- 1Department of Pharmacology, Vanderbilt University School of Medicine, Nashville, TN, United States
- 2Charak Foundation, Orange, CA, United States
- 3Regulatory Wisdom, Delhi, India
- 4Charak Foundation of India, Indore, India
- 5EyeSight Eye Hospital and Retina Centre, Indore, India
- 6Division of Nephrology, Hypertension and Kidney Transplantation, University of California, Irvine, CA, United States
Hypoxemia in COVID-19 pneumonia is associated with hospitalization, mechanical ventilation, and mortality. COVID-19 patients exhibit marked increases in fatty acid levels and inflammatory lipid mediators, predominantly arachidonic acid metabolites, notably thromboxane B2 >> prostaglandin E2 > prostaglandin D2. Thromboxane A2 increases pulmonary capillary pressure and microvascular permeability, leading to pulmonary edema, and causes bronchoconstriction contributing to ventilation/perfusion mismatch. Prostaglandin D2-stimulated IL-13 production is associated with respiratory failure, possibly due to hyaluronan accumulation in the lungs. Ramatroban is an orally bioavailable, dual thromboxane A2/TP and prostaglandin D2/DP2 receptor antagonist used in Japan for allergic rhinitis. Four consecutive outpatients with COVID-19 pneumonia treated with ramatroban exhibited rapid relief of dyspnea and hypoxemia within 12–36 h and complete resolution over 5 days, thereby avoiding hospitalization. Therefore, ramatroban as an antivasospastic, broncho-relaxant, antithrombotic, and immunomodulatory agent merits study in randomized clinical trials that might offer hope for a cost-effective pandemic treatment.
1 Introduction
After symptomatic SARS-CoV-2 infection, 10–20% of patients require hospitalization for respiratory distress and hypoxemia (Trust for American’s Health, 2021). Currently, anti-SARS-CoV-2 monoclonal antibodies are approved for treatment of ambulatory patients with COVID-19 (NIH, 2021), and antiviral treatments have recently been approved, but they are expensive and effective only early after symptom onset. There is an unmet medical need for inexpensive, safe, orally bioavailable drugs that can reduce hypoxemia, provide symptomatic relief, and minimize hospitalization in patients with COVID-19. Identifying the correct therapeutic target is critical to discovering such a drug.
Lungs in COVID-19 patients with acute respiratory distress syndrome (ARDS) produce proinflammatory lipid mediators with predominance of cyclooxygenase metabolites in plasma and bronchoalveolar lavage fluid (BALF), notably thromboxane B2 (TxB2) >> prostaglandin E2 (PGE2) > prostaglandin D2 (PGD2) (Archambault et al., 2021; Regidor et al., 2021). The massive increase in TxA2 metabolites in BALF (Archambault et al., 2021) and systemically (Regidor et al., 2021) in hospitalized COVID-19 patients (Hottz et al., 2020; Al-Hakeim et al., 2021; Regidor et al., 2021), suggests a critical role for TxA2/TxA2 prostanoid receptors (TP) in COVID-19 respiratory distress. We hypothesized that TxA2/TP-induced selective contraction of pulmonary veins (Kadowitz et al., 1977; Bowers et al., 1979; Walch et al., 2001) elevates pulmonary capillary pressure and contributes to pulmonary edema and hypoxemia in COVID-19 pneumonia (Figure 1). TP signaling leads to selective constriction of intrapulmonary veins and small airways with 10-fold higher potency and greater reduction in luminal area than intrapulmonary arteries (Larsson et al., 2011). High local concentrations of TxA2 can effectively divert pulmonary blood flow, increase microvascular pressure and permeability, and force fluid and plasma proteins into alveoli (Larsson et al., 2011). A selective TP antagonist was previously reported to decrease pulmonary capillary pressure by selectively reducing postcapillary resistance in patients with acute lung injury (Schuster et al., 2001). F2-Isoprostane is also increased in COVID-19 (Kumar et al., 2021) and is known to activate vascular TP receptors. TxA2 and isoprostanes stimulate TP-mediated activation of the TGFβ pathway (Craven et al., 1996), and early, untimely TGFβ responses in SARS-CoV-2 infection limit antiviral function of natural killer (NK) cells and promote progression to severe COVID-19 disease (Witkowski et al., 2021).
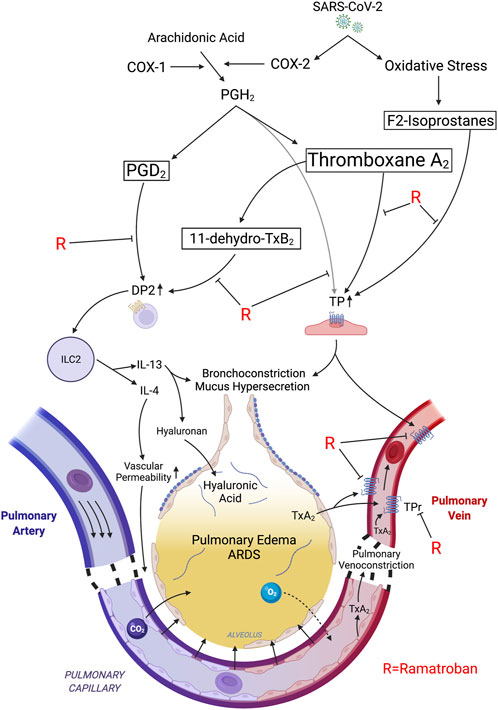
FIGURE 1. Proposed mechanisms of rapid relief in respiratory distress following ramatroban administration during acute SARS-CoV-2 infection. SARS-CoV-2 induced expression of COX-2 generates PGH2 which is converted into thromboxane A2 >> PGD2. Oxidative stress-associated free radicals initiate nonenzymatic peroxidation of arachidonic acid leading to F2-isoprostane generation. PGH2, TxA2, and F2-isoprostanes stimulate thromboxane prostanoid receptors (TP). TP stimulation induces pulmonary venoconstriction leading to an increase in transcapillary pressure in pulmonary microvasculature, and transudation of fluid into the alveoli, thereby causing impaired gas exchange and ARDS. The TxA2/TP axis also induces bronchoconstriction and mucus secretion. TxA2 is rapidly converted to 11-dehydro-TxB2 in the lungs. PGD2 and 11-dehydro-TxB2 stimulate the DP2 receptor on Th2 and ILC2 cells leading to release of type 2 cytokines, IL-4 and IL-13. Both TP activation and IL-4 promote vascular permeability thereby exacerbating fluid transudation, while IL-13 induces hyaluronic acid accumulation and mucus hypersecretion. Ramatroban inhibits the DP2 and TP receptors, thereby promoting pulmonary vasorelaxation and bronchorelaxation and improving capillary barrier function, while attenuating the maladaptive type 2 immune response and mucus secretion, thereby alleviating pulmonary edema and ARDS. Tx, thromboxane; PG, prostaglandin; TP, thromboxane prostanoid receptor; DP2; D-prostanoid receptor 2; Th2; T helper 2; ILC2; innate lymphoid class.
