- 1Unit of Molecular Pharmacology, Department of Applied Research and Technological Development, Fondazione IRCCS Istituto Nazionale dei Tumori, Milan, Italy
- 2Department of Medical Biotechnology and Translational Medicine, University of Milano, Milan, Italy
Curcumin and related compounds are known for the large spectrum of activities. The chemical features of these compounds are important for their biological effects with a key role for the thiol-reactive α−β unsaturated carbonyl groups. Curcumin derivatives may overcome the limitation of the bioavailability of the parent compound, while maintaining the key chemical features responsible for biological activities. Curcumin and related compounds show anti-viral, anti-fungal, anti-microbial and anti-tumor activities. The therapeutic effects of curcumin, used as a supplement in cancer therapy, have been documented in various cancer types, in which inhibition of cell growth and survival pathways, induction of apoptosis and other cell death pathways have been reported. Curcumin-induced apoptosis has been linked both to the intrinsic and extrinsic apoptotic pathways. Necroptosis has also been involved in curcumin-induced toxicity. Among curcumin-induced effects, ferroptosis has also been described. The mechanism of curcumin toxicity can be triggered by reactive oxygen species-mediated endoplasmic reticulum stress. Curcumin targets have been identified in the context of the ubiquitin-proteasome system with evidence of inhibition of the proteasome proteolytic activities and cellular deubiquitinases. Curcumin has recently been shown to act on the tumor microenvironment with effects on cancer-associated fibroblasts and immune cells. The related product caffeic acid phenethyl ester has shown promising preclinical results with an effect on the inflammatory microenvironment. Here, we review the mechanisms underlying curcumin and derivatives toxicity towards cancer cells with particular emphasis on cell death pathways and the ubiquitin-proteasome system.
Introduction
Curcumin and related compounds including caffeic acid phenethyl ester (CAPE), and caffeic acid (Kabala-Dzik et al., 2017) are bioactive compounds mainly derived from natural sources and known for years for their pleiotropic effects (Figure 1). These compounds show anti-viral, anti-fungal, anti-microbial and anti-tumor effects (Chiao et al., 1995; Beauregard et al., 2015; Balaha et al., 2021), mainly ascribable to anti-inflammatory and antioxidant activities. Curcumin product is used as cosmetic additive and dietary supplement and as herbal medicine (Hassanalilou et al., 2019).
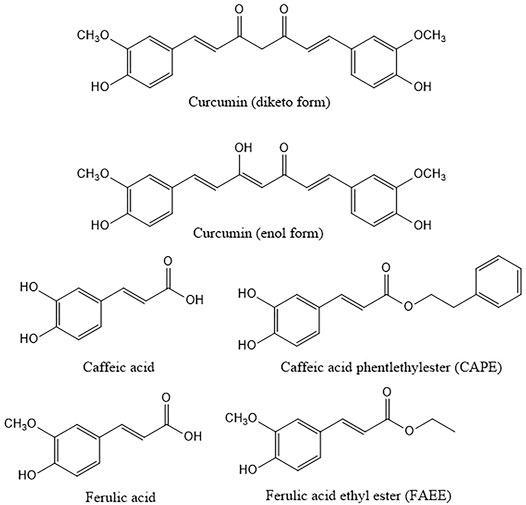
FIGURE 1. Structures of curcumin and the related compounds caffeic, ferulic acid and their ethyl and phenylethyl esters. Curcumin is here represented in both diketo and enol form the latter being the main form present in solution.
Curcumin is a yellow polyphenol component from rhizomes of Curcuma longa extracted for the first time in 1815 by Vogel and Pelletier; the structure was identified in 1973 (Kunnumakkara et al., 2017; Mbese et al., 2019). From a chemical point of view, curcumin is a ß-diketone. It consists of methane with two hydrogens substituted by feruloyl groups, i.e., dipheruloylmethane. The molecule contains a ß-diketone moiety that undergoes keto-enol tautomerization (Nelson et al., 2017). The chemical features of curcumin are important for its biological activity (Giordano and Tommonaro, 2019). Curcumin has been reported to be a pan-assay interference compound (PAINS), that means a compound with possible interference with all assays. Besides, it is an unstable molecule, characterized by reactivity and poor bioavailability. The investigation of stability under physiological and various pH conditions has revealed marked instability (Wang et al., 1997), already in the nineties. Several studies have highlighted the role of the thiol-reactive α, ß unsaturated carbonyl groups of curcumin and derivatives paying attention to the structure activity relationships (Yang et al., 2017). A key role for the methoxy group has also been proposed (Yang et al., 2017).
The search for new delivery systems for curcumin designed to overcome the low bioavailability, poor water solubility and fast metabolism is an active field of investigation (Wong et al., 2019). Liposomal curcumin has shown marked tumor growth inhibitory activity and proapoptotic effects (Feng et al., 2017). Curcumin solid dispersions have also been reported in an attempt to improve curcumin water solubility (Mohamed et al., 2020). In this context, different hydrophilic carriers have been tested and poloxamer 497 has emerged as promising, allowing curcumin to acquire satisfactory water solubility and dissolution; the complex was more effective than curcumin against colorectal cancer cells (Mohamed et al., 2020). Microemulsions are also being tested as drug carrier to improve curcumin topical delivery (Zainuddin et al., 2021). Of note, curcumin containing micelles have been proposed as a therapeutic supplement in COVID-19 patients (Hassaniazad et al., 2020). Protein/polysaccharide nanocomplexes for delivering curcumin are also being developed; for instance, ovalbumin-pullulan nanogels characterized by improved storage stability have been shown to facilitate controlled release (Zeng et al., 2022). In this regard, the different curcumin nanocarriers (nanocurcumin) can be categorized based on the ζ potential and particle size, parameters that may affect the activity in cancer and normal cells with reference to cell death pathways (Shome et al., 2016; Nasery et al., 2020). Besides, Ram and colleague reported a detailed study regarding curcumin-loaded albumin nanoparticles, determining the optimal size for maximum uptake in the target cell (Das et al., 2019). A list of different biopolymer based nanocurcumins reporting their size, ζ Potential and their effects in term of stability, bioavailability and activity on different normal and cancer cell lines and animal models is also reported in an exhaustive review by Nasery et al. (Nasery et al., 2020).
The biological activity of curcumin, observed in spite of its low stability, has been ascribed to degradation products measurable in vivo, such as ferulic acid (Shen and Ji, 2012). The esters of ferulic acid and of its metabolic precursor caffeic acid, i.e., ferulic acid ethyl ester (FAEE) and CAPE are also endowed with biological activities (Sultana et al., 2005; Olgierd et al., 2021). The latter was described for the first time in the eighties (Grunberger et al., 1988). Similarly to curcumin, CAPE has been widely investigated over the years (Chiao et al., 1995; Olgierd et al., 2021). It is a component of propolis, which is obtained from different plant parts (e.g., the bark of conifers) and carried by honeybees to the hives to be mixed with bee wax and bee salivary enzymes (Chiao et al., 1995). The available evidence suggests that it exerts a selective effect on cancer cells (Sari et al., 2020).
