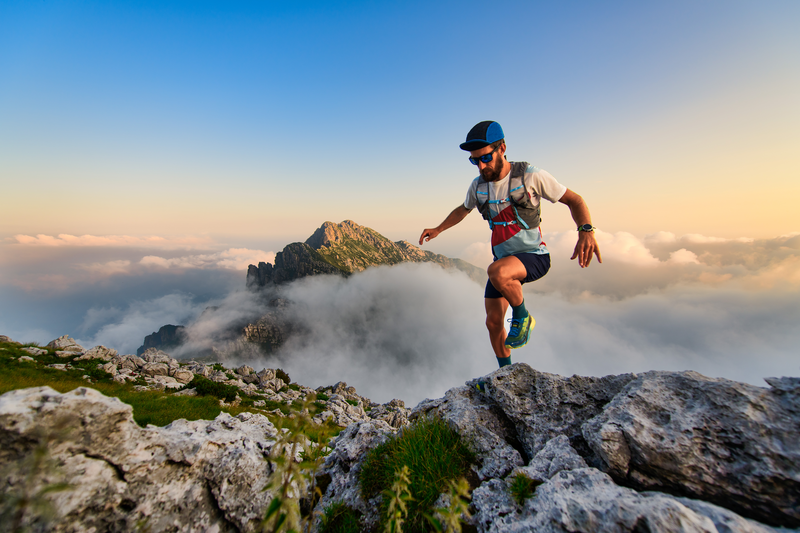
94% of researchers rate our articles as excellent or good
Learn more about the work of our research integrity team to safeguard the quality of each article we publish.
Find out more
MINI REVIEW article
Front. Pharmacol. , 13 May 2022
Sec. Experimental Pharmacology and Drug Discovery
Volume 13 - 2022 | https://doi.org/10.3389/fphar.2022.877543
This article is part of the Research Topic Drug Application and Delivery Targeting Glycolysis-Associated Diseases: Novel Insights and Mechanism View all 4 articles
As a natural sugar, mannose is a type of hexose that is abundant in many different types of fruits. Since mannose is rarely used for glycolysis in mammals, studies on the role of mannose have not attracted much attention. Glycosylation of specific proteins was thought to be the major function of mannose. Surprisingly, during the past few years, mannose was found to be effective in promoting immune tolerance and suppressing inflammatory diseases related to autoimmunity and allergy. Moreover importantly, mannose was also found to be efficient in suppressing tumors by suppressing glycolysis and enhancing chemotherapeutic agents. In this review, we summarize the recent studies of mannose on antitumor properties and anti-inflammatory characteristics. We emphasize that mannose could play a beneficial role in the treatment of a variety of diseases, including cancers and inflammatory diseases, and could be a novel therapeutic strategy that deserves continued evaluation.
In recent years, the general public has paid more and more attention to the relationship between diet and disease. Adjusting diet has also been shown to enhance cancer treatment (Kanarek et al., 2020; Tajan and Vousden, 2020). Among the hexoses people consume on a daily basis, mannose appears to be the most attractive in many ways. Mannose is a hexose, which is a component of many polysaccharides. Mannose is poorly metabolized in the human body, so it is not obvious that mannose enters carbohydrate metabolism after oral administration. Mannose plays an important role in human metabolism, especially in glycosylation of certain proteins (Alton et al., 1998) involved in immune regulation. Congenital glycosylation disorder (CDG) is a kind of disorder that is difficult to diagnose clinically due to its various manifestations and abnormal functions of multiple organ systems (Jaeken and Péanne, 2017; Péanne et al., 2018). CDG-Ib (MPI-CDG) is caused by mutations in mannose phosphate isomerase (MPI) (Freeze and Sharma, 2010). Based on the fact that MPI deletion can lead to liver fibrosis (Janssen et al., 2014), DeRossi et al. conducted experiments with zebrafish as a model, and observed that MPI deletion in zebrafish larvae can lead to liver development defects (DeRossi et al., 2019). They demonstrated that acute and persistent MPI deletions are sufficient to activate hematopoietic stem cells and induce fibrosis in vitro and in whole vertebrate models, suggesting that MPI deletions promote stellate cell activation. Direct production of mannose-6-phosphate based on mannose phosphorylation circumvented the MPI defect (Niehues et al., 1998; Lonlay and Seta, 2009), and together with experimental results, showed that mannose reduced stellate cell activation and fibrosis.
