- 1Departamento de Ciências Fisiológicas, Universidade Estadual de Londrina—UEL, Londrina, Brazil
- 2Departamento de Ciências Patológicas, Universidade Estadual de Londrina- UEL, Londrina, Brazil
Parkinson’s disease (PD) is characterized by the loss of dopaminergic neurons in the substantia nigra, causing motor changes. In addition to motor symptoms, non-motor dysfunctions such as psychological, sensory and autonomic disorders are recorded. Manifestations related to the autonomic nervous system include the cardiovascular system, as postural hypotension, postprandial hypotension, and low blood pressure. One of the mediators involved is the nitric oxide (NO). In addition to the known roles such as vasodilator, neuromodulator, NO acts as an important mediator of the immune response, increasing the inflammatory response provoked by PD in central nervous system. The use of non-specific NOS inhibitors attenuated the neurodegenerative response in animal models of PD. However, the mechanisms by which NO contributes to neurodegeneration are still not well understood. The literature suggest that the contribution of NO occurs through its interaction with superoxides, products of oxidative stress, and blocking of the mitochondrial respiratory chain, resulting in neuronal death. Most studies involving Parkinsonism models have evaluated brain NO concentrations, with little data available on its peripheral action. Considering that studies that evaluated the involvement of NO in the neurodegeneration in PD, through NOS inhibitors administration, showed neuroprotection in rats, it has prompted new studies to assess the participation of NOS isoforms in cardiovascular changes induced by parkinsonism, and thus to envision new targets for the treatment of cardiovascular disorders in PD. The aim of this study was to conduct a literature review to assess available information on the involvement of nitric oxide (NO) in cardiovascular aspects of PD.
Introduction
Parkinson’s disease (PD) is the second most common neurodegenerative disease and affects approximately 1% of the world population aged over 65 years (Lau and Breteler, 2006). It is characterized by the degeneration of dopaminergic neurons in the substantia nigra pars compacta (SNpc) and formation of α-synuclein aggregates, called Lewy bodies. When the neurodegenerative process reduces dopamine levels by about 60%, motor symptoms such as bradykinesia, rigidity and tremor at rest are manifested, mainly (Dauer and Przedborski, 2003). PD also has non-motor symptoms present in the most basal stages of the disease, such as sensory, neuropsychiatric and autonomic disorders, known as dysautonomias (Sian et al., 1999; Rodriguez-Oroz et al., 2009; Schapira et al., 2017)
The worldwide occurrence of Parkinson’s Disease (PD) is estimated between 5 and 35 new cases per 100 thousand inhabitants. The age of onset is usually around 50 years of age, with a higher incidence after the sixth decade of life (Simon et al., 2020). There is a higher prevalence in males, with 134 cases in men and 41 in women per 100,000 inhabitants, aged between 50 and 59 years (Pringsheim et al., 2014). There is also evidence that PD is more prevalent in postmenopausal women compared to premenopausal women of similar age (Ragonese et al., 2006).
With its idiopathic etiology, it is believed that genetic issues are linked to the existence of genes that favor the development of the disease, but acting indirectly. Environmental factors are linked to PD patients who live in rural areas, use well water and are more exposed to pesticides and herbicides (Teive, 2005). Regarding the contribution of brain aging, it would be related to the prevalence of age, associated with progressive neuronal loss (Pereira and Garrett, 2010).
The cardinal signs of PD are: bradykinesia and oligokinesis, observed by slowness and reduction of movements in quantity and amplitude; generalized stiffness; resting tremor and postural instability (Sung and Nicholas, 2013). As the disease progresses, other motor symptoms can be identified, such as reduced facial expression; micrography; disorders in oral communication and swallowing, difficulty in gait, which include freezing, difficulty in changing direction and in overcoming obstacles; postural changes, with a tendency to bend the limbs and respiratory changes, especially due to the decrease in chest expansion (Peterson and Horak, 2016). Motor characteristics of the extrapyramidal region of PD emerge when 30%–50% of the dopamine-producing neurons in the substantia nigra are lost (Fazio et al., 2018). Motor impairment becomes bilateral three to 5 years after the initial diagnosis (Scorza et al., 2001).
Some non-motor symptoms may precede motor dysfunction by several years, such as: neuropsychiatric disorders, sleep disorders, olfactory deficit, anxiety and depression and dysautonomias (Schapira and Tolosa, 2010), but in general, these symptoms occur at any stage of PD, and may vary according to each patient (Jain and Goldstein, 2012).
The autonomic nervous system (ANS), responsible for controlling physiological adjustment parameters, seems to be altered in PD inducing dysautonomias (Azevedo and Cardoso, 2009; Sharabi et al., 2021). A subset of these symptoms are associated with altered function of the ANS, where orthostatic hypotension, hyperhidrosis and gastrointestinal dysfunction are common manifestations in PD that can affect the quality of life of patients and can have an impact even more than the motor symptoms (Teive, 2006). Among the cardiovascular alterations are the orthostatic arterial hypotension (OH), postprandial arterial hypotension, alteration of arterial pressure (BP) variability and, possibly, fatigue and intolerance to physical exercise (Nicaretta et al., 2011; Jain and Goldstein, 2012; Schapira et al., 2017). Those symptoms are quite relevant and impact on the patient’s clinical condition (Sian et al., 1999; Rodriguez-Oroz et al., 2009; Schapira et al., 2017; Balestrino and Schapira, 2020). However, little is know about the origins of those alterations.
