- 1Molecular Neuroinflammation and Neuronal Plasticity Research Laboratory, Research Unit, Hospital Universitario Santa Cristina, Instituto de Investigación Sanitaria-Hospital Universitario de La Princesa, Madrid, Spain
- 2Faculty of Health, University Camilo José Cela, Madrid, Spain
- 3Neuropsychopharmacology Unit, Hospital Doce de Octubre Research Institute (i+12), Madrid, Spain
- 4Genomic Instability Group, Spanish National Cancer Research Centre, Madrid, Spain
- 5Science for Life Laboratory, Division of Genome Biology, Department of Medical Biochemistry and Biophysics, Karolinska Institute, Stockholm, Sweden
- 6UTexas Health San Antonio, Long School of Medicine, San Antonio, TX, United States
- 7Department of Pharmacology and Toxicology, Faculty of Veterinary Medicine, Complutense University of Madrid, Madrid, Spain
Alkylating agents (AAs), are a group of chemo drugs generally divided into six classes; triazenes, nitrogen mustards, nitrosoureas, ethylenimine and methylenamine derivatives, alkyl sulfonates, and platinum-containing antineoplastic agents, which are commonly used, alone or in combination, in high-dose chemotherapy regimens to treat certain types of cancer (Ralhan and Kaur, 2007). These drugs undergo intramolecular cyclization reactions giving rise to highly reactive electrophilic cations, which alkylate nucleophilic sites adding an alkyl group at either oxygen, nitrogen, phosphorous or sulphur atoms on biological molecules (Soll et al., 2017). Consequently, AAs act mainly by inter- and intra-strand cross-linking DNA and RNA and hence inhibiting all cellular reactions using nucleic acid templates such as DNA replication, transcription and translation. Due to its effects on replication, the cytotoxicity induced by AAs is particularly acute in cancer cells or in certain fast-growing normal cells (hematopoietic, reproductive, and endothelial) (Fu et al., 2012). Besides DNA damage, AAs promote molecular changes that can also contribute to cell death such as oxidative stress induction (through glutathione depletion, lipid peroxidation and an overall increase in reactive oxygen and nitrogen species) (Zhang et al., 2021); or the depletion of NAD+ pools upon activation of the DNA repair enzyme Poly (ADP-ribose) polymerase (PARP-1) by AAs (Megnin-Chanet et al., 2010). In addition, events such as inflammation, calcium overload, mitochondrial disruption (Sahu et al., 2021), matrix metalloproteinase-9 (MMP-9) activation (Escalona et al., 2021) and alteration in NF-kB/p53/p38 MAPKs signaling pathways (Tian et al., 2019), among others, can also contribute to the overall toxicity of AAs. On the basis of this complex scenario, it is essential to find a homeostatic balance that allows for the targeted treatment of cancer cells while protecting the normal cells by reducing the side effects of AAs.
In recent years, the ubiquitous methoxyindole melatonin, has received increased attention as an adjuvant supportive treatment in patients of several cancers types (Li et al., 2017). Melatonin is a well characterized antioxidant, anti-inflammatory and free radical scavenger (Reiter et al., 2017). Because of these actions, we have previously hypothesized that melatonin would protect healthy cells from the anticancer chemotherapeutic drug mechlorethamine, by preventing the formation of the guanidine-nitrogen mechlorethamine adducts (Romero et al., 2021) and, therefore, the DNA damage. In addition, pleiotropic melatonin has been shown to protect genome integrity through the modulation of epigenetic mechanisms and recent data seems to indicate that this capacity may represent an interesting opportunity to be explored in the context of genotoxic chemotherapy of oncologic patients (Capote-Moreno et al., 2019). Furthermore, melatonin displays a large number of actions in cancer cells, either through melatonin receptors type-1 (MT1) and receptor type-2 (MT2) which mediate its antiproliferative actions, as well as by reaching intracellular organelles due its high lipophilic nature and eventually activating nuclear receptor-signaling and binding specifically to the ligand-inducible and transcriptionally active Retinoid Z receptor (RZR) or Retinoid Orphan Receptor (ROR) (Liu et al., 2016).
