- 1Department of Pharmacology, Faculty of Medicine, Mashhad University of Medical Sciences, Mashhad, Iran
- 2Pharmacological Research Center of Medicinal Plants, Mashhad University of Medical Sciences, Mashhad, Iran
- 3Department of Internal Medicine, Faculty of Medicine, Mashhad University of Medical Sciences, Mashhad, Iran
- 4Department of Pathology, Faculty of Medicine, Mashhad University of Medical Sciences, Mashhad, Iran
- 5Department of Basic Medical Sciences, College of Medicine, QU Health, Qatar University, Doha, Qatar
- 6Department of Biology, College of Science, United Arab Emirates University, Al-Ain, United Arab Emirates
- 7Isagenix International LLC, Gilbert, AZ, United States
- 8Biotechnology Research Center, Pharmaceutical Technology Institute, Mashhad University of Medical Sciences, Mashhad, Iran
- 9Applied Biomedical Research Center, Mashhad University of Medical Sciences, Mashhad, Iran
- 10School of Medicine, The University of Western Australia, Perth, WA, Australia
- 11Department of Biotechnology, School of Medicine, Mashhad University of Medical Sciences, Mashhad, Iran
Background: Doxorubicin as an anti-cancer drug causes cardiotoxicity, limiting its tolerability and use. The mechanism of toxicity is due to free radical production and cardiomyocytes injury. This research evaluated Rheum turkestanicum (R.turkestanicum) extract against doxorubicin cardiotoxicity due to its considerable in vitro antioxidant activity.
Methods: Male Wistar rats received 2.5 mg/kg doxorubicin intraperitoneally every other day for 2 weeks to create an accumulative dose. R. turkestanicum was administrated at a dose of 100 and 300 mg/kg intraperitoneally from the second week for 7 days. On the 15th day, the animals were anesthetized and blood was collected from cardiac tissue for evaluation of alanine aminotransferase (ALT), cardiac muscle creatinine kinase (CK-MB), troponin T (cTn-T), lactate dehydrogenase (LDH), and B-type natriuretic peptide brain natriuretic peptide. A cardiac homogenate was also collected to determine superoxide dismutase (SOD), catalase Catalase Activity, malondialdehyde (MDA), and thiols. Histopathology was also performed.
Results: Doxorubicin increased all cardiac enzymes and malondialdehyde, correlating with a reduction in SOD, catalase, and thiols. Histopathology revealed extracellular edema, moderate congestion, and hemorrhage of foci. In contrast, administration of R. turkestanicum ameliorated these doxorubicin-induced pathophysiological changes.
Conclusion: This study revealed that the extract ameliorated doxorubicin-induced cardiac toxicity via modulation of oxidative stress-related pathways. Liquid chromatography-mass spectrometry analysis of R. turkestanicum indicated several components with potent pharmacological properties.
Introduction
Doxorubicin is a commonly administered chemotherapeutic agent for a variety of cancers. However, doxorubicin’s utility is restricted due to its associated cardiotoxicity, with a reported incidence of toxicity of approximately 11% (Chatterjee et al., 2010). Additionally, another study showed that 2.2% of 4,000 patients who consumed doxorubicin had symptoms of heart failure (Bennink et al., 2004). This cardiotoxicity involves metabolic activation to a semiquinone, subsequent oxidative stress, and binding of lipid peroxidation products [such as malondialdehyde (MDA)] toward macromolecular targets (Ma et al., 2013). The administration of doxorubicin also attenuates antioxidant enzymes such as catalase (CAT), glutathione peroxidase (GSH), and superoxide dismutase (SOD) (Kosoko et al., 2017). Moreover, doxorubicin stimulates apoptosis in cardiomyocytes resulting in congestive heart failure. Clinically, heart failure is associated with symptoms such as edema, orthopnea, fatigue, and increased venous pressure, which promote cardiac dysfunction (Yancy et al., 20132013). Following heart failure, the level of biomarkers such as lactate dehydrogenase (LDH), creatinine kinase (CK-MB), troponin T, and brain natriuretic peptide (BNP) elevate rapidly (Liquori et al., 2014). Therefore, researchers seek additional protective agents to mitigate doxorubicin’s cardiotoxicity. One such agent, dexrazoxane, can reduce doxorubicin toxicity; however, this drug interacts with the chemotherapeutic benefits of doxorubicin (Swain and Vici, 2004). Researchers are now investigating other natural compounds with lower side effects, including herbal medicines (Hosseini and Sahebkar, 2017). Phytochemical-rich products are candidates for reducing the cardiotoxicity of doxorubicin because of their antioxidant and cardioprotective properties (Hosseini and Sahebkar, 2017). Rheum turkestanicum (R.turkestanicum) belongs to the polygonaceae family. It grows in Asia, especially in the northeast of Iran (Taheri and Assadi, 2013). The studies have reported that R. turkestanicum has beneficial effects in treating diabetes, hypertension, and cancer (Shiezadeh et al., 2013; Moradi et al., 2016; Boroushaki et al., 2019a; Moradzadeh, 2019; Ghorbani et al., 2021). This herb is composed of different chemical components, including anthraquinones (e.g., aloe-emodin, emodin glycosides, physione, and rhein), flavonoids (e.g., epicatechin and quercetin), alkanes (e.g., eicosane and heneicosane), and fatty acids (e.g., linoleic acid and 9-octadecenoic acid) (Hosseini et al., 2017). Therefore, the pharmacological properties of R. turkestanicum can be related to the presence of active ingredients (Ghorbani et al., 2019). One recent study showed R. turkestanicum reduced doxorubicin-induced toxicity in cardiomyocytes (H9c2 cell line) via modulation of oxidative stress (Hosseini and Rajabian, 2016). Also In another study cardioprotective effect of R. turkestanicum against isoprenaline was evaluated which showed anti-oxidant activity of extract reduced isoprenaline-induced cardiac toxicity (Hossini et al., 2022) In the current study, we have evaluated the cardioprotective effects of R. turkestanicum against doxorubicin-induced cardiotoxicity in an animal model.
Materials and Methods
Reagents
Doxorubicin, ketamine hydrochloride/xylazine hydrochloride solution and 2-thiobarbituric acid (TBA) were prepared from Sigma-Aldrich (St. Louis, MO, United States). Pyrogallol and 2,2′ -dinitro-5,5′ -dithiodibenzoic acid (DTNB) were obtained from Cayman (Michigan, United States). Other agents included alanine aminotransferase (ALT) (mancompany, 613,032), muscle, brain creatinine kinase (CK-MB) (Riton, R144), troponin T (Biomerieux, 415,386), lactate dehydrogenase (LDH) (mancompany, 613,036), and B-type natriuretic peptide (BNP) (Sigma Aldrich, RAB0386). CK-MB was measured by VIDAS device according to enzyme-linked fluorescent assay and other biochemical tests were carried out by autoanalyzer Hitachi 902.
