- 1Laboratório de Histotécnica e Cultura Celular, Departamento de Ciências da Vida, Universidade Do Estado da Bahia (UNEB), Salvador, Brazil
- 2Laboratório de Engenharia Tecidual e Imunofarmacologia, Instituto Gonçalo Moniz, Fundação Oswaldo Cruz (FIOCRUZ), Salvador, Brazil
- 3Laboratório de Tecnologia Farmacêutica, Universidade Federal da Paraíba, João Pessoa, Brazil
- 4Instituto Senai de Inovação Em Sistemas Avançados Em Saúde, SENAI/CIMATEC, Salvador, Brazil
Leishmaniasis are endemic diseases caused by different species of intracellular parasites of the genus Leishmania. Due to the high toxicity and drug resistance of current antileishmanial drugs, it is necessary to identify new and more effective drugs. Previously, we investigated the immunomodulatory and anti-Trypanosoma cruzi action of BA5, a derivative of betulinic acid. In the present study, we investigated the in vitro activity of BA5 against different species of Leishmania and their action mechanism. BA5 exhibited low cytotoxicity against macrophages and inhibited the proliferation of promastigote forms of Leishmania amazonensis (IC50 = 4.5 ± 1.1 μM), Leishmania major (IC50 = 3.0 ± 0.8 μM), Leishmania braziliensis (IC50 = 0.9 ± 1.1 μM) and Leishmania infantum (IC50 = 0.15 ± 0.05 μM). Incubation with BA5 reduced the percentage of Leishmania amazonensis-infected macrophages and the number of intracellular parasites (IC50 = 4.1 ± 0.7 μM). To understand the mechanism of action underlying BA5 antileishmanial activity (incubation at IC50/2, IC50 or 2xIC50 values of the drug), we investigated ultrastructural changes by scanning electron microscopy and evaluated cell cycle, membrane mitochondrial potential, and cell death against promastigote forms of Leishmania amazonensis by flow cytometry. Promastigotes incubated with BA5 presented membrane blebbing, flagella damage, increased size, and body deformation. Flow cytometry analysis showed that parasite death is mainly caused by apoptosis-like death, arrested cell cycle in G0/G1 phase and did not alter the membrane mitochondrial potential of Leishmania amazonensis. Surprisingly, the combination of BA5 and amphotericin B, an assay used to determine the degree of drug interaction, revealed synergistic effects (CI = 0.15 ± 0.09) on promastigotes forms of Leishmania amazonensis. In conclusion, BA5 compound is an effective and selective antileishmanial agent.
Introduction
Leishmaniasis is a complex of diseases caused by different species of protozoa of the genus Leishmania. Despite being among the ten most relevant infectious diseases, leishmaniasis is part of a wide group of diseases worldwide neglected (Alvar et al., 2012; Silva et al., 2020). In 2021, WHO published that 54 countries are endemic to Visceral Leishmaniasis (VL) and 53 countries are endemic to Cutaneous Leishmaniasis (CL). Cases have been reported in about 98 countries, and 12 million people approximately have their lives affected by the different clinical spectra of the disease (WHO, 2021).
Clinical manifestations depend on factors inherent to the parasite, the natural resistance of the host and the magnitude of the immune response (Gabriel et al., 2019). After inoculation of promastigote forms through the bite of the insect vector, the parasite is internalized by host defense cells and differentiates into amastigote forms. In this way, the relationship between parasite and the host will determine the course of the disease. The host can be asymptomatic or develop classic skin and mucosal lesions, as well as atypical forms, such as diffuse and disseminated, as well as the visceral manifestation (Gupta et al., 2013; Gabriel et al., 2019). In the Americas, the main species that cause cutaneous leishmaniasis are Leishmania amazonensis and Leishmania braziliensis (Ministério da saúde do Brasil, 2017). On the other hand, visceral leishmaniasis is caused by Leishmania infantum chagasi in Brazil. The variability of species and their clinical outcomes are a challenge for the effective treatment and prophylaxis of the disease (Desjeux, 2004; Burza et al., 2018).
Although the knowledge of cell biology and immunology of leishmaniasis has advanced in recent decades, pharmacotherapy still lacks new alternatives. Pentavalent antimonial has been the first-line drugs since 1960, but they present several limitations such as high toxicity and adverse effects, resistance, the need for hospitalization and treatment failure. The second-line drugs, such as amphotericin B, pentamidine and miltefosine, also have several limitations, like high costs and teratogenicity (Romero and Lopez, 2017; Tiwari et al., 2018).
In this regard, the search for new active compounds plays an important role in the development of new antileishmanial drugs. Betulinic acid is a natural pentacyclic triterpene widely found in the plant kingdom. This compound has raised interest in the scientific community due to its vast number of biological activities, such as antitumor, anti-inflammatory, immunomodulatory, antimicrobial and antiparasitic activities (Takada and Aggarwal, 2003; Chen et al., 2008; Innocente et al., 2012; Sousa et al., 2014). Strategic structural changes of betulinic acid at position C-28 can generate more active molecules than its prototype (Yogeeswari and Sriram, 2005). In a previous study, we tested a series of semi-synthetic molecules derived from betulinic acid against Trypanosoma cruzi, and found compound BA5 active, causing ultrastructural changes in T. cruzi, such as loss of plasma membrane integrity and the appearance of atypical vacuoles, leading to death of the parasite by necrosis (Meira et al., 2016). Additionally, the immunomodulatory activity of BA5 was evaluated on macrophages and lymphocytes, being able to inhibit both the NF-kB and calcineurin pathways (Meira et al., 2017). In the present study, we evaluated the activity of BA5 in vitro against different species of Leishmania, and its mechanisms of action.
Materials and Methods
Drugs
Betulinic acid was extracted from the bark of Ziziphus joazeiro Mart., a native brazilian tree from the Rhamnaceae family, according to the methodology previously described (Barbosa-Filho et al., 1985). The semi-synthetic compound BA5 was prepared from betulinic acid as previously described (Barbosa-Filho et al., 1985) and used in antileishmanial assays (BA5; 94–98% purity by high performance liquid chromatography). Amphotericin B (Gibco Laboratories, Gaithersburg, MD) was used as positive control in antileishmanial assays. Gentian violet (Synth, São Paulo, SP, Brazil) was used as positive control in the cytotoxicity to mammalian cell assays. All compounds were dissolved in dimethyl sulfoxide (DMSO; PanReac, Barcelona, Spain) and diluted in cell culture medium for use in the assays. The final concentration of DMSO was less than 0.1% in all in vitro experiments.
Animals
Male 4–6-weeks old BALB/c were used. All mice were raised and maintained at the animal facilities of the Gonçalo Moniz Institute, Oswaldo Cruz Foundation, Salvador, Brazil in sterilized cages, under a controlled environment and receiving a balanced rodent diet and water ad libitum. All experiments were approved by the local Animal Ethics Committee (Approval number: 004/2019).
Parasites
L. amazonensis (MHOM/BR88/BA-125 Leila strain), L. major (MHOM/RI/WR173), L. braziliensis (MHOM/BR88/BA-3456) promastigotes were cultivated in Schneider (Sigma, St. Louis, MO, United States) medium supplemented with 10% fetal bovine serum (FBS) (Gibco) and 50 μg ml−1 Gentamicin (Sigma). L. infantum (MCAN/BR/89/BA262) promastigotes were cultivated in liver infusion tryptose (LIT) medium supplemented with 20% fetal bovine serum and 50 μg ml−1 Gentamicin, pH 7.2, at 26°C until logarithmic phase. Log phase promastigotes were used to study the effects of the betulinic acid and BA5 derivative.
