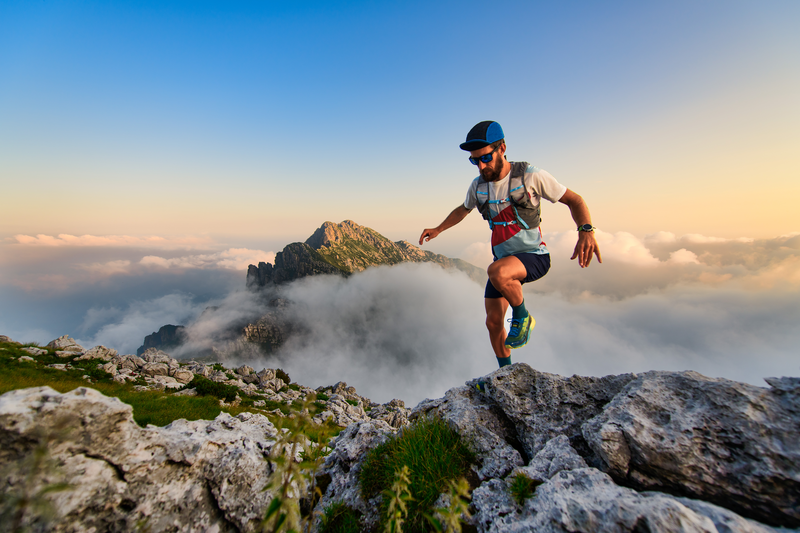
94% of researchers rate our articles as excellent or good
Learn more about the work of our research integrity team to safeguard the quality of each article we publish.
Find out more
ORIGINAL RESEARCH article
Front. Pharmacol. , 25 May 2022
Sec. Gastrointestinal and Hepatic Pharmacology
Volume 13 - 2022 | https://doi.org/10.3389/fphar.2022.894981
This article is part of the Research Topic New Compounds, Novel Targets and Mechanism Study in Inflammation-Associated Liver Diseases View all 10 articles
Background and aims: Saikosaponin d (SSd) has a steroidal structure and significant anti-inflammatory effects. The purpose of this study was to explore the mechanism underlying SSd’s inhibitory effects on liver fibrosis.
Methods: Wild-type and estrogen receptor knockout (ERKO) mice were treated with CCl4 to establish liver fibrosis mouse models. The effects of SSd on hepatic fibrogenesis were studied in these mouse models. Hepatic stellate cells (HSCs) were activated by H2O2 to investigate the potential molecular mechanisms. The establishment of the models and the degrees of inflammation and liver tissue fibrosis were evaluated by detecting changes in serum liver enzymes and liver histopathology. The expression of α-SMA and TGF-β1 was determined by immunohistochemistry. The expression and significance of NLRP3 inflammasome proteins were explored by RT-PCR and Western blotting analyses. The mitochondrial ROS-related indexes were evaluated by MitoSOX Red.
Results: In wild-type and ERKO mice treated with CCl4, the fluorescence expression of mitochondrial ROS was up-regulated, while the mitochondrial membrane potential and ATP content were decreased, suggesting that the mitochondria were damaged. In addition, the expression of NLRP3 inflammatory bodies and fibrosis markers (α-SMA, TGF-β, TIMP-1, MMP-2, and Vimentin) in liver tissue increased. Furthermore, the above indexes showed the same expression trend in activated HSCs. In addition, the peripheral serum ALT and AST levels increased in CCl4-induced liver injury model mice. And HE staining showed a large number of inflammatory cell infiltration in the liver of model mice. Picric acid-Sirius staining and Masson staining showed that there was significant collagen fibrous tissue deposition in mice liver sections. IHC and WB detection confirmed that the expression of α-SMA and TGF-β1 increased. Liver fibrosis scores were also elevated. Then, after SSd intervention, the expression of ROS in wild-type mice and αERKO mice decreased, mitochondrial membrane potential recovered, ATP level increased, NLRP3 inflammasome and fibrosis indexes decreased, liver enzyme levels decreased, and liver pathology showed liver inflammation. The damage and collagen deposition were significantly relieved, the expression of α-SMA and TGF-β1 was decreased, and the fibrosis score was also decreased. More importantly, the effect of SSd in alleviating liver injury and liver fibrosis had no effect on βERKO mice.
Conclusion: SSd alleviated liver fibrosis by negatively regulating the ROS/NLRP3 inflammasome through activating the ERβ pathway. By establishing liver fibrosis models using wild-type and ERKO mice, we demonstrated that SSd could alleviate liver fibrosis by inhibiting the ROS/NLRP3 inflammasome axis through activating the ERβ pathway.
Liver fibrosis is a pathological process through which various chronic liver diseases develop into liver cirrhosis (Pinzani, 2015). Epidemiological studies have shown that various pathogenic factors can trigger liver inflammation, leading to liver cell damage, abnormal proliferation of connective tissue, reduced liver elasticity, and ultimately deteriorated liver tissue structure and functions (Fattovich et al., 2004; Pinzani, 2015; Parola and Pinzani, 2019). Liver fibrosis is reversible. However, once liver cirrhosis develops, liver fibrosis becomes irreversible. Therefore, blocking or reversing liver fibrosis is essential for the treatment of chronic liver diseases (Friedman, 2007; Böttcher and Pinzani, 2017; Parola and Pinzani, 2019). At present, the treatments for liver fibrosis mainly include anti-inflammation, anti-fibrosis, liver protection and other therapies. Although considerable effort has been spent on further understanding the mechanisms of current treatments and exploring effective novel treatments, satisfactory results have not been obtained (Chen et al., 2015; Böttcher and Pinzani, 2017). None of the new methods developed so far is perfect and all of them are far from ready for clinical practice. Therefore, there is still an urgent need to develop novel methods to block or reverse liver fibrosis.
The activation of hepatic stellate cells (HSCs) is a key step during liver fibrosis, which can lead to the production of a large amount of ROS from mitochondria. Overproduction of ROS can in turn promote the activation of HSCs and the NLPR3 inflammasome (Mederacke et al., 2013). According to previous studies, the NLRP3 inflammasome is expressed in many types of liver cells, including HSCs and damaged hepatocytes (Ning et al., 2017; Kim et al., 2018; Mohamed et al., 2018). Anomalous NLRP3 inflammasome activation is associated with the development of many diseases (Zahid et al., 2019). Oxidative stress injury of Mitochondria and activation of NLRP3 inflammatory body play an important role in the development of liver fibrosis. Key components of a functional NLRP3 inflammasome are NLRP3, the adaptor protein ASC and caspase-1. When oxidative stress occurs, NLRP3 recruits ASC and Pro-caspase-1, resulting in activation of Caspase-1 and prohibiting cytokines IL-1β and IL-18 (Martinon et al., 2002; Zahid et al., 2019). It has been widely documented that ROS play an important role in upstream signaling pathways that activate NLRP3 inflammasome (Mederacke et al., 2013; Abais et al., 2015). Recent literature found that NLRP3 inflammasome activation induced by mitochondrial oxidative stress plays an important role in the development of liver fibrosis (Zhao et al., 2019). Physiologically, quiescent HSCs are required for extracellular matrix (ECM) remodeling. Once activated, quiescent HSCs turn into myofibroblasts, accompanied by the overexpression and deposition of ECM. MMP and TIMP proteins play an important role in the process of liver fibrosis and activation of HSCs by regulating the homeostasis and remodeling of ECM. The levels of MMP-2 and TIMP-1 increase during those processes. Studies have confirmed that a reduction in E-Cadherin expression can promote the development of liver fibrosis, renal interstitial fibrosis, peritoneal fibrosis, and pulmonary fibrosis in experiments (Lu et al., 2018). Vimentin, which is mainly expressed in mesenchymal cells, is an intermediate filament protein and an important biomarker of epithelial–mesenchymal transition (EMT) (Cheng et al., 2016). EMT sustains the generation of pathologically “activated” ATII cells that, by secreting pro-fibrotic factors, further amplifies the fibrotic response (Ruaro et al., 2021). When HSCs were activated by lipopolysaccharide (LPS) and H2O2, the expression of NLRP3 inflammatory bodies in HSCs increased (Lin et al., 2018; Zahid et al., 2019).