Several curcumin derivatives are reported in the literature (Kabała-Dzik et al., 2017; Chen et al., 2020) as well as CAPE analogs (Beauregard et al., 2015; Balaha et al., 2021). In the present manuscript we review the available knowledge on the mechanism of action of curcumin and related compounds with major emphasis on novel cell death modes and effect on the ubiquitin-proteasome system (UPS).
Mechanism of Action of Curcumin and Related Compounds
Cell response to stress occurs through different modes including apoptosis. This process which involves an extrinsic and intrinsic pathway provides opportunities to improve the activity of therapeutic agents (Carneiro and El-Deiry, 2020). Indeed, curcumin and related compounds have been regarded as modulators of apoptosis (Reuter et al., 2008) (Figure 2). Recent evidence supports that other less-investigated pathways of cell death, i.e., necroptosis and ferroptosis may play a role in cellular response to curcumin and related compound action (Bertheloot et al., 2021; Lee et al., 2021).
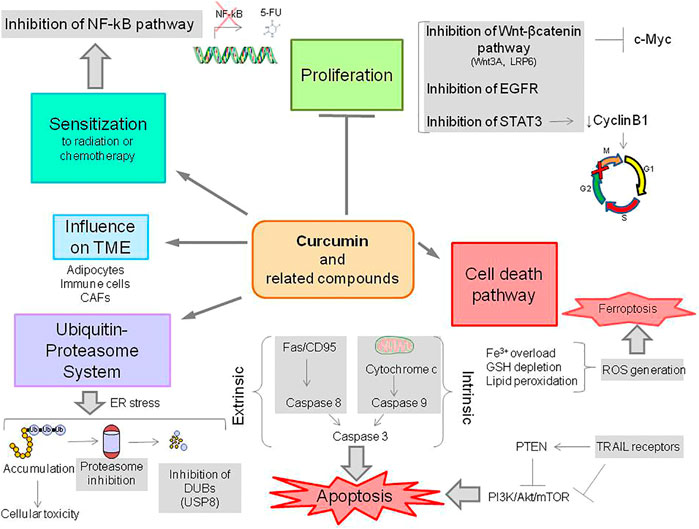
FIGURE 2. Overview of the biological activities of curcumin and related compounds. CAFs, cancer-associated fibroblasts; DUBs, deubiquitinases; ROS, reactive oxygen species; GSH, reduced glutathione; 5-FU, 5-fluorouracil.
Curcumin and derivatives have been proposed as potential therapeutic agents in selected cancer types such as prostate, colon, breast and thyroid cancer (Schwertheim et al., 2017; Mbese et al., 2019) and their anticancer effects have been tested in vitro and in vivo in combination with chemotherapeutic agents and radiotherapy (Yu et al., 2021). Curcumin has shown to amplify the anticancer effects of drugs (e.g., doxorubicin, cisplatin, gefitinib) and radiotherapy (Tan and Norhaizan, 2019; Ashrafizadeh et al., 2020). For instance, curcumin displays a synergistic effect with some chemotherapeutic agents such as 5-fluorouracil and oxaliplatin (Farhood et al., 2019) while protecting normal tissues from cell death, and therefore without side effects.
Inhibition of Cell Growth and Survival Pathways
The action of curcumin and related compounds involves a variety of cellular pathways implicated in key aspects of diseases. Curcumin inhibits the pathway of Nuclear Factor kB (NF-kB), a transcription factor that besides being involved in immune responses and inflammation, acts in regulating genes implicated in cancer development and progression (Dolcet et al., 2005). Curcumin-induced NF-kB inhibition results in sensitization of cancer cells with high expression of NF-kB to radiation or chemotherapy; for example, in cells exposed to 5-fluorouracil induction of high expression of NF-kB has been found in association with resistance to apoptosis and synergism between curcumin and chemotherapy through NF-kB inhibition has been reported for colon and colorectal cancer cell lines (Mortezaee et al., 2019a).
Inhibition of cell proliferation by curcumin has been reported in a variety of cancer cell lines, including non small cell lung cancer cells, in which curcumin-induced ferroptosis (see below) was observed (Tang et al., 2021). In gastric carcinoma cells, curcumin-induced proliferation inhibition was associated with the inhibition of the Wnt/β-catenin signaling pathway (Zheng et al., 2017). This pathway plays a crucial role in development, and mutations of Wnt pathway components are linked to growth-related diseases and cancer (Nusse and Clevers, 2017). Curcumin down-regulated Wnt/β-catenin signaling by suppressing Wnt3a and low-density lipoprotein receptor-related protein 6 (LRP6) phosphorylation - an event critical for the activation of the pathway - as well as downstream target genes contributing to tumor growth, such as c-myc (Zheng et al., 2017).
Curcumin has been shown to down-regulate Epidermal Growth Factor Receptor (EGFR) thereby inhibiting cell proliferation of multiple cancer cells, including lung cancer (Wan Mohd Tajuddin et al., 2019). Of note, a recent in vitro and in silico study suggests that curcumin and its derivatives are potential tyrosine kinase inhibitors that target EGFR (Liang et al., 2021).
The interference of curcumin with multiple cellular targets appears to underlie its growth inhibitory effect because curcumin has also been shown to decrease eukaryotic initiation factor 2 (eIF2) inhibiting translation and protein synthesis, thereby suppressing proliferation (Chen et al., 2010).
The antitumor effects of curcumin have also been associated to inhibition of Signal Transducer And Activator Of Transcription 3 (STAT3) - which is implicated in sustaining proliferation—resulting in downregulation of cyclin B1 and consequently in G2/M phase arrest in lung cancer cells (Wan Mohd Tajuddin et al., 2019).
Cell cycle-related effects of curcumin have been shown in several studies. For instance, in colon cancer cell lines, curcumin reduced cell growth, with induction of G2/M arrest, and partial G1 phase arrest through the inhibition of cyclin D1, induction of reactive oxygen species (ROS) and down-regulation of E2F4 and other cell cycle related genes (cyclin A, p21, p27) (Pricci et al., 2020).
