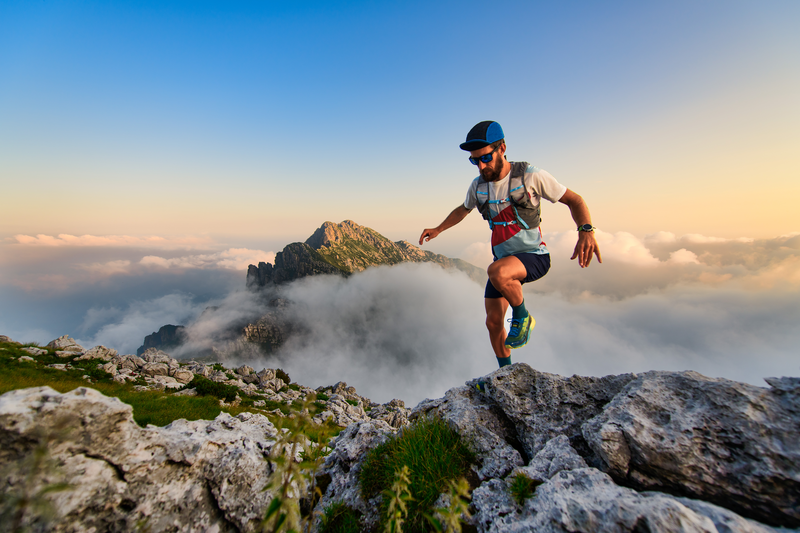
94% of researchers rate our articles as excellent or good
Learn more about the work of our research integrity team to safeguard the quality of each article we publish.
Find out more
REVIEW article
Front. Pharmacol. , 30 May 2022
Sec. Ethnopharmacology
Volume 13 - 2022 | https://doi.org/10.3389/fphar.2022.905898
Nervous system is the most complex system of the human body, hence, the neurological diseases often lack effective treatment strategies. Natural products have the potential to yield unique molecules and produce integrative and synergic effects compared to standard therapy. Mounting evidence has shown that isoflavonoids contained in traditional medicinal plant or dietary supplementation may play a crucial role in the prevention and treatment of neurological diseases due to their pronounced biological activities correlating to nervous system. Formononetin, a non-steroidal isoflavonoid, is a bioactive constituent of numerous medicinal plants such as red clover (Trifolium pratense) and Astragalus membranaceus. Emerging evidence has shown that formononetin possesses considerable anti-inflammatory, antioxidant and anti-cancer effects. This review intends to analyze the neuropharmacological potential of formononetin on the therapy of nervous system disorders. The neuroprotective properties of formononetin are observed in multiple neurological disorders including Alzheimer’s disease, dementia, cerebral ischemia, traumatic brain injury, anxiety, and depression. The beneficial effects of formononetin are achieved partially through attenuating neuroinflammation and oxidative stress via the related signaling pathway. Despite its evident effects in numerous preclinical studies, the definite role of formononetin on humans is still less known. More well-designed clinical trials are required to further confirm the neuroprotective efficacy and safety profile of formononetin before its application in clinic.
Nervous system is the most complex system of the body with quite a few functions still unknown. Neurological diseases are the pathological states that affect either the peripheral nervous system, spinal cord or brain and finally cause functional disorders (Gunata et al., 2020). The etiology is complicated, numerous factors including genetics, trauma, infection, tumor, immunological factors can all lead to the dysfunction of nervous system and cause neurological disorders such as neuropsychiatric disease, neurodevelopment disease and neurodegenerative disease (Upadhyay, 2014). Routine therapies are generally not potent enough in preventing the progression of neuronal diseases, and the cure is even more difficult. Therefore, it is necessary to develop alternative medicaments for treating these diseases.
As an important part of medicine, herbs have been used to treat various diseases for centuries in some Asian countries, especially China and India (Sharma et al., 2018; Wang et al., 2022). However, because of the unclear components and mechanisms, traditional phytomedicine has not been fully admitted and accepted in many countries. With the development of modern medicine, the mysteries surrounding herbs are gradually being uncovered. More and more attention is being focus on the application of herbal medicine in treating complicated diseases because of their distinctive efficacy (Rastogi et al., 2016; Liu et al., 2022). Multiple plants and herbs in traditional Chinese and India Ayurvedic systems (e.g., Panax notoginseng, Shankhapushpi, Guggulu, Yashtimadhu, Centella asiatica) have been shown to be beneficial for controlling the symptoms and related disorders of neurological diseases (Sharma et al., 2019). Compared with the synthetic drugs, which cannot control the gradual progression of neurological diseases in most cases, herbs may have the potential to eliminate the root cause of diseases rather than just alleviate the symptoms because of their synergic and integrative effects. In addition, synthetic drugs often have undesirable but inevitable adverse effects, affecting patients’ compliance and treatment outcome. However, there are no similar concerns about the phytomedicine owing to their natural source and relatively safety profile (Sharma et al., 2018). The comprehensive overview about the beneficial roles of medicinal herbs over synthetic drugs in neurological disorders can refer to these excellent reviews (Sharma et al., 2018; Sharma et al., 2019; Sharma et al., 2021).
Red clovers Trifolium pratense L. is a common perennial herb that has traditionally been used to treat some skin and respiratory diseases (Cassileth, 2010). Recent research has revealed that Trifolium pratense L. possessed more extensive beneficial properties in addition to the above functions, such as preventing the progression of type 2 diabetes and lowering serum lipid and blood pressure (Polak et al., 2015). The products of red clover are also used as dietary supplementation to relieve women’s menopausal symptoms (Andres et al., 2015). Astragalus membranaceus (Huangqi) is also a kind of perennial herb, its rhizome has been used as a traditional Chinese medicine for centuries with multiple benefits for health. The main bioactive components of red clover and Astragalus membranaceus are flavonoids, which can be divided into isoflavones, coumestans and prenylflavonoids from the structure (Krizova et al., 2019). Among the plethora of flavonoids, formononetin has attracted more and more attention because of its antitumor and neuroprotective activities in recent years. Formononetin is a common component of legumes. It is especially rich in red clovers Trifolium pratense L., and Astragalus membranaceus (Huangqi). Formononetin, together with daidzein, biochanin and genistein, belongs to isoflavone phytoestrogens, which are a series of non-steroidal phenolic compounds with estrogen-like biological activities (Mu et al., 2009) (Figure 1). Upon oral administration, formononetin can be hydrolyzed along the whole gastrointestinal tract. After being absorbed in the proximal part of the gut through passive diffusion, formononetin is O-demethylated into daidzein quickly, and then daidzein is conversed into β-glucuronides or sulphate esters by the corresponding transferases with conjugation at one or two (4′ or 7′) locations of the isoflavone ring (Ronis et al., 2006). The conjugates (glucuronides and/or sulfates) are the main form of formononetin in vivo, indicating by the higher plasma concentration time curve (AUC) comparing to free formononetin (Singh et al., 2011).
