- 1Department of General Surgery, The First Affiliated Hospital of Shantou University Medical College, Shantou, China
- 2Guangdong Provincial Key Laboratory for Diagnosis and Treatment of Breast Cancer, Cancer Hospital of Shantou University Medical College, Shantou, China
- 3Department of Physiology/Changjiang Scholar’s Laboratory, Shantou University Medical College, Shantou, China
- 4Department of Pathology, Shantou University Medical College, Shantou, China
Triple-negative breast cancer (TNBC) is the aggressive molecular type of breast carcinoma, with a high metastasis/relapse incidence and cancer-related death rate, due to lack of specific therapeutic targets in the clinic. Exploring potential therapeutic targets or developing novel therapeutic strategies are the focus of intense research to improve the survival and life quality of patients with TNBC. The current study focused on drugs targeting the mTOR signaling pathway by investigating the potential utilization of itraconazole (ITZ) combined with rapamycin in the treatment of TNBC. CCK-8, colony formation and transwell assays were conducted to evaluate the effect of ITZ with rapamycin in combination on MDA-MB-231 and BT-549 TNBC cells. Synergistic inhibition was found in terms of proliferation and motility of TNBC cells. However, apoptosis was not enhanced by the combined treatment of ITZ and rapamycin. Flow cytometry analysis showed that ITZ and/or rapamycin arrested cells in G0/G1 phase and prevented G1/S phase transition. Reduced cyclin D1 protein levels were consistent with G0/G1 phase arrest, especially when resulting from the combination of ITZ with rapamycin. In conclusion, the combination of ITZ with rapamycin is a promising therapeutic strategy for patients with TNBC through synergistically arresting cells in the G0/G1 phase of the cell cycle, rather than inducing apoptosis.
Introduction
Itraconazole (ITZ), a broad-spectrum antifungal agent, has recently been verified as an anti-cancer drug in preclinical and clinical research (Pantziarka et al., 2015). ITZ exerts its antifungal activity through decreasing ergosterol synthesis, which is required for the membrane integrity of fungal cells (Poulsen et al., 2021). Based on its antifungal effects, ITZ is used as a safe and effective long-tern prophylaxis for fungal infections in immunocompromised cancer patients with neutropenia (Muhldorfer and Konig, 1990).
The effects and mechanisms of action for ITZ’s anti-cancer activities have been reported in different types of cancer in vitro and in vivo. Its anti-cancer potential to reverse multi-drug resistance (MDR) has been shown in daunorubicin-resistant P388 leukemia cells (Gupta et al., 1991), adriamycin-resistant K562 leukemia cells (Kurosawa et al., 1996), human breast cancer resistance protein-expressing HEK cells resistant to topotecan (Gupta et al., 2007), P-glycoprotein (MDR1)-overexpressing, multidrug-resistant HeLa cells (Iida et al., 2001), bevacizumab-resistant gastrointestinal cancer (Hara et al., 2016) and metastatic castration-resistant prostate cancer (Antonarakis et al., 2013). It has also been reported that ITZ inhibits tumor growth and angiogenesis or induces apoptosis and autophagy in non-small cell lung cancer (NSCLC) (Aftab et al., 2011; Gerber et al., 2020), gastric cancer (Hu et al., 2017; Lan et al., 2018), cervical cancer (Rojo-Leon et al., 2019), and skin cancer (Liang et al., 2017; Carbone et al., 2018). Importantly, the underlying molecular mechanism of ITZ’s anti-cancer effect has been reported via inhibiting hedgehog signaling, Wnt pathway and/or reducing mTOR expression, recognized as an mTOR inhibitor (Head et al., 2015; Wang et al., 2020).
Growing evidence demonstrates that combined therapeutic strategies with ITZ are promising for cancer patients. Chemotherapy with ITZ has been reported as a promising treatment for patients with unresectable gastric cancer (Sawasaki et al., 2020). A prospective randomized controlled study found that the utilization of ITZ in patients with NSCLC significantly benefits patients in terms of 1-year progression-free survival and overall response rate, although no improvement was found in terms of 1-year overall survival (Mohamed et al., 2021). As arsenic trioxide and ITZ antagonize the hedgehog pathway, Ally et al. proposed a sequential arsenic trioxide and ITZ treatment for metastatic basal cell carcinoma and found that the combined treatment is a feasible strategy for metastatic basal cell carcinoma patients (Ally et al., 2016). For colorectal cancer, ITZ synergistically increases the therapeutic effect of paclitaxel and 99mTc-methoxyisobutylisonitrile accumulation in an HT-29 human colorectal tumor-bearing animal model (Ghadi et al., 2021).
Breast cancer, the most common female malignant tumor, threatens the patients’ health worldwide (Siegel et al., 2021). Among the different molecular types of breast cancer, triple-negative breast cancer (TNBC) is highly aggressive and has a high incidence of metastasis/relapse and increased mortality due to the absence of the estrogen receptor (ER), progesterone receptor (PR) and human epidermal growth factor receptor 2 (HER2) expression, hindering the ability to employ targeted therapy (Cao et al., 2021). Interestingly, for heavily treated patients with TNBC, especially patients with recurring tumors, chemotherapy with ITZ has shown a promising effect in the clinic (Tsubamoto et al., 2014). To evoke drug repurposing and drug combinations, a screening of the most promising drugs with verapamil and ITZ was conducted and identified the combination of ITZ and 5-fluorouracil as the most effective by decreasing cell viability and proliferation (Correia et al., 2018). Recently, EI-Sheridy et al. used miltefosine-modified lipid nanocapsules to develop an ITZ nanoformulation, with a relatively small size and high entrapment efficiency, to enhance the chemotherapeutic efficacy of ITZ, regarding inhibition of tumor growth and cellular proliferation (El-Sheridy et al., 2021). Based on the above evidence, ITZ shows promise as an anti-cancer drug in the treatment of patients with TNBC.
Head et al. focused on the molecular mechanism of ITZ and found the suppressive function of ITZ on the AMPK/Mtor signaling axis (Head et al., 2015). The mTOR signaling pathway is essential for cell proliferation and survival, and in many types of cancers, mTOR is typically abnormally activated due to metabolic changes or mutations in upstream regulatory factors. Not surprisingly, inhibition of the mTOR pathway is a promising strategy for the development of anticancer drugs and has been demonstrated in clinical studies (Ferrari et al., 2022). Rapamycin, another antifungal drug extracted from Streptomyces hygroscopicus, is currently considered as a potent immunosuppressant in clinical and a target for mTOR, a serine/threonine protein kinase (Sabatini, 2017), exerting cytotoxicity against various kinds of cancers (Jelonek et al., 2021). However, Lee et al. demonstrated that rapamycin did not inhibit the activation of downstream effectors of mTOR completely (Lee et al., 2021), leading to the compensatory up-regulation of AKT activity and chemotherapy, limiting the utilization of rapamycin as an independent therapy (Mossmann et al., 2018).