During the last few years, mannose has been shown to have many physiological benefits and applications, such as suppressing inflammation (Zhang et al., 2021), and suppressing tumor growth and metastasis (Gonzalez et al., 2018). Besides suppressing Type 1 diabetes, asthma and macrophage-mediated inflammation (Zhang et al., 2017; Torretta et al., 2020), a most recent study has shown that mannose can suppress experimental autoimmune encephalomyelitis (EAE) by inducing treg cells, suggesting that Mannose may be a potential adjuvant therapy for multiple sclerosis (MS) (Hwang et al., 2021). More importantly, mannose has been determined to be effective in suppressing different kinds of cancers (Gonzalez et al., 2018). This review focuses on the research progress of mannose in treating tumors and inflammation. We emphasize the potential of mannose as a therapeutic agent for the above-mentioned diseases and raise questions to be addressed.
Tumor cells usually have a high demand and affinity for glucose (Bhattacharya et al., 2016). Based on the fact that mannose and glucose are introduced into cells by the same transporters (Thorens and Mueckler, 2010), Gonzalez et al. have proposed that mannose may interfere with glucose uptake by tumor cells, and made relevant experimental verification (Gonzalez et al., 2018). Interestingly, the results showed that mannose treatment did not reduce or even increase the intracellular glucose level, but affected cell growth by interfering with glucose metabolism (Gonzalez et al., 2018). The mechanism at hand is that mannose accumulates in the form of mannose-6-phosphate and the accumulation of M6P suppresses glucose phosphate isomerase (GPI) and other enzymes related to glucose metabolism, which impairs the further metabolism of glucose in glycolysis, tricarboxylic acid cycle, pentose phosphate pathway, and glycan synthesis (Gonzalez et al., 2018). In follow-up experiments, they came up with two important results: First, among various hexoses, only mannose-combined with conventional chemotherapy such as cisplatin and adriamycin can down-regulate Mcl-1 and Bcl-XL protein levels and enhance apoptosis; Second, the inhibitory effect of mannose was negatively correlated with the expression level of mannose phosphate isomerase (PMI) encoded by MPI gene (Figure 1). Therefore, cells with low PMI levels are sensitive to mannose, while cells with high levels are resistant. These results could open up a novel way to fight cancer and provide a safe, simple, potential treatment strategy effectively against many types of cancer by enhancing chemotherapy. However, in terms of the effective dose of mannose, we expect scientists to calculate an appropriate dosage that has maximum effectiveness without any significant side effect. Based on the above mechanisms, a recent study has shown that mannose inhibits cell proliferation and enhances radiation-induced apoptosis in human esophageal squamous carcinoma cells with low MPI expression in a dose-dependent manner (Luo et al., 2022). This suggests that mannose can act as a radiation sensitizer in patients with esophageal squamous cell carcinoma with low MPI expression. There is also a study showed that in the early and middle stages of the development of glioblastoma multiforme (GBM), mannose can be combined with temozolomide concurrent radiotherapy (RT/TMZ) to achieve cure (Liu et al., 2020).
FIGURE 1. Mannose interferes with glucose metabolism in tumor cells with low expression of the MPI gene. Mannose and glucose are transported into cells by the same transporter (GLUT), and transformed by hexokinase (HK) into mannose-6-phosphate (M6P) and glucose-6-phosphate (G6P). In those tumor cells with low mannose phosphate isomerase (MPI) expression, the accumulation of M6P suppresses glucose phosphate isomerase (GPI) and other enzymes related to glucose metabolism, resulting in inhibition of glycolysis and in turn inhibition of the tricarboxylic acid cycle (TCA). In contrast, in tumor cells that express a high level of MPI, mannose could be used for glycolysis efficiently. Recent studies have found that Zn2+ is crucial for mannose phosphate isomerase (PMI) to exert enzymatic activity, and when ZIP10, a transporter of Zn2+, is knocked down, it causes the inactivation of MPI and makes tumor cells become mannose sensitive.
