- Pharmaceutical Research Institute, Albany College of Pharmacy and Health Sciences and Nanopharmaceuticals, LLC, Rensselaer, NY, United States
We have recently reported on the development of fb-PMT (NP751), a conjugate of the thyroid hormone metabolite tetraiodothyroacetic acid (tetrac) and monodisperse polyethylene glycol 36. It exhibited high affinity for thyrointegrin αvβ3 receptor and potent anti-angiogenic and anticancer activity in vivo. The objective of the current study is to determine the pharmacokinetics (PK) of fb-PMT in experimental animals, such as mice, rats, and monkeys. NP751 was quantified using a propylene diamine-modified tetraiodothyroacetic acid (DAT) as an internal standard. The limit of quantification (LOQ) for fb-PMT was 1.5 ng/μL and the recovery efficiency was 93.9% with the developed method. The peak plasma concentration (Cmax) and the area under the curve (AUC) results at different doses in mice, rats and monkeys suggest that pharmacokinetics of NP751 is dose-dependent within the dose ranges administered. Results indicate that NP751 has comparable PK parameters that provides enough exposure as a molecularly tumor targeted molecule in multiple species and is a promising anticancer therapeutic.
Introduction
Anti-cancer therapy has been focused on chemotherapy and radiotherapy to destroy cancer cells (Chen and Kuo, 2017; Mollaei et al., 2021). However, the majority of anti-cancer agents result in serious side effects for the patient (Zhang et al., 2018), thus development of targeted, effective, and safer anti-cancer therapy is needed (Li et al., 2019). Integrin αvβ3 is a potential target for anti-cancer therapy because it is over-expressed in tumor cells and modulates angiogenesis (Davis et al., 2009; Davis and Mousa, 2017; Debreli Coskun et al., 2020). Thus, antagonists of integrin αvβ3 have great potential as cancer therapeutics (Brooks et al., 1994; Mizejewski, 1999; Cox et al., 2010; Desgrosellier and Cheresh, 2010).
We have previously reported that P-bi-TAT, a polydisperse PEG 4000 conjugated to 2 molecules of the αvβ3 active thyroid hormone metabolite tetraiodothyroacetic acid (tetrac) (Davis et al., 2009; Davis and Mousa, 2017; Debreli Coskun et al., 2020), was effective against several different cancer types, with potent effects on solid tumors, (Rajabi et al., 2019; Karakus et al., 2020) and no adverse effects in mice after 28 days of subcutaneous dosing at 30–100 mg/kg (unpublished data). Although the anti-cancer properties of P-bi-TAT were quite promising, the molecule had synthesis scalability issues.
We, therefore, developed a new molecule, fb-PMT, a mono-disperse PEG36 conjugated with tetrac, (Figure 1A) with a readily scalable synthesis and a high affinity for the integrin αvβ3 receptor (IC50 0.23 nM) (Hay et al., 2021). Our previous studies showed the anti-cancer effects in several cancer cell lines including Glioblastoma Multiforme, pancreatic and acute myeloid leukemia cancers in mice (Hay et al., 2021). We also observed no toxicity in mice at high dose 100 mg/kg administrated subcutaneously for 28 days (unpublished data). The high anti-cancer efficacy and safety of NP751 prompted us to investigate it is pharmacokinetic (PK) properties in rodents (mice and rats) and monkeys.
The objective of this study was to develop a quantitative LC-MS/MS method for fb-PMT and to evaluate the PK parameters of fb-PMT, such as peak plasma concentration (Cmax) and area under the curve (AUC), in mice, rats and monkeys. This required a quantitative liquid chromatography-tandem mass spectrometry (LC-MS/MS) method for fb-PMT for bioanalytical assays. Although it had a high molecular weight (Mw 2522.9), we were able to develop a sensitive multiple-reaction-monitoring (MRM) method using a pseudo-molecular ion for monodisperse fb-PMT, in contrast to polydisperse P-bi-TAT, which required collision-induced dissociation (CID), of the precursor ion (Fujioka et al., 2021).
