- 1School of Traditional Chinese Medicine, Beijing University of Chinese Medicine, Beijing, China
- 2Division of Neurobiology, Department of Psychiatry and Behavioral Sciences, Johns Hopkins University School of Medicine, Baltimore, MD, United States
- 3The Solomon H Snyder Department of Neuroscience, Johns Hopkins University School of Medicine, Baltimore, MD, United States
- 4Program in Cellular and Molecular Medicine, Johns Hopkins University School of Medicine, Baltimore, MD, United States
- 5Guangzhou Key Laboratory of Formula-Pattern of Traditional Chinese Medicine, School of Traditional Chinese Medicine, Jinan University, Guangzhou, China
Extracellular vesicles (EVs) are lipid bilayer-delimited particles released by cells, which play an essential role in intercellular communication by delivering cellular components including DNA, RNA, lipids, metabolites, cytoplasm, and cell surface proteins into recipient cells. EVs play a vital role in the pathogenesis of depression by transporting miRNA and effector molecules such as BDNF, IL34. Considering that some herbal therapies exhibit antidepressant effects, EVs might be a practical delivery approach for herbal medicine. Since EVs can cross the blood-brain barrier (BBB), one of the advantages of EV-mediated herbal drug delivery for treating depression with Chinese herbal medicine (CHM) is that EVs can transfer herbal medicine into the brain cells. This review focuses on discussing the roles of EVs in the pathophysiology of depression and outlines the emerging application of EVs in delivering CHM for the treatment of depression.
1 Introduction
1.1 The Potential Application of Extracellular Vesicles for Promoting Herbal Medicine in Treating Depressive Disorder
Characterized by severe and persistent emotional symptoms, cognitive symptoms, and somatic symptoms (Bhatt et al., 2020), depression is negatively impacting more than 264 million people as one of the most prevalent psychiatric disorders (James et al., 2018). The coronavirus disease 2019 (COVID-19) pandemic has also exacerbated the prevalence of depression (Salari et al., 2020). “Depression” can refer to any of several depressive disorders (DD). Thus, we comprehensively included depression-related works of literature by searching Mesh term “depressive disorder” and all entry terms in PubMed. DD requires long-term treatment, placing a heavy burden on public healthcare systems worldwide. While western medicines, such as tricyclic antidepressants (TCAs), are often prescribed for DD, efficacy can vary among individuals, in addition to detrimental impact due to their anticholinergic properties (McClintock et al., 2010) (Prado et al., 2018). Thus, complementary and alternative therapies with fewer adverse effects in treating DD are urgently needed. Traditional Chinese medicine (TCM) treatment includes Chinese herbal medicine (CHM), acupuncture, moxibustion, and naprapathy. The complementary and alternative approach to treating depression is widely applied in China with fewer severe side effects. Many preclinical and clinical studies have demonstrated the antidepressant effects of different Chinese herbal medicine (Wang et al., 2017; Milajerdi et al., 2018; Ruan et al., 2019; Ghasemzadeh Rahbardar and Hosseinzadeh 2020). This paper mainly discusses the potential of herbal therapeutics in TCM for treating DD.
Extracellular vesicles (EVs) are lipid bilayer membrane structures that can carry various nucleic acids, lipids, proteins, and other small metabolisms. All cells, including both prokaryotes and eukaryotes, can release EVs as intercellular communication molecules. EVs play vital roles in interrelated physiological and pathophysiological processes, including intercellular communication in the brain. The classification of different EV types is continuously evolving with advances in relevant research (Théry et al., 2018). For example, a study by E. Cocucci suggested that EVs should be broadly categorized as ectosomes or exosomes based on their size and mechanism of formation (Théry et al., 2018) (see Figure 1). Ectosomes are vesicles shed from the superficies of the plasma membrane by budding outside. These structures can vary in diameter from ∼50 to 1,000 nm and thus include microparticles, microvesicles and large vesicles (Zhang H. et al., 2018). Exosomes originate from endosomes recycled by exocytosis or endocytosis and range from ∼40 to 160 nm in diameter. The formation of exosomes goes through four stages. Firstly, the cup-shaped early-sorting endosome (ESE) consists of soluble proteins related to the extracellular environment and cell surface proteins are formed by endocytosis. Secondly, late-sorting endosomes (LSEs) are matured from ESE. Thirdly, intracellular multivesicular bodies (MVBs) are formed by inward invagination of ESE’s membrane. Finally, MVBs are released by ectocytosis eventually generate exosomes (Kalluri and LeBleu 2020). One hypothesis about the function of EVs proposes that exosomes may take off excessive components in cells to preserve cellular homeostasis (Kalluri and LeBleu 2020). Although the physiological purpose of exosome production remains largely unknown, the studies reviewed in this article indicate that the function, targeting, and particular constituent in exosomes suggest that they could play a significant part by adjusting cell-to-cell communication.
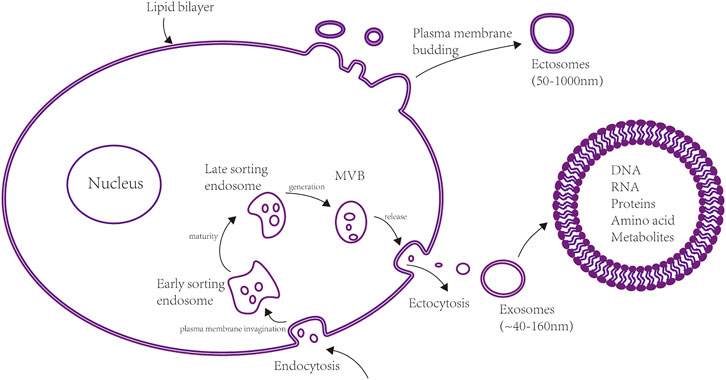
FIGURE 1. Formation mechanisms of two types of extracellular vesicles (EVs). Ectosomes and exosomes are two significant classifications of EVs. Ectosomes are formed by plasma membrane budding, and their diameter range from ∼50 to 1,000 nm. Exosomes range from ∼40 to 160 nm and originate in the endosomal pathway via the formation of early-sorting endosomes (ESEs), late-sorting endosomes (LSEs), and ultimately multivesicular bodies (MVBs). Exosomes are formed when MVBs are released by ectocytosis. The exosome population in cells can be highly heterogeneous. Exosomes exhibit different abilities to produce complicated biological responses in recipient cells depending on their cellular origins and specific content (e.g., amino acids, proteins, lipids, metabolites, cytoplasm).
In this article, we deliberate about the application potential of EVs in herbal therapies for DD by summarizing the body of work available in PubMed published over the last 10 years. Hence, this review provides a reference for further research of EVs, particularly in developing CHM for treating DD.
2 The Pathogenic Role of Extracellular Vesicles in Depression
Depending on the cellular sources, different subcellular components containing DNA, RNA, proteins, lipids, metabolites et al. are delivered into recipient cells by EVs, which can effectively alter the biological response to diseases. The pathogenesis of depression mainly involves synaptic plasticity, oxidative stress, intestinal flora, dysregulation of the hypothalamic pituitary adrenal (HPA) axis, and altered neurotransmitter metabolism and neuroinflammation (Bhatt et al., 2021; Zhang et al., 2021). Signal transmission from one nerve cell to another is essential for synaptic plasticity (Chivet et al., 2012). Given their prominent role in regulating intercellular communication, more and more researches have explored the potential parts of circulating EVs in the etiopathogenesis of depression via the regulation of neurotransmitters. It has been reported that exosomes are associated with cell-to-cell communication, neuroinflammation, neurogenesis and synaptic plasticity in the brain (Saeedi et al., 2019). These pathophysiological changes in the central nervous system (CNS) reflect EVs’ functional potential and emerging significance in developing DD (see Figure 2). In particular, most preclinical studies have focused on the roles of microRNA (miRNA, see Table 1) or protein (Table 2) contents of EVs in DD.
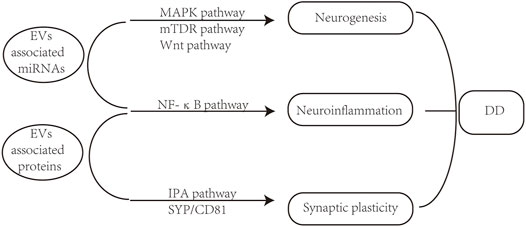
FIGURE 2. EVs associated pathogenic changes in DD. EV associated microRNAs and proteins can regulate neurogenesis, neuroinflammation, and synaptic plasticity in the development of DD.
2.1 Extracellular Vesicle-Associated microRNAs in Depressive Disorders
MiRNAs are small noncoding RNAs(∼22 nucleotides) that perform as post-transcriptional gene regulators through uniting with target messenger RNAs, typically leading to their degradation and subsequent silencing of the target gene (Ramshani et al., 2019). Small (∼30–150 nm), secreted EVs transport miRNAs between cells (Valadi et al., 2007; Mathivanan et al., 2010; Théry et al., 2018), enabling these miRNA cargoes to target genes that directly or indirectly contribute to pathological processes (such as accelerating neuroplasticity and brain development) related to depression. For example, one study showed that exosomes isolated from DD patients could cause depressive-like behaviors in normal mice, while exosomes isolated from healthy volunteers and exosomal miR-139-5p apparently alleviated these behavioral changes (Wei ZX. et al., 2020). In addition, exosomal miR-207 was found to alleviate depressive symptoms of stressed mice through targeting Tril, resulting in inhibition of NF-κB signaling in astrocytes (Li et al., 2020). These findings thus supported a relationship between miRNA-bearing exosomes and depression-like behaviors (Li et al., 2020). Collectively, these findings suggest that miRNA-bearing exosomes can attenuate or exacerbate the pathogenesis of depression, although clinical studies are needed to explore these possibilities in humans (see table 1).
2.2 Extracellular Vesicle-Associated Proteins in Depressive Disorders
Clinical and preclinical proteomics studies have indicated that proteins carried by EVs could potentially serve as biomarkers for depression (Kuwano et al., 2018; Gómez-Molina et al., 2019; Nasca et al., 2020). A study by comparing the proteins in small EVs in two animal models of stress response with depressive-like behaviors has revealed aldolase C, astrocytic GFAP (glial fibrillary acidic protein), synaptophysin (SYP, a synaptic protein), and reelin among the different treatment groups significantly changed (Gómez-Molina et al., 2019; Li et al., 2020). In addition, a study established that SYP, tumor necrosis factor receptor 1 (TNFR1), and interleukin 34 (IL-34) in DD patients’ neuron derived exosomes (NDE) were all positively correlated with the exosomes surface marker cluster of differentiation 81 (CD81) (Kuwano et al., 2018). Another clinical study reported more insulin receptor substrate 1 (IRS-1) in L1 Cell Adhesion Molecule + (L1CAM) exosomes from DD patients. The increased IRS levels in the L1CAM + exosomes were associated with suicidality and anhedonia (Nasca et al., 2020). In addition to screening for EV-associated protein biomarkers of DD, other studies have explored mechanistic connections between MDD and EV protein cargoes. One such study reported that ketamine could suppress the secretion of BDNF and ATP-triggered EV fusion through decreasing astrocytic Ca2+ excitability and elevating the possibility of oping narrow fusion pore (Stenovec et al., 2016). Furthermore, Stenovec et al. found that ketamine can diminish the cytoplasmic mobility of EVs to alter the astroglial ability to regulate extracellular K+ (Stenovec et al., 2020). These cumulative findings suggest that protein-bearing EVs contribute to the development of DD (possibly related to the EV fusion process) and could be potential clinical biomarkers for DD (see Table 2).
