- 1Department of Nephrology, Shaanxi Traditional Chinese Medicine Hospital, Xi’an, China
- 2Key Disciplines of Clinical Pharmacy, Clinical Genetics Laboratory, Affiliated Hospital and Clinical Medical College of Chengdu University, Chengdu, China
- 3School of Food and Bioengineering, Chengdu University, Chengdu, China
Chronic kidney disease (CKD) was a major public health problem worldwide. Renal fibrosis, especially tubulointerstitial fibrosis, is final manifestation of CKD. Many studies have demonstrated that TGF-β/Smad signaling pathway plays a crucial role in renal fibrosis. Therefore, targeted inhibition of TGF-β/Smad signaling pathway can be used as a potential therapeutic measure for tubulointerstitial fibrosis. At present, a variety of targeting TGF-β1 and its downstream Smad proteins have attracted attention. Natural products used as potential therapeutic strategies for tubulointerstitial fibrosis have the characteristics of acting on multiple targets by multiple components and few side effects. With the continuous research and technique development, more and more molecular mechanisms of natural products have been revealed, and there are many natural products that inhibited tubulointerstitial fibrosis via TGF-β/Smad signaling pathway. This review summarized the role of TGF-β/Smad signaling pathway in tubulointerstitial fibrosis and natural products against tubulointerstitial fibrosis by targeting TGF-β/Smad signaling pathway. Additionally, many challenges and opportunities are presented for inhibiting renal fibrosis in the future.
1 Introduction
Chronic kidney disease (CKD) has been recognized as a major public health problem worldwide and the global increase in CKD is associated with the increase in the prevalence of diabetes, hypertension, obesity, and aging (Webster et al., 2017; Hansrivijit et al., 2021). Recent data showed that global prevalence of CKD was estimated to be between 11% and 13% in 2016 (Hill et al., 2016). This number is continuous increase by 2030/2050 (Mantovani and Chiara, 2020). Moreover, with the progression of CKD, it can easily lead to the occurrence of cardiovascular diseases (Ravid et al., 2021). Renal fibrosis, characterized by tubulointerstitial fibrosis and glomerulosclerosis, is the common pathway and inevitable outcome of various progression of CKD to end-stage renal disease (ESRD) and need renal replacement treatments such as dialysis and transplantation (Humphreys, 2018; Aydin et al., 2019; Jain et al., 2019; Carta et al., 2020; Sawhney and Gill, 2020). Regardless of the underlying diseases, it has been demonstrated that patient’s survival is associated with tubulointerstitial fibrosis (Tampe and Zeisberg, 2014) and the extent of tubulointerstitial fibrosis on renal biopsy is thought as a prognostic indicator for CKD treatment (Tang et al., 2019). Fibrosis is a pathological scar formation process (Hu et al., 2020; Miao et al., 2021a). The histopathological characteristics of tubulointerstitial fibrosis are excessive synthesis and deposition of interstitial extracellular matrix (ECM) components, which are association with inflammatory cell infiltration, damage of tubular epithelial cells, activation and proliferation of fibroblasts and rarefaction of the peritubular microvasculature (Ma S.-X. et al., 2018; Humphreys, 2018; Bhargava et al., 2021; Medina Rangel et al., 2021). Renal fibrosis is associated with the activation of transforming growth factor-β (TGF-β)/Smad, Wnt/β-catenin, IκB/NF-κB and Keap1/Nrf2 signaling pathways (Chen DQ. et al., 2017; Chen et al., 2018a), the overexpression of renin-angiotensin system and aryl hydrocarbon receptor (Liu J.-R. et al., 2020; Miao et al., 2020; Miao et al., 2021b) and the dysregulation of metabolites such as amino acids and lipids (Chen et al., 2016a; Chen et al., 2019a; Feng Y. L. et al., 2019; Wang et al., 2021a). The robust pathological markers of CKD progression are the impairment of renal excretion function and the degree of fibrosis or fibroblast number, which are closely related to tubulointerstitial fibrosis (Chen et al., 2019b). The clinical first-line therapy for CKD is using anti-renin-angiotensin system remedies, such as angiotensin-converting enzyme inhibitors or angiotensin II type 1 receptor blockers. These therapies were proven to be beneficial for patients with CKD, protected against proteinuria and renal function decline, and ameliorated the progression to ESRD. However, considerable numbers of patients were still not being adequately treated and often progress toward ESRD. Therefore, it is urgent to find effective drugs to retard tubulointerstitial fibrosis and delay the progression of CKD. However, it is disappointing that no effective and satisfactory therapies in clinic settings have been found until now (Xu BH. et al., 2020).
Members of TGF-β superfamily have a common dimeric structure, which consist of six conserved cysteine residues, including bone morphogenetic protein, growth and differentiation factors, anti-Müllerian hormone, activin, inhibin, nodal and TGF-β (Chen and Ten Dijke, 2016; Hu et al., 2018). Among these effects, TGF-β is a key profibrotic mediator in fibrotic diseases (Hu et al., 2018). TGF-β is a multifunctional regulatory cytokine, which is involved in regulating a broad range of cellular processes, such as proliferation, differentiation, apoptosis, adhesion, migration, wound repair, and the pathogenesis of fibrosis (Meng et al., 2016a). Numerous studies have demonstrated that TGF-β/Smad pathway played a crucial role in renal fibrosis (Meng et al., 2016b; Hu et al., 2018). TGF-β is the major driver of fibrosis in CKD (Meng et al., 2016a) and also exerts protective effects by suppressing inflammation and inducing autophagy (Sureshbabu et al., 2016). Overexpression of TGF-β1 can elicit renal fibrosis by activating both canonica TGF-β/Smad signaling pathways and non-canonical signaling pathways including mitogen-activated protein kinase, p38, Rho-GTPases, phosphatidylinositol-3 kinase, Rac, Cdc42 and integrin linked kinase (Chen et al., 2018b), which results in activation of myofibroblasts, excessive ECM components and ECM degradation inhibition. In TGF-β/Smad signaling pathway, Smad proteins have a complex mechanism, which have competitive effects of profibrosis and antifibrosis. Furthermore, there are complex interactions between TGF-β/Smad signaling pathway and other signaling pathways (Meng et al., 2016b). Therefore, fibrotic mechanism is so complex that it is necessary to further understand the pathogenesis of renal fibrosis regulated by TGF-β/Smad pathway and find possible therapy.
Numerous publications have shown that TGF-β/Smad pathway played a key role in CKD caused by diverse etiologies, such as diabetic nephropathy (Li et al., 2020), hypertensive nephropathy (Liu et al., 2017), IgA nephropathy (Wang P. et al., 2018), post-transplant nephropathy (Wang Z. et al., 2018) and aristolochic acid nephropathy (Song et al., 2019). Moreover, with the gradual loss of renal function, these diseases will inevitably mediate the progression of renal fibrosis. The inhibition of TGF-β1/Smad signaling pathway retarded renal fibrosis in CKD (Meng et al., 2016a). Therefore, targeting TGF-β/Smad pathway against tubulointerstitial fibrosis is a promising therapeutic strategy. Increasing evidence has shown that natural products have been demonstrated to be as a potential therapy in a variety of disease (Chen et al., 2018a; Newman and Cragg, 2020). A number of natural products have been demonstrated to protect against tubulointerstitial fibrosis (Chen et al., 2018a). In this review, we summarized the effect of TGF-β/Smad signaling pathway on the tubulointerstitial fibrosis and highlighted the therapeutic potential of natural products by targeting TGF-β/Smad pathway against tubulointerstitial fibrosis.
2 TGF-β/Smad Signaling Pathway
It is well known that TGF-β/Smad signaling pathway is mainly composed of TGF-β and its receptors, as well as various Smad proteins. TGF-β1, TGF-β2 and TGF-β3 are three isoforms of TGF-β identified in mammals, which have 70-82% amino acid homology and induce different biological reactions (Chen H. et al., 2018; Ma and Meng, 2019). Among them, TGF-β1 can be produced by all types of resident kidney cells and is the most abundant and active isoforms (Meng et al., 2015). TGF-β combines with latency-associated peptide (LAP) that promotes attachment with latent TGF-β binding protein (LTBP) through the formation of disulfide bonds, which is released from LAP and LTBP and becomes active when received various stimulations, such as reactive oxygen species (ROS), integrins, proteases and metalloproteinases (Meng et al., 2013; Meng et al., 2015; Ma and Meng, 2019). TGF-β initiates intracellular signaling by binding to receptor complexes (Chen H. et al., 2018). TGF-β receptors include TGF-β receptors type I (TGFβRI), TGF-β receptors type II (TGFβRII) and TGF-β receptors type III (TGFβRIII). TGF-β1 and TGF-β3 directly bind to TGFβRII, while TGF-β2 needs TGFβRIII to bind to TGFβRII (Yu et al., 2003). Therefore, TGFβRII has high affinity for TGF-β ligands, and TGFβRI does not bind to TGF-β directly. After TGFβRII binds to TGF-β, TGFβRII recruits and activates TGFβRI through phosphorylated the GS domain in TGFβRI (Nakerakanti and Trojanowska, 2012). The TGFβRI and TGFβRII contain serine-threonine protein kinases in their intracellular domains, which can activate intracellular signaling by phosphorylated Smad protein (Blobe et al., 2000). TGFβRIII is the most abundant type, which increases the bioavailability of TGF-β to signal TGF-β receptor by binding TGF-β and then transferring it to its signaling receptors (Blobe et al., 2000; Nakerakanti and Trojanowska, 2012). The Smad family of human includes eight members (Smads 1–8), which are called receptor-regulated Smads (R-Smads). Generally, TGFβRI can phosphorylate and activate Smad2 and Smad3 in the TGF-β/Smad pathway. Smad4, called common mediator Smad (Co-Smad), is a common partner of all of the receptor-activated Smads. It is a specific transcription co-regulatory factor, which can be recruited to Smad transcription complex. Therefore, the trimeric complex formed by phosphorylated R-Smads and Smad4 can be used for transcriptional regulation. Smad6 and Smad7 are called inhibitory Smads (I-Smads), which can interplay with activated receptors and R-Smads to inhibit their activities. Smad7 is mainly involved and acts as a negative feedback regulation loop to regulate the target genes that regulate cell homeostasis (Macias et al., 2015). Therefore, TGF-β and various Smad proteins can interplay each other and work together to regulate various physiological and pathological mechanisms (Figure 1).
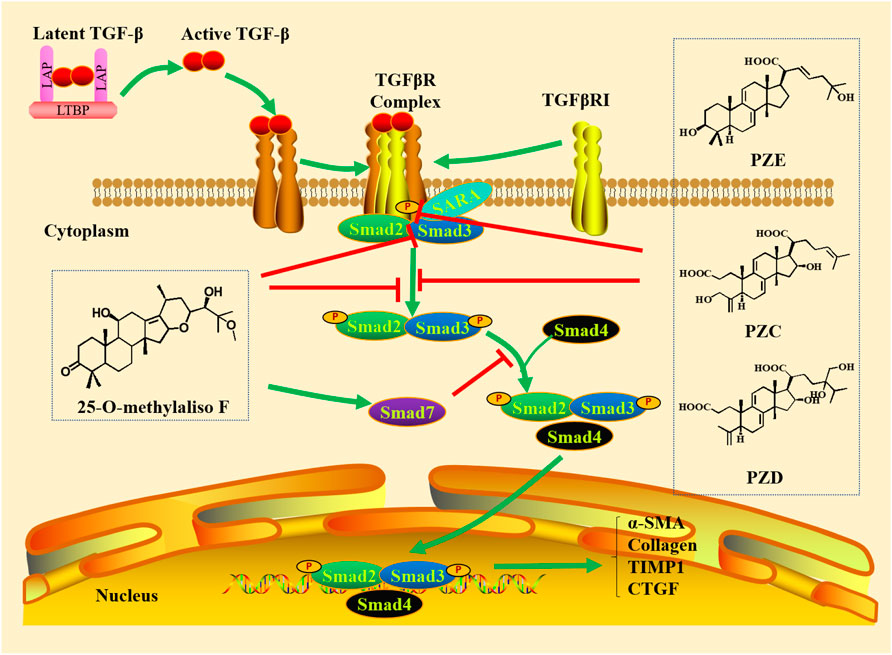
FIGURE 1. Canonical TGF-β/Smad pathway. TGF-β synthesized in precursor form combines with latency-associated peptide (LAP) that promotes attachment with LTBP through the formation of disulfide bonds, which is released from the LAP and LTBP and becomes active when exposed to various stimuli. The active TGF-β binds to TGFβRII, which recruits and activates TGFβRI through phosphorylated the GS domain in TGFβRI. TGFβRI phosphorylates and activates Smad2 and Smad3 (R-Smads). Smad4 combined with phosphorylated R-Smads to form a trimer complex for transcriptional regulation. Smad7 is involved and acts as a negative feedback regulation loop to regulate the target genes that regulate cell homeostasis.