Viability Assay
L. amazonensis, L. major, L. braziliensis and L. infantum chagasi promastigotes (1 × 106 cells/well) were incubated into 96-well plates, cultivated in Schneider (Sigma) medium supplemented with 10% fetal bovine serum (FBS) (Gibco) and μg mL−1 Gentamicin (Sigma). Drugs were added at six concentrations ranging from 1.56 to 50 µM in triplicate, and the plate was incubated for 72 h at 26°C. Amphotericin was added at eight concentrations ranging 0.0156–2.0 µM. Promastigotes viability was measured by twenty µL/well of AlamarBlue (Invitrogen, Carlsbad, CA, United States) during 2 h (L. amazonensis, L. major and L. braziliensis) or 24 h for L. infantum due to slower metabolism (Corral et al., 2013), after which colorimetric readings were performed at 570 and 600 nm.
Cytotoxicity to Mammalian Cell
Peritoneal exudate macrophages were obtained by washing of the peritoneal cavity of BALB/c mice with cold Dulbecco’s Modified Eagle’s Medium (DMEM Life Technologies, GIBCO-BRL), 5 days after injection of 3% thioglycolate in saline (1.5 ml per mice). Cells were added into 96-well plates at a density 1 × 105 cells/well containing DMEM medium supplemented with 10% of fetal bovine serum (FBS; Gibco) and 50 μg ml−1 Gentamicin (Novafarma, Anapolis, Brazil) and incubated for 24 h at 37°C and 5% CO2. Drugs was added in triplicate at eight concentrations ranging from 0.04 to 100 µM and incubated for 72 h. Twenty µL/well of AlamarBlue (Invitrogen) was added to the plates during 10 h. Colorimetric readings were performed at 570 and 600 nm. CC50 values were calculated using data-points gathered from three independent experiments. Gentian violet (Synth, Sao Paulo, Brazil) was used as positive control, at concentrations ranging from 0.04 to 10 µM.
In vitro Macrophage Infection With L. amazonensis
Peritoneal exudate macrophages (5 × 105 cells) were plated onto sterile coverslips in 24-well plates and kept for 24 h. The macrophages were infected with stationary growth phase promastigotes of L. amazonensis at a ratio of 10:1 macrophage at 35°C during 4 h and 5% CO2. Infected macrophages were incubated with different atoxic concentrations with values below the IC50 values of BA (9.4; 4.7; 2.3 µM) and BA5 (15.5; 7.7; 3.8 µM). After 24 h, the cells were fixed in methanol. The percentage of infected macrophages and the number of amastigotes/macrophages were determined by counting 100 cells per slides by counting the slides after Giemsa staining (Sigma) in an optical microscope (Olympus, Tokyo, Japan). Amphotericin B (Gibco) was used as a positive control in this assay.
Annexin V and Propidium Iodide Staining
Promastigotes of L. amazonensis (106 cells/well) were incubated in 24-well plates and incubated with BA and BA5 in different concentrations (IC50 or 2x IC50) for 24 h at 26°C. Parasites were labeled with propidium iodide (PI) and annexin V using the annexin V-fluorescein isothiocyanate (FITC) apoptosis detection kit (Sigma) according to the manufacturer’s instructions. The experiment was performed using a BD FACSCalibur flow cytometer (Becton Dickinson Biosciences, San Jose, CA, United States) by acquiring 10,000 events, and data were analyzed by BD software FlowJo v10 (Tree Star, Ashland, OR).
Cell Cycle Analysis
Promastigotes of L. amazonensis (1 × 107/well) were incubated with BA5 (9.0 and 4.5 μM) for 48 h. Parasites were washed with saline, centrifuged for 10 min at 252.0 g and diluted in the lysis solution containing PI (0.1% Triton X-100 and 2 μg ml−1 propidium iodide in PBS) in the absence of light at 37°C. After 30 min, the samples were acquired on a LSRFortessa flow cytometer (Becton Dickinson Biosciences, San Jose, CA, United States) and analyzed by FlowJo v.10 software (Tree Star).
Analysis of Mitochondrial Membrane Potential
To determine the effect of the compound on mitochondrial membrane potential, L. amazonensis promastigotes were incubated with 9.0 and 4.5 μM of BA5 for 72 h. After the treatment, parasites were incubated with 10 μg/ml of rhodamine 123 (Sigma Aldrich, St. Louis, United States) for 15 min. Methanol was used as negative control. Data acquisition was performed using a LSRFortessa flow cytometer and the analysis was performed by FlowJo v.10 software.
Scanning Microscopy Electronic
L amazonensis promastigotes (1 × 107) were incubated with three concentrations from the IC50 values (2.25, 4.5 and 9.0 μM) of BA5 for 48 h at 26°C. The parasites were fixed in a 2% glutaraldehyde solution and 0.1 M sodium cacodylate buffer for 2 h at room temperature. After fixation, the cells were post-fixed in osmium tetroxide (1%) for 1 h at room temperature. The parasites were placed on glass cover slips with 0.01% poly-L-lysine, dehydrated in graded ethanol (30–100%) and submitted at critical point (replacement of ethanol by CO2) LEICA CPD 030. Samples were metalized with gold and observed in the scanning electron microscope JEOL JSM-6390LV.
Drug Combination Assay
Isobolograms were constructed by the fixed ratio method. Serial double dilutions were performed in triplicate in ratios of 1:1 and 10:1, BA5 and amphotericin B, respectively, using L. amazonensis promastigotes. For each proportion, an IC50 value was calculated for each drug and combination after 72 h of incubation. The fractional inhibitory concentrations (FIC) were calculated by (IC50 when combined/IC50 isolated drug). The FIC values of different ratios were used to construct the isobologram in Graph Pad Prism version 5.01 program (Graph Pad Software, San Diego, CA, United States). The analysis of the combined effects was performed by determining the combination index (CI) as described previously by Chou and Talalay, 2005. CI values were used as cutoff to determine synergism.
Statistical Analysis
One-way analysis of variance and Newman-Keuls multiple comparison tests were employed by using Graph Pad Prism version 5.01 (Graph Pad Software, San Diego, CA, United States). Differences were considered significant when the values were of p < 0.05.
Results
Cytotoxicity and Activity of BA5 Against Promastigote Forms
Betulinic acid and BA5 derivative (Figure 1) presented CC50 values of 18.8 and 31.1 µM, respectively, to mammalian cells. Amphotericin B, the reference antileishmanial drug, presented a CC50 value of 3.3 µM, and gentian violet, a known cytotoxic drug, had a CC50 value of 0.5 μM (Table 1). The effect of BA5 on promastigote forms of different species of leishmania was evaluated at six different concentrations, ranging from 1.56 to 50 μM. As show in the Table 1, BA5 was effective against all tested species. After 72 h of incubation, BA5 inhibited L. amazonensis promastigote proliferation with an IC50 of 4.5 ± 1.1 μM; L. major (IC50 = 3.0 ± 0.8 μM), L. braziliensis (IC50 = 0.9 ± 1.1 μM) and L. infantum (IC50 0.15 ± 0.05 μM). In addition, BA5 was 6.9 times more selective (IS) for L. amazonensis promastigotes, 10.4 times more selective for L. major, 34.5 more selective for L. braziliensis and 207 more selective for L. infantum when compared with mammalian cell. Furthermore, IS of BA5 was higher for L. braziliensis than amphotericin B. Betulinic acid exhibited little or no activity against promastigote forms of different species of leishmania. This prototype was not selective for L. amazonensis (IS = 0.66) and L. braziliensis (IS = 1.1) (Table 1).
