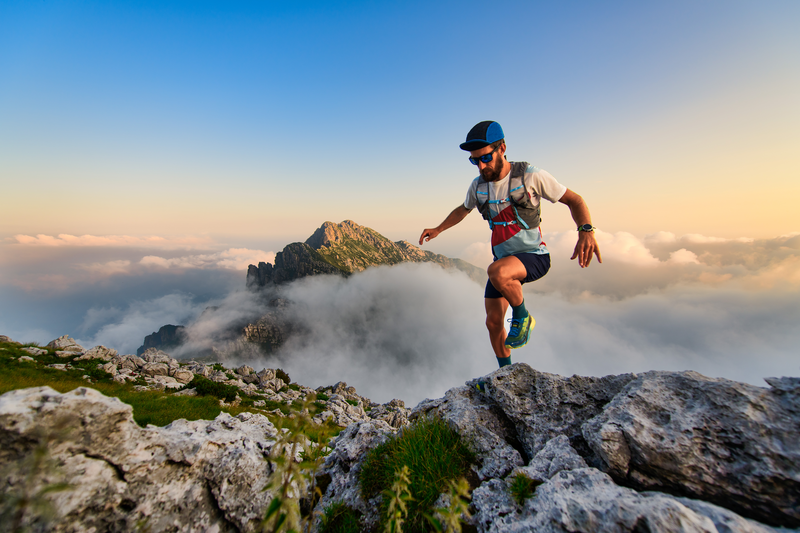
94% of researchers rate our articles as excellent or good
Learn more about the work of our research integrity team to safeguard the quality of each article we publish.
Find out more
ORIGINAL RESEARCH article
Front. Pharmacol. , 06 June 2022
Sec. Ethnopharmacology
Volume 13 - 2022 | https://doi.org/10.3389/fphar.2022.874180
This article is part of the Research Topic Medicinal Plants and Their Active Constituents in the Treatment of Metabolic Syndrome View all 18 articles
Insulin resistance (IR) is a pivotal pathological characteristic that affects the occurrence and development of type 2 diabetes mellitus (T2DM). Thus, the effective control of IR is of great significance for diabetes prevention and treatment. Traditional Chinese medicine (TCM) represents a valuable tool handed down to the world by the Chinese nation and has a long history of use for diabetes clinical therapy. In this study, we focused on a self-drafted TCM-patented formula, Sanghuang Tongxie Formula (SHTXF), which exhibits clinical efficacy in the treatment of diabetes. To explore the effect and molecular mechanism of SHTXF on IR in vivo, Drosophila melanogaster was used and a (Collagen) Cg > InRK1409A diabetic IR fly model was established. SHTXF water extract was found to contribute toward carbohydrate clearance from the circulating system by converting it into triglycerides (TAG), not glycogen, for nutrient storage. In addition, SHTXF activated phosphatidylinositol-3-kinase (PI3K) activity and improved protein kinase B (PKB, also termed Akt) phosphorylation. Finally, SHTXF promoted Drosophila Forkhead Box O (dFoxO) cytoplasmic localization and inhibited its transcriptional activity. Taken together, these findings not only highlight the positive role of SHTXF in ameliorating IR via the PI3K/Akt pathway but also provide potential drug targets and key insights for use in T2DM clinical treatment strategies.
Diabetes mellitus (DM) is a chronic metabolic disorder characterized by sustained hyperglycemia due to insulin resistance (IR) or deficiency. According to the latest data released by the IDF (10th edition), as many as 537 million adults (20–79 years old) worldwide have diabetes in 2021. Among these, type 2 diabetes mellitus (T2DM) accounts for 90%–95% of diabetic patients and is the result of the interaction between genetic and environmental factors (Pearson, 2019; Sun et al., 2022). With the rapid growth of the worldwide incidence, T2DM has become a serious threat to public health. IR is a significant pathological feature of T2DM, occurring throughout the occurrence and development of T2DM (Gutiérrez-Rodelo et al., 2017). The development of insulin resistance typically results in a compensatory increase in endogenous insulin production. The elevated levels of endogenous insulin, an anabolic hormone, are associated with insulin resistance and result in weight gain, which in turn exacerbates insulin resistance. This vicious cycle continues until pancreatic beta-cell activity can no longer adequately meet the insulin demand created by insulin resistance, resulting in hyperglycemia. With the continued mismatch between insulin demand and insulin production, glycemic levels rise to levels consistent with T2DM (Freeman and Pennings, 2020). Therefore, the mitigation, prevention, and control of metabolic abnormalities caused by IR are the main measures for the treatment of T2DM. Although great progress has been made in the study of oral hypoglycemic drugs and insulin, the effective rate of some general medicines for treating diabetes is only 41%, which is far from satisfactory (Nagpal and Bhartia, 2006; Agarwal et al., 2014). In addition, an increasing number of potential toxicities and side effects have been observed in drugs for the treatment of diabetes, including weight gain, bone loss, and increased risk of cardiovascular disease (Valerón and de Pablos-Velasco, 2013; Pang et al., 2015).
Traditional Chinese medicine (TCM) has a long history of use in the prevention and treatment of diabetes in China and other East Asian regions. In contrast to Western medicines, TCM exerts its action via multiple ingredients and multiple target regulatory networks, based on their composition, the functional characteristics of targets, and overall regulation (Tian et al., 2019). Moreover, TCM has the advantages of having low toxicity, fewer side effects, and low cost. Therefore, the research and development of TCM for the prevention and treatment of diabetes is an important area of interest. Sanghuang Tongxie Formula (SHTXF) is a self-drafted patent prescription by Professor Ji-an Li, created based on a combination of the theory of TCM and clinical experience in the treatment of diabetes (Chinese invention patent no. ZL201510724687.8). This formula contains 10 herbal drugs and has the function of clearing the stomach and purging the lung, regulating Qi and turbidity, tonifying the spleen, and benefiting the kidney. The details on the drug composition, as well as the amount of each herb in one dose, are provided in Table 1. In this study, SHTXF was evaluated for its molecular mechanism in the treatment of IR diabetes.
