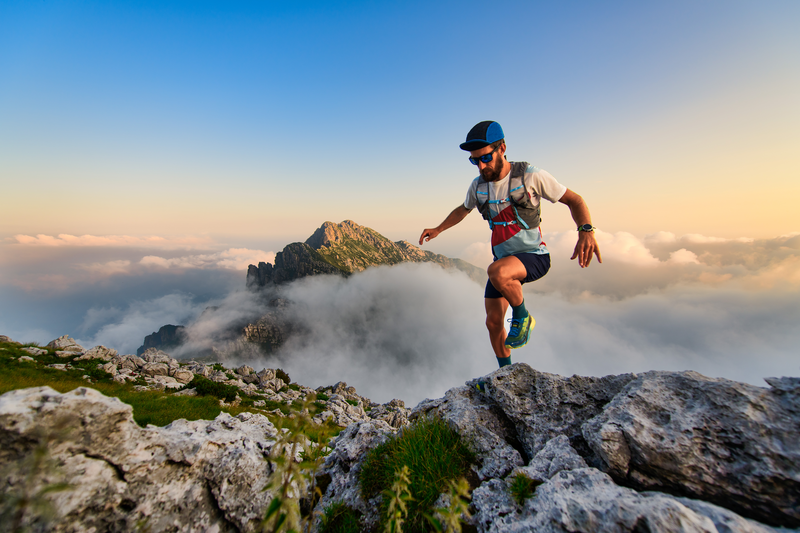
94% of researchers rate our articles as excellent or good
Learn more about the work of our research integrity team to safeguard the quality of each article we publish.
Find out more
BRIEF RESEARCH REPORT article
Front. Pharmacol. , 15 July 2022
Sec. Experimental Pharmacology and Drug Discovery
Volume 13 - 2022 | https://doi.org/10.3389/fphar.2022.896760
This article is part of the Research Topic Drug Repositioning: Current Advances and Future Perspectives, Volume II View all 4 articles
Cisplatin (CDDP) is a well-known chemotherapeutic drug approved for various cancers. However, CDDP accumulates in the inner ear cochlea via organic cation transporter 2 (OCT2) and causes ototoxicity, which is a major clinical limitation. Since lansoprazole (LPZ), a proton pump inhibitor, is known to inhibit OCT2-mediated transport of CDDP, we hypothesized that LPZ might ameliorate CDDP-induced ototoxicity (CIO). To test this hypothesis, we utilized in vivo fluorescence imaging of zebrafish sensory hair cells. The fluorescence signals in hair cells in zebrafish treated with CDDP dose-dependently decreased. Co-treatment with LPZ significantly suppressed the decrease of fluorescence signals in zebrafish treated with CDDP. Knockout of a zebrafish homolog of OCT2 also ameliorated the reduction of fluorescence signals in hair cells in zebrafish treated with CDDP. These in vivo studies suggest that CDDP damages the hair cells of zebrafish through oct2-mediated accumulation and that LPZ protects against CIO, possibly inhibiting the entry of CDDP into the hair cells via oct2. We also evaluated the otoprotective effect of LPZ using a public database containing adverse event reports. The analysis revealed that the incidence rate of CIO was significantly decreased in patients treated with LPZ. We then retrospectively analyzed the medical records of Mie University Hospital to examine the otoprotective effect of LPZ. The incidence rate of ototoxicity was significantly lower in patients co-treated with LPZ compared to those without LPZ. These retrospective findings suggest that LPZ is also protective against CIO in humans. Taken together, co-treatment with LPZ may reduce the risk of CIO.
Cisplatin (CDDP), an inorganic platinum derivative, is one of the most widely used chemotherapeutic drugs against various cancers (Ghosh, 2019). CDDP, however, often causes many adverse events such as ototoxicity, nephrotoxicity, neuropathy, severe nausea and vomiting, and hematological toxicity (Ghosh, 2019). Specifically, hearing impairment is observed in over 60% of patients receiving chemotherapies with CDDP, which limits its clinical application (Karasawa and Steyger, 2015). Hearing impairment can lead to a decrease in quality of life, the development of depression, and poor communication abilities (Li et al., 2014; Nordvik et al., 2018; Bass et al., 2020). There remains an urgent need to develop strategies against CDDP-induced ototoxicity (CIO) (Lanvers-Kaminsky et al., 2017; Freyer et al., 2020; Santos et al., 2020; Yu et al., 2020; Pasquariello et al., 2021).
CDDP is accumulated in the stria vascularis of the cochlea and retained in the cochlea for a long time in humans, resulting in permanent hearing loss (Breglio et al., 2017). Organic cation transporter 2 (OCT2), also known as Solute Carrier Family 22 Member 2 (SLC22A2), is responsible for CDDP-induced ototoxicity via CDDP accumulation in the inner ear cochlea (Lanvers-Kaminsky et al., 2017). The single-nucleotide polymorphism rs316019 in OCT2 (c.808G > T; Ser270Ala), which adversely affects the function of OCT2 (Frenzel et al., 2019), is associated with the protection against CIO (Lanvers-Kaminsky et al., 2015; Langer et al., 2020). CIO is not observed in Oct2 knock-out (KO) mice (Ciarimboli et al., 2010). OCT2 is also involved in CDDP-induced nephrotoxicity (CIN) (Filipski et al., 2009; McSweeney et al., 2021). Considering these findings, we hypothesized that drugs that can inhibit OCT2 may protect against CIO.
Several studies have demonstrated that proton pump inhibitors (PPIs), drugs clinically used to treat and/or prevent gastroesophageal reflux disease and peptic ulcers (Scarpignato et al., 2016; Maret-Ouda et al., 2020), can inhibit OCT2 (Ikemura et al., 2017a). PPIs inhibit OCT2-mediated transport of metformin, a substrate of OCT2 (Nies et al., 2011). PPIs are not the substrates of OCT2 (Nies et al., 2011). Although the underlying mechanisms of how PPIs inhibit OCT2 remain unknown, several studies have demonstrated the protective role of PPIs against CIN. Lansoprazole (LPZ), a clinically used PPI, suppresses CIN through the inhibition of renal OCT2 in rats (Hiramatsu et al., 2020). The incidence rate of CIN is significantly lower in patients co-treated with LPZ compared to those without LPZ (Ikemura et al., 2017b). Pantoprazole, another PPI, also suppresses CIN in humans (Ghonaim et al., 2021). Therefore, we examined the effect of PPIs against CIO using in vivo fluorescence imaging of auditory hair cells in zebrafish and retrospective analyses using a public database, the Food and Drug Administration Adverse Event Reporting System (FAERS), and a database of electronic health records (EHRs) of patients in Mie University Hospital.