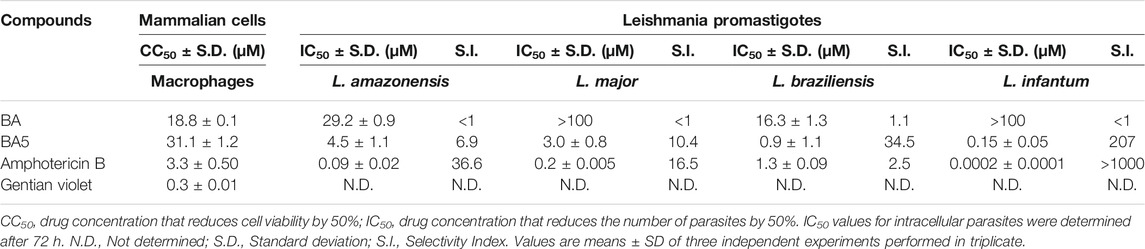
TABLE 1. Cytotoxicity evaluation and antileishmanial activity against promastigotes of L. amazonensis, L. major, L. braziliensis, and L. infantum.
BA5 Reduces the Infection of Macrophages by L. amazonensis
BA and BA5 promoted a significant decrease in the number of L. amazonensis-infected macrophages after 24 h of treatment (Figure 2). BA5 decreased the percentage of infected cells and the number of intracellular parasites at all concentrations tested, in a concentration-dependent manner (Figures 2E,F). BA prototype reduced the number of intracellular forms per macrophage only in the highest concentration tested, presented IC50 value greater than 200 and was not selective against the parasite (SI < 1) (Table 2). BA5 presented an IC50 value of 4.1 ± 0.7 µM (SI = 7.5) and amphotericin B exhibited an IC50 value of 0.05 ± 0.02 µM (SI = 66) (Table 2).
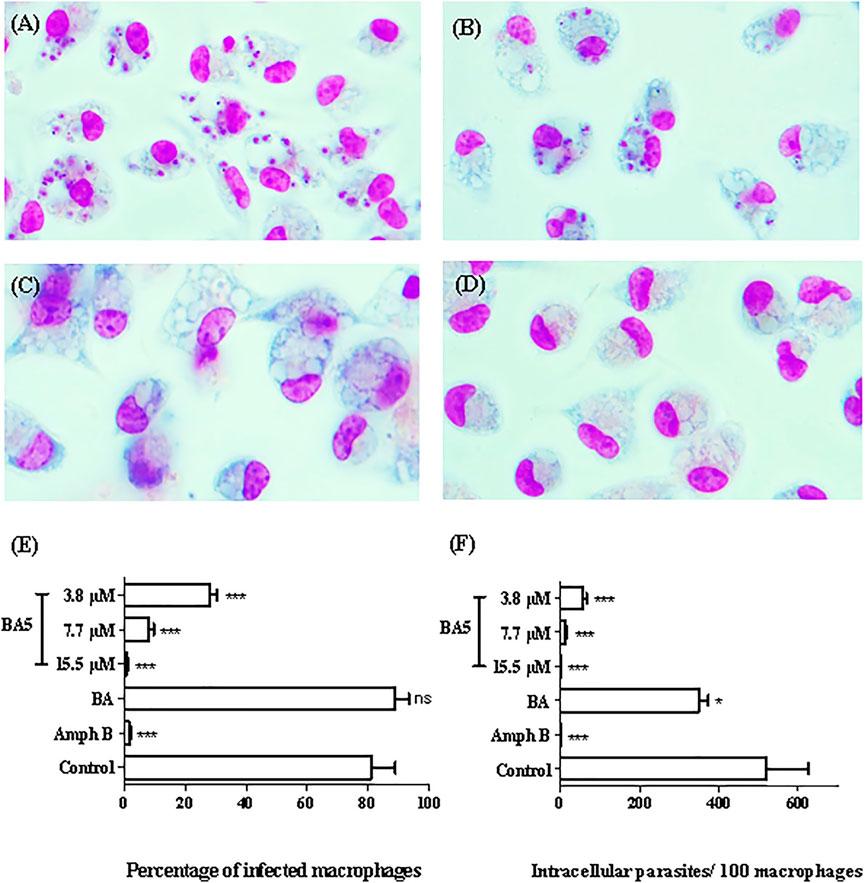
FIGURE 2. In vitro effects of BA and BA5 against intracellular parasites of L. amazonensis. Peritoneal macrophages of BALB/c mice were infected with promastigotes of L. amazonensis at stationary phase (10:1) and were treated with BA or BA5 for 24 h. (A) Untreated control. (B) Treatment with BA at 9.4 µM. (C) BA5 at 15.5 µM. (D) amphotericin B at 1.5 µM. 1000x magnification. The percentage of infection (E) and the number of intracellular parasites per 100 macrophages (F) were determined after 24 h of treatment. Amphotericin B was used as positive control. *p < 0.05; ***p < 0.001.
Ultrastructural Alterations in BA5-Treated Leishmania
After determining the activity against promastigotes and amastigotes forms of Leishmania sp., assays were performed to elucidate a possible mechanism of action of the BA5. First, ultrastructural analysis by scanning electron microscopy (SEM) was used to evaluate the morphology of L. amazonensis promastigotes treated or not with BA5. Untreated promastigotes had the typical elongated shape of the parasite without visible alterations in the plasma membrane or in cell volume (Figure 3A). In contrast, parasites treated for 48 h with BA5 (2.2, 4.5 or 9.0 µM) presented several morphological alterations, such as membrane protrusions resembling surface blebs (Figure 3B), flagella damage, increase in size (Figure 3C), and body deformation (Figure 3D).
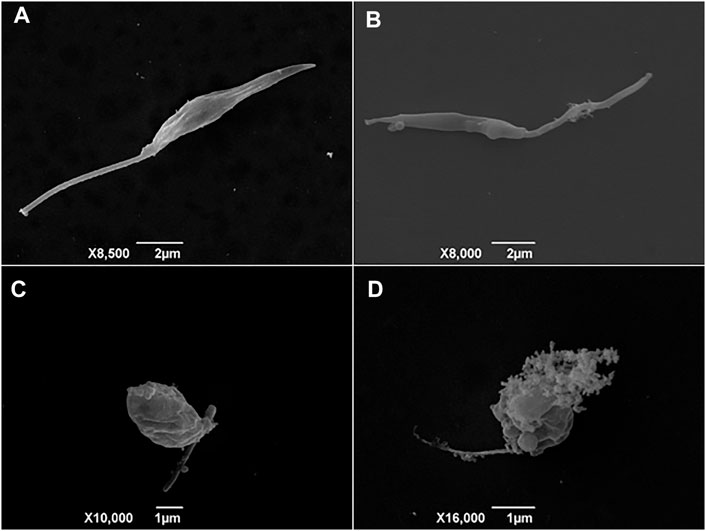
FIGURE 3. Scanning electron microscopy (SEM) analysis of promastigotes of L. amazonensis incubated with BA5. (A) Untreated control cells with normal morphology, (B) parasites treated with IC50/2 value of BA5, (C) parasites treated with IC50 value of BA5, (D) parasites treated with twice the IC50.