Preparation of R. turkestanicum Extract
R. turkestanicum was collected from chenar trees around Khorasan and identified by a botanist. The voucher specimen is 21,377. The root of R. turkestanicum was dried and converted to a fine powder. The hydro-alcoholic extract was obtained by the Soxhlet apparatus. After 48 h, the extract was dried in a water bath and maintained at −18°C until use. The final yield of the extract was determined as 21% (w/w).
Animals
This project was done according to the National Institutes of Health (NIH) Guide for Laboratory Animals and was confirmed by the Animal Ethics Committee of Mashhad University of Medical Sciences, Mashhad, Iran (IR.MUMS.MEDICAL.REC.1399.425). Adult male albino Wistar rats (200–250 g) were housed at the Laboratory Animals Research Center, Mashhad University of Medical Sciences, at 22 ± 4°C with a 12 h dark/light cycle. The animals had free access to standard laboratory chow and tap water ad libitum.
Experimental Protocol
The rats were divided into five groups, with eight rats in each group. Group 1 received normal saline 0.9% intraperitoneally (i.p.) for 14 days. Doxorubicin was administrated i. p. at a dose of 2.5 mg/kg in 6 equal injections for 2 weeks to create a cumulative dose (15 mg/kg body weight) (Arafa et al., 2014). Groups 3 and 4 received R. turkestanicum at doses of 100 and 300 mg/kg (Hosseini et al., 2017; Jahani Yazdi et al., 2020; Hossini et al., 2022) as i. p. one week after doxorubicin administration. R. turkestanicum was injected at a dose of 300 mg/kg to rats in the fifth group without administration of doxorubicin. On the 15th day, ketamine was injected at 75 mg/kg i. p to induce anesthesia. Three ml of blood was collected from the heart, then centrifuged for 10 min at 1000 rpm. The separated serums were kept at −20°C to determine biochemical parameters such as alanine aminotransferase (ALT) (mancompany, 613,032), muscle, and brain creatinine kinase (CK-MB) (Riton, R144), Troponin T (Biomerieux, 415,386), lactate dehydrogenase (LDH), and B-type natriuretic peptide (BNP) (Sigma Aldrich, RAB0386). The isolated cardiac tissue was homogenized in cold KCl solution (1.5%, pH = 7) to give a 10% homogenate, and used for suspension to measure thiol, malondialdehyde (MDA), an anti-oxidant enzyme levels.
Liquid Chromatography-Mass Spectrometry Analysis of R. turkestanicum
According to our previous study, the LC-MS analysis was performed using an AB SCIEX QTRAP (Shimadzu) liquid chromatography coupled with a triple quadrupole mass spectrometer (Hossini et al., 2022). Liquid chromatography separation was performed on a Supelco C18 (15 mm × 2.1 mm × 3 μm) column. MS analysis was carried out in negative and positive ionization modes to monitor as many ions as possible and ensure that the most significant number of metabolites extracted from the sample was detected. The analysis was done at a flow rate of 0.5 ml/min. The gradient analysis started with 95% of 0.2% aqueous formic acid, isocratic conditions were maintained for 1 min, and then a 14-min linear gradient to 40% acetonitrile with 0.2% formic acid was applied. From 15 to 35 min, the acidified acetonitrile was increased to 100%, followed by 5 min of 100% acidified acetonitrile and 5 min at the start conditions to re-equilibrate the column. The mass spectra were acquired in a range of 150–1,000 within 45 min of scan time. Mass feature extraction of the acquired LC-MS data and maximum detection of peaks were done using the MZmine analysis software package, version 2.3.
Lipid Peroxidation Analysis
Lipid peroxidation was assessed via MDA generation as previously reported (Boroushaki et al., 2019a). The samples were mixed with thiobarbituric acid (TBA) (0.67%) and trichloroacetic acid (10%), then boiled for 40 min. HCl and n-butanol were added to cooled samples, centrifuged, and the upper layer was measured spectrophotometrically at 535 nm. MDA concentration (M) was determined as: Absorbance/(1.56*105 cm−1M−1).
Determination of Thiols
Thiol concentrations were determined using DTNB as previously described (11). Briefly, Tris-EDTA buffer (pH = 8.6) was added to 50 µl of the homogenate, then the absorbance was read at 412 nm (A1). The solution was mixed with DTNB and again measured after 15 min (A2). DTNB was applied as blank. Total thiol concentration (mM) = (A2-A1-B)*0.7)/0.05*14.
Determination of Enzyme Markers and Biochemical Parameters
The level of markers such as LDH, CK-MB, cTnT, BNP and ALT was determined according to standard kits and manufacturer’s instructions.
Determination of Catalase Activity (CAT)
Catalase activity was determined according to (Aebi, 1984). This protocol’s based on the constant rate (k) (dimension: s-1, k) of hydrogen peroxide reduction measuring absorbance at 240 nm. The activity of CAT was expressed as K (rate constant) per liter.
Evaluation of Superoxide Dismutase (SOD) Activity
Superoxide dismutase activity was determined colorimetrically according to (20). This procedure measures superoxide production by pyrogallol auto-oxidation, and the prevention of superoxide-dependent reduction of the tetrazolium dye to its Formosan by SOD was evaluated at 570 nm (Madesh and Balasubramanian, 1998).
Histopathological Analysis
The isolated hearts were fixed with 10% formalin solution for histopathological studies. After paraffinization of tissues, the slices of 3 mm thickness were prepared. Hematoxylin and Eosin were used to dye the sections for light microscopic analysis.
Statistical Analyses
Data were analyzed using GraphPad Prism® software version 8 (GraphPad Software, San Diego, CA) and presented as means ± SD. Significance was determined by One-Way ANOVA followed by the Tukey-Kramer test.
Results
LC-MS Analysis of R. turkestanicum Extract
A total of 24 compounds were identified in the hydro-ethanol extract of R. turkestanicum using LC-MS analysis. These compounds include anthraquinones (e.g., emodin, emodin glycosides, physione, rhein, and its derivatives), fatty acids (9-octadecenoic acid), and flavonoids (e.g., epicatechin and quercetin). The extract also contained a high level of glucogallin, a phenolic compound formed from β-D-glucose and gallic acid (Hosseini et al., 2017). Identification of the compounds are shown in Table 1. The total ion chromatograms of R. turkestanicum extract in ESI− mode are shown in Figure 1. The MS spectral data were compared with the reported compounds in some previous literature. Figures 1A–D provide a representative chromatogram.
