- 1Medical Experiment Center, Jiading Branch of Shanghai General Hospital, Shanghai Jiao Tong University School of Medicine, Shanghai, China
- 2Department of Traditional Chinese Medicine, Jiading Branch of Shanghai General Hospital, Shanghai Jiao Tong University School of Medicine, Shanghai, China
- 3Department of Gastroenterology, Longhua Hospital, Shanghai University of Traditional Chinese Medicine, Shanghai, China
- 4Department of Clinical Laboratory, Jiading Branch of Shanghai General Hospital, Shanghai Jiao Tong University School of Medicine, Shanghai, China
- 5Department of Clinical Laboratory and Central Laboratory, Putuo Hospital, Shanghai University of Traditional Chinese Medicine, Shanghai, China
- 6Department of Medical Oncology, Shuguang Hospital, Shanghai University of Traditional Chinese Medicine, Shanghai, China
Methods: Articles on inflammatory tumor microenvironment were retrieved from the Web of Science Core Collection, and the characteristics of the articles were analyzed by CiteSpace software.
Background: The inflammatory tumor microenvironment is an essential feature of the tumor microenvironment. The way in which it promotes or inhibits tumor progression plays an important role in the outcome of a tumor treatment. This research aims to explore a scientific collaboration network, describe evolution of hotspots, and predict future trends through bibliometric analysis.
Results: A total of 3,534 papers published by 390 institutions in 81 countries/regions were screened, and the annual quantity has been increasing rapidly in the past decades. United States was the leading country and has the most productive institutions in this field. The research topics were mainly focused on inflammation and immunity mediated by crucial factors as well as the mechanisms of angiogenesis. Additionally, the development and application of nanoparticles is currently a novel research frontier with bright prospect.
Conclusion: The present scientometric study provides an overview of inflammatory tumor microenvironment research over the previous decades using quantitative and qualitative methods, and the findings of this study can provide references for researchers focusing on tumor treatment.
Introduction
Tumors are a major threat to human health and significantly reduce life expectancy globally (Bray et al., 2021). Tumor occurrence is a complex biological process characterized by several factors, such as inflammation, gene mutation, and immune abnormality (Hanahan and Weinberg, 2011). Previous studies report that inflammation modulates multiple pathological signal networks by affecting the interaction of various cells and molecules in the microenvironment, thus showing a high degree of duality. Inflammation promotes survival during an infection or tissue injury and maintains basic homeostasis under various noxious conditions. This implies that inflammation results in abnormalities in the structure or function of tissues, ultimately promoting the occurrence of diseases, including tumors (Medzhitov, 2010; Nathan and Ding, 2010; Schulz et al., 2019; Olén et al., 2020; Charokopos et al., 2021).
The tumor microenvironment (TME) represents an ecological niche comprising tumor cells, vascular system, extracellular matrix (ECM), tumor-associated immune cells, and signaling molecules (Balkwill et al., 2012). The TME is characterized by hypoxia, acidosis, chronic inflammation, and suppression of immune function (Pérez-Romero et al., 2020). The inflammatory tumor microenvironment is an essential component of the TME and plays a key role in all stages of cancer development, including tumorigenesis, progression, invasion, and metastasis. The formation of the inflammatory tumor microenvironment is caused by external factors, like tobacco (Giotopoulou and Stathopoulos, 2020), alcohol (LoConte et al., 2018), and obesity (Iyengar et al., 2016) as well as other factors, such as gut microbiota (Yang et al., 2021) and exercise (Hojman et al., 2018). Several mechanisms, such as the production of cytokines (Atretkhany et al., 2016), pro-inflammatory mediators (Alspach et al., 2019; Laha et al., 2021), angiogenesis (Zhou et al., 2021), and tissue remodeling (Okayasu et al., 2019), associated with the inflammatory tumor microenvironment have been reported (Comen et al., 2018). However, the main progresses and insights of this field have not been effectively identified and comprehensively explored.
Bibliometric analysis is an important tool for exploring the dynamics of a particular discipline by assessing the research history, turning points, and most prominent research trends. It is an important method for the systematic review of literature and comprises specialized data analysis software. CiteSpace is a scientometric software tool designed for drawing collaborative network maps as well as the analysis of the distribution of co-cited references based on bibliographic databases (Chen, 2017). CiteSpace can intuitively identify the key points and particular trends in the current knowledge compared with other data visualization software (Fan et al., 2018). Knowledge maps generated by CiteSpace are used to visualize the connections between complex silos of information and accurately capture and display disparate pieces of research (Chen, 2017). In recent years, it has been used to explore research progress in several medical fields, such as Alzheimer’s disease (Li et al., 2021), gastrointestinal microbiome (Huang et al., 2019; Yuan et al., 2021), telemedicine (Waqas et al., 2020), and pyroptosis (Ma et al., 2021). The aim of the present study was to explore the scientific collaboration network and research trends on inflammatory tumor microenvironment using Citespace5.7R5W software.
Materials and Methods
Data Sources
A comprehensive search of the Web of Science core collection (SCI-E and SSCI) was performed to retrieve articles published in all languages. The following search strategy was used: TS = (“inflammatory tumor microenvironment”) OR {[TS = (inflammation*)] AND TS = (“tumor microenvironment”)}. Studies published from the inception of the database to 2021 were retrieved. Only research articles and reviews were retrieved. The basic information of these publications, including titles, abstracts, authors, affiliations, keywords, and references, were recorded on November 14, 2021. All valid data were imported to CiteSpace5.7R5W and deduplicated for subsequent visual analysis.
Software Description and Settings
In the knowledge maps generated by CiteSpace, every node represented an entity, and its size was proportional to the frequency. In addition, the link between two nodes represented the relationship between the entities. Different time periods were presented as different colors, from cool (purple) to warm (red), corresponding to the period from the older years (1998) to the recent year (2021). The purple rings outside some nodes referred to high centrality (mainly >0.1) and indicated the outstanding role of the nodes in the corresponding knowledge maps. The silhouette value varied from −1 to 1 and represented the homogeneity of the articles in each cluster. A silhouette value greater than 0.7 indicates that the corresponding cluster is meaningful and credible (Chen, 2006). The analysis of co-citations in CiteSpace can reflect the intellectual landscape of a specific academic field, and the keywords with strong citation bursts indicate emerging research hotspots. CiteSpace was used in the present scientometric study to generate the knowledge maps of countries/regions, authors, keywords, institutions, co-citations, clusters of co-citations, and the timeline view of clusters based on co-citations. Moreover, it was used to detect keywords with strong citation bursts. The details on CiteSpace settings were as follows: link retaining factor (=3), look back years (=5), e for top N (e = 1), time span (1998–2021), years per slice (3), links (strength: cosine, scope: within slices), selection criteria (g-index, k = 25), and pruning (pathfinder-pruning sliced networks). Microsoft Office Excel 2021 was used to manage the data and to generate annual distribution charts based on the number of publications.
Results
Temporal Distribution of the Literature
Relevant literature published every year can reflect the scale and trend of a specific research field. In the current study, a total of 3,534 publications, including 2,316 articles and 1,218 reviews, were retrieved, with no duplicates, and the oldest paper was published in 1998. The publications appeared sporadically before 2006, and the highest number of publications per year was 12 (Figure 1). This indicates that research on inflammatory tumor microenvironment was in its infancy. Since 2007, the number of papers in this field significantly increased and reached a peak in 2020 with 570 publications, which account for 16% of the total number of publications (publications published in 2021 were not fully included). Moreover, the annual citation has significantly increased in the last 10 years, with approximately 10,194.4 times compared with 281.9 times in the earliest 10 years. These findings indicate a surge of interest in the field of inflammatory tumor microenvironment which may be due to in-depth studies of inflammatory factors in the TME.
