- 1Department of Intensive Care Unit, The First Affiliated Hospital of Jinan University, Guangzhou, China
- 2Department of Clinical Research, The First Affiliated Hospital of Jinan University, Guangzhou, China
- 3Department of Orthopaedics, The First Affiliated Hospital of Jinan University, Guangzhou, China
- 4Department of Neurology, The First Affiliated Hospital of Jinan University, Guangzhou, China
- 5Guangdong Provincial Key Laboratory of Traditional Chinese Medicine Informatization, Guangzhou, China
Background: In intensive care units (ICUs), the morbidity and mortality of ventilator-associated pneumonia (VAP) are relatively high, and this condition also increases medical expenses for mechanically ventilated patients, which will seriously affect the prognoses of critically ill patients. The purpose of this study was to determine the impact of bronchoscopy on the prognosis of patients with VAP undergoing invasive mechanical ventilation (IMV).
Methods: This was a retrospective study based on patients with VAP from the Medical Information Mart for Intensive Care IV database. The outcomes were ICU and in-hospital mortality. Patients were divided based on whether or not they had undergone bronchoscopy during IMV. Kaplan-Meier (KM) survival curves and Cox proportional-hazards regression models were used to analyze the association between groups and outcomes. Propensity score matching (PSM) and propensity score based inverse probability of treatment weighting (IPTW) were used to further verify the stability of the results. The effect of bronchoscopy on prognosis was further analyzed by causal mediation analysis (CMA).
Results: This study enrolled 1,560 patients with VAP: 1,355 in the no-bronchoscopy group and 205 in the bronchoscopy group. The KM survival curve indicated a significant difference in survival probability between the two groups. The survival probabilities in both the ICU and hospital were significantly higher in the bronchoscopy group than in the no bronchoscopy group. After adjusting all covariates as confounding factors in the Cox model, the HRs (95% CI) for ICU and in-hospital mortality in the bronchoscopy group were 0.33 (0.20–0.55) and 0.40 (0.26–0.60), respectively, indicating that the risks of ICU and in-hospital mortality were 0.67 and 0.60 lower than in the no-bronchoscopy group. The same trend was obtained after using PSM and IPTW. CMA showed that delta-red blood cell distribution width (RDW) mediated 8 and 7% of the beneficial effects of bronchoscopy in ICU mortality and in-hospital mortality.
Conclusion: Bronchoscopy during IMV was associated with reducing the risk of ICU and in-hospital mortality in patients with VAP in ICUs, and this beneficial effect was partially mediated by changes in RDW levels.
Introduction
Ventilator-associated pneumonia (VAP), defined as infection of the lung parenchyma in patients after at least 48 h of exposure to invasive mechanical ventilation (IMV), (Papazian et al., 2020), is one of the most common infectious diseases in intensive care units (ICUs) (Hunter, 2012), affecting up to 40% of patients on mechanical ventilators (Spalding et al., 2017). Various previous studies found that VAP was associated with longer IMV durations and ICU stays, and also increased antimicrobial use (Hayashi et al., 2013). In some developed countries, VAP was also found to increase the average hospitalization cost of patients by approximately US$ 40,000 (Zimlichman et al., 2013). The mortality rate of patients with VAP may exceed 50% (Ruiz et al., 2000); the results of 58 randomized studies on VAP indicated that the estimated attributable mortality rate was 9% (range 3–17%) (Melsen et al., 2011). VAP has high morbidity and mortality, and also increases the medical expenses of mechanically ventilated patients, which will seriously affect the prognoses of critically ill patients.
Bronchoscopy has been widely used for clinical diagnoses and treatment of respiratory diseases. Airway examination and transbronchial biopsy have greatly improved the diagnosis rates of pulmonary inflammatory (Baselski and Wunderink, 1994; Su et al., 2020) and substantial lung (Navani et al., 2011) diseases, and can also help improve the treatment effect (Pedro et al., 2019). However, currently there is no unified conclusion on whether bronchoscopy can improve the prognoses of patients with VAP. This study used the large public Medical Information Mart for Intensive Care (MIMIC)-IV database as a basis to determine the impact of bronchoscopy on the prognoses of patients with VAP in the ICU.
Methods
Data Source and Population
The MIMIC-IV is a large, free, and open database; the latest version is MIMIC-IV (version 1.0) (Johnson et al., 2021), which contains comprehensive information on approximately 250,000 patients hospitalized from 2008 to 2019, and provides strong data support for clinical studies (Yang et al., 2020; Wu et al., 2021). The database was approved by the Massachusetts Institute of Technology (Cambridge, Mass.) and the Beth Israel Deaconess Medical Center (Boston, Mass.), and consent was obtained for collection of the original data (Zhang et al., 2021). The database also anonymizes patient information, and so informed consent did not need to be obtained. The researchers needed to complete corresponding courses and obtain certificates to access and extract data from this database.
ICU patients diagnosed with VAP in the MIMIC-IV database were included. For patients admitted to the ICU more than once, only information on the first admission was obtained. Patients without a detailed record of ventilator use or who stayed in the ICU for less than 24 h were excluded. Patients were divided based on whether or not they had undergone bronchoscopy during IMV.