So, the current study targets the AKT/mTOR signaling pathway, downstream of ITZ (Head et al., 2015), investigates the anti-cancer effect of monotherapy using ITZ and another drug each, as well as the two in combination, in TNBC cells. Also, the potential molecular mechanism of the therapeutic effect was also evaluated to provide potential drug combinations for patients with TNBC.
Materials and Methods
Chemical Reagents
ITZ (HY-17514) and rapamycin (HY-10219) were purchased from MedChemExpress (United States).
Cell Culture
The human triple-negative breast cancer cell lines MDA-MB-231 and BT-549 were from the cell bank of the Chinese Academy of Sciences (Shanghai). MDA-MB-231 cells were cultured in Dulbecco’s modified Eagle’s medium plus 10% fetal bovine serum (FBS, GIBCO, Brazil) and 1% penicillin-streptomycin antibiotics (Beyotime, China). BT-549 cells were cultured in RPMI 1640 medium (GIBCO, Brazil) plus 10% FBS and 1% penicillin-streptomycin. All cells were cultured in a 37°C incubator containing 5% carbon dioxide.
CCK-8 Assay
Determination of IC50 concentration: 5 × 103 cells were inoculated into each well of a 96-well plate. After 24 h, culture medium containing a series of drug dilutions was changed and cells were further cultured for 48 h. Then, 10 µL CCK-8 reagent (Invigentech, United States) and 100 µL culture medium were added to each well and cells were cultured an additional 2 h before absorbance was measured at 450 nm. Growth inhibition was calculated as Inhibition rate% = (OD value of control group-OD value of experimental group)/(OD value of control group-OD value of the blank) × 100%. The SPSS 19.0 software was used for statistical analysis of data to obtain half maximal growth inhibitory concentration (IC50) value of drugs, each experiment was repeated for three times.
Quantitation of cell proliferation: cells were inoculated at 1 × 103 cells per well into 96-well plates and cultured at 37C, 5% CO2 and allowed to attach for 24 h. Drugs at their half maximal growth inhibitory concentration (IC50) concentrations were added. After 24, 48, 72, 96, and 120 h, 10 μL CCK-8 reagent and 100 μL medium were added for 2 h at 37°C. Then absorbance of each well was measured at 450 nm using a spectrophotometer.
Colony Formation
Cells were inoculated into a 60 mm dish with drug at the IC50 concentration for 48 h. Then, cells were resuspended and seeded at 1 × 103 cells per well in 6-well plates and cultured for 14 days. Cells were fixed with methanol and stained with 0.1% crystal violet. Count the size and number of cell colonies in each group.
Transwell Assay
Chambers with 8-µM pore membranes were used for migration assays. Cells were initially cultured for 48 h in media without and with different concentrations of drugs. Then, cells were digested with pancreatin and diluted in serum-free medium and inoculate into the upper transwell chambers at a density of 2 × 104 cells per chamber. Complete medium was added to the bottom chamber. After 24 or 48 h, migrated cells were stained with 0.1% crystal violet.
Flow Cytometry
For quantitation of apoptosis, Annexin V-FITC Detection Kit (C1063, Beyotime, China) was used and cells were inoculated into a 60 mm dish with drugs at IC50 concentrations for 48 h. After washing the cells twice with PBS, the cells were digested and resuspended in binding buffer containing 5 µL of an Annexin V-FITCAfter incubation at room temperature for 20 min, 10 µL PI was added to the suspension and incubated for another 10 min. Then the degree of apoptosis was detected by flow cytometry (Accuri C6, Becton-Dickinson, United States).
For quantitation of cell cycle phases, Cell cycle Detection Kit (BestBio, China) was applied and cells were incubated with drugs for 24 h, then digested and collected into 70% ethanol at -20°C and allowed to fix for 2 h. The fixed cells were centrifuged at 1000 rpm and then washed with PBS, resuspended in staining buffer (0.5 ml) containing PI (25 µL) and RNase A (10 µL), and incubated at 37°C for 30 min in the dark. Finally, the DNA level was measured by flow cytometry.
Western Blotting
Cell cultures were incubated in 60 mm dishes with different concentrations of drug for 48 h and then washed twice with PBS for digestion and cell collection. The cells were lysed for 30 min in ice-cold RIPA buffer with phenylmethylsulfonyl fluoride and Protein phosphatase inhibitor (Solarbio, China). Then, lysates were ultrasonicated three times (4 s each) and centrifuged at 12,000 rpm for 15 min to remove the precipitate, and the protein concentration was determined using a bicinchoninic acid assay (BCA) kit (GenStar, China). After denaturation, 30 µg of protein was subjected to SDS-polyacrylamide gel electrophoresis, transferred to a polyvinylidene fluoride membrane, and blocked with 5% milk. The PVDF membrane was incubated with primary antibodies overnight to detect the expression of mTOR pathway-related proteins and cycle-related proteins. Primary antibodies and diluted concentration were followed: Akt (1:1000, #4691, CST, United States), p-Akt (1:1000, #4060, CST, United States), mTOR (1:1000, #2983, CST, United States), p-mTOR (1:1000, #5536, CST, United States), p70S6K (1:1000, #9202, CST, United States), p-p70S6K (1:1000, #9205, CST, United States), cyclin D1 (1:1000, #2978, CST, United States), GAPDH (1:1000, #TA309157, ZSGB-BIO, China), p21 (1:1000, #2947, CST, United States), β-Actin (1:1000, #TA-09, ZSGB-BIO, China). After incubation with primary antibody, membranes were washed, then incubated with HRP-conjugated secondary antibody (1:2000) at room temperature for 120 min. Enhanced chemiluminescence chemicals were used to visualize target proteins and chemiluminescence western blotting detection system (Bio-Rad ChemiDoc XRS+, United States) was used for protein detection.
Results
Itraconazole Inhibits the Proliferation and Motility of Triple-Negative Breast Cancer Cells Through Suppressing the AKT/mTOR Pathway
To investigate the potential function and mechanism of ITZ in TNBC, the concentration of ITZ required for treating TNBC cells was determined by CCK-8 assay to identify IC50 for MDA-MB-231 and BT-549 cells. Results showed that with increasing concentration of ITZ, the survival rate of TNBC cells decreased in a dose-dependent manner (Figure 1A), with IC50s of 4.917 μM for MDA-MB-231 and 4.367 μM for BT-549 obtained.