Scientific Co-operation Network Analysis
The 3,534 publications on inflammatory tumor microenvironment research published from 1998 to 2021 were conducted by 390 institutions in 81 countries/regions. The co-occurrence knowledge map of country/region with a density of 0.0623 comprised 81 nodes and 202 edges. The nodes with frequency of over 30 were labeled (Figure 2). The most significant number of studies were conducted in the United States (1,358, 28.90%) and China (766,16.30%), which accounted for approximately half of the total number of articles (Table 1). In addition, other productive countries showing light purple rings which represent active participation in the cooperation among countries included England, Germany, Italy, France, and India. China, Japan, and South Korea are representatives of Asian countries which actively conducted relevant research on inflammatory tumor microenvironment. However, the academic collaborations initiated by them are not as close and frequent as those between European and American countries.
The co-occurrence knowledge map based on institutions whose number of publications was above 25 exhibited 390 nodes and 628 edges, and the density was 0.0083 (Figure 3). It is apparent that the connections between institutions were relatively close, and the distribution of nodes was comparatively uniform. The most productive research institutions were the University of Texas MD Anderson Cancer Center and National Cancer Institute (69, 1.98% respectively), followed by the University of Pittsburgh (66, 1.90%), German Cancer Research Center (57, 1.64%), and Sun Yat-sen University (52, 1.49%) (Table 1). Johns Hopkins University showed the highest centrality, indicating that it had the most active academic collaborations among institutions. Notably, the top 12 institutions were all from the three countries with the highest number of publications (7 came from the United States, 4 were from China, and 1 was from Germany).
The co-occurrence knowledge map based on authors with more than five publications comprised 400 nodes and 644 edges, and the density was 0.0081 (Figure 4). The results showed 4 major collaborating teams that actively participated in the research on inflammatory tumor microenvironment. However, most of the researchers were relatively scattered and lacked stable and intensive collaborations. The four teams shown in different colors appeared at different periods, and the team led by Michael P. Lisanti from England started the earliest collaboration in 2011, whereas Viktor Umansky from Germany started the collaborations in 2011–2018. Jing Wang and Gordon J. Freeman from the United States mediated the close collaborations of the two subgroups (2016–2020). Alberto Mantovani from Italy was the most productive author with 17 papers and led the team with the longest active time span (2008–2018) for collaborations.
Co-citation Analysis
A total of 1,184 papers and 1,522 journals were co-cited by the 3,534 publications on inflammatory tumor microenvironment. The co-occurrence and cluster knowledge map of co-citation was generated, and the references which were co-cited more than 50 times or exhibited purple rings were labeled (Figure 5). The nodes shown in cool and warm colors basically indicated papers cited before and after 2017, and the number of highly cited publications and the overall citation frequency were higher after 2017. Notably, the 4 nodes of references with purple rings, indicating the most frequent and prominent connections with other cited papers, were published by Craig Murdoch, Gregory L. Beatty, Marie Vétizou, and Laura Strauss. The largest 17 clusters based on the co-occurrence of co-citations were obtained, and all silhouette values were greater than 0.7. The 17 clusters were generally divided into three aspects based on log-likelihood ratio, namely: the crucial components or mechanisms in the inflammatory tumor microenvironment (1–4, 6, 8, 9, 10, 13, and 17), condition of tumor (0, 11, and 14), and widely researched tumor types (5 and 7). The timeline view of clusters showed the timepoint of appearance and corresponding duration of clusters more intuitively (Figure 6). After 2004, the number of clusters of co-citations had increased significantly, and the subjects became more detailed and specific, indicating that the scope of research on inflammatory tumor microenvironment became wider and diverse.
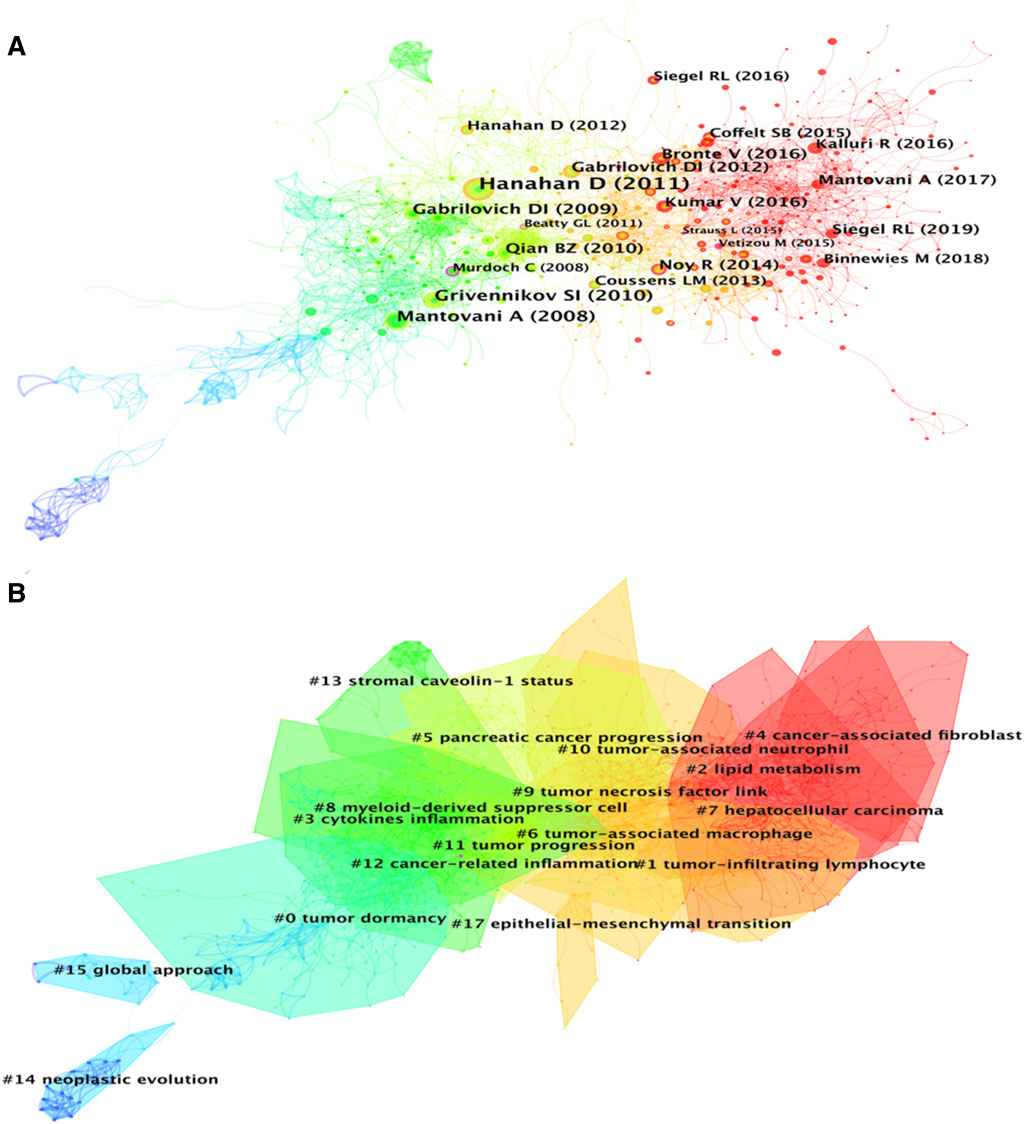
FIGURE 5. (A) Co-citation co-occurrence knowledge map of the inflammatory tumor microenvironment. (B) Co-citation clustering knowledge map of the inflammatory tumor microenvironment.
