- 1Central Laboratory, Guangzhou Panyu Central Hospital, Guangzhou, China
- 2Graduate School, Guangzhou University of Chinese Medicine, Guangzhou, China
- 3Radiology Department, Sun Yat-sen Memorial Hospital, Sun Yat-sen University, Guangzhou, China
Objective: To evaluated the efficacy and safety of noninvasive brain stimulation (NIBS) combined with antidepressants in patients with poststroke depression (PSD).
Methods: Seven databases were searched to identify randomized controlled trials of NIBS combined with antidepressants in the treatment of PSD based on the international classification of diseases (ICD-10) criteria and exclusion criteria. The retrieval time was from the database establishment to 31 October 2021. Two researchers independently screened the identified studies through the search strategy, extracted their characteristics, and evaluated the quality of the included literature. Cochrane Collaboration’s tool was used to assess risk of bias. RevMan 5.3 software was applied for meta-analysis.
Results: A total of 34 randomized controlled trials were included, involving 2,711 patients with PSD. Meta-analysis showed that the total effective rate was higher in the combined therapy than the antidepressant alone [odds ratio (OR): 4.33; 95% confidence interval (CI): 3.07 to 6.11; p < 0.00001]. The Hamilton depressive scale (HAMD) score was significantly lower in repeated transcranial magnetic stimulation (rTMS) (≤10 Hz) combined with antidepressant than in antidepressant alone [standard mean difference (SMD): −1.44; 95% CI: −1.86 to −1.03; p < 0.00001]. No significant difference was seen in rTMS (>10 Hz) combined with antidepressant versus antidepressant alone (SMD: −4.02; 95% CI: −10.43 to 2.39; p = 0.22). In addition, combination therapy more strongly improved the modified Barthel index (MBI) scale than antidepressants [mean difference (MD): 8.29; 95% CI: 5.23–11.35; p < 0.00001]. Adverse effects were not significantly different between two therapies (OR: 1.33; 95% CI: 0.87 to 2.04; p = 0.18).
Conclusion: Low-frequency rTMS (≤10 Hz) combined with antidepressants tends to be more effective than antidepressants alone in patients with PSD, and there are no significant adverse effects. In addition, combined therapy may enhance quality of life after stroke. Combination therapy with high-frequency rTMS (>10 Hz) showed no advantage in treating PSD. The transcranial electrical stimulation (TES) combined with antidepressants might be more effective than antidepressants alone, which are needed to confirm by more clinical trials since the.
Introduction
Stroke is now the third leading cause of death worldwide (Benjamin et al., 2017). About 795,000 people in the United States experience new or recurrent stroke every year and, on average, a person has a stroke every 40 s (Benjamin et al., 2019). In addition to dyskinesia, patients with stroke often have psychological and emotional problems. One of the most common psychiatric complications of stroke is poststroke depression (PSD), which has an incidence in the first year after stroke as high as 33% (Hackett and Pickles, 2014). It severely affects the rehabilitation process after stroke and also exerts a heavy burden on patients’ family and on society. Despite their prevalence, depression and other mood-related deficits generally get the least attention. Accordingly, mood disorders need to be addressed during the rehabilitation process of stroke to improve quality of life.
Antidepressants are currently the mainstay of treatment for PSD, but certain adverse reactions are inevitable (Hackett et al., 2008; Coupland et al., 2011). For example, tricyclic antidepressants (TCAs) and selective serotonin reuptake inhibitors (SSRIs) increase the risk of cardiovascular and anticholinergic adverse effects. Fluoxetine has also been reported to be unable to improve PSD symptoms (Robinson et al., 2000; Rice et al., 2021), and some patients with stroke do not respond to antidepressants (Anderson et al., 2004; Hackett et al., 2008). Thus, an effective combination therapy for PSD is urgently required.
Repeated transcranial magnetic stimulation (rTMS) and transcranial electrical stimulation (TES) have been proven to be effective in boosting upper limb rehabilitation and improving aphasia after stroke (Vines et al., 2008; Szaflarski et al., 2011; Hu et al., 2018; Lefaucheur et al., 2020; Kuzu et al., 2021). More and more clinical trials have recently focused on noninvasive brain stimulation (NIBS) treatment of PSD (George et al., 1995; Jorge et al., 2008; Tang et al., 2018), and most have identified positive effects. We have found that the clinical effect of NIBS combined with antidepressants may be better than that of antidepressants alone, and many studies have also mentioned this possibility (Slotema et al., 2010; Brunoni et al., 2013). The current meta-analysis evaluated the efficacy and safety of NIBS combined with antidepressants in the treatment of PSD to provide evidence-based information for clinical decision-making and guideline recommendations.
Materials and Methods
Search Strategy
Relevant randomized controlled trials (RCTs) of NIBS combined with antidepressants in the treatment of PSD were retrieved from the following databases: PubMed, EMBASE, Web of Science, CNKI, Cochrane Library, Biology Medicine Disc (CBM), and the Wanfang database. The retrieval time was from database establishment to October 2021. Search criteria were formulated according to different databases. The keywords included “noninvasive brain stimulation,” “repeated transcranial magnetic stimulation,” “transcranial direct current stimulation,” “transcranial magnetic stimulation,” “antidepressant,” “antidepressant drugs,” “western medicine,” “after stroke,” “poststroke,” and “depression”. Only English and Chinese articles were considered.
Inclusion Criteria
The literature included conformed to the following inclusion criteria (I) participants: patients were diagnosed with PSD and included those with ischemic stroke and hemorrhagic stroke, with no limit on the degree of depression. The diagnosis of PSD met the international classification of diseases (ICD-10) criteria for organic mental disorder (Brämer, 1988), and the score of Hamilton rating scale for depression (HAMD) exceeds 7 (Hamilton, 1960), the first onset, and the diagnosis of stroke was confirmed by magnetic resonance imaging (MRI) or computed tomography (CT), along with being down in spirits, fatigue and lack of interest. (II) study type: RCT; (III) interventions and comparisons: studies comparing the combination of noninvasive brain stimulation and antidepressants with antidepressants alone, such as fluoxetine, paroxetine, sertraline, fluvoxamine, citalopram, maprotiline, imipramine, amitriptyline, doxepin, and chlorimipramine, with only rTMS and TES chosen as noninvasive brain stimulation in this analysis and no frequency limit in the rTMS; (IV) primary outcomes: total effective rate and Hamilton depressive scale (HAMD) score; and (V) secondary outcomes: adverse effect rate and modified Barthel index (MBI) scale score.
