- 1Department of Anesthesiology, Cathay General Hospital, Taipei, Taiwan
- 2Department of Anesthesiology, Tri-Service General Hospital, Taipei, Taiwan
- 3Grauate Institute of Medical Sciences, National Defense Medical Center, Taipei, Taiwan
Pre-diabetes and diabetes are growing threats to the modern world. Diabetes mellitus (DM) is associated with comorbidities such as hypertension (83.40%), obesity (90.49%), and dyslipidemia (93.43%), creating a substantial burden on patients and society. Reductive and oxidative (Redox) stress level imbalance and inflammation play an important role in DM progression. Various therapeutics have been investigated to treat these neuronal complications. Melatonin and dipeptidyl peptidase IV inhibitors (DPP-4i) are known to possess powerful antioxidant and anti-inflammatory properties and have garnered significant attention in the recent years. In this present review article, we have reviewed the recently published reports on the therapeutic efficiency of melatonin and DPP-4i in the treatment of DM. We summarized the efficacy of melatonin and DPP-4i in DM and associated complications of diabetic neuropathy (DNP) and neuropathic pain. Furthermore, we discussed the mechanisms of action and their efficacy in the alleviation of oxidative stress in DM.
Introduction
Diabetes mellitus (DM) is globally affecting major diseases, and it is a major death-causing disease in well-developed countries, according to the WHO survey (Corriere, Rooparinesingh, & Kalyani, 2013) (Sherr & Lipman, 2015). DM is characterized by a lack of production or cellular uptake of insulin, which depends on various factors (Ostenson, 2001) (S. Dong et al., 2019). In type 2 DM, it is characterized by less secretion of insulin to control the blood sugar in the body (Uno et al., 2018) (Porte, 2001; Reinehr, 2005). DM with other diseases becomes more complicated, and potential treatment procedures are needed to control progression (Harding, Pavkov, Magliano, Shaw, & Gregg, 2019; Pearce, Simo, Lovestam-Adrian, Wong, & Evans, 2019; Y. Zhao, Ye, Boye, Holcombe, & Swindle, 2009). Insulin is an essential enzyme to control glucose levels in the body (E. G. Hong et al., 2007). In the insulin resistance condition, more insulin levels are needed compared with normal conditions to overcome DM (Girgis, Scalley, & Park, 2012; Love et al., 2021; Pang & Narendran, 2008). Type 2 DM occurs in a genetic or acquired manner; in recent reports, authors have notified it is a complex and multifactorial metabolic disease (Smith-Palmer et al., 2014; Q. Q. Yang et al., 2021). Lack of insulin levels impacts the metabolism of carbohydrates, proteins, and lipids to develop hyperglycemia and hyperlipidemia conditions (Lodovici, Bigagli, Bardini, & Rotella, 2009; Sanz-Nogues, Mustafa, Burke, O'Brien, & Coleman, 2020; Y. Zhao et al., 2009). The hyperglycemia and hyperlipidemia conditions advanced complicated diseases such as neuropathy, retinopathy, cognitive defects, Parkinson’s, cardiomyopathy, atherosclerosis, and nephropathy. Type 2 DM is an evolving disease from metabolic disorders to inflammatory complications and further contributes to the development of neuropathic pain and neuropathy, as explained in Figure 1(Kuniss, Freyer, Muller, Kielstein, & Muller, 2019; Nijpels, Beulens, van der Heijden, & Elders, 2019). The long-term innate immune system activation in type 2 DM is due to chronic inflammation, according to recent reports (L. Li et al., 2016; Muggeo, 1998). In type 2 DM, insulin resistance and hyperglycemic conditions lead to the development of the neuronal complications (Cerveny, Leder, & Weart, 1998; Demir, Nawroth, Herzig, & Ustunel, 2021). The major cause of DM is peripheral nervous system (PNS) damage, a common type of nerve damage of the bilateral and symmetric nerves of the feet. The distal to proximal gradient of severity is known as stocking glove neuropathy, which is called DNP (Alam, 2020; Papanas, 2020). DNP is primarily a disorder of sensory nerves. In the early stages of DNP, patients experience positive sensory symptoms in their feet such as pain, tingling, and prickling sensations and negative symptoms such as numbness (P. Li, Yuan, & He, 2019; Maddaloni et al., 2021; Sima, 2008). DNP sensory management may induce pain when the feet are touched (allodynia) and increase the noxious stimuli (hyperglycemia) (Kahl, 2017; Aliabadi, Moradian, Rahmanian, & Mohammadi, 2021; Rumora et al., 2021). In the late stage of the disease, evidence of motor nerve dysfunction was observed. The fact that why sensory nerves are mainly vulnerable in DM is not clear until now compared with motor nerves (C. C. Chao, Tseng, & Hsieh, 2011; Falco et al., 2021; Yu, Zhao, Cao, Zhu, & Li, 2017; B. Q. Zhu, Quinn, & Fritschi, 2018).
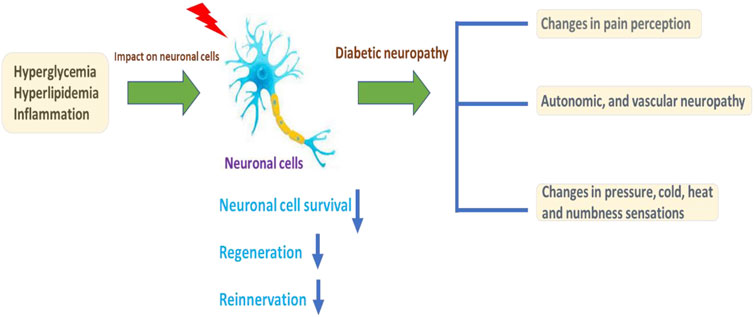
FIGURE 1. Illustration of DM conditions’ influence on the neuronal cells and DNP development, with further cellular consequences.
Melatonin is a hormone secreted from the pineal gland. The plasma concentration of melatonin differs during the day (low) and night (high) (Rocha, Rato, Martins, Alves, & Oliveira, 2015). The plasma concentration of melatonin varies depending on various factors such as light, age, and sex. According to recent reports, the plasma level melatonin concentration (picomolar) is high in nocturnal animals (Cagnacci, 1996; Pohanka, 2013). Melatonin shows various pharmacological effects such as anti-inflammatory, antioxidant, neuroprotection, and inhibitory action in the central and peripheral nervous system (Beyer, Steketee, & Saphier, 1998; J. G.; Lee et al., 2019). These qualities suggest that it may be effective in the treatment of DNP (Xie, Fan, He, & Huang, 2020). Melatonin has low toxic effects, according to recent publications. High dose of 800 mg/kg in rats shows no adverse effects. The aim of this review is to provide an examination of previous reports reporting the actions of melatonin on DNP.
The DPP-4 enzyme rapidly degrades the blood plasma glucagon-like peptide-1 (GLP-1) and influences fasting glycemia in type 2 DM. Therefore, DPP-4i are known as potent antioxidant agents used for prolongation of GLP-1 half-life, increasing the GLP-1 levels and enhancing the secretion of insulin by inhibiting the DPP-4 enzyme to maintain glucose homeostasis. In this review, we focused on DPP-4i and its antioxidant properties for the treatment of DM and its complications.
The rationale design of this narrative review mainly focuses on DM and its complications and treatment with melatonin and DPP-4i for DNP and neuropathic pain. Initially, we have focused on the oxidative stress induction pathways in DM that lead to DNP and neuropathic pain. Furthermore, we explained DM pathogenesis and neuropathic pain mechanisms. Finally, we explained the advantages of using melatonin and DPP-4i for the management of DM and its complications and their potent antioxidant properties. The hypothesis is described with a schematic diagram, as shown in Figure 2.
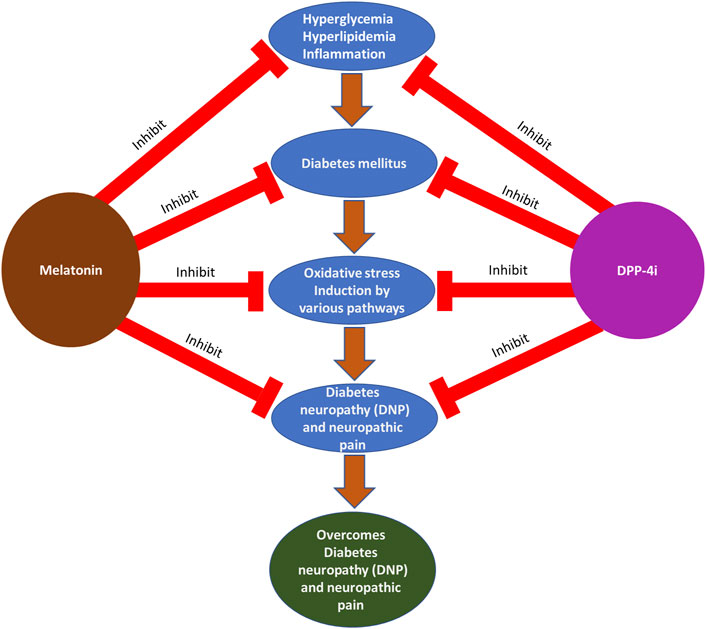
FIGURE 2. Photographical representation of the hypothesis of DM and its complications: treatments with melatonin and DPP-4i at various stages.
Oxidative Stress Mechanistic Features in Diabetic Neuropathy
According to previous reports, low-grade and sub-clinical inflammation and pain depend on the risk factors for diabetes and insulin resistance in hyperglycemia (Figure 3) (Hey-Mogensen et al., 2010; Anthonsen, Larsen, Pedersen, Dalgaard, & Kvetny, 2013; Kassan et al., 2014; Manoharan et al., 2019). The reactive oxygen species (ROS) generation signals for neuropathy associated with DM complications (J. P. Chen, Xu, Liao, & Zhang, 2020; S. H. Lee et al., 2016; Nogueira-Machado et al., 2006; Sommese et al., 2018). The ROS-induced oxidative stress elevated the pro-inflammatory and neuropathic pain-related biomarkers in DM (Turecky, Kupcova, Uhlikova, & Mojto, 2014; Sharifzadeh, Ranjbar, Hosseini, & Khanavi, 2017; Liu et al., 2019). In another report, convincing arguments related to obese patients’ complications with type 2 DM; insulin resistance contributes to the generation and increasing the secretion of tumor necrosis factor–α (TNF-α), which participates in further consequences of neuropathic pain and degeneration of neurons (Ghouini, Rahal, & Djoghlaf, 2016; Z. H.; Yang & Peng, 2010; C. Y.; Zhao, Dai, & Zhou, 2016). The type 2 DM, ROS, and reactive nitrogen species (RNS) are collectively described as oxidative stress-inducing agents to imbalance the cellular redox environment to involve in the neuropathic pain and inflammation (Schofield & Sutherland, 2012; Fagundes-Netto, Anjos, Volpe, & Nogueira-Machado, 2013; Shi, Yang, & Jiang, 2016; Elzinga et al., 2019; Tian et al., 2021). The low and moderate levels of ROS and RNS are very useful for fighting against infections and maintaining cellular structural integrity. The in vivo and clinical studies demonstrate a promising relationship between ROS and neurological complications (Barutta et al., 2011; Mihanfar et al., 2021; Akbarzadeh, Darband, Sadighparvar, and Majidinia; Pulakat and Sumners, 2020; H.; Wu, Wen, Jiang, Liu, & Nie, 2018). ROS complications and oxidative stress lead to the development of unhealthy reactions at the cellular and molecular levels, such as DNA damage, lipid peroxidation, protein degradation, and secretion of antioxidant enzymes. (Kaneto, Katakami, Matsuhisa, & Matsuoka, 2010; Chattopadhyay et al., 2015; Dong et al., 2016a; Panigrahy, Bhatt, & Kumar, 2017). A study reported a relationship between insulin resistance and ROS generation enhancement triggered by treating the pancreatic cells with oxidative stress inducers (Burgos-Moron et al., 2019; De Marañón et al., 2020a; Kassab & Piwowar, 2012; P.; Wang et al., 2018; Wright, Scism-Bacon, & Glass, 2006). Surprisingly, they denoted the cellular events related to ROS enhancement and decreasing the expression of insulin mRNA in the insulin gene (Opara, 2004; Belia et al., 2009; Gonzalez et al., 2011; Yanagi, Monden, Ikeda, Matsumura, & Kasai, 2011). Their studies exploring ROS production inside the cells are strongly involved in insulin resistance in hyperglycemic conditions. In other experimental conditions, long-term exposure of the cells and animals to hyperglycemic conditions could develop insulin resistance, which leads to excess production of ROS (Afanas’ev, 2010; Brown, Bridge, & Kearney, 2021; Mackenzie et al., 2009; Sukotjo, Sargowo, & Wihastuti, 2014). Oxidative stress is known to activate enzymes, such as superoxide dismutase (SOD), glutathione (GPX), catalase (CAT), condensed glutathione, and reduced glutathione (Chang & Chuang, 2010; J.; Chen et al., 2017; Eckers, Altschmied, & Haendeler, 2012). In type 2 DM patients, dropping the daily glucose intake could be a useful strategy to reduce the ROS level (Bunpeng et al., 2022; Boriboonhirunsarn, Boriboonhirunsarn, Sawangpanyangkura, and Tansriratanawong; Z. A.; Ma, Zhao, & Turk, 2012; Nemoto et al., 2007). The development of type 2 DM is well associated with dysglycaemia, chronic sustained hyperglycemia, and acute glycemic fluctuations (W. C. Chao et al., 2015; Dave & Kalia, 2007; Gawlik et al., 2016; Gilardini Montani et al., 2016; Isoni et al., 2009; Mahood, Hussein, & Al-Ahmed, 2018).
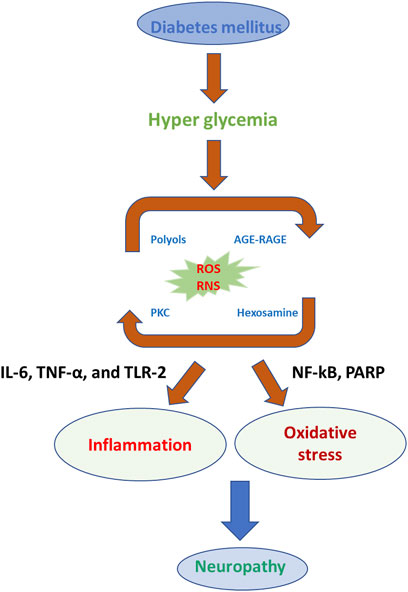
FIGURE 3. Photographical representation of oxidative stress consequences in the development of neuropathy in DM.
The relationship between oxidative stress and DNP, development, and progression requires further elucidation by exploring the detection of biomarkers (Hirao et al., 2010; Khan, Banga, Mashal, & Khan, 2011). In previous reports, oxidative stress as measured by the level of malondialdehyde (MDA) was not related to fasting blood levels (Hayashi, Murakami, Yamamoto, Ono, & Onodera, 1997). MDA was detected in DM, which influences the mitochondrial membrane potential (MMP) and may lead to activation of the electron transport chain complex system to further activate significant pathways for the production of ROS (S. C. W. Tang et al., 2010). In addition, nicotinamide adenine dinucleotide phosphate (NADPH) is the standard complex enzymatic system for the generation of ROS, and it seems like a basic source for glucose-induced ROS production in the tissues/cells of DM prototypes (Jersin et al., 2021; Wong et al., 2010). Besides, xanthine oxidase plays a vital role in the production of ROS, which shows an important role in the development of DM and associated complications (Fidler et al., 2017). In some research studies, glucose and its metabolites react with hydrogen peroxide (H2O2) in the existence of copper (Cu) and iron (Fe) to generate the hydroxyl radicals (.OH) through Fenton-like reaction during auto-oxidation in DM promoting ROS cascade and DM complications (Figure 3) (Gadjeva, Goycheva, Nikolova, & Zheleva, 2017; Guichard, Moreau, Pessayre, Epperson, & Krause, 2008; J. W. ; Kim et al., 2016).
In DM, ROS generation depends on various routes, such as the enhanced formation of advanced glycation end products (AGEs), heightened polyol pathway, and stimulation of protein kinase C (PKC) (Kassab & Piwowar, 2012; Nogueira-Machado et al., 2006). The aldose reductase pathway is a NADPH-dependent enzyme that catalyzes the reduction of glucose into sorbitol (polyol) and goes along with the oxidation of sorbitol to fructose by NAD+-dependent polyol dehydrogenase (Eckers et al., 2012; L. He & Sun, 2021). It is assumed that hyperglycemia in the saturation of hexokinase amounts to over 30% of glucose transferred into the polyol pathway (Hamada, Fujii, & Fukagawa, 2009; Wan et al., 2019). The polyol pathway results from lacking intracellular NADPH and an excess of NADH, which generates the NADH oxidase to produce ROS, causing DNA damage (Babizhayev et al., 2015; Takamura et al., 2008). The polyol pathway works as the most important source of ROS generation in the neuron, retina, and nephron, and sorbitol increase concentration has been associated with neuropathy, retinopathy, and nephropathy of DM (Figure 4) (Eckers et al., 2012; Ojima, Matsui, Maeda, Takeuchi, & Yamagishi, 2012; Ferlita et al., 2019; Darenskaya, Kolesnikova, & Kolesnikov, 2021).
