- 1National Institute of Health and Medical Research (INSERM) U1297, Toulouse, France
- 2Paul Sabatier University, Toulouse, France
- 3Neurobiology of Nutrition and Metabolism Department, Pennington Biomedical Research Center, Louisiana State University System, Baton Rouge, CA, United States
- 4Department of Cardiology, Toulouse University Hospital, Toulouse, France
The regulatory peptide galanin is broadly distributed in the central nervous systems and peripheral tissues where it modulates numerous physiological and pathological processes through binding to its three G-protein-coupled receptors, GalR1-3. However, the function and identity of the galaninergic system in the heart remain unclear. Therefore, we investigated the expression of the galanin receptors in cardiac cells and tissues and found that GalR2 is the dominant receptor subtype in adult mouse hearts, cardiomyocytes and H9C2 cardiomyoblasts. In vivo, genetic suppression of GalR2 promotes cardiac hypertrophy, fibrosis and mitochondrial oxidative stress in the heart. In vitro, GalR2 silencing by siRNA abolished the beneficial effects of galanin on cell hypertrophy and mitochondrial reactive oxygen species (ROS) production. These findings unravel new insights into the role of galaninergic system in the heart and suggest novel therapeutic strategies in heart disease.
Introduction
Heart failure (HF) is a common denominator of acute and chronic heart diseases and one of the leading causes of morbidity and mortality worldwide (Kamran and Tang, 2021). Despite significant advances in treatment and prevention, patients with HF continue to face unacceptably high rates of hospitalization and death reflecting inadequacy of modern therapy strategies. The onset of HF is typically preceded by hypertrophy and mitochondrial defects, the major features of myocardial remodeling, which results in function decline (Sutton and Sharpe, 2000; Konstam et al., 2011; Schiffrin, 2012; Passino et al., 2015). Cardiac hypertrophy is characterized by an increase in cardiomyocyte size and thickening of ventricular walls. Initially, myocyte growth is an adaptive response to maintain cardiac function; however, in settings of sustained stress, hypertrophic remodeling become maladaptive and predispose to cardiovascular morbidity and mortality (Bernardo and McMullen, 2016). Cardiac hypertrophy is closely linked to oxidative stress and mitochondrial damage in HF phenotype (Heineke and Molkentin, 2006), (Rosca et al., 2013). However, the complex molecular and cellular mechanisms underlying abnormal hypertrophic remodeling and mitochondrial homeostasis remain largely obscure.
Galanin is a 29-amino or 30 amino acid peptide naturally present in the tissues and fluids of mammals (Crawley, 1995). Galanin is widely distributed in the central nervous system and peripheral tissues. The amino acid sequence of galanin is very conserved among species (almost 90%), indicating the importance of this peptide (Crawley, 1995). Galanin orchestrates numerous physiological functions, including nociception, sleep regulation, cognition, energy homeostasis, neuroendocrine activities and central cardiovascular control (Crawley, 1995; Díaz-Cabiale et al., 2005; Díaz-Cabiale et al., 2010). Galanin is linked to a number of diseases including epilepsy, depression and eating disorders (McDonald and Crawley, 1997; Lerner et al., 2010; Zhao et al., 2013; Millón et al., 2017). We have recently demonstrated that galanin exhibits cardioprotective properties against ischemia-reperfusion (I/R)-mediated cardiac injury in animals (Pisarenko et al., 2017; Martinelli et al., 2021). Administration of this peptide limited myocardial infarct size and improved metabolic features in vivo (Timotin et al., 2017). Galanin triggers cellular responses by activating three distinct G-protein-coupled receptors, galanin receptor 1 (GalR1), GalR2, and GalR3, which differ in their pharmacology, signaling, and distribution. Among these receptors, GalR1 and GalR3 mainly activate Gi/o types of G proteins, mediating inhibitory actions of galanin (Habert-Ortoli et al., 1994; Burgevin et al., 1995; Wang et al., 1997). In contrast, the GalR2 subtype can transmit either stimulatory effects of galanin, for example on neurotransmitter release, acting via Gq/11 types of G proteins, or it can inhibit neurotransmission via Gi/o types (Fathi et al., 1998; Branchek et al., 2000; Shen et al., 2005). Galanin and its receptors have also been associated with regulation of neurogenesis, stroke-related damage, inflammation, diabetes and cancer (Berger et al., 2005; Lang and Kofler, 2011; Fang et al., 2016). However, the expression and functional activity of galanin receptors in the heart remains largely unexplored.
Here we report that GalR2 is the predominant receptor subtype in the heart that transduces the protective effects of galanin in cardiac cells. Genetic suppression of GalR2 promotes activation of hypertrophic remodeling and mitochondrial oxidative stress in mice.
Materials and Methods
Histology
Hematoxylin-eosin (H&E) and Masson’s Trichrome staining were performed on 10 μm heart cryosections at the Histology Facility according to standard methods. Wheat germ agglutinin (WGA) staining was performed on PFA-fixed cryosections after Triton X-100 permeabilization using Oregon Green-coupled WGA (ThermoFisher Scientific as described before (Cinato et al., 2021). Myocytes cross-sectional area was manually quantified in a blinded manner using ImageJ software on WGA-staining sections. The extent of cardiac structural changes was quantified using ImageJ software.
