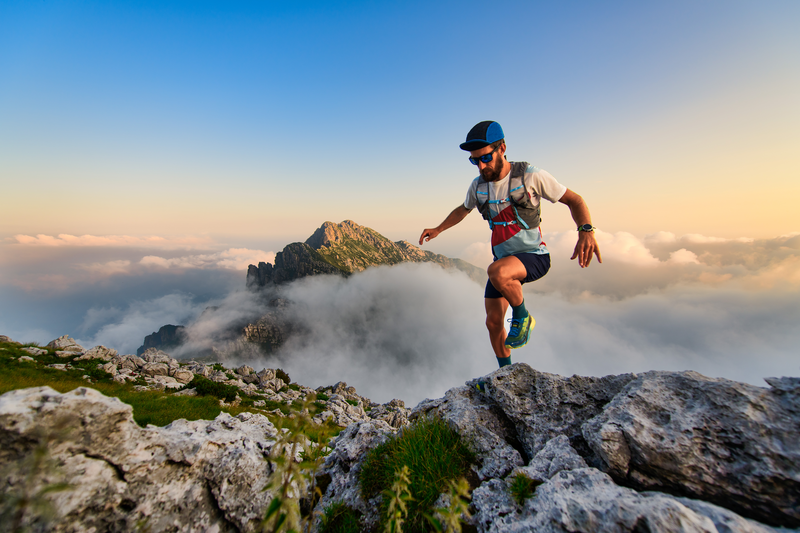
95% of researchers rate our articles as excellent or good
Learn more about the work of our research integrity team to safeguard the quality of each article we publish.
Find out more
ORIGINAL RESEARCH article
Front. Pharmacol. , 29 April 2022
Sec. Respiratory Pharmacology
Volume 13 - 2022 | https://doi.org/10.3389/fphar.2022.898630
This article is part of the Research Topic Drug Prevention and Control of Ventilator-Associated Pneumonia Volume II View all 12 articles
Background: Ventilator-associated pneumonia (VAP) is the most widespread and life-threatening nosocomial infection in intensive care units (ICUs). The duration of antibiotic use is a good predictor of prognosis in patients with VAP, but the ideal duration of antibiotic therapy for VAP in critically ill patients has not been confirmed. Research is therefore needed into the optimal duration of antibiotic use and its impact on VAP.
Methods: The Medical Information Mart for Intensive Care database included 1,609 patients with VAP. Chi-square or Student’s t-tests were used to compare groups, and Cox regression analysis was used to investigate the factors influencing the prognoses of patients with VAP. Nonlinear tests were performed on antibiotic use lasting <7, 7–10, and >10 days. Significant factors were included in the model for sensitivity analysis. For the subgroup analyses, the body mass indexes (BMIs) of patients were separated into BMI <30 kg/m2 and BMI ≥30 kg/m2, with the criterion of statistical significance set at p < 0.05. Restricted cubic splines were used to analyze the relationship between antibiotic use duration and mortality risk in patients with VAP.
Results: In patients with VAP, the effects of antibiotic use duration on the outcomes were nonlinear. Antibiotic use for 7–10 days in models 1–3 increased the risk of antibiotic use by 2.6020-, 2.1642-, and 2.3263-fold relative to for >10 days, respectively. The risks in models 1–3 for <7 days were 2.6510-, 1.9933-, and 2.5151-fold higher than those in models with >10 days of antibiotic use, respectively. These results were robust across the analyses.
Conclusions: The duration of antibiotic treatment had a nonlinear effect on the prognosis of patients with VAP. Antibiotic use durations of <7 days and 7–10 days both presented risks, and the appropriate duration of antibiotic use can ensure the good prognosis of patients with VAP.
Ventilator-associated pneumonia (VAP) is one of the most prominent hospital-acquired diseases in intensive care units (ICUs), and has a substantial risk of adverse effects (Xu et al., 2021). The prevalence of VAP is decreasing according to a hospital report from the United States Healthcare Safety Network, with about 10% of patients who require mechanical ventilation being diagnosed with VAP, a rate that has remained steady over the last decade. Each patient incurs additional VAP-related costs of approximately US$ 40,000 (Kalil et al., 2016). In the United States, epidemiological studies have confirmed that the incidence of VAP ranges from 2 to 16 per 1,000 ventilator days (Torres et al., 2017). VAP is recognized as pneumonia that occurs at least 48 h following endotracheal intubation or mechanical ventilation and is marked by infection-related symptoms such as widespread fever, new or progressive pulmonary infiltrations, and changes in the white blood cell count (Grief and Loza, 2018; Galhardo et al., 2020). VAP is associated with difficulty in weaning, prolonged mechanical ventilation and hospitalization times, and increased hospitalization costs for patients, which increase the economic costs to the health care system and consume huge medical resources (Wu et al., 2019; Pozuelo-Carrascosa et al., 2020).
Antibiotics are widely used to treat infectious diseases, and China is one of the world’s major producers and consumers of antibiotics. Antibiotics are used by about half of all hospital outpatients in China, according to linked publications, and antibiotic prescriptions account for about half of all medications dispensed by hospitals. The top five antibiotics taken by Chinese in 2013 were cephalexin, amoxicillin, ofloxacin, tetracycline, and norfloxacin (Qiao et al., 2018). Antibiotic resistance is higher in China than in Western countries, and drug-resistant microorganisms are becoming more common (Ding et al., 2019).