Materials and methods
Chemicals and biologicals
fb-PMT was synthesized and purified as described earlier at a contract manufacturing organization (Dalton Pharma Services, Toronto, CANADA) under Good Manufacturing Practices (GMP) (Hay et al., 2021). Diamino-propane-tetraiodothyroacetic acid (DAT) was synthesized by formerly DPX Fine Chemicals, Patheon-ThermoFisher (Regensburg, Germany). Acetonitrile, methanol, water, and formic acid were LC-MS grade and purchased from Sigma-Aldrich (St. Louis, MO, United States). Male and female C57BL/6 mice, 4–5 weeks old, weighing 20–22 g and Sprague Dawley Rats, weighing 150–200 g were purchased from Taconic Laboratories (Germantown, NY, United States). Monkey plasma samples were purchased from Bioreclamation IVT (New York, NY).
fb-PMT (NP751)-treatment in mice
fb-PMT-treatment of mice was carried out in the animal facility of the Veterans Affairs (VA) Medical Center (Albany, NY), and the experimental protocol was approved by the Institutional Animal Care and Use Committee of the VA. Mice were housed under controlled conditions (temperature: 20–24°C; humidity: 60–70%). Mice were allowed to acclimatize for at least 1 week prior to the start of treatments. fb-PMT (1, 3, 6, 10 mg/kg body weight) was administered subcutaneously to 4 mice, and plasma was collected at 0.5, 1, 2, 3, 4, 5, 6, 8, 10, 12, 16, 20 and 24 h. Plasma samples were stored at −80°C for further analysis.
fb-PMT-treatment in rats
The rat study was conducted by Inotiv (Lafayette, IN United States). Briefly, fb-PMT (5, 15, 30 mg/kg body weight) was administered subcutaneously to male and female Sprague Dawley rats (n = 3 each) daily for 14 days. Samples were collected at the following target timepoints on Day 1: pre-dose, 0.5, 1, 3, 6, 9, 12 and 24 h following the completion of dosing. Samples were collected at the following target timepoints on Day 28: pre-dose, 0.5, 1, 3, 6, 9, 12 and 24 h following the completion of dosing. Plasma samples were stored at -80°C for further analysis.
fb-PMT-treatment in monkeys
The monkey study was conducted by a contract research organization. Briefly, fb-PMT (2.5, 7.5, 15 mg/kg body weight) was administered subcutaneously to male and female cynomolgus monkeys (n = 2 each) daily for 28 days. Samples were collected at the following target timepoints on Day 1: pre-dose, 0.5, 1, 3, 6, 9, 12 and 24 h following the completion of dosing. Samples were collected at the following target timepoints on Day 28: pre-dose, 0.5, 1, 3, 6, 9, 12 and 24 h following the completion of dosing. Plasma samples were stored at -80 °C for further analysis.
Preparation of plasma samples with liquid-liquid extraction (LLE)
50 μL of mouse, rat, or monkey plasma with or without fb-PMT and an internal standard (IS) of DAT (150 ng/ml) were vortex-mixed with 1 ml of acetonitrile/water (80/20 v/v) for 30 min. After centrifugation, solvent was evaporated under a nitrogen stream at 50°C. Extracts were reconstituted with 50 μL of acetonitrile/water (80/20 v/v).
LC-MS/MS instrumentation
An API-4000 mass spectrometer (Sciex, Framingham, MA) equipped with Shimadzu UPLC system (Kyoto, Japan) was used for LC-MS/MS analyses. A Kinetex 2.6 μm Biphenyl 100 LS column (50 × 2.1 mm, Phenomenex, Torrance, CA) was used for reversed-phase separation. Mobile phases were (A) water containing 0.1% formic acid and 5% acetonitrile and (B) acetonitrile with 0.1% formic acid. The flow rate was 0.4 ml/min, and the gradient was linear from 25% B to 95% B for 2–4 min. The oven temperature was 40°C and the injection volume was 5 μL. Electro-spray ionization (ESI) was used in positive MRM mode. Mass transitions for analytes were Q1/Q3: 841.991/109.26 (fb-PMT), 862.9/845.7 (IS). The operative parameters of the mass spectrometer were as follows: declustering potentials (DP): 56 V; entrance potentials (EP): 10; collision energies (CE): 90 eV; collision cell exit potential (CXP), 6 V; curtain gas (CUR), 10 psi; gas 1 (GS1, nebulizer gas) 40 psi; gas 2 (GS2, heater gas) 40 psi; ion spray voltage (IS), 5500 V; temperature (TEM), 500 °C; collision activate dissociation (CAD) gas: 9 psi; dwell time: 150 ms. Nitrogen was used for the curtain, source, and exhaust gases. A standard curve was obtained using standard solutions of fb-PMT with concentrations of 3000, 1000, 300, 100, 30, 10, 3, and 1 ng/ml.