Mutant Mice Deficient for GalR2
Mice aged 8–10 months deficient for the GalR2 gene (GalR2mut) were derived from the colony of Marina Picciotto (Yale University, New Haven, CT, United States), where they were backcrossed onto a C57Bl/6J background for at least 10 generations as described previously (Hobson et al., 2006; Einstein et al., 2013). Homozygotes mice were used in the study. Breeding and experimental procedures using GalR2 wild type and GalR2mut mice were approved by the Institutional Animal Care and Use Committee at the Pennington Biomedical Research Center.
Cell Culture, Transfection and Treatments
The rat embryonic cardiomyoblastic cell line H9C2 (ATCC) was cultured as described (Tronchere et al., 2017) in DMEM medium supplemented with 10% FBS and 1% penicillin-streptomycin in a 37°C, 5% CO2 incubator. siRNA transfection was performed with Lipofectamine RNAiMAX (Life Technologies) according to manufacturer’s instructions. Briefly, cells seeded in 500 µl medium in 24-well plates were transfected using 45 pmol siRNA with 1 µl LipoRNAiMAX in 100 µL OptiMEM. Twenty-4 hours later, cells were pre-treated with 10 nM galanin for 30 min and then stimulated with 5 µM isoproterenol (ISO) for 4 h (to assess mitochondrial O2− production) or for 48 h to address cell hypertrophic response.
Molecular Biology
siRNA against GalR2 were from Sigma and as follows: 5′-GCTCTGGTCTCCAAGCATT-3′ and 5′-GCUCUGGUCUCCAAGCAUU-3′. siRNA universal negative controls were from Sigma. Gene expression was assessed using quantitative polymerase chain reaction as described (Cinato et al., 2021). Briefly, total RNAs were isolated from rat embryonic cardiomyoblastic cell line H9C2 or mice heart using the RNeasy mini kit (Qiagen). Total RNAs (300 ng) were reverse transcribed using High Capacity cDNA Reverse Transcription Kit (Applied Biosystems) in the presence of random hexamers. The expression of target mRNA was normalized to HPRT (Figures 1, 3), β2-MG (Supplementary Figure S1) or RPLPO (Figures 2, 4) mRNA expression. Primers for qRT-PCR used in this study are as detailed in Table 1.
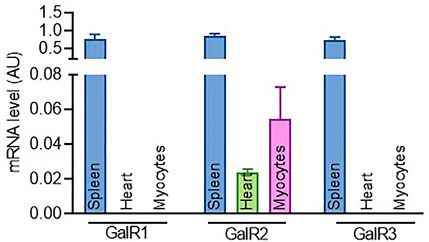
FIGURE1. Expression profiling of GalR1, GalR2 and GalR3 in cardiac tissues and cells. The expression level of GalR1, GalR2 and GalR3 mRNA in mouse spleen, heart and primary cardiomyocytes. Data are presented as the mean ± SEM from at least three independent experiments.
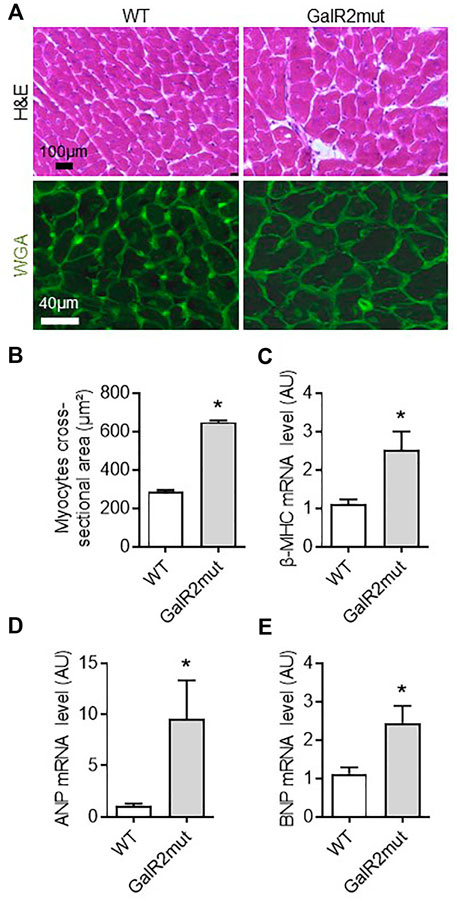
FIGURE 2. Cardiac hypertrophic phenotype in GalR2 deficient mice. Representative images of cardiac sections stained with H&E and WGA (A) and quantification of the cross-sectional area of cardiomyocytes from A in WT and GalR2mut mice (B), n = 5–6 mice. Myocardial mRNA levels of the hypertrophic markers (β-MHC, ANP, and BNP) in WT and GalR2-Mut mice (C–E), n = 7–9 mice. Data are presented as the mean ± SEM, *p < 0.05 vs. WT.
Evaluation of Mitochondrial ROS Production
Mitochondrial O2− production was measured using MitoSOX Red indicator (Life Technologies) as described (Tronchere et al., 2017). Briefly, cells were loaded with MitoSOX (2 µm) for 30min, washed in PBS, PFA-fixed, mounted on glass slide using MOWIOL and imaged on a Zeiss LSM780 confocal microscope. Frozen heart cryosections were hydrated in PBS and incubated with MitoSOX (5 µm) for 30 min at 37°C in a humidified chamber, quickly washed and imaged on a Zeiss LSM780 confocal microscope. The MitoSOX fluorescence intensity was quantified using Image J software on thresholded images.
