- 1Departments of Physiology, Institute of Biomedical Informatics, Bioinformatics Center, Henan Provincial Engineering Center for Tumor Molecular Medicine, School of Basic Medical Sciences, Academy for Advanced Interdisciplinary Studies, Henan University, Kaifeng, China
- 2Department of Preventive Medicine, Institute of Biomedical Informatics, Bioinformatics Center, Henan Provincial Engineering Center for Tumor Molecular Medicine, School of Basic Medical Sciences, Academy for Advanced Interdisciplinary Studies, Henan University, Kaifeng, China
Esophageal cancer (EC) is one of the most common malignancies of digestive tracts with poor five-year survival rate. Hence, it is very significant to further investigate the occurrence and development mechanism of esophageal cancer, find more effective biomarkers and promote early diagnosis and effective treatment. Long non-coding RNAs (lncRNAs) are generally defined as non-protein-coding RNAs with more than 200 nucleotides in length. Existing researches have shown that lncRNAs could act as sponges, guides, scaffolds, and signal molecules to influence the oncogene or tumor suppressor expressions at transcriptional, post-transcriptional, and protein levels in crucial cellular processes. Currently, the dysregulated lncRNAs are reported to involve in the pathogenesis and progression of EC. Importantly, targeting EC-related lncRNAs through genome editing, RNA interference and molecule drugs may be one of the most potential therapeutic methods for the future EC treatment. In this review, we summarized the biological functions and molecular mechanisms of lncRNAs, including oncogenic lncRNAs and tumor suppressor lncRNAs in EC. In addition, we generalized the excellent potential lncRNA candidates for diagnosis, prognosis and therapy in EC. Finally, we discussed the current challenges and opportunities of lncRNAs for EC.
1 Introduction
Esophageal cancer (EC), as a malignancy of the digestive tracts, is one of the most common cancers and ranks 10th in terms of morbidity and sixth in mortality across the world in 2020 cancer statistics (Sung et al., 2021). EC is typically characterized by progressive dysphagia, which tends to occur in middle-aged and elderly people especially male (Falk, 2009; Massey, 2011). There are two main histologic subtypes of EC: esophageal squamous cell carcinoma (ESCC) and esophageal adenocarcinoma (EAC). EAC is more common in western countries, while the proportion of ESCC patients is increasing in Asian countries, which also gradually occupies the main position worldwide. Meanwhile, ESCC is the second most common primary cancer in head and neck areas (Chuang et al., 2008). Compared with other HNSCC (head and neck squamous cell carcinoma) subtypes, such as oral, dental, and maxillofacial squamous cell carcinoma, ESCC has a higher incidence rate and lower survival rate (Nikbakht et al., 2020). Due to the late appearance of obvious clinical symptoms, EC is commonly diagnosed in advanced clinical stages with low five-year survival rate (less than 20%) (Dubecz et al., 2012). At present, the main therapeutic regimen for esophageal cancer is individualized and comprehensive, which is based on surgery, but the patient outcome is not satisfactory (Deng and Lin, 2018; Watanabe et al., 2020). Therefore, to further explore the occurrence and development mechanism of esophageal cancer, find more effective biomarkers, and promote early diagnosis and effective treatment, are greatly needed.
The ENCODE project showed that about 70% of the human genome is transcribed (Tay et al., 2009). However, only 1–2% RNAs encode proteins, and a majority of the human genome is transcribed into non-coding RNAs (Al-Tobasei et al., 2016). There are many types of non-coding RNAs, including microRNA (miRNA), long non-coding RNAs (lncRNAs), small nuclear RNA (snRNA), circular RNAs (circRNAs) and so on (Abraham and Meltzer, 2017; Wu and Kuo, 2020). Although most non-coding RNAs remain unstudied, there are still many ncRNAs that have been revealed to play important roles in normal cellular function and disease development (Slack and Chinnaiyan, 2019; Toden and Goel, 2022). For example, some small non-coding RNAs which are stable in blood could be used for non-invasive cancer screening (Toiyama et al., 2013; Imaoka et al., 2016). Besides, some diseases, like myotonic dystrophy, can be treated by targeting some non-coding RNAs (Wheeler et al., 2012; Levin, 2019).
LncRNAs are defined as non-coding RNAs with greater than 200 nucleotides (Cui et al., 2019). Studies showed that lncRNAs play vital roles in regulating gene expression at transcriptional, post-transcriptional and epigenetic levels in multiple cellular processes in tumors (Slack and Chinnaiyan, 2019). Interestingly, some lncRNAs have been reported to possess the small open reading frame (sORF), which could encode the small peptides to regulate multiple signaling pathways (Chen et al., 2021). Meanwhile, lncRNAs have the potential to be the biomarkers and therapeutic targets for various cancers (Dong et al., 2018). Abnormal expressions of lncRNAs were also found in the occurrence and progression of EC, and some of them were even detected in body fluid for potential biomarker (Pardini et al., 2019). Here, we summarized the biological functions of lncRNAs in the pathogenesis and progression of EC, and discussed the values of lncRNAs as biomarkers for early diagnosis, prognosis and treatment targets in EC.
2 Functions of lncRNAs
Growing studies showed that lncRNAs can be involved in different biological processes, for instance, epigenetic regulation, stem cell differentiation, inflammation-related diseases and tumorigenesis by mediating the transcription and translation process of protein-related coding genes (Shi et al., 2013; Hon et al., 2017; Bao et al., 2019; Zhu et al., 2019). We summarized the specific regulation mechanisms of lncRNAs in Figure 1 (Camier et al., 1990; Houseley et al., 2008; Tripathi et al., 2010; Cesana et al., 2011; Wang and Chang, 2011; Steck et al., 2012; Hu et al., 2014; Yuan et al., 2014; Yin et al., 2015; Nachtergaele and He, 2017; Wang et al., 2019c; Liu et al., 2021a; Liu et al., 2021b; Pietropaolo et al., 2021; Song et al., 2021; Zhang et al., 2021).
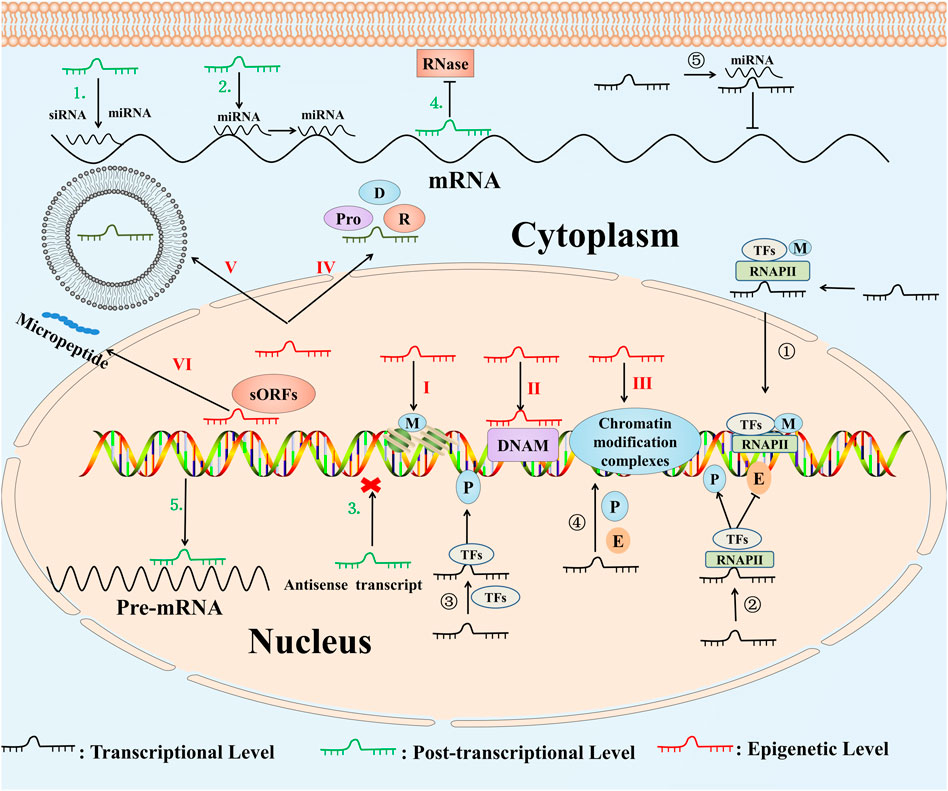
FIGURE1. Functional molecular mechanisms of lncRNAs. ①LncRNAs change the location and modification of TFs (Hu et al., 2014). ②LncRNAs regulate the combination of the TFs, RNA polymerase Ⅱ and the promoter, enhancer (Wang and Chang, 2011). ③LncRNAs interact with DNA as a platform for TFs (Yin et al., 2015). ④LncRNAs act as competitive endogenous RNAs (Cesana et al., 2011). ⑤LncRNAs promoter may act as cis-acting enhancer elements (Liu et al., 2021b). 1) LncRNAs act as the precursor for siRNAs or miRNAs (Steck et al., 2012). 2) LncRNAs regulate the distribution of miRNA (Nachtergaele and He, 2017). 3) LncRNAs act as antisense transcripts (Liu et al., 2021a). 4) LncRNAs form the double-stranded RNA complex by binding to mRNAs (Yuan et al., 2014). 5) LncRNAs regulate the selective splicing process of pre-mRNAs (Tripathi et al., 2010). I. LncRNAs regulate histone modification through affecting the modification factors (Houseley et al., 2008). II. LncRNAs bind to DNA modification to modify the DNA methylation (Song et al., 2021). III. LncRNAs bind to chromatin modification complexes to affect chromatin structure and remodeling (Pietropaolo et al., 2021). IV. LncRNAs locate molecules by binding to DNA, RNA and proteins as a “guide” (Camier et al., 1990). V. LncRNAs participate in the formation of exosomes (Zhang et al., 2021). VI. LncRNAs that contain sORFs can encode small peptide (Wang et al., 2019c). Abbreviations: P, promoter; E, enhancer; M, modification; Pro, protein; D, DNA; R, RNA; miRNA, microRNA; TFs, transcription factors; siRNA, small-interfering RNA; RNAPII, RNA polymerase II; DNAM, DNA modification; sORFs, small open reading frames.
At transcription level, lncRNA targets the transcription factors to regulate gene transcription through trans-activation behaviors, thus affecting the gene activation, repression, cell proliferation and apoptosis (Melendez-Zajgla and Maldonado, 2021). Besides, lncRNAs could bind to DNA strand or some proteins, affecting the localization of transcription factors, decreasing the transcript elongation through inhibiting the activation of elongation factors, and inhibiting polymerase II activity via targeting the trans region (Kapranov et al., 2007).
At post-transcriptional level, lncRNAs function as the regulators of some miRNAs to regulate the gene expression, or as the competitive endogenous RNAs (ceRNAs) to regulate the expression of corresponding gene by binding miRNAs (Maass et al., 2014). For example, lncRNAs regulate the dosage compensation effect, genomic imprinting, DNA methylation, and small polypeptides coding (Sleutels et al., 2002; Ponting et al., 2009; Cai et al., 2021). Interestingly, small polypeptides encoded by small open reading frames enriched in lncRNA transcripts have been reported to play important roles in circulating extracellular vesicles (Cai et al., 2021), cancer development (Cao et al., 2021), and neuronal differentiation (Douka et al., 2021). However, the functions of most lncRNAs are still unclear and require further comprehensive study.
3 LncRNAs Related to Esophageal Cancer
Many kinds of cancers, including EC, are associated with the imbalance of lncRNAs (Leucci et al., 2016; Hua et al., 2018; Lv et al., 2022). A study, which analyzed the lncRNAs expression profile in more than 200 EC samples from Gene Expression Omnibus (GEO) and The Cancer Genome Atlas (TCGA), demonstrated that thousands of lncRNAs were differentially expressed between EC and normal tissues, and many of them are related with patient survival time (Liu et al., 2018). LncRNAs could be divided into oncogenic lncRNAs and tumor suppressive lncRNAs based on their functions in EC, as shown in Figure 2 (St Laurent et al., 2015).