In vivo, formononetin exerts estrogen-like effects by binding to estrogen receptors (ER) and regulating gene expression of down-stream targets. Like other isoflavones, the binding of formononetin with ER is subtype-selective, the binding affinity to ER β is much higher than ERα (Vitale et al., 2013). It has been proved that formononetin is beneficial for attenuating osteoporosis (Ye et al., 2006), cardiovascular diseases (Teede et al., 2001), relieving menopausal symptoms (Lethaby et al., 2007), exerting antitumor and neuroprotective effects (Tay et al., 2019). In view of the shortcomings of chemical drugs for neurological disorders, the good neuroprotective effects of formononetin make it possible to be an alternative medicament for treating these diseases. Thus, an urgent need to understand the neuropharmacological function of formononetin has emerged. Recently, a paper reviewed the signaling pathways under the neuroprotective effects of formononetin, which mainly focused on the molecular targets of formononetin (Geng and Jiang, 2022). In this review, we primarily summarized the biological and pharmacological activities of formononetin in treating neurological disorders, that is, the therapeutic potential of formononetin in preclinical or clinical studies. We also compared the neuroprotective effects of formononetin with other isoflavone phytoestrogens, discussed the pharmacokinetic characteristics and safety profile of formononetin. The synthesis of these scientific evidences about neuroprotective properties of formononetin could facilitate future research to further explore the feasibility of formononetin in clinical therapy.
Alzheimer’s disease (AD) is a progressive neurodegenerative disorder that accounts for most of the dementia among elderly population. With the coming of aging society, it has been estimated that there will be around 14 million cases of AD by 2060 (Alzheimer’s, 2016). We can foresee that AD will emerge as a major public health issue and bring heavy social burden in the near future. Although the etiology of AD is still obscure, a mounting body of evidence has demonstrated that extracellular deposition of fibrillar β-amyloid (Aβ) peptide and the aggregation of neurofibrillary tangles containing hyper-phosphorylated tau are the main pathological hallmarks of AD (Yankner et al., 1990; Zlokovic, 2005). The therapeutic approaches targeting Aβ peptide or/and neurofibrillary tangles may be effective in controlling the symptoms of AD.
Some studies have investigated the role of formononetin in cellular or animal models of AD. In cultured HT22 hippocampal neuronal cells, formononetin reduced Aβ25-35-induced cell apoptosis via activating phosphatidylinositol 3-kinase/protein kinase B (PI3K/Akt) pathway. It also increased the activity of α-secretase, which cleaved amyloid precursor protein (APP) into soluble amyloid precursor protein a (sAPPα), and then inhibited the accumulation of amyloid plaques (Chen et al., 2017). The effect of formononetin on α-secretase was also demonstrated by using hypoxic human-APP Swedish mutation cell (N2a-APP cell) as an in vitro model of AD-like pathology (Sun et al., 2012). Formononetin protected cells against hypoxia-induced neurotoxicity by increasing α-secretase activity and promoting sAPPα secretion via upregulating A-Disintegrin-And-Metalloproteinase 10 (ADAM10) expression at the transcriptional level (Sun et al., 2012).
In vivo, formononetin was demonstrated to be neuroprotective in the APP/PS1 mice (a mouse model of AD), shown by marked functional improvements in spatial learning and memory in Morris water maze test (Fei et al., 2018). The study of molecular mechanisms revealed that formononetin promoted cerebral Aβ clearance in a low-density lipoprotein receptor-associated protein 1 (LRP1)-dependent pathway and inhibited Aβ accumulation via suppressing receptors for advanced glycation-end products (RAGE)/nuclear transcription factor-κB (NF-κB) inflammatory pathway. Another study examined the effects of formononetin on the cognitive function of high-fat diet-induced mice, which mimicked the presymptomatic AD stage (Fu et al., 2019). At the dose of 20 and 40 mg/kg, formononetin significantly attenuated the learning and memory deficits of model mice through inhibiting tau hyperphosphorylation mediated by the activation of glycogen synthase kinase-3β (GSK-3β) pathway in the hippocampus.
AD is a special type of dementia. Besides AD, dementia can also be caused by some other factors. Formononetin was also shown to produce neuroprotective effects against dementia induced by specific drugs. Shaza et al found that formononetin markedly increased the discrimination ratio of novel object recognition test in the demented mice induced by scopolamine, and its efficacy was comparable to donepezil. Further research revealed that formononetin inhibited the elevation of dopamine (DA), norepinephrine (NA) and malondialdehyde (MDA) levels, reduction of glutathione (GSH) and acetylcholine (ACh) levels, enhancement of acetylcholinesterase (AChE) activity in the brain of scopolamine-treated mice. Formononetin seemed to play a neuroprotective role in scopolamine-induced dementia through regulating brain AChE activity and suppressing oxidative stress (Aly et al., 2021). Diabetes is a risk factor for the dysfunction of nervous system. The protective effects of formononetin have been examined in a mouse model of diabetic cognitive dysfunction induced by intraperitoneal injection of streptozotocin (STZ). Formononetin attenuated the decline of learning and memory abilities in STZ-treated mice through suppressing inflammatory response mediated by HMGB1/TLR4/NF-κB signaling in the hippocampus (Wang et al., 2018).
Estrogen deficiency is the initial factor of cognitive decline in menopausal women. In a small-sample clinical trial, Howes et al. (2004) assessed the effects of dietary supplementation with isoflavones from red clover on cognitive function in 30 postmenopausal women. Formononetin was the main component of the isoflavones (each tablet containing formononetin 25 mg, biochanin 2.5 mg and less than 1 mg of daidzein and genistein). However, they found that isoflavone supplementation with two tablets a day for 6 months did not appear to have significant effects on cognitive function in postmenopausal women (Howes et al., 2004). The negative results of this trial may be related to the following aspects: First, the six-month testing period may not be too long enough to assess the effect of dietary isoflavones, as all the included women had been postmenopausal for at least 5 years and never received therapy; Second, the dose of isoflavones may not be higher enough to produce sufficient effect as the estrogenic capacity of these phytoestrogens are lower compared to endogenous estrogens. Third, the sample size of this trail was small, some uncontrollable variables may bring deviation to the results and then cover the real effect of the testing substance. Therefore, further larger-sample size and well-designed clinical trials are still needed to clarify the effect of formononetin on cognitive function.
Cerebral ischemia is a devastating neurological dysfunction caused by reduced or cut-off of blood flowing to the brain, and is the second leading cause of death worldwide (Hankey, 2017). The protective effects of formononetin against cerebral ischemia have been examined by multiple in vitro and in vivo studies. Formononetin protected PC12 cells from oxygen-glucose deprivation/reperfusion (OGD/R) injury by increasing the expression of growth-associated protein 43 (GAP-43) and brain-derived neurotrophic factor (BDNF) (Yan et al., 2019). Formononetin was also shown to protect primary neurons against OGD-induced neurotoxicity by upregulating neuroglobin expression in cAMP response element-binding protein (CREB)-dependent pathway (Liu et al., 2016).
In vivo, the neuroprotective effect of formononetin against ischemic stroke was tested in rats subjected to middle cerebral artery occlusion (MCAO). Formononetin promoted the functional recovery of MCAO rats by facilitating the formation of dendritic spines and enhancing synaptic plasticity, which may be mediated by the phosphatidylinositol 3-kinase/protein kinase B/extracellular regulated protein kinases (PI3K/AKT/ERK) signaling pathway (Wu et al., 2020). Another study found that sulphonated formononetin alleviated the brain edema, reduced cerebral infarct volume and improved the neurological function of cerebral ischemia rats by inhibiting cell apoptosis and promoting cerebrovascular angiogenesis (Zhu et al., 2014). Liang et al. (2014) also demonstrated that formononetin protected rats from the cerebral ischemia through inhibiting the function of pro-apoptosis pathway and promoting the function of anti-apoptosis molecules (Liang et al., 2014). Yu et al. (2022) found that formononetin significantly alleviated the neurological deficit and the pathological state of brain tissues in MCAO rats by attenuating inflammatory pathways and reducing the production of pro-inflammatory cytokines (Yu et al., 2022). The above studies suggest that formononetin is a natural compound with the potential to protect brain against ischemic injury.