Data Extraction
Data with less than 20% missing values in the database were extracted using Structured Query Language. The included demographic information were age, sex, body mass index (BMI), and race. Other information included the first care unit, disease severity [ Acute Physiology Score III (APSIII)], interventional therapy [vasopressor use, continuous renal replacement therapy (CRRT) use, and duration of IMV], major comorbidities [sepsis, myocardial infarction (MI), congestive heart failure (CHF), hypertension, cerebrovascular disease (CD), chronic pulmonary disease (CPD), liver disease (LD), renal disease (RD), diabetes, and malignancy]; microbiology; results of the first laboratory tests after ICU admission [white blood cells (WBC), neutrophils, lymphocytes, basophils, eosinophils, monocytes, red blood cells (RBC), hematocrit, hemoglobin, mean corpuscular volume (MCV), mean corpuscular hemoglobin (MCH), mean corpuscular hemoglobin concentration (MCHC), red blood cell distribution width (RDW), platelets, anion gap (AG), bicarbonate, calcium total, calcium free, magnesium phosphate, chloride, sodium, potassium, base excess (BE), calculated total CO2, pH, PaCO2, PaO2, lactate, creatinine, blood urea nitrogen (BUN), glucose, international normalized ratio (INR), prothrombin time (PT), partial thromboplastin time (PTT), aspartate aminotransferase (AST), alanine aminotransferase (ALT), alkaline phosphatase (AP), lactate dehydrogenase (LDH), total bilirubin, and albumin], and vital signs within 24 h of ICU admission [mean heart rate (mHR), mean value of mean arterial pressure (mMAP), mean respiratory rate (mRR), mean temperature (mT), mean SpO2(mSpO2), urine output].
The outcomes of this study were ICU and in-hospital mortality.
Statistical Analyses
In this study, Pearson’s correlation coefficient was adopted to determine the correlation between characteristic variables and to remove strongly correlated variables (Zhang et al., 2022). The following variables had coefficients higher than 0.6, so were removed since they were considered strongly correlated: RBC, hematocrit, MCV, chloride, BE, calculated total CO2, pH, BUN, PT, AST, and LD (Supplementary Figure S1). For other variables with less than 20% of missing values, the “mice” package of R software was used to perform multiple imputation.
The data for continuous variables were represented as mean and standard-deviation or median and interquartile (IQR) values, while those for categorical data were represented as frequencies. The Mann-Whitney U test was used for continuous variables, and the χ2 test or Fisher’s exact test was used for categorical variables.
The Kaplan-Meier (KM) method draws a cumulative incidence curve, indicating the occurrence of ICU and in-hospital deaths in different groups of patients, and the differences in risk between the groups were compared using log-rank tests. Furthermore, after adjusting different covariates, two Cox proportional-hazards models were constructed to analyze the influence of the relationship between bronchoscopy and outcomes. There were no adjustments for covariates in model I. In model II, all variables, including age, sex, race, BMI, first care unit, APSIII, vasopressors, CRRT, duration of IMV, sepsis, MI, CHF, hypertension, CD, CPD, LD, RD, diabetes, malignancy, WBC, neutrophils, lymphocytes, basophils, eosinophils, monocytes, hemoglobin, MCH, MCHC, RDW, platelet, AG, bicarbonate, calcium total, calcium free, magnesium phosphate, sodium, potassium, PaCO2, PaO2, lactate, creatinine, glucose, INR, PTT, ALT, AP, bilirubin total, albumin, mHR, mMAP, mRR, mT, mSpO2, urine output were adjusted for confounding factors. In the multivariate COX regression, we also evaluated the multicollinearity between the variables using variance inflation factors (VIF). Supplementary Table S1 revealed that the VIF of each variable was less than 4, indicating that there was no multicollinearity between them.
To ensure that the results were stable and reliable, we further adjusted for covariates using propensity score matching (PSM) and propensity score-based inverse probability of treatment (IPTW) after analyzing the original population. Multivariate logistic regression model was used to estimate patient propensity scores by using one-to-one nearest neighbor matching with a caliper width of 0.05. The IPTW model was created using estimated propensity scores as weights. Differences in baseline levels between the two groups were evaluated using p values. Then, COX regression was performed on the matched population and the weighted population, respectively.
Causal mediation analysis (CMA) can distinguish the total effect of treatment into direct effects and indirect effects, If the independent variable X has a certain influence on the dependent variable Y through a certain variable M, then M is called the mediating variable of X and Y (Imai et al., 2010). In the present study, we hypothesized that changes in a particular indicator were mediating variables, that is, we assumed that bronchoscopy might lead to changes in that indicator and that such changes were associated with the prognosis of VAP patients. The average causal mediating effect (ACME), average direct effect (ADE) and total effect obtained through CMA can help us verify the above conjecture. Finally, WBC, platelets and RDW, three indicators that were further investigated for the presence of mediating effects because of their low absence rate after the cessation of IMV. Their change level was expressed as the difference between the corresponding first examination results and recorded as delta-WBC, delta-RDW, delta-platelet.