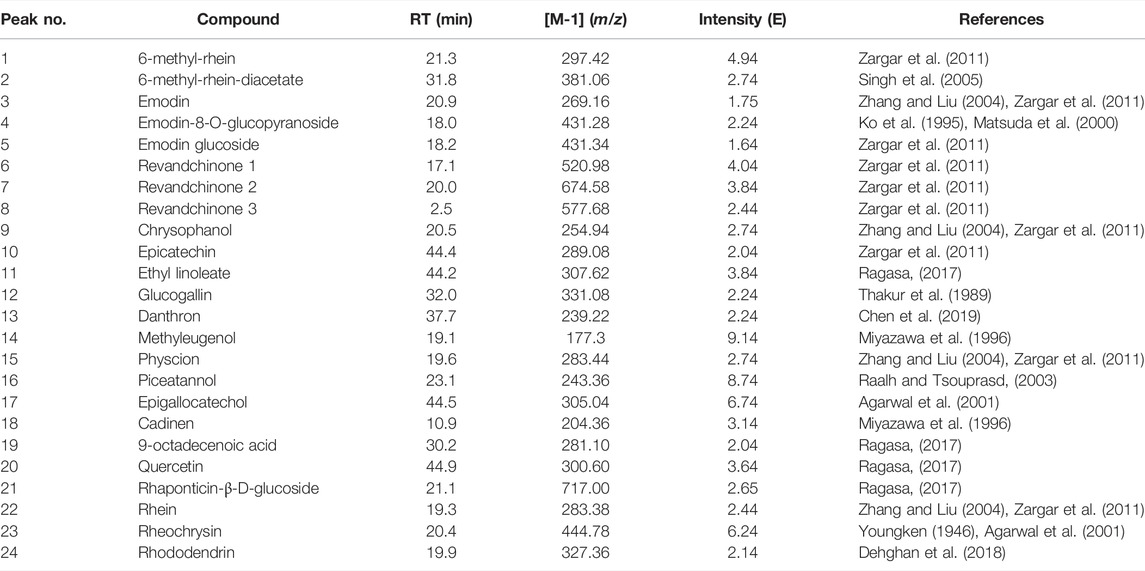
TABLE 1. Peak assignment of metabolites in the hydro-ethanol extract of R.turkestanicum using LC-MS in the negative mode (Hossini et al., 2022).
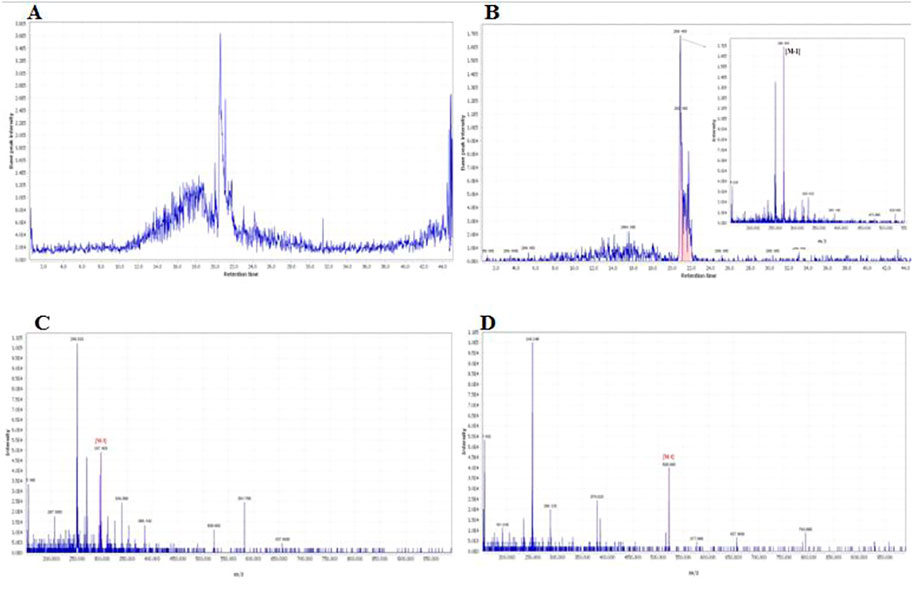
FIGURE 1. Chromatogram and corresponding mass adducts which was reported in our recent work (Hossini et al., 2022). (A) The total ion chromatogram of R.turkestanicum using LC-MS in the positive mode. (B) Chromatogram of emodin and corresponding mass adduct, [M-H], at m/z 269.16. (C) Mass spectra of 6-methyl-rhein, [M-H], at m/z 297.42. (D) Mass spectra of revandchinone 1, [M-H], at m/z 520.98.
Effect of R.turkestanicum on Cardiac Parameters
Our results revealed that doxorubicin increased the level of BNP, CK-MB, cTnT, LDH and ALT significantly in comparison with the control group (p < 0.001) (Figure 3 ). At the dose of 100 mg/kg, R. turkestanicum significantly reduced levels of BNP (p < 0.05), CK-MB (p < 0.05), cTn-T (p < 0.01), and ALT (p < 0.05). The higher dose of the extract (300 mg/kg) further abrogated doxorubicin-induced elevations of marker enzymes, including BNP, cTn-T, CK-MB and ALT (p < 0.001), and LDH (p < 0.01) in comparison with doxorubicin alone (Figure 2). R. turkestanicum alone had no effect on any of these cardiac enzymes.
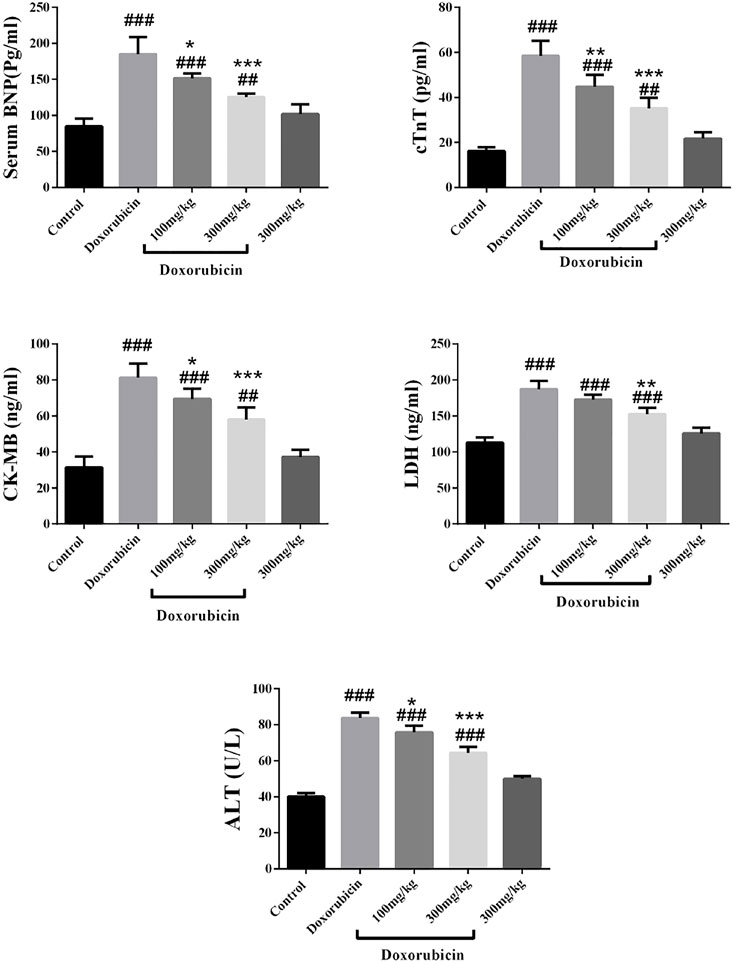
FIGURE 2. Effect of R. turkestanicum on the level of serum BNP, cTnT, CK-MB, LDH and ALT. Data were expressed as mean ± SD. ##p < 0.01, ###p < 0.001 in comparison with the control group. *p < 0.05, **p < 0.01, ***p < 0.001 in comparison with doxorubicin.
