- 1School of Pharmacy, Nanjing University of Chinese Medicine, Nanjing, China
- 2Jiangsu Engineering Research Center for Efficient Delivery System of TCM, Nanjing, China
- 3Jiangsu Key Laboratory of Pediatric Respiratory Disease, Institute of Pediatrics, Nanjing University of Chinese Medicine, Nanjing, China
Aconiti Lateralis Radix Praeparata (Fuzi in Chinese) is a traditional herbal medicine widely used in China and other Asian countries. In clinical practice, it is often used to treat heart failure, rheumatoid arthritis, and different kinds of pains. Fuzi extract and its active ingredients exert considerable anticancer, anti-inflammatory, and analgesic effects. The main chemical substances of Fuzi include alkaloids, polysaccharides, flavonoids, fatty acids, and sterols. Among of them, alkaloids and polysaccharides are responsible for the anticancer efficacy. Most bioactive alkaloids in Fuzi possess C19 diterpenoid mother nucleus and these natural products show great potential for cancer therapy. Moreover, polysaccharides exert extraordinary tumor-suppressive functions. This review comprehensively summarized the active ingredients, antineoplastic effects, and molecular mechanisms of Fuzi by searching PubMed, Web of Science, ScienceDirect, and CNKI. The anticancer effects are largely attributed to inducing apoptosis and autophagy, inhibiting proliferation, migration and invasion, regulating body immunity, affecting energy metabolism, as well as reversing multidrug resistance. Meanwhile, several signaling pathways and biological processes are mainly involved, such as NF-κB, EMT, HIF-1, p38 MAPK, PI3K/AKT/mTOR, and TCA cycle. Collectively, alkaloids and polysaccharides in Fuzi might serve as attractive therapeutic candidates for the development of anticancer drugs. This review would lay a foundation and provide a basis for further basic research and clinical application of Fuzi.
Introduction
With the incidence increasing year by year, malignant tumor has become one of the main factors that jeopardize human health. Currently, researchers are working on pathophysiology of cancer and seeking effective treatments, intending to continuously advance the progress of cancer therapy (Bray et al., 2018; Lee et al., 2018). Surgery, chemotherapy, and radiation are common methods to treat cancer. However, these treatments are often accompanied with adverse reactions and complications, such as fatigue, nausea, and pain. In addition, some commonly used chemotherapeutic drugs also have drug resistance, which hinders the treatment process (Gainor et al., 2016; Gainor et al., 2017). For the past few years, natural compounds extracted from plants have become more and more popular owing to their excellent anticancer effect and low toxicity. Many researchers are also committed to exploring natural compounds with anticancer activity as substitutes for chemotherapy (Guo et al., 2019; Rashid et al., 2019; Liu et al., 2021; Singh et al., 2021).
Fuzi is the processed product of the daughter root of Aconitum carmichaeli Debx, which has hot-natured and pungent in flavor recorded in Shennong’s Classic of Materia Medica firstly. Fuzi has been used as a traditional Chinese medicine (TCM) in China for centuries and offers therapeutic potential for heart failure, rheumatoid arthritis, gastroenteritis, depression, and other diseases (Wu et al., 2018; Meng-Qi Yang et al., 2019; Zhang et al., 2021). Constituents such as alkaloids, polysaccharides, flavonoids, fatty acids, ceramides, and trace elements are the material basis for Fuzi to exert a variety of functions (Wu et al., 2014). Studies indicated that alkaloids and polysaccharides in Fuzi possessed anticancer activity, which could availably induce tumor cell apoptosis, restrain cell proliferation, and regulate immunity. In addition, other compounds such as deltoin, sitosterol, neokadsuranic acid B, and 11,14-eicosadienoic acid were speculated to possess the potential to target PI3K/AKT pathway for anticancer effects under the prediction of network pharmacology and molecular docking (Xin Yang et al., 2019). In view of this, Fuzi alkaloids and polysaccharides have sparked increasing interest in the application of cancer therapy. A comprehensive perception of anticancer mechanisms is a prerequisite to the design of rational therapeutics. Therefore, the mechanisms account for the anticancer efficacies on Fuzi alkaloids and polysaccharides are required to be discussed and concluded. This review would provide a new recognition of Fuzi in treating cancer and be of great significance for guiding clinical medication and developing novel antineoplastic drugs.
Fuzi Alkaloids
Pharmacological studies indicate that alkaloids in Fuzi have the effects of anti-inflammation, anticancer, immunoregulation, etc. (Li et al., 2019). The alkaloids in Fuzi are mainly diterpenoid ones, which are classified into three categories according to the number of carbon atoms on the mother nucleus: C18, C19, and C20 diterpenoid alkaloids (Zhu, 2008). Studies have demonstrated that diterpenoid alkaloids exert anticancer effects.
C18 Diterpenoid Alkaloids
Lappaconitine, a kind of C18 diterpenoid alkaloids, inhibited the proliferation of lung cancer A549 cells and induced cell apoptosis (Sheng et al., 2014; Fang, 2018). In vivo investigation revealed that the tumor inhibitory rate of mice bearing liver cancer is 11.20–53.08%; meanwhile that of mice bearing S180 sarcoma cells was 29.81–53.96% after the administration of lappaconitine (Lin et al., 2005). Besides, lappaconitine was widely used in the analgesia of various cancers with high safety and no addiction (Zhang et al., 2016; Sun, 2017). Figure 1 displays the structural formula of lappaconitine and the mother nucleus of C18 diterpenoid alkaloids in Fuzi.
C19 Diterpenoid Alkaloids
Most of the constituents with antineoplastic efficacies in Fuzi are C19 diterpenoid alkaloids. Apart from the bioactivity, these compounds are also poisonous, which lead to cardiotoxicity and neurotoxicity. Three main C19-diester-diterpenoid alkaloids (DDAs) were responsible for the toxicity of Fuzi, namely, aconitine, mesaconitine, and hypaconitine. Studies have shown that the median lethal dose (LD50) of single-dose oral aconitine in mice is 1.0–1.8 mg/kg, while the LD50 of mesaconitine and hypaconitine are 1.9 and 5.8 mg/kg, respectively. The dose–effect relationship of DDAs on clinical therapy remains to be further studied (Wada et al., 2005; Singhuber et al., 2009). So far, nearly 80 kinds of C19 diterpenoid alkaloids have been extracted (Tang et al., 2017; Zhang et al., 2020). The mother nucleus of C19 diterpenoid alkaloids is shown in Figure 2, and natural C19 diterpenoid alkaloids with anticancer activity are listed in Table 1. As shown in Table 1, the common ingredients of Fuzi, such as aconitine, mesaconitine, hypaconitine, benzoylaconitine, and neoline, have been proved to exhibit anticancer functions. Furthermore, the derivatives of C19 diterpenoid alkaloids could also repress the growth of tumor cells (Ren et al., 2017). The antiproliferative effects of C19 diterpene alkaloids and their derivatives on different tumor cell lines are summarized in Table 2. Among the tested cells, the HepG2 cells were highly sensitive to C19 diterpenoid alkaloids as well as their derivatives. By analyzing the structure–activity relationship, it was found that the alkaloids with stronger cytotoxicity, such as aconitine, mesaconitine, hypaconitine, crassicauline A, oxonitine, and dexyaconitine, were associated with the acetoxy group (OAc) on the substituent of R6, suggesting that the positions and species of substituents on the mother nucleus were closely related to the antitumor activity of C19 diterpenoid alkaloid.
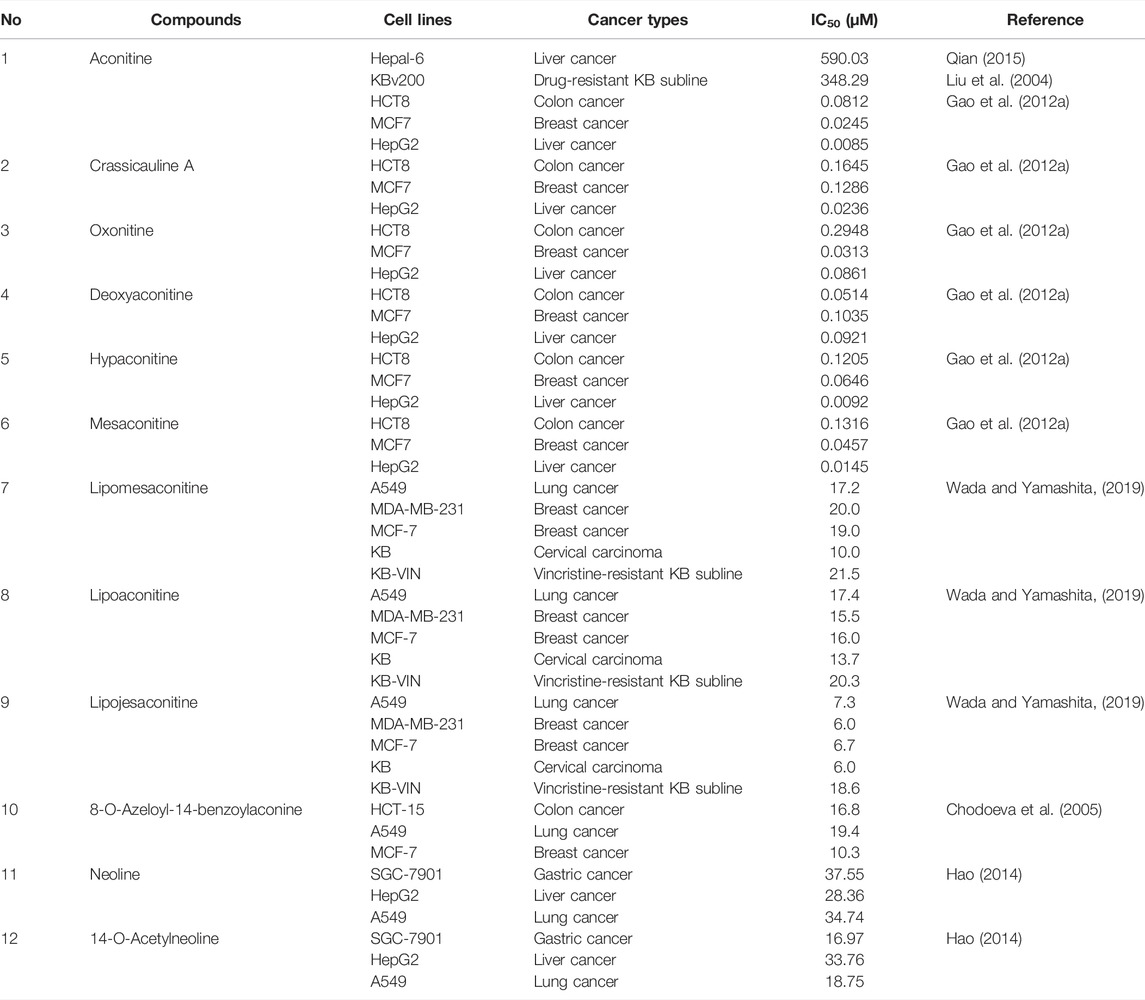
TABLE 2. Cytotoxicity of C19 diterpenoid alkaloids and their derivatives in different cancer cell lines.