There is strong clinical evidence that germ cells are especially sensitive after administration of AAs, which leading to DNA damage and consequent induction of cell death (Meistrich, 2020). In this context, ovarian protection has been shown with melatonin mitigating cisplatin-induced follicle loss (Jang et al., 2016) and reducing nitro-oxidative stress and apoptosis (Moradi et al., 2021). Likewise, the use of amifostine, a radioprotective agent, in combination with melatonin, may be the “two better than one” against the production of reactive oxygen and nitrogen species (RONS) and DNA damage triggered by cisplatin in germinal epithelium cells (Eren et al., 2020). Therefore, the strategy of synergistic effect based on two antioxidants, amifostine and melatonin, could be attractive to ameliorate the AAs-induced side-effects.
Nutritional interventions may play an important role both in the growth and development of tumor cells and in the treatments with established chemotherapy regimens to maximize the drug efficacy and tolerability. Thus, it has recently been reported that metabolic changes associated with fasting has led to a reduction the toxic effects of chemotherapy while enhancing therapeutic efficacy (De Groot et al., 2020). The involvement of melatonin in this scenario is promising, since it has been well documented that melatonin regulates glucose homeostasis in peripheral tissues, from cytosolic glycolysis to mitochondrial oxidative phosphorylation (Bazwinsky-Wutschke et al., 2014; Hevia et al., 2015). It is widely known that glucose levels are fundamental for the survival and growth of many types of cancer. In this sense, has been recently documented the ability of melatonin to reduce the glucose uptake in tumor cells (Rodriguez et al., 2021). Nevertheless, the molecular and cellular mechanisms underlying melatonin´s actions need to be further exploration.
Important questions still need to be addressed regarding the combined use of chemotherapies and melatonin. When would it be reasonable to use melatonin as a supplement with a chemotherapy? Melatonin production declines with age in humans which may is thought to contribute to numerous dysfunctions and make organs more vulnerable to the development of pathologies, including cancer (Hill et al., 2013). Among others, the decline in the biosynthesis of melatonin with age has been suggested as one of the major contributors to immunosenescence and the development of neoplastic diseases (Schernhammer and Schulmeister, 2004). When melatonin is exogenously administered, all subcellular pools of melatonin are increased, with lipid-rich membranes possibly being a major reservoir for this protective molecule. As a result of its ability to enter healthy cells, it can act as a protective agent against toxic chemotherapies. The therapeutic use of this indoleamine as an adjuvant with conventional chemotherapeutic regimen could be a strategy for reducing the molecular damage to non-tumor cells resulting from conventional radio/chemotherapeutics, and enhancing the efficacy of the treatments designed to kill cancer cells.
Protecting normal cells from the side effects of chemotherapy has gained significant interest. In this regard, we recently proposed a strategy based on a combined treatment of chemotherapeutic drugs plus melatonin (Gil-Martin et al., 2019). In this context, protective profile of melatonin versus AAs has been achieved in different models (see Table 1). The hypothesis is that melatonin might limit the toxic-side effects of the chemotherapy in normal cells thereby allowing higher doses of the drugs. Importantly, a large number of studies in both animals and humans have uncovered no acute or chronic toxicities of melatonin; and the drug is widely considered to be safe even at high doses (Andersen et al., 2016; Menczel Schrire et al., 2021). This information is crucial for the clinical management of cancer patients. The optimal melatonin dosing regimens in chemotherapy-treated patients is currently under investigation, and it is possible be that the doses required may be substantially higher than those found at physiological levels, i.e., exceed those used to mitigate sleep disturbances. In this context, it is possible than an oral dose of 1 mg/kg b.w would be required.
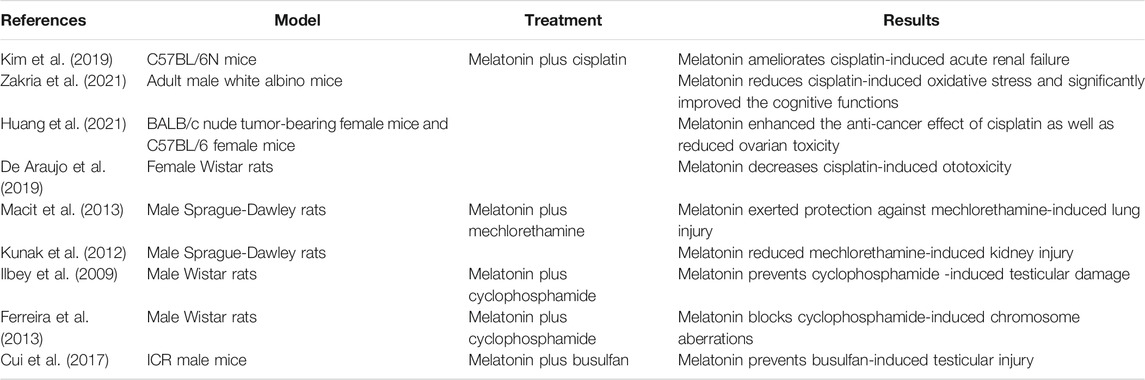
TABLE 1. Summary of in vivo studies including melatonin as an adjuvant against AAs-induced toxicity.