All animal experiments examined in the present study were approved by the Mie University Review Board for Animal Investigation (no. 2020–19) and conformed to the ethical guidelines established by the committee. The clinical study was conducted in accordance with the Declaration of Helsinki and was approved by the Ethics Committee of Mie University Graduate School of Medicine and Faculty of Medicine (no. 2021–170). Informed consent was obtained from participants through an opt-out method, because the data were collected retrospectively from electronic medical records.
Yo-Pro-1 (Catalog number: Y3603) was purchased from Invitrogen (Tokyo, Japan). CDDP, cimetidine (CMD), and LPZ were purchased from Tokyo Chemical Industry Co., Ltd (Tokyo, Japan). Stock solutions of Yo-Pro-1 and LPZ were prepared by dissolving in dimethyl sulfoxide. Other chemicals were dissolved in 0.3 × Danieau’s solution (19.3 mM NaCl, 0.23 mM KCl, 0.13 mM MgSO4, 0.2 mM Ca(NO3)2, and 1.7 mM HEPES, pH 7.2). These solutions were diluted in 0.3 × Danieau’s solution to obtain the indicated concentrations. Lissamine-labeled control morpholino with no known target gene was purchased from Gene Tools (Philomath, OR, United States) and diluted with distilled water to prepare the stock solution.
Zebrafish were maintained as described previously (Nishimura et al., 2016). Briefly, zebrafish were raised at 28.5 ± 0.5°C with a 14 h/10 h light/dark cycle. Embryos were obtained via natural mating and cultured in 0.3 × Danieau’s solution.
There are two oct2 genes (zgc:64,076 and zgc:175,176) in zebrafish according to the database of genetic and genomic data specialized for zebrafish (https://zfin.org/). CRISPR RNA (crRNA) targeting zgc:64,076 or zgc:175,176, trans-activating crRNA (tracrRNA), and recombinant Cas9 protein were obtained from FASMAC (Kanagawa, Japan) (sequences shown in Supplementary Table S1). Crispant zebrafish for zgc:64,076 or zgc:175,176 were generated according to methods described previously (Adachi et al., 2021). Briefly, the larvae exhibiting bright fluorescence of lissamine were selected at 1 day-post fertilization (dpf). Genomic DNA was extracted from zebrafish embryos injected with crRNA targeting zgc:64,076 or zgc:175,176 (crispants) or without crRNA (wild type, WT). Short fragments of the zgc:64,076 and zgc:175,176 gene encompassing the sites targeted by crRNAs were amplified from genomic DNA using the primers shown in Supplementary Table S1. Three-step PCR was carried out, followed by electrophoresis in 10% polyacrylamide gels (FUJIFILM Wako Pure Chemical, Osaka, Japan) and visualization by ethidium bromide staining.
Several studies have demonstrated that the loss of sensory hair cells can be caused by CDDP exposure within 4 h at 1,000 μM and 24 h at 50 μM (Ou et al., 2007; Domarecka et al., 2020). In this study, we wanted to analyze the effects of protectants at relatively early stages of CIO. Zebrafish larvae at 5 dpf were incubated in 0.3× Danieau’s solution containing CDDP (0, 50, 100, 250 μM, 500 μM, or 1,000 μM) alone for 2 h or co-treated with CDDP (0 or 250 μM) and CMD (0 or 1 mM) or LPZ (0, 0.1, 0.25, or 0.5 μM) for 2 h.
Sensory hair cells of zebrafish areanalogous to those of mammals (Lush and Piotrowski, 2014). Sensory hair cells express purinergic receptor P2X ligand-gated ion channel 7 (P2X7), which is activated by ATP secreted from supporting cells under physiological conditions (Zhao et al., 2005). Yo-Pro-1, a fluorescent cyanine dye, can permeate the cells via activated P2X7 (Virginio et al., 1999). Therefore, sensory hair cells of zebrafish can be visualized by vital staining with Yo-Pro-1 (Santos et al., 2006; Sasagawa et al., 2016). In this study, zebrafish larvae at 5 dpf were immersed in medium containing 2 μM of Yo-Pro-1 for 30 min. After staining, zebrafish were transferred to 0.3 × Danieau’s solution containing 2-phenoxyethanol (500 ppm) to be anesthetized and then transferred to glass slides. A few drops of 3% low-melting agarose solution were placed over the larvae, and the zebrafish were immediately oriented sideways dorsal-side-up. The zebrafish were then observed using an epifluorescence microscope (SZX7, Olympus, Tokyo, Japan) with the SZX-MGFP filter (Ex 475/30 nm, Em 510 nm). The 16-bit fluorescence images were converted to 256-gray-scale (0–255) images in Fiji (Schindelin et al., 2012). Considering the previous studies (Chiu et al., 2008; Sasagawa et al., 2016), we measured the fluorescence signal of three neuromasts (MI1, MI2, and O2) (Raible and Kruse, 2000). Neuromasts are composed of different types of cells, including hair cells and supporting cells, which are important for hearing and balance in zebrafish (Froehlicher et al., 2009). The sum of the fluorescent areas of these neuromasts (shown in small rectangles in each figure) with signals over the threshold (25 in the 256-gray scale) was calculated and reported as the fluorescent area of hair cells in a zebrafish.
Zebrafish were harvested at 5 dpf and stored in RNA-later (Applied Biosystems, Foster City, CA, United States). Total RNA was extracted using an RNeasy Mini kit (Qiagen, Germantown, MD, United States) according to the manufacturer’s protocol. cDNA was generated using a ReverTra Ace qPCR RT Kit (Toyobo, Osaka, Japan) and qPCR was performed using QuantStudio3 real-time PCR machine (Applied Biosystems, Thermo Fisher, United States) with THUNDERBIRD SYBR qPCR Mix (Toyobo). The thermal cycling conditions were 95°C for 60 s, followed by 40 cycles of 95 °C for 15 s, 60°C for 15 s, and 72°C for 45 s. Expression of zgc:64,076 and zgc:175,176 mRNA was normalized to that of the eukaryotic translation elongation factor 1 alpha 2 (ef1a) to correct for variability in the initial template concentration and reverse transcription efficiency. qPCR primer sequences are shown in Supplementary Table S1.