Insulin resistance is a key risk factor and an important source of T2DM, the development of which involves the dysregulation of various signaling pathways, such as the PI3K/Akt, protein kinase C (PKC), AMP-activated protein kinase (AMPK), and nuclear factor κB (NF-κB) pathway (Yaribeygi et al., 2019). PI3K/Akt signaling is a highly conserved and important cellular transduction pathway involved in the regulation of glucose transport, glycogen synthesis, glycolysis and gluconeogenesis, protein synthesis, and lipolysis, playing a vital role in cell migration, differentiation, and apoptosis (Huang et al., 2018). For the past two decades, Drosophila melanogaster has been shown to be an appropriate model organism for T2DM modeling (Musselman et al., 2011; Pasco and Léopold, 2012) in view of the conserved insulin-like peptides (dILPs)/insulin receptor (InR)/insulin receptor substrate (IRS) module and downstream components involved in glycolipid homeostasis. Thanks to powerful genetic tools and reduced genome redundancy (Reiter et al., 2001; Garofalo, 2002), flies have been found to have a nutrition-sensing mode and intracellular signaling pathway (e.g., PI3K/Akt axis) similar to that of mammals at the cellular level. Phosphatidylinositol-3-kinase (PI3K) phosphorylates the D3 hydroxyl group of the inositol ring on PI to form the second messenger phosphatidylinositol-3,4,5-triphosphate (PIP3), which promotes protein kinase B (Akt/PKB) recruitment at the cell membrane and induces allosteric activation (Britton et al., 2002). Finally, activated Akt can lead to the phosphorylation of a large number of important substrates, including the transcription factor FoxO, to execute specific physiological activities (Klöting and Blüher, 2005).
In this study, we established a Cg > InRK1409A diabetes fly model to analyze the effects of SHTXF extract on InR/PI3K/Akt-mediated IR in Drosophila, providing a potential molecular mechanism and theoretical basis for SHTXF in the clinical treatment of T2DM.
To prepare Sanghuang Tongxie Formula (SHTXF), ten herbs (Morus alba L. [Moraceae] and Coptis chinensis Franch. [Ranunculaceae], Magnolia officinalis Rehder & E. H. Wilson [Magnoliaceae], Pueraria montana var. Thomsoni (Benth.) M.R.Almeida [Fabaceae], Astragalus mongholicus Bunge [Fabaceae], Cornus officinalis Siebold & Zucc. [Cornaceae], Raphanus raphanistrum subsp. Sativus (L.). Domina [Brassicaceae], Anemarrhena asphodeloides Bunge [Asparagaceae], Polygonatum odoratum (Mill.) Druce [Asparagaceae], and Atractylodes lancea (Thunb.) DC. [Asteraceae]) (Figure 1) were purchased from Beijing Tongrentang Tangshan Chain Store Drug Store Co., Ltd., and authenticated by Prof. Chunyu Tian at the College of Traditional Chinese Medicine, North China University of Science and Technology. The manufacturer, batch number, and weight of each crude herb used for each dose are listed in Table 1.
FIGURE 1. Composition of Sanghuang Tongxie Formula. The photographs show the composition of SHTXF (Sanghuang Tongxie formula). Morus alba L. [Moraceae] Sangbaipi, (A), Coptis chinensis Franch. [Ranunculaceae] [Huanglian, (B)], Magnolia officinalis Rehder and E. H. Wilson [Magnoliaceae] [Houpo, (C)], and Pueraria montana var. Thomsoni (Benth.) M.R.Almeida [Fabaceae] [Gegen, (D)], Astragalus mongholicus Bunge [Fabaceae] [Huangqi, (E)], Cornus officinalis Siebold & Zucc. [Cornaceae] [Shanzhuyu, (F)], Raphanus raphanistrum subsp. Sativus (L.) Domin [Brassicaceae] [Laifuzi, (G)], Anemarrhena asphodeloides Bunge [Asparagaceae]; Zhimu, (H), Polygonatum odoratum (Mill.) Druce [Asparagaceae] [Yuzhu, (I)], and Atractylodis rhizama [Cangzhu, (J)].
Combined drugs of one dose (75 g) were weighed and soaked in 1.5 L of double-distilled water (ddH2O) for 12 h (Wang et al., 2020), followed by heating for 2 h. Next, the extract was separated and centrifuged at 4,200 × g for 30 min at 4°C, and the residue was collected to repeat the abovementioned steps. The two supernatants were then mixed and filtered by vacuum filtration with a 0.45-µm filter. Finally, the pumped liquid was placed in a beaker with medium fire to obtain a stock extract at a concentration of 0.5 g/ml, when the volume of the liquid reached 150 ml (Supplementary Figure S1). The prepared extract was directly mixed in the regular food to obtain a final concentration of 0.0125, 0.025, 0.05, or 0.1 g/ml SHTXF medium. Water alone was used as a control food.
Standard chemicals (berberine, magnolol, and morroniside) were purchased from the National Institutes for Food and Drug Control (NIFDC). Briefly, the quality of SHTXF was determined using a high-performance liquid chromatography (HPLC) system equipped with a UV-visible detector (Kamal et al., 2015). The analysis was performed using a Shimadzu LC-20A instrument. All chromatographic separations were carried out on an Agilent Eclipse XDB-C18 column (4.6 mm × 250 mm, 5 μm) at 35°C. The mobile phase for berberine was acetonitrile (A) and 0.1% phosphoric acid (B) (A: B = 50:50) by adding 0.1 g of sodium dodecyl sulfonate per 100 ml. A gradient system consisting of methanol (C) and water (D) (C:D = 78:22) was used to elute the magnolol. The mobile phase of morroniside was acetonitrile (E) and 0.3% phosphoric acid solution (F), and the elution gradients were 0–20 min (E: 7%, F: 93%) and 20–50 min (E: 7%–20%, F: 93%–80%).
Flies were maintained on a diet of cornmeal and sucrose medium (100°ml medium preparation: 5 g of sucrose, 1 g of agar, 6 g of cornflour, 3 g of yeast, 0.6 ml of propionic acid, and 100 ml of ultra-pure water) at 25°C in a 12 h light-dark cycle incubator with 50%–60% relative humidity. The Drosophila stocks used included: w1118 (#3605), Cg-Gal4 (#7011), UAS-InRK1409A (#8253), tGPH (#8164), and dFoxO-GFP (#37585) obtained from Bloomington Drosophila stock center (BDSC) in Indiana University. The crossing scheme for all the groups is shown in Supplementary Figure S2. For all fly crossing assays, healthy, unmated male and female parents were randomly assigned to different groups.
Early third-instar larvae were starved for 2 h under adverse food conditions (0.8% agar in PBS) and then transferred to fresh dye-containing food (0.5% Brilliant Blue FCF) for 20 min. After feeding, the larvae were washed with phosphate-buffered saline (PBS), dried on tissue paper, and homogenized in an Eppendorf (EP) tube containing 100 µl of lysis buffer using a micro high-speed homogenizer (HR-68; Shanghai Hutong Industrial Co., Ltd.). After boiling and centrifuging for 3 min, followed by clearing by a high-speed refrigerated centrifuge (5430R; Eppendorf), 2 µl of the supernatant was analyzed with a spectrophotometer at 630 nm. A small amount of dye-containing food was weighed, processed as described above, and used as the standard to calculate the amount of ingested food (Matsuda et al., 2015).
