inhibitors. Cells 7 (9), 115. doi:10.3390/cells7090115
Pricci, M., Girardi, B., Giorgio, F., Losurdo, G., Ierardi, E., and Di Leo, A. (2020). Curcumin and Colorectal Cancer: From Basic to Clinical Evidences. Int. J. Mol. Sci. 21 (7), 2364. doi:10.3390/ijms21072364
Price-Troska, T., Yang, Z. Z., Diller, D., Bayden, A., Jarosinski, M., Audie, J., et al. (2019). Inhibiting IL-2 Signaling and the Regulatory T-Cell Pathway Using Computationally Designed Peptides. Investig. New Drugs 37 (1), 9–16. doi:10.1007/s10637-018-0606-9
Purandare, A. V., McDevitt, T. M., Wan, H., You, D., Penhallow, B., Han, X., et al. (2012). Characterization of BMS-911543, a Functionally Selective Small-Molecule Inhibitor of JAK2. Leukemia 26 (2), 280–288. doi:10.1038/leu.2011.292
Qiu, Q., Feng, Q., Tan, X., and Guo, M. (2019). JAK3-selective Inhibitor Peficitinib for the Treatment of Rheumatoid Arthritis. Expert Rev. Clin. Pharmacol. 12 (6), 547–554. doi:10.1080/17512433.2019.1615443
Qiu, X., Tian, Y., Liang, Z., Sun, Y., Li, Z., and Bian, J. (2019). Recent Discovery of Phosphoinositide 3-kinase γ Inhibitors for the Treatment of Immune Diseases and Cancers. Future Med. Chem. 11 (16), 2151–2169. doi:10.4155/fmc-2019-0010
Quijia, C. R., and Chorilli, M. (2021). Piperine for Treating Breast Cancer: A Review of Molecular Mechanisms, Combination with Anticancer Drugs, and Nanosystems. Phytother. Res. doi:10.1002/ptr.7291
Quintás-Cardama, A., and Verstovsek, S. (2013). Molecular Pathways: Jak/STAT Pathway: Mutations, Inhibitors, and Resistance. Clin. Cancer Res. 19 (8), 1933–1940. doi:10.1158/1078-0432.CCR-12-0284
Rajendrasozhan, S., Hwang, J. W., Yao, H., Kishore, N., and Rahman, I. (2010). Anti-inflammatory Effect of a Selective IkappaB Kinase-Beta Inhibitor in Rat Lung in Response to LPS and Cigarette Smoke. Pulm. Pharmacol. Ther. 23, 172–181. doi:10.1016/j.pupt.2010.01.002
Reilly, S. M., Chiang, S. H., Decker, S. J., Chang, L., Uhm, M., Larsen, M. J., et al. (2013). An Inhibitor of the Protein Kinases TBK1 and IKK-Ɛ Improves Obesity-Related Metabolic Dysfunctions in Mice. Nat. Med. 19 (3), 313–321. doi:10.1038/nm.3082
Ren, D., Villeneuve, N. F., Jiang, T., Wu, T., Lau, A., Toppin, H. A., et al. (2011). Brusatol Enhances the Efficacy of Chemotherapy by Inhibiting the Nrf2-Mediated Defense Mechanism. Proc. Natl. Acad. Sci. U. S. A. 108 (4), 1433–1438. doi:10.1073/pnas.1014275108
Ren, Y. X., Yang, J., Sun, R. M., Zhang, L. J., Zhao, L. F., Li, B. Z., et al. (2016). Viral IL-10 Down-Regulates the "MHC-I Antigen Processing Operon" through the NF-Κb Signaling Pathway in Nasopharyngeal Carcinoma Cells. Cytotechnology 68 (6), 2625–2636. doi:10.1007/s10616-016-9987-9
Reynaert, N. L., Ckless, K., Korn, S. H., Vos, N., Guala, A. S., Wouters, E. F., et al. (2004). Nitric Oxide Represses Inhibitory kappaB Kinase through S-Nitrosylation. Proc. Natl. Acad. Sci. U. S. A. 101 (24), 8945–8950. doi:10.1073/pnas.0400588101
Richardson, B. G., Jain, A. D., Speltz, T. E., and Moore, T. W. (2015). Non-electrophilic Modulators of the Canonical Keap1/Nrf2 Pathway. Bioorg Med. Chem. Lett. 25 (11), 2261–2268. doi:10.1016/j.bmcl.2015.04.019
Robinson, M. F., Damjanov, N., Stamenkovic, B., Radunovic, G., Kivitz, A., Cox, L., et al. (2020). Efficacy and Safety of PF-06651600 (Ritlecitinib), a Novel JAK3/TEC Inhibitor, in Patients with Moderate-To-Severe Rheumatoid Arthritis and an Inadequate Response to Methotrexate. Arthritis Rheumatol. 72 (10), 1621–1631. doi:10.1002/art.41316
Rosenkranz, S., Feldman, J., McLaughlin, V., Rischard, F., White, J., Ebrahimi, R., et al. (2017). The ARROW Study: a Phase 2, Prospective, Randomized, Double-Blind, Placebo-Controlled Study of Selonsertib in Subjects with Pulmonary Arterial Hypertension. Eur. Respir. J. 50 (Suppl. 61), OA1983. doi:10.1183/1393003.congress-2017.oa1983
Ross, Y., and Magrey, M. (2021). Use of Upadacitinib in the Treatment of Psoriatic Arthritis. Immunotherapy 13 (18), 1549–1554. doi:10.2217/imt-2021-0130
Rynhoud, H., Gibson, J. S., Meler, E., and Soares Magalhães, R. J. (2021). The Association between the Use of Oclacitinib and Antibacterial Therapy in Dogs with Allergic Dermatitis: A Retrospective Case-Control Study. Front. Vet. Sci. 8, 631443. doi:10.3389/fvets.2021.631443
Sachse, F., Becker, K., Basel, T. J., Weiss, D., and Rudack, C. (2011). IKK-2 Inhibitor TPCA-1 Represses Nasal Epithelial Inflammation In Vitro. Rhinology 49, 168–173. doi:10.4193/Rhino10.099
Saeed, M. E. M., Krishna, S., Greten, H. J., Kremsner, P. G., and Efferth, T. (2016). Antischistosomal Activity of Artemisinin Derivatives In Vivo and in Patients. Pharmacol. Res. 110, 216–226. doi:10.1016/j.phrs.2016.02.017
Sampaio, O. M., Vieira, L. C. C., Bellete, B. S., King-Diaz, B., Lotina-Hennsen, B., Veiga, T. A. M., et al. (2018). Evaluation of Alkaloids Isolated from Ruta Graveolens as Photosynthesis Inhibitors. Molecules 23 (10), 2693. doi:10.3390/molecules23102693