Bupleurum is a traditional Chinese medicine that has been used for the treatment of liver disorders and other common diseases in China for centuries. As an active component of Bupleurum, saikosaponin d (SSd) plays a significant role in anti-inflammation, anti-liver fibrosis, anti-lipid peroxidation, inhibiting the activation of HSCs, and reducing matrix production (Dang et al., 2007; Fan et al., 2007). SSd is a potential treatment for liver fibrosis. Our previous research proved that SSd has estrogenic effects in vivo and in vitro and is an estrogen receptor (ER) modulator (Que et al., 2018). In addition, no obvious hepatotoxicity was observed for SSd at an effective drug dose (Wang et al., 2010). Research on the anti-fibrosis effects of estrogen confirmed that ERs are expressed in hepatocytes, sinusoidal endothelial cells, Kupffer cells and stellate cells, and that estrogen can improve the microcirculation of damaged liver, inhibit the proliferation of activated HSCs and the synthesis of collagen, promote the activity of antioxidant enzyme GPx in hepatocytes, prevent lipid peroxidation, regulate the expression of cytokines such as transforming growth factor, and inhibit the occurrence and development of liver fibrosis (Zhang et al., 2016; Lee et al., 2019). Studies have shown that the inhibition of NLRP3 inflammasome depends on the blockade of ERα in colitis (Gao et al., 2020). Zhang et al. demonstrated that estrogen exerted its anti-fibrosis effects via ERβ rather than ERα or GPER (Zhang et al., 2018). However, it is unclear whether SSd or estrogen alleviates liver fibrosis by inhibiting the expression of NLRP3 inflammasome proteins via activating the ERβ pathway.
In this study, we established models of CCl4-induced liver injure and fibrosis in wild-type and ERKO mice to investigate the molecular mechanism underlying SSd’s anti-fibrosis effects. Specifically, we focused on the expression of protein components of the NLRP3 inflammasome and the role of ERs.
Male specific-pathogen-free (SPF) C57BL/6 mice (6–8 weeks, 20 ± 2 g) were purchased from Shanghai SLAC Laboratory Animal Co., Ltd (Shanghai, China). After purchase, the mice were raised in the animal room without specific pathogens (SPF) in Shanghai Municipal Hospital of Traditional Chinese Medicine. With 5 mice in each cage, they were allowed free access to foods and drinks. Throughout 1 week when the mice were fed adaptively, the temperature was maintained at 20–25°C and the humidity was restricted to the range of 40–70%. All the animal experiments were performed in compliance with local and national guidelines. These experiments approved by the animal experimental ethics and welfare committee of Shanghai Municipal Hospital of Traditional Chinese Medicine (No. 2015032).
For establishment of liver fibrosis mouse models, we injected male wild-type and ER-α and -β knockout mice (αERKO and βERKO mice, respectively, TOS160106CC1, Cyagen Biosciences) subcutaneously twice weekly for 6 weeks with 5 ml/kg body weight carbon tetrachloride (CCl4) diluted 1:4 in vegetable oil. Mice injected with only vegetable oil served as a vehicle control group. To investigate the effect of SSd and the relationship between mitochondrial ROS level and the expression of NLRP3 inflammasome proteins, C57BL/6 male mice were injected subcutaneously with SSd (China National Institute for the Control of Pharmaceutical and Biological Products, China) at a dosage of 2 mg/kg body weight twice a week for 6 weeks. Thus, the following groups (10 mice per group) were established in this study (Pinzani, 2015): vehicle control group (Fattovich et al., 2004); CCl4-induced hepatic fibrosis model group (Parola and Pinzani, 2019); CCl4+SSd group: hepatic fibrosis mice treated with SSd.
Pre-treatment of samples for determination of serum ALT and AST levels: About 500 µl blood was collected from eyeballs of mice and placed in a 1.5 ml apical centrifuge tube at room temperature for 1–2 h, then centrifuged at 4°C at 3,000 rpm for 10 min. The upper serum was collected and placed in a new centrifuge tube for analysis, or stored in a −80°C freezer. ALT and AST analysis kits (Nanjing Jiancheng Biology Research Institute Co., Ltd.) were used to determine their serum levels.
Liver tissue sections were prepared, fixed in 4% paraformaldehyde at room temperature overnight, and subjected to hematoxylin-eosin (H&E) staining, Masson’s trichrome staining and picric acid-Sirius red staining. The stage of liver fibrosis was assessed according to the Ishak score.
Paraffin-embedded sections were baked in a thermostat for 2 h, dewaxed and rehydrated with xylene and ethanol, incubated in 3% H2O2 solution at room temperature for 10 min to block endogenous peroxidase activity, antigen-retrieved with citric acid buffer, incubated for 30 min with 2% goat serum, incubated with primary antibodies against α-SMA and TGF-β1 overnight at 4°C, washed with PBS, incubated with polymer auxiliaries 20 min at 37°C, and incubated with a biotinylated secondary antibody for 2 h at room temperature. The sections were visualized with DBA and the nuclei were stained with hematoxylin. Sectioning, dehydration and mounting were performed according to routine protocols and the sections were observed under a microscope (AE41, McAudi Industrial Group Co., Ltd.).
HSCs-LX2 (Shanghai Fanling Biotechnology Co., Ltd., Shanghai, China) were cultured in DMEM at 37°C with 5% CO2. After seeding in 6 well plates, HSCs were treated with H2O2 at a concentration of 200 μmol/L for 4 h. To investigate the role of ERs in SSd’s anti-fibrotic effects, HSCs-LX2 were treated with MPP (1 μM) (Tocris Bioscience Co., Ltd. UK) or THC (1 μM) (Tocris Bioscience Co., Ltd. UK) for 30 min, subsequently with SSd (5 μM) for 24 h, and finally incubated with H2O2 (200 μM) for 4 h in complete DMEM.
After being extracted from liver tissues and cells, protein samples were separated with SDS-PAGE, and electroblotted onto polyvinylidene difluoride membranes. After blocking with 5% skimmed milk, the membranes were incubated with primary antibodies, then with an appropriate horseradish peroxidase-labeled secondary antibody (1:2000; CST signaling, United States). Specific bands were detected by an enhanced chemiluminescence assay. Primary antibodies and their working concentrations are listed in Supplementary Table S1.
The TRIzol (Invitrogen,United States) reagent was applied for total RNA extraction. Reverse transcription and quantitative RT-PCR were performed with the PrimeScript® RT reagent kit (TaKaRa, Japan) and SYBR Premix Ex Taq (TaKaRa, Japan). The primers were synthesized by Shanghai Shenggong Biology Co., Ltd. Expression levels of genes relative to β-actin mRNA levels in each sample were calculated according to the 2−ΔΔCt method. The primer sequences are shown in Supplementary Table S2, S3.
To determine the level of mitochondrial ROS, a working solution of the probe (5 μM, Beyotime Institute of Biotechnology) was added into cells and incubated in the dark. The cells were washed with preheated PBS, re-stained with a working solution of Hoechst33342 (10 μg/ml, Beyotime Institute of Biotechnology), washed with PBS again, and observed under a fluorescence microscope (DMI3000B, Leica Corp.).
The mitochondria isolation solution was added to liver tissues for grinding. The liquid phase was centrifuged, and the precipitate, which contained mitochondria from liver tissues, was retained. The precipitate was resuspended with mitochondria storage solution, mixed with MitoSOX Red (5 μM, Beyotime Institute of Biotechnology), and incubated at 37°C for 30 min. The fluorescence intensity was determined by a fluorescence microplate reader (DMI3000B, Leica Corp.).
HSCs-LX2 were seeded into 96-well plates at a density of 1 × 104 cells per well and cultured in an incubator at 37°C with 5% CO2. H2O2 (at a concentration of 200 μM) was added into the cells for a 4-h incubation. The viability of HSCs-LX2 was determined by CCK-8 assays (Shanghai Yisheng Bio, China). MDA is an important product of lipid peroxidation, the level of which reflects the degree of lipid peroxidation. Therefore, we evaluated the degree of lipid peroxidation using an MDA assay kit (Beyotime Institute of Biotechnology) according to the manufacturer’s instructions.
The JC-1 probe (Beyotime Institute of Biotechnology) was used for mitochondrial membrane potential measurement. Briefly, cells were treated with JC-1 probe monomers according to the manufacturer’s instructions. Then, nuclei were visualized by DAPI staining (Beyotime Institute of Biotechnology) in the dark. The ratio of red and green fluorescence signal intensities of JC-1 probe monomers was calculated.