Previous reports suggest that infection causes one-third to one-half of all VAP-related deaths, with more fatalities for Pseudomonas aeruginosa and Acinetobacter species (Garnacho-Montero et al., 2005; Micek et al., 2015; Lima et al., 2020). Antibiotic resistance has also increased in common associated pathogens, and VAP risk is time-dependent, potentially leading to significant time-dependent biases, making it more difficult to empirically determine the duration of antibiotic use for these infections (Torres et al., 2017; Fernando et al., 2020; Bassetti et al., 2022). Pathogen diagnosis in patients with VAP typically requires an invasive quantitative culture of the lower respiratory tract using endotracheal aspirates, bronchoalveolar lavage fluid, protective specimen brushes, or semiquantitative noninvasive breath sampling (Arthur et al., 2016). However, due to the stringent growth requirements for detecting pathogens or other aspects of culture methods, important pathogens might not be detected, and it may be difficult to distinguish detected microorganisms from colonizing bacteria and actual pathogens.
While treatment is empirical until definitive results are available, the emergence of multidrug-resistant organisms, particularly in ICUs, necessitates determining the optimal antibiotic use duration (Timsit et al., 2017; Cilloniz et al., 2020; Yoo et al., 2020). In ICU patients, VAP is also the leading cause of antibiotic use (Koulenti et al., 2017), and guidelines recommend that adequate doses should be prescribed to patients with VAP to ensure early, appropriate, broad-spectrum antibiotic treatment, and to optimize the antibacterial treatment effect on patients with VAP by starting at the correct time. Appropriate and adequate treatment has been a consistent factor related to mortality rates (Guidelines for the management of, 2005). However, the importance of immediately administering antibiotics must always be matched against unnecessary hazards from antibiotics such as resistance and secondary infections (Lu et al., 2011).
Obese patients [body mass index (BMI) ≥30 kg/m2] had significantly lower 90-days mortality than nonobese patients (BMI <30 kg/m2) in a 2021 study (Nseir et al., 2021). BMI was also linked to a higher incidence of ICU-acquired infections. In obese patients, continuous antibiotics infusion can optimize the duration of time-dependent antibiotic exposure above the minimum inhibitory concentration (MIC), maximizing apoptosis (De Pascale et al., 2015). In patients with VAP, the optimal duration of antibiotic use could be used as a predictor for swift clinical assessments and prognostic research. It will be important to arrange innovative treatment strategies for patients with VAP if this indicator could effectively predict the probability of negative outcomes. Therefore, a more-thorough and precise insight into the role of an appropriate duration of antibiotic use in the prognosis of patients with VAP is critical.
The purpose of this study was to explore the effect of appropriate timing of antibiotic administration on the survival of VAP patients by applying restricted cubic splines (RCSs) to data in the Medical Information Market for Intensive Care (MIMIC) database.
The Computational Physiology Laboratory of Massachusetts Institute of Technology, Beth Israel Deacon Medical Center, and Philips Healthcare jointly published the MIMIC-IV database (version 1.0) for the period of 2008–2019.
We received permission from an institutional review board of the Massachusetts Institute of Technology and Beth Israel Deaconess Medical Center for a longitudinal single-center database of clinical information on ICU patients, and data extraction from and access to the database were implemented (Yang et al., 2020). Dr. Yixian Xu, who completed the National Institutes of Health’s online training course, extracted the data (certification number: 39194349).
Patients with VAP were identified in the MIMIC-IV database. This study gathered data on the demographics, laboratory tests, medications, vital signs, surgical procedures, and other personal information of patients with VAP (Wu et al., 2021). We extracted the data of 1,609 patients with VAP from the MIMIC-IV database for inclusion in this study. All patients were older than 18 years and had been admitted to the hospital for the first time. Figure 1 illustrates the exclusion and inclusion criteria of patients in this study.
The following variables were extracted from the records of the patients with VAP in the MIMIC-IV database: 1) Demographic data and vital signs at hospital admission [length of hospital stay, serum creatinine (SCR), antibiotic use days, urine output, mean blood pressure (MBP), diastolic blood pressure (DBP), admission age, temperature, and BMI]; 2) laboratory test data [red blood cell distribution width (RDW), hematocrit, hemoglobin, red blood cell (RBC), alanine aminotransferase (ALT), platelet count, bicarbonate, basophils, base excess, and total CO2]; 3) drugs and surgical operations [norepinephrine, vasopressin, cefazolin, meropenem, vancomycin oral liquid, tracheostomy, continuous invasive mechanical ventilation for less than 96 consecutive hours (CIMV), hemodialysis, gastric catheterization (GC), percutaneous (endoscopic) gastrostomy (PEG), performance of urinary filtration (POUF), respiratory ventilation for more than 96 consecutive hours (RV), temporary tracheostomy (TT), and venous catheterization for renal dialysis; and 4) complications and severity scoring systems [chronic kidney disease (CKD), Charlson Comorbidity Index (CCI), Sequential Organ Failure Assessment (SOFA), Acute Physiology Score-III (APSIII), Simplified Acute Physiology Score-II (SAPSII), Glasgow Coma Scale (GCS), Logistic Organ Dysfunction Score (LODS), and Model for End-stage Liver (MELD)]. The impact of antibiotic use duration on the survival of patients with VAP was investigated. The endpoint for this study was survival at discharge.