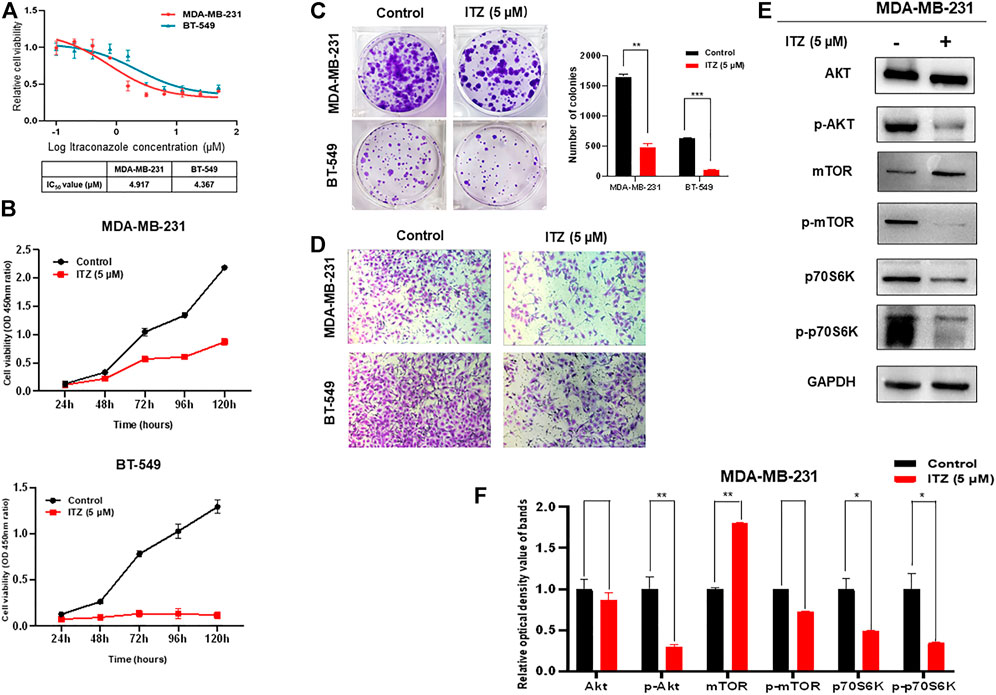
FIGURE 1. Effect of ITZ on TNBC cells. (A) Proliferation of TNBC cells was dose-dependently inhibited by varying concentrations of ITZ (B) The proliferation rate of TNBC cells was suppressed by ITZ in time-dependent manner (C) Colony formation assay showing ITZ treatment reduces both the number and size of colonies formed by both MDA-MB-231 and BT-549 cells, quantified and analyzed in histogram (D) Transwell assay showing the motility of MDA-MB-231 and BT-549 cells was dramatically inhibited by ITZ treatment (E,F) Activity of the AKT/mTOR signaling pathway was decreased by ITZ treatment. The quantified results from Western blot (E) were analyzed with statistical significance in histogram (F). *p < 0.05, **p < 0.01, ***p < 0.001.
The anti-cancer activities of ITZ were examined by measuring the effects of ITZ on the proliferation and motility of TNBC cells. In the CCK-8 assay, TNBC cells were exposed with or without ITZ at the IC50 concentration for 24, 48, 72, 96, and 120 h. ITZ treatment inhibited the proliferation of both MDA-MB-231 and BT-549 cells (Figure 1B). Colony formation was also decreased by ITZ, compared with the control group, in both MDA-MB-231 and BT-549 cells (Figure 1C). Furthermore, transwell assays suggested that treatment with ITZ suppressed the migratory ability of MDA-MB-231 and BT-549 cells (Figure 1D).
To explore the underlying mechanism of ITZ in TNBC cells, protein levels of AKT/mTOR signaling pathway components were examined by western blotting. Interestingly, the activated forms of AKT and mTOR were decreased with ITZ treatment, while the expression levels of AKT and mTOR were not suppressed in the ITZ-treated groups. To confirm the involvement of the AKT/mTOR signaling pathway, phosphorylation of the mTOR downstream target p70S6K was also examined. Phosphorylation of p70S6K was inhibited in the ITZ-treated groups, consistent with reduced p-AKT and p-mTOR levels (Figures 1E,F).
Inhibition of the AKT/mTOR Pathway by Rapamycin Inhibits the Proliferation and Motility of Triple-Negative Breast Cancer Cells
The ability of rapamycin to inhibit growth of TNBC cells was also determined by CCK-8 assay, which showed IC50s of 12.2 μM for MDA-MB-231 and 15.9 μM for BT-549 cells (Figure 2A). Not surprisingly, the proliferation and colony formation of both MDA-MB-231 and BT-549 cells were suppressed by rapamycin treatment at the IC50 concentration (Figures 2B,C). The number of migratory cells in the transwell assay was lower in the rapamycin-treated group than that in the control (Figure 2D). As an mTOR inhibitor, rapamycin treatment of TNBC cells resulted in low activity of the AKT/mTOR signaling pathway, as demonstrated by decreased expression of p-AKT, p-mTOR, and p-p70S6K (Figures 2E,F).
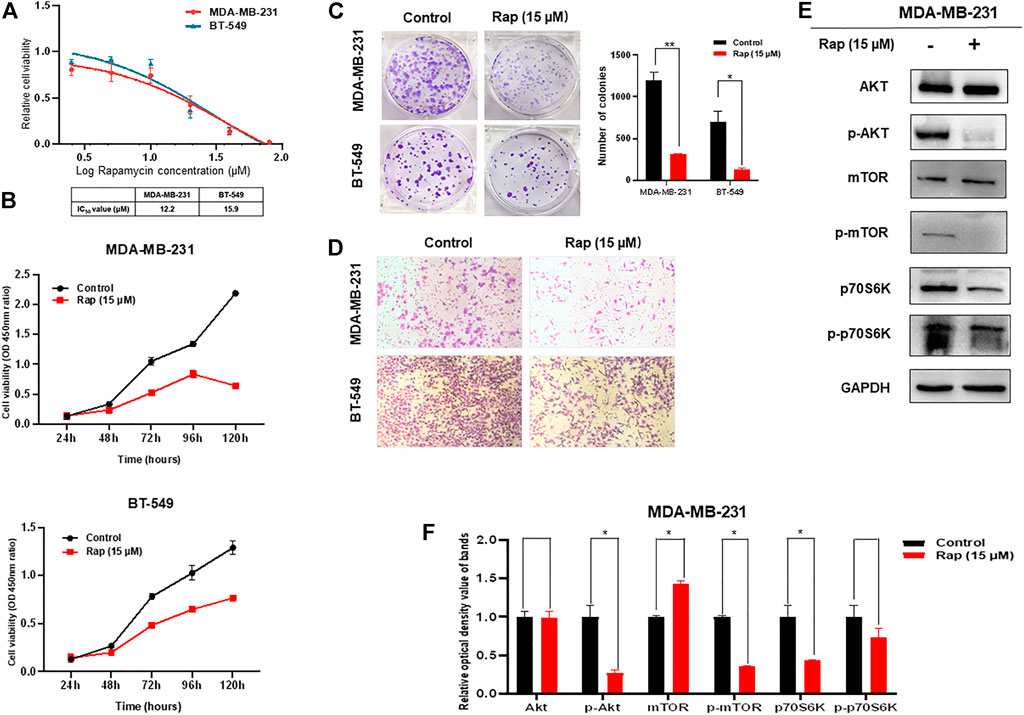
FIGURE 2. Effect of rapamycin on TNBC cells. (A) Viability of TNBC cells treated with varying concentrations of rapamycin (B) Proliferation of TNBC cells was inhibited by rapamycin in time-dependent manner (C) Colony formation of both MDA-MB-231 and BT-549 cells were suppressed by rapamycin treatment, analyzing in histogram (D) Motility of MDA-MB-231 and BT-549 cells was dramatically inhibited by rapamycin treatment (E,F) Rapamycin treatment decreased the activity of the AKT/mTOR signaling pathway. The quantified results from Western blot (E) were analyzed with statistical significance in histogram (F). *p < 0.05, **p < 0.01, ***p < 0.001.
