- 1Department of Biology, Animal Physiology and Neurobiology Section, KU Leuven, Leuven, Belgium
- 2Centre of Environment Climate Change and Public Health, RUSA, Utkal University, Bhubaneswar, India
- 3Chemistry Laboratory of Natural Products, Graduate Program in Animal Science and Biotechnology Applied to Agriculture, Paranaense University, Umuarama, Brazil
- 4Division of Microbiology and NCDs, ICMR-Regional Medical Research Centre, Bhubaneswar, India
- 5Department of Pharmacy, School of Pharmacy and Medicine, College of Medicine and Health Sciences, University of Rwanda, Huye, Rwanda
Tetradenia riparia Hochsteter codd. (Lamiaceae) in its native African continent, is considered one of the most popular aromatic medicinal plants. In folk medicine it may be used as an infusion to treat respiratory problems, cough, headache, stomach pain, diarrhea, fever, malaria, and dengue; and in the form of compresses it is applied for the relief of headaches and toothaches. The species T. riparia has been researched for decades to isolate and identify chemical constituents present in extracts or essential oil obtained from the leaves, floral buds, or stems of this plant. The present study reviews the scientific literature on ethnomedicinal, phytochemical, and pharmacological aspects of T. riparia. We discuss issues related to the botanical and geographical description of the species, ethnobotanical uses, phytochemical studies on its essential oil and extracts, and biological activities of T. riparia. Several compounds have already been isolated from leaves, such as ibozol, 7α-hydroxyroileanone, 1′,2′-dideacetylboronolide, 8(14),15-sandaracopimaradiene-7α,18-diol; 5,6-dihydro-α-pyrone and α-pyrone. Terpenes predominated in the essential oil, comprising monoterpenes, sesquiterpenes, diterpenes, hydrocarbons, and oxygenates. Most phytocompounds were isolated from the leaves and flower buds, namely fenchone, 14-hydroxy-9-epi (E)-caryophyllene, 9β, 13β-epoxy-7-abietene, and 6,7-dehydroroileanone. These compounds provide the species a high pharmacological potential, with antimicrobial, antioxidant, antitumor, analgesic, anti-leishmania, anti-tuberculosis, and anti-parasitic activities. Therefore, this species is a promising herbal medicine.
1 Introduction
The species T. riparia is native to the African continent, where is considered one of the most popular aromatic medicinal plants. It is usually planted close to homes to ward off mosquitoes, illustrating the repellent potential of its essential oil (Van Puyvelde et al., 1986). In folk medicine, its leaves are used to treat several diseases such as malaria, angina, yaws, helminths, dental abscesses, gastroenteritis, several types of fevers, headaches and other pains. Its leaves are also used for the conservation of foodstuffs in traditional silos (Van Puyvelde et al., 1986).
The essential oil produced from its leaves and flower buds has an orange color and consists mostly of terpenoid compounds such as monoterpenes, sesquiterpenes, and diterpenes (in each case as hydrocarbons or oxygenated), whose concentration varies with the seasons (Gazim et al., 2010; Zardeto-Sabec et al., 2020).
Studies with T. riparia have demonstrated many biological activities, such as antimicrobial (Gazim et al., 2010; York et al., 2012; Elaka et al., 2020; Scanavacca et al., In Press), antioxidant (Fernandez et al., 2017), antitumor (Gazim et al., 2014), analgesic (Gazim et al., 2010), anti-leishmania (Cardoso et al., 2015; Demarchi et al., 2015; Leitão et al., 2020), antituberculosis (Baldin et al., 2018), antiparasitic (de Melo et al., 2015), as well as acaricidal and larvicidal (Lorenzi and Matos, 2008; Gazim et al., 2011; Fernandez et al., 2014; Zardeto-Sabec et al., 2020).
Notwithstanding extensive traditional reports on the use of this plant, little progress was made on its bioactivity during 1990–2015. Its phytochemistry was extensively described during 1980–1990, but scientific evidence for its use in various diseases is scarce, both in vitro and in vivo. Thus, the present review aims to summarize the ethnomedicinal, phytochemical and pharmacological aspects of T. riparia. It covers the ethnobotany, chemo-profiling, and biological evaluation (in vitro and in vivo) of T. riparia extracts and essential oils, as well as compounds therein, with a critical discussion of their toxicity, structure activity relationship, computational investigation, as well as suggestions for further basic and clinical research.
2 Botanical Description, Geographic Distribution
Species of the genus Tetradenia are generally aromatic shrubs 1–3 m high, dioecious, soft and very branched. The stems are brittle, semisucculent, semi-juicy, aromatic, rather stout, at first 4-angled and glandular-pubescent, becoming terete and glabrous with age; the bark is pale brown. The leaves are petiolate, ovate-oblong to round, with glandular trichomes distributed on both surfaces (Codd, 1983, Phillipson and Steyn 2008), as can be seen in Figure 1A. The epidermis is uniseriate, with irregular cells, the cells on the adaxial surface being larger than the epidermal cells on the abaxial surface, surrounded by a cuticle and containing glandular (capitate and peltate) and non-glandular trichomes (Martins et al., 2008). Flowering occurs only in subtropical, temperate and frost-free areas (Blythe et al., 2020). The flower buds (Figure 1B) begin to appear in winter (June in the Southern hemisphere), with opening of the flowers in July. The inflorescences appear in large, branched terminal panicles (Figure 1C) that are white to pale mauve in color (Zardeto-Sabec et al., 2020). T. riparia has eight synonyms: Basilicum myriostachyum (Benth.) Kuntze, Basilicum riparium (Hochst.) Kuntze, Gumira ferruginea (A.Rich.) Kuntze, Iboza riparia (Hochst.) N.E.Br., Moschosma myriostachyum Benth., Moschosma riparium Hochst., Plectranthus riparius Hochst., Premna ferrugínea A. Rich. (Powo, 2022).
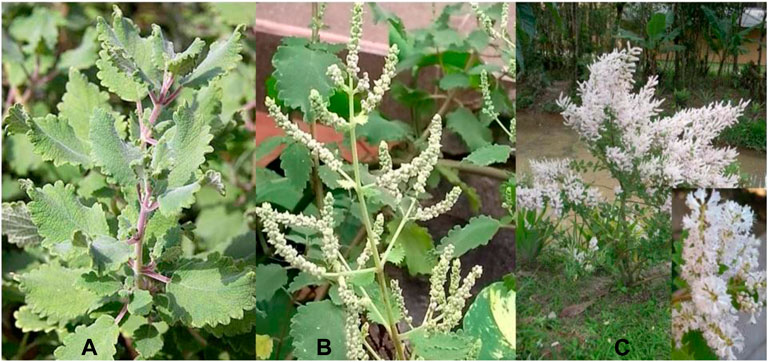
FIGURE 1. T. riparia culture planted in the medicinal garden of Paranaense University. Umuarama, Parana, Brazil. (A): leaves (B): Flower buds (C) Open flowers. Source: the authors.
In South Africa, where it is native, T. riparia is one of the most popular aromatic medicinal plants and is usually planted close to homes to repel mosquitoes (Van Puyvelde et al., 1986 and 1987; Weaver et al., 1994; Campbell et al., 1997; Van Puyvelde and de Kimpe, 1998). The typical range of T. riparia is at lower elevations, from near sea-level, or in more humid habitats (Phillipson and Steyn, 2008), such as along river banks, forest margins, dry wooded valleys and hillsides (Njau and Ndakidemi, 2017). However, it is well adapted in tropical regions such as Brazil. In this country, it is found in more humid areas such as the northern region (Godoy et al., 1999), but also in regions with lower humidity, such as the southern region (Gazim et al., 2010). The vegetative propagation of the T. riparia species occurs by cuttings of woody material, coming from the same matrix, with the objective of maintaining in the seedlings the genetic characteristics of the matrix plants, such as uniformity and precocity in production (Pinheiro et al., 2021). In Brazil, the population does not use it as a medicinal plant, but as an ornamental; thus, it is found in parks and gardens (Zelnik et al., 1978). The natural distribution ranges from South Africa to Angola, Botswana, KwaZulu-Natal, Malawi, Mozambique, Namibia, Northern Provinces, Swaziland, and Zimbabwe. It was introduced in Honduras (Figure 2), from where it was probably distributed throughout the American continent (Powo, 2022).
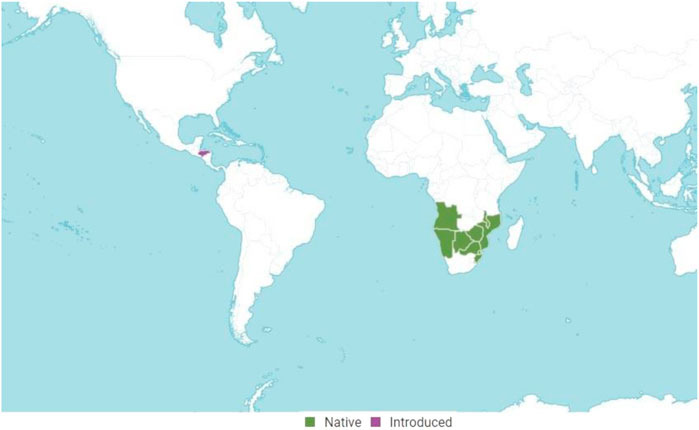
FIGURE 2. Geographical distribution of T. riparia (Source: Plants of the World Online, Royal Botanic Gardens, Kew. 2022. Licensed under Creative Commons Attribution CC BY).
3 Ethnobotanical Uses
The medicinal value of T. riparia, popularly known as umuaravumba, is well known among the native people of Rwanda, and, accordingly, several medicinal applications have been reported in Rwanda but also elsewhere in eastern Africa (Van Puyvelde et al., 1981). Rwandese people often cultivate the plant around their houses, and use it as a remedy against a wide range of diseases including malaria, angina, yaws, helminthic diseases, gastroenteritis, gonorrhea, diarrhea, dental abscesses, headache, and several kinds of fevers and aches (Van Puyvelde et al., 1986). There are also reports about its use to treat toothaches and diseases caused by worms, bacteria, or fungi (de Melo et al., 2015). In addition to being used as a medicinal plant, the leaves are also used to conserve food in traditional silos, as well as in the dry storage of crops, in order to repel insects (Van Puyvelde et al., 1986). The application in the conservation of grains during storage in Rwanda was validated by Weaver et al. (1994), using the essential oil extracted from the leaves on Zabrotes subfasciatus, a beetle that infests the bean grains (see item 5.6 Insecticidal activity on Zabrotes subfasciatus).
According to Lorenzi and Matos (2008), T. riparia is traditionally used as an infusion, for the treatment of respiratory problems, cough, headache, stomach pain, diarrhea, fever, malaria. and dengue, and in the form of compresses, applied to relieve headaches and toothaches. In addition, it is used as an antiseptic. Table 1 summarizes various parts of T. riparia used by indigenous populations throughout different regions of the world.
In Brazil, T. riparia is widely distributed in the Sao Paulo (Zelnik et al., 1978), Amazonas (Godoy et al., 1999), and Parana (Gazim et al., 2010) states, where it is popularly known as incense, lavender, lemongrass, mist plume, or false myrrh. It was introduced as an exotic ornamental plant, and is cultivated in parks, residential gardens, and vegetable gardens due to the intense and pleasant aroma it exudes. Despite this, there are no reports of its use in folk medicine in this country (Lorenzi and Matos, 2008).
4 Chemo-Profiling
4.1 T. riparia Leaf Extracts
The first phytochemical investigation of T. riparia was carried out by Zelnik et al. (1978), from a cultivated specimens from Sao Paulo, Brazil. An extract was obtained from the dried leaves of Iboza riparia using acetone (Me2CO), and chromatographed on silica gel using hexane-benzene (C6H6) (1:1) as an eluent. The compounds 7α-hydroxyroyleanone (Figure 3A) and sitosterol were isolated initially. Further elution with a mixture of hexane-benzene:Me2CO (19:1) yielded the ibozol compound. By a percolation extraction technique, Van Puyvelde et al. (1981) extracted dried powder from the leaves of T. riparia with chloroform (CHCl3). The extract was filtered and evaporated in vacuo at 40°C (135 g), and submitted to chromatography on a silica gel column in C6H6 (C6H6-CHCl3-methanol (MeOH) gradient). The fractions eluted with C6H6-CHCI3 (25:75) contained a mixture of sterols: sitosterol (65%), stigmasterol (30%), and campesterol (5%) (Figure 3B). The fractions eluted with CHCI3-MeOH (93:7) yielded 1,2-dideacetylboronolide. De Kimpe et al. (1982) isolated the diterpenediol (14),15-sandaracopimaradiene-7α,18-diol from a chloroform extract obtained from the leaves of T. riparia. The compounds were purified by chromatography on a silica gel column in benzene (C6H6-CHCl3-MeOH gradient). Colorless crystals were observed after the elution of fractions with CHCl3-MeOH (97:3).