Statistical Analysis in in Vivo and in vitro Studies
Data are expressed as mean ± SEM. Comparison between two groups was performed by a two-tailed unpaired Mann-Whitney test while comparison of multiple groups was performed by Kruskal–Wallis one-way ANOVA followed by a Dunn’s post hoc test using GraphPad Prism version 9.3.1 (GraphPad Software, Inc.).
Results
GalR2 Expression Predominates in Cardiac Tissues and Cells
To gain insights into the role of the galaninergic system in cardiac cells, we first examined the expression of GalR1, GalR2 and GalR3 in tissues from adult mice and mouse cardiac myocytes. As shown in Figure 1, while the expression of the three galanin receptors was readily detected in spleen, only GalR2 mRNA could be detected in mice heart and primary cardiomyocytes. In addition, we found that GalR2 mRNA is present in H9C2 rat cardiomyoblasts (Supplementary Figure S1A).
GalR2 Deficiency Promotes Cardiac Hypertrophic Remodeling in Mice
To further decipher the role of GalR2 in cardiac phenotype in vivo, we examined whether loss of GalR2 could affect hypertrophy, a hallmark of myocardial remodeling in mice. As shown in Figures 2A–C, mice deficient for GalR2 (GalR2mut) promoted cardiac hypertrophic phenotype as evidenced by cross sectional area in H&E and WGA-stained heart sections as compared with control WT mice. GalR2mut-dependent hypertrophy was confirmed by the expression of hypertrophic markers including β-MHC, ANP and BNP (Figures 2C–E).
GalR2 Suppression Mediates Abnormal Mitochondrial Metabolism in Mice
Mitochondria are an important source of ROS in cardiac cells (Rosca et al., 2013). Therefore, we next examined myocardial mitochondrial ROS level in cardiac tissue from GalR2 deficient mice using MitoSOX Red, a fluorogenic dye highly selective for detection of superoxide production. As shown in Figure 3A, cardiac tissue from GalR2mut mice displayed elevated levels of mitochondrial ROS compared to WT mice heart. These findings suggest that GalR2 deficiency promotes cardiac hypertrophic remodeling and mitochondrial oxidative stress in the heart. In addition to mitochondrial ROS status, we measured myocardial expression of genes related to mitochondrial biogenesis. As shown in Figure 3B, we found that GalR2 deficiency in mice evokes a downregulation of genes linked to mitochondrial biogenesis including nuclear respiratory factor 1 (NRF1), ATP6 and COX1, suggesting that the GalR2 pathway regulates mitochondrial biogenesis in the heart.
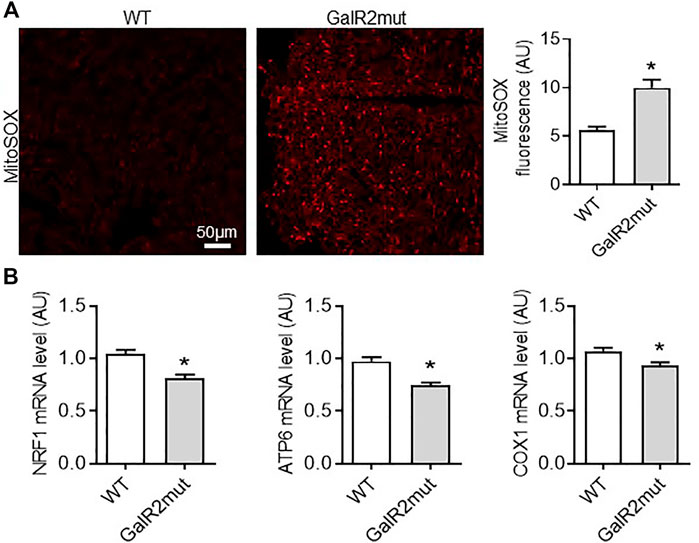
FIGURE 3. Cardiac mitochondrial ROS status in GalR2 deficient mice. (A) Representative images and quantification of cardiac sections stained with MitoSox Red from WT and GalR2mut mice, n = 4–6 mice. (B) Myocardial mRNA levels of NRF1, ATP6 and COX1 in WT and GalR2mut mice, n = 5–7. Data represents the mean ± SEM. *p < 0.05 vs. WT.
GalR2 Deficiency Favors Myocardial Fibrotic Remodeling in Mice
To further characterize the cardiac phenotype of GalR2 deficient mice, we analyzed the collagen deposition and localization on left ventricles from GalR2mut mice. As shown in Figure 4A, loss of GalR2 in mice promoted collagen accumulation in interstitium and perivascular regions within the left ventricle as evidenced by Masson’s trichrome and WGA-staining. Consistent with the reactive tissue fibrosis in mice deficient for GalR2, we demonstrated increased expression of α-smooth muscle actin (α-SMA), a biomarker for myofibroblast differentiation, in GalR2mut hearts compared to WT hearts (Figure 4A). Moreover, fibrosis signatures of heart tissue from GalR2mut mice was confirmed by the up-regulation of pro-fibrotic genes including Col I, Col III and TGFβ (Figure 4B). These findings suggest that GalR2 deficiency promotes cardiac fibrotic remodeling in the left ventricle.
