- 1Department of Food Science and Engineering, Ningbo University, Ningbo, China
- 2Department of Chemical Biology, Ernest Mario School of Pharmacy, Rutgers The State University of New Jersey, Piscataway, NJ, United States
Coronavirus disease 2019 (COVID-19) can disrupt the gut microbiota balance, and patients usually have intestinal disorders. The intestine is the largest immune organ of the human body, and gut microbes can affect the immune function of the lungs through the gut-lung axis. Many lines of evidence support the role of beneficial bacteria in enhancing human immunity, preventing pathogen colonization, and thereby reducing the incidence and severity of infection. In this article, we review the possible approach of modulating microbiota to help prevent and treat respiratory tract infections, including COVID-19, and discuss the possibility of using probiotics and prebiotics for this purpose. We also discuss the mechanism by which intestinal micro-flora regulate immunity and the effects of probiotics on the intestinal micro-ecological balance. Based on this understanding, we propose the use of probiotics and prebiotics to modulate gut microbiota for the prevention or alleviation of COVID-19 through the gut-lung axis.
Introduction
Coronavirus disease 2019 (COVID-19) continues to spread worldwide, seriously threatening human health and profoundly impacting the global economy. Through the tremendous efforts of scientists around the world, the vaccines are available now. But with the constant emergence of variants of SARS-CoV-2, there is still a long way to go for the prevention and control of COVID-19. Many patients with COVID-19 showed dysbiosis in the intestinal tract, with a decrease in beneficial bacteria such as Bifidobacteria, suggesting the need to evaluate the patients’ gastrointestinal function (Chen and Vitetta, 2021; Livanos et al., 2021). It is known that the dysbiosis of the human gut microbiota is associated with various health conditions, including respiratory tract infections (RTI) via the gut-lung axis (Li et al., 2020). Therefore, the use of nutritional support and probiotics are recommended in patients with COVID-19 to regulate the balance of intestinal microbiota and reduce the risk of secondary infection (Badi et al., 2021; Sajdel-Sulkowska, 2021).
The immune system depends on adequate diet and nutrition to prevent infection. For instance, adequate protein intake is essential for optimal antibody production (Verduci and Köglmeier, 2021). Insufficiencies of micronutrients such as vitamin D and vitamin C are associated with an increased risk of infection (Jabczyk et al., 2021). Many recent meta-analyses have found that there is a positive correlation between vitamin D deficiency and the severity of COVID-19, and vitamin D supplementation can be used to prevent or reduce the severity of the disease (Meltzer, et al., 2020; Hadizadeh, 2021; Ma, et al., 2021). Intestinal malnutrition affects the local mucosa and indirectly decreases the immune response on the surface of the lung mucosa, increasing susceptibility to systemic inflammation (Rytter et al., 2014). Therefore, improving the nutritional status of patients and enhancing the body’s immunity through modulating microbiota is of significance for the treatment of new coronavirus pneumonia. To date, several clinical trials have successfully managed COVID-19 with probiotics as adjunctive therapy (Baindara et al., 2021; Pawar et al., 2021; Wu et al., 2021). In this article, we discuss the possible use of probiotics and prebiotics in the prevention of viral infection. In addition, the mechanism of action of probiotics against COVID-19 is discussed from the perspective of the gut-lung axis.
Mechanism of Interaction of Gut-Lung Axis
Gut Microbiota
A person has about 104 microorganisms in the gut, including bacteria, fungi, and viruses (Barko et al., 2018). Intestinal micro-organisms play an important role in the digestion and absorption of nutrients and promote the establishment of the immune system (Kolodziejczyk et al., 2019). A host with no gut microbiome cannot develop a functioning immune system, and the intestinal flora plays an essential role in maintaining the health of the body (Lerner et al., 2017). The host depends on the intestinal microflora to produce different metabolites through anaerobic fermentation (Jang et al., 2020). The monolayer of epithelial cells in the mucosa allows the passage of microbial metabolites and this interaction between microbes and the host cells affects the immune response and disease development (Nasr et al., 2020).
The mammalian gastrointestinal tract is not only the largest food digestion, absorption and metabolism organ in the body, but also the largest immunity organ. There is a large and relatively stable microbial community in the mammalian intestine (Le et al., 2020). The intestinal microflora starts to establish in the early life and affect the physiological function of the host (Bernardeau et al., 2017). After the outbreak of COVID-19, it was observed that critical COVID-19 patients with intestinal flora disorders were more susceptible to secondary infection and death (Spagnolello et al., 2021). Whether manipulating intestinal microflora can prevent and alleviate COVID-19 is an important topic for investigation.
Lung Microbiota
The respiratory tract microbiota, similar to the intestinal microbes, mainly include Bacteroides, Firmicutes, and Proteobacteria, but their relative abundance is much lower, and the species diversity is also lower (Magryś, 2021). The microbial flora in the lungs migrated from the oral cavity, and spread along the mucosa most commonly by micro-aspiration of stomach contents (Ryck et al., 2014). The number and composition of the microbiota are affected by mucus-ciliary clearance or host defense mechanisms, temperature, oxygen tension, pH, nutrient availability, inflammatory cell activation, and bacterial competition (Yang et al., 2019). Airway microbiota were altered in patients with burns and inhalation injury, and the abundance of Streptococcaceae and Enterobacteriaceae increased by 30% in hypoxemia patients compared to patients with the partial pressure of arterial oxygen/fraction of inspired oxygen (PaO2/FiO2) ratio of more than 300; among the patients with PaO2/FiO2 ratio ≤300, Prevotella melaninogenica and Corynebacterium genus-level were significantly enriched (Walsh et al., 2017; Walton et al., 2021). Furthermore, PaO2/FiO2 < 274 mmHg is considered to be a reliable prognostic biomarker for COVID-19 patients (Sinatti et al., 2021). In a recent study in mice, it was found that after a respiratory infection, the relative abundance of Bacteroides increased and Firmicutes decreased (Groves et al., 2018). Changes in the lung microbiome may cause specific host immune responses and affect the prognosis of disease (Sommariva et al., 2020; Zhou et al., 2021).
The lung and airway microbiome directly affect immunity to disease and can change local immunity/inflammation during disease progression (Healy et al., 2021). RTI virus infection can directly cause immune damage to the respiratory tract and intestinal mucosa (Gautier et al., 2021). Lactobacillus rhamnosus GG (LGG) can maintain intestinal barrier homeostasis by enhancing intestinal mucin expression/barrier formation, reducing cell apoptosis and improving cell proliferation (Khailova et al., 2017). These may be the mechanisms for the protective effect of LGG on pneumonia in patients. Whether LGG has a protective effect on the intestines in COVID-19 patients remains to be determined.
The Gut-Lung Axis
In recent years, hypotheses, such as “gut-liver” axis, “gut-brain” axis, “gut-heart” axis and “gut-kidney” axis, have been proposed (Galdeano et al., 2019). The “gut-lung” axis has been proposed to explain the relationship between intestinal flora and lung diseases (Standen et al., 2015). The intestinal micro-ecology can affect not only intestinal immunity but also extraintestinal immunity (Taghinezhad-S et al., 2021).
T-helper (Th)17 cells provide protection in barrier tissues but may also contribute to immune pathology (Bacher et al., 2019). Intestinal segmented filamentous bacteria can stimulate the body to produce Th17 immune cells and reduce the infection rate and mortality of Streptococcus pneumonia (Tanabe, 2013; Gauguet et al., 2015). In patients with respiratory disease, increased Th17 cytokine levels correlate with exaggerated inflammation and lung damage (Iwanaga and Kolls, 2019). Mice inoculated with Lactobacillus johnsonii can significantly reduce the lungs Th2 type inflammatory response (Segal et al., 2016), and Bacteroides can increase the number of regulatory T (Treg) cells and reduce the occurrence of inflammation (Samuelson et al., 2015). In addition, it has been reported that immunity cells can migrate through the blood to the lungs and intestines, forming another mechanism for the connection between the lungs and the intestines (Reibman et al., 2008).
Microbial invasion is the most common cause of RTI (Dickson et al., 2014). The respiratory tract has the physiological function of exchanging gas with the outside world and is exposed to the external environment. When microorganisms break through the respiratory defense, it would cause host respiratory infection (Turck et al., 2019). RTI can cause changes in the intestinal flora and intestinal functions (He et al., 2017). The intestine can alleviate the symptoms of RTI via recovering the microbiota, increasing the production of short-chain fatty acids (SCFAs), and improving the immunity (Liu et al., 2018). In addition to the main symptoms of cough, fever, chest tightness and fatigue, patients with COVID-19 (especially severe patients) may also have gastrointestinal symptoms (diarrhea, nausea, vomiting, etc.) (Yeoh et al., 2021). Therefore, a health gut micro-ecology plays an important role for COVID-19 prevention.
The Relationship Between COVID-19 and Gut-Lung Axis
RTI is a disease with high morbidity and mortality (Wu et al., 2015). Studies have shown that lung damage during influenza virus infection is accompanied by gut damage, which is not directly caused by enteroviruses (Xu et al., 2021).
Changes of Intestinal Microflora After Respiratory Virus Infection
The lung is an organ constantly exposed to micro-organisms through inhalation or subclinical micro-aspiration (Santacroce et al., 2020). Influenza infection can affect the composition of the intestinal flora, and the dysbiosis of intestinal flora may reduce the host’s antiviral immune response, thereby aggravating the lung damage caused by the infection (Marsland et al., 2015). For example, changes in the number of actinomycetes due to gut environment may aggravate the damage in inflammatory bowel disease (Lin et al., 2017). The gut microbiota plays a vital role in the response of the lung to bacterial infections (Sundararaman et al., 2020). P. aeruginosa, K. pneumoniae, and S. pneumoniae are associated with increased morbidity and mortality during the acute pulmonary infection (Brown et al., 2017). Gut-lung interaction affects the role of bacteria in respiratory diseases, and the immune dialogue is a two-way process (Darbandi et al., 2021).
In a recent cross-sectional prospective study on 30 COVID-19 patients with 10 healthy volunteers as a control group, it was found that the increase of intestinal Proteobacteria and the decrease of Firmicute, and these changes may have triggered an abnormal mucosal immune response (Khan et al., 2021). The imbalance of the intestinal microbiota is an important predisposing factor of the severity of COVID-19 disease and the depletion of B. plebeians and F. prausnitzii in the guts further aggravates the situation by causing cytokine overproduction and dysregulated inflammation (Khan et al., 2021). In addition, three bacterial members from the Firmicutes phylum, the genus Coprobacillus, the species Clostridium ramosum and C. hathewayi, were positively associated with COVID-19 disease severity (Zuo et al., 2020). In many COVID-19 patients, the abundance of fecal Bacteroides dorei, Bacteroides thetaiotaomicron, Bacteroides massiliensis, and Bacteroides ovatus, were inversely correlated with SARS-CoV-2 load in fecal samples in many patients (Zuo et al., 2021). These studies illustrate the intestinal microflora changes can be caused by respiratory virus infection, and some intestinal microflora may affect SARS-CoV-2 infection (Goossens et al., 2020).
