- 1Department of Pharmacy, Xiangya Hospital, Central South University, Changsha, China
- 2Xiangya School of Pharmaceutical Sciences, Central South University, Changsha, China
- 3National Clinical Research Center for Geriatric Disorders, Xiangya Hospital, Central South University, Changsha, China
- 4Hunan Carnation Biotechnology Co. LTD, Changsha, China
- 5Hainan Nova Doctor Group Co. Ltd, Haikou, China
- 6Hunan Sheng Bao Biological Technology Co., Ltd (in Yinfeng Biological Group., Ltd), Changsha, China
Stem cell therapy is a thriving topic of interest among researchers and clinicians due to evidence of its effectiveness and promising therapeutic advantage in numerous disease conditions as presented by novel biomedical research. However, extensive clinical application of stem cells is limited by its storage and transportation. The emergence of cryopreservation technology has made it possible for living organs, tissues, cells and even living organisms to survive for a long time at deep low temperatures. During the cryopreservation process, stem cell preparations are subject to three major damages: osmotic damage, mechanical damage, and peroxidative damage. Therefore, Assessing the effectiveness and safety of stem cells following cryopreservation is fundamental to the quality control of stem cell preparations. This article presents the important biosafety and quality control parameters to be assessed during the manufacturing of clinical grade stem cell products, highlights the significance of preventing cryodamage. and provides a reference for protocols in the quality control of stem cell preparations.
1 Introduction
Stem cells are widely studied in the field of biology, and their potential in regenerative medicine and therapy is receiving increasing attention (Krishnan et al., 2021). According to the National Institutes of Health Research Administration’s Clinical Research Registry (https://www.clinicaltrials.gov/), as of March 2021, a total of 1,216 mesenchymal stem cells (MSC) clinical research projects were registered worldwide, with 251 in China, accounting for 20.7% of the global total. Stem cells have been broadly classified as adult stem cells (ASCs) and Embryonic stem cells (ESCs) depending on their origin (Pilbauerová and Suchánek, 2018). Stem cell preparations are various types of products obtained by isolating and culturing stem cells from human tissues or healthy donor tissues. Stem cells can be obtained from a variety of tissues such as bone marrow (Tajima et al., 2015), adipose (Yang et al., 2010), umbilical cord (Katheria et al., 2020), umbilical blood (Gil-Kulik et al., 2020), skeletal muscle (Schüler et al., 2021), dental pulp, placenta, amniotic fluid and amniotic membrane (Zare et al., 2021). Stem cell therapy has evolved from basic laboratory research to progressive application in difficult clinical conditions (Chu et al., 2020). Stem cell therapy is the process of implanting human stem cells of autologous or allogeneic origin into the human body after in vitro manipulation for disease treatment. Such in vitro manipulation includes processes such as isolation, purification, expansion, modification of stem cells, establishment of stem cells (lines), induction of differentiation, freezing (cryopreservation) and recovery after freezing (Hao et al., 2020a). The cell preparation techniques and treatment protocols are diverse, complex and specific to each cell type. However, being a novel biotherapeutic product, all stem cell preparations are subject to similar developmental processes ranging from preparation, in vitro testing, in vivo animal testing, to clinical trials and clinical application via implantation into humans. At each stage of development, relevant studies and quality control used must be performed to ascertain cell quality, safety and biological effects.
Cryopreservation which is basically the storage of biological materials at very low temperatures so as to conserve their viability has become an integral technique in most experimental and clinical protocols including stem cell transplantation (Hao et al., 2020a). Reports obtained from assessing the benefits of cryopreservation in stem cell therapy reveals that the process preserves the quality of the cells from the point of collection, transportation to final implantation without the loss of vital anatomical and physiological properties. In the same vein, it allows ample time for the screening of donors and recipients for markers like human leukocyte antigen (HLA) which may interfere with optimal therapeutic outcome.
Also, multiple passages during culturing and aging of stem cells can lead to reduced cell differentiation potential and genetic alterations (Martin-Piedra et al., 2014), of which cryopreservation becomes the reliable technique to apply in preventing these deleterious effects. None the less, cells are susceptible to damage during freezing, and there are three main types of freezing damage: mechanical damage, osmotic damage, and oxidative damage (Pegg, 2015). Mechanical damage is the irreversible damage to cell membranes and organelles caused by the formation of ice crystals from extracellular and intracellular solutes in cells at low temperatures (Yang et al., 2017). When the extracellular fluid freezes, there is an increase in solute concentration, resulting in cell damage by osmotic dehydration. This event is termed osmotic damage (Finger and Bischof, 2018). On the other hand, oxidative damages are caused by reactive oxygen species (ROS) generated during cryopreservation (Evangelista-Vargas and Santiani, 2017). Ideally, avoiding these undesirable effects is the aim of majority of research studies on cryopreservation as these damages often lead to irreversible harm ranging from the loss of vital functions to even cell death (Calmels et al., 2003) (Figure 1). It is therefore extremely important to explore the cryopreservation of stem cells and its preparations for the establishment of medical cell or tissue banks and the development of clinical regenerative medicine. It is also urgent to develop new approaches for stem cell cryopreservation by analyzing the mechanism underlying cell cryoinjury and optimizing the existing deep cryogenic techniques and methods.
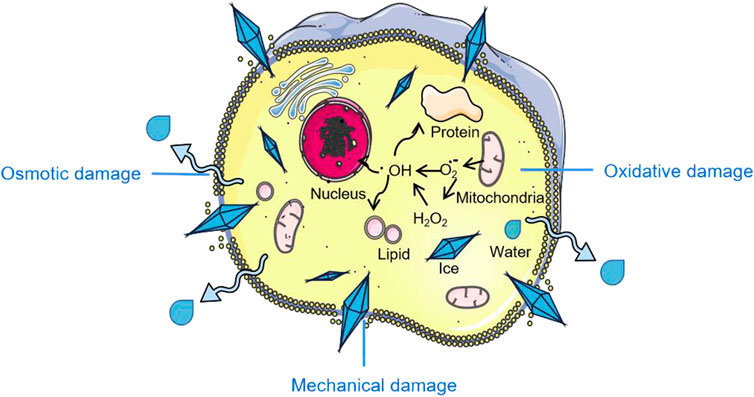
FIGURE 1. Cryopreservation induced stem cell damage. Parts of the figure are adapted from SMART–Servier Medical Art, Servier: https://smart.servier.com.
