- 1Department of Gastroenterology, The First Affiliated Hospital of China Medical University, Shenyang, China
- 2The Second Clinical College, China Medical University, Shenyang, China
- 3Department of Clinical Laboratory, Affiliated Hospital of Guilin Medical University, Guilin, China
- 4Department of Gastroenterology, Shengjing Hospital of China Medical University, Shenyang, China
- 5Department of Geriatric Medicine, The First Affiliated Hospital of China Medical University, Shenyang, China
Allicin is the main active ingredient in freshly-crushed garlic and some other allium plants, and its anticancer effect on cancers of digestive system has been confirmed in many studies. The aim of this review is to summarize epidemiological studies and in vitro and in vivo investigations on the anticancer effects of allicin and its secondary metabolites, as well as their biological functions. In epidemiological studies of esophageal cancer, liver cancer, pancreatic cancer, and biliary tract cancer, the anticancer effect of garlic has been confirmed consistently. However, the results obtained from epidemiological studies in gastric cancer and colon cancer are inconsistent. In vitro studies demonstrated that allicin and its secondary metabolites play an antitumor role by inhibiting tumor cell proliferation, inducing apoptosis, controlling tumor invasion and metastasis, decreasing angiogenesis, suppressing Helicobacter pylori, enhancing the efficacy of chemotherapeutic drugs, and reducing the damage caused by chemotherapeutic drugs. In vivo studies further demonstrate that allicin and its secondary metabolites inhibit cancers of the digestive system. This review describes the mechanisms against cancers of digestive system and therapeutic potential of allicin and its secondary metabolites.
1 Introduction
Global cancer statistics in 2020 showed that digestive system cancers, such as colorectal cancer, liver cancer, gastric cancer, esophageal cancer, pancreatic cancer, and cholangiocarcinoma, usually have a high risk of morbidity and mortality, and digestive system cancer is considered the leading cause of cancer-related death in the world (Sung et al., 2021). Recent reports have shown that the incidence rate and mortality rate of digestive system cancer are still rising, and it has become a global health problem that seriously threatens human health (Smyth et al., 2017; Bray et al., 2018; Villanueva, 2019; Sung et al., 2021). At the same time, some studies have shown that digestive system cancers have some pathogenesis factors in common (Zhang et al., 2018; Wang et al., 2020). Therefore, comparing the pathogenesis of various digestive system cancers and the effect of anticancer agents on cancers of the digestive system may provide a basis for the discovery of more effective digestive system anti-tumor therapies. The current therapeutic strategies for digestive system cancer mainly include surgery, radiotherapy, chemotherapy and immunotherapy, which are often accompanied by many disadvantages, such as drug resistance, risk of recurrence, poor prognosis, and high cost (Pennathur et al., 2013; Gravitz, 2014; Razumilava and Gores, 2014; Dekker et al., 2019; Mizrahi et al., 2020; Smyth et al., 2020). As a result, new therapies are needed to better control digestive system cancer. To reduce the adverse effects of current major therapies, researchers have focused on natural products.
Allicin (diallyl thiosulfinate) is a natural product formed chemically on crushing a garlic clove (Sarvizadeh et al., 2021). It is unstable and transforms into a variety of bioactive secondary metabolites (Sarvizadeh et al., 2021). Allicin and its secondary metabolites are organosulfur compounds (OSCs) with a variety of biological activities, including anticancer, antioxidation, and antipathogenic activities, and their effects act on cancers of digestive system have attracted extensive attention (Min and ZhuBo, 2011; El-Saber Batiha et al., 2020). Previous studies have shown that the OSCs act against digestive system cancers by suppressing proliferation, inducing apoptosis, and inhibiting invasion, metastasis, and angiogenesis of the tumor (Sarvizadeh et al., 2021). In addition, these OSCs have been found to enhance the efficacy of chemotherapeutic drugs and reduce the side effects caused by traditional therapies under certain conditions (Zhang et al., 2020). Although the research results of many studies are promising, the findings of some experiments are controversial. For example, the results of some epidemiological investigations are inconsistent in the study of allicin intervention in gastric cancer (Kim et al., 2018; Li WQ. et al., 2019). Therefore, to further explore the anticancer effect of allicin and its possible clinical application, we searched for articles on related investigations up to March 2022 in the PubMed and Web of Science databases to systematically summarize the biological functions of these compounds in vivo and in vitro and to clarify the mechanism of the anticancer effect of allicin and its secondary metabolites on cancers of the digestive system. The aim of this review is to provide a convenient reference for researchers seeking to carry out further research.
2 Allicin
2.1 Chemical Structure and Formation of Allicin
Allicin is the primary product formed on crushing a garlic clove. It is an electrophilic thioallyl ester of allylsulfinic acid with a pungent smell reminiscent of a pizza parlour (Borlinghaus et al., 2014). Cavallito and Bailey firstly isolated and described the properties of allicin in 1944, and Stoll and Seebeck determined its structure in 1948 (Cavallito C. J. and Bailey J. H., 1944; Stoll and Seebeck, 1948). In nature allicin is produced via an enzymatic reaction after plant tissue damage (Borlinghaus et al., 2014). Alliin [(+)-S-allyl-l-cysteine sulfoxide] is the precursor of allicin and is one of the major S-alk(en)yl-l-cysteine sulfoxides identified in allium plants (Stoll and Seebeck, 1948). S-alk(en)yl-l-cysteine sulfoxides are nonvolatile sulfur storage compounds giving rise to the different odor, flavor and biological activities in allium plants (Rose et al., 2005). They biosynthesized via a series of reactions as follows: S-alk(en)ylation of the cysteine residue of glutathione, followed by transpeptidation to remove the glycyl residue, then proceeding oxidation and loss of the glutamyl group to form the parental S-alk(en)yl-l-cysteine sulfoxides (Rose et al., 2005). Moreover, they can also alternatively biosynthesized via direct S-alk(en)ylation of cysteine or thioalk(en)ylation of O-acetyl serine followed by oxidation (Rose et al., 2005). Under the catalysis of alliinase [EC 4.4.1.4] and the presence of the cofactor pyridoxal 5′-phosphate, S-alk(en)yl-l-cysteine sulfoxides hydrolyze and produce pyruvate, ammonia, and sulfenic acids (Block, 1992). Sulfenic acid (RSOH) synthesized from S-alk(en)yl-l-cysteine sulfoxides are highly reactive and thus converted into thiosulfinates by self-condensation, and due to different R groups, a variety of thiosulfinates can be produced (Figure 1) (Yoshimoto and Saito, 2019). Thiosulifnates derived from allium plants can be divided into four types: 1) fully saturated, RS(O)SR' (R,R' = Me or Pr); 2) mono- or bis-β,γ-unsaturated thiosulfinates AllS(O)SMe, AllSS(O) Me or AllS(O)SAll; 3) mono-α,β-unsaturated thiosulfinates; and 4) mixed α,β- and β,γ-unsaturated thiosulfinates (Block, 1992). As a major S-alk(en)yl-l-cysteine sulfoxides, the main processes of alliin synthesis are the same as the process described above in general. γ-Glutamylcysteine and glutamylcysteine produced by cysteine are the starting compounds for the synthesis of alliin. With the participation of α-methacrylic acid, both γ-glutamylcysteine and glutamylcysteine can form γ-glutamyl-S-(2-carboxypropyl) cysteine (Rose et al., 2005). γ-Glutamyl-S-(2-carboxypropyl) cysteine undergoes decarboxylation and form γ-glutamyl-S-allylcysteine (Rose et al., 2005). In garlic, γ-glutamyl-S-allylcysteine first undergoes deglutamylation to produce S-allylcysteine, which is catalyzed by recombinant proteins AsGGT1, AsGGT2, and AsGGT3 (Yoshimoto and Saito, 2019). Moreover, S-allylcysteine can also been produced via the process of serine reacting with allylthiol. Then S-allylcysteine undergoes S-oxygenation which is catalyzed by recombinant AsFMO1 protein, to produce alliin (Yoshimoto and Saito, 2019). Alliin is further decomposed into allylsulfonic acid under the catalysis of alliinase, and then the two molecules of allylsulfenic acid can condense spontaneously to produce one molecule of allicin, which is a di-S-β,γ-unsaturated thiosulfinate [AllS(O)SAll] (Block, 1992). The specific synthesis process of alliin and allicin are shown in Figure 2. Allicin can be further decomposed into allylsulfenic acid and thioacrolein (Rose et al., 2005). Thioacrolein can undergo self-condensation by a Diels–Alder reaction to produce the cyclics 2-vinyl-[4H]-1,3-dithin (2VD) and 3-vinyl-[4H]-1,2-dithin (3VD), and allylsulfenic acid can also undergo self-condensation to form allicin again (Block, 1985). Allicin is a liposoluble OSC that is unstable after synthesis, and in vitro, it will immediately decompose into a series of liposoluble organic sulfides, including diallyl disulfide (DADS), diallyl trisulfide (DATS), ajoene, allyl methyl trisulfide (AMTS), dithiins, and diallyl sulfide (DAS) (El-Saber Batiha et al., 2020). In vivo, allicin can also synthesize water-soluble OSCs such as S-allylmercaptocysteine (SAMC) and S-allylmercaptoglutathione (SAMG) by interacting with l-cysteine and glutathione (GSH), respectively (Rouf et al., 2020). The structure of allicin and its related compounds are shown in Figure 3.
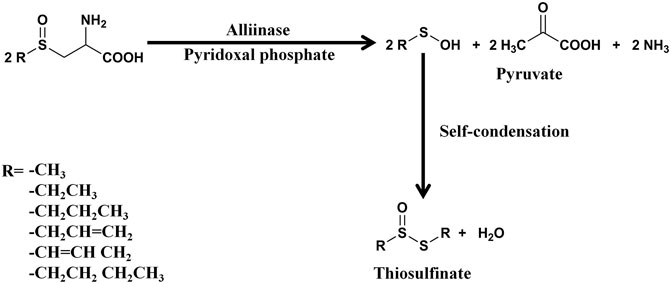
FIGURE 1. The synthesis process of thiosulfinates. One molecule of thiosulfinate is synthesized from two molecules of sulfenic acids, and the figure shows the different R groups of thiosulfinates (Rose et al., 2005).
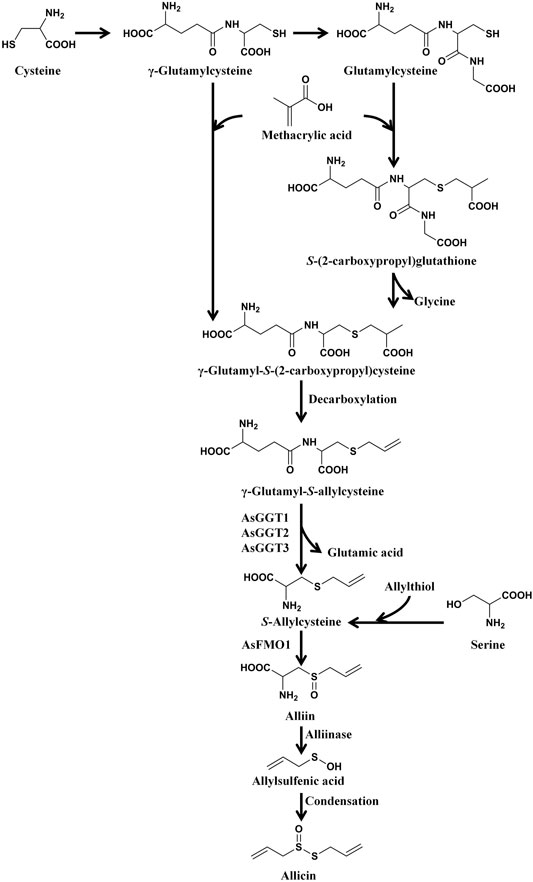
FIGURE 2. The synthesis process of alliin and allicin. Cysteine first synthesizes γ-glutamylcysteine and glutamylcysteine, and then the two compounds react with α-methacrylic acid, and synthesize γ-glutamyl-S (-2-carboxypropyl) cysteine and S-(2-carboxypropyl) glutathione, respectively. Subsequently, S-(2-carboxypropyl) glutathione also forms γ-glutamyl-S (-2-carboxypropyl) cysteine. Then γ-glutamyl-S-(2-carboxypropyl) cysteine undergoes decarboxylation and form γ-glutamyl-S-allylcysteine. γ-Glutamyl-S-allylcysteine first undergoes deglutamylation to produce S-allylcysteine (catalyzed by recombinant AsGGT1, AsGGT2, and AsGGT3), and then undergoes S-oxygenation (catalyzed by recombinant AsFMO1) to produce alliin. S-allylcysteine can also been produced by the process of serine reacting with allylthiol. After synthesis, alliin is further decomposed into allylsulfenic acid under the catalysis of alliinase, and then the two molecules of allylsulfenic acid condense spontaneously to produce one molecule of allicin. (Rose et al., 2005; Yoshimoto and Saito, 2019).
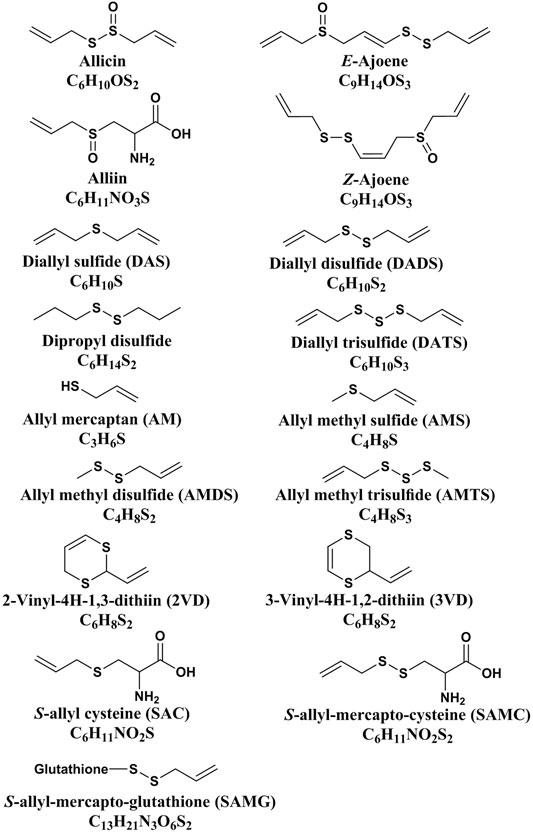
FIGURE 3. The structure of allicin and its related compounds (Zhang et al., 2020).
In addition, allicin can undergo the thiol-disulfide exchange reaction (TDER) with protein thiols, which is considered to be the key to allicin’s biological activity (Poole, 2015). The TDER is that thiols (RSH) react with disulfides (R'SSR') and form a new disulfide (RSSR′) and a new thiol (R'SH) (Rose et al., 2018). In the TDER, though both the divalent sulfur atom and the sulfinyl sulfur atom of allicin are electrophilic, most of the chemistry occurs by nucleophilic attack on the divalent sulfur, with the sulfinyl sulfur being the leaving group when the O-atom is protonated (Borlinghaus et al., 2021). The polarized bond between the O- and S-atoms of the sulfinyl group significantly weakens the disulfide bond in allicin, making it more reactive to target nucleophilic thiol groups than a simple disulfide bond, and the newly formed disulfide bond can be reduced back to a thiol just like other protein disulfide (Borlinghaus et al., 2021). Allicin acts as a sulfenylating agent of thiols to form disulfides with the formation of water. One molecule of allicin can totally react with two molecules of thiols and form two molecules of disulfides (Poole, 2015). This is because the leaving group in the first exchange between thiol and allicin generates allylsulfenic acid, which can also act as an electrophilic sulfenylating agent towards a thiol with the expulsion of water (Poole, 2015). In addition, the allylsulfenic acid can dimerize to generate allicin and water again (Block, 1992). Therefore, allicin is a crucial thiol oxidant participating in the thiol/disulfide homeostasis. Based on the chemistry, allicin can react with protein thiols, modify Cys residues, thereby regulating various metabolic processes in cells and exert its biological activity (Poole, 2015).
In addition to natural plants, allicin and its secondary metabolites also exist in some artificial preparations, such as aged garlic extract (AGE), aged black garlic extract (ABGE), garlic powder (GP), and garlic oil (GO). The commercially available “Allimaxˮ is stabilized allicin, and is extracted from fresh, raw garlic through crushing, filtration and temperature controlled extraction process via a patented aqueous extraction method, and the pure allicin is dissolved in water (Josling, 2001). GP contains alliin and a small amount of oil-soluble sulfur compounds, and its physiological activity is similar to that of fresh garlic (Lawson and Gardner, 2005). The biologically active compounds in GO are oil-soluble sulfur compounds such as DADS and DATS (Amagase et al., 2001). AGE consists of various OSCs, both hydrophilic and hydrophobic, and mainly contains water-soluble compounds (e.g., SAC, SAMC, and GSAC) with a small amount of oil-soluble sulfur compounds (e.g., DASn) (Amagase et al., 2001; Kodera et al., 2020). ABGE is a kind of AGE that is made of aged black garlic, and it plays a role in anticancer activity, regulates lipid metabolism, anti-inflammation, and antioxidant activities and is more potent than other preparations in many of the therapeutic properties of garlic (Dong et al., 2014; Nam et al., 2018; You et al., 2019). As a result, many researchers use these kinds of garlic preparations as materials to study the biological functions of allicin and its secondary metabolites.
2.2 Toxicity
Allicin is a membrane-permeable compound that can enter cells easily and can oxidize cellular thiols such as glutathione or cysteine residues in proteins as well as enzymes containing reactive cysteine since it is a reactive sulfur species (RSS) (Miron et al., 2000; Gruhlke and Slusarenko, 2012). Oxidation of protein thiols results in changes in protein structure, for example through disulfide bond formation (Gruhlke and Slusarenko, 2012), which in turn leads to changes in cell function, which may explain its cytotoxic potential and toxicity on normal cells (Gruhlke et al., 2016).
A randomized controlled trial has shown that high doses of allicin in sensitive people can cause a range of side effects, including insomnia, vomiting, heartburn, dizziness, diarrhea, tachycardia, nausea, bloating, flushing, headache, mild orthostatic hypotension, sweating, offensive body odor, and flatulence (Desai et al., 1990). Another experiment showed that low doses (250, 500 mg/kg/day) (p < 0.02) of garlic endogenous enhanced antioxidant status in mice, but high doses (1,000 mg/kg/day) induced pathological changes in morphology of kidney and liver, indicating dose-related toxicity (Banerjee et al., 2001). Other researches have shown that low doses of garlic are safe, whereas therapeutic doses might cause mild gastrointestinal disorders, while high doses have been reported to cause liver damage (Almogren et al., 2013; Ried and Fakler, 2014). Besides, an in vivo study showed that high doses of raw garlic over a long period of time can lead to weight loss, as well as red blood cell lysis, which could be associated with oxidative hemolysis (Borrelli et al., 2007). Chronic administration of garlic powder (50 mg/d) also resulted in inhibition of spermatogenesis in rats (Dixit and Joshi, 1982). Moreover, intraperitoneal and oral administration of high doses (5 ml garlic juice/kg) led to weight loss, and hepatic and pulmonary toxicity in rats (Nakagawa et al., 1980). Depending on the dose consumed, symptoms of garlic poisoning usually appear a day or several days after taking (Lee et al., 2006).
