- 1Department of Basic Medical Science, Anatomy and Functional Physiology Section, Xiamen Medical College, Xiamen, China
- 2Department of Basic Medical Science, Institute of Respiratory Disease, Xiamen Medical College, Xiamen, China
- 3Department of Anatomy, Kaohsiung Medical University, Kaohsiung, Taiwan
- 4Department of Otorhinolaryngology, the Second Affiliated Hospital of Xiamen Medical College, Xiamen, China
- 5Department of Neurosurgery, Neurological Institute, Neurological Institute, Taipei, Taiwan
Coronavirus disease 2019 (COVID-19) is caused by severe acute respiratory syndrome coronavirus 2 (SARS-CoV-2), which is a positive-strand RNA virus, and has rapidly spread worldwide as a pandemic. The vaccines, repurposed drugs, and specific treatments have led to a surge of novel therapies and guidelines nowadays; however, the epidemic of COVID-19 is not yet fully combated and is still in a vital crisis. In repositioning drugs, natural products are gaining attention because of the large therapeutic window and potent antiviral, immunomodulatory, anti-inflammatory, and antioxidant properties. Of note, the predominant curcumoid extracted from turmeric (Curcuma longa L.) including phenolic curcumin influences multiple signaling pathways and has demonstrated to possess anti-inflammatory, antioxidant, antimicrobial, hypoglycemic, wound healing, chemopreventive, chemosensitizing, and radiosensitizing spectrums. In this review, all pieces of current information related to curcumin-used for the treatment and prevention of severe acute respiratory syndrome coronavirus 2 (SARS-CoV-2) infection through in vitro, in vivo, and in silico studies, clinical trials, and new formulation designs are retrieved to re-evaluate the applications based on the pharmaceutical efficacy of clinical therapy and to provide deep insights into knowledge and strategy about the curcumin’s role as an immune booster, inflammatory modulator, and therapeutic agent against COVID-19. Moreover, this study will also afford a favorable application or approach with evidence based on the drug discovery and development, pharmacology, functional foods, and nutraceuticals for effectively fighting the COVID-19 pandemic.
Introduction
Coronavirus disease 2019 (COVID-19) is an infectious disease that has rapidly spread throughout the world, leading to high mortality rates, and has become a epidemic from the end of 2019. Coronavirus disease (COVID-19), caused by the novel coronavirus—severe acute respiratory syndrome coronavirus 2 (SARS-CoV-2), has surged across the globe, affecting 233 countries or territories, with greater than 337 million confirmed cases and over 5.56 million deaths till January 2022, with the World Health Organization (WHO, 2022) categorizing it as a pandemic (https://covid19.who.int). Infected patients manifest fever, cough, shortness of breath, and lost smell and taste, and critical cases might show acute respiratory infection and multiple organ failure. Probability of these severe indications is further enhanced by age and underlying comorbidities such as diabetes, cardiovascular, or thoracic problems, as well as due to an immunocompromised state (Adhikari et al., 2020). Coronavirus infection, including SARS-CoV, MERS-CoV, and SARS-CoV-2, causes daunting diseases that can be fatal because of lung failure and systemic cytokine storm. The development of coronavirus-evoked pneumonia is associated with excessive inflammatory responses in the lung, releasing extremely high amounts of cytokines known as “cytokine storms,” which result in pulmonary edema, atelectasis, and acute lung injury (ALI) or acute respiratory distress syndrome (ARDS). The pathophysiology of COVID-19 involves the activation of three main pathways: inflammatory, coagulation, and bradykinin cascades (Wiersinga et al., 2020).
SARS-CoV-2 is an enveloped virus belonging to the order Nidovirales, composed of a single-strand, non-segmented, and positive sense RNA genome. SARS-CoV-2 causes COVID-19 that is classified as beta-coronavirus, which primarily occurred and was recorded in December 2019 at Wuhan, China (Godeau et al., 2021). As one member of human coronavirus (HCoV) that has the largest RNA virus genome, the single positive-strand RNA genome of SARS-CoV-2 is approximately 30 kd with two untranslated regions (UTR) linked to the 3′-poly-A tail, and 5′-cap structure, which are crucial for gene transcription and RNA replication. The SARS-CoV-2 genomic structure comprises 5′UTR/NSP/S/3a/E/M/6/7a/7b/8/N/10/3′UTR organization. The non-structural protein (nsp) region of SARS-CoV-2 can be coded and translated as the non-structural proteins 1–16 (nsp1-16), the structural protein regions including spike protein (S), envelop protein (E), membrane protein (M), nucleoprotein (N), and accessory protein regions 3a, 6, 7a, 7b, 8, and 10. Two-thirds of the SARS-CoV-2 genome from the 5′-end contains two open reading frames (ORF), ORF-1a and ORF-1b, which are coded and translated into two long polypeptides, polyprotein 1a (PP1a) and polyprotein 1b (PP1b). The posttranslated cleavage of PP1a and PP1b by two virus-encoded proteases to form 16 NSPs: nsp1-16. Other one-third of the viral genome contains the distinct ORFs derived to form several single-guide RNAs (sgRNAs) that are translated into structural proteins and accessory proteins (Ciotti et al., 2019).
The Pathogenesis of SARS-CoV-2 Infection
The pathogenesis of the infection severity of SARS-CoV-2 encompasses several stages, the suppression of host antiviral and innate immune responses to increase the infected host cells (Shi et al., 2020; Oh and Shin, 2021), the viral replication-induced infected cell oxidative stress (Laforge et al., 2020; Saheb Sharif-Askari et al., 2020), and injury of infected cells expressing and secreting large amounts of various chemokines or cytokines, colony-stimulating factors, interferons (IFNs), interleukins (IL-1, IL-6, IL-8, IL-12), and tumor necrosis factor-α (TNF-α), which cause acute inflammation described as the “cytokine storm symptom” (CSS) (Junqueira et al., 2021; Kunnumakkara et al., 2021). The CSS of serious SARS-CoV-2 infection causes acute lung injury, tissue fibrosis, and pneumonia. Subsequently, CSS with hemophagocytic lymphohistiocytosis (HLH)/macrophage activation syndrome (MAS) may cause serious systemic hyper-inflammation, plasma leakage, peripheral tissue fluid accumulation, and hypotension. The serious pulmonary inflammation, edema, and tissue fluid accumulation in the lung such as ARDS, combined ARDS, HLH, and MAS will affect gas exchange that leads to systemic hypoxemia and multi-organ failure with disseminated intravascular coagulation (DIC) that have resulted in extreme morbidity and mortality (Horowitz and Freeman 2020; Kunnumakkara et al., 2021; Saad and Moussa 2021). Deficiency in red blood cells, serum, and alveolar glutathione levels has been published in the medical literature for ARDS, as well as viral and bacterial pneumonias, resulting from the increased levels of free radical/oxidative stress (Horowitz and Freeman 2020). Even the patients can recover from ARDS of SARS-CoV-2-infected pneumonia, the lung tissue remodeling and pulmonary fibrosis will continue to last and subsequently limit the pulmonary functional recovery (Bazdyrev et al., 2021; Giacomelli et al., 2021). Additionally, the pathophysiology of COVID-19 is highly heterogeneous, and the way of SARS-CoV-2 modulates the different systems of the host remains unidentified, despite recent discoveries such as viral nucleocapsid (N) protein can bind host mRNA to impair host stress response (Nabeel-Shah et al., 2022); viral infection-induced host hypoxia status can modulate ACE2 expression (Prieto-Fernández et al., 2021); and the overexpression of viral nsp9 can reduce the host’s nucleoporin 62 expression to defective nuclear pore complex formation (Makiyama et al., 2021). Remarkably this deadly virus could affect multiple vital organs and systems (blood, lungs, heart, nervous system, and immune system); however, its exact mechanism of pathophysiology remains obscure. Usually depending on the viral load, infected and sick people commonly manifest fever, cough, shortness of breath, coagulopathy, cardiac abnormalities, fatigue, and death. This complex and multifactorial response of COVID-19 requires a comprehensive therapeutic approach, enabling the integration and refinement of therapeutic responses of a given single compound that has several action potentials. With comprehensive interaction (synergism), biosafety of multi-compounds or multiple treatments need to be taken into consideration and can also provide a promising strategy to cure COVID-19 infection. Currently, several available vaccines and drugs are in the process of evaluation of efficacy and safety, and the determination of dosage in the COVID-19 pandemic. Unfortunately, vaccines are on the market from Pfizer (BNT), Moderna, and AstraZeneca (AZ) with limited supply under an Emergency Use Authorization (EUA) by the WHO. In fact, several vaccines filed phase III clinical trials are still underway from other manufacturers (Srivastava et al., 2021). Intensely, BNT and Moderna vaccines have been currently approved by the FDA (Chilamakuri and Agarwal 2021). Within 2 years, there are several mutated variants of SARS-CoV-2 from the origin strain, and their wide dispersion led to multiple waves of outbreaks, especially the mutation on the spike gene of new SARS-CoV-2 variants caused the alteration in several amino acid residues and change the structural conformation of spike protein, decreasing the titer of human antibodies and neutralizing antibodies induced by infection or vaccination (Khateeb et al., 2022; Ma et al., 2022; Tay et al., 2022). New antibody-resistant variants of SARS-CoV-2 in vaccine breakthrough infection can be seen even in people who have received two or three vaccinations within 6 months which shows that the vaccines have failed to fully protect the people from variants of SARS-CoV-2 infection such as Omicron (Khateeb et al., 2022; Servellita et al., 2022; Wang et al., 2022). On the other hand, exploring the repurposing of natural compounds and drugs may provide an alternative approach or strategy against COVID-19. Various repurposing phytochemicals are showing a broad range of antiviral activities, and its different modes of action have been identified (Kumar Verma et al., 2021; Kumar et al., 2022). Repurposing drugs such as Arbidol, hydroxychloroquine, chloroquine, lopinavir, favipiravir, remdesivir, hexamethylene amiloride, dexamethasone, tocilizumab, and INF-β that neutralize antibodies exhibit in vitro anti-coronaviral properties by inhibiting multiple processes in the virus life cycle. Plant-based antiviral compounds such as baicalin, calanolides, curcumin, oxymatrine, matrine, and resveratrol exhibit different modes of action against a wide range of positive-/negative-sense RNA/DNA virus, and future research needs to be conducted to ascertain their role and use in managing SARS-CoV-2 (Rai et al., 2021). Recently, several nutraceuticals have been proven to have an ability of immune-boosting, antiviral, antioxidant, and anti-inflammatory effects in COVID-19 infection (Adhikari et al., 2021; Isidoro et al., 2022), and this scenario will be addressed in the next section.
Prospective of Natural Compounds on COVID-19 Treatment
Remarkably, using herbal natural compounds is explored as a complementary approach to treating various diseases including COVID-19. Using the active constituents of medicinal plants has long been a well-accepted therapeutic treatment strategy, although understanding their complex pharmacological actions is a major challenge as they provide tremendous chemical varieties and frequently exhibit multi-pharmacological functions (Catanzaro et al., 2018). One report has demonstrated that crude extract or pure compounds isolated from several medicinal plants and/or herbs such as Artemisia annua, Agastache rugosa, Astragalus membranaceus, Cassia alata, Ecklonia cava, Gymnema sylvestre, Glycyrrhizae uralensis, Houttuynia cordata, Lindera aggregata, Lycoris radiata, Mollugo cerviana, Polygonum multiflorum, Pyrrosia lingua, Saposhnikoviae divaricata, and Tinospora cordifolia have shown promising inhibitory effects against coronavirus (Adhikari et al., 2021). Moreover, several phytocompounds including acacetin, amentoflavone, allicin, blancoxanthone, curcumin, daidzein, diosmin, epigallocatechin gallate, emodin, hesperidin, herbacetin, hirsutenone, iguesterin, jubanine G, kaempferol, lycorine, pectolinarin, phloroeckol, silvestrol, tanshinone I, taxifolin, rhoifolin, xanthoangelol E, and zingerol isolated from plants could also be considered as potential drug candidates against COVID-19 (Adhikari et al., 2021). In this article, we draw more attention to the ancient active substance, especially curcumin, to exploit all promising mechanisms of the antiviral property or applications in the treatment of COVID-19.
Literature Search
We reviewed all retracted studies up to Feb 2022 that have evaluated the medicinal benefits of curcumin for COVID-19 infection. The following databases were used: Web of Science, SciVerse, Sci-Hub, Google Scholar, Library Genesis, open access library, Public Library of Science, Scientific Research Publishing, Research Gate, Hindawi, ScienceDirect, Scopus, Medscience, Academia, JAMA, PubMed (NCBI), Springer, Directory of Open Access Journals (DOAJ), Elsevier, Wiley, Taylor and Francis, ProQuest, EBSCO, MEDLINE, and SciELO. Thus, case reports, clinical trials, original research, and review articles were also evaluated. We used free text and Medical Subject Heading (MeSH) terms “curcumin,” “COVID-19,” “ACE2,” “Immunomodulation,” “In silico,” “In vitro,” “In vivo,” and “clinical trial.” The search was done with no language restrictions. All cited articles related to COVID-19 and curcumin were categorized according to MeSH or keywords; the portion of each presenting item in this article is shown in Figure 1.
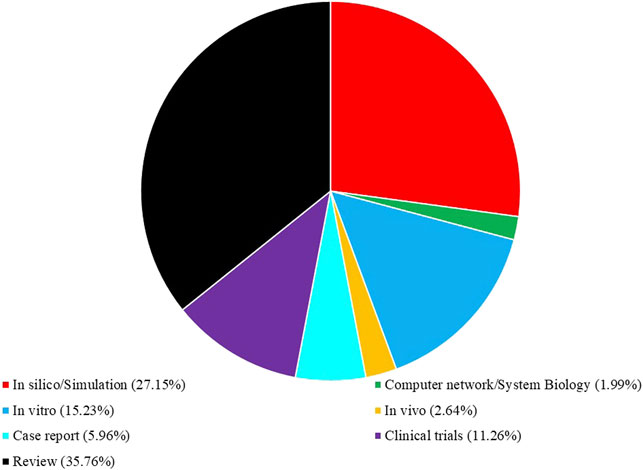
FIGURE 1. Present proportion (%) of cited literature related to COVID-19 and curcumin according to their categories in this review article.
Data Extraction After Search
Data extraction was performed according to the following inclusion and exclusion criteria for the current study: studies examining the effects of curcumin on COVID-19 infection were included in this study. We also considered publications in English language including only English abstract. The authors independently obtained data from each selected publication including the type of study, study population (location, age, gender, and sample size), curcumin dose and measured outcomes, and study results.