For thyroid cancer, one recent study has found that whether the cell line is sensitive to mannose depends mainly on the enzyme activity of PMI (Ma et al., 2021). Structurally, zinc ions (Zn2+) are critical for the binding of the PMI and substrate, so the enzymatic function of PMI depends on Zn2+ concentration (Bangera et al., 2019). ZIP10 is a Zn2+ transporter expressed during early B cell development that transports Zn2+ from outside the cell to the cytoplasm, thereby altering the concentration of Zn2+ within the cell (Miyai et al., 2014). The latest study found that in thyroid cancer cells that are not sensitive to mannose, knocking down ZIP10 can significantly improve their sensitivity to mannose, and M6P accumulation in turn enhances the inhibitory effect of mannose on cellular glycolysis (Ma et al., 2021) (Figure 1). These findings suggest that mannose has potential application value in the treatment of thyroid cancer.
In non-small cell carcinoma (NSCLC), it has been proved that mannose has a significant inhibitory effect on the proliferation, invasion, and metastasis of A549 cells. Mannose was shown to be able to induce G0/G1 arrest by down-regulating the expression of PCNA and up-regulating the expression of p21, both of which are related to the concentration of mannose (Wang et al., 2020). Moreover, mannose can promote the expression of phosphorylated GSK-3β, suppress the activation of ERK, and thereby reduce the expression of β-catenin and SNAIL, which in turn reduce N-cadherin and increases E-cadherin, thereby suppressing tumor metastasis (Wang et al., 2020; Luo et al., 2020) (Figure 2). In terms of mechanism, epithelial-mesenchymal transition (EMT) plays an indispensable role in cancer metastasis (Heerboth et al., 2015). Several transcription factors are upregulated in EMT metastatic cells, represented by SNAIL. SNAIL can be combined with the E-cadherin promoter to suppress the expression of E-cadherin and promote EMT (Al Khatib et al., 2019). Phosphorylation of GSK-3β and phosphorylation and destabilization of β-catenin have significant effects on EMT (Geng et al., 2017; Tian et al., 2020). The activation of ERK promotes the phosphorylation level of β-catenin Tyr654, and the phosphorylation of tyrosine 654 at β-catenin promotes nuclear translocation, which in turn promotes the expression of β-catenin in the nucleus. The phosphorylation of GSK-3β can suppress the function of β-catenin and promote the degradation of β-catenin. Mannose significantly reduces the phosphorylation level of tyrosine 654 at β-catenin in cells in vitro by reducing the phosphorylation level of ERK, and promotes the expression of phosphorylated-GSK-3β (Luo et al., 2020). Wang et al. also found that mannose could exert anticancer effects on A549 and H1299 cells by suppressing PI3K/AKT and ERK signaling pathways (Wang et al., 2020). This implies that mannose can be combined with AKT or ERK targeted therapy to treat lung cancer, but further experiments are needed to explore the molecular mechanism of mannose’s anti-cancer effect. It is worth noting that the suppressed migration induced by mannose is more effective on the migration of carboplatin-treated A549 cells. The last point is that mannose significantly enhances the effect of apoptosis after carboplatin treatment (Sha et al., 2020; Wang et al., 2020). All these findings suggest that mannose may be a selective cancer treatment. However, some problems remain, such as in chronic myeloid leukemia (CML), although D-mannose treatment can inhibit K562 cell growth in vitro, it does not reduce the tumor volume in vivo, and ponatinib and D-mannose treatment show severe drug toxicity in mice (Okabe et al., 2021). This reminds us that some approaches require more evaluation when it comes to targeted therapy for glucose metabolism.
FIGURE 2. Mannose inhibits tumor metastasis by relieving epithelial-mesenchymal transition (EMT). Mannose could reduce the activation of the ERK pathway and increase the phosphorylation of GSK-3β. The phosphorylation of GSK-3β will reduce the phosphorylation level of tyrosine 654 at β-catenin and inhibit the nuclear translocation of β-catenin. The reduction of β-catenin in the nuclear down-regulates SNAIL expression, and the down regulation of SNAIL could promote E-cadherin expression. This regulatory mechanism results in the inhibition of tumor EMT.