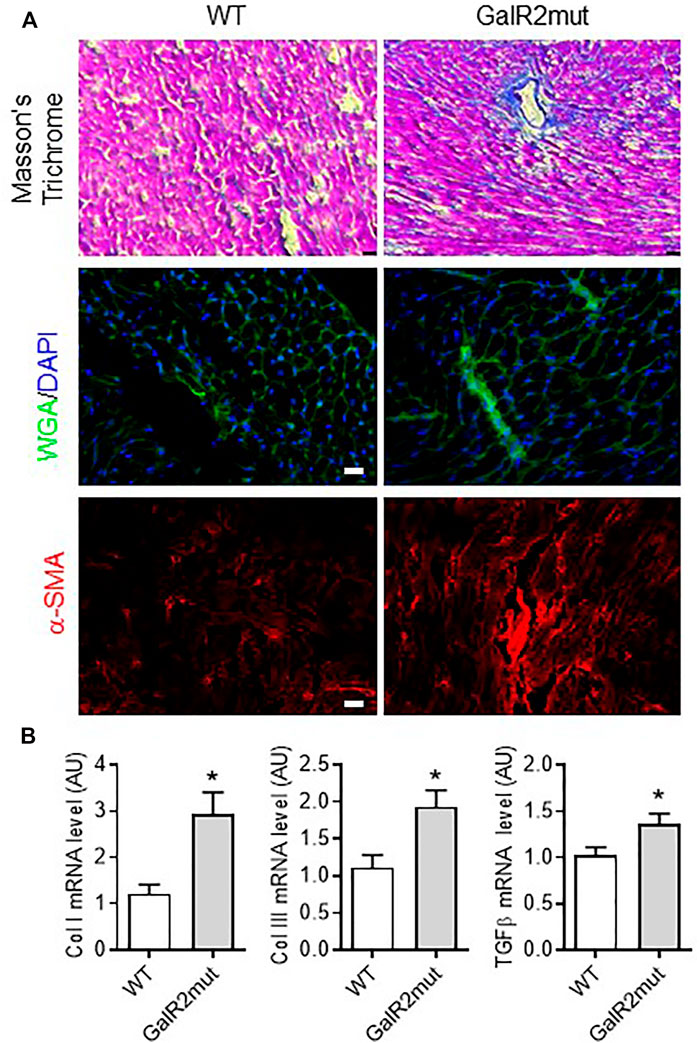
FIGURE 4. Cardiac fibrotic remodeling in GalR2 mutant mice. (A) Representative images of Masson’s Trichrome, WGA- or α-SMA immunolabelling or staining to depict fibrotic remodeling in GalR2mut hearts. (B) Myocardial mRNA levels of profibrotic factors Col I, Col III and TGFβ in WT and GalR2mut mice, n = 5–10. Data represents the mean ± SEM. *p < 0.05 vs. WT.
Galanin Orchestrates Cell Hypertrophic and ROS-Associated Cell Responses via GalR2
We have recently demonstrated that galanin inhibits mitochondrial ROS production and hypertrophy in cardiomyoblasts (Martinelli et al., 2021; Timotin et al., 2017). Therefore, we next examined whether the siRNA-mediated knockdown of GalR2 could affect galanin-mediated effects on cardiomyoblast hypertrophy and ROS accumulation induced by isoproterenol (ISO) treatment. In H9C2 cells, efficient GalR2 knocck-down was demonstrated by qPCR (Supplementary Figure S1B). As shown in Figure 5, galanin attenuated ISO-induced hypertrophy in control siRNA-transfected cells, whereas these effects were abolished in the GalR2 siRNA-transfected cells. Furthermore, galanin-induced effects on ROS generation following ISO treatment were suppressed in GalR2 siRNA transfected H9C2 cells (Figure 6). These data suggest that galanin drives hypertrophic responses and ROS production through GalR2.
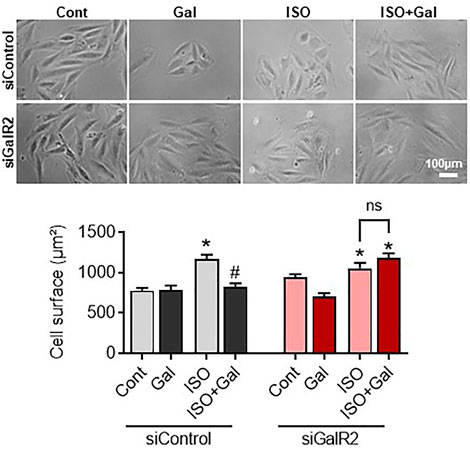
FIGURE 5. Galanin dictates cell hypertrophic responses via GalR2. Representative images and cell surface quantification of H9C2 cardiomyoblasts transfected with control siRNA (siControl) or GalR2 siRNA (siGalR2) under isoproterenol-induced stress (ISO). Data represents the mean ± SEM from three independent experiments. *p < 0.05 vs. control; #p < 0.05 vs. ISO.
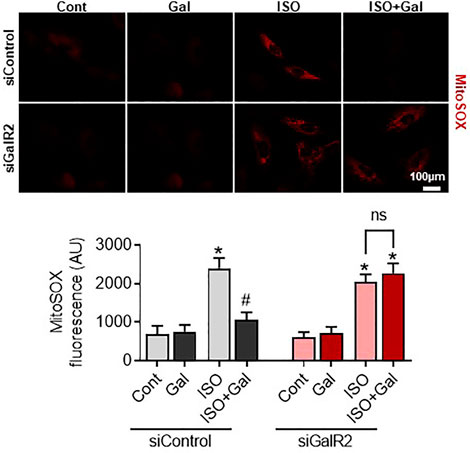
FIGURE 6. Galanin decreases mitochondrial ROS production via GalR2. Representative images and quantification of MitoSox Red staining in H9C2 cells transfected with control siRNA (siControl) or GalR2 siRNA (siGalR2) under isoproterenol-induced stress (ISO). Data represents the mean ± SEM from three independent experiments. *p < 0.05 vs. control; #p < 0.05 vs. ISO.
