- 1Department of Pharmacy, Affiliated Hospital of Inner Mongolia Medical University, Hohhot, China
- 2College of Pharmacy, Inner Mongolia Medical University, Hohhot, China
- 3Department of Natural Medicinal Chemistry, College of Pharmacy, Inner Mongolia Medical University, Hohhot, China
- 4Engineering Technology Research Center of Pharmacodynamic Substance and Quality Control of Mongolian Medicine in Inner Mongolia, Hohhot, China
- 5Center for New Drug Safety Evaluation and Research, Inner Mongolia Medical University, Hohhot, China
- 6Department of Psychiatry, Inner Mongolia Mental Health Center, Hohhot, China
Background: Jaranol has shown a wide range of pharmacological activities; however, no study has yet examined in vivo toxicity. The study aimed to investigate the oral acute and sub-acute toxicity of jaranol in mice.
Methods: The acute toxicity was determined by a single oral dose of jaranol (2000 mg/kg). Therein animal behaviour and mortality rate were observed for 14 days. The jaranol (50, 100 and 200 mg/kg BW·d−1) was given by gavage for 28 days daily in the sub-acute study. The mouse body weight (BW), organ weight, food, water intake, biochemical, haematological parameters, and histopathology were studied in acute and sub-acute toxicity.
Results: During the acute toxicity test, a single oral dose (2000 mg/kg) jaranol did not cause significant alteration in majority of the hematological indices. However, jaranol decreased the level of serum alanine aminotransferase and aspartate aminotransferase. Those results showed that the oral lethal dose 50 (LD50) of jaranol was higher than 2000 mg/kg BW, regardless of sex. In repeated daily oral doses (50, 100 and 200 mg/kg BW·d−1), no mortality was recorded in the various experimental groups. The jaranol reduced body weight gain (200 mg/kg BW·d−1), the relative spleen weight (all doses) and serum alanine aminotransferase activity (200 mg/kg BW·d−1). On the other hand, jaranol significantly elevated red blood cell count (100 and 200 mg/kg BW·d−1) and serum creatinine levels (200 mg/kg BW·d−1). Histological study revealed that spleen bleeding was identified in 200 mg/kg jaranol-treated mice.
Conclusion: Jaranol was relatively safe in Kunming Mice when repetitively administered orally in small doses for a prolonged period of time. We recommend more chronic toxicity studies and clinical trials on jaranol to ensure that its use is free of potential toxicity to humans.
1 Introduction
Traditional medicine still plays an important role in delaying, preventing and treating diseases worldwide, especially in developing countries (Yuan et al., 2016). Terpenoids, alkaloids, flavonoids, phenolics and other ingredients from medicinal herbs could regulate multiple human physiological functions by complex mechanisms. Among these active ingredients, plant-derived flavonoids, including isoflavones, are attracting growing interest due to their diverse biological functions. The plant-derived flavonoids are widely found in fruits, vegetables, nuts and medicinal herbs (Lin et al., 2021). On one hand, flavonoids are important active ingredients of multiple traditional Chinese medicines and have diverse biological functions, including anti-oxidant, anti-inflammatory and anti-carcinogenic effects (Perez-Vizcaino and Fraga 2018). On the other hand, it was reported that flavonoids have potential flavonoid/phenolic toxicity, including pro-oxidant activity and mitochondrial toxicity (Said Ahmad et al., 1992; Galati and O'Brien 2004). Previous studies showed that flavonoids were autoxidized in the presence of O2 and transition metals such as copper (Cu) and iron (Fe), which led to the generation of reactive oxygen species (ROS) and phenoxyl radicals that can damage DNA, lipids, and other biological molecules (Decker 1997). In addition, ROS and phenoxyl radicals from flavonoids are an important cause of mitochondrial dysfunction related to multiple human diseases (Babu et al., 2013; Agrawal and Jha 2020). The toxic effect of flavonoids has a certain significance in cancer therapy, but this also acts as an obstacle for their application in the treatment of other diseases.
Jaranol, a plant-derived flavonoid, could be extracted from various traditional medicinal herbs, such as licorice (Glycyrrhiza spp.), Psychotria serpens and Siparuna cristata (Chen 2011; Xue et al., 2013). Some studies illustrated that jaranol had a potential role in inhibiting SARS-CoV-2 replication (Leal et al., 2021) and exerting anti-cancer (Woo et al., 2017), alpha-glucosidase inhibitory (Dao et al., 2021), anti-bacterial (Zhang et al., 2019), and anti-oxidant activities in vitro (Quek et al. 2021). At present, no studies evaluating the effect of jaranol in vivo were found from a PubMed search with the keywords jaranol or kumatakenin. To reveal jaranol candidate targets and mechanisms, a network pharmacology-based strategy was performed by our team. The results showed that nicotinamide adenine dinucleotide phosphate (NADPH) oxidase 4 (NOX4) was a putative target regulated by jaranol, while NOX4 played a critical role in the pathophysiology of cardiovascular diseases, such as hypertension, cardiac hypertrophy, heart failure and ischemia-reperfusion injury, by promoting ROS generation (Cave et al., 2006). Therefore, it was speculated that jaranol had a protective effect on cardiovascular disease. Although Jing-Ting Huang’s study investigated the pharmacokinetics of jaranol in rats, the pharmacodynamics and toxicity of jaranol remain unclear (Huang et al., 2017). In the current study, the aim was to evaluate the acute and sub-acute toxicity of jaranol in mice, which will provide a critical basis for advanced preclinical studies on the pharmacodynamics and mechanism of jaranol.