3 Role of TGF-β/Smad Signaling Pathway in Tubulointerstitial Fibrosis
3.1 Role of TGF-β in Tubulointerstitial Fibrosis
TGF-β is closely related ligands that have pleiotropic activity on most cell types of the body and play an important role in maintaining homeostasis in normal tissues. TGF-β1 is a major or predominant isoform involved in fibrosis and the effects of TGF-β2 and TGF-β3 may be partially mediated by TGF-β1. The overexpression of TGF-β1 is causally related to the progression of renal fibrosis caused by aging, diabetes, hypertension, obstruction, ischemia and toxin-induced injury (Gifford et al., 2021; Ma et al., 2022). TGF-β1 upregulation has been confirmed in patients and animal models with CKD (Hu et al., 2018). In addition, urinary TGF-β1 level, which reflects the degree of renal pathology and the development of CKD (Lawson et al., 2016), is positive correlated with renal fibrosis (Meng et al., 2015) and can be used as a clinical marker of renal fibrosis. Targeted TGF-β1 gene leads to multifocal and mixed inflammatory cell reaction in mice and conditional deletion of TGFβRII leads to kidney inflammation in mice (Meng et al., 2012a), which indicate that TGF-β1 is an effective anti-inflammatory molecule. However, blocking TGF-β1 signaling could eliminate its profibrotic effect, but inhibit its anti-inflammatory effect and thereby promote renal fibrosis (Sureshbabu et al., 2016). In addition, TGF-β1 could induce autophagy, promoting collagen degradation and cell protection for renal cell survival to prevent and treat renal fibrosis (Sureshbabu et al., 2016). Therefore, TGF-β is closely related to the formation of tubulointerstitial fibrosis and targeted inhibition of TGF-β overexpression is an important strategy for the treatment of tubulointerstitial fibrosis.
3.2 Role of Smad Proteins in Tubulointerstitial Fibrosis
3.2.1 Role of Smad2 and Smad3 Proteins in Tubulointerstitial Fibrosis
It is well known that Smad2 and Smad3 are two important downstream genes of canonical TGF-β signaling that are activated in fibrotic kidneys (Jiang et al., 2019; Li et al., 2019). It is now well documented that Smad3 and Smad3-dependent non-coding RNAs are activated by TGF-β1 to transcriptionally and differentially regulate renal inflammation and fibrosis (Gu et al., 2020). The expression levels of TGF-β1 and Smad3 are elevated and pathological staining results show obvious fibrosis of renal tissues in rats with unilateral ureteral obstruction (UUO) (Tian et al., 2021). However, mice lacking Smad3 expression protected against renal fibrosis following UUO by blocking epithelial-mesenchymal transition (EMT) and eliminating monocyte influx and collagen deposition, and the cultured primary renal tubular epithelial cells from mice lacking Smad3 also revealed that Smad3 is critical for TGF-β1-mediated EMT. In addition, TGF-β/Smad3 pathway could be activated in patients with type 2 diabetic nephropathy. It was found that Smad3 wild-type db/db and Smad3+/− db/db mice suffered from diabetic kidney injury, such as the decrease of renal function, a marked deposition of collagen I and collagen IV, and an increased macrophages in kidney. However, there was no evidence between renal fibrosis and inflammation response in Smad3 knockout db/db mice (Xu M. et al., 2020). Smad3 can also promote renal fibrosis by incorporating collagen promoter region and inhibiting ECM degradation via the induction of tissue inhibitor of matrix metalloproteinases (TIMP) while increasing the activity of matrix metalloproteinase-1 (MMP-1) (Gu et al., 2020). These facts indicate that Smad3 is the core of the pathogenesis of tubulointerstitial fibrosis (Sato et al., 2003). By contrast, Smad2 has renal protective effect. Despite the embryonic lethality of the Smad2 gene knockout mice (Gu et al., 2020), Smad2 conditional deletion from renal tubular epithelial cells and fibroblasts has been reported to significantly enhance renal fibrosis by increasing the expression of collagen I, collagen III, and TIMP-1 as well as decreasing the expression of MMP-2 to TGF-β1, which are due to the fact that Smad2 deletion promotes Smad3 phosphorylation, promoter activity, Smad3 binding to collagen promoter (COL1A2) (Meng et al., 2010). Therefore, targeted regulation of Smad3 expression is a potential target for treatment of tubulointerstitial fibrosis.
3.2.2 Role of Smad4 Protein in Tubulointerstitial Fibrosis
Smad4 is a common Smad protein, which forms heteromeric complex with Smad2/3 and translocates into nucleus to mediate expression of downstream target genes (Meng et al., 2012b). It has been found that Smad4 could enhance Smad3-induced renal fibrosis by transcription and inhibit NF-κB-induced renal inflammation by Smad7-dependent mechanism, thereby exerting multiple effects (Lan, 2011). In streptozotocin-treated rats, the increased expression of TGF-β1 and Smad4 may lead to transcriptional regulation of target genes, which contributes to the progression of renal fibrosis (Yang et al., 2007). It is reported that siRNA Smad4 can inhibit renal fibrosis and α-smooth muscular actin (α-SMA)-positive myofibroblasts in mice induced by single intraperitoneal injection of folic acid (Morishita et al., 2014). The mechanism of inhibiting fibrosis of which may be explained by the fact that Smad4 deletion affects Smad3-mediated promoter activity and the combination of Smad3 and COL1A2 promoter, but does not affect p-Smad3 and nuclear translocation (Meng et al., 2016b). Furthermore, the destruction of Smad4 represses Smad7 transcription, thereby inhibiting NF-κB activation and promoting kidney inflammation as evidenced by inflammatory cells infiltration, upregulation of interleukin-1β (IL-1β), tumor necrosis factor-α (TNF-α), monocyte chemoattratctant protein-1 (MCP-1), and intercellular adhesion molecule-1 (ICAM-1) in conditional Smad4 knockout UUO mice (Meng et al., 2016a). Therefore, Smad4 has an important effect on renal fibrosis (Gu et al., 2020). The double action mechanism of Smad4 on kidney determines that it may not be an important anti-fibrosis target.
3.2.3 Role of Smad7 Protein in Tubulointerstitial Fibrosis
Smad7 is a negative regulator and plays a negative role in renal fibrosis and inflammation (Meng et al., 2015; Gu et al., 2020). It is found that UUO model mice with Smad7 gene destruction can exhibit TGF-β/Smad2/3-dependent severe tubulointerstitial fibrosis by upregulating intrarenal TGF-β1 and connective tissue growth factor (CTGF) and activating Smad2/3, and sustain NF-κB activation, thereby enhancing intrarenal inflammation, including macrophage infiltration and expression upregulation of MCP-1, TNF-α, osteopontin and ICAM-1. The immunoreactivity for p-Smad2 and p-Smad3 are gradually increased in renal fibrosis, and Smad7 degradation and ubiquitination activities are significantly increased in UUO kidneys in mice, which indicate that decreased Smad7 protein caused by enhanced ubiquitin-dependent degradation leads to a pathogenic effect on renal fibrosis (Fukasawa et al., 2004). In streptozotocin-induced rats, insulin can upregulate Smad7 protein expression in the kidneys of diabetic rats, reduce ECM deposition, and delay the progress of diabetic nephropathy after controlling blood glucose, which may be related to the decrease of TGF-β1 and Smurf2 expression and attenuation of Smad7 ubiquitination in renal tissue (Wang YZ. et al., 2013). Therefore, during the process of fibrosis formation, Smad3 is generally activated whereas Smad7 is degraded by ubiquitin-proteasome degradation mechanisms. The imbalance between Smad3 and Smad7 is an key mechanism in renal fibrosis, which causes activation and accumulation of myofibroblasts, the excessive production of ECM and the reduction of ECM degradation (Meng et al., 2015). The latest study demonstrated that Latent TGF-β1 improved diabetic kidney disease through regulating Arkadia/Smad7 signaling (Wu et al., 2021). Therefore, targeting Smad3 inhibition and Smad7 promotion are important potential targets for treatment of tubulointerstitial fibrosis.
4 Crosstalk Between TGF-β1/Smad and Long Non-Coding RNA in Tubulointerstitial Fibrosis
Long non-coding RNAs (lncRNAs) are an emerging class of non-coding RNAs, which are more than 200 nucleotides in length (Luo et al., 2020; Wang et al., 2021b). lncRNAs can regulate various biological functions at epigenetic, transcriptional and post-transcriptional levels (Huang, 2018) and bind DNA, RNA and protein (Wang Y.-N. et al., 2021). Mechanism studies have shown that TGF-β1/Smad3 is able to promote renal inflammation and fibrosis by regulating lncRNAs (Tang et al., 2017). Erbb4-IR is a Smad3-dependent lncRNA that promotes renal fibrosis by suppressing miR-29b of type 2 diabetic nephropathy in db/db mice (Sun X. et al., 2018) and is upregulated in the obstructed kidneys of UUO mice (Feng et al., 2018). Smad7 is a target gene of Erbb4-IR. The overexpression of Erbb4-IR can lead to renal fibrosis by downregulating Smad7 (Feng et al., 2018). The lncRNA9884 is a novel Smad3-dependent lncRNA, which is associated with diabetic renal injury. It can be significantly upregulated and enhance MCP-1-dependent renal inflammation in the diabetic kidney of db/db mice (Zhang YY. et al., 2019). lncRNA AT-rich interactive domain 2-IR (Arid2-IR) is identified as a Smad3-associated lncRNA in UUO kidney disease, the increasing of which can contribute to ECM production in diabetic kidney disease (Yang et al., 2019). Additionally, the overexpression of lncRNA-ATB can activate the TGF-β/Smad2/3 pathway, thus promoting inflammation, cell apoptosis and senescence in the TGF-β1-induced HK-2 cells, which indicates that silencing lncRNA-ATB is a new therapeutic strategy for intervention of renal fibrosis (Sun et al., 2020). All these evidence indicates that targeted regulation of lncRNAs involved in TGF-β/Smad pathway could improve tubulointerstitial fibrosis (Figure 2).
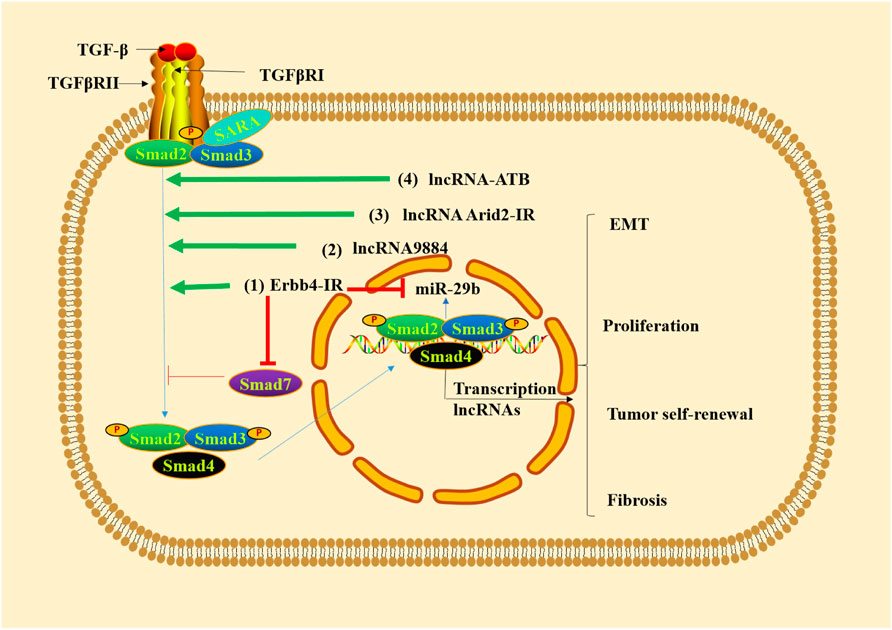
FIGURE 2. TGF-β1/Smad3 signaling regulates lncRNAs in renal fibrosis. (1) Erbb4-IR promotes renal fibrosis by suppressing miR-29b and downregulating Smad7. (2) lncRNA9884 is significantly upregulated in renal fibrosis. (3) The increased lncRNA Arid2-IR leads to ECM production in renal fibrosis. (4) lncRNA-ATB activates TGF-β/Smad2/3 pathway, thus promoting inflammation, cell apoptosis and senescence.