The larvae were collected, rinsed with phosphate-buffered saline (PBS), and dried on tissue paper. The cuticle was carefully torn to release hemolymph on the parafilm membrane. Two microliters of hemolymph were collected using a micropipette, diluted 20 times with cold PBS, centrifuged for 3 min, and cleared by a high-speed refrigerated centrifuge. The supernatant was boiled at 75°C for 10 min and cleared by centrifugation. Next, glucose was measured after 15 min of incubation at 37°C using HK reagent (G3293; Sigma). A plate reader (M200PRD; Tecan) was used to measure absorbance at 340 nm.
Trehalose was converted using porcine trehalase (T8778; Sigma) overnight at 37°C, and the total amount of glucose was measured in the same way. The hemolymph trehalose concentration was determined by subtracting the value of free glucose from the untreated sample.
Six third-instar larvae were collected and rinsed several times with PBS to remove traces of food. The frozen samples were homogenized using a pellet pestle in 200 µl of cold 0.05% PBST (PBS + 0.05% Tween-20) on ice, immediately heat-inactivated at 75°C for 10 min, and then cooled to room temperature (RT). This was followed by homogenization in an EP tube containing 100 µl of lysis buffer using a high-speed homogenizer. Next, 10 μl of homogenate were centrifuged for 3 min, cleared by a high-speed refrigerated centrifuge, and used for the measurement of free glycerol. Ten microliters of homogenate were used to determine the protein content using a bicinchoninic acid assay (BCA) protein assay kit (P0010; Beyotime).
Ten microliters of the homogenate were mixed with 10 µl of a triglyceride reagent (T2449; Sigma) including lipase for hydrolysis of triglycerides to glycerol, incubated at 37°C for 60 min, centrifuged for 3 min, and cleared by a high-speed refrigerated centrifuge. Ten microliters of the supernatant were used for the measurement of triglycerides (TAG) using free glycerol reagent (F6428; Sigma) at 540 nm. A triolein-equivalent glycerol standard (G7793; Sigma) was used as the standard. The TAG concentration for each sample was determined by subtracting the values of free glycerol from the untreated samples.
Five microliters of the sample were incubated with 10 µl of buffer A [5 mM Tris-HCl (pH 6.6), 137 mM NaCl, and 2.7 mM KCl] containing amyloglucosidase (10115-1 g; Sigma) at 50°C for 60 min to digest glycogen. Ten microliters of the sample were incubated with 5 µl of buffer A without enzymes in parallel for the determination of glucose levels. Glycogen was used as a standard (10901393001; Sigma). The glycogen concentration for each sample was determined by subtracting the values of free glucose in the untreated samples. The amounts of TAG and glycogen per fly were normalized to soluble protein levels per fly (Li and Tennessen, 2017).
For Nile red staining, larvae were quickly inverted and dissected in 0.3% PBST. The tissues were incubated for 5 min with 0.001% Nile Red (72485; Sigma) in 0.3% PBST and washed twice with PBS at room temperature. The stained samples were mounted in 75% glycerol for microscopic analysis.
The larval discs were dissected and fixed in 4% formaldehyde for 20 min at room temperature (RT). After several washes with 0.3% (v/v) PBST, the discs were stained with primary antibodies at 4°C overnight and then with secondary antibodies at RT for 2 h. The following antibodies were used: mouse anti-Dlg1 (1:100) (4F3 anti-discs large; Developmental Studies Hybridoma Bank), rabbit anti-Akt (1:400) [4,691; cell signaling technology (CST)], rabbit anti-p-Akt (1:400) (4,060; CST), goat anti-mouse-cyanine3 (Cy3) (1:1000) (A10521; Life Technologies), and goat anti-rabbit Alexa 488 (1:1000) (4,412; CST). Vectashield medium (H-1500; Vector Laboratories) with DAPI (4’,6-diamidino-2-phenylindole) was used for mounting.
Whole larvae were lysed in radioimmunoprecipitation assay (RIPA) buffer (P0013; Beyotime) containing phosphatase inhibitor cocktail A (P1082; Beyotime) and phenylmethanesulfonyl fluoride (PMSF). Equal amounts of protein (10–30 µg, measured by BCA assay) were separated by sodium dodecyl sulfate-polyacrylamide gel electrophoresis (SDS-PAGE), transferred to a polyvinylidene fluoride (PVDF) membrane, and subjected to the standard western blot protocol, as previously described (Roth et al., 2018). The antibodies used in this study were rabbit anti-Akt (1:1000) (4,691; CST), rabbit anti-p-Akt (1:1000) (4,060; CST), rabbit anti-α-tubulin (1:1000) (2,125; CST), and goat anti-rabbit IgG (H + L, HRP) (1:10000) (5220-0336; Sera Care).
TRIzol (Invitrogen) and PureLinkTM RNA Mini Kit (12183018A; Life Technologies) were used to isolate total RNA from third-instar larvae of the indicated groups, followed by qRT-PCR as previously described (Wang et al., 2003). The relative amounts of transcripts were calculated using the comparative CT method with ribosomal protein 49 (rp49) as the reference gene. The following primers were used:
For rp49 Sense: 5’- TCCTACCAGTTCAAGATGAC—3’
Antisense: 5’- TCCTACCAGTTCAAGATGAC—3’
For 4EBP Sense: 5’- TGATCACCAGGAAGGTTGTCATCTC—3’
Antisense: 5’- GAGCCACGGAGATTCTTCATGAAAG—3’
All data were verified using at least three independent experiments. The results were processed using GraphPad Prism 8.0 software as bar graphs. One-way analysis of variance (ANOVA) with Bonferroni’s multiple comparison test was employed for statistical analysis. The p value was set at p < 0.05, and the center value was considered the mean. The error bars indicate standard deviation. “ns” denotes “not significant” values (p ≥ 0.05); ∗p < 0.05; ∗∗p < 0.01; ∗∗∗p < 0.001, and ∗∗∗∗p < 0.0001.