2 Materials and Methods
2.1 Chemicals and Reagents
Jaranol (NO.3301-49-3) was obtained from Alfabiotech (Chengdu, China). Jaranol is a yellow powder with a final purity of at least 98% and stored at 4°C. The chromatograms, LC/MS spectrum and nuclear magnetic resonance spectrum of jaranol were shown in Supplementary Figures S1–S3. Before gavage, jaranol was dissolved in 0.5% carboxymethyl cellulose sodium (CMC-Na) to prepare a suspension.
2.2 Experimental Animals
Five-week-old Kunming mice were obtained from the Laboratory Animal Centre of Inner Mongolia University (License ID: SCXK 2016-0001, Hohhot, China). The animals were housed under 12 h light/dark cycles at 23–25°C in a 40%–70% relative humidity environment. All animals had free access to water and food during the experimental periods. The animals were acclimatized to the laboratory conditions for a week prior to experiments. The animal experiments were performed in accordance with the Principles of Laboratory Animal Care and approved by the ethical committee of experimental animal care at Inner Mongolia Medical University.
2.3 Acute Toxicity Study
It was done as per Organization for Economic Co-operation and Development (OECD) guidelines 423 for acute toxicity studies. Twelve mice were divided into 2 groups of 6 mice each (three males and three females), and the mice were fasted for 24 h prior to experiments. One group was given a single dose of 2,000 mg/kg body weight (BW) jaranol by gavage. Six other mice (three males and three females) received 0.5% CMC-Na with 1 ml/100 g BW as a control. The animals were observed for any clinical signs at least once during the first 30 min, periodically during the first 24 h, with special attention given during the first 4 h, and daily thereafter, for a total of 14 days. Main clinical signs included toxicity, mortality, behavioral pattern, and physical appearance, as well as other adverse effects, for example lethargy, diarrhea, tremors, salivation, etc.
2.4 Sub-Acute Toxicity Study
Sub-acute toxicity study of jaranol in mice was performed as indicated by a slightly modified OECD guidelines 407. Twenty-four mice were divided into 4 groups (three males and three females) to evaluate sub-acute toxicity of Jaranol (Ishtiaq et al., 2017). The experimental groups were given jaranol at graded dose of 50, 100 or 200 mg/kg BW by gavage for 28 consecutive days. The doses were selected according to Schedule Y (The Drugs and Cosmetics Rules, 1945; India) on the basis of ED50 value (Rayees et al., 2013). The control treatment was 0.5% CMC-Na with 1 ml/100 g BW as a control. The mice were weighed weekly and observed for toxic reactions and mortality. Food intake and water consumption were recorded weekly. At the end of the experiments, the mice were anaesthetized with 2% tribromoethanol (T484020, Sigma–Aldrich, St. Louis, MO, United States, 3 ml/20 g BW). Previous study showed that white blood cells parameters, total red blood cells and platelets, hematocrit, and hemoglobin concentration were equivalent for cardiac and retro-orbital bleeding. Therefore, retro-orbital bleeding was selected in this study (Hoggatt et al., 2016). Blood was collected retro-orbitally into tubes containing EDTA (ethylenediaminetetraacetic acid) for hematological study and without EDTA for biological study, and then mice were sacrificed. Mouse major organs, including the heart, kidney, spleen, lung and liver, were harvested to calculate organ coefficients. The organ coefficients of each animal were calculated by the following formula: organ coefficient (g/100 g) = [organ wet weight (g)/body weight (g)] × 100.
2.5 Hematological Study
Blood samples were collected into tubes containing EDTA and were sent to Lab -Test Biotechnology Ltd., Co (Beijing, China) for analyzing hematological parameters using an automatic veterinary blood cell analyzer (BC-5000Vet, CBIO Science and Technology, Beijing, China). Hematological parameters included WBC (white blood cells), NETU (neutrophils), LYMPH (lymphocytes), MONO (monocytes), EO (eosinophil), BASO (basophils), NEU (neutrophils), RBC (red blood cell), HGB (hemoglobin), HCT (hematocrit), MCV (mean corpuscular volume), MCH (melanin-concentrating hormone), MCHC (mean corpuscular hemoglobin concentration), RDW-CV (red cell distribution width-coefficient of variation), RDW-SD (red cell distribution width-standard deviation), PLT (platelet), MPV (mean platelet volume), PDW (platelet distribution width), and PCT (procalcitonin).