Data on patient demographics and administration information, drug/biologic information, and adverse events from July 2014 to December 2020 were obtained from the FAERS database. Duplicate reports were excluded in accordance with the FDA recommendations. Data analyses were performed using ACCESS® 2019 software (Microsoft, Redmond, WA). Data associated with CDDP administration were extracted. Disease names were defined using the Medical Dictionary for Regulatory Activities (MedDRA/J) version 24.0. The standardized MedDRA Query (SMQ) was used for searching for hearing impairment (SMQ code: 20,000,171). The effect of PPIs on CDDP-associated hearing impairment was evaluated using the reporting odds ratio (ROR). To calculate the ROR, CDDP-associated hearing impairment and all other reported adverse events associated with CDDP were defined as “cases” and “non-cases,” respectively. The RORs were calculated from two-by-two contingency tables of counts with or without PPI. RORs were expressed as point estimates with 95% confidence interval (CI). Although stratification based on age, gender, and primary diseases can be done using the FAERS database (Nagashima et al., 2016; Hosoya et al., 2022), we did not perform the stratified analysis due to the small number of the CIO cases treated with PPIs.
Clinical data were collected from EHRs of patients who received CDDP in the Department of Otorhinolaryngology-Head and Neck Surgery or the Department of Medical Oncology of Mie University Hospital during January 2010 and April 2021. Among 463 hospitalized adult patients treated with CDDP, 340 patients treated with ≥300 mg of total CDDP dose for cancers in the lung, esophagus, stomach, kidney, mammary glands, thymus, and reproductive tissues were enrolled in this study. Patients were excluded if they were defined as having hearing loss before CDDP treatment (n = 5), ear disease (n = 6), prescribed aminoglycoside antibiotics (n = 1), and PPIs prescribed other than LPZ (n = 39). Ototoxicity was defined as hearing loss, sensorineural hearing loss, or tinnitus following CDDP treatment.
The results are expressed as the mean ± standard deviation. Differences between two groups were assessed using unpaired t-tests. Statistical comparisons for multiple groups were performed using one-way analysis of variance followed by Dunnett’s test or Tukey’s multiple comparison test. Statistical comparisons between two groups in clinical data were performed using the Mann-Whitney U test and Fisher’s exact test for continuous and categorical variables, respectively. All statistical analyses were performed using GraphPad Prism 9 (GraphPad Software Inc. San Diego, CA). The significance was established at a p value < 0.05.
We first evaluated the ototoxicity of CDDP in zebrafish by in vivo fluorescence imaging of sensory hair cells stained with Yo-Pro-1. The in vivo imaging revealed that the fluorescence signals of hair cells in zebrafish were decreased dependent on the dose of CDDP (Figures 1A,B). Because the fluorescence of hair cells in zebrafish treated with 250 μM CDDP was around 50% (Figure 1B), we selected this dose to examine the otoprotective effects of CMD and LPZ against the ototoxicity of CDDP.
FIGURE 1. In vivo fluorescence imaging of hair cells in zebrafish to assess CDDP-induced ototoxicity and otoprotective effects of cimetidine and lansoprazole. (A,B) Zebrafish were treated with CDDP at indicated doses for 2 h, followed by staining with Yo-Pro-1 of sensory hair cells. The fluorescence signals of three neuromasts including hair cells shown in the white rectangles were calculated and are shown as the percentage of the signal in zebrafish without CDDP treatment. (C–F) Zebrafish were treated with or without cimetidine (CMD, (C,D) or lansoprazole (LPZ, (E,F) to examine their effect on the decrease of fluorescence signals in hair cells in zebrafish exposed to CDDP. Quantitative analyses were performed as described above.
Previous studies have demonstrated that CMD inhibits OCT2 and suppresses both CIO and CIN in rodents (Okuda et al., 1996; Ciarimboli et al., 2010). CMD is a substrate of OCT2 and works as a competitive inhibitor of other OCT2 substrates (Urakami et al., 1998; Harper and Wright, 2013; Yin et al., 2016). Consistent with these studies, co-treatment with 1 mM of CMD significantly suppressed the decrease of fluorescence signals in hair cells in zebrafish treated with 250 μM of CDDP (Figures 1C,D).
We then examined the effect of LPZ on the decrease of fluorescence signals in hair cells in zebrafish treated with 250 μM of CDDP. As shown in Figures 1D,E, LPZ dose-dependently inhibited the decrease of fluorescence signals in hair cells in zebrafish treated with 250 μM of CDDP. These results suggest that LPZ ameliorates CIO through inhibition of OCT2.
To examine the role of oct2 in CIO in zebrafish, we generated two crispant zebrafish with KO of zgc:64,076 or zgc:175,176, zebrafish homologs of OCT2. To KO zgc:64,076, we designed crRNA targeting the sequence including the protospacer adjacent motif located behind the start codon in the first exon, and then injected the crRNA with tracrRNA and Cas9 protein into zebrafish embryos. At 1 dpf, the genomes were extracted, and PCR analysis was performed using primers to amplify the genomic regions around the sequences targeted by the crRNA. As shown in Figure 2A, extra bands (shown in red bracket) were observed below the main PCR products (black arrowhead) in the crispant but not WT zebrafish, suggesting that KO of zgc:64,076 were generated in the crispant zebrafish. There were no external abnormalities in the zgc:64,076 crispant zebrafish (Figure 2B). In vivo fluorescence imaging of zebrafish hair cells revealed that CDDP treatment caused a decrease of fluorescence signals in hair cells in WT but not zgc:64,076 crispant zebrafish (Figures 2C,D).
FIGURE 2. In vivo fluorescence imaging of hair cells in crispant zebrafish to assess the involvement of oct2 in the decrease of fluorescence signals caused by CDDP. Crispant zebrafish with knockout (KO) of zgc:64,076 or zgc:175,176, zebrafish homologs of OCT2, were generated using CRISPR-Cas9 technology. (A,E) Electrophoresis of genomic PCR around the sequences targeted by genome editing of zgc:64,076 (A) or zgc:175,176 (E). (B,F) Bright field imaging of both wild-type (WT) and crispant zebrafish for zgc:64,076 (B) and zgc:175,176 (F). C, D, G, and (H) WT and crispant zebrafish for zgc:64,076 (C,D) and zgc:175,176 (G,H) were treated with or without CDDP, followed by staining with Yo-Pro-1 of sensory hair cells. The fluorescence signals of three neuromasts including hair cells shown in the white rectangles were calculated and are shown as the percentage of the signal of WT zebrafish without CDDP treatment.
