- 1Key Laboratory of Chemical Biology (Ministry of Education), School of Pharmaceutical Science, Cheeloo College of Medicine, Shandong University, Ji’nan, China
- 2Shandong Cancer Hospital and Institute, Shandong First Medical University, Jinan, China
Cyclin-dependent kinase 2 (CDK2) regulates the progression of the cell cycle and is critically associated with tumor growth. Selective CDK2 inhibition provides a potential therapeutic benefit against certain tumors. Purines and related heterocycle (e.g., R-Roscovitine) are important scaffolds in the development of CDK inhibitors. Herein, we designed a new series of 2-aminopurine derivatives based on the fragment-centric pocket mapping analysis of CDK2 crystal structure. Our results indicated that the introduction of polar substitution at the C-6 position of purine would be beneficial for CDK2 inhibition. Among them, compound 11l showed good CDK2 inhibitory activity (IC50 = 19 nM) and possessed good selectivity against other CDKs. Further in vitro tests indicated that compound 11l possesses anti-proliferation activity in triple-negative breast cancer (TNBC) cells. Moreover, molecular dynamics simulation suggested the favorable binding mode of compound 11l, which may serve as a new lead compound for the future development of CDK2 selective inhibitors.
1 Introduction
Cyclin-dependent kinases (CDKs) are essential kinases that drive cell cycle transformation and transcriptional regulation (Wood and Endicott, 2018; Rice, 2019). CDKs involve in a variety of biological processes, including cell metabolism, differentiation, and development. Human CDKs are mainly divided into two categories: 1) One group is involved in cell cycle regulation and related to mitosis, and the subtypes are CDK1, 2, 3, 4, and 6. 2) Another group is mainly involved in transcriptional regulation, regulating phosphorylation of RNA polymerase II, and the subtypes are CDK7, 8, 9, and 11 (Satyanarayana and Kaldis, 2009). Other subtypes, such as CDK5, have long been thought to be neuron-specific kinases that play an important role in cellular activity (survival, motility, etc.) (Roufayel and Murshid, 2019). Heretofore, several CDK4/6 inhibitors (e.g., Palbociclib (Fry et al., 2004), Ribociclib (Tripathy et al., 2017), and Abemaciclib (Lee et al., 2019)) have been approved by the FDA for the treatment of breast cancer and other solid tumors (Yuan et al., 2021). However, the long-term use of CDK4/6 inhibitors results in drug resistance and poor therapeutic effect on Rb-deficient tumors, especially some malignant tumors, which limits the clinical application of CDK4/6 inhibitors (Braal et al., 2021; Gomatou et al., 2021; Julve et al., 2021).
CDK2 plays a key role in cell cycle regulation (Aleem et al., 2004; Tadesse et al., 2019a; Volkart et al., 2019). CDK2 forms a complex with Cyclin E and then phosphorylates Rb, therefore activates E2F (Narasimha et al., 2014). CDK2-Cyclin A complex promotes cells to pass through the S/G2 checkpoint (Kimball and Webster, 2001). CDK2 also controls the phosphorylation of many transcription factors including Smad3 (Liu, 2006), FoxM1, FoxO1 (Adams, 2001), NFY, B-Myb (Joaquin and Watson, 2003), Myc (Hydbring and Larsson, 2010) and promotes the cell cycle. In addition, CDK2 also plays an important role in DNA replication (Fagundes and Teixeira, 2021), adaptive immune response, cell differentiation (Adams, 2001), and apoptosis (Golsteyn, 2005; Satyanarayana and Kaldis, 2009). CDK2 is an important regulatory factor of various carcinogenic signaling pathways (Jin et al., 2020). The overexpression of CDK2 and its related Cyclin A or Cyclin E is closely related to the development of tumors (Sviderskiy et al., 2020). Especially, the inhibition of CDK2 is a potential therapeutic strategy for those tumors that are considered to be ineffective by CDK4/6 inhibitors (Pandey et al., 2019; Tadesse et al., 2020). Inhibition of CDK2 resulted in increased Smad3 activity and decreased triple-negative breast cancer (TNBC) cell migration (Tarasewicz et al., 2014). Recently, CDK2 has been found to mediate phosphorylation of EZH2, which drives tumorigenesis of TNBC (Nie et al., 2019a). Nowadays, CDK2 has been recognized as a potential target for anticancer drug development (Chohan et al., 2015; Zhang et al., 2015; Sánchez-Martínez et al., 2019).
Previously, we have reported a series of purine-2,6-diamine derivatives as CDK2 selective inhibitors (Figure 1) (Wang et al., 2013). We also developed purine-8-one derivatives that displayed good antitumor activities (Lu et al., 2019). In 2016, Coxon et al. designed a series of 6-substituted 2-arylaminopurines, which also possessed good CDK2 selectivity (Figure 1) (Coxon et al., 2017). Based on the crystal structures of the CDK2-inhibitor 73 complex (PDB: 5NEV), we performed fragment-centric topographic mapping using AlphaSpace and analyzed the binding pocket of 73. As shown in Figure 2, we identified an unoccupied polar pocket (pocket 5) besides the biphenyl group of 73. Moreover, the partially polar binding pocket (pocket 2, nonpolar rate = 73%) for the biphenyl group is also not fully occupied (occupancy = 79%). Based on the structural analysis above, we designed a new series of 2-arylaminopurines by introducing various substitutions in the C-6 position of the purine scaffold to further explore the structure–activity relationships.
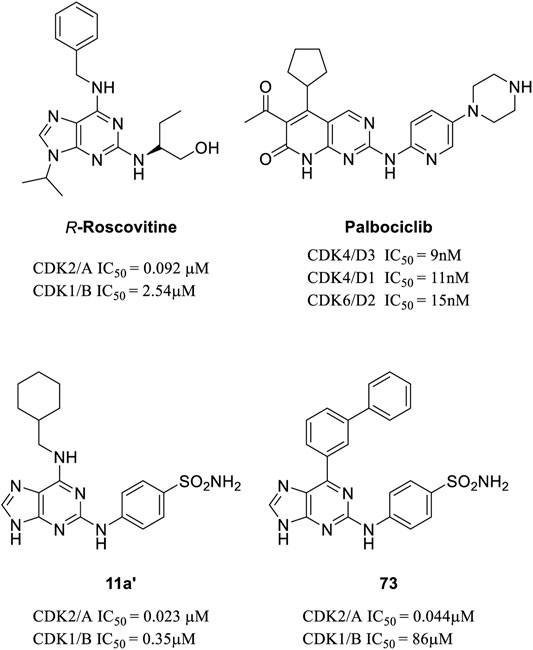
FIGURE 1. Reported CDK2 inhibitors from literature and our previous work: R-Roscovitine (Meijer et al., 1997), Palbociclib (Fry et al., 2004), compound 11a’ (Wang et al., 2013), and compound 73 (Coxon et al., 2017).
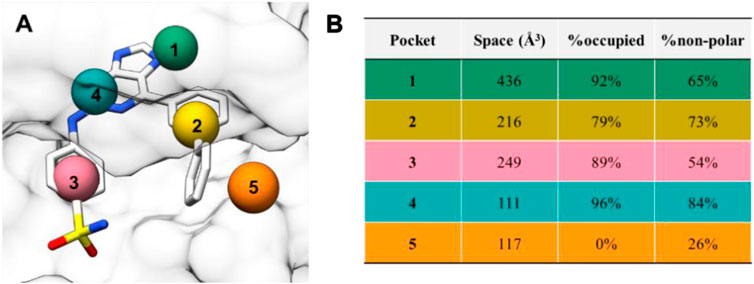
FIGURE 2. (A) Calculated binding pockets of compound 73 in CDK2. Pockets are represented using spheres located at the centroid of each alpha-cluster. (B) The table presents pocket features including space, occupancy, and nonpolar rate.
2 Results and Discussion
2.1 Chemistry
The synthesis routes of compounds 5a-5k are depicted in Scheme 1. The 6-substituted purine derivatives were synthesized from the THP-protected 2,6-dichloropurine via a Suzuki coupling reaction with aryl boric acid or aryl pinacol boric acid ester. Coupling by Buchwald-Harwting Reaction with 3-Nitroaniline, employing Pd(OAc)2/Xantphos afforded the THP-protected 2-aminopurine derivates in excellent yield. Then the N9-THP group was removed under the acidic condition to give the final compound.

SCHEME 1. Synthetic route of target compounds 5a-5k. Reagents and conditions: (A) 3,4-dihydro-2H-pyran, DL-Camphorsulfonic acid, EA, 65°C, 18 h; (B) aryl borate ester, Pd(PPh3)4, K2CO3, 1,4-dioxane/H2O = 4:1, 80°C, 9 h; (C) 3-Nitroaniline, Pd(OAc)2, Xantphos, Cs2CO3, 1,4-dioxane, 100°C, 9 h; (D) HCl/EA, rt, 4 h; (E) LiOH, THF/H2O = 4:1, rt, 4 h.
And the synthesis routes of compounds 11a-11r are depicted in Scheme 2. Ortho- or para-bromo benzylamine were protected by the Boc group, respectively. Then, through the Miyaura borylation reaction, the Boc-protected aryl borate esters were prepared. And then, similar to the synthetic route of Scheme 1, compounds 10a-10r were obtained by Suzuki coupling, Buchwald-Harwting Coupling (Yin et al., 2002), and the removal of protection groups.

SCHEME 2. Synthetic route of target compounds 11a-11r. Reagents and conditions: (A) (Boc)2O, K2CO3, DCM, rt, 4 h; (B) Bis(pinacolato)diboron, Pd (dppf)2Cl2, KOAc, DMSO, 80°C, 9 h; (C) 2, Pd(PPh3)4, K2CO3, 1,4-dioxane:H2O = 4:1, 80°C, 9 h; (D) substituted anilines, Pd(OAc)2, Xantphos, Cs2CO3, 1,4-dioxane, 100°C, 9 h; (E) HCl/EA, rt, 4 h.
2.2 CDK2 Inhibitory Activities
All compounds were screened for CDK2 inhibitory activities at 0.5 μM. Compounds with inhibition rates higher than 50% were further tested at different concentrations to determine IC50 values. And results are summarized in Table 1. The 6-position benzene substituted purine derivative (5a) showed good potency against CDK2 (IC50 = 0.31 μM). When the benzene ring at the C6 position of compound 5a was changed to naphthalene ring (5b), pyrrole ring (5c), benzo[d][1,3]dioxole (5d), and thiophene (5e), the CDK2 inhibitory activity decreased (Table 1). Among these compounds, 5e was inactive, 5b and 5d showed weak activity against CDK2, whereas 5c exhibited a 37% inhibition rate at 0.5 μM. Then, we sought to investigate the impacts of different substituted benzenes at the C-6 position. As shown in Table 1, the introduction of methyl formate (5h and 5k), fluorine (5i), or nitro (5f) substituted the benzene-abolished CDK2 inhibitory activity. Interestingly, the meta-substituted carboxylic group (5g) is beneficial for CDK2 inhibition, whereas the para-substituted carboxylic group leads to a compound with low activity (5j). The introduction of phenylamino or benzylamine group (11a-11d) in the C-6 position increases the CDK2 inhibitory activity. Compound 11a (IC50 = 0.31 μM) exhibited a similar CDK2 inhibitory activity with 5a. Importantly, compound 11c (IC50 = 0.11 μM) possessed a better CDK2 inhibitory activity than 5a. When the pare-amino group was changed to meta-amino group (11d), its CDK2 inhibitory activity was decreased slightly (IC50 = 0.23 μM). The above SAR result is consistent with our hypothesis that the introduction of a polar group at the C-6 site would be beneficial for binding against CDK2 protein. Next, we sought to optimize the R2 substitutions and got compound 11f-11r. When we introduced different substituents to the benzene ring, such as the electron-donating methyl group (11i) and tert-butyl group (11j), the activity decreased obviously. The biphenyl group (11h) seems to be too bulky to occupy the active site and cause a decrease in activity. The introduction of fluorine (11n and 11o), sulfonamide groups (11l, 11p, and 11q), and pyridine group (11r) is beneficial for CDK2 inhibition, and compound 11l exhibited the best activity (IC50 = 0.019 μM).
2.3 Isoform Selectivity
Three potent CDK2 inhibitors (11c, 11l, and 11p) were further selected to evaluate their inhibitory activities against other CDKs isoforms. As shown in Table 2, compounds 11c, 11l, and 11p showed potent activity against CDK1 (IC50 = 0.12–0.24 μM), weak activity against CDK6 (IC50 = 2.2–4.8 μM) and are nearly inactive against CDK8 (inhibition rate <20% @ 5 μM). Compounds 11l and 11p possess good selectivity for CDK2 over CDK6 and CDK8 (more than 140-fold), whereas their selectivity against CDK1 is lower (4.6 to 6.3-fold). Compared with compounds 11l and 11p, compound 11c is a less selective CDK2 inhibitor (2-fold for CDK1, 18-fold for CDK6, more than 42-fold for CDK8). Taking the above results together, our newly designed compound 11l is a potent and selective CDK2 inhibitor.
2.4 Anti-Triple-Negative Breast Cancer Activity of Selected Compounds
Previous studies have proved that CDK2 plays a critical role in breast cancer progression by phosphorylating and activating hormone receptors (Pierson-Mullany and Lange, 2004; Tadesse et al., 2019b). In triple-negative breast cancer (TNBC), inhibition of CDK2 has shown synergistic effects with chemotherapy and radiotherapy (Deans et al., 2006; Rao et al., 2017; Nie et al., 2019b; Zhu et al., 2022). In the current study, we further investigated the antitumor activity of three compounds using MDA-MB-231 cells, which were derived from TNBC patients. As shown in Figure 3A, compounds 11c, 11l, and 11p (IC50 = 8.11–15.66 μM) exhibited better anti-proliferation activities than R-Roscovitine (IC50 = 24.07 μM) in MDA-MB-231 cells. Furthermore, we also evaluated the cytotoxicity of compound 11l in human embryonic kidney cell (293T) using the MTT assay. This compound showed low cytotoxicity with an IC50 value higher than 100 μM.