Patients were divided into two groups based on whether they survived during their hospitalization. Median and IQR values and mean ± SD values were used to summarize the nonnormally and normally distributed continuous variables, respectively. Student’s t-test, the Mann-Whitney U test, one-way ANOVA, and the Kruskal-Wallis H test were used to make comparisons of nonnormally distributed statistical data. The Kolmogorov-Smirnov test was used to determine if continuous variables were normally distributed. Categorical variables are expressed as percentages or numbers. We excluded confounding variables and outliers with considerable effects, and applied Chi-square tests to categorical variables and Student’s t-tests to continuous variables, with the exceptions of survival status and length of hospital stay.
Before performing the multivariate analysis, a univariate analysis was needed for each factor to determine if the covariance inclusion criteria were met to ascertain variables that affect VAP prognosis. Finally, the duration of antibiotic use was divided into >10, 7–10, and <7 days. A nonlinear test was used to determine whether the duration of antibiotics had a nonlinear effect on VAP prognosis. For subgroup analysis, the patients were divided into nonobese and obese groups.
Cox regression was performed, significant variables were added, three models were constructed, and the sensitivity models were tested. In the Cox regression analysis, model 1 only included the duration of antibiotic use, model 2 included some other variables, and model 3 included all key variables. Restricted cubic splines are good at dealing with the nonlinear relationship between continuous variables and response variables, and they can locate crucial key points. p < 0.05 was considered statistically significant. However, using data that had more than 20% missing or data that had missing points, we used the “vim” (Templ et al., 2012) and “MICE” (Zhang, 2016) (R software packages for multiple imputations using Templ’s method for exploration and data visualization. Excel and R (ggplot2, RMS package) were used for the statistical analysis.
Of the 1,609 patients with VAP, 368 died in the hospital. Variables from both groups were displayed and compared, and patients were separated into two groups depending on their survival status. Patients who survived at a median age of 63 (51, 73) days were younger than those who died at 70 (60, 80) years. Surviving patients were less likely to develop complications such as hypertension, chronic obstructive pulmonary disease (COPD), and diabetes than those who died. All variables affected VAP prognosis differently in this study. The baseline characteristics, laboratory parameters, and vital signs of survivors and deceased patients during hospitalization are listed in Table 1.
In the univariate Cox regression analysis, model 3 included all variables for which p < 0.05. The results indicated that CKD, norepinephrine, vasopressin, cefazolin, tracheostomy, GC, PEG, POUF, TT, antibiotic use duration, APSIII, CCI, SCR, GCS score, SOFA score, LODS, MELD score, SAPSII, BMI, urine output, DBP, MBP, temperature, admission age, hemoglobin, RBC, RDW, ALT, platelet count, bicarbonate, and basophils were prognostic factors for patients with VAP (all p < 0.05, Table 2).
RCSs were used to perform the association analysis between duration of antibiotic use and risk of all-cause mortality from VAP. The RCS test yielded statistically significant findings (Table 3). Model 1 included univariate (antibiotic use duration) analyses, as illustrated in Figure 2A. The hazard ratios (HRs) of model 1 were 2.6020 (95% confidence interval [CI] = 1.8940– 3.5750, p < 0.001) and 2.6510 (95% CI = 1.9880–3.5360, p < 0.01) for antibiotic use durations of 7–10 and <7 days, respectively. Model 2 included CKD, norepinephrine, vasopressin, cefazolin, tracheostomy, GC, PEG, POUF, TT, SCR, BMI, urine output, DBP, MBP, antibiotic use duration, temperature, admission age, hemoglobin, RBC, RDW, ALT, platelet count, bicarbonate, and basophils (Figure 2B). Model 2 had HRs of 2.1642 (95% CI = 1.5394–3.0426, p < 0.001) and 1.9933 (95% CI = 1.4077–2.8226, p < 0.001) for antibiotic use durations of 7–10 and <7 days, respectively. As demonstrated in Figure 2C, the significant variables from the Cox univariate regression analysis were incorporated into Model 3. Model 3 had HRs of 2.3263 (95% CI = 1.6375–3.3047, p < 0.001) and 2.5151 (95% CI = 1.7424–3.6307, p < 0.001) for antibiotic use durations of 7–10 and <7 days, respectively. In all models, the prognostic curves for VAP were nonlinear for antibiotic use duration. the antibiotic use duration curve showed a significant change trend at around 12 days (Figure 2, all p < 0.001). Notably, as the adjustment variable increased, the risk for antibiotic use for ≤10 days was more pronounced, and statistically significant that for >10 days.
TABLE 3. Cox regression analyses of the relationship between the duration of antibiotic use and VAP prognosis.
FIGURE 2. The effect of different doses of the duration of antibiotic use on the prognosis of VAP. (A) Univariate. (B) Adjusted for CKD, Norepinephrine, Vasopressin, CefazoLIN, Tracheostomy, GC, PEG, POUF, TT, SCR, BMI, Urineoutput, DBP, MBP, Antibiotics day, Temperature, Admission age, Hemoglobin, RBC, RDW, ALT, Platelet Count, Bicarbonate and Basophils. (C) Adjusted for Antibiotics day, CKD, Norepinephrine, Vasopressin, CefazoLIN, Tracheostomy, GC, PEG, POUF, TT, APSIII, Charlson comorbidity index, SCR, GCS, SOFA, LODS, MELD, SAPSII, BMI, Urineoutput, DBP, MBP, Temperature, Admission age, Hemoglobin, RBC, RDW, ALT, Platelet Count, Bicarbonate and Basophils.