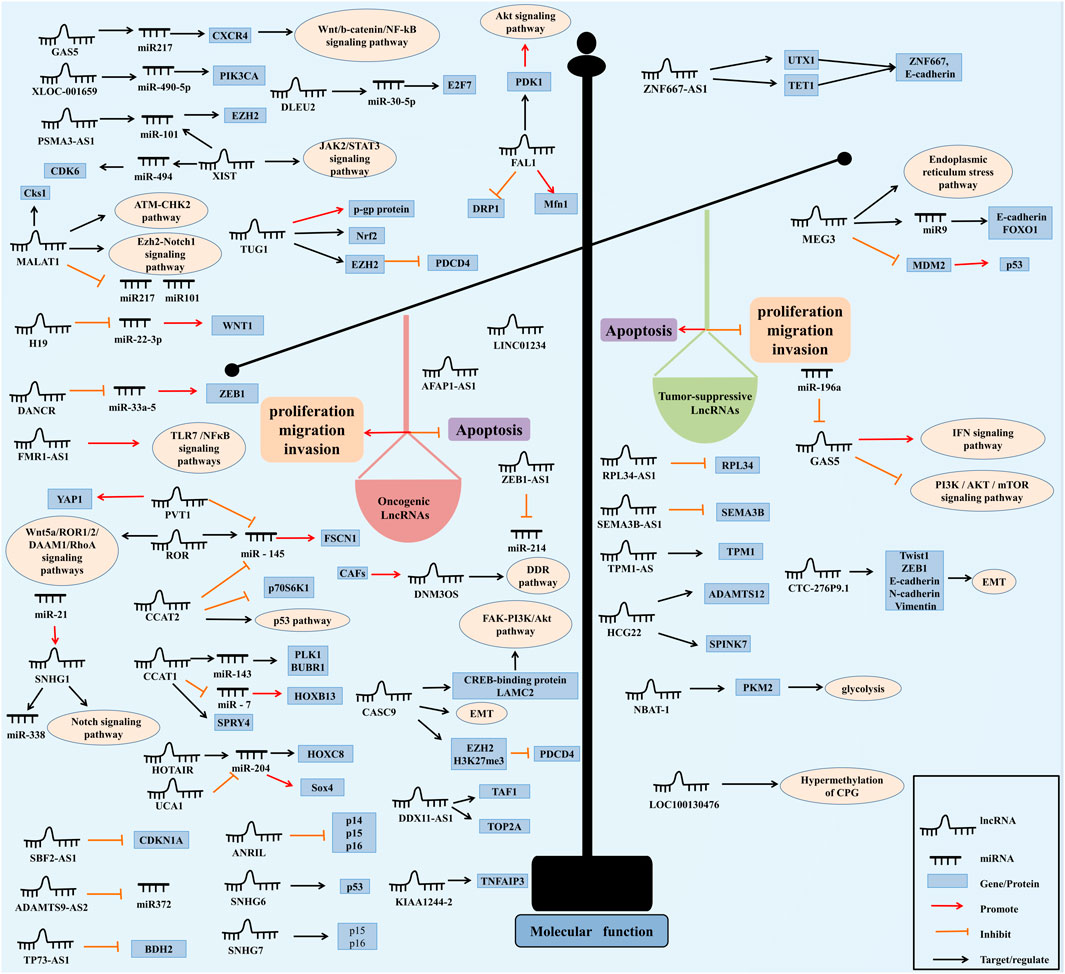
FIGURE 2. The molecular functions of lncRNAs in EC. LncRNAs function as oncogenes or tumor suppressors. On the left, lncRNAs act as oncogenes and are overexpressed in EC. On the contrary in right, lncRNAs act as tumor suppressors and are down-regulated in EC. As reported for the functional mechanisms, lncRNAs can be divided into the cis-acting lncRNAs and trans-acting lncRNAs. Cis-acting lncRNAs can regulate the chromatin state and/or expression of nearby genes: 1) the lncRNA transcript regulates the expression of nearby genes through recruiting regulatory factors (Kopp and Mendell, 2018); 2) the transcription or splicing-dependent regulation of the lncRNA execute the gene-regulation that is independent of the sequence of the RNA transcript but with structural functions (Engreitz et al., 2016); 3) Cis regulation depends on DNA elements within the lncRNA promoter (Dimitrova et al., 2014). And trans-acting lncRNAs leave the site of transcription and execute the functions: 1) lncRNAs regulate gene expression and chromatin states at genomic loci far distant from their transcription region (Rinn et al., 2007); 2) lncRNAs influence the nuclear organization and structure (Clemson et al., 2009); 3) lncRNAs interact with other proteins and/or RNA molecules (Hafner et al., 2010). In addition, lncRNA can be classified into following three categories by genic position: 1) intronic lncRNA, lncRNA within introns: mainly produced in the intron region of the coding gene (Rinn and Chang, 2012); 2) intergenic lncRNA, also known as lincRNA, mainly derived from the intergenic region of two coding genes (Ransohoff et al., 2018); 3) antisense lncRNA, mainly produced in the antisense chain of the coding gene (Rinn and Chang, 2012).
3.1 Oncogenic lncRNAs
As illustrated in Table 1, these lncRNAs are upregulated in EC and facilitate the malignant biological properties of EC cells. Here, we discussed some lncRNAs in more detail according to the oncogenic mechanisms of action in EC.
3.1.1 Oncogenic lncRNAs Involving Wnt Signaling Pathway
Wnt signaling is one of the crucial pathways regulating cell biology (Clevers and Nusse, 2012). The dysregulation of Wnt signaling has been described in various cancers, including lung cancer (Stewart, 2014), colorectal cancer (Zhan et al., 2017), etc. Currently, several studies revealed that lncRNAs can affect the malignant behaviors of EC through Wnt signaling pathway.
Small nucleolar RNA host gene 16 (SNHG16), Colon cancer-associated transcript 2 (CCAT2) and FEZ family zinc finger 1 antisense RNA 1 (FEZF1-AS1) were upregulated in EC cells and the knockdown of them inhibited the malignant behaviors of EC via the reduction of β-catenin expression in Wnt signaling pathway (Cai et al., 2015; Xu et al., 2018b; Han et al., 2018; Yang et al., 2019a; Wang and Wang, 2019). Ma et al. (2021) reported that lncRNA VPS9D1 antisense RNA1 (VPS9D1-AS1) was overexpressed in ESCC cell lines and tissues compared with normal controls. Silencing of VPS9D1-AS1 could inhibit the proliferation, invasion, and migration ability in vitro. Mechanically, VPS9D1-AS1 knockdown can inhibit the Wnt/β-catenin pathways through regulating the expression of β-catenin and c-Myc (Ma et al., 2021). Guo et al. (2021) revealed that DDX11 antisense RNA 1 (DDX11-AS1) overexpression enhanced cell proliferation, invasion, migration, and epithelial - mesenchymal transition (EMT) process of EC through activating the Wnt/β-catenin pathway via targeting miR-30d-5p. Meanwhile, DDX11-AS1 could act as a ceRNA through sponging miR-30d-5p, thus upregulating the expression of SNAI1 and Zinc Finger E-Box Binding Homeobox 2 (ZEB2) (Guo et al., 2021). You et al. (2019) found that LncRNA HERES, as an oncogene, can activate Wnt signaling by interacting with enhancer of zeste homolog 2 (EZH2) via its G-quadruple structure-like motif. Additionally, TUG1 exerts oncogenic ability though activating the Wnt/β-catenin pathway via miR-148a-3p/myeloid cell leukemia-1 (MCL-1) axis (Tang et al., 2020). Similarly, lncRNA growth arrest-specific transcript 5 (GAS5), as a molecular sponge, can regulate miR-301a, thus enhancing C-X-C chemokine receptor 4 (CXCR4) expression and activating NF-κB pathways and the Wnt/β-catenin pathway in EC (Li et al., 2018). Yang et al. (2021) found that knockdown of CCAT2 inhibited the mRNA and protein levels of wnt-induced-secreted-protein-1 (WISP1), β-catenin, and the mRNA levels of their downstream target genes in ESCC. Similarly, HOX transcript antisense intergenic RNA (HOTAIR) directly inhibited the Wnt inhibitory factor 1 (WIF-1) expression by promoting the histone methylation on H3K27 in the promoter region, thus activating the Wnt/β-catenin signaling pathway in ESCC (Ge et al., 2013).
Given the above, many lncRNAs play an important role in the occurrence and development of EC by regulating the Wnt signaling pathway.
3.1.2 Oncogenic lncRNAs Involving PI3K/AKT Signaling Pathway
PI3K/AKT signaling pathway has been reported to be frequently activated in a variety of tumors and has been considered as a potential therapeutic target (Aoki and Fujishita, 2017). Here, we showed that some lncRNAs can regulate the EC progression through PI3K/AKT signaling pathway.
LINC00152 was up-regulated in EC tissues, and knockdown of LINC00152 significantly inhibited the cell proliferation of EC by decreasing the expression levels of PI3K and AKT (Ding et al., 2020). Another study showed that LncRNA HLA complex P5 (HCP5) promoted malignant behaviors of ESCC through regulating the miR-139-5p/phosphodiesterase 4A (PDE4A) axis and activating the PI3K-AKT-mammalian target of rapamycin (mTOR) signaling pathway (Xu et al., 2021b). Similarly, overexpression of LINC01518, PTCSC1, LINC01617, and methyltransferase like 3 (METTL3) can also enhance the malignant behaviors of EC cells through activating the AKT pathway (Zhang et al., 2018a; Zhang et al., 2019b; Liu et al., 2020c; Hou et al., 2020). Liang et al. (2018) found that focally amplified lncRNA (FAL1) promoted the proliferation of EC cells by stimulating the AKT pathway via regulating the 3-phosphoinositide-dependent kinase 1 (PDK1). Importantly, EIF3J-AS1, as an oncogene, can sponge the miR-373-3p to up-regulate mRNA level of AKT1 in EC (Wei et al., 2020). Another study showed that ESCC-related lncRNAs transcript 1 (ESSCAL-1) downregulation can induce the cell apoptosis by the downregulation of the PI3K/AKT signaling via miR-590-3p/apolipoprotein mRNA-editing enzyme catalytic polypeptide 3 protein G (APOBEC3G) axis (Liu et al., 2020a). Xu et al. (2021a) reported that KCNQ1 overlapping transcript 1 (KCNQ1OT1) can activate the PI3K/AKT pathway via inhibiting the repression of miR-133b and indirectly upregulating epidermal growth factor receptor (EGFR). In addition, LINC00184 regulated the glycolysis and mitochondrial oxidative phosphorylation (OXPHOS) of EC cells through inducing the phosphorylation of Akt (Li et al., 2019).
In conclusion, numerous lncRNA dysregulation participates in the occurrence and development of EC through affecting the PI3K/AKT pathway.
3.1.3 Oncogenic lncRNAs Involving TGF-β Signaling Pathway
TGF-β signaling pathway has been showed to regulate numerous cellular functions, including cell proliferation, apoptosis, invasion, and migration (Colak and Ten Dijke, 2017). Current studies revealed that some lncRNAs can regulate the EC progression by affecting the TGF-β signaling pathway.
Zhu et al. (2021) found that lncRNA FAM225A facilitated the ESCC development through sponging miR-197-5p, thus upregulating the non-POU domain-containing octamer-binding protein (NONO) expression and activating the transforming growth factor-β (TGF-β) pathway in ESCC cells. Another study revealed that NCK1-AS1 may promote ESCC progression by upregulating the expression of TGF-β1 (Fu et al., 2022). Similarly, ANRIL knockdown inhibit EC cell proliferation by increasing the expression of p15 via TGFβ1 (Chen et al., 2014).
In brief, some lncRNAs can regulate the TGF-βpathway to affect the EC development.
3.1.4 Oncogenic lncRNAs Involving Other Regulatory Mechanisms
We also discussed lncRNAs promote the tumorigenesis and development of EC through other regulatory mechanisms. Here, we mainly discussed the HOX transcript antisense intergenic RNA (HOTAIR), MALAT1 LINC01535 and BRAF-activated non-coding RNA (BANCR), which have got wide attention in multiple studies.
Several studies have revealed that HOTAIR, as a competing endogenous RNA, plays the oncogenic role through the lncRNA-miRNA-mRNA function network in EC. Ren et al. (2016) reported that HOTAIR was abnormally increased in ESCC cells and could facilitate ESCC occurrence through sponging miR-1 and upregulating CCND1. Xu et al. found that HOTAIR may promote epithelial–mesenchymal transition (EMT) through acting as the miR-148a sponge to facilitate the expression of snail2 (Xu and Zhang, 2017). It was reported that the up-regulation of homeobox C8 (HOXC8) was observed in a variety of cancer types and involved in tumor formation (Li et al., 2020a; Jiang et al., 2021). Importantly, Wang et al. (2019a) showed that HOTAIR could regulate HOXC8 by specifically binding to miR-204 as a competing endogenous RNA. CC motif chemokine ligand 18 (CCL18) has crucial roles in tumor progression and metastasis (Cardoso et al., 2021). Wang et al. (2019e) revealed that HOTAIR upregulated by CCL18 advanced malignant progression of ESCC through miR-130a-5p-zinc finger E-box binding homeobox 1 (ZEB1) axis. Hexokinase 2 (HK2) expression is increased in tumors and contributes to aerobic glycolysis. Ma et al. (2017) found that HOTAIR promoted HK2 expression by sponging miR-125/miR-143, thus facilitating the tumorigenesis of ESCC.
Researchers have also revealed that MALAT1 played a crucial role in the tumorigenesis and development of EC. MALAT1 was significantly up-regulated in EC tissues and cells than the normal controls (Huang et al., 2016). A study showed that the up-regulation of MALAT1 in advanced stage ESCC tissues may facilitate ESCC growth by the ataxia-telangiectasia mutated (ATM)/checkpoint kinase 2 (CHK2) pathway dephosphorylation (Hu et al., 2015). MALAT1 knockdown suppressed the invasion, migration and EMT of EC. Mechanically, MALAT1 could target and sponge the miR-1-3p, thus affecting the coronin-1C (CORO1C)/tropomyosin3 (TPM3) pathway in EC (Li et al., 2020c). Besides, knockdown of MALAT1 inhibited the proliferation, migration and tumor sphere formation of tumors cells by decreasing the expression of β-catenin, Lin28 and EZH2 in ESCC (Wang et al., 2016a). Another study found that MALAT1 could promote the EMT and metastasis of EC cells through Ezh2-Notch1 signaling pathway (Chen et al., 2018). Importantly, down-regulation of MALAT1 by miR-101 and miR-217 through a post-transcriptional regulation mechanism inhibited proliferation, invasion, and migration of ESCC cells (Wang et al., 2015). Subsequently, Wang et al. (2021b) found that N 6-methyladenosine (m6A) modification of MALAT1 promotes the metastasis ability of ESCC through reshaping nuclear speckles (NSs). The transcription events in downstream activated by NSs is partially intermediated through the binding of YTH-domain-containing protein 1 (YTHDC1) onto MALAT1-Δm6A. Interestingly, artificially tethering YTHDC1 onto MALAT1-Δm6A ESCC cells leads to the restoring of migration ability (Wang et al., 2021b), indicating that post-transcriptional modification may affect the oncogenic activity of MALAT1 in EC.