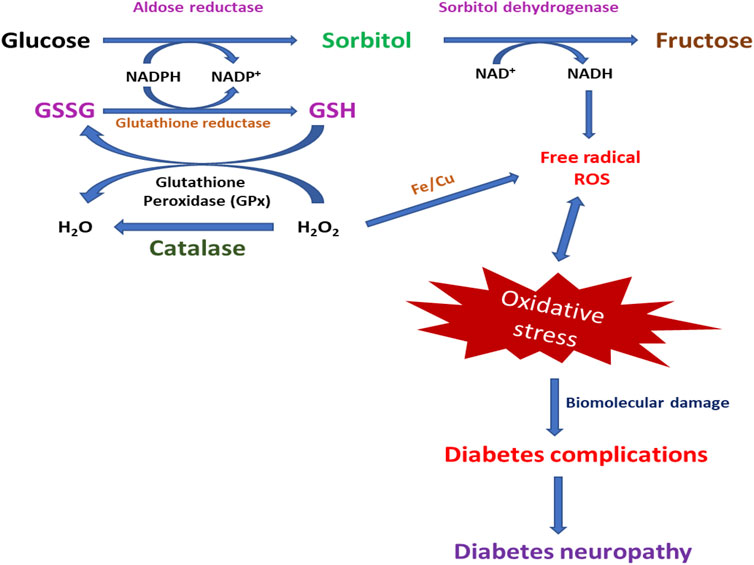
FIGURE 4. Photographical demonstration of mechanisms of oxidative stress-induced DNP. Oxidative stress-activated pathways for the generation of DNP.
AGE development glucose can delightedly act in response with free amino groups of protein to form Schiff bases. These Schiff bases go through complex reactions to form advanced AGEs, which cause tissue destruction through the formation of cross-links that alter the protein structure and function and relations with cell surface receptors on endothelial cells and macrophages, which contributes to the activation of cell signaling and gene expression that induce oxidative stress and inflammation in DM (De Oliveira, Colette, Monnier, Descomps, & Pares-Herbute, 2005; Spadaccio et al., 2014; Dai, Chen, & Chai, 2019).
PKC-dependent stimulation of NADPH oxidase by high glucose levels can promote ROS formation in aortic endothelial cells, smooth muscle, and renal mesangial cells. Laboratory evidence indicates the NADPH oxidase-dependent production of ROS in DNP. Some reports, explained the detailed relationship between retinopathy and DNP by increased levels of NADPH, which leads to the generation of ROS and causes retinopathy and neuropathic complications in DM (Rolo & Palmeira, 2006; Kizub, Klymenko, & Soloviev, 2014; Karunakaran et al., 2015; Panigrahy et al., 2017). Relationships among PKC, NADPH, and ROS generation through different ways in DM are explained through possible examples (M. H. Kim et al., 2017; H. X. Wang et al., 2013). The activation of endothelial nitric oxide (eNOS), NADPH oxidase, vascular endothelial growth factor (VEGF), endothelin-1 (ET-1), transforming growth factor-β (TGF-β), phospholipase A2 (PLA2), and nuclear factor kappa beta (NF-κB) leads to the generation of ROS in DM (F. He et al., 2021; Yao et al., 2021). In another research, diacylglycerol-dependent stimulation of PKC affects the gene expression of key proteins related to blood flow, capillary occlusion, inflammation, and damage of cellular macromolecules in DM. The DM-associated complications, such as high glucose levels, can stimulate ROS generation through PKC and NADPH-dependent pathways in various cells, such as aortic endothelial cells, smooth muscle cells, and renal mesangial cells (N. Jiao et al., 2020; Toma, Sanda, Deleanu, Stancu, & Sima, 2016). In the phagocytic cells, the NADPH oxidase-dependent pathway plays as primary enzyme compared with non-phagocytic cells (Guichard et al., 2008; Sedeek et al., 2010; Kassan et al., 2014). In another research, NADPH oxidase levels in DM animal models are compared with non-DM animals as an indicator of the neuropathy and coronary artery disease treatments (Fagundes-Netto et al., 2013; H. X.; Wang et al., 2013; Xing et al., 2017). Recent studies have shown a relationship between the NADPH-dependent production of ROS in DM, which leads to neuropathy and further consequences (San Martin et al., 2007; Sedeek et al., 2010; Dong et al., 2016b). The activation of antioxidant enzyme production in high glucose levels in DM causes oxidative stress. At cellular levels, we detected high levels of polyunsaturated fatty acids, ferrous ions, and molecular oxygen in reported scientific reports as huge problems in DM. The high levels of glucose and oxidative stress complications play a significant role in the DNP progression and its further complications. Cells have different protection systems to prevent or scavenge the overproduction of ROS and oxidative stress, which includes antioxidant enzymes such as SOD, GPX, and CAT, and overcome oxidative stress and prolongs cell surveillance and participates in the cellular signaling pathways for recovery from DNP (Afanas’ev, 2010; J.; Jiao et al., 2012; Y. H.; Ma, Li, Yin, & Li, 2015; Tseng, Vong, Kwan, Lee, & Hoi, 2016; Yuan et al., 2010).
SOD participates in scavenging the superoxide radical by promoting its conversion to H2O2, while GPX detoxifies H2O2 and lipid peroxides (Song et al., 2018; Liao et al., 2019; Dworzanski et al., 2020). Another enzyme, CAT, accelerates the decomposition of H2O2 into water and molecular oxygen (Yan, Chen, & Zheng, 2017; B. B.; Zhao et al., 2021). Excessive blood glucose can hinder the antioxidant defense system, which influences the actions of antioxidant cascade enzymes in DM conditions (H. Q. Tang et al., 2021; Waldman et al., 2018). In some reports, authors documented changes in the antioxidant defense system in DM as a considerable decline in the SOD enzymatic activity in red blood cells in DM in vivo models (Ding et al., 2019; Q.; Lu et al., 2018). The reduction of the SOD activity in DM conditions could be due to oxidative stress-induced inactivation. The enhancement of H2O2 levels is due to inactivation of SOD, inactivation by glycosylation of SOD, and loss of Cu2+ co-factor necessary for the enzymatic activity can be decreased (Bhattamisra, Koh, Lim, Choudhury, & Pandey, 2021; O. H.; Lee et al., 2009; Liao et al., 2019).
The relationship between oxidative stress and inflammation is an essential physiological response of the body to various disease states, such as pathogen invasion, tissue damage/injury, and irritants. The inflammatory response affects the cell’s infiltration and subsequent activation of the cell’s innate and active immune system at the site of injury by secreting inflammatory mediators’ cytokines (Bagi, Feher, & Beleznai, 2009; J. Wang & Guo, 2019). The scientific reports provide evidence that enhanced the trigger of inflammatory mediators in DM conditions due to high levels of glucose-negotiated oxidative stress. The DM pathophysiology is commonly associated with chronic inflammation and oxidative stress. Complex interactions with these pathways consist of positive feedback mechanisms for both mutual expansions (Figure 5) (Strollo et al., 2013; Dong et al., 2016a). Inflammation is the key factor for the immune responses to eradicate pathogens from the cells and repair the cells to a normal state. Innate immune cells produce pro-inflammatory cytokines and chemokines that promote the production of ROS and RNS. Pro-inflammatory cytokines can ultimately aggravate oxidative stress by encouraging macrophages, which are known to play a key role in eliminating the pathogen via the production of ROS (De Marañón et al., 2020b; P.; Wang et al., 2018). It is significant to note that prolonged inflammation is a pathological condition established by tissue damage, fibrosis, and cell damage. The inflammatory conditions contribute and produce their cellular side effects through the generation of ROS, reduction in antioxidants, and enhanced stress-activated kinases (de Maranon, Diaz-Pozo, et al., 2020; Yanagi et al., 2011). At the cellular level, ROS can activate transcription factors such as NF-κB, which influence the secretion of pro-inflammatory cytokines. Under oxidative stress conditions, activation of the immune system commonly has a short life because of intrinsic negative feedback mechanisms such as enhanced generation of the antioxidant cascade system and anti-inflammatory cytokines. Eventually, in chronic DM conditions, activation of the oxidative system and inflammation serve as positive influences to control DM (Drougard et al., 2013; Rovira-Llopis et al., 2013).
Inflammation plays a crucial role in the pathogenesis of type 2 DM in understanding the inflammatory induction factors. According to recent studies, the relationship between type 2 DM and elevation in the levels of sialic acid, C-reactive protein, and interleukin-6 (IL-6) is expected to accelerate the progression of type 2 DM (Belia et al., 2009; Mushtaq, Ali, Altaf, Abdullah, & Murtaza, 2015). Other studies have shown that enhanced levels of inflammatory biomarkers anticipate insulin resistance and progression of type 2 DM (Sukotjo et al., 2014; Brown et al., 2021). In another study, the connection between fasting insulin levels and C-reactive protein (CRP) levels in the plasma shows that insulin resistance and inflammatory methods are connected (Mackenzie et al., 2009; Cantley & Biden, 2013). Nonetheless, the mechanisms of chronic inflammation’s contribution to development of type 2 DM are not clearly understood yet. However, adipose tissue produces important pro-inflammatory cytokines, tumor necrosis factor, and inflammatory biomarkers, which are associated with body fat mass, suggesting that triggered innate immunity and inflammation factors in the pathogenesis of type 2 DM (Caton et al., 2013; Nokkaew et al., 2021).
Pathogenesis of Diabetic Neuropathy Pain
Similar to the central nervous system (CNS), the PNS is comprised of 12 cranial nerves and 31 pairs of spinal nerves. The PNS is composed of neurons and supporting glial cells (Schwann cells) (Selvarajah, Wilkinson, Griffiths, Emery, & Tesfaye, 2008; Qureshi & Ali, 2021). Commonly, efferent axons from motor neurons transport information from the CNS to muscles and glands. The afferent axons from motor neurons transport information from peripheral sensory receptors to the CNS (El-Salhy, 2002; Kempler et al., 2017). The position of the neuron cell body is also important; sensory neurons, specifically dorsal root ganglion (DRG) neurons, are located outside of the blood–nerve barrier, as do the peripheral sensory receptors, whereas motor neurons are located within the ventral horn of the spinal cord under the protection of the blood–brain barrier (Rondon et al., 2010; Iyer & Tanenberg, 2013).
The DRG neurons are exposed to systemic metabolic and hypoxic stressors, making them much more vulnerable to injury. The anatomy of the sensory system beyond the blood–brain barrier may also explain its exceptional vulnerability (Eaton et al., 2003; Selvarajah, Wilkinson, Gandhi, Griffiths, & Tesfaye, 2011). In the PNS, thin unmyelinated axons known as C fibers carry information for the automatic nervous systems, as well as afferent impulses in response to extreme conditions such as temperature and mechanical force damage to tissue (Mert et al., 2009; Ozkul et al., 2010). Unmyelinated C fibers and small myelinated fibers help with cold detection, which is crucial to the ability to perceive pain, ulcers, and amputation. Simultaneously, developing new ideas into how specific genes, significant pathways, and axonal bioenergy influence injury in DNP, new findings target the pathophysiological processes of causing pain and understanding DNP (Ozkul et al., 2010; Singh, Bansal, Kuhad, Kumar, & Chopra, 2020). Neuropathic pain is a common feature and helps in significantly disabling DNP, pain caused by lesions, or affecting the somatosensory system. The essential unique feature of DNP versus other pain is the paradoxical sensory loss, and with or without the pain sensory hypersensitivity theory, the various sensory modalities in the affected area (Tsuda, Masuda, Tozaki-Saitoh, & Inoue, 2013; Feldman, Nave, Jensen, & Bennett, 2017). The DNP form of pain is different to the chronic inflammatory pain and idiopathic pain where the somatosensory nervous system is undamaged (Inoue & Tsuda, 2009; J. Y.; Zhao et al., 2018). In DM, hyperglycemic conditions promote the various significant pathways in the production of inflammatory cytokines that initially cause neuroinflammation. Later, neuroinflammation conditions show impact on sensory fibers and ion channels, leading to nerve injury and abnormal neuropathic pain.
The signs and symptoms of DNP show sensory loss with less sensitivity to touch, hot and cold temperatures, needle prick, and pain hypersensitivity with hyperalgesia or allodynia (Tavakoli, Mojaddidi, Fadavi, & Malik, 2008). The circulation of symptoms and signs varies on the nerves affected in DNP, in which most common forms are distal symmetric polyneuropathy and pain, and sensory findings are observed in the feet, toe, and occasionally also in the finger of hands (Ambrose & Golightly, 2015; Prajapati, Filippi, & Sears, 2021). Pain has diverse features such as burning, stinging, and shooting. Deep aching types are the most common ones within the region of damaged nerve fibers. This problem remains unsolved as to why some patients develop NP and others remain without pain, even if it is likely to relate to complex interactions between the genotype and environment. Recent two studies demonstrated the significant link between the heritability and environmental factors role. Also, respective huge genome-wide linked studies aids in exploring the relationship between the genotype and occurring DNP (Tsuda, Inoue, & Salter, 2005; Tsuda, 2019). Even though neuronal hyperexcitability is a primary factor in DNP conditions, the clinical studies showing such hyperexcitability are not always present in DNP. In recent studies, relating discussions of patients with clinical examinations in T2DM explains a phenotypic multiplicity in patients with and without pain. Their studies explain that increased sensitivity to thermal and mechanical stimuli is a good selective feature between DNP patients with and without neuropathic pain (Tavakoli & Malik, 2008; Suryavanshi & Kulkarni, 2017). It is convincing that the predominance of sensory loss versus the presence of hyperalgesia and allodynia may explain the failure to find clear characteristic qualities in painful versus painless DNP (Obrosova, 2009; Afrazi & Esmaeili-Mahani, 2014) (Figure 6).
Psychological issues are also critical features to the development of DNP and initially may cause damage to peripheral and brain networks’ subserving pain. Its variations fluctuate among individuals and are expected to have a significant part in the appearance of pain (Q. X. Hong, Xu, Dai, & Zhao, 2016; Tesfaye & Selvarajah, 2012). Some studies show a positive correlation between both neuropathy harshness and poor glycemic control with both the consequence and intensity of DNP pain, relation with genetic predisposition that accurate ion channel variants may be inclined to the progression of painful DNP (P. P. Lu et al., 2020; Lukic, Humpert, Nawroth, & Bierhaus, 2008). Sensory neuron hyperexcitability in the form of impulsive movement and altered incentive response purpose is reported both in patients and in experimental models of DNP. Sensory neuron excitability is administered by the design of expression, trafficking, and function of liganded voltage-gated ion channels. The ion channels are critical for the preliminary transduction of sensory stimuli, action potential production, and propagation, and finally, all neurotransmitters release within the dorsal horn of the spinal cord (Becker, Benromano, Shahar, Nevo, & Pick, 2014; Daugherty, Marquez, Calcutt, & Schubert, 2018). The selective appearance of many of these ion channels by sensory neurons means that they are important for analgesic progress. Painful DNP reveals common pathophysiological mechanisms with other types of neuropathic pains for dysregulation of ion channel manifestation (Kashyap & Farrugia, 2011; Park, Kang, Jeon, Kim, & Lee, 2019).
The cellular significance of the central application of the peripheral neuronal activity is generally displayed by an overstated response to synaptic inputs, a reduction in the threshold to activate neurons, an augmented response to suprathreshold stimuli, and an extension of accessible fields (Alaverdyan & Vartanyan, 2012; Deli, Bosnyak, Pusch, Komoly, & Feher, 2013). Previous reports suggested that these central mechanisms can impact on painful DNP; streptozocin (STZ) rats’ extensive dynamic range of dorsal horn neurons show Rac-1-mediated dendritic spine morphology that is related to natural activity and hyperexcitability to peripheral stimuli (Gao et al., 2019; X. H.; Ma et al., 2020). The reversal of these fluctuations using a Rac-1 inhibitor blocks hypersensitivity both at the electrophysiological and behavioral level. The dorsal horn of the spinal cord is below the control of a descending pain modulatory system that can either inhibit or enable transmission of nociceptive information (Johnson, Ryals, & Wright, 2007; X. Q.; Ma et al., 2019). At the clinical level, conditioned pain modulation is a technique that is used to test these descending controls; importantly, habituated pain variation is reduced in some patients with DNP (Fischer, Tan, & Waxman, 2009). Unusual signaling between neurons and glia also plays a role in pain in the framework of maladaptive plasticity. Following interrupting nerve injury, microglia within the dorsal horn of the spinal cord release factors such as the brain-derived neurotrophic factor (BDNF), which results in the augmentation of nociceptive synaptic processing and thus gating of neuropathic pain (Paulson, Wiley, & Morrow, 2007). Although DNP is related to a gentler rate of de-afforestation associated with traumatic neuropathy, there is also a suggestion for a role of microglia in the progression of neuropathic pain. A significant deliberation is that recent studies report that microglia are essential for the development of powered pain hypersensitivity, following traumatic nerve injury in male but not female rats (Johnson et al., 2007; X. Q.; Ma et al., 2019). In other reports, gender-specific effects have not been studied in the experimental models of painful DNP and highlight the importance of gender when exploring pathophysiological pain mechanisms. Oligodendrocytes have also been associated with showing a role in the central pain mechanism; loss of oligodendrocytes in the spinal cord leads to both an excessive pain response and axon loss in the spinal cord dorsal horn with injury to the spinothalamic tract. Currently, unknown damage to oligodendrocytes in the CNS also contributes to pain in DNP. Recent studies suggest that separate CNS mechanisms independent of a peripherally determined central sensitization may be complicated in pain, following glial damage (Taliyan & Sharma, 2012; Byrne, Cheetham, Vickers, & Chapman, 2015; Yamashita et al., 2019; Jiang, Chen, Chen, Jiao, & Wang, 2021).