Effect of R.turkestanicum on Oxidative Stress
As shown in Figure 3, overall, doxorubicin increased oxidative stress while simultaneously reducing antioxidant defenses. Specifically, the cardiotoxic drug increased MDA production (p < 0.001) and reduced thiols (p < 0.001), and enzymatic activities of SOD and catalase (p < 0.001) compared to control. Administration of R. turkestanicum ameliorated doxorubicin-induced changes. At dosages of both 100 mg/kg and 300 mg/kg, the extract prevented oxidative stress (p < 0.05 and p < 0.001, respectively) and restored thiol content (p < 0.05 and p < 0.001, respectively). Similarly, both dosages of R. turkestanicum significantly increased both SOD (p < 0.001 at 300 mg/kg) and catalase (p < 0.01 and p < 0.001 at 100 mg/kg and 300 mg/kg, respectively). The extract alone had no effect on either biomarkers of oxidative stress and antioxidant defenses.
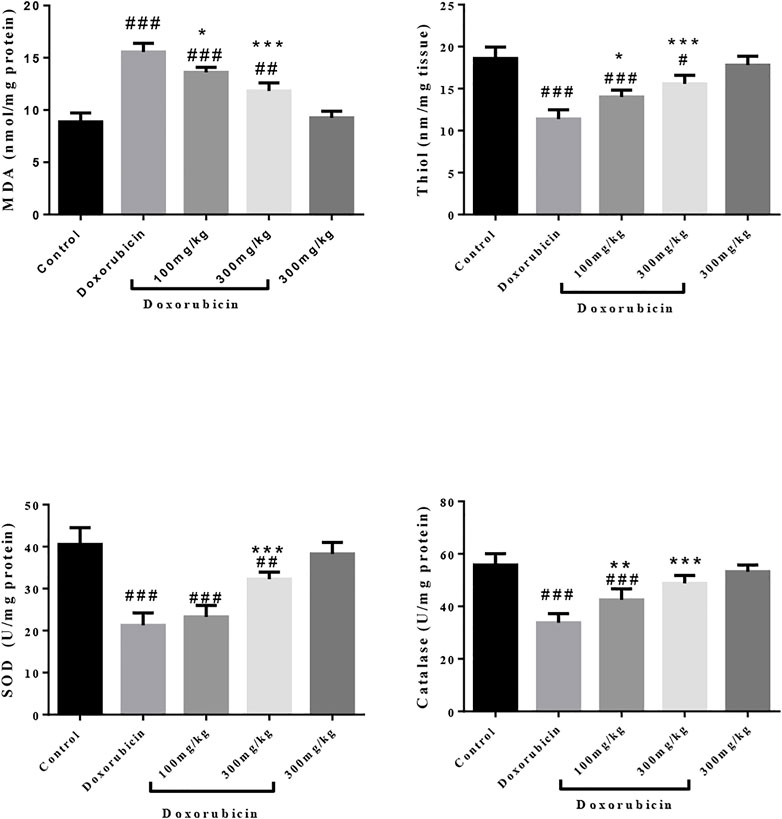
FIGURE 3. Effect of R. turkestanicum on oxidative stress and antioxidant enzymes. Data were expressed as mean ± SD. #p < 0.05, ##p < 0.01, ###p < 0.001 in comparison with the control group. *p < 0.05, **p < 0.01, and ***p < 0.001 in comparison with doxorubicin.
Histopathological Analysis
Microscopic examination of the doxorubicin-treated group showed mild to moderate degrees of mostly extracellular edema and moderate congestion and small foci of hemorrhage (Figure 4). The tissue changes in groups 3 and 4 (doxorubicin + extract), included mild edema and mild congestion. These changes were similar but lessened at the 300 mg/kg dose. Again, R. turkestanicum alone had no effect on histopathology.
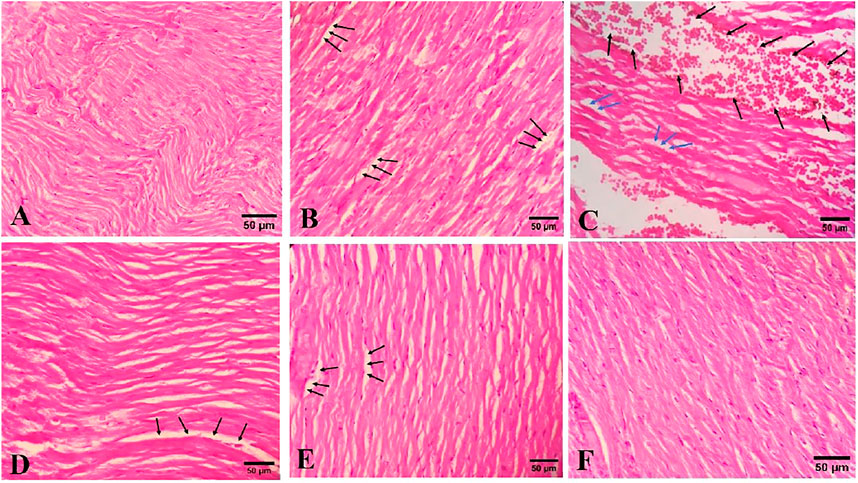
FIGURE 4. The effects of R. turkestanicum on cardiac histopathology. (A) (H&E, ×400) Control group shows normal cardiac tissue, (B) (H&E ×400) doxorubicin group shows edema (black arrows) and (C) (H&E ×400) doxorubicin group indicates edema (blue arrows) and hemorrhage (black arrows), (D) (H&E ×400) R.turkestanicum + doxorubicin (100 mg/kg) shows mild edema (black arrows), (E) (H&E ×400) R.turkestanicum + doxorubicin (300 mg/kg) also exhibits mild edema (black arrows), and (F) (H&E ×400) R.turkestanicum (300 mg/kg) shows no obvious pathological changes and is similar to control group.
Discussion
Although doxorubicin is a drug of choice for various cancer treatments, its cardiotoxicity restricts its clinical utility. The primary finding of this study is that R. turkestanicum reduced doxorubicin-induced cardiotoxicity by modulating oxidative stress and antioxidant defenses. The extract also reduced the drug’s effect on pathological abnormalities.
Several mechanisms may be responsible for doxorubicin-induced cardiotoxicity. These mechanisms include mitochondrial injury, ROS generation, intracellular Ca+2 dysregulation, inflammatory cytokine production, and myocyte damage (Rawat et al., 2021; Sheibani et al., 2022; Wu et al., 2022).