\Some peptides act as regulators of innate immunity, activating macrophages and monocytes, thereby releasing chemicals with potential tumor-killing effects (Kager et al., 2010). As an excellent carrier of peptide drugs, liposomes can protect the structure and biological activity of drugs (Kumar Giri et al., 2016). Tumor epitope peptides can be recognized by dendritic cells (DCs) and processed into MHC-I complexes to specifically activate CD8+ T cells into cytotoxic T cells (CTLs) to achieve targeted killing effects (Wculek et al., 2020). The mannose receptor (MR) is a member of the C-type lectin superfamily, which is mainly present on the membrane surface of macrophages and DC cells, and plays an important role in antigen presentation (Martinez-Pomares, 2012). Therefore, recently, a relevant research team has developed a mannose modified liposome-epitope peptide drug delivery system, in which mannose improves the bonding rate of liposomes and DC cells, improves the targeting efficiency of epitope peptide liposomes to DC cells, and significantly inhibits the hematogenic spread of lung metastasis of triple negative breast cancer (TNBC) in animal experiments, and has good biosecurity (Yu et al., 2021). In addition, a research team has developed a doxorubicin (DOX)-based mannose nanogel (DM NG), which induces immunogenic cell death (ICD), stimulates the phagocytosis of DC cells, and promotes specific T cell anti-tumor immunity (Ma et al., 2022). The study also showed that the mannose released by DM NG interferes with glucose metabolism and thus regulates tumor metabolism, ultimately leading to apoptosis of cancer cells. It is also pointed out that this strategy can systematically modulate the tumor microenvironment (TME) and improve the immunosuppressive tumor microenvironment (ITM).
Besides the anticancer effects, mannose has been proven to suppress inflammation by suppressing glycolysis, promoting TGF-β activation and inducing Treg cells (Zhang et al., 2017; Torretta et al., 2020). Since it has been well summarized in the recent past that mannose is a novel suppressor of inflammation (Zhang et al., 2021), we only emphasize the point that mannose treatment could be a safe and promising strategy to suppress and to treat autoimmunity and asthma. More than that, mannose could also suppress wounding-induced inflammation in skin (Jokela et al., 2013). Hyaluronic acid (HA) is the only non-sulfated glycosaminoglycan in the extracellular matrix (Genasetti et al., 2008). It is involved in endothelial cell proliferation, migration, neovascularization, and leukocyte recruitment, and its metabolism is significantly altered in inflammation (Tammi et al., 2005). During inflammatory stimulation, the HA receptor,CD44, on the surface of leukocytes binds to the HA cable structure (La Motte et al., 1999; La Motte et al., 2003). In vitro, it has been reported that mannose consumes UDP GlcNAc to reduce the synthesis of HA (Jokela et al., 2008). Tiina et al. found that mannose also reduced the content of HA in vivo and prevented the binding of leukocytes to HA on the cell surface (Jokela et al., 2013). Not only that, they also found IL-1β induces the HA shell in keratinocytes to reconstitute the cable structure, and mannose can inhibit the binding of HA dependent monocytes to keratinocytes. They also found that mannose could suppress the function of dermal fibroblasts, and this potential anti fibrosis effect needs to be verified by further in vivo experiments.
Mannose is used in a variety of daily processes mainly because of its low heat and non-toxic characteristics (Wu et al., 2019). It also has the potential for clinical application in drug therapy (Wei et al., 2020). Mannose has shown anti-tumor effects against a variety of tumors, and it is safe in humans at certain concentrations (Gonzalez et al., 2018). In terms of dosage, a person would need to ingest very large amounts of mannose to match the amounts that were given to the mice in the experiments. Therefore, it is critical to find a way to effectively use mannose for human disease treatment.
In addition, related studies have shown that mannose is a biomarker of insulin resistance, which may help in the early identification of patients with insulin resistance and who are more prone to diabetic complications (Ferrannini et al., 2020). It was also found that plasma mannose levels increased in patients with insulin resistance independently of obesity (Mardinoglu et al., 2017). They confirmed that elevated plasma mannose levels were a strong marker for the risk of chronic diseases such as cardiovascular disease and type 2 diabetes and may contribute to their development, although the exact mechanism remains unclear (Mardinoglu et al., 2017). These evidence shows that mannose might have important applications in disease screening and detection.
