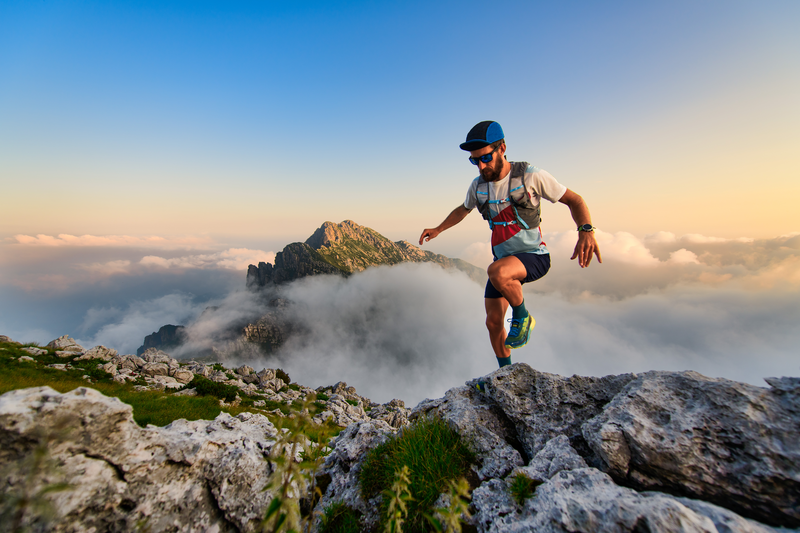
95% of researchers rate our articles as excellent or good
Learn more about the work of our research integrity team to safeguard the quality of each article we publish.
Find out more
REVIEW article
Front. Pharmacol. , 19 July 2022
Sec. Ethnopharmacology
Volume 13 - 2022 | https://doi.org/10.3389/fphar.2022.909363
This article is part of the Research Topic Metabolism of Herbs/Natural Products and Pharmacodynamic or Toxic Mechanisms View all 12 articles
Panax ginseng C.A. Mey. has a history of more than 4000 years and is widely used in Asian countries. Modern pharmacological studies have proved that ginsenosides and their compounds have a variety of significant biological activities on specific diseases, including neurodegenerative diseases, certain types of cancer, gastrointestinal disease, and metabolic diseases, in which most of the interest has focused on ginsenoside Rd. The evidentiary basis showed that ginsenoside Rd ameliorates ischemic stroke, nerve injury, cancer, and other diseases involved in apoptosis, inflammation, oxidative stress, mitochondrial damage, and autophagy. In this review, we summarized available reports on the molecular biological mechanisms of ginsenoside Rd in neurological diseases, cancer, metabolic diseases, and other diseases. We also discussed the main biotransformation pathways of ginsenoside Rd obtained by fermentation.
1) Approximately 120 studies on the use of ginsenoside Rd for the treatment of multiple diseases have been published.
2) This is the first review to report about the biotransformation, pharmacokinetics, and pharmacological effects of ginsenoside Rd.
3) The potential pharmacological mechanisms of ginsenoside Rd have been documented.
4) No specific reviews have been conducted by now.
Panax ginseng C.A. Mey. is a well-known herbal medicine widely used in China, Korea, Japan, and other East Asian countries. At present, the ginseng root and its extract are the most widely used herbal medicine. Modern pharmacological studies have proved that ginsenosides are the main active ingredient of ginseng and have a wide range of pharmacological effects, such as anti-inflammatory (Xu et al., 2021; Yi, 2021), anticancer (Zhang et al., 2021a), and anti-viral (Kang et al., 2021), regulate immunity (Kang et al., 2021), metabolism (Wang et al., 2021a), and improve cardiovascular system (Wang et al., 2021b; Sarhene et al., 2021) and nervous system (Brioschi Guevara et al., 2021) function, whereas most attention has been focused on the ginsenoside Rd.
Ginsenoside Rd, a natural compound extracted from the root of Panax ginseng C.A. Mey., is one of the protopanaxadiol (PPD)-type ginsenosides, while the proportion of ginsenoside Rd in ginseng is very low (Liu et al., 2020a). Interestingly, the promising effects of the pretreatment and treatment of ginsenoside Rd on neurological diseases, cancer, gastrointestinal disease, and metabolic diseases have been studied extensively in in vivo and in vitro models (Guo et al., 2021; Chen et al., 2022; Zhou et al., 2022).
Existing studies related to ginsenoside Rd have shown that various ginsenosides, such as Rb1, Rb2, and Rc, can be transformed into ginsenoside Rd after absorption and metabolism in vivo (Park et al., 2010; Shin and Oh, 2016). In addition, Rd can be prepared in a variety of ways based on the in-depth study of biotransformation and the development of modern fermentation technology (He et al., 2019). Based on the above results, we summarized the biotransformation process of other ginsenosides into Rd, thereby hoping to play a positive role in the large-scale industrial production of Rd. In this study, the biotransformation sources, pharmacokinetics, pharmacological effects, and molecular mechanisms of ginsenoside Rd on various systemic diseases in recent years were reviewed, and their therapeutic potential was discussed.
Multiple studies have confirmed that ginsenosides can be transformed into ginsenoside Rd using enzymes and bacterial communities and can promote the transformation of ginsenoside Rd into other metabolites (He et al., 2019). We summarized the precursors, metabolites, and transformation conditions of ginsenoside Rd (Table 1) (Figure 1).
Ginsenoside Rd can be synthesized from ginsenoside Rb1 by the hydrolysis of glucose at C-20 (Akter and Huq, 2018). The β-glucosidase produced by pectinase (Fang et al., 2020), Dekkera anomala YAE-1 (Renchinkhand et al., 2020), Paenibacillus sp. MBT213 (Renchinkhand et al., 2017), Flavobacterium johnsoniae (Hong et al., 2012), Leuconostoc mesenteroides DC102 (Quan et al., 2011), and Lactobacillus brevis (Zhong et al., 2016) is able to hydrolyze ginsenoside Rb1 (Rb1) and convert it to ginsenoside Rd during the fermentation of the ginseng. In addition, Aspergillus niger strain TH-10 (Feng et al., 2016), Paecilomyces bainier 229-7 (Ye et al., 2010; Ye et al., 2012), Thermus caldophilus GK24 (Son et al., 2008), Microbacterium trichothecenolyticum (Kim et al., 2013a), Cladosporium fulvum (Zhao et al., 2009), and Aspergillus versicolor (Lin et al., 2015) have shown similar effects as those of hydrolases in Rb1.
The α-L-arabinosidase (AbpBs) from Caldicellulosiruptor saccharolyticus (Shin et al., 2013), Thermotoga thermarum DSM 5069 (Xie et al., 2016a), Leuconostoc sp. 22-3 (Liu et al., 2013), and Bacillus subtilis (Zhang et al., 2021b) converts ginsenoside Rc (Rc) into ginsenoside Rd by attacking the C-20 position of α-linked arabinoside, thereby releasing arabinose (Liu et al., 2013; Zhang et al., 2021b). AbpBs can promote the biotransformation of ginsenoside Rb2 (Rb2) to ginsenoside Rd by attacking C-20, thereby releasing arabinoside (Kim et al., 2020). In addition, enzymes PgUGT74AE2 and PgUGT94Q2, which participate in ginsenoside biosynthesis, transfer two glucose groups from UDP-glucose (UDP-Glc) to the C3 hydroxyl group of ginsenoside compound K (CK) to form ginsenoside Rd (Jung et al., 2014).
β-glucosidase cleaves the glycoside at the C-3 position of ginsenoside Rd and produces the ginsenoside compound CK (Renchinkhand et al., 2020). Ginsenoside M1 is formed by the hydrolysis of the C-3 glucose group in ginsenoside Rd by snailase (Renchinkhand et al., 2017).
Intestinal flora can promote the metabolic transformation of ginseng extract and Rb1 into ginsenoside Rd in rats and can enter the blood for absorption in rats (Kim et al., 2014a). Ginsenoside Rd is distributed in various organs, with the highest content in the lungs, followed by the liver, kidney, heart, and intestine, and the lowest content in the brain (Sun et al., 2012). After taking urine 0–24 h after oral administration and intravenous administration, liquid chromatography-mass spectrometry (LC-MS) is used to confirm that oxidation and glycosylation (Yang et al., 2006a; Yang et al., 2007a) are the main metabolic pathways of ginsenoside Rd in rats. The absolute bioavailability of Rd in dogs is 0.26% (Wang et al., 2007). As in clinical trials, ginsenoside Rd shows linear pharmacokinetics, is well tolerated in the dose range of 10–75 mg after an intravenous administration, and is slowly cleared from plasma, and the elimination rate does not change after repeated administration (Zeng et al., 2010).
In ischemic stroke, ginsenoside Rd plays a neuroprotective role by restoring mitochondrial function, reducing neuronal apoptosis, and eliminating neuroinflammation (Figure 2). As for the therapeutic window study, ginsenoside Rd shows an obvious neuroprotective effect in the middle cerebral artery occlusion (MCAO) model (Ye et al., 2011a). Importantly, the results of a clinical trial showed that ginsenoside Rd has a positive effect on the prognosis of acute ischemic stroke (Liu et al., 2012).