Combined Treatment of Itraconazole and Rapamycin Suppresses Triple-Negative Breast Cancer Cells Synergistically
To investigate the potential function of combined ITZ with rapamycin in TNBC cells, CCK-8, colony formation and transwell assays were used to evaluate the malignant behavior of TNBC cells. In Figure 3A, the proliferation of both MDA-MB-231 and BT-549 cells was suppressed in both the ITZ- and rapamycin-treated groups, compared with the control groups, while the combined treatment inhibited the proliferation of TNBC cells dramatically compared to treatment with either drug alone. Consistently, colony formation was decreased by ITZ or rapamycin treatment alone, but the decline was even greater in the combined treatment groups than that in the groups treated with either drug alone (Figures 3B,C). Regarding the migratory ability of TNBC cells, transwell assays showed that the inhibition of TNBC cell motility was the most obvious in the combined treatment of ITZ and rapamycin rather than treatment with ITZ or rapamycin alone (Figure 3D).
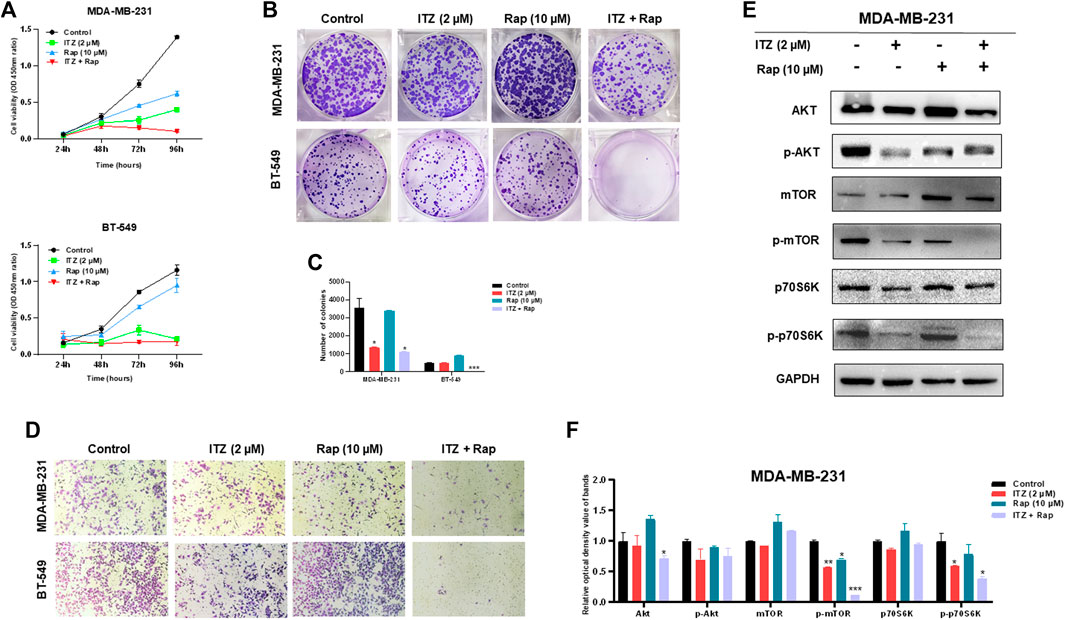
FIGURE 3. Combined effect of ITZ and rapamycin in TNBC cells. (A,B) Proliferation (A) and colony formation (B) of both MDA-MB-231 and BT-549 cells were suppressed by treatment with ITZ, rapamycin and ITZ + rapamycin (C) The quantified results of colony formation in TNBC cell treated with control, monotherapy and combined therapy (D) Motility of MDA-MB-231 and BT-549 cells was dramatically inhibited by treatment with ITZ, rapamycin and ITZ + rapamycin (E) AKT/mTOR signaling pathway activity was decreased by treatment with ITZ and rapamycin alone and combined (F) The quantified results from Western blot were analyzed with statistical significance in histogram. *p < 0.05, **p < 0.01, ***p < 0.001.
The activity of the AKT/mTOR signaling pathway was also evaluated by the expression of biomarkers. In Figures 3E,F, although the expression level of AKT, mTOR, and p70S6K was not decreased following treatment with ITZ and rapamycin alone or in combination, their activated forms, i.e. p-mTOR, and p-p70S6K, were decreased in the single drug-treated groups, and were even lower in the combined treatment group.
Itraconazole and Rapamycin Induce Triple-Negative Breast Cancer Cell Apoptosis Seperately
In order to investigate the combined effect of ITZ and rapamycin in TNBC, the percentage of apoptotic cells was evaluated by flow cytometry after ITZ and rapamycin treatment. Apoptosis was induced by ITZ treatment and rapamycin treatment alone in MDA-MB-231 cells (Figure 4A), whereas for BT-549 cells, ITZ but not rapamycin induced apoptosis (Figure 4A).