2.6 Biochemical Parameters
Blood samples were also collected into tubes without EDTA. The samples were then centrifuged at 5,000 RPM for 10 min and the serum was then removed and stored at −80 °C until analysis. Serum samples were analyzed by an automatic biochemical analyzer (Ichem-340, ICUBIO, Shenzhen, China) to obtain biochemical parameters, including LDL-C (low-density lipoprotein cholesterol), HDL-C (high-density lipoprotein cholesterol), CHO (cholesterol), ALT (alanine aminotransferase), AST (aspartate aminotransferase), T-Bil (total bilirubin), and Cre (Creatinine).
2.7 Histopathology
The histological study of the heart, kidney, spleen, lung and liver were performed as described previously (Leong et al., 2018). Briefly, tissues were fixed in 10% paraformaldehyde and then embedded in paraffin wax after the samples were dehydrated in graded alcohol. Samples were cut transversely into 5 μm sections. Serial sections were stained with a hematoxylin-eosin (HE) staining kit (Yuanye Bio-Technology, R20570, Shanghai, China) to evaluate tissue damage. At least two pathologists (L-TL and D-Y) scored them simultaneously under microscope and awarded a graded expert staining score from 1 to 5 points based on staining quality. The scoring criteria as following: 5, excellent staining; 4, pass with minor remark; 3, deficiency without affecting clinical output; 2, incorrect staining with clinical output affected; and 1, failed staining, impossible to interpret (Keppens et al., 2020).
2.8 Statistical Analyses
The statistical software IBM SPSS 20 (SPSS Inc., Chicago, IL) was used to perform the statistical analysis. All data were presented as the mean ± SD. Statistical differences were calculated with the 2-tailed Student t test when comparing 2 conditions, and ANOVA was used when comparing >2 conditions. For parametric data with equal variance, one-way ANOVA with Tukey post-hoc test was used. For parametric data with unequal variance, one-way ANOVA with the Games-Howell post-hoc test was used (Liu et al., 2020b). Data were considered statistically significant at p < 0.05.
3 Results
3.1 Acute Toxicity Study
During the acute toxicity test, there were no signs of toxicity observed in the autonomic and central nervous systems, skin (fur), water consumption and food intake after administration of jaranol at a single dose of 2,000 mg/kg BW for 14 days (Supplementary Figure S4). No mortality was recorded during this time. No alterations were found in organ coefficient, hematological parameters, kidney function and lipid profile. Adverse effects of jaranol on mouse liver were observed in acute toxicity, which illustrated by a significant decrease in serum alanine aminotransferase and aspartate aminotransferase level of jaranol-treated mice compared to control. However, we observed no significant change in the macroscopic and microscopic structure of the liver between jaranol-treated mice and control. Those results showed that the oral lethal dose 50 (LD50) of jaranol was higher than 2,000 mg/kg BW, regardless of sex (Supplementary Tables S1–S4).
3.2 Sub-Acute Toxicity Study
3.2.1 Effects of Jaranol on Body Weight, Food and Water Intake
The chemical structure of jaranol is shown in Figure 1A. To evaluate the sub-acute toxicity of jaranol in mice, mice were administered 50, 100 or 200 mg/kg BW jaranol per day by gavage for 28 days. No mortality or toxic signs were observed during the study of sub-acute toxicity. A gradual significant increase in body weight was found in the control group, and body weight gain was significantly inhibited in 200 mg/kg BW jaranol-treated mice (Figure 1B). During the experiment, food intake and water consumption were recorded weekly. The results showed no significant change in the food intake and water consumption of the mice in all groups (Figure 1C).