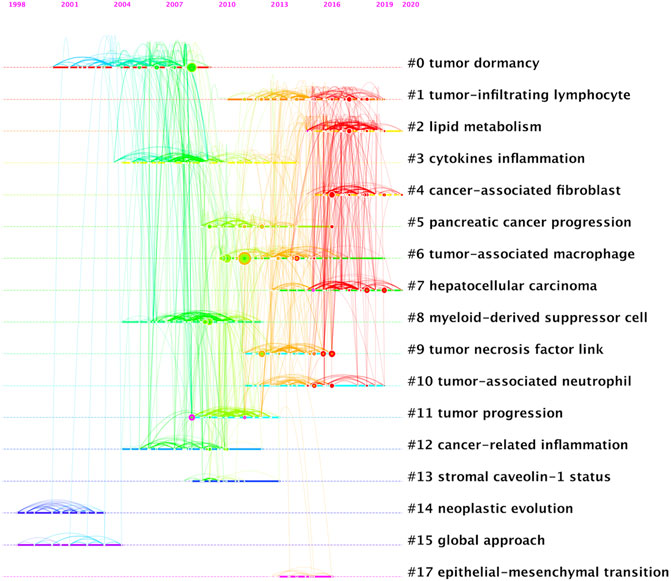
FIGURE 6. Timeline view of co-citation clustering knowledge map of the inflammatory tumor microenvironment.
Highly co-cited publications represent the fundamental theories, key turning points, or significant findings of a specific field. In this study, the basic information of the top 10 co-cited publications are listed in Table 2, and the most highly co-cited publication with 250 times was published in 2011. This paper systematically explored the characteristics of tumors, including sustaining proliferative signaling, evading growth suppressors, resisting cell death, enabling replicative immortality, inducing angiogenesis, activating invasion and metastasis, reprogramming of energy metabolism, and evading immune destruction (Hanahan and Weinberg, 2011). The 2nd and 4th highest co-cited publications, with 135 and 123 citations, respectively, provide details on the relationship between inflammation and tumors from different perspectives (Mantovani et al., 2008; Grivennikov et al., 2010). Notably, 5 of the top 10 co-cited references focused on the role of tumor-associated macrophages (TAMs) or myeloid-derived suppressor cells (MDSCs) in the inflammatory tumor microenvironment, indicating that the synergistic tumor-promoting relationship between immune factors and inflammatory factors has been widely and deeply explored by researchers (Gabrilovich and Nagaraj, 2009; Qian and Pollard, 2010; Gabrilovich et al., 2012; Noy and Pollard, 2014; Bronte et al., 2016). Additionally, 2 of the top 10 co-cited references were epidemiological survey reports on tumors conducted globally and in the United States (Bray et al., 2018; Siegel et al., 2019). This implies that the data and changes in tumor epidemiology are also one of the key areas that researchers pay attention to.
The analysis of the sources of academic literature helps in discovering high-impact journals in a certain research field. The results of the present study showed that most highly cited publications on inflammatory tumor microenvironment were published in top journals, such as Nature, Cell, and Cancer Research. Cancer Research (2,889) was the journal with the highest number of citations, followed by Nature (2,540), Proceedings of the National Academy of Sciences of the United States of America (2,358), Cell (2,319), and Clinical Cancer Research (2,163). Notably, eight journals with more than 2,000 citations belonged to Q1, 4 journals were focused on oncology, and the scope of three journals was multidisciplinary sciences.
Keywords and Citation Bursts
A keyword co-occurrence knowledge map was also generated (Figure 7). Keywords with frequency above 100 were labeled, and the keywords with the top 25 strongest bursts were identified (Figure 8). The keyword co-occurrence knowledge map comprised 726 nodes and 430 edges, and the density was 0.0042. The highest landmark nodes, such as suppressor cell, cancer, regulatory T cell, NF kappa B (nuclear factor-κB, NF-κB), angiogenesis, macrophage, and dendritic cell, represented the crucial components of the inflammatory tumor microenvironment. The turning points with higher centrality included inflammation, cancer cell, carcinoma, inhibition, gene, melanoma, and differentiation, indicating that they had a high number of connections with other keywords in the domain.
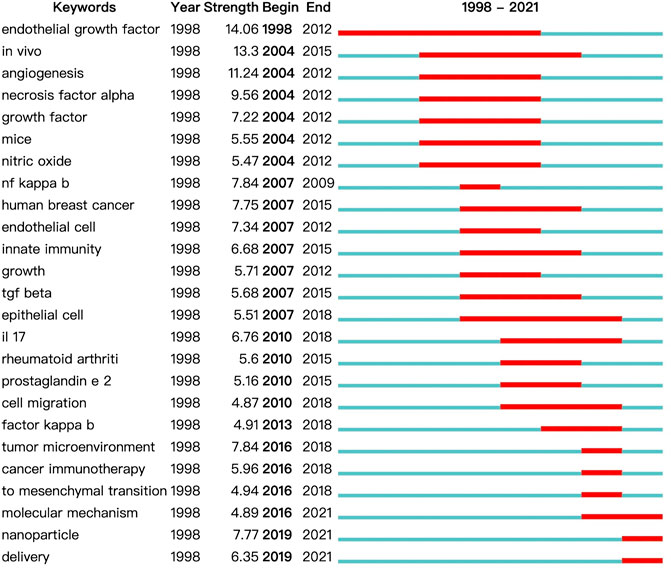
FIGURE 8. Top 25 keywords with the strongest citation bursts on inflammatory tumor microenvironment.
A map based on the top 25 keywords with the strongest citation bursts was generated, in which the blue part indicated the time interval, and the red part indicated the duration of citation burst, thus showing the shift of research hotspots over time. The noticeable keywords in the early stage (1998–2006) included endothelial growth factor (generally refers to vascular endothelial growth factor, VEGF), in vivo, angiogenesis, necrosis factor alpha (generally refers to TNF-α), growth factor, mice, and nitric oxide. Moreover, innate immunity, including the release of NF-κB, transforming growth factor β (TGF-β), interleukin 17 (IL-17), and prostaglandin e 2 (PGE2), and cell migration were the main areas of focus in the middle stage (2007–2015). In recent years (2016–2021), tumor microenvironment, cancer immunotherapy, mesenchymal transition (generally refers to epithelial–mesenchymal transition, EMT), molecular mechanism, nanoparticle, and delivery were the main research hotspots.
Discussion
Inflammatory factors are crucial components in the TME and play a vital role in the occurrence and development of tumors. However, the mechanisms underlying the role of inflammatory factors in tumors have not been fully elucidated. In the present study, the visualization software CiteSpace was used to analyze different aspects of articles or reviews on inflammatory tumor microenvironment. A significant increase in the number of publications in the past 15 years indicate that researchers have paid more attention to the important role of the inflammatory tumor microenvironment than ever before and are actively exploring its role and application prospects in tumor prevention and treatment.
The Research Entities of the Inflammatory Tumor Microenvironment
Academic collaborations initiated by Asian countries represented by China and Japan are relatively less compared with those initiated by European and American countries represented by the United States and Germany. This difference may be attributed to the fact that Asian countries participated in this research relatively later compared with other Western countries. Therefore, it is imperative for these countries to conduct international academic exchanges to achieve novel research breakthroughs. United States and China are the world’s largest developed and developing countries in the world, respectively, and the two countries published a higher number of papers compared with other productive countries. Notably, the gap in the number of publications between productive institutions is not as large as that between productive countries.