C20 Diterpenoid Alkaloids
C20 diterpenoid alkaloid is another kind of alkaloid in Fuzi. Songorine, a natural C20 diterpenoid alkaloid found in Fuzi, has been proved to be antineoplastic. Studies revealed that songorine suppressed the proliferation of HepG2 cells effectively and increased apoptosis at both early and late stages (Sun et al., 2018). Moreover, songorine manifested other biological functions such as analgesic, anti-arrhythmic, and anti-inflammatory activities (Khan et al., 2018). Figure 3 shows the structural formula of songorine and two types of mother nucleus of C20 diterpenoid alkaloids in Fuzi. Based on the structure of natural C20 diterpenoid alkaloids, researchers synthesized a series of derivatives and tested their antiproliferative activity on different tumor cell lines. The results revealed that most derivatives exhibited considerable cytotoxic effects (Table 3). Malignant glioma A172 cells and lung cancer A549 cells were highly sensitive to most of C20 diterpenoid alkaloid derivatives. Of note, certain synthetic alkaloid derivatives displayed stronger anticancer activity than the natural ones. It was suggested that diterpenoid alkaloids demonstrated great potentiality in cancer treatment.
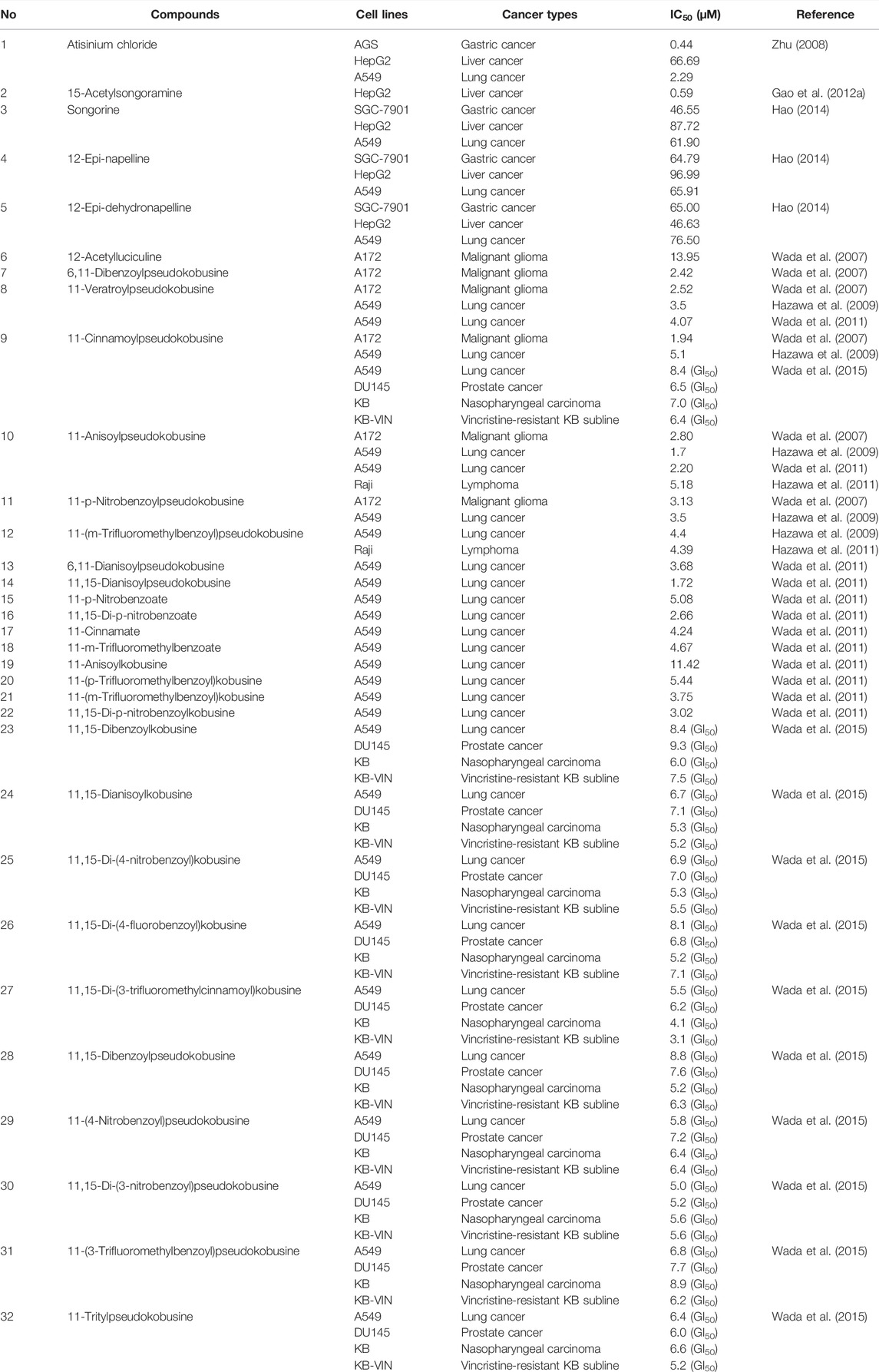
TABLE 3. Cytotoxicity of C20 diterpenoid alkaloids and their derivatives in different cancer cell lines.
Non-Diterpenoid Alkaloids
Apart from C18, C19, and C20 diterpenoid alkaloids, other types of alkaloids in Fuzi such as higenamine and salsolinol also manifested antitumor biological function. Higenamine enhanced the anticancer effects of cucurbitacin B in breast cancer by suppressing the interaction of protein kinase B (AKT) and cyclin-dependent kinase 2 (CDK2) (Jin et al., 2018). In colorectal cancer-bearing nude mice, higenamine inhibited tumor volume by inducing apoptosis (Song et al., 2020). Salsolinol, a water-soluble alkaloid in Fuzi, induced apoptosis of human neuroblastoma SH-SY5Y cells. After incubation with salsolinol, the levels of Bcl-2 decreased, while protein Bax and the release of cytochrome C increased, which led to mitochondrial dysfunction and apoptosis (Wanpen et al., 2007). Figure 4 displays the structures of higenamine and salsolinol.
Fuzi Polysaccharides (FPS)
Polysaccharide is another key component of Fuzi, responsible for anti-inflammatory, anticancer, immunoregulatory, and cholesterol-lowering efficacies (Liu et al., 2019). FPS is mainly composed of glucose, galacturonic acid, galactose, arabinose, and mannose (Lu et al., 2011; Xu et al., 2014). One neutral FPS (FPS1) with 97% purity was isolated from Fuzi. FPS1 was composed of glucan as confirmed by acid hydrolysis, thin-layer chromatography, and infrared spectroscopy (Ruan et al., 2000). In addition, a water-soluble FPS (FPS2) was identified as a (1→3)-branched α-(1→6)-D-glucan. Through the study of structure–activity relationship, it was found that β-glucans and α-glucans consisting of (1→3)-glucopyranosyl units with (1→6)-linked side chains could activate leukocytes, stimulate the phagocytosis, and potentiate host response against many diseases such as cancer and infection. Likewise, in FPS2, α-glucans with similar linkage patterns displayed immunostimulatory activities as well (Zhao et al., 2006).
So far, most studies considered that FPS exerted the antineoplastic effects indirectly by enhancing the immunity capacity. When FPS was used in combination with chemotherapeutic drugs, it exhibited synergism and increased potencies to promote the apoptosis of tumor cells (Dong et al., 2003a; Dong et al., 2003b; Gao et al., 2012b). FPS enhanced the anticancer activity of adriamycin long circulating liposome in treating liver cancer H22 mice by promoting lymphocyte proliferation and potentiating the cytotoxic index of natural killer (NK) cells (Dong et al., 2006). Furthermore, FPS prevented the proliferation and migration of tumor cells via restraining the synthesis of glycosyltransferase and matrix metalloproteinases (Gao et al., 2016; An et al., 2019).
Anticancer Mechanisms of Fuzi Alkaloids and Polysaccharides
Induce Apoptosis and Autophagy
As we know, apoptosis is crucial to the development of the body and the stability of internal environment. When carcinogenic driving factors lead to excessive cell proliferation, it usually triggers apoptosis (Green and Evan, 2002). Apoptosis is also called programmed cell death. The process of apoptosis is actually a cascade amplification process of irreversible hydrolysis of substrate by cysteinyl aspartate specific proteinase (caspase) (McIlwain et al., 2015), which could be divided into two major categories (intrinsic pathway and extrinsic pathway) (Carneiro and El-Deiry, 2020). As a lysosome degradation pathway with the function of nutritional circulation and metabolic adaptation, autophagy is considered to be involved in the regulation of cancer (Amaravadi et al., 2019). In many cases, autophagy is closely related to apoptosis, and autophagy-related genes are also involved in the process of apoptosis (Maheswari et al., 2018). Inducing apoptosis and autophagy is one of the primary mechanisms responsible for the anticancer effect of Fuzi alkaloids and polysaccharides.
Mitochondrial-Mediated Apoptosis
Mitochondria are the regulatory center of apoptosis. There is usually an increase in mitochondrial outer membrane permeability in the last step of apoptosis, accompanied by the release of cytochrome C (Cyto C) (Jin and El-Deiry, 2005; Kalkavan and Green, 2018). Mitochondrial-mediated apoptosis pathway (intrinsic pathway) is usually accompanied by changes in Bax, Bcl-2, Cyto C, ROS, caspase-3, etc. Bcl-2 and Bax are anti- and pro-apoptotic members of Bcl-2 family respectively, which could affect caspase cascade pathways in apoptosis. Cyto C is a key protein encoded by nuclear genes and its release is regulated by the members of Bcl-2 family (Li et al., 1997; Chao and Korsmeyer, 1998; Martinou and Youle, 2011). Aconitine promoted the release of Cyto C by directly increasing the production of reactive oxygen species (ROS) in mitochondria and ultimately induced cell apoptosis. This was demonstrated by the increased expression of caspase-3, caspase-7, Bax/Bcl-2, and PARP in HepG2 cells (Qi et al., 2018). Salsolinol (250 and 500 μM) caused the release of mitochondrial Cyto C in human neuroblastoma SH-SY5Y cells. At the same time, increased levels of Bax and decreased Bcl-2 were detected by western blotting analysis (Wanpen et al., 2007). Moreover, salsolinol enhanced the activity of caspase-3 in a time-dependent manner in SH-SY5Y cells (Jantas et al., 2008). NF-κB is a downstream gene of tumor necrosis factor α (TNF-α) pathway and participates in cell apoptosis (Covert et al., 2005). Studies demonstrated that a series of processes, such as inflammation, angiogenesis, invasion, and cellular metabolism, were controlled by the NF-κB pathway (Taniguchi and Karin, 2018). The activation of the pathway was observed in most kinds of cancers (Yu et al., 2020). The NF-κB pathway was involved in the apoptosis induced by Fuzi alkaloids. With the increment of aconitine, the expression of NF-κB and Bcl-2 decreased gradually, while the protein levels of Bax, caspase-3, caspase-9, PARP, and Cyto C increased (Ji et al., 2016). The research indicated that inhibiting the NF-κB pathway initiated the apoptosis program. Therefore, NF-κB might serve as a promising target in cancer therapy.