Dipeptidyl Peptidase IV Inhibitors and Glucagon-Like Receptor Agonists in Diabetic Neuropathy Management
DPP-4 inhibitors are a class of oral hypoglycemics that block the enzyme DPP-4 for treating diabetes. Blocking DPP-4 activates the stimulators of pancreatic insulin secretion, thereby decreasing blood glucose levels (Rosenstock et al., 2006; Jackiewicz & Katarzynska, 2018). On the other hand, increasing studies support the idea that DPP-4 might also be involved in the development of neurological disorders with a neuroinflammatory component, potentially through its non-incretin activities and its inhibition, which is shown to have protective effects on central and peripheral neuropathies such as Parkinson’s disease, Alzheimer’s disease, and DNP (Gong et al., 2014; Civantos et al., 2017). DPP-4i inhibitors have also shown a promising potential in the alleviation of kidney, brain, and heart diseases through their antioxidant functions. Some recent studies have shown that DPP-4i is expressed in the spinal cord and brain regions during neuropathic pain, which is known to activate mitogen-activated protein kinase (MAPK) pathways by ROS generation (Jing, Zou, Wang, Cai, & Tang, 2021; C. H.; Lee et al., 2018). Its inhibition alleviated hyperalgesia through the selective blocking of glial cell activation. Apart from the deactivation of glial cells, DPP-4 inhibition with drugs such as tripeptide isoleucin-prolin-isoleucin, alogliptin, and the antidiabetic drug vildagliptin is reported to possess antihyperalgesic effects through opioid-dependent and opioid-independent mechanisms in inflammatory and neuropathic pain animal models (Xu et al., 2017; B.; Zhu et al., 2014). Oral administration of DPP-4i such as teneligliptin (TEN) is known to possess analgesic effects in humans against thermal pain. In addition, TEN is also known to enhance glutathione antioxidant production within the cells (Fan et al., 2015; Yoon, Kim, & Song, 2020; Kuthati, Rao, Busa, & Wong, 2021). Recently, several reports have concluded that activation of GLP-1R can suppress neuroinflammation and central sensitization, thereby attenuating neuropathic pain in animal models (Xu et al., 2017; B.; Zhu et al., 2014). Furthermore, in the CNS, GLP-1R is constrained to the microglial cells of the dorsal horn (Jing et al., 2021). The GLP-1R expression is known to decrease after nerve injury and is one of the important factors in microglial cell activation (C. H. Lee et al., 2018). Exenatide, a GLP-1R agonist, is effective in the alleviation of oxaliplatin-induced peripheral neuropathy. The analgesic properties were reversed by either the administration of GLP-1 antagonists or GLP-1 gene knockdown in the animal models of neuropathic pain (Gong et al., 2014). Exendin-4, an agonist of GLP-1, alleviated pain-induced cognitive impairment by suppressing neuroinflammation in neuropathic pain rats (Cui, Fong, Zhang, Xia, & Zhan, 2020). Additionally, DPP-4i such as sitagliptin and GLP-1 analog liraglutide are shown to induce axonal regrowth and locomotor functional repair through the restoration of spinal GLP-1R levels in SCI rats (Han et al., 2020). Recently, we have reported the analgesic effects of DPP-4 TEN against partial sciatic nerve transection–induced NP through the suppression of spinal astrocytes and restoration of spinal GLP-1R (Nakashima, Kaneto, Shimoda, Kimura, & Kaku, 2018). Though several studies have reported the efficacy of DPP-I’s and GLP-1R’s in neuropathic pain animal models using sciatic nerve injury, there are very few studies that have analyzed the efficacy of these compounds in diabetic neuropathic pain animal models (Han et al., 2020). DM conditions have various cellular environments, such as enhanced oxidative stress, AGEs, neuronal damage conditions, and decreased neuronal growth, synaptic plasticity, and neurogenesis. The DPP-4i and GLP-1 used in the treatment reversed all those conditions and reduced diabetes neuropathy and pain. There is a need to pursue future research in this direction to determine if this class of drugs has any beneficial effects over other types of diabetic drugs for DNP management (Figure 7) (H. Y. Wu, Mao, Fan, & Wang, 2017).
Melatonin and its Functions in Diabetic Neuropathy
Melatonin is a broad-spectrum antioxidant with remarkable therapeutic effects against ROS-related diseases through the regulation of oxidative stress (X. X. Zhang et al., 2020). Melatonin has an easier access to subcellular organelles such as the mitochondria, the primary sites for ROS production due to their lipophilic and hydrophilic characteristics (Reiter et al., 2003; Kuthati, Busa, Davuluri, & Wong, 2019). Melatonin can induce antioxidant effects either through direct free radical scavenging or through the synthesis of antioxidant enzymes (Posa, De Gregorio, Gobbi, & Comai, 2018). Melatonin has possible therapeutic effects for treating neurodegenerative diseases, cardiovascular diseases, and many other clinical complications (Amer, Othamn, & El-Missiry, 2021). Melatonin also shows immunomodulatory consequences which depend on its ability to augment the cytokine levels and antioxidant properties (Edizer et al., 2019; Kuthati et al., 2020).
It is estimated that 50% of diabetic patients suffer from DNP, with symptoms involving pain, foot ulcers, and amputation. Oxidative stress and neuroinflammation are known to play an important role in the pathogenesis of DNP (Liang et al., 2020). The high prevalence of DNP in diabetic patients results from multiple reasons. First, diabetic patients have enormous demand for brain oxygen and polyunsaturated fatty acids (PUFAs), resulting in incompetent antioxidant capability within the brain, making it vulnerable to ROS (Onphachanh et al., 2017). In addition, hyperglycemia initiates hippocampal and cortical neuronal degeneration, leading to amputation. DM is also known to damage somatic and autonomous nervous systems, leading to DNP and diminishing life quality.
Effect of Melatonin Treatment on Diabetic Neuropathy in Experimental Findings
As oxidative stress plays an important role in the initiation and progression of DNP; melatonin can be considered as an effective adjuvant in the management of DNP (J. L. Zhang, Hui, Zhou, & Hou, 2018). Melatonin administration is shown to inhibit DNP symptoms through the increase of motor nerve conduction velocity (MNCV) and sciatic nerve diameter (J. L. Zhang et al., 2018). The antihyperalgesic effects of melatonin are medicated through the inhibition of the L-arginine–NO pathway (Kandemir, Guntekin, Tosun, Korucuk, & Bozdemir, 2018). In addition, the analgesic effects of melatonin are corroborated by the melatonin type 2 (MT2) receptor actions through the modulation of brainstem descending pathways (Posa et al., 2018). Melatonin is known to ameliorate hippocampal injury and DNP in STZ-induced diabetic rats. Melatonin is known to alleviate oxidative stress-triggered calcium signaling through the expression of transient receptor potential (TRP), melastatin 2 (TRPM2), and TRP vanilloid type 1 (TRPV1) in the hippocampus (Kahya, Naziroglu, & Ovey, 2017). Melatonin is also known to minimize myelin sheath vacuolization, separation of axon-myelin sheaths, and degeneration of fibers. Though melatonin does not affect the insulin levels directly in DNP rats, it is known to increase brain antioxidant levels and potentiate the beneficial effects of insulin in DNP rats (Gurel-Gokmen et al., 2018; Liang et al., 2020).
The modulative effects of melatonin on inflammation and autophagy are one of the significant mechanisms through which melatonin alleviates DNP (Jangra, Datusalia, Khandwe, & Sharma, 2013). Melatonin promotes the expression of nuclear factor erythroid-2 related factor 2 (Nrf2), which consequently activates phase II antioxidant enzymes such as heme oxygenase-1 (HO-1) and offers protection from oxidative damage (Ahmadi & Ashrafizadeh, 2020; Aliyev, Panieri, Stepanic, Gurer-Orhan, & Saso, 2021). Melatonin is also known to stimulate PTEN-induced putative kinase 1 (PINK1 expression) via an MT2/Akt/NF-κB pathway. The activation of PINK1 is known to aid in the prevention of neuronal cell death under high glucose conditions (Onphachanh et al., 2017). Melatonin is also known to ameliorate diabetes-induced erectile dysfunction in rats through the reduction of oxidative stress and p38/MAPK levels (Sotolongo, Ghiso, & Rostagno, 2020). Additionally, melatonin is also shown to be effective in alleviating diabetes-induced retinal neuropathy through the upregulation of glutamate cysteine ligase in DNP rats through the activation of Nrf2 in the nucleus and stimulation of Akt phosphorylation (Shieh, Wu, Cheng, & Cheng, 2009). In addition, melatonin treatment has also been shown to inhibit the production of pro-inflammatory cytokines through the NF-κB pathway (Tiong, Ng, Koh, Ponnudurai, & Chye, 2019; Nopparat et al., 2021).
One of the most commonly used models for DM is STZ injection in animals (O'Brien et al., 2018). STZ is an antibiotic generated by Streptomyces achromogenes that selectively damages the pancreatic β cells (Bour-Jordan et al., 2013; O'Brien, Sakowski, & Feldman, 2014), leading to hypoinsulinemia and hyperglycemia (Ozaki, Terayama, & Matsuura, 2018). The existing literature suggests that DM induces changes in the CNS and PNS, such as DRG and superior cervical ganglion (SCG). Furthermore, it is also known to inhibit the Na+K+ ATPase pump activity (Pham, Matsumura, Katano, Funatsu, & Ito, 2019). DM is also known to decrease the total area of the pineal gland and reduce the diameter of pinealocytes (Sevak & Goyal, 1996; Yadav, Nagori, & Desai, 2014). Some studies have reported the drastic reduction of pineal melatonin levels after DM induction through pinealocyte alteration (Sevak & Goyal, 1996; Yadav et al., 2014). In contrast, other studies have reported the reduction of melatonin through hyperglycemic signaling and Na+-K+ ATPase activity without the alteration of pinealocytes in DM rats (Pillai, Subramanian, & Kandaswamy, 2013).
Several studies have described the effects of melatonin treatment on DM and DNP using different approaches. Melatonin is known to suppress the upregulation of oxygen and nitrogen free radicals in DM (Adeghate, Rashed, Rajbandari, & Singh, 2006; Silva-dos-Santos et al., 2020). Melatonin is also known to suppress the overexpression of DM-induced inflammatory biomarkers’ levels including TNF-α, IL-6, iNOS, and leptin (Adeghate et al., 2006; Silva-dos-Santos et al., 2020).
DM is known to damage various regions of the brain, including the hippocampus, cortex, and cerebellum, due to the excessive oxidative stress (do Prado et al., 2020; Tong & Cheng, 2005). Melatonin is shown to protect the brain from DM-stimulated glial cell activation and ROS in STZ-induced diabetic rats (Barber et al., 2000, Antonetti, Gardner, and Penn State Retina Res, 2000; Renno, Alkhalaf, Afsari, Abd-El-Basset, & Mousa, 2008) (La Morgia et al., 2010; Galley et al., 2017). In another study, diabetes induction in astrocyte cell culture with high glucose supplementation resulted in an enhanced expression of cytokines and ROS. Addition of melatonin to the cell culture medium resulted in the attenuation of cytokine and ROS levels (Maher, Saleh, Elguindy, Hashem, & Yacout, 2020). One recent study explored the effect of melatonin on central neuropathy by inducing DM through STZ injection in Wistar rats. DM rats displayed neurodegeneration, microglial, and astrocyte activation. Treatment with melatonin abrogated these effects, although melatonin alone did not show any hypoglycemic effect in DM rats (Metwally, Ebraheim, & Galal, 2018). Tiong et al. investigated the antioxidant properties of melatonin at the mitochondrial level in Schwann cells cultured in high glucose. Melatonin is shown to offer protection from glucose-induced mitochondrial membrane depolarization (Tiong et al., 2019; J.; Wang et al., 2012).
Negi and colleagues investigated the protective effects of melatonin and nicotinamide combinational therapy in DM rats on neuronal function, biochemical, and behavioral parameters. Melatonin alone or in combination with nicotinamide improved DM-induced loss of the motor nerve conduction velocity, sciatic nerve blood flow, and pain sensitivity (Negi, Kumar, Kaundal, Gulati, & Sharma, 2010). Melatonin is also known to halt DM-induced activation of the NF-κB cascade of pro-inflammatory cytokines and cyclooxygenase-2 in the sciatic nerve of Sprague–Dawley rats (Seyit, Degirmenci, & Oguzhanoglu, 2016). Melatonin modulates neuroinflammation by lowering the activation of NF-κB and Nrf2 pathways, which may be responsible for its neuroprotective effects in DNP (Seyit et al., 2016).
Orally administered melatonin is shown to alleviate formalin-induced nociception at a low dose, whereas high doses are reported to have anti-allodynia and anti-hyperalgesic effects in DM rats (Arreola-Espino et al., 2007). Moreover, it was observed that K-185 blocked melatonin’s anti-nociception, while naltrindole and naltrexone lowered the antinociceptive effects of melatonin (Arreola-Espino et al., 2007). Previously, melatonin was not known to interact with opioid receptors; in contrast, Arreola and team reported interactions between melatonin and opioid receptors (Arreola-Espino et al., 2007). DM is known to reduce rotarod performance. Treatment with melatonin is shown to improve the rotarod performance. Neurochemical tests have suggested that DM enhances the levels of MDA, acetylcholinesterase, and glutamate in the hippocampus, while treatment with melatonin reverses all changes (Che et al., 2020). One recent report concluded that melatonin is effective in the amelioration of DM-induced PNS neuropathy in Sprague–Dawley rats. The author reported augmented deposition of collagen, oxidative stress, and phosphorylated p38 protein in DNP rats, which were reversed by melatonin (Areti, Komirishetty, Akuthota, Malik, & Kumar, 2017).
Melatonin alone or in combination with drugs such as gabapentin enhanced the hepatic gene expression of peroxisome proliferator–activated receptor and mitochondrial transcription factor-A. Thus, melatonin shows protective effects against DNP in experimental animal studies through multiple pathways (Chahbouni et al., 2017; Juybari et al., 2019). One recent randomized double-blind clinical trial evaluated the potency of melatonin as an adjuvant to pregabalin for pain reduction in painful DNP. Melatonin treatment displayed a decrease in pain sensitivity and a pain-related sleep interference score compared with control (Do Amaral, Andrade-Silva, Kuwabara, & Cipolla-Neto, 2019; Shokri, Sajedi, Mohammadi, & Mehrpooya, 2021).
Conclusion
This comprehensive review gives a full insight into DM and its complications with detailed molecular mechanisms, pathogenesis, and the ROS generation pathways. DNP and neuropathic pain are the most prevalent complications in DM patients, which is a challenging socioeconomic burden to the world. ROS plays a vital role in the oxidative stress at a cytopathic level, leading to the progression of DNP and neuropathic pain. Gaining knowledge regarding the molecular and biochemical mechanisms of abnormalities related to oxidative stress’s role in DNP and neuropathic pain is essential for the development of new pharmaceutical agents. We have explained various metabolic abnormalities caused by hyperlipidemia, hyperglycemia, polyol signaling pathway, AGEs formation, PKC pathway, and hemosome pathway for enhanced generation of ROS, which subsequently accelerates the oxidative stress in DNP and neuropathic pain. Oxidative stress potentiates the abnormalities of these metabolic pathways, which impact cell mitochondrial membrane damage, neuronal cell damage, lipid peroxidation, and irregular genetic modification of genes in the antioxidant defense system, causing pain and damage to neuronal function. We believe that all the topics discussed in this review, with clear mechanisms and pathogenesis roles of oxidative stress in the progression of DNP, may be promising subjects for further studies on DNP, which open up new platforms for the proper management of DNP and neuropathic pain. Therefore, the inhibition of ROS generation and the evacuation of excessive ROS in neuronal cells could protect the neuronal cells from hyperglycemia-mediated oxidative stress impairments. Due to the nature of the antioxidant properties of melatonin and DPP-4i drugs, these drugs could be ideal for the treatment of DNP and neuropathic pain complications with various mechanisms of action. We explained various mechanisms through which melatonin and DPP-4i drugs can alleviate DNP and neuropathic pain. According to our knowledge, very little research was conducted on DM, DNP, and neuropathic pain using melatonin and DPP-4i. We strongly suggest future researchers focus their research on these drugs alone or in combination with other drugs for the management of DM to limit the adverse effects. In our opinion, even though all in vitro and in vivo studies provide useful information and hints for clinical trials with melatonin and DPP-4i or its combinational use, extensive work in clinical studies is still essential, and it may be possible to employ multi-functional drugs with a different mechanism of action to fight these multifactorial complications in DM of DNP and neuropathic pain.
Author Contributions
Conceptualization: PB and C-SW. Methodology, validation, formal analysis and investigation: PB, NH, YK and C-SW. Writing—original draft preparation: PB, YK and C-SW. Writing—review and editing: PB and C-SW. Supervision: C-SW. Funding acquisition: C-SW. All authors have read and approved the final manuscript.
Funding
This research was funded by the Ministry of Science and Technology (Taiwan) with grants MOST 107-2314-B281-002-MY3, MOST 110-2314-B-281-007, and the Cathay Research Grant CGH-MR-A10905.
Conflict of Interest
The authors declare that the research was conducted in the absence of any commercial or financial relationships that could be construed as a potential conflict of interest.
Publisher’s Note
All claims expressed in this article are solely those of the authors and do not necessarily represent those of their affiliated organizations, or those of the publisher, the editors, and the reviewers. Any product that may be evaluated in this article, or any claim that may be made by its manufacturer, is not guaranteed or endorsed by the publisher.