Intracellular ATP levels were measured with an assay kit (Beyotime Institute of Biotechnology) in accordance with the manufacturer’s instructions.
All data are expressed as means ± SEM. Student’s t-test was used to compare the differences between the means of two groups. One-way analysis of variance (ANOVA) with post hoc Fisher’s least significant difference tests was performed for comparisons among multiple groups using SPSS 22.0 (SPSS Inc., Chicago, United States). A p value of less than 0.05 was considered statistically significant.
To investigate the effect of SSd on liver injury, we established a mouse model of CCL4-induced liver injury and liver fibrosis. Unlike to the control group, the AST and ALT levels of the model group increased significantly (Figures 1A,B). The liver fibrosis score of the model group was also meaningfully higher than that of the control group (Figure 1C). Liver injury and fibrosis was occurred as shown by H&E staining (inflammatory cell infiltration and lobular disorder), Masson’s trichrome staining (blue color was indicative of collagen deposition), Picrosirius red staining (red color was indicative of collagen deposition) and the IHC of α-SMA and TNF-β1 (Figures 1D–F). On the contrary, the intervention of SSd significantly promoted the recovery of liver function and the normal liver structure. First of all, we also observed that the activities of liver enzymes AST and ALT were lower in the SSd group than in the control group, indicating an improved liver function (Figures 1A,B). Furthermore, the liver fibrosis score also decreased in the treatment group compared with the model group (Figure 1C). H&E staining displayed significantly reduced proliferation of fibrous tissues, significantly decreased inflammatory cell infiltration, and not obvious fat infiltration in the liver of model mice treated with SSd (Figure 1E). Masson staining revealed that the deposition of collagen fibers was reduced in the SSd intervention group (Figure 1E). Picric acid-Sirius red staining exhibited only a few collagen fibers in the vascular wall of the portal area after SSd intervention (Figure 1E). Correspondingly, the expression levels of α-SMA and TGF-β1 in the portal area and liver tissues of the SSd intervention group were significantly decreased compared with the model group (Figures 1D,E), suggesting that SSd treatment reduced hepatic inflammation and damages in the model mice.
FIGURE 1. SSd alleviated CCL4-induced liver injury and liver fibrosis. (A,B) the changes of serological ALT and AST concentration, n = 6. (C) Liver Fibrosis Score, n = 6. (D–F) liver histological determination (H&E) and IHC of α-SMA and TNF-β (×200; scale bar: 50 μm). Data are expressed in mean ± SEM; **p < 0.01, ****p < 0.0001, versus control group; ##p < 0.01, ####p < 0.0001, AND versus CCL4 treated group.
Compared with untreated mice, the intensity of MitoSOX Red fluorescence in the SSd intervention group was decreased (Figure 2A). Determination of mitochondrial membrane potential showed that the ratio of JC-1 monomers with red fluorescence was higher in the SSd group than in the model group, and a similar trend was observed for intracellular ATP content (Figures 2B,C). As illustrated in Figures 2D,E, the expression levels of NLRP3 inflammasome, pro-IL-1β, IL-1β, and IL-18 in model mice were decreased significantly after treatment with SSd. Altogether, these data suggested that the effect of SSd on NLRP3 inflammasome activation in liver fibrosis was likely mediated through the regulation of mitochondrial ROS level. Taken together, these data suggested that SSd had inhibitory effects on the activation of the NLRP3 inflammatory proteins and mitochondrial oxidative stress damage in a model of liver fibrosis.
FIGURE 2. SSd inhibited mitochondrial dysfunctions and NLRP3 inflammasome activation. (A) MitoSOX Red Mitochondrial Superoxide Indicator detection, n = 6. (B) mitochondrial JC-1 fluorescence expression, n = 6. (C) ATP content, n = 6. (D,E) relative mRNA and proteins expression of inflammasome, n = 3. Data are expressed in mean ± SEM; **p < 0.01, CCL4 group versus control group; ##p < 0.01, CCl4+SSd versus CCL4 treated group.
To investigate whether SSd can protect the mitochondrial functions of activated HSCs in vitro, activated HSCs were treated with SSd. It was found that both MDA content and mitochondrial ROS level in HSCs were significantly decreased after SSd treatment (Figures 3A,B). Conversely, both intracellular ATP content and the mitochondrial proportion of JC-1 monomers with red fluorescence were increased after SSd treatment (Figures 3C,D). The effects of SSd treatment on the expression of NLRP3 inflammatory and fibro-genic proteins in activated HSCs were also examined. The results showed that SSd inhibited the expression of α-SMA, NLRP3 inflammasome, pro-IL-1β, IL-1β, and IL-18 (Figures 3E–H). Collectively, these results indicated that SSd alleviated H2O2-induced mitochondrial dysfunctions, as well as in reducing the expression of inflammatory factors in HSCs.
FIGURE 3. SSd alleviated H2O2-induced mitochondrial dysfunction and NLRP3 activation in HSCs (A) the content of MDA in HSCs after SSd intervention combination with H2O2 or not, n = 6. (B) MitoSOX Red fluorescence images (the heavier the red, the more damage, scale bar, 52 μm). (C) ATP content, shows the degree of damage to mitochondria in HSCs, n = 6. (D) JC-1 fluorescence images (the more JC-1 monomers with green, the more damage to mitochondria, scale bar, 52 μm). (E,F) RT-PCR and WB was employed to examine the expression of inflammasome, n = 3. (G,H) α-SMA protein expression, n = 3. Data are expressed in mean ± SEM; **p < 0.01, H2O2 treated group versus control group; ##p < 0.01, H2O2+SSd group versus H2O2 treated group.
Mitochondrial damages were also present in the CCL4-induced ERKO mice model. Compared with wild-type mice, mitochondrial ROS production was significantly upregulated, while the proportion of JC-1 monomers with red fluorescence and ATP content in the mitochondria were decreased in wild-type and ERKO mice treated by CCL4 (Figures 4A–C). After intervention with SSd, the above changes were reversed in wild-type and αERKO mice, but not in βERKO mice (Figures 4A–C). NLRP3 related mRNA and proteins were further examined in the liver of wild-type and ERKO mice treated with CCL4 alone or in combination with SSd. The results indicated that SSd effectively prevented CCl4-induced increase in NLRP3 inflammasome, pro-IL-1β, IL-1β, and IL-18 levels in wild-type mice and αERKO mice, but not in βERKO mice (Figures 4D,E).
FIGURE 4. Deficiency of ERβ, but not ERα, blocked the inhibitory effect of SSd against CCl4-induced mitochondrial dysfunction and NLRP3 activation in the liver (A). ROS fluorescence expression in the CCl4-induced ERKO mouse model combine with SSd or not, n = 6. (B) the mitochondrial JC-1 fluorescence expression in liver tissue, n = 6. (C) ATP content, n = 6. (D,E) inflammasome complex expression was tested by western blot experiment and PT-PCR in each group, n = 3. Data are expressed in mean ± SEM; **p < 0.01, ***p < 0.001, ****p < 0.0001, CCl4 treated versus control in WT and ERKO mice; ##p < 0.01, ###p < 0.001, ####p < 0.0001, SSd + CCl4 group versus CCl4 group in WT mice; ΔP <0.05, ΔΔP <0.01, ΔΔΔP <0.001, ΔΔΔΔP <0.0001, SSd + CCl4 group versus CCl4 group in αERKO mice.
SSd has estrogen-like effects in vivo and in vitro, and is an estrogen receptor modulator (Wang et al., 2010). It was hypothesized that SSd would have a therapeutic effect on liver injure and fibrosis. After CCl4 administration, increased ALT and AST activities were observed in both wild-type and ERKO mice, which could be significantly inhibited by SSd intervention in both wild-type and αERKO mice, but not in βERKO model mice (Figures 5A,B). Additionally, the H&E staining results revealed that SSd significantly reduced the necrotic area and the number of inflammatory cells in the liver of wild-type and αERKO liver fibrosis mice compared with the control group (Figure 5C). However, these changes were not observed in βERKO liver injure mice, suggesting that SSd blocked the inhibitory effects of SSd against CCL4-induced liver injury through the ERβ pathway.