Endoplasmic reticulum (ER) dysfunction is to blame for ER-dominated apoptosis to a large extent. In cells, protein synthesis, processing, and modification depend heavily on ER. However, under the condition of viral infection and pH imbalance, the accumulation and aggregation of unfolded proteins would cause severe ER stress (ERS) (Hu et al., 2019). The apoptosis induced by C/EBP homologous protein (CHOP) pathway is a critical manner of ERS-mediated apoptosis, which is mainly regulated by kinase and transcription factors such as PKR-like endoplasmic reticulum kinase (PERK), eukaryotic translation initiation factor 2α (eIF2α), activating transcription factor 6 (ATF6), inositol-requiring enzyme-1 (IRE1), and X-box binding protein 1 (XBP1) (Oyadomari and Mori, 2004; Tabas and Ron, 2011; Sanchez-Lopez et al., 2013). The triggering of CHOP pathway is closely associated with mitochondria-mediated apoptosis, including increasing the expression of Bim and decreasing the expression of Bcl-2 (Hu et al., 2019). It was found that aconitine and quercetin increased apoptosis in human cervical cancer HeLa cells. The two components synergistically activated the glucose-regulated protein 78 (GRP78), a maker of ERS, eventually inducing ERS-mediated apoptosis by upregulating the PERK/eIF2α/ATF4/CHOP pathway (Li et al., 2018).
Death Receptor-Mediated Apoptosis
Death receptors on cell membranes play a crucial role in apoptosis. They induce apoptosis by binding to relevant ligands and transmitting apoptotic signals that eventually cause tumor cell death. Accordingly, the death receptor-mediated pathways could be applied to the field of tumor therapy (Yang, 2020). Death receptors are the initial part of extrinsic apoptosis, including Fas, TNF-R1/TNF-R2, and DR4/DR5, etc. (Locksley et al., 2001; MacEwan, 2002; Ashkenazi and Salvesen, 2014). Exogenous death receptor-mediated pathway initiates apoptosis by activating Fas, TNF-R, and other receptors on the cell membrane. When the death receptor binds to its ligand, the apoptosis signal can be transmitted to adaptor protein such as FADD, and then FADD binds to the initial protein caspase-8 or caspase-10, promoting the release of Cyto C and further triggering the cascade of caspase proteolysis (Lavrik et al., 2005; Billen et al., 2008; Walczak, 2013). Aconitine upregulated the expression of DR5 and TNF-R1 by targeting the p38 MAPK, accordingly activating Bax in A549 cells in a concentration-dependent manner, revealing that aconitine exerted the anticancer effects through the death receptor-mediated apoptosis (Fan et al., 2016). Studies showed that p53 gene could induce apoptosis, regulate cell cycle, and repair DNA damage (Zhao et al., 2021). It is noteworthy that p53 could directly participate in the transcription and regulation of death receptors, such as Fas and DR5 (El-Deiry, 1998). Mesaconitine induced apoptosis of leukemia daunorubicin-resistant cells, whose mechanism might be related to the upregulation of p53 and caspase-3 (Guan et al., 2017). S180 sarcoma and H22 liver cancer mice experiments showed that both crude and acidic FPS inhibited neoplasm tissues growth significantly. Further research revealed that the two FPS increased tumor cell apoptosis by upregulating the expression of p53 and Fas (Dong et al., 2003a).
Autophagy
Autophagy is a defense and stress-regulation mechanism of the body, which is essential for maintaining cell homeostasis. Autophagy would be triggered in states of excessive hunger, nutritional deficiency, oxidative stress, etc. (Amaravadi et al., 2016). Beclin-1 is a critical regulator of autophagy, which mainly participates in the formation of autophagosomes and could be blocked by Bcl-2 (Mariño et al., 2014). Studies indicated that oncogenic proteins inhibited the occurrence of autophagy. On the contrary, oncosuppressor proteins could activate autophagy (Galluzzi et al., 2015). The process of autophagy was initiated by the phosphorylated Beclin-1 gene, and then gene LC3 would be transformed into the membrane type LC3-II, eventually substrate p62 protein would be degraded (Onorati et al., 2018; Levine and Kroemer, 2019). It was reported that benzoylaconitine induced autophagy and apoptosis of human lung cancer A549 cells by upregulating Beclin-1, LC3-Ⅱ, Bax, and caspase-3, meanwhile downregulating p62 and Bcl-2 (Shao et al., 2019). The results suggested that Fuzi alkaloids could promote the expression of autophagy and pro-apoptotic-related proteins, also inhibit the anti-apoptotic protein such as Bcl-2 to conduct autophagy and apoptosis.
Inhibit Proliferation, Migration, and Invasion
Proliferation, migration, and invasion are basic biological characteristics of malignancies. Uncontrolled proliferation, rapid migration, and excessive invasion of tumor cells are leading causes for poor prognosis and high recurrence. Accordingly, inhibition of these pathological processes would contribute to the anticancer efficacies of Fuzi. Studies have demonstrated that several processes and targets were involved in these pathological processes, including p38 MAPK, AKT, EMT, β3GnT8, and MMPs.
p38 MAPK
Mitogen-activated protein kinase (MAPK), composed of three kinase members, p38 MAPK, ERK, and JNK, could be activated by a variety of mitogens and sequentially induced cells enter into the division cycle. Researches revealed that p38 MAPK pathway took part in the regulation of cell proliferation, migration, and apoptosis (Martínez-Limón et al., 2020). MAPKAPK, a downstream target of p38 MAPK, directly induced phosphorylation of heat shock protein 27 (HSP27) and regulated cell migration, proliferation and apoptosis. Evidence was provided that p38 MAPK played a pivotal part in the occurrence and progression of cancer. Aconitine could significantly reduce the phosphorylation of p38, MAPKAPK, and HSP27 in liver cancer cells, indicating that aconitine suppressed the proliferation of MHCC97 cells by restraining the activation of p38 MAPK pathway (Xiong et al., 2018).
AKT
AKT, a serine/threonine kinase, is involved in cell growth, proliferation, apoptosis, survival, and glycogen metabolism (Datta et al., 1999). As an oncogenic protein, the activation of AKT is a general molecular biological feature in cancer (Hennessy et al., 2005; Shaw and Cantley, 2006; Song et al., 2019). Studies found that higenamine combined with cucurbitacin B blocked breast cancer cells in the G2/M phase. Furthermore, combination of the two ingredients decreased the expression of AKT and cell-cycle-related protein CDK2, indicating that higenamine possibly suppressed the proliferation of tumor cells by regulating the AKT signaling pathway negatively (Jin et al., 2018).
EMT
Epithelial–mesenchymal transition (EMT) refers to a biological process of epithelial cells transforming into cells with mesenchymal phenotype by specific procedures, which is linked to tumor invasion and migration. EMT is related to many mediators, such as transforming growth factor-β1 (TGF-β1), snail homolog 1 (Snail), and NF-κB pathways (Feng et al., 2020). Hypaconitine could significantly suppress TGF-β1-induced EMT in lung cancer A549 cells. Further investigation verified that hypaconitine attenuated nuclear translocation of NF-κB and reduced the expression of Snail, which showed a similar inhibitory effect on the NF-κB inhibitor. In the meantime, a significant decrease was observed in cell adhesion, invasion, and migration (Feng et al., 2017). The results suggested that hypoaconitine showed its anticancer potential via suppressing EMT.
β3GnT8
Studies observed that the aberrant glycosylation on the surface of tumor cells is a common feature of tumor malignant transformation and metastasis, which usually involves the modifications of terminal sialylation, fucosylation, O-glycan truncation, etc. (Bresalier et al., 1998; Pinho and Reis, 2015; Cagnoni et al., 2016; Girotti et al., 2020). Deregulation of glycosyltransferase in tumor cells leads to an altered glycan pattern of numerous proteins, resulting in abnormal glycosylation of proteins, and ultimately leading to the dysfunction of proteins, which might be involved in the metastasis of tumor cells (Oliveira-Ferrer et al., 2017). β1, 3-N-acetylglucosminyltransferase 8 (β3GnT8) is a glycosyltransferase that could catalyze the synthesis of polylactosamine chains and promote cell glycosylation. The polylactosamine chains and their related structures participated in the invasion and migration of tumor cells (Liu, 2017). It was found that FPS directly targeted the β3GnT8. The malignancy of tumor-bearing mice decreased along with a reduced expression of β3GnT8 and polylactosamine in liver cancer SK-HEP-1 cells after treatment with FPS, indicating that FPS inhibited the migration and invasion through downregulating β3GnT8 (Gao et al., 2016).
MMPs
Members of matrix metalloproteinases (MMPs) family mainly catalyze the proteolytic activities and thereby aid the breakdown of extracellular matrix (ECM), which is a vital tissue barrier for tumor metastasis (Vandenbroucke and Libert, 2014). MMPs are upregulated through all stages of cancer that could degrade various proteins in ECM and destroy the histological barrier against cell invasion (Isaacson et al., 2017). To date, 23 MMP family members have been found in humans. Numerous studies focus on the design of targeted antineoplastic agents by inhibiting MMPs (Cathcart et al., 2015; Das et al., 2021). MMP-2 and MMP-14, two members in the MMPs family, could degrade ECM, promote vascular proliferation, enhance cell migration and invasion, and are considered potential biomarkers of certain cancers (Alaseem et al., 2019; Karamanou et al., 2020). FPS reduced the tumor weight and blocked the expression of MMP-2 and MMP-14 in mice transplanted with gastric cancer, suggesting that FPS exerted anticancer effect by downregulating MMP-2 and MMP-14 (An et al., 2019).
The involved mechanisms of inducing apoptosis and autophagy, inhibiting cell proliferation, migration, and invasion through different molecular pathways by Fuzi alkaloids and FPS are illustrated in Figure 5.
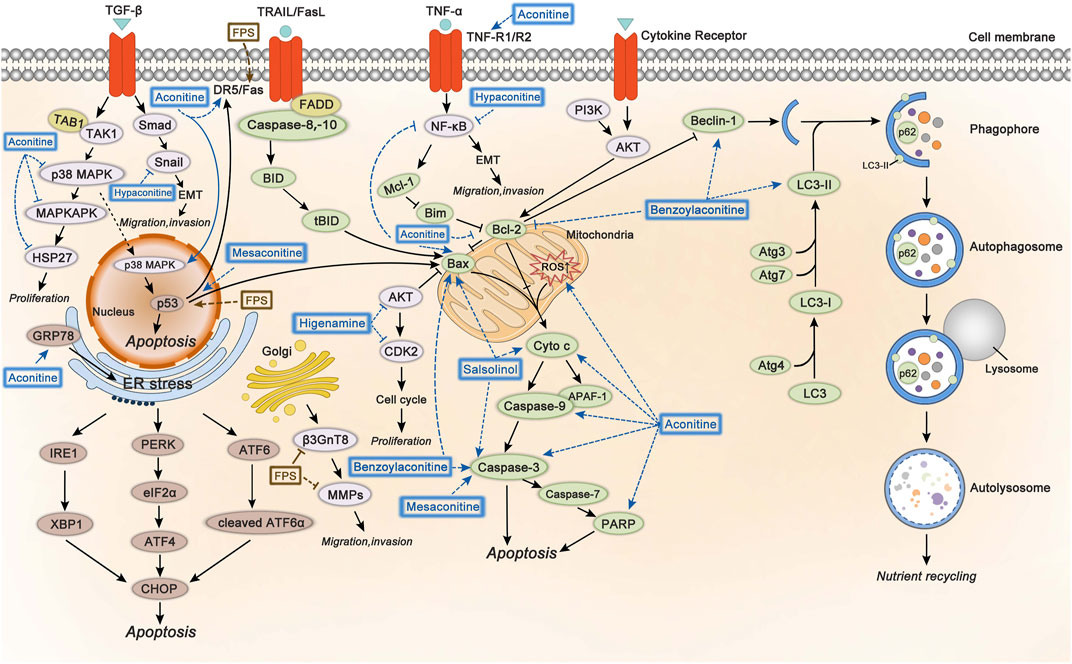
FIGURE 5. The molecular mechanisms of Fuzi against cancer. 1) Fuzi induced cell autophagy and conducted apoptosis by targeting mitochondrial-mediated pathway and death receptor-mediated pathway. 2) Fuzi inhibited cell proliferation, migration, and invasion via regulating p38 MAPK, AKT, EMT, β3GnT8 and MMPs. The blue and brown solid arrows indicated direct targets of Fuzi alkaloids and FPS respectively. Correspondingly, the blue and brown dotted ones indicated downstream effects induced by Fuzi alkaloids and FPS.