The findings showed that the 4 most active academic communities in the field of inflammatory tumor microenvironment were from European and American countries. Furthermore, the main research directions and academic achievements of these countries were highly representative and influential. The team led by Michael P. Lisanti mainly focused on integrating autophagy and metabolism in the TME (Martinez-Outschoorn et al., 2011a). This team proposed the concept of “the autophagic tumor stroma model of cancer metabolism”, also known as “reverse Warburg effect”, that indicates that stromal catabolism promotes the anabolic growth of tumor cells by modulating autophagy and mitophagy and eventually promoting tumor progression and metastasis (Pavlides et al., 2010; Martinez-Outschoorn et al., 2011a; Martinez-Outschoorn et al., 2011b). The team led by Viktor Umansky mainly conducted research on myeloid-derived suppressor cells (MDSCs). The crucial role of MDSCs in the TME was explored in these studies (Umansky et al., 2014; Umansky et al., 2016). In addition, the team explored new mechanisms underlying the role of MDSCs (Umansky et al., 2017; Schröder et al., 2018) and the mechanisms regulated by drugs, such as metformin (Qin et al., 2018) and paclitaxel (Sevko et al., 2013). Alberto Mantovani conducted studies on inflammatory factors, such as TAMs (Galdiero et al., 2013; Bonavita et al., 2015), which are polypeptide messengers of inflammation in the TME (Campesato et al., 2017; Galdiero et al., 2018). The two teams under Jing Wang and Gordon J. Freeman mainly focused on the mechanisms underlying the functions of inflammatory factors in lung cancer—for instance, the findings showed that STK11/LKB1 deficiency promotes the recruitment of neutrophil and increases the release of proinflammatory cytokine to the lung tumor microenvironment (Koyama et al., 2016). Moreover, they report that epithelial signal transducer and activator of transcription 3 (STAT3) signaling plays a key sex-specific role in K-ras mutant lung adenocarcinomas (Caetano et al., 2018).
The general status of research on inflammatory tumor microenvironment inferred by combining the directions of influential authors led to themes of top co-cited references (including MDSCs and TAMs), labels of co-citation clusters [including tumor infiltrating lymphocytes, cancer-associated fibroblasts (CAFs), TAMs, and MDSCs], high-frequency keywords (including IL-6, NF-κB, and TNF-α), and the top keywords with high citation bursts (including VEGF, angiogenesis, TNF-α, NF-κβ, IL-17, innate immunity, nanoparticle, and delivery). These areas indicate the influence of inflammatory factors on immunity and the mechanisms of angiogenesis. Moreover, it is also prompted that studies on nanoparticles (NPs) have significantly increased in the recent past.
Immunity Effect of Inflammatory Tumor Microenvironment
Chronic inflammation and immune disorders are important features of the TME which may be mediated simultaneously by several factors in the TME, although the composition of the TME differs based on cell types and tissues. Studies on the molecular functions of these crucial factors within the TME may provide effective avenues for alleviating tumor progression and provide a basis for the development of therapies targeting the TME. NF-κB is a transcription factor implicated in the pathogenesis of autoimmune diseases, chronic inflammation, and various types of tumors. The canonical NF-κB pathway is activated by multiple signals, such as cytokine receptors, Toll-like receptors as well as T and B cell receptors (Liu et al., 2017). The uncontrolled activation of NF-κB in the TME is mainly induced by the degradation of its inhibitors, such as the inhibitors of NF–κB (IκB) proteins (Taniguchi and Karin, 2018). The pro-tumorigenic and immunosuppressive functions of NF-κβ are associated with various components in the TME, including TAMs, MDSCs, CAFs, TNF, and IL-6 (Grivennikov et al., 2010; Grivennikov and Karin, 2011; Barnabei et al., 2021). TAM is one of the most well-studied myeloid cells in the TME and exhibits M1-like characteristics, such as initiating type I inflammatory responses in the tumor formation stage and M2-like phenotype that inhibits inflammation and the remodeling of ECM in the tumor progression stage (Martinez and Gordon, 2014). The studies report that inflammatory response in tumors is like an unresolved wound healing response. In addition, the diverse roles (such as promoting tumor cell migration and invasion) that TAMs plays in the TME are similar to the roles played by macrophages under physiological conditions (Schäfer and Werner, 2008; Okabe and Medzhitov, 2016). In addition to molecular mechanisms, various studies have explored therapies targeting TAMs. The accumulation of TAMs through recruitment can be blocked by inhibiting CCR2 or CXCR4 (Kioi et al., 2010; Qian et al., 2011). MDSCs are immature myeloid cells, and the CD11b+Ly6G-Ly6Chi subtype has significant immunosuppressive properties (Cassetta et al., 2019). M-MDSCs accumulate in the tumor site under the influence of CCL2, CCL5, CXCL8, and CXCL12, resulting in long-term immunosuppression and interference of the balance of the body. Furthermore, M-MDSCs promote the progression of chronic inflammation through various mechanisms, such as inducing the secretion of high levels of immunosuppressive cytokines (such as nitric oxide, TGF-β, and IL-10), upregulating the expression of PD-L1 and CTLA-4 receptors, activating NF-κB and STAT3 as well as promoting the production of regulatory T cells (Tregs) (Gabrilovich et al., 2012; Shrihari, 2017; Li et al., 2020). Inducing the benign differentiation of MDSCs (into antigen-presenting cells and interferon-γ+ T cells) is a novel strategy for tumor treatment (Lin et al., 2017; Choi et al., 2019). TGF-β is a potent pleiotropic polypeptide cytokine that regulates cell growth, differentiation, and immune function in tissues and systemic processes, such as chronic inflammation and tumor progression. Cancer cells exhibit intact TGF-β-mediated cellular response, such as the promotion of Treg differentiation, thus strengthening immunosuppression within the TME and ultimately promoting tumor progression while evading the growth-inhibitory effects of TGF-β (Drabsch and ten Dijke, 2012; Kubiczkova et al., 2012; Massagué, 2012; Gallo-Oller et al., 2020). IL-17 is the main effector of Th17 and is stimulated by TGF-β which helps to promote the differentiation of Th17 (Su et al., 2010). The overall pro-tumor or anti-tumor impact of IL-17 varies with the specific microenvironment background and mainly depends on other cellular sources of cytokine, such as neutrophils, γδ T cells, and natural killer cells (Maniati et al., 2010).
Angiogenesis in the Inflammatory Tumor Microenvironment
Angiogenesis is the formation of new blood vessels and regulated by several molecules. Despite the beneficial effect of angiogenesis on tissue growth and regeneration, it plays a major role in tumor growth and metastasis. The specific mechanisms of angiogenesis have been widely explored by researchers in the field of TME. Hypoxia is a key characteristic of the TME. It is the main factor that induces tumor angiogenesis because tumor cells, under a hypoxic environment, can secrete VEGF-A, which combines with VEGF-receptor (VEGFR) 2 to promote the formation of micro-vessels (LaGory and Giaccia, 2016). Endothelial cells (ECs) are important components of the vascular barrier and are transformed from quiescent to motile status through the induction of soluble VEGF-A. This change promotes cracking of the surrounding ECM and the formation of new vessels (Treps et al., 2016). Several pro-inflammatory cytokines in the TME play key roles in promoting angiogenesis—for instance, IL-22 plays its role by inducing EC proliferation, survival, and chemotaxis (Choi et al., 2019), whereas IL-9 activates the STAT3 pathway (He et al., 2019). One of the major pathways involved in angiogenesis is the VEGF/VEGFR axis comprising various ligands and receptors (Ferrara et al., 2003). The activation of VEGF/VEGFR triggers multiple signaling networks that promote EC survival, migration, and differentiation, which are dependent on angiogenesis (Jakobsson et al., 2010; Krueger et al., 2011), and increases microvascular permeability, leading to tumor metastasis (Harney et al., 2015). VEGF/VEGFR, ECs, and EMT play key roles in angiogenesis. Thus, their potential in the development of anti-angiogenesis therapy has been widely explored, and several therapies are under clinical use (Carmeliet and Jain, 2011; Wang et al., 2015; Treps et al., 2016).