BA5 Induces Apoptosis Like-Death in L. amazonensis Promastigotes
Because the formation of blebbing in the membrane, cell rounding, and flagella damage generally culminates in the formation of apoptotic bodies (Basmaciyan and Casanova, 2019), we evaluated the mechanism by which compound BA5 could cause parasite death. Promastigotes were double-stained with Annexin-V-FITC and propidium iodide (PI) for flow cytometry analysis. Untreated cells were Annexin-V and PI-negative, demonstrating cell viability. The percentage of promastigotes positive only for annexin-V was 27.3% after treatment with IC50/2 value of BA5, 51.25% when cells were treated with the IC50 value of BA5 and 54.45% when cells were treated with 2x IC50 of BA5. This data suggests that these cells were in early stages of apoptosis-like death. No significant difference in the number of necrotic cells was observed after treatment when compared to untreated controls (Figure 4).
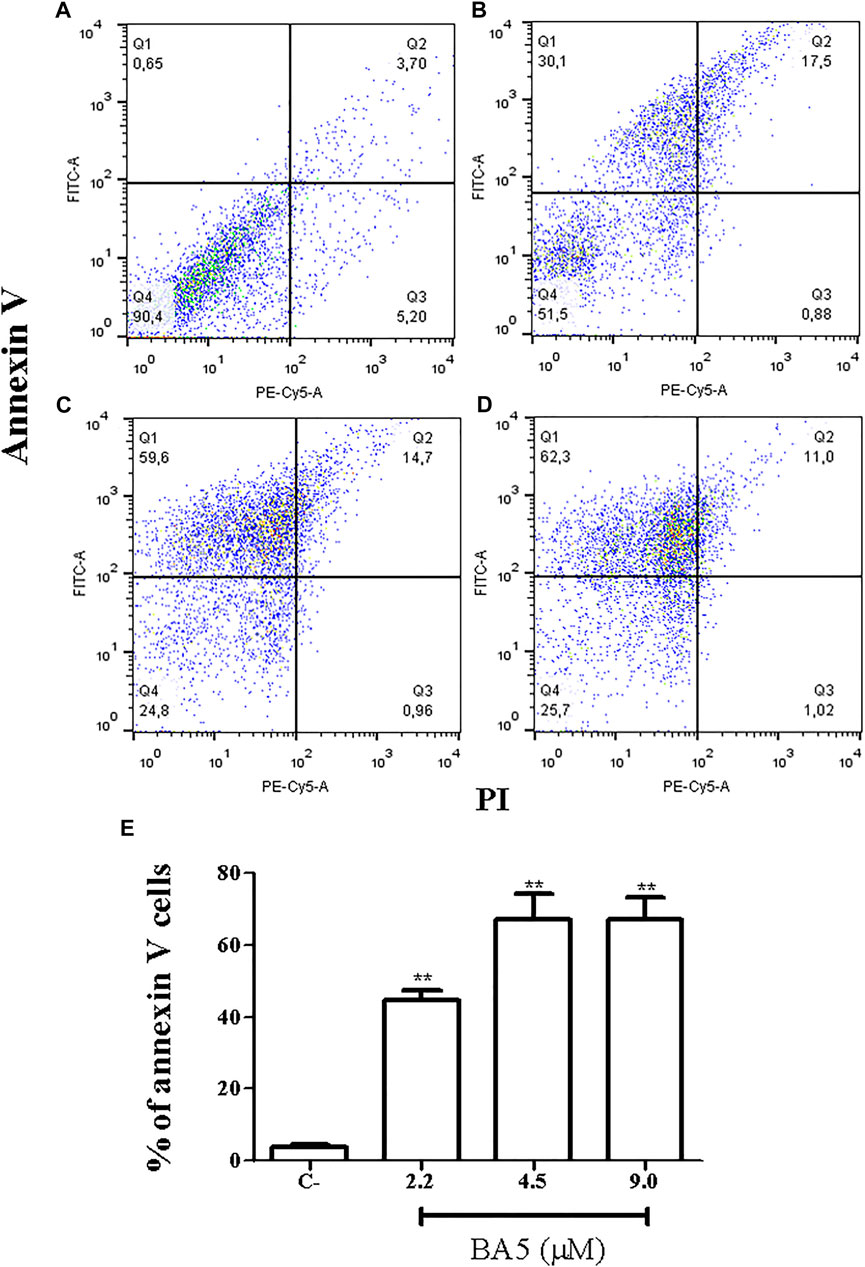
FIGURE 4. Flow cytometry analysis of cell death pattern. L. amazonensis promastigotes were treated with BA5 and incubated with propidium iodide (PI) and annexin V after 48 h of incubation. (A) Untreated promastigotes (B) promastigotes treated with 2.2 µM of BA5 (C) promastigotes treated with 4.5 µM of BA5 (D) promastigotes treated with 9.0 µM of BA5 (E) Percentage of stained cells for annexin V after 48 h of treatment with BA5. Values represent the means ± S.E.M. of three determinations obtained in one of two experiments performed. **p < 0.01 compared to stimulated and untreated cells.
BA5 Acts Independently of Mitochondrial Membrane Depolarization
To better understand the pathways that lead to apoptosis-like death, the mitochondria potential of L. amazonensis was evaluated by flow cytometry, after BA5 treatment and incubation with rhodamine123. As shown in Figure 5, the intensity of rhodamine123 was not significantly altered by incubation with BA5 at IC50 and 2xIC50 values of the drug. Amphotericin B and methanol, two known drugs able to induce mitochondrial alterations, reduced the intensity of the rhodamine 123.
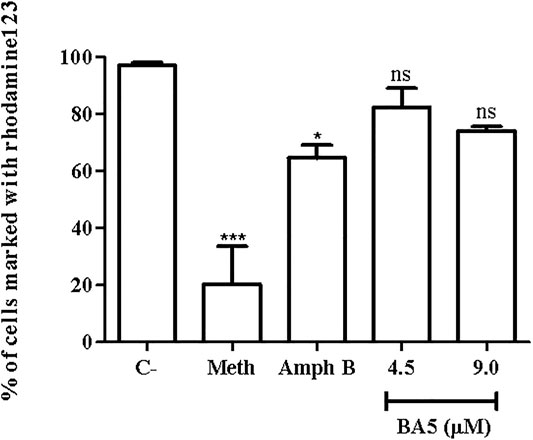
FIGURE 5. Mitochondrial membrane potential of L. amazonensis promastigotes incubated with BA5. Promastigotes were incubated or not with BA5 at concentrations of IC50 and 2x IC50 and amphotericin B (IC50). Methanol (Meth) was used as positive control. After 72 h of incubation, parasites were marked with rhodamine123. The samples were acquired in a LSRFortessa flow cytometer and analyzed by FlowJo software (50,000 events were collected and analyzed). ***p < 0.001, compared to untreated group.