Abbreviations
AGES, advanced glycation end products; BDNF, brain-derived neurotrophic factor; Cu, copper; CAT, catalase; CRP, C-reactive protein; CNS, central nervous system; DM, diabetes mellitus; DPP-4i, dipeptidyl peptidase IV inhibitors; DRG, dorsal root ganglion; DNP, diabetes neuropathy; eNOS, endothelial nitric oxide; ET-1, endothelin-1; Fe, iron; GLP-1R, glucagon-like receptor agonists; GPX, glutathione; GFAP, glial fibrillary acidic protein; OH, hydroxyl radicals; H2O2, hydrogen peroxide; HO-1, heme oxygenase-1; IL-6, interleukin-6; MNCV, motor nerve conduction velocity ; MT2, melatonin type-2 receptor; MMP, mitochondrial membrane potential; MDA, malondialdehyde; MAPK, mitogen-activated protein kinase; NF-kB, nuclear factor kappa beta; Nrf2, nuclear factor erythroid 2- related factor 2; NADPH, nicotinamide adenine dinucleotide phosphate; PNS, peripheral nervous system; PUFAs, polyunsaturated fatty acids; PLA2, phospholipase A2; PKC, protein kinase C; Redox, reductive and oxidative; ROS, reactive oxygen species; RNS, reactive nitrogen species; SOD, superoxide dismutase; STZ, streptozocin; SCG, superior cervical ganglion; TNF-α, tumor necrosis factor–α; TGF-β, transforming growth factor-β; TEN, teneligliptin; TRP, transient receptor potential; TRPM2, melastatin 2; TRPV1, TRP vanilloid type 1; VEGF, vascular endothelial growth factor.
References
Adeghate, E., Rashed, H., Rajbandari, S., and Singh, J. (2006). Pattern of Distribution of Calcitonin Gene-Related Peptide in the Dorsal Root Ganglion of Animal Models of Diabetes Mellitus. Ann. N. Y. Acad. Sci. 1084, 296–303. doi:10.1196/annals.1372.030
Afanas'ev, I. (2010). Signaling of Reactive Oxygen and Nitrogen Species in Diabetes Mellitus. Oxid Med. Cel Longev 3 (6), 361–373. doi:10.4161/oxim.3.6.14415
Afrazi, S., and Esmaeili-Mahani, S. (2014). Allopregnanolone Suppresses Diabetes-Induced Neuropathic Pain and Motor Deficit through Inhibition of GABAA Receptor Down-Regulation in the Spinal Cord of Diabetic Rats. Iran J. Basic Med. Sci. 17 (5), 312–317. Retrieved from <Go to ISI>://WOS:000345354000001.
Ahmadi, Z., and Ashrafizadeh, M. (2020). Melatonin as a Potential Modulator of Nrf2. Fundam. Clin. Pharmacol. 34 (1), 11–19. doi:10.1111/fcp.12498
Alam, U. (2020). Diabetic Neuropathy Collection: Treatment of Diabetic Neuropathy. Diabetes Ther. 11 (4), 765–772. doi:10.1007/s13300-020-00784-3
Alaverdyan, A. R., and Vartanyan, G. S. (2012). The Effects of Protein Kinase C Inhibitors on the Contents of Several Transcription and Apoptosis Modulating Factors in the Spinal Cords of Rats with Diabetic Neuropathy. Neurochem. J. 6 (3), 223–226. doi:10.1134/s1819712412030026
Aliabadi, D. A., Moradian, N., Rahmanian, E., and Mohammadi, M. (2021). Prevalence of Neuropathy in Patients with Type 2 Diabetes in Iran : A Systematic Review and Meta-Analysis. Wien Klin Wochenschr 133 (5-6), 222–228. doi:10.1007/s00508-020-01784-w
Amaral, F. G. D., Andrade-Silva, J., Kuwabara, W. M. T., and Cipolla-Neto, J. (2019). New Insights into the Function of Melatonin and its Role in Metabolic Disturbances. Expert Rev. Endocrinol. Metab. 14 (4), 293–300. doi:10.1080/17446651.2019.1631158
Ambrose, K. R., and Golightly, Y. M. (2015). Physical Exercise as Non-pharmacological Treatment of Chronic Pain: Why and when. Best Pract. Res. Clin. Rheumatol. 29 (1), 120–130. doi:10.1016/j.berh.2015.04.022
Amer, M. E., Othamn, A. I., and El-Missiry, M. A. (2021). Melatonin Ameliorates Diabetes-Induced Brain Injury in Rats. Acta Histochem. 123 (2), 151677. doi:10.1016/j.acthis.2020.151677
Anthonsen, S., Larsen, J., Pedersen, P. L., Dalgaard, L. T., and Kvetny, J. (2013). Basal and T₃-induced ROS Production in Lymphocyte Mitochondria Is Increased in Type 2 Diabetic Patients. Horm. Metab. Res. 45 (4), 261–266. doi:10.1055/s-0032-1327590
Areti, A., Komirishetty, P., Akuthota, M., Malik, R. A., and Kumar, A. (2017). Melatonin Prevents Mitochondrial Dysfunction and Promotes Neuroprotection by Inducing Autophagy during Oxaliplatin-Evoked Peripheral Neuropathy. J. Pineal Res. 62 (3), 1. doi:10.1111/jpi.12393
Arreola-Espino, R., Urquiza-Marín, H., Ambriz-Tututi, M., Araiza-Saldaña, C. I., Caram-Salas, N. L., Rocha-González, H. I., et al. (2007). Melatonin Reduces Formalin-Induced Nociception and Tactile Allodynia in Diabetic Rats. Eur. J. Pharmacol. 577 (1-3), 203–210. doi:10.1016/j.ejphar.2007.09.006
Babizhayev, M. A., Strokov, I. A., Nosikov, V. V., Savel'yeva, E. L., Sitnikov, V. F., Yegorov, Y. E., et al. (2015). The Role of Oxidative Stress in Diabetic Neuropathy: Generation of Free Radical Species in the Glycation Reaction and Gene Polymorphisms Encoding Antioxidant Enzymes to Genetic Susceptibility to Diabetic Neuropathy in Population of Type I Diabetic Patients. Cell Biochem Biophys 71 (3), 1425–1443. doi:10.1007/s12013-014-0365-y
Bagi, Z., Feher, A., and Beleznai, T. (2009). Preserved Coronary Arteriolar Dilatation in Patients with Type 2 Diabetes Mellitus: Implications for Reactive Oxygen Species. Pharmacol. Rep. 61 (1), 99–104. doi:10.1016/s1734-1140(09)70011-8
Barber, A. J., Antonetti, D. A., Gardner, T. W., and Penn State Retina Res, G. (2000). Altered Expression of Retinal Occludin and Glial Fibrillary Acidic Protein in Experimental Diabetes. The Penn State Retina Research Group. Invest. Ophthalmol. Vis. Sci. 41 (11), 3561–3568. Retrieved from <Go to ISI>://WOS:000089567600045.
Barutta, F., Piscitelli, F., Pinach, S., Bruno, G., Gambino, R., Rastaldi, M. P., et al. (2011). Protective Role of Cannabinoid Receptor Type 2 in a Mouse Model of Diabetic Nephropathy. Diabetes 60 (9), 2386–2396. doi:10.2337/db10-1809
Becker, M., Benromano, T., Shahar, A., Nevo, Z., and Pick, C. G. (2014). Changes in the Basal Membrane of Dorsal Root Ganglia Schwann Cells Explain the Biphasic Pattern of the Peripheral Neuropathy in Streptozotocin-Induced Diabetic Rats. J. Mol. Neurosci. 54 (4), 704–713. doi:10.1007/s12031-014-0424-2
Behram Kandemir, Y., Guntekin, Ü., Tosun, V., Korucuk, N., and Bozdemir, M. N. (2018). Melatonin Protects against Streptozotocin-Induced Diabetic Cardiomyopathy by the Phosphorylation of Vascular Endothelial Growth Factor-A (VEGF-A). Cel Mol Biol (Noisy-le-grand) 64 (14), 47–52. doi:10.14715/cmb/2018.64.14.8
Belia, S., Santilli, F., Beccafico, S., De Feudis, L., Morabito, C., Davi, G., et al. (2009). Oxidative-induced Membrane Damage in Diabetes Lymphocytes: Effects on Intracellular Ca(2 +) Homeostasis. Free Radic. Res. 43 (2), 138–148. doi:10.1080/10715760802629588
Beyer, C. E., Steketee, J. D., and Saphier, D. (1998). Antioxidant Properties of Melatonin-Aan Emerging Mystery. Biochem. Pharmacol. 56 (10), 1265–1272. doi:10.1016/s0006-2952(98)00180-4
Bhattamisra, S. K., Koh, H. M., Lim, S. Y., Choudhury, H., and Pandey, M. (2021). Molecular and Biochemical Pathways of Catalpol in Alleviating Diabetes Mellitus and its Complications. Biomolecules 11 (2), 323. doi:10.3390/biom11020323
Bour-Jordan, H., Thompson, H. L., Giampaolo, J. R., Davini, D., Rosenthal, W., and Bluestone, J. A. (2013). Distinct Genetic Control of Autoimmune Neuropathy and Diabetes in the Non-obese Diabetic Background. J. Autoimmun. 45, 58–67. doi:10.1016/j.jaut.2013.06.005
Brown, O. I., Bridge, K. I., and Kearney, M. T. (2021). Nicotinamide Adenine Dinucleotide Phosphate Oxidases in Glucose Homeostasis and Diabetes-Related Endothelial Cell Dysfunction. Cells 10 (9), 2315. doi:10.3390/cells10092315
Bunpeng, N., Boriboonhirunsarn, D., Boriboonhirunsarn, C., Sawangpanyangkura, T., and Tansriratanawong, K. (2022). Association between Gestational Diabetes Mellitus and Periodontitis via the Effect of Reactive Oxygen Species in Peripheral Blood Cells. J. Periodontol. 1, 1. doi:10.1002/jper.21-0455
Burgos-Morón, E., Abad-Jiménez, Z., Marañón, A. M., Iannantuoni, F., Escribano-López, I., López-Domènech, S., et al. (2019). Relationship between Oxidative Stress, ER Stress, and Inflammation in Type 2 Diabetes: The Battle Continues. J. Clin. Med. 8 (9), 1385. doi:10.3390/jcm8091385
Byrne, F. M., Cheetham, S., Vickers, S., and Chapman, V. (20152015). Characterisation of Pain Responses in the High Fat Diet/Streptozotocin Model of Diabetes and the Analgesic Effects of Antidiabetic Treatments. J. Diabetes Res. 2015, 1–13. doi:10.1155/2015/752481
Cagnacci, A. (1996). Melatonin in Relation to Physiology in Adult Humans. J. Pineal Res. 21 (4), 200–213. doi:10.1111/j.1600-079X.1996.tb00287.x
Cantley, J., and Biden, T. J. (2013). Sweet and Sour β-cells: ROS and Hif1α Induce Warburg-like Lactate Production during Type 2 Diabetes. Diabetes 62 (6), 1823–1825. doi:10.2337/db13-0272
Caton, P. W., Richardson, S. J., Kieswich, J., Bugliani, M., Holland, M. L., Marchetti, P., et al. (2013). Sirtuin 3 Regulates Mouse Pancreatic Beta Cell Function and Is Suppressed in Pancreatic Islets Isolated from Human Type 2 Diabetic Patients. Diabetologia 56 (5), 1068–1077. doi:10.1007/s00125-013-2851-y
Cerveny, J. D., Leder, R. D., and Weart, C. W. (1998). Issues Surrounding Tight Glycemic Control in People with Type 2 Diabetes Mellitus. Ann. Pharmacother. 32 (9), 896–905. doi:10.1345/aph.17375
Chahbouni, M., López, M. D. S., Molina-Carballo, A., de Haro, T., Muñoz-Hoyos, A., Fernández-Ortiz, M., et al. (2017). Melatonin Treatment Reduces Oxidative Damage and Normalizes Plasma Pro-inflammatory Cytokines in Patients Suffering from Charcot-Marie-Tooth Neuropathy: A Pilot Study in Three Children. Molecules 22 (10), 1728. doi:10.3390/molecules22101728
Chang, Y. C., and Chuang, L. M. (2010). The Role of Oxidative Stress in the Pathogenesis of Type 2 Diabetes: from Molecular Mechanism to Clinical Implication. Am. J. Transl Res. 2 (3), 316–331. Retrieved from <Go to ISI>://WOS:000208694300009.
Chao, C. C., Tseng, M. T., and Hsieh, S. T. (2011). Pathophysiology of Neuropathic Pain in Type 2 Diabetes: Skin Denervation and Contact Heat Evoked Potentials. J. Peripher. Nervous Syst. 16, S21. Retrieved from <Go to ISI>://WOS:000293510300047.
Chao, W. C., Yen, C. L., Wu, Y. H., Chen, S. Y., Hsieh, C. Y., Chang, T. C., et al. (2015). Increased Resistin May Suppress Reactive Oxygen Species Production and Inflammasome Activation in Type 2 Diabetic Patients with Pulmonary Tuberculosis Infection. Microbes Infect. 17 (3), 195–204. doi:10.1016/j.micinf.2014.11.009
Chattopadhyay, M., Khemka, V. K., Chatterjee, G., Ganguly, A., Mukhopadhyay, S., and Chakrabarti, S. (2015). Enhanced ROS Production and Oxidative Damage in Subcutaneous white Adipose Tissue Mitochondria in Obese and Type 2 Diabetes Subjects. Mol. Cel Biochem 399 (1-2), 95–103. doi:10.1007/s11010-014-2236-7
Che, H., Li, H., Li, Y., Wang, Y. Q., Yang, Z. Y., Wang, R. L., et al. (2020). Melatonin Exerts Neuroprotective Effects by Inhibiting Neuronal Pyroptosis and Autophagy in STZ-Induced Diabetic Mice. FASEB J. 34 (10), 14042–14054. doi:10.1096/fj.202001328R
Chen, J., Chernatynskaya, A. V., Li, J. W., Kimbrell, M. R., Cassidy, R. J., Perry, D. J., et al. (2017). T Cells Display Mitochondria Hyperpolarization in Human Type 1 Diabetes. Sci. Rep. 7, 10835. doi:10.1038/s41598-017-11056-9
Chen, J. P., Xu, H. Y., Liao, L., and Zhang, Z. (2020). Resolvin D2 Prevents Inflammation and Oxidative Stress in the Retina of Streptozocin-Induced Diabetic Mice. Int. J. Clin. Exp. Pathol. 13 (8), 1986–1994. Retrieved from <Go to ISI>://WOS:000573059000005.
Chen, L., Wan, Z.-H., Zhang, Y.-J., Guo, Y.-L., Li, G.-H., Wu, D., et al. (2019). G Protein-Coupled Receptor Kinase 2 Inhibition Improves Erectile Function through Amelioration of Endothelial Dysfunction and Oxidative Stress in a Rat Model of Type 2 Diabetes. Asian J. Androl. 21 (1), 74–79. doi:10.4103/aja.aja_69_18
Civantos, E., Bosch, E., Ramirez, E., Zhenyukh, O., Egido, J., Lorenzo, O., et al. (2017). Sitagliptin Ameliorates Oxidative Stress in Experimental Diabetic Nephropathy by Diminishing the miR-200a/Keap-1/Nrf2 Antioxidant Pathway. Diabetes Metab. Syndr. Obes. 10, 207–222. doi:10.2147/dmso.s132537
Corriere, M., Rooparinesingh, N., and Kalyani, R. R. (2013). Epidemiology of Diabetes and Diabetes Complications in the Elderly: An Emerging Public Health Burden. Curr. Diab Rep. 13 (6), 805–813. doi:10.1007/s11892-013-0425-5
Cui, S. S., Feng, X. B., Zhang, B. H., Xia, Z. Y., and Zhan, L. Y. (2020). Exendin-4 Attenuates Pain-Induced Cognitive Impairment by Alleviating Hippocampal Neuroinflammation in a Rat Model of Spinal Nerve Ligation. Neural Regen. Res. 15 (7), 1333–1339. doi:10.4103/1673-5374.272620
Dai, J., Chen, H., and Chai, Y. (2019). Advanced Glycation End Products (AGES) Induce Apoptosis of Fibroblasts by Activation of NLRP3 Inflammasome via Reactive Oxygen Species (ROS) Signaling Pathway. Med. Sci. Monit. 25, 7499–7508. doi:10.12659/msm.915806
Darenskaya, M. A., Kolesnikova, L. I., and Kolesnikov, S. I. (2021). Oxidative Stress: Pathogenetic Role in Diabetes Mellitus and its Complications and Therapeutic Approaches to Correction. Bull. Exp. Biol. Med. 171 (2), 179–189. doi:10.1007/s10517-021-05191-7
Daugherty, D. J., Marquez, A., Calcutt, N. A., and Schubert, D. (2018). A Novel Curcumin Derivative for the Treatment of Diabetic Neuropathy. Neuropharmacology 129, 26–35. doi:10.1016/j.neuropharm.2017.11.007
Dave, G. S., and Kalia, K. (2007). Hyperglycemia Induced Oxidative Stress in Type-1 and Type-2 Diabetic Patients with and without Nephropathy. Cel Mol Biol (Noisy-le-grand) 53 (5), 68–78. doi:10.1170/t820
De Marañon, A. M., Iannantuoni, F., Abad-Jiménez, Z., Canet, F., Díaz-Pozo, P., López-Domènech, S., et al. (2020b). Relationship between PMN-Endothelium Interactions, ROS Production and Beclin-1 in Type 2 Diabetes. Redox Biol. 34, 101563. doi:10.1016/j.redox.2020.101563
De Marañón, A. M., Díaz-Pozo, P., Iannantuoni, F., Canet, F., Vezza, T., Abad-Jiménez, Z., et al. (2020a). Good Glycaemic Control Reduces Carotid-Intima-media Thickness, Inflammation Markers and ROS Production in Type 2 Diabetes. Free Radic. Biol. Med. 159, S89. doi:10.1016/j.freeradbiomed.2020.10.230
De Oliveira, C., Colette, C., Monnier, L., Descomps, B., and Pares-Herbute, N. (2005). Insulin Alters Nuclear Factor-lambdaB and Peroxisome Proliferator-Activated Receptor-Gamma Protein Expression Induced by Glycated Bovine Serum Albumin in Vascular Smooth-Muscle Cells. J. Lab. Clin. Med. 145 (3), 144–150. doi:10.1016/j.lab.2004.12.006
Deli, G., Bosnyak, E., Pusch, G., Komoly, S., and Feher, G. (2013). Diabetic Neuropathies: Diagnosis and Management. Neuroendocrinology 98 (4), 267–280. doi:10.1159/000358728
Demir, S., Nawroth, P. P., Herzig, S., and Ekim Üstünel, B. (2021). Emerging Targets in Type 2 Diabetes and Diabetic Complications. Adv. Sci. (Weinh) 8 (18), e2100275. doi:10.1002/advs.202100275
Ding, X., Jian, T., Wu, Y., Zuo, Y., Li, J., Lv, H., et al. (2019). Ellagic Acid Ameliorates Oxidative Stress and Insulin Resistance in High Glucose-Treated HepG2 Cells via miR-223/keap1-Nrf2 Pathway. Biomed. Pharmacother. 110, 85–94. doi:10.1016/j.biopha.2018.11.018
do Prado, F. C., Vieira, W. F., Fernandes de Magalhães, S., Bonet, I. J. M., Tambeli, C. H., and Parada, C. A. (2020). The Onset Speed of Hyperglycemia Is Important to the Development of Neuropathic Hyperalgesia in Streptozotocin-Induced Diabetic Rats. Eur. J. Neurosci. 52 (6), 3642–3651. doi:10.1111/ejn.14722
Dong, K., Ni, H., Wu, M., Tang, Z., Halim, M., and Shi, D. (2016a). ROS-mediated Glucose Metabolic Reprogram Induces Insulin Resistance in Type 2 Diabetes. Biochem. Biophys. Res. Commun. 476 (4), 204–211. doi:10.1016/j.bbrc.2016.05.087
Dong, K., Wu, M., Liu, X., Huang, Y., Zhang, D., Wang, Y., et al. (2016b). Glutaredoxins Concomitant with Optimal ROS Activate AMPK through S-Glutathionylation to Improve Glucose Metabolism in Type 2 Diabetes. Free Radic. Biol. Med. 101, 334–347. doi:10.1016/j.freeradbiomed.2016.10.007
Dong, S., Lau, H., Chavarria, C., Alexander, M., Cimler, A., Elliott, J. P., et al. (2019). Effects of Periodic Intensive Insulin Therapy: An Updated Review. Curr. Ther. Res. Clin. Exp. 90, 61–67. doi:10.1016/j.curtheres.2019.04.003
Drougard, A., Duparc, T., Fournel, A., Cani, P. D., Valet, P., and Knauf, C. (2013). Central Apelin Generates a Hypothalamic ROS Production that Controls Hepatic Glucose Metabolism during the Establishment of Type 2 Diabetes. Diabetologia 56, S49. Retrieved from <Go to ISI>://WOS:000329196900104.
