- 1Department of Oral Surgery and Oral Medicine, Institute of Clinical Odontology, University of Oslo, Oslo, Norway
- 2Institute of Oral Biology, University of Oslo, Oslo, Norway
- 3Section for Pharmaceutics and Social Pharmacy, Department of Pharmacy, The Faculty of Mathematics and Natural Sciences, University of Oslo, Oslo, Norway
The unique properties and applications of nanotechnology in targeting drug delivery, cosmetics, fabrics, water treatment and food packaging have received increased focus the last two decades. The application of nanoparticles in medicine is rapidly evolving, requiring careful investigation of toxicity before clinical use. Chitosan, a derivative of the natural polysaccharide chitin, has become increasingly relevant in modern medicine because of its unique properties as a nanoparticle. Chitosan is already widely used as a food additive and in food packaging, bandages and wound dressings. Thus, with an increasing application worldwide, cytotoxicity assessment of nanoparticles prepared from chitosan is of great interest. The purpose of this review is to provide an updated status of cytotoxicity studies scrutinizing the safety of chitosan nanoparticles used in biomedical research. A search in Ovid Medline from 23 March 1998 to 4 January 2022, with the combination of the search words Chitosan or chitosan, nanoparticle or nano particle or nanosphere or nanocapsule or nano capsule, toxicology or toxic or cytotoxic and mucosa or mucous membrane resulted in a total of 88 articles. After reviewing all the articles, those involving non-organic nanoparticles and cytotoxicity assays conducted exclusively on nanoparticles with anti-tumor effect (i.e., having cytotoxic effect) were excluded, resulting in 70 articles. Overall, the chitosan nanoparticles included in this review seem to express low cytotoxicity regardless of particle composition or cytotoxicity assay and cell line used for testing. Nonetheless, all new chitosan derivatives and compositions are recommended to undergo careful characterization and cytotoxicity assessment before being implemented on the market.
1 Nanotechnology and Chitosan
Nanotechnology is rapidly expanding, and the global nanotechnology market has increased its market value by tenfold, from 1.8 billion USD in 2020 to an expected level of more than 33 billion USD in 2030 (Divyanshi Tewari, 2019). Properties of nanomaterials may differ from bulk material, because of their small size, large surface area and polydispersity. Compared to bulk particles with <1% of total atoms on the surface, >80% of total atoms are on the surface of nanoparticles (NPs), offering new biological properties (Singh, 2016). Thus, the surface atoms will influence particle properties and size, and lead to shape-dependent physicochemical properties (Singh, 2016).
Chitosan, a derivative of the natural polysaccharide chitin, is the second most abundant polysaccharide in the world, after cellulose. Because of properties like biocompatibility, biodegradability, antibacterial effect and muco-adhesion, chitosan is widely used in food, cosmetics, fabrics, water treatment and biomedical applications (Elieh-Ali-Komi and Hamblin, 2016). The United States Food and Drug Administration (US-FDA) and EU have approved chitosan as a food additive, fat absorption material and wound dressing (Mohammed et al., 2017). Chitosan and its derivatives are found in several products on the market today, such as food additives (LipoSan UltraTM, Primex), cosmetics (ChitoCareTM, Primex), antibacterial agents (ChitocellTM, ChitoTech), haemostatic dressings (AxiostatTM, Axio), wound healing materials (OpticellTM, Medline) and oral solutions (MoisynTM, Prisyna). The global chitosan market is estimated to have an annually growth of 25% between 2020 and 2027, which will result in a market size of 29 billion USD in 2027 (Grand View Research, 2020).
Previous in vivo toxicity studies on chitosan as bulk material show low toxicity, but nanoparticles possess new biological properties such as high surface-to-area ratio, thus new safety evaluations are called for. The purpose of this review is to provide an updated status on the toxicity of chitosan nanoparticles used in biomedical research.
A search in Ovid Medline, a search engine specialized for biomedical research, at 4 January 2022 with the search words chitosan showed 24,793 results, after specifying the search by combining the words Chitosan or chitosan, nanoparticle or nano particle or nanosphere or nanocapsule or nano capsule, toxicology or toxic or cytotoxic and mucosa or mucous membrane, the result was 88 articles. After applying the exclusion criteria non-organic nanoparticles and studies that evaluated cytotoxicity only of nanoparticles incorporated with anti-tumor effect (i.e., having cytotoxic effect), a set of 70 articles remained to be included and discussed in this overview.
2 Chitin, Chitosan and Chitosan Nanoparticles
Chitin is a natural polysaccharide consisting of the two monosaccharides N-acetyl-D-glucosamine and D-glucosamine, connected by β-1,4-glycosidic bonds. Chitin is mainly found in oceans as a constituent of shells and crustaceans, but is also found in insects, algae, bacteria and fungi. Chitin has a supporting function in cell walls and exhibits many of the same functions as cellulose. The most common sources of commercial chitin are crab and shrimp shells, and it can therefore conveniently be prepared from wastes of seafood processing industries. The content of chitin ranges from 6 to 72% in crustacean shells, crabs and shrimps, dependent on the species (van den Broek et al., 2020). The isolation of chitin is relatively time and energy consuming and is environmentally polluting as it involves hazardous chemicals. The shell isolation process may vary depending on species, but consists mainly of washing, drying, demineralization with hydrochloric acid (HCl) and deproteination with sodium hydroxide before removing pigments (Kurita, 2001). Chitin is insoluble in many solvents, and great attention has been given to convert chitin into more soluble derivatives, the simplest modification being N-deacetylation, which converts chitin into chitosan (Figure 1).
Chitosan has the ability to interact electrostatically with negatively charged molecules, such as cells, nanoparticles, lipids, drugs and polymers because of the functional amino groups on the surface of the molecule (Nurunnabi et al., 2017). The pKa of chitosan is 6.3 and consequently it is soluble in acidic solutions and insoluble in basic conditions, and at pH 6.0–6.5 chitosan will self-aggregate (Kumar et al., 2004). Since only the non-acetylated amino groups are able to bind protons, the solubility of chitosan is mainly dependent on the degree of deacetylation (number of glucosamine units after deacetylation), but also on the ionic strength and the distribution of acetyl groups along the chain (Berth and Dautzenberg, 2002). The reactivity of chitosan is mainly affected by the molecular weight, degree of deacetylation and pH (Jana and Jana, 2020).
Nanoparticles are particles of small size, from 1 to 100 nanometers (nm), but the term is often used for larger particle sizes described in nm. Active substances encapsulated in nanoparticles are concealed from its surroundings, and can be transported incognito to specific sites, depending on the nanoparticle surface properties. Chitosan nanoparticles are especially interesting because of their mucoadhesive properties, positive surface charge and ability to open tight junctions between cells (Liu et al., 2008; Nurunnabi et al., 2017). In medical research, chitosan nanoparticles are promising agents as targeted delivery vehicles for drugs, adjuvants and delivery carriers for vaccines (Prabaharan and Mano, 2005; Amidi et al., 2010). Chitosan nanoparticles are of great interest as oral drug carriers for proteins, as they are capable of preventing enzymatic degradation in the gastrointestinal system and facilitating mucoadhesion to the intestinal mucus layer (Janes et al., 2001; Amidi et al., 2010). Several articles in this review investigated the use of chitosan nanoparticles in ocular-targeted drug delivery, drug delivery over the blood-brain barrier, targeted delivery of bio-imaging markers and vaccination by oral- and intranasal administration (de Campos et al., 2004; Amidi et al., 2006; Borges et al., 2006; Diebold et al., 2007; Sayin et al., 2008; Saremi et al., 2011; Cheng et al., 2012; Patel et al., 2012; Chen et al., 2013a; Dehghan et al., 2013; Ye et al., 2013; Zhao et al., 2014; Liu et al., 2015; da Silva et al., 2016; Shah et al., 2016; Bor et al., 2017; Jin et al., 2017; Pandit et al., 2017; Shi et al., 2017; Zhao et al., 2017; Çelik Tekeli et al., 2018; Cole et al., 2018; Tandberg et al., 2018; Bento et al., 2019; Sinani et al., 2019; Tzeyung et al., 2019). A considerable amount of research on chitosan nanoparticles in cancer medicine has also been conducted, in order to decrease the side effects by encapsulating chemotherapeutics in chitosan nanoparticles, and to enhance the oral bioavailability of anti-cancer drugs (Akhlaghi et al., 2010; Guo et al., 2013; Battogtokh and Ko, 2014; Jain et al., 2015; Khan et al., 2019). Chitosan can act as coating material together with other materials or be the core material in the nanoparticle itself (nanosphere or nanocapsule).
