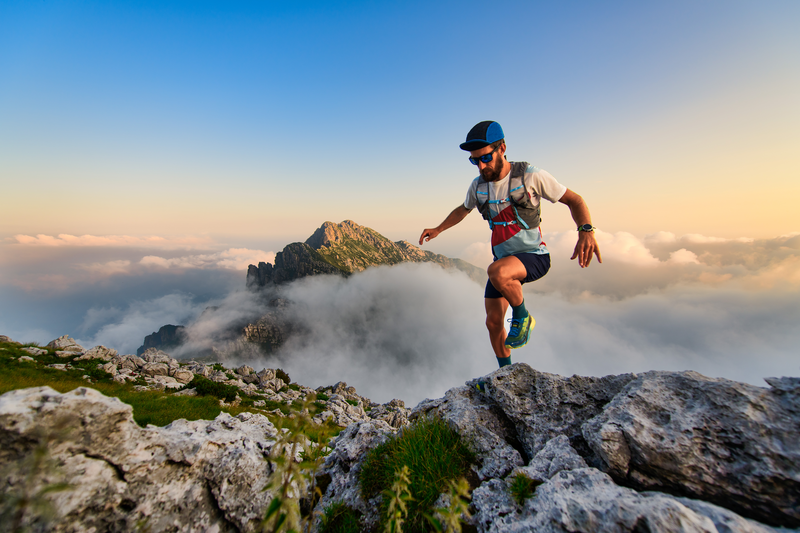
94% of researchers rate our articles as excellent or good
Learn more about the work of our research integrity team to safeguard the quality of each article we publish.
Find out more
SYSTEMATIC REVIEW article
Front. Pharmacol. , 28 June 2022
Sec. Pharmacology of Infectious Diseases
Volume 13 - 2022 | https://doi.org/10.3389/fphar.2022.900551
This article is part of the Research Topic Women in Pharmacology of Infectious Diseases: 2021 View all 5 articles
Introduction: Protein binding can diminish the pharmacological effect of beta-lactam antibiotics. Only the free fraction has an antibacterial effect. The aim of this systematic literature review was to give an overview of the current knowledge of protein binding of cephalosporins in human body fluids as well as to describe patient characteristics influencing the level of protein binding.
Method: A systematic literature search was performed in Embase, Medline ALL, Web of Science Core Collection and the Cochrane Central Register of Controlled Trials with the following search terms: “protein binding,” “beta-lactam antibiotic,” and “body fluid.” Only studies were included where protein binding was measured in humans in vivo.
Results: The majority of studies reporting protein binding were performed in serum or plasma. Other fluids included pericardial fluid, blister fluid, bronchial secretion, pleural exudate, wound exudate, cerebrospinal fluid, dialysate, and peritoneal fluid. Protein binding differs between diverse cephalosporins and between different patient categories. For cefazolin, ceftriaxone, cefpiramide, and cefonicid a non-linear pattern in protein binding in serum or plasma was described. Several patient characteristics were associated with low serum albumin concentrations and were found to have lower protein binding compared to healthy volunteers. This was for critically ill patients, dialysis patients, and patients undergoing cardiopulmonary bypass during surgery. While mean/median percentages of protein binding are lower in these patient groups, individual values may vary considerably. Age is not likely to influence protein binding by itself, however limited data suggest that lower protein binding in newborns. Obesity was not correlated with altered protein binding.
Discussion/Conclusion: Conclusions on protein binding in other body fluids than blood cannot be drawn due to the scarcity of data. In serum and plasma, there is a large variability in protein binding per cephalosporin and between different categories of patients. Several characteristics were identified which lead to a lower protein binding. The finding that some of the cephalosporins display a non-linear pattern of protein binding makes it even more difficult to predict the unbound concentrations in individual patients. Taken all these factors, it is recommended to measure unbound concentrations to optimize antibiotic exposure in individual patients.
Systematic Review Registration: PROSPERO, identifier (CRD42021252776).
Cephalosporins are a frequently used class of beta-lactam antibiotics in hospitalised patients. They are used for a broad spectrum of indications and in many types of patients, from peri-operative prophylactic use in relatively healthy patients to therapeutic use in critically ill septic patients. The efficacy of the cephalosporins is best correlated to the “time that the free (f) concentration is above the minimum inhibitory concentration (MIC) of the pathogen (fT > MIC).” The fT > MIC is one of the pharmacokinetic (PK)/pharmacodynamic (PD)-indices to describe antimicrobial effect. Importantly, all these indices are based on free concentrations, i.e., the fraction of the total concentration that is not bound to proteins. This underlines the importance of protein binding for antimicrobial efficacy.
Protein binding can decrease the pharmacological effect of beta-lactam antibiotics, since only the unbound fraction is pharmacologically active (Craig and Kunin, 1976; Merrikin et al., 1983; Beer et al., 2009; Briscoe et al., 2012). The binding to proteins depends on the number of binding sites as well as binding affinity in relation to the antibiotic concentration. Besides its impact on the antibacterial effect, protein binding also affects the pharmacokinetics, since it has an influence on drug clearance and distribution (Roberts et al., 2013).
It is essential that antibiotics distribute throughout the body since most infections do not occur in the bloodstream. However, only the unbound fraction is able to penetrate into the extravascular space (Tan and Salstrom, 1977). From the extravascular space, the drug will distribute into tissues or be metabolised or excreted from the body (Wise, 1983). The unbound blood concentration will equilibrate with unbound concentration in the extravascular space (Bergogne-Bérézin, 2002). Therefore, it is hypothesised that unbound concentrations reflect the active concentrations at the site of infection.
The level of protein binding is often regarded as a fixed percentage per antibiotic, i.e., independent of the patient disease state and antibiotic concentrations. With increasing knowledge, we nowadays know that protein binding is often more complex. Some antibiotics/drugs display a concentration-dependent protein binding. As a result, proteins are being saturated in high concentrations of antibiotics, resulting in an increased unbound fraction. However, the opposite effect was also shown with lower unbound fractions at high antibiotic concentrations (Bulik et al., 2010). In specific clinical conditions, protein binding might change, such as in critically ill patients or in dialysis patients (Steele et al., 1979; Wilkes et al., 2019; Jager et al., 2020).
Since protein binding is crucial for antibacterial efficacy as well as distribution to the site of infection, the aim of our systematic literature review was to give an overview of the current knowledge on protein binding of cephalosporins in human body fluids as well as to describe characteristics influencing the level of protein binding based on clinical studies in humans.
We followed the PRISMA Statement as a guideline. This systematic review is registered under PROSPERO CRD42021252776.
The final database consisted of articles, which were included in three stages. First, on 12 April 2021, studies were identified by searching four databases (Embase, Medline ALL, Web of Science Core Collection and Cochrane Central Register of Controlled Trials) with no restriction on publication date. Duplicates were removed. The search strategy was developed by a biomedical information specialist from the medical library at the Erasmus University Medical Centre (Supplementary Appendix S1). The following search terms were used for the search strategy: protein binding and beta-lactam antibiotic and body fluid. The second stage consisted of an additional search (December 2021) to check the results of the first search for completeness. This search was performed in Pubmed using the name of the specific cephalosporin and unbound concentration as search terms. The third stage was performed during data extraction and consisted of checking the reference lists of the selected articles for missing articles.
Studies were included if they reported protein binding of cephalosporins in human body fluids. Included were human studies on cephalosporins in blood or other body fluids, measuring and reporting protein binding or measuring both total and unbound concentrations in actual study settings. Reviews, in vitro studies, animal studies, studies in tissues, conference papers and abstracts, and articles not in English were excluded. The primary outcome measure was the degree of protein binding of a beta-lactam antibiotic in a body fluid. In this review, we focus on cephalosporins, one class of beta-lactam antibiotic, as information on protein-binding of these antibiotics was most important for clinical care.
In the first stage, two reviewers screened the references independently according to the method of Bramer et al. (2017). The articles were included based on title and abstract (CJ and PB). In case of uncertainty based on title and abstract, the articles were included for full-text screening. Subsequently, full-texts were screened (CJ and BD). The reasons for exclusion after screening the full-text were noted. For the second and third stages, one reviewer screened the abstracts and full-texts (AM). A second reviewer screened the selected full-texts (CJ). In case of a disagreement between the reviewers, consensus was reached through discussion or if necessary, a third reviewer was consulted to make the final decision (BK).
One reviewer (CJ) extracted data from the included articles. The following data were collected from the included studies in a data extraction form: 1) which antibiotic was used, 2) body fluid in which protein binding was measured, 3) population in which protein binding was measured, 4) number of participants, 5) dosage, 6) time point in which protein binding was measured, 7) sampling method, 8) analytical method, 9) percentage of protein binding, and (10) study type. A second reviewer (AM) checked the data extracted by the first reviewer. In case of a disagreement between the reviewers, consensus was reached through discussion or if necessary, a third reviewer was consulted to make the final decision (BK). With the final dataset the article selection was checked for duplicate publications.
The degree of protein binding was noted as a percentage. If an article noted the unbound fraction, this was converted to percentage of protein binding using the following equation:
Various patterns of protein binding have been described. The degree of protein binding is often reported as a single value. In this situation the unbound fraction remains the same, irrespective of the total concentration. This so called “linear protein binding” is caused by large binding capacities of proteins or low binding affinities of drugs for proteins. At high total concentrations, proteins are still not saturated (Deitchman et al., 2018). However, in clinical practice with an increasing concentration, proteins might become saturated. This results in concentrations dependency and a non-linear protein binding. The most common pattern of non-linear protein binding has an increased unbound fraction with high total concentrations due to saturated binding sites on the proteins (typical non-linear protein binding). However, also the opposite pattern has been described: a high unbound fraction at low concentrations (atypical non-linear protein binding) (Muralidharan et al., 2005; Zhou et al., 2017). This has been described for the tetracyclines. An even more complex pattern has been described for tigecycline, which is U-shaped (Mukker et al., 2014; Dorn et al., 2018). This atypical pattern was linked to divalent metal ion chelation (Singh et al., 2016). The different patterns of protein binding are shown in Figure 1.