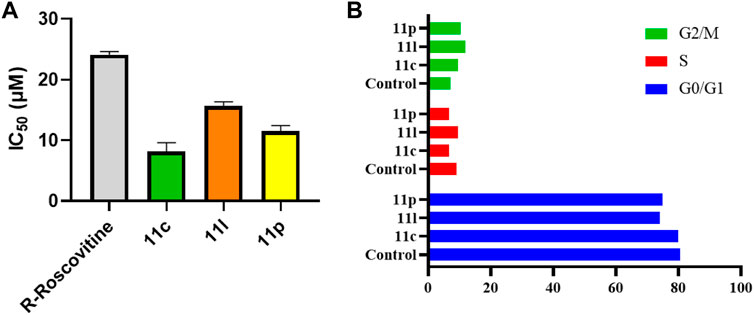
FIGURE 3. (A) Ani-proliferation activities of compounds 11c, 11l, and 11p against MDA-MB-231 cells. R-Roscovitine was employed as the positive control. (B) Impacts of compounds 11c, 11l, and 11p on the cell cycle of MDA-MB-231 cells.
To explore the mechanism of action of our newly designed compound, we further investigated their effects on the cell cycle regulation. As shown in Figure 3B, treatment of compounds 11c, 11l, and 11p increased the percentage of cells in the G2/M phase, compared with the negative control group. The results above suggested that our newly designed CDK2 inhibitors are potential antitumor agents for the treatment of TNBC.
2.5 Molecular Dynamics Simulation
To further decipher the binding mode of 11l, we performed 100 ns molecular dynamics (MD) simulation based on the docking result. As shown in Figure 4, the RMSD values of the protein–ligand complex are within 4 Å, while the RMSD values of compound 11l are within 1.5 Å, indicating that the simulation system is stable during MD simulation. Then we extracted the representative binding mode from the MD trajectory and analyzed the key interacting residues. As shown in Figure 4C, compound 11l forms multiple hydrogen bond interactions with surrounding residues in CDK2. The sulfonamide group forms hydrogen bonds with the side chain and backbone nitrogen of Lys90 and His85. The benzyl amine group of 11l locates at the entrance of the ATP-binding pocket, and form hydrogen bonds with Lys34, Glu52, and Ala145, respectively. The key hydrogen bonds were listed in Table 3. The hydrogen bond between His85/Lys34 and 11l is the most stable hydrogen bond interaction occupation values of 0.57 and 0.4, respectively (Table 3). The results above revealed the most favorable binding mode as well as key interactions of compound 11l with CDK2, which would be helpful for further structural optimization.
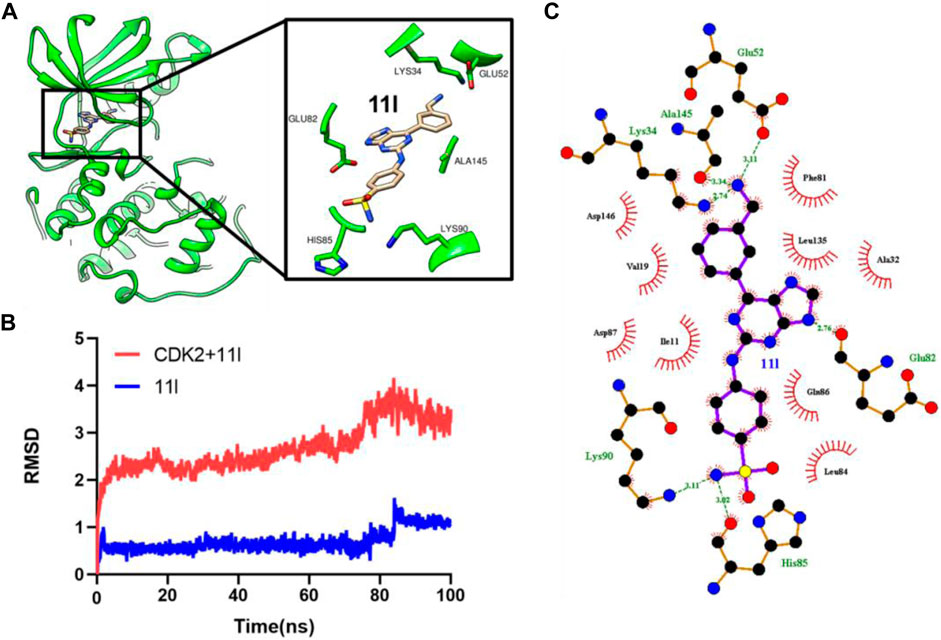
FIGURE 4. (A) Predicted binding mode of 11l in CDK2 from MD simulation. The key residues of CDK2 are highlighted and colored in green. (B) RMSD values of protein–ligand complex and 11l during MD simulation. (C) Interacting residues between 11l and CDK2.
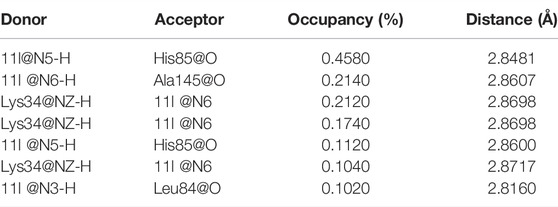
TABLE 3. Statistical analysis of the hydrogen bond interactions between 11l and CDK2 during MD simulation.
3 Conclusion
In the current study, we designed a series of 2-aminopurine derivatives as new CDK2 inhibitors based on the fragment-centric pocket mapping of crystal structure. As expected, the introduction of polar groups in the C-6 position of the purine scaffold is beneficial for CDK2 inhibition. Among them, compound 11l (IC50 = 0.019 μM) exhibited higher CDK2 inhibitory activity against CDK2 than known inhibitor R-Roscovitine (IC50 = 0.073 μM). Moreover, 11l also possessed good selectivity against other CDK isoforms and showed better anti-proliferation activity in MDA-MB-231 cells than R-Roscovitine. Molecular dynamics simulation further suggested the binding mode of 11l with CDK2, which would be helpful for the future development of more potent and selective CDK2 inhibitors.
4 Experimental Section
4.1 Chemistry
Chemical reagents were purchased commercially and were used without further purification. All reactions with air- or moisture-sensitive reagents were carried out under nitrogen and solvents were also dried before use. Reactions were monitored by thin-layer chromatography with preparative silica gel GF254 plates (UV lamp. or iodine), and column chromatography was performed on silica gel. The 1H-NMR spectra were obtained at 400 MHz. For 1H NMR spectra, chemical shifts were given in parts per million (ppm) and were referenced to tetramethylsilane (TMS) peak as an internal standard or the residual solvent peak. 13C NMR spectra were recorded at 101 MHz. Chemical shifts were reported in ppm and were referenced to the appropriate residual solvent peak. Splitting patterns were designed as s, singlet; d, doublet; t, triplet; m, multiplet. High-resolution mass spectrometry (HRMS) data were recorded with a 1200RRLC-6520 Accurate-Mass Q-TOF LC/MS system at the Shandong Analysis and Test Center.
4.1.1 Preparation of 2,6-dichloro-9-(tetrahydro-2H-pyran-2-yl)-9H-purine (2)
2,6-dichloropurine (5.29 mmol) and DL-Camphorsulfonic acid (0.05 mmol) were dissolved in ethyl acetate (20 ml), and heated to 65°C. 3,4-2H-dihydropyran (5.29 mmol) was added slowly and then the reaction mixture was stirred for 18 h at 65°C. After the completion, the reaction mixture is poured into H2O (20 ml), extracted twice with ethyl acetate (50 ml), washed with brine, and dried with anhydrous Mg2SO4. The crude product was concentrated and purified by silica gel chromatography to obtain compound 2. White solid; Yield: 70%; m.p.: 93–95°C; 1H NMR (600 MHz, CDCl3) δ 8.33 (s, 1H), 5.76 (dd, J = 10.8, 2.4 Hz, 1H), 4.21–4.11 (m, 1H), 3.78 (td, J = 11.8, 2.6 Hz, 1H), 2.21–2.15 (m, 1H), 2.13–2.06 (m, 1H), 2.02–1.93 (m, 1H), 1.87–1.72 (m, 2H), 1.71–1.66 (m, 1H).
4.1.2 General Method for the Preparation of Compounds 3a-3f, 3h, 3i, 3k, 9a-9e
Tert-butyl (4-(2-chloro-9-(tetrahydro-2H-pyran-2-yl)-9H-purin-6-yl)benzyl) carbamate (9d). Compounds 8d (4.5 mmol), compound 2 (4.5 mmol), Pd (PPh3)4 (0.05 mmol), and K2CO3 (13.5 mmol) were mixed in a two-neck flask. Under the protection of N2, the solution of 1,4-dioxane and water (4:1) was added and the mixture reacted at 80°C for 12 h. After the completion, the reaction mixture was filtered through a pad of Celite. Spinned the filtrate dry and then dissolved it with ethyl acetate (15 ml) and water (20 ml), extracted twice with ethyl acetate (50 ml), washed with brine, and dried with anhydrous Mg2SO4. The crude product was concentrated and purified by silica gel chromatography (eluting with petroleum ether/ethyl acetate 3/1 to 1/1) to obtain compound 9d. White solid; Yield: 75%; m.p.: 175–177°C; 1H NMR (400 MHz, CDCl3) δ 8.76 (d, J = 7.9 Hz, 2H), 8.32 (s, 1H), 7.46 (d, J = 7.9 Hz, 2H), 5.83 (d, J = 10.4 Hz, 1H), 4.92 (s, 1H), 4.41 (d, J = 5.0 Hz, 2H), 4.20 (d, J = 11.3 Hz, 1H), 3.81 (t, J = 11.0 Hz, 1H), 2.18 (d, J = 12.4 Hz, 1H), 2.08 (s, 1H), 1.99 (dd, J = 22.9, 11.4 Hz, 1H), 1.80 (td, J = 22.9, 12.2 Hz, 2H), 1.68 (d, J = 9.8 Hz, 1H), 1.48 (s, 9H).
Compounds 3a-3f, 3h, 3i, 3k, 9a-9c, 9e were synthesized following the procedure described above.
2-chloro-6-phenyl-9-(tetrahydro-2H-pyran-2-yl)-9H-purine (3a). Light yellow solid; Yield: 95%; m.p.: 132–134°C; 1H NMR (600 MHz, CDCl3) δ 8.86–8.73 (m, 2H), 8.32 (s, 1H), 7.60–7.50 (m, 2H), 5.84 (dd, J = 10.8, 2.4 Hz, 1H), 4.27–4.15 (m, 1H), 3.81 (td, J = 11.8, 2.5 Hz, 1H), 2.18 (dd, J = 12.5, 2.0 Hz, 1H), 2.13–2.06 (m, 1H), 2.00 (ddd, J = 23.5, 12.5, 4.0 Hz, 1H), 1.89–1.73 (m, 2H), 1.68 (d, J = 12.1 Hz, 1H).
2-chloro-6-(naphthalen-1-yl)-9-(tetrahydro-2H-pyran-2-yl)-9H-purine (3b). White solid; Yield: 67%; m.p.: 149–151°C; 1H NMR (400 MHz, CDCl3) δ 8.31 (s, 1H), 8.30–8.24 (m, 1H), 8.03 (t, J = 7.6 Hz, 2H), 7.97–7.89 (m, 1H), 7.66–7.60 (m, 1H), 7.57–7.49 (m, 2H), 5.88 (d, J = 10.6 Hz, 1H), 4.21 (d, J = 11.2 Hz, 1H), 3.83 (t, J = 11.5 Hz, 1H), 2.11 (dt, J = 11.4, 9.6 Hz, 3H), 1.89–1.65 (m, 3H).
Tert-butyl 2-(2-chloro-9-(tetrahydro-2H-pyran-2-yl)-9H-purin-6-yl)-1H-pyrrole-1-carboxylate (3c). White solid; Yield: 60%; m.p.: 142–144°C; 1H NMR (400 MHz, CDCl3) δ 8.24 (s, 1H), 7.50 (s, 1H), 7.27 (s, 1H), 7.11 (d, J = 1.6 Hz, 1H), 6.36 (s, 1H), 5.79 (d, J = 10.7 Hz, 1H), 4.19 (d, J = 11.1 Hz, 1H), 3.78 (d, J = 11.3 Hz, 1H), 2.30–1.93 (m, 3H), 1.76 (ddd, J = 34.6, 22.7, 11.2 Hz, 3H).
6-(benzo[d][1,3]dioxol-5-yl)-2-chloro-9-(tetrahydro-2H-pyran-2-yl)-9H-purine (3d). White solid; Yield: 74%; m.p.: 177–179°C; 1H NMR (400 MHz, CDCl3) δ 8.53 (d, J = 8.3 Hz, 1H), 8.30 (d, J = 17.9 Hz, 2H), 6.98 (d, J = 8.3 Hz, 1H), 6.06 (s, 2H), 5.81 (d, J = 10.5 Hz, 1H), 4.19 (d, J = 11.4 Hz, 1H), 3.80 (t, J = 11.2 Hz, 1H), 2.17 (d, J = 12.1 Hz, 1H), 2.08 (s, 1H), 1.98 (dd, J = 24.3, 13.3 Hz, 1H), 1.79 (td, J = 23.0, 12.0 Hz, 2H), 1.68 (d, J = 9.4 Hz, 1H).
2-chloro-9-(tetrahydro-2H-pyran-2-yl)-6-(thiophen-3-yl)-9H-purine(3e). Yellow solid; Yield:98%; m.p.:156–158°C; 1H NMR (400 MHz, CDCl3) δ 8.97–8.90 (m, 1H), 8.31–8.23 (m, 2H), 7.45 (q, J = 4.55, 4.04 Hz, 1H), 5.81 (d, J = 10.49 Hz, 1H), 4.19 (d, J = 10.81 Hz, 1H), 3.80 (t, J = 11.05 Hz, 1H), 2.23–1.96 (m, 3H), 1.78 (dt, J = 24.39, 11.95 Hz, 3H).
2-chloro-6-(3-nitrophenyl)-9-(tetrahydro-2H-pyran-2-yl)-9H-purine (3f). White solid; Yield: 57%; m.p.: 105–107°C; 1H NMR (400 MHz, CDCl3) δ 9.72 (s, 1H), 9.18 (d, J = 7.8 Hz, 1H), 8.40 (d, J = 3.5 Hz, 2H), 7.74 (t, J = 8.0 Hz, 1H), 5.85 (d, J = 10.4 Hz, 1H), 4.21 (d, J = 11.0 Hz, 1H), 3.82 (t, J = 10.9 Hz, 1H), 2.21 (d, J = 12.5 Hz, 1H), 2.11 (d, J = 6.4 Hz, 1H), 2.02 (dd, J = 12.4, 9.3 Hz, 1H), 1.87–1.64 (m, 3H).
