- 1Institute of Liver Diseases, Shuguang Hospital Affiliated to Shanghai University of Traditional Chinese Medicine, Shanghai, China
- 2Central Laboratory, Baoshan District Hospital of Integrated Traditional Chinese and Western Medicine of Shanghai, Shanghai, China
- 3Shanghai Key Laboratory of Traditional Chinese Clinical Medicine, Shanghai, China
- 4Key Laboratory of Liver and Kidney Diseases, Shanghai University of Traditional Chinese Medicine, Ministry of Education, Shanghai, China
Non-alcoholic fatty liver disease (NAFLD) is a liver disorder characterized by abnormal accumulation of hepatic fat and inflammatory response with complex pathogenesis. Over activation of the pyrin domain-containing protein 3 (NLRP3) inflammasome triggers the secretion of interleukin (IL)-1β and IL-18, induces pyroptosis, and promotes the release of a large number of pro-inflammatory proteins. All of which contribute to the development of NAFLD. There is a great deal of evidence indicating that plant-derived active ingredients are effective and safe for NAFLD management. This review aims to summarize the research progress of 31 active plant-derived components (terpenoids, flavonoids, alkaloids, and phenols) that alleviate lipid deposition, inflammation, and pyroptosis by acting on the NLRP3 inflammasome studied in both in vitro and in vivo NAFLD models. These studies confirmed that the NLRP3 inflammasome and its related genes play a key role in NAFLD amelioration, providing a starting point for further study on the correlation of plant-derived compounds treatment with the NLRP3 inflammasome and NAFLD.
1 Introduction
Non-alcoholic fatty liver disease (NAFLD), defined as the presence of substantial lipid deposits in the liver not resulting from alcohol consumption, covers a spectrum of disorders. These include non-alcoholic fatty liver (NAFL), non-alcoholic steatohepatitis (NASH), NASH-associated fibrosis, cirrhosis, and liver cancer. NAFLD has replaced viral hepatitis as the world’s most common liver disorder, accounting for about 25% of the global incidence of liver disease (Younossi et al., 2016). Patients with NAFLD have a significantly increased risk of cancer (Kanwal et al., 2018; Desai et al., 2019). Insulin resistance, nutritional imbalances, genetic factors, and intestinal microbiota induce the occurrence and development of NAFLD (Buzzetti et al., 2016). Increasing evidence indicates that inflammatory signaling pathways are associated in NAFLD progression. NLRP3 inflammasome acts as a sensor for multiple danger signals, which include damage-related molecular patterns (DAMPs) of endogenous or exogenous pathogen signals and pathogen-associated molecular patterns (PAMPs)-induced aseptic inflammation (Prochnicki et al., 2016). The NLRP3 inflammasome plays a key role in NAFLD development (Wu et al., 2015; Yang G. et al., 2016; Wei et al., 2019).
The pathogenesis of NAFLD is complex, and the pathogenesis and disease processes in different patients are diverse. It is known that the NLRP3 inflammasome plays a major role in inflammation because the inflammatory process is blunted under experimental conditions that inhibit the NLRP3 inflammasome (Di Virgilio 2007). It is expressed in hepatic hepatocytes, myofibroblasts, endothelial cells, epithelial cells, stellate cells, and Kupffer cells (KCs) (Negash and Gale 2015). Meanwhile, NLRP3 inflammasome-related components increase during liver damage (Ganz et al., 2011). At present, no drug has been approved by the Food and Drug Administration (FDA) for the treatment of NAFLD. A number of studies have shown that NLRP3 inflammasome-related inhibitors have therapeutic effects on NAFLD. For example, MCC950, the most widely studied inhibitor, specifically inhibits the assembly of the NLRP3 inflammasome and has a strong inhibitory effect on the inflammatory progression of NAFLD (Mridha et al., 2017). Many plant-derived active ingredients also inhibit NLRP3 inflammasome activation or assembly.
Plant-derived compounds exhibit a rich breadth of structures, diverse biological activities, and high safety. Many have lipid-lowering, hypoglycemic, anti-inflammatory, and hepatoprotective effects and show significant inhibitory activities on NLRP3 inflammasome, making them valuable resources for the development of new NAFLD drugs. Therefore, screening of NLRP3 inhibitors from plant-derived compounds to treat NAFLD has sparked wide interest in the scientific community. In this review, we discussed the plant-derived active ingredients that regulate the NLRP3 inflammasome to improve NAFLD in vivo and in vitro, including terpenoids, flavonoids, phenols, and alkaloids. This information may provide a reference for further research and innovative drug development for the prevention and treatment of NAFLD.
2 An overview of NLRP3 inflammasome
The NLRP3 inflammasome is one of the most significant multimeric protein complexes involved in immune system functions and is widely regarded as the key mechanistic effector of intracellular inflammation (Chen et al., 2015). It consists of the sensor moiety NLRP3, the adaptor protein ASC, and the effector protease caspase-1 (procaspase-1). NLRP3 is a multi-protein complex consisting of three domains: the central nucleotide-binding domain (NACHT), the carboxy-terminal leucine-rich repeat sequence (LRR), and the amino-terminal pyrin domain (PYD). NLRP3 contains a PYD, ASC contains PYD and CARD domains, which interact with ASC through PYD after activation, and the CARD domain of ASC recruits the CARD domain of procaspase-1, which contains CARD and caspase-1 domains in the inactive state. The tight connection of these three proteins forms the NLRP3-ASC-procaspase-1 complex, also known collectively as the NLRP3 inflammasome (Schroder et al., 2010). The precursor molecule procaspase-1 cleaved itself to form active caspase-1, including two subunits, p20 and p10, which promoted the shearing of pro-IL-1β and pro-IL-18 into mature IL-1β and IL-18, leading to inflammation (Haneklaus and O'Neill 2015). Although a moderate inflammatory response helps to resist the invasion of pathogenic microorganisms, the NLRP3 inflammasome can cause great damage to the body if it is over-activated, resulting in excessive release of cytokines such as IL-1β and IL-18, and the resulting “cytokine storm” inflammatory cascade.
Activation of the NLRP3 inflammasome involves initiation and activation steps. The initiation step (Signal 1) is provided by an inflammatory stimulant that stimulates the toll-like receptor (TLR) and then induces nuclear factor kappa-B (NF-κB) activation. NF-κB further promotes the expression of NLRP3, pro-IL-1β, and pro-IL-18. The activation step (Signal 2) includes ion channel opening caused by activation of purinergic receptor X7 (P2X7) on the cell surface or release of intracellular ATP, triggering intracellular K+ outflow and Ca2+ influx (Munoz-Planillo et al., 2013; Kelley et al., 2019), damaged mitochondria releasing large amounts of reactive oxygen species (ROS) (Cruz et al., 2007; Zhou et al., 2010; Zhou et al., 2011), phagocytic irritants such as amyloid-beta leading to lysosome rupture and cathepsin B release (Campden and Zhang 2019; Chevriaux et al., 2020; Svadlakova et al., 2020), which induce activation of the NLRP3 inflammasome. Activation of the NLRP3 inflammasome can lead to recruitment of ASC and caspase-1, promotion of self-oligomerization, self-shearing and activation of caspase-1, and lysis of pro-IL-1β and pro-IL-18 to form mature IL-1β and IL-18 and exert inflammatory effects (Shao et al., 2019).
3 The NLRP3 inflammasome and NAFLD
3.1 The NLRP3 inflammasome has been confirmed to be involved in the development of NAFLD
In recent years, the role of the NLRP3 inflammasome activation in NAFLD/NASH has received considerable attention. In a liver sample from 77 full-spectrum NAFLD patients, mRNA and protein levels of NLRP3 and IL-18 were significantly higher than those in non-NASH patients, and IL-1β mRNA expression was associated with collagen-1A1, a pro-fibrosis gene expressed by activated hepatic stellate cells (HSCs) (Wree et al., 2014). In another study, NLRP3 inflammasome-associated genes (ASC, caspase-1, and NLRP3) were significantly upregulated in patients with NASH (Csak et al., 2011).
In animal studies, hepatic NLRP3, ASC, procaspase-1, and pro-IL-1β mRNA expression increased in methionine-choline deficient diet (MCD)-fed mice (Csak et al., 2011). Interestingly, MCD-induced NASH in NLRP3−/− mice showed that serum alanine transaminase (ALT), aspartate transaminase (AST), IL-1β, and IL-18 levels were lower than those in non-knockout mice and significantly reduced hepatocyte inflammation, hepatomegaly, and liver fibrosis (Cai et al., 2017). Another study using NLRP3−/−, ASC−/−, and caspase-1−/− mice also found that hepatic steatosis and inflammation in NASH were indeed associated with activation of the NLRP3 inflammasome (Henao-Mejia et al., 2012). The NLRP3 inhibitor MCC950 can significantly block NLRP3 expression and ameliorate NASH pathology and lipid deposition in MCD-fed and foz/foz-induced NASH mouse models and hepatic NLRP3, procaspase-1, active caspase-1, pro-IL-1β, and IL-1β levels were significantly decreased (Mridha et al., 2017).
In in vitro experiments, MCC950 reduced IL-1β release by KCs and bone marrow-derived macrophages (BMDMs) and eliminated associated neutrophil migration (Mridha et al., 2017). NLRP3 deletion inhibited the upregulation of NLRP3, ASC, and caspase-1 mRNA and protein expression levels in palmitic acid (PA)-treated KCs (Csak et al., 2011). Similarly, in HepG2 and L02 cells, inhibition of NLRP3 activity reduced uric acid-induced lipid accumulation (Wan et al., 2016).
These clinical and basic studies suggest that activation of the NLRP3 inflammasome may promote the pathological progression of NAFLD.
3.2 NLRP3 inflammasome regulates NAFLD by mediating inflammatory response and pyroptosis
Activated NLRP3 inflammasome releases inflammatory particles and induces pyroptosis, which is significantly associated with NAFLD.
Studies have shown that activated NLRP3 inflammasomes result in caspase-1 activation (Qiu et al., 2018; Yu et al., 2019; Shi et al., 2020). Activated caspase-1 performs two important functions. First, it promotes the conversion of pro-IL-1β and pro-IL-18 to IL-1β and IL-18. The persistence of this process leads to the secretion of large amounts of IL-1β and IL-18 and the initiation of pyroptosis, all of which are major contributors to the progress of inflammatory diseases. These processes spread the inflammatory process to the outside of the cell and release a large number of inflammasome particles, which aggravate the inflammatory response of the liver and promote the occurrence of NAFLD (Henao-Mejia et al., 2012). Second, pyroptosis depends on the inflammasome-mediated activation of caspase-1. Membrane insertion of the pyroptosis medium Gasdermin D (GSDMD) leads to the formation of plasma membrane pores, causing intracellular protein release, ion decompensation, water influx, and cell swelling. Active caspase-1 promotes the cleavage of GSDMD, then GSDMD binds to phosphatidylserine and phosphatidylinositol on the cell membrane and forms membrane pores that signal the beginning of cell death. These pores allow the introduction of a large number of active inflammatory cytokines and DAMPs into the extracellular environment, where they are recognized by inflammatory cells and lead to pyroptosis (Swanson et al., 2019). That study found that the expression of GSDMD and its pyrogenic-induced fragment GSDMD-N were upregulated in NAFLD/NASH liver tissues, and the increased level of GSDMD-N protein in the liver was correlated with NAFLD activity score and fibrosis (Xu B. et al., 2018). This suggests a correlation between the expression of the NLRP3 inflammasome-GSDMD-pyroptosis triplet and the development of NASH.
Hepatocyte pyroptosis and the continuous release of inflammatory factors induce the formation of an inflammatory fibrotic microenvironment in the liver, which promotes the development of NAFLD (Gaul et al., 2021) (Figure 1).