The Cox regression analysis revealed that BMI was a predictive factor for patients with VAP. Tables 4, 5 list the baseline characteristics of each subgroup and the Cox regression analysis findings, with the outcomes of both groups being nonlinear (p < 0.05). Figure 3 depicts the nonlinear curve comparison between the two groups. The curve of antibiotic use time was higher in the nonobese than the obese group. This result reflects that patients with VAP and low BMI have a higher risk from an antibiotic use duration >10 days than those with high BMI. These results were the same in all models.
FIGURE 3. The effect of the duration of antibiotic use on the prognosis of VAP between different BMI groups. (A) Low-BMI group (BMI<30 kg/m2). (B) High-BMI group (BMI ≥30 kg/m2).
VAP is a primary risk factor for mortality among critically ill patients. Treatment strategies may benefit from objective prognostic assessment tools (International Symposium on Intensive Care, 2016). Due to the obvious severity of VAP and the relative vulnerability of critically ill patients, timely and appropriate antimicrobial therapy is critical for reducing its burden, and infection control is implemented by avoiding prolonged ventilation with adequate sedation and weaning and avoiding endotracheal tubes. Biofilm formation, microaspiration of subglottic secretions, and bacterial translocation from the stomach to the upper respiratory tract and oropharynx are the basis for colonization (Martin-Loeches et al., 2018). Some studies have classified VAP into early-onset and late-onset types based on time of admission, but the bacteriological differences between the two are unclear. As an outcome, antibiotic selection based on pneumonia onset may result in over-and undertreatment of broad-spectrum drugs, so determining the perfect duration of antibiotic use for patients with VAP is critical for ensuring good clinical outcomes (Nair and Niederman, 2015; Torres et al., 2017).
Antibiotic stewardship methods in China include bacterial resistance surveillance at the hospital level, as local antibiotic resistance statistics are crucial for guiding rational antibiotic usage, and the development of antibiotic resistance is closely related to the duration of antibiotic use. In this research, a reliable predictive model for the duration of antibiotic treatment in patients with ventilator-associated pneumonia was developed, which can assist clinicians in making decisions about the prognosis of VAP patients and the optimal duration of antibiotic treatment, improving the patient’s chance of survival and reducing the risk of social labor loss cost and national medical resources (Hu et al., 2018; Chen et al., 2021).
Globally, overuse and inappropriate use of antibiotics is widely known as the primary cause of antibiotic resistance, as well as prolonging hospital stays, increasing treatment costs, and mortality associated with infectious diseases, acute upper respiratory infections, and ventilator-related complications. The most prevalent illnesses linked to antibiotic misuse are acute upper respiratory tract infection and ventilator-associated pneumonia. Antibiotic resistance has posed a serious threat to the effectiveness of existing antibiotics and their prescription regimens, affecting people of all socioeconomic backgrounds in community-acquired and nosocomial infections, as well as high health-care costs that lead to chronic poverty, whether untreated or treated. Excessive risk of morbidity and mortality. Appropriate antibiotic treatment length can effectively prevent drug resistance, better reduce human capital loss, mitigate economic shocks suffered by poor and developing societies and countries, and lower the risk of medical poverty traps (Ahmad and Khan, 2019; Zhao et al., 2020).
The bactericidal effect of antibiotics has been observed to depend on the time when the MIC is exceeded, and it is recommended to reach a time where the MIC is 100% or even higher. It is reasonable to apply a positive PharmacoKinetics and PharmacoDynamics (PK/PD) target in patients with VAP. In patients with respiratory tract infection, the 30-days survival rate of those receiving long-term β-lactam infusion was dramatically higher than that of patients receiving an intermittent bolus, and antibiotics had a considerable degree of concentration-dependent apoptosis characteristics as well as time-dependent characteristics (Timsit et al., 2017). The optimal duration of antibiotic use should therefore be invoked as a prognostic indicator to detect the clinical outcomes of patients with VAP.
A Cox proportional-hazards regression model fitted with RCS was used in this study to explore the link between the duration of antibiotic use and the risk of all-cause mortality. Compared with a duration of antibiotic use >10 days, patients with VAP and a duration of ≤10 days had a higher mortality risk. The Cox multivariate analysis indicated that the duration of antibiotic use was a prognostic factor for patients with VAP (p < 0.001). All prediction curves for antibiotic use time in RCS had similar performances. Based on antibiotic use of >10 days, this study set a long-term antibiotic range within which the risk of VAP was lowest. Notably, the effect of antibiotic use duration on VAP prognosis was nonlinear with statistical significance (p < 0.001). When performing sensitivity analyses, all models with antibiotic use ≤10 days also had a higher mortality risk than those with >10 days. For models 1-3, the risks of using antibiotics for 7–10 days were 2.6020-, 2.1642-, and 2.3263-fold higher than for an antibiotic use duration of >10 days, respectively, while the risks of model 1–3 with an antibiotic use duration of <7 days were 2.6020-, 2.1642-, and 2.3263-fold higher, respectively.