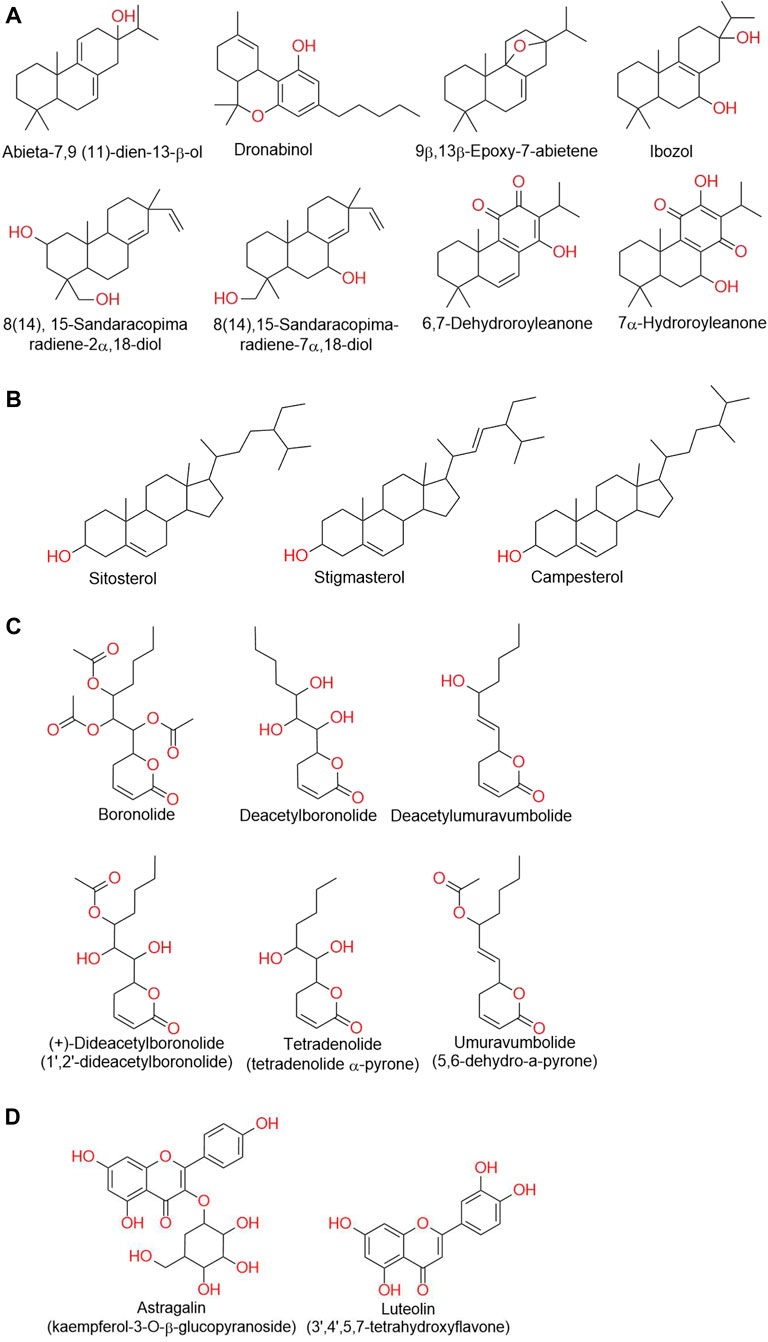
FIGURE 3. (A) Chemical structure of abietane and royleanone classes of isolated diterpenes from T. riparia. (B) Chemical structure of phytosterols isolated from T. riparia. (C) Chemical structure of α, β-unsaturated δ-lactone moiety-bearing phytoconstituents isolated from T. riparia. (D) Chemical structure of flavonoids isolated from T. riparia.
Van Puyvelde et al. (1987) isolated 8(14),15-sandaracopimaradiene-2α,18-diol from T. riparia leaves. The leaves were dried (522 g), pulverized, and extracted in a percolator with petrol (12 L). The extract was filtered, concentrated in vacuo, and extracted with MeOH-H2O (9:1). The petrol phase was then evaporated in vacuo to obtain a brown-green syrup, which was extracted with MeOH-H2O. After MeOH evaporation, the aqueous phase was extracted with CHCl3, resulting, after evaporation in vacuo, in a brown residue. Twelve g of the CHCl3 extract was adsorbed on silica gel, and eluted with an n-hexane-toluene-CHCl3-ethyl acetate (EtOAc)-MeOH gradient. The compound 8(14),15-Sandaracopimaradiene-2α,18-diol was isolated from the EtOAc fraction. Davies-Coleman and Rivett, (1995), isolated the compound 5,6-dihydro-α-pyrone (umuravumbolide) from the dry leaves from a T. riparia culture located in Pongola valley, Africa. The Soxhlet extract was prepared using Me2CO. A pale-yellow gum (0.15 g) was obtained, which showed one main spot on TLC. Flash chromatography was performed using silica gel in EtOAc-hexane (1:1), and the desacetyllumuravumbolide compound was isolated (Figure 3C).
Van Puyvelde et al. (1979) collected the leaves of T. riparia in the “commune” of Huye, Rwanda. The leaves were dried and pulverized and extracted with methanol (Soxhlet) for 40 h. The extract was filtered and concentrated in vacuo at 40°C, yielding the crude extract, which was solubilized in 2% citric acid. The suspension was filtered, defatted with petroleum ether (5 × 600 ml), and extracted with chloroform, (5 × 300 ml). The chloroform, phase was evaporated to a brown oil (6.8 g), which was chromatographed on a silica gel column in benzene (320 g with benzene-chloroform-methanol gradient). The fractions eluted with chloroform, yielded upon evaporating 1.3 g of the compound identified as umuravumbolide (5,6-dihydro-6-(3-acetoxy-1-heptenyl)-2-pyrone), a new α-pyrone from T. riparia. The fractions eluted with chloroform-methanol (99:l) afforded upon evaporation 1.15 g of a compound identified as deacetylumuravumbolide (5,6-dihydro-6- (3-hydroxy-1-heptenyl)-2-pyrone). The fractions eluted with chloroform-methanol (19:1) yielded upon evaporation 616 mg of a compound identified as deacetylboronolide (5,6-dihydro-6-(1,2,3- trihydroxyheptyl)-2-pyrone) (Figure 3C).
A new α-pyrone, tetradenolide, identified as 5,6-dihydro-6-(1,2-dihydroxyhexyl)-2-pyrone, was isolated from T. riparia leaves by Van Puyvelde and de Kimpe (1998). The authors used 713 g of dry leaves and carried out successive extractions with n-hexane and CHCl3. The CHCl3 fraction was extracted with MeOH-H2O (9:1). The H2O phase was first extracted with CHCl3 resulting in a CHCl2 extract, and then, with EtOAc. The EtOAc extract was chromatographed on silica gel and eluted stepwise in a gradient of hexane-EtOAc-MeOH. The tetradenolide compound (Figure 3C), was isolated from the fraction eluted with CHCl3-MeOH (19:1).
Fernandez et al. (2017) investigated the chemical composition of a crude extract obtained from the dried leaves of T. riparia, collected in Umuarama, Parana, Brazil. The powder obtained (230 g) was subjected to a dynamic maceration process with solvent, renewed with 70% ethyl alcohol (v/v) until the plant material was exhausted. The filtrate was then concentrated under reduced pressure in a rotary evaporator at 40 oC to obtain the crude extract. Two g of crude extract was subjected to chromatography on a silica gel column, and eluted in a gradient of hexane-dichloromethane-ethyl acetate-methanol. The fraction dichloromethane-hexane (9:1) yielded the diterpene abieta-7,9 (11)-dien-13-β-ol. The fraction dichloromethane–ethyl acetate (1:1) yielded the ibozol compound. In the fraction ethyl acetate, a mixture was found of two diterpenoids: 8(14),5- sandaracopimaradiene-2α, 18-diol and 8(14),5-sandaracopimaradiene-7α, 18-diol; in the ethyl acetate–methanol (8:2) fraction, a mixture of three compounds was found. The first compound was identified as boronolide, an a-pyrone. The second compound was identified as luteolin, a flavone (Figure 3D). The third compound was identified as astragalin, a flavonol.
From the available evidence, it is clear that different groups have isolated different compounds from T. riparia. To what extent this is due to differences in starting material (different plant parts, differences in collection and processing), in extraction methods, or in geographical and growth conditions remains to be clarified.
4.2 T. riparia Essential Oil
4.2.1 Physicochemical Characteristics
The essential oil extracted from the stems of T. riparia has a characteristic scent of incense, and its color ranges from a light orange (Figure 4), intensifying to a darker orange in the leaves (Figure 4), to a reddish-orange in the flower buds (Figure 4).
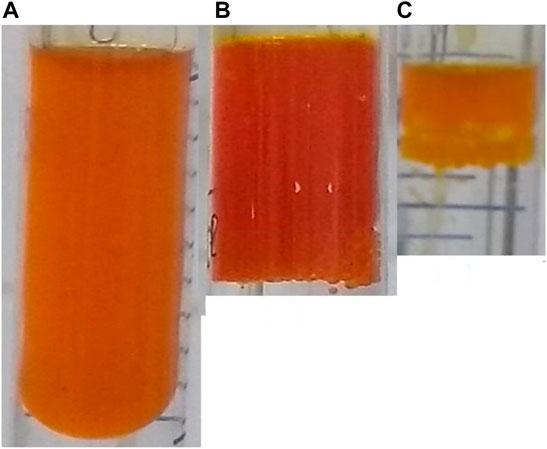
FIGURE 4. Essential oil obtained by hydrodistillation of T. riparia leaves (A), flower buds (B), and stems (C)—Source: Chemical Laboratory of Natural Products-Paranaense University-UNIPAR, Brazil.
Physicochemical analysis was standardized, and the quality of essential oils was evaluated. The determination of density, refractive index, and rotating power are parameters used to detect adulterations in the essential oils (Gil, 2007). The determination of essential oils yield within the plant is essential for carrying out biological tests, its application in products, and commercialization. According to the European Pharmacopoeia, the minimum extraction yield of essential oils for the development of products and application is 2 ml/kg (European Pharmacopoeia, 2013). Physicochemical indexes of T. riparia essential oil are shown in Table 2.
The same study by Gazim et al. (2010) analyzed the yield (g %) of the essential oil under different climatic seasons; the lowest oil yield occurred in plants harvested in spring (0.168 g ± 0.02), and the highest yield was observed in plants harvested in winter (0.265 g ± 0.025), whereas the oil content of plants harvested in summer and autumn remained close, (0.215 g ± 0.007 and 0.237 g ± 0.011, respectively).
4.2.2 Chemical Composition of Essential Oil
T. riparia essential oil has a complex composition of terpenoids, with numerous compounds present in small concentrations. Terpenes are the majority class, represented by monoterpene, sesquiterpene, and diterpene hydrocarbons and oxygenates (Weaver et al., 1994). However, the concentration of these terpenoids varies according to factors such as the location of the crop implantation, climate, altitude, soil, and collection time. Therefore, we reviewed the literature to compare the chemical composition of T. riparia essential oil from different locations: Africa (South Africa and Kenya) (Campbell et al., 1997; Omolo et al., 2004), South America (Brazil) (Godoy et al., 1999; Gazim et al., 2011 and, 2014; Araújo et al., 2018), and North America (Poplarville, MI, United States) (Blythe et al., 2020); and the chemical identification of the essential oil is shown in Table 3 and Figure 5.