5 Natural Products as an Alternative Therapy Against Tubulointerstitial Fibrosis
5.1 The Isolated Compounds Against Tubulointerstitial Fibrosis
Traditional Chinese medicines (TCMs), a unique medical system with the remarkable feature of the use of multicomponent drugs, could hit multiple targets through multiple components (Chen et al., 2018a; Wang YN. et al., 2021). It pursues an overall therapeutic effect with a multi-ingredient treatment in the form of combination drug formulas in an attempt to improve therapeutic efficacy and lower drug side-effects and could also be an effective approach of reducing drug resistance (Jiang, 2005). Therefore, the studies of TCMs have attracted attention owing to its superiority in the treatment of refractory diseases (Chen et al., 2016b; Li et al., 2021a; Shao G. et al., 2021; Wang Y. W. et al., 2021; Yang and Wu, 2021). A variety of compounds isolated from natural products have been protected against renal fibrosis (Feng et al., 2019b; Luo et al., 2021; Shao M. et al., 2021; Zhao et al., 2011; Zhou F. et al., 2021). Therefore, natural products have attracted more and more attention around the world and are committed to the development of new drugs. With the continuous research and the development of technique, more and more molecular mechanisms of natural products have been revealed, and there are many natural products that inhibited tubulointerstitial fibrosis through TGF-β/Smad signaling pathway (Table 1) (Chen et al., 2018a; Hu et al., 2018).
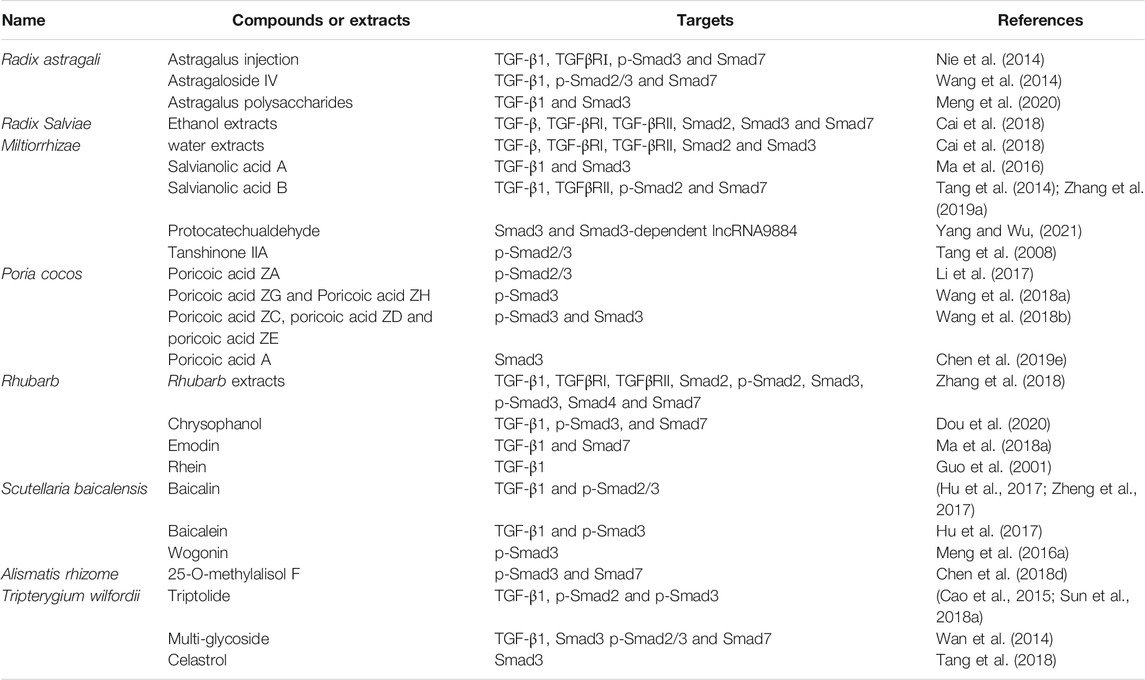
TABLE 1. Summary of targets for natural products and their isolated compounds or extracts to exert anti-tubulointerstitial fibrosis effects through TGF-β/Smad signaling pathway.
Radix Astragali is used for treating kidney diseases (Zhang et al., 2014). Astragalus injection could mitigate diabetic nephropathy by increasing Smad7 expression, inhibiting the expression of TGFβRΙ, Smad3 and its phosphorylation as well as TGF-β1 mRNA levels (Nie et al., 2014). Astragaloside IV (AS-IV) could significantly mitigate tubulointerstitial fibrosis in the UUO rats and TGF-β1-stimulated NRK-49F cells. AS-IV could upregulate Smad7 expression, thereby blocking the upregulated protein expression of TGF-β1 and p-Smad2/3 (Wang et al., 2014). Astragalus polysaccharides could reduce the levels of creatinine and urea and inhibit collagen I, collagen III, collagen IV, α-SMA, TGF-β1 and Smad3 in rats treated by a high-fat diet with streptozotocin (Meng et al., 2020).
Radix Salviae Miltiorrhizae is usually used to treat cardiovascular diseases, but a number of studies have shown that it possessed protective effects on CKD (Cai et al., 2018). The ethanol and water extracts of Radix Salviae Miltiorrhizae prevented EMT through regulating NADPH oxidase/ROS/ERK and TGF-β/Smad signaling pathways in adenine-induced HK-2 rats (Cai et al., 2018). Several studies have demonstrated that salvianolic acid A and salvianolic acid B repressed renal fibrosis by modulating TGF-β1/Smad pathway. Salvianolic acid A reduced the expressions of TGF-β1 and Smad3 (Ma et al., 2016), while salvianolic acid B downregulated the expressions of TGF-β1 and p-Smad2 and preserved Smad7 expression (Zhang X. et al., 2019). Bioinformatics analysis revealed that TGFβRII might be a direct target of the miR-106b-25 cluster (miR-25, miR-93 and miR-106b). During the formation of EMT induced by TGF-β1, these miRNAs were found to be significantly downregulated, which were upregulated by salvianolic acid B treatment in a dose-dependent manner (Tang et al., 2014). This showed that salvianolic acid B could act on TGF-β receptor by targeting the specific miRNAs to retard renal fibrosis. Protocatechualdehyde, a phenolic acid, improved renal function and pathological changes via regulating Smad3 expression and NF-κB signaling in UUO-induced obstruction kidney. It also suppressed Smad3-dependent lncRNA9884 expression and inhibited inflammation by lncRNA9884/MCP-1 pathway (Yang et al., 2021). Tanshinone IIA, an active ingredient of Radix Salviae Miltiorrhizae, exerted its inhibitory effect on tubulointerstitial fibrosis by downregulating p-Smad2/3 expression in renal interstitial fibroblasts induced by TGF-β1 (Tang et al., 2008).
Poria cocos used in TCMs in China and Japan possessed the effects of diuretic, antiinflammation, antioxidative, antifibrosis and antihyperlipidemia (Wang Y. et al., 2013; Miao et al., 2015; Chen et al., 2016c). Earlier findings demonstrated the diuretic effect of the extracts of the surface layer of Poria cocos (Zhao et al., 2012; Feng et al., 2013). Further studies demonstrated that these extracts protected against tubulointerstitial fibrosis in adenine- and nephrectomized-induced chronic renal failure (Zhao et al., 2013a; Zhao et al., 2013b; Zhao et al., 2013c; Feng et al., 2019c). A number of poricoic acids, such as secolanostane tetracyclic triterpenoids and lanostane tetracyclic triterpenoids isolated and identified from Poria cocos protected against renal fibrosis by regulating Wnt1/β-catenin, IκB/NF-κB, and Keap1/Nrf2 pathways (Wang et al., 2017; Chen et al., 2019c; Wang et al., 2020). Poricoic acid A, a major component of Poria cocos, has been demonstrated to protect against renal fibrosis (Chen et al., 2019d; Chen et al., 2020a; Chen et al., 2020b). Previous study showed that poricoic acid A ameliorated AKI-to-CKD continuum by inhibiting Smad3 phosphorylation of renal tissues in renal ischemia-reperfusion injury rats (Chen et al., 2019e). The latest study demonstrated that poricoic acid A inhibited TGF-β1-induced renal fibrosis and proliferation through Smad3 and MAPK pathways (Li et al., 2021b).
New poricoic acid ZA protected against renal fibrosis through suppressing p-Smad2/3 through inhibiting Smad2/3-TGFβRI interaction (Li et al., 2017). Poricoic acid ZG and Poricoic acid ZH exerted their antifibrotic effects by selectively repressing the p-Smad3 via inhibiting the interactions of SARA with TGF-β1 and Smad3 (Wang et al., 2018a). Moreover, Poricoic acid ZC, poricoic acid ZD and poricoic acid ZE not only specific repressed p-Smad3 via blocking interplay between TGFβRI with Smad3, but also selectively suppressed Smad3 expression (Wang et al., 2018a). Structure-function analysis revealed that poricoic acid ZC and poricoic acid ZD, showed a strong inhibitory effect compared with poricoic acid ZE (Wang et al., 2018a). Therefore, compounds to the secolanostance skeleton showed strong bioactivity compared with lanostance skeleton.
Rhubarb is one of the most commonly used natural products, which contains a variety of chemical components that exhibit a variety of pharmacological activities, such as antitumor, antiinflammatory, lipid-lowing, hepatoprotective and renoprotective effects (Chen et al., 2015; Cao et al., 2017). Our earlier several publications showed that Rhubarb can ameliorate tubulointerstitial fibrosis in rat with adenine-induced CKD (Zhang et al., 2015; Zhang et al., 2016). Our studies show that Rhubarb extracts can suppress TGF-β/Smad3 signaling pathway by reducing the protein expressions of TGF-β1, TGFβRI, TGFβRII, Smad2, p-Smad2, Smad3, p-Smad3, and Smad4 and preserving expression of Smad7 protein (Zhang et al., 2018). Some studies have shown that chrysophanol could inhibit tubulointerstitial fibrosis by downregulating expression of TGF-β1 and p-Smad3 and preserving expression of Smad7 without affecting Smad2, Smad4 and TGF-β receptors in UUO mice (Dou et al., 2020). Mechanistically, chrysophanol repressed interaction between Smad3 and TGFβRI to suppress expression of p-Smad3 (Dou et al., 2020). These studies indicated that chrysophanol alleviated tubulointerstitial fibrosis by repressing Smad3 phosphorylation. In addition, emodin ameliorated renal fibrosis in rats via downregulating expression of TGF-β1 and Smurf2 and preserving Smad7 expression (Ma L. et al., 2018). Moreover, rhein inhibited cell hypertrophy and ECM accumulation mediated by TGF-β1 (Guo et al., 2001), which indicated that rhein exerted renoprotective effect, possibly by inhibiting the overexpression of TGF-β1. Rhubarb and astragalus capsule has been treated patients with CKD for decades. Recently, in vivo study showed that Rhubarb and astragalus capsule bunted tubulointerstitial fibrosis in the UUO rats by suppressing apoptosis via inhibiting TGF-β1/p38 MAPK pathway (Zeng et al., 2020). In vitro study demonstrated that drug-containing serum of rhubarb-astragalus capsule retarded EMT in the TGF-β1-induced HK-2 cells by downregulating TGF-β1/p38MAPK/Smad2/3 signaling axis (Qin et al., 2021).
Scutellaria baicalensis showed effects of cleaning away heat and dampness, fire and detoxification. Its extracts and compounds from Scutellaria baicalensis showed many pharmacological activities (Zhao et al., 2019). Baicalein showed a stronger inhibitory effect on cell proliferation, collagen synthesis, TGF-β1 and p-Smad3 upregulation than baicalin in the TGF-β1-induced NRK-49F cells (Hu et al., 2017). Furthermore, Baicalin has been demonstrated to decrease TGF-β1 level in serum and obstructed kidney, and repressed p-Smad2/3 in obstructed kidney of UUO rats (Zheng et al., 2017). In addition, wogonin inhibited upregulation of mRNA and protein of collagen I and α-SMA induced by TGF-β1 compared with wogonoside. Mechanistically, wogonin reduced p-Smad3 expression but showed weakened effect on non-canonical TGF-β pathway, such as p38, extracellular signal-regulated kinase 1/2 and mitogen-activated protein kinase pathways (Meng et al., 2016a).