Notwithstanding the enormous efforts and advancements in cancer research, Cancer is persistently among the high mortality diseases where the therapeutic efficacy of conventional chemotherapeutics is limited by factors including toxic side effects and the reoccurrence of tumor. To this end, researchers are on deck seeking to discover better approaches in cancer treatment. One of such is stem-cell based therapy which capitalizes on the desirable features of various stem cells with possible modifications to enhance their anti-tumor potential (Han et al., 2018). In addition to being the active therapeutic agents, stem cells can serve as drug carriers in targeted delivery (Wang et al., 2018), be applied in immuno-modulation following radio (chemo) therapy (Abraham et al., 2022), replace damaged organs through tissue regeneration (Grayson et al., 2015), and provide suitable models for research to aid better understanding and development of novel cancer therapies (Jo H et al., 2021).
This paper summarizes the major cryodamages encountered during the cryopreservation of stem cells and approaches used to tackle them, discusses the factors responsible for cytotoxicity of stem cell preparations in clinical applications, their biosafety concerns and recent techniques used in quality evaluation. This article also provides reference for the quality testing protocols used in the quality control of stem cell preparations, and suggests directions to consider for future quality control and cryopreservation research.
2 Cryopreservation of Stem Cells
Cryoinjury is the irreversible damage that cells may suffer during freezing or thawing process (Ross-Rodriguez et al., 2010), and is mainly classified as osmotic damage, mechanical damage, and oxidative damage. In osmotic damage, the extracellular ice formed during slow freezing causes an osmotically driven removal of water from the intracellular space. The resulting hypertonicity is capable of causing cell death (Yousefian et al., 2018). Mechanical and structural cell damage occurs when the cells are cooled rapidly giving insufficient time for intracellular fluid to exit the cells. As a result, the cell experiences detrimental ice nucleation and recrystallization (Bakhach, 2009). Lastly, Oxidative damage is the injury inflicted by ROS produced during cryopreservation, often amounting to the oxidation of lipids, proteins and nucleic acids (Chen and Li, 2020; Liu et al., 2021a) (Figure 1).
Conventionally, the steps in stem cell cryopreservation are as follows: the stem cells of interest are harvested, carefully washed and resuspended in suitable media, then cryopreservation solution containing one or more cryoprotectants (CPAs) is then added (Wang et al., 2022). Till date, Dimethyl sulfoxide (DMSO) is the CPA of choice in stem cell cryopreservation commonly applied at a final concentration of 5–10% (v/v) (Yamatoya et al., 2022). The vial containing the cells and survival promoting additives is later frozen to −80°C using controlled rate freezers usually operated at optimal cooling rates of −1 to −3°C per minute. Finally, the frozen sample is placed in a liquid nitrogen tank mostly at −196°C for long-term preservation (Calmels et al., 2003). On demand, the stem cells are unfrozen by rapid thawing in a in a 37°C water bath and the CPA is removed before administration to patients. Adverse drug reaction monitoring following stem cell implantation procedures has documented some unwanted reactions including symptoms like abdominal cramps, diarrhea, nausea, flushing, and life-threatening events such as acute renal failure, respiratory depression and cardiac arrhythmias (Shu et al., 2014). Some of the observed adverse effects has been linked to toxicity caused by traces of DMSO retained in the infusion (Ataseven et al., 2017; Maral et al., 2018). Although the mechanisms underlying the adverse effects from infusing cryopreserved stem cell products are not completely unraveled, other contributing factors besides the toxicity of DMSO include cell aggregation and presence of apoptotic debris (Schlegel et al., 2009), volume of infused suspension and presence of unnucleated cells (Rohner et al., 2019), hypothermic condition of the cell suspension, and electrolyte imbalance (Calmels et al., 2003).
Predictably, decreasing the quantity of DMSO added during cryopreservation would diminish unwanted side effects. CPAs can be classified as permeable and non-permeable based on their ability or inability to cross the cellular membrane. Generally, permeable CPAs like DMSO are more toxic compared to non-permeable CPAs at equivalent concentrations (Raju et al., 2021). Several studies have shown that the inclusion of trehalose (Zhang et al., 2003), sucrose (Pan et al., 2017), polyampholytes (Matsumura et al., 2010) and antifreeze proteins (AFPs) (Shaliutina-Kolešová et al., 2019) during cryopreservation can greatly reduce the working concentration of DMSO, thereby reducing the cytotoxicity caused by DMSO. Another potent strategy is to develop alternatives to DMSO which should ideally prevent cryodamage and promote survival of the cryopreserved material and concomitantly be biocompatible. CPAs like trehalose and sucrose are suitable candidates as they were found to promote cryopreservation outcome in hematopoietic stem cells by maintaining the CD45+/34+ cell population and retaining cell clonogenicity and viability (Rodrigues et al., 2008).
3 Key Aspects of Quality Control of Stem Cell Preparation
Stem cell products should be generated in compliance with Good Manufacturing Practices (GMP). There are no uniform global guidelines for the production and clinical application of stem cell products. The United States Food and Drug Administration (USFDA), the European Medicines Agency (EMA), the Japanese Pharmaceuticals and Medical Devices Agency (PMDA), and other regulatory agencies currently provide GMPs to promote the safe use of therapies for patients. In addition, China has successively proposed general requirements for stem cells, which is applicable to stem cell research and production (Hao et al., 2020a; Hao et al., 2020b; Zhang et al., 2022). The following are the key aspects of quality control of stem cell preparation summarized in Table 1.
3.1 Analysis of Cell Characteristics
Some important methods used to characterize and identify stem cell types include short tandem repeat (STR) typing, morphological examinations, identification of markers, analysis of in vitro differentiation and teratoma formation (Zhang et al., 2022). As made known by science, there is yet a great number of undiscovered truths in stem cell research. For instance, new stem cell markers are being identified which could be beneficial in understanding and classifying stem cells. Also, the variations observed in expression of stem cell markers has been attributed to cell isolation and culturing techniques, cell age, culture medium composition, and stage of cell differentiation. Tapia et al. reports varied cell differentiation and expression of stemness markers in human induced pluripotent stem cells (hiPSCs) cells generated with different reprogramming methods (Tapia and Schöler, 2016). Also, the presence of certain growth factors and removal of oxygen from the growth media has induced alterations in stem cell markers (Hagmann et al., 2013).
3.2 Analysis of Biological Safety
3.2.1 Tumorigenicity
The tumorigenicity of stem cells is one of the main factors hindering their clinical use (Yasuda and Sato, 2015). Tumors can be generated through a number of pathways one of which is the malignancy of stem cells induced during proliferation and differentiation (Miyawaki et al., 2017). Additionally, teratomas can originate from undifferentiated stem cells, especially for human induced pluripotent stem cells (hiPSCs) (Yuan, 2015).