In the subgroup analyses, the nonobese and obese groups also had a higher risk from antibiotic use ≤10 days compared with >10 days. According to Pugh et al., the VAP recurrence rate was higher for a short course of 8 days than for a longer course of 12–15 days in patients with VAP from nonfermenting Gram-negative bacilli organisms. This increased recurrence risk did not appear to be linked to increased mortality, the need for prolonged mechanical ventilation, or the time spent in the ICU (Pugh et al., 2015). Insufficient empirical antibiotic treatment has been linked to increased mortality risk (Hanes et al., 2002), and Rhodes et al. found that patients with VAP are a changing population at risk of antibiotic resistance and an underdosing response to altered antibiotic pharmacokinetic characteristics (Rhodes et al., 2018). Initial empirical treatments for VAP should therefore use antibiotics to cover all possible pathogens, including Acinetobacter baumannii, Pseudomonas aeruginosa, Stenotrophomonas maltophilia, and Methicillin-resistant Staphylococcus aureus, and pathogen identification and drug susceptibility assessments should be carried out as soon as possible. Trials to ensure the adequate coverage and appropriate dose and duration of treatment could reduce the mortality from VAP (Wang et al., 2018; Gore et al., 2020).
De Oliveira noted that across all age groups, obese patients were not more likely to develop pulmonary complications than those with normal BMI (De Oliveira et al., 2017). Wardell et al. found that obesity was not necessarily associated with poor prognoses among critically ill patients (Wardell et al., 2015). This result was consistent with recent research that confirmed the so-called obesity paradox, whereby possibly because adipose tissue appears as an active participant in regulating physiological and pathological processes in hypermetabolic states, adipocytes can not only secrete various hormones and bioactive peptides but also produce and release pro- and anti-inflammatory factors for immune regulation. This may attenuate inflammatory responses during acute disease and improve survival (Fantuzzi, 2005; Torres et al., 2019).
This study explored the relationship between duration of antibiotic use and VAP and performed subgroup analyses to identify a cutoff of approximately 12 days for the prediction curve of antibiotic use duration and quantified the risk across the curve, and also found that obesity was associated with reduced ICU mortality in patients with VAP. Future research should explore other approaches for determining the optimal duration of antibiotic use to help clinicians make better decisions for patients with VAP in ICUs.
This study had some limitations. First, the data for this study originated in the MIMIC database. There was a potential risk of bias because most participants in this database were white Americans, which restricts the generalizability of the conclusions. Second, some patient indicators were not completely reported, which resulted in information leakage. Third, the severity of VAP could not be precisely determined. Fourth, the retrospective design of the study meant that some other biases were undoubtedly present. Fifth, patient functional outcomes and post-discharge disposition for ventilator-associated pneumonia were unknown due to a lack of long-term follow-up procedures in the MIMIC-IV database. Despite these limitations, we believe that determining the optimal antibiotic duration can help to better understand the prognosis of patients with VAP in ICUs.
Considering that patients with VAP are more likely to have unfavorable clinical outcomes, selecting the appropriate antibiotic use duration is critical. VAP patients have a certain risk of death in the ICU, and our study found that the duration of antibiotic use had a nonlinear effect on the prognosis of VAP patients, with the lowest risk of in-hospital mortality in VAP when the duration of antibiotic use was 12 days, which may be a turning point in the prognosis of VAP patients. And so an accurate and reliable prognostic model for the best antibiotic duration to forecast VAP still needs to be developed.
In the furture, we will to keep researching the impact of antibiotic use duration on VAP. In order to develop a predictive model with accurate thresholds, sensitivity, and selectivity, as well as a high level of reliability, to help clinicians make concise management and treatment decisions for the prognosis of and maximizing the survival chances of patients with VAP.
The raw data supporting the conclusions of this article will be made available by the authors, without undue reservation.
YX and DH performed the statistical analysis and data interpretation. JL and HW contributed to the study concept and study design and contributed equally. FX performed literature research and data extraction. SS and XZ were responsible for the quality control of data and algorithms. All authors contributed to the writing of the manuscript and approved the final version.
The study was supported by Guangdong Provincial Key Laboratory of Traditional Chinese Medicine Informatization (2021B1212040007); National Natural Science Foundation, China (Grant No. 82071340); National foreign expert project of the Ministry of science and technology, China (Grant No. G20200019047).
The authors declare that the research was conducted in the absence of any commercial or financial relationships that could be construed as a potential conflict of interest.
All claims expressed in this article are solely those of the authors and do not necessarily represent those of their affiliated organizations, or those of the publisher, the editors and the reviewers. Any product that may be evaluated in this article, or claim that may be made by its manufacturer, is not guaranteed or endorsed by the publisher.