Transport Carriers Regulating the Inflammatory Tumor Microenvironment
Transport carriers, such as proteins and carbohydrates, play an essential role in the metabolism and activities of various cells and subcellular organelles as well as the interactions between them. The formation and function of the inflammatory tumor microenvironment are likewise closely related to the involvement of transport carriers. As novel delivery carriers for several therapeutic agents, NPs have been in the spotlight as effective tools to improve tumor treatment outcomes in recent years. NPs are specifically used to circumvent several limitations, including the reduction of the concentration of the drug reaching the tumor site (Chopdey et al., 2015) and multidrug resistance induced by low-dose drug stimulation (Thakkar et al., 2020) in clinical tumor treatments. NPs can be divided into organic and inorganic according to the difference in composition and structure. Organic NPs, such as liposomes and micelles, are characterized by high drug delivery efficiency and less toxicity, while inorganic NPs, such as gold NPs and magnetic NPs, can reach specific sites of action (Ashfaq et al., 2017; Tang et al., 2021). Various NPs have been engineered individually to target various components in the TME, including CAFs and TAMs, owing to the role of the TME in tumor initiation, progression, metastasis, and therapeutic response (Zhu et al., 2013; Miao and Huang, 2015; Ji et al., 2016) as well as to physiological conditions, such as acidic and hypoxic state (Crayton and Tsourkas, 2011; Yang et al., 2019). In addition to targeting the TME, advanced NPs can be used to reinforce T-cell function, owing to their high effective cellular uptake, and controlled drug release (Wang et al., 2021; Zuo et al., 2021). Despite challenges, such as safety and clinical translation, the application of nanotechnology represented by nanoparticles in tumor therapy is still very promising and worthy of further exploration by researchers.
Limitations
To our knowledge, this study is the first attempt to use bibliometric methods to explore the research status of the inflammatory tumor microenvironment. While achieving meaningful and visual findings, this paper also has some limitations: first, only publications from the Web of Science Core Collection database were retrieved due to the format requirement of CiteSpace software; second, the relevant publication for 2021 was not fully included because the database was incomplete at the time the data was retrieved.
Conclusion
The present study was a systematic analysis of the inflammatory tumor microenvironment using bibliometric method. With the help of information visualization, we were able to identify research foci and overall trends in the field and offer gathered information to future researchers. Research on inflammatory tumor microenvironment has significantly increased in the past 2 decades. In addition, numerous studies would continue to contribute to the reinforcement of the theoretical basis of inflammation targeting.
Data Availability Statement
The original contributions presented in the study are included in the article/supplementary material, further inquiries can be directed to the corresponding author.
Author Contributions
YZ, LH, and ZW contributed to writing, conception, figure, and revision. QT and HS contributed to writing and revising the draft.
Funding
This work was supported by the National Natural Science Foundation of China (grant numbers 81973808 and 82174459), the Science Foundation for Shanghai Committee of Science Project (grant numbers 21S21901400, 19411972400, 19401972000 and 19ZR1447800), the Clinical Research Plan of SHDC (grant number SHDC2020CR4047), the Shanghai Municipal Health Commission (grant number 202140198) and the Natural Science Research Foundation of Jiading District (grant numbers JDKW-2021-0023 and JDKW-2021-0029).
Conflict of Interest
The authors declare that the research was conducted in the absence of any commercial or financial relationships that could be construed as a potential conflict of interest.
Publisher’s Note
All claims expressed in this article are solely those of the authors and do not necessarily represent those of their affiliated organizations or those of the publisher, the editors, and the reviewers. Any product that may be evaluated in this article or claim that may be made by its manufacturer is not guaranteed or endorsed by the publisher.
References
Alspach, E., Lussier, D. M., and Schreiber, R. D. (2019). Interferon γ and its Important Roles in Promoting and Inhibiting Spontaneous and Therapeutic Cancer Immunity. Cold Spring Harb Perspect. Biol. 11, a028480. doi:10.1101/cshperspect.a028480
Ashfaq, U. A., Riaz, M., Yasmeen, E., and Yousaf, M. Z. (2017). Recent Advances in Nanoparticle-Based Targeted Drug-Delivery Systems against Cancer and Role of Tumor Microenvironment. Crit. Rev. Ther. Drug Carrier Syst. 34, 317–353. doi:10.1615/CritRevTherDrugCarrierSyst.2017017845
Atretkhany, K. N., Drutskaya, M. S., Nedospasov, S. A., Grivennikov, S. I., and Kuprash, D. V. (2016). Chemokines, Cytokines and Exosomes Help Tumors to Shape Inflammatory Microenvironment. Pharmacol. Ther. 168, 98–112. doi:10.1016/j.pharmthera.2016.09.011
Balkwill, F. R., Capasso, M., and Hagemann, T. (2012). The Tumor Microenvironment at a Glance. J. Cel Sci 125, 5591–5596. doi:10.1242/jcs.116392
Barnabei, L., Laplantine, E., Mbongo, W., Rieux-Laucat, F., and Weil, R. (2021). NF-κB: At the Borders of Autoimmunity and Inflammation. Front. Immunol. 12, 716469. doi:10.3389/fimmu.2021.716469
Bonavita, E., Galdiero, M. R., Jaillon, S., and Mantovani, A. (2015). Phagocytes as Corrupted Policemen in Cancer-Related Inflammation. Adv. Cancer Res. 128, 141–171. doi:10.1016/bs.acr.2015.04.013
Bray, F., Ferlay, J., Soerjomataram, I., Siegel, R. L., Torre, L. A., and Jemal, A. (2018). Global Cancer Statistics 2018: GLOBOCAN Estimates of Incidence and Mortality Worldwide for 36 Cancers in 185 Countries. CA Cancer J. Clin. 68, 394–424. doi:10.3322/caac.21492