Determination of recovery efficiency
Recovery efficiency was calculated with the response of extracts from spiked plasma sample (sample) and blank plasma sample (blank) with spike after extract as follows:
Recovery efficiency (%) = Response in sample/Response in blank * 100
Determination of limit of quantification (LOQ) and limit of detection (LOD)
The limit of quantification (LOQ) and the limit of detection (LOD) was determined using data of six injections of a standard solution (9.8 ng/ml). LOQ and LOD were calculated using the standard deviations (SD) of signals for the analyte and the slope of linear regression curve as follows:
LOQ = 10 x SD/slope
LOD = 3 x SD/slope
Statistical analysis
It was performed with GraphPad Prism 7 software. Data are presented as mean ± SD. For comparison between groups, ANOVA was used. *p < 0.05 were considered statistically significant.
Results
Development of bioanalytical method for fb-PMT
The ionization of fb-PMT was studied in positive and negative modes. A deprotonated pseudo-molecular ion [M-H]- of fb-PMT was detected in negative mode (data not shown). The MW of fb-PMT calculated with the m/z of the deprotonated pseudo-molecular ion was consistent with the theoretical values. While observing the molecular ion was useful for confirming molecular weight, the intensity of the deprotonated molecular ion [M-H]- was very weak, so it was not applicable to MRM.
In the positive ion mode, a triply charged ion of fb-PMT at m/z 842.0 was detected, which was much stronger than singly or doubly charged ions (Supplementary Figure S1). The MRM method was optimized using the strongest ion as a precursor ion and a fluorobenzyl cation as a product ion (Figure 1B). Under the LC-MS/MS conditions, the retention time for fb-PMT was 3.8 min. The LC-MS/MS method for fb-PMT standard solutions with IS was linear (r = 0.9923) from a concentration of 9.8–2500 ng/ml (Supplementary Figure S2). The LOQ for fb-PMT was estimated to be 8.8 ng/ml under the current conditions. Accuracy was 100.4%; and recovery was 75.1%. The linearity, recovery, accuracy and LOQ were sufficient for the PK study.
The time course of fb-PMT concentrations in mouse plasma is shown in Figure 2. fb-PMT plasma Cmax and AUC dose relationship is shown in Supplementary Figure S3. The PK and TK parameters in mice are shown in Table 1.
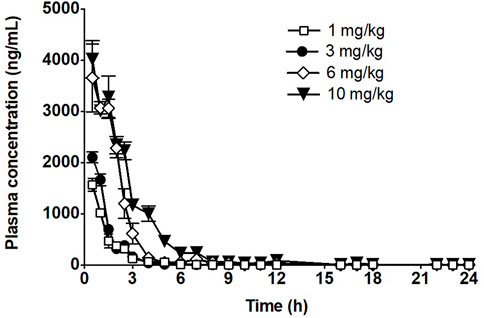
FIGURE 2. Time course of fb-PMT in mouse plasma following subcutaneous administration of fb-PMT. Data represent mean levels ±SD, (Hay et al., 2021).
The time courses of fb-PMT concentrations in rat plasma are shown in Figure 3 (Day1) and Figure 4 (Day28). The fb-PMT plasma Cmax and AUC dose relationship is shown in Supplementary Figure S4. The PK (Day1) and TK (Day28) parameters in rats are shown in Table 2.
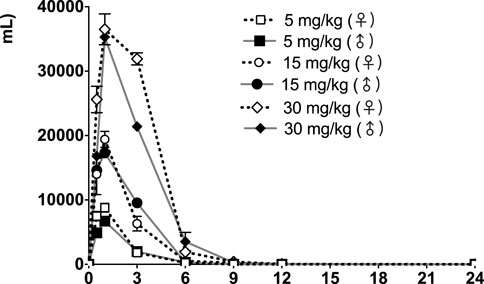
FIGURE 3. fb-PMT concentration (ng/ml) in the plasma versus time following subcutaneous administration of fb-PMT in male and female Sprague Dawley rats on Day 1. Low dose 5 mg/kg; Medium dose 15 mg/kg; High dose 30 mg/kg. Data represent mean levels ± SD.