Exclusion Criteria
Exclusion criteria of this study were as follows (I) language: non-English or non-Chinese studies; (II) study type: not RCTs, such as animal experiments, reviews, retrospective studies, case reports, conference, and comments; (III) duplicate records, those with incomplete, unclear or inconsistent outcomes, or those with missing information that could not be obtained from the authors; and (IV) studies without a control group or with placebo stimulation or NIBS at a different frequency to the control group.
Data Extraction and Management
Two researchers independently searched and browsed the databases according to the retrieval strategy and then carefully read the full article and extracted the characteristics of the included literature. The following information on the included literature was recorded: authors’ names, publication year, sample size, participant age, intervention, control, outcome indicators, and stimulation frequency, intensity, orientation, control, and duration. Any disagreements were negotiated and discussed with a third researcher.
Quality Assessment
Cochrane Collaboration’s risk of bias tool was used to assess the quality of the included studies. The tool considers six items: selection bias, performance bias, detection bias, attrition bias, reporting bias, and other biases. Each item was judged as one of three levels: low risk, unclear risk, or high risk.
Statistical Analysis
We used RevMan 5.3 software to perform this meta-analysis. The weighted mean difference was used for continuous variables, whereas the odds ratio (OR) was used for dichotomous variables. All data were calculated with 95% confidence intervals (95% CIs). Heterogeneity analysis and sensitivity analysis were also performed using RevMan 5.3. The random-effects model was selected if significant heterogeneity was identified (p < 0.05 or I2>50%). Subgroup analysis and investigation of heterogeneity in subgroups were conducted when necessary. The fixed-effects model was selected if the heterogeneity was low (p ≥ 0.05 or I2 ≤ 50%). Reporting bias was assessed by funnel plot, with dissymmetry indicating significant reporting bias in the analysis.
Results
Selection of Results
A total of 555 records were identified in the electronic databases. Of these, 248 records remained after the two researchers read the titles. After the deletion of duplicates and exclusion of 71 studies due to inconsistent primary standards after abstract screening, the full text of 50 articles were read for further assessment. Finally, 34 studies were selected for analysis. Figure 1 shows the flow diagram of the article selection.
The 34 selected studies (Sun and Song, 2013; Hm, 2014; Ma and Ma, 2015; Wang and Ding, 2015; Xing and Wang, 2016; Tan and Zhou, 2017; Wang and Wen, 2017; Yang and Shi, 2018; Zhu, 2018; Wang and Li, 2019; Zhang, 2019; Wang and Qin, 2020; Wang and Wu, 2020; Yang and Hu, 2020; Wei, 2021) included a total of 2,784 patients, with 1,391 patients in the NIBS combined with antidepressant group and 1,393 patients in the antidepressant alone group. The basic characteristics of the included studies are summarized in Supplementary Table S1, including the authors’ names, publication year, sample size, participant age, type of stroke, intervention, control, outcome indicators, and stimulation frequency, intensity, orientation, control, and duration. There was no significant difference in the baseline data between the two groups. The quality assessment of the included studies is shown in Figures 2, 3.
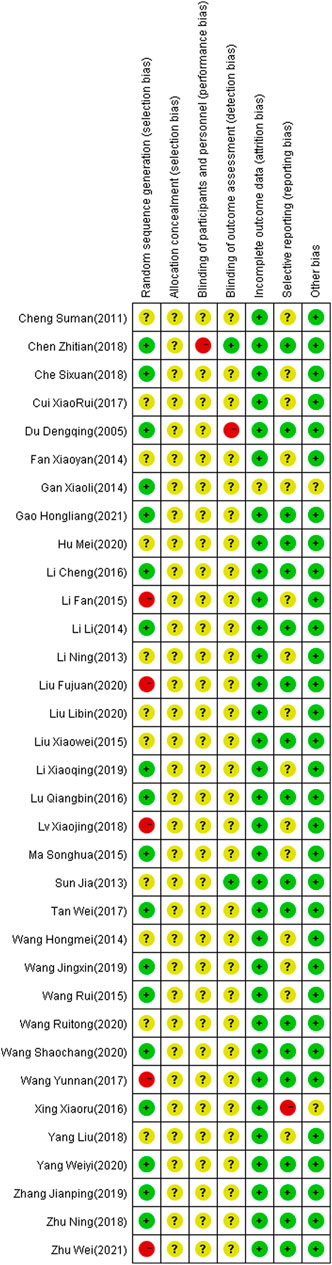
FIGURE 3. Summary of risk of bias of included studies. Red, high risk; green, low risk; yellow, unclear risk.
Meta-Analysis Results
The main indicators were the HAMD score and the total effective rate after treatment. The secondary outcome indicators were the MBI score and adverse effects after treatment.
HAMD Score
Thirty-four of the selected studies (Wei, 2021; Zhu, 2018; Zhang, 2019; Yang and Hu, 2020; Yang and Shi, 2018; Xing and Wang, 2016; Wang and Wen, 2017; Wang and Qin, 2020; Wang and Wu, 2020; Wang and Ding, 2015; Wang and Li, 2019; Hm, 2014; Tan and Zhou, 2017; Sun and Song, 2013; Ma and Ma, 2015; Xj, 2018; Lu and Yang, 2016; Liu, 2015; Liu and Wang, 2020; Li and Chen, 2019; Li and Pan, 2013; L, 2014; Li, 2017; Li and Liang, 2016; Hu and Chen, 2020; Hl, 2021; Gan and Wang, 2015; Xy, 2014; Du, 2005; Xr, 2017; Cheng, 2011; Tian, 2018; Che and Chang, 2018), which involved 2,711 patients, reported the HAMD score as an outcome indicator. Because heterogeneity test analysis showed that there was significant heterogeneity among the included articles (I2 = 96%, p < 0.00001), a random-effects model was used to combine results. Subgroup analysis was performed according to intervention frequency and antidepressant category (Zhu, 2018; Zhang, 2019; Yang and Hu, 2020; Yang and Shi, 2018; Xj, 2018; Wei, 2021; Wang and Wen, 2017; Ma and Ma, 2015; Liu and Wang, 2020; Li, 2017; Li and Liang, 2016; L, 2014; Hu and Chen, 2020; Hm, 2014; Gan and Wang, 2015; Du, 2005; Cheng, 2011; Che and Chang, 2018; Fj, 2020). The meta-analysis showed that the difference was significant (SMD: −1.44; 95% CI: −1.86 to −1.03; p < 0.00001) (Figure 4). However, studies using rTMS combined with fluoxetine with a frequency exceeding 10 Hz showed no significant effect after treatment (SMD: −4.02; 95% CI: −10.43 to –2.39; p = 0.22).