In conclusion, mannose therapy is expected to be a promising strategy for the treatment of cancer and inflammatory diseases, although it is still needed to fully elucidate the mechanism and accurately evaluate and determine its effective dose in humans.
FN wrote the manuscript. YS, HL, JZ and XM edited the manuscript. DZ supervised the work, and edited the manuscript. All authors contributed to the article and approved it for publication.
This work was supported by the National Natural Science Foundation of China (NO. 82171829), the Key Project of the Science and Technology Department of Sichuan Province (NO. 2022YFH0100), the 1·3·5 Project for Disciplines of Excellence, West China Hospital, Sichuan University (NO. ZYYC21012), and the Fundamental Research Funds for the Central Universities (20822041E4084).
The authors declare that the research was conducted in the absence of any commercial or financial relationships that could be construed as a potential conflict of interest.
All claims expressed in this article are solely those of the authors and do not necessarily represent those of their affiliated organizations, or those of the publisher, the editors and the reviewers. Any product that may be evaluated in this article, or claim that may be made by its manufacturer, is not guaranteed or endorsed by the publisher.
DZ sincerely wants to commemorate Dr. Sang-A Park, who passed away suddenly on January 22, 2018.
Al Khatib, A. M., Mărgăritescu, C., Taisescu, O., Andreiana, B. C., Florescu, M. M., and Ciurea, R. N. (2019). Immunoexpression of E-Cadherin, Snail and Twist in Colonic Adenocarcinomas. Rom. J. Morphol. Embryol. 60, 531
Alton, G., Hasilik, M., Niehues, R., Panneerselvam, K., Etchison, J. R., Fana, F., et al. (1998). Direct Utilization of Mannose for Mammalian Glycoprotein Biosynthesis. Glycobiology 8, 285–295. doi:10.1093/glycob/8.3.285
Bangera, M., Gowda K, G., Sagurthi, S. R., and Murthy, M. R. N. (2019). Structural and Functional Insights into Phosphomannose Isomerase: the Role of Zinc and Catalytic Residues. Acta Crystallogr. D. Struct. Biol. 75, 475–487. doi:10.1107/S2059798319004169
Bhattacharya, B., Mohd Omar, M. F., and Soong, R. (2016). The Warburg Effect and Drug Resistance. Br. J. Pharmacol. 173, 970–979. doi:10.1111/bph.13422
de La Motte, C. A., Hascall, V. C., Calabro, A., Yen-Lieberman, B., and Strong, S. A. (1999). Mononuclear Leukocytes Preferentially Bind via CD44 to Hyaluronan on Human Intestinal Mucosal Smooth Muscle Cells after Virus Infection or Treatment with poly(I.C). J. Biol. Chem. 274, 30747–30755. doi:10.1074/jbc.274.43.30747
de la Motte, C. A., Hascall, V. C., Drazba, J., Bandyopadhyay, S. K., and Strong, S. A. (2003). Mononuclear Leukocytes Bind to Specific Hyaluronan Structures on Colon Mucosal Smooth Muscle Cells Treated with Polyinosinic Acid:polycytidylic Acid: Inter-alpha-trypsin Inhibitor Is Crucial to Structure and Function. Am. J. Pathol. 163, 121–133. doi:10.1016/S0002-9440(10)63636-X
de Lonlay, P., and Seta, N. (2009). The Clinical Spectrum of Phosphomannose Isomerase Deficiency, with an Evaluation of Mannose Treatment for CDG-Ib. Biochim. Biophys. Acta 1792, 841–843. doi:10.1016/j.bbadis.2008.11.012