3.2.2 Sterility
The microbial contamination of stem cell products (Hartnett et al., 2021) including bacterial, fungal, mycoplasma and bacterial endotoxin contaminations is responsible for a significant number of adverse reaction cases (Golay et al., 2018). Therefore, microbiological testing prior to clinical application is pertinent for preventing unwanted effects. The recently developed and frequently applied BacT/Alert 3D automated culture system is one of the innovations to aid fast and efficient sterility testing; it requires short incubation period and does not differ significantly from pharmacopeial methods in terms of detection capacity (England et al., 2019). Rapid mycoplasma detection methods include nucleic acid amplification technology and mycoplasma metabolic enzyme activity detection (Zhang et al., 2022).
3.2.3 Pathogenic Factors
A combination of in vivo and in vitro methods should be used to test for human- and animal-derived specific pathogenic factors based on the characteristics of each stem cell preparation. If bovine serum has been used, testing for bovine-derived specific viruses shall be performed; if pig-derived materials such as trypsin are used, testing for at least pig-derived microviruses shall be performed (Zhang et al., 2022).
3.3 Analysis of Biological Activity
The biological effectiveness of various types of stem cells can be basically categorized based on their ability to induce differentiation, immunomodulatory ability and tissue regeneration (Li et al., 2021). Biological potency assay include: secretion of relevant bioactive substances (Matluobi et al., 2018) (e.g., recombinant proteins, glycoproteins or lipoproteins, growth factors, enzymes and cytokines), extracellular matrix/structures, cellular interactions (e.g., immune activation or inhibition), migration differentiation or self-renewal potential of cells (Hao et al., 2020a). To evaluate the immune activity of stem cells, (a combination of) methods like quantitative ribonucleic acid (RNA) analysis, marker assays, secreted protein analysis and immune cell response analysis have been suggested. Furthermore, instrumentations such as immunofluorescence staining, morphological observation, flow cytometry analysis and electrophysiological analysis are applicable in this respect (Rohner et al., 2019).
For MSCs, regardless of their origin, the differentiation ability of multiple cell types (e.g., adipocytes, chondrocytes, osteoblasts, etc.) should be tested in vitro to determine their multipotency of cell differentiation (Lee et al., 2015). For undifferentiated ESCs and iPSCs, the pluripotency of cell differentiation must be measured by their ability to form embryoid bodies in vitro or teratomas in severe combined immunodeficiency disease (SCID) mice model (Li et al., 2013). In addition to this, biological effects tests relevant to confirming the intended therapeutic activity of stem cells should be performed as specific biological activity assays.
4 Future Research and Directions For Quality Control
4.1 Efficient Cryopreservation Solutions
The use of efficient and low toxicity cryopreservation protocols to reduce cryogenic damage would guarantee the effectiveness of stem cell preparation applications post-thaw. Since different cryoprotectants have different protection mechanisms, a mixture of cryoprotectants is usually used to maximize cell survival (Tao et al., 2020). The combination of cryoprotectants can reduce the concentration of a single CPA used, thus reducing cytotoxicity (Elliott et al., 2017). The addition of trehalose (Yamatoya et al., 2022), sucrose (Shu et al., 2014), polyampholytes (Maral et al., 2018) and antifreeze proteins (Shaliutina-Kolešová et al., 2019) during cryopreservation can greatly minimize the required amount of DMSO, thereby reducing cytotoxicity.
4.2 Elimination of Residual Undifferentiated Stem Cells
Residual undifferentiated hiPSCs pose tumorigenic risk, and methods to eliminate these undifferentiated hiPSCs are crucial to ensuring safety (Blum and Benvenisty, 2008). The introduction of suicide genes (Yagyu et al., 2015), addition of plasma-activated medium (Matsumoto et al., 2016), cell sorting using antibodies against hiPSC surface antigens11 and, the use chemical inhibitors (Ben-David et al., 2013) are useful techniques for separating undifferentiated stem cells. Nevertheless, none of these methods have attained application in clinical grade stem cell production due to high cost, low specificity and retainment of residue in the final product (Mao et al., 2017). Consequently, researchers are beset with the task of discovering new effective ways to eliminate undifferentiated hiPSCs. In this light, Takunori et al. presented in 2018 evidence supporting the ability of high L-alanine concentrations to selectively eliminate undifferentiated hiPSCs through a novel pathway (Nagashima et al., 2018).
This method may contribute to the development of a low-cost, safe, and practical method to eliminate residual undifferentiated hiPSCs.
4.3 The Integrity of Mitochondrial DNA in Pluripotent Stem Cells
Mitochondrial DNA mutations occur at a high rate, causing several debilitating and life-threatening diseases like Kearns–Sayre syndrome and Pearson syndrome (Greaves and Taylor, 2006). According to Prigione et al., pluripotency caused mitochondrial DNA mutations originally absent from the parent PSCs (Prigione et al., 2011). Furthermore, fluctuations in the number of mitochondrial copies have been detected during prolonged culturing of iPSCs isolated from donors presenting with different maladies like lactic acidosis, mitochondrial myopathy, encephalopathy and stroke-like episodes (Gherghiceanu and Popescu, 2010). These reports confirm the need to conduct more research geared at identifying other implacable factors, understanding the mechanism (s) behind these DNA alterations and also to assess the integrity of mitochondrial DNA and genomic DNA before the use of stem cells in humans.
4.4 New Cytogenetic Techniques
Presently, an array of sensitive cytogenetic tools such as fluorescent in situ hybridization, comparative genomic hybridization, Giemsa (GTG) karyotyping and whole genome sequencing have been developed to assess the genomic integrity of stem cells (Rohani et al., 2018). Single-cell genome sequencing can rapidly and efficiently detect genetic heterogeneity in large cell samples (Bardy et al., 2016). Single-cell RNA-seq has been used in combination with electrophysiology to evaluate the activity of human iPSCs derived neurons (Bardy et al., 2016). Similarly, single cell sequencing has been applied in the long-term monitoring of trends in cancer development, progression and diversity among populations (Navin, 2015). A collaborative paper published by the International Stem Cell Banking Initiative point out the importance of stem cell genetic integrity, and also mention that other cytogenetic techniques like FISH, SKY, CGH arrays, and whole-genome sequencing would be useful to identify information that GTG karyotyping cannot acquire (Andrews et al., 2015). It is unclear how essential it is to perform more thorough epigenetic screening of pluripotent stem cells prior to clinical application. Report of variable loss of genomic imprinting across lines in iPSCs suggest that standardized epigenetic quality control tests would be beneficial (Pasque et al., 2018).