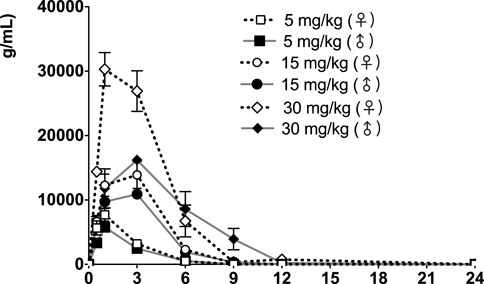
FIGURE 4. fb-PMT concentration (ng/ml) in the plasma versus time following subcutaneous administration of fb-PMT in male and female Sprague Dawley rats on Day 28. Low dose 5 mg/kg; Medium dose 15 mg/kg; High dose 30 mg/kg. Data represent mean levels ± SD.
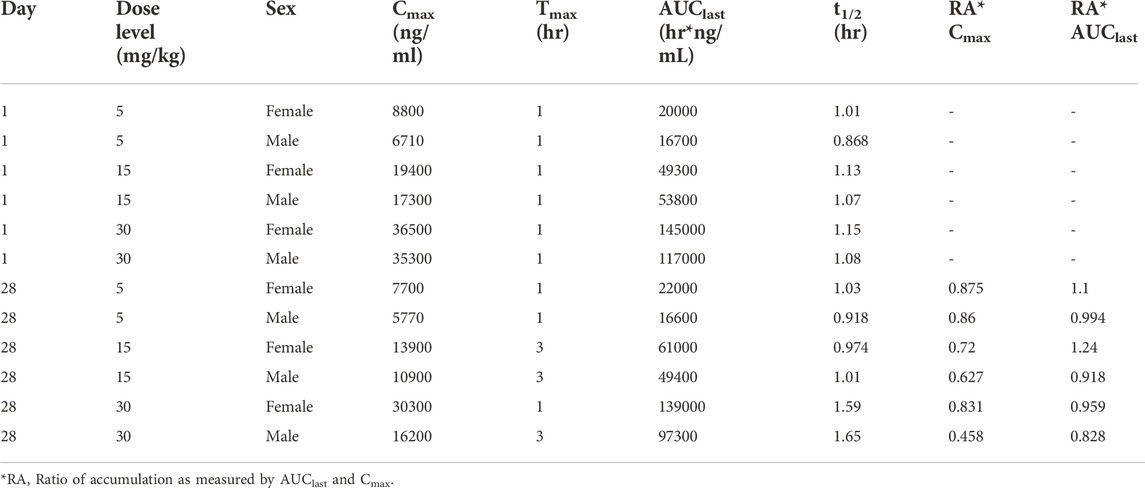
TABLE 2. Pharmacokinetic (Day 1) and toxicokinetic (Day 28) parameters of fb-PMT in male and female Sprague Dawley rats.
The time course of fb-PMT concentrations in monkey plasma is shown in Figures 5, 6. The fb-PMT plasma Cmax and AUC dose relationship is shown in Supplementary Figure S5. The PK and TK parameters in monkeys are shown in Table 3.
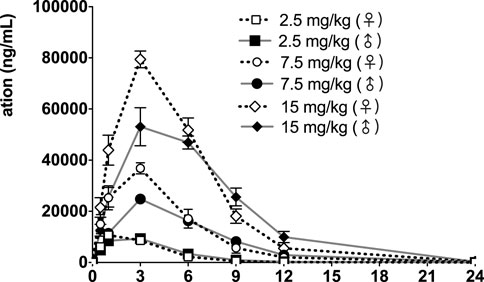
FIGURE 5. fb-PMT concentration (ng/ml) in the plasma versus time following subcutaneous administration of fb-PMT in male and female monkeys on Day 1. Low dose 2.5 mg/kg; Medium dose 7.5 mg/kg; High dose 15 mg/kg. Data represent mean levels ± SD.