Pharmaceutical Potential of Curcumin
For centuries, curcumin chemically known as (1,7-bis(4-hydroxy-3-methoxyphenyl)-1,6-heptadiene-3,5-dione) or diferuloylmethane, is a yellowish pigment and a phenolic component present in the rhizome of turmeric (Curcuma longa Linn) and other Curcuma spp., and has been consumed as a flavoring and therapeutic medicinal natural compound. Turmeric grows primarily in Asian countries, particularly India (Yousefian et al., 2019). Curcumin is a plant of the ginger family Zingiberaceae with the related compounds demethoxycurcumin, bis-demethoxycurcumin, and cyclo-curcumin, which are referred to as curcuminoids (Priyadarsini 2014). In C. longa, the crude extract curcuminoid makes up 1–6% of turmeric by weight, distributed in 60–70% curcumin (CUR), 20–27% demethoxycurcumin (DMC), and 10–15% bis-demethoxycurcumin (BDMC) (Nelson et al., 2017), whereas commercially available curcumin contains about 77% in curcuminoids (Esatbeyoglu et al., 2012). Curcuminoids vary in potency, effectiveness, and stability, that is, the relative potency for suppression of TNF-induced nuclear factor kappa B (NF-κB) activation was Cur > DMC > BDMC (Sandur et al., 2007). An extensive spectrum of pharmacological and physiological actions of curcumin according to an effective and safe substance has been previously documented in the literature. Numerous studies have shown that curcumin has a broad variety of medicinal functions, including anti-inflammatory (Ghandadi and Sahebkar 2017; Gorabi et al., 2021), antiangiogenic (Sahebkar 2010; Hewlings and Kalman 2017), antidiabetic (Panahi et al., 2018; Riyaphan et al., 2018; Huang et al., 2019), antimicrobial (Praditya et al., 2019; Zahedipour et al., 2020), and antitumor properties (Thiyagarajan et al., 2013; Lin et al., 2020a; Mohajeri et al., 2020). It has also been proven that curcumin confers the therapeutic benefits in inflammatory disorders, neoplasms, neurodegenerative disorders, rheumatologic diseases, and cardiovascular diseases (CVDs) (Aggarwal and Harikumar 2009; Velmurugan et al., 2018). Of note, there is scientific evidence of a beneficial impact on human health in functional foods produced by incorporating plant parts that have known or unknown bioactive components like flavonoids, phenolic acid, and alkaloids with various biological properties as aforesaid (Tsuda 2018; Xu et al., 2018; Roy et al., 2022). However, curcumin has some limitations in terms of physicochemical properties such as very low aqueous solubility, high degradation, and limited activity only in acidic pH, which leads to a decrease in bioavailability (Wan et al., 2012; Peng et al., 2018; Dei-Cas and Ghidoni 2019). Importantly, curcumin has been demonstrated to impede inflammation, oxidative stress, cancer cell proliferation, and cell death and abrogate infections caused by bacteria, fungi, and viruses (Omosa et al., 2017; Fu et al., 2021). Additionally, curcumin treatment supports total white blood cell counts and increases the levels of antioxidant indicators, circulating levels of antibodies to sheep red blood cells (SRBC), and plaque-forming cells (PFC) in the spleens of mice (Antony et al., 1999; Shakeri et al., 2017; Afolayan et al., 2018). Furthermore, it also enhances the phagocytic action of macrophages in various animal models including mouse (Wang et al., 2016; Sohn et al., 2021), ferret (Huang et al., 2021) and hamster (Lee et al., 2022; Seldeslachts et al., 2022). As mentioned before, curcumin is a polyphenolic compound, and the activity associated with its polyphenolic chemical structure varies depending on its concentration as well as the cell types and their status (sometimes it can act as a reactive oxygen species (ROS) scavenger, sometimes it can increase ROS production and cause apoptosis, etc.) (Balasubramanyam et al., 2003; Kim et al., 2016; Lin S.-R. et al., 2017; Larasati et al., 2018). From a molecular point of view, curcumin may mediate its pharmacological activities through Janus kinase/signal transducers and activators of transcription (JAK-STAT) (Rajasingh et al., 2006; Salehi et al., 2019), NF-κB (Marquardt et al., 2015; Mortezaee et al., 2019), protein kinase B (AKT or PKB) (Wang et al., 2019), transforming growth factor β (TGF-β) (Li et al., 2013; Thacker and Karunagaran 2015), and mammalian target of rapamycin (mTOR) (Lin J. et al., 2017; Thiyagarajan et al., 2018). In particular, the transcription factors nuclear factor E2-related factor 2 (Nrf2) and NF-κB can regulate 1) the inhibition of the transcription factor NF-κB mediates anti-inflammation, and 2) the induction of Nrf2 signaling pathways promotes antioxidant defense mechanisms and production of phase II enzymes (Dai et al., 2018; Esatbeyoglu et al., 2012). Anticarcinogenic effects of curcumin are also related to an increase in the p53 levels and thus in pro-apoptotic Bax and cytochrome C. The suppression of proliferation and a cell cycle arrest can also be modulated by curcumin through a p53-independent pathway such as the inhibition of NF-κB inhibitor α (IκBα), B-cell lymphoma 2 (Bcl-2), B-cell lymphoma-extra large (Bcl-xl), cyclin D1, and IL-6. Moreover, apoptosis can be initiated by curcumin by the increased cleavage of poly (ADP-ribose) polymerase (PARP) (Xu and Liu 2017; Esatbeyoglu et al., 2012). In vitro and in vivo studies of influenza A virus infection have shown curcumin treatment could suppress influenza A virus absorption and replication by activation of the Nrf2 pathway to suppress the NF-κB pathway and inflammatory cytokines to attenuate the oxidative stress and symptoms (Dai et al., 2018).
Challenges of Curcumin on COVID-19 Treatment
Several lines of evidence suggest curcumin as a talented prophylactic and therapeutic candidate for COVID-19 in the clinic and public health settings. First, curcumin exerts antiviral activity against many types of enveloped viruses, including SARS-CoV-2, through multiple mechanisms: direct interaction with viral membrane proteins, disruption of the viral envelope; inhibition of viral proteases, and induction of host antiviral responses. Second, curcumin protects from lethal pneumonia and ARDS via targeting NF-κB, inflammasomes, IL-6 trans-signal, and high-mobility group box 1 (HMGB1) pathways. Third, curcumin is basically recognized as safe and well-tolerated in both healthy and diseased human subjects (Miryan et al., 2021; Thimmulappa et al., 2021).
According to the aforementioned understanding about the COVID-19 pandemic and pharmacological merits of curcumin, the therapeutic use in SARS-CoV-2 infection remains to be explored. In this context, curcumin/curcuminoids have been shown to possess beneficial effects on the progression of inflammatory diseases including COVID-19 based on numerous action mechanisms including antiviral, anti-inflammatory, anticoagulant, antiplatelet, and cytoprotective (Babaei et al., 2020; Rattis et al., 2021). These investigations and many other effects of curcumin make it a promising agent in the adjuvant treatment of COVID-19; however, all current entities related to curcumin used for proposed treatment and prevention of COVID-19 infection are mostly based on in vitro, in vivo, and in silico studies, clinical trials, and new formulation designs. In fact, there is no direct use in the SARS-CoV-2-infected patients, so it is necessary to re-evaluate the efficacy of curcumin and provide deep insights into knowledge and strategy about curcumin’s role as an immune booster, inflammatory modulator, and therapeutic agent against COVID-19.
Curcumin as the Anti-Inflammatory Drug Candidate and Immune Modulator
There is a great variation in the clinical symptoms of SARS-CoV-2 infection from asymptomatic infection, upper respiratory tract infection, pneumonia, ARDS to multi-organ dysfunction. Although the patients recover from ARDSs of serious COVID-19 pneumonia, their lung parenchyma tissue remodeling and pulmonary fibrosis will continue to suffer and limit the pulmonary functional recovery of the patients, indicating that anti-inflammation will be one of the most important focuses on the treatment of SARS-CoV-2 infection. As an inflammatory response, NAcht leucine-rich repeat protein 3 (NLRP3) inflammasomes induce the production of several cytokines, which have been confirmed to play major roles in the pathogenesis of several viral diseases, including COVID-19 (Freeman and Swartz 2020; Zhao M. et al., 2021; Junqueira et al., 2021; Pan et al., 2021). The key molecular mediators of inflammation include pro-inflammatory cytokines such as TNF-α; chemokines; inflammatory enzymes such as cyclooxygenase-1, and -2 (COX-1, COX-2), matrix metalloproteinase-9 (MMP-9), and 5-lipoxygenase (5-LOX); transcription factors such as signal transducer and activator of transcription 3 (STAT3) and NF-κB; and ILs, for example, IL-1, IL-6, and IL-8 (Ghasemi et al., 2019; Norooznezhad et al., 2020), and notably the inhibition on those proteins or pathways can be considered as the major therapy targets for anti-inflammatory treatments of COVID-19. On the other hand, the cellular oxidative stress could be attenuated by the Nrf2 and heme oxygenase-1 (HO-1) pathway, which helps in decreasing and maintaining the redox balance in cells thereby reducing inflammation (Yang et al., 2009; Ahmed et al., 2017; Zhang et al., 2021); the activation and increase in Nrf2 expression can suppress and downregulate NLRP3 inflammasomes (Hennig et al., 2018; Yarmohammadi et al., 2021); and the activation of Nrf2 relative pathways can be a strategy to attenuate the infective symptoms of COVID-19. Experimentally, in vitro curcumin treatment as an Nrf2 activator can stimulate the Nrf2 signaling pathway to deter the NF-κB pathway and suppress viral replication in the influenza A virus infection with an IC50 of 140.67 μg/ml. In vivo, rodents treated with curcumin (50 mg/kg) significantly promoted Nrf2 expression to scavenge ROS that protects cells against oxidative stress and lung injury (Dai et al., 2018; Lin and Yao, 2020). In in vivo experiments, gavage with curcumin (40–50 μg/ml) inhibited the activation of NLRP3 by triggering the SIRT1/Nrf2 pathway to abrogate the downstream cytokine expression, such as IL-1β, IL-6, IL-18, and TNF-α (Yin et al., 2018; Yin et al., 2020). For virus-induced inflammation, curcumin treatment can revoke pro-inflammatory cytokine production, such as IL-1β, IL-6, and TNF-α via the inhibition on the NF-κB signaling pathway to hinder NLRP3 expression (Sordillo and Helson 2015; Xu and Lin 2017; Saeedi-Boroujeni et al., 2021). Based on the anti-inflammatory dosage of curcumin in rodent experiments and the Guidance for Industry of the FDA (https://www.fda.gov/media/72309/download), we found that the effective dose for human adults (70 kg) is about 450–550 mg/day using the surface area-to-body weight relative relationship.
The anti-inflammation of curcumin suppresses the expressions of inflammatory mediators not only by triggering the SIRT1/Nrf2 pathway but also activating PPARγ, IL-10, and AMPK pathways. Through the activation of IL-10, AMPK, and PPARγ pathways, curcumin can downregulate NF-κB, COX-2, inducible nitric oxide synthase (iNOS) expression, and prostaglandin E2 (PGE2) levels (Zhai et al., 2015; Kumar et al., 2017), and through the activation of the PPARγ pathway, curcumin can obstruct NF-κB, STAT, activator protein 1 (AP-1), and mitogen-activated protein kinases (MAPK) (Carvalho et al., 2021). The inhibition of NF-κB can decrease the expression of several genes, including COX-2, MMP-9, IL-8, iNOS, and TNF-α (Bengmark 2006). Through the activation of the AMPK pathway, curcumin can hinder mTOR, p38, p53, and COX-2 pathway (Thiyagarajan et al., 2018; Soltani et al., 2019), and through IL-10, curcumin can also increase the HO-1 expression and modulate the immune response (Naito et al., 2014). Based on the aforementioned studies, indirect anti-inflammatory investigations including signaling pathways are affected by curcumin, but very few results are directly pertaining to the SARS-CoV-2 treatment, and interestingly, there is still promising beneficial potential for treatment and prevention of COVID-19 in the future.
In Vitro and in Vivo Validations of Curcumin Use in SARS-CoV-2 Infection
According to the quick spread and high mortality of COVID-19, several clinical trials of the antiviral drugs or formulations applied for COVID-19 treatment were conducted or recruited without passing the in vitro and in vivo tests. Only very few in vitro-tested models of curcumin for COVID-19 treatment were investigated using Vero E6 cells or human Calu-3 cells that were infected with SARS-CoV-2 (Bormann et al., 2021). The data revealed that in vitro models treated with curcumin, nano-curcumin formulation, or turmeric root extracts could neutralize and decrease the SARS-CoV-2 viral RNA level at low subtoxic concentrations (Guijarro-Real et al., 2021; Pourhajibagher et al., 2021). Another biochemical and enzyme activity tests showed that the turmeric root extract can significantly constrain the main protease (Mpro) activity of SARS-CoV-2 (Dound et al., 2021). Within the past 3 months, the results of a new in vitro study by Bahun et al. (2022) showed the IC50 of curcumin on the SARS-CoV-2 protease 3CLpro is 11.9 μM, but in another in vitro study by Marín-Palma et al. (2021), results illustrated that curcumin has the EC50 from 1.14 μg/ml to 6.03 μg/ml in the antiviral effects of several different SARS-CoV-2 variants, whereas the data revealed curcumin has a cytotoxic effects with the CC50 about 16.5 μg/ml, which is equal to 6.1 μM. The results of the two in vitro studies demonstrate that curcumin has the potential to inhibit the SARS-CoV-2 infection, but its effective and cytotoxicity doses are very close and have some overlapping that needs additional studies to evaluate its practical safe dose and treatment mode of its application for COVID-19 treatment. Due to urgent demands for curing or preventing COVID-19, there were several clinical trials based on the application of curcumin on COVID-19 treatment that are either under recruitment or completed, but only few non-human in vivo animal studies that are simulated to apply a curcumin formulation on COVID-19 therapy has been published. One study used human beta-coronavirus to mimic the SARS-CoV-2 infection (Dound and Sehgal 2021), and the result showed the application of a curcumin-based herbal formulation could significantly increase CD4+ and CD8+ cell count in blood and plasma IgG and IgM levels in virus-infected animals. Mounting evidence obtained from preclinical studies using animal models of lethal pneumonia shows curcumin exerts the protective effects by regulating the expression of both pro- and anti-inflammatory factors such as IL-6, IL-8, IL-10, and COX-2, promoting the apoptosis of polymorphonuclear leukocytes (PMNs) and scavenging ROS which exacerbates the inflammatory response (Liu and Ying, 2020).
In Silico Study of Curcumin on Viral Proteins
There are several major viral site targets for COVID-19 therapy or prevention simulated by in silico studies. The molecular docking results revealed that curcumin has multiple targets with potential to bind with five viral proteins of SARS-CoV-2, and those candidate proteins contain S protein, main protease (Mpro), RNA-dependent RNA polymerase (RdRp, nsp12), nucleic acid-binding protein (nsp9), and RNA uridylate-specific endoribonuclease (nsp15). In silico studies showed curcumin can bind and act on the host proteins that participate SARS-CoV-2 infection, such as angiotensin-converting enzyme 2 (ACE2), and several proteins applied for intracellular signal transduction pathways and modulate cytokines secretion (Noor et al., 2021).
There are two domains, S1 and S2, which consist the spike protein of SARS-CoV-2 (Walls et al., 2020), and 319–541 aa of S1 is known as the receptor-binding domain (RBD) (Trigueiro-Louro et al., 2020). Within the RBD of S1, 437–508 aa are known as an ACE2 receptor-binding motif (RBM) (Rath and Kumar 2020). The COVID-19 virus entry into host cells is initiated by binding of S protein to the host cell surface ACE2 as its target receptor (Yan et al., 2020), and the RBD is the virus–host binding spots (Veeramachaneni et al., 2021). In silico simulation data exhibited that curcumin has a high binding affinity to the RBD and ACE2 (Babaeekhou et al., 2021; Nag et al., 2021; Umashankar et al., 2021). The binding of amino acid residues to curcumin presented near the RBM of S1 protein, and some curcuminoids have stable interactions with key spot residues for the binding of ACE2 comprising the glycosylation site (Babaeekhou et al., 2021), suggesting the potential efficacy of curcumin/curcuminoids in hindering the formation of S protein–ACE2 complex (Jena et al., 2021). A recent genome sequencing study also indicated that the spread of double mutations at E484Q/L452R, T478K/L452R, and F490S/L452Q of RBD has the latent potential for the enhancement of viral mutated S protein (Sm) and host ACE2 to form Sm–ACE2 binding (Aggarwal et al., 2021), and concurrently, that may cause more and effective infection of SARS-CoV-2 mutants. Interestingly, the molecular docking results showed that curcumin and piperine have been demonstrated not only high binding potential with native S protein but also similar or more stability of binding potential with Sm or/and Sm–ACE2 complex (Nag et al., 2021).
The nsp12 is the RNA-dependent RNA polymerase (RdRp) of SARS-CoV-2, and its binding with nsp7 and nsp8 to form the nsp12–nsp7–nsp8 complex is the central component for viral replication and transcription (Gao et al., 2020; Peng et al., 2020). The inhibition of RdRp could abrogate SARS-CoV-2 replication; thus, this nsp12–nsp7–nsp8 complex is recognized as a potential target for COVID-19 treatment (Khan et al., 2020; Begum et al., 2021; Ruan et al., 2021). Compared with antiviral drugs (favipiravir and remdesivir), the results of molecular docking and molecular dynamic simulation showed curcumin/curcuminoids have a good binding affinity and stability with RdRp–RNA complex of SARS-CoV-2 (Kumar Verma et al., 2021; Singh et al., 2021) and also showed they have higher potential to be developed as viral replication inhibitors of COVID-19.
The genome of the COVID-19 virus is encoded in two protease enzymes, main protease (3CLPRO or Mpro-nsp12) and papain-like protease (PLpro, nsp3), which are involved in the proteolytic processing viral polyproteins into functional proteins for viral replication and genomic expression within the host cells (Das et al., 2021). The proteolytic cleavage by viral proteases at the posttranslational stage plays the crucial role in the life cycle of SARS-CoV-2. Mpro as a main protease can catalyze more than 11 proteolytic cleavage sites for nsp generation and without human homologs (Dai et al., 2020); therefore, Mpro is considered to be an ideal antiviral target for COVID-19 treatment (Li and Kang 2020). Results of in silico studies show that curcumin/curcuminoids can form strong bonds with the active site of SARS-CoV-2 Mpro (Ibrahim et al., 2020; Bahun et al., 2022). Due to high binding affinity and its binding with the interface region of Mpro may cause the protein conformational changes, indicating that curcumin/curcuminoids could be the potential ligands for COVID-19 therapy (Ibrahim et al., 2020; Li and Kang 2020; Kumar M. et al., 2021; Mahmud et al., 2021a; Babaeekhou et al., 2021; Teli et al., 2021; Halder et al., 2022). One more study demonstrated that demethoxycurcumin and bisdemethoxycurcumin had an optimum binding affinity with COVID-19 Mpro by molecular modeling and showed the stable state by molecular dynamic (MD) simulation assay, suggesting these could be one of the potential ligands for COVID-19 therapy (Mulu et al., 2021). Unfortunately, there are only two reports with in vitro validation of the inhibitory and selectivity effects of curcumin on SARS-CoV-2 infection to confirm its powerful potential on COVID-19 therapy (Kandeil et al., 2021; Bahun et al., 2022).