DeRossi, C., Bambino, K., Morrison, J., Sakarin, I., Villacorta-Martin, C., Zhang, C., et al. (2019). Mannose Phosphate Isomerase and Mannose Regulate Hepatic Stellate Cell Activation and Fibrosis in Zebrafish and Humans. Hepatology 70, 2107–2122. doi:10.1002/hep.30677
Ferrannini, E., Bokarewa, M., Brembeck, P., Baboota, R., Hedjazifar, S., Andersson, K., et al. (2020). Mannose Is an Insulin-Regulated Metabolite Reflecting Whole-Body Insulin Sensitivity in Man. Metabolism 102, 153974. doi:10.1016/j.metabol.2019.153974
Freeze, H. H., and Sharma, V. (2010). Metabolic Manipulation of Glycosylation Disorders in Humans and Animal Models. Semin. Cell Dev. Biol. 21, 655–662. doi:10.1016/j.semcdb.2010.03.011
Genasetti, A., Vigetti, D., Viola, M., Karousou, E., Moretto, P., Rizzi, M., et al. (2008). Hyaluronan and Human Endothelial Cell Behavior. Connect. Tissue Res. 49, 120–123. doi:10.1080/03008200802148462
Geng, F., Jiang, Z., Song, X., Zhou, H., and Zhao, H. (2017). Mdig Suppresses Epithelial-Mesenchymal Transition and Inhibits the Invasion and Metastasis of Non small Cell Lung Cancer via Regulating GSK-3β/β-Catenin Signaling. Int. J. Oncol. 51, 1898–1908. doi:10.3892/ijo.2017.4154
Gonzalez, P. S., O'Prey, J., Cardaci, S., Barthet, V. J. A., Sakamaki, J. I., Beaumatin, F., et al. (2018). Mannose Impairs Tumour Growth and Enhances Chemotherapy. Nature 563, 719–723. doi:10.1038/s41586-018-0729-3
Heerboth, S., Housman, G., Leary, M., Longacre, M., Byler, S., Lapinska, K., et al. (2015). EMT and Tumor Metastasis. Clin. Transl. Med. 4, 6. doi:10.1186/s40169-015-0048-3
Hwang, D., Boehm, A., Rostami, A., Zhang, G. X., and Ciric, B. (2022). Oral D-Mannose Treatment Suppresses Experimental Autoimmune Encephalomyelitis via Induction of Regulatory T Cells. J. Neuroimmunol. 362, 577778. doi:10.1016/j.jneuroim.2021.577778
Jaeken, J., and Péanne, R. (2017). What Is New in CDG? J. Inherit. Metab. Dis. 40, 569–586. doi:10.1007/s10545-017-0050-6
Janssen, M. C., de Kleine, R. H., van den Berg, A. P., Heijdra, Y., van Scherpenzeel, M., Lefeber, D. J., et al. (2014). Successful Liver Transplantation and Long-Term Follow-Up in a Patient with MPI-CDG. Pediatrics 134, e279–83. doi:10.1542/peds.2013-2732
Jokela, T. A., Jauhiainen, M., Auriola, S., Kauhanen, M., Tiihonen, R., Tammi, M. I., et al. (2008). Mannose Inhibits Hyaluronan Synthesis by Down-Regulation of the Cellular Pool of UDP-N-Acetylhexosamines. J. Biol. Chem. 283, 7666–7673. doi:10.1074/jbc.M706001200
Jokela, T. A., Kuokkanen, J., Kärnä, R., Pasonen-Seppänen, S., Rilla, K., Kössi, J., et al. (2013). Mannose Reduces Hyaluronan and Leukocytes in Wound Granulation Tissue and Inhibits Migration and Hyaluronan-dependent Monocyte Binding. Wound Repair Regen. 21, 247–255. doi:10.1111/wrr.12022
Kager, L., Pötschger, U., and Bielack, S. (2010). Review of Mifamurtide in the Treatment of Patients with Osteosarcoma. Ther. Clin. Risk Manag. 6, 279–286. doi:10.2147/tcrm.s5688
Kanarek, N., Petrova, B., and Sabatini, D. M. (2020). Dietary Modifications for Enhanced Cancer Therapy. Nature 579, 507–517. doi:10.1038/s41586-020-2124-0
Kumar Giri, T., Giri, A., Kumar Barman, T., and Maity, S. (2016). Nanoliposome Is a Promising Carrier of Protein and Peptide Biomolecule for the Treatment of Cancer. Anticancer Agents Med. Chem. 16, 816–831. doi:10.2174/1871520616666151116121821
