- 1Zhejiang Zhenyuan Pharmaceutical Co., Ltd., Shaoxing, China
- 2State Key Laboratory of Bioactive Substances and Function of Natural Medicine, Institute of Materia Medica, Chinese Academy of Medical Sciences, Beijing, China
- 3First Clinical Division, Peking University Hospital of Stomatology, Beijing, China
- 4Department of Neurosurgery, Peking Union Medical College Hospital, Chinese Academy of Medicine Sciences & Peking Union Medical College, Beijing, China
- 5Beijing Key Laboratory of Traditional Chinese Medicine Basic Research on Prevention and Treatment of Major Diseases, Experimental Research Center, China Academy of Chinese Medical Sciences, Beijing, China
- 6State Key Laboratory of Coal Resources and Safety Mining, China University of Mining and Technology, Beijing, China
- 7School of Chemical and Environmental Engineering, China University of Mining and Technology, Beijing, China
- 8Research Unit of Digestive Tract Microecosystem Pharmacology and Toxicology, Chinese Academy of Medical Sciences, Beijing, China
Chronic pain is one of the most prevalent health problems. The establishment of chronic pain is complex. Current medication for chronic pain mainly dependent on anticonvulsants, tricyclic antidepressants and opioidergic drugs. However, they have limited therapeutic efficacy, and some even with severe side effects. We turned our interest into alkaloids separated from traditional Chinese medicine (TCM), that usually act on multiple drug targets. In this article, we introduced the best-studied analgesic alkaloids derived from TCM, including tetrahydropalmatine, aloperine, oxysophocarpine, matrine, sinomenine, ligustrazine, evodiamine, brucine, tetrandrine, Stopholidine, and lappaconitine, focusing on their mechanisms and potential clinical applications. To better describe the mechanism of these alkaloids, we adopted the concept of drug-cloud (dCloud) theory. dCloud illustrated the full therapeutic spectrum of multitarget analgesics with two dimensions, which are “direct efficacy”, including inhibition of ion channels, activating γ-Aminobutyric Acid/opioid receptors, to suppress pain signal directly; and “background efficacy”, including reducing neuronal inflammation/oxidative stress, inhibition of glial cell activation, restoring the balance between excitatory and inhibitory neurotransmission, to cure the root causes of chronic pain. Empirical evidence showed drug combination is beneficial to 30–50% chronic pain patients. To promote the discovery of effective analgesic combinations, we introduced an ancient Chinese therapeutic regimen that combines herbal drugs with “Jun”, “Chen”, “Zuo”, and “Shi” properties. In dCloud, “Jun” drug acts directly on the major symptom of the disease; “Chen” drug generates major background effects; “Zuo” drug has salutary and supportive functions; and “Shi” drug facilitates drug delivery to the targeted tissue. Subsequently, using this concept, we interpreted the therapeutic effect of established analgesic compositions containing TCM derived analgesic alkaloids, which may contribute to the establishment of an alternative drug discovery model.
1 Introduction
1.1 Background
1.1.1 Social Impact of Chronic Pain
Chronic pain refers to pain that recurs over 3 months, which includes scenarios such as neuropathic pain, fibromyalgia, cancer pain and chronic arthritis pain (Treede et al., 2019). Anyone can suffer from chronic pain due to illness, trauma or surgery. Unfortunately, regardless of the substantial medical progress has been made in recent decades, management of chronic pain remained to be one of the most challenging clinical demands. In China, chronic pain affects around 400 million widely distributed patients (Zheng et al., 2020). However, less than 60% of them sought for medical treatment and of only 20% of the patients who received therapy reached sufficient pain relief (Chen et al., 2016; Zheng et al., 2020).
At an individual level, chronic pain limits the mobility and restricts patients’ essential daily activities such as eating, sleeping, and exercising, rendering many of them losing working abilities. In long term, patients’ quality of life is reduced, with impairment in their mental health (Mills et al., 2019). For instance, from 28.6 to 70% of fibromyalgia patients suffering from major depression, which is much higher than that of healthy population (5%) (Alciati et al., 2012; Gracely et al., 2012). At a society level, chronic pain imposed a huge challenge to the public healthcare system. A study in 2021 showed that in the United States, pain induced productivity loss was estimated to be $296 billion per year, which is greater than cardiovascular disease, cancer, or diabetes (Yong et al., 2021).
1.2 Pain Spots in Pain Medicine
1.2.1 Current Medication Is Unsatisfactory
Currently, treatments for chronic pain are dependent on anticonvulsants, tricyclic antidepressants, and opioidergic drugs.
Anticonvulsants (such as gabapentin and pregabalin) are the first-line choice for chronic pain management. However, their therapeutic efficacy is unsatisfactory: for traumatic nerve injury, pain was moderately relieved in less than 50% of the patients (Gordh et al., 2008); for mixed nerve pain, significant pain relief was achieved in 21% of patients (Serpell, 2002); for banded neuralgia, effectiveness was recorded in 32–34% of patients (Rice and Maton, 2001). In addition, incidences of neurological and systemic adverse reactions of gabapentin and pregabalin have been increasingly reported in recent years, and these adverse reactions were positively correlated with drug dosages (Chen and Chen, 2014).
Tricyclic antidepressants (TCAs), such as amitriptyline and nortriptyline, block the reuptake of norepinephrine (NA) and serotonin (5-HT) in nerve endings, to increase the available amount of inter-synaptic NA and 5-HT in key brain areas and thus modulate pain signals (Bair et al., 2003; Tamano et al., 2016). TCAs have been widely used as off-label drugs for many chronic pain conditions, although its exact analgesic mechanism (which may involve descending noradrenergic inhibitory pathway) remained to be largely unclear (Bair et al., 2003; Obata, 2017). In most studies, TCAs exhibit only limited potency with modest symptom improvements (Moore et al., 2012). Additionally, their usage is often restricted by bothersome anticholinergic side effects (including sedation, drowsiness, dry mouth, and constipation) (Till et al., 2019). Thus, in practice, TCAs are not universally applicable, but favoring a portion of patients who have comorbid psychological conditions (Till et al., 2019).
On the other hand, opioids can induce strong analgesic effects. But as a trade-off, they have crucial side effects, including tolerance, dependence, and addiction, limiting their usage as a long-term solution (Birnbaum et al., 2011; Vowles et al., 2015; Mercadante et al., 2019). Furthermore, inadequate restrictions on prescriptions of opioids (mainly as pain killer) in recent years, has led to an ongoing opioid crisis especially in western countries (Birnbaum et al., 2011). In the United States, drug overdoses resulted in 70,237 deaths during 2017 (surpassed the number of deaths caused by gun crimes or car accidents); among these, 47,600 (67.8%) involved opioids, with an increasing rate of 12.0% (from 2016 to 2017) (Scholl et al., 2018).
1.2.2 Failed Drug Discovery Model
There are currently about 3,500 clinical trials with chronic pain as indication has been registered worldwide (2021, https://clinicaltrials.gov). However, the path to new drug registration was not smooth. During 2001 to 2009, only few analgesics were approved by the United States Food and Drug Administration (FDA), which are mainly new formulations of existing analgesics (such as slow-release version of opioids) (Kinch, 2015). In 2018, FDA approved a total of 59 new drugs and therapies (highest numbers that breaking all-time records), with three pain killers approved for migraine (Kinch and Griesenauer, 2019). In 2019, FDA approved 48 novel drugs, with two pain drugs approved for migraine (Ebied et al., 2020). In 2020, FDA approved 53 novel therapeutics (the second highest total ever), with two pain drugs approved for migraine, and one for acute pain (Mullard, 2021). To some extent, the lack of “First in Class” or “Me-Only” pain killers manifested the frustration of current drug discovery model (Csermely et al., 2005; Kinch and Griesenauer, 2019; Ebied et al., 2020; Mullard, 2021).
In the current model, as a standard route, scientists first identify genes associated with the disease through literature search, and determine the biological target based on one of the relevant genes, then use the computer-assisted tools to design molecules that could interact with the target, and finally perform preclinical and clinical studies to validate if the compound could meet the requirements for new drug registration (Csermely et al., 2005).
1.3 Deep Reasoning of Conundrum
1.3.1 Chronic Pain Mechanism Is Complex
Resembling cancer/metabolic diseases, chronic pain also has multi-facet major symptoms with complicated root causes. So far, the pathology of chronic pain is still not entirely clear, but mechanisms of inflammation, oxidative stress, glial cell activation, and dysregulation of neurotransmitters are involved (Ji et al., 2016; Yang and Chang, 2019; Kaushik et al., 2020).
The development and maintenance of chronic pain is illustrated in a self-reinforcing vicious cycle (Figure 1), in which acute pain eventually undergoing a chronification process and become increasingly robust.
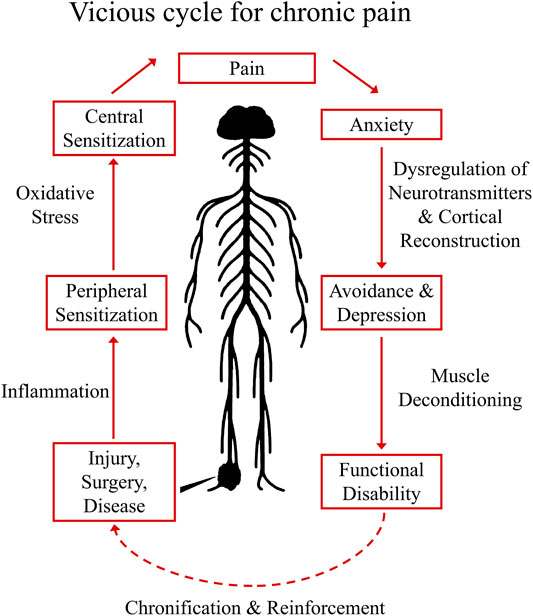
FIGURE 1. Vicious Cycle for Chronic Pain Establishment. The establishment of chronic pain usually starts with injury, surgery, or diseases that could harm the sensory circuit of the nervous system. When these situations were left to be treated, concomitant local inflammation will sensitize peripheral nociceptors (peripheral sensitization), and with accumulated oxidative stress in the CNS, central sensitization (sensitized pain pathway in the spinal cord) may occur, enhancing the pain perception to an intolerable degree (eventually pain symptoms become a disease). In cognitive part, sustained pain could generate anxiety, the pathological sign of chronic pain at this stage may involve dysregulation of neurotransmitters in the CNS and cortical reconstruction, subsequently result in depression and avoidance behaviors. In long term, symptoms of muscle spam and deconditioning could also occur, causing functional disability. In this vicious cycle, the pain syndrome eventually undergoing a chronification/reinforcement process and becoming increasingly robust.
Establishment of chronic pain usually starts with injury, surgery, inflammation, or diseases that harm the sensory circuit of the nervous system. These insults induce the release of neurotransmitters, lipid mediators, fragments of the complement system, neurotrophic factors, cytokines and chemokines (Silva et al., 2017). When these situations are not well managed, local inflammation will sensitize peripheral nociceptors causing peripheral sensitization (Silva et al., 2017). Sensitized primary afferents are responsible for releasing neurotransmitters (including glutamate, substance P, calcitonin gene–related peptide, and brain-derived growth factor) that gradually produce a state of neuronal hyperactivity in the spinal cord and brain known as central sensitization. In this state, spontaneous pain could occur and non-noxious mechanical pressures could be magnified to intolerable pain signals (Ji et al., 2018). When it comes to cognitive functions, sustained pain could produce anxiety, which under certain circumstances may result in depression with the pathological sign of dysregulated neurotransmission and cortical reconstruction (Bair et al., 2003; Holmes et al., 2013). In long term, symptoms of muscle spam and deconditioning could also occur, causing functional disability that further decrease the quality of life of patients.
1.3.2 The Dilemma of Single Target Drugs
Classical drug discovery approach has been mainly focused on the screening/designing of compounds selectively against one specific molecular target. These single target drugs (STDs) have been proven to be effective against many types of disease characterized by one predominant alteration and low potential of molecular evolution (Kong WJ. et al., 2020). Few examples of STDs include cyclooxygenase inhibitors that diminish the prostaglandin synthesis for treatment of inflammation and pain, sodium channel blockers for local anesthesia, and beta blockers/angiotension converting enzyme inhibitors for high blood pressure.
However, agents that affect one target only might not always affect complex systems (Csermely et al., 2005). The limitation of STDs can be seen in some challenging clinical situations due to mutation of the target (invading virus, microbes, tumors etc.). For instance, high frequencies of genomic mutations In RNA viruses (such as in HIV or COVID-19) could eventually induce variants that lose the targeted structure, rendering STDs ineffective. Similar scenario applies to many types of tumors (Csermely et al., 2005), under chemotherapy, tumor cells are also subject to high rate of mutations that could enable them to escape from the attacking sites of STDs.
The other confounding factor is alteration of the innate systems (immune system, metabolic system, nervous system etc.), to generate “compensatory” functions that are different enough to not respond to the same drug (Csermely et al., 2005). In chronic diseases (such as metabolic diseases, auto-inflammatory diseases and neurodegenerative diseases), usually there are not only one pathological mechanism that presides the whole duration of the disease. In some cases, the progression of the disease could profoundly modulate the innate systems, to generate robust trends of cellular outputs that unable to be reversed by STDs (Csermely et al., 2005).
For instance, in type II diabetes, STDs could improve high blood sugar/insulin resistance, but not good at controlling the established peripheral damage and associated complications (Tripathi and Srivastava, 2006); Similarly, in rheumatoid arthritis, STDs usually produce poor efficacy on accumulated bone erosion, sensory dysfunctions and comorbidities (Schett and Gravallese, 2012; Coutant and Miossec, 2020); Likewise, in Parkinson’s disease, the pathological mechanism at “subclinical” period is dissimilar to the phase of Parkinsonism when dopaminergic neuronal damage is irreversible by STDs (Gaig and Tolosa, 2009).
All these scenarios demonstrate that in challenging clinical situations (especially in chronic diseases), mutations and pathological and physiological changes across multi-systems/multi-organs, drive effective treatments to cover not only the symptoms, but also the root causes of the disease (Pang et al., 2012).
2 Introduction of Traditional Chinese Medicine Derived Analgesic Alkaloids
Facing the insufficiency of therapeutic measures for chronic pain, finding new treatments with stronger analgesic effect but lower side effects, becomes a global mission with ever increasing urgency. In such regard, differed from the STD approach, we turned our interest into traditional Chinese medicine (TCM), which harbors a rich source of drug candidates and usually acting on multiple targets (Zhu et al., 2017; Wang et al., 2019). Among all these potential candidates, many natural alkaloids has been report with analgesic potency (Zhu et al., 2017; Jiang W. et al., 2020). In following paragraph, we will introduce the best-studied analgesic alkaloids derived from TCM.
2.1 Tetrahydropalmatine
Tetrahydropalmatine (THP) was originally extracted from the botanic Corydalis yanhusuo W.T.Wang [Papaveraceae; Corydalis rhizoma] (Bory et al., 2010), cultivated in the Zhejiang, Jiangxi, and Anhui provinces of China. Corydalis yanhusuo W.T.Wang [Papaveraceae; Corydalis rhizoma] was recorded in the first Chinese medical classic, “Shennong Herbal Classic” (compiled in 200 A. D.), listed as a medium-grade drug, and used for invigorating blood circulation, reinforcing vital energy, and relieving pain (Table 1).
As the effective component of Corydalis yanhusuo W.T.Wang [Papaveraceae; Corydalis rhizoma], THP also has sedative, hypnotic and especially analgesic effects (Ren et al., 2003) in a variety of pain models, including formalin induced pain (Dosage: 20, 200 nmol) (Kang et al., 2016), bone cancer pain (Dosage: 20, 40, 60 mg/kg) (Zhang MY. et al., 2015) and neuropathic pain induced by sciatic nerve chronic constriction (Dosage: 20 nmol for intraperitoneal injection and 2 nmol for intrathecal injection; Positive control: BD1047 and gabapentin) (Kang et al., 2016) or oxaliplatin (Dosage: 1, 2, 4 mg/kg, Positive control: SCH23390) (Guo et al., 2014). In addition, THP could significantly reverse opioid dependence in rats addicted to morphine (Dosage: 1.25, 2.5, 5 mg/kg; Negative control: morphine) (Jiang WN. et al., 2020). THP has been clinically used in China as analgesics, sedatives, or hypnotics (Ren et al., 2003), and applied for arrhythmia and hypertension treatments (Ren et al., 2003).
2.2 Aloperine
Aloperine (Table 1) is a quinolizidine alkaloid isolated from Sophora alopecuroides L. [Fabaceae; Sophorae alopecuroides herba] in 1989 (Du and Li, 1989). In TCM, Sophora alopecuroides L. [Fabaceae; Sophorae alopecuroides herba] was first mentioned in “Shennong’s Classic of Materia Medica”, and believed to have functions to soothe the five viscera (liver, heart, spleen, lung, and kidney), detoxicate circulation system and stabilize consciousness (Wang et al., 2020). Modern pharmacological studies revealed that aloperine possesses antitumor, antibacterial, antiviral, anti-oxidative, anti-inflammatory, and analgesic properties (Xu et al., 2014; Zhou et al., 2020).
Using experimental animal models, aloperine was found to be effective against inflammatory pain in acetic acid-induced writhing test, formalin test, mouse ear swelling test, carrageenan-induced paw edema test (Dosage: 20, 40, 80 mg/kg; Positive control: aspirin or morphine) (Yang et al., 2015a), and neuropathic pain induced by chronic constriction injury (Dosage: 20, 40, 80 mg/kg; Positive control: pregabalin) (Xu et al., 2014). Clinically, aloperine has been used to treat autoimmune diseases, including rheumatoid arthritis, lupus erythematosus, and eczema, in China (Jin et al., 2015; Wang et al., 2020).
2.3 Oxysophocarpine
Similar as aloperine, oxysophocarpine (Table 1) is also extracted from Sophora alopecuroides L. [Fabaceae; Sophorae alopecuroides herba] (Du and Li, 1989). Oxysophocarpine has favorable anti-inflammatory and anti-oxidative properties. It could protect neurons going through cell death after oxygen and glucose deprivation (Zhu QL. et al., 2014; Lu et al., 2017; Zhao et al., 2018). Pretreatment with oxysophocarpine could protect against lung injury (Gao et al., 2017).
In addition, oxysophocarpine is an analgesic agent against acute pain in tail-flick or hot-plate test (Dosage: 10, 40, 80 mg/kg; Positive control: morphine) (Xu et al., 2013), inflammatory pain (mechanical allodynia) in carrageenan-induced paw edema model (Dosage: 10, 40, 80 mg/kg; Positive control: aspirin) (Yang et al., 2015b) or formalin-induced pain test (Dosage: 10, 40, 80 mg/kg; Positive control: morphine or aspirin) (Xu et al., 2013), and abdominal pain in acetic acid-induced abdominal constriction model (Dosage: 10, 40, 80 mg/kg; Positive control: morphine or aspirin) (Xu et al., 2013). By so far, we have not found any documentation on well-designed clinical studies of oxysophocarpine yet.
