- 1Department of Pharmacodynamics, College of Pharmacy, University of Florida, Gainesville, FL, United States
- 2Department of Pharmaceutical Sciences, School of Pharmacy, Texas Tech University Health Sciences Center, Amarillo, TX, United States
Cannabinoids, including those found in cannabis, have shown promise as potential therapeutics for numerous health issues, including pathological pain and diseases that produce an impact on neurological processing and function. Thus, cannabis use for medicinal purposes has become accepted by a growing majority. However, clinical trials yielding satisfactory endpoints and unequivocal proof that medicinal cannabis should be considered a frontline therapeutic for most examined central nervous system indications remains largely elusive. Although cannabis contains over 100 + compounds, most preclinical and clinical research with well-controlled dosing and delivery methods utilize the various formulations of Δ9-tetrahydrocannabinol (THC) and cannabidiol (CBD), the two most abundant compounds in cannabis. These controlled dosing and delivery methods are in stark contrast to most clinical studies using whole plant cannabis products, as few clinical studies using whole plant cannabis profile the exact composition, including percentages of all compounds present within the studied product. This review will examine both preclinical and clinical evidence that supports or refutes the therapeutic utility of medicinal cannabis for the treatment of pathological pain, neurodegeneration, substance use disorders, as well as anxiety-related disorders. We will predominately focus on purified THC and CBD, as well as other compounds isolated from cannabis for the aforementioned reasons but will also include discussion over those studies where whole plant cannabis has been used. In this review we also consider the current challenges associated with the advancement of medicinal cannabis and its derived potential therapeutics into clinical applications.
1 Introduction
Interest in cannabinoids has continued to grow as they steadily show increased potential as therapeutics for treating a diverse range of diseases and illnesses. Therapeutic actions of these cannabinoids are in part the result of two identified cannabinoid receptors, both of which are G protein-coupled receptors. Cannabinoid 1 receptors (CB1R) appear in high densities among presynaptic neurons within the central nervous system (CNS), particularly among GABAergic interneurons, and peripheral neurons as well as on astrocytes and oligodendrocytes (Huang et al., 2001; Katona et al., 2001; Ohno-Shosaku et al., 2001; Szabo et al., 2005). The behavioral effects of cannabinoid consumption, termed as “cannabimimetic” behavioral effects, are mediated by neuronal CB1R. Such cannabimimetic effects include acute antinociception, decreased locomotion, catalepsy, and hypothermia (Little et al., 1988; Ledent et al., 1999; Grim et al., 2016). CB1R are also associated with anti-inflammatory mechanisms, contributing to therapeutic prospects of CB1R agonists (Richardson et al., 1998; Kraus et al., 2009; Newton et al., 2009). CB2 receptors (CB2R) are expressed by immune cells including microglia, astrocytes, oligodendrocytes (Munro et al., 1993;Galiegue et al., 1995; Schatz et al., 1997; Carayon et al., 1998), and discrete neuronal populations (Onaivi et al., 2008a) within the brainstem (Van Sickle et al., 2005), and the hippocampus (Stempel et al., 2016). Unlike CB1R agonism, CB2R agonism does not result in the cannabimimetic effects observed with CB1R agonists whilst still producing anti-inflammatory signaling cascades (Rahn et al., 2011). Despite the current, vast library of synthetic cannabinoid ligands generated, clinical research has extensively utilized variations of Δ9-tetrahydrocannabinol (THC) and cannabidiol (CBD) due to their well-controlled dosing and delivery methods. Within this review, we report on the use of THC (a non-selective CB1R/CB2R agonist) and its clinically approved synthetic formulations, dronabinol and nabilone, THC/CBD formulations as nabiximols and medicinal cannabis, and CBD (limited agonism at CB1R/CB2R) and its clinically approved formulation, Epidiolex. Not discussed at length within this review, it should be noted that medicinal cannabis contains over 100 different compounds, including acid phytocannabinoids, cannabigerol, as well as cannabis-related terpenoids (Russo, 2018). Each of these cannabis compounds has its own pharmacology which can include activity at receptors not discussed within this review, and these compounds may modify resultant THC, CBD activity as well as overall medicinal cannabis therapeutic potency.
The therapeutic actions of CBD are generally attributed to non-CB1R/ CB2R activity, including partial agonist activity at the 5-HT1A receptor, although potential endocannabinoid modulatory effects of CBD cannot be eliminated (De Gregorio et al., 2019; King et al., 2017). Serotonin 5-HT1A receptors are G protein-coupled receptors located on presynaptic serotonergic neurons and postsynaptic non-serotonergic neurons, astrocytes, oligodendrocytes, and microglia, with a high density of distribution within limbic brain areas (Barnes & Sharp, 1999; Riad et al., 1991, 2000). Additionally, 5-HT1A receptors are also expressed within primary afferent neurons and their peripheral terminals (Björk et al., 1992; Granados-Soto et al., 2010; Laporte et al., 1995; Perrin et al., 2011). Figure 1shows the spatial distribution of CB1R, CB2R, and 5-HT1A receptors within the brain. Agonism of 5-HT1A receptors has been shown to inhibit nociception (Gjerstad et al., 1996; Bardin et al., 2003; Haleem & Nawaz, 2017), exhibit neuroprotective effects (Miyazaki et al., 2013; Isooka et al., 2020; Kikuoka et al., 2020), and alleviate the severity of several anxiety disorders (Sussman and Joffe, 1998; Campos and Guimarães, 2008).
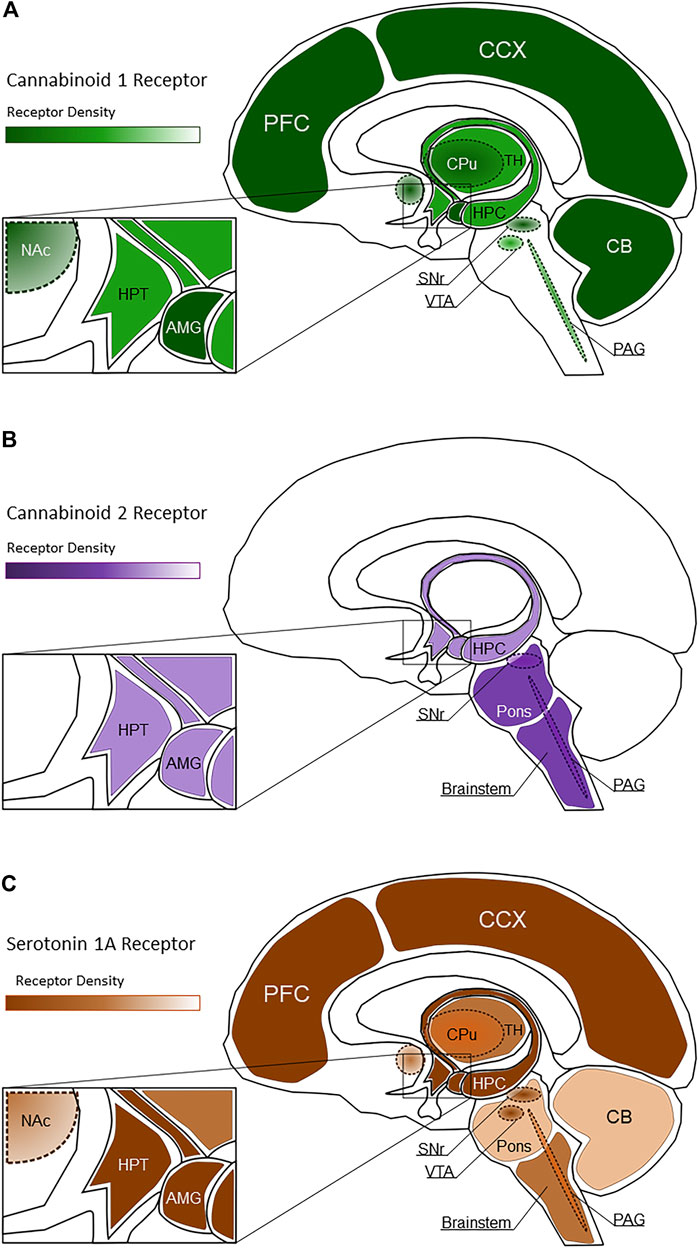
FIGURE 1. Spatial distribution of CB1R [(A), green shading], CB2R [(B), purple shading], and 5-HT1A receptors [(C), brown shading] within healthy brain regions. Lighter shaded regions represent low receptor density while darker shaded regions represent high receptor density. In these images, PFC, Prefrontal Cortex; CCX, Cerebral Cortex; CB, Cerebellum; CPu, Caudate Putamen; HPC, Hippocampus; TH, Thalamus; HPT, Hypothalamus; Nac, Nucleus Accumbens; SNr, Substantia Nigra pars compacta; VTA, Ventral Tegmental Area; PAG, Periaqueductal gray; AMG, Amygdala.
The diverse physiological activity resultant of these cannabinoids indicates a wide breadth of potential therapeutic indications. As medicinal cannabis related clinical research has predominately focused on CNS-related diseases, such as neurodegeneration and neurological disorders, pain, substance use disorders, and anxiety disorders, this review will first examine evidence that supports or refutes the therapeutic utility of cannabinoids for the treatment of neurodegenerative disease, pain, mood disorders, and substance use disorders. Table 1 summarizes these clinical studies. Not discussed at length within this review, medical cannabis has also shown promise in treating other CNS-related diseases, such as brain tumors and gliomas (Russo, 2018). Despite continued growing interest and an increasing trove of preclinical research that exemplifies the therapeutic potential of cannabinoids, the development of viable, approved therapeutics remains elusive due to various challenges in formulation and bioavailability, efficacy, and tolerability. In this review we will address some of the challenges and considerations within the cannabinoid field that may be important in advancing such therapeutics into the clinic while presenting recent findings that provide a more up to date understanding of where the field currently lies regarding the therapeutic viability of cannabinoids.
2 Cannabinoid Involvement in Central Nervous System Diseases and Disorders
2.1 Neurodegenerative and Neurological Diseases
Neurodegenerative and neurological diseases that commonly afflict those in mid to late life have steadily become a common cause of mortality worldwide as elderly populations have continued to grow. Epidemiological reviews of these neurodegenerative diseases show that associated deaths have increased within the last 25 years, having increased worldwide by more than 35% (Group GNDC, 2017).
2.1.1 Alzheimer’s Disease
Alzheimer’s disease (AD) is the most common neurodegenerative disease that contributes to approximately 60–80% of all dementia cases globally (Erkkinen et al., 2018) and is characterized by the formation of β-amyloid plaques, phosphorylated tau proteins, formation of neurofibrillary tangles, glial activation, and neuronal death (Scheltens et al., 2021). Structural imaging of AD brains observed atrophy of the hippocampus and in later stages, the frontal cortex, areas with high density distribution of CB1R (Glass et al., 1997; Biegon & Kerman, 2001; Erkkinen et al., 2018). However, such atrophy is not necessarily correlative of observed deficits in declarative memory and recall (Nelson et al., 2009; Iqbal et al., 2010; Aschenbrenner et al., 2018).
Alterations in CB1R expression resultant of AD maintains itself as a point of contention. Studies have reported considerable decreases in CB1R expression within post-mortem AD patient brain tissues (Ramírez et al., 2005), particularly those compared to age-matched controls (Solas et al., 2013). Others found no change regarding distribution or expression of CB1R within the hippocampus and cortex (Benito et al., 2003; Lee et al., 2010; Mulder et al., 2011; Ahmad et al., 2014). CB2R expression has been found to be significantly increased with the accumulation of b-amyloid plaques (Benito et al., 2003; Ramirez et al., 2005; Solas et al., 2013), even in instances where plaque accumulation did not induce cognitive impairment (Solas et al., 2013). Increasing evidence supports a potential contributory role of the serotonergic system in AD. Serotonin 5-HT1A receptors are expressed in the hippocampus and are involved with memory processing and learning (Ögren et al., 2008; Muzerelle et al., 2016; Solís-Guillén et al., 2021).
Preclinical studies have shown marked decreases in 5-HT1A receptor expression across various regions sampled from human AD brains (Bowen et al., 1983; Cross et al., 1984; Lai et al., 2003). 5-HT1A receptors as a target for alleviating cognitive dysfunction has shown promise. Continued research with 5-HT1A receptor antagonists, such as lecozotan, enhanced cognitive function in a rat model of scopolamine induced amnesia (Skirzewski et al., 2010), as well as enhanced cognitive performance in aged rhesus monkeys and reversed cognitive deficits associated with cholinergic lesions in marmosets (Schechter et al., 2005). As partial agonists buspirone and tandospirone both improved AD patient mood and behavior, further research into 5-HT1A modulation, via CBD or other cannabinoids with serotonergic activity, may provide promising therapeutics able to alleviate AD associated cognitive dysfunction, behavioral decline and memory impairment (Salzman, 2001; Sato et al., 2007). Indeed, in accordance with CBD’s serotonergic activity profile, CBD has shown utility in animal AD models. Utilizing intracerebroventricular administration of beta-amyloid in mice to simulate cognitive impairment associated with AD, it was shown that intraperitoneal administration of CBD was able to modulate beta-amyloid activation of microglia and restore cognitive function as indicated by decreased latencies in the Morris water maze compared to vehicle controls (Martín-Moreno et al., 2011). Furthermore, THC exhibits neuroprotective effects when administered within a transgenic, beta-amyloid expressing AD mouse model with observed reductions in neuronal loss and reduced accumulation of b-amyloid compared to vehicle controls (Franke et al., 2019).
Recent clinical studies have demonstrated potential therapeutic benefit with nabilone for the treatment of neurodegenerative and neuroinflammatory diseases. In a double-blind, randomized cross-over AD study, markers for oxidative stress and neuroinflammation, such as tumor necrosis factor-α, were decreased following nabilone administration (1–2 mg), indicating a correlative association with reductions in agitation and decreased inflammation markers (Ruthirakuhan et al., 2020).
2.1.2 Parkinson’s Disease
Parkinson’s disease (PD) is the second most common neurodegenerative disease after AD, with an estimated prevalence of 572 per 100,000 among those aged 45 years and older (Marras et al., 2018), and doubling of such instances within the next 2 decades is expected (Dorsey et al., 2018). Characterized by the loss of dopaminergic neurons within the substantia nigra pars compacta, the resulting loss of dopaminergic striatal input nigra leads to the hallmark observable changes of PD. These changes include reductions in motor function such as resting tremor, bradykinesia, postural instability, and rigidity (Davie, 2008) as well as cognitive impairment, mood disorders, and pain sensory disturbances (Erkkinen et al., 2018). While instances of PD are thought to be sporadic, genetic mutations are heavily linked to PD onset, including missense mutations with genes PARK1, PARK2, and PARK7 (Blauwendraat et al., 2020). Proinflammatory signaling is thought to play a role in disease progression as well (for reviews see Wang et al., 2015; Klein and Westenberger, 2012), providing therapeutic potential to cannabinoids and their anti-inflammatory nature.
Research has shown that CB1R mRNA expression is decreased within preclinical rat models of toxin induced PD (Silverdale et al., 2001; Walsh et al., 2010) and genetic mouse models of PD (García-Arencibia et al., 2009). However, decreases in mRNA expression observed in genetic mouse models were shown to be reversed in later disease stages with increased CB1R mRNA expression (García-Arencibia et al., 2009). Significant reductions in CB1R expression within the ventral mesencephalic region are observed in early-stage PD patients compared to healthy controls (Van Laere et al., 2012). Similarly, CB1R mRNA was found to be reduced in the caudate nucleus, anterior dorsal putamen, and the external globus pallidus in human post-mortem brain tissues taken from PD patients (Hurley et al., 2003; Van Laere et al., 2012). However, up regulation of both CB1R and CB2R expression has been observed within the substantia nigra in human post-mortem striatal brain tissues taken from medicated PD patients (Navarrete et al., 2018). Serotonergic systems are affected alongside the dopaminergic denervation associated with PD with observed decreases in serotonin and dopamine concentration in cerebrospinal fluid and human striatum (Tohgi et al., 1992; Kish et al., 2008; Politis & Niccolini, 2015). Using positron emission tomography, it was found that 5-HT1A receptor binding was reduced significantly in PD patients compared to healthy controls, with a significant correlation between reduction in binding and tremor severity (Doder et al., 2003).
Blocking serotonergic signaling with the 5-HT1A agonist buspirone was found to reduce the development of l-DOPA-induced dyskinesia in a 6-hydroxydopamine (6-OHDA) lesion model of PD in rats (Eskow et al., 2007). Using the same 6-OHDA lesion model to induce dopamine depletion associated with PD in rats, it was found that CBD was able to recover dopamine levels when given immediately after lesion induction (García-Arencibia et al., 2009). However, the same study had found that administration of CBD 1 week after the lesion did not affect dopamine levels. Preclinical research studying the effects of THC in PD models have reported potential neuroprotective effects. Utilizing an in vitro model of PD with SH-SY5Y cells and PD relevant toxins, THC was shown to have an active neuroprotective effect that mitigated cell death following exposure to toxins that generate free radicals and inhibit mitochondrial function (Carroll et al., 2012). THC treatment within a marmoset PD model was also shown to improve locomotor activity associated with spontaneous exploratory behavior and complex tasks requiring hand-eye coordination (van Vliet et al., 2008). Neuroprotective effects were observed following daily intraperitoneal administration of THC over the course of 2 weeks within a preclinical rat model of toxin induced PD, with THC having reduced dopaminergic loss (Lastres-Becker et al., 2005).
Clinical studies utilizing CBD administration in PD patients have observed a reduction in the occurrence of psychotic symptoms that include sleep disturbances, hallucinations, and delusions (Zuardi et al., 2009), reduced tremor amplitude (de Faria et al., 2020), and an overall observed improvement in patient well-being and motor function (Chagas et al., 2014). A recent clinical trial utilizing CBD (Epidiolex) found similar improvements in PD associated symptoms with patients experiencing good tolerability with no major adverse effects with the 5–25 mg/kg/day dosing schedule (Leehey et al., 2020). A phase II, randomized, placebo-controlled, double-blind study to examine the effectiveness of nabilone to impact non-motor adverse effects related to PD has recently concluded and had found that PD patients given nabilone responded positively to doses up to 1 mg with good tolerability and with no major adverse effects reported (Peball et al., 2020). Clinical assessment surveys and self-scoring methods from this study indicated that patients receiving nabilone experienced improvements to non-motor adverse effects as opposed to the placebo arm, which reported increased disturbances resultant of non-motor adverse effects.
2.1.3 Huntington’s Disease
Huntington’s disease (HD) is a rare genetic neurodegenerative disorder resultant of excessive extension of CAG repeats within the huntingtin gene. Symptoms of HD include alterations in movement, mood, and cognition (see McColgan and Tabrizi, 2018) with an estimated prevalence of approximately 3 cases per 100,000 (Erkkinen et al., 2018). Cardinal features of HD include neuronal death and neuroinflammation in the striatum, globus pallidus, substantia nigra, and cerebral cortex (Hickey and Chesselet, 2003), with advanced stages of HD exhibiting wide-spread neuronal death among the cerebellum, hippocampus, and brain stem (Erkkinen et al., 2018).
Preclinical studies utilizing genetic mouse models of HD observed decreases in CB1R mRNA within the striatum, cortex, and hippocampus in initial phases of HD (Denovan-Wright and Robertson, 2000; McCaw et al., 2004; Dowie et al., 2009). In rat preclinical studies utilizing a pharmacological model of HD, both CB1R mRNA and protein were decreased in the caudate putamen, basal ganglia, globus pallidus, and substantia nigra (Lastres-Becker et al., 2001, 2002), though administration of substances that increased endocannabinoid activity was found to have activated the decreased population of CB1R and improve subject motor function (Lastres-Becker et al., 2002). Utilizing quantitative autoradiography in post-mortem brain tissue sections from HD patients, a significant loss in CB1R protein was observed within the globus pallidus, and substantia nigra (Glass et al., 1993; Richfield & Herkenham, 1994). Conversely, reductions in CB1R expression have been accompanied by increased expression of CB2R among astrocytes and microglia in preclinical rat pharmacological models of HD (Fernández-Ruiz et al., 2007; Basavarajappa et al., 2017). Post-mortem brain tissues from HD patients and transgenic mice models also exhibit increased CB2R expression within the caudate putamen as well as in striatal microglia (Palazuelos et al., 2008). Disruption of the serotonergic system has been observed in striatal samples from HD patient brains where serotonin transporter protein was found in increased concentrations compared to age matched healthy controls and early-stage HD brain samples (He et al., 2019). In brains taken from a transgenic HD mouse, binding analyses of 5-HT1A receptors found reduced binding of 5-HT1A receptor agonists among hippocampal and striatal regions of the brain, indicating further disruption of the serotonergic system within HD (Yohrling IV et al., 2002).
Within a rat pharmacological model of HD, THC produced neuroprotective effects, which further suggests that THC may have therapeutic potential, as well as lends additional credence that cannabinoid receptor dysfunction may be involved in HD etiology (Lastres-Becker et al., 2004). In a preclinical study utilizing a rat model of striatal atrophy, CBD administration was able to reverse neurodegeneration following a 5 mg/kg/day dosing schedule over 5 days, and the authors found that these effects were likely the result of the intrinsic antioxidant potential held by CBD (Sagredo et al., 2007).