Theken and FitzGerald proposed early administration of a TxA2 receptor antagonist as an antithrombotic agent and a D-prostanoid receptor 2 (DP2, formerly referred to as CRTH2) antagonist to boost interferon lambda (IFN-λ) in the upper respiratory tract, thereby limiting SARS-CoV-2 replication and transmission (Sposito et al., 2021; Theken and Fitzgerald, 2021). Ramatroban, the only dual TxA2/TP and PGD2/DP2 receptor antagonist available for clinical study, has been proposed as an antithrombotic and immunomodulator agent in COVID-19 (Gupta and Chiang, 2020; Gupta et al., 2020). In their report showing very high levels of TxB2 >> PGD2 in BALF, Archambault and colleagues also suggested ramatroban to block the deleterious effects of TxA2 and PGD2 in COVID-19 (Archambault et al., 2021). Ramatroban has an established safety profile, having been prescribed for over 20 years in Japan for treatment of allergic rhinitis (Ishizuka et al., 2004; Uller et al., 2007). We report here a small case series of four consecutive COVID-19 patients with worsening respiratory distress and hypoxemia who were treated with ramatroban. Surprisingly, this led to rapid improvement in both respiratory distress and hypoxemia, thereby avoiding hospitalization and promoting recovery from acute disease.
1.1 The First Case of Severe COVID-19 Pneumonia Treated with Ramatroban
S.D., an 87-year-old Indian lady, experienced sudden onset of fever, cough, diarrhea, anorexia, profound weakness, and slight shortness of breath, 10 days after a 2-h flight from New Delhi to Indore, Madhya Pradesh, India. The index patient had received the first dose of COVAXIN, a whole virion inactivated vaccine against SARS-CoV-2, 30 days prior to beginning of symptoms. On examination the patient was fully alert, oriented, and able to make intelligent conversation but lay listlessly in bed unable to ambulate. Patient weighed 42 kg and exhibited severe preexisting muscle wasting and marked kyphosis. Vital signs revealed temperature 102°F, heart rate 100 per minute, blood pressure 90/60 mm of Hg, and respiratory rate 22 per minute. Mucosa were moist, and mild pallor was present. There was no jugular venous distention or pedal edema. Chest examination revealed bilateral coarse rales especially prominent at both lung bases but no wheezes. Abdomen, cardiovascular, and neurological examinations were unremarkable. Patient was not taking any medications.
1.1.1 Past Medical History
Past medical history included hypertension for over 40 years, thyrotoxicosis for over 30 years treated with radioiodine therapy in 1999, severe osteoporosis with kyphosis, bladder suspension surgery in 1999, coronary artery disease leading to acute myocardial infarction and cardiac arrest in 2015 which required coronary angioplasty and stent placement, and chronic kidney disease with estimated glomerular filtration rate of about 20 ml/min (Table 2).
1.1.2 Investigations
Nasopharyngeal and oropharyngeal swabs were positive for SARS-CoV-2 infection by RNA PCR with cycle threshold (Ct range) < 20 cycles. Pulse oximetry revealed oxygen saturation of about 85–88%. Patient was admitted on 9 April 2021 to Medanta Hospital, Indore. CT scan revealed moderate multifocal, patchy ground glass opacities, and consolidation. There was septal thickening in the central and peripheral subpleural aspect of both lung parenchyma. Serial laboratory examinations during the course of the illness are listed in Table 1.
1.1.3 Hospital Course
During the hospital stay, the patient was treated with high-flow nasal oxygen, prophylactic low-molecular weight heparin, intravenous remdesivir, antibiotics, and methylprednisolone. Patient continued to have fever, cough, shortness of breath, diarrhea, and profound weakness during the hospital stay. SpO2 on room air ranged between 82 and 86% (Table 2). After a hospital stay of 5 days, the patient was discharged upon her request on 14 April 2021. Discharge medications included oral oseltamivir, doxycycline, vitamin C, aspirin 75 mg once a day, 5 mg prednisolone, vitamin D3, and nebulization with budesonide and salbutamol twice daily. Continued supportive management with betadine gargles, steam inhalation, and breathing exercises was advised.
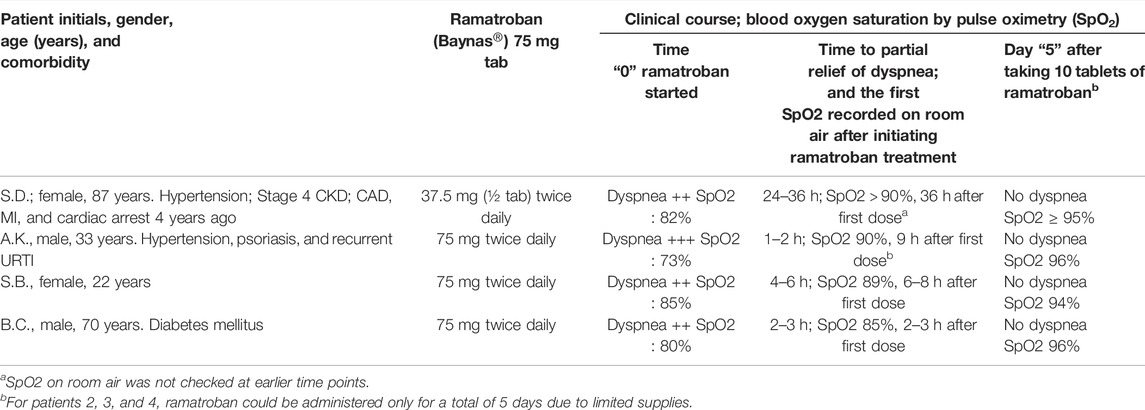
TABLE 2. Clinical course of COVID-19 patients with acute respiratory distress treated with ramatroban.