4.2.2.1 Chemical Composition and Seasonal Variation
Two studies were conducted to evaluate the influence of abiotic factors on the chemical composition of T. riparia essential oil. The first, carried out by Araújo et al. (2018), evaluated the influence of the level of shading on the chemical composition of T. riparia leaves essential oil. The authors demonstrated that the concentrations of the sesquiterpene hydrocarbon 14-hydroxy-9-epi (E)—caryophyllene and the oxygenated monoterpene fenchone were influenced by the level of shading where the plant is growing. Two compounds were identified in all different levels of light applied; 14-hydroxy-9-epi (E)—caryophyllene and fenchone. The former was the major compound (16.48%) in plants grown under 30% shading, followed by those grown under 80% shading (16.42%); plants grown under 50% shading or full sunlight showed the lowest content of this component with 16.41% and 16.03%, respectively. Fenchone content was the highest (9.93%) in plants grown under 30% shading, followed by those grown in full Sun, 50% or 80% shading, with levels of 7.90, 7.78, and 3.59%, respectively.
Gazim et al. (2010) evaluated the chemical composition of T. riparia essential oil collected in spring, summer, autumn, and winter in the northwest region of the Parana State, Brazil. Leaves collected in winter have a higher percentage of calyculone (24.70%), abietadiene (13.54%), and viridiflorol (4.20%). Leaves collected in autumn showed higher percentages of ledol (8.74%) and cis-muurolol-5-en-4-α-ol (13.78%), and leaves collected in spring or summer had higher percentages of fenchone (12 0.67%), 14-hydroxy-9-epi-caryophyllene (24.36%), and α-cadinol (8.33%).
5 Pharmacology and Bioactivity
5.1 Antimicrobial Activity of Crude Extract
York, et al. (2012), investigated the antimicrobial effects of 30 plant species, among them T. riparia, used for the treatment of respiratory infections by the population of a rural region of Maputaland, Kwazulu-Natal, South Africa. In vitro minimal inhibitory concentration (MIC) assays were performed with the organic extract (dichloromethane: methanol), aqueous extract and essential oil obtained from the leaves of T. riparia. These were tested against Cryptococcus neoformans (ATCC 14116), Klebsiella pneumoniae (ATCC 13883), Moraxella catarrhalis (ATCC 23246), Mycobacterium smegmatis (ATCC 14468) and Staphylococcus aureus (ATCC 6538). The organic extract and essential oil were active against fungi Cryptococcus neoformans with MIC of 0.60 mg/ml and 0.83 mg/ml, respectively. The organic extract was also active against Moraxella catarrhalis (MIC of 0.10 mg/ml) and Staphylococcus aureus (MIC of 0.03 mg/ml).
Fernandez et al. (2017) investigated the antibacterial potential of crude extract and fractions of T. riparia leaves by a broth microdilution method. The crude extract was fractionated, and the ibozol compound isolated from the dichloromethane:ethyl acetate (1:1) fraction. This compound showed high activity against S. aureus (MIC of 1.95 μg/ml). From the ethyl acetate fraction, 8(14),5-sandaracopimaradiene-2α, 18-diol and 8(14),5-sandaracopimaradiene-7α,18-diol were isolated, which showed activity against the S. aureus (0.98 μg/ml) and Enterococcus faecalis and Bacillus cereus (31.2 μg/ml).
Kakande et al. (2019), evaluated the antifungal potential of a T. riparia leaves crude alcoholic extract against the fungi Trichophyton tonsurans, Trichophyton mentagrophyte, and Microsporum audouinii; they found MICs ranging from 62.5 to 250 mg/ml, and a minimum fungicidal concentration (MFC) ranging from 125 to 500 mg/ml. This expands the range of pharmacological applications of T. riparia, indicating potential for the control of Trichophyton, which causes skin, nails, and hair dermatophytosis.
Elaka et al. (2020), investigated the antibacterial potential of a T. riparia leaves dichloromethane: methanol (1:1) extract. The extract was obtained by percolation, and tested against S. aureus ATCC 25923, E. coli ATCC25922, and P. aeruginosa ATCC 9027). The strongest activity was found against E. coli (MIC of 125 μg/ml).
Friedrich et al. (2020) studied strawberries coated with a film based on cassava starch, gelatin, and sorbitol, and containing T. riparia leaves crude extract at concentrations ranging from 500 to 1,000 μg/ml. Incorporation of the crude extract into the film inhibited the development of microbial colonies by 98% over 5 days, thus indicating the ability of the crude extract to preserve stored strawberries.
5.2 Antimicrobial Activity of Essential Oil
The essential oil from T. riparia leaves harvested in the summer showed activity against S. aureus (MIC of 15.6 μg/ml), B. subtilis (7.8 μg/ml), E. faecalis (62.5 μg/ml), P. aeruginosa and E. coli (both 125 μg/ml) as well as antifungal activity against Candida albicans (31.2 μg/ml) (Gazim et al., 2010).
Baldin et al. (2018) analyzed the anti-Mycobacterium tuberculosis activity of the essential oil and the isolated compound 6,7-dehydroroyleanone obtained from T. riparia leaves. Both showed activity against Mycobacterium tuberculosis H37Rv, and a similar MIC was found for clinical isolates (between 31.2 and 62.5 μg/ml).
In a study carried out by Scanavacca et al. (In Press), T. riparia leaves essential oil showed high antimicrobial activity against all bacteria evaluated, with MICs ranging from 0.05 to 0.60 mg/ml, similar to the MIC for streptomycin (0.05–0.125 mg/ml) and ampicillin (0.10–0.30 mg/ml). Gram-positive bacteria showed greater sensitivity to the essential oil: B. cereus (0.05 mg/ml), Listeria monocytogenes (0.05 mg/ml), and S. aureus (0.05 mg/ml), while P. aeruginosa was less sensitive (MIC of 0.60 mg/ml). The essential oil also inhibited the growth of fungal strains with MICs ranging from 0.06 to 10.0 mg/ml, which is more potent than the positive controls bifonazole (0.10–0.20 mg/ml) and ketoconazole (0.15–2.50 mg/ml). The most sensitive fungus was Aspergillus versicolor (0.06 mg/ml), followed by Penicillium ochrochloron (0.50 mg/ml).
5.3 Antimicrobial Activity With Special Reference to Multi Drug-Resistant Pathogens
5.3.1 Antimycobacterial Activity
Van Puyvelde et al. (1994) studied the antimycobacterial activity of 8(14),15-sandaracopimaradiene, 7α,18-diol isolated using bioassay-guided purification from leaves of T. riparia (MIC, 25–100 μg/ml). Later, Baldin et al. (2018) also obtained a similar MIC value (31.25 μg/ml) against Mycobacterium tuberculosis H37Rv and several resistant clinical isolates for a different compound (the diterpene 6,7-dehydroroyl) from the essential oil. The compound 6,7-dehydroroyleanone displays moderate activity against multidrug-resistant isolates, with little cytotoxicity to murine macrophages.
5.3.2 Anti-Dermatophytic Activity
Endo et al. (2015) tested T. riparia crude extract against several dermatophytes (Trichophyton rubrum, T. mentagrophytes and Microsporum gypseum) using a microdilution method (MIC and MFC) as well as (fluorescence and scanning electron) microscopy. Hydroalcoholic leaf extract showed strong activity against all three test strains (MIC ranging from 62.5 to 125 μg/ml while MFC 62.5–250 μg/ml). Concentrations of 31.2 and 62.5 μg/ml caused a reduction in hyphal growth, and irregular patterns as well as ungerminated conidia were observed with fluorescence microscopy (Figure 6). Strong inhibition of hyphal growth with irregular growth patterns were also observed using scanning electron microscopy (Endo et al., 2015).
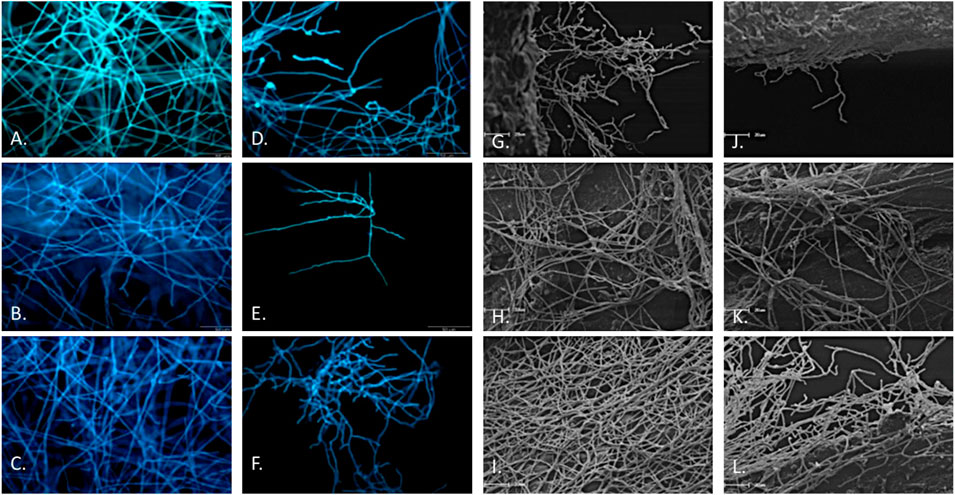
FIGURE 6. Fluorescence microscopy. (A–C) Control cells of T. rubrum, T. mentagrophytes and M. gypseum, respectively. (D–F) T. rubrum, T. mentagrophytes and M. gypseum treated with 31.2, 62.5, and 31.2 μg/ml of T. riparia extract, respectively. Scanning Electron Microscopy, (G–I) Control cells of T. rubrum, T. mentagrophytes and M. gypseum, respectively. (J–L) T. rubrum, T. mentagrophytes and M. gypseum treated with one-fold sub-MIC concentrations of T. riparia extract (adapted from Endo et al., 2015 1).
5.3.2.1 Anti-Biofilm Activity
T. riparia extract had strong effects against pre-formed S. aureus (both methicillin resistant-MRSA and methicillin sensitive-MSSA) biofilms, with BIC50 values of 30–90 μg/ml, which were less than the MIC values of 31.2–125 μg/ml. SEM micrographs showed a strong reduction of the number of cells and disruption of organized structure of S. aureus ATCC 29213 biofilms when treated at a concentration of 250 μg/ml (Endo et al., 2018).
Costa et al. (2015) found that an hydroalcoholic extract of T. riparia inhibited C. albicans biofilm at a concentration of 62.5 μg/ml which was more effective compared with standard fluconazole (MIC >1,000 μg/ml). while less effective than nystatin (MIC = 7.8 μg/ml).
Van Puyvelde et al. (2021) reported the activity of dichloromethane and ethyl acetate fractions obtained from T. riparia leaves extracts against foodborne pathogens Shigella sonnei, Salmonella enterica, E. coli, Micrococcus luteus, S. aureus, and E. faecalis. In the dichloromethane fraction, the compound 8(14),15-sandaracopimaradiene-7α,18-diol was identified, which showed activity against bacteria with IC50 ranging from 11.2 to 212.5 μg/ml. In the ethyl acetate fraction, the compounds deacetylumuravumbolide and umuravumbolide were found, which showed a moderate activity with IC50 between 212.9 and 637.7 μg/ml and 176.1–521.4 μg/ml, respectively. This study reported that 8(14),15-sandaracopimaradiene-7α, 18-diol is bactericidal against S. aureus, and also has antibiofilm activity (BIC50, 8.8 ± 1.5 μg/ml) similar to planktonic activity (MIC50, 11.4 ± 2.8 μg/ml).