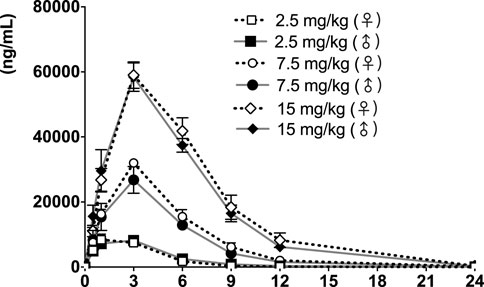
FIGURE 6. fb-PMT concentration (ng/ml) in the plasma versus time following subcutaneous administration of fb-PMT in male and female monkeys on Day 28. Low dose 2.5 mg/kg; Medium dose 7.5 mg/kg; High dose 15 mg/kg. Data represent mean levels ±SD.
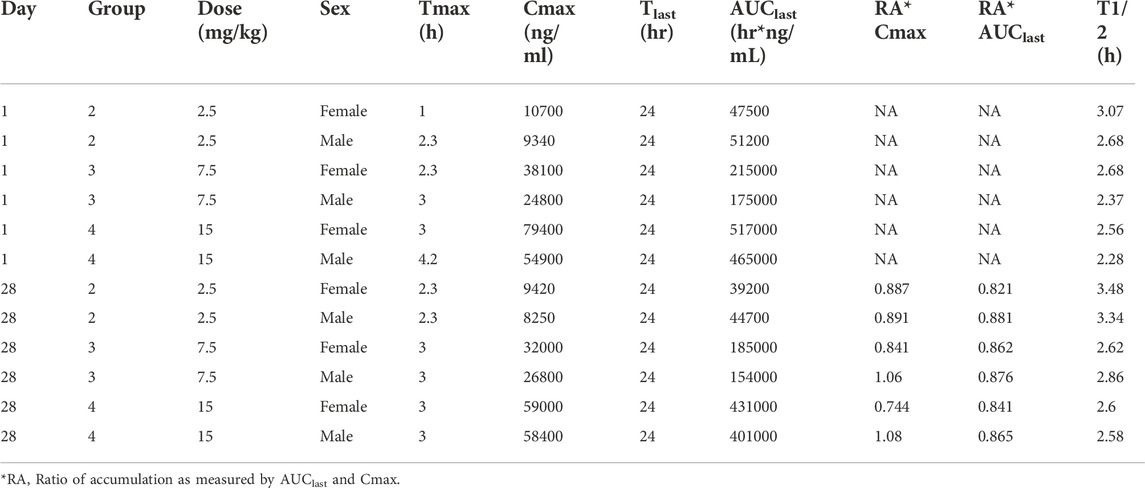
TABLE 3. Pharmacokinetic (Day 1) and Toxicokinetic (Day 28) parameters of fb-PMT Following Single and Multiple SC Doses of fb-PMT in Female and Male Cynomolgus Monkeys.
Discussion
Development of bioanalytical method for fb-PMT
fb-PMT has both hydrophilic and lipophilic moieties and high affinity for the integrin αvβ3 receptor, resulting in favorable pharmaceutical properties as an anticancer therapeutic. Although its molecular weight is less than 3000, which is the maximum range of typical MSD, it shows predominantly multiple-charged ions in the positive mode. The result is consistent with the chemical properties of PEG conjugates, which tend to coordinate with cations in aqueous solutions. As expected, a fluorobenzyl cation exhibited the strongest product ion; we used this to develop the sensitive MRM method for fb-PMT. The LOQ and recovery of the bioanalytical method was satisfactory under those conditions. A variety of polydisperse PEGylated drug conjugates have been reported; however, PK studies have not been reported, most likely because of their poor sensitivity with traditional LC-MS/MS methods due to their polydispersity (Yin et al., 2020). Yin et al. used LC-TOFMS coupled with pre-column derivatization and MSALL technique for PEGylated gemcitabine (Mw 70–76 kDa) and conducted its PK study in rats (Yin et al., 2020). The monodisperse fb-PMT conjugate is more readily analyzed in a conventional LC-MS/MS system.
PK of fb-PMT in mice
Following SC administration, fb-PMT was rapidly distributed into systemic circulation resulting in quantifiable plasma concentrations at 1 h post-dose in all measured mice, and generally remained quantifiable through 12 h. Mean time to peak concentration (Tmax) occurred 1 h post-dose at all four doses tested. Following Tmax, plasma fb-PMT concentrations decreased in a multiphasic manner with mean T1/2 values ranging 0.8–1.5 h across all dose levels. Across the dose range (1–10 mg/kg), mean peak (Cmax) exposures to fb-PMT and mean total (AUC) exposures increased in a dose related manner (R2 = 0.908 and 0.965, respectively).