Nitric oxide (NO) is a small and highly diffuse molecule that, despite having a half-life of only a few seconds, has an ambiguous action as an important physiological mediator, acting as a vasodilator and immune response mediator, and cytotoxic mediator, exacerbating the inflammatory response caused by PD (Doherty, 2011; Rochette et al., 2013). Considering the involvement of this mediator in the central alterations evaluated in animal models of parkinsonism, the question of its possible involvement in the cardiovascular dysfunctions of PD arises, since NO is a modulator of several neurotransmitter systems, such as dopaminergic, cholinergic and adrenergic (Paredes-Rodriguez et al., 2020). Studies in this direction have been initiated and may provide greater support for the use of new therapies.
Animal Models of Parkinsonism
The study of Parkinson’s disease (PD) is possible through the induction of the disease in animal models, such as rats and mice. To simulate the main characteristics, pharmacological models such as reserpine and haloperidol, and neurotoxic models such as 6-hydroxydopamine (6-OHDA), 1-methyl-4-phenyl-1,2,3,6-tetrahydropyridine (MPTP), rotenone, paraquat, lipopolysaccharide (LPS) and methamphetamine are utilized (Blum et al., 2001). Neurotoxic models damage the nigrostriatal pathway by infusing neurotoxins into different areas unilaterally or bilaterally in the substantia nigra pars compacta (SNpc), medial forebrain bundle or striatum. 6-OHDA and MPTP are the most used experimental models, since their induction causes the degeneration of 60%–70% of the nigrostriatal pathway, which corresponds to the onset of motor symptoms. Due to the complexity of PD, there is no experimental model that presents all the characteristics of the disease (Duty and Jenner, 2011; Jackson-Lewis et al., 2012).
6-OHDA is unable to cross the blood-brain barrier, so it is administered directly into the SNpc or striatum via stereotaxic surgery. The neurotoxin binds to dopamine transporters and is carried to dopaminergic neurons, accumulating in the cytosol and causing the formation of reactive oxygen species that lead to neuronal death. Additionally, 6-OHDA can accumulate in the mitochondria, where it binds to complex I of the electron transport chain and inhibits flow (Blandini et al., 2008).
Cardiovascular and Autonomic Dysfunction and Parkinson’s Disease
Cardiovascular alterations such as orthostatic hypotension, heart rate variability, modifications in cardiogram parameters and baroreflex dysfunction can appear in both the early and late stages of PD, worsening as the disease progresses. Cardiovascular abnormalities can also appear as a side effect of PD treatment by L-DOPA leading to a decrease in blood pressure aggravating the orthostatic hypotension. This side effect limits the therapeutic use of L-DOPA in geriatric patients with PD and can contribute to the number of hospital admissions (Cuenca–Bermejo et al., 2021). It is estimated that 80% of PD patients have heart rate and blood pressure abnormalities (Gibbons et al., 2017). Orthostatic hypotension affects 40% of PD patients and causes dizziness and syncope, increasing the risk of falls and injuries. These changes seem to be due to noradrenergic cardiac denervation, extracardiac noradrenergic denervation and baroreflex insufficiency (Jain and Goldstein, 2012; Paredes-Rodriguez et al., 2020). Changes in HR appear to be related to dysfunctional parasympathetic responses, while the inability to regulate BP is related to the loss of sympathetic regulation (Jain and Goldstein, 2012).
Heart rate variability assesses the modulation of the autonomic nervous system on the cardiovascular system and works as an indicator of health (Vanderlei et al., 2009). Patients with PD have a reduction in heart rate variability (HRV) and lower LF values than patients in the control group (Solla et al., 2015; Salsone et al., 2016; Strano et al., 2016). Sorensen and colleagues observed attenuated sympathetic activity in patients with PD, with a reduction in the components related to the sympathetic system in HRV (Sorensen et al., 2013). Evidence also shows loss of sympathetic noradrenergic nerves in the heart and kidneys of patients with PD (Goldstein, 2007).
In experimental models, male rats with bilateral induction of the neurotoxin 6-hydroxydopamine (6-OHDA) directly in the SNpc leads to a decrease in baseline parameters of mean arterial pressure (MAP) and heart rate (HR), which are accompanied by a reduction in modulation systolic blood pressure (Ariza et al., 2015b). Baroreflex and chemoreflex responses are altered in these animals (Ariza et al., 2015a). Bilateral injection also caused a drop in the night/day cycle change of heart rate and weakened phenylephrine-induced bradycardia, suggesting a drop in heart rate (Metzger and Emborg, 2019). Furthermore, rats with 6-OHDA parkinsonism show a decreased response to alpha-adrenergic blocker, suggesting an impaired sympathetic vascular synaptic transmission (Ariza et al., 2015b). Bilateral 6-OHDA animals and Prolopa control animals presented a lower cardiovascular compensation during head up tilt, suggesting a possible autonomic impairment in parkinsonism induced by 6-OHDA (Silva et al., 2015). Also, in the 6-OHDA injury model, lower baroreflex sensitivity and decreased number of cardiovascular neurons in the brainstem were observed (Falquetto et al., 2017). Recent literature has described altered central and peripheral mechanisms affecting the feedback-controlled loops that comprise the reflex arc in patients with PD and animal models (Sabino-Carvalho et al., 2021).