One small scale pilot study has indicated potential therapeutic capacity of nabilone in HD, having observed improvements to motor skills and participant cognition (Curtis et al., 2009). A case report also observed that medicinal cannabis and nabilone were able to improve patient motor function and cognitive behavior, though no measured responses were taken and reports were anecdotal (Curtis & Rickards, 2006) In contrast, clinical trials have observed either no significant difference in motor function and cognition with nabiximols compared to placebo controls (Lopez-Sendon Moreno et al., 2016), failure to provide symptomatic protection with CBD (Consroe et al., 1991), or significant increases in involuntary movements with nabilone (Müller-Vahl et al., 1999).
2.1.4 Multiple Sclerosis
Multiple sclerosis (MS) is a debilitating neurodegenerative disease largely affecting individuals in early adult life with an increasing prevalence of 1 per 3,000 individuals, or approximately 2.8 million people worldwide (Walton et al., 2020). Characterized pathologically by hallmarks that include inflammation, axonal and neuronal loss, demyelination, and astrocytic gliosis within the brain stem and spinal cord (Goldenberg, 2012; Thompson et al., 2018), MS is physiologically characterized by episodes of sensory and motor impairments driven largely by neurodegeneration.
Preclinical studies utilizing experimental autoimmune encephalomyelitis MS models have observed reduced expression of CB1R among the striatum and cortex of rats (Berrendero et al., 2001), and mice deficient in CB1R used in experimental autoimmune encephalomyelitis MS models exhibit enhanced neurodegeneration compared to control subjects (Pryce et al., 2003). Similarly, studies utilizing human post-mortem CNS tissue samples have observed increased expression of CB1R among cortical neurons, oligodendrocytes, oligodendrocyte precursor cells and macrophages near plaques associated with MS (Benito et al., 2007; Palazuelos et al., 2008). Likewise, CB2R receptor expression and density were found to have been increased in MS, particularly in T-lymphocytes, astrocytes, microglia, and macrophages near active plaques (Yiangou et al., 2006; Benito et al., 2007). Utilizing photon emission tomography, patients with MS were found to have lower availability of serotonin transporters throughout the limbic system, a factor that may contribute to the psychiatric symptoms associated with MS, as well as disturbed modulation of the immune system (Hesse et al., 2014). CBD has been shown to provide therapeutic benefits for the treatment of MS, though further research is needed to understand the mechanisms driving such activity. Preclinical studies utilizing experimental autoimmune encephalomyelitis in mice have found that CBD administration ameliorated the severity of MS symptoms when given during disease onset (Kozela et al., 2011), inhibited production of pro-inflammatory cytokines (Giacoppo et al., 2017), and attenuated the infiltration of CD4+ T cells and macrophages into the central nervous system (Constantinescu et al., 2011; Giacoppo et al., 2017).
Although nabiximols administration has not been found to improve cognitive function of patients (Rekand, 2014; Lopez-Sendon Moreno et al., 2016; Riva et al., 2019), the THC:CBD spray combination has not been associated with cognitive decline in long-term use (Rekand, 2014), a salient concern, given that long-term THC use has been linked to poor cognitive health (Crean et al., 2011). Compared to placebo controls, early clinical trials demonstrated that nabiximols displayed efficacy in the alleviation of MS-associated spasticity and reduced both spasm number instances and severity during treatment (Collin et al., 2007; Novotna et al., 2011; Wade et al., 2004, 2006). A more recent clinical trial utilizing nabiximols for the treatment of symptoms associated with MS observed superior improvement of MS induced spasticity compared to adjustments in first-line anti-spasticity medication alone (Markova et al., 2019). Similar improvements have been reported with clinical trials utilizing 10 mg dronabinol, where reductions in spasticity and improved ambulation (University of California, Davis, 2018) and modest improvements in pain assessments (Svendsen et al., 2004) were observed. While these studies report good tolerability with no major adverse effects, dronabinol was found to have no improvements on cognitive function and indications of worsening cognitive function with time (University of California, Davis, 2018). Whole cannabis extract was also found to have relieved muscle stiffness experienced by MS patients (Zajicek et al., 2012). Finally, medicinal cannabis was found to have slightly reduced fatigue among MS patients compared to age/sex matched controls with no record of cannabis use (Rudroff, 2020). Though this comparative observational study reports good tolerability as well, there is no mention of total cannabis consumption among patients. These findings are summarized in Table 1.
2.1.5 Amyotrophic Lateral Sclerosis
Amyotrophic lateral sclerosis (ALS) is a neurodegenerative disease with a prevalence between 4.1 and 8.4 per 100,000 persons, characterized by gradual loss of muscle control resultant of increasing muscle weakness and wasting (Longinetti & Fang, 2019). This results in the progressive loss of the ability to chew, swallow, talk and breathe, ultimately leading to death (Zarei et al., 2015). Portions of those with ALS will also experience frontotemporal dementia and changes in behavior and cognition as the disease progresses (Masrori & Damme, 2020). While 90–95% of all ALS cases are sporadic with unknown etiology (Zarei et al., 2015) and pathogenesis is not completely understood, it is thought that mechanisms associated with excitotoxicity, oxidative stress, and neuroinflammation are implicated with ALS onset (Rao & Weiss, 2004; Liu & Wang, 2017; Batra et al., 2019).
Preclinical research utilizing a genetic mouse model of ALS presents conflicting observations regarding CB1R. Compared to healthy controls, reductions in spinal cord motor neuron CB1R expression has been observed in the early, pre-symptomatic stage in a mouse ALS model, with an elevation of expression observed in the symptomatic stage (Zhao et al., 2008). The authors suggest that this may be indicative of a neuroprotective action compensating for initial losses in CB1R, though ultimately, expression of CB1R was reduced in end-stage ALS mouse models, suggesting continued declination in neuronal health (Zhao et al., 2008). Another preclinical study utilizing genetic ablation of CB1R in a genetic mouse model of ALS observed an extension of life span compared to wild type subjects (Bilsland et al., 2006). However, ablation also resulted in significant motor neuron death and decreased survival of remaining motor neurons. An immunocytochemistry analysis of post-mortem spinal cord tissue taken from ALS patients observed increased CB2R immunoreactivity within areas exhibiting neuronal degeneration (Yiangou et al., 2006). Further analyses will be needed to understand the role of cannabinoid receptors in ALS experienced by humans. Motor neurons preferentially affected in ALS are densely innervated by 5-HT expressing neurons; their degeneration may provide the pathological link to the spasticity commonly seen with ALS (Sandyk, 2006; Dentel et al., 2013). In ALS patient brainstem samples, it was found that there was severe degeneration of serotonergic neurons compared to healthy controls (Dentel et al., 2013).
Studies utilizing a transgenic mouse model of ALS have observed that mice treated with THC (Raman et al., 2004; Weydt et al., 2005), experienced delayed disease progression and prolonged survival. An in vitro component to one such study found that THC effectively reduced oxidative stress and minimized excitotoxicity within mouse spinal cord cultures (Raman et al., 2004), suggesting that THC possesses potential neuroprotective effects which may be beneficial in treating ALS.
Current clinical research into the use of cannabinoids in ALS is limited, though previously mentioned clinical trials in other neurodegenerative diseases suggest therapeutic potential in ALS. Observations gathered from patient surveys suggest that medicinal cannabis provides therapeutic relief of symptoms of ALS such as pain, spasticity, and excessive drooling, however these observations are limited by the comparatively small number (10% of those surveyed) of those having used cannabis recently at the time of survey (Amtmann et al., 2004). A randomized placebo-controlled clinical study utilizing nabiximols in patients with ALS report reductions in spasticity symptoms with no report of major adverse effects (Riva et al., 2019). Finally, a clinical study investigating the efficacy of THC in mitigating cramping associated with ALS observed no subjective improvement of cramp intensity among ALS patients, though THC was well tolerated with no major adverse effects reported with 10 mg daily oral administrations (Weber et al., 2010). These findings are summarized in Table 1.
2.1.6 Epilepsy
Seizures are the result of abnormal synchronous neuronal excitation within the brain with etiologies including genetic predisposition, injury, brain tumors, and neurodegenerative diseases (Falco-Walter, 2020). The prevalence of epilepsy is between 50.4 to 81.7 per 100,000 persons annually and continues to rise as advances in healthcare lead to increased survivability of traumatic head injuries, stroke, and increased lifespans (GBD 2016 Epilepsy Collaborators, 2019).
As discussed above cannabinoid compounds have exhibited antispastic capacity in a range of neurodegenerative disease states that gives further support to the use of cannabinoids for the treatment of epileptic convulsions. THC-related anticonvulsant activity is likely the result of CB1R stimulation. Preclinical evidence suggests that the endogenous cannabinoid system contributes to the regulation of seizure frequency. Mice lacking functional CB1R or mice that have genetic alterations in endogenous cannabinoid system activity which lead to decreased CB1R tone are characterized to be seizure prone (Clement et al., 2003; Marsicano et al., 2003). THC completely abolished spontaneous seizures within a rat model of epilepsy, while CB1R antagonism with SR141716A increased seizure duration and frequency (Wallace et al., 2003). The same study also revealed that CB1R expression was significantly increased within epileptic hippocampi (Wallace et al., 2003). CBD-related anticonvulsant activity is likely due to its 5-HT agonist properties, actions at voltage-gated sodium ion channels, as well as its ability to modulate intracellular calcium storage. In vitro studies, CBD selectively inhibited aberrant sodium currents in mutated sodium ion channel expressing cells and had no effect on normal sodium channel activity (Patel et al., 2016). In a mouse ex vivo epilepsy model CBD pre-exposure blocked aberrant hippocampal nerve firing, and these protective effects were inhibited by either a reduction in serotonin tone, or pharmacologically, by a calcium store antagonist (Maggio et al., 2018).
Clinical research has yielded promising results with the use of cannabinoids for treating epilepsy. However, current interest has been focused largely on CBD due to good tolerability and lack of psychoactive effects (Jones et al., 2010). CBD utilization within clinical trials of treatment-resistant epilepsy (Devinsky et al., 2016; Gaston et al., 2019) and Dravet syndrome (Devinsky et al., 2018, 2019) with clinically approved Epidiolex was found have significantly reduced the occurrence and duration of epileptic seizures. Long term safety and quality of life studies with Epidiolex also indicated that CBD provides effective long-term treatment with good tolerability and improves patient quality of life (Gaston et al., 2019; Laux et al., 2019).
2.1.7 Increasing Prevalence Requires Further Research
The prevalence of neurodegenerative and neurological disease continues to rise globally as improvements in healthcare result in improved survivability of many previously fatal diseases and longer life spans. However, with increases by more than 35% in death rates among those with neurodegenerative diseases within the past 25 years, therapeutics are needed urgently. Many of the pathologies discussed above lack any current clinically approved cures or treatments, with the current extent of our therapies only providing symptomatic relief. With endocannabinoid targets such as CB1R and CB2R and serotonergic involvement with 5-HT1A, development of cannabinoid-based therapeutics shows promise and further research and development is critical considering our aging population.
2.2 Pain
Pathological pain is a substantial component of many chronic illnesses and diseases and can be divided into pain that arises from inflammatory insults, known as inflammatory pain, and pain that is the result of nerve injury, known as neuropathic pain. Both inflammatory and neuropathic pain alter neuronal processing and immune cell function. These alterations ultimately lead to perceived pain with a prevalence of 6.9–10% for neuropathic pain, with much higher estimates for inflammatory pain as it is ubiquitous in many disease states (van Hecke et al., 2014). Diseases with exhibitions of neuropathic pain include diabetes, neurodegeneration, human immunodeficiency virus, and chemotherapy induced peripheral neuropathy. Cancer itself has both inflammatory and neuropathic components that contribute to pain perception, with common cancers such as breast, prostate, kidney, and lung cancers resulting in metastasis to bone that further drive and contribute to pathological pain in cancer (Mantyh, 2014).
2.2.1 Endocannabinoid Targets in Pain
There is continued interest in the endocannabinoid system and its involvement in pain modulation (see Donvito et al., 2018). This is due to the extensive expression of CB1R throughout the CNS in pain relevant regions such as afferent nerve fibers (Hohmann et al., 1999; Morisset and Urban, 2001), spinal cord interneurons (Jennings et al., 2001), trigeminal sensory neurons (Price et al., 2003), and neurons within the periaqueductal grey (Mailleux and Vanderhaeghen, 1992). As for the periphery, CB1R is observed on peripheral nociceptors (Richardson et al., 1998) and the dorsal root ganglia (Hohmann and Herkenham, 1999). As for CB2R, these cannabinoid receptors are expressed in peripheral macrophages and lymphocytes (Munro et al., 1993; Bouaboula et al., 1993; Galiegue et al., 1995) as well as astrocytes, oligodendrocytes, and microglia within the central nervous system (Zhang et al., 2003; Maresz et al., 2005; Beltramo et al., 2006; Racz et al., 2008), suggesting potential mediation of inflammatory pain with cannabinoids. The spatial distribution of CB1R and CB2R within the CNS are shown in Figure 1. CB2R may also be implicated with neuropathic pain, as CB2R has observed expression among sensory neurons of the dorsal horn after sciatic nerve section or spinal nerve ligation in rats (Wotherspoon et al., 2005). 5-HT1A receptors are expressed in areas relevant to pain signaling and transmission such as primary afferent neurons, peripheral terminals, astrocytes, oligodendrocytes, and microglia (Björk et al., 1992; Laporte et al., 1995; Granados-Soto et al., 2010; Perrin et al., 2011). The spatial distribution of 5-HT1A receptors within the CNS is shown in Figure 1.
2.2.2 Preclinical Studies in Pain
Preclinical studies assessing CBD for pain relief have painted a promising picture for the cannabinoid. Though as discussed in the next section, expectations should be tempered as the translational efficacy of cannabinoids into humans is unclear. CBD has been found to exert analgesic effects in animal models of neuropathic pain, such as surgically induced nerve injury and chemotherapy induced neuropathy (Harris et al., 2016; Ward et al., 2011, Ward et al., 2014), though effects are dependent on dose and route of administration (Costa et al., 2007; Abraham et al., 2020). While the therapeutic window of THC is limited by its psychoactive side effects, various studies utilizing combinations of THC and CBD have found improved efficacy in low dose administrations to treat pain. In models of either surgically induced nerve injury (Casey et al., 2017; Linher-Melville et al., 2020) or chemotherapy induced neuropathy (King et al., 2017), 1:1 THC + CBD combinations exhibited greater efficacy at low doses that were ineffective with either THC or CBD alone.
2.2.3 Clinical Trials in Pain
Despite these preclinical studies suggesting therapeutic potential of cannabinoids as analgesics, review of recent clinical trials in various pain pathologies suggests an inconclusive viability of cannabinoids as a therapeutic for pain. Within a double-blind placebo-controlled study in MS patients experiencing neuropathic pain, THC (dronabinol), taken orally up to a maximum dose of 15.9 mg over 16 weeks, decreases patient reported pain measurements, though no significant difference from placebo was ever observed (Schimrigk et al., 2017). Studies in safety also found that whole cannabis was able to alleviate chronic pain whilst improving patient quality of life with both acute and long-term administrations (Ware et al., 2010, 2015). An open label, long-term efficacy and safety portion of this clinical trial also observed maintained decreases in patient reported pain intensities with low occurrences of serious adverse effects being reported (Schimrigk et al., 2017). A clinical trial utilizing THC (dronabinol) for pain relief in patients with noncardiac chest pains observed that 10 mg oral administrations improved patient pain thresholds significantly compared to placebo with good tolerability and with no major adverse effects being reported (Malik et al., 2017). Clinical trials utilizing nabiximols have observed pain relief in patients experiencing pain resultant of a range of pathologies including MS (Notcutt et al., 2004; Rog et al., 2005; Kavia et al., 2010), peripheral neuropathy (Nurmikko et al., 2007), and cancer (Johnson et al., 2010; Portenoy et al., 2012; Fallon et al., 2017; Lichtman et al., 2018). A clinical trial with nabiximols in patients experiencing chronic pain associated with late-stage cancer observed a 15.5% improvement among patient reported perceptions of pain (Lichtman et al., 2018). An identical companion study to this clinical trial once again observed similar improvements with nabiximols (Fallon et al., 2017). It should be noted that patient pools utilized cohorts from both the United States and Eastern Europe, with significant improvements in pain relief compared to placebo among American patients and general improvements among Eastern Europeans, though these effects were not significant compared to placebo in Eastern European cohorts (Fallon et al., 2017; Lichtman et al., 2018). It should be noted in these studies the Eastern European cohort was sicker than the American cohort, suggesting that patient selection criteria may have contributed to the divergent study findings. Additionally, nabiximols administration did meet several secondary endpoints associated with quality of life, which, as suggested by the authors, may indicate therapeutic utility in cancer pain as an adjuvant therapeutic with a low opioid dose (Lichtman et al., 2018). In a small double-blinded, placebo-controlled, crossover design clinical study, oral CBD had no effect on muscle damage markers or muscle soreness in exercised untrained men (Cochrane-Snyman et al., 2021). A recent double-blind, placebo controlled clinical study in an emergency room setting found that orally administered CBD was equal to placebo and did not adequately control acute non-traumatic low back pain (Bebee et al., 2021). Although these above clinical studies suggest CBD may not be a frontline analgesic, further clinical studies examining various route of administration and dosing strategies are needed. Additionally, it is unknown if the other compounds in medicinal cannabis may yield enhanced utility of these cannabinoids in clinical pain management settings.
2.2.4 Opioids and Cannabinoids for Pain
The ability of medical cannabis to augment the analgesic potency of opioids without additional enhancement of opioid associated side effects is an area of growing research interest with recent clinical trials yielding mixed results. Clinical pain research suggests that medicinal cannabis or cannabinoids for chronic pain may yield opioid sparing effects, a major consideration given the interest in minimizing opioid use for severe pain to avoid opioid tolerance, dependance risk, and side effects such as somnolence and respiratory depression. Studies utilizing smoked or oral medicinal cannabis among habitual opioid using, chronic pain patient cohorts observed improvements to quality of life, pain, and opioid prescription cessation (Haroutounian et al., 2016; Capano et al., 2020). Indeed, following 6 months of opioid/cannabis cotreatments, prescribed morphine use was found to have dropped significantly compared to baseline usage among patients with chronic pains, with reductions observed earlier at 3 months opioid/cannabis cotreatment (O’Connell et al., 2019). Similar reductions in prescribed, opioid use were also observed over a 21-month period with 83.8% of patients (N = 37) reporting reduced prescribed daily opioid dosage and 40.5% of patients ceasing opioid prescriptions altogether (Vigil et al., 2017). A clinical trial utilizing healthy participants with no prior indication of pathological pain had observed that orally administered THC (dronabinol, 5 mg) was not able enhance the analgesic effects of oxycodone in coadministrations and reported an increase in both abuse and impairment related effects associated with opioid use (Babalonis et al., 2019). A similar study in healthy subjects also reported that THC (dronabinol, max 10 mg) had no consistent dose-effect relationship with the opioid agonist hydromorphone in measures of both acute and chronic pain, though significant analgesia in acute pain with hydropmorphone and 2.5 mg dronabinol compared to placebo was observed (Dunn et al., 2021). Additionally, a clinical trial utilizing healthy cannabis smokers found that the combination of oxycodone (2.5 mg) and cannabis (cigarettes, 5.6% THC) was not able to provide analgesia in measures of acute pain and increased abuse-related subjective effects but did increase pain thresholds and tolerance (Cooper et al., 2018). This discrepancy may be due to the contributing pharmacological effects of the other compounds found in cannabis rather than THC alone, though more work is needed to explore the complex pharmacology between cannabinoids and opioids.
2.2.5 Disconnect Between Preclinical and Clinical Research Findings
Pain is a substantial component of many chronic illnesses and diseases that range from cancer to diabetes. With extensive expression of CB1R and CB2R and 5-HT receptors, cannabinoid compounds have great potential as novel pain therapeutics for pathologies such as chemotherapy induced peripheral neuropathy, cancer, and neurodegenerative diseases. Current preclinical literature shows promise with cannabinoids being able to effectively alleviate pain across different animal pain models. However, clinical research suggests that more work is needed to examine dose, pain indication, and route of administration questions, given that many studies observe general, but not significant, improvements in pain when compared to proven analgesics such as oxycodone and other opioids. Despite this, these studies and others still report improvements in patient reported assessments of pain and quality of life compared to placebo controls. As such, further clinical research is warranted to determine whether cannabinoids can provide effective pain relief alone or as an adjunctive therapeutic in human pain pathologies.
2.3 Addiction and Substance Use Disorders
From alcohol to opioids, addiction can occur with a variety of psychoactive drugs and consists of use disorders characterized by heavy consumption, loss of intake control, and withdrawal experiences (Zou et al., 2017). Most of these addictive substances can result in elevations of extracellular dopamine that, with time, downregulate the expression of dopamine receptors and negatively affect dopaminergic neurons, like those found in the mesocorticolimbic pathway (Wise & Robble, 2020).