3 Cytotoxicity Measurements
Cytotoxicity studies are divided into in vitro- and in vivo studies, depending on whether the study is performed on cultured cells or tissues in the laboratory or in live animals, respectively. Some of the factors that influence the choice of cytotoxicity methods are exposure duration, amount and frequency of substance exposure, the type of exposed tissues and results from previous toxicity studies. It is generally accepted that animal testing should be replaced with in vitro studies as far as possible for ethical considerations, but it may still be necessary to evaluate animal testing in specific end-points. The most used in vitro cytotoxicity methods in the included studies are different assays based on colorimetric readings of cell activity, with the MTT-assay (3-(4,5-dimethylthiazol-2-yl)-2,5-diphenyltetrazolium bromide reagent assay) being the far most frequently used. For the in vivo studies, clinical investigation such as weight, appetite and behavior, in addition to macroscopic and histologic assessment of the test animals, are the most frequently used methods.
4 Results of Cytotoxicity Measurements on Chitosan Containing Nanoparticles
Cytotoxicity studies regarding nanoparticles containing chitosan are presented below. The nanoparticle composition and chitosan type vary significantly in the selection of articles. Four different nanoparticle structures frequently mentioned in the articles are illustrated in Figure 2. In the following presentation, the articles are categorized into sections according to the nanoparticle composition. Each chapter includes a summary of the main findings concerning cytotoxicity of the specific group of nanoparticles, and a table of the main features from articles included in the section. The tables are sorted by molecular weight (MW), from small to large, and by study design (in vitro/in vivo). The first two sections present chitosan as a nanoparticle, with and without tripolyphosphate (TPP) as crosslinker, which constitute the major group in this review. The two next sections present chitosan in combination with liposomes and nanoparticles coated with chitosan. The following three sections include three of the most common derivatives of chitosan; carboxymethylated-, quaternizied- and thiolated chitosan. The last section describes other derivatives and complexes of chitosan nanoparticles.
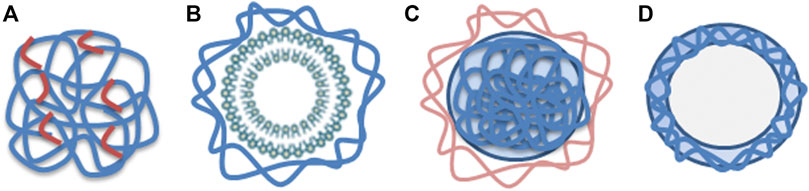
FIGURE 2. (A) Nanosphere composed of chitosan (blue) with crosslinkers (red), (B) Liposome (green) with chitosan coating (blue), (C) Chitosan nanoparticle (blue) covered with other substance (light brown) such as proteins or polymers, (D) Nanocapsule made of chitosan (blue).
4.1 Chitosan Nanoparticles With Tripolyphosphate as Crosslinker
For cytotoxicity of chitosan nanoparticles with TPP as crosslinker 25 articles were retrieved, one in vivo-, four ex vivo- and 20 in vitro studies. The main findings from the articles concerning the chitosan nanoparticles with TPP as crosslinker are presented in Table 1. Four of the articles investigated the cytotoxicity of chitosan nanoparticles using Caco-2 cells (human colorectal adenocarcinoma cells) and the MTT-assay. All studies showed good cell viability (>80%) for particles ranging from 126 to 1,000 nm (Zheng et al., 2011; Loh et al., 2012; Jain et al., 2015; Je et al., 2017). In one of the studies, the cell viability was lower in pH 6 than in pH 7.4. The surface charge was approximately the same, but the particle size was significantly smaller in pH 6 (25 ± 7 nm, 5.3 ± 2.8 mV) than in pH 7.4 (333 ± 43 nm, 3.3 ± 0.4 mV) (Loh et al., 2012). The authors suggested that particle size had more influence on the cytotoxicity in Caco-2 cells than the positive surface charge, because of easier cellular uptake of small particles than larger ones (Loh et al., 2012). This is in accordance with Zheng et al. (2011) who showed that chitosan nanoparticles as compared to chitosan molecules accumulated to a higher extent intracellularly, but in spite of high intracellular concentration of chitosan nanoparticles, the Caco-2 cells showed good viability. Another study reported no difference in cytotoxicity when comparing chitosan nanoparticles of increasing size from 200 to 1,000 nm (Je et al., 2017). These results may indicate that size-dependent cytotoxicity may be more profound when considering nanoparticles in the lower range (<200 nm) (Rejman et al., 2004). But the suggestion may be reserved for Caco-2 cells, as another study of Loh et al. (2010) showed >90% cell viability for human liver cells (BHAL) after incubation with chitosan nanoparticles of 18 and 25 nm.
No toxicity or structural damage was detected in any of the ex vivo studies (Onnainty et al., 2016; Shah et al., 2016; Wardani et al., 2018; Tzeyung et al., 2019). One of them demonstrated that an active ingredient (hydrochlorothiazid) became less toxic when incorporated into chitosan nanoparticles, compared to the free form (Onnainty et al., 2016), and another study showed that chitosan nanoparticles exhibited a protective effect against free radicals (Wardani et al., 2018). An in vivo study in mice demonstrated that the chitosan nanoparticles were well tolerated, as no inflammation or pathological changes were detected (Sonaje et al., 2009).
All 25 articles, except one, showed that chitosan nanoparticles (126–1,000 nm) expressed low cytotoxicity (>80% viability) in concentrations ranging from 0.01 to 10,000 µg/ml when evaluated in vitro. In the in vivo study, up to 100 mg/kg of chitosan nanoparticles were assessed as safe. The only work that showed a somewhat low cell viability was a study where Calu-6 cells were incubated with dry powder chitosan nanoparticles of 250 and 500 µg/ml for 24 h (Dehghan et al., 2013). The Calu-6 cell line is from anaplastic carcinoma with unknown origin, probably the lung. When comparing this finding with the results from another cancer cell line from lungs (Calu-3), the chitosan nanoparticles showed low cytotoxicity at 4 h, and even lower at 48 h (Ye et al., 2013). Recovery of cell viability was also observed in another study, where the cell viability of Caco-2 cells increased from 30% to >80% after 48 h of incubation (Loh et al., 2012). The potential recovery of the Calu-6 cells is not possible to assess because the cells were not incubated for more than 24 h.
4.2 Chitosan Nanoparticles Without Tripolyphosphate as Crosslinker
Five articles evaluated the cytotoxicity of chitosan nanoparticles without TPP as crosslinker, consisting of four in vitro- and three in vivo studies. See Table 2 for main findings and details from the articles on chitosan nanoparticles without TPP as crosslinker. All in vitro studies demonstrated good cell viability and low cytotoxicity (Borges et al., 2006; Zhao et al., 2014; Bento et al., 2019; Mumuni et al., 2020). In one of the studies, the nanoparticle-exposed cells showed higher metabolic activity compared to the control, but no cytotoxicity up to 2 mg/ml was detected (Borges et al., 2006).
Zhao et al. (2014) performed a randomized controlled trial (RCT) to evaluate the in vivo safety of the Newcastle-disease-virus-F-gene-DNA vaccine encapsulated in chitosan nanoparticles. Thirty chickens were observed for 3 weeks, showing no clinical symptoms, nervous signs or histopathological changes. The nanoparticles were therefore considered safe (Zhao et al., 2014). This is in agreement with a second RCT where growth and health performance of the Nile tilapia (fish fingerlings) were investigated after adding chitosan and thymol to a basal fish diet (Abd El-Naby et al., 2020). After 70 days, there were no significant changes in survival rate in any of the groups, compared to the control group.
4.3 Chitosan in Combination With Liposomes
Liposomes are small artificial sphere-shaped vesicles consisting of one or more phospholipid bilayers. The phospholipids may be derived from natural compounds such as soya and egg, or tissue from bovines, or they can be synthetic. The properties of the liposomes depend on the lipid components. Thus, qualities such as charge, permeability and stability can be engineered. Liposomes have the ability to encapsulate both hydrophilic and hydrophobic substances due to their unique composition with both hydrophilic and hydrophobic parts (Akbarzadeh et al., 2013). Chitosan can interact spontaneously with negatively charged liposomes due to functional amino groups on the chitosan molecule, and by such coat the liposomes (Pistone et al., 2017a).