2.4 Matrine
Matrine (Table 1) is a quinolizidine alkaloid purified from the root of Sophora flavescens Ait. [Fabaceae; Sophorae flavescentis radix] (Lai et al., 2003). The dried root of Sophora flavescens Ait. [Fabaceae; Sophorae flavescentis radix] was firstly listed in “Shennong’s Classic of Materia Medica”. The root of Sophora flavescens Ait. [Fabaceae; Sophorae flavescentis radix] is bitter in taste, cold in nature and has beneficial properties in relieving rheumatism, improving eyesight, and nourishing the liver and gall (He et al., 2015). Matrine has displayed a broad scope of biological characteristics, including anticancer, anti-inflammatory, anti-allergic, antiviral, antifibrotic, and analgesic effects (Zhang et al., 2020a; You et al., 2020). The spectrum of treatable diseases extends to many systems, such as nervous system, circulatory system, and immune system.
Specifically, matrine could generate antinociceptive effects on variety of pain models, including acute pain in tail-flick test (Dosage: 30 mg/kg; Positive control: pentazocine) (Kamei et al., 1997), abdominal pain in acetic acid-induced abdominal contraction test (Dosage: 1, 3, 10 mg/kg; Positive control: pentazocine) (Kamei et al., 1997; Dai et al., 2021), and neuropathic pain induced by vincristine (Dosage: 15, 30, 60 mg/kg; Positive control: pregabalin) (Dun et al., 2014) or chronic constriction injury (Dosage: 7.5, 15, 30 mg/kg) (Wang et al., 2013a). In China, matrine has been used for clinical conditions of atrophic vaginitis and bacterial vaginitis (Zhang and Shen, 2021), since it can reduce infections, vaginal itching and swelling. Albeit, matrine induces severe side effects, including hepatotoxicity, neurotoxicity, and reproductive and developmental toxicity, thereby limited its clinical usage (You et al., 2020).
2.5 Sinomenine
Sinomenine (Table 1) is purified from the root of climbing plant Sinomenium acutum (Thunb.) Rehd. et Wils. [Menispermaceae; Sinomenii caulis] (Yamasaki, 1976), which was recorded in the “Compendium of Materia Medica” as an herbal medicine for treatment of various autoimmune diseases, such as rheumatoid arthritis (Zhao et al., 2012). Sinomenine’s pharmacological profile includes immuno-suppression, arthritis amelioration, and protection against hepatitis (Zhao et al., 2012). In addition, evidences revealed sinomenine’s efficacy in alleviating arthritic pain (Xu et al., 2008), and pain in many types of neuralgia, such as sciatic neuritis, lumbalgia and muscular rheumatism (Yamasaki, 1976).
In previous study, we and others had demonstrated that sinomenine possessed a broad spectrum of analgesic effects against acute pain (Dosage: 10, 20, 40 mg/kg) (Gao et al., 2013; Zhu Q. et al., 2014), formalin induced pain (Dosage: 25, 50, 75 mg/kg) (Lee et al., 2017), Freund’s complete adjuvant (CFA) induced inflammatory pain (Dosage: 30, 40 mg/kg) (Li et al., 2017; Yuan et al., 2018), rhuematoid arthritic pain (Model: collagen type II antibody induced arthritis model; Dosage: 10, 20, 40, 80 mg/kg) (Gao et al., 2015), incisional pain (Dosage: 5, 10, 20, 40, 80 mg/kg; Positive control: ligustrazine, paracetamol) (Zhu et al., 2016; Gao et al., 2021), cancer-induced bone pain (Dosage: 10, 20, 40 mg/kg) (Chen SP. et al., 2018), and peripheral (Model: Photochemically induced sciatic nerve injury; Dosage: 10, 20, 40 mg/kg) (Gao et al., 2013) and central neuropathic pain (Model: Photochemically-induced spinal cord injury; Dosage: 10, 20, 40 mg/kg) (Gao et al., 2013). Furthermore, repeated administration of sinomenine did not generate tolerance, but rather increased the basal level of pain threshold (Gao et al., 2014; Gao et al., 2019), suggesting sinomenine’s potential as a long-term therapeutic agent.
Treatment of rheumatoid arthritis is one of the major clinical applications of sinomenine. Compared with nonsteroidal anti-inflammatory drugs, sinomenine was superior in amelioration of morning stiffness, painful joints and erythrocyte sedimentation rate (Xu et al., 2008). In addition, sinomenine is being used for detoxification of heroin addicts (Liu Z. et al., 2007), treatment of patients with systemic lupus erythematosus (Shi et al., 2008; Zeng and Tong, 2020), and decreasing proteinuria and hematuria excretions in patients with glomerular disease (Yin et al., 2016).
2.6 Ligustrazine
Ligustrazine (Table 1) is purified from Ligusticum chuanxiong Hort. [Apiaceae; Chuanxiong rhizoma] (Li et al., 2011), which is recorded in the “Shennong Herbal Classic” and mainly distributed in Sichuan province (China). The rhizome of Ligusticum chuanxiong Hort. [Apiaceae; Chuanxiong rhizoma] is warm in property and pungent in flavor. In a long history, it has been used as folk remedies (for improving blood circulation to protect cardio-cerebrovascular system) and widely applied in food preparation for health promotion (Chen Z. et al., 2018). Ligustrazine has displayed anti-inflammatory, antioxidant, neuroprotective, antifibrotic, antibacterial, and antinociceptive effects (Ozaki, 1992; Jiang and Wang, 2016).
In previous study, we have demonstrated that ligustrazine could effectively reverse carrageenan induced inflammatory pain (Dosage: 10, 20, 80 mg/kg; Positive control: sinomenine, paracetamol) (Gao et al., 2021), incisional pain (Dosage: 10, 20, 80 mg/kg; Positive control: sinomenine, paracetamol) (Gao et al., 2021), peripheral (Model: photochemically induced sciatic nerve injury; Dosage: 10, 20, 80 mg/kg) and central neuropathic pain (Model: photochemically induced spinal cord injury; Dosage: 10, 20, 80 mg/kg) (Gao et al., 2019). By so far, ligustrazine is clinically applied in China for treatment of ischemic cerebrovascular disease (Li et al., 2009), myocardial infarction (Li et al., 2009), pulmonary hypertension and rheumatoid arthritis (Zhang C. et al., 2019).
2.7 Evodiamine
Evodiamine (Table 1) is the active component extracted from the fruit of Tetradium ruticarpum (A.Juss.) Hart. [Rutaceae; Tetradii ruticarpi fructus] (Moon et al., 1999), which was first recorded in “Shennong’s Classic of Materia Medica”, and has been used as a traditional herbal medicine for more than 2000 years. According to TCM, Tetradium ruticarpum (A.Juss.) Hart. [Rutaceae; Tetradii ruticarpi fructus] can disperse cold and relieve pain, arrest vomiting, protect the spleen, help Yang and stop diarrhea (Sun et al., 2020). As the effective component of Tetradium ruticarpum (A.Juss.) Hart. [Rutaceae; Tetradii ruticarpi fructus], evodiamine has been reported to exert beneficial effects on atherosclerosis, cardiomyopathy, and ischaemic and valvular heart disease (Li and Wang, 2020). In addition, evodiamine was found to have anti-tumor effects due to its ability in inducing apoptosis, arresting cell cycle, suppressing angiogenesis, and inhibiting tumor migration (Li and Wang, 2020).
In the cell model of transmembrane transportation, evodiamine could easily pass through the blood brain barrier (Sun et al., 2020). Such distribution pattern correlated with evodiamine’s neuroprotective role via regulating neurotrophic factor, and suppressing inflammatory events (Li and Wang, 2020), implying its therapeutic potential for various clinical pain situations. Indeed, researchers have demonstrated evodiamine’s analgesic effect in hot plate test (Dosage: 5 mg/kg) and neuropathic pain model induced by paclitaxel (Dosage: 5 mg/kg) (Wu and Chen, 2019). In clinic, evodiamine is effective in suppressing inflammation in skin and mucosa. It can be used for treating patients with erythromelalgia or Raynaud’s syndrome (Yarosh et al., 2006), and prevention of oral mucosal disorders such as periodontitis, or gingivitis (Zeligs, 2006).
2.8 Brucine
Brucine (Table 1), is one of the main bioactive and toxic constituents isolated from Strychnos nux-vomica L. [Loganiaceae; Strychni semen] (Tang et al., 2009), which is often used for treating swelling and pain (Guo et al., 2018). Modern pharmacology studies demonstrated brucine’s anticancer effect on many types of tumors, including liver cancer, breast cancer, colon cancer and multiple myeloma (Ma et al., 2013; Lu et al., 2020).
Researchers also validated brucine’s anti-inflammatory and analgesic effects. Brucine was found to induce analgesia in hot-plate test (Dosage: 10.3, 14.7, 20, 30 mg/kg; Positive control: pethidine) (Yin et al., 2003), tail-flick test (Dosage: 10 mg/kg) (Yu et al., 2019), acetic acid-induced writhing test (Dosage: 3.75, 7.5, 15, 30 mg/kg), formalin test (Dosage: 7.5, 15, 30 mg/kg; Positive control: indomethacin), carrageenan-induced inflammatory pain model (Dosage: 15, 30 mg/kg; Positive control: indomethacin), adjuvant-induced arthritis model (Dosage: 15, 30 mg/kg) (Yin et al., 2003), and chronic constriction injury (CCI) model of peripheral neuropathic pain (Dosage: 30 mg/kg; Positive control: gabapentin) (Yu et al., 2019). Clinical applications of brucine are closely related to its analgesic and anti-inflammatory properties. It is typically effective for the treatment of rheumatism, and traumatic pain (Lu et al., 2020). However, brucine showed moderate toxicity on nervous system, immune system, urinary system, and digestive systems (Lu et al., 2020).
2.9 Tetrandrine
Tetrandrine (Table 1) is a natural bisbenzylisoquinoline alkaloid purified from the root of Stephania tetrandra S. Moore [Menispermaceae; Stephaniae tetrandrae radix] (Chen and Chen, 1935), which was firstly recorded in “Shennong’s Classic of Materia Medica”. Stephania tetrandra S. Moore [Menispermaceae; Stephaniae tetrandrae radix] has a diuretic effect and is used in China as a folk remedy for malaria, edema, wet beriberi, dysuria, eczema and inflamed sores (Jiang Y. et al., 2020). As the effective component of Stephania tetrandra S. Moore [Menispermaceae; Stephaniae tetrandrae radix], tetrandrine exhibited a variety of therapeutic effects. For instance, anti-tumor properties of tetrandrine had been reported comprehensively based on in vitro and in vivo studies, against a wide range of cancers (Luan et al., 2020). It has been also found that tetrandrine could exert antimicrobial activity by preventing drug efflux in clinical isolates of treatment-resistant Mycobacterium tuberculosis (Zhang Z. et al., 2015). Tetrandrine also displayed antioxidant, anti-inflammatory, anti-allergic, antidiabetic, immunosuppressant, and analgesic effects (Xie et al., 2002; Bhagya and Chandrashekar, 2016).
The antinociceptive effect was validated using animal models of hot-plate test (Dosage: 15, 30, 45 mg/kg; Positive control: morphine) and acetic acid-induced abdominal constriction test (Dosage: 15, 30, 45 mg/kg; Positive control: morphine) (Zhao et al., 2014). Clinically, tetrandrine has been applied to treat arthritis, arrhythmia, hypertension, silicosis, pneumoconiosis, hepatic fibrosis, and portal hypertensive gastropathy (Kong X. et al., 2020). However, the safety, bioavailability, and pharmacokinetic parameters of tetrandrine are still not well studied, especially in clinical settings (Luan et al., 2020).
2.10 Stopholidine
Stopholidine (Table 1) is the active component extracted from the botanic Stephania japonica (Thunb.) Miers [Menispermaceae; Stephaniae japonicae folium et rhizome] (Brochmann-Hanssen and Richte, 1975), which is traditional used in China for curing asthma, dysmenorrhea, and leprosy (Desgrouas et al., 2014). In the past decades, Chinese researchers have made a great effort to explore the mechanisms of Stopholidine and its potential utility in treating drug abuse, schizophrenia, and pain (Mo et al., 2007; Chu et al., 2008). The evidence of Stopholidine could produce antinociception is primarily revealed by animal model of hot-plate test (Dosage: 20 mg/kg) (Chen et al., 1986). Stopholidine was further shown to have promising potential for clinical use in pain management (Chu et al., 2008). However, the clinical application of stopholidine is still rare and predominantly restricted to treat patients with schizophrenia. Clinical studies revealed that stopholidine has a therapeutic value in schizophrenic patients without produce significant side effects (Mo et al., 2007). Stopholidine also relieved the motor symptoms of Parkinson’s disease when co-administered with Levodopa (Yang et al., 2007).
2.11 Lappaconitine
Lappaconitine (Table 1) is a naturally occurring alkaloid purified from the root of Aconitum sinomontanum Nakai [Ranunculaceae; Aconiti sinomontani radix] (Bessonova et al., 1990), which by TCM theory possesses a hot and dry nature to resist cold, and being applied as painkillers or antirheumatic agents (Ono and Satoh, 1988; Nyirimigabo et al., 2015). Similarly, lappaconitine is reported to have mixed pharmacological properties, including producing antirheumatic, antiarrhythmic, antiepileptic, and analgesic effects (Nyirimigabo et al., 2015). When examined after oral or subcutaneous administration to rodents in the hot-plate, tail-immersion, or acetic acid-induced writhing tests, lappaconitine exhibited a strong antinociceptive activity, which was even comparable to morphine (Ono and Satoh, 1988). Lappaconitine is also shown to be effective in animal models of acetic acid-induced writhing test (Ono and Satoh, 1988) and CCI model of peripheral neuropathic pain (Dosage: 4 mg/kg) (Ou et al., 2011). In clinical settings, lappaconitine has been successfully used for post-operative analgesia with epidural injection, and generated desired analgesic effects (Chen et al., 1996).
3 dCloud Theory
To better understand the integrated analgesic properties of TCM derived alkaloids, we introduced the notion of “drug cloud” (dCloud) (Kong WJ. et al., 2020), to illustrate their full therapeutic spectrum with two dimensions, which are “direct efficacy” on symptoms and “background efficacy” on the root causes of pain (Figure 2).
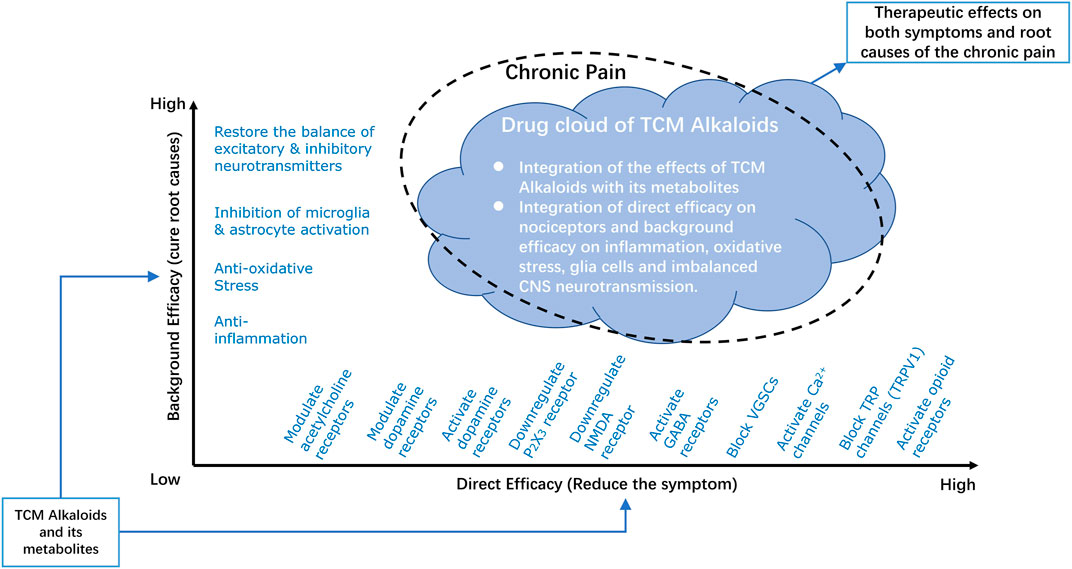
FIGURE 2. Drug Cloud of TCM derived Analgesic Alkaloids. The integrated analgesic properties of TCM derived alkaloids are illustrated by introducing the notion of drug cloud. The drug cloud could be described from two dimensions, which are direct efficacy and background efficacy. Direct efficacy refers to direct actions of TCM derived alkaloids on nociceptors or pain relaying network through activation/inhibition of ion channels or regulation the expression of certain receptors, such as by downregulating P2X3 or NMDA receptors, activating dopamine, GABA, or opioid receptors, and blocking VGSCs, Ca2+, or TRP channels. Background efficacy refers to the ability of TCM derived alkaloids to reverse the root causes of the chronic pain disease, including inhibition of inflammation and oxidative stress, inhibition of microglia and astrocyte activities and restore the balance between excitatory and inhibitory neurotransmitters. The chemical basis of drug cloud is TCM derived alkaloids together with its metabolites. By integration of the direct efficacy and background efficacy, this chemical basis of TCM derived alkaloids could have profound therapeutic effects on both symptoms and root causes of chronic pain. NMDA, N-methyl-d-aspartate; GABA, γ-amino butyric acid; VGSCs, voltage-gated sodium channels; TRP, transient receptor potential; TRPV1, transient receptor potential vanilloid receptor 1.
3.1 Direct Efficacy to Treat Symptoms
Direct efficacy refers to direct actions of TCM derived alkaloids on nociceptors or pain relaying networks in the peripheral nervous system (PNS) and central nervous system (CNS), to suppress pain signal directly (Table 2). Typical direct efficacy can be generated by inhibition of certain ion channels or modulating the expression of receptors that are key to the synaptic transmission.
3.1.1 Inhibition of Sodium or Transient Receptor Potential Channels
Direct therapeutic efficacy can be achieved through inhibition of ion channels such as blocking voltage gated sodium channels (VGSCs) (Ameri, 1998; Lee et al., 2017; Yu et al., 2019) or transient receptor potential (TRP) channels, especially transient receptor potential vanilloid 1 (TRPV1) (Pearce et al., 2004).
3.1.2 Activation of Dopamine, GABA or Acetylcholine Receptors
Activating the receptors of neurotransmitters such as dopamine (Chu et al., 2008; Liu et al., 2019), or γ-aminobutyric acid (GABA) (Zhu Q. et al., 2014), could also generate direct efficacy against pain. In addition, acetylcholine receptors have emerged as a novel therapeutic target for pain in recent years. Allosteric modulators and silent agonists for acetylcholine receptors have shown to have potential for reducing chronic pain (Toma et al., 2020).