5.3.2.2 Synergy Studies
Endo et al. (2018) combined a hydroalcoholic extract from the leaves of T. riparia with the standard drug penicillin against 13 multidrug resistant/sensitive S. aureus strains and found synergistic effects against 69.2% of the isolates. From the checkerboard assay, synergy was found with five isolates such as MRSA 78 (Fractional Inhibitory Concentration Index, FICI = 0.14), MRSA 81 (FICI = 0.24), MRSA 83 (FICI = 0.18), MSSA 97 (FICI = 0.26), and MSSA 170 (FICI = 0.25). Although this is a very interesting findings, the authors did not identify which bioactive plant compounds are responsible for the synergy. Later, Van Puyvelde et al. (2021) useded bioassay-guided purification with S. aureus as model organism, and obtained 8(14),15-sandaracopimaradiene-7α, 18-diol as the major compound responsible for the bactericidal as well as antibiofilm activity. This research needs to be followed up further to combine this compound with penicillin to study synergy. Costa et al. (2015) also observed synergy when T. riparia extract was combined with nystatin against C. albicans (FICI = 0.24). This was also a preliminary study, which needs further exploration with the active anticandidal plant compounds combined with various antifungal agents. Supplementary Table S1, summarizes list of antimicrobial compounds reported from various studies, including isolation and identification techniques.
5.4 Anti-tumor and Antioxidant Activity of Essential Oil From T. riparia Leaves
Essential oil from T. riparia leaves and two compounds (9β, 13β-epoxy-7-abieethane and 6,7-dehydroroileanone) were evaluated for cytotoxic potential by a 3-(4,5-dimethylthiazol-2-yl)-2,5-diphenyl-2H-tetrazolium bromide (MTT) assay, using tumor cells MDA-MB-435 (human breast carcinoma), HCT-8 (human colon), SF-295 (human glioblastoma) and HL-60 (human promyelocytic leukemia). The essential oil and compound 9β, 13β-epoxy-7-abietane showed high cytotoxic activity on the cell lines SF-295 (78.06 and 94.80%), HCT-8 (85.00 and 86.54%), and MDA -MB-435 (59.48 and 45.43%). Moreover, the cytotoxicity of the essential oil and the isolated compound yielding a selectivity index (SI) of 1.9 for the essential oil and 7.9 for 6,7-dehydroroyleanone. The selectivity index was defined as the ratio between the cytotoxicity of the compound for tumor cells and its activity on non-tumor mammalian cells (VERO). The higher this index, the greater the specificity of these molecules for the tumor cells tested, thus indicating grater specificity of the isolated compound 6,7-dehydroroyleanone for tumor cells.
The authors also investigated the antioxidant activity of the essential oil and isolated compounds by the 2,2-diphenyl-1-picryl-hydrazyl (DPPH) and β-carotene-linoleic acid assays. The compound 6,7-dehydroroileanone strongly regenerated the DPPH radical with IC50 = 0.01 μg/ml. The essential oil and 6,7-dehydroroileanone also inhibited oxidation in the β-carotene linoleic acid test with 130.1% for the essential oil and 109.6% for the compound 6,7-dehydroroileanone. Thus, 9β, 13β-epoxy-7-abietene showed high cytotoxic potential and 6,7-dehydroroileanone high antioxidant potential (Gazim et al., 2014).
5.5 Analgesic Activity of T. riparia Leaves Essential Oil
Analgesic effects were studied of the essential oil from T. riparia leaves harvested during different seasons: spring, summer, autumn, and winter. An oral dose of 200 mg/kg caused analgesia in mice, inhibiting acetic acid-induced contortions by 38.94–46.13%. This effect showed no seasonal variation (Gazim et al., 2010).
5.6 Antiparasitic Activity of T. riparia Leaves
The vermicidal action of essential oil from T. riparia leaves was investigated by de Melo et al. (2015) at two concentrations: 50 and 100 μg/ml on Schistosoma mansoni worms. All worms died after 24 h of incubation with 100 μg/ml. At 50 μg/ml, the oil reduced the motor activity of the adult worm after periods of more than 72 h of incubation. Also, after 120 h of incubation, there was a slight decrease in the number of eggs produced by adult Schistosoma mansoni worms and a dose-dependent reduction in egg development.
The leishmanicidal action of the essential oil from the leaves of T. riparia was investigated by Cardoso et al. (2015). The oil inhibited the growth of Leishmania (L.) amazonensis promastigotes after 24 h of treatment. The inhibitory concentrations (IC50) were 15.47 ± 4.6 ng/ml for oil samples obtained in the spring, 15.67 ± 1.70 ng/ml in the summer, 15.66 ± 2.22 in the autumn and 13.31 ± 0.85 ng/ml in the winter.
In the same study, the essential oil of T. riparia obtained in different seasons also inhibited the survival of intracellular L. amazonensis amastigotes at concentrations of 30 (p < 0.001) and 3 ng/ml (p < 0 0.05). The strongest effects were observed at concentration of 30 ng/ml, with inhibition of parasite growth of 43.53, 32.03, 40.54, and 52.49% for the oils obtained in spring, summer, autumn, and winter, respectively (Cardoso et al., 2015).
Another study with L. amazonensis demonstrated that 30 ng/ml of the essential oil from T. riparia leaves induced 50% amastigote death after 24 h of incubation. For the infected and untreated macrophages, the infection index was 112 for each macrophage (Demarchi et al., 2015), with an effective concentration of 30 ng/ml (IC50) and an LD50 of 0.5 μg/ml. Transmission electronic microscopy revealed modifications of the morphology of L. amazonensis promastigotes with ultrastructural changes such as “cytoplasm vacuolization, membranous profiles inside the organelle, lipid vesicles, and membrane blebbing that suggested autophagy, thickening of the kinetoplast, chromatin condensation, and nuclear fragmentation” (Figure 7) (Demarchi et al., 2015). The same study, demonstrated that the essential oil of T. riparia had no cytotoxicity in murine macrophages at 30 ng/ml (>95% viable cells); however, 0.2 μg/ml had a cytotoxic effect of 50%. The authors suggest that the essential oil in high doses is cytotoxic to macrophages, while lower doses are effective against the parasite. Thus, the dose of the essential oil and the route of administration need to be evaluated under specific conditions.
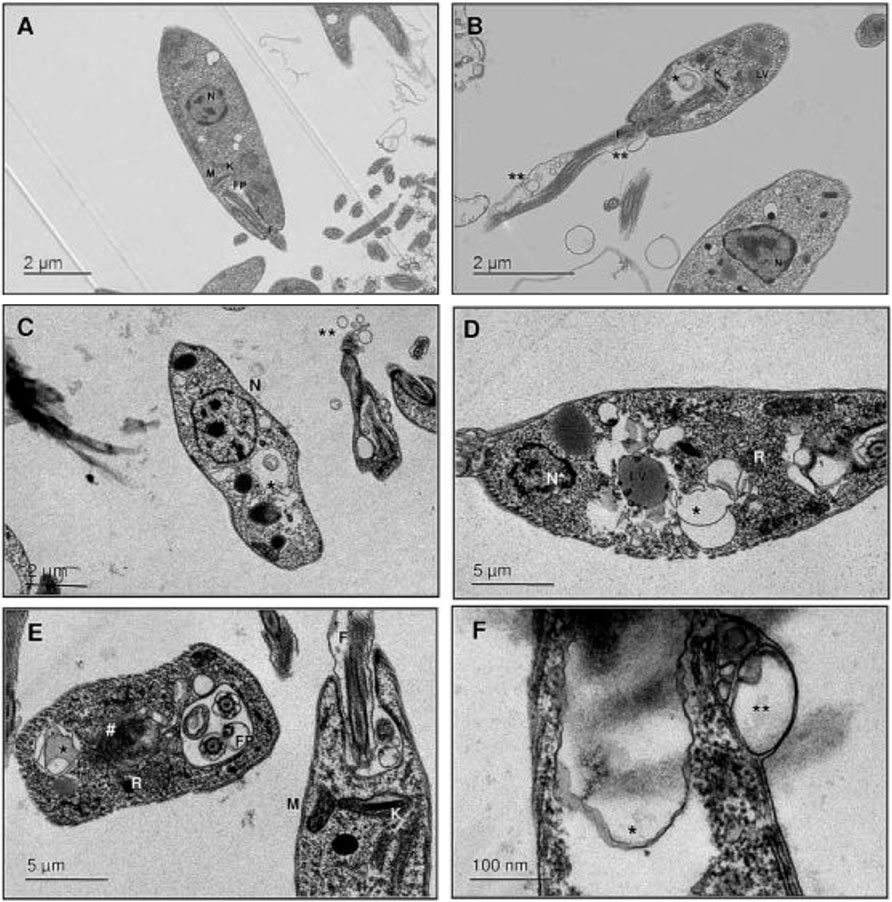
FIGURE 7. Transmission electron microscopy of L. amazonensis treated with T. riparia essential oil (TrEO) for 24 h. (A) Leishmania promastigotes. (B–F) Promastigotes treated with TrEO (30 ng/ml). N, nucleus; N*, abnormal chromatin condensation nuclear alterations; K, kinetoplast; M, mitochondria; FP, flagellar pocket; F, flagellum; V, vacuoles; LV, lipid vesicles; R, myelin-like figure appears in close association with the flagellar pocket membrane; *membranous profiles; **blebbing; #mitochondrial swelling (Adopted from Demarchi et al., 2015 2).
T. riparia essential oil stimulated pro-inflammatory cytokine expression in macrophages; the effects varied according to the incubation time. After 3 h, the mRNA expression of interleukin-1β (IL-1β), IL-10, IL-12, IL-17, and IFN-γ was detected, but only IL-1β expression remained high after 6 h. Tumor necrosis factor α (TNF-α), IL-18, and IL-33 mRNA expression was unchanged at 3 or 6 h. The essential oil modulated the synthesis of cytokines up to 24 h. Cytokine production, e.g. of IL-10, IL-4 and IL-5, was also induced by infection with L. amazonensis, which may be prevented by treatment with the essential oil. Cell proliferative mediators and granulocyte-macrophage colony-stimulating factors were also inhibited by treatment with T. riparia essential oil. The results suggest that the essential oil reduced the expression of cytokines related to the infection progress, and increased IFN-γ (Demarchi et al., 2015).
Lorenzi and Matos (2008) showed that the essential oil from T. riparia leaves has moderate antimalarial activity against the malaria parasite Plasmodium falciparum.
Van Puyvelde et al. (2018), isolated 8(14), 15-sandaracopimaradieno-7α, 18-diol from T. riparia leaves, and found anthelmintic activity against the model nematode Caenorhabditis elegans (wild type and mutants) with an IC50 of 5.4 ± 0.9 μg/ml. The anthelmintic activity of this diterpenediol validates the use of T. riparia for worm infection by Rwandese tribes in Africa. Moreover, 8(14),15-sandaracopimaradiene-7α,18-diol had similar potency against a Slo-1 mutant of C. elegans (Slo-1 is an orthologue of mammalian BK channels), suggesting that this channel is not the molecular target.
5.7 Insecticide and Acaricide Activity of the Essential Oil From T. riparia Leaves
Lorenzi and Matos (2008) showed that the essential oil from T. riparia leaves has insect repellent action, in particular against the species Anopheles gambiae.
Fernandez et al. (2014) analyzed the activity of T. riparia leaves essential oil against Aedes aegypti larvae, using essential oil obtained at different times (spring, summer, autumn and winter). Larvae were exposed for 24 h to concentrations ranging from 2,500 to 3,125 μg/ml, and the larvicidal activity was measured by calculating the lethal dose (LD50) using the Probit test. Larvicidal activity (mainly the LD50) varied with the seasons (78.72; 83.29 and 123.02 μg/ml for autumn, spring and summer, respectively; and lowest larvicidal activity in winter (2,619.79 μg/ml).
The percentage mortality of Rhipicephalus (Boophilus) microplus mite larvae exposed to different concentrations of essential oil from T. riparia leaves was measured by the larval immersion test (LIT). At concentrations of 100, 50, and 25% the essential oil showed maximum efficacy with a mortality rate of 100% of the larvae. At dilutions ranging from 12.5% to 0.014%, larval mortality ranged from 97.6 to 10.60%, respectively (Gazim et al., 2011).