5 Conclusion
Quality control of stem cells and stem cell-based medicines have received widespread attention, and the development of cryopreservation technology has provided a technical guarantee for the application of stem cell preparations. The main risks faced by patients with stem cell preparations are the occurrence of allergic or immune reactions, the formation of tumors, and the occurrence of microbial contamination. These risk-posing factors should be eliminated or reduced to tolerable limits in the preclinical stages by extensive in vivo/in vitro evaluation to improve the efficacy and safety of stem cells. To ensure the safety and efficacy of stem cell products, each batch of stem cell preparation shall meet the existing stem cell quality requirements covering cell identification, viability and growth activity, purity and homogeneity, sterility testing and mycoplasma testing, detection of endogenous pathogenic factors, endotoxin testing, abnormal immunological response, tumorigenicity, biological potency testing, residual amount of culture medium and other added components. These strict standards of quality control must be adhered to before stem cells can be used for clinical applications, and such standards must be harmonized and monitored globally to ensure uniformity in the grade of clinically applicable stem cell products. Advanced techniques like single-cell genome sequencing of large samples may provide better understanding of genomic integrity in stem cell lines. Also, these evaluations should be conducted post-cryopreservation during the formulation and testing phases to ensure there is no cryodamage. Furthermore, cryopreservation protocols should be reviewed and tailored to each stem cell, putting into key consideration its efficacy and safety. In any case, more clinical resources and research studies should be targeted at further optimizing the quality control of stem cells before venturing further into application of stem cell therapies.
Data Availability Statement
All data used to support the findings of this review are included within the article.
Author Contributions
Writing—original draft preparation, JX and ME. Writing—review and editing, HZ, XB, YL, GZ, and DL. Supervision and approval, JX and ST.
Funding
The work is supported by the following projects: Changsha Natural Science Foundation of 2020 (kq2007039); National Clinical Research Center for Geriatric Disorders, Xiangya Hospital (XYYYJSTG-15); Parallel and Distributed Processing for the Stable Support Project of the National Defense Science and Technology Key Laboratory (WDZC20205500121); Key Research Project of Ningxia Hui Autonomous Region in 2021 (Major Project) (2021BEG01001); Natural Science Foundation of Hunan Province (No. 2021JJ31043); Postgraduate Innovative Project of Central South University (No. 207211045).
Conflict of Interest
HZ, XB and YL are employed by Hunan Carnation Biotechnology Co. LTD, Changsha and Hainan Nova Doctor Group Co., Ltd., Haikou. GZ and DL are employed by Hunan Sheng Bao Biological Technology Co., Ltd (in Yinfeng Biological Group., Ltd), Changsha.
The remaining authors declare that the research was conducted in the absence of any commercial or financial relationships that could be construed as a potential conflict of interest.
Publisher’s Note
All claims expressed in this article are solely those of the authors and do not necessarily represent those of their affiliated organizations, or those of the publisher, the editors and the reviewers. Any product that may be evaluated in this article, or claim that may be made by its manufacturer, is not guaranteed or endorsed by the publisher.
Supplementary Material
The Supplementary Material for this article can be found online at: https://www.frontiersin.org/articles/10.3389/fphar.2022.907943/full#supplementary-material
References
Abraham, I., Goyal, A., Deniz, B., Moran, D., Chioda, M., MacDonald, K. M., et al. (2022). Budget Impact Analysis of Trilaciclib for Decreasing the Incidence of Chemotherapy-Induced Myelosuppression in Patients with Extensive-Stage Small Cell Lung Cancer in the United States. J. Manag. Care Spec. Pharm. 28 (4), 435–448. doi:10.18553/jmcp.2022.21379
Ain, Q. U., Woo, Y. S., Chung, J. Y., and Kim, Y.-H. (2018). Regeneration of Anti-hypoxic Myocardial Cells by Transduction of Mesenchymal Stem Cell-Derived Exosomes Containing Tat-Metallothionein Fusion Proteins. Macromol. Res. 26 (8), 709–716. doi:10.1007/s13233-018-6101-5
Andrews, P. W., Baker, D., Benvinisty, N., Miranda, B., Bruce, K., Brüstle, O., et al. (2015). Points to Consider in the Development of Seed Stocks of Pluripotent Stem Cells for Clinical Applications: International Stem Cell Banking Initiative (ISCBI). Regen. Med. 10 (2 Suppl. l), 1–44. doi:10.2217/rme.14.93
Andrews, P. W., Ben-David, U., Benvenisty, N., Coffey, P., Eggan, K., Knowles, B. B., et al. (2017). Assessing the Safety of Human Pluripotent Stem Cells and Their Derivatives for Clinical Applications. Stem Cel Rep. 9 (1), 1–4. doi:10.1016/j.stemcr.2017.05.029
Ataseven, E., Tüfekçi, Ö., Yilmaz, Ş., Güleryüz, H., and Ören, H. (2017). Neurotoxicity Associated with Dimethyl Sulfoxide Used in Allogeneic Stem Cell Transplantation. J. Pediatr. Hematol. Oncol. 39 (5), e297–e9. doi:10.1097/MPH.0000000000000784
Bakhach, J. (2009). The Cryopreservation of Composite Tissues: Principles and Recent Advancement on Cryopreservation of Different Type of Tissues. Organogenesis 5 (3), 119–126. doi:10.4161/org.5.3.9583