The nsp9 is single-strand RNA-binding protein of SARS-CoV (nsp9–SARS) which was found to be essential for viral replication (Frieman et al., 2012). nsp9 of SARS-CoV-2 can bind to the nidovirus RdRp-associated nucleotidyltransferase (NiRAN) domain relating the viral replication and transcription (Kumar M. et al., 2021), and it was also considered to be the therapy target for COVID-19 treatment (Zhang et al., 2020; Kumar et al., 2021b). There were several studies to screen the potential candidate compounds to bind or act on nsp9 by in silico tools (Barros et al., 2020; Chandra et al., 2021; Junior et al., 2021), and one in vitro cellular assay showed the inhibition on nsp9 can reduce the viral replication of SARS-CoV-2 (Littler et al., 2021). The molecular docking results showed that curcumin can bind to the ligand binding site of nsp9, and curcumin can form about 11 interaction sites with nsp9 (Kumar et al., 2021b).
The nsp15 of SARS-CoV-2 is a RNA uridylate-specific endoribonuclease (NendoU) and a conserved protein in coronavirus (Bai et al., 2021). nsp15 can degrade negative-strand viral RNA to protect virus from the host immune responses (Hackbart et al., 2020; Zhao et al., 2020; Gao et al., 2021), and the inhibition of nsp15 can also promote viral elimination by the host immune system (Kumar et al., 2021c). There were several in silico-based studies on nsp15 to find potential antiviral compounds or candidates (Mahmud et al., 2021b; Canal et al., 2021; Quimque et al., 2021; Zrieq et al., 2021), and only few studies have conducted further validation of the antiviral effects by in vitro or in vivo study (Canal et al., 2021; Kumar et al., 2021c). The molecular docking result showed curcumin can bind to nsp15, which might have the potential to inhibit SARS-CoV-2 replication (Kumar et al., 2021d).
Gaining deep insights into all those viral protein in silico studies of SARS-CoV-2, we infer that curcumin can bind and interact with several target viral proteins that assist in viral attachment (S protein), replication (nsp12, nsp9), posttranslational protein cleavage/modification (Mpro), and host immunity evasion (nsp15), suggesting that curcumin/curminoids provide a promising hit against COVID-19 even without any additional demonstration (in vitro and in vivo validation). To meet the urgent demand for managing the COVID-19 pandemic, curcumin use will be the first priority with high biosafety because the toxicity of curcumin is very low even in high amounts up to 12–18 g/day (Fu et al., 2021).
In Silico Study of Curcumin on Host Proteins Associated With COVID-19 Infection
As SARS-CoV-2 is a single-strand RNA virus, its mutation may quickly and easily evade the host immune system or targeting drugs; therefore, targeting on the inhibition of host proteins that participate in the viral infections will have the stable therapeutic advantages for COVID-19 treatment or prevention. The viral infection of SARS-CoV-2 is initiated by its S protein binding to ACE2 of the host cellular surface (Alcocer-Díaz-Barreiro et al., 2020; Jahanafrooz et al., 2022). Several in silico studies showed curcumin can bind to the S protein and ACE2 to restrict viral entry (Maurya et al., 2020; Nag et al., 2021). The molecular docking results showed that curcumin not only exhibits high interaction with ACE2 but also has the most potent binding with Sm and Sm–ACE2 complex (Nag et al., 2021), indicating that curcumin can be applied in treatments for different SARS-CoV-2 mutated strand infections. The human serine protease serine 2 (TMPRSS2) is a transmembrane protease of host cells; it cleaves and activates the S protein of SARS-CoV-2 to bind with ACE2 on the initiated stage of the viral infection (Singh et al., 2020) and reveals that TMPRSS2 is a suitable target for COVID-19 therapy. TMPRSS2 genes may be co-expressed with SARS-CoV-2 cell receptor genes ACE2 and basigin (BSG), but only TMPRSS2 is demonstrated to have tissue-specific expression in alveolar cells (Brooke and Prischi 2020). Acetaminophen (paracetamol) and curcumin can downregulate the expression of TMPRSS2 in human cells (Zarubin et al., 2020). In addition to the major mode of viral infection by S protein binding to ACE2, SARS-CoV-2 can infect host cells by endocytosis and follow the proteolytic activation by cathepsin L (Jackson et al., 2021; Takeda 2021). Based on the MD simulations, curcumin was found to be the inhibitor of TMPRSS2 (Umadevi et al., 2020; Jackson et al., 2021), and in vitro results showed curcumin treatment can also impede the activity of cathepsin L (Goc et al., 2021; Oso et al., 2022). Another key host cell membrane protein in SARS-CoV-2 infection is the glucose-regulating protein 78 (GRP78) receptor. GRP78, also termed as a HSP5A or binding immunoglobulin protein (Bip), is one member of the 70-kDa heat shock protein (HSP70) family and functions as the endoplasmic reticulum (ER)-resident molecular chaperone for the clearance of misfolded proteins (Dessie and Malik 2021). An intracellular ER stress increase may upregulate the GRP78 expression and induce GRP78 re-localization to the cell membrane as cell surface GRP78 (Elfiky et al., 2021). The cellular surface GRP78 can associate with the major histocompatibility complex class I (MHC-I) that may aid SARS-CoV-2 to get into the host cells for starting an infection or help viral release from infected host cells (Ha et al., 2020; Gonzalez-Gronow et al., 2021). In silico data showed curcumin could interact with the S protein binding site and ATPase domain of GRP78 (Allam et al., 2020; Sudeep et al., 2020), suggesting that curcumin can assist in preventing COVID-19 viral attachment and enter host cells by binding to and inhibiting GRP78 of host cell surface.
In silico studies of curcumin/curcuminoids on the viral proteins and host proteins with SARS-CoV-2 infection are given in Table 1. It was deems the same as the viral site; all in silico results of the host proteins on SARS-CoV-2 infection show that curcumin can bind and interact with several targeting host proteins that play roles in viral binding (ACE2) and S protein activation (TMPRSS2, cathepsin L), and aid viral entry host cells (GRP78). Multiple targeting effects of curcumin on the site of virus and host cells during SARS-CoV-2 infective stages show its high potential to be applied for COVID-19 prevention or therapy, but until now, validations by in vitro or/and in vivo studies are still few or nil, and strikingly, it is only applicable as a co-supplement or adjuvant for therapy; nowadays, filing for approval by the FDA to become a real anti-COVID-19 medicine is most challenging.
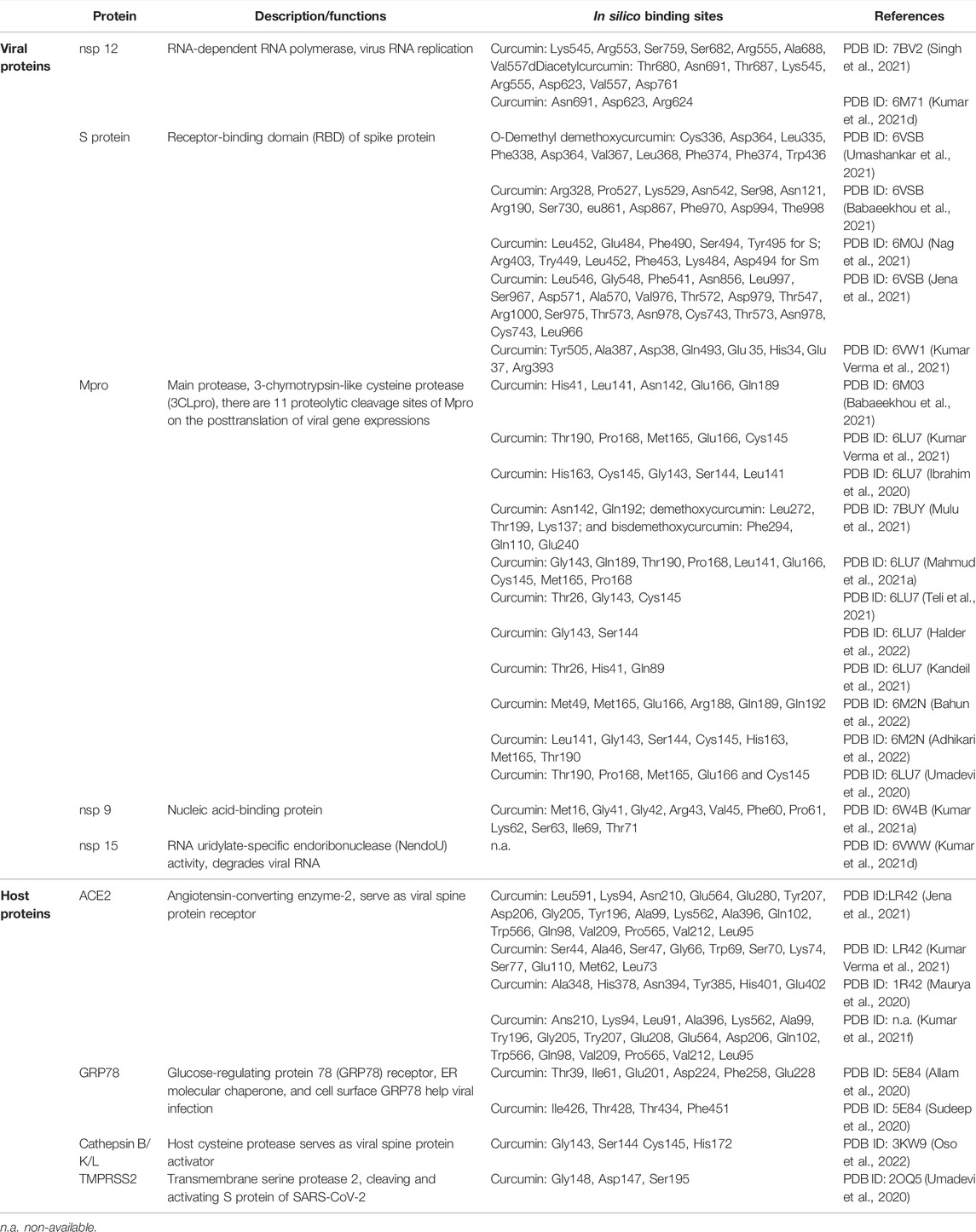
TABLE 1. In silico studies of curcumin/curcuminoids on the viral proteins and host proteins with SARS-CoV-2 infection.
Clinical Trials of Curcumin on COVID-19 Treatment
Remarkably, there are numerous reports of in silico, in vitro, and in vivo studies demonstrating that curcumin has the therapeutic potential against the COVID-19 based on its anti-inflammation, antioxidant, and antiviral effects. In this section, we have reviewed many clinical trial applications and published reports regarding the antiviral potential of curcumin/curcuminoids on COVID-19 therapy from PubMed and clinicaltrials.gov. In all these clinical trials, curcumin/curcuminoids formulations are used as a therapeutic co-supplement in the treatment of COVID-19 patients (Table 2). Because of the drawback of poor bioavailability of curcumin, several strategies are applied to improve the oral bioavailability of curcumin in clinical trials containing nano-delivery systems (Hatamipour et al., 2019), with addition of adjuvants (Tabanelli et al., 2021) and new formulation (Flory et al., 2021). Inferiorly, the metabolic rate of curcumin is high in the intestine and liver, which causes its poor bioavailability. Extraordinarily, piperine is an inhibitor of glucuronidation in the liver and intestine (Kaur et al., 2018). Therefore, the combination of curcumin and 1% piperine is a potential option for the management of COVID-19 based on several properties including antiviral, anti-inflammatory, immunomodulatory, anti-fibrotic, and antioxidant effects (Miryan et al., 2021). In other clinical trials, results of non-COVID-19 treatment revealed that co-supplementation of curcumin/curcuminoids with 1% piperine could significantly improve the bioavailability (Panahi et al., 2015a; Shoba et al., 1998), and this formulation provides more antioxidant (Panahi et al., 2016a,b) and anti-inflammatory effects (Panahi et al., 2015b; Rahimnia et al., 2015). Different ayurvedic therapeutic agents (C. Longa L, green tea, and Piper nigrum) suppress the entry of virus into host cells, transmission of pathogens, and concurrently promote the immunity. Curcumin and piperine (1-piperoylpiperidine) interact with each other and form a π–π intermolecular complex which enhances the bioavailability of curcumin by inhibiting glucuronidation of curcumin in the liver (Kumar G. et al., 2021). The dose of curcumin from two clinical trial applications and one report for COVID-19 treatment is 1,000 mg/day curcumin plus 0.5–1% piperine (Askari et al., 2021; Pawar et al., 2021). When compared with the control group, the clinical results indicated that patients with the manifestation of mild, moderate, and severe symptoms orally receiving curcumin with piperine formulations as the adjuvant symptomatic therapy for COVID-19 infection have better clinical outcomes, and it can also reduce morbidity and mortality (Miryan et al., 2020).
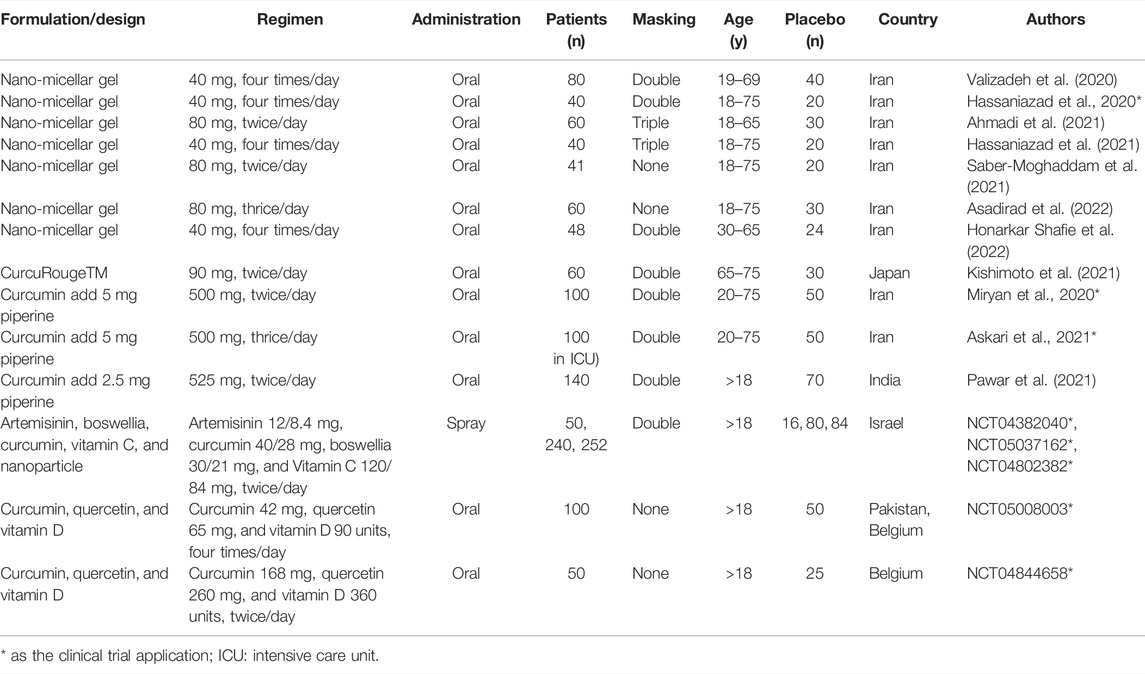
TABLE 2. Clinical trial of curcumin on COVID-19 treatment of PubMed and ClinicalTrial.gov.