PK of fb-PMT in rats
Following daily SC administration for 28 days, fb-PMT was rapidly distributed into systemic circulation resulting in quantifiable plasma concentrations at 0.5 h post-dose in all measured rats on both Day 1 and Day 28, and generally remained quantifiable through 12 h. Predose fb-PMT concentrations were not observed at all dose levels on Day 1, and at the 5 and 15 mg/kg dose levels on Day 28. There were quantifiable pre-dose fb-PMT concentrations at the 30 mg/kg dose level across both sexes on Day 28. Composite mean time to peak concentration (Tmax) occurred 1 h post-dose on Day 1 and 1–3 h post-dose on Day 28. Elevated Day 28 plasma concentrations at the 12 h relative to the 9 h timepoint were observed for the females administered 5 and 30 mg/kg.
Following daily SC administration of fb-PMT for 28 days, female and male fb-PMT plasma concentrations declined in a multiphasic manner with individual composite mean half-life (T1/2) values ranging from 0.87 to 1.7 h. Female composite mean peak (Cmax) and total (AUClast) exposures to fb-PMT were comparable (within 2-fold) to the male exposures on Day 1 and Day 28. Across the dose range (5–30 mg/kg), composite mean peak (Cmax) and total (AUClast) exposures to fb-PMT generally increased in a dose proportional manner on Day 1 and Day 28. Accumulation was not observed based on RA of Cmax and AUClast, which ranged from 0.458 to 0.875 and 0.828 to 1.24, respectively.
Tmax was 1 h on Day 1 and ranged 1–3 h on Day 28 at three doses in female and male rats. T1/2 ranged 0.868–1.15 h at three doses in female and male rats on Day 1 (Table 2). T1/2 at low and mid doses ranged 0.918 to 1.03 on Day 28; and T1/2 at the high dose on Day 28 were slightly longer than T1/2 on Day 1 by 38 and 53% (Table 2).
PK of fb-PMT in monkeys
Following daily SC administration of fb-PMT for 28 days, fb-PMT was rapidly distributed into systemic circulation resulting in quantifiable plasma concentrations at 0.5 h post-dose in all monkeys on both Day 1 and Day 28 and remained quantifiable through 24 h. Quantifiable pre-dose fb-PMT concentrations were not observed at all dose levels on Day 1. Quantifiable pre-dose fb-PMT concentrations were observed at all dose levels on Day 28. Individual time to peak concentration (Tmax) occurred 1–6 h post-dose on Day 1 and occurred 1–3 h post-dose on Day 28. Following Tmax, plasma fb-PMT concentrations decreased in a multiphasic manner with mean T1/2 values ranging 2.28–3.48 h across all genders and dose levels on Days 1 and 28.
Female mean peak (Cmax) and total (AUClast) exposures to fb-PMT were comparable (within 2-fold) to the male exposures on Day 1 and Day 28. Across the dose range (2.5–15 mg/kg), mean peak (Cmax) exposures to fb-PMT increased in a dose proportional manner and mean total (AUClast) exposures to fb-PMT generally increased in a dose proportional manner on Day 1 and Day 28. Accumulation was not observed based on Ratio of accumulation (RA) of Cmax and AUC, which ranged from 0.744 to 1.08 and 0.821 to 0.862, respectively (Table 3).
fb-PMT showed favorable PK parameters without accumulation and differences among different genders (Table 3). A previous study showed that fb-PMT was distributed in the brain after 1 h of the treatment in mice (Hay et al., 2021). The result is consistent with our previous results of the biodistribution of P-bi-TAT (Fujioka et al., 2021) and Fleming et al.‘s results of the chemotherapy drug Camptothecin conjugated with PEG3400 in rats (Fleming et al., 2004; Haverstick et al., 2007). The half-lives of fb-PMT are shorter than the half-lives of P-bi-TAT in rats and in monkeys (Fujioka et al., 2021). Meanwhile, the potency of fb-PMT is similar to that of P-bi-TAT (Rajabi et al., 2019). The high potency and a shorter T1/2 of fb-PMT compared to P-bi-TAT may result in the same pharmacological effects with less accumulation into off-target tissues (Mollaei et al., 2021).