1.1.4 Postdischarge Course
On April 15, the day after discharge from the hospital, the patient had fever with a temperature of 101°F. Pulse oximetry revealed an oxygen saturation (SpO2) of 82–84% on room air, and patient was continued on oxygen. Patient was profoundly weak and unable to get out of bed without assistance. At this time all drugs including low-dose aspirin were discontinued, and the patient was started on ramatroban (Baynas®, 75 mg tablet) in a dose of one-half tablet (37.5 mg) orally twice daily. The patient was continued on oxygen using a nasal cannula and SpO2 was not checked on room air. After about 36 h, having received three one-half doses of ramatroban, there was noticeable improvement in her general condition, and SpO2 increased to 90% on room air. The dose of ramatroban was increased to 37.5 mg in the morning and 75 mg at bedtime. Patient had complete resolution of cough and diarrhea over the next 3 days and started ambulating independently without assistance. Ramatroban was discontinued after 2 weeks due to nonavailability, and the patient was switched to 75 mg aspirin daily. Patient had recovered almost completely by 22 April 2021 and gradually recovered fully over the next 3–4 weeks to baseline status. On 10 October 2021, 6 months after the acute COVID-19, a high-resolution, noncontrast CT scan demonstrated nonhomogenous ground glass pattern with normal lung volumes and absence of lung fibrosis. Patient continues to be asymptomatic.
1.2 Case 2
A.K., a 33-year-old business manager in New Delhi, developed sore throat, cough, loss of smell, altered taste, loss of appetite, high grade fever (104–106°F), profound weakness, and severe body aches around 17 April 2021. A.K. had not received the COVID-19 vaccine. Patient has past medical history of mild hypertension, psoriasis and psoriatic arthropathy treated with homeopathy, nasal polyposis, and recurrent upper respiratory infections every winter for the past several years. Nasopharyngeal and oropharyngeal swabs taken the next day were positive for SARS-CoV-2 infection by RNA PCR with cycle threshold (Ct range) of 21 cycles.
On 18 April 2021, the patient developed progressive shortness of breath and was started on oral favipiravir, hydroxychloroquine, doxycycline, and multivitamins. SpO2 checked in the morning was about 90%, declining to 82–85% by the evening. The shortness of breath worsened around midnight and patient could not catch his breath, was unable to speak, and was very anxious and restless. The SpO2 was 73% (Table 2). Patient could not be transferred to a COVID hospital due to nonavailability of hospital beds.
Desperate attempts to secure an oxygen cylinder failed. Ramatroban was rushed to patient’s home by Uber and first dose of 75 mg was taken at 1:30 a.m. on the morning of April 19th. The “breathing improved in 25–30 min,” and the patient calmed down and fell asleep at 3 a.m. Pulse oximetry remained disconnected while the patient was sleeping so as not to disturb him. Patient woke up at 11 a.m. at which time SpO2 on room air was 88–90%. On April 20th, oral temperature was 101°F and SpO2 was 90–92%. Ramatroban was administered in a dose of 75 mg twice daily for a total of 5 days with continued improvement (Table 2). On the 25th of April, patient noticed that the sputum was streaked with blood and oral acetylcysteine was started. A chest CT on April 27th revealed ground glass opacities involving bilateral lung fields with mild interstitial thickening giving the appearance of crazy-paving pattern. There were scattered areas of bronchopneumonic changes and consolidation involving both lungs. A few small fibrotic bands were noted in both lower lobes. The patient had made a near complete recovery by May 5th and resumed work on May 10th. Patient continued to have altered taste and smell 7 months after the acute illness, but by one year was back to normal.
1.3 Case 3
S.B., a 22-year-old, healthy lady in New Delhi developed fever, cough, loss of smell and taste, and body aches due to COVID-19. S.B. had not received COVID vaccination. S.B. was treated with favipiravir, steroids, and multivitamins. Patient experienced progressively worsening shortness of breath and SpO2 dropped to 85% on room air. Patient was prescribed Ramatroban 75 mg twice daily. Within 6–8 h after taking the first dose of ramatroban, respiratory distress improved and the SpO2 increased to 89%.
The next day SpO2 increased to 90–91%. There was progressive improvement with complete resolution of respiratory symptoms over the next 5 days. On day 5, the SpO2 was 94% on room air (Table 2). Patient has made a complete recovery from acute COVID-19.
1.4 Case 4
B.C., a 70-year-old man living in a rural area of Bihar, India, developed high grade fever and cough presumably secondary to SARS-CoV-2 infection. Patient has a history of diabetes mellitus controlled with diet. B.C. was not taking any medications and had only received one dose of COVAXIN vaccine for COVID-19. Patient developed shortness of breath with SpO2 measuring about 80% on room air. Two to three hours after taking 75 mg ramatroban, respiratory distress and cough improved and the SpO2 increased to 85%. After a total of 10 tablets taken over 5 days, dyspnea had resolved and SpO2 increased to 96% on room air (Table 2). Patient has made a complete recovery from acute COVID-19.
1.5 Discussion
We present the first reported cases of COVID-19 treated with ramatroban (Baynas®), a dual antagonist of the TxA2/TP and PGD2/DP2 receptors. All four COVID-19 patients were characterized by respiratory distress that was new in onset or had worsened (Table 2). Despite presenting with severe hypoxemia, gas exchange rapidly improved in all four patients. They were able to avoid hospitalization and recovered without any further need for supplemental oxygen or corticosteroids.
Relief of hypoxemia is a key success factor in COVID-19 treatment. The rapidity of improvement following oral ramatroban is surprising. For COVID-19 patients needing supplemental oxygen outside the intensive care unit in Germany, Daher reported, “The single most outstanding finding of this study is the length of hospitalization and the need of supplemental oxygen: patients were treated for 12 days and needed oxygen therapy for 8 days on average.” Compared to patients being hospitalized for severe influenza, “patients with COVID-19 seem to need a significantly longer hospital stay and are longer on oxygen therapy” (Daher et al., 2021). In India, the PLACID trial evaluated convalescent plasma in patients with moderate COVID-19 and found “median (interquartile range) total days of respiratory support of 9 (6–13) n = 227 without and 10 (6–13); n = 224 with convalescent plasma.” This trial included younger patients; median age in years was 52 (42–60). (Agarwal et al., 2020). More rapid improvement in hypoxemia and relief of respiratory distress with ramatroban is consistent with acute relaxation of vascular and airway smooth muscles. We hypothesize that blocking TxA2/TP inhibits pulmonary venous constriction, lowers pulmonary capillary pressure, and relaxes bronchial smooth muscle. We envision a TP-dependent pressure gradient across the pulmonary microvasculature that forces fluid from the vascular compartment into the alveoli, which together with a TP-dependent increase in microvascular permeability overwhelms lymphatics and floods the small airways, leading to pulmonary edema (Figure 1).