We also analyzed the impact of bronchoscopy on the prognoses of patients from different subgroups. The subgroups included age (dichotomized at 65 years), sex, type of first care unit, APSIII (dichotomized at the median of 69), and all comorbidities. The interactions between subgroups were further analyzed.
A two-tailed probability value of p < 0.05 was considered statistically significant. All statistical analyses in this study were performed using R software (version 4.1.0).
Results
Baseline Characteristics
This study enrolled 1,560 patients with VAP: 1,355 in the no-bronchoscopy group and 205 in the bronchoscopy group (Figure 1). The median (IQR) ages of the patients in these two groups were 64.00 years (53.00–75.00 years) and 62.00 years (50.00–74.00 years), respectively; there were more male patients in the two groups (62.7 and 65.9%, respectively); the median (IQR) APSIII were 69.00 (51.00–90.00) and 70.00 (56.00–91.00), respectively; the median (IQR) durations of IMV were 136.00 h (60.50–253.50 h) and 145.00 h (59.00–286.00 h), respectively; and sepsis was the most common complication, present in 96.5 and 97.6% of patients in the two groups, respectively. The remaining baseline characteristics of the patients are listed in detail in Table 1.
Survival Analysis and Cox Proportional-Hazards Regression Model
The KM survival curve in Figure 2 indicated a significant difference in survival probability between the two groups. The survival probability in the ICU and in hospital was significantly higher in the bronchoscopy group than in the no-bronchoscopy group (Figure 2). The results of the log-rank test indicated that the mortality risks in the ICU and in hospital differed between the two groups.
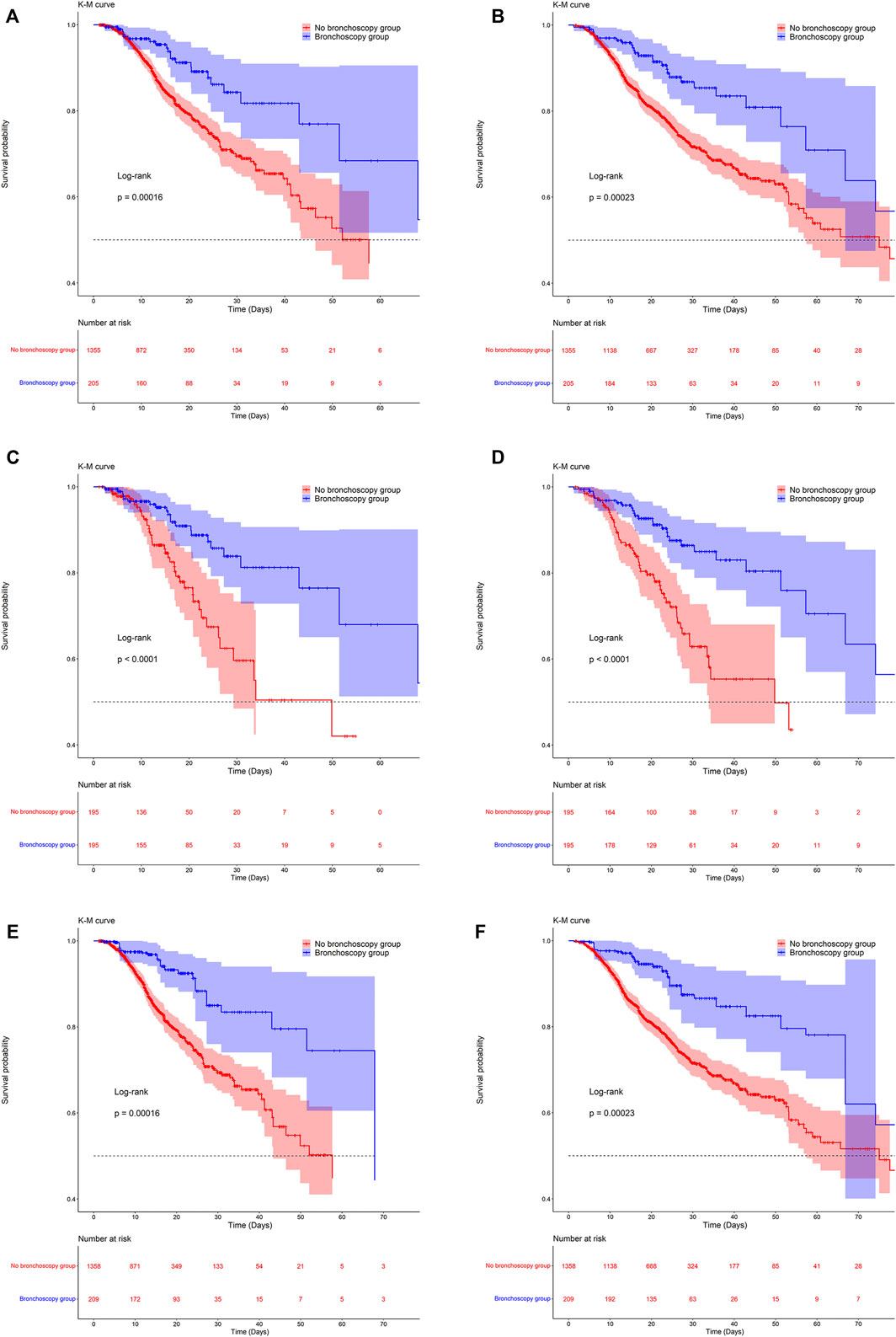
FIGURE 2. Kaplan-Meier survival curves between groups. (A,C,E) are the ICU mortality risk for the original population, the PSM population and the IPTW population; (B,D,F) are the in-hospital mortality risk for the original population, the PSM population and the IPTW population.