In the MPTP model, in mice, attenuation of baroreflex sensitivity was observed, associated with loss of TH + neurons and decrease of catecholamines in the brainstem. There was an increase in HR and a decrease in HRV power. Regarding the frequency domains, an increase in sympathetic tone and a decrease in parasympathetic tone were observed (Liu et al., 2020).
Nitric Oxide and Parkinson’s Disease
NO is synthesized by nitric oxide synthase (NOS) through the oxidation of L-Arginine. The enzyme is found into constitutive NOS (cNOS), whose activation depends on calcium, and inducible NOS (iNOS), dependent on cytokines. cNOS is expressed at basal levels and has two isoforms: endothelial NOS (eNOS) and neuronal NOS (nNOS). Among the functions of eNOS are vasodilation, inhibition of platelet adhesion and aggregation in blood vessels, inhibition of vascular inflammation and leukocyte adhesion, while nNOS regulates synaptic transmission in the central nervous system (CNS) and is present in smooth muscles promoting vasoregulation. The expression of iNOS occurs specially under inflammatory conditions through immunological or microbial stimuli (Förstermann and Sessa, 2012).
Post mortem analyses, clinical findings and experimental models of parkinsonism suggest the involvement of NO in the neurodegeneration observed in PD. Barthwal et al. (2001) demonstrated that the use of a non-specific inhibitor for NO (Nw-nitro-arginine-methyl-ester or L-NAME) results in a decrease in the neurodegenerative response after 6-OHDA injury in rats (Barthwal et al., 2001). In the MPTP model, inhibitors of nNOS—7-Nitroindazole (7-NI) and of iNOS—S-methylisothiourea (SMT) were effective in protecting against the neurotoxic effects of the lesion, suggesting the participation of NO in PD (Schulz et al., 1995; Di Monte et al., 1996; Aras et al., 2014). The administration of GW274150 [(2-((1-iminoethyl)amino)ethyl]-L-homocysteine - selective iNOS inhibitor) after unilateral 6-OHDA injury significantly attenuated the loss of DA in the striatum of Sprague-Dawley rats (Broom et al., 2011). Furthermore, the increased degeneration of dopaminergic neurons in 6-OHDA-injured rats leads to a decrease in the concentration of nNOS in the substantia nigra of these animals (Czarnecka et al., 2013), while MPTP injury in mice results in an increase in iNOS (Liberatore et al., 1999). Literature showed differences in the effects of MPTP toxicity between males and females, with males having a faster decrease in DA and later, females having better recovery from this decrease. Furthermore, males were the first to show increased expression of iNOS and nNOS in the striatum (Joniec et al., 2009)
Another factor that suggests the involvement of NOS is the correlation between alterations in the genes responsible for iNOS and nNOS and the risk of developing PD (Levecque et al., 2003). Regarding peripheral NO, Çubukçu et al. (2016) demonstrate a decrease in plasma NO concentration, without a decrease in NOS activity in patients with PD. Thus, evidence indicates that NOS appear to participate in the neurodegeneration of dopaminergic neurons in animal models of PD. Therefore, the use of NOS inhibitors in the treatment of PD has been discussed (Broom et al., 2011).
The mechanisms by which NO contributes to neurodegeneration are not well understood. It is known that the induction of PD is related to increased oxidative stress in the brain, which leads to damage to the neurons of the substantia nigra (Dias et al., 2013). Studies suggest that the combination of NO with superoxides formed through the process of oxidative stress results in the formation of peroxynitrites (ONOO-), a molecule that is highly harmful to nervous tissue. In addition, NO has a high affinity for the heme group present in oxygen-carrying proteins, causing a delay in the functioning of the mitochondrial respiratory chain and consequently neuronal death (McDonald and Murad, 1996; Przedborski et al., 1996). Oxidative stress, in turn, decreases the availability of NO in endothelial cells and the central nervous system (Tieu et al., 2003). NO appears to be also involved in the dopamine metabolism by a common toxic pathway that involves mitochondrial compromise, nitroxidative stress and GSH depletion and that may be operating and contributing to the neurodegeneration observed in this disease (Nunes and Laranjinha, 2021).
Nitric Oxide in Cardiovascular Dysfunction in Parkinson's Disease
Peripherally, NO acts as a potent vasodilator to increase blood flow, and it synthesis and bioavailability by the vascular endothelium contributes to greater vasodilation for adaptation in different conditions by the cardiovascular system (Green et al., 2004; McAllister and Laughlin, 2006; Di Francescomarino et al., 2009). Changes in its production can hamper hemodynamic responses and compromise cardiovascular health.