Zardeto-Sabec et al. (2020) evaluated the essential oil of T. riparia leaves or flower buds against Rhipicephalus sanguineus tick larvae. Larvae were exposed for 24 h to concentrations ranging from 50,000–0.47 mg/ml, and the larvicidal activity was measured by calculating lethal concentration (LC) using the Probit test. The LCs of the oils that killed 99.9% of the larvae (LC99.9) were 9.98 ± 0.10 mg/ml for the essential oil of the leaves, and 20.12 ± 0.54 mg/ml for that of the flower buds. The authors also studied the mechanism of action of the essential oil, evaluating the inhibitory potential on the enzyme acetylcholinesterase (AChE), whose inhibition doses were 0.70 mg/ml for the essential oil of the leaves and 1.40 mg/ml for that of the flower buds. The insecticidal action of T. riparia leaves essential oil was evaluated by Weaver et al. (1994). The oil was diluted at concentrations of 396, 791, 1,583, and 3,165 μg/cm2, applied to filter paper, and placed inside a Petri dish containing adult Zabrotes subfasciatus insects (0–2 days post-emergence from the bean, 5 males and 5 females). Insects that were moribund or died after oil exposure within 24 h were pooled to calculate the percentage of incapacitation by Probit analysis. The authors also sprayed the oil at different concentrations on beans containing eggs and larvae of Z. subfasciatus. The essential oil interfered with the reproduction of adult females (EC50 of 72 μg/cm2). Eggs were also sensitive to oil with an EC50 of 50 μg/cm2. The activity of the oil on the larvae was lower, as they were inside the bean grains, making it difficult for the oil to penetrate, with an EC50 of approximately 3,980 μg/cm2. These results validate the popular use of T. riparia leaves in grain storage silos, helping the preservation of grains during storage.
This bibliographic review verified that T. riparia has wide biological activities. Although investigations began 50 years ago, there is still much to learn about this plant. Its flower buds and stems have not yet been thoroughly investigated from a phytochemical and bioactivity point of view, opening new perspectives for biological assays.
6 Computational Investigation (in Silico Studies)
Although several compounds have been isolated from T. riparia, the bioactivity of only a few were documented. Only five compounds were identified through bioassay-guided purification based on multiple biological activities. In an attempt to close this gap, molecular docking was employed to assess potential antibacterial (anti-biofilm), anticancer (anti-inflammatory) and antiparasitic (anti-Leishmania) mechanisms using three putative target enzymes: the biofilm-associated Staphylococcus aureus sortase A (SaSrtA) (Thappeta et al., 2020), the inflammatory and cancer-associated human cyclooxygenase-2 (hCOX-2) (Méric et al., 2006) and the Leishmania infantum trypanothione reductase (LiTH) as an antiparasitic target (Saccoliti et al., 2017). The crystallographic structure of each enzyme was retrieved from the protein data bank (PDB): SaSrtA (PBD ID: 1T2W), hCOX-2(PDB ID: 5IKT) and LiTH (PDB ID: 2JK6). All twenty isolated phytochemicals from Tetradenia were used as ligands for docking study. Three-dimensional structures of both target and ligand were saved in .pdb file format for virtual screening with the software PyRx-AutoDock (Swain et al., 2021a). The protein-ligand interactions were visualized using Discovery Studio Visualizer software (Sahoo et al., 2021). Furthermore, the structural-activity relationship (SAR) between each phytochemical and its biological activity was explored mainly the software ChemDraw 18.0 (Swain et al., 2021b).
The docking score (kcal/mol) of each phytochemical against the three individual target enzymes was recorded (Table 4 and Figure 8). All phytochemicals exhibited docking scores between −5 and −9 kcal/mol. All three-sterol classes of compounds, campesterol (−7.8 kcal/mol), stigmasterol (−7.8 kcal/mol), sitosterol (−7.6 kcal/mol) and flavonoid luteolin (−7.5 kcal/mol) had higher scores than other terpenes against SaSrtA. On the other hand, astragalin and luteolin (−8.4 kcal/mol) together with stigmasterol and 13-epimanoyloxide (−8 kcal/mol) exhibited the highest scores against hCOX-2. Finally, stigmasterol (−9 kcal/mol), astragalin (−7.9 kcal/mol), luteolin (−7.6 kcal/mol) and 9β,13β-epoxy-7-abietene (−7.3 kcal/mol) showed the highest docking scores against LiTH. Based on the average docking score against three targets, stigmasterol (−8.26 kcal/mol), luteolin (−7.83 kcal/mol), astragalin (−7.66 kcal/mol), sitosterol (−7.53 kcal/mol) were the four most potent candidates, and their interactions with the three potential target enzymes are shown in Figure 8. However, often the docking score of a compound shows little difference between the three (unrelated) targets (Table 4), suggesting that the predicted interaction is rather non-selective. Nonetheless, bioinformatics tools play an increasing role as a guide in contemporary drug discovery and development by assessing possible biological activity targeting. This helps to reduce time and resources devoted to experimental testing (Romano and Tatonetti, 2019; Sahoo et al., 2021).
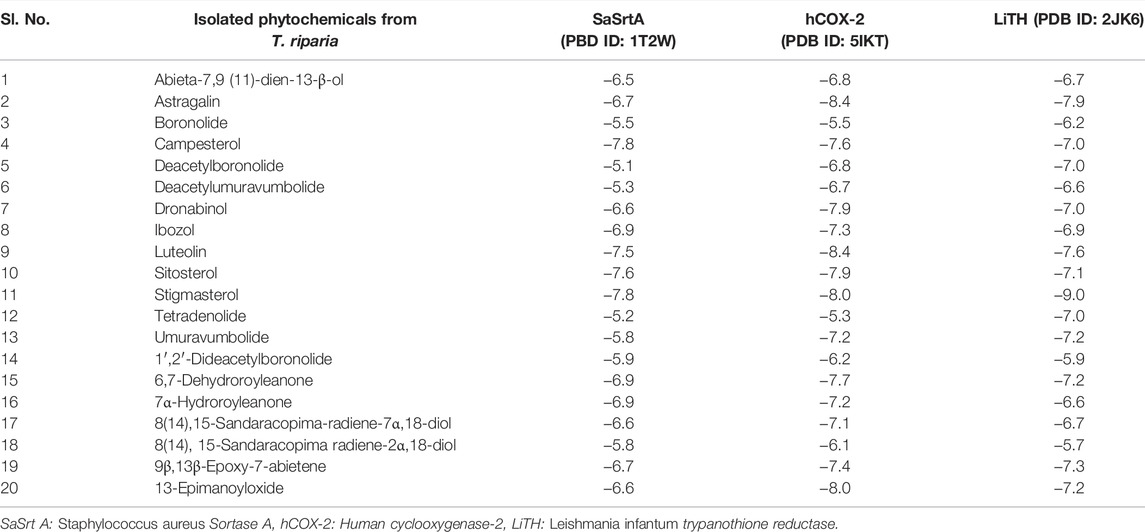
TABLE 4. Molecular docking study of phytochemicals from T. riparia against three target enzymes potentially important for the antibiofilm, anticancer and antiparasitic activity.
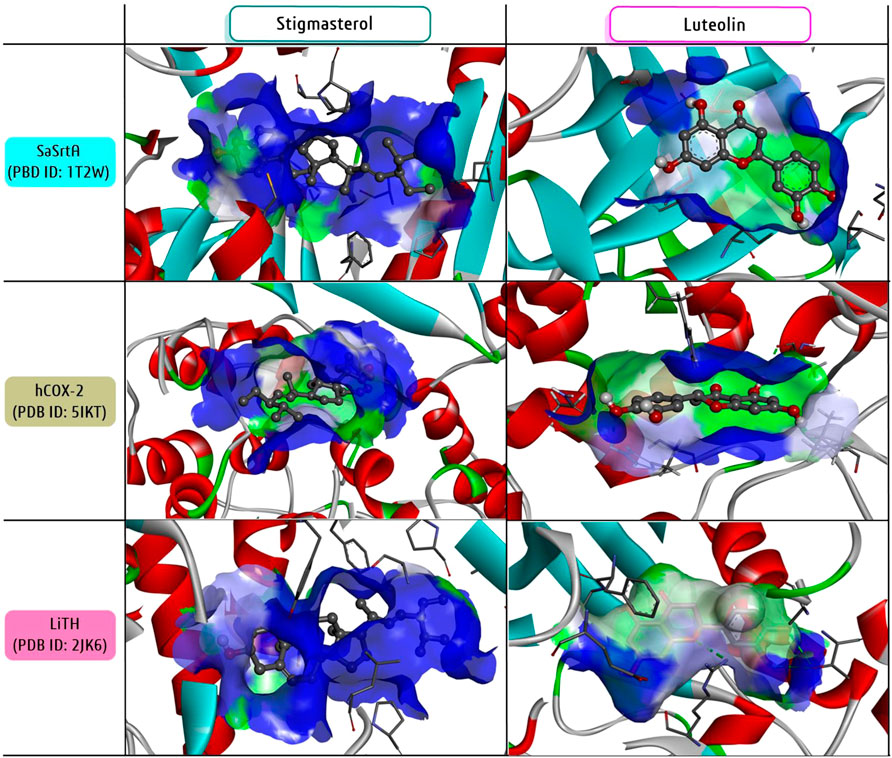
FIGURE 8. Protein-ligand interactions of two most potential candidates, stigmasterol and luteolin against selected three candidates. The molecular interactions were presented using the software Discovery studio visualizer.
7 Structure-Activity Relationship
The structure-activity relationship (SAR) between each phytochemical in relation to its biological activity was analyzed mainly by the ChemDraw 18.0 software (Swain et al., 2021b). Three six-member fused ring royleanone diterpene derivatives, 6,7-dehydroroyleanone and 7α-hydroroyleanone have similar in structure, but the carbonyl (C=O) attachment at C-11 instead of C-13 in 7a-hydroroyleanone reduces the activity against LiTH more than for the other two targets (Figure 9A). Among three abietene derivatives, the one with a single hydroxy (-OH) groups (abieta-7,9 (11)-dien-13-β-ol) exhibited a comparatively lower docking score than the other two derivatives. At the same time the methoxymethane (CH3-O-CH3 or C2H6O) in 9β,13β-Epoxy-7-abietene was comparative potential to double hydroxy contained ibozol (Figure 9B) and overall, all are potential against hCOX-2. Similarly, methoxymethane with methyl or methoxy ethane (C3H8O) at the C-3 position in 8(14),15-sandaracopimaradiene-7α,18-diol showed reasonably higher biological activity than methoxymethane at same C-3 with additional -OH group at the C-1 position in 8(14), 15-sandaracopimaradiene-2α,18-diol (Figure 9C). Between two α-pyrone derivatives, the presence of hydroxy at the C-10 position in deacetylumuravumbolide exhibited a lesser docking score than methyl acetate (C3H6O2) attached umuravumbolide at the same C-10 position (Figure 9D). Overall due to the presence of -OH and C=O functional groups, they combined higher effectiveness against hCOX-2 other targets.
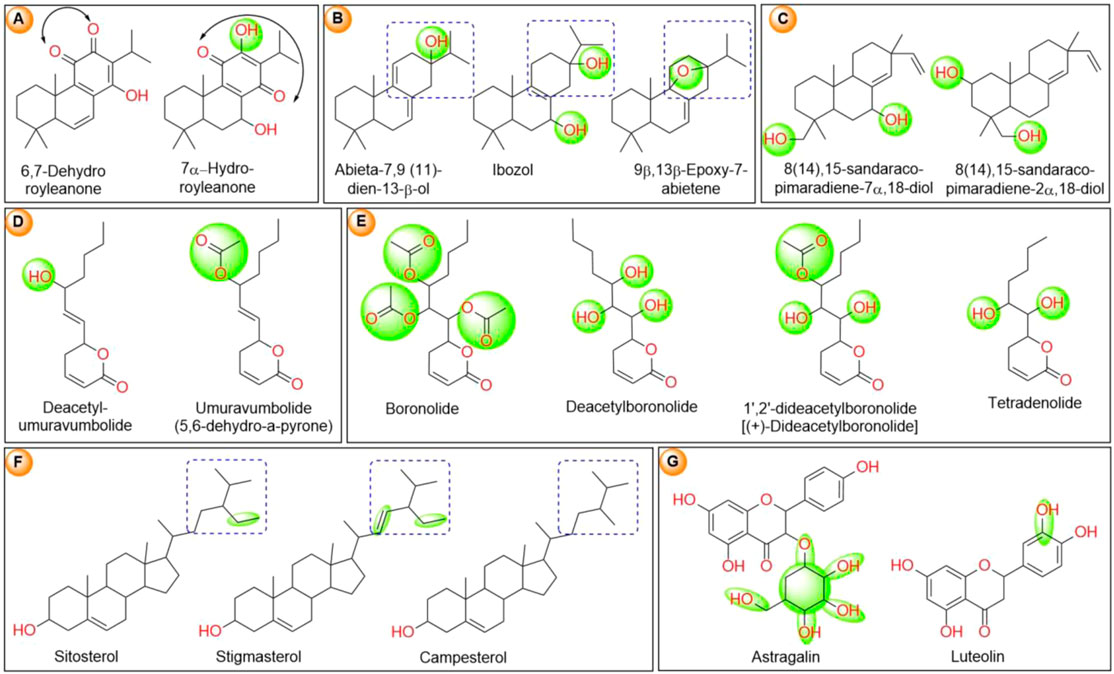
FIGURE 9. (A–G). Structure-activity relationship analysis among phytochemicals reported from T. riparia with respect to biological activity in the form of generated docking score. The chemical structures are presented with the ChemDraw 18.0 software.