Furthermore, curcumin incorporated with a nano-delivery system can enhance water solubility, decrease degradation, control slow release, and increase absorptive efficiency (Chen et al., 2020; Li et al., 2020; Quispe et al., 2021), thereby increasing circulation time, pharmacokinetics, and biodistribution. Cumulative evidence unveiled that encapsulated curcumin with a nano-delivery system has more significant effects than native curcumin in the clinical trials for the treatment in metabolic syndrome to decrease triglyceride levels in serum (Bateni et al., 2021); in coronary elective angioplasty treatment to improve total cholesterol, triglycerides, and antioxidative capacity (Helli et al., 2021); and in knee osteoarthritis treatment to improve the severity of symptoms (Hashemzadeh et al., 2020). Curcumin is re-proposed as a potential antiviral key for the treatment of SARS-CoV-2 based on its relation to the infection pathways. Moreover, the use of curcumin-loaded nanocarriers for increasing its bioavailability and therapeutic efficiency was highlighted in several clinical trials (Dourado et al., 2021; Hassaniazad et al., 2021; Tahmasebi et al., 2021a; Tahmasebi et al., 2021b; Valizadeh et al., 2020; Hassaniazad et al., 2020; Ahmadi et al., 2021; Saber-Moghaddam et al., 2021; Asadirad et al., 2022; Honarkar Shafie, et al., 2022). Additionally, the potential of the nanostructured systems and their synergistic action with curcumin on the molecular targets for viral infections have been explored (Hassaniazad et al., 2020). One report illustrates that the administration of nano-curcumin can accelerate recovery from the acute inflammatory phase of COVID-19 by mediating inflammatory immune responses (decrease in serum IFN-γ and IL-17, TBX21 mRNA; increase in serum IL-4 and TGF-β, FOXP3 mRNA) (Ahmadi et al., 2021). COVID-19 patients receiving nano-curcumin supplements could significantly increase the O2 saturation and decrease the scores of the Wisconsin Upper Respiratory System Survey (WURSS-24) in the third domain, fourth domain, and total score, indicating nano-curcumin supplementation could help decrease hypoxia and moderate the symptoms induced by SARS-CoV-2 infection (Honarkar Shafie, et al., 2022). After nano-curcumin treatment, a significant reduction in the frequency of Th17 cells, downregulation of Th17 cell-related factors, and decreased levels of Th17 cell-related cytokines were found in mild and severe COVID-19 patients, implying that curcumin could be a potential modulatory compound in improving the patient’s inflammatory condition (Hassaniazad et al., 2020; Saber-Moghaddam et al., 2021). In all these clinical trials of COVID-19 treatment, nano-micelle curcumin was applied with the dose of 160 or 240 mg/day, and the dosage was lower than that of native curcumin treatment. Positively, hospitalized COVID-19 patients with oral administration of nano-curcumin formulation can modulate the inflammatory cytokine expression and secretion, such as IFN-γ, IL-1β, IL-6, and TNF-α (Kishimoto et al., 2021; Asadirad et al., 2022); regulate inflammatory immune responses to accelerate the recovery of the acute inflammatory phase; and shorten total recovery time (Ahmadi et al., 2021; Saber-Moghaddam et al., 2021). Additionally, oral spray with nano-curcumin formulation was developed and has entered phase III clinical trial for COVID-19 treatment in Israel (NCT04382040, NCT05037162, NCT04802382). Moreover, a new modified starch–curcumin formulation could increase curcumin absorption and distribution in blood circulation over 150 times compared to oral administration of native curcumin. This result showed this can significantly improve the anti-inflammatory effects and reduce the neutrophil/lymphocyte ratio with the administration of lower dose (Kishimoto et al., 2021).
Clinical trials of curcumin for COVID-19 treatment from PubMed and ClinicalTrial.gov are listed in Table 2. There are some points worth noting from all those clinical trials with curcumin formulations as the co-supplementation in COVID-19 treatment. As the sample size of those clinical trials was too small, their results cannot be representative and promising. Within 2 years from 2019, the mutants of SARS-CoV-2 (Sm) varied and increased, and the outbreak of the mutated strands was faster; consequently, patients diagnosed with COVID-19 were infected by different viral variants with different clinical symptoms, severity, and mortality. These several viral variants of COVID-19 spread simultaneously around the world, but most clinical trials with curcumin applications in COVID-19 treatment did not easily establish the sample criteria for the patient’s viral mutants. There some pieces of evidence show that curcumin has a significant inhibitory effect on cytochrome P450 (CYP450) enzymes (Mashayekhi-Sardoo et al., 2021; Sharma et al., 2022), which play important roles in the phase 1 metabolism of several clinical drugs, such as steroids (Zhao N. et al., 2021). Generally, steroids are widely used for anti-inflammation in the clinical treatment of COVID-19 (Lin et al., 2021). Therefore, the safety of curcumin or curcuminoids was approved by the U.S. FDA, but the inhibitory effect of curcumin on CYP450 isoenzymes still needs more studies to investigate the interaction between curcumin and clinical drugs for treating COVID-19.
Promises and Perils of Curcuminoids on the Remedy of COVID-19
Curcumin attenuates oxidative stress and inflammation and regulates inflammatory and pro-inflammatory pathways associated with most chronic diseases (Quiñonez-Flores et al., 2016), and it presents future perspectives regarding the usage of curcumin as an immunomodulatory drug. Paradoxically, curcumin (along with many other dietary polyphenols), as abovementioned, can target multiple organs or cell lineages without a known receptor or a defined target. This, along with other chemical features of curcumin, has generated enormous difficulties in the detailed dissection of mechanistic pathways underlying its biomedical functions. Indeed, these features have been causing some confusion among drug developers and have been heavily debated recently (Jin et al., 2018; Heger 2017; Nelson et al., 2017). Taken together, the pharmaceutical potential of curcumin and curcuminoids in the remedy of COVID-19 is depicted in Figure 2. The underlying mechanisms of curcumin and curcuminoids on the viral protein and host cell proteins in combating COVID-19 infection are also illustrated, including proposed signaling pathways of stimulation and inhibition from both viral and host cellular sites. Recently, with the awareness of the immunomodulatory properties of curcumin, curcumin was successfully added to different food matrices to formulate functional foods that can improve human body immunity to abate the outbreak of SARS-CoV-2 (reviewed in Tripathy et al., 2021). Further investigation is needed to unequivocally determine whether curcumin might provide a therapeutic benefit in various diseases, particularly COVID-19. The utilization of purified active compounds like curcumin at higher than normal doses warrants additional scrutiny, including an accurate determination of the appropriate dose, dosing regimen, duration of treatment, and further clarification of the mechanism(s) of action as it pertains to viral infection. With regard to the treatment of COVID-19 infection or any other viral infection disease in which curcumin is administered via the oral route, another important issue concerns the increase in the bioavailability of curcumin. Despite its efficacy and safety, the therapeutic potential of curcumin is indeed still debated due to relatively poor bioavailability in humans including its poor solubility in water, chemical instability, and a low pharmacokinetic profile (Anand et al., 2007). Importantly, the oral bioavailability of curcumin is low due to a relatively low absorption by the small intestine coupled with an extensive reductive and conjugative metabolism in the liver and elimination through the gall bladder. Moreover, although curcumin is orally administered at a high dose, only a small quantity is detected in the blood plasma that is rapidly metabolized and excreted via faces and urine (Niu et al., 2012). Aforementioned limitations have restricted curcumin’s therapeutic effectiveness in treating human diseases. Numerous studies have been conducted under heat and pressure with various formulations (amorphous) (Tran et al., 2019; Sunagawa et al., 2021) to increase the aqueous solubility and bioavailability of curcumin, which demonstrated that curcumin supplemented with nanoparticles of active substances or nano-formulations had enhanced bioavailability, controlled release, and increased stability. Additionally, lipid-based delivery systems and the encapsulation are mainly used for the enrichment of food products with health-promoting compounds. Notably, the delivery systems of curcumin (such as particles, micelles, emulsions, and liposomes) have a demonstrably positive effect on augment bioavailability (Chebl et al., 2017; Guerrero et al., 2018; Tan et al., 2018).
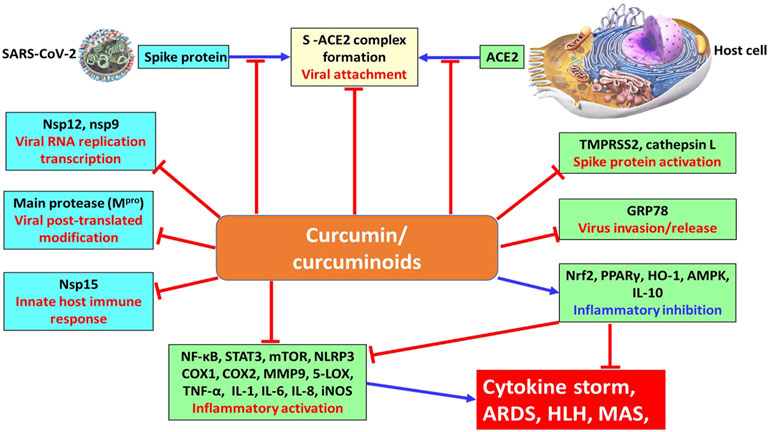
FIGURE 2. Underlying mechanism of curcumin and curcuminoids on the viral protein and host cell proteins for combating the COVID-19 pandemic. stimulation; inhibition.
Conclusion
Curcumin is a bioactive phytochemical that can be utilized as a nutraceutical or pharmaceutical in functional foods, supplements, and medicines. There are several limitations of curcumin use like low solubility and fast metabolism which restrict its absorption in the gastrointestinal tract and lead to poor oral bioavailability. Curcumin has multiple effects on the antioxidative stress, anti-inflammation, antiviral infections, but it has some inhibitory effects on the drug metabolism which need to be further clarified. To overcome these limitations, various curcumin formulations such as encapsulation in edible nanoparticles or microparticles to enhance its water dispersibility, chemical stability, and bioavailability are applied. Clinical trials of curcumin indicate safety, tolerability, and non-toxicity. However, the efficacy is questionable because of the small number of patients enrolled in each study. The challenges concerning research on curcumin’s health benefits are given as follows: clarifying the relationship between curcumin’s health benefits and the immunomodulation particularly in treatment of COVID-19 and conducting further human trials in which multiple research groups use the same samples and conditions. For the application of curcumin in COVID-19 treatment, further studies are still needed to optimize or improve its bioavailability following oral administration and to explore the degree of influence or interference of curcumin on clinical drugs metabolism. Moreover, this study after re-evaluation can afford a favorable application or approach of curcumin with evidence based on the drug discovery and development, pharmacology, functional foods, and nutraceuticals for effectively fighting COVID-19. Overall, curcumin is a promising ingredient of novel functional foods with protective efficacy in preventing or reducing the manifestation or complications of COVID-19 infection.
Author Contributions
W-YH and JW conceived the articles collections and data analysis. M-JT, LH, and NK participated and designed the flow of article. Y-SF and C-FW prepared the manuscript draft and critically reviewed the manuscript. All authors have reviewed and approved the submitting version of manuscript.
Funding
The financial support received from the Xiamen Medical College (K2019-01) and Xiamen Medical College (K2019-03).
Conflict of Interest
The authors declare that the research was conducted in the absence of any commercial or financial relationships that could be construed as a potential conflict of interest.
Publisher’s Note
All claims expressed in this article are solely those of the authors and do not necessarily represent those of their affiliated organizations, or those of the publisher, the editors, and the reviewers. Any product that may be evaluated in this article, or claim that may be made by its manufacturer, is not guaranteed or endorsed by the publisher.
Acknowledgments
The authors sincerely appreciate Professor Max K Leong and Mr. Ting-Hsu Chen who provide technical assist in molecular docking. We also thank Professor, MD, PhD. Henrich Cheng for his help in consulting clinical trial.
References
Adhikari, B., Marasini, B. P., Rayamajhee, B., Bhattarai, B. R., Lamichhane, G., Khadayat, K., et al. (2021). Potential Roles of Medicinal Plants for the Treatment of Viral Diseases Focusing on COVID ‐19: A Review. Phytotherapy Res. 35 (3), 1298–1312. doi:10.1002/ptr.6893
Adhikari, N., Banerjee, S., Baidya, S. K., Ghosh, B., and Jha, T. (2022). Ligand-based Quantitative Structural Assessments of SARS-CoV-2 3CLpro Inhibitors: An Analysis in Light of Structure-Based Multi-Molecular Modeling Evidences. J. Mol. Struct. 1251, 132041. doi:10.1016/j.molstruc.2021.132041
Adhikari, S. P., Meng, S., Wu, Y. J., Mao, Y. P., Ye, R. X., Wang, Q. Z., et al. (2020). Epidemiology, Causes, Clinical Manifestation and Diagnosis, Prevention and Control of Coronavirus Disease (COVID-19) during the Early Outbreak Period: a Scoping Review. Infect. Dis. Poverty 9 (1), 29. doi:10.1186/s40249-020-00646-x
Afolayan, F. I. D., Erinwusi, B., and Oyeyemi, O. T. (2018). Immunomodulatory Activity of Curcumin-Entrapped Poly D,l-Lactic-Co-Glycolic Acid Nanoparticles in Mice. Integr. Med. Res. 7 (2), 168–175. doi:10.1016/j.imr.2018.02.004
Aggarwal, A., Naskar, S., Maroli, N., Gorai, B., Dixit, N. M., and Maiti, P. K. (2021). Mechanistic Insights into the Effects of Key Mutations on SARS-CoV-2 RBD-ACE2 Binding. Phys. Chem. Chem. Phys. 23, 26451–26458. doi:10.1039/d1cp04005g
Aggarwal, B. B., and Harikumar, K. B. (2009). Potential Therapeutic Effects of Curcumin, the Anti-inflammatory Agent, against Neurodegenerative, Cardiovascular, Pulmonary, Metabolic, Autoimmune and Neoplastic Diseases. Int. J. Biochem. Cel Biol 41 (1), 40–59. doi:10.1016/j.biocel.2008.06.010