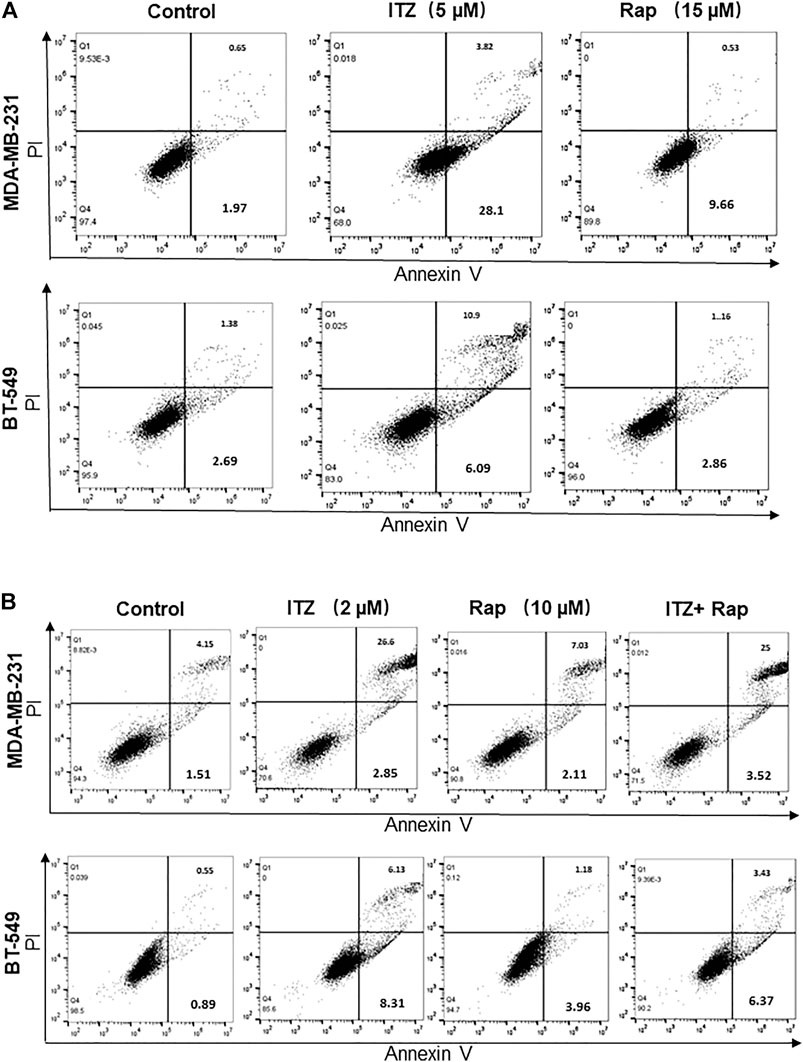
FIGURE 4. Apoptosis induced by ITZ and/or rapamycin in TNBC cells. (A) The percentage of apoptotic cells with or without ITZ or rapamycin treatment (B) The combined treatment of TNBC cells induced apoptosis without a synergistic effect.
For the combined treatment of ITZ and rapamycin, the cells were collected after a 48 h treatment and analyzed by flow cytometry. Although in the ITZ-treated group, apoptosis was induced accordingly, the percentage of apoptotic cells in the combined treatment group was not increased compared with the single drug groups (Figure 4B), indicating that the synergistic inhibition of ITZ and rapamycin on TNBC cells was not through inducing apoptosis.
Synergistic Inhibition of Itraconazole in Combination With Rapamycin Is due to Cell Cycle Arrest
As apoptosis was not synergistically induced by ITZ and rapamycin in combination, the question arose as to how the proliferation and motility of TNBC cells was inhibited. Cell cycle analysis of TNBC cells was conducted to investigate the underlying mechanism. Interestingly, the proportion of TNBC cells in G0/G1 phase increased with ITZ and rapamycin treatment alone, but was especially high in the combined treatment group (Figure 5A).
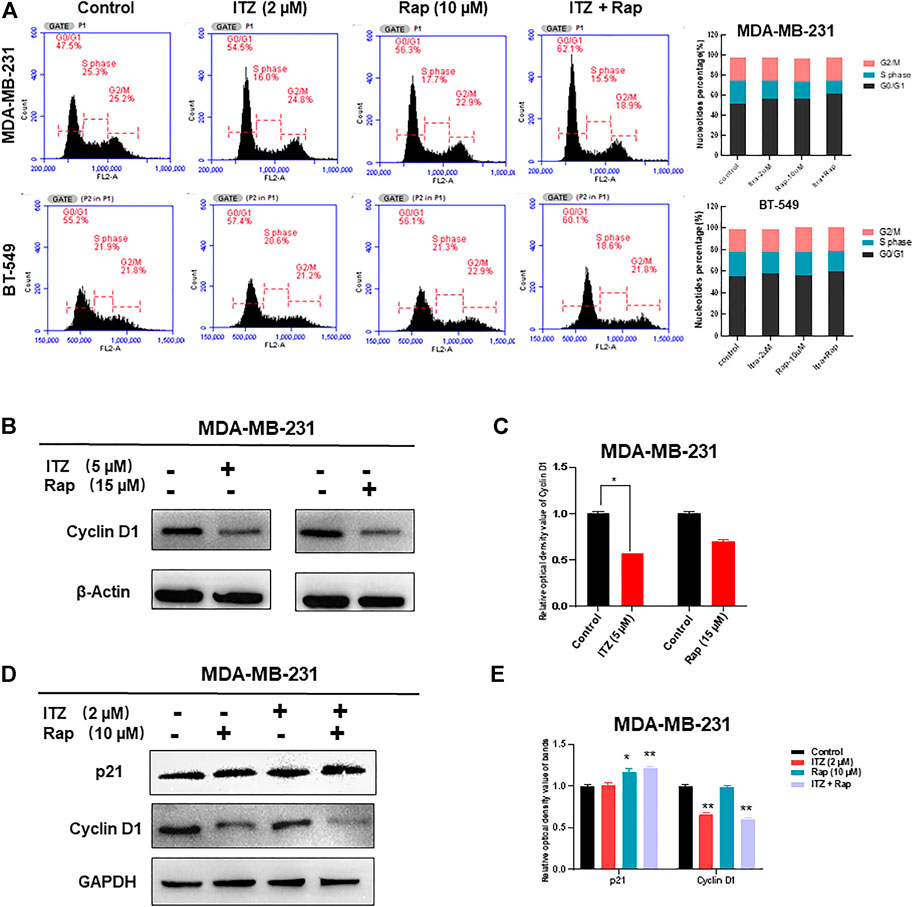
FIGURE 5. Cell cycle arrest caused by synergism between ITZ and rapamycin in TNBC cells. (A) ITZ and rapamycin caused cell cycle arrest in G0/G1 phase to inhibit the malignant behavior of TNBC cells (B,C) Expression of cyclin D1 was suppressed by ITZ (5 μM) or rapamycin (15 μM) treatment, analyzed in histogram (C) (D,E) Expression of cyclin D1 was decreased by the synergistic effect of ITZ (2 μM) combined with rapamycin (10 μM) in TNBC cells, whereas p21 accumulated in the combined group, analyzed in histogram (E). *p < 0.05, **p < 0.01, ***p < 0.001.