To date, few studies have evaluated NO in the cardiovascular system of animals with parkinsonism de Jager et al. (2018) observed differences in tissue concentrations of nitrite in sedentary animals or animals submitted to physical training before 6-OHDA injury, with NO being increased in the aorta of 6-OHDA animals compared to their controls. However, inhibition of NOS by L-NAME administration promoted a smaller increase in MAP in 6-OHDA animals, suggesting that eNOS nitrergic tonus is lower in animals injured by 6-OHDA than in Sham animals (de Jager et al., 2018). In this sense, it is possible that different isoforms are participating in these responses. Ongoing studies assess which isoform may be involved in vascular alterations in 6-OHDA animals and may contribute to explain the basal hypotension and sympathetic decrease in male rats.
Data from our group using female rats observed that adult females do not present the cardiovascular dysfunction observed by males, who have hypotension compared to their control group. However, when the females are submitted to ovariectomy they show basal hypotension, and a higher concentration of nitrite in the aorta, suggesting a role for NO associated with ovarian hormones at the vascular level (de Campos et al., 2020). Our group has studied the possible mechanisms of gender involvement in the cardiovascular dysfunctions of PD, including redox balance.
Regarding the central control of the cardiovascular system, it was observed that nNOS is increased in the paraventricular nucleus of the hypothalamus, an area of cardiovascular control, leading to an increase in GABAergic tone on cardiovascular function and contributing to the lower basal BP values in male 6-OHDA injured rats (Turossi Amorim et al., 2019). By this way, the decreased baseline blood pressure in animals with Parkinsonism by 6-OHDA may be due to a central effect mediated by the NO in the PVN. However, we do not rule out the hypothesis of peripheral mechanisms participating in these cardiovascular dysfunctions (Figure 1). The study of these mechanisms is important to focus on future therapeutic targets for patients with PD.
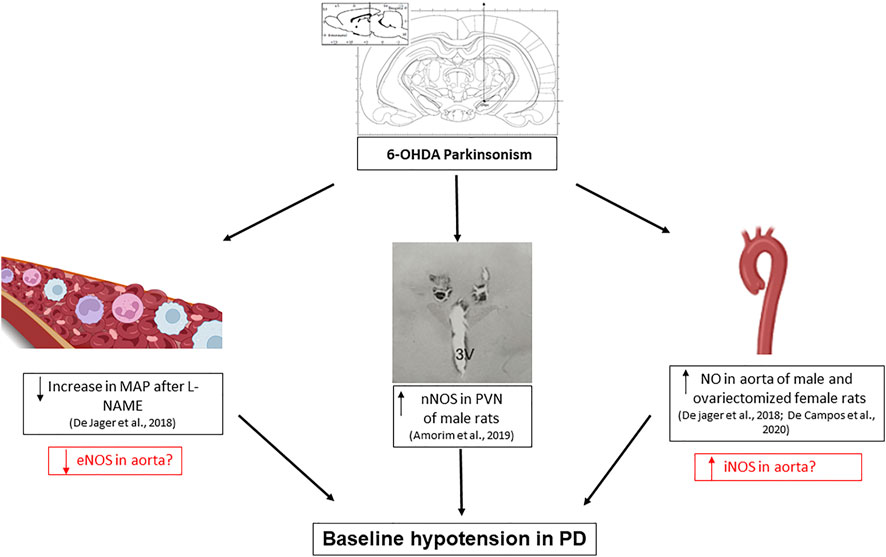
FIGURE 1. Scheme of changes involving nitric oxide alterations found in the cardiovascular system of rats induced to Parkinsonism using the bilateral model of substantia nigra pars compacta lesion with 6-hydroxydopamine (6-OHDA). The increased production of nitrite in the aorta of male and ovariectomized female rats, associated with an increase in nNOS activity in the region of the paraventricular nucleus of the hypothalamus (PVN), and a smaller increase in mean arterial pressure (MAP) after intravenous administration of L-NAME, suggests the involvement of the 3 isoforms of NO-synthase (NOS) in blood pressure dysfunction in PD. The boxes in red are questions and possible hypotheses. The central illustrations were taken from the cited articles by Ariza et al., 2015b and Turossi Amorim et al., 2019. The lateral illustrations were taken from BioRender.
Conclusion
The findings so far regarding the participation of NO in the cardiovascular dysfunctions in PD show that hypothalamic nuclei that participate in tonic autonomic and cardiovascular control seem to be a target for NO alterations. In addition, still initial data have suggested the participation of NO peripherally (blood vessels), which brings us perspectives about possible new treatments for the cardiovascular dysfunctions in PD, especially related to hypotension.
Author Contributions
Conception and design: MM-P. Drafting of the manuscript: MM-P, LJ, BC, LB, PT, and PP-F. Final revision: MM-P, LJ, BC, LB, PT, and PP-F. All authors read and approved the final version of the manuscript.
Conflict of Interest
The authors declare that the research was conducted in the absence of any commercial or financial relationships that could be construed as a potential conflict of interest.
Publisher’s Note
All claims expressed in this article are solely those of the authors and do not necessarily represent those of their affiliated organizations, or those of the publisher, the editors and the reviewers. Any product that may be evaluated in this article, or claim that may be made by its manufacturer, is not guaranteed or endorsed by the publisher.