2.3.1 Endocannabinoid Targets in Addiction and Substance Use Disorders
In addition to dopaminergic receptors, CB1R are expressed in abundance throughout this pathway in brain regions involved in reward signaling such as the ventral tegmental area, nucleus accumbens, amygdala, pre-frontal cortex, and hippocampus (Oleson et al., 2021). Preclinical studies utilizing either CB1R antagonists or deletion of the receptor have observed reduced motivation for the consumption and self-administration of ethanol in rat and mouse alcohol dependence models (Gallate et al., 2004; Thanos et al., 2005; Femenía et al., 2010). Rimonabant, the CB1R inverse agonist/functional CB1R antagonist, was shown to reduce conditioned place preference and self-administration of alcohol (Arnone et al., 1997), heroin (De Vries et al., 2003), and nicotine (Cohen et al., 2005; Robinson et al., 2018). While serious psychiatric side effects such as anxiety, depression, and suicide ideation have prevented rimonabant from passing clinical trials (Manzanares et al., 2018), it supports the notion that CB1R antagonism may allow for the attenuation of substance use disorders. CB2R in substance use disorders may also provide a potential target with cannabinoid therapeutics as research suggests their potential role in modulating behaviors associated with addiction (Onaivi et al., 2008b; Agudelo et al., 2013; Galaj et al., 2020). Similarly, given the role that the serotonergic system plays in both motivational and reinforcement processes, serotonergic modulation may provide a solution to alleviating substance use disorders (Müller & Homberg, 2015; Yagishita, 2020). Research shows that extracellular serotonin is acutely increased following administration of morphine (Tao & Auerbach, 1994; Fadda et al., 2005) and alcohol (Yoshimoto et al., 1992; Bare et al., 1998; Thielen et al., 2002). Additionally, chronic administration of psychoactive substances such as morphine, ethanol, and cocaine have been found to reduce the basal levels of extracellular serotonin within the brain, potentially resulting in increased sensitivity (Pelloux et al., 2012; Müller & Homberg, 2015). Preclinical research focused on 5-HT1A receptor modulation in the context of drug reward and addictive behaviors found CBD decreased morphine-induced reward facilitation in an operant behavioral paradigm within rats that was mediated through 5-HT1A receptor activation in the dorsal raphe nucleus (Katsidoni et al., 2013). Though further studies are required, current research has provided proof of concept regarding the treatment of drug dependency and use disorders with cannabinoids, suggesting their use as an alternative or co-adjuvant therapeutic.
2.3.2 Alcohol Use Disorder
US Food and Drug Administration approval for drugs in the treatment of alcohol use disorder has not occurred since 2004 with the approval of acamprosate. Preclinical research has shown that CBD may hold particular promise as an alcohol use disorder therapeutic. Activity at CB2R may provide for an initial target in therapeutic development with cannabinoids. An early study utilizing the CB2R agonist, JWH 015 in stressed mice observed enhanced alcohol preference compared to controls (Onaivi et al., 2008a). Upregulation of CB2R was also observed in dendritic cells from patients with alcohol abuse disorders (Agudelo et al., 2013). Preclinical studies utilizing mice in the two-bottle choice paradigm and oral ethanol self-administration demonstrated that systemic CBD administration significantly reduced both ethanol consumption and preference, suggesting that CBD can reduce the motivational properties of ethanol (Viudez-Martínez et al., 2018). The same study found that CBD administration prevented relapse in oral ethanol self-administration (Viudez-Martínez et al., 2018). Contrary to the potential benefits observed with CBD, THC has been found to reinstate alcohol seeking behavior in abstinent rats (McGregor et al., 2005). Utilizing a beer (4.5% ethanol v/v) self-administration paradigm, the study observed that intraperitoneal THC administration significantly reinstated responding previously reinforced with beer. However, both sucrose trained subjects and beer trained subjects had self-administration responses reinstated with THC administration (McGregor et al., 2005).
2.3.3 Opioid Use Disorder
Preclinical studies with cannabinoids as a therapeutic for opioid use disorders are largely motivated by the neurobiological interactions between the cannabinoid and opioid systems (Rodríguez et al., 2001; Schoffelmeer et al., 2006). A preclinical study in rats utilizing a self-administration, drug-seeking behavior model found that CBD inhibited reinstatement of cue-induced heroin seeking behavior, though such effects were not observed with drug seeking behavior initiated by a priming dose of heroin (Ren et al., 2009). A similar study utilizing a conditioned place preference paradigm in mice with morphine treatment found that CBD decreased the establishment of opioid reward as indicated by an attenuation of morphine place preference (Markos et al., 2018). In contrast, some research suggests that THC may not be a viable therapeutic for treating opioid use disorders, though use in treating withdrawal symptoms show promise. It has been observed that subjects pre-exposed in adolescence shown marked opiate sensitivity with higher consumption of heroin and upward shifts in self-administration acquisition (Ellgren et al., 2007). Similar studies observing the effects of systemic THC administration in cannabinoid-opioid system interactions have reported similar results with enhanced opioid intake in operant behavioral studies (Vela et al., 1998; Solinas et al., 2004). THC may not be without therapeutic opioid use disorder utility. Current research, though limited, demonstrates that systemic THC administration inhibits symptoms (jumping, rearing, wet shakes, diarrhea) associated with naloxone-induced opioid withdrawal (Hine et al., 1975; Bhargava, 1976).
2.3.4 Tobacco Use Disorder
While currently limited, there is increasing evidence that cannabinoid compounds are able to modulate tobacco use disorder. However, some studies are seemingly contradictory, and have left the exact therapeutic utility of cannabinoids for the treatment of tobacco use disorder unclear. A small-scale pilot study in treatment-seeking smokers had found that use of a CBD inhaler resulted in reduced self-reported smoking compared to placebo treatment over a 7-day period, although cravings for cigarettes remained unchanged (Morgan et al., 2013). Acute administration of THC has been found to attenuate the somatic and motivational manifestations of nicotine withdrawal in mice, though it is unlikely a result of the compensatory changes on CB1R density following chronic nicotine exposure (Balerio et al., 2004). A similar study assessing nicotine and THC coadministration in mice found enhancement of both the expression of nicotine withdrawal symptoms and nicotine induced conditioned place preference (Valjent et al., 2002). Further research will be required to elucidate any potential therapeutics for nicotine use disorders.
2.3.5 Cocaine Use Disorder
With no currently approved therapeutics for psychostimulant addiction, the use of cannabinoids as a treatment for cocaine addiction has garnered interest despite a small body of literature. Recent preclinical work with CBD administration in mice observed reduced CB1R expression within the nucleus accumbens with simultaneous increases in CB2R expression (Calpe-López et al., 2019). These results are intriguing and raise the possibility that although CBD may not act directly via CB1R or CB2R based upon binding affinity, it may alter cannabinoid receptor tone. An earlier study utilizing JWH133, a CB2R agonist, found that CB2R agonism was able to dose dependently inhibit cocaine-enhanced locomotion and cocaine self-administration in mice (Xi et al., 2011). Such effects were not observed in CB2R knockout mice and were blocked with AM630, a CB2R antagonist, suggesting a role for CB2R in modulating cocaine-induced rewarding and locomotor enhancing effects (Xi et al., 2011). A similar study conducted last year in mice also reported similar benefits, as CBD prevented behavioral alterations associated with cocaine addiction that included locomotor stimulation and memory deficits related to cocaine withdrawal (Ledesma et al., 2021). Finally, in a rat cocaine self-administration model, it was observed that CBD reduced cocaine self-administration, and these effects are blocked following CB2R and 5-HT1A antagonist administration (Galaj et al., 2020).
2.3.6 Considerations of Cannabis Dependence and Therapeutic Capacity
As mentioned earlier, the two primary constituents of cannabis are THC and CBD, with THC having psychoactive properties and marked effects on dopamine release like other drugs of addiction. Indeed, clinical research shows that acute administration of THC does elicit dopamine release within the striatum (Bossong et al., 2015; Bloomfield et al., 2016), with such effects being dose dependent. Chronic cannabis use is also associated with increased risk of substance use disorder development and development of withdrawal behaviors that include irritability, anxiety, depression, fever, and tremors (Katz et al., 2014; Volkow et al., 2014). Furthermore, utilization of THC in anxiolytic therapies is limited due to psychoactive sequelae, risk of abuse, and anxiogenic effects (Kayser et al., 2020b; García-Gutiérrez et al., 2020). While the therapeutic window of THC is limited by its psychoactive side effects, various studies utilizing combinations of THC and CBD have found improved efficacy in low dose administrations to treat pain, anxiety, and depression. Although the negative attributes of cannabis are largely attributed to THC, as described above, CBD continues to draw attention as an anxiolytic and analgesic. The minor components of cannabis may also prove to be beneficial in either the selective development of whole cannabis therapeutics or as isolated cannabinoid compound mixtures. This rationale is due to the discovery that cannabis terpenoids and minor phytocannabinoids exhibit therapeutic capacity in a variety of pathologies, including epilepsy, neurodegenerative disease, and traumatic brain injuries (Russo & Marcu, 2017; Russo, 2018).
2.4 Anxiety Disorders
Early epidemiological studies observing the prevalence of mood disorders had found that anxiety disorders are highly prevalent within the United States (Weissman, 1988; Stein et al., 2017). Anxiety disorders to date are maintained as the most common mood-related disorders (Vos et al., 2015; Penninx et al., 2021) both within the United States and worldwide. Psychological symptoms of common anxiety disorders include frequent and prolonged states of amplified fear and/or anxiety (Giacobbe & Flint, 2018).
2.4.1 Endocannabinoid Targets in Anxiety
Brain regions relevant in feelings of anxiety and fear include the prefrontal cortex, hippocampus, amygdala, hypothalamic nuclei, and the bed nucleus of the stria terminalis (Lafenetre et al., 2007), regions with notable expression of neuronal CB1R. Additionally, CB2R present within the periphery and the CNS, have been implicated in both anxiety disorders and anxiety regulation (Garcia-Gutierrez and Manzanares, 2011; Liu et al., 2017; Patel et al., 2017). Like CB1R, 5-HT1A receptors are present in high densities throughout the CNS in areas associated with emotional control and anxiety, including regions such as the hippocampus, amygdala, and cerebral cortex (Mestikawy et al., 1991; Wang et al., 2009; Marcinkiewcz et al., 2016). While their direct role in anxiety onset is unclear, the contribution of the serotonergic system is evident as 5-HT1A receptor knockout mice exhibit increased anxiety-like behavior in assays such as the elevated-plus maze and open-field test, both of which provide face and predictive validity in human models of anxiety (Hirshfeld et al., 1992; Graeff et al., 1998; Lesch, 2005).
2.4.2 Cannabinoid Consideration for Anxiety
Primary first line pharmacotherapeutics for the treatment of anxiety are serotonergic, which include selective serotonin reuptake inhibitors (SSRIs) and azapirones like buspirone. These therapeutics are generally well tolerated with short-term adverse effects that include nausea, diarrhea, and constipation. However, more problematic adverse effects include sexual dysfunction (Jing & Straw-Wilson, 2016), suicide ideation in pediatric patients (Hammell et al., 2016), and serotonin syndrome (Volpi-Abadie et al., 2013) with SSRIs and the development of buspirone induced movement disorders (Rissardo & Caprara, 2020). While the utilization of THC in anxiolytic therapies is limited due to psychoactive sequelae, risk of abuse, and anxiogenic effects (Kayser et al., 2020b; García-Gutiérrez et al., 2020), CBD continues to draw increasing attention in its use as an anxiolytic as work continues in developing therapeutics that can mimic the beneficial effects of current first line anxiety therapeutics while having improved side effect profiles over SSRIs and buspirone. CBD has been indicated as a potential treatment of a range of anxiety disorders that include both generalized anxiety disorder (GAD) and social anxiety disorder (SAD) as well as the excessive anxiety associated with post-traumatic stress disorder (PTSD) and obsessive-compulsive disorder (OCD) (Micale et al., 2013; Blessing et al., 2015).
2.4.3 Preclinical Studies in Anxiety
Preclinical literature regarding CBD in rodent models of generalized anxiety suggest CBD’s efficacy in minimizing anxiety associated behaviors relevant in GAD, SAD, PTSD, and OCD. Studies utilizing CBD in elevated plus and elevated T mazes with rodents have observed anxiolytic effects following both acute systemic administration (Campos et al., 2012; Campos et al., 2013a; Campos et al., 2013b) and acute local administrations in areas such as the amygdala central nucleus (Hsiao et al., 2012), bed nucleus of the stria terminalis (Gomes et al., 2011), and the intra-dorsal periaqueductal gray (Soares et al., 2010). Anxiolytic effects of CBD in these models are presented as a bell-shaped dose-response curve, with anxiolytic effects generally observed at moderate doses; 2.5–10.0 mg/kg in rats (Guimarães et al., 1990), 1 and 10 mg/kg in mice (Onaivi et al., 1990). Chronic administrations of CBD have also been found to produce anxiolytic effects in mice with the open-field test (Long et al., 2010), though contrasting results from a later study show that chronic CBD had no such effect in the elevated plus maze (Schiavon et al., 2016). Despite these mixed results and considering current preclinical evidence, use of CBD as an anxiolytic appears favorable with an improved side effect profile and no risk of anxiogenic effects (Garakani et al., 2020).
2.4.4 Clinical Trials in Anxiety
Secondary outcomes of clinical trials utilizing dronabinol (Malik et al., 2017), nabilone (John Redmond et al., 2008), and oral titrations of THC (Attal et al., 2004) all have reported general improvements to patient anxiety alongside their primary outcomes on pain relief. Studies assessing cannabinoid/opioid cotreatments also observed improvements to patient quality of life with secondary outcomes looking at measures of anxiety (Haroutounian et al., 2016; Capano et al., 2020). Direct assessments of patient anxiety provide clinical evidence that suggests that CBD has potential as a treatment for anxiety disorders, though such studies have generally focused on acute administrations utilizing small subject sizes often in healthy patients (Blessing et al., 2015; García-Gutiérrez et al., 2020). Clinical studies utilizing the simulation public speaking test had found that acute oral administration of CBD capsules reduced subjective (visual analog mood scale) and physiological (blood pressure, heart rate) measures of stress in healthy patients (Zuardi et al., 2009; Bergamaschi et al., 2011). Of these studies, a treatment naïve patient group with SAD was given CBD and had also exhibited indications of reduced anxiety, both subjective and physiological (Bergamaschi et al., 2011). Clinical studies assessing the anxiolytic properties of cannabinoids in PTSD and chronic pain pathologies have also observed general improvements in patients having consumed whole cannabis products (Greer et al., 2014; Bonn-Miller et al., 2021) or CBD alone (Elms et al., 2019) as indicated by lowered scores in clinician administered posttraumatic stress scales and PTSD checklists which assess emotional response and cognitive function. Instances of self-reported anxiety associated with OCD were found to have been no different than placebo after administration of cannabis high in either CBD (0.4% THC/10.4% CBD) or THC (7.0% THC/0.18% CBD) (Kayser et al., 2020a). Another retrospective clinical study had found that general anxiety experienced by patients was reduced following continued CBD administration using the Hamilton anxiety rating scale, though it should be noted that this study utilized open-label treatment for patients without a comparison group (Shannon et al., 2019). These studies support the potential for CBD as a treatment for anxiety disorders, especially when paired with preclinical findings. However, larger clinical trials assessing both acute and chronic dosing in additional anxiety disorders are needed.
2.4.5 CBD, But Not THC for Anxiolytic Development
Anxiety disorders are highly prevalent within the United States and is maintained as the most common mood-related disorder worldwide. First line therapeutics for the treatment of anxiety include SSRIs and buspirone and while generally tolerated, these therapeutics are associated with problematic adverse effects that include sexual dysfunction and suicide ideation in pediatric patients. Therefore, development of cannabinoid-based anxiolytics would provide a potentially safer alternative to current therapies. It should be noted though that the psychoactive components of cannabis, such as THC, are generally anxiogenic at higher doses and while clinical research has indicated anxiolytic effects at low doses, THC alone seems to have fallen out of favor in anxiolytic development. With indications from both preclinical and clinical research, CBD may prove to be an effective cannabinoid in relieving anxiety in patients and further development of cannabinoid-based anxiolytics is warranted.
3 Considerations for Cannabinoid Administration and Formulation
Cannabinoids exhibit particular characteristics that must be considered for both compound formulation and routes of administration as the pharmacokinetics and effects observed are heavily dependent on these (Lucas et al., 2018). Cannabinoids such as THC exhibit high lipophilicity, low aqueous solubility, and susceptibility to degradation via light, heat, and auto-oxidation (Grotenhermen, 2003). Interest in cannabinoid formulation, delivery strategies, and utilization of optimal routes of administration continues to grow in parallel with interests in the use of cannabinoids for potential therapeutic applications. Formulation strategies have been developed to overcome challenges brought upon by characteristics such as high lipophilicity in other compounds, though these strategies require testing in cannabinoids to determine if they would provide favorable pharmacokinetic improvements in items such as distribution and bioavailability (Kumari et al., 2010; Allen and Cullis, 2013; Dengler et al., 2013).
3.1 Oral Administration
Current clinically approved cannabinoids such as nabilone, dronabinol, and cannabidiol (Epidiolex) utilize oral administration and is the most prevalent route of administration for therapeutic applications. In the case of dronabinol, an exciting opportunity presents itself where direct comparisons can be made between the capsule and liquid formulation of the cannabinoid regarding efficacy. Companion clinical trials aiming to assess potential differences between these capsule and liquid formulations had observed a large, though insignificant, difference in dronabinol absorption times with 4.25 mg liquid formulations being superior to 5 mg capsules (Parikh et al., 2016; Oh et al., 2017). However, peak serum concentration was higher for both dronabinol and its metabolite, 11-OH-Δ9-THC, with the capsule formulation (Parikh et al., 2016; Oh et al., 2017). This disparity among formulations may likely be the result of hydrophobic drugs being less bioavailable when delivered in oil-based formulations (MacGregor et al., 1997). Indeed, both THC and CBD oral administrations in sesame oil exhibit poor bioavailability, as low as 6% in humans (Agurell et al., 1981; Huestis, 2005), likely resulting from variable absorption and extensive first pass metabolism (Lucas et al., 2018). Utilization of a self-emulsifying drug delivery system (SEDDS) could provide a more desirable endpoint for compound bioavailability as the mixing oils, surfactants, solvents, and other excipients can improve the oral bioavailability of lipophilic compounds. SEDDS may provide a viable solution to the challenges brought upon by the inherent lipophilicity of cannabinoids as patents filed by Murty Pharmaceuticals show a growing body of research that supports this drug delivery system (Murty and Murty, 2012). A recent clinical study utilizing a SEDDS-CBD oral administration (standardized to 25 mg) in healthy volunteers has observed significant improvements across pharmacokinetic parameters, including increased CBD plasma values, enhanced bioavailability, and fast absorption with no safety concerns being noted (Knaub et al., 2019). Ultimately, these oral formulations could provide symptomatic relief over prolonged periods (Lucas et al., 2018), making them suitable for continued administrations for the chronic symptomatic relief.
3.2 Nasal and Oral Mucosal Administrations
Alternative routes of administration can provide methods of circumventing variable absorption and extensive first pass metabolism. Both the oral mucosa and nasal cavity provide attractive targets for alternative routes of administration due to thin layering coupled with extensive vascularization. An assessment report conducted by the Australian Department of Health’s Therapeutic Goods Administration for nabiximols surmised that oromucosal formulations of nabiximols are rapidly absorbed, resulting in higher plasma concentrations of THC and CBD compared to oral formulations (Therapeutic Goods Administration, 2013). Though administration via the nasal mucosal membrane provides favorable absorption rates, current formulations are not as attractive given patient reluctance, formulation safety concerns, and nasal spray particle size (Therapeutic Goods Administration, 2013). It is likely that these issues associated with nasal administration has resulted in few recent developments regarding intranasal formulations (Bryson & Sharma, 2017; Bruni et al., 2018). Current oromucosal formulations of cannabinoids are therefore preferable in providing rapid, potentially therapeutic effects in a manner that is comfortable to patients and is more likely to be self-administered.
3.3 Pulmonary Administration
Among the possible routes of administration utilized for cannabinoids, pulmonary administration of cannabis is likely the most well-known route of administration among the general population. Like nasal and oral mucosa, pulmonary administration is highly effective given the high bioavailability, rapid onset, and avoidance of first-pass metabolism this route provides (Grotenhermen, 2003). However, critical issues associated with both intrapatient and interpatient variability arise given the inherent variability with pulmonary administration without the use of standardized methods. These include variations in inhalation depth, irritation or discomfort, technique and experience, and pharmacokinetic parameters such as maximum plasma concentration (Ohlsson et al., 1982; Hunault et al., 2010; Solowij et al., 2014; Lucas et al., 2018). Factors such as these could ultimately affect the efficacy of inhaled cannabis or cannabinoids and should be considered with concerns of dosing frequency and of side effects such as intoxication and cognitive function with psychoactive components like THC. Much interest surrounds the development of a standardized system or device that can deliver a metered dose of inhaled cannabinoid as a result. Comparatively, cannabinoid vaporization has grown in popularity due to ease of use and relative safety compared traditional combustion methods, such as with cannabis cigarettes (Gieringer et al., 2004). However, a standardized methodology has yet to be developed to account for sources of variability such as inhalation depth, though some have presented method proposals (Solowij et al., 2014; Lanz et al., 2016) and metered inhalation device patents (Davidson et al., 2018). A clinical study utilizing this metered inhaler observed that the product was able to administer consistent doses (15.1 ± 0.1 mg) of cannabis (19.9% THC, 0.1% CBD, 0.2% cannabinol) that provided effective neuropathic pain relief in patients (Eisenberg et al., 2014).
3.4 Topical Applications
Like mucosal and pulmonary administration, topical administration of cannabinoids provides an avoidance of first-pass metabolism, steady administration over time, and consistent dosing. However, due to the hydrophobicity of cannabinoids, diffusion across the skin is limited and such topical formulations require enhancements to permeation (Challapalli & Stinchcomb, 2002; Lodzki et al., 2003). Current applications for topical cannabinoids, though almost exclusively CBD, range from treating inflammatory dermatological disorders to localized pain relief among instances of arthritis and joint pain, though continued research suggests potential benefit in neuropathic pains (Baswan et al., 2020; D’Andre et al., 2021; Hammell et al., 2016; Xu et al., 2020). Similar to other aspects of cannabinoids as potential therapeutics, advances in formulation and optimization of administration drives further interest research into cannabinoids, though more work is needed.