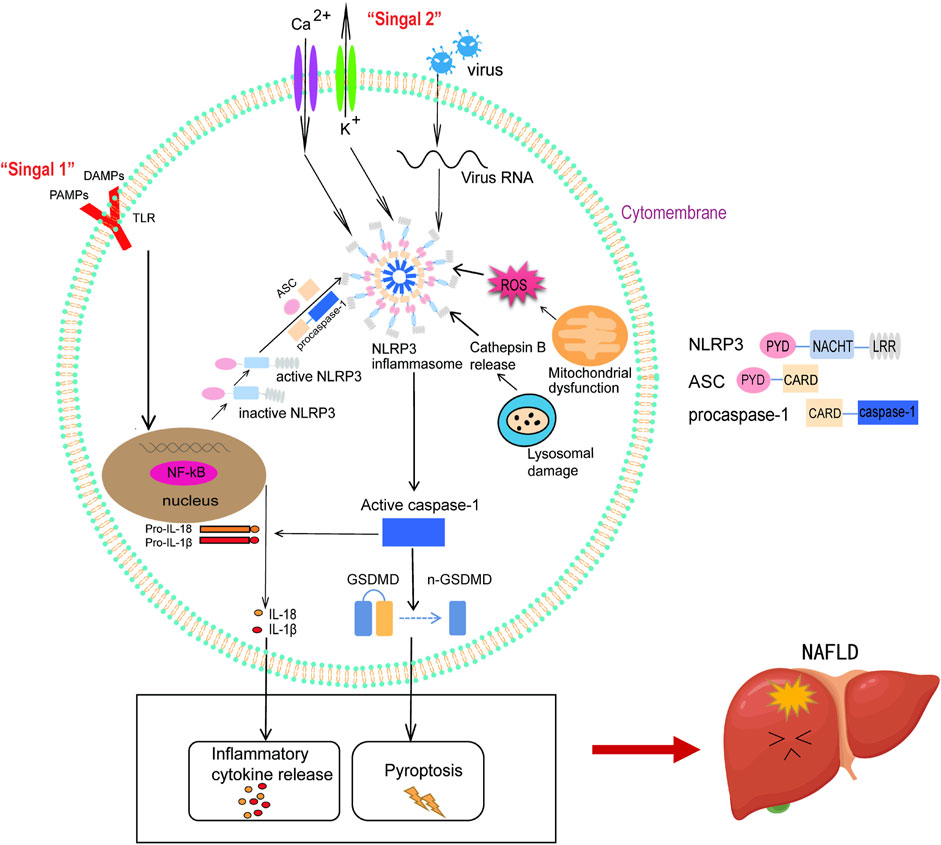
FIGURE 1. Activation of NLRP3 inflammasome mediates the development of NAFLD. The activation of NLRP3 inflammasome includes initiation and activation steps. During the initiation step, TLR is stimulated by extracellular signalling molecules, leading to NF-κB activation, which activates NLRP3 and promotes the expression of pro-IL-1β and pro-IL-18 (Signal 1). During the activation step, stimulation such as K+ outflow accompanied Ca2+ influx, ROS release, mitochondrial dysfunction, and lysosome damage induce the assembly of NLRP3 inflammasome (Signal 2). After NLRP3 inflammasome activation, activated caspase-1 converts pro-IL-1β and pro-IL-18 into mature IL-1β and IL-18, leading to the release of inflammatory cytokines. Activated caspase-1 dissociates GSDMD, releases its N-terminal, and forms pores in the plasma membrane, stimulating the occurrence of pyroptosis. The interaction between inflammatory factors and pyroptosis induces the inflammatory fibrosis microenvironment in the liver and promotes the occurrence and development of NAFLD.
4 Plant-derived active ingredients treat NAFLD by regulating NLRP3 inflammasome
Here, we described plant-derived active ingredients that improve NAFLD by inhibiting the NLRP3 inflammasome in a variety of cell and animal models and the mechanisms underlying these inhibitory effects. These active ingredients are mainly isolated or derived from plants or traditional Chinese herbs and can be roughly classified as terpenoids, flavonoids, alkaloids, and phenols (Table 1).
4.1 Terpenoids
Terpenoids are natural products with a wide distribution, diverse structures, and various biological activities. They are composed of isoprene and play important roles in the metabolism of all organisms (Bouvier et al., 2005). Terpenoids have been proven to have antioxidant and anti-inflammatory effects and can be used as therapeutic drugs for many inflammatory diseases (Grassmann 2005; Kim et al., 2020). Many plant-derived terpenoids exert anti-NASH effects by inhibiting NLRP3 inflammasome activation.
4.1.1 Antcin A
Antcin A, a terpenoid isolated from Antrodia camphorata, is a classical, traditional Chinese medicine used for NAFLD therapy. Antcin A has strong anti-inflammatory and hepatoprotective effects (Xu G. B. et al., 2018). In vivo and in vitro studies have shown that antcin A could ameliorate liver lipid deposition, reduce ALT and AST levels, and inhibit the expression levels of NLRP3 inflammasome (including NLRP3, ASC, and caspase-1) and pyroptosis-associated proteins (GSDMD-FL and GSDMD-NT). The cell membrane pore permeability and the number of pyroptotic cells significantly decreased after treatment with Antcin A. It worked by binding to NLRP3 and inhibiting the assembly and activation of the NLRP3 inflammasome, further mediating the inflammatory response and pyroptosis and thereby improving NASH (Ruan et al., 2021).
4.1.2 Ginseng saponins, Rh1/Rg2/Rg1
Ginseng saponins can be divided into Rg1, Re, Rg2, Rh1, Rf, Rb1, Rb2, Rd, Rg3, Rh2, and Ro. Substantial evidence suggests that ginseng saponins have antioxidant, anti-inflammatory, and anti-pyroptosis properties (Wong et al., 2015; Xu Y. et al., 2018). Rh1/Rg2 extenuated hepatic lipid and fibrosis and reduced lipogenesis-related, fibrosis-related, and inflammatory genes in fast food diet (FFD)-fed male C57BL/6J mice. In addition, Rh1/Rg2 inhibited the NLRP3 inflammasome and the release of IL-1β. In vitro experiments have shown the exertion of anti-NASH effects by maintaining mitochondrial homeostasis and inhibiting NLRP3 inflammasome activation (Wang et al., 2021). Another study showed that ginsenoside Rg1 also alleviated this phenotype and reduced NLRP3, IL-1β, and cleaved IL-1β expression in NASH model mice by inhibiting inflammasome activation (Xu B. et al., 2018).
4.1.3 Andrographolide (Andro)
Andro, a natural terpenoid from Andrographis paniculata, possesses a wide range of bioactive compounds, including anti-inflammatory, antitumor, anti-diabetic, anti-bacterial, anti-malarial, and hepatoprotective properties (Lim et al., 2012; Jayakumar et al., 2013). In vivo experiments have shown that it could reduce liver fat content, alleviate the expression of liver fibrosis indicators and autophagy, and inhibit the expression of NLRP3, ASC, caspase-1, and IL-1β. In vitro studies also have detailed good anti-inflammatory and anti-fibrosis effects of Andro and its anti-NASH effects were mainly regulated by NF-κB-dependent NLRP3 inflammasome (Cabrera et al., 2017). One study found that the oral bioavailability of 14-Deoxy-11, 12-Didehydroandrographolid, a derivative of Andro was higher than Andro (Guan et al., 2011; Chen et al., 2014). It could lower ALT and hepatic total cholesterol (TC) levels and reduce the expression of NLRP3 and related inflammatory factors by increasing antioxidant and anti-inflammatory activities in NAFLD mice (Liu Y. T. et al., 2020).
4.1.4 β-patchoulene (β-PAE)
β-PAE, a natural terpenoid of Pogostemonis herba, has anti-inflammatory, antioxidant, and hepatoprotective effects (Wu et al., 2018; Liu Y. et al., 2020). Studies have shown that β-PAE ameliorates serum lipid and liver transaminase in a high-fat diet (HFD)-induced NASH model. In addition, β-PAE reduced the expression of thioredoxin interaction protein (TXNIP), NLRP3, ASC, and cleaved-caspase-1 and inhibited the release of IL-Iβ. TXNIP is a key modulator of the redox system that inhibits TXN (thioredoxin) to cause cellular oxidative stress (Zhou et al., 2010). β-PAE reduced the levels of ROS and TXNIP, futher inhibited NLRP3 inflammasome activation (Luo et al., 2021).
4.1.5 Sweroside
Sweroside, a plant-derived terpenoid, can effectively alleviate liver fibrosis and liver injury-related diseases (Yang Q. L. et al., 2016; Gong et al., 2020) and has been shown to delay the pathological progression of NASH, such as liver inflammatory, lipid accumulation, and fibrosis in vivo. This was accompanied by inhibitory effects on the hepatic NLRP3 inflammasome. Additionally, it has been shown to inhibit inflammasome activation as NLRP3, ASC, procaspase-1, caspase-1, and IL-1β reduced in the BMDMs inflammatory model induced by lipopolysaccharide (LPS), adenosine triphosphate (ATP), and nigericin (Yang et al., 2020). These results indicated that targeting the NLRP3 inflammasome with sweroside could be beneficial for improving NASH symptoms.
4.1.6 Carnosic acid (CA)
CA is a natural terpenoid isolated from rosemary and common sages. It has been shown that CA can reduce TC, triglyceride (TG), ALT, AST, and insulin resistance, inhibit the expression of pro-inflammatory factors and adipogenic genes, and improve the expression of myristoylated alanine-rich protein kinase C substrate (MARCKS). MARCKS is a major protein kinase C (PKC) substrate in many different cell types, which has been investigated in LPS-induced disease from inflammation-related signaling pathways (Yin et al., 2016). The protein expression of NLRP3 and caspase-1 were upregulated in the MARCKS-deficient mice. MARCKS deficiency induced pro-inflammatory cytokine secretion, including IL-1β, IL-18, and tumor necrosis factor (TNF)-α, and induced NLRP3 inflammasome activation in vivo. This study suggested that the mechanism of CA treatment for NASH is to inhibit the activation of the NLRP3 inflammasome signaling pathway by increasing the expression of MARCKS (Song et al., 2018).
4.1.7 Gardenoside
Gardenoside is the most effective bioactive molecule of the medicinal plant Gardenia jasminoides Ellis and has been shown to inhibit inflammatory cytokines and hepatocyte steatosis induced by free fatty acids (FFA), which play an important role in NAFLD (Liang et al., 2015). Gardenoside can ameliorate lipid deposition and liver fibrosis, and has been shown to decrease the expression levels of dipeptidyl peptidase-4 (DPP4), CCCTC-binding factor (CTCF), NLRP3, ASC, caspase-1 p20, GSDMD-N, and IL-1β in vitro and in vivo NAFLD models. DPP4, an aminopeptidase, regulates the activity of a variety of biological peptides and participates in a variety of pathological and physiological processes (Lambeir et al., 2003). CTCF is a gene known for its chromatin-organizing and transcription factor properties (Braccioli and de Wit 2019). Importantly, CTCF or DPP4 silencing showed effects similar to those of Gardenoside treatment, whereas CTCF overexpression offset this trend. Compared with the NAFLD model group, the number of apoptotic cells decreased and the cell membrane integrity of hepatocytes improved significantly in the gardenoside group. These results suggested that gardenoside inhibited the activation of NLRP3 inflammasome -mediated inflammation and pyroptosis via the CTCF/DPP4 axis (Shen et al., 2021).