Five papers concerning the cytotoxicity of liposomes in combination with chitosan were identified; three in vitro studies (Adamczak et al., 2016; Klemetsrud et al., 2018; Khan et al., 2019) and two with both in vivo and in vitro studies (Diebold et al., 2007; Chen et al., 2013a). Cytotoxicity studies regarding nanoparticles with liposomes and chitosan are displayed in Table 3. All the studies used different cell lines and test animals. Four of the articles concluded with low toxicity, high degree of biocompatibility and good tolerance (Diebold et al., 2007; Chen et al., 2013a; Adamczak et al., 2016; Khan et al., 2019). On the contrary, one of the papers demonstrated 10% cell viability after incubation with chitosan coated liposomes (chitosan conc. 0.5%) (Klemetsrud et al., 2018). Interestingly, in another paper, the same nanoparticles but with lower concentration of chitosan (0.1%) showed no reduction in cell viability using both confluent and diluted cell samples in three different cell viability tests (Adamczak et al., 2016). In both papers, the coating of the liposomes was achieved by adding the negatively charged liposomes dropwise into the positively charged chitosan solution, inducing spontaneous formation of chitosan-coated liposomes. Due to up-concentration of the samples in one of the studies the chitosan concentration ended up much higher than in the other. The cell viability results may therefore reflect the chitosan concentration and the amount of potential free chitosan instead of the toxicity of the chitosan coated liposomes.
In one of the in vivo studies, the passage of fluorescently labelled chitosan/DNA liposomes were traced at different time intervals after intranasal administration in mice (Figure 3). The experiment disclosed nanoparticle clearance via the digestive tract, and no distribution to other organs except the lung was detected (Chen et al., 2013a).
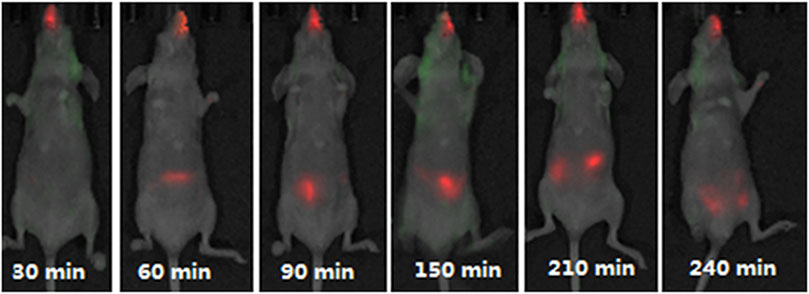
FIGURE 3. Fluorescence detected in mice after intranasal administration of Cy5.5-marked anionic liposome/chitosan/DNA nanoparticles at different time intervals. Figure adopted from Chen et al. (2013) (https://creativecommons.org/licenses/by/4.0/).
4.4 Nanoparticles Coated With Chitosan
Four in vitro and one in vivo study on the cytotoxicity of nanoparticles coated with chitosan were found. The main results from each study are listed in Table 4. Three of the in vitro studies investigated poly (lactic-co-glycolid acid) (PLGA) nanoparticles coated with chitosan. Different cell lines and test animals were used, and the results showed low cytotoxicity and non-irritant properties (Guo et al., 2013; Pandit et al., 2017; Lima et al., 2018). Two of the studies (Guo et al., 2013; Lima et al., 2018) had somewhat conflicting results regarding the non-cytotoxic concentrations (30 vs. 500 µg/ml), but considering that different cell lines were used and that the nanoparticles in one of the studies were loaded with ferulic acid while the others were not, the observed differences should not be overemphasized.
In the in vivo study, no mortality or pathological abnormalities were observed in adult zebrafish after injection with bacterial membrane vesicles coated with chitosan (cMVs) (Tandberg et al., 2018).
4.5 Derivatives of Chitosan
Several chitosan derivatives have been designed to meet desired requirements and to alter the properties of chitosan. Better solubility and mucoadhesion are the most common requirements. The main drawback of chitosan use has been the low solubility at pH > 6, as this limits its use as a nanocarrier in applications that involve higher pH. Mucoadhesion is also a desirable feature for a nanocarrier for local drug delivery, as it increases the residence time of drugs at the site of action, minimizes the degradation of drugs in various sites and gives the opportunity for a sustained drug release (Ways T. et al., 2018).
4.5.1 Carboxymethyl Chitosan
To increase its water solubility, chitosan can be chemically modified into carboxymethyl chitosan (CMC) by incorporating negatively charged carboxyl groups to C-6 hydroxyl groups or the NH2 group of the glucosamine units, as seen in Figure 4. CMC derivatives are regarded as polyampholytic since they contain both cationic and anionic groups (Chen et al., 2013b). The interest in CMC is rapidly increasing, especially in the biomedical and pharmaceutical field due to its antimicrobial and antioxidant properties. Also in cosmetics, CMC is highly interesting because of the moisturizing and protective effects (Shariatinia, 2018).
Six articles with five in vitro and two in vivo studies were identified. The main features of the cytotoxicity studies carried out on nanoparticles containing CMC is seen in Table 5. Cytotoxicity of the same nanoparticles were investigated in three of the six papers, using different cell lines and experimental animals (Chakraborty et al., 2010; Chakraborty et al., 2011; Chakraborty et al., 2012). The other three articles used different cell lines and investigated nanoparticles with chitosan of various molecular weights (Liu et al., 2012; Liu et al., 2013; Li et al., 2021). All nanoparticles showed more than 80% cell viability for all tested concentrations, and results from the two in vivo studies showed no tissue damage or acute toxicity for any of the tested concentrations (up to 1,000 mg/kg).
4.5.2 Quaternized Chitosan
Quaternized chitosan is another large group of chitosan derivatives. Both the hydrophilic and mucoadhesive properties of chitosan are improved by quaternization of the primary amino groups. Quaternization of chitosan conserves its positive charge at neutral pH, thus increasing solubility significantly in a much broader pH and concentration range, compared to unmodified chitosan (Kotzé et al., 1999; Thanou et al., 2001). The simplest form of quaternized chitosan is N,N,N-trimethyl chitosan (TMC).
Seven papers that investigated different quaternized chitosan nanoparticles and their cytotoxicity were identified, four with in vivo studies (Liu et al., 2015; Jin et al., 2017; Zhao et al., 2017; Yan et al., 2020), two with both ex vivo and in vitro studies (Amidi et al., 2006; Yin et al., 2009) and one in vitro study using three different cell lines (Facchinatto et al., 2021). An overview of cytotoxicity studies concerning nanoparticles with quaternized chitosan is seen in Table 6.
The three in vitro and ex vivo studies showed no cytotoxicity in the specific cell lines or after injection of nanoparticles to the ileal loop of rats. One of the studies measured the reversibility of the ciliary beat frequency in chicken embryo trachea after incubation with TMC. A cilio-inhibiting (25%–75%) effect was seen for the highest concentration (40 mg/ml), but after a concentration adjustment to meet the natural environment (8 mg/ml), the results turned to cilio-friendly (>70%) (Amidi et al., 2006). For all three studies, the nanoparticles showed less cytotoxicity than free TMC.
The four in vivo studies showed no obvious toxicity, no pathological changes and no difference in hematological or biochemical parameters from the control group, indicating high level of safety when nanoparticles were administrated intranasally, orally or intramuscularly to mice, rats and chickens (Liu et al., 2015; Jin et al., 2017; Zhao et al., 2017; Yan et al., 2020). In one of the studies, TMC nanoparticles loaded with low molecular weight heparin (LMWH) reversed a drug-induced colitis in mice when the mice were treated orally for 15 days, while mice treated with free LMWH showed no signs of recovery (Yan et al., 2020).
4.5.3 Thiolated Chitosan
Thiolated chitosan is synthesized by covalently coupling sulfhydryl bearing agents such as cysteine, thioglycolic acid or glutathione onto the backbone of chitosan. Thiolated chitosan improves the mucoadhesion properties by forming disulfide units both with glycoproteins of the mucus substrate and the polymer chains (Chen et al., 2013b). The improved mucoadhesive properties make thiolated chitosan attractive for oral delivery of macromolecules. Improved mucoadhesive properties, in combination with permeation properties, enhance the bioavailability of drugs by prolonged residence time and controlled release of the drug (Sakloetsakun et al., 2010; Millotti et al., 2011). As seen in Table 7, the majority of cytotoxicity studies conducted on thiolated chitosan nanoparticles are transmucosal studies with Caco-2 cells.