BA5 Induces Cell Cycle Arrest in L. amazonensis Promastigotes
Next, flow cytometric analysis after cell permeabilization and labelling with PI was used for quantification of nuclear DNA of parasites. Promastigotes of L. amazonensis treated with BA5 and amphotericin B with IC50 and 2xIC50 values were marked with PI and analyzed by flow cytometry. Figure 6 shows the distribution of cellular DNA through the cell cycle of the parasites in the absence and presence of the tested compounds. A significant increase in population of cells in pre-phase G0 and a significant decrease in population of cells in G2/M were observed in cells treated with IC50 value (Figure 6B), and 2x IC50 value (Figure 6C) concentrations of BA5, compared to untreated control (Figure 6A), 24 h after incubation.
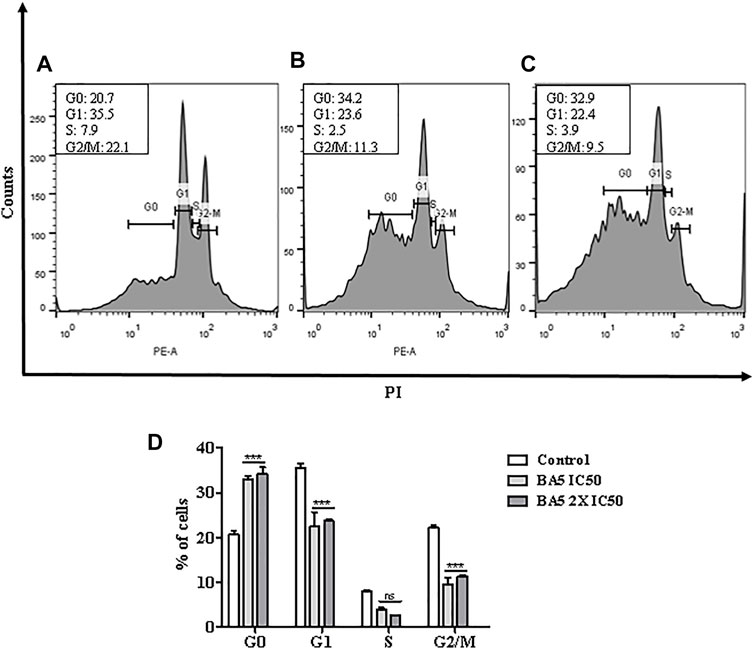
FIGURE 6. Analysis of cell cycle progression after treatment with BA5 using propidium iodide by flow cytometry. The distribution and percentage of parasites in pre-phase G0, G1, S and G2/M phase of the cell cycle are indicated. Cells treated with IC50 value (B), and 2x IC50 value (C) concentrations of BA5, compared to untreated control (A). (D) Percentage of cells in different phases of cell cycle. Values represent the means ± S.E.M. of three determinations obtained in one of two experiments performed. ***p < 0.001 compared to stimulated and untreated cells.
Synergistic Effects of BA5 and Amphotericin B
The antileishmanial effect of BA5 and amphotericin B combination was investigated on promastigote forms of L. amazonensis. The combination of the drugs reduced the IC50 values of amphotericin B by seven times and decreased the IC50 values of BA5 by 50 times compared to each drug separately. The combination index values (0.15 ± 0.09 µM) Table 3 associated with a concave isobologram revealed that BA5 and amphotericin B have synergistic effects (Figure 7).
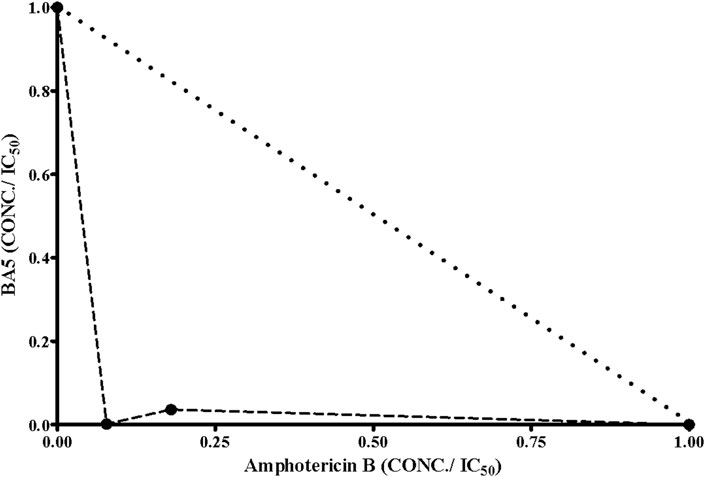
FIGURE 7. Isobologram showing the synergistic effects between BA5 and amphotericin B on L. amazonensis promastigotes. Broken lines correspond to the predicted positions of the experimental points for additive effects.
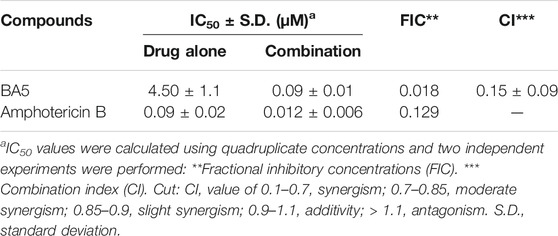
TABLE 3. Concentration reductions and combination rates by BA5 and amphotericin B on L. amazonensis promastigotes.
Discussion
The search for molecules of natural origin has intensified and played an important role in the development of new drugs (Newman and Cragg, 2016). Betulinic acid is a molecule in the class of lupane-type pentacyclic triterpenes found in all parts of higher plants. This molecule has a vast number of activities described in the literature, such as antitumor, antimalarial, anti-HIV, analgesic, anti-inflammatory, and bactericidal (Fujioka et al., 1994; Kim et al., 2001; Ali-Seyed et al., 2016; Li et al., 2017). Previous studies have shown that substitution on the carboxyl group can generate more potent molecules than the prototype, aiming at different pharmacological targets (Krogh et al., 1999; Chandramu et al., 2003; Barbosa Filho et al., 2004; Mullauer et al., 2010; Halder et al., 2018; Mehrizi et al., 2018). A recent work from our group reported that the insertion of amines at C-28 in BA increased the anti-T. cruzi activity by inducing ultrastructural changes in the parasite (Meira et al., 2016). This is the first report, however, regarding the contribution of the incorporation of amides on C-28 as drug design strategy to enhance the antileishmanial activity.
Structural changes in betulinic acid prototype are associated with reduction in the cytotoxicity of the new compounds (Alakurtti et al., 2010; Newman and Cragg, 2016; Mehrizi et al., 2019). In agreement with these studies, we observed that the BA5 derivative is less cytotoxic (CC50 = 31.1 µM) than their prototype (CC50 = 18.8 µM). Furthermore, BA5 was less cytotoxic than amphotericin B (CC50 = 3.3 µM). These data reinforce the importance of structural chemical modifications in reducing cytotoxicity and enhancing the practical applicability of the compounds in medicinal chemistry.
Previous reports showed the activity of betulinic acid derivatives against Leishmania sp promastigotes (Magaraci et al., 2003; Dominguez-Carmona et al., 2010). Heterocyclic derivatives of betulinic acid showed activity against L. donovani with IC50 values of 8.9–30 µM and carbamate derivatives against L. infantum with IC50 values of 25.8 µM (Alakurtti et al., 2010; Sousa et al., 2014). Dominguez-Carmona et al., 2010, reported activities of an acetate derivative against L. amazonensis (IC50 = 44.9 µM). In our study, the addition of amines in the C-28 of BA5 optimized the effects of the molecule in relation to the prototype and showed better antileishmanial activity than other triterpenes.