3.5 Standardized Oral Administrations Over Non Standardized Pulmonary Administrations
Pharmacokinetic characteristics of cannabinoids is dependent on route of administration. While clinically approved cannabinoids such as dronabinol utilize oral administrations, general consumption of cannabis is primarily pulmonary with inhalation of cannabis smoke. However, unlike these oral administrations with a standardized formulation and administration method, pulmonary administrations of cannabis introduce numerous variables that could introduce interpatient and interpatient variability. These same factors can also directly affect the kinetics of the cannabinoid. While standardization and development of inhalation devices are being developed, current oral administrations, either through ingestion or through the oral mucosa, provide ease of use among patients and standardization to ensure consistency in dosing.
4 Discussion
The field of cannabinoid research continues to experience advancements as interest in therapeutic applications continuously grows, whether that be from urgent needs in replacement therapeutics or the development of a therapeutic the first of its kind. This review of current and past studies finds that preclinical research indicates therapeutic potential for cannabis, THC, and CBD mediated through either CB1R, CB2R, 5-HT1A, or a variable combination of these receptors. Clinical research utilizing cannabinoids within instances of neurodegenerative disease, pain, addiction, and anxiety suggest both tolerability and therapeutic potential either alone or in combination with current therapeutics. However, preclinical literature dominates, and additional clinical studies are required to clarify these therapeutic indications before definitive declarations can be made. Further advancement of cannabinoids to the clinical setting is dependent on these clinical trials. There still exists a wide gap between the purported and anecdotal medicinal cannabis uses and specific therapeutic indications irrefutably supported by strong scientific evidence. One possible explanation for this disparity may lie in the complex pharmacological nature of cannabis. Although this review focuses on THC and CBD, there are over 100 different compounds in cannabis including minor cannabinoids, cannabis terpenoids, and phytocannabinoids which have additional pharmacological and biological activity. Additional work in the field of medicinal cannabis to identify the exact composition of studied strains, including minor cannabinoid and terpenoid profiles and concentrations, which can vary dramatically between different cannabis strains, is sparse. This information is desperately needed within the field to study interactive effects between minor cannabinoids, terpenoids, as well as THC and CBD. Indeed, it may be that the interactive pharmacological profiles of minor cannabinoids and terpenoids may underlie at least some of the purported medicinal cannabis benefits that have so far been elusive to definitively confirm.
Author Contributions
Wrote or contributed to the writing of the manuscript: YTO, LRM and JLW.
Funding
This work was supported by the National Institute on Drug Abuse DA25267 and DA48353 (LRM). JLW received funding from the 2021 Research Grants Program of the Consortium for Medical Marijuana Clinical Outcomes Research, which is funded through State of Florida appropriations.
Conflict of Interest
The authors declare that the research was conducted in the absence of any commercial or financial relationships that could be construed as a potential conflict of interest.
Publisher’s Note
All claims expressed in this article are solely those of the authors and do not necessarily represent those of their affiliated organizations, or those of the publisher, the editors and the reviewers. Any product that may be evaluated in this article, or claim that may be made by its manufacturer, is not guaranteed or endorsed by the publisher.
Abbreviations
AD, alzheimer’s disease; ALS, amyotrophic lateral sclerosis; CBD, cannabidiol; CB1R, cannabinoid 1 receptor; CB2R, cannabinoid 2 receptor; CNS, central nervous system; GAD, generalized anxiety disorder; HD, Huntington’s disease; MS, multiple sclerosis; OCD, obsessive-compulsive disorder; PD, Parkinson’s disease; PTSD, post-traumatic stress disorder; 5-HT1A, serotonin 1a; SEDDS, Self-emulsifying drug delivery system; SSRIs, selective serotonin reuptake inhibitors; SAD, social anxiety disorder; THC, Δ9-tetrahydrocannabinol.
References
Abraham, A. D., Leung, E. J. Y., Wong, B. A., Rivera, Z. M. G., Kruse, L. C., Clark, J. J., et al. (2020). Orally Consumed Cannabinoids Provide Long-Lasting Relief of Allodynia in a Mouse Model of Chronic Neuropathic Pain. Neuropsychopharmacology 45 (7), 1105–1114. doi:10.1038/s41386-019-0585-3
Agudelo, M., Yndart, A., Morrison, M., Figueroa, G., Muñoz, K., Samikkannu, T., et al. (2013). Differential Expression and Functional Role of Cannabinoid Genes in Alcohol Users. Drug Alcohol Depend 133 (2), 789–793. doi:10.1016/j.drugalcdep.2013.08.023
Agurell, S., Carlsson, S., Lindgren, J. E., Ohlsson, A., Gillespie, H., and Hollister, L. (1981). Interactions of delta 1-tetrahydrocannabinol with Cannabinol and Cannabidiol Following Oral Administration in Man. Assay of Cannabinol and Cannabidiol by Mass Fragmentography. Experientia 37 (10), 1090–1092. doi:10.1007/BF02085029
Ahmad, R., Goffin, K., Van den Stock, J., De Winter, F. L., Cleeren, E., Bormans, G., et al. (2014). In Vivo type 1 Cannabinoid Receptor Availability in Alzheimer's Disease. Eur. Neuropsychopharmacol. 24 (2), 242–250. doi:10.1016/j.euroneuro.2013.10.002
Allen, T. M., and Cullis, P. R. (2013). Liposomal Drug Delivery Systems: From Concept to Clinical Applications. Adv. Drug Deliv. Rev. 65 (1), 36–48. doi:10.1016/j.addr.2012.09.037
Amtmann, D., Weydt, P., Johnson, K. L., Jensen, M. P., and Carter, G. T. (2004). Survey of Cannabis Use in Patients with Amyotrophic Lateral Sclerosis. Am. J. Hosp. Palliat. Care 21 (2), 95–104. doi:10.1177/104990910402100206
Arnone, M., Maruani, J., Chaperon, F., Thiébot, M. H., Poncelet, M., Soubrié, P., et al. (1997). Selective Inhibition of Sucrose and Ethanol Intake by SR 141716, an Antagonist of central Cannabinoid (CB1) Receptors. Psychopharmacology (Berl) 132 (1), 104–106. doi:10.1007/s002130050326
Aschenbrenner, A. J., Gordon, B. A., Benzinger, T. L. S., Morris, J. C., and Hassenstab, J. J. (2018). Influence of Tau PET, Amyloid PET, and Hippocampal Volume on Cognition in Alzheimer Disease. Neurology 91 (9), e859. doi:10.1212/WNL.0000000000006075
Attal, N., Brasseur, L., Guirimand, D., Clermond-Gnamien, S., Atlami, S., and Bouhassira, D. (2004). Are Oral Cannabinoids Safe and Effective in Refractory Neuropathic Pain? Eur. J. Pain 8 (2), 173–177. doi:10.1016/S1090-3801(03)00084-3
Babalonis, S., Lofwall, M. R., Sloan, P. A., Nuzzo, P. A., Fanucchi, L. C., and Walsh, S. L. (2019). Cannabinoid Modulation of Opioid Analgesia and Subjective Drug Effects in Healthy Humans. Psychopharmacology (Berl) 236 (11), 3341–3352. doi:10.1007/s00213-019-05293-1
Balerio, G. N., Aso, E., Berrendero, F., Murtra, P., and Maldonado, R. (2004). Delta9-tetrahydrocannabinol Decreases Somatic and Motivational Manifestations of Nicotine Withdrawal in Mice. Eur. J. Neurosci. 20 (10), 2737–2748. doi:10.1111/j.1460-9568.2004.03714.x
Bardin, L., Tarayre, J. P., Malfetes, N., Koek, W., and Colpaert, F. C. (2003). Profound, Non-opioid Analgesia Produced by the High-Efficacy 5-HT(1A) Agonist F 13640 in the Formalin Model of Tonic Nociceptive Pain. Pharmacology 67 (4), 182–194. doi:10.1159/000068404
Bare, D. J., McKinzie, J. H., and McBride, W. J. (1998). Development of Rapid Tolerance to Ethanol-Stimulated Serotonin Release in the Ventral Hippocampus. Alcohol. Clin. Exp. Res. 22 (6), 1272–1276. doi:10.1111/j.1530-0277.1998.tb03908.x
Barnes, N. M., and Sharp, T. (1999). A Review of central 5-HT Receptors and Their Function. Neuropharmacology 38 (8), 1083–1152. doi:10.1016/S0028-3908(99)00010-6
Basavarajappa, B. S., Shivakumar, M., Joshi, V., and Subbanna, S. (2017). Endocannabinoid System in Neurodegenerative Disorders. J. Neurochem. 142 (5), 624–648. doi:10.1111/jnc.14098
Baswan, S. M., Klosner, A. E., Glynn, K., Rajgopal, A., Malik, K., Yim, S., et al. (2020). Therapeutic Potential of Cannabidiol (CBD) for Skin Health and Disorders. Ccid 13, 927–942. doi:10.2147/CCID.S286411
Batra, G., Jain, M., Singh, R. S., Sharma, A. R., Singh, A., Prakash, A., et al. (2019). Novel Therapeutic Targets for Amyotrophic Lateral Sclerosis. Indian J. Pharmacol. 51 (6), 418–425. doi:10.4103/ijp.IJP_823_19
Bebee, B., Taylor, D. M., Bourke, E., Pollack, K., Foster, L., Ching, M., et al. (2021). The CANBACK Trial: a Randomised, Controlled Clinical Trial of Oral Cannabidiol for People Presenting to the Emergency Department with Acute Low Back Pain. Med. J. Aust. 214 (8), 370–375. doi:10.5694/mja2.51014
Beltramo, M., Bernardini, N., Bertorelli, R., Campanella, M., Nicolussi, E., Fredduzzi, S., et al. (2006). CB2 Receptor-Mediated Antihyperalgesia: Possible Direct Involvement of Neural Mechanisms. Eur. J. Neurosci. 23 (6), 1530–1538. doi:10.1111/j.1460-9568.2006.04684.x
Benito, C., Núñez, E., Tolón, R. M., Carrier, E. J., Rábano, A., Hillard, C. J., et al. (2003). Cannabinoid CB2 Receptors and Fatty Acid Amide Hydrolase Are Selectively Overexpressed in Neuritic Plaque-Associated Glia in Alzheimer's Disease Brains. J. Neurosci. 23 (35), 11136–11141. doi:10.1523/JNEUROSCI.23-35-11136.2003
Benito, C., Romero, J. P., Tolón, R. M., Clemente, D., Docagne, F., Hillard, C. J., et al. (2007). Cannabinoid CB1 and CB2 Receptors and Fatty Acid Amide Hydrolase Are Specific Markers of Plaque Cell Subtypes in Human Multiple Sclerosis. J. Neurosci. 27 (9), 2396–2402. doi:10.1523/JNEUROSCI.4814-06.2007
Bergamaschi, M. M., Queiroz, R. H., Chagas, M. H., de Oliveira, D. C., De Martinis, B. S., Kapczinski, F., et al. (2011). Cannabidiol Reduces the Anxiety Induced by Simulated Public Speaking in Treatment-Naïve Social Phobia Patients. Neuropsychopharmacology 36 (6), 1219–1226. doi:10.1038/npp.2011.6
Berrendero, F., Sánchez, A., Cabranes, A., Puerta, C., Ramos, J. A., García-Merino, A., et al. (2001). Changes in Cannabinoid CB(1) Receptors in Striatal and Cortical Regions of Rats with Experimental Allergic Encephalomyelitis, an Animal Model of Multiple Sclerosis. Synapse 41 (3), 195–202. doi:10.1002/syn.1075
Bhargava, H. N. (1976). Inhibition of Naloxone-Induced Withdrawal in Morphine Dependent Mice by 1-trans-delta9-tetrahydrocannabinol. Eur. J. Pharmacol. 36 (1), 259–262. doi:10.1016/0014-2999(76)90283-1
Biegon, A., and Kerman, I. A. (2001). Autoradiographic Study of Pre- and Postnatal Distribution of Cannabinoid Receptors in Human Brain. NeuroImage 14 (6), 1463–1468. doi:10.1006/nimg.2001.0939
Bilsland, L. G., Dick, J. R., Pryce, G., Petrosino, S., Di Marzo, V., Baker, D., et al. (2006). Increasing Cannabinoid Levels by Pharmacological and Genetic Manipulation Delay Disease Progression in SOD1 Mice. FASEB J. 20 (7), 1003–1005. doi:10.1096/fj.05-4743fje
Björk, L., Fredriksson, A., Hacksell, U., and Lewander, T. (1992). Effects of (R)-8-OH-DPAT and the Enantiomers of UH-301 on Motor Activities in the Rat: Antagonism of (R)-8-OH-DPAT-induced Effects. Eur. Neuropsychopharmacol. 2 (2), 141–147. doi:10.1016/0924-977X(92)90024-3
Blauwendraat, C., Nalls, M. A., and Singleton, A. B. (2020). The Genetic Architecture of Parkinson's Disease. Lancet Neurol. 19 (2), 170–178. doi:10.1016/S1474-4422(19)30287-X
Blessing, E. M., Steenkamp, M. M., Manzanares, J., and Marmar, C. R. (2015). Cannabidiol as a Potential Treatment for Anxiety Disorders. Neurotherapeutics 12 (4), 825–836. doi:10.1007/s13311-015-0387-1
Bloomfield, M. A., Ashok, A. H., Volkow, N. D., and Howes, O. D. (2016). The Effects of Δ9-tetrahydrocannabinol on the Dopamine System. Nature 539 (7629), 369–377. doi:10.1038/nature20153
Bonn-Miller, M. O., Sisley, S., Riggs, P., Yazar-Klosinski, B., Wang, J. B., Loflin, M. J. E., et al. (2021). The Short-Term Impact of 3 Smoked Cannabis Preparations versus Placebo on PTSD Symptoms: A Randomized Cross-Over Clinical Trial. PLoS One 16 (3), e0246990. doi:10.1371/journal.pone.0246990
Bossong, M. G., Mehta, M. A., van Berckel, B. N., Howes, O. D., Kahn, R. S., and Stokes, P. R. (2015). Further Human Evidence for Striatal Dopamine Release Induced by Administration of ∆9-tetrahydrocannabinol (THC): Selectivity to Limbic Striatum. Psychopharmacology (Berl) 232 (15), 2723–2729. doi:10.1007/s00213-015-3915-0
Bouaboula, M., Rinaldi, M., Carayon, P., Carillon, C., Delpech, B., Shire, D., et al. (1993). Cannabinoid-receptor Expression in Human Leukocytes. Eur. J. Biochem. 214 (1), 173–180. doi:10.1111/j.1432-1033.1993.tb17910.x
Bowen, D. M., Allen, S. J., Benton, J. S., Goodhardt, M. J., Haan, E. A., Palmer, A. M., et al. (1983). Biochemical Assessment of Serotonergic and Cholinergic Dysfunction and Cerebral Atrophy in Alzheimer's Disease. J. Neurochem. 41 (1), 266–272. doi:10.1111/j.1471-4159.1983.tb11838.x
Bruni, N., Della Pepa, C., Oliaro-Bosso, S., Pessione, E., Gastaldi, D., and Dosio, F. (2018). Cannabinoid Delivery Systems for Pain and Inflammation Treatment. Molecules 23 (10), 2478. doi:10.3390/molecules23102478
Bryson, N., and Sharma, A. C. (2017). Nasal Cannabidiol Compositions (World Intellectual Property Organization Patent No. WO2017208072A2). Availableat: https://patents.google.com/patent/WO2017208072A2/en.
Calpe-López, C., García-Pardo, M. P., and Aguilar, M. A. (2019). Cannabidiol Treatment Might Promote Resilience to Cocaine and Methamphetamine Use Disorders: A Review of Possible Mechanisms. Molecules 24 (14), 2583. doi:10.3390/molecules24142583
Campos, A. C., de Paula Soares, V., Carvalho, M. C., Ferreira, F. R., Vicente, M. A., Brandão, M. L., et al. (2013a). Involvement of Serotonin-Mediated Neurotransmission in the Dorsal Periaqueductal gray Matter on Cannabidiol Chronic Effects in Panic-like Responses in Rats. Psychopharmacology (Berl) 226 (1), 13–24. doi:10.1007/s00213-012-2878-7
Campos, A. C., Ferreira, F. R., and Guimarães, F. S. (2012). Cannabidiol Blocks Long-Lasting Behavioral Consequences of Predator Threat Stress: Possible Involvement of 5HT1A Receptors. J. Psychiatr. Res. 46 (11), 1501–1510. doi:10.1016/j.jpsychires.2012.08.012
Campos, A. C., and Guimarães, F. S. (2008). Involvement of 5HT1A Receptors in the Anxiolytic-like Effects of Cannabidiol Injected into the Dorsolateral Periaqueductal gray of Rats. Psychopharmacology (Berl) 199 (2), 223–230. doi:10.1007/s00213-008-1168-x
Campos, A. C., Ortega, Z., Palazuelos, J., Fogaça, M. V., Aguiar, D. C., Díaz-Alonso, J., et al. (2013b). The Anxiolytic Effect of Cannabidiol on Chronically Stressed Mice Depends on Hippocampal Neurogenesis: Involvement of the Endocannabinoid System. Int. J. Neuropsychopharmacol. 16 (6), 1407–1419. doi:10.1017/S1461145712001502
Capano, A., Weaver, R., and Burkman, E. (2020). Evaluation of the Effects of CBD Hemp Extract on Opioid Use and Quality of Life Indicators in Chronic Pain Patients: A Prospective Cohort Study. Postgrad. Med. 132 (1), 56–61. doi:10.1080/00325481.2019.1685298
Carayon, P., Marchand, J., Dussossoy, D., Derocq, J. M., Jbilo, O., Bord, A., et al. (1998). Modulation and Functional Involvement of CB2 Peripheral Cannabinoid Receptors during B-Cell Differentiation. Blood 92 (10), 3605–3615. doi:10.1182/blood.V92.10.3605
Carroll, C. B., Zeissler, M.-L., Hanemann, C. O., and Zajicek, J. P. (2012). Δ9-tetrahydrocannabinol (Δ9-THC) Exerts a Direct Neuroprotective Effect in a Human Cell Culture Model of Parkinson's Disease. Neuropathol. Appl. Neurobiol. 38 (6), 535–547. doi:10.1111/j.1365-2990.2011.01248.x
Casey, S. L., Atwal, N., and Vaughan, C. W. (2017). Cannabis Constituent Synergy in a Mouse Neuropathic Pain Model. Pain 158 (12), 2452–2460. doi:10.1097/j.pain.0000000000001051
Chagas, M. H., Zuardi, A. W., Tumas, V., Pena-Pereira, M. A., Sobreira, E. T., Bergamaschi, M. M., et al. (2014). Effects of Cannabidiol in the Treatment of Patients with Parkinson's Disease: an Exploratory Double-Blind Trial. J. Psychopharmacol. 28 (11), 1088–1098. doi:10.1177/0269881114550355
Challapalli, P. V., and Stinchcomb, A. L. (2002). In Vitro experiment Optimization for Measuring Tetrahydrocannabinol Skin Permeation. Int. J. Pharm. 241 (2), 329–339. doi:10.1016/s0378-5173(02)00262-4
Clement, A. B., Hawkins, E. G., Lichtman, A. H., and Cravatt, B. F. (2003). Increased Seizure Susceptibility and Proconvulsant Activity of Anandamide in Mice Lacking Fatty Acid Amide Hydrolase. J. Neurosci. 23 (9), 3916–3923. doi:10.1523/jneurosci.23-09-03916.2003
Cochrane-Snyman, K. C., Cruz, C., Morales, J., and Coles, M. (2021). The Effects of Cannabidiol Oil on Noninvasive Measures of Muscle Damage in Men. Med. Sci. Sports Exerc. 53 (7), 1460–1472. doi:10.1249/MSS.0000000000002606
Cohen, C., Perrault, G., Griebel, G., and Soubrié, P. (2005). Nicotine-associated Cues Maintain Nicotine-Seeking Behavior in Rats Several Weeks after Nicotine Withdrawal: Reversal by the Cannabinoid (CB1) Receptor Antagonist, Rimonabant (SR141716). Neuropsychopharmacology 30 (1), 145–155. doi:10.1038/sj.npp.1300541
Collin, C., Davies, P., Mutiboko, I. K., and Ratcliffe, S.Sativex Spasticity in MS Study Group (2007). Randomized Controlled Trial of Cannabis-Based Medicine in Spasticity Caused by Multiple Sclerosis. Eur. J. Neurol. 14 (3), 290–296. doi:10.1111/j.1468-1331.2006.01639.x
Colwill, A. C., Alton, K., Bednarek, P. H., Bayer, L. L., Jensen, J. T., Garg, B., et al. (2020). Cannabinoids for Pain Control During Medical Abortion: A Randomized Controlled Trial. Obstet Gynecol 135, 1289–1295.