Acknowledgments
We thank Superintendência de Ciência, Tecnologia e Ensino Superior (SETI), Fundação Araucária (FA), Universidade Estadual de Londrina (PROPPG), and Programa de Pós-graduação em Patologia Experimental, for assistance in paying the publication fee for the journal.
References
Aras, S., Tanriover, G., Aslan, M., Yargicoglu, P., and Agar, A. (2014). The Role of Nitric Oxide on Visual-Evoked Potentials in MPTP-Induced Parkinsonism in Mice. Neurochem. Int. 72 (1), 48–57. doi:10.1016/j.neuint.2014.04.014
Ariza, D., Lopes, F. N. C., Crestani, C. C., and Martins-Pinge, M. C. (2015a). Chemoreflex and Baroreflex Alterations in Parkinsonism Induced by 6-OHDA in Unanesthetized Rats. Neurosci. Lett. 607, 77–82. doi:10.1016/j.neulet.2015.09.024
Ariza, D., Sisdeli, L., Crestani, C. C., Fazan, R., and Martins-Pinge, M. C. (2015b). Dysautonomias in Parkinson's Disease: Cardiovascular Changes and Autonomic Modulation in Conscious Rats after Infusion of Bilateral 6-OHDA in Substantia Nigra. Am. J. Physiol. Heart Circ. Physiol. 308 (3), H250–H257. doi:10.1152/ajpheart.00406.2014
Azevedo, L. L. D., and Cardoso, F. (2009). Ação da levodopa e sua influência na voz e na fala de indivíduos com doença de Parkinson The action of levodopa and its influence on voice and speech of patients. Rev. Soc. Bras. Fonoaudiol. 14 (8), 136–141. doi:10.1590/s1516-80342009000100021
Balestrino, R., and Schapira, A. H. V. (2020). Parkinson Disease. Eur. J. Neurol. 27 (1), 27–42. doi:10.1111/ene.14108
Barthwal, M. K., Srivastava, N., and Dikshit, M. (2001). Role of Nitric Oxide in a Progressive Neurodegeneration Model of Parkinson's Disease in the Rat. Redox Rep. 6 (5), 297–302. doi:10.1179/135100001101536436
Blandini, F., Armentero, M. T., and Martignoni, E. (2008). The 6-hydroxydopamine Model: News from the Past. Park. Relat. Disord. 14 (Suppl. 2), S124–S129. doi:10.1016/j.parkreldis.2008.04.015
Blum, D., Torch, S., Lambeng, N., Nissou, M., Benabid, A. L., Sadoul, R., et al. (2001). Molecular Pathways Involved in the Neurotoxicity of 6-OHDA, Dopamine and MPTP: Contribution to the Apoptotic Theory in Parkinson's Disease. Prog. Neurobiol. 65 (2), 135–172. doi:10.1016/S0301-0082(01)00003-X
Broom, L., Marinova-Mutafchieva, L., Sadeghian, M., Davis, J. B., Medhurst, A. D., and Dexter, D. T. (2011). Neuroprotection by the Selective iNOS Inhibitor GW274150 in a Model of Parkinson Disease. Free Radic. Biol. Med. 50 (5), 633–640. doi:10.1016/j.freeradbiomed.2010.12.026
Çubukçu, H. C., Yurtdaş, M., Durak, Z. E., Aytaç, B., Güneş, H. N., Çokal, B. G., et al. (2016). Oxidative and Nitrosative Stress in Serum of Patients with Parkinson's Disease. Neurol. Sci. 37 (11), 1793–1798. doi:10.1007/s10072-016-2663-1
Cuenca-Bermejo, L., Almela, P., Navarro-Zaragoza, J., Fernández Villalba, E., González-Cuello, A. M., Laorden, M. L., et al. (2021). Cardiac Changes in Parkinson's Disease: Lessons from Clinical and Experimental Evidence. Int. J. Mol. Sci. 22 (24), 13488. doi:10.3390/ijms222413488
Czarnecka, A., Lenda, T., Domin, H., Konieczny, J., Smiałowska, M., and Lorenc-Koci, E. (2013). Alterations in the Expression of nNOS in the Substantia Nigra and Subthalamic Nucleus of 6-OHDA-Lesioned Rats: The Effects of Chronic Treatment with L-DOPA and the Nitric Oxide Donor, Molsidomine. Brain Res. 1541, 92–105. doi:10.1016/j.brainres.2013.10.011
Dauer, W., and Przedborski, S. (2003). Parkinson's Disease: Mechanisms and Models. Neuron 39 (6), 889–909. doi:10.1016/S0896-6273(03)00568-3
de Campos, B. H., de Jager, L., Reginato, G. S., Pereira, R. S., Crestani, C. C., Pinge-Filho, P., et al. (2020). Cardiovascular Evaluation of Female Rats with 6-OHDA-Induced Parkinsonism: Possible Protection by Ovarian Hormones and Participation of Nitric Oxide. Life Sci. 259, 118259. doi:10.1016/j.lfs.2020.118259
de Jager, L., Amorim, E. D. T., Lucchetti, B. F. C., Lopes, F. N. C., Crestani, C. C., Pinge-Filho, P., et al. (2018). Nitric Oxide Alterations in Cardiovascular System of Rats with Parkinsonism Induced by 6-OHDA and Submitted to Previous Exercise. Life Sci. 204, 78–86. doi:10.1016/j.lfs.2018.05.017‘
de Lau, L. M., and Breteler, M. M. (2006). Epidemiology of Parkinson's Disease. Lancet Neurology 5, 525–535. doi:10.1016/S1474-4422(06)70471-9
