- 1Department of Pharmacy, The Second Xiangya Hospital, Central South University, Changsha, China
- 2Hunan Provincial Engineering Research Centre of Translational Medicine and Innovative Drug, Changsha, China
- 3Institute of Clinical Pharmacy, Central South University, Changsha, China
Cancer has posed a major threat to human life and health with a rapidly increasing number of patients. The complexity and refractory of tumors have brought great challenges to tumor treatment. In recent years, nanomaterials and nanotechnology have attracted more attention and greatly improved the efficiency of tumor therapies and significantly prolonged the survival period, whether for traditional tumor treatment methods such as radiotherapy, or emerging methods, such as phototherapy and immunotherapy, sonodynamic therapy, chemodynamic therapy and RNA interference therapeutics. Various monotherapies have obtained positive results, while combination therapies are further proposed to prevent incomplete eradication and recurrence of tumors, strengthen tumor killing efficacy with minimal side effects. In view of the complementary promotion effects between different therapies, it is vital to utilize nanomaterials as the link between monotherapies to achieve synergistic performance. Further development of nanomaterials with efficient tumor-killing effect and better biosafety is more in line with the needs of clinical treatment. In a word, the development of nanomaterials provides a promising way for tumor treatment, and here we will review the emerging nanomaterials towards radiotherapy, phototherapy and immunotherapy, and summarized the developed nanocarriers applied for the tumor combination therapies in the past 5 years, besides, the advances of some other novel therapies such as sonodynamic therapy, chemodynamic therapy, and RNA interference therapeutics have also been mentioned.
Introduction
Cancer has severely threatened human life worldwide, and malignant tumors are still a serious problem that needs to be solved urgently (Siegel et al., 2020). Traditional tumor treatment methods mainly include surgery, chemotherapy and radiotherapy, while some limitations are also manifested, such as the damage to surrounding tissues, poor effect on hypoxic tumors, possible wound complications, and inconvenience of treatment (Kaur and Asea, 2012; Sundaram et al., 2020). Research on development of tumor therapies is aimed to better eradicate tumors while minimizing side effects.
The application of nanomaterials has further optimized traditional tumor therapies and provided more options for emerging tumor therapies such as phototherapy, immunotherapy, sonodynamic therapy, chemodynamic therapy and RNA interference (RNAi) therapeutics (Song et al., 2017; Hou et al., 2018). The unique properties of nanomaterials made them applicative for tumor diagnose and treatment (He et al., 2015). The nano-scale size facilitates the penetration cross biological barriers, increases the drug delivery efficiency and controls the release behavior, thus achieving better therapeutic effects (Sun et al., 2014). In addition, appropriate nanomaterials can be effectively targeted to the tumor site and reduce systemic toxicity; Modified nanomaterials have been widely applied for both traditional and novel tumor therapies due to the specific physicochemical properties and targeting ability (Sun et al., 2014; Aikins et al., 2020). Nanocarriers have also been constructed to overcome hypoxia of the tumor microenvironment to obtain more efficient tumor killing effects (Abbasi et al., 2016; Meng et al., 2018). Nevertheless, although monotherapy strategies such as radiotherapy and phototherapy are proven effective methods for tumor treatment, there may be a potential risk of tumor recurrence or the refractory of deep-seated tumors. Based on this, tumor combination therapy has been proposed to eradicate tumors synergistically and effectively in a complementary manner, minimizing systemic toxicity and side effects as much as possible. Studies have demonstrated that radiotherapy can break through the limitation of insufficient tissue penetration of phototherapy. In turn, photothermal therapy can promote oxygen perfusion to relieve the hypoxic environment of tumors, which is beneficial to the efficiency of oxygen-dependent therapies such as radiotherapy, photodynamic therapy and sonodynamic therapy. In addition, a large amount of evidence has shown that the therapies can induce the release of tumor-associated antigens locally to activate the immune response, therefore, combination therapies with immunotherapy are a smart approach to achieve synergistic tumor treatment effects (Hou et al., 2018; Chen et al., 2019; Galon and Bruni, 2019). On this basis, recent research has also focused on the development of nanomaterials for the construction of carriers for combination therapy.
The review is aim to outline the recent advances in nanomaterial-mediated radiotherapy, phototherapy, and immunotherapy, and some other therapies (sonodynamic therapy, chemodynamic therapy, and RNAi therapeutics). Besides, we will present innovate strategies for combined tumor treatment based on nanomaterials in the past 5 years.
Application of Nanomaterials in Radiotherapy
Radiotherapy utilizes high-dose radiation to induce DNA destruction and generate free radicals to kill tumor cells, including internal and external radiotherapy, usually in conjunction with chemotherapy or surgery (Sundaram et al., 2020; Zeng et al., 2021). However, traditional radiotherapy causes irreversible damage to surrounding normal tissues due to the large amount of radiation. Besides, the hypoxic environment limits oxygen-dependent DNA damage, which in turn leads to tumor resistance to radiation (Jarosz-Biej et al., 2019). Hypoxia also protects dormant cancer stem cells, allowing them to retain the potential for proliferation and differentiation, thus leading to a potential risk of recurrence (Wang et al., 2019a). Therefore, it is necessary to improve the sensitivity of tumor cells to irradiation, overcome the hypoxic environment at the tumor site and increase oxidative stress, strengthen the killing effect on tumor cells and alleviate its side effects on normal tissues (Figure 1).
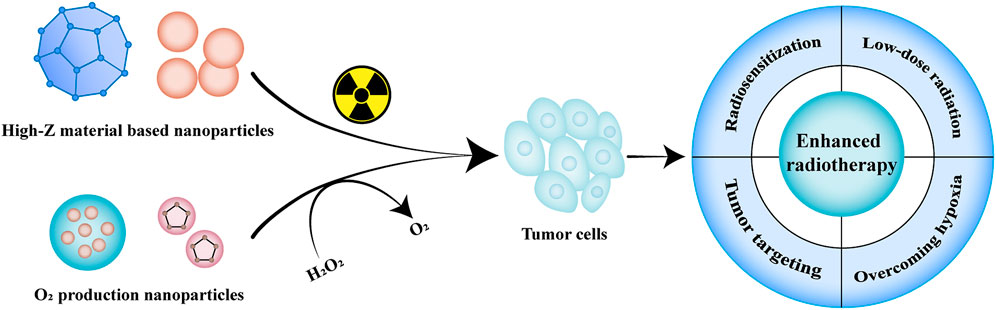
FIGURE 1. Illustration of nanomaterials used in radiotherapy. The commonly used nanomaterials in radiotherapy mainly include high-Z materials-based nanoparticles and oxygen-producing nanoparticles, which can effectively enhance the accumulation at the tumor site, improve the sensitivity of radiotherapy, overcome the hypoxic environment of tumors and enhance the radiotherapy efficiency.
High-Z materials such as gold (Dong et al., 2020), silver (Liu Z. et al., 2018), platinum (Li et al., 2019) and gadolinium (Du and Sun, 2020) have been widely reported to be used as radiosensitizers and accelerate the generation of reactive oxygen species (ROS) through photoelectric effect, Auger electronics and Compton effect (Sundaram et al., 2020). Lan et al. developed a metal-organic frameworks (MOF) hierarchically composed of Hf-based secondary building units and Ir-based bridging ligands, encapsulated (P2W18O62)6– (W18) polyoxometalates through electrostatic adsorption to further enhance the sensitivity of radiotherapy (Lan et al., 2019). The hierarchical structure facilitated the generation of hydroxyl radical (•OH), singlet oxygen (1O2) and superoxide (O2−), which is an excellent radio-enhancer for the treatment of MC38 and CT26 tumor bearing mice (Lan et al., 2019).
Among high-Z materials, gold-based nanoparticles (AuNPs) have received extensive attention due to the good biocompatibility and surface modifiability. Studies have further enhanced its superiority in radiotherapy sensitization by changing the size, structure or surface properties (Yang et al., 2014; Dong et al., 2020). Dong et al. prepared gold sub-nanoparticles encapsulating cell cycle regulator α-difluoromethylornithine, and modified them with arginine-glycine-aspartic acid (RGD) penetrating peptide to promote its penetration of the blood-brain barrier. The results showed enhanced sensitivity to radiation and significant therapeutic effects in low-dose radiation (Dong et al., 2021). AuNPs can also be modified to improve targeting ability. A conjugated complex composed of AuNPs and a plectin-1 targeting peptide showed more aggregation at the tumor site, and induced tumor cell apoptosis with better biosafety (Dong et al., 2020).
Hypoxia of the tumor microenvironment hinders radiation therapy sensitivity and the formation of ROS. Hypoxia-inducible factor 1 (HIF-1) is stably expressed under hypoxic conditions, which has been shown to participate in the proliferation and metastasis of tumor cells by regulating its downstream signaling molecules (Meng et al., 2018; Sundaram et al., 2020). Based on this, studies utilized nanocarriers combined with tumor oxygenation agents such as catalase and MnO2 to decompose the over-expressed H2O2 into oxygen, thereby alleviating the tumor hypoxia and enhancing the sensitivity of radiotherapy (Abbasi et al., 2016; Song et al., 2016). Meng et al. encapsulated the HIF-1 inhibitor acriflavine into MnO2 nanoparticles to generate oxygen in tumor tissues and improve the effect of radiotherapy. On the other hand, acriflavine inhibited the expression of HIF-1 and down-regulated its downstream signaling molecules such as vascular endothelial growth factor and glucose transporter, showing an 84.70% tumor inhibition rate in abscopal tumors (Meng et al., 2018).