Bardy, C., van den Hurk, M., Kakaradov, B., Erwin, J. A., Jaeger, B. N., Hernandez, R. V., et al. (2016). Predicting the Functional States of Human iPSC-Derived Neurons with Single-Cell RNA-Seq and Electrophysiology. Mol. Psychiatry 21 (11), 1573–1588. doi:10.1038/mp.2016.158
Ben-David, U., Gan, Q. F., Golan-Lev, T., Arora, P., Yanuka, O., Oren, Y. S., et al. (2013). Selective Elimination of Human Pluripotent Stem Cells by an Oleate Synthesis Inhibitor Discovered in a High-Throughput Screen. Cell Stem Cell 12 (2), 167–179. doi:10.1016/j.stem.2012.11.015
Blau, I. W., Basara, N., Serr, A., Seidl, C., Seifried, E., Fuchs, M., et al. (1999). A Second Unrelated Bone Marrow Transplant: Successful Quantitative Monitoring of Mixed Chimerism Using a Highly Discriminative PCR-STR System. Clin. Lab. Haematol. 21 (2), 133–138. doi:10.1046/j.1365-2257.1999.00188.x
Blum, B., and Benvenisty, N. (2008). The Tumorigenicity of Human Embryonic Stem Cells. Adv. Cancer Res. 100, 133–158. doi:10.1016/S0065-230X(08)00005-5
Calmels, B., Houzé, P., Hengesse, J. C., Ducrot, T., Malenfant, C., and Chabannon, C. (2003). Preclinical Evaluation of an Automated Closed Fluid Management Device: Cytomate, for Washing Out DMSO from Hematopoietic Stem Cell Grafts after Thawing. Bone Marrow Transpl. 31 (9), 823–828. doi:10.1038/sj.bmt.1703905
Chen, W., and Li, D. (2020). Reactive Oxygen Species (ROS)-Responsive Nanomedicine for Solving Ischemia-Reperfusion Injury. Front. Chem. 8, 732. doi:10.3389/fchem.2020.00732
Chu, D. T., Phuong, T. N. T., Tien, N. L. B., Tran, D. K., Thanh, V. V., Quang, T. L., et al. (2020). An Update on the Progress of Isolation, Culture, Storage, and Clinical Application of Human Bone Marrow Mesenchymal Stem/Stromal Cells. Int. J. Mol. Sci. 21 (3), 708. doi:10.3390/ijms21030708
Daekee, K., Mi-Jung, H., Minjun, J., Hee-Jin, A., Kwang-Won, S., and Kyung-Sun, K. (2019). Generation of Genetically Stable Human Direct-Conversion-Derived Neural Stem Cells Using Quantity Control of Proto-Oncogene Expression. Mol. Ther. Nucleic Acids 14, 388–397. doi:10.1016/j.omtn.2018.12.009
Dutta, R., Malakar, D., Khate, K., Sahu, S., Akshey, Y., and Mukesh, M. (2011). A Comparative Study on Efficiency of Adult Fibroblast, Putative Embryonic Stem Cell and Lymphocyte as Donor Cells for Production of Handmade Cloned Embryos in Goat and Characterization of Putative ntES Cells Obtained from These Embryos. Theriogenology 76 (5), 851–863. doi:10.1016/j.theriogenology.2011.03.025
Elliott, G. D., Wang, S., and Fuller, B. J. (2017). Cryoprotectants: A Review of the Actions and Applications of Cryoprotective Solutes that Modulate Cell Recovery from Ultra-low Temperatures. Cryobiology 76, 74–91. doi:10.1016/j.cryobiol.2017.04.004
England, M. R., Stock, F., Gebo, J. E. T., Frank, K. M., and Lau, A. F. (2019). Comprehensive Evaluation of Compendial USP, BacT/Alert Dual-T, and Bactec FX for Detection of Product Sterility Testing Contaminants. J. Clin. Microbiol. 57 (2), e01548. doi:10.1128/JCM.01548-18
Evangelista-Vargas, S., and Santiani, A. (2017). Detection of Intracellular Reactive Oxygen Species (Superoxide Anion and Hydrogen Peroxide) and Lipid Peroxidation during Cryopreservation of Alpaca Spermatozoa. Reprod. Domest. Anim. 52 (5), 819–824. doi:10.1111/rda.12984
Fendrik, A. J., Romanelli, L., and Rotondo, E. (2019). Stochastic Cell Renewal Process and Lengthening of Cell Cycle. Phys. Biol. 17 (1), 016004. doi:10.1088/1478-3975/ab576c
Fernandez-Muñoz, B., Garcia-Delgado, A. B., Arribas-Arribas, B., and Sanchez-Pernaute, R. (2021). Human Neural Stem Cells for Cell-Based Medicinal Products. Cells 10 (9), 2377. doi:10.3390/cells10092377
Finger, E. B., and Bischof, J. C. (2018). Cryopreservation by Vitrification: a Promising Approach for Transplant Organ Banking. Curr. Opin. Organ. Transpl. 23 (3), 353–360. doi:10.1097/MOT.0000000000000534
Gherghiceanu, M., and Popescu, L. M. (2010). Cardiomyocyte Precursors and Telocytes in Epicardial Stem Cell Niche: Electron Microscope Images. J. Cel Mol. Med. 14 (4), 871–877. doi:10.1111/j.1582-4934.2010.01060.x
Gil-Kulik, P., Świstowska, M., Kondracka, A., Chomik, P., Krzyżanowski, A., Kwaśniewska, A., et al. (2020). Increased Expression of BIRC2, BIRC3, and BIRC5 from the IAP Family in Mesenchymal Stem Cells of the Umbilical Cord Wharton's Jelly (WJSC) in Younger Women Giving Birth Naturally. Oxid. Med. Cel Longev. 2020, 9084730. doi:10.1155/2020/9084730
Golay, J., Pedrini, O., Capelli, C., Gotti, E., Borleri, G., Magri, M., et al. (2018). Utility of Routine Evaluation of Sterility of Cellular Therapy Products with or without Extensive Manipulation: Best Practices and Clinical Significance. Cytotherapy 20 (2), 262–270. doi:10.1016/j.jcyt.2017.11.009
Gowing, G., Shelley, B., Staggenborg, K., Hurley, A., Avalos, P., Victoroff, J., et al. (2014). Glial Cell Line-Derived Neurotrophic Factor-Secreting Human Neural Progenitors Show Long-Term Survival, Maturation into Astrocytes, and No Tumor Formation Following Transplantation into the Spinal Cord of Immunocompromised Rats. Neuroreport 25 (6), 367–372. doi:10.1097/WNR.0000000000000092
Grayson, W. L., Bunnell, B. A., Martin, E., Frazier, T., Hung, B. P., and Gimble, J. M. (2015). Stromal Cells and Stem Cells in Clinical Bone Regeneration. Nat. Rev. Endocrinol. 11 (3), 140–150. doi:10.1038/nrendo.2014.234