Ahmadi, R., Salari, S., Sharifi, M. D., Reihani, H., Rostamiani, M. B., Behmadi, M., et al. (2021). Oral Nano-Curcumin Formulation Efficacy in the Management of Mild to Moderate Outpatient COVID-19: A Randomized Triple-Blind Placebo-Controlled Clinical Trial. Food Sci. Nutr. 9 (8), 4068–4075. doi:10.1002/fsn3.2226
Ahmed, S. M., Luo, L., Namani, A., Wang, X. J., and Tang, X. (2017). Nrf2 Signaling Pathway: Pivotal Roles in Inflammation. Biochim. Biophys. Acta Mol. Basis Dis. 1863 (2), 585–597. doi:10.1016/j.bbadis.2016.11.005
Alcocer-Díaz-Barreiro, L., Cossio-Aranda, J., Verdejo-Paris, J., Odin-de-los-Ríos, M., Galván-Oseguera, H., Álvarez-López, H., et al. (2021). COVID-19 Y El Sistema Renina, Angiotensina, Aldosterona. Una Relación Compleja. Acm 90 (Suppl. l), 19–25. doi:10.24875/ACM.M20000063
Allam, L., Ghrifi, F., Mohammed, H., El Hafidi, N., El Jaoudi, R., El Harti, J., et al. (2020). Targeting the GRP78-Dependant SARS-CoV-2 Cell Entry by Peptides and Small Molecules. Bioinform. Biol. Insights 14, 1177932220965505. doi:10.1177/1177932220965505
Anand, P., Kunnumakkara, A. B., Newman, R. A., and Aggarwal, B. B. (2007). Bioavailability of Curcumin: Problems and Promises. Mol. Pharm. 4 (6), 807–818. doi:10.1021/mp700113r
Antony, S., Kuttan, R., and Kuttan, G. (1999). Immunomodulatory Activity of Curcumin. Immunol. Invest. 28 (5-6), 291–303. doi:10.3109/08820139909062263
Asadirad, A., Nashibi, R., Khodadadi, A., Ghadiri, A. A., Sadeghi, M., Aminian, A., et al. (2022). Antiinflammatory Potential of Nano‐curcumin as an Alternative Therapeutic Agent for the Treatment of Mild‐to‐moderate Hospitalized COVID‐19 Patients in a Placebo‐controlled Clinical Trial. Phytotherapy Res. 36, 1023–1031. doi:10.1002/ptr.7375
Askari, G., Alikiaii, B., Soleimani, D., Sahebkar, A., Mirjalili, M., Feizi, A., et al. (2021). Effect of Curcumin-Pipeine Supplementation on Clinical Status, Mortality Rate, Oxidative Stress, and Inflammatory Markers in Critically Ill ICU Patients with COVID-19: a Structured Summary of a Study Protocol for a Randomized Controlled Trial. Trials 22 (1), 434. doi:10.1186/s13063-021-05372-9
Babaeekhou, L., Ghane, M., and Abbas-Mohammadi, M. (2021). In Silico targeting SARS-CoV-2 Spike Protein and Main Protease by Biochemical Compounds. Biologia 76, 3547–3565. doi:10.1007/s11756-021-00881-z
Babaei, F., Nassiri-Asl, M., and Hosseinzadeh, H. (2020). Curcumin (A Constituent of Turmeric): New Treatment Option against COVID-19. Food Sci. Nutr. 8 (10), 5215–5227. doi:10.1002/fsn3.1858
Bahun, M., Jukić, M., Oblak, D., Kranjc, L., Bajc, G., Butala, M., et al. (2022). Inhibition of the SARS-CoV-2 3CLpro Main Protease by Plant Polyphenols. Food Chem. 373 (Pt B), 131594. doi:10.1016/j.foodchem.2021.131594
Bai, C., Zhong, Q., and Gao, G. F. (2021). Overview of SARS-CoV-2 Genome-Encoded Proteins. Sci. China Life Sci. 65, 280–294. doi:10.1007/s11427-021-1964-4
Balasubramanyam, M., Koteswari, A. A., Kumar, R. S., Monickaraj, S. F., Maheswari, J. U., and Mohan, V. (2003). Curcumin-induced Inhibition of Cellular Reactive Oxygen Species Generation: Novel Therapeutic Implications. J. Biosci. 28 (6), 715–721. doi:10.1007/bf02708432
Barros, R. O., Junior, F. L. C. C., Pereira, W. S., Oliveira, N. M. N., and Ramos, R. M. (2020). Interaction of Drug Candidates with Various SARS-CoV-2 Receptors: An In Silico Study to Combat COVID-19. J. Proteome Res. 19 (11), 4567–4575. doi:10.1021/acs.jproteome.0c00327
Bateni, Z., Rahimi, H. R., Hedayati, M., Afsharian, S., Goudarzi, R., and Sohrab, G. (2021). The Effects of Nano-Curcumin Supplementation on Glycemic Control, Blood Pressure, Lipid Profile, and Insulin Resistance in Patients with the Metabolic Syndrome: A Randomized, Double-Blind Clinical Trial. Phytother Res. 35 (7), 3945–3953. doi:10.1002/ptr.7109
Bazdyrev, E., Rusina, P., Panova, M., Novikov, F., Grishagin, I., and Nebolsin, V. (2021). Lung Fibrosis after COVID-19: Treatment Prospects. Pharmaceuticals (Basel) 14 (8), 807. doi:10.3390/ph14080807
Begum, F., Srivastava, A. K., and Ray, U. (2021). Repurposing Nonnucleoside Antivirals against SARS-CoV2 NSP12 (RNA Dependent RNA Polymerase): In Silico-molecular Insight. Biochem. Biophys. Res. Commun. 571, 26–31. doi:10.1016/j.bbrc.2021.07.050
Bengmark, S. (2006). Curcumin, an Atoxic Antioxidant and Natural NFkappaB, Cyclooxygenase-2, Lipooxygenase, and Inducible Nitric Oxide Synthase Inhibitor: a Shield against Acute and Chronic Diseases. JPEN J. Parenter. Enteral Nutr. 30 (1), 45–51. doi:10.1177/014860710603000145
Bormann, M., Alt, M., Schipper, L., van de Sand, L., Le-Trilling, V. T. K., Rink, L., et al. (2021). Turmeric Root and its Bioactive Ingredient Curcumin Effectively Neutralize SARS-CoV-2 In Vitro. Viruses 13 (10), 1914. doi:10.3390/v13101914
Brooke, G. N., and Prischi, F. (2020). Structural and Functional Modelling of SARS-CoV-2 Entry in Animal Models. Sci. Rep. 10 (1), 15917. doi:10.1038/s41598-020-72528-z
Canal, B., Fujisawa, R., McClure, A. W., Deegan, T. D., Wu, M., Ulferts, R., et al. (2021). Identifying SARS-CoV-2 Antiviral Compounds by Screening for Small Molecule Inhibitors of Nsp15 Endoribonuclease. Biochem. J. 478 (13), 2465–2479. doi:10.1042/BCJ20210199
Carvalho, M. V. d., Gonçalves-de-Albuquerque, C. F., and Silva, A. R. (2021). PPAR Gamma: From Definition to Molecular Targets and Therapy of Lung Diseases. Ijms 22 (2), 805. doi:10.3390/ijms22020805
Catanzaro, M., Corsini, E., Rosini, M., Racchi, M., and Lanni, C. (2018). Immunomodulators Inspired by Nature: a Review on Curcumin and Echinacea. Molecules 23 (11), 2778. doi:10.3390/molecules23112778
Chandra, A., Gurjar, V., Ahmed, M. Z., Alqahtani, A. S., Qamar, I., and Singh, N. (2021). Exploring Potential Inhibitor of SARS-CoV2 Replicase from FDA Approved Drugs Using Insilico Drug Discovery Methods. J. Biomol. Struct. Dyn., 1–8. doi:10.1080/07391102.2020.1871416
Chebl, M., Moussa, Z., Peurla, M., and Patra, D. (2017). Polyelectrolyte Mediated Nano Hybrid Particle as a Nano-Sensor with Outstandingly Amplified Specificity and Sensitivity for Enzyme Free Estimation of Cholesterol. Talanta 169, 104–114. doi:10.1016/j.talanta.2017.03.070
Chen, Y., Lu, Y., Lee, R. J., and Xiang, G. (2020). Nano Encapsulated Curcumin: and its Potential for Biomedical Applications. Int. J. Nanomedicine 15, 3099–3120. doi:10.2147/IJN.S210320
Chilamakuri, R., and Agarwal, S. (2021). COVID-19: Characteristics and Therapeutics. Cells 10 (2), 206. doi:10.3390/cells10020206
Ciotti, M., Angeletti, S., Minieri, M., Giovannetti, M., Benvenuto, D., Pascarella, S., et al. (2019). COVID-19 Outbreak: an Overview. chemotherapy 64 (5-6), 215–223. doi:10.1159/000507423
Dai, J., Gu, L., Su, Y., Wang, Q., Zhao, Y., Chen, X., et al. (2018). Inhibition of Curcumin on Influenza A Virus Infection and Influenzal Pneumonia via Oxidative Stress, TLR2/4, p38/JNK MAPK and NF-Κb Pathways. Int. Immunopharmacol 54, 177–187. doi:10.1016/j.intimp.2017.11.009
Dai, W., Zhang, B., Jiang, X. M., Su, H., Li, J., Zhao, Y., et al. (2020). Structure-based Design of Antiviral Drug Candidates Targeting the SARS-CoV-2 Main Protease. Science 368 (6497), 1331–1335. doi:10.1126/science.abb4489
Das, A., Ahmed, R., Akhtar, S., Begum, K., and Banu, S. (2021). An Overview of Basic Molecular Biology of SARS-CoV-2 and Current COVID-19 Prevention Strategies. Gene Rep. 23, 101122. doi:10.1016/j.genrep.2021.101122
Dei Cas, M., and Ghidoni, R. (2019). Dietary Curcumin: Correlation between Bioavailability and Health Potential. Nutrients 11 (9), 2147. doi:10.3390/nu11092147
Dessie, G., and Malik, T. (2021). Role of Serine Proteases and Host Cell Receptors Involved in Proteolytic Activation, Entry of SARS-CoV-2 and its Current Therapeutic Options. Infect. Drug Resist. 14, 1883–1892. doi:10.2147/IDR.S308176
Dound, Y. A., and Sehgal, R. (2021). Preclinical Efficacy and Safety Studies of Formulation SSV-003, a Potent Anti-viral Herbal Formulation. J. Exp. Pharmacol. 13, 913–921. doi:10.2147/JEP.S310452
Dourado, D., Freire, D. T., Pereira, D. T., Amaral-Machado, L., N Alencar, É., de Barros, A. L. B., et al. (2021). Will Curcumin Nanosystems Be the Next Promising Antiviral Alternatives in COVID-19 Treatment Trials? Biomed. Pharmacother. 139, 111578. doi:10.1016/j.biopha.2021.111578
Elfiky, A. A., Ibrahim, I. M., Amin, F. G., Ismail, A. M., and Elshemey, W. M. (2021). COVID-19 and Cell Stress. Adv. Exp. Med. Biol. 1318, 169–178. doi:10.1007/978-3-030-63761-3_10
Esatbeyoglu, T., Huebbe, P., Ernst, I. M., Chin, D., Wagner, A. E., and Rimbach, G. (2012). Curcumin--from Molecule to Biological Function. Angew. Chem. Int. Ed. Engl. 51, 5308–5332. doi:10.1002/anie.201107724
FDA (2022). Guidance for Industry of FDA. Availableat: https://www.fda.gov/media/72309/download (Accessed Jan 21, 2022).
Flory, S., Sus, N., Haas, K., Jehle, S., Kienhöfer, E., Waehler, R., et al. (2021). Increasing Post‐Digestive Solubility of Curcumin Is the Most Successful Strategy to Improve its Oral Bioavailability: A Randomized Cross‐Over Trial in Healthy Adults and In Vitro Bioaccessibility Experiments. Mol. Nutr. Food Res 65, 2100613. doi:10.1002/mnfr.202100613
Freeman, T. L., and Swartz, T. H. (2020). Targeting the NLRP3 Inflammasome in Severe COVID-19. Front. Immunol. 11, 1518. doi:10.3389/fimmu.2020.01518
Frieman, M., Yount, B., Agnihothram, S., Page, C., Donaldson, E., Roberts, A., et al. (2012). Molecular Determinants of Severe Acute Respiratory Syndrome Coronavirus Pathogenesis and Virulence in Young and Aged Mouse Models of Human Disease. J. Virol. 86 (2), 884–897. doi:10.1128/JVI.05957-11
Fu, Y. S., Chen, T. H., Weng, L., Huang, L., Lai, D., and Weng, C. F. (2021). Pharmacological Properties and Underlying Mechanisms of Curcumin and Prospects in Medicinal Potential. Biomed. Pharmacother. 141, 111888. doi:10.1016/j.biopha.2021.111888
Gao, B., Gong, X., Fang, S., Weng, W., Wang, H., Chu, H., et al. (2021). Inhibition of Anti-viral Stress Granule Formation by Coronavirus Endoribonuclease Nsp15 Ensures Efficient Virus Replication. Plos Pathog. 17 (2), e1008690. doi:10.1371/journal.ppat.1008690
Gao, Y., Yan, L., Huang, Y., Liu, F., Zhao, Y., Cao, L., et al. (2020). Structure of the RNA-dependent RNA Polymerase from COVID-19 Virus. Science 368 (6492), 779–782. doi:10.1126/science.abb7498
Ghandadi, M., and Sahebkar, A. (2017). Curcumin: an Effective Inhibitor of Interleukin-6. Curr. Pharm. Des. 23 (6), 921–931. doi:10.2174/1381612822666161006151605
Ghasemi, F., Bagheri, H., Barreto, G. E., Read, M. I., and Sahebkar, A. (2019). Effects of Curcumin on Microglial Cells. Neurotox Res. 36 (1), 12–26. doi:10.1007/s12640-019-00030-0
Giacomelli, C., Piccarducci, R., Marchetti, L., Romei, C., and Martini, C. (2021). Pulmonary Fibrosis from Molecular Mechanisms to Therapeutic Interventions: Lessons from post-COVID-19 Patients. Biochem. Pharmacol. 193, 114812. doi:10.1016/j.bcp.2021.114812
Goc, A., Sumera, W., Rath, M., and Niedzwiecki, A. (2021). Phenolic Compounds Disrupt Spike-Mediated Receptor-Binding and Entry of SARS-CoV-2 Pseudo-virions. PLoS One 16 (6), e0253489. doi:10.1371/journal.pone.0253489
Godeau, D., Petit, A., Richard, I., Roquelaure, Y., and Descatha, A. (2021). Return-to-work, Disabilities and Occupational Health in the Age of COVID-19. Scand. J. Work Environ. Health 47 (5), 408–409. doi:10.5271/sjweh.3960
Gonzalez-Gronow, M., Gopal, U., Austin, R. C., and Pizzo, S. V. (2021). Glucose-regulated Protein (GRP78) Is an Important Cell Surface Receptor for Viral Invasion, Cancers, and Neurological Disorders. IUBMB Life 73 (6), 843–854. doi:10.1002/iub.2502
Gorabi, A. M., Razi, B., Aslani, S., Abbasifard, M., Imani, D., Sathyapalan, T., et al. (2021). Effect of Curcumin on Proinflammatory Cytokines: A Meta-Analysis of Randomized Controlled Trials. Cytokine 143, 155541. doi:10.1016/j.cyto.2021.155541
Guerrero, S., Inostroza-Riquelme, M., Contreras-Orellana, P., Diaz-Garcia, V., Lara, P., Vivanco-Palma, A., et al. (2018). Curcumin-loaded Nanoemulsion: a New Safe and Effective Formulation to Prevent Tumor Reincidence and Metastasis. Nanoscale 10, 22612–22622. doi:10.1039/c8nr06173d
Guijarro-Real, C., Plazas, M., Rodríguez-Burruezo, A., Prohens, J., and Fita, A. (2021). Potential In Vitro Inhibition of Selected Plant Extracts against SARS-CoV-2 Chymotripsin-like Protease (3CLPro) Activity. Foods 10 (7), 1503. doi:10.3390/foods10071503
Ha, D. P., Van Krieken, R., Carlos, A. J., and Lee, A. S. (2020). The Stress-Inducible Molecular Chaperone GRP78 as Potential Therapeutic Target for Coronavirus Infection. J. Infect. 81 (3), 452–482. doi:10.1016/j.jinf.2020.06.017
Hackbart, M., Deng, X., and Baker, S. C. (2020). Coronavirus Endoribonuclease Targets Viral Polyuridine Sequences to Evade Activating Host Sensors. Proc. Natl. Acad. Sci. U S A. 117 (14), 8094–8103. doi:10.1073/pnas.1921485117
Halder, P., Pal, U., Paladhi, P., Dutta, S., Paul, P., Pal, S., et al. (2022). Evaluation of Potency of the Selected Bioactive Molecules from Indian Medicinal Plants with MPro of SARS-CoV-2 through In Silico Analysis. J. Ayurveda Integr. Med. 13 (2), 100449. doi:10.1016/j.jaim.2021.05.003
Hanafy, N. A. N., and El-Kemary, M. A. (2022). Silymarin/curcumin Loaded Albumin Nanoparticles Coated by Chitosan as Muco-Inhalable Delivery System Observing Anti-inflammatory and Anti COVID-19 Characterizations in Oleic Acid Triggered Lung Injury and In Vitro COVID-19 experiment. Int. J. Biol. Macromol 198, 101–110. doi:10.1016/j.ijbiomac.2021.12.073
Hashemzadeh, K., Davoudian, N., Jaafari, M. R., and Mirfeizi, Z. (2020). The Effect of Nanocurcumin in Improvement of Knee Osteoarthritis: a Randomized Clinical Trial. Curr. Rheumatol. Rev. 16 (2), 158–164. doi:10.2174/1874471013666191223152658
Hassaniazad, M., Eftekhar, E., Inchehsablagh, B. R., Kamali, H., Tousi, A., Jaafari, M. R., et al. (2021). A Triple-Blind, Placebo-Controlled, Randomized Clinical Trial to Evaluate the Effect of Curcumin-Containing Nanomicelles on Cellular Immune Responses Subtypes and Clinical Outcome in COVID-19 Patients. Phytother Res. 35 (11), 6417–6427. doi:10.1002/ptr.7294