Alismatis rhizoma exhibited lipid-lowing, diuretic and renoprotective activities (Tian et al., 2014; Miao et al., 2017). Our previous findings demonstrated the diuretic and anti-diuretic effects of Alismatis rhizome (Chen et al., 2014; Feng et al., 2014). Further studies demonstrated that these extracts protected against tubulointerstitial fibrosis in rats with adenine-induced CKD (Dou et al., 2018). Alisol B 23-acetate, as a major component of Alismatis rhizoma, ameliorated renal fibrosis by modulating renin–angiotensin system and gut microbiota–kidney signaling axis (Chen H. et al., 2020). Our previous study identified new compound 25-O-methylalisol F as a novel RAS inhibitor (Chen et al., 2018b). It could attenuate upregulated p-Smad3 and downregulated Smad7 without affecting the expression of phosphorylated Smad2, PI3K, ERK1/2 and p38 and expression of Smad4 in the TGF-β1- and angiotensin II-mediated NRK-52E and NRK-49F cells. It also specific repressed the combination of Smad3 with TGFβRI and Smad3 with SARA without affecting interaction of Smad2, TGFβRI and SARA (Chen et al., 2018b).
Tripterygium wilfordii is considered as an effective drug for treating diabetic nephropathy. Although it causes side effects, such as hepatic impairment, gastrointestinal reactions, menstrual disorders and reproductive effects, it is feasible to use it cautiously and appropriately to treat disease (Huang et al., 2020). Triptolide repressed proliferation, mediated apoptosis and led to cell cycle arrest in human mesangial cells (Sun SF. et al., 2018). Triptolide could upregulate Ski protein expression and downregulate protein expressions of α-SMA, TGF-β1 and p-Smad2/3 (Cao et al., 2015; Sun SF. et al., 2018). In addition, multi-glycoside of Tripterygium wilfordii could reduce ECM deposition and glomerulosclerosis by regulating mRNA or protein expressions of TGF-β1, Smad3, p-Smad2/3 and Smad7 in adriamycin-induced nephropathy rats (Wan et al., 2014). Moreover, celastrol could inhibit UUO- or TGF-β1-induced activation of Smad3, which can be candidate drug against renal fibrosis (Tang et al., 2018).
Compared with experimental researches, a number of studies have demonstrated that TCMs showed beneficial effects on patients with CKD (Chen et al., 2018a). Meta-analysis analysis showed that ligustrazine injection lowered the levels of creatinine and urea in serum as well as proteinuria, urine microalbumin and albumin excretion rate in patients with diabetic nephropathy including 858 treatment patients and 787 controls from 25 randomized controlled trial centers (Wang et al., 2012). In addition, ligustrazine blunted contrast-induced nephropathy in 148 patients with unstable angina (Ye et al., 2017). Recently, a randomized controlled trial showed Bupi yishen formula mitigated patients with non-diabetic stage 4 CKD (Mao et al., 2020). Moreover, a retrospective population-based cohort study showed that Chinese herbal medicine, such as Salviae miltiorrhizae and Jia-Wei-Xiao-Yao-San, mitigated CKD in patients with chronic hepatitis C (Chang et al., 2019). So far, although TCMs have been used for treating patients with CKD, few study demonstrated that TCMs were applied to treatment kidney diseases through targeting TGF-β/Smad signaling pathway.
5.2 The Traditional Chinese Medicinal Prescriptions Against Tubulointerstitial Fibrosis
There were a number of traditional Chinese medicinal prescriptions that inhibited renal fibrosis by regulating TGF-β/Smad pathway (Chen et al., 2018a; Yang et al., 2021). Oryeongsan (Wulingsan) is frequently used to treat nephrosis, dropsy and uremia. Oryeongsan could improve renal function, reduce mesangial expansion and downregulate expression of TGF-β1, Smad2, Smad4, Smad7 and collagen IV in the db/db mice (Yoon et al., 2014). You-gui Pill is treated to warm and recuperate “kidney-yang,” which could ameliorate tubulointerstitial fibrosis by downregulating translocation of p-Smad2/3 (Wang et al., 2015). Shenqiwan could ameliorate renal fibrosis by inhibiting mRNA and protein expressions of p-Smad2/3 via upregulating Smad7 in adenine-induced rats (Chen H. et al., 2017). HuangQi decoction inhibited protein expressions of α-SMA, collagen I, collagen III and collagen IV through downregulating the expressions of TGF-β1, TGFβRI, TGFβRII, Smad4, Smad2/3, p-Smad2/3 and preserved Smad7 protein expression in the UUO mice (Zhao et al., 2016). Fu-Fang-Jin-Qian-Cao granules, widely used to treat nephrolithiasis, alleviated renal EMT and fibrosis induced by calcium oxalate via downregulating protein expressions of TGF-β1, TGFβRI, TGFβRII, p-Smad3 and p-Smad2, and preserving Smad7 protein expressions (Liu W. et al., 2020). Bu-Shen-Jiang-Ya decoction can relieve renal fibrosis in Dahl salt-sensitive rats by inhibiting protein expressions of TGF-β1 and Smad2/3 (Liu WR. et al., 2020). Uremic Clearance Granules attenuated renal dysfunction and renal fibrosis by downregulating protein expressions of TGF-β1, TGFβRI, p-Smad2/3 and Smad4, and preserving Smad7 protein expression in the kidney of rats induced by adenine and UUO (Huang et al., 2014). Tangshen formula has been demonstrated to slow diabetic kidney disease in humans and animals. The latest findings indicated that Tangshen formula retarded renal fibrosis in rats with diabetic kidney disease by repressing TGF-β1/Smad3 signaling and lncRNA MEG3 expression (Zhou X. F. et al., 2021).
There are a variety of natural products and Chinese medicine compounds formed by different compatibility compositions of natural products. Further in-depth studies of the underlying mechanism of natural products through TGF-β/Smad signaling pathway is beneficial to the popularization and application of natural products.
6 Conclusion
The common pathway of progression of various CKD is renal fibrosis, so making every effort to prevent progression of renal fibrosis can effectively reduce global economic burden. In the early development of fibrosis, inhibiting or eliminating pathogenical factors and the use of antifibrotic agents can partially or completely inhibit renal fibrosis. However, due to the strong compensation of glomerular filtration function, the renal function may be unaffected or slightly damaged, so the patients often have no symptoms, thereby missing the optimal treatment opportunity. In view of the important role of TGF-β/Smad pathway in renal fibrosis, inhibition of renal fibrosis by targeting potential targets of this pathway will be an attractive therapeutic approach. TGF-β1 is considered to be the key mediator involved in fibrosis. Smad3 is the core of the pathogenesis of interstitial fibrosis. Smad2 and Smad7 have the renal protective effect. Smad4 enhances Smad3-mediated renal fibrosis by transcription and inhibits NF-κB-driven inflammation via a Smad7-dependent molecular mechanism. Therefore, targeting TGF-β1 and its receptors as well as its downstream Smad proteins and Smad-dependent lncRNAs is regarded as one of the feasible therapeutic strategies.
Currently, the renin-angiotensin system blockade is central to the treatment of patients with CKD, the renoprotective effects of which are aimed at preventing or slowing the progression of ESRD (Lee et al., 2015), but its efficacy is limited. Furthermore, the incidence of CKD increases year by year and has reached epidemic proportions (Wang et al., 2021a). It means that we will face great challenges to find more effective drugs for treatment of renal fibrosis. Meanwhile, natural products have unique advantages in inhibiting renal fibrosis and many natural products have been proved to target TGF-β/Smad signaling pathway to treat renal fibrosis, which means that we will have more opportunities to explore new treatments for renal fibrosis. Although there is a long way to go to suppress renal fibrosis in the future, we are confident that the more frustrated we are on this road, the more courageous we are, and we firmly believe that new treatments will always be found.
Data Availability Statement
The raw data supporting the conclusions of this article will be made available by the authors, without undue reservation.
Author Contributions
X-YY and Y-YZ were responsible for the conception. X-YY, QS and Y-YZ wrote the manuscript. Y-MZ and LZ revised the manuscript. All authors read and approved the final manuscript.
Funding
The authors are grateful for the financial support provided by National Natural Science Foundation of China (Nos. 82174366, 81872985) and Shaanxi Key Science and Technology Plan Project (No. 2019ZDLSF04-04-02).
Conflict of Interest
The authors declare that the research was conducted in the absence of any commercial or financial relationships that could be construed as a potential conflict of interest.
Publisher’s Note
All claims expressed in this article are solely those of the authors and do not necessarily represent those of their affiliated organizations, or those of the publisher, the editors, and the reviewers. Any product that may be evaluated in this article, or claim that may be made by its manufacturer, is not guaranteed or endorsed by the publisher.