The Sanghuang Tongxie Formula (SHTXF) is composed of 10 herbal medicines, including Morus alba L. [Moraceae] (Sangbaipi) and Coptis chinensis Franch. [Ranunculaceae] (Huanglian), Magnolia officinalis Rehder and E. H. Wilson [Magnoliaceae] (Houpo), Pueraria montana var. Thomsoni (Benth.) M.R.Almeida [Fabaceae] (Gegen), Astragalus mongholicus Bunge [Fabaceae] (Huangqi), Cornus officinalis Siebold & Zucc. [Cornaceae] (Shanzhuyu), and Raphanus raphanistrum subsp. Sativus (L.) Domin [Brassicaceae] (Laifuzi), Anemarrhena asphodeloides Bunge [Asparagaceae]; Zhimu, Polygonatum odoratum (Mill.) Druce [Asparagaceae] (Yuzhu), and Atractylodes lancea (Thunb.) DC. [Asteraceae] (Cangzhu) (Figure 1; Table 1). To examine the quality of SHTXF, three representative compounds were selected as the quality standards. When the SHTXF high-performance liquid chromatography (HPLC) chromatogram was compared with the standard control (Supplementary Figure S3), we observed distinguished test peaks of berberine, magnolol, and morroniside in the chemical fingerprints of the SHTXF aqueous extract (Figure 2). Additionally, the proportions of the three ingredients determined by HPLC were 1.4%, 0.34%, and 0.022%, respectively (Table 2). This suggests that the prepared SHTXF contains the necessary active components and is suitable for further study.
FIGURE 2. HPLC chromatogram of SHTXF. Three major compounds (berberine, magnolol, and morroniside) of SHTXF were identified and compared with the standards using HPLC. The peaks of berberine [(A), 26 nm], magnolol [(B), 294 nm], and morroniside [(C), 240 nm] are indicated by red arrows.
To better dissect the effect of drugs on IR, we employed the GAL4/upstream activation sequence (Gal4/UAS) system and generated an IR model in Drosophila third-instar larvae (Brand and Perrimon, 1993), which was achieved by expressing a dominant-negative (DN) form of Drosophila InR (carrying the amino acid replacement K1409A, InRK1409A, FBtp0018596, http://flybase.org/reports/FBtp0018596) under the control of the fat body (equivalent to mammalian adipose and liver tissues)-specific Collagen (Cg) promoter (FBti0027802, https://flybase.org/reports/FBti0027802) (Wu et al., 2005; Hennig et al., 2006). Misexpression of the InR antimorphic allele (UAS-InRK1409A)-driven Cg-Gal4 (Cg > InRK1409A) interfered with the physiological function of endogenous InR and undermined the downstream response of the insulin signaling cascade. As a result, both the hemolymph glucose level and the hemolymph trehalose (a glucose disaccharide that constitutes the major form of circulating sugar in insects) were significantly increased by 67.57% and 40.00%, respectively, in the larval stage (Figures 3A,B, Supplementary Figure S2). This suggests that fat body impairment in insulin signaling triggers insulin resistance (IR) and diabetic phenotypes.
FIGURE 3. SHTXF reduced the increased level of glucose and trehalose in Cg > InRK1409A files. (A,B) Assays for circulating the metabolites were performed on the wandering third-instar larvae of indicated groups: hemolymph glucose [(A), 10–12 larvae per pool, n = 5], hemolymph trehalose [(B), 6–8 larvae per pool, n = 5]. (C) Measurement of 20-min food intake by early third-instar larvae as calculated by blue food ingestion (6–8 larvae per pool, n ≥ 3). (D) Amount of protein indicates the soluble fraction in 0.05% PBST after heat inactivation (3 larvae per pool, n = 5). Error bars indicate the standard deviation (SD). One-way ANOVA with Bonferroni’s multiple comparison test was used to compute the p values: ****p < 0.0001, ***p < 0.001, and **p < 0.01. ns, no significant difference.
To determine whether there was an inhibitory effect, we prepared feeding media consisting of SHTXF aqueous extract and raised flies with genotype Cg > InRK1409A from the egg stage to the third-instar larval stage. The results showed that the dominant-negative InR-induced elevated circulating glucose was significantly suppressed by SHTXF extract at the concentrations of 0.025, 0.05, and 0.1, but not 0.0125 g/ml (Figure 3A). For circulating trehalose, SHTXF at a concentration of 0.025 g/ml displayed a stronger inhibitory effect than 0.0125 and 0.05 g/ml, while the concentrations of 0.1 g/ml did not show an obvious inhibitory effect (Figure 3B). Next, to exclude the possibility that changes in feeding rate may interfere with the metabolic phenotypes, we evaluated the level of food intake and observed a normal ingestion rate of drug-addled groups or compared with that of the control group (Figure 3C).
The protein content of larvae in each group was similar, with no significant statistical difference (Figure 3D). In addition, the hyperglycemic diabetic phenotypes of Cg > InRK1409A larvae were notably inhibited by treatment with metformin (10 mM, a positive control) (Shirazi et al., 2014), which is one of the most popular oral glucose-lowering medications and is widely considered to be the optimal initial therapy for patients with T2DM (Figures 3A,B) (Lv and Guo, 2020). Collectively, SHTXF displayed a dose-dependent effect on Cg > InRK1409A-triggered diabetic high circulating sugar in the Drosophila larval stage.
Consistent with the widely investigated roles of the insulin pathway in promoting anabolism, the downregulation of insulin signal activity by expressing InRK1409A in the fat body reduced the total body storage sugar glycogen level by 17.48% during development compared with the Cg-Gal4/+ genetically matched controls (Supplementary Figure S4). However, we found that neither SHTXF (0.0125 and 0.025 g/ml) nor metformin significantly altered the overall larval glycogen content in the Cg > InRK1409A background. Intriguingly, SHTXF extract at a concentration of 0.05 g/ml or 0.1 g/ml even decreased the level of stored glycogen (Supplementary Figure S4).
To examine the effect of SHTXF on lipid metabolism, we first checked the whole body TAG content and found that fat body showed low activity of the insulin pathway (Cg > InRK1409A) decreased the storage lipid TAG level by 15.67% compared with the control (Figure 4A). Moreover, the reduced TAG level in the IR model was restored by treatment with metformin or 0.025 g/ml SHTXF (Figure 4A). Taken together, these data indicate that SHTXF may contribute toward carbohydrate clearance from the circulatory system by converting it into TAG, not glycogen, for nutrient storage. Ultimately, a concentration of 0.025 g/ml for the SHTXF water extract was chosen, which showed a strong inhibitory effect, to further explore the mechanisms.