Liu, F., Xu, X., Li, C., Li, C., Li, Y., Yin, S., et al. (2020). Mannose Synergizes with Chemoradiotherapy to Cure Cancer via Metabolically Targeting HIF-1 in a Novel Triple-Negative Glioblastoma Mouse Model. Clin. Transl. Med. 10, e226. doi:10.1002/ctm2.226
Luo, H., Wang, X., Wang, Y., Dan, Q., and Ge, H. (2022). Mannose Enhances the Radio-Sensitivity of Esophageal Squamous Cell Carcinoma with Low MPI Expression by Suppressing Glycolysis. Discov. OncOncology 13, 1. doi:10.1007/s12672-021-00447-0
Luo, Q., Li, B., and Li, G. (2020). Mannose Suppresses the Proliferation and Metastasis of Lung Cancer by Targeting the ERK/GSK-3β/β-Catenin/SNAIL Axis. Onco Targets Ther. 13, 2771–2781. doi:10.2147/OTT.S241816
Ma, S., Wang, N., Liu, R., Zhang, R., Dang, H., Wang, Y., et al. (2021). ZIP10 Is a Negative Determinant for Anti-tumor Effect of Mannose in Thyroid Cancer by Activating Phosphate Mannose Isomerase. J. Exp. Clin. Cancer Res. 40, 387. doi:10.1186/s13046-021-02195-z
Ma, X., Yang, S., Zhang, T., Wang, S., Yang, Q., Xiao, Y., et al. (2022). Bioresponsive Immune-Booster-Based Prodrug Nanogel for Cancer Immunotherapy. Acta Pharm. Sin. B 12, 451–466. doi:10.1016/j.apsb.2021.05.016
Mardinoglu, A., Stančáková, A., Lotta, L. A., Kuusisto, J., Boren, J., Blüher, M., et al. (2017). Plasma Mannose Levels Are Associated with Incident Type 2 Diabetes and Cardiovascular Disease. Cell Metab. 26, 281–283. doi:10.1016/j.cmet.2017.07.006
Martinez-Pomares, L. (2012). The Mannose Receptor. J. Leukoc. Biol. 92, 1177–1186. doi:10.1189/jlb.0512231
Miyai, T., Hojyo, S., Ikawa, T., Kawamura, M., Irié, T., Ogura, H., et al. (2014). Zinc Transporter SLC39A10/ZIP10 Facilitates Antiapoptotic Signaling during Early B-Cell Development. Proc. Natl. Acad. Sci. U. S. A. 111, 11780–11785. doi:10.1073/pnas.1323549111
Niehues, R., Hasilik, M., Alton, G., Körner, C., Schiebe-Sukumar, M., Koch, H. G., et al. (1998). Carbohydrate-deficient Glycoprotein Syndrome Type Ib. Phosphomannose Isomerase Deficiency and Mannose Therapy. J. Clin. Invest. 101, 1414–1420. doi:10.1172/JCI2350
Okabe, S., Tanaka, Y., and Gotoh, A. (2021). Effect of D-Mannose on Philadelphia Chromosome-Positive Leukemia Cells. Cbm, 1–10. doi:10.3233/CBM-210141
Péanne, R., de Lonlay, P., Foulquier, F., Kornak, U., Lefeber, D. J., Morava, E., et al. (2018). Congenital Disorders of Glycosylation (CDG): Quo Vadis? Eur. J. Med. Genet. 61, 643–663. doi:10.1016/j.ejmg.2017.10.012
Sha, J., Cao, D., Cui, R., Xia, L., Hua, X., Lu, Y., et al. (2020). Mannose Impairs Lung Adenocarcinoma Growth and Enhances the Sensitivity of A549 Cells to Carboplatin. Cancer Manag. Res. 12, 11077–11083. doi:10.2147/CMAR.S278673
Tajan, M., and Vousden, K. H. (2020). Dietary Approaches to Cancer Therapy. Cancer Cell 37, 767–785. doi:10.1016/j.ccell.2020.04.005
Tammi, R., Pasonen-Seppänen, S., Kolehmainen, E., and Tammi, M. (2005). Hyaluronan Synthase Induction and Hyaluronan Accumulation in Mouse Epidermis Following Skin Injury. J. Invest. Dermatol 124, 898–905. doi:10.1111/j.0022-202X.2005.23697.x
Thorens, B., and Mueckler, M. (2010). Glucose Transporters in the 21st Century. Am. J. Physiol. Endocrinol. Metab. 298, E141–E145. doi:10.1152/ajpendo.00712.2009