LINC01535 promoted the proliferation and decreased the apoptosis in ESCC cells via activating the JAK/STAT3 pathway (Fang et al., 2020). Another study showed that small nucleolar RNA host gene 20 (SNHG20) played the oncogenic role through enhancing the growth and metastasis of ESCC through the ataxia telangiectasia-mutated kinase (ATM)-JAK-programmed cell death 1 ligand 1 (PD-L1) axis (Zhang et al., 2019a).
BRAF-activated non-coding RNA (BANCR) was revealed to enhance the cell proliferation, invasion and migration of ESCC by Raf/MEK/ERK signaling (Yu et al., 2021). Song et al. (2020) also found that BANCR can promote the malignant behaviors of ESCC and BANCR downregulation inhibited the ESCC development by inactivating the insulin like growth factor 1 receptor (IGF1R)/Raf/MEK/ERK axis by sponging the miR-338-3p.
To sum up, the oncogenic effects of lncRNAs in EC are multi-channel through various cell pathways.
3.2 Tumor-Suppressive lncRNAs
Deregulation of tumor suppressors plays important roles during carcinogenesis and tumor progression. In-depth understanding of tumor suppressors can serve new ideas for tumor-targeted therapy. It can promote EC occurrence and development once the tumor suppressive lncRNAs are downregulated. We summarize the information about tumor suppressive lncRNAs in Table 2. In this section, we discussed the tumor-suppressive role of some lncRNAs according to the mechanisms of action in EC.
3.2.1 Tumor-Suppressive lncRNAs Involving Wnt Signaling Pathway
Overexpression of lncRNA-neighboring enhancer of FOXA2 (NEF), growth-arrest associated lncRNA 1 (GASL1), LINC00675 inhibited cell proliferation, invasion and migration through decreasing the expression of β-catenin in ESCC (Zhang et al., 2018b; Zhong et al., 2018; Ren et al., 2021). Urothelial carcinoma-associated (UCA1) expression was significantly lower in ESCC tissues than adjacent normal tissues. And mRNA microarray analysis indicated the overexpression of UCA1 can inhibit the Wnt signaling pathway through reducing the β-catenin (active form) levels (Wang et al., 2016b). Another study displayed that miR-4261, which could promote ESCC cell function in vitro, is one of maternally expressed gene 3 (MEG3) targets. The anti-tumor effect of MEG3 in ESCC was related to MEG3-miR-4261 axis regulating the dickkopf-2 (DKK2) and Wnt/β-catenin signaling (Ma et al., 2019).
3.2.2 Tumor-Suppressive lncRNAs Involving Other Regulatory Mechanisms
Here, we discussed other regulatory mechanisms lncRNAs, which can inhibit the tumorigenesis and development of EC. We mainly discussed the regulatory mechanisms regulated by MEG3 and GAS5 in EC.
The results of Li’s study proposed that MEG3 may inhibit EMT and EC cell function through downregulating phosphoserine aminotransferase 1 (PSAT1) and restraining the PSAT1 dependent GSK-3β/Snail signaling pathway (Li et al., 2020b). In addition, MEG3 was found to be significantly reduced in ESCC tissues, which was mediated by DNA methylation. And ectopic expression of MEG3 could promote ESCC cell apoptosis and inhibit cell proliferation and metastasis. Tumor protein 53 (TP53) is one of best-known tumor suppressors. One way of MEG3 inhibiting EC is by inducing p53 activation due to mouse double minute 2 (MDM2) downregulation (Lv et al., 2016). Moreover, lower expression of MEG3 predicted shorter survival by analyzing EC dataset from TCGA (Lv et al., 2016). Huang et al. (2017) also discovered MEG3 expression was diminished in ESCC tissue. MEG3 could suppress EC cell growth and induce apoptosis through activating endoplasmic reticulum stress pathway because the overexpression of MEG3 increased endoplasmic reticulum stress - related proteins (ATF6, GRP78, CHOP, PERK, IRE1, and cleaved caspase-3) expression (Huang et al., 2017). Dong et al. (2017) observed that there were hypermethylation of MEG3 both in ESCC tissue and EC cell lines, and it is crucial to MEG3 gene silencing. They also found that MEG3 may regulate forkhead box other 1 (FOXO1) and E-cadherin expression through competitively binding miR-9 (Dong et al., 2017).
In Wang’s study, GAS5 was lower in tumor tissues than that in healthy tissues, and the serum GAS5 level in EC patients was significantly lower than that in normal controls. Overexpression of GAS5 inhibited EC cell proliferation and migration by inactivating PI3K/AKT/mTOR signal pathway (Wang et al., 2018). Huang et al. (2018) found that tumor-suppressive lncRNA GAS5 was regulated by interferon (IFN) responses through the JAK/STAT signaling pathway. A study discovered the level of NBAT-1 was lower in EC tissue than adjacent normal tissues. And NBAT-1 overexpression could inhibit EC cell proliferation and tumor glycolysis partially by reducing pyruvate kinase M2 (PKM2) protein expression (Zhao et al., 2019). According to Ke et al. (2018)’s study, they also found that the expression of GAS5 was downregulated in ESCC tissue and ESCC cell. GAS5 overexpression may suppress ESCC cell function through inducing cell cycle arrest at G2/M stage by activating the ATM-CHK2 pathway and affecting EMT-associated proteins (Ke et al., 2018).
4 LncRNAs in Diagnosis, Prognosis and Therapy of Esophageal Cancer
LncRNAs play crucial roles in both cellular physiological and pathological processes, including in the pathogenesis of various tumors and often reveal a higher cell/tissue specificity than mRNA (Derrien et al., 2012; De Falco et al., 2021; Turai et al., 2021), so they were suggested as potential valuable diagnostic, prognostic factors even therapeutic targets. Below, we summarize the excellent potential lncRNA candidates for diagnosis, prognosis and therapy in EC.
4.1 LncRNAs in Esophageal Cancer Diagnosis
The treatment effect is not satisfactory for EC patients with advanced stages who have lost the opportunity of surgical treatment. To improve the therapy efficacy, it is urgent to find more excellent EC biomarkers for early diagnosis. We have summarized the present EC-related lncRNA diagnostic biomarkers in Table 3.
Most of the lncRNAs with diagnostic potential are located in tumor tissues. Compared with the diagnostic markers derived from tumor tissues, the markers in peripheral blood are easy to be obtained and non-invasive, which are more suitable for early screening and early diagnosis of tumors. Tong et al. (2015) identified three stable plasma ESCC-related lncRNAs. By receiver operating characteristic curve (ROC) analysis, plasma lncRNA POU3F3 could be used as a promising biomarker for the diagnosis of ESCC. A study found serum level of lncRNA HOTAIR was increased in ESCC patients, and it was positively associated with the expression of HOTAIR in ESCC tissues. Through ROC analysis, it was detected that the serum level of HOTAIR can distinguish ESCC patients from healthy controls. The results indicated that serum HOTAIR could be a promising diagnostic indicator of ESCC (Wang et al., 2017). Small nucleolar RNA host gene 1 (SNHG1) has abnormal expression in many cancers and plays a key role in ESCC (Zhang et al., 2017; Luo et al., 2020). Luo et al. (2020) discovered that SNHG1 can promote ESCC cells proliferation, and its expression level in frozen cancer tissues is significantly related to its serum level. Combined with the results of ROC analysis, it was showed that SNHG1 can be applied as a potential diagnostic biomarker for ESCC patients. In ESCC, GAS5 is considered to be a tumor suppressor (Huang et al., 2018). GAS5 serum level in EC patients was lower compared within healthy controls, and it was decreased with the primary tumor stage (T stage) increasing. Combined with the results of AUC analysis, serum GAS5 may have diagnostic values for EC (Wang et al., 2018). LncRNA PGM5 antisense RNA 1 (PGM5-AS1) was markedly decreased in ESCC cell lines, plasma and tissues. And plasma PGM5-AS1 level was promising in diagnosis of ESCC (Zhihua et al., 2019).
In summary, because of the characteristics and important functions, lncRNAs have important significance and great potential for EC diagnosis.
4.2 LncRNAs in Esophageal Cancer Prognosis
Recent studies have found that several lncRNAs have characteristic prognostic functions in EC (Yang et al., 2019b). Owing to the important roles in tumorigenesis and progression, combined with the stability and specificity, lncRNAs have been considered as promising biomarkers for evaluating the prognosis of EC patients, such as HOTAIR, MALAT1, non-coding RNA activated by DNA damage (NORAD), LincRNA-uc002yug.2, and long non-coding RNA activated by transforming growth factor-β (lncRNA-ATB) (Wu et al., 2014; Khorkova et al., 2015). We summarized the lncRNA prognostic biomarkers for EC in Table 4.
As previously mentioned, HOTAIR is an important oncogenic lncRNA and has great potential for EC diagnosis. At the same time, it is also an important prognostic biomarker for EC. Kaplan–Meier survival curves showed, compared with patients with low HOTAIR expression, patients with high HOTAIR expression had noticeably shorter survival times (HR value >1). The results indicate that HOTAIR is an unfavorable factor for the prognosis of EC and can be used as a prognostic biomarker for EC (Ge et al., 2013; Li et al., 2013). LncRNA MALAT1 is located on chromosome 11q13.1 and its expression is conserved in multiple species (Ji et al., 2003; Huang et al., 2016). MALAT1 is involved in multiple biological functions and overexpressed in many cancers, such as liver cancer, lung cancer, renal cancer, gastric cancer (Ji et al., 2003; Su et al., 2021). MALAT1 acts on proliferation, metastasis, cell cycle and drug resistance in these cancer cells (Su et al., 2021). Compared with the expression in adjacent non-carcinoma tissues, MALAT1 expression is higher in EC tissues and correlates with unfavorable prognosis (Wang et al., 2019b; Syllaios et al., 2021). MALAT1 promotes EC cell proliferation, migration and invasion (Wang et al., 2019b; Syllaios et al., 2021). According to the multivariate analysis of prognostic factors (HR,6.638, 95% CI,2.948–14.947), MALAT1 was significantly related to the prognosis of EC patients and could be applied as an independent factor to EC prognosis (Huang et al., 2016). NORAD, a 5300 nt lncRNA and annotated in RefSeq as LINC00657, is a highly conserved lncRNA. It takes a primary part in regulating both ploidy and chromosomal stability in diploid cells. And the loss of NORAD function can result in chromosomal instability (Lee et al., 2016). Importantly, lncRNA NORAD is overexpressed in many cancers, including breast cancer, gastric cancer, bladder cancer, liver cancer, and so on (Soghli et al., 2021). Studies showed that NORAD could act as a ceRNA (Yang et al., 2019c). A meta-analysis reported that higher NORAD expression was significantly related with poorer overall survival in cancers (Wang et al., 2021a). And its expression level was appreciably higher in ESCC tissues than that in adjacent normal tissues. The multivariate analysis indicated that NORAD was an independent predictor of ESCC overall survival, which showed that NORAD is a potential ESCC prognostic marker (Wu et al., 2017).
In addition, many lncRNAs, such as LincRNA-uc002yug.2, ATB, are shown to be potential prognostic biomarkers for EC by the Multivariate Cox regression model (Table 4). Whether these lncRNAs can be further applied in clinic needs further verification, and the combined application of multiple lncRNAs is an important direction for future research.
4.3 LncRNAs in Esophageal Cancer Therapy
Therapy resistance leads to poor treatment efficacy and high recurrence rate of cancers to result in dismal prognosis. Resistance to cancer therapy ordinarily stems from deregulation of signaling pathways. LncRNAs are involved in many these pathways (Askarian-Amiri et al., 2016; Majidinia and Yousefi, 2016; Borkiewicz et al., 2021). For example, exogenous lncRNA UCA1 increased the invasiveness of cancer cells and worked in cisplatin resistance to bladder cancer therapy (Wang et al., 2008). LncRNA HOTAIR overexpression increased breast cancer cell proliferation and contributed to tamoxifen resistance in breast cancer (Xue et al., 2016). We summarized the lncRNAs involved in resistance of EC therapy (Table 5).
MALAT1 is a potential prognostic biomarker for EC. Knockdown of MALAT1 could enhance the radiosensitivity and chemosensitivity of ESCC cells (Yao et al., 2019). And MALAT1 overexpression inhibited the viability decrease and apoptosis increase of EC cells which were induced by irradiation (Li et al., 2017).
H19 is an oncogenic lncRNA and takes part in the tumorigenesis and progression of EC (Huang et al., 2015). Radioresistance is a main factor limiting the efficacy of radiotherapy for EC. H19 was upregulated in an ESCC radioresistant cell line. And H19 knockdown downregulated Wnt1 through upregulating miR-22-3p expression, then resulted in the inhibition of radioresistance, proliferation and migration in radioresistant ESCC cell (Luo et al., 2019).
