- Institute for Clinical and Experimental Surgery, Saarland University, Homburg, Germany
Despite careful preoperative planning, surgical flaps are prone to ischemic tissue damage and ischemia–reperfusion injury. The resulting wound breakdown and flap necrosis increase both treatment costs and patient morbidity. Hence, there is a need for strategies to promote flap survival and prevent ischemia-induced tissue damage. Phytochemicals, defined as non-essential, bioactive, and plant-derived molecules, are attractive candidates for perioperative treatment as they have little to no side effects and are well tolerated by most patients. Furthermore, they have been shown to exert beneficial combinations of pro-angiogenic, anti-inflammatory, anti-oxidant, and anti-apoptotic effects. This review provides an overview of bioactive phytochemicals that have been used to increase flap survival in preclinical animal models and discusses the underlying molecular and cellular mechanisms.
Introduction
The preparation of tissue flaps is one of the central surgical techniques in plastic surgery, with numerous options and variations in use, covering a broad spectrum of indications. The primary goal of flap surgery is usually to reconstruct missing tissue and to restore the form and function of the body, but secondary benefits can contribute to the decision to perform flap surgery. For instance, well-perfused muscle tissue can be a crucial factor in combatting tissue infections, such as osteomyelitis, in addition to the obliteration of the dead space and the reconstruction of the soft tissue coverage (Zhang et al., 2021).
Flap surgery is, however, associated with several complications, such as wound dehiscence, wound breakdown, or partial necrosis, with an incidence of up to ∼50% (Fischer et al., 2013). These ischemia-induced complications are favored by the fragile microcirculation of tissue flaps. In fact, during flap elevation, a large portion of the perfusing vessels is severed, for e.g., when an adipocutaneous flap is dissected from the underlying richly perfused fascia. Accordingly, the tissue has to adapt to the changed blood supply and the decreased arterial inflow. Flap areas distal to this inflow are left ischemic when capillary perfusion pressure is no longer maintained above the critical level, which triggers cellular hypoxic signaling pathways, leading to apoptosis or necrosis (Kalogeris et al., 2014; Lucas, 2017). In the case of random pattern flaps, blood perfusion is provided by the dermal plexus and/or musculocutaneous arterioles passing through the flaps’ base. Accordingly, the flaps are planned, considering certain length-to-width ratios depending on their tissue composition and preparation site (Saint-Cyr et al., 2009; Memarzadeh et al., 2016). Additional factors that contribute to the changes in tissue perfusion after flap elevation are manifold and include the effects of sympathetic denervation, local inflammatory changes, elevated interstitial pressure, and the neovascularization of the flap from the wound bed (Lucas, 2017). Therefore, mechanisms other than ischemic cell death can contribute to flap tissue damage. For instance, the changed flow rates and pro-inflammatory mediators cause a prothrombotic environment that can lead to capillary obstruction, both by microthrombosis and by vessel occlusion from the invading immune cells (Menger et al., 2003).
During free flap transfer, the tissue additionally has to endure a period of complete ischemia, while the flap is moved from the donor to the recipient site and vascular anastomosis is performed. After the reestablishment of blood perfusion, tissue reoxygenation can paradoxically result in additional cell damage, also referred to as ischemia–reperfusion injury (IRI), due to reactive oxygen species (ROS) formation and leukocytic inflammation (Siemionow and Arslan, 2004). To overcome ischemic cell damage and flap tissue IRI, several approaches have been developed in the past decades. In addition to invasive strategies, such as surgical delay Reinisch (1974) and ischemic preconditioning Zahir et al., (1998), pharmacological treatment with phytochemicals is an emerging concept to improve tissue survival.
Phytochemicals are defined as bioactive plant-derived compounds that are not considered essential to the human diet (Liu, 2013). Compounds derived from mushrooms or mycelia are usually also included in this general category, even though they are not plants. Although rapidly increasing experimental evidence suggests the efficacy of phytochemical compounds for the prevention and therapy of various pathologies (George et al., 2021; Ismaeel et al., 2021), their potential is still underestimated in clinical practice. Particularly in flap surgery, phytochemicals may be highly promising to prevent ischemic tissue damage because they promote the formation of new microvessels (Thangapazham et al., 2016) and are capable of modulating anti-inflammatory, anti-oxidant, and anti-apoptotic signaling pathways (Figure 1) (Haidarali et al., 2015; Zhang et al., 2015; Al-Ishaq et al., 2020). Furthermore, they have been shown to modulate the tissue microenvironment (Cheng et al., 2016), i.e., the tissue’s exact molecular and cellular composition, including growth factors and cytokines, all cell types, and the extracellular matrix. Of interest, this tissue microenvironment is also tightly associated with flap survival (Vogt et al., 2005; Pei et al., 2020). In line with this view, we provide in the present review for the first time an up-to-date overview of experimental studies analyzing the beneficial effects of phytochemicals on blood perfusion and tissue survival of surgical flaps.
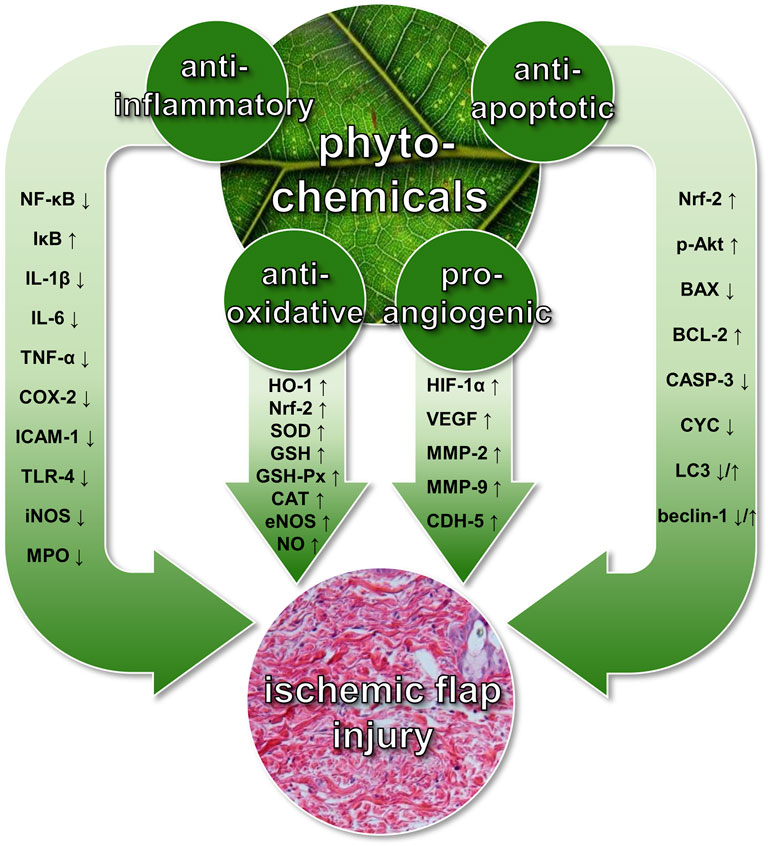
FIGURE 1. Beneficial effects of phytochemicals on ischemic flap injury. These include pro-angiogenic, anti-inflammatory, anti-oxidant, and anti-apoptotic mechanisms, which are mediated by the up- or downregulation of various signaling molecules. P-Akt = phosphorylated protein kinase B; BAX = B-cell lymphoma 2-associated X protein; BCL-2 = B-cell lymphoma 2; CASP-3 = caspase-3; CAT = catalase; CDH-5 = cadherin-5; COX-2 = cyclooxygenase-2; CYC = cytochrome C; eNOS = endothelial nitric oxidase synthase; GSH = glutathione; GSH-Px = glutathione peroxidase; HIF-1α = hypoxia-inducible factor-1α; HO-1 = hemeoxygenase-1; ICAM-1 = intercellular adhesion molecule-1; IL-1β/IL-6 = interleukin-1β/interleukin-6; iNOS = inducible nitric oxide synthase; IκB = nuclear factor of kappa light polypeptide gene enhancer in B-cells inhibitor; LC3 = microtubule-associated proteins 1A/1B light chain 3B; MMP-2/MMP-9 = matrix metalloproteinase-2/matrix metalloproteinase-9; MPO = myeloperoxidase; NF-κB = nuclear factor kappa-light-chain-enhancer of activated B cells; NO = nitric oxide; Nrf-2 = nuclear factor erythroid 2-related factor-2; SOD = superoxide dismutase; TLR-4 = toll-like receptor-4; TNF-α = tumor necrosis factor-α; VEGF = vascular endothelial growth factor.