Total Effect Rate
Seventeen of the included studies (Zhu, 2018; Zhang, 2019; Xy, 2014; Xing and Wang, 2016; Wei, 2021; Hm, 2014; Wang and Qin, 2020; Wang and Li, 2019; Tian, 2018; Lu and Yang, 2016; Liu and Wang, 2020; Li and Chen, 2019; Hl, 2021; Li and Liang, 2016; Cheng, 2011; L, 2014; Fj, 2020), which involved 1,406 patients, reported the total effect rate as an outcome indicator. There was significant heterogeneity among the included articles (I2 = 0%, p < 0.00001). Accordingly, the fixed-effects model was used to combine results. The meta-analysis showed that the difference was significant (OR: 4.33; 95% CI: 3.07 to 6.11; p < 0.00001) (Figure 5).
MBI Score
Seven of the selected studies (Xy, 2014; Xj, 2018; Tan and Zhou, 2017; Li and Pan, 2013; L, 2014; Hl, 2021; Cheng, 2011), involving 572 patients, reported the MBI score as an outcome indicator. The included articles showed significant heterogeneity (I2 = 86%, p < 0.00001) and the random-effects model was therefore used to combine results. The meta-analysis showed that the difference was significant (MD: 8.29; 95% CI: 5.23 to 11.35; p < 0.00001) (Figure 6).
Adverse Effect Rate
The adverse effect rate was reported as an outcome indicator in 12 of the included studies (Zhu, 2018; Zhang, 2019; Yang and Hu, 2020; Wang and Wu, 2020; Tian, 2018; Sun and Song, 2013; Liu, 2015; Liu and Wang, 2020; Li and Liang, 2016; L, 2014; Hl, 2021; Fj, 2020), which involved 981 patients. Because heterogeneity test analysis showed that there was significant heterogeneity among the included articles (I2 = 47%, p = 0.04), the fixed-effects model was used to combine results. The meta-analysis showed that there was no significant difference in the adverse effect rate between the two groups (OR = 1.33; 95% CI: 0.87–2.04, p = 0.18) (Figure 7).
Adverse reactions mainly included behavioral toxicity, nervous system abnormalities, and cardiovascular system abnormalities. Behavioral toxicity included somnolence and epilepsy. Nervous system abnormalities commonly included headache. Digestive system abnormalities included nausea, vomiting, and indigestion. In the NIBS combined with antidepressant group, 36 patients had headache, three had insomnia, three had thirst, eight had nausea, 12 had vomiting, and two had cardiovascular system abnormalities. In the antidepressant group, four patients had headaches, three had insomnia, four had thirst, five had nausea, 13 had vomiting, one had fatigue, and one had cardiovascular system abnormalities.
Sensitivity Analysis
Sensitivity analyses of each outcome indicator were performed by excluding single articles one-by-one to test the effect of each study on the pooled effect size. In the meta-analysis of the HAMD score, the heterogeneity decreased from 92% to 34% after deleting the study by Liu FJ from 2020 (Fj, 2020). The results showed that this heterogeneity was mainly due to this study. There was no qualitative change in the combined effect for all outcome indicators. Thus, the pooled results of the included studies were steady.
Publication Bias
Funnel plot analysis was used to analyze the publication bias of the HAMD score, total effect rate, and adverse effects. There was no obvious publication bias in the studies of the total effect rate and adverse effects. The poor symmetry of the funnel plot indicated the existence of a publication bias due to the study by Liu LB from 2020 (Liu and Wang, 2020). After deleting this study, the combined effect was not changed but the total heterogeneity decreased to 79%. The publication bias results for the HAMD score analysis are shown in Figure 8.
Discussion
Our meta-analysis included 34 studies of the effects of NIBS combined with antidepressants for patients with PSD. The results showed that the combination of NIBS and antidepressants might have a better effect on PSD and could improve the depression scale score and quality of life compared with antidepressants alone. It is well known that guidelines recommend rTMS for the treatment of major depression, and many meta-analyses have shown that TMS intervention with PSD was positive (Shen et al., 2017; Liu et al., 2019; Shao et al., 2021), but a growing number of studies have recommended multi-module combination therapy and population-specific personalized treatment (Wang et al., 2019; Nestor and Blumberger, 2020), which warrants further research on the frequency and site of TMS intervention. The use of low-frequency TMS by Daniel R Schaffer significantly improved depression with cognitive impairment, suggesting that low-frequency TMS is more effective in specific populations (Schaffer et al., 2021). Compared with previous reviews (Bucur and Papagno, 2018; Liu et al., 2019), our analysis had the following advantages (I) combination NIBS and antidepressant therapy; (II) internationally recognized depression assessment scales; (III) inconsistent results with previous studies due to negative outcomes of high-frequency TMS combined with antidepressants; and (IV) the inclusion of more than 30 studies. There have been no studies evaluating NIBS in combination with antidepressants for PSD, although combination therapy is more clinically appropriate. Therefore, this meta-analysis may have a greater reference value than previous reviews.
According to the results of this analysis, combined NIBS and antidepressant therapy reduced the HAMD score of PSD more than antidepressants alone. However, this result was highly heterogeneous. We grouped the studies by a variety of clinically relevant factors, including age, intervention frequency and intensity, drug type, type of stroke, and stimulation orientation and duration. In the final analysis, rTMS combined with fluoxetine (less than 1 Hz and between 5 and 10 Hz) was more effective than fluoxetine alone, but the effect was not better with a frequency exceeding 10 Hz. TES combined with antidepressants improved the HAMD score more than antidepressants alone, although only two included articles examined this combination. After deleting the study by Liu FJ from 2020 (Fj, 2020) due to its high heterogeneity, rTMS combined with paroxetine was also more effective in reducing the HAMD score. This previous study by Liu FJ (Fj, 2020) was probably a retrospective study due to its vague description and was excluded from the pooled effect. Martijn (Arns et al., 2010) also commented that there were possibly differential effects of different rTMS stimulation frequencies, although many searches concluded that high-frequency rTMS has the same effect as low-frequency rTMS or antidepressants (Berlim et al., 2013).