Conclusion
A quantitative bioanalytical method for fb-PMT was developed using LC-MS/MS and LLE. The LOQ for fb-PMT was 1.5 ng/μL and the recovery efficiency was 93.9% with the developed method. The bioanalytical method was used in fb-PMT PK studies to generate PK and TK parameters in mice, rats, and monkeys. The results of this study and the previous study of biodistribution of fb-PMT suggest that fb-PMT is a promising candidate as an anticancer drug. Additional studies, including efficacy in multiple cancer models, accumulation in organs, and metabolism and pharmacodynamics are planned. Furthermore, biodistribution and elimination kinetics will be determined in future studies.
Ethical Approval Statement: All animal studies were conducted at the animal facility of the Veteran Affairs (VA) Medical Center, Albany, NY, United States in accordance with current institutional guidelines for humane animal treatment and approved by the VA IACUC (protocol number 545017).
Data availability statement
The original contributions presented in the study are included in the article/Supplementary Materials, further inquiries can be directed to the corresponding author.
Ethics statement
The animal study was reviewed and approved by the VA IACUC and Inotiv IACUC.
Author contributions
KF contributed to the analytical and bioanalytical method development, validation and drafted the manuscript; KG contributed to the animal studies for PK and biodistribution; and SM contributed the study design, concepts, data review, supervision, and edits of the manuscript. BH designed and did the initial synthesis of fb-PMT and edits of the manuscript.
Acknowledgments
Special thanks to both NanoPharmaceuticals LLC and from the Pharmaceutical Research Institute for their funding. Special thanks to PRI chemists and biologists for their teamwork efforts. We appreciate the Inotiv team in generating TK data with fb-PMT in rat and monkey.
Conflict of interest
SM is a founder and shareholder of Nanopharmaceuticals LLC.
The remaining authors declare that the research was conducted in the absence of any commercial or financial relationships that could be construed as a potential conflict of interest.
Publisher’s note
All claims expressed in this article are solely those of the authors and do not necessarily represent those of their affiliated organizations, or those of the publisher, the editors and the reviewers. Any product that may be evaluated in this article, or claim that may be made by its manufacturer, is not guaranteed or endorsed by the publisher.
Supplementary material
The Supplementary Material for this article can be found online at: https://www.frontiersin.org/articles/10.3389/fphar.2022.902141/full#supplementary-material
References
Brooks, P. C., Clark, R. A., and Cheresh, D. A. (1994). Requirement of vascular integrin alpha v beta 3 for angiogenesis. Science 264, 569–571. doi:10.1126/science.7512751
Chen, H. H. W., and Kuo, M. T. (2017). Improving radiotherapy in cancer treatment: Promises and challenges. Oncotarget 8 (37), 62742–62758. doi:10.18632/oncotarget.18409
Cox, D., Brennan, M., and Moran, N. (2010). Integrins as therapeutic targets: Lessons and opportunities. Nat. Rev. Drug Discov. 9, 804–820. doi:10.1038/nrd3266
Davis, P. J., Davis, F. B., and Mousa, S. A. (2009). Thyroid hormone-induced angiogenesis. Curr. Cardiol. Rev. 5 (1), 12–16. doi:10.2174/157340309787048158
Davis, P. J., and Mousa, S. A. (2017). Anti-angiogenesis Strategies in cancer therapeutics. Amsterdam: Elsevier Science, 133–146. Chapter 9 - Tetraiodothyroacetic Acid at Integrin αvβ3: A Model of Pharmaceutical Anti-Angiogenesis.
Debreli Coskun, M., Sudha, T., Bharali, D. J., Celikler, S., Davis, P. J., and Mousa, S. A. (2020). αvβ3 integrin antagonists Enhance chemotherapy response in an Orthotopic pancreatic cancer model. Front. Pharmacol. 11, 95. doi:10.3389/fphar.2020.00095
Desgrosellier, J. S., and Cheresh, D. A. (2010). Integrins in cancer: Biological implications and therapeutic opporyunities. Nat. Rev. Cancer 11, 727–731.