FIGURE 5. Deficiency of ERβ, but not ERα, blocked the inhibitory effect of SSd against CCL4-induced liver injury (A,B) plasma ALT and AST levels were tested after CCl4 treatment in WT and ERKO mice treated with SSd or without SSd, n = 6. (C) liver injury was detected by HE (×200; scale bar: 50 μm). Data are expressed in mean ± SEM; **p < 0.01, CCl4 treated versus control in WT and ERKO mice; ##p < 0.01, SSd + CCl4 group versus CCl4 group in WT mice; ΔΔP <0.01, SSd + CCl4 group versus CCl4 group in αERKO mice.
Consistent with the blood biochemistry results, SSd treatment failed to prevent liver fibrosis induced by CCl4 in βERKO mice. RT-PCR and WB analyses indicated that liver fibrosis-related factors (including α-SMA, TGF-β1, TIMP-1, MMP-2, and Vimentin) were significantly downregulated, while E-cadherin was significantly upregulated in wild-type and αERKO mice after SSd treatment (Figures 6A,B). Liver fibrosis scores of wild type mice and αERKO mice were also significantly lower than that of the control mice after SSd treatment (Figure 6C). Furthermore, liver sections from CCl4-induced wild-type and ERKO mice with or without SSd treatment were analyzed by Masson’s trichrome staining, Picric acid-Sirius red staining and IHC. It was observed that SSd effectively decreased collagen accumulation and the expression of α-SMA and TGF-β1 as compared with controls group in wild-type and αERKO mice (Figures 6D–G). No significant changes were observed in βERKO mice after SSd treatment, suggesting that SSd inhibited CCl4-induced liver fibrosis via the ERβ pathway.
FIGURE 6. Deficiency of ERβ, but not ERα, blocks the inhibitory effect of SSd against CCl4-induced liver fibrosis (A,B). α-SMA, TGF-β1, TIMP-1, MMP-2, E-cadherin, and Vimentin mRNA and protein expression were tested by RT-PCR and WB in liver tissues, n = 3. (C) liver fibrosis scores in each group, n = 6. (D,E) Liver fibrosis was detected by Masson staining, picric acid-Sirius staining (×200; scale bar: 50 μm). (F,G) IHC detection of the expression of α-SMA, TGF-β1 (×200; scale bar: 100 μm). Data are expressed in mean ± SEM; *p < 0.05, **p < 0.001, ****p < 0.0001, CCl4 treated versus control in WT and ERKO mice; #p < 0.05, ##p < 0.01, ####p < 0.0001, SSd + CCl4 group versus CCl4 group in WT mice; ΔP <0.05, ΔΔP <0.01, ΔΔΔP <0.0001, SSd + CCl4 group versus CCl4 group in αERKO mice.
To better understand the molecular mechanism underlying SSd’s effects on liver fibrosis, we attempted to link the ER pathway with the impaired activation of HSCs. First, ER blockers alone or in combination with SSd were used to treat HSCs (normal or activated). It was found that ER inhibitors alone had no significant effect on HSCs compared with the control group. Interestingly, when combined with SSd, ERα inhibitor MPP decreased ROS production, increased the proportion of JC-1 monomers with red fluorescence and intracellular ATP content, which were similar to the therapeutic effects of SSd alone (Figures 7A–C). However, treatment with the combination of SSd and ERβ inhibitor THC showed no significant effect (Figures 7A–C). Furthermore, the levels of NLRP3 related proteins were assessed, which shown that treatment with the combination of SSd and ERα blocker notably decreased the levels of NLRP3, pro-IL-1β and IL-1β proteins, the degrees of which were comparable to those caused by SSd treatment alone (Figure 7D). Consistent with these results, RT-PCR analysis revealed that the combination of ERα inhibitor and SSd inhibited the expression of inflammasome genes in HSCs (Figure 7E).
FIGURE 7. Specific antagonist of ERβ, but not ERα, blocked the inhibitory effect of SSd against H2O2-induced mitochondrial dysfunction and NLRP3 activation in HSCs (A). ROS production in HSCs in each group in estrogen receptor blockers alone or in combination with SSd treated group in HSCs (normal or activated state) (Scale bar, 52 μm). (B) JC-1 membrane potential (Scale bar, 52 μm). (C) ATP content, n = 6. (D,E) inflammasome complex expression was tested by PT-PCR and western blot experiment. in each group, n = 3. Data are expressed in mean ± SEM; **p < 0.01, ****p < 0.0001, H2O2, H2O2 +MPP, and H2O2 +THC group versus control group; #p < 0.05, ##p < 0.01, ####p < 0.0001, SSd + H2O2 group versus H2O2 group; ΔP <0.05, ΔΔP <0.01, ΔΔΔΔP <0.0001, MPP + SSd + H2O2 group versus MPP + H2O2 group.
To explore the biomechanism underlying SSd’s inhibitory effects on ECM deposition and EMT in activated HSCs, we tested the expression levels of relative indicators. The results showed that the combination of SSd and MPP significantly impaired the expression of α-SMA, TGF-β, TIMP-1, MMP-2, and vimentin, and improved the expression of E-cadherin in HSCs exposed to H2O2, while no therapeutic effect was observed in the SSd + THC intervention group (Figures 8A,B). Our results indicated that SSd-induced changes in expression of fibrosis-related factors depended on the activation of the ERβ pathway.
FIGURE 8. Specific antagonist of ERβ, but not ERα, blocked the inhibitory effect of SSd against H2O2-induced extracellular matrix deposition and EMT in HSCs (A,B) the indicators of extracellular matrix deposition and EMT (α-SMA, TGF-β1, TIMP-1, MMP-2, Vimentin, and Ecadherin) expression in HSCs were detected by RT-PCR and WB, n = 3. Data are expressed in mean ± SEM; **p < 0.01, H2O2, H2O2 +MPP, and H2O2 +THC group versus control group; #p < 0.05, ##p < 0.01, SSd + H2O2 group versus H2O2 group; ΔP <0.05, ΔΔP <0.01, MPP + SSd + H2O2 group versus MPP + H2O2 group.
Our research confirmed the important role of SSd in the treatment of liver fibrosis. First, the application of SSd reduced mitochondria damage, activation of the NLRP3 inflammasome and inhibited HSCs activation and proliferation and liver injure and fibrosis. Second, the ERβ pathway played an important role in anti-liver fibrosis effects about SSd.
Subcutaneous CCl4 administration has been successfully applied to induce liver injury and cirrhosis, with histological changes and serum ALT levels being similar to those of human hepatic diseases. Persistent hepatocyte damages, excessive ECM deposition, and abnormal proliferation of connective tissue in the liver can lead to liver fibrosis (Hernandez-Gea and Friedman, 2011). HSCs activation, a key event in the occurrence of liver fibrosis, is the source of myofibroblasts and the final target of various fibrosis-inducing factors (Mederacke et al., 2013; Ray, 2014). Fortunately, EMT can be reversed into mesenchymal-epithelial transformation (MET), promoting the repair and reversal of hepatic fibrosis (Cicchini et al., 2015; Yang et al., 2020). Our experimental results showed that SSd intervention accelerated the degradation of deposited ECM, inhibited and reversed the process of EMT, and had anti-fibrosis effects. Early interventions can effectively alleviate liver fibrosis. Radix Bupleuri, the dry root of Bupleurum Chinense DC, has been characterized as a hepatoprotective herbal medicine that can treat various liver diseases, such as chronic hepatic inflammation, liver cirrhosis, hepatocellular carcinoma, and viral hepatitis (Li et al., 2018; Tian et al., 2019). SSd is one of the pharmacologically active compounds of Bupleurum. Recently, the anti-inflammatory, anti-fibrotic, anti-tumor, and hepatoprotective effects of SSd observed both in vivo and in vitro have attracted widespread attention (Xie et al., 2012; Yuan et al., 2017; Cui et al., 2019). A previous study showed that SSd could prevent hepatic inflammation and liver injury in acetaminophen-induced mice (Liu et al., 2014). The study showed that SSd reduced the infiltration and proliferation of CD8+ T cells, increased the expression of anti-inflammatory cytokines, inhibited the expression of TGF-β1, and ultimately alleviated inflammation and fibrosis in a mouse model of glomerulonephritis (Li et al., 2005). Furthermore, studies have shown that SSd can inhibit the activation of HSCs by regulating intracellular inflammatory responses, thereby eventually inhibiting liver fibrosis (Chen et al., 2013). Our previous studies also confirmed that SSd had a protective effect on CCl4-induced acute liver injury (Chen et al., 2020). CCl4-induced chronic liver injury and liver fibrosis model mice were treated with SSd, and found that liver injury and liver fibrosis were significantly alleviated by SSd treatment compared with the control group.