Consroe, P., Laguna, J., Allender, J., Snider, S., Stern, L., Sandyk, R., et al. (1991). Controlled Clinical Trial of Cannabidiol in Huntington's Disease. Pharmacol. Biochem. Behav. 40 (3), 701–708. doi:10.1016/0091-3057(91)90386-g
Constantinescu, C. S., Farooqi, N., O'Brien, K., and Gran, B. (2011). Experimental Autoimmune Encephalomyelitis (EAE) as a Model for Multiple Sclerosis (MS). Br. J. Pharmacol. 164 (4), 1079–1106. doi:10.1111/j.1476-5381.2011.01302.x
Cooper, Z. D., Bedi, G., Ramesh, D., Balter, R., Comer, S. D., and Haney, M. (2018). Impact of Co-administration of Oxycodone and Smoked Cannabis on Analgesia and Abuse Liability. Neuropsychopharmacology 43 (10), 2046–2055. doi:10.1038/s41386-018-0011-2
Costa, B., Trovato, A. E., Comelli, F., Giagnoni, G., and Colleoni, M. (2007). The Non-psychoactive Cannabis Constituent Cannabidiol Is an Orally Effective Therapeutic Agent in Rat Chronic Inflammatory and Neuropathic Pain. Eur. J. Pharmacol. 556 (1–3), 75–83. doi:10.1016/j.ejphar.2006.11.006
Crean, R. D., Crane, N. A., and Mason, B. J. (2011). An Evidence Based Review of Acute and Long-Term Effects of Cannabis Use on Executive Cognitive Functions. J. Addict. Med. 5 (1), 1–8. doi:10.1097/ADM.0b013e31820c23fa
Cross, A. J., Crow, T. J., Ferrier, I. N., Johnson, J. A., Bloom, S. R., and Corsellis, J. A. (1984). Serotonin Receptor Changes in Dementia of the Alzheimer Type. J. Neurochem. 43 (6), 1574–1581. doi:10.1111/j.1471-4159.1984.tb06081.x
Curtis, A., Mitchell, I., Patel, S., Ives, N., and Rickards, H. (2009). A Pilot Study Using Nabilone for Symptomatic Treatment in Huntington's Disease. Mov Disord. 24 (15), 2254–2259. doi:10.1002/mds.22809
Curtis, A., and Rickards, H. (2006). Nabilone Could Treat Chorea and Irritability in Huntington's Disease. J. Neuropsychiatry Clin. Neurosci. 18 (4), 553–554. doi:10.1176/jnp.2006.18.4.553
D'Andre, S., McAllister, S., Nagi, J., Giridhar, K. V., Ruiz-Macias, E., and Loprinzi, C. (2021). Topical Cannabinoids for Treating Chemotherapy-Induced Neuropathy: A Case Series. Integr. Cancer Ther. 20, 15347354211061739. doi:10.1177/15347354211061739
Davidson, P., Almog, S., and Kindler, S. (2018). Methods, Devices and Systems for Pulmonary Delivery of Active Agents (United States Patent No. US10118006B2). Availableat: https://patents.google.com/patent/US10118006B2/en.
Davie, C. A. (2008). A Review of Parkinson's Disease. Br. Med. Bull. 86, 109–127. doi:10.1093/bmb/ldn013
de Faria, S. M., de Morais Fabrício, D., Tumas, V., Castro, P. C., Ponti, M. A., Hallak, J. E., et al. (2020). Effects of Acute Cannabidiol Administration on Anxiety and Tremors Induced by a Simulated Public Speaking Test in Patients with Parkinson's Disease. J. Psychopharmacol. 34 (2), 189–196. doi:10.1177/0269881119895536
De Gregorio, D., McLaughlin, R. J., Posa, L., Ochoa-Sanchez, R., Enns, J., Lopez-Canul, M., et al. (2019). Cannabidiol Modulates Serotonergic Transmission and Reverses Both Allodynia and Anxiety-like Behavior in a Model of Neuropathic Pain. Pain 160 (1), 136–150. doi:10.1097/j.pain.0000000000001386
De Vries, T. J., Homberg, J. R., Binnekade, R., Raasø, H., and Schoffelmeer, A. N. M. (2003). Cannabinoid Modulation of the Reinforcing and Motivational Properties of Heroin and Heroin-Associated Cues in Rats. Psychopharmacology (Berl) 168 (1–2), 164–169. doi:10.1007/s00213-003-1422-1
Dengler, E. C., Liu, J., Kerwin, A., Torres, S., Olcott, C. M., Bowman, B. N., et al. (2013). Mesoporous Silica-Supported Lipid Bilayers (Protocells) for DNA Cargo Delivery to the Spinal Cord. J. Control. Release 168 (2), 209–224. doi:10.1016/j.jconrel.2013.03.009
Denovan-Wright, E. M., and Robertson, H. A. (2000). Cannabinoid Receptor Messenger RNA Levels Decrease in a Subset of Neurons of the Lateral Striatum, Cortex and hippocampus of Transgenic Huntington's Disease Mice. Neuroscience 98 (4), 705–713. doi:10.1016/S0306-4522(00)00157-3
Dentel, C., Palamiuc, L., Henriques, A., Lannes, B., Spreux-Varoquaux, O., Gutknecht, L., et al. (2013). Degeneration of Serotonergic Neurons in Amyotrophic Lateral Sclerosis: A Link to Spasticity. Brain 136 (Pt 2), 483–493. doi:10.1093/brain/aws274
Devinsky, O., Marsh, E., Friedman, D., Thiele, E., Laux, L., Sullivan, J., et al. (2016). Cannabidiol in Patients with Treatment-Resistant Epilepsy: An Open-Label Interventional Trial. Lancet Neurol. 15 (3), 270–278. doi:10.1016/S1474-4422(15)00379-8
Devinsky, O., Nabbout, R., Miller, I., Laux, L., Zolnowska, M., Wright, S., et al. (2019). Long-term Cannabidiol Treatment in Patients with Dravet Syndrome: An Open-Label Extension Trial. Epilepsia 60 (2), 294–302. doi:10.1111/epi.14628
Devinsky, O., Patel, A. D., Thiele, E. A., Wong, M. H., Appleton, R., Harden, C. L., et al. GWPCARE1 Part A Study Group (2018). Randomized, Dose-Ranging Safety Trial of Cannabidiol in Dravet Syndrome. Neurology 90 (14), e1204–e1211. doi:10.1212/WNL.0000000000005254
Doder, M., Rabiner, E. A., Turjanski, N., Lees, A. J., and Brooks, D. J. (2003). Tremor in Parkinson's Disease and Serotonergic Dysfunction: an 11C-WAY 100635 PET Study. Neurology 60 (4), 601–605. doi:10.1212/01.WNL.0000031424.51127.2B
Donvito, G., Nass, S. R., Wilkerson, J. L., Curry, Z. A., Schurman, L. D., Kinsey, S. G., et al. (2018). The Endogenous Cannabinoid System: A Budding Source of Targets for Treating Inflammatory and Neuropathic Pain. Neuropsychopharmacology 43 (1), 52–79. doi:10.1038/npp.2017.204
Dorsey, E. R., Sherer, T., Okun, M. S., and Bloem, B. R. (2018). The Emerging Evidence of the Parkinson Pandemic. J. Parkinsons Dis. 8 (Suppl. 1), S3. doi:10.3233/JPD-181474
Dowie, M. J., Bradshaw, H. B., Howard, M. L., Nicholson, L. F., Faull, R. L., Hannan, A. J., et al. (2009). Altered CB1 Receptor and Endocannabinoid Levels Precede Motor Symptom Onset in a Transgenic Mouse Model of Huntington's Disease. Neuroscience 163 (1), 456–465. doi:10.1016/j.neuroscience.2009.06.014
Dunn, K. E., Bergeria, C. L., Huhn, A. S., Speed, T. J., Mun, C. J., Vandrey, R., et al. (2021). Within-subject, Double-Blinded, Randomized, and Placebo-Controlled Evaluation of the Combined Effects of the Cannabinoid Dronabinol and the Opioid Hydromorphone in a Human Laboratory Pain Model. Neuropsychopharmacology 46 (8), 1451–1459. doi:10.1038/s41386-021-01007-4
Eisenberg, E., Ogintz, M., and Almog, S. (2014). The Pharmacokinetics, Efficacy, Safety, and Ease of Use of a Novel Portable Metered-Dose Cannabis Inhaler in Patients with Chronic Neuropathic Pain: A Phase 1a Study. J. Pain Palliat. Care Pharmacother. 28 (3), 216–225. doi:10.3109/15360288.2014.941130
el Mestikawy, S., Fargin, A., Raymond, J. R., Gozlan, H., and Hnatowich, M. (1991). The 5-HT1A Receptor: An Overview of Recent Advances. Neurochem. Res. 16 (1), 1–10. doi:10.1007/BF00965820
Ellgren, M., Spano, S. M., and Hurd, Y. L. (2007). Adolescent Cannabis Exposure Alters Opiate Intake and Opioid Limbic Neuronal Populations in Adult Rats. Neuropsychopharmacology 32 (3), 607–615. doi:10.1038/sj.npp.1301127
Elms, L., Shannon, S., Hughes, S., and Lewis, N. (2019). Cannabidiol in the Treatment of Post-Traumatic Stress Disorder: A Case Series. J. Altern. Complement. Med. 25 (4), 392–397. doi:10.1089/acm.2018.0437
Erkkinen, M. G., Kim, M. O., and Geschwind, M. D. (2018). Clinical Neurology and Epidemiology of the Major Neurodegenerative Diseases. Cold Spring Harb Perspect. Biol. 10 (4). doi:10.1101/cshperspect.a033118
Eskow, K. L., Gupta, V., Alam, S., Park, J. Y., and Bishop, C. (2007). The Partial 5-HT(1A) Agonist Buspirone Reduces the Expression and Development of L-DOPA-Induced Dyskinesia in Rats and Improves L-DOPA Efficacy. Pharmacol. Biochem. Behav. 87 (3), 306–314. doi:10.1016/j.pbb.2007.05.002
Fadda, P., Scherma, M., Fresu, A., Collu, M., and Fratta, W. (2005). Dopamine and Serotonin Release in Dorsal Striatum and Nucleus Accumbens Is Differentially Modulated by Morphine in DBA/2J and C57BL/6J Mice. Synapse 56 (1), 29–38. doi:10.1002/syn.20122
Falco-Walter, J. (2020). Epilepsy-Definition, Classification, Pathophysiology, and Epidemiology. Semin. Neurol. 40 (6), 617–623. doi:10.1055/s-0040-1718719
Fallon, M. T., Albert Lux, E., McQuade, R., Rossetti, S., Sanchez, R., Sun, W., et al. (2017). Sativex Oromucosal spray as Adjunctive Therapy in Advanced Cancer Patients with Chronic Pain Unalleviated by Optimized Opioid Therapy: Two Double-Blind, Randomized, Placebo-Controlled Phase 3 Studies. Br. J. Pain 11 (3), 119–133. doi:10.1177/2049463717710042
FemenÃa, T., GarcÃa-Gutiérrez, M. a. S., and Manzanares, J. (2010). CB1 Receptor Blockade Decreases Ethanol Intake and Associated Neurochemical Changes in Fawn-Hooded Rats. Alcohol. Clin. Exp. Res. 34 (1), 131–141. doi:10.1111/j.1530-0277.2009.01074.x
Fernández-Ruiz, J., Romero, J., Velasco, G., Tolón, R. M., Ramos, J. A., and Guzmán, M. (2007). Cannabinoid CB2 Receptor: A New Target for Controlling Neural Cell Survival? Trends Pharmacol. Sci. 28 (1), 39–45. doi:10.1016/j.tips.2006.11.001
Franke, T., Irwin, C., Beindorff, N., Bouter, Y., and Bouter, C. (2019). Effects of Tetrahydrocannabinol Treatment on Brain Metabolism and Neuron Loss in a Mouse Model of Sporadic Alzheimer's Disease. Nuklearmedizin - NuclearMedicine 58 (2), P94. doi:10.1055/s-0039-1683689
Galaj, E., Bi, G. H., Yang, H. J., and Xi, Z. X. (2020). Cannabidiol Attenuates the Rewarding Effects of Cocaine in Rats by CB2, 5-HT1A and TRPV1 Receptor Mechanisms. Neuropharmacology 167, 107740. doi:10.1016/j.neuropharm.2019.107740
Galiègue, S., Mary, S., Marchand, J., Dussossoy, D., Carrière, D., Carayon, P., et al. (1995). Expression of Central and Peripheral Cannabinoid Receptors in Human Immune Tissues and Leukocyte Subpopulations. Eur. J. Biochem. 232 (1), 54–61. doi:10.1111/j.1432-1033.1995.tb20780.x
Gallate, J. E., Mallet, P. E., and McGregor, I. S. (2004). Combined Low Dose Treatment with Opioid and Cannabinoid Receptor Antagonists Synergistically Reduces the Motivation to Consume Alcohol in Rats. Psychopharmacology (Berl) 173 (1), 210–216. doi:10.1007/s00213-003-1694-5
Garakani, A., Murrough, J. W., Freire, R. C., Thom, R. P., Larkin, K., Buono, F. D., et al. (2020). Pharmacotherapy of Anxiety Disorders: Current and Emerging Treatment Options. Front. Psychiatry 11, 595584. doi:10.3389/fpsyt.2020.595584
García-Arencibia, M., García, C., Kurz, A., Rodríguez-Navarro, J. A., Gispert-Sánchez, S., Mena, M. A., et al. (2009). “Cannabinoid CB1 Receptors Are Early DownRegulated Followed by a Further UpRegulation in the Basal Ganglia of Mice with Deletion of Specific Park Genes,” in Birth, Life and Death of Dopaminergic Neurons in the Substantia Nigra. Editors G. Giovanni, V. Di Matteo, and E. Esposito (Springer), 269–275. doi:10.1007/978-3-211-92660-4_22
García-Gutiérrez, M. S., Navarrete, F., Gasparyan, A., Austrich-Olivares, A., Sala, F., and Manzanares, J. (2020). Cannabidiol: A Potential New Alternative for the Treatment of Anxiety, Depression, and Psychotic Disorders. Biomolecules 10 (11), 1575. doi:10.3390/biom10111575
García-Gutiérrez, M. S., and Manzanares, J. (2011). Overexpression of CB2 Cannabinoid Receptors Decreased Vulnerability to Anxiety and Impaired Anxiolytic Action of Alprazolam in Mice. J. Psychopharmacol. 25 (1), 111–120. doi:10.1177/0269881110379507
Gaston, T. E., Szaflarski, M., Hansen, B., Bebin, E. M., and Szaflarski, J. P.UAB CBD Program (2019). Quality of Life in Adults Enrolled in an Open-Label Study of Cannabidiol (CBD) for Treatment-Resistant Epilepsy. Epilepsy Behav. 95, 10–17. doi:10.1016/j.yebeh.2019.03.035
GBD 2016 Epilepsy Collaborators (2019). Global, Regional, and National burden of Epilepsy, 1990-2016: a Systematic Analysis for the Global Burden of Disease Study 2016. Lancet Neurol. 18 (4), 357–375. doi:10.1016/S1474-4422(18)30454-X
Giacobbe, P., and Flint, A. (2018). Diagnosis and Management of Anxiety Disorders. Continuum (Minneap Minn) 24 (3), 893–919. doi:10.1212/CON.0000000000000607
Giacoppo, S., Pollastro, F., Grassi, G., Bramanti, P., and Mazzon, E. (2017). Target Regulation of PI3K/Akt/mTOR Pathway by Cannabidiol in Treatment of Experimental Multiple Sclerosis. Fitoterapia 116, 77–84. doi:10.1016/j.fitote.2016.11.010
Gieringer, D., St. Laurent, J., and Goodrich, S. (2004). Cannabis Vaporizer Combines Efficient Delivery of THC with Effective Suppression of Pyrolytic Compounds. J. Cannabis Ther. 4 (1), 7–27. doi:10.1300/J175v04n01_02
Gjerstad, J., Tjolsen, A., and Hole, K. (1996). The Effect of 5-HT1A Receptor Stimulation on Nociceptive Dorsal Horn Neurones in Rats. Eur. J. Pharmacol. 318 (2–3), 315–321. doi:10.1016/s0014-2999(96)00819-9
Glass, M., Dragunow, M., and Faull, R. L. (1997). Cannabinoid Receptors in the Human Brain: A Detailed Anatomical and Quantitative Autoradiographic Study in the Fetal, Neonatal and Adult Human Brain. Neuroscience 77 (2), 299–318. doi:10.1016/S0306-4522(96)00428-9
Glass, M., Faull, R. L., and Dragunow, M. (1993). Loss of Cannabinoid Receptors in the Substantia Nigra in Huntington's Disease. Neuroscience 56 (3), 523–527. doi:10.1016/0306-4522(93)90352-g
Gomes, F. V., Resstel, L. B., and Guimarães, F. S. (2011). The Anxiolytic-like Effects of Cannabidiol Injected into the Bed Nucleus of the Stria Terminalis Are Mediated by 5-HT1A Receptors. Psychopharmacology (Berl) 213 (2–3), 465–473. doi:10.1007/s00213-010-2036-z
Graeff, F. G., Netto, C. F., and Zangrossi, H. (1998). The Elevated T-Maze as an Experimental Model of Anxiety. Neurosci. Biobehav Rev. 23 (2), 237–246. doi:10.1016/S0149-7634(98)00024-4
Granados-Soto, V., Argüelles, C. F., Rocha-González, H. I., Godínez-Chaparro, B., Flores-Murrieta, F. J., and Villalón, C. M. (2010). The Role of Peripheral 5-HT1A, 5-HT1B, 5-HT1D, 5-HT1E and 5-HT1F Serotonergic Receptors in the Reduction of Nociception in Rats. Neuroscience 165 (2), 561–568. doi:10.1016/j.neuroscience.2009.10.020
Greer, G. R., Grob, C. S., and Halberstadt, A. L. (2014). PTSD Symptom Reports of Patients Evaluated for the New Mexico Medical Cannabis Program. J. Psychoactive Drugs 46 (1), 73–77. doi:10.1080/02791072.2013.873843
Grim, T. W., Morales, A. J., Gonek, M. M., Wiley, J. L., Thomas, B. F., Endres, G. W., et al. (2016). Stratification of Cannabinoid 1 Receptor (CB1R) Agonist Efficacy: Manipulation of CB1R Density through Use of Transgenic Mice Reveals Congruence between In Vivo and In Vitro Assays. J. Pharmacol. Exp. Ther. 359 (2), 329–339. doi:10.1124/jpet.116.233163
Grotenhermen, F. (2003). Pharmacokinetics and Pharmacodynamics of Cannabinoids. Clin. Pharmacokinet. 42 (4), 327–360. doi:10.2165/00003088-200342040-00003
Guimarães, F. S., Chiaretti, T. M., Graeff, F. G., and Zuardi, A. W. (1990). Antianxiety Effect of Cannabidiol in the Elevated Plus-Maze. Psychopharmacology (Berl) 100 (4), 558–559. doi:10.1007/BF02244012
Haleem, D. J., and Nawaz, S. (2017). Inhibition of Reinforcing, Hyperalgesic, and Motor Effects of Morphine by Buspirone in Rats. J. Pain 18 (1), 19–28. doi:10.1016/j.jpain.2016.10.001
Hammell, D. C., Zhang, L. P., Ma, F., Abshire, S. M., McIlwrath, S. L., Stinchcomb, A. L., et al. (2016). Transdermal Cannabidiol Reduces Inflammation and Pain-Related Behaviours in a Rat Model of Arthritis. Eur. J. Pain 20 (6), 936–948. doi:10.1002/ejp.818
Haroutounian, S., Ratz, Y., Ginosar, Y., Furmanov, K., Saifi, F., Meidan, R., et al. (2016). The Effect of Medicinal Cannabis on Pain and Quality-Of-Life Outcomes in Chronic Pain: A Prospective Open-Label Study. Clin. J. Pain 32 (12), 1036–1043. doi:10.1097/AJP.0000000000000364
Harris, H. M., Sufka, K. J., Gul, W., and ElSohly, M. A. (2016). Effects of Delta-9-Tetrahydrocannabinol and Cannabidiol on Cisplatin-Induced Neuropathy in Mice. Planta Med. 82 (13), 1169–1172. doi:10.1055/s-0042-106303
He, Y., Suofu, Y., Yablonska, S., Wang, X., Larkin, T. M., Kim, J., et al. (2019). Increased Serotonin Transporter Expression in Huntington's Disease Patients Is Not Consistently Replicated in Murine Models. J. Huntingtons Dis. 8 (4), 449–457. doi:10.3233/JHD-180318
Hesse, S., Moeller, F., Petroff, D., Lobsien, D., Luthardt, J., Regenthal, R., et al. (2014). Altered Serotonin Transporter Availability in Patients with Multiple Sclerosis. Eur. J. Nucl. Med. Mol. Imaging 41 (5), 827–835. doi:10.1007/s00259-013-2636-z
Hickey, M. A., and Chesselet, M. F. (2003). Apoptosis in Huntington's Disease. Prog. Neuropsychopharmacol. Biol. Psychiatry 27 (2), 255–265. doi:10.1016/S0278-5846(03)00021-6
Hill, M. N., Campolongo, P., Yehuda, R., and Patel, S. (2017). Integrating Endocannabinoid Signaling and Cannabinoids into the Biology and Treatment of Posttraumatic Stress Disorder. Neuropsychopharmacol. 43, 80–102.