Sanda, T., Asamitsu, K., Ogura, H., Iida, S., Utsunomiya, A., Ueda, R., et al. (2006). Induction of Cell Death in Adult T-Cell Leukemia Cells by a Novel IkappaB Kinase Inhibitor. Leukemia 20, 590–598. doi:10.1038/sj.leu.2404129
Sanda, T., Iida, S., Ogura, H., Asamitsu, K., Murata, T., Bacon, K. B., et al. (2005). Growth Inhibition of Multiple Myeloma Cells by a Novel IkappaB Kinase Inhibitor. Clin. Cancer Res. 11, 1974–1982. doi:10.1158/1078-0432.CCR-04-1936
Santarpia, L., Lippman, S. M., and El-Naggar, A. K. (2012). Targeting the MAPK-RAS-RAF Signaling Pathway in Cancer Therapy. Expert Opin. Ther. Targets 16 (1), 103–119. doi:10.1517/14728222.2011.645805
Sarker, D., Dawson, N. A., Aparicio, A. M., Dorff, T. B., Pantuck, A. J., Vaishampayan, U. N., et al. (2021). A Phase I, Open-Label, Dose-Finding Study of GSK2636771, a PI3Kβ Inhibitor, Administered with Enzalutamide in Patients with Metastatic Castration-Resistant Prostate Cancer. Clin. Cancer Res. 27, 5248–5257. doi:10.1158/1078-0432.CCR-21-1115
Schattenberg, J. M., Wörns, M. A., Zimmermann, T., He, Y. W., Galle, P. R., and Schuchmann, M. (2012). The Role of Death Effector Domain-Containing Proteins in Acute Oxidative Cell Injury in Hepatocytes. Free Radic. Biol. Med. 52 (9), 1911–1917. doi:10.1016/j.freeradbiomed.2012.02.049
Schopf, L., Savinainen, A., Anderson, K., Kujawa, J., DuPont, M., Silva, M., et al. (2006). IKKbeta Inhibition Protects against Bone and Cartilage Destruction in a Rat Model of Rheumatoid Arthritis. Arthritis Rheum. 54, 3163–3173. doi:10.1002/art.22081
Schroeder, M. A., Khoury, H. J., Jagasia, M., Ali, H., Schiller, G. J., Staser, K., et al. (2020). A Phase 1 Trial of Itacitinib, a Selective JAK1 Inhibitor, in Patients with Acute Graft-Versus-Host Disease. Blood Adv. 4 (8), 1656–1669. doi:10.1182/bloodadvances.2019001043
Schust, J., Sperl, B., Hollis, A., Mayer, T. U., and Berg, T. (2006). Stattic: a Small-Molecule Inhibitor of STAT3 Activation and Dimerization. Chem. Biol. 13 (11), 1235–1242. doi:10.1016/j.chembiol.2006.09.018
Schuster, S., McGeough, M. D., Johnson, C. D., Zagorska, A., Budas, G., Hoffman, H. M., et al. (2017). Apoptosis Signal-Regulating Kinase 1 (ASK1) Inhibition Reduces Liver Fibrosis and Apoptosis in a NLRP3 Mutant Model of NASH. J. Hepatol. 66 (1), S608–S609. doi:10.1016/s0168-8278(17)31654-9
Seitz, T., Hackl, C., Freese, K., Dietrich, P., Mahli, A., Thasler, R. M., et al. (2021). Xanthohumol, a Prenylated Chalcone Derived from Hops, Inhibits Growth and Metastasis of Melanoma Cells. Cancers (Basel) 13 (3), 511. doi:10.3390/cancers13030511
Shankar, E., Zhang, A., Franco, D., and Gupta, S. (2017). Betulinic Acid-Mediated Apoptosis in Human Prostate Cancer Cells Involves P53 and Nuclear Factor-Kappa B (NF-Κb) Pathways. Molecules 22 (2). doi:10.3390/molecules22020264
Shapiro, G. I., LoRusso, P., Cho, D. C., Musib, L., Yan, Y., Wongchenko, M., et al. (2021). A Phase Ib Open-Label Dose Escalation Study of the Safety, Pharmacokinetics, and Pharmacodynamics of Cobimetinib (GDC-0973) and Ipatasertib (GDC-0068) in Patients with Locally Advanced or Metastatic Solid Tumors. Investig. New Drugs 39 (1), 163–174. doi:10.1007/s10637-020-00975-6
Shapiro, G. I., LoRusso, P., Kwak, E., Pandya, S., Rudin, C. M., Kurkjian, C., et al. (2020). Phase Ib Study of the MEK Inhibitor Cobimetinib (GDC-0973) in Combination with the PI3K Inhibitor Pictilisib (GDC-0941) in Patients with Advanced Solid Tumors. Investig. New Drugs 38 (2), 419–432. doi:10.1007/s10637-019-00776-6
Shu, Y. S., Tao, W., Miao, Q. B., Zhu, Y. B., and Yang, Y. F. (2014). Improvement of Ventilation-Induced Lung Injury in a Rodent Model by Inhibition of Inhibitory κB Kinase. J. Trauma Acute Care Surg. 76, 1417–1424. doi:10.1097/TA.0000000000000229
Siddiquee, K., Zhang, S., Guida, W. C., Blaskovich, M. A., Greedy, B., Lawrence, H. R., et al. (2007). Selective Chemical Probe Inhibitor of Stat3, Identified through Structure-Based Virtual Screening, Induces Antitumor Activity. Proc. Natl. Acad. Sci. U. S. A. 104, 7391–7396. doi:10.1073/pnas.0609757104
Silva, S. D., Jara, Z. P., and Peres, R. (2017). Temporal Changes in Cardiac Oxidative Stress, Infammation and Remodeling Induced by Exercise in Hypertension Role for Local Angiotensin II Reduction [J]. PLoS One 12 (12), e0189535. doi:10.1371/journal.pone.0189535
Singh, A., Venkannagari, S., Oh, K. H., Zhang, Y. Q., Rohde, J. M., Liu, L., et al. (2016). Small Molecule Inhibitor of NRF2 Selectively Intervenes Therapeutic Resistance in KEAP1-Deficient NSCLC Tumors. ACS Chem. Biol. 11 (11), 3214–3225. doi:10.1021/acschembio.6b00651
Singh, S., and Aggarwal, B. B. (1995). Protein-tyrosine Phosphatase Inhibitors Block Tumor Necrosis Factor-dependent Activation of the Nuclear Transcription Factor NF-Kappa B. J. Biol. Chem. 270, 10631–10639. doi:10.1074/jbc.270.18.10631