Prostate cancer associated transcripts 1 (PCAT-1) was first discovered in patients with prostate cancer by transcript sequencing and was identified as a transcriptional repressor (Prensner et al., 2011). LncRNA PCAT-1 is up-regulated and could play an oncogenic role in multiple cancers, such as prostate cancer, ESCC, gastric cancer, ovarian cancer (Prensner et al., 2011; Shi et al., 2015; Bi et al., 2017; Ding et al., 2019). PCAT-1 expression is remarkably increased in ESCC tissues, which is significantly related to tumor invasion (Shi et al., 2015; Razavi and Ghorbian, 2019). And it was found that PCAT-1 facilitated ESCC progression by PCAT-1/miR-508-3p/ANXA10 axis in cell experiments (Zang et al., 2019). When PCAT-1 was inhibited, the chemosensitivity of EC to cisplatin was increased (Zhen et al., 2018).
Taurine up-regulated gene 1 (TUG1) is a lncRNA which is abnormally expressed in various tumors (Da et al., 2021). It was found that TUG1 could function as ceRNA to specifically sponging microRNAs to regulate gene expression and was involved in oncogenesis and development of many malignant tumors, such as gastric cancer (Ren et al., 2017), osteosarcoma (Sheng and Li, 2019). And TUG1 could regulate resistance and sensitivity of some cancers to chemotherapeutic drugs, such as bladder cancer (Yu et al., 2019), cervical cancer (Wei et al., 2019) and EC. TUG1, as an oncogenic lncRNA in EC, is significantly upregulated in EC and promotes EC development (Jin et al., 2020; Tang et al., 2020; Zong et al., 2020). TUG1 is also related to radiotherapy resistance and chemotherapy resistance of ESCC. Patients with high TUG1 expression displayed more resistance to chemotherapy (platinum-based chemotherapy combined with paclitaxel or 5-fluo-rouracil) compared with low TUG1 expression group (Jiang et al., 2016). Mechanically, TUG1 can promote cisplatin resistance in ESCC through upregulating Nrf2 or epigenetically suppressing PDCD4 expression via EZH2 (Xu et al., 2018a; Zhang et al., 2019c). In addition, TUG1 can increase radiotherapy resistance of ESCC by reducing miR-144-3p and regulating MET/EGFR/AKT axis (Wang et al., 2020).
Notably, lncRNA POU3F3 was reported to promote ESCC cell proliferation and cisplatin resistance through exosomal POU3F3-induced transformation of normal fibroblasts to cancer-associated fibroblasts in ESCC (Tong et al., 2020). However, studies about exosomal lncRNAs and chemoresistance are relatively scarce, especially those about the association between lncRNAs and multidrug resistance in EC.
In summary, recent advances have found that many lncRNAs participate in the drug resistance of EC. However, the exact role of lncRNAs is unclear in the entire regulatory network of drug resistance, and exploring the underlying mechanism will help us to reverse drug resistance of EC via using lncRNA-targeting strategy.
5 Conclusion and Future Perspectives
With the high-speed development of high-throughput sequencing technology, Genome Map and bioinformatics, a great deal of lncRNAs have been discovered. LncRNA is involved in various processes such as epigenetic regulation, chromatin remodeling and gene expression regulation (Shi et al., 2013; Beermann et al., 2016; Quinn and Chang, 2016). Many human diseases, including cancers, are related to the dysregulation of lncRNA (Bhan et al., 2017; Chi et al., 2019). Increasing evidence indicates that lncRNAs are involved in regulating the carcinogenesis and progression of EC. Importantly, these dyregulated lncRNAs may be useful for the early diagnosis, prognosis, and treatment of EC.
Nowadays, the researches of lncRNA mainly focus on several aspects: identification and classification of lncRNA; the interaction between lncRNA and other factors; the roles of lncRNA in major diseases; the potential of lncRNA as biomarkers and drug targets of diseases. The role of lncRNA in cancers is a hotspot. In this paper, we mainly summarized the biological functions and molecular mechanisms of lncRNAs. At the same time, we reviewed the main oncogenic lncRNAs and suppressive lncRNAs in EC, and lncRNAs related to EC diagnosis, treatment, and prognosis. The previous studies have shown that lncRNAs play important roles in the occurrence and development of EC and hold much promise as novel biomarkers and therapeutic targets for EC.
However, the research on lncRNAs in EC is still in initial phase and faced with a number of challenges especially in clinical application. Moreover, several novel mechanisms of lncRNAs in EC should be focused on in the future. Currently, despite the dysregulated lncRNAs seem to be important in the tumorigenesis or progression, there are still some questions required to be answered by further studies before their clinical translation. First, although multiple screening wet methods (microarrays, next-generation sequencing and qRT-PCR) have been used to identify the cancer-associated lncRNAs, more effective bioinformatics tools need to be developed. OSescc for identifying the prognosis-related genes in ESCC patients (Wang et al., 2019d), have been developed in recent years, however available clinical lncRNA testing method has not been seen to now. Second, how do lncRNAs co-regulate with other functional molecules (such as miRNAs, circRNAs, proteins) in the pathogenesis of EC? For example, the disordered regulatory networks of lncRNAs-miRNAs-mRNAs can induce the occurrence and development of EC (Karreth and Pandolfi, 2013). Third, the small peptides encoded by ncRNAs have been recurrently reported (Wang et al., 2019c). It will be very interesting to explore whether these lncRNAs encode small peptides, which is the direct regulator for malignant behaviors of EC.
In addition, increasing evidences showed that EC-derived exosomes may be useful for the early detection of EC. RNA sequencing revealed that dysregulated exosomal lncRNAs are associated with early-stage ESCC (Tian et al., 2020). Some lncRNA-based signature shows the higher efficiency of the prognosis of EC. Liu et al. (2020b) reported a toll-like receptor (TLR)-related four-lncRNA signature as a prognostic biomarker in EC. Moreover, several effective machine learning methods have been developed to identify and predict lncRNAs in various organisms (Xu et al., 2021c). For instance, Liu et al. (2022) constructed an immune-associated lncRNA signature to predict the clinical outcome of colorectal cancer patients based on machine learning-based methods. Zhang et al. (2018c) constructed the CRlncRC, a machine learning-based method for identifying the cancer-related lncRNAs. Therefore, the application of machine learning-based lncRNAs in the diagnosis of EC will be interesting.
In brief, lncRNAs might be promising biomarkers or therapeutic targets for clinical application for EC after in-depth basic and clinical investigations.
Author Contributions
Conceptualization, YH and XG. Writing—original draft preparation, YH, GZ, XS, and XW. Writing—review and editing, YH, GZ, YW, LZ, and XG. Funding acquisition, YH and XG. All authors have read and agreed to the published version of the manuscript.
Funding
This work was supported by the National Natural Science Foundation of China (No. U2004136), supporting program for Central Plain Young Top Talents (No. ZYQR201912176), supporting grant of Henan University (Nos. 2018YLJC01, 2019YLXKJC01 and 2020YLZDYJ14), program for Science and Technology Development in Henan Province (Nos. 212102310158 and 212102310709), Program from Academy for advanced interdisciplinary studies of Henan University (No. Y21008L).
Conflict of Interest
The authors declare that the research was conducted in the absence of any commercial or financial relationships that could be construed as a potential conflict of interest.
Publisher’s Note
All claims expressed in this article are solely those of the authors and do not necessarily represent those of their affiliated organizations, or those of the publisher, the editors and the reviewers. Any product that may be evaluated in this article, or claim that may be made by its manufacturer, is not guaranteed or endorsed by the publisher.
References
Abraham, J. M., and Meltzer, S. J. (2017). Long Noncoding RNAs in the Pathogenesis of Barrett's Esophagus and Esophageal Carcinoma. Gastroenterology 153 (1), 27–34. doi:10.1053/j.gastro.2017.04.046
Al-Tobasei, R., Paneru, B., and Salem, M. (2016). Genome-Wide Discovery of Long Non-coding RNAs in Rainbow Trout. PLoS One 11 (2), e0148940. doi:10.1371/journal.pone.0148940
Aoki, M., and Fujishita, T. (2017). Oncogenic Roles of the PI3K/AKT/mTOR Axis. Curr. Top. Microbiol. Immunol. 407, 153–189. doi:10.1007/82_2017_6
Askarian-Amiri, M. E., Leung, E., Finlay, G., and Baguley, B. C. (2016). The Regulatory Role of Long Noncoding RNAs in Cancer Drug Resistance. Methods Mol. Biol. 1395, 207–227. doi:10.1007/978-1-4939-3347-1_12
Bao, Z., Yang, Z., Huang, Z., Zhou, Y., Cui, Q., and Dong, D. (2019). LncRNADisease 2.0: an Updated Database of Long Non-coding RNA-Associated Diseases. Nucleic Acids Res. 47 (D1), D1034–D1037. doi:10.1093/nar/gky905
Beermann, J., Piccoli, M. T., Viereck, J., and Thum, T. (2016). Non-coding RNAs in Development and Disease: Background, Mechanisms, and Therapeutic Approaches. Physiol. Rev. 96 (4), 1297–1325. doi:10.1152/physrev.00041.2015
Bhan, A., Soleimani, M., and Mandal, S. S. (2017). Long Noncoding RNA and Cancer: A New Paradigm. Cancer Res. 77 (15), 3965–3981. doi:10.1158/0008-5472.CAN-16-2634
Bi, M., Yu, H., Huang, B., and Tang, C. (2017). Long Non-coding RNA PCAT-1 Over-expression Promotes Proliferation and Metastasis in Gastric Cancer Cells through Regulating CDKN1A. Gene 626, 337–343. doi:10.1016/j.gene.2017.05.049
Borkiewicz, L., Kalafut, J., Dudziak, K., Przybyszewska-Podstawka, A., and Telejko, I. (2021). Decoding LncRNAs. Cancers 13 (11), 2643. doi:10.3390/cancers13112643
Cai, T., Zhang, Q., Wu, B., Wang, J., Li, N., Zhang, T., et al. (2021). LncRNA-encoded Microproteins: A New Form of Cargo in Cell Culture-Derived and Circulating Extracellular Vesicles. J. Extracell Vesicles 10 (9), e12123. doi:10.1002/jev2.12123
Cai, Y., He, J., and Zhang, D. (2015). Long Noncoding RNA CCAT2 Promotes Breast Tumor Growth by Regulating the Wnt Signaling Pathway. Onco Targets Ther. 8, 2657–2664. doi:10.2147/OTT.S90485
Camier, S., Baker, R. E., and Sentenac, A. (1990). On the Flexible Interaction of Yeast Factor Tau with the Bipartite Promoter of tRNA Genes. Nucleic Acids Res. 18 (15), 4571–4578. doi:10.1093/nar/18.15.4571
Cao, Y., Yang, R., Lee, I., Zhang, W., Sun, J., Meng, X., et al. (2021). Prediction of LncRNA-Encoded Small Peptides in Glioma and Oligomer Channel Functional Analysis Using In Silico Approaches. PLoS One 16 (3), e0248634. doi:10.1371/journal.pone.0248634
Cardoso, A. P., Pinto, M. L., Castro, F., Costa, Â. M., Marques-Magalhães, Â., Canha-Borges, A., et al. (2021). The Immunosuppressive and Pro-tumor Functions of CCL18 at the Tumor Microenvironment. Cytokine Growth Factor. Rev. 60, 107–119. doi:10.1016/j.cytogfr.2021.03.005
Cesana, M., Cacchiarelli, D., Legnini, I., Santini, T., Sthandier, O., Chinappi, M., et al. (2011). A Long Noncoding RNA Controls Muscle Differentiation by Functioning as a Competing Endogenous RNA. Cell 147 (2), 358–369. doi:10.1016/j.cell.2011.09.028
Chen, D., Zhang, Z., Mao, C., Zhou, Y., Yu, L., Yin, Y., et al. (2014). ANRIL Inhibits p15(INK4b) through the TGFβ1 Signaling Pathway in Human Esophageal Squamous Cell Carcinoma. Cell Immunol 289 (1-2), 91–96. doi:10.1016/j.cellimm.2014.03.015
Chen, M., Xia, Z., Chen, C., Hu, W., and Yuan, Y. (2018). LncRNA MALAT1 Promotes Epithelial-To-Mesenchymal Transition of Esophageal Cancer through Ezh2-Notch1 Signaling Pathway. Anticancer Drugs 29 (8), 767–773. doi:10.1097/CAD.0000000000000645
Chen, Y., Long, W., Yang, L., Zhao, Y., Wu, X., Li, M., et al. (2021). Functional Peptides Encoded by Long Non-coding RNAs in Gastrointestinal Cancer. Front. Oncol. 11, 777374. doi:10.3389/fonc.2021.777374
Chi, Y., Wang, D., Wang, J., Yu, W., and Yang, J. (2019). Long Non-coding RNA in the Pathogenesis of Cancers. Cells 8 (9). doi:10.3390/cells8091015
Chuang, S. C., Scelo, G., Tonita, J. M., Tamaro, S., Jonasson, J. G., Kliewer, E. V., et al. (2008). Risk of Second Primary Cancer Among Patients with Head and Neck Cancers: A Pooled Analysis of 13 Cancer Registries. Int. J. Cancer 123 (10), 2390–2396. doi:10.1002/ijc.23798
Clemson, C. M., Hutchinson, J. N., Sara, S. A., Ensminger, A. W., Fox, A. H., Chess, A., et al. (2009). An Architectural Role for a Nuclear Noncoding RNA: NEAT1 RNA Is Essential for the Structure of Paraspeckles. Mol. Cel 33 (6), 717–726. doi:10.1016/j.molcel.2009.01.026
Clevers, H., and Nusse, R. (2012). Wnt/β-catenin Signaling and Disease. Cell 149 (6), 1192–1205. doi:10.1016/j.cell.2012.05.012
Colak, S., and Ten Dijke, P. (2017). Targeting TGF-β Signaling in Cancer. Trends Cancer 3 (1), 56–71. doi:10.1016/j.trecan.2016.11.008
Cui, Y. S., Song, Y. P., and Fang, B. J. (2019). The Role of Long Non-coding RNAs in Multiple Myeloma. Eur. J. Haematol. 103 (1), 3–9. doi:10.1111/ejh.13237
Da, M., Zhuang, J., Zhou, Y., Qi, Q., and Han, S. (2021). Role of Long Noncoding RNA Taurine-Upregulated Gene 1 in Cancers. Mol. Med. 27 (1), 51. doi:10.1186/s10020-021-00312-4
De Falco, V., Napolitano, S., Esposito, D., Guerrera, L. P., Ciardiello, D., Formisano, L., et al. (2021). Comprehensive Review on the Clinical Relevance of Long Non-coding RNAs in Cutaneous Melanoma. Int. J. Mol. Sci. 22 (3). doi:10.3390/ijms22031166
Deng, W., and Lin, S. H. (2018). Advances in Radiotherapy for Esophageal Cancer. Ann. Transl Med. 6 (4), 79. doi:10.21037/atm.2017.11.28
Derrien, T., Johnson, R., Bussotti, G., Tanzer, A., Djebali, S., Tilgner, H., et al. (2012). The GENCODE V7 Catalog of Human Long Noncoding RNAs: Analysis of Their Gene Structure, Evolution, and Expression. Genome Res. 22 (9), 1775–1789. doi:10.1101/gr.132159.111
Dimitrova, N., Zamudio, J. R., Jong, R. M., Soukup, D., Resnick, R., Sarma, K., et al. (2014). LincRNA-p21 Activates P21 in Cis to Promote Polycomb Target Gene Expression and to Enforce the G1/S Checkpoint. Mol. Cel 54 (5), 777–790. doi:10.1016/j.molcel.2014.04.025
Ding, C., Wei, R., Rodríguez, R. A., and Del Mar Requena Mullor, M. (2019). LncRNA PCAT-1 Plays an Oncogenic Role in Epithelial Ovarian Cancer by Modulating cyclinD1/CDK4 Expression. Int. J. Clin. Exp. Pathol. 12 (6), 2148.