Table 2 lists the results for the Cox proportional-hazards model. In two models with no adjustment for confounders, or adjustment for all confounders, the hazard ratio (HR) was significantly lower than one for the bronchoscopy group compared with the no-bronchoscopy group. In other words, compared with patients in the no-bronchoscopy group, those in the bronchoscopy group had a lower risk of ICU and in-hospital mortality. In model II, after adjusting for all covariates as confounding factors, the HR (95% CI) values for ICU and in-hospital mortality in the bronchoscopy group were 0.33 (0.20–0.55) and 0.40 (0.26–0.60), respectively, indicating that the risks of ICU and in-hospital mortality were 0.67 and 0.60 lower than in the no-bronchoscopy group. (Table 2).
Propensity Score Matching and Inverse Probability of Treatment Weighing
After PSM and IPTW, there was no significant difference in baseline levels between the two groups (Supplementary Table S2). Kaplan-Meier survival curves of matched and weighted populations are consistent with the original population (Figure 2). Univariate and multivariate COX regressions were then performed on the matched and weighted populations, respectively, yielding results consistent with the original population (Table 2). After multivariate COX regression, HR (95% CI) values for ICU mortality in the bronchoscopy group were 0.33 (0.17,0.64) and 0.26 (0.14,0.50), and in-hospital mortality the HR (95% CI) values of 0.33 (0.20,0.58) and 0.35 (0.21,0.57), respectively.
Causal Mediation Analysis
In the CMA analysis, after analyzing the three indicators, only the changes of RDW were significant. Figures 3A, B showed that in terms of in-hospital mortality of VAP patients, delta-RDW mediated 8% (95% CI:1–26%; p = 0.02) of the beneficial effect of bronchoscopy (ACME:p = 0.02). At the same time, Figures 3C, D implied that in terms of ICU mortality, delta-RDW mediated 7% (95% CI: 1–21%; p = 0.02) of the beneficial effects of bronchoscopy (ACME:p = 0.02).
Subgroup Analysis
There was no significant difference between the subgroups of in-hospital mortality, meaning that no interaction was present. However, bronchoscopy was associated with a reduced risk of ICU mortality in MI and CPD populations, with HRs (95% CI) of 0.21 (0.05–0.86) and 0.13 (0.05–0.34), respectively. Meanwhile, patients without LD were more likely to benefit from bronchoscopy than those with LD (HR = 0.29, 95% CI = 0.16–0.54) (Table 3).
Discussion
Few previous studies have investigated the prognostic effects of bronchoscopy among patients with VAP, with many studying the value of bronchoscopy for diagnosing or preventing VAP (Timsit et al., 2001), or limited to specific populations such as trauma or pediatric patients (Bush, 2003; Nannapaneni et al., 2021). The present study included patients with VAP from the large public MIMIC-IV database as the study population. In this present study, we took the VAP patients in the large public database MIMIC-IV as the study population, whether it was univariate analysis, or adjusted for a number of confounding factors such as demography, intervention, disease severity score, complications, vital signs, laboratory examination indicators, etc., and reached a consistent conclusion, that is, in patients with IMV in ICU, the risk of ICU mortality and in-hospital mortality in VPA patients who underwent bronchoscopy were significantly lower than those who did not receive bronchoscopy. In addition, after PSM and IPTW, the trend was consistent with that of the original population, which proved that our results were robust and reliable.
We believe that most VAP patients in the ICU are critically ill patients with severe diseases such as sepsis (Markwart et al., 2020), with unclear self-consciousness (Hübscher and Isenmann, 2016) and poor sputum expectoration ability (Tang et al., 2011), causing large amounts of sputum or respiratory secretions cannot be discharged and block the trachea, aggravating the condition. At the same time, there are a large number of inflammatory factor secretions in the patient’s airway, forming a cascade amplification effect, which can spread to cause systemic inflammation (Zaragoza et al., 2020), which seriously affects the patient’s prognosis. While bronchoscopy can go deep into the lower respiratory tract and directly reach the lungs with the most severe disease (Estella, 2012). When patients are undergoing IMV, bronchoscopy can not only help suck out the obstructions such as sputum and foreign bodies in the trachea, remove secretions, but also repeatedly suction and wash the lungs, which is of great significance for reducing inflammation and improving lung ventilation.