Doxorubicin is metabolized via several oxidative/reductive enzymes into a semiquinone, which increases the generation of superoxide radicals via redox cycling. Relatedly, doxorubicin facilitates oxidative stress by impairing antioxidant defense enzymes such as SOD and catalase. Concomitantly, this oxidative stress is associated with a depletion of glutathione (GSH), the primary thiol antioxidant within cells. Reduced GSH levels are also important in the doxorubicin-induced downregulation of GSH-Px 4 (Tadokoro et al., 2020). Restoration of GSH status protects against the drug’s cardiotoxicity (Mohamed et al., 2000). Interestingly, Hosseini et al. reported that R. turkestanicum prevented doxorubicin-induced cardiotoxicity, similar to intervention with N-acetylcysteine, a structurally related non-protein thiol antioxidant (Hosseini and Rajabian, 2016). Maintenance of GSH-dependent antioxidant defenses and various other compounds, including carvedilol, omega-3 fatty acids, and dexrazoxane, attenuate some of the drugs’ toxicity (Takemura and Fujiwara, 2007).
Doxorubicin preferentially accumulates in the cardiac mitochondria and is associated with cardiac toxicity. For example, doxorubicin significantly reduces mitochondrial complex 1 activity and can promote apoptosis (Marcillat et al., 1989; Basit et al., 2017; Rawat et al., 2021). Additionally, the expression of PARP1 in cardiomyocytes impairs mitochondrial function (Wen et al., 2018). Therefore, stabilizing the mitochondria can prevent doxorubicin-induced cardiotoxicity (Montaigne et al., 2010). Indeed, recent studies have reported that natural products may be appropriate alternatives for chemical agents in various diseases (Momtazi et al., 2017; Bagherniya et al., 2018; Alidadi et al., 2020; Soltani et al., 2021). One such natural product, R. turkestanicum, belongs to the Polygonaceae family and is composed of active ingredients with various pharmacological and biochemical properties (Ghorbani et al., 2019). The current study supports an earlier report that R. turkestanicum can reduce doxorubicin-induced toxicity in myocytes (Hosseini and Rajabian, 2016). In that study, the extract’s protective effects against doxorubicin were evaluated in vivo. Our findings confirm doxorubicin-induced cardiotoxicity via mechanisms involving elevated oxidative stress and reduced antioxidant defenses (Songbo et al., 2019). Other studies have found that R. turkestanicum could decrease oxidative stress in endothelial cells (Hosseini et al., 2020).
Patients receiving doxorubicin exhibit left ventricular dysfunction demonstrated by elevated CK-MB and BNP activities (Pongprot et al., 2012). Additionally, animal studies have reported increased CK-MB, LDH, BNP, and cTnT activities following doxorubicin administration (Arafa et al., 2014; Kulkarni and Swamy, 2015; Nugraha et al., 2020; Syahputra et al., 2021). Moreover, previous research has also reported that doxorubicin elevates ALT (Barakat et al., 2018); our findings support these previous ones of doxorubicin-induced clinical enzyme alterations. Our findings also support that R. turkestanicum protects against doxorubicin-induced cardiotoxicity by restoring cellular antioxidant defenses and as an antioxidant in various cells and toxicity models (Hosseini et al., 2018; Boroushaki et al., 2019a; Boroushaki et al., 2019b; Hosseini et al., 2020). The protection by the plant is also associated with improvements in LDH and creatinine phosphokinase activities (Hosseini et al., 2017; Hossini et al., 2022). The protective effect of R. turkestanicum observed in our study is potentially attributed to the presence of specific plant bioactives. Specifically, R. turkestanicum is rich in molecules offering cellular and tissue protection, including emodin, rhein, epicatechin, and others, as we show in Table 1 and as reported by others Dehghan et al., 2018. As one example of these bioactives, it was reported that chrysophanol could improve cardiac function via inhibition of apoptosis, modulation of oxidative stress, and prevention of c-Jun N-terminal kinase (JNK 1/2) activation (Xie and Li, 2020). Additionally, chrysophanol reduced doxorubicin-induced cardiotoxicity by suppressing mitochondrial swelling, mitochondrial depolarization, and PARP1 inhibition (Lu et al., 2019). Epicatechin reduces blood pressure in hypertensive patients and infarct size (Yamazaki et al., 2008; Yamazaki et al., 2010). Another bioactive in R. turkestanicum, rhein, had demonstrated cytoprotection against oxidative stress-induced endothelial cell injury (Zhong et al., 2012). Finally, the cardioprotective effects of emodin and quercetin have been reported (Wu et al., 2007; Dong et al., 2014) and include the potential modulation by emodin of doxorubicin-induced cardiotoxicity via reduced Toll-like receptor four and P38 mitogen activating protein kinase (P38 MAPK) expression (Zhang et al., 2016).
Conclusion
The protective effect of R. turkestanicum against doxorubicin-induced cardiotoxicity is attributed, at least in part, to the plant’s antioxidant properties and enhancement of cardiac tissue antioxidant defenses. However, our study has some limitations, such as the lack of cardiac function evaluation by echocardiography and the unknown mechanistic effects of co-administration of R. turkestanicum and doxorubicin. These considerations will be necessary in future studies. Future studies are also warranted to test the impact of extract upon oral administration, and perform quantitative analysis of R. turkestanicum extract and identify the main active phytochemicals responsible for the plant’s cardioprotection.
Data Availability Statement
The raw data supporting the conclusion of this article will be made available by the authors, upon a reasonable request.
Ethics Statement
The animal study was approved by the Animal Ethics Committee of Mashhad University of Medical Sciences, Mashhad, Iran (IR.MUMS.MEDICAL.REC.1399.425).
Author Contributions
Conceptualization: AH. Supervision: AH, AS, AE, and YA. Investigation: AH, M-KS, AR, and SB-N. Writing-original draft: AH, M-KS, AR, and SB-N. Writing-review and editing: EG, AS, AE, and YA. Approval of the final version: All authors.
Funding
Mashhad University of Medical Sciences (AH) and UAEU Program for Advanced Research, grant number 31S398-UPAR (YA).
Conflict of Interest
EG is employed by Isagenix International, LLC.
The remaining authors declare that the research was conducted in the absence of any commercial or financial relationships that could be construed as a potential conflict of interest.
Publisher’s Note
All claims expressed in this article are solely those of the authors and do not necessarily represent those of their affiliated organizations, or those of the publisher, the editors and the reviewers. Any product that may be evaluated in this article, or claim that may be made by its manufacturer, is not guaranteed or endorsed by the publisher.
Acknowledgments
The authors would like to thank the Vice Chancellery for Research and Technology, Mashhad University of Medical Sciences, Mashhad, Iran.
References
Aebi, H. (1984). Catalase In Vitro. Methods Enzymol. 105, 121–126. doi:10.1016/s0076-6879(84)05016-3
Agarwal, S. K., Singh, S. S., Lakshmi, V., Verma, S., and Kumar, S. (2001). Chemistry and Pharmacology of Rhubarb (Rheum Species)—A Review. J. Sci. Industrial Res. 60 (1), 1–9.