BA5 was able to inhibit macrophage infection and the number of intracellular forms of L. amazonensis with a reduced IC50 value (4.1 ± 0.7). Other studies demonstrated that treatment with alkaloid derivatives of the betulinic acid reduced in 83% the number of infected macrophages by L. amazonensis, using higher drug concentrations, with an IC50 value of 210 µM (Moraes et al., 2015). Furthermore, incubation with nanoparticle-loaded betulinic acid reduced the number of macrophages infected by L. major (81%), improving its activity and reducing toxic effects (Mehrizi et al., 2019).
It is suggested that the higher SI, more effective and safer a drug would be during in vivo treatment (Pritchett et al., 2014). In this study, the SI value of amphotericin was higher than BA5. Amphotericin B presents an elevated cost, high toxicity and its use requires hospitalization of patients. This drug present difficult structural changes in the liposomal molecule to reduce toxicity (Filippin and Souza, 2006), whereas BA5 is a prototype for the design and its selectivity can be increased with conformational alterations (Meira et al., 2016).
Apoptosis is an important event in the context of the host’s immune response and in the successful establishment of infection by leishmania. The survival of these parasites within macrophages is a crucial issue in the pathogenesis of the disease in the mammalian host (Aliança et al., 2017). Despite being an event markedly of multicellular organisms, currently, there are studies in the literature that suggest a mechanism similar to apoptosis in single-celled eukaryotes. In trypanosomatids, regulated cell death is shown to be advantageous to prevent the activation of the immune system and, therefore, the survival of intracellular parasites (Baréa et al., 2018). Flow cytometry analysis demonstrated that BA5 acts to induce cell death by apoptosis in parasites. These data were confirmed when we evaluated the morphology of the parasites treated with BA5. Similar morphological changes, such as flagellar damage, appearance of blebs and increase in the size of L. amazonensis, have previously been associated with induced apoptotic death. Some changes were seen in the same species of parasites treated with a series of triazine hybrids and with a calpain inhibitor (Marinõ et al., 2014; Baréa et al., 2018). In addition, the treatment of promastigotes of L. amazonensis with BA5 induce changes in the cell cycle of the parasites with arrested in the G0/G1 phase and a significant decrease in population of cells in G2/M (Meira et al., 2017). Altogether, these results suggest that BA5-induced apoptosis may have led to DNA degradation.
The mitochondria play an important role in cell death by apoptosis (Koonin and Aravind, 2002). Rhodamine 123 is a cationic lipophilic dye that is readily sequestered by active mitochondria without cytotoxic effects. Additionally, this dye can be used to assay mitochondrial membrane potential in populations of apoptotic cells (Mehta and Shaha, 2004; Marinho et al., 2014). Reports indicate that the mitochondria integrity is a good indicator of structural changes in the kinetoplastid parasite (Silva et al., 2020). To elucidate the mechanism of cell death possibly induced by BA5, we evaluated the potential of mitochondrial membrane. BA5 did not induce alterations in membrane potential in L. amazonensis promastigotes, suggesting that the action of the compound is independent of this pathway. Moreover, Basmaciyan and Casanova, 2019 demonstrated that mitochondrial depolarization when preceded by transient hyperpolarization in leishmania, and the loss of plasma membrane integrity is not specific apoptosis markers.
Drug combination is an alternative applied in the clinic for treatment of leishmaniasis that have advantages over current monotherapy. Amphotericin B is a second-choice drug for the treatment of leishmaniasis in many places around the world. Combined drug therapy can be an important tool for reducing toxic effects, as well as reducing the duration of treatment and improving treatment compliance by the patient (Bastos et al., 2016; Roatt et al., 2020). In this regard, some studies were caried out with the aim to associating promising compounds to amphotericin B (Marinho et al., 2014; Mehrizi et al., 2019; Roatt et al., 2020; Silva et al., 2020). In this work, BA5 was shown to increase the activity of the reference drug (CI = 0.15; synergistic action), showing a promising profile for drug combination. This result encourages further investigations since the combination of drugs is becoming increasingly attractive to combat parasitic diseases. This semi-synthetic derivative was able to prevent the parasite development and invasion into host cells during T. cruzi infection, with synergistic activity when used in combination to benznidazole (Meira et al., 2016). Our research group also reported the activity of BA5 increasing the immunosuppressive effect of dexamethasone. BA5 presented synergistic effects with dexamethasone on the inhibition of lymphocyte proliferation, suggesting a promising profile for drug combination therapeutic schemes (Meira et al., 2017).
In conclusion, this work showed that BA5 has antileishmanial activity against different species causative of cutaneous and visceral leishmaniasis. BA5 presents low cytotoxicity, in vitro activity against parasite proliferation and macrophage infection by leishmania. Although its mechanism of action still needs further evaluation, it was found that BA5 promotes cell death due to apoptosis and arrest the cell cycle progression. Therefore, BA5 may be a suitable candidate for antileishmanial drug development, alone or in combination with other drugs.
Data Availability Statement
The original contributions presented in the study are included in the article/Supplementary Material, further inquiries can be directed to the corresponding author.
Ethics Statement
The animal study was reviewed and approved by Gonçalo Moniz Institute, Oswaldo Cruz Foundation.
Author Contributions
GE and SM conceptualized the project. B-FJ and MD were responsible for synthesis of compounds. MT, SD, TJ, and DJ conducted the biological assays. All authors co-wrote the first draft of the manuscript and proofread the submitted manuscript.
Funding
This work was supported by grants from Conselho Nacional de Desenvolvimento Científico e Tecnológico (CNPq), Programa de apoio a Núcleos de Excelência (PRONEX), and Fundação de Amparo à Pesquisa do Estado da Bahia (FAPESB).
Conflict of Interest
The authors declare that the research was conducted in the absence of any commercial or financial relationships that could be construed as a potential conflict of interest.
Publisher’s Note
All claims expressed in this article are solely those of the authors and do not necessarily represent those of their affiliated organizations, or those of the publisher, the editors and the reviewers. Any product that may be evaluated in this article, or claim that may be made by its manufacturer, is not guaranteed or endorsed by the publisher.
References
Alakurtti, S., Heiska, T., Kiriazis, A., Sacerdoti-Sierra, N., Jaffe, C. L., and Yli-Kauhaluoma, J. (2010). Synthesis and Anti-leishmanial Activity of Heterocyclic Betulin Derivatives. Bioorg. Med. Chem. 18, 1573–1582. doi:10.1016/j.bmc.2010.01.003
Ali-Seyed, M., Jantan, I., Vijayaraghavan, K., and Bukhari, S. N. A. (2016). Betulinic Acid: Recent Advances in Chemical Modifications, Effective Delivery, and Molecular Mechanisms of a Promising Anticancer Therapy. Chem. Biol. Drug Des. 87, 517–536. doi:10.1111/cbdd.12682
Aliança, A. S. D. S., Oliveira, A. R., Feitosa, A. P. S., Ribeiro, K. R. C., de Castro, M. C. A. B., Leite, A. C. L., et al. (2017). In Vitro evaluation of Cytotoxicity and Leishmanicidal Activity of Phthalimido-Thiazole Derivatives. Eur. J. Pharm. Sci. 105, 1–10. doi:10.1016/j.ejps.2017.05.005
Alvar, J., Vélez, I. D., Bern, C., Herrero, M., Desjeux, P., Cano, J., et al. (2012). Leishmaniasis Worldwide and Global Estimates of its Incidence. PLoS One 7, e35671. doi:10.1371/journal.pone.0035671
Barbosa-Filho, J. M., Lima, S. A., Camorim, E. L., de Sena, K. X. F., Almeida, J. R. G., da-Cunha, V. L., et al. (2004). Botanical Study, Phytochemistry and Antimicrobial Activity of Tabebuia Aurea. Phyton 73, 221–228.