Methyl 3-(2-chloro-9-(tetrahydro-2H-pyran-2-yl)-9H-purin-6-yl)benzoate (3h). White solid; Yield: 42%; m.p.: 110–112°C; 1H NMR (400 MHz, CDCl3) δ 9.42 (s, 1H), 9.00 (d, J = 7.8 Hz, 1H), 8.35 (s, 1H), 8.22 (d, J = 7.7 Hz, 1H), 7.64 (t, J = 7.8 Hz, 1H), 5.84 (d, J = 10.4 Hz, 1H), 4.20 (d, J = 10.0 Hz, 1H), 3.81 (t, J = 11.3 Hz, 1H), 2.19 (d, J = 12.5 Hz, 1H), 2.09 (d, J = 6.4 Hz, 1H), 1.98 (dd, J = 16.8, 7.0 Hz, 1H), 1.90–1.71 (m, 3H).
2-chloro-6-(4-fluorophenyl)-9-(tetrahydro-2H-pyran-2-yl)-9H-purine (3i). Oil; Yield: 88%. The product was used for the next step without purification.
Methyl 4-(2-chloro-9-(tetrahydro-2H-pyran-2-yl)-9H-purin-6-yl)benzoate (3k). Oil; Yield: 80%; 1H NMR (400 MHz, CDCl3) δ 8.87 (d, J = 8.0 Hz, 2H), 8.36 (s, 1H), 8.20 (d, J = 8.0 Hz, 2H), 5.84 (d, J = 10.3 Hz, 1H), 4.20 (d, J = 10.7 Hz, 1H), 3.97 (s, 3H), 3.81 (t, J = 11.0 Hz, 1H), 2.19 (d, J = 12.3 Hz, 1H), 2.10 (d, J = 6.7 Hz, 1H), 2.03–1.94 (m, 1H), 1.79 (dt, J = 23.7, 11.7 Hz, 2H), 1.69 (d, J = 9.7 Hz, 1H).
Tert-butyl (3-(2-chloro-9-(tetrahydro-2H-pyran-2-yl)-9H-purin-6-yl)phenyl)carbamate (9a). White solid; Yield: 58%; m.p.: 158–160°C; 1H NMR (400 MHz, CDCl3) δ 8.53 (s, 1H), 8.47 (d, J = 7.8 Hz, 1H), 8.32 (s, 1H), 7.84 (s, 1H), 7.49 (t, J = 8.0 Hz, 1H), 6.75 (s, 1H), 5.82 (d, J = 10.2 Hz, 1H), 4.19 (d, J = 12.4 Hz, 1H), 3.80 (t, J = 10.8 Hz, 1H), 2.17 (d, J = 12.9 Hz, 1H), 2.09 (d, J = 10.1 Hz, 1H), 2.02–1.96 (m, 1H), 1.88–1.74 (m, 2H), 1.70 (d, J = 11.3 Hz, 1H), 1.54 (s, 9H).
Tert-butyl (4-(2-chloro-9-(tetrahydro-2H-pyran-2-yl)-9H-purin-6-yl)phenyl)carbamate (9b). White solid; Yield: 62%; m.p.: 199–201°C; 1H NMR (400 MHz, CDCl3) δ 8.79 (d, J = 7.9 Hz, 2H), 8.29 (s, 1H), 7.54 (d, J = 8.2 Hz, 2H), 6.70 (s, 1H), 5.82 (d, J = 10.6 Hz, 1H), 4.19 (d, J = 10.9 Hz, 1H), 3.80 (t, J = 11.2 Hz, 1H), 2.16 (d, J = 12.5 Hz, 1H), 2.06 (d, J = 10.4 Hz, 1H), 1.98 (dd, J = 22.7, 12.0 Hz, 1H), 1.87–1.72 (m, 2H), 1.67 (d, J = 10.1 Hz, 1H), 1.54 (s, 9H).
Tert-butyl (3-(2-chloro-9-(tetrahydro-2H-pyran-2-yl)-9H-purin-6-yl)benzyl)carbamate (9c). White solid; Yield: 60%; mp: 102–104°C; 1H NMR (400 MHz, CDCl3) δ 8.74 (d, J = 7.2 Hz, 1H), 8.64 (s, 1H), 8.31 (s, 1H), 7.56–7.46 (m, 2H), 5.83 (d, J = 10.5 Hz, 1H), 4.97 (s, 1H), 4.45 (d, J = 4.3 Hz, 2H), 4.20 (d, J = 10.8 Hz, 1H), 3.81 (t, J = 10.9 Hz, 1H), 2.18 (d, J = 12.5 Hz, 1H), 2.08 (s, 1H), 1.99 (d, J = 11.0 Hz, 1H), 1.80 (td, J = 23.3, 12.3 Hz, 2H), 1.68 (d, J = 10.7 Hz, 1H), 1.24 (s, 9H).
Tert-butyl (4-(2-chloro-9-(tetrahydro-2H-pyran-2-yl)-9H-purin-6-yl)benzyl)carbamate (9d). White solid; Yield: 75%; m.p.: 175–177°C; 1H NMR (400 MHz, CDCl3) δ 8.76 (d, J = 7.9 Hz, 2H), 8.32 (s, 1H), 7.46 (d, J = 7.9 Hz, 2H), 5.83 (d, J = 10.4 Hz, 1H), 4.92 (s, 1H), 4.41 (d, J = 5.0 Hz, 2H), 4.20 (d, J = 11.3 Hz, 1H), 3.81 (t, J = 11.0 Hz, 1H), 2.18 (d, J = 12.4 Hz, 1H), 2.08 (s, 1H), 1.99 (dd, J = 22.9, 11.4 Hz, 1H), 1.80 (td, J = 22.9, 12.2 Hz, 2H), 1.68 (d, J = 9.8 Hz, 1H), 1.48 (s, 9H).
Tert-butyl (3-(2-chloro-9-(tetrahydro-2H-pyran-2-yl)-9H-purin-6-yl)phenethyl) carbamate (9e). Yellow solid; Yield: 65%; m.p.: 149–151°C; 1H NMR (400 MHz, CDCl3) δ 8.69 (d, J = 7.7 Hz, 1H), 8.57 (s, 1H), 8.31 (s, 1H), 7.50 (t, J = 7.7 Hz, 1H), 7.39 (d, J = 7.3 Hz, 1H), 5.83 (d, J = 10.4 Hz, 1H), 4.60 (s, 1H), 4.20 (d, J = 12.4 Hz, 1H), 3.81 (t, J = 11.0 Hz, 1H), 3.46 (d, J = 5.7 Hz, 2H), 2.94 (t, J = 6.7 Hz, 2H), 2.18 (d, J = 12.5 Hz, 1H), 2.08 (s, 1H), 2.00 (dd, J = 24.6, 13.5 Hz, 1H), 1.80 (td, J = 23.3, 12.3 Hz, 2H), 1.68 (d, J = 10.7 Hz, 1H), 1.43 (s, 9H).
4.1.3 General Method for the Preparation of Compounds 4a-4f, 4h, 4i, 4k, 10a-10r
Tert-butyl (4-(2-((4-fluorophenyl)amino)-9-(tetrahydro-2H-pyran-2-yl)-9H-purin-6-yl)benzyl)carbamate (10o). Compound 9d (1.0 mmol), 4-fluoroaniline (2 mmol), Pd(OAc)2 (0.05 mmol), Xantphos (0.10 mmol), and Cs2CO3 (13.5 mmol) were mixed in a two-neck flask. Under the protection of N2, the anhydrous 1,4-dioxane was added and the mixture reacted at 100°C for 18 h. After the completion, the reaction mixture was filtered through a pad of Celite. Spinned the filtrate dry and then dissolved it with ethyl acetate (15 ml) and water (20 ml), extracted twice with ethyl acetate (50 ml), washed with brine, and dried with anhydrous Mg2SO4. The crude product was concentrated and purified by silica gel chromatography (eluting with dichloromethane/menthol 100/1 to 40/1) to obtain compounds 10o.
Compounds 4a-4f, 4h, 4i, 4k, 10a-10n, and 10p-10r were synthesized following the procedure described above.
N-(3-nitrophenyl)-6-phenyl-9-(tetrahydro-2H-pyran-2-yl)-9H-purin-2-amine (4a). Light yellow solid; Yield: 76%; m.p.: 164–166°C; 1H NMR (400 MHz, DMSO-d6) δ 10.29 (s, 1H), 9.38 (s, 1H), 8.85 (d, J = 7.2 Hz, 2H), 8.61 (s, 1H), 8.01 (d, J = 8.1 Hz, 1H), 7.81 (d, J = 7.9 Hz, 1H), 7.60 (t, J = 8.5 Hz, 4H), 5.73 (d, J = 10.9 Hz, 1H), 4.10 (d, J = 11.4 Hz, 1H), 3.76 (dd, J = 15.6, 6.5 Hz, 1H), 2.41 (dd, J = 21.4, 10.6 Hz, 1H), 2.08 (d, J = 11.3 Hz, 2H), 1.84–1.58 (m, 3H).
6-(naphthalen-1-yl)-N-(3-nitrophenyl)-9-(tetrahydro-2H-pyran-2-yl)-9H-purin-2-amine(4b). White solid; Yield: 81%. m.p.: 190–192°C; 1H NMR (400 MHz, DMSO-d6) δ 10.42 (s, 1H), 9.30 (s, 1H), 8.53 (s, 1H), 8.21 (d, J = 8.4 Hz, 1H), 8.14 (d, J = 8.2 Hz, 1H), 8.05 (t, J = 8.0 Hz, 2H), 7.97 (d, J = 7.0 Hz, 1H), 7.80 (d, J = 8.0 Hz, 1H), 7.70 (t, J = 7.6 Hz, 1H), 7.57 (ddd, J = 25.1, 12.7, 7.3 Hz, 3H), 5.78 (d, J = 10.9 Hz, 1H), 4.12 (d, J = 11.1 Hz, 1H), 3.80 (dd, J = 15.8, 6.6 Hz, 1H), 2.45 (d, J = 9.5 Hz, 1H), 2.10 (t, J = 14.2 Hz, 2H), 1.87–1.60 (m, 3H).
Tert-butyl 2-(2-((3-nitrophenyl)amino)-9-(tetrahydro-2H-pyran-2-yl)-9H-purin-6-yl)-1H-pyrrole-1-carboxylate (4c). White solid; Yield: 80%; m.p.: 185–187°C; 1H NMR (400 MHz, DMSO-d6) δ 10.27 (s, 1H), 9.26 (s, 1H), 8.51 (d, J = 12.6 Hz, 1H), 8.01 (d, J = 8.3 Hz, 1H), 7.79 (d, J = 8.1 Hz, 1H), 7.63–7.52 (m, 2H), 6.86 (s, 1H), 6.44 (s, 1H), 5.70 (d, J = 10.8 Hz, 1H), 4.09 (d, J = 11.0 Hz, 1H), 3.75 (dd, J = 15.5, 6.6 Hz, 1H), 2.43 (d, J = 10.7 Hz, 1H), 2.04 (d, J = 9.4 Hz, 2H), 1.85–1.70 (m, 1H), 1.65 (d, J = 15.4 Hz, 2H), 1.23 (s, 9H).
6-(benzo[d][1,3]dioxol-5-yl)-N-(3-nitrophenyl)-9-(tetrahydro-2H-pyran-2-yl)-9H-purin-2-amine (4d). Yellow solid; Yield: 65%; m.p.: 207–209°C; 1H NMR (400 MHz, DMSO-d6) δ 10.20 (s, 1H), 9.34 (s, 1H), 8.58 (d, J = 10.4 Hz, 2H), 8.36 (s, 1H), 7.97 (d, J = 8.3 Hz, 1H), 7.80 (d, J = 8.1 Hz, 1H), 7.59 (t, J = 8.1 Hz, 1H), 7.15 (d, J = 8.2 Hz, 1H), 6.16 (s, 2H), 5.71 (d, J = 10.8 Hz, 1H), 4.09 (d, J = 11.4 Hz, 1H), 3.73 (d, J = 8.7 Hz, 1H), 2.39 (dd, J = 22.0, 10.6 Hz, 1H), 2.06 (d, J = 10.5 Hz, 2H), 1.84–1.57 (m, 3H).
N-(3-nitrophenyl)-9-(tetrahydro-2H-pyran-2-yl)-6-(thiophen-3-yl)-9H-purin-2-amine (4e). Light yellow solid; Yield: 60%; m.p.: 208–210°C; 1H NMR (400 MHz, DMSO-d6) δ 10.23 (s, 1H), 9.39 (t, J = 2.12 Hz, 1H), 8.96 (dd, J = 2.93, 0.97 Hz, 1H), 8.59 (s, 1H), 8.27 (dd, J = 5.07, 0.89 Hz, 1H), 8.00 (dd, J = 8.17, 1.38 Hz, 1H), 7.87–7.77 (m, 2H), 7.59 (t, J = 8.17 Hz, 1H), 5.71 (dd, J = 10.91, 1.69 Hz, 1H), 4.09 (d, J = 11.31 Hz, 1H), 3.74 (td, J = 11.36, 3.74 Hz, 1H), 2.40 (ddd, J = 16.28, 12.65, 4.22 Hz, 1H), 2.07 (d, J = 10.72 Hz, 2H), 1.82–1.59 (m, 3H).
N,6-bis(3-nitrophenyl)-9-(tetrahydro-2H-pyran-2-yl)-9H-purin-2-amine (4f). Yellow solid; Yield: 77%; The product was put into the next step without purification.
Methyl 3-(2-((3-nitrophenyl)amino)-9-(tetrahydro-2H-pyran-2-yl)-9H-purin-6-yl) benzoate (4h). Yellow solid; Yield: 40%; m.p.: 222–224°C; 1H NMR (400 MHz, DMSO-d6) δ 10.35 (d, J = 9.6 Hz, 1H), 9.41 (s, 1H), 9.31 (s, 1H), 9.11 (d, J = 6.7 Hz, 1H), 8.65 (d, J = 6.2 Hz, 1H), 8.17 (d, J = 6.5 Hz, 1H), 8.04 (d, J = 7.4 Hz, 1H), 7.85–7.68 (m, 2H), 7.67–7.54 (m, 1H), 5.75 (d, J = 10.1 Hz, 1H), 4.11 (d, J = 11.3 Hz, 1H), 3.75 (d, J = 9.5 Hz, 1H), 2.42 (d, J = 12.0 Hz, 1H), 2.08 (t, J = 11.5 Hz, 2H), 1.84–1.60 (m, 3H).