Regulate Immunity
The immune escape of tumor refers to the phenomenon that tumor cells escape the recognition and attack of immune system through a variety of mechanisms, so as to survive and proliferate in the body. Some cytokines, such as interleukin-2 (IL-2), IL-6, and IL-12, are able to enhance immune response. In contrast, regulatory T cells (Tregs) could rapidly detect and inhibit IL-2 or other cytokines in the early stage of the immune response to suppress autoimmunity, which might hinder the anticancer immunity (Wing et al., 2019). Therefore, inhibiting the production of Tregs is one of the methods of anticancer immunotherapy. Fuzi aqueous extract has been proved to enhance the anticancer effect of radiotherapy in the treatment of lung cancer. The experimental data indicated that ionizing radiation affected the anticancer immune response of mice. However, after the administration of Fuzi aqueous extract, the levels of IL-2, IL-6, and IL-12 in mice serum increased, which might arouse the immune response. Moreover, Fuzi aqueous extract reduced the radiation-induced production of IL-10, TGF-β, and Tregs, revealing the mechanism of Fuzi in modulating immunity and inhibiting tumor growth (Zhang et al., 2017). Another research showed that, in gastric cancer-bearing mice, aconitine was involved in the intervention of Tregs by regulating the prostaglandin E2/cyclooxygenase-2 (PGE2/COX-2) pathway. It was noteworthy that high-dose aconitine reduced PGE2 and Tregs significantly, whose effect was more obvious than that of the celecoxib-positive group. These results suggested that aconitine exerted antitumor efficacy by reversing immune escape through regulating Tregs and mediating the PGE2/COX-2 pathway (Cheng, 2019).
Cancer immunotherapy aims to promote tumor-specific T-cell response. As antigen-presenting cells, dendritic cells (DC) allow antigens to be recognized by CD4+ T cells and CD8+ T cells, and then CD4+ T cells transmit information to CD8+ T cells and facilitate the differentiation of effector T cells to kill tumor cells (Borst et al., 2018). Aconitine possessed a direct anticancer activity, but it also had the drawback of damaging immunity to a certain extent (Qian, 2015). FPS increased the number of macrophages, CD4+, and CD8+ T cells in the spleen of Hepa1-6 tumor-bearing mice, indicating FPS had immune-improving functions. The results suggested that FPS combined with Fuzi alkaloids might achieve better anticancer efficacy (Qian, 2015). Treatment with FPS induced differentiation of peripheral blood monocytes to DC and expressed its mature phenotypes, which acted as the second signal to activate T lymphocytes and stimulated tumor immunity (Gao L. L. et al., 2012). The synergic effect of FPS and adriamycin was evaluated in H22 tumor-bearing mice. The killing activity of NK cells and lymphocyte transformation rate were dramatically improved; meanwhile the expression of IL-2 and IL-12 in splenic lymphocytes increased, indicating that FPS enhanced the anticancer effect of adriamycin by strengthening the immune system (Dong et al., 2003b; Dong et al., 2006). The anticancer immunomodulatory mechanism of Fuzi is shown in Figure 6.
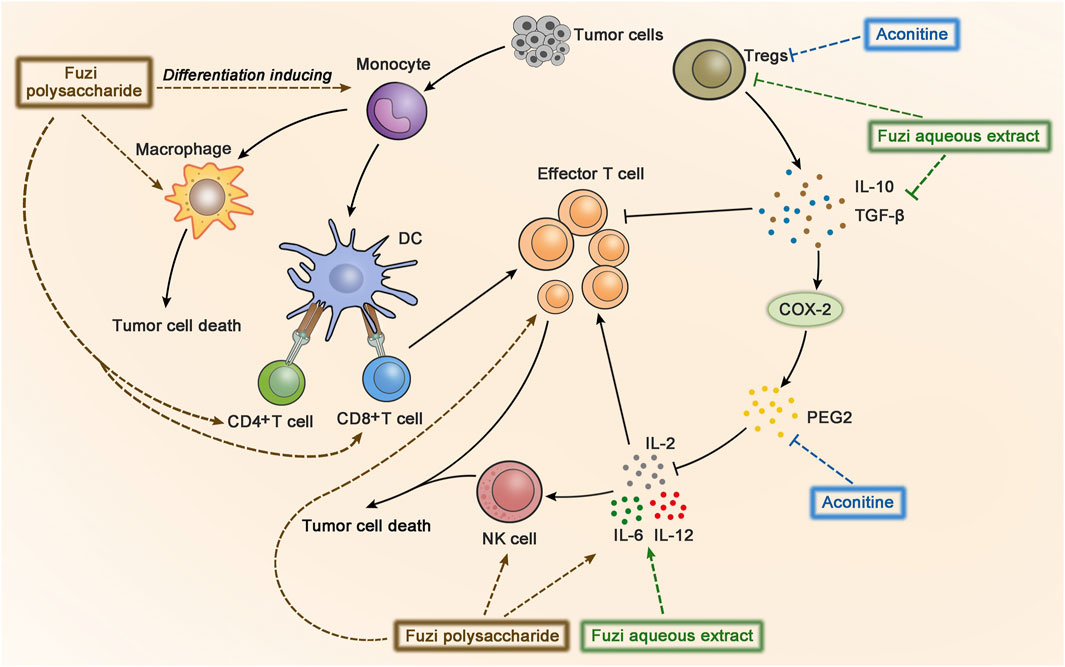
FIGURE 6. The anticancer immunomodulatory mechanism of Fuzi. Fuzi induced peripheral blood monocytes to differentiate into DC, activated T lymphocytes, and increased the expression of macrophages, CD4+, CD8+ T cells, NK cells, IL2, IL-12, IL-6, etc. Besides, Fuzi reduced the production of IL-10, TGF-β, Treg, and arrested PGE2/COX-2 pathway. The blue, brown and green dotted arrows indicated the influence induced by alkaloids, FPS and Fuzi aqueous extract respectively.
Affect Energy Metabolism
The abnormal energy metabolism is one of the major characteristics of tumor cells (Hanahan and Weinberg, 2011). The main sources of biological energy depend on central carbon metabolism, including aerobic respiration and glycolysis. In the presence of adequate oxygen, pyruvate, transformed from glucose, is further oxidatively phosphorylated by mitochondrial respiration. However, under hypoxic conditions, pyruvate would be reduced to lactate. Tumor cells tend to obtain ATP through glycolysis even under aerobic conditions, this phenomenon is called Warburg effect (Warburg et al., 1927). PI3K/AKT/mTOR and HIF-1 pathways would influence glycolysis and participate in the energy metabolism of tumor cells. The PI3K/AKT/mTOR pathway could activate glucose transporters on cell membrane and the metabolic enzyme hexokinase 2 (HK2) in glycolysis, sequentially increase glucose uptake and glycolysis rate (Gottlob et al., 2001; Roberts et al., 2013; Abdel-Wahab et al., 2019). The HIF-1α could induce the activation of lactate dehydrogenase A (LDHA) and pyruvate dehydrogenase kinase 1 (PDK1) to catalyze the conversion of pyruvate to lactate, accordingly promote glycolysis to produce ATP in the anoxic tumor microenvironment (Valvona et al., 2016; Zhou et al., 2018; Nagao et al., 2019).
Mitochondria are the most important organelle of energy metabolism. Besides supplying energy for life, mitochondria also regulate cell death, control redox reactions, and provide substrates for anabolism (Porporato et al., 2018). Angiogenesis in the center of many solid tumors is poor, resulting in limited supply of glucose and oxygen. However, electron transport chain (ETC) in the mitochondria could function even under hypoxia, allowing the tumor cells to breathe and produce ATP (Vasan et al., 2020). Complex I, II, III, and IV, located on the mitochondrial inner membrane, are key components of ETC to transfer electrons. Complex I and II respectively oxidize the NADH and FADH2 to NAD+ (nicotinamide adenine dinucleotide) and FAD+ (flavin adenine dinucleotide), which keep TCA cycle running. Complex I, II, III, and IV transfer electrons to oxygen, discharge protons into the gap of mitochondrial membrane, and eventually generate ATP by ATP synthase (Letts and Sazanov, 2017). Regulation of cellular metabolism might be a significant clue in developing antineoplastic agents (Stuart et al., 2014; Wheaton et al., 2014; Kuntz et al., 2017). Figure 7 shows glycolysis, TCA cycle, and relevant pathways involved in cellular energy metabolism.
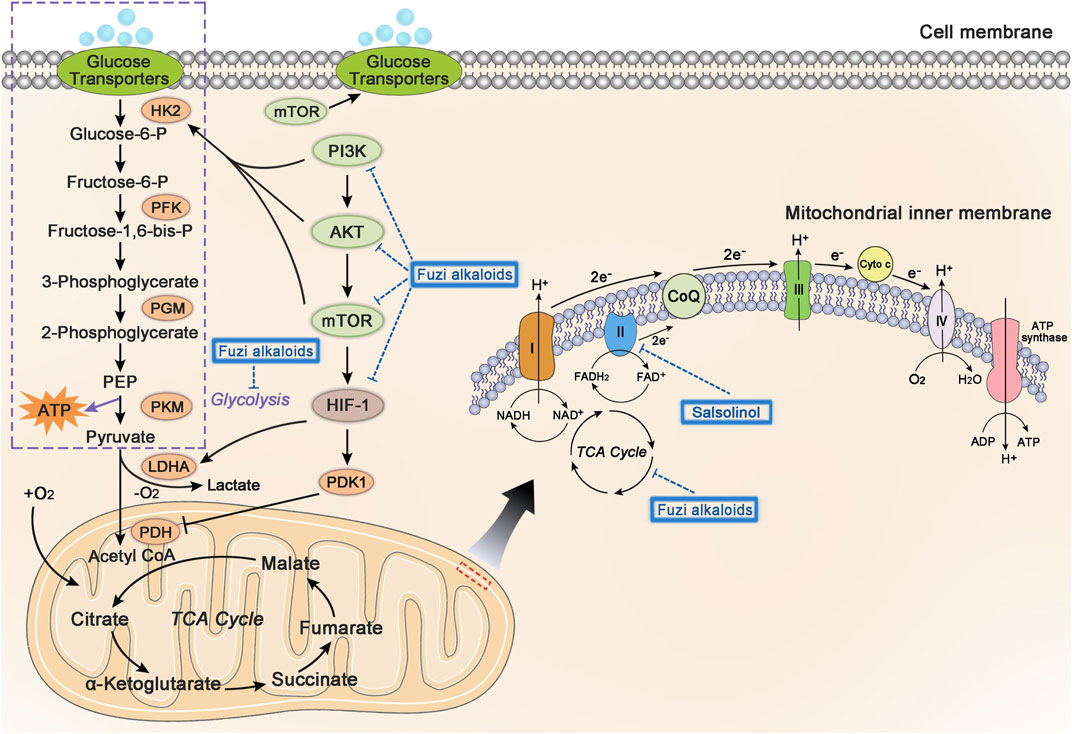
FIGURE 7. The influence of Fuzi alkaloids on cancer energy metabolism. Fuzi regulated the energy supply of cancer cells, as well as induced cell death by inhibiting mitochondrial complex II, PI3K/AKT/mTOR pathway, HIF-1 pathway, and central carbon metabolism. The blue dotted arrow indicated the effect induced by Fuzi alkaloids.