Introductory Remarks
In the context of phytochemical flap treatment, we have mainly identified alkaloid, terpenoid, and phenolic compounds. Alkaloids are a group of cyclic nitrogen-containing compounds that are found in over 20% of plant species (Kennedy and Wightman, 2011). Many poisons, neurotoxins, and traditional psychedelics (e.g., atropine and scopolamine), and also most social drugs (e.g., nicotine, caffeine, and cocaine) consumed by humans are part of this chemical group (Zenk and Juenger, 2007). Terpenoids are a group of lipid-soluble compounds that are present in Ginkgo biloba and ginseng extracts. They have been shown to act synergistically, which may explain the fact that it is difficult to identify a major single active component in many terpenoid-containing herbal extracts, complicating their standardization (Kennedy and Wightman, 2011). Last, phenolics are ubiquitously found across the plant kingdom. Flavonoids, a subgroup of phenolics, are particularly well represented in this review because they are well-known anti-oxidant and anti-inflammatory mediators (Panche et al., 2016).
Most phytochemicals do not only target one specific mechanism within the cell but rather modulate several pathways at once with synergistic effects adding up to the overall outcome. This pleiotropic mode of action makes it difficult to trace all altered mechanisms and to identify the primary cause for the observed outcome. In line with this fact, almost all addressed studies describe various effects of the examined phytochemical compounds without specifying one dominant mechanism. These effects are summarized for isolated compounds or whole plant extracts in Table 1. However, to improve the readability of the present review and to avoid unnecessary repetitions, individual phytochemicals may only be discussed in the context of one selected mode of action. Furthermore, compounds, such as sea buckthorn extract or acai seed extract, have been included in this review for the sake of comprehensiveness, even though the corresponding studies include little or even no analysis to clarify the underlying mechanisms of flap necrosis reduction (Emsen, 2005; Coelho da Mota et al., 2018).
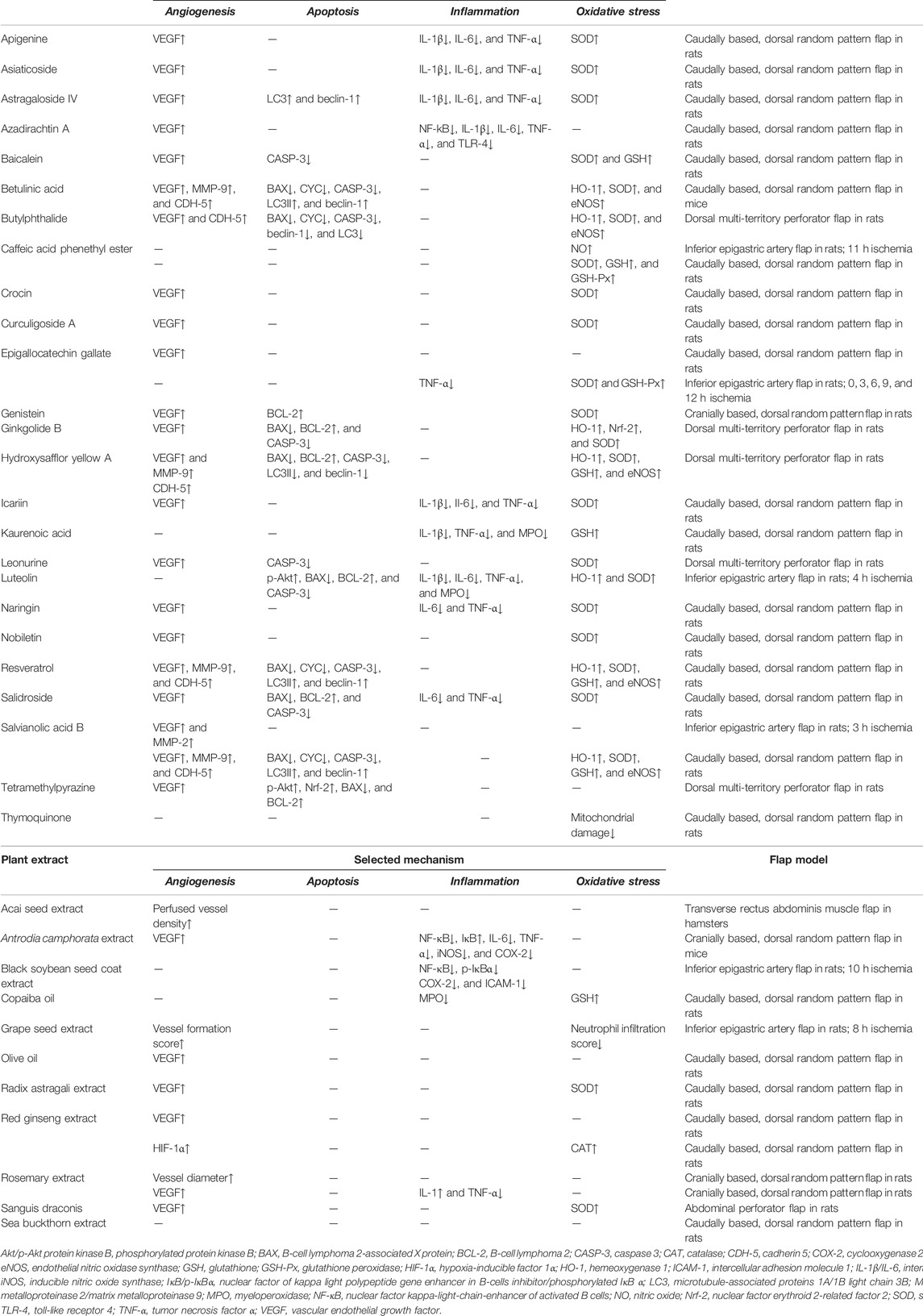
TABLE 1. Overview of isolated phytochemical compounds and whole plant extracts that have been evaluated in experimental flap models. Availability and selected mechanisms mediating their effects are listed.
Similarly, as few experimental flap models (Figure 2) have been redundantly used to analyze the effects of phytochemicals; these models are not discussed for every individual study included in this review but are listed in Table 1. Of note, studies focusing on IRI and its prevention are typically conducted in models with a period of total ischemia and subsequent reperfusion, such as the axial epigastric island flap (Figure 2A) (Petry and Wortham, 1984; Padubidri and Browne, 1997). Moreover, many studies are performed on well-established random pattern flap models, which are simple to prepare and are characterized by high reproducibility (Figure 2B) (McFarlane et al., 1965; Schmauss et al., 2018). A third popular flap preparation is based on the deep circumflex iliac artery as the axial vessel and contains several perforasomes to simulate the physiology of perforator flaps (Figure 2C) (Saint-Cyr et al., 2009; Wang et al., 2017). However, even if the same experimental flap model is applied in individual studies, differences in lab animals, surgical techniques, flap dimensions, or duration of ischemia are likely to influence flap survival rates. Hence, direct comparisons between individual studies should be considered with caution.
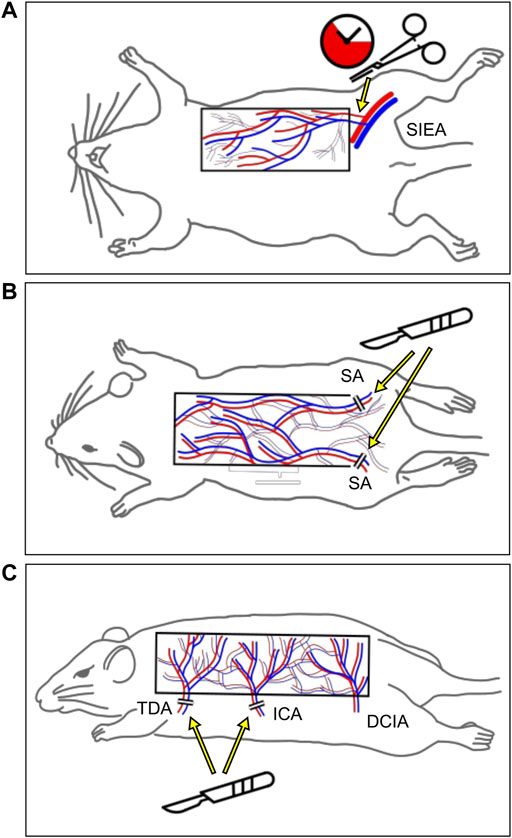
FIGURE 2. Schematic of the three most frequently used animal flap models. (A) The superficial inferior epigastric artery flap enables the transient interruption of the blood flow into the flap with a clap. (B) The random pattern “McFarlane” flap is designed on the dorsum of the animal. In the majority of studies, the flap base is located caudally and both sacral arteries (SAs) are ligated to ensure distal flap necrosis. (C) The more recently established multi-territory dorsal perforator flap includes the supply area of the thoracodorsal (TDA), intercostal (ICA), and deep circumflex iliac artery (DCIA). By raising the flap on the vascular axis of the DCIA, this model simulates the physiology of perforator flaps.
Effects of Phytochemicals on Surgical Flaps
We have identified four general mechanisms underlying the beneficial effects of phytochemicals on blood perfusion and tissue survival of surgical flaps. These include pro-angiogenic, anti-inflammatory, anti-oxidant, and anti-apoptotic mechanisms, as outlined in the following sections.