SARS-COV-2 virus enters the cell using angiotensin-converting enzyme 2 (ACE2) as a receptor, which is abundantly expressed on the cell surface of glandular epithelial cilia and gastric and intestinal epithelial cells (Liang et al., 2020; Shang et al., 2020). The high expression of ACE2 in the epithelial cells of the small intestine makes them highly susceptible to SARS-CoV-2 infection (Yeung et al., 2012). SARS-CoV-2 may disrupt the function of ACE2, leading to intestinal malabsorption, secretion imbalance, stimulation of the enteric nervous system, and diarrhea (Wong et al., 2020). Meanwhile, cytokines are exaggeratedly released, promoting the recruitment of other cells, and leading to a huge inflammatory response (Lee et al., 2020). ACE2 also modulates innate immunity and influences the composition of the gut microbiota (Hashimoto et al., 2012; Penninger et al., 2021). Viral RNA was detected in fecal samples from 50% of COVID-19 patients, reflecting gastrointestinal infection (Cheung et al., 2020). The different changes of gut-lung axis after SARS-CoV-2 infection are illustrated in Figure 1.
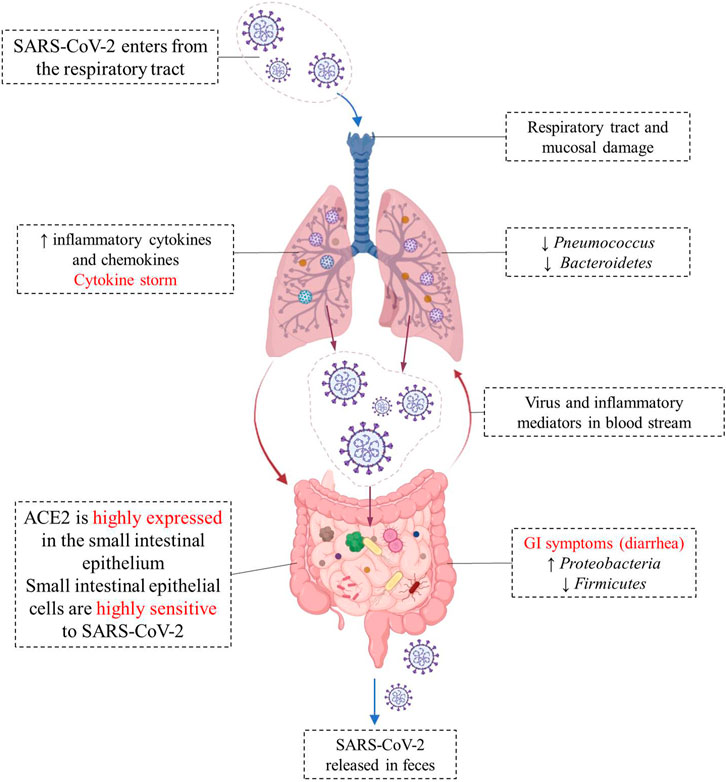
FIGURE 1. Different changes in the “gut-lung axis” after infection with COVID-19. Respiratory tract infection increases the production of cytokines and chemokines and causes changes in the microbial composition of microflora. Changes in the lung microbiome may cause specific host immune responses. The high expression of ACE2 in the small intestine makes small intestinal epithelial cells highly sensitive to SARS-CoV-2, and the viral infection leads to intestinal malabsorption, secretion imbalance, and diarrhea. SARS-CoV-2 RNA has been detected in fecal sample of COVID-19 patients.
The Role of Gut-Lung Axis Microbiota in Immune System Regulation
Changes in the intestinal microbiome often alter in the respiratory tract immune response and homeostasis (Selwal et al., 2021). Microbes can regulate the lungs’ immune response by producing bacterial ligands and metabolites and affect the final composition of the lung flora (Budden et al., 2017). SCFAs-producing gut microbiota can deliver SCFAs to the lungs, thereby facilitating the generation of signals that initiate lung immunity (Liu et al., 2021). Specific gut microbes (such as Staphylococcus, Streptococcus, Lactobacillus, and Bifidobacterium) can affect respiratory diseases such as asthma, chronic obstructive pulmonary disease, and influenza virus infection (Chung, 2017). For example, Lactobacillus paracasei consumption can allow an early activation of pro-inflammatory cytokines (IL-1α, IL-1β) and a massive recruitment of immune cells in the lungs, mice fed Lactobacillus paracasei showed reduced susceptibility to influenza infection (Belkacem et al., 2017). Critically ill patients with COVID-19 disease can cause overall changes in the intestinal mucus layer (decreased thickness, reduced lumen coverage, poor adhesion) and intestinal barrier function (El-Sayed et al., 2021). Under the immunosuppressive environment, activation of the Th1 and Th17 cell immune responses by muramyl dipeptide and tuftsin fusion protein (MT) could result in the better suppression of Treg cells among CD4+T cells, Th17 cells generated following the MT induction enhanced the specific immune response at the mucosal surface (Jiang et al., 2014). Interleukin (IL)-17 plays an important role in mediating host defense against viruses and chronic lung disease (Gurczynski and Moore, 2018). IL-17 can lead to a significant increase in neutrophil and promote pulmonary fibrosis (Gasse et al., 2011). γδ T cells are tissue-resident cells that produce IL-17, and the microbiota-derived metabolites (particularly propionate) that inhibit intestinal γδ T cell production of IL-17 and IL-22 (Dupraz et al., 2021). In COVID-19 patients, the increase of IL-17 was positively correlated with the increase of lung injury, which suggested that IL-17 could be used as a biomarker of disease severity (Pacha et al., 2020). Therefore, targeting IL-17 is an effective strategy to prevent COVID-19 and alleviate the damage of SARS-CoV-2 in immunology.
The gut has long been thought to be the driving force behind multiple organ dysfunction syndromes (Klingensmith and Coopersmith, 2016). The balance of gut microbes plays a crucial role in the body’s immune system, and if this balance is upset, the immune system may collapse or spin out of control (Thirumdas et al., 2021). Intestinal microbiota disorders are associated with RTI, and the health of the microbiota is related to the conditions of other organs or tissues, including the lungs (Tao et al., 2020). SCFAs produced by the intestinal microbial fiber fermentation, support immune function by serving as signaling molecules on resident antigen-presenting cells to attenuate the inflammatory response (Wang et al., 2019). Intestinal microbes also induce immune tolerance by inhibiting unnecessary inflammatory reaction mechanisms through host adaptive immune evolution (Simčič et al., 2019; Hamzelou, 2020). Recent investigations showed that gut-mucosal immunity and gut flora play a role in the pathogenesis of HIV infection. Probiotics have been shown as a novel strategy to attenuate or prevent gastrointestinal involvement and to improve gut-mucosal immunity in HIV-infected subjects (Ceccarelli et al., 2019). A stable and nutritious intestinal micro-environment is a key factor in maintaining a healthy gut-lung axis. However, the blind use of conventional probiotics for COVID-19 is not recommended until we have further understanding of the pathogenesis of SARS-CoV-2 and its effect on gut microbiota, as the rationale for using probiotics in COVID-19 is derived from indirect evidence (Mak et al., 2020).
Probiotics and COVID-19
The “gut-lung axis” concept can be explored for the prevention and treatment of COVID-19 with probiotics. Treatment of respiratory virus infection involves antagonism of respiratory viruses and regulation of cellular immunity and humoral immunity (Zelaya et al., 2020). In addition, probiotics may also be used to help regulate and maintain the balance of intestinal micro-ecology, thereby reducing the incidence of secondary bacterial infections.
Application of Probiotics for the Prevention and Treatment of Respiratory Diseases
Beneficial bacteria interact with the mucosal surface to stimulate the immune response and reject pathogens from the intestinal epithelium through competitive rejection (Littman and Pamer, 2011). In addition, after oral administration of probiotics, the probiotics are believed to affect the relationship between commensal microorganisms and mucosal immunity, changing the basic and induced inflammatory balance in response to viral infections (Hardy et al., 2013). In a recent clinical trial of 70 COVID-19 patients, 42 received standard medical treatment, and 28 received additional oral beneficial bacterial treatment (including Streptococcus thermophilus DSM 32345, Lactobacillus acidophilus DSM 32241, Lactobacillus brevis DSM 27961, and other bacteria). The results showed that the estimated risk of respiratory failure in patients receiving oral bacterial treatment was 8 times lower. Among patients who did not receive oral bacterial treatment, the prevalence and mortality of patients transferred to the intensive care unit were higher (d'Ettorre et al., 2020).
In some COVID-19 patients, the intestinal Bifidobacteria and Lactobacilli were significantly reduced, this is could be an indicator of their weak immunity (Walton et al., 2021). In a meta-analysis of 20 randomized controlled trials, the effects of probiotics (especially Lactobacillus and Bifidobacterium strains) on the duration of acute RTI in otherwise healthy children and adults were analyzed. These results showed that the consumption of probiotics significantly reduced the duration of RTI disease onset, the number of days each person was ill, and the number of days absent/work/school. Children treated with probiotics had a lower risk of requiring antibiotic prescriptions than children who were not treated (King et al., 2014). In addition, on a total of 58 patients hospitalized with COVID-19, 24 received oral probiotic therapy (including Bifidobacterium, Lactobacillus, and Streptococcus) during hospitalization, while 34 received only standard care (no supplementation with oral bacteria). The results showed that patients taking probiotics had increased serum arginine, asparagine, and lactate levels, which could prevent the development of chronic fatigue by better-utilizing glucose and energy pathways (Santinelli et al., 2022). As secondary infections may be a serious issue in COVID-19, in addition to anti-inflammatory regimens, treatment with probiotics may be an adjuvant or alternative modality. However, the specific types and dosage of probiotics needs to be studied in future clinical trials.
Probiotics Enhance Intestinal Immunity and Prevent Viral Immune Injury
Probiotics can be used to treat a variety of diseases, including viral infections (Kanauchi et al., 2018). The use of probiotics can also suppress inflammatory cytokines and help clear viral infections to minimize lung damage (Schiavi et al., 2016). Beneficial bacteria from probiotic supplements can stick to the surface of the epithelium, block the attachment of viruses by their spatial position, cover receptor sites in a nonspecific way, or compete for specific receptors (Etienne-Mesmin et al., 2019).
Bifidobacteria is mainly used to prevent and treat human intestinal diseases, because it can be colonized in the intestines (Salminen and Isolauri, 2006). Adhesion to the intestinal mucosa is the prerequisite for Bifidobacteria colonization and persistence in the gastrointestinal tract (Zhang et al., 2020). The attachment of Bifidobacteria, causes secretion of Bifidobacteria adhesin, which may bind to the receptor proteins of intestinal epithelial cells, and inhibit the colonization of pathogenic microorganisms (Westermann et al., 2016). A review of a total of 33 clinical trials related to viral respiratory infections found that beneficial bacteria from probiotic treatment also induced mucosal regeneration and intestinal mucins binding to viruses, lowered viral adhesion to epithelial cells and viral replication, and reduced the risk or duration of respiratory tract infection (Lehtoranta et al., 2014). In another study, among COVID-19 patients who received oral Bifidobacteria treatment, the interleukin-6 levels decreased and the mortality rate was decreased to 5% (from as 25%) (Bozkurt and Bilen, 2021). This approach should be further explored for treatment of hospitalized patients with moderate/severe COVID-19.