We also generated zgc:175,176 crispant zebrafish using CRISPR-Cas9 technology. Genomic PCR analysis confirmed KO of zgc:175,176 in the crispant zebrafish (Figure 2E). No external abnormalities were observed in the zgc:175,176 crispant zebrafish (Figure 2F). In vivo fluorescence imaging of zebrafish hair cells, however, revealed that CDDP treatment caused a decrease in fluorescence signals in hair cells in both WT and zgc:175,176 crispant zebrafish (Figures 2G,H). These results are consistent with the result showing that the expression of zgc:64,076 was significantly higher than that of zgc:175,176 in zebrafish at 5 dpf (Supplementary Figure S1) and suggest that zgc:64,076 is mainly involved in the CDDP-induced hair cell damage.
To examine the effect of LPZ on the incidence of CIO in humans, we analyzed the FAERS database that has been successfully used for drug repositioning (Zamami et al., 2021). We extracted 29,976 patients who experienced ototoxicity in relation to CDDP treatment. We then analyzed the reporting ratio of CIO, the ROR, and the 95% CI (Table 1). The reporting ratio of CIO in patients with LPZ (1.0%) was significantly lower than that in patients without LPZ (2.7%, p = 0.039). The ROR of CIO in patients treated with LPZ was 0.37 (95% CI: 0.14–0.99). In addition, the reporting ratio of CIO in patients treated with PPIs other than LPZ (i.e., omeprazole and esomeprazole) was also significantly lower than in that in patients treated without these PPIs (Table 1), suggesting that the protective effects of LPZ against CIO may be a class effect of PPIs.
We then validated the effect of LPZ on the incidence of CIO using the EHR of Mie University Hospital. According to the inclusion and exclusion criteria, we enrolled 289 patients in this study. Table 2 shows a comparison of patient characteristics between patients with ototoxicity and without ototoxicity. The rate of concomitant LPZ in patients without ototoxicity (88 out of 260 patients, 34%) was significantly higher than that in patients with ototoxicity (2 out of 29 patients, 7%, p = 0.002). Taken together, these results suggest that LPZ may be protective against CIO in humans.
TABLE 2. Comparison of characteristics between CDDP-treated patients with ototoxicity and without ototoxicity in EHR of Mie University Hospital.
In this study, we demonstrated that co-treatment with LPZ or KO of zgc:64,076, a homolog of OCT2, suppressed the decrease of fluorescence signals in sensory hair cells in zebrafish treated with CDDP. We also found that the incidence rate of CIO was significantly lower in patients treated with LPZ compared to those without LPZ.
The inhibitory effect of PPIs on OCT2 and the protective effects of PPIs against CIN have been demonstrated in several studies (Nies et al., 2011; Hacker et al., 2015; Ikemura et al., 2017b; Gao et al., 2019; Gao et al., 2020; Hiramatsu et al., 2020; Ismail et al., 2020; Ghonaim et al., 2021; McSweeney et al., 2021). A variant of human OCT2 rs316019, which inhibits the function of OCT2 (Frenzel et al., 2019), is associated with protection against both CIN (Iwata et al., 2012) and CIO (Lanvers-Kaminsky et al., 2015; Langer et al., 2020). KO of Oct2 is also protective against CIO (Ciarimboli et al., 2010). These findings suggest that inhibition of OCT2 is a promising approach against both CIN and CIO (Lanvers-Kaminsky et al., 2017; Gessner et al., 2019; McSweeney et al., 2021; Pasquariello et al., 2021). Both PPIs and CMD can inhibit multidrug and toxin extrusion 1 (MATE1), which is a key transporter for the excretion of CDDP from renal tubular epithelial cells (Nakamura et al., 2010; Yin et al., 2016; Guo et al., 2018). However, PPIs are not the substrates of OCT2, suggesting that the inhibition of MATE1 by PPIs may not have a significant impact on the excretion of CDDP (Nies et al., 2011; Hiramatsu et al., 2020). Consistent with this idea, the incidence of CIN in head and neck cancer patients treated with pantoprazole (PTZ), which is a PPI known to inhibit OCT2 (Nies et al., 2011; Hacker et al., 2015), is significantly lower in those without PTZ (Ghonaim et al., 2021). It should be noted, however, that PTZ is not protective against CIN and CIO in patients diagnosed with osteosarcoma treated with CDDP, methotrexate, and doxorubicin (Fox et al., 2018). Antioxidant signaling may also be involved in the protective effect of LPZ against CIO. CDDP generates oxidative stress which leads to apoptosis and necroptosis of auditory hair cells (Ramkumar et al., 2021). LPZ can exert antioxidant effects through activation of nuclear factor erythroid 2-related factor 2 (Takagi et al., 2009). Immunohistochemical analysis of zebrafish neuromasts using antibodies against hair cell markers, cleaved caspase 3, and annexin V (Shahab et al., 2021; Warchol et al., 2021) should be done to elucidate the protective mechanism of LPZ against CIO.
In this study, we found that the incidence of CIO in the FAERS database was significantly lower in patients treated with LPZ, omeprazole (OMZ), or esomeprazole, but not PTZ. Although these PPIs inhibit OCT2, there are several differences among them (Nies et al., 2011; Hacker et al., 2015). In human embryonic kidney cells (HEK293) stably overexpressing OCT2, OMZ inhibits the uptake of metformin, 4-(4-(dimethylamino)styryl)-N-methylpyridinium iodide (ASP+), and N-methyl-4-phenylpyridinium iodide (MPP+), which are substrates of OCT2 (Hacker et al., 2015). In the same cell line, LPZ inhibits the uptake of metformin and ASP+, whereas PTZ inhibits the uptake of metformin and MPP+ (Hacker et al., 2015). The IC50 of LPZ to OCT3 is smaller than that of LPZ to OCT2, whereas the IC50s of OMZ and PTZ to OCT2 are smaller than those to OCT3 in HEK293 cells stably overexpressing one of these OCTs (Nies et al., 2011). These findings suggest that the inhibition of CDDP uptake into cells through OCT2 may differ among these PPIs.