3.1.3 Downregulation of P2X3 and NMDA Receptors
ATP facilitates generation and transmission of the neuropathic pain in dorsal root ganglion (DRG) via the P2X receptors, especially the subtype P2X3. Usually, P2X3 receptor expression is upregulated under neuropathic pain conditions (Ou et al., 2011). Therefore, downregulation of P2X3 receptor may reverse the established trend and generate direct efficacy against chronic pain (Gao et al., 2008; Ou et al., 2011; Rao et al., 2017). Furthermore, downregulating the expression of NMDA receptors could prevent the development of central sensitization, and produce direct antinociceptive effect (Dai et al., 2017).
3.1.4 Activating Opioid μ-Receptor
Receptor phosphorylation is thought to be a pivotal event in agonist regulation of the opioid μ-receptor. The activation/phosphorylation of opioid μ-receptor could be considered as one form of the direct efficacy induced by TCM derived alkaloids (Higashiyama et al., 2005).
3.2 Background Efficacy to Treat Root Causes
Background efficacy refers to the ability of TCM derived alkaloids to reverse the root causes of the chronic pain (Table 2), including inhibition of inflammation (Ozaki, 1992; Shaheen et al., 2005) and oxidative stress (Shi et al., 1995; Xu et al., 2014), suppression of microglia/astrocyte activations (Lin et al., 2008; Xue et al., 2008; Zhang MY. et al., 2015), and restoring the balance between excitatory and inhibitory neurotransmission (Xiang and Jiang, 2013; Danduga et al., 2018).
3.2.1 Anti-Inflammation
Inflammation in the nervous system is one root cause of chronic pain. When local inflammation was not well managed, inflammatory mediators (e.g. TNF) released by immune cells (e.g., microglia cells) will sensitize nociceptors (Ji et al., 2016; Jiang W. et al., 2020), and produce persistent pain. In accordance, a number of studies have shown that TNF neutralization antibodies can suppress chronic pain (Marchand et al., 2005; Leung and Cahill, 2010). Thus, anti-inflammation could be a mechanism for background efficacy.
3.2.2 Anti-Oxidation
Oxidative stress emerges when an imbalance exists between free radical formation and the capability of cells to clear them. Under environmental challenge (such as ultraviolet exposure or ischemic damage), the content of free radicals will sharply rise to destroy cellular structures (Pizzino et al., 2017).
Oxidative stress contributes to the establishment and maintenance of chronic pain by exacerbating inflammation and neuropathy (Inoue and Tsuda, 2018; Kaushik et al., 2020). During disease progression, reactive oxygen species (ROS) were accumulated in the CNS, which subsequently activate NMDA receptors, thereby promoting central sensitization (Woolf, 1983). Treatment with ROS scavengers could reverse this effect (Gao et al., 2007), and reduce allodynia induced by nerve damage (Guedes et al., 2008). In addition, superoxide dismutase analogs showed similar effects (reducing inflammation and hyperalgesia) by eliminating ROS (Gao et al., 2007).
3.2.3 Downregulation of Glial Cell Activity
Glial cells (microglial cells and astrocytes), are the most abundant and widely distributed cells which comprises around 90% of the total cell composition in the CNS (Yang and Zhou, 2019). Glial cells are responsible for maintaining a stable environment (clearing the toxic chemicals) and supplying neurons with nutrition. microglial cells serve as the resident macrophages of the spinal cord and brain, spinal microglia have been implicated in the pathogenesis of neuropathic pain after nerve injury (Jessen, 2004; Ji et al., 2016). While astrocytes perform critical functions such as neurotransmitter recycling, formation of the blood-brain barrier, regulation of extracellular ion concentration, and modulation of synaptic transmission. Nerve injury induces myriad changes in astrocytes that lead to enhanced pain (Ji et al., 2016).
Upon activation, glial cells will produce inflammatory factors such as ATP, TNF and interleukin-1β (IL-1β) (Jiang et al., 2020). These inflammatory mediators can dramatically increase the environmental glutamate levels and regulate glutamate transporter in microglial cells (Zumkehr et al., 2018), further enhance the responsiveness of postsynaptic glutamate receptors (such as NMDA and AMPA receptors), and escalate the excitability of the secondary neurons (Yunus, 2015). Glial cell activation eventually results in central sensitization, thereby promoting persistent pain states (Ji et al., 2013; Yunus, 2015). Thereby, enduring beneficial effect could be generated by suppressing the glial cell activity.
3.2.4 Restoring the Homeostasis of Neurotransmitters in Central Nervous System
Excitatory and inhibitory neurotransmitters (including glutamate, dopamine, serotonin, and GABA etc.), play important roles in maintaining the normal function of the CNS and supporting a rich set of functions for cell–cell communication (Hyman, 2005). These neurotransmitters are not only involved in the sensory process but also associated with the affective component of pain (Yam et al., 2018).
Homeostasis between excitatory and inhibitory neurotransmission is dysregulated when chronic pain occurred. For instance, the results of a study using proton magnetic resonance spectroscopy revealed the abnormal increase of glutamate concentration in the CNS in patients with chronic back pain and fibromyalgia (Tzschentke and Schmidt, 2003). On the other hand, in most clinical reports, central GABA concentration in chronic pain patients was not altered (Peek et al., 2020).
As an important root cause of chronic pain, dysregulation of neurotransmitters in the CNS could be detrimental and even result in cortical reconstruction (Elman and Borsook, 2016). Animal study indicated the inhibitory GABA could be converted to a paradoxical excitatory neurotransmitter, following neuronal damage (Hökfelt et al., 1994; Ben-Ari et al., 2012). These reorganizational processes usually produce profound impacts on the sensory system as well as contributed to the establishment of concomitant symptoms such as anxiety and depression (Nutt, 2008). Thus, restoring the homeostasis of neurotransmitters is key to cure the root causes of chronic pain.
3.3 Chemical Basis of dCloud
TCM derived alkaloids and its metabolites constituted the chemical basis of drug cloud. By integration of the direct efficacy and background efficacy, chemical basis of TCM derived alkaloids could have profound therapeutic effects on both symptoms and root causes of chronic pain.
4 Direct and Background Efficacy of Traditional Chinese Medicine Alkaloids
4.1 Tetrahydropalmatine
Direct Efficacy
Tetrahydropalmatine (Table 2) generates direct antinociceptive effects via activating the dopamine D1 and D2 receptors (Model: partial sciatic nerve ligation model; Dosage: 10 mg/kg; Positive control: SCH23390, quinpirole) (Liu et al., 2019). However, there are also controversial results indicated that tetrahydropalmatine could block dopamine D2 receptors in the midbrain to regulate nociception (Model: dopamine D2 receptor knockout mice; Dosage: 200 mg/kg; Control: wild type and vehicle) (Wang L. et al., 2016). Further, low dose of tetrahydropalmatine could significantly reduce anxiety in mice, by activation of GABAA receptor (Dosage: 1 mg/kg) (Leung et al., 2003).
Background Efficacy
Tetrahydropalmatine (Table 2) inhibits the production of proinflammatory mediators such as TNF, IL-1β and IL-18 (Model: Lipopolysaccharide-stimulated THP-1 cells, bone cancer pain model; Dosage: 0.2, 1, 2 mM, 60 mg/kg; Control: blank, naïve and sham operation) (Oh et al., 2010); Zhang MY. et al., 2015). Besides to decrease the accumulation of inflammatory mediators, it could also reduce the generation of oxidative stress (Model: model of myocardial ischaemia-reperfusion injury; Dosage: 20 mg/kg; Control: vehicle and sham operation) (Han et al., 2012). In addition, tetrahydropalmatine suppressed tumor cell implantation induced activation of microglial cells, which contributed to the attenuation of bone cancer pain (Dosage: 60 mg/kg; Control: vehicle and sham operation) (Zhang MY. et al., 2015). As to restore the balance between excitatory and inhibitory neurotransmission, tetrahydropalmatine markedly reduced the brain concentrations of glutamate, and the ratio of glutamate/GABA in mice with cerebral ischemia (Dosage: 14, 28, 56 mg/kg) (Zhang et al., 2004). Further, the effect of tetrahydropalmatine on opioid dependence, is related to its ability to reverse the abnormal decrease of dopamine (in morphine-dependent rats) and regulate the expression of dopamine receptors (Model: conditioned place preference model; Dosage: 1.25, 2.5 mg/kg) (Jiang WN. et al., 2020).
4.2 Aloperine
Background Efficacy
Aloperine (Table 2) can inhibit nuclear factor kappa-B (NF-κB) pathway and downregulate the subsequent expression of proinflammatory cytokines (Model: chronic constriction injury model; Dosage: 80 mg/kg; Control: sham operation) (Xu et al., 2014). Aloperine can also reduce the production of ROS while upregulate superoxide dismutase (SOD) to suppress oxidative stress (Model: chronic constriction injury model; Dosage: 80 mg/kg; Control: sham operation) (Xu et al., 2014).
4.3 Oxysophocarpine
Background Efficacy
Oxysophocarpine (Table 2) exhibited anti-inflammatory effects by suppressing the release of proinflammatory cytokines, such as TNF, IL-1β, IL-6 (Model: carrageenan-induced inflammatory pain model; Dosage: 80 mg/kg; Control: vehicle and sham operation) (Yang et al., 2015b). After the treatment with oxysophocarpine, the xylene-included ear swelling, and carrageenan-induced paw edema decreased as the result of inhibition of prostaglandin E2 (PGE2) (Dosage: 80 mg/kg; Control: vehicle and sham operation) (Yang et al., 2015b). Oxysophocarpine also exerted antioxidant activity by activation of nuclear factor-erythroid 2-related factor 2 (Nrf2), the essential transcription factor that regulates an array of detoxifying and antioxidant defense gene expression (Dosage: 1, 2, μM; Control: blank) (Gao et al., 2017). In addition, oxysophocarpine increased the expression of GABAAα1 receptor in mice to restore the balance between excitatory and inhibitory neurotransmission (Dosage: 80 mg/kg) (Xu et al., 2013).
4.4 Matrine
Direct Efficacy
Matrine (Table 2) could presynaptically activate acetylcholine receptors in the CNS (Model: hot-plate test; Dosage: 10 mg/kg) (Yin and Zhu, 2005). It could also activate κ-opioid receptors and μ-opioid receptors (Model: acetic acid-induced abdominal constriction test; Dosage: 30 mg/kg) (Kamei et al., 1997) to generate direct efficacy on pain symptoms.
Background Efficacy
Matrine (Table 2) exhibited anti-inflammatory properties by inhibiting the production of inflammatory cytokines such as TNF, IL-1β, IL-6 and IL-8 (Model: cultured HaCaT cells and fibroblasts, vincristine-induced neuropathic pain model; Dosage: 10, 50, 100 μg/ml, 60 mg/kg) (Hu et al., 1996; Liu JY. et al., 2007; Gong et al., 2016). Matrine also attenuated the vincristine induced oxidative stress, manifested by reduction of malondialdehyde, total antioxidant capacity, and total calcium (Dosage: 15, 30, 60 mg/kg; Positive control: pregabalin) (Gong et al., 2016). In addition, antiepileptic potential of matrine is realized via regulation of GABA and glutamate levels in the CNS, to promote inhibitory neurotransmission while decreasing glutamate toxicity (Model: maximal electroshock-induced seizures in mice; Dosage: 7.5, 15, 30, 60 mg/kg) (Xiang and Jiang, 2013).
4.5 Sinomenine
Direct Efficacy
Whole-cell patch clamp recordings of mouse DRG neurons show that sinomenine (Table 2) can dose-dependently reduce voltage-gated sodium currents, thereby reducing pain signal input in the peripheral (Dosage: 3mM, 0.1, 1, 10 mM; Control: blank) (Lee et al., 2017). In addition, the use of GABAA receptor antagonist bicuculine blocked analgesic effect of sinomenine (Zhu Q. et al., 2014), indicating sinomenine’s antinociceptive effect is mediated by the activation of GABAA receptors (Model: chronic constriction injury model; Dosage: 40 mg/kg; Control: vehicle) (Zhu Q. et al., 2014). Besides, sinomenine is able to down-regulate the expression of P2X3 receptor in DRG to suppress diabetic neuropathic pain (Model: type 2 diabetic model rats; Dosage: 40 mg/kg) (Rao et al., 2017). Further, sinomenine has an ability to activate opioid μ-receptor (Model: Chinese hamster ovary cell transfected with opioid μ-receptor; Dosage: 1, 10 μM) (Wang et al., 2008), without generating tolerance (Gao et al., 2014).
Background Efficacy
Sinomenine (Table 2) significantly reduced the expression of various factors related to inflammation, including p38 MAPK, NF-κB, c-fos, p-CAMKII, COX-2, p-CREB, TLR4 and IL-17A (Model: cultured dorsal root ganglia cell line, spinal nerve ligation model, cancer-induced bone pain model; Dosage: 800 μmol/L, 20, 40 mg/kg; Control: sham operation and vehicle; Positive control: KN93, SB203580 and oxycodone) (Chen SP. et al., 2018; Wang et al., 2021). Sinomenine can also inhibit the expression of transcription factor NF-κB, to further reduce the production of TNF, IL-1β, IL-6, INF-γ, IL-4, and IL-8, thereby alleviates neurogenic inflammation (Model: collagen-induced arthritis in mice, Freund’s complete adjuvant-induced adjuvant arthritis rats model; Dosage: 30, 100, 300 mg/kg; Positive control: dexamethasone) (Wang Y. et al., 2005; Li et al., 2006; Feng et al., 2007). In addition, sinomenine inhibited the expression of pain-inducing PGE2 (Model: mesencephalic neuron-glia cultures and reconstituted cultures Dosage: 10−5 M, 10−10 M, 10−14 M; Control: blank) (Qian et al., 2007).
As for antioxidant activities, sinomenine may upregulate Nrf2 (Model: spinal cord injury model; Dosage: 40 mg/kg) (Zhang L. et al., 2019) and nicotinamide adenine dinucleotide phosphate oxidase (NADPH) (Model: mesencephalic neuron-glia cultures and reconstituted cultures; Dosage: 10−5 M, 10−10 M, 10−14 M; Control: blank) (Qian et al., 2007), thereby reducing the production of ROS, to play a neuroprotective effect. Besides, by inhibiting the JAK2/STAT3 (Model: cancer-induced bone pain model; Dosage: 40 mg/kg; Control: sham operation and vehicle) (Chen SP. et al., 2018) or P38 MAPK pathway (Model: cultured dorsal root ganglia cell line, spinal nerve ligation model; Dosage: 800 μmol/L, 20 mg/kg; Positive control: KN93, SB203580 and oxycodone) (Wang et al., 2021), sinomenine can suppress the activation of microglia/astrocytes, thereby reducing the production of inflammatory mediators and ATP.
Further, sinomenine has the ability to down-regulate the excessive levels of glutamate in extracellular fluid of CNS, which helps to reconstruct the imbalanced excitatory and inhibitory neurotransmission, in rats with neuropathic pain (Model: spared sciatic nerve injury rat model; Dosage: 20, 40 mg/kg; Positive control: gabapentin) (Li et al., 2012).
4.6 Ligustrazine
Direct Efficacy
The direct analgesic effect of ligustrazine (Table 2) on neuropathic pain is mediated by suppressing the expression of P2X3 receptor in primary afferents (Model: chronic constriction injury model; Dosage: 100 mg/kg; Control: sham operation) (Gao et al., 2008).
Background Efficacy
Ligustrazine (Table 2) inhibited the edema induced by carrageenan, the increase of the dye leakage induced by acetic acid and the granuloma formation induced by cotton pellet (Model: hind-paw edema test, acetic acid-induced vascular permeability test, cotton pellet granuloma inhibition test; Dosage: 30, 100 mg/kg; Positive control: indomethacin) (Ozaki Y, 1992). Ligustrazine could selectively suppress c-Jun N-terminal kinase (JNK) activity, and decrease the expression of MMP-2/9 to generate antinociceptive effect in CCI-induced neuropathic pain model (Dosage: 30 mg/kg) (Jiang et al., 2017). In addition, ligustrazine attenuated neuropathic pain by inhibition of JAK/STAT3 pathway and subsequently reversed the elevated levels of TNF-α, IL-1β, and IL-2 in a rat model of CCI (Dosage: 100 mg/kg; Control: sham operation) (Wang S. et al., 2016).
Ligustrazine can also decrease hydroxyl radicals in hypothalamic regions of the brain to generate antioxidant activities (Model: microdialysis in the hypothalamus of rabbit brain; Dosage: 10, 20, 40 mg/kg) (Chang et al., 2013). Besides, ligustrazine attenuated the activation of microglia/astrocyte in the spinal cord and reduced neuroinflammation (Model: chronic constriction injury model; Dosage: 30 mg/kg) (Jiang et al., 2017; Wang et al., 2017). Further, ligustrazine increased GABA levels while decreasing glutamate levels in the CNS to generate neuroprotective activities and reconstruct the balance between excitatory and inhibitory neurotransmission (Model: 3-NP neurotoxin model; Dosage: 40, 80 mg/kg; Control: blank) (Chang et al., 2013; Danduga et al., 2018).
4.7 Evodiamine
Direct Efficacy
Evodiamine (Table 2) functions as an agonist for the vanilloid receptor TRPV1, to generate direct efficacy (Model: stably transfected CHO-VR1 cells; Dosage: 0.1–10μM; Positive control: [3H] RTX) (Pearce et al., 2004).
Background Efficacy
Evodiamine (Table 2) attenuated adjuvant-induced arthritis in rats by inhibiting synovial inflammation and restoring the Th17/Treg balance (Dosage: 10, 20, 40 mg/kg) (Zhang et al., 2020b). Evodiamine also ameliorated paclitaxel-induced neuropathic pain by inhibiting inflammatory mediators including IL-1β, IL-6, TNF-α and MCP-1 (Dosage: 5 mg/kg; Control: vehicle) (Wu and Chen, 2019). Increasing number of studies have demonstrated that evodiamine meliorate inflammatory response through AKT/Nrf2/HO-1 activation and regulating NF-κB signaling pathways (Model: RAW264.7 cells, lipopolysaccharide -stimulated BV-2 cells; Dosage: 0.3, 1, 3, 10, 20 μM; Control: blank) (Liu et al., 2009; Meng et al., 2021).
In addition, evodiamine ameliorates paclitaxel-induced neuropathic pain by maintaining mitochondrial anti-oxidant functions to reduce oxidative stress (Dosage: 5 mg/kg; Control: vehicle) (Wu and Chen, 2019). Evodiamine suppressed over-activated microglias via regulation of environmental inflammatory response (Model: lipopolysaccharide -stimulated BV-2 cells; Dosage: 10, 20μM; Control: blank) (Meng et al., 2021). Further, evodiamine enhanced the expression of GABAA receptor subunits in the hypothalamus and reduced caffeine-induced sleep disturbances (Dosage: 10, 20 mg/kg) (Ko et al., 2018).
4.8 Brucine
Direct Efficacy
Electrophysiological results show that brucine (Table 2) can directly inhibit the excitability of tetrodotoxin-sensitive and tetrodotoxin-resistant sodium channels in DRG neurons (Model: chronic constriction injury model; Dosage: 30 mg/kg; Positive control: gabapentin) (Yu et al., 2019).