Di Francescomarino, S., Sciartilli, A., Di Valerio, V., Di Baldassarre, A., and Gallina, S. (2009). The Effect of Physical Exercise on Endothelial Function. Sports Med. 39 (10), 797–812. doi:10.2165/11317750-000000000-00000
Di Monte, D. A., Royland, J. E., Jakowec, M. W., and Langston, J. W. (1996). Role of Nitric Oxide in Methamphetamine Neurotoxicity: Protection by 7-nitroindazole, an Inhibitor of Neuronal Nitric Oxide Synthase. J. Neurochem. 67 (6), 2443–2450. doi:10.1046/j.1471-4159.1996.67062443.x
Dias, V., Junn, E., and Mouradian, M. M. (2013). The Role of Oxidative Stress in Parkinson's Disease. J. Park. Dis. 3 (4), 461–491. doi:10.3233/JPD-130230
Doherty, G. H. (2011). Nitric Oxide in Neurodegeneration: Potential Benefits of Non-steroidal Anti-inflammatories. Neurosci. Bull. 27 (6), 366–382. doi:10.1007/s12264-011-1530-6
Duty, S., and Jenner, P. (2011). Animal Models of Parkinson's Disease: a Source of Novel Treatments and Clues to the Cause of the Disease. Br. J. Pharmacol. 164 (4), 1357–1391. doi:10.1111/j.1476-5381.2011.01426.x
Falquetto, B., Tuppy, M., Potje, S. R., Moreira, T. S., Antoniali, C., and Takakura, A. C. (2017). Cardiovascular Dysfunction Associated with Neurodegeneration in an Experimental Model of Parkinson's Disease. Brain Res. 1657, 156–166. doi:10.1016/j.brainres.2016.12.008
Fazio, P., Svenningsson, P., Cselényi, Z., Halldin, C., Farde, L., and Varrone, A. (2018). Nigrostriatal Dopamine Transporter Availability in Early Parkinson's Disease. Mov. Disord. 33 (4), 592–599. doi:10.1002/mds.27316
Forstermann, U., and Sessa, W. C. (2012). Nitric Oxide Synthases: Regulation and Function. Eur. Heart J. 33 (7), 829–837. doi:10.1093/eurheartj/ehr304
Gibbons, C. H., Simon, D. K., Huang, M., Tilley, B., Aminoff, M. J., Bainbridge, J. L., et al. (2017). Autonomic and Electrocardiographic Findings in Parkinson's Disease. Aut. Neurosci. 205, 93–98. doi:10.1016/j.autneu.2017.04.002.Autonomic
Goldstein, D. S. (2007). Cardiac Denervation in Patients with Parkinson Disease. Clevel. Clin. J. Med. 74 (Suppl. 1), S91. doi:10.3949/ccjm.74.Suppl_1.S91
Green, D. J., Maiorana, A., O'Driscoll, G., and Taylor, R. (2004). Effect of Exercise Training on Endothelium-Derived Nitric Oxide Function in Humans. J. Physiol. 561 (1), 1–25. doi:10.1113/jphysiol.2004.068197
Jackson-Lewis, V., Blesa, J., and Przedborski, S. (2012). Animal Models of Parkinson's Disease. Park. Relat. Disord. 18 (Suppl. 1), S183–S185. doi:10.1016/S1353-8020(11)70057-8
Jain, S., and Goldstein, D. S. (2012). Cardiovascular Dysautonomia in Parkinson Disease: From Pathophysiology to Pathogenesis. Neurobiol. Dis. 46, 572–580. doi:10.1016/j.nbd.2011.10.025
Joniec, I., Ciesielska, A., Kurkowska-Jastrzebska, I., Przybylkowski, A., Czlonkowska, A., and Czlonkowski, A. (2009). Age- and Sex-Differences in the Nitric Oxide Synthase Expression and Dopamine Concentration in the Murine Model of Parkinson's Disease Induced by 1-Methyl-4-Phenyl-1,2,3,6-Tetrahydropyridine. Brain Res. 1261, 7–19. doi:10.1016/j.brainres.2008.12.081
Levecque, C., Elbaz, A., Clavel, J., Richard, F., Vidal, J. S., Amouyel, P., et al. (2003). Association between Parkinson's Disease and Polymorphisms in the nNOS and iNOS Genes in a Community-Based Case-Control Study. Hum. Mol. Genet. 12 (1), 79–86. doi:10.1093/hmg/ddg009
Liberatore, G. T., Jackson-Lewis, V., Vukosavic, S., Mandir, A. S., Vila, M., McAuliffe, W. G., et al. (1999). Inducible Nitric Oxide Synthase Stimulates Dopaminergic Neurodegeneration in the MPTP Model of Parkinson Disease. Nat. Med. 5 (12), 1403–1409. doi:10.1038/70978
Liu, X., Wei, B., Bi, Q., Sun, Q., Li, L., He, J., et al. (2020). MPTP-induced Impairment of Cardiovascular Function. Neurotox. Res. 38 (1), 27–37. doi:10.1007/s12640-020-00182-4
McAllister, R. M., and Laughlin, M. H. (2006). Vascular Nitric Oxide: Effects of Physical Activity, Importance for Health. Essays Biochem. 42, 119–131. doi:10.1042/bse0420119
McDonald, L. J., and Murad, F. (1996). Nitric Oxide and Cyclic GMP Signaling. Proc. Soc. Exp. Biol. Med. 211 (1), 1–6. doi:10.1177/15353702062310090110.3181/00379727-211-43950a
Metzger, J. M., and Emborg, M. E. (2019). Autonomic Dysfunction in Parkinson Disease and Animal Models. Clin. Auton. Res. 29 (4), 397–414. doi:10.1007/s10286-018-00584-7
Nicaretta, D. H., Rosso, A. L., and Mattos, J. P. D. (2011). Disautonomia na Doença de Parkinson, Revisão da literatura. Rev. Bras. Neurol. 47 (4), 25–29.