RDW is a parameter that reflects the heterogeneity of red blood cells in the blood, and is also a new marker of inflammation (Hou et al., 2018). At present, there are a lot of published evidences indicating that there is an inseparable connection between RDW and inflammatory response (Fava et al., 2019; Jandaghian et al., 2021). The inflammatory response causes red blood cell maturation disorder through iron metabolism disorder and erythropoietin destruction, resulting in immature red blood cells into the bloodstream, but also reduce the survival rate of red blood cells, resulting in the mixing of red blood cell volume in circulation and other ways to change RDW (Salvagno et al., 2015). And some other studies have also shown that RDW is related to some conventional inflammatory markers such as C-reactive protein, erythrocyte sedimentation rate, tumor necrosis factor-α and interleukin-6 (He et al., 2018; Fava et al., 2019). In the CMA analysis, we have demonstrated that the beneficial effects of bronchoscopy on VAP patients are partially mediated by changes in RDW levels. Bronchoscopy is also essential for clinical targeted antimicrobial therapy to control inflammation, as it helps to obtain more accurate etiology, resulting in better diagnostic information. The findings of Christopher et al. showed that diagnostic bronchial therapy can help reduce the use of antibiotics and shorten the length of hospital stay (Guidry et al., 2014). Another retrospective study also indicated that the correct use of bronchoscopy can help intensive care clinicians formulate specific antibacterial treatments (Allen et al., 1994). Therefore, we can think that the reason why bronchoscopy can improve the prognosis of VAP patients is that whether it is aspiration of sputum or getting more accurate pathogens to help the adjustment of antibiotics, they all help to improve the inflammatory response of patients to some extent. Some scholars’ study has shown that early bronchoscopy is associated with a lower 90-days mortality rate in mechanically ventilated patients, which also supports our findings (Lee et al., 2015).
The results of the present subgroup analysis indicated no significant interaction between the subgroups regarding in-hospital mortality, indicating that whether the ICU patient population with different characteristics underwent bronchial examination was consistent with the in-hospital mortality risk. Among the outcomes for ICU mortality, after bronchoscopy, patients with MI or CPD had a lower mortality risk than those who did not undergo bronchoscopy. When bronchoscopy is performed on patients in the ICU without LD, the mortality risk was lower than for those with LD. We will explain it step by step, chronic obstructive pulmonary disease and bronchiectasis are common chronic lung diseases chronic obstructive pulmonary disease and bronchiectasis are common chronic lung diseases (Raherison and Girodet, 2009; Hill et al., 2019), such patients are prone to infection and inflammation due to dysfunctional airway clearance and defense, and long-term chronic inflammation leads to bronchial epithelial cell degeneration, necrosis, bronchial scarring, distortion, impaired lung function, and sputum accumulation, which are more likely to occur in the presence of acute inflammation (Tantucci and Modina, 2012; Hill et al., 2019). There is research evidence that the hemodynamic changes in patients with MI will lead to varying degrees of pulmonary function changes (Hales and Kazemi, 1977), and there is a pathophysiological basis for the interaction between some chronic lung diseases and MI (Goedemans et al., 2020). Therefore, we can consider that when VAP is present, bronchoscopy is more likely to benefit in patients with chronic inflammatory disease of the lungs and varying degrees of changes in lung function. The liver can synthesize and remove most coagulation factors, plasminogen, etc., and so LD can lead to coagulation and anticoagulation balance disorders (Amitrano et al., 2002; Monroe and Hoffman, 2009). One of the main complications of bronchoscopy is tracheal mucosal bleeding, so patients without LD are more likely to benefit from bronchoscopy than those with LD.
Strengths and Limitations
This research study was the first to explore the entire patients with VAP in the ICU from MIMIC-IV database, the large data sample provides a solid basis for the results. Furthermore, PSM and IPTW further confirmed the results. We also found that alterations in RDW mediated part of the positive effect of bronchoscopy on the prognosis of VAP patients by CMA methods. The technology for bronchoscopy is currently quite mature, and the results of this study provide evidence to support the guidance of clinicians for using this technique. Of course, our research still had certain limitations. First of all, because the specific diagnosis time of patients is not recorded in the analyzed database, this study cannot determine the duration between bronchoscopy and VAP, only how bronchoscopy impacts the prognoses of patients with VAP who received mechanical ventilation. Because of this we were also unable to analyze the specific antibiotics for VAP treatment, and so we did not consider the impact of antibiotic use on the outcome. Second, although we adopted multivariate analysis to control for confounding factors that may affect the results as much as possible, there are still potential confounding factors that cannot be corrected in a retrospective study. Finally, the interaction between subgroups needs to be analyzed prospective in a larger sample of people for further confirmation.
Conclusion
Bronchoscopy during MV was associated with reducing the risk of ICU and in-hospital mortality in patients with VAP in ICUs, and this beneficial effect was partially mediated by changes in RDW levels.
Data Availability Statement
Publicly available datasets were analyzed in this study. This data can be found here: The data were available on the MIMIC-IV website at https://mimic.physionet.org/, https://doi.org/10.13026/a3wn-hq05.
Author Contributions
LZ created the study protocol, performed the statistical analyses and wrote the first manuscript draft. SL conceived the study and wrote the first manuscript draft. SY assisted with manuscript revision and data confirmation. XL assisted with the study design. JiL assisted with data collection. YL and TH guided the literature review. JuL and HY conceptualized the research aims. All authors read and approved the final manuscript.
