- 1Department of Craniofacial Diagnostics and Biosciences, Faculty of Dentistry, Universiti Kebangsaan Malaysia, Kuala Lumpur, Malaysia
- 2Centre for Diagnostic, Therapeutic and Investigative Studies, Universiti Kebangsaan Malaysia, Kuala Lumpur, Malaysia
Periodontitis is an oral inflammatory process involving the periodontium, which is mainly caused by the invasion of periodontopathogenic microorganisms that results in gingival connective tissue and alveolar bone destruction. Metabolic products of the oral pathogens and the associated host immune and inflammatory responses triggered are responsible for the local tissue destruction. Numerous studies in the past decades have demonstrated that natural polyphenols are capable of modulating the host inflammatory responses by targeting multiple inflammatory components. The proposed mechanism by which polyphenolic compounds exert their great potential is by regulating the immune cell, proinflammatory cytokines synthesis and gene expression. However, due to its low absorption and bioavailability, the beneficial effects of these substances are very limited and it hampers their use as a therapeutic agent. To address these limitations, targeted delivery systems by nanoencapsulation techniques have been explored in recent years. Nanoencapsulation of polyphenolic compounds with different carriers is an efficient and promising approach to boost their bioavailability, increase the efficiency and reduce the degradability of natural polyphenols. In this review, we focus on the effects of different polyphenolic substances in periodontal inflammation and to explore the pharmaceutical significance of polyphenol-loaded nanoparticles in controlling periodontitis, which may be useful for further enhancement of their efficacy as therapeutic agents for periodontal disease.
Introduction
Periodontitis contributes to a significant public health problem globally due to its high prevalence, economic impact and health consequences (Dom et al., 2012; Marcenes et al., 2013). As a common complex inflammatory disease of the oral cavity, it has been estimated that severe periodontitis affects 11% of the world population in last decades (Kassebaum et al., 2014). Increasing burden of severe periodontitis is suggested to be partly due to an increase in life expectancy in the growing world population. In addition, recent study has reported that half of the adult population worldwide have at least one tooth with apical periodontitis, which is usually asymptomatic and underestimated (Tibúrcio-Machado et al., 2021). Apart from the local effects on the dentition and tooth-supporting tissues, oral diseases may also have an impact on systemic health (Scannapieco and Cantos 2016).
Diseases of the periodontium such as gingivitis and periodontitis are preventable with the application of correct preventive strategies. Nonsurgical treatment modalities remain the gold standard for managing periodontal diseases. Dental cleaning, gingival scaling/root planing (SRP) and proper oral hygiene are among the periodontal treatments available that aim to reduce inflammation, pocket depth and clinical attachment gain (Plessas 2014). In scaling and root planning procedure, antibiotics have been used as adjunct microbial therapy in periodontal pockets through systemic or topical administration (Leszczyńska et al., 2011). According to literature, deep periodontal pockets pose a great challenge for nonsurgical periodontal treatment and in cases where surgical therapy cannot be undertaken, SRP alone may not be sufficient. Bacteria that penetrate the gingival tissue may not be eliminated by mechanical instrumentation hence local and systemic antibiotics are used as an adjunctive modality (Ahad et al., 2016).
The application of non-surgical or surgical periodontal therapy in certain cases as well as antimicrobial therapy however is not wholly successful. In addition to its unwanted side effects, the rise of antimicrobial resistance among patients with severe periodontitis necessitates new strategies in managing periodontal inflammation (Kwon et al., 2021). Based on a recent report in the management of periodontitis, the use of the highest dosage of antibiotic for the shortest duration of time should be considered by clinicians in order to reduce the risk of antibiotic resistance (McGowan et al., 2018). Other major disadvantages that are related to systemic administration are the availability of insufficient concentration of the drug in gingival crevicular fluid and disturbance of intestinal microflora related to the use of antibiotics. Recent studies have also suggested the use of antimicrobial photodynamic therapy (aPDT) as an adjunct to nonsurgical treatment of deep periodontal pockets, however controversial results were reported. Available evidence on aPDT is still scarce due to the low number of controlled studies and high heterogeneity in the study outcome (Salvi et al., 2020; Zhao et al., 2021).
Natural compounds with the capability to modulate host inflammatory response have gained considerable research attention (Palaska et al., 2013). Host modulation therapy (HMT) using anti-inflammatory and antioxidant agents has been investigated and explored as a non-invasive therapeutic approach for periodontitis (Preshaw 2018; Sulijaya et al., 2019). Plant-derived polyphenols have been known to possess antimicrobial, anti-inflammatory, immunomodulatory and antioxidant properties contributing to its benefits in human health. Such characteristics have been reported as the biological mechanisms involve in reducing the initiation and progression of periodontal inflammation. In vitro and in vivo studies demonstrated that polyphenol possesses an antimicrobial and immunomodulatory potential in treating and preventing periodontitis (Bunte et al., 2019). However, the use of systemic route administration in particular may yield limited results on account of poor pharmacokinetics and pharmacodynamics properties of polyphenol (Hoda et al., 2019). Despite its great potential, polyphenolic compounds have been associated with limited bioavailability mainly due to its low solubility, poor stability in the gastrointestinal tract (GIT) and low intestinal permeability as well as its extremely short plasma half-life (Lu et al., 2016).
Besides continuing the search for new agents that have anti-inflammatory or bone-regeneration properties, the development of slow-release agents is highly desirable to prevent this prevalent and costly disease. The economic burden of periodontitis is highly associated with its prevalence and cost of treatment and in fact is comparable with that of other chronic diseases (Mohd-Dom et al., 2014; Mohd Dom et al., 2016). One of the strategies to overcome the limitations related to polyphenol bioavailability and to enhance its therapeutic applications is by incorporating them into nanoparticles (Conte et al., 2016; Abdul Rahim et al., 2019). The therapeutic potential of polyphenol in managing periodontal inflammation from recently published studies is discussed and the effects of different polyphenol-loaded nanoparticles are highlighted in this present review.
Host Immune and Inflammatory Responses in Periodontal Inflammation
Gingivitis and periodontitis are the two most common gum diseases that affect periodontal tissues and the supporting structures of a tooth (Hasturk et al., 2012). Gingivitis is most commonly caused by bacterial plaque accumulation on the tooth surface resulting in gum inflammation. In gingivitis, the inflammatory condition is restricted to the soft-tissue area of the gingival epithelium and connective tissue without affecting the deeper compartments of the periodontium. Nonetheless, gingivitis may progress and develop into periodontitis, and therefore it is also considered as the prerequisite for the onset of periodontitis (Murukami et al., 2018). Periodontitis is a chronic multifactorial inflammatory disease characterized by progressive destruction of the periodontal supporting tissue including periodontal ligament and alveolar bone. It is indicated by gingival inflammation, clinical attachment loss, alveolar bone loss that could be assessed by radiography, presence of periodontal pocket and gingival bleeding (Papapanou et al., 2018).
Activation of Host Immune and Inflammatory Responses
Inflammation is an innate immune response of the body intended to eliminate the initial cause of tissue injury. Inflammatory responses play a major role in our body defence system against pathogens that also involve wound healing. Hence, inflammation is vital for our health and survival. The mouth provides a suitable environment for the growth of microorganisms. Thus, bacteria are present constantly in the mouth and some of these bacteria can trigger inflammatory response and implicated in oral disease. Most of the cases in periodontal disease are originated from bacterial dental plaque or biofilm accumulated on the teeth that induce host periodontal tissues inflammatory response (Hajishengallis et al., 2020). Both chronic gingivitis and periodontitis are chronic lesions that display inflammation and attempts at healing (Hasan and Palmer 2014).
The earliest process of inflammation involves its response against the invading microorganisms. In a susceptible host, invasion of periodontal pathogens particularly a group of specific Gram-negative anaerobic species in subgingival dental biofilm including Porphyromonas gingivalis, Tannerella forsythia, and Treponema denticola may result in chronic inflammation. These complex bacteria are predominantly found in deep periodontal pockets of patients with periodontitis (Kwon et al., 2021). The major component of the most Gram-negative bacteria present in the oral biofilm particularly lipopolysachharide (LPS) along with other virulence factors cause stimulation of mast cells and the release of vasoactive amines that cause vasodilation of blood vessels. The release of preformed tumour necrosis factor α (TNF-α) causes a subsequent release of inflammatory mediators in the gingival tissue (Hasturk et al., 2012; Yucel-Lindberg & Båge 2013). The chemoattractant proteins (chemokines) generated at this stage results in the initiation of the first line of defence, which are neutrophils that move and migrate to the site of microbial invasion. This process leads to the release of lysosomal enzymes that may also contribute to tissue degradation (Ge et al., 2015). Neutrophil infiltration is then followed by the activation of macrophages. Macrophages activation play a critical role in the elimination of the invaded bacteria, recruitment of other cells to the site of infection, removal of neutrophils excess, production of cytokines and chemokines and activation of lymphocyte-mediated adaptive immunity (Hasturk et al., 2012).
As lymphocytes and macrophages further invade the tissue, collagen content in gingiva is degraded but the bone at the site of lesion may still be intact. At this point, the associated damage is still reversible as it is still possible for gingival tissues to undergo repair and remodelling. In complete resolution with healing, the outcome of the inflammatory process can be restricted or cleared by the role of neutrophils and macrophages. The destructive inflammatory lesion that results in loss of local collagen due to accumulation of polymicrobial biofilm at the gingiva of the teeth is reversible upon resolution of the inflammation (Van Dyke et al., 2020). At this stage, the process of fibrosis and scar tissue formation may limit the infection but failure to clear the infection might establish a chronic inflammatory lesion.
Progression From Gingivitis to Periodontitis
It is noted that gingivitis is a major risk factor for periodontitis and it may develop into periodontitis in disease-susceptible individuals in which the host response is ineffective and dysregulated (Yucel-Lindberg and Båge 2013; Hajishengallis 2015). The pathogenesis of periodontal disease can be generally categorized into four stages, based on histopathological examination of the development of periodontal inflammation due to plaque accumulation. These stages are called 1) the initial, 2) the early, 3) the established, and 4) the advanced lesions. The advanced lesion which is also known as the destructive phase is clinically recognised as periodontitis where the inflammation extends deeper, with the formation of periodontal pocket, clinical attachment loss, collagen and bone loss (Hasan and Palmer 2014). The “established lesion” can persist for many years and the progression to an “advanced lesion” marks the transition from chronic and successful defence mechanism to destructive immunopathological mechanism or periodontitis. Recently, a new periodontitis classification scheme has been adopted according to the staging and grading system. Classification of periodontitis based on stages is dependent upon the severity of disease at presentation and the complexity of disease management. Meanwhile, classification of periodontitis based on grades reflect biologic features of the disease including analysis of the rate of periodontitis progression, risk for further progression, possibility of poor treatment outcomes and assessment of the risk that the disease or its treatment could affect the systemic health of the patient (Papapanou et al., 2018).