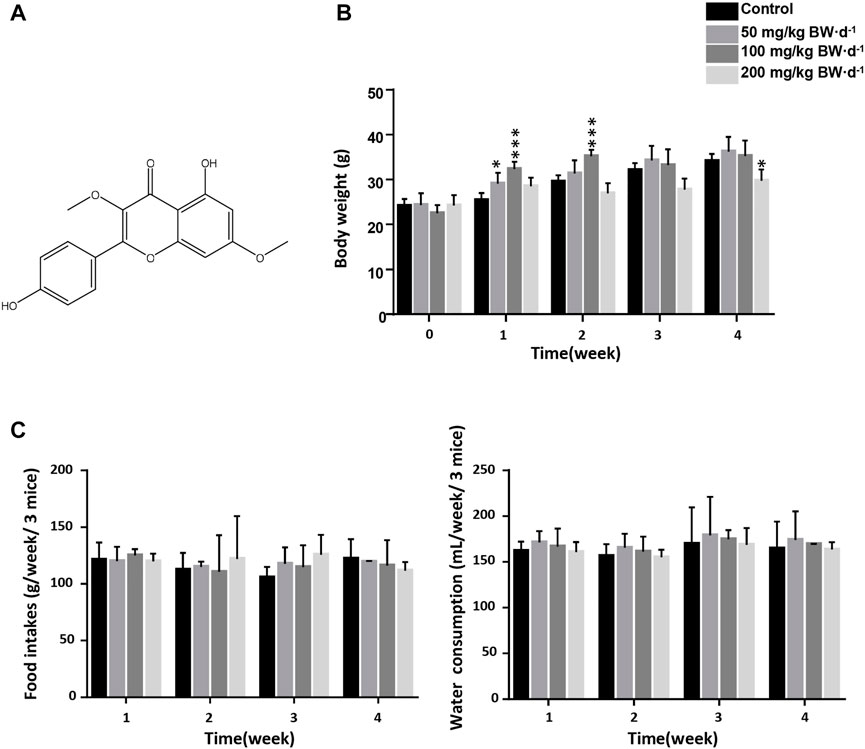
FIGURE 1. Effect of daily oral administration of jaranol on body weight, food intake and water consumption in mice. (A) Chemical structure of jaranol. (B) Mouse body weights of different groups (n = 6). One-way One-way ANOVA followed by Tukey post-hoc test, compared with Control, *p < 0.05, ***p < 0.001. (C) Food intake and water consumption in mice, n = 2 cage, 3 mice/cage.
3.3 Effects of Jaranol on Organ Coefficients
After the mice were treated by jaranol for 28 days, mouse major organs, including the heart, kidney, spleen, lung and liver, were harvested and weighted, and then the organ coefficient was calculated. Organ weight data was shown in Supplementary Table S5. A significant decrease in the ratio of spleen weight to body weight was found in all jaranol-treated mice, and 200 mg/kg BW·d−1 jaranol-treated mice had a significant decrease in the ratio of liver weight to body weight compared to control. No statistically significant difference in the ratio of heart, kidney and lung weight to body weight was found between the control and jaranol-treated mice (Table 1). Female mice administered jaranol at a dosage of 200 mg/kg BW·d−1 by gavage for 28 days had a significant increase in the ratio of lung weight to body weight compared to control. Except for lung, the effect of jaranol on organ coefficient were no any differences between the sexes (Supplementary Table S6).
3.4 Effects of Jaranol on Hematological Parameters
To further evaluate the sub-acute toxicity of jaranol, mouse hematological parameters were detected by an automatic veterinary blood cell analyzer after treatment for 28 days. Our results showed that 50 mg/kg BW·d−1 jaranol treatment by gavage had no significant effect on hematological parameters compared with the control. Mice administered by jaranol at a dosage of 100 mg/kg BW·d−1 by gavage for 28 days had a significant increase in RBCs, while MCV and MCH levels were significantly decreased compared to the control. Meanwhile, a significant increase in RBCs was also found in 200 mg/kg BW·d−1 jaranol-treated mice compared to control mice (Table 2). In conclusion, we considered that 100 and 200 mg/kg BW·d−1 jaranol treatment by gavage for 28 days had a significant effect on RBCs in mice. For female mice, high dose (100 and 200 mg/kg BW·d−1) jaranol administered by gavage for 28 days had a significant effect on neutrophil and lymphocyte count, the effects of jaranol on others hematological parameters were not significantly differential between the sexes (Supplementary Table S7).
3.5 Effects of Jaranol on Liver and Kidney Function
Serum ALT, AST and T-Bil levels were measured by an automatic biochemical analyzer to evaluate mouse liver function. The results showed a significant decrease in serum ALT levels in mice that received jaranol treatment at dose of 200 mg/kg BW·d−1 for 28 days compared with control, especially for male mice. Serum AST and T-Bil levels had not significantly difference between control and jaranol-treated mice (Table 3 and Supplementary Table S8). For kidney function, mice administered 200 mg/kg BW·d−1 jaranol treatment for 28 days had a significant increase in serum Cre levels compared with control (Table 3). The effects of jaranol on kidney function were not significantly difference between the sexes (Supplementary Table S8). These results indicate that chronic (28 days) oral administration of jaranol (200 mg/kg BW·d−1) has an effect on liver and kidney function.
3.6 Effects of Jaranol on the Lipid Profile
After jaranol treatment for 28 days, serum lipid profile was detected by an automatic biochemical analyzer. The effects of jaranol on the lipid profile are presented in Table 4. The results showed that no significant change in serum HDL-C, LDL-C or CHO levels was observed between control and jaranol-treated mice after treatment for 28 days. Moreover, the effects of jaranol on lipid profile had not significantly difference between the sexes (Supplementary Table S9).