2.3 Biological Function of Allicin and its Secondary Metabolites
2.3.1 Anticancer
An early study in 1960 showed that tumor growth could be inhibited by intraperitoneal injection of tumor cells into mice after culturing ascites with allicin in vitro (Dipaolo and Carruthers, 1960). Allicin can induce cancer cells to form apoptotic bodies and DNA ladders (Oommen et al., 2004). Furthermore, it has been found that allicin can induce redox shifts in cultured human cells, which results in the activation of the mitochondrial apoptotic pathway (Miron et al., 2008). In addition, studies found that NF-E2-related factor-2 (Nrf2) can mediate the apoptosis induced by allicin in colonic cancer cells, although Nrf2 is usually considered an anti-apoptotic factor that upregulates the anti-apoptotic protein Bcl-2 (Bat-Chen et al., 2010; Niture and Jaiswal, 2012). As the precursor of allicin, alliin shows anti-proliferative potential in a human gastric adenocarcinoma cell line, and it has been confirmed that it does not affect the growth of normal intestinal cells when inducing apoptosis of gastric cancer cells (Mansingh et al., 2018). In addition, DADS has been proven to inhibit human esophageal xenograft tumors through various pathways, such as RAF/MEK/ERK and mitochondria-dependent pathways (Yin et al., 2014). DATS works against tumors by blocking the cell cycle, inhibiting tumor cell proliferation, and inhibiting angiogenesis (Jiang X. et al., 2017; Wei et al., 2017). Z-ajoene can inhibit the growth of tumor cells by resisting proliferation, stimulating apoptosis, and increasing the production of peroxide, caspase-3-like, and caspase-8 (Li et al., 2002; Bayan et al., 2014). SAMC and SAC are also two essential anticancer allicin secondary metabolites that play antitumor roles by inhibiting tumor cell proliferation and inducing apoptosis (Xu et al., 2014; Zhang et al., 2014; Xiao et al., 2018).
Allicin not only protects against tumors but also alleviates the adverse effects of anticancer treatment and enhances the chemotherapeutic response under certain conditions. For instance, allicin has a protective effect against liver toxicity induced by As2O3 (arsenic trioxide), an effective therapeutic agent for acute promyelocytic leukemia, through the activation of the Nrf2 signaling pathway involving KLF9 in rats (Yang et al., 2017). In addition, a study suggests the beneficial role of allicin as an adjuvant to TAM (tamoxifen, which is widely used for the treatment of hormone-dependent breast cancer) in cancer treatment by alleviating liver injury (Suddek, 2014).
We summarize the effects of allicin and its secondary metabolites against digestive system cancer in Table 1. In addition to allicin, DATS has also shown noticeable anti-cancer effects in digestive system. Therefore, in sections 4.2 and 4.3 of this review, we compare the anti-cancer effects, stability, action targets and specific mechanisms of the two compounds in detail.
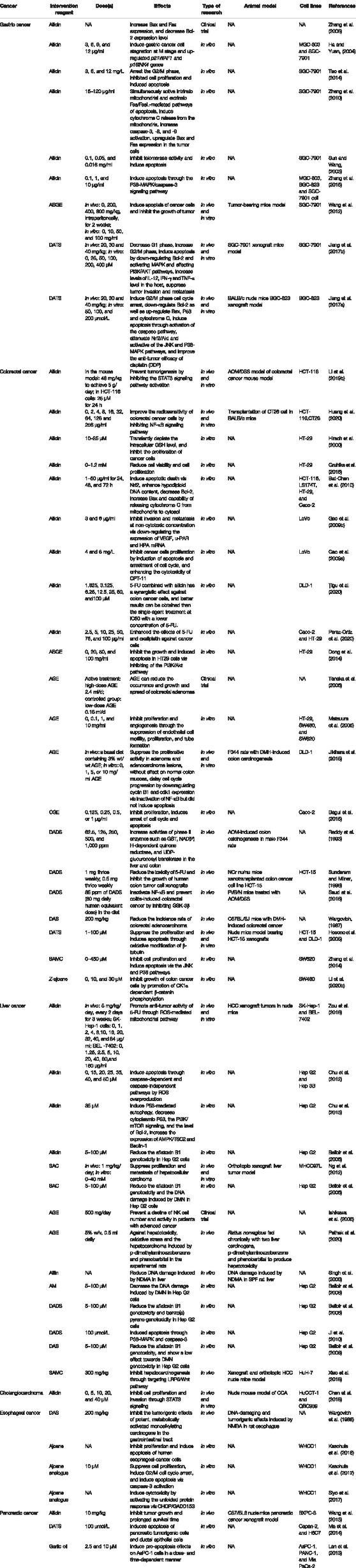
TABLE 1. Summary of mechanisms of allicin and its secondary metabolites against cancers of digestive system.
2.3.2 Against Pathogenic Organisms
Garlic has long been used as an antimicrobial agent, and allicin is considered to be the primary substance that contributes to garlic’s antimicrobial activity (Cavallito C. J. and Bailey J. H., 1944). In addition to having a wide range of antibacterial and antifungal properties, allicin has also been found to have antiviral and antiparasitic effects (Guo, 2014).
2.3.2.1 Antibacterial Effect
Allicin can inhibit the growth of both Gram-negative and Gram-positive bacteria, G(-) bacteria such as Bacillus spp. and Streptococcus spp. and G(+) bacteria such as Salmonella typhimurium, Agrobacterium tumefaciens, Escherichia coli K12, Vibrio cholera, Pseudomonas syringae (various pathovars) (Cavallito C. J. and Bailey J. H., 1944; Small et al., 1947; Feldberg et al., 1988; Curtis et al., 2004; Leng et al., 2011). In preclinical trials, allicin has been proven to protect against Helicobacter pylori (HP), which is considered to be an important factor for gastritis, peptic ulcers and gastric cancer, but clinical studies have shown that taking fresh oral garlic and using garlic oil cannot improve HP infection in most conditions (Graham et al., 1999; Aydin et al., 2000; Leontiev et al., 2018). A meta-analysis showed that allicin can enhance the effect of anti-HP when combined with front-line treatment, PPI triple therapy or bismuth-containing quadruple therapy (Si XB. et al., 2019). Allicin can also protect against some drug-resistant strains, such as Staphylococcus aureus (NBRC 12732) and Staphylococcus aureus (clinical isolates) (Cutler and Wilson, 2004; Fujisawa et al., 2009). In addition, allicin has also been found to enhance the antimicrobial effect when treated combined with other antibiotics (Choo et al., 2020).
The antibacterial activity is based on allicin’s two essential features: entering the bacterial cell and killing it (Borlinghaus et al., 2014). Due to its lipophilic character, allicin can easily diffuse across both natural and artificial phospholipid membranes, which means that allicin can easily enter the cell (Miron et al., 2000). It is noteworthy that similar to penicillin, citrinin, gliotoxin, clavacin and pyocyanines, the antibacterial activity of allicin can be suppressed by interacting with cysteine (Cavallito CJ. and Bailey JH., 1944). It has been reported that allicin inhibits the proliferation of bacteria by its–S(O)–S–group because it can react with the sulfhydryl group of cellular proteins to form mixed disulfides (Kyung, 2012). In addition, allicin can inhibit the synthesis of DNA, RNA, and protein in bacteria, and the inhibition effect on RNA synthesis is more significantly (Feldberg et al., 1988). Diallyl polysulfides are the decomposition products of allicin. Studies have shown that diallyl polysulfides can modify bacterial cell membrane or cell wall by reacting with sulfhydryl groups to disrupt the composition and integrity of bacterial cell membrane or cell wall (Lu et al., 2011).
2.3.2.2 Antifungal Effect
It has been reviewed that allicin can inhibit the growth of various fungi ranging from yeasts to filamentous fungi, while in another review, allicin has been suggested to be the main antifungal compound in garlic; moreover, the secondary metabolites of allicin can stimulate cellular immunity and have better effects than conventional chemotherapy in the antifungal process (Davis, 2005; Choo et al., 2020). In addition, studies have shown that allicin can protect against Candida albicans, and its potency is comparable to that of fluconazole both in vitro and in a systemic candidiasis mouse model (Khodavandi et al., 2011). Recently, allicin has been reported to show more effective inhibition of the growth of yeast BY4742 cells than of bacteria (Leontiev et al., 2018). YKL071w gene in S. cerevisiae is highly induced by allicin and other thiol-reactive compounds, and in silico analysis revealed multiple Yap1p binding motifs in the YKL071w promoter sequence (Yu et al., 2010). Allicin has been found to directly activate Yap1p, which is considered to be the central regulator of the S. cerevisiae oxidative stress response, target at the C-term C598 and C620 residues to play an antifungal role (Gruhlke et al., 2017). The antifungal effect of allicin is closely related to its chemical structure. The reactivity of thiosulfinates towards thiol-groups is important for their antimicrobial activity (Small et al., 1947; Wills, 1956). The chemical basis of the reaction is that electron-withdrawing effect of the O-atom creates an electrophilic sulfur centre which reacts readily with thiols, thereby forming an S-thioallyl adduct (Leontiev et al., 2018). Therefore, allicin affects the fungal enzymes and proteins with thiol-groups through its proteotoxicity. Moreover, it has also been found that combining allicin with antifungal agents can enhance the antifungal effect; for example, allicin synergizes with amphotericin B against Candida albicans by enhancing its oxidative damage effect (Shen et al., 1996; An et al., 2009).
2.3.2.3 Antiviral Effect
The antiviral activity of allicin and its secondary metabolites, such as ajoene, DATS, DAS, and DADS, has been proven both in vivo and in vitro (Weber et al., 1992; Walder et al., 1997; Fang et al., 1999; Liu et al., 2004; Terrasson et al., 2007; Hall et al., 2017; Wang et al., 2017). The crude extract of garlic and thiosulfinates have been found to be more active on the envelope virus (herpes simplex virus-1 and 2, parainfluenza-3, vaccinia virus, vesicular stomatitis virus) than non-enveloped virus (human rhinovirus-2) (Weber et al., 1992). The molecular mechanism is that OSCs react with the thiol group in various active viral proteins or enzymes that are crucial for microbial surveillance and fusion (Ankri and Mirelman, 1999; Jain et al., 2007). Allicin has been found to inhibit the viral RNA polymerase by react with the thiol groups, and has been proven to protect against REV in specific pathogen-free chickens by reducing the immunosuppression induced by REV through the ERK/mitogen-activated protein kinase pathway (Schafer and Buettner, 2001; Wang et al., 2017). DATS, the secondary metabolite of allicin, has been found to fight against CMV both in vivo and in vitro by inhibiting viral replication and reducing the DNA load of HCMV in mice (Liu et al., 2004; Zhen et al., 2006).
2.3.2.4 Antiparasitic Effect
Allicin also plays a role in inhibiting the growth of various kinds of parasites, such as Schistosome, Babesia, Theileria equi, Plasmodium falciparum, and Trypanosoma brucei (Waag et al., 2010; Salama et al., 2014; Metwally et al., 2018). In a study conducted by Coppi et al., allicin was shown to inhibit malaria infection by inhibiting both sporozoite infectivity and erythrocytic stages (Coppi et al., 2006). Their studies showed that allicin can inhibit the cleavage of CSP, which is the main surface protein of Plasmodium sporozoites and has cell invasion activity (Coppi et al., 2005; Coppi et al., 2006). In addition, the studies conducted by Salama et al. show that allicin has a potent effect against Babesia parasites, and it is speculated that the inhibition may occur at the invasion step (Salama et al., 2014). Moreover, they found that combining allicin with diminazene aceturate improves antiparasitic effect (Salama et al., 2014). Recently, a study found that mice treated with allicin can increase the expression of IL-13, which is a cytokine that has an antiparasitic effect, and this study indicated that allicin protects against Schistosomiasis through its anti-inflammatory and immunoregulatory effects (Metwally et al., 2018).
2.3.3 Affect Gut Microbiota
In addition to against bacteria, allicin has also been shown to modulate the composition of gut microbiota (GM) and increase the diversity of beneficial bacteria in animal models (Guillamón et al., 2021). Allicin has been found to play a role in improving intestinal epithelial barrier function and preventing barrier damage via a microbiota-regulated short-chain fatty acid-TLR4/MyD88/NF-κB cascade response in an acrylamide-induced rat model (Yuan et al., 2021; Gao et al., 2022). Researchers suggested that allicin could block intestinal bacterial translocation by increasing the immunologic barrier function of mesenteric lymph nodes by modulating dendritic cell maturation (Zhang Y. et al., 2017). Moreover, in alcoholic hepatic steatosis mice, allicin has been found to modulate the GM and improve the CD14-TLR4 pathway to alleviate inflammation in the liver (Panyod et al., 2020).
2.3.4 Antioxidation
As early as 2006, through detailed kinetic and mechanistic studies, allicin was confirmed to have strong antioxidant properties (Okada et al., 2006). Allicin is a reactive sulfur species (RSS) and a potent thiol-trapping reagent, rapidly reacting with glutathione (GSH) to yield S-allylmercaptoglutathione (GSSA) (Borlinghaus et al., 2021). Thus, allicin depletes the intracellular GSH pool and reacts with cysteine thiols available in proteins through S-thioallylation (Borlinghaus et al., 2021). This reaction is the key to the biological activity of allicin, and the reversible oxidation and reduction of protein-thiols is the core of many processes in cells (Schafer and Buettner, 2001). However, some studies have found opposite results (Horev-Azaria et al., 2009; Izigov et al., 2011). Allicin has been observed to up-regulate the intracellular glutathione level, which may be related to the antioxidant and SH-modifying properties of its derivatives, S-allylmercaptocysteine (CSSA), and S-allylmercaptoglutathione (GSSA) (Horev-Azaria et al., 2009; Izigov et al., 2011). More research is needed to explain the paradoxical effects. Allicin is often described as an antioxidant, with the reasons from following two aspects (Borlinghaus et al., 2021). First, chemically, allicin readily undergoes a Cope elimination reaction at room temperature to form allylsulfenic acid (Vaidya et al., 2009). Allylsulfenic acid is a very potent antioxidant, and two molecules of allylsulfenic acid can be converted into allicin again by self-condensation (Block, 1992). Secondly, allicin induces mild oxidative stress in cells which activates oxidative stress protection responses and makes cells more resistant to subsequent greater oxidative damage (Borlinghaus et al., 2021). In a hypertrophic heart mouse model, the clearance of intracellular ROS by allicin was measured, and has been shown to reduce the production of ROS and block ROS-dependent ERK1/2, JNK1/2, AKT, NF-κB and Smad signaling, which leads to the inhibition of hypertrophy (Liu et al., 2010). Another study showed that allicin can stimulate the inhibition of acrylamide-induced oxidation by regulating the mitogen-activated protein kinase (MAPK) pathway in BRL-3A cells (Hong et al., 2019). The antioxidant properties of allicin have been used to protect human umbilical vein endothelial cells (HUVECs) from oxidative stress and senescence induced by hydrogen peroxide and to improve the quality of aged oocytes in vitro (Zhang M. et al., 2017; Park et al., 2019). Allicin was also used to protect neurons from glutamate-induced oxidative stress, which suggested that allicin may be an effective treatment strategy for spinal cord injury (Liu et al., 2015). In addition, allicin can protect nucleus pulpotheca cells from oxidative stress and mitochondrial dysfunction induced by advanced oxidative protein products by inhibiting the P38-MAPK pathway (Xiang et al., 2020). Moreover, allicin has been shown to attenuate depressive like behaviors triggered by long-term high-fat diet consumption, which is associated with sustained oxidative stress damage and insulin resistance (Gao et al., 2019b). It has been found to improve the behaviors by inhibiting ROS production and oxidative stress, improving mitochondrial function, regulating autophagy, and reducing insulin resistance in the hippocampus via optimization of the NOX/Nrf2 imbalance (Gao et al., 2019b).
2.3.5 Anti-Inflammatory
Allicin is the compound responsible for the anti-inflammatory effects of garlic (Shin et al., 2013). It has been found to reduce inflammation caused by diabetic macroangiopathy through both the Nrf2 and NF-κB pathways in mice (Li CL. et al., 2020). In another study, allicin was shown to improve osteoarthritis by downregulating PI3K/Akt/NF-κB signaling (Qian et al., 2018). It can also significantly alleviate the inflammation caused by trinitrophenylsulfuric acid by inhibiting the expression of the P38 and JNK pathways and NF-κB (Li et al., 2015). Allicin protected against inflammation by inhibiting ROS production and regulating autophagy in a mouse model infected with Aspergillus fumigatus, and it has also been found to improve inflammation and oxidative stress in a rabbit model infected with Pasteurella multocida type B (Alam et al., 2018; Dai et al., 2020). Moreover, allicin has been proven to play a hepatoprotective role against acetaminophen (APAP)-induced liver injury by reducing oxidative stress, inhibiting inflammatory pathways, and inhibiting hepatocyte apoptosis (Samra et al., 2020). Allicin could also protect against acute murine malaria infection through enhancement of the host innate and adaptive immune responses (Feng et al., 2012). In terms of psychotherapy, allicin can reduce the apoptosis of hippocampal neurons by inhibiting neuroinflammation and the NLRP3 inflammasome, thereby alleviating the depression-like behavior induced by chronic social failure stress (Gao et al., 2019a). In addition to allicin, its secondary metabolite Z-ajoene has also been found to have anti-inflammatory effects. Z-ajoene has been found to inhibit the pro-inflammatory cytokine (including IL-1 β, IL-12 and IL-6 β), and up regulate the anti-inflammatory cytokine (IL = 10) (Hitchcock et al., 2021). Moreover, it is reported that Z-ajoene or its analogue dansyl-ajoene was found to decrease phosphorylation and nuclear translocation of STAT3, and to covalently modify the protein by S-thiolation at Cys108, Cys367, and Cys687 (Hitchcock et al., 2021). In the same study, Z-Ajoene was also found to inhibit the activity of cyclooxygenase 2 (COX2) in a dose-dependently and non-competitively manner, which may be attributed to the S-thiolation at Cys9 and Cys299 (Hitchcock et al., 2021). It is reported that the anti-inflammatory effect of allicin is related to its anti-cancer effect (Schäfer and Kaschula, 2014). OSCs in garlic has been shown to inhibit the tumor-mediated pro-inflammatory activity by modulating the cytokine pattern in a way that leads to an overall inhibition of NF-κB (Schäfer and Kaschula, 2014). NF-κB is the central regulator of pro-inflammatory gene expression, and acting as the molecular link between inflammation and tumor promotion and progression (Ide and Lau, 2001). Therefore, OSCs in garlic are considered to inhibit tumor via acting as immune modulators that can shift the balance from a pro-inflammatory and immunosuppressive environment to an enhanced anti-tumor response (Schäfer and Kaschula, 2014) (Figure 4).