Nine articles concerning cytotoxicity of thiolated chitosan nanoparticles were identified, containing nine in vitro studies and two ex vivo studies, while five of these involved the use of Caco-2 cells (Akhlaghi et al., 2010; Sakloetsakun et al., 2010; Millotti et al., 2011; Pradines et al., 2015a; Noi et al., 2018). All five of these studies showed low cytotoxicity of the thiolated chitosan containing nanoparticles, with the exception of one study that compared non-crosslinked thiolated chitosan nanoparticles to crosslinked thiolated chitosan nanoparticles (Noi et al., 2018). The non-crosslinked as compared to the crosslinked thiolated chitosan nanoparticles expressed very variable cell viability. When the thiolated chitosan nanoparticles were crosslinked, the cell viability increased considerably. The reason for these results may be due to the positively charged surface of the amino group in the non-crosslinked thiolated chitosan that can bind to the negatively charged cell membrane in a cytotoxic manner. In the crosslinked thiolated chitosan, the positively charged surface is neutralized, and the formulation is therefore less cytotoxic. These results are in accordance with previous studies where free chitosan exhibited higher cytotoxicity than crosslinked chitosan, because the charge density of chitosan is reduced by TPP (Pistone et al., 2017b).
Three of the in vitro studies also concluded with no, or reduced, cytotoxicity of thiolated chitosan compared to unthiolated chitosan (Akhlaghi et al., 2010; Millotti et al., 2011; Patel et al., 2012). One of the authors explained the results by referring to the higher solubility of thiolated chitosan, and therefore faster removal from the site of application, compared to non-thiolated chitosan (Patel et al., 2012). One of the ex vivo studies showed that the herb extract Centella asiatica demonstrated corrosive action comparable to the positive control (isopropyl alcohol) when it was exposed to the nasal mucosa of goats (Haroon et al., 2021). When the same extract was loaded into thiolated chitosan nanoparticles, no erosion or necrosis was detected, and the same results were seen for the unloaded nanoparticles.
In another study, three different cell lines were exposed to chitosan- and thiolated-chitosan coated PIBCA (poly (isobytylcyanoacrylate)) nanoparticles (Pradines et al., 2015a). Both nanoparticles expressed high cytotoxicity towards HeLa cells, but the reason was assumed to be the core nanoparticle (PIBCA) because the same cytotoxicity profile was seen in uncoated PIBCA nanoparticles. The same nanoparticles were investigated in situ using pig vaginal mucosa, with no toxicity detected (Pradines et al., 2015b).
4.6 Other Derivatives and Complexes With Chitosan
Eight papers concerning the cytotoxicity of other complexes of chitosan nanoparticles were obtained, five in vitro studies (Müller et al., 2000; Cheng et al., 2012; Battogtokh and Ko, 2014; Nguyen et al., 2015; Lim et al., 2018), and three in vivo studies (Yan et al., 2019; Mosa et al., 2020; Thai et al., 2020). The complexes in this section are nanoparticles made of chitosan and an active ingredient such as a contrast agent or curcumin, and solid lipid nanoparticles (SLNs) which are hydrophobic nanoparticles based on solid lipid components (Müller et al., 2000). One of the papers investigate the chitosan derivative glycol chitosan and one investigates chitosan nanoparticles with unknown specifications. An overview of the papers on cytotoxicity of nanoparticles of other derivatives and complexes of chitosan, with main findings, is seen in Table 8. The five in vitro studies used different cell lines, but they all expressed high cell viability when incubated with the chitosan nanoparticles. The three in vivo studies also indicated low toxicity to rats and mice, with no histological changes compared to the negative control, as seen in Figure 5 (Thai et al., 2020). No alterations in hematological or biochemical parameters compared to the control were detected in any of the in vivo studies (Yan et al., 2019; Mosa et al., 2020; Thai et al., 2020). The medial lethal dose (LD50) of lovastatin loaded nanoparticles was greater than 5,000 mg/kg when administrated orally to mice, and therefore considered nontoxic (Thai et al., 2020).
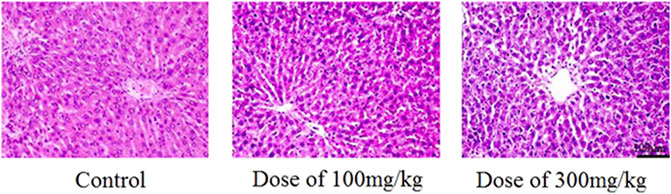
FIGURE 5. Histological HE-staining of liver from rats after 28 days of oral treatment with and without Alginate/Chitosan/Lovastatin nanoparticles, in two different concentrations (100 and 300 mg/kg). Figure adopted from Thai et al. (2020) (https://creativecommons.org/licenses/by/4.0/).
In addition to expressing low toxicity in several in vivo studies, chitosan nanoparticles (280 mg/kg bw) showed an anti-inflammatory activity by significantly reducing the gastric toxic effect induced by hydroxyapatite nanoparticles in rats (Mosa et al., 2020).
5 Discussion
In this overview, 55 papers with in vitro studies were identified involving nanoparticles that were exposed to more than 30 different cell lines. Only two studies showed somewhat reduced cell viability after incubation with chitosan nanoparticles (Dehghan et al., 2013; Klemetsrud et al., 2018). Several of the papers demonstrated that chitosan in nanoparticle form was less cytotoxic than chitosan in free form (Amidi et al., 2006; Yin et al., 2009; Pistone et al., 2017b; Facchinatto et al., 2021). The active ingredient (clotrimazole and hydrochlorothiazide) also showed less cytotoxicity when incorporated in chitosan nanoparticles (Onnainty et al., 2016; Facchinatto et al., 2021). Reduced toxicity of the active ingredient (Centella asiatica) was also seen after incorporation into chitosan nanoparticles ex vivo (Haroon et al., 2021).
Regarding the in vivo studies, all 17 studies showed low toxicity of chitosan nanoparticles independent of administration method, even in high doses (5,000 mg/kg bw). In one of the studies, chitosan nanoparticles even significantly reduced several of the toxic parameters induced by hydroxyapatite NPs (Mosa et al., 2020), and in another study the chitosan nanoparticles exhibited a protective effect against free radicals (Wardani et al., 2018).
The available data regarding the cytotoxicity of chitosan nanoparticles are challenging to compare and summarize due to the vast variation of several factors, such as chitosan properties (molecular weight and deacetylation degree), chitosan derivatives, nanoparticle composition, cell lines, experimental animals and cytotoxicity assays. Several of the collected papers lack details on chitosan properties, such as molecular weight and deacetylation degree, which makes it difficult to draw clear conclusions when it comes to chitosan properties and cytotoxicity.
The pH seems to be an important parameter to consider when evaluating the cytotoxicity, because of its ability to influence particle size and zeta potential. This was demonstrated by Loh et al. (2012) where the viability of Caco-2 cells dropped from 80% to 20% for the same nanoparticles in pH 7.4 and 6.0, respectively. As an example, the pH in the gastrointestinal tract varies from 1 to 8. Therefore, it may be necessary to evaluate the cytotoxicity of nanoparticles in a wide range of pH dependent on the desired exposure route (Jana and Jana, 2020).
Considering the majority of in vitro studies, their shortcomings, such as lack of biologic complexity, should be considered and the cytotoxicity results interpreted thereafter. Additionally, the various cell lines may demonstrate different sensitivity towards the same chitosan nanoparticles, as observed in Loh et al. (2010), Loh et al. (2012) where the same nanoparticles showed low viability in Caco-2 cells but good viability in BHAL cells. This was also the case with Klemetsrud et al. (2018) and Adamczak et al. (2016) where the same nanoparticles expressed different cell cytotoxicity towards two different cell lines. The nanoparticles expressed low cytotoxicity towards the mucin producing HT29-MTX cell line, compared to the non-mucin producing TR146 cell line. But the results could also be due to different concentrations of chitosan. In vitro models containing multiple cell layers and mucous-like features may mimic biological complexity in a more realistic manner, such as EpiskinTM (L’Oréal) or 3D cell culture models (Teubl et al., 2013; De Souza, 2018). Either way, choosing a relevant cell line to the area of use should give results that are more applicable to the final use.
In summary, in spite of all the challenges with comparing the results from different tests and methods, the majority of chitosan nanoparticles demonstrated low cytotoxicity regardless of particle composition, derivatives, cytotoxicity assay, cell lines and animals used in both in vitro and in vivo studies. Furthermore, chitosan-based nanoparticles have been shown to be less cytotoxic compared to free chitosan, which should strengthen the hypothesis that chitosan nanoparticles are safe. In view of the fact that free chitosan is already on the marked, with increasing demand worldwide, chitosan nanoparticles seem to be a safe and upcoming product. Considering the extensive variation of chitosan and nanoparticle composition in this review, thorough cytotoxicity evaluation should still be performed for all new chitosan-containing nanoparticles in medicine.
Author Contributions
JF authored the draft, and all authors contributed to the manuscript revision, and read and approved the submitted version.
Conflict of Interest
The authors declare that the research was conducted in the absence of any commercial or financial relationships that could be construed as a potential conflict of interest.