Dworzański, J., Strycharz-Dudziak, M., Kliszczewska, E., Kiełczykowska, M., Dworzańska, A., Drop, B., et al. (2020). Glutathione Peroxidase (GPx) and Superoxide Dismutase (SOD) Activity in Patients with Diabetes Mellitus Type 2 Infected with Epstein-Barr Virus. Plos One 15 (3), e0230374. doi:10.1371/journal.pone.0230374
Eaton, S. E., Harris, N. D., Ibrahim, S., Patel, K. A., Selmi, F., Radatz, M., et al. (2003). Increased Sural Nerve Epineurial Blood Flow in Human Subjects with Painful Diabetic Neuropathy. Diabetologia 46 (7), 934–939. doi:10.1007/s00125-003-1127-3
Eckers, A., Altschmied, J., and Haendeler, J. (2012). [Oxidative Stress in Endothelial Cells and in Diabetes Type 2]. Z. Gerontol. Geriatr. 45 (2), 90–94. doi:10.1007/s00391-011-0277-z
El-Salhy, M. (2002). The Possible Role of the Gut Neuroendocrine System in Diabetes Gastroenteropathy. Histol. Histopathol 17 (4), 1153–1161. Retrieved from <Go to ISI>://WOS:000178234100017. doi:10.14670/HH-17.1153
Elzinga, S., Murdock, B. J., Guo, K., Hayes, J. M., Tabbey, M. A., Hur, J., et al. (2019). Toll-like Receptors and Inflammation in Metabolic Neuropathy; a Role in Early versus Late Disease? Exp. Neurol. 320, 112967. doi:10.1016/j.expneurol.2019.112967
Fagundes-Netto, F. S., Anjos, P. M., Volpe, C. M., and Nogueira-Machado, J. A. (2013). The Production of Reactive Oxygen Species in TLR-Stimulated Granulocytes Is Not Enhanced by Hyperglycemia in Diabetes. Int. Immunopharmacol 17 (3), 924–929. doi:10.1016/j.intimp.2013.09.018
Falco, P., Galosi, E., Fasolino, A., Di Pietro, G., De Stefano, G., Di Lionardo, A., et al. (2021). Neuropathic Pain Mechanisms in Painful Polyneuropathies. Learnings from Type 2 Diabetes and TTR-FAP. Eur. J. Neurol. 28, 491. Retrieved from <Go to ISI>://WOS:000663065901079.
Fan, H., Gong, N., Li, T. F., Ma, A. N., Wu, X. Y., Wang, M. W., et al. (2015). The Non-peptide GLP-1 Receptor Agonist WB4-24 Blocks Inflammatory Nociception by Stimulating β-endorphin Release from Spinal Microglia. Br. J. Pharmacol. 172 (1), 64–79. doi:10.1111/bph.12895
Feldman, E. L., Nave, K. A., Jensen, T. S., and Bennett, D. L. H. (2017). New Horizons in Diabetic Neuropathy: Mechanisms, Bioenergetics, and Pain. Neuron 93 (6), 1296–1313. doi:10.1016/j.neuron.2017.02.005
Ferlita, S., Yegiazaryan, A., Noori, N., Lal, G., Nguyen, T., To, K., et al. (2019). Type 2 Diabetes Mellitus and Altered Immune System Leading to Susceptibility to Pathogens, Especially Mycobacterium tuberculosis. J. Clin. Med. 8 (12), 2219. doi:10.3390/jcm8122219
Fidler, T. P., Rowley, J. W., Araujo, C., Boudreau, L. H., Marti, A., Souvenir, R., et al. (2017). Superoxide Dismutase 2 Is Dispensable for Platelet Function. Thromb. Haemost. 117 (10), 1859–1867. doi:10.1160/th17-03-0174
Fischer, T. Z., Tan, A. M., and Waxman, S. G. (2009). Thalamic Neuron Hyperexcitability and Enlarged Receptive fields in the STZ Model of Diabetic Pain. Brain Res. 1268, 154–161. doi:10.1016/j.brainres.2009.02.063
Gadjeva, V. G., Goycheva, P., Nikolova, G., and Zheleva, A. (2017). Influence of Glycemic Control on Some Real-Time Biomarkers of Free Radical Formation in Type 2 Diabetic Patients: An EPR Study. Adv. Clin. Exp. Med. 26 (8), 1237–1243. doi:10.17219/acem/68988
Galley, H. F., McCormick, B., Wilson, K. L., Lowes, D. A., Colvin, L., and Torsney, C. (2017). Melatonin Limits Paclitaxel-Induced Mitochondrial Dysfunction In Vitro and Protects against Paclitaxel-Induced Neuropathic Pain in the Rat. J. Pineal Res. 63 (4), e12444. doi:10.1111/jpi.12444
Gao, Z., Wei, H., Chen, Z., Jalava, N., Koivisto, A., and Pertovaara, A. (2019). Ongoing Pain in Streptozotocin Model of Diabetes in the Rat: Correlation with Cutaneous Cheminociception. J. Physiol. Pharmacol. 70 (6), 969–978. doi:10.26402/jpp.2019.6.14
Gawlik, K., Naskalski, J. W., Fedak, D., Pawlica-Gosiewska, D., Grudzień, U., Dumnicka, P., et al. (2016). Markers of Antioxidant Defense in Patients with Type 2 Diabetes. Oxidative Med. Cell Longevity 2016, 1–6. doi:10.1155/2016/2352361
Ghouini, A., Rahal, L., and Djoghlaf, D. E. (2016). Screening of Insulin Resistance in Subjects at Risk for Type 2 Diabetes. Acta Physiol. 217, 109. Retrieved from <Go to ISI>://WOS:000379514000248.
Gilardini Montani, M. S., Granato, M., Cuomo, L., Valia, S., Di Renzo, L., D'Orazi, G., et al. (2016). High Glucose and Hyperglycemic Sera from Type 2 Diabetic Patients Impair DC Differentiation by Inducing ROS and Activating Wnt/β-Catenin and P38 MAPK. Biochim. Biophys. Acta 1862 (4), 805–813. doi:10.1016/j.bbadis.2016.01.001
Girgis, C. M., Scalley, B. D., and Park, K. E. (2012). Utility of the Estimated Glucose Disposal Rate as a Marker of Microvascular Complications in Young Adults with Type 1 Diabetes. Diabetes Res. Clin. Pract. 96 (3), E70–E72. doi:10.1016/j.diabres.2012.02.004
Gong, N., Xiao, Q., Zhu, B., Zhang, C. Y., Wang, Y. C., Fan, H., et al. (2014). Activation of Spinal Glucagon-like Peptide-1 Receptors Specifically Suppresses Pain Hypersensitivity. J. Neurosci. 34 (15), 5322–5334. doi:10.1523/jneurosci.4703-13.2014
Gonzalez, C. D., Lee, M. S., Marchetti, P., Pietropaolo, M., Towns, R., Vaccaro, M. I., et al. (2011). The Emerging Role of Autophagy in the Pathophysiology of Diabetes Mellitus. Autophagy 7 (1), 2–11. doi:10.4161/auto.7.1.13044
Guichard, C., Moreau, R., Pessayre, D., Epperson, T. K., and Krause, K. H. (2008). NOX Family NADPH Oxidases in Liver and in Pancreatic Islets: a Role in the Metabolic Syndrome and Diabetes? Biochem. Soc. Trans. 36, 920–929. doi:10.1042/bst0360920
Gurel-Gokmen, B., Ipekci, H., Oktay, S., Alev, B., Ustundag, U. V., Ak, E., et al. (2018). Melatonin Improves Hyperglycemia Induced Damages in Rat Brain. Diabetes Metab. Res. Rev. 34 (8), e3060. doi:10.1002/dmrr.3060
Hamada, Y., Fujii, H., and Fukagawa, M. (2009). Role of Oxidative Stress in Diabetic Bone Disorder. Bone 45 (Suppl. 1), S35–S38. doi:10.1016/j.bone.2009.02.004
Han, W., Li, Y., Cheng, J., Zhang, J., Chen, D., Fang, M., et al. (2020). Sitagliptin Improves Functional Recovery via GLP-1r-Induced Anti-apoptosis and Facilitation of Axonal Regeneration after Spinal Cord Injury. J. Cel Mol Med 24 (15), 8687–8702. doi:10.1111/jcmm.15501
Harding, J. L., Pavkov, M. E., Magliano, D. J., Shaw, J. E., and Gregg, E. W. (2019). Global Trends in Diabetes Complications: a Review of Current Evidence. Diabetologia 62 (1), 3–16. doi:10.1007/s00125-018-4711-2
Hayashi, T., Murakami, M., Yamamoto, S., Ono, K., and Onodera, T. (1997). Dimethylthiourea Reduces Pancreatic Islet-Cell Damage in DBA/1 Sucking Mice with Reovirus Type-2 Infection. J. Comp. Pathol. 117 (4), 329–338. doi:10.1016/s0021-9975(97)80080-1
He, F., Huang, Y., Song, Z., Zhou, H. J., Zhang, H., Perry, R. J., et al. (2021). Mitophagy-mediated Adipose Inflammation Contributes to Type 2 Diabetes with Hepatic Insulin Resistance. J. Exp. Med. 218 (3), e20201416. doi:10.1084/jem.20201416
He, L., and Sun, Y. (2021). The Potential Role of Keap1-Nrf2 Pathway in the Pathogenesis of Alzheimer's Disease, Type 2 Diabetes, and Type 2 Diabetes-Related Alzheimer's Disease. Metab. Brain Dis. 36 (7), 1469–1479. doi:10.1007/s11011-021-00762-z
Hey-Mogensen, M., Højlund, K., Vind, B. F., Wang, L., Dela, F., Beck-Nielsen, H., et al. (2010). Effect of Physical Training on Mitochondrial Respiration and Reactive Oxygen Species Release in Skeletal Muscle in Patients with Obesity and Type 2 Diabetes. Diabetologia 53 (9), 1976–1985. doi:10.1007/s00125-010-1813-x
Hirao, K., Maruyama, T., Ohno, Y., Hirose, H., Shimada, A., Takei, I., et al. (2010). Association of Increased Reactive Oxygen Species Production with Abdominal Obesity in Type 2 Diabetes. Obes. Res. Clin. Pract. 4 (2), E83–E90. doi:10.1016/j.orcp.2009.09.004
Hong, E. G., Jung, D. Y., Ko, H. J., Zhang, Z., Ma, Z., Jun, J. Y., et al. (2007). Nonobese, Insulin-Deficient Ins2Akita Mice Develop Type 2 Diabetes Phenotypes Including Insulin Resistance and Cardiac Remodeling. Am. J. Physiol. Endocrinol. Metab. 293 (6), E1687–E1696. doi:10.1152/ajpendo.00256.2007
Hong, Q. X., Xu, S. Y., Dai, S. H., and Zhao, W. X. (2016). Expression Profiling of Spinal Genes in Peripheral Neuropathy Model Rats with Type 2 Diabetes Mellitus. Int. J. Clin. Exp. Med. 9 (3), 6376–6384. Retrieved from <Go to ISI>://WOS:000377959900125.
Inoue, K., and Tsuda, M. (2009). Microglia and Neuropathic Pain. Glia 57 (14), 1469–1479. doi:10.1002/glia.20871
Isoni, C. A., Borges, E. A., Veloso, C. A., Mattos, R. T., Chaves, M. M., and Nogueira-Machado, J. A. (2009). cAMP Activates the Generation of Reactive Oxygen Species and Inhibits the Secretion of IL-6 in Peripheral Blood Mononuclear Cells from Type 2 Diabetic Patients. Oxid Med. Cel Longev 2 (5), 317–321. doi:10.4161/oxim.2.5.9657
Iyer, S., and Tanenberg, R. J. (2013). Pharmacologic Management of Diabetic Peripheral Neuropathic Pain. Expert Opin. Pharmacother. 14 (13), 1765–1775. doi:10.1517/14656566.2013.811490
Jackiewicz, A., and Katarzyńska, J. (2018). Dipeptidyl Peptidase IV: An Attractive Target for the Development of Effective Diagnostic and Therapeutic Strategies. Postepy Hig Med. Dosw 72, 417–441. doi:10.5604/01.3001.0012.0538
Jangra, A., Datusalia, A. K., Khandwe, S., and Sharma, S. S. (2013). Amelioration of Diabetes-Induced Neurobehavioral and Neurochemical Changes by Melatonin and Nicotinamide: Implication of Oxidative Stress-PARP Pathway. Pharmacol. Biochem. Behav. 114-115, 43–51. doi:10.1016/j.pbb.2013.10.021
Jersin, R. Å., Tallapragada, D. S. P., Madsen, A., Skartveit, L., Fjære, E., McCann, A., et al. (2021). Role of the Neutral Amino Acid Transporter SLC7A10 in Adipocyte Lipid Storage, Obesity, and Insulin Resistance. Diabetes 70 (3), 680–695. doi:10.2337/db20-0096
Jiang, Z., Chen, Z., Chen, Y., Jiao, J., and Wang, Z. (2021). Retracted Article: Involvement of Pro-inflammatory Cytokines in Diabetic Neuropathic Pain via central PI3K/Akt/mTOR Signal Pathway. Arch. Physiol. Biochem. 127 (6), (I)-(IX)–IX. doi:10.1080/13813455.2019.1651869
Jiao, J., Dou, L., Li, M., Lu, Y., Guo, H. B., Man, Y., et al. (2012). NADPH Oxidase 2 Plays a Critical Role in Dysfunction and Apoptosis of Pancreatic β-cells Induced by Very Low-Density Lipoprotein. Mol. Cel Biochem 370 (1-2), 103–113. doi:10.1007/s11010-012-1402-z
Jiao, N., Chen, Y., Zhu, Y., Wang, W., Liu, M., Ding, W., et al. (2020). Protective Effects of Catalpol on Diabetes Mellitus-Induced Male Reproductive Damage via Suppression of the AGEs/RAGE/Nox4 Signaling Pathway. Life Sci. 256, 116736. doi:10.1016/j.lfs.2019.116736
Jing, F., Zou, Q., Wang, Y., Cai, Z., and Tang, Y. (2021). Activation of Microglial GLP-1R in the Trigeminal Nucleus Caudalis Suppresses central Sensitization of Chronic Migraine after Recurrent Nitroglycerin Stimulation. J. Headache Pain 22 (1), 1. doi:10.1186/s10194-021-01302-x
Johnson, M. S., Ryals, J. M., and Wright, D. E. (2007). Diabetes-induced Chemogenic Hypoalgesia Is Paralleled by Attenuated Stimulus-Induced Fos Expression in the Spinal Cord of Diabetic Mice. J. Pain 8 (8), 637–649. doi:10.1016/j.jpain.2007.04.004
Juybari, K. B., Hosseinzadeh, A., Ghaznavi, H., Kamali, M., Sedaghat, A., Mehrzadi, S., et al. (2019). Melatonin as a Modulator of Degenerative and Regenerative Signaling Pathways in Injured Retinal Ganglion Cells. Curr. Pharm. Des. 25 (28), 3057–3073. doi:10.2174/1381612825666190829151314
Kahl, S. (2017). Diabetische Neuropathie bei Jugendlichen mit Typ-1- und Typ-2-Diabetes. Diabetologe 13 (6), 448–449. doi:10.1007/s11428-017-0245-3
Kahya, M. C., Nazıroğlu, M., and Övey, İ. S. (2017). Modulation of Diabetes-Induced Oxidative Stress, Apoptosis, and Ca2+ Entry through TRPM2 and TRPV1 Channels in Dorsal Root Ganglion and Hippocampus of Diabetic Rats by Melatonin and Selenium. Mol. Neurobiol. 54 (3), 2345–2360. doi:10.1007/s12035-016-9727-3
Kaneto, H., Katakami, N., Matsuhisa, M., and Matsuoka, T.-a. (2010). Role of Reactive Oxygen Species in the Progression of Type 2 Diabetes and Atherosclerosis. Mediators Inflamm. 2010, 1–11. doi:10.1155/2010/453892
Karunakaran, U., Park, S. J., Jun, D. Y., Sim, T., Park, K.-G., Kim, M. O., et al. (2015). Non-receptor Tyrosine Kinase Inhibitors Enhances β-cell Survival by Suppressing the PKCδ Signal Transduction Pathway in Streptozotocin - Induced β-cell Apoptosis. Cell Signal. 27 (6), 1066–1074. doi:10.1016/j.cellsig.2015.01.018
Kashyap, P., and Farrugia, G. (2011). Oxidative Stress: Key Player in Gastrointestinal Complications of Diabetes. Neurogastroenterol Motil. 23 (2), 111–114. doi:10.1111/j.1365-2982.2010.01659.x
Kassab, A., and Piwowar, A. (2012). Cell Oxidant Stress Delivery and Cell Dysfunction Onset in Type 2 Diabetes. Biochimie 94 (9), 1837–1848. doi:10.1016/j.biochi.2012.01.020
Kassan, M., Choi, S. K., Galán, M., Lee, Y. H., Trebak, M., and Matrougui, K. (2014). Enhanced P22phox Expression Impairs Vascular Function through P38 and ERK1/2 MAP Kinase-dependent Mechanisms in Type 2 Diabetic Mice. Am. J. Physiol. Heart Circ. Physiol. 306 (7), H972–H980. doi:10.1152/ajpheart.00872.2013
Kempler, P., Czupryniak, L., Garcia-Verdugo, R., Gurieve, I., Mankovskys, B. N., Manske, C., et al. (2017). Perspectives in Diabetic Neuropathy: Update on Diagnostic Criteria and Treatment Options. Diabetes Stoffwechsel Und Herz 26 (5), 279–285. Retrieved from <Go to ISI>://WOS:000412793800005.