Pro-Angiogenic Mechanisms
Angiogenesis, i.e., the development of new microvessels from pre-existing ones, is of major importance for flap survival. This process is regulated by a balanced interplay of pro- and anti-angiogenic factors and requires the close interaction of endothelial cells and perivascular cells (Chiaverina et al., 2019). Blood vessel development is typically driven by tissue exposure to hypoxia or inflammatory cytokines in the peripheral flap tissue (Schürmann et al., 2014). Under ischemic conditions, hypoxia-inducible factor (HIF)-1α is no longer degraded by proteasomes and translocates into the nucleus, in which it induces the expression of vascular endothelial growth factor (VEGF), the central cytokine stimulating angiogenesis (Zimna and Kurpisz, 2015). Almost all studies presented in this review (Table 1) reported an increased VEGF expression in flap tissue, including studies focusing on crocin, curculigoside A, epigallocatechin gallate, nobiletin, olive oil, rosemary extract, or sanguis draconis (Cheon et al., 2012; Kirkil et al., 2016; Zhang et al., 2016; Chen T. et al., 2018; İnce et al., 2018; Cai et al., 2020; Jiang et al., 2020). However, only Korean red ginseng extract has also been proven to upregulate HIF-1α as the underlying mechanism (Yun et al., 2017).
In vitro, it has been shown that the phytochemical radix astragali extract stimulates endothelial cell proliferation and motility via the VEGF axis (Zhang et al., 2009). In line with these findings, Cai et al. (2015) could increase the survival of random pattern flaps in radix astragali extract-treated rats. In a follow-up study using the identical flap model, Lin et al. (2017) suggested that this effect may be mediated by astragaloside IV as one of the pro-angiogenic molecules in this root extract.
Additional examples of phytochemicals with a high pro-angiogenic activity promoting flap survival are resveratrol (Ciloglu et al., 2014; Lin J. et al., 2019), betulinic acid (Li et al., 2019), salvianolic acid B (Lay et al., 2003; Lin J. et al., 2018), and hydroxysafflor yellow A (Zhang C. et al., 2020). All of these compounds simultaneously increase the expression of VEGF and matrix metalloproteinases (MMPs). During the process of angiogenesis, the latter enzyme group is responsible for the degradation of the basement membrane surrounding each blood vessel, allowing new vessel sprouts to form (Quintero-Fabián et al., 2019).
Anti-Inflammatory Mechanisms
In response to tissue injury, cytokines are crucial in initiating and sustaining an inflammatory reaction with the goal of reestablishing tissue integrity (Zhang et al., 2006). However, in the case of ischemic flaps, this inflammatory reaction rather exacerbates the stress on the hypoxic tissue by releasing ROS and increasing endothelial permeability, resulting in the formation of tissue edema (van den Heuvel et al., 2009). The release of inflammatory cytokines and the invasion of immune cells, particularly neutrophilic granulocytes, is therefore a hallmark of flap-associated IRI (Hernandez et al., 1987).
Several studies reported a downregulation of the expression of the pro-inflammatory transcription factor nuclear factor (NF)-κB, for instance, after the application of Antrodia camphorata extract or black soybean seed coat extract (Kim et al., 2012; Tsai et al., 2015). Other works analyzed the expression and secretion of inflammatory cytokines in flap tissue after the administration of phytochemicals. As expected, the analyzed phytochemicals exerted a suppressive effect on these cytokines, which was associated with an improved flap survival (Table 1). For instance, tumor necrosis factor (TNF)-α and interleukin (IL)-6 serum levels were significantly lower in random pattern flaps and axial epigastric island flaps of salidroside-, luteolin-, and naringin-pretreated rats when compared to controls (Deheng et al., 2016; Cheng L. et al., 2017; Chen G. et al., 2018). Moreover, apigenine, asiaticoside, icariin, and astragaloside IV inhibited inflammation in ischemic random pattern flaps, as demonstrated by a reduced expression of TNF-α, IL-1β, and IL-6 (Lin et al., 2017; Huang et al., 2019; Feng et al., 2021; Ma et al., 2021). Another compound that suppresses tissue inflammation following flap elevation is azadirachtin A. In fact, He et al. (2020) demonstrated a markedly reduced secretion of TNF-α and IL-1β in random pattern flap tissue of azadirachtin A-treated animals. Of interest, the authors additionally found a downregulation of toll-like receptor (TLR)-4, which is a central receptor known to trigger tissue injury during IRI by aggravating the inflammatory response (Lee et al., 2016).
Anti-Oxidant Mechanisms
ROS formation by ischemic cells or invading neutrophilic granulocytes play a detrimental role in damaging flap tissue (Suzuki et al., 1991). While a certain amount of ROS is necessary for adequate cellular signaling and the regulation of apoptosis, the excessive presence of ROS and other free radicals can damage the tissue (Sies, 2015). Once the anti-oxidant defenses of the cells are exhausted, ROS start to disrupt physiological cellular mechanisms by causing DNA strand breaks or by oxidizing, thus inactivating proteins (Neri et al., 2015).
It is well known that phytochemicals exert anti-oxidant effects by functioning as free radical scavengers or by modulating signaling pathways responsible for ROS homeostasis and anti-oxidant gene expression (Zhang et al., 2015; Cheng YT. et al., 2017). The latter mechanism is mainly mediated by the transcription factor nuclear factor erythroid 2-related factor (Nrf)-2, which regulates the expression of anti-oxidant response elements, such as hemeoxygenase (HO)-1 and superoxide dismutase (SOD)-1 (Sotolongo et al., 2020). Several studies reported that resveratrol increases the expression of HO-1 in an Nrf-2-dependent manner (Gao et al., 2018; Zhang Y. et al., 2020; Lian et al., 2020). In line with this finding, the administration of resveratrol elevated the expression of HO-1 and SOD-1 in random pattern flaps of rats, which was associated with a lower apoptosis rate (Ciloglu et al., 2014; Lin J. et al., 2019). Moreover, luteolin and ginkgolide B triggered the gene expression of Nrf-2, leading to elevated HO-1 mRNA levels and higher viability of surgical flaps (Chen G. et al., 2018; Lin D. et al., 2019). A significant upregulation of HO-1 in flaps has also been observed after the administration of betulinic acid, salvianolic acid B, and hydroxysafflor yellow A (Lin J. et al., 2018; Li et al., 2019; Zhang C. et al., 2020). HO-1 also modulates the expression of the enzyme endothelial nitric oxide synthase (eNOS) (Luo et al., 2018). This induction of eNOS counteracts ROS, which consume its product, the vasodilator NO. In addition to its anti-oxidant effects, NO also inhibits the endothelial adhesion of leukocytes and their subsequent tissue invasion (Kubes et al., 1991; Ma et al., 1993). These beneficial effects can be observed after the application of betulinic acid, butylphthalide, hxydroxysafflor yellow A, resveratrol, and salvianolic acid B to flap tissue (Lin J. et al., 2018, Lin et al., 2019 J.; Li et al., 2019, 2021; Zhang C. et al., 2020).
Another anti-oxidant enzyme that is upregulated after phytochemical application is glutathione peroxidase (GSH-Px) (Table 1). Its upregulation or the upregulation of the substrate glutathione (GSH) can be observed after the administration of many phytochemicals discussed in this review, including caffeic acid phenethyl ester, kaurenoic acid, and copaiba oil (Aydogan et al., 2007; De Lima Silva et al., 2009; de Lima Silva et al., 2015).
Anti-Apoptotic Mechanisms
Several phytochemicals exert their tissue-protective effects on ischemic flap tissue by modulating signaling pathways of apoptosis. One of them is tetramethylpyrazine (TMP), an alkaloid compound that has long been used in traditional Chinese medicine for the treatment of cardiovascular and cerebrovascular diseases (Donkor et al., 2016). Qing et al., (2019) reported that TMP attenuates apoptosis in the ischemic areas of dorsal multi-territory perforator flaps in rats due to a decreased ratio of the pro-apoptotic protein B-cell lymphoma protein (BCL)-2-associated X protein (BAX) and the anti-apoptotic protein BCL-2. In addition, the authors detected reduced protein kinase B (Akt) phosphorylation, indicating that TMP may suppress cell death by interfering with this major pro-apoptotic pathway (Qing et al., 2019).
Chen G. et al., (2018) found that the administration of luteolin, a naturally occurring polyphenol found in several vegetables, ameliorates IRI-induced flap tissue injury by inhibition of acute inflammation and lowering oxidative stress levels. The investigation of the underlying signaling revealed that luteolin preconditioning decreases the cytotoxic effect of H2O2 in keratinocytes (Chen G. et al., 2018). This is due to increased phosphorylation of Akt, an upregulated expression of BCL-2, and a downregulated expression of BAX (Chen G. et al., 2018). Similarly, the phenolic compound salidroside improved the survival of random pattern skin flaps in rats (Deheng et al., 2016). This was associated with an enhanced BCL-2 expression and diminished expression of BAX and cleaved caspase (CASP)-3 in the flap tissue (Deheng et al., 2016). Moreover, flaps in baicalein- and leonurine-treated animals exhibited decreased levels of CASP-3 (Lin R. et al., 2018; Lin et al., 2020).