References
Aydin, Z., Karadag, S., Ozturk, S., Gursu, M., Uzun, S., Cebeci, E., et al. (2019). Evaluation of the Relationship between Advanced Oxidation End Products and Inflammatory Markers in Maintenance Hemodialysis Patients. Jna 1 (2), 24–30. doi:10.14302/issn.2574-4488.jna-19-3112
Bhargava, V., Singh, K., Meena, P., and Sanyal, R. (2021). Nephrogenic Systemic Fibrosis: A Frivolous Entity. World J. Nephrol. 10 (3), 29–36. doi:10.5527/wjn.v10.i3.29
Blobe, G. C., Schiemann, W. P., and Lodish, H. F. (2000). Role of Transforming Growth Factor Beta in Human Disease. N. Engl. J. Med. 342 (18), 1350–1358. doi:10.1056/nejm200005043421807
Cai, H., Su, S., Li, Y., Zeng, H., Zhu, Z., Guo, J., et al. (2018). Protective Effects of Salvia Miltiorrhiza on Adenine-Induced Chronic Renal Failure by Regulating the Metabolic Profiling and Modulating the NADPH oxidase/ROS/ERK and TGF-β/Smad Signaling Pathways. J. Ethnopharmacol 212, 153–165. doi:10.1016/j.jep.2017.09.021
Cao, Y., Huang, X., Fan, Y., and Chen, X. (2015). Protective Effect of Triptolide against Glomerular Mesangial Cell Proliferation and Glomerular Fibrosis in Rats Involves the TGF-β1/Smad Signaling Pathway. Evidence-Based Complement. Altern. Med. 2015, 1–10. doi:10.1155/2015/814089
Cao, Y. J., Pu, Z. J., Tang, Y. P., Shen, J., Chen, Y. Y., Kang, A., et al. (2017). Advances in Bio-Active Constituents, Pharmacology and Clinical Applications of Rhubarb. Chin. Med. 12, 36. doi:10.1186/s13020-017-0158-5
Carta, P., Lorenzo, D. M., Luciano, M., Aida, L., Caroti, L., and Cirami, L. (2020). Malignancies after Renal Transplantation: A Single center Retrospective Study. J. Nephrol. Hypertens. 3 (1), 1009. doi:10.12659/AOT.890706
Chang, C.-P., Su, Y.-C., Lin, M.-C., and Huang, S.-T. (2019). Chinese Herbal Medicine Ameliorated the Development of Chronic Kidney Disease in Patients with Chronic Hepatitis C: a Retrospective Population-Based Cohort Study. Evidence-Based Complement. Altern. Med. 2019, 1–11. doi:10.1155/2019/5319456
Chen, D. Q., Cao, G., Chen, H., Argyopoulos, C. P., Yu, H., Su, W., et al. (2019c). Identification of Serum Metabolites Associating with Chronic Kidney Disease Progression and Anti-fibrotic Effect of 5-methoxytryptophan. Nat. Commun. 10 (1), 1476. doi:10.1038/s41467-019-09329-0
Chen, D. Q., Cao, G., Chen, H., Liu, D., Su, W., Yu, X. Y., et al. (2017a). Gene and Protein Expressions and Metabolomics Exhibit Activated Redox Signaling and Wnt/β-Catenin Pathway Are Associated with Metabolite Dysfunction in Patients with Chronic Kidney Disease. Redox Biol. 12, 505–521. doi:10.1016/j.redox.2017.03.017
Chen, D. Q., Cao, G., Zhao, H., Chen, L., Yang, T., Wang, M., et al. (2019b). Combined Melatonin and Poricoic Acid A Inhibits Renal Fibrosis through Modulating the Interaction of Smad3 and β-catenin Pathway in AKI-To-CKD Continuum. Ther. Adv. Chronic Dis. 10, 2040622319869116. doi:10.1177/2040622319869116
Chen, D. Q., Feng, Y. L., Cao, G., and Zhao, Y. Y. (2018a). Natural Products as a Source for Antifibrosis Therapy. Trends Pharmacol. Sci. 39 (11), 937–952. doi:10.1016/j.tips.2018.09.002
Chen, D. Q., Feng, Y. L., Chen, L., Liu, J. R., Wang, M., Vaziri, N. D., et al. (2019a). Poricoic Acid A Enhances Melatonin Inhibition of AKI-To-CKD Transition by Regulating Gas6/AxlNFκB/Nrf2 axis. Free Radic. Biol. Med. 134, 484–497. doi:10.1016/j.freeradbiomed.2019.01.046
Chen, D. Q., Feng, Y. L., Tian, T., Chen, H., Yin, L., Zhao, Y. Y., et al. (2014). Diuretic and Anti-diuretic Activities of Fractions of Alismatis Rhizoma. J. Ethnopharmacol 157, 114–118. doi:10.1016/j.jep.2014.09.022
Chen, D. Q., Hu, H. H., Wang, Y. N., Feng, Y. L., Cao, G., and Zhao, Y. Y. (2018b). Natural Products for the Prevention and Treatment of Kidney Disease. Phytomedicine 50, 50–60. doi:10.1016/j.phymed.2018.09.182
Chen, D. Q., Wang, Y. N., Vaziri, N. D., Chen, L., Hu, H. H., and Zhao, Y. Y. (2020a). Poricoic Acid A Activates AMPK to Attenuate Fibroblast Activation and Abnormal Extracellular Matrix Remodelling in Renal Fibrosis. Phytomedicine 72, 153232. doi:10.1016/j.phymed.2020.153232
Chen, D. Q., Wu, X. Q., Chen, L., Hu, H. H., Wang, Y. N., and Zhao, Y. Y. (2020b). Poricoic Acid A as a Modulator of TPH-1 Expression Inhibits Renal Fibrosis via Modulating Protein Stability of β-catenin and β-catenin-mediated Transcription. Ther. Adv. Chronic Dis. 11, 2040622320962648. doi:10.1177/2040622320962648
Chen, H., Cao, G., Chen, D. Q., Wang, M., Vaziri, N. D., Zhang, Z. H., et al. (2016b). Metabolomics Insights into Activated Redox Signaling and Lipid Metabolism Dysfunction in Chronic Kidney Disease Progression. Redox Biol. 10, 168–178. doi:10.1016/j.redox.2016.09.014
Chen, H., Tian, T., Miao, H., and Zhao, Y. Y. (2016c). Traditional Uses, Fermentation, Phytochemistry and Pharmacology of Phellinus Linteus: A Review. Fitoterapia 113, 6–26. doi:10.1016/j.fitote.2016.06.009
Chen, H., Wang, M. C., Chen, Y. Y., Chen, L., Wang, Y. N., Vaziri, N. D., et al. (2020c). Alisol B 23-acetate Attenuates CKD Progression by Regulating the Renin-Angiotensin System and Gut-Kidney axis. Ther. Adv. Chronic Dis. 11, 2040622320920025. doi:10.1177/2040622320920025
Chen, H., Yang, T., Wang, M. C., Chen, D. Q., Yang, Y., and Zhao, Y. Y. (2018c). Novel RAS Inhibitor 25-O-Methylalisol F Attenuates Epithelial-To-Mesenchymal Transition and Tubulo-Interstitial Fibrosis by Selectively Inhibiting TGF-β-Mediated Smad3 Phosphorylation. Phytomedicine 42, 207–218. doi:10.1016/j.phymed.2018.03.034
Chen, H., Chen, L., Tang, D.-D., Chen, D.-Q., Miao, H., Zhao, Y.-Y., et al. (2016a). Metabolomics Reveals Hyperlipidemic Biomarkers and Antihyperlipidemic Effect of Poria Cocos. Cmb 4 (2), 104–115. doi:10.2174/2213235X04999160603155430
Chen, H., Xu, Y., Yang, Y., Zhou, X., Dai, S., and Li, C. (2017b). Shenqiwan Ameliorates Renal Fibrosis in Rats by Inhibiting TGF-β1/Smads Signaling Pathway. Evidence-Based Complement. Altern. Med. 2017, 1–9. doi:10.1155/2017/7187038
Chen, H., Yuan, B., Miao, H., Tan, Y., Bai, X., Zhao, Y.-Y., et al. (2015). Urine Metabolomics Reveals New Insights into Hyperlipidemia and the Therapeutic Effect of Rhubarb. Anal. Methods R. Soc. Chem. 7 (7), 3113–3123. doi:10.1039/C5AY00023H
Chen, L., Cao, G., Wang, M., Feng, Y. L., Chen, D. Q., Vaziri, N. D., et al. (2019d). The Matrix Metalloproteinase-13 Inhibitor Poricoic Acid ZI Ameliorates Renal Fibrosis by Mitigating Epithelial-Mesenchymal Transition. Mol. Nutr. Food Res. 63 (13).e1900132. doi:10.1002/mnfr.201900132
Chen, L., Chen, D. Q., Liu, J. R., Zhang, J., Vaziri, N. D., Zhuang, S., et al. (2019e). Unilateral Ureteral Obstruction Causes Gut Microbial Dysbiosis and Metabolome Disorders Contributing to Tubulointerstitial Fibrosis. Exp. Mol. Med. 51 (3), 1–18. doi:10.1038/s12276-019-0234-2
Chen, L., Yang, T., Lu, D. W., Zhao, H., Feng, Y. L., Chen, H., et al. (2018d). Central Role of Dysregulation of TGF-β/Smad in CKD Progression and Potential Targets of its Treatment. Biomed. Pharmacother. 101, 670–681. doi:10.1016/j.biopha.2018.02.090
Chen, W., and Ten Dijke, P. (2016). Immunoregulation by Members of the TGFβ Superfamily. Nat. Rev. Immunol. 16 (12), 723–740. doi:10.1038/nri.2016.112
Dou, F., Ding, Y., Wang, C., Duan, J., Wang, W., Xu, H., et al. (2020). Chrysophanol Ameliorates Renal Interstitial Fibrosis by Inhibiting the TGF-β/Smad Signaling Pathway. Biochem. Pharmacol. 180, 114079. doi:10.1016/j.bcp.2020.114079
Dou, F., Miao, H., Wang, J. W., Chen, L., Wang, M., Chen, H., et al. (2018). An Integrated Lipidomics and Phenotype Study Reveals Protective Effect and Biochemical Mechanism of Traditionally Used Alisma Orientale Juzepzuk in Chronic Kidney Disease. Front. Pharmacol. 9, 53. doi:10.3389/fphar.2018.00053
Feng, M., Tang, P. M., Huang, X. R., Sun, S. F., You, Y. K., Xiao, J., et al. (2018). TGF-β Mediates Renal Fibrosis via the Smad3-Erbb4-IR Long Noncoding RNA Axis. Mol. Ther. 26 (1), 148–161. doi:10.1016/j.ymthe.2017.09.024
Feng, Y. L., Cao, G., Chen, D. Q., Vaziri, N. D., Chen, L., Zhang, J., et al. (2019b). Microbiome-metabolomics Reveals Gut Microbiota Associated with Glycine-Conjugated Metabolites and Polyamine Metabolism in Chronic Kidney Disease. Cell Mol Life Sci 76 (24), 4961–4978. doi:10.1007/s00018-019-03155-9
Feng, Y. L., Chen, H., Chen, D. Q., Vaziri, N. D., Su, W., Ma, S. X., et al. (2019c). Activated NF-κB/Nrf2 and Wnt/β-Catenin Pathways Are Associated with Lipid Metabolism in CKD Patients with Microalbuminuria and Macroalbuminuria. Biochim. Biophys. Acta Mol. Basis Dis. 1865 (9), 2317–2332. doi:10.1016/j.bbadis.2019.05.010
Feng, Y. L., Chen, H., Tian, T., Chen, D. Q., Zhao, Y. Y., and Lin, R. C. (2014). Diuretic and Anti-diuretic Activities of the Ethanol and Aqueous Extracts of Alismatis Rhizoma. J. Ethnopharmacol 154 (2), 386–390. doi:10.1016/j.jep.2014.04.017
Feng, Y. L., Lei, P., Tian, T., Yin, L., Chen, D. Q., Chen, H., et al. (2013). Diuretic Activity of Some Fractions of the Epidermis of Poria Cocos. J. Ethnopharmacol 150 (3), 1114–1118. doi:10.1016/j.jep.2013.10.043
Feng, Y. L., Chen, D. Q., Vaziri, N. D., Guo, Y., and Zhao, Y. Y. (2019a). Small Molecule Inhibitors of Epithelial‐mesenchymal Transition for the Treatment of Cancer and Fibrosis. Med. Res. Rev. 40, 54–78. doi:10.1002/med.21596
Fukasawa, H., Yamamoto, T., Togawa, A., Ohashi, N., Fujigaki, Y., Oda, T., et al. (2004). Down-regulation of Smad7 Expression by Ubiquitin-dependent Degradation Contributes to Renal Fibrosis in Obstructive Nephropathy in Mice. Proc. Natl. Acad. Sci. U S A. 101 (23), 8687–8692. doi:10.1073/pnas.0400035101
Gifford, C. C., Tang, J., Costello, A., Khakoo, N. S., Nguyen, T. Q., Goldschmeding, R., et al. (2021). Negative Regulators of TGF-Β1 Signaling in Renal Fibrosis; Pathological Mechanisms and Novel Therapeutic Opportunities. Clin. Sci. (Lond) 135 (2), 275–303. doi:10.1042/cs20201213
Gu, Y. Y., Liu, X. S., Huang, X. R., Yu, X. Q., and Lan, H. Y. (2020). Diverse Role of TGF-β in Kidney Disease. Front Cel Dev Biol 8, 123. doi:10.3389/fcell.2020.00123
Guo, X. H., Liu, Z. H., Dai, C. S., Li, H., Liu, D., and Li, L. S. (2001). Rhein Inhibits Renal Tubular Epithelial Cell Hypertrophy and Extracellular Matrix Accumulation Induced by Transforming Growth Factor Beta1. Acta Pharmacol. Sin 22 (10), 934–938.