Discussion
Myocardial hypertrophic remodeling is the most common structural aberration linked to HF and sudden cardiac death (Konstam et al., 2011). In the hypertrophied hearts, mitochondrial ROS overproduction is associated with maladaptive ventricular reprogramming and decline in cardiac function. Despite the progress in understanding the pathophysiology of cardiac remodeling processes leading to HF, treatment options remain limited due to a lack of insight into the mechanisms orchestrating myocardial damage. Here, we report that galanin governs hypertrophic and ROS-associated responses to stress via GalR2. We provide the first evidence that genetic suppression of GalR2 promotes cardiac hypertrophy, fibrosis and mitochondrial oxidative stress in vivo. These findings provide new insights into the role of galaninergic system in cardiac remodeling and open news therapeutic avenues in heart protection.
Galanin, a multifaceted bioactive peptide, is widely distributed throughout the central and peripheral nervous system and some peripheral tissues (Crawley, 1995). Most of the biological functions of galanin remain unclear; however, galanin is mainly involved in modulating cognition, feeding, memory and neuroendocrine control (McDonald and Crawley, 1997; Díaz-Cabiale et al., 2005; Díaz-Cabiale et al., 2010; Lerner et al., 2010; Millón et al., 2017). We recently reported that galanin induces cardioprotective effects against myocardial I/R injury in animals (Pisarenko et al., 2017; Martinelli et al., 2021). Administration of this peptide prevented cardiomyocyte apoptosis and improved metabolic status of the myocardium in vivo (Timotin et al., 2017). The molecular actions of galanin are mediated through three galanin receptors subtypes, GalR1, GalR2 and GalR3 belonging to G protein coupled receptors, and signaling via multiple transduction pathways, including inhibition of cyclic AMP/protein kinase A (GalR1, GalR3) and stimulation of phospholipase C (GalR2) (Howard et al., 1997; Wang et al., 1997). In the present study, we found that GalR2 is the predominant receptor expressed in mouse cardiac cells and tissues. Comparative analysis of mRNA levels of galanin receptor family revealed that cultured myocytes and heart tissues express three galanin receptor subtypes with the prevailing pattern of GalR2 subtype. These data suggest that GalR2 could be involved in the function of galanin in cardiac cells. Previous study reported that GalR2 transcript is widely distributed in both central and peripheral tissues, whereas the expression of GalR1 is more restricted to brain and spinal cord (Kerr et al., 2015). The rat model showed the detectable levels of GalR2 mRNA in the heart demonstrating the significant role of GalR2 in the regulating cardiovascular responses (Kerr et al., 2015). Pharmacological or genetic manipulation of specific receptor subtypes and subsequent phenotypic changes should provide further insight into the relevant GalRs involved in cardiovascular control.
While the anatomical distribution and the distinct galanin receptor subtypes have been described, it remains unclear which galanin receptor or combination of galanin receptors may be mediating the cardiovascular effects of galanin. Previously, the experiments with knockout mice have explored the role of the GalR2 receptor in the anxiety-related behaviors, learning and memory, feeding, and analgesia (Bailey et al., 2007; Lu et al., 2008; Saar et al., 2011; Kerr et al., 2015). Despite the powerful assumption that would implicate galanin/GalR2 axis in the regulation of various complex behaviors, analysis of the GalR2 null mutants did not reveal a discernible phenotype. Gottsch et al. reported that GalR2 deficient mice exhibit normal growth, reproduction, body weight regulation, learning and memory, and susceptibility to seizure induction (Gottsch et al., 2005). It is possible that developmental mechanisms compensate for the congenital lack of GalR2 signaling or that redundant pathways mask the phenotype of the null mutation in GalR2. Alternatively, it is conceivable that GalR2 plays only a subtle role in these complex behaviors and that genetic deletion of GalR2 primes a phenotype that falls below detectable limits of the assays used to analyze these physiological processes. The present experiments elucidated the role of GalR2 in cardiac structural alterations and oxidative stress status in mice. Phenotypic analysis of mice deficient for GalR2 reveals a role for GalR2 in coordination of cardiac hypertrophy, fibrosis and mitochondrial ROS status. We found that suppression of GalR2 induces myocyte hypertrophy and abnormal expression of hypertrophic markers including ANP, BNP and β-MHC. Furthermore, we demonstrated that loss of GalR2 in mice leads to abnormal collagen accumulation within the myocardium and cardiac fibroblast differentiation into myofibroblasts reflecting fibrotic remodeling in GalR2mut mice. Importantly, we also shown that GalR2 deficiency in mice evokes a downregulation of NRF1, ATP6 and COX1, suggesting that GalR2 may have a role in the regulation of cardiac mitochondrial bioenergetic function. These data strongly suggest that GalR2 alone is sufficient to mediate perturbations in mitochondrial metabolic status and cardiac remodeling processes including hypertrophy and fibrosis. Further studies will be required to determine the exact functions of GalR2 in cardiovascular homeostasis, and whether it is indeed part of a pathway that could coordinate myocardial mitochondrial function.