Hassaniazad, M., Inchehsablagh, B. R., Kamali, H., Tousi, A., Eftekhar, E., Jaafari, M. R., et al. (2020). The Clinical Effect of Nano Micelles Containing Curcumin as a Therapeutic Supplement in Patients with COVID-19 and the Immune Responses Balance Changes Following Treatment: A Structured Summary of a Study Protocol for a Randomised Controlled Trial. Trials 21 (1), 876. doi:10.1186/s13063-020-04824-y
Hatamipour, M., Sahebkar, A., Alavizadeh, S. H., Dorri, M., and Jaafari, M. R. (2019). Novel Nanomicelle Formulation to Enhance Bioavailability and Stability of Curcuminoids. Iran J. Basic Med. Sci. 22 (3), 282–289. doi:10.22038/ijbms.2019.32873.7852
Heger, M. (2017). Drug Screening: Don't Discount All Curcumin Trial Data. Nature 543 (7643), 40. doi:10.1038/543040c
Helli, B., Gerami, H., Kavianpour, M., Heybar, H., Hosseini, S. K., and Haghighian, H. K. (2021). Curcumin Nanomicelle Improves Lipid Profile, Stress Oxidative Factors and Inflammatory Markers in Patients Undergoing Coronary Elective Angioplasty; a Randomized Clinical Trial. Emiddt 21, 2090–2098. doi:10.2174/1871530321666210104145231
Hennig, P., Garstkiewicz, M., Grossi, S., Di Filippo, M., French, L. E., and Beer, H. D. (2018). The Crosstalk between Nrf2 and Inflammasomes. Int. J. Mol. Sci. 19 (2), 562. doi:10.3390/ijms19020562
Hewlings, S. J., and Kalman, D. S. (2017). Curcumin: a Review of its Effects on Human Health. Foods 6 (10), 92. doi:10.3390/foods6100092
Honarkar Shafie, E., Taheri, F., Alijani, N., Okhovvat, A. R., Goudarzi, R., Borumandnia, N., et al. (2022). Effect of Nanocurcumin Supplementation on the Severity of Symptoms and Length of Hospital Stay in Patients with COVID‐19: A Randomized Double‐blind Placebo‐controlled Trial. Phytotherapy Res. 36, 1013–1022. doi:10.1002/ptr.7374
Horowitz, R. I., and Freeman, P. R. (2020). Three Novel Prevention, Diagnostic, and Treatment Options for COVID-19 Urgently Necessitating Controlled Randomized Trials. Med. Hypotheses 143, 109851. doi:10.1016/j.mehy.2020.109851
Huang, P. K., Lin, S. R., Chang, C. H., Tsai, M. J., Lee, D. N., and Weng, C. F. (2019). Natural Phenolic Compounds Potentiate Hypoglycemia via Inhibition of Dipeptidyl Peptidase IV. Sci. Rep. 9 (1), 15585. doi:10.1038/s41598-019-52088-7
Huang, Y., Skarlupka, A. L., Jang, H., Blas-Machado, U., Holladay, N., Hogan, R. J., et al. (2021). SARS-CoV-2 and Influenza A Virus Co-infections in Ferrets. J. Virol. doi:10.1128/JVI.01791-21
Ibrahim, M. A. A., Abdelrahman, A. H. M., Hussien, T. A., Badr, E. A. A., Mohamed, T. A., El-Seedi, H. R., et al. (2020). In Silico drug Discovery of Major Metabolites from Spices as SARS-CoV-2 Main Protease Inhibitors. Comput. Biol. Med. 126, 104046. doi:10.1016/j.compbiomed.2020.104046
Isidoro, C., Chiung-Fang Chang, A., and Sheen, L. Y. (2022). Natural Products as a Source of Novel Drugs for Treating SARS-CoV2 Infection. J. Tradit Complement. Med. 12 (1), 1–5. doi:10.1016/j.jtcme.2022.02.001
Jackson, C. B., Farzan, M., Chen, B., and Choe, H. (2021). Mechanisms of SARS-CoV-2 Entry into Cells. Nat. Rev. Mol. Cel Biol 23, 3–20. doi:10.1038/s41580-021-00418-x
Jahanafrooz, Z., Chen, Z., Bao, J., Li, H., Lipworth, L., and Guo, X. (2022). An Overview of Human Proteins and Genes Involved in SARS-CoV-2 Infection. Gene 808, 145963. doi:10.1016/j.gene.2021.145963
Jena, A. B., Kanungo, N., Nayak, V., Chainy, G. B. N., and Dandapat, J. (2021). Catechin and Curcumin Interact with S Protein of SARS-CoV2 and ACE2 of Human Cell Membrane: Insights from Computational Studies. Sci. Rep. 11 (1), 2043. doi:10.1038/s41598-021-81462-7
Jin, T., Song, Z., Weng, J., and Fantus, I. G. (2018). Curcumin and Other Dietary Polyphenols: Potential Mechanisms of Metabolic Actions and Therapy for Diabetes and Obesity. Am. J. Physiol. Endocrinol. Metab. 314 (3), E201–E205. doi:10.1152/ajpendo.00285.2017
Junior, N. N., Santos, I. A., Meireles, B. A., Nicolau, M. S. A. P., Lapa, I. R., Aguiar, R. S., et al. (2021). In Silico evaluation of Lapachol Derivatives Binding to the Nsp9 of SARS-CoV-2. J. Biomol. Struct. Dyn., 1–15. doi:10.1080/07391102.2021.1875050
Junqueira, C., Crespo, Â., Ranjbar, S., Lewandrowski, M., Ingber, J., de Lacerda, L. B., et al. (2021). SARS-CoV-2 Infects Blood Monocytes to Activate NLRP3 and AIM2 Inflammasomes, Pyroptosis and Cytokine Release. Res. Sq Rs 3, rs-153628. doi:10.21203/rs.3.rs-153628/v1
Kandeil, A., Mostafa, A., Kutkat, O., Moatasim, Y., Al-Karmalawy, A. A., Rashad, A. A., et al. (2021). Bioactive Polyphenolic Compounds Showing strong Antiviral Activities against Severe Acute Respiratory Syndrome Coronavirus 2. Pathogens 10 (6), 758. doi:10.3390/pathogens10060758
Kaur, H., He, B., Zhang, C., Rodriguez, E., Hage, D. S., and Moreau, R. (2018). Piperine Potentiates Curcumin-Mediated Repression of mTORC1 Signaling in Human Intestinal Epithelial Cells: Implications for the Inhibition of Protein Synthesis and TNFα Signaling. J. Nutr. Biochem. 57, 276–286. doi:10.1016/j.jnutbio.2018.04.010
Khan, A., Khan, M., Saleem, S., Babar, Z., Ali, A., Khan, A. A., et al. (2020). Phylogenetic Analysis and Structural Perspectives of RNA-dependent RNA-Polymerase Inhibition from SARs-CoV-2 with Natural Products. Interdiscip. Sci. 12 (3), 335–348. doi:10.1007/s12539-020-00381-9
Khateeb, D., Gabrieli, T., Sofer, B., Hattar, A., Cordela, S., Chaouat, A., et al. (2022). SARS-CoV-2 Variants with Reduced Infectivity and Varied Sensitivity to the BNT162b2 Vaccine Are Developed during the Course of Infection. Plos Pathog. 18 (1), e1010242. doi:10.1371/journal.ppat.1010242
Kim, B., Kim, H. S., Jung, E. J., Lee, J. Y., K Tsang, B., Lim, J. M., et al. (2016). Curcumin Induces ER Stress-Mediated Apoptosis through Selective Generation of Reactive Oxygen Species in Cervical Cancer Cells. Mol. Carcinog 55 (5), 918–928. doi:10.1002/mc.22332
Kishimoto, A., Imaizumi, A., Wada, H., Yamakage, H., Satoh-Asahara, N., Hashimoto, T., et al. (2021). Newly Developed Highly Bioavailable Curcumin Formulation, curcuRougeTM, Reduces Neutrophil/lymphocyte Ratio in the Elderly: a Double-Blind, Placebo-Controlled Clinical Trial. J. Nutr. Sci. Vitaminol (Tokyo) 67 (4), 249–252. doi:10.3177/jnsv.67.249
Kumar, G., Kumar, D., and Singh, N. P. (2021e). Therapeutic Approach against 2019-nCoV by Inhibition of ACE-2 Receptor. Drug Res. (Stuttg) 71 (4), 213–218. doi:10.1055/a-1275-0228
Kumar, M., Sodhi, K. K., and Singh, D. K. (2021a). Addressing the Potential Role of Curcumin in the Prevention of COVID-19 by Targeting the Nsp9 Replicase Protein through Molecular Docking. Arch. Microbiol. 203 (4), 1691–1696. doi:10.1007/s00203-020-02163-9
Kumar, P., Barua, C. C., Sulakhiya, K., and Sharma, R. K. (2017). Curcumin Ameliorates Cisplatin-Induced Nephrotoxicity and Potentiates its Anticancer Activity in SD Rats: Potential Role of Curcumin in Breast Cancer Chemotherapy. Front. Pharmacol. 8, 132. doi:10.3389/fphar.2017.00132
Kumar, S., Kashyap, P., Chowdhury, S., Kumar, S., Panwar, A., and Kumar, A. (2021d). Identification of Phytochemicals as Potential Therapeutic Agents that Binds to Nsp15 Protein Target of Coronavirus (SARS-CoV-2) that Are Capable of Inhibiting Virus Replication. Phytomedicine 85, 153317. doi:10.1016/j.phymed.2020.153317
Kumar, S., Sharma, P. P., Upadhyay, C., Kempaiah, P., Rathi, B., and Poonam, M. (2021b). Multi-targeting Approach for Nsp3, Nsp9, Nsp12 and Nsp15 Proteins of SARS-CoV-2 by Diosmin as Illustrated by Molecular Docking and Molecular Dynamics Simulation Methodologies. Methods 195, 44–56. doi:10.1016/j.ymeth.2021.02.017
Kumar, S., Gupta, Y., Zak, S. E., Upadhyay, C., Sharma, N., Herbert, A. S., et al. (2021c). A Novel Compound Active against SARS-CoV-2 Targeting Uridylate-specific Endoribonuclease (NendoU/NSP15): In Silico and In Vitro Investigations. RSC Med. Chem. 12 (10), 1757–1764. doi:10.1039/d1md00202c
Kumar, S., Kovalenko, S., Bhardwaj, S., Sethi, A., Gorobets, N. Y., Desenko, S. M., et al. (2022). Drug Repurposing against SARS-CoV-2 Using Computational Approaches. Drug Discov. Today S1359-6446 (22), 00044–00047. doi:10.1016/j.drudis.2022.02.004
Kumar Verma, A., Kumar, V., Singh, S., Goswami, B. C., Camps, I., Sekar, A., et al. (2021). Repurposing Potential of Ayurvedic Medicinal Plants Derived Active Principles against SARS-CoV-2 Associated Target Proteins Revealed by Molecular Docking, Molecular Dynamics and MM-PBSA Studies. Biomed. Pharmacother. 137, 111356. doi:10.1016/j.biopha.2021.111356
Kunnumakkara, A. B., Rana, V., Parama, D., Banik, K., Girisa, S., Henamayee, S., et al. (2021). COVID-19, Cytokines, Inflammation, and Spices: How Are They Related? Life Sci. 284, 119201. doi:10.1016/j.lfs.2021.119201
Laforge, M., Elbim, C., Frère, C., Hémadi, M., Massaad, C., Nuss, P., et al. (2020). Tissue Damage from Neutrophil-Induced Oxidative Stress in COVID-19. Nat. Rev. Immunol. 20 (9), 515–516. doi:10.1038/s41577-020-0407-1
Larasati, Y. A., Yoneda-Kato, N., Nakamae, I., Yokoyama, T., Meiyanto, E., and Kato, J. Y. (2018). Curcumin Targets Multiple Enzymes Involved in the ROS Metabolic Pathway to Suppress Tumor Cell Growth. Sci. Rep. 8 (1), 2039. doi:10.1038/s41598-018-20179-6
Lee, H., Lee, T. Y., Jeon, P., Kim, N., Kim, J. W., Yang, J. S., et al. (2022). J2N-k Hamster Model Simulates Severe Infection Caused by Severe Acute Respiratory Syndrome Coronavirus 2 in Patients with Cardiovascular Diseases. J. Virol. Methods 299, 114306. doi:10.1016/j.jviromet.2021.114306
Li, Q., and Kang, C. (2020). Progress in Developing Inhibitors of SARS-CoV-2 3C-like Protease. Microorganisms 8 (8), 1250. doi:10.3390/microorganisms8081250
Li, R., Wang, Y., Liu, Y., Chen, Q., Fu, W., Wang, H., et al. (2013). Curcumin Inhibits Transforming Growth Factor-Β1-Induced EMT via PPARγ Pathway, Not Smad Pathway in Renal Tubular Epithelial Cells. PLoS One 8 (3), e58848. doi:10.1371/journal.pone.0058848
Li, Z., Shi, M., Li, N., and Xu, R. (2020). Application of Functional Biocompatible Nanomaterials to Improve Curcumin Bioavailability. Front. Chem. 8, 589957. doi:10.3389/fchem.2020.589957
Lin, C. Y., and Yao, C. A. (2020). Potential Role of Nrf2 Activators with Dual Antiviral and Anti-inflammatory Properties in the Management of Viral Pneumonia. Infect. Drug Resist. 13, 1735–1741. doi:10.2147/IDR.S256773
Lin, J., Huo, X., and Liu, X. (2017). "mTOR Signaling Pathway": A Potential Target of Curcumin in the Treatment of Spinal Cord Injury. Biomed. Res. Int. 2017, 1634801. doi:10.1155/2017/1634801
Lin, S.-R., Fu, Y.-S., Tsai, M.-J., Cheng, H., and Weng, C.-F. (2017). Natural Compounds from Herbs that Can Potentially Execute as Autophagy Inducers for Cancer Therapy. Ijms 18 (7), 1412. doi:10.3390/ijms18071412
Lin, S. R., Chang, C. H., Hsu, C. F., Tsai, M. J., Cheng, H., Leong, M. K., et al. (2020a). Natural Compounds as Potential Adjuvants to Cancer Therapy: Preclinical Evidence. Br. J. Pharmacol. 177 (6), 1409–1423. doi:10.1111/bph.14816
Lin, Z., Phyu, W. H., Phyu, Z. H., and Mon, T. Z. (2021). The Role of Steroids in the Management of COVID-19 Infection. Cureus 13 (8), e16841. doi:10.7759/cureus.16841
Littler, D. R., Liu, M., McAuley, J. L., Lowery, S. A., Illing, P. T., Gully, B. S., et al. (2021). A Natural Product Compound Inhibits Coronaviral Replication In Vitro by Binding to the Conserved Nsp9 SARS-CoV-2 Protein. J. Biol. Chem. 297 (6), 101362. doi:10.1016/j.jbc.2021.101362
Liu, Z., and Ying, Y. (2020). The Inhibitory Effect of Curcumin on Virus-Induced Cytokine Storm and its Potential Use in the Associated Severe Pneumonia. Front Cel Dev Biol 8, 479. doi:10.3389/fcell.2020.00479
Ma, W., Yang, J., Fu, H., Su, C., Yu, C., Wang, Q., et al. (2022). Genomic Perspectives on the Emerging SARS-CoV-2 Omicron Variant. Genomics, Proteomics & Bioinformatics S1672-0229 (22), 00002–X. doi:10.1016/j.gpb.2022.01.001
Mahmud, S., Uddin, M. A. R., Paul, G. K., Shimu, M. S. S., Islam, S., Rahman, E., et al. (2021b). Virtual Screening and Molecular Dynamics Simulation Study of Plant-Derived Compounds to Identify Potential Inhibitors of Main Protease from SARS-CoV-2. Brief Bioinform 22 (2), 1402–1414. doi:10.1093/bib/bbaa428
Mahmud, S., Elfiky, A. A., Amin, A., Mohanto, S. C., Rahman, E., Acharjee, U. K., et al. (2021a). Targeting SARS-CoV-2 Nonstructural Protein 15 Endoribonuclease: an In Silico Perspective. Future Virol. 16, 467–474. doi:10.2217/fvl-2020-0233
Makiyama, K., Hazawa, M., Kobayashi, A., Lim, K., Voon, D. C., and Wong, R. W. (2022). NSP9 of SARS-CoV-2 Attenuates Nuclear Transport by Hampering Nucleoporin 62 Dynamics and Functions in Host Cells. Biochem. Biophys. Res. Commun. 586, 137–142. doi:10.1016/j.bbrc.2021.11.046
Marín-Palma, D., Tabares-Guevara, J. H., Zapata-Cardona, M. I., Flórez-Álvarez, L., Yepes, L. M., Rugeles, M. T., et al. (2021). Curcumin Inhibits In Vitro SARS-CoV-2 Infection in Vero E6 Cells through Multiple Antiviral Mechanisms. Molecules 26 (22), 6900. doi:10.3390/molecules26226900
Marquardt, J. U., Gomez-Quiroz, L., Arreguin Camacho, L. O., Pinna, F., Lee, Y. H., Kitade, M., et al. (2015). Curcumin Effectively Inhibits Oncogenic NF-Κb Signaling and Restrains Stemness Features in Liver Cancer. J. Hepatol. 63 (3), 661–669. doi:10.1016/j.jhep.2015.04.018
Mashayekhi-Sardoo, H., Mashayekhi-Sardoo, A., Roufogalis, B. D., Jamialahmadi, T., and Sahebkar, A. (2021). Impact of Curcumin on Microsomal Enzyme Activities: Drug Interaction and Chemopreventive Studies. Curr. Med. Chem. 28 (34), 7122–7140. doi:10.2174/0929867328666210329123449
Maurya, V. K., Kumar, S., Prasad, A. K., Bhatt, M. L. B., and Saxena, S. K. (2020). Structure-based Drug Designing for Potential Antiviral Activity of Selected Natural Products from Ayurveda against SARS-CoV-2 Spike Glycoprotein and its Cellular Receptor. Virusdisease 31 (2), 179–193. doi:10.1007/s13337-020-00598-8
Miryan, M., Bagherniya, M., Sahebkar, A., Soleimani, D., Rouhani, M. H., Iraj, B., et al. (2020). Effects of Curcumin-Piperine Co-supplementation on Clinical Signs, Duration, Severity, and Inflammatory Factors in Patients with COVID-19: a Structured Summary of a Study Protocol for a Randomised Controlled Trial. Trials 21 (1), 1027. doi:10.1186/s13063-020-04924-9
Miryan, M., Soleimani, D., Askari, G., Jamialahmadi, T., Guest, P. C., Bagherniya, M., et al. (2021). Curcumin and Piperine in COVID-19: a Promising Duo to the rescue? Adv. Exp. Med. Biol. 1327, 197–204. doi:10.1007/978-3-030-71697-4_16