Existing studies found that Fuzi alkaloids regulated cellular metabolism by affecting ETC in the mitochondria. Salsolinol blocked the energy supply of SH-SY5Y cells and induced cell death. It was noteworthy that adding d-glucose to enhance glycolysis could not prevent the cytotoxicity of salsolinol on SH-SY5Y cells. Further research demonstrated that salsolinol inhibited the activity of complex II (succinate-Q reductase) and caused a rapid depletion in intracellular ATP, which might account for the cytotoxic effects of salsolinol (Storch et al., 2000). Moreover, network pharmacology predicted that Fuzi alkaloids might exert antitumor effects by suppressing the PI3K/AKT/mTOR pathway, HIF-1 pathway, and central carbon metabolism in cancer (Lu et al., 2021) (Figure 7). The evidence proved that energy metabolism and its relevant signal pathways might be involved in the anticancer process of Fuzi alkaloids.
Reverse Multidrug Resistance
Multidrug resistance (MDR) is a principal reason of the therapeutic failure. P-glycoprotein (P-gp) and breast cancer resistance protein (BCRP) located on the cell membrane control the absorption, distribution, and excretion of various chemicals. These transporters protect cancer cells from high doses of intracellular drugs and lead to the occurrence of MDR (Bukowski et al., 2020). Many inhibitors, such as verapamil and cyclosporine A, are designed to target P-gp. However, due to the low affinity of these inhibitors to P-gp, high concentrations are required and side effects would ensue (Höllt et al., 1992; List et al., 2001; Waghray and Zhang, 2018). Aconitine was shown to reverse the MDR of KBV200 cells, accordingly the inhibitory rate by aconitine reached 56.15% at 50 μg/ml. The combined use of aconitine and vincristine achieved a better reversal effect of MDR, largely due to downregulating P-gp expression (Liu et al., 2004).
The anticancer active compounds of Fuzi and their mechanisms are summarized in Table 4.
Conclusion
Natural plants have attracted significant interest ascribed to their anticancer properties. In this review, we rounded up the available evidence on the antineoplastic efficacies of Fuzi. As mentioned above, Fuzi alkaloids and polysaccharides showed tumor-suppressive effects in vitro and in vivo by inducing apoptosis and autophagy, inhibiting cell proliferation, migration and invasion, regulating immunity, affecting energy metabolism, and reversing MDR. Several signaling pathways and biological processes were involved in these pharmacological functions, such as NF-κB, EMT, HIF-1, p38 MAPK, PI3K/AKT/mTOR, and TCA cycle. The anticancer molecular mechanism of Fuzi alkaloids and polysaccharides concluded in this review could lay a foundation for further basic research and clinical application.
Outstanding achievements have been obtained in the anticancer chemical elements and pharmacological mechanism of Fuzi. Nevertheless, there are some deficiencies in current research. Nowadays, researchers focus on alkaloid monomers, crude polysaccharide or Fuzi extract. Other constituents such as flavonoids, ceramides and fatty acids are seldomly investigated about their anticancer activity. According to the characteristics of multi-components and multi-targets of TCM, the combination efficacy and network regulation mechanism of bioactive constituents in Fuzi remain to be further studied. With the applications of diversified techniques, specific mechanisms such as drug ligand-receptor interactions and conformational changes of targets are expected to be clarified. To sum up, Fuzi and its active ingredients would serve as attractive therapeutic candidates for the development of anticancer drugs.
Author Contributions
WZ conceived and designed the manuscript. CL and WZ wrote the manuscript. LD and SC assisted with the manuscript preparation. LD, JS and YF revised and polished the manuscript. All authors contributed to the article and approved the submitted version.
Funding
This work was supported by the National Natural Science Foundation of China (82104688), Natural Science Foundation of Jiangsu Province (BK20190800), Natural Science Foundation of the Jiangsu Higher Education Institutions of China (19KJB360004), and Natural Science Foundation of Nanjing University of Chinese Medicine (XPT82104688).
Conflict of Interest
The authors declare that the research was conducted in the absence of any commercial or financial relationships that could be construed as a potential conflict of interest.
Publisher’s Note
All claims expressed in this article are solely those of the authors and do not necessarily represent those of their affiliated organizations, or those of the publisher, the editors, and the reviewers. Any product that may be evaluated in this article, or claim that may be made by its manufacturer, is not guaranteed or endorsed by the publisher.
References
Abdel-Wahab, A. F., Mahmoud, W., and Al-Harizy, R. M. (2019). Targeting Glucose Metabolism to Suppress Cancer Progression: Prospective of Anti-glycolytic Cancer Therapy. Pharmacol. Res. 150, 104511. doi:10.1016/j.phrs.2019.104511
Alaseem, A., Alhazzani, K., Dondapati, P., Alobid, S., Bishayee, A., and Rathinavelu, A. (2019). Matrix Metalloproteinases: A Challenging Paradigm of Cancer Management. Semin. Cancer Biol. 56, 100–115. doi:10.1016/j.semcancer.2017.11.008
Amaravadi, R., Kimmelman, A. C., and White, E. (2016). Recent Insights into the Function of Autophagy in Cancer. Genes Dev. 30 (17), 1913–1930. doi:10.1101/gad.287524.116
Amaravadi, R. K., Kimmelman, A. C., and Debnath, J. (2019). Targeting Autophagy in Cancer: Recent Advances and Future Directions. Cancer Discov. 9 (9), 1167–1181. doi:10.1158/2159-8290.CD-19-0292
An, Z. X., He, Y. L., Wang, M., and Chen, Y. J. (2019). Effect of Aconiti Lateralis Radix Praeparata Polysaccharide on Expressions of MMP-2, MMP-14 in Gastric Cancer Xenografts in Nude Mice. Chin. J. Exp. Traditional Med. Formulae 25 (23), 79–85. doi:10.13422/j.cnki.syfjx.20191621
Ashkenazi, A., and Salvesen, G. (2014). Regulated Cell Death: Signaling and Mechanisms. Annu. Rev. Cel Dev Biol 30, 337–356. doi:10.1146/annurev-cellbio-100913-013226
Billen, L. P., Shamas-Din, A., and Andrews, D. W. (2008). Bid: a Bax-like BH3 Protein. Oncogene 27 (Suppl. 1), S93–S104. doi:10.1038/onc.2009.47
Borst, J., Ahrends, T., Bąbała, N., Melief, C. J. M., and Kastenmüller, W. (2018). CD4+ T Cell Help in Cancer Immunology and Immunotherapy. Nat. Rev. Immunol. 18 (10), 635–647. doi:10.1038/s41577-018-0044-0
Bray, F., Ferlay, J., Soerjomataram, I., Siegel, R. L., Torre, L. A., and Jemal, A. (2018). Global Cancer Statistics 2018: GLOBOCAN Estimates of Incidence and Mortality Worldwide for 36 Cancers in 185 Countries. CA Cancer J. Clin. 68 (6), 394–424. doi:10.3322/caac.21492
Bresalier, R. S., Mazurek, N., Sternberg, L. R., Byrd, J. C., Yunker, C. K., Nangia-Makker, P., et al. (1998). Metastasis of Human colon Cancer Is Altered by Modifying Expression of the Beta-Galactoside-Binding Protein Galectin 3. Gastroenterology 115 (2), 287–296. doi:10.1016/s0016-5085(98)70195-7
Bukowski, K., Kciuk, M., and Kontek, R. (2020). Mechanisms of Multidrug Resistance in Cancer Chemotherapy. Int. J. Mol. Sci. 21 (9), 3233. doi:10.3390/ijms21093233
Cagnoni, A. J., Pérez Sáez, J. M., Rabinovich, G. A., and Mariño, K. V. (2016). Turning-Off Signaling by Siglecs, Selectins, and Galectins: Chemical Inhibition of Glycan-dependent Interactions in Cancer. Front. Oncol. 6, 109. doi:10.3389/fonc.2016.00109
Carneiro, B. A., and El-Deiry, W. S. (2020). Targeting Apoptosis in Cancer Therapy. Nat. Rev. Clin. Oncol. 17 (7), 395–417. doi:10.1038/s41571-020-0341-y
Cathcart, J., Pulkoski-Gross, A., and Cao, J. (2015). Targeting Matrix Metalloproteinases in Cancer: Bringing New Life to Old Ideas. Genes Dis. 2 (`1), 26–34. doi:10.1016/j.gendis.2014.12.002
Chao, D. T., and Korsmeyer, S. J. (1998). BCL-2 Family: Regulators of Cell Death. Annu. Rev. Immunol. 16, 395–419. doi:10.1146/annurev.immunol.16.1.395
Cheng, C. (2019). Intervention of Aconitine on Treg in Gastric Cancer-Bearing Mice by Regulating PGE2/COX2 Pathway. Master’s thesis. Hefei: Anhui University of Chinese Medicine.
Chodoeva, A., Bosc, J. J., Guillon, J., Decendit, A., Petraud, M., Absalon, C., et al. (2005). 8-O-Azeloyl-14-benzoylaconine: A New Alkaloid from the Roots of Aconitum Karacolicum Rapcs and its Antiproliferative Activities. Bioorg. Med. Chem. 13 (23), 6493–6501. doi:10.1016/j.bmc.2005.07.015
Covert, M. W., Leung, T. H., Gaston, J. E., and Baltimore, D. (2005). Achieving Stability of Lipopolysaccharide-Induced NF-kappaB Activation. Science 309 (5742), 1854–1857. doi:10.1126/science.1112304
Das, S., Amin, S. A., and Jha, T. (2021). Inhibitors of Gelatinases (MMP-2 and MMP-9) for the Management of Hematological Malignancies. Eur. J. Med. Chem. 223, 113623. doi:10.1016/j.ejmech.2021.113623
Datta, S. R., Brunet, A., and Greenberg, M. E. (1999). Cellular Survival: a Play in Three Akts. Genes Dev. 13 (22), 2905–2927. doi:10.1101/gad.13.22.2905
Dong, L. F., Zhang, Y. J., Liu, J. S., Song, S. X., Hou, J., and Lu, Z. J. (2006). Anti-tumor Effect of Monkshood Polysaccharide with Adriamycin Long Circulating Temperature-Sensitive Liposome and its Mechanism. Xi Bao Yu Fen Zi Mian Yi Xue Za Zhi 22 (04), 458–462. doi:10.3321/j.issn:1007-8738.2006.04.015
Dong, L. F., Liu, J. S., Miao, Z. H., Han, C. Z., Zheng, X. L., and Han, Y. Q. (2003a). Antineoplastic Effect of Monkshood Polysaccharide on Mice Transplanted with Tumor Lines of H22 and S180. J. Basic Chin. Med. 2003 (09), 14–17. doi:10.3969/j.issn.1006-3250.2003.09.007
Dong, L. F., Liu, J. S., Song, S. X., Wang, H. M., and Lu, Z. J. (2003b). Cooperative Effects of Monkshood Polysaccharide with Adriamycin Magnetic Albumin Microsphere Targeting Therapy on Tumor. J. China Pharm. Univ. 2003 (06), 68–72. doi:10.3321/j.issn:1000-5048.2003.06.016
El-Deiry, W. S. (1998). Regulation of P53 Downstream Genes. Semin. Cancer Biol. 8 (5), 345–357. doi:10.1006/scbi.1998.0097
Fan, Y., Jiang, Y., Liu, J., Kang, Y., Li, R., and Wang, J. (2016). The Anti-tumor Activity and Mechanism of Alkaloids from Aconitum Szechenyianum Gay. Bioorg. Med. Chem. Lett. 26 (2), 380–387. doi:10.1016/j.bmcl.2015.12.006
Fang, C. X. (2018). Study on the Hydrosolube Constituents of Fuzi and the Cardiotoxicity on Zebrafish. Master’s thesis. Chengdu: Chengdu University of Traditional Chinese Medicine.