Another mechanism, by which phytochemicals counteract apoptosis, is the modulation of autophagy. As a highly conserved survival mechanism of all eukaryotic cells, autophagy primarily acts as an adaptive response to cellular stressors, such as nutrient deprivation and/or ischemia (Webster, 2012). During autophagy, the cell starts to degrade and recycle macromolecules, including proteins, lipids, and carbohydrates, for the synthesis of essential components and as an energy supply (Glick et al., 2010). The beneficial effect of autophagy on flap survival could be observed in a study by Lin J. et al., (2018), who investigated the effects of salvianolic acid B on random pattern skin flap necrosis. They found an upregulated expression of microtubule-associated protein 1A/1B-light chain 3 (LC3)-II as well as beclin-1 and vacuolar sorting protein (VSP) 34 in areas of ischemia, indicating higher numbers of autophagosomes and autolysosomes (Lin J. et al., 2018). More importantly, they detected a suppression of classical apoptosis markers, such as CASP-3, cytochrome C (CYC), and BAX. This may be explained by the fact that autophagy and apoptosis act complementary to each other. Autophagy blocks the induction of apoptosis, while caspase activation downregulates the autophagic process (Mariño et al., 2014). Similarly, studies evaluating the phytochemicals such as betulinic acid, resveratrol, and astragaloside IV for the prevention of flap necrosis found that upregulating autophagy plays a central role in increasing tissue survival (Lin et al., 2017, Lin et al., 2019 J.; Li et al., 2019). These compounds enhanced the expression of LC3-II and beclin-1 and decreased ischemic cell death within surgical flaps. These findings demonstrate the beneficial effect of phytochemical-induced autophagy on flap tissue survival. In contrast, the treatment of rat multi-territory perforator flaps with hydroxysafflor yellow A and butylphthalide increased flap tissue survival and downregulated the autophagy markers LC3 and beclin-1 (Zhang C. et al., 2020; Li et al., 2021). Nonetheless, both compounds also suppressed apoptotic cell death, as shown by a reduced number of CASP-3-positive cells in the ischemic tissue. Hence, the authors speculated that excessive autophagy may lead to self-digestion and eventually cell death (Zhang C. et al., 2020). Taken together, these contradictory results indicate that additional studies are required to further unravel the effects of phytochemical-induced autophagy on ischemic flap injury.
Discussion
The medicinal use of phytochemicals has a long history dating back more than 5,000 years. Phytochemicals are still present as health resources today because particularly in developing countries they are more readily available than prescription drugs (Aggarwal et al., 2007). Accordingly, around 80% of people worldwide are still relying on herbal medicines for some part of primary healthcare (Ekor, 2014). Moreover, more and more phytochemicals are also used in Western medicine as useful and effective therapeutic substances (Siddiqui et al., 2015).
Many phytochemical compounds have been shown to effectively improve flap survival in preclinical animal studies, as outlined in the present review. However, the exact mechanisms underlying this beneficial effect usually remain elusive. This is most probably due to the fact that phytochemicals typically exhibit a pleiotropic spectrum of activity, simultaneously targeting different molecular and cellular processes. Although this may contribute to their efficiency, it makes it difficult to develop approved clinical treatment regimens because it hampers the assessment of the clinical response to their application and associated side effects by clear readout parameters. Hence, extensive preclinical studies focusing on the identification of the phytochemicals’ main mode of action in highly standardized in vitro and in vivo settings are largely required. Moreover, robust data on the pharmacokinetics and pharmacodynamics of individual phytochemicals need to be assessed. In addition, it is important to analyze possible interactions between phytochemicals and conventional drugs, as their combined use may bear the risk of unwanted complications (Nowack, 2008; Li et al., 2017). On the other hand, the supplementation of pharmacotherapy with phytochemicals offers the opportunity to improve the efficiency of current treatment modalities while reducing the dosage of individual components and thus their side effects. In this context, it may also be beneficial to evaluate different routes of phytochemical administration. For instance, the topical application of phytochemicals on flap tissue may help prevent systemic side effects. For this purpose, future studies should clarify which phytochemicals fulfill the biopharmaceutical requirements for effective topical drug delivery, particularly through the skin barrier (Gugleva et al., 2021).
Clinical studies focusing on phytochemicals in flap surgery are still rare, even though the favorable risk–benefit profile of phytochemicals would make them good candidates for the treatment of patients (Wahab et al., 2021). Indeed, from the compounds addressed, only three have been tested in human studies in the context of flap surgery, i.e., salvianolic acid B, TMP, and hydroxysafflor yellow A (Chen et al., 2010; Ma et al., 2010; Shining and Zha, 2011). Interestingly, the studies on hydroxysafflor yellow and TMP focused on the positive effect of the compounds on blood rheology, while the blood flow characteristics were not analyzed in the respective preclinical studies (Ma et al., 2010; Shining and Zha, 2011). Similarly, Chen et al., (2010) speculated that the effect of Salvia miltiorrhiza extract and its component salvianolic acid B is largely based on the inhibition of platelet aggregation and increase in prothrombin time. This observation highlights the fact that it is difficult to identify and monitor all involved pathways due to the pleiotropic mode of action of phytochemicals.
Furthermore, many phytochemicals remain largely unstandardized. In fact, source plants can markedly differ in their phytochemical content (Chen et al., 2019). In addition, the variable composition of available products, including teas or extracts, makes it difficult to obtain consistent data on their toxicological risk profile (Collins et al., 2020). Hence, whenever possible, the use of phytochemicals with a well-defined content, such as Ginkgo biloba extract EGb 761 (Bekerecioǧlu et al., 1998; Christen and Maixent, 2002; Sambuy et al., 2012) or purified plant compounds, may contribute to the generation of robust data and thus the establishment of evidence-based phytochemical treatment strategies. In fact, such standardized or purified compounds may be crucial to prove the efficiency and safety in comparison to conventional therapeutic approaches in randomized controlled multicenter trials. In this context, it should also be considered that many phytochemical compounds may not be patentable or their patents have expired a long time ago. Hence, there is no incentive for the pharmaceutical industry to drive the development in this field or even to conduct expensive clinical trials. Therefore, the synthesis of chemical analogs of phytochemicals may be an alternative route to consider. This may also increase their therapeutic potential, as it would overcome the limited availability of phytochemicals in their natural sources (Mora-Pale et al., 2013).
The generally missing translation of preclinical studies into human trials may be caused by several critical factors, such as the poor solubility and stability of phytochemicals and their lack of selectivity. For instance, low solubility may cause poor absorption into targeted cells. Moreover, the activity of phytochemical compounds gets compromised due to the methods of their extraction and purification. Phytochemicals usually also exhibit a comparatively low bioavailability that complicates their application (Holst and Williamson, 2008). Modifications in the extraction/purification protocols for phytochemicals or the use of natural bioavailability enhancers may help to overcome these problems (Singh et al., 2019; Asuzu et al., 2022). In addition, there are several promising strategies for targeted drug delivery, such as the generation of micronized and encapsulated formulations or the binding of phytochemicals to nanocarriers (Smoliga and Blanchard, 2014). In fact, there has been a continually increasing interest in generating nanoformulations of phytochemicals by using liposomes, micelles, nanoemulsions, and nanoparticles to maximize their therapeutic potential (Singh et al., 2019). However, unresolved issues related to nanotechnology, such as the toxicity of nanomaterials, their degradability, stability, and selectivity, also need to be carefully evaluated before using them for the application of phytochemicals (Sharifi et al., 2012; Allen, 2019).
Another important factor, which may crucially determine the successful integration of phytochemical treatment into perioperative routines, is the time point of treatment application. For instance, Fáber et al. (2018) reported that the administration of genistein starting 3 days prior to flap elevation is more effective than an administration starting the day of flap elevation. Similarly, the administration of thymoquinone before and after flap elevation decreases flap necrosis more effectively than isolated pre- or postoperative treatment (Kocak et al., 2016). These findings clearly demonstrate that the identification of the most effective treatment sequence is of major importance for the clinical application of phytochemicals in flap surgery.
Taken together, a rapidly increasing number of preclinical studies suggest that phytochemicals bear great potential for the improvement of surgical flap survival due to their pleiotropic, tissue-protective effects. The next important step toward the clinical application of such phytochemicals in flap surgery is the evaluation of their efficiency and safety in prospective, randomized clinical trials. If this succeeds, the application of phytochemicals may markedly prevent ischemic flap complications and improve the overall outcome of flap-based reconstructive procedures in future clinical practice.
Author Contributions
AW conducted the literary research. The first draft of the manuscript was written by AW, EA, and ML. The draft was revised and corrected by MM. All authors read and approved the final manuscript.
Conflict of Interest
The authors declare that the research was conducted in the absence of any commercial or financial relationships that could be construed as a potential conflict of interest.