Mohajeri, M., Bianconi, V., Ávila-Rodriguez, M. F., Barreto, G. E., Jamialahmadi, T., Pirro, M., et al. (2020). Curcumin: a Phytochemical Modulator of Estrogens and Androgens in Tumors of the Reproductive System. Pharmacol. Res. 156, 104765. doi:10.1016/j.phrs.2020.104765
Mortezaee, K., Salehi, E., Mirtavoos-Mahyari, H., Motevaseli, E., Najafi, M., Farhood, B., et al. (2019). Mechanisms of Apoptosis Modulation by Curcumin: Implications for Cancer Therapy. J. Cel Physiol 234 (8), 12537–12550. doi:10.1002/jcp.28122
Mulu, A., Gajaa, M., Woldekidan, H. B., and W/Mariam, J. F. (2021). The Impact of Curcumin Derived Polyphenols on the Structure and Flexibility COVID-19 Main Protease Binding Pocket: a Molecular Dynamics Simulation Study. PeerJ 9, e11590. doi:10.7717/peerj.11590
Nabeel-Shah, S., Lee, H., Ahmed, N., Burke, G. L., Farhangmehr, S., Ashraf, K., et al. (2022). SARS-CoV-2 Nucleocapsid Protein Binds Host mRNAs and Attenuates Stress Granules to Impair Host Stress Response. iScience 25 (1), 103562. doi:10.1016/j.isci.2021.103562
Nag, A., Paul, S., Banerjee, R., and Kundu, R. (2021). In Silico study of Some Selective Phytochemicals against a Hypothetical SARS-CoV-2 Spike RBD Using Molecular Docking Tools. Comput. Biol. Med. 137, 104818. doi:10.1016/j.compbiomed.2021.104818
Naito, Y., Takagi, T., and Higashimura, Y. (2014). Heme Oxygenase-1 and Anti-inflammatory M2 Macrophages. Arch. Biochem. Biophys. 564, 83–88. doi:10.1016/j.abb.2014.09.005
Nelson, K. M., Dahlin, J. L., Bisson, J., Graham, J., Pauli, G. F., and Walters, M. A. (2017). The Essential Medicinal Chemistry of Curcumin. J. Med. Chem. 60, 1620–1637. doi:10.1021/acs.jmedchem.6b00975
Niu, Y., Ke, D., Yang, Q., Wang, X., Chen, Z., An, X., et al. (2012). Temperature-dependent Stability and DPPH Scavenging Activity of Liposomal Curcumin at pH 7.0. Food Chem. 135 (3), 1377–1382. doi:10.1016/j.foodchem.2012.06.018
Noor, H., Ikram, A., Rathinavel, T., Kumarasamy, S., Nasir Iqbal, M., and Bashir, Z. (2021). Immunomodulatory and Anti-cytokine Therapeutic Potential of Curcumin and its Derivatives for Treating COVID-19 - a Computational Modeling. J. Biomol. Struct. Dyn., 1–16. doi:10.1080/07391102.2021.1873190
Norooznezhad, F., Rodriguez-Merchan, E. C., Asadi, S., and Norooznezhad, A. H. (2020). Curcumin: Hopeful Treatment of Hemophilic Arthropathy via Inhibition of Inflammation and Angiogenesis. Expert Rev. Hematol. 13 (1), 5–11. doi:10.1080/17474086.2020.1685867
Oh, S. J., and Shin, O. S. (2021). SARS-CoV-2 Nucleocapsid Protein Targets RIG-I-like Receptor Pathways to Inhibit the Induction of Interferon Response. Cells 10 (3), 530. doi:10.3390/cells10030530
Omosa, L. K., Midiwo, J. O., and Kuete, V. (2017). “Curcuma Longa,” in Medicinal Spices and Vegetables from Africa, Chapter 19: Curcuma Longa. Editor V. Kuete (Academic Press), 425–435. 978-0-12-809286-6. doi:10.1016/b978-0-12-809286-6.00019-4
Oso, B. J., Adeoye, A. O., and Olaoye, I. F. (2022). Pharmacoinformatics and Hypothetical Studies on Allicin, Curcumin, and Gingerol as Potential Candidates against COVID-19-Associated Proteases. J. Biomol. Struct. Dyn. 40 (1), 389–400. doi:10.1080/07391102.2020.1813630
Pan, P., Shen, M., Yu, Z., Ge, W., Chen, K., Tian, M., et al. (2021). SARS-CoV-2 N Protein Promotes NLRP3 Inflammasome Activation to Induce Hyperinflammation. Nat. Commun. 12 (1), 4664. doi:10.1038/s41467-021-25015-6
Panahi, Y., Alishiri, G. H., Parvin, S., and Sahebkar, A. (2016a). Mitigation of Systemic Oxidative Stress by Curcuminoids in Osteoarthritis: Results of a Randomized Controlled Trial. J. Diet. Suppl. 13 (2), 209–220. doi:10.3109/19390211.2015.1008611
Panahi, Y., Badeli, R., Karami, G. R., and Sahebkar, A. (2015a). Investigation of the Efficacy of Adjunctive Therapy with Bioavailability-Boosted Curcuminoids in Major Depressive Disorder. Phytother Res. 29 (1), 17–21. doi:10.1002/ptr.5211
Panahi, Y., Ghanei, M., Hajhashemi, A., and Sahebkar, A. (2016b). Effects of Curcuminoids-Piperine Combination on Systemic Oxidative Stress, Clinical Symptoms and Quality of Life in Subjects with Chronic Pulmonary Complications Due to Sulfur Mustard: a Randomized Controlled Trial. J. Diet. Suppl. 13 (1), 93–105. doi:10.3109/19390211.2014.952865
Panahi, Y., Hosseini, M. S., Khalili, N., Naimi, E., Majeed, M., and Sahebkar, A. (2015b). Antioxidant and Anti-inflammatory Effects of Curcuminoid-Piperine Combination in Subjects with Metabolic Syndrome: a Randomized Controlled Trial and an Updated Meta-Analysis. Clin. Nutr. 34 (6), 1101–1108. doi:10.1016/j.clnu.2014.12.019
Panahi, Y., Khalili, N., Sahebi, E., Namazi, S., Simental-Mendía, L. E., Majeed, M., et al. (2018). Effects of Curcuminoids Plus Piperine on Glycemic, Hepatic and Inflammatory Biomarkers in Patients with Type 2 Diabetes Mellitus: a Randomized Double-Blind Placebo-Controlled Trial. Drug Res. (Stuttg) 68 (7), 403–409. doi:10.1055/s-0044-101752
Pawar, K. S., Mastud, R. N., Pawar, S. K., Pawar, S. S., Bhoite, R. R., Bhoite, R. R., et al. (2021). Oral Curcumin with Piperine as Adjuvant Therapy for the Treatment of COVID-19: a Randomized Clinical Trial. Front. Pharmacol. 12, 669362. doi:10.3389/fphar.2021.669362
Peng, Q., Peng, R., Yuan, B., Zhao, J., Wang, M., Wang, X., et al. (2020). Structural and Biochemical Characterization of the Nsp12-Nsp7-Nsp8 Core Polymerase Complex from SARS-CoV-2. Cell Rep 31 (11), 107774. doi:10.1016/j.celrep.2020.107774
Peng, S., Li, Z., Zou, L., Liu, W., Liu, C., and McClements, D. J. (2018). Improving Curcumin Solubility and Bioavailability by Encapsulation in Saponin-Coated Curcumin Nanoparticles Prepared Using a Simple pH-Driven Loading Method. Food Funct. 9 (3), 1829–1839. doi:10.1039/c7fo01814b
Pourhajibagher, M., Azimi, M., Haddadi-Asl, V., Ahmadi, H., Gholamzad, M., Ghorbanpour, S., et al. (2021). Robust Antimicrobial Photodynamic Therapy with Curcumin-Poly (Lactic-co-glycolic Acid) Nanoparticles against COVID-19: A Preliminary In Vitro Study in Vero Cell Line as a Model. Photodiagnosis Photodyn Ther. 34, 102286. doi:10.1016/j.pdpdt.2021.102286
Praditya, D., Kirchhoff, L., Brüning, J., Rachmawati, H., Steinmann, J., and Steinmann, E. (2019). Anti-infective Properties of the golden Spice Curcumin. Front. Microbiol. 10, 912. doi:10.3389/fmicb.2019.00912
Prieto-Fernández, E., Egia-Mendikute, L., Vila-Vecilla, L., Bosch, A., Barreira-Manrique, A., Lee, S. Y., et al. (2021). Hypoxia Reduces Cell Attachment of SARS-CoV-2 Spike Protein by Modulating the Expression of ACE2, Neuropilin-1, Syndecan-1 and Cellular Heparan Sulfate. Emerging Microbes & Infections 10 (1), 1065–1076. doi:10.1080/22221751.2021.1932607
Priyadarsini, K. I. (2014). The Chemistry of Curcumin: from Extraction to Therapeutic Agent. Molecules 19, 20091–20112. doi:10.3390/molecules191220091
Quimque, M. T. J., Notarte, K. I. R., Fernandez, R. A. T., Mendoza, M. A. O., Liman, R. A. D., Lim, J. A. K., et al. (2021). Virtual Screening-Driven Drug Discovery of SARS-CoV2 Enzyme Inhibitors Targeting Viral Attachment, Replication, post-translational Modification and Host Immunity Evasion Infection Mechanisms. J. Biomol. Struct. Dyn. 39 (12), 4316–4333. doi:10.1080/07391102.2020.1776639
Quiñonez-Flores, C. M., González-Chávez, S. A., Del Río Nájera, D., and Pacheco-Tena, C. (2016). Oxidative Stress Relevance in the Pathogenesis of the Rheumatoid Arthritis: A Systematic Review. Biomed. Res. Int. 2016, 1–14. doi:10.1155/2016/6097417
Quispe, C., Cruz-Martins, N., Manca, M. L., Manconi, M., Sytar, O., Hudz, N., et al. (2021). Nano-derived Therapeutic Formulations with Curcumin in Inflammation-Related Diseases. Oxid Med. Cel Longev 2021, 3149223. doi:10.1155/2021/3149223
Rahimnia, A. R., Panahi, Y., Alishiri, G., Sharafi, M., and Sahebkar, A. (2015). Impact of Supplementation with Curcuminoids on Systemic Inflammation in Patients with Knee Osteoarthritis: Findings from a Randomized Double-Blind Placebo-Controlled Trial. Drug Res. (Stuttg) 65 (10), 521–525. doi:10.1055/s-0034-1384536
Rai, P. K., Mueed, Z., Chowdhury, A., Deval, R., Kumar, D., Kamal, M. A., et al. (2022). Current Overviews on COVID-19 Management Strategies. Cpb 23, 361–387. doi:10.2174/1389201022666210509022313
Rajasingh, J., Raikwar, H. P., Muthian, G., Johnson, C., and Bright, J. J. (2006). Curcumin Induces Growth-Arrest and Apoptosis in Association with the Inhibition of Constitutively Active JAK-STAT Pathway in T Cell Leukemia. Biochem. Biophys. Res. Commun. 340 (2), 359–368. doi:10.1016/j.bbrc.2005.12.014
Rath, S. L., and Kumar, K. (2020). Investigation of the Effect of Temperature on the Structure of SARS-CoV-2 Spike Protein by Molecular Dynamics Simulations. Front. Mol. Biosci. 7, 583523. doi:10.3389/fmolb.2020.583523
Rattis, B. A. C., Ramos, S. G., and Celes, M. R. N. (2021). Curcumin as a Potential Treatment for COVID-19. Front. Pharmacol. 12, 675287. doi:10.3389/fphar.2021.675287
Riyaphan, J., Jhong, C.-H., Lin, S.-R., Chang, C.-H., Tsai, M.-J., Lee, D.-N., et al. (2018). Hypoglycemic Efficacy of Docking Selected Natural Compounds against α-Glucosidase and α-Amylase. Molecules 23 (9), 2260. doi:10.3390/molecules23092260
Roy, S., Priyadarshi, R., Ezati, P., and Rhim, J. W. (2022). Curcumin and its Uses in Active and Smart Food Packaging Applications - a Comprehensive Review. Food Chem. 375, 131885. doi:10.1016/j.foodchem.2021.131885
Ruan, Z., Liu, C., Guo, Y., He, Z., Huang, X., Jia, X., et al. (2021). SARS-CoV-2 and SARS-CoV: Virtual Screening of Potential Inhibitors Targeting RNA-dependent RNA Polymerase Activity (NSP12). J. Med. Virol. 93 (1), 389–400. doi:10.1002/jmv.26222
Saad, N., and Moussa, S. (2021). Immune Response to COVID-19 Infection: a Double-Edged Sword. Immunol. Med. 44 (3), 187–196. doi:10.1080/25785826.2020.1870305
Saber‐Moghaddam, N., Salari, S., Hejazi, S., Amini, M., Taherzadeh, Z., Eslami, S., et al. (2021). Oral Nano‐curcumin Formulation Efficacy in Management of Mild to Moderate Hospitalized Coronavirus Disease ‐19 Patients: An Open Label Nonrandomized Clinical Trial. Phytotherapy Res. 35, 2616–2623. doi:10.1002/ptr.7004
Saeedi-Boroujeni, A., Mahmoudian-Sani, M. R., Bahadoram, M., and Alghasi, A. (2021). COVID-19: a Case for Inhibiting NLRP3 Inflammasome, Suppression of Inflammation with Curcumin? Basic Clin. Pharmacol. Toxicol. 128 (1), 37–45. doi:10.1111/bcpt.13503
Saheb Sharif-Askari, N., Saheb Sharif-Askari, F., Mdkhana, B., Hussain Alsayed, H. A., Alsafar, H., Alrais, Z. F., et al. (2021). Upregulation of Oxidative Stress Gene Markers during SARS-COV-2 Viral Infection. Free Radic. Biol. Med. 172, 688–698. doi:10.1016/j.freeradbiomed.2021.06.018
Sahebkar, A. (2010). Molecular Mechanisms for Curcumin Benefits against Ischemic Injury. Fertil. Steril 94 (5), e75–e76. doi:10.1016/j.fertnstert.2010.07.1071
Salehi, B., Stojanović-Radić, Z., Matejić, J., Sharifi-Rad, M., Anil Kumar, N. V., Martins, N., et al. (2019). The Therapeutic Potential of Curcumin: A Review of Clinical Trials. Eur. J. Med. Chem. 163, 527–545. doi:10.1016/j.ejmech.2018.12.016
Sandur, S. K., Pandey, M. K., Sung, B., Ahn, K. S., Murakami, A., Sethi, G., et al. (2007). Curcumin, Demethoxycurcumin, Bisdemethoxycurcumin, Tetrahydrocurcumin and Turmerones Differentially Regulate Anti-inflammatory and Anti-proliferative Responses through a ROS-independent Mechanism. Carcinogenesis 28 (8), 1765–1773. doi:10.1093/carcin/bgm123
Seldeslachts, L., Cawthorne, C., Kaptein, S. F., Boudewijns, R., Thibaut, H. J., Sanchez Felipe, L., et al. (2022). Use of Micro-computed Tomography to Visualize and Quantify COVID-19 Vaccine Efficiency in Free-Breathing Hamsters. Methods Mol. Biol. 2410, 177–192. doi:10.1007/978-1-0716-1884-4_8
Servellita, V., Morris, M. K., Sotomayor-Gonzalez, A., Gliwa, A. S., Torres, E., Brazer, N., et al. (2022). Predominance of Antibody-Resistant SARS-CoV-2 Variants in Vaccine Breakthrough Cases from the San Francisco Bay Area, California. Nat. Microbiol. 7, 277–288. doi:10.1038/s41564-021-01041-4
Shakeri, F., Soukhtanloo, M., and Boskabady, M. H. (2017). The Effect of Hydro-Ethanolic Extract of Curcuma Longa Rhizome and Curcumin on Total and Differential WBC and Serum Oxidant, Antioxidant Biomarkers in Rat Model of Asthma. Iran J. Basic Med. Sci. 20 (2), 155–165. doi:10.22038/ijbms.2017.8241
Sharma, A. K., Kapoor, V. K., and Kaur, G. (2022). Herb-drug Interactions: a Mechanistic Approach. Drug Chem. Toxicol. 45 (2), 594–603. doi:10.1080/01480545.2020.1738454
Shi, M., Wang, L., and Fontana, P. (2020). SARS-CoV-2 Nsp1 Suppresses Host but Not Viral Translation through a Bipartite Mechanism. bioRxiv 2020, 302901. doi:10.1101/2020.09.18.302901
Shoba, G., Joy, D., Joseph, T., Majeed, M., Rajendran, R., and Srinivas, P. S. (1998). Influence of Piperine on the Pharmacokinetics of Curcumin in Animals and Human Volunteers. Planta Med. 64 (4), 353–356. doi:10.1055/s-2006-957450
Singh, N., Decroly, E., Khatib, A. M., and Villoutreix, B. O. (2020). Structure-based Drug Repositioning over the Human TMPRSS2 Protease Domain: Search for Chemical Probes Able to Repress SARS-CoV-2 Spike Protein Cleavages. Eur. J. Pharm. Sci. 153, 105495. doi:10.1016/j.ejps.2020.105495
Singh, R., Bhardwaj, V. K., and Purohit, R. (2021). Potential of Turmeric-Derived Compounds against RNA-dependent RNA Polymerase of SARS-CoV-2: An In-Silico Approach. Comput. Biol. Med. 139, 104965. doi:10.1016/j.compbiomed.2021.104965
Sohn, S. Y., Hearing, J., Mugavero, J., Kirillov, V., Gorbunova, E., Helminiak, L., et al. (2021). Interferon-lambda Intranasal protection and Differential Sex Pathology in a Murine Model of SARS-CoV-2 Infection. mBio 12 (6), e0275621. doi:10.1128/mBio.02756-21
Soltani, A., Salmaninejad, A., Jalili-Nik, M., Soleimani, A., Javid, H., Hashemy, S. I., et al. (2019). 5'-Adenosine Monophosphate-Activated Protein Kinase: A Potential Target for Disease Prevention by Curcumin. J. Cel Physiol 234 (3), 2241–2251. doi:10.1002/jcp.27192
Sordillo, P. P., and Helson, L. (2015). Curcumin Suppression of Cytokine Release and Cytokine Storm. A Potential Therapy for Patients with Ebola and Other Severe Viral Infections. In Vivo 29 (1), 1–4.