Background Efficacy
In formalin test, brucine (Table 2) showed inhibitory effect on carrageenan-induced rat paw edema. It significantly inhibited the release of PGE2 in the inflammatory tissue, reduced acetic acid-induced vascular permeability and the content of 6-keto-PGF1a in blood plasma of CFA induced arthritis rats (Dosage: 15, 30 mg/kg; Positive control: acetylsalicylic acid) (Yin et al., 2003).
In addition, brucine could reduce the content of 5-HT in CFA-induced arthritis rat’s blood plasma, while increase the content of 5-hydroxytryindole-3-acetic acid (5-HIAA) accordingly (Dosage: 15, 30 mg/kg; Positive control: acetylsalicylic acid) (Yin et al., 2003). Besides, in vitro study showed that brucine has GABA antagonistic property by effectively reversing the inhibitory action of GABA on 35S-TBPS binding (Model: iontophoretical test on population spikes in the rat hippocampus; Dosage: 0.1 M) (Dalkara et al., 1986).
4.9 Tetrandrine
Direct Efficacy
Voltage-dependant L-type and T-type calcium channel blockers prevent the movement of Ca2+ across the channels, thus controlling signaling events. Tetrandrine (Table 2) acts on both L and T-type Ca2+ channels to inhibit Ca2+ mediated signaling events in ventricular cells (Liu et al., 1992).
Background Efficacy
Tetrandrine (Table 2) is an anti-inflammatory and immunosuppressive agent (Xie et al., 2002). Tetrandrine inhibits the production and activation of interleukins, TNF, prostaglandins, COX-2 and T cells under experimental conditions to reduce inflammation (Bhagya and Chandrashekar, 2016). Antinociceptive effect of tetrandrine on LPS-induced hyperalgesia is mediated by the inhibition of IKKβ phosphorylation and the COX-2/PGE2 pathway in mice (Dosage: 1 × 10−8, 1 × 10−7, 1 × 10−6 mol/L; Control: blank) (Zhao et al., 2014).
In addition, tetrandrine has antioxidant activities. Tetrandrine efficiently eliminated hydroxyl radicals and caused a significant inhibition on freshly fractured quartz-induced lipid peroxidation (Dosage: 60 mM) (Shi et al., 1995). Tetrandrine also scavenged superoxide radicals produced from xanthine/xanthine oxidase (Model: freshly fractured quartz-induced lipid peroxidation; Dosage: 60 mM) (Shi et al., 1995), reduced hexose-monophosphate shunt activity, and inhibited superoxide anion generation (Seow et al., 1988). In addition, tetrandrine inhibited the H2O2-induced generation of ROS in cultured rat cerebellar granule neurons (Dosage: 0.1, 1, 10 μM; Positive control: MK-801, l-NAME, verapamil) (Koh et al., 2003), and exhibited protective effects on hydrogen peroxide-induced oxidative neuronal cell damage in cultured rat cerebellar granule cells (Dosage: 0.1, 1, 10μM; Positive control: MK-801, l-NAME, verapamil) (Koh et al., 2003).
Besides, tetrandrine suppressed lipopolysaccharide-induced microglial activation by inhibiting NF-κB pathway (Dosage: 25, 50 μM; Control: blank) (Xue et al., 2008), and inhibited LPS-induced astrocyte activation via modulating IKKs-IκBα-NF-κB signaling pathway (Dosage: 15, 30 μM; Control: blank) (Lin et al., 2008). Further, tetrandrine could inhibit the H2O2-induced elevation of glutamate release in cultured rat cerebellar granule neurons to restore balance between excitatory and inhibitory neurotransmission (Dosage: 0.1, 1, 10 μM; Positive control: MK-801, l-NAME, verapamil) (Koh et al., 2003).
4.10 Stopholidine
Direct Efficacy
Stopholidine (Table 2) possesses dopamine D1 partial agonistic and D2 antagonistic properties in the nigrostriatal and mesocorticolimbic DAergic pathways, and can effectively restore the imbalanced functional linkage between D1 and D2 receptors in neurological disorders (Model: heroin-induced reinstatement model; Dosage: 2.5, 5.0, 10.0 mg/kg; Control: Vehicle) (Yang et al., 2007; Chu et al., 2008; Ma et al., 2014).
Background Efficacy
Stopholidine (Table 2) exhibits neuroprotective effects through an anti-oxidative mechanism (Yang et al., 2007), in which stopholidine not only protects striatal cells against transient cerebral ischemic injury, but also scavenges hydroxyl free radicals and maintains neuronal survival following exposure to H2O2 induced neurotoxicity (Yang et al., 2007).
4.11 Lappaconitine
Direct Efficacy
Electrophysiological studies have revealed lappaconitine (Table 2) possesses strong antinociceptive property due to a use-dependent inhibition of the voltage-gated sodium channels (Ameri, 1998). Analgesic effect of lappaconitine also involves the reduction of P2X3 receptor expression and sensitization in rat DRG neurons following CCI (Dosage: 4 mg/kg; Control: sham operation) (Ou et al., 2011).
Background Efficacy
There are results indicating lappaconitine (Table 2) exhibited significant anti-inflammatory and anti-oxidant activities (Model: 1,1-diphenyl-2-picryl hydrazyl free radical scavenging activity; Dosage: 100 μg/ml, 1 mM; Control: blank) (Shaheen et al., 2005).
5 Drug Combination as a New Therapeutic Approach
The failure of the single target drug discovery model advocates innovation ideas to be adopted in identifying new chronic pain treatment strategies. Natural analgesics introduced in this article is a good resource. But, their potency could be limited, confining their application as mono-therapies. Fortunately, progresses are made in recent years, to demonstrate combinational approach works to improve the clinical efficacy of potential medication (Dale and Stacey, 2016). Empirical evidence shows that 30–50% of the chronic pain patients found beneficial in using drug combinations to control their pain symptoms (Dale and Stacey, 2016).
Therefore, efficient identification of effective combinations could serve as an alternative drug discovery route. In this regard, an ancient Chinese concept could be introduced to treat chronic diseases from a holistic point of view, focusing on promoting the quality of life, instead of completely eliminating the symptom. Simply speaking, the strategy is to combine herbal drugs with different properties (into TCM formulas), to exert effects of mutual reinforcement and detoxification (Chen and Du, 2019). So far, nearly 100,000 formulas have been reported and used in TCM (Zhou and Chen, 2014). Depends on the intrinsic traits of different herbal medicines, TCM formula is a therapeutic regimen containing components of “Jun” (Emperor), “Chen” (Minister), “Zuo” (Assistant), and “Shi” (Delivering Servant) (Zhou and Chen, 2014; Chen, 2016).
“Jun” drug refers to drugs that act on symptom-specific targets in disease-specific tissue (the internal cause) to generate direct efficacy on the major symptom of the disease (in case of chronic pain, blocking ion channels, or directly silencing the signals from pain-relaying neuronal circuits), usually with fast onset but also contribute to majority of the side effects (of the drug combination). “Chen” drug refers to drugs that act on targets against major root causes (both the internal and external causes of disease), to generate primary background effects (in case of chronic pain, suppressing glial cell activation, enhance inhibitory neurotransmission, and to have anti-inflammatory/anti-oxidant effects). The efficacy of “Chen” drug usually accumulates with repeated dosages.
Supplementing “Jun” and “Chen” drugs, “Zuo” drug refers to drugs (usually without toxicity) that act on the external cause of the disease to exhibit salutary and supportive functions, generating desirable protective effects to strengthen the overall health (such as by regulating the composition of gut microbiota or boosting the immune system to combat illness), and in certain cases can mitigate the toxicity (of “Jun” or “Chen” drugs), or improve the compliance (such as by improving flavors of taste). “Shi” drug is the element that acts on absorption/circulation systems (also the external cause of the disease) to facilitate drug (“Jun” or “Chen” drugs) delivery to targeted tissue/organs. Typical “Shi” drug is borneol, which is believed to facilitate the drug penetration across the blood brain barrier to reach CNS (Zhao et al., 2021). In TCM, at least one “Jun” drug is indispensable for the combination therapy to be functional (Wang and Yang, 2013).
The theory of “Jun”, “Chen”, “Zuo”, “Shi” is also compatible with the theory dCloud, as illustrated in Figure 3. Combining “Jun”, “Chen”, “Zuo”, and “Shi” drugs, following benefits could be realized (Zhou and Chen, 2014): 1) Increased efficacy on symptoms of chronic disease; 2) Minimized effective dosages; 3) Increased the compliance with mitigation of side effects; 4) Prolonged efficacy over long term usage with less chance of developing tolerance; 5) Restoring the healthy physiological balance to facilitate the overall health.
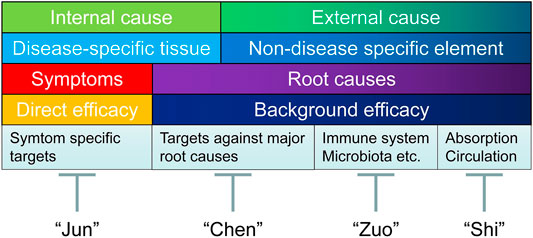
FIGURE 3. Holistic treatment strategy integrating dCloud and TCM combinational treatment regimen. Above figure illustrated a holistic treatment strategy integrating dCloud and TCM combinational treatment regimen combing herbal drugs with “Jun”, “Chen”, “Zuo”, and “Shi” properties, to exert effects of mutual reinforcement and detoxification. “Jun” drug refers to drugs that act on symptom-specific targets in disease-specific tissue (the internal cause) to generate direct efficacy on the major symptom of the disease (in case of chronic pain, blocking ion channels, or directly silencing the signals from pain-relaying neuronal circuits), usually with fast onset but also contribute to majority of the side effects (of the drug combination). “Chen” drug refers to drugs that act on targets against major root causes (both the internal and external causes of disease), to generate primary background effects (in case of chronic pain, suppressing microglial cell activation, restoring the balance between excitatory and inhibitory neurotransmission, and to have anti-inflammatory/anti-oxidant properties). The efficacy of “Chen” drug usually accumulates with repeated dosages. Supplementing “Jun” and “Chen” drugs, “Zuo” drug refers to drugs acts on the external cause of the disease to have salutary and supportive functions, that often generating desirable protective effects or to strengthen the overall health (such as by regulating the composition of gut microbiota or boosting the immune system to combat illness), and in certain cases can mitigate the toxicity (of “Jun” or “Chen” drugs), or improve the compliance (such as by improving flavors of taste). “Shi” drug is the element that acts on absorption/circulation systems (also the external cause of the disease) to promote drug (“Jun” or “Chen” drugs) delivery to targeted tissue/organs. Typical “Shi " drug is borneol, which is believed to facilitate the drug penetration across the blood brain barrier to reach CNS. In TCM, at least one form of “Jun” drug is indispensable for the combination therapy to be functional.
In light of the above-mentioned concept of “Jun”, “Chen”, “Zuo”, and “Shi”, we interpreted relevant studies, validating drug combinations integrating TCM derived analgesic alkaloids with other components, including natural products, analgesic compounds and other alkaloids (Table 3).
5.1 Tetrahydropalmatine + Angelica dahurica
As mentioned, tetrahydropalmatine has direct antinociceptive efficacy by activating dopamine or GABA receptors (Leung et al., 2003; Liu et al., 2019). It also reduced the brain ratio of glutamate/GABA to restore imbalanced neurotransmission (Zhang et al., 2004), and have anti-inflammatory and anti-oxidant activities (Han et al., 2012; Zhang MY. et al., 2015).
On the other hand, Angelica dahurica (Fisch, ex Hoffm.) Benth. et Hook. f. [Apiaceae; angelicae dahuricae radix] is known to have natures of activating blood to promote circulation, nourishing liver blood, and driving away cold to relieve pain. Besides, it might increase the plasma concentration of co-applied tetrahydropalmatine (Model: acetic acid-induced writhing test; Dosage: Corydalis alkaloid 17 mg/kg, Angelica dahurica 40 mg/kg, volatile oil 0.05 mg/kg) (Liao et al., 2010).
In this combination, tetrahydropalmatine bares the properties of “Jun” and “Chen” drug (by inducing direct and background effects), while Angelica dahurica (Fisch, ex Hoffm.) Benth. et Hook. f. [Apiaceae; angelicae dahuricae radix] could be “Zuo” and “Shi” drug (by strengthen overall health and improve drug exposure). In practice, Angelica dahurica (Fisch, ex Hoffm.) Benth. et Hook.f. [Apiaceae; angelicae dahuricae radix] enhanced the analgesic effects of tetrahydropalmatine in acetic acid writhing test and hot plate test, and increased the plasma concentration of tetrahydropalmatine (Liao et al., 2010).
5.2 Matrine + Paracetamol
As described above, through modulating opioid or acetylcholine receptors, matrine can generate direct antinociceptive effects (Kamei et al., 1997). It can also produce neuroprotective effects by suppressing the release of inflammatory cytokines (Hu et al., 1996; Liu JY. et al., 2007), reducing the total antioxidant capacity (Gong et al., 2016), or regulating GABA and glutamate levels to diminish the over-excitated neurotransmission (Xiang and Jiang, 2013).
Paracetamol (also known as acetaminophen), could suppress the production of COX dependent PGE2 and preventing the sensitizing of nociceptors (Graham et al., 2013).
In this combination, matrine could be considered as the “Jun” and “Chen” drug, while paracetamol also bares the property of “Chen” drug. In practice, it has been reported that there is a synergistic interaction between matrine and paracetamol in the acetic acid writhing test in mice (Dai et al., 2021).
5.3 Oxymatrine + Sodium Ferulate
Similar to matrine, oxymatrine also possess direct antinociceptive effects, and also produces anti-inflammatory and anti-oxidant background effects (Model: synovial tissues gained from RA patients, chronic constrictive injury model; Dosage: 10, 50, 100 μM, 80, 160 mg/kg; Control: blank, sham operation) (Wang et al., 2013b; Liang et al., 2018; Lan et al., 2020).
On the other hand, sodium ferulate induces vasodilation, promotes blood circulation, and has anti-thrombosis, anti-inflammatory, and anti-oxidant effects (Model: xylene-induced mouse ear edema model, carrageenan-induced rat paw edema model, lipopolysaccharide-activated RAW 264.7 cells; Dosage: 7.7, 15.4, 30.9 mg/kg, 50, 100, 200, 400 μmol/L; Positive control: dexamethasone) (Wang and Ou-Yang, 2005; Yuan et al., 2011). In addition, sodium ferulate is also frequently used for strengthen the body’s immunity (Hu et al., 2017).
In this combination, oxymatrine could be considered as the “Jun” drug, while sodium ferulate is suitable to be both “Chen” and “Zuo” drug. In practice, oxymatrine combined with sodium ferulate produced synergistic analgesic effect in acetic acid writhing test and formalin test (Dosage for sodium ferulate + oxymatrine: 24.5 + 49.0, 49.0 + 98.0, 98.0 + 196.0 μmol/kg; Positive control: diclofenac) (Liu et al., 2010).
5.4 Evodiamine + Rutaecarpine + Ginsenoside-Rg1 + Ginsenoside-Rb
Evodiamine activates TRPV1 to produce direct antinociceptive effects (Pearce et al., 2004). It also inhibits the release of inflammatory cytokines and ROS to reduce oxidative stress (Wu and Chen, 2019). In addition, evodiamine could suppress neuroinflammation caused by over-activated microglias (Meng et al., 2021), and restore the imbalanced neurotransmission via enhancing the expression of GABAA receptor subunits in the CNS (Ko et al., 2018).
Rutaecarpine is believed that have property of dispelling cold and relieving pain. Evodipine can inhibit the expression of COX-2 and inducible nitric oxide synthase (iNOS) in a dose-dependent manner, further reducing the release of PGE2 (Liu et al., 2009).
Ginsenosides are a set of steroid compounds, mainly exist in ginseng genus of medicinal botanics. Ginsenosides can increase the vitality and quantity of NK cells, B cells, and bone marrow cells. At the same time, ginsenosides can also increase the concentration of immune mediators such as IL-2 and IFN-γ (Lee et al., 2004; Lv et al., 2014). Ginsenoside-Rg1 and ginsenoside-Rb, could boost the immune system and strengthen the overall health, protecting the host from cancer cell invasion and metastasis (Model: murine macrophage-like RAW264.7 cells and human THP-1 monocyte; Dosage: 5, 10, 20, 40, 60, 80, 100, 120 μM; Control: blank) (Li et al., 2018; Ren et al., 2020). They can also promote the resolution of inflammation, and accelerate wound recovery (Model: spinal cord injury model; Dosage: 10 mg/kg; Control: vehicle) (Ahmed et al., 2016; Gao et al., 2020; Xu et al., 2020).
In this combination, evodiamine is both the “Jun” and “Chen” drug, while, rutaecarpine is “Chen” drug, and ginsenoside-Rg1 with ginsenoside-Rb are “Zuo” drugs. In practice, it has been demonstrated that there is a synergistic interaction between evodiamine, rutaecarpine, ginsenoside-Rg1, and ginsenoside-Rb1 for the treatment of migraine (Wu et al., 2016).
5.5 Ligustrazine + Ketamine
As mentioned, ligustrazine suppresses the expression of P2X3 receptor in primary afferents to directly modulate nociception (Gao et al., 2008). It also exhibited anti-inflammatory and anti-oxidant properties, and attenuate activation of microglia and astrocytes (Chang et al., 2013; Wang S. et al., 2016; Jiang et al., 2017). In addition, ligustrazine increased GABA levels while decreased glutamate levels in the CNS to generate neuroprotective activity (Danduga et al., 2018).
Ketamine is used to treat various chronic pain syndromes, especially those that have a neuropathic component (Niesters et al., 2014). Low dose ketamine produces strong analgesia in neuropathic pain states, presumably by inhibition of the NMDA receptor, though other mechanisms are possibly involved, including enhancement of descending inhibition (Niesters et al., 2014).
In this combination, Ketamine is the “Jun” drug, while ligustrazine is the both the “Jun” and “Chen” drug. In practice, there is an additive interaction between ligustrazine and ketamine in analgesic effect against acute pain (Model: heat radiation-induced tail-flick test) in normal mice (Liu et al., 2018).
5.6 Sinomenine + Ligustrazine
As stated, sinomenine is an analgesic alkaloid that can act on both the peripheral and the central nervous system. It can generate direct analgesic effect by blocking ion channels to stop the firing of pain-relaying neurons (Lee et al., 2017), as well as activating GABAA receptor or opioid μ-receptor (Wang et al., 2008; Zhu et al., 2016), and down-regulating the expression of P2X3 receptor in DRG (Rao et al., 2017). Besides, sinomeine also has profound anti-inflammatory and anti-oxidant activities, and it inhibits activation of microglial cells (Qian et al., 2007; Wang et al., 2021). In addition, sinomenine might mitigate the glutamate toxicity in neuropathic states, by reducing the excessive levels of glutamate in CNS (Li et al., 2012).