Alidadi, M., Jamialahmadi, T., Cicero, A. F. G., Bianconi, V., Pirro, M., Banach, M., et al. (2020). The Potential Role of Plant-Derived Natural Products in Improving Arterial Stiffness: A Review of Dietary Intervention Studies. Trends Food Sci. Technol. 99, 426–440. doi:10.1016/j.tifs.2020.03.026
Arafa, M. H., Mohammad, N. S., Atteia, H. H., and Abd-Elaziz, H. R. (2014). Protective Effect of Resveratrol against Doxorubicin-Induced Cardiac Toxicity and Fibrosis in Male Experimental Rats. J. Physiol. Biochem. 70 (3), 701–711. doi:10.1007/s13105-014-0339-y
Bagherniya, M., Nobili, V., Blesso, C. N., and Sahebkar, A. (2018). Medicinal Plants and Bioactive Natural Compounds in the Treatment of Non-alcoholic Fatty Liver Disease: A Clinical Review. Pharmacol. Res. 130, 213–240. doi:10.1016/j.phrs.2017.12.020
Barakat, B. M., Ahmed, H. I., Bahr, H. I., and Elbahaie, A. M. (2018). Protective Effect of Boswellic Acids against Doxorubicin-Induced Hepatotoxicity: Impact on Nrf2/HO-1 Defense Pathway. Oxid. Med. Cell Longev. 2018, 8296451. doi:10.1155/2018/8296451
Basit, F., van Oppen, L. M., Schöckel, L., Bossenbroek, H. M., van Emst-de Vries, S. E., Hermeling, J. C., et al. (2017). Mitochondrial Complex I Inhibition Triggers a Mitophagy-dependent ROS Increase Leading to Necroptosis and Ferroptosis in Melanoma Cells. Cell Death Dis. 8 (3), e2716. doi:10.1038/cddis.2017.133
Bennink, R. J., van den Hoff, M. J., van Hemert, F. J., de Bruin, K. M., Spijkerboer, A. L., Vanderheyden, J. L., et al. (2004). Annexin V Imaging of Acute Doxorubicin Cardiotoxicity (Apoptosis) in Rats. J. Nucl. Med. 45 (5), 842–848.
Boroushaki, M. T., Fanoudi, S., Mollazadeh, H., Boroumand-Noughabi, S., and Hosseini, A. (2019). Reno-protective Effect of Rheum Turkestanicum against Gentamicin-Induced Nephrotoxicity. Iran. J. Basic Med. Sci. 22 (3), 328–333. doi:10.22038/ijbms.2019.31552.7597
Boroushaki, M. T., Fanoudi, S., Rajabian, A., Boroumand, S., Aghaee, A., and Hosseini, A. (2019). Evaluation of Rheum Turkestanicum in Hexachlorobutadien-Induced Renal Toxicity. Drug Res. (Stuttg) 69 (8), 434–438. doi:10.1055/a-0821-5653
Chatterjee, K., Zhang, J., Honbo, N., and Karliner, J. S. (2010). Doxorubicin Cardiomyopathy. Cardiology 115 (2), 155–162. doi:10.1159/000265166
Chen, H., Zhao, C., He, R., Zhou, M., Liu, Y., Guo, X., et al. (2019). Danthron Suppresses Autophagy and Sensitizes Pancreatic Cancer Cells to Doxorubicin. Toxicol Vitro 54, 345–353. doi:10.1016/j.tiv.2018.10.019
Dehghan, H., Salehi, P., and Amiri, M. S. (2018). Bioassay-guided Purification of α-amylase, α-glucosidase Inhibitors and DPPH Radical Scavengers from Roots of Rheum Turkestanicum. Industrial Crops Prod. 117, 303–309. doi:10.1016/j.indcrop.2018.02.086
Dong, Q., Chen, L., Lu, Q., Sharma, S., Li, L., Morimoto, S., et al. (2014). Quercetin Attenuates Doxorubicin Cardiotoxicity by Modulating Bmi-1 Expression. Br. J. Pharmacol. 171 (19), 4440–4454. doi:10.1111/bph.12795
Ghorbani, A., Amiri, M. S., and Hosseini, A. (2019). Pharmacological Properties of Rheum Turkestanicum Janisch. Heliyon 5 (6), e01986. doi:10.1016/j.heliyon.2019.e01986
Ghorbani, A., Hosseini, A., Mirzavi, F., Hooshmand, S., Amiri, M. S., Jafarian, A. H., et al. (2021). Protective Effects of Rheum Turkestanicum Janischagainst Diethylnitrosamine-Induced Hepatocellular Carcinoma in Rats. Res. Square. doi:10.21203/rs.3.rs-528331/v1
Hosseini, A., Fanoudi, S., Mollazadeh, H., Aghaei, A., and Boroushaki, M. T. (2018). Protective Effect of Rheum Turkestanicum against Cisplatin by Reducing Oxidative Stress in Kidney Tissue. J. Pharm. Bioallied Sci. 10 (2), 66–71. doi:10.4103/JPBS.JPBS_9_18
Hosseini, A., Mollazadeh, H., Amiri, M. S., Sadeghnia, H. R., and Ghorbani, A. (2017). Effects of a Standardized Extract of Rheum Turkestanicum Janischew Root on Diabetic Changes in the Kidney, Liver and Heart of Streptozotocin-Induced Diabetic Rats. Biomed. Pharmacother. 86, 605–611. doi:10.1016/j.biopha.2016.12.059
Hosseini, A., and Sahebkar, A. (2017). Reversal of Doxorubicin-Induced Cardiotoxicity by Using Phytotherapy: a Review. J. Pharmacopuncture 20 (4), 243–256. doi:10.3831/KPI.2017.20.030
Hosseini, A., Sheikh, S., Soukhtanloo, M., Malaekeh-Nikouei, B., and Rajabian, A. (2020). The Effect of Hydro-Alcoholic Extract of Rheum Turkestanicum Roots against Oxidative Stress in Endothelial Cells. Int. J. Prev. Med. 11, 122. doi:10.4103/ijpvm.IJPVM_386_19
Hosseini, A., and Rajabian, A. (2016). Protective Effect of Rheum Turkestanikum Root against Doxorubicin-Induced Toxicity in H9c2 Cells. Rev. Bras. Farmacogn. 26, 347–351. doi:10.1016/j.bjp.2016.02.004
Hossini, A., Rajabian, A., Sobhanifar, M. A., Alavi, M. S., Taghipour, Z., Hasanpour, M., et al. (2022). Attenuation of Isoprenaline-Induced Myocardial Infarction by Rheum Turkestanicum. Biomed. Pharmacother. 148, 112775. doi:10.1016/j.biopha.2022.112775
Jahani Yazdi, A., Javanshir, S., Soukhtanloo, M., Jalili-Nik, M., Jafarian, A. H., Iranshahi, M., et al. (2020). Acute and Sub-acute Toxicity Evaluation of the Root Extract of Rheum Turkestanicum Janisch. Drug Chem. Toxicol. 43 (6), 609–615. doi:10.1080/01480545.2018.1561713
Ko, S. K., Whang, W. K., and Kim, I. H. (1995). Anthraquinone and Stilbene Derivatives from the Cultivated Korean Rhubarb Rhizomes. Arch. Pharm. Res. 18 (4), 282–288. doi:10.1007/bf02976414
Kosoko, A., Olurinde, O., and Oyinloye, O. (2017). Attenuation of Doxorubicin-Induced Oxidative Stress and Organ Damage in Experimental Rats by Theobroma Cacao Stem Bark. Jocamr 2, 1–27. doi:10.9734/jocamr/2017/30604
Kulkarni, J., and Swamy, A. V. (2015). Cardioprotective Effect of Gallic Acid against Doxorubicin-Induced Myocardial Toxicity in Albino Rats. Indian J. Health Sci. Biomed. Res. (KLEU) 8 (1), 28. doi:10.4103/2349-5006.158219
Liquori, M. E., Christenson, R. H., Collinson, P. O., and Defilippi, C. R. (2014). Cardiac Biomarkers in Heart Failure. Clin. Biochem. 47 (6), 327–337. doi:10.1016/j.clinbiochem.2014.01.032
Lu, J., Li, J., Hu, Y., Guo, Z., Sun, D., Wang, P., et al. (2019). Chrysophanol Protects against Doxorubicin-Induced Cardiotoxicity by Suppressing Cellular PARylation. Acta Pharm. Sin. B 9 (4), 782–793. doi:10.1016/j.apsb.2018.10.008
Ma, J., Wang, Y., Zheng, D., Wei, M., Xu, H., and Peng, T. (2013). Rac1 Signalling Mediates Doxorubicin-Induced Cardiotoxicity through Both Reactive Oxygen Species-dependent and -independent Pathways. Cardiovasc Res. 97 (1), 77–87. doi:10.1093/cvr/cvs309
Madesh, M., and Balasubramanian, K. A. (1998). Microtiter Plate Assay for Superoxide Dismutase Using MTT Reduction by Superoxide. Indian J. Biochem. Biophys. 35 (3), 184–188.