Activation of Apoptosis and Other Cell Death Pathways
The contribution of the extrinsic and intrinsic apoptotic pathways to curcumin-induced apoptosis has been recognized in different cellular models. Activation of extrinsic or intrinsic pathways are not necessarily mutually exclusive and they may be both activated by curcumin in some cell types. The predominant apoptotic pathway targeted by curcumin may differ between cell type, differentiation stage or curcumin concentration. Curcumin has been shown to regulate the expression of TRAIL receptors, a phenomenon associated with regulation of phosphatase and tensin homolog (PTEN) and inhibition of PI3K/Akt resulting in apoptosis (Mortezaee et al., 2019b). In gastric cancer cell lines, curcumin exerts apoptotic effects through activation of the intrinsic apoptotic pathway promoting the release of cytochrome c from the mitochondria to the cytoplasm, leading to Bcl-2 decrease, Bax increase, caspase-9 and caspase-3 activation and Poly (ADP-ribose) polymerase (PARP) cleavage (Hassanalilou et al., 2019).
Induction of the intrinsic and extrinsic apoptosis pathways by curcumin occur via suppression of COX2 through deregulation of NF-kB, downregulation of Bcl-2 and upregulation of Bax and Bad proteins in lung cancer cells; suppression of the PI3K/Akt/mTOR pathway and enhanced Fas/CD95 expression and caspase-8 activity (extrinsic apoptotic pathway activation) have been observed (Wan Mohd Tajuddin et al., 2019). Curcumin-induced apoptosis has also been related to accumulation of ceramide in the membrane as a consequence of enhanced ROS production in human colon cancer cells (Mortezaee et al., 2019a).
Curcumin induces pro-apoptotic proteins while reducing antiapoptotic proteins in cancer cells, also promotes ROS production to induce apoptosis. Curcumin can also trigger endoplasmic reticulum (ER) stress response by inhibiting proteasomal activity, accumulating cytosolic Ca2+, and disrupting disulfide bonds (Reuter et al., 2008).
Besides apoptosis cells can activate additional types of cell death. Ferroptosis is a form of programmed cell death characterized by lipid peroxidation and is inducible by iron and the accumulation of ROS (Jiang et al., 2021). The available evidence suggests that curcumin action is at least in part mediated by ferroptosis. Indeed, in curcumin/curcumin analog-treated cells iron overload was observed together with reduced glutathione depletion and lipid peroxidation (Li et al., 2020; Lin et al., 2021). The phenomenon has been described to be associated with curcumin tumor growth inhibitory effect in various cancer types such as glioblastoma and lung cancer (Chen et al., 2020; Tang et al., 2021). The ferroptotic effect induced by curcumin seems to be direct or indirect, e.g. mediated by excessive authophagy (Tang et al., 2021). A protective effect of curcumin against iron toxicity and Erastin-induced ferroptosis was observed in murine MIN5 pancreatic beta cells., likely dependent on the modulation of the expression and activity of iron metabolism factors such as iron regulatory proteins (IRPs) and transferring receptor one mRNA (TfR1); cotreatment of curcumin and erastin reversed the cell death triggered by erastin and suppressed iron accumulation (Kose et al., 2019).
A cytotoxic effect has also been referred for nanocurcumin, which in general appears to be more potent than curcumin (Table 1).
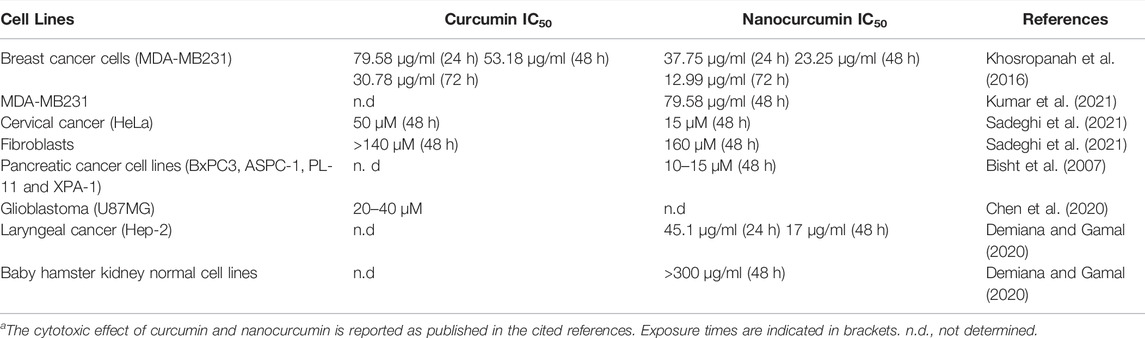
TABLE 1. Cyototoxicity of curcumin and nanocurcumina.
Regarding curcumin capability to activate cell death, the engagement of various pathways has been recently reported (Yu et al., 2021). Besides, in the literature it is widely reported that normal cells are less sensitive to curcumin treatment than cancer cells and curcumin does not induce any apoptotic death in normal cells and tissues (Syng-Ai et al., 2004). However, under selected conditions curcumin may induce cell death in normal cells in dose-dependent manner. For instance, in human osteoblast cells, treatment with curcumin (12.5–25 µM) can activate apoptosis with activation of caspase-3, c-Jun NH2-terminal Kinase (JNK) and PARP cleavage, while treatment with curcumin at higher concentrations (50–200 µM) triggers necrotic cell death. In fact, curcumin affects intracellular ATP pool and ROS generation, involved in the switching between apoptosis and necrosis. (Chan et al., 2006).
The different effects of curcumin in normal cells or cancer cells have not been fully clarified, but some reasons have been suggested, such as a higher uptake and lower glutathione levels in tumor cells as compared to normal cells as well as costitutive NF-kB activation in most tumor cells (Syng-Ai et al., 2004; Shishodia et al., 2005; Kunwar et al., 2008).
Inhibition of the Ubiquitin-Proteasome System
The UPS consists of enzymes that conjugate ubiquitin (Ub) to/from target proteins upstream of the 26S proteasome. Ub can be conjugated to target proteins as a monomer or as an Ub chain variable in length and type. Lys48-linked Ub chains target proteins to proteasomal degradation, which occurs after an ATP-dependent unfolding of the poly-ubiquitinated substrate that is translocated into the 20S catalytic core, thus becoming available to proteases. The conjugation of Ub is a controlled event involving Ub-activating, -conjugating and ligating enzymes. The reverse reaction is highly regulated and is mediated by a class of enzymes known as deubiquitinases (DUBs) that remove Ub from target proteins (Clague et al., 2019). Cancer cells display high rate of protein synthesis and depend on the UPS for their homeostasis. Indeed, protein degradation participates in the regulation of multiple functions (e.g., signal transduction, transcription, cell cycle) by controlling regulatory protein levels and prevents the accumulation of damaged/misfolded proteins in the cells. Three DUBs are proteasome-associated and their inhibition results in accumulation of poly-ubiquitinated proteins and cell toxicity (D'Arcy et al., 2011). Conversely, several non proteasome-associated DUBs remove Ub from cell survival, DNA damage repair- and apoptosis-related proteins (Clague et al., 2019).