Nanomaterials Used in Photothermal Therapy and Photodynamic Therapy
Photothermal therapy and photodynamic therapy are currently the most common phototherapy for tumor treatment. Photothermal therapy is to gather materials with high light-to-heat conversion efficiency in the tumor tissue and heat it to ablate cancer cells under the irradiation of external light source (Hou et al., 2018). And photodynamic therapy utilizes a specific wavelength of light to irradiate a photosensitizer at the tumor site and generate ROS to kill tumor cells. Compared with traditional tumor therapy, both photothermal and photodynamic therapy can be applied accurately and effectively with less side effects and greatly reduce the patient’s pain in a noninvasive way (Figure 2) (Li et al., 2020).
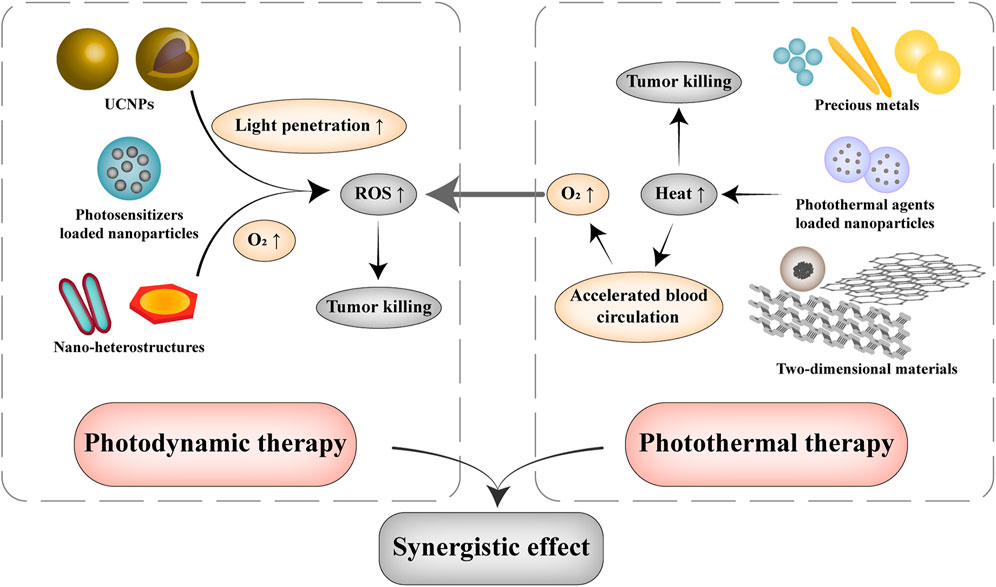
FIGURE 2. Illustration of nanomaterials used in photothermal therapy and photodynamic therapy. Photodynamic therapy stimulates photosensitizers to produce ROS in an oxygen-dependent manner. UCNPs are applied to enhance the tissue permeability of light by converting NIR into UV or visible light, and nano heterostructures have been proposed for the simultaneous generation of ROS and O2 through electron-hole separation under normoxia or hypoxia condition. Photothermal therapy uses NIR to excite nanomaterials with good light-to-heat conversion capabilities for tumor ablation, which can effectively accelerate the blood circulation and increase the oxygen concentration of tumor tissue, which in turn facilitates the performance of photodynamic therapy.
Photothermal agents are materials with good light-to-heat conversion capabilities, mainly including noble metals (gold (Wang et al., 2013), silver (Park et al., 2020), platinum (Ma et al., 2020), etc.), two-dimensional materials (graphene (Chen et al., 2016), black phosphorus (BP) (Geng et al., 2019), transition metal-based materials (Xie et al., 2019), etc.), and some organic small molecule photothermal agents (porphyrin, phthalocyanine, cyanine, etc.) (Lv et al., 2020). The current research aims to develop materials with excellent biocompatibility in addition to photothermal performance. Most metal-free 2D materials such as graphene oxide and BP have great biocompatibility and biodegradability, and have attracted more attention as candidate materials for photothermal therapy in recent years (Liu S. et al, 2020). Graphene has been a hotspot of scientific research since its discovery. Recently, graphene derivatives have been favored due to its better drug loading efficiency and photothermal conversion capacity (Chen et al., 2016). It is proved that folic acid coupled chitosan functionalized graphene oxide (FA-CS-GO) can completely destroy cancer cells under near-infrared (NIR) light irradiation in vitro, and showed excellent anti-tumor effect in vivo with no recurrence in 20 days (Jun et al., 2020). Besides, BP has been considered as a promising photothermal material, but its rapid degradation in the physiological environment limits its application. Based on this, Geng et al. assembled NIR-II responsive carbon dots on BP nanosheets to construct a hybrid photothermal agent, which improved the stability of BP and showed synergistic photothermal effect, attained higher light-to-heat conversion efficiency in the NIR-I and NIR-II window (77.3 and 61.4%, respectively), and the deep tumors were eradicated under the 1,064 nm laser (Geng et al., 2019). Shao et al. prepared a core-shell structured nanospheres with black phosphorous quantum dots (BPQDs) encapsulated in poly (lactic-co-glycolic acid) (PLGA), and the nanospheres achieved a better passive targeting effect, retained good photothermal performance with excellent stability (Shao et al., 2016).
As for photodynamic therapy, there have been many studies on nanocarriers loaded photosensitizers such as photofrin (Kano et al., 2013), 5-aminolevulinic acid (Zhang et al., 2015) and chlorine e6 (Ce6) (Yang et al., 2019) to improve delivery efficiency and enhance tumor killing effects, but the hypoxia microenvironment greatly limits the therapeutic efficiency. In addition to the strategies of delivering MnO2 and catalase to increase the production of oxygen, studies have indicated that nano-heterostructure can promote electron-hole separation and the accumulation of ROS, which is considered an effective material for photodynamic therapy (Cheng et al., 2020). Zhang et al. designed a bismuth sulfide (Bi2S3)-bismuth (Bi) Z-type heterostructure with a good electron-hole separation ability, simultaneously generated ROS and O2 under NIR irradiation, improved photodynamic therapy efficiency with excellent biocompatibility and biodegradability (Cheng et al., 2020). Qiu et al. also prepared bismuth/bismuth oxide (Bi/BiOx) lateral nano-heterostructures based on the regioselective oxidation strategy. Under 660 nm laser irradiation, 1O2 can be effectively generated under normal oxygen conditions, and cytotoxic ⋅OH and H2 can be generated under hypoxic conditions, showing synergetic photodynamic performance on tumor elimination (Qiu et al., 2021).
Furthermore, studies used up-conversion nanomaterials (UCNPs) to emit visible light under NIR excitation and stimulate photosensitizers to effectively produce ROS, overcome the limitation of weak tissue penetration of visible light, and improve the efficacy of photodynamic therapy (Liang et al., 2020a; Liu et al., 2020b). Liu et al. constructed a Nd3+ ion-doped UCNPs surface-modified porphyrin-like metal-organic framework and functionalized it to target the mitochondria to achieve enhanced photodynamic therapy under the trigger of NIR irradiation (Liu et al., 2020c). Liang et al. developed UCNPs modified with oleic acid-polyamide dendrimers to form hydrophobic and hydrophilic pockets on the surface of nanoparticles through click reaction, loaded with photosensitizer Ce6 and catalase, which can effectively overcome tumor hypoxia and improve photodynamic therapy efficiency, indicating significant anti-tumor effect through synergistic mitochondrial targeting modification (Liang et al., 2020b).
In addition, photodynamic and photothermal therapy are usually applied in combination due to their synergistic tumor killing effect (Figure 2). Photothermal therapy generates heat locally can not only ablate tumor cells, but also accelerate blood circulation and accumulate oxygen in the tumor’s hypoxic microenvironment to provide suitable conditions for photodynamic therapy (Yang et al., 2021). Guan et al. prepared covalent organic framework nanoparticles with a particle size of ∼140 nm that co-loaded the photosensitizer porphyrin and the photothermal agent naphthalocyanine. The temperature increase led by the photothermal effect significantly enhanced the sensitivity of photodynamic therapy by destroying lysosomes and mitochondria (Guan et al., 2019). Yang et al. synthesized TiO2@RP nanorods with red phosphorus (RP) as the shell and TiO2 as the core. After being irradiated with 808 nm NIR light for 5 min, the nanorods can reach 39.3–44.1°C and induce the production of 1O2, effectively killing cancer cells in deep tissues of renal cell carcinoma (Yang et al., 2021).
Nanomaterial-Mediated Immunotherapy
Immunotherapy is based on the regulation of the immune system, activating a long-term immune response against malignant tumor cells, thereby eradicating tumors and inhibiting tumor metastasis (Riley et al., 2019). Tumor associate antigens derived from necrotic or apoptotic tumor cells can be taken up and processed by antigen-presenting cells, presented to T cells in lymph nodes, inducing the activation of immature T cells to effector T cells. T cell-mediated cellular immune response is the key to tumor immunity (Song et al., 2017).
Tumor vaccines can effectively trigger potent immune responses for tumor prevention and treatment specifically. Nanomaterials are applied to co-deliver antigens and adjuvants (Zhu et al., 2018), increase the uptake of antigen-presenting cells, improve the ability of cross presentation, or directly target lymph nodes to induce strong effective immune response (Li H. et al, 2017; Zhong et al., 2019a). Zhu et al. co-encapsulated B16 melanoma-derived tyrosinase-related protein 2 (Trp2) peptides and toll-like receptor agonists (CpG oligonucleotides and monophosphoryl lipid A) in a mesoporous silica vector, which improved the efficiency of antigen delivery to dendritic cells, effectively activated T cell-specific immune responses and prolonged the survival period of tumor-bearing mice significantly (Zhu et al., 2018). Li et al. prepared micelles composed of polycaprolactone-polyethyleneimine and polycaprolactone-polyethylene glycol, and co-loaded Trp2 and the adjuvant CpG oligodeoxynucleotide, which showed higher activity of cytotoxic T lymphocytes (CTLs) and stronger anti-tumor ability in B16F10 melanoma mice compared with the mixture of free Trp2 and CpG (Li M. et al, 2017).