6-(4-fluorophenyl)-N-(3-nitrophenyl)-9-(tetrahydro-2H-pyran-2-yl)-9H-purin-2-amine (4i). Yellow solid; Yield: 69%; m.p.: 206–208°C; 1H NMR (400 MHz, DMSO-d6) δ 10.30 (s, 1H), 9.34 (s, 1H), 8.97–8.87 (m, 2H), 8.62 (s, 1H), 8.01 (d, J = 8.4 Hz, 1H), 7.82 (d, J = 8.1 Hz, 1H), 7.60 (t, J = 8.1 Hz, 1H), 7.47 (t, J = 8.4 Hz, 2H), 5.73 (d, J = 10.8 Hz, 1H), 4.10 (d, J = 11.3 Hz, 1H), 3.74 (d, J = 8.6 Hz, 1H), 2.47–2.34 (m, 1H), 2.08 (d, J = 11.4 Hz, 2H), 1.75 (s, 1H), 1.65 (s, 2H).
Methyl 4-(2-((3-nitrophenyl)amino)-9-(tetrahydro-2H-pyran-2-yl)-9H-purin-6-yl) benzoate (4k). Yellow oil; Yield: 41%; 1H NMR (400 MHz, DMSO-d6) δ 10.38 (s, 1H), 9.36 (s, 1H), 8.96 (d, J = 7.9 Hz, 2H), 8.66 (s, 1H), 8.19 (d, J = 8.0 Hz, 2H), 8.00 (d, J = 8.0 Hz, 1H), 7.82 (d, J = 8.1 Hz, 1H), 7.61 (t, J = 8.1 Hz, 1H), 5.74 (d, J = 10.9 Hz, 1H), 4.10 (d, J = 10.7 Hz, 1H), 3.92 (s, 3H), 3.74 (d, J = 9.5 Hz, 1H), 2.47–2.35 (m, 1H), 2.09 (d, J = 11.0 Hz, 2H), 1.83–1.59 (m, 3H).
Tert-butyl(3-(2-((3-nitrophenyl)amino)-9-(tetrahydro-2H-pyran-2-yl)-9H-purin-6-yl)phenyl)carbamate (10a). Yellow solid; Yield: 22%; m.p.: 192–194°C; 1H NMR (400 MHz, DMSO-d6) δ 10.25 (s, 1H), 9.54 (s, 1H), 9.23 (s, 1H), 8.90 (s, 1H), 8.60 (s, 1H), 8.44 (d, J = 7.7 Hz, 1H), 8.17 (d, J = 8.1 Hz, 1H), 7.81 (d, J = 8.1 Hz, 1H), 7.54 (m, J = 32.5, 16.0, 8.0 Hz, 3H), 5.73 (d, J = 10.9 Hz, 1H), 4.10 (d, J = 10.9 Hz, 1H), 3.75 (t, J = 10.8 Hz, 1H), 2.41 (dd, J = 20.7, 11.2 Hz, 1H), 2.07 (d, J = 9.6 Hz, 2H), 1.76 (d, J = 9.2 Hz, 1H), 1.64 (s, 2H), 1.50 (s, 9H).
Tert-butyl(4-(2-((3-nitrophenyl)amino)-9-(tetrahydro-2H-pyran-2-yl)-9H-purin-6-yl)phenyl)carbamate (10b). Yellow solid; Yield: 68%; m.p.: 220–222°C; 1H NMR (400 MHz, DMSO-d6) δ 10.22 (s, 1H), 9.74 (s, 1H), 9.41 (s, 1H), 8.80 (d, J = 8.2 Hz, 2H), 8.57 (s, 1H), 7.98 (d, J = 8.2 Hz, 1H), 7.80 (d, J = 8.0 Hz, 1H), 7.70 (d, J = 8.3 Hz, 2H), 7.59 (t, J = 8.2 Hz, 1H), 5.72 (d, J = 10.9 Hz, 1H), 4.09 (d, J = 11.5 Hz, 1H), 3.75 (t, J = 10.8 Hz, 1H), 2.39 (dd, J = 21.4, 10.8 Hz, 1H), 2.06 (d, J = 10.7 Hz, 2H), 1.84–1.60 (m, 3H), 1.51 (s, 9H).
Tert-butyl(3-(2-((3-nitrophenyl)amino)-9-(tetrahydro-2H-pyran-2-yl)-9H-purin-6-yl)benzyl)carbamate (10c). Yellow solid; Yield: 77%; m.p.: 163–165°C; 1H NMR (400 MHz, DMSO-d6) δ 10.29 (s, 1H), 9.36 (s, 1H), 8.75 (d, J = 7.8 Hz, 1H), 8.67 (s, 1H), 8.59 (s, 1H), 8.03 (d, J = 8.3 Hz, 1H), 7.81 (d, J = 8.0 Hz, 1H), 7.65–7.54 (m, 2H), 7.47 (d, J = 6.9 Hz, 2H), 5.74 (d, J = 11.0 Hz, 1H), 4.27 (d, J = 5.5 Hz, 2H), 4.10 (d, J = 11.4 Hz, 1H), 3.74 (d, J = 9.2 Hz, 1H), 2.40 (dd, J = 31.6, 20.3 Hz, 1H), 2.08 (d, J = 9.8 Hz, 2H), 1.73 (d, J = 14.4 Hz, 1H), 1.66 (d, J = 15.1 Hz, 2H), 1.35 (d, J = 40.1 Hz, 9H).
Tert-butyl(4-(2-((3-nitrophenyl)amino)-9-(tetrahydro-2H-pyran-2-yl)-9H-purin-6-yl)benzyl)carbamate (10d). Yellow solid; Yield: 53%; m.p.: 195–197°C; 1H NMR (400 MHz, DMSO-d6) δ 10.27 (s, 1H), 9.40 (s, 1H), 8.80 (d, J = 7.8 Hz, 2H), 8.61 (s, 1H), 7.99 (d, J = 8.1 Hz, 1H), 7.81 (d, J = 8.0 Hz, 1H), 7.60 (t, J = 7.9 Hz, 1H), 7.56–7.40 (m, 3H), 5.73 (d, J = 10.9 Hz, 1H), 4.25 (d, J = 5.8 Hz, 2H), 4.10 (d, J = 11.6 Hz, 1H), 3.75 (t, J = 8.7 Hz, 1H), 2.47–2.32 (m, 1H), 2.05 (t, J = 19.1 Hz, 2H), 1.69 (m, J = 38.5, 16.9 Hz, 3H), 1.38 (d, J = 36.5 Hz, 9H).
Tert-butyl(3-(2-((3-nitrophenyl)amino)-9-(tetrahydro-2H-pyran-2-yl)-9H-purin-6-yl)phenethyl)carbamate (10e). Yellow solid; Yield: 73%; m.p.: 104–106°C; 1H NMR (400 MHz, CDCl3) δ 9.52 (s, 1H), 8.69 (d, J = 7.3 Hz, 1H), 8.55 (s, 1H), 8.13 (s, 1H), 7.87 (d, J = 7.4 Hz, 1H), 7.60 (s, 1H), 7.47 (dd, J = 19.6, 7.2 Hz, 3H), 7.37 (d, J = 6.3 Hz, 1H), 5.79 (d, J = 9.8 Hz, 1H), 4.68 (s, 1H), 4.23 (d, J = 11.8 Hz, 1H), 3.89 (t, J = 11.4 Hz, 1H), 3.48 (s, 2H), 2.96 (s, 2H), 2.17 (dd, J = 19.4, 10.6 Hz, 3H), 1.85 (ddd, J = 36.7, 24.7, 12.0 Hz, 2H), 1.71 (d, J = 12.5 Hz, 1H), 1.43 (s, 9H).
Tert-butyl(3-(2-(phenylamino)-9-(tetrahydro-2H-pyran-2-yl)-9H-purin-6-yl)benzyl)carbamate (10f). Yellow solid; Yield: 62%; m.p.: 110–112°C; 1H NMR (400 MHz, DMSO-d6) δ 9.68 (s, 1H), 8.70 (d, J = 7.7 Hz, 1H), 8.64 (s, 1H), 8.51 (s, 1H), 7.93 (d, J = 7.9 Hz, 2H), 7.54 (dd, J = 15.2, 7.3 Hz, 2H), 7.44 (d, J = 7.3 Hz, 1H), 7.35 (t, J = 7.5 Hz, 2H), 6.96 (t, J = 7.2 Hz, 1H), 5.69 (d, J = 10.8 Hz, 1H), 4.25 (d, J = 5.7 Hz, 2H), 4.08 (d, J = 11.0 Hz, 1H), 3.70 (s, 1H), 2.39 (dd, J = 22.1, 10.9 Hz, 1H), 2.03 (d, J = 11.2 Hz, 2H), 1.77 (s, 1H), 1.63 (s, 2H), 1.49–1.17 (s, 9H).
Tert-butyl(3-(2-([1,1′-biphenyl]-4-ylamino)-9-(tetrahydro-2H-pyran-2-yl)-9H-purin-6-yl)benzyl)carbamate(10h). Yellow solid; Yield:77%; m.p.: 120; 1H NMR (400 MHz, DMSO-d6) δ 9.82 (s, 1H), 8.72 (d, J = 7.8 Hz, 1H), 8.65 (s, 1H), 8.53 (s, 1H), 8.04 (d, J = 8.1 Hz, 2H), 7.69 (d, J = 7.7 Hz, 4H), 7.60–7.41 (m, 5H), 7.31 (t, J = 7.0 Hz, 1H), 5.71 (d, J = 10.7 Hz, 1H), 4.26 (d, J = 5.6 Hz, 2H), 4.09 (d, J = 9.9 Hz, 1H), 3.73 (s, 1H), 2.42–2.32 (m, 1H), 2.05 (d, J = 11.9 Hz, 2H), 1.79 (s, 1H), 1.64 (s, 2H), 1.38 (s, 9H).
Tert-butyl(3-(9-(tetrahydro-2H-pyran-2-yl)-2-(p-tolylamino)-9H-purin-6-yl)benzyl)carbamate(10i). Yellow solid; Yield: 60; m.p.:106–108°C; 1H NMR (400 MHz, DMSO-d6) δ 9.57 (s, 1H), 8.68 (d, J = 7.6 Hz, 1H), 8.63 (s, 1H), 8.48 (s, 1H), 7.81 (d, J = 7.9 Hz, 2H), 7.53 (dd, J = 16.2, 8.2 Hz, 2H), 7.44 (d, J = 7.6 Hz, 1H), 7.15 (d, J = 7.9 Hz, 2H), 5.67 (d, J = 10.8 Hz, 1H), 4.25 (d, J = 5.7 Hz, 2H), 4.07 (d, J = 11.3 Hz, 1H), 3.72 (d, J = 13.1 Hz, 1H), 2.39 (dd, J = 22.0, 11.7 Hz, 1H), 2.28 (s, 3H), 2.02 (d, J = 11.0 Hz, 2H), 1.76 (s, 1H), 1.63 (s, 2H), 1.48–1.19 (s, 9H).
Tert-butyl(3-(2-((4-(tert-butyl)phenyl)amino)-9-(tetrahydro-2H-pyran-2-yl)-9H-purin-6-yl)benzyl)carbamate (10j). Oil; Yield: 64%; 1H NMR (400 MHz, DMSO-d6) δ 9.54 (s, 1H), 8.67 (d, J = 7.6 Hz, 1H), 8.64 (s, 1H), 8.47 (s, 1H), 7.84 (d, J = 8.0 Hz, 2H), 7.54 (t, J = 7.7 Hz, 1H), 7.44 (d, J = 7.4 Hz, 2H), 7.36 (d, J = 8.1 Hz, 2H), 5.69 (d, J = 10.9 Hz, 1H), 4.25 (d, J = 5.3 Hz, 2H), 4.07 (d, J = 11.2 Hz, 1H), 3.72 (s, 1H), 2.45–2.33 (m, 1H), 2.03 (d, J = 11.2 Hz, 2H), 1.78 (s, 1H), 1.63 (s, 2H), 1.40 (s, 9H), 1.30 (s, 9H).
Tert-butyl 4-(4-((6-(3-(((tert-butoxycarbonyl)amino)methyl)phenyl)-9-(tetrahydro-2H-pyran-2-yl)-9H-purin-2-yl)amino)phenyl)piperazine-1-carboxylate (10k). Yellow solid; Yield: 72%; m.p.: 120–124°C; 1H NMR (400 MHz, DMSO-d6) δ 9.45 (s, 1H), 8.67 (d, J = 7.3 Hz, 1H), 8.61 (s, 1H), 8.45 (s, 1H), 7.78 (d, J = 8.2 Hz, 2H), 7.49 (dt, J = 25.1, 7.7 Hz, 3H), 6.98 (d, J = 8.4 Hz, 2H), 5.66 (d, J = 11.0 Hz, 1H), 4.24 (d, J = 5.4 Hz, 2H), 4.07 (d, J = 11.0 Hz, 1H), 3.70 (s, 1H), 3.48 (s, 4H), 3.03 (s, 4H), 2.43–2.30 (m, 1H), 2.02 (d, J = 10.2 Hz, 2H), 1.76 (s, 1H), 1.63 (s, 2H), 1.35 (dd, J = 55.6, 18.5 Hz, 18H).
Tert-butyl(3-(2-((4-sulfamoylphenyl)amino)-9-(tetrahydro-2H-pyran-2-yl)-9H-purin-6-yl)benzyl)carbamate (10l). Yellow solid; Yield: 35%; m.p.: 145–147°C; 1H NMR (400 MHz, DMSO-d6) δ 10.12 (s, 1H), 8.76–8.62 (m, 2H), 8.57 (s, 1H), 8.09 (d, J = 8.3 Hz, 2H), 7.80 (d, J = 8.3 Hz, 2H), 7.57 (t, J = 7.6 Hz, 1H), 7.47 (t, J = 8.2 Hz, 2H), 7.16 (s, 2H), 5.73 (d, J = 10.7 Hz, 1H), 4.26 (d, J = 5.5 Hz, 2H), 4.09 (d, J = 11.2 Hz, 1H), 3.74 (s, 1H), 2.47–2.32 (m, 1H), 2.05 (d, J = 10.6 Hz, 2H), 1.80 (s, 1H), 1.65 (s, 2H), 1.36 (d, J = 36.9 Hz, 9H).
Tert-butyl(3-(2-((4-fluorophenyl)amino)-9-(tetrahydro-2H-pyran-2-yl)-9H-purin-6-yl)benzyl)carbamate (10n). Yellow solid; Yield: 52%; m.p.: 159–161°C; 1H NMR (400 MHz, DMSO-d6) δ 9.57 (s, 1H), 8.68 (d, J = 7.6 Hz, 1H), 8.63 (s, 1H), 8.48 (s, 1H), 7.81 (d, J = 7.9 Hz, 2H), 7.53 (dd, J = 16.2, 8.2 Hz, 2H), 7.44 (d, J = 7.6 Hz, 1H), 7.15 (d, J = 7.9 Hz, 2H), 5.67 (d, J = 10.8 Hz, 1H), 4.25 (d, J = 5.7 Hz, 2H), 4.07 (d, J = 11.3 Hz, 1H), 3.72 (d, J = 13.1 Hz, 1H), 2.39 (dd, J = 22.0, 11.7 Hz, 1H), 2.28 (s, 3H), 2.02 (d, J = 11.0 Hz, 2H), 1.76 (s, 1H), 1.63 (s, 2H), 1.48–1.19 (s, 9H).