Nunes, C., and Laranjinha, J. (2021). Nitric Oxide and Dopamine Metabolism Converge via Mitochondrial Dysfunction in the Mechanisms of Neurodegeneration in Parkinson's Disease. Arch. Biochem. Biophys. 704, 108877. doi:10.1016/j.abb.2021.108877
Paredes-Rodriguez, E., Vegas-Suarez, S., Morera-Herreras, T., De Deurwaerdere, P., and Miguelez, C. (2020). The Noradrenergic System in Parkinson's Disease. Front. Pharmacol. 11, 435. doi:10.3389/fphar.2020.00435
Pereira, D., and Garrett, C. (2010). Fatores de risco na doença de Parkinson. Acta Med. Port. 23 (1), 15–24.
Peterson, D. S., and Horak, F. B. (2016). Neural Control of Walking in People with Parkinsonism. Physiol. (Bethesda) 31 (2), 95–107. doi:10.1152/physiol.00034.2015
Pringsheim, T., Jette, N., Frolkis, A., and Steeves, T. D. (2014). The Prevalence of Parkinson's Disease: a Systematic Review and Meta-Analysis. Mov. Disord. 29 (13), 1583–1590. doi:10.1002/mds.25945
Przedborski, S., Jackson-Lewis, V., Yokoyama, R., Shibata, T., Dawson, V. L., and Dawson, T. M. (1996). Role of Neuronal Nitric Oxide in 1-Methyl-4-Phenyl-1,2,3,6-Tetrahydropyridine (MPTP)-induced Dopaminergic Neurotoxicity. Proc. Natl. Acad. Sci. U. S. A. 93 (10), 4565–4571. doi:10.1073/pnas.93.10.4565
Ragonese, P., D'Amelio, M., Callari, G., Salemi, G., Morgante, L., and Savettieri, G. (2006). Age at Menopause Predicts Age at Onset of Parkinson's Disease. Mov. Disord. 21 (12), 2211–2214. doi:10.1002/mds.21127
Rochette, L., Lorin, J., Zeller, M., Guilland, J. C., Lorgis, L., Cottin, Y., et al. (2013). Nitric Oxide Synthase Inhibition and Oxidative Stress in Cardiovascular Diseases: Possible Therapeutic Targets? Pharmacol. Ther. 140 (3), 239–257. doi:10.1016/j.pharmthera.2013.07.004
Rodriguez-Oroz, M. C., Jahanshahi, M., Krack, P., Litvan, I., Macias, R., Bezard, E., et al. (2009). Initial Clinical Manifestations of Parkinson's Disease: Features and Pathophysiological Mechanisms. Lancet Neurol. 8 (12), 1128–1139. doi:10.1016/S1474-4422(09)70293-5
Sabino-Carvalho, J. L., Falquetto, B., Takakura, A. C., and Vianna, L. C. (2021). Baroreflex Dysfunction in Parkinson's Disease: Integration of Central and Peripheral Mechanisms. J. Neurophysiol. 125 (4), 1425–1439. doi:10.1152/jn.00548.2020
Salsone, M., Nistico', R., Vescio, B., Novellino, F., Morelli, M., Lupo, A., et al. (2016). Heart Rate Variability in Patients with Essential Tremor: A Cross Sectional Study. Park. Relat. Disord. 33, 134–137. doi:10.1016/j.parkreldis.2016.09.027
Schapira, A. H., and Tolosa, E. (2010). Molecular and Clinical Prodrome of Parkinson Disease: Implications for Treatment. Nat. Rev. Neurol. 6 (6), 309–317. doi:10.1038/nrneurol.2010.52
Schapira, A. H. V., Chaudhuri, K. R., and Jenner, P. (2017). Non-motor Features of Parkinson Disease. Nat. Rev. Neurosci. 18 (7), 435–450. doi:10.1038/nrn.2017.62
Schulz, J. B., Matthews, R. T., Muqit, M. M., Browne, S. E., and Beal, M. F. (1995). Inhibition of Neuronal Nitric Oxide Synthase by 7-Nitroindazole Protects against MPTP-Induced Neurotoxicity in Mice. J. Neurochem. 64 (2), 936–939. doi:10.1046/j.1471-4159.1995.64020936.x
Scorza, F. A., Henriques, L. D., and Albuquerque, M. (2001). Doença de Parkinson: tratamento medicamentoso e seu impacto na reabilitação de seus portadores. Mundo Saúde 25 (4), 365–370.