The exact factors that are responsible for the progression of periodontal diseases are unknown but chronic periodontal diseases involve the interaction of several components, which are the bacterial product or the pathogenicity factor, various cell populations and inflammatory mediators (Yucel-Lindberg and Båge 2013). Interaction between the host and microflora with time may result in dysbiosis of microbiome and dysregulation of host inflammation (Hajishengallis 2015). Colonization of Gram-negative bacteria up to 80% in the gingival sulcus during the establishment of periodontal disease form subgingival plaque, leading to periodontal pockets formation and gum recession (Palaska et al., 2013). Among the major pathogens, A. actinomycetemcomitants are more commonly detected in high levels in patients with aggressive periodontitis (Teles et al., 2013). The growth of pathogenic microbes within the dental plaque produces substances that could exacerbate inflammation, which may then lead to tissue destruction and even tooth loss. The immune-inflammatory mechanism in periodontal diseases is in part controlled by an individual’s susceptibility and interaction with environmental factors (Lamont et al., 2018).
The destruction of periodontal tissue and bone resorption are contributed by cellular activation, inflammatory mediators including cytokines, chemokines, prostaglandins and proteolytic enzymes particularly matrix metalloproteinases (MMP). The progression of periodontitis involves the release of prominent cytokines such as TNF-α, which is also involved at the early stage of inflammatory cascade, and IL-1 that are produced by the B-cell/plasma cell (Pan et al., 2019). These two cytokines may induce a number of inflammatory mediators such as IL-6, IL-8, MMP and prostaglandin E2 (PGE2), which is the most prominent prostaglandin implicated in the pathogenesis of periodontitis. IL-1 exerts different biologic effects on different cells and is an important biological mediator of autoimmune and inflammatory diseases. It plays a crucial role in both innate and adaptive immunity. Among first members of IL-1 identified include IL-1α and IL-1β (Dinarello 2018). 11 known members of IL-1 includes molecules with agonist activity such as IL-1α, IL-1β, IL-18, IL-33, IL-36α, IL-36β, and IL-36γ), receptor antagonists of IL-1Ra, IL-36Ra and two anti-inflammatory cytokines which are IL-37, and IL-38 (Dinarello 2018).
Dental biofilm dysbiosis will stimulate the release of IL-1 proinflammatory cytokines including IL-1α, IL-1β, IL-18, IL-36 from the oral junctional epithelium. These proinflammatory signals combined with bacterial products in the periodontal tissues in turn will stimulate both innate and adaptive immunity response. Hence, both complexes will promote release of inflammatory cell mediators into the periodontium (Papathanasiou et al., 2020). Virulence properties of periodontal pathogens such as lipopolysaccharides, stimulates the release of both IL-1α and IL-1β form oral epithelial cells hence resulting in periodontal destruction. Recently, it has been demonstrated that host defense peptides (HDPs) released from the gingival epithelium leading to accumulation of mast cells (MCs) that induces release of more pro-inflammatory cytokines. Therefore, enhancing further breakdown of periodontal tissue (Chompunud et al., 2020). IL-1β upregulates MMPs secretion hence contributing to augmented vasodilation, chemotaxis of inflammatory cells and degradation of collagen. In addition, osteoclastogenesis is also enhanced leading to elevated activity of bone resorption. A study reported that within gingival biopsies of active periodontal disease, there was reduced expression of inflammasome regulators while expression of messenger RNA (mRNA), NLRP3 and IL-1β were amplified (Aral et al., 2020).
Dendritic cells (DCs) release IL-18 that stimulates differentiation of T helper 1 cell (Th1) and T helper 17 cell (Th17) which in turn upregulates the release of IL-17, TNF-α, and IL-1β, hence contributing to further periodontal destruction. Elevated release of IL-1 proinflammatory has been associated with increased receptor activator of nuclear factor-κB ligand (RANKL) release, thus inducing osteoclasts progenitors responsible for alveolar bone resorption in periodontitis. However, IL-1 family members possessing anti-inflammatory properties play a defence role in periodontitis. Here, they will alleviate the magnitude of periodontal inflammation (Papathanasiou et al., 2020). The production of PGE2 by the immune cells, fibroblasts and other resident gingival cells is associated with the formation of osteoclast via RANKL upregulation and osteoprotegerin (OPG) inhibition (Belibasakis & Guggenheim 2011).
Since the development of periodontitis is mediated by bacterial-induced inflammation that lead to an excessive host response, the use of conventional mechanical therapy and pharmacological adjuncts is therefore plausible to control the inflammation (Bartold and Van Dyke 2013). Evidence showed that, adjunctive antibiotics and other anti-inflammatory agents can be effective to inhibit or eliminate periodontopathogenic microorganisms and modulate the inflammatory response of the tissue (Da Rocha Júnior et al., 2015). The use of nonsurgical periodontal therapy with or without antimicrobials remains as standard of care that could mechanically removes dental biofilm but targeting only microbes does not equally favourable in all periodontal patients (Preshaw 2018). In addition, the use of adjunctive antibiotics is associated with the unwanted side effects and should only be recommended for progressive disease (Tilakaratne and Soory 2014). Some of the side effects associated with the most commonly used antibiotics in managing periodontal disease include nephritis, gastrointestinal problems, increased risk of allergy, allergic signs on the skin, disturbance in the nervous system and electrolytes imbalance (Heta and Robo 2018).
This is where other agents with similar properties and biological effects with fewer side effects can take its place in improving and managing periodontitis. In fact, One of the promising therapeutic approaches in managing periodontitis particularly in individuals with a higher risk for periodontitis is by modulation of host inflammatory mediators. The term ‘host modulation therapy’ was initially introduced by Golub et al., 30 years ago (Golub et al., 1998). In the last decades, the efficacy of HMT using anti-inflammatory and antioxidant agents in periodontitis was explored. The two major HMT categories accepted include firstly the modulation of the host’s inflammatory response via inhibition or resolution and secondly modulation of host’s pathologic collagenolytic response within the periodontal soft tissue along with alveolar bone (Van Dyke, 2017; Golub et al., 2018). The adjunctive use of host modulatory agents has been postulated to have a positive implication on the progression of periodontal disease particularly in susceptible patients and in individuals whom conventional therapeutic approach is ineffective (Balta et al., 2021). Recently, polyphenols have been documented to cause immunomodulatory effects by downregulating the proinflammatory cytokines, IL-1 and IFN-γ which could be beneficial to be used as adjunct therapeutic approaches in reducing the burden of various inflammatory diseases (Shakoor et al., 2021).
Role of Polyphenolic Compounds in Managing Periodontal Inflammation
In recent years, polyphenolic compounds are among plant-derived phytochemicals that have gained remarkable attention due to their low toxicity, compared to allopathic drugs in the treatment of inflammatory diseases (Bellik et al., 2013). Polyphenols are secondary reactive metabolites, and they are abundant in plant-derived food, particularly fruits, seed and leaves. Polyphenols represent a wide variety of active compounds that are divided into several major categories based on the number of phenol rings and their structural elements (Belščak-Cvitanović et al., 2018). They are sub-categorized into phenolic acids (hydrobenzoic and hydroxycinnamic acid), flavonoids (flavones, flavonols, flavanols, isoflavones, flavanones, anthocyanins), stilbenes (resveratrol, piceatannol), lignans (sesamol, pinoresinol, sinol, enterodiol), and others including tannins (hydrolysable, non-hydrolysable, and condensed tannins), lignins, xanthones, chromones, anthraquinones (Singla et al., 2019).
Preservation and maintenance of periodontal health is an important component of oral and overall health (Baehni et al., 2010). A substantial number of studies have been done to ascertain the use of polyphenol as an adjunct to managing inflammatory conditions and hence support its role in the prevention and treatment of periodontal disease. Basically, these natural compounds regulate the inflammatory signalling by modifying the expression of several pro-inflammatory genes in addition to their antioxidative potential (Yahfoufi et al., 2018). In vitro and in vivo studies have demonstrated that the immune modulatory effect of polyphenols is particularly contributed by its potential in modulating immune cells populations, cytokines production and pro-inflammatory genes expression.
Apart from that, a number of studies supported the antimicrobial effect of polyphenols against a variety of pathogens including periodontal pathogens in complex biofilms. Ideally, prevention and treatment of periodontal diseases should also consist of strategies to eliminate or reduce these biofilms. Dietary polyphenols have been reported to have bacteriostatic/bactericidal activity against microbial species such as P. gingivalis (Basu et al., 2018). Since periodontal illnesses are inflammatory diseases of bacterial origin, anti-inflammatory and anti-microbial properties of polyphenols may anticipate various biological mechanisms for reducing the initiation and progression of periodontitis. In this review, we presented several polyphenolic compounds that have been reported to have remarkable anti-inflammatory properties particularly in an experimental model of periodontitis. Antibacterial properties of the selected polyphenolic compounds were also described in the current review.
Quercetin
Quercetin is categorized as a flavonol, one of the six subclasses of flavonoids compounds (Li et al., 2016). The antimicrobial and anti-inflammatory properties of quercetin have been found to be effective to restrict inflammatory reaction in periodontitis. During acute and chronic inflammation in periodontitis, high amount of TNF-α generated by activated macrophages may lead to periodontal tissues degeneration (Noh et al., 2013). A study by Xiong et al. (2019) has demonstrated that all three doses of quercetin (5, 10 and 20 μM) attenuated the production of inflammatory mediators including TNF-α, IL-1β, IL-6, and IL-8 in Porphyromonas gingivalis (P. gingivalis) LPS-treated human gingival fibroblast. In addition, quercetin has been found to suppress LPS-induced nuclear factor kappa-B (NF-κB) activation in a dose-dependent manner (Xiong et al., 2019). Suppression of these inflammatory mediators and its signalling pathway could restrict the initiation and progression of periodontal disease (Muniz et al., 2015).
In an earlier study, Cheng et al. (2010) investigated the effect of quercetin on experimental periodontitis induced by LPS injection and silk ligation (Cheng et al., 2010). The study has reported that 5 days of oral quercetin treatment at the dose of 75 mg/kg reduced LPS-induced osteoclast formation, ligature-enhanced periodontal inflammation and subsequent alveolar bone loss. Inflammation was induced in the quercetin-administered group but not severe enough to cause alveolar bone loss as evidenced from bone micro-computerized tomography (μ−CT) evaluation. Quercetin decreased the area of inflammatory cell infiltration in connective tissue and narrowed connective tissue attachment. However, the findings also reported a similar attachment loss in both ligation and the ligation-plus-quercetin groups. The results indicated that alveolar bone loss may be prevented in the experimental periodontitis but quercetin was unable to prevent attachment loss, which questioned the beneficial effects of quercetin. The author postulated that the study duration might not be sufficient to observe the effect of quercetin on long-term attachment loss in chronic periodontitis.
In another study, the anti-inflammatory properties of 100 mg/kg quercetin were evaluated using a mouse periodontitis model induced by inoculation of A. actinomycetemcomitans (Napimoga et al., 2013). Subcutaneous treatment with quercetin has been found to reduce gingival pro-inflammatory cytokines (IL-1, TNF-α, IL-17), and down-regulate adhesion molecule ICAM-1 and osteoclastogenic cytokine RANKL production in A. actinomycetemcomitans-induced alveolar bone loss. Alteration in cell-mediated and humoral levels of adaptive immune defence is thought to be involved in the development of experimental bacterial-immune inflammation in periodontal tissue. The potential of quercetin in reducing bone loss was also demonstrated in a study by Taskan and Gevrek (2020). Osteoblastic activity was increased while osteoclastic activity, apoptosis and inflammation were decreased in ligature-induced periodontitis rats treated with quercetin. A study by Demkovych et al. (2021) was carried out to investigate the effects of quercetin on adaptive immunity in relation to the development of experimental bacterial-immune periodontitis. Intramuscular injection of water-soluble quercetin at a dose of 100 mg/kg for 7 days was found to normalize the cellular adaptive immunity indices and reverse the inflammatory process in the periodontal complex.