Publisher’s Note
All claims expressed in this article are solely those of the authors and do not necessarily represent those of their affiliated organizations, or those of the publisher, the editors, and the reviewers. Any product that may be evaluated in this article, or claim that may be made by its manufacturer, is not guaranteed or endorsed by the publisher.
References
Aggarwal, B. B., Sundaram, C., Malani, N., and Ichikawa, H. (2007). Curcumin: The Indian Solid Gold. Adv. Exp. Med. Biol. 595, 1–75. doi:10.1007/978-0-387-46401-5_1
Al-Ishaq, R. K., Overy, A. J., and Büsselberg, D. (2020). Phytochemicals and Gastrointestinal Cancer: Cellular Mechanisms and Effects to Change Cancer Progression. Biomolecules 10 (1), 105. doi:10.3390/biom10010105
Allen, C. (2019). The Question of Toxicity of Nanomaterials and Nanoparticles. J. Control. Release 304, 288. doi:10.1016/j.jconrel.2019.06.008
Aslan, C., Melikoglu, C., Ocal, I., Saglam, G., Sutcu, R., and Hosnuter, M. (2014). Effect of Epigallocatechin Gallate on Ischemia-Reperfusion Injury: An Experimental Study in a Rat Epigastric Island Flap. Int. J. Clin. Exp. Med. 7, 57–66.
Asuzu, P. C., Trompeter, N. S., Cooper, C. R., Besong, S. A., and Aryee, A. N. A. (2022). Cell Culture-Based Assessment of Toxicity and Therapeutics of Phytochemical Antioxidants. Molecules 27 (3), 1087. doi:10.3390/molecules27031087
Aydogan, H., Gurlek, A., Parlakpinar, H., Askar, I., Bay-Karabulut, A., Aydogan, N., et al. (2007). Beneficial Effects of Caffeic Acid Phenethyl Ester (CAPE) on the Ischaemia-Reperfusion Injury in Rat Skin Flaps. J. Plast. Reconstr. Aesthet. Surg. 60, 563–568. doi:10.1016/j.bjps.2006.01.030
Bekerecioglu, M., Tercan, M., and Irfan, O. (1998). THE EFFECT of GINGKO BILOBA EXTRACT (Egb 761) AS A FREE RADICAL SCAVENGER on the SURVIVAL of SKIN FLAPS IN RATS: A Comparative Study. Scand. J. Plast. Reconstr. Surg. Hand Surg. 32, 135–139. doi:10.1080/02844319850158741
Bilen, B. T., Kilinç, H., Alaybeyoglu, N., Celik, M., Iraz, M., Sezgin, N., et al. (2006). Effect of Caffeic Acid Phenethyl Ester on Survival of Axial Pattern Flaps in Rats with Ischaemia-Reperfusion Injuries. Scand. J. Plast. Reconstr. Surg. Hand Surg. 40, 73–78. doi:10.1080/02844310500443030
Cai, L., Cao, B., and Lin, D. (2015). Effects of Traditional Chinese Medicine Huangqi Injection (Radix Astragali) on Random Skin Flap Survival in Rats. J. Reconstr. Microsurg 31, 565–570. doi:10.1055/s-0035-1555142
Cai, L., Xie, L., and Dong, Q. (2020). Crocin Enhances the Viability of Random Pattern Skin Flaps: Involvement of Enhancing Angiogenesis and Inhibiting Oxidative Stress. Am. J. Transl Res. 12, 2929–2938.
Chen, G., Shen, H., Zang, L., Su, Z., Huang, J., Sun, Y., et al. (2018a). Protective Effect of Luteolin on Skin Ischemia-Reperfusion Injury through an AKT-dependent Mechanism. Int. J. Mol. Med. 42, 3073–3082. doi:10.3892/ijmm.2018.3915
Chen, J., Lv, Q., Yu, M., Zhang, X., and Gou, J. (2010). Randomized Clinical Trial of Chinese Herbal Medications to Reduce Wound Complications after Mastectomy for Breast Carcinoma. Br. J. Surg. 97, 1798–1804. doi:10.1002/bjs.7227
Chen, T., Tu, Q., Cheng, L., Li, Z., and Lin, D. (2018b). Effects of Curculigoside A on Random Skin Flap Survival in Rats. Eur. J. Pharmacol. 834, 281–287. doi:10.1016/j.ejphar.2018.07.030
Chen, W., Balan, P., and Popovich, D. G. (2019). Analysis of Ginsenoside Content (Panax Ginseng) from Different Regions. Molecules 24 (19), 3491. doi:10.3390/molecules24193491
Cheng, L., Chen, T., Tu, Q., Li, H., Feng, Z., Li, Z., et al. (2017a). Naringin Improves Random Skin Flap Survival in Rats. Oncotarget 8, 94142–94150. doi:10.18632/oncotarget.21589
Cheng, Y. T., Lu, C. C., and Yen, G. C. (2017b). Phytochemicals Enhance Antioxidant Enzyme Expression to Protect against NSAID-Induced Oxidative Damage of the Gastrointestinal Mucosa. Mol. Nutr. Food Res. 61 (6). doi:10.1002/mnfr.201600659
Cheng, Y. T., Yang, C. C., and Shyur, L. F. (2016). Phytomedicine-Modulating Oxidative Stress and the Tumor Microenvironment for Cancer Therapy. Pharmacol. Res. 114, 128–143. doi:10.1016/j.phrs.2016.10.022
Cheon, Y. W., Tark, K. C., and Kim, Y. W. (2012). Better Survival of Random Pattern Skin Flaps through the Use of Epigallocatechin Gallate. Dermatol. Surg. 38, 1835–1842. doi:10.1111/j.1524-4725.2012.02566.x
Chiaverina, G., di Blasio, L., Monica, V., Accardo, M., Palmiero, M., Peracino, B., et al. (2019). Dynamic Interplay between Pericytes and Endothelial Cells during Sprouting Angiogenesis. Cells 8, 1109. doi:10.3390/cells8091109
Christen, Y., and Maixent, J. M. (2002). What Is Ginkgo Biloba Extract EGb 761? an Overview-Ffrom Molecular Biology to Clinical Medicine. Cell Mol. Biol. (Noisy-le-Grand, France) 48 (6), 601–611.
Ciloglu, N. S., Zeytin, K., and Aker, F. (2014). The Effects of Resveratrol on Flap Survival in Diabetic Rats. J. Plast. Surg. Hand Surg. 48, 234–237. doi:10.3109/2000656X.2013.863777
Coelho da Mota, D. S., Sicuro, F. L., Resende, A. C., de Moura, R. S., Bottino, D. A., and Bouskela, E. (2018). Effects of Açaí and Cilostazol on Skin Microcirculation and Viability of TRAM Flaps in Hamsters. J. Surg. Res. 228, 253–262. doi:10.1016/j.jss.2018.03.014
Collins, B. J., Kerns, S. P., Aillon, K., Mueller, G., Rider, C. V., DeRose, E. F., et al. (2020). Comparison of Phytochemical Composition of Ginkgo Biloba Extracts Using a Combination of Non-targeted and Targeted Analytical Approaches. Anal. Bioanal. Chem. 412, 6789–6809. doi:10.1007/s00216-020-02839-7
De Lima Silva, J. J., Guimarães, S. B., Da Silveira, E. R., De Vasconcelos, P. R., Lima, G. G., Torres, S. M., et al. (2009). Effects of Copaifera Langsdorffii Desf. On Ischemia-Reperfusion of Randomized Skin Flaps in Rats. Aesthet. Plast Surg 33, 104–109. doi:10.1007/s00266-008-9263-2
Deheng, C., Kailiang, Z., Weidong, W., Haiming, J., Daoliang, X., Ningyu, C., et al. (2016). Salidroside Promotes Random Skin Flap Survival in Rats by Enhancing Angiogenesis and Inhibiting Apoptosis. J. Reconstr. Microsurg 32, 580–586. doi:10.1055/s-0036-1584205
Donkor, P. O., Chen, Y., Ding, L., and Qiu, F. (2016). Locally and Traditionally Used Ligusticum Species - A Review of Their Phytochemistry, Pharmacology and Pharmacokinetics. J. Ethnopharmacol 194, 530–548. doi:10.1016/j.jep.2016.10.012
Ekor, M. (2014). The Growing Use of Herbal Medicines: Issues Relating to Adverse Reactions and Challenges in Monitoring Safety. Front. Pharmacol. 4, 177. doi:10.3389/fphar.2013.00177
Emsen, I. M. (2005). A New Agent for Flap Survival - Hippophae Rhamnoides L. (Sea Buckthorn): An Experimental Study in Rats. Can. J. Plast. Surg. 13, 195–198. doi:10.1177/229255030501300404
Fáber, L., Kováč, I., Mitrengová, P., Novotný, M., Varinská, L., Vasilenko, T., et al. (2018). Genistein Improves Skin Flap Viability in Rats: A Preliminary In Vivo and In Vitro Investigation. Molecules 23, 1637. doi:10.3390/molecules23071637