Several reports have provided evidence that curcumin acts as a proteasome inhibitor. Indeed, inhibition of proteasomal function by curcumin has been associated with apoptosis induction through the mitochondrial pathway (Jana et al., 2004). Curcumin inhibited different enzymatic proteasomal activities such as chymotrypsin, trypsin, and post-glutamyl peptidyl-like protease activity, both in murine neuroblastoma and human cancer cells (Jana et al., 2004). Induction of stress response by curcumin has also been related to inhibition of proteasome, preventing NF-kB activation (Dikshit et al., 2006). In silico docking studies and nucleophilic susceptibility assays have shown that the carbonyl carbons of curcumin undergoes nucleophilc attach by the hydroxyl group of the N terminal threonine of the chymothrypsin-like subunit of the proteasome, thereby providing a mechanistic interpretation of the proteasome inhibitory action (Milacic et al., 2008). Consistently, curcumin treatment of colon cancer cells results in proteasome inhibition, accumulation of ubiquitinated proteins and induction of apoptosis, features also observed in tumors from xenografted mice (Milacic et al., 2008).
Besides inhibition of the 20S proteasome proteolytic activity, curcumin has been shown to inhibit cellular DUBs (Si et al., 2007), suggesting an impact of this compound on various components of the UPS. Indeed, a pharmacophore model has been proposed according to which curcumin beta-carbon may inhibit ubiquitin-isopeptidase activity (Mullally and Fitzpatrick, 2002). The chemical requirements for inhibition of DUBs are shared by curcumin and most related compounds including CAPE. In fact, a recent study support that CAPE can inihibit the DUB ubiquitin-specific protease 8, but not proteasome associated DUBs in cell-free assays, suggesting partial selectivity (Colombo et al., 2022). CAPE, similarly to what observed for curcumin, has also been shown to reduce 26S proteasome proteolytic activities, mainly affecting chymotrypsin-like activity (Matsunaga et al., 2019). In addition, the interference of curcumin with the proteasome activity has also been linked to a direct inhibition of dual-specificity tyrosine-regulated kinase two which phosphorylates the RPT3 subunit (Banerjee et al., 2018) implying the multimodal nature of proteasome inhibition by curcumin.
Effects on Tumor Microenvironment
The tumor microenvironment (TME) contributes to response to antitumor therapy. Indeed, the role of key players present in the tumor stroma such as fibroblasts, immune cells, the extracellular matrix and extracellular molecules has been widely investigated leading to the evidence that all these components participate in modulation of tumor cell response to treatment (Wu and Dai, 2017).
The antitumor effects of curcumin have been defined in a large number of in vitro and in vivo studies, mainly addressing curcumin direct activity on tumor cells with less attention to the TME. A recent study has highlighted the effects of curcumin on prostate cancer-associated fibroblasts (CAFs) in which a reduction of mitochondrial membrane potential and the promotion of the intrinsic apoptotic pathway was observed, together with cell cycle arrest (Zeng et al., 2020). Curcumin pro-apoptotic effect appeared to occur via ROS generation and ER stress involving the ROS-dependent RNA-dependent protein kinase -like ER kinase (PERK)-eIF2α-ATF4 axis (Zeng et al., 2020).
An action of curcumin on adipocytes has been reported. The treatment of preadipocytes with curcumin has a dual effect (Wu et al., 2019). Whereas a high concentration of curcumin induces apoptosis through the activation of both intrinsic and extrinsic apoptosis, a low concentration inhibits adipocyte differentiation through inhibition of cell cycle regulators and down-regulation of adipogenic transcription factors (e.g., PPARγ) (Wu et al., 2019).
Curcumin effects on immune cells have been described, with curcumin ability to regulate immune responses reducing apoptosis in natural killer cells and increasing their cytotoxic activity against cancer cells (Fiala, 2015). In addition, curcumin can potentiate cytotoxic T Lymphocites action against cancer cells and induce polarization of tumor associated macrophages towards inflammatory macrophages, thereby regulating the TME in favor of antitumor immunity (Bhattacharyya et al., 2010; Mukherjee et al., 2018).
The capability of curcumin to interfere with the microenvironment is supported by a study in which curcumin antioxidant action improves stem cell transplantation efficiency. The excess of ROS can damage the mitochondria changing the permeability with release of proapoptotic factors in the cytosol; ROS can also promote lipid peroxidation, increasing levels of malondialdehyde thereby damaging the cell membrane. Curcumin can modulate the expression of oxidative stress regulatory proteins such as SOD2 and GPX1 decreasing intracellular ROS levels (Tan et al., 2021), thereby protecting human periodontal ligament stem cells mitochondria from damage and apoptosis by reducing ROS levels and stimulating the ERK pathway (Tan et al., 2021).
Finally, curcumin related compounds such as CAPE appear also to be endowed with the capability to interfere with the TME as reported in a study showing CAPE inhibition of breast cancer cell proliferation in inflammatory microenvironment associated with activation of apoptosis and inhibition of the TLR4 signaling pathway (Chang et al., 2017).
Conclusion and Perspectives
Curcumin has been widely studied as anti-aging and chemopreventive agent (Pricci et al., 2020; Zia et al., 2021). Overall, the efficacy of curcumin has been ascribed to the modulation of signaling pathways associated with tumor development and progression via interaction with a variety of proteins involved in proliferation, apoptosis, and inflammation. Opposite effects are described depending on the context (e.g., normal versus tumor cells) (Tan et al., 2021).
A very promising aspect of research on curcumin appears the investigation of strategies to improve the molecule drawbacks or the use of alternative related compounds such as CAPE which seems to be more stable. In this context, the effects of curcumin related compounds on the TME and not only on tumor cells appears attractable. The bioavailability of curcumin, which per se cannot be considered a drug in cancer therapy, but a supplement, can be improved by encapsulation in micelles as well as in other types of nanoparticles (Tagde et al., 2021). In this context, the toxicity of surfactants used for such formulations has to be taken into account.
The identification of novel targets for curcumin and related compounds in the UPS and the elucidation of the precise mechanisms of interaction with the proteasome open new avenues to the understanding of the tumor-related effects of these compounds.
Author Contributions
PP, MC, DC, and CC conceived the study. MC, DC, and PP wrote the manuscript. MC, CC, and DC prepared the Figures. All authors contributed to manuscript revision and read and approved the submitted version.
Funding
Funding by Associazione Italiana per la Ricerca sul Cancro (AIRC), grant number 24725.