It was suggested that the inhibition of periodontal pathogen virulent factor could hamper the progression of periodontitis, prevent and control periodontal inflammation. The effect of quercetin on P. gingivalis virulent pathogenicity was demonstrated in a study by He et al. (2020). The study found that quercetin inhibits virulence and physiological properties of P. gingivalis as indicated by gingipain, hemolytic and hemagglutination activity. Quercetin also could modulate cell surface hydrophobicity, aggregation, biofilm formation and virulence gene expression. These findings suggested that quercetin might be beneficial in the treatment of periodontitis as it could impair the pathogenicity of P. gingivalis, a keystone pathogen for periodontal disease. Table 1 summarized the effects of quercetin in periodontal inflammation, in vivo and in vitro.
Resveratrol
Resveratrol (trans-3,4,5-trihydroxystilbene) is a common stilbene found in berries, grape skin and in other plants (Gambini et al., 2015). Resveratrol has attracted substantial attention, as it possesses remarkable biological properties and this could be due to its molecular structure that confers its ability to bind to many biomolecules. Based on its promising anti-inflammatory and antioxidant properties, resveratrol has been studied for its prophylaxis and therapeutic potential in controlling periodontal disease. Resveratrol was shown to have a favourable effect on vascular inflammation induced by periodontal pathogen, P. gingivalis as it was able to inhibit NF-κB-dependent cell adhesion molecules in monocyte adhesion to the endothelium (Park et al., 2009). Casati et al. (2013) studied the effect of resveratrol that was administered continually on the progression of experimental periodontitis. Findings of the study showed that bone-loss value in ligated molars and unligated teeth in the control group were higher than in the treatment group that was administered with 10 mg/kg resveratrol for 30 days (19 days before periodontitis induction and 11 days after ligature placement). Resveratrol treated group also showed lower concentration of IL-17 in the gingival tissue. Modulation of IL-17 levels in gingival tissue that presents ligature-induced experimental periodontitis suggests the possible biologic mechanism of resveratrol during periodontal inflammation.
It is known that periodontitis is a multicomponent disorder that affects the supporting structures of the teeth including periodontal ligament and the alveolar bone. Bone loss values were found insignificant between resveratrol, curcumin and the combined groups in a study of continuous curcumin and resveratrol administration against the progression of experimental periodontitis. When compared with the placebo group, the concentration of IL-1 was lower in the combined group as revealed by immune-enzymatic assays. However, resveratrol, curcumin and combined groups showed higher IL-4 levels when compared to placebo group. The reduction in IFN-γ level was only observed in the resveratrol group and the difference in TNF-α levels among groups were not significant. Whether agents were added singly or in combination, both poplyphenolic compounds were able to attenuate alveolar bone loss in the experimental model of periodontitis. Nonetheless, the effects were neither synergistic nor additive (Corrêa et al., 2017). In another study, resveratrol derivative-rich melinjo seed extract was found to have a potent impact on inflammation-induced bone loss in a murine model of established periodontitis as indicated by a reduction in osteoclast differentiation (Ikeda et al., 2018) The production of IL-1β in gingival tissue was reduced but no significant changes in IL-6, TNF-α, and IL-17 levels were observed.
The antibacterial effects of resveratrol against periodontal pathogens P. gingivalis, T. forsythia and A. actinomycetemcomitans were studied by Cirano et al. (2016) in experimental model of periodontitis. The study however found that resveratrol does not exert positive effects on microbiological outcomes suggesting other mechanisms could contribute to its promising effect in controlling periodontitis. Nevertheless, in a recent study, resveratrol has been found to prevent biofilm formation and inhibit the virulence properties of P. gingivalis by reducing the expression of virulence factor genes including fimbriae and proteinases (Kugaji et al., 2019). The effect of resveratrol in in vivo and in vitro model of periodontal inflammation has been summarized in Table 2.
Curcumin
Curcumin, a yellow-coloured compound with low molecular weight is the main polyphenols extracted from the rhizome of turmeric plant, Curcuma longa L., (family: Zingiberaceae) (Wilken et al., 2011). This natural polyphenol has attracted considerable attention due to its nontoxicity and it has been used to treat various inflammatory diseases since long ago. Curcumin (diferuloylmethane) makes up 2–5% of turmeric and no adverse effects were found when it is given even as high as 8 g/day (Bhatia et al., 2014). Therapeutic potential of curcumin was observed in controlling inflammation and bone resorption in periodontitis. The antioxidant, antimicrobial, anti-inflammatory and analgesic properties of curcumin make them a suitable candidate for the management of periodontal diseases (Forouzanfar et al., 2020).
The possible mechanisms of curcumin involved in the suppression of periodontal disease reported in the existing studies are mainly based on its antibacterial and anti-inflammatory properties. In vitro studies have shown that curcumin can inhibit various periodontal pathogens growth such as A. actinomycetemcomitans, F. nucleatum, and P. gingivalis and the formation of biofilms (Shahzad et al., 2015). Curcumin has been found to have anti-biofilm and high antibacterial activity against P. gingivalis, which is considered as the main pathogen and major colonizer in host tissues (Shahzad et al., 2015; Izui et al., 2021; Kumbar et al., 2021). On the other hand, curcumin has low antibacterial activity against S. mitis, which is a part of the normal flora in the oral cavity and exert no threat to oral health. This result suggested that curcumin may have selective antimicrobial properties. In gene expression studies done by Kumbar et al. (2021), the virulence of P. gingivalis was reduced by curcumin as indicated by a reduce expression of genes coding for major virulence factors including adhesions and proteinases.
The occurrence and development of periodontitis involve the production of a vast number of inflammatory mediators. A recent study carried out by Xiao et al. (2018) showed that the production of IL-β and TNF-α were attenuated in rat gingival fibroblasts supplemented with 10 and 20 μM curcumin. The ratio of OPG/RANKL and the activation of NF-κB induced by LPS in vitro were also inhibited. In the same study, an in vivo ligation-induced experimental periodontitis showed that curcumin at the dose of 30 and 100 μg/g could alleviate gingival inflammation and modulated collagen fibre and alveolar bone loss as observed in histological and micro-CT results. An earlier study by Zhou et al. (2013) reported that intra-gastric curcumin administration at the dose of 100 mg/kg for 30 days could reduce alveolar bone loss in ligature-induced experimental periodontitis through the suppression of RANKL/RANK/OPG expression and its inflammatory properties (Zhou et al., 2013). Moderate bone resorption and root exposure, and mild bone loss were observed microscopically in curcumin treated animals. The expression of TNF-α and IL-6 in the gingival tissues of experimental rats treated with curcumin was significantly lower than the experimental periodontitis animal. In line with this study, several other studies also reported that curcumin can modulate the inflammatory response, suppress the pro-inflammatory cytokines particularly TNF-α and IL-6 in ligature-induced experimental periodontitis rat model (Guimarães et al., 2011) and LPS-induced periodontitis rat model (Guimaraes et al., 2012). Though curcumin is effective in inhibiting cytokine gene expression at mRNA and protein levels, the inhibition of NF-κB in the gingival tissue was only observed in the lower dose of curcumin (30 mg/kg), whereas p38 MAPK activation was not affected in both doses. Alveolar bone resorption was not prevented by daily dose of intragastric curcumin administration (30 and 50 mg/kg) for 15 days but its potential anti-inflammatory effect suggests their therapeutic potential in periodontal disease. Table 3 summarized the effects of curcumin in periodontal inflammation, in vivo and in vitro.
Proanthocyanidins
Proanthocyanidins are condensed tannins that take the form if oligomers or polymers of monomeric flavan-3-ols produced as an end product of flavonoid biosynthesis pathway (Rauf et al., 2019). The flavan-3-ols are catechin, epicatechin or their substituted derivatives. It is termed as condensed tannins for its capability to form insoluble complexes with carbohydrates and proteins (Balalaie et al., 2018). Proanthocyanidins are highly hydroxylated structures that is categorized according to the number of hydroxyl substitutions in the B ring in which one hydroxyl substitution refers to propelargonidin, two hydroxyl substitution refers to procyanidin and three hydroxyl substitution refers to prodelphinidin. The therapeutic potential of proanthocyanidins emerges from their unique chemical structure (Luca et al., 2020).
The antibacterial activity of proanthocyanidins has been substantially reported in literature (Nawrot-Hadzik et al., 2021). A study by Savickiene et al. (2018) revealed that proanthocyanidins had a unique antibacterial property that could selectively targets the keystone periodontal pathogens viability such as P. gingivalis while preserving the beneficial oral commensal S. salivarius. Lagha et al. (2018) reported the antibacterial and anti-biofilm effects of proanthocyanidins against A. actinomycetemcomitans. The treatment with proanthocyanidins reduced the growth of A. actinomycetemcomitans and resulted in a loss of bacterial viability as indicated by the damage to the bacterial cell membrane. Proanthocyanidins also possessed an anti-biofilm activity against P. aeruginosa as reported by Ulrey et al. (2014) and its anti-virulence potential was further investigated in a study by Maisuria et al. (2016).
Apart from its ability to inhibit biofilm formation and adhesion of periodontopathogenic bacteria, the therapeutic effect of proanthocyanidins with regards to periodontal disease include its potential to inhibit cytokine production by immune and mucosal cells and its capability to inhibit MMP production (Bonifait and Grenier 2010). Their capability to inhibit MMP and dentin cross-linker activity have been reported as an additional notable benefit of proanthocyanidins (Balalalie et al., 2018). The anti-inflammatory properties of proanthocyanidins were revealed by the attenuation of pro-inflammatory cytokines secretion (IL-1β, TNF-α, IL-6) as well as MMP-3 and MMP-9 secretion by macrophages stimulated with A. actinomycetemcomitans (Lagha et al., 2018). In another recent study, Jekabsone et al. (2019) demonstrated a strong antibacterial, anti-inflammatory and gingival tissue protecting properties of proanthocyanidins in periodontitis mimicking condition. Proanthocyanidins fraction has been found to have a stronger efficiency in suppressing caspases as indicated by the level of caspase-3 and caspase-8, and preventing mediator release as indicated by IL-8 and PGE2 secretion from gingival fibroblast, and IL-6 secretion from peripheral blood mononuclear cells. In an earlier study, proanthocyanidins-enriched cranberry fraction has been shown to inhibit the production of MMP-3 and MMP-9 in LPS-induced gingival fibroblasts (Bodet et al., 2007). Gingival fibroblasts, the most abundant cells found in periodontal tissues are actively involved in the host inflammatory response to oral pathogens and is known to mediate local tissue destruction in periodontal disease. The productions of IL-6, IL-8 and PGE2 by gingival fibroblast stimulated by LPS were inhibited with the treatment of proanthocyanidins-enriched cranberry fraction (Bodet et al., 2007). The effects of proanthocyanidins in in vivo and in vitro model of periodontal inflammation has been summarized in Table 4.