In Ca2+ influx and mitochondrial dysfunction, ginsenoside Rd, a potential Ca2+ channel blocker (Li et al., 2010), significantly reduces the burst of glutamate by increasing the expression of glutamate transporter-1 (GLT-1) and inhibits the channels of Ca2+ influx (Zhang et al., 2013a) to protect the rat hippocampal neurons (Zhang et al., 2012a). Similar to a calcineurin inhibitor, ginsenoside Rd exerts a neuroprotective effect by inhibiting the elevation of N-methyl-D-aspartate (NMDA) receptors and the hyperphosphorylation of the N-methyl-D-aspartate receptor 2B (NR2B) subunit in the MCAO model and oxygen–glucose deprivation (OGD) cultured neurons (Xie et al., 2016b; Zhang et al., 2020a). Ginsenoside Rd pretreatment exerts neuroprotective effects by inhibiting the Ca2+ overload and specificity attenuated the expression of transient receptor potential melastatin (TRPM) 7 and acid-sensing ion channel (ASIC) 1a while promoting ASIC2a expression following focal ischemia (Zhang et al., 2012b). Remarkably, the results of a clinical trial based on Ca2+ disorder and subsequent neurotoxicity induced by acuteischemic stroke, ginsenoside Rd can be considered a calcium channel antagonist and a neuroprotectant (Liu et al., 2009). As for mitochondrial dysfunction, ginsenoside Rd markedly protects the mitochondria, as indicated by regulating enzyme activity, reducing mitochondrial hydrogen peroxide production and depolarizing mitochondrial membrane potential (MMP), decreasing reactive oxygen species (ROS) production in isolated mitochondria from Sprague–Dawley (SD) rats (Ye et al., 2011b), and reducing the mitochondrial DNA (mtDNA) and nuclear DNA (nDNA) damage and cell apoptosis in MCAO-induced ischemic stroke model (Hu et al., 2013; Yang et al., 2016). These findings are also confirmed in primary cultured hippocampal neuron cells (Ye et al., 2009). In addition, in elderly stroke mice, ginsenoside Rd can play an equivalent neuroprotective role in elderly transient focal ischemic mice by regulating lipid peroxide accumulation, mitochondrial complex activity, and MMP (Ye et al., 2011c).
As far as apoptosis is concerned, ginsenoside Rd may reduce cerebral ischemia-induced tau phosphorylation by decreasing the activity of glycogen synthase kinase-3β (GSK-3β) and enhancing the activity of protein kinase B (PKB/AKT) (Zhang et al., 2014). In PC12 cells with OGD/reperfusion (OGD/R) and SD rats with ischemia/reperfusion (I/R) injury, ginsenoside Rd significantly limits the expression of vascular endothelial growth factor (VEGF), brain-derived neurotrophic factor (BDNF), and the phosphatidylinositol 3-kinase (PI3K)/AKT and ERK1/2 pathways (Liu et al., 2015a). As a neuroprotective agent ginsenoside Rd also prevents trimethyltin (TMT)-induced neurotoxicity and significantly reduces neuronal loss in TMT-induced hippocampal dysfunction and active astrocytes via regulation of B-cell lymphoma-2 (Bcl-2), Bcl-2-like protein 4, and caspase-3 (Hou et al., 2017). Taken together, ginsenoside Rd has neuroprotective effects via mitogen-activated protein kinase (MAPK)/ERK-, PI3K/AKT, PI3K/AKT/GSK-3β, and ERK1/2-dependent pathways.
For inflammation, ginsenoside Rd inhibits ischemic stroke-indeced neuronal death and inflammation by inhibiting cleaved poly adenosine diphosphate-ribose polymerase-1(PARP-1) activity, levels of poly (ADP-ribose), sequential apoptosis-inducing factor (AIF) translocation, and nuclear factor kappa-light-chain-enhancer of activated B cells (NF-κB) nuclear accumulation (Hu et al., 2013). Postischemic syntheses of two damaging enzymes, cyclooxygenase-2 (COX-2) and inducible nitric oxide synthase (iNOS), are also significantly inhibited by ginsenoside Rd treatment. Ginsenoside Rd reduces free radical generation during I/R and suppresses oxidative damage and inflammatory injury (Ye et al., 2011d). As a proteasome-related compound, ginsenoside Rd protects against MCAO-induced ischemic brain injury by inhibiting the proteasome activity and NF-κB/matrix metalloproteinase-9 (MMP-9) signal pathway (Zhang et al., 2020b). Ginsenoside Rd inhibits MCAO-induced microglial activation, decreases the expression levels of nuclear factor of kappa light polypeptide gene enhancer in B cell inhibitor, alpha (IκBα) phosphorylation and NF-κB nuclear translocation within a short time, and has fewer side effects than glucocorticoids (Zhang et al., 2016).
Ginsenoside Rd has a significant neuroprotective effect on a variety of neurological diseases, which may be related to its promotion of stem cell proliferation (Shi et al., 2005) and differentiation into astrocytes (Lin et al., 2012). Ginsenoside Rd may promote neurite outgrowth by upregulating growth-associated protein of 43 kDa (GAP-43) expression via ERK- and ARK-dependent signaling pathways in NGF-induced PC12 cells (Wu et al., 2016).
In H2O2-induced PC12 cells, ginsenoside Rd, as a neuroprotective agent, has neuroprotective effects on neurodegenerative diseases (Ye et al., 2008). In the converting monocyte phenotype and macrophages of the Guillain–Barre syndrome (GBS) mouse model, ginsenoside Rd attenuates experimental autoimmune neuritis (Ren et al., 2021). Ginsenoside Rd can regulate MMP by decreasing intracellular ROS and enhancing the activity of antioxidant enzymes and mitochondrial complex, thereby increasing intracellular ATP levels and ultimately reducing 1-methyl-4-phenylpyridinium (MPP+)-induced cell death in Parkinson’s disease (PD) (Liu et al., 2015b). Meanwhile, in the Aβ25–35-induced neuronal damage model, apoptosis and oxidative stress are ameliorated by ginsenoside Rd by regulating antioxidant capacity and the production of apoptotic proteins (Liu et al., 2015c). Learning and memory abilities can be improved in ginsenoside Rd-pretreated APP transgenic mice by significantly suppressing the NF-κB pathway to reduce the generation of proinflammatory factors (Liu et al., 2015d). Ginsenoside Rd-mediated neuroprotective effects against Alzheimer’s disease (AD) progression play a significant role in Neuro2a cells (Kim et al., 2014b). Ginsenoside Rd pretreatment can inhibit tau protein phosphorylation by maintaining a balance of GSK-3β, cyclin-dependent kinase 5 (CDK5/P25), and protein phosphatase 2A (PP-2A) (Li et al., 2013) to inhibit tau phosphorylation of tau protein at Ser199/202, Ser396, or Ser404 in okadaic acid-induced rats, APP transgenic mice, and cortical neurons to increase PP-2A activity for protection against AD (Li et al., 2011a; Li et al., 2021), respectively. Moreover, ginsenoside Rd increases the soluble amyloid-β precursor protein α (sAPPα) level and reduces extracellular Aβ to enhance the cognitive and memory functions of ovariectomy rats (Yan et al., 2017).
In experimental autoimmune encephalomyelitis, ginsenoside Rd exerts a neuroprotective role by regulating the immune response and inflammatory reaction via a signal pathway of IFN-g/IL-4, BDNF/NGF (Zhu et al., 2014), and Foxp3/RORγt/JAK2/STAT3 (Jin et al., 2020a). In spinal cord injury (SCI) models, ginsenoside Rd shows anti-inflammatory effects consistent with dexamethasone that could significantly decrease the biomarkers of apoptosis, inflammation, oxidative damage factor, and repaired damaged mitochondria; particularly, there is no obvious difference in terms of dexamethasone in anti-inflammatory (Zhou et al., 2014; Cong and Chen, 2016), and these effects depended on the ASK1/JNK pathway (Wang et al., 2014). In the pathology of noise-induced hearing loss (NIHL), ginsenoside Rd could alleviate the apoptosis and oxidative stress damage on neuron cells by activating the SIRT1/PGC-1α signaling pathway (Chen et al., 2020). In addition, ginsenoside Rd treatment effectively eliminates the oxidative injury and the production of proinflammatory factors and peroxides in the chronic restraint stress (CRS) paradigm (Wang et al., 2020). Ginsenoside Rd pretreatment may be neuroprotective in old rats following acute Pb exposure through limited microglial activation and maintained neural stem cell proliferation (Wang et al., 2013a).
To summarize, ginsenoside Rd can play a significant role in neuron damage by inhibiting the production of excitatory amino acids, reducing the intracellular Ca2+ influx mediated by the NMDA pathway, changing the neurotoxicity of Ca2+ to mitochondrial function damage, and regulating apoptosis-inducing and neuroinflammatory factors (Table 2).
TABLE 2. Summary of the neuroprotective effects and mechanism of ginsenoside Rd in animal and cell models.
As indicated in Table 3 and Figure 3, ginsenoside Rd can inhibit the proliferation of various cancer cells by participating in the apoptotic pathway. As a potential therapeutic and specific 26S proteasome inhibitor, ginsenoside Rd plays an important role in anticancer therapy by targeting 26S proteasome (Chang et al., 2008).
TABLE 3. Summary of the effects and mechanisms of ginsenoside Rd on cell and animal models of multiple cancers.
Ginsenoside Rd can appreciably inhibit the proliferation of gastric cancer cells and can stimulate apoptosis by downregulating cyclin D1, thereby inducing cell cycle arrest in the G0/G1 phase and enhancing the expression of caspase-3 and caspase-9 and the ratio of Bax/Bcl-2 (Tian et al., 2020). After heat processing, the anticancer activity of deglycosylated Rd could be improved via the apoptotic pathway for AGS cells (Kim et al., 2013b).