Skvara, H., Dawid, M., Kleyn, E., Wolff, B., Meingassner, J. G., Knight, H., et al. (2008). The PKC Inhibitor AEB071 May Be a Therapeutic Option for Psoriasis. J. Clin. Investig. 118 (9), 3151–3159. doi:10.1172/JCI35636
Sohn, S. J., Lewis, G. M., and Winoto, A. (2008). Non-redundant Function of the MEK5-ERK5 Pathway in Thymocyte Apoptosis. EMBO J. 27 (13), 1896–1906. doi:10.1038/emboj.2008.114
Sommers, C. D., Thompson, J. M., Guzova, J. A., Bonar, S. L., Rader, R. K., Mathialagan, S., et al. (2009). Novel Tight-Binding Inhibitory Factor-kappaB Kinase (IKK-2) Inhibitors Demonstrate Target-specific Anti-inflammatory Activities in Cellular Assays and Following Oral and Local Delivery in an In Vivo Model of Airway Inflammation. J. Pharmacol. Exp. Ther. 330 (2), 377–388. doi:10.1124/jpet.108.147538
Sommers, C. D., Thompson, J. M., Guzova, J. A., Bonar, S. L., Rader, R. K., Mathialagan, S., et al. (2009). Novel Tight-Binding Inhibitory Factor-kappaB Kinase (IKK-2) Inhibitors Demonstrate Target-specific Anti-inflammatory Activities in Cellular Assays and Following Oral and Local Delivery in an In Vivo Model of Airway Inflammation. J. Pharmacol. Exp. Ther. 330 (2), 377–388. doi:10.1124/jpet.108.147538
Sommers, C. D., Thompson, J. M., Guzova, J. A., Bonar, S. L., Rader, R. K., Mathialagan, S., et al. (2009). Novel Tight-Binding Inhibitory Factor-kappaB Kinase (IKK-2) Inhibitors Demonstrate Target-specific Anti-inflammatory Activities in Cellular Assays and Following Oral and Local Delivery in an In Vivo Model of Airway Inflammation. J. Pharmacol. Exp. Ther. 330, 377–388. doi:10.1124/jpet.108.147538
Son, Y. K., Hong, D. H., Kim, D. J., Firth, A. L., and Park, W. S. (2011). Direct Effect of Protein Kinase C Inhibitors on Cardiovascular Ion Channels. BMB Rep. 44 (9), 559–565. doi:10.5483/bmbrep.2011.44.9.559
Song, K. W., Edgar, K. A., Hanan, E. J., Hafner, M., Oeh, J., Merchant, M., et al. (20212000). RTK-dependent Inducible Degradation of Mutant PI3Kα Drives GDC-0077 (Inavolisib) Efficacy, 12, 204–219. doi:10.1158/2159-8290.CD-21-0072RTK-dependent Inducible Degradation of Mutant PI3Kα Drives GDC-0077 (Inavolisib) EfficacyCancer Discov.
Sordi, R., Chiazza, F., Johnson, F. L., Patel, N. S., Brohi, K., Collino, M., et al. (2015). Inhibition of IκB Kinase Attenuates the Organ Injury and Dysfunction Associated with Hemorrhagic Shock. Mol. Med. 21, 563–575. doi:10.2119/molmed.2015.00049
Spreafico, A., and Mackay, H. J. (2013). Current Phase II Clinical Data for Ridaforolimus in Cancer. Expert Opin. Investig. Drugs 22 (11), 1485–1493. doi:10.1517/13543784.2013.831404
Sreenivasan, Y., Sarkar, A., and Manna, S. K. (2003). Mechanism of Cytosine Arabinoside-Mediated Apoptosis: Role of Rel A (P65) Dephosphorylation. Oncogene 22, 4356–4369. doi:10.1038/sj.onc.1206486
Srivastava, R. K., Li, C., Weng, Z., Agarwal, A., Elmets, C. A., Afaq, F., et al. (2016). Defining Cutaneous Molecular Pathobiology of Arsenicals Using Phenylarsine Oxide as a Prototype. Sci. Rep. 6, 34865. doi:10.1038/srep34865
Starosyla, S. A., Volynets, G. P., Lukashov, S. S., Gorbatiuk, O. B., Golub, A. G., Bdzhola, V. G., et al. (2015). Identification of Apoptosis Signalregulating Kinase 1 (ASK1) Inhibitors Among the Derivatives Ofbenzothiazol-2-Yl-3-Hydroxy-5-Phenyl-1,5-Dihydro-Pyrrol-2-One. Bioorg Med. Chem. 23 (10), 2489–2497. doi:10.1016/j.bmc.2015.03.056
Stebbins, J. L., De, S. K., and Machleidt, T. (2008). Identification of a New JNK Inhibitor Targeting the JNK-JIP Interaction Site. Proc. Natl. Acad. Sci. U. S. A. 105, 16809–16813. doi:10.1073/pnas.0805677105
Sun, J. Y., Hou, Y. J., Yin, Y. B., Wang, F. Z., Yang, M. F., Zhang, Y. Y., et al. (2020). CCT128930 Induces G1-phase Arrest and Apoptosis and Synergistically Enhances the Anticancer Efficiency of VS5584 in Human Osteosarcoma Cells. Biomed PharmacotherErratum Biomed Pharmacother 130132, 110544110931. doi:10.1016/j.biopha.2020.110544
Sun, M., Shi, Y., Dang, U. J., and Di Pasqua, A. J. (2019). Phenethyl Isothiocyanate and Cisplatin Co-encapsulated in a Liposomal Nanoparticle for Treatment of Non-Small Cell Lung Cancer. Molecules 24 (4), 801. doi:10.3390/molecules24040801
Sung, B., Pandey, M. K., Ahn, K. S., Yi, T., Chaturvedi, M. M., Liu, M., et al. (2008). Anacardic Acid (6-nonadecyl Salicylic Acid), an Inhibitor of Histone Acetyltransferase, Suppresses Expression of Nuclear Factor-kappaB-Regulated Gene Products Involved in Cell Survival, Proliferation, Invasion, and Inflammation through Inhibition of the Inhibitory Subunit of Nuclear Factor-kappaBalpha Kinase, Leading to Potentiation of Apoptosis. Blood 111, 4880–4891. doi:10.1182/blood-2007-10-117994
Sunwoo, J. B., Chen, Z., Dong, G., Yeh, N., Crowl Bancroft, C., Sausville, E., et al. (2001). Novel Proteasome Inhibitor PS-341 Inhibits Activation of Nuclear Factor-Kappa B, Cell Survival, Tumor Growth, and Angiogenesis in Squamous Cell Carcinoma. Clin. Cancer Res. 7, 1419–1428.