3.7 Effects of Jaranol on Histopathology
At the end of treatment, major organs, including the heart, liver, spleen, lung and kidney, were subjected to histopathological evaluation to provide evidence supporting the findings of the biochemical assessments. Graded expert staining score was obtained by two pathologists to evaluate staining quality, which was shown in Supplementary Table S10. According to the results, no significant change was found in the histopathological examination of the lung, heart, liver, and kidneys of the jaranol-treated groups compared to control (Figure 2). The macroscopic evaluations showed that no lesions, inflammation or pathological changes were determined in the lung, spleen, heart, liver and kidneys due to the jaranol administration. Moreover, the histopathological evaluation of kidneys, spleen, liver, lung and heart showed no necrosis and were consistent to control. However, histological study revealed the spleen bleeding was identified in jaranol-treated mice at dose of 200 mg/kg BW·d−1 by gavage for 28 days (Figure 2).
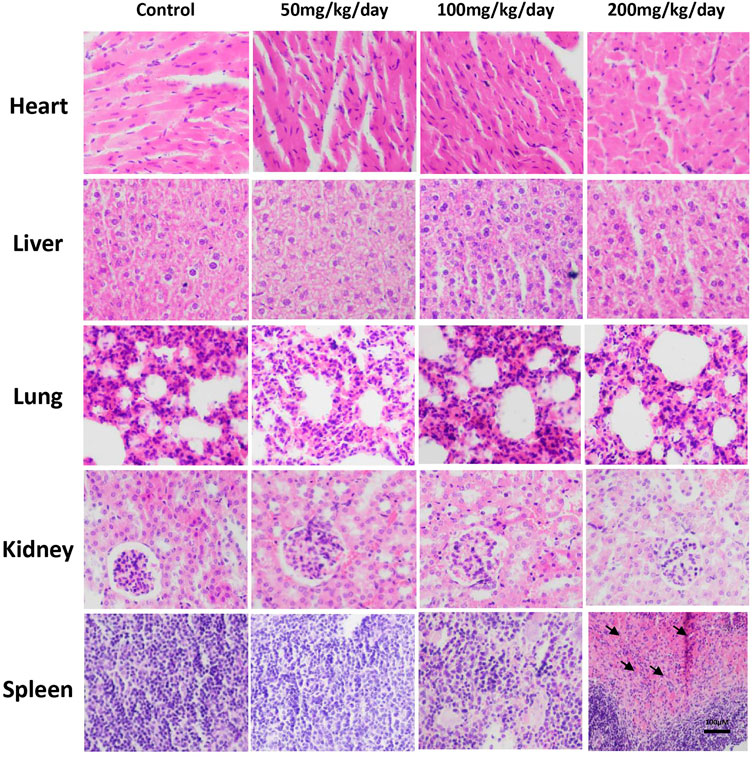
FIGURE 2. Histopathological study of mouse major organs following oral administration of jaranol for 28 days. Scale bar = 100 μM (black arrow: bleeding).
4 Discussion
Previous studies indicated that flavonoids had a significant effect on increasing RBCs in normal and diabetic rat, which was consistent with our results (Mahmoud 2013; Gao et al., 2017). Indeed, Ken Y Z Zheng’s study showed that flavonoids from Radix Astragali could promote the expression of erythropoietin in vitro, and erythropoietin is an important hormone that could stimulate stem cells in the bone marrow to produce red blood cells (Ohlsson and Aher 2006; Zheng et al., 2011). In addition, the effect of jaranol on RBCs may be attributed to its anti-oxidant activity. It was reported that flavonoids could decrease lipid peroxidation levels, which leads to hemolysis of erythrocytes (Mahmoud et al., 2012). In our study, a significant increase in RBC counts was found in jaranol-treated mice; however, MCH and MCHC levels were not significantly differential compared to the control mice. These results illustrate that the effect of jaranol on RBC counts was related to its anti-oxidant activity. The detailed mechanism needs further research.
Our results showed that 200 mg/kg BW·d−1 jaranol-treated mice had a significantly decreased serum ALT level. However, it was not clear whether decreased serm ALT levels could reflect the protective and adverse effects of jaranol on liver function under normal pathological conditions. Similar results were also found in other studies (Gao et al., 2017; Erdenetsogt et al., 2020). Previous studies showed that flavonoids were mainly metabolized by phase I and phase II metabolism in the liver and gastrointestinal tract. Although flavonoids have been reported to have a protective effect on multiple liver injuries, intake of flavonoids at high dosages causes a liver metabolic burden (Liu et al., 2020a). In addition, flavonoids could regulate the activity of hepatic cytochrome CYP450, and cytochrome CYP450 signaling was involved in the pathological process of liver injury (Ekstrand et al., 2015; Li et al., 2021). At present, there are no reports detailing kidney injury induced by flavonoids. Some studies have shown that kidney injury is improved by the administration of flavonoids from different sources (Park et al., 2020). However, flavonoids could reduce the elimination of nephron cardiovascular toxins by impairing renal function (Vargas et al., 2018). In this study, a significant change in serum Cre levels was found in 200 mg/kg BW·d−1 jaranol-treated mice, which may be attributed to jaranol-induced alterations to the kidney elimination process.