Peptides produced by some beneficial bacteria (such as Lactobacillus and Paenibacillus) have been shown to bind to ACE2 in vitro, and may block the binding of SARS-CoV-2 to target cells (Minato et al., 2020). Beneficial bacteria have also been shown to trigger antiviral immunity to promote the elimination of viruses (Din et al., 2021). Intranasal pre- and post-treatment of neonatal mice with LGG significantly improved mice survival after influenza virus infection (Kumova et al., 2019). LGG pretreatment was shown to improve immune gene transcriptional responses during early infection. Recent studies indicated that Lactobacillus gassier could be used as an auxiliary nutritional therapy in purine management, to reduce virus replication and help the treatment of COVID-19 (Morais et al., 2020). Figure 2 illustrates that beneficial bacteria originated from oral probiotics may block the binding of SARS-CoV-2 to the host cells, reduce virus replication, and increase the host’s immunity to the virus.
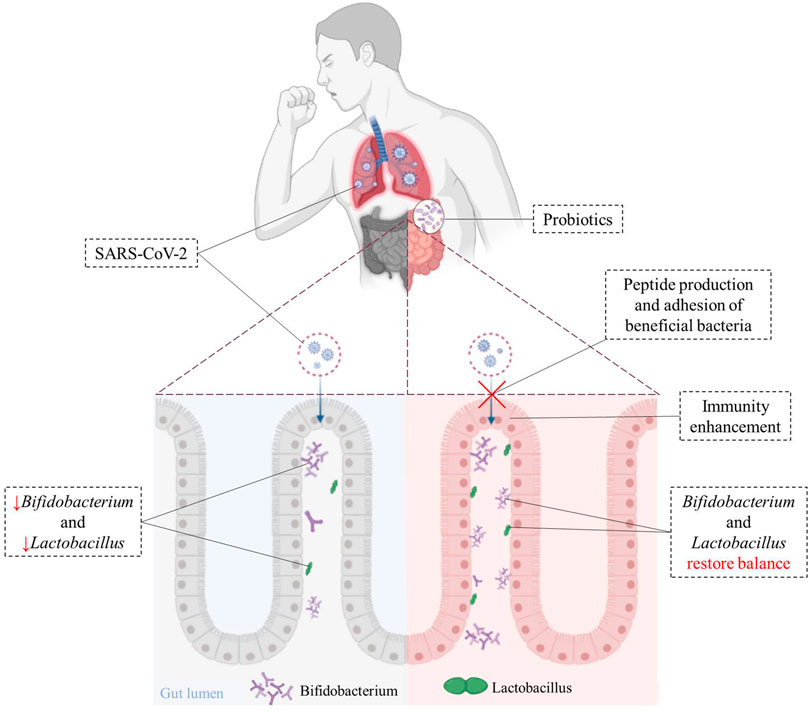
FIGURE 2. Immunoregulatory effects of probiotics on cells after SARS-CoV-2 infection. Beneficial bacteria from oral probiotics can block the binding of SARS-CoV-2 to the host cells, and helps improve gut dysbiosis caused by SARS-CoV-2 and hastens recovery in patients. The use of probiotics can suppress inflammatory-cytokines and help clear viral infections.
Overall, probiotics can improve the immune response to infections and this activity may also apply to SARS-CoV-2 infection. The effects of probiotics have a high degree of strain specificity. The transfer of sufficient numbers of bacteria to the effect site in the intestine is crucial for the successful treatment of COVID-19 patients. More laboratory and clinical studies are needed in this area.
Proper Use of Probiotics
Although probiotics are known to be safety, they should be used with caution in patients with severely impaired immune function and premature infants (Sabina, 2014). LGG has been shown to cause bacteremia in some immunocompromised patients (Gupta and Garg, 2009). The dose-effect of Lactobacillus Acidophilus NCFM strain on the T cell immune response of rotavirus vaccination in a sterile pig model showed that probiotics may be ineffective or even harmful if not used in the proper dose (Wen et al., 2012). For patients critically ill, at risk of fungal infection or with central venous catheters the use of Saccharomyces boulardii should be avoided (Boyanova and Mitov, 2012). It has also been reported that LGG causes sepsis in cardiac surgery patients (Kochan et al., 2011).
The long-term effects of probiotic use are unknown and need to be studied in randomized controlled trials. Individualized and specific probiotic administration methods may be necessary, because empirical supplementation of probiotics may not produce uniform and lasting effects on the mucosa (Celiberto et al., 2018). Probiotic dietary supplements are usually made by preservation of bacterial strains, mainly through lyophilization or microencapsulation, and marketed in the form of tablets, capsules and freeze-dried formulations (Salehi et al., 2020). SARS-CoV-2 infected patients may present lesions in the lungs compromising their gas exchange capability. In vitro data suggested that the probiotic formulation SLAB51 can reduce nitric oxide synthesis in intestinal cells (Ceccarelli et al., 2021). Studies have shown that 108–109 CFU/g is an ideal probiotic dose for health benefits (Gurram et al., 2021). Certain strains of lactic acid bacteria modulate both innate and acquired immunity when administered at an adjusted dose of 109 colony forming units (CFU)/day (Roobab et al., 2020). A study has proposed that the number of probiotics required must be consumed in a sufficient amount (>7 log CFU) to protect and treat respiratory infections, including COVID-19 (Olaimat et al., 2020). For patients with COVID-19, probiotics can be used to maintain the intestinal microecological balance and prevent secondary bacterial infections (Oliveira et al., 2021). More research is required into these types of bacteria to determine their effectiveness as probiotic formulations.
Probiotics can alter the composition of intestinal flora, improve the immune function, and subside intestinal inflammatory response (Plaza-Diaz et al., 2019). To date, no published studies have reported the use of probiotics as an add-on therapy for the treatment of COVID-19. Scientists and clinicians around the world are also studying the relationship between the gut microbiome and susceptibility to COVID-19 and evaluating the effects of various probiotic strains in reducing viral load through different mechanisms. The application of oral probiotics in the clinical treatment of COVID-19 still needs to be studied further in large-scale randomized clinical trials. In addition to clinical data, eating patterns, genetic associations, and environmental exposure should also be considered.
Potential Application of Prebiotics for the Prevention of COVID-19
The definition of prebiotics has been revised several times. The International Scientific Association for Probiotics and Prebiotics (ISAPP) proposed in 2017 that the definition be revised to “a substrate that is selectively utilized by host microorganisms conferring a health benefit” (Gibson, et al., 2017). Prebiotics can alter the composition of the intestinal flora, and provide energy for the growth of beneficial bacteria (Calatayud et al., 2021). In addition, prebiotics can improve the function of the intestinal barrier and stimulate the production of metabolites beneficial to the host (Davani-Davari et al., 2019). Foods containing prebiotics, such as fiber, oligosaccharides, and polyphenols, can enhance the growth of beneficial bacteria (Parnell and Reimer, 2012; Lordan et al., 2020; Wiciński et al., 2020). For example, inulin-enriched diet stimulated the growth of Bifidobacterium and Bacteroides (Trompette et al., 2018); and whole grain cereals can modify bacterial profiles increasing relative quantities of Bifidobacterium and Lactobacilli (Tuohy et al., 2012). In principle, prebiotics should reduce the risk of a person to contract COVID-19 or alleviate the syndromes (Gasmi et al., 2020; Batista et al., 2021). However, more laboratory and human studies are needed to test this hypothesis.
Fermentable dietary fiber can promote the production of SCFAs (Koh et al., 2016), which enhance intestinal barrier function (Makki et al., 2018). Improving gut barrier function reduces the entry of harmful microorganisms and their metabolites into the host circulatory system (Knudsen et al., 2018; Loo et al., 2020). Fermentable dietary fiber has been shown to alter the number of gut bacteria in rodents and the concentration of SCFAs in their fecal (Cui et al., 2019). Dietary fermentable fiber and SCFAs could balance innate and adaptive immunity, thereby promoting resolution of influenza virus infection (Trompette et al., 2018). Mice fed a high-fiber diet and butyrate exhibited reduced neutrophil infiltration, enhanced T cell bioenergetics, relieved lung inflammation, and facilitated influenza virus clearance (Visan, 2018).
Oligosaccharides can fight against pathogenic viruses by the direct interaction with the virus (negative charge on the surface of viruses), inhibiting the invasion and adsorption of viruses, and also hindering the obstruction of the viral transcription and replication via direct interference (Iravani and Varma, 2021). A recent study investigated the antiviral effects of resveratrol oligosaccharides for SARS-CoV-2. Human MRC5 lung cells infected with SARS-CoV-2 were incubated with various concentrations of resveratrol oligosaccharides. This result indicated that resveratrol oligosaccharides at 5 and 10% effectively decreased the infection of the MRC5 cells by SARS-CoV-2 (Hamada et al., 2021). Dietary intervention with fructans and galactooligosaccharide (GOS), leads to a higher abundance of Bifidobacterium and Lactobacillus (So et al., 2018). GOS cannot be digested by humans but can be fermented by commensal bacteria in the intestines (Wilson and Whelan, 2017). GOS has shown to be a substrate of commensal bacteria Lactobacilli and increase the abundance of Lactobacilli and Bifidobacteria (Gonai et al., 2017). In a human study, three dose levels of fructo-oligosaccharides (FOS) (2.5, 5, and 10 g/day) or placebo (maltodextrin 10 g/day) were given to 80 participants. The results showed that the consumption of FOS increased the relative abundance of Bifidobacteria and Lactobacilli (Tandon et al., 2019). After 8 weeks of prebiotic (oligofructose) treatment to mice under physiological stress (high-fat diet), the modulation of the taxa and functional gut microbiota was found to be associated with the beneficial effects on obesity and related metabolic disorders such as a decrease in inflammation, a decrease in plasma leptin levels and an improvement of glucose homeostasis (Everard et al., 2014). In a recent randomized controlled trial on overweight or obese children, compared to children given placebo, those received prebiotic oligofructose-enriched inulin lost 3.1% of weight. The species of Bifidobacterium increased significantly, while the number of Bacteroides vulgaris decreased significantly (Nicolucci et al., 2017).