Greaves, L. C., and Taylor, R. W. (2006). Mitochondrial DNA Mutations in Human Disease. IUBMB Life 58 (3), 143–151. doi:10.1080/15216540600686888
Guo, C. J., Gao, Y., Hou, D., Shi, D. Y., Tong, X. M., Shen, D., et al. (2011). Preclinical Transplantation and Safety of HS/PCs Expanded from Human Umbilical Cord Blood. World J. Stem Cell 3 (5), 43–52. doi:10.4252/wjsc.v3.i5.43
Hagmann, S., Moradi, B., Frank, S., Dreher, T., Kämmerer, P. W., Richter, W., et al. (2013). FGF-2 Addition during Expansion of Human Bone Marrow-Derived Stromal Cells Alters MSC Surface Marker Distribution and Chondrogenic Differentiation Potential. Cell Prolif. 46 (4), 396–407. doi:10.1111/cpr.12046
Han, S. W., Kim, Y. Y., Kang, W. J., Kim, H. C., Ku, S. Y., Kang, B. C., et al. (2018). The Use of Normal Stem Cells and Cancer Stem Cells for Potential Anti-cancer Therapeutic Strategy. Tissue Eng. Regen. Med. 15 (4), 365–380. doi:10.1007/s13770-018-0128-8
Hao, J., Ma, A., Wang, L., Cao, J., Chen, S., Wang, L., et al. (2020). General Requirements for Stem Cells. Cel Prolif. 53 (12), e12926. doi:10.1111/cpr.12926
Hao, J., Cao, J., Wang, L., Ma, A., Chen, S., Ding, J., et al. (2020). Requirements for Human Embryonic Stem Cells. Cel Prolif. 53 (12), e12925. doi:10.1111/cpr.12925
Hartnett, K. P., Powell, K. M., Rankin, D., Gable, P., Kim, J. J., Spoto, S., et al. (2021). Investigation of Bacterial Infections Among Patients Treated with Umbilical Cord Blood-Derived Products Marketed as Stem Cell Therapies. JAMA Netw. Open 4 (10), e2128615. doi:10.1001/jamanetworkopen.2021.28615
Huang, W., Bei, L., and Eklund, E. A. (2013). Fas-associated Phosphatase 1 Mediates Fas Resistance in Myeloid Progenitor Cells Expressing the Bcr-Abl Oncogene. Leuk. Lymphoma 54 (3), 619–630. doi:10.3109/10428194.2012.720979
Jo H, H., Brito, S., Kwak, B. M., Park, S., Lee, M. G., and Bin, B. H. (2021). Applications of Mesenchymal Stem Cells in Skin Regeneration and Rejuvenation. Int. J. Mol. Sci. 22 (5), 2410. doi:10.3390/ijms22052410
Jo HY, H. Y., Seo, H. H., Gil, D., Park, Y., Han, H. J., Han, H. W., et al. (2021). Single-Cell RNA Sequencing of Human Pluripotent Stem Cell-Derived Macrophages for Quality Control of the Cell Therapy Product. Front. Genet. 12, 658862. doi:10.3389/fgene.2021.658862
Kamalifar, S., Azarpira, N., Sadeghi, L., Ghorbani-Dalini, S., Nekoei, S. M., Aghdaie, M. H., et al. (2020). ROCK Y-27632 Inhibitor, Ascorbic Acid, and Trehalose Increase Survival of Human Wharton Jelly Mesenchymal Stem Cells after Cryopreservation. Exp. Clin. Transpl. 18 (4), 505–511. doi:10.6002/ect.2017.0101
Kang, P. J., Zheng, J., Lee, G., Son, D., Kim, I. Y., Song, G., et al. (2019). Glycine Decarboxylase Regulates the Maintenance and Induction of Pluripotency via Metabolic Control. Metab. Eng. 53, 35–47. doi:10.1016/j.ymben.2019.02.003
Katheria, A. C., Amino, R., Konop, J. M., Orona, A. J., Kim, E., Liu, Y., et al. (2020). Stem Cell Composition of Umbilical Cord Blood Following Milking Compared with Delayed Clamping of the Cord Appears Better Suited for Promoting Hematopoiesis. J. Pediatr. 216, 222–226. doi:10.1016/j.jpeds.2019.07.043
Krishnan, M., Kumar, S., Kangale, L. J., Ghigo, E., and Abnave, P. (2021). The Act of Controlling Adult Stem Cell Dynamics: Insights from Animal Models. Biomolecules 11 (5), 667. doi:10.3390/biom11050667
Lee, J., Abdeen, A. A., Kim, A. S., and Kilian, K. A. (2015). Influence of Biophysical Parameters on Maintaining the Mesenchymal Stem Cell Phenotype. ACS Biomater. Sci. Eng. 1 (4), 218–226. doi:10.1021/ab500003s
Li, G., Chunxu, Y., and Guisheng, L. (2013). Efficient P53 Gene Targeting by Homologous Recombination in Rat-Induced Pluripotent Stem Cells. Cel Prolif. 46 (1), 1–9. doi:10.1111/cpr.12000
Li, H., Wang, W., and Chang, J. (2021). Calcium Silicate Enhances Immunosuppressive Function of MSCs to Indirectly Modulate the Polarization of Macrophages. Regen. Biomater. 8 (6), rbab056. doi:10.1093/rb/rbab056
Liu, X., Xu, Y., Liu, F., Pan, Y., Miao, L., Zhu, Q., et al. (2021). The Feasibility of Antioxidants Avoiding Oxidative Damages from Reactive Oxygen Species in Cryopreservation. Front. Chem. 9, 648684. doi:10.3389/fchem.2021.648684
Liu, X., Hu, Y., Pan, Y., Fang, M., Tong, Z., Sun, Y., et al. (2021). Exploring the Application and Mechanism of Sodium Hyaluronate in Cryopreservation of Red Blood Cells. Mater. Today Bio 12, 100156. doi:10.1016/j.mtbio.2021.100156
Mao, D., Ando, S., Sato, S. I., Qin, Y., Hirata, N., Katsuda, Y., et al. (2017). A Synthetic Hybrid Molecule for the Selective Removal of Human Pluripotent Stem Cells from Cell Mixtures. Angew. Chem. Int. Ed. Engl. 56 (7), 1765–1770. doi:10.1002/anie.201610284
Maral, S., Albayrak, M., Pala, C., Yildiz, A., Sahin, O., and Ozturk, H. B. (2018). Dimethyl Sulfoxide-Induced Tonic-Clonic Seizure and Cardiac Arrest during Infusion of Autologous Peripheral Blood Stem Cells. Cell Tissue Bank 19 (4), 831–832. doi:10.1007/s10561-018-9718-x
Martin-Piedra, M. A., Garzon, I., Oliveira, A. C., Alfonso-Rodriguez, C. A., Carriel, V., Scionti, G., et al. (2014). Cell Viability and Proliferation Capability of Long-Term Human Dental Pulp Stem Cell Cultures. Cytotherapy 16 (2), 266–277. doi:10.1016/j.jcyt.2013.10.016
Matluobi, D., Araghi, A., Maragheh, B. F. A., Rezabakhsh, A., Soltani, S., Khaksar, M., et al. (2018). Carvacrol Promotes Angiogenic Paracrine Potential and Endothelial Differentiation of Human Mesenchymal Stem Cells at Low Concentrations. Microvasc. Res. 115, 20–27. doi:10.1016/j.mvr.2017.08.003