From another similar type of α-pyrone derivatives, boronolide, deacetylboronolide, 1′,2′-dideacetylboronolide and tetradenolide substituted by distinct -OH and C3H6O2 groups are not significantly influenced in biological activity; however, all four candidates were comparatively higher docking score against potential against LiTH (Figure 9E). From three steroid classes of constitutes, the presence of extra methyl group or 3-ethyl-4-methylpent-1-ene functional attachment in stigmasterol enhanced the biological activity mainly against LiTH than 3-ethyl-2-methyl pentane presented sitosterol and 2,3-dimethyl pentane presented campesterol (Figure 9F). Further, structural comparison between isolated two polyphenolic classes of compounds, astragalin (kaempferol-O-glucoside) and luteolin, the attachment of extract glucose at C-8 in astragalin showed similar activity with the extra -OH group at C-15 in the tetrahydroxyflavone moieties of luteolin (Figure 9G). However, the same astragalin has significantly lesser antibiofilm activity than luteolin. Thus, the position and substituted functional groups really influence the biological activity and the biological activity also varies by phytochemicals class (Swain et al., 2021a; Sahoo et al., 2021).
8 In vivo and Isolated Organ Studies
The methanolic extract from fruit, leaf, stem and root of T. riparia were tested at different concentrations on smooth (guinea pig ileum), skeletal (toad rectus abdominis), and uterine (non-pregnant guinea pig) muscle (Chagnon, 1984) . Only the leaf extract contracted the ileum (at 0.05 μg/ml). All extracts except that of the root contracted uterine muscle (at 500 μg/ml). Leaf extract contracted the skeletal muscle (at 50 μg/ml), whereas stem and fruit extract inhibited (both at 50 μg/ml) the contraction induced by acetylcholine (1 μg/ml), while root extract had no effect (up to 500 μg/ml). In the same study, hyper- and hypotensive effects of the extracts were tested in vivo in urethane-anesthetised rabbits, but no activity was observed from any extract at the dose used (5 mg/kg IV) (Chagnon, 1984). The diterpenediol from T. riparia has also been shown to possess papaverine-like antispasmodic activity on histamine, methacholine, and barium chloride-induced contractions of guinea pig ileum, as well as on noradrenaline-induced contractions of rabbit aorta (Van Puyvelde et al., 1987).
9 Toxicology
T. riparia taken in self-administered over-dosage of hot water extract as a remedy for cold or flu is reported in some cases of poisoning that occurred in adult males in South Africa during 18 years of clinical practice amongst Zulu communities. The symptoms included a severe toxic inflammatory response of mucous membranes, conspicuous at all body orifices, as well as profuse salivation. In more severe cases this went on to tissue necrosis and large-scale sloughing; In all cases of terminal illness, urine and stools consisted of almost pure blood; they were dark in color and contained shreds of exfoliated mucous membrane; The patients who were fatally ill went into anuria during the last 24–48 h, but one man recovered after 24 h of anuria (Bodenstein, 1977; Hutchings, 1996; Duke, 2002; Njau and Ndakidemi, 2017). No toxicity of methanolic leaf or stem extracts was observed upon intraperitoneal injection in mice at 1 g/kg (Chagnon, 1984). The cytotoxicity of essential oils and crude extracts from leaves, flower buds and stems of T. riparia was determined in Vero cells, with GI50 ranging from 143.00 ± 11.00 to 190.00 ± 15.00 μg/mL. As a positive control, ellipticin with GI50: 1.41 ± 0.06 μg/ml was used. The results indicated that the essential oil and the crude extract are non-toxic (unpublished data).
10 Conclusion
T. riparia is one of the most commonly used medicinal plants by indigenous communities of Africa. Commonly, it is planted close to homes to ward off mosquitoes and is traditionally used to treat several diseases including respiratory problems, cough, headache, stomach pain, diarrhea, fever, malaria and dengue etc. The active compound 8(14),15- sandaracopimaradiene-7α, 18-diol was isolated on several occasions through bioassay-guided purification, and has demonstrated multiple bioactivities, such as antispasmodic, anthelmintic and antimicrobial against mostly Gram-positive bacteria, including M. tuberculosis and S. aureus (both planktonic and biofilm). Other major bioactive compounds are 6,7-dehydroroyleanone (antimicrobial, antiparasitic), ibozol (antimicrobial, antitumor) and abieta-7,9(11)-dien-13-β-ol (antimicrobial), which was also documented in several experimental investigations. Moreover, essential oils demonstrated multiple bioactivities with major active compounds 6,7-dehydroroyleanone and 9β, 13β-epoxy-7-abietene. When all the isolated constituents were subjected to molecular docking using putative target enzymes: sitosterol, stigmasterol, luteolin and astragalin had the highest scores, but show little selectivity for any of the targets. This suggests that the have another mechanism of action. Based on in vitro evidence and calculated docking scores, 13-epimanoyloxide (−8 kcal/mol) against human cyclooxygenase-2 and 9β,13β-epoxy-7-abietene (−7.3 kcal/mol) against Leishmania infantum trypanothione reductase deserve further follow-up study in vivo. From our review it is clear that crude extracts have multiple in vitro effects, such as anticancer, analgesic, acaricide, insecticidal etc., but in most cases additional work is needed to isolate and characterize the active compounds. Further follow-up studies are also necessary to elucidate the mechanism of action and SAR. Also, pharmacokinetic and further toxicity studies will be required to assess their potential as drug candidates.
Author Contributions
Conceptualization, SP; methodology, SS; software, SS, validation, ZG, MM, and LV; formal analysis, SP, SS, and ZG; investigation, ZG, SS, MB, and JS; resources, ZG, SS, MB, and JS; data curation, ZG, MM, MB, and JS; writing—original draft preparation, ZG, SS, and SP; writing—review and editing, SP and WL; visualization, SS; supervision, WL; project administration, SP; funding acquisition, SP. All authors have read and agreed to the published version of the manuscript.
Conflict of Interest
The authors declare that the research was conducted in the absence of any commercial or financial relationships that could be construed as a potential conflict of interest.
Publisher’s Note
All claims expressed in this article are solely those of the authors and do not necessarily represent those of their affiliated organizations, or those of the publisher, the editors and the reviewers. Any product that may be evaluated in this article, or claim that may be made by its manufacturer, is not guaranteed or endorsed by the publisher.
Acknowledgments
The author ZG thank Universidade Paranaense, Coordenação de Aperfeiçoamento de Pessoal de Nível Superior–Brazil (CAPES), Conselho Nacional de Desenvolvimento Científico e Tecnológico (CNPq) and Fundação Araucária for the financial support and the fellowship. SP is thankful to the RUSA 2.0 for supporting the Centre of Excellence in Environment, Climate Change and Public Health (ECCPH), Utkal University. LP and WL largely funded themselves. All the authors of this manuscript are thankful to their respective Institution for their support in the preparation and publication of this manuscript.
Supplementary Material
The Supplementary Material for this article can be found online at: https://www.frontiersin.org/articles/10.3389/fphar.2022.896078/full#supplementary-material
Abbreviations
Me2CO, Acetone; ATCC, American Type Culture Collection (Manassas, Virginia, United States); C6H6, Benzene; BGF, Bioassay-guided fractionation; CHCl3, Chloroform; EtOAc, Ethyl acetate; FICI, Fractional Inhibitory Concentration Index; IC, Inhibitory concentration; IR, Infrared spectroscopy; MS, Mass spectral analysis; MeOH, Methanol; MIC, Minimal inhibitory concentration; MBC, Minimum bactericidal concentration; NMR, Nuclear magnetic resonance; SGCC, Silica gel column chromatography; TLC, Thin layer chromatography.
Footnotes
1Reproduced from “Endo EH, Costa GM, Nakamura TU, Nakamura CV, Dias Filho BP. Antidermatophytic activity of hydroalcoholic extracts from Rosmarinus officinalis and Tetradenia riparia. J Mycol Med. 2015 December; 25(4):274-279. doi: 10.1016/j.mycmed. 2015.09.003”. Copyright © 2015 Elsevier Masson SAS. All rights reserved.
2Reproduced from “Demarchi IG, Thomazella MV, de Souza Terron M, Lopes L, Gazim ZC, Cortez DA, Donatti L, Aristides SM, Silveira TG, Lonardoni MV. Antileishmanial activity of essential oil and 6,7-dehydroroyleanone isolated from Tetradenia riparia. Exp Parasitol. 2015 October; 157:128-137. doi: 10.1016/j.exppara. 2015.06.014”. Copyright © 2015 Elsevier Inc. All rights reserved.
References
Araújo, L. L. N., Melo, H. C., Paula, J. R., Alves, F. R. R., and Portes, T. A. (2018). Yield and Composition of the Essential Oil of Tetradenia Riparia (Hochst) Codd (Lamiaceae) Cultivated under Different Shading Levels. Planta daninha. 36, e0181647451–9. doi:10.1590/S0100-83582018360100066
Baldin, V. P., Scodro, R. B. L., Lopes-Ortiz, M. A., de Almeida, A. L., Gazim, Z. C., Ferarrese, L., et al. (2018). Anti-Mycobacterium tuberculosis Activity of Essential Oil and 6,7-dehydroroyleanone Isolated from Leaves of Tetradenia Riparia (Hochst.) Codd (Lamiaceae). Phytomedicine 147, 34–39. doi:10.1016/j.phymed.2018.04.043
Bekalo, T. H., Woodmatas, S. D., and Woldemariam, Z. A. (2009). An Ethnobotanical Study of Medicinal Plants Used by Local People in the Lowlands of Konta Special Woreda, Southern Nations, Nationalities and Peoples Regional State, Ethiopia. J. Ethnobiol. Ethnomed 5 (26), 26–15. doi:10.1186/1746-4269-5-26
Blythe, E. K., Tabanca, N., Demirci, B., and Kendra, P. E. (2020). Chemical Composition of Essential Oil from Tetradenia Riparia and its Attractant Activity for Mediterranean Fruit Fly, Ceratitis Capitata. Nat. Product. Commun. 15 (9), 1934578X2095395–6. doi:10.1177/1934578X20953955
Boily, Y., and Van Puyvelde, L. (1986). Screening of Medicinal Plants of Rwanda (Central Africa) for Antimicrobial Activity. J. Ethnopharmacol. 16 (1), 1–13. doi:10.1016/0378-8741(86)90062-0
Campbell, W. E., Gammon, D. W., Smith, P., Abrahams, M., and Purves, T. D. (1997). Composition and Antimalarial Activity In Vitro of the Essential Oil of Tetradenia Riparia. Planta Med. 63 (03), 270–272. doi:10.1055/s-2006-957672
Cardoso, B. M., de Mello, T. F., Lopes, S. N., Demarchi, I. G., Lera, D. S., Pedroso, R. B., et al. (2015). Antileishmanial Activity of the Essential Oil from Tetradenia Riparia Obtained in Different Seasons. Mem. Inst. Oswaldo Cruz 110 (8), 1024–1034. doi:10.1590/0074-02760150290
Chagnon, M. (1984). Inventaire pharmacologique general des plantes medicinales rwandaises. J. Ethnopharmacol. 12 (3), 239–251. doi:10.1016/0378-8741(84)90053-9
Codd, L. E. (1983). The Genus Tetradenia Benth. (Lamiaceae). I. African Species. Bothalia 14 (2), 177–183. doi:10.4102/abc.v14i2.1156
Coopoosamy, R., and Naidoo, K. (2011). Assessing the Potential of Tetradenia Riparia in Treatment of Common Skin Conditions in Rural Communities of South Africa. Afr. J. Microbiol. Res. 519, 2942–2945. doi:10.5897/AJMR11.396
Costa, G. M., Endo, E. H., Cortez, D. A. G., Ueda-Nakamura, T., Nakamura, C. V., and Prado Dias Filho, B. P. D. (2015). Effect of Plant Extracts on Planktonic Growth and Biofilm of Staphylococcus aureus and. Candida Albicans. Int. J. Curr. Microbiol. App. Sci. 4 (6), 908–917.