Polyphenols, found in many foods and beverages are known to affect gut microbiota in favor of the growth of beneficial bacteria (Jayabalan et al., 2014; Singh et al., 2019; Ma and Chen, 2020; Plamada and Vodnar, 2021). Many studies have shown tea polyphenols can improve the intestinal microecology by regulating the diversity and quantity of intestinal flora (Chen and Yang, 2019; Yan et al., 2020; Sun et al., 2021). Herein, some of these studies are used as examples to illustrate the prebiotic activities of polyphenols. (-)-Epigallocatechin gallate (EGCG), the most abundant and biologically active catechin in green tea, has been shown to significantly increase the production of SCFAs and the population of the Bifidobacterium (Zhang et al., 2013). Oral administration of green tea extract (equivalent to EGCG doses of 300–400 mg/kg) in drinking water to mice, almost completely prevented interstitial and peribronchial fibrosis (Donà et al., 2003). EGCG has shown anti-viral activities including the inhibition of SARS-CoV-2 infection (Ohgitani et al., 2021; Tallei et al., 2021). For example, following the infection of H9N2 swine influenza virus in mice, daily administration of EGCG (10 mg/kg, i. g.) for 5 days significantly prolongs mouse survival and reduces the death rate from 65 to 35% (Xu et al., 2017). With demonstrated activities in alleviating pulmonary fibrosis in rodent models and humans, EGCG and green tea extract may be useful for the prevention and treatment of pulmonary fibrosis in COVID-19 patients (Zhang et al., 2021).
Many epidemiological studies have shown that tea consumption is associated with lower incidence of obesity, metabolic syndrome, and related diseases (Yang and Zhang, 2019). Similar beneficial activity has also been shown for dietary fiber and oligosaccharides (Shoaib et al., 2016; Holscher, 2017; Myhrstad et al., 2020). High body mass index has been recognized as an important risk factor for COVID-19 developing into a critical state, especially abdominal obesity (Földi et al., 2021), and obesity is associated with an increase in Th17 and IL-17 (Tarantino et al., 2014). Therefore, weight loss and improved metabolic health may help better cope with COVID-19, whether regular drinking tea (and the required amount) can reduce the risk of COVID-19 infection and related syndromes needs to be further investigated.
Conclusion
In this article, we illustrate the importance of gut microbiota in the body’s immune system and anti-viral functions. The gut microbiome also affects lung health through the gut-lung axis. Viral infection, such as SARS-CoV-2 infections in the lung, can also cause intestinal microbiota dysbiosis. Therefore, maintaining a healthy intestinal microbiota with a healthy diet is important. The use of probiotics and prebiotics to enhance the development of healthy microbiota is a promising approach to prevent and alleviate viral infection. This includes the prevention and treatment of COVID-19, to alleviate associated symptoms and prevent secondary infections. Some published successful examples and mechanisms of action in this area are discussed; however, more studies are needed to substantiate this approach. It is also important to determine the specific probiotics or prebiotics and the optimal dosage to be used for the purpose of prevention and for adjuvant therapy in COVID-19 patients. In a practical sense, studies on the effects of probiotics and prebiotics on common influenza virus caused respiratory diseases are easier and may have a broader application. Such studies may also help us to deal with COVID-19. During SARS-CoV-2 infection in the respiratory tract and gastrointestinal mucosa, many disorders occur. Detailed multi-omics studies may be needed to analyze the changes in the gut and lung during and after the infection.
Author Contributions
LX: conceptualization, validation, and writing–original draft. CY: supervision. YL: validation and writing–original draft. XZ: supervision, writing–review, and editing. All authors contributed to the article and approved the submitted version.
Funding
This work was sponsored by Zhejiang Provincial Key Research and Development Program (2020C02037) and the Ningbo Natural Science Foundation (2021J107).
Conflict of Interest
The authors declare that the research was conducted in the absence of any commercial or financial relationships that could be construed as a potential conflict of interest.
Publisher’s Note
All claims expressed in this article are solely those of the authors and do not necessarily represent those of their affiliated organizations, or those of the publisher, the editors and the reviewers. Any product that may be evaluated in this article, or claim that may be made by its manufacturer, is not guaranteed or endorsed by the publisher.
References
Ahmadi Badi, S., Tarashi, S., Fateh, A., Rohani, P., Masotti, A., and Siadat, S. D. (2021). From the Role of Microbiota in Gut-Lung Axis to SARS-CoV-2 Pathogenesis. Mediators Inflamm. 2021, 1–12. doi:10.1155/2021/6611222
Bacher, P., Hohnstein, T., Beerbaum, E., Röcker, M., Blango, M. G., Kaufmann, S., et al. (2019). Human Anti-fungal Th17 Immunity and Pathology Rely on Cross-Reactivity against Candida Albicans. Cell 176 (6), 1340–e15. doi:10.1016/j.cell.2019.01.041
Baindara, P., Chakraborty, R., Holliday, Z. M., Mandal, S. M., and Schrum, A. G. (2021). Oral Probiotics in Coronavirus Disease 2019: Connecting the Gut-Lung Axis to Viral Pathogenesis, Inflammation, Secondary Infection and Clinical Trials. New Microbes New Infect. 40, 100837. doi:10.1016/j.nmni.2021.100837
Barko, P. C., Mcmichael, M. A., Swanson, K. S., and Williams, D. A. (2018). The Gastrointestinal Microbiome: A Review. J. Vet. Intern. Med. 32 (1), 9–25. doi:10.1111/jvim.14875
Batista, K. S., de Albuquerque, J. G., Vasconcelos, M. H. A., Bezerra, M. L. R., da Silva Barbalho, M. B., Pinheiro, R. O., et al. (2021). Probiotics and Prebiotics: Potential Prevention and Therapeutic Target for Nutritional Management of COVID-19? Nutr. Res. Rev., 1–18. doi:10.1017/S0954422421000317
Belkacem, N., Serafini, N., Wheeler, R., Derrien, M., Boucinha, L., Couesnon, A., et al. (2017). Lactobacillus Paracasei Feeding Improves Immune Control of Influenza Infection in Mice. PLoS One 12 (9), e0184976. doi:10.1371/journal.pone.0184976
Bernardeau, M., Lehtinen, M. J., Forssten, S. D., and Nurminen, P. (2017). Importance of the Gastrointestinal Life Cycle of Bacillus for Probiotic Functionality. J. Food Sci. Technol. 54 (8), 2570–2584. doi:10.1007/s13197-017-2688-3
Boyanova, L., and Mitov, I. (2012). Coadministration of Probiotics with Antibiotics: Why, when and for How Long? Expert Rev. Anti Infect. Ther. 10 (4), 407–409. doi:10.1586/eri.12.24
Bozkurt, H. S., and Bilen, Ö. (2021). Oral Booster Probiotic Bifidobacteria in SARS-COV-2 Patients. Int. J. Immunopathol. Pharmacol. 35, 20587384211059677–20587384211059678. doi:10.1177/20587384211059677
Brown, R. L., Sequeira, R. P., and Clarke, T. B. (2017). The Microbiota Protects against Respiratory Infection via GM-CSF Signaling. Nat. Commun. 8 (1), 1512. doi:10.1038/s41467-017-01803-x
Budden, K. F., Gellatly, S. L., Wood, D. L., Cooper, M. A., Morrison, M., Hugenholtz, P., et al. (2017). Emerging Pathogenic Links between Microbiota and the Gut-Lung Axis. Nat. Rev. Microbiol. 15 (1), 55–63. doi:10.1038/nrmicro.2016.142
Calatayud, M., Van den Abbeele, P., Ghyselinck, J., Marzorati, M., Rohs, E., and Birkett, A. (2021). Comparative Effect of 22 Dietary Sources of Fiber on Gut Microbiota of Healthy Humans In Vitro. Front. Nutr. 8, 700571. doi:10.3389/fnut.2021.700571
Ceccarelli, G., Marazzato, M., Celani, L., Lombardi, F., Piccirilli, A., Mancone, M., et al. (2021). Oxygen Sparing Effect of Bacteriotherapy in COVID-19. Nutrients 13 (8), 2898. doi:10.3390/nu13082898
Ceccarelli, G., Statzu, M., Santinelli, L., Pinacchio, C., Bitossi, C., Cavallari, E. N., et al. (2019). Challenges in the Management of HIV Infection: Update on the Role of Probiotic Supplementation as a Possible Complementary Therapeutic Strategy for cART Treated People Living with HIV/AIDS. Expert Opin. Biol. Ther. 19 (9), 949–965. doi:10.1080/14712598.2019.1638907
Celiberto, L. S., Pinto, R. A., Rossi, E. A., Vallance, B. A., and Cavallini, D. C. U. (2018). Isolation and Characterization of Potentially Probiotic Bacterial Strains from Mice: Proof of Concept for Personalized Probiotics. Nutrients 10 (11), 1684. doi:10.3390/nu10111684
Chen, J., and Vitetta, L. (2021). Modulation of Gut Microbiota for the Prevention and Treatment of COVID-19. Jcm 10 (13), 2903. doi:10.3390/jcm10132903
Chen, T., and Yang, C. S. (2019). Biological Fates of Tea Polyphenols and Their Interactions with Microbiota in the Gastrointestinal Tract: Implications on Health Effects. Crit. Rev. Food Sci. Nutr. 60 (16), 2691–2709. doi:10.1080/10408398.2019.1654430