The antigen captured by dendritic cells are presented to the major histocompatibility complex class I (MHCI) or major histocompatibility complex class II (MHCII) molecules extracellularly (Song et al., 2017). For tumor immunotherapy, it is vital that exogenous antigens escape from lysosomes and activate CD8+T cells through the MHCI pathway through cross presentation (Du et al., 2020). Suitable nanomaterials are applied according to the unique acidic environment of the lysosome to promote the escape of antigen from the lysosome and enhance cross presentation (Li H. et al, 2017; Du et al., 2020). The most commonly used methods are cationic polymer modifications such as polyethylenimine (Li M. et al, 2017), 1,2-dioleoyl-3-trimethylammonium-propane (Zhang et al., 2019) and dimethyl dioctadecylammonium bromide (Xia et al., 2018), inducing the proton sponge effect to cause the destruction of the endosomal membrane, thereby increasing the endosomal escape of the antigen (Du et al., 2020). Recent studies have shown that pH-sensitive materials can also promote cross presentation by inducing endosomal membrane fusion and transferring antigens through the cytosol (Zhong et al., 2019b; Du et al., 2020). MOFs have attracted attention due to their excellent drug-carrying capacity and pH-sensitive properties. Zhong et al. used zeolite imidazole skeleton (ZIF-8), a pH-sensitive degradable MOF, co-loaded antigen and CpG for subcutaneous injection, which decomposed in the lysosome to release the antigen into the cytoplasm and enhanced cross presentation, remarkably inhibiting EG7-OVA tumor growth (Zhong et al., 2019a). In another study, the MOF composed of guanine monophosphate and lanthanide ions can be actively internalized by antigen presenting cells, release the antigen and promote its escape from the lysosome, significantly improving the CTL response (Duan et al., 2017).
Although tumor vaccines have shown excellent anti-tumor effects, the immunosuppressive microenvironment presents a major challenge for tumor immunotherapy, and immune checkpoint inhibitors have been considered as a promising strategy in recent years (Song et al., 2017). Blocking the immune checkpoints facilitates the recognition of T cells and initiates an effective anti-tumor immune response, thus preventing tumor immune escape and improving the efficiency of anti-tumor immunotherapy (Song et al., 2017; Chen et al., 2018). The most studied inhibitory receptors are cytotoxic T lymphocyte-associated protein 4 (CTLA-4) and programmed death 1 (PD-1). CTLA-4 is expressed on activated T cells and interacts with costimulatory molecules on antigen presenting cells to impede T cell activation (Ruan et al., 2019). And PD-1 is up-regulated on activated T cells, interacts with the corresponding ligand PD-L1 on tumor cells to inhibit the function of T cells. Anti-CTLA-4 monoclonal antibody ipilimumab and anti-PD-1/PD-L1 antibodies such as pembrolizumab and avelumab have been approved by the United States Food and Drug Administration (FDA) for clinical cancer treatment (Chen et al., 2018; Ruan et al., 2019). Studies have shown that combining tumor vaccines with immune checkpoint inhibitors can effectively combat tumors and prevent the recurrence (Kuai et al., 2017; Kim et al., 2020). Kim et al. used biocompatible phospholipid nanoparticles to co-encapsulate tumor antigens and CpG adjuvants, which showed strong anti-tumor effects in the prevention and treatment of EG7 tumor models, but the vaccine induced T-cell exhaustion by increasing PD-L1 expression, leading to tumor recurrence. Combining anti-PD-1 therapy with nano-vaccine suppressed tumor recurrence and showed a synergistic anti-tumor effect (Kim et al., 2020).
Developed Nanomaterials Towards the Tumor Combination Therapies
Nanomaterials have exhibited great potential in tumor treatment and obtained remarkable results in both traditional and emerging therapies. However, there are still some limitations in monotherapy such as the inability to completely eliminate the tumor or the potential risk of tumor recurrence. The application of nanomaterials in combination therapy is emphasized to achieve synergistic effects for tumor treatment (Figure 3).
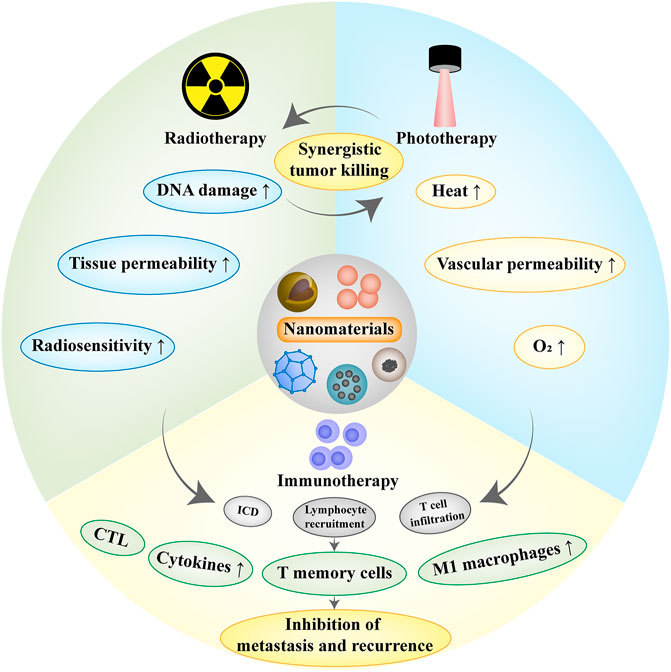
FIGURE 3. Schematic diagram of tumor combination therapies. Radiotherapy can break through the limitation of insufficient tissue penetration of phototherapy. In turn, photothermal therapy can improve vascular permeability and increase O2 concentration, which facilitates the performance of radiotherapy. Radiotherapy/phototherapy can induce immunogenic cell death (ICD), release tumor-associated antigens locally and increase T cell infiltration, and the combination with immunotherapy can effectively inhibit tumor metastasis and recurrence.
Radiotherapy Combined With Phototherapy
NIR light used in photothermal therapy has a limited penetration depth and fails to eradicate tumor cells in the deep tissue. Relatively, radiotherapy has a stronger penetrating ability, which can effectively break through the restriction while also improving the photothermal conversion capability (Zheng et al., 2021). At the same time, photothermal therapy can improve oxygen perfusion and relieve hypoxic environment, thus amplifying the effect of radiotherapy (Luo et al., 2020). Research has shown that nanomaterials based on high-Z element can simultaneously promote photothermal therapy and radiotherapy. Zheng designed a W18O49 nanosphere which showed excellent radiation sensitization and photothermal performance, promoted the production of 1O2 and ⋅OH, increased radiation-induced DNA damage and significantly inhibited tumor cell proliferation and metastasis (Zheng et al., 2021). In addition, the combined application with nano-heterostructure is conducive to the production of ROS. Cai et al. prepared a copper sulfide nano-heterostructure modified by platinum gold nanoparticles with enhanced light-to-heat conversion capacity, leading to efficient glutathione clearance and formation of ROS with the co-excitation of NIR and X-ray. The nano-heterostructure can effectively remove tumors and inhibit tumor recurrence, exerting the synergistic anti-tumor effect of radiotherapy and photothermal therapy (Cai et al., 2021). Similarly, Huang et al. developed a dumbbell-shaped heterostructure based on copper selenide-gold nanoparticles for enhanced radiophotothermal therapy. The heterostructure possesses excellent photothermal conversion efficiency due to enhanced localized surface plasmon resonance and also has better X-ray attenuation based on the synergy of multiple elements, indicating a remarkable tumor cell elimination effect (Huang et al., 2019).
The combination of radiotherapy and photodynamic therapy can also solve the limitation of tissue penetration to eliminate deep-seated tumors. Studies have demonstrated that the strategy of combining high-Z materials and photosensitizers has achieved remarkable results. Sun et al. successfully synthesized polymer nanodots based on gadolinium ions and the clinically used photosensitizer Rose Bengal through solvothermal reaction. The nanodots induced the generation efficiency of 1O2 increased by 1.9 times compared with Rose Bengal, significantly inhibiting tumor growth with a tumor suppression rate of 98.8% without systemic toxicity or long-term side effects (Sun et al., 2020). Another promising approach is to combine scintillator materials and photosensitizers to activate radiotherapy and photodynamic therapy under the same ionizing radiation. Scintillators are developed to absorb X-rays and convert them into photons to activate photosensitizers to generate ROS, providing a new strategy for treatment of deep tumors (Zhong et al., 2019b). Ahmad et al. prepared a scintillating nanoparticle CeF3:Gd3+, Tb3+coated with mesoporous silica and loaded Bengal Rose for X-ray-induced photodynamic therapy. Low-dose X-ray irradiation confirmed a better tumor regression effect of synergistic therapies relative to radiotherapy alone by down-regulating the amino acids involved in protein and DNA synthesis (Ahmad et al., 2019). Shi et al. prepared mesoporous zinc gallium germanate long afterglow nanoparticles (Zn3Ga2GeO8:Cr3+, Yb3+, Er3+), which can penetrate muscle tissue to achieve effective afterglow imaging in mice. Under X-ray irradiation, the nanoparticles emitted NIR long afterglow thus activating the photosensitizer to kill cancer cells and effectively inhibiting the growth of liver tumors in situ (Shi et al., 2020). Zhong et al. prepared a rod-like structure of NaCeF4: Gd, Tb nano scintillation crystals to enhance the local radiation intensity, which was also activated as an X-ray responsive photosensitizer to generate a large amount of O2− and ⋅OH (Zhong et al., 2019b).