This study has some limitations. First, the protective role of LPZ against the ototoxicity caused by CDDP at relatively low doses and long exposure remains to be elucidated. Second, the localization of zgc:64,076 and zgc:175,176 in zebrafish sensory hair cells is currently unknown. Third, crispants, CRISPR-generated F0 mutants, for zgc:64,076 or zgc:175,176 were used to examine the role of zebrafish OCT2 homologs in CIO. Although crispant zebrafish have been successfully used to examine the functions of gene of interest (Watson et al., 2020; Kroll et al., 2021), a validation using homozygous germline mutants for the expression of zebrafish OCT2 homologs and protection against CIO is important to strengthen the findings of this study. Fourth, although we demonstrated that the incidence rate of CIO was significantly lower in patients treated with LPZ compared to those treated without LPZ in the EHRs of Mie University Hospital, the number of patients with CIO (n = 29) was relatively low among the total number of patients (n = 260). Additional real-world evidence among general populations is required to confirm whether LPZ or other PPIs can protect against CIO (Rajan et al., 2022). Fifth, we were not able to analyze the effect of LPZ on the survival of patients treated with CDDP. Whether these PPIs negatively affect the uptake of CDDP into tumor cells and affect patient survival should be clarified (Sprowl et al., 2013; Kichenadasse et al., 2021).
To our knowledge, however, this is the first study to demonstrate a protective effect of LPZ against CIO, and warrants further investigation to clarify the mechanisms underlying this protection.
The raw data supporting the conclusion of this article will be made available by the authors, without undue reservation.
The studies involving human participants were reviewed and approved by the Ethics Committee of Mie University Graduate School of Medicine and Faculty of Medicine. The ethics committee waived the requirement of written informed consent for participation. The animal study was reviewed and approved by the Mie University Review Board for Animal Investigation.
EW, KI, MO, and YN contributed to conception and design of the study. EW performed the experiments and the statistical analysis. TM, KT, and ST contributed to the analysis of EHR of Mie University Hospital. EW, KI, MO, and YN wrote the first draft of the manuscript. TM, KT, and ST wrote sections of the manuscript. All authors contributed to manuscript revision and read and approved the submitted version.
This work was supported in part by JSPS KAKENHI (21K06689 to KI and 19K07318 to YN). We thank Junko Koiwa and Rie Ikeyama for experimental and secretarial support, respectively.
The authors declare that the research was conducted in the absence of any commercial or financial relationships that could be construed as a potential conflict of interest.
All claims expressed in this article are solely those of the authors and do not necessarily represent those of their affiliated organizations, or those of the publisher, the editors and the reviewers. Any product that may be evaluated in this article, or claim that may be made by its manufacturer, is not guaranteed or endorsed by the publisher.
The Supplementary Material for this article can be found online at: https://www.frontiersin.org/articles/10.3389/fphar.2022.896760/full#supplementary-material
ASP+: 4-(4-(dimethylamino)styryl)-N-methylpyridinium iodide; CDDP, Cisplatin; CMD, cimetidine; CI, confidence interval; CIN, CDDP-induced nephrotoxicity; CIO, CDDP-induced ototoxicity; crRNA, CRISPR RNA; dpf, day-post fertilization; EHRs, electronic health records; FAERS, the Food and Drug Administration Adverse Event Reporting System; KO, knock-out; LPZ, lansoprazole; MATE1, multidrug and toxin extrusion 1; MPP+: N-methyl-4-phenylpyridinium iodide; OCT2, organic cation transporter 2; ROR, reporting odds ratio.
Adachi, Y., Higuchi, A., Wakai, E., Shiromizu, T., Koiwa, J., and Nishimura, Y. (2021). Involvement of Homeobox Transcription Factor Mohawk in Palatogenesis. Congenit. Anomalies 62, 27–37. doi:10.1111/cga.12451
Bass, J. K., Liu, W., Banerjee, P., Brinkman, T. M., Mulrooney, D. A., Gajjar, A., et al. (2020). Association of Hearing Impairment with Neurocognition in Survivors of Childhood Cancer. JAMA Oncol. 6, 1363–1371. doi:10.1001/jamaoncol.2020.2822
Breglio, A. M., Rusheen, A. E., Shide, E. D., Fernandez, K. A., Spielbauer, K. K., McLachlin, K. M., et al. (2017). Cisplatin is Retained in the Cochlea Indefinitely Following Chemotherapy. Nat. Commun. 8, 1654. doi:10.1038/s41467-017-01837-1
Chiu, L. L., Cunningham, L. L., Raible, D. W., Rubel, E. W., and Ou, H. C. (2008). Using the Zebrafish Lateral Line to Screen for Ototoxicity. J. Assoc. Res. Otolaryngol. 9, 178–190. doi:10.1007/s10162-008-0118-y
Ciarimboli, G., Deuster, D., Knief, A., Sperling, M., Holtkamp, M., Edemir, B., et al. (2010). Organic Cation Transporter 2 Mediates Cisplatin-Induced Oto- and Nephrotoxicity and is a Target for Protective Interventions. Am. J. Pathol. 176, 1169–1180. doi:10.2353/ajpath.2010.090610