Swahn, B. M., Huerta, F., and Kallin, E. (2005). Design and Synthesis of 6-anilinoindazoles as Selective Inhibitors of C-Jun N-Terminal Kinase-3. Bioorg Med. Chem. Lett. 15, 5095–5099. doi:10.1016/j.bmcl.2005.06.083
Swahn, B. M., Xue, Y., and Arzel, E. (2006). Design and Synthesis of 20-Anilino-4,40-Bipyridines as Selective Inhibitors of C-Jun N-Terminal Kinase-3. Bioorg Med. Chem. Lett. 16, 1397–1401. doi:10.1016/j.bmcl.2005.11.039
Syam, Y. M., Anwar, M. M., Kotb, E. R., Elseginy, S. A., Awad, H. M., and Awad, G. E. A. (2019). Development of Promising Thiopyrimidine-Based Anti-cancer and Antimicrobial Agents: Synthesis and QSAR Analysis. Mini Rev. Med. Chem. 19 (15), 1255–1275. doi:10.2174/1389557518666180330110828
Takakura, A., Nelson, E. A., Haque, N., Humphreys, B. D., Zandi-Nejad, K., Frank, D. A., et al. (2011). Pyrimethamine Inhibits Adult Polycystic Kidney Disease by Modulating STAT Signaling Pathways. Hum. Mol. Genet. 20 (21), 4143–4154. doi:10.1093/hmg/ddr338
Takeda, T., Tsubaki, M., Sakamoto, K., Ichimura, E., Enomoto, A., Suzuki, Y., et al. (3062). Mangiferin, a Novel Nuclear Factor Kappa B-Inducing Kinase Inhibitor, Suppresses Metastasis and Tumor Growth in a Mouse Metastatic Melanoma Model. Toxicol. Appl. Pharmacol. 306, 105–112. doi:10.1016/j.taap.2016.07.005
Talpaz, M., and Kiladjian, J. J. (2021). Fedratinib, a Newly Approved Treatment for Patients with Myeloproliferative Neoplasm-Associated Myelofibrosis. Leukemia 35 (1), 1–17. doi:10.1038/s41375-020-0954-2
Tam, C. S., and Verstovsek, S. (2013). Investigational Janus Kinase Inhibitors. Expert Opin. Investig. Drugs 22 (6), 687–699. doi:10.1517/13543784.2013.774373
Tanaka, A., Muto, S., Konno, M., Itai, A., and Matsuda, H. (2006). A New IkappaB Kinase Beta Inhibitor Prevents Human Breast Cancer Progression through Negative Regulation of Cell Cycle Transition. Cancer Res. 66, 419–426. doi:10.1158/0008-5472.CAN-05-0741
Tang, X., Wang, H., Fan, L., Wu, X., Xin, A., Ren, H., et al. (2011). Luteolin Inhibits Nrf2 Leading to Negative Regulation of the Nrf2/ARE Pathway and Sensitization of Human Lung Carcinoma A549 Cells to Therapeutic Drugs. Free Radic. Biol. Med. 50 (11), 1599–1609. doi:10.1016/j.freeradbiomed.2011.03.008
Taniguchi, K., and Karin, M. (2018). NF-κB, Inflammation, Immunity and Cancer: Coming of Age. Nat. Rev. Immunol. 18 (5), 309–324. doi:10.1038/nri.2017.142
Tarumoto, T., Nagai, T., Ohmine, K., Miyoshi, T., Nakamura, M., Kondo, T., et al. (2004). Ascorbic Acid Restores Sensitivity to Imatinib via Suppression of Nrf2-dependent Gene Expression in the Imatinib-Resistant Cell Line. Exp. Hematol. 32 (4), 375–381. doi:10.1016/j.exphem.2004.01.007
Tauber, A. L., Schweiker, S. S., and Levonis, S. M. (2020). From Tea to Treatment; Epigallocatechin Gallate and its Potential Involvement in Minimizing the Metabolic Changes in Cancer. Nutr. Res. 74, 23–36. doi:10.1016/j.nutres.2019.12.004
Terao, Y., Suzuki, H., Yoshikawa, M., Yashiro, H., Takekawa, S., Fujitani, Y., et al. (2012). Design and Biological Evaluation of Imidazo[1,2-A]pyridines as Novel and Potent ASK1 Inhibitors. Bioorg Med. Chem. Lett. 22 (24), 7326–7329. doi:10.1016/j.bmcl.2012.10.084
Tesch, G. H., Ma, F. Y., Han, Y., Liles, J. T., Breckenridge, D. G., and Nikolic Paterson, D. J. (2015). ASK1 Inhibitor Halts Progression of Diabeticnephropathy in Nos3-Deficient Mice. Diabetes 64 (11), 3903–3913. doi:10.2337/db15-0384
Thoreen, C. C., Kang, S. A., Chang, J. W., Liu, Q., Zhang, J., Gao, Y., et al. (2009). An ATP-Competitive Mammalian Target of Rapamycin Inhibitor Reveals Rapamycin-Resistant Functions of mTORC1. J. Biol. Chem. 284, 8023–8032. doi:10.1074/jbc.M900301200
Tian, H., Ji, C., Liu, C., Kong, L., Cheng, Y., and Huang, G. C. (2013). Benzoheterocyclic Compounds and Use Thereof. Pat. EP 2804855 A4, 25.