Feng, H. T., Zhao, W. W., Lu, J. J., Wang, Y. T., and Chen, X. P. (2017). Hypaconitine Inhibits TGF-Β1-Induced Epithelial-Mesenchymal Transition and Suppresses Adhesion, Migration, and Invasion of Lung Cancer A549 Cells. Chin. J. Nat. Med. 15 (06), 427–435. doi:10.1016/S1875-5364(17)30064-X
Feng, Y. L., Chen, D. Q., Vaziri, N. D., Guo, Y., and Zhao, Y. Y. (2020). Small Molecule Inhibitors of Epithelial-Mesenchymal Transition for the Treatment of Cancer and Fibrosis. Med. Res. Rev. 40 (1), 54–78. doi:10.1002/med.21596
Gainor, J. F., Dardaei, L., Yoda, S., Friboulet, L., Leshchiner, I., Katayama, R., et al. (2016). Molecular Mechanisms of Resistance to First- and Second-Generation ALK Inhibitors in ALK-Rearranged Lung Cancer. Cancer Discov. 6 (10), 1118–1133. doi:10.1158/2159-8290.CD-16-0596
Gainor, J. F., Tseng, D., Yoda, S., Dagogo-Jack, I., Friboulet, L., Lin, J. J., et al. (2017). Patterns of Metastatic Spread and Mechanisms of Resistance to Crizotinib in ROS1-Positive Non-small-cell Lung Cancer. JCO Precis Oncol. 2017 (2017), 1–13. PO.17.00063. doi:10.1200/po.17.00063
Galluzzi, L., Pietrocola, F., Bravo-San Pedro, J. M., Amaravadi, R. K., Baehrecke, E. H., Cecconi, F., et al. (2015). Autophagy in Malignant Transformation and Cancer Progression. EMBO J. 34 (7), 856–880. doi:10.15252/embj.201490784
Gao, F., Li, Y. Y., Wang, D., Huang, X., and Liu, Q. (2012a). Diterpenoid Alkaloids from the Chinese Traditional Herbal “Fuzi” and Their Cytotoxic Activity. Molecules 17 (5), 5187–5194. doi:10.3390/molecules17055187
Gao, L. L., Zeng, S. P., and Pan, L. T. (2012b). Induction of Differentiation of Dendritic Cells Derived from Hepatocellular Carcinoma by Fuzi Polysaccharice. Chin. J. Clin. Oncol. 39 (13), 882–885+894. doi:10.3969/j.issn.1000-8179.2012.13.002
Gao, J. D., Li, Y. J., Chen, J. L., Wu, S. L., and Liu, C. L. (2016). Effects of Compound Aconitum Polysaccharide on Glycosylation of Tumor Cells. Zhongguo Zhong Xi Yi Jie He Za Zhi 36 (09), 1103–1106. doi:10.7661/CJIM.2016.09.1103
Girotti, M. R., Salatino, M., Dalotto-Moreno, T., and Rabinovich, G. A. (2020). Sweetening the Hallmarks of Cancer: Galectins as Multifunctional Mediators of Tumor Progression. J. Exp. Med. 217 (2), e20182041. doi:10.1084/jem.20182041
Gottlob, K., Majewski, N., Kennedy, S., Kandel, E., Robey, R. B., and Hay, N. (2001). Inhibition of Early Apoptotic Events by Akt/PKB Is Dependent on the First Committed Step of Glycolysis and Mitochondrial Hexokinase. Genes Dev. 15 (11), 1406–1418. doi:10.1101/gad.889901
Green, D. R., and Evan, G. I. (2002). A Matter of Life and Death. Cancer Cell 1 (1), 19–30. doi:10.1016/s1535-6108(02)00024-7
Guan, X., Yang, T. H., Zhang, C. R., and Fen, S. (2017). Induction Effect of Mesaconitine on Apoptosis of K562 and K562/DNR Cells and Related Mechanism. Acta Medicinae Universitatis Scientiae et Technologiae Huazhong 46 (03), 281–284. doi:10.3870/j.issn.1672-0741.2017.03.008
Guo, C., He, J., Song, X., Tan, L., Wang, M., Jiang, P., et al. (2019). Pharmacological Properties and Derivatives of Shikonin-A Review in Recent Years. Pharmacol. Res. 149, 104463. doi:10.1016/j.phrs.2019.104463
Hanahan, D., and Weinberg, R. A. (2011). Hallmarks of Cancer: the Next Generation. Cell 144 (5), 646–674. doi:10.1016/j.cell.2011.02.013
Hao, W. J. (2014). Study on the Chemical Constituents of the Alkaloids from the Root of Aconitum Flavum Hand.-Mazz and its Anti-tumor Activities. Master’s thesis. Ningxia: Ningxia Medical University.
Hazawa, M., Wada, K., Takahashi, K., Mori, T., Kawahara, N., and Kashiwakura, I. (2009). Suppressive Effects of Novel Derivatives Prepared from Aconitum Alkaloids on Tumor Growth. Invest. New Drugs 27 (2), 111–119. doi:10.1007/s10637-008-9141-4
Hazawa, M., Takahashi, K., Wada, K., Mori, T., Kawahara, N., and Kashiwakura, I. (2011). Structure-activity Relationships between the Aconitum C20-Diterpenoid Alkaloid Derivatives and the Growth Suppressive Activities of Non-hodgkin's Lymphoma Raji Cells and Human Hematopoietic Stem/progenitor Cells. Invest. New Drugs 29 (1), 1–8. doi:10.1007/s10637-009-9327-4
Hennessy, B. T., Smith, D. L., Ram, P. T., Lu, Y., and Mills, G. B. (2005). Exploiting the PI3K/AKT Pathway for Cancer Drug Discovery. Nat. Rev. Drug Discov. 4 (12), 988–1004. doi:10.1038/nrd1902
Höllt, V., Kouba, M., Dietel, M., and Vogt, G. (1992). Stereoisomers of Calcium Antagonists Which Differ Markedly in Their Potencies as Calcium Blockers Are Equally Effective in Modulating Drug Transport by P-Glycoprotein. Biochem. Pharmacol. 43 (12), 2601–2608. doi:10.1016/0006-2952(92)90149-d
Hu, H., Tian, M., Ding, C., and Yu, S. (2019). The C/EBP Homologous Protein (CHOP) Transcription Factor Functions in Endoplasmic Reticulum Stress-Induced Apoptosis and Microbial Infection. Front. Immunol. 9, 3083. doi:10.3389/fimmu.2018.03083
Isaacson, K. J., Martin Jensen, M., Subrahmanyam, N. B., and Ghandehari, H. (2017). Matrix-metalloproteinases as Targets for Controlled Delivery in Cancer: An Analysis of Upregulation and Expression. J. Control. Release 259, 62–75. doi:10.1016/j.jconrel.2017.01.034
Jantas, D., Pytel, M., Mozrzymas, J. W., Leskiewicz, M., Regulska, M., Antkiewicz-Michaluk, L., et al. (2008). The Attenuating Effect of Memantine on Staurosporine-, Salsolinol- and Doxorubicin-Induced Apoptosis in Human Neuroblastoma SH-Sy5y Cells. Neurochem. Int. 52 (4-5), 864–877. doi:10.1016/j.neuint.2007.10.003
Ji, B. L., Xia, L. P., Zhou, F. X., Mao, G. Z., and Xu, L. X. (2016). Aconitine Induces Cell Apoptosis in Human Pancreatic Cancer via NF-Κb Signaling Pathway. Eur. Rev. Med. Pharmacol. Sci. 20 (23), 4955–4964.
Jin, Z., and El-Deiry, W. S. (2005). Overview of Cell Death Signaling Pathways. Cancer Biol. Ther. 4 (2), 139–163. doi:10.4161/cbt.4.2.1508
Jin, Z. Q., Hao, J., Yang, X., He, J. H., Liang, J., Yuan, J. W., et al. (2018). Higenamine Enhances the Antitumor Effects of Cucurbitacin B in Breast Cancer by Inhibiting the Interaction of AKT and CDK2. Oncol. Rep. 40 (4), 2127–2136. doi:10.3892/or.2018.6629
Kalkavan, H., and Green, D. R. (2018). MOMP, Cell Suicide as a BCL-2 Family Business. Cell Death Differ 25 (1), 46–55. doi:10.1038/cdd.2017.179
Karamanou, K., Franchi, M., Vynios, D., and Brézillon, S. (2020). Epithelial-to-mesenchymal Transition and Invadopodia Markers in Breast Cancer: Lumican a Key Regulator. Semin. Cancer Biol. 62, 125–133. doi:10.1016/j.semcancer.2019.08.003
Khan, H., Nabavi, S. M., Sureda, A., Mehterov, N., Gulei, D., Berindan-Neagoe, I., et al. (2018). Therapeutic Potential of Songorine, a Diterpenoid Alkaloid of the Genus Aconitum. Eur. J. Med. Chem. 153, 29–33. doi:10.1016/j.ejmech.2017.10.065
Kuntz, E. M., Baquero, P., Michie, A. M., Dunn, K., Tardito, S., Holyoake, T. L., et al. (2017). Targeting Mitochondrial Oxidative Phosphorylation Eradicates Therapy-Resistant Chronic Myeloid Leukemia Stem Cells. Nat. Med. 23 (10), 1234–1240. doi:10.1038/nm.4399
Lavrik, I., Golks, A., and Krammer, P. H. (2005). Death Receptor Signaling. J. Cel Sci 118 (Pt 2), 265–267. doi:10.1242/jcs.01610
Lee, Y. T., Tan, Y. J., and Oon, C. E. (2018). Molecular Targeted Therapy: Treating Cancer with Specificity. Eur. J. Pharmacol. 834, 188–196. doi:10.1016/j.ejphar.2018.07.034
Letts, J. A., and Sazanov, L. A. (2017). Clarifying the Supercomplex: the Higher-Order Organization of the Mitochondrial Electron Transport Chain. Nat. Struct. Mol. Biol. 24 (10), 800–808. doi:10.1038/nsmb.3460
Levine, B., and Kroemer, G. (2019). Biological Functions of Autophagy Genes: A Disease Perspective. Cell 176 (1-2), 11–42. doi:10.1016/j.cell.2018.09.048
Li, P., Nijhawan, D., Budihardjo, I., Srinivasula, S. M., Ahmad, M., Alnemri, E. S., et al. (1997). Cytochrome C and dATP-dependent Formation of Apaf-1/caspase-9 Complex Initiates an Apoptotic Protease cascade. Cell 91 (4), 479–489. doi:10.1016/s0092-8674(00)80434-1
Li, X. M., Liu, J., Pan, F. F., Shi, D. D., Wen, Z. G., and Yang, P. L. (2018). Quercetin and Aconitine Synergistically Induces the Human Cervical Carcinoma HeLa Cell Apoptosis via Endoplasmic Reticulum (ER) Stress Pathway. PloS one 13 (1), e0191062. doi:10.1371/journal.pone.0191062
Li, S., Li, R., Zeng, Y., Meng, X. L., Wen, C. B., and Zheng, S. C. (2019). Chemical Components and Pharmacological Action of Aconiti Radix. Zhongguo Zhong Yao Za Zhi 44 (12), 2433–2443. doi:10.19540/j.cnki.cjcmm.20190221.004
Lin, N., Xiao, L. Y., Lin, P. Y., Zhang, D., and Chen, Q. W. (2005). Experimental Study on Antitumor Effect of Lappaconitine Hydrobromide. Traditional Chin. Med. Res. 18 (10), 16–18. doi:10.3969/j.issn.1001-6910.2005.10.010
List, A. F., Kopecky, K. J., Willman, C. L., Head, D. R., Persons, D. L., Slovak, M. L., et al. (2001). Benefit of Cyclosporine Modulation of Drug Resistance in Patients with Poor-Risk Acute Myeloid Leukemia: a Southwest Oncology Group Study. Blood 98 (12), 3212–3220. doi:10.1182/blood.v98.12.3212
Liu, C. L. (2017). The Function of β3GnT8 and Polylactosamine in the Malignant Phenotype of Liver Cancer. Dissertation. Suzhou: Soochow University.