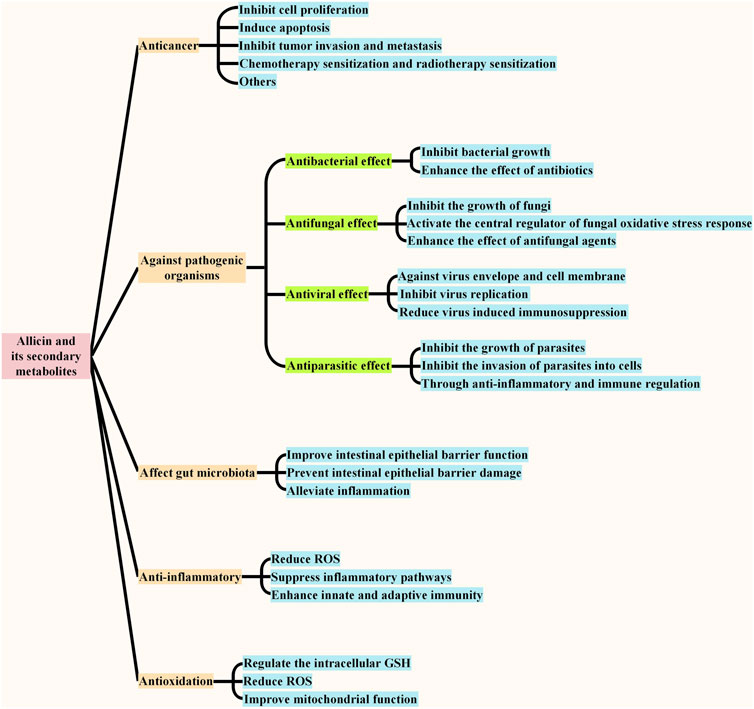
FIGURE 4. | Biological functions of allicin and its secondary metabolites. The main physiological functions of allicin and its secondary metabolites include anticancer, acting against pathogenic organisms, affecting gut microbiota, antioxidant and anti-inflammatory, and the effects against pathogenic organisms include anti-bacterial effect, anti-fungal effect, anti-viral effect, and anti-parasitic effect. The main effect of each function is summarized in the figure.
3 Effect of Allicin on Cancers of the Digestive System
3.1 Epidemiological Studies
Many epidemiological studies have been conducted to explore the effect of allium vegetables intake on digestive system cancers in the population. Though many studies have shown that the consumption of allium vegetables can reduce the risk of digestive system cancers, some studies have shown conflicting results (Kim et al., 2018; Zhou et al., 2020). We have discussed conclusions of these studies and the possible causes of the conflicting results, and summarized them as follows.
3.1.1 Gastric Cancer
A recent Italian case-control study that contained 230 cases and 547 controls suggests that high allium vegetable intake is associated with reduced gastric cancer risk (the group treated with high garlic intake: OR = 0.69, 95% CI: 0.41–1.15; the group treated with more than 2 portions of onion per week: OR = 0.59, 95% CI: 0.25–1.41; the group treated with frequent use of both onion and garlic: OR = 0.70, 95% CI: 0.39–1.28) (Turati et al., 2015). In addition, the meta-analysis conducted by the same research team contains 22 case-control and four cohort studies, and the results suggested that high allium vegetable intake is associated with reduced gastric cancer risk, and the pooled RR for the highest versus lowest garlic intake was 0.60 (95% CI: 0.47–0.76) (based on 12 case-control studies) (Turati et al., 2015).
A blinded randomized placebo-controlled trial conducted in Linqu County, Shandong Province, China, compared the anti-gastric cancer effects of three interventions, including anti-HP treatment with amoxicillin and omeprazole for 2 weeks and garlic (extract and oil) and vitamin (C, E, and selenium) supplementation for 7.3 years (1995–2003) (including 3,365 residents of a high-risk region for gastric cancer in total) (Li WQ. et al., 2019). The results indicated that anti-HP treatment for 2 weeks and garlic or vitamin supplementation for 7 years can significantly reduce the risk of death due to gastric cancer for more than 22 years, and garlic supplementation showed favorable persistent effects on gastric cancer incidence and mortality during the extended follow-up of 14.7 years, which became apparent after approximately 12 years of supplementation (Li WQ. et al., 2019). Moreover, no interaction was found between HP therapy and supplementation, and combination therapy may further reduce the incidence rate and mortality of gastric cancer (Li WQ. et al., 2019).
Although many previous studies suggest that garlic consumption can reduce the risk of gastric cancer, evidence from two large prospective US cohort studies is inconsistent (Kim et al., 2018). This study suggests that there is no evidence that a high intake of garlic can reduce gastric cancer risk (Kim et al., 2018). However, because the actual active principle (allicin and its secondary products) in garlic, varies with the preparation and processing methods of garlic, the anticancer potency of different garlic preparations (including raw garlic, cooked garlic, aged garlic, garlic powder, garlic oil, garlic extract) is different (Ramirez et al., 2021). Therefore, it is understandable that epidemiological studies have reached contradictory conclusions.
3.1.2 Colorectal Cancer
According to the World Cancer Research Fund/American Institute for Cancer Research (WCRF/AICR) evidence for diet, nutrition, physical activity, and colon cancer risk, garlic intake can probably decrease the risks of colon cancer, although this effect is classified as “limited-no conclusion” based on the updated evidence (Clinton et al., 2020). A recent meta-analysis of garlic intake and colorectal cancer risk consisting of 8 case–control and four cohort studies suggested that an increase in garlic intake led to a decrease in CRC risk (RR = 0.80, 95% CI: 0.69–0.91) (Zhou et al., 2020). A hospital-based matched case-control study conducted in northeastern China showed that consuming allium vegetables (such as garlic, garlic stalks, leek, onion, etc.) was associated with a reduced risk of CRC both in men and women (aORs = 0.21 when comparing high total allium intake and low total allium intake, 95% CI: 0.14–0.30, p < 0.001); however, this association was not significant among patients suffering from distal colon cancer (aOR = 0.53, 95% CI: 0.27–1.05, p = 0.248) (Wu et al., 2019).
However, a meta-analysis including eight studies of allium vegetable effects and five studies of garlic supplementation effects showed that increased intake of allium vegetables did not decrease the risk for CRC (RR = 1.06, 95% CI: 0.96–1.17, p = 0.26), and in the subgroup analysis of the study, the researchers found that increased intake of allium vegetables was marginally associated with increased colon cancer risk in women (RR = 1.23, 95% CI: 1.01–1.50, p = 0.05). In addition, they found that using garlic supplementation can increase the risk of CRC (RR = 1.18, 95% CI: 1.02–1.36, p = 0.03), but external validation is needed (Zhu et al., 2014). Moreover, a “use-no use” meta-analysis based on two studies showed that there was no statistically significant association between a higher garlic supplementation intake and colorectal cancer risk increase (RR = 1.24, 95% CI: 0.99–1.54) (Dorant et al., 1996; Satia et al., 2009; Heine-Bröring et al., 2015). A pooled analysis of seven cohort studies and seven case-control studies concluded that garlic consumption is not related to a reduced CRC risk (OR = 0.93, 95% CI: 0.82–1.06, p = 0.281; I2 = 83.6%, p ≤ 0.001) (Chiavarini et al., 2016). Another meta-analysis of five prospective cohort studies also concluded that there was no significant association between the consumption of either raw or cooked garlic (RR = 1.06, 95% CI: 0.95–1.19) or garlic supplementation (RR = 1.12, 95% CI: 0.96–1.31) and CRC risk (Hu et al., 2014).
3.1.3 Esophageal Cancer
Epidemiological research on the association of garlic consumption with esophageal cancer is very limited (Kim and Kwon, 2009). A study including 395 cases (median age: 60 years) and 1,066 controls (median age: 60 years) suggested that the increase in garlic consumption was inversely correlated with the risk of esophageal cancer (OR = 0.43) (Galeone et al., 2006). An epidemiological study conducted in a Chinese smoking and drinking esophageal cancer population suggests that high raw garlic intake can reduce the risk of esophageal cancer and may act as a potential prevention factor among high-risk smokers and drinkers for esophageal cancer in the Chinese population (Jin et al., 2019). In addition, a meta-analysis of observational studies suggests that there is a moderate inverse association between allium vegetable intake and the risk of squamous cell carcinoma of the upper aerodigestive tract in case-control studies (total allium: 0.79 (95% CI 0.56–1.11), garlic: 0.74 (95% CI 0.57–0.95), and onion: 0.72 (95% CI 0.57–0.91) for the highest versus the lowest consumption) (Guercio et al., 2016).
3.1.4 Other Digestive System Cancers
To explore the preventive factors for liver cancer, a large population-based case-control study conducted in Jiangsu, China, interviewed 2011 incident liver cancer cases and 7,933 randomly selected population controls from 2003 to 2010 and concluded that after controlling for known risk factors and potential confounders, such as virus infection and drinking, compared to patients who ingested no raw garlic or did so less than twice per week, those who ate raw garlic twice or more per week had reduced rates of liver cancer [adjusted odds ratio (aOR) = 0.77, 95% CI 0.62–0.96] (Liu et al., 2019). In their stratified analyses, the researchers also found that the high intake of raw garlic was inversely related to liver cancer among people without a family history of liver cancer, hepatitis B surface antigen (HBsAg)-negative individuals, frequent alcohol drinkers, and patients who had a history of eating mold-contaminated food or drinking raw water; moreover, they also identified potential additive interactions between low raw garlic intake and HBV infection or heavy alcohol drinking (Liu et al., 2019). The authors suggested that raw garlic might be used as a dietary intervention for reducing liver cancer in the Chinese population (Liu et al., 2019).
Another epidemiological study showed that allium plant intake is also associated with pancreatic cancer risk. The study included 532 cases and 1,701 age- and sex-matched controls from 1995 to 1999 and showed that the risk of pancreatic cancer was inversely associated with the consumption of total and specific vegetables and fruits, and for onions and garlic, the OR and 95% CI for the highest versus the lowest quartile were 0.46 and 0.33–0.63, respectively (Chan et al., 2005).
A population-based case-control study in Shanghai, China, assessed the relationship between diet and biliary tract cancer (Nelson et al., 2017). The researchers collected food frequency questionnaire data from 225 gallbladder cancer cases, 190 extrahepatic bile duct cancer cases, and 68 ampullae of Vater cancer cases and created 39 food groups. The allium food group, which consisted of onions, garlic, and shallots, showed an inverse association with gallbladder cancer (OR = 0.81, 95% CI 0.68–0.97) (Nelson et al., 2017). In addition, the protective effects of allium plants such as onion and garlic toward gallbladder cancer have also been observed in a case-control study including 1,170 histologically confirmed cases and 2,525 group-matched visitor controls in India (Mhatre et al., 2020).
3.1.5 Conclusion for Epidemiological Studies
To sum up, the current epidemiological study on the association between allium vegetables and digestive system tumors is mainly concentrated on gastric cancer and colorectal cancer. Relatively few studies have been done on other digestive cancers, such as esophageal, liver, cholangiocarcinoma and pancreatic cancer. The evidences of epidemiological studies are not certain that the increase of allium vegetable consumption is inversely associated with the risk of gastric cancer and colorectal cancer. This is because different allium preparations have different active principle which leads to various effects. Therefore, some studies have reached contradictory results. Further study on the association between consumption of different allium vegetable preparations and the risk of gastric cancer and colorectal cancer is needed. Taking garlic as an example, studies on the association between the consumption of different garlic preparations (including raw garlic, cooked garlic, aged garlic, and garlic supplements) and the risk of gastric and colon cancer should be further conducted. The epidemiological evidence of esophageal cancer, liver cancer, cholangiocarcinoma, and pancreatic cancer has drawn a relatively consistent conclusion that the increase in consumption of allium vegetables is inversely associated with cancer risk. This may be because the epidemiological studies on the association between allium vegetables and these cancers are relatively few, and more epidemiological evidence is needed to prove the reliability of the conclusion.
3.2 In vitro Studies
3.2.1 Gastric Cancer
3.2.1.1 Inhibition of Tumor Cell Proliferation
A study clarified the effect of allicin on the cell cycle of human gastric cancer cells and its possible mechanism by treating gastric cancer cell lines MGC-803 and SGC-7901 (two human gastric cancer cell lines) with allicin (Ha and Yuan, 2004). The results showed that allicin induced gastric cancer cell stagnation at the M stage, which may be related to the upregulation of p21WAF1 and p16INK4 genes (Ha and Yuan, 2004). Another study showed that allicin has an obvious inhibitory effect on the proliferation of gastric cancer cells by arresting the G2/M phase of the cell cycle of the SGC-7901 cell line (Tao et al., 2014).
A study focused on the effect of DATS toward the SGC-7901 cell line found that DATS can inhibit the growth of SGC-7901 cells and induce cell cycle arrest (Jiang X. et al., 2017). This study showed a significant decrease in the G1 phase (p < 0.05) and a corresponding increase in the G2/M phase (p < 0.05) of SGC-7901 cells treated with 200 μM DATS for 24 h compared with the control group (Jiang X. et al., 2017). Another study proved the antitumor effect of DATS in BGC-823 cells (a human gastric cancer cell line). The result showed that DATS stabilized the cell cycle at G2 phase through regulation of intracellular cyclin levels (significant accumulation of cyclin A2 and B1) to suppress cell viability (Jiang XY. et al., 2017).
3.2.1.2 Induction of Apoptosis
Allicin has been found to inhibit the activity of telomerase and induce apoptosis of gastric cancer SGC-7901 cancer cells and has also been shown to induce apoptosis by arresting the G2/M phase (Sun and Wang, 2003). A study exploring the potential mechanism of allicin in gastric cancer cells showed that allicin induced SGC-7901 cancer cells apoptosis by simultaneously activating intrinsic mitochondria and extrinsic Fas/FasL-mediated apoptosis (Zhang et al., 2010). The specific effects on the two pathways were to induce the release of cytochrome C from mitochondria and increase the activation of caspase-3, -8, and -9 at the molecular level and upregulate the expression of Bax and Fas in tumor cells (Zhang et al., 2010). Moreover, in MGC-803 human gastric carcinoma cells, allicin was found to induce apoptosis of tumor cells by increasing the expression of P38 and cleaved caspase-3 through the P38 MAPK/caspase-3 signaling pathway (Zhang et al., 2015). Although these studies showed the ability of allicin to induce apoptosis of gastric cancer cells and its corresponding signal transduction mechanism, they did not clarify the specific molecular mechanism of allicin induced apoptosis of gastric cancer cells. However, studies focus on other cancers has illustrated the molecular mechanism of allicin against cancer, which may provide direction for further study of the molecular mechanism of allicin against gastric cancer (Miron et al., 2008; Li et al., 2018). Under physiological conditions, allicin can easily penetrate the cell membrane and react with sulfhydryl groups (e.g., GSH cysteine residues) to produce oxidized biomolecules. In leukemia cells (HL-60 and U937), the reaction of allicin and GSH is the trigger of apoptosis (Li et al., 2018). Allicin (5 µM) rapidly moved inside the cells and oxidized GSH in glutathione disulfide (GSSG), which lead to the GSH/GSSG ratio imbalance and cellular reduction potential subsequently decrease, then causing mitochondrial damage and starting the intrinsic apoptotic pathway (Miron et al., 2008).
Allicin exerts its anti-cancer activity via the thiol-disulfide exchange reaction (TDER) with protein thiols (Poole, 2015). DATS can also undergo TDER with protein thiols, and additionally produce H2S gas via reacting with the cellular thiol glutathione, which can regulate various processes in the cells (Rose et al., 2018). DATS can induce apoptosis of cancer cells by regulating apoptosis-related proteins as well as activating MAPK and affecting PI3K/AKT pathways in cells (Jiang X. et al., 2017). The results showed that DATS not only downregulated the anti-apoptotic factor Bcl-2 but also increased the expression of P53 and cytochrome C (apoptosis markers) (Jiang X. et al., 2017). Furthermore, DATS has been found to activate three MAPK pathways, including the ERK, JNK, and P38 pathways, in SGC-7901 cells (Jiang X. et al., 2017). In another gastric cancer cell line, BGC-823, the growth inhibitory effect of DATS has also been suggested to correlate with apoptosis due to the obvious apoptosis sub-G1 peak in cell cycle analysis (Jiang XY. et al., 2017). In this study, DATS significantly downregulated Bcl-2 and upregulated Bax and the levels of P53 and cytochrome C (Jiang XY. et al., 2017). The activation of cysteine proteases is an important pathway in cell apoptosis, and this study demonstrated that DATS can induce apoptosis in BGC-823 cells through activation of the caspase pathway, attenuation of Nrf2/Akt, and activation of the JNK and P38-MAPK pathways (Olsson and Zhivotovsky, 2011; Jiang XY. et al., 2017).
3.2.1.3 Anti-Helicobacter pylori
At present, the research on the anti-HP effect of allicin is still limited. A study focused on the effect of garlic oil on HP in the environment of artificial gastric juice both alone and in the presence of other substances such as mucus, peptone, rapeseed oil, dextrin, and simulated meal mixtures, and the researchers concluded that although the anti-HP activity of garlic oil was affected by food materials and mucin, it still retained high activity under simulated gastric conditions (O'Gara et al., 2008).
3.2.2 Colorectal Cancer
3.2.2.1 Inhibition of Tumor Cell Proliferation
The antiproliferation effect of allicin may be attributed to the ability of allicin to instantaneously deplete intracellular GSH levels (Hirsch et al., 2000). It is reported that allicin can easily penetrate the cell membrane and react with the cellular thiol glutathione via thiolysis exchange producing S-allylmercaptogluthione, to transiently deplete the intracellular GSH level, and induce the inhibition of cell cycle progression and growth arrest (Hirsch et al., 2000). The same study also reported that the extent of reduction in GSH level was related to allicin-induced growth inhibition (Hirsch et al., 2000). Allicin has been reported to induce ROS in cultured cancer cells in a dose-dependent manner (Schäfer and Kaschula, 2014). However, GSH in cancer cells quenches ROS produced by allicin, and the rapid reduction in GSH may allow the excess of allicin to react directly with different thiol-containing molecules in the cell that are usually protected by GSH (Hirsch et al., 2000). Allicin has been found to reduced human colon cancer cell activity and inhibited cell proliferation in a concentration-dependent manner on HT-29 cell line (Gruhlke et al., 2016). It has been shown to have a time- and dose-dependent cytostatic effect on the proliferation of HCT-116, LS174T, and Caco-2 colon cancer cell lines, at concentrations ranging from 6.2 to 310 µM (Bat-Chen et al., 2010). It has also been observed to block the cell cycle to exert it s anti-proliferation effect on LoVo human colon cancer cells (Gao et al., 2009a).
Accumulating evidence has shown that ABGE might prove beneficial in preventing or inhibiting oncogenesis through inhibiting the growth of cancer cells and inducing apoptosis, and the mechanism is to inhibit the PI3K/Akt pathway (Dong et al., 2014). ABGE upregulates PTEN, downregulates Akt and p-Akt expression, and suppresses the downstream target of the PI3K/Akt pathway (70-kDa ribosomal protein S6 kinase 1) at mRNA and protein levels (Dong et al., 2014). AGE has also been shown not only to significantly inhibit the proliferation of human colorectal carcinoma cell lines, including HT29, SW480, and SW620 cells, but also to suppress the growth of endothelial cells, including ECV304 cells and TRLECs (transformed rat lung endothelial cells), which indicates that AGE can inhibit angiogenesis (Matsuura et al., 2006). Compared with endothelial cells, colorectal carcinoma cells seemed to be suppressed at slightly lower concentrations of AGE (Matsuura et al., 2006). Finally, the researchers concluded that AGE can directly suppress the proliferation of colorectal carcinoma cells and inhibit tumor angiogenesis (Matsuura et al., 2006). Furthermore, the molecular mechanism of the antitumor proliferation effect of AGE was explored in another study. The results showed that AGE delayed cell cycle progression by downregulating cyclin B1 and CDK1 expression of NF-κB in human colorectal cancer cells but did not induce apoptosis (Jikihara et al., 2015). Another study investigated the effects of CGE on the proliferation of the human cancer cell lines and mouse macrophage cell line (TIB-71), including hepatic (Hep-G2), colon (Caco-2), prostate (PC-3), and breast (MCF-7) cell lines (Bagul et al., 2015). The authors found that for Hep-G2, MCF-7, TIB-71, and PC-3 cells, the inhibition of cell proliferation reached 80–90% (treated with 0.125, 0.25, 0.5, or 1 μg/ml of CGE), while for the Caco-2 cells, the inhibition was only 40–55% (treated with 0.25, 0.5, or 1 μg/ml) (Bagul et al., 2015). However, in the coculture study of Caco-2 and TIB-71 cells, the proliferation inhibition rate for Caco-2 cells was 90%, compared to 40–55% when cultured separately (Bagul et al., 2015).