Traumatic brain injury (TBI) is a kind of brain damage resulted from the external physical force (Larsson et al., 2013). It is a main cause of brain dysfunction, characterized by structural damage and cell death of the brain, which may result in permanent disability or death (Levy et al., 2005; Durand et al., 2017). The phytomedicine may be effective in managing post-traumatic symptoms.
In rat TBI model, formononetin ameliorated the brain damage by repairing neuronal ultrastructural organization and promoting cortical proliferation in a dose-dependent manner, which was consistent with the functional improvements, such as reduction of injure scores and improvement of neurological symptoms. The levels of interleukin-6 (IL6) and tumor necrosis factor (TNF-α) in the serum were increased, while the levels of interleukin-10 (IL10) in serum and cortical neurons were decreased in the TBI rats. The above changes were reversed following the treatment with formononetin. The neuroprotective effects of formononetin against TBI were achieved by activating IL10-based pathway and suppressing neuroinflammatory reaction in the cortex nearing lesioned tissue (Li et al., 2018). Another study found that pretreatment with formononetin for 5 days remarkably prevented pathological lesions and neuronal apoptosis, attenuated brain edema and improved the neurological scores of TBI rats. Formononetin inhibited the downregulation of heme oxygenase-1 (HO-1) and upregulation of BACH1, which was a core transcriptional repressor protein in the nuclear factor erythroid 2-related factor 2 (Nrf2)/HO-1 signaling pathway. The neuroprotective effects of formononetin was achieved through attenuating the oxidative stress via activation of Nrf2-dependent antioxidant pathway (Li et al., 2017).
The anti-infammatory and antioxidant effects of formononetin were also verified by other studies. Li et al. (2014) found that the expression of glutathione peroxidase (GSH-Px), superoxide dismutase (SOD) and Nrf2 were decreased, whereas the concentrations of MDA, TNF-α, IL-6 and expression of cyclooxygenase-2 (COX-2) were elevated in the lesioned brain region of the TBI rats. Formononetin treatment reversed above alterations induced by TBI. Formononetin also attenuated the cellular pathological alterations in the lesioned area of brain and increased the neural cell number, alleviated hydrocephalus and neurological abnormalities in TBI rats. It was confirmed again that formononetin protected the brain against TBI at least partially through elevating the activities of antioxidant cascade and inhibiting the inflammatory response in the injured area (Li et al., 2014).
Anxiety and depression are two common mental disorders affecting millions of people globally. The current antidepressant drugs have poor efficacy along with many side effects and cannot meet clinical needs (Dinoff et al., 2017). In the complete Freund’s adjuvant (CFA)-induced mouse model of anxiety, formononetin increased the time spent in the open arms of elevated plus maze test and the central area of open field test compared with the model mice, indicating that formononetin possessed anxiolytic effects. The mechanism study showed that formononetin attenuated inflammation and neuronal hyperexcitability in the basolateral amygdala, which contributed to its anxiolytic effects (Wang et al., 2019). Recently, a study evaluated the antidepressant effect of formononetin on chronic corticosterone-treated mice. The results demonstrated that formononetin alleviated depression-like behaviors of model mice reflected by increasing the sucrose preference index and reducing immobility time in the forced swimming test. Mechanism study revealed that formononetin enhanced the expression level of the glucocorticoid receptor and BDNF, attenuated neuronal impairment and promoted neurogenesis in the hippocampus (Zhang et al., 2022). The above researches laid a foundation for further studying the effects of formononetin on mental illness.
Although neuroinflammation is not a specific disease, it is the pathological state accompanied by various neurological disorders. As the innate immune cells of the central nervous system, microglia is also the mediator of neuroinflammation. Activated microglia promoted the production of TNF-α, IL-6, IL-1β, which are pro-inflammatory cytokines.
The effects of formononetin on neuroinflammation were investigated in lipopolysaccharide (LPS)-stimulated BV2 mouse microglia cell line. Formononetin was found to reduce the level of TNF-α, IL-6, IL-1β significantly and also inhibit COX-2-mediated prostaglandin E2 (PGE2) production and inducible nitric oxide synthase (iNOS)-mediated nitrite production. Mechanisms research revealed that formononetin exerted the anti-neuroinflammation effects through inhibiting NF-κB signalling pathway. Formononetin also showed protective effects in HT22 hippocampal neurons against the neurotoxicity induced by microglia conditioned medium, which may be through the mechanisms involving estrogen receptor β (El-Bakoush and Olajide, 2018). In rat mesencephalic neuron-glia co-cultures, formononetin prevented reduction of dopamine uptake and loss of dopaminergic neurons via suppressing microglia activation and production of pro-inflammatory and pro-oxidant factors (Chen et al., 2008).
In vivo, formononetin significantly inhibited the production of TNF-α and IL-1β in the hippocampus of high-fat diet-induced mice in a dose-dependent manner. For the mechanisms underlying it, Fu et al revealed that formononetin activated anti-inflammatory Nrf-2/HO-1 signaling pathway, and inhibited the pro-inflammatory NF-κB signaling pathway (Fu et al., 2019). The above results suggested that the anti-neuroinflammatory effect of formononetin is one important mechanism underlying its neuroprotective property.
Peripheral neuropathy is a common comorbidity of type 2 diabetes mellitus (T2DM), with more than half of diabetic patients developing neuropathy within few years after diagnosis (Khawaja et al., 2018). It is an important reason for the decline of life quality and amputation of foot or toe. In the rat model of T2DM induced by high-fat diet and streptozotocin administration, formononetin improved the nerve conduction, inhibited thermal hyperalgesia and mechanical allodynia markedly. Mechanism research found that formononetin may ameliorate hyperglycemia-induced neuropathic condition in T2DM rats by activating sirtuin1(SIRT1) and nerve growth factor (NGF) pathway in sciatic nerve tissue (Oza and Kulkarni, 2020). Another study found that formononetin alleviated oxaliplatin-induced peripheral neuropathy by inhibiting nociceptive sensations to cold and mechanical stimuli. Mechanism study revealed that formononetin prevented oxaliplatin-induced neuronal apoptosis via activating Nrf2/glutathione S-transferase pi 1 (GSTP1) signaling pathway (Fang et al., 2020).
Formononetin markedly reduced the number of abdominal writhes and produced evident antinociceptive effects in acetic acid writhing test. In the glutamate-induced nociceptive model, formononetin produced analgesic effect by inhibiting the licking response in the glutamate-injected paw of mice. Formononetin also suppressed oedema response and leukocyte migration caused by carrageenan, and played a significant anti-inflammatory role (Lima Cavendish et al., 2015). However, in the complete Freund’s adjuvant (CFA)-induced chronic inflammatory pain model, formononetin administration remarkably alleviated pain-related anxiety-like behaviors but did not inhibit the mechanical allodynia and thermal hyperalgesia of model mice (Wang et al., 2019). The discrepancy may be due to the different methods in inducing pain models and/or the dosage/duration of formononetin administration.