The most important protein for protein binding of antibiotics is albumin. Therefore, a decreased level of albumin is thought to significantly reduce the level of protein binding. Several clinical characteristics are known to influence the level of albumin. Low levels can be caused by specific situations such as systemic inflammatory response syndromes, hepatic diseases, aging, malnutrition, malignancy, burns, pregnancy, diabetes mellitus, infection, or pulmonary oedema. Otherwise, the albumin level can be within the normal range, but binding is altered due to displacement from protein binding sites by for example, bilirubin, urea or co-administered drugs (Tillement et al., 1978; Roberts et al., 2013).
An effect of temperature as well as pH on protein binding has been described previously. However, the physiological variation of both temperature and pH are limited (Hinderling and Hartmann, 2005). Therefore, the influence of both factors might be relevant in in vitro experiments, but not in a clinical setting (Kochansky et al., 2008).
Interaction with co-administered drugs might also influence protein binding. If two highly protein bound drugs are administered simultaneously, competition for the binding capacity will occur since there is a limited amount of protein available. This will lead to a higher free fraction of the drugs compared to the situation after administration of the drugs alone.
Especially in hydrophilic drugs, an increased unbound concentration might result in an increased clearance. In addition, a change in protein binding is a major factor influencing the volume of distribution because only the unbound fraction is able to penetrate into the extravascular space (Tan and Salstrom, 1977; Nix et al., 1991). The change in volume of distribution is linearly correlated with the unbound fraction (Nix et al., 1991).
The changes in pharmacokinetics, as described, might result in an altered half-life, (unbound) concentrations in steady state, penetration into tissues and bioavailability (Schmidt et al., 2010). The degree and pattern of protein binding is likely to change the unbound concentrations over time during a dosing interval. These unbound concentrations are the parameters of interest to determine the clinical efficacy as well as potential toxicity. Drugs with an increased unbound concentration might be more effective at the beginning of the dosing interval. However, as a result of the increased clearance with the increased unbound concentrations, the antibiotic might be cleared faster with consequently inadequate concentrations at the end of the dosing interval. The magnitude of these changes might differ per antibiotic and in special populations. Some changes might lead to the need for altered drug dosing.
Overall, changes in protein binding are assumed to be of relevance mainly in highly protein bound drugs, since changes for those drugs will have a larger effect. Highly protein bound is defined as 90%–99.9% protein binding (Wright et al., 1996). A non-linear pattern of protein binding complicates the interpretation, since the unbound concentration-time profile will be less predictable (Deitchman et al., 2018). Notably, to predict the efficacy of an antibiotic dosing regimen, the unbound concentration-time profile needs to be correlated with the minimum target concentration required for antibacterial effect.
In the first stage a total of 3,142 studies were identified. After duplicate removal (N = 844), 2,298 studies were screened on title and abstract, resulting in 194 primary studies. The full-text was not available for one of them. Of the remaining studies, 128 were excluded for the following reasons: not in English, double publication, did not measure protein binding, did measure protein binding but did not mention it, measured protein binding in body tissue, in animals or in vitro, review and not cephalosporins (Figure 2). The remaining 66 studies were included in the systematic review. During the second stage, 157 studies were screened of which 24 were eligible for inclusion. From the third stage, two studies were included out of 12 screened articles. This resulted in a total of 92 eligible for inclusion for this systematic review.
FIGURE 2. Flowchart of the identification of studies via databases and registers (N = number of articles).
Table 1 gives an overview of the studies in which protein binding of cephalosporins was determined. The compromised version of the data extraction is available in this article whereas the full version of the data extraction is available in Supplementary Appendix S2.
The included studies represented the protein binding of 23 different cephalosporins in 11 different body fluids (N = 117): plasma (N = 62), serum (N = 38), pericardial fluid (N = 4), blister fluid (N = 3) wound exudate (N = 2), bronchial secretion (N = 2), pleural exudate (N = 2), dialysate (N = 1), peritoneal fluid (N = 1), cerebrospinal fluid (N = 1), and cord serum (N = 1). Some studies reported the protein binding in more than one fluid. The protein binding of cefazolin and ceftriaxone was studied most extensively. Protein binding was mostly determined in blood serum or blood plasma compared to other body.
Overall, the degree in protein binding is variable between the different cephalosporins (see examples in Figure 3). Even between studies of an individual cephalosporin, there is considerable variability. As can be seen in Table 1, several cephalosporins are highly protein bound, such as cefonicid (mean 82%–98%, four studies) (Dudley et al., 1986; Ackerman et al., 1988; Trang et al., 1989; Swanson et al., 1991) and cefoperazone in adults (mean 89%–92%, four studies) (Lam et al., 1988; Kalman et al., 1992; Kan et al., 2020; Rao et al., 2020). Also, ceftriaxone is generally highly bound to proteins, although there is a large variability between studies. Some cephalosporins show a consistently low protein binding, such as cefuroxime (mean 16%–33%, five studies) (Foord, 1976; Verhagen et al., 1994; Mand'ák et al., 2007; Aalbers et al., 2015; van Raaij et al., 2020), cefotaxime (mean 8%–41%, four studies) (Scaglione et al., 1990; Seguin et al., 2009; Dahyot-Fizelier et al., 2013; Aardema et al., 2020) and ceftazidime (mean 0%–21%, six studies) (Van Dalen et al., 1986; Lam et al., 1988; Matzke et al., 2000a; Berkhout et al., 2003; Isla et al., 2007; Schieser et al., 2021).
FIGURE 3. Protein binding of different cephalosporins in different populations N, number of participants; CABG, coronary artery bypass graft; CVVHD, continuous venovenous hemodialysis; CVVH, continuous venovenous hemofiltration; ECMO, extracorporeal membrane oxygenation.
In some studies the pattern of protein binding was mentioned as well. For the concentration range as achieved in clinical patients, the protein binding was found to be linear for ceforanide (DiPiro et al., 1985), cefamandole (Berkhout et al., 2003), cefmenoxime (Reitberg et al., 1984), cefotaxime (Scaglione et al., 1990; Dahyot-Fizelier et al., 2013), cefluprenam (Nakashima et al., 1994), cefotetan (Carver et al., 1989), and cefoxitin (Carver et al., 1989). However, for some cephalosporins a typical non-linear pattern was found. A clear example hereof is cefazolin. As shown in Figure 4, the protein binding for the peak-concentrations is consistently lower in five studies as compared to the through-concentrations (Vella-Brincat et al., 2007; Roberts et al., 2015; Lantinga et al., 2016; Dhanani et al., 2019; Dorn et al., 2019). This non-linear behavior was shown for different categories of patients. Other cephalosporins with a non-linear pattern are cefonicid (Dudley et al., 1986; Ackerman et al., 1988; Trang et al., 1989), cefpiramide (Conte, 1987; Demotes-Mainard et al., 1991), and ceftriaxone (Stoeckel et al., 1984; Popick et al., 1987; Toth et al., 1991; Bourget et al., 1993; Matzke et al., 2000b; Fukumoto et al., 2009; Tsai et al., 2016; Bos et al., 2018; Grégoire et al., 2019; Herrera-Hidalgo et al., 2020; Gijsen et al., 2021; Hartman et al., 2021; Kan et al., 2021). An atypical non-linear pattern was not found for any of the cephalosporins.
FIGURE 4. Peak and trough concentrations of cefazolin in different populations N, number of participants; ECMO, extracorporeal membrane oxygenation.
An important factor determining the degree of protein binding is the serum albumin level. A low level of serum albumin is expected to result in a lower protein binding. Correlations between the albumin concentrations and protein binding were seen for cefazolin, ceftizoxime, ceftriaxone, and cefoperazone (Ohashi et al., 1986; Fortunato et al., 1993; Allegaert et al., 2009; Himebauch et al., 2014; Schleibinger et al., 2015; de Cock et al., 2017; Asada et al., 2018; Kan et al., 2020; Booke et al., 2021; Hartman et al., 2021). However, the studies of Toth et al. (1991) and Tsai et al. (2016) showed no correlation between the albumin concentration and the protein binding of ceftriaxone. For cefonicid and cefmonoxime, no correlation was seen between albumin concentrations and protein binding (Reitberg et al., 1984; Trang et al., 1989).
Several medical characteristics are known to be associated with a low serum albumin level and are therefore expected to result in lower degrees of protein binding. Examples will be described.
Patients on dialysis are known to have low albumin concentrations. This hypoalbuminemia is multifactorial and caused by malnutrition, exogenous albumin loss, and volume expansion (Yeun and Kaysen, 1998). Other factors such as the type of dialyses, surface area, type of membrane, blood flow and dialysate flow might also influence protein binding (Garcia et al., 1979a). This is in line with the results found for the different cephalosporins. Protein binding in several patients on peritoneal dialysis with a peritonitis has been described. Mean or median values for the protein binding of cefazolin was found to be between 64% and 82% (Roberts et al., 2015; Parker et al., 2016; Roberts et al., 2016), while in healthy volunteers these values were higher 84%–89% (Craig et al., 1973; Ohashi et al., 1986). In a 25-year old patient on extracorporeal membrane oxygenation (ECMO) the protein binding was even lower (52%–60%) (Dhanani et al., 2019). For cefalothin, the protein binding for patients on peritoneal dialysis with a peritonitis was found to be 47%–51% (Parker et al., 2016; Roberts et al., 2016) as compared to healthy volunteers of mean 65.2% (Craig et al., 1973) (Figure 3). For patients on hemodialysis, a lower degree in protein binding has also been shown. For example, protein binding for cefoxitin was mean 41.5% in patients on hemodialysis (Garcia et al., 1979a), compared healthy volunteers (mean 52%) (Carver et al., 1989). The same applies to ceftriaxone [mean 43% for dialysis patients (Matzke et al., 2000b) and 90%–93% for healthy volunteers (Mimoz et al., 2000; Neves et al., 2018; Herrera-Hidalgo et al., 2020)].
Critically ill patients are another important group. For several cephalosporins low protein binding has been described in this patient category. Cefazolin protein binding in critically ill adults was found to be 60%–77% (Roberts et al., 2015). This is lower compared to healthy volunteers (84%–89%) (Craig et al., 1973; Ohashi et al., 1986). In critically ill patients on ECMO its protein binding was even lower (mean 51%) (Booke et al., 2021). Also for ceftriaxone several studies have been performed in critically ill adults, all resulting in a lower protein binding compared to healthy volunteers (Schleibinger et al., 2015; Tsai et al., 2016; Bos et al., 2018; Gijsen et al., 2021; Meenks et al., 2021; Ulldemolins et al., 2021). However, also within this category of patients the degree of protein binding varies considerably, with values from 50% to 94%. Tsai et al. (2016) concluded that the protein binding of ceftriaxone was not correlated with albumin, but with hyperbilirubinemia and diabetes mellitus.