3.2.2.2 Induction of Apoptosis
Allicin has been shown not only to block the cell cycle but also to induce cell apoptosis in LoVo human colon cancer cells (Gao et al., 2009a). Moreover, a study investigated the effects of OSCs in garlic, including allicin, and its secondary metabolites, on human Caco-2 and HT-29 colon carcinoma cell lines and showed that OSC-induced cell death in the two cell lines in the following order: allicin < DAS = DADS < DATS (Caco-2 cell line) and allicin = DAS < DADS < DATS (HT-29 cell line) (Jakubíková and Sedlák, 2006). It was concluded that the number of sulfur atoms in OSCs was correlated with their ability to induce apoptosis, and the result also supported the role of redox-sensitive “sulfhydryl switches”, which are commonly triggered by disulfide bond formation and can also be controlled by S-thiolation, in maintaining the intracellular redox milieu (Jakubíková and Sedlák, 2006). Allicin reacts with protein thiols via undergoing TDER and modifies the cell protein to exert its anti-cancer activity (Poole, 2015). A study proved the cytotoxic effects of allicin purified from fresh garlic cloves on HCT116 colon cancer cells and showed that allicin induced apoptosis via a mechanism associated with transactivation of the transcription factor Nrf2 and was characterized by decreasing Bcl-2 levels, increasing Bax levels, enhancing hypodiploid DNA content, and enhancing the ability to release cytochrome C from mitochondria to the cytosol (Bat-Chen et al., 2010). In addition to allicin, as mentioned above, DATS also reacts with protein thiols via TDER and additionally produce H2S. It acts as a fast H2S donor in the cell, and H2S has been reported that can induce apoptosis of cancer cells (Liang et al., 2015; Lee et al., 2017). As a gas, H2S can react quickly, which also explained why DATS is the most active OSC in inducing apoptosis in previous studies. Moreover, in HT-29 colon cancer cells, DATS has been found to oxidative modify thiols of specific cysteine residues (Cys12 β and Cys354 β) in β-tubulin molecules, thereby inducing rapid microtubule disassembly, and inducing apoptosis of HT-29 colon cancer cells (Hosono et al., 2008).
3.2.2.3 Inhibition of Tumor Invasion and Metastasis
Allicin has been shown to inhibit the invasion and metastasis of LoVo human colon cancer cells by downregulating the expression of VEGF, u-PAR, and HPA mRNA (Gao et al., 2009b). VEGF is an important tumor angiogenesis factor related to tumor vascularization, metastasis, and growth; in addition, both u-PAR and HPA can also promote tumor invasion and metastasis (Andreasen et al., 2000; Boyd and Nakajima, 2004; Gao et al., 2009b). Therefore, allicin decreases the expression of VEGF, u-PAR, and HPA, indicating that it can inhibit the invasion and metastasis of tumors. AGE can inhibit cell motility and invasion by enhancing the adhesion of endothelial cells to collagen and fibronectin and inhibit angiogenesis via the suppression of endothelial cell motility and proliferation, as well as the tube (which can later become blood vessels) formation of endothelial cell (Matsuura et al., 2006). The study found that AGEs could inhibit the invasiveness of SW480 and SW620 cells but had no effect on the invasive activity of HT29 cells, suggesting that AGEs’ anti-invasiveness appears to be dependent on the type of cancer cell (Matsuura et al., 2006).
3.2.2.4 Chemosensitization
It has been reported that allicin can enhance the cytotoxicity of CPT-11 (an inhibitor of Topoisomerase I, which is used as first-line chemotherapy for advanced or metastatic colorectal cancers) (Gao et al., 2009a; Si J. et al., 2019). Allicin has also been found to increase the impact of 5-FU and oxaliplatin (500 µM) in decreasing the viability of colon cancer cells, has a better effect than standard 5-FU and oxaliplatin chemotherapy, and reduces the clinical cost of treatment at the same time (Perez-Ortiz et al., 2020).
3.2.3 Liver Cancer
3.2.3.1 Induction of Autophagy in Cancer Cells
Allicin can induce P53-mediated cell autophagy and inhibit the viability of the human hepatocellular carcinoma cell line HepG2 (Chu et al., 2012). The P53 protein is a tumor suppressor protein, and it has been found to participate in cellular autophagy in various pathways and ultimately result in the modulation of mTOR, AMPK, and TSC2 (Chu et al., 2012). Autophagy regulation of P53 has a dual effect, promoting autophagy in the nucleus while suppressing autophagy in the cytoplasm (Sui et al., 2011). Allicin has been found to decrease the levels of cytoplasmic P53, Bcl-2, and the PI3K/mTOR signaling pathway and to increase the expression of the AMPK/TSC2 and Beclin-1 signaling pathways in HepG2 cells, which can also induce the degradation of mitochondria in cancer cells, therefore, allicin promote the autophagy of cancer cells (Chu et al., 2012). It has also been reported that allicin could regulate autophagic cell death pathway through p53 gene in both transcription and translation levels, and the existence of p53 may guide cancer cells to autophagy or lead other cancer cells without p53 to apoptosis (Chu et al., 2013).
3.2.3.2 Protection Effect on Hepatocytes and Chemosensitization Effect on Cancer Cells
Allicin and secondary metabolites can protect cells from damage caused by some carcinogens or chemotherapy. The antigenotoxic activity of several OSCs in garlic (including allicin, DAS, DADS, SAC, and AM) has been assessed in the human hepatocellular carcinoma cell line HepG2 by using the comet assay, and it has been found that all the studied OSCs were shown to reduce the genotoxicity of the direct-acting compounds hydrogen peroxide and methyl methanesulfonate (Belloir et al., 2006). In addition, except for AM, all the studied OSCs were found to significantly decrease the genotoxicity of aflatoxin B1 (Belloir et al., 2006). Another study showed that allicin can also enhance the cytotoxicity induced by 5-fluorouracil (5-FU) (anticancer drug) in HCC cells by the ROS-mediated mitochondrial pathway, and this may provide a novel chemotherapy regimen for HCC (Zou et al., 2016).
3.2.4 Other Digestive System Cancers
Allicin has been shown to suppress the proliferation of cholangiocarcinoma cells by activating the caspase cascade, inducing apoptosis, and reducing the expression of STAT3 downstream proteins such as Bcl-2 (Chen et al., 2018). In addition to cholangiocarcinoma, OSCs have also been found to induce apoptosis in pancreatic cancer cells. Garlic oil has been observed to inhibit the proliferation of pancreatic cancer cell lines, including AsPC-1, PANC-1, and Mia PaCa-2 cells, and a study suggested that garlic oil could induce a pro-apoptosis effect on AsPC-1 cells (Lan et al., 2013). One of the main components of garlic oil, DATS, has been shown to induce apoptosis in pancreatic cancer cells (Capan-2) as well as nontumorigenic pancreatic ductal epithelial cells (H6C7), and it has been observed that DATS could increase the G2/M phase in the cell cycle through increasing cyclin B1 and p21 levels and decreasing cyclin D1 level (Ma et al., 2014).
3.2.5 Stability
To better understand the anticancer effect of allicin and DATS, we should also pay attention to their stability. Under the influence of temperature, allicin can easily degrade to form a variety of compounds, including DATS, ajoenes and vinyldithiins (El-Saber Batiha et al., 2020). Allicin has been observed to be more stable in protic polar methanol than in aprotic polar ethyl acetate. It has also been reported that about 90% of the allicin remained in the stimulated intestinal fluid (pH = 7.5) and simulated gastric fluid (pH = 1.2) after incubation at 37 °C for 5 h, while only a small amount of allicin could be detected after incubation in blood for 5 min (Freeman and Koder, 1995). It is also reported that the biological and chemical half-lives of allicin in aqueous and ethanolic solutions at room temperature are about 6 and 11 days, respectively, while in vegetable oil, allicin is unstable with an activity half-life of 0.8 h and a chemical half-life of 3.1 h (Fujisawa et al., 2008). Moreover, it has been shown that acidic media were more conducive than neutral or alkaline media to stabilize thiosulfinates (e.g. allicin), and allicin formed at an optimum pH of 4.5–5.0 (Lawson and Hughes, 1992; Shen et al., 2002). DATS is the secondary metabolite of allicin, and has also been found to degrades rapidly under normal conditions, therefore, some suitable DATS delivery systems have been conducted to better evaluate its anti-cancer effect (Ju et al., 2010; Janik-Hazuka et al., 2021).
3.2.6 Conclusion of in Vitro Studies
To sum up, both allicin and DATS have showed anti-digestive system cancer activity. They exert anti-tumor activity mainly by inhibiting proliferation, inducing apoptosis, suppressing migration and metastasis, and enhancing chemosensitivity of the tumor cells. In vitro evidences have shown that allicin is mainly against gastric cancer, colorectal cancer, liver cancer and cholangiocarcinoma, while DATS is mainly against gastric cancer, colorectal cancer and pancreatic cancer. To date, there is no evidence of allicin and DATS against esophageal cancer in vitro. It is reported that ajoene, another secondary product of allicin, and its analogues have anti-esophageal cancer effects (Kaschula et al., 2011). The basis of allicin and DATS exerting their anti-cancer ability is the thiol-disulfide exchange reaction. Allicin quickly moves inside the cells and reacts with protein thiol groups to modify cell protein and affect signaling, while DATS not only reacts with protein thiols but also intracellularly produces H2S via TDER, and then exerts subsequent effects on a series of processes such as modifying cell protein, signaling, and metabolism through H2S.
The specific targets of allicin on digestive system cancer are summarized as follows. Firstly, allicin can inhibit the proliferation of cancer cells by exhausting GSH in cells. It can also block the cell cycle and inhibit cell proliferation by up-regulating p21WAF1 and p16INK4 genes. Secondly, allicin has been found to induce both extrinsic and intrinsic apoptosis by up-regulating the P38-MAPK pathway, transactivating Nrf2, and down-regulating the STAT3 pathway. It can also inhibit telomerase activity to induce apoptosis. Thirdly, allicin has also been shown to induce P53-mediated autophagy by down-regulating the PI3K/mTOR signaling pathway and up-regulating the AMPK/TSC2 and Beclin-1 signaling pathways. Moreover, allicin can inhibit tumor angiogenesis, migration, and metastasis by down-regulating VEGF, u-PAR, and HPA. Finally, it has a chemosensitization effect when treated with chemotherapeutic drugs including CPT-11, 5-FU, and oxaliplatin. (Figure 5). In vitro evidences showed that the specific anti-cancer effect of DATS is mainly reflected in inhibiting proliferation and inducing apoptosis. It arrests the cell cycle to G2/M phase by regulating cyclin and p21 levels, so as to inhibit cell proliferation. DATS has been found to induce apoptosis via activating three MAPK pathways (including the ERK, JNK, and P38 pathways), and attenuating Nrf2/Akt pathway, as well as regulating apoptosis-related proteins. Moreover, it has also been shown to inducing apoptosis via rapid microtubule disassembly by modifying thiols of Cys12 β and Cys354 β in β-tubulin molecules. (Figure 6). AGE has also been shown to suppress proliferation, inhibit tumor angiogenesis, and inhibit tumor invasion and metastasis. In conclusion, the anti-tumor effect of allicin, DATS, and garlic extract on digestive system in vitro is noteworthy.
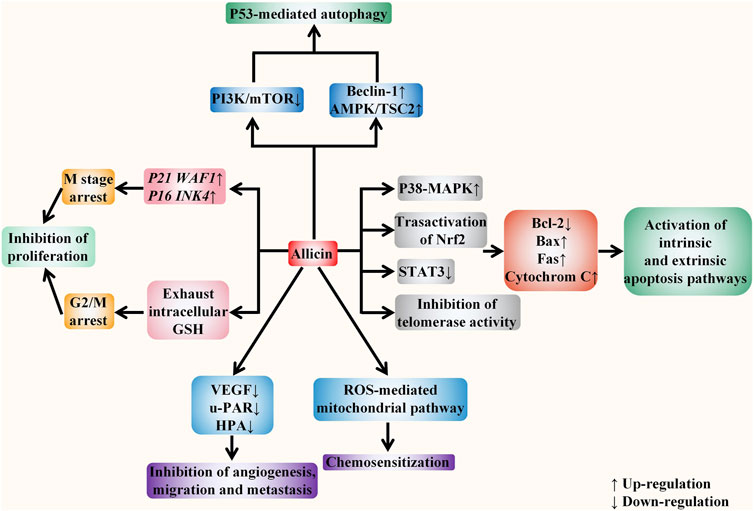
FIGURE 5. Allicin acts against digestive system cancers in vitro. The anticancer effect of allicin in vitro is mainly reflected in five aspects, including inhibiting proliferation, inducing apoptosis, inducing autophagy, inhibiting angiogenesis, invasion, and metastasis, and enhancing the sensitivity of tumor chemotherapy. It depletes intracellular glutathione (GSH) and up-regulates p21WAF1 and p16INK4 genes to block the cell cycle and inhibit cell proliferation. It activates both endogenous and exogenous apoptotic pathways by regulating intracellular signaling pathways, inhibiting telomerase activity, and regulating the activity of apoptosis-related proteins. In addition, it also down-regulates the PI3K/mTOR signaling pathway and up-regulates the AMPK/TSC2 and Beclin-1 signaling pathways to induce the P53 mediated autophagy. It inhibits tumor angiogenesis, migration, and metastasis by down-regulating VEGF, u-PAR, and HPA. Moreover, via ROS -mediated mitochondrial pathway, it exerts the chemosensitization effect towards tumor cells.
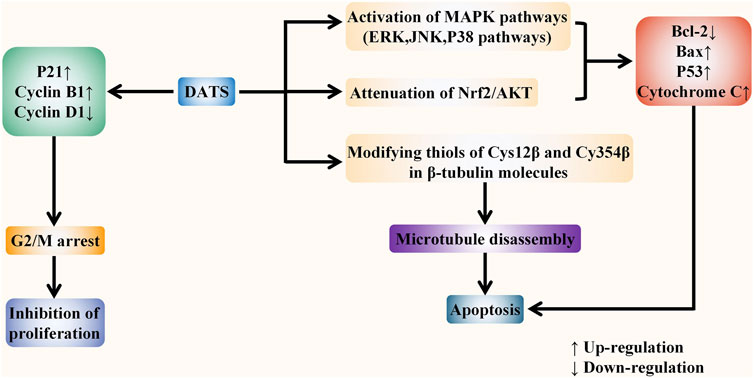
FIGURE 6. DATS fights against digestive system cancers in vitro. DATS exerts the anti-tumor role mainly in two ways: inhibiting proliferation and inducing apoptosis. DATS can inhibit cell proliferation by inducing G2/M phase arrest via regulating the levels of P21 and Cyclin. In addition, DATS can activate three MAPK pathways and attenuate Nrf2/Akt pathway to regulate apoptosis-related proteins. Moreover, it has also been shown to induce rapid microtubule disassembly by modifying thiols of Cys12 β and Cys354 β in β-tubulin molecules, thereby inducing apoptosis.
3.3 In vivo Studies
3.3.1 Gastric Cancer
In SGC-7901 xenograft mice, it has been found that DATS can significantly suppress tumor growth, which was manifested in the following aspects (Jiang X. et al., 2017). Firstly, it significantly decreased the volume (p < 0.001) and the weight (p < 0.001) of the tumor (Jiang X. et al., 2017). Secondly, it stimulated the immune response of the host organism by increasing the levels of IL-12, IFN-γ, and TNF-α, which can also be found in mice without xenograft (Jiang X. et al., 2017). Thirdly, it also blocked proliferation, as well as inducing apoptosis of tumors through activating MAPK pathways (Jiang X. et al., 2017). Moreover, it suppressed tumor invasion and metastasis by regulating the expression of MMP-9 (participating in remodeling extracellular matrix) and E-cadherin (a cell adhesion junction molecule), without significant side effects in major organs of the SGC-7901 xenograft mice (Jiang X. et al., 2017). The antitumor effect of DATS has also been proved in a BALB/c nude mouse BGC-823 xenograft model. The results suggested that DATS can slow tumor growth and reduce the weight and volume of tumors compared to the control group (p < 0.01 for both), and it can induce apoptosis and downregulate Ki-67 (proliferation marker) expression in tumor tissues (Jiang XY. et al., 2017). The study also showed that DATS can significantly activate kinases such as P38 and JNK/MAPK and attenuates the Nrf2/Akt pathway in the BGC-823 xenograft mouse model (Jiang XY. et al., 2017). Moreover, the study indicated that DATS can improve the antitumor efficacy of cisplatin (DDP, a chemotherapeutic agent widely used in the treatment of solid malignant tumors) in the treatment of gastric cancer without notable side effects (Jiang XY. et al., 2017).
A clinical trial focused on the effect of local application of allicin under gastroscope among patients with gastric adenocarcinoma showed that local application of allicin could inhibit cell proliferation and induce apoptosis of progressive gastric carcinoma through increasing Bax and Fas expression and decreasing the Bcl-2 expression level (Zhang et al., 2008). The clinical trial results of allicin against HP are controversial. Although the anti-H. pylori activity of garlic powder and garlic oil has been proved in an in vitro study, subsequent clinical studies failed to confirm this activity (McNulty et al., 2001; Si XB. et al., 2019). However, allicin has been found to improve HP eradication, healing of ulcers, and remission of symptoms and was suggested to have potential as an add-on therapy (Si XB. et al., 2019). In addition, ABGE has been proved to induce dose-dependent apoptosis in SGC-7901 human gastric cancer cells, and further study in tumor-bearing mice revealed that ABGE could significantly inhibit the growth of the tumor due to its antioxidant and immunomodulatory effects (Wang et al., 2012).
3.3.2 Colorectal Cancer
DATS is also considered to be a potential anti-colorectal cancer agent. Based on the fact that DATS can inhibit the growth of tumor cells in vitro by blocking the cell cycle, destroying the cell microtubule network, increasing caspase-3 activity, and inducing apoptosis, the researchers conducted in vivo experiments, and the results showed that DATS can also suppress tumor growth in nude mice bearing HCT-15 xenografts (Hosono et al., 2005). Allicin has been proved to promote HCT116 cell apoptosis and suppress cancer cell survival and proliferation by inhibiting the STAT3 signal, and this result has also been confirmed in the azoxymethane methane/sodium dextran sulfate (AOM/DSS) model of colorectal cancer mice (Li X. et al., 2019). In addition, a further study found that allicin could improve the radiosensitivity of colorectal cancer cells by inhibiting the NF-κB signaling pathway in the BALB/c mouse model, which suggests that allicin may be used as a potential sensitizer in clinical tumor radiotherapy (Huang et al., 2020). In addition to treatment with allicin or its secondary metabolites alone, a double-blind randomized clinical trial suggested that using AGE can reduce the occurrence, growth, and spread of colorectal adenomas (Tanaka et al., 2006).