Tibbles, L. A., Ing, Y. L., Kiefer, F., Chan, J., Iscove, N., Woodgett, J. R., et al. (1996). MLK-3 Activates the SAPK/JNK and P38/RK Pathways via SEK1 and MKK3/6. EMBO J. 15 (24), 7026–7035. doi:10.1002/j.1460-2075.1996.tb01094.x
Townsend, R. M., Postelnek, J., Susulic, V., McIntyre, K. W., Shuster, D. J., Qiu, Y., et al. (2004). A Highly Selective Inhibitor of IkappaB Kinase, BMS-345541, Augments Graft Survival Mediated by Suboptimal Immunosuppression in a Murine Model of Cardiac Graft Rejection. Transplantation 77, 1090–1094. doi:10.1097/01.tp.0000118407.05205.05
Trengove, M. C., and Ward, A. C. (2013). SOCS Proteins in Development and Disease. Am. J. Clin. Exp. Immunol. 2 (1), 1–29.
Tsuchida, K., Tsujita, T., Hayashi, M., Ojima, A., Keleku-Lukwete, N., Katsuoka, F., et al. (2017). Halofuginone Enhances the Chemo-Sensitivity of Cancer Cells by Suppressing NRF2 Accumulation. Free Radic. Biol. Med. 103, 236–247. doi:10.1016/j.freeradbiomed.2016.12.041
Tuli, H. S., Joshi, R., Aggarwal, D., Kaur, G., Kaur, J., Kumar, M., et al. (2021). Molecular Mechanisms Underlying Chemopreventive Potential of Butein: Current Trends and Future Perspectives. Chem. Biol. Interact. 350, 109699. doi:10.1016/j.cbi.2021.109699
Tyner, J. W., Bumm, T. G., Deininger, J., Wood, L., Aichberger, K. J., Loriaux, M. M., et al. (2010). CYT387, a Novel JAK2 Inhibitor, Induces Hematologic Responses and Normalizes Inflammatory Cytokines in Murine Myeloproliferative Neoplasms. Blood 115, 5232–5240. doi:10.1182/blood-2009-05-223727
Tzoneva, R., Stoyanova, T., Petrich, A., Popova, D., Uzunova, V., Momchilova, A., et al. (2020). Effect of Erufosine on Membrane Lipid Order in Breast Cancer Cell Models. Biomolecules 10 (5), 802. doi:10.3390/biom10050802
Uchihara, Y., Ohe, T., Mashino, T., Kidokoro, T., Tago, K., Tamura, H., et al. (2019). N-acetyl Cysteine Prevents Activities of STAT3 Inhibitors, Stattic and BP-1-102 Independently of its Antioxidant Properties. Pharmacol. Rep. 71 (6), 1067–1078. doi:10.1016/j.pharep.2019.05.021
Uckun, F. M., Saund, S., Windlass, H., and Trieu, V. (2021). Repurposing Anti-malaria Phytomedicine Artemisinin as a COVID-19 Drug. Front. Pharmacol. 12, 649532. doi:10.3389/fphar.2021.649532
Umezawa, K. (2006). Inhibition of Tumor Growth by NF-kappaB Inhibitors. Cancer Sci. 97, 990–995. doi:10.1111/j.1349-7006.2006.00285.x
Uota, S., Zahidunnabi Dewan, M., Saitoh, Y., Muto, S., Itai, A., Utsunomiya, A., et al. (2012). An IκB Kinase 2 Inhibitor IMD-0354 Suppresses the Survival of Adult T-Cell Leukemia Cells. Cancer Sci. 103, 100–106. doi:10.1111/j.1349-7006.2011.02110.x
Uprety, D., and Adjei, A. A. (2020). KRAS: From Undruggable to a Druggable Cancer Target. Cancer Treat. Rev. 89, 102070. doi:10.1016/j.ctrv.2020.102070
Vakana, E., Pratt, S., Blosser, W., Dowless, M., Simpson, N., Yuan, X. J., et al. (300912020). A panRAF Inhibitor, Has Significant Anti-tumor Activity in BRAF and KRAS Mutant Preclinical Models of Colorectal Cancer. Oncotarget 8 (6), 9251–9266. doi:10.18632/oncotarget.14002
Valencia-Sanchez, C., and Carter, J. L. (2020). An Evaluation of Dimethyl Fumarate for the Treatment of Relapsing Remitting Multiple Sclerosis. Expert Opin. Pharmacother. 21 (12), 1399–1405. doi:10.1080/14656566.2020.1763304
Vellasamy, S., Murugan, D., Abas, R., Alias, A., Seng, W. Y., and Woon, C. K. (2021). Biological Activities of Paeonol in Cardiovascular Diseases: A Review. Molecules 26 (16), 4976. doi:10.3390/molecules26164976
Verhoef, T. I., Redekop, W. K., Darba, J., Geitona, M., Hughes, D. A., Siebert, U., et al. (2010). A Systematic Review of Cost-Effectiveness Analyses of Pharmacogenetic-Guided Dosing in Treatment with Coumarin Derivatives. Pharmacogenomics 11 (7), 989–1002. doi:10.2217/pgs.10.74
Victoriano, A. F., Asamitsu, K., Hibi, Y., Imai, K., Barzaga, N. G., and Okamoto, T. (2006). Inhibition of Human Immunodeficiency Virus Type 1 Replication in Latently Infected Cells by a Novel IkappaB Kinase Inhibitor. Antimicrob. Agents Chemother. 50, 547–555. doi:10.1128/AAC.50.2.547-555.2006
Vidy, A., El Bougrini, J., Chelbi-Alix, M. K., and Blondel, D. (2007). The Nucleocytoplasmic Rabies Virus P Protein Counteracts Interferon Signaling by Inhibiting Both Nuclear Accumulation and DNA Binding of STAT1. J. Virol. 81 (8), 4255–4263. doi:10.1128/JVI.01930-06
Villarino, A. V., Kanno, Y., Ferdinand, J. R., and O'Shea, J. J. (2015). Mechanisms of Jak/STAT Signaling in Immunity and Disease. J. Immunol. 194 (1), 21–27. doi:10.4049/jimmunol.1401867
Vodanovic-Jankovic, S., Hari, P., Jacobs, P., Komorowski, R., and Drobyski, W. R. (2006). NF-kappaB as a Target for the Prevention of Graft-Versus-Host Disease: Comparative Efficacy of Bortezomib and PS-1145. Blood 107, 827–834. doi:10.1182/blood-2005-05-1820
Wakabayashi, N., Dinkova-Kostova, A. T., Holtzclaw, W. D., Kang, M. I., Kobayashi, A., Yamamoto, M., et al. (2004). Protection against Electrophile and Oxidant Stress by Induction of the Phase 2 Response: Fate of Cysteines of the Keap1 Sensor Modified by Inducers. Proc. Natl. Acad. Sci. U. S. A. 101 (7), 2040–2045. doi:10.1073/pnas.0307301101
Wallace, D. J., Furie, R. A., Tanaka, Y., Kalunian, K. C., Mosca, M., Petri, M. A., et al. (2018). Baricitinib for Systemic Lupus Erythematosus: a Double-Blind, Randomised, Placebo-Controlled, Phase 2 Trial. Lancet 392 (10143), 222–231. doi:10.1016/S0140-6736(18)31363-1
Wallace, E., Lyssikatos, J., Blake, J., Marlow, A., Greschuk, J., Yeh, T., et al. (2009). “AZD8330 (ARRY-424704): Preclinical Evaluation of a Potent, Selective MEK 1/2 Inhibitor Currently in Phase I Trials,” in Proceedings of the AACR (Denver, CO, USA, 18–22.