Marcillat, O., Zhang, Y., and Davies, K. J. (1989). Oxidative and Non-oxidative Mechanisms in the Inactivation of Cardiac Mitochondrial Electron Transport Chain Components by Doxorubicin. Biochem. J. 259 (1), 181–189. doi:10.1042/bj2590181
Matsuda, H., Kageura, T., Morikawa, T., Toguchida, I., Harima, S., and Yoshikawa, M. (2000). Effects of Stilbene Constituents from Rhubarb on Nitric Oxide Production in Lipopolysaccharide-Activated Macrophages. Bioorg Med. Chem. Lett. 10 (4), 323–327. doi:10.1016/s0960-894x(99)00702-7
Miyazawa, M., Minamino, Y., and Kameoka, H. (1996). Volatile Components of the Rhizomes ofRheum Palmatum L. Flavour Fragr. J. 11 (1), 57–60. doi:10.1002/(sici)1099-1026(199601)11:1<57::aid-ffj549>3.0.co;2-k
Mohamed, H. E., El-Swefy, S. E., and Hagar, H. H. (2000). The Protective Effect of Glutathione Administration on Adriamycin-Induced Acute Cardiac Toxicity in Rats. Pharmacol. Res. 42 (2), 115–121. doi:10.1006/phrs.1999.0630
Momtazi, A. A., Banach, M., Pirro, M., Katsiki, N., and Sahebkar, A. (2017). Regulation of PCSK9 by Nutraceuticals. Pharmacol. Res. 120, 157–169. doi:10.1016/j.phrs.2017.03.023
Montaigne, D., Marechal, X., Baccouch, R., Modine, T., Preau, S., Zannis, K., et al. (2010). Stabilization of Mitochondrial Membrane Potential Prevents Doxorubicin-Induced Cardiotoxicity in Isolated Rat Heart. Toxicol. Appl. Pharmacol. 244 (3), 300–307. doi:10.1016/j.taap.2010.01.006
Moradi, M.-T., Asadi-Samani, M., and Bahmani, M. (2016). Hypotensive Medicinal Plants According to Ethnobotanical Evidence of Iran: A Systematic Review. Int. J. PharmTech Res. 9 (5), 416–426.
Moradzadeh, M. (2019). Rheum Turkestanicum Induced Apoptosis through ROS without a Differential Effect on Human Leukemic Cells. Jundishapur J. Nat. Pharm. Prod. 14, e12198. doi:10.5812/jjnpp.12198
Nugraha, S. E., Yuandani, E., Nasution, E. S., and Syahputra, R. A. (2020). Investigation of Phytochemical Constituents and Cardioprotective Activity of Ethanol Extract of Beetroot (Beta Vulgaris. L) on Doxorubicin Induced Toxicity in Rat. Rjc 13 (02), 973–978. doi:10.31788/rjc.2020.1325601
Pongprot, Y., Sittiwangkul, R., Charoenkwan, P., and Silvilairat, S. (2012). Use of Cardiac Markers for Monitoring of Doxorubixin-Induced Cardiotoxicity in Children with Cancer. J. Pediatr. Hematol. Oncol. 34 (8), 589–595. doi:10.1097/MPH.0b013e31826faf44
Raalh, A., and Tsouprasd, G. (2003). “Hydroxystilbenes in the Roots of Rheum Rhaponticum,” in Proceedings of the Estonian Academy of Sciences, Chemistry (Estonian Academy Publishers).
Rawat, P. S., Jaiswal, A., Khurana, A., Bhatti, J. S., and Navik, U. (2021). Doxorubicin-induced Cardiotoxicity: An Update on the Molecular Mechanism and Novel Therapeutic Strategies for Effective Management. Biomed. Pharmacother. 139, 111708. doi:10.1016/j.biopha.2021.111708
Sheibani, M., Azizi, Y., Shayan, M., Nezamoleslami, S., Eslami, F., Farjoo, M. H., et al. (2022). Doxorubicin-Induced Cardiotoxicity: An Overview on Pre-clinical Therapeutic Approaches. Cardiovasc. Toxicol. 22 (4), 292–310. doi:10.1007/s12012-022-09721-1
Shiezadeh, F., Mousavi, S. H., Amiri, M. S., Iranshahi, M., Tayarani-Najaran, Z., and Karimi, G. (2013). Cytotoxic and Apoptotic Potential of Rheum Turkestanicum Janisch Root Extract on Human Cancer and Normal Cells. Iran. J. Pharm. Res. 12 (4), 811–819.
Singh, S. S., Pandey, S., Singh, R., and Agarwal, S. K. (2005). 1, 8-Dihydroxyanthraquinone Derivatives from Rhizomes of Rheum Emodi Wall. Indian J. Chem. Sect. B 44 (7).