The pharmacological activities of formononetin against nervous system related disorders are shown in Table 1.
Apart from formononetin, other multiple isoflavones are also commonly used as health supplementation for their beneficial properties (Messina, 2010; Zhang et al., 2019). These isoflavones can bind to estrogen receptors (ER) and display estrogen-like effects although their binding affinities are relatively lower than 17β-estradiol (Cederroth and Nef, 2009). Several studies compared the neuroprotective effects of formononetin with other isoflavone phytoestrogens. The protective effects of formononetin and other four isoflavones (daidzein, calycosin, pratensein and irilone) were examined with the model of LPS-induced dopaminergic neurodegeneration (Chen et al., 2008). All five isoflavones promoted the survival of dopaminergic neurons, suppressed LPS-induced microglia activation and lowered the production of TNF-α, nitric oxide and superoxide. As for the neuroprotective potency, it decreased gradually in the order of pratensein, daidzein, calycosin, formononetin, irilone at the same concentration (Chen et al., 2008). Yu et al. (2005) investigated the neuroprotective effects of formononetin, ononin and calycosin using PC12 cells, and found that they attenuated glutamate-induced cell death, with an estimated 50% effective concentration (EC50) of 0.027 μg/ml, 0.047 μg/ml and 0.031 μg/ml, respectively (Yu et al., 2005). In addition, both formononetin and calycosin significantly increased the activity of SOD and GSH-Px as well as the level of GSH. Formononetin had a greater effect on GSH-Px and GSH, while calycosin had a greater effect on SOD (Yu et al., 2009). Recently, another study investigated the beneficial effects of formononetin, daidzein (the metabolite of formononetin) and calycosin (another major isoflavone from Astragali Radix) in cerebral ischemia-reperfusion injury (Gu et al., 2021). The results showed that they protected the primary cultured neurons against oxygen-glucose deprivation plus reoxygenation (OGD/RO) or L-glutamate treatment with an efficacy order: daidzein > formononetin > calycosin (Gu et al., 2021). Their neuroprotective effects were also evaluated by in vivo middle cerebral artery occlusion (MCAO) model. It was found that daidzein, formononetin, calycosin at higher dose (60 μmol/kg) reduced the infarct volumes significantly to 13.84 ± 0.95%, 10.76 ± 4.21% and 10.99 ± 6.07%, respectively, compared with vehicle treatment group (27.93% ± 7.1%) (Gu et al., 2021). However, the overall pharmacological outcome of these isoflavones in the “real world” application may be affected by multiple factors, such as the concentration/dose, physical condition of the user, age, sex, food, and so on. Therefore, the neuroprotective efficacy of these isoflavone phytoestrogens are not set in stone and may be varying under different conditions.
The lipophilicity is an important factor that determines the absorption, distribution, accumulation and elimination of drugs in vivo. The logarithm of octanol/water partition coefficient is commonly used as the physico-chemical parameter to characterize lipophilicity, which is usually determined by the true partition coefficient (log P) value and the distribution coefficient (log DpH). Formononetin shows favorable log P and log D7.4 value regarding drug-likeness and Lipinski’s rule of five, suggesting that it can passively permeate through biological membranes easily (Gampe et al., 2022). In the central nervous system, lipophilicity affects the amount of drugs passing through the blood-brain barrier (BBB). As for evaluating the BBB permeability, the results of parallel artificial membrane permeability assay (PAMPA) shows that formononetin has a good permeation capability (Gampe et al., 2022), which is the foundation of exerting protective effects against neurological disorders.
Upon oral administration, formononetin is rapidly absorbed and it shows a better permeability in the large intestine segments than small intestine segments. However, the small intestine is still the main site for absorbing formononetin since it is much longer than large intestine, resulting in a larger absorption area and longer residence period (Luo et al., 2018). The plasma concentration of formononetin reaches peak 30–60 min after oral administration, its plasma protein binding rate is about 95% at the physiological concentration (Singh et al., 2011). The maximum plasma concentration (Cmax) of formononetin ranged from 62 to 302 nM after being taken orally at the dose of 20–50 mg/kg, while it could reach nearly 1,303 nM and 16,957 nM after being administered intravenously at the dose of 4 and 10 mg/kg, respectively (Singh et al., 2011; Luo et al., 2018). The apparent volume of distribution (Vd) of formononetin is 14.16 L/kg in rats, which is significantly higher than the total volume of bodily fluids, suggesting an extensive tissue distribution of formononetin (Singh et al., 2011). The liver is the main place of formononetin metabolism, it is demethylated to daidzein by cytochrome P450, and both formononetin and its metabolite daidzein then subjected to phase II conjugation reactions (glucuronidation and/or sulfation) (Chen et al., 2005; Singh et al., 2011), which are the main existing form in plasma after oral and intravenous administration. Formononetin is eliminated with a half-life of nearly 2 h (Luo et al., 2018), and found to have a high systemic total body clearance (CLTotal) (5.13 L/h/kg in rats), indicating that formononetin is a high extraction compound. Formononetin has a moderate bioavailability of 21.8% upon oral administration at 20 mg/kg, which is higher than many other flavonoids and isoflavones (Luo et al., 2018). It is mainly due to the structure of methylated isoflavone, which confers formononetin a slower glucuronidation and higher gut permeability compared with other unmethylated ones, resulting in a higher bioavailability and tissue distribution (Jia et al., 2004; Walle et al., 2007).
The bioavailability of formononetin may be lower compared with many other chemical drugs for treating neurological disorders. However, long-term dietary supplementation may still raise significant concentrations in plasma and nervous system, and then produce corresponding pharmacological effects (D'Archivio et al., 2010; Di Lorenzo et al., 2021). Moreover, the metabolites of formononetin also have certain biological activities and may become an important source of cellular aglycones upon enzymatic hydrolysis at the target site (Zhang et al., 2007; Barnes et al., 2011). Then the synergistic effects between formononetin and its metabolites may further strengthen the pharmacological functions and endow it good neuroprotective properties.
The acute toxicity of formononetin has been investigated by intravenous administration of sodium formononetin-3-sulphonate (Sul-F), a water-soluble derivate of formononetin, in rats and dogs. Sul-F at the dosage of 2000 mg/kg showed no significant body weight change, apparent toxic effects or mortality in rats during the following 2 weeks period. However, non-metabolic Sul-F was found after urine volatilization at the first day after Sul-F administration (Li et al., 2015). In the dogs, 1000 mg/kg Sul-F had no effect on the results of electrocardiogram examinations, ophthalmic examinations, and body weight. However, the transient vomiting was observed at 15–20 min after administration of Sul-F. Pathological examination showed that Sul-F had no evident effects on the main organs such as brain, heart, liver, kidney, stomach, spleen, and intestines. As for the biochemical index, the decrease of phosphocreatine kinase (CK) and the increase of total protein (TP) level were observed in Sul-F treated dogs at the end of the experiment (Li et al., 2015).