The results of 17 studies (Zhu, 2018; Zhang, 2019; Xy, 2014; Xing and Wang, 2016; Wei, 2021; Wang and Qin, 2020; Hm, 2014; Hl, 2021; Wang and Li, 2019; Tian, 2018; Lu and Yang, 2016; Liu and Wang, 2020; Li and Chen, 2019; Li and Liang, 2016; Cheng, 2011; L, 2014; Fj, 2020) also indicated that NIBS combined with antidepressants was better than antidepressants alone regarding the total effect rate. Moreover, for the MBI score, seven studies (Xy, 2014; Xj, 2018; Tan and Zhou, 2017; Li and Pan, 2013; L, 2014; Hl, 2021; Cheng, 2011) showed that the combination therapy has potential benefits in patients with PSD. Combination therapy may be able to improve quality of life after stroke. Since a few included articles reported MBI scores, the meta-regression did not be conducted. Subgroup analyses were added based on clinical characteristics, including frequency, intensity and location of intervention, degree of depression, and course of disease. Heterogeneity still could not decrease to a reasonable range. We used sensitivity analysis to find no articles causing high heterogeneity, and adopted a random effect model. This result is stable and conservative. Some studies (Zhu, 2018; Zhang, 2019; Yang and Hu, 2020; Ma and Ma, 2015; L, 2014; Fj, 2020) have reported headache, nausea, vomiting, insomnia, thirst, and fatigue in both control and experimental groups. The adverse reactions may be caused by antidepressants. Twelve studies (Zhu, 2018; Zhang, 2019; Yang and Hu, 2020; Wang and Wu, 2020; Tian, 2018; Sun and Song, 2013; Liu, 2015; Liu and Wang, 2020; Li and Liang, 2016; L, 2014; Hl, 2021; Fj, 2020) demonstrated consistent and stable results in adverse reaction rates. This suggests that combined NIBS and antidepressant therapy is safe.
There is still a contradiction between the advantages and disadvantages of the different frequencies of NIBS, and the effects of different frequencies of NIBS are still disputed. Different frequencies of rTMS have been shown to reduce fluorodeoxy glucose F18 (18F-FDG) uptake in the dorsal cortical region while simultaneously increasing 18F-FDG uptake in the ventral region (Parthoens et al., 2016). The rTMS decreased glucose metabolism in the stimulated temporal region, with increases in the bilateral precentral, ipsilateral superior and midfrontal, prefrontal, and cingulate gyri. This suggests that 1 Hz rTMS could induce cortical regulation and extensive changes in the neural network through long-range neuronal connectivity (Lee et al., 2013). Studies have also shown that low-intensity TMS mainly stimulates low-threshold inhibitory neurons (Duan et al., 2018). High-frequency TMS caused greater activation than low-frequency TMS in normal humans. However, oxidative stress, lipid peroxidation, and protein oxidation were found in the neural tissue of stroke patients. Any of these pathophysiological processes may be related to PSD (Nabavi et al., 2015). Kimbrell et al. (1999) also reported that the antidepressant response to rTMS might depend on the pretreatment cerebral metabolism and the stimulation frequency. Thus, it is possible that patients with PSD are more sensitive to low-frequency TMS. Due to abnormal expression of amine neurotransmitters and cytokine expression after stroke, the combination of antidepressants with rTMS may be more effective with the mild stimulation of low-frequency TMS.
The mechanism of PSD is still unclear, which may involve neurobiological pathways, inflammation and apoptosis mechanisms (Robinson and Jorge, 2016; Medeiros et al., 2020). Robinson (Robinson et al., 1984; Narushima et al., 2003) suggested that lesions in the left frontal lobe or left basal ganglia were associated with PSD. And focal brain stimulation using rTMS was only effective when administered to the left dorsolateral prefrontal cortex in patients with vascular depression (Jorge et al., 2008). A meta-analysis (Carson et al., 2000) showed that stroke site was not associated with depression, and a study (Wei et al., 2015) suggested a significant association between stroke in the right hemisphere and the incidence of depression. Some studies have hypothesized that stroke lesion area is related to depression degree, which could be explained by some pro-inflammatory factors (Spalletta et al., 2006). For example, the increase of IFN-γleads to the cascade reaction of other pro-inflammatory cytokines IL-6, IL-1βand TNF-α, which aggravates depression. Secondly, IFN-γcan affect the HPA axis (Capuron et al., 2003), leading to increased adrenocortical hormone and cortisol levels, resulting in increased reactive oxides (Altieri et al., 2012; Ferrari and Villa, 2017), which further cause cell death and damage. Proinflammatory factors also stimulate the activity of indoleamine 2, 3-dioxygenase, which degrades tryptophan, the biological precursor of serotonin, into a toxic metabolite (Bansal and Kuhad, 2016). Compared with common depression, PSD is associated with focal ischemia, which leads to programmed cell death, cell swelling, or cell necrosis and a series of complex events related to cellular and molecular mechanisms (Brouns and De Deyn, 2009). Whether the neuroanatomical location of stroke affects depression remains controversial. It remains unknown whether the severity of stroke is positively correlated with the severity of depression, or whether there are differences in depression at different times after stroke. It is hoped that more RCTs will be designed in this direction in the future.
Limitations and Prospects
This meta-analysis emphasized the clinical efficacy and depression improvement of combination therapy in PSD patients but also examined quality of life and safety. However, all included RCTs were from China, which may indicate publication bias. Funnel plot analysis revealed that a study by Liu LB (Liu and Wang, 2020) had significant publication bias due to selective reporting of outcomes. Accordingly, the result should be treated with caution. This meta-analysis was not registered and there may be a small deviation, but we still strictly followed the procedures of systematic evaluation. In addition, some indicators were significantly heterogeneous. Therefore, caution is required for these findings. More basic studies are needed to determine the mechanism underlying the effect of low-frequency TMS combined with antidepressants on depression after stroke. Moreover, large multicenter studies are needed to assess the best frequency and type of depression drugs to promote the final translation of combination treatment into daily clinical practice and guidelines.
Conclusion
Our analysis demonstrate that low-frequency rTMS(10 ≤ Hz) combined with antidepressants tends to be more effective than antidepressants alone in patients with PSD and there are no significant adverse effects. In addition, combined therapy may boost quality of life after stroke. Combination therapy with high-frequency rTMS (>10 Hz) showed no advantage in treating PSD. The transcranial electrical stimulation (TES) combined with antidepressants may be more effective than antidepressants alone. More randomized controlled studies with detailed design for different stroke periods, depression levels and stroke location are needed to verify this conclusion.
Data Availability Statement
The original contributions presented in the study are included in the article/Supplementary Material, further inquiries can be directed to the corresponding authors.
Author Contributions
HC conceived the conception and design of the study. JL did the search of studies, performed the meta-analytic statistics. JL, JF, and YJ did the search of studies, performed the data extraction and prepared the tables and figures. JH was involved in the study selection and did the data extraction. HZ prepared the references and provided the detailed critical comments. JL and JF wrote the manuscript. All authors approved the final version of the manuscript.