Patients with liver cirrhosis might also have lower values for the protein binding of cephalosporins. This could be due to less efficient removal of cumulated endogenous binding inhibitors in patients with cirrhosis and ascites (Stoeckel et al., 1984). This was confirmed for cefazolin, which was bound for mean 72.3% in cirrhotic patients (Ohashi et al., 1986). Also for ceftriaxone protein binding was lower (83.9% vs. 90%–93% in healthy volunteers) (Stoeckel et al., 1984; Mimoz et al., 2000; Neves et al., 2018; Herrera-Hidalgo et al., 2020) as well as for cefpiramide (89.6 vs. 98.1 in healthy volunteers) (Demotes-Mainard et al., 1991). For cefalothin there was not a significant difference in protein binding between patients with cirrhosis (73.4%) and controls (75.9%) (Ohashi et al., 1986).
Pregnancy is also described as to be associated with a low albumin level. Limited data are available on the protein binding during pregnancy. Allegaert et al. (2009) showed a reduced protein binding of 75% in 30 pregnant women treated with cefazolin as compared to 84%–89% protein binding in healthy volunteers (Craig et al., 1973; Ohashi et al., 1986; Allegaert et al., 2009). However, in a study with ceftriaxone the protein binding was not reduced and found to be 92% in pregnant women with chorioamnionitis or pyelonephritis (Bourget et al., 1993).
Cardiopulmonary bypass (CPB) led to differences in protein binding during the procedure (Hollis et al., 2015; de Cock et al., 2017; Asada et al., 2018). Cefazolin protein binding was shown to be low (53%–55%) during CBP (Hollis et al., 2015; Asada et al., 2018). One study compared the binding during CBP with the binding preoperative in the same group of patients and found different values of 55% during CBP and 79% pre-CBP (Asada et al., 2018). For cefuroxime the data were not consistent. Mand’ák et al. (2007) showed a low protein binding of 11%–17% during the procedure as compared to 27% before the onset of the study, while another study found no difference in protein binding due to CBP (Aalbers et al., 2015).
Patients with viral, bacterial or fungal infections might also have hypoalbuminemia (Wiedermann, 2021). Studies on protein binding on cephalosporins in patients with infections without other causes of hypoalbuminemia were not frequently performed. For cefazolin two studies were performed in infectious patients. Protein binding in patients treated with cefazolin for an infection was 81.3% for through-concentrations and 68.7% for the peak-concentrations (Vella-Brincat et al., 2007). This was not different from a study in obese and non-obese patients reporting a median protein binding of 79% (Van Kralingen et al., 2011). Also, in patients with an acute exacerbation of chronic bronchitis there was not a relevant reduction in protein binding as compared to volunteers for both cefodizime and ceftriaxone (Scaglione et al., 1997a; Herrera-Hidalgo et al., 2020). In patients with hepatitis, cefazolin protein binding was 84.7% versus 88.6% in controls (Ohashi et al., 1986). These studies indicate that a profound reduction in protein binding caused by infection itself has not been proven yet.
Many physiological changes take place during development from newborn to adult. Therefore, differences in protein binding as compared to adults might be expected. Unfortunately, limited data are available for newborns and children. From the available data it becomes clear that protein binding might be reduced in children, especially newborns (Deguchi et al., 1988; Kan et al., 2020). A study in children from 2 months to 4 years of age treated with ceftriaxone for meningitis (Hoshino et al., 2010) can be compared to data in adults with suspected in meningitis (Grégoire et al., 2019). In children the protein binding was 81.8% ± 8.4% and in adults the median value was higher (92.4%), however the range in adults was larger (51%–98%). The cefazolin protein binding in neonates in Intensive Care Unit (ICU) was found to be lower (49%) (Deguchi et al., 1988), while values for adults in the ICU were around 60%–77% (Roberts et al., 2015). A study on cefoperazone compared three age groups: newborns (30.1–42.3 weeks), infants (0.1–2.0 years) and children (2.2–9.0 years) (Kan et al., 2020). They found the lowest protein binding in newborns (74.5% ± 9.1%), and higher protein binding for infants (82.2% ± 7.1%) and even higher values for children (87.5% ± 3.2%) (Figure 3). Differences were also correlated to the levels of albumin.
One study determined the protein binding in cord blood. Lower protein binding in cord serum than in maternal serum was seen for ceftizoxime (21.9% vs. 57.8%). As arterial cord blood originates from the fetus, the lower binding of combined arterial/venous cord blood is in line with the lower protein binding found in newborns and the lower protein binding as described in pregnant women. It could also be due to difference in protein milieu or high level of bilirubin in cord serum competing for binding sites and an increase in available albumin in maternal serum (Fortunato et al., 1993).
The influence of several other factors has been studied as well, but the influence on protein binding was not present or not relevant. For cefazolin several studies investigated whether there was a differences between (morbidly) obese and non—obese patients (Van Kralingen et al., 2011; Hites et al., 2016; Palma et al., 2018; Dorn et al., 2019). It was concluded that obesity did not influence protein binding. The influence of various degrees of renal impairment (with the exception of dialysis) did not influence protein binding (Craig et al., 1973; Brodwall et al., 1977). Small differences are possibly related to differences in protein levels rather than renal function (Garcia et al., 1979b). For cefazolin and cefalothin has been shown that hepatitis did not have an effect on protein binding (Ohashi et al., 1986). Patients undergoing elective surgery are also studied and found to be relatively similar to healthy volunteers (Scaglione et al., 1997b; Douglas et al., 2011). Age, as the only factor, will likely not influence protein binding (Faulkner et al., 1988), however small differences might be present and they might be caused by a lower protein level in the elderly (Luderer et al., 1984).
The protein binding in pericardial fluid was determined for cefazolin, cefradine, cefalothin, and cefapirin. The protein binding of cefazolin in pericardial fluid in patients undergoing heart surgery was slightly equal to binding in serum of healthy volunteers (83.8% and 83.9%/88.6% respectively) (Craig et al., 1973; Nightingale et al., 1980; Ohashi et al., 1986). For cefradine, the protein binding in pericardial fluid in patients undergoing heart surgery was <10%, which was lower than that of serum seen in a patient with continuous ambulatory peritoneal dialysis for end-stage renal failure with and were therefore expected to be lower than in healthy volunteers (Nightingale et al., 1980; Martea et al., 1987). The protein binding of cefalothin in pericardial fluid of pediatric patients (65.2%) was lower than that in serum of healthy adult volunteers (83.9%/88.6%) (Craig et al., 1973; Green et al., 1981; Ohashi et al., 1986). The protein binding of cefapirin in pericardial fluid of pediatric patients was 36.7% (Green et al., 1981). This could not be compared to the protein binding of blood since we could not include a study which determined the protein binding in blood.
Whether the protein binding in blister fluid is lower, equal to or higher than in blood differs per cephasporin. For cefoxitin, cefuroxime and cefodizime the protein binding in blister fluid was determined.
The protein binding of cefoxitin in blister fluid of healthy volunteers might be slightly higher than that in serum (59% vs. 52%) (Wise et al., 1980; Carver et al., 1989). However, the study of Garcia et al. (1979b) showed a protein binding of 73.22% in plasma which was much higher than that of Carver et al. (1989) (52%). The protein binding of cefuroxime in blister fluid was 34% (Wise et al., 1980) and this is equal to that in serum of healthy males (33%) (Foord, 1976), but higher compared to another study with healthy volunteers (17.2%) (Verhagen et al., 1994). The protein binding of cefodizime in skin blister fluid was significantly lower than in serum of the same healthy subjects (61.6% vs. 81%) (Schafer-Korting et al., 1986).
The protein binding of cefodizime in bronchial secretion (68.1%–73.1%) was lower than that in serum (81.1%–84%) of the same subjects. This was also the case for ceftriaxone 78.4%–80.8% (bronchial secretion) vs. 92.5%–94% (serum) (Scaglione et al., 1997a). One study determined the protein binding of cefotaxime and ceftriaxone in pleural exudate. The protein binding was lower than in serum of the same subjects (Scaglione et al., 1990). For cefotaxime the difference was smaller (7.64% vs. 9.93%) than for ceftriaxone (60.56% vs. 70.17%). However, the protein binding of cefotaxime in serum and plasma differed in various populations (Scaglione et al., 1990; Seguin et al., 2009; Dahyot-Fizelier et al., 2013; Aardema et al., 2020).
Protein binding in wound exudate was lower than in serum. The protein binding of cefazolin in wound exudate in burn and trauma patients was significantly lower than that in serum of healthy volunteers (44.6% vs. 83.9%/88.6%) (Craig et al., 1973; Ohashi et al., 1986; Rowan et al., 2017). There was also a potential lower protein binding for cefonicid seen in wound drainage of oncologic patients undergoing head and neck surgery compared to their protein binding in serum (85% vs. 89%) (Swanson et al., 1991). However, protein binding in serum differs from other studies which determined protein binding in healthy volunteers (Dudley et al., 1986; Ackerman et al., 1988; Trang et al., 1989). This could be due to the advanced age, nutritional status, and illness of the oncologic patients (Swanson et al., 1991).
The protein binding of ceftriaxone in cerebrospinal fluid (18.8% ± 6.21%) was significantly lower than in serum/plasma (ranging from 32.3% to 95%) (Toth et al., 1991; Hoshino et al., 2010; Ebisch et al., 2020).
The protein binding of cefradine in dialysate from peritoneal dialysis was significantly lower than that in plasma of a patient with continuous ambulatory peritoneal dialysis for end-stage renal failure with peritonitis (6.1% vs. 29.1%) (Martea et al., 1987).
The protein binding of cefotaxime in peritoneal fluid was lower (12.9%) than that in plasma in critically ill patients with secondary peritonitis (17.4%) (Seguin et al., 2009). However, this protein binding in serum is lower than other studies (Dahyot-Fizelier et al., 2013; Aardema et al., 2020).
In this systematic review, data on protein binding of cephalosporins in human fluids were reviewed. Most studies analyzed protein binding in serum or plasma and only a few in other body fluids, such as wound exudate or pericardial fluid.