In this combination, sinomenine together with ligustrazine are both “Jun” and “Chen” drugs. Ligustrazine failed to perform as a “Shi” drug for sinomenine since co-applied ligustrazine (i.v.) could not enhance sinomenine’s penetration through BBB into the brain (Gao et al., 2021). In practice, sinomenine facilitated the analgesic efficacy of ligustrazine (in a synergistic way) on inflammatory pain, postoperative pain (Gao et al., 2021), and peripheral or central neuropathic pain in rodent models (Gao et al., 2019).
5.7 Sinomenine + Paracetamol
In this combination, sinomenine is both the “Jun” and “Chen” drug, while paracetamol has the property of “Chen” drug. In practice, the overall efficacy of this combination varies between pain models and pain modalities. Sinomenine combined with paracetamol generated synergistic effect in reducing heat but not mechanical hypersensitivity in carrageenan induced inflammatory pain model in mice (Gao et al., 2021). And surprisingly, there is a significant infra-additive interaction between sinomenine and acetaminophen in rat model of incisional pain, which could be related to pharmacokinetic interaction between the two drugs (Zhu et al., 2016; Gao et al., 2021).
5.8 Sinomenine + Gabapentin
Gabapentin was firstly developed as an antiepileptic drug, increasing the efficacy of the inhibitory neurotransmitter GABA. However, its principal analgesic mechanism is through modulating the activities of the alpha 2-delta subunit of L-type voltage-regulated calcium channels rather than solely enhancing GABAergic neurotransmission (Striano and Striano, 2008).
In this combination, gabapentin is the “Jun” drug, while sinomenine functions as the “Jun” and “Chen” drug. In practice, sinominine plus gabapentin generated synergistic effects on peripheral and central neuropathic pain in animal models (Gao et al., 2019).
5.9 Sinominine + Tetrahydropalmatine
In this combination, sinomenine together with tetrahydropalmatine both function as the “Jun” and “Chen” drugs. In practice, we found an additive interaction between sinomenine and tetrahydropalmatine in rat model of central neuropathic pain (Unpublished data).
5.10 Sinominine + Dextromethorphan
Dextromethorphan is a weak NMDA antagonist (Stahl, 2019) and commonly used as antitussive agent (Nguyen et al., 2016). It has beneficial effects across a variety of neurological disorders including traumatic brain injury, seizure, pain, methotrexate neurotoxicity, Parkinson’s disease and autism (Nguyen et al., 2016).
In this combination, sinomenine is both the “Jun” and “Chen” drug, while dextromethorphan is considered as the “Chen” drug. In practice, surprisingly, we found dextromethorphan did not enhance the analgesic effects of sinomenine in rodents with peripheral or central neuropathic pain. In addition, dextromethorphan alone did not possess analgesic efficacy in these models (Unpublished data).
5.11 Sinomenine + Panax notoginseng Saponins
Panax notoginseng saponins are the main ingredients of Panax notoginseng (Burk) F. H. Chen [Araliaceae; notoginseng radix et rhizoma], being classified into four types: protopanaxadiol, protopanaxatriol, ocotilloltype, and oleanolic acid constituents. Panax notoginseng saponins are also the main effective constituents of Xuesaitong Injection, which is widely used in the treatment of cerebral ischemic stroke and cardiovascular disease in China (Zhang X. et al., 2015). Panax notoginseng saponins have neuroprotective and anti-oxidant activities via reducing the levels of ROS, malondialdehyde and nitric oxide (Model: cisplatin-induced nephrotoxicity model; Dosage: 31.35 mg/kg; Control: vehicle) (Li et al., 2020a), while increasing the levels of superoxide dismutase, catalase and glutathione (Qu et al., 2020). Panax notoginseng saponins are also found to be able to modulate gut microbiota and boost the immune system to strengthen the overall health (Model: lincomycin hydrochloride-induced microbial intestinal disorder model; Dosage: 20, 60 mg/kg) (Sun et al., 2003; Song and Hu, 2009; Bai et al., 2021).
In this combination, sinomenine is both the “Jun” and “Chen” drug, while panax notoginseng saponins is both the “Chen” and “Zuo” drug. In practice, panax notoginseng saponins did not enhance the analgesic effects of sinomenine in rats with central neuropathic pain. In addition, panax notoginseng saponins alone did not possess analgesic efficacy in this model (Unpublished data).
5.12 Stopholidine + Dolantin
As mentioned, stopholidine could modulate the activity of dopamine receptors which may contribute to its direct effects on nociception (Yang et al., 2007; Chu et al., 2008; Ma et al., 2014). In addition, Stopholidine exhibits neuroprotective effects through an anti-oxidative mechanism (Yang et al., 2007).
Dolantin was developed as an anti-spasmodic analgesic in 1939 (Ngan Kee, 1998). It is the first synthetic opioid, which mainly activating the opioid μ receptors, with a rapid onset and short duration of action (Thigpen et al., 2019). Clinically, dolantin is used for moderate to severe pain relief, such as for post-traumatic pain. Even evidences has challenged dolantin’s analgesic benefits (with concerns have been raised about its safety profile), the drug remains frequently used (Ching Wong and Cheung, 2020).
In this combination, dolantin is the “Jun” drug, while Stopholidine might be the “Jun” and “Chen” drug. In practice, systemically applied Stephenidine (2 mg/kg, i. p.) can enhance dolantin (15 mg/kg, i. p.) induced analgesia in hot plate test (in mice) by 175%, to generate synergistic effect (Bian et al., 1985).
6 Conclusion
The dilemma for drug development in chronic pain filed represents the typical failure of the current drug discovery mode, which mainly focused on validating one drug target, and designing molecules solely act on that target (Csermely et al., 2005). Differed from classical STDs, TCM analgesic alkaloids have different pattern of action. Specifically, through pleiotropic activities on multiple targets and pathways essential to pathogenesis and progression of chronic pain, they could provide treatments both on symptoms/signs and root causes. As natural compounds, TCM analgesic alkaloids usually possess moderate potency, but their long-term adverse effects are lower especially compared with opioids (Du et al., 2016), thus are potential drug candidates.
In TCM, normally drugs are combinedly used to boost the overall efficacy (Chen and Du, 2019). The same philosophy could be applied in the identification of effective therapeutic combinations between TCM analgesic alkaloids and other established treatments. In this article, we provided examples on how TCM analgesic alkaloids could enhance the therapeutic potency of other drugs on chronic pain, provided a basis for future investigation to identify and evaluate the most promising combinations.
As the booming in the application of computer and artificial intelligence (AI) technologies, traditional industries are being transformed. We are facing an era that our existing mode of the drug discovery to be modulated by AI based technologies (such as deep learning algorithm), especially in the drug designing process (Hessler and Baringhaus, 2018). For instance, new technologies might enable the chemists to deliberate a drug design that rationally assembles a so called “fusion compound” by assembling functional groups associated with both symptoms/signs and root causes. Integrating these approaches with comprehensive pharmaceutical and pharmacological studies may revolutionize the current mode of drug discovery.
We see in recent years, the number of approved TCM drugs dropped rapidly in China. We believe that this is related to the current evaluation standard of the Chinese authority (Center for Drug Evaluation), solely focusing on the short-term improvement on symptoms. While TCM often has low toxicity, and by targeting the root causes of the disease, bring beneficial outcome in long-term including less adverse events and a higher quality of life, especially for chronic diseases. The current situation call for the establishment of innovative standards for evaluating TCM drugs. Such as by setting up new criterion for long-term safety testing and patient’s quality of life assessment. This is also the area that future studies could help to provide scientific evidence in framing these protocols and eventually facilitate clinical translation.
Author Contributions
TG, WJ, MT, LY, XZ, and JG, have major contributions for accomplishing the research study; All authors involved in preparing the manuscript; All authors approved the final version of the manuscript.
Funding
This study was supported by National Key Technologies R & D Program of China (2018YFA0108602), Open fund of the state key laboratory coal resources and safe mining (SKLCRSM21KFA11), CAMS Innovation Fund for Medical Sciences (2017-I2M-B&R-07, 2019-I2M-5-055), Fundamental Research Funds for the Central Universities (3332018086), and The Drug Innovation Major Project (2018ZX09711001-011-003).
Conflict of Interest
WJ and LY were employed by Zhejiang Zhenyuan Pharmaceutical Co., Ltd.
The remaining authors declare that the research was conducted in the absence of any commercial or financial relationships that could be construed as a potential conflict of interest.
Publisher’s Note
All claims expressed in this article are solely those of the authors and do not necessarily represent those of their affiliated organizations, or those of the publisher, the editors and the reviewers. Any product that may be evaluated in this article, or claim that may be made by its manufacturer, is not guaranteed or endorsed by the publisher.
References
Ahmed, T., Raza, S. H., Maryam, A., Setzer, W. N., Braidy, N., Nabavi, S. F., et al. (2016). Ginsenoside Rb1 as a Neuroprotective Agent: A Review. Brain Res. Bull. 125, 30–43. doi:10.1016/j.brainresbull.2016.04.002
Alciati, A., Sgiarovello, P., Atzeni, F., and Sarzi-Puttini, P. (2012). Psychiatric Problems in Fibromyalgia: Clinical and Neurobiological Links between Mood Disorders and Fibromyalgia. Reumatismo 64, 268–274. doi:10.4081/reumatismo.2012.268
Ameri, A. (1998). The Effects of Aconitum Alkaloids on the Central Nervous System. Prog. Neurobiol. 56, 211–235. doi:10.1016/s0301-0082(98)00037-9
Bai, X., Fu, R., Duan, Z., Liu, Y., Zhu, C., and Fan, D. (2021). Ginsenoside Rh4 Alleviates Antibiotic-Induced Intestinal Inflammation by Regulating the TLR4-MyD88-MAPK Pathway and Gut Microbiota Composition. Food Funct. 12, 2874–2885. doi:10.1039/d1fo00242b
Bair, M. J., Robinson, R. L., Katon, W., and Kroenke, K. (2003). Depression and Pain Comorbidity: a Literature Review. Arch. Intern. Med. 163, 2433–2445. doi:10.1001/archinte.163.20.2433
Ben-Ari, Y., Khalilov, I., Kahle, K. T., and Cherubini, E. (2012). The GABA Excitatory/inhibitory Shift in Brain Maturation and Neurological Disorders. Neuroscientist 18, 467–486. doi:10.1177/1073858412438697
Bessonova, I. A., Samusenko, L. N., Yunusov, M. S., and Kondrat'ev, V. G. (1990). Alkaloids of Aconitum Coreanum. IV. 14-Hydroxy-2-Isobutyrylhetisine N-Oxide. Chem. Nat. Compd. 26, 318–320. doi:10.1007/bf00597859
Bhagya, N., and Chandrashekar, K. R. (2016). Tetrandrine--A Molecule of Wide Bioactivity. Phytochemistry 125, 5–13. doi:10.1016/j.phytochem.2016.02.005
Bian, C., Duan, S., Xing, S., Yu, Y., Qin, W., Jin, G., et al. (1985). The Interaction between Cephalomantine and Analgesics. Pharmacol. Clin. Chin. Materia Medica 00, 88–89. (in Chinese).
Birnbaum, H. G., White, A. G., Schiller, M., Waldman, T., Cleveland, J. M., and Roland, C. L. (2011). Societal Costs of Prescription Opioid Abuse, Dependence, and Misuse in the United States. Pain Med. 12, 657–667. doi:10.1111/j.1526-4637.2011.01075.x
Bory, S., Bun, S. S., Baghdikian, B., Mabrouki, F., Cheng, S. K., Elias, R., et al. (2010). Simultaneous HPLC Determination of Three Bioactive Alkaloids in the Asian Medicinal Plant Stephania Rotunda. Nat. Prod. Commun. 5 (6), 877–882. doi:10.1177/1934578x1000500611
Brochmann-Hanssen, E., and Richter, W. J. (1975). Opium Alkaloids XV: Isolation of Stepholidine. J. Pharm. Sci. 64 (6), 1040–1041. doi:10.1002/jps.2600640637
Chang, C. H., Huang, W. T., Kao, C. H., Chen, S. H., and Lin, C. H. (2013). Tetramethylpyrazine Decreases Hypothalamic Glutamate, Hydroxyl Radicals and Prostaglandin-E2 and Has Antipyretic Effects. Inflamm. Res. 62, 527–535. doi:10.1007/s00011-013-0606-3
Chen, B., Li, L., Donovan, C., Gao, Y., Ali, G., Jiang, Y., et al. (2016). Prevalence and Characteristics of Chronic Body Pain in China: a National Study. Springerplus 5, 938. doi:10.1186/s40064-016-2581-y
Chen, K. K., and Chen, A. L. (1935). The Alkaloids of Han-Fang-Chi. J. Biol. Chem. 109, 681–685. doi:10.1016/s0021-9258(18)75199-5
Chen, L. F., Gao, J. Z., and Wang, F. C. (1986). Analgesic and Antipyretic Effects of L-Stepholidine without Addiction. Zhongguo Yao Li Xue Bao 7, 311–314. (in Chinese).
Chen, M., and Du, G. (2019). On Compatibility of Formulas. Shandong J. Traditional Chin. Med. 38, 916–920. (in Chinese). doi:10.16295/j.cnki.0257-358x.2019.10.004
Chen, M. G., Wang, Q. H., and Lin, W. (1996). Clinical Study in Epidural Injection with Lappaconitine Compound for Post-operative Analgesia. Zhongguo Zhong Xi Yi Jie He Za Zhi 16, 525–528. (in Chinese).
Chen, S. P., Sun, J., Zhou, Y. Q., Cao, F., Braun, C., Luo, F., et al. (2018). Sinomenine Attenuates Cancer-Induced Bone Pain via Suppressing Microglial JAK2/STAT3 and Neuronal CAMKII/CREB Cascades in Rat Models. Mol. Pain 14, 1744806918793232. doi:10.1177/1744806918793232
Chen, Y., and Chen, C. (2014). The Intolerance and Addiction of Pregabalin. J. Int. Neurology Neurosurg. 41, 189–192. (in Chinese). doi:10.16636/j.cnki.jinn.2014.02.026
Chen, Y. (2016). The Analysis of the Effective Application of the Theory of Jun Chen Zuo Shi in Modern Clinical Prescriptions. Inn. Mong. J. Traditional Chin. Med. 35, 78–79. (in Chinese). doi:10.16040/j.cnki.cn15-1101.2016.12.070
Chen, Z., Zhang, C., Gao, F., Fu, Q., Fu, C., He, Y., et al. (2018). A Systematic Review on the Rhizome of Ligusticum Chuanxiong Hort. (Chuanxiong). Food Chem. Toxicol. 119, 309–325. doi:10.1016/j.fct.2018.02.050
Ching Wong, S. S., and Cheung, C. W. (2020). Analgesic Efficacy and Adverse Effects of Meperidine in Managing Postoperative or Labor Pain: A Narrative Review of Randomized Controlled Trials. Pain Physician 23, 175–201.
Chu, H., Jin, G., Friedman, E., and Zhen, X. (2008). Recent Development in Studies of Tetrahydroprotoberberines: Mechanism in Antinociception and Drug Addiction. Cell. Mol. Neurobiol. 28, 491–499. doi:10.1007/s10571-007-9179-4
Coutant, F., and Miossec, P. (2020). Evolving Concepts of the Pathogenesis of Rheumatoid Arthritis with Focus on the Early and Late Stages. Curr. Opin. Rheumatol. 32, 57–63. doi:10.1097/BOR.0000000000000664
Csermely, P., Agoston, V., and Pongor, S. (2005). The Efficiency of Multi-Target Drugs: the Network Approach Might Help Drug Design. Trends Pharmacol. Sci. 26, 178–182. doi:10.1016/j.tips.2005.02.007
Dai, G., Li, B., Xu, Y., Li, Z., Mo, F., and Wei, C. (2021). Synergistic Interaction between Matrine and Paracetamol in the Acetic Acid Writhing Test in Mice. Eur. J. Pharmacol. 895, 173869. doi:10.1016/j.ejphar.2021.173869
Dai, W. L., Yan, B., Jiang, N., Wu, J. J., Liu, X. F., Liu, J. H., et al. (2017). Simultaneous Inhibition of NMDA and mGlu1/5 Receptors by Levo-Corydalmine in Rat Spinal Cord Attenuates Bone Cancer Pain. Int. J. Cancer. 141, 805–815. doi:10.1002/ijc.30780
Dale, R., and Stacey, B. (2016). Multimodal Treatment of Chronic Pain. Med. Clin. North Am. 100, 55–64. doi:10.1016/j.mcna.2015.08.012
Dalkara, T., Saederup, E., Squires, R. F., and Krnjevic, K. (1986). Iontophoretic Studies on Rat hippocampus with Some Novel GABA Antagonists. Life Sci. 39, 415–422. doi:10.1016/0024-3205(86)90521-7
Danduga, R. C. S. R., Dondapati, S. R., Kola, P. K., Grace, L., Tadigiri, R. V. B., and Kanakaraju, V. K. (2018). Neuroprotective Activity of Tetramethylpyrazine against 3-nitropropionic Acid Induced Huntington's Disease-like Symptoms in Rats. Biomed. Pharmacother. 105, 1254–1268. doi:10.1016/j.biopha.2018.06.079
Desgrouas, C., Taudon, N., Bun, S. S., Baghdikian, B., Bory, S., Parzy, D., et al. (2014). Ethnobotany, Phytochemistry and Pharmacology of Stephania Rotunda Lour. J. Ethnopharmacol. 154, 537–563. doi:10.1016/j.jep.2014.04.024
Du, B., and Li, J. (1989). Study on Alkaloids of Sophora Sphaerocarpa Root. Northwest Pharm. J. 4, 15–17. (in Chinese) CNKI:SUN:XBYZ.0.1989-02-008.
Du, G. H., Yuan, T. Y., Du, L. D., and Zhang, Y. X. (2016). The Potential of Traditional Chinese Medicine in the Treatment and Modulation of Pain. Adv. Pharmacol. 75, 325–361. doi:10.1016/bs.apha.2016.01.001
Ebied, A. M., Patel, K. H., and Cooper-DeHoff, R. M. (2020). New Drugs Approved in 2019. Am. J. Med. 133, 675–678. doi:10.1016/j.amjmed.2020.01.030
Elman, I., and Borsook, D. (2016). Common Brain Mechanisms of Chronic Pain and Addiction. Neuron 89, 11–36. doi:10.1016/j.neuron.2015.11.027
Feng, H., Yamaki, K., Takano, H., Inoue, K., Yanagisawa, R., and Yoshino, S. (2007). Effect of Sinomenine on Collagen-Induced Arthritis in Mice. Autoimmunity 40, 532–539. doi:10.1080/08916930701615159
Gaig, C., and Tolosa, E. (2009). When Does Parkinson's Disease Begin? Mov. Disord. 24 (Suppl. 2), S656–S664. doi:10.1002/mds.22672
Gao, J., Li, Y., Wang, Q., Ma, X., and Zhang, Y. (2017). Oxysophocarpine Inhibits Lung Injury Induced by Respiratory Syncytial Virus. Am. J. Transl. Res. 9, 4083–4093.