The Subchronic toxicity of formononetin has also been assessed using its water-soluble derivate, Sul-F. Dogs were administrated with Sul-F at dose of 0, 33.3, 100, and 300 mg/kg/day for 90 days followed by a four-week recovery period. No mortality, ophthalmic abnormalities, changes of body weight, evident alterations of biochemical and histopathological parameters were observed except that transient vomiting and recoverable vascular stimulation were detected in the group of 300 mg/kg/day (Li et al., 2016).
Although there is still no study reporting the LD50 of formononetin, it is not difficult to get the conclusion that formononetin has a good safety profile from the above acute and subchronic toxicity studies, because the dose above 300 mg/kg is very high and it only elicit transient and mild adverse effects, with no apparent toxicity being reported. Even so, further detailed and more rigorously designed studies are still needed to uncover other potential toxic and side effects of formononetin.
Increasing evidences have suggested that phytoestrogens exert neuroprotective effects in neurological disorders. As an important phytoestrogen and also the main bioactive component of dietary foods such as soybean, alfalfa beans and chickpeas, formononetin may be a suitable candidate for the treatment of neurological disorders with a good safety profile.
In this review, the beneficial neuropharmacological effects of formononetin in both in vitro and in vivo studies were summarized. Formononetin showed promising effects in a series of neurological disorders such as AD, pathological pain, TBI, anxiety/depression, cerebral ischemia, dementia, and so on. The protective efficacy of formononetin was compared with some other isoflavone phytoestrogen, the pharmacokinetic characteristics and safety profile of formononetin were also discussed in this paper. Numerous molecular targets and pharmacological mechanisms are involved in the neuroprotective property of formononetin. The potential molecular targets and signaling pathways underlying neuroprotective effects of formononetin are briefly summarized in Figure 2 and Figure 3, respectively. Actually, some signaling pathways modulated by formononetin had been summarized in a recent paper, which mainly focused on the biological mechanisms of formononetin at the molecular level (Geng and Jiang, 2022). Our review primarily discussed the pharmacological effects and important pharmacological parameters of formononetin based on diseases, not just only signaling pathways. They complement each other and will facilitate future studies to further investigate the neuroprotective properties of formononetin.
FIGURE 3. The graphical summary of signaling pathways underlying the neuroprotective effects of formononetin. It benefits multiple aspects of nervous system, such as promoting neurosurvival, neurogenesis and synaptic function via modulation PI3K/AKT pathway, ERK pathway, PKA/CREB pathway. Formononetin attenuates neuroinflammation through inhibiting HMGB1/TLR4/NF-κB pathway and RAGE pathway. It can also inhibit oxidative stress and neuronal apoptosis through activating Nrf2 pathway. In addition, SIRT1 is also involved in the neuroprotective effects of formononetin. PI3K, phosphatidylinositol 3-kinase; AKT, protein kinase B; GSK3β, glycogen synthase kinase-3β; ADAM10, A-Disintegrin-And -Metalloproteinase 10; APP, amyloid precursor protein; AC, adenylate cyclase; cAMP, cyclic adenosine monophosphate; PKA, protein kinase A; CREB, cAMP response element-binding protein; TRK, receptor tyrosine kinase; HMGB1, high mobility group box-1 protein; TLR4, toll-like receptor 4; RAGE, receptors for advanced glycation-end products; NF-κB, nuclear factor kappa-B; Nrf2, nuclear factor erythroid 2-related factor 2; HO-1, heme oxygenase-1; GPX, glutathione peroxidase; CAT, catalase; SOD, superoxide dismutase.
Although multiple preclinical studies have demonstrated independently that formononetin possessed therapeutic potentials against a wide range of neurological disorders, it also has many problems that remain to be solved. First, formononetin is absorbed rapidly and undergoes extensive metabolism upon administration, the metabolites may have varying bioactivities and pharmacokinetic properties, which will affect the overall pharmacological outcomes. It is essential to determine the amount of formononetin and its bioactive metabolites reaching the target tissues. However, few studies have attempted to investigate formononetin and its metabolites qualitatively and quantitatively in vivo. Therefore, future studies should focus more on clarifying the pharmacokinetic characteristics of the parent molecule and its metabolites, and evaluating their influence on the overall bioactivity of formononetin. Second, the safety profile of formononetin should be further confirmed. Although the acute and subchronic toxicity of formononetin have been assessed, the data comes from the same laboratory, it would be more convincing if there were data from multiple different laboratories. On the other hand, there is no data on the long-term safety of formononetin, which needs to be fully elucidated to ensure that this bioactive isoflavone is safe for clinical development. Third, the lack of clinical trials on the therapeutic effects of formononetin is another important limitation. So, detailed and well-designed clinical studies are needed in future research to assess the efficacy and safety of formononetin in treating neurological disorders. This is crucial because the pharmacodynamic and pharmacokinetic properties of formononetin may be diverse between animals and humans, as well as the different physiological states of the body.
Taken together, the promising neuropharmacological effects of formononetin strongly indicate that it may be a potential candidate for treating neurological diseases, though some clinical aspects, such as bioavailability, appropriate dose, tolerability, and efficacy should be further clarified before expanding formononetin treatment into humans.
JT conceptualized the story, searched the literature and wrote the original draft. X-QW searched the literature, wrote and edited the original draft. ZT supervised, wrote and edited the original draft. All the authors approved the content of the manuscript.
This study is supported by National Natural Science Foundation of China (No. 81801329), Chongqing Natural Science Foundation (No. cstc2021jcyj-msxmX0610), Science and technology research project of Chongqing Education Commission (No. KJQN202100213), Fundamental Research Funds for the Central Universities (No. SWU-KQ22018) and Guangdong Basic and Applied Basic Research Foundation (No. 2020A1515110564).
The authors declare that the research was conducted in the absence of any commercial or financial relationships that could be construed as a potential conflict of interest.
All claims expressed in this article are solely those of the authors and do not necessarily represent those of their affiliated organizations, or those of the publisher, the editors and the reviewers. Any product that may be evaluated in this article, or claim that may be made by its manufacturer, is not guaranteed or endorsed by the publisher.
Aly, S. H., Elissawy, A. M., Fayez, A. M., Eldahshan, O. A., Elshanawany, M. A., and Singab, A. N. B. (2021). Neuroprotective Effects of Sophora Secundiflora, Sophora tomentosa Leaves and Formononetin on Scopolamine-Induced Dementia. Nat. Prod. Res. 35 (24), 5848–5852. doi:10.1080/14786419.2020.1795853
Alzheimer's, A. (2016). 2016 Alzheimer's Disease Facts and Figures. Alzheimers Dement. 12 (4), 459–509. doi:10.1016/j.jalz.2016.03.001
Andres, S., Hansen, U., Niemann, B., Palavinskas, R., and Lampen, A. (2015). Determination of the Isoflavone Composition and Estrogenic Activity of Commercial Dietary Supplements Based on Soy or Red Clover. Food Funct. 6 (6), 2017–2025. doi:10.1039/c5fo00308c
Barnes, S., Prasain, J., D'Alessandro, T., Arabshahi, A., Botting, N., Lila, M. A., et al. (2011). The Metabolism and Analysis of Isoflavones and Other Dietary Polyphenols in Foods and Biological Systems. Food Funct. 2 (5), 235–244. doi:10.1039/c1fo10025d
Cassileth, B. (2010). Complementary Therapies, Herbs, and Other OTC Agents: Red Clover (Trifolium Pratense). Oncol. Willist. Park) 24 (10), 960.