Funding
This work was supported by the Science and Technology Program of Panyu (Nos. 2019-Z04-07, 2019-Z04-35, 2019-Z04-84, and 2020-Z04-054) and the Science and Technology Project of Guangzhou Health Commission (No. 20211A011114).
Conflict of Interest
The authors declare that the research was conducted in the absence of any commercial or financial relationships that could be construed as a potential conflict of interest.
Publisher’s Note
All claims expressed in this article are solely those of the authors and do not necessarily represent those of their affiliated organizations, or those of the publisher, the editors and the reviewers. Any product that may be evaluated in this article, or claim that may be made by its manufacturer, is not guaranteed or endorsed by the publisher.
Acknowledgments
Thanks to Professor Shuaishuai Liu and Professor Quoqi Shi from the School of Foreign Languages, Guangzhou University of Chinese Medicine for their knowledge of writing.
Supplementary Material
The Supplementary Material for this article can be found online at: https://www.frontiersin.org/articles/10.3389/fphar.2022.887115/full#supplementary-material
References
Altieri, M., Maestrini, I., Mercurio, A., Troisi, P., Sgarlata, E., Rea, V., et al. (2012). Depression after Minor Stroke: Prevalence and Predictors. Eur. J. Neurol. 19, 517–521. doi:10.1111/j.1468-1331.2011.03583.x
Anderson, C. S., Hackett, M. L., and House, A. O. (2004). Interventions for Preventing Depression after Stroke. Cochrane database Syst. Rev. 2004, CD003689.
Arns, M., Spronk, D., and Fitzgerald, P. B. (2010). Potential Differential Effects of 9 Hz rTMS and 10 Hz rTMS in the Treatment of Depression. Brain Stimul. 3, 124–126. doi:10.1016/j.brs.2009.07.005
Bansal, Y., and Kuhad, A. (2016). Mitochondrial Dysfunction in Depression. Curr. Neuropharmacol. 14, 610–618. doi:10.2174/1570159x14666160229114755
Benjamin, E. J., Blaha, M. J., Chiuve, S. E., Cushman, M., Das, S. R., Deo, R., et al. (2017). Heart Disease and Stroke Statistics-2017 Update: A Report from the American Heart Association. Circulation 135, E146–E603. doi:10.1161/CIR.0000000000000485
Benjamin, E. J., Muntner, P., Alonso, A., Bittencourt, M. S., Callaway, C. W., Carson, A. P., et al. (2019). Heart Disease and Stroke Statistics-2019 Update: A Report from the American Heart Association. Circulation 139, e56–e528. doi:10.1161/CIR.0000000000000659
Berlim, M. T., Van Den Eynde, F., and Jeff Daskalakis, Z. (2013). Clinically Meaningful Efficacy and Acceptability of Low-Frequency Repetitive Transcranial Magnetic Stimulation (rTMS) for Treating Primary Major Depression: A Meta-Analysis of Randomized, Double-Blind and Sham-Controlled Trials. Neuropsychopharmacology 38, 543–551. doi:10.1038/npp.2012.237
Brämer, G. R. (1988). International Statistical Classification of Diseases and Related Health Problems. Tenth Revision. World Health Stat. Q. 41, 32–36.
Brouns, R., and De Deyn, P. P. (2009). The Complexity of Neurobiological Processes in Acute Ischemic Stroke. Clin. Neurol. Neurosurg. 111, 483–495. doi:10.1016/j.clineuro.2009.04.001
Brunoni, A. R., Valiengo, L., Baccaro, A., Zanão, T. A., De Oliveira, J. F., Goulart, A., et al. (2013). The Sertraline vs. Electrical Current Therapy for Treating Depression Clinical Study: Results from a Factorial, Randomized, Controlled Trial. Jama Psychiatry 70, 383–391. doi:10.1001/2013.jamapsychiatry.32
Bucur, M., and Papagno, C. (2018). A Systematic Review of Noninvasive Brain Stimulation for Post-stroke Depression. J. Affect Disord. 238, 69–78. doi:10.1016/j.jad.2018.05.026
Capuron, L., Raison, C. L., Musselman, D. L., Lawson, D. H., Nemeroff, C. B., and Miller, A. H. (2003). Association of Exaggerated HPA axis Response to the Initial Injection of Interferon-Alpha with Development of Depression during Interferon-Alpha Therapy. Am. J. Psychiatry 160, 1342–1345. doi:10.1176/appi.ajp.160.7.1342
Carson, A. J., Machale, S., Allen, K., Lawrie, S. M., Dennis, M., House, A., et al. (2000). Depression after Stroke and Lesion Location: a Systematic Review. Lancet 356, 122–126. doi:10.1016/S0140-6736(00)02448-X
Che, L. Y., and Chang, X. (2018). Effects of Fluoxetine Combined with Repeated Transcranial Magnetic Stimulation on Neurological Function and Serum Hs-CRP in Patients with Acute Cerebral Infarction. Chongqing Med. Sci. 47, 2349–2352. doi:10.3969/j.issn.1671-8348.2018.17.026
Cheng, C. J. (2011). Efficacy of Fluoxetine Combined with Transcranial Magnetic Stimulation in the Treatment of Post-stroke Depression. Chin. J. Rehabilitation Med. 26, 980–981. doi:10.3969/j.issn.1001-1242.2011.10.023
Coupland, C., Dhiman, P., Morriss, R., Arthur, A., Barton, G., and Hippisley-Cox, J. (2011). Antidepressant Use and Risk of Adverse Outcomes in Older People: Population Based Cohort Study. Bmj 343, d4551. doi:10.1136/bmj.d4551
Du, W. Y. (2005). Living Ability and Cognitive Function Ameliorated by Low Frequency Repetitive Transcranial Magnetic Stimulation in Patients with Post-stroke Depression:comparison with Drug Plus Psychological Treatment. Chin. J. Clin. Rehabilitation 16, 22–23.
Duan, X., Yao, G., Liu, Z., Cui, R., and Yang, W. (2018). Mechanisms of Transcranial Magnetic Stimulation Treating on Post-stroke Depression. Front. Hum. Neurosci. 12, 215. doi:10.3389/fnhum.2018.00215
Ferrari, F., and Villa, R. F. (2017). The Neurobiology of Depression: an Integrated Overview from Biological Theories to Clinical Evidence. Mol. Neurobiol. 54, 4847–4865. doi:10.1007/s12035-016-0032-y
Fj, L. (2020). Effect of Repetitive Transcranial Magnetic Stimulation and Paroxetine Hydrochloride on the Efficacy and Complications of Patients with Post-Stroke Depression. Reflexology Rehabilitation Med. 1, 133–135.