For serum and plasma, the degree of protein binding was variable. As expected, there was a large variability between the different cephalosporins. Even for a specific cephalosporin, the protein binding differs between different categories of patients and even within the categories there was a considerable amount of variability. For ceftriaxone, for example, a group of eight patients on hemodialysis displayed a mean protein binding of 43% (Matzke et al., 2000b), which was considerably lower compared to healthy volunteers (mean/median 90%–95%) (Stoeckel et al., 1984; Neves et al., 2018; Herrera-Hidalgo et al., 2020). However, even within the group of dialysis patients the protein binding ranged approximately from 13% to 73% (Matzke et al., 2000b). This shows that based on the mean or median of the groups it is possible to identify categories of patients with expected lower protein binding, but on individual level the percentage is still unpredictable.
Several patient characteristics were identified with overall lower protein binding compared to healthy volunteers. This is mainly caused by a concomitant hypoalbuminemia. These characteristics include critically ill patients, patients on (all types) of dialysis and during a CPB (Garcia et al., 1979a; Mand'ák et al., 2007; Parker et al., 2016; Bos et al., 2018; Aardema et al., 2020; Ebisch et al., 2020). Although studies in children are scarce, available data suggests that protein binding might be lower in children compared to adults, especially in newborns (Kan et al., 2020). Comparison between the different categories of patients is sometimes complicated by the fact that multiple factors are present at the same time, such as a critically ill patients on dialysis in a malnutrition state. These findings are in line with current reports in the literature (Roberts et al., 2013).
Studies on protein binding in other body fluids are scarce. In most studies values in fluids other than blood were lower compared to those in serum or plasma. However, given the low quantity of data and the high variability in serum and plasma, no conclusions can be drawn about other body fluids than serum of plasma.
A limitation of the review is that the literature search had to be performed in several steps. The original search was quite broad including all beta-lactams to make sure we did not miss one of the cephalosporins. However, after the systematic search there were missing articles. Protein binding is often reported in studies while it is not the purpose of the specific studies. This is most likely the reason why some articles were missing in the original systematic search. We corrected this manually by searching for studies reporting unbound concentrations of the cephalosporins found in the systematic search as well as checking the references lists of the included articles. By using this method we were able to add 26 articles to the selection.
Except from differences between patients or patient groups, also other factors might contribute to the variability in protein binding as found in our review. One of these factors is the non-linear protein binding, as found for f.e. ceftriaxone. Despite the different percentages of protein binding dependent on the concentration, many studies only report a single percentage as mean with variability. Part of the variability might therefore be explained by the total concentration and thereby also sampling time in relation to the dose. Another factor might be the method used to determine the protein binding. Most of the studies used equilibrium dialysis (ED) or ultrafiltration (UF) as method for the separation of free drug from the protein bound. ED is accepted as the “golden standard” to determine the free fraction of a drug. This method is based on the diffusion of free drug through the a permeable membrane until there is an equilibrium between both compartments. The protein bound fraction cannot pass this membrane. ED is often performed at 37°C to simulate in vivo conditions. ED is a time consuming procedure because of the long equilibrium time (3–48 h for conventional equilibrium dialysis, 2–6 h for rapid ED). UF is much quicker. It separates bound and unbound drug from each other through a filter membrane with centrifugal force. Disadvantages are that there is non-specific binding to this membrane and temperature cannot be controlled (de Boer and Meijering, 2019). The non-specific binding makes it extra important that validation was done prior to the analysis.
In this review only studies measuring protein binding after administering the antibiotic to humans were included. So, studies using human samples to determine protein binding after adding antibiotics in the laboratory were excluded. As a result only clinically achievable concentration ranges were included and artificial influences, such as temperature and pH (Hinderling and Hartmann, 2005), were excluded. Interindividual differences can also be determined when using samples instead of adding the drug to a body fluid (Wright et al., 1996). Kratzer et al. (2019) found differences in the protein binding of ceftolozane and tazobactam between in vitro and ex vivo plasma samples. Also in plasma of dogs differences were seen in in vitro and ex vivo samples (Stegemann et al., 2006). However, for voriconazole there were no differences in the protein binding between in vitro experiments and samples from patients (Vanstraelen et al., 2014).
A more in-depth evaluation of the data also showed great differences in protein binding, as becomes clear from the following examples. The protein binding of ceftazidime was low with a range of 0%–31% (Van Dalen et al., 1986), while the protein binding of cefpiramide and cefodizim was higher (77%–99.3% and 81%–85.45% respectively) (Schafer-Korting et al., 1986; Conte, 1987; Demotes-Mainard et al., 1994; Scaglione et al., 1997b). When also taking into account different patient populations even more variability was described, such as within critically ill patients. The range in protein binding of ceftriaxone in these patients varied between 26.3% and 95% (Wong et al., 2018; Ebisch et al., 2020). Cefuroxime also showed a great variation in protein binding in critically ill patients (0.25%–72.64%) (van Raaij et al., 2020). As mentioned earlier, patients on hemodialysis showed lower protein binding (8%) for ceftriaxone compared to healthy volunteers (protein binding 90%–95%) (Stoeckel et al., 1984; Matzke et al., 2000b; Neves et al., 2018; Herrera-Hidalgo et al., 2020). This was also seen for cefepime in patients on continuous venovenous hemofiltration and continuous venovenous hemodialysis. Cefepime was 21% bound to proteins in patients the renal replacement therapies, which was lower compared to other patients where cefepime showed a median protein binding of 39% (Isla et al., 2005; Al-Shaer et al., 2020).
The percentage of protein binding is important in clinical practice, since the effectivity of an antibiotic is correlated to the unbound concentration-time profile. The protein binding in individual patients is hardy predictable, especially in patients with multiple characteristics known to influence protein binding. Several characteristics seems to result in a lower protein binding, but the complex interaction between a reduced protein binding and increased drug clearance results in unpredictable concentration-time profiles. The increased clearance at the beginning of the dosing interval might lead to too low unbound concentrations at through levels. To avoid this, it might be beneficial to extent the duration of infusion on cephalosporins with typical non-linear protein binding. An example hereof is cefazolin used as perioperative prophylaxis. Especially during surgery the concentrations needs to remain adequate over the entire dosing interval. Continuous infusion might therefore be beneficial to prevent subtherapeutic concentrations at the end of the dosing interval.
Therapeutic drug monitoring (TDM) is increasingly used, also for the cephalosporins, to guide individual dosing regimen to optimize the exposure. However, most TDM is currently based on total drug concentrations, that are subsequently corrected for the protein binding using a fixed percentage. Considering the fact that most clinical patients have lower protein binding compared to healthy volunteers, the use of a fixed percentage based on studies in healthy volunteers, will result in an increased exposure based on unbound concentrations in severely ill patients as compared to healthy volunteers. Based on the overview in this review it became clear that the variability between patients and for non-linear drugs also with different concentrations, leads to the recommendation to determine unbound concentrations to be used in TDM for individual patients.
A larger variability was found in the protein binding in serum or plasma between different categories of patients as well as within a category of patients, than was usually account for in clinical practice. The differences described in in protein binding in serum or plasma might be of clinical relevance, since only the free fraction is able to enter the extravascular space where most infections are and bound antibiotic is inactive (Craig and Kunin, 1976; Merrikin et al., 1983; Beer et al., 2009). Several characteristics were identified, such as critically ill patients and patients on dialysis, which are prone to having a low protein binding. The typical non-linear pattern of protein binding as found for several cephalosporins even complicates the possibility to predict the unbound concentration-time profile in individual patients even more. Taken all these factors, it is recommended to measure unbound concentrations to perform TDM to optimize antibiotic exposure in individual patients.
Conclusions on protein binding in different body fluids other than blood cannot be drawn due to the paucity of data. More research is needed to determine the relationship between protein binding in blood and protein binding in other body fluids.
CJ, AM, and BK: conception and design. CJ, BK, and AM: analysis, interpretation of the data, and wrote the first draft of the manuscript. PB, BC, and CJ: abstract screening. All authors contributed to subsequent drafts and gave final approval of the version to be published.
The authors declare that the research was conducted in the absence of any commercial or financial relationships that could be construed as a potential conflict of interest.
All claims expressed in this article are solely those of the authors and do not necessarily represent those of their affiliated organizations, or those of the publisher, the editors and the reviewers. Any product that may be evaluated in this article, or claim that may be made by its manufacturer, is not guaranteed or endorsed by the publisher.
The authors wish to thank W. Bramer from the Erasmus MC Medical Library for the search strategy.