3 Herbal Therapies for Depressive Disorders
Herbal therapies are an integral component of traditional Chinese medicines (TCM). Currently, herbal therapies are widely used in China as essential alternative medicine and have been reported to ameliorate clinical symptoms of COVID-19 (Hu et al., 2021). Herbal remedies can be taken in many forms in TCM, and studies into their mechanisms of action and therapeutic efficacy are typically categorized by whether they are administered as herbal formulas (multiple herbs prescriptions), individual herbs, or specific phytochemicals (bioactive herbal constituents) (Hirshler and Doron 2017; Lin et al., 2019). Below, we discuss the antidepressant effects of these three types of herbal therapies.
3.1 Herbal Formulas for Treating Depressive Disorders
Numerous preclinical and clinical studies of herbal formulas have described the antidepressant effects of herbs such as Yueju (Ren and Chen 2017), Chai Hu Shu Gan San (Sun et al., 2018), or lily bulb and Rehmannia Decoction (Chi et al., 2019). The antidepressant mechanisms differ among these herbal formulas. For example, Bangpungtongsung-San was shown to reduce levels of nitric oxide (NO), inducible nitric oxide synthase (iNOS), cyclooxygenase (COX)-2, tumor necrosis factor-α (TNF-α), interleukin-1β (IL-1β), and interleukin-6 (IL-6) in a dose-dependent manner via decreased expression of nuclear factor (NF)-κB p65, which suggested that its antidepressant effects were likely related to the suppression of neuroinflammation (Park et al., 2020). By contrast, the antidepressant mechanisms of Jiaweisinisan appeared to be associated with regulating immune-mediated inflammation, cell apoptosis and synaptic transmission (Chen et al., 2020). In addition, Xiaoyaosan exhibited synergistic antidepression effects by adjusting Caspase-3 and Nitric oxide synthase-3 (Liu et al., 2021). These studies provide mechanistic evidence that at least partially explains the therapeutic effects of these herbal formulas, although further analytical chemistry is needed to narrow down the contributions of each herbal component.
3.2 Individual Herbs for Treating Depressive Disorders
While herbal formulas comprised of multiple herbal components are commonly prescribed for DD, several herbal therapies reported to provide antidepressant effects use individual herbs, such as Cistanche (Wang et al., 2017), rosemary (Ghasemzadeh Rahbardar and Hosseinzadeh 2020), Angelicae Sinensis Radix (Gong et al., 2019). Senegenin (Li H. et al., 2017), Panax ginseng (Wang W. et al., 2018), Lonicera japonica Thunb (Liu et al., 2019), Polygonum aviculare L. (Park et al., 2018), Hemerocallis citrina (Li CF. et al., 2017), Ginkgo (Zhao et al., 2015) and Armillaria mellea (Vahl) P. Kumm. (Lin et al., 2021). exert the antidepression effect through inhibiting neuroinflammation. Lycium barbarum deploys a protective effect on depression by promoting neurogenesis (Po et al., 2017). Baicalin exerts an antidepressant effect through enhancing neuronal differentiation (Zhang R. et al., 2019). Perilla frutescens (Ji et al., 2014a), Tribulus terrestris (Wang Z. et al., 2013), and Rehmannia glutinosa Libosch (Wang JM. et al., 2018) alleviate depression by regulating neuroendocrine. Angelicae Sinensis Radix manifests an antidepression effect by modulating the hematological anomalies (Gong et al., 2019). Agarwood exhibits the antidepressive effect by suppressing the HPA axis (Wang S. et al., 2018). Here we listed herbs that were reported to be effective in treating depression published in the past 10 years (see Table 3).
3.3 Phytochemicals for Treating Depressive Disorders
Although many herbs can exhibit various biological responses, the specific molecular mechanisms of these activities are still mainly uncharacterized. Because of the complexity of multiple chemicals and their efficacies, few herbal pharmacokinetic parameters have been applied successfully for therapeutic monitoring. From the herbal formulas to the individual phytochemicals, the object of study becomes more precise. Because the structure of phytochemicals is explicit, it is gained more and more attention recently. As chemical compounds produced by herbs, phytochemicals can be used as the basic unit of herbal research. Table 4 presents antidepressant mechanisms of reported phytochemicals in recently 10 years (see Table 4).
4 Extracellular Vesicles and Herbal Therapies
Herbal formulas are composed of various herbs, and the individual herb is composed of a variety of phytochemicals. Due to the complex composition of herbal formulae and individual herbs, it is challenging to use EVs to deliver herbal formulas. There are studies using EVs to deliver phytochemicals. A study reported that EVs packaged with curcumin preserve mice from septic shock provoked by lipopolysaccharide (LPS), and it also shown EVs can increase their bioavailability stability and solubility when served as vehicles of curcumin (Sun et al., 2010). Another study reported daily intranasal delivery of curcumin-loaded EVs diminished experimental autoimmune encephalomyelitis, whose mechanism may resulted from increasing induction of apoptosis in microglial cells (Zhuang et al., 2011). These studies demonstrate the potential of EVs for delivering phytochemicals.
In addition, the EVs secreted from cells treated with herb and herb-derived EVs exhibit a therapeutic effect. Ruan et al. found Suxiao Jiuxin pill promotes cardiac mesenchymal stem cells (CMSC) secret exosome through a GTPase-dependent pathway (Ruan et al., 2018a). Exosomes extracted from Suxiao Jiuxin pill-treated CMSC can also decline the expression of H3K27 demethylase UTX, furthermore, enhance cardiomyocyte proliferation (Ruan et al., 2018b). Besides EVs secreted by cells treated with herbal formulas, the EVs isolated from plant samples also had therapeutic functions (Kim et al., 2021). Vesicles derived from plants are structural units composed of various primary and secondary metabolites, which play a synergistic role in biological transport and pharmacodynamics (Cao et al., 2019b). Zhang et al. reported that plant cell secrets, EVs, and plant-derived EVs could be a new therapeutic method against diseases (Zhang et al., 2016c). For example, EVs-liked ginseng-derived nanoparticles (GDNPs) can be recognized and internalized with macrophages and induce M1-type polarization of macrophages to inhibit melanoma growth in mice (Cao et al., 2019c). Exosomes derived from ginseng can promote the neural differentiation of bone marrow derived mesenchymal stem cells (Xu et al., 2021). In addition, the targeting specificity of plant-derived EVs can also be improved by modifying their surface. For example, folate-conjugated arrowtail pRNA-3WJ were reported to facilitate the binding and uptake of ginger-derived exosome-like nanovesicles to NK cells (Li et al., 2018).
Moreover, EVs are used as biomarkers in herbal research. For example, Platelet-derived microvesicles (PMVs) were the indicator of platelets activation in a study that explores Tanshinone IIA’s function in a cluster of differentiation 36 (CD36) and mitogen-activated protein kinase kinase 4/c-Jun NH 2 terminal kinase (MKK4/JNK2) signaling pathway (Wang H. et al., 2020). Tanshinone IIA also elicited its impacts by the eicosanoid metabolism pathway and provoking endothelial microparticles production (Liu et al., 2011). Macropinocytosis is known to be a form of actin-dependent endocytosis, which is an endocytic procedure that typifies the engulfment of macropinosomes. Macropinosomes are large vesicles that consist of extracellular fluid. Tubeimoside-1 (TBM1), a low toxic triterpenoid saponin isolated from Bolbostemma paniculatum (Maxim.), efficiently lead to in vitro and in vivo micropinocytosis, which is able to traffic small molecules into colorectal cancer (CRC) cells (Gong et al., 2018). Another study demonstrated that matrine could induce macropinocytosis and the regulation of adenosine triphosphate (ATP) metabolism (Zhang B. et al., 2018). In Fructus Meliae Toosendan -induced liver injury mice, serum exosomal miR-222 and miR-370-3p were reported as significantly downregulated miRNAs (Zheng et al., 2018; Yu et al., 2020). By suppressing TGF1 exosomes transferring from Glomerular mesangial cells to glomerular endothelial cells, Tongxinluo can impede renal fibrosis in diabetic nephropathy (Wu et al., 2017). Buyang Huanwu Decoction can enhance angiogenic by elevating miRNA-126 levels in mesenchymal stem cell secreted exosomes (Yang et al., 2015).
5 Future Perspectives
5.1 Extracellular Vesicles: A New Delivery Approach for Treatments of Depression?
Blood-brain barrier (BBB) restricts the substances passing between the CNS and the vascular circulation system, thereby protecting the CNS from exposure to overactive immune responses or toxic substances (Obermeier et al., 2013; Andreone et al., 2015). Since the substrates from the blood to the CNS is controlled by the BBB (Kadry et al., 2020), effective drug transfer to the brain poses a challenge for treating CNS disorders, including neurodegenerative diseases, stroke, autoimmune diseases, or neuropsychiatric diseases like DD (Abbott et al., 2006; Upadhyay 2014). Almost all large molecule biologics and about 98% of small molecule drugs cannot traverse the BBB (Pardridge 2012). Nevertheless, the BBB permits transmembrane diffusion of lipid soluble (lipophilic) molecules smaller than 400 Da and can selectively transport some compounds into and out of the brain (Sanchez-Covarrubias et al., 2014). In this context, EVs could have advantages as drug vehicles, such as their small size, low immunogenicity, and ability to cross the BBB carrying cellular components or pharmacological agents (see Figure 3). Since EVs have the regenerative ability, they can also be exploited to potentially inhibit ongoing neurodegenerative processes associated with DD (Bhatt et al., 2021). Previous researches have established the successful transmission of exosomes to the brain in mice via intranasal injection or intravenous administration (Zhuang et al., 2011; Yuan et al., 2017). Another study also showed that exosomes could pass over the BBB and communicate bi-directionally between the brain and the rest of body (Bhatt et al., 2021). Despite the expected benefits of EVs for the treatment of DD, precise mechanisms of action and routes of delivery still require careful and rigorous investigation (Bhatt et al., 2021).
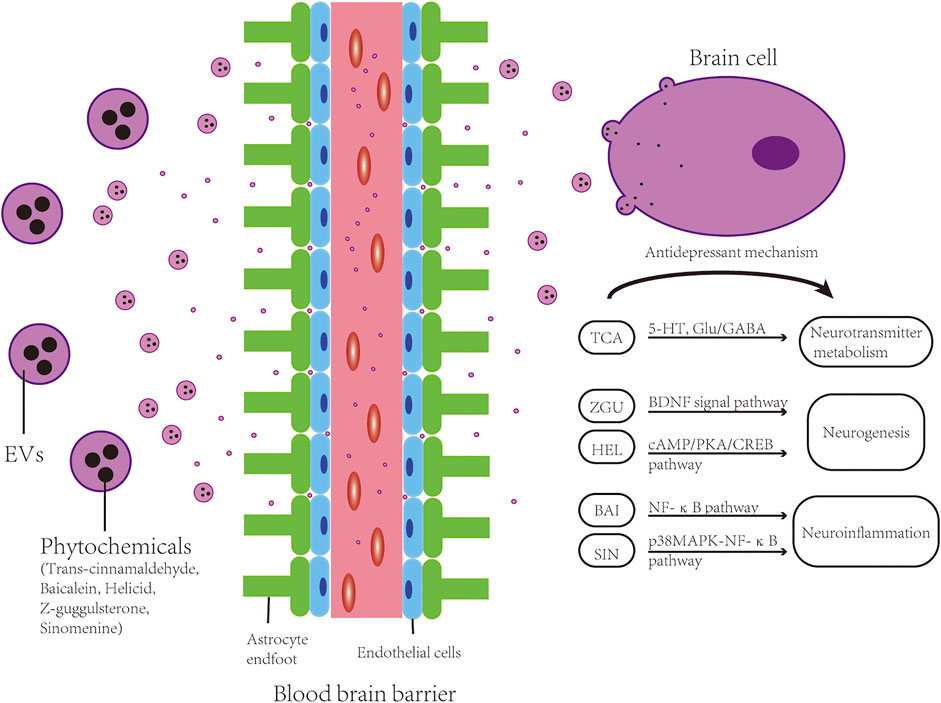
FIGURE 3. EVs for DD treatment by drug delivery. Phytochemicals such as Trans-cinnamaldehyde (TCA), Baicalein (BAI), Helicid (HEL), Z-guggulsterone (ZGU) and Sinomenine (SIN) can be packaged into extracellular vesicles and conveyed through the BBB to the brain cells (neurons and neuroglial cells), and exert antidepressant effect by regulating neuroinflammation, neurogenesis and neurotransmitter metabolism through a variety of pathways.