5 Conclusion
A study of acute toxicity indicated that a single administration of jaranol by the oral route did not cause any lethality or any changes in general behavior in mice. However, regarding sub-acute toxicity, the results showed that jaranol treatment at a dosage of 200 mg/kg BW·d−1 by gavage had a significant effect on RBCs and liver and kidney function. This studies are the first studies of this nature with jaranol, and provide important toxicological information to be used for further preclinical studies on the pharmacodynamics and mechanism of action.
Data Availability Statement
The original contributions presented in the study are included in the article/Supplementary Materials, further inquiries can be directed to the corresponding authors.
Ethics Statement
The animal study was reviewed and approved by the Inner Mongolia Medical University.
Author Contributions
TL, YZ, YD, and YX worked on experimental design, conduction of the experiments, data analysis and drafted the manuscript. XJ, JP, and JL conducted experiments. TL, LZ, and YD worked on experimental design, data analysis, and manuscript preparation.
Funding
National Natural Science Foundation of China (81960048, 82160058), the Natural Science Foundation of Inner Mongolia Autonomous Region (2019BS08003), a PhD Initial Funding Project of the Affiliated Hospital of Inner Mongolia Medical University (NYFY BS 202114), and the Million Project of Inner Mongolia Medical University Science and Technology [YKD2018KJBW(LH)026].
Conflict of Interest
The authors declare that the research was conducted in the absence of any commercial or financial relationships that could be construed as a potential conflict of interest.
Publisher’s Note
All claims expressed in this article are solely those of the authors and do not necessarily represent those of their affiliated organizations, or those of the publisher, the editors and the reviewers. Any product that may be evaluated in this article, or claim that may be made by its manufacturer, is not guaranteed or endorsed by the publisher.
Supplementary Material
The Supplementary Material for this article can be found online at: https://www.frontiersin.org/articles/10.3389/fphar.2022.903232/full#supplementary-material
References
Agrawal, I., and Jha, S. (2020). Mitochondrial Dysfunction and Alzheimer's Disease: Role of Microglia. Front. Aging Neurosci. 12, 252. doi:10.3389/fnagi.2020.00252
Babu, S., Uppu, S., Claville, M. O., and Uppu, R. M. (2013). Prooxidant Actions of Bisphenol A (BPA) Phenoxyl Radicals: Implications to BPA-Related Oxidative Stress and Toxicity. Toxicol. Mech. Methods 23 (4), 273–280. doi:10.3109/15376516.2012.753969
Cave, A. C., Brewer, A. C., Narayanapanicker, A., Ray, R., Grieve, D. J., Walker, S., et al. (2006). NADPH Oxidases in Cardiovascular Health and Disease. Antioxid. Redox Signal 8 (5-6), 691–728. doi:10.1089/ars.2006.8.691
Chen, C. Y. (2011). TCM Database@Taiwan: the World's Largest Traditional Chinese Medicine Database for Drug Screening In Silico. PLoS One 6 (1), e15939. doi:10.1371/journal.pone.0015939
Dao, T. B., Nguyen, T. M., Nguyen, V. Q., Tran, T. M., Tran, N. M., Nguyen, C. H., et al. (2021). Flavones from Combretum Quadrangulare Growing in Vietnam and Their Alpha-Glucosidase Inhibitory Activity. Molecules 26 (9). doi:10.3390/molecules26092531
Decker, E. A. (1997). Phenolics: Prooxidants or Antioxidants? Nutr. Rev. 55 (11 Pt 1), 396–398. doi:10.1111/j.1753-4887.1997.tb01580.x
Ekstrand, B., Rasmussen, M. K., Woll, F., Zlabek, V., and Zamaratskaia, G. (2015). In Vitro gender-dependent Inhibition of Porcine Cytochrome P450 Activity by Selected Flavonoids and Phenolic Acids. Biomed. Res. Int. 2015, 387918. doi:10.1155/2015/387918
Erdenetsogt, U., Nadmid, S., Paulus, C., Chanagsuren, G., Dolgor, E., Gotov, C., et al. (2020). Bioactive Flavonoids from Plant Extract of Pyrethrum Pulchrum and its Acute Toxicity. Nat. Prod. Res., 1–4. doi:10.1080/14786419.2020.1806271