Nanoparticles as Potential Delivery System of Polyphenol
Despite promising biological activities of natural polyphenol, several limitations have been addressed in relation to their bioavailability. The absorption of polyphenolic compounds is negatively affected by its molecular size and their pharmacokinetics is modified by pre-systemic metabolism and gastric environment, which is highly acidic (Neves et al., 2012). Polyphenols were metabolized extensively during their transport across the small intestine and liver causing remarkable alteration of the redox potential. Consequently, the small proportion of polyphenolic compounds that are available following oral administration limits the activity and beneficial health effects of polyphenols. For instance, only a trace amount of curcumin available in blood plasma even after high dose intake, and after rapid metabolization of orally administered curcumin, they form several reduced products in the intestine and is most excreted in the urine and faeces (Niu et al., 2012). Low solubility and stability, short biological half-life and rapid elimination of polyphenolic compounds hinder their clinical application and thus lead the way towards the establishment of systems that could deliver these substances effectively.
The potency of many species of medicinal plants is determined by the availability of the active compounds. Recent research has proposed to combine herbal medicine with nanotechnology because nanoencapsulated particles are expected to potentiate the action of the plant extracts, reduce the required dose and undesirable effects as well as improving its activity (Bonifácio et al., 2014). Nanomedicine is an on-going research area that applies nanotechnology to medical intervention for prevention, diagnosis and treatment of diseases (Chang et al., 2015). Nanotechnology involved the production, processing, and application of particles with diameter ranging from 1 to 1,000 nm (Etheridge et al., 2013). Encapsulation is a process that incorporates an active compound or substances within a carrier material or another substance (Nedovic et al., 2011). The goal of encapsulation is to reduce the damage of sensitive and labile bioactive agents and to protect them from unwanted circumstances (Jafari 2017). Nanoencapsulation is then can be defined as a process of encapsulating a substance within another material at sizes on the nano-scale.
The incorporation with nanoparticles would basically protect the substances against chemical and enzymatic degradation (Muhamad et al., 2014). Nanoparticles have been used in advanced drug delivery systems as the size and surface characteristics of nanoparticles can be manipulated easily which prompt the use for passive and active drug targeting. In addition, nanoscale drug delivery systems could also enhance the solubility of hydrophobic compounds in aqueous medium. Since the biodistribution and clearance of substances from the body can be altered, the therapeutic efficacy can be increased and reduction in side effects can be achieved by the use of nanoparticles as carrier (Liang et al., 2017). Collectively, the nanoencapsulation process aims to enhance the properties of active compounds and to transport them to the target destinations more effectively (Esfanjani and Jafari 2016). Basically, the drug is made active in the targeted area at pre-determined release rate over a period of time (Harini & Kaarthikeyan 2014).
The process of encapsulating one compound to another involves several methods according to their chemical, physical and physiochemical properties (Conte et al., 2016). Chemical nanoencapsulation refers to polymerization of monomers through the addition of a cross-linker in the external phase while physical nanoencapsulation involves the interaction of the vector material with the encapsulated molecules when both are aerosolized or atomized (Wais et al., 2016). Physiochemical processes involve the formation of stable nanometre size drug nano-suspensions or nanoparticles through the reduction of particle size (Merisko-Liversidge & Liversidge 2011). Nanocarriers for phenolic compounds can be roughly divided into polysaccharide- and protein-based delivery systems (Milinčić et al., 2019). Substances such as cyclodectrins, polymeric nanoparticles, nanomicelles, food-protein nanoparticles, zein nanoparticles, gelatin nanoparticles and films, chitosan, lipid nanocarriers, or protein-polysaccharide complex nanoparticles are suitable to be used as carriers for nanoencapsulation of polyphenolic compounds. The interactions between polyphenol and nanocarrier can improve its bioavailability, prevent extensive degradation in the GIT, enhance its delivery to the targeted sites, or even provide stability during the storage or processing. Hence, the application of polyphenol-loaded nanoparticles is an interesting means to improve their overall activity.
Nanoparticles Mediated Delivery in Managing Periodontal Inflammation
Nanoencapsulation of polyphenolic compounds could overcome the drawbacks related to its instability, limited bioavailability and short half-life in vivo and in vitro (Fang and Bhandari 2010). The most frequently encapsulated polyphenols reported are quercetin, catechins, epigallocatechin, epigallocatechin-gallate (ECGC), curcumin, eugenol and tea polyphenols (Milinčić et al., (2019). The incorporation of these polyphenols with nanoparticles could be one of the promising approaches to enhance their efficacy as therapeutic agents for managing periodontal diseases.
Rutin is a glycoside that comprise of flavonolic aglycone quercetin along disaccharide rutinose (Ganeshpurkar and Saluja 2017). Rutin or rutin glycoside of quercetin has been reported to have a number of pharmacological activities including anti-inflammatory and antioxidant properties (Wang et al., 2019). The mechanism in which it inhibits oxidative stress and inflammatory reactions in animal models is through the regulation of MAPK pathway (Ganeshpurkar and Saluja 2017). Xu et al. (2020) evaluated the therapeutic effect of local rutin application on gingiva of periodontitis rats. In the study, rutin was incorporated with poly-lactic-co-glycolic acid (PLGA) nanoparticles by chemical precipitation method to improve its bioavailability and to make it more targeted. PLGA is among the widely used biodegradable organic polymer as it has a good biocompatibility, non-toxic and has passed FDA certification. Findings of the study showed that local application of rutin-loaded PLGA nanospheres inhibit the inflammatory reaction in LPS-induced periodontitis that may be due to downstream target effect of rutin combined with prostaglandin endoperoxide synthase 2 and downregulation of NFκBIα.
In a recent study by Wang et al. (2019), the incorporation of quercetin onto nano-octahedral ceria by chemical bonding has been found to be efficient in reprogramming pro-inflammatory macrophages to the anti-inflammatory phenotype that eventually could alleviate inflammation. Post subgingival injection of quercetin-loaded nanoceria was also found efficient to decrease local periodontal inflammation in a rat model of periodontitis induced by P. gingivalis injection. This study discovered that quercetin and cerium oxide (CeO2) nanoparticles (nanoceria) have synergistic and intense regulation on host immunity against periodontal disease. It is well documented that macrophage serves as the first line of host immune defense against periodontal pathogen infection as it is involved during the onset and resolution of inflammation (Yu et al., 2016). This nanocomposite was able to modulate the phenotypic switch of macrophages by inhibition of M1 (pro-inflammatory) polarization and also promotion of M2 (anti-inflammatory) polarization. Previously, quercetin has been proven to be able to modulate macrophage that eventually gives rise to efficient anti-inflammatory activity (Li et al., 2016; Hu et al., 2019). In the recent study, CeO2 nanoparticles were shown to inhibit the polarization of M1 macrophage by suppressing the inflammatory cytokines expression and arresting NF-κB signal pathway (Selvaraj et al., 2015). It has been reported that quercetin could also drive M2 phenotype macrophage polarization (Tan et al., 2020) and this is crucial as M2 macrophage activation could down-regulate gingival inflammation, prevent alveolar bone loss and more interestingly promote periodontal tissue regeneration. Incorporation of quercetin with cerium oxide nanoparticle may exhibit great potential in treating periodontitis.
Though resveratrol is well tolerated by humans, it is rapidly metabolized, leading to a short half-life and insubstantial effectiveness (Cottart et al., 2010). Researchers have mainly focused on increasing the absorption of resveratrol by increasing the residence time and lengthening its activity by incorporating it in biopolymers and lipids (Augustin et al., 2013; Sessa et al., 2014). A study by Giménez-Siurana et al. (2020) evaluated the therapeutic potential of silk fibroin nanoparticles loaded with resveratrol in diabetic-induced periodontitis. The link between periodontitis and other systemic diseases such as diabetes has been widely reported. Proinflammatory factors specifically IL-6, IL-1β and TGF-1β are implicated in both diseases. In the recent study, the levels of IL-1β and IL-6 were significantly decreased with the administration of resveratrol-loaded silk fibroin nanoparticles in the experimental animal. The reduction of these two significant proinflammatory cytokines indicates the recovery from periodontitis (Corrêa et al., 2017). This effect is contributed not solely by silk fibroin nanoparticles but also due to the anti-inflammatory activity exhibited by polyphenol resveratrol that has undergone an encapsulation process (Giménez-Siurana et al., 2020).
The therapeutic potential of curcumin on pathologic bone resorption in vivo may be dependent on the dose, route of administration and the type of experimental model used (De Almeida Brandão et al., 2019). The heterogeneity of findings associated with the use of natural curcumin may also be contributed by other variables such as the source, type of vehicle and the pharmacokinetic-related issues including their short half-life and low absorption rate in the GIT. Different encapsulation techniques such as curcumin-based nanoparticles formula and curcumin structure modification are among the latest approaches that can be applied to increase the bioavailability of curcumin in vivo but studies are still scarce. The use of alternative vehicles such as lipid-, chitosan- or hydrolysed corn protein associated nanoparticles formulation can be used to improve the pharmacodynamics and biological properties of curcumin (Shome et al., 2016; Wang et al., 2016). A study by Zambrono et al. (2018) reported the effect of curcumin-loaded nanoparticle in LPS-induced model of experimental periodontal disease but instead of using systemic route, this study investigates the local administration of polylactic acid and co-glycolic acid nanoencapsulated curcumin. These locally administered nanoparticles showed 15 times increase of curcumin half-life in the plasma of rats (Khalil et al., 2013). Apart from that, the application of nanoparticles also enables chemical modification that could specify its absorption in the given tissue or cell type and modify their absorption process to avoid macropinocytosis and liposome degradation or allow the tracking of its sub-cellular localization by the covalent binding with fluorescent molecules (Paka and Ramassamy 2017). Local application of nanocurcumin by direct injection into the gingival tissues twice a week in the LPS-induced model was shown to inhibit inflammatory bone resorption indicated by micro-CT analysis (Zambrano et al., 2018). This finding is explained by the reduction of osteoclast numbers, neutrophils (PMNs) and mononuclear cells numbers as indicated by histomorphometric analysis. The reduction of inflammatory cells infiltration suggests the anti-inflammatory effect of local nanocurcumin administration and this result is further supported by the attenuation of both signalling pathways in the gingival tissues, the p38 MAPK and NF-κB. In the earlier studies, systemic administration of curcumin in lipid vehicles was found to inhibit NF-κB activation but not p38 MAPK in LPS-induced experimental periodontitis (Guimarães et al., 2011; Guimaraes et al., 2012).