In non-small-cell lung cancer (NSCLC), ginsenoside Rd, as a therapeutic drug, inhibits the nuclear factor erythroid 2-associated factor 2 (NRF2) pathway, and the synergistic effect of ginsenoside Rd in A549 and cisplatin (DDP)-resistant A549 cell lines (A549/DDP) can be weakened by knocking out NRF2 (Chian et al., 2019). As for glioblastoma, ginsenoside Rd decreases the proliferation of human glioma U251 cells and promotes apoptosis by downregulating the expression of hTERT and Bcl-2, upregulating the expression of the caspase-3 level, and inhibiting the telomerase activity of U251 cells (Gu et al., 2019). Ginsenoside Rd inhibits the proliferation and migration of glioblastoma cells by decreasing the expression of tumor-suppressor Mir-144-5p and promoting the expression of the target of Mir-144-5p toll-like receptor 2 (Liu et al., 2020b). In colorectal cancer cells, ginsenoside Rd, a therapeutic agent, targets epidermal growth factor receptor (EGFR)/SOX2 signaling (Phi et al., 2019).
Ginsenoside Rd also plays a crucial role in breast cancer. In MDA-MB-231 cell xenografted mice, ginsenoside Rd treatment inhibits the activation of PI3K, AKT, mammalian target of rapamycin (mTOR), and p70S6K in cells and decreases the expression of hypoxia-inducible factor 1-α (HIF1-α) (Zhang et al., 2017). In MCF-7 cells, ginsenoside Rd inhibits the proliferation of MCF-7 cells by enhancing caspase-3 activity, mitochondrial depolarization, and sub-G1 populations (Kim, 2013). In 4T1 cells, the expression of Mir-18a and Smad2 decreases with ginsenoside Rd treatment (Wang et al., 2016). Furthermore, ginsenoside Rd promotes the ubiquitination of MDR1 and inhibits doxorubicin resistance in MCF-7/ADR cells (Pokharel et al., 2010). In cervical cancer, ginsenoside Rd treatment in HeLa cells upregulates Bax expression, downregulates Bcl-2 expression, decreases the mitochondrial transmembrane potential, activates the caspase-3 pathway, significantly inhibits proliferation, and induces apoptosis (Yang et al., 2006b).
Finally, in HepG2 cells and the HepG2 cell-injected nude mice-induced hepatocellular carcinoma model, the combination of CA4P and ginsenoside Rd has synergistic antitumor effects via the PI3K/AKT/mTOR signaling pathway-related inhibition of HIF-1α (Yang et al., 2021a). HepG2 cells treated with ginsenoside Rd noticeably promoted matrix metalloproteinases’ (MMPs) activation, and MAPK signaling pathways were involved in cancer cell migration, thereby suggesting that ginsenoside Rd inhibits the activity of HepG2 cells in a dose-dependent and time-dependent manner (Yoon et al., 2012).
In a sodium dextran sulfate (DSS)-induced colitis model, ginsenoside Rd reduces DSS-induced colonic pathology via the adenosine 5′-monophosphate-activated protein kinase/Unc-51 like autophagy activating kinase 1 (AMPK/ULK1)-induced autophagy signaling pathway and the inhibition of the production of proinflammatory cytokines (IL-1β, TNF-α, and IL-6) in serum and colon tissues (Liu et al., 2018). In irradiation-induced intestinal epithelial cells, ginsenoside Rd reduces apoptosis by activating a pathway of PI3K/AKT, inactivates MEK, and inhibits a mitochondria/caspase pathway (Tamura et al., 2008). Meanwhile, in 2,4,6-trinitrobenzenesulfonic acid (TNBS)-induced ulcerative colitis model, ginsenoside Rd showed obvious anti-inflammatory activity by inhibiting neutrophil infiltration, regulating apoptosis signal and oxidative stress (Yang et al., 2012a), reduced the accumulation of leukocytes, and downregulated multiple proinflammatory cytokines (Yang et al., 2012b).
Laboratory data of ginsenoside Rd suggest that it has effects on multiple metabolic diseases. The browning of white adipose tissue induced by cold stress and cAMP levels are increased by ginsenoside Rd. In particular, Rd alleviates obesity and insulin resistance by upregulating thermogenesis through the cAMP/protein kinase A (PKA) signaling pathway (Yao et al., 2020). In fast-food diet-induced non-alcoholic fatty liver disease (NAFLD), fermented ginsenoside Rd with Cordyceps militaris regulates lipid metabolism and the inflammatory response via mTORC1 signaling (Choi et al., 2019). Ginsenoside Rd inhibits the progress of the death of islet transplantation by decreasing the apoptosis of the islet cells (Kaviani et al., 2019). In the atherosclerosis process, ginsenoside Rd decreases oxidized low-density lipoprotein (Ox-LDL) and cholesterol by inhibiting Ca2+ influx (Li et al., 2011b). In diabetic db/db mice and mesangial cells, pectin-lyase-modified ginsenoside Rd relieves diabetic nephropathy via alleviated ROS production (Jung et al., 2021).
Ginsenoside Rd has positive effects on skin injury, osteoporosis, kidney injury, vessel injury, heart injury, lung injury, aging, and inflammation. In animal wound models, ginsenoside Rd significantly increases wound healing by promoting the proliferation and migration level of keratinocyte progenitor cells (KPCs) and human dermal fibroblasts (HDFs) (Kim et al., 2013c). Ginsenoside Rd also has a positive effect on rejection caused by a transplant skin allograft (Wang et al., 2012a). Beyond that, ginsenoside Rd, as an antiosteoporotic agent, promotes differentiation and mineralization in osteoblastic MC3T3-E1 cells (Kim et al., 2012). In animal models of renal I/R injury and cultured proximal tubule cells, ginsenoside Rd has a protective effect by inhibiting inflammation and regulating biochemical indexes of renal function (Yokozawa et al., 1998; Ren et al., 2016). In addition, ginsenoside Rd downregulates NF-κB and the expression of iNOS and COX-2 in lipopolysaccharide (LPS)-induced Institute of Cancer Research (ICR) mice, and RAW264.7 cells were suppressed (Kim et al., 2013d). In the nicotine-induced vascular endothelial injury model, ginsenoside Rd plays an important role in the prevention of cardiovascular diseases via participation in NO signaling and regulates platelet and vascular function (Zhang et al., 2020c). Ginsenoside Rd upregulates Cyto C release and caspase-9/caspase-3 activation and decreases the MMP and the ratio of Bcl-2/Bax via the mitochondria-dependent pathway in H2O2-induced apoptosis in basilar artery smooth muscle cells (BASMCs) (Li et al., 2012). Furthermore, ginsenoside Rd could relieve the cisplatin-induced kidney injury (Yokozawa and Liu, 2000; Yokozawa and Dong, 2001) and kidney proximal tubules cephaloridine injury under cephaloridine treatment (Yokozawa and Dong, 2001). In an adrenocorticotrophic hormone (ACTH)–induced corticosterone secretion cell model, ginsenoside Rd inhibits ACTH-induced corticosterone production by inhibiting the MC2R-cAMP/PKA/cyclic AMP response element binding (CREB) pathway in adrenocortical cells (Jin et al., 2020b). In myocardial I/R-induced rats and simulated I/R-induced primary neonatal rat cardiomyocyte models, ginsenoside Rd promotes cardioprotection via the activation of AKT/GSK-3b signaling (Wang et al., 2013b). In addition, ginsenoside Rd can protect against LPS-induced acute lung injury by inhibiting the PI3K/AKT signaling pathway (Yang et al., 2021b). Other studies have indicated that ginsenoside Rd can significantly enhance the survival time of Caenorhabditis elegans via lipid metabolism and the activation of the stress response signaling pathway (Yu et al., 2021) and can alleviate the oxidative damage caused by aging in senescence-accelerated mice (Yokozawa et al., 2004). Finally, the anti-inflammatory activity of ginsenoside Rd is well documented, is considered to be associated with its antioxidant effects (Kim et al., 2007; Zhang et al., 2013b), and selectively produces prostaglandin E2 (PGE2) by activating the CCAAT/enhancer binding protein (C/EBP) and CREB to express COX-2 (Jeong et al., 2007). Ginsenoside Rd exerts anti-inflammation effects in carrageenan-induced inflammation rats via the inhibition of the NF-κB signaling pathway (Wang et al., 2012b) and in ovalbumin-induced allergic rhinitis mice by regulating multiple inflammatory factors (Kim et al., 2019) and elicits a Th1 and Th2 immune responses (Yang et al., 2007b). Ginsenoside Rd enhances the Th1 response to surface mannan extract in mice, which protects mice from disseminated candida infection by stimulating higher titers of Th1 antibodies and a Th1-dominated immune response (Han and Rhew, 2013).
As a widely used herbal medicine, ginseng appears in the form of dietary supplements nowadays. Available evidence suggests that the antiapoptotic, antioxidant, and anti-inflammatory activities, which suppress the calcium influx of ginsenoside Rd, may have an important role in the neuroprotective and anticancer effects. Ginsenoside Rd play a crucial role in neuroprotective, anticancer effects, metabolism, and other diseases by regulating PI3K/AKT, inhibiting Cyto C released and caspase activation, and regulating the release of inflammatory factors, which play a crucial role in neuroprotective, anticancer effects, metabolism, and other diseases.
In addition, ginsenoside Rd has potential therapeutic effects on regulating metabolism and in multiorgan protection. However, attributable to the shortage of clinical studies on ginsenoside Rd, it is difficult to make a clear decision. In addition to exploring its various activities, it is suggested to verify existing activities in a deeper mechanism, design clinical trials to prove its safety and effectiveness, and obtain a more extensive clinical application.
JnL, QH, and YY collected, analyzed, and reviewed the literature and wrote the main manuscript; PJ, JC, ME, ZZ, HQ, JaL, and ZC added/checked references and assembled figures/tables; DZ and LZ revised the manuscript; and XL and LZ designed and supervised the final version of the manuscript. All authors have read and agreed to the published version of the manuscript.
This work was supported by the National Natural Science Foundation of China (U19A2013, 82104432), the National Key Research and Development Program of China (2017YFC1702103), and the Science and Technology Development Plan Project of Jilin Province (202002053JC, 20200201419JC).