Gan, L. Q., and Wang, X. J. (2015). Clinical Observation of Transcranial Electrical Stimulation Combined with Dalixin in the Treatment of Post-stroke Depression. Chin. J. Clin. Physicians 43, 61–62. doi:10.3969/j.issn.2095—8552.2015.02.019
George, M. S., Wassermann, E. M., Williams, W. A., Callahan, A., Ketter, T. A., Basser, P., et al. (1995). Daily Repetitive Transcranial Magnetic Stimulation (Rtms) Improves Mood in Depression. Neuroreport 6, 1853–1856. doi:10.1097/00001756-199510020-00008
Hackett, M. L., and Pickles, K. (2014). Part I: Frequency of Depression after Stroke: an Updated Systematic Review and Meta-Analysis of Observational Studies. Int. J. Stroke 9, 1017–1025. doi:10.1111/ijs.12357
Hackett, M. L., Anderson, C. S., House, A., and Xia, J. (2008). Interventions for Treating Depression after Stroke. Cochrane Database Syst. Rev. 2008, Cd003437. doi:10.1002/14651858.CD003437.pub3
Hamilton, M. (1960). A Rating Scale for Depression. J. Neurol. Neurosurg. Psychiatry 23, 56–62. doi:10.1136/jnnp.23.1.56
Hl, G. (2021). Effect of High Frequency Transcranial Magnetic Stimulation on Depressive Symptoms and Neurological Deficits in Patients with Stroke Treated on Duloxetine. Acad. J. Guangzhou Med. Univ. 49, 60–63. doi:10.3969/j.issn.2095-9664.2021.03.12
Hm, W. (2014). Clinical Effect of Transcranial Magnetic Stimulation on Post-stroke Depressive Disorder. Med. Health Care 10, 20–21.
Hu, L. M., and Chen, Y. Q. (2020). Application of Repetitive Transcranial Magnetic Stimulation Combined with Paroxetine Hydrochloride in Patients with Post-stroke Depression. Neurological psychiatric Disord. 27, 63–65. doi:10.3969/j.issn.1674-4721.2020.18.018
Hu, X. Y., Zhang, T., Rajah, G. B., Stone, C., Liu, L. X., He, J. J., et al. (2018). Effects of Different Frequencies of Repetitive Transcranial Magnetic Stimulation in Stroke Patients with Non-fluent Aphasia: a Randomized, Sham-Controlled Study. Neurol. Res. 40, 459–465. doi:10.1080/01616412.2018.1453980
Jorge, R. E., Moser, D. J., Acion, L., and Robinson, R. G. (2008). Treatment of Vascular Depression Using Repetitive Transcranial Magnetic Stimulation. Arch. Gen. Psychiatry 65, 268–276. doi:10.1001/archgenpsychiatry.2007.45
Kimbrell, T. A., Little, J. T., Dunn, R. T., Frye, M. A., Greenberg, B. D., Wassermann, E. M., et al. (1999). Frequency Dependence of Antidepressant Response to Left Prefrontal Repetitive Transcranial Magnetic Stimulation (rTMS) as a Function of Baseline Cerebral Glucose Metabolism. Biol. Psychiatry 46, 1603–1613. doi:10.1016/s0006-3223(99)00195-x
Kuzu, O., Adiguzel, E., Kesikburun, S., Yasar, E., and Yilmaz, B. (2021). The Effect of Sham Controlled Continuous Theta Burst Stimulation and Low Frequency Repetitive Transcranial Magnetic Stimulation on Upper Extremity Spasticity and Functional Recovery in Chronic Ischemic Stroke Patients. J. Stroke & Cerebrovasc. Dis. 30. doi:10.1016/j.jstrokecerebrovasdis.2021.105795
L, L. (2014). Effect of Fluoxetine Capsule Combined with Repeated Transcranial Magnetic Stimulation on Post-stroke Depression. Chin. J. Pract. Nerv. Dis. 17, 105–106. doi:10.3969/j.issn.1673-5110.2014.20.077
Lee, M., Kim, S. E., Kim, W. S., Han, J., Kim, H. J., Kim, H. J., et al. (2013). Cortico-cortical Modulation Induced by 1-Hz Repetitive Transcranial Magnetic Stimulation of the Temporal Cortex. J. Clin. Neurol. 9, 75–82. doi:10.3988/jcn.2013.9.2.75
Lefaucheur, J. P., Aleman, A., Baeken, C., Benninger, D. H., Brunelin, J., Di Lazzaro, V., et al. (2020). Evidence-based Guidelines on the Therapeutic Use of Repetitive Transcranial Magnetic Stimulation (rTMS): An Update (2014-2018). Clin. Neurophysiol. 131, 474–528. doi:10.1016/j.clinph.2019.11.002
Li, C., Li, X., Liang, H., Yan, N., and Wang, Y. (2016). Repetitive Transcranial Magnetic Stimulation for Treatment of Post-stroke Depression of Liver-Qi Stagnation Type in 33 Cases. Rehabil. Med. 26, 14–18+23. doi:10.3724/sp.j.1329.2016.06014
Li, H. Y., and Pan, W. M. (2013). A Study of Efficacy Mirtazapine Merge rTMS to Treatment of Post-stroke Depression. Chin. J. Trauma Disabil. Med. 21, 48–50. doi:10.3969/j.issn.1673-6567.2013.06.027
Li, L. X., and Chen, L. J. (2019). Effect of Repeated Transskull Magnetic Stimulation Combined with Antidepressants on Post-stroke Depression and its Effect on Sleep Quality. World J. Sleep Med. 6, 718–719. doi:10.3969/j.issn.2095-7130.2019.06.010
Li, Y. L. (2017). Clinical Effect of Repeated Transcranial Magnetic Stimulation Combined with Fluoxetine on Post-stroke Depression. J. Clin. Psychiatry 27, 144. doi:10.3969/j.issn.1005-3220.2017.02.026
Liu, C., Wang, M., Liang, X., Xue, J., and Zhang, G. (2019). Efficacy and Safety of High-Frequency Repetitive Transcranial Magnetic Stimulation for Poststroke Depression: A Systematic Review and Meta-Analysis. Arch. Phys. Med. Rehabil. 100, 1964–1975. doi:10.1016/j.apmr.2019.03.012
Liu, X. Q. (2015). Effect of Repeated Transcranial Magnetic Stimulation on Post-stroke Depression. Chin. J. Gerontology 35, 5621–5622. doi:10.3969/j.issn.1005-9202.2015.19.119
Liu, Z. X., and Wang, L. J. (2020). Clinical Study of Transcranial Magnetic Stimulation in the Treatment of Post-stroke Depression. Chin. Community Dr. 36, 82–84. doi:10.3969/j.issn.1007-614x.2020.24.040
Lu, Z. H., and Yang, J. S. (2016). Low Frequency Repetitive Transcranial Magnetic Stimulation for Post-stroke Depression: a Controlled Study. Hainan Med. 27, 1963–1965. doi:10.3969/j.issn.1003-6350.2016.12.022
Ma, S. X., and Ma, D. (2015). Early Effect of Transcranial Magnetic Stimulation Combined with Fluoxetine in Patients with Depression after Acute Cerebral Infarction. Med. J. Commun. 260, 257–258.