Activation of the NLRP3 inflammasome has been shown to cause liver injury and hepatic fibrosis in various acute and chronic liver diseases (Wree et al., 2014). Key components of a functional NLRP3 inflammasome are NLRP3, the adaptor protein ASC and caspase-1. NLRP3 recruits ASC and procaspase-1, which results in caspase-1 activation and processing of cytoplasmic targets, including the pro-inflammatory cytokines IL-1β and IL-18, whose purpose being to drive the inflammatory process and restore homeostasis. Mostly, priming is provided by signals of tissue damage activation downstream of pattern recognition receptors and is thought to be required both for NLRP3 and pro-IL-1β induction (Haneklaus and O'Neill, 2015). Active caspase-1 cleaves the cytokines pro-IL-1β and pro-IL-18 into their mature and biologically active forms (Martinon et al., 2002). Maria et al. introduced an NLRP3−/− mouse model and confirmed that the NLRP3 inflammasome could directly activate HSCs and trigger liver fibrosis (Inzaugarat et al., 2019). Our experimental results confirmed that the NLRP3 inflammasome was overexpressed and activated during the process of liver fibrosis, suggesting that the increased levels of NLRP3 inflammasome proteins were associated with liver injury, liver fibrosis and HSCs activation. According to a previous study, mitochondrial ROS is the main activator of the NLRP3 inflammasome (Scambler et al., 2019). ROS can induce chronic inflammation and fibrous hyperplasia, leading to the activation of HSCs and finally promoting fibrosis (Dickie et al., 2012; Chen et al., 2019). In addition, oxidative stress significantly contributes to HSCs activation and fibrosis (Lin et al., 2020). Consistent with the findings in literature, our analyses of mitochondrial functions in vivo and in vitro confirmed that the production of mitochondrial ROS increased in CCl4-induced liver fibrosis mice and H2O2-induced activated HSCs. Therefore, reducing oxidative stress and eliminating ROS have been promising treatment strategies for liver fibrosis. SSd possesses anti-oxidative stress effects. According to a previous study, SSd could prevent oxidative damage, oxidative stress, and cytotoxicity in neural cells (Lin et al., 2016). SSd can reverse the impaired hepatic activity of superoxide dismutase, improve liver antioxidant capacity, inhibit lipid peroxidation, eliminate ROS, and ultimately prevent oxidative stress and liver injury (Fan et al., 2007). It was found that SSd significantly protected HL-7702 cells against CCl4-mediated oxidative stress and inhibited NLRP3 inflammasome-induced inflammation (Lin et al., 2018). Consistent with these findings, our study also confirmed that mitochondrial oxidative stress could activate HSCs and the NLRP3 inflammasome, which played an important role in the development of liver fibrosis. In this study, it was also focused on the correlation between SSd treatment and activation of the ROS/NLRP3 inflammasome axis. It was found that SSd treatment inhibited mitochondrial oxidative stress, reduced the production of mitochondrial ROS, downregulated the expression of NLRP3 inflammasome proteins and pro-IL-1β, IL-1β, and IL-18 induction, and eventually protected liver from injury and fibrosis.
Estradiol acts by binding to and activating different subtypes of estrogen receptors, including the two classical receptors ERα and ERβ, and the G protein-coupled estrogen receptor on the plasma membrane (Revankar et al., 2005; Dahlman-Wright et al., 2006). ERα and ERβ receptors can be detected in many tissues, but their distributions and expression levels are different. In liver cells, ERα is the predominant ER subtype, while ERβ is expressed in a variety of tissues during human fetal development; HSCs have been demonstrated to possess functional ERβ but not ERα; these findings suggest different organ-specific roles for the ERs (Brandenberger et al., 1997; Zhou et al., 2001; Gao et al., 2008). Some studies have shown that the function of E2 in preventing liver inflammation is mediated by ERα (Evans et al., 2002). Preclinical findings in rodents demonstrate that endogenous estradiol can prevent steatosis, insulin resistance, and fibrosis in liver diseases by activating the ER signal pathways (Grossmann et al., 2019). ERβ2 can lead to suppression of the ERα signaling (Zhao et al., 2007). ERβ may inhibit EMT (Mak et al., 2010) and effectively improve cirrhosis with portal hypertension in ovariectomized rats (Zhang et al., 2016). Zhang et al. found that selective agonists for ERβ exert antifibrogenic effects by inhibiting the activation and proliferation of HSCs (Zhang et al., 2018). These effects were obviously mediated by ERβ, but not ERα or GPER. SSd has estrogen-like effects in vivo and in vitro, and is an ER modulator (Wang et al., 2010). Liu et al. demonstrated that SSd improved memory in rats by modulating ERα activation, but not E2 level or ERβ activity, in the hippocampus (Liu et al., 2019). Que et al. found that SSd could inhibit oxidative stress-induced activation of HSCs, an effect that depended on the regulation of ERβ (21). Therefore, it can be seen that SSd activates estrogen ligands in difference organs and tissues with different functions. To this end, we introduced ERKO mice to verify that the anti-fibrosis effects of SSd were mediated by the ERβ pathway.
Studies have shown that E2 is a strong endogenous antioxidant that inhibits oxidative stress-induced lipid peroxidation and ROS production in rat liver mitochondria (Yagi, 1997; Omoya et al., 2001). Simvastatin could activate the expression of ERα and ERβ, thereby inhibiting the activation of the NLRP3 inflammasome and decreasing the levels of pro-inflammatory mediators (Menze et al., 2021). 17β-estradiol can reduce NLRP3 inflammasome activation to abrogate airway inflammation (Cheng et al., 2019). However, it has also been reported that E2 significantly inhibited the malignant behaviors of hepatocellular carcinoma cells through E2/ERβ pathway-mediated upregulation of the NLRP3 inflammasome (Wei et al., 2015). So far, a variety of estrogen receptor modulators have been found, such as soybean isoflavones, lignans, stilbenes, resveratrol, ginsenosides and SSd (Chan et al., 2002; Dixon, 2004). However, studies have shown that phytoestrogens have different affinities and transactivating activities for ERα and ERβ. For example, genistein and coumestrol preferentially interact with and activate ERβ rather than ERα to mediate estrogenic effects (Gutendorf and Westendorf, 2001). In addition, a previous study showed that SSd could upregulate the expression of ERα in the hippocampus of ovariectomized rats, but had no significant effect on the expression of ERβ (Liu et al., 2019). Moreover, they can inhibit the expression of NLRP3, HSCs activation, and liver fibrosis by acting on different estrogen ligands. Soybean isoflavones can alleviate DSS-induced colitis by inhibiting the ERα pathway and downregulating the subsequent activation of the NLRP3 inflammasome (Gao et al., 2020). Phytoestrogen calycosin inhibits the activation, proliferation, and migration of HSCs induced by TGF-β1 via downregulating ERβ (Deng et al., 2018). Comprehensive studies have shown that the mechanism of interaction between estrogen regulators and ligands is complex, and a specific regulator may even have opposite effects under different circumstances. Therefore, we used ER knockout mice to carry out experiments, and obtained strong evidence to support that SSd has ERβ pathway-dependent anti-liver fibrosis effects, such as inhibiting the expression of NLRP3 inflammasome proteins. By treating HSCs with ERα and ERβ blockers and establishing αERKO and βERKO knockout liver fibrosis mouse models, we found that the experimental results obtained from mouse and cellular model groups were not significantly different from those obtained from the THC intervention group and the βERKO mouse group, while there were significant differences between the MPP intervention group, the αERKO mouse group and the control group. These findings suggested that SSd inhibited HSCs activation through the ERβ pathway, and alleviated liver injury and liver fibrosis by negatively regulating the ROS/NLRP3 inflammasome. Thus, we confirmed a new molecular mechanism underlying SSd’s anti-fibrosis effects—by negatively regulating the ROS/NLRP3 inflammasome through activating the ERβ pathway.
Collectively, the results of our study demonstrate SSd suppresses hepatic injure and fibrosis by exerting inhibiting mitochondrial injury effect that inhibit the activation of NLRP3 inflammasome, which in turn leads to inhibition of HSCs activation and proliferation. This suggests SSd may be an effective therapeutic agent for the treatment of hepatic fibrosis.