Ding, Y., Guo, H., Zhu, L., Xu, L., Pei, Q., and Cao, Y. (2020). LINC00152 Knock-Down Suppresses Esophageal Cancer by EGFR Signaling Pathway. Open Med. (Wars) 15, 126–133. doi:10.1515/med-2020-0019
Dong, D., Mu, Z., Zhao, C., and Sun, M. (2018). ZFAS1: a Novel Tumor-Related Long Non-coding RNA. Cancer Cel Int 18, 125. doi:10.1186/s12935-018-0623-y
Dong, Z., Zhang, A., Liu, S., Lu, F., Guo, Y., Zhang, G., et al. (2017). Aberrant Methylation-Mediated Silencing of lncRNA MEG3 Functions as a ceRNA in Esophageal Cancer. Mol. Cancer Res. 15 (7), 800–810. doi:10.1158/1541-7786.MCR-16-0385
Douka, K., Birds, I., Wang, D., Kosteletos, A., Clayton, S., Byford, A., et al. (2021). Birds, ICytoplasmic Long Noncoding RNAs Are Differentially Regulated and Translated during Human Neuronal Differentiation. RNA 27 (9), 1082–1101. doi:10.1261/rna.078782.121
Dubecz, A., Gall, I., Solymosi, N., Schweigert, M., Peters, J. H., Feith, M., et al. (2012). Temporal Trends in Long-Term Survival and Cure Rates in Esophageal Cancer: a SEER Database Analysis. J. Thorac. Oncol. 7 (2), 443–447. doi:10.1097/JTO.0b013e3182397751
Engreitz, J. M., Haines, J. E., Perez, E. M., Munson, G., Chen, J., Kane, M., et al. (2016). Local Regulation of Gene Expression by lncRNA Promoters, Transcription and Splicing. Nature 539 (7629), 452–455. doi:10.1038/nature20149
Falk, G. W. (2009). Risk Factors for Esophageal Cancer Development. Surg. Oncol. Clin. N. Am. 18 (3), 469–485. doi:10.1016/j.soc.2009.03.005
Fang, Y., Zhang, S., Yin, J., Shen, Y. X., Wang, H., Chen, X. S., et al. (2020). LINC01535 Promotes Proliferation and Inhibits Apoptosis in Esophageal Squamous Cell Cancer by Activating the JAK/STAT3 Pathway. Eur. Rev. Med. Pharmacol. Sci. 24 (7), 3694–3700. doi:10.26355/eurrev_202004_20832
Fu, X., Chen, X., Si, Y., Yao, Y., Jiang, Z., and Chen, K. (2022). Long Non-coding RNA NCK1-AS1 Is Overexpressed in Esophageal Squamous Cell Carcinoma and Predicts Survival. Bioengineered 13 (4), 8302–8310. doi:10.1080/21655979.2022.2038449
Ge, X. S., Ma, H. J., Zheng, X. H., Ruan, H. L., Liao, X. Y., Xue, W. Q., et al. (2013). HOTAIR, a Prognostic Factor in Esophageal Squamous Cell Carcinoma, Inhibits WIF-1 Expression and Activates Wnt Pathway. Cancer Sci. 104 (12), 1675–1682. doi:10.1111/cas.12296
Guo, Y., Sun, P., Guo, W., Yin, Q., Han, J., Sheng, S., et al. (2021). LncRNA DDX11 Antisense RNA 1 Promotes EMT Process of Esophageal Squamous Cell Carcinoma by Sponging miR-30d-5p to Regulate SNAI1/ZEB2 Expression and Wnt/β-Catenin Pathway. Bioengineered 12 (2), 11425–11440. doi:10.1080/21655979.2021.2008759
Hafner, M., Landthaler, M., Burger, L., Khorshid, M., Hausser, J., Berninger, P., et al. (2010). Transcriptome-wide Identification of RNA-Binding Protein and microRNA Target Sites by PAR-CLIP. Cell 141 (1), 129–141. doi:10.1016/j.cell.2010.03.009
Han, G. H., Lu, K. J., Wang, P., Ye, J., Ye, Y. Y., and Huang, J. X. (2018). LncRNA SNHG16 Predicts Poor Prognosis in ESCC and Promotes Cell Proliferation and Invasion by Regulating Wnt/β-Catenin Signaling Pathway. Eur. Rev. Med. Pharmacol. Sci. 22 (12), 3795–3803. doi:10.26355/eurrev_201806_15262
Hon, C. C., Ramilowski, J. A., Harshbarger, J., Bertin, N., Rackham, O. J., Gough, J., et al. (2017). An Atlas of Human Long Non-coding RNAs with Accurate 5' Ends. Nature 543 (7644), 199–204. doi:10.1038/nature21374
Hou, H., Zhao, H., Yu, X., Cong, P., Zhou, Y., Jiang, Y., et al. (2020). METTL3 Promotes the Proliferation and Invasion of Esophageal Cancer Cells Partly through AKT Signaling Pathway. Pathol. Res. Pract. 216 (9), 153087. doi:10.1016/j.prp.2020.153087
Houseley, J., Rubbi, L., Grunstein, M., Tollervey, D., and Vogelauer, M. (2008). A ncRNA Modulates Histone Modification and mRNA Induction in the Yeast GAL Gene Cluster. Mol. Cel 32 (5), 685–695. doi:10.1016/j.molcel.2008.09.027
Hu, L., Wu, Y., Tan, D., Meng, H., Wang, K., Bai, Y., et al. (2015). Up-regulation of Long Noncoding RNA MALAT1 Contributes to Proliferation and Metastasis in Esophageal Squamous Cell Carcinoma. J. Exp. Clin. Cancer Res. 34, 7. doi:10.1186/s13046-015-0123-z
Hu, X., Feng, Y., Zhang, D., Zhao, S. D., Hu, Z., Greshock, J., et al. (2014). A Functional Genomic Approach Identifies FAL1 as an Oncogenic Long Noncoding RNA that Associates with BMI1 and Represses P21 Expression in Cancer. Cancer Cell 26 (3), 344–357. doi:10.1016/j.ccr.2014.07.009
Hua, J. T., Ahmed, M., Guo, H., Zhang, Y., Chen, S., Soares, F., et al. (2018). Risk SNP-Mediated Promoter-Enhancer Switching Drives Prostate Cancer through lncRNA PCAT19. Cell 174 (3), 564–e18. doi:10.1016/j.cell.2018.06.014
Huang, C., Cao, L., Qiu, L., Dai, X., Ma, L., Zhou, Y., et al. (2015). Upregulation of H19 Promotes Invasion and Induces Epithelial-To-Mesenchymal Transition in Esophageal Cancer. Oncol. Lett. 10 (1), 291–296. doi:10.3892/ol.2015.3165
Huang, C., Yu, Z., Yang, H., and Lin, Y. (2016). Increased MALAT1 Expression Predicts Poor Prognosis in Esophageal Cancer Patients. Biomed. Pharmacother. 83, 8–13. doi:10.1016/j.biopha.2016.05.044
Huang, J., Li, Y., Lu, Z., Che, Y., Sun, S., Mao, S., et al. (2018). Long Non‐coding RNA GAS 5 Is Induced by Interferons and Plays an Antitumor Role in Esophageal Squamous Cell Carcinoma. Cancer Med. 7, 3157–3167. doi:10.1002/cam4.1524
Huang, Z. L., Chen, R. P., Zhou, X. T., Zhan, H. L., Hu, M. M., Liu, B., et al. (2017). Long Non-coding RNA MEG3 Induces Cell Apoptosis in Esophageal Cancer through Endoplasmic Reticulum Stress. Oncol. Rep. 37 (5), 3093–3099. doi:10.3892/or.2017.5568
Imaoka, H., Toiyama, Y., Fujikawa, H., Hiro, J., Saigusa, S., Tanaka, K., et al. (2016). Circulating microRNA-1290 as a Novel Diagnostic and Prognostic Biomarker in Human Colorectal Cancer. Ann. Oncol. 27 (10), 1879–1886. doi:10.1093/annonc/mdw279
Ji, P., Diederichs, S., Wang, W., Böing, S., Metzger, R., Schneider, P. M., et al. (2003). MALAT-1, a Novel Noncoding RNA, and Thymosin Beta4 Predict Metastasis and Survival in Early-Stage Non-small Cell Lung Cancer. Oncogene 22 (39), 8031–8041. doi:10.1038/sj.onc.1206928
Jiang, L., Wang, W., Li, G., Sun, C., Ren, Z., Sheng, H., et al. (2016). High TUG1 Expression Is Associated with Chemotherapy Resistance and Poor Prognosis in Esophageal Squamous Cell Carcinoma. Cancer Chemother. Pharmacol. 78 (2), 333–339. doi:10.1007/s00280-016-3066-y
Jiang, Y., Wang, Z., Ying, C., Hu, J., Zeng, T., and Gao, L. (2021). FMR1/circCHAF1A/miR-211-5p/HOXC8 Feedback Loop Regulates Proliferation and Tumorigenesis via MDM2-dependent P53 Signaling in GSCs. Oncogene 40 (24), 4094–4110. doi:10.1038/s41388-021-01833-2
Jin, G., Yang, Y., Tuo, G., Wang, W., and Zhu, Z. (2020). LncRNA TUG1 Promotes Tumor Growth and Metastasis of Esophageal Squamous Cell Carcinoma by Regulating XBP1 via Competitively Binding to miR-498. Neoplasma 67 (4), 751–761. doi:10.4149/neo_2020_190805N717
Kapranov, P., Cheng, J., Dike, S., Nix, D. A., Duttagupta, R., Willingham, A. T., et al. (2007). RNA Maps Reveal New RNA Classes and a Possible Function for Pervasive Transcription. Science 316 (5830), 1484–1488. doi:10.1126/science.1138341
Karreth, F. A., and Pandolfi, P. P. (2013). ceRNA Cross-Talk in Cancer: when Ce-Bling Rivalries Go Awry. Cancer Discov. 3 (10), 1113–1121. doi:10.1158/2159-8290.CD-13-0202
Ke, K., Sun, Z., and Wang, Z. (2018). Downregulation of Long Non-coding RNA GAS5 Promotes Cell Proliferation, Migration and Invasion in Esophageal Squamous Cell Carcinoma. Oncol. Lett. 16 (2), 1801–1808. doi:10.3892/ol.2018.8797
Khorkova, O., Hsiao, J., and Wahlestedt, C. (2015). Basic Biology and Therapeutic Implications of lncRNA. Adv. Drug Deliv. Rev. 87, 15–24. doi:10.1016/j.addr.2015.05.012
Kopp, F., and Mendell, J. T. (2018). Functional Classification and Experimental Dissection of Long Noncoding RNAs. Cell 172 (3), 393–407. doi:10.1016/j.cell.2018.01.011
Lee, S., Kopp, F., Chang, T. C., Sataluri, A., Chen, B., Sivakumar, S., et al. (2016). Noncoding RNA NORAD Regulates Genomic Stability by Sequestering PUMILIO Proteins. Cell 164 (1-2), 69–80. doi:10.1016/j.cell.2015.12.017
Leucci, E., Vendramin, R., Spinazzi, M., Laurette, P., Fiers, M., Wouters, J., et al. (2016). Melanoma Addiction to the Long Non-coding RNA SAMMSON. Nature 531 (7595), 518–522. doi:10.1038/nature17161
Levin, A. A. (2019). Treating Disease at the RNA Level with Oligonucleotides. N. Engl. J. Med. 380 (1), 57–70. doi:10.1056/NEJMra1705346
Li, M., Cai, J., Han, X., and Ren, Y. (2020a). Downregulation of circNRIP1 Suppresses the Paclitaxel Resistance of Ovarian Cancer via Regulating the miR-211-5p/HOXC8 Axis. Cancer Manag. Res. 12, 9159–9171. doi:10.2147/CMAR.S268872
Li, M. K., Liu, L. X., Zhang, W. Y., Zhan, H. L., Chen, R. P., Feng, J. L., et al. (2020b). Long Non coding RNA MEG3 Suppresses Epithelial to mesenchymal Transition by Inhibiting the PSAT1 dependent GSK 3β/Snail Signaling Pathway in Esophageal Squamous Cell Carcinoma. Oncol. Rep. 44 (5), 2130–2142. doi:10.3892/or.2020.7754
Li, Q., Dai, Z., Xia, C., Jin, L., and Chen, X. (2020c). Suppression of Long Non-coding RNA MALAT1 Inhibits Survival and Metastasis of Esophagus Cancer Cells by Sponging miR-1-3p/CORO1C/TPM3 axis. Mol. Cel Biochem 470 (1-2), 165–174. doi:10.1007/s11010-020-03759-x