Herbal compounds are derived from diverse natural products. Since Chinese herbal concoctions are complex and undefined mixtures, it is challenging to demonstrate which component of the herbal therapy is responsible for a given effect (Corson and Crews 2007; Xu 2011). In particular, small phytochemicals could serve as viable cargoes for EV delivery (Liu et al., 2021) (Li et al., 2021). Indeed, studies exploring the application of EVs as vehicles for drug delivery have already begun. For example, curcumin-loaded EVs were found to protect mice from lipopolysaccharide (LPS)- induced septic shock (Sun et al., 2010). However, very few studies have examined DD treatment with phytochemical-loaded EVs, suggesting great potential for this line of research. For further references of phytochemical-loaded EVs research of DD, we screened potential phytochemicals from Table 4 by Lipinski’s rule of five, the rule of thumb to evaluate if a chemical compound has chemical properties and physical properties would make it an orally active drug in humans (see Table 5).
Besides serving as cargoes for EV delivery, herbs can also be applied to be the vehicle of EV. Distinct from artificially fabricated liposomes, plant-derived nanovector was reported to transport chemotherapeutic agents through mammalian hindrances such as BBB, and refrain from inflammatory response or necrosis (Wang Q. et al., 2013). Moreover, the lipid bilayer structure of plant-derived nanovector can protect the cargo from the enzymatic decomposition of proteinases and nucleases (Wang et al., 2015). Since plants do not retain zoonotic or human pathogens, plant-derived EVs take advantage of non-immunogenic and innocuous compared with mammalian cell-derived EVs(Schuh et al., 2019; Dad et al., 2021). On the other side, plant-derived EVs do not have cell targeting specificity because they have no ligands in comparison to mammalian cell-derived EVs. Previous studies reported that plant-derived EVs arrive at the liver and intestines through their natural biodistribution properties (Wang B. et al., 2014; Zhuang et al., 2015; Zhang et al., 2016b). Fortunately, plant-derived EVs can obtain specific cellular targeting by modification (Wang Q. et al., 2013).
5.2 Herb-Derived Extracellular Vesicles: Emerging Therapeutics for Depression?
As mentioned before, plant-derived EVs are beneficial to be the vehicle of phytochemicals since they are innocuous, low immunogenicity, and editable for target specificity. They can also promote cellular uptake and have higher stability in the GI tract (GIT) (Fujita et al., 2018), and the versatile therapeutic potential of plant-derived EVs rooted in their active source plants (Mu et al., 2014). Moreover, EVs extracted from the plant have been reported to be introduced via oral (Wang B. et al., 2014; Zhang et al., 2017), intravenous (Li et al., 2018), intramuscular, and intranasal administration (Wang Q. et al., 2013; Ju et al., 2013). This is another advantage of herb-derived EVs compared with Chinese herb decoction because the component complexity is always troubling applying effective Chinese herb to intramuscular, intravenous, and intranasal administration. These characteristics above make herb-derived EVs attractive to be an emerging therapeutic. Although many research have explained the anti-depressant mechanism of Chinese herbs (see table 3), few studies explored the effect of Chinese herb-derived EVs in treating depression, which is an exciting direction required to be followed.
5.3 Extracellular Vesicles: Potential Biomarkers for Diagnostic Depression
The unique property of EVs that can easily traverse BBB makes EVs a potential early diagnostic marker of CNS disorders like depression (Chen et al., 2016; Yao et al., 2018; Cufaro et al., 2019). Candidate protein biomarkers and potential diagnostic miRNAs for DD have been suggested (Al Shweiki et al., 2017; Tavakolizadeh et al., 2018; Saeedi et al., 2019). Besides miRNAs and proteins, exosomes as nanocarriers own the potential to be diagnostic biomarkers in various CNS disorders including DD (Perets et al., 2018; Wallensten et al., 2021).
The reasons why exosomes have the potential to be clinical diagnostics and biomarker are as follow (Kanninen et al., 2016): Firstly, exosomal contents can be changed along with disease conditions, which can reflect the dynamic state of disease in real-time; Secondly, exosomes can be easily extracted non-invasively from biological fluids (Bhatt et al., 2021), which is particular important because non-invasive availability is beneficial to early diagnosis of DD; Thirdly, exosomal contents are protected by the membranous structure, which keeps off the degradation of potential biomarkers (Kanninen et al., 2016); Fourthly, exosomes are very stable and can be preserved for prolonged periods (Grapp et al., 2013), making their clinical application feasible; Fifthly, exosomes can express their original cellular surface markers, so that they can be traced to their origin; Last but not least, since exosomes are able to pass over the BBB, which provide information of CNS cells that is hard to obtain without invasive techniques (Boukouris and Mathivanan 2015; Kawikova and Askenase 2015; Lin et al., 2015; Aryani and Denecke 2016). Because exosomes are distributed in all biological fluids and all cells can secret them, their biogenesis enables the arresting of the complex extracellular and intracellular molecular cargo (Kalluri and LeBleu 2020), rendering exosome-based liquid biopsy attractive in diagnosing the prognosis of DD. Liquid biopsies can allow us to understand the pathophysiology change of DD and diagnose the progressive disorders in the early stages (Topuzoğlu and Ilgın 2020). Moreover, studies relating the biomarkers associated with EVs in the context of DD still need more exploration. However, with the utility of liquid biopsy in diagnosing the prognosis of DD, the multicomponent analysis of EVs in the future may determine the disease progression and response to treatment.
5.4 Extracellular Vesicles: A Connection Bridge Between Herbal Therapies for Depression and Metabolomics, Proteomics, Transcriptomics and Epigenetics Studies
Metabolomics is a discipline to obtain all information of metabolites in a biological sample and would give mechanistic insights into the etiology of DD (Nedic Erjavec et al., 2018; Du et al., 2022). For example, nine potential biomarkers involved the depression pathogenesis were identified based on metabolomics analysis by comparing the rats’ serum metabolites of CUMS(chronic unpredictable mild stress) model group and Xiao-Chai-Hu-Tang group (Xiong et al., 2016). Proteomics includes all levels of protein composition, structure, and activity exploration of proteomes. Shweiki et al. summarized 42 differentially regulated proteins in DD and discussed the diagnostic potential of the biomarker candidates and their association with the suggested pathologies (Al Shweiki et al., 2017). Transcriptomics is the study associated with the process of all RNA transcripts during the biological process of transcription, and many transcriptomics studies provide insight into DD (Belzeaux et al., 2018; Cho et al., 2019; Rainville et al., 2021). By transferring key miRNAs, exosomes from the neuron, astrocyte, and neural progenitor cell exhibited significant efficiency in promoting neurogenesis (Takeda and Xu 2015; You et al., 2020; Yuan et al., 2021). Xu et al. systematically identified the miRNAs of exosomes from the juice of ginseng by transcriptomic technology, and found 44 kinds of miRNAs perfectly match to the ginseng genome database (Xu et al., 2021). Epigenetics covers heritable phenotype changes that are not involved in alterations of the DNA sequence, which is associated with DD reported by numerous studies (Yeshurun and Hannan 2019; Wheater et al., 2020; Xu et al., 2020). As discussed above, EVs are ideal herbal drug carriers due to their remarkable biocompatibility. Moreover, since DNA, RNA, lipids, proteins, cytoplasm, and metabolites are delivered by EVs, it can be taken as the critical point connecting herbal therapies to metabolomics, proteomics, transcriptomics and epigenetics in DD (see Figure 4).
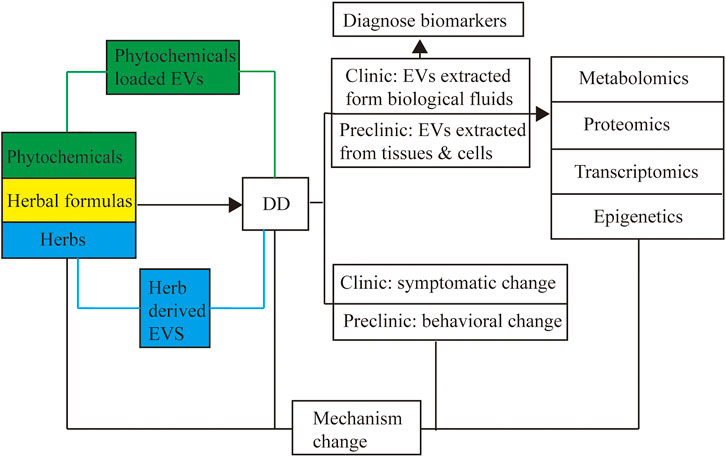
FIGURE 4. EVs application for CHM. Combined with metabolomics, proteomics, transcriptomics, and epigenetics, extracellular vesicles can be applied to explore the mechanism when treating DD with herbal formulas and act as the potential diagnose biomarkers in the clinic and preclinic studies.
6 Conclusion
Although CHM has been applied in China for thousands of years to help people fight many diseases, and some of Chines herbal original phytochemicals such as artemisinin have already been proved effective, composition complexity still remains a strenuous challenge for the mechanistic studies of CHM. Opportunely, the cargos and ligands of EVs can be determined by metabolomics, proteomics, and transcriptomics technologies, which means that the composition of herb-derived EVs can be specified for further mechanism study. Once the composition is precise, it can also be applied to different delivery routes such as intravenous or intranasal administration, which used to be limited to explore by the composition complexity of CHM. In addition, non-immunogenic, innocuous, and target-specific features make herb-derived EVs attractive to be therapeutic agents.
EVs can serve as drug vehicles for phytochemicals and biomarkers in developing the treatment for DD. Trials in intranasal administration of EVs indicate their significance in CNS diseases and show high promise to be a new medical way to transfer phytochemicals across the BBB. Since there are no specific biomarkers available for DD, the diagnosis has to depend on the combination of psychiatric evaluation, physical exam and lab tests. However, combined with metabolomics, proteomics, transcriptomics, and epigenetics technologies, the specifically altered contents in EVs from DD patients can be measured.
Even though EVs own promising advantages for delivering CHM, especially effective phytochemicals for treating DD, the components complexity of herbs and herbal formulas makes it challenging to be delivered by EVs. Moreover, there are few studies on pharmacological functions and in vivo transport pathways of CHM-derived EVs, which need more exploration before clinical practice. Therefore, the CHM study of EVs is still in the initial stage. More in-depth study in different CHM-derived EVs will be helpful to explain the complicated pharmacology of CHM and develop a new administration mode.
This review has summarized the reported effective CHM for treating DD and the advantages of EVs in facilitating CHM for DD treatment. Currently, few studies have been focused on herb-derived EVs in treating DD, which is exciting but remains to be explored in this area.
Author Contributions
QW completed the literature review and wrote the review, W-ZD thoroughly reviewed and edited the review, J-BC extracted helpful information from included studies, X-PZ helped with the abstract, X-JL classified the pieces of literature, Y-YL helped check the writing of the essay, ZX helped with the tables and the revision of the whole manuscript, Q-YM and J-XC, as primary reviewers screened titles and abstracts for eligibility. All authors read and approved the final manuscript.
Funding
This research work and publication were financially supported by Key Program of National Natural Science Foundation of China (No. 81630104), National Natural Science Foundation of China (No. 81973748, No. 82174278), Youth Science Fundation Project of National Natural Science Foundation of China (No. 81803972).