4.1.8 Glycyrrhizin (GL)
GL, derived from the traditional Chinese medicine licorice, is widely used for treating liver disease (Wang H. et al., 2017). In an MCD-induced NAFLD model study, GL reduced the lipid levels in the liver and serum, reduced the expression of apoptosis marker genes, regulated FFA synthesis, and inhibited liver fibrosis. The expression levels of TLR, NLRP3, ASC, caspase-1, and IL-1β were reduced after treatment with GL. This study confirmed that GL plays a therapeutic role in NASH by inhibiting the TLR/NLRP3 inflammasome signalling pathway (Yan et al., 2018).
4.2 Flavonoids
Flavonoids are naturally existing and broadly distributed secondary metabolites of plants with a variety of biological functions, including antibacterial, antioxidant, anti-inflammatory, and anti-fibrosis activities. Flavonoids can inhibit the production of inflammatory mediators such as IL-1β, IL-18, TNF-α, and IL-6 (Yi, 2018). An increasing number of flavonoids have been reported to show anti-inflammatory and anti-fibrotic activities through the inhibition of NLRP3 inflammasome activation.
4.2.1 Naringenin
Naringenin is mainly found in plants, especially citrus fruits such as grapefruit (Orhan, Nabavi et al., 2015), and has anti-inflammatory (Jin et al., 2017), antioxidant (Zhao et al., 2017), lipid-lowering (Mulvihill et al., 2009), and immunomodulatory effects (Zeng et al., 2018). Naringenin reduced lipid accumulation and inflammation in the liver of MCD-induced mice and decreased the expression of NF-κB, NLRP3, IL-1β, and IL-18, but did not further extenuate lipid accumulation in the livers of NLRP3−/− mice. Meanwhile, similar results have been obtained in vitro. The study concluded that naringenin prevents NAFLD by downregulating the NF-κB/NLRP3 signaling pathway (Wang Y. et al., 2020).
4.2.2 Apigenin (API)
API, a naturally existing bioflavonoid, is commonly found in fruits, herbs, and vegetables (Miean and Mohamed 2001). API has distinct pharmacological activities, such as antioxidant, anti-inflammatory activity (Lee et al., 2007), antitumor (Shao et al., 2013), and antidepressant effects (Li et al., 2016). Many studies have indicated that API has anti-obesity and anti-diabetic activity (Ono and Fujimori 2011; Kim et al., 2014). The potential mechanism of these therapeutic effects was attributed to the regulation of xanthine oxidase (XO) by API, which further regulated NLRP3 inflammasome activation and the release of inflammatory cytokines IL-1β and IL-18. These results indicated that API ameliorated HFD-induced NAFLD by regulating the XO/NLRP3 pathway (Lv et al., 2019).
4.2.3 Echinatin (Echi)
Echi, a bioactive flavonoid of Glycyrrhiza plants (licorice), has antioxidant and anti-inflammatory activities (Fu et al., 2013; Tian et al., 2016). Echi ameliorated lipid deposition, fibrosis, and inflammation in an MCD-induced NASH mouse model. This study showed that Echi treatment had a beneficial effect on the level of ALT, AST, caspase-1, IL-1β, TNF-α, and lipid steatosis and fibrosis in the liver in MCD-induced NASH, which is similar to NLRP3 inhibitors, MCC950. These findings suggested that Echi could alleviate liver inflammation and the NASH pathology via inhibition of NLRP3 inflammasome. Further research showed that Echi exerts its inhibitory effect on the NLRP3 inflammasome by binding to heat-shock protein 90 (HSP90), a molecular chaperone family member that is crucial for the correct folding of many newly synthesized peptides and rematuration of denatured/misfolded client proteins, suppressing the ATPase activity of NLRP3 (Nizami et al., 2021), and destroying the association between cochaperone solanidine galactosyltransferases (SGT1) and HSP90-NLRP3 (Xu et al., 2021).
4.2.4 Licochalcone E
Licochalcone E, a flavonoid isolated from licorice, possesses various biological activities, especially anti-diabetic effects (Park et al., 2012). Licochalcone E significantly raised the consumption of glucose and reduced the concentrations of TC and TG in PA-treated HepG2 cells. Further, licochalcone E depressed the expression of NLRP3, caspase-1, and IL-1β. Meanwhile, molecular simulations exhibited that licochalcone E has a high binding affinity for the NLPR3 inflammasome and inhibits the NLPR3 signalling pathway (Cao et al., 2021).
4.2.5 Silybin
Silybin, a natural flavonoid from milk thistle (silybum marianum), has been shown to have significant anticancer, anti-diabetic, and beneficial liver effects (Ferenci et al., 1989; Huseini et al., 2006; Hawke et al., 2010; Serviddio et al., 2014). The present study demonstrated that silybin reduced NLRP3, ASC, and the secretion of IL-1β. The sirtuin type2 (SIRT2) protein is the third type of nicotbambeadenbe dinuclotide (NAD+) dependent histone deacetylases, and its activity and expression level are closely related to intracellular NAD + content (Wang et al., 2019). The NAD+/SIRT2 pathway is a crucial mediator of silybin blocking NLRP3 inflammasome activation in NAFLD, and the anti-inflammatory effects of silybin were impeded by silencing SIRT2 or the SIRT2 inhibitor AGK2. These results suggested that silybin constrains NLRP3 inflammasome assembly through the NAD+/SIRT2 pathway in mice with NAFLD (Zhang et al., 2018).
4.2.6 Baicalin (BA)
BA, a primary bioactive flavonoid ingredient that originates from the root of Scutellaria baicalensis Georgi, has anti-inflammatory and antioxidant properties and extenuates cell lipotoxicity (Zhang Y. et al., 2017; Kwak et al., 2017). This study showed that BA inhibited the expression of NLRP3 and decreased the production of IL-1β and IL-6. A further mechanistic study indicated that BA alleviated PA-induced cytotoxicity in AML-12 cells via the inhibition of ER stress and TXNIP/NLRP3 inflammasome activation (Zhang J. et al., 2017).
4.2.7 Cyanidin-3-O-glucoside (C3G)
C3G, one of the most plentiful anthocyanins belonging to the flavonoid family (Galvano et al., 2004), ameliorates oxidative stress and hepatic steatosis in mice (Guo et al., 2012). C3G lessened body weight, liver weight, TG, and lipid droplets in HFD-induced NAFLD. Meanwhile, C3G reduced the expression of NLRP3, caspase-1, IL-1β, and IL-18. Mechanistic studies showed that C3G promoted PINK1 (PTEN-induced kinase 1)/Parkin expression and increased PINK1-mediated mitophagy. The PINK1/Parkin pathway is considered to be the main pathway of mitochondrial autophagy (Nguyen et al., 2016). The knockdown of hepatic PINK1 canceled the mitophagy-inducing effect of C3G, which blunted the useful effects of C3G on oxidative stress, NLRP3 inflammasome activation, glucose metabolism, and hepatic steatosis (Zhu et al., 2012).
4.2.8 Mangiferin (Man)
Man is particularly abundant in the leaves of mangoes and has anti-oxidative, anti-inflammatory, anticancer, and anti-diabetic activities (Yon, Teramoto et al., 2005; Gold-Smith, Fernandez et al., 2016; Saha, Sadhukhan et al., 2016). A study indicated that body weight, liver weight, TC, TG, ALT, and AST were reduced after treatment with Man, while NLRP3, caspase-1, IL-1β, and GSDMD expression were downregulated in vivo and in vitro. These results indicated that the anti-NAFLD activity of Man is mediated by its anti-inflammatory effects via NLRP3 inflammasome inhibition (Yong, Ruiqi et al., 2021).
4.2.9 Nobiletin (Nob)
Nob, a polymethoxylated flavonoid present in citrus peels, has antioxidant, antitumor, and anti-lipotoxic properties (Nemoto, Ikeda et al., 2013; Gajaria, Patel et al., 2015; Kwon, Jung et al., 2015; Peng, Li et al., 2018). Nob decreased the expression of NLRP3, caspase-1, IL-1β, and IL-18 in AML-12 cells. Furthermore, Nob improved PA-induced lipotoxicity by inhibiting NLRP3 inflammasome activation in a SIRT1-dependent manner (Peng, Li et al., 2018).
4.3 Alkaloids
Alkaloids are widely distributed in various plants and are a kind of organic secondary metabolites containing nitrogen with various structures, which have a wide variety of bioactive roles, including antioxidant, anti-inflammatory, antitumor, and immunoregulatory activities (Liu, Yang et al., 2019). Several studies have shown that the effects of alkaloids on NASH were mediated by the inhibition of NLRP3 inflammasome activation.
4.3.1 Berberine (BBR)
BBR is an isoquinoline alkaloid that is derived from diverse herbal plants, including Coptis chinensis. Studies have shown that BBR has anti-lipogenic, anti-inflammatory, anti-fibrotic, and antitumor effects (Luo, Tian et al., 2019). BBR significantly reduced ALT, AST, TC, and TG levels. BBR works by enhancing the permeability of intestinal mucosa and balancing intestinal innate immune constituents. Research has shown that the expressions of NOD1, NOD2, NLRP3, caspase-1, and the release of IL-1β, IL-18, and TNF-α were upregulated in NAFLD intestine and reduced after treatment of BBR. The mechanism may be inhibition of the NOD/NLRP3 inflammasome axis (Wang Q. et al., 2020). Another study suggested that BBR significantly ameliorated lipid accumulation, lipid peroxides, TNF-α expression, inhibited NLRP3 expression, caspase-1 activity, and GSDMD-N expression with a decrease in ROS levels and TXNIP expression in vivo and in vitro. These results suggested that BBR suppressed NLRP3 inflammasome activation and pyroptosis via the ROS/TXNIP axis to ameliorate NASH (Mai et al., 2020).
4.3.2 Dimethylenetetrahydroberberine (DMTHB)
DMTHB, an innovative berberine derivative, has a similar structure to dimethyleneberberine (DMB), a natural mitochondria-targeting antioxidant that exhibits significant anti-inflammatory and antioxidant pharmacological activities (Zhang et al., 2015; Qiang et al., 2016). DMTHB suppressed AST and ALT, hepatic TG and TC, and indicators related to hepatic fibrosis in the MCD-fed model. In addition, further results have shown that DMTHB inhibited the release of IL-1β by suppressing the expression of NLRP3, ASC, and caspase-1. The anti-NASH mechanism was regarded to involve DMTHB, which could target the NLRP3 inflammasome to suppress NLRP3 inflammasome activation (Zhang et al., 2021).
4.3.3 Matrine (Mtr)
Mtr is originally derived from the plant Sophora flavescens and possesses potent hepatoprotective (Liu et al., 2003) and anti-inflammatory effects (Zhang et al., 2011). It was verified that Mtr reduced AST, ALT, and collagen-1 and inhibited the expression of hepatic TNF-α and NLRP3 in the MCD-fed model. The anti-NASH was related to the downregulation of NLRP3 in the NASH model (Mahzari et al., 2019).
4.3.4 Betaine
Betaine, a naturally occurring compound in common foods, has been used to treat NAFLD (Sookoian et al., 2017; Veskovic et al., 2019; Veskovic et al., 2020; Chen et al., 2021). Betaine could lower serum lipid, hepatic lipid, and blood glucose and reduce the levels of NLRP3, ASC, caspase-1, and IL-1β. Betaine ameliorated hepatic lipid accumulation, gluconeogenesis, and inflammation by inhibiting the activation of the hepatic NF-κB/NLRP3 inflammasome pathway in fructose-fed NAFLD rats (Ge et al., 2016).
4.4 Phenols
Phenolic compounds are secondary metabolites synthesized in the process of plant growth as important plant components. They have excellent lipid-lowering and anti-inflammatory properties, improve mitochondrial function, effectively inhibit lipid oxidation, and treat NASH and other inflammatory diseases (Lee et al., 2011; Rafiei et al., 2019).