In addition, it is demonstrated that the combination of photothermal and X-ray-induced photodynamic therapy has a better therapeutic effect, as photothermal therapy is believed to enhance the permeability of nanoparticles in tumors and increase oxygen accumulation, which is conducive to improving the efficacy of oxygen-dependent treatments such as photodynamic therapy and radiotherapy (Guo et al., 2017; Luo et al., 2020). Based on this, Luo et al. conjugated europium complex scintillators and mesoporous silica coated gold nanorods, loaded with photosensitizer hematoporphyrin to applied for the synergistic treatment of radiotherapy/photodynamic/photothermal therapy. The nanoparticle has strong NIR absorption and X-ray conversion efficiency, showed excellent photothermal effect and radiosensitization ability, and realized the effective treatment for deep tumor with minimal side effects upon low-dose laser and X-rays (Luo et al., 2020). Guo et al. constructed bovine serum albumin (BSA)-encapsulated BiOI@Bi2S3 semiconductor heterojunction nanoparticles, in which bismuth and iodine were used as radiotherapy sensitizers due to the strong X-ray attenuation ability, and the electron-hole pairs and heterojunction structure in semiconductors can promote the generation of ROS through the photodynamic therapy process. Besides, bismuth sulfide was used as a photothermal agent to kill hypoxic cells insensitive to radiotherapy, improved the hypoxic environment of tumors and achieved a synergistic tumor-inhibiting effect superior to monotherapy (Guo et al., 2017).
Radiotherapy/Phototherapy Combined With Immunotherapy
Currently, immunotherapy has received a lot of attention and showed a gratifying rapid development. However, the therapeutic effect is unsatisfactory in the treatment of “cold tumors” (Bonaventura et al., 2019). “Hot tumors” and “cold tumors” refer to tumors with or without lymphocytes infiltration and inflammation respectively. Cold tumors greatly limit the effectiveness of tumor immunotherapy, especially the application of immune checkpoint inhibitors (Galon and Bruni, 2019). In recent years, it has been discovered that tumor therapies such as radiotherapy, chemotherapy or phototherapy can stimulate the immune system by inducing immunogenic cell death to release tumor-related antigens and damage-related molecular patterns, recruit lymphocytes and enhance T cell infiltration by destroying tumor microenvironment and turn cold tumors into hot tumors, which facilitates the induction of stronger T cell immune responses to attack tumors cells and inhibit tumor metastasis and recurrence (Bonaventura et al., 2019; Galon and Bruni, 2019). Therefore, the combination of multiple tumor therapies is considered a promising strategy to achieve synergistic anti-tumor effects and nanomaterial-based strategies have been proven to achieve remarkable therapeutic outcomes (Table 1).
Studies have shown that combining radiotherapy enhancement methods with immune adjuvants or immune checkpoint inhibitors can obtain ideal anti-tumor effects (Ni et al., 2019; Patel et al., 2019). Chen et al. prepared a PLGA nanoparticle (PLGA-R837@Cat) that co-loaded catalase and Toll-like receptor-7 agonist (R837), which can effectively decompose H2O2 and increase the oxygen in the tumor to enhance efficacy of radiotherapy. Radiotherapy based on PLGA-R837@Cat induced the immunogenic death of tumor cells and triggered a stronger tumor immune response with the assistance of R837. Furthermore, the results proved that the combination therapy with anti-CTLA-4 antibody can effectively inhibit the growth of distant tumors (Chen et al., 2019). Huang et al. synthesized the triangular star tellurium nanomaterials and modified RGD polypeptide to target the tumor, which increased the accumulation of ROS and the efficacy of radiotherapy. Combined treatment with anti-PD-1 enhanced the infiltration of cytotoxic T lymphocytes in tumor tissues, promoted the polarization of protumorigenic M2 phenotype macrophages to tumoricidal M1 phenotype macrophages, and the proportion of M2 phenotype macrophages decreased from 24.46 to 4.66%, effectively inhibiting the growth of distal tumors (Huang et al., 2020).
Phototherapy combined with immunotherapy is also considered to be a promising strategy (Chen et al., 2021; Feng et al., 2021). The application of nanomaterials with high light-to-heat conversion efficiency in combination with immune adjuvants or immune checkpoint inhibitors has shown excellent tumor treatment effects (Ming et al., 2020; Fang et al., 2021). Ming et al. utilized the photothermal material Pd nanosheets to deliver CpG ODNs, which effectively inhibited tumors under NIR irradiation. The levels of TNF-α and IL-6 were significantly increased, and a strong CTL response was induced, which greatly improved the survival rate of tumor-bearing mice (Ming et al., 2020). Fang et al. designed a two-dimensional material FePSe3 wrapped in colon CT26 membrane, loaded with anti-PD-1 polypeptides, which showed significant accumulation at the tumor site, promoted the maturation of dendritic cells and the activation of T cells, remarkably prolonged the survival period of mice under the irradiation of infrared laser (Fang et al., 2021).
As for photodynamic therapy combined immunotherapy, Meng et al. prepared a light-triggered in situ gelation system for tumor treatment: modified catalase with photosensitizer Ce6 and mixed with polymeric matrix polyethylene glycol diacrylate, and R837 was added as an immune adjuvant. After injection into the tumor, it was irradiated with 660 nm light to form an in-situ hydrogel for multiple rounds of photodynamic therapy. In combination with CTLA-4 inhibitors, it further inhibited the growth of distant tumors, and also provided effective long-term immune memory protection to avoid tumor recurrence (Meng et al., 2019). Wang et al. designed pH-sensitive nanoparticles through the reaction of dextran and hyaluronidase, which can rapidly degrade hyaluronic acid in the extracellular matrix by releasing hyaluronidase in the acidic microenvironment of the tumor, thereby alleviating the hypoxic environment. It was proved that the nanoparticles can improve the efficacy of subsequent photodynamic therapy of Ce6@liposome and further enhance the infiltration of CTLs in tumor tissues after combining with anti-PD-L1, showing a significantly stronger anti-tumor effect and effectively inhibiting the growth of distant tumors. (Wang et al., 2019b). Shao et al. prepared a core-shell nanostructure formed by porphyrinic MOFs and UCNPs. Besides, a hypoxia-activated prodrug tilapazamin was loaded to achieve an excellent synergistic therapeutic effect on CT26 tumor-bearing mice (Shao et al., 2020).
Given that photothermal and photodynamic therapy have a great synergistic effect, its further combination with immunotherapy has also obtained ideal results. Yan et al. prepared NaGdF4: Yb/Er upconversion layer-coated polydopamine (PDA) nanoparticles, and modified the photosensitizer Ce6 to maximize the synergistic effect of phototherapy under 980 nm laser irradiation. Higher concentrations of cytokines such as IL-6 and TNF-α and lower level of IL-10 were induced, which proved the activation of a stronger immune response and the reduction of M2 suppressive macrophages, respectively. When combined with anti-PD-1 treatment, the survival rate of mice reached up to 77.8%, significantly higher than the group without PD-1 blockade antibody. Besides, the activation of CTLs and T memory cells can inhibit tumor metastasis and recurrence effectively (Yan et al., 2019). Jin et al. constructed a corn-like Au/Ag nanorod irradiated with a 1,064 nm laser to induce ICD of tumor cells. Combined with anti-CTLA-4 treatment can induce a strong immune memory effect, which was an effective method to reverse the immunosuppressive cold tumor microenvironment and prevent the tumor from recurring (Jin et al., 2021).
Other Tumor Therapies Based on Nanomaterials
Sonodynamic Therapy
Sonodynamic therapy uses ultrasound to focus acoustic energy on tumor tissue, activating sonosensitizers to generate ROS and trigger anti-tumor effects. Various nanocarriers have been constructed to deliver small molecule sonosensitizers such as porphyrin, hematoporphyrin monomethyl ether, and rose bengal to enhance the accumulation at tumor sites (Liang et al., 2020a). Besides, inorganic sonosensitizers are favored due to the better physicochemical properties. TiO2 is a traditional sonosensitizer and its modification has always been the focus of research (Çeşmeli and Biray Avci, 2019). The combination of modified TiO2 and noble metals can effectively prevent the recombination of electrons and holes generated by ultrasound excitation. ROS generation efficiency of Au-TiO2 nanosheets is significantly higher than that of TiO2 itself (Cao et al., 2019). In addition, the combination of BP and Au was also found to improve the efficiency of sonodynamic therapy, inhibiting tumor growth with less side effects (Ouyang et al., 2018).
Ultrasound poses stronger penetration ability through biological tissues to achieve better therapeutic effect on deep tumors, which can effectively overcome the limitation of insufficient tissue penetration of phototherapy. Besides, some sonosensitizers or nanomaterials can be activated by ultrasound and laser at the same time, so as to achieve synergistic therapeutic effect, which is a novel therapeutic strategy to provide a promising solution for the treatment of deep-seated tumors (Liang et al., 2020b). Liang et al. synthesized a complex composed of CuS and Pt, and the hollow cavity of CuS could be loaded with the sonosensitizer tetra (4-aminophenyl) porphyrin. Furthermore, the deposition of Pt enhanced the photothermal performance and catalyzed the generation of O2 from H2O2 to accumulate sufficient ROS and induce tumor cell apoptosis. Besides, the heat generated by the laser can accelerate the catalytic activity of Pt and increase the oxygen level, which can further promote sonodynamic therapy efficacy and achieve synergistic tumor killing effect (Liang et al., 2019). In addition, sonodynamic therapy can also induce the release of damage-related molecular pattern molecules and trigger anti-tumor immune responses. Yue et al. prepared a liposome containing sonosensitizer hematoporphyrin monomethyl ether and immune adjuvant R837, which elicited strong anti-tumor immune responses, and the combination with anti-PD-L1 checkpoint blocker induced a potent memory immune response (Yue et al., 2019).