Galati, G., and O'Brien, P. J. (2004). Potential Toxicity of Flavonoids and Other Dietary Phenolics: Significance for Their Chemopreventive and Anticancer Properties. Free Radic. Biol. Med. 37 (3), 287–303. doi:10.1016/j.freeradbiomed.2004.04.034
Gao, Y., Wang, Y., Wang, K., Zhu, J., Li, G., Tian, J., et al. (2017). Acute and a 28-day Repeated-Dose Toxicity Study of Total Flavonoids from Clinopodium Chinense (Benth.) O. Ktze in Mice and Rats. Regul. Toxicol. Pharmacol. 91, 117–123. doi:10.1016/j.yrtph.2017.10.027
Hoggatt, J., Hoggatt, A. F., Tate, T. A., Fortman, J., and Pelus, L. M. (2016). Bleeding the Laboratory Mouse: Not All Methods Are Equal. Exp. Hematol. 44 (2), 132–e1. doi:10.1016/j.exphem.2015.10.008
Huang, J. T., Cheng, Y. Y., Lin, L. C., and Tsai, T. H. (2017). Structural Pharmacokinetics of Polymethoxylated Flavones in Rat Plasma Using HPLC-MS/MS. J. Agric. Food Chem. 65 (11), 2406–2413. doi:10.1021/acs.jafc.6b05390
Ishtiaq, S., Akram, M., Kamran, S. H., Hanif, U., Afridi, M. S. K., Sajid-Ur-Rehman, R., et al. (2017). Acute and Sub-acute Toxicity Study of a Pakistani Polyherbal Formulation. BMC Complement. Altern. Med. 17 (1), 387. doi:10.1186/s12906-017-1889-7
Keppens, C., von der Thüsen, J., Pauwels, P., Ryska, A., 't Hart, N., Schuuring, E., et al. (2020). Staining Performance of ALK and ROS1 Immunohistochemistry and Influence on Interpretation in Non-small-cell Lung Cancer. J. Mol. Diagn 22 (12), 1438–1452. doi:10.1016/j.jmoldx.2020.09.006
Leal, C. M., Leitao, S. G., Sausset, R., Mendonca, S. C., Nascimento, P. H. A., de Araujo, R. C. C. F., et al. (2021). Flavonoids from Siparuna Cristata as Potential Inhibitors of SARS-CoV-2 Replication. Rev. Bras. Farmacogn., 1–9. doi:10.1007/s43450-021-00162-5
Leong, Y. H., Isa, A. S. M., Mohamed Mahmood, M., Moey, C. E. J., Utar, Z., Soon, Y. I., et al. (2018). Acute and Repeated Dose 28-day Oral Toxicity of Poly(3-Hydroxybutyrate-Co-4-Hydroxybutyrate) Nanoparticles in Sprague-Dawley Rats. Regul. Toxicol. Pharmacol. 95, 280–288. doi:10.1016/j.yrtph.2018.03.011
Li, S., Wang, X., Xiao, Y., Wang, Y., Wan, Y., Li, X., et al. (2021). Curcumin Ameliorates Mercuric Chloride-Induced Liver Injury via Modulating Cytochrome P450 Signaling and Nrf2/HO-1 Pathway. Ecotoxicol. Environ. Saf. 208, 111426. doi:10.1016/j.ecoenv.2020.111426
Lin, S., Wade, J. D., and Liu, S. (2021). De Novo Design of Flavonoid-Based Mimetics of Cationic Antimicrobial Peptides: Discovery, Development, and Applications. Acc. Chem. Res. 54 (1), 104–119. doi:10.1021/acs.accounts.0c00550
Liu, T., Tan, F., Long, X., Pan, Y., Mu, J., Zhou, X., et al. (2020a). Improvement Effect of Lotus Leaf Flavonoids on Carbon Tetrachloride-Induced Liver Injury in Mice. Biomedicines 8 (2). doi:10.3390/biomedicines8020041
Liu, T., Wen, H., Li, H., Xu, H., Xiao, N., Liu, R., et al. (2020b). Oleic Acid Attenuates Ang II (Angiotensin II)-Induced Cardiac Remodeling by Inhibiting FGF23 (Fibroblast Growth Factor 23) Expression in Mice. Hypertension 75 (3), 680–692. doi:10.1161/HYPERTENSIONAHA.119.14167
Mahmoud, A. M., Ashour, M. B., Abdel-Moneim, A., and Ahmed, O. M. (2012). Hesperidin and Naringin Attenuate Hyperglycemia-Mediated Oxidative Stress and Proinflammatory Cytokine Production in High Fat Fed/streptozotocin-Induced Type 2 Diabetic Rats. J. Diabetes Complicat. 26 (6), 483–490. doi:10.1016/j.jdiacomp.2012.06.001
Mahmoud, A. M. (2013). Hematological Alterations in Diabetic Rats - Role of Adipocytokines and Effect of Citrus Flavonoids. EXCLI J. 12, 647–657. doi:10.3923/pjn.2013.736.747
Ohlsson, A., and Aher, S. M. (2006). Early Erythropoietin for Preventing Red Blood Cell Transfusion in Preterm And/or Low Birth Weight Infants. Cochrane Database Syst. Rev. 3, CD004863. doi:10.1002/14651858.CD004863.pub2