Hine, B., Friedman, E., Torrelio, M., and Gershon, S. (1975). Morphine-Dependent Rats: Blockade of Precipitated Abstinence by Tetrahydrocannabinol. Science 187, 443–445. doi:10.1126/science.1167428
Hirshfeld, D. R., Rosenbaum, J. F., Biederman, J., Bolduc, E. A., Faraone, S. V., Snidman, N., et al. (1992). Stable Behavioral Inhibition and its Association with Anxiety Disorder. J. Am. Acad. Child. Adolesc. Psychiatry 31 (1), 103–111. doi:10.1097/00004583-199201000-00016
Hohmann, A. G., Briley, E. M., and Herkenham, M. (1999). Pre- and Postsynaptic Distribution of Cannabinoid and Mu Opioid Receptors in Rat Spinal Cord. Brain Res. 822 (1), 17–25. doi:10.1016/S0006-8993(98)01321-3
Hohmann, A. G., and Herkenham, M. (1999). Cannabinoid Receptors Undergo Axonal Flow in Sensory Nerves. Neuroscience 92 (4), 1171–1175. doi:10.1016/S0306-4522(99)00220-1
Hsiao, Y. T., Yi, P. L., Li, C. L., and Chang, F. C. (2012). Effect of Cannabidiol on Sleep Disruption Induced by the Repeated Combination Tests Consisting of Open Field and Elevated Plus-Maze in Rats. Neuropharmacology 62 (1), 373–384. doi:10.1016/j.neuropharm.2011.08.013
Huang, S. M., Bisogno, T., Petros, T. J., Chang, S. Y., Zavitsanos, P. A., Zipkin, R. E., et al. (2001). Identification of a New Class of Molecules, the Arachidonyl Amino Acids, and Characterization of One Member that Inhibits Pain. J. Biol. Chem. 276 (46), 42639–42644. doi:10.1074/jbc.M107351200
Huestis, M. A. (2005). “Pharmacokinetics and Metabolism of the Plant Cannabinoids, Δ9-Tetrahydrocannibinol, Cannabidiol and Cannabinol,” in Cannabinoids. Editor R. G. Pertwee (Springer), 657–690. doi:10.1007/3-540-26573-2_23
Hunault, C. C., van Eijkeren, J. C., Mensinga, T. T., de Vries, I., Leenders, M. E., and Meulenbelt, J. (2010). Disposition of Smoked Cannabis with High Δ(9)-tetrahydrocannabinol Content: a Kinetic Model. Toxicol. Appl. Pharmacol. 246 (3), 148–153. doi:10.1016/j.taap.2010.04.019
Hurley, M. J., Mash, D. C., and Jenner, P. (2003). Expression of Cannabinoid CB1 Receptor mRNA in Basal Ganglia of normal and Parkinsonian Human Brain. J. Neural Transm. (Vienna) 110 (11), 1279–1288. doi:10.1007/s00702-003-0033-7
Iqbal, K., Liu, F., Gong, C. X., and Grundke-Iqbal, I. (2010). Tau in Alzheimer Disease and Related Tauopathies. Curr. Alzheimer Res. 7 (8), 656–664. doi:10.2174/156720510793611592
Isooka, N., Miyazaki, I., Kikuoka, R., Wada, K., Nakayama, E., Shin, K., et al. (2020). Dopaminergic Neuroprotective Effects of Rotigotine via 5-HT1A Receptors: Possibly Involvement of Metallothionein Expression in Astrocytes. Neurochem. Int. 132, 104608. doi:10.1016/j.neuint.2019.104608
Jennings, E. A., Vaughan, C. W., and Christie, M. J. (2001). Cannabinoid Actions on Rat Superficial Medullary Dorsal Horn Neurons In Vitro. J. Physiol. 534 (3), 805–812. doi:10.1111/j.1469-7793.2001.00805.x
Jing, E., and Straw-Wilson, K. (2016). Sexual Dysfunction in Selective Serotonin Reuptake Inhibitors (SSRIs) and Potential Solutions: A Narrative Literature Review. Ment. Health Clin. 6 (4), 191–196. doi:10.9740/mhc.2016.07.191
Johnson, J. R., Burnell-Nugent, M., Lossignol, D., Ganae-Motan, E. D., Potts, R., and Fallon, M. T. (2010). Multicenter, Double-Blind, Randomized, Placebo-Controlled, Parallel-Group Study of the Efficacy, Safety, and Tolerability of THC:CBD Extract and THC Extract in Patients with Intractable Cancer-Related Pain. J. Pain Symptom Manage. 39 (2), 167–179. doi:10.1016/j.jpainsymman.2009.06.008
Jones, N. A., Hill, A. J., Smith, I., Bevan, S. A., Williams, C. M., Whalley, B. J., et al. (2010). Cannabidiol Displays Antiepileptiform and Antiseizure Properties In Vitro and In Vivo. J. Pharmacol. Exp. Ther. 332 (2), 569–577. doi:10.1124/jpet.109.159145
Katona, I., Rancz, E. A., Acsady, L., Ledent, C., Mackie, K., Hajos, N., et al. (2001). Distribution of CB1 Cannabinoid Receptors in the Amygdala and Their Role in the Control of GABAergic Transmission. J. Neurosci. 21 (23), 9506–9518. doi:10.1523/JNEUROSCI.21-23-09506.2001
Katsidoni, V., Anagnostou, I., and Panagis, G. (2013). Cannabidiol Inhibits the Reward-Facilitating Effect of Morphine: Involvement of 5-HT1A Receptors in the Dorsal Raphe Nucleus. Addict. Biol. 18 (2), 286–296. doi:10.1111/j.1369-1600.2012.00483.x
Katz, G., Lobel, T., Tetelbaum, A., and Raskin, S. (2014). Cannabis Withdrawal - A New Diagnostic Category in DSM-5. Isr. J. Psychiatry Relat. Sci. 51 (4), 270–275.
Kavia, R. B., De Ridder, D., Constantinescu, C. S., Stott, C. G., and Fowler, C. J. (2010). Randomized Controlled Trial of Sativex to Treat Detrusor Overactivity in Multiple Sclerosis. Mult. Scler. 16 (11), 1349–1359. doi:10.1177/1352458510378020
Kayser, R. R., Haney, M., Raskin, M., Arout, C., and Simpson, H. B. (2020a). Acute Effects of Cannabinoids on Symptoms of Obsessive-Compulsive Disorder: A Human Laboratory Study. Depress. Anxiety 37 (8), 801–811. doi:10.1002/da.23032
Kayser, R. R., Raskin, M., Snorrason, I., Hezel, D. M., Haney, M., and Simpson, H. B. (2020b). Cannabinoid Augmentation of Exposure-Based Psychotherapy for Obsessive-Compulsive Disorder. J. Clin. Psychopharmacol. 40 (2), 207–210. doi:10.1097/JCP.0000000000001179
Kikuoka, R., Miyazaki, I., Kubota, N., Maeda, M., Kagawa, D., Moriyama, M., et al. (2020). Mirtazapine Exerts Astrocyte-Mediated Dopaminergic Neuroprotection. Sci. Rep. 10 (1), 20698. doi:10.1038/s41598-020-77652-4
King, K. M., Myers, A. M., Soroka-Monzo, A. J., Tuma, R. F., Tallarida, R. J., Walker, E. A., et al. (2017). Single and Combined Effects of Δ9 -tetrahydrocannabinol and Cannabidiol in a Mouse Model of Chemotherapy-Induced Neuropathic Pain. Br. J. Pharmacol. 174 (17), 2832–2841. doi:10.1111/bph.13887
Kish, S. J., Tong, J., Hornykiewicz, O., Rajput, A., Chang, L. J., Guttman, M., et al. (2008). Preferential Loss of Serotonin Markers in Caudate versus Putamen in Parkinson's Disease. Brain 131 (Pt 1), 120–131. doi:10.1093/brain/awm239
Klein, C., and Westenberger, A. (2012). Genetics of Parkinson's Disease. Cold Spring Harb Perspect. Med. 2 (1), a008888. doi:10.1101/cshperspect.a008888
Knaub, K., Sartorius, T., Dharsono, T., Wacker, R., Wilhelm, M., and Schön, C. (2019). A Novel Self-Emulsifying Drug Delivery System (SEDDS) Based on VESIsorb® Formulation Technology Improving the Oral Bioavailability of Cannabidiol in Healthy Subjects. Molecules 24 (16), 2967. doi:10.3390/molecules24162967
Kozela, E., Lev, N., Kaushansky, N., Eilam, R., Rimmerman, N., Levy, R., et al. (2011). Cannabidiol Inhibits Pathogenic T Cells, Decreases Spinal Microglial Activation and Ameliorates Multiple Sclerosis-like Disease in C57BL/6 Mice. Br. J. Pharmacol. 163 (7), 1507–1519. doi:10.1111/j.1476-5381.2011.01379.x
Kraus, J., Börner, C., and Höllt, V. (2009). 22. Cannabinoid and Opioid-Mediated Increase in Cyclic AMP Inhibits T Cell Receptor-Triggered Signaling. Brain Behav. Immun. 23 (23), S14. doi:10.1016/j.bbi.2009.05.025
Kumari, A., Yadav, S. K., and Yadav, S. C. (2010). Biodegradable Polymeric Nanoparticles Based Drug Delivery Systems. Colloids Surf. B Biointerfaces 75 (1), 1–18. doi:10.1016/j.colsurfb.2009.09.001
Lafenêtre, P., Chaouloff, F., and Marsicano, G. (2007). The Endocannabinoid System in the Processing of Anxiety and Fear and How CB1 Receptors May Modulate Fear Extinction. Pharmacol. Res. 56 (5), 367–381. doi:10.1016/j.phrs.2007.09.006
Lai, M. K., Tsang, S. W., Francis, P. T., Esiri, M. M., Keene, J., Hope, T., et al. (2003). Reduced Serotonin 5-HT1A Receptor Binding in the Temporal Cortex Correlates with Aggressive Behavior in Alzheimer Disease. Brain Res. 974 (1), 82–87. doi:10.1016/S0006-8993(03)02554-X
Lanz, C., Mattsson, J., Soydaner, U., and Brenneisen, R. (2016). Medicinal Cannabis: In Vitro Validation of Vaporizers for the Smoke-free Inhalation of Cannabis. PLOS ONE 11 (1), e0147286. doi:10.1371/journal.pone.0147286
Laporte, A. M., Fattaccini, C. M., Lombard, M. C., Chauveau, J., and Hamon, M. (1995). Effects of Dorsal Rhizotomy and Selective Lesion of Serotonergic and Noradrenergic Systems on 5-HT1A, 5-HT1B, and 5-HT3 Receptors in the Rat Spinal Cord. J. Neural Transm. Gen. Sectgeneral Section 100 (3), 207–223. doi:10.1007/BF01276459
Lastres-Becker, I., Bizat, N., Boyer, F., Hantraye, P., Fernández-Ruiz, J., and Brouillet, E. (2004). Potential Involvement of Cannabinoid Receptors in 3-nitropropionic Acid Toxicity In Vivo. NeuroReport 15 (15), 2375–2379. doi:10.1097/00001756-200410250-00015
Lastres-Becker, I., Fezza, F., Cebeira, M., Bisogno, T., Ramos, J. A., Milone, A., et al. (2001). Changes in Endocannabinoid Transmission in the Basal Ganglia in a Rat Model of Huntington's Disease. Neuroreport 12 (10), 2125–2129. doi:10.1097/00001756-200107200-00017
Lastres-Becker, I., Hansen, H. H., Berrendero, F., De Miguel, R., Pérez-Rosado, A., Manzanares, J., et al. (2002). Alleviation of Motor Hyperactivity and Neurochemical Deficits by Endocannabinoid Uptake Inhibition in a Rat Model of Huntington's Disease. Synapse 44 (1), 23–35. doi:10.1002/syn.10054
Lastres-Becker, I., Molina-Holgado, F., Ramos, J. A., Mechoulam, R., and Fernández-Ruiz, J. (2005). Cannabinoids Provide Neuroprotection against 6-hydroxydopamine Toxicity In Vivo and In Vitro: Relevance to Parkinson's Disease. Neurobiol. Dis. 19 (1), 96–107. doi:10.1016/j.nbd.2004.11.009
Laux, L. C., Bebin, E. M., Checketts, D., Chez, M., Flamini, R., Marsh, E. D., et al. (2019). Long-term Safety and Efficacy of Cannabidiol in Children and Adults with Treatment Resistant Lennox-Gastaut Syndrome or Dravet Syndrome: Expanded Access Program Results. Epilepsy Res. 154, 13–20. doi:10.1016/j.eplepsyres.2019.03.015
Ledent, C., Valverde, O., Cossu, G., Petitet, F., Aubert, J. F., Beslot, F., et al. (1999). Unresponsiveness to Cannabinoids and Reduced Addictive Effects of Opiates in CB1 Receptor Knockout Mice. Science 283 (5400), 401–404. doi:10.1126/science.283.5400.401
Ledesma, J. C., Manzanedo, C., and Aguilar, M. A. (2021). Cannabidiol Prevents Several of the Behavioral Alterations Related to Cocaine Addiction in Mice. Prog. Neuropsychopharmacol. Biol. Psychiatry 111, 110390. doi:10.1016/j.pnpbp.2021.110390
Lee, J. H., Agacinski, G., Williams, J. H., Wilcock, G. K., Esiri, M. M., Francis, P. T., et al. (2010). Intact Cannabinoid CB1 Receptors in the Alzheimer's Disease Cortex. Neurochem. Int. 57 (8), 985–989. doi:10.1016/j.neuint.2010.10.010
Leehey, M. A., Liu, Y., Hart, F., Epstein, C., Cook, M., Sillau, S., et al. (2020). Safety and Tolerability of Cannabidiol in Parkinson Disease: An Open Label, Dose-Escalation Study. Cannabis Cannabinoid Res. 5 (4), 326–336. doi:10.1089/can.2019.0068
Lesch, K. P. (2005). Serotonergic Gene Inactivation in Mice: Models for Anxiety and Aggression? Novartis Found. Symp. 268, 111–170.
Levin, D. N., Dulberg, Z., Chan, A. W., Hare, G. M., Mazer, C. D., and Hong, A. (2017). A Randomized-Controlled Trial of Nabilone for the Prevention of Acute Postoperative Nausea and Vomiting in Elective Surgery. Can J Anaesth. 64, 385–395.
Lichtman, A. H., Lux, E. A., McQuade, R., Rossetti, S., Sanchez, R., Sun, W., et al. (2018). Results of a Double-Blind, Randomized, Placebo-Controlled Study of Nabiximols Oromucosal Spray as an Adjunctive Therapy in Advanced Cancer Patients with Chronic Uncontrolled Pain. J. Pain Symptom Manage. 55 (2), 179–e1. e1. doi:10.1016/j.jpainsymman.2017.09.001
Linher-Melville, K., Zhu, Y. F., Sidhu, J., Parzei, N., Shahid, A., Seesankar, G., et al. (2020). Evaluation of the Preclinical Analgesic Efficacy of Naturally Derived, Orally Administered Oil Forms of Δ9-tetrahydrocannabinol (THC), Cannabidiol (CBD), and Their 1:1 Combination. PLoS One 15 (6), e0234176. doi:10.1371/journal.pone.0234176
Lintzeris, N., Bhardwaj, A., Mills, L., Dunlop, A., Copeland, J., McGregor, I., et al. (2019). Nabiximols for the Treatment of Cannabis Dependence: A Randomized Clinical Trial. JAMA Intern Med. 179, 1242–1253. doi:10.1001/jamainternmed.2019.1993
Little, P. J., Compton, D. R., Johnson, M. R., Melvin, L. S., and Martin, B. R. (1988). Pharmacology and Stereoselectivity of Structurally Novel Cannabinoids in Mice. J. Pharmacol. Exp. Ther. 247 (3), 1046–1051.
Liu, J., and Wang, F. (2017). Role of Neuroinflammation in Amyotrophic Lateral Sclerosis: Cellular Mechanisms and Therapeutic Implications. Front. Immunol. 8, 1005. doi:10.3389/fimmu.2017.01005
Liu, Q. R., Canseco-Alba, A., Zhang, H. Y., Tagliaferro, P., Chung, M., Dennis, E., et al. (2017). Cannabinoid Type 2 Receptors in Dopamine Neurons Inhibits Psychomotor Behaviors, Alters Anxiety, Depression and Alcohol Preference. Sci. Rep. 7 (1), 17410. doi:10.1038/s41598-017-17796-y
Lodzki, M., Godin, B., Rakou, L., Mechoulam, R., Gallily, R., and Touitou, E. (2003). Cannabidiol-transdermal Delivery and Anti-inflammatory Effect in a Murine Model. J. Control. Release 93 (3), 377–387. doi:10.1016/j.jconrel.2003.09.001
Long, L. E., Chesworth, R., Huang, X. F., McGregor, I. S., Arnold, J. C., and Karl, T. (2010). A Behavioural Comparison of Acute and Chronic Delta9-tetrahydrocannabinol and Cannabidiol in C57BL/6JArc Mice. Int. J. Neuropsychopharmacol. 13 (7), 861–876. doi:10.1017/S1461145709990605
Longinetti, E., and Fang, F. (2019). Epidemiology of Amyotrophic Lateral Sclerosis: An Update of Recent Literature. Curr. Opin. Neurol. 32 (5), 771–776. doi:10.1097/WCO.0000000000000730
López-Sendón Moreno, J. L., García Caldentey, J., Trigo Cubillo, P., Ruiz Romero, C., García Ribas, G., Alonso Arias, M. A. A., et al. (2016). A Double-Blind, Randomized, Cross-Over, Placebo-Controlled, Pilot Trial with Sativex in Huntington's Disease. J. Neurol. 263 (7), 1390–1400. doi:10.1007/s00415-016-8145-9
Lucas, C. J., Galettis, P., and Schneider, J. (2018). The Pharmacokinetics and the Pharmacodynamics of Cannabinoids. Br. J. Clin. Pharmacol. 84 (11), 2477–2482. doi:10.1111/bcp.13710
MacGregor, K. J., Embleton, J. K., Lacy, J. E., Perry, E. A., Solomon, L. J., Seager, H., et al. (1997). Influence of Lipolysis on Drug Absorption from the Gastro-Intestinal Tract. Adv. Drug Deliv. Rev. 25 (1), 33–46. doi:10.1016/S0169-409X(96)00489-9
Maggio, N., Shavit Stein, E., and Segal, M. (2018). Cannabidiol Regulates Long Term Potentiation Following Status Epilepticus: Mediation by Calcium Stores and Serotonin. Front. Mol. Neurosci. 11, 32. doi:10.3389/fnmol.2018.00032
Mailleux, P., and Vanderhaeghen, J. J. (1992). Distribution of Neuronal Cannabinoid Receptor in the Adult Rat Brain: A Comparative Receptor Binding Radioautography and In Situ Hybridization Histochemistry. Neuroscience 48 (3), 655–668. doi:10.1016/0306-4522(92)90409-U
Malik, Z., Bayman, L., Valestin, J., Rizvi-Toner, A., Hashmi, S., and Schey, R. (2017). Dronabinol Increases Pain Threshold in Patients with Functional Chest Pain: A Pilot Double-Blind Placebo-Controlled Trial. Dis. Esophagus 30 (2), 1–8. doi:10.1111/dote.12455
Mantyh, P. W. (2014). Bone Cancer Pain: From Mechanism to Therapy. Curr. Opin. Support. Palliat. Care 8 (2), 83–90. doi:10.1097/SPC.0000000000000048
Manzanares, J., Cabañero, D., Puente, N., García-Gutiérrez, M. S., Grandes, P., and Maldonado, R. (2018). Role of the Endocannabinoid System in Drug Addiction. Biochem. Pharmacol. 157, 108–121. doi:10.1016/j.bcp.2018.09.013
Marcinkiewcz, C. A., Mazzone, C. M., D'Agostino, G., Halladay, L. R., Hardaway, J. A., DiBerto, J. F., et al. (2016). Serotonin Engages an Anxiety and Fear-Promoting Circuit in the Extended Amygdala. Nature 537 (7618), 97–101. doi:10.1038/nature19318
Maresz, K., Carrier, E. J., Ponomarev, E. D., Hillard, C. J., and Dittel, B. N. (2005). Modulation of the Cannabinoid CB2 Receptor in Microglial Cells in Response to Inflammatory Stimuli. J. Neurochem. 95 (2), 437–445. doi:10.1111/j.1471-4159.2005.03380.x
Markos, J. R., Harris, H. M., Gul, W., ElSohly, M. A., and Sufka, K. J. (2018). Effects of Cannabidiol on Morphine Conditioned Place Preference in Mice. Planta Med. 84 (4), 221–224. doi:10.1055/s-0043-117838
Markovà, J., Essner, U., Akmaz, B., Marinelli, M., Trompke, C., Lentschat, A., et al. (2019). Sativex® as Add-On Therapy vs. Further Optimized First-Line ANTispastics (SAVANT) in Resistant Multiple Sclerosis Spasticity: a Double-Blind, Placebo-Controlled Randomised Clinical Trial. Int. J. Neurosci. 129 (2), 119–128. doi:10.1080/00207454.2018.1481066
Marras, C., Beck, J. C., Bower, J. H., Roberts, E., Ritz, B., Ross, G. W., et al. (2018). Prevalence of Parkinson's Disease across North America. NPJ Parkinsons Dis. 4, 21. doi:10.1038/s41531-018-0058-0
Marsicano, G., Goodenough, S., Monory, K., Hermann, H., Eder, M., Cannich, A., et al. (2003). CB1 Cannabinoid Receptors and On-Demand Defense against Excitotoxicity. Science 302 (5642), 84–88. doi:10.1126/science.1088208
Martín-Moreno, A. M., Reigada, D., Ramírez, B. G., Mechoulam, R., Innamorato, N., Cuadrado, A., et al. (2011). Cannabidiol and Other Cannabinoids Reduce Microglial Activation In Vitro and In Vivo: Relevance to Alzheimer's Disease. Mol. Pharmacol. 79 (6), 964–973. doi:10.1124/mol.111.071290
Masrori, P., and Van Damme, P. (2020). Amyotrophic Lateral Sclerosis: A Clinical Review. Eur. J. Neurol. 27 (10), 1918–1929. doi:10.1111/ene.14393
McCaw, E. A., Hu, H., Gomez, G. T., Hebb, A. L., Kelly, M. E., and Denovan-Wright, E. M. (2004). Structure, Expression and Regulation of the Cannabinoid Receptor Gene (CB1) in Huntington's Disease Transgenic Mice. Eur. J. Biochem. 271 (23–24), 4909–4920. doi:10.1111/j.1432-1033.2004.04460.x
McColgan, P., and Tabrizi, S. J. (2018). Huntington's Disease: a Clinical Review. Eur. J. Neurol. 25 (1), 24–34. doi:10.1111/ene.13413
McGregor, I. S., Dam, K. D., Mallet, P. E., and Gallate, J. E. (2005). Delta9-THC Reinstates Beer- and Sucrose-Seeking Behaviour in Abstinent Rats: Comparison with Midazolam, Food Deprivation and Predator Odour. Alcohol Alcohol 40 (1), 35–45. doi:10.1093/alcalc/agh113
Micale, V., Di Marzo, V., Sulcova, A., Wotjak, C. T., and Drago, F. (2013). Endocannabinoid System and Mood Disorders: Priming a Target for New Therapies. Pharmacol. Ther. 138 (1), 18–37. doi:10.1016/j.pharmthera.2012.12.002
Miyazaki, I., Asanuma, M., Murakami, S., Takeshima, M., Torigoe, N., Kitamura, Y., et al. (2013). Targeting 5-HT(1A) Receptors in Astrocytes to Protect Dopaminergic Neurons in Parkinsonian Models. Neurobiol. Dis. 59, 244–256. doi:10.1016/j.nbd.2013.08.003
Morgan, C. J., Das, R. K., Joye, A., Curran, H. V., and Kamboj, S. K. (2013). Cannabidiol Reduces Cigarette Consumption in Tobacco Smokers: Preliminary Findings. Addict. Behav. 38 (9), 2433–2436. doi:10.1016/j.addbeh.2013.03.011
Morisset, V., and Urban, L. (2001). Cannabinoid-Induced Presynaptic Inhibition of Glutamatergic EPSCs in Substantia Gelatinosa Neurons of the Rat Spinal Cord. J. Neurophysiol. 86 (1), 40–48. doi:10.1152/jn.2001.86.1.40
Mulder, J., Zilberter, M., Pasquaré, S. J., Alpár, A., Schulte, G., Ferreira, S. G., et al. (2011). Molecular Reorganization of Endocannabinoid Signalling in Alzheimer's Disease. Brain 134 (4), 1041–1060. doi:10.1093/brain/awr046
Müller, C. P., and Homberg, J. R. (2015). The Role of Serotonin in Drug Use and Addiction. Behav. Brain Res. 277, 146–192. doi:10.1016/j.bbr.2014.04.007
Müller-Vahl, K. R., Schneider, U., and Emrich, H. M. (1999). Nabilone Increases Choreatic Movements in Huntington’s Disease. Movement Disord. Official J. Movement Disord. Soc. 14 (6), 1038–1040. doi:10.1002/1531-8257(199911)14:6<1038::aid-mds1024>3.0.co;2-7
Munro, S., Thomas, K. L., and Abu-Shaar, M. (1993). Molecular Characterization of a Peripheral Receptor for Cannabinoids. Nature 365 (6441), 61–65. doi:10.1038/365061a0
Murty, R. B., and Murty, S. B. (2012). An Improved Oral Dosage Form of Tetrahydrocannabinol and a Method of Avoiding And/or Suppressing Hepatic First Pass Metabolism via Targeted Chylomicron/lipoprotein Delivery (World Intellectual Property Organization Patent No. WO2012033478A1). Availableat: https://patents.google.com/patent/WO2012033478A1/en.