Chemoresistance and tumor relapse represent two important challenges for increasing the quality and life expectancy of cancer patients. Tumor cells often develop resistance to several chemotherapeutic drugs through various mechanisms such as dampened apoptosis, drug efflux through ATP-binding cassette (ABC) transporters, alterations in pro-survival signaling pathways or improved DNA repair mechanism (Mollaei et al., 2021). Thus, for therapeutic scheduling several chemotherapeutic drugs may have to be used concurrently to reduce chemoresistance, increasing side effects. In this regard, certain evidences suggest that melatonin may not only reinforce the therapeutic effect of chemotherapy modalities but also reduce chemoresistance. For instance, in malignant glioma cells melatonin downregulates the overexpressed ABC transporter (Martin et al., 2013). In addition, activation of the nuclear factor erythroid 2-related factor 2 (NRF2) signaling is involved in the development of chemoresistance and melatonin upregulates NRF2 through its specific receptors MT1 and MT2, SIRT1 and PI3K/Akt pathways in non-tumor cells. In contrast, melatonin has been shown to inhibit glucocorticoid-induced kinase 1 (SGK1)-mediated NRF2 upregulation in tumor cells (Wang et al., 2019). Finally, melatonin was shown overcome cisplatin chemoresistance by inhibiting expression of Wnt/β-catenin response genes and enhancing the efficacy of this alkylant agent (Zhang et al., 2020).
A current focus of our group is to develop high dose melatonin formulations that will be beneficial for its use in cancer patients by mitigating its side-effects on normal cells and enabling a higher dosing of the chemotherapy. We are currently working on the pharmacokinetics characteristics, that should help us direct the adjuvant use of orally and/or parenterally administered melatonin in cancer patients undertreatment with AAs. Ultimately, our purpose is to help deciphering the potential of melatonin to maximize the drug efficacies while mitigating chemotherapeutic drug-induced toxicity and side effects in cancer patients.
Author Contributions
All authors listed have made a substantial, direct, and intellectual contribution to the work and approved it for publication. AR conceived the idea and he has made substantial contributions to conception, design, supervision and writing-original draft preparation and acquisition of funding. OF-C, JE and RJR writing-original draft preparation and critically revised the manuscript. FL-M contributed to critically revised the manuscript. All co-authors read and approved the final version of the manuscript.
Funding
AR thanks Office Weapons and Material of the Spanish Ministry of Defense (DGAM) for MELVES project and UCJC (QUIMELTER and ONCOMEL) for its continued support; grant CPII19/00005 from Instituto de Salud Carlos III (ISCIII, Spain) and Fondo Europeo para el Desarrollo Regional (FEDER) to JE.
Conflict of Interest
The authors declare that the research was conducted in the absence of any commercial or financial relationships that could be construed as a potential conflict of interest.
Publisher’s Note
All claims expressed in this article are solely those of the authors and do not necessarily represent those of their affiliated organizations, or those of the publisher, the editors and the reviewers. Any product that may be evaluated in this article, or claim that may be made by its manufacturer, is not guaranteed or endorsed by the publisher.