Tian, S., Peng, P., Li, J., Deng, H., Zhan, N., Zeng, Z., et al. (2020). SERPINH1 Regulates EMT and Gastric Cancer Metastasis via the Wnt/β-Catenin Signaling Pathway. Aging (Albany NY) 12, 3574–3593. doi:10.18632/aging.102831
Torretta, S., Scagliola, A., Ricci, L., Mainini, F., Di Marco, S., Cuccovillo, I., et al. (2020). D-mannose Suppresses Macrophage IL-1β Production. Nat. Commun. 11, 6343. doi:10.1038/s41467-020-20164-6
Wang, Y., Xie, S., and He, B. (2020). Mannose Shows Antitumour Properties against Lung Cancer via Inhibiting Proliferation, Promoting Cisplatin-mediated Apoptosis and Reducing Metastasis. Mol. Med. Rep. 22, 2957–2965. doi:10.3892/mmr.2020.11354
Wculek, S. K., Cueto, F. J., Mujal, A. M., Melero, I., Krummel, M. F., and Sancho, D. (2020). Dendritic Cells in Cancer Immunology and Immunotherapy. Nat. Rev. ImmunolImmunology 20, 7–24. doi:10.1038/s41577-019-0210-z
Wei, Z., Huang, L., Cui, L., and Zhu, X. (2020). Mannose: Good Player and Assister in Pharmacotherapy. Biomed. Pharmacother. 129, 110420. doi:10.1016/j.biopha.2020.110420
Wu, H., Zhang, W., and Mu, W. (2019). Recent Studies on the Biological Production of D-Mannose. Appl. Microbiol. Biotechnol. 103, 8753–8761. doi:10.1007/s00253-019-10151-3
Yu, J., Wang, S., Qi, J., Yu, Z., Xian, Y., Liu, W., et al. (2021). Mannose-modified Liposome Designed for Epitope Peptide Drug Delivery in Cancer Immunotherapy. Int. Immunopharmacol. 101, 108148. doi:10.1016/j.intimp.2021.108148
Zhang, D., Chia, C., Jiao, X., Jin, W., Kasagi, S., Wu, R., et al. (2017). D-mannose Induces Regulatory T Cells and Suppresses Immunopathology. Nat. Med. 23, 1036–1045. doi:10.1038/nm.4375
Keywords: mannose, treg cells, autoimmune disorders, tumor therapy, mannose phosphate isomerase, glycolysis
Citation: Nan F, Sun Y, Liang H, Zhou J, Ma X and Zhang D (2022) Mannose: A Sweet Option in the Treatment of Cancer and Inflammation. Front. Pharmacol. 13:877543. doi: 10.3389/fphar.2022.877543
Received: 16 February 2022; Accepted: 29 April 2022;
Published: 13 May 2022.
Edited by:
Wei Zhao, City University of Hong Kong, Hong Kong SAR, ChinaReviewed by:
Marcelina Janik, Jagiellonian University, PolandCopyright © 2022 Nan, Sun, Liang, Zhou, Ma and Zhang. This is an open-access article distributed under the terms of the Creative Commons Attribution License (CC BY). The use, distribution or reproduction in other forums is permitted, provided the original author(s) and the copyright owner(s) are credited and that the original publication in this journal is cited, in accordance with accepted academic practice. No use, distribution or reproduction is permitted which does not comply with these terms.
*Correspondence: Dunfang Zhang, aXpkZkAxNjMuY29t
Disclaimer: All claims expressed in this article are solely those of the authors and do not necessarily represent those of their affiliated organizations, or those of the publisher, the editors and the reviewers. Any product that may be evaluated in this article or claim that may be made by its manufacturer is not guaranteed or endorsed by the publisher.
Research integrity at Frontiers
Learn more about the work of our research integrity team to safeguard the quality of each article we publish.