Funding
This work was supported by the National Natural Science Foundation of China (No. 82072232; 81871585), the Natural Science Foundation of Guangdong Province (No. 2018A030313058), Technology and Innovation Commission of Guangzhou Science, China (No. 201804010308), Guangdong Provincial Key Laboratory of Traditional Chinese Medicine Informatization (2021B1212040007).
Conflict of Interest
The authors declare that the research was conducted in the absence of any commercial or financial relationships that could be construed as a potential conflict of interest.
Publisher’s Note
All claims expressed in this article are solely those of the authors and do not necessarily represent those of their affiliated organizations, or those of the publisher, the editors and the reviewers. Any product that may be evaluated in this article, or claim that may be made by its manufacturer, is not guaranteed or endorsed by the publisher.
Supplementary Material
The Supplementary Material for this article can be found online at: https://www.frontiersin.org/articles/10.3389/fphar.2022.868920/full#supplementary-material
References
Allen, R. M., Dunn, W. F., and Limper, A. H. (1994). Diagnosing Ventilator-Associated Pneumonia: the Role of Bronchoscopy. Mayo Clin. Proc. 69 (10), 962–968. doi:10.1016/s0025-6196(12)61821-7
Amitrano, L., Guardascione, M. A., Brancaccio, V., and Balzano, A. (2002). Coagulation Disorders in Liver Disease. Semin. Liver Dis. 22 (1), 83–96. doi:10.1055/s-2002-23205
Baselski, V. S., and Wunderink, R. G. (1994). Bronchoscopic Diagnosis of Pneumonia. Clin. Microbiol. Rev. 7 (4), 533–558. doi:10.1128/cmr.7.4.533
Bush, A. (2003). Bronchoscopy in Paediatric Intensive Care. Paediatr. Respir. Rev. 4 (1), 67–73. doi:10.1016/s1526-0542(02)00313-5
Estella, A. (2012). Analysis of 208 Flexible Bronchoscopies Performed in an Intensive Care Unit. Med. Intensiva 36 (6), 396–401. doi:10.1016/j.medin.2011.11.005
Fava, C., Cattazzo, F., Hu, Z. D., Lippi, G., and Montagnana, M. (2019). The Role of Red Blood Cell Distribution Width (RDW) in Cardiovascular Risk Assessment: Useful or Hype? Ann. Transl. Med. 7 (20), 581. doi:10.21037/atm.2019.09.58
Goedemans, L., Bax, J. J., and Delgado, V. (2020). COPD and Acute Myocardial Infarction. Eur. Respir. Rev. 29 (156), 190139. doi:10.1183/16000617.0139-2019
Guidry, C. A., Mallicote, M. U., Petroze, R. T., Hranjec, T., Rosenberger, L. H., Davies, S. W., et al. (2014). Influence of Bronchoscopy on the Diagnosis of and Outcomes from Ventilator-Associated Pneumonia. Surg. Infect. (Larchmt) 15 (5), 527–532. doi:10.1089/sur.2013.142
Hales, C. A., and Kazemi, H. (1977). Clinical Significance of Pulmonary Function Tests. Pulmonary Function after Uncomplicated Myocardial Infarction. Chest 72 (3), 350–358. doi:10.1378/chest.72.3.350
Hayashi, Y., Morisawa, K., Klompas, M., Jones, M., Bandeshe, H., Boots, R., et al. (2013). Toward Improved Surveillance: The Impact of Ventilator-Associated Complications on Length of Stay and Antibiotic Use in Patients in Intensive Care Units. Clin. Infect. Dis. 56 (4), 471–477. doi:10.1093/cid/cis926
He, Y., Liu, C., Zeng, Z., Ye, W., Lin, J., and Ou, Q. (2018). Red Blood Cell Distribution Width: A Potential Laboratory Parameter for Monitoring Inflammation in Rheumatoid Arthritis. Clin. Rheumatol. 37 (1), 161–167. doi:10.1007/s10067-017-3871-7
Hill, A. T., Sullivan, A. L., Chalmers, J. D., Soyza, A. D., Elborn, S. J., Floto, A. R., et al. (2019). British Thoracic Society Guideline for Bronchiectasis in Adults. Thorax. 74, 1–69. doi:10.1136/thoraxjnl-2018-212463
Hou, P., Xue, H. P., Mao, X. E., Li, Y. N., Wu, L. F., and Liu, Y. B. (2018). Inflammation Markers are Associated with Frailty in Elderly Patients with Coronary Heart Disease. Aging (Albany NY) 10 (10), 2636–2645. doi:10.18632/aging.101575
Hübscher, A., and Isenmann, S. (2016). Delirium: Concepts, Etiology, and Clinical Management. Fortschr. Neurol. Psychiatr. 84 (4), 233–244. doi:10.1055/s-0042-104502
Imai, K., Keele, L., and Tingley, D. (2010). A General Approach to Causal Mediation Analysis. Psychol. Methods 15 (4), 309–334. doi:10.1037/a0020761