3.3.3 Liver Cancer
Both allicin and DATS have been shown to exert anti-hepatocellular carcinoma effect in the mice model (Zhang et al., 2006; Zhang et al., 2007). On BALB/c mice xenografted with BEL7402 hepatocellular carcinoma cells, it was found that allicin can up-regulate the expression of Bax and FasL in a dose-dependent manner to induce the apoptosis of tumor cells (Zhang et al., 2006). Allicin has also been shown to suppress the growth of tumor volume in a dose-dependent manner, and at the concentration of 5 mg/ml, allicin showed a stronger effect on inhibiting the growth of tumor volume than that of the positive control group (treated with 0.2 mg/mLl adriamycin) (Zhang et al., 2006). Moreover, it was also observed that combination of low-dose allicin (1 mg/ml) and adriamycin (0.2 mg/ml) has better inhibitory effect on tumor than single drug treatment of high-dose allicin (5 mg/ml), suggesting the ability of allicin to be used as an adjuvant compound in chemotherapy. In addition, allicin has alos been found to promote the antitumor activity of 5-FU toward HCC xenograft tumors in nude mice by increasing intracellular ROS level, reducing mitochondrial membrane potential (ΔΨm), activating caspase-3 and PARP, and downregulating Bcl-2 (Zou et al., 2016).
On orthotopic transplantation HepG2 hepatocellular carcinoma model in nude mice, hepatic targeted polybutylcyanoacrylate nanoparticles of diallyl trisulfide (DATS-PBCA-NP) showed significant anti-tumor effect with good prolonged release effect and hepatic-targeted activity (Zhang et al., 2007). Treatment with DATS-PBCA-NP can dramatically decrease the expression of Bcl-2 protein without significantly changing the expression of Fas, FasL and Bax protein, causing a decrease in Bcl-2/Bax ratio, thus leading to the predominance of pro-apoptosis protein in the ratio between pro- and anti-apoptosis subsets, then resulting in apoptosis (Zhang et al., 2007).
OSCs in garlic have also been found to resist drug-induced liver cancer and liver injury. AGE has been shown to against hepatotoxicity, oxidative stress, and hepatocarcinoma induced by p-dimethylaminoazobenzene and phenobarbital in experimental rats (Pathak et al., 2020). Garlic powder has been found to reduce the DNA damage of rat liver caused by N-nitrosodimethylamine (NDMA) and aflatoxin B1, and the experimental results showed that the increase in alliin content in garlic powder is closely related to the proportional decrease in DNA alteration induced by NDMA but is not related to the decrease in liver DNA damage caused by aflatoxin B1 (Singh et al., 2006).
3.3.4 Other Digestive System Cancers
Allicin has been shown to inhibit the proliferation, migration, invasion, and epithelial-mesenchymal transformation (EMT) of cholangiocarcinoma in an in vivo model (Chen et al., 2018). In C57/BL6 nude mouse pancreatic cancer xenograft models, researchers found that treatment with allicin alone or in combination with recombinant interleukin-2 could inhibit the progression of pancreatic tumors, and they found that the combination treatment could significantly inhibit the growth of xenografts and prolong the survival time through the activation of CD4 T, CD8 T, and NK cells (Wang et al., 2013). In addition to the antitumor effect, it has been proved that AGE can improve immune function in patients with gastrointestinal cancer (Ishikawa et al., 2006). The study included 42 patients with liver cancer, seven patients with pancreatic cancer, and one patient with colon cancer and found that treatment with AGE significantly increased both the number and the activity of NK cells without adverse effects (Ishikawa et al., 2006).
3.3.5 Conclusion of in Vivo Studies
In mice models, allicin can inhibit gastric cancer, colorectal cancer, liver cancer, cholangiocarcinoma, and pancreatic cancer, while DATS can inhibit gastric cancer, colon cancer, and liver cancer. To sum up, previous studies have shown that both allicin and DATS could play an anti-tumor role in mice models via reducing the weight and volume of the tumor, inducing apoptosis and proliferation, suppressing invasion and metastasis, and improving the chemosensitivity of the tumor. In addition, allicin has been found to enhance the tumor radiosensitivity, as well as activate CD4 T, CD8 T, and NK cells in combination with recombinant interleukin-2. Moreover, DATS also exert it anti-cancer ability via stimulate the host immune response. The effects of allicin and DATS against digestive system cancer have been shown in Figure 7. This is roughly consistent with in vitro evidences.
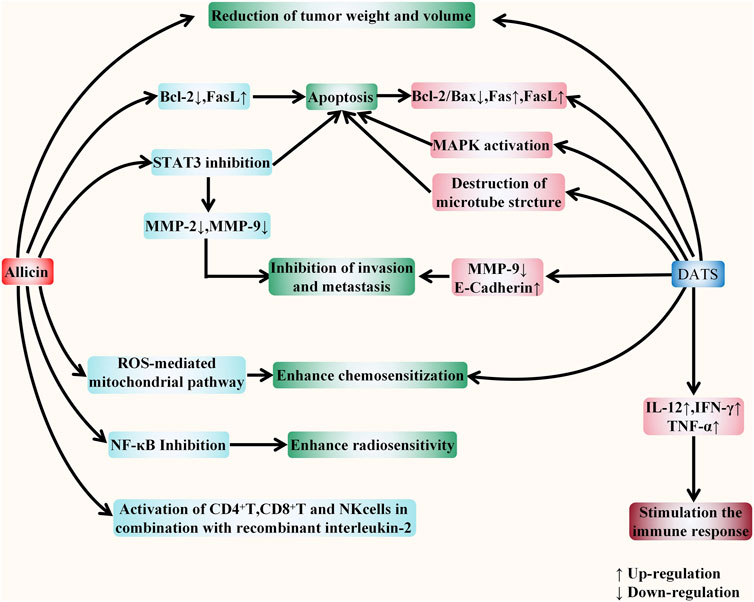
FIGURE 7. The comparison between the effects of allicin and DATS against digestive system cancers in vivo. The figure summarizes the different anticancer effects of allicin and DATS in vivo. Firstly, allicin and DATS have four consistent anticancer effects, including reducing tumor weight and volume, inducing apoptosis, inhibiting tumor invasion and metastasis, and enhancing tumor cell chemosensitivity. Both compounds can induce apoptosis by regulating apoptosis-related proteins. In addition, allicin can also induce apoptosis, inhibit tumor invasion and metastasis by inhibiting STAT3, and enhance tumor chemosensitivity through ROS-mediated mitochondrial pathway. However, DATS induces apoptosis by activating MAPK pathway and destroying cell microstructure, and inhibit invasion and metastasis by down regulating MMP-9 and up regulating E-cadherin. Allicin also has two more effects: enhancing the radiotherapy sensitivity of tumor cells and activating T cells and NK cells with the participation of recombinant interleukin-2. DATS has one more effect: increasing the expression of cytokines, including IL-12 and IFN-γ, TNF-α, to stimulate the immune response of the host.
4 Conclusion
This review describes the synthesis process, structure, toxicity, and biological effects of allicin (also mentions the structure and biological effects of some allicin secondary metabolites e.g., DATS, Z-ajoene.) in section 2. Based on the chemical structure and biological effects, we summarize the role of allicin, its secondary metabolite DATS and garlic extracts (including AGE, ABGE etc.) in cancers of digestive system from the evidence of epidemiology, in vitro and in vivo. Among them, there are relatively more studies on gastric cancer, colorectal cancer and liver cancer, while relatively few studies on other digestive system cancers.
To date, allicin, DATS, and garlic extract have been shown certain anti-cancer effects on gastric cancer, colorectal cancer, liver cancer, cholangiocarcinoma, and pancreatic cancer. Their various biological activities, including anti-inflammatory, anti-oxidation, antibacterial, and anti-virus effects, also promote the anti-cancer effect. In general, the thiol-disulfide exchange reaction is the key reaction for allicin and DATS to exert their biological activities. Allicin regulates signaling and various metabolic processes in the cell by reacting with GSH and the thiols available in proteins through S-thioallylation. DATS reacts with cellular thiol glutathione through the thiol-disulfide exchange reaction and produces H2S gas to exert various regulatory functions. Base on this chemical mechanism, allicin and DATS play an anti-cancer role mainly by inhibiting tumor proliferation, inducing apoptosis, inhibiting tumor invasion and metastasis, and enhancing the chemosensitivity of tumor cells. However, this reaction is not selective, allicin can also react with protein thiols in normal cells, which indicates the cytotoxic potential and toxicity of normal cells.
Both in vivo and in vitro, allicin not only showed a direct anti-tumor effect on the digestive system but also showed a sensitization effect toward radiotherapy and chemotherapy, as well as protecting normal cells against carcinogens. However, there is no FDA-approved drug containing purified allicin. The data of allicin clinical trials are relatively lacking, and more patient-centered, multi-center and large-scale studies are needed to determine the efficacy of allicin and its secondary metabolites. Although many garlic preparations have been proved to have antitumor effects, the main components of each preparation are different, so their anticancer effects may be quite different. However, at present, there are few clinical studies on the difference of anticancer effect of allicin and its secondary metabolites, so it is difficult to determine which OSC plays the greatest anticancer effect in clinical application. In addition, the dosage and the administration method of allicin in the experiment is in lack of a unified standard. Although allicin can be used as a drug everyday by ingesting together with food, further research is needed to achieve the precise treatment of allicin against digestive system tumors. In conclusion, allicin and its secondary metabolites have shown remarkable anticancer potential, but it is still far away from its clinical application as a drug, and more experiments are needed to achieve its precise treatment of digestive system cancers.
Author Contributions
YZ drafted the manuscript, performed the selection and organization of literature, and prepared the figures. XL helped to organize references and prepare the table. BW, WL, JuZ, JiZ, MW, and LS helped to revise the manuscript. BC carried out the design of this review and revised the manuscript. All authors contributed to this manuscript and approved the final manuscript.
Funding
This work was supported by the a grant from Higher education Innovative talent support project of Liao Ning Province (2018-478). This work was also supported by a grant from Shenyang science and technology plan fund project (20-205-4-094). This work was also supported by Natural Science Foundation of Guangxi (No. 2021GXNSFAA325001).
Conflict of Interest
The authors declare that the research was conducted in the absence of any commercial or financial relationships that could be construed as a potential conflict of interest.
Publisher’s Note
All claims expressed in this article are solely those of the authors and do not necessarily represent those of their affiliated organizations, or those of the publisher, the editors and the reviewers. Any product that may be evaluated in this article, or claim that may be made by its manufacturer, is not guaranteed or endorsed by the publisher.
Acknowledgments
The authors would like to thank the First Affiliated Hospital and Shengjing Hospital of China Medical University and the Second Clinical College of China Medical University for providing the research facilities and support to conduct this study.
References
Alam, R. T. M., Fawzi, E. M., Alkhalf, M. I., Alansari, W. S., Aleya, L., and Abdel-Daim, M. M. (2018). Anti-Inflammatory, Immunomodulatory, and Antioxidant Activities of Allicin, Norfloxacin, or Their Combination against Pasteurella Multocida Infection in Male New Zealand Rabbits. Oxid. Med. Cell Longev. 2018, 1780956. doi:10.1155/2018/1780956
Almogren, A., Shakoor, Z., and Adam, M. H. (2013). Garlic and Onion Sensitization Among Saudi Patients Screened for Food Allergy: a Hospital Based Study. Afr. Health Sci. 13 (3), 689–693. doi:10.4314/ahs.v13i3.24
Amagase, H., Petesch, B. L., Matsuura, H., Kasuga, S., and Itakura, Y. (2001). Intake of Garlic and its Bioactive Components. J. Nutr. 131 (3s), 955S–62S. doi:10.1093/jn/131.3.955S
An, M., Shen, H., Cao, Y., Zhang, J., Cai, Y., Wang, R., et al. (2009). Allicin Enhances the Oxidative Damage Effect of Amphotericin B against Candida Albicans. Int. J. Antimicrob. Agents 33 (3), 258–263. doi:10.1016/j.ijantimicag.2008.09.014
Andreasen, P. A., Egelund, R., and Petersen, H. H. (2000). The Plasminogen Activation System in Tumor Growth, Invasion, and Metastasis. Cell Mol. Life Sci. 57 (1), 25–40. doi:10.1007/s000180050497
Ankri, S., and Mirelman, D. (1999). Antimicrobial Properties of Allicin from Garlic. Microbes Infect. 1 (2), 125–129. doi:10.1016/s1286-4579(99)80003-3
Aydin, A., Ersöz, G., Tekesin, O., Akçiçek, E., and Tuncyürek, M. (2000). Garlic Oil and Helicobacter pylori Infection. Am. J. Gastroenterol. 95 (2), 563–564. doi:10.1111/j.1572-0241.2000.t01-1-01812.x
Bagul, M., Kakumanu, S., and Wilson, T. A. (2015). Crude Garlic Extract Inhibits Cell Proliferation and Induces Cell Cycle Arrest and Apoptosis of Cancer Cells In Vitro. J. Med. Food 18 (7), 731–737. doi:10.1089/jmf.2014.0064
Banerjee, S. K., Maulik, M., Manchanda, S. C., Dinda, A. K., Das, T. K., and Maulik, S. K. (2001). Garlic-induced Alteration in Rat Liver and Kidney Morphology and Associated Changes in Endogenous Antioxidant Status. Food Chem. Toxicol. 39 (8), 793–797. doi:10.1016/s0278-6915(01)00018-7
Bat-Chen, W., Golan, T., Peri, I., Ludmer, Z., and Schwartz, B. (2010). Allicin Purified from Fresh Garlic Cloves Induces Apoptosis in Colon Cancer Cells via Nrf2. Nutr. Cancer 62 (7), 947–957. doi:10.1080/01635581.2010.509837
Bayan, L., Koulivand, P. H., and Gorji, A. (2014). Garlic: a Review of Potential Therapeutic Effects. Avicenna J. Phytomed 4 (1), 1–14.
Belloir, C., Singh, V., Daurat, C., Siess, M. H., and Le Bon, A. M. (2006). Protective Effects of Garlic Sulfur Compounds against DNA Damage Induced by Direct- and Indirect-Acting Genotoxic Agents in HepG2 Cells. Food Chem. Toxicol. 44 (6), 827–834. doi:10.1016/j.fct.2005.11.005
Block, E. (1985). The Chemistry of Garlic and Onions. Sci. Am. 252 (3), 114–119. doi:10.1038/scientificamerican0385-114
Block, E. (1992). The Organosulfur Chemistry of the GenusAllium - Implications for the Organic Chemistry of Sulfur. Angew. Chem. Int. Ed. Engl. 31 (9), 1135–1178. doi:10.1002/anie.199211351
Borlinghaus, J., Albrecht, F., Gruhlke, M. C., Nwachukwu, I. D., and Slusarenko, A. J. (2014). Allicin: Chemistry and Biological Properties. Molecules 19 (8), 12591–12618. doi:10.3390/molecules190812591
Borlinghaus, J., Foerster, J., Kappler, U., Antelmann, H., Noll, U., Gruhlke, M., et al. (2021). Allicin, the Odor of Freshly Crushed Garlic: A Review of Recent Progress in Understanding Allicin's Effects on Cells. Molecules 26 (6), 1505. doi:10.3390/molecules26061505
Borrelli, F., Capasso, R., and Izzo, A. A. (2007). Garlic (Allium Sativum L.): Adverse Effects and Drug Interactions in Humans. Mol. Nutr. Food Res. 51 (11), 1386–1397. doi:10.1002/mnfr.200700072
Boyd, D. D., and Nakajima, M. (2004). Involvement of Heparanase in Tumor Metastases: a New Target in Cancer Therapy? J. Natl. Cancer Inst. 96 (16), 1194–1195. doi:10.1093/jnci/djh256
Bray, F., Ferlay, J., Soerjomataram, I., Siegel, R. L., Torre, L. A., and Jemal, A. (2018). Global Cancer Statistics 2018: GLOBOCAN Estimates of Incidence and Mortality Worldwide for 36 Cancers in 185 Countries. CA Cancer J. Clin. 68 (6), 394–424. doi:10.3322/caac.21492
Cavallito, C. J., and Bailey, J. H. (1944b). Preliminary Note on the Inactivation of Antibiotics. Science 100 (2600), 390. doi:10.1126/science.100.2600.390
Cavallito, C. J., and Bailey, J. H. (1944a). Allicin, the Antibacterial Principle of Allium Sativum. I. Isolation, Physical Properties and Antibacterial Action. J. Am. Chem. Soc. 66 (11), 1950–1951. doi:10.1021/ja01239a048
Chan, J. M., Wang, F., and Holly, E. A. (2005). Vegetable and Fruit Intake and Pancreatic Cancer in a Population-Based Case-Control Study in the San Francisco Bay Area. Cancer Epidemiol. Biomarkers Prev. 14 (9), 2093–2097. doi:10.1158/1055-9965.EPI-05-0226
Chen, H., Zhu, B., Zhao, L., Liu, Y., Zhao, F., Feng, J., et al. (2018). Allicin Inhibits Proliferation and Invasion In Vitro and In Vivo via SHP-1-Mediated STAT3 Signaling in Cholangiocarcinoma. Cell Physiol. Biochem. 47 (2), 641–653. doi:10.1159/000490019
Chiavarini, M., Minelli, L., and Fabiani, R. (2016). Garlic Consumption and Colorectal Cancer Risk in Man: a Systematic Review and Meta-Analysis. Public Health Nutr. 19 (2), 308–317. doi:10.1017/S1368980015001263
Choo, S., Chin, V. K., Wong, E. H., Madhavan, P., Tay, S. T., Yong, P. V. C., et al. (2020). Review: Antimicrobial Properties of Allicin Used Alone or in Combination with Other Medications. Folia Microbiol. (Praha) 65 (3), 451–465. doi:10.1007/s12223-020-00786-5
Chu, Y. L., Ho, C. T., Chung, J. G., Raghu, R., Lo, Y. C., and Sheen, L. Y. (2013). Allicin Induces Anti-human Liver Cancer Cells through the P53 Gene Modulating Apoptosis and Autophagy. J. Agric. Food Chem. 61 (41), 9839–9848. doi:10.1021/jf403241s
Chu, Y. L., Ho, C. T., Chung, J. G., Rajasekaran, R., and Sheen, L. Y. (2012). Allicin Induces P53-Mediated Autophagy in Hep G2 Human Liver Cancer Cells. J. Agric. Food Chem. 60 (34), 8363–8371. doi:10.1021/jf301298y
Clinton, S. K., Giovannucci, E. L., and Hursting, S. D. (2020). The World Cancer Research Fund/American Institute for Cancer Research Third Expert Report on Diet, Nutrition, Physical Activity, and Cancer: Impact and Future Directions. J. Nutr. 150 (4), 663–671. doi:10.1093/jn/nxz268
Coppi, A., Cabinian, M., Mirelman, D., and Sinnis, P. (2006). Antimalarial Activity of Allicin, a Biologically Active Compound from Garlic Cloves. Antimicrob. Agents Chemother. 50 (5), 1731–1737. doi:10.1128/AAC.50.5.1731-1737.2006
Coppi, A., Pinzon-Ortiz, C., Hutter, C., and Sinnis, P. (2005). The Plasmodium Circumsporozoite Protein Is Proteolytically Processed during Cell Invasion. J. Exp. Med. 201 (1), 27–33. doi:10.1084/jem.20040989
Curtis, H., Noll, U., Störmann, J., and Slusarenko, A. J. (2004). Broad-spectrum Activity of the Volatile Phytoanticipin Allicin in Extracts of Garlic (Allium Sativum L.) against Plant Pathogenic Bacteria, Fungi and Oomycetes. Physiological Mol. Plant Pathology 65 (2), 79–89. doi:10.1016/j.pmpp.2004.11.006
Cutler, R. R., and Wilson, P. (2004). Antibacterial Activity of a New, Stable, Aqueous Extract of Allicin against Methicillin-Resistant Staphylococcus aureus. Br. J. Biomed. Sci. 61 (2), 71–74. doi:10.1080/09674845.2004.11732646
Dai, J., Chen, Y., and Jiang, F. (2020). Allicin Reduces Inflammation by Regulating ROS/NLRP3 and Autophagy in the Context of A. fumigatus Infection in Mice. Gene 762, 145042. doi:10.1016/j.gene.2020.145042
Davis, S. R. (2005). An Overview of the Antifungal Properties of Allicin and its Breakdown Products-Tthe Possibility of a Safe and Effective Antifungal Prophylactic. Mycoses 48 (2), 95–100. doi:10.1111/j.1439-0507.2004.01076.x
Dekker, E., Tanis, P. J., Vleugels, J. L. A., Kasi, P. M., and Wallace, M. B. (2019). Colorectal Cancer. Lancet 394 (10207), 1467–1480. doi:10.1016/S0140-6736(19)32319-0
Desai, H. G., Kalro, R. H., and Choksi, A. P. (1990). Effect of Ginger & Garlic on DNA Content of Gastric Aspirate. Indian J. Med. Res. 92, 139–141.