Domarecka, E., Skarzynska, M., Szczepek, A. J., and Hatzopoulos, S. (2020). Use of Zebrafish Larvae Lateral Line to Study Protection against Cisplatin-Induced Ototoxicity: A Scoping Review. Int. J. Immunopathol. Pharmacol. 34, 2058738420959554. doi:10.1177/2058738420959554
Filipski, K. K., Mathijssen, R. H., Mikkelsen, T. S., Schinkel, A. H., and Sparreboom, A. (2009). Contribution of Organic Cation Transporter 2 (OCT2) to Cisplatin-Induced Nephrotoxicity. Clin. Pharmacol. Ther. 86, 396–402. doi:10.1038/clpt.2009.139
Fox, E., Levin, K., Zhu, Y., Segers, B., Balamuth, N., Womer, R., et al. (2018). Pantoprazole, an Inhibitor of the Organic Cation Transporter 2, Does Not Ameliorate Cisplatin-Related Ototoxicity or Nephrotoxicity in Children and Adolescents with Newly Diagnosed Osteosarcoma Treated with Methotrexate, Doxorubicin, and Cisplatin. Oncologist 23, 762–e79. doi:10.1634/theoncologist.2018-0037
Frenzel, D., Köppen, C., Bauer, O. B., Karst, U., Schröter, R., Tzvetkov, M. V., et al. (2019). Effects of Single Nucleotide Polymorphism Ala270Ser (rs316019) on the Function and Regulation of hOCT2. Biomolecules 9, 578. doi:10.3390/biom9100578
Freyer, D. R., Brock, P. R., Chang, K. W., Dupuis, L. L., Epelman, S., Knight, K., et al. (2020). Prevention of Cisplatin-Induced Ototoxicity in Children and Adolescents with Cancer: A Clinical Practice Guideline. Lancet Child. Adolesc. Health 4, 141–150. doi:10.1016/s2352-4642(19)30336-0
Froehlicher, M., Liedtke, A., Groh, K. J., Neuhauss, S. C., Segner, H., and Eggen, R. I. (2009). Zebrafish (Danio R) Neuromast: Promising Biological Endpoint Linking Developmental and Toxicological Studies. Aquat. Toxicol. 95, 307–319. doi:10.1016/j.aquatox.2009.04.007
Gao, H., Wang, X., Qu, X., Zhai, J., Tao, L., Zhang, Y., et al. (2020). Omeprazole Attenuates Cisplatin-Induced Kidney Injury through Suppression of the TLR4/NF-κB/NLRP3 Signaling Pathway. Toxicology 440, 152487. doi:10.1016/j.tox.2020.152487
Gao, H., Zhang, S., Hu, T., Qu, X., Zhai, J., Zhang, Y., et al. (2019). Omeprazole Protects against Cisplatin-Induced Nephrotoxicity by Alleviating Oxidative Stress, Inflammation, and Transporter-Mediated Cisplatin Accumulation in Rats and HK-2 Cells. Chem. Biol. Interact. 297, 130–140. doi:10.1016/j.cbi.2018.11.008
Gessner, A., König, J., and Fromm, M. F. (2019). Clinical Aspects of Transporter-Mediated Drug-Drug Interactions. Clin. Pharmacol. Ther. 105, 1386–1394. doi:10.1002/cpt.1360
Ghonaim, E., El-Haggar, S., and Gohar, S. (2021). Possible Protective Effect of Pantoprazole against Cisplatin-Induced Nephrotoxicity in Head and Neck Cancer Patients: A Randomized Controlled Trial. Med. Oncol. 38, 108. doi:10.1007/s12032-021-01558-y
Ghosh, S. (2019). Cisplatin: The First Metal Based Anticancer Drug. Bioorg Chem. 88, 102925. doi:10.1016/j.bioorg.2019.102925
Guo, D., Yang, H., Li, Q., Bae, H. J., Obianom, O., Zeng, S., et al. (2018). Selective Inhibition on Organic Cation Transporters by Carvedilol Protects Mice from Cisplatin-Induced Nephrotoxicity. Pharm. Res. 35, 204. doi:10.1007/s11095-018-2486-2
Hacker, K., Maas, R., Kornhuber, J., Fromm, M. F., and Zolk, O. (2015). Substrate-Dependent Inhibition of the Human Organic Cation Transporter OCT2: A Comparison of Metformin with Experimental Substrates. PLoS One 10, e0136451. doi:10.1371/journal.pone.0136451
Harper, J. N., and Wright, S. H. (2013). Multiple Mechanisms of Ligand Interaction with the Human Organic Cation Transporter, OCT2. Am. J. Physiol. Ren. Physiol. 304, F56–F67. doi:10.1152/ajprenal.00486.2012
Hiramatsu, S. I., Ikemura, K., Fujisawa, Y., Iwamoto, T., and Okuda, M. (2020). Concomitant Lansoprazole Ameliorates Cisplatin-Induced Nephrotoxicity by Inhibiting Renal Organic Cation Transporter 2 in Rats. Biopharm. Drug Dispos. 41, 239–247. doi:10.1002/bdd.2242
Hosoya, R., Ishii-Nozawa, R., Kurosaki, K., and Uesawa, Y. (2022). Analysis of Factors Associated with Hiccups Using the FAERS Database. Pharmaceuticals 15, 27. doi:10.3390/ph15010027
Ikemura, K., Hiramatsu, S., and Okuda, M. (2017). Drug Repositioning of Proton Pump Inhibitors for Enhanced Efficacy and Safety of Cancer Chemotherapy. Front. Pharmacol. 8, 911. doi:10.3389/fphar.2017.00911
Ikemura, K., Oshima, K., Enokiya, T., Okamoto, A., Oda, H., Mizuno, T., et al. (2017). Co-Administration of Proton Pump Inhibitors Ameliorates Nephrotoxicity in Patients Receiving Chemotherapy with Cisplatin and Fluorouracil: A Retrospective Cohort Study. Cancer Chemother. Pharmacol. 79, 943–949. doi:10.1007/s00280-017-3296-7
Ismail, R. S., El-Awady, M. S., and Hassan, M. H. (2020). Pantoprazole Abrogated Cisplatin-Induced Nephrotoxicity in Mice via Suppression of Inflammation, Apoptosis, and Oxidative Stress. Naunyn Schmiedeb. Arch. Pharmacol. 393, 1161–1171. doi:10.1007/s00210-020-01823-3
Iwata, K., Aizawa, K., Kamitsu, S., Jingami, S., Fukunaga, E., Yoshida, M., et al. (2012). Effects of Genetic Variants in SLC22A2 Organic Cation Transporter 2 and SLC47A1 Multidrug and Toxin Extrusion 1 Transporter on Cisplatin-Induced Adverse Events. Clin. Exp. Nephrol. 16, 843–851. doi:10.1007/s10157-012-0638-y