Li, W., Huang, K., Wen, F., Cui, G., Guo, H., He, Z., et al. (2019). LINC00184 Silencing Inhibits Glycolysis and Restores Mitochondrial Oxidative Phosphorylation in Esophageal Cancer through Demethylation of PTEN. EBioMedicine 44, 298–310. doi:10.1016/j.ebiom.2019.05.055
Li, W., Zhao, W., Lu, Z., Zhang, W., and Yang, X. (2018). Long Noncoding RNA GAS5 Promotes Proliferation, Migration, and Invasion by Regulation of miR-301a in Esophageal Cancer. Oncol. Res. 26 (8), 1285–1294. doi:10.3727/096504018X15166193231711
Li, X., Wu, Z., Mei, Q., Li, X., Guo, M., Fu, X., et al. (2013). Long Non-coding RNA HOTAIR, a Driver of Malignancy, Predicts Negative Prognosis and Exhibits Oncogenic Activity in Oesophageal Squamous Cell Carcinoma. Br. J. Cancer 109 (8), 2266–2278. doi:10.1038/bjc.2013.548
Li, Z., Zhou, Y., Tu, B., Bu, Y., Liu, A., and Kong, J. (2017). Long Noncoding RNA MALAT1 Affects the Efficacy of Radiotherapy for Esophageal Squamous Cell Carcinoma by Regulating Cks1 Expression. J. Oral Pathol. Med. 46 (8), 583–590. doi:10.1111/jop.12538
Liang, X. S., Sun, Y., and Liu, T. (2018). Long Non-coding RNA FAL1 Regulated Cell Proliferation through Akt Pathway via Targeting PDK1 in Esophageal Cancer Cells. Eur. Rev. Med. Pharmacol. Sci. 22 (16), 5214–5222. doi:10.26355/eurrev_201808_15719
Liu, B., Xiang, W., Liu, J., Tang, J., Wang, J., Liu, B., et al. (2021a). The Regulatory Role of Antisense lncRNAs in Cancer. Cancer Cel Int 21 (1), 459. doi:10.1186/s12935-021-02168-4
Liu, J., Mayekar, M. K., Wu, W., Yan, M., Guan, H., Wang, J., et al. (2020a). Long Non-coding RNA ESCCAL-1 Promotes Esophageal Squamous Cell Carcinoma by Down Regulating the Negative Regulator of APOBEC3G. Cancer Lett. 493, 217–227. doi:10.1016/j.canlet.2020.09.001
Liu, J., Wang, Y., Chu, Y., Xu, R., Zhang, D., and Wang, X. (2020b). Identification of a TLR-Induced Four-lncRNA Signature as a Novel Prognostic Biomarker in Esophageal Carcinoma. Front Cel Dev Biol 8, 649. doi:10.3389/fcell.2020.00649
Liu, S., Liu, J., Yang, X., Jiang, M., Wang, Q., Zhang, L., et al. (2021b). Cis -acting Lnc-Cxcl2 Restrains Neutrophil-Mediated Lung Inflammation by Inhibiting Epithelial Cell CXCL2 Expression in Virus Infection. Proc. Natl. Acad. Sci. U.S.A. 118 (41), 76118. doi:10.1073/pnas.2108276118
Liu, T., Liang, X., Yang, S., and Sun, Y. (2020c). Long Noncoding RNA PTCSC1 Drives Esophageal Squamous Cell Carcinoma Progression through Activating Akt Signaling. Exp. Mol. Pathol. 117, 104543. doi:10.1016/j.yexmp.2020.104543
Liu, W., Zhang, Y., Chen, M., Shi, L., Xu, L., and Zou, X. (2018). A Genome-wide Analysis of Long Noncoding RNA Profile Identifies Differentially Expressed lncRNAs Associated with Esophageal Cancer. Cancer Med. 7 (8), 4181–4189. doi:10.1002/cam4.1536
Liu, Z., Liu, L., Weng, S., Guo, C., Dang, Q., Xu, H., et al. (2022). Machine Learning-Based Integration Develops an Immune-Derived lncRNA Signature for Improving Outcomes in Colorectal Cancer. Nat. Commun. 13 (1), 816. doi:10.1038/s41467-022-28421-6
Luo, D., Huang, Z., Lv, H., Wang, Y., Sun, W., and Sun, X. (2020). Up-Regulation of MicroRNA-21 Indicates Poor Prognosis and Promotes Cell Proliferation in Esophageal Squamous Cell Carcinoma via Upregulation of lncRNA SNHG1. Cancer Manag. Res. 12, 1–14. doi:10.2147/CMAR.S221731
Luo, W., Liu, W., Yao, J., Zhu, W., Zhang, H., Sheng, Q., et al. (2019). Downregulation of H19 Decreases the Radioresistance in Esophageal Squamous Cell Carcinoma Cells. Onco Targets Ther. 12, 4779–4788. doi:10.2147/OTT.S203235
Lv, D., Sun, R., Yu, Q., and Zhang, X. (2016). The Long Non-coding RNA Maternally Expressed Gene 3 Activates P53 and Is Downregulated in Esophageal Squamous Cell Cancer. Tumor Biol. 37, 16259–16267. doi:10.1007/s13277-016-5426-y
Lv, T., Jin, Y., Miao, Y., Xu, T., Jia, F., Feng, H., et al. (2022). LncRNA PVT1 Promotes Tumorigenesis of Glioblastoma by Recruiting COPS5 to Deubiquitinate and Stabilize TRIM24. Mol. Ther. Nucleic Acids 27, 109–121. doi:10.1016/j.omtn.2021.11.012
Ma, J., Fan, Y., Feng, T., Chen, F., Xu, Z., Li, S., et al. (2017). HOTAIR Regulates HK2 Expression by Binding Endogenous miR-125 and miR-143 in Oesophageal Squamous Cell Carcinoma Progression. Oncotarget 8 (49), 86410–86422. doi:10.18632/oncotarget.21195
Ma, J., Li, T. F., Han, X. W., and Yuan, H. F. (2019). Downregulated MEG3 Contributes to Tumour Progression and Poor Prognosis in Oesophagal Squamous Cell Carcinoma by Interacting with miR-4261, Downregulating DKK2 and Activating the Wnt/β-Catenin Signalling. Artif. Cell Nanomed Biotechnol 47 (1), 1513–1523. doi:10.1080/21691401.2019.1602538
Ma, L., Yan, W., Sun, X., and Chen, P. (2021). Long Noncoding RNA VPS9D1-AS1 Promotes Esophageal Squamous Cell Carcinoma Progression via the Wnt/β-Catenin Signaling Pathway. J. Cancer 12 (22), 6894–6904. doi:10.7150/jca.54556
Maass, P. G., Luft, F. C., and Bähring, S. (2014). Long Non-coding RNA in Health and Disease. J. Mol. Med. (Berl) 92 (4), 337–346. doi:10.1007/s00109-014-1131-8
Majidinia, M., and Yousefi, B. (2016). Long Non-coding RNAs in Cancer Drug Resistance Development. DNA Repair (Amst) 45, 25–33. doi:10.1016/j.dnarep.2016.06.003
Massey, S. (2011). Esophageal Cancer and Palliation of Dysphagia. Clin. J. Oncol. Nurs. 15 (3), 327–329. doi:10.1188/11.CJON.327-329
Melendez-Zajgla, J., and Maldonado, V. (2021). The Role of lncRNAs in the Stem Phenotype of Pancreatic Ductal Adenocarcinoma. Int. J. Mol. Sci. 22 (12), 126374. doi:10.3390/ijms22126374
Nachtergaele, S., and He, C. (2017). The Emerging Biology of RNA post-transcriptional Modifications. RNA Biol. 14 (2), 156–163. doi:10.1080/15476286.2016.1267096
Nikbakht, H. A., Sahraian, S., Ghaem, H., Javadi, A., Janfada, M., Hassanipour, S., et al. (2020). Trends in Mortality Rates for Gastrointestinal Cancers in Fars Province, Iran (2005-2015). J. Gastrointest. Cancer 51 (1), 63–69. doi:10.1007/s12029-019-00204-1
Pardini, B., Sabo, A. A., Birolo, G., and Calin, G. A. (2019). Noncoding RNAs in Extracellular Fluids as Cancer Biomarkers: The New Frontier of Liquid Biopsies. Cancers (Basel) 11 (8), 1170. doi:10.3390/cancers11081170
Pietropaolo, V., Prezioso, C., and Moens, U. (2021). Role of Virus-Induced Host Cell Epigenetic Changes in Cancer. Int. J. Mol. Sci. 22 (15), 158346. doi:10.3390/ijms22158346
Ponting, C. P., Oliver, P. L., and Reik, W. (2009). Evolution and Functions of Long Noncoding RNAs. Cell 136 (4), 629–641. doi:10.1016/j.cell.2009.02.006
Prensner, J. R., Iyer, M. K., Balbin, O. A., Dhanasekaran, S. M., Cao, Q., Brenner, J. C., et al. (2011). Transcriptome Sequencing across a Prostate Cancer Cohort Identifies PCAT-1, an Unannotated lincRNA Implicated in Disease Progression. Nat. Biotechnol. 29 (8), 742–749. doi:10.1038/nbt.1914
Quinn, J. J., and Chang, H. Y. (2016). Unique Features of Long Non-coding RNA Biogenesis and Function. Nat. Rev. Genet. 17 (1), 47–62. doi:10.1038/nrg.2015.10
Ransohoff, J. D., Wei, Y., and Khavari, P. A. (2018). The Functions and Unique Features of Long Intergenic Non-coding RNA. Nat. Rev. Mol. Cel Biol 19 (3), 143–157. doi:10.1038/nrm.2017.104
Razavi, M., and Ghorbian, S. (2019). Up-regulation of Long Non-coding RNA-PCAT-1 Promotes Invasion and Metastasis in Esophageal Squamous Cell Carcinoma. EXCLI J. 18, 422–428. doi:10.17179/excli2018-1847
Ren, K., Li, Y., Lu, H., Li, Z., Li, Z., Wu, K., et al. (2016). Long Noncoding RNA HOTAIR Controls Cell Cycle by Functioning as a Competing Endogenous RNA in Esophageal Squamous Cell Carcinoma. Transl Oncol. 9 (6), 489–497. doi:10.1016/j.tranon.2016.09.005
Ren, K., Li, Z., Li, Y., Zhang, W., and Han, X. (2017). Long Noncoding RNA Taurine-Upregulated Gene 1 Promotes Cell Proliferation and Invasion in Gastric Cancer via Negatively Modulating miRNA-145-5p. Oncol. Res. 25 (5), 789–798. doi:10.3727/096504016X14783677992682
Ren, Y., Guo, T., Xu, J., Liu, Y., and Huang, J. (2021). The Novel Target of Esophageal Squamous Cell Carcinoma: lncRNA GASL1 Regulates Cell Migration, Invasion and Cell Cycle Stagnation by Inactivating the Wnt3a/β-Catenin Signaling. Pathol. Res. Pract. 217, 153289. doi:10.1016/j.prp.2020.153289
Rinn, J. L., and Chang, H. Y. (2012). Genome Regulation by Long Noncoding RNAs. Annu. Rev. Biochem. 81, 145–166. doi:10.1146/annurev-biochem-051410-092902
Rinn, J. L., Kertesz, M., Wang, J. K., Squazzo, S. L., Xu, X., Brugmann, S. A., et al. (2007). Functional Demarcation of Active and Silent Chromatin Domains in Human HOX Loci by Noncoding RNAs. Cell 129 (7), 1311–1323. doi:10.1016/j.cell.2007.05.022
Sheng, K., and Li, Y. (2019). LncRNA TUG1 Promotes the Development of Osteosarcoma through RUNX2. Exp. Ther. Med. 18 (4), 3002–3008. doi:10.3892/etm.2019.7880
Shi, W. H., Wu, Q. Q., Li, S. Q., Yang, T. X., Liu, Z. H., Tong, Y. S., et al. (2015). Upregulation of the Long Noncoding RNA PCAT-1 Correlates with Advanced Clinical Stage and Poor Prognosis in Esophageal Squamous Carcinoma. Tumour Biol. 36 (4), 2501–2507. doi:10.1007/s13277-014-2863-3
Shi, X., Sun, M., Liu, H., Yao, Y., and Song, Y. (2013). Long Non-coding RNAs: a New Frontier in the Study of Human Diseases. Cancer Lett. 339 (2), 159–166. doi:10.1016/j.canlet.2013.06.013