Liu, X. Q., Chen, X. Y., Wang, Y. Z., Yuan, S. J., and Tang, Y. (2004). Study on Reversing Multi-Drug Tolerance of KBV200 Cell by Aconitine. J. Basic Chin. Med. 10, 55–57.
Liu, S., Zhang, X., Wang, H., Zeng, Z., Zhang, J., and Zuo, A. (2019). Research Progress on Polysaccharides of Aconitum Medico-Plants. China Pharmaceuticals 28 (03), 1–6. doi:10.3969/j.issn.1006-4931.2019.03.001
Liu, K., Zhang, X., Xie, L., Deng, M., Chen, H., Song, J., et al. (2021). Lupeol and its Derivatives as Anticancer and Anti-inflammatory Agents: Molecular Mechanisms and Therapeutic Efficacy. Pharmacol. Res. 164, 105373. doi:10.1016/j.phrs.2020.105373
Locksley, R. M., Killeen, N., and Lenardo, M. J. (2001). The TNF and TNF Receptor Superfamilies: Integrating Mammalian Biology. Cell 104 (4), 487–501. doi:10.1016/s0092-8674(01)00237-9
Lu, Y., Bu, H., Yang, L., Li, X., and Li, F. (2011). Comparasion of Polysaccharides in Parent Root, Daughter Root and Rootlet of Aconitum Carmichaeli. Zhongguo Zhong Yao Za Zhi 36 (09), 1154–1157. doi:10.4268/cjcmm20110909
Lu, C. Y., Ding, M. L., Cai, S. H., Xie, H., Zhang, W., and Di, L. Q. (2021). Analysis of Alkaloids in Aconiti Lateralis Radix Praeparata and Prediction of Their Mechanism in the Treatment of Cancer. J. Nanjing Univ. Traditional Chin. Med. 37 (05), 720–729. doi:10.14148/j.issn.1672-0482.2021.0720
MacEwan, D. J. (2002). TNF Ligands and Receptors-Aa Matter of Life and Death. Br. J. Pharmacol. 135 (4), 855–875. doi:10.1038/sj.bjp.0704549
Maheswari, U., Ghosh, K., and Sadras, S. R. (2018). Licarin A Induces Cell Death by Activation of Autophagy and Apoptosis in Non-small Cell Lung Cancer Cells. Apoptosis 23 (3-4), 210–225. doi:10.1007/s10495-018-1449-8
Mariño, G., Niso-Santano, M., Baehrecke, E. H., and Kroemer, G. (2014). Self-consumption: the Interplay of Autophagy and Apoptosis. Nat. Rev. Mol. Cel Biol 15 (2), 81–94. doi:10.1038/nrm3735
Martínez-Limón, A., Joaquin, M., Caballero, M., Posas, F., and de Nadal, E. (2020). The P38 Pathway: From Biology to Cancer Therapy. Int. J. Mol. Sci. 21 (6), 1913. doi:10.3390/ijms21061913
Martinou, J. C., and Youle, R. J. (2011). Mitochondria in Apoptosis: Bcl-2 Family Members and Mitochondrial Dynamics. Dev. Cel 21 (1), 92–101. doi:10.1016/j.devcel.2011.06.017
McIlwain, D. R., Berger, T., and Mak, T. W. (2015). Caspase Functions in Cell Death and Disease. Cold Spring Harb Perspect. Biol. 7 (4), a026716. doi:10.1101/cshperspect.a026716
Meng-Qi Yang, M. Q., Song, Y. M., Gao, H. Y., and Xue, Y. T. (2019). Efficacy and Safety of Fuzi Formulae on the Treatment of Heart Failure as Complementary Therapy: A Systematic Review and Meta-Analysis of High-Quality Randomized Controlled Trials. Evid. Based Complement. Alternat Med. 2019, 9728957. doi:10.1155/2019/9728957
Nagao, A., Kobayashi, M., Koyasu, S., Chow, C. C. T., and Harada, H. (2019). HIF-1-Dependent Reprogramming of Glucose Metabolic Pathway of Cancer Cells and its Therapeutic Significance. Int. J. Mol. Sci. 20 (2), 238. doi:10.3390/ijms20020238
Oliveira-Ferrer, L., Legler, K., and Milde-Langosch, K. (2017). Role of Protein Glycosylation in Cancer Metastasis. Semin. Cancer Biol. 44, 141–152. doi:10.1016/j.semcancer.2017.03.002
Onorati, A. V., Dyczynski, M., Ojha, R., and Amaravadi, R. K. (2018). Targeting Autophagy in Cancer. Cancer 124 (16), 3307–3318. doi:10.1002/cncr.31335
Oyadomari, S., and Mori, M. (2004). Roles of CHOP/GADD153 in Endoplasmic Reticulum Stress. Cel Death Differ 11 (4), 381–389. doi:10.1038/sj.cdd.4401373
Pinho, S. S., and Reis, C. A. (2015). Glycosylation in Cancer: Mechanisms and Clinical Implications. Nat. Rev. Cancer 15 (9), 540–555. doi:10.1038/nrc3982
Porporato, P. E., Filigheddu, N., Pedro, J. M. B., Kroemer, G., and Galluzzi, L. (2018). Mitochondrial Metabolism and Cancer. Cell Res 28 (3), 265–280. doi:10.1038/cr.2017.155
Qi, X., Wang, L., Wang, H., Yang, L., Li, X., and Wang, L. (2018). Aconitine Inhibits the Proliferation of Hepatocellular Carcinoma by Inducing Apoptosis. Int. J. Clin. Exp. Pathol. 11 (11), 5278–5289.
Qian, Z. (2015). The Effect and Preliminary Mechanism Study on Monkshood Polysaccharide Combined with Aconitine to the Hepatocellualar Carcinoma Cell. Master’s thesis. Nanjing: Nanjing University of Chinese Medicine.
Rashid, H. U., Xu, Y., Muhammad, Y., Wang, L., and Jiang, J. (2019). Research Advances on Anticancer Activities of Matrine and its Derivatives: An Updated Overview. Eur. J. Med. Chem. 161, 205–238. doi:10.1016/j.ejmech.2018.10.037
Ren, M.-Y., Yu, Q.-T., Shi, C.-Y., and Luo, J.-B. (2017). Anticancer Activities of C18-, C19-, C20-, and Bis-Diterpenoid Alkaloids Derived from Genus Aconitum. Molecules 22 (2), 267. doi:10.3390/molecules22020267
Roberts, D. J., Tan-Sah, V. P., Smith, J. M., and Miyamoto, S. (2013). Akt Phosphorylates HK-II at Thr-473 and Increases Mitochondrial HK-II Association to Protect Cardiomyocytes. J. Biol. Chem. 288 (33), 23798–23806. doi:10.1074/jbc.M113.482026
Ruan, Q. P., Gao, C. J., Li, H. L., and Zhou, L. (2000). Isolation, Purification and Physicochemical Properties of the Polysaccharide F1 from Aconitum Carmichaeli Debx. Nat. Product. Res. Dev. 2000 (05), 46–49. doi:10.16333/j.1001-6880.2000.05.012
Sanchez-Lopez, E., Zimmerman, T., Gomez del Pulgar, T., Moyer, M. P., Lacal Sanjuan, J. C., and Cebrian, A. (2013). Choline Kinase Inhibition Induces Exacerbated Endoplasmic Reticulum Stress and Triggers Apoptosis via CHOP in Cancer Cells. Cell Death Dis 4 (11), e933. doi:10.1038/cddis.2013.453
Shao, X., Han, B., Jiang, X. H., Li, F., He, M., and Liu, F. (2019). Effects of Benzoylaconitine on Autophagy and Apoptosis of Human Lung Cancer Cell Line A549. China Pharm. 30 (20), 2782–2788. doi:10.6039/j.issn.1001-0408.2019.20.10
Shaw, R. J., and Cantley, L. C. (2006). Ras, PI(3)K and mTOR Signalling Controls Tumour Cell Growth. Nature 441 (7092), 424–430. doi:10.1038/nature04869
Sheng, L. H., Xu, M., Xu, L. Q., and Xiong, F. (2014). Cytotoxic Effect of Lappaconitine on Non-small Cell Lung Cancer In Vitro and its Molecular Mechanism. Zhong Yao Cai 37 (05), 840–843. doi:10.13863/j.issn1001-4454.2014.05.026
Singh, S., Meena, A., and Luqman, S. (2021). Baicalin Mediated Regulation of Key Signaling Pathways in Cancer. Pharmacol. Res. 164, 105387. doi:10.1016/j.phrs.2020.105387
Singhuber, J., Zhu, M., Prinz, S., and Kopp, B. (2009). Aconitum in Traditional Chinese Medicine: a Valuable Drug or an Unpredictable Risk? J. Ethnopharmacol. 126, 18–30. doi:10.1016/j.jep.2009.07.031
Song, M., Bode, A. M., Dong, Z., and Lee, M. H. (2019). AKT as a Therapeutic Target for Cancer. Cancer Res. 79 (6), 1019–1031. doi:10.1158/0008-5472.CAN-18-2738
Song, L., Jia, Q., Zhou, Y. Y., Wu, Y. Y., Chen, W. X., Wang, A. Y., et al. (2020). Analyze and Verify the Potential Anti-tumor Mechanism of Higenamine in Aconitum Carmichaeli Debx Based on the Biological Databases. J. Nanjing Univ. Traditional Chin. Med. 36 (05), 655–660. doi:10.14148/j.issn.1672-0482.2020.0655
Storch, A., Kaftan, A., Burkhardt, K., and Schwarz, J. (2000). 1-Methyl-6,7-dihydroxy-1,2,3,4-tetrahydroisoquinoline (Salsolinol) Is Toxic to Dopaminergic Neuroblastoma SH-Sy5y Cells via Impairment of Cellular Energy Metabolism. Brain Res. 855 (1), 67–75. doi:10.1016/s0006-8993(99)02272-6
Stuart, S. D., Schauble, A., Gupta, S., Kennedy, A. D., Keppler, B. R., Bingham, P. M., et al. (2014). A Strategically Designed Small Molecule Attacks Alpha-Ketoglutarate Dehydrogenase in Tumor Cells through a Redox Process. Cancer Metab. 2 (1), 4. doi:10.1186/2049-3002-2-4
Sun, J. R., Qiu, Z. J., Wang, D. H., Zhang, B., and Yuan, J. F. (2018). Anti-tumor Activity of 3-acetylaconitine and Songorine from Aconitum Szechenyianum Gay. Fine Chemicals 35 (07), 1163–1169. doi:10.13550/j.jxhg.20170615
Sun, M. L. (2017). Mechanism Underlying Lappaconitine Antinociception in Chronic Pain. Master’s thesis. Shanghai: Shanghai Jiao Tong University.