Cederroth, C. R., and Nef, S. (2009). Soy, Phytoestrogens and Metabolism: A Review. Mol. Cell. Endocrinol. 304 (1-2), 30–42. doi:10.1016/j.mce.2009.02.027
Chen, H. Q., Wang, X. J., Jin, Z. Y., Xu, X. M., Zhao, J. W., and Xie, Z. J. (2008). Protective Effect of Isoflavones from Trifolium Pratense on Dopaminergic Neurons. Neurosci. Res. 62 (2), 123–130. doi:10.1016/j.neures.2008.07.001
Chen, J., Wang, S., Jia, X., Bajimaya, S., Lin, H., Tam, V. H., et al. (2005). Disposition of Flavonoids via Recycling: Comparison of Intestinal versus Hepatic Disposition. Drug Metab. Dispos. 33 (12), 1777–1784. doi:10.1124/dmd.105.003673
Chen, L., Ou, S., Zhou, L., Tang, H., Xu, J., and Guo, K. (2017). Formononetin Attenuates Aβ25-35-Induced Cytotoxicity in HT22 Cells via PI3K/Akt Signaling and Non-amyloidogenic Cleavage of APP. Neurosci. Lett. 639, 36–42. doi:10.1016/j.neulet.2016.12.064
D'Archivio, M., Filesi, C., Varì, R., Scazzocchio, B., and Masella, R. (2010). Bioavailability of the Polyphenols: Status and Controversies. Int. J. Mol. Sci. 11 (4), 1321–1342. doi:10.3390/ijms11041321
Di Lorenzo, C., Colombo, F., Biella, S., Stockley, C., and Restani, P. (2021). Polyphenols and Human Health: The Role of Bioavailability. Nutrients 13 (1), 273. doi:10.3390/nu13010273
Dinoff, A., Herrmann, N., and Lanctôt, K. L. (2017). Ceramides and Depression: A Systematic Review. J. Affect Disord. 213, 35–43. doi:10.1016/j.jad.2017.02.008
Durand, E., Chevignard, M., Ruet, A., Dereix, A., Jourdan, C., and Pradat-Diehl, P. (2017). History of Traumatic Brain Injury in Prison Populations: A Systematic Review. Ann. Phys. Rehabil. Med. 60 (2), 95–101. doi:10.1016/j.rehab.2017.02.003
El-Bakoush, A., and Olajide, O. A. (2018). Formononetin Inhibits Neuroinflammation and Increases Estrogen Receptor Beta (ERβ) Protein Expression in BV2 Microglia. Int. Immunopharmacol. 61, 325–337. doi:10.1016/j.intimp.2018.06.016
Fang, Y., Ye, J., Zhao, B., Sun, J., Gu, N., Chen, X., et al. (2020). Formononetin Ameliorates Oxaliplatin-Induced Peripheral Neuropathy via the KEAP1-NRF2-GSTP1 axis. Redox Biol. 36, 101677. doi:10.1016/j.redox.2020.101677
Fei, H. X., Zhang, Y. B., Liu, T., Zhang, X. J., and Wu, S. L. (2018). Neuroprotective Effect of Formononetin in Ameliorating Learning and Memory Impairment in Mouse Model of Alzheimer's Disease. Biosci. Biotechnol. Biochem. 82 (1), 57–64. doi:10.1080/09168451.2017.1399788
Fu, X., Qin, T., Yu, J., Jiao, J., Ma, Z., Fu, Q., et al. (2019). Formononetin Ameliorates Cognitive Disorder via PGC-1α Pathway in Neuroinflammation Conditions in High-Fat Diet-Induced Mice. CNS Neurol. Disord. Drug Targets 18 (7), 566–577. doi:10.2174/1871527318666190807160137
Gampe, N., Dávid, D. N., Takács-Novák, K., Backlund, A., and Béni, S. (2022). In Vitro and In Silico Evaluation of Ononis Isoflavonoids as Molecules Targeting the Central Nervous System. PLoS One 17 (3), e0265639. doi:10.1371/journal.pone.0265639
Geng, L.-M., and Jiang, J.-G. (2022). The Neuroprotective Effects of Formononetin: Signaling Pathways and Molecular Targets. J. Funct. Foods 88, 104911. doi:10.1016/j.jff.2021.104911
Gu, Y., Chen, X., Fu, S., Liu, W., Wang, Q., Liu, K.-J., et al. (2021). Astragali Radix Isoflavones Synergistically Alleviate Cerebral Ischemia and Reperfusion Injury via Activating Estrogen Receptor-Pi3k-Akt Signaling Pathway. Front. Pharmacol. 12, 533028. doi:10.3389/fphar.2021.533028
Gunata, M., Parlakpinar, H., and Acet, H. A. (2020). Melatonin: A Review of its Potential Functions and Effects on Neurological Diseases. Rev. Neurol. Paris. 176 (3), 148–165. doi:10.1016/j.neurol.2019.07.025
Hankey, G. J. (2017). The Role of Nutrition in the Risk and Burden of Stroke: An Update of the Evidence. Stroke 48 (11), 3168–3174. doi:10.1161/STROKEAHA.117.016993
Howes, J. B., Bray, K., Lorenz, L., Smerdely, P., and Howes, L. G. (2004). The Effects of Dietary Supplementation with Isoflavones from Red Clover on Cognitive Function in Postmenopausal Women. Climacteric 7 (1), 70–77. doi:10.1080/13697130310001651490
Jia, X., Chen, J., Lin, H., and Hu, M. (2004). Disposition of Flavonoids via Enteric Recycling: Enzyme-Transporter Coupling Affects Metabolism of Biochanin A and Formononetin and Excretion of Their Phase II Conjugates. J. Pharmacol. Exp. Ther. 310 (3), 1103–1113. doi:10.1124/jpet.104.068403
Khawaja, N., Abu-Shennar, J., Saleh, M., Dahbour, S. S., Khader, Y. S., and Ajlouni, K. M. (2018). The Prevalence and Risk Factors of Peripheral Neuropathy Among Patients with Type 2 Diabetes Mellitus; the Case of Jordan. Diabetol. Metab. Syndr. 10, 8. doi:10.1186/s13098-018-0309-6
Krizova, L., Dadakova, K., Kasparovska, J., and Kasparovsky, T. (2019). Isoflavones. Mol. 24 (6). doi:10.3390/molecules24061076
Larsson, J., Björkdahl, A., Esbjörnsson, E., and Sunnerhagen, K. S. (2013). Factors Affecting Participation after Traumatic Brain Injury. J. Rehabil. Med. 45 (8), 765–770. doi:10.2340/16501977-1184
Lethaby, A. E., Brown, J., Marjoribanks, J., Kronenberg, F., Roberts, H., and Eden, J. (2007). Phytoestrogens for Vasomotor Menopausal Symptoms. Cochrane Database Syst. Rev. 17 (4), CD001395. doi:10.1002/14651858.CD001395.pub3
Levy, M., Berson, A., Cook, T., Bollegala, N., Seto, E., Tursanski, S., et al. (2005). Treatment of Agitation Following Traumatic Brain Injury: a Review of the Literature. NeuroRehabilitation 20 (4), 279–306. doi:10.3233/nre-2005-20405
Li, C., Li, G., Gao, Y., Sun, C., and Wang, X. (2016). A 90-day Subchronic Toxicity Study with Sodium Formononetin-3'-Sulphonate (Sul-F) Delivered to Dogs via Intravenous Administration. Regul. Toxicol. Pharmacol. 77, 87–92. doi:10.1016/j.yrtph.2016.02.016
Li, G., Yang, M., Hao, X., Li, C., Gao, Y., and Tao, J. (2015). Acute Toxicity of Sodium Formononetin-3'-Sulphonate (Sul-F) in Sprague-Dawley Rats and Beagle Dogs. Regul. Toxicol. Pharmacol. 73 (2), 629–633. doi:10.1016/j.yrtph.2015.09.010
Li, Z., Dong, X., Zhang, J., Zeng, G., Zhao, H., Liu, Y., et al. (2014). Formononetin Protects TBI Rats against Neurological Lesions and the Underlying Mechanism. J. Neurol. Sci. 338 (1-2), 112–117. doi:10.1016/j.jns.2013.12.027
Li, Z., Wang, Y., Zeng, G., Zheng, X., Wang, W., Ling, Y., et al. (2017). Increased miR-155 and Heme Oxygenase-1 Expression Is Involved in the Protective Effects of Formononetin in Traumatic Brain Injury in Rats. Am. J. Transl. Res. 9 (12), 5653–5661.