U-46619, a PGH2 analog TP agonist, at 1 nM reduced guinea-pig pulmonary venous and airways luminal areas by 50% with little or no change in arterial luminal area (Larsson et al., 2011). Higher concentrations collapsed both pulmonary veins and airways, indicating that subnanomolar concentrations of the more potent TxA2 could produce meaningful increases in airway tone and pulmonary venous resistance (Larsson et al., 2011). This is consistent with the measured effect of ifetroban, a selective TP antagonist which reduced pulmonary venous resistance and capillary pressure in patients with acute lung injury (Schuster et al., 2001). Moreover, TP antagonism prevented hypoxemia in a lethal porcine septic shock model (Slotman et al., 1984), attenuated airway mucus hyperproduction induced by cigarette smoke (An et al., 2013), and reduced pulmonary edema in mouse models of acute lung injury (Kobayashi et al., 2016). In the patient cases presented here, we hypothesize that ramatroban enhanced pulmonary blood flow, reduced pulmonary capillary pressures, improved ventilation-perfusion matching, promoted resolution of edema, reduced bronchoconstriction and airway mucus hyperproduction, and improved gas exchange, thereby mitigating SARS-CoV-2 respiratory distress and hypoxemia (Figure 1 and Table 3).
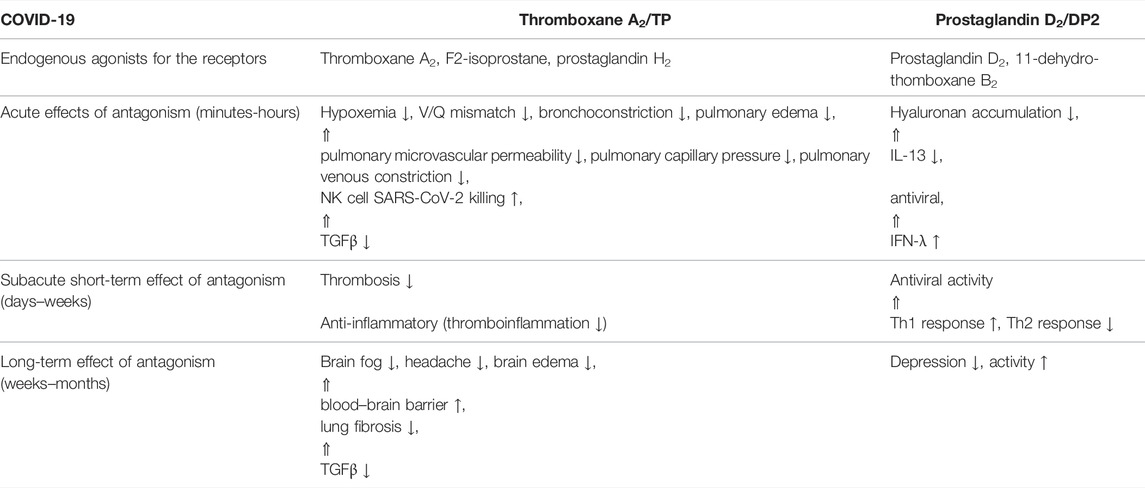
TABLE 3. Proposed effect of antagonizing thromboxane A2/TP and prostaglandin D2/DP2 signaling by ramatroban in patients with COVID-19.
Lung TxA2 generation is sufficiently elevated in symptomatic COVID-19 that TP activation may affect other critical organ functions. For example, vascular effects might include vasospasm and thrombosis resulting in renal injury, angina, arrhythmias, myocardial infarction, and/or stroke (Bauer et al., 2014). In the cerebral circulation, TP activation can increase blood–brain barrier permeability (Zhao et al., 2017), which may contribute to headache and brain fog in COVID-19. The potential of TP blockade to affect the lungs and other critical organs during acute illness and during convalescence or long COVID merits focused research.
PGD2/DP2 signaling promotes allergic inflammation by stimulating Th2 and innate lymphocyte class 2 (ILC2) cells as in asthma (Figure 1) (Xue et al., 2005; Xue et al., 2014). The maladaptive immune response in COVID-19 is characterized by a shift from Th1 to Th2 with basophilia, eosinophilia, lymphopenia, and an increase in plasma levels of type 2 cytokines produced by Th2 cells, including IL-4 and IL-13 (Lucas et al., 2020; Perlman, 2020; Yang et al., 2020). IL-4 is known to impair the barrier function of endothelial cells, leading to microvascular leakage and edema formation (Figure 1) (Skaria et al., 2016). IL-13 increases hyaluronan accumulation in mouse lungs (Donlan et al., 2021) and mucus overproduction in cultured human bronchial epithelial cells (Tanabe et al., 2008). This is correlated with ARDS, need for mechanical ventilation, acute kidney injury (AKI), and mortality in COVID-19 (Gómez-Escobar et al., 2021). Whether ramatroban inhibits inflammation and hyaluronan accumulation in ARDS remains to be investigated.
The beneficial effects of ramatroban may be additionally attributed to enhanced antiviral activity due to TxA2/TP and PGD2/DP2 antagonism. First, TxA2/TP activation stimulates activation of the TGF-β pathway (Craven et al., 1996), and early, untimely TGF-β responses in SARS-CoV-2 infection limit antiviral function of natural killer (NK) cells (Witkowski et al., 2021). Second, TxA2/TP activation may directly modulate natural cytotoxic effector cell function (Rola-Pleszczynski et al., 1985). Third, PGD2/DP2 signaling may suppress innate mucosal antiviral responses by inhibiting expression of interferon (IFN)-λ, the first line of defense against viruses at mucosal surfaces. Notably, IFN-λ is markedly suppressed in the upper respiratory tract in COVID-19 patients (Broggi et al., 2020). Increased expression of phospholipase A2 group IID and PGD2 in the elderly may further suppress IFN-λ expression (Werder et al., 2018), thereby impairing antiviral responses and contributing to increased morbidity and mortality observed consistently in the elderly (Sposito et al., 2021). Expression of nasal and pharyngeal PGD2 and DP2 in SARS-CoV-2 infection remains to be investigated even though there is significant elevation of PGD2 in bronchoalveolar lavage fluid and human lung epithelial cells (Archambault et al., 2021; Ricke-Hoch et al., 2021) and expression of PGD2 synthase and DP2 in COVID-19 kidneys (Diao et al., 2021).