Hansrivijit, P., Chen, Y. J., Lnu, K., Trongtorsak, A., Puthenpura, M. M., Thongprayoon, C., et al. (2021). Prediction of Mortality Among Patients with Chronic Kidney Disease: A Systematic Review. World J. Nephrol. 10 (4), 59–75. doi:10.5527/wjn.v10.i4.59
Hill, N. R., Fatoba, S. T., Oke, J. L., Hirst, J. A., O'Callaghan, C. A., Lasserson, D. S., et al. (2016). Global Prevalence of Chronic Kidney Disease - A Systematic Review and Meta-Analysis. PLoS One 11 (7). e0158765. doi:10.1371/journal.pone.0158765
Hu, H. H., Cao, G., Wu, X. Q., Vaziri, N. D., and Zhao, Y. Y. (2020). Wnt Signaling Pathway in Aging-Related Tissue Fibrosis and Therapies. Ageing Res. Rev. 60, 101063. doi:10.1016/j.arr.2020.101063
Hu, H. H., Chen, D. Q., Wang, Y. N., Feng, Y. L., Cao, G., Vaziri, N. D., et al. (2018). New Insights into TGF-β/Smad Signaling in Tissue Fibrosis. Chem. Biol. Interact 292, 76–83. doi:10.1016/j.cbi.2018.07.008
Hu, Q., Gao, L., Peng, B., and Liu, X. (2017). Baicalin and Baicalein Attenuate Renal Fibrosis In Vitro via Inhibition of the TGF-Β1 Signaling Pathway. Exp. Ther. Med. 14 (4), 3074–3080. doi:10.3892/etm.2017.4888
Huang, W. J., Liu, W. J., Xiao, Y. H., Zheng, H. J., Xiao, Y., Jia, Q., et al. (2020). Tripterygium and its Extracts for Diabetic Nephropathy: Efficacy and Pharmacological Mechanisms. Biomed. Pharmacother. 121, 109599. doi:10.1016/j.biopha.2019.109599
Huang, Y. (2018). The Novel Regulatory Role of lncRNA-miRNA-mRNA axis in Cardiovascular Diseases. J. Cel Mol Med 22 (12), 5768–5775. doi:10.1111/jcmm.13866
Huang, Y. R., Wei, Q. X., Wan, Y. G., Sun, W., Mao, Z. M., Chen, H. L., et al. (2014). Ureic Clearance Granule, Alleviates Renal Dysfunction and Tubulointerstitial Fibrosis by Promoting Extracellular Matrix Degradation in Renal Failure Rats, Compared with Enalapril. J. Ethnopharmacol 155 (3), 1541–1552. doi:10.1016/j.jep.2014.07.048
Humphreys, B. D. (2018). Mechanisms of Renal Fibrosis. Annu. Rev. Physiol. 80, 309–326. doi:10.1146/annurev-physiol-022516-034227
Jain, D., Haddad, D. B., and Goel, N. (2019). Choice of Dialysis Modality Prior to Kidney Transplantation: Does it Matter? World J. Nephrol. 8 (1), 1–10. doi:10.5527/wjn.v8.i1.1
Jiang, M., Fan, J., Qu, X., Li, S., Nilsson, S. K., Sun, Y. B. Y., et al. (2019). Combined Blockade of Smad3 and JNK Pathways Ameliorates Progressive Fibrosis in Folic Acid Nephropathy. Front. Pharmacol. 10, 880. doi:10.3389/fphar.2019.00880
Jiang, W. Y. (2005). Therapeutic Wisdom in Traditional Chinese Medicine: a Perspective from Modern Science. Discov. Med. 5 (11), 455–461. doi:10.1016/j.tips.2005.09.006
Lan, H. Y. (2011). Diverse Roles of TGF-β/Smads in Renal Fibrosis and Inflammation. Int. J. Biol. Sci. 7 (7), 1056–1067. doi:10.7150/ijbs.7.1056
Lawson, J. S., Syme, H. M., Wheeler-Jones, C. P., and Elliott, J. (2016). Urinary Active Transforming Growth Factor β in Feline Chronic Kidney Disease. Vet. J. 214, 1–6. doi:10.1016/j.tvjl.2016.02.004
Lee, S. Y., Kim, S. I., and Choi, M. E. (2015). Therapeutic Targets for Treating Fibrotic Kidney Diseases. Transl Res. 165 (4), 512–530. doi:10.1016/j.trsl.2014.07.010
Li, J., Sun, Y. B. Y., Chen, W., Fan, J., Li, S., Qu, X., et al. (2020). Smad4 Promotes Diabetic Nephropathy by Modulating Glycolysis and OXPHOS. EMBO Rep. 21 (2), e48781. doi:10.15252/embr.201948781
Li, Q., Ming, Y., Jia, H., and Wang, G. (2021b). Poricoic Acid A Suppresses TGF-Β1-Induced Renal Fibrosis and Proliferation via the PDGF-C, Smad3 and MAPK Pathways. Exp. Ther. Med. 21 (4), 289. doi:10.3892/etm.2021.9720
Li, Q., Xing, C., and Yuan, Y. (2021a). Mitochondrial Targeting of Herbal Medicine in Chronic Kidney Disease. Front. Pharmacol. 12, 632388. doi:10.3389/fphar.2021.632388
Li, S., Xu, P., Han, L., Mao, W., Wang, Y., Luo, G., et al. (2017). Disease-syndrome Combination Modeling: Metabolomic Strategy for the Pathogenesis of Chronic Kidney Disease. Sci. Rep. 7 (1), 8830. doi:10.1038/s41598-017-09311-0
Li, S., Yu, L., He, A., and Liu, Q. (2019). Klotho Inhibits Unilateral Ureteral Obstruction-Induced Endothelial-To-Mesenchymal Transition via TGF-β1/Smad2/Snail1 Signaling in Mice. Front. Pharmacol. 10, 348. doi:10.3389/fphar.2019.00348
Liu, J.-R., Miao, H., Deng, D.-Q., Vaziri, N. D., Li, P., and Zhao, Y.-Y. (2020a). Gut Microbiota-Derived Tryptophan Metabolism Mediates Renal Fibrosis by Aryl Hydrocarbon Receptor Signaling Activation. Cell. Mol. Life Sci. 78, 909–922. doi:10.1007/s00018-020-03645-1
Liu, W., Li, Y., Xiong, X., Chen, Y., Qiao, L., Wang, J., et al. (2020b). Traditional Chinese Medicine Protects against Hypertensive Kidney Injury in Dahl Salt-Sensitive Rats by Targeting Transforming Growth Factor-β Signaling Pathway. Biomed. Pharmacother. 131, 110746. doi:10.1016/j.biopha.2020.110746
Liu, W. R., Lu, H. T., Zhao, T. T., Ding, J. R., Si, Y. C., Chen, W., et al. (2020c). Fu-Fang-Jin-Qian-Cao Herbal Granules Protect against the Calcium Oxalate-Induced Renal EMT by Inhibiting the TGF-Β/smad Pathway. Pharm. Biol. 58 (1), 1115–1122. doi:10.1080/13880209.2020.1844241
Liu, Z., Huang, X. R., Chen, H. Y., Fung, E., Liu, J., and Lan, H. Y. (2017). Deletion of Angiotensin-Converting Enzyme-2 Promotes Hypertensive Nephropathy by Targeting Smad7 for Ubiquitin Degradation. Hypertension 70 (4), 822–830. doi:10.1161/hypertensionaha.117.09600
Luo, B., He, Z., Huang, S., Wang, J., Han, D., Xue, H., et al. (2020). Long Non-coding RNA 554 Promotes Cardiac Fibrosis via TGF-Β1 Pathway in Mice Following Myocardial Infarction. Front. Pharmacol. 11, 585680. doi:10.3389/fphar.2020.585680
Luo, L. P., Suo, P., Ren, L. L., Liu, H. J., Zhang, Y., and Zhao, Y. Y. (2021). Shenkang Injection and its Three Anthraquinones Ameliorates Renal Fibrosis by Simultaneous Targeting IƙB/NF-Ƙb and Keap1/Nrf2 Signaling Pathways. Front. Pharmacol. 12, 800522. doi:10.3389/fphar.2021.800522
Ma, G., Chen, F., Liu, Y., Zheng, L., Jiang, X., Tian, H., et al. (2022). Nur77 Ameliorates Age-Related Renal Tubulointerstitial Fibrosis by Suppressing the TGF-β/Smads Signaling Pathway. Faseb j. 36 (2),e22124. doi:10.1096/fj.202101332R
Ma, L., Li, H., Zhang, S., Xiong, X., Chen, K., Jiang, P., et al. (2018a). Emodin Ameliorates Renal Fibrosis in Rats via TGF-β1/Smad Signaling Pathway and Function Study of Smurf 2. Int. Urol. Nephrol. 50 (2), 373–382. doi:10.1007/s11255-017-1757-x
Ma, S.-X., Shang, Y.-Q., Zhang, H.-Q., and Su, W. (2018b). Action Mechanisms and Therapeutic Targets of Renal Fibrosis. Jna 1 (2), 4–14. doi:10.14302/issn.2574-4488.jna-18-2443
Ma, T. T., and Meng, X. M. (2019). TGF-β/Smad and Renal Fibrosis. Adv. Exp. Med. Biol. 1165, 347–364. doi:10.1007/978-981-13-8871-2_16
Ma, Z., Tang, Y., Zhong, L., Yu, K., and He, L. (2016). Anti-fibrosis and Relative Mechanism of Salvianolic Acid A on Rat Model with Renal Fibrosis. Int. J. Clin. Exp. Med. 9 (7), 12713–12720.
Macias, M. J., Martin-Malpartida, P., and Massagué, J. (2015). Structural Determinants of Smad Function in TGF-β Signaling. Trends Biochem. Sci. 40 (6), 296–308. doi:10.1016/j.tibs.2015.03.012
Mantovani, A., and Zusi, C. (2020). PNPLA3 Gene and Kidney Disease. Explor Med. 1, 42–50. doi:10.37349/emed.2020.00004
Mao, W., Yang, N., Zhang, L., Li, C., Wu, Y., Ouyang, W., et al. (2020). Bupi Yishen Formula versus Losartan for Non-diabetic Stage 4 Chronic Kidney Disease: a Randomized Controlled Trial. Front. Pharmacol. 11, 627185. doi:10.3389/fphar.2020.627185
Medina Rangel, P. X., Priyadarshini, A., and Tian, X. (2021). New Insights into the Immunity and Podocyte in Glomerular Health and Disease: From Pathogenesis to Therapy in Proteinuric Kidney Disease. Integr. Med. Nephrol. Androl. 8, 5. doi:10.4103/imna.imana_26_21
Meng, X., Wei, M., Wang, D., Qu, X., Zhang, K., Zhang, N., et al. (2020). Astragalus Polysaccharides Protect Renal Function and Affect the TGF-β/Smad Signaling Pathway in Streptozotocin-Induced Diabetic Rats. J. Int. Med. Res. 48 (5), 300060520903612. doi:10.1177/0300060520903612
Meng, X. M., Chung, A. C., and Lan, H. Y. (2013). Role of the TGF-Β/bmp-7/Smad Pathways in Renal Diseases. Clin. Sci. (Lond) 124 (4), 243–254. doi:10.1042/cs20120252
Meng, X. M., Huang, X. R., Chung, A. C., Qin, W., Shao, X., Igarashi, P., et al. (2010). Smad2 Protects against TGF-beta/Smad3-Mediated Renal Fibrosis. J. Am. Soc. Nephrol. 21 (9), 1477–1487. doi:10.1681/asn.2009121244
Meng, X. M., Huang, X. R., Xiao, J., Chen, H. Y., Zhong, X., Chung, A. C., et al. (2012b). Diverse Roles of TGF-β Receptor II in Renal Fibrosis and Inflammation In Vivo and In Vitro. J. Pathol. 227 (2), 175–188. doi:10.1002/path.3976
Meng, X. M., Huang, X. R., Xiao, J., Chung, A. C., Qin, W., Chen, H. Y., et al. (2012a). Disruption of Smad4 Impairs TGF-β/Smad3 and Smad7 Transcriptional Regulation during Renal Inflammation and Fibrosis In Vivo and In Vitro. Kidney Int. 81 (3), 266–279. doi:10.1038/ki.2011.327
Meng, X. M., Nikolic-Paterson, D. J., and Lan, H. Y. (2016a). TGF-β: the Master Regulator of Fibrosis. Nat. Rev. Nephrol. 12 (6), 325–338. doi:10.1038/nrneph.2016.48
Meng, X. M., Ren, G. L., Gao, L., Li, H. D., Wu, W. F., Li, X. F., et al. (2016b). Anti-fibrotic Effect of Wogonin in Renal Tubular Epithelial Cells via Smad3-dependent Mechanisms. Eur. J. Pharmacol. 789, 134–143. doi:10.1016/j.ejphar.2016.07.014
Meng, X. M., Tang, P. M., Li, J., and Lan, H. Y. (2015). TGF-β/Smad Signaling in Renal Fibrosis. Front. Physiol. 6, 82. doi:10.3389/fphys.2015.00082