Feng, X., Huang, D., Lin, D., Zhu, L., Zhang, M., Chen, Y., et al. (2021). Effects of Asiaticoside Treatment on the Survival of Random Skin Flaps in Rats. J. Invest. Surg. 34, 107–117. doi:10.1080/08941939.2019.1584255
Fischer, J. P., Sieber, B., Nelson, J. A., Cleveland, E., Kovach, S. J., Wu, L. C., et al. (2013). Comprehensive Outcome and Cost Analysis of Free Tissue Transfer for Breast Reconstruction: An Experience with 1303 Flaps. Plast. Reconstr. Surg. 131, 195–203. doi:10.1097/PRS.0b013e318277856f
Gao, Y., Fu, R., Wang, J., Yang, X., Wen, L., and Feng, J. (2018). Resveratrol Mitigates the Oxidative Stress Mediated by Hypoxic-Ischemic Brain Injury in Neonatal Rats via Nrf2/ho-1 Pathway. Pharm. Biol. 56, 440–449. doi:10.1080/13880209.2018.1502326
George, B. P., Chandran, R., and Abrahamse, H. (2021). Role of Phytochemicals in Cancer Chemoprevention: Insights. Antioxidants (Basel) 10 (9), 1455. doi:10.3390/antiox10091455
Glick, D., Barth, S., and Macleod, K. F. (2010). Autophagy: Cellular and Molecular Mechanisms. J. Pathol. 221, 3–12. doi:10.1002/path.2697
Gugleva, V., Ivanova, N., Sotirova, Y., and Andonova, V. (2021). Dermal Drug Delivery of Phytochemicals with Phenolic Structure via Lipid-Based Nanotechnologies. Pharmaceuticals (Basel) 14 (9), 837. doi:10.3390/ph14090837
Haidarali, S., Patil, C. R., Ojha, S., Mohanraj, R., Arya, D. S., and Goyal, S. N. (2015). Targeting Apoptotic Pathways in Myocardial Infarction: Attenuated by Phytochemicals. Cardiovasc. Hematol. Agents Med. Chem. 12, 72–85. doi:10.2174/1871525713666150123152711
He, J. B., Fang, M. J., Ma, X. Y., Li, W. J., and Lin, D. S. (2020). Angiogenic and Anti-inflammatory Properties of Azadirachtin A Improve Random Skin Flap Survival in Rats. Exp. Biol. Med. (Maywood) 245, 1672–1682. doi:10.1177/1535370220951896
Hernandez, L. A., Grisham, M. B., Twohig, B., Arfors, K. E., Harlan, J. M., and Granger, D. N. (1987). Role of Neutrophils in Ischemia-Reperfusion-Induced Microvascular Injury. Am. J. Physiol. 253, H699–H703. doi:10.1152/ajpheart.1987.253.3.h699
Holst, B., and Williamson, G. (2008). Nutrients and Phytochemicals: from Bioavailability to Bioefficacy beyond Antioxidants. Curr. Opin. Biotechnol. 19, 73–82. doi:10.1016/j.copbio.2008.03.003
Huang, G., Lin, Y., Fang, M., and Lin, D. (2019). Protective Effects of Icariin on Dorsal Random Skin Flap Survival: An Experimental Study. Eur. J. Pharmacol. 861, 172600. doi:10.1016/j.ejphar.2019.172600
İnce, B., Bilgen, F., Gündeşlioğlu, A. Ö., Dadacı, M., and Kozacıoğlu, S. (2016). Use of Systemic Rosmarinus Officinalis to Enhance the Survival of Random-Pattern Skin Flaps. Balkan Med. J. 33, 645–651. doi:10.5152/balkanmedj.2016.150981
İnce, B., Dadacı, M., Kılınç, İ., Oltulu, P., Yarar, S., and Uyar, M. (2018). Effect of Cineole, Alpha-Pinene, and Camphor on Survivability of Skin Flaps. Turk J. Med. Sci. 48, 644–652. doi:10.3906/sag-1704-166
Ismaeel, A., Greathouse, K. L., Newton, N., Miserlis, D., Papoutsi, E., Smith, R. S., et al. (2021). Phytochemicals as Therapeutic Interventions in Peripheral Artery Disease. Nutrients 13 (7), 2143. doi:10.3390/nu13072143
Jiang, R., Lin, C., Jiang, C., Huang, Z., Gao, W., and Lin, D. (2020). Nobiletin Enhances the Survival of Random Pattern Skin Flaps: Involvement of Enhancing Angiogenesis and Inhibiting Oxidative Stress. Int. Immunopharmacol 78, 106010. doi:10.1016/j.intimp.2019.106010
Kalogeris, T., Bao, Y., and Korthuis, R. J. (2014). Mitochondrial Reactive Oxygen Species: A Double Edged Sword in Ischemia/reperfusion vs Preconditioning. Redox Biol. 2, 702–714. doi:10.1016/j.redox.2014.05.006
Karaaslan, O., Ulusoy, M. G., Kankaya, Y., Tiftikcioglu, Y. O., Kocer, U., Kankaya, D., et al. (2010). Protective Effect of Grape Seed Extract against Ischaemia/reperfusion Injury in a Rat Epigastricflap Model. J. Plast. Reconstr. Aesthet. Surg. 63, 705–710. doi:10.1016/j.bjps.2009.01.018
Kennedy, D. O., and Wightman, E. L. (2011). Herbal Extracts and Phytochemicals: Plant Secondary Metabolites and the Enhancement of Human Brain Function. Adv. Nutr. 2, 32–50. doi:10.3945/an.110.000117
Kim, H. J., Xu, L., Chang, K. C., Shin, S. C., Chung, J. I., Kang, D., et al. (2012). Anti-inflammatory Effects of Anthocyanins from Black Soybean Seed Coat on the Keratinocytes and Ischemia-Reperfusion Injury in Rat Skin Flaps. Microsurgery 32, 563–570. doi:10.1002/micr.22019
Kırkıl, C., Yiğit, M. V., Özercan, İ. H., Aygen, E., Gültürk, B., and Artaş, G. (2016). The Effect of Ozonated Olive Oil on Neovascularizatıon in an Experimental Skin Flap Model. Adv. Skin Wound Care 29, 322–327. doi:10.1097/01.ASW.0000484172.04260.46
Kocak, O. F., Bozan, N., Oksuz, M., Yuce, S., Demir, C. Y., Bulut, G., et al. (2016). The Effect of the Active Ingredient Thymoquinone on Flap Viability in Random Pattern Flaps in Rats. J. Membr. Biol. 249, 513–522. doi:10.1007/s00232-016-9893-2
Kubes, P., Suzuki, M., and Granger, D. N. (1991). Nitric Oxide: An Endogenous Modulator of Leukocyte Adhesion. Proc. Natl. Acad. Sci. U S A. 88, 4651–4655. doi:10.1073/pnas.88.11.4651
Lay, I. S., Hsieh, C. C., Chiu, J. H., Shiao, M. S., Lui, W. Y., and Wu, C. W. (2003). Salvianolic Acid B Enhances In Vitro Angiogenesis and Improves Skin Flap Survival in Sprague-Dawley Rats. J. Surg. Res. 115, 279–285. doi:10.1016/S0022-4804(03)00226-9
Lee, S. M., Hutchinson, M., and Saint, D. A. (2016). The Role of Toll-like Receptor 4 (TLR4) in Cardiac Ischaemic-Reperfusion Injury, Cardioprotection and Preconditioning. Clin. Exp. Pharmacol. Physiol. 43, 864–871. doi:10.1111/1440-1681.12602
Li, B., Chen, Z., Luo, X., Zhang, C., Chen, H., Wang, S., et al. (2021). Butylphthalide Inhibits Autophagy and Promotes Multiterritory Perforator Flap Survival. Front. Pharmacol. 11. doi:10.3389/fphar.2020.612932
Li, J., Bao, G., ALyafeai, E., Ding, J., Li, S., Sheng, S., et al. (2019). Betulinic Acid Enhances the Viability of Random-Pattern Skin Flaps by Activating Autophagy. Front. Pharmacol. 10, 1017. doi:10.3389/fphar.2019.01017
Li, Y., Revalde, J., and Paxton, J. W. (2017). The Effects of Dietary and Herbal Phytochemicals on Drug Transporters. Adv. Drug Deliv. Rev. 116, 45–62. doi:10.1016/j.addr.2016.09.004
Lian, N., Zhang, S., Huang, J., Lin, T., and Lin, Q. (2020). Resveratrol Attenuates Intermittent Hypoxia-Induced Lung Injury by Activating the Nrf2/ARE Pathway. Lung 198 (2), 323–331. doi:10.1007/s00408-020-00321-w
Lin, D., Wu, H., Zhou, Z., Tao, Z., Gao, W., and Jia, T. (2020). The Effect of Leonurine on Multiterritory Perforator Flap Survival in Rats. J. Surg. Res. 245, 453–460. doi:10.1016/j.jss.2019.07.085
Lin, D., Wu, H., Zhou, Z., Tao, Z., Jia, T., and Gao, W. (2019a). Ginkgolide B Improves Multiterritory Perforator Flap Survival by Inhibiting Endoplasmic Reticulum Stress and Oxidative Stress. J. Invest. Surg. 34, 610–616. doi:10.1080/08941939.2019.1676483
Lin, J., Lin, R., Li, S., Wu, H., Ding, J., Xiang, G., et al. (2019b). Protective Effects of Resveratrol on Random-Pattern Skin Flap Survival: An Experimental Study. Am. J. Transl Res. 11, 379–392.