Interestingly, 11-dehydro-TxB2 (11dhTxB2), a major stable metabolite of thromboxane A2, serves as a full agonist of DP2 receptors (Böhm et al., 2004). Urinary 11dhTxB2 levels are markedly increased in COVID-19 and correlate with length of hospitalization, mechanical ventilation, and mortality (Tantry et al., 2021). In rabbits infused with TxB2, 11dhTxB2 was a major metabolite, and enzymatic conversion of TxB2 to 11dhTxB2 was not detected in blood cells or plasma (Westlund et al., 1986). The dehydrogenase catalyzing formation of 11dhTxB2 was tissue bound with the highest activity in lung (Westlund et al., 1986). The above suggests that elevated lung TxA2 is converted to 11dhTxB2, which may exert local or systemic effects via DP2. In a neonatal mouse model of severe respiratory syncytial virus-induced bronchiolitis, treatment with a DP2 antagonist decreased viral load and improved morbidity associated with upregulating interferon (IFN)-λ expression (Werder et al., 2018; Theken and Fitzgerald, 2021). Whether ramatroban enhances NK cell and IFN-λ responses and reduces SARS-CoV-2 viral load remains to be investigated.
Currently, there is no treatment for long-haul COVID symptoms following recovery from acute illness. Long-haul COVID is often characterized by neuropsychiatric manifestations including “brain fog,” anxiety or depression, fatigue and problems with mobility, dyspnea, in part due to lung fibrosis and lung diffusion impairment, and microvascular thrombosis persisting for >4 months in about 25% of patients (Huang et al., 2021; Townsend et al., 2021). Despite persistence of ground glass opacities 6 months later in patient 1, lung fibrosis was not detected. This is consistent with the antifibrotic effect of ramatroban in an animal model of silicosis that is associated with markedly increased pulmonary thromboxane A2 and PGD2 (Pang et al., 2021). Moreover, in well-established animal models of depression, elevation in PGD2 mediates depression-like behavior, while ramatroban restores object exploration and social interaction (Onaka et al., 2015). The above suggests that ramatroban may help prevent and/or treat certain long-haul COVID symptoms (Table 3).
This report has several limitations. Only 4 patients could be treated with ramatroban, and the duration of treatment was brief due to very limited availability of the drug in India. Only the first patient had laboratory studies performed. Patients 2, 3, and 4 were not examined by a physician and the clinical course was reported by patients or their relatives.
During the ongoing pandemic, there is an unmet need for a drug that can provide rapid relief of respiratory symptoms and hypoxemia, halt progression of disease, and minimize hospitalization, which is associated with poor outcomes for the patient and added burden on the healthcare system. Ramatroban (Baynas®, Bayer Yakuhin, Ltd., Japan) has been safely used for the treatment of allergic rhinitis in Japan since 2000 (Ishizuka et al., 2004). The usual adult oral dose of 75 mg twice daily achieves an average plasma concentration of about 0.1 mg/L or 240 nM which is sufficient to inhibit pulmonary venous constriction, platelet activation, and release of type 2 cytokines (Table 3).
Bleeding has emerged as a significant complication in hospitalized COVID-19 patients. The overall and major bleeding rates were 4.8% (95% CI, 2.9–7.3) and 2.3% (95% CI, 1.0–4.2), respectively, among 144 patients with moderate to severe COVID-19 (Al-Samkari et al., 2020). Major bleeding occurred in 3.8% of the patients assigned to therapeutic-dose anticoagulation with heparin and in 2.3% of those assigned to usual-care pharmacologic thromboprophylaxis (The REMAP-CAP et al., 2021). In the 4-patient case series reported here, only one patient (Case 2, A.K.) experienced any evidence of bleeding. He reported blood-tinged sputum >24 h after the last dose of ramatroban, but that was not clinically significant. Because ramatroban’s plasma half-life is about 2 h, platelet-dependent hemostasis is unlikely to be continuously impaired with 75-mg doses given about 12 h apart (Ishizuka et al., 2004; Nippon Shinyaku Co. Ltd, 2009).
The rapid and salutary responses to ramatroban reported here, and its diverse actions targeting the major pathobiologic mechanisms underlying COVID-19 (Table 1 and Figure 1), coupled with its oral bioavailability and an excellent safety profile make ramatroban an attractive therapeutic agent to test in randomized controlled clinical trials.
Data Availability Statement
The original contributions presented in the study are included in the article/Supplementary Material, and further inquiries can be directed to the corresponding authors.
Ethics Statement
Ethical review and approval was not required for the study on human participants in accordance with the local legislation and institutional requirements. The patients/participants provided their written informed consent to participate in this study.
Author Contributions
KC and AG conceptualized the study. The data were provided by authors RK, AdA, and AsA. MLO expanded and refocused scientific rationale. KC and AG prepared the first draft of the manuscript which was subsequently edited, reviewed, and approved by all authors.
Conflict of Interest
MLO has filed a patent claiming thromboxane receptor antagonists for the treatment of COVID-19. AG and KC have filed a patent on the use of a dual receptor antagonist of thromboxane A2 /TP and PGD2–DP2 receptors for treatment of maladaptive immune response or thrombotic diathesis. RK was employed by Regulatory Wisdom.
The remaining authors declare that the research was conducted in the absence of any commercial or financial relationships that could be construed as a potential conflict of interest.
Publisher’s Note
All claims expressed in this article are solely those of the authors and do not necessarily represent those of their affiliated organizations, or those of the publisher, the editors, and the reviewers. Any product that may be evaluated in this article, or claim that may be made by its manufacturer, is not guaranteed or endorsed by the publisher.