Tert-butyl(3-(2-((4-(N-methylsulfamoyl)phenyl)amino)-9-(tetrahydro-2H-pyran-2-yl)-9H-purin-6-yl)benzyl)carbamate (10p). Yellow solid; Yield: 30%; 1H NMR (400 MHz, DMSO-d6) δ 10.21 (s, 1H), 8.70 (d, J = 7.5 Hz, 1H), 8.65 (s, 1H), 8.59 (s, 1H), 8.14 (d, J = 8.3 Hz, 2H), 7.76 (d, J = 8.3 Hz, 2H), 7.62–7.43 (m, 3H), 7.23 (d, J = 5.0 Hz, 1H), 5.74 (d, J = 10.9 Hz, 1H), 4.26 (d, J = 5.8 Hz, 2H), 4.08 (d, J = 11.3 Hz, 1H), 3.75 (s, 1H), 2.46–2.30 (m, 4H), 2.04 (dd, J = 24.5, 13.0 Hz, 2H), 1.80 (s, 1H), 1.64 (s, 2H), 1.40 (s, 9H).
Tert-butyl(3-(2-((4-(N,N-dimethylsulfamoyl)phenyl)amino)-9-(tetrahydro-2H-pyran-2-yl)-9H-purin-6-yl)benzyl)carbamate (10q). Yellow solid; Yield: 57%; m.p.: 123–125°C; 1H NMR (400 MHz, DMSO-d6) δ 10.27 (s, 1H), 8.71 (d, J = 7.7 Hz, 1H), 8.62 (d, J = 15.9 Hz, 2H), 8.20 (d, J = 8.1 Hz, 2H), 7.74 (d, J = 8.1 Hz, 2H), 7.57 (t, J = 7.6 Hz, 1H), 7.54–7.42 (m, 2H), 5.74 (d, J = 10.8 Hz, 1H), 4.25 (d, J = 5.5 Hz, 2H), 4.08 (d, J = 11.1 Hz, 1H), 3.74 (d, J = 10.8 Hz, 1H), 2.61 (s, 6H), 2.37 (dd, J = 22.3, 11.4 Hz, 1H), 2.05 (d, J = 10.0 Hz, 2H), 1.80 (s, 1H), 1.64 (s, 2H), 1.40 (s, 9H).
Tert-butyl(3-(2-(pyridin-3-ylamino)-9-(tetrahydro-2H-pyran-2-yl)-9H-purin-6-yl)benzyl)carbamate (10r). Yellow solid; Yield: 46%; 1H NMR (400 MHz, DMSO-d6) δ 9.89 (s, 1H), 9.01 (s, 1H), 8.67 (d, J = 7.6 Hz, 1H), 8.63 (s, 1H), 8.54 (s, 1H), 8.42 (d, J = 8.2 Hz, 1H), 8.17 (s, 1H), 7.56 (t, J = 7.5 Hz, 1H), 7.50 (s, 1H), 7.45 (d, J = 7.5 Hz, 1H), 7.40 (d, J = 6.4 Hz, 1H), 5.70 (d, J = 10.7 Hz, 1H), 4.25 (d, J = 5.7 Hz, 2H), 4.08 (d, J = 11.2 Hz, 1H), 3.71 (t, J = 8.4 Hz, 1H), 2.38 (dd, J = 22.4, 11.3 Hz, 1H), 2.04 (d, J = 10.4 Hz, 2H), 1.78 (m, 1H), 1.63 (m, 2H), 1.35 (s, 9H).
4.1.4 General Method for the Preparation of Compounds 5a-5f, 5h, 5i, 5k, 11a-11r
6-(4-(aminomethyl)phenyl)-N-(4-fluorophenyl)-9H-purin-2-amine hydrochloride (11o). Compounds 10o (1.0 mmol) were dissolved in HCl saturated ethyl acetate solution (15 ml) and stirred at room temperature for 4 h and then filtered to get compounds 11o. Light yellow solid; Yield: 90%; m.p.: >300°C; 1H NMR (400 MHz, DMSO-d6) δ 9.56 (s, 1H), 8.80 (d, J = 8.06 Hz, 2H), 8.36 (d, J = 14.57 Hz, 6H), 7.91–7.82 (m, 3H), 7.69 (d, J = 8.22 Hz, 3H), 7.16 (t, J = 8.90 Hz, 2H), 4.14 (q, J = 5.94 Hz, 3H), 4.10 (s, 16H).13C NMR (101 MHz, DMSO-d6) δ 155.46, 155.24, 152.79, 148.27, 144.12, 140.34, 137.29, 136.04, 129.84, 129.59, 125.56, 117.53, 42.49. HRMS (AP-ESI) m/z Calcd for C18H15FN6 [M + H]+ 335.1415, found: 335.1418.
Compounds 5a-5f, 5h, 5i, 5k, 11a-11n, and 11p-11r were synthesized following the procedure described above.
N-(3-nitrophenyl)-6-phenyl-9H-purin-2-amine (5a). Light yellow solid; Yield: 85%; m.p.: 201–203°C; 1H NMR (400 MHz, DMSO-d6) δ 10.18 (s, 1H), 9.18 (s, 1H), 8.80 (d, J = 7.1 Hz, 2H), 8.53 (s, 1H), 8.12 (d, J = 8.2 Hz, 1H), 7.80 (d, J = 8.0 Hz, 1H), 7.61 (dd, J = 12.3, 7.1 Hz, 4H); 13C NMR (101 MHz, DMSO-d6) δ 155.77, 155.21, 153.43, 148.72, 143.21, 143.07, 136.26, 131.34, 130.09, 129.82, 128.99, 126.03, 124.61, 115.39, 112.23. HRMS (AP-ESI) m/z Calcd for C17H12N6O2 [M + H]+ 333.1095, found: 333.1090.
6-(naphthalen-1-yl)-N-(3-nitrophenyl)-9H-purin-2-amine (5b). Light yellow solid. Yield: 92%; m.p.: >300°C; 1H NMR (400 MHz, DMSO-d6) δ 10.31 (s, 1H), 8.98 (s, 1H), 8.54 (s, 1H), 8.18 (dd, J = 20.0, 9.5 Hz, 3H), 8.07 (d, J = 8.0 Hz, 1H), 7.95 (d, J = 7.0 Hz, 1H), 7.77 (d, J = 8.3 Hz, 1H), 7.71 (t, J = 7.5 Hz, 1H), 7.56 (m, J = 15.1, 14.2, 7.2 Hz, 3H). 13C NMR (101 MHz, DMSO-d6) δ 156.27, 148.72, 143.93, 142.79, 133.86, 130.85, 130.67, 130.20, 129.36, 128.80, 127.23, 126.72, 126.19, 125.70, 124.73, 115.71, 112.46. HRMS (AP-ESI) m/z Calcd for C21H14N6O2 [M + H]+ 383.1251, found: 383.1250.
N-(3-nitrophenyl)-6-(1H-pyrrol-2-yl)-9H-purin-2-amine hydrochloride (5c). Yellow solid; Yield: 91%; m.p.: 236°C (Dec.); 1H NMR (400 MHz, DMSO-d6) δ 11.41 (s, 1H), 9.87 (s, 1H), 9.14 (s, 1H), 8.31 (s, 1H), 8.15 (d, J = 8.1 Hz, 1H), 7.77 (d, J = 8.0 Hz, 1H), 7.58 (t, J = 8.1 Hz, 1H), 7.42 (s, 1H), 7.20 (s, 1H), 6.35 (s, 1H); 13C NMR (101 MHz, DMSO-d6) δ 155.98, 154.38, 148.73, 147.23, 143.24, 142.59, 130.08, 128.69, 124.54, 124.45, 122.78, 115.11, 113.89, 112.32, 110.54. HRMS (AP-ESI) m/z Calcd for C15H11N7O2 [M + H]+ 322.1047, found: 322.1044.
6-(benzo[d][1,3]dioxol-5-yl)-N-(3-nitrophenyl)-9H-purin-2-amine (5d). Yellow solid; Yield: 97%; m.p.: 260°C (Dec.); 1H NMR (400 MHz, DMSO-d6) δ 10.12 (s, 1H), 9.14 (s, 1H), 8.75–8.44 (m, 2H), 8.33 (s, 1H), 8.08 (d, J = 8.2 Hz, 1H), 7.79 (d, J = 8.0 Hz, 1H), 7.59 (t, J = 8.1 Hz, 1H), 7.16 (d, J = 8.2 Hz, 1H), 6.17 (s, 2H); 13C NMR (101 MHz, DMSO-d6) δ 155.92, 154.27, 152.61, 150.62, 148.62, 148.32, 143.28, 142.42, 130.16, 128.88, 125.40, 124.89, 121.08, 115.92, 112.53, 109.00, 108.95, 102.33. HRMS (AP-ESI) m/z Calcd for C18H12N6O4 [M + H]+ 377.0993, found: 377.0993.
N-(3-nitrophenyl)-6-(thiophen-3-yl)-9H-purin-2-amine (5e). Yellow solid; Yield: 93%; m.p.:> 280°C; 1H NMR (400 MHz, DMSO-d6) δ 10.09 (s, 1H), 9.22 (s, 1H), 8.97 (s, 1H), 8.40 (s, 1H), 8.28 (d, J = 5.02 Hz, 1H), 8.09 (d, J = 8.04 Hz, 1H), 7.79 (d, J = 5.09 Hz, 2H), 7.59 (t, J = 8.13 Hz, 1H). 13C NMR (101 MHz, DMSO-d6) δ 155.89, 154.93, 149.24, 148.66, 143.55, 142.89, 138.35, 131.01, 130.14, 127.79, 127.55, 124.73, 115.54, 112.32. HRMS (AP-ESI) m/z Calcd for C15H10N6O2S [M + H]+ 339.0659, found: 339.0661.
N,6-bis(3-nitrophenyl)-9H-purin-2-amine (5f). Brown solid; Yield: 84%; m.p.: >300°C; 1H NMR (400 MHz, DMSO-d6) δ 13.44 (s, 1H), 10.29 (s, 1H), 9.79 (s, 1H), 9.34 (d, J = 7.7 Hz, 1H), 9.12 (s, 1H), 8.57–8.45 (m, 2H), 8.20 (d, J = 8.0 Hz, 1H), 7.98 (t, J = 8.0 Hz, 1H), 7.86 (d, J = 7.9 Hz, 1H), 7.66 (t, J = 8.1 Hz, 1H). 13C NMR (101 MHz, DMSO-d6) δ 155.81, 154.95, 152.96, 147.88, 144.25, 140.57, 135.49, 135.07, 132.55, 130.41, 129.76, 129.62, 125.58, 117.81, 42.73. HRMS (AP-ESI) m/z Calcd for C17H11N7O4 [M + H]+ 378.0945, found: 378.0964.
Methyl 3-(2-((3-nitrophenyl)amino)-9H-purin-6-yl)benzoate (5h). Light yellow solid; Yield: 53%; m.p.: 257°C; 1H NMR (400 MHz, DMSO-d6) δ 10.20 (s, 1H), 9.45 (s, 1H), 9.19–9.04 (m, 2H), 8.46 (s, 1H), 8.17 (d, J = 8.0 Hz, 2H), 7.84–7.77 (m, 2H), 7.60 (t, J = 8.1 Hz, 1H), 3.93 (s, 3H); 13C NMR (101 MHz, DMSO-d6) δ 166.51, 155.96, 155.30, 152.22, 148.69, 143.74, 142.84, 136.50, 134.22, 131.87, 130.66, 130.34, 130.15, 129.64, 124.81, 115.67, 112.48, 52.84. HRMS (AP-ESI) m/z Calcd for C19H14N6O4 [M + H]+ 391.1149, found: 391.1147.
6-(4-fluorophenyl)-N-(3-nitrophenyl)-9H-purin-2-amine (5i). Yellow solid; Yield: 97%; m.p.: 245–247°C; 1H NMR (400 MHz, DMSO-d6) δ 10.13 (s, 1H), 9.13 (s, 1H), 8.97–8.89 (m, 2H), 8.41 (s, 1H), 8.11 (d, J = 8.1 Hz, 1H), 7.79 (d, J = 7.9 Hz, 1H), 7.59 (t, J = 8.1 Hz, 1H), 7.46 (t, J = 8.3 Hz, 2H); 13C NMR (101 MHz, DMSO-d6) δ 165.58, 163.10, 155.97, 154.99, 152.26, 148.67, 143.51, 142.77, 132.21, 132.12, 130.17, 124.77, 116.26, 116.05, 115.67, 112.41. HRMS (AP-ESI) m/z Calcd for C17H11FN6O2 [M + H]+ 351.1000, found: 351.0998.
Methyl 4-(2-((3-nitrophenyl)amino)-9H-purin-6-yl)benzoate (5k). Light yellow solid; Yield: 95%; m.p.; 212–214°C; 1H NMR (400 MHz, DMSO-d6) δ 10.26 (s, 1H), 9.17 (s, 1H), 8.93 (d, J = 8.1 Hz, 2H), 8.57 (s, 1H), 8.18 (d, J = 8.0 Hz, 2H), 8.11 (d, J = 8.1 Hz, 1H), 7.80 (d, J = 7.9 Hz, 1H), 7.60 (t, J = 8.1 Hz, 1H), 3.92 (s, 3H). 13C NMR (101 MHz, DMSO-d6) δ 166.37, 155.88, 155.66, 151.78, 148.72, 144.04, 142.88, 140.37, 131.73, 130.15, 129.89, 129.76, 125.53, 124.73, 115.59, 112.35, 52.81. HRMS (AP-ESI) m/z Calcd for C19H14N6O4 [M + H]+ 391.1149, found: 391.1148.
6-(3-aminophenyl)-N-(3-nitrophenyl)-9H-purin-2-amine hydrochloride (11a). Yellow brown solid; Yield: 90%; mp: >300°C; 1H NMR (400 MHz, DMSO-d6) δ 13.16 (s, 1H), 10.05 (s, 1H), 9.16 (s, 1H), 8.34 (s, 1H), 8.17–8.09 (m, 2H), 8.05 (d, J = 7.7 Hz, 1H), 7.78 (d, J = 8.0 Hz, 1H), 7.58 (dd, J = 18.0, 9.8 Hz, 1H), 7.24 (t, J = 7.8 Hz, 1H), 6.78 (d, J = 7.8 Hz, 1H), 5.26 (s, 2H).