The Supplementary Material for this article can be found online at: https://www.frontiersin.org/articles/10.3389/fphar.2022.900551/full#supplementary-material
Aalbers, M., ter Horst, P. G., Hospes, W., Hijmering, M. L., and Spanjersberg, A. J. (2015). Targeting Cefuroxime Plasma Concentrations during Coronary Artery Bypass Graft Surgery with Cardiopulmonary Bypass. Int. J. Clin. Pharm. 37 (4), 592–598. doi:10.1007/s11096-015-0101-8
Aardema, H., Bult, W., van Hateren, K., Dieperink, W., Touw, D. J., Alffenaar, J. C., et al. (2020). Continuous versus Intermittent Infusion of Cefotaxime in Critically Ill Patients: a Randomized Controlled Trial Comparing Plasma Concentrations. J. Antimicrob. Chemother. 75 (2), 441–448. doi:10.1093/jac/dkz463
Ackerman, B. H., Trang, J. M., Nelson, C. L., Pearce, C. E., Manning, J. T., and Harrison, B. H. (1988). Disposition of Cefonicid in Orthopedic Surgery Patients. Drug Intell. Clin. Pharm. 22 (11), 864–868. doi:10.1177/106002808802201104
Allegaert, K., Van Mieghem, T., Verbesselt, R., Vanhole, C., Devlieger, R., Cossey, V., et al. (2009). Cefazolin Plasma Protein Binding Saturability during Pregnancy. Methods Find. Exp. Clin. Pharmacol. 31 (1), 25–28. doi:10.1358/mf.2009.31.1.1338413
Al-Shaer, M. H., Alghamdi, W. A., Graham, E., and Peloquin, C. A. (2020). Meropenem, Cefepime, and Piperacillin Protein Binding in Patient Samples. Ther. Drug Monit. 42 (1), 129–132. doi:10.1097/FTD.0000000000000675
Asada, M., Nagata, M., Mizuno, T., Uchida, T., Kurashima, N., Takahashi, H., et al. (2018). Effects of Cardiopulmonary Bypass on the Disposition of Cefazolin in Patients Undergoing Cardiothoracic Surgery. Pharmacol. Res. Perspect. 6 (6), e00440. doi:10.1002/prp2.440
Barbour, A., Schmidt, S., Sabarinath, S. N., Grant, M., Seubert, C., Skee, D., et al. (2009). Soft-Tissue Penetration of Ceftobiprole in Healthy Volunteers Determined by In Vivo Microdialysis. Antimicrob. Agents Chemother. 53 (7), 2773–2776. doi:10.1128/AAC.01409-08
Beer, J., Wagner, C. C., and Zeitlinger, M. (2009). Protein Binding of Antimicrobials: Methods for Quantification and for Investigation of its Impact on Bacterial Killing. Aaps J. 11 (1), 1–12. doi:10.1208/s12248-008-9072-1
Bergogne-Bérézin, E. (2002). Clinical Role of Protein Binding of Quinolones. Clin. Pharmacokinet. 41 (10), 741–750.doi:10.2165/00003088-200241100-00004
Berkhout, J., Visser, L. G., van den Broek, P. J., van de Klundert, J. A., and Mattie, H. (2003). Clinical Pharmacokinetics of Cefamandole and Ceftazidime Administered by Continuous Intravenous Infusion. Antimicrob. Agents Chemother. 47 (6), 1862–1866. doi:10.1128/aac.47.6.1862-1866.2003
Booke, H., Frey, O. R., Röhr, A. C., Chiriac, U., Zacharowski, K., Holubec, T., et al. (2021). Excessive Unbound Cefazolin Concentrations in Critically Ill Patients Receiving Veno-Arterial Extracorporeal Membrane Oxygenation (vaECMO): an Observational Study. Sci. Rep. 11 (1), 16981. doi:10.1038/s41598-021-96654-4
Bos, J. C., Prins, J. M., Mistício, M. C., Nunguiane, G., Lang, C. N., Beirão, J. C., et al. (2018). Pharmacokinetics and Pharmacodynamic Target Attainment of Ceftriaxone in Adult Severely Ill Sub-saharan African Patients: A Population Pharmacokinetic Modelling Study. J. Antimicrob. Chemother. 73 (6), 1620–1629. doi:10.1093/jac/dky071
Bourget, P., Fernandez, H., Quinquis, V., and Delouis, C. (1993). Pharmacokinetics and Protein Binding of Ceftriaxone during Pregnancy. Antimicrob. Agents Chemother. 37 (1), 54–59. doi:10.1128/aac.37.1.54
Bramer, W. M., Milic, J., and Mast, F. (2017). Reviewing Retrieved References for Inclusion in Systematic Reviews Using EndNote. J. Med. Libr. Assoc. 105 (1), 84–87. doi:10.5195/jmla.2017.111
Briscoe, S. E., McWhinney, B. C., Lipman, J., Roberts, J. A., and Ungerer, J. P. (2012). A Method for Determining the Free (Unbound) Concentration of Ten Beta-Lactam Antibiotics in Human Plasma Using High Performance Liquid Chromatography with Ultraviolet Detection. J. Chromatogr. B Anal. Technol. Biomed. Life Sci. 907, 178–184. doi:10.1016/j.jchromb.2012.09.016
Brodwall, E. K., Bergan, T., and Orjavik, O. (1977). Kidney Transport of Cefazolin in Normal and Impaired Renal Function. J. Antimicrob. Chemother. 3 (6), 585–592. doi:10.1093/jac/3.6.585
Bulik, C. C., Wiskirchen, D. E., Shepard, A., Sutherland, C. A., Kuti, J. L., and Nicolau, D. P. (2010). Tissue Penetration and Pharmacokinetics of Tigecycline in Diabetic Patients with Chronic Wound Infections Described by Using In Vivo Microdialysis. Antimicrob. Agents Chemother. 54 (12), 5209–5213. doi:10.1128/AAC.01051-10
Carver, P. L., Nightingale, C. H., and Quintiliani, R. (1989). Pharmacokinetics and Pharmacodynamics of Total and Unbound Cefoxitin and Cefotetan in Healthy Volunteers. J. Antimicrob. Chemother. 23 (1), 99–106. doi:10.1093/jac/23.1.99
Conte, J. E. (1987). Pharmacokinetics of Cefpiramide in Volunteers with Normal or Impaired Renal Function. Antimicrob. Agents Chemother. 31 (10), 1585–1588. doi:10.1128/aac.31.10.1585
Craig, W. A., and Kunin, C. M. (1976). Significance of Serum Protein and Tissue Binding of Antimicrobial Agents. Annu. Rev. Med. 27, 287–300. doi:10.1146/annurev.me.27.020176.001443
Craig, W. A., Welling, P. G., Jackson, T. C., and Kunin, C. M. (1973). Pharmacology of Cefazolin and Other Cephalosporins in Patients with Renal Insufficiency. J. Infect. Dis. 128, S347–5. doi:10.1093/infdis/128.supplement_2.s347
Dahyot-Fizelier, C., Frasca, D., Grégoire, N., Adier, C., Mimoz, O., Debaene, B., et al. (2013). Microdialysis Study of Cefotaxime Cerebral Distribution in Patients with Acute Brain Injury. Antimicrob. Agents Chemother. 57 (6), 2738–2742. doi:10.1128/AAC.02570-12
de Boer, T., and Meijering, H. (2019). “Equilibrium Dialysis, Ultracentrifugation, and Ultrafiltration in LC‐MS Bioanalysis,” in Sample Preparation in LC‐MS Bioanalysis, 45–51. doi:10.1002/9781119274315.ch3
de Cock, P. A., Mulla, H., Desmet, S., De Somer, F., McWhinney, B. C., Ungerer, J. P., et al. (2017). Population Pharmacokinetics of Cefazolin before, during and after Cardiopulmonary Bypass to Optimize Dosing Regimens for Children Undergoing Cardiac Surgery. J. Antimicrob. Chemother. 72 (3), 791–800. doi:10.1093/jac/dkw496
Deguchi, Y., Koshida, R., Nakashima, E., Watanabe, R., Taniguchi, N., Ichimura, F., et al. (1988). Interindividual Changes in Volume of Distribution of Cefazolin in Newborn Infants and its Prediction Based on Physiological Pharmacokinetic Concepts. J. Pharm. Sci. 77 (8), 674–678. doi:10.1002/jps.2600770807
Deitchman, A. N., Singh, R. S. P., and Derendorf, H. (2018). Nonlinear Protein Binding: Not what You Think. J. Pharm. Sci. 107 (7), 1754–1760. doi:10.1016/j.xphs.2018.03.023
Demotes-Mainard, F., Vinçon, G., Amouretti, M., Dumas, F., Necciari, J., Kieffer, G., et al. (1991). Pharmacokinetics and Protein Binding of Cefpiramide in Patients with Alcoholic Cirrhosis. Clin. Pharmacol. Ther. 49 (3), 263–269. doi:10.1038/clpt.1991.27
Demotes-Mainard, F., Vinçon, G., Labat, L., Amouretti, M., Necciari, J., Kieffer, G., et al. (1994). Cefpiramide Kinetics and Plasma Protein Binding in Cholestasis. Br. J. Clin. Pharmacol. 37 (3), 295–297. doi:10.1111/j.1365-2125.1994.tb04278.x
Dhanani, J. A., Lipman, J., Pincus, J., Townsend, S., Livermore, A., Wallis, S. C., et al. (2019). Pharmacokinetics of Total and Unbound Cefazolin during Veno-Arterial Extracorporeal Membrane Oxygenation: A Case Report. Chemotherapy 64 (3), 115–118. doi:10.1159/000502474
DiPiro, J. T., Bayoumi, S. M., Vallner, J. J., Nesbit, R. R., Gokhale, R., and Rissing, J. P. (1985). Intraoperative Ceforanide Pharmacokinetics and Protein Binding. Antimicrob. Agents Chemother. 27 (4), 487–490. doi:10.1128/aac.27.4.487
Dorn, C., Kratzer, A., Liebchen, U., Schleibinger, M., Murschhauser, A., Schlossmann, J., et al. (2018). Impact of Experimental Variables on the Protein Binding of Tigecycline in Human Plasma as Determined by Ultrafiltration. J. Pharm. Sci. 107 (2), 739–744. doi:10.1016/j.xphs.2017.09.006
Dorn, C., Kratzer, A., Schiesser, S., Kees, F., Wrigge, H., and Simon, P. (2019). Determination of Total or Free Cefazolin and Metronidazole in Human Plasma or Interstitial Fluid by HPLC-UV for Pharmacokinetic Studies in Man. J. Chromatogr. B Anal. Technol. Biomed. Life Sci. 1118-1119, 51–54. doi:10.1016/j.jchromb.2019.04.025
Douglas, A., Udy, A. A., Wallis, S. C., Jarrett, P., Stuart, J., Lassig-Smith, M., et al. (2011). Plasma and Tissue Pharmacokinetics of Cefazolin in Patients Undergoing Elective and Semielective Abdominal Aortic Aneurysm Open Repair Surgery. Antimicrob. Agents Chemother. 55 (11), 5238–5242. doi:10.1128/AAC.05033-11
Dudley, M. N., Shyu, W. C., Nightingale, C. H., and Quintiliani, R. (1986). Effect of Saturable Serum Protein Binding on the Pharmacokinetics of Unbound Cefonicid in Humans. Antimicrob. Agents Chemother. 30 (4), 565–569. doi:10.1128/aac.30.4.565
Ebisch, R. M. F., Meenks, S. D., Foudraine, N., Janssen, P. K. C., and le Noble, J. L. M. L. (2020). Ceftriaxone Dosing in a Critically Ill Patient with Hypoalbuminemia during Continuous Venous Hemofiltration: Emphasis on Unbound Pharmacokinetics. J. Clin. Pharmacol. 60 (1), 140–142. doi:10.1002/jcph.1503
Faulkner, R. D., Bohaychuk, W., Lanc, R. A., Haynes, J. D., Desjardins, R. E., Yacobi, A., et al. (1988). Pharmacokinetics of Cefixime in the Young and Elderly. J. Antimicrob. Chemother. 21 (6), 787–794. doi:10.1093/jac/21.6.787
Foord, R. D. (1976). Cefuroxime: Human Pharmacokinetics. Antimicrob. Agents Chemother. 9 (5), 741–747. doi:10.1128/aac.9.5.741
Fortunato, S. J., Welt, S. I., and Stewart, J. T. (1993). Differential Protein Binding of Ceftizoxime in Cord versus Maternal Serum. Am. J. Obstet. Gynecol. 168 (3 I), 914–915. doi:10.1016/s0002-9378(12)90844-3
Fukumoto, K., Aida, S., Oishi, T., and Ueno, K. (2009). Pharmacokinetics of Ceftriaxione, a Third-Generation Cephalosporin, in Pediatric Patients. Biol. Pharm. Bull. 32 (7), 1139–1141. doi:10.1248/bpb.32.1139
Garcia, M. J., Dominguez-Gil, A., Tabernero, J. M., and Bondia Román, A. (1979a). Pharmacokinetics of Cefoxitin in Patients Undergoing Hemodialysis. Int. J. Clin. Pharmacol. Biopharm. 17 (8), 366–370.