Chemodynamic Therapy
Chemodynamic therapy is to activate the Fenton/Fenton-like reaction in the tumor microenvironment to generate ROS and kill tumor cells. Chemodynamic therapy can also generate O2 at tumor sites without tissue depth limitations (Xin et al., 2021). Nanomaterials with high catalytic efficiency and excellent biocompatibility have been applied in chemodynamic therapy, including iron-based nanomaterials, other metal-based nanomaterials such as manganese (Ding et al., 2020) and molybdenum (Dhas et al., 2021), and some organic nanomaterials such as MOF (Zhang S. et al, 2020). Copper-based nanomaterials have received extensive attention in recent years due to the low cost and easy availability (Ai et al., 2021). Cu-MOF nanoparticles can be rapidly degraded after exposure to the tumor microenvironment, release Cu2+ to deplete glutathione and generate highly cytotoxic ⋅OH to enhance the chemodynamic therapy effect (Zhang K. et al, 2020). Similarly, Ma et al. designed a Zn2+/Cu2+ co-doped MOF loaded with cisplatin and achieved excellent tumor inhibitory effect (Ma et al., 2021). The combination of chemodynamic therapy and other therapies has been found to obtain a synergistic effect, and the generated ROS and O2 can effectively overcome tumor hypoxia and enhance the efficacy of radiotherapy and photodynamic therapy (Liu S. et al, 2020). In addition, chemodynamic therapy combined with photothermal therapy has great potential to improve the therapeutic performance: the temperature increase induced by photothermal therapy promotes the production of ROS, which in turn enhances tumor cell sensitization, thus achieving a stronger anti-tumor effect (Zhang S. et al, 2020). Studies have also shown that further combination of checkpoint blockade therapy can successfully inhibit distant tumor growth and cancer metastasis (Hu et al., 2020).
Nano-Based Delivery of RNA Interference Therapeutics
RNA interference (RNAi) is an important gene expression regulation method, which is a gene silencing process induced by endogenous or artificially transfected small interfering double-stranded RNA (Xin et al., 2017). A major challenge for RNAi therapeutics is the construction of efficient vectors, and various cationic polymer nanoparticles and lipid-based carriers have been applied for RNAi delivery (Xin et al., 2017). More effective delivery systems are needed to specifically target tumors according to the tumor microenvironment. Gold nanoclusters are favored due to the unique ultra-small size, which can increase the aggregation of nerve growth factor small interfering RNA (NGF siRNA) at tumor sites, and effectively interfere with the NGF gene to inhibit tumor development (Lei et al., 2017). Xu et al. synthesized a pH-responsive siRNA nanoparticle, which was modified with polyethylene glycol to attain long-circulating effect, and could rapidly release siRNA in the tumor microenvironment, thereby leading to effective gene silencing and significantly inhibiting the growth of prostate cancer (Xu et al., 2017). In addition, the development of multifunctional nanocarriers and combination therapies can achieve better therapeutic effects. Liu et al. synthesized a siRNA-loaded amorphous iron oxide nanoparticle, which can inhibit the up-regulation of monocarboxylic acid transporter in tumor cells to induce cellular acidosis, and catalyze H2O2 to generate ROS through Fenton-like reaction, thereby killing tumor cells and inhibiting tumor growth (Liu Y. et al, 2018).
Conclusion and Prospect
Here we reviewed the progress of nanomaterials used in radiotherapy, phototherapy, immunotherapy and some other therapies such as sonodynamic therapy, chemodynamic therapy and RNAi therapeutics in the past 5 years, listed some creative designs of nanocarriers and summarized the major development direction of tumor combination therapies. The application of nanomaterials has been a promising strategy in both traditional and emerging therapies, and a variety of suitable nanomaterial-based carriers with specific properties have been gradually developed to effectively accumulate at the tumor site and overcome the adverse tumor microenvironment, which have shown great potential for improving the efficiency of tumor treatment. Recent studies have been focused on the development of the combination of multiple therapies in order to maximize the therapeutic effect. The combination of radiotherapy and phototherapy can effectively combat deep-seated tumors with minimal side effects, and the oxygen perfusion lead by photothermal therapy is conducive to oxygen-dependent therapies such as radiotherapy and photodynamic therapy. In addition, various therapies have been proved to activate the immune response to a certain extent in the process of tumor treatment. Therefore, combining immunotherapy can synergistically strengthen the therapeutic outcome and dramatically prevent tumor metastasis and recurrence.
Despite satisfactory progress in the development and application of nanomaterials, some challenges remain to be addressed. The clinical translation of nanomaterials is the most critical issue for tumor treatment, so the materials should possess excellent biocompatibility and biodegradability, and FDA-approved materials ought to be prioritized for consideration. Similarly, the scale-up process from the laboratory to the clinic usually requires optimization or changes of the preparation method, so the efforts should be made to establish nanocarriers that facilitate the scale-up production while ensuring the effectiveness of tumor treatment. Besides, the toxicity and safety of nanomaterials are still pressing issues, and research should give more consideration to the long-term and potential toxicity of nanomaterials. In addition, it is of great importance to develop nanomaterials with specific physicochemical properties to meet the needs of different tumor therapies. Currently combination therapy is favored due to its synergistic anti-tumor efficacy, accordingly, nanomaterials with multiple functional properties are gradually developed to simplify the design of the carriers and achieve better therapeutic effect. It is vital to explore multifunctional nanomaterials to trigger multiple therapeutic effects in further research. The complexity of the tumor microenvironment presents a great challenge for the design of nanocarriers. With the continuous deepening of current research, the relationship between tumor therapies and its further mechanism has gradually become clear. According to the actual tumor conditions, suitable carriers can be designed to reverse unfavorable microenvironment to enhance the therapeutic outcome through monotherapies or combination therapies. The development of nanomaterials with suitable properties and biosafety to achieve remarkable therapeutic effect is the vital topic for tumor treatment in the future.
Author Contributions
XH: wrote the manuscript and drew the drawings. JW and DX: read and improved the scientific quality. YY: read and improved the scientific quality; supervision and correction of the manuscript. All authors contributed to the article and approved the submitted version.
Conflict of Interest
The authors declare that the research was conducted in the absence of any commercial or financial relationships that could be construed as a potential conflict of interest.
The reviewer (HDL) declared a past co-authorship with the author(s) (DX) to the handling editor.
Publisher’s Note
All claims expressed in this article are solely those of the authors and do not necessarily represent those of their affiliated organizations, or those of the publisher, the editors, and the reviewers. Any product that may be evaluated in this article, or claim that may be made by its manufacturer, is not guaranteed or endorsed by the publisher.