Tabas, I., and Ron, D. (2011). Integrating the Mechanisms of Apoptosis Induced by Endoplasmic Reticulum Stress. Nat. Cel Biol 13 (3), 184–190. doi:10.1038/ncb0311-184
Tang, M., Zhao, L. C., Xu, M., Leng, J., Tang, N., Hu, Z. Y., et al. (2017). Chemical Constituents and Pharmacological Activities of Aconiti Lateralis Radix Praeparata. Guihaia 37 (12), 1614–1627. doi:10.11931/guihaia.gxzw201704033
Taniguchi, K., and Karin, M. (2018). NF-κB, Inflammation, Immunity and Cancer: Coming of Age. Nat. Rev. Immunol. 18 (5), 309–324. doi:10.1038/nri.2017.142
Valvona, C. J., Fillmore, H. L., Nunn, P. B., and Pilkington, G. J. (2016). The Regulation and Function of Lactate Dehydrogenase A: Therapeutic Potential in Brain Tumor. Brain Pathol. 26 (1), 3–17. doi:10.1111/bpa.12299
Vandenbroucke, R. E., and Libert, C. (2014). Is There new hope for Therapeutic Matrix Metalloproteinase Inhibition? Nat. Rev. Drug Discov. 13 (12), 904–927. doi:10.1038/nrd4390
Vasan, K., Werner, M., and Chandel, N. S. (2020). Mitochondrial Metabolism as a Target for Cancer Therapy. Cell Metab 32 (3), 341–352. doi:10.1016/j.cmet.2020.06.019
Wada, K., Nihira, M., Hayakawa, H., Tomita, Y., Hayashida, M., and Ohno, Y. (2005). Effects of Long-Term Administrations of Aconitine on Electrocardiogram and Tissue Concentrations of Aconitine and its Metabolites in Mice. Forensic Sci. Int. 148, 21–29. doi:10.1016/j.forsciint.2004.04.016
Wada, K., Hazawa, M., Takahashi, K., Mori, T., Kawahara, N., and Kashiwakura, I. (2007). Inhibitory Effects of Diterpenoid Alkaloids on the Growth of A172 Human Malignant Cells. J. Nat. Prod. 70 (12), 1854–1858. doi:10.1021/np070270w
Wada, K., Hazawa, M., Takahashi, K., Mori, T., Kawahara, N., and Kashiwakura, I. (2011). Structure-activity Relationships and the Cytotoxic Effects of Novel Diterpenoid Alkaloid Derivatives against A549 Human Lung Carcinoma Cells. J. Nat. Med. 65 (1), 43–49. doi:10.1007/s11418-010-0452-3
Wada, K., Ohkoshi, E., Zhao, Y., Goto, M., Morris-Natschke, S. L., and Lee, K. H. (2015). Evaluation of Aconitum Diterpenoid Alkaloids as Antiproliferative Agents. Bioorg. Med. Chem. Lett. 25 (7), 1525–1531. doi:10.1016/j.bmcl.2015.02.018
Wada, K., and Yamashita, H. (2019). Cytotoxic Effects of Diterpenoid Alkaloids against Human Cancer Cells. Molecules 24 (12), 2317. doi:10.3390/molecules24122317
Waghray, D., and Zhang, Q. (2018). Inhibit or Evade Multidrug Resistance P-Glycoprotein in Cancer Treatment. J. Med. Chem. 61 (12), 5108–5121. doi:10.1021/acs.jmedchem.7b01457
Walczak, H. (2013). Death Receptor-Ligand Systems in Cancer, Cell Death, and Inflammation. Cold Spring Harb Perspect. Biol. 5 (5), a008698. doi:10.1101/cshperspect.a008698
Wanpen, S., Kooncumchoo, P., Shavali, S., Govitrapong, P., and Ebadi, M. (2007). Salsolinol, an Endogenous Neurotoxin, Activates JNK and NF-kappaB Signaling Pathways in Human Neuroblastoma Cells. Neurochem. Res. 32 (3), 443–450. doi:10.1007/s11064-006-9246-0
Warburg, O., Wind, F., and Negelein, E. (1927). THE METABOLISM OF TUMORS IN THE BODY. J. Gen. Physiol. 8 (6), 519–530. doi:10.1085/jgp.8.6.519
Wheaton, W. W., Weinberg, S. E., Hamanaka, R. B., Soberanes, S., Sullivan, L. B., Anso, E., et al. (2014). Metformin Inhibits Mitochondrial Complex I of Cancer Cells to Reduce Tumorigenesis. Elife 3, e02242. doi:10.7554/eLife.02242
Wing, J. B., Tanaka, A., and Sakaguchi, S. (2019). Human FOXP3+ Regulatory T Cell Heterogeneity and Function in Autoimmunity and Cancer. Immunity 50 (2), 302–316. doi:10.1016/j.immuni.2019.01.020
Wu, K. H., Tang, L. Y., Wang, Z. J., Xu, Y. L., and Zhou, X. D. (2014). Advances in Studies on Chemical Constituents and Bioactivities of Aconitum Carmichaeli. Chin. J. Exp. Traditional Med. Formulae 20 (02), 212–220. doi:10.11653/syfj2014020212
Wu, J. J., Guo, Z. Z., Zhu, Y. F., Huang, Z. J., Gong, X., Li, Y. H., et al. (2018). A Systematic Review of Pharmacokinetic Studies on Herbal Drug Fuzi: Implications for Fuzi as Personalized Medicine. Phytomedicine 44, 187–203. doi:10.1016/j.phymed.2018.03.001
Xin Yang, X., Li, Y. H., Pan, S. J., Li, Y. F., Chen, X. Y., and Yang, C. F. (2019). Screening of Antitumor Active Components and Key Targets of Aconiti Lateralis Radix Praeparata-Pinelliae Rhizoma Based on PI3K/Akt Pathway. Chin. J. Exp. Traditional Med. Formulae 25 (10), 170–179. doi:10.13422/j.cnki.syfjx.20191026
Xiong, H. S., Jiang, C., Gao, R., and Chen, L. H. (2018). Regulatory Effects and Mechanism of Aconitine on Proliferation, Invasion and Migration of Hepatoma Carcinoma Cell MHCC97. Chin. J. Immunol. 34 (05), 688–692. doi:10.3969/j.issn.1000-484X.2018.05.009
Xu, L., Geng, Z., Luo, F. L., and Guo, L. (2014). Analysis of Polysaccharide Content and Monosaccharide Composition in Different Processed Products of Aconite. Lishizhen Med. Materia Med. Res. 25 (06), 1388–1390. doi:10.3969/j.issn.1008-0805.2014.06.041
Yang, H. Y. (2020). Expression of TRAIL in Esophageal Squamous Cell Carcinoma Promotes Tumor Progression. Master’s thesis. Zhengzhou: Zhengzhou University.
Yu, H., Lin, L., Zhang, Z., Zhang, H., and Hu, H. (2020). Targeting NF-Κb Pathway for the Therapy of Diseases: Mechanism and Clinical Study. Signal. Transduct Target. Ther. 5 (1), 209. doi:10.1038/s41392-020-00312-6
Zhang, Q., Xu, B., Jia, Q., and Li, Y. M. (2016). Research Progress on Chemical Constituents and Pharmacological Activities of C18-Diterpenoid Alkaloids. Chin. Traditional Patent Med. 38 (05), 1109–1114. doi:10.3969/j.issn.1001-1528.2016.05.030
Zhang, Q., Chen, X., Luo, Y., Ren, H., and Qiao, T. (2017). Fuzi Enhances Anti-tumor Efficacy of Radiotherapy on Lung Cancer. J. Cancer 8 (19), 3945–3951. doi:10.7150/jca.22162
Zhang, X. C., Zheng, Q. G., Yang, J. H., Rong, R., and Yang, Y. (2020). Research Progress on Structure and Activity of C19 Diterpeneoid Alkaloids from Aconiti Lateralis Radix Praeparata. Chin. Traditional Herbal Drugs 51 (02), 531–541. doi:10.7501/j.issn.0253-2670.2020.02.033
Zhang, K., Liu, Y., Lin, X., Yang, J., and Wu, C. (2021). Assessment of Reproductive Toxicity and Genotoxicity of Aconiti Lateralis Radix Praeparata and its Processed Products in Male Mice. J. Ethnopharmacol 275, 114102. doi:10.1016/j.jep.2021.114102
Zhao, C., Li, M., Luo, Y., and Wu, W. (2006). Isolation and Structural Characterization of an Immunostimulating Polysaccharide from Fuzi, Aconitum Carmichaeli. Carbohydr. Res. 341 (4), 485–491. doi:10.1016/j.carres.2005.11.032
Zhao, X., Sun, W., Ren, Y., and Lu, Z. (2021). Therapeutic Potential of P53 Reactivation in Cervical Cancer. Crit. Rev. Oncol. Hematol. 157, 103182. doi:10.1016/j.critrevonc.2020.103182
Zhou, L., Wang, Y., Zhou, M., Zhang, Y., Wang, P., Li, X., et al. (2018). HOXA9 Inhibits HIF-1α-Mediated Glycolysis through Interacting with CRIP2 to Repress Cutaneous Squamous Cell Carcinoma Development. Nat. Commun. 9 (1), 1480. doi:10.1038/s41467-018-03914-5
Zhu, T. (2008). Studies on the Antitumor Constituents of Aconitum Vaginatum. Master’s thesis. Wuhan: Huazhong University of Science and Technology.
Keywords: Aconiti Lateralis Radix Praeparata, Fuzi, alkaloid, polysaccharide, anticancer, mechanism
Citation: Zhang W, Lu C, Cai S, Feng Y, Shan J and Di L (2022) Aconiti Lateralis Radix Praeparata as Potential Anticancer Herb: Bioactive Compounds and Molecular Mechanisms. Front. Pharmacol. 13:870282. doi: 10.3389/fphar.2022.870282
Received: 06 February 2022; Accepted: 18 March 2022;
Published: 18 May 2022.
Edited by:
Linlin Lu, Guangzhou University of Chinese Medicine, ChinaReviewed by:
Mansi Wu, Jinan University, ChinaWei Huang, Chinese Academy of Medical Sciences and Peking Union Medical College, China
Copyright © 2022 Zhang, Lu, Cai, Feng, Shan and Di. This is an open-access article distributed under the terms of the Creative Commons Attribution License (CC BY). The use, distribution or reproduction in other forums is permitted, provided the original author(s) and the copyright owner(s) are credited and that the original publication in this journal is cited, in accordance with accepted academic practice. No use, distribution or reproduction is permitted which does not comply with these terms.
*Correspondence: Wen Zhang, d2VuemhhbmdAbmp1Y20uZWR1LmNu; Liuqing Di, ZGlsaXVxaW5nQG5qdWNtLmVkdS5jbg==
†These authors have contributed equally to this work and share first authorship