The authors declare that the research was conducted in the absence of any commercial or financial relationships that could be construed as a potential conflict of interest.
All claims expressed in this article are solely those of the authors and do not necessarily represent those of their affiliated organizations or those of the publisher, the editors, and the reviewers. Any product that may be evaluated in this article or claim that may be made by its manufacturer is not guaranteed or endorsed by the publisher.
Akter, S., and Huq, M. A. (2018). Biological Synthesis of Ginsenoside Rd Using Paenibacillus Horti Sp. Nov. Isolated from Vegetable Garden. Curr. Microbiol. 75, 1566–1573. doi:10.1007/s00284-018-1561-6
Brioschi Guevara, A., Bieler, M., Altomare, D., Berthier, M., Csajka, C., Dautricourt, S., et al. (2021). Protocols for Cognitive Enhancement. A User Manual for Brain Health Services-Part 5 of 6. Alz Res. Ther. 13, 172. doi:10.1186/s13195-021-00844-1
Chang, T. L., Ding, H. Y., and Kao, Y. W. (2008). Role of Ginsenoside Rd in Inhibiting 26S Proteasome Activity. J. Agric. Food Chem. 56, 12011–12015. doi:10.1021/jf801427e
Chen, X. M., Ji, S. F., Liu, Y. H., Xue, X. M., Xu, J., Gu, Z. H., et al. (2020). Ginsenoside Rd Ameliorates Auditory Cortex Injury Associated with Military Aviation Noise-Induced Hearing Loss by Activating SIRT1/PGC-1α Signaling Pathway. Front. Physiol. 11, 788. doi:10.3389/fphys.2020.00788
Chen, Y. Y., Liu, Q. P., An, P., Jia, M., Luan, X., Tang, J. Y., et al. (2022). Ginsenoside Rd: A Promising Natural Neuroprotective Agent. Phytomedicine 95, 153883. doi:10.1016/j.phymed.2021.153883
Chian, S., Zhao, Y., Xu, M., Yu, X., Ke, X., Gao, R., et al. (2019). Ginsenoside Rd Reverses Cisplatin Resistance in Non-small-cell Lung Cancer A549 Cells by Downregulating the Nuclear Factor Erythroid 2-related Factor 2 Pathway. Anticancer Drugs 30, 838–845. doi:10.1097/CAD.0000000000000781
Choi, S. Y., Park, J. S., Shon, C. H., Lee, C. Y., Ryu, J. M., Son, D. J., et al. (2019). Fermented Korean Red Ginseng Extract Enriched in Rd and Rg3 Protects against Non-alcoholic Fatty Liver Disease through Regulation of mTORC1. Nutrients 11. doi:10.3390/nu11122963
Cong, L., and Chen, W. (2016). Neuroprotective Effect of Ginsenoside Rd in Spinal Cord Injury Rats. Basic Clin. Pharmacol. Toxicol. 119, 193–201. doi:10.1111/bcpt.12562
Fang, H., Wei, Y., Li, Y., and Zhou, G. (2020). One-Pot Process for the Production of Ginsenoside Rd by Coupling Enzyme-Assisted Extraction with Selective Enzymolysis. Biol. Pharm. Bull. 43, 1443–1447. doi:10.1248/bpb.b19-01127
Feng, L., Xu, C., Li, Z., Li, J., Dai, Y., Han, H., et al. (2016). Microbial Conversion of Ginsenoside Rd from Rb1 by the Fungus Mutant Aspergillus niger Strain TH-10a. Prep. Biochem. Biotechnol. 46, 336–341. doi:10.1080/10826068.2015.1031391
Gu, B., Wang, J., Song, Y., Wang, Q., and Wu, Q. (2019). The Inhibitory Effects of Ginsenoside Rd on the Human Glioma U251 Cells and its Underlying Mechanisms. J. Cell Biochem. 120, 4444–4450. doi:10.1002/jcb.27732
Guo, Y. X., Zhang, Y., Gao, Y. H., Deng, S. Y., Wang, L. M., Li, C. Q., et al. (2021). Role of Plant-Derived Natural Compounds in Experimental Autoimmune Encephalomyelitis: A Review of the Treatment Potential and Development Strategy. Front. Pharmacol. 12, 639651. doi:10.3389/fphar.2021.639651
Han, Y., and Rhew, K. Y. (2013). Ginsenoside Rd Induces Protective Anti-Candida Albicans Antibody through Immunological Adjuvant Activity. Int. Immunopharmacol. 17, 651–657. doi:10.1016/j.intimp.2013.08.003
He, Y., Hu, Z., Li, A., Zhu, Z., Yang, N., Ying, Z., et al. (2019). Recent Advances in Biotransformation of Saponins. Molecules 24. doi:10.3390/molecules24132365
Hong, H., Cui, C. H., Kim, J. K., Jin, F. X., Kim, S. C., and Im, W. T. (2012). Enzymatic Biotransformation of Ginsenoside Rb1 and Gypenoside XVII into Ginsenosides Rd and F2 by Recombinant β-glucosidase from Flavobacterium Johnsoniae. J. Ginseng Res. 36, 418–424. doi:10.5142/jgr.2012.36.4.418
Hou, J., Xue, J., Lee, M., and Sung, C. (2017). Ginsenoside Rd as a Potential Neuroprotective Agent Prevents Trimethyltin Injury. Biomed. Rep. 6, 435–440. doi:10.3892/br.2017.864
Hu, G., Wu, Z., Yang, F., Zhao, H., Liu, X., Deng, Y., et al. (2013). Ginsenoside Rd Blocks AIF Mitochondrio-Nuclear Translocation and NF-Κb Nuclear Accumulation by Inhibiting poly(ADP-Ribose) Polymerase-1 after Focal Cerebral Ischemia in Rats. Neurol. Sci. 34, 2101–2106. doi:10.1007/s10072-013-1344-6
Jeong, H. G., Pokharel, Y. R., Han, E. H., and Kang, K. W. (2007). Induction of Cyclooxygenase-2 by Ginsenoside Rd via Activation of CCAAT-Enhancer Binding Proteins and Cyclic AMP Response Binding Protein. Biochem. Biophys. Res. Commun. 359, 51–56. doi:10.1016/j.bbrc.2007.05.034
Jin, B., Zhang, C., Geng, Y., and Liu, M. (2020). Therapeutic Effect of Ginsenoside Rd on Experimental Autoimmune Encephalomyelitis Model Mice: Regulation of Inflammation and Treg/Th17 Cell Balance. Mediat. Inflamm. 2020, 8827527. doi:10.1155/2020/8827527
Jin, W., Ma, R., Zhai, L., Xu, X., Lou, T., Huang, Q., et al. (2020). Ginsenoside Rd Attenuates ACTH-Induced Corticosterone Secretion by Blocking the MC2R-cAMP/PKA/CREB Pathway in Y1 Mouse Adrenocortical Cells. Life Sci. 245, 117337. doi:10.1016/j.lfs.2020.117337
Jung, E., Pyo, M. K., and Kim, J. (2021). Pectin-Lyase-Modified Ginseng Extract and Ginsenoside Rd Inhibits High Glucose-Induced ROS Production in Mesangial Cells and Prevents Renal Dysfunction in Db/db Mice. Molecules 26. doi:10.3390/molecules26020367
Jung, S. C., Kim, W., Park, S. C., Jeong, J., Park, M. K., Lim, S., et al. (2014). Two Ginseng UDP-Glycosyltransferases Synthesize Ginsenoside Rg3 and Rd. Plant Cell Physiol. 55, 2177–2188. doi:10.1093/pcp/pcu147
Kang, N., Gao, H., He, L., Liu, Y., Fan, H., Xu, Q., et al. (2021). Ginsenoside Rb1 Is an Immune-Stimulatory Agent with Antiviral Activity against Enterovirus 71. J. Ethnopharmacol. 266, 113401. doi:10.1016/j.jep.2020.113401
Kaviani, M., Keshtkar, S., Azarpira, N., Hossein Aghdaei, M., Geramizadeh, B., Karimi, M. H., et al. (2019). Cytoprotective Effects of Ginsenoside Rd on Apoptosis-Associated Cell Death in the Isolated Human Pancreatic Islets. EXCLI J. 18, 666–676. doi:10.17179/excli2019-1698
Kim, B. J. (2013). Involvement of Melastatin Type Transient Receptor Potential 7 Channels in Ginsenoside Rd-Induced Apoptosis in Gastric and Breast Cancer Cells. J. Ginseng Res. 37, 201–209. doi:10.5142/jgr.2013.37.201
Kim, D. H., Chung, J. H., Yoon, J. S., Ha, Y. M., Bae, S., Lee, E. K., et al. (2013). Ginsenoside Rd Inhibits the Expressions of iNOS and COX-2 by Suppressing NF-Κb in LPS-Stimulated RAW264.7 Cells and Mouse Liver. J. Ginseng Res. 37, 54–63. doi:10.5142/jgr.2013.37.54
Kim, D. Y., Park, Y. G., Quan, H. Y., Kim, S. J., Jung, M. S., and Chung, S. H. (2012). Ginsenoside Rd Stimulates the Differentiation and Mineralization of Osteoblastic MC3T3-E1 Cells by Activating AMP-Activated Protein Kinase via the BMP-2 Signaling Pathway. Fitoterapia 83, 215–222. doi:10.1016/j.fitote.2011.10.017
Kim, H., Kim, J. H., Lee, P. Y., Bae, K. H., Cho, S., Park, B. C., et al. (2013). Ginsenoside Rb1 Is Transformed into Rd and Rh2 by Microbacterium Trichothecenolyticum. J. Microbiol. Biotechnol. 23, 1802–1805. doi:10.4014/jmb.1307.07049
Kim, H. I., Kim, J. K., Kim, J. Y., Han, M. J., and Kim, D. H. (2019). Fermented Red Ginseng and Ginsenoside Rd Alleviate Ovalbumin-Induced Allergic Rhinitis in Mice by Suppressing IgE, Interleukin-4, and Interleukin-5 Expression. J. Ginseng Res. 43, 635–644. doi:10.1016/j.jgr.2019.02.006
Kim, J. H., Oh, J. M., Chun, S., Park, H. Y., and Im, W. T. (2020). Enzymatic Biotransformation of Ginsenoside Rb2 into Rd by Recombinant α-L-Arabinopyranosidase from Blastococcus Saxobsidens. J. Microbiol. Biotechnol. 30, 391–397. doi:10.4014/jmb.1910.10065
Kim, K. A., Yoo, H. H., Gu, W., Yu, D. H., Jin, M. J., Choi, H. L., et al. (2014). Effect of a Soluble Prebiotic Fiber, NUTRIOSE, on the Absorption of Ginsenoside Rd in Rats Orally Administered Ginseng. J. Ginseng Res. 38, 203–207. doi:10.1016/j.jgr.2014.03.003
Kim, M. S., Yu, J. M., Kim, H. J., Kim, H. B., Kim, S. T., Jang, S. K., et al. (2014). Ginsenoside Re and Rd Enhance the Expression of Cholinergic Markers and Neuronal Differentiation in Neuro-2a Cells. Biol. Pharm. Bull. 37, 826–833. doi:10.1248/bpb.b14-00011
Kim, N. D., Pokharel, Y. R., and Kang, K. W. (2007). Ginsenoside Rd Enhances Glutathione Levels in H4IIE Cells via NF-kappaB-dependent Gamma-Glutamylcysteine Ligase Induction. Pharmazie 62, 933–936.