Muzerelle, A., Scotto-Lomassese, S., Bernard, J. F., Soiza-Reilly, M., and Gaspar, P. (2016). Conditional Anterograde Tracing Reveals Distinct Targeting of Individual Serotonin Cell Groups (B5-B9) to the Forebrain and Brainstem. Brain Struct. Funct. 221 (1), 535–561. doi:10.1007/s00429-014-0924-4
Navarrete, F., García-Gutiérrez, M. S., Aracil-Fernández, A., Lanciego, J. L., and Manzanares, J. (2018). Cannabinoid CB1 and CB2 Receptors, and Monoacylglycerol Lipase Gene Expression Alterations in the Basal Ganglia of Patients with Parkinson's Disease. Neurotherapeutics 15 (2), 459–469. doi:10.1007/s13311-018-0603-x
Nelson, P. T., Braak, H., and Markesbery, W. R. (2009). Neuropathology and Cognitive Impairment in Alzheimer Disease: A Complex but Coherent Relationship. J. Neuropathol. Exp. Neurol. 68 (1), 1–14. doi:10.1097/NEN.0b013e3181919a48
Newton, C. A., Chou, P. J., Perkins, I., and Klein, T. W. (2009). CB(1) and CB(2) Cannabinoid Receptors Mediate Different Aspects of delta-9-tetrahydrocannabinol (THC)-induced T Helper Cell Shift Following Immune Activation by Legionella pneumophila Infection. J. Neuroimmune Pharmacol. 4 (1), 92–102. doi:10.1007/s11481-008-9126-2
Notcutt, W., Price, M., Miller, R., Newport, S., Phillips, C., Simmons, S., et al. (2004). Initial Experiences with Medicinal Extracts of Cannabis for Chronic Pain: Results from 34 'N of 1' Studies. Anaesthesia 59 (5), 440–452. doi:10.1111/j.1365-2044.2004.03674.x
Novotna, A., Mares, J., Ratcliffe, S., Novakova, I., Vachova, M., Zapletalova, O., et al. and the S. S. S. (2011). A Randomized, Double-Blind, Placebo-Controlled, Parallel-Group, Enriched-Design Study of Nabiximols* (Sativex(®) ), as Add-On Therapy, in Subjects with Refractory Spasticity Caused by Multiple Sclerosis. Eur. J. Neurol. 18 (9), 1122–1131. doi:10.1111/j.1468-1331.2010.03328.x
Nurmikko, T. J., Serpell, M. G., Hoggart, B., Toomey, P. J., Morlion, B. J., and Haines, D. (2007). Sativex Successfully Treats Neuropathic Pain Characterised by Allodynia: A Randomised, Double-Blind, Placebo-Controlled Clinical Trial. Pain 133 (1–3), 210–220. doi:10.1016/j.pain.2007.08.028
O'Connell, M., Sandgren, M., Frantzen, L., Bower, E., and Erickson, B. (2019). Medical Cannabis: Effects on Opioid and Benzodiazepine Requirements for Pain Control. Ann. Pharmacother. 53 (11), 1081–1086. doi:10.1177/1060028019854221
Ögren, S. O., Eriksson, T. M., Elvander-Tottie, E., D’Addario, C., Ekström, J. C., Svenningsson, P., et al. (2008). The Role of 5-HT1A Receptors in Learning and Memory. Behav. Brain Res. 195 (1), 54–77. doi:10.1016/j.bbr.2008.02.023
Oh, D. A., Parikh, N., Khurana, V., Cognata Smith, C., and Vetticaden, S. (2017). Effect of Food on the Pharmacokinetics of Dronabinol Oral Solution versus Dronabinol Capsules in Healthy Volunteers. Clin. Pharmacol. 9, 9–17. doi:10.2147/CPAA.S119676
Ohlsson, A., Lindgren, J. E., Wahlén, A., Agurell, S., Hollister, L. E., and Gillespie, H. K. (1982). Single Dose Kinetics of Deuterium Labelled delta 1-tetrahydrocannabinol in Heavy and Light Cannabis Users. Biomed. Mass. Spectrom. 9 (1), 6–10. doi:10.1002/bms.1200090103
Ohno-Shosaku, T., Maejima, T., and Kano, M. (2001). Endogenous Cannabinoids Mediate Retrograde Signals from Depolarized Postsynaptic Neurons to Presynaptic Terminals. Neuron 29 (3), 729–738. doi:10.1016/S0896-6273(01)00247-1
Oleson, E. B., Hamilton, L. R., and Gomez, D. M. (2021). Cannabinoid Modulation of Dopamine Release during Motivation, Periodic Reinforcement, Exploratory Behavior, Habit Formation, and Attention. Front. Synaptic Neurosci. 13, 660218. doi:10.3389/fnsyn.2021.660218
Onaivi, E. S., Carpio, O., Ishiguro, H., Schanz, N., Uhl, G. R., and Benno, R. (2008a). Behavioral Effects of Cb2 Cannabinoid Receptor Activation and its Influence on Food and Alcohol Consumption. Ann. N. Y Acad. Sci. 1139, 426–433. doi:10.1196/annals.1432.035
Onaivi, E. S., Green, M. R., and Martin, B. R. (1990). Pharmacological Characterization of Cannabinoids in the Elevated Plus Maze. J. Pharmacol. Exp. Ther. 253 (3), 1002–1009.
Onaivi, E. S., Ishiguro, H., Gong, J. P., Patel, S., Meozzi, P. A., Myers, L., et al. (2008b). Functional Expression of Brain Neuronal CB2 Cannabinoid Receptors Are Involved in the Effects of Drugs of Abuse and in Depression. Ann. N. Y Acad. Sci. 1139, 434–449. doi:10.1196/annals.1432.036
Palazuelos, J., Davoust, N., Julien, B., Hatterer, E., Aguado, T., Mechoulam, R., et al. (2008). The CB(2) Cannabinoid Receptor Controls Myeloid Progenitor Trafficking: Involvement in the Pathogenesis of an Animal Model of Multiple Sclerosis. J. Biol. Chem. 283 (19), 13320–13329. doi:10.1074/jbc.M707960200
Parikh, N., Kramer, W. G., Khurana, V., Cognata Smith, C., and Vetticaden, S. (2016). Bioavailability Study of Dronabinol Oral Solution versus Dronabinol Capsules in Healthy Volunteers. Clin. Pharmacol. 8, 155–162. doi:10.2147/CPAA.S115679
Patel, R. R., Barbosa, C., Brustovetsky, T., Brustovetsky, N., and Cummins, T. R. (2016). Aberrant Epilepsy-Associated Mutant Nav1.6 Sodium Channel Activity Can Be Targeted with Cannabidiol. Brain 139 (Pt 8), 2164–2181. doi:10.1093/brain/aww129
Patel, S., Hill, M. N., Cheer, J. F., Wotjak, C. T., and Holmes, A. (2017). The Endocannabinoid System as a Target for Novel Anxiolytic Drugs. Neurosci. Biobehav Rev. 76, 56–66. doi:10.1016/j.neubiorev.2016.12.033
Peball, M., Krismer, F., Knaus, H. G., Djamshidian, A., Werkmann, M., Carbone, F., et al. (2020). Non-Motor Symptoms in Parkinson's Disease Are Reduced by Nabilone. Ann. Neurol. 88 (4), 712–722. doi:10.1002/ana.25864
Pelloux, Y., Dilleen, R., Economidou, D., Theobald, D., and Everitt, B. J. (2012). Reduced Forebrain Serotonin Transmission Is Causally Involved in the Development of Compulsive Cocaine Seeking in Rats. Neuropsychopharmacology 37 (11), 2505–2514. doi:10.1038/npp.2012.111
Penninx, B. W., Pine, D. S., Holmes, E. A., and Reif, A. (2021). Anxiety Disorders. The Lancet 397 (10277), 914–927. doi:10.1016/S0140-6736(21)00359-7
Perrin, F. E., Gerber, Y. N., Teigell, M., Lonjon, N., Boniface, G., Bauchet, L., et al. (2011). Anatomical Study of Serotonergic Innervation and 5-HT(1A) Receptor in the Human Spinal Cord. Cell Death Dis 2, e218. doi:10.1038/cddis.2011.98
Politis, M., and Niccolini, F. (2015). Serotonin in Parkinson's Disease. Behav. Brain Res. 277, 136–145. doi:10.1016/j.bbr.2014.07.037
Portenoy, R. K., Ganae-Motan, E. D., Allende, S., Yanagihara, R., Shaiova, L., Weinstein, S., et al. (2012). Nabiximols for Opioid-Treated Cancer Patients with Poorly-Controlled Chronic Pain: A Randomized, Placebo-Controlled, Graded-Dose Trial. J. Pain 13 (5), 438–449. doi:10.1016/j.jpain.2012.01.003
Price, T. J., Helesic, G., Parghi, D., Hargreaves, K. M., and Flores, C. M. (2003). The Neuronal Distribution of Cannabinoid Receptor Type 1 in the Trigeminal Ganglion of the Rat. Neuroscience 120 (1), 155–162. doi:10.1016/S0306-4522(03)00333-6
Pryce, G., Ahmed, Z., Hankey, D. J., Jackson, S. J., Croxford, J. L., Pocock, J. M., et al. (2003). Cannabinoids Inhibit Neurodegeneration in Models of Multiple Sclerosis. Brain 126 (10), 2191–2202. doi:10.1093/brain/awg224
Racz, I., Nadal, X., Alferink, J., Baños, J. E., Rehnelt, J., Martín, M., et al. (2008). Crucial Role of CB(2) Cannabinoid Receptor in the Regulation of central Immune Responses during Neuropathic Pain. J. Neurosci. 28 (46), 12125–12135. doi:10.1523/JNEUROSCI.3400-08.2008
Rahn, E. J., Thakur, G. A., Wood, J. A., Zvonok, A. M., Makriyannis, A., and Hohmann, A. G. (2011). Pharmacological Characterization of AM1710, a Putative Cannabinoid CB2 Agonist from the Cannabilactone Class: Antinociception without central Nervous System Side-Effects. Pharmacol. Biochem. Behav. 98 (4), 493–502. doi:10.1016/j.pbb.2011.02.024
Raman, C., McAllister, S. D., Rizvi, G., Patel, S. G., Moore, D. H., and Abood, M. E. (2004). Amyotrophic Lateral Sclerosis: Delayed Disease Progression in Mice by Treatment with a Cannabinoid. Comp. Study 5 (1), 33–39. doi:10.1080/14660820310016813
Ramirez, B. G., Blázquez, C., Pulgar, T. G. del., Guzmán, M., and Ceballos, M. L. de. (2005). Prevention of Alzheimer's Disease Pathology by Cannabinoids: Neuroprotection Mediated by Blockade of Microglial Activation. J. Neurosci. 25 (8), 1904–1913. doi:10.1523/JNEUROSCI.4540-04.2005
Rao, S. D., and Weiss, J. H. (2004). Excitotoxic and Oxidative Cross-Talk between Motor Neurons and Glia in ALS Pathogenesis. Trends Neurosci. 27 (1), 17–23. doi:10.1016/j.tins.2003.11.001
Redmond, W. J., Goffaux, P., Potvin, S., and Marchand, S. (2008). Analgesic and Antihyperalgesic Effects of Nabilone on Experimental Heat Pain. Curr. Med. Res. Opin. 24 (4), 1017–1024. doi:10.1185/030079908X280635
Rekand, T. (2014). THC:CBD Spray and MS Spasticity Symptoms: Data from Latest Studies. Eur. Neurol. 71 (Suppl. 1), 4–9. doi:10.1159/000357742
Ren, Y., Whittard, J., Higuera-Matas, A., Morris, C. V., and Hurd, Y. L. (2009). Cannabidiol, a Nonpsychotropic Component of Cannabis, Inhibits Cue-Induced Heroin Seeking and Normalizes Discrete Mesolimbic Neuronal Disturbances. J. Neurosci. 29 (47), 14764–14769. doi:10.1523/JNEUROSCI.4291-09.2009
Riad, M., Garcia, S., Watkins, K. C., Jodoin, N., Doucet, E., Langlois, X., et al. (2000). Somatodendritic Localization of 5-HT1A and Preterminal Axonal Localization of 5-HT1B Serotonin Receptors in Adult Rat Brain. J. Comp. Neurol. 417 (2), 181–194. doi:10.1002/(SICI)1096-9861(20000207)417:2<181::AID-CNE4>3.0.CO;2-A
Riad, M., Mestikawy, S. E., Verge, D., Gozlan, H., and Hamon, M. (1991). Visualization and Quantification of central 5-HT1A Receptors with Specific Antibodies. Neurochem. Int. 19 (4), 413–423. doi:10.1016/0197-0186(91)90058-L
Richardson, J. D., Kilo, S., and Hargreaves, K. M. (1998). Cannabinoids Reduce Hyperalgesia and Inflammation via Interaction with Peripheral CB1 Receptors. Pain 75 (1), 111–119. doi:10.1016/S0304-3959(97)00213-3
Richfield, E. K., and Herkenham, M. (1994). Selective Vulnerability in Huntington's Disease: Preferential Loss of Cannabinoid Receptors in Lateral Globus Pallidus. Ann. Neurol. 36 (4), 577–584. doi:10.1002/ana.410360406
Rissardo, J. P., and Caprara, A. L. F. (2020). Buspirone-associated Movement Disorder: A Literature Review. Prague Med. Rep. 121 (1), 5–24. doi:10.14712/23362936.2020.1
Riva, N., Mora, G., Sorarù, G., Lunetta, C., Ferraro, O. E., Falzone, Y., et al. (2019). Safety and Efficacy of Nabiximols on Spasticity Symptoms in Patients with Motor Neuron Disease (CANALS): A Multicentre, Double-Blind, Randomised, Placebo-Controlled, Phase 2 Trial. Lancet Neurol. 18 (2), 155–164. doi:10.1016/S1474-4422(18)30406-X
Robinson, J. D., Cinciripini, P. M., Karam-Hage, M., Aubin, H. J., Dale, L. C., Niaura, R., et al. (2018). Pooled Analysis of Three Randomized, Double-Blind, Placebo Controlled Trials with Rimonabant for Smoking Cessation. Addict. Biol. 23 (1), 291–303. doi:10.1111/adb.12508
Rodrı́guez, J. J., Mackie, K., and Pickel, V. M. (2001). Ultrastructural Localization of the CB1 Cannabinoid Receptor in μ-Opioid Receptor Patches of the Rat Caudate Putamen Nucleus. J. Neurosci. 21 (3), 823–833. doi:10.1523/JNEUROSCI.21-03-00823.2001
Rog, D. J., Nurmikko, T. J., Friede, T., and Young, C. A. (2005). Randomized, Controlled Trial of Cannabis-Based Medicine in central Pain in Multiple Sclerosis. Neurology 65 (6), 812–819. doi:10.1212/01.wnl.0000176753.45410.8b
Rudroff, T. (2020). Medical Marijuana and its Effects on Motor Function in People with Multiple Sclerosis: An Observational Case-Control Study (Clinical Trial Registration No. NCT02898974). Availableat: https://clinicaltrials.gov/ct2/show/NCT02898974.