Tea is very rich in polyphenolic compounds mainly flavonoids including epicatechin (EC), epigallocatechin, epicatechin-3-gallate (ECG) and apigallocatechin-3-gallate (EGCG) (Kanwar et al., 2012). In dentistry, the use of catechin for the treatment of dental caries, periodontal and pulp diseases have been documented (Azmi et al., 2020). However, like other polyphenolic compounds, issues on its poor stability and low operational bioactivities lead to the unsatisfactory effect of tea polyphenol. Since previous methods of encapsulating tea polyphenol require tedious procedure, Tian et al. (2021) have developed a one-step polyphenolic condensation reaction that functionalized EGCG, the green tea derivative nanoparticles. The potent antioxidant capacity of EGCG-based nanoparticles was found to improve the chemical stability of epigallocatechin gallate. In addition, EGCG-based nanoparticles also provide more effective ROS scavenging activity and the expression of pro-inflammatory cytokines is down-regulated by reprogramming macrophages from pro-inflammatory M1 to anti-inflammatory M2 phenotype (Tian et al., 2021). In vivo findings showed that subgingival injection of EGCG nanoparticles could inhibit the alveolar bone loss and reduce osteoclastic activity in ligature-induced chronic periodontitis model in rats. EGCG nanoparticles have been found to remove ∼50% ROS in vivo more efficiently and safely. Down-regulation of the inflammatory cytokines and stimulation of macrophages differentiation to anti-inflammatory phenotype eventually prevent alveolar bone loss. In light of these findings, the development of ECGC-based nanomaterial provides better biocompatibility and anticipates an effective antioxidant defence mechanism for the treatment of chronic periodontitis. Table 5 summarizes recent studies on the effects of nanopolyphenols/nanoencapsulated polyphenols in the application of periodontal inflammation. Figure 1 illustrate the potential effect of polyphenol nanoencapsulation on the main cellular pathways involved in periodontal inflammation.
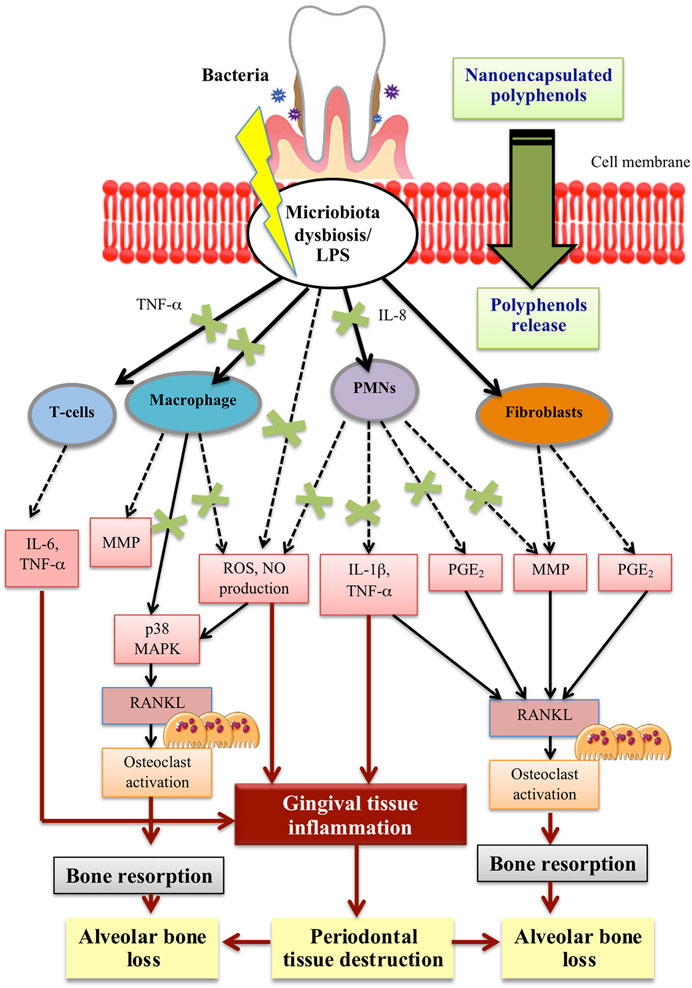
FIGURE 1. Diagrammatic representation of the nanoencapsulated polyphenols effect on the main cellular pathways involve in periodontal inflammation.
Conclusion
In the recent decades, phytochemicals, particularly polyphenols have been reported to have a remarkable therapeutic effect in preventing and/or treating inflammatory diseases. From the preclinical studies, polyphenol showed potential to modulate host immune and inflammatory profile in periodontal disease. However, poor water solubility, stability and bioavailability render the biological effects of polyphenol and limit their future clinical application. These drawbacks can be tackled by the application of nanosize delivery systems, which could increase the solubility and stability of phytochemicals and eventually improve their absorption. In addition, this delivery system could protect the substances from untimely enzymatic degradation or metabolism in the body and hence lengthen their circulation time. This review on the application of polyphenol-loaded nanoparticles may be useful for the enhancement of phytochemical efficacy as therapeutic agents in managing periodontal disease. A number of recent studies have investigated the pharmaceutical significance and therapeutic applicability of nanoparticles advance delivery system in improving and enhancing in vitro and in vivo performance of polyphenolic compounds, but much has yet to be explored. Comparative studies on advance delivery systems for the delivery of polyphenolic compounds and studies on safety/toxicity profile of polyphenol-loaded nanoparticles are warranted to bring the anti-inflammatory phytochemicals closer to the clinical applications.
Author Contributions
PJ and SB contributed toward conceptualization, planning and writing the paper. NN, NM, and NI contributed toward conceptualization and editing of the manuscript.
Conflict of Interest
The authors declare that the research was conducted in the absence of any commercial or financial relationships that could be construed as a potential conflict of interest.
Publisher’s Note
All claims expressed in this article are solely those of the authors and do not necessarily represent those of their affiliated organizations, or those of the publisher, the editors and the reviewers. Any product that may be evaluated in this article, or claim that may be made by its manufacturer, is not guaranteed or endorsed by the publisher.
Acknowledgments
We thank Ministry of Higher Education Malaysia for providing the Fundamental Research Grant Scheme (FRGS) for this study (FRGS/1/2019/STG03/UKM/01/3).
References
A, D. S., Calarco, A., Napoletano, A., Valentino, A., Margarucci, S., Di Cristo, F., et al. (2016). Polyphenols Nanoencapsulation for Therapeutic Applications. J. Biomol. Res. Ther. 5, 2. doi:10.4172/2167-7956.1000139
Abdul Rahim, R., Jayusman, P. A., Muhammad, N., Ahmad, F., Mokhtar, N., Naina Mohamed, I., et al. (2019). Recent Advances in Nanoencapsulation Systems Using PLGA of Bioactive Phenolics for Protection against Chronic Diseases. Int. J. Environ. Res. Public Health 16 (24), 4962. doi:10.3390/ijerph16244962
Ahad, A., Lamba, A. K., Faraz, F., Tandon, S., Chawla, K., and Yadav, N. (2016). Effect of Antimicrobial Photodynamic Therapy as an Adjunct to Nonsurgical Treatment of Deep Periodontal Pockets: A Clinical Study. J. Lasers Med. Sci. 7 (4), 220–226. doi:10.15171/jlms.2016.39
Aral, K., Berdeli, E., Cooper, P. R., Milward, M. R., Kapila, Y., Karadede Ünal, B., et al. (2020). Differential Expression of Inflammasome Regulatory Transcripts in Periodontal Disease. J. Periodontol. 91 (5), 606–616. doi:10.1002/JPER.19-0222
Augustin, M. A., Sanguansri, L., and Lockett, T. (2013). Nano- and Micro-encapsulated Systems for Enhancing the Delivery of Resveratrol. Ann. N. Y. Acad. Sci. 1290 (1), 107–112. doi:10.1111/nyas.12130
Baehni, P., Tonetti, M. S., and Periodontology, G. O. T. E. W. O. (2010). Conclusions and Consensus Statements on Periodontal Health, Policy and Education in Europe: a Call for Action-Cconsensus View 1. Consensus Report of the 1st European Workshop on Periodontal Education. Eur. J. Dent. Educ. 14, 2–3. doi:10.1111/j.1600-0579.2010.00619.x
Balalaie, A., Rezvani, M. B., and Mohammadi Basir, M. (2018). Dual Function of Proanthocyanidins as Both MMP Inhibitor and Crosslinker in Dentin Biomodification: A Literature Review. Dent. Mat. J. 37 (2), 173–182. doi:10.4012/dmj.2017-062
Balta, M. G., Papathanasiou, E., Blix, I. J., and Van Dyke, T. E. (2021). Host Modulation and Treatment of Periodontal Disease. J. Dent. Res. 100 (8), 798–809. doi:10.1177/0022034521995157
Bartold, P. M., and Van Dyke, T. E. (2013). Periodontitis: a Host-Mediated Disruption of Microbial Homeostasis. Unlearning Learned Concepts. Periodontol. 2000 62 (1), 203–217. doi:10.1111/j.1600-0757.2012.00450.x
Basu, A., Masek, E., and Ebersole, J. L. (2018). Dietary Polyphenols and Periodontitis-A Mini-Review of Literature. Molecules 23 (7), 1786. doi:10.3390/molecules23071786
Belibasakis, G. N., and Guggenheim, B. (2011). Induction of Prostaglandin E(2) and Interleukin-6 in Gingival Fibroblasts by Oral Biofilms. FEMS Immunol. Med. Microbiol. 63 (3), 381–386. doi:10.1111/j.1574-695X.2011.00863.x
Bellik, Y., Boukraâ, L., Alzahrani, H. A., Bakhotmah, B. A., Abdellah, F., Hammoudi, S. M., et al. (2013). Molecular Mechanism Underlying Anti-inflammatory and Anti-allergic Activities of Phytochemicals: an Update. Molecules 18 (1), 322–353. doi:10.3390/molecules18010322
Belščak-Cvitanović, A., Durgo, K., Huđek, A., Bačun-Družina, V., and Komes, D. (2018). Overview of Polyphenols and Their Properties. In Polyphenols Properties, Recovery, and Applications. Elsevier, 3–44.
Ben Lagha, A., Lebel, G., and Grenier, D. (2018). Dual Action of Highbush Blueberry Proanthocyanidins on Aggregatibacter Actinomycetemcomitans and the Host Inflammatory Response. BMC Complement. Altern. Med. 18 (1), 10–14. doi:10.1186/s12906-017-2072-x
Bhatia, M., Urolagin, S. S., Pentyala, K. B., Urolagin, S. B., K B, M., and Bhoi, S. (2014). Novel Therapeutic Approach for the Treatment of Periodontitis by Curcumin. J. Clin. Diagn. Res. 8 (12), ZC65–9. doi:10.7860/jcdr/2014/8231.5343
Bodet, C., Chandad, F., and Grenier, D. (2007). Cranberry Components Inhibit Interleukin-6, Interleukin-8, and Prostaglandin E Production by Lipopolysaccharide-Activated Gingival Fibroblasts. Eur. J. Oral Sci. 115 (1), 64–70. doi:10.1111/j.1600-0722.2007.00415.x
Bonifait, L., and Grenier, D. (2010). Cranberry Polyphenols: Potential Benefits for Dental Caries and Periodontal Disease. J. Can. Dent. Assoc. 76, a130.