Lin, J., Lin, R., Li, S., Wu, H., Ding, J., Xiang, G., et al. (2018a). Salvianolic Acid B Promotes the Survival of Random-Pattern Skin Flaps in Rats by Inducing Autophagy. Front. Pharmacol. 9, 1178. doi:10.3389/fphar.2018.01178
Lin, R., Chen, H., Callow, D., Li, S., Wang, L., Li, S., et al. (2017). Multifaceted Effects of Astragaloside IV on Promotion of Random Pattern Skin Flap Survival in Rats. Am. J. Transl Res. 9, 4161–4172.
Lin, R., Lin, J., Li, S., Ding, J., Wu, H., Xiang, G., et al. (2018b). Effects of the Traditional Chinese Medicine Baicalein on the Viability of Random Pattern Skin Flaps in Rats. Drug Des. Devel Ther. 12, 2267–2276. doi:10.2147/DDDT.S173371
Liu, R. H. (2013). Health-promoting Components of Fruits and Vegetables in the Diet. Adv. Nutr. 4, 384S–92S. doi:10.3945/an.112.003517
Lucas, J. B. (2017). The Physiology and Biomechanics of Skin Flaps. Facial Plast. Surg. Clin. North. Am. 25, 303–311. doi:10.1016/j.fsc.2017.03.003
Luo, W., Wang, Y., Yang, H., Dai, C., Hong, H., Li, J., et al. (2018). Heme Oxygenase-1 Ameliorates Oxidative Stress-Induced Endothelial Senescence via Regulating Endothelial Nitric Oxide Synthase Activation and Coupling. Aging (Albany NY) 10, 1722–1744. doi:10.18632/aging.101506
Ma, W. L., Cheng, C. S., and Zhao, Z. W. (2010). Perioperative Intervention of Tetramethylpyrazine on Free Skin Flap Transplantation. Zhongguo Zhong yi yan jiu yuan zhu ban 30 (7), 714–716.
Ma, X. L., Weyrich, A. S., Lefer, D. J., and Lefer, A. M. (1993). Diminished Basal Nitric Oxide Release after Myocardial Ischemia and Reperfusion Promotes Neutrophil Adherence to Coronary Endothelium. Circ. Res. 72, 403–412. doi:10.1161/01.res.72.2.403
Ma, X., Lin, Y., Liu, y., Li, W., He, J., Fang, M., et al. (2021). Effects of Apigenin Treatment on Random Skin Flap Survival in Rats. Front. Pharmacol. 12, 625733. doi:10.3389/fphar.2021.625733
Mariño, G., Niso-Santano, M., Baehrecke, E. H., and Kroemer, G. (2014). Self-consumption: The Interplay of Autophagy and Apoptosis. Nat. Rev. Mol. Cel Biol 15, 81–94. doi:10.1038/nrm3735
McFarlane, R. M., DeYoung, G., Henry, R. A., and McFarlane, R. M. (1965). The Design of a Pedicle Flap in the Rat to Study Necrosis and its Prevention. Plast. Reconstr. Surg. 35, 177–182. doi:10.1097/00006534-196502000-00007
Memarzadeh, K., Sheikh, R., Blohmé, J., Torbrand, C., and Malmsjö, M. (2016). Perfusion and Oxygenation of Random Advancement Skin Flaps Depend More on the Length and Thickness of the Flap Than on the Width to Length Ratio. Eplasty 16, e12.
Menger, M. D., Laschke, M. W., Amon, M., Schramm, R., Thorlacius, H., Rücker, M., et al. (2003). Experimental Models to Study Microcirculatory Dysfunction in Muscle Ischemia?reperfusion and Osteomyocutaneous Flap Transfer. Langenbeck's Arch. Surg. 388, 281–290. doi:10.1007/s00423-003-0426-y
Mora-Pale, M., Sanchez-Rodriguez, S. P., Linhardt, R. J., Dordick, J. S., and Koffas, M. A. (2013). Metabolic Engineering and In Vitro Biosynthesis of Phytochemicals and Non-natural Analogues. Plant Sci. 210, 10–24. doi:10.1016/j.plantsci.2013.05.005
Neri, M., Fineschi, V., Di Paolo, M., Pomara, C., Riezzo, I., Turillazzi, E., et al. (2015). Cardiac Oxidative Stress and Inflammatory Cytokines Response after Myocardial Infarction. Curr. Vasc. Pharmacol. 13, 26–36. doi:10.2174/15701611113119990003
Nowack, R. (2008). Herb-drug Interactions in Nephrology: Documented and Theoretical. Clin. Nephrol. 69, 319–325. doi:10.5414/CNP69319
Padubidri, A. N., and Browne, E. (1997). Modification in Flap Design of the Epigastric Artery Flap in Rats-Aa New Experimental Flap Model. Ann. Plast. Surg. 39, 500–504. doi:10.1097/00000637-199711000-00010
Panche, A. N., Diwan, A. D., and Chandra, S. R. (2016). Flavonoids: An Overview. J. Nutr. Sci. 5, e47. doi:10.1017/jns.2016.41
Pei, Y., Zhang, L., Mao, X., Liu, Z., Cui, W., Sun, X., et al. (2020). Biomaterial Scaffolds for Improving Vascularization during Skin Flap Regeneration. Chin. J. Plast. Reconstr. Surg. 2 (2), 109–119. doi:10.1016/s2096-6911(21)00021-2
Petry, J. J., and Wortham, K. A. (1984). The Anatomy of the Epigastric Flap in the Experimental Rat. Plast. Reconstr. Surg. 74, 410–413. doi:10.1097/00006534-198409000-00014
Qing, L., Wu, P., Zhou, Z., Yu, F., and Tang, J. (2019). Tetramethylpyrazine Improved the Survival of Multiterritory Perforator Flaps by Inducing Angiogenesis and Suppressing Apoptosis via the Akt/Nrf2 Pathway. Drug Des. Devel Ther. 13, 1437–1447. doi:10.2147/DDDT.S195090
Quintero-Fabián, S., Arreola, R., Becerril-Villanueva, E., Torres-Romero, J. C., Arana-Argáez, V., Lara-Riegos, J., et al. (2019). Role of Matrix Metalloproteinases in Angiogenesis and Cancer. Front. Oncol. 9, 1370. doi:10.3389/fonc.2019.01370
Reinisch, J. F. (1974). The Pathophysiology of Skin Flap Circulation. The Delay Phenomenon. Plast. Reconstr. Surg. 54, 585–598. doi:10.1097/00006534-197411000-00010
Saint-Cyr, M., Wong, C., Schaverien, M., Mojallal, A., and Rohrich, R. J. (2009). The Perforasome Theory: Vascular Anatomy and Clinical Implications. Plast. Reconstr. Surg. 124, 1529–1544. doi:10.1097/PRS.0b013e3181b98a6c
Sambuy, M. T., Costa, A. C., Cohen, C., and Chakkour, I. (2012). Effect of Ginkgo Biloba Extract (GbE-761) on the Survival of Fasciocutaneous Flaps in Rats. Phytother Res. 26, 299–302. doi:10.1002/ptr.3661
Schmauss, D., Weinzierl, A., Schmauss, V., and Harder, Y. (2018). Common Rodent Flap Models in Experimental Surgery. Eur. Surg. Res. 59, 255–264. doi:10.1159/000492414
Schürmann, C., Schmidt, N., Seitz, O., Pfeilschifter, J., and Frank, S. (2014). Angiogenic Response Pattern during normal and Impaired Skin Flap Re-integration in Mice: A Comparative Study. J. Craniomaxillofac. Surg. 42, 1710–1716. doi:10.1016/j.jcms.2014.06.004
Sharifi, S., Behzadi, S., Laurent, S., Forrest, M. L., Stroeve, P., and Mahmoudi, M. (2012). Toxicity of Nanomaterials. Chem. Soc. Rev. 41, 2323–2343. doi:10.1039/c1cs15188f
Shi, N. N., Cheng, C. S., and Zha, Z. Q. (2011). Clinical Study of Safflower Injection in Treating and Preventing the Vascular Crisis after Free Flap Transplantation. Zhongguo Zhong yi yan jiu yuan zhu ban 31 (10), 1322–1327.