Russo, E. B. (2018). Cannabis Therapeutics and the Future of Neurology. Front. Integr. Neurosci. 12, 51. doi:10.3389/fnint.2018.00051
Russo, E. B., and Marcu, J. (2017). Cannabis Pharmacology: The Usual Suspects and a Few Promising Leads. Adv. Pharmacol. 80, 67–134. doi:10.1016/bs.apha.2017.03.004
Ruthirakuhan, M., Herrmann, N., Andreazza, A. C., Verhoeff, N. P. L. G., Gallagher, D., Black, S. E., et al. (2020). Agitation, Oxidative Stress, and Cytokines in Alzheimer Disease: Biomarker Analyses from a Clinical Trial with Nabilone for Agitation. J. Geriatr. Psychiatry Neurol. 33 (4), 175–184. doi:10.1177/0891988719874118
Sagredo, O., Ramos, J. A., Decio, A., Mechoulam, R., and Fernández-Ruiz, J. (2007). Cannabidiol Reduced the Striatal Atrophy Caused 3-nitropropionic Acid In Vivo by Mechanisms Independent of the Activation of Cannabinoid, Vanilloid TRPV1 and Adenosine A2A Receptors. Eur. J. Neurosci. 26 (4), 843–851. doi:10.1111/j.1460-9568.2007.05717.x
Salzman, C. (2001). Treatment of the Agitation of Late-Life Psychosis and Alzheimer's Disease. Eur. Psychiatry 16 (S1), 25s–28S. doi:10.1016/S0924-9338(00)00525-3
Sandyk, R. (2006). Serotonergic Mechanisms in Amyotrophic Lateral Sclerosis. Int. J. Neurosci. 116 (7), 775–826. doi:10.1080/00207450600754087
Sato, S., Mizukami, K., and Asada, T. (2007). A Preliminary Open-Label Study of 5-HT1A Partial Agonist Tandospirone for Behavioural and Psychological Symptoms Associated with Dementia. Int. J. Neuropsychopharmacol. 10 (2), 281–283. doi:10.1017/S1461145706007000
Schatz, A. R., Lee, M., Condie, R. B., Pulaski, J. T., and Kaminski, N. E. (1997). Cannabinoid Receptors CB1 and CB2: A Characterization of Expression and Adenylate Cyclase Modulation within the Immune System. Toxicol. Appl. Pharmacol. 142 (2), 278–287. doi:10.1006/taap.1996.8034
Schechter, L. E., Smith, D. L., Rosenzweig-Lipson, S., Sukoff, S. J., Dawson, L. A., Marquis, K., et al. (2005). Lecozotan (SRA-333): A Selective Serotonin 1A Receptor Antagonist that Enhances the Stimulated Release of Glutamate and Acetylcholine in the Hippocampus and Possesses Cognitive-Enhancing Properties. J. Pharmacol. Exp. Ther. 314 (3), 1274–1289. doi:10.1124/jpet.105.086363
Scheltens, P., De Strooper, B., Kivipelto, M., Holstege, H., Chételat, G., Teunissen, C. E., et al. (2021). Alzheimer's Disease. The Lancet 397 (10284), 1577–1590. doi:10.1016/S0140-6736(20)32205-4
Schiavon, A. P., Bonato, J. M., Milani, H., Guimarães, F. S., and Weffort de Oliveira, R. M. (2016). Influence of Single and Repeated Cannabidiol Administration on Emotional Behavior and Markers of Cell Proliferation and Neurogenesis in Non-stressed Mice. Prog. Neuropsychopharmacol. Biol. Psychiatry 64, 27–34. doi:10.1016/j.pnpbp.2015.06.017
Schimrigk, S., Marziniak, M., Neubauer, C., Kugler, E. M., Werner, G., and Abramov-Sommariva, D. (2017). Dronabinol Is a Safe Long-Term Treatment Option for Neuropathic Pain Patients. Eur. Neurol. 78 (5–6), 320–329. doi:10.1159/000481089
Schoffelmeer, A. N., Hogenboom, F., Wardeh, G., and De Vries, T. J. (2006). Interactions between CB1 Cannabinoid and Mu Opioid Receptors Mediating Inhibition of Neurotransmitter Release in Rat Nucleus Accumbens Core. Neuropharmacology 51 (4), 773–781. doi:10.1016/j.neuropharm.2006.05.019
Shannon, S., Lewis, N., Lee, H., and Hughes, S. (2019). Cannabidiol in Anxiety and Sleep: A Large Case Series. Perm J. 23, 18–041. doi:10.7812/TPP/18-041
Silverdale, M. A., McGuire, S., McInnes, A., Crossman, A. R., and Brotchie, J. M. (2001). Striatal Cannabinoid CB1 Receptor mRNA Expression Is Decreased in the Reserpine-Treated Rat Model of Parkinson's Disease. Exp. Neurol. 169 (2), 400–406. doi:10.1006/exnr.2001.7649
Skirzewski, M., Hernandez, L., Schechter, L. E., and Rada, P. (2010). Acute Lecozotan Administration Increases Learning and Memory in Rats without Affecting Anxiety or Behavioral Depression. Pharmacol. Biochem. Behav. 95 (3), 325–330. doi:10.1016/j.pbb.2010.02.008
Soares, Vde. P., Campos, A. C., Bortoli, V. C., Zangrossi, H., Guimarães, F. S., and Zuardi, A. W. (2010). Intra-dorsal Periaqueductal gray Administration of Cannabidiol Blocks Panic-like Response by Activating 5-HT1A Receptors. Behav. Brain Res. 213 (2), 225–229. doi:10.1016/j.bbr.2010.05.004
Solas, M., Francis, P. T., Franco, R., and Ramirez, M. J. (2013). CB2 Receptor and Amyloid Pathology in Frontal Cortex of Alzheimer's Disease Patients. Neurobiol. Aging 34 (3), 805–808. doi:10.1016/j.neurobiolaging.2012.06.005
Solinas, M., Panlilio, L. V., and Goldberg, S. R. (2004). Exposure to delta-9-tetrahydrocannabinol (THC) Increases Subsequent Heroin Taking but Not Heroin's Reinforcing Efficacy: a Self-Administration Study in Rats. Neuropsychopharmacology 29 (7), 1301–1311. doi:10.1038/sj.npp.1300431
Solís-Guillén, R., Leopoldo, M., Meneses, A., and Centurión, D. (2021). Activation of 5-HT1A and 5-HT7 Receptors Enhanced a Positively Reinforced Long-Term Memory. Behav. Brain Res. 397, 112932. doi:10.1016/j.bbr.2020.112932
Solowij, N., Broyd, S. J., van Hell, H. H., and Hazekamp, A. (2014). A Protocol for the Delivery of Cannabidiol (CBD) and Combined CBD and ∆9-tetrahydrocannabinol (THC) by Vaporisation. BMC Pharmacol. Toxicol. 15, 58. doi:10.1186/2050-6511-15-58
Stein, D. J., Scott, K. M., de Jonge, P., and Kessler, R. C. (2017). Epidemiology of Anxiety Disorders: From Surveys to Nosology and Back. Dialogues Clin. Neurosci. 19 (2), 127–136. doi:10.31887/DCNS.2017.19.2/dstein
Stempel, A. V., Stumpf, A., Zhang, H. Y., Özdoğan, T., Pannasch, U., Theis, A. K., et al. (2016). Cannabinoid Type 2 Receptors Mediate a Cell Type-specific Plasticity in the Hippocampus. Neuron 90 (4), 795–809. doi:10.1016/j.neuron.2016.03.034
Sussman, N., and Joffe, R. T. (1998). Antidepressant Augmentation: Conclusions and Recommendations. J. Clin. Psychiatry 59 (Suppl. 5), 70–73.
Svendsen, K. B., Jensen, T. S., and Bach, F. W. (2004). Does the Cannabinoid Dronabinol Reduce central Pain in Multiple Sclerosis? Randomised Double Blind Placebo Controlled Crossover Trial. BMJ 329 (7460), 253. doi:10.1136/bmj.38149.566979.AE
Szabó, Á., Helyes, Z., Sándor, K., Bite, A., Pintér, E., Németh, J., et al. (2005). Role of Transient Receptor Potential Vanilloid 1 Receptors in Adjuvant-Induced Chronic Arthritis: In Vivo Study Using Gene-Deficient Mice. J. Pharmacol. Exp. Ther. 314 (1), 111–119. doi:10.1124/jpet.104.082487
Tao, R., and Auerbach, S. B. (1994). Increased Extracellular Serotonin in Rat Brain after Systemic or Intraraphe Administration of Morphine. J. Neurochem. 63 (2), 517–524. doi:10.1046/j.1471-4159.1994.63020517.x
Thanos, P. K., Dimitrakakis, E. S., Rice, O., Gifford, A., and Volkow, N. D. (2005). Ethanol Self-Administration and Ethanol Conditioned Place Preference Are Reduced in Mice Lacking Cannabinoid CB1 Receptors. Behav. Brain Res. 164 (2), 206–213. doi:10.1016/j.bbr.2005.06.021
Thielen, R. J., Bare, D. J., McBride, W. J., Lumeng, L., and Li, T. K. (2002). Ethanol-stimulated Serotonin Release in the Ventral hippocampus: An Absence of Rapid Tolerance for the Alcohol-Preferring P Rat and Insensitivity in the Alcohol-Nonpreferring NP Rat. Pharmacol. Biochem. Behav. 71 (1), 111–117. doi:10.1016/S0091-3057(01)00633-5
Thompson, A. J., Baranzini, S. E., Geurts, J., Hemmer, B., and Ciccarelli, O. (2018). Multiple Sclerosis. Lancet 391 (10130), 1622–1636. doi:10.1016/S0140-6736(18)30481-1
Tohgi, H., Abe, T., Takahashi, S., Kimura, M., Takahashi, J., and Kikuchi, T. (1992). Concentrations of Serotonin and its Related Substances in the Cerebrospinal Fluid in Patients with Alzheimer Type Dementia. Neurosci. Lett. 141 (1), 9–12. doi:10.1016/0304-3940(92)90322-x
Trigo, J. M., Soliman, A., Quilty, L. C., Fischer, B., Rehm, J., Selby, P., et al. (2018). Nabiximols Combined with Motivational Enhancement/Cognitive Behavioral Therapy for the Treatment of Cannabis Dependence: A Pilot Randomized Clinical Trial. PLoS One, e0190768
University of California, Davis (2018). Cannabis for Spasticity in Multiple Sclerosis: A Placebo-Controlled Study (Clinical Trial Registration No. NCT00682929). Availableat: https://clinicaltrials.gov/ct2/show/NCT00682929.
Valjent, E., Mitchell, J. M., Besson, M. J., Caboche, J., and Maldonado, R. (2002). Behavioural and Biochemical Evidence for Interactions between Delta 9-tetrahydrocannabinol and Nicotine. Br. J. Pharmacol. 135 (2), 564–578. doi:10.1038/sj.bjp.0704479
van Hecke, O., Austin, S. K., Khan, R. A., Smith, B. H., and Torrance, N. (2014). Neuropathic Pain in the General Population: A Systematic Review of Epidemiological Studies. Pain 155 (4), 654–662. doi:10.1016/j.pain.2013.11.013
Van Laere, K., Casteels, C., Lunskens, S., Goffin, K., Grachev, I. D., Bormans, G., et al. (2012). Regional Changes in Type 1 Cannabinoid Receptor Availability in Parkinson's Disease In Vivo. Neurobiol. Aging 33 (3), 620–8620. e8. doi:10.1016/j.neurobiolaging.2011.02.009
Van Sickle, M. D., Duncan, M., Kingsley, P. J., Mouihate, A., Urbani, P., Mackie, K., et al. (2005). Identification and Functional Characterization of Brainstem Cannabinoid CB2 Receptors. Science 310 (5746), 329–332. doi:10.1126/science.1115740
van Vliet, S. A., Vanwersch, R. A., Jongsma, M. J., Olivier, B., and Philippens, I. H. (2008). Therapeutic Effects of Delta9-THC and Modafinil in a Marmoset Parkinson Model. Eur. Neuropsychopharmacol. 18 (5), 383–389. doi:10.1016/j.euroneuro.2007.11.003
Vela, G., Martín, S., García-Gil, L., Crespo, J. A., Ruiz-Gayo, M., Fernández-Ruiz, J. J., et al. (1998). Maternal Exposure to delta9-tetrahydrocannabinol Facilitates Morphine Self-Administration Behavior and Changes Regional Binding to central Mu Opioid Receptors in Adult Offspring Female Rats. Brain Res. 807 (1), 101–109. doi:10.1016/S0006-8993(98)00766-5
Vigil, J. M., Stith, S. S., Adams, I. M., and Reeve, A. P. (2017). Associations between Medical Cannabis and Prescription Opioid Use in Chronic Pain Patients: A Preliminary Cohort Study. PLOS ONE 12 (11), e0187795. doi:10.1371/journal.pone.0187795
Viudez-Martínez, A., García-Gutiérrez, M. S., Navarrón, C. M., Morales-Calero, M. I., Navarrete, F., Torres-Suárez, A. I., et al. (2018). Cannabidiol Reduces Ethanol Consumption, Motivation and Relapse in Mice. Addict. Biol. 23 (1), 154–164. doi:10.1111/adb.12495
Volkow, N. D., Baler, R. D., Compton, W. M., and Weiss, S. R. (2014). Adverse Health Effects of Marijuana Use. N. Engl. J. Med. 370 (23), 2219–2227. doi:10.1056/NEJMra1402309
Volpi-Abadie, J., Kaye, A. M., and Kaye, A. D. (2013). Serotonin Syndrome. Ochsner J. 13 (4), 533–540.
Vos, T., Barber, R. M., Bell, B., Bertozzi-Villa, A., Biryukov, S., Bolliger, I., et al. (2015). Global, Regional, and National Incidence, Prevalence, and Years Lived with Disability for 301 Acute and Chronic Diseases and Injuries in 188 Countries, 1990-2013: a Systematic Analysis for the Global Burden of Disease Study 2013. Lancet 386 (9995), 743–800. doi:10.1016/S0140-6736(15)60692-4
Wade, D. T., Makela, P., Robson, P., House, H., and Bateman, C. (2004). Do cannabis-based Medicinal Extracts Have General or Specific Effects on Symptoms in Multiple Sclerosis? A Double-Blind, Randomized, Placebo-Controlled Study on 160 Patients. Mult. Scler. 10 (4), 434–441. doi:10.1191/1352458504ms1082oa
Wade, D. T., Makela, P. M., House, H., Bateman, C., and Robson, P. (2006). Long-term Use of a Cannabis-Based Medicine in the Treatment of Spasticity and Other Symptoms in Multiple Sclerosis. Mult. Scler. 12 (5), 639–645. doi:10.1177/1352458505070618
Wallace, M. J., Blair, R. E., Falenski, K. W., Martin, B. R., and DeLorenzo, R. J. (2003). The Endogenous Cannabinoid System Regulates Seizure Frequency and Duration in a Model of Temporal Lobe Epilepsy. J. Pharmacol. Exp. Ther. 307 (1), 129–137. doi:10.1124/jpet.103.051920
Walsh, S., Mnich, K., Mackie, K., Gorman, A. M., Finn, D. P., and Dowd, E. (2010). Loss of Cannabinoid CB1 Receptor Expression in the 6-Hydroxydopamine-Induced Nigrostriatal Terminal Lesion Model of Parkinson's Disease in the Rat. Brain Res. Bull. 81 (6), 543–548. doi:10.1016/j.brainresbull.2010.01.009
Walton, C., King, R., Rechtman, L., Kaye, W., Leray, E., Marrie, R. A., et al. (2020). Rising Prevalence of Multiple Sclerosis Worldwide: Insights from the Atlas of MS, Third Edition. Mult. Scler. 26 (14), 1816–1821. doi:10.1177/1352458520970841
Wang, H. T., Han, F., and Shi, Y. X. (2009). Activity of the 5-HT1A Receptor Is Involved in the Alteration of Glucocorticoid Receptor in hippocampus and Corticotropin-Releasing Factor in Hypothalamus in SPS Rats. Int. J. Mol. Med. 24 (2), 227–231. doi:10.3892/ijmm_00000225
Wang, Q., Liu, Y., and Zhou, J. (2015). Neuroinflammation in Parkinson's Disease and its Potential as Therapeutic Target. Transl Neurodegener 4 (1), 19. doi:10.1186/s40035-015-0042-0
Ward, S. J., McAllister, S. D., Kawamura, R., Murase, R., Neelakantan, H., and Walker, E. A. (2014). Cannabidiol Inhibits Paclitaxel-Induced Neuropathic Pain through 5-HT(1A) Receptors without Diminishing Nervous System Function or Chemotherapy Efficacy. Br. J. Pharmacol. 171 (3), 636–645. doi:10.1111/bph.12439
Ward, S. J., Ramirez, M. D., Neelakantan, H., and Walker, E. A. (2011). Cannabidiol Prevents the Development of Cold and Mechanical Allodynia in Paclitaxel-Treated Female C57Bl6 Mice. Anesth. Analg 113 (4), 947–950. doi:10.1213/ANE.0b013e3182283486
Ware, M. A., Wang, T., Shapiro, S., Collet, J. P., Boulanger, A., Esdaile, J. M., et al. (2015). Cannabis for the Management of Pain: Assessment of Safety Study (COMPASS). J. Pain 16 (12), 1233–1242. doi:10.1016/j.jpain.2015.07.014
Ware, M. A., Wang, T., Shapiro, S., Robinson, A., Ducruet, T., Huynh, T., et al. (2010). Smoked Cannabis for Chronic Neuropathic Pain: A Randomized Controlled Trial. CMAJ 182 (14), E694–E701. doi:10.1503/cmaj.091414
Weber, M., Goldman, B., and Truniger, S. (2010). Tetrahydrocannabinol (THC) for Cramps in Amyotrophic Lateral Sclerosis: A Randomised, Double-Blind Crossover Trial. J. Neurol. Neurosurg. Psychiatry 81 (10), 1135–1140. doi:10.1136/jnnp.2009.200642
Weissman, M. M. (1988). The Epidemiology of Anxiety Disorders: Rates, Risks and Familial Patterns. J. Psychiatr. Res. 22 (Suppl. 1), 99–114. doi:10.1016/0022-3956(88)90071-4
Weydt, P., Hong, S., Witting, A., Möller, T., Stella, N., and Kliot, M. (2005). Cannabinol Delays Symptom Onset in SOD1 (G93A) Transgenic Mice without Affecting Survival. Amyotroph. Lateral Scler. Other Mot. Neuron Disord 6 (3), 182–184. doi:10.1080/14660820510030149
Wise, R. A., and Robble, M. A. (2020). Dopamine and Addiction. Annu. Rev. Psychol. 71, 79–106. doi:10.1146/annurev-psych-010418-103337
Wotherspoon, G., Fox, A., McIntyre, P., Colley, S., Bevan, S., and Winter, J. (2005). Peripheral Nerve Injury Induces Cannabinoid Receptor 2 Protein Expression in Rat Sensory Neurons. Neuroscience 135 (1), 235–245. doi:10.1016/j.neuroscience.2005.06.009
Xi, Z. X., Peng, X. Q., Li, X., Song, R., Zhang, H. Y., Liu, Q. R., et al. (2011). Brain Cannabinoid CB₂ Receptors Modulate Cocaine's Actions in Mice. Nat. Neurosci. 14 (9), 1160–1166. doi:10.1038/nn.2874
Xu, D. H., Cullen, B. D., Tang, M., and Fang, Y. (2020). The Effectiveness of Topical Cannabidiol Oil in Symptomatic Relief of Peripheral Neuropathy of the Lower Extremities. Curr. Pharm. Biotechnol. 21 (5), 390–402. doi:10.2174/1389201020666191202111534
Yagishita, S. (2020). Transient and Sustained Effects of Dopamine and Serotonin Signaling in Motivation-Related Behavior. Psychiatry Clin. Neurosci. 74 (2), 91–98. doi:10.1111/pcn.12942
Yiangou, Y., Facer, P., Durrenberger, P., Chessell, I. P., Naylor, A., Bountra, C., et al. (2006). COX-2, CB2 and P2X7-Immunoreactivities Are Increased in Activated Microglial Cells/macrophages of Multiple Sclerosis and Amyotrophic Lateral Sclerosis Spinal Cord. BMC Neurol. 6, 12. doi:10.1186/1471-2377-6-12
Yohrling IV, G. J., Jiang, G. C., DeJohn, M. M., Robertson, D. J., Vrana, K. E., and Cha, J. H. (2002). Inhibition of Tryptophan Hydroxylase Activity and Decreased 5-HT1A Receptor Binding in a Mouse Model of Huntington's Disease. J. Neurochem. 82 (6), 1416–1423. doi:10.1046/j.1471-4159.2002.01084.x
Yoshimoto, K., McBride, W. J., Lumeng, L., and Li, T. K. (1992). Alcohol Stimulates the Release of Dopamine and Serotonin in the Nucleus Accumbens. Alcohol 9 (1), 17–22. doi:10.1016/0741-8329(92)90004-T
Zajicek, J. P., Hobart, J. C., Slade, A., Barnes, D., and Mattison, P. G., and MUSEC Research Group. (2012). Multiple Sclerosis and Extract of Cannabis: Results of the MUSEC Trial. J. Neurol. Neurosurg. Psychiatry 83 (11), 1125–1132. doi:10.1136/jnnp-2012-302468
Zarei, S., Carr, K., Reiley, L., Diaz, K., Guerra, O., Altamirano, P. F., et al. (2015). A Comprehensive Review of Amyotrophic Lateral Sclerosis. Surg. Neurol. Int. 6, 171. doi:10.4103/2152-7806.169561
Zhang, J., Hoffert, C., Vu, H. K., Groblewski, T., Ahmad, S., and O'Donnell, D. (2003). Induction of CB2 Receptor Expression in the Rat Spinal Cord of Neuropathic but Not Inflammatory Chronic Pain Models. Eur. J. Neurosci. 17 (12), 2750–2754. doi:10.1046/j.1460-9568.2003.02704.x
Zhao, P., Ignacio, S., Beattie, E. C., and Abood, M. E. (2008). Altered Presymptomatic AMPA and Cannabinoid Receptor Trafficking in Motor Neurons of ALS Model Mice: Implications for Excitotoxicity. Eur. J. Neurosci. 27 (3), 572–579. doi:10.1111/j.1460-9568.2008.06041.x
Zou, Z., Wang, H., d'Oleire Uquillas, F., Wang, X., Ding, J., and Chen, H. (2017). Definition of Substance and Non-substance Addiction. Adv. Exp. Med. Biol. 1010, 21–41. doi:10.1007/978-981-10-5562-1_2
Keywords: cannabinoid 1 receptor, cannabinoid 2 receptor, serotonin 1a receptor, clinical research, addiction, pain, neurodegeneration, anxiety
Citation: Ortiz YT, McMahon LR and Wilkerson JL (2022) Medicinal Cannabis and Central Nervous System Disorders. Front. Pharmacol. 13:881810. doi: 10.3389/fphar.2022.881810
Received: 23 February 2022; Accepted: 05 April 2022;
Published: 21 April 2022.
Edited by:
Shimon Ben -Shabat, Ben-Gurion University of the Negev, IsraelReviewed by:
Ethan Budd Russo, CReDO Science, United StatesMaria Scherma, University of Cagliari, Italy
Copyright © 2022 Ortiz, McMahon and Wilkerson. This is an open-access article distributed under the terms of the Creative Commons Attribution License (CC BY). The use, distribution or reproduction in other forums is permitted, provided the original author(s) and the copyright owner(s) are credited and that the original publication in this journal is cited, in accordance with accepted academic practice. No use, distribution or reproduction is permitted which does not comply with these terms.
*Correspondence: Jenny L. Wilkerson, amVubnkud2lsa2Vyc29uQHR0dWhzYy5lZHU=