Kim, W. K., Song, S. Y., Oh, W. K., Kaewsuwan, S., Tran, T. L., Kim, W. S., et al. (2013). Wound-healing Effect of Ginsenoside Rd from Leaves of Panax Ginseng via Cyclic AMP-dependent Protein Kinase Pathway. Eur. J. Pharmacol. 702, 285–293. doi:10.1016/j.ejphar.2013.01.048
Kim, Y. J., Yamabe, N., Choi, P., Lee, J. W., Ham, J., and Kang, K. S. (2013). Efficient Thermal Deglycosylation of Ginsenoside Rd and its Contribution to the Improved Anticancer Activity of Ginseng. J. Agric. Food Chem. 61, 9185–9191. doi:10.1021/jf402774d
Li, J., Xie, Z. Z., Tang, Y. B., Zhou, J. G., and Guan, Y. Y. (2011). Ginsenoside-Rd, a Purified Component from Panax Notoginseng Saponins, Prevents Atherosclerosis in apoE Knockout Mice. Eur. J. Pharmacol. 652, 104–110. doi:10.1016/j.ejphar.2010.11.017
Li, L., Li, T., Tian, X., and Zhao, L. (2021). Ginsenoside Rd Attenuates Tau Phosphorylation in Olfactory Bulb, Spinal Cord, and Telencephalon by Regulating Glycogen Synthase Kinase 3β and Cyclin-dependent Kinase 5. Evid. Based Complement. Altern. Med. 2021, 4485957. doi:10.1155/2021/4485957
Li, L., Liu, J., Yan, X., Qin, K., Shi, M., Lin, T., et al. (2011). Protective Effects of Ginsenoside Rd against Okadaic Acid-Induced Neurotoxicity In Vivo and In Vitro. J. Ethnopharmacol. 138, 135–141. doi:10.1016/j.jep.2011.08.068
Li, L., Liu, Z., Liu, J., Tai, X., Hu, X., Liu, X., et al. (2013). Ginsenoside Rd Attenuates Beta-Amyloid-Induced Tau Phosphorylation by Altering the Functional Balance of Glycogen Synthase Kinase 3beta and Protein Phosphatase 2A. Neurobiol. Dis. 54, 320–328. doi:10.1016/j.nbd.2013.01.002
Li, S. Y., Wang, X. G., Ma, M. M., Liu, Y., Du, Y. H., Lv, X. F., et al. (2012). Ginsenoside-Rd Potentiates Apoptosis Induced by Hydrogen Peroxide in Basilar Artery Smooth Muscle Cells through the Mitochondrial Pathway. Apoptosis 17, 113–120. doi:10.1007/s10495-011-0671-4
Li, X. Y., Liang, J., Tang, Y. B., Zhou, J. G., and Guan, Y. Y. (2010). Ginsenoside Rd Prevents Glutamate-Induced Apoptosis in Rat Cortical Neurons. Clin. Exp. Pharmacol. Physiol. 37, 199–204. doi:10.1111/j.1440-1681.2009.05286.x
Lin, F., Guo, X., and Lu, W. (2015). Efficient Biotransformation of Ginsenoside Rb1 to Rd by Isolated Aspergillus versicolor, Excreting β-glucosidase in the Spore Production Phase of Solid Culture. Ant. Van Leeuwenhoek 108, 1117–1127. doi:10.1007/s10482-015-0565-5
Lin, T., Liu, Y., Shi, M., Liu, X., Li, L., Liu, Y., et al. (2012). Promotive Effect of Ginsenoside Rd on Proliferation of Neural Stem Cells In Vivo and In Vitro. J. Ethnopharmacol. 142, 754–761. doi:10.1016/j.jep.2012.05.057
Liu, C., Wang, J., Yang, Y., Liu, X., Zhu, Y., Zou, J., et al. (2018). Ginsenoside Rd Ameliorates Colitis by Inducing P62-Driven Mitophagy-Mediated NLRP3 Inflammasome Inactivation in Mice. Biochem. Pharmacol. 155, 366–379. doi:10.1016/j.bcp.2018.07.010
Liu, G. M., Lu, T. C., Sun, M. L., Jia, W. Y., Ji, X., and Luo, Y. G. (2020). Ginsenoside Rd Inhibits Glioblastoma Cell Proliferation by Up-Regulating the Expression of miR-144-5p. Biol. Pharm. Bull. 43, 1534–1541. doi:10.1248/bpb.b20-00338
Liu, H., Lu, X., Hu, Y., and Fan, X. (2020). Chemical Constituents of Panax Ginseng and Panax Notoginseng Explain Why They Differ in Therapeutic Efficacy. Pharmacol. Res. 161, 105263. doi:10.1016/j.phrs.2020.105263
Liu, J., Yan, X., Li, L., Li, Y., Zhou, L., Zhang, X., et al. (2015). Ginsenoside Rd Improves Learning and Memory Ability in APP Transgenic Mice. J. Mol. Neurosci. 57, 522–528. doi:10.1007/s12031-015-0632-4
Liu, J. F., Yan, X. D., Qi, L. S., Li, L., Hu, G. Y., Li, P., et al. (2015). Ginsenoside Rd Attenuates Aβ25-35-Induced Oxidative Stress and Apoptosis in Primary Cultured Hippocampal Neurons. Chem. Biol. Interact. 239, 12–18. doi:10.1016/j.cbi.2015.06.030
Liu, Q. M., Jung, H. M., Cui, C. H., Sung, B. H., Kim, J. K., Kim, S. G., et al. (2013). Bioconversion of Ginsenoside Rc into Rd by a Novel α-L-arabinofuranosidase, Abf22-3 from Leuconostoc Sp. 22-3: Cloning, Expression, and Enzyme Characterization. Ant. Van Leeuwenhoek 103, 747–754. doi:10.1007/s10482-012-9856-2
Liu, X., Wang, L., Wen, A., Yang, J., Yan, Y., Song, Y., et al. (2012). Ginsenoside-Rd Improves Outcome of Acute Ischaemic Stroke - a Randomized, Double-Blind, Placebo-Controlled, Multicenter Trial. Eur. J. Neurol. 19, 855–863. doi:10.1111/j.1468-1331.2011.03634.x
Liu, X., Xia, J., Wang, L., Song, Y., Yang, J., Yan, Y., et al. (2009). Efficacy and Safety of Ginsenoside-Rd for Acute Ischaemic Stroke: a Randomized, Double-Blind, Placebo-Controlled, Phase II Multicenter Trial. Eur. J. Neurol. 16, 569–575. doi:10.1111/j.1468-1331.2009.02534.x
Liu, X. Y., Zhou, X. Y., Hou, J. C., Zhu, H., Wang, Z., Liu, J. X., et al. (2015). Ginsenoside Rd Promotes Neurogenesis in Rat Brain after Transient Focal Cerebral Ischemia via Activation of PI3K/Akt Pathway. Acta Pharmacol. Sin. 36, 421–428. doi:10.1038/aps.2014.156
Liu, Y., Zhang, R. Y., Zhao, J., Dong, Z., Feng, D. Y., Wu, R., et al. (2015). Ginsenoside Rd Protects SH-Sy5y Cells against 1-Methyl-4-Phenylpyridinium Induced Injury. Int. J. Mol. Sci. 16, 14395–14408. doi:10.3390/ijms160714395
Park, C. S., Yoo, M. H., Noh, K. H., and Oh, D. K. (2010). Biotransformation of Ginsenosides by Hydrolyzing the Sugar Moieties of Ginsenosides Using Microbial Glycosidases. Appl. Microbiol. Biotechnol. 87, 9–19. doi:10.1007/s00253-010-2567-6
Phi, L. T. H., Sari, I. N., Wijaya, Y. T., Kim, K. S., Park, K., Cho, A. E., et al. (2019). Ginsenoside Rd Inhibits the Metastasis of Colorectal Cancer via Epidermal Growth Factor Receptor Signaling Axis. IUBMB Life 71, 601–610. doi:10.1002/iub.1984
Pokharel, Y. R., Kim, N. D., Han, H. K., Oh, W. K., and Kang, K. W. (2010). Increased Ubiquitination of Multidrug Resistance 1 by Ginsenoside Rd. Nutr. Cancer 62, 252–259. doi:10.1080/01635580903407171
Quan, L. H., Piao, J. Y., Min, J. W., Kim, H. B., Kim, S. R., Yang, D. U., et al. (2011). Biotransformation of Ginsenoside Rb1 to Prosapogenins, Gypenoside XVII, Ginsenoside Rd, Ginsenoside F2, and Compound K by Leuconostoc Mesenteroides DC102. J. Ginseng Res. 35, 344–351. doi:10.5142/jgr.2011.35.3.344
Ren, K., Jin, C., Ma, P., Ren, Q., Jia, Z., and Zhu, D. (2016). Ginsenoside Rd Alleviates Mouse Acute Renal Ischemia/reperfusion Injury by Modulating Macrophage Phenotype. J. Ginseng Res. 40, 196–202. doi:10.1016/j.jgr.2015.12.003
Ren, K., Li, S., Ding, J., Zhao, S., Liang, S., Cao, X., et al. (2021). Ginsenoside Rd Attenuates Mouse Experimental Autoimmune Neuritis by Modulating Monocyte Subsets Conversion. Biomed. Pharmacother. 138, 111489. doi:10.1016/j.biopha.2021.111489