4.4.1 Cannabidiol (CBD)
CBD, a non-psychoactive compound extracted from Cannabis sativa, possesses a wide range of pharmacological functions, including antioxidant and anti-inflammatory functions, cardiac and neural protection, and anti-epileptic potential (Yang et al., 2014; Wang Y. et al., 2017). A previous study showed that CBD alleviated hepatic steatosis and oxidative stress and significantly decreased the expression of NF-κB, NLRP3, ASC, procaspase-1, caspase-1 p20, GSDMD, and cleaved GSDMD both in vivo and in vitro. The anti-NASH mechanism of CBD was related to the suppression of the NF-κB/NLRP3 inflammasome pathway (Huang et al., 2019; Jiang et al., 2021).
4.4.2 Salvianolic acid A (SalA)
SalA, a highly efficacious phenolic acid, is a water-soluble compound isolated from Radix Salvia miltiorrhiza. Many studies have shown that SalA exerts various pharmacological properties, such as antioxidant, anti-inflammatory, anti-fibrotic, anti-carcinogenic (Zhang et al., 2014; Dai et al., 2015; Wang et al., 2015), and anti-apoptotic properties (Qiang et al., 2014). It reduced the levels of hepatic pro-inflammatory cytokines (IL-1β, TNF-α, and IL-6) and lipid accumulation (TG and TC) in the NAFLD model. This study concluded that TXNIP and NLRP3 inflammasome activation is involved in the pathogenesis of NAFLD. SalA extenuated hepatic lipid accumulation and inflammation in HFD-induced NAFLD, and these protective effects were associated with the TXNIP/NLRP3 pathway (Ding et al., 2016).
4.4.3 Dieckol (DK)
DK, a natural phenolic compound found in Laminaria japonica, decreases hepatic steatosis by alleviating hepatic inflammation (Byun et al., 2021). The accumulation of TG and histological NAFLD score in the liver were reduced by DK in the HFD-induced NAFLD model. In addition, DK could decrease the expression of NLRP3, ASC, caspase-1, GSDMD, and cleaved-GSDMD to improve NAFLD in vivo. The pyroptotic cell numbers in the liver were increased by HFD and decreased after treatment with DK. Above all were related to decreased formation of the NLRP3 inflammasome and inhibition of pyroptosis (Oh et al., 2021).
4.4.4 Polydatin
Polydatin is extracted from Polygonum cuspidatum (Siebold & Zucc) and has been used in traditional Chinese medicine to prevent liver disorders associated with oxidative stress, inflammation, and lipid deposition in patients and experimental animals (Li et al., 2018; Chen et al., 2019). Polydatin downregulated NLRP3, ASC, caspase-1, IL-1β, TG, and TC in the livers of fructose-fed rats. It was verified that the mechanism for NASH involves blocking ROS-driven TXNIP to suppress NLRP3 inflammasome activation (Zhao et al., 2018).
4.4.5 Resveratrol
Resveratrol, a polyphenol present in grapes, mulberries, and red wine, exerts antioxidant and anti-inflammatory effects (Szkudelski and Szkudelska 2011). Resveratrol significantly reduced serum glucose and TG content. In addition, resveratrol treatment reduced the levels of pro-inflammatory markers such as IL-1, IL-6, and TNF-α. Futher mechnism research showed that these improvements were related to suppresse the SIRT/NLRP3 inflammasome pathway (Yang and Lim 2014).
4.4.6 Magnolol (MG)
MG, a major bioactive compound isolated from Magnolia officinalis, has been shown to possess anti-inflammatory, anti-oxidative, and hepatoprotective properties (Tian et al., 2018; Zhang et al., 2019). MG reduced serum TG and TC content, decreased the expression of NLRP3, ASC, and caspase-1, and reduced the production of IL-1β, IL-18, IL-6, and TNF-α in vivo and in vitro. Another study showed that MG inhibited hepatic steatosis-induced NLRP3 inflammasome activation through the restoration of autophagy to promote Heme Oxygenase-1 (HO-1) signalling. HO-1 is an anti-inflammatory, antioxidant and neuroprotective inducible enzyme (Campbell et al., 2021), which is capable of ameliorating oxidative stress and inflammatory responses (Kuo et al., 2020).
4.4.7 Salidroside (SAL)
SAL, a phenylpropanoid glycoside compound, is the major active ingredient of Rhodiola rosea. Earlier studies have shown that salidroside has pharmacological properties, including antioxidant and metabolic regulation, and exerts therapeutic effects on type 2 diabetes (Zheng et al., 2015; Wu et al., 2016). This study indicated that salidroside defends against NAFLD by alleviating hepatic lipid metabolism, reducing the release of inflammatory factors, and inhibiting NLRP3 inflammasome activation in vivo and in vitro, which were related to the regulation of the TXNIP/NLRP3 pathways (Zheng et al., 2018).
5 Conclusion and perspectives
We have summarized the latest research results on the improvement of NAFLD afforded by the regulation of NLRP3 by plant-derived active ingredients. First, we systematically discussed the mechanism of the NLRP3 inflammasome in the development of NAFLD, which mainly participates in the occurrence and development of NAFLD by mediating the inflammatory response and pyroptosis. We then described in detail the effects of these plant-derived compounds on the phenotype of the NAFLD model in vitro and in vivo. In addition, we summarized their effect on the NLRP3 inflammasome and its related indicators, procaspase-1, active caspase-1, ASC, pro-IL-1β, and IL-1β, and pyroptosis-related indicators, as well as the mechanism by which these compounds inhibit the NLRP3 inflammasome to improve NAFLD.
The NLRP3 inflammasome plays an important role in the development and progression of NAFLD. Excitingly, many plant-derived active ingredients can improve NAFLD by inhibiting NLRP3 inflammasome. Therefore, identifying NLRP3 inhibitors in plant-derived active compounds is of great significance for drug discovery as they are mostly safe, widely distributed, and available. These compounds included terpenoids, flavonoids, phenols, and alkaloids. Antcin A, a terpenoid, can improve NAFLD by binding to NLRP3 to inhibit both the inflammatory response and pyroptosis. Gardenoside, mangiferin, berberine, and dieckol can also inhibit inflammatory response and pyroptosis. We suspect that these five compounds may elicit a better anti-NAFLD effect in combination because of synergistic inhibitory effects. Glycyrrhizin inhibits NLRP3 initiation through the TLR/NLRP3 pathway, and de novo inhibition of NLRP3 may explain why glycyrrhizin exerts a strong anti-inflammatory effect. Naringin and antcin A have inhibitory effects on NLRP3 inflammasome assembly, which could provide a reference for the development of inhibitors of NLRP3 assembly. Most of these compounds ameliorated NAFLD by acting on NF-κB/NLRP3 and TXNIP/NLRP3 upstream signals or by combating oxidative stress by promoting NF-E2-related factor 2 (Nrf2). Nrf2 is an essential transcription factor that regulates an array of detoxifying and antioxidant defense gene expression in the liver (Beyer and Werner 2008). Berberine and ginsenoside have been studied the most. DMTHB, a derivative of berberine, has a significant effect on improving NAFLD models in vitro and in vivo. The 31 plant-derived compounds mentioned herein exhibit inhibitory effects on NLRP3, serving as a reference for the development of treatments for NAFLD.
Nevertheless, much work remains to be done to screen for natural drugs that are effective against NAFLD. Our analysis found that most of the current studies are limited to descriptive findings, and only a few studies have explored the potential interaction between these compounds and NLRP3, whereas most of the studies lack in-depth mechanistic research. For example, one included study of using resveratrol in treating NAFLD, only the effects of resveratrol on the expressions of NLRP3, caspase-1 and ASC proteins were observed, but the mechanism was not further explored. In addition, in a study on mangiferin inhibiting pyroptosis, the authors only showed the effect of mangiferin on cell pyrosis marker GSDMD, but did not observe the pathological manifestation of pyroptosis. Therefore, future studies on these mechanisms in depth and comprehensiveness will contribute to a better understanding of the complex mechanisms of NLRP3 inflammasome activation. All of the compounds mentioned herein inhibit NLRP3, but further research is needed to reveal whether this relationship is direct. Although the binding site of NLRP3 inflammasome-associated proteins to a natural product has been identified, further studies are needed to uncover the mechanism of action of potential NLRP3 inflammasome inhibitors and to screen for molecules that can selectively antagonize NLRP3 for NAFLD treatment. The large dose difference of compounds used in different studies may lead to off-target effects and false-positive results. Therefore, standardization of NLRP3 specific inhibitory dose is of great significance for the safety and efficacy of future NAFLD drug studies. Although many biological effects of these plant-derived active ingredients have been confirmed in cell and animal models in the treatment of NAFLD, insufficient clinical trials have been conducted to confirm the efficacy of these plant-derived substances for NAFLD, and further clinical trials are warranted.
In summary, we have discussed plant-derived active ingredients that can improve NASH by mediating NLRP3 to alleviate the inflammatory response or pyroptosis. In light of the collected data described herein, we also propose relevant suggestions and improvement measures, which are of significance for the development of NAFLD treatment drugs.
Author contributions
QF conceived this study. QH was responsible for organizing the literature and drawing chemical formulas. QH, XX, QS, ZA, and XG were in charge of consulting the literature. QH wrote the manuscript and drew the figures. QF supervised the study and gave final approval of the version to be published. All authors critically participated in the discussion and commented on the manuscript.
Funding
This work was supported by the National Natural Science Foundation of China (No.82174040 to QF), the Shanghai Shenkang 3-year action plan (No. SHDC2020CR4051 to QF), the Shanghai Science and Technology Development Funds (No. 19401972100 to QF), the District level Medical and Health Key Project of Shanghai Baoshan District Science (No. 21-E-63 to XG) and the Innovative Projects of Shanghai University of Traditional Chinese Medicine (No. Y2021030 to ZA).
Conflict of interest
The authors declare that the research was conducted in the absence of any commercial or financial relationships that could be construed as a potential conflict of interest.
Publisher’s note
All claims expressed in this article are solely those of the authors and do not necessarily represent those of their affiliated organizations, or those of the publisher, the editors and the reviewers. Any product that may be evaluated in this article, or claim that may be made by its manufacturer, is not guaranteed or endorsed by the publisher.