Slack, F. J., and Chinnaiyan, A. M. (2019). The Role of Non-coding RNAs in Oncology. Cell 179 (5), 1033–1055. doi:10.1016/j.cell.2019.10.017
Sleutels, F., Zwart, R., and Barlow, D. P. (2002). The Non-coding Air RNA Is Required for Silencing Autosomal Imprinted Genes. Nature 415 (6873), 810–813. doi:10.1038/415810a
Soghli, N., Yousefi, T., Abolghasemi, M., and Qujeq, D. (2021). NORAD, a Critical Long Non-coding RNA in Human Cancers. Life Sci. 264, 118665. doi:10.1016/j.lfs.2020.118665
Song, H., Chen, L., Liu, W., Xu, X., Zhou, Y., Zhu, J., et al. (2021). Depleting Long Noncoding RNA HOTAIR Attenuates Chronic Myelocytic Leukemia Progression by Binding to DNA Methyltransferase 1 and Inhibiting PTEN Gene Promoter Methylation. Cell Death Dis 12 (5), 440. doi:10.1038/s41419-021-03637-4
Song, W., Wang, K., Yang, X., Dai, W., and Fan, Z. (2020). Long Non coding RNA BANCR Mediates Esophageal Squamous Cell Carcinoma Progression by Regulating the IGF1R/Raf/MEK/ERK Pathway via miR 338 3p. Int. J. Mol. Med. 46 (4), 1377–1388. doi:10.3892/ijmm.2020.4687
St Laurent, G., Wahlestedt, C., and Kapranov, P. (2015). The Landscape of Long Noncoding RNA Classification. Trends Genet. 31 (5), 239–251. doi:10.1016/j.tig.2015.03.007
Steck, E., Boeuf, S., Gabler, J., Werth, N., Schnatzer, P., Diederichs, S., et al. (2012). Regulation of H19 and its Encoded microRNA-675 in Osteoarthritis and under Anabolic and Catabolic In Vitro Conditions. J. Mol. Med. (Berl) 90 (10), 1185–1195. doi:10.1007/s00109-012-0895-y
Stewart, D. J., Chang, D. W., Ye, Y., Spitz, M., Lu, C., Shu, X., et al. (2014). Wnt Signaling Pathway Pharmacogenetics in Non-small Cell Lung Cancer. Pharmacogenomics J. 14 (1), 509–522. doi:10.1093/jnci/djt35610.1038/tpj.2014.21
Su, K., Wang, N., Shao, Q., Liu, H., Zhao, B., and Ma, S. (2021). The Role of a ceRNA Regulatory Network Based on lncRNA MALAT1 Site in Cancer Progression. Biomed. Pharmacother. 137, 111389. doi:10.1016/j.biopha.2021.111389
Sung, H., Ferlay, J., Siegel, R. L., Laversanne, M., Soerjomataram, I., Jemal, A., et al. (2021). Global Cancer Statistics 2020: GLOBOCAN Estimates of Incidence and Mortality Worldwide for 36 Cancers in 185 Countries. CA Cancer J. Clin. 71 (3), 209–249. doi:10.3322/caac.21660
Syllaios, A., Moris, D., Karachaliou, G. S., Sakellariou, S., Karavokyros, I., Gazouli, M., et al. (2021). Pathways and Role of MALAT1 in Esophageal and Gastric Cancer. Oncol. Lett. 21 (5), 343. doi:10.3892/ol.2021.12604
Tang, Y., Yang, P., Zhu, Y., and Su, Y. (2020). LncRNA TUG1 Contributes to ESCC Progression via Regulating miR-148a-3p/MCL-1/Wnt/β-Catenin axis In Vitro. Thorac. Cancer 11 (1), 82–94. doi:10.1111/1759-7714.13236
Tay, S. K., Blythe, J., and Lipovich, L. (2009). Global Discovery of Primate-specific Genes in the Human Genome. Proc. Natl. Acad. Sci. U S A. 106 (29), 12019–12024. doi:10.1073/pnas.0904569106
Tian, L., Yang, L., Zheng, W., Hu, Y., Ding, P., Wang, Z., et al. (2020). RNA Sequencing of Exosomes Revealed Differentially Expressed Long Noncoding RNAs in Early-Stage Esophageal Squamous Cell Carcinoma and Benign Esophagitis. Epigenomics 12 (6), 525–541. doi:10.2217/epi-2019-0371
Toden, S., and Goel, A. (2022). Non-coding RNAs as Liquid Biopsy Biomarkers in Cancer. Br. J. Cancer 126, 351–360. doi:10.1038/s41416-021-01672-8
Toiyama, Y., Takahashi, M., Hur, K., Nagasaka, T., Tanaka, K., Inoue, Y., et al. (2013). Serum miR-21 as a Diagnostic and Prognostic Biomarker in Colorectal Cancer. J. Natl. Cancer Inst. 105 (12), 849–859. doi:10.1093/jnci/djt101
Tong, Y., Yang, L., Yu, C., Zhu, W., Zhou, X., Xiong, Y., et al. (2020). Tumor-Secreted Exosomal lncRNA POU3F3 Promotes Cisplatin Resistance in ESCC by Inducing Fibroblast Differentiation into CAFs. Mol. Ther. Oncolytics 18, 1–13. doi:10.1016/j.omto.2020.05.014
Tong, Y. S., Wang, X. W., Zhou, X. L., Liu, Z. H., Yang, T. X., Shi, W. H., et al. (2015). Identification of the Long Non-coding RNA POU3F3 in Plasma as a Novel Biomarker for Diagnosis of Esophageal Squamous Cell Carcinoma. Mol. Cancer 14, 3. doi:10.1186/1476-4598-14-3
Tripathi, V., Ellis, J. D., Shen, Z., Song, D. Y., Pan, Q., Watt, A. T., et al. (2010). The Nuclear-Retained Noncoding RNA MALAT1 Regulates Alternative Splicing by Modulating SR Splicing Factor Phosphorylation. Mol. Cel 39 (6), 925–938. doi:10.1016/j.molcel.2010.08.011
Turai, P. I., Nyírő, G., Butz, H., Patócs, A., and Igaz, P. (2021). MicroRNAs, Long Non-coding RNAs, and Circular RNAs: Potential Biomarkers and Therapeutic Targets in Pheochromocytoma/Paraganglioma. Cancers (Basel) 13 (7), 1522. doi:10.3390/cancers13071522
Wang, A. H., Tan, P., Zhuang, Y., Zhang, X. T., Yu, Z. B., and Li, L. N. (2019a). Down-regulation of Long Non-coding RNA HOTAIR Inhibits Invasion and Migration of Oesophageal Cancer Cells via Up-Regulation of microRNA-204. J. Cel Mol Med 23 (10), 6595–6610. doi:10.1111/jcmm.14502
Wang, C., Zhang, Q., Hu, Y., Zhu, J., and Yang, J. (2019b). Emerging Role of Long Non-coding RNA MALAT1 in Predicting Clinical Outcomes of Patients with Digestive System Malignancies: A Meta-Analysis. Oncol. Lett. 17 (2), 2159–2170. doi:10.3892/ol.2018.9875
Wang, F., Li, X., Xie, X., Zhao, L., and Chen, W. (2008). UCA1, a Non-protein-coding RNA Up-Regulated in Bladder Carcinoma and Embryo, Influencing Cell Growth and Promoting Invasion. FEBS Lett. 582 (13), 1919–1927. doi:10.1016/j.febslet.2008.05.012
Wang, G., Sun, J., Zhao, H., and Li, H. (2018). Long Non-coding RNA (lncRNA) Growth Arrest Specific 5 (GAS5) Suppresses Esophageal Squamous Cell Carcinoma Cell Proliferation and Migration by Inactivating Phosphatidylinositol 3-kinase (PI3K)/AKT/Mammalian Target of Rapamycin (mTOR) Signaling Pathway. Med. Sci. Monit. 24, 7689–7696. doi:10.12659/MSM.910867
Wang, J., Zhu, S., Meng, N., He, Y., Lu, R., and Yan, G. R. (2019c). ncRNA-Encoded Peptides or Proteins and Cancer. Mol. Ther. 27 (10), 1718–1725. doi:10.1016/j.ymthe.2019.09.001
Wang, K. C., and Chang, H. Y. (2011). Molecular Mechanisms of Long Noncoding RNAs. Mol. Cel 43 (6), 904–914. doi:10.1016/j.molcel.2011.08.018
Wang, P., Yang, Z., Ye, T., Shao, F., Li, J., Sun, N., et al. (2020). lncTUG1/miR-144-3p Affect the Radiosensitivity of Esophageal Squamous Cell Carcinoma by Competitively Regulating C-MET. J. Exp. Clin. Cancer Res. 39 (1), 7. doi:10.1186/s13046-019-1519-y
Wang, Q., Wang, F., Lv, J., Xin, J., Xie, L., Zhu, W., et al. (2019d). Interactive Online Consensus Survival Tool for Esophageal Squamous Cell Carcinoma Prognosis Analysis. Oncol. Lett. 18 (2), 1199–1206. doi:10.3892/ol.2019.10440
Wang, Q., Zhang, Q., Wang, J., Feng, W., Chen, Y., Liu, J., et al. (2021a). Prognostic and Clinicopathological Role of Long Noncoding RNA NORAD in Various Cancers: a Meta-Analysis. Biomark Med. 15 (6), 427–436. doi:10.2217/bmm-2020-0566
Wang, W., He, X., Zheng, Z., Ma, X., Hu, X., Wu, D., et al. (2017). Serum HOTAIR as a Novel Diagnostic Biomarker for Esophageal Squamous Cell Carcinoma. Mol. Cancer 16 (1), 75. doi:10.1186/s12943-017-0643-6
Wang, W., Wu, D., He, X., Hu, X., Hu, C., Shen, Z., et al. (2019e). CCL18-induced HOTAIR Upregulation Promotes Malignant Progression in Esophageal Squamous Cell Carcinoma through the miR-130a-5p-ZEB1 axis. Cancer Lett. 460, 18–28. doi:10.1016/j.canlet.2019.06.009
Wang, W., Zhu, Y., Li, S., Chen, X., Jiang, G., Shen, Z., et al. (2016a). Long Noncoding RNA MALAT1 Promotes Malignant Development of Esophageal Squamous Cell Carcinoma by Targeting β-catenin via Ezh2. Oncotarget 7 (18), 25668–25682. doi:10.18632/oncotarget.8257
Wang, X., Gao, Z., Liao, J., Shang, M., Li, X., Yin, L., et al. (2016b). lncRNA UCA1 Inhibits Esophageal Squamous-Cell Carcinoma Growth by Regulating the Wnt Signaling Pathway. J. Toxicol. Environ. Health A. 79 (9-10), 407–418. doi:10.1080/15287394.2016.1176617
Wang, X., Li, M., Wang, Z., Han, S., Tang, X., Ge, Y., et al. (2015). Silencing of Long Noncoding RNA MALAT1 by miR-101 and miR-217 Inhibits Proliferation, Migration, and Invasion of Esophageal Squamous Cell Carcinoma Cells. J. Biol. Chem. 290 (7), 3925–3935. doi:10.1074/jbc.M114.596866
Wang, X., Liu, C., Zhang, S., Yan, H., Zhang, L., Jiang, A., et al. (2021b). N6-methyladenosine Modification of MALAT1 Promotes Metastasis via Reshaping Nuclear Speckles. Dev. Cel 56 (5), 702–e8. doi:10.1016/j.devcel.2021.01.015
Wang, X., and Wang, X. (2019). Long Non-coding RNA colon Cancer-Associated Transcript 2 May Promote Esophageal Cancer Growth and Metastasis by Regulating the Wnt Signaling Pathway. Oncol. Lett. 18 (2), 1745–1754. doi:10.3892/ol.2019.10488
Watanabe, M., Otake, R., Kozuki, R., Toihata, T., Takahashi, K., Okamura, A., et al. (2020). Recent Progress in Multidisciplinary Treatment for Patients with Esophageal Cancer. Surg. Today 50 (1), 12–20. doi:10.1007/s00595-019-01878-7
Wei, W. T., Wang, L., Liang, J. X., Wang, J. F., Li, Q., and Zeng, J. (2020). LncRNA EIF3J-AS1 Enhanced Esophageal Cancer Invasion via Regulating AKT1 Expression through Sponging miR-373-3p. Sci. Rep. 10 (1), 13969. doi:10.1038/s41598-020-70886-2
Wei, X., Zhou, Y., Qiu, J., Wang, X., Xia, Y., and Sui, L. (2019). Low Expression of TUG1 Promotes Cisplatin Sensitivity in Cervical Cancer by Activating the MAPK Pathway. J. BUON 24 (3), 1020.