Sharabi, Y., Vatine, G. D., and Ashkenazi, A. (2021). Parkinson's Disease outside the Brain: Targeting the Autonomic Nervous System. Lancet Neurol. 20 (10), 868–876. doi:10.1016/S1474-4422(21)00219-2
Sian, J., Gerlach, M., Youdim, M. B., and Riederer, P. (1999). Parkinson's Disease: a Major Hypokinetic Basal Ganglia Disorder. J. Neural Transm. (Vienna) 106 (5–6), 443–476. doi:10.1007/s007020050171
Silva, A. S., Ariza, D., Dias, D. P., Crestani, C. C., and Martins-Pinge, M. C. (2015). Cardiovascular and Autonomic Alterations in Rats with Parkinsonism Induced by 6-OHDA and Treated with L-DOPA. Life Sci. 127, 82–89. doi:10.1016/j.lfs.2015.01.032
Simon, D. K., Tanner, C. M., and Brundin, P. (2020). Parkinson Disease Epidemiology, Pathology, Genetics, and Pathophysiology. Clin. Geriatr. Med. 36 (1), 1–12. doi:10.1016/j.cger.2019.08.002
Solla, P., Cadeddu, C., Cannas, A., Deidda, M., Mura, N., Mercuro, G., et al. (2015). Heart Rate Variability Shows Different Cardiovascular Modulation in Parkinson's Disease Patients with Tremor Dominant Subtype Compared to Those with Akinetic Rigid Dominant Subtype. J. Neural Transm. (Vienna) 122 (10), 1441–1446. doi:10.1007/s00702-015-1393-5
Sorensen, G. L., Mehlsen, J., and Jennum, P. (2013). Reduced Sympathetic Activity in Idiopathic Rapid-Eye-Movement Sleep Behavior Disorder and Parkinson's Disease. Auton. Neurosci. 179 (1–2), 138–141. doi:10.1016/j.autneu.2013.08.067
Strano, S., Fanciulli, A., Rizzo, M., Marinelli, P., Palange, P., Tiple, D., et al. (2016). Cardiovascular Dysfunction in Untreated Parkinson's Disease: A Multi-Modality Assessment. J. Neurol. Sci. 370, 251–255. doi:10.1016/j.jns.2016.09.036
Sung, V. W., and Nicholas, A. P. (2013). Nonmotor Symptoms in Parkinson's Disease: Expanding the View of Parkinson's Disease beyond a Pure Motor, Pure Dopaminergic problemNeurologic Clinics. Neurol. Clin. 31, S1–S16. doi:10.1016/j.ncl.2013.04.013
Teive, H. A. G. (2006). “Neuroproteção: fatos, mitos e quimeras,” in Teive HAG. Doença de Parkinson: estratégias atuais de tratamento. Editors L. A. F. Andrade, R. E. Barbosa, and F. Cardoso (São Paulo: Segmento Farma), 17–35.
Tieu, K., Ischiropoulos, H., and Przedborski, S. (2003). Nitric Oxide and Reactive Oxygen Species in Parkinson's Disease. IUBMB Life 55 (6), 329–335. doi:10.1080/1521654032000114320
Turossi Amorim, E. D., de Jager, L., Martins, A. B., Rodrigues, A. T., Cruz Lucchetti, B. F., Ariza, D., et al. (2019). Glutamate and GABA Neurotransmission Are Increased in Paraventricular Nucleus of Hypothalamus in Rats Induced to 6-OHDA Parkinsonism: Involvement of nNOS. Acta Physiol. (Oxf) 226 (3), e13264–16. doi:10.1111/apha.13264
Keywords: arterial pressure, heart rate, hypotension, 6-OHDA, NO-synthase, parkinsonism
Citation: Martins-Pinge MC, de Jager L, de Campos BH, Bezerra LO, Turini PG and Pinge-Filho P (2022) Nitric Oxide Involvement in Cardiovascular Dysfunctions of Parkinson Disease. Front. Pharmacol. 13:898797. doi: 10.3389/fphar.2022.898797
Received: 17 March 2022; Accepted: 20 June 2022;
Published: 11 July 2022.
Edited by:
Nidhi Agarwal, Jamia Hamdard University, IndiaReviewed by:
José Ronaldo dos Santos, Federal University of Sergipe, BrazilCopyright © 2022 Martins-Pinge, de Jager, de Campos, Bezerra, Turini and Pinge-Filho. This is an open-access article distributed under the terms of the Creative Commons Attribution License (CC BY). The use, distribution or reproduction in other forums is permitted, provided the original author(s) and the copyright owner(s) are credited and that the original publication in this journal is cited, in accordance with accepted academic practice. No use, distribution or reproduction is permitted which does not comply with these terms.
*Correspondence: Marli Cardoso Martins-Pinge, martinspinge@uel.br