Karasawa, T., and Steyger, P. S. (2015). An Integrated View of Cisplatin-Induced Nephrotoxicity and Ototoxicity. Toxicol. Lett. 237, 219–227. doi:10.1016/j.toxlet.2015.06.012
Kichenadasse, G., Miners, J. O., Mangoni, A. A., Karapetis, C. S., Hopkins, A. M., and Sorich, M. J. (2021). Proton Pump Inhibitors and Survival in Patients with Colorectal Cancer Receiving Fluoropyrimidine-Based Chemotherapy. J. Natl. Compr. Canc Netw. 19, 1037–1044. doi:10.6004/jnccn.2020.7670
Kroll, F., Powell, G. T., Ghosh, M., Gestri, G., Antinucci, P., Hearn, T. J., et al. (2021). A Simple and Effective F0 Knockout Method for Rapid Screening of Behaviour and Other Complex Phenotypes. eLife 10, e59683. doi:10.7554/eLife.59683
Langer, T., Clemens, E., Broer, L., Maier, L., Uitterlinden, A. G., de Vries, A. C. H., et al. (2020). Usefulness of Current Candidate Genetic Markers to Identify Childhood Cancer Patients at Risk for Platinum-Induced Ototoxicity: Results of the European PanCareLIFE Cohort Study. Eur. J. Cancer 138, 212–224. doi:10.1016/j.ejca.2020.07.019
Lanvers-Kaminsky, C., Sprowl, J. A., Malath, I., Deuster, D., Eveslage, M., Schlatter, E., et al. (2015). Human OCT2 Variant c.808G>T Confers Protection Effect against Cisplatin-Induced Ototoxicity. Pharmacogenomics 16, 323–332. doi:10.2217/pgs.14.182
Lanvers-Kaminsky, C., Zehnhoff-Dinnesen, A. A., Parfitt, R., and Ciarimboli, G. (2017). Drug-Induced Ototoxicity: Mechanisms, Pharmacogenetics, and Protective Strategies. Clin. Pharmacol. Ther. 101, 491–500. doi:10.1002/cpt.603
Li, C. M., Zhang, X., Hoffman, H. J., Cotch, M. F., Themann, C. L., and Wilson, M. R. (2014). Hearing Impairment Associated with Depression in US Adults, National Health and Nutrition Examination Survey 2005-2010. JAMA Otolaryngol. Head. Neck Surg. 140, 293–302. doi:10.1001/jamaoto.2014.42
Lush, M. E., and Piotrowski, T. (2014). Sensory Hair Cell Regeneration in the Zebrafish Lateral Line. Dev. Dyn. 243, 1187–1202. doi:10.1002/dvdy.24167
Maret-Ouda, J., Markar, S. R., and Lagergren, J. (2020). Gastroesophageal Reflux Disease: A Review. JAMA 324, 2536–2547. doi:10.1001/jama.2020.21360
McSweeney, K. R., Gadanec, L. K., Qaradakhi, T., Ali, B. A., Zulli, A., and Apostolopoulos, V. (2021). Mechanisms of Cisplatin-Induced Acute Kidney Injury: Pathological Mechanisms, Pharmacological Interventions, and Genetic Mitigations. Cancers 13, 1572. doi:10.3390/cancers13071572
Nagashima, T., Shirakawa, H., Nakagawa, T., and Kaneko, S. (2016). Prevention of Antipsychotic-Induced Hyperglycaemia by Vitamin D: A Data Mining Prediction Followed by Experimental Exploration of the Molecular Mechanism. Sci. Rep. 6, 26375. doi:10.1038/srep26375
Nakamura, T., Yonezawa, A., Hashimoto, S., Katsura, T., and Inui, K. (2010). Disruption of Multidrug and Toxin Extrusion MATE1 Potentiates Cisplatin-Induced Nephrotoxicity. Biochem. Pharmacol. 80, 1762–1767. doi:10.1016/j.bcp.2010.08.019
Nies, A. T., Hofmann, U., Resch, C., Schaeffeler, E., Rius, M., and Schwab, M. (2011). Proton Pump Inhibitors Inhibit Metformin Uptake by Organic Cation Transporters (OCTs). PLoS One 6, e22163. doi:10.1371/journal.pone.0022163
Nishimura, Y., Inoue, A., Sasagawa, S., Koiwa, J., Kawaguchi, K., Kawase, R., et al. (2016). Using Zebrafish in Systems Toxicology for Developmental Toxicity Testing. Congenit. Anom. (Kyoto) 56, 18–27. doi:10.1111/cga.12142
Nordvik, Ø., Laugen Heggdal, P. O., Brännström, J., Vassbotn, F., Aarstad, A. K., and Aarstad, H. J. (2018). Generic Quality of Life in Persons with Hearing Loss: A Systematic Literature Review. BMC Ear Nose Throat Disord. 18, 1. doi:10.1186/s12901-018-0051-6
Okuda, M., Saito, H., Urakami, Y., Takano, M., and Inui, K. (1996). cDNA Cloning and Functional Expression of a Novel Rat Kidney Organic Cation Transporter, OCT2. Biochem. Biophys. Res. Commun. 224, 500–507. doi:10.1006/bbrc.1996.1056
Ou, H. C., Raible, D. W., and Rubel, E. W. (2007). Cisplatin-Induced Hair Cell Loss in Zebrafish (Danio R) Lateral Line. Hear Res. 233, 46–53. doi:10.1016/j.heares.2007.07.003
Pasquariello, K. Z., Dey, J. M., and Sprowl, J. A. (2021). Current Understanding of Membrane Transporters as Regulators or Targets for Cisplatin-Induced Hearing Loss. Mol. Pharmacol. 100, 348–355. doi:10.1124/molpharm.121.000274
Raible, D. W., and Kruse, G. J. (2000). Organization of the Lateral Line System in Embryonic Zebrafish. J. Comp. Neurol. 421, 189–198. doi:10.1002/(sici)1096-9861(20000529)421:2<189::aid-cne5>3.0.co;2-k
Rajan, P., Iglay, K., Rhodes, T., Girman, C. J., Bennett, D., and Kalantar-Zadeh, K. (2022). Risk of Bias in Non-Randomized Observational Studies Assessing the Relationship between Proton-Pump Inhibitors and Adverse Kidney Outcomes: A Systematic Review. Ther. Adv. Gastroenterol. 15, 17562848221074183. doi:10.1177/17562848221074183
Ramkumar, V., Mukherjea, D., Dhukhwa, A., and Rybak, L. P. (2021). Oxidative Stress and Inflammation Caused by Cisplatin Ototoxicity. Antioxidants 10, 1919. doi:10.3390/antiox10121919
Santos, F., MacDonald, G., Rubel, E. W., and Raible, D. W. (2006). Lateral Line Hair Cell Maturation is a Determinant of Aminoglycoside Susceptibility in Zebrafish (Danio R). Hear Res. 213, 25–33. doi:10.1016/j.heares.2005.12.009