Davies-Coleman, M. T., and Rivett, D. E. A. (1995). Structure of the 5,6-Dihydro-α-Pyrone, Umuravumbolide. Phytochemistry 38 (3), 791–792. doi:10.1016/0031-9422(95)93874-f
de Melo, N. I., Mantovani, A. L., de Oliveira, P. F., Groppo, M., Filho, A. A., Rodrigues, V., et al. (2015). Antischistosomal and Cytotoxic Effects of the Essential Oil of Tetradenia Riparia (Lamiaceae). Nat. Prod. Commun. 10 (9), 1627–1630. doi:10.1177/1934578x1501000934
Demarchi, I. G., Terron, M. d.-S., Thomazella, M. V., Pedroso, R. B., Gazim, Z. C., Cortez, D. A. G., et al. (2015). Immunomodulatory Activity of Essential Oil fromTetrania riparia(Hochstetter) Codd in Murine Macrophages. Flavour Fragr. J. 30 (6), 428–438. doi:10.1002/ffj.3284
Dunkel, F. V., Weaver, D., Van Puyvelde, L., Cusker, J. L., and Serugendo, A. (1991). “Population Suppression Effects of Rwandan Medicinal Plant, Tetradenia Riparia (Hochst.) Codd (Lamiaceae) on Stored Grain and Bean Insects,” in Proceedings of the Fifth International Working Conference on Stored-Product Protection, Bordeaux, France, September 1990 (Paris: INRA), 1589–1598.
Elaka, I. K., Kapepula, P. M., Ngombe, N. K., Mpoyi, J. M., Lukusa, D. M., Muabilwa, M. M., et al. (2020). Microscopic Features, Antioxidant and Antibacterial Capacities of Plants of the Congolese Cosmetopoeia, Raw Materials of Cosmeceuticals. Jbm 08 (9), 149–166. doi:10.4236/jbm.2020.89013
Endo, E. H., Costa, G. M., Nakamura, T. U., Nakamura, C. V., and Dias Filho, B. P. (2015). Antidermatophytic Activity of Hydroalcoholic Extracts from Rosmarinus Officinalis and Tetradenia Riparia. J. Mycol. Med. 25 (4), 274–279. doi:10.1016/j.mycmed.2015.09.003
Endo, E. H., Costa, G. M., Makimori, R. Y., Ueda-Nakamura, T., Nakamura, C. V., and Dias Filho, B. P. (2018). Anti-biofilm Activity of Rosmarinus Officinalis, Punica Granatum and Tetradenia Riparia against Methicillin-Resistant Staphylococcus aureus (MRSA) and Synergic Interaction with Penicillin. J. Herb. Med. 14, 48–54. doi:10.1016/j.hermed.2018.07.001
Fernandez, A. C. A. M., Rosa, M. F., Fernandez, C. M. M., C Bortolucci, W., Melo, U. Z., Siqueira, V. L. D., et al. (2017). Antimicrobial and Antioxidant Activities of the Extract and Fractions of Tetradenia Riparia (Hochst.) Codd (Lamiaceae) Leaves from Brazil. Curr. Microbiol. 74 (12), 1453–1460. doi:10.1007/s00284-017-1340-9
Fernandez, C. M. M., Barba, E. L., Fernandez, A. C. M., Cardoso, B. K., Borges, I. B., Takemura, O. S., et al. (2014). Larvicidal Activity of Essential Oil fromTetradenia Ripariato Control ofAedes aegyptiLarvae in Function of Season Variation. J. Essent. Oil Bear. Plants 17 (5), 813–823. doi:10.1080/0972060X.2014.892841
Friedrich, J. C. C., Silva, O. A., Faria, M. G. I., Colauto, N. B., Gazzin, Z. C., Colauto, G. A. L., et al. (2020). Improved Antioxidant Activity of a Starch and Gelatin-Based Biodegradable Coating Containing Tetradenia Riparia Extract. Int. J. Biol. Macromol. 165, 1038–1046. doi:10.1016/j.ijbiomac.2020.09.143
Gahamanyi, N., Munyaneza, E., Dukuzimana, E., Tuyiringire, N., Pan, C. H., and Komba, E. V. G. (2021). Ethnobotany, Ethnopharmacology, and Phytochemistry of Medicinal Plants Used for Treating Human Diarrheal Cases in Rwanda: A Review. Antibiot. (Basel) 10, 1231. doi:10.3390/antibiotics10101231
Gairola, S., Naidoo, Y., Bhatt, A., and Nicholas, A. (2009). An Investigation of the Foliar Trichomes of Tetradenia Riparia (Hochst.) Codd [Lamiaceae]: An Important Medicinal Plant of Southern Africa. Flora - Morphol. Distribution, Funct. Ecol. Plants 204, 325–330. doi:10.1016/j.flora.2008.04.002
Gazim, Z. C., Amorim, A. C., Hovell, A. M., Rezende, C. M., Nascimento, I. A., Ferreira, G. A., et al. (2010). Seasonal Variation, Chemical Composition, and Analgesic and Antimicrobial Activities of the Essential Oil from Leaves of Tetradenia Riparia (Hochst.) Codd in Southern Brazil. Molecules 15 (8), 5509–5524. doi:10.3390/molecules15085509
Gazim, Z. C., Demarchi, I. G., Lonardoni, M. V., Amorim, A. C., Hovell, A. M., Rezende, C. M., et al. (2011). Acaricidal Activity of the Essential Oil from Tetradenia Riparia (Lamiaceae) on the Cattle Tick Rhipicephalus (Boophilus) Microplus (Acari; Ixodidae). Exp. Parasitol. 129 (2), 175–178. doi:10.1016/j.exppara.2011.06.011
Gazim, Z. C., Rodrigues, F., Amorin, A. C., de Rezende, C. M., Soković, M., Tešević, V., et al. (2014). New Natural Diterpene-type Abietane from Tetradenia Riparia Essential Oil with Cytotoxic and Antioxidant Activities. Molecules 19 (1), 514–524. doi:10.3390/molecules19010514
Gil, E. S. (2007). Controle Físico-químico de qualidade de medicamentos. São Paulo: Pharmabooks, 370–371.
Githinji, C. W., and Kokwaro, J. O. (1993). Ethnomedicinal Study of Major Species in the Family Labiatae from Kenya. J. Ethnopharmacol. 39, 197–203. doi:10.1016/0378-8741(93)90036-5
Godoy, R. L. O., Koketsu, M., Gonçalves, S. L., Lopes, D., Sá Sobrinho, A. F., and Torquilho, H. S. (1999). Essential Oil ofMoschosma ripariumHochst. (Lamiaceae) from Manaus, Amazonas, Brazil. J. Essent. Oil Res. 11 (3), 321–323. doi:10.1080/10412905.1999.9701144
Hakizamungu, E., van Puyvelde, L., and Wéry, M. (1992). Screening of Rwandese Medicinal Plants for Anti-trichomonas Activity. J. Ethnopharmacol. 36 (2), 143–146. doi:10.1016/0378-8741(92)90014-i
Hakizamungu, E., Van Puyvelde, L., Wéty, M., and Schamp, N. (1988). Active Principles ofTetradenia Riparia III. Anti-trichomonas Activity of 8(14), 15-sandaracopimaradiene-7α, 18-diol. Phytother. Res. 2 (4), 207–208. doi:10.1002/ptr.2650020414
Hamill, F. A., Apio, S., Mubiru, N. K., Mosango, M., Bukenya-Ziraba, R., Maganyi, O. W., et al. (2000). Traditional Herbal Drugs of Southern Uganda, I. J. Ethnopharmacol. 70 (3), 281–300. doi:10.1016/s0378-8741(00)00180-x
Hutchings, A. (1996). Zulu Medicinal Plants: An Inventory. Pietermaritzburg: University of Natal Press.
Kakande, T., Batunge, Y., Eilu, E., Shabohurira, A., Abimana, J., Akinola, S. A., et al. (2019). Prevalence of Dermatophytosis and Antifungal Activity of Ethanolic Crude Leaf Extract of Tetradenia Riparia against Dermatophytes Isolated from Patients Attending Kampala International University Teaching Hospital, Uganda. Dermatol Res. Pract. 2019, 1–13. doi:10.1155/2019/9328621
Kamatenesi-Mugisha, M., and Oryem-Origa, H. (2007). Medicinal Plants Used to Induce Labour during Childbirth in Western Uganda. J. Ethnopharmacol. 109, 1–9. doi:10.1016/j.jep.2006.06.011
Leitão, G., Pinheiro, A., Rezende, V., Silva, R., Paula, L., Soares, D., et al. (2020). Unexpected Rearrangement and Aromatization on Dehydration Reaction of the Bioactive Diterpenequinone 7α-Hydroxyroyleanone. J. Braz. Chem. Soc. 31 (3), 597–602. doi:10.21577/0103-5053.20190223
Lorenzi, H., and Matos, F. J. (2008). Plantas medicinais no Brasil: nativas e exóticas. Nova Odessa: Instituto Plantarum.
Martins, M. B. G., Martins, R. G. M., and Cavalheiro, J. A. (2008). Chemical and Antibacterial Study of Tetradenia Riparia Leaves. Rev. Biociências 14 (2), 127–140.
Méric, J. B., Rottey, S., Olaussen, K., Soria, J. C., Khayat, D., Rixe, O., et al. (2006). Cyclooxygenase-2 as a Target for Anticancer Drug Development. Crit. Rev. Oncol. Hematol. 59 (1), 51–64. doi:10.1016/j.critrevonc.2006.01.003
Nabukenya, I., Rubaire-Akiiki, C., Olila, D., Ikwap, K., and Höglund, J. (2014). Ethnopharmacological Practices by Livestock Farmers in Uganda: Survey Experiences from Mpigi and Gulu Districts. J. Ethnobiol. Ethnomed. 10, 9. doi:10.1186/1746-4269-10-9
Nalule, A. S., Mbaria, J. M., Olila, D., and Kimenju, J. W. (2011). Ethnopharmacological Practices in Management of Livestock Helminthes by Pastoral Communities in the Drylands of Uganda. Livest. Res. Rural Dev. 23 (2), 1–27.
Namukobe, J., Kasenene, J. M., Kiremire, B. T., Byamukama, R., Kamatenesi-Mugisha, M., Krief, S., et al. (2011). Traditional Plants Used for Medicinal Purposes by Local Communities Around the Northern Sector of Kibale National Park, Uganda. J. Ethnopharmacol. 136 (1), 236–245. doi:10.1016/j.jep.2011.04.044
Ngezahayo, J., Havyarimana, F., Hari, L., Stévigny, C., and Duez, P. (2015). Medicinal Plants Used by Burundian Traditional Healers for the Treatment of Microbial Diseases. J. Ethnopharmacol. 173, 338–351. doi:10.1016/j.jep.2015.07.028
Njau, E.-F., Alcorn, J.-M., Buza, J., Chirino-Trejo, M., and Ndakidemi, P. (2014). Antimicrobial Activity of Tetradenia Riparia (Hochst.) Lamiaceae, a Medicinal Plant from Tanzania. Ejmp 4 (12), 1462–1478. doi:10.9734/EJMP/2014/11370
Njau, E.-F. A., and Ndakidemi, P. A. (2017). The Genus Tetradenia (Lamiaceae): A Review of its Ethnomedicinal, Botanical, Chemical and Pharmacological Activities. Ijb 9 (4), 35–41. doi:10.5539/ijb.v9n4p35
Njau, E. A. (2001). “An Ethnobotanical Study of Medicinal Plants Used by the Maasai People of Manyara, Arusha-Tanzania,” in Master’s Thesis, School of Graduate Studies (Addis Ababa: Addis Ababa University-Ethiopia), 95.