Renchinkhand, G., Cho, S. H., Park, Y. W., Song, G. Y., and Nam, M. S. (2020). Biotransformation of Major Ginsenoside Rb1 to Rd by Dekkera Anomala YAE-1 from Mongolian Fermented Milk (Airag). J. Microbiol. Biotechnol. 30, 1536–1542. doi:10.4014/jmb.2004.04022
Renchinkhand, G., Cho, S. H., Urgamal, M., Park, Y. W., Nam, J. H., Bae, H. C., et al. (2017). Characterization of Paenibacillus Sp. MBT213 Isolated from Raw Milk and its Ability to Convert Ginsenoside Rb1 into Ginsenoside Rd from Panax Ginseng. Korean J. Food Sci. Anim. Resour. 37, 735–742. doi:10.5851/kosfa.2017.37.5.735
Sarhene, M., Ni, J. Y., Duncan, E. S., Liu, Z., Li, S., Zhang, J., et al. (2021). Ginsenosides for Cardiovascular Diseases; Update on Pre-clinical and Clinical Evidence, Pharmacological Effects and the Mechanisms of Action. Pharmacol. Res. 166, 105481. doi:10.1016/j.phrs.2021.105481
Shi, Q., Hao, Q., Bouissac, J., Lu, Y., Tian, S., and Luu, B. (2005). Ginsenoside-Rd from Panax Notoginseng Enhances Astrocyte Differentiation from Neural Stem Cells. Life Sci. 76, 983–995. doi:10.1016/j.lfs.2004.07.026
Shin, K. C., Lee, G. W., and Oh, D. K. (2013). Production of Ginsenoside Rd from Ginsenoside Rc by α-L-arabinofuranosidase from Caldicellulosiruptor Saccharolyticus. J. Microbiol. Biotechnol. 23, 483–488. doi:10.4014/jmb.1211.11012
Shin, K. C., and Oh, D. K. (2016). Classification of Glycosidases that Hydrolyze the Specific Positions and Types of Sugar Moieties in Ginsenosides. Crit. Rev. Biotechnol. 36, 1036–1049. doi:10.3109/07388551.2015.1083942
Son, J. W., Kim, H. J., and Oh, D. K. (2008). Ginsenoside Rd Production from the Major Ginsenoside Rb(1) by Beta-Glucosidase from Thermus Caldophilus. Biotechnol. Lett. 30, 713–716. doi:10.1007/s10529-007-9590-4
Sun, D., Wang, B., Shi, M., Zhang, Y. X., Zhou, L. F., Liu, Z. R., et al. (2012). Pharmacokinetic, Tissue Distribution and Excretion of Ginsenoside-Rd in Rodents. Phytomedicine 19, 369–373. doi:10.1016/j.phymed.2011.08.061
Tamura, T., Cui, X., Sakaguchi, N., and Akashi, M. (2008). Ginsenoside Rd Prevents and Rescues Rat Intestinal Epithelial Cells from Irradiation-Induced Apoptosis. Food Chem. Toxicol. 46, 3080–3089. doi:10.1016/j.fct.2008.06.011
Tian, Y. Z., Liu, Y. P., Tian, S. C., Ge, S. Y., Wu, Y. J., and Zhang, B. L. (2020). Antitumor Activity of Ginsenoside Rd in Gastric Cancer via Up-Regulation of Caspase-3 and Caspase-9. Pharmazie 75, 147–150. doi:10.1691/ph.2020.9931
Wang, B., Feng, G., Tang, C., Wang, L., Cheng, H., Zhang, Y., et al. (2013). Ginsenoside Rd Maintains Adult Neural Stem Cell Proliferation during Lead-Impaired Neurogenesis. Neurol. Sci. 34, 1181–1188. doi:10.1007/s10072-012-1215-6
Wang, B., Zhu, Q., Man, X., Guo, L., and Hao, L. (2014). Ginsenoside Rd Inhibits Apoptosis Following Spinal Cord Ischemia/reperfusion Injury. Neural Regen. Res. 9, 1678–1687. doi:10.4103/1673-5374.141802
Wang, F., Park, J. S., Ma, Y., Ma, H., Lee, Y. J., Lee, G. R., et al. (2021). Ginseng Saponin Enriched in Rh1 and Rg2 Ameliorates Nonalcoholic Fatty Liver Disease by Inhibiting Inflammasome Activation. Nutrients 13. doi:10.3390/nu13030856
Wang, H., Jiang, N., Lv, J., Huang, H., and Liu, X. (2020). Ginsenoside Rd Reverses Cognitive Deficits by Modulating BDNF-dependent CREB Pathway in Chronic Restraint Stress Mice. Life Sci. 258, 118107. doi:10.1016/j.lfs.2020.118107
Wang, L., Zhang, Y., Chen, J., Li, S., Wang, Y., Hu, L., et al. (2012). Immunosuppressive Effects of Ginsenoside-Rd on Skin Allograft Rejection in Rats. J. Surg. Res. 176, 267–274. doi:10.1016/j.jss.2011.06.038
Wang, L., Zhang, Y., Wang, Z., Li, S., Min, G., Wang, L., et al. (2012). Inhibitory Effect of Ginsenoside-Rd on Carrageenan-Induced Inflammation in Rats. Can. J. Physiol. Pharmacol. 90, 229–236. doi:10.1139/y11-127
Wang, P., Du, X., Xiong, M., Cui, J., Yang, Q., Wang, W., et al. (2016). Ginsenoside Rd Attenuates Breast Cancer Metastasis Implicating Derepressing microRNA-18a-Regulated Smad2 Expression. Sci. Rep. 6, 33709. doi:10.1038/srep33709
Wang, Q., Fu, W., Yu, X., Xu, H., Sui, D., and Wang, Y. (2021). Ginsenoside Rg2 Alleviates Myocardial Fibrosis by Regulating TGF-β1/Smad Signalling Pathway. Pharm. Biol. 59, 106–113. doi:10.1080/13880209.2020.1867197
Wang, W., Wang, G. J., Xie, H. T., Sun, J. G., Zhao, S., Jiang, X. L., et al. (2007). Determination of Ginsenoside Rd in Dog Plasma by Liquid Chromatography-Mass Spectrometry after Solid-phase Extraction and its Application in Dog Pharmacokinetics Studies. J. Chromatogr. B Anal. Technol. Biomed. Life Sci. 852, 8–14. doi:10.1016/j.jchromb.2006.12.046
Wang, Y., Li, X., Wang, X., Lau, W., Wang, Y., Xing, Y., et al. (2013). Ginsenoside Rd Attenuates Myocardial Ischemia/reperfusion Injury via Akt/GSK-3β Signaling and Inhibition of the Mitochondria-dependent Apoptotic Pathway. PLoS One 8, e70956. doi:10.1371/journal.pone.0070956
Wu, S. D., Xia, F., Lin, X. M., Duan, K. L., Wang, F., Lu, Q. L., et al. (2016). Ginsenoside-Rd Promotes Neurite Outgrowth of PC12 Cells through MAPK/ERK- and PI3K/AKT-dependent Pathways. Int. J. Mol. Sci. 17. doi:10.3390/ijms17020177
Xie, J., Zhao, D., Zhao, L., Pei, J., Xiao, W., Ding, G., et al. (2016). Characterization of a Novel Arabinose-Tolerant α-L-arabinofuranosidase with High Ginsenoside Rc to Ginsenoside Rd Bioconversion Productivity. J. Appl. Microbiol. 120, 647–660. doi:10.1111/jam.13040
Xie, Z., Shi, M., Zhang, C., Zhao, H., Hui, H., and Zhao, G. (2016). Ginsenoside Rd Protects against Cerebral Ischemia-Reperfusion Injury via Decreasing the Expression of the NMDA Receptor 2B Subunit and its Phosphorylated Product. Neurochem. Res. 41, 2149–2159. doi:10.1007/s11064-016-1930-0
Xu, X. F., Qu, W. J., Jia, Z., Han, T., Liu, M. N., Bai, Y. Y., et al. (2021). Effect of Cultivation Ages on Anti-inflammatory Activity of a New Type of Red Ginseng. Biomed. Pharmacother. 136, 111280. doi:10.1016/j.biopha.2021.111280
Yan, X., Hu, G., Yan, W., Chen, T., Yang, F., Zhang, X., et al. (2017). Ginsenoside Rd Promotes Non-amyloidogenic Pathway of Amyloid Precursor Protein Processing by Regulating Phosphorylation of Estrogen Receptor Alpha. Life Sci. 168, 16–23. doi:10.1016/j.lfs.2016.11.002
Yang, B., Wang, R., Ji, L. L., Li, X. P., Li, X. H., Zhou, H. G., et al. (2021). Exploration of the Function of Ginsenoside RD Attenuates Lipopolysaccharide-Induced Lung Injury: A Study of Network Pharmacology and Experimental Validation. Augusta, Ga: Shock.