Conflict of Interest
The authors declare that the research was conducted in the absence of any commercial or financial relationships that could be construed as a potential conflict of interest.
Publisher’s Note
All claims expressed in this article are solely those of the authors and do not necessarily represent those of their affiliated organizations, or those of the publisher, the editors and the reviewers. Any product that may be evaluated in this article, or claim that may be made by its manufacturer, is not guaranteed or endorsed by the publisher.
Acknowledgments
The authors would like to acknowledge AIRC for funding a fellowship (“Le Falchette di AIRC”) to CC.
References
Ashrafizadeh, M., Najafi, M., Makvandi, P., Zarrabi, A., Farkhondeh, T., and Samarghandian, S. (2020). Versatile Role of Curcumin and its Derivatives in Lung Cancer Therapy. J. Cell Physiol. 235 (12), 9241–9268. doi:10.1002/jcp.29819
Balaha, M., De Filippis, B., Cataldi, A., and di Giacomo, V. (2021). CAPE and Neuroprotection: A Review. Biomolecules 11 (2), 176. doi:10.3390/biom11020176
Banerjee, S., Ji, C., Mayfield, J. E., Goel, A., Xiao, J., Dixon, J. E., et al. (2018). Ancient Drug Curcumin Impedes 26S Proteasome Activity by Direct Inhibition of Dual-Specificity Tyrosine-Regulated Kinase 2. Proc. Natl. Acad. Sci. U. S. A. 115 (32), 8155–8160. doi:10.1073/pnas.1806797115
Beauregard, A. P., Harquail, J., Lassalle-Claux, G., Belbraouet, M., Jean-Francois, J., Touaibia, M., et al. (2015). CAPE Analogs Induce Growth Arrest and Apoptosis in Breast Cancer Cells. Molecules 20 (7), 12576–12589. doi:10.3390/molecules200712576
Bertheloot, D., Latz, E., and Franklin, B. S. (2021). Necroptosis, Pyroptosis and Apoptosis: an Intricate Game of Cell Death. Cell Mol. Immunol. 18 (5), 1106–1121. doi:10.1038/s41423-020-00630-3
Bhattacharyya, S., Md Sakib Hossain, D., Mohanty, S., Sankar Sen, G., Chattopadhyay, S., Banerjee, S., et al. (2010). Curcumin Reverses T Cell-Mediated Adaptive Immune Dysfunctions in Tumor-Bearing Hosts. Cell Mol. Immunol. 7 (4), 306–315. doi:10.1038/cmi.2010.11
Bisht, S., Feldmann, G., Soni, S., Ravi, R., Karikar, C., Maitra, A., et al. (2007). Polymeric Nanoparticle-Encapsulated Curcumin ("nanocurcumin"): a Novel Strategy for Human Cancer Therapy. J. Nanobiotechnology 5 (5), 3. doi:10.1186/1477-3155-5-3
Carneiro, B. A., and El-Deiry, W. S. (2020). Targeting Apoptosis in Cancer Therapy. Nat. Rev. Clin. Oncol. 17 (7), 395–417. doi:10.1038/s41571-020-0341-y
Chan, W. H., Wu, H. Y., and Chang, W. H. (2006). Dosage Effects of Curcumin on Cell Death Types in a Human Osteoblast Cell Line. Food Chem. Toxicol. 44 (8), 1362–1371. doi:10.1016/j.fct.2006.03.001
Chang, H., Wang, Y., Yin, X., Liu, X., and Xuan, H. (2017). Ethanol Extract of Propolis and its Constituent Caffeic Acid Phenethyl Ester Inhibit Breast Cancer Cells Proliferation in Inflammatory Microenvironment by Inhibiting TLR4 Signal Pathway and Inducing Apoptosis and Autophagy. BMC Complement. Altern. Med. 17 (1), 471. doi:10.1186/s12906-017-1984-9
Chen, L., Tian, G., Shao, C., Cobos, E., and Gao, W. (2010). Curcumin Modulates Eukaryotic Initiation Factors in Human Lung Adenocarcinoma Epithelial Cells. Mol. Biol. Rep. 37 (7), 3105–3110. doi:10.1007/s11033-009-9888-5
Chen, T. C., Chuang, J. Y., Ko, C. Y., Kao, T. J., Yang, P. Y., Yu, C. H., et al. (2020). AR Ubiquitination Induced by the Curcumin Analog Suppresses Growth of Temozolomide-Resistant Glioblastoma through Disrupting GPX4-Mediated Redox Homeostasis. Redox Biol. 30, 101413. doi:10.1016/j.redox.2019.101413
Chiao, C., Carothers, A. M., Grunberger, D., Solomon, G., Preston, G. A., and Barrett, J. C. (1995). Apoptosis and Altered Redox State Induced by Caffeic Acid Phenethyl Ester (CAPE) in Transformed Rat Fibroblast Cells. Cancer Res. 55 (16), 3576–3583.