13C NMR (101 MHz, DMSO-d6) δ 155.67, 149.17, 148.74, 143.18, 136.84, 130.13, 129.36, 124.53, 117.78, 116.99, 115.26, 112.19. HRMS (AP-ESI) m/z Calcd for C17H13N7O2 [M + H]+ 348.1203, found: 348.1200.
6-(4-aminophenyl)-N-(3-nitrophenyl)-9H-purin-2-amine hydrochloride (11b). Yellow solid; Yield: 85%; m.p.: >300°C; 1H NMR (400 MHz, DMSO-d6) δ 10.46 (s, 1H), 9.13 (s, 1H), 8.74 (d, J = 7.7 Hz, 3H), 8.08 (d, J = 8.1 Hz, 1H), 7.83 (d, J = 8.1 Hz, 1H), 7.62 (t, J = 8.1 Hz, 1H), 7.32 (d, J = 7.7 Hz, 2H); 13C NMR (101 MHz, DMSO-d6) δ 155.36, 154.78, 151.97, 148.69, 143.66, 142.35, 131.41, 130.30, 124.96, 120.21, 116.05, 112.60, 40.60, 40.40, 40.19, 39.98, 39.77, 39.56, 39.35. HRMS (AP-ESI) m/z Calcd for C17H13N7O2 [M + H]+ 348.1203, found: 348.1205.
6-(3-(aminomethyl)phenyl)-N-(3-nitrophenyl)-9H-purin-2-amine hydrochloride (11c). White solid; Yield: 81%; m.p.: 236–238°C; 1H NMR (400 MHz, DMSO-d6) δ 10.20 (s, 1H), 9.29 (s, 1H), 8.84 (d, J = 31.7 Hz, 2H), 8.52 (s, 4H), 8.07 (d, J = 8.2 Hz, 1H), 7.81 (d, J = 8.1 Hz, 1H), 7.76 (s, 1H), 7.70 (t, J = 7.6 Hz, 1H), 7.61 (t, J = 8.1 Hz, 1H), 4.19 (d, J = 5.4 Hz, 2H); 13C NMR (101 MHz, DMSO-d6) δ 156.32, 152.77, 148.70, 143.88, 142.63, 135.05, 132.47, 130.37, 130.21, 129.83, 129.59, 124.92, 115.85, 112.51, 42.76. HRMS (AP-ESI) m/z Calcd for C18H15N7O2 [M + H]+ 362.1360, found: 362.1358.
6-(4-(aminomethyl)phenyl)-N-(3-nitrophenyl)-9H-purin-2-amine hydrochloride (11d). Light yellow solid; Yield: 92%; m.p.: >300°C; 1H NMR (400 MHz, DMSO-d6) δ 10.24 (s, 1H), 9.23 (s, 1H), 8.82 (d, J = 7.7 Hz, 2H), 8.63 (s, 4H), 8.08 (d, J = 8.1 Hz, 1H), 7.79 (dd, J = 16.3, 7.9 Hz, 3H), 7.60 (t, J = 8.1 Hz, 1H). 13C NMR (101 MHz, DMSO-d6) δ 156.07, 154.90, 152.60, 148.64, 143.76, 142.66, 137.67, 135.36, 130.16, 129.72, 129.68, 124.84, 122.81, 115.77, 112.43, 42.34. HRMS (AP-ESI) m/z Calcd for C18H15N7O2 [M + H]+ 362.1360, found: 362.1356.
6-(3-(2-aminoethyl)phenyl)-N-(3-nitrophenyl)-9H-purin-2-amine hydrochloride (11e). Yellow solid; Yield: 54%; mp: >230°C; 1H NMR (400 MHz, DMSO-d6) δ 10.24 (s, 1H), 9.21 (s, 1H), 8.77–8.52 (m, 3H), 8.19 (s, 3H), 8.11 (d, J = 8.2 Hz, 1H), 7.81 (d, J = 7.9 Hz, 1H), 7.61 (dd, J = 12.0, 7.7 Hz, 2H), 7.53 (d, J = 7.4 Hz, 1H), 3.23–3.00 (m, 4H); 13C NMR (101 MHz, DMSO-d6) δ 156.41, 154.21, 153.39, 153.02, 148.60, 146.95, 143.64, 142.37, 138.68, 138.57, 135.14, 132.34, 130.19, 129.84, 129.59, 128.16, 124.99, 116.04, 112.60, 40.25 33.35. HRMS (AP-ESI) m/z Calcd for C19H17N7O2 [M + H]+ 376.1516, found: 376.1520.
6-(3-(aminomethyl)phenyl)-N-phenyl-9H-purin-2-amine hydrochloride (11f). Light yellow solid; Yield: 92%; m.p.: 203–205°C; 1H NMR (400 MHz, DMSO-d6) δ 9.97 (s, 1H), 9.09 (s, 1H), 8.77 (s, 3H), 8.58 (s, 1H), 8.48 (d, J = 7.5 Hz, 1H), 7.87 (d, J = 7.8 Hz, 2H), 7.81 (d, J = 7.4 Hz, 1H), 7.69 (t, J = 7.5 Hz, 1H), 7.34 (t, J = 7.4 Hz, 2H), 7.00 (t, J = 7.1 Hz, 1H), 4.18 (d, J = 4.5 Hz, 2H); 13C NMR (101 MHz, DMSO-d6) δ 157.16, 154.55, 152.94, 143.13, 140.84, 135.06, 132.67, 130.59, 129.66, 129.44, 129.07, 122.15, 119.43, 42.69. HRMS (AP-ESI) m/z Calcd for C18H16N6 [M + H]+ 317.1509, found: 317.1507.
6-(4-(aminomethyl)phenyl)-N-phenyl-9H-purin-2-amine hydrochloride (11g). Light yellow solid; Yield: 92%; m.p.: 200–202°C; 1H NMR (400 MHz, DMSO-d6) δ 9.53 (s, 1H), 8.81 (d, J = 8.09 Hz, 2H), 8.36 (s, 1H), 7.87 (d, J = 8.05 Hz, 2H), 7.70 (d, J = 8.21 Hz, 2H), 7.32 (t, J = 7.85 Hz, 2H), 6.95 (t, J = 7.27 Hz, 1H), 4.15 (q, J = 5.90 Hz, 2H).13C NMR (101 MHz, DMSO-d6) δ 156.05, 155.04, 152.76, 144.44, 143.60, 137.54, 136.35, 135.56, 129.73, 129.66, 127.06, 123.46, 117.98, 42.38. HRMS (AP-ESI) m/z Calcd for C18H16N6 [M + H]+ 317.1509, found: 317.1507.
N-([1,1′-biphenyl]-4-yl)-6-(3-(aminomethyl)phenyl)-9H-purin-2-amine hydrochloride (11h). Yellow solid; Yield: 89%, m.p.: 210°C (Dec.); 1H NMR (400 MHz, DMSO-d6) δ 9.80 (s, 1H), 8.75 (d, J = 7.7 Hz, 2H), 8.57 (s, 1H), 8.49 (s, 3H), 8.00 (d, J = 7.8 Hz, 2H), 7.70 (dt, J = 13.3, 7.0 Hz, 6H), 7.45 (t, J = 7.2 Hz, 2H), 7.32 (t, J = 7.4 Hz, 1H), 4.18 (d, J = 5.2 Hz, 2H). 13C NMR (101 MHz, DMSO-d6) δ 157.13, 154.45, 153.07, 143.16, 140.41, 135.09, 133.75, 132.71, 130.59, 129.69, 129.49, 129.34, 128.34, 127.29, 127.22, 126.60, 124.40, 119.66, 42.69. HRMS (AP-ESI) m/z Calcd for C24H20N6 [M + H]+ 393.1822, found: 393.1826.
6-(3-(aminomethyl)phenyl)-N-(p-tolyl)-9H-purin-2-amine hydrochloride (11i). Light yellow solid, Yield: 90%; m.p.: >300°C; 1H NMR (400 MHz, DMSO-d6) δ 9.85 (s, 1H), 9.03 (s, 1H), 8.72 (s, 3H), 8.59 (s, 1H), 8.50 (d, J = 7.5 Hz, 1H), 7.73 (dq, J = 23.2, 7.6 Hz, 4H), 7.16 (d, J = 7.9 Hz, 2H), 4.18 (d, J = 5.0 Hz, 2H), 2.29 (s, 3H). 13C NMR (101 MHz, DMSO-d6) δ 157.20, 154.70, 152.84, 143.01, 138.28, 135.16, 135.03, 132.59, 131.02, 130.57, 129.65, 129.48, 119.57, 60.23, 42.72.HRMS (AP-ESI) m/z Calcd for C19H18N6 [M + H]+ 331.1666, found: 331.1667.
6-(3-(aminomethyl)phenyl)-N-(4-(tert-butyl)phenyl)-9H-purin-2-amine hydrochloride (11j). Yellow solid; Yield: 64%; 260°C (Dec.); 1H NMR (400 MHz, DMSO-d6) δ 9.50 (s, 1H), 8.78–8.69 (m, 2H), 8.48 (d, J = 9.8 Hz, 4H), 7.77 (d, J = 7.9 Hz, 2H), 7.74–7.65 (m, 2H), 7.34 (d, J = 8.0 Hz, 2H), 4.16 (d, J = 5.2 Hz, 3H), 1.31 (d, J = 12.0 Hz, 9H); 13C NMR (101 MHz, DMSO-d6) δ 157.29, 154.55, 152.88, 144.65, 143.05, 138.07, 135.06, 134.95, 132.70, 130.59, 129.68, 129.42, 125.68, 119.45, 42.68, 34.42, 31.78. HRMS (AP-ESI) m/z Calcd for C22H24N6 [M + H]+ 373.2135, found: 373.213.
6-(3-(aminomethyl)phenyl)-N-(4-(piperazin-1-yl)phenyl)-9H-purin-2-amine hydrochloride (11k). Yellow solid; Yield: 91%; m.p.: >300°C; 1H NMR (400 MHz, DMSO-d6) δ 9.68 (s, 1H), 9.42 (s, 2H), 8.78 (s, 1H), 8.69–8.53 (m, 4H), 7.83–7.72 (m, 3H), 7.68 (t, J = 7.7 Hz, 1H), 7.08 (d, J = 8.3 Hz, 2H), 4.17 (d, J = 5.2 Hz, 2H), 3.27 (s, 4H), 1.99 (s, 4H). 13C NMR (101 MHz, DMSO-d6) δ 157.79, 156.51, 154.95, 152.82, 142.99, 134.96, 132.41, 130.49, 129.62, 129.52, 120.64, 117.93, 47.38, 42.79. HRMS (AP-ESI) m/z Calcd for C22H24N8 [M + H]+ 401.2197, found: 401.2193.
4-((6-(3-(aminomethyl)phenyl)-9H-purin-2-yl)amino)benzenesulfonamide hydrochloride (11l). Yellow solid; Yield: 96%;1H NMR (400 MHz, DMSO-d6) δ 10.04 (s, 2H), 8.79 (s, 3H), 8.73–8.59 (m, 1H), 8.50 (d, J = 5.42 Hz, 2H), 8.44 (s, 7H), 8.09–8.02 (m, 4H), 7.82–7.63 (m, 7H), 7.20 (s, 3H), 4.18 (d, J = 6.00 Hz, 4H), 4.03 (t, J = 6.87 Hz, 1H), 2.00 (d, J = 1.84 Hz, 1H), 1.26–1.14 (m, 1H). 13C NMR (101 MHz, DMSO-d6) δ 156.14, 152.84, 144.57, 143.72, 136.21, 134.93, 132.10, 130.39, 129.81, 129.53, 127.11, 117.89, 42.89. HRMS (AP-ESI) m/z Calcd for C18H17N7O2S [M + H]+ 396.1237, found: 396.1234.
N1-(6-(3-(aminomethyl)phenyl)-9H-purin-2-yl)benzene-1,3-diamine dihydrochloride (11m). Yellow solid; Yield: 96%; m.p.: >300°C; 1H NMR (400 MHz, DMSO-d6) δ 10.52 (s, 2H), 9.95 (s, 1H), 8.84 (d, J = 6.78 Hz, 1H), 8.73 (s, 1H), 8.54 (s, 3H), 8.22 (s, 1H), 7.73 (ddd, J = 25.27, 17.03, 7.59 Hz, 3H), 7.43 (t, J = 8.13 Hz, 1H), 6.99 (d, J = 7.67 Hz, 1H). 13C NMR (101 MHz, DMSO-d6) δ 156.47, 152.82, 143.75, 142.57, 135.93, 135.01, 132.49, 132.38, 130.37, 130.26, 130.15, 129.55, 118.43, 115.95, 113.36, 42.78. HRMS (AP-ESI) m/z Calcd for C18H17N7 [M + H]+ 332.1618, found: 332.1621.
6-(3-(aminomethyl)phenyl)-N-(4-fluorophenyl)-9H-purin-2-amine hydrochloride (11n). Light yellow solid; Yield: 99%; m.p.: >300°C; 1H NMR (400 MHz, DMSO-d6) δ 9.93 (s, 1H), 8.99 (s, 1H), 8.72 (s, 3H), 8.59 (s, 1H), 8.52 (d, J = 7.3 Hz, 1H), 7.87 (dd, J = 7.6, 5.3 Hz, 3H), 7.79 (d, J = 7.4 Hz, 1H), 7.69 (t, J = 7.7 Hz, 2H), 7.20 (t, J = 8.5 Hz, 3H), 4.18 (d, J = 5.2 Hz, 2H); 13C NMR (101 MHz, DMSO-d6) δ 158.88, 157.13, 156.51, 154.70, 153.03, 143.05, 137.39, 135.37, 135.02, 132.53, 130.49, 129.62, 129.52, 121.07, 120.99, 115.68, 115.46, 60.23, 42.72. HRMS (AP-ESI) m/z Calcd for C18H15FN6 [M + H]+ 335.1415, found: 335.1418.