FIGURE 4. SHTXF rescues the IR-induced lipid homeostasis disorder. (A) Assays for stored triglycerides (TAG) contents of Drosophila larval stage, which are presented relative to the protein contents of the tissue sample to normalize the weight difference between control and other indicated groups (3 larvae per pool, n = 5). (B–E) Nile red staining of lipid storage droplets in the larval fat body adipose tissue. (F–H) Quantification of lipid droplet size (F), lipid droplets number (G), and total area of lipid droplets in Cg-Gal4/+ (control) larvae and Cg > InRK1409A larvae in normal, metformin-, or SHTXF-added diets (n = 10). The error bars indicate the standard deviation. p values were calculated by one-way ANOVA followed by Bonferroni’s multiple comparison test: ****p < 0.0001, ***p < 0.001, and *p < 0.05. ns, no significant difference. Scale bar, 20 µm (B–E).
To further determine the role of SHTXF in modulating lipid storage, we analyzed the distribution and lipid content in the fat body visualized by Nile Red staining. Consistent with a previous study (Fischer et al., 2017; Lourido et al., 2021), we observed that Cg > InRK1409A-promoted IR enlarged the fat body cell lipid droplet size and reduced the lipid droplet number, total area of lipid droplets, and cell size when compared with the control (Figures 4B,C,F–H; Supplementary Figure S5). SHTXF partially reversed the increase in the size of lipid droplets and the decrease in lipid droplet number, total area, and cell size (Figures 4E–H; Supplementary Figure S5). Except for lipid droplet size, metformin displayed a similar effect on IR-mediated lipid storage disorder as SHTXF (Figures 4D,F–H; Supplementary Figure S5). These results indicate that SHTXF reverses insulin resistance-induced lipid homeostasis.
As phosphoinositide 3-kinase (PI3K) plays a pivotal role in the insulin pathway, we introduced an in situ fluorescent reporter termed tGPH (a fusion protein containing a tubulin promoter, GFP, and PH domain) (Britton et al., 2002), which recognizes PI (3,4,5)P3 and visualizes PI3K activation by observing cell membrane-associated GFP, to check whether insulin signaling was truly impaired in IR diabetic files and the effect of SHTXF. Consistent with our previous work, compared with the control, the fat body cells of the IR model showed decreased cell membrane localization, indicating reduced PI3K activity and the downstream signaling cascade (Figures 5A–A’’’,B–B’’’). While Cg > InRK1409A flies were raised on metformin- or SHTXF-added medium, the GFP signal of fat body cells was recruited to the membrane, indicating that PI3K activity was restored (Figures 5C–C’’’,D–D’’’). These results indicate that SHTXF may improve PI3K activity to ameliorate insulin resistance.
FIGURE 5. SHTXF improves the PI3K activity in vivo. The representative merged fluorescence micrographs show the third-instar larval fat body carrying the tGPH reporter stained with anti-Dlg, which outlines the cell membranes. The individual channels detect only Dlg signal [red, (A’–D’)], and only tGPH [green, (A’’’–D’’’)]. Nuclei were labeled with DAPI [blue, (A’–D’)]. Scale bar, 20 μm. Genotypes: (A) Cg-Gal4/+; tGPH/+ and (B–D) Cg-Gal4/+; tGPH/UAS-InRK1409A.
To further identify the effect of SHTXF on insulin resistance, we monitored Akt phosphorylation using an in vivo immunostaining assay and a western blotting (WB) assay in vitro. As expected, the misexpression of InRK1409A in the larval fat body (Cg > InRK1409A) led to reduced Akt Ser505 (equivalent to Ser473 in human Akt1) phosphorylation (p-Akt) in this organ compared with the control (Figures 6A–A’’’,B–B’’’,E,F), while total Akt levels were unchanged (Figure 6E; Supplementary Figure 6A–A’’’,B–B’’’). The decreased p-Akt level in the Cg > InRK1409A IR model was largely rescued by SHTXF or moderately inhibited by metformin (Figures 6C–C’’’,D–D’’’,E,F). However, the expression of total Akt protein was unaffected by SHTXF or metformin (Supplementary Figures S6C–C’’’,D–D’’’). Taken together, these data confirmed that SHTXF enhanced Akt phosphorylation.
FIGURE 6. SHTXF increases the level of Akt phosphorylation. (A–D) Merged fluorescence micrographs of Drosophila third-instar larval fat body are shown. The individual channels detect only Dlg [red, (A’–D’)], only DAPI [blue, (A’’–D’’)], and only p-Akt [green, (A”’–D”’), fluorescent dots]. (E) Western blot analysis of whole-body extracts from third-instar larvae for phosphorylated Akt, total Akt, and α-tubulin (9 larvae per time). α-tubulin was used as a loading control. Groups (from left to right): Control (Cg-Gal4/+), Model (Cg-Gal4/+; UAS-InRK1409A/+), Metformin (Cg-Gal4/+; UAS-InRK1409A/+ files treated with 10 mM metformin), SHTXF (Cg-Gal4/+; UAS-InRK1409A/+ files treated with 0.025 g/ml SHTXF). (F) Statistical analysis of the p-Akt/Akt relative level from three independent experiments is shown in (E) (n = 3). The error bars indicate the standard deviation. One-way ANOVA with Bonferroni’s multiple comparison test was used to compute p values: ****p < 0.0001, ***p < 0.001, and *p < 0.05. Scale bar, 20 μm (A–D). Genotypes: (A) Cg-Gal4/+ and (B–D) Cg-Gal4/+; UAS-InRK1409A/+.
To address whether SHTXF modulates the nuclear–cytoplasmic shuttling of Drosophila FoxO (dFoxO), we examined the subcellular localization of the dFoxO-GFP fusion protein in fat body cells (Wu et al., 2015). We observed that the nuclear localization of dFoxO-GFP was significantly increased when InRK1409A was expressed in fat body cells by Cg-Gal4 (Figures 7A–A’’’,B–B’’’). SHTXF, similar to metformin, hindered the nuclear localization of dFoxO-GFP (Figures 7C–C’’’,D–D’’’). In addition, to monitor dFoxO activity directly, we detected the transcription of the well-characterized dFoxO target gene 4E-BP using a quantitative reverse transcription-polymerase chain reaction (qRT-PCR) assay. Compared to the control, the upregulated 4E-BP mRNA level in Cg > InRK1409A larvae was appreciably blocked by SHTXF and metformin (Figure 7E), indicating that SHTXF promotes dFoxO cytoplasmic localization and inhibits its transcriptional activity.