Publisher’s Note
All claims expressed in this article are solely those of the authors and do not necessarily represent those of their affiliated organizations, or those of the publisher, the editors, and the reviewers. Any product that may be evaluated in this article, or claim that may be made by its manufacturer, is not guaranteed or endorsed by the publisher.
Conflict of Interest
The authors declare that the research was conducted in the absence of any commercial or financial relationships that could be construed as a potential conflict of interest.
The reviewer YJ declared a shared affiliation with the author(s) QW and WD to the handling editor at the time of review.
References
Abbott, N. J., Rönnbäck, L., and Hansson, E. (2006). Astrocyte-endothelial Interactions at the Blood-Brain Barrier. Nat. Rev. Neurosci. 7, 41–53. doi:10.1038/nrn1824
Al Shweiki, M. R., Oeckl, P., Steinacker, P., Hengerer, B., Schönfeldt-Lecuona, C., and Otto, M. (2017). Major Depressive Disorder: Insight into Candidate Cerebrospinal Fluid Protein Biomarkers from Proteomics Studies. Expert Rev. Proteomics 14, 499–514. doi:10.1080/14789450.2017.1336435
Andreone, B. J., Lacoste, B., and Gu, C. (2015). Neuronal and Vascular Interactions. Annu. Rev. Neurosci. 38, 25–46. doi:10.1146/annurev-neuro-071714-033835
Aryani, A., and Denecke, B. (2016). Exosomes as a Nanodelivery System: a Key to the Future of Neuromedicine? Mol. Neurobiol. 53, 818–834. doi:10.1007/s12035-014-9054-5
Belzeaux, R., Lin, R., Ju, C., Chay, M. A., Fiori, L. M., Lutz, P. E., et al. (2018). Transcriptomic and Epigenomic Biomarkers of Antidepressant Response. J. Affect. Disord. 233, 36–44. doi:10.1016/j.jad.2017.08.087
Bhatt, S., Kanoujia, J., Dhar, A. K., Arumugam, S., Silva, A. K. A., and Mishra, N. (2021). Exosomes: A Novel Therapeutic Paradigm for the Treatment of Depression. Curr. Drug Targets 22, 183–191. doi:10.2174/1389450121999201006193005
Bhatt, S., Nagappa, A. N., and Patil, C. R. (2020). Role of Oxidative Stress in Depression. Drug Discov. Today 25, 1270–1276. doi:10.1016/j.drudis.2020.05.001
Bian, X., Liu, X., Liu, J., Zhao, Y., Li, H., Cai, E., et al. (2018). Study on Antidepressant Activity of Chiisanoside in Mice. Int. Immunopharmacol. 57, 33–42. doi:10.1016/j.intimp.2018.02.007
Boukouris, S., and Mathivanan, S. (2015). Exosomes in Bodily Fluids Are a Highly Stable Resource of Disease Biomarkers. Proteomics Clin. Applclin. Appl. 9, 358–367. doi:10.1002/prca.201400114
Cao, L. H., Qiao, J. Y., Huang, H. Y., Fang, X. Y., Zhang, R., Miao, M. S., et al. (2019a). PI3K-AKT Signaling Activation and Icariin: The Potential Effects on the Perimenopausal Depression-like Rat Model. Molecules 24. doi:10.3390/molecules24203700
Cao, M., Yan, H., Han, X., Weng, L., Wei, Q., Sun, X., et al. (2019c). Ginseng-derived Nanoparticles Alter Macrophage Polarization to Inhibit Melanoma Growth. J. Immunother. Cancer 7, 326. doi:10.1186/s40425-019-0817-4
Cao, M., Yan, H. H., Han, X., Weng, L., Wei, Q., Sun, X., et al. (2019b). Astrocytes at the Hub of the Stress Response: Potential Modulation of Neurogenesis by miRNAs in Astrocyte-Derived Exosomes. J. Ethnopharmacol. 7, 144–158. 10.1016/j.jad.2020.05.017. doi:10.1186/s40425-019-0817-4
Chen, C. C., Liu, L., Ma, F., Wong, C. W., Guo, X. E., Chacko, J. V., et al. (2016). Elucidation of Exosome Migration across the Blood-Brain Barrier Model In Vitro. Cell. Mol. Bioeng. 9, 509–529. doi:10.1007/s12195-016-0458-3
Chen, J., Huang, Y., Li, L., Niu, J., Ye, W., Wang, Y., et al. (2020). Antidepressant Pathways of the Chinese Herb Jiaweisinisan through Genetic Ontology Analysis. J. Integr. Neurosci. 19, 385–395. doi:10.31083/j.jin.2020.02.1246
Chen, Z., Huang, C., He, H., and Ding, W. (2017). 2, 3, 5, 4'-Tetrahydroxystilbene-2-O-β-D-Glucoside Prevention of Lipopolysaccharide-Induced Depressive-like Behaviors in Mice Involves Neuroinflammation and Oxido-Nitrosative Stress Inhibition. Behav. Pharmacol. 28, 365–374. doi:10.1097/FBP.0000000000000307
Chi, X., Wang, S., Baloch, Z., Zhang, H., Li, X., Zhang, Z., et al. (2019). Research Progress on Classical Traditional Chinese Medicine Formula Lily Bulb and Rehmannia Decoction in the Treatment of Depression. Biomed. Pharmacother. 112, 108616. doi:10.1016/j.biopha.2019.108616
Chivet, M., Hemming, F., Pernet-Gallay, K., Fraboulet, S., and Sadoul, R. (2012). Emerging Role of Neuronal Exosomes in the central Nervous System. Front. Physiol. 3, 145. doi:10.3389/fphys.2012.00145
Cho, J. H., Irwin, M. R., Eisenberger, N. I., Lamkin, D. M., and Cole, S. W. (2019). Transcriptomic Predictors of Inflammation-Induced Depressed Mood. Neuropsychopharmacology 44, 923–929. doi:10.1038/s41386-019-0316-9
Choi, J. L., Kao, P. F., Itriago, E., Zhan, Y., Kozubek, J. A., Hoss, A. G., et al. (2017). miR-149 and miR-29c as Candidates for Bipolar Disorder Biomarkers. Am. J. Med. Genet. B Neuropsychiatr. Genet. 174, 315–323. doi:10.1002/ajmg.b.32518
Corson, T. W., and Crews, C. M. (2007). Molecular Understanding and Modern Application of Traditional Medicines: Triumphs and Trials. Cell 130, 769–774. doi:10.1016/j.cell.2007.08.021
Cufaro, M. C., Pieragostino, D., Lanuti, P., Rossi, C., Cicalini, I., Federici, L., et al. (2019). Extracellular Vesicles and Their Potential Use in Monitoring Cancer Progression and Therapy: The Contribution of Proteomics. J. Oncol. 2019, 1639854. doi:10.1155/2019/1639854
Dad, H. A., Gu, T. W., Zhu, A. Q., Huang, L. Q., and Peng, L. H. (2021). Plant Exosome-like Nanovesicles: Emerging Therapeutics and Drug Delivery Nanoplatforms. Mol. Ther. 29, 13–31. doi:10.1016/j.ymthe.2020.11.030
Du, H. X., Chen, X. G., Zhang, L., Liu, Y., Zhan, C. S., Chen, J., et al. (2019). Microglial Activation and Neurobiological Alterations in Experimental Autoimmune Prostatitis-Induced Depressive-like Behavior in Mice. Neuropsychiatr. Dis. Treat. 15, 2231–2245. doi:10.2147/NDT.S211288
Du, Y., Dong, J. H., Chen, L., Liu, H., Zheng, G. E., Chen, G. Y., et al. (2022). Metabolomic Identification of Serum Exosome-Derived Biomarkers for Bipolar Disorder. Oxid. Med. Cell. Longev. 2022, 5717445. doi:10.1155/2022/5717445
Fan, C., Song, Q., Wang, P., Li, Y., Yang, M., Liu, B., et al. (2018). Curcumin Protects against Chronic Stress-Induced Dysregulation of Neuroplasticity and Depression-like Behaviors via Suppressing IL-1β Pathway in Rats. Neuroscience 392, 92–106. doi:10.1016/j.neuroscience.2018.09.028
Fujita, D., Arai, T., Komori, H., Shirasaki, Y., Wakayama, T., Nakanishi, T., et al. (2018). Apple-Derived Nanoparticles Modulate Expression of Organic-Anion-Transporting Polypeptide (OATP) 2B1 in Caco-2 Cells. Mol. Pharm. 15, 5772–5780. doi:10.1021/acs.molpharmaceut.8b00921
Geng, J., Liu, J., Yuan, X., Liu, W., and Guo, W. (2019). Andrographolide Triggers Autophagy-Mediated Inflammation Inhibition and Attenuates Chronic Unpredictable Mild Stress (CUMS)-induced Depressive-like Behavior in Mice. Toxicol. Appl. Pharmacol. 379, 114688. doi:10.1016/j.taap.2019.114688
Ghasemzadeh Rahbardar, M., and Hosseinzadeh, H. (2020). Therapeutic Effects of Rosemary (Rosmarinus Officinalis L.) and its Active Constituents on Nervous System Disorders. Iran. J. Basic Med. Sci. 23, 1100–1112. doi:10.22038/ijbms.2020.45269.10541
Gómez-Molina, C., Sandoval, M., Henzi, R., Ramírez, J. P., Varas-Godoy, M., Luarte, A., et al. (2019). Small Extracellular Vesicles in Rat Serum Contain Astrocyte-Derived Protein Biomarkers of Repetitive Stress. Int. J. Neuropsychopharmacol. 22, 232–246. doi:10.1093/ijnp/pyy098
Gong, W., Zhu, S., Chen, C., Yin, Q., Li, X., Du, G., et al. (2019). The Anti-depression Effect of Angelicae Sinensis Radix Is Related to the Pharmacological Activity of Modulating the Hematological Anomalies. Front. Pharmacol. 10, 192. doi:10.3389/fphar.2019.00192
Gong, X., Sun, R., Gao, Z., Han, W., Liu, Y., Zhao, L., et al. (2018). Tubeimoside 1 Acts as a Chemotherapeutic Synergist via Stimulating Macropinocytosis. Front. Pharmacol. 9, 1–10. doi:10.3389/fphar.2018.01044
Grapp, M., Wrede, A., Schweizer, M., Hüwel, S., Galla, H. J., Snaidero, N., et al. (2013). Choroid Plexus Transcytosis and Exosome Shuttling Deliver Folate into Brain Parenchyma. Nat. Commun. 4, 2123. doi:10.1038/ncomms3123
Guo, L. T., Wang, S. Q., Su, J., Xu, L. X., Ji, Z. Y., Zhang, R. Y., et al. (2019). Baicalin Ameliorates Neuroinflammation-Induced Depressive-like Behavior through Inhibition of Toll-like Receptor 4 Expression via the PI3K/AKT/FoxO1 Pathway. J. Neuroinflammation 16, 95. doi:10.1186/s12974-019-1474-8
Hirshler, Y., and Doron, R. (2017). Neuroplasticity-related Mechanisms Underlying the Antidepressant-like Effects of Traditional Herbal Medicines. Eur. Neuropsychopharmacol. 27, 945–958. doi:10.1016/j.euroneuro.2017.07.008
Hu, K., Guan, W. J., Bi, Y., Zhang, W., Li, L., Zhang, B., et al. (2021). Efficacy and Safety of Lianhuaqingwen Capsules, a Repurposed Chinese Herb, in Patients with Coronavirus Disease 2019: A Multicenter, Prospective, Randomized Controlled Trial. Phytomedicine 85, 153242. doi:10.1016/j.phymed.2020.153242