Khan, M. W., Banga, K., Mashal, S. N., and Khan, W. A. (2011). Detection of Autoantibodies against Reactive Oxygen Species Modified Glutamic Acid Decarboxylase-65 in Type 1 Diabetes Associated Complications. BMC Immunol. 12, 19. doi:10.1186/1471-2172-12-19
Kim, J. W., Park, S. Y., You, Y. H., Ham, D. S., Lee, S. H., Yang, H. K., et al. (2016). Suppression of ROS Production by Exendin-4 in PSC Attenuates the High Glucose-Induced Islet Fibrosis. Plos One 11 (12), e0163187. doi:10.1371/journal.pone.0163187
Kim, M. H., Kim, E. H., Jung, H. S., Yang, D., Park, E. Y., and Jun, H. S. (2017). EX4 Stabilizes and Activates Nrf2 via PKCδ, Contributing to the Prevention of Oxidative Stress-Induced Pancreatic Beta Cell Damage. Toxicol. Appl. Pharmacol. 315, 60–69. doi:10.1016/j.taap.2016.12.005
Kizub, I. V., Klymenko, K. I., and Soloviev, A. I. (2014). Protein Kinase C in Enhanced Vascular Tone in Diabetes Mellitus. Int. J. Cardiol. 174 (2), 230–242. doi:10.1016/j.ijcard.2014.04.117
Kuniss, N., Freyer, M., Müller, N., Kielstein, V., and Müller, U. A. (2019). Expectations and Fear of Diabetes-Related Long-Term Complications in People with Type 2 Diabetes at Primary Care Level. Acta Diabetol. 56 (1), 33–38. doi:10.1007/s00592-018-1217-9
Kuthati, Y., Busa, P., Goutham Davuluri, V. N., and Wong, C. S. (2019). Manganese Oxide Nanozymes Ameliorate Mechanical Allodynia in a Rat Model of Partial Sciatic Nerve-Transection Induced Neuropathic Pain. Int. J. Nanomedicine 14, 10105–10117. doi:10.2147/ijn.s225594
Kuthati, Y., Navakanth Rao, V., Busa, P., Tummala, S., Davuluri Venkata Naga, G., and Wong, C. S. (2020). Scope and Applications of Nanomedicines for the Management of Neuropathic Pain. Mol. Pharm. 17 (4), 1015–1027. doi:10.1021/acs.molpharmaceut.9b01027
Kuthati, Y., Rao, V. N., Busa, P., and Wong, C. S. (2021). Teneligliptin Exerts Antinociceptive Effects in Rat Model of Partial Sciatic Nerve Transection Induced Neuropathic Pain. Antioxidants (Basel) 10 (9), 1438. doi:10.3390/antiox10091438
La Morgia, C., Ross-Cisneros, F. N., Sadun, A. A., Hannibal, J., Munarini, A., Mantovani, V., et al. (2010). Melanopsin Retinal Ganglion Cells Are Resistant to Neurodegeneration in Mitochondrial Optic Neuropathies. Brain 133, 2426–2438. doi:10.1093/brain/awq155
Lee, C. H., Jeon, S. J., Cho, K. S., Moon, E., Sapkota, A., Jun, H. S., et al. (2018). Activation of Glucagon-like Peptide-1 Receptor Promotes Neuroprotection in Experimental Autoimmune Encephalomyelitis by Reducing Neuroinflammatory Responses. Mol. Neurobiol. 55 (4), 3007–3020. doi:10.1007/s12035-017-0550-2
Lee, J. G., Woo, Y. S., Park, S. W., Seog, D. H., Seo, M. K., and Bahk, W. M. (2019). Erratum: Lee, J.G., et al. The Neuroprotective Effects of Melatonin: Possible Role in the Pathophysiology of Neuropsychiatric Disease. Brain Sci. 9 (10), 341. doi:10.3390/brainsci9120341
Lee, O.-H., Kwon, Y. I., Hong, H. D., Park, C. S., Lee, B. Y., and Kim, Y. C. (2009). Production of Reactive Oxygen Species and Changes in Antioxidant Enzyme Activities during Differentiation of 3T3-L1 Adipocyte. J. Korean Soc. Appl. Biol. Chem. 52 (1), 70–75. doi:10.3839/jksabc.2009.012
Lee, S. H., Du, J., Stitham, J., Atteya, G., Lee, S., Xiang, Y., et al. (2016). Inducing Mitophagy in Diabetic Platelets Protects against Severe Oxidative Stress. EMBO Mol. Med. 8 (7), 779–795. doi:10.15252/emmm.201506046
Li, L., ZHeng-Qing, Y., Juan-Yu, H., Jian-Yong, X., Fan, L., Guang-Chun, Z., et al. (2016). Association between Interleukin-19 and Angiopoietin-2 with Vascular Complications in Type 2 Diabetes. J. Diabetes Investig. 7 (6), 895–900. doi:10.1111/jdi.12519
Li, P., Yuan, X. H., and He, L. (2019). Clinical Analysis of Type 2 Diabetes Combined with Peripheral Neuropathy. Acta Med. Mediterranea 35 (2), 957–960. doi:10.19193/0393-6384_2019_2_145
Liang, F., Wang, J., Zhu, X., Wang, Z., Zheng, J., Sun, Z., et al. (2020). Melatonin Alleviates Neuronal Damage after Intracerebral Hemorrhage in Hyperglycemic Rats. Drug Des. Devel Ther. 14, 2573–2584. doi:10.2147/dddt.s257333
Liao, Z., Zhang, J., Liu, B., Yan, T., Xu, F., Xiao, F., et al. (2019). Polysaccharide from Okra (Abelmoschus Esculentus (L.) Moench) Improves Antioxidant Capacity via PI3K/AKT Pathways and Nrf2 Translocation in a Type 2 Diabetes Model. Molecules 24 (10), 1906. doi:10.3390/molecules24101906
Liu, C., Whitener, R. L., Lin, A., Xu, Y., Chen, J., Savinov, A., et al. (2019). Neutrophil Cytosolic Factor 1 in Dendritic Cells Promotes Autoreactive CD8+ T Cell Activation via Cross-Presentation in Type 1 Diabetes. Front. Immunol. 10, 952. doi:10.3389/fimmu.2019.00952
Lodovici, M., Bigagli, E., Bardini, G., and Rotella, C. M. (2009). Lipoperoxidation and Antioxidant Capacity in Patients with Poorly Controlled Type 2 Diabetes. Toxicol. Ind. Health 25 (4-5), 337–341. doi:10.1177/0748233709106464
Love, K. M., Barrett, E. J., Malin, S. K., Reusch, J. E. B., Regensteiner, J. G., and Liu, Z. (2021). Diabetes Pathogenesis and Management: the Endothelium Comes of Age. J. Mol. Cel Biol 13 (7), 500–512. doi:10.1093/jmcb/mjab024
Lu, P. P., Chen, M. H., Dai, G. C., Li, Y. J., Shi, L., and Rui, Y. F. (2020). Understanding Cellular and Molecular Mechanisms of Pathogenesis of Diabetic Tendinopathy. World J. Stem Cell 12 (11), 1255–1275. doi:10.4252/wjsc.v12.i11.1255
Lu, Q., Zhou, Y., Hao, M., Li, C., Wang, J., Shu, F., et al. (2018). The mTOR Promotes Oxidative Stress-Induced Apoptosis of Mesangial Cells in Diabetic Nephropathy. Mol. Cel Endocrinol 473, 31–43. doi:10.1016/j.mce.2017.12.012
Lukic, I. K., Humpert, P. M., Nawroth, P. P., and Bierhaus, A. (2008). “The RAGE Pathway,”. Editors E. Schleicher, V. Somoza, and P. Shieberle, 1126, 76–80. doi:10.1196/annals.1433.059Maillard React. Recent Adv. Food Biomed. Sci.
Ma, X., Liu, S., Liu, D., Wang, Q., Li, H., and Zhao, Z. (2020). Exercise Intervention Attenuates Neuropathic Pain in Diabetes via Mechanisms of Mammalian Target of Rapamycin (mTOR). Arch. Physiol. Biochem. 126 (1), 41–48. doi:10.1080/13813455.2018.1489851
Ma, X. Q., Qin, J., Li, H. Y., Yan, X. L., Zhao, Y., and Zhang, L. J. (2019). Role of Exercise Activity in Alleviating Neuropathic Pain in Diabetes via Inhibition of the Pro-inflammatory Signal Pathway. Biol. Res. Nurs. 21 (1), 14–21. doi:10.1177/1099800418803175
Ma, Z. A., Zhao, Z., and Turk, J. (2012). Mitochondrial Dysfunction Andβ-Cell Failure in Type 2 Diabetes Mellitus. Exp. Diabetes Res. 2012, 1–11. doi:10.1155/2012/703538
Mackenzie, R. M., Hamilton, C. A., Miller, W. H., Salt, I. P., Murphy, M. P., Delles, C., et al. (2009). MITOQ10 Reduces Mitochondrial Reactive Oxygen Species (ROS) Production and AMP-Activated Protein Kinase (AMPK) Activity in Vessels from Patients with Type 2 Diabetes and Coronary Artery Disease (CAD). J. Hum. Hypertens. 23 (10), 691. Retrieved from <Go to ISI>://WOS:000269871500019.
Maddaloni, E., Moretti, C., Del Toro, R., Sterpetti, S., Ievolella, M. V., Arnesano, G., et al. (2021). Risk of Cardiac Autonomic Neuropathy in Latent Autoimmune Diabetes in Adults Is Similar to Type 1 Diabetes and Lower Compared to Type 2 Diabetes: A Cross-Sectional Study. Diabet Med. 38 (2), e14455. doi:10.1111/dme.14455
Maher, A. M., Saleh, S. R., Elguindy, N. M., Hashem, H. M., and Yacout, G. A. (2020). Exogenous Melatonin Restrains Neuroinflammation in High Fat Diet Induced Diabetic Rats through Attenuating Indoleamine 2,3-dioxygenase 1 Expression. Life Sci. 247, 117427. doi:10.1016/j.lfs.2020.117427
Mahood, R. A. H., Hussein, M. H., and Al-Ahmed, H. (2018). Oxidative Stress and DNA Damage in Type 2 Diabetes Mellitus Patients in Population of Baghdad. Biosci. Res. 15 (1), 310–315. Retrieved from <Go to ISI>://WOS:000441152200036.
Manoharan, B., Bobby, Z., Dorairajan, G., Jacob, S. E., Gladwin, V., Vinayagam, V., et al. (2019). Increased Placental Expressions of Nuclear Factor Erythroid 2-related Factor 2 and Antioxidant Enzymes in Gestational Diabetes: Protective Mechanisms against the Placental Oxidative Stress? Eur. J. Obstet. Gynecol. Reprod. Biol. 238, 78–85. doi:10.1016/j.ejogrb.2019.05.016
Mert, T., Gunay, I., Ocal, I., Guzel, A. I., Inal, T. C., Sencar, L., et al. (2009). Macrophage Depletion Delays Progression of Neuropathic Pain in Diabetic Animals. Naunyn Schmiedebergs Arch. Pharmacol. 379 (5), 445–452. doi:10.1007/s00210-008-0387-3
Metwally, M. M. M., Ebraheim, L. L. M., and Galal, A. A. A. (2018). Potential Therapeutic Role of Melatonin on STZ-Induced Diabetic central Neuropathy: A Biochemical, Histopathological, Immunohistochemical and Ultrastructural Study. Acta Histochem. 120 (8), 828–836. doi:10.1016/j.acthis.2018.09.008
Mihanfar, A., Akbarzadeh, M., Ghazizadeh Darband, S., Sadighparvar, S., and Majidinia, M. (2021). SIRT1: a Promising Therapeutic Target in Type 2 Diabetes Mellitus. Arch. Physiol. Biochem. 1, 1–16. doi:10.1080/13813455.2021.1956976
Muggeo, M. (1998). Accelerated Complications in Type 2 Diabetes Mellitus: The Need for Greater Awareness and Earlier Detection. Diabet Med. 15 (12), S60–S62. doi:10.1002/(sici)1096-9136(1998120)15:4+<s60::aid-dia736>3.3.co;2-a
Mushtaq, S., Ali, T., Altaf, F., Abdullah, M., and Murtaza, I. (2015). Stress-responsive Factor Regulation in Patients Suffering from Type 2 Diabetes and Myocardial Infarction. Turk J. Med. Sci. 45 (1), 148–152. doi:10.3906/sag-1308-25
Nakashima, K., Kaneto, H., Shimoda, M., Kimura, T., and Kaku, K. (2018). Pancreatic Alpha Cells in Diabetic Rats Express Active GLP-1 Receptor: Endosomal Co-localization of GLP-1/GLP-1R Complex Functioning through Intra-islet Paracrine Mechanism. Sci. Rep. 8, 3725. doi:10.1038/s41598-018-21751-w
Negi, G., Kumar, A., Kaundal, R. K., Gulati, A., and Sharma, S. S. (2010). Functional and Biochemical Evidence Indicating Beneficial Effect of Melatonin and Nicotinamide Alone and in Combination in Experimental Diabetic Neuropathy. Neuropharmacology 58 (3), 585–592. doi:10.1016/j.neuropharm.2009.11.018
Nemoto, M., Nishimura, R., Sasaki, T., Hiki, Y., Miyashita, Y., Nishioka, M., et al. (2007). Genetic Association of Glutathione Peroxidase-1 with Coronary Artery Calcification in Type 2 Diabetes: a Case Control Study with Multi-Slice Computed Tomography. Cardiovasc. Diabetol. 6, 23. doi:10.1186/1475-2840-6-23
Nijpels, G., Beulens, J. W., van der Heijden, A. A., and Elders, P. J. (2019). Innovations in Personalised Diabetes Care and Risk Management. Eur. J. Prev. Cardiol. 26 (2_Suppl. L), 125–132. doi:10.1177/2047487319880043
Nogueira-Machado, J. A., Lima e Silva, F. C., Cunha, E. P., Calsolari, M. R., Costa, D. C., Perilo, C. S., et al. (2006). Modulation of the Production of Reactive Oxygen Species (ROS) by cAMP-Elevating Agents in Granulocytes from Diabetic Patients: an Akt/PKB-dependent Phenomenon. Diabetes Metab. 32 (4), 331–335. doi:10.1016/s1262-3636(07)70287-2
Nokkaew, N., Mongkolpathumrat, P., Junsiri, R., Jindaluang, S., Tualamun, N., Manphatthanakan, N., et al. (2021). p38 MAPK Inhibitor (SB203580) and Metformin Reduces Aortic Protein Carbonyl and Inflammation in Non-obese Type 2 Diabetic Rats. Indian J. Clin. Biochem. 36 (2), 228–234. doi:10.1007/s12291-019-0815-9
Nopparat, C., Chaopae, W., Boontem, P., Sopha, P., Wongchitrat, P., and Govitrapong, P. (2021). Melatonin Attenuates High Glucose-Induced Changes in Beta Amyloid Precursor Protein Processing in Human Neuroblastoma Cells. Neurochem. Res. 1, 1. doi:10.1007/s11064-021-03290-5
O'Brien, P. D., Hinder, L. M., Rumora, A. E., Hayes, J. M., Dauch, J. R., Backus, C., et al. (2018). Juvenile Murine Models of Prediabetes and Type 2 Diabetes Develop Neuropathy. Dis. Model. Mech. 11 (12), dmm037374. doi:10.1242/dmm.037374
O'Brien, P. D., Sakowski, S. A., and Feldman, E. L. (2014). Mouse Models of Diabetic Neuropathy. ILAR J. 54 (3), 259–272. doi:10.1093/ilar/ilt052
Obrosova, I. G. (2009). Diabetic Painful and Insensate Neuropathy: Pathogenesis and Potential Treatments. Neurotherapeutics 6 (4), 638–647. doi:10.1016/j.nurt.2009.07.004
Ojima, A., Matsui, T., Maeda, S., Takeuchi, M., and Yamagishi, S. (2012). Glucose-dependent Insulinotropic Polypeptide (GIP) Inhibits Signaling Pathways of Advanced Glycation End Products (AGEs) in Endothelial Cells via its Antioxidative Properties. Horm. Metab. Res. 44 (7), 501–505. doi:10.1055/s-0032-1312595
Onphachanh, X., Lee, H. J., Lim, J. R., Jung, Y. H., Kim, J. S., Chae, C. W., et al. (2017). Enhancement of High Glucose-Induced PINK1 Expression by Melatonin Stimulates Neuronal Cell Survival: Involvement of MT2 /Akt/NF-Κb Pathway. J. Pineal Res. 63 (2), 1. doi:10.1111/jpi.12427
Opara, E. C. (2004). Role of Oxidative Stress in the Etiology of Type 2 Diabetes and the Effect of Antioxidant Supplementation on Glycemic Control. J. Investig. Med. 52 (1), 19–23. Retrieved from <Go to ISI>://WOS:000188254600012. doi:10.1136/jim-52-01-22
Ostenson, C. G. (2001). The Pathophysiology of Type 2 Diabetes Mellitus: an Overview. Acta Physiol. Scand. 171 (3), 241–247. doi:10.1046/j.1365-201x.2001.00826.x
Ozaki, K., Terayama, Y., and Matsuura, T. (2018). Extended Duration of Hyperglycemia Result in Human-like Corneal Nerve Lesions in Mice with Alloxan- and Streptozotocin-Induced Type 1 Diabetes. Invest. Ophthalmol. Vis. Sci. 59 (15), 5868–5875. doi:10.1167/iovs.18-25693
Ozkul, A., Ayhan, M., Yenisey, C., Akyol, A., Guney, E., and Ergin, F. A. (2010). The Role of Oxidative Stress and Endothelial Injury in Diabetic Neuropathy and Neuropathic Pain. Neuro Endocrinol. Lett. 31 (2), 261–264. Retrieved from <Go to ISI>://WOS:000277702100016.