Garcia, M. J., Dominguez-Gil, A., Tabernero, J. M., and Sanchez Tomero, J. A. (1979b). Pharmacokinetics of Cefoxitin in Patients with Normal or Impaired Renal Function. Eur. J. Clin. Pharmacol. 16 (2), 119–124. doi:10.1007/bf00563118
Gijsen, M., Dreesen, E., Van Daele, R., Annaert, P., Debaveye, Y., Wauters, J., et al. (2021). Pharmacokinetic/Pharmacodynamic Target Attainment Based on Measured versus Predicted Unbound Ceftriaxone Concentrations in Critically Ill Patients with Pneumonia: An Observational Cohort Study. Antibiot. (Basel) 10 (5), 557. doi:10.3390/antibiotics10050557
Grégoire, M., Dailly, E., Le Turnier, P., Garot, D., Guimard, T., Bernard, L., et al. (2019). High-Dose Ceftriaxone for Bacterial Meningitis and Optimization of Administration Scheme Based on Nomogram. Antimicrob. Agents Chemother. 63 (9), e00634–00619. doi:10.1128/AAC.00634-19
Green, E. R., Subramanian, S., Faden, H., Quintiliani, R., and Nightingale, C. H. (1981). A Comparison of the Penetration Characteristics of Cephapirin and Cephalothin into Right Atrial Appendage, Muscle, Fat, and Pericardial Fluid of Pediatric Patients Undergoing Open-Heart Operation. Ann. Thorac. Surg. 31 (2), 155–160. doi:10.1016/s0003-4975(10)61535-4
Hartman, S. J. F., Upadhyay, P. J., Hagedoorn, N. N., Mathôt, R. A. A., Moll, H. A., van der Flier, M., et al. (2021). Current Ceftriaxone Dose Recommendations Are Adequate for Most Critically Ill Children: Results of a Population Pharmacokinetic Modeling and Simulation Study. Clin. Pharmacokinet. 60 (10), 1361–1372. doi:10.1007/s40262-021-01035-9
Heinemeyer, G., Link, J., Weber, W., Meschede, V., and Roots, I. (1990). Clearance of Ceftriaxone in Critical Care Patients with Acute Renal Failure. Intensive Care Med. 16 (7), 448–453. doi:10.1007/bf01711224
Herrera-Hidalgo, L., de Alarcón, A., López-Cortes, L. E., Luque-Márquez, R., López-Cortes, L. F., Gutiérrez-Valencia, A., et al. (2020). Is Once-Daily High-Dose Ceftriaxone Plus Ampicillin an Alternative for Enterococcus faecalis Infective Endocarditis in Outpatient Parenteral Antibiotic Therapy Programs? Antimicrob. Agents Chemother. 65 (1), e02099–02020. doi:10.1128/AAC.02099-20
Himebauch, A. S., Nicolson, S. C., Sisko, M., Moorthy, G., Fuller, S., Gaynor, J. W., et al. (2014). Skeletal Muscle and Plasma Concentrations of Cefazolin during Cardiac Surgery in Infants. J. Thorac. Cardiovasc Surg. 148 (6), 2634–2641. doi:10.1016/j.jtcvs.2014.06.064
Hinderling, P. H., and Hartmann, D. (2005). The pH Dependency of the Binding of Drugs to Plasma Proteins in Man. Ther. Drug Monit. 27 (1), 71–85. doi:10.1097/00007691-200502000-00014
Hites, M., Deprez, G., Wolff, F., Ickx, B., Verleije, A., Closset, J., et al. (2016). Evaluation of Total Body Weight and Body Mass Index Cut-Offs for Increased Cefazolin Dose for Surgical Prophylaxis. Int. J. Antimicrob. Agents 48 (6), 633–640. doi:10.1016/j.ijantimicag.2016.08.019
Hollis, A. L., Heil, E. L., Nicolau, D. P., Odonkor, P., Dowling, T. C., and Thom, K. A. (2015). Validation of a Dosing Strategy for Cefazolin for Surgery Requiring Cardiopulmonary Bypass. Surg. Infect. (Larchmt) 16 (6), 829–832. doi:10.1089/sur.2014.250
Hoshino, T., Ishiwada, N., and Kohno, Y. (2010). Free Concentration and Protein-Binding Ratio of Ceftriaxone in Cerebrospinal Fluid in Paediatric Patients with Purulent Meningitis Caused by Haemophilus Influenzae Type B. Int. J. Antimicrob. Agents 35 (5), 512–513. doi:10.1016/j.ijantimicag.2010.01.001
Isla, A., Gascón, A. R., Maynar, J., Arzuaga, A., Toral, D., and Pedraz, J. L. (2005). Cefepime and Continuous Renal Replacement Therapy (CRRT): In Vitro Permeability of Two CRRT Membranes and Pharmacokinetics in Four Critically Ill Patients. Clin. Ther. 27 (5), 599–608. doi:10.1016/j.clinthera.2005.05.004
Isla, A., Gascón, A. R., Maynar, J., Arzuaga, A., Sánchez-Izquierdo, J. A., and Pedraz, J. L. (2007). In Vitro AN69 and Polysulphone Membrane Permeability to Ceftazidime and In Vivo Pharmacokinetics during Continuous Renal Replacement Therapies. Chemotherapy 53 (3), 194–201. doi:10.1159/000100864
Jager, N. G. L., van Hest, R. M., Xie, J., Wong, G., Ulldemolins, M., Brüggemann, R. J. M., et al. (2020). Optimization of Flucloxacillin Dosing Regimens in Critically Ill Patients Using Population Pharmacokinetic Modelling of Total and Unbound Concentrations. J. Antimicrob. Chemother. 75 (9), 2641–2649. doi:10.1093/jac/dkaa187
Kalman, D., Barriere, S. L., and Johnson, B. L. (1992). Pharmacokinetic Disposition and Bactericidal Activities of Cefepime, Ceftazidime, and Cefoperazone in Serum and Blister Fluid. Antimicrob. Agents Chemother. 36 (2), 453–457. doi:10.1128/aac.36.2.453
Kan, M., Wu, Y. E., Li, X., Dong, Y. N., Du, B., Guo, Z. X., et al. (2020). An Adapted LC-MS/MS Method for the Determination of Free Plasma Concentration of Cefoperazone in Children: Age-dependent Protein Binding. J. Chromatogr. B Anal. Technol. Biomed. Life Sci. 1144, 122081. doi:10.1016/j.jchromb.2020.122081
Kan, M., Shi, H. Y., Han, B., Wu, Y. E., Li, Q., Guo, Z. X., et al. (2021). Prediction of Unbound Ceftriaxone Concentration in Children: Simple Bioanalysis Method and Basic Mathematical Equation. Antimicrob. Agents Chemother. 65 (1), e00779–00720.