Cheung, K. S., Hung, I. F. N., Chan, P. P. Y., Lung, K. C., Tso, E., Liu, R., et al. (2020). Gastrointestinal Manifestations of SARS-CoV-2 Infection and Virus Load in Fecal Samples from a Hong Kong Cohort: Systematic Review and Meta-Analysis. Gastroenterology 159 (1), 81–95. doi:10.1053/j.gastro.2020.03.065
Chung, K. F. (2017). Airway Microbial Dysbiosis in Asthmatic Patients: A Target for Prevention and Treatment? J. Allergy Clin. Immunol. 139 (4), 1071–1081. doi:10.1016/j.jaci.2017.02.004
Chunxi, L., Haiyue, L., Yanxia, L., Jianbing, P., and Jin, S. (2020). The Gut Microbiota and Respiratory Diseases: New Evidence. J. Immunol. Res. 2020, 1–12. doi:10.1155/2020/2340670
Cui, J., Lian, Y., Zhao, C., Du, H., Han, Y., Gao, W., et al. (2019). Dietary Fibers from Fruits and Vegetables and Their Health Benefits via Modulation of Gut Microbiota. Compr. Rev. Food Sci. Food Saf. 18 (5), 1514–1532. doi:10.1111/1541-4337.12489
Darbandi, A., Asadi, A., Ghanavati, R., Afifirad, R., Emamie, A. D., Kakanj, M., et al. (2021). Corrigendum to 'The Effect of Probiotics on Respiratory Tract Infection with Special Emphasis on COVID-19: Systemic Review 2010-20' [International Journal of Infectious Diseases 105 (April 2021) 91-104]. Int. J. Infect. Dis. 110, 337. doi:10.1016/j.ijid.2021.08.002
Davani-Davari, D., Negahdaripour, M., Karimzadeh, I., Seifan, M., Mohkam, M., Masoumi, S. J., et al. (2019). Prebiotics: Definition, Types, Sources, Mechanisms, and Clinical Applications. Foods 8 (3), 92. doi:10.1016/j.ijid.2021.08.00210.3390/foods8030092
de Oliveira, G. L. V., Oliveira, C. N. S., Pinzan, C. F., de Salis, L. V. V., and Cardoso, C. R. B. (2021). Microbiota Modulation of the Gut-Lung Axis in COVID-19. Front. Immunol. 12, 635471. doi:10.3389/fimmu.2021.635471
Dickson, R. P., Martinez, F. J., and Huffnagle, G. B. (2014). The Role of the Microbiome in Exacerbations of Chronic Lung Diseases. Lancet 384 (9944), 691–702. doi:10.1016/S0140-6736(14)61136-3
Din, A. U., Mazhar, M., Waseem, M., Ahmad, W., Bibi, A., Hassan, A., et al. (2021). SARS-CoV-2 Microbiome Dysbiosis Linked Disorders and Possible Probiotics Role. Biomed. Pharmacother. 133, 110947. doi:10.1016/j.biopha.2020.110947
Donà, M., Dell'Aica, I., Calabrese, F., Benelli, R., Morini, M., Albini, A., et al. (2003). Neutrophil Restraint by Green Tea: Inhibition of Inflammation, Associated Angiogenesis, and Pulmonary Fibrosis. J. Immunol. 170 (8), 4335–4341. doi:10.4049/jimmunol.170.8.4335
Dupraz, L., Magniez, A., Rolhion, N., Richard, M. L., Da Costa, G., Touch, S., et al. (2021). Gut Microbiota-Derived Short-Chain Fatty Acids Regulate IL-17 Production by Mouse and Human Intestinal γδ T Cells. Cell Rep 36 (1), 109332. doi:10.1016/j.celrep.2021.109332
El-Sayed, A., Aleya, L., and Kamel, M. (2021). Microbiota's Role in Health and Diseases. Environ. Sci. Pollut. Res. 28 (28), 36967–36983. doi:10.1007/s11356-021-14593-z
Etienne-Mesmin, L., Chassaing, B., Desvaux, M., De Paepe, K., Gresse, R., Sauvaitre, T., et al. (2019). Experimental Models to Study Intestinal Microbes-Mucus Interactions in Health and Disease. FEMS Microbiol. Rev. 43 (5), 457–489. doi:10.1093/femsre/fuz013
Ettorre, G., Ceccarelli, G., Marazzato, M., Campagna, G., Pinacchio, C., Alessandri, F., et al. (2020). Challenges in the Management of SARS-CoV2 Infection: The Role of Oral Bacteriotherapy as Complementary Therapeutic Strategy to Avoid the Progression of COVID-19. Front. Med. (Lausanne) 7, 389. doi:10.3389/fmed.2020.00389
Everard, A., Lazarevic, V., Gaïa, N., Johansson, M., Ståhlman, M., Backhed, F., et al. (2014). Microbiome of Prebiotic-Treated Mice Reveals Novel Targets Involved in Host Response during Obesity. ISME J. 8 (10), 2116–2130. doi:10.1038/ismej.2014.45
Fijan, S. (2014). Microorganisms with Claimed Probiotic Properties: An Overview of Recent Literature. Ijerph 11 (5), 4745–4767. doi:10.3390/ijerph110504745
Földi, M., Farkas, N., Kiss, S., Dembrovszky, F., Szakács, Z., Balaskó, M., et al. (2021). Visceral Adiposity Elevates the Risk of Critical Condition in COVID-19: A Systematic Review and Meta-Analysis. Obesity (Silver Spring) 29 (3), 521–528. doi:10.1002/oby.23096
Gasmi, A., Noor, S., Tippairote, T., Dadar, M., Menzel, A., and Bjørklund, G. (2020). Individual Risk Management Strategy and Potential Therapeutic Options for the COVID-19 Pandemic. Clin. Immunol. 215, 108409. doi:10.1016/j.clim.2020.108409
Gasse, P., Riteau, N., Vacher, R., Michel, M. L., Fautrel, A., di Padova, F., et al. (2011). IL-1 and IL-23 Mediate Early IL-17A Production in Pulmonary Inflammation Leading to Late Fibrosis. PloS one 6 (8), e23185. doi:10.1371/journal.pone.0023185
Gauguet, S., D'Ortona, S., Ahnger-Pier, K., Duan, B., Surana, N. K., Lu, R., et al. (2015). Intestinal Microbiota of Mice Influences Resistance to Staphylococcus aureus Pneumonia. Infect. Immun. 83 (10), 4003–4014. doi:10.1128/IAI.00037-15
Gautier, T., David-Le Gall, S., Sweidan, A., Tamanai-Shacoori, Z., Jolivet-Gougeon, A., Loréal, O., et al. (2021). Next-Generation Probiotics and Their Metabolites in COVID-19. Microorganisms 9 (5), 941. doi:10.3390/microorganisms9050941
Gibson, G. R., Hutkins, R., Sanders, M. E., Prescott, S. L., Reimer, R. A., Salminen, S. J., et al. (2017). Expert Consensus Document: The International Scientific Association for Probiotics and Prebiotics (ISAPP) Consensus Statement on the Definition and Scope of Prebiotics. Nat. Rev. Gastroenterol. Hepatol. 14 (8), 491–502. doi:10.1038/nrgastro.2017.75
Gonai, M., Shigehisa, A., Kigawa, I., Kurasaki, K., Chonan, O., Matsuki, T., et al. (2017). Galacto-Oligosaccharides Ameliorate Dysbiotic Bifidobacteriaceae Decline in Japanese Patients with Type 2 Diabetes. Benef. Microbes 8 (5), 705–716. doi:10.3920/BM2016.0230
Goossens, G. H., Dicker, D., Farpour-Lambert, N. J., Frühbeck, G., Mullerova, D., Woodward, E., et al. (2020). Obesity and COVID-19: A Perspective from the European Association for the Study of Obesity on Immunological Perturbations, Therapeutic Challenges, and Opportunities in Obesity. Obes. Facts 13 (4), 439–452. doi:10.1159/000510719
Groves, H. T., Cuthbertson, L., James, P., Moffatt, M. F., Cox, M. J., and Tregoning, J. S. (2018). Respiratory Disease Following Viral Lung Infection Alters the Murine Gut Microbiota. Front. Immunol. 9 (9), 182. doi:10.3389/fimmu.2018.00182
Gupta, V., and Garg, R. (2009). Probiotics. Indian J. Med. Microbiol. 27 (3), 202–209. doi:10.4103/0255-0857.53201
Gurczynski, S. J., and Moore, B. B. (2018). IL-17 in the Lung: The Good, the Bad, and the Ugly. Am. J. Physiol. Lung Cel. Mol. Physiol. 314 (1), L6–L16. doi:10.1152/ajplung.00344.2017
Gurram, S., Jha, D. K., Shah, D. S., Kshirsagar, M. M., and Amin, P. D. (2021). Insights on the Critical Parameters Affecting the Probiotic Viability during Stabilization Process and Formulation Development. AAPS PharmSciTech 22 (5), 156. doi:10.1208/s12249-021-02024-8
Hadizadeh, F. (2021). Supplementation with Vitamin D in the COVID-19 Pandemic? Nutr. Rev. 79 (2), 200–208. doi:10.1093/nutrit/nuaa081
Hamada, H., Hamada, H., Shimoda, K., Kuboki, A., Iwaki, T., Kiriake, Y., et al. (2021). Resveratrol Oligosaccharides (Gluco-Oligosaccharides) Effectively Inhibit SARS-CoV-2 Infection: Glycoside (Polysaccharide) Approach for Treatment of COVID-19. Nat. Product. Commun. 16 (5), 1934578. doi:10.1177/1934578X211012903
Hamzelou, J. (2020). Does A Cell Protein Explain COVID-19 Severity? New Sci. 246 (3277), 9. doi:10.1016/S0262-4079(20)30705-3
Hardy, H., Harris, J., Lyon, E., Beal, J., and Foey, A. D. (2013). Probiotics, Prebiotics and Immunomodulation of Gut Mucosal Defences: Homeostasis and Immunopathology. Nutrients 5 (6), 1869–1912. doi:10.3390/nu5061869
Hashimoto, T., Perlot, T., Rehman, A., Trichereau, J., Ishiguro, H., Paolino, M., et al. (2012). ACE2 Links Amino Acid Malnutrition to Microbial Ecology and Intestinal Inflammation. Nature 487 (7408), 477–481. doi:10.1038/nature11228
He, Y., Wen, Q., Yao, F., Xu, D., Huang, Y., and Wang, J. (2017). Gut-Lung Axis: The Microbial Contributions and Clinical Implications. Crit. Rev. Microbiol. 43 (1), 81–95. doi:10.1080/1040841X.2016.1176988
Healy, C., Munoz-Wolf, N., Strydom, J., Faherty, L., Williams, N. C., Kenny, S., et al. (2021). Nutritional Immunity: The Impact of Metals on Lung Immune Cells and the Airway Microbiome during Chronic Respiratory Disease. Respir. Res. 22 (1), 133. doi:10.1186/s12931-021-01722-y
Holscher, H. D. (2017). Dietary Fiber and Prebiotics and the Gastrointestinal Microbiota. Gut Microbes 8 (2), 172–184. doi:10.1080/19490976.2017.1290756