Medeiros, G. C., Roy, D., Kontos, N., and Beach, S. R. (2020). Post-stroke Depression: A 2020 Updated Review. Gen. Hosp. Psychiatry 66, 70–80. doi:10.1016/j.genhosppsych.2020.06.011
Nabavi, S. F., Dean, O. M., Turner, A., Sureda, A., Daglia, M., and Nabavi, S. M. (2015). Oxidative Stress and Post-stroke Depression: Possible Therapeutic Role of Polyphenols? Curr. Med. Chem. 22, 343–351. doi:10.2174/0929867321666141106122319
Narushima, K., Kosier, J. T., and Robinson, R. G. (2003). A Reappraisal of Poststroke Depression, Intra- and Inter-hemispheric Lesion Location Using Meta-Analysis. J. Neuropsychiatry Clin. Neurosci. 15, 422–430. doi:10.1176/jnp.15.4.422
Nestor, S. M., and Blumberger, D. M. (2020). Mapping Symptom Clusters to Circuits: Toward Personalizing TMS Targets to Improve Treatment Outcomes in Depression. Am. J. Psychiatry 177, 373–375. doi:10.1176/appi.ajp.2020.20030271
Parthoens, J., Verhaeghe, J., Servaes, S., Miranda, A., Stroobants, S., and Staelens, S. (2016). Performance Characterization of an Actively Cooled Repetitive Transcranial Magnetic Stimulation Coil for the Rat. Neuromodulation 19, 459–468. doi:10.1111/ner.12387
Rice, D. R., Okeng'o, K., Massawe, E., Ismail, S., Mworia, N. A., Chiwanga, F., et al. (2021). Efficacy of Fluoxetine for Post-Ischemic Stroke Depression in Tanzania. J. stroke Cerebrovasc. Dis. official J. Natl. Stroke Assoc. 31, 106181. doi:10.1016/j.jstrokecerebrovasdis.2021.106181
Robinson, R. G., and Jorge, R. E. (2016). Post-stroke Depression: A Review. Am. J. Psychiatry 173, 221–231. doi:10.1176/appi.ajp.2015.15030363
Robinson, R. G., Kubos, K. L., Starr, L. B., Rao, K., and Price, T. R. (1984). Mood Disorders in Stroke Patients. Importance of Location of Lesion. Brain 107 ( Pt 1) (Pt 1), 81–93. doi:10.1093/brain/107.1.81
Robinson, R. G., Schultz, S. K., Castillo, C., Kopel, T., Kosier, J. T., Newman, R. M., et al. (2000). Nortriptyline versus Fluoxetine in the Treatment of Depression and in Short-Term Recovery after Stroke: A Placebo-Controlled, Double-Blind Study. Am. J. Psychiatry 157, 351–359. doi:10.1176/appi.ajp.157.3.351
Schaffer, D. R., Okhravi, H. R., and Neumann, S. A. (2021). Low-Frequency Transcranial Magnetic Stimulation (LF-TMS) in Treating Depression in Patients with Impaired Cognitive Functioning. Arch. Clin. Neuropsychol. 36, 801–814. doi:10.1093/arclin/acaa095
Shao, D., Zhao, Z. N., Zhang, Y. Q., Zhou, X. Y., Zhao, L. B., Dong, M., et al. (2021). Efficacy of Repetitive Transcranial Magnetic Stimulation for Post-stroke Depression: a Systematic Review and Meta-Analysis of Randomized Clinical Trials. Braz J. Med. Biol. Res. 54, e10010. doi:10.1590/1414-431X202010010
Shen, X., Liu, M., Cheng, Y., Jia, C., Pan, X., Gou, Q., et al. (2017). Repetitive Transcranial Magnetic Stimulation for the Treatment of Post-stroke Depression: A Systematic Review and Meta-Analysis of Randomized Controlled Clinical Trials. J. Affect Disord. 211, 65–74. doi:10.1016/j.jad.2016.12.058
Slotema, C. W., Blom, J. D., Hoek, H. W., and Sommer, I. E. (2010). Should We Expand the Toolbox of Psychiatric Treatment Methods to Include Repetitive Transcranial Magnetic Stimulation (rTMS)? A Meta-Analysis of the Efficacy of rTMS in Psychiatric Disorders. J. Clin. Psychiatry 71, 873–884. doi:10.4088/JCP.08m04872gre
Spalletta, G., Bossù, P., Ciaramella, A., Bria, P., Caltagirone, C., and Robinson, R. G. (2006). The Etiology of Poststroke Depression: a Review of the Literature and a New Hypothesis Involving Inflammatory Cytokines. Mol. Psychiatry 11, 984–991. doi:10.1038/sj.mp.4001879
Sun, W. H., and Song, Q. Y. (2013). Effect of Repeated Transcranial Magnetic Stimulation on Post-stroke Depression. Chin. J. Integr. Traditional West. Med. Cardio-cerebrovascular Dis. 11, 321–322. doi:10.3969/j.issn.1672-1349.2013.03.037
Szaflarski, J. P., Vannest, J., Wu, S. W., Difrancesco, M. W., Banks, C., and Gilbert, D. L. (2011). Excitatory Repetitive Transcranial Magnetic Stimulation Induces Improvements in Chronic Post-stroke Aphasia. Med. Sci. Monit. 17, CR132–9. doi:10.12659/msm.881446
Tan, Y. H., and Zhou, Y. (2017). A Controlled Study of the Effects of Repeated Transcranial Magnetic Stimulation on Patients with Post-stroke Depression. Shanxi Med. J. 46, 936–938. doi:10.3969/J.issn.0253-9926.2017.08.029
Tang, Y., Chen, A., Zhu, S., Yang, L., Zhou, J., Pan, S., et al. (2018). Repetitive Transcranial Magnetic Stimulation for Depression after Basal Ganglia Ischaemic Stroke: Protocol for a Multicentre Randomised Double-Blind Placebo-Controlled Trial. BMJ Open 8, e018011. doi:10.1136/bmjopen-2017-018011
Tian, C. Z. (2018). Clinical Study of rTMS Combined with Paroxetine in the Intervention of Post-stroke Depression. Lanzhou, China: Gansu University of Traditional Chinese Medicine.