Jandaghian, S., Vaezi, A., Manteghinejad, A., Nasirian, M., Vaseghi, G., and Haghjooy Javanmard, S. (2021). Red Blood Cell Distribution Width (RDW) as a Predictor of In-Hospital Mortality in COVID-19 Patients; A Cross Sectional Study. Arch. Acad. Emerg. Med. 9 (1), e67. doi:10.22037/aaem.v9i1.1325
Johnson, A., Bulgarelli, L., Pollard, T., Horng, S., Celi, L. A., and Mark, R. (2021). MIMIC-IV (Version 1.0). PhysioNet. https://physionet.org/content/mimiciv/1.0/. doi:10.13026/s6n6-xd98
Lee, H. W., Min, J., Park, J., Lee, Y. J., Kim, S. J., Park, J. S., et al. (2015). Clinical Impact of Early Bronchoscopy in Mechanically Ventilated Patients with Aspiration Pneumonia. Respirology 20 (7), 1115–1122. doi:10.1111/resp.12590
Markwart, R., Saito, H., Harder, T., Tomczyk, S., Cassini, A., Fleischmann-Struzek, C., et al. (2020). Epidemiology and Burden of Sepsis Acquired in Hospitals and Intensive Care Units: A Systematic Review and Meta-Analysis. Intensive Care Med. 46 (8), 1536–1551. doi:10.1007/s00134-020-06106-2
Melsen, W. G., Rovers, M. M., Koeman, M., and Bonten, M. J. (2011). Estimating the Attributable Mortality of Ventilator-Associated Pneumonia from Randomized Prevention Studies. Crit. Care Med. 39 (12), 2736–2742. doi:10.1097/CCM.0b013e3182281f33
Monroe, D. M., and Hoffman, M. (2009). The Coagulation Cascade in Cirrhosis. Clin. Liver Dis. 13, 1–9. doi:10.1016/j.cld.2008.09.014
Nannapaneni, S., Silvis, J., Curfman, K., Chung, T., Simunich, T., Morrissey, S., et al. (2021). Bronchoscopy Decreases Ventilator-Associated Pneumonia in Trauma Patients. Am. Surg. 88, 31348211058639. doi:10.1177/00031348211058639
Navani, N., Booth, H. L., Kocjan, G., Falzon, M., Capitanio, A., Brown, J. M., et al. (2011). Combination of Endobronchial Ultrasound-Guided Transbronchial Needle Aspiration with Standard Bronchoscopic Techniques for the Diagnosis of Stage I and Stage II Pulmonary Sarcoidosis. Respirology 16 (3), 467–472. doi:10.1111/j.1440-1843.2011.01933.x
Papazian, L., Klompas, M., and Luyt, C. E. (2020). Ventilator-associated Pneumonia in Adults: a Narrative Review. Intensive Care Med. 46 (5), 888–906. doi:10.1007/s00134-020-05980-0
Pedro, C., Melo, N., Novais E Bastos, H., Magalhães, A., Fernandes, G., Martins, N., et al. (2019). Role of Bronchoscopic Techniques in the Diagnosis of Thoracic Sarcoidosis. J. Clin. Med. 8 (9), 1327. doi:10.3390/jcm8091327
Raherison, C., and Girodet, P. O. (2009). Epidemiology of COPD. Eur. Respir. Rev. 18 (114), 213–221. doi:10.1183/09059180.00003609
Ruiz, M., Torres, A., Ewig, S., Marcos, M. A., Alcón, A., Lledó, R., et al. (2000). Noninvasive versus Invasive Microbial Investigation in Ventilator-Associated Pneumonia: Evaluation of Outcome. Am. J. Respir. Crit. Care Med. 162 (1), 119–125. doi:10.1164/ajrccm.162.1.9907090
Salvagno, G. L., Sanchis-Gomar, F., Picanza, A., and Lippi, G. (2015). Red Blood Cell Distribution Width: A Simple Parameter with Multiple Clinical Applications. Crit. Rev. Clin. Lab. Sci. 52 (2), 86–105. doi:10.3109/10408363.2014.992064
Spalding, M. C., Cripps, M. W., and Minshall, C. T. (2017). Ventilator-Associated Pneumonia: New Definitions. Crit. Care Clin. 33 (2), 277–292. doi:10.1016/j.ccc.2016.12.009
Su, Z. Q., Ye, T. S., Chen, D. F., Deng, X. L., Chen, H. J., and Li, S. Y. (2020). The Role of Bronchoscopy in COVID-19. Respiration 99 (8), 697–698. doi:10.1159/000507402
Tang, P., Chan, H. K., Rajbhandari, D., and Phipps, P. (2011). Method to Introduce Mannitol Powder to Intubated Patients to Improve Sputum Clearance. J. Aerosol Med. Pulm. Drug Deliv. 24 (1), 1–9. doi:10.1089/jamp.2010.0825
Tantucci, C., and Modina, D. (2012). Lung Function Decline in COPD. Int. J. Chron. Obstruct Pulmon Dis. 7, 95–99. doi:10.2147/COPD.S27480
Timsit, J. F., Cheval, C., Gachot, B., Bruneel, F., Wolff, M., Carlet, J., et al. (2001). Usefulness of a Strategy Based on Bronchoscopy with Direct Examination of Bronchoalveolar Lavage Fluid in the Initial Antibiotic Therapy of Suspected Ventilator-Associated Pneumonia. Intensive Care Med. 27 (4), 640–647. doi:10.1007/s001340000840