Wan, X., and Helman, L. J. (2007). The Biology behind mTOR Inhibition in Sarcoma. Oncologist 12 (8), 1007–1018. doi:10.1634/theoncologist.12-8-1007
Wang, C., Shu, L., Zhang, C., Li, W., Wu, R., Guo, Y., et al. (2018). Histone Methyltransferase Setd7 Regulates Nrf2 Signaling Pathway by Phenethyl Isothiocyanate and Ursolic Acid in Human Prostate Cancer Cells. Mol. Nutr. Food Res. 62 (18), e1700840. doi:10.1002/mnfr.201700840
Wang, G., Tian, W., Liu, Y., Ju, Y., Shen, Y., Zhao, S., et al. (2016). Visfatin Triggers the Cell Motility of Non-small Cell Lung Cancer via Up-Regulation of Matrix Metalloproteinases. Basic Clin. Pharmacol. Toxicol. 119 (6), 548–554. doi:10.1111/bcpt.12623
Wang, H., Liu, K., Geng, M., Gao, P., Wu, X., Hai, Y., et al. (2013). RXRα Inhibits the NRF2-ARE Signaling Pathway through a Direct Interaction with the Neh7 Domain of NRF2. Cancer Res. 73 (10), 3097–3108. doi:10.1158/0008-5472.CAN-12-3386
Wang, K. S., Li, J., Wang, Z., Mi, C., Ma, J., Piao, L. X., et al. (2017). Artemisinin Inhibits Inflammatory Response via Regulating NF-Κb and MAPK Signaling Pathways. Immunopharmacol. Immunotoxicol. 39 (1), 28–36. doi:10.1080/08923973.2016.1267744
Wang, X. J., Hayes, J. D., Henderson, C. J., and Wolf, C. R. (2007). Identification of Retinoic Acid as an Inhibitor of Transcription Factor Nrf2 through Activation of Retinoic Acid Receptor Alpha. Proc. Natl. Acad. Sci. U. S. A. 104 (49), 19589–19594. doi:10.1073/pnas.0709483104
Wang, X., Yang, Y., An, Y., and Fang, G. (2019). The Mechanism of Anticancer Action and Potential Clinical Use of Kaempferol in the Treatment of Breast Cancer. Biomed. Pharmacother. 117, 109086. doi:10.1016/j.biopha.2019.109086
Watanabe, K., Nakagawa, H., and Tsurufuji, S. (1986). Vascular Permeability Changes by Proteinase Inhibitors in Carrageenin-Induced Inflammation in Rats. Agents Actions 17 (5-6), 472–477. doi:10.1007/BF01965516
Webster, W. S., and Leibovich, B. C. (2005). Exisulind in the Treatment of Prostate Cancer. Expert Rev. Anticancer Ther. 5 (6), 957–962. doi:10.1586/14737140.5.6.957
Weichhart, T. (2018). mTOR as Regulator of Lifespan, Aging, and Cellular Senescence: A Mini-Review. Gerontology 64 (2), 127–134. doi:10.1159/000484629
Wellbrock, C., Karasarides, M., and Marais, R. (2004). The RAF Proteins Take Centre Stage. Nat. Rev. Mol. Cell. Biol. 5 (11), 875–885. doi:10.1038/nrm1498
Wieczorek, M., Ginter, T., Brand, P., Heinzel, T., and Krämer, O. H. (2012). Acetylation Modulates the STAT Signaling Code. Cytokine Growth Factor Rev. 23 (6), 293–305. doi:10.1016/j.cytogfr.2012.06.005
Winter-Vann, A. M., Baron, R. A., Wong, W., dela Cruz, J., York, J. D., Gooden, D. M., et al. (2005). A Small-Molecule Inhibitor of Isoprenylcysteine Carboxyl Methyltransferase with Antitumor Activity in Cancer Cells. Proc. Natl. Acad. Sci. U. S. A. 102 (12), 4336–4341. doi:10.1073/pnas.0408107102
Wong, A. L., Soo, R. A., Tan, D. S., Lee, S. C., Lim, J. S., Marban, P. C., et al. (2015). Phase I and Biomarker Study of OPB-51602, a Novel Signal Transducer and Activator of Transcription (STAT) 3 Inhibitor, in Patients with Refractory Solid Malignancies. Ann. Oncol. 26 (5), 998–1005. doi:10.1093/annonc/mdv026
Wu, Q., Wu, W., Jacevic, V., Franca, T. C. C., Wang, X., and Kuca, K. (2020). Selective Inhibitors for JNK Signalling: a Potential Targeted Therapy in Cancer. J. Enzyme Inhib. Med. Chem. 35 (1), 574–583. doi:10.1080/14756366.2020.1720013
Wu, Y. H., Wu, Y. R., Li, B., and Yan, Z. Y. (2020). Cryptotanshinone: A Review of its Pharmacology Activities and Molecular Mechanisms. Fitoterapia 145, 104633. doi:10.1016/j.fitote.2020.104633
Wu, Y. X., Wang, Y. Y., Gao, Z. Q., Chen, D., Liu, G., Wan, B. B., et al. (2021). Ethyl Ferulate Protects against Lipopolysaccharide-Induced Acute Lung Injury by Activating AMPK/Nrf2 Signaling Pathway. Acta Pharmacol. Sin. 42 (12), 2069–2081. doi:10.1038/s41401-021-00742-0
Xie, J., Lai, Z., Zheng, X., Liao, H., Xian, Y., Li, Q., et al. (2021). Apoptotic Activities of Brusatol in Human Non-small Cell Lung Cancer Cells: Involvement of ROS-Mediated Mitochondrial-dependent Pathway and Inhibition of Nrf2-Mediated Antioxidant Response. Toxicology 451, 152680. doi:10.1016/j.tox.2021.152680
Xie, Y., Ramachandran, A., Breckenridge, D. G., Liles, J. T., Lebofsky, M., Farhood, A., et al. (2015). Inhibitor of Apoptosis Signalregulating Kinase 1 Protects against Acetaminophen-Induced Liver Injury. Toxicol. Appl. Pharmacol. 286 (1), 1–9. doi:10.1016/j.taap.2015.03.019