Publisher’s Note
All claims expressed in this article are solely those of the authors and do not necessarily represent those of their affiliated organizations, or those of the publisher, the editors and the reviewers. Any product that may be evaluated in this article, or claim that may be made by its manufacturer, is not guaranteed or endorsed by the publisher.
References
Abd El-Naby, A. S., Al-Sagheer, A. A., Negm, S. S., and Naiel, M. A. E. (2020). Dietary Combination of Chitosan Nanoparticle and Thymol Affects Feed Utilization, Digestive Enzymes, Antioxidant Status, and Intestinal Morphology of Oreochromis niloticus. Aquaculture 515, 734577. doi:10.1016/j.aquaculture.2019.734577
Adamczak, M. I., Hagesaether, E., Smistad, G., and Hiorth, M. (2016). An In Vitro Study of Mucoadhesion and Biocompatibility of Polymer Coated Liposomes on HT29-MTX Mucus-Producing Cells. Int. J. Pharm. 498 (1), 225–233. doi:10.1016/j.ijpharm.2015.12.030
Akbarzadeh, A., Rezaei-Sadabady, R., Davaran, S., Joo, S. W., Zarghami, N., Hanifehpour, Y., et al. (2013). Liposome: Classification, Preparation, and Applications. Nanoscale Res. Lett. 8 (1), 102. doi:10.1186/1556-276X-8-102
Akhlaghi, S. P., Saremi, S., Ostad, S. N., Dinarvand, R., and Atyabi, F. (2010). Discriminated Effects of Thiolated Chitosan-Coated pMMA Paclitaxel-Loaded Nanoparticles on Different normal and Cancer Cell Lines. Nanomedicine 6 (5), 689–697. doi:10.1016/j.nano.2010.01.011
Ali, M. S., Metwally, A. A., Fahmy, R. H., and Osman, R. (2020). Chitosan-Coated Nanodiamonds: Mucoadhesive Platform for Intravesical Delivery of Doxorubicin. Carbohydr. Polym. 245, 116528. doi:10.1016/j.carbpol.2020.116528
Amidi, M., Mastrobattista, E., Jiskoot, W., and Hennink, W. E. (2010). Chitosan-Based Delivery Systems for Protein Therapeutics and Antigens. Adv. Drug Deliv. Rev. 62 (1), 59–82. doi:10.1016/j.addr.2009.11.009
Amidi, M., Romeijn, S. G., Borchard, G., Junginger, H. E., Hennink, W. E., and Jiskoot, W. (2006). Preparation and Characterization of Protein-Loaded N-Trimethyl Chitosan Nanoparticles as Nasal Delivery System. J. Control. Release 111 (1–2), 107–116. doi:10.1016/j.jconrel.2005.11.014
Arancibia, R., Maturana, C., Silva, D., Tobar, N., Tapia, C., Salazar, J. C., et al. (2013). Effects of Chitosan Particles in Periodontal Pathogens and Gingival Fibroblasts. J. Dent Res. 92, 740–745. doi:10.1177/0022034513494816
Battogtokh, G., and Ko, Y. T. (2014). Self-assembled Chitosan-Ceramide Nanoparticle for Enhanced Oral Delivery of Paclitaxel. Pharm. Res. 31 (11), 3019–3030. doi:10.1007/s11095-014-1395-2
Bento, D., Jesus, S., Lebre, F., Gonçalves, T., and Borges, O. (2019). Chitosan Plus Compound 48/80: Formulation and Preliminary Evaluation as a Hepatitis B Vaccine Adjuvant. Pharmaceutics 11 (2), 09. doi:10.3390/pharmaceutics11020072
Berth, G., and Dautzenberg, H. (2002). The Degree of Acetylation of Chitosans and its Effect on the Chain Conformation in Aqueous Solution. Carbohydr. Polym. 47 (1), 39–51. doi:10.1016/s0144-8617(00)00343-x
Bor, G., Üçüncü, M., Emrullahoğlu, M., Tomak, A., and Şanlı-Mohamed, G. (2017). BODIPY-Conjugated Chitosan Nanoparticles as a Fluorescent Probe. Drug Chem. Toxicol. 40 (4), 375–382. doi:10.1080/01480545.2016.1238481
Borges, O., Cordeiro-da-Silva, A., Romeijn, S. G., Amidi, M., de Sousa, A., Borchard, G., et al. (2006). Uptake Studies in Rat Peyer’s Patches, Cytotoxicity and Release Studies of Alginate Coated Chitosan Nanoparticles for Mucosal Vaccination. J. Control. Release 114 (3), 348–358. doi:10.1016/j.jconrel.2006.06.011
Çelik Tekeli, M., Yücel, Ç., Ünal, S., and Aktaş, Y. (2018). Development and Characterization of Insulin-Loaded Liposome-Chitosan-Nanoparticle (LCS-NP) Complex and Investigation of Transport Properties through a Pancreatic Beta Tc Cell Line. Turk J. Pharm. Sci. 15 (1), 91–96. doi:10.4274/tjps.70783
Chakraborty, S. P., Mahapatra, S. K., Sahu, S. K., Pramanik, P., and Roy, S. (2011). Antioxidative Effect of Folate-Modified Chitosan Nanoparticles. Asian Pac. J. Trop. Biomed. 1 (1), 29–38. doi:10.1016/S2221-1691(11)60064-6
Chakraborty, S. P., Sahu, S. K., Mahapatra, S. K., Santra, S., Bal, M., Roy, S., et al. (2010). Nanoconjugated Vancomycin: New Opportunities for the Development of Anti-VRSA Agents. Nanotechnology 21 (10), 105103. doi:10.1088/0957-4484/21/10/105103
Chakraborty, S. P., Sahu, S. K., Pramanik, P., and Roy, S. (2012). Biocompatibility of Folate-Modified Chitosan Nanoparticles. Asian Pac. J. Trop. Biomed. 2 (3), 215–219. doi:10.1016/S2221-1691(12)60044-6
Chen, L., Zhu, J., Li, Y., Lu, J., Gao, L., Xu, H., et al. (2013). Enhanced Nasal Mucosal Delivery and Immunogenicity of Anti-caries DNA Vaccine through Incorporation of Anionic Liposomes in Chitosan/DNA Complexes. PLoS One 8 (8), e71953. doi:10.1371/journal.pone.0071953
Chen, M. C., Mi, F. L., Liao, Z. X., Hsiao, C. W., Sonaje, K., Chung, M. F., et al. (2013). Recent Advances in Chitosan-Based Nanoparticles for Oral Delivery of Macromolecules. Adv. Drug Deliv. Rev. 65 (6), 865–879. doi:10.1016/j.addr.2012.10.010
Cheng, J. J., Zhu, J., Liu, X. S., He, D. N., Xu, J. R., Wu, L. M., et al. (2012). Gadolinium-Chitosan Nanoparticles as a Novel Contrast Agent for Potential Use in Clinical Bowel-Targeted MRI: a Feasibility Study in Healthy Rats. Acta Radiol. 53 (8), 900–907. doi:10.1258/ar.2012.110017
Cole, H., Bryan, D., Lancaster, L., Mawas, F., and Vllasaliu, D. (2018). Chitosan Nanoparticle Antigen Uptake in Epithelial Monolayers Can Predict Mucosal but Not Systemic In Vivo Immune Response by Oral Delivery. Carbohydr. Polym. 190, 248–254. doi:10.1016/j.carbpol.2018.02.084
da Silva, S. B., Ferreira, D., Pintado, M., and Sarmento, B. (2016). Chitosan-Based Nanoparticles for Rosmarinic Acid Ocular Delivery--In Vitro Tests. Int. J. Biol. Macromol. 84, 112–120. doi:10.1016/j.ijbiomac.2015.11.070
de Campos, A. M., Diebold, Y., Carvalho, E. L., Sánchez, A., and Alonso, M. J. (2004). Chitosan Nanoparticles as New Ocular Drug Delivery Systems: In Vitro Stability, In Vivo Fate, and Cellular Toxicity. Pharm. Res. 21 (5), 803–810. doi:10.1023/b:pham.0000026432.75781.cb
Dehghan, S., Tavassoti Kheiri, M., Tabatabaiean, M., Darzi, S., and Tafaghodi, M. (2013). Dry-Powder Form of Chitosan Nanospheres Containing Influenza Virus and Adjuvants for Nasal Immunization. Arch. Pharm. Res. 36 (8), 981–992. doi:10.1007/s12272-013-0043-4
Diebold, Y., Jarrín, M., Sáez, V., Carvalho, E. L., Orea, M., Calonge, M., et al. (2007). Ocular Drug Delivery by Liposome-Chitosan Nanoparticle Complexes (LCS-NP). Biomaterials 28 (8), 1553–1564. doi:10.1016/j.biomaterials.2006.11.028
Divyanshi Tewari, S. B. (2019). Nanotechnology Market by Type (Nanodevices and Nanosensors) and Application (Electronics, Energy, Chemical Manufacturing, Aerospace & Defense, Healthcare, and Others): Global Opportunity Analysis and Industry Forecast, 2018–2025: Allied Market Research. Portland, OR: Allied Market Research. Available at: https://www.alliedmarketresearch.com/nanotechnology-market.