References
Andersen, L. P., Gögenur, I., Rosenberg, J., and Reiter, R. J. (2016). The Safety of Melatonin in Humans. Clin. Drug Investig. 36 (3), 169–175. doi:10.1007/s40261-015-0368-5
Bazwinsky-Wutschke, I., Bieseke, L., Mühlbauer, E., and Peschke, E. (2014). Influence of Melatonin Receptor Signalling on Parameters Involved in Blood Glucose Regulation. J. Pineal Res. 56 (1), 82–96. doi:10.1111/jpi.12100
Capote-Moreno, A., Ramos, E., Egea, J., López-Muñoz, F., Gil-Martín, E., and Romero, A. (2019). Potential of Melatonin as Adjuvant Therapy of Oral Cancer in the Era of Epigenomics. Cancers (Basel) 11 (11), 1712. doi:10.3390/cancers11111712
Cui, Y., Ren, L., Li, B., Fang, J., Zhai, Y., He, X., et al. (2017). Melatonin Relieves Busulfan-Induced Spermatogonial Stem Cell Apoptosis of Mouse Testis by Inhibiting Endoplasmic Reticulum Stress. Cell Physiol Biochem 44 (6), 2407–2421. doi:10.1159/000486165
De Araujo, J. G., Serra, L. S. M., Lauand, L., Kückelhaus, S. A. S., and Sampaio, A. L. L. (2019). Protective Effect of Melatonin on Cisplatin-Induced Ototoxicity in Rats. Anticancer Res. 39 (5), 2453–2458. doi:10.21873/anticanres.13364
De Groot, S., Lugtenberg, R. T., Cohen, D., Welters, M. J. P., Ehsan, I., Vreeswijk, M. P. G., et al. (2020). Fasting Mimicking Diet as an Adjunct to Neoadjuvant Chemotherapy for Breast Cancer in the Multicentre Randomized Phase 2 DIRECT Trial. Nat. Commun. 11 (1), 3083. doi:10.1038/s41467-020-16138-3
Eren, H., Mercantepe, T., Tumkaya, L., Mercantepe, F., Dil, E., Horsanali, M. O., et al. (2020). Evaluation of the Protective Effects of Amifostine and Melatonin against Cisplatin Induced Testis Injury via Oxidative Stress and Apoptosis in Rats. Exp. Mol. Pathol. 112, 104324. doi:10.1016/j.yexmp.2019.104324
Escalona, R. M., Kannourakis, G., Findlay, J. K., and Ahmed, N. (2021). Expression of TIMPs and MMPs in Ovarian Tumors, Ascites, Ascites-Derived Cells, and Cancer Cell Lines: Characteristic Modulatory Response before and after Chemotherapy Treatment. Front. Oncol. 11, 796588. doi:10.3389/fonc.2021.796588
Ferreira, S. G., Peliciari-Garcia, R. A., Takahashi-Hyodo, S. A., Rodrigues, A. C., Amaral, F. G., Berra, C. M., et al. (2013). Effects of Melatonin on DNA Damage Induced by Cyclophosphamide in Rats. Braz. J. Med. Biol. Res. 46 (3), 278–286. doi:10.1590/1414-431x20122230
Fu, D., Calvo, J. A., and Samson, L. D. (2012). Balancing Repair and Tolerance of DNA Damage Caused by Alkylating Agents. Nat. Rev. Cancer 12 (2), 104–120. doi:10.1038/nrc3185
Gil-Martín, E., Egea, J., Reiter, R. J., and Romero, A. (2019). The Emergence of Melatonin in Oncology: Focus on Colorectal Cancer. Med. Res. Rev. 39 (6), 2239–2285. doi:10.1002/med.21582
Hevia, D., González-Menéndez, P., Quiros-González, I., Miar, A., Rodríguez-García, A., Tan, D. X., et al. (2015). Melatonin Uptake through Glucose Transporters: a New Target for Melatonin Inhibition of Cancer. J. Pineal Res. 58 (2), 234–250. doi:10.1111/jpi.12210
Hill, S. M., Cheng, C., Yuan, L., Mao, L., Jockers, R., Dauchy, B., et al. (2013). Age-related Decline in Melatonin and its MT1 Receptor Are Associated with Decreased Sensitivity to Melatonin and Enhanced Mammary Tumor Growth. Curr. Aging Sci. 6 (1), 125–133. doi:10.2174/1874609811306010016
Huang, J., Shan, W., Li, N., Zhou, B., Guo, E., Xia, M., et al. (2021). Melatonin Provides protection against Cisplatin-Induced Ovarian Damage and Loss of Fertility in Mice. Reprod. Biomed. Online 42 (3), 505–519. doi:10.1016/j.rbmo.2020.10.001