Li, Z., Zeng, G., Zheng, X., Wang, W., Ling, Y., Tang, H., et al. (2018). Neuroprotective Effect of Formononetin against TBI in Rats via Suppressing Inflammatory Reaction in Cortical Neurons. Biomed. Pharmacother. 106, 349–354. doi:10.1016/j.biopha.2018.06.041
Liang, K., Ye, Y., Wang, Y., Zhang, J., and Li, C. (2014). Formononetin Mediates Neuroprotection against Cerebral Ischemia/reperfusion in Rats via Downregulation of the Bax/Bcl-2 Ratio and Upregulation PI3K/Akt Signaling Pathway. J. Neurol. Sci. 344 (1-2), 100–104. doi:10.1016/j.jns.2014.06.033
Lima Cavendish, R., de Souza Santos, J., Belo Neto, R., Oliveira Paixão, A., Valéria Oliveira, J., Divino de Araujo, E., et al. (2015). Antinociceptive and Anti-inflammatory Effects of Brazilian Red Propolis Extract and Formononetin in Rodents. J. Ethnopharmacol. 173, 127–133. doi:10.1016/j.jep.2015.07.022
Liu, J., Gao, L. D., Fu, B., Yang, H. T., Zhang, L., Che, S. Q., et al. (2022). Efficacy and Safety of Zicuiyin Decoction on Diabetic Kidney Disease: A Multicenter, Randomized Controlled Trial. Phytomedicine 100, 154079. doi:10.1016/j.phymed.2022.154079
Liu, N., Yu, Z., Gao, X., Song, Y. S., Yuan, J., Xun, Y., et al. (2016). Establishment of Cell-Based Neuroglobin Promoter Reporter Assay for Neuroprotective Compounds Screening. CNS Neurol. Disord. Drug Targets 15 (5), 629–639. doi:10.2174/1871527315666160321105612
Luo, L. Y., Fan, M. X., Zhao, H. Y., Li, M. X., Wu, X., and Gao, W. Y. (2018). Pharmacokinetics and Bioavailability of the Isoflavones Formononetin and Ononin and Their In Vitro Absorption in Ussing Chamber and Caco-2 Cell Models. J. Agric. Food Chem. 66 (11), 2917–2924. doi:10.1021/acs.jafc.8b00035
Messina, M. (2010). A Brief Historical Overview of the Past Two Decades of Soy and Isoflavone Research. J. Nutr. 140 (7), 1350S–4S. doi:10.3945/jn.109.118315
Mu, H., Bai, Y. H., Wang, S. T., Zhu, Z. M., and Zhang, Y. W. (2009). Research on Antioxidant Effects and Estrogenic Effect of Formononetin from Trifolium Pratense (Red Clover). Phytomedicine 16 (4), 314–319. doi:10.1016/j.phymed.2008.07.005
Oza, M. J., and Kulkarni, Y. A. (2020). Formononetin Ameliorates Diabetic Neuropathy by Increasing Expression of SIRT1 and NGF. Chem. Biodivers. 17 (6), e2000162. doi:10.1002/cbdv.202000162
Polak, R., Phillips, E. M., and Campbell, A. (2015). Legumes: Health Benefits and Culinary Approaches to Increase Intake. Clin. Diabetes 33 (4), 198–205. doi:10.2337/diaclin.33.4.198
Rastogi, S., Pandey, M. M., and Rawat, A. K. (2016). Traditional Herbs: a Remedy for Cardiovascular Disorders. Phytomedicine 23 (11), 1082–1089. doi:10.1016/j.phymed.2015.10.012
Ronis, M. J., Little, J. M., Barone, G. W., Chen, G., Radominska-Pandya, A., and Badger, T. M. (2006). Sulfation of the Isoflavones Genistein and Daidzein in Human and Rat Liver and Gastrointestinal Tract. J. Med. Food 9 (3), 348–355. doi:10.1089/jmf.2006.9.348
Sharma, R., Kabra, A., Rao, M. M., and Prajapati, P. K. (2018). Herbal and Holistic Solutions for Neurodegenerative and Depressive Disorders: Leads from Ayurveda. Curr. Pharm. Des. 24 (22), 2597–2608. doi:10.2174/1381612824666180821165741
Sharma, R., Kuca, K., Nepovimova, E., Kabra, A., Rao, M. M., and Prajapati, P. K. (2019). Traditional Ayurvedic and Herbal Remedies for Alzheimer's Disease: from Bench to Bedside. Expert Rev. Neurother. 19 (5), 359–374. doi:10.1080/14737175.2019.1596803
Sharma, R., Garg, N., Verma, D., Rathi, P., Sharma, V., Kuca, K., et al. (2021). Indian Medicinal Plants as Drug Leads in Neurodegenerative Disorders. Nutraceuticals in Brain Health and Beyond. 2021, 31–45. doi:10.1016/B978-0-12-820593-8.00004-5
Singh, S. P., WahajuddinTewari, D., Tewari, D., Pradhan, T., and Jain, G. K. (2011). PAMPA Permeability, Plasma Protein Binding, Blood Partition, Pharmacokinetics and Metabolism of Formononetin, a Methoxylated Isoflavone. Food Chem. Toxicol. 49 (5), 1056–1062. doi:10.1016/j.fct.2011.01.012
Sun, M., Zhou, T., Zhou, L., Chen, Q., Yu, Y., Yang, H., et al. (2012). Formononetin Protects Neurons against Hypoxia-Induced Cytotoxicity through Upregulation of ADAM10 and sAβPPα. J. Alzheimers Dis. 28 (4), 795–808. doi:10.3233/JAD-2011-110506
Tay, K. C., Tan, L. T., Chan, C. K., Hong, S. L., Chan, K. G., Yap, W. H., et al. (2019). Formononetin: A Review of its Anticancer Potentials and Mechanisms. Front. Pharmacol. 10, 820. doi:10.3389/fphar.2019.00820
Teede, H. J., Dalais, F. S., Kotsopoulos, D., Liang, Y. L., Davis, S., and McGrath, B. P. (2001). Dietary Soy Has Both Beneficial and Potentially Adverse Cardiovascular Effects: a Placebo-Controlled Study in Men and Postmenopausal Women. J. Clin. Endocrinol. Metab. 86 (7), 3053–3060. doi:10.1210/jcem.86.7.7645
Upadhyay, R. K. (2014). Drug Delivery Systems, CNS Protection, and the Blood Brain Barrier. Biomed. Res. Int. 2014, 869269. doi:10.1155/2014/869269
Vitale, D. C., Piazza, C., Melilli, B., Drago, F., and Salomone, S. (2013). Isoflavones: Estrogenic Activity, Biological Effect and Bioavailability. Eur. J. Drug Metab. Pharmacokinet. 38 (1), 15–25. doi:10.1007/s13318-012-0112-y