Abbreviations
Tx, thromboxane; 11dhTxB2, 11-dehydro-thromboxane B2; TP, thromboxane prostanoid receptor; PG, prostaglandin; COX, cyclooxygenase; DP2, D prostanoid receptor 2; URTI, upper respiratory tract infection; BALF, bronchoalveolar lavage fluid; SARS-CoV-2, severe acute respiratory syndrome coronavirus 2; COVID-19, coronavirus disease 2019; RSV, respiratory syncytial virus; IL, interleukin; MDSC, monocyte–macrophage-derived suppressor cell; IFN, interferon; ARDS, acute respiratory distress syndrome; SpO2, blood oxygen saturation by pulse oximetry; TGF-β, transforming growth factor beta; NK cells, natural killer cells; NETs, neutrophil extracellular traps.
References
Agarwal, A., Mukherjee, A., Kumar, G., Chatterjee, P., Bhatnagar, T., and Malhotra, P. (2020). Convalescent Plasma in the Management of Moderate Covid-19 in Adults in India: Open Label Phase II Multicentre Randomised Controlled Trial (PLACID Trial). BMJ 371, m3939. doi:10.1136/bmj.m3939
Al-Hakeim, H. K., Al-Hamami, S. A., Almulla, A. F., and Maes, M. (2021). Increased Serum Thromboxane A2 and Prostacyclin but Lower Complement C3 and C4 Levels in COVID-19: Associations with Chest CT Scan Anomalies and Lowered Peripheral Oxygen Saturation. COVID 1 (2), 489–502. doi:10.3390/covid1020042
Al-Samkari, H., Karp Leaf, R. S., Dzik, W. H., Carlson, J. C. T., Fogerty, A. E., Waheed, A., et al. (2020). COVID-19 and Coagulation: Bleeding and Thrombotic Manifestations of SARS-CoV-2 Infection. Blood 136 (4), 489–500. doi:10.1182/blood.2020006520
An, J., Li, J. Q., Wang, T., Li, X. O., Guo, L. L., Wan, C., et al. (2013). Blocking of Thromboxane A₂ Receptor Attenuates Airway Mucus Hyperproduction Induced by Cigarette Smoke. Eur. J. Pharmacol. 703 (1-3), 11–17. doi:10.1016/j.ejphar.2013.01.042
Archambault, A. S., Zaid, Y., Rakotoarivelo, V., Turcotte, C., Doré, É., Dubuc, I., et al. (2021). High Levels of Eicosanoids and Docosanoids in the Lungs of Intubated COVID-19 Patients. FASEB J. 35 (6), e21666. doi:10.1096/fj.202100540r
Bauer, J., Ripperger, A., Frantz, S., Ergün, S., Schwedhelm, E., and Benndorf, R. A. (2014). Pathophysiology of Isoprostanes in the Cardiovascular System: Implications of Isoprostane-Mediated Thromboxane A2 Receptor Activation. Br. J. Pharmacol. 171 (13), 3115–3131. doi:10.1111/bph.12677
Böhm, E., Sturm, G. J., Weiglhofer, I., Sandig, H., Shichijo, M., McNamee, A., et al. (2004). 11-Dehydro-thromboxane B2, a Stable Thromboxane Metabolite, Is a Full Agonist of Chemoattractant Receptor-Homologous Molecule Expressed on TH2 Cells (CRTH2) in Human Eosinophils and Basophils. J. Biol. Chem. 279 (9), 7663–7670. doi:10.1074/jbc.m310270200
Bowers, R. E., Ellis, E. F., Brigham, K. L., and Oates, J. A. (1979). Effects of Prostaglandin Cyclic Endoperoxides on the Lung Circulation of Unanesthetized Sheep. J. Clin. Invest. 63 (1), 131–137. doi:10.1172/JCI109266
Broggi, A., Ghosh, S., Sposito, B., Spreafico, R., Balzarini, F., Lo Cascio, A., et al. (2020). Type III Interferons Disrupt the Lung Epithelial Barrier upon Viral Recognition. Science 369 (6504), 706–712. doi:10.1126/science.abc3545
Craven, P. A., Studer, R. K., and DeRubertis, F. R. (1996). Thromboxane/prostaglandin Endoperoxide-Induced Hypertrophy of Rat Vascular Smooth Muscle Cells Is Signaled by Protein Kinase C-dependent Increases in Transforming Growth Factor-Beta. Hypertension 28 (2), 169–176. doi:10.1161/01.HYP.28.2.169
Daher, A., Balfanz, P., Aetou, M., Hartmann, B., Müller-Wieland, D., Müller, T., et al. (2021). Clinical Course of COVID-19 Patients Needing Supplemental Oxygen outside the Intensive Care Unit. Sci. Rep. 11 (1), 2256. doi:10.1038/s41598-021-81444-9
Diao, B., Wang, C., Wang, R., Feng, Z., Zhang, J., Yang, H., et al. (2021). Human Kidney Is a Target for Novel Severe Acute Respiratory Syndrome Coronavirus 2 Infection. Nat. Commun. 12 (1), 2506. doi:10.1038/s41467-021-22781-1
Donlan, A. N., Sutherland, T. E., Marie, C., Preissner, S., Bradley, B. T., Carpenter, R. M., et al. (2021). IL-13 Is a Driver of COVID-19 Severity. JCI Insight 6 (15), e150107. doi:10.1172/jci.insight.150107
Gómez-Escobar, L. G., Hoffman, K. L., Choi, J. J., Borczuk, A., Salvatore, S., Alvarez-Mulett, S. L., et al. (2021). Cytokine Signatures of End Organ Injury in COVID-19. Sci. Rep. 11 (1), 12606. doi:10.1038/s41598-021-91859-z
Gupta, A., and Chander Chiang, K. (2020). Prostaglandin D2 as a Mediator of Lymphopenia and a Therapeutic Target in COVID-19 Disease. Med. Hypotheses 143, 110122. doi:10.1016/j.mehy.2020.110122