Huang, Q., Ye, X., Wang, L., and Pan, J. (2019). Salvianolic Acid B Abolished Chronic Mild Stress-Induced Depression through Suppressing Oxidative Stress and Neuro-Inflammation via Regulating NLRP3 Inflammasome Activation. J. Food Biochem. 43, e12742. doi:10.1111/jfbc.12742
James, S. L., Abate, D., Abate, K. H., Abay, S. M., Abbafati, C., Abbasi, N., et al. (2018). Global, Regional, and National Incidence, Prevalence, and Years Lived with Disability for 354 Diseases and Injuries for 195 Countries and Territories, 1990-2017: A Systematic Analysis for the Global Burden of Disease Study 2017. Lancet 392, 1789–1858. doi:10.1016/S0140-6736(18)32279-7
Ji, W. W., Li, R. P., Li, M., Wang, S. Y., Zhang, X., Niu, X. X., et al. (2014a). Antidepressant-like Effect of Essential Oil of Perilla Frutescens in a Chronic, Unpredictable, Mild Stress-Induced Depression Model Mice. Chin. J. Nat. Med. 12, 753–759. doi:10.1016/S1875-5364(14)60115-1
Ji, W. W., Wang, S. Y., Ma, Z. Q., Li, R. P., Li, S. S., Xue, J. S., et al. (2014b). Effects of Perillaldehyde on Alternations in Serum Cytokines and Depressive-like Behavior in Mice after Lipopolysaccharide Administration. Pharmacol. Biochem. Behav. 116, 1–8. doi:10.1016/j.pbb.2013.10.026
Jiang, C. Y., Qin, X. Y., Yuan, M. M., Lu, G. J., and Cheng, Y. (2018). 2,3,5,4'-Tetrahydroxystilbene-2-O-beta-D-glucoside Reverses Stress-Induced Depression via Inflammatory and Oxidative Stress Pathways. Oxid. Med. Cell. Longev. 2018, 9501427. doi:10.1155/2018/9501427
Jiang, N., Lv, J., Wang, H., Huang, H., Wang, Q., Lu, C., et al. (2020). Ginsenoside Rg1 Ameliorates Chronic Social Defeat Stress-Induced Depressive-like Behaviors and Hippocampal Neuroinflammation. Life Sci. 252, 117669. doi:10.1016/j.lfs.2020.117669
Ju, S., Mu, J., Dokland, T., Zhuang, X., Wang, Q., Jiang, H., et al. (2013). Grape Exosome-like Nanoparticles Induce Intestinal Stem Cells and Protect Mice from DSS-Induced Colitis. Mol. Ther. 21, 1345–1357. doi:10.1038/mt.2013.64
Kadry, H., Noorani, B., and Cucullo, L. (2020). A Blood-Brain Barrier Overview on Structure, Function, Impairment, and Biomarkers of Integrity. Fluids Barr. CNS 17, 69. doi:10.1186/s12987-020-00230-3
Kalluri, R., and LeBleu, V. S. (2020). The Biology, Function, and Biomedical Applications of Exosomes. Science 367, eaau6977. doi:10.1126/science.aau6977
Kang, A., Xie, T., Zhu, D., Shan, J., Di, L., and Zheng, X. (2017). Suppressive Effect of Ginsenoside Rg3 against Lipopolysaccharide-Induced Depression-like Behavior and Neuroinflammation in Mice. J. Agric. Food Chem. 65, 6861–6869. doi:10.1021/acs.jafc.7b02386
Kanninen, K. M., Bister, N., Koistinaho, J., and Malm, T. (2016). Exosomes as New Diagnostic Tools in CNS Diseases. Biochim. Biophys. Acta 1862, 403–410. doi:10.1016/j.bbadis.2015.09.020
Kawikova, I., and Askenase, P. W. (2015). Diagnostic and Therapeutic Potentials of Exosomes in CNS Diseases. Brain Res. 1617, 63–71. doi:10.1016/j.brainres.2014.09.070
Kim, J., Li, S., Zhang, S., and Wang, J. (2022). Plant-derived Exosome-like Nanoparticles and Their Therapeutic Activities. Asian J. Pharm. Sci. 17, 53–69. doi:10.1016/j.ajps.2021.05.006
Kuwano, N., Kato, T. A., Mitsuhashi, M., Sato-Kasai, M., Shimokawa, N., Hayakawa, K., et al. (2018). Neuron-related Blood Inflammatory Markers as an Objective Evaluation Tool for Major Depressive Disorder: An Exploratory Pilot Case-Control Study. J. Affect. Disord. 240, 88–98. doi:10.1016/j.jad.2018.07.040
Li, C. F., Chen, X. Q., Chen, S. M., Chen, X. M., Geng, D., Liu, Q., et al. (2017a). Evaluation of the Toxicological Properties and Anti-inflammatory Mechanism of Hemerocallis Citrina in LPS-Induced Depressive-like Mice. Biomed. Pharmacother. 91, 167–173. doi:10.1016/j.biopha.2017.04.089
Li, D., Wang, Y., Jin, X., Hu, D., Xia, C., Xu, H., et al. (2020). NK Cell-Derived Exosomes Carry miR-207 and Alleviate Depression-like Symptoms in Mice. J. Neuroinflammation 17, 126. doi:10.1186/s12974-020-01787-4
Li, H., Lin, S., Qin, T., Li, H., Ma, Z., and Ma, S. (2017c). Senegenin Exerts Anti-depression Effect in Mice Induced by Chronic Un-predictable Mild Stress via Inhibition of NF-Κb Regulating NLRP3 Signal Pathway. Int. Immunopharmacol. 53, 24–32. doi:10.1016/j.intimp.2017.10.001
Li, H. Y., Zhao, Y. H., Zeng, M. J., Fang, F., Li, M., Qin, T. T., et al. (2017b). Saikosaponin D Relieves Unpredictable Chronic Mild Stress Induced Depressive-like Behavior in Rats: Involvement of HPA axis and Hippocampal Neurogenesis. Psychopharmacology (Berl) 234, 3385–3394. doi:10.1007/s00213-017-4720-8
Li, J., Huang, S., Huang, W., Wang, W., Wen, G., Gao, L., et al. (2017d). Paeoniflorin Ameliorates Interferon-Alpha-Induced Neuroinflammation and Depressive-like Behaviors in Mice. Oncotarget 8, 8264–8282. doi:10.18632/oncotarget.14160
Li, X., Qin, X. M., Tian, J. S., Gao, X. X., Du, G. H., and Zhou, Y. Z. (2021). Integrated Network Pharmacology and Metabolomics to Dissect the Combination Mechanisms of Bupleurum Chinense DC-Paeonia Lactiflora Pall Herb Pair for Treating Depression. J. Ethnopharmacol. 264, 113281. doi:10.1016/j.jep.2020.113281
Li, X. Y., Qi, W. W., Zhang, Y. X., Jiang, S. Y., Yang, B., Xiong, L., et al. (2019). Helicid Ameliorates Learning and Cognitive Ability and Activities cAMP/PKA/CREB Signaling in Chronic Unpredictable Mild Stress Rats. Biol. Pharm. Bull. 42, 1146–1154. doi:10.1248/bpb.b19-00012
Li, Y. C., Wang, L. L., Pei, Y. Y., Shen, J. D., Li, H. B., Wang, B. Y., et al. (2015). Baicalin Decreases SGK1 Expression in the hippocampus and Reverses Depressive-like Behaviors Induced by Corticosterone. Neuroscience 311, 130–137. doi:10.1016/j.neuroscience.2015.10.023
Li, Z., Wang, H., Yin, H., Bennett, C., Zhang, H. G., and Guo, P. (2018). Arrowtail RNA for Ligand Display on Ginger Exosome-like Nanovesicles to Systemic Deliver siRNA for Cancer Suppression. Sci. Rep. 8, 14644. doi:10.1038/s41598-018-32953-7
Liang, J. Q., Liao, H. R., Xu, C. X., Li, X. L., Wei, Z. X., Xie, G. J., et al. (2020). Serum Exosome-Derived miR-139-5p as a Potential Biomarker for Major Depressive Disorder. Neuropsychiatr. Dis. Treat. 16, 2689–2693. doi:10.2147/NDT.S277392
Lin, J., Li, J., Huang, B., Liu, J., Chen, X., Chen, X. M., et al. (2015). Exosomes: Novel Biomarkers for Clinical Diagnosis. ScientificWorldJournal 2015, 657086. doi:10.1155/2015/657086
Lin, J., Song, Z., Chen, X., Zhao, R., Chen, J., Chen, H., et al. (2019). Trans-cinnamaldehyde Shows Anti-depression Effect in the Forced Swimming Test and Possible Involvement of the Endocannabinoid System. Biochem. Biophys. Res. Commun. 518, 351–356. doi:10.1016/j.bbrc.2019.08.061
Lin, Y. E., Wang, H. L., Lu, K. H., Huang, Y. J., Panyod, S., Liu, W. T., et al. (2021). Water Extract of Armillaria Mellea (Vahl) P. Kumm. Alleviates the Depression-like Behaviors in Acute- and Chronic Mild Stress-Induced Rodent Models via Anti-inflammatory Action. J. Ethnopharmacol. 265, 113395. doi:10.1016/j.jep.2020.113395
Liu, B., Xu, C., Wu, X., Liu, F., Du, Y., Sun, J., et al. (2015). Icariin Exerts an Antidepressant Effect in an Unpredictable Chronic Mild Stress Model of Depression in Rats and Is Associated with the Regulation of Hippocampal Neuroinflammation. Neuroscience 294, 193–205. doi:10.1016/j.neuroscience.2015.02.053
Liu, F. G., Hu, W. F., Wang, J. L., Wang, P., Gong, Y., Tong, L. J., et al. (2017a). Z-guggulsterone Produces Antidepressant-like Effects in Mice through Activation of the BDNF Signaling Pathway. Int. J. Neuropsychopharmacol. 20, 485–497. doi:10.1093/ijnp/pyx009
Liu, J. Q., Lee, T. F., Miedzyblocki, M., Chan, G. C., Bigam, D. L., and Cheung, P. Y. (2011). Effects of Tanshinone IIA, a Major Component of Salvia Miltiorrhiza, on Platelet Aggregation in Healthy Newborn Piglets. J. Ethnopharmacol. 137, 44–49. doi:10.1016/j.jep.2011.03.047
Liu, P., Bai, X., Zhang, T., Zhou, L., Li, J., and Zhang, L. (2019). The Protective Effect of Lonicera japonica Polysaccharide on Mice with Depression by Inhibiting NLRP3 Inflammasome. Ann. Transl. Med. 7, 811. doi:10.21037/atm.2019.12.64
Liu, S., Xu, S., Wang, Z., Guo, Y., Pan, W., and Shen, Z. (2018). Anti-Depressant-Like Effect of Sinomenine on Chronic Unpredictable Mild Stress-Induced Depression in a Mouse Model. Med. Sci. Monit. 24, 7646–7653. doi:10.12659/MSM.908422
Liu, X. J., Wang, Y. Z., Wei, F. X., Lv, M., Qu, P., Chen, S. J., et al. (2021). The Synergistic Anti-depression Effects of Different Efficacy Groups of Xiaoyaosan as Demonstrated by the Integration of Network Pharmacology and Serum Metabolomics. J. Pharm. Biomed. Anal. 197, 113949. doi:10.1016/j.jpba.2021.113949
Liu, Y. M., Shen, J. D., Xu, L. P., Li, H. B., Li, Y. C., and Yi, L. T. (2017b). Ferulic Acid Inhibits Neuro-Inflammation in Mice Exposed to Chronic Unpredictable Mild Stress. Int. Immunopharmacol. 45, 128–134. doi:10.1016/j.intimp.2017.02.007