Bunte, O., Groote, J. F., Keiren, J. J. A., Laveaux, M., Neele, T., De Vink, E. P., et al. (2019). “The mCRL2 Toolset for Analysing Concurrent Systems,” in 25th International Conference on Tools and Algorithms for the Construction and Analysis of Systems conference series, TACAS 2019 held as part of the 22nd European Joint Conferences on Theory and Practice of Software, (HW Den Haag, Netherlands: ETAPS), 21–39. doi:10.1007/978-3-030-17465-1_2
Casati, M. Z., Algayer, C., Cardoso da Cruz, G., Ribeiro, F. V., Casarin, R. C., Pimentel, S. P., et al. (2013). Resveratrol Decreases Periodontal Breakdown and Modulates Local Levels of Cytokines during Periodontitis in Rats. J. Periodontol. 84 (10), e58–64. doi:10.1902/jop.2013.120746
Cekici, A., Kantarci, A., Hasturk, H., and Van Dyke, T. E. (2014). Inflammatory and Immune Pathways in the Pathogenesis of Periodontal Disease. Periodontol. 2000 64 (1), 57–80. doi:10.1111/prd.12002
Chang, E. H., Harford, J. B., Eaton, M. A., Boisseau, P. M., Dube, A., Hayeshi, R., et al. (2015). Nanomedicine: Past, Present and Future - A Global Perspective. Biochem. Biophys. Res. Commun. 468 (3), 511–517. doi:10.1016/j.bbrc.2015.10.136
Cheng, W. C., Huang, R. Y., Chiang, C. Y., Chen, J. K., Liu, C. H., Chu, C. L., et al. (2010). Ameliorative Effect of Quercetin on the Destruction Caused by Experimental Periodontitis in Rats. J. Periodontal Res. 45 (6), 788–795. doi:10.1111/j.1600-0765.2010.01301.x
Chompunud Na Ayudhya, C., Roy, S., Thapaliya, M., Ali, H., and Ali, H. (2020). Roles of a Mast Cell-specific Receptor MRGPRX2 in Host Defense and Inflammation. J. Dent. Res. 99 (8), 882–890. doi:10.1177/0022034520919107
Cirano, F. R., Casarin, R. C., Ribeiro, F. V., Casati, M. Z., Pimentel, S. P., Taiete, T., et al. (2016). Effect of Resveratrol on Periodontal Pathogens during Experimental Periodontitis in Rats. Braz. Oral Res. 30 (1), e128–7. doi:10.1590/1807-3107BOR-2016.vol30.0128
Corrêa, M. G., Pires, P. R., Ribeiro, F. V., Pimentel, S. Z., Casarin, R. C., Cirano, F. R., et al. (2017). Systemic Treatment with Resveratrol And/or Curcumin Reduces the Progression of Experimental Periodontitis in Rats. J. Periodontal Res. 52 (2), 201–209. doi:10.1111/jre.12382
Cottart, C. H., Nivet-Antoine, V., Laguillier-Morizot, C., and Beaudeux, J. L. (2010). Resveratrol Bioavailability and Toxicity in Humans. Mol. Nutr. Food Res. 54 (1), 7–16. doi:10.1002/mnfr.200900437
Da Rocha, H. A., Silva, C. F., Santiago, F. L., Martins, L. G., Dias, P. C., and De Magalhães, D. (2015). Local Drug Delivery Systems in the Treatment of Periodontitis: a Literature Review. J. Int. Acad. Periodontol. 17 (3), 82–90.
de Almeida Brandão, D., Spolidorio, L. C., Johnson, F., Golub, L. M., Guimarães-Stabili, M. R., and Rossa, C. (2019). Dose-response Assessment of Chemically Modified Curcumin in Experimental Periodontitis. J. Periodontol. 90 (5), 535–545. doi:10.1002/JPER.18-0392
Demkovych, A., Bondarenko, Y., Shcherba, V., Luchynskyi, V., Vitkovskyy, V., and Machogan, V. (2021). Quercetin Effects on Adaptive Immune Response in Experimental Periodontitis of Bacterial-Immune Genesis. Pharmacia 68, 877. doi:10.3897/pharmacia.68.e70883
Dinarello, C. A. (2018). Overview of the IL-1 Family in Innate Inflammation and Acquired Immunity. Immunol. Rev. 281 (1), 8–27. doi:10.1111/imr.12621
Etheridge, M. L., Campbell, S. A., Erdman, A. G., Haynes, C. L., Wolf, S. M., and Mccullough, J. (2013). The Big Picture on Nanomedicine: the State of Investigational and Approved Nanomedicine Products. Nanomedicine 9 (1), 1–14. doi:10.1016/j.nano.2012.05.013
Fang, Z., and Bhandari, B. (2010). Encapsulation of Polyphenols - a Review. Trends Food Sci. Technol. 21 (10), 510–523. doi:10.1016/j.tifs.2010.08.003
Faridi Esfanjani, A., and Jafari, S. M. (2016). Biopolymer Nano-Particles and Natural Nano-Carriers for Nano-Encapsulation of Phenolic Compounds. Colloids Surf. B Biointerfaces 146, 532–543. doi:10.1016/j.colsurfb.2016.06.053
Forouzanfar, F., Forouzanfar, A., Sathyapalan, T., Orafai, H. M., and Sahebkar, A. (2020). Curcumin for the Management of Periodontal Diseases: a Review. Curr. Pharm. Des. 26 (34), 4277–4284. doi:10.2174/1381612826666200513112607
Gambini, J., Inglés, M., Olaso, G., Lopez-Grueso, R., Bonet-Costa, V., Gimeno-Mallench, L., et al. (2015). Properties of Resveratrol:In VitroandIn VivoStudies about Metabolism, Bioavailability, and Biological Effects in Animal Models and Humans. Oxidative Med. Cell. Longev. 2015, 1–13. doi:10.1155/2015/837042
Ganeshpurkar, A., and Saluja, A. K. (2017). The Pharmacological Potential of Rutin. Saudi Pharm. J. 25 (2), 149–164. doi:10.1016/j.jsps.2016.04.025
Ge, W., Li, D., Gao, Y., and Cao, X. (2015). The Roles of Lysosomes in Inflammation and Autoimmune Diseases. Int. Rev. Immunol. 34 (5), 415–431. doi:10.3109/08830185.2014.936587
Giménez-Siurana, A., Gómez García, F., Pagan Bernabeu, A., Lozano-Pérez, A. A., Aznar-Cervantes, S. D., Cenis, J. L., et al. (2020). Chemoprevention of Experimental Periodontitis in Diabetic Rats with Silk Fibroin Nanoparticles Loaded with Resveratrol. Antioxidants 9 (1), 85. doi:10.3390/antiox9010085
Golub, L. M., Lee, H. M., Ryan, M. E., Giannobile, W. V., Payne, J., and Sorsa, T. (1998). Tetracyclines Inhibit Connective Tissue Breakdown by Multiple Non-antimicrobial Mechanisms. Adv. Dent. Res. 12, 12–26. doi:10.1177/08959374980120010501
Golub, L. M., Gu, Y., Tenenbaum, H., Goldberg, M., and Sorsa, T. (2018). A “Point by Point” Statement on Periodontal Host Modulation Therapy:SAFETY FIRST! Oral Health, 1–8.
Guimarães, M. R., Coimbra, L. S., De Aquino, S. G., Spolidorio, L. C., Kirkwood, K. L., and Rossa, C. (2011). Potent Anti-inflammatory Effects of Systemically Administered Curcumin Modulate Periodontal Disease In Vivo. J. Periodontal Res. 46 (2), 269–279. doi:10.1111/j.1600-0765.2010.01342.x
Guimarães, M. R., De Aquino, S. G., Coimbra, L. S., Spolidorio, L. C., Kirkwood, K. L., and Rossa, C. (2012). Curcumin Modulates the Immune Response Associated with LPS-Induced Periodontal Disease in Rats. Innate Immun. 18 (1), 155–163. doi:10.1177/1753425910392935
Hajishengallis, G., Chavakis, T., and Lambris, J. D. (2020). Current Understanding of Periodontal Disease Pathogenesis and Targets for Host-Modulation Therapy. Periodontol. 2000 84 (1), 14–34. doi:10.1111/prd.12331
Hajishengallis, G. (2015). Periodontitis: from Microbial Immune Subversion to Systemic Inflammation. Nat. Rev. Immunol. 15 (1), 30–44. doi:10.1038/nri3785
Harini, G., Kaarthikeyan, G., and Kaarthikeyan, G. (2014). Advanced Drug Delivery Systems in Treating Periodontal Diseases-A Review. Iosrjdms 13 (1), 27–32. doi:10.9790/0853-13182732
Hasan, A., and Palmer, R. M. (2014). A Clinical Guide to Periodontology: Pathology of Periodontal Disease. Br. Dent. J. 216 (8), 457–461. doi:10.1038/sj.bdj.2014.299
Hasturk, H., Kantarci, A., and Van Dyke, T. E. (2012). Oral Inflammatory Diseases and Systemic Inflammation: Role of the Macrophage. Front. Immunol. 3, 118. doi:10.3389/fimmu.2012.00118
He, Z., Zhang, X., Song, Z., Li, L., Chang, H., Li, S., et al. (2020). Quercetin Inhibits Virulence Properties of Porphyromas Gingivalis in Periodontal Disease. Sci. Rep. 10 (1), 18313–13. doi:10.1038/s41598-020-74977-y
Heta, S., and Robo, I. (2018). The Side Effects of the Most Commonly Used Group of Antibiotics in Periodontal Treatments. Med. Sci. (Basel) 6 (1), 6. doi:10.3390/medsci6010006
Hoda, M., Hemaiswarya, S., and Doble, M. (2019). “Pharmacokinetics and Pharmacodynamics of Polyphenols,” in Role of Phenolic Phytochemicals in Diabetes Management (Singapore: Springer), 159–173. doi:10.1007/978-981-13-8997-9_7
Hu, Y., Gui, Z., Zhou, Y., Xia, L., Lin, K., and Xu, Y. (2019). Quercetin Alleviates Rat Osteoarthritis by Inhibiting Inflammation and Apoptosis of Chondrocytes, Modulating Synovial Macrophages Polarization to M2 Macrophages. Free Radic. Biol. Med. 145, 146–160. doi:10.1016/j.freeradbiomed.2019.09.024
Ikeda, E., Ikeda, Y., Wang, Y., Fine, N., Sheikh, Z., Viniegra, A., et al. (2018). Resveratrol Derivative-Rich Melinjo Seed Extract Induces Healing in a Murine Model of Established Periodontitis. J. Periodontol. 89 (5), 586–595. doi:10.1002/JPER.17-0352
Izui, S., Sekine, S., Murai, H., Takeuchi, H., and Amano, A. (2021). Inhibitory Effects of Curcumin against Cytotoxicity of Porphyromonas Gingivalis Outer Membrane Vesicles. Arch. Oral Biol. 124, 105058. doi:10.1016/j.archoralbio.2021.105058
Jafari, S. M. (2017). “An Overview of Nanoencapsulation Techniques and Their Classification,” in Nanoencapsulation Technologies for the Food and Nutraceutical Industries (Elsevier), 1–34. doi:10.1016/b978-0-12-809436-5.00001-x