Wheeler, T. M., Leger, A. J., Pandey, S. K., MacLeod, A. R., Nakamori, M., Cheng, S. H., et al. (2012). Targeting Nuclear RNA for In Vivo Correction of Myotonic Dystrophy. Nature 488 (7409), 111–115. doi:10.1038/nature11362
Wu, X., Lim, Z. F., Li, Z., Gu, L., Ma, W., Zhou, Q., et al. (2017). NORAD Expression Is Associated with Adverse Prognosis in Esophageal Squamous Cell Carcinoma. Oncol. Res. Treat. 40 (6), 370–374. doi:10.1159/000464465
Wu, Y. Y., and Kuo, H. C. (2020). Functional Roles and Networks of Non-coding RNAs in the Pathogenesis of Neurodegenerative Diseases. J. Biomed. Sci. 27 (1), 49. doi:10.1186/s12929-020-00636-z
Wu, Z., Liu, X., Liu, L., Deng, H., Zhang, J., Xu, Q., et al. (2014). Regulation of lncRNA Expression. Cell Mol Biol Lett 19 (4), 561–575. doi:10.2478/s11658-014-0212-6
Xu, C., Guo, Y., Liu, H., Chen, G., Yan, Y., and Liu, T. (2018a). TUG1 Confers Cisplatin Resistance in Esophageal Squamous Cell Carcinoma by Epigenetically Suppressing PDCD4 Expression via EZH2. Cell Biosci 8, 61. doi:10.1186/s13578-018-0260-0
Xu, F., and Zhang, J. (2017). Long Non-coding RNA HOTAIR Functions as miRNA Sponge to Promote the Epithelial to Mesenchymal Transition in Esophageal Cancer. Biomed. Pharmacother. 90, 888–896. doi:10.1016/j.biopha.2017.03.103
Xu, H., Miao, J., Liu, S., Liu, H., Zhang, L., and Zhang, Q. (2021a). Long Non-coding RNA KCNQ1 Overlapping Transcript 1 Promotes the Progression of Esophageal Squamous Cell Carcinoma by Adsorbing microRNA-133b. Clinics (Sao Paulo) 76, e2175. doi:10.6061/clinics/2021/e2175
Xu, J., Ma, J., Guan, B., Li, J., Wang, Y., and Hu, S. (2021b). LncRNA HCP5 Promotes Malignant Cell Behaviors in Esophageal Squamous Cell Carcinoma via the PI3K/AKT/mTOR Signaling. Cell Cycle 20 (14), 1374–1388. doi:10.1080/15384101.2021.1944512
Xu, L., Jiao, S., Zhang, D., Wu, S., Zhang, H., and Gao, B. (2021c). Identification of Long Noncoding RNAs with Machine Learning Methods: a Review. Brief. Funct. Genomics 20 (3), 174–180. doi:10.1093/bfgp/elab017
Xu, L. J., Yu, X. J., Wei, B., Hui, H. X., Sun, Y., Dai, J., et al. (2018b). Long Non-coding RNA DUXAP8 Regulates Proliferation and Invasion of Esophageal Squamous Cell Cancer. Eur. Rev. Med. Pharmacol. Sci. 22 (9), 2646–2652. doi:10.26355/eurrev_201805_14959
Xue, X., Yang, Y. A., Zhang, A., Fong, K. W., Kim, J., Song, B., et al. (2016). LncRNA HOTAIR Enhances ER Signaling and Confers Tamoxifen Resistance in Breast Cancer. Oncogene 35 (21), 2746–2755. doi:10.1038/onc.2015.340
Yang, C., Li, F., Zhou, W., and Huang, J. (2021). Knockdown of Long Non-coding RNA CCAT2 Suppresses Growth and Metastasis of Esophageal Squamous Cell Carcinoma by Inhibiting the β-catenin/WISP1 Signaling Pathway. J. Int. Med. Res. 49 (5), 3000605211019938. doi:10.1177/03000605211019938
Yang, L., Ye, Y., Chu, J., Jia, J., Qu, Y., Sun, T., et al. (2019a). Long Noncoding RNA FEZF1-AS1 Promotes the Motility of Esophageal Squamous Cell Carcinoma through Wnt/β-Catenin Pathway. Cancer Manag. Res. 11, 4425–4435. doi:10.2147/CMAR.S196004
Yang, W., Han, Y., Zhao, X., Duan, L., Zhou, W., Wang, X., et al. (2019b). Advances in Prognostic Biomarkers for Esophageal Cancer. Expert Rev. Mol. Diagn. 19 (2), 109–119. doi:10.1080/14737159.2019.1563485
Yang, Z., Zhao, Y., Lin, G., Zhou, X., Jiang, X., and Zhao, H. (2019c). Noncoding RNA Activated by DNA Damage (NORAD): Biologic Function and Mechanisms in Human Cancers. Clin. Chim. Acta 489, 5–9. doi:10.1016/j.cca.2018.11.025
Yao, Q., Yang, J., Liu, T., Zhang, J., and Zheng, Y. (2019). Long Noncoding RNA MALAT1 Promotes the Stemness of Esophageal Squamous Cell Carcinoma by Enhancing YAP Transcriptional Activity. FEBS Open Bio 9 (8), 1392–1402. doi:10.1002/2211-5463.12676
Yin, Y., Yan, P., Lu, J., Song, G., Zhu, Y., Li, Z., et al. (2015). Opposing Roles for the lncRNA Haunt and its Genomic Locus in Regulating HOXA Gene Activation during Embryonic Stem Cell Differentiation. Cell Stem Cell 16 (5), 504–516. doi:10.1016/j.stem.2015.03.007
You, B. H., Yoon, J. H., Kang, H., Lee, E. K., Lee, S. K., and Nam, J. W. (2019). HERES, a lncRNA that Regulates Canonical and Noncanonical Wnt Signaling Pathways via Interaction with EZH2. Proc. Natl. Acad. Sci. U S A. 116 (49), 24620–24629. doi:10.1073/pnas.1912126116
Yu, G., Zhou, H., Yao, W., Meng, L., and Lang, B. (2019). lncRNA TUG1 Promotes Cisplatin Resistance by Regulating CCND2 via Epigenetically Silencing miR-194-5p in Bladder Cancer. Mol. Ther. Nucleic Acids 16, 257–271. doi:10.1016/j.omtn.2019.02.017
Yu, X., Huang, M., and Yang, G. (2021). Long Non coding RNA BANCR Promotes Proliferation, Invasion and Migration in Esophageal Squamous Cell Carcinoma Cells via the Raf/MEK/ERK Signaling Pathway. Mol. Med. Rep. 23 (6), 12104. doi:10.3892/mmr.2021.12104
Yuan, J. H., Yang, F., Wang, F., Ma, J. Z., Guo, Y. J., Tao, Q. F., et al. (2014). A Long Noncoding RNA Activated by TGF-β Promotes the Invasion-Metastasis cascade in Hepatocellular Carcinoma. Cancer Cell 25 (5), 666–681. doi:10.1016/j.ccr.2014.03.010
Zang, B., Zhao, J., and Chen, C. (2019). LncRNA PCAT-1 Promoted ESCC Progression via Regulating ANXA10 Expression by Sponging miR-508-3p. Cancer Manag. Res. 11, 10841–10849. doi:10.2147/CMAR.S233983
Zhan, T., Rindtorff, N., and Boutros, M. (2017). Wnt Signaling in Cancer. Oncogene 36 (11), 1461–1473. doi:10.1038/onc.2016.304
Zhang, C., Jiang, F., Su, C., Xie, P., and Xu, L. (2019a). Upregulation of Long Noncoding RNA SNHG20 Promotes Cell Growth and Metastasis in Esophageal Squamous Cell Carcinoma via Modulating ATM‐JAK‐PD‐L1 Pathway. J. Cell. Biochem. 120, 11642–11650. doi:10.1002/jcb.28444
Zhang, D., Yang, W., Wang, S., Wang, F., Liu, D., Dong, J., et al. (2018a). Long Non-coding RNA LINC01617 Promotes Proliferation and Metastasis of Esophageal Cancer Cells through AKT Pathway. Gene 677, 308–316. doi:10.1016/j.gene.2018.08.054
Zhang, D., Zhang, H., Wang, X., Hu, B., Zhang, F., Wei, H., et al. (2019b). LINC01518 Knockdown Inhibits Tumorigenicity by Suppression of PIK3CA/Akt Pathway in Oesophageal Squamous Cell Carcinoma. Artif. Cell Nanomed Biotechnol 47 (1), 4284–4292. doi:10.1080/21691401.2019.1699815
Zhang, J., Hu, S. L., Qiao, C. H., Ye, J. F., Li, M., Ma, H. M., et al. (2018b). LncRNA-NEF Inhibits Proliferation, Migration and Invasion of Esophageal Squamous-Cell Carcinoma Cells by Inactivating Wnt/β-Catenin Pathway. Eur. Rev. Med. Pharmacol. Sci. 22 (20), 6824–6831. doi:10.26355/eurrev_201810_16150
Zhang, W., Wang, Q., Yang, Y., Zhou, S., Zhang, P., and Feng, T. (2021). The Role of Exosomal lncRNAs in Cancer Biology and Clinical Management. Exp. Mol. Med. 53 (11), 1669–1673. doi:10.1038/s12276-021-00699-4
Zhang, X., Wang, J., Li, J., Chen, W., and Liu, C. (2018c). CRlncRC: a Machine Learning-Based Method for Cancer-Related Long Noncoding RNA Identification Using Integrated Features. BMC Med. Genomics 11 (Suppl. 6), 120. doi:10.1186/s12920-018-0436-9
Zhang, Y., Jin, X., Wang, Z., Zhang, X., Liu, S., and Liu, G. (2017). Downregulation of SNHG1 Suppresses Cell Proliferation and Invasion by Regulating Notch Signaling Pathway in Esophageal Squamous Cell Cancer. Cancer Biomark 21 (1), 89–96. doi:10.3233/CBM-170286
Zhang, Z., Xiong, R., Li, C., Xu, M., and Guo, M. (2019c). LncRNA TUG1 Promotes Cisplatin Resistance in Esophageal Squamous Cell Carcinoma Cells by Regulating Nrf2. Acta Biochim. Biophys. Sin (Shanghai) 51 (8), 826–833. doi:10.1093/abbs/gmz069
Zhao, B., Cao, P., Hu, S., Li, F., Kong, K., and Zu, Y. (2019). LncRNA-NBAT-1 Modulates Esophageal Cancer Proliferation via PKM2. Am. J. Transl Res. 11 (9), 5978–5987.
Zhen, Q., Gao, L. N., Wang, R. F., Chu, W. W., Zhang, Y. X., Zhao, X. J., et al. (2018). LncRNA PCAT-1 Promotes Tumour Growth and Chemoresistance of Oesophageal Cancer to Cisplatin. Cell Biochem Funct 36 (1), 27–33. doi:10.1002/cbf.3314
Zhihua, Z., Weiwei, W., Lihua, N., Jianying, Z., and Jiang, G. (2019). p53-induced Long Non-coding RNA PGM5-AS1 Inhibits the Progression of Esophageal Squamous Cell Carcinoma through Regulating miR-466/PTEN axis. IUBMB Life 71 (10), 1492–1502. doi:10.1002/iub.2069
Zhong, Y. B., Shan, A. J., Lv, W., Wang, J., and Xu, J. Z. (2018). Long Non-coding RNA LINC00675 Inhibits Tumorigenesis and EMT via Repressing Wnt/β-Catenin Signaling in Esophageal Squamous Cell Carcinoma. Eur. Rev. Med. Pharmacol. Sci. 22 (23), 8288–8297. doi:10.26355/eurrev_201812_16526
Zhu, J., Yu, W., Wang, Y., Xia, K., Huang, Y., Xu, A., et al. (2019). lncRNAs: Function and Mechanism in Cartilage Development, Degeneration, and Regeneration. Stem Cel Res Ther 10 (1), 344. doi:10.1186/s13287-019-1458-8
Zhu, P., Huang, H., Gu, S., Liu, Z., Zhang, X., Wu, K., et al. (2021). Long Noncoding RNA FAM225A Promotes Esophageal Squamous Cell Carcinoma Development and Progression via Sponging MicroRNA-197-5p and Upregulating NONO. J. Cancer 12 (4), 1073–1084. doi:10.7150/jca.51292
Keywords: long non-coding RNAs, esophageal cancer, biological function, clinical application, cancer therapy
Citation: Han Y, Zhao G, Shi X, Wang Y, Wen X, Zhang L and Guo X (2022) The Emerging Role of Long Non-Coding RNAs in Esophageal Cancer: Functions in Tumorigenesis and Clinical Implications. Front. Pharmacol. 13:885075. doi: 10.3389/fphar.2022.885075
Received: 27 February 2022; Accepted: 12 April 2022;
Published: 13 May 2022.
Edited by:
Nicola Amodio, Magna Græcia University, ItalyReviewed by:
Marco Bocchetti, University of Campania Luigi Vanvitelli, ItalyPrashanth N. Suravajhala, Amrita Vishwa Vidyapeetham University, India
Copyright © 2022 Han, Zhao, Shi, Wang, Wen, Zhang and Guo. This is an open-access article distributed under the terms of the Creative Commons Attribution License (CC BY). The use, distribution or reproduction in other forums is permitted, provided the original author(s) and the copyright owner(s) are credited and that the original publication in this journal is cited, in accordance with accepted academic practice. No use, distribution or reproduction is permitted which does not comply with these terms.
*Correspondence: Xiangqian Guo, eHFndW9AaGVudS5lZHUuY24=
†These authors have contributed equally to this work