Elieh-Ali-Komi, D., and Hamblin, M. R. (2016). Chitin and Chitosan: Production and Application of Versatile Biomedical Nanomaterials. Int. J. Adv. Res. 4 (3), 411–427.
Facchinatto, W. M., Galante, J., Mesquita, L., Silva, D. S., Martins Dos Santos, D., Moraes, T. B., et al. (2021). Clotrimazole-loaded N-(2-hydroxy)-propyl-3-trimethylammonium, O-Palmitoyl Chitosan Nanoparticles for Topical Treatment of Vulvovaginal Candidiasis. Acta Biomater. 125, 312–321. doi:10.1016/j.actbio.2021.02.029
Grand View Research (2020). Chitosan Market Size, Share & Trends Analysis Report by Application (Pharmaceutical & Biomedical, Water Treatment, Cosmetics, Food & Beverage). By Region (APAC, North America, Europe, MEA), and Segment Forecasts, 2020–2027. Available at: https://www.grandviewresearch.com/industry-analysis/global-chitosan-market.
Grenha, A., Grainger, C. I., Dailey, L. A., Seijo, B., Martin, G. P., Remuñán-López, C., et al. (2007). Chitosan Nanoparticles Are Compatible with Respiratory Epithelial Cells In Vitro. Eur. J. Pharm. Sci. 31 (2), 73–84. doi:10.1016/j.ejps.2007.02.008
Guo, M., Rong, W. T., Hou, J., Wang, D. F., Lu, Y., Wang, Y., et al. (2013). Mechanisms of Chitosan-Coated Poly(lactic-Co-Glycolic Acid) Nanoparticles for Improving Oral Absorption of 7-Ethyl-10-Hydroxycamptothecin. Nanotechnology 24 (24), 245101. doi:10.1088/0957-4484/24/24/245101
Haroon, H. B., Mukherjee, D., Anbu, J., and Teja, B. V. (2021). Thiolated Chitosan-Centella Asiatica Nanocomposite: A Potential Brain Targeting Strategy through Nasal Route. AAPS PharmSciTech 22 (8), 251. doi:10.1208/s12249-021-02131-6
Jain, A., Jain, S., Jain, R., and Kohli, D. V. (2015). Coated Chitosan Nanoparticles Encapsulating Caspase 3 Activator for Effective Treatment of Colorectral Cancer. Drug Deliv. Transl Res. 5 (6), 596–610. doi:10.1007/s13346-015-0255-x
Jana, S., and Jana, S. (2020). Functional Chitosan: Drug Delivery and Biomedical Applications. Cham, Switzerland: Springer.
Janes, K. A., Calvo, P., and Alonso, M. J. (2001). Polysaccharide Colloidal Particles as Delivery Systems for Macromolecules. Adv. Drug Deliv. Rev. 47 (1), 83–97. doi:10.1016/s0169-409x(00)00123-x
Je, H. J., Kim, E. S., Lee, J. S., and Lee, H. G. (2017). Release Properties and Cellular Uptake in Caco-2 Cells of Size-Controlled Chitosan Nanoparticles. J. Agric. Food Chem. 65 (50), 10899–10906. doi:10.1021/acs.jafc.7b03627
Jin, Z., Li, D., Dai, C., Cheng, G., Wang, X., and Zhao, K. (2017). Response of Live Newcastle Disease Virus Encapsulated in N-2-Hydroxypropyl Dimethylethyl Ammonium Chloride Chitosan Nanoparticles. Carbohydr. Polym. 171, 267–280. doi:10.1016/j.carbpol.2017.05.022
Khan, M. M., Madni, A., Torchilin, V., Filipczak, N., Pan, J., Tahir, N., et al. (2019). Lipid-Chitosan Hybrid Nanoparticles for Controlled Delivery of Cisplatin. Drug Deliv. 26 (1), 765–772. doi:10.1080/10717544.2019.1642420
Klemetsrud, T., Kjøniksen, A. L., Hiorth, M., Jacobsen, J., and Smistad, G. (2018). Polymer Coated Liposomes for Use in the Oral Cavity - A Study of the In Vitro Toxicity, Effect on Cell Permeability and Interaction with Mucin. J. Liposome Res. 28 (1), 62–73. doi:10.1080/08982104.2016.1255640
Kotzé, A. F., Thanou, M. M., Lueben, H. L., De Boer, A. G., Verhoef, J. C., and Junginger, H. E. (1999). Enhancement of Paracellular Drug Transport with Highly Quaternized N‐trimethyl Chitosan Chloride in Neutral Environments: In Vitro Evaluation in Intestinal Epithelial Cells (Caco‐2). J. Pharm. Sci. 88 (2), 253–257.
Kumar, M. N., Muzzarelli, R. A., Muzzarelli, C., Sashiwa, H., and Domb, A. J. (2004). Chitosan Chemistry and Pharmaceutical Perspectives. Chem. Rev. 104 (12), 6017–6084. doi:10.1021/cr030441b
Kurita, K. (2001). Controlled Functionalization of the Polysaccharide Chitin. Prog. Polym. Sci. 26 (9), 1921–1971. doi:10.1016/s0079-6700(01)00007-7
Li, Q., Wang, W., Hu, G., Cui, X., Sun, D., Jin, Z., et al. (2021). Evaluation of Chitosan Derivatives Modified Mesoporous Silica Nanoparticles as Delivery Carrier. Molecules 26 (9), 24. doi:10.3390/molecules26092490
Lim, L. M., Tran, T. T., Long Wong, J. J., Wang, D., Cheow, W. S., and Hadinoto, K. (2018). Amorphous Ternary Nanoparticle Complex of Curcumin-Chitosan-Hypromellose Exhibiting Built-In Solubility Enhancement and Physical Stability of Curcumin. Colloids Surf. B Biointerfaces 167, 483–491. doi:10.1016/j.colsurfb.2018.04.049
Lima, I. A., Khalil, N. M., Tominaga, T. T., Lechanteur, A., Sarmento, B., and Mainardes, R. M. (2018). Mucoadhesive Chitosan-Coated PLGA Nanoparticles for Oral Delivery of Ferulic Acid. Artif. Cell Nanomed Biotechnol 46 (Suppl. 2), 993–1002. doi:10.1080/21691401.2018.1477788
Liu, Q., Zheng, X., Zhang, C., Shao, X., Zhang, X., Zhang, Q., et al. (2015). Antigen-Conjugated N-Trimethylaminoethylmethacrylate Chitosan Nanoparticles Induce strong Immune Responses after Nasal Administration. Pharm. Res. 32 (1), 22–36. doi:10.1007/s11095-014-1441-0
Liu, Y., Di Zang, H., Kong, M., Ma, F. K., Dang, Q. F., Cheng, X. J., et al. (2012). In Vitro evaluation of Mucoadhesion and Permeation Enhancement of Polymeric Amphiphilic Nanoparticles. Carbohydr. Polym. 89 (2), 453–460. doi:10.1016/j.carbpol.2012.03.028
Liu, Y., Kong, M., Cheng, X. J., Wang, Q. Q., Jiang, L. M., and Chen, X. G. (2013). Self-Assembled Nanoparticles Based on Amphiphilic Chitosan Derivative and Hyaluronic Acid for Gene Delivery. Carbohydr. Polym. 94 (1), 309–316. doi:10.1016/j.carbpol.2012.12.058
Liu, Z., Jiao, Y., Wang, Y., Zhou, C., and Zhang, Z. (2008). Polysaccharides-Based Nanoparticles as Drug Delivery Systems. Adv. Drug Deliv. Rev. 60 (15), 1650–1662. doi:10.1016/j.addr.2008.09.001
Loh, J. W., Saunders, M., and Lim, L. Y. (2012). Cytotoxicity of Monodispersed Chitosan Nanoparticles against the Caco-2 Cells. Toxicol. Appl. Pharmacol. 262 (3), 273–282. doi:10.1016/j.taap.2012.04.037
Loh, J. W., Yeoh, G., Saunders, M., and Lim, L. Y. (2010). Uptake and Cytotoxicity of Chitosan Nanoparticles in Human Liver Cells. Toxicol. Appl. Pharmacol. 249 (2), 148–157. doi:10.1016/j.taap.2010.08.029
Millotti, G., Perera, G., Vigl, C., Pickl, K., Sinner, F. M., and Bernkop-Schnürch, A. (2011). The Use of Chitosan-6-Mercaptonicotinic Acid Nanoparticles for Oral Peptide Drug deliveryDrug Delivery: Special Issue - Chitosan in Drug Delivery. Drug Deliv. 18 (3), 190–197. doi:10.3109/10717544.2010.522611
Mohammed, M. A., Syeda, J. T. M., Wasan, K. M., and Wasan, E. K. (2017). An Overview of Chitosan Nanoparticles and its Application in Non-parenteral Drug Delivery. Pharmaceutics 9 (4), 20. doi:10.3390/pharmaceutics9040053
Mosa, I. F., Abd, H. H., Abuzreda, A., Assaf, N., and Yousif, A. B. (2020). Bio-Evaluation of the Role of Chitosan and Curcumin Nanoparticles in Ameliorating Genotoxicity and Inflammatory Responses in Rats' Gastric Tissue Followed Hydroxyapatite Nanoparticles’ Oral Uptake. Toxicol. Res. 9 (4), 493–508. doi:10.1093/toxres/tfaa054
Müller, R. H., Mäder, K., and Gohla, S. (2000). Solid Lipid Nanoparticles (SLN) for Controlled Drug Delivery – A Review of the State of the Art. Eur. J. Pharm. Biopharm. 50 (1), 161–177.