Santos, N. A. G. D., Ferreira, R. S., and Santos, A. C. D. (2020). Overview of Cisplatin-Induced Neurotoxicity and Ototoxicity, and the Protective Agents. Food Chem. Toxicol. 136, 111079. doi:10.1016/j.fct.2019.111079
Sasagawa, S., Nishimura, Y., Koiwa, J., Nomoto, T., Shintou, T., Murakami, S., et al. (2016). In Vivo Detection of Mitochondrial Dysfunction Induced by Clinical Drugs and Disease-Associated Genes Using a Novel Dye ZMJ214 in Zebrafish. ACS Chem. Biol. 11, 381–388. doi:10.1021/acschembio.5b00751
Scarpignato, C., Gatta, L., Zullo, A., and Blandizzi, C. (2016). Effective and Safe Proton Pump Inhibitor Therapy in Acid-Related Diseases - A Position Paper Addressing Benefits and Potential Harms of Acid Suppression. BMC Med. 14, 179. doi:10.1186/s12916-016-0718-z
Schindelin, J., Arganda-Carreras, I., Frise, E., Kaynig, V., Longair, M., Pietzsch, T., et al. (2012). Fiji: An Open-Source Platform for Biological-Image Analysis. Nat. Methods 9, 676–682. doi:10.1038/nmeth.2019
Shahab, M., Rosati, R., Meyers, D., Sheilds, J., Crofts, E., Baker, T. R., et al. (2021). Cisplatin-Induced Hair Cell Loss in Zebrafish Neuromasts is Accompanied by Protein Nitration and Lmo4 Degradation. Toxicol. Appl. Pharmacol. 410, 115342. doi:10.1016/j.taap.2020.115342
Sprowl, J. A., van Doorn, L., Hu, S., van Gerven, L., de Bruijn, P., Li, L., et al. (2013). Conjunctive Therapy of Cisplatin with the OCT2 Inhibitor Cimetidine: Influence on Antitumor Efficacy and Systemic Clearance. Clin. Pharmacol. Ther. 94, 585–592. doi:10.1038/clpt.2013.145
Takagi, T., Naito, Y., and Yoshikawa, T. (2009). The Expression of Heme Oxygenase-1 Induced by Lansoprazole. J. Clin. Biochem. Nutr. 45, 9–13. doi:10.3164/jcbn.SR09-2810.3164/jcbnSR09-28
Urakami, Y., Okuda, M., Masuda, S., Saito, H., and Inui, K. I. (1998). Functional Characteristics and Membrane Localization of Rat Multispecific Organic Cation Transporters, OCT1 and OCT2, Mediating Tubular Secretion of Cationic Drugs. J. Pharmacol. Exp. Ther. 287, 800–805.
Virginio, C., MacKenzie, A., North, R. A., and Surprenant, A. (1999). Kinetics of Cell Lysis, Dye Uptake and Permeability Changes in Cells Expressing the Rat P2X7 Receptor. J. Physiol. 519 (Pt 2), 335–346. doi:10.1111/j.1469-7793.1999.0335m.x
Warchol, M. E., Schrader, A., and Sheets, L. (2021). Macrophages Respond Rapidly to Ototoxic Injury of Lateral Line Hair Cells but Are Not Required for Hair Cell Regeneration. Front. Cell. Neurosci. 14, 613246. doi:10.3389/fncel.2020.613246
Watson, C. J., Monstad-Rios, A. T., Bhimani, R. M., Gistelinck, C., Willaert, A., Coucke, P., et al. (2020). Phenomics-Based Quantification of CRISPR-Induced Mosaicism in Zebrafish. Cell Syst. 10, 275–286. e5. doi:10.1016/j.cels.2020.02.007
Yin, J., Duan, H., and Wang, J. (2016). Impact of Substrate-Dependent Inhibition on Renal Organic Cation Transporters hOCT2 and hMATE1/2-K-Mediated Drug Transport and Intracellular Accumulation. J. Pharmacol. Exp. Ther. 359, 401–410. doi:10.1124/jpet.116.236158
Yu, D., Gu, J., Chen, Y., Kang, W., Wang, X., and Wu, H. (2020). Current Strategies to Combat Cisplatin-Induced Ototoxicity. Front. Pharmacol. 11, 999. doi:10.3389/fphar.2020.00999
Zamami, Y., Hamano, H., Niimura, T., Aizawa, F., Yagi, K., Goda, M., et al. (2021). Drug-Repositioning Approaches Based on Medical and Life Science Databases. Front. Pharmacol. 12, 752174. doi:10.3389/fphar.2021.752174
Keywords: cisplatin, ototoxicity, proton pump inhibitor, organic cation transporter 2, zebrafish, genome editing, adverse event, electronic health record
Citation: Wakai E, Ikemura K, Mizuno T, Takeuchi K, Tamaru S, Okuda M and Nishimura Y (2022) Repositioning of Lansoprazole as a Protective Agent Against Cisplatin-Induced Ototoxicity. Front. Pharmacol. 13:896760. doi: 10.3389/fphar.2022.896760
Received: 15 March 2022; Accepted: 14 June 2022;
Published: 15 July 2022.
Edited by:
Santiago Gómez-Ruiz, Rey Juan Carlos University, SpainReviewed by:
Giuliano Ciarimboli, University of Münster, GermanyCopyright © 2022 Wakai, Ikemura, Mizuno, Takeuchi, Tamaru, Okuda and Nishimura. This is an open-access article distributed under the terms of the Creative Commons Attribution License (CC BY). The use, distribution or reproduction in other forums is permitted, provided the original author(s) and the copyright owner(s) are credited and that the original publication in this journal is cited, in accordance with accepted academic practice. No use, distribution or reproduction is permitted which does not comply with these terms.
*Correspondence: Yuhei Nishimura, eXVoZWlAbWVkLm1pZS11LmFjLmpw
Disclaimer: All claims expressed in this article are solely those of the authors and do not necessarily represent those of their affiliated organizations, or those of the publisher, the editors and the reviewers. Any product that may be evaluated in this article or claim that may be made by its manufacturer is not guaranteed or endorsed by the publisher.
Research integrity at Frontiers
Learn more about the work of our research integrity team to safeguard the quality of each article we publish.