Xing, J., Yang, J., Gu, Y., and Yi, J. (2021). Research Update on the Anticancer Effects of Buparlisib. Oncol. Lett. 21 (4), 266. doi:10.3892/ol.2021.12527
Xu, D., and Qu, C. K. (2008). Protein Tyrosine Phosphatases in the JAK/STAT Pathway. Front. Biosci. 13, 4925–4932. doi:10.2741/3051
Yamaji, M., Ota, A., Wahiduzzaman, M., Karnan, S., Hyodo, T., Konishi, H., et al. (2017). Novel ATP-Competitive Akt Inhibitor Afuresertib Suppresses the Proliferation of Malignant Pleural Mesothelioma Cells. Cancer Med. 6 (11), 2646–2659. doi:10.1002/cam4.1179
Yamamoto, Y., Yin, M. J., Lin, K. M., and Gaynor, R. B. (1999). Sulindac Inhibits Activation of the NF-kappaB Pathway. J. Biol. Chem. 274, 27307–27314. doi:10.1074/jbc.274.38.27307
Yang, J., Fan, G. H., Wadzinski, B. E., Sakurai, H., and Richmond, A. (2001). Protein Phosphatase 2A Interacts with and Directly Dephosphorylates RelA. J. Biol. Chem. 276, 47828–47833. doi:10.1074/jbc.M106103200
Yang, J., Lin, Y., Guo, Z., Cheng, J., Huang, J., Deng, L., et al. (2001). The Essential Role of MEKK3 in TNF-Induced NF-kappaB Activation. Nat. Immunol. 2 (7), 620–624. doi:10.1038/89769
Yang, J. Y., Li, M., Zhang, C. L., and Liu, D. (2021). Pharmacological Properties of Baicalin on Liver Diseases: a Narrative Review. Pharmacol. Rep. 73 (5), 1230–1239. doi:10.1007/s43440-021-00227-1
Yang, K., Tang, X. J., Xu, F. F., Liu, J. H., Tan, Y. Q., Gao, L., et al. (2020). PI3K/mTORC1/2 Inhibitor PQR309 Inhibits Proliferation and Induces Apoptosis in Human Glioblastoma Cells. Oncol. Rep. 43 (3), 773–782. doi:10.3892/or.2020.7472
Yao, J., Kong, W., and Jiang, J. (2015). Learning from Berberine: Treating Chronic Diseases through Multiple Targets. Sci. China Life Sci. 58 (9), 854–859. doi:10.1007/s11427-013-4568-z
Yao, K., Cho, Y. Y., Bode, A. M., Vummenthala, A., Park, J. G., Liu, K., et al. (2009). A Selective Small-Molecule Inhibitor of C-Jun N-Terminal Kinase 1. FEBS Lett. 583 (13), 2208–2212. doi:10.1016/j.febslet.2009.06.017
Yin, M. J., Yamamoto, Y., and Gaynor, R. B. (1998). The Anti-inflammatory Agents Aspirin and Salicylate Inhibit the Activity of I(kappa)B Kinase-Beta. Nature 396, 77–80. doi:10.1038/23948
Yin, Z., Zhang, J., Chen, L., Guo, Q., Yang, B., Zhang, W., et al. (2020). Anticancer Effects and Mechanisms of Action of Plumbagin: Review of Research Advances. Biomed. Res. Int., 20206940953. doi:10.1155/2020/6940953
You, H., Xu, D., Zhao, J., Li, J., Wang, Q., Tian, X., et al. (2020). JAK Inhibitors: Prospects in Connective Tissue Diseases. Clin. Rev. Allergy Immunol. 59 (3), 334–351. doi:10.1007/s12016-020-08786-6
Younossi, Z. M., Stepanova, M., Lawitz, E., Charlton, M., Loomba, R., Myers, R. P., et al. (2018). Improvement of Hepatic Fibrosis and Patient-Reported Outcomes in Non-alcoholic Steatohepatitis Treated with Selonsertib. Liver Int. 38 (10), 1849–1859. doi:10.1111/liv.13706
Yousefnia, S. (2021). Mechanistic Effects of Arsenic Trioxide on Acute Promyelocytic Leukemia and Other Types of Leukemias. Cell. Biol. Int. 45 (6), 1148–1157. doi:10.1002/cbin.11563
Yu, K., Toral-Barza, L., Shi, C., Zhang, W. G., Lucas, J., Shor, B., et al. (2009). Biochemical, Cellular, and In Vivo Activity of Novel ATP-Competitive and Selective Inhibitors of the Mammalian Target of Rapamycin. Cancer Res. 69, 6232–6240. doi:10.1158/0008-5472.CAN-09-0299
Zang, X., Cheng, M., Zhang, X., and Chen, X. (2021). Quercetin Nanoformulations: a Promising Strategy for Tumor Therapy. Food Funct. 12 (15), 6664–6681. doi:10.1039/d1fo00851j
Zarnegar, B. J., Wang, Y., Mahoney, D. J., Dempsey, P. W., Cheung, H. H., He, J., et al. (2008). Noncanonical NF-kappaB Activation Requires Coordinated Assembly of a Regulatory Complex of the Adaptors cIAP1, cIAP2, TRAF2 and TRAF3 and the Kinase NIK. Nat. Immunol. 9 (12), 1371–1378. doi:10.1038/ni.1676
Zarubin, T., and Han, J. (2005). Activation and Signaling of the P38 MAP Kinase Pathway. Cell. Res. 15 (1), 11–18. doi:10.1038/sj.cr.7290257
Zeke, A., Misheva, M., Reményi, A., and Bogoyevitch, M. A. (2016). JNK Signaling: Regulation and Functions Based on Complex Protein-Protein Partnerships. Microbiol. Mol. Biol. Rev. 80 (3), 793–835. doi:10.1128/MMBR.00043-14
Zeng, G., Lian, C., Yang, P., Zheng, M., Ren, H., and Wang, H. (2019). E3-ubiquitin Ligase TRIM6 Aggravates Myocardial Ischemia/reperfusion Injury via Promoting STAT1-dependent Cardiomyocyte Apoptosis. Aging (Albany NY) 11 (11), 3536–3550. doi:10.18632/aging.101995