Siddiqui, J. A., Singh, A., Chagtoo, M., Singh, N., Godbole, M. M., and Chakravarti, B. (2015). Phytochemicals for Breast Cancer Therapy: Current Status and Future Implications. Curr. Cancer Drug Targets 15, 116–135. doi:10.2174/1568009615666141229152256
Siemionow, M., and Arslan, E. (2004). Ischemia/reperfusion Injury: A Review in Relation to Free Tissue Transfers. Microsurgery 24, 468–475. doi:10.1002/micr.20060
Sies, H. (2015). Oxidative Stress: A Concept in Redox Biology and Medicine. Redox Biol. 4, 180–183. doi:10.1016/j.redox.2015.01.002
Silva, J. J., Pompeu, D. G., Ximenes, N. C., Duarte, A. S., Gramosa, N. V., Carvalho, Kde. M., et al. (2015). Effects of Kaurenoic Acid and Arginine on Random Skin Flap Oxidative Stress, Inflammation, and Cytokines in Rats. Aesthet. Plast Surg 39, 971–977. doi:10.1007/s00266-015-0559-8
Singh, V. K., Arora, D., Ansari, M. I., and Sharma, P. K. (2019). Phytochemicals Based Chemopreventive and Chemotherapeutic Strategies and Modern Technologies to Overcome Limitations for Better Clinical Applications. Phytother Res. 33, 3064–3089. doi:10.1002/ptr.6508
Smoliga, J. M., and Blanchard, O. (2014). Enhancing the Delivery of Resveratrol in Humans: If Low Bioavailability Is the Problem, what Is the Solution? Molecules 19, 17154–17172. doi:10.3390/molecules191117154
Sotolongo, K., Ghiso, J., and Rostagno, A. (2020). Nrf2 Activation through the PI3K/GSK-3 axis Protects Neuronal Cells from Aβ-Mediated Oxidative and Metabolic Damage. Alzheimers Res. Ther. 12, 13. doi:10.1186/s13195-019-0578-9
Suzuki, S., Yoshioka, N., Isshiki, N., Hamanaka, H., and Miyachi, Y. (1991). Involvement of Reactive Oxygen Species in post-ischaemic Flap Necrosis and its Prevention by Antioxidants. Br. J. Plast. Surg. 44, 130–134. doi:10.1016/0007-1226(91)90047-N
Tekin, H., Tekin, G., Bulut, G., Sargon, M. F., and Işik, D. (2017). Investigating the Effect of Korean Red Ginseng on the Viability of Random-Pattern Skin Flaps in Rats. Ann. Plast. Surg. 79, e1. doi:10.1097/SAP.0000000000000976
Thangapazham, R. L., Sharad, S., and Maheshwari, R. K. (2016). Phytochemicals in Wound Healing. Adv. Wound Care (New Rochelle) 5, 230–241. doi:10.1089/wound.2013.0505
Tsai, T. C., Tung, Y. T., Kuo, Y. H., Liao, J. W., Tsai, H. C., Chong, K. Y., et al. (2015). Anti-inflammatory Effects of Antrodia Camphorata, a Herbal Medicine, in a Mouse Skin Ischemia Model. J. Ethnopharmacol 159, 113–121. doi:10.1016/j.jep.2014.11.015
van den Heuvel, M. G., Buurman, W. A., Bast, A., and van der Hulst, R. R. (2009). Review: Ischaemia-Reperfusion Injury in Flap Surgery. J. Plast. Reconstr. Aesthet. Surg. 62, 721–726. doi:10.1016/j.bjps.2009.01.060
Vogt, P. M., Boorboor, P., Vaske, B., Topsakal, E., Schneider, M., and Muehlberger, T. (2005). Significant Angiogenic Potential Is Present in the Microenvironment of Muscle Flaps in Humans. J. Reconstr. Microsurg 21, 517–523. doi:10.1055/s-2005-922429
Wahab, M. S. A., Zaini, M. H., Ali, A. A., Sahudin, S., Mehat, M. Z., Hamid, H. A., et al. (2021). The Use of Herbal and Dietary Supplement Among Community-Dwelling Elderly in a Suburban Town of Malaysia. BMC Complement. Med. Ther. 21, 110. doi:10.1186/s12906-021-03287-1
Wang, L., Zhou, Z. W., Yang, L. H., Tao, X. Y., Feng, X. L., Ding, J., et al. (2017). Vasculature Characterization of a Multiterritory Perforator Flap: An Experimental Study. J. Reconstr. Microsurg 33, 292–297. doi:10.1055/s-0036-1598011
Webster, K. A. (2012). Mitochondrial Membrane Permeabilization and Cell Death during Myocardial Infarction: Roles of Calcium and Reactive Oxygen Species. Future Cardiol. 8, 863–884. doi:10.2217/fca.12.58
Yun, I. S., Kim, Y. S., Roh, T. S., Lee, W. J., Park, T. H., Roh, H., et al. (2017). The Effect of Red Ginseng Extract Intake on Ischemic Flaps. J. Invest. Surg. 30, 19–25. doi:10.1080/08941939.2016.1215577
Zahir, K. S., Syed, S. A., Zink, J. R., Restifo, R. J., and Thomson, J. G. (1998). Ischemic Preconditioning Improves the Survival of Skin and Myocutaneous Flaps in a Rat Model. Plast. Reconstr. Surg. 102, 140–142. doi:10.1097/00006534-199807000-00022
Zenk, M. H., and Juenger, M. (2007). Evolution and Current Status of the Phytochemistry of Nitrogenous Compounds. Phytochemistry 68, 2757–2772. doi:10.1016/j.phytochem.2007.07.009
Zhang, C., Shao, Z., Chen, Z., Lin, C., Hu, S., Lou, Z., et al. (2020a). Hydroxysafflor Yellow A Promotes Multiterritory Perforating Flap Survival: an Experimental Study. Am. J. Transl Res. 12 (8), 4781–4794.
Zhang, F., Hu, E. C., Topp, S., Lei, M., Chen, W., and Lineaweaver, W. C. (2006). Proinflammatory Cytokines Gene Expression in Skin Flaps with Arterial and Venous Ischemia in Rats. J. Reconstr. Microsurg 22, 641–647. doi:10.1055/s-2006-956238
Zhang, X., Yang, X., Chen, Y., Wang, G., Ding, P., Zhao, Z., et al. (2021). Clinical Study on Orthopaedic Treatment of Chronic Osteomyelitis with Soft Tissue Defect in Adults. Int. Wound J. doi:10.1111/iwj.13729
Zhang, Y., Cai, X., Shen, L., Huang, X., Wang, X., Lan, Y., et al. (2016). Effects of Sanguis Draconis on Perforator Flap Survival in Rats. Molecules 21 (10), 1262. doi:10.3390/molecules21101262
Zhang, Y., Hu, G., Lin, H. C., Hong, S. J., Deng, Y. H., Tang, J. Y., et al. (2009). Radix Astragali Extract Promotes Angiogenesis Involving Vascular Endothelial Growth Factor Receptor-Related Phosphatidylinositol 3-kinase/akt-dependent Pathway in Human Endothelial Cells. Phytother Res. 23, 1205–1213. doi:10.1002/ptr.2479
Zhang, Y., Zhu, X. B., Zhao, J. C., Gao, X. F., Zhang, X. N., and Hou, K. (2020b). Neuroprotective Effect of Resveratrol against Radiation after Surgically Induced Brain Injury by Reducing Oxidative Stress, Inflammation, and Apoptosis through NRf2/HO-1/nf-Κb Signaling Pathway. J. Biochem. Mol. Toxicol. 34 (12), e22600. doi:10.1002/jbt.22600
Zhang, Y. J., Gan, R. Y., Li, S., Zhou, Y., Li, A. N., Xu, D. P., et al. (2015). Antioxidant Phytochemicals for the Prevention and Treatment of Chronic Diseases. Molecules 20, 21138–21156. doi:10.3390/molecules201219753
Keywords: phytochemicals, nutraceuticals, herbal medicine, flap, necrosis, ischemia–reperfusion injury
Citation: Weinzierl A, Ampofo E, Menger MD and Laschke MW (2022) Tissue-Protective Mechanisms of Bioactive Phytochemicals in Flap Surgery. Front. Pharmacol. 13:864351. doi: 10.3389/fphar.2022.864351
Received: 31 January 2022; Accepted: 24 March 2022;
Published: 25 April 2022.
Edited by:
Ning Liu, Tulane University, United StatesReviewed by:
Yinghua Jiang, Tulane University, United StatesCopyright © 2022 Weinzierl, Ampofo, Menger and Laschke. This is an open-access article distributed under the terms of the Creative Commons Attribution License (CC BY). The use, distribution or reproduction in other forums is permitted, provided the original author(s) and the copyright owner(s) are credited and that the original publication in this journal is cited, in accordance with accepted academic practice. No use, distribution or reproduction is permitted which does not comply with these terms.
*Correspondence: Andrea Weinzierl, YW5kcmVhd2VpbnppZXJsQGljbG91ZC5jb20=