Yang, L., Deng, Y., Xu, S., and Zeng, X. (2007). In Vivo pharmacokinetic and Metabolism Studies of Ginsenoside Rd. J. Chromatogr. B Anal. Technol. Biomed. Life Sci. 854, 77–84. doi:10.1016/j.jchromb.2007.04.014
Yang, L., Xu, S. J., Zeng, X., Liu, Y. M., Deng, S. G., Wu, Z. F., et al. (2006). Determination of Ginsenoside Rd and its Metabolites in Rat Urine by LC-MS. Yao Xue Xue Bao 41, 742–746.
Yang, L. X., Zhang, X., and Zhao, G. (2016). Ginsenoside Rd Attenuates DNA Damage by Increasing Expression of DNA Glycosylase Endonuclease VIII-like Proteins after Focal Cerebral Ischemia. Chin. Med. J. Engl. 129, 1955–1962. doi:10.4103/0366-6999.187851
Yang, X., Gao, M., Miao, M., Jiang, C., Zhang, D., Yin, Z., et al. (2021). Combining Combretastatin A4 Phosphate with Ginsenoside Rd Synergistically Inhibited Hepatocellular Carcinoma by Reducing HIF-1α via PI3K/AKT/mTOR Signalling Pathway. J. Pharm. Pharmacol. 73, 263–271. doi:10.1093/jpp/rgaa006
Yang, X. L., Guo, T. K., Wang, Y. H., Gao, M. T., Qin, H., and Wu, Y. J. (2012). Therapeutic Effect of Ginsenoside Rd in Rats with TNBS-Induced Recurrent Ulcerative Colitis. Arch. Pharm. Res. 35, 1231–1239. doi:10.1007/s12272-012-0714-6
Yang, X. L., Guo, T. K., Wang, Y. H., Huang, Y. H., Liu, X., Wang, X. X., et al. (2012). Ginsenoside Rd Attenuates the Inflammatory Response via Modulating P38 and JNK Signaling Pathways in Rats with TNBS-Induced Relapsing Colitis. Int. Immunopharmacol. 12, 408–414. doi:10.1016/j.intimp.2011.12.014
Yang, Z., Chen, A., Sun, H., Ye, Y., and Fang, W. (2007). Ginsenoside Rd Elicits Th1 and Th2 Immune Responses to Ovalbumin in Mice. Vaccine 25, 161–169. doi:10.1016/j.vaccine.2006.05.075
Yang, Z. G., Sun, H. X., and Ye, Y. P. (2006). Ginsenoside Rd from Panax Notoginseng Is Cytotoxic towards HeLa Cancer Cells and Induces Apoptosis. Chem. Biodivers. 3, 187–197. doi:10.1002/cbdv.200690022
Yao, L., Han, Z., Zhao, G., Xiao, Y., Zhou, X., Dai, R., et al. (2020). Ginsenoside Rd Ameliorates High Fat Diet-Induced Obesity by Enhancing Adaptive Thermogenesis in a cAMP-dependent Manner. Obes. (Silver Spring) 28, 783–792. doi:10.1002/oby.22761
Ye, L., Zhang, C., Li, J., Shi, X., and Feng, M. (2012). Effects of External Calcium on the Biotransformation of Ginsenoside Rb1 to Ginsenoside Rd by Paecilomyces Bainier 229-7. World J. Microbiol. Biotechnol. 28, 857–863. doi:10.1007/s11274-011-0882-4
Ye, L., Zhou, C. Q., Zhou, W., Zhou, P., Chen, D. F., Liu, X. H., et al. (2010). Biotransformation of Ginsenoside Rb1 to Ginsenoside Rd by Highly Substrate-Tolerant Paecilomyces Bainier 229-7. Bioresour. Technol. 101, 7872–7876. doi:10.1016/j.biortech.2010.04.102
Ye, R., Han, J., Kong, X., Zhao, L., Cao, R., Rao, Z., et al. (2008). Protective Effects of Ginsenoside Rd on PC12 Cells against Hydrogen Peroxide. Biol. Pharm. Bull. 31, 1923–1927. doi:10.1248/bpb.31.1923
Ye, R., Kong, X., Yang, Q., Zhang, Y., Han, J., Li, P., et al. (2011). Ginsenoside Rd in Experimental Stroke: Superior Neuroprotective Efficacy with a Wide Therapeutic Window. Neurotherapeutics 8, 515–525. doi:10.1007/s13311-011-0051-3
Ye, R., Kong, X., Yang, Q., Zhang, Y., Han, J., and Zhao, G. (2011). Ginsenoside Rd Attenuates Redox Imbalance and Improves Stroke Outcome after Focal Cerebral Ischemia in Aged Mice. Neuropharmacology 61, 815–824. doi:10.1016/j.neuropharm.2011.05.029
Ye, R., Li, N., Han, J., Kong, X., Cao, R., Rao, Z., et al. (2009). Neuroprotective Effects of Ginsenoside Rd against Oxygen-Glucose Deprivation in Cultured Hippocampal Neurons. Neurosci. Res. 64, 306–310. doi:10.1016/j.neures.2009.03.016
Ye, R., Yang, Q., Kong, X., Han, J., Zhang, X., Zhang, Y., et al. (2011). Ginsenoside Rd Attenuates Early Oxidative Damage and Sequential Inflammatory Response after Transient Focal Ischemia in Rats. Neurochem. Int. 58, 391–398. doi:10.1016/j.neuint.2010.12.015
Ye, R., Zhang, X., Kong, X., Han, J., Yang, Q., Zhang, Y., et al. (2011). Ginsenoside Rd Attenuates Mitochondrial Dysfunction and Sequential Apoptosis after Transient Focal Ischemia. Neuroscience 178, 169–180. doi:10.1016/j.neuroscience.2011.01.007
Yi, Y. S. (2021). New Mechanisms of Ginseng Saponin-Mediated Anti-inflammatory Action via Targeting Canonical Inflammasome Signaling Pathways. J. Ethnopharmacol. 278, 114292. doi:10.1016/j.jep.2021.114292
Yokozawa, T., and Dong, E. (2001). Role of Ginsenoside-Rd in Cisplatin-Induced Renal Injury: Special Reference to DNA Fragmentation. Nephron 89, 433–438. doi:10.1159/000046116
Yokozawa, T., Liu, Z. W., and Dong, E. (1998). A Study of Ginsenoside-Rd in a Renal Ischemia-Reperfusion Model. Nephron 78, 201–206. doi:10.1159/000044911
Yokozawa, T., and Liu, Z. W. (2000). The Role of Ginsenoside-Rd in Cisplatin-Induced Acute Renal Failure. Ren. Fail 22, 115–127. doi:10.1081/jdi-100100858
Yokozawa, T., and Owada, S. (1999). Effect of Ginsenoside-Rd in Cephaloridine-Induced Renal Disorder. Nephron 81, 200–207. doi:10.1159/000045277
Yokozawa, T., Satoh, A., and Cho, E. J. (2004). Ginsenoside-Rd Attenuates Oxidative Damage Related to Aging in Senescence-Accelerated Mice. J. Pharm. Pharmacol. 56, 107–113. doi:10.1211/0022357022449
Yoon, J. H., Choi, Y. J., Cha, S. W., and Lee, S. G. (2012). Anti-metastatic Effects of Ginsenoside Rd via Inactivation of MAPK Signaling and Induction of Focal Adhesion Formation. Phytomedicine 19, 284–292. doi:10.1016/j.phymed.2011.08.069
Yu, X., Li, H., Lin, D., Guo, W., Xu, Z., Wang, L., et al. (2021). Ginsenoside Prolongs the Lifespan of C. elegans via Lipid Metabolism and Activating the Stress Response Signaling Pathway. Int. J. Mol. Sci. 22. doi:10.3390/ijms22189668
Zeng, X., Deng, Y., Feng, Y., Liu, Y., Yang, L., Huang, Y., et al. (2010). Pharmacokinetics and Safety of Ginsenoside Rd Following a Single or Multiple Intravenous Dose in Healthy Chinese Volunteers. J. Clin. Pharmacol. 50, 285–292. doi:10.1177/0091270009344334
Zhang, B., Hu, X., Wang, H., Wang, R., Sun, Z., Tan, X., et al. (2020). Effects of a Dammarane-type Saponin, Ginsenoside Rd, in Nicotine-Induced Vascular Endothelial Injury. Phytomedicine 79, 153325. doi:10.1016/j.phymed.2020.153325
Zhang, C., Du, F., Shi, M., Ye, R., Cheng, H., Han, J., et al. (2012). Ginsenoside Rd Protects Neurons against Glutamate-Induced Excitotoxicity by Inhibiting Ca(2+) Influx. Cell Mol. Neurobiol. 32, 121–128. doi:10.1007/s10571-011-9742-x
Zhang, C., Liu, X., Xu, H., Hu, G., Zhang, X., Xie, Z., et al. (2020). Protopanaxadiol Ginsenoside Rd Protects against NMDA Receptor-Mediated Excitotoxicity by Attenuating Calcineurin-Regulated DAPK1 Activity. Sci. Rep. 10, 8078. doi:10.1038/s41598-020-64738-2
Zhang, E., Shi, H., Yang, L., Wu, X., and Wang, Z. (2017). Ginsenoside Rd Regulates the Akt/mTOR/p70S6K Signaling Cascade and Suppresses Angiogenesis and Breast Tumor Growth. Oncol. Rep. 38, 359–367. doi:10.3892/or.2017.5652
Zhang, G., Xia, F., Zhang, Y., Zhang, X., Cao, Y., Wang, L., et al. (2016). Ginsenoside Rd Is Efficacious against Acute Ischemic Stroke by Suppressing Microglial Proteasome-Mediated Inflammation. Mol. Neurobiol. 53, 2529–2540. doi:10.1007/s12035-015-9261-8
Zhang, H., Park, S., Huang, H., Kim, E., Yi, J., Choi, S. K., et al. (2021). Anticancer Effects and Potential Mechanisms of Ginsenoside Rh2 in Various Cancer Types (Review). Oncol. Rep. 45. doi:10.3892/or.2021.7984