Dipaolo, J. A., and Carruthers, C. (1960). The Effect of Allicin from Garlic on Tumor Growth. Cancer Res. 20, 431–434.
Dixit, V. P., and Joshi, S. (1982). Effects of Chronic Administration of Garlic (Allium Sativum Linn) on Testicular Function. Indian J. Exp. Biol. 20 (7), 534–536.
Dong, M., Yang, G., Liu, H., Liu, X., Lin, S., Sun, D., et al. (2014). Aged Black Garlic Extract Inhibits HT29 Colon Cancer Cell Growth via the PI3K/Akt Signaling Pathway. Biomed. Rep. 2 (2), 250–254. doi:10.3892/br.2014.226
Dorant, E., van den Brandt, P. A., and Goldbohm, R. A. (1996). A Prospective Cohort Study on the Relationship between Onion and Leek Consumption, Garlic Supplement Use and the Risk of Colorectal Carcinoma in The Netherlands. Carcinogenesis 17 (3), 477–484. doi:10.1093/carcin/17.3.477
El-Saber Batiha, G., Magdy Beshbishy, A., G Wasef, L., Elewa, Y. H. A., A Al-Sagan, A., Abd El-Hack, M. E., et al. (2020). Chemical Constituents and Pharmacological Activities of Garlic (Allium Sativum L.): A Review. Nutrients 12 (3). doi:10.3390/nu12030872
Fang, F., Li, H., Cui, W., and Dong, Y. (1999). Treatment of Hepatitis Caused by Cytomegalovirus with Allitridin Injection-Aan Experimental Study. J. Tongji Med. Univ. 19 (4), 271–274. doi:10.1007/BF02886960
Feldberg, R. S., Chang, S. C., Kotik, A. N., Nadler, M., Neuwirth, Z., Sundstrom, D. C., et al. (1988). In Vitro mechanism of Inhibition of Bacterial Cell Growth by Allicin. Antimicrob. Agents Chemother. 32 (12), 1763–1768. doi:10.1128/aac.32.12.1763
Feng, Y., Zhu, X., Wang, Q., Jiang, Y., Shang, H., Cui, L., et al. (2012). Allicin Enhances Host Pro-inflammatory Immune Responses and Protects against Acute Murine Malaria Infection. Malar. J. 11, 268. doi:10.1186/1475-2875-11-268
Freeman, F., and Kodera, Y. (1995). Garlic Chemistry: Stability of S-(2-Propenyl)-2-Propene-1-sulfinothioate (Allicin) in Blood, Solvents, and Simulated Physiological Fluids. J. Agric. Food Chem. 43 (9), 2332–2338. doi:10.1021/jf00057a004
Fujisawa, H., Suma, K., Origuchi, K., Kumagai, H., Seki, T., and Ariga, T. (2008). Biological and Chemical Stability of Garlic-Derived Allicin. J. Agric. Food Chem. 56 (11), 4229–4235. doi:10.1021/jf8000907
Fujisawa, H., Watanabe, K., Suma, K., Origuchi, K., Matsufuji, H., Seki, T., et al. (2009). Antibacterial Potential of Garlic-Derived Allicin and its Cancellation by Sulfhydryl Compounds. Biosci. Biotechnol. Biochem. 73 (9), 1948–1955. doi:10.1271/bbb.90096
Galeone, C., Pelucchi, C., Levi, F., Negri, E., Franceschi, S., Talamini, R., et al. (2006). Onion and Garlic Use and Human Cancer. Am. J. Clin. Nutr. 84 (5), 1027–1032. doi:10.1093/ajcn/84.5.1027
Gao, J., Song, G., Shen, H., Wu, Y., Zhao, C., Zhang, Z., et al. (2022). Allicin Improves Intestinal Epithelial Barrier Function and Prevents LPS-Induced Barrier Damages of Intestinal Epithelial Cell Monolayers. Front. Immunol. 13, 847861. doi:10.3389/fimmu.2022.847861
Gao, W., Wang, W., Liu, G., Zhang, J., Yang, J., and Deng, Z. (2019a). Allicin Attenuated Chronic Social Defeat Stress Induced Depressive-like Behaviors through Suppression of NLRP3 Inflammasome. Metab. Brain Dis. 34 (1), 319–329. doi:10.1007/s11011-018-0342-z
Gao, W., Wang, W., Zhang, J., Deng, P., Hu, J., Yang, J., et al. (2019b). Allicin Ameliorates Obesity Comorbid Depressive-like Behaviors: Involvement of the Oxidative Stress, Mitochondrial Function, Autophagy, Insulin Resistance and NOX/Nrf2 Imbalance in Mice. Metab. Brain Dis. 34 (5), 1267–1280. doi:10.1007/s11011-019-00443-y
Gao, Y., Liu, Y., Cao, W., Deng, Z., Liu, H., Xu, L., et al. (2009a). Allicin Enhances Cytotoxicity of CPT-11 to Colon Cancer LoVo Cell In Vitro. Zhongguo Zhong Yao Za Zhi 34 (23), 3092–3095.
Gao, Y., Liu, Y. Q., Cao, W. K., Chen, X. F., Wan, Y. Y., Heng, C., et al. (2009b). Effects of Allicin on Invasion and Metastasis of Colon Cancer LoVo Cell Line In Vitro. Zhonghua Yi Xue Za Zhi 89 (20), 1382–1386.
Graham, D. Y., Anderson, S. Y., and Lang, T. (1999). Garlic or Jalapeño Peppers for Treatment of Helicobacter pylori Infection. Am. J. Gastroenterol. 94 (5), 1200–1202. doi:10.1111/j.1572-0241.1999.01066.x
Gruhlke, M. C., Nicco, C., Batteux, F., and Slusarenko, A. J. (2016). The Effects of Allicin, a Reactive Sulfur Species from Garlic, on a Selection of Mammalian Cell Lines. Antioxidants (Basel) 6 (1). doi:10.3390/antiox6010001
Gruhlke, M. C., and Slusarenko, A. J. (2012). The Biology of Reactive Sulfur Species (RSS). Plant Physiol. Biochem. 59, 98–107. doi:10.1016/j.plaphy.2012.03.016
Gruhlke, M. C. H., Schlembach, I., Leontiev, R., Uebachs, A., Gollwitzer, P. U. G., Weiss, A., et al. (2017). Yap1p, the Central Regulator of the S. cerevisiae Oxidative Stress Response, Is Activated by Allicin, a Natural Oxidant and Defence Substance of Garlic. Free Radic. Biol. Med. 108, 793–802. doi:10.1016/j.freeradbiomed.2017.05.004
Guercio, V., Turati, F., La Vecchia, C., Galeone, C., and Tavani, A. (2016). Allium Vegetables and Upper Aerodigestive Tract Cancers: a Meta-Analysis of Observational Studies. Mol. Nutr. Food Res. 60 (1), 212–222. doi:10.1002/mnfr.201500587
Guillamón, E., Andreo-Martínez, P., Mut-Salud, N., Fonollá, J., and Baños, A. (2021). Beneficial Effects of Organosulfur Compounds from Allium cepa on Gut Health: A Systematic Review. Foods 10 (8), 1680. doi:10.3390/foods10081680
Guo, Y. (2014). Experimental Study on the Optimization of Extraction Process of Garlic Oil and its Antibacterial Effects. Afr. J. Tradit. Complement. Altern. Med. 11 (2), 411–414. doi:10.4314/ajtcam.v11i2.27
Ha, M. W., and Yuan, Y. (2004). Allicin Induced Cell Cycle Arrest in Human Gastric Cancer Cell Lines. Zhonghua Zhong Liu Za Zhi 26 (10), 585–589.
Hall, A., Troupin, A., Londono-Renteria, B., and Colpitts, T. M. (2017). Garlic Organosulfur Compounds Reduce Inflammation and Oxidative Stress during Dengue Virus Infection. Viruses 9 (7). doi:10.3390/v9070159
Heine-Bröring, R. C., Winkels, R. M., Renkema, J. M., Kragt, L., van Orten-Luiten, A. C., Tigchelaar, E. F., et al. (2015). Dietary Supplement Use and Colorectal Cancer Risk: a Systematic Review and Meta-Analyses of Prospective Cohort Studies. Int. J. Cancer 136 (10), 2388–2401. doi:10.1002/ijc.29277
Hirsch, K., Danilenko, M., Giat, J., Miron, T., Rabinkov, A., Wilchek, M., et al. (2000). Effect of Purified Allicin, the Major Ingredient of Freshly Crushed Garlic, on Cancer Cell Proliferation. Nutr. Cancer 38 (2), 245–254. doi:10.1207/s15327914nc382_14
Hitchcock, J. K., Mkwanazi, N., Barnett, C., Graham, L. M., Katz, A. A., Hunter, R., et al. (2021). The Garlic Compound Z-Ajoene, S-Thiolates COX2 and STAT3 and Dampens the Inflammatory Response in RAW264.7 Macrophages. Mol. Nutr. Food Res. 65 (3), e2000854. doi:10.1002/mnfr.202000854
Hong, Y., Nan, B., Wu, X., Yan, H., and Yuan, Y. (2019). Allicin Alleviates Acrylamide-Induced Oxidative Stress in BRL-3A Cells. Life Sci. 231, 116550. doi:10.1016/j.lfs.2019.116550
Horev-Azaria, L., Eliav, S., Izigov, N., Pri-Chen, S., Mirelman, D., Miron, T., et al. (2009). Allicin Up-Regulates Cellular Glutathione Level in Vascular Endothelial Cells. Eur. J. Nutr. 48 (2), 67–74. doi:10.1007/s00394-008-0762-3
Hosono, T., Fukao, T., Ogihara, J., Ito, Y., Shiba, H., Seki, T., et al. (2005). Diallyl Trisulfide Suppresses the Proliferation and Induces Apoptosis of Human Colon Cancer Cells through Oxidative Modification of Beta-Tubulin. J. Biol. Chem. 280 (50), 41487–41493. doi:10.1074/jbc.M507127200
Hosono, T., Hosono-Fukao, T., Inada, K., Tanaka, R., Yamada, H., Iitsuka, Y., et al. (2008). Alkenyl Group Is Responsible for the Disruption of Microtubule Network Formation in Human Colon Cancer Cell Line HT-29 Cells. Carcinogenesis 29 (7), 1400–1406. doi:10.1093/carcin/bgn124
Hu, J. Y., Hu, Y. W., Zhou, J. J., Zhang, M. W., Li, D., and Zheng, S. (2014). Consumption of Garlic and Risk of Colorectal Cancer: an Updated Meta-Analysis of Prospective Studies. World J. Gastroenterol. 20 (41), 15413–15422. doi:10.3748/wjg.v20.i41.15413
Huang, W. L., Wu, S. F., Xu, S. T., Ma, Y. C., Wang, R., Jin, S., et al. (2020). Allicin Enhances the Radiosensitivity of Colorectal Cancer Cells via Inhibition of NF-Κb Signaling Pathway. J. Food Sci. 85 (6), 1924–1931. doi:10.1111/1750-3841.15156
Ide, N., and Lau, B. H. (2001). Garlic Compounds Minimize Intracellular Oxidative Stress and Inhibit Nuclear Factor-Kappa B Activation. J. Nutr. 131 (3s), 1020S–6S. doi:10.1093/jn/131.3.1020S
Ishikawa, H., Saeki, T., Otani, T., Suzuki, T., Shimozuma, K., Nishino, H., et al. (2006). Aged Garlic Extract Prevents a Decline of NK Cell Number and Activity in Patients with Advanced Cancer. J. Nutr. 136 (3 Suppl. l), 816S–820S. doi:10.1093/jn/136.3.816S
Izigov, N., Farzam, N., and Savion, N. (2011). S-allylmercapto-N-acetylcysteine Up-Regulates Cellular Glutathione and Protects Vascular Endothelial Cells from Oxidative Stress. Free Radic. Biol. Med. 50 (9), 1131–1139. doi:10.1016/j.freeradbiomed.2011.01.028
Jain, S., McGinnes, L. W., and Morrison, T. G. (2007). Thiol/disulfide Exchange Is Required for Membrane Fusion Directed by the Newcastle Disease Virus Fusion Protein. J. Virol. 81 (5), 2328–2339. doi:10.1128/JVI.01940-06
Jakubíková, J., and Sedlák, J. (2006). Garlic-derived Organosulfides Induce Cytotoxicity, Apoptosis, Cell Cycle Arrest and Oxidative Stress in Human Colon Carcinoma Cell Lines. Neoplasma 53 (3), 191–199.
Janik-Hazuka, M., Kamiński, K., Kaczor-Kamińska, M., Szafraniec-Szczęsny, J., Kmak, A., Kassassir, H., et al. (2021). Hyaluronic Acid-Based Nanocapsules as Efficient Delivery Systems of Garlic Oil Active Components with Anticancer Activity. Nanomaterials 11 (5), 1354. doi:10.3390/nano11051354
Ji, C., Ren, F., Ma, H., and Xu, M. (2010). The Roles of p38MAPK and Caspase-3 in DADS-Induced Apoptosis in Human HepG2 Cells. J. Exp. Clin. Cancer Res. 29, 50. doi:10.1186/1756-9966-29-50
Jiang, X., Zhu, X., Huang, W., Xu, H., Zhao, Z., Li, S., et al. (2017b). Garlic-derived Organosulfur Compound Exerts Antitumor Efficacy via Activation of MAPK Pathway and Modulation of Cytokines in SGC-7901 Tumor-Bearing Mice. Int. Immunopharmacol. 48, 135–145. doi:10.1016/j.intimp.2017.05.004
Jiang, X. Y., Zhu, X. S., Xu, H. Y., Zhao, Z. X., Li, S. Y., Li, S. Z., et al. (2017a). Diallyl Trisulfide Suppresses Tumor Growth through the Attenuation of Nrf2/Akt and Activation of P38/JNK and Potentiates Cisplatin Efficacy in Gastric Cancer Treatment. Acta Pharmacol. Sin. 38 (7), 1048–1058. doi:10.1038/aps.2016.176
Jikihara, H., Qi, G., Nozoe, K., Hirokawa, M., Sato, H., Sugihara, Y., et al. (2015). Aged Garlic Extract Inhibits 1,2-Dimethylhydrazine-Induced Colon Tumor Development by Suppressing Cell Proliferation. Oncol. Rep. 33 (3), 1131–1140. doi:10.3892/or.2014.3705
Jin, Z. Y., Wallar, G., Zhou, J. Y., Yang, J., Han, R. Q., Wang, P. H., et al. (2019). Consumption of Garlic and its Interactions with Tobacco Smoking and Alcohol Drinking on Esophageal Cancer in a Chinese Population. Eur. J. Cancer Prev. 28 (4), 278–286. doi:10.1097/CEJ.0000000000000456
Josling, P. (2001). Preventing the Common Cold with a Garlic Supplement: a Double-Blind, Placebo-Controlled Survey. Adv. Ther. 18 (4), 189–193. doi:10.1007/BF02850113
Ju, X., Zhang, S., Wang, Q., Li, X., and Yang, P. (2010). Preparation and Stability of Diallyl Trisulfide Self-Assembled Micellar Injection. PDA J. Pharm. Sci. Technol. 64 (2), 92–96.