Gao, T., Hao, J., Wiesenfeld-Hallin, Z., Wang, D. Q., and Xu, X. J. (2013). Analgesic Effect of Sinomenine in Rodents after Inflammation and Nerve Injury. Eur. J. Pharmacol. 721, 5–11. doi:10.1016/j.ejphar.2013.09.062
Gao, T., Li, T., Jiang, W., Fan, W., Xu, X. J., Zhao, X., et al. (2020). Antinociceptive Effects of Sinomenine Combined with Ligustrazine or Paracetamol in Animal Models of Incisional and Inflammatory Pain. Front. Physiol. 11, 523769. doi:10.3389/fphys.2020.523769
Gao, T., Shi, T., Wang, D. Q., Wiesenfeld-Hallin, Z., and Xu, X. J. (2014). Repeated Sinomenine Administration Alleviates Chronic Neuropathic Pain-like Behaviours in Rodents without Producing Tolerance. Scand. J. Pain. 5, 249–255. doi:10.1016/j.sjpain.2014.05.006
Gao, T., Shi, T., Wiesenfeld-Hallin, Z., Li, T., Jiang, J. D., and Xu, X. J. (2019). Sinomenine Facilitates the Efficacy of Gabapentin or Ligustrazine Hydrochloride in Animal Models of Neuropathic Pain. Eur. J. Pharmacol. 854, 101–108. doi:10.1016/j.ejphar.2019.03.061
Gao, T., Shi, T., Wiesenfeld-Hallin, Z., Svensson, C. I., and Xu, X. J. (2015). Sinomenine Alleviates Mechanical Hypersensitivity in Mice with Experimentally Induced Rheumatoid Arthritis. Scand. J. Pain. 7, 9–14. doi:10.1016/j.sjpain.2014.12.003
Gao, X., Kim, H. K., Chung, J. M., and Chung, K. (2007). Reactive Oxygen Species (ROS) Are Involved in Enhancement of NMDA-Receptor Phosphorylation in Animal Models of Pain. Pain 131, 262–271. doi:10.1016/j.pain.2007.01.011
Gao, Y., Li, J., Wang, J., Li, X., Li, J., Chu, S., et al. (2020). Ginsenoside Rg1 Prevent and Treat Inflammatory Diseases: A Review. Int. Immunopharmacol. 87, 106805. doi:10.1016/j.intimp.2020.106805
Gao, Y., Xu, C., Liang, S., Zhang, A., Mu, S., Wang, Y., et al. (2008). Effect of Tetramethylpyrazine on Primary Afferent Transmission Mediated by P2X3 Receptor in Neuropathic Pain States. Brain Res. Bull. 77, 27–32. doi:10.1016/j.brainresbull.2008.02.026
Gong, S. S., Li, Y. X., Zhang, M. T., Du, J., Ma, P. S., Yao, W. X., et al. (2016). Neuroprotective Effect of Matrine in Mouse Model of Vincristine-Induced Neuropathic Pain. Neurochem. Res. 41, 3147–3159. doi:10.1007/s11064-016-2040-8
Gordh, T. E., Stubhaug, A., Jensen, T. S., Arnèr, S., Biber, B., Boivie, J., et al. (2008). Gabapentin in Traumatic Nerve Injury Pain: a Randomized, Double-Blind, Placebo-Controlled, Cross-Over, Multi-Center Study. Pain 138, 255–266. doi:10.1016/j.pain.2007.12.011
Gracely, R. H., Ceko, M., and Bushnell, M. C. (2012). Fibromyalgia and Depression. Pain Res. Treat. 2012, 486590. doi:10.1155/2012/486590
Graham, G. G., Davies, M. J., Day, R. O., Mohamudally, A., and Scott, K. F. (2013). The Modern Pharmacology of Paracetamol: Therapeutic Actions, Mechanism of Action, Metabolism, Toxicity and Recent Pharmacological Findings. Inflammopharmacology 21, 201–232. doi:10.1007/s10787-013-0172-x
Guedes, R. P., Araújo, A. S., Janner, D., Belló-Klein, A., Ribeiro, M. F., and Partata, W. A. (2008). Increase in Reactive Oxygen Species and Activation of Akt Signaling Pathway in Neuropathic Pain. Cell. Mol. Neurobiol. 28, 1049–1056. doi:10.1007/s10571-008-9279-9
Guo, R., Wang, T., Zhou, G., Xu, M., Yu, X., Zhang, X., et al. (2018). Botany, Phytochemistry, Pharmacology and Toxicity of Strychnos Nux-Vomica L.: A Review. Am. J. Chin. Med. 46, 1–23. doi:10.1142/S0192415X18500015
Guo, Z., Man, Y., Wang, X., Jin, H., Sun, X., Su, X., et al. (2014). Levo-tetrahydropalmatine Attenuates Oxaliplatin-Induced Mechanical Hyperalgesia in Mice. Sci. Rep. 4, 3905. doi:10.1038/srep03905
Haiyan, W., Yuxiang, L., Linglu, D., Tingting, X., Yinju, H., Hongyan, L., et al. (2013a). Antinociceptive Effects of Matrine on Neuropathic Pain Induced by Chronic Constriction Injury. Pharm. Biol. 51, 844–850. doi:10.3109/13880209.2013.767363
Han, Y., Zhang, W., Tang, Y., Bai, W., Yang, F., Xie, L., et al. (2012). l-Tetrahydropalmatine, an Active Component of Corydalis Yanhusuo W.T. Wang, Protects against Myocardial Ischaemia-Reperfusion Injury in Rats. PloS one 7, e38627. doi:10.1371/journal.pone.0038627
He, X., Fang, J., Huang, L., Wang, J., and Huang, X. (2015). Sophora Flavescens Ait.: Traditional Usage, Phytochemistry and Pharmacology of an Important Traditional Chinese Medicine. J. Ethnopharmacol. 172, 10–29. doi:10.1016/j.jep.2015.06.010
Hessler, G., and Baringhaus, K. H. (2018). Artificial Intelligence in Drug Design. Molecules 23, 2520. doi:10.3390/molecules23102520
Higashiyama, K., Takeuchi, Y., Yamauchi, T., Imai, S., Kamei, J., Yajima, Y., et al. (2005). Implication of the Descending Dynorphinergic Neuron Projecting to the Spinal Cord in the (+)-matrine- and (+)-Allomatrine-Induced Antinociceptive Effects. Biol. Pharm. Bull. 28, 845–848. doi:10.1248/bpb.28.845
Hökfelt, T., Zhang, X., and Wiesenfeld-Hallin, Z. (1994). Messenger Plasticity in Primary Sensory Neurons Following Axotomy and its Functional Implications. Trends. Neurosci. 17, 22–30. doi:10.1016/0166-2236(94)90031-0
Holmes, A., Christelis, N., and Arnold, C. (2013). Depression and Chronic Pain. Med. J. Aust. 199, S17–S20. doi:10.5694/mja12.10589
Hu, B., Song, J. T., Ji, X. F., Liu, Z. Q., Cong, M. L., and Liu, D. X. (2017). Sodium Ferulate Protects against Angiotensin II-Induced Cardiac Hypertrophy in Mice by Regulating the MAPK/ERK and JNK Pathways. Biomed. Res. Int. 2017, 3754942. doi:10.1155/2017/3754942
Hu, Z. L., Zhang, J. P., Qian, D. H., Lin, W., Xie, W. F., Zhang, X. R., et al. (1996). Effects of Matrine on Mouse Splenocyte Proliferation and Release of Interleukin-1 and -6 from Peritoneal Macrophages In Vitro. Zhongguo Yao Li Xue Bao 17, 259–261.
Inoue, K., and Tsuda, M. (2018). Microglia in Neuropathic Pain: Cellular and Molecular Mechanisms and Therapeutic Potential. Nat. Rev. Neurosci. 19, 138–152. doi:10.1038/nrn.2018.2
Jessen, K. R. (2004). Glial Cells. Int. J. Biochem. Cell. Biol. 36, 1861–1867. doi:10.1016/j.biocel.2004.02.023
Ji, R. R., Berta, T., and Nedergaard, M. (2013). Glia and Pain: Is Chronic Pain a Gliopathy? Pain 154 (Suppl. 1), S10–S28. S10–S28. doi:10.1016/j.pain.2013.06.022
Ji, R. R., Chamessian, A., and Zhang, Y. Q. (2016). Pain Regulation by Non-neuronal Cells and Inflammation. Science 354, 572–577. doi:10.1126/science.aaf8924
Ji, R. R., Nackley, A., Huh, Y., Terrando, N., and Maixner, W. (2018). Neuroinflammation and Central Sensitization in Chronic and Widespread Pain. Anesthesiology 129, 343–366. doi:10.1097/ALN.0000000000002130
Jiang, L., Pan, C. L., Wang, C. Y., Liu, B. Q., Han, Y., Hu, L., et al. (2017). Selective Suppression of the JNK-Mmp2/9 Signal Pathway by Tetramethylpyrazine Attenuates Neuropathic Pain in Rats. J. Neuroinflammation. 14, 174. doi:10.1186/s12974-017-0947-x
Jiang, W., Fan, W., Gao, T., Li, T., Yin, Z., Guo, H., et al. (2020). Analgesic Mechanism of Sinomenine against Chronic Pain. Pain Res. Manag. 2020, 1876862. doi:10.1155/2020/1876862
Jiang, W. N., Jing, X., Li, M., Deng, H., Jiang, T., Xiong, K. Z., et al. (2020). Corydaline and L-Tetrahydropalmatine Attenuate Morphine-Induced Conditioned Place Preference and the Changes in Dopamine D2 and GluA1 AMPA Receptor Expression in Rats. Eur. J. Pharmacol. 884, 173397. doi:10.1016/j.ejphar.2020.173397
Jiang, Y., Liu, M., Liu, H., and Liu, S. (2020). A Critical Review: Traditional Uses, Phytochemistry, Pharmacology and Toxicology of Stephania Tetrandra S. Moore (Fen Fang Ji). Phytochem. Rev. 19, 449–489. 1–41. Advance online publication. doi:10.1007/s11101-020-09673-w
Jiang, Y., and Wang, D. (2016). Research Progress on Pharmacological Effect of Tetramethylpyrazine. Mod. Chin. Med. 18, 1364–1370. (in Chinese). doi:10.13313/j.issn.1673-4890.2016.10.028
Jin, S., Jin, D., Wang, W., and Zhou, L. (2015). The Progress in Research of Pharmacology of Aloperine. Pharmacol. Clin. Chin. Materia Medica 31, 214–217. (in Chinese). doi:10.13412/j.cnki.zyyl.2015.03.077
Kamei, J., Xiao, P., Ohsawa, M., Kubo, H., Higashiyama, K., Takahashi, H., et al. (1997). Antinociceptive Effects of (+)-matrine in Mice. Eur. J. Pharmacol. 337, 223–226. doi:10.1016/s0014-2999(97)01273-9
Kang, D. W., Moon, J. Y., Choi, J. G., Kang, S. Y., Ryu, Y., Park, J. B., et al. (2016). Antinociceptive Profile of Levo-Tetrahydropalmatine in Acute and Chronic Pain Mice Models: Role of Spinal Sigma-1 Receptor. Sci. Rep. 6, 37850. doi:10.1038/srep37850
Kaushik, A. S., Strath, L. J., and Sorge, R. E. (2020). Dietary Interventions for Treatment of Chronic Pain: Oxidative Stress and Inflammation. Pain Ther. 9, 487–498. doi:10.1007/s40122-020-00200-5
Kinch, M. S. (2015). An Analysis of FDA-Approved Drugs for Pain and Anesthesia. Drug Discov. Today. 20, 3–6. doi:10.1016/j.drudis.2014.09.002
Kinch, M. S., and Griesenauer, R. H. (2019). 2018 in Review: FDA Approvals of New Molecular Entities. Drug Discov. Today. 24, 1710–1714. doi:10.1016/j.drudis.2019.05.022
Ko, Y. H., Shim, K. Y., Lee, S. Y., and Jang, C. G. (2018). Evodiamine Reduces Caffeine-Induced Sleep Disturbances and Excitation in Mice. Biomol. Ther. Seoul. 26, 432–438. doi:10.4062/biomolther.2017.146
Koh, S. B., Ban, J. Y., Lee, B. Y., and Seong, Y. H. (2003). Protective Effects of Fangchinoline and Tetrandrine on Hydrogen Peroxide-Induced Oxidative Neuronal Cell Damage in Cultured Rat Cerebellar Granule Cells. Planta. Med. 69, 506–512. doi:10.1055/s-2003-40647
Kong, W. J., Vernieri, C., Foiani, M., and Jiang, J. D. (2020). Berberine in the Treatment of Metabolism-Related Chronic Diseases: A Drug Cloud (dCloud) Effect to Target Multifactorial Disorders. Pharmacol. Ther. 209, 107496. doi:10.1016/j.pharmthera.2020.107496
Kong, X., Zuo, H., and Li, Y. (2020). Pharmacological Effects and Clinical Application of Tetrandrine: Research Advances. J. Int. Pharm. Res. 47, 496–501. (in Chinese). doi:10.13220/j.cnki.jipr.2020.07.002
Lai, J. P., He, X. W., Jiang, Y., and Chen, F. (2003). Preparative Separation and Determination of Matrine from the Chinese Medicinal Plant Sophora Flavescens Ait by Molecularly Imprinted Solid-phase Extraction. Anal. Bioanal. Chem. 375 (2), 264–269. doi:10.1007/s00216-002-1675-2
Lan, X., Zhao, J., Zhang, Y., Chen, Y., Liu, Y., and Xu, F. (2020). Oxymatrine Exerts Organ- and Tissue-Protective Effects by Regulating Inflammation, Oxidative Stress, Apoptosis, and Fibrosis: From Bench to Bedside. Pharmacol. Res. 151, 104541. doi:10.1016/j.phrs.2019.104541
Lee, E. J., Ko, E., Lee, J., Rho, S., Ko, S., Shin, M. K., et al. (2004). Ginsenoside Rg1 Enhances CD4(+) T-Cell Activities and Modulates Th1/Th2 Differentiation. Int. Immunopharmacol. 4, 235–244. doi:10.1016/j.intimp.2003.12.007
Lee, J. Y., Yoon, S. Y., Won, J., Kim, H. B., Kang, Y., and Oh, S. B. (2017). Sinomenine Produces Peripheral Analgesic Effects via Inhibition of Voltage-Gated Sodium Currents. Neuroscience 358, 28–36. doi:10.1016/j.neuroscience.2017.06.024
Leung, L., and Cahill, C. M. (2010). TNF-alpha and Neuropathic Pain-Aa Review. J. Neuroinflammation. 7, 27. doi:10.1186/1742-2094-7-27
Leung, W. C., Zheng, H., Huen, M., Law, S. L., and Xue, H. (2003). Anxiolytic-like Action of Orally Administered Dl-Tetrahydropalmatine in Elevated Plus-Maze. Prog. Neuropsychopharmacol. Biol. Psychiatry. 27, 775–779. doi:10.1016/s0278-5846(03)00108-8
Li, H., Huang, N., Zhu, W., Wu, J., Yang, X., Teng, W., et al. (2018). Modulation the Crosstalk between Tumor-Associated Macrophages and Non-small Cell Lung Cancer to Inhibit Tumor Migration and Invasion by Ginsenoside Rh2. BMC Cancer 18, 579. doi:10.1186/s12885-018-4299-4
Li, M., and Wang, C. (2020). Traditional Uses, Phytochemistry, Pharmacology, Pharmacokinetics and Toxicology of the Fruit of Tetradium Ruticarpum: A Review. J. Ethnopharmacol. 263, 113231. doi:10.1016/j.jep.2020.113231
Li, P., Zhang, M., Wang, D., Xu, S., and Xu, X. (2012). Effects of Sinomenine on Analgesia and Exciting Amino Acid Neurotransmitters in Brain of SSNI Rat Model. Chin. Pharmacol. Bull. 28, 1365–1369. (in Chinese). doi:10.3969/j.issn.1001-1978.2012.10.009
Li, Q., Liang, X., Yang, Y., Zeng, X., Zhong, X., and Huang, C. (2020). Panax Notoginseng Saponins Ameliorate Cisplatin-Induced Mitochondrial Injury via the HIF-1α/mitochondria/ROS Pathway. FEBS Open Bio 10, 118–126. doi:10.1002/2211-5463.12760
Li, Q., Li, B., and Liu, H. (2009). Pharmacological Effect and Clinical Application of Ligustrazine Injection. Med. Recapitulate 15, 1402–1405. (in Chinese) CNKI:SUN:YXZS.0.2009-09-043.