APSIII, acute physiology score-III; ALT, alanine aminotransferase; BMI, body mass index; CIMV, continuous invasive mechanical ventilation for less than 96 consecutive hours; CKD, chronic kidney disease; CCI, charlson comorbidity index; CI, confidence interval; DBP, diastolic blood pressure; GC, gastric catheterization; GCS, Glasgow Coma Scale; HR, hazard ratio; ICU, intensive care unit; LODS, logistic organ dysfunction score; MELD, model for end-stage liver; MIMIC-IV, medical information mart for intensive care IV; MIC, minimum inhibitory concentration; MBP, mean blood pressure; PEG, percutaneous (endoscopic) gastrostomy; POUF, performance of urinary filtration; PK/PD, pharmacokinetics and pharmacodynamics; RV, respiratory ventilation for more than 96 consecutive hours; RCS, restricted cubic spline; RBC, red blood cell; RDW, red blood cell distribution width; SCR, serum creatinine; SOFA, sequential organ failure assessment; SAPSII, simplified acute physiology score-II; TT, temporary tracheostomy; VAP, ventilator-associated pneumonia.
36th International Symposium on Intensive Care and Emergency Medicine : Brussels, Belgium. 15-18 March 2016. 2016. Crit. Care. (2016) 20Suppl. 20:94. doi:10.1186/s13054-016-1208-6
Ahmad, M., and Khan, A. U. Global Economic Impact of Antibiotic Resistance: A Review. J. Glob. Antimicrob. Resist. (2019) 19:313–316. doi:10.1016/j.jgar.2019.05.024
Arthur, L. E., Kizor, R. S., Selim, A. G., van Driel, M. L., and Seoane, L. Antibiotics for Ventilator-Associated Pneumonia. Cochrane Database Syst. Rev. (2016) 10:CD004267. doi:10.1002/14651858.CD004267.pub4
Bassetti, M., Mularoni, A., Giacobbe, D. R., Castaldo, N., and Vena, A. New Antibiotics for Hospital-Acquired Pneumonia and Ventilator-Associated Pneumonia. Semin. Respir. Crit. Care Med. (2022). doi:10.1055/s-0041-1740605
Chen, Q., Li, D., Beiersmann, C., Neuhann, F., Moazen, B., Lu, G., et al. Risk Factors for Antibiotic Resistance Development in Healthcare Settings in China: a Systematic Review. Epidemiol. Infect. e141. doi:10.1017/S0950268821001254
Cilloniz, C., Liapikou, A., and Torres, A. Advances in Molecular Diagnostic Tests for Pneumonia. Curr. Opin. Pulm. Med. (2020) 26:241–248. doi:10.1097/MCP.0000000000000668
De Oliveira, G. S., McCarthy, R. J., Davignon, K., Chen, H., Panaro, H., and Cioffi, W. G. Predictors of 30-Day Pulmonary Complications after Outpatient Surgery: Relative Importance of Body Mass Index Weight Classifications in Risk Assessment. J. Am. Coll. Surg., 225. (2017) 312, e7. doi:10.1016/j.jamcollsurg.2017.04.013
De Pascale, G., Fortuna, S., Tumbarello, M., Cutuli, S. L., Vallecoccia, M., Spanu, T., et al. Linezolid Plasma and Intrapulmonary Concentrations in Critically Ill Obese Patients with Ventilator-Associated Pneumonia: Intermittent vs Continuous Administration. Intensive Care Med. (2015) 41:103–110. doi:10.1007/s00134-014-3550-y
Ding, G., Vinturache, A., and Lu, M. Addressing Inappropriate Antibiotic Prescribing in China. CMAJ. (2019) 191:E149–E150. doi:10.1503/cmaj.181417
Fantuzzi, G. Adipose Tissue, Adipokines, and Inflammation. J. Allergy Clin. Immunol., 115. (2005) 911, 920. doi:10.1016/j.jaci.2005.02.023
Fernando, S. M., Tran, A., Cheng, W., Klompas, M., Kyeremanteng, K., Mehta, S., et al. Diagnosis of Ventilator-Associated Pneumonia in Critically Ill Adult Patients-A Systematic Review and Meta-Analysis. Intensive Care Med. (2020) 46:1170–1179. doi:10.1007/s00134-020-06036-z
Galhardo, L. F., Ruivo, G. F., Santos, F. O., Ferreira, T. T., Santos, J., Eão, M. VP.L, et al. Impact of Oral Care and Antisepsis on the Prevalence of Ventilator-Associated Pneumonia. Oral Health Prev. Dent. (2020) 18:331–336.