4-((6-(3-(aminomethyl)phenyl)-9H-purin-2-yl)amino)-N-methylbenzenesulfonamide hydrochloride (11p). Yellow solid; Yield: 91%, m.p.: >300°C; 1H NMR (400 MHz, DMSO-d6) δ 10.16 (s, 1H), 8.77–8.71 (m, 1H), 8.63 (s, 0H), 8.56 (s, 1H), 8.14–8.07 (m, 1H), 7.73 (dt, J = 14.44, 7.08 Hz, 2H), 4.74 (s, 9H), 4.18 (q, J = 5.95 Hz, 1H), 2.41 (s, 3H). 13C NMR (101 MHz, DMSO-d6) δ 156.04, 152.84, 145.17, 136.21, 134.93, 132.09, 130.79, 130.35, 129.88, 129.55, 128.28, 118.04, 42.89, 29.18. HRMS (AP-ESI) m/z Calcd for C19H19N7O2S [M + H]+ 409.4680, found: 409.4675.
4-((6-(3-(aminomethyl)phenyl)-9H-purin-2-yl)amino)-N,N-dimethylbenzene sulfonamide hydrochloride (11q). Yellow solid; Yield: 91%, m.p.: >300°C; 1H NMR (400 MHz, DMSO-d6) δ 10.34 (s, 1H), 8.86 (s, 1H), 8.67 (t, J = 11.0 Hz, 5H), 8.16 (d, J = 8.1 Hz, 2H), 7.86–7.67 (m, 4H), 4.19 (d, J = 5.2 Hz, 2H), 2.61 (s, 6H). 13C NMR (101 MHz, DMSO-d6) δ 156.20, 155.13, 152.97, 145.64, 143.91, 135.76, 135.03, 132.38, 130.39, 129.77, 129.62, 129.24, 126.10, 118.14, 42.78, 38.18. HRMS (AP-ESI) m/z Calcd for C20H21N7O2S [M + H]+ 424.1550, found: 424.1554.
6-(3-(aminomethyl)phenyl)-N-(4-(piperazin-1-yl)phenyl)-9H-purin-2-amine dihydrochloride (11r). Yellow solid; Yield: 49%; m.p.: >300°C; 1H NMR (400 MHz, DMSO-d6) δ 10.70 (s, 1H), 9.73 (s, 1H), 8.86 (d, J = 7.1 Hz, 1H), 8.72 (s, 1H), 8.67 (d, J = 8.6 Hz, 1H), 8.54 (d, J = 7.2 Hz, 4H), 8.08–8.01 (m, 1H), 7.75 (d, J = 7.3 Hz, 1H), 7.69 (t, J = 7.6 Hz, 1H), 4.24 (d, J = 5.4 Hz, 2H). 13C NMR (101 MHz, DMSO-d6) δ 152.82, 142.99, 134.96, 132.41, 130.49, 129.62, 129.52, 120.64, 117.93, 47.38, 42.79.HRMS (AP-ESI) m/z Calcd for C17H15N7 [M + H]+ 318.1462, found: 318.1457.
4.1.5 General Method for the Preparation of Compounds 5g, 5j
3-(2-((3-nitrophenyl)amino)-9H-purin-6-yl)benzoic acid (5g). To a solution of compound 5h (0.48 mmol) in THF/H2O solution (4:1, 10 ml), LiOH (1.5 mmol) was added, and the mixture was stirred at rt for 4 h. The mixture was adjusted to around pH 2 with HCl solution (2M), and the mixture was extracted twice with ethyl acetate (50 ml), washed with brine, and dried with anhydrous Mg2SO4. The solution was concentrated to get compound 5g. White solid; Yield: 69%; m.p.:>300°C; 1H NMR (400 MHz, DMSO-d6) δ 13.30 (s, 1H), 13.15 (s, 1H), 10.19 (s, 1H), 9.51 (s, 1H), 9.11 (d, J = 7.8 Hz, 1H), 9.08 (s, 1H), 8.42 (s, 1H), 8.16 (dd, J = 16.5, 7.9 Hz, 2H), 7.80 (d, J = 8.0 Hz, 1H), 7.75 (t, J = 7.6 Hz, 1H), 7.60 (t, J = 8.1 Hz, 1H). 13C NMR (101 MHz, DMSO-d6) δ 167.63, 155.84, 155.28, 152.52, 148.72, 143.46, 142.97, 136.56, 133.80, 131.96, 131.76, 131.12, 130.84, 130.14, 129.38, 126.06, 124.70, 115.53, 112.38. HRMS (AP-ESI) m/z Calcd for C18H12N6O4 [M + H]+ 377.0993, found: 377.0998.
Compound 5j was synthesized following the procedure described above.
4-(2-((3-nitrophenyl)amino)-9H-purin-6-yl)benzoic acid (5j).Yellow solid; Yield: 97%; m.p.: >300°C; 1H NMR (400 MHz, DMSO-d6) δ 13.31 (s, 2H), 10.21 (s, 1H), 10.17 (s, 2H), 9.17 (s, 2H), 9.00 (t, J = 8.00 Hz, 4H), 8.42 (s, 2H), 8.17 (t, J = 7.15 Hz, 4H), 8.11 (d, J = 8.38 Hz, 2H), 7.80 (d, J = 8.20 Hz, 2H), 7.60 (t, J = 8.27 Hz, 2H), 7.56–7.47 (m, 1H). 13C NMR (101 MHz, DMSO-d6) δ 166.40, 155.80, 155.55, 148.74, 143.86, 142.94, 140.53, 131.68, 130.68, 130.16, 129.90, 129.76, 124.70, 115.54, 112.31, 52.81. HRMS (AP-ESI) m/z Calcd for C18H12N6O4 [M + H]+ 377.0993, found: 377.0998.
4.1.6 General Method for the Preparation of Compounds 7a-7e
Tert-butyl (4-bromophenethyl)carbamate (7d). Compounds 6d (2 mmol) and Di-tert-butyl dicarbonate (2.4 mmol) were dissolved in dichloromethane (25 ml). K2CO3 (6 mmol) was added and stirred for 4 h at room temperature. After the completion, the reaction mixture is extracted with ethyl acetate (15 ml x 3), washed with water, 1M citric acid solution, and brine, and dried with anhydrous Mg2SO4. The crude product were concentrated and purified by silica gel chromatography to obtain compound 7d. Oil; Yield: 90%; m.p.: 44–46°C; 1H NMR (400 MHz, CDCl3) δ 7.36 (d, J = 7.7 Hz, 2H), 7.20–7.10 (m, 2H), 4.56 (s, 1H), 3.36 (d, J = 6.2 Hz, 2H), 2.77 (t, J = 6.5 Hz, 2H), 1.44 (s, 9H).
Compounds 7a-7c and 7e were synthesized following the procedure described above.
Tert-butyl (3-bromophenyl)carbamate (7a). White solid; Yield: 70%; m.p.: 85–87°C; 1H NMR (400 MHz, CDCl3) δ 7.67 (s, 1H), 7.21 (d, J = 7.1 Hz, 1H), 7.18–7.10 (m, 2H), 6.49 (s, 1H), 1.52 (s, 9H).
Tert-butyl (4-bromophenyl)carbamate (7b). White solid; Yield: 77%; m.p.: 62–64°C; 1H NMR (400 MHz, CDCl3) δ 7.39 (d, J = 8.3 Hz, 2H), 7.25 (d, J = 7.2 Hz, 2H), 6.46 (s, 1H), 1.51 (s, 9H).
Tert-butyl (3-bromobenzyl)carbamate (7c). White solid; Yield: 60%; m.p.: 55–57°C; 1H NMR (400 MHz, CDCl3) δ 7.43 (s, 1H), 7.39 (d, J = 6.4 Hz, 1H), 7.20 (d, J = 6.0 Hz, 2H), 4.86 (s, 1H), 4.29 (d, J = 5.1 Hz, 2H), 1.46 (s, 9H).
Tert-butyl (3-bromophenethyl)carbamate (7e). White solid; Yield: 100%; m.p.: 44–46°C; 1H NMR (400 MHz, CDCl3) δ 7.36 (d, J = 7.7 Hz, 2H), 7.20–7.10 (m, 2H), 4.56 (s, 1H), 3.36 (d, J = 6.2 Hz, 2H), 2.77 (t, J = 6.5 Hz, 2H), 1.44 (s, 9H).
4.1.7 General Method for the Preparation of Compounds 8a-8e
Tert-butyl(4-(4,4,5,5-tetramethyl-1,3,2-dioxaborolan-2-yl)benzyl)carbamate (8d). Compound 7d (4.5 mmol), bis(pinacolato)diboron (4.5 mmol), Pd (dppf)2Cl2 (0.05 mmol), and KOAc (13.5 mmol) were mixed in a two-neck flask. Under the protection of N2, anhydrous DMSO (10 ml) was added and the mixture reacted at 80°C for 12 h. After the completion, the reaction mixture was filtered through a pad of Celite. Spinned the filtrate dry and then dissolved it with ethyl acetate (15 ml) and water (20 ml), extracted twice with ethyl acetate (50 ml), washed with brine, and dried with anhydrous Mg2SO4. The crude product was concentrated and purified by silica gel chromatography to obtain compounds 8d. Oil; Yield: 90%; 1H NMR (400 MHz, CDCl3) δ 7.83 (d, J = 7.4 Hz, 2H), 7.09 (d, J = 7.4 Hz, 2H), 2.56 (s, 2H), 1.37 (s, 9H), 1.34 (s, 12H).
Compounds 8a-8c and 8e were synthesized following the procedure described above.
Tert-butyl (3-(4,4,5,5-tetramethyl-1,3,2-dioxaborolan-2-yl)phenyl)carbamate (8a). White solid; Yield: 93%; m.p.: 108–110°C; 1H NMR (400 MHz, CDCl3) δ 7.61 (s, 2H), 7.47 (d, J = 7.2 Hz, 1H), 7.31 (t, J = 7.8 Hz, 1H), 6.46 (s, 1H), 1.51 (s, 9H), 1.33 (s, 12H).
Tert-butyl (4-(4,4,5,5-tetramethyl-1,3,2-dioxaborolan-2-yl)phenyl)carbamate (8b). White solid; Yield: 63%. The product is put into the next step without purification.
Tert-butyl (3-(4,4,5,5-tetramethyl-1,3,2-dioxaborolan-2-yl)benzyl)carbamate (8c). White solid; Yield:70%; 1H NMR (400 MHz, DMSO-d6) δ 7.57 (s, 1H), 7.52 (d, J = 6.4 Hz, 1H), 7.40 (s, 1H), 7.34 (t, J = 8.1 Hz, 2H), 4.12 (d, J = 5.8 Hz, 2H), 1.39 (s, 9H), 1.29 (s, 12H).
Tert-butyl (3-(4,4,5,5-tetramethyl-1,3,2-dioxaborolan-2-yl)phenethyl)carbamate (8e). Oil; Yield: 58%; 1H NMR (400 MHz, CDCl3) δ 7.67 (d, J = 6.6 Hz, 1H), 7.64 (s, 1H), 7.38–7.28 (m, 2H), 4.52 (s, 1H), 3.38 (d, J = 6.1 Hz, 2H), 2.80 (t, J = 6.6 Hz, 2H), 1.43 (s, 9H), 1.35 (s, 12H).
4.2 Cyclin-Dependent Kinases Inhibition Test
Experiments were carried out using the Kinase-Glo® Luminescent Kinase Assays as described previously (Kashem et al., 2007). Briefly, all enzymatic reactions were conducted at 30°C for 40 min. The 50 µl reaction mixture contains 40 mM Tris, pH 7.4, 10 mM MgCl2, 0.1 mg/ml BSA, 1 mM DTT, 10 µM ATP, 0.2 μg/ml CDKs, and 100 μM lipid substrate. The compounds were diluted with 10% DMSO and then 5 µl of the dilution was removed and put into the subsequent reaction. The kinase activities were measured by detecting the content of remaining ATP. The luminescent signal was correlated with the amount of residual ATP and negatively correlated with the amount of kinase activity. The IC50 values were calculated using Prism GraphPad software.
4.3 Anti-proliferation Test
Standard MTT (thiazolyl blue; 3-[4,5-dimethylthiazol-2-yl]-2,5-diphenyltetrazolium bromide) assays were performed as 5 mg/ml. Briefly, MDA-MB-231 or 293T cells were seeded into 96-well plates and incubated for 24 h at 37°C. All compounds were dissolved in DMSO, and a gradient dilution series were prepared in 100 μl of cell medium, added to cells (in triplicates), and incubated for 48 h at 37°C with 5% CO2. MTT was added (5 mg/ml, 20 μl) to each plate and these mixtures were incubated for another 4 h. Then, the medium was removed, and the mixture was completely dissolved in DMSO (200 μL) after shaking for 10 min. The absorbance was recorded at 490 nm (detection wavelength) and 630 nm (reference wavelength) and inhibition rates were calculated to determine IC50 values.
4.4 Cell Cycles
MDA-MB-231 cells were seeded in six-well plates and incubated with 20 μM compounds 11c, 11l, 11p, and vehicle (0.2% DMSO) for 24 h. Subsequently, cells were centrifugated and washed with cold PBS buffer. After the centrifugation, the supernatants were removed, and the cells were resuspended in PBS buffer. Then, 10 μl of PI were added and the cells were incubated in the dark for 15 min at room temperature. The stained cells were analyzed by a flow cytometer (BD Accuri C6).
4.5 Molecular Dynamics Simulation
Based on the crystal structure of CDK2–inhibitor complex (PDB: 5NEV), we performed molecular docking used by AutoDock Vina to obtain the initial structure complex for molecular dynamics simulation. Molecular dynamics simulations of CDK2-11l complex were carried out employing Amber16 package. The Amber14SB force field was used for proteins, and the TIP3P model was used for water molecules. The partial charge of 11l was assigned using AM1-BCC methods via antechamber. The system was neutralized with Cl-counterions and solvated in a rectangular periodic box with explicit TIP3P water using AmberTools17. The solvation system consists of ∼30,000 atoms. The Particle-mesh Ewald method for nonbonded interactions is used for MD simulation. After a series of minimization and equilibration, standard molecular dynamics simulations were performed on the GPU using the CUDA version of PMEMD (Particle Mesh Ewald Molecular Dynamics) for 50 ns with periodic boundary conditions. The SHAKE algorithm is used to constrain all the bonds involving hydrogen atoms. A time step of 2 fs was used and the system temperature was controlled at 300K using the Berendsen thermostat method. The snapshots were saved every 10 ps for analysis. All other parameters are default.
Data Availability Statement
The original contributions presented in the study are included in the article/Supplementary Material, further inquiries can be directed to the corresponding authors.
Author Contributions
HL and YZ performed synthetic work and wrote the manuscript. ZZ designed target compound and performed molecular docking and molecular dynamics simulation. JD performed in vitro biological experiments. XY performed data analysis. XH and HF designed and supervised the study, revised manuscript and provided materials. HL, YZ, and ZZ contributed equally to this work.