FIGURE 7. SHTXF remedies Cg > InRK1409A-triggered dFoxO nuclear localization and transcriptional activity. (A–D) Representative merged fluorescence micrographs showing the third-instar larval fat body carrying dFoxO-GFP reporter. The individual channels detect only Dlg [red, (A’–D’)], only DAPI [blue, (A’’–D’’)], and only dFoxO-GFP [green, (A”’–D”’)]. (E) Histogram shows the 4EBP mRNA transcription level as measured by qRT-PCR. Total RNA of Drosophila third-instar larvae was extracted and normalized for cDNA synthesis. Groups (from left to right): control (Cg-Gal4/+), model (Cg-Gal4/+; UAS-InRK1409A/+), metformin (Cg-Gal4/+; UAS-InRK1409A/+ files treated with 10 mM metformin), SHTXF (Cg-Gal4/+; UAS-InRK1409A/+ files treated with 0.025 g/ml SHTXF) (n = 3). The error bars indicate the standard deviation from three independent experiments. The p values were calculated by one-way ANOVA and Bonferroni’s multiple comparison test: ****p < 0.0001, ***p < 0.001 and *p < 0.05. Scale bar, 20 μm (A–D). Genotypes: (A) Cg-Gal4/+; dFoxO-GFP/+ and (B–D) Cg-Gal4/+; dFoxO-GFP/UAS-InRK1409A.
Drosophila melanogaster has become an ideal model organism for the study of disorders of glucose and lipid metabolism caused by insulin resistance (IR), thanks to the presence of evolutionarily conserved metabolic signaling pathways (insulin and insulin-like growth factor signaling pathways) and hormone regulation processes (the antagonistic regulation of insulin-like peptides and adipokine hormones). More importantly, the successful application of the GAL4/UAS binary expression system has made it possible to reveal complex gene functions (Musselman et al., 2018). As only one InR and insulin receptor substrate (IRS, encoded by chico) is expressed in Drosophila, we employed the GAL4/UAS system to specifically express the dominant-negative form of InR (UAS-InRK1409A) in the fly fat body (Cg-Gal4) by genetic hybridization (Supplementary Figure S2). Logically, the misexpression of the InR antimorphic allele interferes with the physiological functions of endogenous InR and reduces the transduction activity of insulin signaling, resulting in IR, and establishing the Cg > InRK1409A diabetic model. A previous study reported that a high-sugar diet (HSD) could induce obesity and insulin resistance in wild-type Drosophila with type 2 diabetes (Pasco and Léopold, 2012). However, the Cg > InRK1409A IR model generated in our study did not present an adiposity phenotype with reduced total TAG content (Figure 4A). Given the complicated relationship between fat and IR during diabetes occurrence and development, applying the Cg > InRK1409A model could better elucidate the roles of IR in diabetes and screen potential drugs or ingredients.
The sugars in Drosophila mainly participate in nutrient circulation in the form of glucose (monosaccharide) and trehalose (disaccharide), provide energy for growth and development through glycolysis, and store energy in the form of glycogen (Heydemann, 2016). As a major nutrient, lipids store energy, participate in cell membrane structure, and synthesize hormones and vitamins, which are essential for normal life activities. When apolipoprotein binds to its receptor, triglycerides (TAG) are generated and enter the body’s adipocytes to form lipid droplets and organelles which are common in animal and plant cells that store neutral fat (Heier and Kühnlein, 2018). Therefore, we analyzed glucose and lipid metabolism in fruit flies fed SHTXF aqueous extract. As a result, it was found that SHTXF may contribute to sugar clearance from the circulating system by transforming it into TAG, but not glycogen, for nutrient storage. Moreover, our results showed that SHTXF could improve the activity of PI3K, promote the phosphorylation of Akt, and impede dFoxO transcriptional activity. Overall, these results indicate that SHTXF activates the central factors of the PI3K/Akt signaling pathway to ameliorate IR.
As a self-drafted patent prescription based on TCM theory and clinical experience, SHTXF contains 10 herbs and has shown a notable effect on the treatment of IR diabetes with its active ingredients (Chinese invention patent No. ZL201510724687.8). Coptis chinensis Franch. [Ranunculaceae] (Huanglian) has been used for centuries as an anti-diabetic drug in TCM, and its main bioactive base berberine can reduce blood glucose, regulate lipids, and improve insulin resistance to alleviate T2DM (Abd El-Wahab et al., 2013). Berberine has been found to show potential in acting on block lipid transport-1 (BLT1), modulating the leukotriene B4-Block lipid transport-1 (LTB4-BLT1) axis to ameliorate insulin resistance and inflammation. It has also been found to increase the peroxisome proliferator-activated receptor γ co-activator 1α (PGC1α), activate the phosphorylation of adenosine monophosphate (AMP), protein kinase (p-AMPK), and liver kinase B1 (p-LKB1), and reduce the adenosine monophosphate/adenosine triphosphate (AMP/ATP) ratio rate, thereby reversing fructose-induced insulin resistance (Li et al., 2020; Gong et al., 2021). In addition, magnolol exerts antioxidant activity by inhibiting the liver activity of cytochromeP450, family2, subfamily E, and polypeptide1 (CYP2E1) to improve glucose tolerance, thereby reducing IR and improving glucose and lipid metabolism disorders (Wang et al., 2014). Cornus officinalis Siebold & Zucc. [Cornaceae] extract can promote the expression of peroxisome proliferator-activated receptor γ (PPAR-γ) in adipocytes, increase the expression of recombinant glucose transporter 4 (GLUT4) and adiponectin, improve insulin resistance, and increase glucose utilization, ultimately achieving the effect of lowering the blood sugar levels (Kim et al., 2009). Thus, SHTXF treatment in diabetic IR appears to be the result of multiple factors, multiple pathways, and multi-target regulatory networks.
Based on previous research, our work focuses on the positive effect of SHTXF on IR by modulating the InR/PI3K/Akt signaling pathway, not only indicating potential drug targets but also providing key insights for the clinical treatment of T2DM and related metabolic diseases.
The original contributions presented in the study are included in the article/Supplementary Material, further inquiries can be directed to the corresponding authors.
XC, XL, CW, and J-aL conceived and designed the experiments. XC, XL, BZ, ZW, J-aL, and YB conducted the experiments. HC, XG, and CT supervised and advised on the study. XC, XL, CW, and J-aL analyzed the data and wrote the manuscript. All authors approved the final manuscript for publication.
This work was supported by the Science and Technology Partnership Program, Ministry of Science and Technology of China (No. KY201904005), Tangshan Science and Technology Innovation Team Training Program (No. 19130205C) to J-aL, and the Science and Technology Project of Hebei Education Department (No. BJ2019040) and Tangshan Science and Technology Project (No. 21130230C) to CW.