Iravani, S., and Varma, R. S. (2021). Important Roles of Oligo- and Polysaccharides against SARS-Cov-2: Recent Advances. Appl. Sci. 11 (8), 3512. doi:10.3390/app11083512
Iwanaga, N., and Kolls, J. K. (2019). Updates on T Helper Type 17 Immunity in Respiratory Disease. Immunology 156 (1), 3–8. doi:10.1111/imm.13006
Jabczyk, M., Nowak, J., Hudzik, B., and Zubelewicz-Szkodzińska, B. (2021). Diet, Probiotics and Their Impact on the Gut Microbiota during the COVID-19 Pandemic. Nutrients 13 (9), 3172. doi:10.3390/nu13093172
Jang, E. Y., Hong, K. B., Chang, Y. B., Shin, J., Jung, E. Y., Jo, K., et al. (2020). In Vitro Prebiotic Effects of Malto-Oligosaccharides Containing Water-Soluble Dietary Fiber. Molecules 25 (21), 5201. doi:10.3390/molecules25215201
Jayabalan, R., Malbaša, R. V., Lončar, E. S., Vitas, J. S., and Sathishkumar, M. (2014). A Review on Kombucha Tea-Microbiology, Composition, Fermentation, Beneficial Effects, Toxicity, and Tea Fungus. Compr. Rev. Food Sci. Food Saf. 13 (4), 538–550. doi:10.1111/1541-4337.12073
Jiang, X., Yu, M., Qiao, X., Liu, M., Tang, L., Jiang, Y., et al. (2014). Up-Regulation of MDP and Tuftsin Gene Expression in Th1 and Th17 Cells as an Adjuvant for an Oral Lactobacillus Casei Vaccine against Anti-transmissible Gastroenteritis Virus. Appl. Microbiol. Biotechnol. 98 (19), 8301–8312. doi:10.1007/s00253-014-5893-2
Kanauchi, O., Andoh, A., AbuBakar, S., and Yamamoto, N. (2018). Probiotics and Paraprobiotics in Viral Infection: Clinical Application and Effects on the Innate and Acquired Immune Systems. Curr. Pharm. Des. 24 (6), 710–717. doi:10.2174/1381612824666180116163411
Khailova, L., Baird, C. H., Rush, A. A., Barnes, C., and Wischmeyer, P. E. (2017). Lactobacillus Rhamnosus GG Treatment Improves Intestinal Permeability and Modulates Inflammatory Response and Homeostasis of Spleen and Colon in Experimental Model of Pseudomonas aeruginosa Pneumonia. Clin. Nutr. 36 (6), 1549–1557. doi:10.1016/j.clnu.2016.09.025
Khan, M., Mathew, B. J., Gupta, P., Garg, G., Khadanga, S., Vyas, A. K., et al. (2021). Gut Dysbiosis and IL-21 Response in Patients with Severe COVID-19. Microorganisms 9 (6), 1292. doi:10.3390/microorganisms9061292
King, S., Glanville, J., Sanders, M. E., Fitzgerald, A., and Varley, D. (2014). Effectiveness of Probiotics on the Duration of Illness in Healthy Children and Adults Who Develop Common Acute Respiratory Infectious Conditions: A Systematic Review and Meta-Analysis. Br. J. Nutr. 112 (1), 41–54. doi:10.1017/S0007114514000075
Klingensmith, N. J., and Coopersmith, C. M. (2016). The Gut as the Motor of Multiple Organ Dysfunction in Critical Illness. Crit. Care Clin. 32 (2), 203–212. doi:10.1016/j.ccc.2015.11.004
Kochan, P., Chmielarczyk, A., Szymaniak, L., Brykczynski, M., Galant, K., Zych, A., et al. (2011). Lactobacillus Rhamnosus Administration Causes Sepsis in A Cardiosurgical Patient--Is the Time Right to Revise Probiotic Safety Guidelines? Clin. Microbiol. Infect. 17 (10), 1589–1592. doi:10.1111/j.1469-0691.2011.03614.x
Koh, A., De Vadder, F., Kovatcheva-Datchary, P., and Bäckhed, F. (2016). From Dietary Fiber to Host Physiology: Short-Chain Fatty Acids as Key Bacterial Metabolites. Cell 165 (6), 1332–1345. doi:10.1016/j.cell.2016.05.041
Kolodziejczyk, A. A., Zheng, D., and Elinav, E. (2019). Diet-Microbiota Interactions and Personalized Nutrition. Nat. Rev. Microbiol. 17 (12), 742–753. doi:10.1038/s41579-019-0256-8
Kumova, O. K., Fike, A. J., Thayer, J. L., Nguyen, L. T., Mell, J. C., Pascasio, J., et al. (2019). Lung Transcriptional Unresponsiveness and Loss of Early Influenza Virus Control in Infected Neonates Is Prevented by Intranasal Lactobacillus Rhamnosus GG. Lactobacillus Rhamnosus Ggplos Pathog. 15 (10), e1008072. doi:10.1371/journal.ppat.1008072
Le, V., Quinn, T. P., Tran, T., and Venkatesh, S. (2020). Deep in the Bowel: Highly Interpretable Neural Encoder-Decoder Networks Predict Gut Metabolites from Gut Microbiome. BMC Genomics 21 (4), 256. doi:10.1186/s12864-020-6652-7
Lee, S., Channappanavar, R., and Kanneganti, T. D. (2020). Coronaviruses: Innate Immunity, Inflammasome Activation, Inflammatory Cell Death, and Cytokines. Trends Immunol. 41 (12), 1083–1099. doi:10.1016/j.it.2020.10.005
Lehtoranta, L., Pitkäranta, A., and Korpela, R. (2014). Probiotics in Respiratory Virus Infections. Eur. J. Clin. Microbiol. Infect. Dis. 33 (8), 1289–1302. doi:10.1007/s10096-014-2086-y
Lerner, A., Neidhöfer, S., and Matthias, T. (2017). The Gut Microbiome Feelings of the Brain: A Perspective for Non-microbiologists. Microorganisms 5 (4), 66. doi:10.3390/microorganisms5040066
Liang, W., Feng, Z., Rao, S., Xiao, C., Xue, X., Lin, Z., et al. (2020). Diarrhoea May Be Underestimated: A Missing Link in 2019 Novel Coronavirus. Gut 69 (6), 1141–1143. doi:10.1136/gutjnl-2020-320832
Lin, K., Lin, S., Lin, A. N., Lin, T., Htun, Z. M., and Reddy, M. (2017). A Rare Thermophilic Bug in Complicated Diverticular Abscess. Case. Rep. Gastroenterol. 11, 569–575. doi:10.1159/000480072
Littman, D. R., and Pamer, E. G. (2011). Role of the Commensal Microbiota in Normal and Pathogenic Host Immune Responses. Cell Host Microbe 10 (4), 311–323. doi:10.1016/j.chom.2011.10.004
Liu, Q., Tian, X., Maruyama, D., Arjomandi, M., and Prakash, A. (2021). Lung Immune Tone via Gut-Lung axis: Gut-Derived LPS and Short-Chain Fatty Acids' Immunometabolic Regulation of Lung IL-1β, FFAR2, and FFAR3 Expression. Am. J. Physiol. Lung Cel Mol Physiol 321 (1), L65–L78. doi:10.1152/ajplung.00421.2020
Liu, T. H., Zhang, X. M., Han, N. P., Liu, Y., Wu, Y. Y., Li, X. Y., et al. (20182018). Regulation Effect of a Chinese Herbal Formula on Flora and Mucosal Immune Secretory Immunoglobulin A in Rats. Evid. Based Complement. Alternat Med. 2018, 4821821. doi:10.1155/2018/4821821
Livanos, A. E., Jha, D., Cossarini, F., Gonzalez-Reiche, A. S., Tokuyama, M., Aydillo, T., et al. (2021). Intestinal Host Response to SARS-CoV-2 Infection and COVID-19 Outcomes in Patients with Gastrointestinal Symptoms. Gastroenterology 160 (7), 2435–2450. doi:10.1053/j.gastro.2021.02.056
Loo, Y. T., Howell, K., Chan, M., Zhang, P., and Ng, K. (2020). Modulation of the Human Gut Microbiota by Phenolics and Phenolic Fiber‐rich Foods. Compr. Rev. Food Sci. Food Saf. 19 (4), 1268–1298. doi:10.1111/1541-4337.12563
Lordan, C., Thapa, D., Ross, R. P., and Cotter, P. D. (2020). Potential for Enriching Next-Generation Health-Promoting Gut Bacteria through Prebiotics and Other Dietary Components. Gut Microbes 11 (1), 1–20. doi:10.1080/19490976.2019.1613124
Ma, G., and Chen, Y. (2020). Polyphenol Supplementation Benefits Human Health via Gut Microbiota: A Systematic Review via Meta-Analysis. J. Funct. Foods 66, 103829. doi:10.1016/j.jff.2020.103829
Ma, H., Zhou, T., Heianza, Y., and Qi, L. (2021). Habitual Use of Vitamin D Supplements and Risk of Coronavirus Disease 2019 (COVID-19) Infection: a Prospective Study in UK Biobank. Am. J. Clin. Nutr. 113 (5), 1275–1281. doi:10.1093/ajcn/nqaa381
Magryś, A. (2021). Microbiota: A Missing Link in the Pathogenesis of Chronic Lung Inflammatory Diseases. Pol. J. Microbiol. 70 (1), 25–32. doi:10.33073/pjm-2021-013
Mak, J. W. Y., Chan, F. K. L., and Ng, S. C. (2020). Probiotics and COVID-19: One Size Does Not Fit All. Lancet Gastroenterol. Hepatol. 5 (7), 644–645. doi:10.1016/S2468-1253(20)30122-9
Makki, K., Deehan, E. C., Walter, J., and Bäckhed, F. (2018). The Impact of Dietary Fiber on Gut Microbiota in Host Health and Disease. Cell Host Microbe 23 (6), 705–715. doi:10.1016/j.chom.2018.05.012
Maldonado Galdeano, C., Cazorla, S. I., Lemme Dumit, J. M., Vélez, E., and Perdigón, G. (2019). Beneficial Effects of Probiotic Consumption on the Immune System. Ann. Nutr. Metab. 74 (2), 115–124. doi:10.1159/000496426
Marsland, B. J., Trompette, A., and Gollwitzer, E. S. (2015). The Gut-Lung Axis in Respiratory Disease. Ann. Am. Thorac. Soc. 12, S150–S156. doi:10.1513/AnnalsATS.201503-133AW
Meltzer, D. O., Best, T. J., Zhang, H., Vokes, T., Arora, V., and Solway, J. (2020). Association of Vitamin D Status and Other Clinical Characteristics with COVID-19 Test Results. JAMA Netw. Open 3 (9), e2019722. doi:10.1001/jamanetworkopen.2020.19722
Minato, T., Nirasawa, S., Sato, T., Yamaguchi, T., Hoshizaki, M., Inagaki, T., et al. (2020). B38-CAP Is A Bacteria-Derived ACE2-like Enzyme that Suppresses Hypertension and Cardiac Dysfunction. Nat. Commun. 11 (11), 1058. doi:10.1038/s41467-020-14867-z
Morais, A. H. A., Passos, T. S., Maciel, B. L. L., and da Silva-Maia, J. K. (2020). Can Probiotics and Diet Promote Beneficial Immune Modulation and Purine Control in Coronavirus Infection? Nutrients 12 (6), 1737. doi:10.3390/nu12061737
Myhrstad, M. C. W., Tunsjø, H., Charnock, C., and Telle-Hansen, V. H. (2020). Dietary Fiber, Gut Microbiota, and Metabolic Regulation-Current Status in Human Randomized Trials. Nutrients 12 (3), 859. doi:10.3390/nu12030859