Recently, we demonstrated that galanin plays a critical role in cardiac cell survival in the failing heart (Martinelli et al., 2021), however, the receptor subtypes that mediate the protective effects of galanin in cardiac cells are unclear. Here we report that galanin protects against hypertrophy and mitochondrial oxidative stress through GalR2-dependent pathway. Using siRNA knockdown in cardiomyoblasts, we show that galanin can reverse the effects of galanin on isoproterenol-induced hypertrophy and mitochondrial ROS generation. These findings imply that galanin dictates anti-hypertrophic and anti-oxidant effects through GalR2 pathway. Our findings are consistent with previous studies demonstrating that GalR2 is the predominant receptor subtype that transduces the neuroprotective effects of galanin (Elliott-Hunt et al., 2007). Respectively, GalR2-specific agonist would have utility in various forms of brain or heart damage, either reducing or minimizing cell death.
In summary, these findings unravel new insights into the role of galanin/GalR2 axis in the heart and suggest novel therapeutic strategies in heart disease. We report that GalR2 is the predominant receptor subtype in the myocardium orchestrating the antihypertrophic and ROS-suppressing actions of galanin.
Data Availability Statement
The original contributions presented in the study are included in the article/Supplementary Materials, further inquiries can be directed to the corresponding author.
Ethics Statement
The animal study was reviewed and approved by the INSERM ethics committee.
Author Contributions
OK and HT designed the study, analyzed data, and wrote the manuscript. FB and MC conducted key experiments, data analysis and drafted the manuscript. AT, SK, HL and SK contributed to histological and in vitro experiments. HM, EQC contributed to in vivo study. All authors contributed to the article and approved the submitted version.
Funding
This work was supported by the INSERM and Région Midi-Pyrénées RBIO and ERASMUS MUNDUS MEDEA project, National Institute of Health 1R01AT011683-01 (HM) and T32-DK064584 (EQC).
Conflict of Interest
The authors declare that the research was conducted in the absence of any commercial or financial relationships that could be construed as a potential conflict of interest.
Publisher’s Note
All claims expressed in this article are solely those of the authors and do not necessarily represent those of their affiliated organizations, or those of the publisher, the editors and the reviewers. Any product that may be evaluated in this article, or claim that may be made by its manufacturer, is not guaranteed or endorsed by the publisher.
Supplementary Material
The Supplementary Material for this article can be found online at: https://www.frontiersin.org/articles/10.3389/fphar.2022.869179/full#supplementary-material
References
Bailey, K. R., Pavlova, M. N., Rohde, A. D., Hohmann, J. G., and Crawley, J. N. (2007). Galanin Receptor Subtype 2 (GalR2) Null Mutant Mice Display an Anxiogenic-like Phenotype Specific to the Elevated Plus-Maze. Pharmacol. Biochem. Behav. [Internet 86 (1), 8–20. doi:10.1016/j.pbb.2006.11.024 Available at: https://pubmed.ncbi.nlm.nih.gov/17257664/(cited Jan 31, 2022).
Berger, A., Santic, R., Hauser-Kronberger, C., Schilling, F. H., Kogner, P., Ratschek, M., et al. (2005). Galanin and Galanin Receptors in Human Cancers. Neuropeptides 39 (3), 353–359. doi:10.1016/j.npep.2004.12.016
Bernardo, B. C., and McMullen, J. R. (2016). Molecular Aspects of Exercise-Induced Cardiac Remodeling. Cardiol. Clin. 34, 515–530. doi:10.1016/j.ccl.2016.06.002
Branchek, T. A., Smith, K. E., Gerald, C., and Walker, M. W. (2000). Galanin Receptor Subtypes. Trends Pharmacol. Sci. 21 (3), 109–117. doi:10.1016/s0165-6147(00)01446-2
Burgevin, M. C., Loquet, I., Quarteronet, D., and Habert-Ortoli, E. (1995). Cloning, Pharmacological Characterization, and Anatomical Distribution of a Rat cDNA Encoding for a Galanin Receptor. J. Mol. Neurosci. 6 (1), 33–41. doi:10.1007/BF02736757
Cinato, M., Guitou, L., Saidi, A., Timotin, A., Sperazza, E., Duparc, T., et al. (2021). Apilimod Alters TGFβ Signaling Pathway and Prevents Cardiac Fibrotic Remodeling. Theranostics 11 (13), 6491–6506. doi:10.7150/thno.55821
Crawley, J. N. (1995). Biological Actions of Galanin. Regul. Peptides 59, 1–16. doi:10.1016/0167-0115(95)00083-n
Díaz-Cabiale, Z., Parrado, C., Narváez, M., Millón, C., Puigcerver, A., Fuxe, K., et al. (2010). Neurochemical Modulation of central Cardiovascular Control: the Integrative Role of Galanin. Exp. Suppl. 102, 113–131. doi:10.1007/978-3-0346-0228-0_9
Díaz-Cabiale, Z., Parrado, C., Vela, C., Razani, H., Coveñas, R., Fuxe, K., et al. (2005). Role of Galanin and Galanin(1-15) on central Cardiovascular Control. Neuropeptides 39 (3), 185–190. doi:10.1016/j.npep.2004.12.009
Einstein, E. B., Asaka, Y., Yeckel, M. F., Higley, M. J., and Picciotto, M. R. (2013). Galanin-induced Decreases in Nucleus Accumbens/striatum Excitatory Postsynaptic Potentials and Morphine Conditioned Place Preference Require Both Galanin Receptor 1 and Galanin Receptor 2. Eur. J. Neurosci. [Internet 37 (9), 1541–1549. doi:10.1111/ejn.12151 Available at: http://www.ncbi.nlm.nih.gov/pubmed/23387435 (cited Jun 14, 2018)
Elliott-Hunt, C. R., Pope, R. J. P., Vanderplank, P., and Wynick, D. (2007). Activation of the Galanin Receptor 2 (GalR2) Protects the hippocampus from Neuronal Damage. J. Neurochem. [Internet 100 (3), 780–789. doi:10.1111/j.1471-4159.2006.04239.x Available at: https://pubmed.ncbi.nlm.nih.gov/17263796/(cited Jan 31, 2022).