Concomitant with cell cycle arrest in G0/G1 phase, the number of TNBC cells entering S and G2 phases was decreased accordingly. To confirm the synergistic effect of ITZ and rapamycin on blocking cell cycling, cell cycle-related proteins were detected by western blotting, which showed that treatment with ITZ (5 μM) or rapamycin (15 μM) alone suppressed the expression of cyclin D1, an indicator of G1/S phase transition (Figures 5B,C). Importantly, upon combined treatment with ITZ (2 μM) and rapamycin (10 μM), the expression of cyclin D1 was the lowest compared with control and single drug-treated groups (Figures 5D,E).
Discussion
For patients with TNBC, chemotherapy has been the main therapeutic strategy due to the lack of targeted therapy. However, chemo-resistance, distant metastasis and relapse seriously affect the quality of life and survival of patients with TNBC (Shi et al., 2018). Drug combinations or drug repurposing have the potential to treat TNBC and/or reduce the amount of drug and related toxicity. Important signaling pathways, such as NF-κB, PI3K/Akt/mTOR, Notch 1, Wnt/β-catenin, and YAP, have been considered as therapeutic targets for patients with cancer (Lee et al., 2020). The current study proposes a novel combination of ITZ and rapamycin, both of which target the mTOR signaling pathway.
First, the optimal concentration used for TNBC cells was evaluated by CCK-8 assay. Interestingly, although both MDA-MB-231 and BT-549 cells are TNBC cell lines, the tolerance to ITZ and rapamycin are quite different with different IC50 values. For other types of breast cancer cell lines, the sensitivity to ITZ and rapamycin can be different based on the exposure time (Tengku Din et al., 2014; Ozates et al., 2021). Our results show that at the IC50 concentrations for ITZ and rapamycin, the proliferation and motility of both MDA-MB-231 and BT-549 cells are inhibited.
Cai et al. investigated the influence of different triazole antifungal drugs on the pharmacokinetics of cyclophosphamide, and all tested triazoles, including ITZ, increased the pharmacokinetics of cyclophosphamide in cancer patients, as well as the area under the plasma concentration-time curve of cyclophosphamide (Cai et al., 2020). Importantly, it has been reported that combination with ITZ treatment enhanced the toxicity due to chemotherapy in patients with Hodgkin’s lymphoma, causing severe myelosuppression and neurotoxicity after concurrent administration (Bashir et al., 2006). However, our combined treatment used lower concentrations of ITZ and rapamycin than the IC50, with the hypothesis that ITZ would enhance the cytotoxicity of rapamycin in TNBC cells. As expected, synergistic inhibition was observed to occur through suppressing the AKT/mTOR signaling pathway.
Further investigation focused on the mechanism of synergism in TNBC cells. Both apoptosis and cell cycle arrest are involved in this process. Flow cytometric analyses were conducted in this study. Interestingly, no enhanced induction of apoptosis was found upon combined treatment, indicating other mechanisms are involved in the inhibition of malignant behavior. Buczacki et al. demonstrated that ITZ could cause cycling and dormant cells to switch to global senescence in CRC models (Buczacki et al., 2018). Consistent with this, either ITZ or rapamycin treatment induced cell cycle arrest. Moreover, combined treatment with ITZ and rapamycin enhanced cell cycle arrest in TNBC cells through or partially p21 protein and decreasing cyclin D1 levels.
Nevertheless, attention also should be paid to side effects due to ITZ-enhanced toxicity of other compounds. In adult acute lymphoblastic leukemia, ITZ was found to enhance vindesine neurotoxicity (Chen et al., 2007). Recently, Foroughinia et al. reported vincristine-induced seizures potentiated by ITZ following chemotherapy of diffuse large B-cell lymphoma (Foroughinia et al., 2012), calling for attention to the need to evaluate the benefits and risks of patients before clinical use.
Conclusion
Combination of ITZ with rapamycin exerts an antitumor effect on TNBC cells through arresting cell cycling in G0/G1 phase, rather than synergistically inducing apoptosis.
Data Availability Statement
The raw data supporting the conclusion of this article will be made available by the authors, without undue reservation.
Author Contributions
JL and W-JC: conceptualization. H-TW, C-LL, Z-XF, and W-TL: organization of the database. H-TW, and C-LL: performance of most of the experiments. Z-XF, W-JC, and W-TL: performance of some of the experiments. H-TW and C-LL: writing–original draft preparation. JL: writing–review and editing. JL: supervision and project administration. JL and H-TW: funding acquisition. All authors have read and agreed to the published version of the manuscript.
Funding
This work was supported by the National Natural Science Foundation of China (No. 81501539), the Natural Science Foundation of Guangdong Province (Nos. 2021A1515012180 and 2016A030312008), 2021 Science and Technology Special Project of Guangdong Province, China (No. 210715216902829), Special Grant for Key Area Programs of Guangdong Department of Education (No. 2021ZDZX2004), and “Dengfeng Project” for the construction of high-level hospital in Guangdong Province—The First Affiliated Hospital of Shantou University College Supporting Funding (No. 202003-10).
Conflict of Interest
The authors declare that the research was conducted in the absence of any commercial or financial relationships that could be construed as a potential conflict of interest.
Publisher’s Note
All claims expressed in this article are solely those of the authors and do not necessarily represent those of their affiliated organizations, or those of the publisher, the editors and the reviewers. Any product that may be evaluated in this article, or claim that may be made by its manufacturer, is not guaranteed or endorsed by the publisher.