References
Beyer, T. A., and Werner, S. (2008). The cytoprotective Nrf2 transcription factor controls insulin receptor signaling in the regenerating liver. Cell Cycle 7 (7), 874–878. doi:10.4161/cc.7.7.5617
Bouvier, F., Rahier, A., and Camara, B. (2005). Biogenesis, molecular regulation and function of plant isoprenoids. Prog. Lipid Res. 44 (6), 357–429. doi:10.1016/j.plipres.2005.09.003
Braccioli, L., and de Wit, E. (2019). Ctcf: A Swiss-army knife for genome organization and transcription regulation. Essays Biochem. 63 (1), 157–165. doi:10.1042/EBC20180069
Buzzetti, E., Pinzani, M., and Tsochatzis, E. A. (2016). The multiple-hit pathogenesis of non-alcoholic fatty liver disease (NAFLD). Metabolism. 65 (8), 1038–1048. doi:10.1016/j.metabol.2015.12.012
Byun, K. A., Oh, S., Son, M., Park, C. H., Son, K. H., Byun, K., et al. (2021). Dieckol decreases caloric intake and attenuates nonalcoholic fatty liver disease and hepatic lymphatic vessel dysfunction in high-fat-diet-fed mice. Mar. Drugs 19 (9), 495. doi:10.3390/md19090495
Cabrera, D., Wree, A., Povero, D., Solis, N., Hernandez, A., Pizarro, M., et al. (2017). Andrographolide ameliorates inflammation and fibrogenesis and attenuates inflammasome activation in experimental non-alcoholic steatohepatitis. Sci. Rep. 7 (1), 3491. doi:10.1038/s41598-017-03675-z
Cai, C., Zhu, X., Li, P., Li, J., Gong, J., Shen, W., et al. (2017). NLRP3 deletion inhibits the non-alcoholic steatohepatitis development and inflammation in kupffer cells induced by palmitic acid. Inflammation 40 (6), 1875–1883. doi:10.1007/s10753-017-0628-z
Campbell, N. K., Fitzgerald, H. K., and Dunne, A. (2021). Regulation of inflammation by the antioxidant haem oxygenase 1. Nat. Rev. Immunol. 21 (7), 411–425. doi:10.1038/s41577-020-00491-x
Campden, R. I., and Zhang, Y. (2019). The role of lysosomal cysteine cathepsins in NLRP3 inflammasome activation. Arch. Biochem. Biophys. 670, 32–42. doi:10.1016/j.abb.2019.02.015
Cao, Y., Si, Y., Li, M., Fan, D., Cao, M., Cheon, S. H., et al. (2021). Licochalcone E improves insulin sensitivity in palmitic acid-treated HepG2 cells through inhibition of the NLRP3 signaling pathway. Int. Immunopharmacol. 99, 107923. doi:10.1016/j.intimp.2021.107923
Chen, L. X., He, H., Xia, G. Y., Zhou, K. L., and Qiu, F. (2014). A new flavonoid from the aerial parts of Andrographis paniculata. Nat. Prod. Res. 28 (3), 138–143. doi:10.1080/14786419.2013.856907
Chen, W., Zhang, X., Xu, M., Jiang, L., Zhou, M., Liu, W., et al. (2021). Betaine prevented high-fat diet-induced NAFLD by regulating the FGF10/AMPK signaling pathway in ApoE(-/-) mice. Eur. J. Nutr. 60 (3), 1655–1668. doi:10.1007/s00394-020-02362-6
Chen, X., Chan, H., Zhang, L., Liu, X., Ho, I. H. T., Zhang, X., et al. (2019). The phytochemical polydatin ameliorates non-alcoholic steatohepatitis by restoring lysosomal function and autophagic flux. J. Cell. Mol. Med. 23 (6), 4290–4300. doi:10.1111/jcmm.14320
Chen, Y., Pitzer, A. L., Li, X., Li, P. L., Wang, L., Zhang, Y., et al. (2015). Instigation of endothelial Nlrp3 inflammasome by adipokine visfatin promotes inter-endothelial junction disruption: Role of HMGB1. J. Cell. Mol. Med. 19 (12), 2715–2727. doi:10.1111/jcmm.12657
Chevriaux, A., Pilot, T., Derangere, V., Simonin, H., Martine, P., Chalmin, F., et al. (2020). Cathepsin B is required for NLRP3 inflammasome activation in macrophages, through NLRP3 interaction. Front. Cell Dev. Biol. 8, 167. doi:10.3389/fcell.2020.00167
Cruz, C. M., Rinna, A., Forman, H. J., Ventura, A. L., Persechini, P. M., Ojcius, D. M., et al. (2007). ATP activates a reactive oxygen species-dependent oxidative stress response and secretion of proinflammatory cytokines in macrophages. J. Biol. Chem. 282 (5), 2871–2879. doi:10.1074/jbc.M608083200
Csak, T., Ganz, M., Pespisa, J., Kodys, K., Dolganiuc, A., Szabo, G., et al. (2011). Fatty acid and endotoxin activate inflammasomes in mouse hepatocytes that release danger signals to stimulate immune cells. Hepatology 54 (1), 133–144. doi:10.1002/hep.24341
Dai, J. P., Zhu, D. X., Sheng, J. T., Chen, X. X., Li, W. Z., Wang, G. F., et al. (2015). Inhibition of Tanshinone IIA, salvianolic acid A and salvianolic acid B on Areca nut extract-induced oral submucous fibrosis in vitro. Molecules 20 (4), 6794–6807. doi:10.3390/molecules20046794
Desai, A., Sandhu, S., Lai, J. P., and Sandhu, D. S. (2019). Hepatocellular carcinoma in non-cirrhotic liver: A comprehensive review. World J. Hepatol. 11 (1), 1–18. doi:10.4254/wjh.v11.i1.1
Di Virgilio, F. (2007). Liaisons dangereuses: P2X(7) and the inflammasome. Trends Pharmacol. Sci. 28 (9), 465–472. doi:10.1016/j.tips.2007.07.002
Ding, C., Zhao, Y., Shi, X., Zhang, N., Zu, G., Li, Z., et al. (2016). New insights into salvianolic acid A action: Regulation of the TXNIP/NLRP3 and TXNIP/ChREBP pathways ameliorates HFD-induced NAFLD in rats. Sci. Rep. 6, 28734. doi:10.1038/srep28734
Ferenci, P., Dragosics, B., Dittrich, H., Frank, H., Benda, L., Lochs, H., et al. (1989). Randomized controlled trial of silymarin treatment in patients with cirrhosis of the liver. J. Hepatol. 9 (1), 105–113. doi:10.1016/0168-8278(89)90083-4
Fu, Y., Chen, J., Li, Y. J., Zheng, Y. F., and Li, P. (2013). Antioxidant and anti-inflammatory activities of six flavonoids separated from licorice. Food Chem. 141 (2), 1063–1071. doi:10.1016/j.foodchem.2013.03.089
Gajaria, T. K., Patel, D. K., Devkar, R. V., and Ramachandran, A. V. (2015). Flavonoid rich extract of Murraya Koenigii alleviates in-vitro LDL oxidation and oxidized LDL induced apoptosis in raw 264.7 Murine macrophage cells. J. Food Sci. Technol. 52 (6), 3367–3375. doi:10.1007/s13197-014-1399-2
Galvano, F., La Fauci, L., Lazzarino, G., Fogliano, V., Ritieni, A., Ciappellano, S., et al. (2004). Cyanidins: Metabolism and biological properties. J. Nutr. Biochem. 15 (1), 2–11. doi:10.1016/j.jnutbio.2003.07.004
Ganz, M., Csak, T., Nath, B., and Szabo, G. (2011). Lipopolysaccharide induces and activates the Nalp3 inflammasome in the liver. World J. Gastroenterol. 17 (43), 4772–4778. doi:10.3748/wjg.v17.i43.4772
Gaul, S., Leszczynska, A., Alegre, F., Kaufmann, B., Johnson, C. D., Adams, L. A., et al. (2021). Hepatocyte pyroptosis and release of inflammasome particles induce stellate cell activation and liver fibrosis. J. Hepatol. 74 (1), 156–167. doi:10.1016/j.jhep.2020.07.041
Ge, C. X., Yu, R., Xu, M. X., Li, P. Q., Fan, C. Y., Li, J. M., et al. (2016). Betaine prevented fructose-induced NAFLD by regulating LXRα/PPARα pathway and alleviating ER stress in rats. Eur. J. Pharmacol. 770, 154–164. doi:10.1016/j.ejphar.2015.11.043
Gold-Smith, F., Fernandez, A., and Bishop, K. (2016). Mangiferin and cancer: Mechanisms of action. Nutrients 8 (7), E396. doi:10.3390/nu8070396
Gong, J., Yang, F., Yang, Q., Tang, X., Shu, F., Xu, L., et al. (2020). Sweroside ameliorated carbon tetrachloride (CCl4)-induced liver fibrosis through FXR-miR-29a signaling pathway. J. Nat. Med. 74 (1), 17–25. doi:10.1007/s11418-019-01334-3
Grassmann, J. (2005). Terpenoids as plant antioxidants. Vitam. Horm. 72, 505–535. doi:10.1016/S0083-6729(05)72015-X
Guan, S. P., Kong, L. R., Cheng, C., Lim, J. C., and Wong, W. S. (2011). Protective role of 14-deoxy-11, 12-didehydroandrographolide, a noncytotoxic analogue of andrographolide, in allergic airway inflammation. J. Nat. Prod. 74 (6), 1484–1490. doi:10.1021/np2002572
Guo, H., Xia, M., Zou, T., Ling, W., Zhong, R., Zhang, W., et al. (2012). Cyanidin 3-glucoside attenuates obesity-associated insulin resistance and hepatic steatosis in high-fat diet-fed and db/db mice via the transcription factor FoxO1. J. Nutr. Biochem. 23 (4), 349–360. doi:10.1016/j.jnutbio.2010.12.013
Haneklaus, M., and O'Neill, L. A. (2015). NLRP3 at the interface of metabolism and inflammation. Immunol. Rev. 265 (1), 53–62. doi:10.1111/imr.12285
Hawke, R. L., Schrieber, S. J., Soule, T. A., Wen, Z., Smith, P. C., Reddy, K. R., et al. (2010). Silymarin ascending multiple oral dosing phase I study in noncirrhotic patients with chronic hepatitis C. J. Clin. Pharmacol. 50 (4), 434–449. doi:10.1177/0091270009347475
Henao-Mejia, J., Elinav, E., Jin, C., Hao, L., Mehal, W. Z., Strowig, T., et al. (2012). Inflammasome-mediated dysbiosis regulates progression of NAFLD and obesity. Nature 482 (7384), 179–185. doi:10.1038/nature10809
Huang, Y., Wan, T., Pang, N., Zhou, Y., Jiang, X., Li, B., et al. (2019). Cannabidiol protects livers against nonalcoholic steatohepatitis induced by high-fat high cholesterol diet via regulating NF-κB and NLRP3 inflammasome pathway. J. Cell. Physiol. 234 (11), 21224–21234. doi:10.1002/jcp.28728
Huseini, H. F., Larijani, B., Heshmat, R., Fakhrzadeh, H., Radjabipour, B., Toliat, T., et al. (2006). The efficacy of Silybum marianum (L.) gaertn. (silymarin) in the treatment of type II diabetes: A randomized, double-blind, placebo-controlled, clinical trial. Phytother. Res. 20 (12), 1036–1039. doi:10.1002/ptr.1988
Jayakumar, T., Hsieh, C. Y., Lee, J. J., and Sheu, J. R. (2013). Experimental and clinical Pharmacology of Andrographis paniculata and its major bioactive phytoconstituent andrographolide. Evid. Based Complement. Altern. Med. 2013, 846740. doi:10.1155/2013/846740
Jiang, X., Gu, Y., Huang, Y., Zhou, Y., Pang, N., Luo, J., et al. (2021). CBD alleviates liver injuries in alcoholics with high-fat high-cholesterol diet through regulating NLRP3 inflammasome-pyroptosis pathway. Front. Pharmacol. 12, 724747. doi:10.3389/fphar.2021.724747