Omolo, M. O., Okinyo, D., Ndiege, I. O., Lwande, W., and Hassanali, A. (2004). Repellency of Essential Oils of Some Kenyan Plants against Anopheles gambiae. Phytochemistry 65 (20), 2797–2802. doi:10.1016/j.phytochem.2004.08.035
Phillipson, P. B., and Steyn, C. F. (2008). Tetradenia (Lamiaceae) in Africa: New Species and New Combinations. Adansonia 30 (1), 177–196.
Pinheiro, M. V. M., Schmidt, D., Thiesen, L. A., Junges, D. L., Diel, M. I., Holz, E., et al. (2021). Propagação De Falsa-Mirra Por Miniestaquia De Diferentes Porções Do Ramo E Tipos De Corte. Rcr 23 (1), 156–170. doi:10.30945/rcr-v23i1.2775
Pooley, E. (1998). A Field Guide to Wild Flowers of KwaZulu-Natal and the Eastern Region. Durban, South Africa: Natal Flora Publications Trust.
Puyvelde, L. V., Kimpe, N. D., Dubé, S., Chagnon-Dubé, M., Boily, Y., Borremans, F., et al. (1981). 1′,2′-Dideacetylboronolide, an α-pyrone from Iboza Riparia. Phytochemistry 20 (12), 2753–2755. doi:10.1016/0031-9422(81)85280-6
Randriamiharisoa, M. N., Kuhlman, A. R., Jeannoda, V., Rabarison, H., Rakotoarivelo, N., Randrianarivony, T., et al. (2015). Medicinal Plants Sold in the Markets of Antananarivo, Madagascar. J. Ethnobiol. Ethnomed 11, 601–613. doi:10.1186/s13002-015-0046-y
Romano, J. D., and Tatonetti, N. P. (2019). Informatics and Computational Methods in Natural Product Drug Discovery: A Review and Perspectives. Front. Genet. 10 (368), 1–29. doi:10.3389/fgene.2019.00368
Sabec, G. Z., Jesus, R. A. d., Oliveira, H. L. M. d., CampoJacomassi, C. F. d. A. A. E., jacomassi, E., Gonçalves, J. E., et al. (2020). Tetradenia Riparia (Lamiaceae) Essential Oil: an Alternative to Rhipicephalus Sanguineus. Aust. J. Crop Sci. 14 (10), 1608–1615. doi:10.21475/ajcs.20.14.10.p2389
Saccoliti, F., Angiulli, G., Pupo, G., Pescatori, L., Madia, V. N., Messore, A., et al. (2017). Inhibition of Leishmania Infantum Trypanothione Reductase by Diaryl Sulfide Derivatives. J. Enzyme Inhib. Med. Chem. 32 (1), 304–310. doi:10.1080/14756366.2016.1250755
Sahoo, A., Fuloria, S., Swain, S. S., Panda, S. K., Sekar, M., Subramaniyan, V., et al. (2021). Potential of Marine Terpenoids against SARS-CoV-2: An In Silico Drug Development Approach. Biomedicines 911, 2–22. doi:10.3390/biomedicines9111505
Scanavacca, J., Bortolucci, W. C., Jacomassi, E., Baretta, I. P., Faria, M. G. I., Fernandez, C. M. M., et al. (In Press). “Antimicrobial activity of Tetradenia riparia leaf essential oil, ” in Bol. latinoam. Caribe plantas. Editors J. L. Martínez (Santiago, Chile : University of Santiago de Chile).
Swain, S. S., Hussain, T., and Pati, S. (2021b). Drug-lead Anti-tuberculosis Phytochemicals: A Systematic Review. Curr. Top. Med. Chem. 21 (20), 1832–1868. doi:10.2174/1568026621666210705170510
Swain, S. S., Panda, S. K., and Luyten, W. (2021a). Phytochemicals against SARS-CoV as Potential Drug Leads. Biomed. J. 44 (1), 74–85. doi:10.1016/j.bj.2020.12.002
Thappeta, K. R. V., Zhao, L. N., Nge, C. E., Crasta, S., Leong, C. Y., Ng, V., et al. (2020). In-silico Identified New Natural Sortase A Inhibitors Disrupt S. aureus Biofilm Formation. Int. J. Mol. Sci. 21 (22), 2–18. doi:10.3390/ijms21228601
Van Puyvelde, L., Aissa, A., Panda, S. K., De Borggraeve, W. M., Mukazayire, M. J., and Luyten, W. (2021). Bioassay-guided Isolation of Antibacterial Compounds from the Leaves of Tetradenia Riparia with Potential Bactericidal Effects on Food-Borne Pathogens. J. Ethnopharmacol. 273, 113956. doi:10.1016/j.jep.2021.113956
Van Puyvelde, L., De Kimpe, N., Ayobangira, F. X., Costa, J., Nshimyumukiza, P., Boily, Y., et al. (1988). Wheat Rootlet Growth Inhibition Test of Rwandese Medicinal Plants: Active Principles of Tetradenia Riparia and Diplolophium Africanum. J. Ethnopharmacol. 24 (2-3), 233–246. doi:10.1016/0378-8741(88)90156-0
Van Puyvelde, L., Geysen, D., Ayobangira, F. X., Hakizamungu, E., Nshimiyimana, A., and Kalisa, A. (1985). Screening of Medicinal Plants of Rwanda for Acaricidal Activity. J. Ethnopharmacol. 13 (2), 209–215. doi:10.1016/0378-8741(85)90008-x
Van Puyvelde, L., Liu, M., Veryser, C., De Borggraeve, W. M., Mungarulire, J., Mukazayire, M. J., et al. (2018). Active Principles of Tetradenia Riparia. IV. Anthelmintic Activity of 8(14),15-Sandaracopimaradiene-7α,18-Diol. J. Ethnopharmacol. 216 (216), 229–232. doi:10.1016/j.jep.2018.01.024
Van Puyvelde, L., Nyirankuliza, S., Panebianco, R., Boily, Y., Geizer, I., Sebikali, B., et al. (1986). Active Principles of Tetradenia Riparia. I. Antimicrobial Activity of 8(14),15-sandaracopimaradiene-7 Alpha,18-Diol. J. Ethnopharmacol. 17 (3), 269–275. doi:10.1016/0378-8741(86)90115-7
Van Puyvelde, L., De Kimpe, N., Borremans, F., Dube, S., Chagnon-Dube, M., Schamp, N., et al. (1982). Isolation and Structural Identification of 8(14),15-sandaracopimaradiene-7.alpha.,18-diol from Iboza Riparia. J. Org. Chem. 47 (19), 3628–3630. doi:10.1021/jo00140a006
Van Puyvelde, L., De Kimpe, N., Borremans, F., Dube, S., Chagnon-Dube, M., Schamp, N., et al. (1982). Isolation and Structural Identification of 8(14),15-sandaracopimaradiene-7.alpha.,18-diol from Iboza Riparia. J. Org. Chem. 47, 3628–3630. doi:10.1021/jo00140a006
Van Puyvelde, L., De Kimpe, N., Borremans, F., Zhang, W., and Schamp, N. (1987). 8(14),15-sandaracopimaradiene-2α,18-diol, a Minor Constituent of the Rwandese Medicinal Plant Tetradenia Riparia. Phytochemistry 26 (2), 493–495. doi:10.1016/s0031-9422(00)81440-5
Van Puyvelde, L., and de Kimpe, N. (1998). Tetradenolide, an α-PYRONE from Tetradenia Riparia. Phytochemistry 49 (4), 1157–1158. doi:10.1016/s0031-9422(98)00112-5
Van Puyvelde, L., Dube, S., Uwimana, E., Uwera, C., Dommisse, R. A., Esmans, E. L., et al. (1979). New α-pyrones from Iboza Riparia. Phytochemistry 18 (7), 1215–1218. doi:10.1016/0031-9422(79)80138-7
Van Puyvelde, L., Ntawukiliyayo, J. D., Portaels, F., and Hakizamungu, E. (1994). In Vitro inhibition of Mycobacteria by Rwandese Medicinal Plants. Phytother. Res. 8, 65–69. doi:10.1002/ptr.2650080202
Van Wyk, B.-E., and Wink, M. (2004). Medicinal Plants of the World. Pretoria, Southern Africa: Briza Publications, Timber Press publisher.
Vlietinck, A. J., Van Hoof, L., Totté, J., Lasure, A., Vanden Berghe, D., Rwangabo, P. C., et al. (1995). Screening of Hundred Rwandese Medicinal Plants for Antimicrobial and Antiviral Properties. J. Ethnopharmacol. 46, 31–47. doi:10.1016/0378-8741(95)01226-4
Wasswa, P., and Olila, D. (2006). The In-Vitro Ascaricidal Activity of Selected Indigenous Medicinal Plants Used in Ethno Veterinary Practices in Uganda. Afr. J. Tradit. Complement. Altern. Med. 3 (2), 94–103.
Watt, J. M., and Breyer-Brandwijk, M. G. (1962). The Medicinal and poisonous plants of Southern and Eastern Africa. London: Livingstone.
Weaver, D. K., Dunkel, F. V., Puyvelde, L., Richards, D. C., and Fitzgerald, G. W. (1994). Toxicity and Protectant Potential of the Essential Oil ofTetradenia riparia(Lamiales, Lamiaceae) againstZabrotes subfasciatus(Col., Bruchidae) Infesting Dried Pinto Beans (Fabales, Leguminosae). J. Appl. Ent. 118 (1-5), 179–196. doi:10.1111/j.1439-0418.1994.tb00793.x
Wintola, O., and Afolayan, A. (2015). An Inventory of Indigenous Plants Used as Anthelmintics in Amathole District Municipality of the Eastern Cape Province, South Africa. Afr. J. Trad. Compl. Alt. Med. 12 (4), 112–121. doi:10.4314/ajtcam.v12i4.17
York, T., Van Vuuren, S. F., and de Wet, H. (2012). An Antimicrobial Evaluation of Plants Used for the Treatment of Respiratory Infections in Rural Maputaland, KwaZulu-Natal, South Africa. J. Ethnopharmacol. 144 (1), 118–127. doi:10.1016/j.jep.2012.08.038
Keywords: ethnopharmacology, traditional folk medicine, diterpenes, 8(14),15sandaracopimaradiene-7 α, 18-diol, 14-hydroxy-9-epi(E)-caryophyllene, 6,7-dehydroroileanone, computational analysis
Citation: Panda SK, Gazim ZC, Swain SS, Bento MCVdA, Sena JdS, Mukazayire MJ, Van Puyvelde L and Luyten W (2022) Ethnomedicinal, Phytochemical and Pharmacological Investigations of Tetradenia riparia (Hochst.) Codd (Lamiaceae). Front. Pharmacol. 13:896078. doi: 10.3389/fphar.2022.896078
Received: 14 March 2022; Accepted: 26 April 2022;
Published: 02 June 2022.
Edited by:
Michał Tomczyk, Medical University of Bialystok, PolandReviewed by:
Flavien Shimira, Çukurova University, TurkeyLyndy Joy McGaw, University of Pretoria, South Africa
Copyright © 2022 Panda, Gazim, Swain, Bento, Sena, Mukazayire, Van Puyvelde and Luyten. This is an open-access article distributed under the terms of the Creative Commons Attribution License (CC BY). The use, distribution or reproduction in other forums is permitted, provided the original author(s) and the copyright owner(s) are credited and that the original publication in this journal is cited, in accordance with accepted academic practice. No use, distribution or reproduction is permitted which does not comply with these terms.
*Correspondence: Sujogya Kumar Panda, c3Vqb2d5YXBhbmRhQHV0a2FsdW5pdmVyc2l0eS5hYy5pbg==
†These authors have contributed equally to this work