Bray, F., Laversanne, M., Weiderpass, E., and Soerjomataram, I. (2021). The Ever-Increasing Importance of Cancer as a Leading Cause of Premature Death Worldwide. Cancer 127, 3029–3030. doi:10.1002/cncr.33587
Bronte, V., Brandau, S., Chen, S. H., Colombo, M. P., Frey, A. B., Greten, T. F., et al. (2016). Recommendations for Myeloid-Derived Suppressor Cell Nomenclature and Characterization Standards. Nat. Commun. 7, 12150. doi:10.1038/ncomms12150
Caetano, M. S., Hassane, M., Van, H. T., Bugarin, E., Cumpian, A. M., McDowell, C. L., et al. (2018). Sex Specific Function of Epithelial STAT3 Signaling in Pathogenesis of K-Ras Mutant Lung Cancer. Nat. Commun. 9, 4589. doi:10.1038/s41467-018-07042-y
Campesato, L. F., Silva, A. P. M., Cordeiro, L., Correa, B. R., Navarro, F. C. P., Zanin, R. F., et al. (2017). High IL-1R8 Expression in Breast Tumors Promotes Tumor Growth and Contributes to Impaired Antitumor Immunity. Oncotarget 8, 49470–49483. doi:10.18632/oncotarget.17713
Carmeliet, P., and Jain, R. K. (2011). Molecular Mechanisms and Clinical Applications of Angiogenesis. Nature 473, 298–307. doi:10.1038/nature10144
Cassetta, L., Baekkevold, E. S., Brandau, S., Bujko, A., Cassatella, M. A., Dorhoi, A., et al. (2019). Deciphering Myeloid-Derived Suppressor Cells: Isolation and Markers in Humans, Mice and Non-human Primates. Cancer Immunol. Immunother. 68, 687–697. doi:10.1007/s00262-019-02302-2
Charokopos, A., Braman, S. S., Brown, S. A. W., Mhango, G., de-Torres, J. P., Zulueta, J. J., et al. (2021). Lung Cancer Risk Among Patients with Asthma-Chronic Obstructive Pulmonary Disease Overlap. Ann. ATS 18, 1894–1900. doi:10.1513/AnnalsATS.202010-1280OC
Chen, C. (2006). CiteSpace II: Detecting and Visualizing Emerging Trends and Transient Patterns in Scientific Literature. J. Am. Soc. Inf. Sci. 57, 359–377. doi:10.1002/asi.20317
Chen, C. (2017). Science Mapping: A Systematic Review of the Literature. J. Data Inf. Sci. 2, 1–40. doi:10.1515/jdis-2017-0006
Choi, J. N., Sun, E. G., and Cho, S. H. (2019). IL-12 Enhances Immune Response by Modulation of Myeloid Derived Suppressor Cells in Tumor Microenvironment. Chonnam Med. J. 55, 31–39. doi:10.4068/cmj.2019.55.1.31
Chopdey, P. K., Tekade, R. K., Mehra, N. K., Mody, N., and Jain, N. K. (2015). Glycyrrhizin Conjugated Dendrimer and Multi-Walled Carbon Nanotubes for Liver Specific Delivery of Doxorubicin. J. Nanosci Nanotechnol 15, 1088–1100. doi:10.1166/jnn.2015.9039
Comen, E. A., Bowman, R. L., and Kleppe, M. (2018). Underlying Causes and Therapeutic Targeting of the Inflammatory Tumor Microenvironment. Front Cel Dev Biol 6, 56. doi:10.3389/fcell.2018.00056
Crayton, S. H., and Tsourkas, A. (2011). pH-Titratable Superparamagnetic Iron Oxide for Improved Nanoparticle Accumulation in Acidic Tumor Microenvironments. Acs Nano 5, 9592–9601. doi:10.1021/nn202863x
Drabsch, Y., and ten Dijke, P. (2012). TGF-β Signalling and its Role in Cancer Progression and Metastasis. Cancer Metastasis Rev. 31, 553–568. doi:10.1007/s10555-012-9375-7
Fan, Y., Yu, G., Yu, J., Sun, J., Wu, Y., Zhao, X., et al. (2018). Research Trends and Hotspots Analysis Related to the Effects of Xenobiotics on Glucose Metabolism in Male Testes. Int. J. Environ. Res. Public Health 15, 1590. doi:10.3390/ijerph15081590
Ferrara, N., Gerber, H. P., and LeCouter, J. (2003). The Biology of VEGF and its Receptors. Nat. Med. 9, 669–676. doi:10.1038/nm0603-669
Gabrilovich, D. I., and Nagaraj, S. (2009). Myeloid-derived Suppressor Cells as Regulators of the Immune System. Nat. Rev. Immunol. 9, 162–174. doi:10.1038/nri2506
Gabrilovich, D. I., Ostrand-Rosenberg, S., and Bronte, V. (2012). Coordinated Regulation of Myeloid Cells by Tumours. Nat. Rev. Immunolimmunology 12, 253–268. doi:10.1038/nri3175
Galdiero, M. R., Bonavita, E., Barajon, I., Garlanda, C., Mantovani, A., and Jaillon, S. (2013). Tumor Associated Macrophages and Neutrophils in Cancer. Immunobiology 218, 1402–1410. doi:10.1016/j.imbio.2013.06.003
Galdiero, M. R., Marone, G., and Mantovani, A. (2018). Cancer Inflammation and Cytokines. Cold Spring Harb Perspect. Biol. 10, 860–867. doi:10.1101/cshperspect.a028662
Gallo-Oller, G., Di Scala, M., Aranda, F., and Dotor, J. (2020). Transforming Growth Factor Beta (TGF-β) Activity in Immuno-Oncology Studies. Methods Enzymol. 636, 129–172. doi:10.1016/bs.mie.2019.06.008
Giotopoulou, G. A., and Stathopoulos, G. T. (2020). Effects of Inhaled Tobacco Smoke on the Pulmonary Tumor Microenvironment. Adv. Exp. Med. Biol. 1225, 53–69. doi:10.1007/978-3-030-35727-6_4
Grivennikov, S. I., Greten, F. R., and Karin, M. (2010). Immunity, Inflammation, and Cancer. Cell 140, 883–899. doi:10.1016/j.cell.2010.01.025
Grivennikov, S. I., and Karin, M. (2011). Inflammatory Cytokines in Cancer: Tumour Necrosis Factor and Interleukin 6 Take the Stage. Ann. Rheum. Dis. 70 Suppl 1, i104–8. doi:10.1136/ard.2010.140145
Hanahan, D., and Weinberg, R. A. (2011). Hallmarks of Cancer: the Next Generation. Cell 144, 646–674. doi:10.1016/j.cell.2011.02.013
Harney, A. S., Arwert, E. N., Entenberg, D., Wang, Y., Guo, P., Qian, B. Z., et al. (2015). Real-Time Imaging Reveals Local, Transient Vascular Permeability, and Tumor Cell Intravasation Stimulated by TIE2hi Macrophage-Derived VEGFA. Cancer Discov. 5, 932–943. doi:10.1158/2159-8290.Cd-15-0012
He, J., Wang, L., Zhang, C., Shen, W., Zhang, Y., Liu, T., et al. (2019). Interleukin-9 Promotes Tumorigenesis through Augmenting Angiogenesis in Non-small Cell Lung Cancer. Int. Immunopharmacol 75, 105766. doi:10.1016/j.intimp.2019.105766
Hojman, P., Gehl, J., Christensen, J. F., and Pedersen, B. K. (2018). Molecular Mechanisms Linking Exercise to Cancer Prevention and Treatment. Cell Metab 27, 10–21. doi:10.1016/j.cmet.2017.09.015
Huang, X., Fan, X., Ying, J., and Chen, S. (2019). Emerging Trends and Research Foci in Gastrointestinal Microbiome. J. Transl Med. 17, 67. doi:10.1186/s12967-019-1810-x
Iyengar, N. M., Gucalp, A., Dannenberg, A. J., and Hudis, C. A. (2016). Obesity and Cancer Mechanisms: Tumor Microenvironment and Inflammation. J. Clin. Oncol. 34, 4270–4276. doi:10.1200/jco.2016.67.4283
Jakobsson, L., Franco, C. A., Bentley, K., Collins, R. T., Ponsioen, B., Aspalter, I. M., et al. (2010). Endothelial Cells Dynamically Compete for the Tip Cell Position during Angiogenic Sprouting. Nat. Cel Biol 12, 943–953. doi:10.1038/ncb2103