Kaschula, C. H., Hunter, R., Cotton, J., Tuveri, R., Ngarande, E., Dzobo, K., et al. (2016). The Garlic Compound Ajoene Targets Protein Folding in the Endoplasmic Reticulum of Cancer Cells. Mol. Carcinog. 55 (8), 1213–1228. doi:10.1002/mc.22364
Kaschula, C. H., Hunter, R., Hassan, H. T., Stellenboom, N., Cotton, J., Zhai, X. Q., et al. (2011). Anti-proliferation Activity of Synthetic Ajoene Analogues on Cancer Cell-Lines. Anticancer Agents Med. Chem. 11 (3), 260–266. doi:10.2174/187152011795347450
Kaschula, C. H., Hunter, R., Stellenboom, N., Caira, M. R., Winks, S., Ogunleye, T., et al. (2012). Structure-activity Studies on the Anti-proliferation Activity of Ajoene Analogues in WHCO1 Oesophageal Cancer Cells. Eur. J. Med. Chem. 50, 236–254. doi:10.1016/j.ejmech.2012.01.058
Khodavandi, A., Alizadeh, F., Harmal, N. S., Sidik, S. M., Othman, F., Sekawi, Z., et al. (2011). Comparison between Efficacy of Allicin and Fluconazole against Candida Albicans In Vitro and in a Systemic Candidiasis Mouse Model. FEMS Microbiol. Lett. 315 (2), 87–93. doi:10.1111/j.1574-6968.2010.02170.x
Kim, H., Keum, N., Giovannucci, E. L., Fuchs, C. S., and Bao, Y. (2018). Garlic Intake and Gastric Cancer Risk: Results from Two Large Prospective US Cohort Studies. Int. J. Cancer 143 (5), 1047–1053. doi:10.1002/ijc.31396
Kim, J. Y., and Kwon, O. (2009). Garlic Intake and Cancer Risk: an Analysis Using the Food and Drug Administration's Evidence-Based Review System for the Scientific Evaluation of Health Claims. Am. J. Clin. Nutr. 89 (1), 257–264. doi:10.3945/ajcn.2008.26142
Kodera, Y., Kurita, M., Nakamoto, M., and Matsutomo, T. (2020). Chemistry of Aged Garlic: Diversity of Constituents in Aged Garlic Extract and Their Production Mechanisms via the Combination of Chemical and Enzymatic Reactions. Exp. Ther. Med. 19 (2), 1574–1584. doi:10.3892/etm.2019.8393
Kyung, K. H. (2012). Antimicrobial Properties of Allium Species. Curr. Opin. Biotechnol. 23 (2), 142–147. doi:10.1016/j.copbio.2011.08.004
Lan, X., Sun, H., Liu, J., Lin, Y., Zhu, Z., Han, X., et al. (2013). Effects of Garlic Oil on Pancreatic Cancer Cells. Asian Pac J. Cancer Prev. 14 (10), 5905–5910. doi:10.7314/apjcp.2013.14.10.5905
Lawson, L. D., and Gardner, C. D. (2005). Composition, Stability, and Bioavailability of Garlic Products Used in a Clinical Trial. J. Agric. Food Chem. 53 (16), 6254–6261. doi:10.1021/jf050536+
Lawson, L. D., and Hughes, B. G. (1992). Characterization of the Formation of Allicin and Other Thiosulfinates from Garlic. Planta Med. 58 (4), 345–350. doi:10.1055/s-2006-961482
Lee, L. S., Andrade, A. S., and Flexner, C. (2006). Interactions between Natural Health Products and Antiretroviral Drugs: Pharmacokinetic and Pharmacodynamic Effects. Clin. Infect. Dis. 43 (8), 1052–1059. doi:10.1086/507894
Lee, Z. W., Teo, X. Y., Song, Z. J., Nin, D. S., Novera, W., Choo, B. A., et al. (2017). Intracellular Hyper-Acidification Potentiated by Hydrogen Sulfide Mediates Invasive and Therapy Resistant Cancer Cell Death. Front. Pharmacol. 8, 763. doi:10.3389/fphar.2017.00763
Leng, B. F., Qiu, J. Z., Dai, X. H., Dong, J., Wang, J. F., Luo, M. J., et al. (2011). Allicin Reduces the Production of α-toxin by Staphylococcus aureus. Molecules 16 (9), 7958–7968. doi:10.3390/molecules16097958
Leontiev, R., Hohaus, N., Jacob, C., Gruhlke, M. C. H., and Slusarenko, A. J. (2018). A Comparison of the Antibacterial and Antifungal Activities of Thiosulfinate Analogues of Allicin. Sci. Rep. 8 (1), 6763. doi:10.1038/s41598-018-25154-9
Li, C., Jing, H., Ma, G., and Liang, P. (2018). Allicin Induces Apoptosis through Activation of Both Intrinsic and Extrinsic Pathways in Glioma Cells. Mol. Med. Rep. 17 (4), 5976–5981. doi:10.3892/mmr.2018.8552
Li, C., Lun, W., Zhao, X., Lei, S., Guo, Y., Ma, J., et al. (2015). Allicin Alleviates Inflammation of Trinitrobenzenesulfonic Acid-Induced Rats and Suppresses P38 and JNK Pathways in Caco-2 Cells. Mediat. Inflamm. 2015, 434692. doi:10.1155/2015/434692
Li, C. L., Liu, X. H., Qiao, Y., Ning, L. N., Li, W. J., Sun, Y. S., et al. (2020a). Allicin Alleviates Inflammation of Diabetic Macroangiopathy via the Nrf2 and NF-kB Pathway. Eur. J. Pharmacol. 876, 173052. doi:10.1016/j.ejphar.2020.173052
Li, H., Jeong, J. H., Kwon, S. W., Lee, S. K., Lee, H. J., and Ryu, J. H. (2020b). Z-ajoene Inhibits Growth of Colon Cancer by Promotion of CK1α Dependent β-Catenin Phosphorylation. Molecules 25 (3). doi:10.3390/molecules25030703
Li, M., Ciu, J. R., Ye, Y., Min, J. M., Zhang, L. H., Wang, K., et al. (2002). Antitumor Activity of Z-Ajoene, a Natural Compound Purified from Garlic: Antimitotic and Microtubule-Interaction Properties. Carcinogenesis 23 (4), 573–579. doi:10.1093/carcin/23.4.573
Li, W. Q., Zhang, J. Y., Ma, J. L., Li, Z. X., Zhang, L., Zhang, Y., et al. (2019a). Effects of Helicobacter pylori Treatment and Vitamin and Garlic Supplementation on Gastric Cancer Incidence and Mortality: Follow-Up of a Randomized Intervention Trial. BMJ 366, l5016. doi:10.1136/bmj.l5016
Li, X., Ni, J., Tang, Y., Wang, X., Tang, H., Li, H., et al. (2019b). Allicin Inhibits Mouse Colorectal Tumorigenesis through Suppressing the Activation of STAT3 Signaling Pathway. Nat. Prod. Res. 33 (18), 2722–2725. doi:10.1080/14786419.2018.1465425
Liang, D., Wu, H., Wong, M. W., and Huang, D. (2015). Diallyl Trisulfide Is a Fast H2S Donor, but Diallyl Disulfide Is a Slow One: The Reaction Pathways and Intermediates of Glutathione with Polysulfides. Org. Lett. 17 (17), 4196–4199. doi:10.1021/acs.orglett.5b01962
Liu, C., Cao, F., Tang, Q. Z., Yan, L., Dong, Y. G., Zhu, L. H., et al. (2010). Allicin Protects against Cardiac Hypertrophy and Fibrosis via Attenuating Reactive Oxygen Species-dependent Signaling Pathways. J. Nutr. Biochem. 21 (12), 1238–1250. doi:10.1016/j.jnutbio.2009.11.001
Liu, S. G., Ren, P. Y., Wang, G. Y., Yao, S. X., and He, X. J. (2015). Allicin Protects Spinal Cord Neurons from Glutamate-Induced Oxidative Stress through Regulating the Heat Shock Protein 70/inducible Nitric Oxide Synthase Pathway. Food Funct. 6 (1), 321–330. doi:10.1039/c4fo00761a
Liu, X., Baecker, A., Wu, M., Zhou, J. Y., Yang, J., Han, R. Q., et al. (2019). Raw Garlic Consumption and Risk of Liver Cancer: A Population-Based Case-Control Study in Eastern China. Nutrients 11 (9). doi:10.3390/nu11092038
Liu, Z. F., Fang, F., Dong, Y. S., Li, G., and Zhen, H. (2004). Experimental Study on the Prevention and Treatment of Murine Cytomegalovirus Hepatitis by Using Allitridin. Antivir. Res. 61 (2), 125–128. doi:10.1016/s0166-3542(03)00087-1
Lu, X., Rasco, B. A., Jabal, J. M., Aston, D. E., Lin, M., and Konkel, M. E. (2011). Investigating Antibacterial Effects of Garlic (Allium Sativum) Concentrate and Garlic-Derived Organosulfur Compounds on Campylobacter Jejuni by Using Fourier Transform Infrared Spectroscopy, Raman Spectroscopy, and Electron Microscopy. Appl. Environ. Microbiol. 77 (15), 5257–5269. doi:10.1128/aem.02845-10
Ma, H. B., Huang, S., Yin, X. R., Zhang, Y., and Di, Z. L. (2014). Apoptotic Pathway Induced by Diallyl Trisulfide in Pancreatic Cancer Cells. World J. Gastroenterol. 20 (1), 193–203. doi:10.3748/wjg.v20.i1.193
Matsuura, N., Miyamae, Y., Yamane, K., Nagao, Y., Hamada, Y., Kawaguchi, N., et al. (2006). Aged Garlic Extract Inhibits Angiogenesis and Proliferation of Colorectal Carcinoma Cells. J. Nutr. 136 (3 Suppl. l), 842s–846s. doi:10.1093/jn/136.3.842S
McNulty, C. A., Wilson, M. P., Havinga, W., Johnston, B., O'Gara, E. A., and Maslin, D. J. (2001). A Pilot Study to Determine the Effectiveness of Garlic Oil Capsules in the Treatment of Dyspeptic Patients with Helicobacter pylori. Helicobacter 6 (3), 249–253. doi:10.1046/j.1523-5378.2001.00036.x
Metwally, D. M., Al-Olayan, E. M., Alanazi, M., Alzahrany, S. B., and Semlali, A. (2018). Antischistosomal and Anti-inflammatory Activity of Garlic and Allicin Compared with that of Praziquantel In Vivo. BMC Complement. Altern. Med. 18 (1), 135. doi:10.1186/s12906-018-2191-z
Mhatre, S., Rajaraman, P., Chatterjee, N., Bray, F., Goel, M., Patkar, S., et al. (2020). Mustard Oil Consumption, Cooking Method, Diet and Gallbladder Cancer Risk in High- and Low-Risk Regions of India. Int. J. Cancer 147 (6), 1621–1628. doi:10.1002/ijc.32952
Min, C., and ZhuBo, L. I. (2011). Advance in Antitumor of Garlic Vegetable-Derived Organosulfur Compounds. Chin. Bull. Life Sci. 23 (5), 487–491.
Miron, T., Rabinkov, A., Mirelman, D., Wilchek, M., and Weiner, L. (2000). The Mode of Action of Allicin: its Ready Permeability through Phospholipid Membranes May Contribute to its Biological Activity. Biochim. Biophys. Acta 1463 (1), 20–30. doi:10.1016/s0005-2736(99)00174-1
Miron, T., Wilchek, M., Sharp, A., Nakagawa, Y., Naoi, M., Nozawa, Y., et al. (2008). Allicin Inhibits Cell Growth and Induces Apoptosis through the Mitochondrial Pathway in HL60 and U937 Cells. J. Nutr. Biochem. 19 (8), 524–535. doi:10.1016/j.jnutbio.2007.06.009
Mizrahi, J. D., Surana, R., Valle, J. W., and Shroff, R. T. (2020). Pancreatic Cancer. Lancet 395 (10242), 2008–2020. doi:10.1016/S0140-6736(20)30974-0
Nakagawa, S., Masamoto, K., Sumiyoshi, H., Kunihiro, K., and Fuwa, T. (1980). Effect of Raw and Extracted-Aged Garlic Juice on Growth of Young Rats and Their Organs after Peroral Administration (Author's Transl). J. Toxicol. Sci. 5 (1), 91–112. doi:10.2131/jts.5.91
Nam, H., Jung, H., Kim, Y., Kim, B., Kim, K. H., Park, S. J., et al. (2018). Aged Black Garlic Extract Regulates Lipid Metabolism by Inhibiting Lipogenesis and Promoting Lipolysis in Mature 3T3-L1 Adipocytes. Food Sci. Biotechnol. 27 (2), 575–579. doi:10.1007/s10068-017-0268-y
Nelson, S. M., Gao, Y. T., Nogueira, L. M., Shen, M. C., Wang, B., Rashid, A., et al. (2017). Diet and Biliary Tract Cancer Risk in Shanghai, China. PloS one 12 (3), e0173935. doi:10.1371/journal.pone.0173935
Ng, K. T., Guo, D. Y., Cheng, Q., Geng, W., Ling, C. C., Li, C. X., et al. (2012). A Garlic Derivative, S-Allylcysteine (SAC), Suppresses Proliferation and Metastasis of Hepatocellular Carcinoma. PloS one 7 (2), e31655. doi:10.1371/journal.pone.0031655
Niture, S. K., and Jaiswal, A. K. (2012). Nrf2 Protein Up-Regulates Antiapoptotic Protein Bcl-2 and Prevents Cellular Apoptosis. J. Biol. Chem. 287 (13), 9873–9886. doi:10.1074/jbc.M111.312694
O'Gara, E. A., Maslin, D. J., Nevill, A. M., and Hill, D. J. (2008). The Effect of Simulated Gastric Environments on the Anti-Helicobacter Activity of Garlic Oil. J. Appl. Microbiol. 104 (5), 1324–1331. doi:10.1111/j.1365-2672.2007.03637.x
Okada, Y., Tanaka, K., Sato, E., and Okajima, H. (2006). Kinetic and Mechanistic Studies of Allicin as an Antioxidant. Org. Biomol. Chem. 4 (22), 4113–4117. doi:10.1039/b611506c
Olsson, M., and Zhivotovsky, B. (2011). Caspases and Cancer. Cell Death Differ. 18 (9), 1441–1449. doi:10.1038/cdd.2011.30
Oommen, S., Anto, R. J., Srinivas, G., and Karunagaran, D. (2004). Allicin (From Garlic) Induces Caspase-Mediated Apoptosis in Cancer Cells. Eur. J. Pharmacol. 485 (1), 97–103. doi:10.1016/j.ejphar.2003.11.059
Panyod, S., Wu, W. K., Lu, K. H., Liu, C. T., Chu, Y. L., Ho, C. T., et al. (2020). Allicin Modifies the Composition and Function of the Gut Microbiota in Alcoholic Hepatic Steatosis Mice. J. Agric. Food Chem. 68 (10), 3088–3098. doi:10.1021/acs.jafc.9b07555
Park, Y. G., Lee, S. E., Yoon, J. W., Kim, E. Y., and Park, S. P. (2019). Allicin Protects Porcine Oocytes against Damage during Aging In Vitro. Mol. Reprod. Dev. 86 (9), 1116–1125. doi:10.1002/mrd.23227
Pathak, S., Catanzaro, R., Vasan, D., Marotta, F., Chabria, Y., Jothimani, G., et al. (2020). Benefits of Aged Garlic Extract in Modulating Toxicity Biomarkers against P-Dimethylaminoazobenzene and Phenobarbital Induced Liver Damage in Rattus norvegicus. Drug Chem. Toxicol. 43 (5), 454–467. doi:10.1080/01480545.2018.1499773
Pennathur, A., Gibson, M. K., Jobe, B. A., and Luketich, J. D. (2013). Oesophageal Carcinoma. Lancet 381 (9864), 400–412. doi:10.1016/S0140-6736(12)60643-6
Perez-Ortiz, J. M., Galan-Moya, E. M., de la Cruz-Morcillo, M. A., Rodriguez, J. F., Gracia, I., Garcia, M. T., et al. (2020). Cost Effective Use of a Thiosulfinate-Enriched Allium Sativum Extract in Combination with Chemotherapy in Colon Cancer. Int. J. Mol. Sci. 21 (8). doi:10.3390/ijms21082766
Poole, L. B. (2015). The Basics of Thiols and Cysteines in Redox Biology and Chemistry. Free Radic. Biol. Med. 80, 148–157. doi:10.1016/j.freeradbiomed.2014.11.013
Qian, Y. Q., Feng, Z. H., Li, X. B., Hu, Z. C., Xuan, J. W., Wang, X. Y., et al. (2018). Downregulating PI3K/Akt/NF-Κb Signaling with Allicin for Ameliorating the Progression of Osteoarthritis: In Vitro and Vivo Studies. Food Funct. 9 (9), 4865–4875. doi:10.1039/c8fo01095a
Rachel Vasanthi, A., Mansingh, D., Dalpati, N., and Sali, V. (2018). Alliin the Precursor of Allicin in Garlic Extract Mitigates Proliferation of Gastric Adenocarcinoma Cells by Modulating Apoptosis. Phcog Mag. 14 (55), 84–91. doi:10.4103/pm.pm_342_17
Ramirez, D. A., Altamirano, J. C., and Camargo, A. B. (2021). Multi-phytochemical Determination of Polar and Non-polar Garlic Bioactive Compounds in Different Food and Nutraceutical Preparations. Food Chem. 337, 127648. doi:10.1016/j.foodchem.2020.127648
Razumilava, N., and Gores, G. J. (2014). Cholangiocarcinoma. Lancet 383 (9935), 2168–2179. doi:10.1016/S0140-6736(13)61903-0
Reddy, B. S., Rao, C. V., Rivenson, A., and Kelloff, G. (1993). Chemoprevention of Colon Carcinogenesis by Organosulfur Compounds. Cancer Res. 53 (15), 3493–3498.
Ried, K., and Fakler, P. (2014). Potential of Garlic (Allium Sativum) in Lowering High Blood Pressure: Mechanisms of Action and Clinical Relevance. Integr. Blood Press Control 7, 71–82. doi:10.2147/ibpc.S51434
Rose, P., Moore, P. K., and Zhu, Y. Z. (2018). Garlic and Gaseous Mediators. Trends Pharmacol. Sci. 39 (7), 624–634. doi:10.1016/j.tips.2018.03.009
Rose, P., Whiteman, M., Moore, P. K., and Zhu, Y. Z. (2005). Bioactive S-alk(en)yl cysteine sulfoxide metabolites in the genus Allium: the chemistry of potential therapeutic agents. Nat. Prod. Rep. 22 (3), 351–368. doi:10.1039/b417639c
Rouf, R., Uddin, S. J., Sarker, D. K., Islam, M. T., Ali, E. S., Shilpi, J. A., et al. (2020). Antiviral Potential of Garlic (Allium Sativum) and its Organosulfur Compounds: A Systematic Update of Pre-clinical and Clinical Data. Trends Food Sci. Technol. 104, 219–234. doi:10.1016/j.tifs.2020.08.006
Salama, A. A., AbouLaila, M., Terkawi, M. A., Mousa, A., El-Sify, A., Allaam, M., et al. (2014). Inhibitory Effect of Allicin on the Growth of Babesia and Theileria Equi Parasites. Parasitol. Res. 113 (1), 275–283. doi:10.1007/s00436-013-3654-2
Samra, Y. A., Hamed, M. F., and El-Sheakh, A. R. (2020). Hepatoprotective Effect of Allicin against Acetaminophen-Induced Liver Injury: Role of Inflammasome Pathway, Apoptosis, and Liver Regeneration. J. Biochem. Mol. Toxicol. 34 (5), e22470. doi:10.1002/jbt.22470
Sarvizadeh, M., Hasanpour, O., Naderi Ghale-Noie, Z., Mollazadeh, S., Rezaei, M., Pourghadamyari, H., et al. (2021). Allicin and Digestive System Cancers: From Chemical Structure to its Therapeutic Opportunities. Front. Oncol. 11, 650256. doi:10.3389/fonc.2021.650256
Satia, J. A., Littman, A., Slatore, C. G., Galanko, J. A., and White, E. (2009). Associations of Herbal and Specialty Supplements with Lung and Colorectal Cancer Risk in the VITamins and Lifestyle Study. Cancer Epidemiol. Biomarkers Prev. 18 (5), 1419–1428. doi:10.1158/1055-9965.EPI-09-0038
Saud, S. M., Li, W., Gray, Z., Matter, M. S., Colburn, N. H., Young, M. R., et al. (2016). Diallyl Disulfide (DADS), a Constituent of Garlic, Inactivates NF-Κb and Prevents Colitis-Induced Colorectal Cancer by Inhibiting GSK-3β. Cancer Prev. Res. (Phila) 9 (7), 607–615. doi:10.1158/1940-6207.Capr-16-0044
Schafer, F. Q., and Buettner, G. R. (2001). Redox Environment of the Cell as Viewed through the Redox State of the Glutathione Disulfide/glutathione Couple. Free Radic. Biol. Med. 30 (11), 1191–1212. doi:10.1016/s0891-5849(01)00480-4
Schäfer, G., and Kaschula, C. H. (2014). The Immunomodulation and Anti-inflammatory Effects of Garlic Organosulfur Compounds in Cancer Chemoprevention. Anti-cancer Agents Med. Chem. 14 (2), 233–240.