Pang, T. T., and Narendran, P. (2008). Addressing Insulin Resistance in Type 1 Diabetes. Diabet Med. 25 (9), 1015–1024. doi:10.1111/j.1464-5491.2008.02493.x
Panigrahy, S. K., Bhatt, R., and Kumar, A. (2017). Reactive Oxygen Species: Sources, Consequences and Targeted Therapy in Type 2 Diabetes. J. Drug Target. 25 (2), 93–101. doi:10.1080/1061186x.2016.1207650
Papanas, N. (2020). Diabetic Neuropathy Collection: Progress in Diagnosis and Screening. Diabetes Ther. 11 (4), 761–764. doi:10.1007/s13300-020-00776-3
Park, S., Kang, H. J., Jeon, J. H., Kim, M. J., and Lee, I. K. (2019). Recent Advances in the Pathogenesis of Microvascular Complications in Diabetes. Arch. Pharm. Res. 42 (3), 252–262. doi:10.1007/s12272-019-01130-3
Paulson, P. E., Wiley, J. W., and Morrow, T. J. (2007). Concurrent Activation of the Somatosensory Forebrain and Deactivation of Periaqueductal gray Associated with Diabetes-Induced Neuropathic Pain. Exp. Neurol. 208 (2), 305–313. doi:10.1016/j.expneurol.2007.09.001
Pearce, I., Simó, R., Lövestam-Adrian, M., Wong, D. T., and Evans, M. (2019). Association between Diabetic Eye Disease and Other Complications of Diabetes: Implications for Care. A Systematic Review. Diabetes Obes. Metab. 21 (3), 467–478. doi:10.1111/dom.13550
Pham, V. M., Matsumura, S., Katano, T., Funatsu, N., and Ito, S. (2019). Diabetic Neuropathy Research: from Mouse Models to Targets for Treatment. Neural Regen. Res. 14 (11), 1870–1879. doi:10.4103/1673-5374.259603
Pillai, S. I., Subramanian, S. P., and Kandaswamy, M. (2013). Evaluation of Antioxidant Efficacy of Vanadium-3-Hydroxyflavone Complex in Streptozotocin-Diabetic Rats. Chem. Biol. Interact 204 (2), 67–74. doi:10.1016/j.cbi.2013.04.012
Pohanka, M. (2013). Impact of Melatonin on Immunity: a Review. Cent. Eur. J. Med. 8 (4), 369–376. doi:10.2478/s11536-013-0177-2
Porte, D. (2001). Clinical Importance of Insulin Secretion and its Interaction with Insulin Resistance in the Treatment of Type 2 Diabetes Mellitus and its Complications. Diabetes Metab. Res. Rev. 17 (3), 181–188. Retrieved from <Go to ISI>://WOS:000169431200003. doi:10.1002/1520-7560(200105/06)17:3<181::aid-dmrr197>3.0.co;2-1
Posa, L., De Gregorio, D., Gobbi, G., and Comai, S. (2018). Targeting Melatonin MT2 Receptors: A Novel Pharmacological Avenue for Inflammatory and Neuropathic Pain. Curr. Med. Chem. 25 (32), 3866–3882. doi:10.2174/0929867324666170209104926
Prajapati, B. B., Filippi, A., and Sears, E. H. (2021). Chronic Joint Pain in a Young Adult with Cystic Fibrosis. Cureus 13 (8), e17229. doi:10.7759/cureus.17229
Pulakat, L., and Sumners, C. (2020). Angiotensin Type 2 Receptors: Painful, or Not? Front. Pharmacol. 11, 571994. doi:10.3389/fphar.2020.571994
Qureshi, Z., and Ali, M. N. (2021). Diabetic Neuropathy Pain Management: A Global Challenge. Curr. Diabetes Rev. 17 (5), e031120187542. doi:10.2174/1573399816666201103142521
Reinehr, T. (2005). Pathophysiologie und Spätfolgen des Diabetes mellitus Typ 2. Monatsschr Kinderheilkd 153 (10), 927–935. doi:10.1007/s00112-005-1224-0
Reiter, R. J., Tan, D. X., Mayo, J. C., Sainz, R. M., Leon, J., and Czarnocki, Z. (2003). Melatonin as an Antioxidant: Biochemical Mechanisms and Pathophysiological Implications in Humans. Acta Biochim. Pol. 50 (4), 1129–1146. Retrieved from <Go to ISI>://WOS:0001877976000170350041129.
Renno, W. M., Alkhalaf, M., Afsari, Z., Abd-El-Basset, E., and Mousa, A. (2008). Consumption of green tea Alters Glial Fibriliary Acidic Protein Immunoreactivity in the Spinal Cord Astrocytes of STZ-Diabetic Rats. Nutr. Neurosci. 11 (1), 32–40. doi:10.1179/147683008x301405
Rocha, C. S., Rato, L., Martins, A. D., Alves, M. G., and Oliveira, P. F. (2015). Melatonin and Male Reproductive Health: Relevance of Darkness and Antioxidant Properties. Curr. Mol. Med. 15 (4), 299–311. doi:10.2174/1566524015666150505155530
Rolo, A. P., and Palmeira, C. M. (2006). Diabetes and Mitochondrial Function: Role of Hyperglycemia and Oxidative Stress. Toxicol. Appl. Pharmacol. 212 (2), 167–178. doi:10.1016/j.taap.2006.01.003
Rondón, L. J., Privat, A. M., Daulhac, L., Davin, N., Mazur, A., Fialip, J., et al. (2010). Magnesium Attenuates Chronic Hypersensitivity and Spinal Cord NMDA Receptor Phosphorylation in a Rat Model of Diabetic Neuropathic Pain. J. Physiol. 588 (21), 4205–4215. doi:10.1113/jphysiol.2010.197004
Rosenstock, J., Brazg, R., Andryuk, P. J., Lu, K., Stein, P., and Sitagliptin Study, G. (2006). Efficacy and Safety of the Dipeptidyl Peptidase-4 Inhibitor Sitagliptin Added to Ongoing Pioglitazone Therapy in Patients with Type 2 Diabetes: A 24-week, Multicenter, Randomized, Double-Blind, Placebo-Controlled, Parallel-Group Study. Clin. Ther. 28 (10), 1556–1568. doi:10.1016/j.clinthera.2006.10.007
Rovira-Llopis, S., Rocha, M., Guzman-Fulgencio, M., Iannantuomi, F., Falcon, R., Jover, A., et al. (2013). Enhanced Levels of Myeloperoxidase in the ROS-Induced Vascular Damage Is Related to Nephropathy in Type 2 Diabetes. Free Radic. Biol. Med. 65, S54. doi:10.1016/j.freeradbiomed.2013.08.093
Rumora, A., Alakwaa, F., Andersen, S., Jorgensen, M., Charles, M., Callaghan, B., et al. (2021). Novel Plasma Metabolomic Signatures Associated with Diabetic Neuropathy in a Cohort with Screen-Tested Type 2 Diabetes: Addition-Denmark. J. Peripher. Nervous Syst. 26 (1), 127. Retrieved from <Go to ISI>://WOS:000626893200034.
San Martín, A., Du, P., Dikalova, A., Lassègue, B., Aleman, M., Góngora, M. C., et al. (2007). Reactive Oxygen Species-Selective Regulation of Aortic Inflammatory Gene Expression in Type 2 Diabetes. Am. J. Physiol. Heart Circ. Physiol. 292 (5), H2073–H2082. doi:10.1152/ajpheart.00943.2006
Sanz-Nogués, C., Mustafa, M., Burke, H., O'Brien, T., and Coleman, C. M. (2020). Knowledge, Perceptions and Concerns of Diabetes -Associated Complications Among Individuals Living with Type 1 and Type 2 Diabetes Mellitus. Healthcare (Basel) 8 (1), 1. doi:10.3390/healthcare8010025
Schofield, C. J., and Sutherland, C. (2012). Disordered Insulin Secretion in the Development of Insulin Resistance and Type 2 Diabetes. Diabet Med. 29 (8), 972–979. doi:10.1111/j.1464-5491.2012.03655.x
Sedeek, M., Callera, G., Montezano, A., Gutsol, A., Heitz, F., Szyndralewiez, C., et al. (2010). Critical Role of Nox4-Based NADPH Oxidase in Glucose-Induced Oxidative Stress in the Kidney: Implications in Type 2 Diabetic Nephropathy. Am. J. Physiol. Ren. Physiol 299 (6), F1348–F1358. doi:10.1152/ajprenal.00028.2010
Selvarajah, D., Wilkinson, I. D., Gandhi, R., Griffiths, P. D., and Tesfaye, S. (2011). Microvascular Perfusion Abnormalities of the Thalamus in Painful but Not Painless Diabetic Polyneuropathy: a Clue to the Pathogenesis of Pain in Type 1 Diabetes. Diabetes Care 34 (3), 718–720. doi:10.2337/dc10-1550
Selvarajah, D., Wilkinson, I. D., Griffiths, P. D., Emery, C. J., and Tesfaye, S. (2008). Raised Thalamic Blood Volume in Painful Diabetic Neuropathy: Clues to the Pathogenesis of Neuropathic Pain in Diabetes? Diabetologia 51, S106. Retrieved from <Go to ISI>://WOS:000258660200242. doi:10.1007/s00125-008-1139-0
Sevak, A. R., and Goyal, R. K. (1996). Effects of Chronic Treatment with Lisinopril on Cardiovascular Complications in Streptozotocin Diabetic and DOCA Hypertensive Rats. Pharmacol. Res. 34 (5-6), 201–209. doi:10.1006/phrs.1996.0089
Seyit, D. A., Degirmenci, E., and Oguzhanoglu, A. (2016). Evaluation of Electrophysiological Effects of Melatonin and Alpha Lipoic Acid in Rats with Streptozotocine Induced Diabetic Neuropathy. Exp. Clin. Endocrinol. Diabetes 124 (5), 300–306. doi:10.1055/s-0042-103750
Sharifzadeh, M., Ranjbar, A., Hosseini, A., and Khanavi, M. (2017). The Effect of Green Tea Extract on Oxidative Stress and Spatial Learning in Streptozotocin-Diabetic Rats. Iran J. Pharm. Res. 16 (1), 201–209. Retrieved from <Go to ISI>://WOS:000396490200018.
Sherr, D., and Lipman, R. D. (2015). The Diabetes Educator and the Diabetes Self-Management Education Engagement: The 2015 National Practice Survey. Diabetes Educ. 41 (5), 616–624. doi:10.1177/0145721715599268
Shi, L. L., Yang, J., and Jiang, H. Y. (2016). Effects of Sitagliptin Phosphate/metformin Hydrochloride Tablets on Insulin Resistance in Obese Patients with Type 2 Diabetes and NAFLD. Diabetes-Metabolism Res. Rev. 32 (Suppl. 2), 45–46. Retrieved from <Go to ISI>://WOS:000386961400081.
Shieh, J. M., Wu, H. T., Cheng, K. C., and Cheng, J. T. (2009). Melatonin Ameliorates High Fat Diet-Induced Diabetes and Stimulates Glycogen Synthesis via a PKCzeta-Akt-GSK3beta Pathway in Hepatic Cells. J. Pineal Res. 47 (4), 339–344. doi:10.1111/j.1600-079X.2009.00720.x
Shokri, M., Sajedi, F., Mohammadi, Y., and Mehrpooya, M. (2021). Adjuvant Use of Melatonin for Relieving Symptoms of Painful Diabetic Neuropathy: Results of a Randomized, Double-Blinded, Controlled Trial. Eur. J. Clin. Pharmacol. 77 (11), 1649–1663. doi:10.1007/s00228-021-03170-5
Silva-dos-Santos, N. M., Oliveira-Abreu, K., Moreira-Junior, L., Santos-Nascimento, T. D., Silva-Alves, K. S. D., Coelho-de-Souza, A. N., et al. (2020). Diabetes Mellitus Alters Electrophysiological Properties in Neurons of superior Cervical Ganglion of Rats. Brain Res. 1729, 146599. doi:10.1016/j.brainres.2019.146599
Sima, A. A. (2008). The Heterogeneity of Diabetic Neuropathy. Front. Biosci. 13, 4809–4816. doi:10.2741/3040
Singh, P., Bansal, S., Kuhad, A., Kumar, A., and Chopra, K. (2020). Naringenin Ameliorates Diabetic Neuropathic Pain by Modulation of Oxidative-Nitrosative Stress, Cytokines and MMP-9 Levels. Food Funct. 11 (5), 4548–4560. doi:10.1039/c9fo00881k
Smith-Palmer, J., Brändle, M., Trevisan, R., Orsini Federici, M., Liabat, S., and Valentine, W. (2014). Assessment of the Association between Glycemic Variability and Diabetes-Related Complications in Type 1 and Type 2 Diabetes. Diabetes Res. Clin. Pract. 105 (3), 273–284. doi:10.1016/j.diabres.2014.06.007
Sommese, L., Benincasa, G., Lanza, M., Sorriento, A., Schiano, C., Lucchese, R., et al. (2018). Novel Epigenetic-Sensitive Clinical Challenges Both in Type 1 and Type 2 Diabetes. J. Diabetes Complications 32 (11), 1076–1084. doi:10.1016/j.jdiacomp.2018.08.012
Song, Y., Ding, W., Bei, Y., Xiao, Y., Tong, H. D., Wang, L. B., et al. (2018). Insulin Is a Potential Antioxidant for Diabetes-Associated Cognitive Decline via Regulating Nrf2 Dependent Antioxidant Enzymes. Biomed. Pharmacother. 104, 474–484. doi:10.1016/j.biopha.2018.04.097
Sotolongo, K., Ghiso, J., and Rostagno, A. (2020). Nrf2 Activation through the PI3K/GSK-3 axis Protects Neuronal Cells from Aβ-Mediated Oxidative and Metabolic Damage. Alzheimers Res. Ther. 12 (1), 13. doi:10.1186/s13195-019-0578-9
Spadaccio, C., De Marco, F., Di Domenico, F., Coccia, R., Lusini, M., Barbato, R., et al. (2014). Simvastatin Attenuates the Endothelial Pro-thrombotic Shift in Saphenous Vein Grafts Induced by Advanced Glycation Endproducts. Thromb. Res. 133 (3), 418–425. doi:10.1016/j.thromres.2013.12.023
Strollo, R., Rizzo, P., Spoletini, M., Landy, R., Hughes, C., Ponchel, F., et al. (2013). HLA-dependent Autoantibodies against post-translationally Modified Collagen Type II in Type 1 Diabetes Mellitus. Diabetologia 56 (3), 563–572. doi:10.1007/s00125-012-2780-1
Sukotjo, C. T., Sargowo, D., and Wihastuti, T. A. (2014). The Possible Reactive Oxygen Species (ROS) Inhibitory Activity of Synthetic Polysaccharide Peptide (PsP) through the Reduction of Foam Cell in Diabetes Mellitus (DM) Type 2. Eur. J. Heart Fail. 16, 24. Retrieved from <Go to ISI>://WOS:000333409300121.