Ilbey, Y. O., Ozbek, E., Simsek, A., Otunctemur, A., Cekmen, M., and Somay, A. (2009). Potential Chemoprotective Effect of Melatonin in Cyclophosphamide- and Cisplatin-Induced Testicular Damage in Rats. Fertil. Steril 92 (3), 1124–1132. doi:10.1016/j.fertnstert.2008.07.1758
Jang, H., Lee, O. H., Lee, Y., Yoon, H., Chang, E. M., Park, M., et al. (2016). Melatonin Prevents Cisplatin-Induced Primordial Follicle Loss via Suppression of PTEN/AKT/FOXO3a Pathway Activation in the Mouse Ovary. J. Pineal Res. 60 (3), 336–347. doi:10.1111/jpi.12316
Kim, J. W., Jo, J., Kim, J. Y., Choe, M., Leem, J., and Park, J. H. (2019). Melatonin Attenuates Cisplatin-Induced Acute Kidney Injury through Dual Suppression of Apoptosis and Necroptosis. Biology (Basel) 8 (3), 64. doi:10.3390/biology8030064
Kunak, Z. I., Macit, E., Yaren, H., Yaman, H., Cakir, E., Aydin, I., et al. (2012). Protective Effects of Melatonin and S-Methylisothiourea on Mechlorethamine Induced Nephrotoxicity. J. Surg. Res. 175 (1), e17–23. doi:10.1016/j.jss.2011.11.002
Li, Y., Li, S., Zhou, Y., Meng, X., Zhang, J. J., Xu, D. P., et al. (2017). Melatonin for the Prevention and Treatment of Cancer. Oncotarget 8 (24), 39896–39921. doi:10.18632/oncotarget.16379
Liu, J., Clough, S. J., Hutchinson, A. J., Adamah-Biassi, E. B., Popovska-Gorevski, M., and Dubocovich, M. L. (2016). MT1 and MT2 Melatonin Receptors: A Therapeutic Perspective. Annu. Rev. Pharmacol. Toxicol. 56, 361–383. doi:10.1146/annurev-pharmtox-010814-124742
Macit, E., Yaren, H., Aydin, I., Kunak, Z. I., Yaman, H., Onguru, O., et al. (2013). The Protective Effect of Melatonin and S-Methylisothiourea Treatments in Nitrogen Mustard Induced Lung Toxicity in Rats. Environ. Toxicol. Pharmacol. 36 (3), 1283–1290. doi:10.1016/j.etap.2013.10.001
Martín, V., Sanchez-Sanchez, A. M., Herrera, F., Gomez-Manzano, C., Fueyo, J., Alvarez-Vega, M. A., et al. (2013). Melatonin-induced Methylation of the ABCG2/BCRP Promoter as a Novel Mechanism to Overcome Multidrug Resistance in Brain Tumour Stem Cells. Br. J. Cancer 108 (10), 2005–2012. doi:10.1038/bjc.2013.188
Mégnin-Chanet, F., Bollet, M. A., and Hall, J. (2010). Targeting poly(ADP-Ribose) Polymerase Activity for Cancer Therapy. Cell Mol Life Sci 67 (21), 3649–3662. doi:10.1007/s00018-010-0490-8
Meistrich, M. L. (2020). Risks of Genetic Damage in Offspring Conceived Using Spermatozoa Produced during Chemotherapy or Radiotherapy. Andrology 8 (3), 545–558. doi:10.1111/andr.12740
Menczel Schrire, Z., Phillips, C. L., Chapman, J. L., Duffy, S. L., Wong, G., D’Rozario, A. L., et al. (2021). Safety of Higher Doses of Melatonin in Adults: A Systematic Review and Meta‐analysis. J. Pineal Res. 72, e12782. doi:10.1111/jpi.12782
Mollaei, M., Hassan, Z. M., Khorshidi, F., and Langroudi, L. (2021). Chemotherapeutic Drugs: Cell Death- and Resistance-Related Signaling Pathways. Are They Really as Smart as the Tumor Cells? Transl Oncol. 14 (5), 101056. doi:10.1016/j.tranon.2021.101056
Moradi, M., Goodarzi, N., Faramarzi, A., Cheraghi, H., Hashemian, A. H., and Jalili, C. (2021). Melatonin Protects Rats Testes against Bleomycin, Etoposide, and Cisplatin-Induced Toxicity via Mitigating nitro-oxidative Stress and Apoptosis. Biomed. Pharmacother. 138, 111481. doi:10.1016/j.biopha.2021.111481
Ralhan, R., and Kaur, J. (2007). Alkylating Agents and Cancer Therapy. Expert Opin. Ther. Patents 17 (9), 1061–1075. doi:10.1517/13543776.17.9.1061