Li, S., Chen, H., Wang, X., Wu, J., Jiang, J., and Wang, Y. (2011). Pharmacokinetic Study of a Novel Stroke Therapeutic, 2-[[(1,1-Dimethylethyl)oxidoimino]methyl]-3,5,6-Trimethylpyrazine, by a Simple HPLC-UV Method in Rats. Eur. J. Drug Metab. Pharmacokinet. 36 (2), 95–101. doi:10.1007/s13318-011-0032-2
Li, S., Han, J., Wang, D. S., Yang, Q., Feng, B., Kang, W. B., et al. (2017). Sinomenine Attenuates Chronic Inflammatory Pain in Mice. Metab. Brain Dis. 32, 211–219. doi:10.1007/s11011-016-9889-8
Li, X. J., Yue, P. Y., Ha, W. Y., Wong, D. Y., Tin, M. M., Wang, P. X., et al. (2006). Effect of Sinomenine on Gene Expression of the IL-1 Beta-Activated Human Synovial Sarcoma. Life Sci. 79, 665–673. doi:10.1016/j.lfs.2006.02.014
Liang, J., Chang, B., Huang, M., Huang, W., Ma, W., Liu, Y., et al. (2018). Oxymatrine Prevents Synovial Inflammation and Migration via Blocking NF-Κb Activation in Rheumatoid Fibroblast-like Synoviocytes. Int. Immunopharmacol. 55, 105–111. doi:10.1016/j.intimp.2017.12.006
Liao, Z. G., Liang, X. L., Zhu, J. Y., Zhao, G. W., Yang, M., Wang, G. F., et al. (2010). Correlation between Synergistic Action of Radix Angelica Dahurica Extracts on Analgesic Effects of Corydalis Alkaloid and Plasma Concentration of Dl-THP. J. Ethnopharmacol. 129, 115–120. doi:10.1016/j.jep.2010.03.005
Lin, S. T., Wang, Y., Xue, Y., Feng, D. C., Xu, Y., and Xu, L. Y. (2008). Tetrandrine Suppresses LPS-Induced Astrocyte Activation via Modulating IKKs-IkappaBalpha-NF-kappaB Signaling Pathway. Mol. Cell. Biochem. 315, 41–49. doi:10.1007/s11010-008-9787-4
Linglu, D., Yuxiang, L., Yaqiong, X., Ru, Z., Lin, M., Shaoju, J., et al. (2014). Antinociceptive Effect of Matrine on Vincristine-Induced Neuropathic Pain Model in Mice. Neurol. Sci. 35, 815–821. doi:10.1007/s10072-013-1603-6
Liu, C., Li, Z., Huang, Z., Zhang, K., Hu, C., Zuo, Z., et al. (2018). Ligustrazine Enhances the Hypnotic and Analgesic Effect of Ketamine in Mice. Biol. Pharm. Bull. 41, 690–696. doi:10.1248/bpb.b17-00869
Liu, H., Sun, Y., Gao, Y., Chen, F., Xu, M., and Liu, Z. (2010). The Analgesic Effect and Mechanism of the Combination of Sodium Ferulate and Oxymatrine. Neurochem. Res. 35, 1368–1375. doi:10.1007/s11064-010-0193-4
Liu, J. Y., Hu, J. H., Zhu, Q. G., Li, F. Q., Wang, J., and Sun, H. J. (2007). Effect of Matrine on the Expression of Substance P Receptor and Inflammatory Cytokines Production in Human Skin Keratinocytes and Fibroblasts. Int. Immunopharmacol. 7, 816–823. doi:10.1016/j.intimp.2007.02.003
Liu, Q. Y., Karpinski, E., and Pang, P. K. (1992). Tetrandrine Inhibits Both T and L Calcium Channel Currents in Ventricular Cells. J. Cardiovasc. Pharmacol. 20, 513–519. doi:10.1097/00005344-199210000-00001
Liu, Y. N., Pan, S. L., Liao, C. H., Huang, D. Y., Guh, J. H., Peng, C. Y., et al. (2009). Evodiamine Represses Hypoxia-Induced Inflammatory Proteins Expression and Hypoxia-Inducible Factor 1alpha Accumulation in RAW264.7. Shock 32, 263–269. doi:10.1097/SHK.0b013e31819940cb
Liu, Y. Y., Wang, T. X., Zhou, J. C., Qu, W. M., and Huang, Z. L. (2019). Dopamine D1 and D2 Receptors Mediate Analgesic and Hypnotic Effects of L-Tetrahydropalmatine in a Mouse Neuropathic Pain Model. Psychopharmacol. Berl. 236, 3169–3182. doi:10.1007/s00213-019-05275-3
Liu, Z., Zheng, J. F., Yang, L. Q., Yi, L., and Hu, B. (2007b). Effects of Sinomenine on NO/nNOS System in Cerebellum and Spinal Cord of Morphine-dependent and Withdrawal Mice. Sheng Li Xue Bao 59, 285–292. (in Chinese) CNKI:SUN:SLXU.0.2007-03-009.
Lu, L., Huang, R., Wu, Y., Jin, J. M., Chen, H. Z., Zhang, L. J., et al. (2020). Brucine: A Review of Phytochemistry, Pharmacology, and Toxicology. Front. Pharmacol. 11, 377. doi:10.3389/fphar.2020.00377
Lu, Y., Lou, J., Liu, X., and Wang, S. (2017). Oxysophocarpine Reduces Oxygen-Glucose Deprivation-Induced Microglial Activation and Injury. Am. J. Transl. Res. 9, 2266–2275.
Luan, F., He, X., and Zeng, N. (2020). Tetrandrine: a Review of its Anticancer Potentials, Clinical Settings, Pharmacokinetics and Drug Delivery Systems. J. Pharm. Pharmacol. 72, 1491–1512. doi:10.1111/jphp.13339
Lv, S., Yi, P. F., Shen, H. Q., Zhang, L. Y., Dong, H. B., Wu, S. C., et al. (2014). Ginsenoside Rh2-B1 Stimulates Cell Proliferation and IFN-γ Production by Activating the P38 MAPK and ERK-dependent Signaling Pathways in CTLL-2 Cells. Immunopharmacol. Immunotoxicol. 36, 43–51. doi:10.3109/08923973.2013.864669
Ma, B., Yue, K., Chen, L., Tian, X., Ru, Q., Gan, Y., et al. (2014). L-stepholidine, a Natural Dopamine Receptor D1 Agonist and D2 Antagonist, Inhibits Heroin-Induced Reinstatement. Neurosci. Lett. 559, 67–71. doi:10.1016/j.neulet.2013.10.066
Ma, Y., Li, Z., Wang, Y., and Feng, J. (2013). Brucine Induces the Apoptosis of U266 Multiple Myeloma Cells by Phosphorylation of C-Jun. Mol. Med. Rep. 7, 481–484. doi:10.3892/mmr.2012.1194
Marchand, F., Perretti, M., and McMahon, S. B. (2005). Role of the Immune System in Chronic Pain. Nat. Rev. Neurosci. 6, 521–532. doi:10.1038/nrn1700
Meng, T., Fu, S., He, D., Hu, G., Gao, X., Zhang, Y., et al. (2021). Evodiamine Inhibits Lipopolysaccharide (LPS)-Induced Inflammation in BV-2 Cells via Regulating AKT/Nrf2-HO-1/NF-κB Signaling Axis. Cell. Mol. Neurobiol. 41, 115–127. doi:10.1007/s10571-020-00839-w
Mercadante, S., Arcuri, E., and Santoni, A. (2019). Opioid-Induced Tolerance and Hyperalgesia. CNS Drugs 33, 943–955. doi:10.1007/s40263-019-00660-0
Mills, S. E. E., Nicolson, K. P., and Smith, B. H. (2019). Chronic Pain: a Review of its Epidemiology and Associated Factors in Population-Based Studies. Br. J. Anaesth. 123, e273–e283. doi:10.1016/j.bja.2019.03.023
Mo, J., Guo, Y., Yang, Y. S., Shen, J. S., Jin, G. Z., and Zhen, X. (2007). Recent Developments in Studies of L-Stepholidine and its Analogs: Chemistry, Pharmacology and Clinical Implications. Curr. Med. Chem. 14, 2996–3002. doi:10.2174/092986707782794050
Moon, T. C., Murakami, M., Kudo, I., Son, K. H., Kim, H. P., Kang, S. S., et al. (1999). A New Class of COX-2 Inhibitor, Rutaecarpine from Evodia Rutaecarpa. Inflamm. Res. 48 (12), 621–625. doi:10.1007/s000110050512
Moore, R. A., Derry, S., Aldington, D., Cole, P., and Wiffen, P. J. (2012). Amitriptyline for Neuropathic Pain and Fibromyalgia in Adults. Cochrane Database Syst. Rev. 12, CD008242. doi:10.1002/14651858.CD008242.pub2
Mullard, A. (2021). 2020 FDA Drug Approvals. Nat. Rev. Drug Discov. 20, 85–90. doi:10.1038/d41573-021-00002-0
Ngan Kee, W. D. (1998). Intrathecal Pethidine: Pharmacology and Clinical Applications. Anaesth. Intensive Care 26, 137–146. doi:10.1177/0310057X9802600202
Nguyen, L., Thomas, K. L., Lucke-Wold, B. P., Cavendish, J. Z., Crowe, M. S., and Matsumoto, R. R. (2016). Dextromethorphan: An Update on its Utility for Neurological and Neuropsychiatric Disorders. Pharmacol. Ther. 159, 1–22. doi:10.1016/j.pharmthera.2016.01.016
Niesters, M., Martini, C., and Dahan, A. (2014). Ketamine for Chronic Pain: Risks and Benefits. Br. J. Clin. Pharmacol. 77, 357–367. doi:10.1111/bcp.12094
Nutt, D. J. (2008). Relationship of Neurotransmitters to the Symptoms of Major Depressive Disorder. J. Clin. Psychiatry. 69 (Suppl. E1), 4–7.
Nyirimigabo, E., Xu, Y., Li, Y., Wang, Y., Agyemang, K., and Zhang, Y. (2015). A Review on Phytochemistry, Pharmacology and Toxicology Studies of Aconitum. J. Pharm. Pharmacol. 67, 1–19. doi:10.1111/jphp.12310
Obata, H. (2017). Analgesic Mechanisms of Antidepressants for Neuropathic Pain. Int. J. Mol. Sci. 18, 2483. doi:10.3390/ijms18112483
Oh, Y. C., Choi, J. G., Lee, Y. S., Brice, O. O., Lee, S. C., Kwak, H. S., et al. (2010). Tetrahydropalmatine Inhibits Pro-inflammatory Mediators in Lipopolysaccharide-Stimulated THP-1 Cells. J. Med. Food. 13, 1125–1132. doi:10.1089/jmf.2009.1388
Ono, M., and Satoh, T. (1988). Pharmacological Studies of Lappaconitine. Analgesic Activities. Arzneimittelforschung 38, 892–895. PMID: 3207434.
Ou, S., Zhao, Y. D., Xiao, Z., Wen, H. Z., Cui, J., and Ruan, H. Z. (2011). Effect of Lappaconitine on Neuropathic Pain Mediated by P2X3 Receptor in Rat Dorsal Root Ganglion. Neurochem. Int. 58, 564–573. doi:10.1016/j.neuint.2011.01.016
Ozaki, Y. (1992). Antiinflammatory Effect of Tetramethylpyrazine and Ferulic Acid. Chem. Pharm. Bull. (Tokyo) 40, 954–956. doi:10.1248/cpb.40.954
Pang, M. H., Kim, Y., Jung, K. W., Cho, S., and Lee, D. H. (2012). A Series of Case Studies: Practical Methodology for Identifying Antinociceptive Multi-Target Drugs. Drug Discov. Today. 17, 425–434. doi:10.1016/j.drudis.2012.01.003
Pearce, L. V., Petukhov, P. A., Szabo, T., Kedei, N., Bizik, F., Kozikowski, A. P., et al. (2004). Evodiamine Functions as an Agonist for the Vanilloid Receptor TRPV1. Org. Biomol. Chem. 2, 2281–2286. doi:10.1039/B404506H
Peek, A. L., Rebbeck, T., Puts, N. A., Watson, J., Aguila, M. R., and Leaver, A. M. (2020). Brain GABA and Glutamate Levels across Pain Conditions: A Systematic Literature Review and Meta-Analysis of 1H-MRS Studies Using the MRS-Q Quality Assessment Tool. NeuroImage 210, 116532. doi:10.1016/j.neuroimage.2020.116532
Pizzino, G., Irrera, N., Cucinotta, M., Pallio, G., Mannino, F., Arcoraci, V., et al. (2017). Oxidative Stress: Harms and Benefits for Human Health. Oxid. Med. Cell. Longev. 2017, 8416763. doi:10.1155/2017/8416763
Qian, L., Xu, Z., Zhang, W., Wilson, B., Hong, J. S., and Flood, P. M. (2007). Sinomenine, a Natural Dextrorotatory Morphinan Analog, Is Anti-inflammatory and Neuroprotective through Inhibition of Microglial NADPH Oxidase. J. Neuroinflammation. 4, 23. doi:10.1186/1742-2094-4-23
Qu, J., Xu, N., Zhang, J., Geng, X., and Zhang, R. (2020). Panax Notoginseng Saponins and Their Applications in Nervous System Disorders: a Narrative Review. Ann. Transl. Med. 8, 1525. doi:10.21037/atm-20-6909
Rao, S., Liu, S., Zou, L., Jia, T., Zhao, S., Wu, B., et al. (2017). The Effect of Sinomenine in Diabetic Neuropathic Pain Mediated by the P2X3 Receptor in Dorsal Root Ganglia. Purinergic Signal 13, 227–235. doi:10.1007/s11302-016-9554-z
Ren, F., Jin, A., and Niu, X. (2003). Pharmacological Effects and Clinical Application of Tetrahydropalmatine, a Plant-Derived Calcium Antagonist. Dig. World Core Med. Journals 2, 672–674. (in Chinese) CNKI:SUN:SJXY.0.2003-03-013.
Ren, Z., Chen, X., Hong, L., Zhao, X., Cui, G., Li, A., et al. (2020). Nanoparticle Conjugation of Ginsenoside Rg3 Inhibits Hepatocellular Carcinoma Development and Metastasis. Small 16, e1905233. doi:10.1002/smll.201905233
Rice, A. S., and Maton, S. (2001). Gabapentin in Postherpetic Neuralgia: a Randomised, Double Blind, Placebo Controlled Study, Postherpetic Neuralgia Study Group. Pain 94, 215–224. doi:10.1016/S0304-3959(01)00407-9
Schett, G., and Gravallese, E. (2012). Bone Erosion in Rheumatoid Arthritis: Mechanisms, Diagnosis and Treatment. Nat. Rev. Rheumatol. 8, 656–664. doi:10.1038/nrrheum.2012.153
Scholl, L., Seth, P., Kariisa, M., Wilson, N., and Baldwin, G. (2018). Drug and Opioid-Involved Overdose Deaths - United States, 2013-2017. MMWR Morb. Mortal. Wkly. Rep. 67, 1419–1427. doi:10.15585/mmwr.mm675152e1
Seow, W. K., Ferrante, A., Li, S. Y., and Thong, Y. H. (1988). Antiphagocytic and Antioxidant Properties of Plant Alkaloid Tetrandrine. Int. Arch. Allergy. Appl. Immunol. 85, 404–409. doi:10.1159/000234542
Serpell, M. G. (2002). Neuropathic Pain Study GroupGabapentin in Neuropathic Pain Syndromes: a Randomised, Double-Blind, Placebo-Controlled Trial. Pain 99, 557–566. doi:10.1016/S0304-3959(02)00255-5
Shaheen, F., Ahmad, M., Khan, M. T., Jalil, S., Ejaz, A., Sultankhodjaev, M. N., et al. (2005). Alkaloids of Aconitum Laeve and Their Anti-inflammatory Antioxidant and Tyrosinase Inhibition Activities. Phytochemistry 66, 935–940. doi:10.1016/j.phytochem.2005.02.010
Shi, X., Mao, Y., Saffiotti, U., Wang, L., Rojanasakul, Y., Leonard, S. S., et al. (1995). Antioxidant Activity of Tetrandrine and its Inhibition of Quartz-Induced Lipid Peroxidation. J. Toxicol. Environ. Health. 46, 233–248. doi:10.1080/15287399509532031
Shi, Y., Meng, R., Li, L., and Wu, C. (2008). Suppressive Effects of SIN on Immunological Function and Cytokine Production by T Cells from the Patients with SLE. Chin. J. Immunol. 24, 800–807. (in Chinese) CNKI:SUN:ZMXZ.0.2008-09-013.
Silva, R. L., Lopes, A. H., Guimarães, R. M., and Cunha, T. M. (2017). CXCL1/CXCR2 Signaling in Pathological Pain: Role in Peripheral and Central Sensitization. Neurobiol. Dis. 105, 109–116. doi:10.1016/j.nbd.2017.06.001
Song, X., and Hu, S. (2009). Adjuvant Activities of Saponins from Traditional Chinese Medicinal Herbs. Vaccine 27, 4883–4890. doi:10.1016/j.vaccine.2009.06.033
Stahl, S. M. (2019). Dextromethorphan/Bupropion: A Novel Oral NMDA (N-Methyl-D-Aspartate) Receptor Antagonist with Multimodal Activity. CNS Spectr. 24, 461–466. doi:10.1017/S1092852919001470
Striano, P., and Striano, S. (2008). Gabapentin: a Ca2+ Channel Alpha 2-delta Ligand Far beyond Epilepsy Therapy. Drugs Today (Barc) 44, 353–368. doi:10.1358/dot.2008.44.5.1186403
Sun, H. X., Pan, H. J., and Pan, Y. J. (2003). Haemolytic Activities and Immunologic Adjuvant Effect of Panax Notoginseng Saponins. Acta Pharmacol. Sin. 24, 1150–1154.
Sun, Q., Xie, L., Song, J., and Li, X. (2020). Evodiamine: A Review of its Pharmacology, Toxicity, Pharmacokinetics and Preparation Researches. J. Ethnopharmacol. 262, 113164. doi:10.1016/j.jep.2020.113164
Tamano, R., Ishida, M., Asaki, T., Hasegawa, M., and Shinohara, S. (2016). Effect of Spinal Monoaminergic Neuronal System Dysfunction on Pain Threshold in Rats, and the Analgesic Effect of Serotonin and Norepinephrine Reuptake Inhibitors. Neurosci. Lett. 615, 78–82. doi:10.1016/j.neulet.2016.01.025
Tang, H., Zhang, L., Li, X., Sun, C., and Ma, P. (2009). The Experimental Study of Different Solvent Extracting Total Alkaloid from Semen Strychni. Guid. J. Tradit. Chin. Med. Pharm. 15 (11), 52–53. doi:10.13862/j.cnki.cn43-1446/r.2009.11.039
Thigpen, J. C., Odle, B. L., and Harirforoosh, S. (2019). Opioids: A Review of Pharmacokinetics and Pharmacodynamics in Neonates, Infants, and Children. Eur. J. Drug. Metab. Pharmacokinet. 44, 591–609. doi:10.1007/s13318-019-00552-0
Till, S. R., As-Sanie, S., and Schrepf, A. (2019). Psychology of Chronic Pelvic Pain: Prevalence, Neurobiological Vulnerabilities, and Treatment. Clin. Obstet. Gynecol. 62, 22–36. doi:10.1097/GRF.0000000000000412
Toma, W., Ulker, E., Alqasem, M., AlSharari, S. D., McIntosh, J. M., and Damaj, M. I. (2020). Behavioral and Molecular Basis of Cholinergic Modulation of Pain: Focus on Nicotinic Acetylcholine Receptors. Curr. Top. Behav. Neurosci. 45, 153–166. doi:10.1007/7854_2020_135
Treede, R. D., Rief, W., Barke, A., Aziz, Q., Bennett, M. I., Benoliel, R., et al. (2019). Chronic Pain as a Symptom or a Disease: the IASP Classification of Chronic Pain for the International Classification of Diseases (ICD-11). Pain 160, 19–27. doi:10.1097/j.pain.0000000000001384
Tripathi, B. K., and Srivastava, A. K. (2006). Diabetes Mellitus: Complications and Therapeutics. Med. Sci. Monit. 12, RA130–47.