Zhang, R., Tan, S. Q., Zhang, B. L., Guo, Z. Y., Tian, L. Y., Weng, P., et al. (2021). Two Key Amino Acids Variant of Alpha-L-Arabinofuranosidase from Bacillus Subtilis Str. 168 with Altered Activity for Selective Conversion Ginsenoside Rc to Rd. Molecules 26, 1733. doi:10.3390/molecules26061733
Zhang, X., Liu, X., Hu, G., Zhang, G., Zhao, G., and Shi, M. (2020). Ginsenoside Rd Attenuates Blood-Brain Barrier Damage by Suppressing Proteasome-Mediated Signaling after Transient Forebrain Ischemia. Neuroreport 31, 466–472. doi:10.1097/WNR.0000000000001426
Zhang, X., Shi, M., Bjørås, M., Wang, W., Zhang, G., Han, J., et al. (2013). Ginsenoside Rd Promotes Glutamate Clearance by Up-Regulating Glial Glutamate Transporter GLT-1 via PI3K/AKT and ERK1/2 Pathways. Front. Pharmacol. 4, 152. doi:10.3389/fphar.2013.00152
Zhang, X., Shi, M., Ye, R., Wang, W., Liu, X., Zhang, G., et al. (2014). Ginsenoside Rd Attenuates Tau Protein Phosphorylation via the PI3K/AKT/GSK-3β Pathway after Transient Forebrain Ischemia. Neurochem. Res. 39, 1363–1373. doi:10.1007/s11064-014-1321-3
Zhang, Y., Zhou, L., Zhang, X., Bai, J., Shi, M., and Zhao, G. (2012). Ginsenoside-Rd Attenuates TRPM7 and ASIC1a but Promotes ASIC2a Expression in Rats after Focal Cerebral Ischemia. Neurol. Sci. 33, 1125–1131. doi:10.1007/s10072-011-0916-6
Zhang, Y. X., Wang, L., Xiao, E. L., Li, S. J., Chen, J. J., Gao, B., et al. (2013). Ginsenoside-Rd Exhibits Anti-inflammatory Activities through Elevation of Antioxidant Enzyme Activities and Inhibition of JNK and ERK Activation In Vivo. Int. Immunopharmacol. 17, 1094–1100. doi:10.1016/j.intimp.2013.10.013
Zhao, X., Wang, J., Li, J., Fu, L., Gao, J., Du, X., et al. (2009). Highly Selective Biotransformation of Ginsenoside Rb1 to Rd by the Phytopathogenic Fungus Cladosporium Fulvum (Syn. Fulvia Fulva). J. Ind. Microbiol. Biotechnol. 36, 721–726. doi:10.1007/s10295-009-0542-y
Zhong, F. L., Ma, R., Jiang, M., Dong, W. W., Jiang, J., Wu, S., et al. (2016). Cloning and Characterization of Ginsenoside-Hydrolyzing β-Glucosidase from Lactobacillus Brevis that Transforms Ginsenosides Rb1 and F2 into Ginsenoside Rd and Compound K. J. Microbiol. Biotechnol. 26, 1661–1667. doi:10.4014/jmb.1605.05052
Zhou, J. S., Wang, J. F., He, B. R., Cui, Y. S., Fang, X. Y., Ni, J. L., et al. (2014). Ginsenoside Rd Attenuates Mitochondrial Permeability Transition and Cytochrome C Release in Isolated Spinal Cord Mitochondria: Involvement of Kinase-Mediated Pathways. Int. J. Mol. Sci. 15, 9859–9877. doi:10.3390/ijms15069859
Zhou, S., Ji, Y., Yao, H., Guo, H., Zhang, Z., Wang, Z., et al. (2022). Application of Ginsenoside Rd in Periodontitis with Inhibitory Effects on Pathogenicity, Inflammation, and Bone Resorption. Front. Cell Infect. Microbiol. 12, 813953. doi:10.3389/fcimb.2022.813953
Zhu, D., Liu, M., Yang, Y., Ma, L., Jiang, Y., Zhou, L., et al. (2014). Ginsenoside Rd Ameliorates Experimental Autoimmune Encephalomyelitis in C57BL/6 Mice. J. Neurosci. Res. 92, 1217–1226. doi:10.1002/jnr.23397
PPD protopanaxadiol
LC-MS liquid chromatography-mass spectrometry
MCAO middle cerebral artery occlusion
GLT-1 glutamate transporter-1
NMDA N-methyl-D-aspartate
NR2B N-methyl-D-aspartate receptor 2B
OGD oxygen–glucose deprivation
TRPM transient receptor potential melastatin
ASIC acid-sensing ion channel
MMP mitochondrial membrane potential
ROS reactive oxygen species
SD Sprague–Dawley
mtDNA mitochondrial DNA
nDNA nuclear DNA
PKB protein kinase B protein kinase B
GSK-3β glycogen synthase kinase-3β
PKB protein kinase B protein kinase B
OGD/R oxygen–glucose deprivation/reperfusion
I/R ischemia/reperfusion
VEGF vascular endothelial growth factor
BDNF brain-derived neurotrophic factor
PI3K phosphatidylinositol 3-kinase
TMT trimethyltin
Bcl-2 B-cell lymphoma-2
MAPK mitogen-activated protein kinase
PARP-1 poly adenosine diphosphate-ribose polymerase-1
AIF apoptosis-inducing factor
NF-κB nuclear factor kappa-light-chain-enhancer of activated B cells
COX-2 cyclooxygenase-2
iNOS inducible nitric oxide synthase
MMP-9 matrix metalloproteinase-9
IκBα nuclear factor of kappa light polypeptide gene enhancer in B cells inhibitor, alpha
GAP-43 growth-associated protein of 43 kDa
GBS Guillain–Barre syndrome
MPP+ 1-methyl-4-phenylpyridinium
PD Parkinson’s disease
AD Alzheimer’s disease
CDK5/P25 cyclin dependent Kinase 5
PP-2A protein phosphatase 2A
sAPPα soluble amyloid-β precursor protein α
SCI spinal cord injury
NIHL noise-induced hearing loss
CRS chronic restraint stress
NSCLC non-small-cell lung cancer
NRF2 nuclear factor erythroid 2-associated factor 2
DDP cisplatin
EGFR epidermal growth factor receptor
mTOR mammalian target of rapamycin
HIF1-α hypoxia-inducible factor 1-α
MMPs matrix metalloproteinases
DSS sodium dextran sulfate
AMPK adenosine 5′-monophosphate-activated protein kinase
ULK1 Unc-51 like autophagy activating kinase 1
TNBS trinitrobenzenesulfonic acid
PKA protein kinase A
NAFLD non-alcoholic fatty liver disease
Ox-LDL oxidation low lipoprotein
KPCs keratinocyte progenitor cells
HDFs human dermal fibroblasts
LPS lipopolysaccharides
ICR Institute of Cancer Research
BASMCs basilar artery smooth muscle cells
ACTH adrenocorticotrophic hormone
PGE2 prostaglandin E2
C/EBP CCAAT/enhancer binding protein
CREB cyclic AMP response element binding protein
Keywords: Panax ginseng C.A. Mey., ginsenoside Rd, biotransformation, pharmacokinetics, molecular mechanisms
Citation: Li J, Huang Q, Yao Y, Ji P, Mingyao E, Chen J, Zhang Z, Qi H, Liu J, Chen Z, Zhao D, Zhou L and Li X (2022) Biotransformation, Pharmacokinetics, and Pharmacological Activities of Ginsenoside Rd Against Multiple Diseases. Front. Pharmacol. 13:909363. doi: 10.3389/fphar.2022.909363
Received: 31 March 2022; Accepted: 01 June 2022;
Published: 19 July 2022.
Edited by:
Qi Wang, Harbin Medical University, ChinaReviewed by:
Hyeong-Geug Kim, Indiana University–Purdue University Indianapolis, United StatesCopyright © 2022 Li, Huang, Yao, Ji, Mingyao, Chen, Zhang, Qi, Liu, Chen, Zhao, Zhou and Li. This is an open-access article distributed under the terms of the Creative Commons Attribution License (CC BY). The use, distribution or reproduction in other forums is permitted, provided the original author(s) and the copyright owner(s) are credited and that the original publication in this journal is cited, in accordance with accepted academic practice. No use, distribution or reproduction is permitted which does not comply with these terms.
*Correspondence: Lei Zhou, emhvdWxlaTIwMjJAMTI2LmNvbQ==; Xiangyan Li, eGlhbmd5YW5fbGkxOTgxQDE2My5jb20=
†These authors have contributed equally to this work
Disclaimer: All claims expressed in this article are solely those of the authors and do not necessarily represent those of their affiliated organizations, or those of the publisher, the editors and the reviewers. Any product that may be evaluated in this article or claim that may be made by its manufacturer is not guaranteed or endorsed by the publisher.
Research integrity at Frontiers
Learn more about the work of our research integrity team to safeguard the quality of each article we publish.