Kochansky, C. J., McMasters, D. R., Lu, P., Koeplinger, K. A., Kerr, H. H., Shou, M., et al. (2008). Impact of pH on Plasma Protein Binding in Equilibrium Dialysis. Mol. Pharm. 5 (3), 438–448. doi:10.1021/mp800004s
Koshida, R., Nakashima, E., Ichimura, F., Nakano, O., Watanabe, R., Taniguchi, N., et al. (1987). Comparative Distribution Kinetics of Cefazolin and Tobramycin in Children. J. Pharmacobiodyn 10 (9), 436–442. doi:10.1248/bpb1978.10.436
Kratzer, A., Schießer, S., Matzneller, P., Wulkersdorfer, B., Zeitlinger, M., Schlossmann, J., et al. (2019). Determination of Total and Free Ceftolozane and Tazobactam in Human Plasma and Interstitial Fluid by HPLC-UV. J. Pharm. Biomed. Anal. 163, 34–38. doi:10.1016/j.jpba.2018.09.044
Lam, Y. W., Duroux, M. H., Gambertoglio, J. G., Barriere, S. L., and Guglielmo, B. J. (1988). Effect of Protein Binding on Serum Bactericidal Activities of Ceftazidime and Cefoperazone in Healthy Volunteers. Antimicrob. Agents Chemother. 32 (3), 298–302. doi:10.1128/aac.32.3.298
Lantinga, M. A., Wijnands, T. F., Te Morsche, R. H., de Sévaux, R. G., Kuipers, S., Allegaert, K., et al. (2016). Hepatic Cyst Penetration of Cefazolin in Patients Receiving Aspiration Sclerotherapy. J. Antimicrob. Chemother. 71 (9), 2547–2552. doi:10.1093/jac/dkw172
Luderer, J. R., Patel, I. H., Durkin, J., and Schneck, D. W. (1984). Age and Ceftriaxone Kinetics. Clin. Pharmacol. Ther. 35 (1), 19–25. doi:10.1038/clpt.1984.3
Mand'ák, J., Pojar, M., Maláková, J., Lonsky, V., Palička, V., and Zivný, P. (2007). Tissue and Plasma Concentrations of Cephuroxime during Cardiac Surgery in Cardiopulmonary Bypass - a Microdialysis Study. Perfusion 22 (2), 129–136. doi:10.1177/0267659107080116
Martea, M., Hekster, Y. A., Vree, T. B., Voets, A. J., and Berden, J. H. (1987). Pharmacokinetics of Cefradine, Sulfamethoxazole and Trimethoprim and Their Metabolites in a Patient with Peritonitis Undergoing Continuous Ambulatory Peritoneal Dialysis. Pharm. Weekbl. Sci. 9 (2), 110–116. doi:10.1007/BF01960745
Matzke, G. R., Frye, R. F., Joy, M. S., and Palevsky, P. M. (2000a). Determinants of Ceftazidime Clearance by Continuous Venovenous Hemofiltration and Continuous Venovenous Hemodialysis. Antimicrob. Agents Chemother. 44 (6), 1639–1644. doi:10.1128/aac.44.6.1639-1644.2000
Matzke, G. R., Frye, R. F., Joy, M. S., and Palevsky, P. M. (2000b). Determinants of Ceftriaxone Clearance by Continuous Venovenous Hemofiltration and Hemodialysis. Pharmacotherapy 20, 635–643. doi:10.1592/phco.20.7.635.35170
Meenks, S., le Noble, J., Foudraine, N., de Vries, F., and Janssen, P. (2021). Liquid Chromatography-Tandem Mass Spectrometry to Monitor Unbound and Total Ceftriaxone in Serum of Critically Ill Patients. Curr. Rev. Clin. Exp. Pharmacol. 16 (4), 341–349. doi:10.2174/1574884715666201228115150
Merrikin, D. J., Briant, J., and Rolinson, G. N. (1983). Effect of Protein Binding on Antibiotic Activity In Vivo. J. Antimicrob. Chemother. 11 (3), 233–238. doi:10.1093/jac/11.3.233
Mimoz, O., Soreda, S., Padoin, C., Tod, M., Petitjean, O., and Benhamou, D. (2000). Ceftriaxone Pharmacokinetics during Iatrogenic Hydroxyethyl Starch-Induced Hypoalbuminemia: A Model to Explore the Effects of Decreased Protein Binding Capacity on Highly Bound Drugs. Anesthesiology 93 (3), 735–743. doi:10.1097/00000542-200009000-00023
Mukker, J. K., Singh, R. P., and Derendorf, H. (2014). Determination of Atypical Nonlinear Plasma−protein-Binding Behavior of Tigecycline Using an In Vitro Microdialysis Technique. J. Pharm. Sci. 103 (3), 1013–1019. doi:10.1002/jps.23872
Muralidharan, G., Micalizzi, M., Speth, J., Raible, D., and Troy, S. (2005). Pharmacokinetics of Tigecycline after Single and Multiple Doses in Healthy Subjects. Antimicrob. Agents Chemother. 49 (1), 220–229. doi:10.1128/AAC.49.1.220-229.2005
Naik, B. I., Roger, C., Ikeda, K., Todorovic, M. S., Wallis, S. C., Lipman, J., et al. (2017). Comparative Total and Unbound Pharmacokinetics of Cefazolin Administered by Bolus versus Continuous Infusion in Patients Undergoing Major Surgery: A Randomized Controlled Trial. Br. J. Anaesth. 118 (6), 876–882. doi:10.1093/bja/aex026
Nakashima, M., Uematsu, T., Nagashima, S., Inaba, H., Hirasawa, T., Tomono, Y., et al. (1994). Phase I Study of E1077, a Novel Parenteral Cephem Antibiotic. J. Clin. Pharmacol. 34 (11), 1053–1059. doi:10.1002/j.1552-4604.1994.tb01980.x
Neves, D. V., Vieira, C. P., Rocha, A., and Lanchote, V. L. (2018). Therapeutic Doses of Eltrombopag Do Not Inhibit Hepatic BCRP in Healthy Volunteers: Intravenous Ceftriaxone as a Model. J. Pharm. Pharm. Sci. 21 (1), 236–246. doi:10.18433/jpps29856
Nightingale, C. H., Klimek, J. J., and Quintiliani, R. (1980). Effect of Protein Binding on the Penetration of Nonmetabolized Cephalosporins into Atrial Appendage and Pericardial Fluids in Open-Heart Surgical Patients. Antimicrob. Agents Chemother. 17 (4), 595–598. doi:10.1128/aac.17.4.595
Nix, D. E., Goodwin, S. D., Peloquin, C. A., Rotella, D. L., and Schentag, J. J. (1991). Antibiotic Tissue Penetration and its Relevance: Impact of Tissue Penetration on Infection Response. Antimicrob. Agents Chemother. 35 (10), 1953–1959. doi:10.1128/aac.35.10.1953
Ohashi, K., Tsunoo, M., and Tsuneoka, K. (1986). Pharmacokinetics and Protein Binding of Cefazolin and Cephalothin in Patients with Cirrhosis. J. Antimicrob. Chemother. 17 (3), 347–351. doi:10.1093/jac/17.3.347
Palma, E. C., Laureano, J. V., de Araújo, B. V., Meinhardt, N. G., Stein, A. T., and Dalla Costa, T. (2018). Fast and Sensitive HPLC/UV Method for Cefazolin Quantification in Plasma and Subcutaneous Tissue Microdialysate of Humans and Rodents Applied to Pharmacokinetic Studies in Obese Individuals. Biomed. Chromatogr. 32 (8), e4254. doi:10.1002/bmc.4254
Parker, S. L., Guerra Valero, Y. C., Roberts, D. M., Lipman, J., Roberts, J. A., and Wallis, S. C. (2016). Determination of Cefalothin and Cefazolin in Human Plasma, Urine and Peritoneal Dialysate by UHPLC-MS/MS: Application to a Pilot Pharmacokinetic Study in Humans. Biomed. Chromatogr. 30 (6), 872–879. doi:10.1002/bmc.3622
Popick, A. C., Crouthamel, W. G., and Bekersky, I. (1987). Plasma Protein Binding of Ceftriaxone. Xenobiotica 17 (10), 1139–1145. doi:10.3109/00498258709167406
Rao, Z., Dang, Z. L., Li, B., Zhu, L., Qin, H. Y., Wu, X. A., et al. (2020). Determination of Total and Unbound Meropenem, Imipenem/Cilastatin, and Cefoperazone/Sulbactam in Human Plasma: Application for Therapeutic Drug Monitoring in Critically Ill Patients. Ther. Drug Monit. 42 (4), 578–587. doi:10.1097/FTD.0000000000000736
Reitberg, D. P., Cumbo, T. J., Smith, I. L., and Schentag, J. J. (1984). Effect of Protein Binding on Cefmenoxime Steady-State Kinetics in Critical Patients. Clin. Pharmacol. Ther. 35 (1), 64–73. doi:10.1038/clpt.1984.10
Roberts, J. A., Pea, F., and Lipman, J. (2013). The Clinical Relevance of Plasma Protein Binding Changes. Clin. Pharmacokinet. 52 (1), 1–8. doi:10.1007/s40262-012-0018-5
Roberts, J. A., Udy, A. A., Jarrett, P., Wallis, S. C., Hope, W. W., Sharma, R., et al. (2015). Plasma and Target-Site Subcutaneous Tissue Population Pharmacokinetics and Dosing Simulations of Cefazolin in Post-trauma Critically Ill Patients. J. Antimicrob. Chemother. 70 (5), 1495–1502. doi:10.1093/jac/dku564
Roberts, D. M., Ranganathan, D., Wallis, S. C., Varghese, J. M., Kark, A., Lipman, J., et al. (2016). Pharmacokinetics of Intraperitoneal Cefalothin and Cefazolin in Patients Being Treated for Peritoneal Dialysis-Associated Peritonitis. Perit. Dial. Int. 36 (4), 415–420. doi:10.3747/pdi.2015.00008
Rowan, M. P., Niece, K. L., Rizzo, J. A., and Akers, K. S. (2017). Wound Penetration of Cefazolin, Ciprofloxacin, Piperacillin, Tazobactam, and Vancomycin during Negative Pressure Wound Therapy. Adv. Wound Care (New Rochelle) 6 (2), 55–62. doi:10.1089/wound.2016.0698
Scaglione, F., Raichi, M., and Fraschini, F. (1990). Serum Protein Binding and Extravascular Diffusion of Methoxyimino Cephalosporins. Time Courses of Free and Total Concentrations of Cefotaxime and Ceftriaxone in Serum and Pleural Exudate. J. Antimicrob. Chemother. 26 Suppl A (Suppl. A), 1–10. doi:10.1093/jac/26.suppl_a.1
Scaglione, F., Demartini, G., Arcidiacono, M. M., Dugnani, S., and Fraschini, F. (1997a). Serum Protein Binding and Extravascular Diffusion of Cefodizime and Ceftriaxone. An In Vivo Study. Clin. Drug Investig. 14 (3), 211–216. doi:10.2165/00044011-199714030-00008
Scaglione, F., De Martini, G., Peretto, L., Ghezzi, R., Baratelli, M., Arcidiacono, M. M., et al. (1997b). Pharmacokinetic Study of Cefodizime and Ceftriaxone in Sera and Bones of Patients Undergoing Hip Arthroplasty. Antimicrob. Agents Chemother. 41 (10), 2292–2294. doi:10.1128/AAC.41.10.2292
Schäfer-Korting, M., Korting, H. C., Maass, L., Klesel, N., Grigoleit, H. G., and Mutschler, E. (1986). Cefodizime Penetration into Skin Suction Blister Fluid Following a Single Intravenous Dose. Eur. J. Clin. Pharmacol. 30 (3), 295–298. doi:10.1007/BF00541531