Wu, W. T., Li, Y. J., Feng, A. Z., Li, L., Huang, T., Xu, A. D., et al. (2021). Data Mining in Clinical Big Data: The Frequently Used Databases, Steps, and Methodological Models. Mil. Med. Res. 8 (1), 44. doi:10.1186/s40779-021-00338-z
Yang, J., Li, Y., Liu, Q., Li, L., Feng, A., Wang, T., et al. (2020). Brief Introduction of Medical Database and Data Mining Technology in Big Data Era. J. Evid. Based Med. 13 (1), 57–69. doi:10.1111/jebm.12373
Zaragoza, R., Vidal-Cortés, P., Aguilar, G., Borges, M., Diaz, E., Ferrer, R., et al. (2020). Update of the Treatment of Nosocomial Pneumonia in the ICU. Crit. Care 24 (1), 383. doi:10.1186/s13054-020-03091-2
Zhang, L., Xu, F., Han, D., Huang, T., Li, S., Yin, H., et al. (2021). Influence of the Trajectory of the Urine Output for 24 H on the Occurrence of AKI in Patients with Sepsis in Intensive Care Unit. J. Transl. Med. 19 (1), 518. doi:10.1186/s12967-021-03190-w
Zhang, Z., Chen, L., Xu, P., and Hong, Y. (2022). Predictive Analytics with Ensemble Modeling in Laparoscopic Surgery: A Technical Note. Laparosc. Endosc. Robotic Surg. 5, 25. doi:10.1016/j.lers.2021.12.003
Zimlichman, E., Henderson, D., Tamir, O., Franz, C., Song, P., Yamin, C. K., et al. (2013). Health Care-Associated Infections: A Meta-Analysis of Costs and Financial Impact on the US Health Care System. JAMA Intern Med. 173 (22), 2039–2046. doi:10.1001/jamainternmed.2013.9763
Glossary
AST aspartate aminotransferase
ALT alanine aminotransferase
AP alkaline phosphatase
APSIII Acute Physiology Score III
AG anion gap
BE base excess
BUN blood urea nitrogen
ACME average causal mediating effect
ADE average direct effect
BMI body mass index
CRRT continuous renal replacement therapy
CHF congestive heart failure
CD cerebrovascular disease
CPD chronic pulmonary disease
LD liver disease
CMA causal mediation analysis
LDH lactate dehydrogenase
IMV invasive mechanical ventilation
ICU intensive care unit
INR international normalized ratio
KM Kaplan-Meier
VIF variance inflation factor
IPTW propensity score-based inverse probability of treatment
MCV mean corpuscular volume
MCH mean corpuscular hemoglobin
MCHC mean corpuscular hemoglobin concentration
MICU Medical Intensive Care Unit
MIMIC Medical Information Mart for Intensive Care
MI myocardial infarction
mHR mean heart rate
mMAP mean value of mean arterial pressure
mRR mean respiratory rate
mT mean temperature
mSpO2 mean SpO2()
PT prothrombin time
PTT partial thromboplastin time
PSM propensity score matching
RD renal disease
RBC red blood cells
RDW red blood cell distribution width
SICU Surgical Intensive Care Unit
VAP: ventilator-associated pneumonia
WBC white blood cells
Keywords: ICU, ventilator-associated pneumonia, bronchoscopy, mortality, causal mediation analysis
Citation: Zhang L, Li S, Yuan S, Lu X, Li J, Liu Y, Huang T, Lyu J and Yin H (2022) The Association Between Bronchoscopy and the Prognoses of Patients With Ventilator-Associated Pneumonia in Intensive Care Units: A Retrospective Study Based on the MIMIC-IV Database. Front. Pharmacol. 13:868920. doi: 10.3389/fphar.2022.868920
Received: 03 February 2022; Accepted: 06 May 2022;
Published: 08 June 2022.
Edited by:
Xian-Tao Zeng, Wuhan University, ChinaReviewed by:
Konlawij Trongtrakul, Chiang Mai University, ThailandYaolong Chen, Lanzhou University, China
Qilin Yang, The Second Affiliated Hospital of Guangzhou Medical University, China
Copyright © 2022 Zhang, Li, Yuan, Lu, Li, Liu, Huang, Lyu and Yin. This is an open-access article distributed under the terms of the Creative Commons Attribution License (CC BY). The use, distribution or reproduction in other forums is permitted, provided the original author(s) and the copyright owner(s) are credited and that the original publication in this journal is cited, in accordance with accepted academic practice. No use, distribution or reproduction is permitted which does not comply with these terms.
*Correspondence: Jun Lyu, lyujun2020@jnu.edu.cn; Haiyan Yin, yinhaiyan1867@126.com
†ORCID: Haiyan Yin, orcid.org/0000-0002-9680-4219
†These authors have contributed equally to this work