Jin, L., Zeng, W., Zhang, F., Zhang, C., and Liang, W. (2017). Naringenin ameliorates acute inflammation by regulating intracellular cytokine degradation. J. Immunol. 199 (10), 3466–3477. doi:10.4049/jimmunol.1602016
Kanwal, F., Kramer, J. R., Mapakshi, S., Natarajan, Y., Chayanupatkul, M., Richardson, P. A., et al. (2018). Risk of hepatocellular cancer in patients with non-alcoholic fatty liver disease. Gastroenterology 155 (6), 1828–1837. e1822. doi:10.1053/j.gastro.2018.08.024
Kelley, N., Jeltema, D., Duan, Y., and He, Y. (2019). The NLRP3 inflammasome: An overview of mechanisms of activation and regulation. Int. J. Mol. Sci. 20 (13), E3328. doi:10.3390/ijms20133328
Kim, M. A., Kang, K., Lee, H. J., Kim, M., Kim, C. Y., Nho, C. W., et al. (2014). Apigenin isolated from Daphne genkwa Siebold et Zucc. inhibits 3T3-L1 preadipocyte differentiation through a modulation of mitotic clonal expansion. Life Sci. 101 (1-2), 64–72. doi:10.1016/j.lfs.2014.02.012
Kim, T., Song, B., Cho, K. S., and Lee, I. S. (2020). Therapeutic potential of volatile terpenes and terpenoids from forests for inflammatory diseases. Int. J. Mol. Sci. 21 (6), E2187. doi:10.3390/ijms21062187
Kuo, N. C., Huang, S. Y., Yang, C. Y., Shen, H. H., and Lee, Y. M. (2020). Involvement of HO-1 and autophagy in the protective effect of magnolol in hepatic steatosis-induced NLRP3 inflammasome activation in vivo and in vitro. Antioxidants (Basel) 9 (10), E924. doi:10.3390/antiox9100924
Kwak, H. J., Yang, D., Hwang, Y., Jun, H. S., and Cheon, H. G. (2017). Baicalein protects rat insulinoma INS-1 cells from palmitate-induced lipotoxicity by inducing HO-1. PLoS One 12 (4), e0176432. doi:10.1371/journal.pone.0176432
Kwon, E. Y., Jung, U. J., Park, T., Yun, J. W., and Choi, M. S. (2015). Luteolin attenuates hepatic steatosis and insulin resistance through the interplay between the liver and adipose tissue in mice with diet-induced obesity. Diabetes 64 (5), 1658–1669. doi:10.2337/db14-0631
Lambeir, A. M., Durinx, C., Scharpe, S., and De Meester, I. (2003). Dipeptidyl-peptidase IV from bench to bedside: An update on structural properties, functions, and clinical aspects of the enzyme DPP IV. Crit. Rev. Clin. Lab. Sci. 40 (3), 209–294. doi:10.1080/713609354
Lee, J. H., Zhou, H. Y., Cho, S. Y., Kim, Y. S., Lee, Y. S., Jeong, C. S., et al. (2007). Anti-inflammatory mechanisms of apigenin: Inhibition of cyclooxygenase-2 expression, adhesion of monocytes to human umbilical vein endothelial cells, and expression of cellular adhesion molecules. Arch. Pharm. Res. 30 (10), 1318–1327. doi:10.1007/BF02980273
Lee, J., Li, Y., Li, C., and Li, D. (2011). Natural products and body weight control. N. Am. J. Med. Sci. 3 (1), 13–19. doi:10.4297/najms.2011.313
Li, R., Li, J., Huang, Y., Li, H., Yan, S., Lin, J., et al. (2018). Polydatin attenuates diet-induced nonalcoholic steatohepatitis and fibrosis in mice. Int. J. Biol. Sci. 14 (11), 1411–1425. doi:10.7150/ijbs.26086
Li, R., Wang, X., Qin, T., Qu, R., and Ma, S. (2016). Apigenin ameliorates chronic mild stress-induced depressive behavior by inhibiting interleukin-1β production and NLRP3 inflammasome activation in the rat brain. Behav. Brain Res. 296, 318–325. doi:10.1016/j.bbr.2015.09.031
Liang, H., Zhang, L., Wang, H., Tang, J., Yang, J., Wu, C., et al. (2015). Inhibitory effect of gardenoside on free fatty acid-induced steatosis in HepG2 hepatocytes. Int. J. Mol. Sci. 16 (11), 27749–27756. doi:10.3390/ijms161126058
Lim, J. C., Chan, T. K., Ng, D. S., Sagineedu, S. R., Stanslas, J., Wong, W. S., et al. (2012). Andrographolide and its analogues: Versatile bioactive molecules for combating inflammation and cancer. Clin. Exp. Pharmacol. Physiol. 39 (3), 300–310. doi:10.1111/j.1440-1681.2011.05633.x
Liu, C., Yang, S., Wang, K., Bao, X., Liu, Y., Zhou, S., et al. (2019). Alkaloids from traditional Chinese medicine against hepatocellular carcinoma. Biomed. Pharmacother. 120, 109543. doi:10.1016/j.biopha.2019.109543
Liu, J., Zhu, M., Shi, R., and Yang, M. (2003). Radix sophorae flavescentis for chronic Hepatitis B: A systematic review of randomized trials. Am. J. Chin. Med. 31 (3), 337–354. doi:10.1142/S0192415X03001107
Liu, Y. T., Chen, H. W., Lii, C. K., Jhuang, J. H., Huang, C. S., Li, M. L., et al. (2020b). A diterpenoid, 14-deoxy-11, 12-didehydroandrographolide, in Andrographis paniculata reduces steatohepatitis and liver injury in mice fed a high-fat and high-cholesterol diet. Nutrients 12 (2), E523. doi:10.3390/nu12020523
Liu, Y., Wu, J., Chen, L., Wu, X., Gan, Y., Xu, N., et al. (2020a). β-patchoulene simultaneously ameliorated dextran sulfate sodium-induced colitis and secondary liver injury in mice via suppressing colonic leakage and flora imbalance. Biochem. Pharmacol. 182, 114260. doi:10.1016/j.bcp.2020.114260
Luo, H., Xu, N., Wu, J., Gan, Y., Chen, L., Guan, F., et al. (2021). β-patchoulene protects against non-alcoholic steatohepatitis via interrupting the vicious circle among oxidative stress, histanoxia and lipid accumulation in rats. Int. Immunopharmacol. 98, 107915. doi:10.1016/j.intimp.2021.107915
Luo, Y., Tian, G., Zhuang, Z., Chen, J., You, N., Zhuo, L., et al. (2019). Berberine prevents non-alcoholic steatohepatitis-derived hepatocellular carcinoma by inhibiting inflammation and angiogenesis in mice. Am. J. Transl. Res. 11 (5), 2668–2682.
Lv, Y., Gao, X., Luo, Y., Fan, W., Shen, T., Ding, C., et al. (2019). Apigenin ameliorates HFD-induced NAFLD through regulation of the XO/NLRP3 pathways. J. Nutr. Biochem. 71, 110–121. doi:10.1016/j.jnutbio.2019.05.015
Mahzari, A., Li, S., Zhou, X., Li, D., Fouda, S., Alhomrani, M., et al. (2019). Matrine protects against MCD-induced development of NASH via upregulating HSP72 and downregulating mTOR in a manner distinctive from metformin. Front. Pharmacol. 10, 405. doi:10.3389/fphar.2019.00405
Mai, W., Xu, Y., Xu, J., Zhao, D., Ye, L., Yu, G., et al. (2020). Berberine inhibits nod-like receptor family pyrin domain containing 3 inflammasome activation and pyroptosis in nonalcoholic steatohepatitis via the ROS/TXNIP Axis. Front. Pharmacol. 11, 185. doi:10.3389/fphar.2020.00185
Miean, K. H., and Mohamed, S. (2001). Flavonoid (myricetin, quercetin, kaempferol, luteolin, and apigenin) content of edible tropical plants. J. Agric. Food Chem. 49 (6), 3106–3112. doi:10.1021/jf000892m
Mridha, A. R., Wree, A., Robertson, A. A. B., Yeh, M. M., Johnson, C. D., Van Rooyen, D. M., et al. (2017). NLRP3 inflammasome blockade reduces liver inflammation and fibrosis in experimental NASH in mice. J. Hepatol. 66 (5), 1037–1046. doi:10.1016/j.jhep.2017.01.022
Mulvihill, E. E., Allister, E. M., Sutherland, B. G., Telford, D. E., Sawyez, C. G., Edwards, J. Y., et al. (2009). Naringenin prevents dyslipidemia, apolipoprotein B overproduction, and hyperinsulinemia in LDL receptor-null mice with diet-induced insulin resistance. Diabetes 58 (10), 2198–2210. doi:10.2337/db09-0634
Munoz-Planillo, R., Kuffa, P., Martinez-Colon, G., Smith, B. L., Rajendiran, T. M., Nunez, G., et al. (2013). K⁺ efflux is the common trigger of NLRP3 inflammasome activation by bacterial toxins and particulate matter. Immunity 38 (6), 1142–1153. doi:10.1016/j.immuni.2013.05.016
Negash, A. A., and Gale, M. (2015). Hepatitis regulation by the inflammasome signaling pathway. Immunol. Rev. 265 (1), 143–155. doi:10.1111/imr.12279
Nemoto, K., Ikeda, A., Yoshida, C., Kimura, J., Mori, J., Fujiwara, H., et al. (2013). Characteristics of nobiletin-mediated alteration of gene expression in cultured cell lines. Biochem. Biophys. Res. Commun. 431 (3), 530–534. doi:10.1016/j.bbrc.2013.01.024
Nguyen, T. N., Padman, B. S., and Lazarou, M. (2016). Deciphering the molecular signals of PINK1/parkin mitophagy. Trends Cell Biol. 26 (10), 733–744. doi:10.1016/j.tcb.2016.05.008
Nizami, S., Arunasalam, K., Green, J., Cook, J., Lawrence, C. B., Zarganes-Tzitzikas, T., et al. (2021). Inhibition of the NLRP3 inflammasome by HSP90 inhibitors. Immunology 162 (1), 84–91. doi:10.1111/imm.13267
Oh, S., Son, M., Byun, K. A., Jang, J. T., Choi, C. H., Son, K. H., et al. (2021). Attenuating effects of dieckol on high-fat diet-induced nonalcoholic fatty liver disease by decreasing the NLRP3 inflammasome and pyroptosis. Mar. Drugs 19 (6), 318. doi:10.3390/md19060318
Ono, M., and Fujimori, K. (2011). Antiadipogenic effect of dietary apigenin through activation of AMPK in 3T3-L1 cells. J. Agric. Food Chem. 59 (24), 13346–13352. doi:10.1021/jf203490a
Orhan, I. E., Nabavi, S. F., Daglia, M., Tenore, G. C., Mansouri, K., Nabavi, S. M., et al. (2015). Naringenin and atherosclerosis: A review of literature. Curr. Pharm. Biotechnol. 16 (3), 245–251. doi:10.2174/1389201015666141202110216
Park, H. G., Bak, E. J., Woo, G. H., Kim, J. M., Quan, Z., Kim, J. M., et al. (2012). Licochalcone E has an antidiabetic effect. J. Nutr. Biochem. 23 (7), 759–767. doi:10.1016/j.jnutbio.2011.03.021
Peng, Z., Li, X., Xing, D., Du, X., Wang, Z., Liu, G., et al. (2018). Nobiletin alleviates palmitic acidinduced NLRP3 inflammasome activation in a sirtuin 1dependent manner in AML12 cells. Mol. Med. Rep. 18 (6), 5815–5822. doi:10.3892/mmr.2018.9615
Prochnicki, T., Mangan, M. S., and Latz, E. (2016). Recent insights into the molecular mechanisms of the NLRP3 inflammasome activation. F1000Res 5, 1469. doi:10.12688/f1000research.8614.1
Qiang, G., Yang, X., Xuan, Q., Shi, L., Zhang, H., Chen, B., et al. (2014). Salvianolic Acid a prevents the pathological progression of hepatic fibrosis in high-fat diet-fed and streptozotocin-induced diabetic rats. Am. J. Chin. Med. 42 (5), 1183–1198. doi:10.1142/S0192415X14500748