Ji, T., Zhao, Y., Ding, Y., Wang, J., Zhao, R., Lang, J., et al. (2016). Transformable Peptide Nanocarriers for Expeditious Drug Release and Effective Cancer Therapy via Cancer-Associated Fibroblast Activation. Angew. Chem. Int. Ed. Engl. 55, 1050–1055. doi:10.1002/anie.201506262
Kioi, M., Vogel, H., Schultz, G., Hoffman, R. M., Harsh, G. R., and Brown, J. M. (2010). Inhibition of Vasculogenesis, but Not Angiogenesis, Prevents the Recurrence of Glioblastoma after Irradiation in Mice. J. Clin. Invest. 120, 694–705. doi:10.1172/jci40283
Koyama, S., Akbay, E. A., Li, Y. Y., Aref, A. R., Skoulidis, F., Herter-Sprie, G. S., et al. (2016). STK11/LKB1 Deficiency Promotes Neutrophil Recruitment and Proinflammatory Cytokine Production to Suppress T-Cell Activity in the Lung Tumor Microenvironment. Cancer Res. 76, 999–1008. doi:10.1158/0008-5472.Can-15-1439
Krueger, J., Liu, D., Scholz, K., Zimmer, A., Shi, Y., Klein, C., et al. (2011). Flt1 Acts as a Negative Regulator of Tip Cell Formation and Branching Morphogenesis in the Zebrafish Embryo. Development 138, 2111–2120. doi:10.1242/dev.063933
Kubiczkova, L., Sedlarikova, L., Hajek, R., and Sevcikova, S. (2012). TGF-β - an Excellent Servant but a Bad Master. J. Transl Med. 10, 183. doi:10.1186/1479-5876-10-183
LaGory, E. L., and Giaccia, A. J. (2016). The Ever-Expanding Role of HIF in Tumour and Stromal Biology. Nat. Cel Biol 18, 356–365. doi:10.1038/ncb3330
Laha, D., Grant, R., Mishra, P., and Nilubol, N. (2021). The Role of Tumor Necrosis Factor in Manipulating the Immunological Response of Tumor Microenvironment. Front. Immunol. 12, 656908. doi:10.3389/fimmu.2021.656908
Li, B. H., Garstka, M. A., and Li, Z. F. (2020). Chemokines and Their Receptors Promoting the Recruitment of Myeloid-Derived Suppressor Cells into the Tumor. Mol. Immunol. 117, 201–215. doi:10.1016/j.molimm.2019.11.014
Li, Y., Fang, R., Liu, Z., Jiang, L., Zhang, J., Li, H., et al. (2021). The Association between Toxic Pesticide Environmental Exposure and Alzheimer's Disease: A Scientometric and Visualization Analysis. Chemosphere 263, 128238. doi:10.1016/j.chemosphere.2020.128238
Lin, Y., Yang, X., Liu, W., Li, B., Yin, W., Shi, Y., et al. (2017). Chemerin Has a Protective Role in Hepatocellular Carcinoma by Inhibiting the Expression of IL-6 and GM-CSF and MDSC Accumulation. Oncogene 36, 3599–3608. doi:10.1038/onc.2016.516
Liu, T., Zhang, L., Joo, D., and Sun, S. C. (2017). NF-κB Signaling in Inflammation. Signal. Transduct Target. Ther. 2, 86. doi:10.1038/sigtrans.2017.23
LoConte, N. K., Brewster, A. M., Kaur, J. S., Merrill, J. K., and Alberg, A. J. (2018). Alcohol and Cancer: A Statement of the American Society of Clinical Oncology. J. Clin. Oncol. 36, 83–93. doi:10.1200/jco.2017.76.1155
Ma, D., Yang, B., Guan, B., Song, L., Liu, Q., Fan, Y., et al. (2021). A Bibliometric Analysis of Pyroptosis from 2001 to 2021. Front. Immunol. 12, 731933. doi:10.3389/fimmu.2021.731933
Maniati, E., Soper, R., and Hagemann, T. (2010). Up for Mischief? IL-17/Th17 in the Tumour Microenvironment. Oncogene 29, 5653–5662. doi:10.1038/onc.2010.367
Mantovani, A., Allavena, P., Sica, A., and Balkwill, F. (2008). Cancer-related Inflammation. Nature 454, 436–444. doi:10.1038/nature07205
Martinez, F. O., and Gordon, S. (2014). The M1 and M2 Paradigm of Macrophage Activation: Time for Reassessment. F1000prime Rep. 6, 13. doi:10.12703/p6-13
Martinez-Outschoorn, U. E., Pavlides, S., Howell, A., Pestell, R. G., Tanowitz, H. B., Sotgia, F., et al. (2011a). Stromal-epithelial Metabolic Coupling in Cancer: Integrating Autophagy and Metabolism in the Tumor Microenvironment. Int. J. Biochem. Cel Biol 43, 1045–1051. doi:10.1016/j.biocel.2011.01.023
Martinez-Outschoorn, U. E., Whitaker-Menezes, D., Lin, Z., Flomenberg, N., Howell, A., Pestell, R. G., et al. (2011b). Cytokine Production and Inflammation Drive Autophagy in the Tumor Microenvironment: Role of Stromal Caveolin-1 as a Key Regulator. Cell Cycle 10, 1784–1793. doi:10.4161/cc.10.11.15674
Massagué, J. (2012). TGFβ Signalling in Context. Nat. Rev. Mol. Cel Biol 13, 616–630. doi:10.1038/nrm3434
Medzhitov, R. (2010). Inflammation 2010: New Adventures of an Old Flame. Cell 140, 771–776. doi:10.1016/j.cell.2010.03.006
Miao, L., and Huang, L. (2015). Exploring the Tumor Microenvironment with Nanoparticles. Cancer Treat. Res. 166, 193–226. doi:10.1007/978-3-319-16555-4_9
Miller, K. D., Nogueira, L., Mariotto, A. B., Rowland, J. H., Yabroff, K. R., Alfano, C. M., et al. (2019). Cancer Treatment and Survivorship Statistics, 2019. CA Cancer J. Clin. 69, 363–385. doi:10.3322/caac.2155110.3322/caac.21565
Nathan, C., and Ding, A. (2010). Nonresolving Inflammation. Cell 140, 871–882. doi:10.1016/j.cell.2010.02.029
Noy, R., and Pollard, J. W. (2014). Tumor-associated Macrophages: from Mechanisms to Therapy. Immunity 41, 49–61. doi:10.1016/j.immuni.2014.06.010
Okabe, Y., and Medzhitov, R. (2016). Tissue Biology Perspective on Macrophages. Nat. Immunol. 17, 9–17. doi:10.1038/ni.3320
Okayasu, I., Ichinoe, M., and Yoshida, T. (2019). Proposal for an Organ-specific Chronic Inflammation-Remodeling-Carcinoma Sequence. GastrointestDisord 1, 341–357. doi:10.3390/gidisord1030028
Olén, O., Erichsen, R., Sachs, M. C., Pedersen, L., Halfvarson, J., Askling, J., et al. (2020). Colorectal Cancer in Ulcerative Colitis: a Scandinavian Population-Based Cohort Study. Lancet 395, 123–131. doi:10.1016/s0140-6736(19)32545-0
Pavlides, S., Tsirigos, A., Vera, I., Flomenberg, N., Frank, P. G., Casimiro, M. C., et al. (2010). Loss of Stromal Caveolin-1 Leads to Oxidative Stress, Mimics Hypoxia and Drives Inflammation in the Tumor Microenvironment, Conferring the "reverse Warburg Effect": a Transcriptional Informatics Analysis with Validation. Cell Cycle 9, 2201–2219. doi:10.4161/cc.9.11.11848
Pérez-Romero, K., Rodríguez, R. M., Amedei, A., Barceló-Coblijn, G., and Lopez, D. H. (2020). Immune Landscape in Tumor Microenvironment: Implications for Biomarker Development and Immunotherapy. Ijms 21, 5521. doi:10.3390/ijms21155521