References
Aftab, B. T., Dobromilskaya, I., Liu, J. O., and Rudin, C. M. (2011). Itraconazole Inhibits Angiogenesis and Tumor Growth in Non-small Cell Lung Cancer. Cancer Res. 71, 6764–6772. doi:10.1158/0008-5472.CAN-11-0691
Ally, M. S., Ransohoff, K., Sarin, K., Atwood, S. X., Rezaee, M., Bailey-Healy, I., et al. (2016). Effects of Combined Treatment with Arsenic Trioxide and Itraconazole in Patients with Refractory Metastatic Basal Cell Carcinoma. JAMA Dermatol. 152, 452–456. doi:10.1001/jamadermatol.2015.5473
Antonarakis, E. S., Heath, E. I., Smith, D. C., Rathkopf, D., Blackford, A. L., Danila, D. C., et al. (2013). Repurposing Itraconazole as a Treatment for Advanced Prostate Cancer: a Noncomparative Randomized Phase II Trial in Men with Metastatic Castration-Resistant Prostate Cancer. Oncologist 18, 163–173. doi:10.1634/theoncologist.2012-314
Bashir, H., Motl, S., Metzger, M. L., Howard, S. C., Kaste, S., Krasin, M. P., et al. (2006). Itraconazole-enhanced Chemotherapy Toxicity in a Patient with Hodgkin Lymphoma. J. Pediatr. Hematol. Oncol. 28, 33–35. doi:10.1097/01.mph.0000196452.91823.f9
Buczacki, S. J. A., Popova, S., Biggs, E., Koukorava, C., Buzzelli, J., Vermeulen, L., et al. (2018). Itraconazole Targets Cell Cycle Heterogeneity in Colorectal Cancer. J. Exp. Med. 215, 1891–1912. doi:10.1084/jem.20171385
Cai, T., Liao, Y., Chen, Z., Zhu, Y., and Qiu, X. (2020). The Influence of Different Triazole Antifungal Agents on the Pharmacokinetics of Cyclophosphamide. Ann. Pharmacother. 54, 676–683. doi:10.1177/1060028019896894
Cao, Y., Chen, C., Tao, Y., Lin, W., and Wang, P. (2021). Immunotherapy for Triple-Negative Breast Cancer. Pharmaceutics 13, 2003. doi:10.3390/pharmaceutics13122003
Carbone, C., Martins-Gomes, C., Pepe, V., Silva, A. M., Musumeci, T., Puglisi, G., et al. (2018). Repurposing Itraconazole to the Benefit of Skin Cancer Treatment: A Combined Azole-DDAB Nanoencapsulation Strategy. Colloids Surf. B Biointerfaces 167, 337–344. doi:10.1016/j.colsurfb.2018.04.031
Chen, S., Wu, D., Sun, A., Qiu, H., Jin, Z., Tang, X., et al. (2007). Itraconazole-enhanced Vindesine Neurotoxicity in Adult Acute Lymphoblastic Leukaemia. Am. J. Hematol. 82, 942. doi:10.1002/ajh.20918
Correia, A., Silva, D., Correia, A., Vilanova, M., Gärtner, F., and Vale, N. (2018). Study of New Therapeutic Strategies to Combat Breast Cancer Using Drug Combinations. Biomolecules 8, 175. doi:10.3390/biom8040175
El-Sheridy, N. A., El-Moslemany, R. M., Ramadan, A. A., Helmy, M. W., and El-Khordagui, L. K. (2021). Enhancing the In Vitro and In Vivo Activity of Itraconazole against Breast Cancer Using Miltefosine-Modified Lipid Nanocapsules. Drug Deliv. 28, 906–919. doi:10.1080/10717544.2021.1917728
Ferrari, P., Scatena, C., Ghilli, M., Bargagna, I., Lorenzini, G., and Nicolini, A. (2022). Molecular Mechanisms, Biomarkers and Emerging Therapies for Chemotherapy Resistant TNBC. Int. J. Mol. Sci. 23, 1665. doi:10.3390/ijms23031665
Foroughinia, F., Baniasadi, S., Seifi, S., and Fahimi, F. (2012). Vincristine-induced Seizure Potentiated by Itraconazole Following RCHOP Chemotherapy for Diffuse Large B-Cell Lymphoma. Curr. Drug Saf. 7, 372–374. doi:10.2174/157488612805076633
Gerber, D. E., Putnam, W. C., Fattah, F. J., Kernstine, K. H., Brekken, R. A., Pedrosa, I., et al. (2020). Concentration-dependent Early Antivascular and Antitumor Effects of Itraconazole in Non-small Cell Lung Cancer. Clin. Cancer Res. 26, 6017–6027. doi:10.1158/1078-0432.CCR-20-1916
Ghadi, M., Hosseinimehr, S. J., Amiri, F. T., Mardanshahi, A., and Noaparast, Z. (2021). Itraconazole Synergistically Increases Therapeutic Effect of Paclitaxel and 99mTc-MIBI Accumulation, as a Probe of P-Gp Activity, in HT-29 Tumor-Bearing Nude Mice. Eur. J. Pharmacol. 895, 173892. doi:10.1016/j.ejphar.2021.173892
Gupta, S., Kim, J., and Gollapudi, S. (1991). Reversal of Daunorubicin Resistance in P388/ADR Cells by Itraconazole. J. Clin. Invest. 87, 1467–1469. doi:10.1172/JCI115154
Gupta, A., Unadkat, J. D., and Mao, Q. (2007). Interactions of Azole Antifungal Agents with the Human Breast Cancer Resistance Protein (BCRP). J. Pharm. Sci. 96, 3226–3235. doi:10.1002/jps.20963
Hara, M., Nagasaki, T., Shiga, K., and Takeyama, H. (2016). Suppression of Cancer-Associated Fibroblasts and Endothelial Cells by Itraconazole in Bevacizumab-Resistant Gastrointestinal Cancer. Anticancer Res. 36, 169–177.