The authors declare that the research was conducted in the absence of any commercial or financial relationships that could be construed as a potential conflict of interest.
All claims expressed in this article are solely those of the authors and do not necessarily represent those of their affiliated organizations, or those of the publisher, the editors, and the reviewers. Any product that may be evaluated in this article, or claim that may be made by its manufacturer, is not guaranteed or endorsed by the publisher.
The authors thank the Bloomington Drosophila Stock Center for fly stocks, Dr. Ying Sun for graph editing, and members of the Wu lab and Li lab for discussion and critical comments.
The Supplementary Material for this article can be found online at: https://www.frontiersin.org/articles/10.3389/fphar.2022.874180/full#supplementary-material
Abd El-Wahab, A. E., Ghareeb, D. A., Sarhan, E. E., Abu-Serie, M. M., and El Demellawy, M. A. (2013). In Vitro biological Assessment of Berberis Vulgaris and its Active Constituent, Berberine: Antioxidants, Anti-acetylcholinesterase, Anti-diabetic and Anticancer Effects. BMC Complement. Altern. Med. 13, 218. doi:10.1186/1472-6882-13-218
Agarwal, A. A., Jadhav, P. R., and Deshmukh, Y. A. (2014). Prescribing Pattern and Efficacy of Anti-diabetic Drugs in Maintaining Optimal Glycemic Levels in Diabetic Patients. J. Basic Clin. Pharm. 5, 79–83. doi:10.4103/0976-0105.139731
Brand, A. H., and Perrimon, N. (1993). Targeted Gene Expression as a Means of Altering Cell Fates and Generating Dominant Phenotypes. Development 118, 401–415. doi:10.1242/dev.118.2.401
Britton, J. S., Lockwood, W. K., Li, L., Cohen, S. M., and Edgar, B. A. (2002). Drosophila's insulin/PI3-Kinase Pathway Coordinates Cellular Metabolism with Nutritional Conditions. Dev. Cel 2, 239–249. doi:10.1016/s1534-5807(02)00117-x
Fischer, Z., Das, R., Shipman, A., Fan, J. Y., Pence, L., Bouyain, S., et al. (2017). A Drosophila Model of Insulin Resistance Associated with the Human TRIB3 Q/R Polymorphism. Dis. Model. Mech. 10, 1453–1464. doi:10.1242/dmm.030619
Garofalo, R. S. (2002). Genetic Analysis of Insulin Signaling in Drosophila. Trends Endocrinol. Metab. 13, 156–162. doi:10.1016/s1043-2760(01)00548-3
Gong, M., Duan, H., Wu, F., Ren, Y., Gong, J., Xu, L., et al. (2021). Berberine Alleviates Insulin Resistance and Inflammation via Inhibiting the LTB4-BLT1 Axis. Front. Pharmacol. 12, 722360. doi:10.3389/fphar.2021.722360
Gutiérrez-Rodelo, C., Roura-Guiberna, A., and Olivares-Reyes, J. A. (2017). Molecular Mechanisms of Insulin Resistance: An Update. Gaceta Med. de Mexico 153, 214–228.
Heier, C., and Kühnlein, R. P. (2018). Triacylglycerol Metabolism in Drosophila melanogaster. Genetics 210, 1163–1184. doi:10.1534/genetics.118.301583
Hennig, K. M., Colombani, J., and Neufeld, T. P. (2006). TOR Coordinates Bulk and Targeted Endocytosis in the Drosophila melanogaster Fat Body to Regulate Cell Growth. J. Cel Biol 173, 963–974. doi:10.1083/jcb.200511140
Heydemann, A. (2016). An Overview of Murine High Fat Diet as a Model for Type 2 Diabetes Mellitus. J. Diabetes Res. 2016, 2902351. doi:10.1155/2016/2902351
Huang, X., Liu, G., Guo, J., and Su, Z. (2018). The PI3K/AKT Pathway in Obesity and Type 2 Diabetes. Int. J. Biol. Sci. 14, 1483–1496. doi:10.7150/ijbs.27173
Kamal, A., Gulfraz, M., Anwar, M. A., and Malik, R. N. (2015). Reverse Phase High Performance Liquid Chromatographic Method Development Based on Ultravioletvisible Detector for the Analysis of 1-hydroxypyrene (PAH Biomarker) in Human Urine. Int. J. Occup. Med. Environ. Health 28, 399–403. doi:10.13075/ijomeh.1896.00325
Kim, H. J., Kim, K. S., Lee, T. J., and Kim, Y. C. (2009). Antidiabetic Effects of Corni Fructus Extract on Blood Glucose and Insulin Resistance in Db/db Mice. Toxicol. Res. 25, 93–99. doi:10.5487/TR.2009.25.2.093
Klöting, N., and Blüher, M. (2005). Extended Longevity and Insulin Signaling in Adipose Tissue. Exp. Gerontol. 40, 878–883. doi:10.1016/j.exger.2005.07.004
Li, H., and Tennessen, J. M. (2017). Methods for Studying the Metabolic Basis of Drosophila Development. Wiley Interdiscip. Rev. Dev. Biol. 6, e280. doi:10.1002/wdev.280
Li, Y., Wang, B., Shen, J., Bai, M., and Xu, E. (2020). Berberine Attenuates Fructose-Induced Insulin Resistance by Stimulating the Hepatic LKB1/AMPK/PGC1α Pathway in Mice. Pharm. Biol. 58, 385–392. doi:10.1080/13880209.2020.1756349
Lourido, F., Quenti, D., Salgado-Canales, D., and Tobar, N. (2021). Domeless Receptor Loss in Fat Body Tissue Reverts Insulin Resistance Induced by a High-Sugar Diet in Drosophila melanogaster. Sci. Rep. 11, 3263. doi:10.1038/s41598-021-82944-4
Lv, Z., and Guo, Y. (2020). Metformin and its Benefits for Various Diseases. Front. Endocrinol. (Lausanne) 11, 191. doi:10.3389/fendo.2020.00191
Matsuda, H., Yamada, T., Yoshida, M., and Nishimura, T. (2015). Flies without Trehalose. J. Biol. Chem. 290, 1244–1255. doi:10.1074/jbc.M114.619411
Musselman, L. P., Fink, J. L., Grant, A. R., Gatto, J. A., Tuthill, B. F., and Baranski, T. J. (2018). A Complex Relationship between Immunity and Metabolism in Drosophila Diet-Induced Insulin Resistance. Mol. Cel Biol 38. doi:10.1128/MCB.00259-17