Clague, M. J., Urbé, S., and Komander, D. (2019). Breaking the Chains: Deubiquitylating Enzyme Specificity Begets Function. Nat. Rev. Mol. Cell Biol. 20 (6), 338–352. doi:10.1038/s41580-019-0099-1
Colombo, D., Gatti, L., Sjöstrand, L., Carenini, N., Costantino, M., Corna, E., et al. (2022). Caffeic Acid Phenethyl Ester Targets Ubiquitin-specific Protease 8 and Synergizes with Cisplatin in Endometrioid Ovarian Carcinoma Cells. Biochem. Pharmacol. 197, 114900. doi:10.1016/j.bcp.2021.114900
D'Arcy, P., Brnjic, S., Olofsson, M. H., Fryknäs, M., Lindsten, K., De Cesare, M., et al. (2011). Inhibition of Proteasome Deubiquitinating Activity as a New Cancer Therapy. Nat. Med. 17 (12), 1636–1640. doi:10.1038/nm.2536
Das, R. P., Gandhi, V. V., Singh, B. G., Kunwar, A., Kumar, N. N., and Priyadarsini, K. I. (2019). Preparation of Albumin Nanoparticles: Optimum Size for Cellular Uptake of Entrapped Drug (Curcumin). Colloids Surfaces A Physicochem. Eng. Aspects 567, 86–95. doi:10.1016/j.colsurfa.2019.01.043
Dikshit, P., Goswami, A., Mishra, A., Chatterjee, M., and Jana, N. R. (2006). Curcumin Induces Stress Response, Neurite Outgrowth and Prevent NF-kappaB Activation by Inhibiting the Proteasome Function. Neurotox. Res. 9 (1), 29–37. doi:10.1007/BF03033305
Dolcet, X., Llobet, D., Pallares, J., and Matias-Guiu, X. (2005). NF-kB in Development and Progression of Human Cancer. Virchows Arch. 446 (5), 475–482. doi:10.1007/s00428-005-1264-9
Farhood, B., Mortezaee, K., Goradel, N. H., Khanlarkhani, N., Salehi, E., Nashtaei, M. S., et al. (2019). Curcumin as an Anti-inflammatory Agent: Implications to Radiotherapy and Chemotherapy. J. Cell Physiol. 234 (5), 5728–5740. doi:10.1002/jcp.27442
Feng, T., Wei, Y., Lee, R. J., and Zhao, L. (2017). Liposomal Curcumin and its Application in Cancer. Int. J. Nanomedicine 12, 6027–6044. doi:10.2147/IJN.S132434
Fiala, M. (2015). Curcumin and Omega-3 Fatty Acids Enhance NK Cell-Induced Apoptosis of Pancreatic Cancer Cells but Curcumin Inhibits Interferon-γ Production: Benefits of Omega-3 with Curcumin against Cancer. Molecules 20 (2), 3020–3026. doi:10.3390/molecules20023020
Giordano, A., and Tommonaro, G. (2019). Curcumin and Cancer. Nutrients 11 (10), 2376. doi:10.3390/nu11102376
Grunberger, D., Banerjee, R., Eisinger, K., Oltz, E. M., Efros, L., Caldwell, M., et al. (1988). Preferential Cytotoxicity on Tumor Cells by Caffeic Acid Phenethyl Ester Isolated from Propolis. Experientia 44 (3), 230–232. doi:10.1007/BF01941717
Hanna, D. H., and Saad, G. R. (2020). Nanocurcumin: Preparation, Characterization and Cytotoxic Effects towards Human Laryngeal Cancer Cells. RSC Adv. 10, 20724–20737. doi:10.1039/D0RA03719B
Hassanalilou, T., Ghavamzadeh, S., and Khalili, L. (2019). Curcumin and Gastric Cancer: a Review on Mechanisms of Action. J. Gastrointest. Cancer 50 (2), 185–192. doi:10.1007/s12029-018-00186-6
Hassaniazad, M., Inchehsablagh, B. R., Kamali, H., Tousi, A., Eftekhar, E., Jaafari, M. R., et al. (2020). The Clinical Effect of Nano Micelles Containing Curcumin as a Therapeutic Supplement in Patients with COVID-19 and the Immune Responses Balance Changes Following Treatment: A Structured Summary of a Study Protocol for a Randomised Controlled Trial. Trials 21 (1), 876. doi:10.1186/s13063-020-04824-y
Jana, N. R., Dikshit, P., Goswami, A., and Nukina, N. (2004). Inhibition of Proteasomal Function by Curcumin Induces Apoptosis through Mitochondrial Pathway. J. Biol. Chem. 279 (12), 11680–11685. doi:10.1074/jbc.M310369200
Jiang, X., Stockwell, B. R., and Conrad, M. (2021). Ferroptosis: Mechanisms, Biology and Role in Disease. Nat. Rev. Mol. Cell Biol. 22 (4), 266–282. doi:10.1038/s41580-020-00324-8
Kabała-Dzik, A., Rzepecka-Stojko, A., Kubina, R., Jastrzębska-Stojko, Ż., Stojko, R., Wojtyczka, R., et al. (2017). Comparison of Two Components of Propolis: Caffeic Acid (CA) and Caffeic Acid Phenethyl Ester (CAPE) Induce Apoptosis and Cell Cycle Arrest of Breast Cancer Cells MDA-MB-231. Molecules 22 (9), 1554. doi:10.3390/molecules22091554
Khosropanah, M. H., Dinarvand, A., Nezhadhosseini, A., Haghighi, A., Hashemi, S., Nirouzad, F., et al. (2016). Analysis of the Antiproliferative Effects of Curcumin and Nanocurcumin in MDA-Mb231 as a Breast Cancer Cell Line. Iran. J. Pharm. Res. 15 (1), 231–239.
Kose, T., Vera-Aviles, M., Sharp, P. A., and Latunde-Dada, G. O. (2019). Curcumin and (-)- Epigallocatechin-3-Gallate Protect Murine MIN6 Pancreatic Beta-Cells against Iron Toxicity and Erastin-Induced Ferroptosis. Pharm. (Basel) 12 (1), 26. doi:10.3390/ph12010026
Kumar, V., Kumar, R., Jain, V. K., and Nagpal, S. (2021). Preparation and Characterization of Nanocurcumin Based Hybrid Virosomes as a Drug Delivery Vehicle with Enhanced Anticancerous Activity and Reduced Toxicity. Sci. Rep. 11, 368. doi:10.1038/s41598-020-79631-1
Kunnumakkara, A. B., Bordoloi, D., Harsha, C., Banik, K., Gupta, S. C., and Aggarwal, B. B. (20171979). Curcumin Mediates Anticancer Effects by Modulating Multiple Cell Signaling Pathways. Clin. Sci. (Lond) 131 (15), 1781–1799. doi:10.1042/CS20160935
Kunwar, A., Barik, A., Mishra, B., Rathinasamy, K., Pandey, R., and Priyadarsini, K. I. (2008). Quantitative Cellular Uptake, Localization and Cytotoxicity of Curcumin in Normal and Tumor Cells. Biochim. Biophys. Acta 1780 (4), 673–679. doi:10.1016/j.bbagen.2007.11.016
Lee, Y. J., Park, K. S., and Lee, S. H. (2021). Curcumin Targets Both Apoptosis and Necroptosis in Acidity-Tolerant Prostate Carcinoma Cells. Biomed. Res. Int. 2021, 8859181. doi:10.1155/2021/8859181
Li, R., Zhang, J., Zhou, Y., Gao, Q., Wang, R., Fu, Y., et al. (2020). Transcriptome Investigation and In Vitro Verification of Curcumin-Induced HO-1 as a Feature of Ferroptosis in Breast Cancer Cells. Oxid. Med. Cell Longev. 2020, 3469840. doi:10.1155/2020/3469840
Liang, Y., Zhao, J., Zou, H., Zhang, J., and Zhang, T. (2021). In Vitro and In Silico Evaluation of EGFR Targeting Activities of Curcumin and its Derivatives. Food Funct. 12 (21), 10667–10675. doi:10.1039/d1fo02002a