Head, S. A., Shi, W., Zhao, L., Gorshkov, K., Pasunooti, K., Chen, Y., et al. (2015). Antifungal Drug Itraconazole Targets VDAC1 to Modulate the AMPK/mTOR Signaling axis in Endothelial Cells. Proc. Natl. Acad. Sci. U S A. 112, E7276–E7285. doi:10.1073/pnas.1512867112
Hu, Q., Hou, Y. C., Huang, J., Fang, J. Y., and Xiong, H. (2017). Itraconazole Induces Apoptosis and Cell Cycle Arrest via Inhibiting Hedgehog Signaling in Gastric Cancer Cells. J. Exp. Clin. Cancer Res. 36, 50. doi:10.1186/s13046-017-0526-0
Iida, N., Takara, K., Ohmoto, N., Nakamura, T., Kimura, T., Wada, A., et al. (2001). Reversal Effects of Antifungal Drugs on Multidrug Resistance in MDR1-Overexpressing HeLa Cells. Biol. Pharm. Bull. 24, 1032–1036. doi:10.1248/bpb.24.1032
Jelonek, K., Zajdel, A., Wilczok, A., Kaczmarczyk, B., Musiał-Kulik, M., Hercog, A., et al. (2021). Comparison of PLA-Based Micelles and Microspheres as Carriers of Epothilone B and Rapamycin. The Effect of Delivery System and Polymer Composition on Drug Release and Cytotoxicity against MDA-MB-231 Breast Cancer Cells. Pharmaceutics 13, 1881. doi:10.3390/pharmaceutics13111881
Kurosawa, M., Okabe, M., Hara, N., Kawamura, K., Suzuki, S., Sakurada, K., et al. (1996). Reversal Effect of Itraconazole on Adriamycin and Etoposide Resistance in Human Leukemia Cells. Ann. Hematol. 72, 17–21. doi:10.1007/BF00663011
Lan, K., Yan, R., Zhu, K., Li, W., Xu, Z., Dang, C., et al. (2018). Itraconazole Inhibits the Proliferation of Gastric Cancer Cells In Vitro and Improves Patient Survival. Oncol. Lett. 16, 3651–3657. doi:10.3892/ol.2018.9072
Lee, Y. T., Chuang, Y. M., and Chan, M. W. Y. (2020). Combinatorial Epigenetic and Immunotherapy in Breast Cancer Management: A Literature Review. Epigenomes 4, 27. doi:10.3390/epigenomes4040027
Lee, B. J., Boyer, J. A., Burnett, G. L., Thottumkara, A. P., Tibrewal, N., Wilson, S. L., et al. (2021). Selective Inhibitors of mTORC1 Activate 4EBP1 and Suppress Tumor Growth. Nat. Chem. Biol. 17, 1065–1074. doi:10.1038/s41589-021-00813-7
Liang, G., Liu, M., Wang, Q., Shen, Y., Mei, H., Li, D., et al. (2017). Itraconazole Exerts its Anti-melanoma Effect by Suppressing Hedgehog, Wnt, and PI3K/mTOR Signaling Pathways. Oncotarget 8, 28510–28525. doi:10.18632/oncotarget.15324
Mohamed, A. W., Elbassiouny, M., Elkhodary, D. A., Shawki, M. A., and Saad, A. S. (2021). The Effect of Itraconazole on the Clinical Outcomes of Patients with Advanced Non-small Cell Lung Cancer Receiving Platinum-Based Chemotherapy: a Randomized Controlled Study. Med. Oncol. 38, 23. doi:10.1007/s12032-021-01475-0
Mossmann, D., Park, S., and Hall, M. N. (2018). mTOR Signalling and Cellular Metabolism Are Mutual Determinants in Cancer. Nat. Rev. Cancer 18, 744–757. doi:10.1038/s41568-018-0074-8
Muhldorfer, S. M., and Konig, H. J. (1990). Prophylaxis of Fungal Infections with Itraconazole in Immunocompromised Cancer Patients. Mycoses 33, 291–295. doi:10.1111/myc.1990.33.6.291
Ozates, N. P., Soğutlu, F., Lerminoglu, F., Demir, B., Gunduz, C., Shademan, B., et al. (2021). Effects of Rapamycin and AZD3463 Combination on Apoptosis, Autophagy, and Cell Cycle for Resistance Control in Breast Cancer. Life Sci. 264, 118643. doi:10.1016/j.lfs.2020.118643
Pantziarka, P., Sukhatme, V., Bouche, G., Meheus, L., and Sukhatme, V. P. (2015). Repurposing Drugs in Oncology (ReDO)-Itraconazole as an Anti-cancer Agent. Ecancermedicalscience 9, 521. doi:10.3332/ecancer.2015.521
Poulsen, J. S., Madsen, A. M., White, J. K., and Nielsen, J. L. (2021). Physiological Responses of Aspergillus niger Challenged with Itraconazole. Antimicrob. Agents Chemother. 65, e02549. doi:10.1128/AAC.02549-20
Rojo-Leon, V., García, C., Valencia, C., Méndez, M. A., Wood, C., and Covarrubias, L. (2019). The E6/E7 Oncogenes of Human Papilloma Virus and Estradiol Regulate Hedgehog Signaling Activity in a Murine Model of Cervical Cancer. Exp. Cel Res. 381, 311–322. doi:10.1016/j.yexcr.2019.05.024
Sabatini, D. M. (2017). Twenty-five Years of mTOR: Uncovering the Link from Nutrients to Growth. Proc. Natl. Acad. Sci. U S A. 114, 11818–11825. doi:10.1073/pnas.1716173114
Sawasaki, M., Tsubamoto, H., Nakamoto, Y., Kakuno, A., and Sonoda, T. (2020). S-1, Oxaliplatin, Nab-Paclitaxel and Itraconazole for Conversion Surgery for Advanced or Recurrent Gastric Cancer. Anticancer Res. 40, 991–997. doi:10.21873/anticanres.14033
Shi, L., Tang, X., Qian, M., Liu, Z., Meng, F., Fu, L., et al. (2018). A SIRT1-Centered Circuitry Regulates Breast Cancer Stemness and Metastasis. Oncogene 37, 6299–6315. doi:10.1038/s41388-018-0370-5
Siegel, R. L., Miller, K. D., Fuchs, H. E., and Jemal, A. (2021). Cancer Statistics, 2021. CA Cancer J. Clin. 71, 7–33. doi:10.3322/caac.21654
Tengku Din, T. A., Seeni, A., Khairi, W. N., Shamsuddin, S., and Jaafar, H. (2014). Effects of Rapamycin on Cell Apoptosis in MCF-7 Human Breast Cancer Cells. Asian Pac. J. Cancer Prev. 15, 10659–10663. doi:10.7314/apjcp.2014.15.24.10659
Tsubamoto, H., Sonoda, T., and Inoue, K. (2014). Impact of Itraconazole on the Survival of Heavily Pre-treated Patients with Triple-Negative Breast Cancer. Anticancer Res. 34, 3839–3844.
Keywords: itraconazole, rapamycin, triple-negative breast cancer, cell cycle, apoptosis
Citation: Wu H-T, Li C-L, Fang Z-X, Chen W-J, Lin W-T and Liu J (2022) Induced Cell Cycle Arrest in Triple-Negative Breast Cancer by Combined Treatment of Itraconazole and Rapamycin. Front. Pharmacol. 13:873131. doi: 10.3389/fphar.2022.873131
Received: 10 February 2022; Accepted: 24 March 2022;
Published: 19 April 2022.
Edited by:
Simona Rapposelli, University of Pisa, ItalyReviewed by:
Zhuo-Xun Wu, St. John’s University, United StatesPaula Rezende-Teixeira, University of São Paulo, Brazil
Copyright © 2022 Wu, Li, Fang, Chen, Lin and Liu. This is an open-access article distributed under the terms of the Creative Commons Attribution License (CC BY). The use, distribution or reproduction in other forums is permitted, provided the original author(s) and the copyright owner(s) are credited and that the original publication in this journal is cited, in accordance with accepted academic practice. No use, distribution or reproduction is permitted which does not comply with these terms.
*Correspondence: Jing Liu, amxpdTEyQHN0dS5lZHUuY24=
†These authors have contributed equally to this work