Nasr, R., Shamseddine, A., Mukherji, D., Nassar, F., and Temraz, S. (2020). The Crosstalk between Microbiome and Immune Response in Gastric Cancer. Int. J. Mol. Sci. 21 (18), 6586. doi:10.3390/ijms21186586
Nicolucci, A. C., Hume, M. P., Martínez, I., Mayengbam, S., Walter, J., and Reimer, R. A. (2017). Prebiotics Reduce Body Fat and Alter Intestinal Microbiota in Children Who Are Overweight or with Obesity. Gastroenterology 153 (3), 711–722. doi:10.1053/j.gastro.2017.05.055
Ohgitani, E., Shin-Ya, M., Ichitani, M., Kobayashi, M., Takihara, T., Kawamoto, M., et al. (2021). Significant Inactivation of SARS-CoV-2 In Vitro by a Green Tea Catechin, a Catechin-Derivative, and Black Tea Galloylated Theaflavins. Molecules 26 (12), 3572. doi:10.3390/molecules26123572
Olaimat, A. N., Aolymat, I., Al-Holy, M., Ayyash, M., Abu Ghoush, M., Al-Nabulsi, A. A., et al. (2020). The Potential Application of Probiotics and Prebiotics for the Prevention and Treatment of COVID-19. NPJ Sci. Food 4, 17. doi:10.1038/s41538-020-00078-9
Pacha, O., Sallman, M. A., and Evans, S. E. (2020). COVID-19: A Case for Inhibiting IL-17? Nat. Rev. Immunol. 20 (6), 345–346. doi:10.1038/s41577-020-0328-z
Parnell, J. A., and Reimer, R. A. (2012). Prebiotic Fiber Modulation of the Gut Microbiota Improves Risk Factors for Obesity and the Metabolic Syndrome. Gut Microbes 3 (1), 29–34. doi:10.4161/gmic.19246
Pawar, K. S., Mastud, R. N., Pawar, S. K., Pawar, S. S., Bhoite, R. R., Bhoite, R. R., et al. (2021). Oral Curcumin with Piperine as Adjuvant Therapy for the Treatment of COVID-19: A Randomized Clinical Trial. Front. Pharmacol. 12, 669362. doi:10.3389/fphar.2021.669362
Penninger, J. M., Grant, M. B., and Sung, J. J. Y. (2021). The Role of Angiotensin Converting Enzyme 2 in Modulating Gut Microbiota, Intestinal Inflammation, and Coronavirus Infection. Gastroenterology 160 (1), 39–46. doi:10.1053/j.gastro.2020.07.067
Plamada, D., and Vodnar, D. C. (2021). Polyphenols-Gut Microbiota Interrelationship: A Transition to a New Generation of Prebiotics. Nutrients 14 (1), 137. doi:10.3390/nu14010137
Plaza-Diaz, J., Ruiz-Ojeda, F. J., Gil-Campos, M., and Gil, A. (2019). Mechanisms of Action of Probiotics. Adv. Nutr. 10 (1), S49–S66. doi:10.1093/advances/nmy063
Reibman, J., Marmor, M., Filner, J., Fernandez-Beros, M. E., Rogers, L., Perez-Perez, G. I., et al. (2008). Asthma Is Inversely Associated with Helicobacter Pylori Status in an Urban Population. PLoS One 3 (12), e4060. doi:10.1371/journal.pone.0004060
Roobab, U., Batool, Z., Manzoor, M. F., Shabbir, M. A., Khan, M. R., and Aadil, R. M. (2020). Sources, Formulations, Advanced Delivery and Health Benefits of Probiotics. Curr. Opin. Food Sci. 32, 17–28. doi:10.1016/j.cofs.2020.01.003
Ryck, T., Grootaert, C., Jaspaert, L., Kerckhof, F. M., Van Gele, M., De Schrijver, J., et al. (2014). Development of an Oral Mucosa Model to Study Host-Microbiome Interactions during Wound Healing. Appl. Microbiol. Biotechnol. 98 (15), 6831–6846. doi:10.1007/s00253-014-5841-1
Rytter, M. J., Kolte, L., Briend, A., Friis, H., and Christensen, V. B. (2014). The Immune System in Children with Malnutrition--A Systematic Review. PLoS One 9 (8), e105017. doi:10.1371/journal.pone.0105017
Sajdel-Sulkowska, E. M. (2021). A Dual-Route Perspective of SARS-CoV-2 Infection: Lung- vs. Gut-specific Effects of ACE-2 Deficiency. Front. Pharmacol. 12, 684610. doi:10.3389/fphar.2021.684610
Salehi, B., Calina, D., Docea, A. O., Koirala, N., Aryal, S., Lombardo, D., et al. (2020). Curcumin's Nanomedicine Formulations for Therapeutic Application in Neurological Diseases. J. Clin. Med. 9 (2), 430. doi:10.3390/jcm9020430
Salminen, S., and Isolauri, E. (2006). Intestinal Colonization, Microbiota, and Probiotics. J. Pediatr. 149 (5), S115–S120. doi:10.1016/j.jpeds.2006.06.062
Samuelson, D. R., Welsh, D. A., and Shellito, J. E. (2015). Regulation of Lung Immunity and Host Defense by the Intestinal Microbiota. Front. Microbiol. 6, 1085. doi:10.3389/fmicb.2015.01085
Santacroce, L., Charitos, I. A., Ballini, A., Inchingolo, F., Luperto, P., De Nitto, E., et al. (2020). The Human Respiratory System and its Microbiome at a Glimpse. Biology (Basel) 9 (10), 318. doi:10.3390/biology9100318
Santinelli, L., Laghi, L., Innocenti, G. P., Pinacchio, C., Vassalini, P., Celani, L., et al. (2021). Oral Bacteriotherapy Reduces the Occurrence of Chronic Fatigue in COVID-19 Patients. Front. Nutr. 8, 756177. doi:10.3389/fnut.2021.756177
Schiavi, E., Gleinser, M., Molloy, E., Groeger, D., Frei, R., Ferstl, R., et al. (2016). The Surface-Associated Exopolysaccharide of Bifidobacterium Longum 35624 Plays an Essential Role in Dampening Host Proinflammatory Responses and Repressing Local TH17 Responses. Appl. Environ. Microbiol. 82 (24), 7185–7196. doi:10.1128/AEM.02238-16
Segal, L. N., Clemente, J. C., Tsay, J. C., Koralov, S. B., Keller, B. C., Wu, B. G., et al. (2016). Enrichment of the Lung Microbiome with Oral Taxa Is Associated with Lung Inflammation of a Th17 Phenotype. Nat. Microbiol. 1, 16031. doi:10.1038/nmicrobiol.2016.31
Selwal, K. K., Selwal, M. K., and Yu, Z. (2021). Mucolytic Bacteria: Prevalence in Various Pathological Diseases. World J. Microbiol. Biotechnol. 37 (10), 176. doi:10.1007/s11274-021-03145-9
Shang, J., Ye, G., Shi, K., Wan, Y., Luo, C., Aihara, H., et al. (2020). Structural Basis of Receptor Recognition by SARS-Cov-2. Nature 581 (7807), 221–224. doi:10.1038/s41586-020-2179-y
Shoaib, M., Shehzad, A., Omar, M., Rakha, A., Raza, H., Sharif, H. R., et al. (2016). Inulin: Properties, Health Benefits and Food Applications. Carbohydr. Polym. 147, 444–454. doi:10.1016/j.carbpol.2016.04.020
Simčič, S., Berlec, A., Stopinšek, S., Štrukelj, B., and Orel, R. (2019). Engineered and Wild-type L Lactis Promote Anti-inflammatory Cytokine Signalling in Inflammatory Bowel Disease Patient's Mucosa. World J. Microbiol. Biotechnol. 35 (3), 45. doi:10.1007/s11274-019-2615-z
Sinatti, G., Santini, S. J., Tarantino, G., Picchi, G., Cosimini, B., Ranfone, F., et al. (2021). PaO2/FiO2 Ratio Forecasts COVID-19 Patients' Outcome Regardless of Age: A Cross-Sectional, Monocentric Study. Intern. Emerg. Med. 1-9. doi:10.1007/s11739-021-02840-7
So, D., Whelan, K., Rossi, M., Morrison, M., Holtmann, G., Kelly, J. T., et al. (2018). Dietary Fiber Intervention on Gut Microbiota Composition in Healthy Adults: A Systematic Review and Meta-Analysis. Am. J. Clin. Nutr. 107 (6), 965–983. doi:10.1093/ajcn/nqy041
Sommariva, M., Le Noci, V., Bianchi, F., Camelliti, S., Balsari, A., Tagliabue, E., et al. (2020). The Lung Microbiota: Role in Maintaining Pulmonary Immune Homeostasis and its Implications in Cancer Development and Therapy. Cell Mol Life Sci 77 (14), 2739–2749. doi:10.1007/s00018-020-03452-8
Spagnolello, O., Pinacchio, C., Santinelli, L., Vassalini, P., Innocenti, G. P., De Girolamo, G., et al. (2021). Targeting Microbiome: An Alternative Strategy for Fighting SARS-Cov-2 Infection. Chemotherapy 66 (1-2), 24–32. doi:10.1159/000515344
Standen, B. T., Rodiles, A., Peggs, D. L., Davies, S. J., Santos, G. A., and Merrifield, D. L. (2015). Modulation of the Intestinal Microbiota and Morphology of Tilapia, Oreochromis niloticus, Following the Application of a Multi-Species Probiotic. Appl. Microbiol. Biotechnol. 99 (20), 8403–8417. doi:10.1007/s00253-015-6702-2
Sun, Q., Cheng, L., Zhang, X., Wu, Z., and Weng, P. (2021). The Interaction between Tea Polyphenols and Host Intestinal Microorganisms: An Effective Way to Prevent Psychiatric Disorders. Food Funct. 12 (3), 952–962. doi:10.1039/d0fo02791j
Sundararaman, A., Ray, M., Ravindra, P. V., and Halami, P. M. (2020). Role of Probiotics to Combat Viral Infections with Emphasis on COVID-19. Appl. Microbiol. Biotechnol. 104 (19), 8089–8104. doi:10.1007/s00253-020-10832-4
Taghinezhad-S, S., Mohseni, A. H., Bermúdez-Humarán, L. G., Casolaro, V., Cortes-Perez, N. G., Keyvani, H., et al. (2021). Probiotic-Based Vaccines May Provide Effective Protection against COVID-19 Acute Respiratory Disease. Vaccines (Basel) 9 (5), 466. doi:10.3390/vaccines9050466
Tallei, T. E., FatimawaliNiode, N. J., Niode, N. J., Idroes, R., Zidan, B. M. R. M., Mitra, S., et al. (2021). A Comprehensive Review of the Potential Use of Green Tea Polyphenols in the Management of COVID-19. Evidence-Based Complement. Altern. Med. 2021, 1–13. doi:10.1155/2021/7170736
Tanabe, S. (2013). The Effect of Probiotics and Gut Microbiota on Th17 Cells. Int. Rev. Immunol. 32 (5-6), 511–525. doi:10.3109/08830185.2013.839665
Tandon, D., Haque, M. M., Gote, M., Jain, M., Bhaduri, A., Dubey, A. K., et al. (2019). A Prospective Randomized, Double-Blind, Placebo-Controlled, Dose-Response Relationship Study to Investigate Efficacy of Fructo-Oligosaccharides (FOS) on Human Gut Microflora. Sci. Rep. 9 (1), 5473. doi:10.1038/s41598-019-41837-3