Mumuni, M. A., Kenechukwu, F. C., Ofokansi, K. C., Attama, A. A., and Díaz, D. D. (2020). Insulin-loaded Mucoadhesive Nanoparticles Based on Mucin-Chitosan Complexes for Oral Delivery and Diabetes Treatment. Carbohydr. Polym. 229, 115506. doi:10.1016/j.carbpol.2019.115506
Nasti, A., Zaki, N. M., de Leonardis, P., Ungphaiboon, S., Sansongsak, P., Rimoli, M. G., et al. (2009). Chitosan/TPP and Chitosan/TPP-Hyaluronic Acid Nanoparticles: Systematic Optimisation of the Preparative Process and Preliminary Biological Evaluation. Pharm. Res. 26 (8), 1918–1930. doi:10.1007/s11095-009-9908-0
Nguyen, M. H., Yu, H., Kiew, T. Y., and Hadinoto, K. (2015). Cost-effective Alternative to Nano-Encapsulation: Amorphous Curcumin-Chitosan Nanoparticle Complex Exhibiting High Payload and Supersaturation Generation. Eur. J. Pharm. Biopharm. 96, 1–10. doi:10.1016/j.ejpb.2015.07.007
Noi, I., Schlachet, V. I., Kumarasamy, M., and Sosnik, A. (2018). Permeability of Novel Chitosan-G-Poly(Methyl Methacrylate) Amphiphilic Nanoparticles in a Model of Small Intestine In Vitro. Polymers 10 (5). doi:10.3390/polym10050478
Nurunnabi, M., Revuri, V., Huh, K. M., and Lee, Y.-k. (2017). “Polysaccharide Based Nano/Microformulation: An Effective and Versatile Oral Drug Delivery System,” in Nanostructures for Oral Medicine. Editors Anderson, E., and Grumezescu, A. M. (Elsevier), 409–433. doi:10.1016/b978-0-323-47720-8.00015-8
Onnainty, R., Schenfeld, E. M., Petiti, J. P., Longhi, M. R., Torres, A., Quevedo, M. A., et al. (2016). Permeability Profiles and Intestinal Toxicity Assessment of Hydrochlorothiazide and its Inclusion Complex with β-Cyclodextrin Loaded into Chitosan Nanoparticles. Mol. Pharm. 13 (11), 3736–3746. doi:10.1021/acs.molpharmaceut.6b00532
Pandit, J., Sultana, Y., and Aqil, M. (2017). Chitosan-coated PLGA Nanoparticles of Bevacizumab as Novel Drug Delivery to Target Retina: Optimization, Characterization, and In Vitro Toxicity Evaluation. Artif. Cell Nanomed. Biotechnol. 45 (7), 1397–1407. doi:10.1080/21691401.2016.1243545
Patel, D., Naik, S., and Misra, A. (2012). Improved Transnasal Transport and Brain Uptake of Tizanidine HCl-Loaded Thiolated Chitosan Nanoparticles for Alleviation of Pain. J. Pharm. Sci. 101 (2), 690–706. doi:10.1002/jps.22780
Pistone, S., Goycoolea, F. M., Young, A., Smistad, G., and Hiorth, M. (2017). Formulation of Polysaccharide-Based Nanoparticles for Local Administration into the Oral Cavity. Eur. J. Pharm. Sci. 96, 381–389. doi:10.1016/j.ejps.2016.10.012
Pistone, S., Rykke, M., Smistad, G., and Hiorth, M. (2017). Polysaccharide-Coated Liposomal Formulations for Dental Targeting. Int. J. Pharm. 516 (1), 106–115. doi:10.1016/j.ijpharm.2016.11.028
Prabaharan, M., and Mano, J. F. (2005). Chitosan-based Particles as Controlled Drug Delivery Systems. Drug Deliv. 12 (1), 41–57. doi:10.1080/10717540590889781
Pradines, B., Bories, C., Vauthier, C., Ponchel, G., Loiseau, P. M., and Bouchemal, K. (2015). Drug-Free Chitosan Coated Poly(isobutylcyanoacrylate) Nanoparticles Are Active against Trichomonas Vaginalis and Non-toxic towards Pig Vaginal Mucosa. Pharm. Res. 32 (4), 1229–1236. doi:10.1007/s11095-014-1528-7
Pradines, B., Lievin-Le Moal, V., Vauthier, C., Ponchel, G., Loiseau, P. M., and Bouchemal, K. (2015). Cell Line-Dependent Cytotoxicity of Poly(isobutylcyanoacrylate) Nanoparticles Coated with Chitosan and Thiolated Chitosan: Insights from Cultured Human Epithelial HeLa, Caco2/TC7 and HT-29/MTX Cells. Int. J. Pharm. 491 (1-2), 17–20. doi:10.1016/j.ijpharm.2015.06.001
Rejman, J., Oberle, V., Zuhorn, I. S., and Hoekstra, D. (2004). Size-Dependent Internalization of Particles via the Pathways of Clathrin- and Caveolae-Mediated Endocytosis. Biochem. J. 377 (Pt 1), 159–169. doi:10.1042/BJ20031253
Sakloetsakun, D., Perera, G., Hombach, J., Millotti, G., and Bernkop-Schnürch, A. (2010). The Impact of Vehicles on the Mucoadhesive Properties of Orally Administrated Nanoparticles: a Case Study with Chitosan-4-Thiobutylamidine Conjugate. AAPS PharmSciTech 11 (3), 1185–1192. doi:10.1208/s12249-010-9479-8
Sampathkumar, K., and Loo, S. C. J. (2018). Targeted Gastrointestinal Delivery of Nutraceuticals with Polysaccharide-Based Coatings. Macromol Biosci. 18 (4), e1700363. doi:10.1002/mabi.201700363
Sang, Z., Qian, J., Han, J., Deng, X., Shen, J., Li, G., et al. (2020). Comparison of Three Water-Soluble Polyphosphate Tripolyphosphate, Phytic Acid, and Sodium Hexametaphosphate as Crosslinking Agents in Chitosan Nanoparticle Formulation. Carbohydr. Polym. 230, 115577. doi:10.1016/j.carbpol.2019.115577
Saremi, S., Atyabi, F., Akhlaghi, S. P., Ostad, S. N., and Dinarvand, R. (2011). Thiolated Chitosan Nanoparticles for Enhancing Oral Absorption of Docetaxel: Preparation, In Vitro and Ex Vivo Evaluation. Int. J. Nanomed. 6, 119–128. doi:10.2147/IJN.S15500
Sayin, B., Somavarapu, S., Li, X. W., Thanou, M., Sesardic, D., Alpar, H. O., et al. (2008). Mono-N-carboxymethyl Chitosan (MCC) and N-Trimethyl Chitosan (TMC) Nanoparticles for Non-invasive Vaccine Delivery. Int. J. Pharm. 363 (1–2), 139–148. doi:10.1016/j.ijpharm.2008.06.029
Shah, B., Khunt, D., Misra, M., and Padh, H. (2016). “Application of Box-Behnken Design for Optimization and Development of Quetiapine Fumarate Loaded Chitosan Nanoparticles for Brain Delivery via Intranasal Route*”. Int. J. Biol. Macromol 89, 206–218. doi:10.1016/j.ijbiomac.2016.04.076
Shariatinia, Z. (2018). Carboxymethyl Chitosan: Properties and Biomedical Applications. Int. J. Biol. Macromol 120, 1406–1419. doi:10.1016/j.ijbiomac.2018.09.131
Shi, L. L., Lu, J., Cao, Y., Liu, J. Y., Zhang, X. X., Zhang, H., et al. (2017). Gastrointestinal Stability, Physicochemical Characterization and Oral Bioavailability of Chitosan or its Derivative-Modified Solid Lipid Nanoparticles Loading Docetaxel. Drug Dev. Ind. Pharm. 43 (5), 839–846. doi:10.1080/03639045.2016.1220571
Shi, Y., Xue, J., Liu, Z., Du, M., Xu, L., Sun, Q., et al. (2018). Polyelectrolyte Complex Nanoparticles Based on Methoxy Poly(Ethylene Glycol)-B-Poly (ε-Caprolactone) Carboxylates and Chitosan for Delivery of Tolbutamide. J. Biomater. Sci. Polym. Ed. 29 (15), 1799–1811. doi:10.1080/09205063.2018.1498720
Silva, D., Arancibia, R., Tapia, C., Acuña-Rougier, C., Diaz-Dosque, M., Cáceres, M., et al. (2013). Chitosan and Platelet-Derived Growth Factor Synergistically Stimulate Cell Proliferation in Gingival Fibroblasts. J. Periodontal Res. 48 (6), 677–686. doi:10.1111/jre.12053
Sinani, G., Sessevmez, M., Gök, M. K., Özgümüş, S., Alpar, H. O., and Cevher, E. (2019). Modified Chitosan-Based Nanoadjuvants Enhance Immunogenicity of Protein Antigens after Mucosal Vaccination. Int. J. Pharm. 569, 118592. doi:10.1016/j.ijpharm.2019.118592
Singh, A. (2016). Engineered Nanoparticles : Structure, Properties and Mechanisms of Toxicity. Boston, MA: Elsevier.