The original contributions presented in the study are included in the article/Supplementary Material, further inquiries can be directed to the corresponding author.
The animal study was reviewed and approved by Shanghai Municipal Hospital of Traditional Chinese Medicine Ethics Committee.
KZ conducted experimental operation, performed data acquisition and analysis and wrote the manuscript. LL made contributions in the revision stage of the article. YZ contributed to the correction of article writing. NZ and MEZ performed part of the experimental operations and data collection. Others had great help to the author in terms of articles logic and writing. YL conceived the study design and is the guarantor of the article.
This work was supported by the National Natural Science Foundation of China (NO. 81573775; NO. 81873157); Shanghai Natural Science Foundation (NO. 22ZR1459400); Future Plan for Traditional Chinese Medicine development of Science and Technology of Shanghai Municipal Hospital of Traditional Chinese Medicine (WL-HBQN-2021005K). The afore-mentioned support agents provided financial support only and had no role in study design, data collection, data analysis, data interpretation, decision to publish, or preparation of the manuscript.
The authors declare that the research was conducted in the absence of any commercial or financial relationships that could be construed as a potential conflict of interest.
All claims expressed in this article are solely those of the authors and do not necessarily represent those of their affiliated organizations, or those of the publisher, the editors and the reviewers. Any product that may be evaluated in this article, or claim that may be made by its manufacturer, is not guaranteed or endorsed by the publisher.
The Supplementary Material for this article can be found online at: https://www.frontiersin.org/articles/10.3389/fphar.2022.894981/full#supplementary-material
CCl4, carbon tetrachloride; ER-β, estrogen receptor β; ERKO, estrogen receptors knockout; HSCs, hepatic stellate cells; mtROS, mitochondrial reactive oxygen species; MitoSOX Red, MitoSOX Red mitochondrial superoxide indication; NLRP3, NLR family, domain containing protein 3; SSd, saikosaponind d.
Abais, J. M., Xia, M., Zhang, Y., Boini, K. M., and Li, P. L. (2015). Redox Regulation of NLRP3 Inflammasomes: ROS as Trigger or Effector? Antioxid. Redox Signal 22 (13), 1111–1129. doi:10.1089/ars.2014.5994
Böttcher, K., and Pinzani, M. (2017). Pathophysiology of Liver Fibrosis and the Methodological Barriers to the Development of Anti-fibrogenic Agents. Adv. drug Deliv. Rev. 121, 3–8. doi:10.1016/j.addr.2017.05.016
Brandenberger, A. W., Tee, M. K., Lee, J. Y., Chao, V., and Jaffe, R. B. (1997). Tissue Distribution of Estrogen Receptors Alpha (ER-Alpha) and Beta (ER-Beta) mRNA in the Midgestational Human Fetus. J. Clin. Endocrinol. Metab. 82 (10), 3509–3512. doi:10.1210/jcem.82.10.4400
Chan, R. Y., Chen, W. F., Dong, A., Guo, D., and Wong, M. S. (2002). Estrogen-like Activity of Ginsenoside Rg1 Derived from Panax Notoginseng. J. Clin. Endocrinol. Metab. 87 (8), 3691–3695. doi:10.1210/jcem.87.8.8717
Chen, M. F., Huang, C. C., Liu, P. S., Chen, C. H., and Shiu, L. Y. (2013). Saikosaponin a and Saikosaponin D Inhibit Proliferation and Migratory Activity of Rat HSC-T6 Cells. J. Med. Food 16 (9), 793–800. doi:10.1089/jmf.2013.2762
Chen, R. J., Wu, H. H., and Wang, Y. J. (2015). Strategies to Prevent and Reverse Liver Fibrosis in Humans and Laboratory Animals. Arch. Toxicol. 89 (10), 1727–1750. doi:10.1007/s00204-015-1525-6
Chen, Y., Que, R., Lin, L., Shen, Y., Liu, J., and Li, Y. (2020). Inhibition of Oxidative Stress and NLRP3 Inflammasome by Saikosaponin-D Alleviates Acute Liver Injury in Carbon Tetrachloride-Induced Hepatitis in Mice. Int. J. Immunopathol. Pharmacol. 34, 2058738420950593. doi:10.1177/2058738420950593
Chen, Y., Zhao, C., Liu, X., Wu, G., Zhong, J., Zhao, T., et al. (2019). Plumbagin Ameliorates Liver Fibrosis via a ROS-Mediated NF-Кb Signaling Pathway In Vitro and In Vivo. Biomed. Pharmacother. 116, 108923. doi:10.1016/j.biopha.2019.108923
Cheng, C., Wu, H., Wang, M., Wang, L., Zou, H., Li, S., et al. (2019). Estrogen Ameliorates Allergic Airway Inflammation by Regulating Activation of NLRP3 in Mice. Biosci. Rep. 39 (1). doi:10.1042/bsr20181117
Cheng, F., Shen, Y., Mohanasundaram, P., Lindström, M., Ivaska, J., Ny, T., et al. (2016). Vimentin Coordinates Fibroblast Proliferation and Keratinocyte Differentiation in Wound Healing via TGF-β-Slug Signaling. Proc. Natl. Acad. Sci. U. S. A. 113 (30), E4320–E4327. doi:10.1073/pnas.1519197113
Cicchini, C., Amicone, L., Alonzi, T., Marchetti, A., Mancone, C., and Tripodi, M. (2015). Molecular Mechanisms Controlling the Phenotype and the EMT/MET Dynamics of Hepatocyte. Liver Int. 35 (2), 302–310. doi:10.1111/liv.12577
Cui, L. H., Li, C. X., Zhuo, Y. Z., Yang, L., Cui, N. Q., and Zhang, S. K. (2019). Saikosaponin D Ameliorates Pancreatic Fibrosis by Inhibiting Autophagy of Pancreatic Stellate Cells via PI3K/Akt/mTOR Pathway. Chem. Biol. Interact. 300, 18–26. doi:10.1016/j.cbi.2019.01.005
Dahlman-Wright, K., Cavailles, V., Fuqua, S. A., Jordan, V. C., Katzenellenbogen, J. A., Korach, K. S., et al. (2006). International Union of Pharmacology. LXIV. Estrogen Receptors. Pharmacol. Rev. 58 (4), 773–781. doi:10.1124/pr.58.4.8
Dang, S. S., Wang, B. F., Cheng, Y. A., Song, P., Liu, Z. G., and Li, Z. F. (2007). Inhibitory Effects of Saikosaponin-D on CCl4-Induced Hepatic Fibrogenesis in Rats. World J. Gastroenterol. 13 (4), 557–563. doi:10.3748/wjg.v13.i4.557
Deng, T., Liu, J., Zhang, M., Wang, Y., Zhu, G., and Wang, J. (2018). Inhibition Effect of Phytoestrogen Calycosin on TGF-Β1-Induced Hepatic Stellate Cell Activation, Proliferation, and Migration via Estrogen Receptor β. Can. J. Physiol. Pharmacol. 96 (12), 1268–1275. doi:10.1139/cjpp-2018-0474
Dickie, L. J., Aziz, A. M., Savic, S., Lucherini, O. M., Cantarini, L., Geiler, J., et al. (2012). Involvement of X-Box Binding Protein 1 and Reactive Oxygen Species Pathways in the Pathogenesis of Tumour Necrosis Factor Receptor-Associated Periodic Syndrome. Ann. Rheum. Dis. 71 (12), 2035–2043. doi:10.1136/annrheumdis-2011-201197
Dixon, R. A. (2004). Phytoestrogens. Annu. Rev. Plant Biol. 55, 225–261. doi:10.1146/annurev.arplant.55.031903.141729