Soltani, S., Boozari, M., Cicero, A. F. G., Jamialahmadi, T., and Sahebkar, A. (2021). Effects of Phytochemicals on Macrophage Cholesterol Efflux Capacity: Impact on Atherosclerosis. Phytother. Res. 35 (6), 2854–2878. doi:10.1002/ptr.6991
Songbo, M., Lang, H., Xinyong, C., Bin, X., Ping, Z., and Liang, S. (2019). Oxidative Stress Injury in Doxorubicin-Induced Cardiotoxicity. Toxicol. Lett. 307, 41–48. doi:10.1016/j.toxlet.2019.02.013
Swain, S. M., and Vici, P. (2004). The Current and Future Role of Dexrazoxane as a Cardioprotectant in Anthracycline Treatment: Expert Panel Review. J. Cancer Res. Clin. Oncol. 130 (1), 1–7. doi:10.1007/s00432-003-0498-7
Syahputra, R. A., Harahap, U., Dalimunthe, A., Pandapotan, M., and Satria, D. (2021). Protective Effect of Vernonia Amygdalina Delile against Doxorubicin-Induced Cardiotoxicity. Heliyon 7 (7), e07434. doi:10.1016/j.heliyon.2021.e07434
Tadokoro, T., Ikeda, M., Ide, T., Deguchi, H., Ikeda, S., Okabe, K., et al. (2020). Mitochondria-dependent Ferroptosis Plays a Pivotal Role in Doxorubicin Cardiotoxicity. JCI insight 5 (9). doi:10.1172/jci.insight.132747
Taheri, G., and Assadi, M. (2013). A Synopsis of the Genus Rheum (Polygonaceae) in Iran with Description of Three New Species. Rostaniha 14 (1), 85–93.
Takemura, G., and Fujiwara, H. (2007). Doxorubicin-induced Cardiomyopathy from the Cardiotoxic Mechanisms to Management. Prog. Cardiovasc Dis. 49 (5), 330–352. doi:10.1016/j.pcad.2006.10.002
Thakur, R., Puri, H. S., and Husain, A. (1989). Major Medicinal Plants of India. Vol. 12. India: Central Institute of medicinal and aromatic plants Lucknow.
Wen, J. J., Yin, Y. W., and Garg, N. J. (2018). PARP1 Depletion Improves Mitochondrial and Heart Function in Chagas Disease: Effects on POLG Dependent mtDNA Maintenance. PLoS Pathog. 14 (5), e1007065. doi:10.1371/journal.ppat.1007065
Wu, B. B., Leung, K. T., and Poon, E. N. (2022). Mitochondrial-Targeted Therapy for Doxorubicin-Induced Cardiotoxicity. Int. J. Mol. Sci. 23 (3), 1912. doi:10.3390/ijms23031912
Wu, Y., Tu, X., Lin, G., Xia, H., Huang, H., Wan, J., et al. (2007). Emodin-mediated Protection from Acute Myocardial Infarction via Inhibition of Inflammation and Apoptosis in Local Ischemic Myocardium. Life Sci. 81 (17-18), 1332–1338. doi:10.1016/j.lfs.2007.08.040
Xie, X. J., and Li, C. Q. (2020). Chrysophanol Protects against Acute Heart Failure by Inhibiting JNK1/2 Pathway in Rats. Med. Sci. Monit. 26, e926392. doi:10.12659/MSM.926392
Yamazaki, K. G., Romero-Perez, D., Barraza-Hidalgo, M., Cruz, M., Rivas, M., Cortez-Gomez, B., et al. (2008). Short- and Long-Term Effects of (-)-epicatechin on Myocardial Ischemia-Reperfusion Injury. Am. J. Physiol. Heart Circ. Physiol. 295 (2), H761–H767. doi:10.1152/ajpheart.00413.2008
Yamazaki, K. G., Taub, P. R., Barraza-Hidalgo, M., Rivas, M. M., Zambon, A. C., Ceballos, G., et al. (2010). Effects of (-)-epicatechin on Myocardial Infarct Size and Left Ventricular Remodeling after Permanent Coronary Occlusion. J. Am. Coll. Cardiol. 55 (25), 2869–2876. doi:10.1016/j.jacc.2010.01.055
Yancy, C. W., Jessup, M., Bozkurt, B., Butler, J., Casey, D. E., Drazner, M. H., et al. (20132013). 2013 ACCF/AHA Guideline for the Management of Heart Failure: Executive Summary: a Report of the American College of Cardiology Foundation/American Heart Association Task Force on Practice Guidelines. Circulation 128 (16), 1810–1852. doi:10.1161/CIR.0b013e31829e8807
Youngken, H. W. (1946). Studies on Indian Rhubarb; Rheum Emodi. J. Am. Pharm. Assoc. Am. Pharm. Assoc. 35 (5), 148–154. doi:10.1002/jps.3030350504
Zargar, B. A., Masoodi, M. H., Ahmed, B., and Ganie, S. A. (2011). Phytoconstituents and Therapeutic Uses of Rheum Emodi Wall. Ex Meissn. Food Chem. 128 (3), 585–589. doi:10.1016/j.foodchem.2011.03.083
Zhang, H. X., and Liu, M. C. (2004). Separation Procedures for the Pharmacologically Active Components of Rhubarb. J. Chromatogr. B Anal. Technol. Biomed. Life Sci. 812 (1-2), 175–181. doi:10.1016/j.jchromb.2004.08.010
Zhang, Y., Lin, C., Yang, X., Wang, Y., Fang, Y.-F., and Wang, F. (2016). Effect of Emodin on the Expression of TLR4 and P38MAPK in Mouse Cardiac Tissues with Viral Myocarditis. Int. J. Clin. Exp. Pathol. 9 (10), 10839–10845.
Keywords: Rheum turkestanicum, chemotherapy, doxorubicin, oxidative stress, herbal medicine, cardiotoxicity
Citation: Hosseini A, Safari M-K, Rajabian A, Boroumand-Noughabi S, Eid AH, Al Dhaheri Y, Gumpricht E and Sahebkar A (2022) Cardioprotective Effect of Rheum turkestanicum Against Doxorubicin-Induced Toxicity in Rats. Front. Pharmacol. 13:909079. doi: 10.3389/fphar.2022.909079
Received: 31 March 2022; Accepted: 23 May 2022;
Published: 08 June 2022.
Edited by:
Vinod Tiwari, Indian Institute of Technology (BHU), IndiaReviewed by:
Alaaeldin Ahmed Hamza, National Organization for Drug Control and Research (NODCAR), EgyptBasel A. Abdel-Wahab, Assiut University, Egypt
Copyright © 2022 Hosseini, Safari, Rajabian, Boroumand-Noughabi, Eid, Al Dhaheri, Gumpricht and Sahebkar. This is an open-access article distributed under the terms of the Creative Commons Attribution License (CC BY). The use, distribution or reproduction in other forums is permitted, provided the original author(s) and the copyright owner(s) are credited and that the original publication in this journal is cited, in accordance with accepted academic practice. No use, distribution or reproduction is permitted which does not comply with these terms.
*Correspondence: Ali H. Eid, QWxpLmVpZEBxdS5lZHUucWE=; Amirhossein Sahebkar, YW1pcl9zYWhlYjIwMDBAeWFob28uY29t, c2FoZWJrYXJhQG11bXMuYWMuaXI=