Funding
This work was supported by the National Natural Science Foundation of China (81874288, 82003590, and 92053105), the Natural Science Foundation of Shandong Province (ZR2019LZL004 and ZR2020QH342), and the Young Scholars Program of Shandong University.
Conflict of Interest
The authors declare that the research was conducted in the absence of any commercial or financial relationships that could be construed as a potential conflict of interest.
Publisher’s Note
All claims expressed in this article are solely those of the authors and do not necessarily represent those of their affiliated organizations, or those of the publisher, the editors and the reviewers. Any product that may be evaluated in this article, or claim that may be made by its manufacturer, is not guaranteed or endorsed by the publisher.
References
Adams, P. D. (2001). Regulation of the Retinoblastoma Tumor Suppressor Protein by Cyclin/cdks. Biochim. Biophys. Acta 1471, M123–M133. doi:10.1016/s0304-419x(01)00019-1
Aleem, E., Berthet, C., and Kaldis, P. (2004). Cdk2 as a Master of S Phase Entry: Fact or Fake? Cell Cycle 3, 35–37. doi:10.4161/cc.3.1.632
Braal, C. L., Jongbloed, E. M., Wilting, S. M., Mathijssen, R. H. J., Koolen, S. L. W., and Jager, A. (2021). Inhibiting CDK4/6 in Breast Cancer with Palbociclib, Ribociclib, and Abemaciclib: Similarities and Differences. Drugs 81, 317–331. doi:10.1007/s40265-020-01461-2
Chohan, T. A., Qian, H., Pan, Y., and Chen, J. Z. (2015). Cyclin-Dependent Kinase-2 as a Target for Cancer Therapy: Progress in the Development of CDK2 Inhibitors as Anti-cancer Agents. Curr. Med. Chem. 22, 237–263. doi:10.2174/0929867321666141106113633
Coxon, C. R., Anscombe, E., Harnor, S. J., Martin, M. P., Carbain, B., Golding, B. T., et al. (2017). Cyclin-Dependent Kinase (CDK) Inhibitors: Structure-Activity Relationships and Insights into the CDK-2 Selectivity of 6-Substituted 2-Arylaminopurines. J. Med. Chem. 60, 1746–1767. doi:10.1021/acs.jmedchem.6b01254
Deans, A. J., Khanna, K. K., Mcnees, C. J., Mercurio, C., Heierhorst, J., and Mcarthur, G. A. (2006). Cyclin-dependent Kinase 2 Functions in normal DNA Repair and Is a Therapeutic Target in BRCA1-Deficient Cancers. Cancer Res. 66, 8219–8226. doi:10.1158/0008-5472.CAN-05-3945
Fagundes, R., and Teixeira, L. K. (2021). Cyclin E/CDK2: DNA Replication, Replication Stress and Genomic Instability. Front. Cel. Dev. Biol. 9, 774845. doi:10.3389/fcell.2021.774845
Fry, D. W., Harvey, P. J., Keller, P. R., Elliott, W. L., Meade, M., Trachet, E., et al. (2004). Specific Inhibition of Cyclin-dependent Kinase 4/6 by PD 0332991 and Associated Antitumor Activity in Human Tumor Xenografts. Mol. Cancer Ther. 3, 1427–1438. doi:10.1158/1535-7163.1427.3.11
Golsteyn, R. M. (2005). Cdk1 and Cdk2 Complexes (Cyclin Dependent Kinases) in Apoptosis: a Role beyond the Cell Cycle. Cancer Lett. 217, 129–138. doi:10.1016/j.canlet.2004.08.005
Gomatou, G., Trontzas, I., Ioannou, S., Drizou, M., Syrigos, N., and Kotteas, E. (2021). Mechanisms of Resistance to Cyclin-dependent Kinase 4/6 Inhibitors. Mol. Biol. Rep. 48, 915–925. doi:10.1007/s11033-020-06100-3
Hydbring, P., and Larsson, L. G. (2010). Cdk2: a Key Regulator of the Senescence Control Function of Myc. Aging (Albany NY) 2, 244–250. doi:10.18632/aging.100140
Jin, X., Ge, L. P., Li, D. Q., Shao, Z. M., Di, G. H., Xu, X. E., et al. (2020). LncRNA TROJAN Promotes Proliferation and Resistance to CDK4/6 Inhibitor via CDK2 Transcriptional Activation in ER+ Breast Cancer. Mol. Cancer 19, 87. doi:10.1186/s12943-020-01210-9
Joaquin, M., and Watson, R. J. (2003). Cell Cycle Regulation by the B-Myb Transcription Factor. Cell Mol. Life Sci. 60, 2389–2401. doi:10.1007/s00018-003-3037-4
Julve, M., Clark, J. J., and Lythgoe, M. P. (2021). Advances in Cyclin-dependent Kinase Inhibitors for the Treatment of Melanoma. Expert Opin. Pharmacother. 22, 351–361. doi:10.1080/14656566.2020.1828348
Kashem, M. A., Nelson, R. M., Yingling, J. D., Pullen, S. S., Prokopowicz, A. S., Jones, J. W., et al. (2007). Three Mechanistically Distinct Kinase Assays Compared: Measurement of Intrinsic ATPase Activity Identified the Most Comprehensive Set of ITK Inhibitors. J. Biomol. Screen. 12, 70–83. doi:10.1177/1087057106296047
Kimball, S. D., and Webster, K. R. (2001). Chapter 14. Cell Cycle Kinases and Checkpoint Regulation in Cancer. Annu. Rep. Med. Chem. Vol. 36, 139–148. ed. A.M. Doherty. doi:10.1016/s0065-7743(01)36054-2
Lee, K. A., Shepherd, S. T., and Johnston, S. R. (2019). Abemaciclib, a Potent Cyclin-dependent Kinase 4 and 6 Inhibitor, for Treatment of ER-Positive Metastatic Breast Cancer. Future Oncol. 15, 3309–3326. doi:10.2217/fon-2019-0169
Liu, F. (2006). Smad3 Phosphorylation by Cyclin-dependent Kinases. Cytokine Growth Factor. Rev. 17, 9–17. doi:10.1016/j.cytogfr.2005.09.010
Lu, M. J., Li, W., Yang, X. Y., and Fang, H. (2019). Synthesis and Antitumor Activity of N9 Position Aromatic Substituted Purine-8-One Derivatives. Chem. J. Chin. Universities 40, 254–261. doi:10.7503/cjcu20180573
Meijer, L., Borgne, A., Mulner, O., Chong, J. P., Blow, J. J., Inagaki, N., et al. (1997). Biochemical and Cellular Effects of Roscovitine, a Potent and Selective Inhibitor of the Cyclin-dependent Kinases Cdc2, Cdk2 and Cdk5. Eur. J. Biochem. 243, 527–536. doi:10.1111/j.1432-1033.1997.t01-2-00527.x
Narasimha, A. M., Kaulich, M., Shapiro, G. S., Choi, Y. J., Sicinski, P., and Dowdy, S. F. (2014). Cyclin D Activates the Rb Tumor Suppressor by Mono-Phosphorylation. Elife 3, e02872. doi:10.7554/eLife.02872
Nie, L., Wei, Y., Zhang, F., Hsu, Y. H., Chan, L. C., Xia, W., et al. (2019a). CDK2-mediated Site-specific Phosphorylation of EZH2 Drives and Maintains Triple-Negative Breast Cancer. Nat. Commun. 10, 5114. doi:10.1038/s41467-019-13105-5
Nie, L., Wei, Y., Zhang, F., Hsu, Y. H., Chan, L. C., Xia, W., et al. (2019b). CDK2-mediated Site-specific Phosphorylation of EZH2 Drives and Maintains Triple-Negative Breast Cancer. Nat. Commun. 10, 5114. doi:10.1038/s41467-019-13105-5
Pandey, K., An, H. J., Kim, S. K., Lee, S. A., Kim, S., Lim, S. M., et al. (2019). Molecular Mechanisms of Resistance to CDK4/6 Inhibitors in Breast Cancer: A Review. Int. J. Cancer 145, 1179–1188. doi:10.1002/ijc.32020
Pierson-Mullany, L. K., and Lange, C. A. (2004). Phosphorylation of Progesterone Receptor Serine 400 Mediates Ligand-independent Transcriptional Activity in Response to Activation of Cyclin-dependent Protein Kinase 2. Mol. Cel Biol. 24, 10542–10557. doi:10.1128/MCB.24.24.10542-10557.2004
Rao, S. S., Stoehr, J., Dokic, D., Wan, L., Decker, J. T., Konopka, K., et al. (2017). Synergistic Effect of Eribulin and CDK Inhibition for the Treatment of Triple Negative Breast Cancer. Oncotarget 8, 83925–83939. doi:10.18632/oncotarget.20202
Rice, A. P. (2019). Roles of CDKs in RNA Polymerase II Transcription of the HIV-1 Genome. Transcription 10, 111–117. doi:10.1080/21541264.2018.1542254
Roufayel, R., and Murshid, N. (2019). CDK5: Key Regulator of Apoptosis and Cell Survival. Biomedicines 7, 88. doi:10.3390/biomedicines7040088
Sánchez-Martínez, C., Lallena, M. J., Sanfeliciano, S. G., and De Dios, A. (2019). Cyclin Dependent Kinase (CDK) Inhibitors as Anticancer Drugs: Recent Advances (2015-2019). Bioorg. Med. Chem. Lett. 29, 126637. doi:10.1016/j.bmcl.2019.126637
Satyanarayana, A., and Kaldis, P. (2009). Mammalian Cell-Cycle Regulation: Several Cdks, Numerous Cyclins and Diverse Compensatory Mechanisms. Oncogene 28, 2925–2939. doi:10.1038/onc.2009.170
Sviderskiy, V. O., Blumenberg, L., Gorodetsky, E., Karakousi, T. R., Hirsh, N., Alvarez, S. W., et al. (2020). Hyperactive CDK2 Activity in Basal-like Breast Cancer Imposes a Genome Integrity Liability that Can Be Exploited by Targeting DNA Polymerase ε. Mol. Cel 80, 682–e7. doi:10.1016/j.molcel.2020.10.016
Tadesse, S., Anshabo, A. T., Portman, N., Lim, E., Tilley, W., Caldon, C. E., et al. (2020). Targeting CDK2 in Cancer: Challenges and Opportunities for Therapy. Drug Discov. Today 25, 406–413. doi:10.1016/j.drudis.2019.12.001
Tadesse, S., Caldon, E. C., Tilley, W., and Wang, S. (2019a). Cyclin-Dependent Kinase 2 Inhibitors in Cancer Therapy: An Update. J. Med. Chem. 62, 4233–4251. doi:10.1021/acs.jmedchem.8b01469
Tadesse, S., Caldon, E. C., Tilley, W., and Wang, S. (2019b). Cyclin-Dependent Kinase 2 Inhibitors in Cancer Therapy: An Update. J. Med. Chem. 62, 4233–4251. doi:10.1021/acs.jmedchem.8b01469
Tarasewicz, E., Rivas, L., Hamdan, R., Dokic, D., Parimi, V., Bernabe, B. P., et al. (2014). Inhibition of CDK-Mediated Phosphorylation of Smad3 Results in Decreased Oncogenesis in Triple Negative Breast Cancer Cells. Cell Cycle 13, 3191–3201. doi:10.4161/15384101.2014.950126
Tripathy, D., Bardia, A., and Sellers, W. R. (2017). Ribociclib (LEE011): Mechanism of Action and Clinical Impact of This Selective Cyclin-dependent Kinase 4/6 Inhibitor in Various Solid Tumors. Clin. Cancer Res. 23, 3251–3262. doi:10.1158/1078-0432.CCR-16-3157
Volkart, P. A., Bitencourt-Ferreira, G., Souto, A. A., and De Azevedo, W. F. (2019). Cyclin-Dependent Kinase 2 in Cellular Senescence and Cancer. A Structural and Functional Review. Curr. Drug Targets 20, 716–726. doi:10.2174/1389450120666181204165344
Wang, J., Wang, Q., Zhang, L., and Fang, H. (2013). Design, Synthesis and Preliminary Biological Evaluation of Purine-2,6-Diamine Derivatives as Cyclin-dependent Kinase (CDK) Inhibitors. Chin. J. Chem. 31, 1181–1191. doi:10.1002/cjoc.201300420
Wood, D. J., and Endicott, J. A. (2018). Structural Insights into the Functional Diversity of the CDK-Cyclin Family. Open Biol. 8, 180112. doi:10.1098/rsob.180112
Yin, J., Zhao, M. M., Huffman, M. A., and Mcnamara, J. M. (2002). Pd-catalyzed N-Arylation of Heteroarylamines. Org. Lett. 4, 3481–3484. doi:10.1021/ol0265923
Yuan, K., Wang, X., Dong, H., Min, W., Hao, H., and Yang, P. (2021). Selective Inhibition of CDK4/6: A Safe and Effective Strategy for Developing Anticancer Drugs. Acta Pharm. Sin. B 11, 30–54. doi:10.1016/j.apsb.2020.05.001
Zhang, J., Wang, Q., Hou, X., and Liu, H. (2015). Rencent Advances in Cyclin-dependent Kinase Inhibitors with Purine Scaffold. Chin. J. Org. Chem. 35, 1022. doi:10.6023/cjoc201410039
Keywords: structure-based drug design, CDK2 inhibitor, purine, anticancer, triple-negative breast cancer
Citation: Liang H, Zhu Y, Zhao Z, Du J, Yang X, Fang H and Hou X (2022) Structure-Based Design of 2-Aminopurine Derivatives as CDK2 Inhibitors for Triple-Negative Breast Cancer. Front. Pharmacol. 13:864342. doi: 10.3389/fphar.2022.864342
Received: 28 January 2022; Accepted: 24 March 2022;
Published: 03 May 2022.
Edited by:
Adriano D. Andricopulo, University of Sao Paulo, BrazilReviewed by:
Wade Russu, The University of the Pacific, United StatesChung-Hang Leung, University of Macau, China
Copyright © 2022 Liang, Zhu, Zhao, Du, Yang, Fang and Hou. This is an open-access article distributed under the terms of the Creative Commons Attribution License (CC BY). The use, distribution or reproduction in other forums is permitted, provided the original author(s) and the copyright owner(s) are credited and that the original publication in this journal is cited, in accordance with accepted academic practice. No use, distribution or reproduction is permitted which does not comply with these terms.
*Correspondence: Xuben Hou, hxb@sdu.edu.cn; Hao Fang, haofangcn@sdu.edu.cn
†These authors have contributed equally to this work