Lin, H., Chen, X., Zhang, C., Yang, T., Deng, Z., Song, Y., et al. (2021). EF24 Induces Ferroptosis in Osteosarcoma Cells through HMOX1. Biomed. Pharmacother. 136, 111202. doi:10.1016/j.biopha.2020.111202
Matsunaga, T., Tsuchimura, S., Azuma, N., Endo, S., Ichihara, K., and Ikari, A. (2019). Caffeic Acid Phenethyl Ester Potentiates Gastric Cancer Cell Sensitivity to Doxorubicin and Cisplatin by Decreasing Proteasome Function. Anticancer Drugs 30 (3), 251–259. doi:10.1097/CAD.0000000000000715
Mbese, Z., Khwaza, V., and Aderibigbe, B. A. (2019). Curcumin and its Derivatives as Potential Therapeutic Agents in Prostate, Colon and Breast Cancers. Molecules 24 (23), 4386. doi:10.3390/molecules24234386
Milacic, V., Banerjee, S., Landis-Piwowar, K. R., Sarkar, F. H., Majumdar, A. P., and Dou, Q. P. (2008). Curcumin Inhibits the Proteasome Activity in Human Colon Cancer Cells In Vitro and In Vivo. Cancer Res. 68 (18), 7283–7292. doi:10.1158/0008-5472.CAN-07-6246
Moballegh Nasery, M., Abadi, B., Poormoghadam, D., Zarrabi, A., Keyhanvar, P., Khanbabaei, H., et al. (2020). Curcumin Delivery Mediated by Bio-Based Nanoparticles: A Review. Molecules 25 (3), 689. doi:10.3390/molecules25030689
Mohamed, J. M. M., Alqahtani, A., Ahmad, F., Krishnaraju, V., and Kalpana, K. (2020). Stoichiometrically Governed Curcumin Solid Dispersion and its Cytotoxic Evaluation on Colorectal Adenocarcinoma Cells. Drug Des. Devel Ther. 14, 4639–4658. doi:10.2147/DDDT.S273322
Mortezaee, K., Najafi, M., Farhood, B., Ahmadi, A., Shabeeb, D., and Musa, A. E. (2019a). NF-κB Targeting for Overcoming Tumor Resistance and Normal Tissues Toxicity. J. Cell Physiol. 234 (10), 17187–17204. doi:10.1002/jcp.28504
Mortezaee, K., Salehi, E., Mirtavoos-Mahyari, H., Motevaseli, E., Najafi, M., Farhood, B., et al. (2019b). Mechanisms of Apoptosis Modulation by Curcumin: Implications for Cancer Therapy. J. Cell Physiol. 234 (8), 12537–12550. doi:10.1002/jcp.28122
Mukherjee, S., Baidoo, J. N. E., Sampat, S., Mancuso, A., David, L., Cohen, L. S., et al. (2018). Liposomal TriCurin, A Synergistic Combination of Curcumin, Epicatechin Gallate and Resveratrol, Repolarizes Tumor-Associated Microglia/Macrophages, and Eliminates Glioblastoma (GBM) and GBM Stem Cells. Molecules 23 (1), 201. doi:10.3390/molecules23010201
Mullally, J. E., and Fitzpatrick, F. A. (2002). Pharmacophore Model for Novel Inhibitors of Ubiquitin Isopeptidases that Induce P53-independent Cell Death. Mol. Pharmacol. 62 (2), 351–358. doi:10.1124/mol.62.2.351
Nelson, K. M., Dahlin, J. L., Bisson, J., Graham, J., Pauli, G. F., and Walters, M. A. (2017). The Essential Medicinal Chemistry of Curcumin. J. Med. Chem. 60 (5), 1620–1637. doi:10.1021/acs.jmedchem.6b00975
Nusse, R., and Clevers, H. (2017). Wnt/β-Catenin Signaling, Disease, and Emerging Therapeutic Modalities. Cell 169 (6), 985–999. doi:10.1016/j.cell.2017.05.016
Olgierd, B., Kamila, Ż., Anna, B., and Emilia, M. (2021). The Pluripotent Activities of Caffeic Acid Phenethyl Ester. Molecules 26 (5), 1335. doi:10.3390/molecules26051335
Pricci, M., Girardi, B., Giorgio, F., Losurdo, G., Ierardi, E., and Di Leo, A. (2020). Curcumin and Colorectal Cancer: From Basic to Clinical Evidences. Int. J. Mol. Sci. 21 (7), 2364. doi:10.3390/ijms21072364
Reuter, S., Eifes, S., Dicato, M., Aggarwal, B. B., and Diederich, M. (2008). Modulation of Anti-apoptotic and Survival Pathways by Curcumin as a Strategy to Induce Apoptosis in Cancer Cells. Biochem. Pharmacol. 76 (11), 1340–1351. doi:10.1016/j.bcp.2008.07.031
Sadeghi, R. V., Parsania, M., Sadeghizadeh, M., Haghighat, S., and Farsani, S. S. M. (2021). The Comparison of Curcumin and Nanocurcumin Effects on the Expression of E6 and E7 Human Papilloma Virus Oncogenes and P53 and pRb Factors in HeLa and Fibroblast Cell Lines. Res. Square [Online ahead of print]. doi:10.21203/rs.3.rs-254954/v1
Sari, A. N., Bhargava, P., Dhanjal, J. K., Putri, J. F., Radhakrishnan, N., Shefrin, S., et al. (2020). Combination of Withaferin-A and CAPE Provides Superior Anticancer Potency: Bioinformatics and Experimental Evidence to Their Molecular Targets and Mechanism of Action. Cancers (Basel) 12 (5), 1160. doi:10.3390/cancers12051160
Schwertheim, S., Wein, F., Lennartz, K., Worm, K., Schmid, K. W., and Sheu-Grabellus, S. Y. (2017). Curcumin Induces G2/M Arrest, Apoptosis, NF-κB Inhibition, and Expression of Differentiation Genes in Thyroid Carcinoma Cells. J. Cancer Res. Clin. Oncol. 143 (7), 1143–1154. doi:10.1007/s00432-017-2380-z
Shen, L., and Ji, H. F. (2012). The Pharmacology of Curcumin: Is it the Degradation Products? Trends Mol. Med. 18 (3), 138–144. doi:10.1016/j.molmed.2012.01.004
Shishodia, S., Amin, H. M., Lai, R., and Aggarwal, B. B. (2005). Curcumin (Diferuloylmethane) Inhibits Constitutive NF-kappaB Activation, Induces G1/S Arrest, Suppresses Proliferation, and Induces Apoptosis in Mantle Cell Lymphoma. Biochem. Pharmacol. 70 (5), 700–713. doi:10.1016/j.bcp.2005.04.043
Shome, S., Talukdar, A. D., Choudhury, M. D., Bhattacharya, M. K., and Upadhyaya, H. (2016). Curcumin as Potential Therapeutic Natural Product: a Nanobiotechnological Perspective. J. Pharm. Pharmacol. 68 (12), 1481–1500. doi:10.1111/jphp.12611
Si, X., Wang, Y., Wong, J., Zhang, J., McManus, B. M., and Luo, H. (2007). Dysregulation of the Ubiquitin-Proteasome System by Curcumin Suppresses Coxsackievirus B3 Replication. J. Virol. 81 (7), 3142–3150. doi:10.1128/JVI.02028-06
Sultana, R., Ravagna, A., Mohmmad-Abdul, H., Calabrese, V., and Butterfield, D. A. (2005). Ferulic Acid Ethyl Ester Protects Neurons against Amyloid Beta- Peptide(1-42)-Induced Oxidative Stress and Neurotoxicity: Relationship to Antioxidant Activity. J. Neurochem. 92 (4), 749–758. doi:10.1111/j.1471-4159.2004.02899.x
Syng-Ai, C., Kumari, A. L., and Khar, A. (2004). Effect of Curcumin on Normal and Tumor Cells: Role of Glutathione and Bcl-2. Mol. Cancer Ther. 3 (9), 1101–1108.