Miao, H., Cao, G., Wu, X. Q., Chen, Y. Y., Chen, D. Q., Chen, L., et al. (2020). Identification of Endogenous 1-aminopyrene as a Novel Mediator of Progressive Chronic Kidney Disease via Aryl Hydrocarbon Receptor Activation. Br. J. Pharmacol. 177 (15), 3415–3435. doi:10.1111/bph.15062
Miao, H., Wu, X. Q., Zhang, D. D., Wang, Y. N., Guo, Y., Li, P., et al. (2021a). Deciphering the Cellular Mechanisms Underlying Fibrosis-Associated Diseases and Therapeutic Avenues. Pharmacol. Res. 163, 105316. doi:10.1016/j.phrs.2020.105316
Miao, H., Zhang, L., Chen, D. Q., Chen, H., Zhao, Y. Y., and Ma, S. C. (2017). Urinary Biomarker and Treatment Mechanism of Rhizoma Alismatis on Hyperlipidemia. Biomed. Chromatogr. 31 (4), e3829. doi:10.1002/bmc.3829
Miao, H., Li, M.-H., Zhang, X., Yuan, S.-J., Ho, C. C., and Zhao, Y.-Y. (2015). The Antihyperlipidemic Effect of Fu-Ling-Pi Is Associated with Abnormal Fatty Acid Metabolism as Assessed by UPLC-HDMS-Based Lipidomics. RSC Adv. 5 (79), 64208–64219. doi:10.1039/C5RA09766E
Miao, H., Wu, X. Q., Wang, Y. N., Chen, D. Q., Chen, L., Vaziri, N. D., et al. (2021b). 1‐Hydroxypyrene Mediates Renal Fibrosis through Aryl Hydrocarbon Receptor Signalling Pathway. Br. J Pharmacol. 179, 103–124. doi:10.1111/bph.15705
Morishita, Y., Yoshizawa, H., Watanabe, M., Ishibashi, K., Muto, S., Kusano, E., et al. (2014). siRNAs Targeted to Smad4 Prevent Renal Fibrosis In Vivo. Sci. Rep. 4, 6424. doi:10.1038/srep06424
Nakerakanti, S., and Trojanowska, M. (2012). The Role of TGF-β Receptors in Fibrosis. Open Rheumatol. J. 6, 156–162. doi:10.2174/1874312901206010156
Newman, D. J., and Cragg, G. M. (2020). Natural Products as Sources of New Drugs over the Nearly Four Decades from 01/1981 to 09/2019. J. Nat. Prod. 83 (3), 770–803. doi:10.1021/acs.jnatprod.9b01285
Nie, Y., Li, S., Yi, Y., Su, W., Chai, X., Jia, D., et al. (2014). Effects of astragalus Injection on the TGFβ/Smad Pathway in the Kidney in Type 2 Diabetic Mice. BMC Complement. Altern. Med. 14, 148. doi:10.1186/1472-6882-14-148
Qin, M. Y., Huang, S. Q., Zou, X. Q., Zhong, X. B., Yang, Y. F., Zhang, Y. T., et al. (2021). Drug-containing Serum of Rhubarb-astragalus Capsule Inhibits the Epithelial-Mesenchymal Transformation of HK-2 by Downregulating TGF-β1/p38MAPK/Smad2/3 Pathway. J. Ethnopharmacol 280, 114414. doi:10.1016/j.jep.2021.114414
Ravid, J. D., Kamel, M. H., and Chitalia, V. C. (2021). Uraemic Solutes as Therapeutic Targets in CKD-Associated Cardiovascular Disease. Nat. Rev. Nephrol. 17 (6), 402–416. doi:10.1038/s41581-021-00408-4
Sato, M., Muragaki, Y., Saika, S., Roberts, A. B., and Ooshima, A. (2003). Targeted Disruption of TGF-beta1/Smad3 Signaling Protects against Renal Tubulointerstitial Fibrosis Induced by Unilateral Ureteral Obstruction. J. Clin. Invest. 112 (10), 1486–1494. doi:10.1172/jci19270
Sawhney, H., and Gill, S. S. (2020). Renal Transplant Recipient Seizure Practical Management. World J. Nephrol. 9 (1), 1–8. doi:10.5527/wjn.v9.i1.1
Shao, G., Zhu, S., and Yang, B. (2021a). Applications of Herbal Medicine to Treat Autosomal Dominant Polycystic Kidney Disease. Front. Pharmacol. 12, 629848. doi:10.3389/fphar.2021.629848
Shao, M., Ye, C., Bayliss, G., and Zhuang, S. (2021b). New Insights into the Effects of Individual Chinese Herbal Medicines on Chronic Kidney Disease. Front. Pharmacol. 12, 774414. doi:10.3389/fphar.2021.774414
Song, M. K., Lee, J. H., Ryoo, I. G., Lee, S. H., Ku, S. K., and Kwak, M. K. (2019). Bardoxolone Ameliorates TGF-Β1-Associated Renal Fibrosis through Nrf2/Smad7 Elevation. Free Radic. Biol. Med. 138, 33–42. doi:10.1016/j.freeradbiomed.2019.04.033
Sun, H., Ke, C., Zhang, L., Tian, C., Zhang, Z., and Wu, S. (2020). Long Non-coding RNA (LncRNA)-ATB Promotes Inflammation, Cell Apoptosis and Senescence in Transforming Growth Factor-Β1 (TGF-Β1) Induced Human Kidney 2 (HK-2) Cells via TGFβ/SMAD2/3 Signaling Pathway. Med. Sci. Monit. 26, e922029. doi:10.12659/msm.922029
Sun, S. F., Tang, P. M. K., Feng, M., Xiao, J., Huang, X. R., Li, P., et al. (2018a). Novel lncRNA Erbb4-IR Promotes Diabetic Kidney Injury in Db/db Mice by Targeting miR-29b. Diabetes 67 (4), 731–744. doi:10.2337/db17-0816
Sun, X., Ning, Y., Li, R., Liu, Y., Sun, W., and Wang, Z. (2018b). Triptolide Inhibits Lipopolysaccharide-Induced Proliferation, Induces Apoptosis and Causes Cell Cycle Arrest of Human Mesangial Cells In Vitro. Int. J. Clin. Exp. Med. 11 (4), 3135–3145. doi:10.1016/j.ejphar.2019.172811
Sureshbabu, A., Muhsin, S. A., and Choi, M. E. (2016). TGF-β Signaling in the Kidney: Profibrotic and Protective Effects. Am. J. Physiol. Ren. Physiol 310 (7), F596–f606. doi:10.1152/ajprenal.00365.2015
Tampe, B., and Zeisberg, M. (2014). Contribution of Genetics and Epigenetics to Progression of Kidney Fibrosis. Nephrol. Dial. Transpl. 29, iv72. doi:10.1093/ndt/gft025
Tang, J., Zhan, C., and Zhou, J. (2008). Effects of Tanshinone IIA on Transforming Growth Factor Beta1-Smads Signal Pathway in Renal Interstitial Fibroblasts of Rats. J. Huazhong Univ. Sci. Technolog Med. Sci. 28 (5), 539–542. doi:10.1007/s11596-008-0511-0
Tang, M., Cao, X., Zhang, K., Li, Y., Zheng, Q. Y., Li, G. Q., et al. (2018). Celastrol Alleviates Renal Fibrosis by Upregulating Cannabinoid Receptor 2 Expression. Cell Death Dis 9 (6), 601. doi:10.1038/s41419-018-0666-y
Tang, P. M., Nikolic-Paterson, D. J., and Lan, H. Y. (2019). Macrophages: Versatile Players in Renal Inflammation and Fibrosis. Nat. Rev. Nephrol. 15 (3), 144–158. doi:10.1038/s41581-019-0110-2
Tang, P. M., Tang, P. C., Chung, J. Y., and Lan, H. Y. (2017). TGF-β1 Signaling in Kidney Disease: From Smads to Long Non-coding RNAs. Noncoding RNA Res. 2 (1), 68–73. doi:10.1016/j.ncrna.2017.04.001
Tang, Q., Zhong, H., Xie, F., Xie, J., Chen, H., and Yao, G. (2014). Expression of miR-106b-25 Induced by Salvianolic Acid B Inhibits Epithelial-To-Mesenchymal Transition in HK-2 Cells. Eur. J. Pharmacol. 741, 97–103. doi:10.1016/j.ejphar.2014.07.051
Tian, F., Zhang, Z. Y., Sun, J., and Han, Y. C. (2021). Expression of miR-207 in Renal Tissue of Renal Fibrosis Rats and its Correlation Analysis with Protein Expression of TGF-Β1 and Smad3. Eur. Rev. Med. Pharmacol. Sci. 25 (2), 787–794. doi:10.26355/eurrev_202101_24641
Tian, T., Chen, H., and Zhao, Y. Y. (2014). Traditional Uses, Phytochemistry, Pharmacology, Toxicology and Quality Control of Alisma Orientale (Sam.) Juzep: a Review. J. Ethnopharmacol 158, 373–387. doi:10.1016/j.jep.2014.10.061
Wan, Y. G., Che, X. Y., Sun, W., Huang, Y. R., Meng, X. J., Chen, H. L., et al. (2014). Low-dose of Multi-Glycoside of Tripterygium Wilfordii Hook. f., a Natural Regulator of TGF-β1/Smad Signaling Activity Improves Adriamycin-Induced Glomerulosclerosis In Vivo. J. Ethnopharmacol 151 (3), 1079–1089. doi:10.1016/j.jep.2013.12.005
Wang, B., Ni, Q., Wang, X., and Lin, L. (2012). Meta-analysis of the Clinical Effect of Ligustrazine on Diabetic Nephropathy. Am. J. Chin. Med. 40 (1), 25–37. doi:10.1142/s0192415x12500036
Wang, L., Cao, A. L., Chi, Y. F., Ju, Z. C., Yin, P. H., Zhang, X. M., et al. (2015). You-gui Pill Ameliorates Renal Tubulointerstitial Fibrosis via Inhibition of TGF-β/Smad Signaling Pathway. J. Ethnopharmacol 169, 229–238. doi:10.1016/j.jep.2015.04.037
Wang, L., Chi, Y. F., Yuan, Z. T., Zhou, W. C., Yin, P. H., Zhang, X. M., et al. (2014). Astragaloside IV Inhibits Renal Tubulointerstitial Fibrosis by Blocking TGF-β/Smad Signaling Pathway In Vivo and In Vitro. Exp. Biol. Med. (Maywood) 239 (10), 1310–1324. doi:10.1177/1535370214532597
Wang, M., Chen, D. Q., Chen, L., Cao, G., Zhao, H., Liu, D., et al. (2018a). Novel Inhibitors of the Cellular Renin-Angiotensin System Components, Poricoic Acids, Target Smad3 Phosphorylation and Wnt/β-Catenin Pathway against Renal Fibrosis. Br. J. Pharmacol. 175 (13), 2689–2708. doi:10.1111/bph.14333
Wang, M., Chen, D. Q., Chen, L., Liu, D., Zhao, H., Zhang, Z. H., et al. (2018b). Novel RAS Inhibitors Poricoic Acid ZG and Poricoic Acid ZH Attenuate Renal Fibrosis via a Wnt/β-Catenin Pathway and Targeted Phosphorylation of Smad3 Signaling. J. Agric. Food Chem. 66 (8), 1828–1842. doi:10.1021/acs.jafc.8b00099
Wang, M., Chen, D. Q., Wang, M. C., Chen, H., Chen, L., Liu, D., et al. (2017). Poricoic Acid ZA, a Novel RAS Inhibitor, Attenuates Tubulo-Interstitial Fibrosis and Podocyte Injury by Inhibiting TGF-β/Smad Signaling Pathway. Phytomedicine 36, 243–253. doi:10.1016/j.phymed.2017.10.008
Wang, M., Hu, H. H., Chen, Y. Y., Chen, L., Wu, X. Q., and Zhao, Y. Y. (2020). Novel Poricoic Acids Attenuate Renal Fibrosis through Regulating Redox Signalling and Aryl Hydrocarbon Receptor Activation. Phytomedicine 79, 153323. doi:10.1016/j.phymed.2020.153323
Wang, P., Luo, M. L., Song, E., Zhou, Z., Ma, T., Wang, J., et al. (2018c). Long Noncoding RNA Lnc-TSI Inhibits Renal Fibrogenesis by Negatively Regulating the TGF-β/Smad3 Pathway. Sci. Transl Med. 10 (462), eaat2039. doi:10.1126/scitranslmed.aat2039
Wang, Y., Wang, Y., Yang, M., and Ma, X. (2021a). Implication of Cellular Senescence in the Progression of Chronic Kidney Disease and the Treatment Potencies. Biomed. Pharmacother. 135, 111191. doi:10.1016/j.biopha.2020.111191
Wang, Y.-N., Yang, C.-E., Zhang, D.-D., Chen, Y.-Y., Yu, X.-Y., Zhao, Y.-Y., et al. (2021c). Long Non-coding RNAs: A Double-Edged Sword in Aging Kidney and Renal Disease. Chemico-Biological Interactions 337, 109396. doi:10.1016/j.cbi.2021.109396
Wang, Y., Li, S., Liu, L., Su, B., Shi, L., Shi, M., et al. (2013a). Effect of Blood Glucose Control on Expression of Smad7 and Renal Fibrosis in Diabetic Rats. Chin. J. Pathophysiology 29 (1), 43–49.