Zerbini, C. A., and Lomonte, A. B. (2012). Tofacitinib for the Treatment of Rheumatoid Arthritis. Expert Rev. Clin. Immunol. 8 (4), 319–331. doi:10.1586/eci.12.19
Zhang, F., Qian, L., Flood, P. M., Shi, J. S., Hong, J. S., and Gao, H. M. (2010). Inhibition of IkappaB Kinase-Beta Protects Dopamine Neurons against Lipopolysaccharide-Induced Neurotoxicity. J. Pharmacol. Exp. Ther. 333, 822–833. doi:10.1124/jpet.110.165829
Zhang, H., Niu, X., and Qian, Z. (2015). The C-Jun N-Terminal Kinase Inhibitor SP600125 Inhibits Human Cytomegalovirus Replication. J. Med. Virol. 87, 2135–2144. doi:10.1002/jmv.24286
Zhang, J., Feng, H., Lv, J., Zhao, L., Zhao, J., and Wang, L. A. (2020). Protective Effect of Coumarin-Pi against T-BHP-Induced Hepatotoxicity by Upregulating Antioxidant Enzymes via Enhanced Nrf2 Signaling. Mol. Cell. Biochem. 475 (1-2), 277–283. doi:10.1007/s11010-020-03880-x
Zhao, X., Wang, J., Deng, Y., Liao, L., Zhou, M., Peng, C., et al. (2021). Quercetin as a Protective Agent for Liver Diseases: A Comprehensive Descriptive Review of the Molecular Mechanism. Phytother. Res. 35 (9), 4727–4747. doi:10.1002/ptr.7104
Zhao, Y., Spigolon, G., and Bonny, C. (2012). The JNK Inhibitor D-JNKI-1 Blocks Apoptotic JNK Signaling in Brain Mitochondria. Mol. Cell. Neurosci. 49, 300–310. doi:10.1016/j.mcn.2011.12.005
Zhong, Y., Zhang, F., Sun, Z., Zhou, W., Li, Z. Y., You, Q. D., et al. (2013). Drug Resistance Associates with Activation of Nrf2 in MCF-7/DOX Cells, and Wogonin Reverses it by Down-Regulating Nrf2-Mediated Cellular Defense Response. Mol. Carcinog. 52 (10), 824–834. doi:10.1002/mc.21921
Zhu, K., Liu, X., Liu, C., Xu, Y., Fu, Y., Dong, W., et al. (2021). AKT Inhibitor AZD5363 Suppresses Stemness and Promotes Anti-cancer Activity of 3,3'-diindolylmethane in Human Breast Cancer Cells. Toxicol. Appl. Pharmacol. 429, 115700. doi:10.1016/j.taap.2021.115700
Zhu, P., Qian, J., Xu, Z., Meng, C., Zhu, W., Ran, F., et al. (2021). Overview of Piperlongumine Analogues and Their Therapeutic Potential. Eur. J. Med. Chem. 220, 113471. doi:10.1016/j.ejmech.2021.113471
Zhuang, S. (2013). Regulation of STAT Signaling by Acetylation. Cell. Signal 25 (9), 1924–1931. doi:10.1016/j.cellsig.2013.05.007
Ziegelbauer, K., Gantner, F., Lukacs, N. W., Berlin, A., Fuchikami, K., Niki, T., et al. (2005). A Selective Novel Low-Molecular-Weight Inhibitor of IkappaB Kinase-Beta (IKK-Beta) Prevents Pulmonary Inflammation and Shows Broad Anti-inflammatory Activity. Br. J. Pharmacol. 145, 178–192. doi:10.1038/sj.bjp.0706176
Zimmermann, G., Papke, B., Ismail, S., Vartak, N., Chandra, A., Hoffmann, M., et al. (2013). Small Molecule Inhibition of the KRAS-PDEδ Interaction Impairs Oncogenic KRAS Signalling. Nature 497 (7451), 638–642. doi:10.1038/nature12205
Zirlik, K., and Veelken, H. (2018). Idelalisib. Idelalisib. Recent Results Cancer Res. 212, 243–264. doi:10.1007/978-3-319-91439-8_12
Zou, L. J., Xiang, Q. P., Xue, X. Q., Zhang, C., Li, C. C., Wang, C., et al. (2019). Y08197 Is a Novel and Selective CBP/EP300 Bromodomain Inhibitor for the Treatment of Prostate Cancer. Acta Pharmacol. Sin. 40 (11), 1436–1447. doi:10.1038/s41401-019-0237-5
—Chalmers, A., Cannon, L., and Akerley, W. (2019). Adverse Event Management in Patients with BRAFV600E-Mutant Non-small Cell Lung Cancer Treated with Dabrafenib Plus Trametinib. Oncologist 24 (7), 963–972. doi:10.1634/theoncologist.2018-0296
Keywords: immunosuppressive agent, jak-stat, NF-κB, PI3K-AKT-mTOR, MAPK, Keap1/Nrf2-ARE
Citation: Xu Z and Chu M (2022) Advances in Immunosuppressive Agents Based on Signal Pathway. Front. Pharmacol. 13:917162. doi: 10.3389/fphar.2022.917162
Received: 10 April 2022; Accepted: 02 May 2022;
Published: 26 May 2022.
Edited by:
Satoshi Kubo, National Institute of Allergy and Infectious Diseases (NIH), United StatesReviewed by:
Hiroyuki Nakamura, National Institute of Dental and Craniofacial Research (NIH), United StatesYun Ma, Institute of Microbiology (CAS), China
Copyright © 2022 Xu and Chu. This is an open-access article distributed under the terms of the Creative Commons Attribution License (CC BY). The use, distribution or reproduction in other forums is permitted, provided the original author(s) and the copyright owner(s) are credited and that the original publication in this journal is cited, in accordance with accepted academic practice. No use, distribution or reproduction is permitted which does not comply with these terms.
*Correspondence: Ming Chu, ZmFtb3VzQGJqbXUuZWR1LmNu