Fleming, A. B., Haverstick, K., and Saltzman, W. M. (2004). In vitro cytotoxicity and in vivo distribution after direct delivery of PEG-camptothecin conjugates to the rat brain. Bioconjug. Chem. 15 (6), 1364–1375. doi:10.1021/bc034180o
Fujioka, K., Godugu, K., and Mousa, S. A. (2021). Pharmacokinetics and biodistribution of a novel anticancer thyrointegrin αvβ3 antagonist: Triazole modified tetraiodothyroacetic acid conjugated to polyethylene glycol (P-bi-TAT). AAPS Open 7, 2. doi:10.1186/s41120-021-00036-z
Haverstick, K., Fleming, A., and Mark Saltzman, W. (2007). Conjugation to increase treatment volume during local therapy: A case study with PEGylated camptothecin. Bioconjug. Chem. 18 (6), 2115–2121. doi:10.1021/bc700214h
Hay, B. A., Godugu, K., Darwish, N. H. E., Fujioka, K., Sudha, T., Karakus, O. O., et al. (2021). New thyrointegrin αvβ3 antagonist with a scalable synthesis, brain Penetration, and potent activity against Glioblastoma Multiforme. J. Med. Chem. 64 (9), 6300–6309. doi:10.1021/acs.jmedchem.1c00350
Karakus, O. O., Godugu, K., Rajabi, M., and Mousa, S. A. (2020). Dual targeting of norepinephrine transporter (net) function and thyrointegrinαvβ3receptors in the treatment of neuroblastoma. J. Med. Chem. 63, 7653–7662. doi:10.1021/acs.jmedchem.0c00537
Li, W., Yalcin, M., Bharali, D. J., Lin, Q., Godugu, K., Fujioka, K., et al. (2019). Pharmacokinetics, biodistribution, and anti-angiogenesis efficacy of Diamino propane tetraiodothyroacetic acid-conjugated Biodegradable Polymeric Nanoparticle. Sci. Rep. 9 (1), 9006. doi:10.1038/s41598-019-44979-6
Mizejewski, G. J. (1999). Role of integrins in cancer: Survey of expression patterns. Proc. Soc. Exp. Biol. Med. 222, 124–138. doi:10.1046/j.1525-1373.1999.d01-122.x
Mollaei, M., Hassan, Z. M., Khorshidi, F., and Langroudi, L. (2021). Chemotherapeutic drugs: Cell death- and resistance-related signaling pathways. Are they really as smart as the tumor cells? Transl. Oncol. 14 (5), 101056. doi:10.1016/j.tranon.2021.101056
Rajabi, M., Kavitha Godugu, K., Sudha, T., Bharali, D. J., and Mousa, S. A. (2019). Triazole modified tetraiodothyroacetic acid conjugated to polyethylene glycol: High affinity thyrointegrin αvβ3 antagonist with potent anticancer Activities in Glioblastoma Multiforme. Bioconjug. Chem. 30 (12), 3087–3097. doi:10.1021/acs.bioconjchem.9b00742
Yin, L., Ren, T., Zhao, S., Shi, M., and Gu, J. (2020). Comparative pharmacokinetic study of PEGylated gemcitabine and gemcitabine in rats by LC-MS/MS coupled with pre-column derivatization and MSALL technique. Talanta 206, 120184. doi:10.1016/j.talanta.2019.120184
Keywords: fb-PMT, NP751, targeted cancer therapeutic, tetrac, thyrointegrin αvβ3 receptor, LC-MS/MS, pharmacokinetic
Citation: Fujioka K, Hay BA, Godugu K and Mousa SA (2022) Pharmacokinetics of fluorobenzyl polyethylene glycol conjugated tetraiodothyroacetic acid (NP751), a novel anticancer thyrointegrin αvβ3 antagonist. Front. Pharmacol. 13:902141. doi: 10.3389/fphar.2022.902141
Received: 22 March 2022; Accepted: 02 November 2022;
Published: 28 November 2022.
Edited by:
Chandraiah Godugu, National Institute of Pharmaceutical Education and Research, IndiaCopyright © 2022 Fujioka, Hay, Godugu and Mousa. This is an open-access article distributed under the terms of the Creative Commons Attribution License (CC BY). The use, distribution or reproduction in other forums is permitted, provided the original author(s) and the copyright owner(s) are credited and that the original publication in this journal is cited, in accordance with accepted academic practice. No use, distribution or reproduction is permitted which does not comply with these terms.
*Correspondence: Shaker A. Mousa, c2hha2VyLm1vdXNhQGFjcGhzLmVkdQ==
†This paper is dedicated to the memory of first author Kazutoshi Fujioka, who passed away earlier this year