Gupta, A., Kalantar-Zadeh, K., and Reddy, S. T. (2020). Ramatroban as a Novel Immunotherapy for COVID-19. J. Mol. Genet. Med. 14 (3). doi:10.37421/jmgm.2020.14.457
Hottz, E. D., Azevedo-Quintanilha, I. G., Palhinha, L., Teixeira, L., Barreto, E. A., Pão, C. R. R., et al. (2020). Platelet Activation and Platelet-Monocyte Aggregate Formation Trigger Tissue Factor Expression in Patients with Severe COVID-19. Blood 136, 1330–1341. doi:10.1182/blood.2020007252
Huang, L., Yao, Q., Gu, X., Wang, Q., Ren, L., Wang, Y., et al. (2021). 1-year Outcomes in Hospital Survivors with COVID-19: a Longitudinal Cohort Study. Lancet 398 (10302), 747–758. doi:10.1016/s0140-6736(21)01755-4
Ishizuka, T., Matsui, T., Okamoto, Y., Ohta, A., and Shichijo, M. (2004). Ramatroban (BAY U 3405): a Novel Dual Antagonist of TXA2 Receptor and CRTh2, a Newly Identified Prostaglandin D2 Receptor. Cardiovasc Drug Rev. 22 (2), 71–90. doi:10.1111/j.1527-3466.2004.tb00132.x
Kadowitz, P. J., Gruetter, C. A., McNamara, D. B., Gorman, R. R., Spannhake, E. W., and Hyman, A. L. (1977). Comparative Effects of Endoperoxide PGH2 and an Analog on the Pulmonary Vascular Bed. J. Appl. Physiol. Respir. Environ. Exerc Physiol. 42 (6), 953–958. doi:10.1152/jappl.1977.42.6.953
Kobayashi, K., Horikami, D., Omori, K., Nakamura, T., Yamazaki, A., Maeda, S., et al. (2016). Thromboxane A2 Exacerbates Acute Lung Injury via Promoting Edema Formation. Sci. Rep. 6 (1), 32109. doi:10.1038/srep32109
Kumar, P., Osahon, O., Vides, D. B., Hanania, N., Minard, C. G., and Sekhar, R. V. (2021). Severe Glutathione Deficiency, Oxidative Stress and Oxidant Damage in Adults Hospitalized with COVID-19: Implications for GlyNAC (Glycine and N-Acetylcysteine) Supplementation. Antioxidants (Basel) 11 (1). doi:10.3390/antiox11010050
Larsson, A. K., Hagfjärd, A., Dahlén, S. E., and Adner, M. (2011). Prostaglandin D₂ Induces Contractions through Activation of TP Receptors in Peripheral Lung Tissue from the guinea Pig. Eur. J. Pharmacol. 669 (1-3), 136–142. doi:10.1016/j.ejphar.2011.07.046
Lucas, C., Wong, P., Klein, J., Castro, T. B. R., Silva, J., Sundaram, M., et al. (2020). Longitudinal Analyses Reveal Immunological Misfiring in Severe COVID-19. Nature 584 (7821), 463–469. doi:10.1038/s41586-020-2588-y
NIH (2021). What's New in the Guidelines [Online]. Available: https://www.covid19treatmentguidelines.nih.gov/about-the-guidelines/whats-new/(Accessed October 30, 2021).
Nippon Shinyaku Co. Ltd (2009). Prostaglandin D2 and Thromboxane A2 Receptor Antagonists; Medicine for Allergic Rhinitis; Baynas Tablets Package Insert [Online]. Available: https://www.google.com/search?q=https%3A%2F%2Fwww.nippon-+shinyaku.co.jp%2Fassets%2Ffiles%2Fpdfs%2Fmedicine%2Fproduct%2Fha%2Fbaynas%2Finterview_baynas_t.pdf&rlz=1C1GCEB_enIN990IN990&oq=https%3A%2F%2Fwww.nippon-+shinyaku.co.jp%2Fassets%2Ffiles%2Fpdfs%2Fmed (Accessed April 27, 2021).
Onaka, Y., Shintani, N., Nakazawa, T., Haba, R., Ago, Y., Wang, H., et al. (2015). CRTH2, a Prostaglandin D2 Receptor, Mediates Depression-Related Behavior in Mice. Behav. Brain Res. 284, 131–137. doi:10.1016/j.bbr.2015.02.013
Pang, J., Qi, X., Luo, Y., Li, X., Shu, T., Li, B., et al. (2021). Multi-omics Study of Silicosis Reveals the Potential Therapeutic Targets PGD2 and TXA2. Theranostics 11 (5), 2381–2394. doi:10.7150/thno.47627
Perlman, S. (2020). COVID-19 Poses a Riddle for the Immune System. Nature 584 (7821), 345–346. doi:10.1038/d41586-020-02379-1
Regidor, P. A., De La Rosa, X., Santos, F. G., Rizo, J. M., Gracia Banzo, R., and Silva, R. S. (2021). Acute Severe SARS COVID-19 Patients Produce Pro-resolving Lipids Mediators and Eicosanoids. Eur. Rev. Med. Pharmacol. Sci. 25 (21), 6782–6796. doi:10.26355/eurrev_202111_27123
Ricke-Hoch, M., Stelling, E., Lasswitz, L., Gunesch, A. P., Kasten, M., Zapatero-Belinchón, F. J., et al. (2021). Impaired Immune Response Mediated by Prostaglandin E2 Promotes Severe COVID-19 Disease. PLOS ONE 16 (8), e0255335. doi:10.1371/journal.pone.0255335
Rola-Pleszczynski, M., Gagnon, L., Bolduc, D., and LeBreton, G. (1985). Evidence for the Involvement of the Thromboxane Synthase Pathway in Human Natural Cytotoxic Cell Activity. J. Immunol. 135 (6), 4114–4119.
Schuster, D. P., Kozlowski, J., and Brimiouelle, S. (2001). “Effect of Thromboxane Receptor Blockade on Pulmonary Capillary Hypertension in Acute Lung Injury,” in 2001 Meeting of the American Thoracic Society (San Francisco, CA: Springer).