Qian, B. Z., Li, J., Zhang, H., Kitamura, T., Zhang, J., Campion, L. R., et al. (2011). CCL2 Recruits Inflammatory Monocytes to Facilitate Breast-Tumour Metastasis. Nature 475, 222–225. doi:10.1038/nature10138
Qian, B. Z., and Pollard, J. W. (2010). Macrophage Diversity Enhances Tumor Progression and Metastasis. Cell 141, 39–51. doi:10.1016/j.cell.2010.03.014
Qin, G., Lian, J., Huang, L., Zhao, Q., Liu, S., Zhang, Z., et al. (2018). Metformin Blocks Myeloid-Derived Suppressor Cell Accumulation through AMPK-DACH1-CXCL1 axis. Oncoimmunology 7, e1442167. doi:10.1080/2162402x.2018.1442167
Schäfer, M., and Werner, S. (2008). Cancer as an Overhealing Wound: an Old Hypothesis Revisited. Nat. Rev. Mol. Cel Biol 9, 628–638. doi:10.1038/nrm2455
Schröder, M., Krötschel, M., Conrad, L., Naumann, S. K., Bachran, C., Rolfe, A., et al. (2018). Genetic Screen in Myeloid Cells Identifies TNF-α Autocrine Secretion as a Factor Increasing MDSC Suppressive Activity via Nos2 Up-Regulation. Sci. Rep. 8, 13399. doi:10.1038/s41598-018-31674-1
Schulz, C., Schütte, K., Mayerle, J., and Malfertheiner, P. (2019). The Role of the Gastric Bacterial Microbiome in Gastric Cancer: Helicobacter pylori and beyond. Therap Adv. Gastroenterol. 12, 1756284819894062. doi:10.1177/1756284819894062
Sevko, A., Michels, T., Vrohlings, M., Umansky, L., Beckhove, P., Kato, M., et al. (20131950). Antitumor Effect of Paclitaxel Is Mediated by Inhibition of Myeloid-Derived Suppressor Cells and Chronic Inflammation in the Spontaneous Melanoma Model. J. Immunol. 190, 2464–2471. doi:10.4049/jimmunol.1202781
Shrihari, T. G. (2017). Dual Role of Inflammatory Mediators in Cancer. Ecancermedicalscience 11, 721. doi:10.3332/ecancer.2017.721
Su, X., Ye, J., Hsueh, E. C., Zhang, Y., Hoft, D. F., and Peng, G. (20101950). Tumor Microenvironments Direct the Recruitment and Expansion of Human Th17 Cells. J. Immunol. 184, 1630–1641. doi:10.4049/jimmunol.0902813
Tang, L., Mei, Y., Shen, Y., He, S., Xiao, Q., Yin, Y., et al. (2021). Nanoparticle-Mediated Targeted Drug Delivery to Remodel Tumor Microenvironment for Cancer Therapy. Int. J. Nanomedicine 16, 5811–5829. doi:10.2147/ijn.S321416
Taniguchi, K., and Karin, M. (2018). NF-κB, Inflammation, Immunity and Cancer: Coming of Age. Nat. Rev. Immunol. 18, 309–324. doi:10.1038/nri.2017.142
Thakkar, S., Sharma, D., Kalia, K., and Tekade, R. K. (2020). Tumor Microenvironment Targeted Nanotherapeutics for Cancer Therapy and Diagnosis: A Review. Acta Biomater. 101, 43–68. doi:10.1016/j.actbio.2019.09.009
Treps, L., Conradi, L. C., Harjes, U., and Carmeliet, P. (2016). Manipulating Angiogenesis by Targeting Endothelial Metabolism: Hitting the Engine rather Than the Drivers-A New Perspective. Pharmacol. Rev. 68, 872–887. doi:10.1124/pr.116.012492
Umansky, V., Blattner, C., Gebhardt, C., and Utikal, J. (2017). CCR5 in Recruitment and Activation of Myeloid-Derived Suppressor Cells in Melanoma. Cancer Immunol. Immunothercii 66, 1015–1023. doi:10.1007/s00262-017-1988-9
Umansky, V., Sevko, A., Gebhardt, C., and Utikal, J. (2014). Myeloid-derived Suppressor Cells in Malignant Melanoma. J. Dtsch Dermatol. Ges 12, 1021–1027. doi:10.1111/ddg.12411
Umansky, V., Blattner, C., Gebhardt, C., and Utikal, J. (2016). The Role of Myeloid-Derived Suppressor Cells (MDSC) in Cancer Progression. Vaccines 4, 36. doi:10.3390/vaccines4040036
Wang, Y., Wang, M., Wu, H. X., and Xu, R. H. (2021). Advancing to the Era of Cancer Immunotherapy. Cancer Commun. 41, 803–829. doi:10.1002/cac2.12178
Wang, Z., Dabrosin, C., Yin, X., Fuster, M. M., Arreola, A., Rathmell, W. K., et al. (2015). Broad Targeting of Angiogenesis for Cancer Prevention and Therapy. Semin. Cancer Biol. 35 Suppl, S224–S243. doi:10.1016/j.semcancer.2015.01.001
Waqas, A., Teoh, S. H., Lapão, L. V., Messina, L. A., and Correia, J. C. (2020). Harnessing Telemedicine for the Provision of Health Care: Bibliometric and Scientometric Analysis. J. Med. Internet Res. 22, e18835. doi:10.2196/18835
Yang, G., Tian, J., Chen, C., Jiang, D., Xue, Y., Wang, C., et al. (2019). An Oxygen Self-Sufficient NIR-Responsive Nanosystem for Enhanced PDT and Chemotherapy against Hypoxic Tumors. Chem. Sci. 10, 5766–5772. doi:10.1039/c9sc00985j
Yang, X., Guo, Y., Chen, C., Shao, B., Zhao, L., Zhou, Q., et al. (2021). Interaction between Intestinal Microbiota and Tumour Immunity in the Tumour Microenvironment. Immunology 164, 476–493. doi:10.1111/imm.13397
Yuan, X., Chang, C., Chen, X., and Li, K. (2021). Emerging Trends and Focus of Human Gastrointestinal Microbiome Research from 2010-2021: a Visualized Study. J. Transl Med. 19, 327. doi:10.1186/s12967-021-03009-8
Zhou, W., Yang, L., Nie, L., and Lin, H. (2021). Unraveling the Molecular Mechanisms between Inflammation and Tumor Angiogenesis. Am. J. Cancer Res. 11, 301–317.
Zhu, S., Niu, M., O'Mary, H., and Cui, Z. (2013). Targeting of Tumor-Associated Macrophages Made Possible by PEG-Sheddable, Mannose-Modified Nanoparticles. Mol. Pharm. 10, 3525–3530. doi:10.1021/mp400216r
Keywords: bibliometric, visualization, hotspot, inflammatory tumor microenvironment, CiteSpace
Citation: Zhang Y, Huo L, Wei Z, Tang Q and Sui H (2022) Hotspots and Frontiers in Inflammatory Tumor Microenvironment Research: A Scientometric and Visualization Analysis . Front. Pharmacol. 13:862585. doi: 10.3389/fphar.2022.862585
Received: 26 January 2022; Accepted: 11 February 2022;
Published: 17 March 2022.
Edited by:
Wei Zhao, City University of Hong Kong, Hong Kong SAR, ChinaReviewed by:
Fengjie Zheng, Beijing University of Chinese Medicine, ChinaSizhi Paul Gao, Memorial Sloan Kettering Cancer Center, United States
Copyright © 2022 Zhang, Huo, Wei, Tang and Sui. This is an open-access article distributed under the terms of the Creative Commons Attribution License (CC BY). The use, distribution or reproduction in other forums is permitted, provided the original author(s) and the copyright owner(s) are credited and that the original publication in this journal is cited, in accordance with accepted academic practice. No use, distribution or reproduction is permitted which does not comply with these terms.
*Correspondence: Hua Sui, c3loMDgwOEAxNjMuY29t
†These authors have contributed equally to this work