Mathivanan, S., Ji, H., and Simpson, R. J. (2010). Exosomes: Extracellular Organelles Important in Intercellular Communication. J. Proteomics 73, 1907–1920. doi:10.1016/j.jprot.2010.06.006
McClintock, S. M., Husain, M. M., Greer, T. L., and Cullum, C. M. (2010). Association between Depression Severity and Neurocognitive Function in Major Depressive Disorder: a Review and Synthesis. Neuropsychology 24, 9–34. doi:10.1037/a0017336
Milajerdi, A., Jazayeri, S., Shirzadi, E., Hashemzadeh, N., Azizgol, A., Djazayery, A., et al. (2018). The Effects of Alcoholic Extract of Saffron (Crocus Satious L.) on Mild to Moderate Comorbid Depression-Anxiety, Sleep Quality, and Life Satisfaction in Type 2 Diabetes Mellitus: A Double-Blind, Randomized and Placebo-Controlled Clinical Trial. Complement. Ther. Med. 41, 196–202. doi:10.1016/j.ctim.2018.09.023
Mizohata, Y., Toda, H., Koga, M., Saito, T., Fujita, M., Kobayashi, T., et al. (2021). Neural Extracellular Vesicle-Derived miR-17 in Blood as a Potential Biomarker of Subthreshold Depression. Hum. Cell 34, 1087–1092. doi:10.1007/s13577-021-00553-9
Mou, Z., Huang, Q., Chu, S. F., Zhang, M. J., Hu, J. F., Chen, N. H., et al. (2017). Antidepressive Effects of Ginsenoside Rg1 via Regulation of HPA and HPG axis. Biomed. Pharmacother. 92, 962–971. doi:10.1016/j.biopha.2017.05.119
Mu, J., Zhuang, X., Wang, Q., Jiang, H., Deng, Z. B., Wang, B., et al. (2014). Interspecies Communication between Plant and Mouse Gut Host Cells through Edible Plant Derived Exosome-like Nanoparticles. Mol. Nutr. Food Res. 58, 1561–1573. doi:10.1002/mnfr.201300729
Nasca, C., Dobbin, J., Bigio, B., Watson, K., de Angelis, P., Kautz, M., et al. (2020). Insulin Receptor Substrate in Brain-Enriched Exosomes in Subjects with Major Depression: on the Path of Creation of Biosignatures of central Insulin Resistance. Mol. Psychiatry 26, 5140–5149. doi:10.1038/s41380-020-0804-7
Nedic Erjavec, G., Konjevod, M., Nikolac Perkovic, M., Svob Strac, D., Tudor, L., Barbas, C., et al. (2018). Short Overview on Metabolomic Approach and Redox Changes in Psychiatric Disorders. Redox Biol. 14, 178–186. doi:10.1016/j.redox.2017.09.002
Obermeier, B., Daneman, R., and Ransohoff, R. M. (2013). Development, Maintenance and Disruption of the Blood-Brain Barrier. Nat. Med. 19, 1584–1596. doi:10.1038/nm.3407
Pardridge, W. M. (2012). Drug Transport across the Blood-Brain Barrier. J. Cereb. Blood Flow Metab. 32, 1959–1972. doi:10.1038/jcbfm.2012.126
Park, B. K., Kim, N. S., Kim, Y. R., Yang, C., Jung, I. C., Jang, I. S., et al. (2020). Antidepressant and Anti-neuroinflammatory Effects of Bangpungtongsung-San. Front. Pharmacol. 11, 958. doi:10.3389/fphar.2020.00958
Park, S. H., Jang, S., Son, E., Lee, S. W., Park, S. D., Sung, Y. Y., et al. (2018). Polygonum Aviculare L. Extract Reduces Fatigue by Inhibiting Neuroinflammation in Restraint-Stressed Mice. Phytomedicine 42, 180–189. doi:10.1016/j.phymed.2018.03.042
Perets, N., Hertz, S., London, M., and Offen, D. (2018). Intranasal Administration of Exosomes Derived from Mesenchymal Stem Cells Ameliorates Autistic-like Behaviors of BTBR Mice. Mol. Autism 9, 57. doi:10.1186/s13229-018-0240-6
Po, K. K., Leung, J. W., Chan, J. N., Fung, T. K., Sánchez-Vidaña, D. I., Sin, E. L., et al. (2017). Protective Effect of Lycium Barbarum Polysaccharides on Dextromethorphan-Induced Mood Impairment and Neurogenesis Suppression. Brain Res. Bull. 134, 10–17. doi:10.1016/j.brainresbull.2017.06.014
Prado, C. E., Watt, S., and Crowe, S. F. (2018). A Meta-Analysis of the Effects of Antidepressants on Cognitive Functioning in Depressed and Non-depressed Samples. Neuropsychol. Rev. 28, 32–72. doi:10.1007/s11065-018-9369-5
Qiu, Z. K., Zhang, G. H., Zhong, D. S., He, J. L., Liu, X., Chen, J. S., et al. (2017). Puerarin Ameliorated the Behavioral Deficits Induced by Chronic Stress in Rats. Sci. Rep. 7, 6266. doi:10.1038/s41598-017-06552-x
Rainville, J. R., Lipuma, T., and Hodes, G. E. (2022). Translating the Transcriptome: Sex Differences in the Mechanisms of Depression and Stress, Revisited. Biol. Psychiatry 91, 25–35. doi:10.1016/j.biopsych.2021.02.003
Ramshani, Z., Zhang, C., Richards, K., Chen, L., Xu, G., Stiles, B. L., et al. (2019). Extracellular Vesicle microRNA Quantification from Plasma Using an Integrated Microfluidic Device. Commun. Biol. 2, 189–9. doi:10.1038/s42003-019-0435-1
Ren, L., and Chen, G. (2017). Rapid Antidepressant Effects of Yueju: A New Look at the Function and Mechanism of an Old Herbal Medicine. J. Ethnopharmacol. 203, 226–232. doi:10.1016/j.jep.2017.03.042
Ruan, J., Liu, L., Shan, X., Xia, B., and Fu, Q. (2019). Anti-depressant Effects of Oil from Fructus Gardeniae via PKA-CREB-BDNF Signaling. Biosci. Rep. 39. doi:10.1042/BSR20190141
Ruan, X. F., Ju, C. W., Shen, Y., Liu, Y. T., Kim, I. M., Yu, H., et al. (2018a). Suxiao Jiuxin Pill Promotes Exosome Secretion from Mouse Cardiac Mesenchymal Stem Cells In Vitro. Acta Pharmacol. Sin. 39, 569–578. doi:10.1038/aps.2018.19
Ruan, X. F., Li, Y. J., Ju, C. W., Shen, Y., Lei, W., Chen, C., et al. (2018b). Exosomes from Suxiao Jiuxin Pill-Treated Cardiac Mesenchymal Stem Cells Decrease H3K27 Demethylase UTX Expression in Mouse Cardiomyocytes In Vitro. Acta Pharmacol. Sin. 39, 579–586. doi:10.1038/aps.2018.18
Saeedi, S., Israel, S., Nagy, C., and Turecki, G. (2019). The Emerging Role of Exosomes in Mental Disorders. Transl PsychiatryPsychiatry 9, 122. doi:10.1038/s41398-019-0459-9
Salari, N., Hosseinian-Far, A., Jalali, R., Vaisi-Raygani, A., Rasoulpoor, S., Mohammadi, M., et al. (2020). Prevalence of Stress, Anxiety, Depression Among the General Population during the COVID-19 Pandemic: a Systematic Review and Meta-Analysis. Glob. Health 16, 57. doi:10.1186/s12992-020-00589-w
Sanchez-Covarrubias, L., Slosky, L. M., Thompson, B. J., Davis, T. P., and Ronaldson, P. T. (2014). Transporters at CNS Barrier Sites: Obstacles or Opportunities for Drug Delivery? Curr. Pharm. Des. 20, 1422–1449. doi:10.2174/13816128113199990463
Schuh, C. M. A. P., Cuenca, J., Alcayaga-Miranda, F., and Khoury, M. (2019). Exosomes on the Border of Species and Kingdom Intercommunication. Transl. Res. 210, 80–98. doi:10.1016/j.trsl.2019.03.008
Song, Y., Sun, R., Ji, Z., Li, X., Fu, Q., and Ma, S. (2018). Perilla Aldehyde Attenuates CUMS-Induced Depressive-like Behaviors via Regulating TXNIP/TRX/NLRP3 Pathway in Rats. Life Sci. 206, 117–124. doi:10.1016/j.lfs.2018.05.038
Stenovec, M., Lasič, E., Božić, M., Bobnar, S. T., Stout, R. F., Grubišić, V., et al. (2016). Ketamine Inhibits ATP-Evoked Exocytotic Release of Brain-Derived Neurotrophic Factor from Vesicles in Cultured Rat Astrocytes. Mol. Neurobiol. 53, 6882–6896. doi:10.1007/s12035-015-9562-y
Stenovec, M., Li, B., Verkhratsky, A., and Zorec, R. (2020). Astrocytes in Rapid Ketamine Antidepressant Action. Neuropharmacology 173, 108158. doi:10.1016/j.neuropharm.2020.108158
Su, J., Pan, Y. W., Wang, S. Q., Li, X. Z., Huang, F., and Ma, S. P. (2020). Saikosaponin-d Attenuated Lipopolysaccharide-Induced Depressive-like Behaviors via Inhibiting Microglia Activation and Neuroinflammation. Int. Immunopharmacol. 80, 106181. doi:10.1016/j.intimp.2019.106181
Sun, D., Zhuang, X., Xiang, X., Liu, Y., Zhang, S., Liu, C., et al. (2010). A Novel Nanoparticle Drug Delivery System: The Anti-inflammatory Activity of Curcumin Is Enhanced when Encapsulated in Exosomes. Mol. Ther. 18, 1606–1614. doi:10.1038/mt.2010.105
Sun, Y., Xu, X., Zhang, J., and Chen, Y. (2018). Treatment of Depression with Chai Hu Shu Gan San: a Systematic Review and Meta-Analysis of 42 Randomized Controlled Trials. BMC Complement. Altern. Med. 18, 66. doi:10.1186/s12906-018-2130-z
Takeda, Y. S., and Xu, Q. (2015). Neuronal Differentiation of Human Mesenchymal Stem Cells Using Exosomes Derived from Differentiating Neuronal Cells. PLoS One 10, e0135111. doi:10.1371/journal.pone.0135111
Tavakolizadeh, J., Roshanaei, K., Salmaninejad, A., Yari, R., Nahand, J. S., Sarkarizi, H. K., et al. (2018). MicroRNAs and Exosomes in Depression: Potential Diagnostic Biomarkers. J. Cell. Biochem. 119, 3783–3797. doi:10.1002/jcb.26599
Théry, C., Witwer, K. W., Aikawa, E., Alcaraz, M. J., Anderson, J. D., Andriantsitohaina, R., et al. (2018). Minimal Information for Studies of Extracellular Vesicles 2018 (MISEV2018): a Position Statement of the International Society for Extracellular Vesicles and Update of the MISEV2014 Guidelines. J. Extracell. Vesicles 7, 1535750. doi:10.1080/20013078.2018.1535750
Topuzoğlu, A., and Ilgın, C. (2020). Mentalexo Approach for Diagnosis of Psychiatric Disorders. Med. Hypotheses 143, 109823. doi:10.1016/j.mehy.2020.109823
Upadhyay, R. K. (2014). Drug Delivery Systems, CNS protection, and the Blood Brain Barrier. Biomed. Res. Int. 2014, 869269. doi:10.1155/2014/869269
Valadi, H., Ekström, K., Bossios, A., Sjöstrand, M., Lee, J. J., and Lötvall, J. O. (2007). Exosome-mediated Transfer of mRNAs and microRNAs Is a Novel Mechanism of Genetic Exchange between Cells. Nat. Cell Biol. 9, 654–659. doi:10.1038/ncb1596