Matsumoto, R., Shimizu, K., Nagashima, T., Tanaka, H., Mizuno, M., Kikkawa, F., et al. (2016). Plasma-activated Medium Selectively Eliminates Undifferentiated Human Induced Pluripotent Stem Cells. Regen. Ther. 5, 55–63. doi:10.1016/j.reth.2016.07.001
Matsumura, K., Bae, J. Y., and Hyon, S. H. (2010). Polyampholytes as Cryoprotective Agents for Mammalian Cell Cryopreservation. Cel Transpl. 19 (6), 691–699. doi:10.3727/096368910X508780
Mendes Oliveira, D., Grillone, K., Mignogna, C., De Falco, V., Laudanna, C., Biamonte, F., et al. (2018). Next-generation Sequencing Analysis of Receptor-type Tyrosine Kinase Genes in Surgically Resected colon Cancer: Identification of Gain-Of-Function Mutations in the RET Proto-Oncogene. J. Exp. Clin. Cancer Res. 37 (1), 84. doi:10.1186/s13046-018-0746-y
Miyawaki, S., Okada, Y., Okano, H., and Miura, K. (2017). Teratoma Formation Assay for Assessing Pluripotency and Tumorigenicity of Pluripotent Stem Cells. Bio Protoc. 7 (16), e2518. doi:10.21769/BioProtoc.2518
Nagashima, T., Shimizu, K., Matsumoto, R., and Honda, H. (2018). Selective Elimination of Human Induced Pluripotent Stem Cells Using Medium with High Concentration of L-Alanine. Sci. Rep. 8 (1), 12427. doi:10.1038/s41598-018-30936-2
Navin, N. E. (2015). The First Five Years of Single-Cell Cancer Genomics and beyond. Genome Res. 25 (10), 1499–1507. doi:10.1101/gr.191098.115
Nomura, Y., Fukui, C., Morishita, Y., and Haishima, Y. (2018). A Biological Study Establishing the Endotoxin Limit for Osteoblast and Adipocyte Differentiation of Human Mesenchymal Stem Cells. Regen. Ther. 8, 46–57. doi:10.1016/j.reth.2018.01.002
Pan, C.-y., Yu, S., Zhang, P.-f., Wang, B., Zhu, Z.-d., Liu, Y.-y., et al. (2017). Effect of Sucrose on Cryopreservation of Pig Spermatogonial Stem Cells. J. Integr. Agric. 16 (5), 1120–1129. doi:10.1016/s2095-3119(16)61489-2
Pasque, V., Karnik, R., Chronis, C., Petrella, P., Langerman, J., Bonora, G., et al. (2018). X Chromosome Dosage Influences DNA Methylation Dynamics during Reprogramming to Mouse iPSCs. Stem Cel Rep. 10 (5), 1537–1550. doi:10.1016/j.stemcr.2018.03.019
Patrick, E. M., Slivka, J. D., Payne, B., Comstock, M. J., and Schmidt, J. C. (2020). Observation of Processive Telomerase Catalysis Using High-Resolution Optical Tweezers. Nat. Chem. Biol. 16 (7), 801–809. doi:10.1038/s41589-020-0478-0
Pegg, D. E. (2015). Principles of Cryopreservation. Methods Mol. Biol. 1257, 3–19. doi:10.1007/978-1-4939-2193-5_1
Pilbauerová, N., and Suchánek, J. (2018). Cryopreservation of Dental Stem Cells. Acta Med. (Hradec Kralove) 61, 1–7. doi:10.14712/18059694.2018.16
Prigione, A., Lichtner, B., Kuhl, H., Struys, E. A., Wamelink, M., Lehrach, H., et al. (2011). Human Induced Pluripotent Stem Cells Harbor Homoplasmic and Heteroplasmic Mitochondrial DNA Mutations while Maintaining Human Embryonic Stem Cell-like Metabolic Reprogramming. Stem Cells 29 (9), 1338–1348. doi:10.1002/stem.683
Raju, R., Bryant, S. J., Wilkinson, B. L., and Bryant, G. (2021). The Need for Novel Cryoprotectants and Cryopreservation Protocols: Insights into the Importance of Biophysical Investigation and Cell Permeability. Biochim. Biophys. Acta Gen. Subj. 1865 (1), 129749. doi:10.1016/j.bbagen.2020.129749
Rodrigues, J. P., Paraguassú-Braga, F. H., Carvalho, L., Abdelhay, E., Bouzas, L. F., and Porto, L. C. (2008). Evaluation of Trehalose and Sucrose as Cryoprotectants for Hematopoietic Stem Cells of Umbilical Cord Blood. Cryobiology 56 (2), 144–151. doi:10.1016/j.cryobiol.2008.01.003
Rohani, L., Johnson, A. A., Naghsh, P., Rancourt, D. E., Ulrich, H., and Holland, H. (2018). Concise Review: Molecular Cytogenetics and Quality Control: Clinical Guardians for Pluripotent Stem Cells. Stem Cell Transl. Med 7 (12), 867–875. doi:10.1002/sctm.18-0087
Rohner, N., Passweg, J. R., Tsakiris, D. A., Halter, J. P., Heim, D., Buser, A. S., et al. (2019). The Value of the post-thaw CD34+ Count with and without DMSO Removal in the Setting of Autologous Stem Cell Transplantation. Transfusion 59 (3), 1052–1060. doi:10.1111/trf.15107
Ross-Rodriguez, L. U., Elliott, J. A., and McGann, L. E. (2010). Investigating Cryoinjury Using Simulations and Experiments: 2. TF-1 Cells during Graded Freezing (Interrupted Slow Cooling without Hold Time). Cryobiology 61 (1), 46–51. doi:10.1016/j.cryobiol.2010.04.005
Sane, M. S., Misra, N., Mousa, O. M., Czop, S., Tang, H., Khoo, L. T., et al. (2018). Cytokines in Umbilical Cord Blood-Derived Cellular Product: a Mechanistic Insight into Bone Repair. Regen. Med. 13 (8), 881–898. doi:10.2217/rme-2018-0102
Sato, Y., Bando, H., Di Piazza, M., Gowing, G., Herberts, C., Jackman, S., et al. (2019). Tumorigenicity Assessment of Cell Therapy Products: The Need for Global Consensus and Points to Consider. Cytotherapy 21 (11), 1095–1111. doi:10.1016/j.jcyt.2019.10.001
Schlegel, P. G., Wölfl, M., Schick, J., Winkler, B., and Eyrich, M. (2009). Transient Loss of Consciousness in Pediatric Recipients of Dimethylsulfoxide (DMSO)-cryopreserved Peripheral Blood Stem Cells Independent of Morphine Co-medication. Haematologica 94 (10), 1473–1475. doi:10.3324/haematol.2009.009860
Schüler, S. C., Dumontier, S., Rigaux, J., and Bentzinger, C. F. (2021). Visualization of the Skeletal Muscle Stem Cell Niche in Fiber Bundles. Curr. Protoc. 1 (10), e263. doi:10.1002/cpz1.263