Vines, B. W., Cerruti, C., and Schlaug, G. (2008). Dual-hemisphere tDCS Facilitates Greater Improvements for Healthy Subjects' Non-dominant Hand Compared to Uni-Hemisphere Stimulation. BMC Neurosci. 9, 103. doi:10.1186/1471-2202-9-103
Wang, G. R., and Ding, J. Y. (2015). The Treatment of Citalopram Combined Transcranial Magnetic Stimulation for Post-stroke Depression. China Med. Eng. 23, 25–26.
Wang, W. Y., and Li, Y. L. (2019). Effect of Low-Frequency Repetitive Transcranial Magnetic stimuIation on Serum Neurotransmitters and Serum Inflammatory Factors in Patients with Post-stoke Depression. J. Clin. Psychosomatic Dis. 25, 5–8. doi:10.3969/j.issn.1672-187X.2019.05.002
Wang, Y. X., and Wen, C. (2017). Analysis of Therapeutic Effect of Repeated Transcranial Magnetic Stimulation on Post-stroke Depression and Sleep Disturbance. Acad. J. Guangzhou Med. Univ. 45, 30–33. doi:10.3969/j.issn.2095-9664.2017.04.08
Wang, Z., Ma, X., and Xiao, C. (2019). Standardized Treatment Strategy for Depressive Disorder. Adv. Exp. Med. Biol. 1180, 193–199. doi:10.1007/978-981-32-9271-0_10
Wang, Z. C., and Qin, X. Y. (2020). Clinical Efficacy and Mechanism of Low Frequency Repetitive Transcranial Magnetic Stimulation Combined with Paroxetine in the Treatment of Post-stroke Depression. Nerve Inj. Funct. Reconstr. 15, 349–351+354. doi:10.16780/j.cnki.sjssgncj.20190548
Wang, Z. H., and Wu, R. Q. (2020). Effects of Repetitive Transcranial Magnetic Stimulation on Mismatch Negativity and Visual P300 in Patients with Post-stroke Depression. J. Clin. Psychiatry 30, 174–177. doi:10.3969/j.issn.1005-3220.2020.03.009
Wei, N., Yong, W., Li, X., Zhou, Y., Deng, M., Zhu, H., et al. (2015). Post-stroke Depression and Lesion Location: a Systematic Review. J. Neurol. 262, 81–90. doi:10.1007/s00415-014-7534-1
Wei, Z. (2021). Clinical Efficacy of Repeated Transcranial Magnetic Stimulation Combined with Paroxetine Hydrochloride in Patients with Post-stroke Depression. Contemp. Med. 27, 163–164. doi:10.3969/j.issn.1009-4393.2021.02.070
Xing, Z. X., and Wang, X. C. (2016). Effects of Low Frequency Repetitive Transcranial Magnetic Stimulation on Cognitive and Daily Life Ability of Patients with Post Stroke Depression. Int. J. Psychiatry 43, 648–650+654.
Xj, L. (2018). Effect of CES Combined with escitaIopram on Quality of Life and Activities of Daily Living of Patients with Depression after Cerebral Infarction. Chin. J. Pract. Neurological Dis. 21, 2529–2534. doi:10.12083/SYSJ.2018.22.535
Xr, C. (2017). Effect of Duloxetine Combined with Repeated Transcranial Magnetic Stimulation in the Treatment of Post-stroke Depression. J. Mudanjiang Med. Coll. 38, 102–103.
Xy, F. (2014). Effect of Duloxetine Combined with Repeated Transcranial Magnetic Stimulation in the Treatment of Post-stroke Depression. Chin. J. Pract. Neuropathy 17, 102–103.
Yang, L. Y., and Shi, W. Z. (2018). The Curative Effect of High-Frequency Transcranial Magnetic Stimulation in Post-stroke Depression. Chin. J. Pract. Nerv. Dis. 21, 72–74. doi:10.12083/SYSJ.2018.01.018
Yang, Z. H., and Hu, X. H. (2020). Effects of Fluoxetine Combined with Repetitive Transcranial Magnetic Stimulation on Cognitive Function, Neurological Function, Serum BDNF, and CRP in Acute Stroke Patients with Post-stroke Depression. Hainan Med. 31, 959–962. doi:10.3969/j.issn.1003-6350.2020.08.003
Zhang, W. Y. (2019). Efficacy of High Frequency Transcranial Magnetic Stimulation in the Treatment of Post-stroke Depression and its Effect on 5-hydroxytryptamine Levels. Nerve Inj. Funct. Reconstr. 14, 645–646. doi:10.16780/j.cnki.sjssgncj.2019.12.015
Keywords: noninvasive brain stimulation, poststroke depression, repeated transcranial magnetic stimulation, antidepressant, depression
Citation: Liang J, Feng J, He J, Jiang Y, Zhang H and Chen H (2022) Effects of Noninvasive Brain Stimulation Combined With Antidepressants in Patients With Poststroke Depression: A Systematic Review and Meta-Analysis. Front. Pharmacol. 13:887115. doi: 10.3389/fphar.2022.887115
Received: 01 March 2022; Accepted: 21 April 2022;
Published: 19 May 2022.
Edited by:
Anwen Shao, Zhejiang University, ChinaReviewed by:
Peidong Huang, Yunnan University of Traditional Chinese Medicine, ChinaYong Xu, First Hospital of Shanxi Medical University, China
Copyright © 2022 Liang, Feng, He, Jiang, Zhang and Chen. This is an open-access article distributed under the terms of the Creative Commons Attribution License (CC BY). The use, distribution or reproduction in other forums is permitted, provided the original author(s) and the copyright owner(s) are credited and that the original publication in this journal is cited, in accordance with accepted academic practice. No use, distribution or reproduction is permitted which does not comply with these terms.
*Correspondence: Jiabin Liang, bGlhbmdqaWFiaW40MjNAMTYzLmNvbQ==; Hanwei Chen, ZG9jdGVyd2VpQHNpbmEuY29t
†These authors have contributed equally to this work and share first authorship