Tzschentke, T. M., and Schmidt, W. J. (2003). Glutamatergic Mechanisms in Addiction. Mol. Psychiatry. 8, 373–382. doi:10.1038/sj.mp.4001269
Vowles, K. E., McEntee, M. L., Julnes, P. S., Frohe, T., Ney, J. P., and van der Goes, D. N. (2015). Rates of Opioid Misuse, Abuse, and Addiction in Chronic Pain: a Systematic Review and Data Synthesis. Pain 156, 569–576. doi:10.1097/01.j.pain.0000460357.01998.f1
Wang, B. H., and Ou-Yang, J. P. (2005). Pharmacological Actions of Sodium Ferulate in Cardiovascular System. Cardiovasc. Drug. Rev. 23, 161–172. doi:10.1111/j.1527-3466.2005.tb00163.x
Wang, H., Li, Y., Dun, L., Xu, Y., Jin, S., Du, J., et al. (2013b). Antinociceptive Effects of Oxymatrine from Sophora Flavescens, through Regulation of NR2B-Containing NMDA Receptor-ERK/CREB Signaling in a Mice Model of Neuropathic Pain. Phytomedicine 20, 1039–1045. doi:10.1016/j.phymed.2013.04.012
Wang, L., Zhang, Y., Wang, Z., Gong, N., Kweon, T. D., Vo, B., et al. (2016b). The Antinociceptive Properties of the Corydalis Yanhusuo Extract. PloS one 11, e0162875. doi:10.1371/journal.pone.0162875
Wang, M. H., Chang, C. K., Cheng, J. H., Wu, H. T., Li, Y. X., and Cheng, J. T. (2008). Activation of Opioid Mu-Receptor by Sinomenine in Cell and Mice. Neurosci. Lett. 443, 209–212. doi:10.1016/j.neulet.2008.07.088
Wang, M., and Yang, W. (2013). The Research on the Compatibility Law of the “Jun”, “Chen”, “Zuo”, and “Shi” in Traditional Chinese Medicine. J. Traditional Chin. Med. 54, 1974–1975. (in Chinese). doi:10.13288/j.11-2166/r.2013.22.022
Wang, R., Deng, X., Gao, Q., Wu, X., Han, L., Gao, X., et al. (2020). Sophora Alopecuroides L.: An Ethnopharmacological, Phytochemical, and Pharmacological Review. J. Ethnopharmacol. 248, 112172. doi:10.1016/j.jep.2019.112172
Wang, S., Li, A., and Guo, S. (2016a). Ligustrazine Attenuates Neuropathic Pain by Inhibition of JAK/STAT3 Pathway in a Rat Model of Chronic Constriction Injury. Pharmazie 71, 408–412. doi:10.1691/ph.2016.6546
Wang, X., Liu, Y., Zhang, H., Jin, J., Ma, Y., and Leng, Y. (2021). Sinomenine Alleviates Dorsal Root Ganglia Inflammation to Inhibit Neuropathic Pain via the P38 MAPK/CREB Signalling Pathway. Eur. J. Pharmacol. 897, 173945. doi:10.1016/j.ejphar.2021.173945
Wang, Y., Fang, Y., Huang, W., Zhou, X., Wang, M., Zhong, B., et al. (2005a). Effect of Sinomenine on Cytokine Expression of Macrophages and Synoviocytes in Adjuvant Arthritis Rats. J. Ethnopharmacol. 98, 37–43. doi:10.1016/j.jep.2004.12.022
Wang, Y. S., Shen, C. Y., and Jiang, J. G. (2019). Antidepressant Active Ingredients from Herbs and Nutraceuticals Used in TCM: Pharmacological Mechanisms and Prospects for Drug Discovery. Pharmacol. Res. 150, 104520. doi:10.1016/j.phrs.2019.104520
Wang, Z., Wang, Q., Wang, C., Xu, X., and Yu, H. (2017). Tetramethylpyrazine Attenuates Periorbital Allodynia and Neuroinflammation in a Model of Traumatic Brain Injury. J. Inflamm. (Lond) 14, 13. doi:10.1186/s12950-017-0161-8
Woolf, C. J. (1983). Evidence for a Central Component of Post-injury Pain Hypersensitivity. Nature 306, 686–688. doi:10.1038/306686a0
Wu, P., and Chen, Y. (2019). Evodiamine Ameliorates Paclitaxel-Induced Neuropathic Pain by Inhibiting Inflammation and Maintaining Mitochondrial Anti-oxidant Functions. Hum. Cell. 32, 251–259. doi:10.1007/s13577-019-00238-4
Wu, Y., Pan, X., Xu, Y., Lu, X., He, S., He, R., et al. (2016). Optimization of Combinations of Ginsenoside-Rg1, Ginsenoside-Rb1, Evodiamine and Rutaecarpine for Effective Therapy of Mouse Migraine. J. Nat. Med. 70, 207–216. doi:10.1007/s11418-015-0960-2
Xiang, J., and Jiang, Y. (2013). Antiepileptic Potential of Matrine via Regulation the Levels of Gamma-Aminobutyric Acid and Glutamic Acid in the Brain. Int. J. Mol. Sci. 14, 23751–23761. doi:10.3390/ijms141223751
Xie, Q. M., Tang, H. F., Chen, J. Q., and Bian, R. L. (2002). Pharmacological Actions of Tetrandrine in Inflammatory Pulmonary Diseases. Acta. Pharmacol. Sin. 23, 1107–1113. PMID: 12466048.
Xu, L., Tang, Y. Y., Ben, X. L., Cheng, M. H., Guo, W. X., Liu, Y., et al. (2020). Ginsenoside Rg1-Induced Activation of Astrocytes Promotes Functional Recovery via the PI3K/Akt Signaling Pathway Following Spinal Cord Injury. Life Sci. 252, 117642. doi:10.1016/j.lfs.2020.117642
Xu, M., Liu, L., Qi, C., Deng, B., and Cai, X. (2008). Sinomenine versus NSAIDs for the Treatment of Rheumatoid Arthritis: a Systematic Review and Meta-Analysis. Planta. Med. 74, 1423–1429. doi:10.1055/s-2008-1081346
Xu, T., Li, Y., Wang, H., Xu, Y., Ma, L., Sun, T., et al. (2013). Oxysophocarpine Induces Anti-nociception and Increases the Expression of GABAAα1 Receptors in Mice. Mol. Med. Rep. 7, 1819–1825. doi:10.3892/mmr.2013.1414
Xu, Y. Q., Jin, S. J., Liu, N., Li, Y. X., Zheng, J., Ma, L., et al. (2014). Aloperine Attenuated Neuropathic Pain Induced by Chronic Constriction Injury via Anti-oxidation Activity and Suppression of the Nuclear Factor Kappa B Pathway. Biochem. Biophys. Res. Commun. 451, 568–573. doi:10.1016/j.bbrc.2014.08.025
Xue, Y., Wang, Y., Feng, D. C., Xiao, B. G., and Xu, L. Y. (2008). Tetrandrine Suppresses Lipopolysaccharide-Induced Microglial Activation by Inhibiting NF-kappaB Pathway. Acta. Pharmacol. Sin. 29, 245–251. doi:10.1111/j.1745-7254.2008.00734.x
Yam, M. F., Loh, Y. C., Tan, C. S., Khadijah Adam, S., Abdul Manan, N., and Basir, R. (2018). General Pathways of Pain Sensation and the Major Neurotransmitters Involved in Pain Regulation. Int. J. Mol. Sci. 19, 2164. doi:10.3390/ijms19082164
Yamasaki, H. (1976). Pharmacology of Sinomenine, an Anti-rheumatic Alkaloid from Sinomenium Acutum. Acta. Med. okayama. 30, 1–20.
Yang, K., Jin, G., and Wu, J. (2007). The Neuropharmacology of (-)-stepholidine and its Potential Applications. Curr. Neuropharmacol. 5, 289–294. doi:10.2174/157015907782793649
Yang, Q. Q., and Zhou, J. W. (2019). Neuroinflammation in the Central Nervous System: Symphony of Glial Cells. Glia 67, 1017–1035. doi:10.1002/glia.23571
Yang, S., and Chang, M. C. (2019). Chronic Pain: Structural and Functional Changes in Brain Structures and Associated Negative Affective States. Int. J. Mol. Sci. 20, 3130. doi:10.3390/ijms20133130
Yang, Y., Jin, S. J., Wang, H. L., Li, Y. X., Du, J., Zhou, R., et al. (2015a). Effects of Aloperine on Acute and Inflammatory Pain Models in Mice. Scand. J. Pain. 8, 28–34. doi:10.1016/j.sjpain.2015.04.001
Yang, Y., Li, Y. X., Wang, H. L., Jin, S. J., Zhou, R., Qiao, H. Q., et al. (2015b). Oxysophocarpine Ameliorates Carrageenan-Induced Inflammatory Pain via Inhibiting Expressions of Prostaglandin E2 and Cytokines in Mice. Planta Med. 81, 791–797. doi:10.1055/s-0035-1546153
Yarosh, D. B., Brown, D. A., and Matthew, C. T. (2006). Biomimetic of Evodia Rutaecarpa Fruit Extract for Amelioration of Inflammation. Washington, DC: U.S. Patent and Trademark Office. U.S. Patent No 5,592,956.
Yin, L. L., and Zhu, X. Z. (2005). The Involvement of Central Cholinergic System in (+)-Matrine-Induced Antinociception in Mice. Pharmacol. Biochem. Behav. 80, 419–425. doi:10.1016/j.pbb.2004.12.008
Yin, Q., Xia, Y., and Wang, G. (2016). Sinomenine Alleviates High Glucose-Induced Renal Glomerular Endothelial Hyperpermeability by Inhibiting the Activation of RhoA/ROCK Signaling Pathway. Biochem. Biophys. Res. Commun. 477, 881–886. doi:10.1016/j.bbrc.2016.06.152
Yin, W., Wang, T. S., Yin, F. Z., and Cai, B. C. (2003). Analgesic and Anti-inflammatory Properties of Brucine and Brucine N-Oxide Extracted from Seeds of Strychnos Nux-Vomica. J. Ethnopharmacol. 88, 205–214. doi:10.1016/s0378-8741(03)00224-1
Yong, R. J., Mullins, P. M., and Bhattacharyya, N. (2021). Prevalence of Chronic Pain Among Adults in the United States. Pain 163, e328–e332. doi:10.1097/j.pain.0000000000002291
Yongjun, Z., Tingjie, Z., Xiaoqiu, Y., Zhiying, F., Feng, Q., Guangke, X., et al. (2020). A Survey of Chronic Pain in China. J. Med. 15, 1730550. doi:10.1080/19932820.2020.1730550
You, L., Yang, C., Du, Y., Wang, W., Sun, M., Liu, J., et al. (2020). A Systematic Review of the Pharmacology, Toxicology and Pharmacokinetics of Matrine. Front. Pharmacol. 11, 01067. doi:10.3389/fphar.2020.01067
Yu, G., Qian, L., Yu, J., Tang, M., Wang, C., Zhou, Y., et al. (2019). Brucine Alleviates Neuropathic Pain in Mice via Reducing the Current of the Sodium Channel. J. Ethnopharmacol. 233, 56–63. doi:10.1016/j.jep.2018.12.045
Yuan, X., Sun, Y., Miao, N., Sun, S., Wang, Y., Hu, Z., et al. (2011). The Synergistic Anti-inflammatory Effect of the Combination of Sodium Ferulate and Oxymatrine and its Modulation on Inflammation-Associated Mediators in RAW 264.7 Cells. J. Ethnopharmacol. 137, 1477–1485. doi:10.1016/j.jep.2011.08.031
Yuan, Y., Zhang, Y., He, X., and Fan, S. (2018). Protective Effects of Sinomenine on CFA-Induced Inflammatory Pain in Rats. Med. Sci. Monit. 24, 2018–2024. doi:10.12659/msm.906726
Yunus, M. B. (2015). Editorial Review: an Update on Central Sensitivity Syndromes and the Issues of Nosology and Psychobiology. Curr. Rheumatol. Rev. 11, 70–85. doi:10.2174/157339711102150702112236
Zeligs, M. A. (2006). Diindolylmethane-based Compositions and Methods of Use Thereof for Promoting Oral Mucosal and Bone Health. U.S. Patent No 2,002,115,708. Washington, DC: U.S. Patent and Trademark Office.
Zeng, M. Y., and Tong, Q. Y. (2020). Anti-inflammation Effects of Sinomenine on Macrophages through Suppressing Activated TLR4/NF-Κb Signaling Pathway. Curr. Med. Sci. 40, 130–137. doi:10.1007/s11596-020-2156-6
Zhang, C., Guan, D., Jiang, M., Liang, C., Li, L., Zhao, N., et al. (2019). Efficacy of Leflunomide Combined with Ligustrazine in the Treatment of Rheumatoid Arthritis: Prediction with Network Pharmacology and Validation in a Clinical Trial. Chin. Med. 14, 26. doi:10.1186/s13020-019-0247-8
Zhang, H., Chen, L., Sun, X., Yang, Q., Wan, L., and Guo, C. (2020a). Matrine: A Promising Natural Product with Various Pharmacological Activities. Front. Pharmacol. 11, 588. doi:10.3389/fphar.2020.00588
Zhang, H., Yin, L., Lu, M., Wang, J., Li, Y. T., Gao, W. L., et al. (2020b). Evodiamine Attenuates Adjuvant-Induced Arthritis in Rats by Inhibiting Synovial Inflammation and Restoring the Th17/Treg Balance. J. Pharm. Pharmacol. 72, 798–806. doi:10.1111/jphp.13238
Zhang, L., Zhang, W., Zheng, B., and Tian, N. (2019). Sinomenine Attenuates Traumatic Spinal Cord Injury by Suppressing Oxidative Stress and Inflammation via Nrf2 Pathway. Neurochem. Res. 44, 763–775. doi:10.1007/s11064-018-02706-z
Zhang, M., and Shen, Y. (2021). Research Progress on Clinical Pharmacological Effects of Matrine and Oxymatrine in Prevention and Treatment of Bacterial Vaginitis. Anti-Infection Pharm. 18, 936–940. (in Chinese). doi:10.13493/j.issn.1672-7878.2021.07-002
Zhang, M. Y., Liu, Y. P., Zhang, L. Y., Yue, D. M., Qi, D. Y., Liu, G. J., et al. (2015). Levo-Tetrahydropalmatine Attenuates Bone Cancer Pain by Inhibiting Microglial Cells Activation. Mediat. InflammInflamm 2015, 752512. doi:10.1155/2015/752512
Zhang, X., Wu, J., and Zhang, B. (2015). Xuesaitong Injection as One Adjuvant Treatment of Acute Cerebral Infarction: A Systematic Review and Meta-Analysis. BMC Complement. Altern. Med. 15, 36. doi:10.1186/s12906-015-0560-4
Zhang, Z., Yan, J., Xu, K., Ji, Z., and Li, L. (2015). Tetrandrine Reverses Drug Resistance in Isoniazid and Ethambutol Dual Drug-Resistant Mycobacterium tuberculosis Clinical Isolates. BMC Infect. Dis. 15, 153. doi:10.1186/s12879-015-0905-0
Zhang, Z. M., Zheng, X. X., Jiang, B., and Zhou, Q. (2004). Effects of L-Tetrahydropalmatine on Concentrations of Neurotransmitter Amino Acids in Mice with Cerebral Ischemia. Zhongguo Zhong Yao Za Zhi 29, 371–373. (in Chinese).
Zhao, H., Luo, F., Li, H., Zhang, L., Yi, Y., and Wan, J. (2014). Antinociceptive Effect of Tetrandrine on LPS-Induced Hyperalgesia via the Inhibition of IKKβ Phosphorylation and the COX-2/PGE₂ Pathway in Mice. PloS one 9, e94586. doi:10.1371/journal.pone.0094586
Zhao, P., Chang, R. Y., Liu, N., Wang, J., Zhou, R., Qi, X., et al. (2018). Neuroprotective Effect of Oxysophocarpine by Modulation of MAPK Pathway in Rat Hippocampal Neurons Subject to Oxygen-Glucose Deprivation and Reperfusion. Cell. Mol. Neurobiol. 38, 529–540. doi:10.1007/s10571-017-0501-5
Zhao, X. X., Peng, C., Zhang, H., and Qin, L. P. (2012). Sinomenium Acutum: a Review of Chemistry, Pharmacology, Pharmacokinetics, and Clinical Use. Pharm. Biol. 50, 1053–1061. doi:10.3109/13880209.2012.656847
Zhao, Y., Guo, Y., Huang, S., Yin, B., and Tang, Y. (2021). Advances in Research on the Parmacological Effects and Molecular Mechanisms of Borneol Guiding Action. J. Nanjing Univ. Traditional Chin. Med. 37, 150–155. (in Chinese). doi:10.14148/j.issn.1672-0482.2021.0150
Zhou, G., and Chen, Z. (2014). Translating the Power of TCM into Patients' Hope. Front. Med. 8, 265–267. doi:10.1007/s11684-014-0329-z
Zhou, H., Li, J., Sun, F., Wang, F., Li, M., Dong, Y., et al. (2020). A Review on Recent Advances in Aloperine Research: Pharmacological Activities and Underlying Biological Mechanisms. Front. Pharmacol. 11, 538137. doi:10.3389/fphar.2020.538137
Zhu, Q., Sun, Y., Mao, L., Liu, C., Jiang, B., Zhang, W., et al. (2016). Antinociceptive Effects of Sinomenine in a Rat Model of Postoperative Pain. Br. J. Pharmacol. 173, 1693–1702. doi:10.1111/bph.13470
Zhu, Q., Sun, Y., Zhu, J., Fang, T., Zhang, W., and Li, J. X. (2014b). Antinociceptive Effects of Sinomenine in a Rat Model of Neuropathic Pain. Sci. Rep. 4, 7270. doi:10.1038/srep07270
Zhu, Q. L., Li, Y. X., Zhou, R., Ma, N. T., Chang, R. Y., Wang, T. F., et al. (2014a). Neuroprotective Effects of Oxysophocarpine on Neonatal Rat Primary Cultured Hippocampal Neurons Injured by Oxygen-Glucose Deprivation and Reperfusion. Pharm. Biol. 52, 1052–1059. doi:10.3109/13880209.2013.877039
Zhu, W., Zhang, Y., Huang, Y., and Lu, L. (2017). Chinese Herbal Medicine for the Treatment of Drug Addiction. Int. Rev. Neurobiol. 135, 279–295. doi:10.1016/bs.irn.2017.02.013
Keywords: analgesics, TCM (trad. Chinese medicine), chronic pain, combinational therapy, alkaloids
Citation: Jiang W, Tang M, Yang L, Zhao X, Gao J, Jiao Y, Li T, Tie C, Gao T, Han Y and Jiang J-D (2022) Analgesic Alkaloids Derived From Traditional Chinese Medicine in Pain Management. Front. Pharmacol. 13:851508. doi: 10.3389/fphar.2022.851508
Received: 10 January 2022; Accepted: 25 April 2022;
Published: 10 May 2022.
Edited by:
Yu Chiang Hung, Kaohsiung Chang Gung Memorial Hospital, TaiwanReviewed by:
Yaswanth Kuthati, Cathay General Hospital, TaiwanXiuli Zhang, Dalian Institute of Chemical Physics (CAS), China
Copyright © 2022 Jiang, Tang, Yang, Zhao, Gao, Jiao, Li, Tie, Gao, Han and Jiang. This is an open-access article distributed under the terms of the Creative Commons Attribution License (CC BY). The use, distribution or reproduction in other forums is permitted, provided the original author(s) and the copyright owner(s) are credited and that the original publication in this journal is cited, in accordance with accepted academic practice. No use, distribution or reproduction is permitted which does not comply with these terms.
*Correspondence: Tianle Gao, tl_gao@hotmail.com; Yanxing Han, hanyanxing@imm.ac.cn; Jian-Dong Jiang, jiang.jdong@163.com
†These authors have contributed equally to this work