Garnacho-Montero, J., Ortiz-Leyba, C., Fernández-Hinojosa, E., Aldabó-Pallás, T., Cayuela, A., Marquez-Vácaro, J. A., et al. Acinetobacter Baumannii Ventilator-Associated Pneumonia: Epidemiological and Clinical Findings. Intensive Care Med. (2005) 31:649–655. doi:10.1007/s00134-005-2598-0
Gore, A., Gauthier, A. G., Lin, M., Patel, V., Thomas, D. D., Ashby, C. R., et al. The Nitric Oxide Donor, (Z)-1-[N-(2-aminoethyl)-N-(2-ammonioethyl)amino]diazen-1-ium-1,2-diolate (DETA-NONOate/D-NO), Increases Survival by Attenuating Hyperoxia-Compromised Innate Immunity in Bacterial Clearance in a Mouse Model of Ventilator-Associated Pneumonia. Biochem. Pharmacol. (2020) 176:113817. doi:10.1016/j.bcp.2020.113817
Grief, S. N., and Loza, J. K. Guidelines for the Evaluation and Treatment of Pneumonia. Prim. Care. (2018) 45:485–503. doi:10.1016/j.pop.2018.04.001
Guidelines for the Management of Adults with Hospital-Acquired, Ventilator-Associated, and Healthcare-Associated Pneumonia. Am. J. Respir. Crit. Care Med. (2005) 171:388–416. doi:10.1164/rccm.200405-644ST
Hanes, S. D., Demirkan, K., Tolley, E., Boucher, B. A., Croce, M. A., Wood, G. C., et al. Risk Factors for Late-Onset Nosocomial Pneumonia Caused by Stenotrophomonas Maltophilia in Critically Ill Trauma Patients. Clin. Infect. Dis. (2002) 35:228–235. doi:10.1086/341022
Hu, F., Zhu, D., Wang, F., and Wang, M. Current Status and Trends of Antibacterial Resistance in China. Clin. Infect. Dis., 67. (2018) 67suppl_2:S128–S134. doi:10.1093/cid/ciy657
Kalil, A. C., Metersky, M. L., Klompas, M., Muscedere, J., Sweeney, D. A., Palmer, L. B., et al. Executive Summary: Management of Adults with Hospital-Acquired and Ventilator-Associated Pneumonia: 2016 Clinical Practice Guidelines by the Infectious Diseases Society of America and the American Thoracic Society. Clin. Infect. Dis., 63. (2016) 575, 82. doi:10.1093/cid/ciw353doi:10.1093/cid/ciw504
Koulenti, D., Tsigou, E., and Rello, J. Nosocomial Pneumonia in 27 ICUs in Europe: Perspectives from the EU-VAP/CAP Study. Eur. J. Clin. Microbiol. Infect. Dis. (2017) 36:1999–2006. doi:10.1007/s10096-016-2703-z
Lima, W. G., Brito, J. C. M., and da Cruz Nizer, W. S. Ventilator-associated Pneumonia (VAP) Caused by Carbapenem-Resistant Acinetobacter Baumannii in Patients with COVID-19: Two Problems, One Solution? Med. Hypotheses. (2020) 144:110139. doi:10.1016/j.mehy.2020.110139
Lu, Q., Yang, J., Liu, Z., Gutierrez, C., Aymard, G., and Rouby, J. J. Nebulized Ceftazidime and Amikacin in Ventilator-Associated Pneumonia Caused by Pseudomonas aeruginosa. Am. J. Respir. Crit. Care Med. (2011) 184:106–115. doi:10.1164/rccm.201011-1894OC
Martin-Loeches, I., Rodriguez, A. H., and Torres, A. New Guidelines for Hospital-Acquired Pneumonia/ventilator-Associated Pneumonia: USA vs. Europe. Curr. Opin. Crit. Care. (2018) 24:347–352. doi:10.1097/MCC.0000000000000535
Micek, S. T., Wunderink, R. G., Kollef, M. H., Chen, C., Rello, J., Chastre, J., et al. An International Multicenter Retrospective Study of Pseudomonas aeruginosa Nosocomial Pneumonia: Impact of Multidrug Resistance. Crit. Care. (2015) 19:219. doi:10.1186/s13054-015-0926-5
Nair, G. B., and Niederman, M. S. Ventilator-associated Pneumonia: Present Understanding and Ongoing Debates. Intensive Care Med. (2015) 41:34–48. doi:10.1007/s00134-014-3564-5
Nseir, S., Le Gouge, A., Pouly, O., Lascarrou, J.-B., Lacherade, J.-C., Mira, J.-P., et al. Relationship between Obesity and Ventilator-Associated Pneumonia. Chest. (2021) 159:2309–2317. doi:10.1016/j.chest.2021.01.081
Pozuelo-Carrascosa, D. P., Herráiz-Adillo, Á., Alvarez-Bueno, C., Añón, J. M., Martínez-Vizcaíno, V., and Cavero-Redondo, I.Herráiz-Adillo Á Subglottic Secretion Drainage for Preventing Ventilator-Associated Pneumonia: an Overview of Systematic Reviews and an Updated Meta-Analysis. Eur. Respir. Rev., 29. (2020) 29155. doi:10.1183/16000617.0107-2019
Pugh, R., Grant, C., Cooke, R. P., and Dempsey, G. Short-course versus Prolonged-Course Antibiotic Therapy for Hospital-Acquired Pneumonia in Critically Ill Adults. Cochrane Database Syst. Rev. (2015) 8:CD007577. doi:10.1002/14651858.CD007577doi:10.1002/14651858.CD007577.pub2
Qiao, M., Ying, G. G., Singer, A. C., and Zhu, Y. G. Review of Antibiotic Resistance in China and its Environment. Environ. Int. (2018) 110:160–172. doi:10.1016/j.envint.2017.10.016
Rhodes, N. J., Cruce, C. E., O'Donnell, J. N., Wunderink, R. G., and Hauser, A. R. Resistance Trends and Treatment Options in Gram-Negative Ventilator-Associated Pneumonia. Curr. Infect. Dis. Rep. (2018) 20:3. doi:10.1007/s11908-018-0609-x
Templ, M., Alfons, A., and Filzmoser, P. Exploring Incomplete Data Using Visualization Techniques. Adv. Data Anal. Classif. (2012) 6:29–47. doi:10.1007/s11634-011-0102-y