Wallensten, J., Nager, A., Åsberg, M., Borg, K., Beser, A., Wilczek, A., et al. (2021). Leakage of Astrocyte-Derived Extracellular Vesicles in Stress-Induced Exhaustion Disorder: a Cross-Sectional Study. Sci. Rep. 11, 2009. doi:10.1038/s41598-021-81453-8
Wang, A. R., Mi, L. F., Zhang, Z. L., Hu, M. Z., Zhao, Z. Y., Liu, B., et al. (2021a). Saikosaponin A Improved Depression-like Behavior and Inhibited Hippocampal Neuronal Apoptosis after Cerebral Ischemia through P-Creb/bdnf Pathway. Behav. Brain Res. 403, 113138. doi:10.1016/j.bbr.2021.113138
Wang, B., Zhuang, X., Deng, Z. B., Jiang, H., Mu, J., Wang, Q., et al. (2014a). Targeted Drug Delivery to Intestinal Macrophages by Bioactive Nanovesicles Released from Grapefruit. Mol. Ther. 22, 522–534. doi:10.1038/mt.2013.190
Wang, D., Wang, H., and Gu, L. (2017). The Antidepressant and Cognitive Improvement Activities of the Traditional Chinese Herb Cistanche. Evid. Based. Complement. Alternat. Med. 2017, 3925903. doi:10.1155/2017/3925903
Wang, F., Wang, J., An, J., Yuan, G., Hao, X., and Zhang, Y. (2018a). Resveratrol Ameliorates Depressive Disorder through the NETRIN1-Mediated Extracellular Signal-Regulated kinase/cAMP Signal Transduction Pathway. Mol. Med. Rep. 17, 4611–4618. doi:10.3892/mmr.2018.8379
Wang, H., Zhang, R., Qiao, Y., Xue, F., Nie, H., Zhang, Z., et al. (2014b). Gastrodin Ameliorates Depression-like Behaviors and Up-Regulates Proliferation of Hippocampal-Derived Neural Stem Cells in Rats: Involvement of its Anti-inflammatory Action. Behav. Brain Res. 266, 153–160. doi:10.1016/j.bbr.2014.02.046
Wang, H., Zhong, L., Mi, S., Song, N., Zhang, W., and Zhong, M. (2020a). Tanshinone IIA Prevents Platelet Activation and Down-Regulates CD36 and MKK4/JNK2 Signaling Pathway. BMC Cardiovasc. Disord. 20, 81–87. doi:10.1186/s12872-019-01289-z
Wang, J. M., Pei, L. X., Zhang, Y. Y., Cheng, Y. X., Niu, C. L., Cui, Y., et al. (2018b). Ethanol Extract of Rehmannia Glutinosa Exerts Antidepressant-like Effects on a Rat Chronic Unpredictable Mild Stress Model by Involving Monoamines and BDNF. Metab. Brain Dis. 33, 885–892. doi:10.1007/s11011-018-0202-x
Wang, M., Yan, S., Zhou, Y., and Xie, P. (2020b). trans-Cinnamaldehyde Reverses Depressive-like Behaviors in Chronic Unpredictable Mild Stress Rats by Inhibiting NF-Κb/nlrp3 Inflammasome Pathway. Evid. Based. Complement. Alternat. Med. 2020, 4572185. doi:10.1155/2020/4572185
Wang, Q., Ren, Y., Mu, J., Egilmez, N. K., Zhuang, X., Deng, Z., et al. (2015). Grapefruit-Derived Nanovectors Use an Activated Leukocyte Trafficking Pathway to Deliver Therapeutic Agents to Inflammatory Tumor Sites. Cancer Res. 75, 2520–2529. doi:10.1158/0008-5472.CAN-14-3095
Wang, Q., Zhuang, X., Mu, J., Deng, Z. B., Jiang, H., Zhang, L., et al. (2013a). Delivery of Therapeutic Agents by Nanoparticles Made of Grapefruit-Derived Lipids. Nat. Commun. 4, 1867. doi:10.1038/ncomms2886
Wang, S., Wang, C., Yu, Z., Wu, C., Peng, D., Liu, X., et al. (2018c). Agarwood Essential Oil Ameliorates Restrain Stress-Induced Anxiety and Depression by Inhibiting HPA Axis Hyperactivity. Int. J. Mol. Sci. 19, 3468. doi:10.3390/ijms19113468
Wang, W., Liu, X., Liu, J., Cai, E., Zhao, Y., Li, H., et al. (2018d). Sesquiterpenoids from the Root of Panax Ginseng Attenuates Lipopolysaccharide-Induced Depressive-like Behavior through the Brain-Derived Neurotrophic Factor/Tropomyosin-Related Kinase B and Sirtuin Type 1/Nuclear Factor-Κb Signaling Pathways. J. Agric. Food Chem. 66, 265–271. doi:10.1021/acs.jafc.7b04835
Wang, Y., Gao, C., Gao, T., Zhao, L., Zhu, S., and Guo, L. (2021b). Plasma Exosomes from Depression Ameliorate Inflammation-Induced Depressive-like Behaviors via Sigma-1 Receptor Delivery. Brain Behav. Immun. 94, 225–234. doi:10.1016/j.bbi.2021.02.004
Wang, Z., Zhang, D., Hui, S., Zhang, Y., and Hu, S. (2013b). Effect of Tribulus Terrestris Saponins on Behavior and Neuroendocrine in Chronic Mild Stress Depression Rats. J. Tradit Chin. Med. 33, 228–232. doi:10.1016/s0254-6272(13)60130-2
Wei, Q., Zhou, W., Zheng, J., Li, D., Wang, M., Feng, L., et al. (2020a). Antidepressant Effects of 3-(3,4-Methylenedioxy-5-Trifluoromethyl Phenyl)-2e-Propenoic Acid Isobutyl Amide Involve TSPO-Mediated Mitophagy Signalling Pathway. Basic Clin. Pharmacol. Toxicol. 127, 380–388. doi:10.1111/bcpt.13452
Wei, Z. X., Xie, G. J., Mao, X., Zou, X. P., Liao, Y. J., Liu, Q. S., et al. (2020b). Exosomes from Patients with Major Depression Cause Depressive-like Behaviors in Mice with Involvement of miR-139-5p-Regulated Neurogenesis. Neuropsychopharmacology 45, 1050–1058. doi:10.1038/s41386-020-0622-2
Wheater, E. N. W., Stoye, D. Q., Cox, S. R., Wardlaw, J. M., Drake, A. J., Bastin, M. E., et al. (2020). DNA Methylation and Brain Structure and Function across the Life Course: A Systematic Review. Neurosci. Biobehav. Rev. 113, 133–156. doi:10.1016/j.neubiorev.2020.03.007
Wu, X. M., Gao, Y. B., Xu, L. P., Zou, D. W., Zhu, Z. Y., Wang, X. L., et al. (2017). Tongxinluo Inhibits Renal Fibrosis in Diabetic Nephropathy: Involvement of the Suppression of Intercellular Transfer of TGF-[Formula: See Text]1-Containing Exosomes from GECs to GMCs. Am. J. Chin. Med. 45, 1075–1092. doi:10.1142/S0192415X17500586
Xia, B., Huang, X., Sun, G., and Tao, W. (2021). Iridoids from Gardeniae Fructus Ameliorates Depression by Enhancing Synaptic Plasticity via AMPA Receptor-mTOR Signaling. J. Ethnopharmacol. 268, 113665. doi:10.1016/j.jep.2020.113665
Xiong, Z., Yang, J., Huang, Y., Zhang, K., Bo, Y., Lu, X., et al. (2016). Serum Metabonomics Study of Anti-depressive Effect of Xiao-Chai-Hu-Tang on Rat Model of Chronic Unpredictable Mild Stress. J. Chromatogr. B Analyt Technol. Biomed. Life Sci. 1029-1030, 28–35. doi:10.1016/j.jchromb.2016.06.044
Xu, J. N., Chen, L. F., Su, J., Liu, Z. L., Chen, J., Lin, Q. F., et al. (2018). The Anxiolytic-like Effects of Ginsenoside Rg3 on Chronic Unpredictable Stress in Rats. Sci. Rep. 8, 7741. doi:10.1038/s41598-018-26146-5
Xu, Q., Jiang, M., Gu, S., Wang, F., and Yuan, B. (2020). Early Life Stress Induced DNA Methylation of Monoamine Oxidases Leads to Depressive-like Behavior. Front. Cell Dev. Biol. 8, 582247. doi:10.3389/fcell.2020.582247
Xu, X. H., Yuan, T. J., Dad, H. A., Shi, M. Y., Huang, Y. Y., Jiang, Z. H., et al. (2021). Plant Exosomes as Novel Nanoplatforms for MicroRNA Transfer Stimulate Neural Differentiation of Stem Cells In Vitro and In Vivo. Nano Lett. 21, 8151–8159. doi:10.1021/acs.nanolett.1c02530
Yang, J., Gao, F., Zhang, Y., Liu, Y., and Zhang, D. (2015). Buyang Huanwu Decoction (BYHWD) Enhances Angiogenic Effect of Mesenchymal Stem Cell by Upregulating VEGF Expression after Focal Cerebral Ischemia. J. Mol. Neurosci. 56, 898–906. doi:10.1007/s12031-015-0539-0
Yang, S. J., Yu, H. Y., Kang, D. Y., Ma, Z. Q., Qu, R., Fu, Q., et al. (2014). Antidepressant-like Effects of Salidroside on Olfactory Bulbectomy-Induced Pro-inflammatory Cytokine Production and Hyperactivity of HPA axis in Rats. Pharmacol. Biochem. Behav. 124, 451–457. doi:10.1016/j.pbb.2014.07.015
Yang, X. H., Song, S. Q., and Xu, Y. (2017). Resveratrol Ameliorates Chronic Unpredictable Mild Stress-Induced Depression-like Behavior: Involvement of the HPA axis, Inflammatory Markers, BDNF, and Wnt/β-Catenin Pathway in Rats. Neuropsychiatr. Dis. Treat. 13, 2727–2736. doi:10.2147/NDT.S150028
Yao, Z. Y., Chen, W. B., Shao, S. S., Ma, S. Z., Yang, C. B., Li, M. Z., et al. (2018). Role of Exosome-Associated microRNA in Diagnostic and Therapeutic Applications to Metabolic Disorders. J. Zhejiang Univ. Sci. B 19, 183–198. doi:10.1631/jzus.B1600490
Yeshurun, S., and Hannan, A. J. (2019). Transgenerational Epigenetic Influences of Paternal Environmental Exposures on Brain Function and Predisposition to Psychiatric Disorders. Mol. Psychiatry 24, 536–548. doi:10.1038/s41380-018-0039-z
You, Y., Borgmann, K., Edara, V. V., Stacy, S., Ghorpade, A., and Ikezu, T. (2020). Activated Human Astrocyte-Derived Extracellular Vesicles Modulate Neuronal Uptake, Differentiation and Firing. J. Extracell. Vesicles 9, 1706801. doi:10.1080/20013078.2019.1706801
Yu, L., Zheng, J., Li, J., Wang, Y., Lu, X., and Fan, X. (2020). Integrating Serum Exosomal microRNA and Liver microRNA Profiles Disclose the Function Role of Autophagy and Mechanisms of Fructus Meliae Toosendan-Induced Hepatotoxicity in Mice. Biomed. Pharmacother. 123, 109709. doi:10.1016/j.biopha.2019.109709
Yuan, D., Zhao, Y., Banks, W. A., Bullock, K. M., Haney, M., Batrakova, E., et al. (2017). Macrophage Exosomes as Natural Nanocarriers for Protein Delivery to Inflamed Brain. Biomaterials 142, 1–12. doi:10.1016/j.biomaterials.2017.07.011
Yuan, P., Ding, L., Chen, H., Wang, Y., Li, C., Zhao, S., et al. (2021). Neural Stem Cell-Derived Exosomes Regulate Neural Stem Cell Differentiation through miR-9-Hes1 Axis. Front. Cell Dev. Biol. 9, 601600. doi:10.3389/fcell.2021.601600