Baréa, P., Barbosa, V. A., Bidóia, D. L., de Paula, J. C., Stefanello, T. F., da Costa, W. F., et al. (2018). Synthesis, Antileishmanial Activity and Mechanism of Action Studies of Novel β-carboline-1,3,5-triazine Hybrids. Eur. J. Med. Chem. 150, 579–590. doi:10.1016/j.ejmech.2018.03.014
Basmaciyan, L., and Casanova, M. (2019). Cell Death in Leishmania. Parasite 26, 71–83. doi:10.1051/parasite/2019071
Burza, S., Croft, S. L., and Boelaert, M. (2018). Leishmaniasis. Lancet 392, 951–970. doi:10.1016/S0140-6736(18)31204-2
Chandramu, C., Manohar, R. D., Krupadanam, D. G., and Dashavantha, R. V. (2003). Isolation, Characterization and Biological Activity of Betulinic Acid and Ursolic Acid from Vitex Negundo L. Phytother Res. 17, 129–134. doi:10.1002/ptr.1088
Chen, Z., Wu, Q., Chen, Y., and He, J. (2008). Effects of Betulinic Acid on Proliferation and Apoptosis in Jurkat Cells and its In Vitro Mechanism. J. Huazhong Univ. Sci. Technol. [Med. Sci. 28, 634–638. doi:10.1007/s11596-008-0604-9
Chou, T. C., and Talalay, P. (2005). Quantitative Analysis of Dose-Effect Relationships: the Combined Effects of Multiple Drugs or Enzyme Inhibitors. Adv. Enzyme Regul. 22, 27–55. doi:10.1016/0065-2571(84)90007-4
Corral, M. J., González, E., Cuquerella, M., and Alunda, J. M. (2013). Improvement of 96-well Microplate Assay for Estimation of Cell Growth and Inhibition of Leishmania with Alamar Blue. J. Microbiol. Methods 94, 111–116. doi:10.1016/j.mimet.2013.05.012
Desjeux, P. (2004). Leishmaniasis: Current Situation and New Perspectives. Comp. Immunol. Microbiol. Infect. Dis. 27, 305–318. doi:10.1016/j.cimid.2004.03.004
Domínguez-Carmona, D. B., Escalante-Erosa, F., García-Sosa, K., Ruiz-Pinell, G., Gutierrez-Yapu, D., Chan-Bacab, M. J., et al. (2010). Antiprotozoal Activity of Betulinic Acid Derivatives. Phytomedicine 17, 379–382. doi:10.1016/j.phymed.2009.08.002
Filippin, F. B., and Souza, L. C. (2006). Eficiência terapêutica das formulações lipídicas de anfotericina B. Rev. Bras. Cienc. Farm. 42, 167–194. doi:10.1590/s1516-93322006000200003
Fujioka, T., Kashiwada, Y., Kilkuskie, R. E., Cosentino, L. M., Ballas, L. M., Jiang, J. B., et al. (1994). Anti-AIDS Agents, 11. Betulinic Acid and Platanic Acid as Anti-HIV Principles from Syzigium Claviflorum, and the Anti-HIV Activity of Structurally Related Triterpenoids. J. Nat. Prod. 57, 243–247. doi:10.1021/np50104a008
Gabriel, Á., Valério-Bolas, A., Palma-Marques, J., Mourata-Gonçalves, P., Ruas, P., Dias-Guerreiro, T., et al. (20192019). Cutaneous Leishmaniasis: The Complexity of Host's Effective Immune Response against a Polymorphic Parasitic Disease. J. Immunol. Res. 2019, 2603730. doi:10.1155/2019/2603730
Gupta, G., Oghumu, S., and Satoskar, A. R. (2013). Mechanisms of Immune Evasion in Leishmaniasis. Adv. Appl. Microbiol. 82, 155–184. doi:10.1016/B978-0-12-407679-2.00005-3
Halder, A., Shukla, D., Das, S., Roy, P., Mukherjee, A., and Saha, B. (2018). Lactoferrin-modified Betulinic Acid-Loaded PLGA Nanoparticles Are strong Anti-leishmanials. Cytokine 110, 412–415. doi:10.1016/j.cyto.2018.05.010
Innocente, A. M., Silva, G. N., Cruz, L. N., Moraes, M. S., Nakabashi, M., Sonnet, P., et al. (2012). Synthesis and Antiplasmodial Activity of Betulinic Acid and Ursolic Acid Analogues. Molecules 17, 12003–12014. doi:10.3390/molecules171012003
Kim, J. Y., Koo, H. M., and Kim, D. S. (2001). Development of C-20 Modified Betulinic Acid Derivatives as Antitumor Agents. Bioorg. Med. Chem. Lett. 11, 2405–2408. doi:10.1016/s0960-894x(01)00460-7
Koonin, E. V., and Aravind, L. (2002). Origin and Evolution of Eukaryotic Apoptosis: the Bacterial Connection. Cell Death Differ 9, 394–404. doi:10.1038/sj.cdd.4400991
Krogh, R., Kroth, R., Berti, C., Madeira, A. O., Souza, M. M., Cechinel-Filho, V., et al. (1999). Isolation and Identification of Compounds with Antinociceptive Action from Ipomoea Pes-Caprae (L.) R. Br. Pharmazie 54, 464–466.
Li, J., Jing, J., Bai, Y., Li, Z., Xing, R., Tan, B., et al. (2017). SH479, a Betulinic Acid Derivative, Ameliorates Experimental Autoimmune Encephalomyelitis by Regulating the T Helper 17/regulatory T Cell Balance. Mol. Pharmacol. 91, 464–474. doi:10.1124/mol.116.107136
Macedo Bastos, M., Villas Bôas Hoelz, L., Boechat, N., and de Oliveira, A. P. (2016). Antileishmanial Chemotherapy: A Literature Review. Rev. Virtual Quim. 8, 2072–2104. doi:10.21577/1984-6835.20160139
Magaraci, F., Jimenez, C. J., Rodrigues, C., Rodrigues, J. C., Braga, M. V., Yardley, V., et al. (2003). Azasterols as Inhibitors of Sterol 24-methyltransferase in Leishmania Species and Trypanosoma Cruzi. J. Med. Chem. 46, 4714–4727. doi:10.1021/jm021114j
Mariño, G., Niso-Santano, M., Baehrecke, E. H., and Kroemer, G. (2014). Self-consumption: the Interplay of Autophagy and Apoptosis. Nat. Rev. Mol. Cel Biol 15, 81–94. doi:10.1038/nrm3735
Mehta, A., and Shaha, C. (2004). Apoptotic Death in Leishmania Donovani Promastigotes in Response to Respiratory Chain Inhibition: Complex II Inhibition Results in Increased Pentamidine Cytotoxicity. J. Biol. Chem. 279, 11798–11813. doi:10.1074/jbc.M309341200
Meira, C. S., Barbosa-Filho, J. M., Lanfredi-Rangel, A., Guimarães, E. T., Moreira, D. R., and Soares, M. B. (2016). Antiparasitic Evaluation of Betulinic Acid Derivatives Reveals Effective and Selective Anti-Trypanosoma Cruzi Inhibitors. Exp. Parasitol. 166, 108–115. doi:10.1016/j.exppara.2016.04.007
Meira, C. S., Espírito Santo, R. F. D., dos Santos, T. B., Orge, I. D., Silva, D. K. C., Guimarães, E. T., et al. (2017). Betulinic Acid Derivative BA5, a Dual NF-kB/calcineurin Inhibitor, Alleviates Experimental Shock and Delayed Hypersensitivity. Eur. J. Pharmacol. 815, 156–165. doi:10.1016/j.ejphar.2017.09.008
Ministério da saúde do Brasil (2017). Manual de Vigilância da Leishmaniose Tegumentar. Available at: https://bvsms.saude.gov.br/bvs/publicacoes/manual_vigilancia_leishmaniose_tegumentar.pdf (Accessed March 10, 2021).