References
Abbasi, A. Z., Gordijo, C. R., Amini, M. A., Maeda, A., Rauth, A. M., DaCosta, R. S., et al. (2016). Hybrid Manganese Dioxide Nanoparticles Potentiate Radiation Therapy by Modulating Tumor Hypoxia. Cancer Res. 76, 6643–6656. doi:10.1158/0008-5472.CAN-15-3475
Ahmad, F., Wang, X., Jiang, Z., Yu, X., Liu, X., Mao, R., et al. (2019). Codoping Enhanced Radioluminescence of Nanoscintillators for X-ray-Activated Synergistic Cancer Therapy and Prognosis Using Metabolomics. ACS Nano 13, 10419–10433. doi:10.1021/acsnano.9b04213
Ai, K., Huang, J., Xiao, Z., Yang, Y., Bai, Y., and Peng, J. (2021). Localized Surface Plasmon Resonance Properties and Biomedical Applications of Copper Selenide Nanomaterials. Mater. Today Chem. 20, 100402. doi:10.1016/j.mtchem.2020.100402
Aikins, M. E., Xu, C., and Moon, J. J. (2020). Engineered Nanoparticles for Cancer Vaccination and Immunotherapy. Acc. Chem. Res. 53, 2094–2105. doi:10.1021/acs.accounts.0c00456
Bonaventura, P., Shekarian, T., Alcazer, V., Valladeau-Guilemond, J., Valsesia-Wittmann, S., Amigorena, S., et al. (2019). Cold Tumors: A Therapeutic Challenge for Immunotherapy. Front. Immunol. 10, 168. doi:10.3389/fimmu.2019.00168
Cai, R., Xiang, H., Yang, D., Lin, K. T., Wu, Y., Zhou, R., et al. (2021). Plasmonic AuPt@CuS Heterostructure with Enhanced Synergistic Efficacy for Radiophotothermal Therapy. J. Am. Chem. Soc. 143, 16113–16127. doi:10.1021/jacs.1c06652
Cao, Y., Wu, T., Dai, W., Dong, H., and Zhang, X. (2019). TiO2 Nanosheets with the Au Nanocrystal-Decorated Edge for Mitochondria-Targeting Enhanced Sonodynamic Therapy. Chem. Mater. 31, 9105–9114. doi:10.1021/acs.chemmater.9b03430
Çeşmeli, S., and Biray Avci, C. (2019). Application of Titanium Dioxide (TiO2) Nanoparticles in Cancer Therapies. J. Drug Target. 27, 762–766. doi:10.1080/1061186X.2018.1527338
Chen, C., Song, M., Du, Y., Yu, Y., Li, C., Han, Y., et al. (2021). Tumor-Associated-Macrophage-Membrane-Coated Nanoparticles for Improved Photodynamic Immunotherapy. Nano Lett. 21, 5522–5531. doi:10.1021/acs.nanolett.1c00818
Chen, Q., Chen, J., Yang, Z., Xu, J., Xu, L., Liang, C., et al. (2019). Nanoparticle-Enhanced Radiotherapy to Trigger Robust Cancer Immunotherapy. Adv. Mater. 31, e1802228. doi:10.1002/adma.201802228
Chen, Q., Wang, C., Chen, G., Hu, Q., and Gu, Z. (2018). Delivery Strategies for Immune Checkpoint Blockade. Adv. Healthc. Mater. 7, e1800424. doi:10.1002/adhm.201800424
Chen, Y. W., Su, Y. L., Hu, S. H., and Chen, S. Y. (2016). Functionalized Graphene Nanocomposites for Enhancing Photothermal Therapy in Tumor Treatment. Adv. Drug Deliv. Rev. 105, 190–204. doi:10.1016/j.addr.2016.05.022
Cheng, Y., Kong, X., Chang, Y., Feng, Y., Zheng, R., Wu, X., et al. (2020). Spatiotemporally Synchronous Oxygen Self-Supply and Reactive Oxygen Species Production on Z-Scheme Heterostructures for Hypoxic Tumor Therapy. Adv. Mater. 32, e1908109. doi:10.1002/adma.201908109
Dhas, N., Kudarha, R., Garkal, A., Ghate, V., Sharma, S., Panzade, P., et al. (2021). Molybdenum-based Hetero-Nanocomposites for Cancer Therapy, Diagnosis and Biosensing Application: Current Advancement and Future Breakthroughs. J. Control. Release 330, 257–283. doi:10.1016/j.jconrel.2020.12.015
Ding, B., Zheng, P., Ma, P., and Lin, J. (2020). Manganese Oxide Nanomaterials: Synthesis, Properties, and Theranostic Applications. Adv. Mater. 32, e1905823. doi:10.1002/adma.201905823
Dong, C. Y., Hong, S., Zheng, D. W., Huang, Q. X., Liu, F. S., Zhong, Z. L., et al. (2021). Multifunctionalized Gold Sub-nanometer Particles for Sensitizing Radiotherapy against Glioblastoma. Small 17, e2006582. doi:10.1002/smll.202006582
Dong, W., Cai, Z., Pang, J., Wang, J., Tang, N., Zhang, W., et al. (2020). Radiotherapy Enhancement for Human Pancreatic Carcinoma Using a Peptide-Gold Nanoparticle Hybrid. J. Biomed. Nanotechnol 16, 352–363. doi:10.1166/jbn.2020.2898
Du, G., and Sun, X. (2020). Engineering Nanoparticulate Vaccines for Enhancing Antigen Cross-Presentation. Curr. Opin. Biotechnol. 66, 113–122. doi:10.1016/j.copbio.2020.06.015
Du, Y., Sun, H., Lux, F., Xie, Y., Du, L., Xu, C., et al. (2020). Radiosensitization Effect of AGuIX, a Gadolinium-Based Nanoparticle, in Nonsmall Cell Lung Cancer. ACS Appl. Mater. Inter. 12, 56874–56885. doi:10.1021/acsami.0c16548
Duan, F., Feng, X., Yang, X., Sun, W., Jin, Y., Liu, H., et al. (2017). A Simple and Powerful Co-delivery System Based on pH-Responsive Metal-Organic Frameworks for Enhanced Cancer Immunotherapy. Biomaterials 122, 23–33. doi:10.1016/j.biomaterials.2017.01.017
Fang, X., Wu, X., Li, Z., Jiang, L., Lo, W. S., Chen, G., et al. (2021). Biomimetic Anti-PD-1 Peptide-Loaded 2D FePSe3 Nanosheets for Efficient Photothermal and Enhanced Immune Therapy with Multimodal MR/PA/Thermal Imaging. Adv. Sci. (Weinh) 8, 2003041. doi:10.1002/advs.202003041
Feng, Y., Xie, X., Zhang, H., Su, Q., Yang, G., Wei, X., et al. (2021). Multistage-responsive Nanovehicle to Improve Tumor Penetration for Dual-Modality Imaging-Guided Photodynamic-Immunotherapy. Biomaterials 275, 120990. doi:10.1016/j.biomaterials.2021.120990
Galon, J., and Bruni, D. (2019). Approaches to Treat Immune Hot, Altered and Cold Tumours with Combination Immunotherapies. Nat. Rev. Drug Discov. 18, 197–218. doi:10.1038/s41573-018-0007-y
Geng, B., Shen, W., Li, P., Fang, F., Qin, H., Li, X. K., et al. (2019). Carbon Dot-Passivated Black Phosphorus Nanosheet Hybrids for Synergistic Cancer Therapy in the NIR-II Window. ACS Appl. Mater. Inter. 11, 44949–44960. doi:10.1021/acsami.9b15569
Guan, Q., Zhou, L. L., Li, Y. A., Li, W. Y., Wang, S., Song, C., et al. (2019). Nanoscale Covalent Organic Framework for Combinatorial Antitumor Photodynamic and Photothermal Therapy. ACS Nano 13, 13304–13316. doi:10.1021/acsnano.9b06467
Guo, Z., Zhu, S., Yong, Y., Zhang, X., Dong, X., Du, J., et al. (2017). Synthesis of BSA-Coated BiOI@Bi2 S3 Semiconductor Heterojunction Nanoparticles and Their Applications for Radio/Photodynamic/Photothermal Synergistic Therapy of Tumor. Adv. Mater. 29. doi:10.1002/adma.201704136
He, W., Ai, K., and Lu, L. (2015). Nanoparticulate X-ray CT Contrast Agents. Sci. China Chem. 58, 753–760. doi:10.1007/s11426-015-5351-8
Hou, X., Tao, Y., Pang, Y., Li, X., Jiang, G., and Liu, Y. (2018). Nanoparticle-based Photothermal and Photodynamic Immunotherapy for Tumor Treatment. Int. J. Cancer 143, 3050–3060. doi:10.1002/ijc.31717
Hu, C., Cai, L., Liu, S., Liu, Y., Zhou, Y., and Pang, M. (2020). Copper-Doped Nanoscale Covalent Organic Polymer for Augmented Photo/Chemodynamic Synergistic Therapy and Immunotherapy. Bioconjug. Chem. 31, 1661–1670. doi:10.1021/acs.bioconjchem.0c00209
Huang, Q., Zhang, S., Zhang, H., Han, Y., Liu, H., Ren, F., et al. (2019). Boosting the Radiosensitizing and Photothermal Performance of Cu2- xSe Nanocrystals for Synergetic Radiophotothermal Therapy of Orthotopic Breast Cancer. ACS Nano 13, 1342–1353. doi:10.1021/acsnano.8b06795
Huang, W., He, L., Ouyang, J., Chen, Q., Liu, C., Tao, W., et al. (2020). Triangle-shaped Tellurium Nanostars Potentiate Radiotherapy by Boosting Checkpoint Blockade Immunotherapy. Matter 3, 1725–1753. doi:10.1016/j.matt.2020.08.027
Jarosz-Biej, M., Smolarczyk, R., Cichoń, T., and Kułach, N. (2019). Tumor Microenvironment as A "Game Changer" in Cancer Radiotherapy. Int. J. Mol. Sci. 20. doi:10.3390/ijms20133212
Jin, L., Shen, S., Huang, Y., Li, D., and Yang, X. (2021). Corn-like Au/Ag Nanorod-Mediated NIR-II Photothermal/photodynamic Therapy Potentiates Immune Checkpoint Antibody Efficacy by Reprogramming the Cold Tumor Microenvironment. Biomaterials 268, 120582. doi:10.1016/j.biomaterials.2020.120582
Jun, S. W., Manivasagan, P., Kwon, J., Nguyen, V. T., Mondal, S., Ly, C. D., et al. (2020). Folic Acid-Conjugated Chitosan-Functionalized Graphene Oxide for Highly Efficient Photoacoustic Imaging-Guided Tumor-Targeted Photothermal Therapy. Int. J. Biol. Macromol 155, 961–971. doi:10.1016/j.ijbiomac.2019.11.055