Zhang, B., Wang, P. P., Hu, K. L., Li, L. N., Yu, X., Lu, Y., et al. (2019a). Antidepressant-Like Effect and Mechanism of Action of Honokiol on the Mouse Lipopolysaccharide (LPS) Depression Model. Molecules 24, 2035. doi:10.3390/molecules24112035
Zhang, B., Wang, X., Li, Y., Wu, M., Wang, S.-Y., and Li, S. (2018a). Matrine Is Identified as a Novel Macropinocytosis Inducer by a Network Target Approach. Front. Pharmacol. 9, 1–11. doi:10.3389/fphar.2018.00010
Zhang, C. Y., Zeng, M. J., Zhou, L. P., Li, Y. Q., Zhao, F., Shang, Z. Y., et al. (2018b). Baicalin Exerts Neuroprotective Effects via Inhibiting Activation of GSK3β/NF-Κb/nlrp3 Signal Pathway in a Rat Model of Depression. Int. Immunopharmacol. 64, 175–182. doi:10.1016/j.intimp.2018.09.001
Zhang, H., Freitas, D., Kim, H. S., Fabijanic, K., Li, Z., Chen, H., et al. (2018c). Identification of Distinct Nanoparticles and Subsets of Extracellular Vesicles by Asymmetric Flow Field-Flow Fractionation. Nat. Cell Biol. 20, 332–343. doi:10.1038/s41556-018-0040-4
Zhang, J. Q., Wu, X. H., Feng, Y., Xie, X. F., Fan, Y. H., Yan, S., et al. (2016a). Salvianolic Acid B Ameliorates Depressive-like Behaviors in Chronic Mild Stress-Treated Mice: Involvement of the Neuroinflammatory Pathway. Acta Pharmacol. Sin. 37, 1141–1153. doi:10.1038/aps.2016.63
Zhang, L., Previn, R., Lu, L., Liao, R. F., Jin, Y., and Wang, R. K. (2018d). Crocin, a Natural Product Attenuates Lipopolysaccharide-Induced Anxiety and Depressive-like Behaviors through Suppressing NF-kB and NLRP3 Signaling Pathway. Brain Res. Bull. 142, 352–359. doi:10.1016/j.brainresbull.2018.08.021
Zhang, M., Viennois, E., Prasad, M., Zhang, Y., Wang, L., Zhang, Z., et al. (2016b). Edible Ginger-Derived Nanoparticles: A Novel Therapeutic Approach for the Prevention and Treatment of Inflammatory Bowel Disease and Colitis-Associated Cancer. Biomaterials 101, 321–340. doi:10.1016/j.biomaterials.2016.06.018
Zhang, M., Viennois, E., Xu, C., and Merlin, D. (2016c). Plant Derived Edible Nanoparticles as a New Therapeutic Approach against Diseases. Tissue barriers 4, e1134415. doi:10.1080/21688370.2015.1134415
Zhang, M., Wang, X., Han, M. K., Collins, J. F., and Merlin, D. (2017). Oral Administration of Ginger-Derived Nanolipids Loaded with siRNA as a Novel Approach for Efficient siRNA Drug Delivery to Treat Ulcerative Colitis. Nanomedicine (Lond) 12, 1927–1943. doi:10.2217/nnm-2017-0196
Zhang, R., Ma, Z., Liu, K., Li, Y., Liu, D., Xu, L., et al. (2019b). Baicalin Exerts Antidepressant Effects through Akt/FOXG1 Pathway Promoting Neuronal Differentiation and Survival. Life Sci. 221, 241–248. doi:10.1016/j.lfs.2019.02.033
Zhang, W. Y., Guo, Y. J., Han, W. X., Yang, M. Q., Wen, L. P., Wang, K. Y., et al. (2019c). Curcumin Relieves Depressive-like Behaviors via Inhibition of the NLRP3 Inflammasome and Kynurenine Pathway in Rats Suffering from Chronic Unpredictable Mild Stress. Int. Immunopharmacol. 67, 138–144. doi:10.1016/j.intimp.2018.12.012
Zhang, X., Du, Q., Liu, C., Yang, Y., Wang, J., Duan, S., et al. (2016d). Rhodioloside Ameliorates Depressive Behavior via Up-Regulation of Monoaminergic System Activity and Anti-inflammatory Effect in Olfactory Bulbectomized Rats. Int. Immunopharmacol. 36, 300–304. doi:10.1016/j.intimp.2016.05.008
Zhang, Y., Long, Y., Yu, S., Li, D., Yang, M., Guan, Y., et al. (2021). Natural Volatile Oils Derived from Herbal Medicines: A Promising Therapy Way for Treating Depressive Disorder. Pharmacol. Res. 164, 105376. doi:10.1016/j.phrs.2020.105376
Zhao, Y., Zhang, Y., and Pan, F. (2015). The Effects of EGb761 on Lipopolysaccharide-Induced Depressive-like Behaviour in C57BL/6J Mice. Cent. Eur. J. Immunol. 40, 11–17. doi:10.5114/ceji.2015.49427
Zheng, J., Yu, L., Chen, W., Lu, X., and Fan, X. (2018). Circulating Exosomal microRNAs Reveal the Mechanism of Fructus Meliae Toosendan-Induced Liver Injury in Mice. Sci. Rep. 8, 2832. doi:10.1038/s41598-018-21113-6
Zhuang, X., Deng, Z. B., Mu, J., Zhang, L., Yan, J., Miller, D., et al. (2015). Ginger-derived Nanoparticles Protect against Alcohol-Induced Liver Damage. J. Extracell. Vesicles 4, 28713. doi:10.3402/jev.v4.28713
Zhuang, X., Xiang, X., Grizzle, W., Sun, D., Zhang, S., Axtell, R. C., et al. (2011). Treatment of Brain Inflammatory Diseases by Delivering Exosome Encapsulated Anti-inflammatory Drugs from the Nasal Region to the Brain. Mol. Ther. 19, 1769–1779. doi:10.1038/mt.2011.164
Glossary
4-HNE 4-hydroxynonenal
5-HIAA 5-hydroxyindoleacetic acid
5-HT 5-hydroxytryptamine
8-OHdG 8-hydroxy-2′-deoxyguanosine
11β-HSD2 11β-hydroxysteroid dehydrogenase-2
ACTH adrenocorticotropic hormone
AKT protein kinase B
ASC Anti-TMS1
ATP adenosine triphosphate
Bax Bcl-2-associated X protein
BBB blood brain barrier
Bcl-2 B-cell lymphoma 2
BDNF brain-derived neurotrophic factor
CA1 the first region in the hippocampal circuit
CAT Catalase
CD36 cluster of differentiation 36
CD81 cluster of differentiation 81
CHM Chinese herbal medicine
CMS chronic mild stress
CMSC cardiac mesenchymal stem cells
CNS central nervous system
CORT CORT
COVID-19 coronavirus disease 2019
COX Cyclooxygenase
CRC colorectal cancer
CRH corticotropin-releasing hormone
CRP C-reactive protein
CRS chronic restraint stress
CSDS Chronic social defeat stress
CUMS chronic unpredictable mild stress
CUS chronic unpredictable stress
DCX doublecortin
DG dentate gyrus
DXM dextromethorphan
EAP experimental autoimmune prostatitis
EVs extracellular vesicles
FGF2 Fibroblast growth factor
FOXG1 Forkhead box transcription factor
FoxO1 forkhead box protein O 1
FST forced swimming test
GDNPs ginseng-derived nanoparticles
GFAP glial fibrillary acidic protein
GluA1 Glutamate Receptor 1
GPx Glutathione peroxidase
GR glucocorticoid receptor
GSH-pX glutathione peroxidase
HPA hypothalamic pituitary adrenal
Iba1 Ionized calcium binding adaptor molecule 1
IBA1 Ionized calcium binding adaptor molecule 1
IDO indoleamine 2,3-dioxygenase
IFN-γ interferon γ
IL-18 interleukin-18
IL-1β interleukin-1β
IL-34 interleukin 34
IL-6 interleukin-6
iNOS inducible nitric oxide synthase
IRS-1 insulin receptor substrate 1
IκB-α inhibitor of κB-α
JNK2 c-Jun NH 2 terminal kinase
KIFC2 Kinesin Family Member C2
Kir4.1 inward rectifying potassium channel
L1CAM L1 Cell Adhesion Molecule
LDHA lactate dehydrogenase A
LH learned helplessness
LPS lipopolysaccharide
Maxim. Bolbostemma paniculatum
MCAO middle cerebral artery occlusion
MDA malondialdehyde
MDD major depressive disorder
miRNAs microRNAs
MKK4 mitogen-activated protein kinase kinase 4
NF-κB nuclear factor kappa-light-chain-enhancer of activated B cells
NLRP3 oligomerization domain-like receptor family pyrin domain-containing 3
nNOS neural nitric oxide synthase
NO nitric oxide
NSCs neural stem cells
NSE Neuron-specific enolase
NT-3 Neurotrophin-3
p-AKT phosphorylation-akt
p-CREB phospho-cAMP response element-binding protein
PDK-1 pyruvate dehydrogenase lipoamide kinase isozyme 1
PI3K phosphoinositide 3-kinase
p-iκB phospho-inhibitor of kappa B
PMVs platelet-derived microvesicles
p-p65 anti-p-NF-κB p65
p-P70S6K Phospho-p70 S6 kinase
PSD-95 Postsynaptic density protein 95
ROS reactive oxide species
RS restraint stress
SDS social defeat stress
SERTs serotonin transporters
SGK1 glucocorticoid-regulated kinase 1
Sig-1R sigma-1 receptor
Sirt 1 sirtuin type 1
SOD superoxide dismutase
SRS spatial restraint stress
TBM1 tubeimoside-1
TCAs tricyclic antidepressants
TLR4 Toll Like Receptor 4
TNFR1 tumor necrosis factor receptor 1
TNF-α TNF-α
TrkB tropomyosin-related kinase B
TSPO translocator protein
Keywords: phytochemials, herbal therapies, extracellular vehicles, exosomes, ectosomes, microvescicles, depressive disorder
Citation: Wu Q, Duan W-Z, Chen J-B, Zhao X-P, Li X-J, Liu Y-Y, Ma Q-Y, Xue Z and Chen J-X (2022) Extracellular Vesicles: Emerging Roles in Developing Therapeutic Approach and Delivery Tool of Chinese Herbal Medicine for the Treatment of Depressive Disorder. Front. Pharmacol. 13:843412. doi: 10.3389/fphar.2022.843412
Received: 25 December 2021; Accepted: 28 February 2022;
Published: 24 March 2022.
Edited by:
Liu Qing-Shan, Minzu University of China, ChinaReviewed by:
Yong Cheng, Minzu University of China, ChinaYan Jouroukhin, Johns Hopkins Medicine, United States
Guangxin Yue, China Academy of Chinese Medical Science, China
Copyright © 2022 Wu, Duan, Chen, Zhao, Li, Liu, Ma, Xue and Chen. This is an open-access article distributed under the terms of the Creative Commons Attribution License (CC BY). The use, distribution or reproduction in other forums is permitted, provided the original author(s) and the copyright owner(s) are credited and that the original publication in this journal is cited, in accordance with accepted academic practice. No use, distribution or reproduction is permitted which does not comply with these terms.
*Correspondence: Qing-Yu Ma, dG1hcWluZ3l1QGpudS5lZHUuY24=; Zhe Xue, eHVlemhlc2FuY3RpdHlAMTYzLmNvbQ==; Jia-Xu Chen, Y2hlbmppYXh1QGhvdG1haWwuY29t