Timsit, J. F., Pilmis, B., and Zahar, J. R. How Should We Treat Hospital-Acquired and Ventilator-Associated Pneumonia Caused by Extended-Spectrum β-Lactamase-Producing Enterobacteriaceae? Semin. Respir. Crit. Care Med.(2017) 38:287–300. doi:10.1055/s-0037-1603112
Torres, A., Niederman, M. S., Chastre, J., Ewig, S., Fernandez-Vandellos, P., Hanberger, H., et al. International ERS/ESICM/ESCMID/ALAT guidelines for the management of hospital-acquired pneumonia and ventilator-associated pneumonia: Guidelines for the management of hospital-acquired pneumonia (HAP)/ventilator-associated pneumonia (VAP) of the European Respiratory Society (ERS), European Society of Intensive Care Medicine (ESICM), European Society of Clinical Microbiology and Infectious Diseases (ESCMID) and Asociación Latinoamericana del Tórax (ALAT). Eur. Respir. J., 50. (2017) 503.doi:10.1183/13993003.00582-2017
Torres, S., Fabersani, E., Marquez, A., and Gauffin-Cano, P. Adipose Tissue Inflammation and Metabolic Syndrome. The Proactive Role of Probiotics. Eur. J. Nutr. (2019) 58:27–43. doi:10.1007/s00394-018-1790-2
Wang, Y., Zhang, R., and Liu, W. Distribution and Drug Resistance of Pathogenic Bacteria in Ventilator-Associated Pneumonia at a Local Hospital of North-eastern China. Infect. Drug Resist. (2018) 11:2249–2255.doi:10.2147/IDR.S172598
Wardell, S., Wall, A., Bryce, R., Gjevre, J. A., Laframboise, K., and Reid, J. K. The Association between Obesity and Outcomes in Critically Ill Patients. Can. Respir. J. (2015) 22:23–30. doi:10.1155/2015/938930
Wu, D., Wu, C., Zhang, S., and Zhong, Y. Risk Factors of Ventilator-Associated Pneumonia in Critically III Patients. Front. Pharmacol. (2019) 10:482. doi:10.3389/fphar.2019.00482
Wu, W.-T., Li, Y.-J., Feng, A.-Z., Li, L., Huang, T., Xu, A.-D., et al. Data Mining in Clinical Big Data: the Frequently Used Databases, Steps, and Methodological Models. Mil. Med Res. (2021) 8:44. doi:10.1186/s40779-021-00338-z
Xu, E., Pérez-Torres, D., Fragkou, P. C., Zahar, J.-R., and Koulenti, D. Nosocomial Pneumonia in the Era of Multidrug-Resistance: Updates in Diagnosis and Management. Microorganisms., 9. (2021) 534 doi:10.3390/microorganisms9030534
Yang, J., Li, Y., Liu, Q., Li, L., Feng, A., Wang, T., et al. Brief Introduction of Medical Database and Data Mining Technology in Big Data Era. J. Evid. Based Med. (2020) 13:57–69. doi:10.1111/jebm.12373
Yoo, I. Y., Huh, K., Shim, H. J., Yun, S. A., Chung, Y. N., Kang, O. K., et al. Evaluation of the BioFire FilmArray Pneumonia Panel for Rapid Detection of Respiratory Bacterial Pathogens and Antibiotic Resistance Genes in Sputum and Endotracheal Aspirate Specimens. Int. J. Infect. Dis. (2020) 95:326–331. doi:10.1016/j.ijid.2020.03.024
Zhang, Z. Multiple Imputation with Multivariate Imputation by Chained Equation (MICE) Package. Ann. Transl Med. (2016) 4:30. doi:10.3978/j.issn.2305-5839.2015.12.63
Keywords: MIMIC-IV1, ventilator-associated pneumonia, restricted cubic splines, duration of antibiotic use, intensive care unit, mortality, prediction
Citation: Xu Y, Han D, Xu F, Shen S, Zheng X, Wang H and Lyu J (2022) Using Restricted Cubic Splines to Study the Duration of Antibiotic Use in the Prognosis of Ventilator-Associated Pneumonia. Front. Pharmacol. 13:898630. doi: 10.3389/fphar.2022.898630
Received: 17 March 2022; Accepted: 12 April 2022;
Published: 29 April 2022.
Edited by:
Zhi Mao, People’s Liberation Army General Hospital, ChinaReviewed by:
Qilin Yang, The Second Affiliated Hospital of Guangzhou Medical University, ChinaCopyright © 2022 Xu, Han, Xu, Shen, Zheng, Wang and Lyu. This is an open-access article distributed under the terms of the Creative Commons Attribution License (CC BY). The use, distribution or reproduction in other forums is permitted, provided the original author(s) and the copyright owner(s) are credited and that the original publication in this journal is cited, in accordance with accepted academic practice. No use, distribution or reproduction is permitted which does not comply with these terms.
*Correspondence: Jun Lyu, bHl1anVuMjAyMEBqbnUuZWR1LmNu; Hao Wang, aGFvd2FuZ0BqbnUuZWR1LmNu
†These authors have contributed equally to this work and share first authorship
Disclaimer: All claims expressed in this article are solely those of the authors and do not necessarily represent those of their affiliated organizations, or those of the publisher, the editors and the reviewers. Any product that may be evaluated in this article or claim that may be made by its manufacturer is not guaranteed or endorsed by the publisher.
Research integrity at Frontiers
Learn more about the work of our research integrity team to safeguard the quality of each article we publish.