Skaria, T., Burgener, J., Bachli, E., and Schoedon, G. (2016). IL-4 Causes Hyperpermeability of Vascular Endothelial Cells through Wnt5A Signaling. PLOS ONE 11 (5), e0156002. doi:10.1371/journal.pone.0156002
Slotman, G. J., Quinn, J. V., Burchard, K. W., and Gann, D. S. (1984). Thromboxane Interaction with Cardiopulmonary Dysfunction in Graded Bacterial Sepsis. J. Trauma 24 (9), 803–810. doi:10.1097/00005373-198409000-00005
Sposito, B., Broggi, A., Pandolfi, L., Crotta, S., Clementi, N., Ferrarese, R., et al. (2021). The Interferon Landscape along the Respiratory Tract Impacts the Severity of COVID-19. Cell 184, 4953–4968. doi:10.1016/j.cell.2021.08.016
Tanabe, T., Fujimoto, K., Yasuo, M., Tsushima, K., Yoshida, K., Ise, H., et al. (2008). Modulation of Mucus Production by Interleukin-13 Receptor Alpha2 in the Human Airway Epithelium. Clin. Exp. Allergy 38 (1), 122–134. doi:10.1111/j.1365-2222.2007.02871.x
Tantry, U. S., Bliden, K. P., Cho, A., Walia, N., Dahlen, J. R., Ens, G., et al. (2021). First Experience Addressing the Prognostic Utility of Novel Urinary Biomarkers in Patients with COVID-19. Open Forum Infect. Dis. 8 (7), ofab274. doi:10.1093/ofid/ofab274
The REMAP-CAPACTIV-4aATTACC Investigators (2021). Therapeutic Anticoagulation with Heparin in Critically Ill Patients with Covid-19. N. Engl. J. Med. 385 (9), 777–789. doi:10.1056/NEJMoa2103417
Theken, K. N., and Fitzgerald, G. A. (2021). Bioactive Lipids in Antiviral Immunity. Science 371 (6526), 237–238. doi:10.1126/science.abf3192
Townsend, L., Fogarty, H., Dyer, A., Martin‐Loeches, I., Bannan, C., Nadarajan, P., et al. (2021). Prolonged Elevation of D‐dimer Levels in Convalescent COVID‐19 Patients Is Independent of the Acute Phase Response. J. Thromb. Haemost. 19 (4), 1064–1070. doi:10.1111/jth.15267
Trust for American’s Health (2021). CDC Data Show High Hospitalization Rates for Diagnosed COVID-19 Patients with Underlying Conditions in the United States [Online]. Available: https://www.tfah.org/wp- content/uploads/2020/04/COVIDunderlyingconditions040320.pdf (Accessed October 30, 2021).
Uller, L., Mathiesen, J. M., Alenmyr, L., Korsgren, M., Ulven, T., Högberg, T., et al. (2007). Antagonism of the Prostaglandin D2 Receptor CRTH2 Attenuates Asthma Pathology in Mouse Eosinophilic Airway Inflammation. Respir. Res. 8 (1), 16. doi:10.1186/1465-9921-8-16
Walch, L., De Montpreville, V., Brink, C., and Norel, X. (2001). Prostanoid EP(1)- and TP-Receptors Involved in the Contraction of Human Pulmonary Veins. Br. J. Pharmacol. 134 (8), 1671–1678. doi:10.1038/sj.bjp.0704423
Werder, R. B., Lynch, J. P., Simpson, J. C., Zhang, V., Hodge, N. H., Poh, M., et al. (2018). PGD2/DP2 Receptor Activation Promotes Severe Viral Bronchiolitis by Suppressing IFN-λ Production. Sci. Transl. Med. 10 (440). doi:10.1126/scitranslmed.aao0052
Westlund, P., Kumlin, M., Nordenström, A., and Granström, E. (1986). Circulating and Urinary Thromboxane B2 Metabolites in the Rabbit: 11-Dehydro-Thromboxane B2 as Parameter of Thromboxane Production. Prostaglandins 31 (3), 413–443. doi:10.1016/0090-6980(86)90106-1
Witkowski, M., Tizian, C., Ferreira-Gomes, M., Niemeyer, D., Jones, T. C., Heinrich, F., et al. (2021). Untimely TGFβ Responses in COVID-19 Limit Antiviral Functions of NK Cells. Nature 600, 295–301. doi:10.1038/s41586-021-04142-6
Xue, L., Gyles, S. L., Wettey, F. R., Gazi, L., Townsend, E., Hunter, M. G., et al. (2005). Prostaglandin D2 Causes Preferential Induction of Proinflammatory Th2 Cytokine Production through an Action on Chemoattractant Receptor-like Molecule Expressed on Th2 Cells. J. Immunol. 175 (10), 6531–6536. doi:10.4049/jimmunol.175.10.6531
Xue, L., Salimi, M., Panse, I., Mjösberg, J. M., McKenzie, A. N., Spits, H., et al. (2014). Prostaglandin D2 Activates Group 2 Innate Lymphoid Cells through Chemoattractant Receptor-Homologous Molecule Expressed on TH2 Cells. J. Allergy Clin. Immunol. 133 (4), 1184–1194. e1187. doi:10.1016/j.jaci.2013.10.056
Yang, L., Liu, S., Liu, J., Zhang, Z., Wan, X., Huang, B., et al. (2020). COVID-19: Immunopathogenesis and Immunotherapeutics. Signal Transduct. Target Ther. 5 (1), 128. doi:10.1038/s41392-020-00243-2
Keywords: SARS-CoV-2, COVID-19, ARDS, hypoxemia, thromboxane A2, ramatroban, pulmonary hypertension, pneumonia
Citation: Ogletree ML, Chander Chiang K, Kulshrestha R, Agarwal A, Agarwal A and Gupta A (2022) Treatment of COVID-19 Pneumonia and Acute Respiratory Distress With Ramatroban, a Thromboxane A2 and Prostaglandin D2 Receptor Antagonist: A Four-Patient Case Series Report. Front. Pharmacol. 13:904020. doi: 10.3389/fphar.2022.904020
Received: 25 March 2022; Accepted: 15 June 2022;
Published: 22 July 2022.
Edited by:
Emanuela Ricciotti, University of Pennsylvania, United StatesCopyright © 2022 Ogletree, Chander Chiang, Kulshrestha, Agarwal, Agarwal and Gupta. This is an open-access article distributed under the terms of the Creative Commons Attribution License (CC BY). The use, distribution or reproduction in other forums is permitted, provided the original author(s) and the copyright owner(s) are credited and that the original publication in this journal is cited, in accordance with accepted academic practice. No use, distribution or reproduction is permitted which does not comply with these terms.
*Correspondence: Martin L. Ogletree, martin.ogletree@vanderbilt.edu; Ajay Gupta, ajayg1@hs.uci.edu
†ORCID: Martin L. Ogletree, orcid.org/0000-0001-5142-1276; Kate Chander Chiang, orcid.org/0000-0003-0999-7516; Ajay Gupta, orcid.org/0000-0001-9182-3499
‡These authors have contributed equally to this work