Walle, T., Ta, N., Kawamori, T., Wen, X., Tsuji, P. A., and Walle, U. K. (2007). Cancer Chemopreventive Properties of Orally Bioavailable Flavonoids-Mmethylated versus Unmethylated Flavones. Biochem. Pharmacol. 73 (9), 1288–1296. doi:10.1016/j.bcp.2006.12.028
Wang, J., Wang, L., Zhou, J., Qin, A., and Chen, Z. (2018). The Protective Effect of Formononetin on Cognitive Impairment in Streptozotocin (STZ)-induced Diabetic Mice. Biomed. Pharmacother. 106, 1250–1257. doi:10.1016/j.biopha.2018.07.063
Wang, Q., Gao, S., Zhang, W., Zhao, Y., He, Y., Sun, W., et al. (2022). Traditional Use and Safety Evaluation of Combination Traditional Chinese Medicine in European Registration: with XiaoYao Tablets as an Example. Pharmazie 77 (3), 125–130. doi:10.1691/ph.2022.1227
Wang, X. S., Guan, S. Y., Liu, A., Yue, J., Hu, L. N., Zhang, K., et al. (2019). Anxiolytic Effects of Formononetin in an Inflammatory Pain Mouse Model. Mol. Brain 12 (1), 36. doi:10.1186/s13041-019-0453-4
Wu, Q.-L., Cheng, Y.-Q., Liu, A.-J., and Zhang, W.-D. (2020). Formononetin Recovered Injured Nerve Functions by Enhancing Synaptic Plasticity in Ischemic Stroke Rats. Biochem. Biophysical Res. Commun. 525, 67–72. doi:10.1016/j.bbrc.2020.02.015
Yan, X., Wang, S., Yu, A., Shen, X., Zheng, H., and Wang, L. (2019). Cell Chromatography-Based Screening of the Active Components in Buyang Huanwu Decoction Promoting Axonal Regeneration. Biomed. Res. Int. 2019, 6970198. doi:10.1155/2019/6970198
Yankner, B. A., Duffy, L. K., and Kirschner, D. A. (1990). Neurotrophic and Neurotoxic Effects of Amyloid Beta Protein: Reversal by Tachykinin Neuropeptides. Science 250 (4978), 279–282. doi:10.1126/science.2218531
Ye, Y. B., Tang, X. Y., Verbruggen, M. A., and Su, Y. X. (2006). Soy Isoflavones Attenuate Bone Loss in Early Postmenopausal Chinese Women : a Single-Blind Randomized, Placebo-Controlled Trial. Eur. J. Nutr. 45 (6), 327–334. doi:10.1007/s00394-006-0602-2
Yu, D., Duan, Y., Bao, Y., Wei, C., and An, L. (2005). Isoflavonoids from Astragalus mongholicus Bunge Protect PC12 Cells from Toxicity Induced by L-Glutamate. J. Ethnopharmacol. 98 (1-2), 89–94. doi:10.1016/j.jep.2004.12.027
Yu, D. H., Bao, Y. M., An, L. J., and Yang, M. (2009). Protection of PC12 Cells against Superoxide-Induced Damage by Isoflavonoids from Astragalus mongholicus Bunge. Biomed. Environ. Sci. 22 (1), 50–54. doi:10.1016/S0895-3988(09)60022-2
Yu, L., Zhang, Y., Chen, Q., He, Y., Zhou, H., Wan, H., et al. (2022). Formononetin Protects against Inflammation Associated with Cerebral Ischemia-Reperfusion Injury in Rats by Targeting the JAK2/STAT3 Signaling Pathway. Biomed. Pharmacother. 149, 112836. doi:10.1016/j.biopha.2022.112836
Zhang, C., Zhu, L., Lu, S., Li, M., Bai, M., Li, Y., et al. (2022). The Antidepressant-like Effect of Formononetin on Chronic Corticosterone-Treated Mice. Brain Res. 1783, 147844. doi:10.1016/j.brainres.2022.147844
Zhang, L., Zuo, Z., and Lin, G. (2007). Intestinal and Hepatic Glucuronidation of Flavonoids. Mol. Pharm. 4 (6), 833–845. doi:10.1021/mp700077z
Zhang, W., Zhu, J. H., Xu, H., Huang, X. P., Liu, X. D., and Deng, C. Q. (2019). Five Active Components Compatibility of Astragali Radix and Angelicae Sinensis Radix Protect Hematopoietic Function against Cyclophosphamide-Induced Injury in Mice and T-BHP-Induced Injury in HSCs. Front. Pharmacol. 10, 936. doi:10.3389/fphar.2019.00936
Zhu, H., Zou, L., Tian, J., Lin, F., He, J., and Hou, J. (2014). Protective Effects of Sulphonated Formononetin in a Rat Model of Cerebral Ischemia and Reperfusion Injury. Planta Med. 80 (4), 262–268. doi:10.1055/s-0033-1360340
Keywords: neurological disorders, formononetin, Alzheimer’s disease, dementia, traumatic brain injury, nociception, neuroinflammation, oxidative stress
Citation: Tian J, Wang X-Q and Tian Z (2022) Focusing on Formononetin: Recent Perspectives for its Neuroprotective Potentials. Front. Pharmacol. 13:905898. doi: 10.3389/fphar.2022.905898
Received: 28 March 2022; Accepted: 16 May 2022;
Published: 30 May 2022.
Edited by:
Simona Federica Spampinato, University of Catania, ItalyReviewed by:
Wai San Cheang, University of Macau, ChinaCopyright © 2022 Tian, Wang and Tian. This is an open-access article distributed under the terms of the Creative Commons Attribution License (CC BY). The use, distribution or reproduction in other forums is permitted, provided the original author(s) and the copyright owner(s) are credited and that the original publication in this journal is cited, in accordance with accepted academic practice. No use, distribution or reproduction is permitted which does not comply with these terms.
*Correspondence: Xing-Qin Wang, bmV1cm93YW5neHFAMTYzLmNvbQ==; Zhen Tian, emhlbnRpYW56aEAxNjMuY29t
Disclaimer: All claims expressed in this article are solely those of the authors and do not necessarily represent those of their affiliated organizations, or those of the publisher, the editors and the reviewers. Any product that may be evaluated in this article or claim that may be made by its manufacturer is not guaranteed or endorsed by the publisher.
Research integrity at Frontiers
Learn more about the work of our research integrity team to safeguard the quality of each article we publish.