Tagde, P., Tagde, P., Islam, F., Tagde, S., Shah, M., Hussain, Z. D., et al. (2021). The Multifaceted Role of Curcumin in Advanced Nanocurcumin Form in the Treatment and Management of Chronic Disorders. Molecules 26 (23), 7109. doi:10.3390/molecules26237109
Tan, B. L., and Norhaizan, M. E. (2019). Curcumin Combination Chemotherapy: The Implication and Efficacy in Cancer. Molecules 24 (14), 2527. doi:10.3390/molecules24142527
Tan, L., Cao, Z., Chen, H., Xie, Y., Yu, L., Fu, C., et al. (2021). Curcumin Reduces Apoptosis and Promotes Osteogenesis of Human Periodontal Ligament Stem Cells under Oxidative Stress In Vitro and In Vivo. Life Sci. 270, 119125. doi:10.1016/j.lfs.2021.119125
Tang, X., Ding, H., Liang, M., Chen, X., Yan, Y., Wan, N., et al. (2021). Curcumin Induces Ferroptosis in Non-small-cell Lung Cancer via Activating Autophagy. Thorac. Cancer 12 (8), 1219–1230. doi:10.1111/1759-7714.13904
Wan Mohd Tajuddin, W. N. B., Lajis, N. H., Abas, F., Othman, I., and Naidu, R. (2019). Mechanistic Understanding of Curcumin's Therapeutic Effects in Lung Cancer. Nutrients 11 (12), 2989. doi:10.3390/nu11122989
Wang, Y. J., Pan, M. H., Cheng, A. L., Lin, L. I., Ho, Y. S., Hsieh, C. Y., et al. (1997). Stability of Curcumin in Buffer Solutions and Characterization of its Degradation Products. J. Pharm. Biomed. Anal. 15 (12), 1867–1876. doi:10.1016/s0731-7085(96)02024-9
Wong, K. E., Ngai, S. C., Chan, K. G., Lee, L. H., Goh, B. H., and Chuah, L. H. (2019). Curcumin Nanoformulations for Colorectal Cancer: A Review. Front. Pharmacol. 10, 152. doi:10.3389/fphar.2019.00152
Wu, L. Y., Chen, C. W., Chen, L. K., Chou, H. Y., Chang, C. L., and Juan, C. C. (2019). Curcumin Attenuates Adipogenesis by Inducing Preadipocyte Apoptosis and Inhibiting Adipocyte Differentiation. Nutrients 11 (10), 2307. doi:10.3390/nu11102307
Wu, T., and Dai, Y. (2017). Tumor Microenvironment and Therapeutic Response. Cancer Lett. 387, 61–68. doi:10.1016/j.canlet.2016.01.043
Yang, H., Du, Z., Wang, W., Song, M., Sanidad, K., Sukamtoh, E., et al. (2017). Structure-Activity Relationship of Curcumin: Role of the Methoxy Group in Anti-inflammatory and Anticolitis Effects of Curcumin. J. Agric. Food Chem. 65 (22), 4509–4515. doi:10.1021/acs.jafc.7b01792
Yu, C., Yang, B., and Najafi, M. (2021). Targeting of Cancer Cell Death Mechanisms by Curcumin: Implications to Cancer Therapy. Basic Clin. Pharmacol. Toxicol. 129 (6), 397–415. doi:10.1111/bcpt.13648
Zainuddin, N., Ahmad, I., Zulfakar, M. H., Kargarzadeh, H., and Ramli, S. (2021). Cetyltrimethylammonium Bromide-Nanocrystalline Cellulose (CTAB-NCC) Based Microemulsions for Enhancement of Topical Delivery of Curcumin. Carbohydr. Polym. 254, 117401. doi:10.1016/j.carbpol.2020.117401
Zeng, Q., Zeng, W., Jin, Y., and Sheng, L. (2022). Construction and Evaluation of Ovalbumin-Pullulan Nanogels as a Potential Delivery Carrier for Curcumin. Food Chem. 367, 130716. doi:10.1016/j.foodchem.2021.130716
Zeng, Y., Du, Q., Zhang, Z., Ma, J., Han, L., Wang, Y., et al. (2020). Curcumin Promotes Cancer-Associated Fibroblasts Apoptosis via ROS-Mediated Endoplasmic Reticulum Stress. Arch. Biochem. Biophys. 694, 108613. doi:10.1016/j.abb.2020.108613
Zheng, R., Deng, Q., Liu, Y., and Zhao, P. (2017). Curcumin Inhibits Gastric Carcinoma Cell Growth and Induces Apoptosis by Suppressing the Wnt/β-Catenin Signaling Pathway. Med. Sci. Monit. 23, 163–171. doi:10.12659/msm.902711
Keywords: curcumin, cancer, cell death, proteasome, deubiquitinases
Citation: Costantino M, Corno C, Colombo D and Perego P (2022) Curcumin and Related Compounds in Cancer Cells: New Avenues for Old Molecules. Front. Pharmacol. 13:889816. doi: 10.3389/fphar.2022.889816
Received: 04 March 2022; Accepted: 06 May 2022;
Published: 24 May 2022.
Edited by:
Patricia Dias Fernandes, Federal University of Rio de Janeiro, BrazilReviewed by:
Shima Tavakol, Iran University of Medical Sciences, IranCopyright © 2022 Costantino, Corno, Colombo and Perego. This is an open-access article distributed under the terms of the Creative Commons Attribution License (CC BY). The use, distribution or reproduction in other forums is permitted, provided the original author(s) and the copyright owner(s) are credited and that the original publication in this journal is cited, in accordance with accepted academic practice. No use, distribution or reproduction is permitted which does not comply with these terms.
*Correspondence: Paola Perego, paola.perego@istitutotumori.mi.it