Kano, A., Taniwaki, Y., Nakamura, I., Shimada, N., Moriyama, K., and Maruyama, A. (2013). Tumor Delivery of Photofrin® by PLL-G-PEG for Photodynamic Therapy. J. Control. Release 167, 315–321. doi:10.1016/j.jconrel.2013.02.016
Kaur, P., and Asea, A. (2012). Radiation-induced Effects and the Immune System in Cancer. Front. Oncol. 2, 191. doi:10.3389/fonc.2012.00191
Kim, Y., Kang, S., Shin, H., Kim, T., Yu, B., Kim, J., et al. (2020). Sequential and Timely Combination of a Cancer Nanovaccine with Immune Checkpoint Blockade Effectively Inhibits Tumor Growth and Relapse. Angew. Chem. Int. Ed. Engl. 59, 14628–14638. doi:10.1002/anie.202006117
Kuai, R., Ochyl, L. J., Bahjat, K. S., Schwendeman, A., and Moon, J. J. (2017). Designer Vaccine Nanodiscs for Personalized Cancer Immunotherapy. Nat. Mater. 16, 489–496. doi:10.1038/nmat4822
Lan, G., Ni, K., Veroneau, S. S., Luo, T., You, E., and Lin, W. (2019). Nanoscale Metal-Organic Framework Hierarchically Combines High-Z Components for Multifarious Radio-Enhancement. J. Am. Chem. Soc. 141, 6859–6863. doi:10.1021/jacs.9b03029
Lei, Y., Tang, L., Xie, Y., Xianyu, Y., Zhang, L., Wang, P., et al. (2017). Gold Nanoclusters-Assisted Delivery of NGF siRNA for Effective Treatment of Pancreatic Cancer. Nat. Commun. 8, 15130. doi:10.1038/ncomms15130
Li, H., Li, Y., Wang, X., Hou, Y., Hong, X., Gong, T., et al. (2017a). Rational Design of Polymeric Hybrid Micelles to Overcome Lymphatic and Intracellular Delivery Barriers in Cancer Immunotherapy. Theranostics 7, 4383–4398. doi:10.7150/thno.20745
Li, M., Li, Y., Peng, K., Wang, Y., Gong, T., Zhang, Z., et al. (2017b). Engineering Intranasal mRNA Vaccines to Enhance Lymph Node Trafficking and Immune Responses. Acta Biomater. 64, 237–248. doi:10.1016/j.actbio.2017.10.019
Li, X., Lovell, J. F., Yoon, J., and Chen, X. (2020). Clinical Development and Potential of Photothermal and Photodynamic Therapies for Cancer. Nat. Rev. Clin. Oncol. 17, 657–674. doi:10.1038/s41571-020-0410-2
Li, Y., Yun, K. H., Lee, H., Goh, S. H., Suh, Y. G., and Choi, Y. (2019). Porous Platinum Nanoparticles as a High-Z and Oxygen Generating Nanozyme for Enhanced Radiotherapy In Vivo. Biomaterials 197, 12–19. doi:10.1016/j.biomaterials.2019.01.004
Liang, S., Deng, X., Chang, Y., Sun, C., Shao, S., Xie, Z., et al. (2019). Intelligent Hollow Pt-CuS Janus Architecture for Synergistic Catalysis-Enhanced Sonodynamic and Photothermal Cancer Therapy. Nano Lett. 19, 4134–4145. doi:10.1021/acs.nanolett.9b01595
Liang, S., Deng, X., Ma, P., Cheng, Z., and Lin, J. (2020b). Recent Advances in Nanomaterial-Assisted Combinational Sonodynamic Cancer Therapy. Adv. Mater. 32, e2003214. doi:10.1002/adma.202003214
Liang, S., Sun, C., Yang, P., Ma, P., Huang, S., Cheng, Z., et al. (2020a). Core-shell Structured Upconversion Nanocrystal-Dendrimer Composite as a Carrier for Mitochondria Targeting and Catalase Enhanced Anti-cancer Photodynamic Therapy. Biomaterials 240, 119850. doi:10.1016/j.biomaterials.2020.119850
Liu, C., Cao, Y., Cheng, Y., Wang, D., Xu, T., Su, L., et al. (2020c). An Open Source and Reduce Expenditure ROS Generation Strategy for Chemodynamic/photodynamic Synergistic Therapy. Nat. Commun. 11, 1735. doi:10.1038/s41467-020-15591-4
Liu, C., Liu, B., Zhao, J., Di, Z., Chen, D., Gu, Z., et al. (2020b). Nd3+ -Sensitized Upconversion Metal-Organic Frameworks for Mitochondria-Targeted Amplified Photodynamic Therapy. Angew. Chem. Int. Ed. Engl. 59, 2634–2638. doi:10.1002/anie.201911508
Liu, S., Pan, X., and Liu, H. (2020a). Two-Dimensional Nanomaterials for Photothermal Therapy. Angew. Chem. Int. Ed. Engl. 59, 5890–5900. doi:10.1002/anie.201911477
Liu, Y., Ji, X., Tong, W. W. L., Askhatova, D., Yang, T., Cheng, H., et al. (2018b). Engineering Multifunctional RNAi Nanomedicine to Concurrently Target Cancer Hallmarks for Combinatorial Therapy. Angew. Chem. Int. Ed. Engl. 57, 1510–1513. doi:10.1002/anie.201710144
Liu, Z., Tan, H., Zhang, X., Chen, F., Zhou, Z., Hu, X., et al. (2018a). Enhancement of Radiotherapy Efficacy by Silver Nanoparticles in Hypoxic Glioma Cells. Artif. Cell Nanomed Biotechnol 46, S922–S30. doi:10.1080/21691401.2018.1518912
Luo, L., Sun, W., Feng, Y., Qin, R., Zhang, J., Ding, D., et al. (2020). Conjugation of a Scintillator Complex and Gold Nanorods for Dual-Modal Image-Guided Photothermal and X-ray-Induced Photodynamic Therapy of Tumors. ACS Appl. Mater. Inter. 12, 12591–12599. doi:10.1021/acsami.0c01189
Lv, S., Miao, Y., Liu, D., and Song, F. (2020). Recent Development of Photothermal Agents (PTAs) Based on Small Organic Molecular Dyes. Chembiochem 21, 2098–2110. doi:10.1002/cbic.202000089
Ma, Y., Su, Z., Zhou, L., He, L., Hou, Z., Zou, J., et al. (2021). Biodegradable Metal‐Organic Framework‐Gated Organosilica for Tumor Microenvironment‐Unlocked Glutathione Depletion‐Enhanced Synergistic Therapy. Adv. Mater., 2107560. doi:10.1002/adma.202107560
Ma, Z., Zhang, Y., Zhang, J., Zhang, W., Foda, M. F., Dai, X., et al. (2020). Ultrasmall Peptide-Coated Platinum Nanoparticles for Precise NIR-II Photothermal Therapy by Mitochondrial Targeting. ACS Appl. Mater. Inter. 12, 39434–39443. doi:10.1021/acsami.0c11469
Meng, L., Cheng, Y., Tong, X., Gan, S., Ding, Y., Zhang, Y., et al. (2018). Tumor Oxygenation and Hypoxia Inducible Factor-1 Functional Inhibition via a Reactive Oxygen Species Responsive Nanoplatform for Enhancing Radiation Therapy and Abscopal Effects. ACS Nano 12, 8308–8322. doi:10.1021/acsnano.8b03590
Meng, Z., Zhou, X., Xu, J., Han, X., Dong, Z., Wang, H., et al. (2019). Light-Triggered In Situ Gelation to Enable Robust Photodynamic-Immunotherapy by Repeated Stimulations. Adv. Mater. 31, e1900927. doi:10.1002/adma.201900927
Ming, J., Zhang, J., Shi, Y., Yang, W., Li, J., Sun, D., et al. (2020). A Trustworthy CpG Nanoplatform for Highly Safe and Efficient Cancer Photothermal Combined Immunotherapy. Nanoscale 12, 3916–3930. doi:10.1039/c9nr09402d
Ni, K., Lan, G., Chan, C., Duan, X., Guo, N., Veroneau, S. S., et al. (2019). Ultrathin Metal-Organic Layer-Mediated Radiotherapy-Radiodynamic Therapy Enhances Immunotherapy of Metastatic Cancers. Matter 1, 1331–1353. doi:10.1016/j.matt.2019.06.007
Ouyang, J., Deng, L., Chen, W., Sheng, J., Liu, Z., Wang, L., et al. (2018). Two Dimensional Semiconductors for Ultrasound-Mediated Cancer Therapy: the Case of Black Phosphorus Nanosheets. Chem. Commun. (Camb) 54, 2874–2877. doi:10.1039/c8cc00392k
Park, T., Lee, S., Amatya, R., Cheong, H., Moon, C., Kwak, H. D., et al. (2020). ICG-loaded PEGylated BSA-Silver Nanoparticles for Effective Photothermal Cancer Therapy. Int. J. Nanomedicine 15, 5459–5471. doi:10.2147/IJN.S255874
Patel, R. B., Ye, M., Carlson, P. M., Jaquish, A., Zangl, L., Ma, B., et al. (2019). Development of an In Situ Cancer Vaccine via Combinational Radiation and Bacterial-Membrane-Coated Nanoparticles. Adv. Mater. 31, e1902626. doi:10.1002/adma.201902626
Qiu, M., Wang, D., Huang, H., Yin, T., Bao, W., Zhang, B., et al. (2021). A Regioselectively Oxidized 2D Bi/BiOx Lateral Nano‐Heterostructure for Hypoxic Photodynamic Therapy. Adv. Mater. 33, 2102562. doi:10.1002/adma.202102562
Riley, R. S., June, C. H., Langer, R., and Mitchell, M. J. (2019). Delivery Technologies for Cancer Immunotherapy. Nat. Rev. Drug Discov. 18, 175–196. doi:10.1038/s41573-018-0006-z
Ruan, H., Bu, L., Hu, Q., Cheng, H., Lu, W., and Gu, Z. (2019). Strategies of Combination Drug Delivery for Immune Checkpoint Blockades. Adv. Healthc. Mater. 8, e1801099. doi:10.1002/adhm.201801099
Shao, J., Xie, H., Huang, H., Li, Z., Sun, Z., Xu, Y., et al. (2016). Biodegradable Black Phosphorus-Based Nanospheres for In Vivo Photothermal Cancer Therapy. Nat. Commun. 7, 12967. doi:10.1038/ncomms12967