Park, E., Hong, K., Kwon, B. M., Kim, Y., and Kim, J. H. (2020). Jaceosidin Ameliorates Insulin Resistance and Kidney Dysfunction by Enhancing Insulin Receptor Signaling and the Antioxidant Defense System in Type 2 Diabetic Mice. J. Med. Food 23 (10), 1083–1092. doi:10.1089/jmf.2020.4739
Perez-Vizcaino, F., and Fraga, C. G. (2018). Research Trends in Flavonoids and Health. Arch. Biochem. Biophys. 646, 107–112. doi:10.1016/j.abb.2018.03.022
Quek, A., Mohd Zaini, H., Kassim, N. K., Sulaiman, F., Rukayadi, Y., Ismail, A., et al. (2021). Oxygen Radical Antioxidant Capacity (ORAC) and Antibacterial Properties of Melicope Glabra Bark Extracts and Isolated Compounds. PLoS One 16 (5), e0251534. doi:10.1371/journal.pone.0251534
Rayees, S., Sharma, R., Singh, G., Najar, I. A., Singh, A., Ahamad, D. B., et al. (2013). Acute, Sub-acute and General Pharmacological Evaluation of 5-(3,4-Methylenedioxyphenyl)-4-Ethyl-2e,4e-Pentadienoic Acid Piperidide (SK-20): a Novel Drug Bioavailability Enhancer. Environ. Toxicol. Pharmacol. 35 (2), 347–359. doi:10.1016/j.etap.2012.08.007
Said Ahmad, M., Fazal, F., Rahman, A., Hadi, S. M., and Parish, J. H. (1992). Activities of Flavonoids for the Cleavage of DNA in the Presence of Cu(II): Correlation with Generation of Active Oxygen Species. Carcinogenesis 13 (4), 605–608. doi:10.1093/carcin/13.4.605
Vargas, F., Romecín, P., García-Guillén, A. I., Wangesteen, R., Vargas-Tendero, P., Paredes, M. D., et al. (2018). Flavonoids in Kidney Health and Disease. Front. Physiol. 9, 394. doi:10.3389/fphys.2018.00394
Woo, J. H., Ahn, J. H., Jang, D. S., Lee, K. T., and Choi, J. H. (2017). Effect of Kumatakenin Isolated from Cloves on the Apoptosis of Cancer Cells and the Alternative Activation of Tumor-Associated Macrophages. J. Agric. Food Chem. 65 (36), 7893–7899. doi:10.1021/acs.jafc.7b01543
Xue, R., Fang, Z., Zhang, M., Yi, Z., Wen, C., and Shi, T. (2013). TCMID: Traditional Chinese Medicine Integrative Database for Herb Molecular Mechanism Analysis. Nucleic Acids Res. 41, D1089–D1095. Database issue. doi:10.1093/nar/gks1100
Yuan, H., Ma, Q., Ye, L., and Piao, G. (2016). The Traditional Medicine and Modern Medicine from Natural Products. Molecules 21 (5). doi:10.3390/molecules21050559
Zhang, X., Wang, L., Mu, H., Wang, D., and Yu, Y. (2019). Synergistic Antibacterial Effects of Buddleja Albiflora Metabolites with Antibiotics against Listeria Monocytogenes. Lett. Appl. Microbiol. 68 (1), 38–47. doi:10.1111/lam.13084
Zheng, K. Y., Choi, R. C., Cheung, A. W., Guo, A. J., Bi, C. W., Zhu, K. Y., et al. (2011). Flavonoids from Radix Astragali Induce the Expression of Erythropoietin in Cultured Cells: a Signaling Mediated via the Accumulation of Hypoxia-Inducible Factor-1α. J. Agric. Food Chem. 59 (5), 1697–1704. doi:10.1021/jf104018u
Keywords: jaranol, acute toxicity, sub-acute toxicity, mice, safety
Citation: Liu T, Zhang Y, Liu J, Peng J, Jia X, Xiao Y, Zheng L and Dong Y (2022) Evaluation of the Acute and Sub-Acute Oral Toxicity of Jaranol in Kunming Mice. Front. Pharmacol. 13:903232. doi: 10.3389/fphar.2022.903232
Received: 24 March 2022; Accepted: 03 June 2022;
Published: 30 June 2022.
Edited by:
Eleonore Fröhlich, Medical University of Graz, AustriaReviewed by:
Mahrous A. Ibrahim, Jouf University, Saudi ArabiaGuangqiu Qin, Guangxi University of Chinese Medicine, China
Salwan Ali Abed, University of Al-Qadisiyah, Iraq
Copyright © 2022 Liu, Zhang, Liu, Peng, Jia, Xiao, Zheng and Dong. This is an open-access article distributed under the terms of the Creative Commons Attribution License (CC BY). The use, distribution or reproduction in other forums is permitted, provided the original author(s) and the copyright owner(s) are credited and that the original publication in this journal is cited, in accordance with accepted academic practice. No use, distribution or reproduction is permitted which does not comply with these terms.
*Correspondence: Yu Dong, dongyu010@126.com; Lanbing Zheng, nmgwdx@sina.com
†These authors have contributed equally to this work