Jekabsone, A., Sile, I., Cochis, A., Makrecka-Kuka, M., Laucaityte, G., Makarova, E., et al. (2019). Investigation of Antibacterial and Antiinflammatory Activities of Proanthocyanidins from pelargonium Sidoides DC Root Extract. Nutrients 11 (11), 2829. doi:10.3390/nu11112829
Kanwar, J., Taskeen, M., Mohammad, I., Huo, C., Chan, T. H., and Dou, Q. P. (2012). Recent Advances on Tea Polyphenols. Front. Biosci. (Elite Ed. 4, 111–131. doi:10.2741/363
Kassebaum, N. J., Bernabé, E., Dahiya, M., Bhandari, B., Murray, C. J., and Marcenes, W. (2014). Global Burden of Severe Periodontitis in 1990-2010: A Systematic Review and Meta-Regression. J. Dent. Res. 93 (11), 1045–1053. doi:10.1177/0022034514552491
Khalil, N. M., Do Nascimento, T. C., Casa, D. M., Dalmolin, L. F., De Mattos, A. C., Hoss, I., et al. (2013). Pharmacokinetics of Curcumin-Loaded PLGA and PLGA-PEG Blend Nanoparticles after Oral Administration in Rats. Colloids Surf. B Biointerfaces 101, 353–360. doi:10.1016/j.colsurfb.2012.06.024
Kugaji, M. S., Kumbar, V. M., Peram, M. R., Patil, S., Bhat, K. G., and Diwan, P. V. (2019). Effect of Resveratrol on Biofilm Formation and Virulence Factor Gene Expression of Porphyromonas Gingivalis in Periodontal Disease. APMIS 127 (4), 187–195. doi:10.1111/apm.12930
Kumbar, V. M., Peram, M. R., Kugaji, M. S., Shah, T., Patil, S. P., Muddapur, U. M., et al. (2021). Effect of Curcumin on Growth, Biofilm Formation and Virulence Factor Gene Expression of Porphyromonas Gingivalis. Odontology 109 (1), 18–28. doi:10.1007/s10266-020-00514-y
Kwon, T., Lamster, I. B., and Levin, L. (2021). Current Concepts in the Management of Periodontitis. Int. Dent. J. 71 (6), 462–476. doi:10.1111/idj.12630
Lamont, R. J., Koo, H., and Hajishengallis, G. (2018). The Oral Microbiota: Dynamic Communities and Host Interactions. Nat. Rev. Microbiol. 16 (12), 745–759. doi:10.1038/s41579-018-0089-x
Leszczyńska, A., Buczko, P., Buczko, W., and Pietruska, M. (2011). Periodontal Pharmacotherapy - an Updated Review. Adv. Med. Sci. 56 (2), 123–131. doi:10.2478/v10039-011-0044-9
Li, Y., Yao, J., Han, C., Yang, J., Chaudhry, M. T., Wang, S., et al. (2016). Quercetin, Inflammation and Immunity. Nutrients 8 (3), 167. doi:10.3390/nu8030167
Liang, J., Yan, H., Puligundla, P., Gao, X., Zhou, Y., and Wan, X. (2017). Applications of Chitosan Nanoparticles to Enhance Absorption and Bioavailability of Tea Polyphenols: A Review. Food Hydrocoll. 69, 286–292. doi:10.1016/j.foodhyd.2017.01.041
Lu, W., Kelly, A. L., and Miao, S. (2016). Emulsion-based Encapsulation and Delivery Systems for Polyphenols. Trends Food Sci. Technol. 47, 1–9. doi:10.1016/j.tifs.2015.10.015
Luca, S. V., Macovei, I., Bujor, A., Miron, A., Skalicka-Woźniak, K., Aprotosoaie, A. C., et al. (2020). Bioactivity of Dietary Polyphenols: The Role of Metabolites. Crit. Rev. Food Sci. Nutr. 60 (4), 626–659. doi:10.1080/10408398.2018.1546669
Maisuria, V. B., Los Santos, Y. L., Tufenkji, N., and Déziel, E. (2016). Cranberry-Derived Proanthocyanidins Impair Virulence and Inhibit Quorum Sensing of Pseudomonas aeruginosa. Sci. Rep. 6 (1), 1–12. doi:10.1038/srep30169
Marcenes, W., Kassebaum, N. J., Bernabé, E., Flaxman, A., Naghavi, M., Lopez, A., et al. (2013). Global Burden of Oral Conditions in 1990-2010: a Systematic Analysis. J. Dent. Res. 92 (7), 592–597. doi:10.1177/0022034513490168
McGowan, K., Mcgowan, T., and Ivanovski, S. (2018). Optimal Dose and Duration of Amoxicillin-Plus-Metronidazole as an Adjunct to Non-surgical Periodontal Therapy: A Systematic Review and Meta-Analysis of Randomized, Placebo-Controlled Trials. J. Clin. Periodontol. 45 (1), 56–67. doi:10.1111/jcpe.12830
Merisko-Liversidge, E., and Liversidge, G. G. (2011). Nanosizing for Oral and Parenteral Drug Delivery: a Perspective on Formulating Poorly-Water Soluble Compounds Using Wet Media Milling Technology. Adv. Drug Deliv. Rev. 63 (6), 427–440. doi:10.1016/j.addr.2010.12.007
Milinčić, D. D., Popović, D. A., Lević, S. M., Kostić, A. Ž., Tešić, Ž. L., Nedović, V. A., et al. (2019). Application of Polyphenol-Loaded Nanoparticles in Food Industry. Nanomaterials 9 (11), 1629. doi:10.1016/j.addr.2010.12.007
Mohd Azmi, F., Mp Sockalingam, S. N., Mohd Said, M., and Irfani Zakaria, A. S. (2020). Clinical Applications of Catechin in Dentistry: A Review. J. Nat. Remedies 20 (1), 2–15. doi:10.18311/jnr/2020/23941
Mohd Dom, T. N., Ayob, R., Abd Muttalib, K., and Aljunid, S. M. (2016). National Economic Burden Associated with Management of Periodontitis in Malaysia. Int. J. Dent. 2016, 1–6. doi:10.1155/2016/1891074
Mohd Dom, T. N., Mohd Said, S., Abdul Aziz, A. F., Abdul Manaf, M. R., and Aljunid, S. M. (2012). Periodontal Disease as an Indicator of Chronic Non-communicable Diseases: Evidence from Literatures. BMC Public Health 12 (2), 1. doi:10.1186/1471-2458-12-S2-A26
Mohd-Dom, T., Ayob, R., Mohd-Nur, A., Abdul-Manaf, M. R., Ishak, N., Abdul-Muttalib, K., et al. (2014). Cost Analysis of Periodontitis Management in Public Sector Specialist Dental Clinics. BMC Oral Health 14 (1), 56–10. doi:10.1186/1472-6831-14-56
Muhamad, I. I., Selvakumaran, S., and Lazim, N. a. M. (2014). Designing Polymeric Nanoparticles for Targeted Drug Delivery System. Nanomed 287, 287.
Muniz, F. W., Nogueira, S. B., Mendes, F. L., Rösing, C. K., Moreira, M. M., De Andrade, G. M., et al. (2015). The Impact of Antioxidant Agents Complimentary to Periodontal Therapy on Oxidative Stress and Periodontal Outcomes: A Systematic Review. Arch. Oral Biol. 60 (9), 1203–1214. doi:10.1016/j.archoralbio.2015.05.007
Murakami, S., Mealey, B. L., Mariotti, A., and Chapple, I. L. C. (2018). Dental Plaque-Induced Gingival Conditions. J. Periodontol. 89, S17–S27. doi:10.1111/jcpe.12937
Napimoga, M. H., Clemente-Napimoga, J. T., Macedo, C. G., Freitas, F. F., Stipp, R. N., Pinho-Ribeiro, F. A., et al. (2013). Quercetin Inhibits Inflammatory Bone Resorption in a Mouse Periodontitis Model. J. Nat. Prod. 76 (12), 2316–2321. doi:10.1021/np400691n
Nawrot-Hadzik, I., Matkowski, A., Hadzik, J., Dobrowolska-Czopor, B., Olchowy, C., Dominiak, M., et al. (2021). Proanthocyanidins and Flavan-3-Ols in the Prevention and Treatment of Periodontitis-Antibacterial Effects. Nutrients 13 (1), 165. doi:10.3390/nu13010165
Nedovic, V., Kalusevic, A., Manojlovic, V., Levic, S., and Bugarski, B. (2011). An Overview of Encapsulation Technologies for Food Applications. Procedia Food Sci. 1, 1806–1815. doi:10.1016/j.profoo.2011.09.265
Neves, A. R., Lucio, M., Lima, J. L., and Reis, S. (2012). Resveratrol in Medicinal Chemistry: a Critical Review of its Pharmacokinetics, Drug-Delivery, and Membrane Interactions. Curr. Med. Chem. 19 (11), 1663–1681. doi:10.2174/092986712799945085
Niu, Y., Ke, D., Yang, Q., Wang, X., Chen, Z., An, X., et al. (2012). Temperature-dependent Stability and DPPH Scavenging Activity of Liposomal Curcumin at pH 7.0. Food Chem. 135 (3), 1377–1382. doi:10.1016/j.foodchem.2012.06.018
Noh, M. K., Jung, M., Kim, S. H., Lee, S. R., Park, K. H., Kim, D. H., et al. (2013). Assessment of IL-6, IL-8 and TNF-α Levels in the Gingival Tissue of Patients with Periodontitis. Exp. Ther. Med. 6 (3), 847–851. doi:10.3892/etm.2013.1222
Paka, G. D., and Ramassamy, C. (2017). Optimization of Curcumin-Loaded PEG-PLGA Nanoparticles by GSH Functionalization: Investigation of the Internalization Pathway in Neuronal Cells. Mol. Pharm. 14 (1), 93–106. doi:10.1021/acs.molpharmaceut.6b00738
Palaska, I., Papathanasiou, E., and Theoharides, T. C. (2013). Use of Polyphenols in Periodontal Inflammation. Eur. J. Pharmacol. 720 (1-3), 77–83. doi:10.1016/j.ejphar.2013.10.047
Pan, W., Wang, Q., and Chen, Q. (2019). The Cytokine Network Involved in the Host Immune Response to Periodontitis. Int. J. Oral Sci. 11 (3), 30–13. doi:10.1038/s41368-019-0064-z
Papapanou, P. N., Sanz, M., Buduneli, N., Dietrich, T., Feres, M., Fine, D. H., et al. (2018). Periodontitis: Consensus Report of Workgroup 2 of the 2017 World Workshop on the Classification of Periodontal and Peri-Implant Diseases and Conditions. J. Clin. Periodontol. 45, S162–S182. doi:10.1111/jcpe.12946
Papathanasiou, E., Conti, P., Carinci, F., Lauritano, D., and Theoharides, T. C. (2020). IL-1 Superfamily Members and Periodontal Diseases. J. Dent. Res. 99 (13), 1425–1434. doi:10.1177/0022034520945209
Park, H. J., Jeong, S. K., Kim, S. R., Bae, S. K., Kim, W. S., Jin, S. D., et al. (2009). Resveratrol Inhibits Porphyromonas Gingivalis Lipopolysaccharide-Induced Endothelial Adhesion Molecule Expression by Suppressing NF-kappaB Activation. Arch. Pharm. Res. 32 (4), 583–591. doi:10.1007/s12272-009-1415-7
Plessas, A. (2014). Nonsurgical Periodontal Treatment: Review of the Evidence. Oral Health Dent. Manag. 13 (1), 71–80.