Shao, Y., Liu, B., Di, Z., Zhang, G., Sun, L. D., Li, L., et al. (2020). Engineering of Upconverted Metal-Organic Frameworks for Near-Infrared Light-Triggered Combinational Photodynamic/Chemo-/Immunotherapy against Hypoxic Tumors. J. Am. Chem. Soc. 142, 3939–3946. doi:10.1021/jacs.9b12788
Shi, T., Sun, W., Qin, R., Li, D., Feng, Y., Chen, L., et al. (2020). X‐Ray‐Induced Persistent Luminescence Promotes Ultrasensitive Imaging and Effective Inhibition of Orthotopic Hepatic Tumors. Adv. Funct. Mater. 30, 2001166. doi:10.1002/adfm.202001166
Siegel, R. L., Miller, K. D., Fidler-Benaoudia, M., Keegan, T. H., Hipp, H. S., and Jemal, A. (2020). Cancer Statistics for Adolescents and Young Adults, 2020. CA Cancer J. Clin. 70, 443–459. doi:10.3322/caac.21637
Song, G., Chen, Y., Liang, C., Yi, X., Liu, J., Sun, X., et al. (2016). Catalase-Loaded TaOx Nanoshells as Bio-Nanoreactors Combining High-Z Element and Enzyme Delivery for Enhancing Radiotherapy. Adv. Mater. 28, 7143–7148. doi:10.1002/adma.201602111
Song, W., Musetti, S. N., and Huang, L. (2017). Nanomaterials for Cancer Immunotherapy. Biomaterials 148, 16–30. doi:10.1016/j.biomaterials.2017.09.017
Sun, T., Zhang, Y. S., Pang, B., Hyun, D. C., Yang, M., and Xia, Y. (2014). Engineered Nanoparticles for Drug Delivery in Cancer Therapy. Angew. Chem. Int. Ed. Engl. 53, 12320–12364. doi:10.1002/anie.201403036
Sun, W., Luo, L., Feng, Y., Qiu, Y., Shi, C., Meng, S., et al. (2020). Gadolinium-Rose Bengal Coordination Polymer Nanodots for MR-/Fluorescence-Image-Guided Radiation and Photodynamic Therapy. Adv. Mater. 32, e2000377. doi:10.1002/adma.202000377
Sundaram, A., Peng, L., Chai, L., Xie, Z., Ponraj, J. S., Wang, X., et al. (2020). Advanced Nanomaterials for Hypoxia Tumor Therapy: Challenges and Solutions. Nanoscale 12, 21497–21518. doi:10.1039/d0nr06271e
Wang, H., Jiang, H., Van De Gucht, M., and De Ridder, M. (2019b). Hypoxic Radioresistance: Can ROS Be the Key to Overcome it? Cancers (Basel) 11. doi:10.3390/cancers11010112
Wang, H., Han, X., Dong, Z., Xu, J., Wang, J., and Liu, Z. (2019a). Hyaluronidase with pH‐responsive Dextran Modification as an Adjuvant Nanomedicine for Enhanced Photodynamic‐Immunotherapy of Cancer. Adv. Funct. Mater. 29, 1902440. doi:10.1002/adfm.201902440
Wang, X., Liu, H., Chen, D., Meng, X., Liu, T., Fu, C., et al. (2013). Multifunctional Fe3O4@P(St/MAA)@chitosan@Au Core/shell Nanoparticles for Dual Imaging and Photothermal Therapy. ACS Appl. Mater. Inter. 5, 4966–4971. doi:10.1021/am400721s
Xia, Y., Wu, J., Du, Y., Miao, C., Su, Z., and Ma, G. (2018). Bridging Systemic Immunity with Gastrointestinal Immune Responses via Oil-In-Polymer Capsules. Adv. Mater. 30, e1801067. doi:10.1002/adma.201801067
Xie, Z., Chen, S., Duo, Y., Zhu, Y., Fan, T., Zou, Q., et al. (2019). Biocompatible Two-Dimensional Titanium Nanosheets for Multimodal Imaging-Guided Cancer Theranostics. ACS Appl. Mater. Inter. 11, 22129–22140. doi:10.1021/acsami.9b04628
Xin, J., Deng, C., Aras, O., Zhou, M., Wu, C., and An, F. (2021). Chemodynamic Nanomaterials for Cancer Theranostics. J. Nanobiotechnol 19, 192. doi:10.1186/s12951-021-00936-y
Xin, Y., Huang, M., Guo, W. W., Huang, Q., Zhang, L. Z., and Jiang, G. (2017). Nano-based Delivery of RNAi in Cancer Therapy. Mol. Cancer 16, 134. doi:10.1186/s12943-017-0683-y
Xu, X., Saw, P. E., Tao, W., Li, Y., Ji, X., Yu, M., et al. (2017). Tumor Microenvironment-Responsive Multistaged Nanoplatform for Systemic RNAi and Cancer Therapy. Nano Lett. 17, 4427–4435. doi:10.1021/acs.nanolett.7b01571
Yan, S., Zeng, X., Tang, Y., Liu, B. F., Wang, Y., and Liu, X. (2019). Activating Antitumor Immunity and Antimetastatic Effect through Polydopamine-Encapsulated Core-Shell Upconversion Nanoparticles. Adv. Mater. 31, e1905825. doi:10.1002/adma.201905825
Yang, C., Zhu, Y., Li, D., Liu, Y., Guan, C., Man, X., et al. (2021). Red Phosphorus Decorated TiO 2 Nanorod Mediated Photodynamic and Photothermal Therapy for Renal Cell Carcinoma. Small 17, 2101837. doi:10.1002/smll.202101837
Yang, J., Teng, Y., Fu, Y., and Zhang, C. (2019). Chlorins e6 loaded silica nanoparticles coated with gastric cancer cell membrane for tumor specific photodynamic therapy of gastric cancer. Int. J. Nanomedicine 14, 5061–5071. doi:10.2147/IJN.S202910
Yang, Y. S., Carney, R. P., Stellacci, F., and Irvine, D. J. (2014). Enhancing Radiotherapy by Lipid Nanocapsule-Mediated Delivery of Amphiphilic Gold Nanoparticles to Intracellular Membranes. ACS Nano 8, 8992–9002. doi:10.1021/nn502146r
Yue, W., Chen, L., Yu, L., Zhou, B., Yin, H., Ren, W., et al. (2019). Checkpoint Blockade and Nanosonosensitizer-Augmented Noninvasive Sonodynamic Therapy Combination Reduces Tumour Growth and Metastases in Mice. Nat. Commun. 10, 2025. doi:10.1038/s41467-019-09760-3
Zeng, L., Cao, Y., He, L., Ding, S., Bian, X. W., and Tian, G. (2021). Metal-ligand Coordination Nanomaterials for Radiotherapy: Emerging Synergistic Cancer Therapy. J. Mater. Chem. B 9, 208–227. doi:10.1039/d0tb02294b
Zhang, K., Meng, X., Yang, Z., Dong, H., and Zhang, X. (2020b). Enhanced Cancer Therapy by Hypoxia-Responsive Copper Metal-Organic Frameworks Nanosystem. Biomaterials 258, 120278. doi:10.1016/j.biomaterials.2020.120278
Zhang, L., Wu, S., Qin, Y., Fan, F., Zhang, Z., Huang, C., et al. (2019). Targeted Codelivery of an Antigen and Dual Agonists by Hybrid Nanoparticles for Enhanced Cancer Immunotherapy. Nano Lett. 19, 4237–4249. doi:10.1021/acs.nanolett.9b00030
Zhang, S., Cao, C., Lv, X., Dai, H., Zhong, Z., Liang, C., et al. (2020a). A H2O2 Self-Sufficient Nanoplatform with Domino Effects for thermal-responsive Enhanced Chemodynamic Therapy. Chem. Sci. 11, 1926–1934. doi:10.1039/c9sc05506a
Zhang, Z., Wang, S., Xu, H., Wang, B., and Yao, C. (2015). Role of 5-aminolevulinic Acid-Conjugated Gold Nanoparticles for Photodynamic Therapy of Cancer. J. Biomed. Opt. 20, 51043. doi:10.1117/1.JBO.20.5.051043
Zheng, N., Zhang, S., Wang, L., Qi, Z., Peng, Q., Jian, L., et al. (2021). Boosting Image-Guiding Radiation Therapy through W18O49 Nanospheres and the Second Near-Infrared Light Irradiation. Nano Res. doi:10.1007/s12274-021-3814-0
Zhong, X., Wang, X., Zhan, G., Tang, Y., Yao, Y., Dong, Z., et al. (2019a). NaCeF4:Gd,Tb Scintillator as an X-ray Responsive Photosensitizer for Multimodal Imaging-Guided Synchronous Radio/Radiodynamic Therapy. Nano Lett. 19, 8234–8244. doi:10.1021/acs.nanolett.9b03682
Zhong, X., Zhang, Y., Tan, L., Zheng, T., Hou, Y., Hong, X., et al. (2019b). An Aluminum Adjuvant-Integrated Nano-MOF as Antigen Delivery System to Induce strong Humoral and Cellular Immune Responses. J. Control. Release 300, 81–92. doi:10.1016/j.jconrel.2019.02.035
Keywords: nanomaterial, radiotherapy, phototherapy, immunotherapy, combination therapies
Citation: Hao X, Wu J, Xiang D and Yang Y (2022) Recent Advance of Nanomaterial-Mediated Tumor Therapies in the Past Five Years. Front. Pharmacol. 13:846715. doi: 10.3389/fphar.2022.846715
Received: 31 December 2021; Accepted: 31 January 2022;
Published: 18 February 2022.
Edited by:
Zeming Liu, Huazhong University of Science and Technology, ChinaReviewed by:
Peng Li, Xinxiang Medical University, ChinaHua De Liao, Central South University, China
Copyright © 2022 Hao, Wu, Xiang and Yang. This is an open-access article distributed under the terms of the Creative Commons Attribution License (CC BY). The use, distribution or reproduction in other forums is permitted, provided the original author(s) and the copyright owner(s) are credited and that the original publication in this journal is cited, in accordance with accepted academic practice. No use, distribution or reproduction is permitted which does not comply with these terms.
*Correspondence: Yongyu Yang, yongyuyang@csu.edu.cn