Suryavanshi, S. V., and Kulkarni, Y. A. (2017). NF-κβ: A Potential Target in the Management of Vascular Complications of Diabetes. Front. Pharmacol. 8, 798. doi:10.3389/fphar.2017.00798
Takamura, T., Misu, H., Matsuzawa-Nagata, N., Sakurai, M., Ota, T., Shimizu, A., et al. (2008). Obesity Upregulates Genes Involved in Oxidative Phosphorylation in Livers of Diabetic Patients. Obesity (Silver Spring) 16 (12), 2601–2609. doi:10.1038/oby.2008.419
Taliyan, R., and Sharma, P. L. (2012). Protective Effect and Potential Mechanism of Ginkgo Biloba Extract EGb 761 on STZ-Induced Neuropathic Pain in Rats. Phytother Res. 26 (12), 1823–1829. doi:10.1002/ptr.4648
Tang, H., Li, K., Zhang, S., Lan, H., Liang, L., Huang, C., et al. (2021). Inhibitory Effect of Paeonol on Apoptosis, Oxidative Stress, and Inflammatory Response in Human Umbilical Vein Endothelial Cells Induced by High Glucose and Palmitic Acid Induced through Regulating SIRT1/FOXO3a/NF-Κb Pathway. J. Interferon Cytokine Res. 41 (3), 111–124. doi:10.1089/jir.2019.0236
Tang, S. C., Leung, J. C., Chan, L. Y., Cheng, A. S., Lan, H. Y., and Lai, K. N. (2010). Renoprotection by Rosiglitazone in Accelerated Type 2 Diabetic Nephropathy: Role of STAT1 Inhibition and Nephrin Restoration. Am. J. Nephrol. 32 (2), 145–155. doi:10.1159/000316056
Tascioglu Aliyev, A., Panieri, E., Stepanić, V., Gurer-Orhan, H., and Saso, L. (2021). Involvement of NRF2 in Breast Cancer and Possible Therapeutical Role of Polyphenols and Melatonin. Molecules 26 (7), 1853. doi:10.3390/molecules26071853
Tavakoli, M., and Malik, R. A. (2008). Management of Painful Diabetic Neuropathy. Expert Opin. Pharmacother. 9 (17), 2969–2978. doi:10.1517/14656560802498149
Tavakoli, M., Mojaddidi, M., Fadavi, H., and Malik, R. A. (2008). Pathophysiology and Treatment of Painful Diabetic Neuropathy. Curr. Pain Headache Rep. 12 (3), 192–197. doi:10.1007/s11916-008-0034-1
Tesfaye, S., and Selvarajah, D. (2012). Advances in the Epidemiology, Pathogenesis and Management of Diabetic Peripheral Neuropathy. Diabetes Metab. Res. Rev. 28 (Suppl. 1), 8–14. doi:10.1002/dmrr.2239
Tian, J., Song, T., Wang, H., Wang, W., Ma, X., and Hu, Y. (2021). Toll-Like Receptor 2 Antagonist Ameliorates Type 2 Diabetes Mellitus Associated Neuropathic Pain by Repolarizing Pro-inflammatory Macrophages. Neurochem. Res. 46 (9), 2276–2284. doi:10.1007/s11064-021-03365-3
Tiong, Y. L., Ng, K. Y., Koh, R. Y., Ponnudurai, G., and Chye, S. M. (2019). Melatonin Prevents Oxidative Stress-Induced Mitochondrial Dysfunction and Apoptosis in High Glucose-Treated Schwann Cells via Upregulation of Bcl2, NF-Κb, mTOR, Wnt Signalling Pathways. Antioxidants (Basel) 8 (7), 198. doi:10.3390/antiox8070198
Toma, L., Sanda, G. M., Deleanu, M., Stancu, C. S., and Sima, A. V. (2016). Glycated LDL Increase VCAM-1 Expression and Secretion in Endothelial Cells and Promote Monocyte Adhesion through Mechanisms Involving Endoplasmic Reticulum Stress. Mol. Cel Biochem 417 (1-2), 169–179. doi:10.1007/s11010-016-2724-z
Tong, Y. C., and Cheng, J. T. (2005). Changes in Bladder Nerve-Growth Factor and P75 Genetic Expression in Streptozotocin-Induced Diabetic Rats. BJU Int. 96 (9), 1392–1396. doi:10.1111/j.1464-410X.2005.05854.x
Tseng, H. H., Vong, C. T., Kwan, Y. W., Lee, S. M., and Hoi, M. P. (2016). TRPM2 Regulates TXNIP-Mediated NLRP3 Inflammasome Activation via Interaction with P47 Phox under High Glucose in Human Monocytic Cells. Sci. Rep. 6, 35016. doi:10.1038/srep35016
Tsuda, M., Inoue, K., and Salter, M. W. (2005). Neuropathic Pain and Spinal Microglia: a Big Problem from Molecules in "small" Glia. Trends Neurosci. 28 (2), 101–107. doi:10.1016/j.tins.2004.12.002
Tsuda, M., Masuda, T., Tozaki-Saitoh, H., and Inoue, K. (2013). Microglial Regulation of Neuropathic Pain. J. Pharmacol. Sci. 121 (2), 89–94. doi:10.1254/jphs.12R14CP
Tsuda, M. (2019). Microglia-Mediated Regulation of Neuropathic Pain: Molecular and Cellular Mechanisms. Biol. Pharm. Bull. 42 (12), 1959–1968. Retrieved from <Go to ISI>://WOS:000500080000001. doi:10.1248/bpb.b19-00715
Tuna Edizer, D., Dönmez, Z., Gül, M., Yiğit, Ö., Yiğitcan, B., Adatepe, T., et al. (2019). Effects of Melatonin and Dexamethasone on Facial Nerve Neurorrhaphy. J. Int. Adv. Otol 15 (1), 43–50. doi:10.5152/iao.2018.3273
Turecký, L., Kupčová, V., Uhlíková, E., and Mojto, V. (2014). Peroxisomal Enzymes in the Liver of Rats with Experimental Diabetes Mellitus Type 2. Physiol. Res. 63, S585–S591. doi:10.33549/physiolres.932918
Uno, S., Imagawa, A., Kozawa, J., Fukui, K., Iwahashi, H., and Shimomura, I. (2018). Complete Loss of Insulin Secretion Capacity in Type 1A Diabetes Patients during Long-Term Follow up. J. Diabetes Investig. 9 (4), 806–812. doi:10.1111/jdi.12763
Waldman, M., Cohen, K., Yadin, D., Nudelman, V., Gorfil, D., Laniado-Schwartzman, M., et al. (2018). Regulation of Diabetic Cardiomyopathy by Caloric Restriction Is Mediated by Intracellular Signaling Pathways Involving 'SIRT1 and PGC-1α'. Cardiovasc. Diabetol. 17, 111. doi:10.1186/s12933-018-0754-4
Wang, H. X., Wu, X. R., Yang, H., Yin, C. L., Shi, L. J., and Wang, X. J. (2013). Urotensin II Inhibits Skeletal Muscle Glucose Transport Signaling Pathways via the NADPH Oxidase Pathway. Plos One 8 (10), e76796. doi:10.1371/journal.pone.0076796
Wang, J., and Guo, H. M. (2019). Astragaloside IV Ameliorates High Glucose-Induced HK-2 Cell Apoptosis and Oxidative Stress by Regulating the Nrf2/ARE Signaling Pathway. Exp. Ther. Med. 17 (6), 4409–4416. doi:10.3892/etm.2019.7495
Wang, J., Li, G., Wang, Z., Zhang, X., Yao, L., Wang, F., et al. (2012). High Glucose-Induced Expression of Inflammatory Cytokines and Reactive Oxygen Species in Cultured Astrocytes. Neuroscience 202, 58–68. doi:10.1016/j.neuroscience.2011.11.062
Wang, P., Lu, Y. C., Wang, J., Wang, L., Yu, H., Li, Y. F., et al. (2018). Type 2 Diabetes Promotes Cell Centrosome Amplification via AKT-ROS-dependent Signalling of ROCK1 and 14-3-3σ. Cell Physiol Biochem 47 (1), 356–367. doi:10.1159/000489812
Wong, W. T., Tian, X. Y., Xu, A., Ng, C. F., Lee, H. K., Chen, Z. Y., et al. (2010). Angiotensin II Type 1 Receptor-dependent Oxidative Stress Mediates Endothelial Dysfunction in Type 2 Diabetic Mice. Antioxid. Redox Signal. 13 (6), 757–768. doi:10.1089/ars.2009.2831
Wright, E., Scism-Bacon, J. L., and Glass, L. C. (2006). Oxidative Stress in Type 2 Diabetes: the Role of Fasting and Postprandial Glycaemia. Int. J. Clin. Pract. 60 (3), 308–314. doi:10.1111/j.1368-5031.2006.00825.x
Wu, H., Wen, F., Jiang, M., Liu, Q., and Nie, Y. (2018). LncRNA uc.48+ Is Involved in the Diabetic Immune and Inflammatory Responses Mediated by P2X7 Receptor in RAW264.7 Macrophages. Int. J. Mol. Med. 42 (2), 1152–1160. doi:10.3892/ijmm.2018.3661
Wu, H. Y., Mao, X. F., Fan, H., and Wang, Y. X. (2017). p38β Mitogen-Activated Protein Kinase Signaling Mediates Exenatide-Stimulated Microglial β-Endorphin Expression. Mol. Pharmacol. 91 (5), 451–463. doi:10.1124/mol.116.107102
Xie, S., Fan, W., He, H., and Huang, F. (2020). Role of Melatonin in the Regulation of Pain. J. Pain Res. 13, 331–343. doi:10.2147/jpr.s228577
Xing, Y., Ji, Q., Li, X., Ming, J., Zhang, N., Zha, D., et al. (2017). Asiaticoside Protects Cochlear Hair Cells from High Glucose-Induced Oxidative Stress via Suppressing AGEs/RAGE/NF-κB Pathway. Biomed. Pharmacother. 86, 531–536. doi:10.1016/j.biopha.2016.12.025
Xu, M., Wu, H. Y., Liu, H., Gong, N., Wang, Y. R., and Wang, Y. X. (2017). Morroniside, a Secoiridoid Glycoside from Cornus Officinalis, Attenuates Neuropathic Pain by Activation of Spinal Glucagon-like Peptide-1 Receptors. Br. J. Pharmacol. 174 (7), 580–590. doi:10.1111/bph.13720
Yadav, S. K., Nagori, B. P., and Desai, P. K. (2014). Pharmacological Characterization of Different Fractions of Calotropis Procera (Asclepiadaceae) in Streptozotocin Induced Experimental Model of Diabetic Neuropathy. J. Ethnopharmacol 152 (2), 349–357. doi:10.1016/j.jep.2014.01.020
Yamashita, A., Matsuoka, Y., Matsuda, M., Kawai, K., Sawa, T., and Amaya, F. (2019). Dysregulation of P53 and Parkin Induce Mitochondrial Dysfunction and Leads to the Diabetic Neuropathic Pain. Neuroscience 416, 9–19. doi:10.1016/j.neuroscience.2019.07.045
Yan, F., Chen, X., and Zheng, X. (2017). Protective Effect of mulberry Fruit Anthocyanin on Human Hepatocyte Cells (LO2) and Caenorhabditis elegans under Hyperglycemic Conditions. Food Res. Int. 102, 213–224. doi:10.1016/j.foodres.2017.10.009
Yanagi, K., Monden, T., Ikeda, S., Matsumura, M., and Kasai, K. (2011). A Crossover Study of Rosuvastatin and Pitavastatin in Patients with Type 2 Diabetes. Adv. Ther. 28 (2), 160–171. doi:10.1007/s12325-010-0098-2
Yang, Q. Q., Sun, J. W., Shao, D., Zhang, H. H., Bai, C. F., and Cao, F. L. (2021). The Association between Diabetes Complications, Diabetes Distress, and Depressive Symptoms in Patients with Type 2 Diabetes Mellitus. Clin. Nurs. Res. 30 (3), 293–301. doi:10.1177/1054773820951933
Yang, Z. H., and Peng, X. D. (2010). Insulin Resistance and Heart Injury in Rats with Insulin Resistance or Type 2 Diabetes Mellitus. Acta Cardiol. 65 (3), 329–335. doi:10.2143/ac.65.3.2050350
Yao, J., Li, Y., Jin, Y., Chen, Y., Tian, L., and He, W. (2021). Synergistic Cardioptotection by Tilianin and Syringin in Diabetic Cardiomyopathy Involves Interaction of TLR4/NF-Κb/nlrp3 and PGC1a/SIRT3 Pathways. Int. Immunopharmacology 96, 107728. doi:10.1016/j.intimp.2021.107728
Yoon, G., Kim, Y. K., and Song, J. (2020). Glucagon-like Peptide-1 Suppresses Neuroinflammation and Improves Neural Structure. Pharmacol. Res. 152, 104615. doi:10.1016/j.phrs.2019.104615
Yu, W., Zhao, G.-q., Cao, R.-j., Zhu, Z.-h., and Li, K. (2017). LncRNA NONRATT021972 Was Associated with Neuropathic Pain Scoring in Patients with Type 2 Diabetes. Behav. Neurol. 2017, 1–6. doi:10.1155/2017/2941297
Yuan, H., Lu, Y., Huang, X., He, Q., Man, Y., Zhou, Y., et al. (2010). Suppression of NADPH Oxidase 2 Substantially Restores Glucose-Induced Dysfunction of Pancreatic NIT-1 Cells. FEBS J. 277 (24), 5061–5071. doi:10.1111/j.1742-4658.2010.07911.x
Yuhong, M., Weizu, L., Yanyan, Y., and Weiping, L. (2015). AST IV Inhibits H2O2-Induced Human Umbilical Vein Endothelial Cell Apoptosis by Suppressing Nox4 Expression through the TGF-β1/Smad2 Pathway. Int. J. Mol. Med. 35 (6), 1667–1674. doi:10.3892/ijmm.2015.2188
Zhang, J. L., Hui, Y., Zhou, F., and Hou, J. Q. (2018). Neuroprotective Effects of Melatonin on Erectile Dysfunction in Streptozotocin-Induced Diabetic Rats. Int. Urol. Nephrol. 50 (11), 1981–1988. doi:10.1007/s11255-018-1989-4
Zhang, X., Zhao, D., Wu, W., Ali Shah, S. Z., Lai, M., Yang, D., et al. (2020). Melatonin Regulates Mitochondrial Dynamics and Alleviates Neuron Damage in Prion Diseases. Aging (Albany NY) 12 (11), 11139–11151. doi:10.18632/aging.103328
Zhao, B., Li, S., Guo, Z., Chen, Z., Zhang, X., Xu, C., et al. (2021). Dopamine Receptor D2 Inhibition Alleviates Diabetic Hepatic Stellate Cells Fibrosis by Regulating the TGF-β1/Smads and NFκB Pathways. Clin. Exp. Pharmacol. Physiol. 48 (3), 370–380. doi:10.1111/1440-1681.13437
Zhao, C. Y., Dai, Q. X., and Zhou, J. (2016). Relationship between Serum Iron and Ferritin and Insulin Resistance in Type 2 Diabetes in Tibetan Adults. Diabetes-Metabolism Res. Rev. 32 (Suppl. 2), 31. Retrieved from <Go to ISI>://WOS:000386961400053.
Zhao, J. Y., Yang, L., Bai, H. H., Liu, J. P., Suo, Z. W., Yang, X., et al. (2018). Inhibition of Protein Tyrosine Phosphatase 1B in Spinal Cord Dorsal Horn of Rats Attenuated Diabetic Neuropathic Pain. Eur. J. Pharmacol. 827, 189–197. doi:10.1016/j.ejphar.2018.03.012
Zhao, Y., Ye, W., Boye, K. S., Holcombe, J. H., and Swindle, R. (2009). Healthcare Charges and Utilization Associated with Diabetic Neuropathy: Impact of Type 1 Diabetes and Presence of Other Diabetes-Related Complications and Comorbidities. Diabet Med. 26 (1), 61–69. doi:10.1111/j.1464-5491.2008.02616.x
Zhu, B., Gong, N., Fan, H., Peng, C. S., Ding, X. J., Jiang, Y., et al. (2014). Lamiophlomis Rotata, an Orally Available Tibetan Herbal Painkiller, Specifically Reduces Pain Hypersensitivity States through the Activation of Spinal Glucagon-like Peptide-1 Receptors. Anesthesiology 121 (4), 835–851. doi:10.1097/aln.0000000000000320
Keywords: diabetes mellitus (DM), neuropathic pain, neuroinflammation, melatonin, redox levels, antioxidant, anti-inflammatory
Citation: Busa P, Kuthati Y, Huang N and Wong C-S (2022) New Advances on Pathophysiology of Diabetes Neuropathy and Pain Management: Potential Role of Melatonin and DPP-4 Inhibitors. Front. Pharmacol. 13:864088. doi: 10.3389/fphar.2022.864088
Received: 28 January 2022; Accepted: 14 March 2022;
Published: 12 April 2022.
Edited by:
Wiebke Kallenborn-Gerhardt, Goethe University Frankfurt, GermanyReviewed by:
Ana Isabel Arroba, Fundación para la gestión de la investigación Biomédica de Cádiz, SpainPranav Kumar Prabhakar, Lovely Professional University, India
Copyright © 2022 Busa, Kuthati, Huang and Wong. This is an open-access article distributed under the terms of the Creative Commons Attribution License (CC BY). The use, distribution or reproduction in other forums is permitted, provided the original author(s) and the copyright owner(s) are credited and that the original publication in this journal is cited, in accordance with accepted academic practice. No use, distribution or reproduction is permitted which does not comply with these terms.
*Correspondence: Chih-Shung Wong, dzgyNTU2QGdtYWlsLmNvbQ==