Qiang, X., Xu, L., Zhang, M., Zhang, P., Wang, Y., Wang, Y., et al. (2016). Demethyleneberberine attenuates non-alcoholic fatty liver disease with activation of AMPK and inhibition of oxidative stress. Biochem. Biophys. Res. Commun. 472 (4), 603–609. doi:10.1016/j.bbrc.2016.03.019
Qiu, T., Pei, P., Yao, X., Jiang, L., Wei, S., Wang, Z., et al. (2018). Taurine attenuates arsenic-induced pyroptosis and nonalcoholic steatohepatitis by inhibiting the autophagic-inflammasomal pathway. Cell Death Dis. 9 (10), 946. doi:10.1038/s41419-018-1004-0
Rafiei, H., Omidian, K., and Bandy, B. (2019). Dietary polyphenols protect against oleic acid-induced steatosis in an in vitro model of NAFLD by modulating lipid metabolism and improving mitochondrial function. Nutrients 11 (3), E541. doi:10.3390/nu11030541
Ruan, S., Han, C., Sheng, Y., Wang, J., Zhou, X., Guan, Q., et al. (2021). Antcin A alleviates pyroptosis and inflammatory response in Kupffercells of non-alcoholic fatty liver disease by targeting NLRP3. Int. Immunopharmacol. 100, 108126. doi:10.1016/j.intimp.2021.108126
Saha, S., Sadhukhan, P., and Sil, P. C. (2016). Mangiferin: A xanthonoid with multipotent anti-inflammatory potential. Biofactors 42 (5), 459–474. doi:10.1002/biof.1292
Schroder, K., Zhou, R., and Tschopp, J. (2010). The NLRP3 inflammasome: A sensor for metabolic danger? Science 327 (5963), 296–300. doi:10.1126/science.1184003
Serviddio, G., Bellanti, F., Stanca, E., Lunetti, P., Blonda, M., Tamborra, R., et al. (2014). Silybin exerts antioxidant effects and induces mitochondrial biogenesis in liver of rat with secondary biliary cirrhosis. Free Radic. Biol. Med. 73, 117–126. doi:10.1016/j.freeradbiomed.2014.05.002
Shao, B. Z., Wang, S. L., Pan, P., Yao, J., Wu, K., Li, Z. S., et al. (2019). Targeting NLRP3 inflammasome in inflammatory bowel disease: Putting out the fire of inflammation. Inflammation 42 (4), 1147–1159. doi:10.1007/s10753-019-01008-y
Shao, H., Jing, K., Mahmoud, E., Huang, H., Fang, X., Yu, C., et al. (2013). Apigenin sensitizes colon cancer cells to antitumor activity of ABT-263. Mol. Cancer Ther. 12 (12), 2640–2650. doi:10.1158/1535-7163.MCT-13-0066
Shen, T., Lei, T., Chen, L., Zhu, B. B., Xu, B. L., Zhang, C. P., et al. (2021). Gardenoside hinders caspase-1-mediated hepatocyte pyroptosis through the CTCF/DPP4 signaling pathway. Front. Physiol. 12, 669202. doi:10.3389/fphys.2021.669202
Shi, H., Zhang, Y., Xing, J., Liu, L., Qiao, F., Li, J., et al. (2020). Baicalin attenuates hepatic injury in non-alcoholic steatohepatitis cell model by suppressing inflammasome-dependent GSDMD-mediated cell pyroptosis. Int. Immunopharmacol. 81, 106195. doi:10.1016/j.intimp.2020.106195
Song, H. M., Li, X., Liu, Y. Y., Lu, W. P., Cui, Z. H., Zhou, L., et al. (2018). Carnosic acid protects mice from high-fat diet-induced NAFLD by regulating MARCKS. Int. J. Mol. Med. 42 (1), 193–207. doi:10.3892/ijmm.2018.3593
Sookoian, S., Puri, P., Castano, G. O., Scian, R., Mirshahi, F., Sanyal, A. J., et al. (2017). Nonalcoholic steatohepatitis is associated with a state of betaine-insufficiency. Liver Int. 37 (4), 611–619. doi:10.1111/liv.13249
Svadlakova, T., Hubatka, F., Turanek Knotigova, P., Kulich, P., Masek, J., Kotoucek, J., et al. (2020). Proinflammatory effect of carbon-based nanomaterials: In vitro study on stimulation of inflammasome NLRP3 via destabilisation of lysosomes. Nanomater. (Basel) 10 (3), E418. doi:10.3390/nano10030418
Swanson, K. V., Deng, M., and Ting, J. P. (2019). The NLRP3 inflammasome: Molecular activation and regulation to therapeutics. Nat. Rev. Immunol. 19 (8), 477–489. doi:10.1038/s41577-019-0165-0
Szkudelski, T., and Szkudelska, K. (2011). Anti-diabetic effects of resveratrol. Ann. N. Y. Acad. Sci. 1215, 34–39. doi:10.1111/j.1749-6632.2010.05844.x
Tian, X. H., Liu, C. L., Jiang, H. L., Zhang, Y., Han, J. C., Liu, J., et al. (2016). Cardioprotection provided by Echinatin against ischemia/reperfusion in isolated rat hearts. BMC Cardiovasc. Disord. 16, 119. doi:10.1186/s12872-016-0294-3
Tian, Y., Feng, H., Han, L., Wu, L., Lv, H., Shen, B., et al. (2018). Magnolol alleviates inflammatory responses and lipid accumulation by AMP-activated protein kinase-dependent peroxisome proliferator-activated receptor alpha activation. Front. Immunol. 9, 147. doi:10.3389/fimmu.2018.00147
Veskovic, M., Labudovic-Borovic, M., Mladenovic, D., Jadzic, J., Jorgacevic, B., Vukicevic, D., et al. (2020). Effect of betaine supplementation on liver tissue and ultrastructural changes in methionine-choline-deficient diet-induced NAFLD. Microsc. Microanal. 26 (5), 997–1006. doi:10.1017/S1431927620024265
Veskovic, M., Mladenovic, D., Milenkovic, M., Tosic, J., Borozan, S., Gopcevic, K., et al. (2019). Betaine modulates oxidative stress, inflammation, apoptosis, autophagy, and Akt/mTOR signaling in methionine-choline deficiency-induced fatty liver disease. Eur. J. Pharmacol. 848, 39–48. doi:10.1016/j.ejphar.2019.01.043
Wan, X., Xu, C., Lin, Y., Lu, C., Li, D., Sang, J., et al. (2016). Uric acid regulates hepatic steatosis and insulin resistance through the NLRP3 inflammasome-dependent mechanism. J. Hepatol. 64 (4), 925–932. doi:10.1016/j.jhep.2015.11.022
Wang, F., Park, J. S., Ma, Y., Ma, H., Lee, Y. J., Lee, G. R., et al. (2021). Ginseng saponin enriched in Rh1 and Rg2 ameliorates nonalcoholic fatty liver disease by inhibiting inflammasome activation. Nutrients 13 (3), 856. doi:10.3390/nu13030856
Wang, H., Fang, Z. Z., Meng, R., Cao, Y. F., Tanaka, N., Krausz, K. W., et al. (2017a). Glycyrrhizin and glycyrrhetinic acid inhibits alpha-naphthyl isothiocyanate-induced liver injury and bile acid cycle disruption. Toxicology 386, 133–142. doi:10.1016/j.tox.2017.05.012
Wang, Q., Ou, Y., Hu, G., Wen, C., Yue, S., Chen, C., et al. (2020a). Naringenin attenuates non-alcoholic fatty liver disease by down-regulating the NLRP3/NF-κB pathway in mice. Br. J. Pharmacol. 177 (8), 1806–1821. doi:10.1111/bph.14938
Wang, X., Wang, C., Zhang, L., Li, Y., Wang, S., Wang, J., et al. (2015). Salvianolic acid A shows selective cytotoxicity against multidrug-resistant MCF-7 breast cancer cells. Anticancer. Drugs 26 (2), 210–223. doi:10.1097/CAD.0000000000000184
Wang, Y., Cui, S., Zheng, J., Li, Y., Li, P., Hou, H., et al. (2020b). Berberine ameliorates intestinal mucosal barrier dysfunction in nonalcoholic fatty liver disease (NAFLD) rats. J. King Saud Univ. - Sci. 32 (5), 2534–2539. doi:10.1016/j.jksus.2020.03.019
Wang, Y., Mukhopadhyay, P., Cao, Z., Wang, H., Feng, D., Hasko, G., et al. (2017b). Cannabidiol attenuates alcohol-induced liver steatosis, metabolic dysregulation, inflammation and neutrophil-mediated injury. Sci. Rep. 7 (1), 12064. doi:10.1038/s41598-017-10924-8
Wang, Y., Yang, J., Hong, T., Chen, X., and Cui, L. (2019). SIRT2: Controversy and multiple roles in disease and physiology. Ageing Res. Rev. 55, 100961. doi:10.1016/j.arr.2019.100961
Wei, Q., Zhu, R., Zhu, J., Zhao, R., and Li, M. (2019). E2-Induced activation of the NLRP3 inflammasome triggers pyroptosis and inhibits autophagy in HCC cells. Oncol. Res. 27 (7), 827–834. doi:10.3727/096504018X15462920753012
Wong, A. S., Che, C. M., and Leung, K. W. (2015). Recent advances in ginseng as cancer therapeutics: A functional and mechanistic overview. Nat. Prod. Rep. 32 (2), 256–272. doi:10.1039/c4np00080c
Wree, A., McGeough, M. D., Pena, C. A., Schlattjan, M., Li, H., Inzaugarat, M. E., et al. (2014). NLRP3 inflammasome activation is required for fibrosis development in NAFLD. J. Mol. Med. 92 (10), 1069–1082. doi:10.1007/s00109-014-1170-1
Wu, D., Yang, X., Zheng, T., Xing, S., Wang, J., Chi, J., et al. (2016). A novel mechanism of action for salidroside to alleviate diabetic albuminuria: Effects on albumin transcytosis across glomerular endothelial cells. Am. J. Physiol. Endocrinol. Metab. 310 (3), E225–E237. doi:10.1152/ajpendo.00391.2015
Wu, J. Z., Liu, Y. H., Liang, J. L., Huang, Q. H., Dou, Y. X., Nie, J., et al. (2018). Protective role of beta-patchoulene from Pogostemon cablin against indomethacin-induced gastric ulcer in rats: Involvement of anti-inflammation and angiogenesis. Phytomedicine 39, 111–118. doi:10.1016/j.phymed.2017.12.024
Wu, X., Zhang, F., Xiong, X., Lu, C., Lian, N., Lu, Y., et al. (2015). Tetramethylpyrazine reduces inflammation in liver fibrosis and inhibits inflammatory cytokine expression in hepatic stellate cells by modulating NLRP3 inflammasome pathway. IUBMB Life 67 (4), 312–321. doi:10.1002/iub.1348
Xu, B., Jiang, M., Chu, Y., Wang, W., Chen, D., Li, X., et al. (2018a). Gasdermin D plays a key role as a pyroptosis executor of non-alcoholic steatohepatitis in humans and mice. J. Hepatol. 68 (4), 773–782. doi:10.1016/j.jhep.2017.11.040
Xu, G. B., Xiao, Y. H., Zhang, Q. Y., Zhou, M., and Liao, S. G. (2018b). Hepatoprotective natural triterpenoids. Eur. J. Med. Chem. 145, 691–716. doi:10.1016/j.ejmech.2018.01.011
Xu, G., Fu, S., Zhan, X., Wang, Z., Zhang, P., Shi, W., et al. (2021). Echinatin effectively protects against NLRP3 inflammasome-driven diseases by targeting HSP90. JCI Insight 6 (2), 134601. doi:10.1172/jci.insight.134601
Xu, Y., Yang, C., Zhang, S., Li, J., Xiao, Q., Huang, W., et al. (2018c). Ginsenoside Rg1 protects against non-alcoholic fatty liver disease by ameliorating lipid peroxidation, endoplasmic reticulum stress, and inflammasome activation. Biol. Pharm. Bull. 41 (11), 1638–1644. doi:10.1248/bpb.b18-00132