Shaliutina-Kolešová, A., Dietrich, M., Xian, M., and Nian, R. (2019). Seminal Plasma Transferrin Effects on Cryopreserved Common Carp Cyprinus carpio Sperm and Comparison with Bovine Serum Albumin and Antifreeze Proteins. Anim. Reprod. Sci. 204, 125–130. doi:10.1016/j.anireprosci.2019.03.013
Shu, Z., Heimfeld, S., and Gao, D. (2014). Hematopoietic SCT with Cryopreserved Grafts: Adverse Reactions after Transplantation and Cryoprotectant Removal before Infusion. Bone Marrow Transpl. 49 (4), 469–476. doi:10.1038/bmt.2013.152
Tajima, S., Tobita, M., Orbay, H., Hyakusoku, H., and Mizuno, H. (2015). Direct and Indirect Effects of a Combination of Adipose-Derived Stem Cells and Platelet-Rich Plasma on Bone Regeneration. Tissue Eng. Part. A. 21 (5-6), 895–905. doi:10.1089/ten.TEA.2014.0336
Tao, Y., Sanger, E., Saewu, A., and Leveille, M. C. (2020). Human Sperm Vitrification: the State of the Art. Reprod. Biol. Endocrinol. 18 (1), 17. doi:10.1186/s12958-020-00580-5
Tapia, N., and Schöler, H. R. (2016). Molecular Obstacles to Clinical Translation of iPSCs. Cell Stem Cell 19 (3), 298–309. doi:10.1016/j.stem.2016.06.017
Wang, X., Gao, J., Ouyang, X., Wang, J., Sun, X., and Lv, Y. (2018). Mesenchymal Stem Cells Loaded with Paclitaxel-Poly(lactic-Co-Glycolic Acid) Nanoparticles for Glioma-Targeting Therapy. Int. J. Nanomedicine 13, 5231–5248. doi:10.2147/IJN.S167142
Wang, J., Shi, X., Xiong, M., Tan, W. S., and Cai, H. (2022). Trehalose Glycopolymers for Cryopreservation of Tissue-Engineered Constructs. Cryobiology 104, 47–55. doi:10.1016/j.cryobiol.2021.11.004
Yagyu, S., Hoyos, V., Del Bufalo, F., and Brenner, M. K. (2015). An Inducible Caspase-9 Suicide Gene to Improve the Safety of Therapy Using Human Induced Pluripotent Stem Cells. Mol. Ther. 23 (9), 1475–1485. doi:10.1038/mt.2015.100
Yahata, N., Matsumoto, Y., Omi, M., Yamamoto, N., and Hata, R. (2017). TALEN-mediated Shift of Mitochondrial DNA Heteroplasmy in MELAS-iPSCs with m.13513G>A Mutation. Sci. Rep. 7 (1), 15557. doi:10.1038/s41598-017-15871-y
Yamatoya, K., Nagai, Y., Teramoto, N., Kang, W., Miyado, K., Nakata, K., et al. (2022). Cryopreservation of Undifferentiated and Differentiated Human Neuronal Cells. Regen. Ther. 19, 58–68. doi:10.1016/j.reth.2021.12.007
Yang, Y., Rossi, F. M., and Putnins, E. E. (2010). Periodontal Regeneration Using Engineered Bone Marrow Mesenchymal Stromal Cells. Biomaterials 31 (33), 8574–8582. doi:10.1016/j.biomaterials.2010.06.026
Yang, J., Pan, C., Zhang, J., Sui, X., Zhu, Y., Wen, C., et al. (2017). Exploring the Potential of Biocompatible Osmoprotectants as Highly Efficient Cryoprotectants. ACS Appl. Mater. Inter. 9 (49), 42516–42524. doi:10.1021/acsami.7b12189
Yasuda, S., and Sato, Y. (2015). Tumorigenicity Assessment of Human Cell-Processed Therapeutic Products. Biologicals 43 (5), 416–421. doi:10.1016/j.biologicals.2015.05.008
Yousefian, I., Emamverdi, M., Karamzadeh-Dehaghani, A., Sabzian-Melei, R., Zhandi, M., and Zare-Shahneh, A. (2018). Attenuation of Cryopreservation-Induced Oxidative Stress by Antioxidant: Impact of Coenzyme Q10 on the Quality of post-thawed Buck Spermatozoa. Cryobiology 81, 88–93. doi:10.1016/j.cryobiol.2018.02.005
Yuan, B. Z. (2015). Establishing a Quality Control System for Stem Cell-Based Medicinal Products in China. Tissue Eng. Part. A. 21 (23-24), 2783–2790. doi:10.1089/ten.TEA.2014.0498
Zare, S., Ahmadi, R., Mohammadnia, A., Nilforoushzadeh, M. A., and Mahmoodi, M. (2021). Biological Characteristics and Optical Reflectance Spectroscopy of Human Placenta Derived Mesenchymal Stem Cells for Application in Regenerative Medicine. J. Lasers Med. Sci. 12, e18. doi:10.34172/jlms.2021.18
Zhang, X. B., Li, K., Yau, K. H., Tsang, K. S., Fok, T. F., Li, C. K., et al. (2003). Trehalose Ameliorates the Cryopreservation of Cord Blood in a Preclinical System and Increases the Recovery of CFUs, Long-Term Culture-Initiating Cells, and Nonobese Diabetic-SCID Repopulating Cells. Transfusion 43 (2), 265–272. doi:10.1046/j.1537-2995.2003.00301.x
Zhang, Y., Peng, L., Xie, C., Lin, B. L., Huang, Y. S., Xie, J. Q., et al. (2011). Safety Evaluation and Quality Control of MSCs from Hepatitis B Patient In Vitro. Zhonghua Gan Zang Bing Za Zhi 19 (6), 445–449. doi:10.3760/cma.j.issn.1007-3418.2011.06.013
Keywords: stem cells, quality control, safety evaluation, cryopreservation, cell-based therapy
Citation: Xie J, Ekpo MD, Xiao J, Zhao H, Bai X, Liang Y, Zhao G, Liu D and Tan S (2022) Principles and Protocols For Post-Cryopreservation Quality Evaluation of Stem Cells in Novel Biomedicine. Front. Pharmacol. 13:907943. doi: 10.3389/fphar.2022.907943
Received: 30 March 2022; Accepted: 13 April 2022;
Published: 03 May 2022.
Edited by:
Zeming Liu, Huazhong University of Science and Technology, ChinaReviewed by:
Yunrun Liu, Hong Kong Baptist University, Hong Kong, SAR ChinaYan Zhang, Hunan Normal University, China
Copyright © 2022 Xie, Ekpo, Xiao, Zhao, Bai, Liang, Zhao, Liu and Tan. This is an open-access article distributed under the terms of the Creative Commons Attribution License (CC BY). The use, distribution or reproduction in other forums is permitted, provided the original author(s) and the copyright owner(s) are credited and that the original publication in this journal is cited, in accordance with accepted academic practice. No use, distribution or reproduction is permitted which does not comply with these terms.
*Correspondence: Jian Xiao, YWRtYW5vYXNAMTYzLmNvbQ==; Songwen Tan, c3RhbjAzMDlAdW5pLnN5ZG5leS5lZHUuYXU=