Evans, M. J., Lai, K., Shaw, L. J., Harnish, D. C., and Chadwick, C. C. (2002). Estrogen Receptor Alpha Inhibits IL-1beta Induction of Gene Expression in the Mouse Liver. Endocrinology 143 (7), 2559–2570. doi:10.1210/endo.143.7.8919
Fan, J., Li, X., Li, P., Li, N., Wang, T., Shen, H., et al. (2007). Saikosaponin-d Attenuates the Development of Liver Fibrosis by Preventing Hepatocyte Injury. Biochem. Cell Biol. 85 (2), 189–195. doi:10.1139/o07-010
Fattovich, G., Stroffolini, T., Zagni, I., and Donato, F. (2004). Hepatocellular Carcinoma in Cirrhosis: Incidence and Risk Factors. Gastroenterology 127 (5 Suppl. 1), S35–S50. doi:10.1053/j.gastro.2004.09.014
Friedman, S. L. (2007). Reversibility of Hepatic Fibrosis and Cirrhosis-Iis it All Hype? Nat. Clin. Pract. Gastroenterol. Hepatol. 4 (5), 236–237. doi:10.1038/ncpgasthep0813
Gao, H., Fält, S., Sandelin, A., Gustafsson, J. A., and Dahlman-Wright, K. (2008). Genome-wide Identification of Estrogen Receptor Alpha-Binding Sites in Mouse Liver. Mol. Endocrinol. 22 (1), 10–22. doi:10.1210/me.2007-0121
Gao, X., Fan, W., Tan, L., Shi, Y., Ding, C., Liu, S., et al. (2020). Soy Isoflavones Ameliorate Experimental Colitis by Targeting ERα/NLRP3 Inflammasome Pathways. J. Nutr. Biochem. 83, 108438. doi:10.1016/j.jnutbio.2020.108438
Grossmann, M., Wierman, M. E., Angus, P., and Handelsman, D. J. (2019). Reproductive Endocrinology of Nonalcoholic Fatty Liver Disease. Endocr. Rev. 40 (2), 417–446. doi:10.1210/er.2018-00158
Gutendorf, B., and Westendorf, J. (2001). Comparison of an Array of In Vitro Assays for the Assessment of the Estrogenic Potential of Natural and Synthetic Estrogens, Phytoestrogens and Xenoestrogens. Toxicology 166, 79–89. doi:10.1016/s0300-483x(01)00437-1
Haneklaus, M., and O'Neill, L. A. (2015). NLRP3 at the Interface of Metabolism and Inflammation. Immunol. Rev. 265 (1), 53–62. doi:10.1111/imr.12285
Hernandez-Gea, V., and Friedman, S. L. (2011). Pathogenesis of Liver Fibrosis. Annu. Rev. Pathol. 6, 425–456. doi:10.1146/annurev-pathol-011110-130246
Inzaugarat, M. E., Johnson, C. D., Holtmann, T. M., McGeough, M. D., Trautwein, C., Papouchado, B. G., et al. (2019). NLR Family Pyrin Domain-Containing 3 Inflammasome Activation in Hepatic Stellate Cells Induces Liver Fibrosis in Mice. Hepatology 69 (2), 845–859. doi:10.1002/hep.30252
Kim, J. W., Roh, Y. S., Jeong, H., Yi, H. K., Lee, M. H., Lim, C. W., et al. (2018). Spliceosome-Associated Protein 130 Exacerbates Alcohol-Induced Liver Injury by Inducing NLRP3 Inflammasome-Mediated IL-1β in Mice. Am. J. Pathol. 188 (4), 967–980. doi:10.1016/j.ajpath.2017.12.010
Lee, Y. H., Son, J. Y., Kim, K. S., Park, Y. J., Kim, H. R., Park, J. H., et al. (2019). Estrogen Deficiency Potentiates Thioacetamide-Induced Hepatic Fibrosis in Sprague-Dawley Rats. Int. J. Mol. Sci. 20 (15). doi:10.3390/ijms20153709
Li, P., Gong, Y., Zu, N., Li, Y., Wang, B., and Shimizu, F. (2005). Therapeutic Mechanism of Saikosaponin-D in Anti-thy1 mAb 1-22-3-induced Rat Model of Glomerulonephritis. Nephron Exp. Nephrol. 101 (4), e111–8. doi:10.1159/000087437
Li, X., Li, X., Huang, N., Liu, R., and Sun, R. (2018). A Comprehensive Review and Perspectives on Pharmacology and Toxicology of Saikosaponins. Phytomedicine 50, 73–87. doi:10.1016/j.phymed.2018.09.174
Lin, L., Gong, H., Li, R., Huang, J., Cai, M., Lan, T., et al. (2020). Nanodrug with ROS and pH Dual-Sensitivity Ameliorates Liver Fibrosis via Multicellular Regulation. Adv. Sci. (Weinh) 7 (7), 1903138. doi:10.1002/advs.201903138
Lin, L., Que, R., Shen, Y., Chen, Y., Yan, N., and Li, Y. (2018). Saikosaponin-d A-lleviates C-arbon-tetrachloride I-nduced A-cute H-epatocellular I-njury by I-nhibiting O-xidative S-tress and NLRP3 I-nflammasome A-ctivation in the HL-7702 C-ell L-ine. Mol. Med. Rep. 17 (6), 7939–7946. doi:10.3892/mmr.2018.8849
Lin, X., Wu, S., Wang, Q., Shi, Y., Liu, G., Zhi, J., et al. (2016). Saikosaponin-D Reduces H2O2-Induced PC12 Cell Apoptosis by Removing ROS and Blocking MAPK-dependent Oxidative Damage. Cell Mol. Neurobiol. 36 (8), 1365–1375. doi:10.1007/s10571-016-0336-5
Liu, A., Tanaka, N., Sun, L., Guo, B., Kim, J. H., Krausz, K. W., et al. (2014). Saikosaponin D Protects against Acetaminophen-Induced Hepatotoxicity by Inhibiting NF-Κb and STAT3 Signaling. Chem. Biol. Interact. 223, 80–86. doi:10.1016/j.cbi.2014.09.012
Liu, L., Yan, J., Ge, F., Xu, X., Lu, J., Shi, H., et al. (2019). Saikosaponin-D I-mproves F-ear M-emory D-eficits in O-variectomized R-ats via the A-ction of E-strogen R-eceptor-α in the hippocampus. Mol. Med. Rep. 20 (1), 332–340. doi:10.3892/mmr.2019.10232
Lu, M., Wu, J., Hao, Z. W., Shang, Y. K., Xu, J., Nan, G., et al. (2018). Basolateral CD147 Induces Hepatocyte Polarity Loss by E-Cadherin ubiquitination and Degradation in Hepatocellular Carcinoma Progress. Hepatology 68 (1), 317–332. doi:10.1002/hep.29798
Mak, P., Leav, I., Pursell, B., Bae, D., Yang, X., Taglienti, C. A., et al. (2010). ERbeta Impedes Prostate Cancer EMT by Destabilizing HIF-1alpha and Inhibiting VEGF-Mediated Snail Nuclear Localization: Implications for Gleason Grading. Cancer Cell 17 (4), 319–332. doi:10.1016/j.ccr.2010.02.030
Martinon, F., Burns, K., and Tschopp, J. (2002). The Inflammasome: a Molecular Platform Triggering Activation of Inflammatory Caspases and Processing of proIL-Beta. Mol. Cell 10 (2), 417–426. doi:10.1016/s1097-2765(02)00599-3
Mederacke, I., Hsu, C. C., Troeger, J. S., Huebener, P., Mu, X., Dapito, D. H., et al. (2013). Fate Tracing Reveals Hepatic Stellate Cells as Dominant Contributors to Liver Fibrosis Independent of its Aetiology. Nat. Commun. 4, 2823. doi:10.1038/ncomms3823
Menze, E. T., Ezzat, H., Shawky, S., Sami, M., Selim, E. H., Ahmed, S., et al. (2021). Simvastatin Mitigates Depressive-like Behavior in Ovariectomized Rats: Possible Role of NLRP3 Inflammasome and Estrogen Receptors' Modulation. Int. Immunopharmacol. 95, 107582. doi:10.1016/j.intimp.2021.107582
Mohamed, I. N., Sarhan, N. R., Eladl, M. A., El-Remessy, A. B., and El-Sherbiny, M. (2018). Deletion of Thioredoxin-Interacting Protein Ameliorates High Fat Diet-Induced Non-alcoholic Steatohepatitis through Modulation of Toll-like Receptor 2-NLRP3-Inflammasome axis: Histological and Immunohistochemical Study. Acta histochem. 120 (3), 242–254. doi:10.1016/j.acthis.2018.02.006
Ning, Z. W., Luo, X. Y., Wang, G. Z., Li, Y., Pan, M. X., Yang, R. Q., et al. (2017). MicroRNA-21 Mediates Angiotensin II-Induced Liver Fibrosis by Activating NLRP3 Inflammasome/IL-1β Axis via Targeting Smad7 and Spry1. Antioxid. Redox Signal 27 (1), 1–20. doi:10.1089/ars.2016.6669