Shen, C., Xiao, H., and Parkin, K. L. (2002). In Vitro stability and Chemical Reactivity of Thiosulfinates. J. Agric. Food Chem. 50 (9), 2644–2651. doi:10.1021/jf011013e
Shen, J., Davis, L. E., Wallace, J. M., Cai, Y., and Lawson, L. D. (1996). Enhanced Diallyl Trisulfide Has In Vitro Synergy with Amphotericin B against Cryptococcus Neoformans. Planta Med. 62 (5), 415–418. doi:10.1055/s-2006-957929
Shin, J. H., Ryu, J. H., Kang, M. J., Hwang, C. R., Han, J., and Kang, D. (2013). Short-term Heating Reduces the Anti-inflammatory Effects of Fresh Raw Garlic Extracts on the LPS-Induced Production of NO and Pro-inflammatory Cytokines by Downregulating Allicin Activity in RAW 264.7 Macrophages. Food Chem. Toxicol. 58, 545–551. doi:10.1016/j.fct.2013.04.002
Si, J., Zhao, X., Gao, S., Huang, D., and Sui, M. (2019a). Advances in Delivery of Irinotecan (CPT-11) Active Metabolite 7-Ethyl-10-Hydroxycamptothecin. Int. J. Pharm. 568, 118499. doi:10.1016/j.ijpharm.2019.118499
Si, X. B., Zhang, X. M., Wang, S., Lan, Y., Zhang, S., and Huo, L. Y. (2019b). Allicin as Add-On Therapy for Helicobacter pylori Infection: A Systematic Review and Meta-Analysis. World J. Gastroenterol. 25 (39), 6025–6040. doi:10.3748/wjg.v25.i39.6025
Singh, V., Belloir, C., Siess, M. H., and Le Bon, A. M. (2006). Inhibition of Carcinogen-Induced DNA Damage in Rat Liver and Colon by Garlic Powders with Varying Alliin Content. Nutr. Cancer 55 (2), 178–184. doi:10.1207/s15327914nc5502_9
Siyo, V., Schäfer, G., Hunter, R., Grafov, A., Grafova, I., Nieger, M., et al. (2017). The Cytotoxicity of the Ajoene Analogue BisPMB in WHCO1 Oesophageal Cancer Cells Is Mediated by CHOP/GADD153. Molecules 22 (6). doi:10.3390/molecules22060892
Small, L. D., Bailey, J. H., and Cavallito, C. J. (1947). Alkyl Thiolsulfinates. J. Am. Chem. Soc. 69 (7), 1710–1713. doi:10.1021/ja01199a040
Smyth, E. C., Lagergren, J., Fitzgerald, R. C., Lordick, F., Shah, M. A., Lagergren, P., et al. (2017). Oesophageal Cancer. Nat. Rev. Dis. Prim. 3, 17048. doi:10.1038/nrdp.2017.48
Smyth, E. C., Nilsson, M., Grabsch, H. I., van Grieken, N. C., and Lordick, F. (2020). Gastric Cancer. Lancet 396 (10251), 635–648. doi:10.1016/S0140-6736(20)31288-5
Stoll, A., and Seebeck, E. (1948). About Alliin, the Genuine Mother Substance of Garlic Oil. Helv. Chim. Acta 31 (1), 189–210. doi:10.1002/hlca.19480310140
Suddek, G. M. (2014). Allicin Enhances Chemotherapeutic Response and Ameliorates Tamoxifen-Induced Liver Injury in Experimental Animals. Pharm. Biol. 52 (8), 1009–1014. doi:10.3109/13880209.2013.876053
Sui, X., Jin, L., Huang, X., Geng, S., He, C., and Hu, X. (2011). p53 Signaling and Autophagy in Cancer: a Revolutionary Strategy Could Be Developed for Cancer Treatment. Autophagy 7 (6), 565–571. doi:10.4161/auto.7.6.14073
Sun, L., and Wang, X. (2003). Effects of Allicin on Both Telomerase Activity and Apoptosis in Gastric Cancer SGC-7901 Cells. World J. Gastroenterol. 9 (9), 1930–1934. doi:10.3748/wjg.v9.i9.1930
Sundaram, S. G., and Milner, J. A. (1996). Diallyl Disulfide Suppresses the Growth of Human Colon Tumor Cell Xenografts in Athymic Nude Mice. J. Nutr. 126 (5), 1355–1361. doi:10.1093/jn/126.5.1355
Sung, H., Ferlay, J., Siegel, R. L., Laversanne, M., Soerjomataram, I., Jemal, A., et al. (2021). Global Cancer Statistics 2020: GLOBOCAN Estimates of Incidence and Mortality Worldwide for 36 Cancers in 185 Countries. CA A Cancer J. Clin. 71 (3), 209–249. doi:10.3322/caac.21660
Tanaka, S., Haruma, K., Yoshihara, M., Kajiyama, G., Kira, K., Amagase, H., et al. (2006). Aged Garlic Extract Has Potential Suppressive Effect on Colorectal Adenomas in Humans. J. Nutr. 136 (3 Suppl. l), 821s–826s. doi:10.1093/jn/136.3.821S
Tao, M., Gao, L., Pan, J., and Wang, X. (2014). Study on the Inhibitory Effect of Allicin on Human Gastric Cancer Cell Line SGC-7901 and its Mechanism. Afr. J. Tradit. Complement. Altern. Med. 11 (1), 176–179. doi:10.4314/ajtcam.v11i1.28
Terrasson, J., Xu, B., Li, M., Allart, S., Davignon, J. L., Zhang, L. H., et al. (2007). Activities of Z-Ajoene against Tumour and Viral Spreading In Vitro. Fundam. Clin. Pharmacol. 21 (3), 281–289. doi:10.1111/j.1472-8206.2007.00470.x
Țigu, A. B., Toma, V. A., Moț, A. C., Jurj, A., Moldovan, C. S., Fischer-Fodor, E., et al. (2020). The Synergistic Antitumor Effect of 5-Fluorouracil Combined with Allicin against Lung and Colorectal Carcinoma Cells. Molecules 25 (8). doi:10.3390/molecules25081947
Turati, F., Pelucchi, C., Guercio, V., La Vecchia, C., and Galeone, C. (2015). Allium Vegetable Intake and Gastric Cancer: a Case-Control Study and Meta-Analysis. Mol. Nutr. Food Res. 59 (1), 171–179. doi:10.1002/mnfr.201400496
Vaidya, V., Ingold, K. U., and Pratt, D. A. (2009). Garlic: Source of the Ultimate Antioxidants-Ssulfenic Acids. Angew. Chem. Int. Ed. Engl. 48 (1), 157–160. doi:10.1002/anie.200804560
Villanueva, A. (2019). Hepatocellular Carcinoma. N. Engl. J. Med. 380 (15), 1450–1462. doi:10.1056/NEJMra1713263
Waag, T., Gelhaus, C., Rath, J., Stich, A., Leippe, M., and Schirmeister, T. (2010). Allicin and Derivates Are Cysteine Protease Inhibitors with Antiparasitic Activity. Bioorg Med. Chem. Lett. 20 (18), 5541–5543. doi:10.1016/j.bmcl.2010.07.062
Walder, R., Kalvatchev, Z., Garzaro, D., Barrios, M., and Apitz-Castro, R. (1997). In Vitro suppression of HIV-1 replication by ajoene [(e)-(z)-4,5,9-trithiadodeca-1,6,11-triene-9 oxide]. Biomed. Pharmacother. 51 (9), 397–403. doi:10.1016/s0753-3322(97)89433-4
Wang, C. J., Wang, C., Han, J., Wang, Y. K., Tang, L., Shen, D. W., et al. (2013). Effect of Combined Treatment with Recombinant Interleukin-2 and Allicin on Pancreatic Cancer. Mol. Biol. Rep. 40 (12), 6579–6585. doi:10.1007/s11033-013-2766-1
Wang, L., Jiao, H., Zhao, J., Wang, X., Sun, S., and Lin, H. (2017). Allicin Alleviates Reticuloendotheliosis Virus-Induced Immunosuppression via ERK/Mitogen-Activated Protein Kinase Pathway in Specific Pathogen-free Chickens. Front. Immunol. 8, 1856. doi:10.3389/fimmu.2017.01856
Wang, N., Zhou, P., Chen, Y., Qu, H., Lu, K., and Xia, J. (2020). MicroRNA-149: A Review of its Role in Digestive System Cancers. Pathol. Res. Pract. 216 (12), 153266. doi:10.1016/j.prp.2020.153266
Wang, X., Jiao, F., Wang, Q. W., Wang, J., Yang, K., Hu, R. R., et al. (2012). Aged Black Garlic Extract Induces Inhibition of Gastric Cancer Cell Growth In Vitro and In Vivo. Mol. Med. Rep. 5 (1), 66–72. doi:10.3892/mmr.2011.588
Wargovich, M. J. (1987). Diallyl Sulfide, a Flavor Component of Garlic (Allium Sativum), Inhibits Dimethylhydrazine-Induced Colon Cancer. Carcinogenesis 8 (3), 487–489. doi:10.1093/carcin/8.3.487
Wargovich, M. J., Woods, C., Eng, V. W., Stephens, L. C., and Gray, K. (1988). Chemoprevention of N-Nitrosomethylbenzylamine-Induced Esophageal Cancer in Rats by the Naturally Occurring Thioether, Diallyl Sulfide. Cancer Res. 48 (23), 6872–6875.
Weber, N. D., Andersen, D. O., North, J. A., Murray, B. K., Lawson, L. D., and Hughes, B. G. (1992). In Vitro virucidal Effects of Allium Sativum (Garlic) Extract and Compounds. Planta Med. 58 (5), 417–423. doi:10.1055/s-2006-961504
Wei, Z., Shan, Y., Tao, L., Liu, Y., Zhu, Z., Liu, Z., et al. (2017). Diallyl Trisulfides, a Natural Histone Deacetylase Inhibitor, Attenuate HIF-1α Synthesis, and Decreases Breast Cancer Metastasis. Mol. Carcinog. 56 (10), 2317–2331. doi:10.1002/mc.22686
Wills, E. D. (1956). Enzyme Inhibition by Allicin, the Active Principle of Garlic. Biochem. J. 63 (3), 514–520. doi:10.1042/bj0630514
Wu, X., Shi, J., Fang, W. X., Guo, X. Y., Zhang, L. Y., Liu, Y. P., et al. (2019). Allium Vegetables Are Associated with Reduced Risk of Colorectal Cancer: A Hospital-Based Matched Case-Control Study in China. Asia Pac J. Clin. Oncol. 15 (5), e132–e141. doi:10.1111/ajco.13133
Xiang, Q., Cheng, Z., Wang, J., Feng, X., Hua, W., Luo, R., et al. (2020). Allicin Attenuated Advanced Oxidation Protein Product-Induced Oxidative Stress and Mitochondrial Apoptosis in Human Nucleus Pulposus Cells. Oxid. Med. Cell Longev. 2020, 6685043. doi:10.1155/2020/6685043
Xiao, J., Xing, F., Liu, Y., Lv, Y., Wang, X., Ling, M. T., et al. (2018). Garlic-derived Compound S-Allylmercaptocysteine Inhibits Hepatocarcinogenesis through Targeting LRP6/Wnt Pathway. Acta Pharm. Sin. B 8 (4), 575–586. doi:10.1016/j.apsb.2017.10.003
Xu, Y. S., Feng, J. G., Zhang, D., Zhang, B., Luo, M., Su, D., et al. (2014). S-allylcysteine, a Garlic Derivative, Suppresses Proliferation and Induces Apoptosis in Human Ovarian Cancer Cells In Vitro. Acta Pharmacol. Sin. 35 (2), 267–274. doi:10.1038/aps.2013.176
Yang, D., Lv, Z., Zhang, H., Liu, B., Jiang, H., Tan, X., et al. (2017). Activation of the Nrf2 Signaling Pathway Involving KLF9 Plays a Critical Role in Allicin Resisting against Arsenic Trioxide-Induced Hepatotoxicity in Rats. Biol. Trace Elem. Res. 176 (1), 192–200. doi:10.1007/s12011-016-0821-1
Yin, X., Zhang, J., Li, X., Liu, D., Feng, C., Liang, R., et al. (2014). DADS Suppresses Human Esophageal Xenograft Tumors through RAF/MEK/ERK and Mitochondria-dependent Pathways. Int. J. Mol. Sci. 15 (7), 12422–12441. doi:10.3390/ijms150712422
Yoshimoto, N., and Saito, K. (2019). S-Alk(en)ylcysteine sulfoxides in the genus Allium: proposed biosynthesis, chemical conversion, and bioactivities. J. Exp. Bot. 70 (16), 4123–4137. doi:10.1093/jxb/erz243
You, B. R., Yoo, J. M., Baek, S. Y., and Kim, M. R. (2019). Anti-inflammatory Effect of Aged Black Garlic on 12-O-Tetradecanoylphorbol-13-Acetate-Induced Dermatitis in Mice. Nutr. Res. Pract. 13 (3), 189–195. doi:10.4162/nrp.2019.13.3.189
Yu, L., Guo, N., Meng, R., Liu, B., Tang, X., Jin, J., et al. (2010). Allicin-induced Global Gene Expression Profile of Saccharomyces cerevisiae. Appl. Microbiol. Biotechnol. 88 (1), 219–229. doi:10.1007/s00253-010-2709-x
Yuan, Y., Lu, L., Bo, N., Chaoyue, Y., and Haiyang, Y. (2021). Allicin Ameliorates Intestinal Barrier Damage via Microbiota-Regulated Short-Chain Fatty Acids-TLR4/MyD88/NF-Κb Cascade Response in Acrylamide-Induced Rats. J. Agric. Food Chem. 69 (43), 12837–12852. doi:10.1021/acs.jafc.1c05014
Zhang, H., Feng, Q., Chen, W. D., and Wang, Y. D. (2018). HGF/c-MET: A Promising Therapeutic Target in the Digestive System Cancers. Int. J. Mol. Sci. 19 (11). doi:10.3390/ijms19113295
Zhang, M., Pan, H., Xu, Y., Wang, X., Qiu, Z., and Jiang, L. (2017a). Allicin Decreases Lipopolysaccharide-Induced Oxidative Stress and Inflammation in Human Umbilical Vein Endothelial Cells through Suppression of Mitochondrial Dysfunction and Activation of Nrf2. Cell Physiol. Biochem. 41 (6), 2255–2267. doi:10.1159/000475640
Zhang, W., Ha, M., Gong, Y., Xu, Y., Dong, N., and Yuan, Y. (2010). Allicin Induces Apoptosis in Gastric Cancer Cells through Activation of Both Extrinsic and Intrinsic Pathways. Oncol. Rep. 24 (6), 1585–1592. doi:10.3892/or_00001021
Zhang, X., Zhu, Y., Duan, W., Feng, C., and He, X. (2015). Allicin Induces Apoptosis of the MGC-803 Human Gastric Carcinoma Cell Line through the P38 Mitogen-Activated Protein Kinase/caspase-3 Signaling Pathway. Mol. Med. Rep. 11 (4), 2755–2760. doi:10.3892/mmr.2014.3109
Zhang, Y., Li, H. Y., Zhang, Z. H., Bian, H. L., and Lin, G. (2014). Garlic-derived Compound S-Allylmercaptocysteine Inhibits Cell Growth and Induces Apoptosis via the JNK and P38 Pathways in Human Colorectal Carcinoma Cells. Oncol. Lett. 8 (6), 2591–2596. doi:10.3892/ol.2014.2579
Zhang, Y., Liu, X., Ruan, J., Zhuang, X., Zhang, X., and Li, Z. (2020). Phytochemicals of Garlic: Promising Candidates for Cancer Therapy. Biomed. Pharmacother. 123, 109730. doi:10.1016/j.biopha.2019.109730
Zhang, Y., Zhang, J., Xu, T., Wu, W., Huang, F. F., Yu, W. Q., et al. (2017b). Allicin Ameliorates Intraintestinal Bacterial Translocation after Trauma/hemorrhagic Shock in Rats: The Role of Mesenteric Lymph Node Dendritic Cell. Surgery 161 (2), 546–555. doi:10.1016/j.surg.2016.08.029
Zhang, Z. D., Li, Y., and Jiao, Z. K. (2008). Effect of Local Application of Allicin via Gastroscopy on Cell Proliferation and Apoptosis of Progressive Gastric Carcinoma. Zhongguo Zhong Xi Yi Jie He Za Zhi 28 (2), 108–110.
Zhang, Z. M., Yang, X. Y., Deng, S. H., Xu, W., and Gao, H. Q. (2007). Anti-tumor Effects of Polybutylcyanoacrylate Nanoparticles of Diallyl Trisulfide on Orthotopic Transplantation Tumor Model of Hepatocellular Carcinoma in BALB/c Nude Mice. Chin. Med. J. Engl. 120 (15), 1336–1342. doi:10.1097/00029330-200708010-00008
Zhang, Z. M., Zhong, N., Gao, H. Q., Zhang, S. Z., Wei, Y., Xin, H., et al. (2006). Inducing Apoptosis and Upregulation of Bax and Fas Ligand Expression by Allicin in Hepatocellular Carcinoma in Balb/c Nude Mice. Chin. Med. J. Engl. 119 (5), 422–425. doi:10.1097/00029330-200603010-00013
Zhen, H., Fang, F., Ye, D. Y., Shu, S. N., Zhou, Y. F., Dong, Y. S., et al. (2006). Experimental Study on the Action of Allitridin against Human Cytomegalovirus In Vitro: Inhibitory Effects on Immediate-Early Genes. Antivir. Res. 72 (1), 68–74. doi:10.1016/j.antiviral.2006.03.017
Zhou, X., Qian, H., Zhang, D., and Zeng, L. (2020). Garlic Intake and the Risk of Colorectal Cancer: A Meta-Analysis. Med. Baltim. 99 (1), e18575. doi:10.1097/md.0000000000018575
Zhu, B., Zou, L., Qi, L., Zhong, R., and Miao, X. (2014). Allium Vegetables and Garlic Supplements Do Not Reduce Risk of Colorectal Cancer, Based on Meta-Analysis of Prospective Studies. Clin. Gastroenterol. Hepatol. 12 (12), 1991–e121. doi:10.1016/j.cgh.2014.03.019
Keywords: allicin, digestive system cancer, gastrointestinal cancer, therapy, allicin secondary metabolites
Citation: Zhou Y, Li X, Luo W, Zhu J, Zhao J, Wang M, Sang L, Chang B and Wang B (2022) Allicin in Digestive System Cancer: From Biological Effects to Clinical Treatment. Front. Pharmacol. 13:903259. doi: 10.3389/fphar.2022.903259
Received: 24 March 2022; Accepted: 23 May 2022;
Published: 13 June 2022.
Edited by:
Haike Antelmann, Freie Universität Berlin, GermanyReviewed by:
Roger Hunter, University of Cape Town, South AfricaAdrian Bogdan Tigu, Iuliu Hațieganu University of Medicine and Pharmacy, Romania
Copyright © 2022 Zhou, Li, Luo, Zhu, Zhao, Wang, Sang, Chang and Wang. This is an open-access article distributed under the terms of the Creative Commons Attribution License (CC BY). The use, distribution or reproduction in other forums is permitted, provided the original author(s) and the copyright owner(s) are credited and that the original publication in this journal is cited, in accordance with accepted academic practice. No use, distribution or reproduction is permitted which does not comply with these terms.
*Correspondence: Bing Chang, CB000216@163.com