Moraes, L. S., Donza, M. R., Rodrigues, A. P., Silva, B. J., Brasil, D. S., Zoghbi, Md., et al. (2015). Leishmanicidal Activity of (+)-Phyllanthidine and the Phytochemical Profile of Margaritaria Nobilis (Phyllanthaceae). Molecules 20, 22157–22169. doi:10.3390/molecules201219829
Mullauer, F. B., Kessler, J. H., and Medema, J. P. (2010). Betulinic Acid, a Natural Compound with Potent Anticancer Effects. Anticancer Drugs 21, 215–227. doi:10.1097/CAD.0b013e3283357c62
Newman, D. J., and Cragg, G. M. (2016). Natural Products as Sources of New Drugs from 1981 to 2014. J. Nat. Prod. 79, 629–661. doi:10.1021/acs.jnatprod.5b01055
Pritchett, J. C., Naesens, L., and Montoya, J. (2014). “Treating HHV-6 Infections,” in Human Herpesviruses HHV-6A, HHV-6B & HHV-7. Editors L. Flamand, I. Lautenschlager, G. Krueger, and D. Ablashi. 3rd ed. (New York, NY: Elsevier), 311–331. doi:10.1016/B978-0-444-62703-2.00019-7
Roatt, B. M., De Oliveira Cardoso, J. M., De Brito, R. C. F., Coura-Vital, W., de Oliveira Aguiar-Soares, R. D., and Reis, A. B. (2020). Recent Advances and New Strategies on Leishmaniasis Treatment. Appl. Microbiol. Biotechnol. 104, 8965–8977. doi:10.1007/s00253-020-10846-y10.1007/s00253-020-10856-w
Romero, A. H., and López, S. E. (2017). In Silico molecular Docking Studies of New Potential 4-Phthalazinyl-Hydrazones on Selected Trypanosoma Cruzi and Leishmania Enzyme Targets. J. Mol. Graph Model. 76, 313–329. doi:10.1016/j.jmgm.2017.07.013
Silva, D. K. C., Teixeira, J. S., Moreira, D. R. M., da Silva, T. F., Barreiro, E. J. L., de Freitas, H. F., et al. (20201995). In Vitro, In Vivo and In Silico Effectiveness of LASSBio-1386, an N-Acyl Hydrazone Derivative Phosphodiesterase-4 Inhibitor, against Leishmania Amazonensis. Front. Pharmacol. 11, 590544. doi:10.3389/fphar.2020.590544
Sousa, M. C., Varandas, R., Santos, R. C., Santos-Rosa, M., Alves, V., and Salvador, J. A. (2014). Antileishmanial Activity of Semisynthetic Lupane Triterpenoids Betulin and Betulinic Acid Derivatives: Synergistic Effects with Miltefosine. PLoS One 9, e89939. doi:10.1371/journal.pone.0089939
Takada, Y., and Aggarwal, B. B. (2003). Betulinic Acid Suppresses Carcinogen-Induced NF-Κb Activation through Inhibition of IκBα Kinase and P65 Phosphorylation: Abrogation of Cyclooxygenase-2 and Matrix Metalloprotease-9. J. Immunol. 171, 3278–3286. doi:10.4049/jimmunol.171.6.3278
Tiwari, N., Gedda, M. R., Tiwari, V. K., Singh, S. P., and Singh, R. K. (2018). Limitations of Current Therapeutic Options, Possible Drug Targets and Scope of Natural Products in Control of Leishmaniasis. Mini Rev. Med. Chem. 18, 26–41. doi:10.2174/1389557517666170425105129
World Health Organization (2021). Neglected Diseases: Leishmaniasis. Available at: http://www.who.int/gho/neglected_diseases/leishmaniasis/en/(Accessed May 20, 2021).
Yogeeswari, P., and Sriram, D. (2005). Betulinic Acid and its Derivatives: a Review on Their Biological Properties. Curr. Med. Chem. 12, 657–666. doi:10.2174/0929867053202214
Zadeh Mehrizi, T., Khamesipour, A., Shafiee Ardestani, M., Ebrahimi Shahmabadi, H., Haji Molla Hoseini, M., Mosaffa, N., et al. (2019). Comparative Analysis between Four Model Nanoformulations of Amphotericin B-Chitosan, Amphotericin B-Dendrimer, Betulinic Acid-Chitosan and Betulinic Acid-Dendrimer for Treatment of Leishmania Major: Real-Time PCR Assay Plus. Int. J. Nanomedicine 14, 7593–7607. doi:10.2147/IJN.S220410
Zadeh Mehrizi, T., Shafiee Ardestani, M., Haji Molla Hoseini, M., Khamesipour, A., Mosaffa, N., and Ramezani, A. (2018). Novel Nanosized Chitosan-Betulinic Acid against Resistant Leishmania Major and First Clinical Observation of Such Parasite in Kidney. Sci. Rep. 8, 11759–11778. doi:10.1038/s41598-018-30103-7
Keywords: leishmaniasis, betulinic acid, antileishmanial drugs, mechanism action, L. amazonensis
Citation: Magalhães TBdS, Silva DKC, Teixeira JdS, De Lima JDT, Barbosa-Filho JM, Moreira DRM, Guimarães ET and Soares MBP (2022) A Betulinic Acid Derivative, BA5, Induces G0/G1 Cell Arrest, Apoptosis Like-Death, and Morphological Alterations in Leishmania sp. Front. Pharmacol. 13:846123. doi: 10.3389/fphar.2022.846123
Received: 30 December 2021; Accepted: 21 February 2022;
Published: 22 March 2022.
Edited by:
John Ogbaji Igoli, Federal University of Agriculture Makurdi (FUAM), NigeriaReviewed by:
Juan Diego Maya, University of Chile, ChileLizandra Guidi Magalhães, University of Franca, Brazil
Copyright © 2022 Magalhães, Silva, Teixeira, De Lima, Barbosa-Filho, Moreira, Guimarães and Soares. This is an open-access article distributed under the terms of the Creative Commons Attribution License (CC BY). The use, distribution or reproduction in other forums is permitted, provided the original author(s) and the copyright owner(s) are credited and that the original publication in this journal is cited, in accordance with accepted academic practice. No use, distribution or reproduction is permitted which does not comply with these terms.
*Correspondence: Elisalva Teixeira Guimarães, etguimaraes@uneb.br