Preshaw, P. M. (2018). Host Modulation Therapy with Anti-inflammatory Agents. Periodontol. 2000 76 (1), 131–149. doi:10.1111/prd.12148
Rauf, A., Imran, M., Abu-Izneid, T., Iahtisham-Ul-Haq, S., Patel, S., Pan, X., et al. (2019). Proanthocyanidins: A Comprehensive Review. Biomed. Pharmacother. 116, 108999. doi:10.1016/j.biopha.2019.108999
Salvi, G. E., Stähli, A., Schmidt, J. C., Ramseier, C. A., Sculean, A., and Walter, C. (2020). Adjunctive Laser or Antimicrobial Photodynamic Therapy to Non-surgical Mechanical Instrumentation in Patients with Untreated Periodontitis: A Systematic Review and Meta-Analysis. J. Clin. Periodontol. 47, 176–198. doi:10.1111/jcpe.13236
Savickiene, N., Jekabsone, A., Raudone, L., Abdelgeliel, A. S., Cochis, A., Rimondini, L., et al. (2018). Efficacy of Proanthocyanidins from Pelargonium Sidoides Root Extract in Reducing P. Gingivalis Viability while Preserving Oral Commensal S. Salivarius. Mater. (Basel) 11 (9), 1499. doi:10.3390/ma11091499
Scannapieco, F. A., and Cantos, A. (2016). Oral Inflammation and Infection, and Chronic Medical Diseases: Implications for the Elderly. Periodontol. 2000 72 (1), 153–175. doi:10.1111/prd.12129
Selvaraj, V., Manne, N. D., Arvapalli, R., Rice, K. M., Nandyala, G., Fankenhanel, E., et al. (2015). Effect of Cerium Oxide Nanoparticles on Sepsis Induced Mortality and NF-Κb Signaling in Cultured Macrophages. Nanomedicine (Lond) 10 (8), 1275–1288. doi:10.2217/nnm.14.205
Sessa, M., Balestrieri, M. L., Ferrari, G., Servillo, L., Castaldo, D., D'Onofrio, N., et al. (2014). Bioavailability of Encapsulated Resveratrol into Nanoemulsion-Based Delivery Systems. Food Chem. 147, 42–50. doi:10.1016/j.foodchem.2013.09.088
Shahzad, M., Millhouse, E., Culshaw, S., Edwards, C. A., Ramage, G., and Combet, E. (2015). Selected Dietary (Poly)phenols Inhibit Periodontal Pathogen Growth and Biofilm Formation. Food Funct. 6 (3), 719–729. doi:10.1039/C4FO01087F
Shakoor, H., Feehan, J., Apostolopoulos, V., Platat, C., Al Dhaheri, A. S., Ali, H. I., et al. (2021). Immunomodulatory Effects of Dietary Polyphenols. Nutrients 13 (3), 728. doi:10.3390/nu13030728
Shome, S., Talukdar, A. D., Choudhury, M. D., Bhattacharya, M. K., and Upadhyaya, H. (2016). Curcumin as Potential Therapeutic Natural Product: a Nanobiotechnological Perspective. J. Pharm. Pharmacol. 68 (12), 1481–1500. doi:10.1111/jphp.12611
Silva, P., Bonifácio, B., Ramos, M., Negri, K., Maria Bauab, T., and Chorilli, M. (2014). Nanotechnology-based Drug Delivery Systems and Herbal Medicines: a Review. Ijn 9, 1. doi:10.2147/IJN.S52634
Singla, R. K., Dubey, A. K., Garg, A., Sharma, R. K., Fiorino, M., Ameen, S. M., et al. (2019). Natural Polyphenols: Chemical Classification, Definition of Classes, Subcategories, and Structures. J. AOAC Int. 102, 1397–1400. doi:10.1093/jaoac/102.5.1397
Sulijaya, B., Takahashi, N., and Yamazaki, K. (2019). Host Modulation Therapy Using Anti-inflammatory and Antioxidant Agents in Periodontitis: A Review to a Clinical Translation. Arch. Oral Biol. 105, 72–80. doi:10.1016/j.archoralbio.2019.07.002
Tan, R. Z., Wang, C., Deng, C., Zhong, X., Yan, Y., Luo, Y., et al. (2020). Quercetin Protects against Cisplatin-Induced Acute Kidney Injury by Inhibiting Mincle/Syk/NF-Κb Signaling Maintained Macrophage Inflammation. Phytother. Res. 34 (1), 139–152. doi:10.1002/ptr.6507
Taskan, M. M., and Gevrek, F. (2020). Quercetin Decreased Alveolar Bone Loss and Apoptosis in Experimentally Induced Periodontitis Model in Wistar Rats. Antiinflamm. Antiallergy Agents Med. Chem. 19 (4), 436–448. doi:10.2174/1871523019666200124114503
Teles, R., Teles, F., Frias-Lopez, J., Paster, B., and Haffajee, A. (2013). Lessons Learned and Unlearned in Periodontal Microbiology. Periodontol. 2000 62 (1), 95–162. doi:10.1111/prd.12010
Tian, M., Chen, G., Xu, J., Lin, Y., Yi, Z., Chen, X., et al. (2022). Epigallocatechin Gallate-Based Nanoparticles with Reactive Oxygen Species Scavenging Property for Effective Chronic Periodontitis Treatment. Chem. Eng. J. 433, 132197. doi:10.1016/j.cej.2021.132197
Tibúrcio-Machado, C. S., Michelon, C., Zanatta, F. B., Gomes, M. S., Marin, J. A., and Bier, C. A. (2021). The Global Prevalence of Apical Periodontitis: a Systematic Review and Meta-Analysis. Int. Endod. J. 54 (5), 712–735. doi:10.1111/iej.13467
Tilakaratne, A., and Soory, M. (2014). Anti-inflammatory Actions of Adjunctive Tetracyclines and Other Agents in Periodontitis and Associated Comorbidities. Open Dent. J. 8, 109–124. doi:10.2174/1874210601408010109
Ulrey, R. K., Barksdale, S. M., Zhou, W., and Van Hoek, M. L. (2014). Cranberry Proanthocyanidins Have Anti-biofilm Properties against Pseudomonas aeruginosa. BMC Complement. Altern. MedAltern. Med. 14 (1), 1–12. doi:10.1186/1472-6882-14-499
Van Dyke, T. E., Bartold, P. M., and Reynolds, E. C. (2020). The Nexus between Periodontal Inflammation and Dysbiosis. Front. Immunol. 11, 511. doi:10.3389/fimmu.2020.00511
Van Dyke, T. E. (2017). Pro-resolving Mediators in the Regulation of Periodontal Disease. Mol. Asp. Med. 58, 21–36. doi:10.1016/j.mam.2017.04.006
Wais, U., Jackson, A. W., He, T., and Zhang, H. (2016). Nanoformulation and Encapsulation Approaches for Poorly Water-Soluble Drug Nanoparticles. Nanoscale 8 (4), 1746–1769. doi:10.1039/C5NR07161E
Wang, T., Ma, X., Lei, Y., and Luo, Y. (2016). Solid Lipid Nanoparticles Coated with Cross-Linked Polymeric Double Layer for Oral Delivery of Curcumin. Colloids Surf. B Biointerfaces 148, 1–11. doi:10.1016/j.colsurfb.2016.08.047
Wang, X., Zhao, W., Chen, Y., Shi, H., Wang, R., Zhong, W., et al. (2019). Preparation and Characterization of PEGylated Poly-L-Lysine-Hydroxycamptothecin Nanoparticles without and with Methotrexate. Nanosci. Nanotechnol. Lett. 11 (6), 795–803. doi:10.1166/nnl.2019.2942
Wilken, R., Veena, M. S., Wang, M. B., and Srivatsan, E. S. (2011). Curcumin: A Review of Anti-cancer Properties and Therapeutic Activity in Head and Neck Squamous Cell Carcinoma. Mol. Cancer 10 (1), 12–19. doi:10.1186/1476-4598-10-12
Xiao, C. J., Yu, X. J., Xie, J. L., Liu, S., and Li, S. (2018). Protective Effect and Related Mechanisms of Curcumin in Rat Experimental Periodontitis. Head. Face Med. 14 (1), 12–18. doi:10.1186/s13005-018-0169-1
Xiong, G., Ji, W., Wang, F., Zhang, F., Xue, P., Cheng, M., et al. (2019). Quercetin Inhibits Inflammatory Response Induced by LPS from Porphyromonas Gingivalis in Human Gingival Fibroblasts via Suppressing NF-Κb Signaling Pathway. Biomed. Res. Int. 2019, 6282635. doi:10.1155/2019/6282635
Xu, X., Li, C., Chen, Q., Sun, W., Xiao, F., Chen, Q., et al. (2020). Therapeutic Effect of Rutin-Loaded Poly Lactic-Co-Glycolic Acid Nanoparticles on Gingiva of Periodontitis Rats. Mat. Express. 10 (12), 2048–2055. doi:10.1166/mex.2020.1867
Yahfoufi, N., Alsadi, N., Jambi, M., and Matar, C. (2018). The Immunomodulatory and Anti-inflammatory Role of Polyphenols. Nutrients 10 (11), 1618. doi:10.3390/nu10111618
Yu, T., Zhao, L., Huang, X., Ma, C., Wang, Y., Zhang, J., et al. (2016). Enhanced Activity of the Macrophage M1/M2 Phenotypes and Phenotypic Switch to M1 in Periodontal Infection. J. Periodontol. 87 (9), 1092–1102. doi:10.1902/jop.2016.160081
Yucel-Lindberg, T., and Båge, T. (2013). Inflammatory Mediators in the Pathogenesis of Periodontitis. Expert Rev. Mol. Med. 15, e7. doi:10.1017/erm.2013.8
Zambrano, L. M. G., Brandao, D. A., Rocha, F. R. G., Marsiglio, R. P., Longo, I. B., Primo, F. L., et al. (2018). Local Administration of Curcumin-Loaded Nanoparticles Effectively Inhibits Inflammation and Bone Resorption Associated with Experimental Periodontal Disease. Sci. Rep. 8 (1), 1–11. doi:10.1038/s41598-018-24866-2
Zhao, Y., Pu, R., Qian, Y., Shi, J., and Si, M. (2021). Antimicrobial Photodynamic Therapy versus Antibiotics as an Adjunct in the Treatment of Periodontitis and Peri-Implantitis: A Systematic Review and Meta-Analysis. Photodiagnosis Photodyn. Ther. 34, 102231. doi:10.1016/j.pdpdt.2021.102231
Keywords: periodonditis, inflammation, nanotechnolody, nanoencapsulation, polyphenol
Citation: Jayusman PA, Nasruddin NS, Mahamad Apandi NI, Ibrahim N and Budin SB (2022) Therapeutic Potential of Polyphenol and Nanoparticles Mediated Delivery in Periodontal Inflammation: A Review of Current Trends and Future Perspectives. Front. Pharmacol. 13:847702. doi: 10.3389/fphar.2022.847702
Received: 03 January 2022; Accepted: 21 June 2022;
Published: 12 July 2022.
Edited by:
Roberto Paganelli, Institute for Advanced Biologic Therapies, ItalyReviewed by:
Francesca Diomede, University of Studies G d'Annunzio Chieti and Pescara, ItalyEvangelos Papathanasiou, Tufts University, United States
Copyright © 2022 Jayusman, Nasruddin, Mahamad Apandi, Ibrahim and Budin. This is an open-access article distributed under the terms of the Creative Commons Attribution License (CC BY). The use, distribution or reproduction in other forums is permitted, provided the original author(s) and the copyright owner(s) are credited and that the original publication in this journal is cited, in accordance with accepted academic practice. No use, distribution or reproduction is permitted which does not comply with these terms.
*Correspondence: Putri Ayu Jayusman, putriayu@ukm.edu.my