Yan, T., Wang, H., Cao, L., Wang, Q., Takahashi, S., Yagai, T., et al. (2018). Glycyrrhizin alleviates nonalcoholic steatohepatitis via modulating bile acids and meta-inflammation. Drug Metab. Dispos. 46 (9), 1310–1319. doi:10.1124/dmd.118.082008
Yang, G., Jang, J. H., Kim, S. W., Han, S. H., Ma, K. H., Jang, J. K., et al. (2020). Sweroside prevents non-alcoholic steatohepatitis by suppressing activation of the NLRP3 inflammasome. Int. J. Mol. Sci. 21 (8), E2790. doi:10.3390/ijms21082790
Yang, G., Lee, H. E., and Lee, J. Y. (2016a). A pharmacological inhibitor of NLRP3 inflammasome prevents non-alcoholic fatty liver disease in a mouse model induced by high fat diet. Sci. Rep. 6, 24399. doi:10.1038/srep24399
Yang, L., Rozenfeld, R., Wu, D., Devi, L. A., Zhang, Z., Cederbaum, A., et al. (2014). Cannabidiol protects liver from binge alcohol-induced steatosis by mechanisms including inhibition of oxidative stress and increase in autophagy. Free Radic. Biol. Med. 68, 260–267. doi:10.1016/j.freeradbiomed.2013.12.026
Yang, Q. L., Yang, F., Gong, J. T., Tang, X. W., Wang, G. Y., Wang, Z. T., et al. (2016b). Sweroside ameliorates alpha-naphthylisothiocyanate-induced cholestatic liver injury in mice by regulating bile acids and suppressing pro-inflammatory responses. Acta Pharmacol. Sin. 37 (9), 1218–1228. doi:10.1038/aps.2016.86
Yang, S. J., and Lim, Y. (2014). Resveratrol ameliorates hepatic metaflammation and inhibits NLRP3 inflammasome activation. Metabolism. 63 (5), 693–701. doi:10.1016/j.metabol.2014.02.003
Yi, Y. S. (2018). Regulatory roles of flavonoids on inflammasome activation during inflammatory responses. Mol. Nutr. Food Res. 62 (13), e1800147. doi:10.1002/mnfr.201800147
Yin, Q., Fang, S., Park, J., Crews, A. L., Parikh, I., Adler, K. B., et al. (2016). An inhaled inhibitor of myristoylated alanine-rich C kinase substrate reverses LPS-induced acute lung injury in mice. Am. J. Respir. Cell Mol. Biol. 55 (5), 617–622. doi:10.1165/rcmb.2016-0236RC
Yon, C., Teramoto, T., Mueller, N., Phelan, J., Ganesh, V. K., Murthy, K. H., et al. (2005). Modulation of the nucleoside triphosphatase/RNA helicase and 5'-RNA triphosphatase activities of Dengue virus type 2 nonstructural protein 3 (NS3) by interaction with NS5, the RNA-dependent RNA polymerase. J. Biol. Chem. 280 (29), 27412–27419. doi:10.1074/jbc.M501393200
Yong, Z., Ruiqi, W., Hongji, Y., Ning, M., Chenzuo, J., Yu, Z., et al. (2021). Mangiferin ameliorates HFD-induced NAFLD through regulation of the AMPK and NLRP3 inflammasome signal pathways. J. Immunol. Res. 2021, 4084566. doi:10.1155/2021/4084566
Younossi, Z. M., Koenig, A. B., Abdelatif, D., Fazel, Y., Henry, L., Wymer, M., et al. (2016). Global epidemiology of nonalcoholic fatty liver disease-Meta-analytic assessment of prevalence, incidence, and outcomes. Hepatology 64 (1), 73–84. doi:10.1002/hep.28431
Yu, X., Hao, M., Liu, Y., Ma, X., Lin, W., Xu, Q., et al. (2019). Liraglutide ameliorates non-alcoholic steatohepatitis by inhibiting NLRP3 inflammasome and pyroptosis activation via mitophagy. Eur. J. Pharmacol. 864, 172715. doi:10.1016/j.ejphar.2019.172715
Zeng, W., Jin, L., Zhang, F., Zhang, C., and Liang, W. (2018). Naringenin as a potential immunomodulator in therapeutics. Pharmacol. Res. 135, 122–126. doi:10.1016/j.phrs.2018.08.002
Zhang, B., Liu, Z. Y., Li, Y. Y., Luo, Y., Liu, M. L., Dong, H. Y., et al. (2011). Antiinflammatory effects of matrine in LPS-induced acute lung injury in mice. Eur. J. Pharm. Sci. 44 (5), 573–579. doi:10.1016/j.ejps.2011.09.020
Zhang, B., Xu, D., She, L., Wang, Z., Yang, N., Sun, R., et al. (2018). Silybin inhibits NLRP3 inflammasome assembly through the NAD(+)/SIRT2 pathway in mice with nonalcoholic fatty liver disease. FASEB J. 32 (2), 757–767. doi:10.1096/fj.201700602R
Zhang, H., Liu, Y. Y., Jiang, Q., Li, K. R., Zhao, Y. X., Cao, C., et al. (2014). Salvianolic acid A protects RPE cells against oxidative stress through activation of Nrf2/HO-1 signaling. Free Radic. Biol. Med. 69, 219–228. doi:10.1016/j.freeradbiomed.2014.01.025
Zhang, J., Chen, Z., Huang, X., Shi, W., Zhang, R., Chen, M., et al. (2019). Insights on the multifunctional activities of magnolol. Biomed. Res. Int. 2019, 1847130. doi:10.1155/2019/1847130
Zhang, J., Zhang, H., Deng, X., Zhang, Y., and Xu, K. (2017a). Baicalin protects AML-12 cells from lipotoxicity via the suppression of ER stress and TXNIP/NLRP3 inflammasome activation. Chem. Biol. Interact. 278, 189–196. doi:10.1016/j.cbi.2017.10.010
Zhang, P., Qiang, X., Zhang, M., Ma, D., Zhao, Z., Zhou, C., et al. (2015). Demethyleneberberine, a natural mitochondria-targeted antioxidant, inhibits mitochondrial dysfunction, oxidative stress, and steatosis in alcoholic liver disease mouse model. J. Pharmacol. Exp. Ther. 352 (1), 139–147. doi:10.1124/jpet.114.219832
Zhang, Y., Liao, P., Zhu, M., Li, W., Hu, D., Guan, S., et al. (2017b). Baicalin attenuates cardiac dysfunction and myocardial remodeling in a chronic pressure-overload mice model. Cell. Physiol. biochem. 41 (3), 849–864. doi:10.1159/000459708
Zhang, Y., Wen, J., Liu, D., Qiu, Z., Zhu, Q., Li, R., et al. (2021). Demethylenetetrahydroberberine alleviates nonalcoholic fatty liver disease by inhibiting the NLRP3 inflammasome and oxidative stress in mice. Life Sci. 281, 119778. doi:10.1016/j.lfs.2021.119778
Zhao, M., Li, C., Shen, F., Wang, M., Jia, N., Wang, C., et al. (2017). Naringenin ameliorates LPS-induced acute lung injury through its anti-oxidative and anti-inflammatory activity and by inhibition of the PI3K/AKT pathway. Exp. Ther. Med. 14 (3), 2228–2234. doi:10.3892/etm.2017.4772
Zhao, X. J., Yu, H. W., Yang, Y. Z., Wu, W. Y., Chen, T. Y., Jia, K. K., et al. (2018). Polydatin prevents fructose-induced liver inflammation and lipid deposition through increasing miR-200a to regulate Keap1/Nrf2 pathway. Redox Biol. 18, 124–137. doi:10.1016/j.redox.2018.07.002
Zheng, T., Yang, X., Li, W., Wang, Q., Chen, L., Wu, D., et al. (2018). Salidroside attenuates high-fat diet-induced nonalcoholic fatty liver disease via AMPK-dependent TXNIP/NLRP3 pathway. Oxid. Med. Cell. Longev. 2018, 8597897. doi:10.1155/2018/8597897
Zheng, T., Yang, X., Wu, D., Xing, S., Bian, F., Li, W., et al. (2015). Salidroside ameliorates insulin resistance through activation of a mitochondria-associated AMPK/PI3K/Akt/GSK3β pathway. Br. J. Pharmacol. 172 (13), 3284–3301. doi:10.1111/bph.13120
Zhou, R., Tardivel, A., Thorens, B., Choi, I., and Tschopp, J. (2010). Thioredoxin-interacting protein links oxidative stress to inflammasome activation. Nat. Immunol. 11 (2), 136–140. doi:10.1038/ni.1831
Zhou, R., Yazdi, A. S., Menu, P., and Tschopp, J. (2011). A role for mitochondria in NLRP3 inflammasome activation. Nature 469 (7329), 221–225. doi:10.1038/nature09663
Zhu, W., Jia, Q., Wang, Y., Zhang, Y., and Xia, M. (2012). The anthocyanin cyanidin-3-O-beta-glucoside, a flavonoid, increases hepatic glutathione synthesis and protects hepatocytes against reactive oxygen species during hyperglycemia: Involvement of a cAMP-PKA-dependent signaling pathway. Free Radic. Biol. Med. 52 (2), 314–327. doi:10.1016/j.freeradbiomed.2011.10.483
Glossary
NAFLD non-alcoholic fatty liver disease
NLRP3 pyrin domain-containing protein 3
IL-1β interleukin (IL)-1β
NAFL non-alcoholic fatty liver
NASH non-alcoholic steatohepatitis
DAMPs damage-related molecular patterns
PAMPs pathogen-associated molecular patterns
KCs Kupffer cells
FDA Food and Drug Administration
TLR toll-like receptor
NF-κB nuclear factor kappa-B
ROS reactive oxygen species
HSCs hepatic stellate cells
ALT alanine transaminase
AST aspartate transaminase
BMDMs bone marrow-derived macrophages
PA palmitic acid
GSDMD Gasdermin D
FFD fast food diet
TC total cholesterol
HFD high-fat diet
TXNIP thioredoxin interaction protein
LPS Lipopolysaccharide
ATP adenosine triphosphate
TG triglyceride
TNF-α tumor necrosis factor -α
FFA free fatty-acids
CTCF CCCTC-binding factor silencing
DPP4 dipeptidyl peptidase-4
MCD methionine-choline deficient diet
XO xanthine oxidase
HSP90 heat-shock protein 90
SGT1 solanidine galactosyltransferases
NAD+ nicotbambeadenbe dinuclotide
SIRT2 sirtuin type2
PINK1 PTEN-induced kinase 1
HO-1 Heme Oxygenase-1
Keywords: non-alcoholic fatty liver disease, NLRP3 inflammasome, plant-derived active ingredients, terpenoids, flavonoids, alkaloids, phenols
Citation: Huang Q, Xin X, Sun Q, An Z, Gou X and Feng Q (2022) Plant-derived bioactive compounds regulate the NLRP3 inflammasome to treat NAFLD. Front. Pharmacol. 13:896899. doi: 10.3389/fphar.2022.896899
Received: 15 March 2022; Accepted: 29 June 2022;
Published: 09 August 2022.
Edited by:
Somasundaram Arumugam, National Institute of Pharmaceutical Education and Research, Kolkata, IndiaReviewed by:
Lei Gao, Southern Medical University, ChinaSabine Klein, University Hospital Frankfurt, Germany
Copyright © 2022 Huang, Xin, Sun, An, Gou and Feng. This is an open-access article distributed under the terms of the Creative Commons Attribution License (CC BY). The use, distribution or reproduction in other forums is permitted, provided the original author(s) and the copyright owner(s) are credited and that the original publication in this journal is cited, in accordance with accepted academic practice. No use, distribution or reproduction is permitted which does not comply with these terms.
*Correspondence: Qin Feng, ZmVuZ3FpbkBzaHV0Y20uZWR1LmNu