Srivastava, A., Gupta, R. C., Doss, R. B., and Lall, R. (2021). Trace Minerals, Vitamins and Nutraceuticals in Prevention and Treatment of COVID-19. J. Dietary Supplements, 1–35. doi:10.1080/19390211.2021.1890662
Sudeep, H. V., Gouthamchandra, K., and Shyamprasad, K. (2020). Molecular Docking Analysis of Withaferin A from Withania Somnifera with the Glucose Regulated Protein 78 (GRP78) Receptor and the SARS-CoV-2 Main Protease. Bioinformation 16 (5), 411–417. doi:10.6026/97320630016411
Sunagawa, Y., Miyazaki, Y., Funamoto, M., Shimizu, K., Shimizu, S., Nurmila, S., et al. (2021). A Novel Amorphous Preparation Improved Curcumin Bioavailability in Healthy Volunteers: a Single-Dose, Double-Blind, Two-Way Crossover Study. J. Funct. Foods 81, 104443. doi:10.1016/j.jff.2021.104443
Tabanelli, R., Brogi, S., and Calderone, V. (2021). Improving Curcumin Bioavailability: Current Strategies and Future Perspectives. Pharmaceutics 13 (10), 1715. doi:10.3390/pharmaceutics13101715
Tahmasebi, S., El-Esawi, M. A., Mahmoud, Z. H., Timoshin, A., Valizadeh, H., Roshangar, L., et al. (2021a). Immunomodulatory Effects of Nanocurcumin on Th17 Cell Responses in Mild and Severe COVID-19 Patients. J. Cel Physiol 236 (7), 5325–5338. doi:10.1002/jcp.30233
Tahmasebi, S., Saeed, B. Q., Temirgalieva, E., Yumashev, A. V., El-Esawi, M. A., Navashenaq, J. G., et al. (2021b). Nanocurcumin Improves Treg Cell Responses in Patients with Mild and Severe SARS-CoV2. Life Sci. 276, 119437. doi:10.1016/j.lfs.2021.119437
Takeda, M. (2021). Proteolytic Activation of SARS‐CoV‐2 Spike Protein. Microbiol. Immunol. 66, 15–23. doi:10.1111/1348-0421.12945
Tan, X., Kim, G., Lee, D., Oh, J., Kim, M., Piao, C., et al. (2018). A Curcumin-Loaded Polymeric Micelle as a Carrier of a microRNA-21 Antisense-Oligonucleotide for Enhanced Anti-tumor Effects in a Glioblastoma Animal Model. Biomater. Sci. 6 (2), 407–417. doi:10.1039/c7bm01088e
Tay, J. H., Porter, A. F., Wirth, W., and Duchene, S. (2022). The Emergence of SARS-CoV-2 Variants of Concern Is Driven by Acceleration of the Substitution Rate. Mol. Biol. Evol. Msac013 39. doi:10.1093/molbev/msac013
Teli, D. M., Shah, M. B., and Chhabria, M. T. (2020). In Silico screening of Natural Compounds as Potential Inhibitors of SARS-CoV-2 Main Protease and Spike RBD: Targets for COVID-19. Front. Mol. Biosci. 7, 599079. doi:10.3389/fmolb.2020.599079
Thacker, P. C., and Karunagaran, D. (2015). Curcumin and Emodin Down-Regulate TGF-β Signaling Pathway in Human Cervical Cancer Cells. PLoS One 10 (3), e0120045. doi:10.1371/journal.pone.0120045
Thimmulappa, R. K., Mudnakudu-Nagaraju, K. K., Shivamallu, C., Subramaniam, K. J. T., Radhakrishnan, A., Bhojraj, S., et al. (2021). Antiviral and Immunomodulatory Activity of Curcumin: a Case for Prophylactic Therapy for COVID-19. Heliyon 7 (2), e06350. doi:10.1016/j.heliyon.2021.e06350
Thiyagarajan, V., Lee, K. W., Leong, M. K., and Weng, C. F. (2018). Potential Natural mTOR Inhibitors Screened by In Silico Approach and Suppress Hepatic Stellate Cells Activation. J. Biomol. Struct. Dyn. 36 (16), 4220–4234. doi:10.1080/07391102.2017.1411295
Thiyagarajan, V., Lin, S. H., Chia, Y. C., and Weng, C. F. (2013). A Novel Inhibitor, 16-Hydroxy-Cleroda-3,13-Dien-16,15-Olide, Blocks the Autophosphorylation Site of Focal Adhesion Kinase (Y397) by Molecular Docking. Biochim. Biophys. Acta 1830 (8), 4091–4101. doi:10.1016/j.bbagen.2013.04.027
Tran, P. H. L., Duan, W., Lee, B. J., and Tran, T. T. D. (2019). Modulation of Drug Crystallization and Molecular Interactions by Additives in Solid Dispersions for Improving Drug Bioavailability. Curr. Pharm. Des. 25 (18), 2099–2107. doi:10.2174/1381612825666190618102717
Trigueiro-Louro, J., Correia, V., Figueiredo-Nunes, I., Gíria, M., and Rebelo-de-Andrade, H. (2020). Unlocking COVID Therapeutic Targets: a Structure-Based Rationale against SARS-CoV-2, SARS-CoV and MERS-CoV Spike. Comput. Struct. Biotechnol. J. 18, 2117–2131. doi:10.1016/j.csbj.2020.07.017
Tripathy, S., Verma, D. K., Thakur, M., Patel, A. R., Srivastav, P. P., Singh, S., et al. (2021). Curcumin Extraction, Isolation, Quantification and its Application in Functional Foods: A Review with a Focus on Immune Enhancement Activities and COVID-19. Front. Nutr. 8, 747956. doi:10.3389/fnut.2021.747956
Tsuda, T. (2018). Curcumin as a Functional Food-Derived Factor: Degradation Products, Metabolites, Bioactivity, and Future Perspectives. Food Funct. 9 (2), 705–714. doi:10.1039/c7fo01242j
Umadevi, P., Manivannan, S., Fayad, A. M., and Shelvy, S. (2020). In Silico analysis of Phytochemicals as Potential Inhibitors of Proteases Involved in SARS-CoV-2 Infection. J. Biomol. Struct. Dyn. 29, 1–9. doi:10.1080/07391102.2020.1866669
Umashankar, V., Deshpande, S. H., Hegde, H. V., Singh, I., and Chattopadhyay, D. (2021). Phytochemical Moieties from Indian Traditional Medicine for Targeting Dual Hotspots on SARS-CoV-2 Spike Protein: an Integrative In-Silico Approach. Front. Med. (Lausanne) 8, 672629. doi:10.3389/fmed.2021.672629
Valizadeh, H., Abdolmohammadi-Vahid, S., Danshina, S., Ziya Gencer, M., Ammari, A., Sadeghi, A., et al. (2020). Nano-curcumin Therapy, a Promising Method in Modulating Inflammatory Cytokines in COVID-19 Patients. Int. Immunopharmacol 89 (Pt B), 107088. doi:10.1016/j.intimp.2020.107088
Veeramachaneni, G. K., Thunuguntla, V. B. S. C., Bobbillapati, J., and Bondili, J. S. (2021). Structural and Simulation Analysis of Hotspot Residues Interactions of SARS-CoV 2 with Human ACE2 Receptor. J. Biomol. Struct. Dyn. 39 (11), 4015–4025. doi:10.1080/07391102.2020.1773318
Velmurugan, B. K., Rathinasamy, B., Lohanathan, B. P., Thiyagarajan, V., and Weng, C. F. (2018). Neuroprotective Role of Phytochemicals. Molecules 23 (10), 2485. doi:10.3390/molecules23102485
Walls, A. C., Park, Y. J., Tortorici, M. A., Wall, A., McGuire, A. T., and Veesler, D. (2020). Structure, Function, and Antigenicity of the SARS-CoV-2 Spike Glycoprotein. Cell 181 (2), 281–e6. doi:10.1016/j.cell.2020.02.058
Wan, S., Sun, Y., Qi, X., and Tan, F. (2012). Improved Bioavailability of Poorly Water-Soluble Drug Curcumin in Cellulose Acetate Solid Dispersion. AAPS PharmSciTech 13 (1), 159–166. doi:10.1208/s12249-011-9732-9
Wang, C., Song, X., Shang, M., Zou, W., Zhang, M., Wei, H., et al. (2019). Curcumin Exerts Cytotoxicity Dependent on Reactive Oxygen Species Accumulation in Non-small-cell Lung Cancer Cells. Future Oncol. 15 (11), 1243–1253. doi:10.2217/fon-2018-0708
Wang, J., Kang, Y.-X., Pan, W., Lei, W., Feng, B., and Wang, X.-J. (2016). Enhancement of Anti-inflammatory Activity of Curcumin Using Phosphatidylserine-Containing Nanoparticles in Cultured Macrophages. Ijms 17 (5), 969. doi:10.3390/ijms17060969
Wang, M., Zhang, L., Li, Q., Wang, B., Liang, Z., Sun, Y., et al. (2022). Reduced Sensitivity of the SARS-CoV-2 Lambda Variant to Monoclonal Antibodies and Neutralizing Antibodies Induced by Infection and Vaccination. Emerg. Microbes Infect. 11 (1), 18–29. doi:10.1080/22221751.2021.2008775
WHO (2022). WHO Coronavirus (COVID-19) Dashboard. Availableat: https://covid19.who.int (Accessed Jan 21, 2022).
Wiersinga, W. J., Rhodes, A., Cheng, A. C., Peacock, S. J., and Prescott, H. C. (2020). Pathophysiology, Transmission, Diagnosis, and Treatment of Coronavirus Disease 2019 (COVID-19): a Review. JAMA 324 (8), 782–793. doi:10.1001/jama.2020.12839
Xu, X. Y., Meng, X., Li, S., Gan, R. Y., Li, Y., and Li, H. B. (2018). Bioactivity, Health Benefits, and Related Molecular Mechanisms of Curcumin: Current Progress, Challenges, and Perspectives. Nutrients 10 (10), 1553. doi:10.3390/nu10101553
Xu, Y., and Liu, L. (2017). Curcumin Alleviates Macrophage Activation and Lung Inflammation Induced by Influenza Virus Infection through Inhibiting the NF-Κb Signaling Pathway. Influenza Other Respir. Viruses 11 (5), 457–463. doi:10.1111/irv.12459
Yan, R., Zhang, Y., Li, Y., Xia, L., Guo, Y., and Zhou, Q. (2020). Structural Basis for the Recognition of SARS-CoV-2 by Full-Length Human ACE2. Science 367 (6485), 1444–1448. doi:10.1126/science.abb2762
Yang, C., Zhang, X., Fan, H., and Liu, Y. (2009). Curcumin Upregulates Transcription Factor Nrf2, HO-1 Expression and Protects Rat Brains against Focal Ischemia. Brain Res. 1282, 133–141. doi:10.1016/j.brainres.2009.05.009
Yarmohammadi, F., Hayes, A. W., and Karimi, G. (2021). Protective Effects of Curcumin on Chemical and Drug-Induced Cardiotoxicity: a Review. Naunyn Schmiedebergs Arch. Pharmacol. 394 (7), 1341–1353. doi:10.1007/s00210-021-02072-8
Yin, H., Guo, Q., Li, X., Tang, T., Li, C., Wang, H., et al. (2018). Curcumin Suppresses IL-1β Secretion and Prevents Inflammation through Inhibition of the NLRP3 Inflammasome. J.I. 200 (8), 2835–2846. doi:10.4049/jimmunol.1701495
Yin, Y., Wu, X., Peng, B., Zou, H., Li, S., Wang, J., et al. (2020). Curcumin Improves Necrotising Microscopic Colitis and Cell Pyroptosis by Activating SIRT1/NRF2 and Inhibiting the TLR4 Signalling Pathway in Newborn Rats. Innate Immun. 26 (7), 609–617. doi:10.1177/1753425920933656
Yousefian, M., Shakour, N., Hosseinzadeh, H., Hayes, A. W., Hadizadeh, F., and Karimi, G. (2019). The Natural Phenolic Compounds as Modulators of NADPH Oxidases in Hypertension. Phytomedicine 55, 200–213. doi:10.1016/j.phymed.2018.08.002
Zahedipour, F., Hosseini, S. A., Sathyapalan, T., Majeed, M., Jamialahmadi, T., Al-Rasadi, K., et al. (2020). Potential Effects of Curcumin in the Treatment of COVID-19 Infection. Phytother Res. 34 (11), 2911–2920. doi:10.1002/ptr.6738
Zarubin, A., Stepanov, V., Markov, A., Kolesnikov, N., Marusin, A., Khitrinskaya, I., et al. (2020). Structural Variability, Expression Profile, and Pharmacogenetic Properties of TMPRSS2 Gene as a Potential Target for COVID-19 Therapy. Genes 12 (1), 19. doi:10.3390/genes12010019
Zhai, X., Qiao, H., Guan, W., Li, Z., Cheng, Y., Jia, X., et al. (2015). Curcumin Regulates Peroxisome Proliferator-Activated Receptor-γ Coactivator-1α Expression by AMPK Pathway in Hepatic Stellate Cells In Vitro. Eur. J. Pharmacol. 746, 56–62. doi:10.1016/j.ejphar.2014.10.055
Zhang, C., Chen, Y., Li, L., Yang, Y., He, J., Chen, C., et al. (2020). Structural Basis for the Multimerization of Nonstructural Protein Nsp9 from SARS-CoV-2. Mol. Biomed. 1 (1), 5. doi:10.1186/s43556-020-00005-0
Zhang, M., Zhang, X., Tian, T., Zhang, Q., Wen, Y., Zhu, J., et al. (2022). Anti-inflammatory Activity of Curcumin-Loaded Tetrahedral Framework Nucleic Acids on Acute Gouty Arthritis. Bioact Mater. 8, 368–380. doi:10.1016/j.bioactmat.2021.06.003
Zhao, J., Sun, L., Zhao, Y., Feng, D., Cheng, J., and Zhang, G. (2021). Coronavirus Endoribonuclease Ensures Efficient Viral Replication and Prevents Protein Kinase R Activation. J. Virol. 95 (7), 02103–02120. doi:10.1128/JVI.02103-20
Zhao, M., Ma, J., Li, M., Zhang, Y., Jiang, B., Zhao, X., et al. (2021a). Cytochrome P450 Enzymes and Drug Metabolism in Humans. Int. J. Mol. Sci. 22 (23), 12808. doi:10.3390/ijms222312808
Zhao, N., Di, B., and Xu, L. L. (2021b). The NLRP3 Inflammasome and COVID-19: Activation, Pathogenesis and Therapeutic Strategies. Cytokine Growth Factor. Rev. 61, 2–15. doi:10.1016/j.cytogfr.2021.06.002
Zrieq, R., Ahmad, I., Snoussi, M., Noumi, E., Iriti, M., Algahtani, F. D., et al. (2021). Tomatidine and Patchouli Alcohol as Inhibitors of SARS-CoV-2 Enzymes (3CLpro, PLpro and NSP15) by Molecular Docking and Molecular Dynamics Simulations. Int. J. Mol. Sci. 22 (19), 10693. doi:10.3390/ijms221910693
Keywords: curcumin/curcuminoids, COVID-19, immunomodulation, nutraceuticals, inflammation, antioxidant, chemosensitizing, oxidative stress
Citation: Fu Y-S, Ho W-Y, Kang N, Tsai M-J, Wu J, Huang L and Weng C-F (2022) Pharmaceutical Prospects of Curcuminoids for the Remedy of COVID-19: Truth or Myth. Front. Pharmacol. 13:863082. doi: 10.3389/fphar.2022.863082
Received: 26 January 2022; Accepted: 01 March 2022;
Published: 14 April 2022.
Edited by:
Zheng Zeng, Peking University First Hospital, ChinaReviewed by:
Lihua Song, Beijing University of Chemical Technology, ChinaDuygu Agagündüz, Gazi University, Turkey
Copyright © 2022 Fu, Ho, Kang, Tsai, Wu, Huang and Weng. This is an open-access article distributed under the terms of the Creative Commons Attribution License (CC BY). The use, distribution or reproduction in other forums is permitted, provided the original author(s) and the copyright owner(s) are credited and that the original publication in this journal is cited, in accordance with accepted academic practice. No use, distribution or reproduction is permitted which does not comply with these terms.
*Correspondence: Ching-Feng Weng, cfwengcf@gmail.com, cfweng-cfweng@hotmail.com