Schießer, S., Hitzenbichler, F., Kees, M. G., Kratzer, A., Lubnow, M., Salzberger, B., et al. (2021). Measurement of Free Plasma Concentrations of Beta-Lactam Antibiotics: An Applicability Study in Intensive Care Unit Patients. Ther. Drug Monit. 43 (2), 264–270. doi:10.1097/FTD.0000000000000827
Schleibinger, M., Steinbach, C. L., Töpper, C., Kratzer, A., Liebchen, U., Kees, F., et al. (2015). Protein Binding Characteristics and Pharmacokinetics of Ceftriaxone in Intensive Care Unit Patients. Br. J. Clin. Pharmacol. 80 (3), 525–533. doi:10.1111/bcp.12636
Schmidt, S., Gonzalez, D., and Derendorf, H. (2010). Significance of Protein Binding in Pharmacokinetics and Pharmacodynamics. J. Pharm. Sci. 99 (3), 1107–1122. doi:10.1002/jps.21916
Seguin, P., Verdier, M. C., Chanavaz, C., Engrand, C., Laviolle, B., Donnio, P. Y., et al. (2009). Plasma and Peritoneal Concentration Following Continuous Infusion of Cefotaxime in Patients with Secondary Peritonitis. J. Antimicrob. Chemother. 63 (3), 564–567. doi:10.1093/jac/dkn522
Singh, R. S. P., Mukker, J. K., Deitchman, A. N., Drescher, S. K., and Derendorf, H. (2016). Role of Divalent Metal Ions in Atypical Nonlinear Plasma Protein Binding Behavior of Tigecycline. J. Pharm. Sci. 105 (11), 3409–3414. doi:10.1016/j.xphs.2016.07.013
Steele, W. H., Lawrence, J. R., Elliott, H. L., and Whiting, B. (1979). Alterations of Phenytoin Protein Binding with In Vivo Haemodialysis in Dialysis Encephalopathy. Eur. J. Clin. Pharmacol. 15 (1), 69–71. doi:10.1007/BF00563560
Stegemann, M. R., Sherington, J., and Blanchflower, S. (2006). Pharmacokinetics and Pharmacodynamics of Cefovecin in Dogs. J. Vet. Pharmacol. Ther. 29 (6), 501–511. doi:10.1111/j.1365-2885.2006.00801.x
Stoeckel, K., Tuerk, H., Trueb, V., and McNamara, P. J. (1984). Single-dose Ceftriaxone Kinetics in Liver Insufficiency. Clin. Pharmacol. Ther. 36 (4), 500–509. doi:10.1038/clpt.1984.210
Swanson, D., Maxwell, R. A., Johnson, J. T., Wagner, R. L., and Yu, V. L. (1991). Cefonicid versus Clindamycin Prophylaxis for Head and Neck Surgery in a Randomized, Double-Blind Trial, with Pharmacokinetic Implications. Antimicrob. Agents Chemother. 35 (7), 1360–1364. doi:10.1128/aac.35.7.1360
Tan, J. S., and Salstrom, S. J. (1977). Levels of Carbenicillin, Ticarcillin, Cephalothin, Cefazolin, Cefamandole, Gentamicin, Tobramycin, and Amikacin in Human Serum and Interstitial Fluid. Antimicrob. Agents Chemother. 11 (4), 698–700. doi:10.1128/aac.11.4.698
Tillement, J. P., Lhoste, F., and Giudicelli, J. F. (1978). Diseases and Drug Protein Binding. Clin. Pharmacokinet. 3 (2), 144–154. doi:10.2165/00003088-197803020-00004
Toth, A., Abdallah, H. Y., Venkataramanan, R., Teperman, L., Halsf, G., Rabinovitch, M., et al. (1991). Pharmacokinetics of Ceftriaxone in Liver-Transplant Recipients. J. Clin. Pharmacol. 31 (8), 722–728. doi:10.1002/j.1552-4604.1991.tb03767.x
Trang, J. M., Monson, T. P., Ackerman, B. H., Underwood, F. L., Manning, J. T., and Kearns, G. L. (1989). Effect of Age and Renal Function on Cefonicid Pharmacokinetics. Antimicrob. Agents Chemother. 33 (2), 142–146. doi:10.1128/aac.33.2.142
Tsai, D., Stewart, P., Goud, R., Gourley, S., Hewagama, S., Krishnaswamy, S., et al. (2016). Total and Unbound Ceftriaxone Pharmacokinetics in Critically Ill Australian Indigenous Patients with Severe Sepsis. Int. J. Antimicrob. Agents 48 (6), 748–752. doi:10.1016/j.ijantimicag.2016.09.021
Ulldemolins, M., Bastida, C., Llauradó-Serra, M., Csajka, C., Rodríguez, A., Badia, J. R., et al. (2021). Once-daily 1 G Ceftriaxone Optimizes Exposure in Patients with Septic Shock and Hypoalbuminemia Receiving Continuous Veno-Venous Hemodiafiltration. Eur. J. Clin. Pharmacol. 77 (8), 1169–1180. doi:10.1007/s00228-021-03100-5
Van Dalen, R., Vree, T. B., Baars, A. M., and Termond, E. (1986). Dosage Adjustment for Ceftazidime in Patients with Impaired Renal Function. Eur. J. Clin. Pharmacol. 30 (5), 597–605. doi:10.1007/BF00542421
Van Kralingen, S., Taks, M., Diepstraten, J., Van De Garde, E. M., Van Dongen, E. P., Wiezer, M. J., et al. (2011). Pharmacokinetics and Protein Binding of Cefazolin in Morbidly Obese Patients. Eur. J. Clin. Pharmacol. 67 (10), 985–992. doi:10.1007/s00228-011-1048-x
van Raaij, J. J., Mabelis, N. J. D., Shudofsky, K. N., Meenks, S. D., le Noble, J. L. M. L., and Janssen, P. K. C. (2020). Quantification of Total and Unbound Cefuroxime in Plasma by Ultra-performance Liquid Chromatography Tandem Mass Spectrometry in a Cohort of Critically Ill Patients with Hypoalbuminemia and Renal Failure. J. Clin. Lab. Anal. 34 (3), e23100. doi:10.1002/jcla.23100
Vanstraelen, K., Wauters, J., De Loor, H., Vercammen, I., Annaert, P., Lagrou, K., et al. (2014). Protein-binding Characteristics of Voriconazole Determined by High-Throughput Equilibrium Dialysis. J. Pharm. Sci. 103 (8), 2565–2570. doi:10.1002/jps.24064
Vella-Brincat, J. W., Begg, E. J., Kirkpatrick, C. M., Zhang, M., Chambers, S. T., and Gallagher, K. (2007). Protein Binding of Cefazolin Is Saturable In Vivo Both between and within Patients. Br. J. Clin. Pharmacol. 63 (6), 753–757. doi:10.1111/j.1365-2125.2006.02827.x
Verhagen, C. A., Mattie, H., and Van Strijen, E. (1994). The Renal Clearance of Cefuroxime and Ceftazidime and the Effect of Probenecid on Their Tubular Excretion. Br. J. Clin. Pharmacol. 37 (2), 193–197. doi:10.1111/j.1365-2125.1994.tb04260.x
Wiedermann, C. J. (2021). Hypoalbuminemia as Surrogate and Culprit of Infections. Int. J. Mol. Sci. 22 (9), 4496. doi:10.3390/ijms22094496
Wilkes, S., van Berlo, I., Ten Oever, J., Jansman, F., and Ter Heine, R. (2019). Population Pharmacokinetic Modelling of Total and Unbound Flucloxacillin in Non-critically Ill Patients to Devise a Rational Continuous Dosing Regimen. Int. J. Antimicrob. Agents 53 (3), 310–317. doi:10.1016/j.ijantimicag.2018.11.018
Wise, R., Gillett, A. P., Cadge, B., Durham, S. R., and Baker, S. (1980). The Influence of Protein Binding upon Tissue Fluid Levels of Six Beta-Lactam Antibiotics. J. Infect. Dis. 142 (1), 77–82. doi:10.1093/infdis/142.1.77
Wise, R. (1983). Protein Binding of Beta-Lactams: the Effects on Activity and Pharmacology Particularly Tissue Penetration. I. J. Antimicrob. Chemother. 12 (1), 105–118. doi:10.1093/jac/12.2.105
Wong, G., Briscoe, S., McWhinney, B., Ally, M., Ungerer, J., Lipman, J., et al. (2018). Therapeutic Drug Monitoring of β-lactam Antibiotics in the Critically Ill: Direct Measurement of Unbound Drug Concentrations to Achieve Appropriate Drug Exposures. J. Antimicrob. Chemother. 73 (11), 3087–3094. doi:10.1093/jac/dky314
Wright, J. D., Boudinot, F. D., and Ujhelyi, M. R. (1996). Measurement and Analysis of Unbound Drug Concentrations. Clin. Pharmacokinet. 30 (6), 445–462. doi:10.2165/00003088-199630060-00003
Yates, R. A., Adam, H. K., Donnelly, R. J., Houghton, H. L., Charlesworth, E. A., and Laws, E. A. (1983). Pharmacokinetics and Tolerance of Single Intravenous Doses of Cefotetan Disodium in Male Caucasian Volunteers. J. Antimicrob. Chemother. 11 Suppl (Suppl. l), 185–191. doi:10.1093/jac/11.suppl_a.185
Yeun, J. Y., and Kaysen, G. A. (1998). Factors Influencing Serum Albumin in Dialysis Patients. Am. J. Kidney Dis. 32, S118–S125. doi:10.1016/s0272-6386(98)70174-x
Keywords: cephalosporins, protein binding, human, body fluids, pharmacokinetics, systematic review
Citation: Jongmans C, Muller AE, Van Den Broek P, Cruz De Almeida BDM, Van Den Berg C, Van Oldenrijk J, Bos PK and Koch BCP (2022) An Overview of the Protein Binding of Cephalosporins in Human Body Fluids: A Systematic Review. Front. Pharmacol. 13:900551. doi: 10.3389/fphar.2022.900551
Received: 20 March 2022; Accepted: 18 May 2022;
Published: 28 June 2022.
Edited by:
Elena Schneider-Futschik, The University of Melbourne, AustraliaReviewed by:
Ashok K. Shakya, Al-Ahliyya Amman University, JordanCopyright © 2022 Jongmans, Muller, Van Den Broek, Cruz De Almeida, Van Den Berg, Van Oldenrijk, Bos and Koch. This is an open-access article distributed under the terms of the Creative Commons Attribution License (CC BY). The use, distribution or reproduction in other forums is permitted, provided the original author(s) and the copyright owner(s) are credited and that the original publication in this journal is cited, in accordance with accepted academic practice. No use, distribution or reproduction is permitted which does not comply with these terms.
*Correspondence: B. C. P. Koch, Yi5rb2NoQGVyYXNtdXNtYy5ubA==
Disclaimer: All claims expressed in this article are solely those of the authors and do not necessarily represent those of their affiliated organizations, or those of the publisher, the editors and the reviewers. Any product that may be evaluated in this article or claim that may be made by its manufacturer is not guaranteed or endorsed by the publisher.
Research integrity at Frontiers
Learn more about the work of our research integrity team to safeguard the quality of each article we publish.