Wang, Y. N., Hu, H. H., Zhang, D. D., Wu, X. Q., Liu, J. L., Guo, Y., et al. (2021d). The Dysregulation of Eicosanoids and Bile Acids Correlates with Impaired Kidney Function and Renal Fibrosis in Chronic Renal Failure. Metabolites 11 (2), 127. doi:10.3390/metabo11020127
Wang, Y. W., Zeng, Q., and Yu, R. H. (2021e). Treatment of Membranoproliferative Glomerulonephritis with Traditional Chinese Medicine and Rituximab: A Case Report. Integr. Med. Nephrol. Androl. 8, 3. doi:10.4103/imna.imna_10_21
Wang, Y. Z., Zhang, J., Zhao, Y. L., Li, T., Shen, T., Li, J. Q., et al. (2013b). Mycology, cultivation, traditional uses, phytochemistry and pharmacology of Wolfiporia cocos (Schwein.) Ryvarden et Gilb.: a review. J. Ethnopharmacol 147 (2), 265–276. doi:10.1016/j.jep.2013.03.027
Wang, Y., Zhou, X., Luo, M., and Zhao, T. (2021b). Xiaochaihu Decoction in Diabetic Kidney Disease: A Study Based on Network Pharmacology and Molecular Docking Technology. Integr. Med. Nephrol. Androl. 8, 13. doi:10.4103/imna.imna_21_21
Wang, Z., Fei, S., Suo, C., Han, Z., Tao, J., Xu, Z., et al. (2018d). Antifibrotic Effects of Hepatocyte Growth Factor on Endothelial-To-Mesenchymal Transition via Transforming Growth Factor-Beta1 (TGF-β1)/Smad and Akt/mTOR/P70S6K Signaling Pathways. Ann. Transpl. 23, 1–10. doi:10.12659/aot.906700
Webster, A. C., Nagler, E. V., Morton, R. L., and Masson, P. (2017). Chronic Kidney Disease. Lancet 389 (10075), 1238–1252. doi:10.1016/s0140-6736(16)32064-5
Wu, W., Huang, X. R., You, Y., Xue, L., Wang, X. J., Meng, X., et al. (2021). Latent TGF-Β1 Protects against Diabetic Kidney Disease via Arkadia/Smad7 Signaling. Int. J. Biol. Sci. 17 (13), 3583–3594. doi:10.7150/ijbs.61647
Xu, B. H., Sheng, J., You, Y. K., Huang, X. R., Ma, R. C. W., Wang, Q., et al. (2020a). Deletion of Smad3 Prevents Renal Fibrosis and Inflammation in Type 2 Diabetic Nephropathy. Metabolism 103, 154013. doi:10.1016/j.metabol.2019.154013
Xu, M., Li, S., Wang, J., Huang, S., Zhang, A., Zhang, Y., et al. (2020b). Cilomilast Ameliorates Renal Tubulointerstitial Fibrosis by Inhibiting the TGF-Β1-Smad2/3 Signaling Pathway. Front. Med. (Lausanne) 7, 626140. doi:10.3389/fmed.2020.626140
Yang, J., Li, J., Tan, R., He, X., Lin, X., Zhong, X., et al. (2021). Protocatechualdehyde Attenuates Obstructive Nephropathy through Inhibiting lncRNA9884 Induced Inflammation. Phytother Res. 35 (3), 1521–1533. doi:10.1002/ptr.6919
Yang, Q., Xie, R. J., Yang, T., Fang, L., Han, B., Zhang, G. Z., et al. (2007). Transforming Growth Factor-Beta1 and Smad4 Signaling Pathway Down-Regulates Renal Extracellular Matrix Degradation in Diabetic Rats. Chin. Med. Sci. J. 22 (4), 243–249.
Yang, Y. L., Hu, F., Xue, M., Jia, Y. J., Zheng, Z. J., Li, Y., et al. (2019). Early Growth Response Protein-1 Upregulates Long Noncoding RNA Arid2-IR to Promote Extracellular Matrix Production in Diabetic Kidney Disease. Am. J. Physiol. Cel Physiol 316 (3), C340–c352. doi:10.1152/ajpcell.00167.2018
Yang, Y., and Wu, C. (2021). Traditional Chinese Medicine in Ameliorating Diabetic Kidney Disease via Modulating Gut Microbiota. Integr. Med. Nephrol. Androl. 8, 8. doi:10.4103/imna.imna_28_21
Ye, Z., Lu, H., Su, Q., Xian, X., and Li, L. (2017). Effect of Ligustrazine on Preventing Contrast-Induced Nephropathy in Patients with Unstable Angina. Oncotarget 8 (54), 92366–92374. doi:10.18632/oncotarget.21310
Yoon, J. J., Lee, Y. J., Kang, D. G., and Lee, H. S. (2014). Protective Role of Oryeongsan against Renal Inflammation and Glomerulosclerosis in Db/db Mice. Am. J. Chin. Med. 42 (6), 1431–1452. doi:10.1142/s0192415x14500906
Yu, L., Border, W. A., Huang, Y., and Noble, N. A. (2003). TGF-beta Isoforms in Renal Fibrogenesis. Kidney Int. 64 (3), 844–856. doi:10.1046/j.1523-1755.2003.00162.x
Zeng, X., Cai, G., Liang, T., Li, Q., Yang, Y., Zhong, X., et al. (2020). Rhubarb and Astragalus Capsule Attenuates Renal Interstitial Fibrosis in Rats with Unilateral Ureteral Obstruction by Alleviating Apoptosis through Regulating Transforming Growth Factor Beta1 (TGF-Β1)/p38 Mitogen-Activated Protein Kinases (P38 MAPK) Pathway. Med. Sci. Monit. 26, e920720. doi:10.12659/msm.920720
Zhang, H. W., Lin, Z. X., Xu, C., Leung, C., and Chan, L. S. (2014). Astragalus (A Traditional Chinese Medicine) for Treating Chronic Kidney Disease. Cochrane Database Syst. Rev. 10, Cd008369. doi:10.1002/14651858.CD008369.pub2
Zhang, X., Zhu, Y.-m., Tang, B.-l., Ren, Y.-n., Tao, S.-j., Yang, J.-r., et al. (2019a). Ameliorative Effect of Salvianolic Acid B on Renal Fibrosis in Diabetic Rats and Potential Mechanisms Implicated. Chin. Pharmacol. Bull. 35 (1), 51–55. doi:10.3969/j.issn.1001-1978.2019.01.012
Zhang, Y. Y., Tang, P. M., Tang, P. C., Xiao, J., Huang, X. R., Yu, C., et al. (2019b). LRNA9884, a Novel Smad3-dependent Long Noncoding RNA, Promotes Diabetic Kidney Injury in Db/db Mice via Enhancing MCP-1-dependent Renal Inflammation. Diabetes 68 (7), 1485–1498. doi:10.2337/db18-1075
Zhang, Z. H., Li, M. H., Liu, D., Chen, H., Chen, D. Q., Tan, N. H., et al. (2018). Rhubarb Protect Against Tubulointerstitial Fibrosis by Inhibiting TGF-β/Smad Pathway and Improving Abnormal Metabolome in Chronic Kidney Disease. Front. Pharmacol. 9, 1029. doi:10.3389/fphar.2018.01029
Zhang, Z. H., Vaziri, N. D., Wei, F., Cheng, X. L., Bai, X., and Zhao, Y. Y. (2016). An Integrated Lipidomics and Metabolomics Reveal Nephroprotective Effect and Biochemical Mechanism of Rheum Officinale in Chronic Renal Failure. Sci. Rep. 6, 22151. doi:10.1038/srep22151
Zhang, Z. H., Wei, F., Vaziri, N. D., Cheng, X. L., Bai, X., Lin, R. C., et al. (2015). Metabolomics Insights into Chronic Kidney Disease and Modulatory Effect of Rhubarb against Tubulointerstitial Fibrosis. Sci. Rep. 5 (5), 14472. doi:10.1038/srep14472
Zhao, J., Wang, L., Cao, A. L., Jiang, M. Q., Chen, X., Wang, Y., et al. (2016). HuangQi Decoction Ameliorates Renal Fibrosis via TGF-β/Smad Signaling Pathway In Vivo and In Vitro. Cell Physiol Biochem 38 (5), 1761–1774. doi:10.1159/000443115
Zhao, T., Tang, H., Xie, L., Zheng, Y., Ma, Z., Sun, Q., et al. (2019). Scutellaria Baicalensis Georgi. (Lamiaceae): a Review of its Traditional Uses, Botany, Phytochemistry, Pharmacology and Toxicology. J. Pharm. Pharmacol. 71 (9), 1353–1369. doi:10.1111/jphp.13129
Zhao, Y. Y., Feng, Y. L., Bai, X., Tan, X. J., Lin, R. C., and Mei, Q. (2013c). Ultra Performance Liquid Chromatography-Based Metabonomic Study of Therapeutic Effect of the Surface Layer of Poria Cocos on Adenine-Induced Chronic Kidney Disease Provides New Insight into Anti-fibrosis Mechanism. PLoS One 8 (3), e59617. doi:10.1371/journal.pone.0059617
Zhao, Y. Y., Feng, Y. L., Du, X., Xi, Z. H., Cheng, X. L., and Wei, F. (2012). Diuretic Activity of the Ethanol and Aqueous Extracts of the Surface Layer of Poria Cocos in Rat. J. Ethnopharmacol 144 (3), 775–778. doi:10.1016/j.jep.2012.09.033
Zhao, Y. Y., Lei, P., Chen, D. Q., Feng, Y. L., and Bai, X. (2013b). Renal Metabolic Profiling of Early Renal Injury and Renoprotective Effects of Poria Cocos Epidermis Using UPLC Q-TOF/HSMS/MSE. J. Pharm. Biomed. Anal. 81-82, 202–209. doi:10.1016/j.jpba.2013.03.028
Zhao, Y. Y., Li, H. T., Feng, Y. L., Bai, X., and Lin, R. C. (2013a). Urinary Metabonomic Study of the Surface Layer of Poria Cocos as an Effective Treatment for Chronic Renal Injury in Rats. J. Ethnopharmacol 148 (2), 403–410. doi:10.1016/j.jep.2013.04.018
Zhao, Y. Y., Zhang, L., Mao, J. R., Cheng, X. H., Lin, Y., et al. (2011). Ergosta-4,6,8(14),22-tetraen-3-one Isolated from Polyporus Umbellatus Prevents Early Renal Injury in Aristolochic Acid-Induced Nephropathy Rats. J. Pharm. Pharmacol. 63 (12), 1581–1586. doi:10.1111/j.2042-7158.2011.01361.x
Zheng, L., Zhang, C., Li, L., Hu, C., Hu, M., Sidikejiang, N., et al. (2017). Baicalin Ameliorates Renal Fibrosis via Inhibition of Transforming Growth Factor β1 Production and Downstream Signal Transduction. Mol. Med. Rep. 15 (4), 1702–1712. doi:10.3892/mmr.2017.6208
Zhou, F., Zou, X., Zhang, J., Wang, Z., Yang, Y., and Wang, D. (2021a). Jian-Pi-Yi-Shen Formula Ameliorates Oxidative Stress, Inflammation, and Apoptosis by Activating the Nrf2 Signaling in 5/6 Nephrectomized Rats. Front. Pharmacol. 12, 630210. doi:10.3389/fphar.2021.630210
Keywords: chronic kidney disease, renal fibrosis, TGF-β/Smad signaling pathway, tubulointerstitial fibrosis, natural products
Citation: Yu X-Y, Sun Q, Zhang Y-M, Zou L and Zhao Y-Y (2022) TGF-β/Smad Signaling Pathway in Tubulointerstitial Fibrosis. Front. Pharmacol. 13:860588. doi: 10.3389/fphar.2022.860588
Received: 23 January 2022; Accepted: 28 February 2022;
Published: 24 March 2022.
Edited by:
Jianping Chen, Shenzhen Traditional Chinese Medicine Hospital, ChinaReviewed by:
Liang Ma, Sichuan University, ChinaXiao-Liang Zhang, Southeast University, China
Xuemei Zhang, Fudan University, China
Copyright © 2022 Yu, Sun, Zhang, Zou and Zhao. This is an open-access article distributed under the terms of the Creative Commons Attribution License (CC BY). The use, distribution or reproduction in other forums is permitted, provided the original author(s) and the copyright owner(s) are credited and that the original publication in this journal is cited, in accordance with accepted academic practice. No use, distribution or reproduction is permitted which does not comply with these terms.
*Correspondence: Xiao-Yong Yu, gub70725@126.com; Liang Zou, zouliangcdu@126.com; Ying-Yong Zhao, zhaoyybr@gmail.com