Musselman, L. P., Fink, J. L., Narzinski, K., Ramachandran, P. V., Hathiramani, S. S., Cagan, R. L., et al. (2011). A High-Sugar Diet Produces Obesity and Insulin Resistance in Wild-type Drosophila. Dis. Model. Mech. 4, 842–849. doi:10.1242/dmm.007948
Nagpal, J., and Bhartia, A. (2006). Quality of Diabetes Care in the Middle- and High-Income Group Populace: the Delhi Diabetes Community (DEDICOM) Survey. Diabetes Care 29, 2341–2348. doi:10.2337/dc06-0783
Pang, B., Zhao, L. H., Zhou, Q., Zhao, T. Y., Wang, H., Gu, C. J., et al. (2015). Application of Berberine on Treating Type 2 Diabetes Mellitus. Int. J. Endocrinol. 2015, 905749. doi:10.1155/2015/905749
Pasco, M. Y., and Léopold, P. (2012). High Sugar-Induced Insulin Resistance in Drosophila Relies on the Lipocalin Neural Lazarillo. PLoS One 7, e36583. doi:10.1371/journal.pone.0036583
Pearson, E. R. (2019). Type 2 Diabetes: a Multifaceted Disease. Diabetologia 62, 1107–1112. doi:10.1007/s00125-019-4909-y
Reiter, L. T., Potocki, L., Chien, S., Gribskov, M., and Bier, E. (2001). A Systematic Analysis of Human Disease-Associated Gene Sequences in Drosophila melanogaster. Genome Res. 11, 1114–1125. doi:10.1101/gr.169101
Roth, S. W., Bitterman, M. D., Birnbaum, M. J., and Bland, M. L. (2018). Innate Immune Signaling in Drosophila Blocks Insulin Signaling by Uncoupling PI(3,4,5)P3 Production and Akt Activation. Cell Rep 22, 2550–2556. doi:10.1016/j.celrep.2018.02.033
Shirazi, F., Farmakiotis, D., Yan, Y., Albert, N., Do, K. A., Kim-Anh, D., et al. (2014). Diet Modification and Metformin Have a Beneficial Effect in a Fly Model of Obesity and Mucormycosis. PLoS One 9, e108635. doi:10.1371/journal.pone.0108635
Sun, H., Saeedi, P., Karuranga, S., Pinkepank, M., Ogurtsova, K., Duncan, B. B., et al. (2022). IDF Diabetes Atlas: Global, Regional and Country-Level Diabetes Prevalence Estimates for 2021 and Projections for 2045. Diabetes Res. Clin. Pract. 183, 109119. doi:10.1016/j.diabres.2021.109119
Tian, J., Jin, D., Bao, Q., Ding, Q., Zhang, H., Gao, Z., et al. (2019). Evidence and Potential Mechanisms of Traditional Chinese Medicine for the Treatment of Type 2 Diabetes: A Systematic Review and Meta-Analysis. Diabetes Obes. Metab. 21, 1801–1816. doi:10.1111/dom.13760
Valerón, P. F., and de Pablos-Velasco, P. L. (2013). Limitations of Insulin-Dependent Drugs in the Treatment of Type 2 Diabetes Mellitus. Med. Clin. (Barc) 141 Suppl 2 (Suppl. 2), 20–25. doi:10.1016/S0025-7753(13)70059-9
Wang, J. J., Zhao, R., Liang, J. C., and Chen, Y. (2014). The Antidiabetic and Hepatoprotective Effects of Magnolol on Diabetic Rats Induced by High-Fat Diet and Streptozotocin. Yao Xue Xue Bao 49, 476–481.
Wang, M. C., Bohmann, D., and Jasper, H. (2003). JNK Signaling Confers Tolerance to Oxidative Stress and Extends Lifespan in Drosophila. Dev. Cel 5, 811–816. doi:10.1016/s1534-5807(03)00323-x
Wang, S., Wu, F., Ye, B., Zhang, S., Wang, X., Xu, Q., et al. (2020). Effects of Xuefu Zhuyu Decoction on Cell Migration and Ocular Tumor Invasion inDrosophila. Biomed. Res. Int. 2020, 1–13. doi:10.1155/2020/5463652
Wu, C., Chen, Y., Wang, F., Chen, C., Zhang, S., Li, C., et al. (2015). Pelle Modulates dFoxO-Mediated Cell Death in Drosophila. Plos Genet. 11, e1005589. doi:10.1371/journal.pgen.1005589
Wu, Q., Zhang, Y., Xu, J., and Shen, P. (2005). Regulation of Hunger-Driven Behaviors by Neural Ribosomal S6 Kinase in Drosophila. Proc. Natl. Acad. Sci. U S A. 102, 13289–13294. doi:10.1073/pnas.0501914102
Keywords: Sanghuang Tongxie formula, insulin resistance, Drosophila, PI3K/AKT signaling, T2DM
Citation: Cao X, La X, Zhang B, Wang Z, Li Y, Bo Y, Chang H, Gao X, Tian C, Wu C and Li J-a (2022) Sanghuang Tongxie Formula Ameliorates Insulin Resistance in Drosophila Through Regulating PI3K/Akt Signaling. Front. Pharmacol. 13:874180. doi: 10.3389/fphar.2022.874180
Received: 11 February 2022; Accepted: 11 April 2022;
Published: 06 June 2022.
Edited by:
Zhiling Yu, Hong Kong Baptist University, Hong Kong SAR, ChinaCopyright © 2022 Cao, La, Zhang, Wang, Li, Bo, Chang, Gao, Tian, Wu and Li. This is an open-access article distributed under the terms of the Creative Commons Attribution License (CC BY). The use, distribution or reproduction in other forums is permitted, provided the original author(s) and the copyright owner(s) are credited and that the original publication in this journal is cited, in accordance with accepted academic practice. No use, distribution or reproduction is permitted which does not comply with these terms.
*Correspondence: Chenxi Wu, Y2hlbnhpLnd1QG5jc3QuZWR1LmNu; Ji-an Li, bG55eUB2aXAuc2luYS5jb20=
†These authors have contributed equally to this work
Disclaimer: All claims expressed in this article are solely those of the authors and do not necessarily represent those of their affiliated organizations, or those of the publisher, the editors and the reviewers. Any product that may be evaluated in this article or claim that may be made by its manufacturer is not guaranteed or endorsed by the publisher.
Research integrity at Frontiers
Learn more about the work of our research integrity team to safeguard the quality of each article we publish.