Fang, P., Shi, M., Zhu, Y., Bo, P., and Zhang, Z. (2016). Type 2 Diabetes Mellitus as a Disorder of Galanin Resistance. Exp. Gerontol. 73, 72–77. doi:10.1016/j.exger.2015.11.007
Fathi, Z., Battaglino, P. M., Iben, L. G., Li, H., Baker, E., Zhang, D., et al. (1998). Molecular Characterization, Pharmacological Properties and Chromosomal Localization of the Human GALR2 Galanin Receptor. Brain Res. Mol. Brain Res. 58 (1), 156–169. doi:10.1016/s0169-328x(98)00116-8
Gottsch, M. L., Zeng, H., Hohmann, J. G., Weinshenker, D., Clifton, D. K., and Steiner, R. A. (2005). Phenotypic Analysis of Mice Deficient in the Type 2 Galanin Receptor (GALR2). Mol. Cel Biol [Internet 25 (11), 4804–4811. doi:10.1128/mcb.25.11.4804-4811.2005 Available at: https://pubmed.ncbi.nlm.nih.gov/15899880/(cited Jan 31, 2022).
Habert-Ortoli, E., Amiranoff, B., Loquet, I., Laburthe, M., and Mayaux, J. F. (1994). Molecular Cloning of a Functional Human Galanin Receptor. Proc. Natl. Acad. Sci. U S A. 91 (October), 9780–9783. doi:10.1073/pnas.91.21.9780
Heineke, J., and Molkentin, J. D. (2006). Regulation of Cardiac Hypertrophy by Intracellular Signalling Pathways. Nat. Rev. Mol. Cel Biol 7, 589–600. doi:10.1038/nrm1983
Hobson, S-A., Holmes, F. E., Kerr, N. C. H., Pope, R. J. P., and Wynick, D. (2006). Mice Deficient for Galanin Receptor 2 Have Decreased Neurite Outgrowth from Adult Sensory Neurons and Impaired Pain-like Behaviour. J. Neurochem. [Internet 99 (3), 1000–1010. Available at: http://doi.wiley.com/10.1111/j.1471-4159.2006.04143.x (cited Apr 11, 2018).
Howard, A. D., Tan, C., Shiao, L. L., Palyha, O. C., McKee, K. K., Weinberg, D. H., et al. (1997). Molecular Cloning and Characterization of a New Receptor for Galanin. FEBS Lett. 405 (3), 285–290. doi:10.1016/s0014-5793(97)00196-8
Kamran, H., and Tang, W. H. W. (2021). Medical Management of Acute Heart Failure. Fac. Rev. [Internet 10. doi:10.12703/r/10-82 Available at: https://pubmed.ncbi.nlm.nih.gov/35028647/(cited Jan 31, 2022).
Kerr, N., Holmes, F. E., Hobson, S-A., Vanderplank, P., Leard, A., Balthasar, N., et al. (2015). The Generation of Knock-In Mice Expressing Fluorescently Tagged Galanin Receptors 1 and 2. Mol. Cel Neurosci [Internet 68, 258–271. doi:10.1016/j.mcn.2015.08.006 Available at: http://linkinghub.elsevier.com/retrieve/pii/S1044743115300129
Konstam, M. A., Kramer, D. G., Patel, A. R., Maron, M. S., and Udelson, J. E. (2011). Left Ventricular Remodeling in Heart Failure: Current Concepts in Clinical Significance and Assessment. JACC Cardiovasc. Imaging 4 (1), 98–108. doi:10.1016/j.jcmg.2010.10.008
Lang, R., and Kofler, B. (2011). The Galanin Peptide Family in Inflammation. Neuropeptides 45, 1–8. doi:10.1016/j.npep.2010.10.005
Lerner, J. T., Sankar, R., and Mazarati, A. M. (2010). Galanin and Epilepsy. Experientia Supplementum,Galanin. 102, 183–194. doi:10.1007/978-3-0346-0228-0_13
Lu, X., Ross, B., Sanchez-Alavez, M., Zorrilla, E. P., and Bartfai, T. (2008). Phenotypic Analysis of GalR2 Knockout Mice in Anxiety- and Depression-Related Behavioral Tests. Neuropeptides [Internet 42 (4), 387–397. doi:10.1016/j.npep.2008.04.009 Available at: https://pubmed.ncbi.nlm.nih.gov/18554714/(cited Jan 31, 2022).
Martinelli, I., Timotin, A., Moreno-Corchado, P., Marsal, D., Kramar, S., Loy, H., et al. (2021). Galanin Promotes Autophagy and Alleviates Apoptosis in the Hypertrophied Heart through FoxO1 Pathway. Redox Biol. [Internet 40, 101866. doi:10.1016/j.redox.2021.101866 Available at: https://linkinghub.elsevier.com/retrieve/pii/S2213231721000148 (cited Jan 22, 2021)
McDonald, M. P., and Crawley, J. N. (1997). Galanin-acetylcholine Interactions in Rodent Memory Tasks and Alzheimer's Disease. J. Psychiatry Neurosci. 22, 303–317.