Sonaje, K., Lin, Y. H., Juang, J. H., Wey, S. P., Chen, C. T., and Sung, H. W. (2009). In Vivo evaluation of Safety and Efficacy of Self-Assembled Nanoparticles for Oral Insulin Delivery. Biomaterials 30 (12), 2329–2339. doi:10.1016/j.biomaterials.2008.12.066
Tandberg, J., Lagos, L., Ropstad, E., Smistad, G., Hiorth, M., and Winther-Larsen, H. C. (2018). The Use of Chitosan-Coated Membrane Vesicles for Immunization against Salmonid Rickettsial Septicemia in an Adult Zebrafish Model. Zebrafish 15 (4), 372–381. doi:10.1089/zeb.2017.1556
Teubl, B. J., Absenger, M., Fröhlich, E., Leitinger, G., Zimmer, A., and Roblegg, E. (2013). The Oral Cavity as a Biological Barrier System: Design of an Advanced Buccal In Vitro Permeability Model. Eur. J. Pharm. Biopharm. 84 (2), 386–393. doi:10.1016/j.ejpb.2012.10.021
Thai, H., Thuy Nguyen, C., Thi Thach, L., Thi Tran, M., Duc Mai, H., Thi Thu Nguyen, T., et al. (2020). Characterization of Chitosan/alginate/lovastatin Nanoparticles and Investigation of Their Toxic Effects In Vitro and In Vivo. Sci. Rep. 10 (1), 909. doi:10.1038/s41598-020-57666-8
Thanou, M., Verhoef, J. C., and Junginger, H. E. (2001). Oral Drug Absorption Enhancement by Chitosan and its Derivatives. Adv. Drug Deliv. Rev. 52 (2), 117–126. doi:10.1016/s0169-409x(01)00231-9
Tzeyung, A. S., Md, S., Madheswaran, T., Alhakamy, N. A., Aldawsari, H. M., and Radhakrishnan, A. K. (2019). Fabrication, Optimization, and Evaluation of Rotigotine-Loaded Chitosan Nanoparticles for Nose-To-Brain Delivery. Pharmaceutics 11 (1), 10. doi:10.3390/pharmaceutics11010026
van den Broek, L., LAMvd, B., and Boeriu, C. G. (2020). Chitin and Chitosan : Properties and Applications. Hoboken, New Jersey ;,West Sussex, England: Wiley.
Wardani, G., Eraiko, K., Koerniasari K, K., and Sudjarwo, S. A. (2018). Protective Activity of Chitosan Nanoparticle against Cadmium Chloride Induced Gastric Toxicity in Rat. Jyp 10 (3), 303–307. doi:10.5530/jyp.2018.10.67
Ways T, M., Lau, W., and Khutoryanskiy, V. (2018). Chitosan and its Derivatives for Application in Mucoadhesive Drug Delivery Systems. Polymers 10 (3), 267.
Wei, W., Behloul, N., Wang, W., Baha, S., Liu, Z., Shi, R., et al. (2021). Chitosan Nanoparticles Loaded with Truncated ORF2 Protein as an Oral Vaccine Candidate against Hepatitis. E. Macromol. 21 (4), e2000375. doi:10.1002/mabi.202000375
Yan, C., Gu, J., Lv, Y., Shi, W., Huang, Z., and Liao, Y. (2019). 5β-Cholanic Acid/Glycol Chitosan Self-Assembled Nanoparticles (5β-CHA/GC-NPs) for Enhancing the Absorption of FDs and Insulin by Rat Intestinal Membranes. AAPS PharmSciTech 20 (1), 30. doi:10.1208/s12249-018-1242-6
Yan, Y., Sun, Y., Wang, P., Zhang, R., Huo, C., Gao, T., et al. (2020). Mucoadhesive Nanoparticles-Based Oral Drug Delivery Systems Enhance Ameliorative Effects of Low Molecular Weight Heparin on Experimental Colitis. Carbohydr. Polym. 246, 116660. doi:10.1016/j.carbpol.2020.116660
Ye, Y., Xu, Y., Liang, W., Leung, G. P., Cheung, K. H., Zheng, C., et al. (2013). DNA-loaded Chitosan Oligosaccharide Nanoparticles with Enhanced Permeability across Calu-3 Cells. J. Drug Target. 21 (5), 474–486. doi:10.3109/1061186X.2013.766885
Yin, L., Ding, J., He, C., Cui, L., Tang, C., and Yin, C. (2009). Drug Permeability and Mucoadhesion Properties of Thiolated Trimethyl Chitosan Nanoparticles in Oral Insulin Delivery. Biomaterials 30 (29), 5691–5700. doi:10.1016/j.biomaterials.2009.06.055
Zhao, K., Li, S., Li, W., Yu, L., Duan, X., Han, J., et al. (2017). Quaternized Chitosan Nanoparticles Loaded with the Combined Attenuated Live Vaccine against Newcastle Disease and Infectious Bronchitis Elicit Immune Response in Chicken after Intranasal Administration. Drug Deliv. 24 (1), 1574–1586. doi:10.1080/10717544.2017.1388450
Zhao, K., Zhang, Y., Zhang, X., Li, W., Shi, C., Guo, C., et al. (2014). Preparation and Efficacy of Newcastle Disease Virus DNA Vaccine Encapsulated in Chitosan Nanoparticles. Int. J. Nanomed. 9, 389–402. doi:10.2147/IJN.S54226
Keywords: chitosan, nanoparticles, drug carriers, drug delivery systems, cytotoxicity, cell viability, nanocapsule
Citation: Frigaard J, Jensen JL, Galtung HK and Hiorth M (2022) The Potential of Chitosan in Nanomedicine: An Overview of the Cytotoxicity of Chitosan Based Nanoparticles. Front. Pharmacol. 13:880377. doi: 10.3389/fphar.2022.880377
Received: 21 February 2022; Accepted: 08 April 2022;
Published: 04 May 2022.
Edited by:
Manuela Banciu, Babeș-Bolyai University, RomaniaReviewed by:
Paulo Cesar Morais, Catholic University of Brasilia (UCB), BrazilYu Zhao, Nankai University, China
Copyright © 2022 Frigaard, Jensen, Galtung and Hiorth. This is an open-access article distributed under the terms of the Creative Commons Attribution License (CC BY). The use, distribution or reproduction in other forums is permitted, provided the original author(s) and the copyright owner(s) are credited and that the original publication in this journal is cited, in accordance with accepted academic practice. No use, distribution or reproduction is permitted which does not comply with these terms.
*Correspondence: Julie Frigaard, anVsaWVtZkB1aW8ubm8=