Omoya, T., Shimizu, I., Zhou, Y., Okamura, Y., Inoue, H., Lu, G., et al. (2001). Effects of Idoxifene and Estradiol on NF-kappaB Activation in Cultured Rat Hepatocytes Undergoing Oxidative Stress. Liver 21 (3), 183–191. doi:10.1034/j.1600-0676.2001.021003183.x
Parola, M., and Pinzani, M. (2019). Liver Fibrosis: Pathophysiology, Pathogenetic Targets and Clinical Issues. Mol. Asp. Med. 65, 37–55. doi:10.1016/j.mam.2018.09.002
Pinzani, M. (2015). Pathophysiology of Liver Fibrosis. Dig. Dis. 33 (4), 492–497. doi:10.1159/000374096
Que, R., Shen, Y., Ren, J., Tao, Z., Zhu, X., and Li, Y. (2018). Estrogen Receptor-β-dependent E-ffects of S-aikosaponin-d on the S-uppression of O-xidative S-tress-induced R-at H-epatic S-tellate C-ell A-ctivation. Int. J. Mol. Med. 41 (3), 1357–1364. doi:10.3892/ijmm.2017.3349
Ray, K. (2014). Liver: Hepatic Stellate Cells Hold the Key to Liver Fibrosis. Nat. Rev. Gastroenterol. Hepatol. 11 (2), 74. doi:10.1038/nrgastro.2013.244
Revankar, C. M., Cimino, D. F., Sklar, L. A., Arterburn, J. B., and Prossnitz, E. R. (2005). A Transmembrane Intracellular Estrogen Receptor Mediates Rapid Cell Signaling. Science 307 (5715), 1625–1630. doi:10.1126/science.1106943
Ruaro, B., Salton, F., Braga, L., Wade, B., Confalonieri, P., Volpe, M. C., et al. (2021). The History and Mystery of Alveolar Epithelial Type II Cells: Focus on Their Physiologic and Pathologic Role in Lung. Int. J. Mol. Sci. 22 (5). doi:10.3390/ijms22052566
Scambler, T., Jarosz-Griffiths, H. H., Lara-Reyna, S., Pathak, S., Wong, C., Holbrook, J., et al. (2019). ENaC-mediated Sodium Influx Exacerbates NLRP3-dependent Inflammation in Cystic Fibrosis. eLife 8, e49248. doi:10.7554/eLife.49248
Tian, Y. D., Lin, S., Yang, P. T., Bai, M. H., Jin, Y. Y., Min, W. L., et al. (2019). Saikosaponin-d Increases the Radiosensitivity of Hepatoma Cells by Adjusting Cell Autophagy. J. Cancer 10 (20), 4947–4953. doi:10.7150/jca.30286
Wang, P., Ren, J., Tang, J., Zhang, D., Li, B., and Li, Y. (2010). Estrogen-like Activities of Saikosaponin-D In Vitro: a Pilot Study. Eur. J. Pharmacol. 626 (2-3), 159–165. doi:10.1016/j.ejphar.2009.09.047
Wei, Q., Guo, P., Mu, K., Zhang, Y., Zhao, W., Huai, W., et al. (2015). Estrogen Suppresses Hepatocellular Carcinoma Cells through ERβ-Mediated Upregulation of the NLRP3 Inflammasome. Lab. Invest. 95 (7), 804–816. doi:10.1038/labinvest.2015.63
Wree, A., Eguchi, A., McGeough, M. D., Pena, C. A., Johnson, C. D., Canbay, A., et al. (2014). NLRP3 Inflammasome Activation Results in Hepatocyte Pyroptosis, Liver Inflammation, and Fibrosis in Mice. Hepatology 59 (3), 898–910. doi:10.1002/hep.26592
Xie, J. Y., Di, H. Y., Li, H., Cheng, X. Q., Zhang, Y. Y., and Chen, D. F. (2012). Bupleurum Chinense DC Polysaccharides Attenuates Lipopolysaccharide-Induced Acute Lung Injury in Mice. Phytomedicine 19 (2), 130–137. doi:10.1016/j.phymed.2011.08.057
Yagi, K. (1997). Female Hormones Act as Natural Antioxidants-Aa Survey of Our Research. Acta Biochim. Pol. 44 (4), 701–709. doi:10.18388/abp.1997_4372
Yang, J., Antin, P., Berx, G., Blanpain, C., Brabletz, T., Bronner, M., et al. (2020). Guidelines and Definitions for Research on Epithelial-Mesenchymal Transition. Nat. Rev. Mol. Cell Biol. 21 (6), 341–352. doi:10.1038/s41580-020-0237-9
Yuan, B., Yang, R., Ma, Y., Zhou, S., Zhang, X., and Liu, Y. (2017). A Systematic Review of the Active Saikosaponins and Extracts Isolated from Radix Bupleuri and Their Applications. Pharm. Biol. 55 (1), 620–635. doi:10.1080/13880209.2016.1262433
Zahid, A., Li, B., Kombe, A. J. K., Jin, T., and Tao, J. (2019). Pharmacological Inhibitors of the NLRP3 Inflammasome. Front. Immunol. 10, 2538. doi:10.3389/fimmu.2019.02538
Zhang, B., Zhang, C. G., Ji, L. H., Zhao, G., and Wu, Z. Y. (2018). Estrogen Receptor β Selective Agonist Ameliorates Liver Cirrhosis in Rats by Inhibiting the Activation and Proliferation of Hepatic Stellate Cells. J. Gastroenterol. Hepatol. 33 (3), 747–755. doi:10.1111/jgh.13976
Zhang, C. G., Zhang, B., Deng, W. S., Duan, M., Chen, W., and Wu, Z. Y. (2016). Role of Estrogen Receptor β Selective Agonist in Ameliorating Portal Hypertension in Rats with CCl4-Induced Liver Cirrhosis. World J. Gastroenterol. 22 (18), 4484–4500. doi:10.3748/wjg.v22.i18.4484
Zhao, C., Matthews, J., Tujague, M., Wan, J., Ström, A., Toresson, G., et al. (2007). Estrogen Receptor Beta2 Negatively Regulates the Transactivation of Estrogen Receptor Alpha in Human Breast Cancer Cells. Cancer Res. 67 (8), 3955–3962. doi:10.1158/0008-5472.Can-06-3505
Zhao, Y., Wang, Z., Feng, D., Zhao, H., Lin, M., Hu, Y., et al. (2019). p66Shc Contributes to Liver Fibrosis through the Regulation of Mitochondrial Reactive Oxygen Species. Theranostics 9 (5), 1510–1522. doi:10.7150/thno.29620
Keywords: saikosaponin d, estrogen receptors, mitochondrial reactive oxygen species, nodlike receptor protein 3 inflammasome, liver fibrosis
Citation: Zhang K, Lin L, Zhu Y, Zhang N, Zhou M and Li Y (2022) Saikosaponin d Alleviates Liver Fibrosis by Negatively Regulating the ROS/NLRP3 Inflammasome Through Activating the ERβ Pathway. Front. Pharmacol. 13:894981. doi: 10.3389/fphar.2022.894981
Received: 12 March 2022; Accepted: 09 May 2022;
Published: 25 May 2022.
Edited by:
Lei Zhang, Anhui Medical University, ChinaCopyright © 2022 Zhang, Lin, Zhu, Zhang, Zhou and Li. This is an open-access article distributed under the terms of the Creative Commons Attribution License (CC BY). The use, distribution or reproduction in other forums is permitted, provided the original author(s) and the copyright owner(s) are credited and that the original publication in this journal is cited, in accordance with accepted academic practice. No use, distribution or reproduction is permitted which does not comply with these terms.
*Correspondence: Yong Li, bGl5b25nQHNodXRjbS5lZHUuY24=
†ORCID: Kehui Zhang, orcid.org/0000-0002-3603-6553
‡These authors have contributed equally to this work
Disclaimer: All claims expressed in this article are solely those of the authors and do not necessarily represent those of their affiliated organizations, or those of the publisher, the editors and the reviewers. Any product that may be evaluated in this article or claim that may be made by its manufacturer is not guaranteed or endorsed by the publisher.
Research integrity at Frontiers
Learn more about the work of our research integrity team to safeguard the quality of each article we publish.