Millón, C., Flores-Burgess, A., Narváez, M., Borroto-Escuela, D. O., Gago, B., Santín, L., et al. (2017). The Neuropeptides Galanin and Galanin(1-15) in Depression-like Behaviours. Neuropeptides 64, 39–45. doi:10.1016/j.npep.2017.01.004
Passino, C., Barison, A., Vergaro, G., Gabutti, A., Borrelli, C., Emdin, M., et al. (2015). Markers of Fibrosis, Inflammation, and Remodeling Pathways in Heart Failure. Clin. Chim. Acta 443, 29–38. doi:10.1016/j.cca.2014.09.006
Pisarenko, O., Timotin, A., Sidorova, M., Studneva, I., Shulzhenko, V., Palkeeva, M., et al. (2017). Cardioprotective Properties of N-Terminal Galanin Fragment (2-15) in Experimental Ischemia/reperfusion Injury. Oncotarget [Internet 8 (60), 101659–101671. Available at: http://www.oncotarget.com/fulltext/21503 (cited Dec 22, 2017).
Rosca, M. G., Tandler, B., and Hoppel, C. L. (2013). Mitochondria in Cardiac Hypertrophy and Heart Failure. J. Mol. Cel Cardiol 55, 31–41. doi:10.1016/j.yjmcc.2012.09.002 Available at: https://linkinghub.elsevier.com/retrieve/pii/S0022282812003355
Saar, I., Runesson, J., McNamara, I., Järv, J., Robinson, J. K., and Langel, Ü. (2011). Novel Galanin Receptor Subtype Specific Ligands in Feeding Regulation. Neurochem. Int. [Internet 58 (6), 714–720. doi:10.1016/j.neuint.2011.02.012 Available at: https://pubmed.ncbi.nlm.nih.gov/21333705/(cited Jan 31, 2022).
Schiffrin, E. L. (2012). Vascular Remodeling in Hypertension. Hypertension 59, 367–374. doi:10.1161/hypertensionaha.111.187021
Shen, P. J., Yuan, C. G., Ma, J., Cheng, S., Yao, M., Turnley, A. M., et al. (2005). Galanin in Neuro(glio)genesis: Expression of Galanin and Receptors by Progenitor Cells In Vivo and In Vitro and Effects of Galanin on Neurosphere Proliferation. Neuropeptides 39, 201–205. doi:10.1016/j.npep.2004.12.021
Sutton, M. G., and Sharpe, N. (2000). Left Ventricular Remodeling after Myocardial Infarction: Pathophysiology and Therapy. Circulation 101 (25), 2981–2988. doi:10.1161/01.cir.101.25.2981
Timotin, A., Pisarenko, O., Sidorova, M., Studneva, I., Shulzhenko, V., Palkeeva, M., et al. (2017). Myocardial protection from Ischemia/reperfusion Injury by Exogenous Galanin Fragment. Oncotarget [Internet 8 (13), 21241–21252. Available at: http://www.oncotarget.com/abstract/15071 (cited Feb 23, 2017).
Tronchere, H., Cinato, M., Timotin, A., Guitou, L., Villedieu, C., Thibault, H., et al. (2017). Inhibition of PIKfyve Prevents Myocardial Apoptosis and Hypertrophy through Activation of SIRT3 in Obese Mice. EMBO Mol. Med. [Internet 9, 770–785. doi:10.15252/emmm.201607096 Available at: https://www-ncbi-nlm-nih-gov.gate2.inist.fr/pmc/articles/PMC5452048/pdf/EMMM-9-770.pdf (cited Jun 8, 2017)
Wang, S., Hashemi, T., He, C., Strader, C., and Bayne, M. (1997). Molecular Cloning and Pharmacological Characterization of a New Galanin Receptor Subtype. Mol. Pharmacol. 52 (3), 337–343. doi:10.1124/mol.52.3.337
Keywords: galanin, galanin receptors, oxidative stress, hypertrophy, cardiac remodeling
Citation: Boal F, Cinato M, Timotin A, Münzberg H, Qualls-Creekmore E, Kramar S, Loi H, Roncalli J, Keita S, Tronchere H and Kunduzova O (2022) Galanin Regulates Myocardial Mitochondrial ROS Homeostasis and Hypertrophic Remodeling Through GalR2. Front. Pharmacol. 13:869179. doi: 10.3389/fphar.2022.869179
Received: 04 February 2022; Accepted: 01 March 2022;
Published: 31 March 2022.
Edited by:
Vadim V. Sumbayev, University of Kent, United KingdomReviewed by:
Vyacheslav Dyachuk, Karolinska Institutet (KI), SwedenElizaveta Fasler-Kan, Bern University Hospital, Switzerland
Daria M. Potashnikova, Lomonosov Moscow State University, Russia
Copyright © 2022 Boal, Cinato, Timotin, Münzberg, Qualls-Creekmore, Kramar, Loi, Roncalli, Keita, Tronchere and Kunduzova. This is an open-access article distributed under the terms of the Creative Commons Attribution License (CC BY). The use, distribution or reproduction in other forums is permitted, provided the original author(s) and the copyright owner(s) are credited and that the original publication in this journal is cited, in accordance with accepted academic practice. No use, distribution or reproduction is permitted which does not comply with these terms.
*Correspondence: Oksana Kunduzova, Oxana.Koundouzova@inserm.fr
†These authors have contributed equally to this work