Tao, C., Zhang, Q., Zeng, W., Liu, G., and Shao, H. (2020). The Effect of Antibiotic Cocktails on Host Immune Status Is Dynamic and Does Not Always Correspond to Changes in Gut Microbiota. Appl. Microbiol. Biotechnol. 104 (11), 4995–5009. doi:10.1007/s00253-020-10611-1
Tarantino, G., Costantini, S., Finelli, C., Capone, F., Guerriero, E., La Sala, N., et al. (2014). Is Serum Interleukin-17 Associated with Early Atherosclerosis in Obese Patients? J. Transl. Med. 12, 214. doi:10.1186/s12967-014-0214-1
Thirumdas, R., Kothakota, A., Pandiselvam, R., Bahrami, A., and Barba, F. J. (2021). Role of Food Nutrients and Supplementation in Fighting against Viral Infections and Boosting Immunity: A Review. Trends Food Sci. Technol. 110, 66–77. doi:10.1016/j.tifs.2021.01.069
Trompette, A., Gollwitzer, E. S., Pattaroni, C., Lopez-Mejia, I. C., Riva, E., Pernot, J., et al. (2018). Dietary Fiber Confers Protection against Flu by Shaping Ly6c- Patrolling Monocyte Hematopoiesis and CD8+ T Cell Metabolism. Immunity 48 (5), 992–e8. doi:10.1016/j.immuni.2018.04.022
Tuohy, K. M., Conterno, L., Gasperotti, M., and Viola, R. (2012). Up-Regulating the Human Intestinal Microbiome Using Whole Plant Foods, Polyphenols, And/or Fiber. J. Agric. Food Chem. 60 (36), 8776–8782. doi:10.1021/jf2053959
Turck, D., Turck, D., Castenmiller, J., De Henauw, S., Hirsch-Ernst, K. I., Kearney, J., et al. (2019). Nutrimune and Immune Defence against Pathogens in the Gastrointestinal and Upper Respiratory Tracts: Evaluation of a Health Claim Pursuant to Article 14 of Regulation (EC) No 1924/2006. EFSA J. 17 (4), e05656. doi:10.2903/j.efsa.2019.5656
Verduci, E., and Köglmeier, J. (2021). Immunomodulation in Children: The Role of the Diet. J. Pediatr. Gastroenterol. Nutr. 73 (3), 293–298. doi:10.1097/MPG.0000000000003152
Walsh, D. M., McCullough, S. D., Yourstone, S., Jones, S. W., Cairns, B. A., Jones, C. D., et al. (2017). Alterations in Airway Microbiota in Patients with PaO2/FiO2 Ratio ≤ 300 after Burn and Inhalation Injury. PloS one 12 (3), e0173848. doi:10.1371/journal.pone.0173848
Walton, G. E., Gibson, G. R., and Hunter, K. A. (2021). Mechanisms Linking the Human Gut Microbiome to Prophylactic and Treatment Strategies for COVID-19. Br. J. Nutr. 126 (2), 219–227. doi:10.1097/MPG.000000000000315210.1017/S0007114520003980
Wang, M., Wichienchot, S., He, X., Fu, X., Huang, Q., and Zhang, B. (2019). In Vitro Colonic Fermentation of Dietary Fibers: Fermentation Rate, Short-Chain Fatty Acid Production and Changes in Microbiota. Trends Food Sci. Tech. 88, 1–9. doi:10.1016/j.tifs.2019.03.005
Wen, K., Li, G., Bui, T., Liu, F., Li, Y., Kocher, J., et al. (2012). High Dose and Low Dose Lactobacillus Acidophilus Exerted Differential Immune Modulating Effects on T Cell Immune Responses Induced by an Oral Human Rotavirus Vaccine in Gnotobiotic Pigs. Vaccine 30 (6), 1198–1207. doi:10.1016/j.vaccine.2011.11.107
Westermann, C., Gleinser, M., Corr, S. C., and Riedel, C. U. (2016). A Critical Evaluation of Bifidobacterial Adhesion to the Host Tissue. Front. Microbiol. 7, 1220. doi:10.3389/fmicb.2016.01220
Wiciński, M., Gębalski, J., Mazurek, E., Podhorecka, M., Śniegocki, M., Szychta, P., et al. (2020). The Influence of Polyphenol Compounds on Human Gastrointestinal Tract Microbiota. Nutrients 12 (2), 350. doi:10.3390/nu12020350
Wilson, B., and Whelan, K. (2017). Prebiotic Inulin-type Fructans and Galacto-Oligosaccharides: Definition, Specificity, Function, and Application in Gastrointestinal Disorders. J. Gastroenterol. Hepatol. 32, 64–68. doi:10.1111/jgh.13700
Wong, S. H., Lui, R. N., and Sung, J. J. (2020). COVID-19 and the Digestive System. J. Gastroenterol. Hepatol. 35 (5), 744–748. doi:10.1111/jgh.15047
Wu, A., Budge, P. J., Williams, J., Griffin, M. R., Edwards, K. M., Johnson, M., et al. (2015). Incidence and Risk Factors for Respiratory Syncytial Virus and Human Metapneumovirus Infections Among Children in the Remote Highlands of Peru. PLoS One 10 (6), e0130233. doi:10.1371/journal.pone.0130233
Wu, C., Xu, Q., Cao, Z., Pan, D., Zhu, Y., Wang, S., et al. (2021). The Volatile and Heterogeneous Gut Microbiota Shifts of COVID-19 Patients over the Course of A Probiotics-Assisted Therapy. Clin. Transl. Med. 11 (12), e643. doi:10.1002/ctm2.643
Xu, M. J., Liu, B. J., Wang, C. L., Wang, G. H., Tian, Y., Wang, S. H., et al. (2017). Epigallocatechin-3-Gallate Inhibits TLR4 Signaling through the 67-Kda Laminin Receptor and Effectively Alleviates Acute Lung Injury Induced by H9N2 Swine Influenza Virus. Int. Immunopharmacol. 52, 24–33. doi:10.1016/j.intimp.2017.08.023
Xu, Y., Zhu, J., Feng, B., Lin, F., Zhou, J., Liu, J., et al. (2021). Immunosuppressive Effect of Mesenchymal Stem Cells on Lung and Gut CD8 + T Cells in Lipopolysaccharide‐induced Acute Lung Injury in Mice. Cell prolif 54 (5), e13028. doi:10.1111/cpr.13028
Yan, R., Ho, C. T., and Zhang, X. (2020). Interaction between Tea Polyphenols and Intestinal Microbiota in Host Metabolic Diseases from the Perspective of the Gut-Brain Axis. Mol. Nutr. Food Res. 64 (14), e2000187. doi:10.1002/mnfr.202000187
Yang, B., Zhang, Y., Li, B., Zou, Y., and Xiao, C. (2019). Fine Particulate Matter Alters the Microecology of the Murine Respiratory Tract. Environ. Sci. Pollut. Res. Int. 26 (9), 8623–8632. doi:10.1007/s11356-019-04372-2
Yang, C. S., and Zhang, J. (2019). Studies on the Prevention of Cancer and Cardiometabolic Diseases by Tea: Issues on Mechanisms, Effective Doses, and Toxicities. J. Agric. Food Chem. 67 (19), 5446–5456. doi:10.1021/acs.jafc.8b05242
Yeoh, Y. K., Zuo, T., Lui, G. C., Zhang, F., Liu, Q., Li, A. Y., et al. (2021). Gut Microbiota Composition Reflects Disease Severity and Dysfunctional Immune Responses in Patients with COVID-19. Gut 70 (4), 698–706. doi:10.1136/gutjnl-2020-323020
Yeung, M. L., Teng, J. L. L., Jia, L., Zhang, C., Huang, C., Cai, J.-P., et al. (2021). Soluble ACE2-Mediated Cell Entry of SARS-Cov-2 via Interaction with Proteins Related to the Renin-Angiotensin System. Cell 184 (8), 2212–2228. doi:10.1016/j.cell.2021.02.053
Zelaya, H., Laiño, J., Villena, J., Marranzino, G., Alvarez, S., and Agüero, G. (2020). Lactobacillus Casei CRL431 Modulates Hemostatic Activation Induced by Protein Malnourishment and Pneumococcal Respiratory Infection. Appl. Microbiol. Biotechnol. 104 (24), 10669–10683. doi:10.1007/s00253-020-10957-6
Zhang, G., Zhao, J., Wen, R., Zhu, X., Liu, L., and Li, C. (2020). 2'-Fucosyllactose Promotes Bifidobacterium Bifidum DNG6 Adhesion to Caco-2 Cells. J. Dairy Sci. 103 (11), 9825–9834. doi:10.3168/jds.2020-18773
Zhang, X., Zhu, X., Sun, Y., Hu, B., Sun, Y., Jabbar, S., et al. (2013). Fermentation In Vitro of EGCG, GCG and EGCG3"Me Isolated from Oolong Tea by Human Intestinal Microbiota. Food Res. Int. 54 (2), 1589–1595. doi:10.1016/j.foodres.2013.10.005
Zhang, Z., Zhang, X., Bi, K., He, Y., Yan, W., Yang, C. S., et al. (2021). Potential Protective Mechanisms of Green Tea Polyphenol EGCG against COVID-19. Trends Food Sci. Technol. 114, 11–24. doi:10.1016/j.tifs.2021.05.023
Zhou, A., Lei, Y., Tang, L., Hu, S., Yang, M., Wu, L., et al. (2021). Gut Microbiota: The Emerging Link to Lung Homeostasis and Disease. J. Bacteriol. 203 (4), e00454. doi:10.1128/JB.00454-20
Zuo, T., Liu, Q., Zhang, F., Lui, G. C., Tso, E. Y., Yeoh, Y. K., et al. (2021). Depicting SARS-CoV-2 Faecal Viral Activity in Association with Gut Microbiota Composition in Patients with COVID-19. Gut 70 (2), 276–284. doi:10.1136/gutjnl-2020-322294
Keywords: COVID-19, gut microbiota, probiotics, gut-lung axis, prebiotics
Citation: Xu L, Yang CS, Liu Y and Zhang X (2022) Effective Regulation of Gut Microbiota With Probiotics and Prebiotics May Prevent or Alleviate COVID-19 Through the Gut-Lung Axis. Front. Pharmacol. 13:895193. doi: 10.3389/fphar.2022.895193
Received: 13 March 2022; Accepted: 31 March 2022;
Published: 25 April 2022.
Edited by:
Stefano Fiorucci, University of Perugia, ItalyReviewed by:
Giovanni Tarantino, University of Naples Federico II, ItalyGabriella d’Ettorre, Sapienza University of Rome, Italy
Copyright © 2022 Xu, Yang, Liu and Zhang. This is an open-access article distributed under the terms of the Creative Commons Attribution License (CC BY). The use, distribution or reproduction in other forums is permitted, provided the original author(s) and the copyright owner(s) are credited and that the original publication in this journal is cited, in accordance with accepted academic practice. No use, distribution or reproduction is permitted which does not comply with these terms.
*Correspondence: Chung S. Yang, Y3N5YW5nQHBoYXJtYWN5LnJ1dGdlcnMuZWR1; Xin Zhang, emhhbmd4aW5AbmJ1LmVkdS5jbg==