Reiter, R. J., Rosales-Corral, S. A., Tan, D. X., Acuna-Castroviejo, D., Qin, L., Yang, S. F., et al. (2017). Melatonin, a Full Service Anti-cancer Agent: Inhibition of Initiation, Progression and Metastasis. Int. J. Mol. Sci. 18 (4), 843. doi:10.3390/ijms18040843
Rodríguez, C., Puente-Moncada, N., Reiter, R. J., Sánchez-Sánchez, A. M., Herrera, F., Rodríguez-Blanco, J., et al. (2021). Regulation of Cancer Cell Glucose Metabolism Is Determinant for Cancer Cell Fate after Melatonin Administration. J. Cel Physiol 236 (1), 27–40. doi:10.1002/jcp.29886
Romero, A., Ramos, E., López-Muñoz, F., De Los Ríos, C., Egea, J., Gil-Martín, E., et al. (2021). Toxicology of Blister Agents: Is Melatonin a Potential Therapeutic Option. Diseases 9 (2), 27. doi:10.3390/diseases9020027
Sahu, K., Langeh, U., Singh, C., and Singh, A. (2021). Crosstalk between Anticancer Drugs and Mitochondrial Functions. Curr. Res. Pharmacol. Drug Discov. 2, 100047. doi:10.1016/j.crphar.2021.100047
Schernhammer, E. S., and Schulmeister, K. (2004). Melatonin and Cancer Risk: Does Light at Night Compromise Physiologic Cancer protection by Lowering Serum Melatonin Levels? Br. J. Cancer 90 (5), 941–943. doi:10.1038/sj.bjc.6601626
Soll, J. M., Sobol, R. W., and Mosammaparast, N. (2017). Regulation of DNA Alkylation Damage Repair: Lessons and Therapeutic Opportunities. Trends Biochem. Sci. 42 (3), 206–218. doi:10.1016/j.tibs.2016.10.001
Tian, M., Tian, D., Qiao, X., Li, J., and Zhang, L. (2019). Modulation of Myb-Induced NF-kB -STAT3 Signaling and Resulting Cisplatin Resistance in Ovarian Cancer by Dietary Factors. J. Cel Physiol 234 (11), 21126–21134. doi:10.1002/jcp.28715
Wang, M., Xue, Y., Shen, L., Qin, P., Sang, X., Tao, Z., et al. (2019). Inhibition of SGK1 Confers Vulnerability to Redox Dysregulation in Cervical Cancer. Redox Biol. 24, 101225. doi:10.1016/j.redox.2019.101225
Zakria, M., Ahmad, N., Al Kury, L. T., Alattar, A., Uddin, Z., Siraj, S., et al. (2021). Melatonin Rescues the Mice Brain against Cisplatin-Induced Neurodegeneration, an Insight into Antioxidant and Anti-inflammatory Effects. Neurotoxicology 87, 1–10. doi:10.1016/j.neuro.2021.08.010
Zhang, J., Xie, T., Zhong, X., Jiang, H. L., Li, R., Wang, B. Y., et al. (2020). Melatonin Reverses Nasopharyngeal Carcinoma Cisplatin Chemoresistance by Inhibiting the Wnt/β-Catenin Signaling Pathway. Aging (Albany NY) 12 (6), 5423–5438. doi:10.18632/aging.102968
Keywords: melatonin, alkylating agents, toxicity, inflammation, oxidative stress, molecular therapeutics, cancer, drug safety
Citation: Egea J, López-Muñoz F, Fernández-Capetillo O, Reiter RJ and Romero A (2022) Alkylating Agent-Induced Toxicity and Melatonin-Based Therapies. Front. Pharmacol. 13:873197. doi: 10.3389/fphar.2022.873197
Received: 10 February 2022; Accepted: 28 February 2022;
Published: 23 March 2022.
Edited by:
Lei Xi, Virginia Commonwealth University, United StatesReviewed by:
Shahanshah Khan, University of Texas Southwestern Medical Center, United StatesCelal Guven, Niğde Ömer Halisdemir University, Turkey
Copyright © 2022 Egea, López-Muñoz, Fernández-Capetillo, Reiter and Romero. This is an open-access article distributed under the terms of the Creative Commons Attribution License (CC BY). The use, distribution or reproduction in other forums is permitted, provided the original author(s) and the copyright owner(s) are credited and that the original publication in this journal is cited, in accordance with accepted academic practice. No use, distribution or reproduction is permitted which does not comply with these terms.
*Correspondence: Alejandro Romero, manarome@ucm.es